- 1Molecular Biology Laboratory, Department of Biochemistry, Federal University of Pernambuco - UFPE, Recife, PE, Brazil
- 2Department of Civil and Environmental Engineering, Federal University of Pernambuco - UFPE, Recife, PE, Brazil
- 3Amity School of Earth & Environmental Sciences, Amity University Punjab (AUP), Mohali, India
- 4Department of Environmental Studies, Delhi College of Arts and Commerce, University of Delhi, New Delhi, India
Introduction: Microplastics (MPs) are widely distributed in the environment, causing damage to biota and human health. Due to their physicochemical characteristics, they become resistant particles to environmental degradation, leading to their accumulation in large quantities in the terrestrial ecosystem. Thus, there is an urgent need for measures to mitigate such pollution, with biological degradation being a viable alternative, where bacteria play a crucial role, demonstrating high efficiency in degrading various types of MPs. Therefore, the study aimed to identify bacteria with the potential for MP biodegradation and the enzymes produced during the process.
Methods: The methodology used followed the Preferred Reporting Items for Systematic Reviews and Meta-Analyses (PRISMA) protocol.
Results and Discussion: The research yielded 68 eligible studies, highlighting bacteria from the genera Bacillus, Pseudomonas, Stenotrophomonas, and Rhodococcus as the main organisms involved in MP biodegradation. Additionally, enzymes such as hydrolases and alkane hydroxylases were emphasized for their involvement in this process. Thus, the potential of bacterial biodegradation is emphasized as a promising pathway to mitigate the environmental impact of MPs, highlighting the relevance of identifying bacteria with biotechnological potential for large-scale applications in reducing MP pollution.
1 Introduction
The microplastics (MPs) are considered emerging contaminants due to their occurrence in different environmental compartments, including atmospheric, aquatic, and terrestrial. They are defined as plastic particles ranging in size from 1 μm to 5 mm and are found in various types, sizes, shapes, and primary and secondary polymeric compositions (Miri et al., 2022; Thakur et al., 2023).
Microplastics (MPs) are considered harmful to wildlife and humans due to their persistent properties and bioaccumulation. This is attributed to the addition of various substances during their manufacturing process, such as pigments, plasticizers, and flame retardants. Additionally, due to their chemical-physical characteristics, they exhibit high durability, requiring an extended period for degradation in the environment (Cai et al., 2023; Niu et al., 2023).
Therefore, the production of plastics in the industry has been going on since the 1950s, with annual production reaching around 2 million tons, so that in 2015 this production rose significantly to 380 million tons per year. As a result, looking back from 1950 to 2015, approximately more than 7,800 million tons of plastics were produced, resulting in approximately 6,300 million tons of waste. Over the past 70 years, global plastic production has increased from 1.5 million tons to approximately 359.0 million tons, with an estimated projection of reaching 500.0 million tons by 2025. This trend raises significant concerns within civil society, as MPs are primarily generated through the degradation of larger polymers, a process influenced by physical, chemical, or biological factors (Cverenkárová et al., 2021; Torena et al., 2021; Villalobos et al., 2022; Osman et al., 2023). As microplastics increasingly contaminate the environment, the food chain has also been significantly impacted. Plastic contamination has occurred in invertebrates such as polychaetes, 51 crustaceans, echinoderms, bivalves, and vertebrates, including fish, seabirds, and mammals. These particles have entered the food chain either directly or through trophic transfer. Indeed, one of the main concerns arising from microplastic contamination is its bioaccumulative effect in the digestive tract (Cverenkárová et al., 2021).
Microplastics (MPs) enter the environment through various pathways due to poor management and dumping practices. However, there are mechanisms that can be employed to control their presence in the environment, such as biological, thermal, and photocatalytic degradation. Biological degradation occurs through the use of different types of microorganisms, as some have the potential to be employed in bioremediation processes (Park and Kim, 2019).
These microorganisms are widely distributed in nature, with abundance among bacteria due to their rapid reproduction, diverse nutritional capabilities, strong adaptability, and significant potential for degrading MPs. They demonstrate high efficiency in degrading MPs such as Polyethylene terephthalate (PET), Polyethylene (PE), and Polypropylene (PP) in the natural environment (Yuan et al., 2020; Li et al., 2022). Although polymers have a relatively simple chemical structure, they are known for their high resistance to biodegradation, especially due to their hydrophobic structure, high molecular weight, and lack of a favorable functional group. Consequently, when present in the environment in combination with biotic and abiotic factors, they can undergo transformations leading to the formation of alcoholic or carbonyl groups. This process increases plastic hydrophilicity and provides anchors that facilitate the attachment of bacterial species (Villalobos et al., 2022; Pathak, 2023; Thakur et al., 2023).
Thus, exploring the capability of bacteria and the interaction between bacterial enzymes and microplastics is crucial for obtaining and identifying key microorganisms with potential for bioremediation through the biodegradation of synthetic polymers. Therefore, the present study aims to identify the main bacteria that demonstrate viability for the biodegradation of MPs in various environments, as well as the enzymes produced during the degradation process.
2 Materials and methods
2.1 Protocol
This systematic review was conducted in accordance with the Preferred Reporting Items for Systematic Reviews and Meta-Analyses (PRISMA) protocol, organized into the respective phases of planning, execution, and data reporting.
2.2 Eligibility criteria
For the conduct of this investigation, the PECO strategy was employed: Population—Microorganisms, Exposure—Microplastics, Comparison—Not applicable, and Outcomes—Potential of bacteria for microplastic biodegradation.
Thus, according to the aforementioned strategy, studies that considered the key microorganisms involved in microplastic biodegradation were deemed eligible without restrictions on the year and/or language. Consequently, the exclusion criteria encompassed studies and editorial files, typical discussion documents, comments, letters, reviews, studies with incomplete or insufficient data regarding methodology and microorganism identification, as well as duplicates and titles that did not align with the proposed theme.
2.3 Information and research sources
Searches were conducted in the electronic databases PubMed, Medline, and LILACS. Subsequently, the definition of Medical Subject Headings (MeSH) and Health Sciences Descriptors (Decs) descriptors and synonyms, in addition to keywords and Boolean operators, was carried out for the composition of the controlled search strategy. Thus, the terms “Microplastics” AND “Bacteria” AND “Ecosystem” AND “Environment” AND “Biodegradation” AND “Bioremediation” were obtained.
2.4 Articles selection
For study selection, two reviewers participated independently and blindly, resulting in the following stages for the inclusion and exclusion of studies. The first stage involved title analysis, excluding duplicates. The second stage involved discussing eligibility criteria separately according to the PECO strategy, enabling the exclusion of studies not related to the proposed strategy. The third stage consisted of eliminating studies after reading the abstracts, which could not provide sufficient information and data for the fulfillment of the current proposal.
2.5 Data collection process
Subsequently, following the selection of studies, information from the main data of eligible studies was extracted using a form created by the authors with predefined items. The key items included: first author, year of publication, genus and species of the isolated microorganism, source of microorganisms, type of microplastics, and microbial enzymes with potential for microplastic biodegradation. The aforementioned data was then tabulated in an Excel spreadsheet, and any additional calculations and necessary tabulations were performed by two researchers.
2.6 Bias risk
The publication bias was assessed using the Joanna Briggs Institute’s (JBI) critical appraisal checklist for qualitative research (Lockwood et al., 2017). This checklist involves three respective classifications: High, Moderate, and Low. A High-risk rating results in more than 49% scoring “yes,” Moderate involves achieving 50–69% scoring “yes,” and Low consists of a score of “yes” ≥ 70%. According to this assessment, studies with a high risk of publication bias will be excluded.
3 Results
In this systematic search, initially, 954 studies were found. Of these, 58 were excluded due to duplication, 685 due to title, 69 due to abstract, and 74 did not meet eligibility criteria, resulting in a total of 68 eligible studies for systematic review. Figure 1 presents the flowchart demonstrating the main quantitative and qualitative data of the excluded and included articles.
According to the eligible studies, the first analysis was conducted to identify the pre-dominance of microorganisms with the potential for microplastic biodegradation. Table 1 presents the main genera and species of microorganisms and their action in the biodegradation of different types of microplastics and the main enzymes analysis related to the degradation of microplastics.
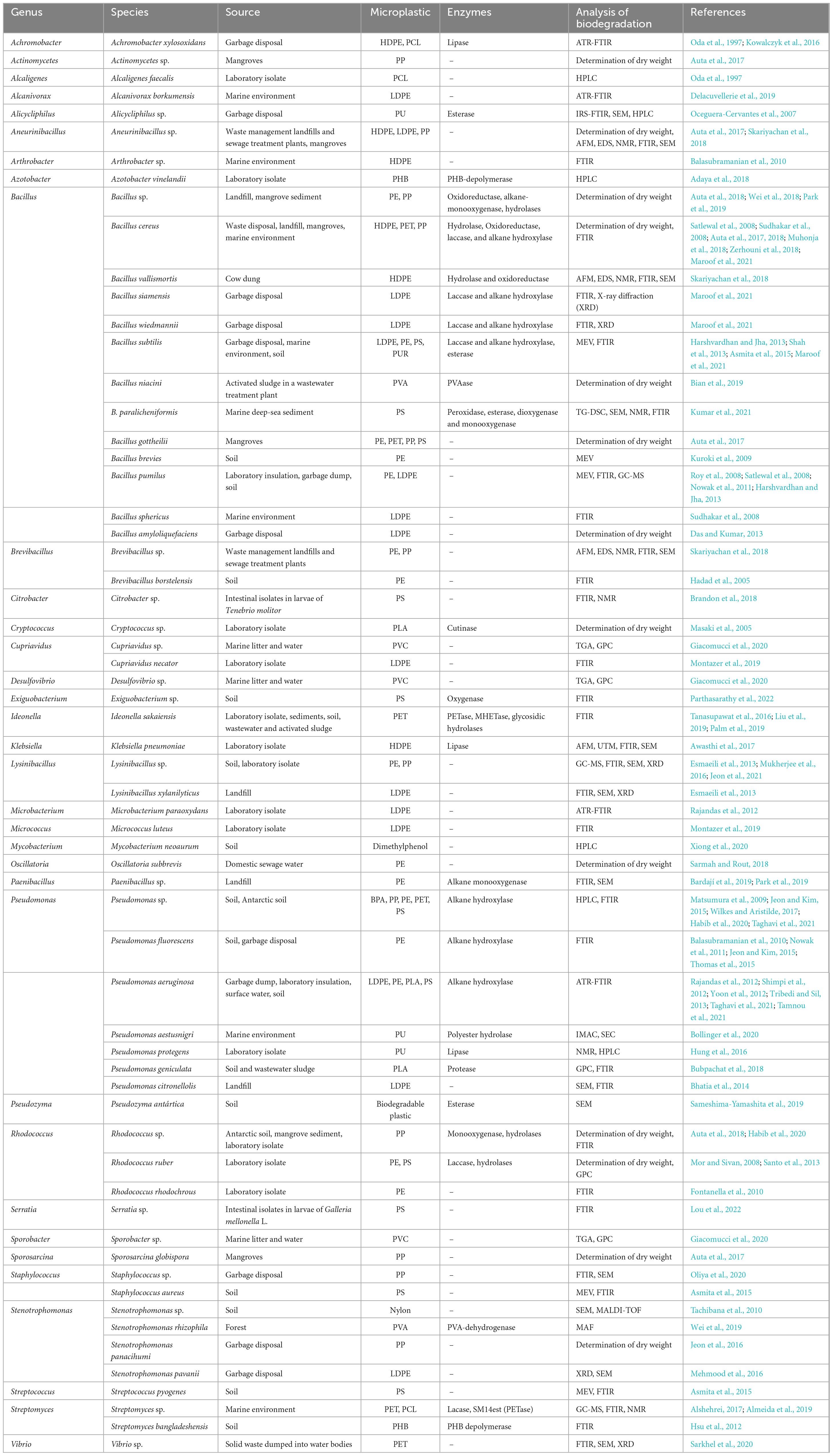
Table 1. Qualitative synthesis of the main genera and species of microorganisms with potential for microplastic biodegradation.
The literature describes different types of microplastics (MPs), and for this study, the biodegradation actions were investigated for the following types: Polyethylene (PE), Poly-propylene (PP), Polyvinyl chloride (PVC), Polyethylene terephthalate (PET), Polystyrene (PS), High-density polyethylene (HDPE), Low-density polyethylene (LDPE), Polycaprolactone (PCL), Polyhydroxybutyrate (PHB), Polylactic acid (PLA), Bisphenol (Dimethylphenol, BPA), Polyurethane (PU), Biodegradable plastic, Nylon, and Polyvinyl acetate (PVA).
Also for this study, biodegradation analysis actions were investigated: attenuated total reflectance Fourier transform infrared spectroscopy (ATR-FTIR) as well as electron microscope (SEM), Fourier transform infrared spectroscopy (IRS-FTIR), and gas chromatography-mass spectrometry analyses of hydroform (GC-MS), high-performance liquid chromatography (HPLC), atomic force microscopy (AFM), energy dispersive spectroscopy (EDS), nuclear magnetic resonance (NMR), X-ray diffraction (XRD), Fourier transform infrared spectroscopy (FTIR), scanning electron microscopy (SEM), thermogravimetry and differential scanning calorimetry (TG-DSC), nuclear magnetic resonance (NMR), gel permeation chromatography (GPC), thermogravimetric analysis (TGA), universal tensile machine (UTM), atomic force microscope (AFM), immobilized metal ion affinity chromatography (IMAC), size exclusion chromatography (SEC), gel permeation chromatography (GPC), matrix assisted laser desorption/ionization time of flight (MALDI TOF), mobile amorphous fraction (MAF) and determination of dry weight.
A total of 34 different bacterial genera and 63 species were observed. The most frequently found genera were Bacillus (n = 20), Pseudomonas (n = 14), Stenotrophomonas (n = 4), Rhodococcus (n = 3), and their respective species. For the genus Bacillus, the following species were identified: Bacillus sp., B. cereus, B. vallismortis, B. siamensis, B. wiedmannii, B. subtilis, B. niacini, B. paralicheniformis, B. gottheilii, B. brevies, B. pumilus, B. sphericus, and B. amyloliquefaciens. For the genus Pseudomonas, the identified species included Pseudomonas sp., P. fluorescens, P. aeruginosa, P. aestusnigri, P. protegens, P. geniculata, and P. citronellolis. Stenotrophomonas genus included the species Stenotrophomonas sp., S. rhizophila, S. panacihumi, and S. pavanii. For the genus Rhodococcus, the identified species were Rhodococcus sp., R. ruber, and R. rhodochrous.
In the qualitative synthesis regarding the main enzymes found with biodegradation activities, hydrolases and alkane hydroxylase were described as more abundant, especially for the genera Bacillus, Pseudomonas, Rhodococcus, and Ideonella.
Regarding the bias risk from the JBI checklist, it was observed that the majority of responses to the critical appraisal questionnaire from the 68 studies consisted of > 80% “Yes” responses. This indicates that the eligible studies in this investigation had a low risk of bias, meaning they demonstrated high methodological quality.
4 Discussion
The degradation of MPs in the environment is considered an integrated process, involving biological, physical, and chemical actions. Studies have shown that biodegradation has been the most frequent and represents a future perspective for reducing these pollutants in aquatic and terrestrial environments, known as bioremediation (Yuan et al., 2020).
Thus, this work aimed to conduct a literature review on the main bacteria and microbial enzymes involved in the degradation of MPs.
Approximately 80% of commercially marketed plastic materials are obtained from thermoplastic polymers, named for their ability to change from a solid to a viscous state when subjected to high temperatures. The main industrial polymers derived from these thermoplastics and marketed worldwide are Polyethylene, Polypropylene, Polyvinyl chloride, Polyethylene terephthalate, and Polystyrene (Kotova et al., 2021).
This review found that the degradation of MPs through microbial biodegradation can occur in various sediments, including wastewater, landfill deposits, sanitary landfills, sewage residues, soil, among others (Yuan et al., 2020). This occurs because MPs represent a favorable compartment for bacterial colonization and growth, mainly by providing carbon as an energy source (Rujnić-Sokele and Pilipović, 2017). Therefore, studying pure cultures of bacterial isolates is advantageous most of the time, as it enables a controlled analysis of the metabolic pathways of these respective MPs degrading organisms. In this study, the main species and bacterial enzymes (Table 1) involved in this process of MP degradation can be observed, although this data is still not sufficient to understand the entire degradation mechanism (Bacha et al., 2021).
Over the years, an increase in the number of bacterial species with the potential for MP degradation has been observed. The most reported genera are Bacillus, Pseudomonas, Stenotrophomonas, and Rhodococcus (Auta et al., 2018; Wei et al., 2018; Amobonye et al., 2021; Li et al., 2023; Thakur et al., 2023).
The action of these bacteria occurs mainly by forming pores and irregularities on the surfaces of MPs, making them rough with various grooves and fissures, as well as by gaining the ability to adhere, colonize, and damage the MPs (Du et al., 2021; Golmohammadi et al., 2023).
Auta et al. (2017, 2018), used isolates from the genera Rhodococcus sp. and Bacillus sp. and detected a weight reduction of PP by 6.4 and 4.0%, respectively, after a period of just over a month of incubation with the MPs. Additionally, the authors found that the species B. cereus and B. gottheilii showed degradative capacity for PE of 1.6 and 6.2%, for PET of 6.6 and 3.0%, and for PS of 7.4 and 5.8%, respectively. In this perspective, Shimpi et al. (2012) identified a biodegradative capacity of 10% for PS and PLA by the species P. aeruginosa.
The studies demonstrate that these microorganisms not only cause changes in the appearance of MPs but also enable conformational changes in their structures, especially in the functional groups, in addition to reducing the molecular weight and tensile properties, as seen in the work of Yuan et al. (2020) using Stenotrophomonas maltophilia.
Biodegradation of some plastic materials such as PVC and PET is challenging because PVC contains various additives in its composition, such as plasticizers, heat stabilizers, flame retardants, and/or biocides, resulting in a total weight of approximately 50–75% of the final material. PET, due to its high content of aromatic terephthalate elements, limits the mobility of the polymeric chains, making it highly resistant to degradation by bacteria (Kotova et al., 2021).
Thus, it can be observed that the respective studies addressed have shown a more significant effect on the degradation of modified plastics such as PS, PE, and PLA, which can be explained by these plastic materials presenting better biodegradability (Chandra and Singh, 2020; Yuan et al., 2020).
Initially, the biodegradation of MPs by bacteria occurs from the degradation of larger polymer structures to smaller particles, consequently, degradation into oligomers, dimers, and monomers, finally leading to mineralization through microbial biomass. Therefore, this decomposition is aided by a diversity of enzymes that produce intermediate products (Miri et al., 2022).
Such bacterial enzymes with the potential for biodegradation are demonstrated in the studies of Shahnawaz et al. (2019), Taniguchi et al. (2019), and Sol et al. (2020). These studies reinforce that extracellular enzymes are the most studied in the literature, such as esterases, lipases, lignin peroxidases, laccases, depolymerases, cutinases, and manganese peroxidases, as they increase the hydrophilicity of MPs, allowing the conversion of carboxylic and/or alcoholic groups and significantly improving bacterial attachment and the degradation of these compounds.
Thus, the biodegradation of microplastics by bacteria through enzymes is capable of digesting these particles into carbon sources, thus changing the structure, function, molecular weight, etc., making it less toxic to the environment. Therefore, the main products obtained after mineralization by biodegradation of microplastics by bacteria are CO2 and H2O molecules (Anand et al., 2023).
The biodegradation of MPs by bacteria has been significantly reported in several studies as a bioremediation factor for the elimination of these compounds in the environment, as plastic materials have been increasingly used extensively and indiscriminately, causing pollution in terrestrial and aquatic environments and even impacting the public and health due to its cumulative effect. Thus, promising biotechnological techniques such as biodegradation of MPs by bacteria, however, is a challenging approach, given its high cost, since the species of bacteria and their main enzymes involved in the degradation process is still considered a high-quality treatment. Therefore, studies have intensified so that this biotechnological tactic can be incorporated into practice in order to reduce its cost, be reproducible and apply it appropriately on a large scale. Therefore, even though it is a methodology with a future perspective, it is still necessary at present for there to be a worldwide economy of polymers so that it can be directed toward a green and sustainable environmental future.
5 Conclusion
The findings in this investigation highlighted that the genera Bacillus, Pseudomonas, Stenotrophomonas, and Rhodococcus, along with their corresponding species and enzymes—hydroxylases, lipases, proteases, esterases, hydrolases, and laccases—were the main ones reported in the scientific literature regarding the potential for MP biodegradation. This indicates that these microorganisms can act as functional agents in reducing MPs.
Therefore, studies like this emphasize the importance of conducting further research, especially considering the establishment of protocols with experiments under real environmental conditions. This is crucial so that, in the future, the interaction of bacteria with MPs holds practical and biotechnological value on a large scale, aiming to reduce the impacts caused by these compounds in the environment.
Data availability statement
The datasets presented in this study can be found in online repositories. The names of the repository/repositories and accession number(s) can be found below: PubMed, Medline, and LILACS.
Author contributions
MS: Writing – original draft, Writing – review & editing. KS: Formal Analysis, Investigation, Methodology, Validation, Writing – original draft, Writing – review & editing. FM: Writing – original draft, Writing – review & editing. LA: Writing – original draft, Writing – review & editing. RS: Writing – original draft, Writing – review & editing. RB: Writing – original draft, Writing – review & editing. MO: Supervision, Writing – original draft, Writing – review & editing.
Funding
The authors declare financial support was received for the research, authorship, and/or publication of this article. This research was funded by Coordination for the Improvement of Higher Education Personnel (CAPES/Brazil—Proc. no 88887.500819/2020-00).
Acknowledgments
The authors would like to thank Francisco Henrique Santana da Silva for the critical review of the manuscript and translation.
Conflict of interest
The authors declare that the research was conducted in the absence of any commercial or financial relationships that could be construed as a potential conflict of interest.
Publisher’s note
All claims expressed in this article are solely those of the authors and do not necessarily represent those of their affiliated organizations, or those of the publisher, the editors and the reviewers. Any product that may be evaluated in this article, or claim that may be made by its manufacturer, is not guaranteed or endorsed by the publisher.
References
Adaya, L., Millán, M., Peña, C., Jendrossek, D., Espín, G., Tinoco-Valencia, R., et al. (2018). Inactivation of an intracellular poly-3-hydroxybutyrate depolymerase of Azotobacter vinelandii allows to obtain a polymer of uniform high molecular mass. Appl. Microbiol. Biotechnol. 102, 2693–2707. doi: 10.1007/s00253-018-8806-y
Almeida, E. L., Rincón, A. F. C., Jackson, S. A., and Dobson, A. D. (2019). In silico screening and heterologous expression of a polyethylene terephthalate hydrolase (PETase)-like enzyme (SM14est) with polycaprolactone (PCL)-degrading activity, from the marine sponge-derived strain Streptomyces sp. SM14. Front. Microbiol. 10:2187. doi: 10.3389/fmicb.2019.02187
Alshehrei, F. (2017). Biodegradation of synthetic and natural plastic by microorganisms. J. Appl. Environ. Microbiol. 5, 8–19.
Amobonye, A., Bhagwat, P., Singh, S., and Pillai, S. (2021). Plastic biodegradation: frontline microbes and their enzymes. Sci. Total Environ. 759:143536. doi: 10.1016/j.scitotenv.2020.143536
Anand, U., Dey, S., Bontempi, E., Ducoli, S., Vethaak, A. D., Dey, A., et al. (2023). Biotechnological methods to remove microplastics: a review. Environ. Chem. Lett. 21, 1787–1810. doi: 10.1007/s10311-022-01552-4
Asmita, K., Shubhamsingh, T., and Tejashree, S. (2015). Isolation of plastic degrading micro-organisms from soil samples collected at various locations in Mumbai, India. Int. Res. J Environ. Sci. 4, 77–85.
Auta, H. S., Emenike, C. U., Jayanthi, B., and Fauziah, S. H. (2018). Growth kinetics and biodeterioration of polypropylene microplastics by Bacillus sp. and Rhodococcus sp. isolated from mangrove sediment. Mar. Pollut. Bull. 127, 15–21. doi: 10.1016/j.marpolbul.2017.11.036
Auta, S. H., Emenike, C. U., and Fauziah, S. H. (2017). Screening for polypropylene degradation potential of bacteria isolated from mangrove ecosystems in Peninsular Malaysia. IJBBB 7, 245–251. doi: 10.17706/ijbbb.2017.7.4.245-251
Awasthi, S., Srivastava, P., Singh, P., Tiwary, D., and Mishra, P. K. (2017). Biodegradation of thermally treated high-density polyethylene (HDPE) by Klebsiella pneumoniae CH001. 3 Biotech 7:332. doi: 10.1007/s13205-017-0959-3
Bacha, A. U. R., Nabi, I., and Zhang, L. (2021). Mechanisms and the engineering approaches for the degradation of microplastics. Acs EST Eng. 1, 1481–1501. doi: 10.1021/acsestengg.1c00216
Balasubramanian, V., Natarajan, K., Hemambika, B., Ramesh, N., Sumathi, C. S., Kottaimuthu, R., et al. (2010). High-density polyethylene (HDPE)-degrading potential bacteria from marine ecosystem of Gulf of Mannar. India. Lett. Appl. Microbiol. 51, 205–211. doi: 10.1111/j.1472-765X.2010.02883.x
Bardají, D. K. R., Furlan, J. P. R., and Stehling, E. G. (2019). Isolation of a polyethylene degrading Paenibacillus sp. from a landfill in Brazil. Arch. Microbiol. 201, 699–704. doi: 10.1007/s00203-019-01637-9
Bhatia, M., Girdhar, A., Tiwari, A., and Nayarisseri, A. (2014). Implications of a novel Pseudomonas species on low density polyethylene biodegradation: an in vitro to in silico approach. SpringerPlus 3, 1–10. doi: 10.1186/2193-1801-3-497
Bian, H., Cao, M., Wen, H., Tan, Z., Jia, S., and Cui, J. (2019). Biodegradation of polyvinyl alcohol using cross-linked enzyme aggregates of degrading enzymes from Bacillus niacini. Int. J. Biol. Macromol. 124, 10–16. doi: 10.1016/j.ijbiomac.2018.11.204
Bollinger, A., Thies, S., Knieps-Grünhagen, E., Gertzen, C., Kobus, S., Höppner, A., et al. (2020). A novel polyester hydrolase from the marine bacterium Pseudomonas aestusnigri–structural and functional insights. Front. Microbiol. 11:114. doi: 10.3389/fmicb.2020.00114
Brandon, A. M., Gao, S. H., Tian, R., Ning, D., Yang, S. S., Zhou, J., et al. (2018). Biodegradation of polyethylene and plastic mixtures in mealworms (larvae of Tenebrio molitor) and effects on the gut microbiome. Environ. Sci. Technol. 52, 6526–6533. doi: 10.1021/acs.est.8b02301
Bubpachat, T., Sombatsompop, N., and Prapagdee, B. (2018). Isolation and role of polylactic acid-degrading bacteria on degrading enzymes productions and PLA biodegradability at mesophilic conditions. Poly. Degradation Stabil. 152, 75–85. doi: 10.1016/j.polymdegradstab.2018.03.023
Cai, Z., Li, M., Zhu, Z., Wang, X., Huang, Y., Li, T., et al. (2023). Biological degradation of plastics and microplastics: a recent perspective on associated mechanisms and influencing factors. Microorganisms 11:1661. doi: 10.3390/microorganisms11071661
Chandra, P., and Singh, D. P. (2020). “Microplastic degradation by bacteria in aquatic ecosystem,” in Microorganisms for Sustainable Environment and Health, eds P. Chowdhary, A. Raj, D. Y. Verma, and Y. Akhter (Amsterdam: Elsevier). doi: 10.1016/B978-0-12-819001-2.00022-X
Cverenkárová, K., Valachovičová, M., Mackul’ak, T., Žemlička, L., and Bírošová, L. (2021). Microplastics in the food chain. Life 11:1349. doi: 10.3390/life11121349
Das, M. P., and Kumar, S. (2013). Influence of cell surface hydrophobicity in colonization and biofilm formation on LDPE biodegradation. Int. J. Pharm. Pharm. Sci. 5, 690–694.
Delacuvellerie, A., Cyriaque, V., Gobert, S., Benali, S., and Wattiez, R. (2019). The plastisphere in marine ecosystem hosts potential specific microbial degraders including Alcanivorax borkumensis as a key player for the low-density polyethylene degradation. J. Hazardous Mater. 380:120899. doi: 10.1016/j.jhazmat.2019.120899
Du, H., Xie, Y., and Wang, J. (2021). Microplastic degradation methods and corresponding degradation mechanism: research status and future perspectives. J. Hazardous Mater. 418:126377. doi: 10.1016/j.jhazmat.2021.126377
Esmaeili, A., Pourbabaee, A. A., Alikhani, H. A., Shabani, F., and Esmaeili, E. (2013). Biodegradation of low-density polyethylene (LDPE) by mixed culture of Lysinibacillus xylanilyticus and Aspergillus niger in soil. PLoS One 8:e71720. doi: 10.1371/journal.pone.0071720
Fontanella, S., Bonhomme, S., Koutny, M., Husarova, L., Brusson, J. M., Courdavault, J. P., et al. (2010). Comparison of the biodegradability of various polyethylene films containing pro-oxidant additives. Poly. Degradation Stabil. 95, 1011–1021. doi: 10.1016/j.polymdegradstab.2010.03.009
Giacomucci, L., Raddadi, N., Soccio, M., Lotti, N., and Fava, F. (2020). Biodegradation of polyvinyl chloride plastic films by enriched anaerobic marine consortia. Mar. Environ. Res. 158:104949. doi: 10.1016/j.marenvres.2020.104949
Golmohammadi, M., Musavi, S. F., Habibi, M., Maleki, R., Golgoli, M., Zargar, M., et al. (2023). Molecular mechanisms of microplastics degradation: a review. Separation Purification Technol. 309:122906. doi: 10.1016/j.seppur.2022.122906
Habib, S., Iruthayam, A., Abd Shukor, M. Y., Alias, S. A., Smykla, J., and Yasid, N. A. (2020). Biodeterioration of untreated polypropylene microplastic particles by Antarctic bacteria. Polymers 12:2616. doi: 10.3390/polym12112616
Hadad, D., Geresh, S., and Sivan, A. (2005). Biodegradation of polyethylene by the thermophilic bacterium Brevibacillus borstelensis. J. Appl. Microbiol. 98, 1093–1100. doi: 10.1111/j.1365-2672.2005.02553.x
Harshvardhan, K., and Jha, B. (2013). Biodegradation of low-density polyethylene by marine bacteria from pelagic waters, Arabian Sea, India. Mar. Pollut. Bull. 77, 100–106. doi: 10.1016/j.marpolbul.2013.10.025
Hsu, K. J., Tseng, M., Don, T. M., and Yang, M. K. (2012). Biodegradation of poly (β-hydroxybutyrate) by a novel isolate of Streptomyces bangladeshensis 77T-4. Bot. Stud. 53, 307–313.
Hung, C. S., Zingarelli, S., Nadeau, L. J., Biffinger, J. C., Drake, C. A., Crouch, A. L., et al. (2016). Carbon catabolite repression and Impranil polyurethane degradation in Pseudomonas protegens strain Pf-5. Appl. Environ. Microbiol. 82, 6080–6090. doi: 10.1128/AEM.01448-16
Jeon, H. J., and Kim, M. N. (2015). Functional analysis of alkane hydroxylase system derived from Pseudomonas aeruginosa E7 for low molecular weight polyethylene biodegradation. Int. Biodeterioration Biodegr. 103, 141–146. doi: 10.1016/j.ibiod.2015.04.024
Jeon, J. M., Park, S. J., Choi, T. R., Park, J. H., Yang, Y. H., and Yoon, J. J. (2021). Biodegradation of polyethylene and polypropylene by Lysinibacillus species JJY0216 isolated from soil grove. Poly. Degradation Stabil. 191:109662. doi: 10.1016/j.polymdegradstab.2021.109662
Jeon, Y. D., Jeong, W. Y., Kim, M. H., Jung, I. Y., Ahn, M. Y., Ann, H. W., et al. (2016). Risk factors for mortality in patients with Stenotrophomonas maltophilia bacteremia. Medicine 95:e4375. doi: 10.1097/MD.0000000000004375
Kotova, I. B., Taktarova, Y. V., Tsavkelova, E. A., Egorova, M. A., Bubnov, I. A., Malakhova, D. V., et al. (2021). Microbial degradation of plastics and approaches to make it more efficient. Microbiology 90, 671–701. doi: 10.1134/S0026261721060084
Kowalczyk, A., Chyc, M., Ryszka, P., and Latowski, D. (2016). Achromobacter xylosoxidans as a new microorganism strain colonizing high-density polyethylene as a key step to its biodegradation. Environ. Sci. Pollut. Res. 23, 11349–11356. doi: 10.1007/s11356-016-6563-y
Kumar, A. G., Hinduja, M., Sujitha, K., Rajan, N. N., and Dharani, G. (2021). Biodegradation of polystyrene by deep-sea Bacillus paralicheniformis G1 and genome analysis. Sci. Total Environ. 774:145002. doi: 10.1016/j.scitotenv.2021.145002
Kuroki, R., Kawakami, K., Qin, L., Kaji, C., Watanabe, K., Kimura, Y., et al. (2009). Nosocomial bacteremia caused by biofilm-forming Bacillus cereus and Bacillus thuringiensis. Int. Med. 48, 791–796. doi: 10.2169/internalmedicine.48.1885
Li, C., Cui, Q., Li, Y., Zhang, K., Lu, X., and Zhang, Y. (2022). Effect of LDPE and biodegradable PBAT primary microplastics on bacterial community after four months of soil incubation. J. Hazardous Mater. 429:128353. doi: 10.1016/j.jhazmat.2022.128353
Li, S., Yang, Y., Yang, S., Zheng, H., Zheng, Y., Jun, M., et al. (2023). Recent advances in biodegradation of emerging contaminants-microplastics (MPs): feasibility, mechanism, and future prospects. Chemosphere 331:138776. doi: 10.1016/j.chemosphere.2023.138776
Liu, C., Shi, C., Zhu, S., Wei, R., and Yin, C. C. (2019). Structural and functional characterization of polyethylene terephthalate hydrolase from Ideonella sakaiensis. Biochem. Biophys. Res. Commun. 508, 289–294. doi: 10.1016/j.bbrc.2018.11.148
Lockwood, C., Porrit, K., Munn, Z., Rittenmeyer, L., Salmond, S., Bjerrum, M., et al. (2017). “Chapter 2: Systematic reviews of qualitative evidence,” in Joanna Briggs Institute Reviewer’s Manual. The Joanna Briggs Institute, eds E. Aromataris and Z. Munn (JBI). doi: 10.46658/JBIMES-20-03
Lou, H., Fu, R., Long, T., Fan, B., Guo, C., Li, L., et al. (2022). Biodegradation of polyethylene by Meyerozyma guilliermondii and Serratia marcescens isolated from the gut of waxworms (larvae of Plodia interpunctella). Sci. Total Environ. 853:158604. doi: 10.1016/j.scitotenv.2022.158604
Maroof, L., Khan, I., Yoo, H. S., Kim, S., Park, H. T., Ahmad, B., et al. (2021). Identification and characterization of low density polyethylene-degrading bacteria isolated from soils of waste disposal sites. Environ. Eng. Res. 26:2020167. doi: 10.4491/eer.2020.167
Masaki, K., Kamini, N. R., Ikeda, H., and Iefuji, H. (2005). Cutinase-like enzyme from the yeast Cryptococcus sp. strain S-2 hydrolyzes polylactic acid and other biodegradable plastics. Appl. Environ. Microbiol. 71, 7548–7550. doi: 10.1128/AEM.71.11.7548-7550.2005
Matsumura, Y., Hosokawa, C., Sasaki-Mori, M., Akahira, A., Fukunaga, K., Ikeuchi, T., et al. (2009). Isolation and characterization of novel bisphenol-A-degrading bacteria from soils. Biocontrol Sci. 14, 161–169. doi: 10.4265/bio.14.161
Mehmood, C. T., Qazi, I. A., Hashmi, I., Bhargava, S., and Deepa, S. (2016). Biodegradation of low density polyethylene (LDPE) modified with dye sensitized titania and starch blend using Stenotrophomonas pavanii. Int. Biodeterior. Biodegradation 113, 276–286. doi: 10.1016/j.ibiod.2016.01.025
Miri, S., Saini, R., Davoodi, S. M., Pulicharla, R., Brar, S. K., and Magdouli, S. (2022). Biodegradation of microplastics: better late than never. Chemosphere 286:131670. doi: 10.1016/j.chemosphere.2021.131670
Montazer, Z., Habibi Najafi, M. B., and Levin, D. B. (2019). Microbial degradation of low-density polyethylene and synthesis of polyhydroxyalkanoate polymers. Can. J. Microbiol. 65, 224–234. doi: 10.1139/cjm-2018-0335
Mor, R., and Sivan, A. (2008). Biofilm formation and partial biodegradation of polystyrene by the actinomycete Rhodococcus ruber: biodegradation of polystyrene. Biodegradation 19, 851–858. doi: 10.1007/s10532-008-9188-0
Muhonja, C. N., Makonde, H., Magoma, G., and Imbuga, M. (2018). Biodegradability of polyethylene by bacteria and fungi from Dandora dumpsite Nairobi-Kenya. PLoS One 13:e0198446. doi: 10.1371/journal.pone.0198446
Mukherjee, S., Chowdhuri, U. R., and Kundu, P. P. (2016). Bio-degradation of polyethylene waste by simultaneous use of two bacteria: Bacillus licheniformis for production of bio-surfactant and Lysinibacillus fusiformis for bio-degradation. RSC Adv. 6, 2982–2992. doi: 10.1039/C5RA25128A
Niu, L., Chen, Y., Li, Y., Wang, Y., Shen, J., Wang, L., et al. (2023). Diversity, abundance and distribution characteristics of potential polyethylene and polypropylene microplastic degradation bacterial communities in the urban river. Water Res. 232:119704. doi: 10.1016/j.watres.2023.119704
Nowak, B., Pająk, J., Drozd-Bratkowicz, M., and Rymarz, G. (2011). Microorganisms participating in the biodegradation of modified polyethylene films in different soils under laboratory conditions. Int. Biodeterioration Biodegr. 65, 757–767. doi: 10.1016/j.ibiod.2011.04.007
Oceguera-Cervantes, A., Carrillo-García, A., López, N., Bolanos-Nunez, S., Cruz-Gómez, M. J., Wacher, C., et al. (2007). Characterization of the polyurethanolytic activity of two Alicycliphilus sp. strains able to degrade polyurethane and N-methylpyrrolidone. Appl. Environ. Microbiol. 73, 6214–6223. doi: 10.1128/AEM.01230-07
Oda, Y., Oida, N., Urakami, T., and Tonomura, K. (1997). Polycaprolactone depolymerase produced by the bacterium Alcaligenes faecalis. FEMS Microbiol. Lett. 152, 339–343. doi: 10.1111/j.1574-6968.1997.tb10449.x
Oliya, P., Singh, S., Goel, N., Singh, U. P., and Srivastava, A. K. (2020). Polypropylene degradation potential of microbes isolated from solid waste dumping site. Pollut. Res. 39, 268–277.
Osman, A. I., Hosny, M., Eltaweil, A. S., Omar, S., Elgarahy, A. M., Farghali, M., et al. (2023). Microplastic sources, formation, toxicity and remediation: a review. Environ. Chem. Lett. 21, 2129–2169. doi: 10.1007/s10311-023-01593-3
Palm, G. J., Reisky, L., Böttcher, D., Müller, H., Michels, E. A., Walczak, M. C., et al. (2019). Structure of the plastic-degrading Ideonella sakaiensis MHETase bound to a substrate. Nat. Commun. 10:1717. doi: 10.1038/s41467-019-09326-3
Park, S. H., Kwon, S., Lee, C. W., Kim, C. M., Jeong, C. S., Kim, K. J., et al. (2019). Crystal structure and functional characterization of a xylose isomerase (PbXI) from the Psychrophilic Soil Microorganism, Paenibacillus sp. J. Microbiol. Biotechnol. 29, 244–255. doi: 10.4014/jmb.1810.10057
Park, S. Y., and Kim, C. G. (2019). Biodegradation of micro-polyethylene particles by bacterial colonization of a mixed microbial consortium isolated from a landfill site. Chemosphere 222, 527–533. doi: 10.1016/j.chemosphere.2019.01.159
Parthasarathy, A., Miranda, R. R., Eddingsaas, N. C., Chu, J., Freezman, I. M., Tyler, A. C., et al. (2022). Polystyrene degradation by Exiguobacterium sp. RIT 594: preliminary evidence for a pathway containing an atypical oxygenase. Microorganisms 10:1619. doi: 10.3390/microorganisms10081619
Pathak, V. M. (2023). Exploitation of bacterial strains for microplastics (LDPE) biodegradation. Chemosphere 316:137845. doi: 10.1016/j.chemosphere.2023.137845
Rajandas, H., Parimannan, S., Sathasivam, K., Ravichandran, M., and Yin, L. S. (2012). A novel FTIR-ATR spectroscopy based technique for the estimation of low-density polyethylene biodegradation. Poly. Testing 31, 1094–1099. doi: 10.1016/j.polymertesting.2012.07.015
Roy, P. K., Titus, S., Surekha, P., Tulsi, E., Deshmukh, C., and Rajagopal, C. (2008). Degradation of abiotically aged LDPE films containing pro-oxidant by bacterial consortium. Poly. Degradation Stabil. 93, 1917–1922. doi: 10.1016/j.polymdegradstab.2008.07.016
Rujnić-Sokele, M., and Pilipović, A. (2017). Challenges and opportunities of biodegradable plastics: a mini review. Waste Manag. Res. 35, 132–140. doi: 10.1177/0734242X16683272
Sameshima-Yamashita, Y., Ueda, H., Koitabashi, M., and Kitamoto, H. (2019). Pretreatment with an esterase from the yeast Pseudozyma antarctica accelerates biodegradation of plastic mulch film in soil under laboratory conditions. J. Biosci. Bioeng. 127, 93–98. doi: 10.1016/j.jbiosc.2018.06.011
Santo, M., Weitsman, R., and Sivan, A. (2013). The role of the copper-binding enzyme–laccase–in the biodegradation of polyethylene by the actinomycete Rhodococcus ruber. Int. Biodeterioration Biodegr. 84, 204–210. doi: 10.1016/j.ibiod.2012.03.001
Sarkhel, R., Sengupta, S., Das, P., and Bhowal, A. (2020). Comparative biodegradation study of polymer from plastic bottle waste using novel isolated bacteria and fungi from marine source. J. Poly. Res. 27, 1–8. doi: 10.1007/s10965-019-1973-4
Sarmah, P., and Rout, J. (2018). Phytochemical screening and antioxidant activity of a cyanobacterium, Oscillatoria limosa isolated from polythene surface in domestic sewage water. J. Algal Biomass Utilization 9, 48–54.
Satlewal, A., Soni, R., Zaidi, M. G. H., Shouche, Y., and Goel, R. (2008). Comparative biodegradation of HDPE and LDPE using an indigenously developed microbial consortium. J. Microbiol. Biotechnol. 18, 477–482.
Shah, Z., Krumholz, L., Aktas, D. F., Hasan, F., Khattak, M., and Shah, A. A. (2013). Degradation of polyester polyurethane by a newly isolated soil bacterium, Bacillus subtilis strain MZA-75. Biodegradation 24, 865–877. doi: 10.1007/s10532-013-9634-5
Shahnawaz, M., Sangale, M. K., and Ade, A. B. (2019). Bioremediation Technology for Plastic Waste. Berlin: Springer. doi: 10.1007/978-981-13-7492-0
Shimpi, N., Borane, M., Mishra, S., and Kadam, M. (2012). Biodegradation of polystyrene (PS)-poly (lactic acid)(PLA) nanocomposites using Pseudomonas aeruginosa. Macromol. Res. 20, 181–187. doi: 10.1007/s13233-012-0026-1
Skariyachan, S., Patil, A. A., Shankar, A., Manjunath, M., Bachappanavar, N., and Kiran, S. (2018). Enhanced polymer degradation of polyethylene and polypropylene by novel thermophilic consortia of Brevibacillus sps. and Aneurinibacillus sp. screened from waste management landfills and sewage treatment plants. Poly. Degradation Stability 149, 52–68. doi: 10.1016/j.polymdegradstab.2018.01.018
Sol, D., Laca, A., Laca, A., and Díaz, M. (2020). Approaching the environmental problem of microplastics: importance of WWTP treatments. Sci. Total Environ. 740:140016. doi: 10.1016/j.scitotenv.2020.140016
Sudhakar, M., Doble, M., Murthy, P. S., and Venkatesan, R. (2008). Marine microbe-mediated biodegradation of low-and high-density polyethylenes. Int. Biodeterioration Biodegradation 61, 203–213. doi: 10.1016/j.ibiod.2007.07.011
Tachibana, K., Hashimoto, K., Yoshikawa, M., and Okawa, H. (2010). Isolation and characterization of microorganisms degrading nylon 4 in the composted soil. Poly. Degradation Stabil. 95, 912–917. doi: 10.1016/j.polymdegradstab.2010.03.031
Taghavi, N., Singhal, N., Zhuang, W. Q., and Baroutian, S. (2021). Degradation of plastic waste using stimulated and naturally occurring microbial strains. Chemosphere 263:127975. doi: 10.1016/j.chemosphere.2020.127975
Tamnou, E. B. M., Arfao, A. T., Nougang, M. E., Metsopkeng, C. S., Ewoti, O. V. N., Moungang, L. M., et al. (2021). Biodegradation of polyethylene by the bacterium Pseudomonas aeruginosa in acidic aquatic microcosm and effect of the environmental temperature. Environ. Challenges 3:100056. doi: 10.1016/j.envc.2021.100056
Tanasupawat, S., Takehana, T., Yoshida, S., Hiraga, K., and Oda, K. (2016). Ideonella sakaiensis sp. nov., isolated from a microbial consortium that degrades poly (ethylene terephthalate). Int. J. Syst. Evol. Microbiol. 66, 2813–2818. doi: 10.1099/ijsem.0.001058
Taniguchi, I., Yoshida, S., Hiraga, K., Miyamoto, K., Kimura, Y., and Oda, K. (2019). Biodegradation of PET: current status and application aspects. Acs Catalysis 9, 4089–4105. doi: 10.1021/acscatal.8b05171
Thakur, B., Singh, J., Singh, J., Angmo, D., and Vig, A. P. (2023). Biodegradation of different types of microplastics: molecular mechanism and degradation efficiency. Sci. Total Environ. 877:162912. doi: 10.1016/j.scitotenv.2023.162912
Thomas, B. T., Olanrewaju-Kehinde, D. S. K., Popoola, O. D., and James, E. S. (2015). Degradation of plastic and polythene materials by some selected microorganisms isolated from soil. World Appl. Sci. J. 33, 1888–1891.
Torena, P., Alvarez-Cuenca, M., and Reza, M. (2021). Biodegradation of polyethylene terephthalate microplastics by bacterial communities from activated sludge. Can. J. Chem. Eng. 99, S69–S82. doi: 10.1002/cjce.24015
Tribedi, P., and Sil, A. K. (2013). Bioaugmentation of polyethylene succinate-contaminated soil with Pseudomonas sp. AKS2 results in increased microbial activity and better polymer degradation. Environ. Sci. Pollut. Res. 20, 1318–1326. doi: 10.1007/s11356-012-1080-0
Villalobos, N. F., Costa, M. C., and Marín-Beltrán, I. (2022). A community of marine bacteria with potential to biodegrade petroleum-based and biobased microplastics. Mar. Pollut. Bull. 185:114251. doi: 10.1016/j.marpolbul.2022.114251
Wei, R., Breite, D., Song, C., Gräsing, D., Ploss, T., Hille, P., et al. (2019). Biocatalytic degradation efficiency of postconsumer polyethylene terephthalate packaging determined by their polymer microstructures. Adv. Sci. 6:1900491. doi: 10.1002/advs.201900491
Wei, Y., Fu, J., Wu, J., Jia, X., Zhou, Y., Li, C., et al. (2018). Bioinformatics analysis and characterization of highly efficient polyvinyl alcohol (PVA)-degrading enzymes from the novel PVA degrader Stenotrophomonas rhizophila QL-P4. Appl. Environ. Microbiol. 84:e01898-17. doi: 10.1128/AEM.01898-17
Wilkes, R. A., and Aristilde, L. (2017). Degradation and metabolism of synthetic plastics and associated products by Pseudomonas sp.: capabilities and challenges. J. Appl. Microbiol. 123, 582–593. doi: 10.1111/jam.13472
Xiong, L. B., Liu, H. H., Song, X. W., Meng, X. G., Liu, X. Z., Ji, Y. Q., et al. (2020). Improving the biotransformation of phytosterols to 9α-hydroxy-4-androstene-3, 17-dione by deleting embC associated with the assembly of cell envelope in Mycobacterium neoaurum. J. Biotechnol. 323, 341–346. doi: 10.1016/j.jbiotec.2020.09.019
Yoon, M. G., Jeon, H. J., and Kim, M. N. (2012). Biodegradation of polyethylene by a soil bacterium and AlkB cloned recombinant cell. J. Bioremed. Biodegrad. 3, 1–8.
Yuan, J., Ma, J., Sun, Y., Zhou, T., Zhao, Y., and Yu, F. (2020). Microbial degradation and other environmental aspects of microplastics/plastics. Sci. Total Environ. 715:136968. doi: 10.1016/j.scitotenv.2020.136968
Keywords: bacteria, bioremediation, synthetic polymers, microorganisms, xenobiotic
Citation: da Silva MRF, Souza KS, Motteran F, de Araújo LCA, Singh R, Bhadouria R and de Oliveira MBM (2024) Exploring biodegradative efficiency: a systematic review on the main microplastic-degrading bacteria. Front. Microbiol. 15:1360844. doi: 10.3389/fmicb.2024.1360844
Received: 24 December 2023; Accepted: 14 February 2024;
Published: 18 March 2024.
Edited by:
Ranjith Kumavath, Pondicherry University, IndiaReviewed by:
Shrikant D. Khandare, National Institute of Ocean Technology, IndiaSurjit Singh, Sister Nivedita University, India
Copyright © 2024 da Silva, Souza, Motteran, de Araújo, Singh, Bhadouria and de Oliveira. This is an open-access article distributed under the terms of the Creative Commons Attribution License (CC BY). The use, distribution or reproduction in other forums is permitted, provided the original author(s) and the copyright owner(s) are credited and that the original publication in this journal is cited, in accordance with accepted academic practice. No use, distribution or reproduction is permitted which does not comply with these terms.
*Correspondence: Milena Roberta Freire da Silva, bWlsZW5hLmZyZWlyZUB1ZnBlLmJy