- 1Department of Plant Pathology, Faculty of Agriculture Sciences, Quaid-e-Azam Campus, University of the Punjab, Lahore, Pakistan
- 2Botany and Microbiology Department, College of Science, King Saud University, Riyadh, Saudi Arabia
- 3Facultad de Ciencias Agrotecnológicas, Universidad Autónoma de Chihuahua, Chihuahua, Mexico
- 4Plant Production Department, College of Food and Agricultural Sciences, King Saud University, Riyadh, Saudi Arabia
Soil degradation has been accelerated by the use of chemical pesticides and poor agricultural practices, which has had an impact on crop productivity. Recently, there has been a lot of interest in the use of eco-friendly biochar applications to enhance soil quality and sequester carbon in sustainable agriculture. This study aimed to determine the individual and combined effects of Leaf Waste Biochar (LWB) and the bio-control agent Trichoderma harzianum (BCA) on the development of bacterial wilt in eggplants (Solanum melongena) caused by Ralstonia solanacearum (RS). The effects of LWB and BCA on eggplant physiology and defense-related biochemistry were comprehensively examined. Inoculated (+RS) and un-inoculated (–RS) eggplants were grown in potting mixtures containing 3% and 6% (v/v) LWB, both with and without BCA. The percentage disease index was considerably reduced (90%) in plants grown in the 6% LWB+ BCA amended treatments. Moreover, the plants grown in LWB and inoculated with BCA had higher phenolics, flavonoids and peroxidase contents compared to the non-amended control. The level of NPK was significantly increased (92.74% N, 76.47% P, 53.73% K) in the eggplants cultivated in the 6% LWB + BCA composition. This study has shown that the association of T. harzianum with biochar improved plant growth and reduced R. solanacearum induced wilt. Furthermore, the combined impact of biochar and T. harzianum was greater in terms of wilt suppression and increase in plant physiological measurements when the biochar concentration was 6%. Biochar and bio-control agents triggered biochemical alterations, thus enhancing the management of disease-infested soils.
1 Introduction
Although global food production has increased dramatically in recent decades, approximately one billion people still suffer from malnutrition (Semida et al., 2019). There is a growing global interest in decreasing food losses and wastage to improve food security (Alexander et al., 2017). Plant diseases, a major cause of crop losses, pose a significant risk to global food security and account for 10 to 16% of annual crop losses (Bag et al., 2017). Phyto-pathogenic diseases continue to threaten Pakistan's economy, causing billions of dollars in losses (Ali et al., 2018).
Solanum melongena L., commonly known as eggplant, brinjal, and aubergine, belongs to the Solanaceae family. This family renowned for its nutritional advantages due to the rich presence of bioactive substances, including vitamins, minerals, phenolics, dry matter content, and macronutrients. The primary source of eggplant's health benefits appears to be the secondary metabolites, which include glycoalkaloids, antioxidant chemicals, and vitamins (Saha et al., 2023). Recent reports have shown a strong link between the frequent consumption of phytochemicals and disease prevention (Sharma and Kaushik, 2021). Ralstonia solanacearum is known to have a significant impact on economically important crops, such as potato, tomato, tobacco, chili, and eggplant, by causing wilt. The bacterial wilt is most prevalent during the vegetative growth stage (Hussain and Abid, 2011). This disease is one of the major causes of significantly low eggplants yields in Pakistan; therefore, control measures should be adopted to prevent the spread and severity of diseases. In different parts of the world, the causal agent of bacterial wilt is responsible for massive losses in a variety of plants, particularly those belonging to the Solanaceae family (Kongkiattikajorn and Thepa, 2007). It is estimated that Ralstonia solanacearum costs the global economy approximately $1 billion annually (Champoiseau et al., 2009). Biochar, a carbon-rich product, is produced from organic feedstock using thermal combustion techniques with limited oxygen (Širić et al., 2023). The International Biochar project claims that biochar is a pyrolysis by-product with a high carbon composition. Biomass is pyrolyzed under anaerobic (oxygen-controlled) conditions to produce biochar. Pyrolysis involves heating a variety of organic wastes to temperatures ranging from 200 to 900°C (Širić et al., 2022). The application of Biochar results in plant development and the reduction of plant diseases (Elad et al., 2011). Biochar improves water retention, enzymatic activity, and cation exchange capacity in soil, reducing salinity stress and promoting the growth of plants and the survival of beneficial microbes (Patel et al., 2017). According to Graber et al. (2010), the population of Trichoderma spp. increased in soils receiving biochar compared to control soils. Hu et al. (2014) also documented alterations in the fungal community after applying biochar to the soil, showing a proportion of Trichoderma spp. that was 14.5 % greater than in soil that had not been amended with biochar.
Trichoderma spp. are beneficial fungi that act as bio-control agents against plant pathogens and promote plant development (da Silva et al., 2016). The fungal symbiosis increases the surface area of roots, leading to increased absorption and subsequently enhancing resistance to plant pathogens (Oskiera et al., 2017). Trichoderma also promotes soil preservation by boosting seed germination rates, reducing the effects of abiotic interference, and building a systemic defense that improving soil productivity (Das et al., 2022). Studies have demonstrated the advantages of applying biochar to soil. Patel et al. (2017) use Trichoderma as a tool to encourage plant growth and biological control. Trichoderma thrives in soil with an abundance of organic materials. The application of Trichoderma harzianum in conjunction with organic waste resulted in higher levels of accessible Phosphorus (P) and promoted soil carbon fixation (Khomari et al., 2018). According to Muter et al. (2017), the treatment of biochar together with Trichoderma improved the germination of maize seeds and biomass production.
To protect the environment from toxic agrochemicals and crops from phytopathogen-induced damages, it is necessary to devise new disease management strategies. Therefore, this research was conducted to investigate the relationship between biochar and T. harzianum while considering the plant response against invading Ralstonia solanacearum. Additionally, it would be beneficial to understand the synergistic potential of biochar and BCA on the growth and defense-related biochemical parameters of eggplant.
2 Materials and methods
2.1 Biochar production
The leaves of Syzygium cumini were pyrolyzed at 450°C to create leaf waste biochar (LWB). TLUD (Top-lit Updraft), the portable kiln technique, was used to make biochar with a few modifications (Aftab et al., 2022). The primary burner is a large drum filled with leaf waste. Airflow is controlled by creating holes in the base of the primary burner and using an adapter and chimney. Bricks were used to elevate the burner allow air to enter through the holes. After pyrolysis, tap water was sprayed on the biochar to reduce the temperature, and a sieve was used to powder the biochar.
2.2 Soil preparation and experimental plan
The BD (bulk density) of the formalin-sterilized soil was 1.30 g/cm−3. The soil substrate was classified as sandy loam, containing 5% clay, 41% silt, and 52.2% sand (>63 mm) (Rasool et al., 2021). Different volumetric concentrations (3% and 6%) of biochar were used as soil amendments either inoculated or uninoculated with R. solanacearum in the experimental setup. The experimental setup consisted of the following treatment plan: (a) only soil, (b) 3% leaf waste biochar (LWB), and (c) 6% LWB amended soil substrate for eggplant cultivation. The plants were either inoculated with or without the bio-control agent Trichoderma harzianum (+BCA and –BCA, respectively), as well as infected (+RS) with Ralstonia solanacearum or remained un-inoculated (–RS). Each treatment had 10 replicates assigned to it in a completely randomized (CRD) experimental design. The characteristics of soil, leaf waste biochar and compost are shown in Table 1.
2.3 Isolation of pathogen and acquisition of Trichoderma harzianum
Approximately 10 cm long wilted plant stem segments were chopped into small pieces after being superficially cleaned with 70% ethanol. The cut pieces were then shaken for 5 min at room temperature with 5 sterilized distilled water. Each bacterial suspension was streaked separately onto the bacterial growth medium, ensuring even distribution, and incubated at 28°C for 24 h (Hugh and Leifson, 1953). To get pure cultures, one colony that appeared smooth, circular and dirty white from each bacterial culture isolated from the soil and stem was re-streaked on nutrient agar medium in a sterilized environment. The pathogen inoculum was prepared by stirring bacterial cells in LB broth at 37°C for 48 h (Kim et al., 2016). The Trichoderma harzianum culture was provided by the First Fungal Culture Bank of Pakistan (FCBP), Department of Plant Pathology, University of the Punjab (FCBP-SF-1277), w in collaboration with the World Culture Collection Center. Malt extracts agar (MEA) was used for the multiplication of Trichoderma spp. (Sunesson et al., 1995).
2.4 Ralstonia solanacearum inoculum preparation
The inoculum of R. solanacearum was prepared by stirring bacterial cells in LB broth at 37°C for 48 h (Kim et al., 2016). The final bacterial culture concentration was adjusted to 108 CFU/mL (OD600 = 0.8) for inoculation. The concentration was adjusted by calculating the optical density using a spectrophotometer.
2.5 Application of bio-control agent (T. harzianum)
Trichoderma harzianum was cultured on MEA media plates at 25°C for 7 days to create a conidial suspension. The conidia were collected from the surface of the plates and then suspended in 0.05% Tween 20 after being cleaned with sterile distilled water. The fungal spore count was increased to 105 spores per mL (Khaledi and Taheri, 2016). The eggplant seeds were surface-sterilized using 50% commercial bleach (3.8% NaOCl) (Davoudpour et al., 2020). The seeds were then submerged in a 10 mL suspension of 105 mL−1 spores, agitated with an electric shaker for 0.5 h at room temperature, and allowed to air dry in a laminar flow hood. Methyl cellulose was used to keep the conidia attached to the seed surface. Methylcellulose is typically used as a sticker in bio-control agent formulations at a rate of 2.5% (Larena et al., 2010), and it was mixed with the conidial suspension. Additionally, methylcellulose acts as a shield, protecting the bio-control agent from abrasive physical or chemical treatment. For the soil application of the bio-control agent Trichoderma spore suspension, it was applied to already prepared biochar mixed soil pots (Khaledi and Taheri, 2016).
2.6 Inoculation of pathogen
The roots of eggplant seedlings were soaked in a bacterial culture for up to 3 min before being planted in pots (Yanti et al., 2017).
2.7 Eggplant growth assessment
Agronomic plant physiological variables, such as plant height, root length, above- and below-ground biomass dry weights, were measured 40 days after inoculation. The separated eggplant stems and roots were dried in a 70°C oven until they reached a constant weight (Carter et al., 1994).
2.8 Biochemical analysis of eggplant
Total phenolic contents were calculated using the modified Folin-Ciocalteu technique (Singleton and Rossi, 1965). The extracts (1 mL) were mixed with Na2CO3, the Folin-Ciocalteu reagent (0.5 mL), and the reagent. The absorbance value at 760 nm was determined after 10 min of incubation using a spectrophotometer (Perkin Elmer Lambda-EZ200). Gallic acid equivalent (GAE) was used to express the overall phenolic content of the samples.
The total flavonoid contents of the crude extract were determined using the aluminum chloride (AlCl3) colorimetric method (Kaur and Kapoor, 2002). After being homogenized in 3 mL of 80% aqueous ethanol, 250 mg of eggplant leaf tissue was kept at 37°C in the absence of light for 40 min. The supernatant was collected after centrifugation at 10,000 rpm and mixed with 4.3 mL each of 80% aqueous ethanol, 10% aluminum nitrate and 1 M sodium acetate. The absorbance reading was noted at 495 nm following 30 min of dark incubation (Chang et al., 2002). The amount of total flavonoids in the tissue sample was estimated as mg/g of fresh weight of eggplant leaf tissue.
Peroxidase activity was measured by taking 0.5 mL of enzyme extract in a mixture containing 2 mL of phosphate buffer, 1 mL of 0.05 mol L-1 H2O2 and 1 mL of pyrogallol. Then the mixture was incubated at 25 °C. H2SO4 (2.5 mol L-1) was added to stop the reaction. The reading was taken at a wavelength of 420 nm against a pyrogallol-removed blank.
2.9 Nutrient content determination
To determine the amounts of nitrogen, phosphate, and potassium in eggplant shoots, we used the Kjeldhal technique (Estefan, 2013). The plants were dried and prepared for examination before being powdered. The substances were then digested to determine the amount of N. We digested 0.5 g of the material with 10 mL of H2SO4 at 420°C for 2 h, using K2SO4 and CuSO4 as catalysts in a 9:1 ratio. To measure the total P and K in the plant material (Estefan, 2013), we used the ICARDA manual wet-digestion method. Spectrophotometers and flame photometers were used to measure the amounts of P and K, respectively.
2.10 Disease assessment
Following a modified method of Winsted and Kelman, we recorded disease rating for evaluating disease symptoms and level of infection in plants (Winstead, 1952). The disease evaluation scale is given in Table 2.
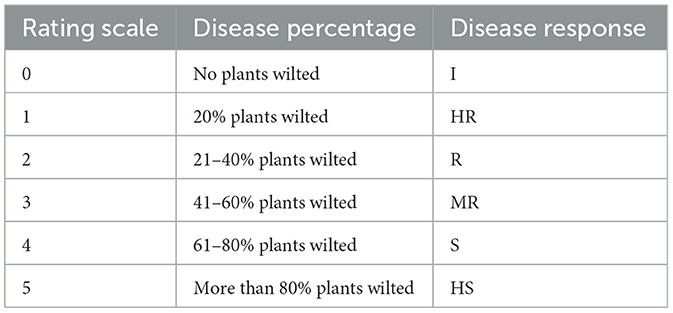
Table 2. Disease rating scale for Ralstonia solanacearum (Kaur and Kapoor, 2002).
For the determination of disease incidence, prevalence, and percent disease index, a disease evaluation was done using the following formula (Wheeler, 1969):
Disease incidence of bacterial wilt was calculated 40 days after inoculation as percentage of diseased plants in treatment by using the following formula (Awan et al., 2018; Awan and Shoaib, 2019):
The following formula was used to compute the percentage of protection provided by biochar, where A represents the PDI of untreated control plants and B represents the PDI of treated plants (Attia et al., 2022):
2.11 Statistical analysis
The data were analyzed using the software Statistix 8.1 to determine the homogeneity of variance. Subsequently, the treatments underwent a three-way analysis of variance (ANOVA), with the factors of analysis being soil composition, Trichoderma harzianum and R. solanacearum. The means were then compared using Tukey's HSD all-pairwise comparisons test (P ≤ 0.05).
3 Results
3.1 Plant growth-related agronomical parameters
Both the bio-control agent and soil substrate composition had a significant impact on the evaluated agronomic parameters of eggplant under Ralstonia solanacearum stress. However, the root and shoot lengths, as well as the dry weights, were strongly influenced by the interaction between soil composition (association with T. harzianum) and R. solanacearum (Table 3). Generally, the pathogen inoculation had an inhibitory effect on eggplant physiological parameters. However, 3-way (BCA × SC × RS) significant interactive effect was recorded in shoot dry weight. Results of three-way ANOVA are summarized as P values in Table 3.
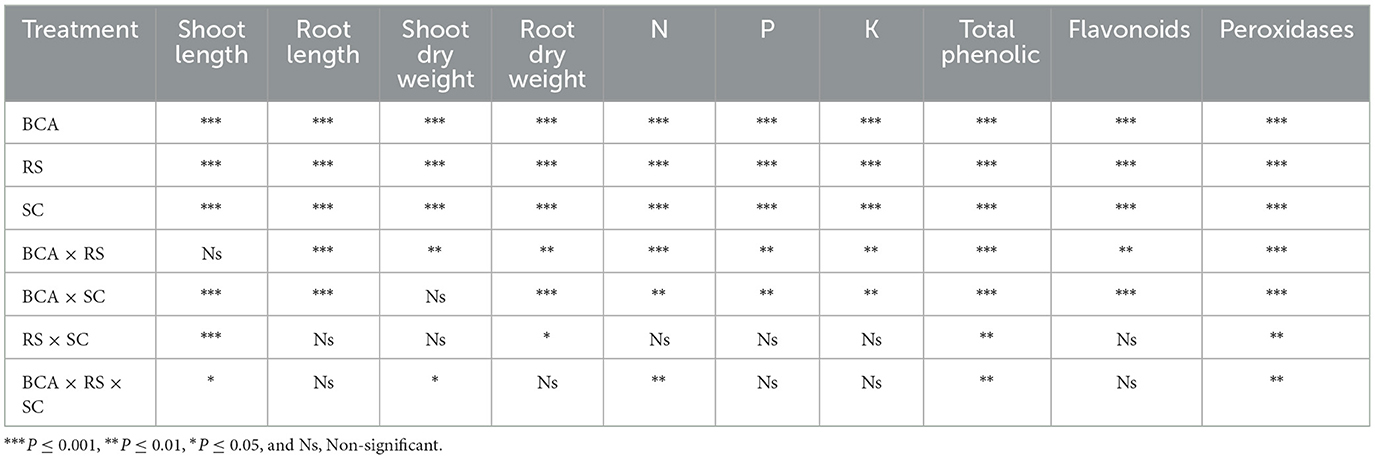
Table 3. The level of significance of the relationship between Trichoderma harzianum (BCA), Ralstonia solanacearum (RS), and soil substrate composition on eggplant development and physiological parameters was determined by the results of a three-way ANOVA.
3.1.1 Shoot length of eggplants
Plant height was significantly reduced in all treatments inoculated with R. solanacearum (Table 3). However, the highest plant shoot length (32.10 cm) was observed in the un-inoculated (–RS) treatment, where 6% LWB soil amendment was used in association with T. harzianum treatment. On the other hand, the lowest shoot length (16.50 cm) was recorded in the non-amended soil control group under disease stress (+RS) (Table 4), whereas in the non-amended soil control group under pathogen stress, there was an increase of 52.12% and 72.72% for plants grown in 3% and 6% LWB, respectively. There was an increase of 79.69% in the shoot length of plants grown the in 6% LWB amended treatment, which received both the pathogen and the bio-control agent.
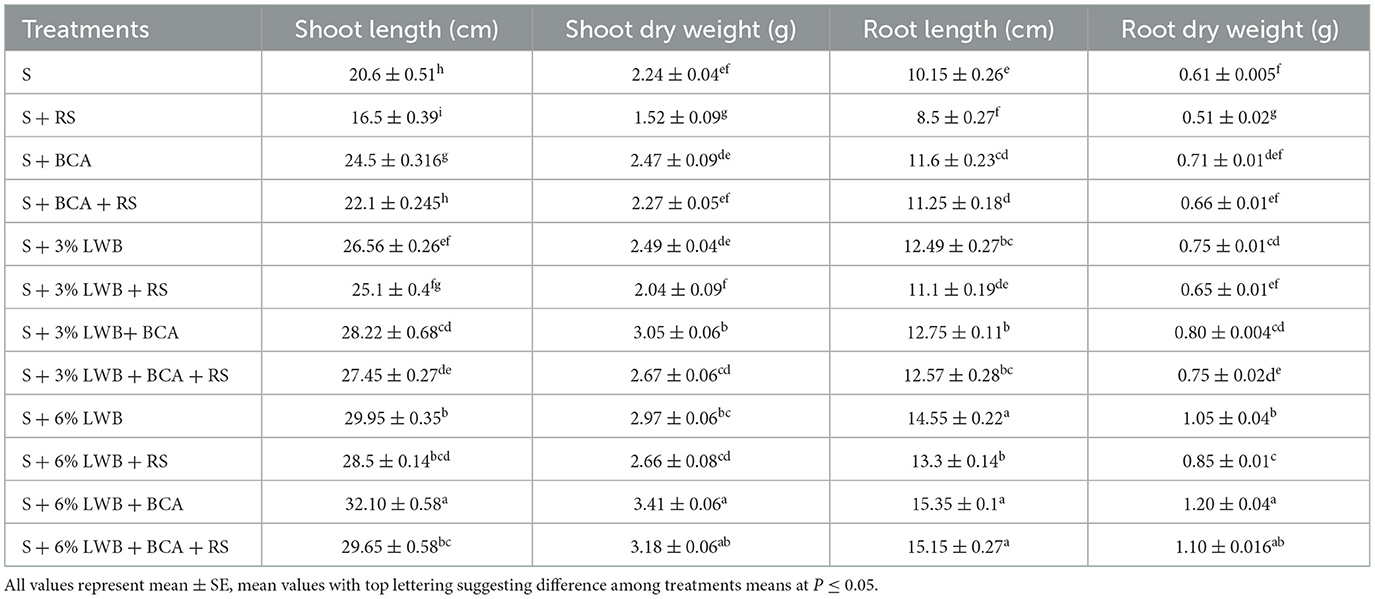
Table 4. Effect of leaf waste biochar (LWB) and Ralstonia solanacearum (RS) on plant physiological parameters of eggplant cultivated in following soil compositions: only soil (S), soil with 3% leaf waste biochar, and soil with 6% leaf waste biochar associated with and without bio-control agent (+ and –BCA, respectively) either inoculated (+RS) or un-inoculated (–RS).
3.1.2 Root length of eggplants
The highest root length (14.95 and 15.19 cm) was recorded in the treatment where 6% leaf waste biochar was amended in association with T. harzianum (S+6%LWB+BCA), whether infected (+RS) or uninfected (–RS) with R. solanacearum, respectively (Table 4). In the 3% LWB amended treatment, there was an increase of 35.29% (–BCA+RS) and 47.88% (+BCA+RS) compared to their respective non-amended soil control. However, the lowest root length (8.50 and 10.15 cm) was recorded in soil only potting media (control treatment) in the –RS and +RS treatments, respectively.
3.1.3 Shoot dry weight
The shoot dry weight of eggplants was significantly reduced under bacterial wilt stress. While in 6% LWB, there was an increase of 16.71% and 11.81% in shoot dry weight in comparison with 3% LWB amended treatment association with (+BCA) or without (BCA) bio-control agent Trichoderma harzianum, respectively (Table 4). In the presence of R. solanacearum, the highest shoot dry weight (1.10 g) was observed in 6% biochar associated with BCA treatment (S+ 6% LWB+ BCA+ RS). On the other hand, the lowest shoot dry weight (1.52 g) was recorded in the non-amended soil control.
3.1.4 Root dry weight
With or without biochar and the bio-control agent (T. harzianum), the inoculation of Ralstonia solanacearum resulted in a decrease in the root dry weights of eggplant in all treatments (Table 4). The highest root (1.10 and 1.20 g) dry weight was noted in 6% LWB amended soil associated with T. harzianum treatment, whether infected (+RS) or un-infected (–RS) with pathogen, which was 60% >3% LWB amended soil associated with T. harzianum treatment under pathogen stress (S+ 3% LWB+ BCA+ RS), whereas the lowest root dry weight (0.51 g) was recoded in soil in the absence of LWB and BCA under R. solanacearum stress.
3.2 Disease assessment
The response of the eggplant to R. solanacearum inoculation varied from resistant to highly susceptible with respect to soil composition (Table 5). Plants grown in soil treated with Ralstonia solanacearum (S+RS) showed a highly susceptible response to bacterial wilt, with the highest PDI of 88% and 100% disease incidence. The highest plant defense response against the development of bacterial wilt was observed in the treatment containing 6% biochar and T. harzianum (S+6% LWB+ RS+ BCA), with minimum (8%) recorded PDI, DS (8.81%) and DI (20%). In association with the bio-control agent, 6LWB provided maximum (90%) protection against bacterial wilt on the eggplants, while it was at 63% in case of plants grown in 3% LWB (Table 5).
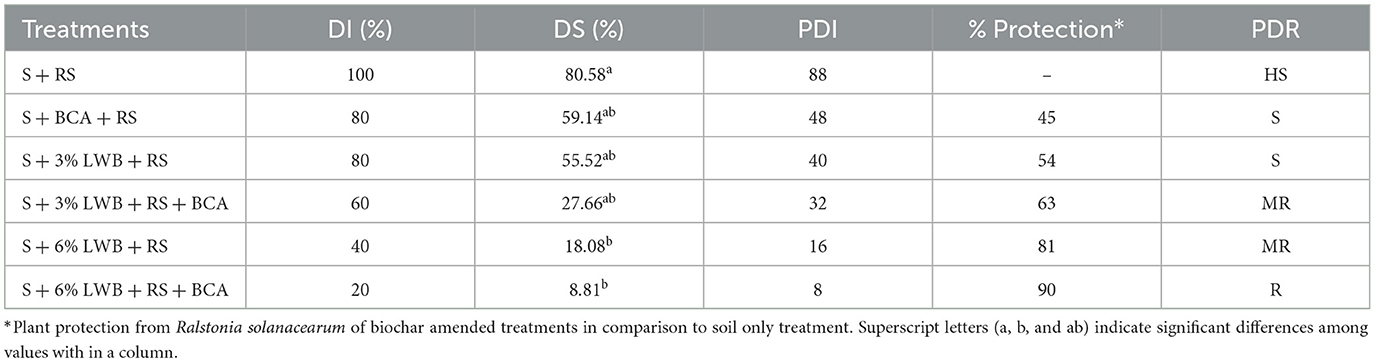
Table 5. Estimation of disease incidence (DI), disease severity, percent disease index (PDI), percentage protection and plant disease response (PDR) parameters for bacterial wilt of egg-plants cultivated in different soil substrate compositions in association with bio-control agent (BCA) Trichoderma harzianum.
3.3 Nutritional content of eggplants
There was a significant 3-way (SC × BCA × RS) interaction between soil composition with the bio-control agent, and R. solanacearum on the N, P, and K contents of eggplants (Table 3). Growing eggplants in 6%LWB+BCA amended soil with or without R. solanacearum stress had a positive impact on the nutritional content of the plants.
The effect of soil composition in association with the bio-control agent and R. solanacearum was significant (P ≤ 0.01) for nitrogen (N) content of eggplants (Table 3). Figure 1A shows a higher percentage of N content in 6% the leaf waste biochar treatment, with or without the bio-control agent. Highest nitrogen (2.28 and 2.39 %) content was observed in the 6% biochar amendment along with BCA treatment, whether infected (+RS) or uninfected (-RS), respectively (Figure 1A). Among all biochar amended treatments associated with the bio-control agent, there was an increase of 24.59 and 22.56% of plant N content in the 6% LWB containing soil as compared to the 3% LWB amended soil, whether infected (+RS) or uninfected (–RS), respectively. The lowest nitrogen content (1.24%) was recorded in the non-amended soil control under pathogen stress.
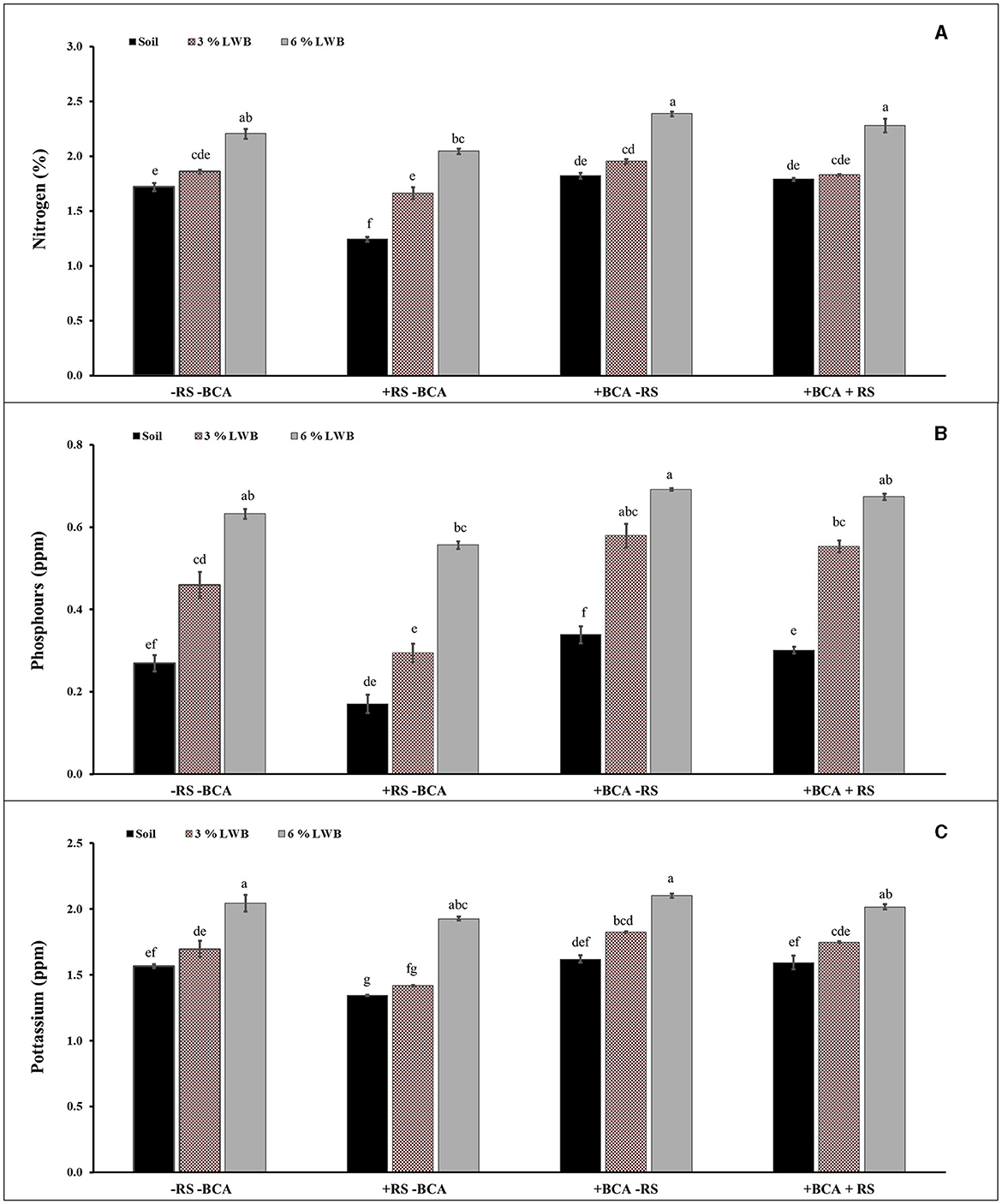
Figure 1. Effect of leaf waste biochar and Ralstonia solanacearum on nitrogen (A), phosphorus (B), and potassium (C) content of eggplant cultivated in the following soil compositions: only soil, soil with 3% LWB, and soil with 6% LWB associated with and without bio-control agent (+ and –BCA, respectively), whether infected (+RS) or non-infected (–RS). All values represent mean ±SE, and bars with top lettering suggest the difference among treatments at the P ≤ 0.05.
A significant (P ≤ 0.01) interaction effect of BCA × RS and BCA × SC was observed in the case of phosphorus content (ppm) (Table 3). The highest significant Phosphorus content was observed in plant grown in soil amended 6% LWB in association with bio-control agent either infected or uninfected with R. solanacearum (0.67 and 0.69 ppm), respectively (Figure 1B). Whereas in remaining (3% LWB) biochar amended treatments inoculated with R. solanacearum, both with or without bio-control agent the phosphorus content was significantly reduced 21.81% and 18.96%, respectively, in comparison with plants grown in 6% LWB. However, the lowest (0.17 ppm) P content was observed in R. solanacearum infected plants raised in non-amended soil only treatment. Under the pathogen stress, there was a significant increase of 76.47% of phosphorus contents in plant grown in soil amended with 6% LWB in the presence of bio-control agent, when compared with non-amended soil control.
Bacterial wilt infection in eggplant had a significant impact on reducing the K content (Table 3). Eggplants in soil amended with 6% LWB associated with biocontrol agent had shown the highest (2.06 ppm) K contents. The association of biocontrol agent T. harzianum also significantly influenced the potassium content in eggplants. The lowest (1.34 ppm) K content was recorded in non-amended soil control under R. solanacearum stress (Figure 1C). The K contents among all the treatments were recorded from lowest to highest in the following order: soil<3% LWB<6% LWB, either inoculated (+RS) or non-inoculated (–RS).
3.4 Biochemical analysis
The association of a bio-control agent with biochar had a significant impact on the biochemical content in eggplants. A three-way strong interactive effect was observed between the bio-control agent, different soil compositions and R. solanacearum, which influenced the production of biochemicals in eggplants (Table 3). The phenolic content increased (12.73%) significantly in the 6% biochar-amended treatment compared to plants in the 3%LWB co-inoculated (BCA+RS) (Figure 2A) treatment. In plants grown in the 6% LWB without BCA association (6% LWB-BCA), there was an increase of 7.19% (in comparison with 3%LWB-BCA) under disease stress (+RS). Ralstonia solanacearum significantly increases the total phenolic content of eggplants, with a maximum of 18.51% in plants grown under the 6% LWB amended treatment, compared to the non-amended soil only treatment (Figure 2A).
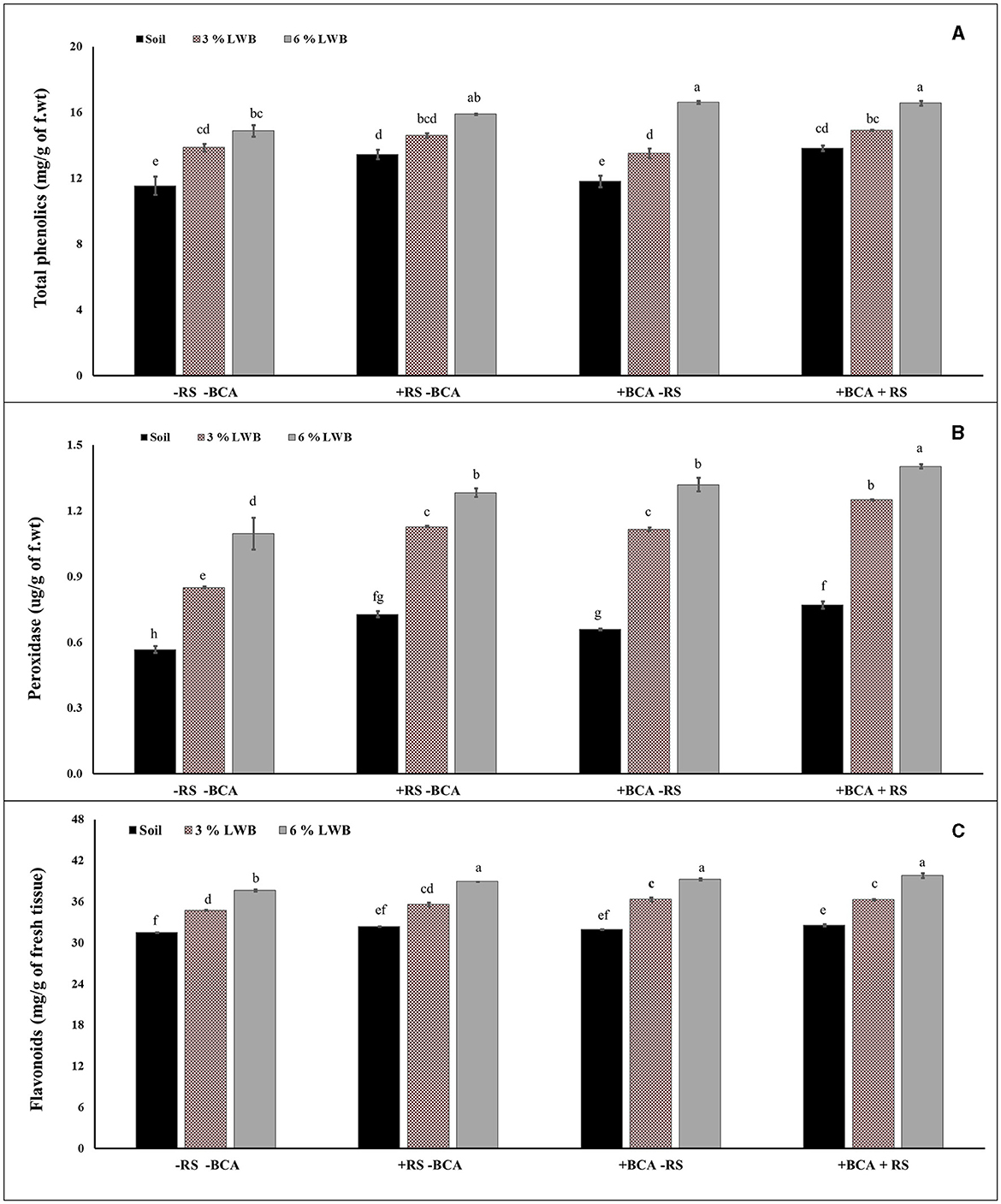
Figure 2. Effect of leaf waste biochar and Ralstonia solanacearum on total phenolics (A), peroxidases (B), and flavonoids (C) content of eggplant cultivated in following soil compositions: soil, soil with 3% LWB, and soil with 6% LWB associated with and without the bio-control agent (+ and –BCA, respectively), whether infected (+RS) or uninfected (–RS). All values represent mean ±SE, and bars with top lettering suggest the difference among treatments at P ≤ 0.05.
The combined effect of T. harzianum and biochar significantly increased the production of peroxidase. Among the biochar amended treatments, the highest (1.42 ug/g of f.wt) peroxidase activity was observed in eggplants grown in 6% leaf waste biochar amended co-inoculated (BCA + RS) treatment, whereas the lowest (0.57 ug/g of f.wt) peroxidase activity was recorded for non-amended soil control treatment in the absence of pathogesn (Figure 2B). However, there was a significant difference in peroxidase production between the 3% and 6% biochar amended treatments and soil composition in the presence and absence of R. solanacearum and T. harzianum.
The highest concentration of flavonoids was recorded in plants grown in the 6% LWB treatment in association with T. harzianum, whether they were inoculated (+RS) or un-inoculated (–RS) (39.81 and 39.25 mg/g of fresh tissue, respectively) (Figure 2C). In the absence of biochar and the bio-control agent, flavonoids' concentrations were found to be the lowest (31.49 mg/g of fresh tissue) in soil only treatment along with pathogens. The flavonoid content increased significantly in co-inoculated plants (+RS+BCA) with the increase in biochar concentration, that is, 9.72% increase was recorded in plants grown in the 6% LWB amended soil substrate compared to the respective 3%LWB counterpart.
4 Discussion
Plant diseases are primarily responsible for the decline in both the quality and quantity of agricultural products (Savary et al., 2019). In order to move toward a sustainable agricultural system in the 21st century, it is essential to thoroughly assess different crop production strategies. Sustainable agriculture aims to meet current needs without jeopardizing the ability of future generations to satisfy their own needs. By understanding ecosystem services, sustainable agriculture aim to create a more environmentally pleasant world. Due to plant pathogens induced reduction in the quantity and quality of agricultural products, agri-business is severely hampered (Savary et al., 2012). In a recent study, Širić et al. (2022) found that the cauliflower plants benefited from both PGPR and biochar, in terms of growth improvement and yield enhancement. Microorganisms in the PGPR-based bio-fertilizer adhere to the biochar particles. The biochar particles serve as a host, giving the microorganisms a carbon niche for their better growth and development (Tao et al., 2018).
Despite biochar being used for a long time to improve soil quality, researchers have recently developed a specific interest in its potential for enhancing plant growth and carbon sequestration (Lehmann et al., 2011). The application of biochar can change how plants react to disease stress (Graber et al., 2014) in addition to having an effect on crop yield (Kloss et al., 2014). In the past, biochar has been shown to reduce bacterial and fungal soil-borne diseases in tomato plants. However, no research has been conducted to evaluate the effect of biochar on bacterial wilt in eggplants. To our knowledge, the findings presented are the first to demonstrate the impact of biochar (with or without a bio-control agent) on the development of a soil-borne pathogen (R. solanacearum) in eggplants.
It is widely known that bio-control agents, such as Bacillus subtilis (Shoaib et al., 2019; Attia et al., 2020), Trichoderma spp. (Freeman et al., 2004), Penicillium axalicum (De Cal et al., 2008), and others can induce resistance in plants against various pathogens, including R. solanacearum, A. solani, Colletotrichum acutatum, and Botrytis cinerea. In a recent study by Rasool et al. (2021), it was found that the application of biochar along with PGPR (plant growth promoting rhizobacteria) had a reducing effect on the incidence and severity of solani. They attributed the decrease in disease development in tomatoes to the up-regulation of defense-related gene expression.
Trichoderma harzianum is a free-living organism that exhibits antagonistic action against several phyto-pathogenic fungi and bacteria on roots and in plant tissues (Rajendiran et al., 2010). Globally, T. harzianum is one of the most extensively researched and utilized bio-control agents for plant diseases. According to Graber et al. (2010), the population of Trichoderma spp. increased in soils treated with biochar compared to control soils. Hu et al. (2014) also observed changes in the fungal community after the application of biochar to the soil, with the proportion of Trichoderma being 14.5% higher in soils with biochar compared to those without. The connection between T. harzianum and root leads to an increase in the area of root absorption which in turn enhances nutrient absorption and then increases the pathogen resistance factor (Oskiera et al., 2017). Similarly, the present results indicated that the biochar along with T. harzianum improves t plant's ability to cope with R. solanacearum. Trichoderma associated with higher (6%) concentration of biochar had a strong impact on eggplants compared to plants grown in 3% leaf waste biochar amended soil. The advantages of applying biochar to soil along with the use of Trichoderma as a tool to promote plant growth and biological control have been documented (Patel et al., 2017). The application of Trichoderma harzianum along with organic waste resulted in levels of accessible P and promoted soil carbon fixation (Khomari et al., 2018). According to Muter et al. (2017), the treatment of biochar together with Trichoderma improved the germination of maize seeds and produced higher plants. Biochar in combination with vermi-compost known to have stimulating effect on plant growth as well as on the fungal and bacterial populations in the soil (Wang et al., 2021).
The highest concentration of nitrogen in plants was attributed to increased availability and reduced leaching of mineral nutrients. According to Chan et al. (2007), the use of biochar together with mineral fertilizers had a positive effect on nitrogen uptake. Prior research by Doan et al. (2015) reported beneficial effects of increasing nitrogen availability on the development and production of dry matter in maize plants. Several studies have demonstrated that the application of organic fertilizers, such as biochar and compost, along with mineral fertilizers stimulated plant growth and improved the efficiency of the fertilizer (Alburquerque et al., 2013). Previous studies had also shown the combined use of spent mushroom substrate biochar and PGPR improved the nutrients contents of cauliflower (Širić et al., 2022).
According to a study by Agegnehu et al. (2016), biochar and compost application serve as a source of transferable and easily accessible phosphorus. Due to phosphorus precipitating as dicalcium phosphate, the calcareous soils are phosphorus deficient (Abbas et al., 2010). However, soil biochar amendments enhance phosphorus availability, thus, resulting into improved plant growth (Nigussie et al., 2012). Similar to this, Steiner et al. (2008) has shown that the addition of compost and biochar enhanced crop stand, growth, and yield, which resulted in increased plant absorption of potash. Increased nitrogen and phosphorus availability may be directly or indirectly responsible for the rising potassium content in plants and soil. It can be believed that the increased nutritional concentration in biochar results from the saturation of nutrients during the pyrolysis of biomass.
In addition to harming the environment and human health, the over use of agricultural pesticides also speed-up the process of evolution of pathogenic strains, making them immune to commonly employed disease management techniques (Schmitz et al., 2014). The highest amounts of defense-related bio-chemicals were found in eggplants grown in soil that contained both biochar and Trichoderma. Phenolics in plants function by boosting the level of defense related proteins, while inducing structural alterations like lignification of the cell wall, as well as reduction in reactive oxygen species induced stress (Ozdal et al., 2013; Kaur et al., 2017). According to reports, tomato plant defense against early blight is boosted by the formation of phenolics and antioxidants (Awan et al., 2018). According to the present findings, R. solanacearum infection on eggplants in the biochar soil amendment (with and without BCA) also considerably influenced the levels of catalase and peroxidase synthesis, which in turn helped to mitigate the stress by bacterial infection (Attia et al., 2022). Biochar and PGPR are known to interact with crop plants biochemical responses toward biotic and abiotic stresses. Lalay et al. (2022) studied the combined application of biochar and Pseudomonas sp. influenced the concentrations of anthocyanins, carotenoids, chlorophyll pigments and catalases in Brassica napus L.
The results of this study have greatly expanded the understanding of how biochar contributes to the development of disease resistance and the transformation of waste products into carbon-rich soil amendments. Organic amendments in association with bio-control agent plausibly increased the soil's organic carbon contents and eventually rendered the soil structure permeable. Biochar addition plausibly influenced the roots to grow deeper into the soil to acquire more nutrients. These findings may also be utilized to create organic materials to replace traditional plant protection practices, for preventing bacterial wilt in eggplants.
5 Conclusions
Biochar-based organic soil amendments can strengthen plant defense and manage emerging biotic threats. Eggplant's reaction to the invasive R. solanacearum was affected by the presence of biochar in soil substrate. The comparison revealed that T. harzianum alone and in combination with 6% leaf waste biochar was the most efficient in suppressing R. solanacearum. Using biochar with association of T. harzianum increased microbial activity in the soil, which activated plant growth and inhibited further disease progression. The physico-chemical properties of biochar might be influenced by the raw material and temperature used for pyrolysis, which in turn impact how plants will react to phyto-pathogens (biochemically and physiologically). We anticipate greater interest in biochar in the near future from the standpoint of the agricultural industry and an effective waste management plan. To understand the mechanism behind induced resistance, future studies should focus at the molecular plant response to the biochar application. There is also need to standardize the effective doze for various biochars classified on the basis of feedstock to extract the full benefits under field conditions.
Data availability statement
The original contributions presented in the study are included in the article/supplementary material, further inquiries can be directed to the corresponding author.
Author contributions
CA: Writing—review & editing, Writing—original draft, Software, Methodology, Investigation, Formal analysis, Data curation. AA: Writing—review & editing, Visualization, Supervision, Conceptualization. MH: Writing—review & editing, Visualization, Validation, Supervision, Conceptualization. MA: Writing—review & editing, Methodology, Investigation, Formal analysis, Data curation. AH: Writing—review & editing, Resources, Project administration, Funding acquisition. GA-Q: Writing—review & editing, Resources, Project administration, Funding acquisition. EA: Writing—review & editing, Resources, Project administration, Funding acquisition.
Funding
The author(s) declare that financial support was received for the research, authorship, and/or publication of this article. The authors would like to extend their sincere appreciation to the Researchers Supporting Project Number (RSP2024R134), King Saud University, Riyadh, Saudi Arabia.
Acknowledgments
The authors are grateful to the Researchers Supporting Project Number (RSP2024R134), King Saud University, Riyadh, Saudi Arabia.
Conflict of interest
The authors declare that the research was conducted in the absence of any commercial or financial relationships that could be construed as a potential conflict of interest.
Publisher's note
All claims expressed in this article are solely those of the authors and do not necessarily represent those of their affiliated organizations, or those of the publisher, the editors and the reviewers. Any product that may be evaluated in this article, or claim that may be made by its manufacturer, is not guaranteed or endorsed by the publisher.
References
Abbas, G., Hassan, G., Ali, M. A., Aslam, M., and Abbas, Z. (2010). Response of wheat to different doses of ZnSO4 under Thal desert environment. Pak. J. Bot. 42, 4079–4085.
Aftab, Z. E. H., Aslam, W., Aftab, A., Shah, A. N., Akhter, A., Fakhar, U., et al. (2022). Incorporation of engineered nanoparticles of biochar and fly ash against bacterial leaf spot of pepper. Sci. Rep. 12:8561. doi: 10.1038/s41598-022-10795-8
Agegnehu, G., Bass, A. M., Nelson, P. N., and Bird, M. I. (2016). Benefits of biochar, compost and biochar–compost for soil quality, maize yield and greenhouse gas emissions in a tropical agricultural soil. Sci. Total Environ. 543, 295–306. doi: 10.1016/j.scitotenv.2015.11.054
Alburquerque, J. A., Salazar, P., Barrón, V., Torrent, J., del Campillo, MdC, Gallardo, A., et al. (2013). Enhanced wheat yield by biochar addition under different mineral fertilization levels. Agron. Sustain. Dev. 33, 475–484. doi: 10.1007/s13593-012-0128-3
Alexander, P., Brown, C., Arneth, A., Finnigan, J., Moran, D., Rounsevell, M. D., et al. (2017). Losses, inefficiencies and waste in the global food system. Agric. Syst. 153, 190–200. doi: 10.1016/j.agsy.2017.01.014
Ali, M. A., Abbas, A., Atiq, M., Rajput, N. A., Naveed, K., Javed, N., et al. (2018). “Plant diseases of major crops and the way forward for their management,” in Developing Sustainable Agriculture in Pakistan (CRC Press), 389–416. doi: 10.1201/9781351208239-20
Attia, M. S., El-Sayyad, G. S., Abd Elkodous, M., and El-Batal, A. I. (2020). The effective antagonistic potential of plant growth-promoting rhizobacteria against Alternaria solani-causing early blight disease in tomato plant. Sci. Hortic. 266:109289. doi: 10.1016/j.scienta.2020.109289
Attia, M. S., Hashem, A. H., Badawy, A. A., and Abdelaziz, A. M. (2022). Biocontrol of early blight disease of eggplant using endophytic Aspergillus terreus: improving plant immunological, physiological and antifungal activities. Botan. Stud. 63:26. doi: 10.1186/s40529-022-00357-6
Awan, Z. A., and Shoaib, A. (2019). Combating early blight infection by employing Bacillus subtilis in combination with plant fertilizers. Curr. Plant Biol. 20:100125. doi: 10.1016/j.cpb.2019.100125
Awan, Z. A., Shoaib, A., and Khan, K. A. (2018). Variations in total phenolics and antioxidant enzymes cause phenotypic variability and differential resistant response in tomato genotypes against early blight disease. Sci. Hortic. 239, 216–223. doi: 10.1016/j.scienta.2018.05.044
Bag, M., Yadav, M., and Mukherjee, A. (2017). Changing disease scenario with special emphasis on false smut of rice. SATSA Mukhapatra-Annual. Tech. Issue. 21, 219–224.
Carter, J., Gardner, W., and Gibson, A. (1994). Improved growth and yield of Faba beans (Vicia faba cv. Fiord) by inoculation with strains of Rhizobium leguminosarum biovar viciae in acid soils in south west Victoria Australian. J. Agric. Res. 45, 613–623. doi: 10.1071/AR9940613
Champoiseau, P. G., Jones, J. B., and Allen, C. (2009). Ralstonia solanacearum race 3 biovar 2 causes tropical losses and temperate anxieties. Plant Health Prog. 10:35. doi: 10.1094/PHP-2009-0313-01-RV
Chan, K., Van Zwieten, L., Meszaros, I., Downie, A., and Joseph, S. (2007). Agronomic values of greenwaste biochar as a soil amendment. Soil Res. 45, 629–634. doi: 10.1071/SR07109
Chang, C. C., Yang, M. H., Wen, H. M., and Chern, J. C. (2002). Estimation of total flavonoid content in propolis by two complementary colorimetric methods. J. Food Drug Anal. 10. doi: 10.38212/2224-6614.2748
da Silva, J. A. T., de Medeiros, E. V., da Silva, J. M., Tenório, DdA, Moreira, K. A., Nascimento TCEd, S., et al. (2016). Trichoderma aureoviride URM 5158 and Trichoderma hamatum URM 6656 are biocontrol agents that act against cassava root rot through different mechanisms. J. Phytopathol. 164, 1003–1011. doi: 10.1111/jph.12521
Das, P. P., Singh, K. R., Nagpure, G., Mansoori, A., Singh, R. P., Ghazi, I. A., et al. (2022). Plant-soil-microbes: A tripartite interaction for nutrient acquisition and better plant growth for sustainable agricultural practices. Environ. Res. 214:113821. doi: 10.1016/j.envres.2022.113821
Davoudpour, Y., Schmidt, M., Calabrese, F., Richnow, H. H., and Musat, N. (2020). High resolution microscopy to evaluate the efficiency of surface sterilization of Zea Mays seeds. PLoS ONE 15:e0242247. doi: 10.1371/journal.pone.0242247
De Cal, A., Redondo, C., Sztejnberg, A., and Melgarejo, P. (2008). Biocontrol of powdery mildew by Penicillium oxalicum in open-field nurseries of strawberries. Biol. Control. 47, 103–107. doi: 10.1016/j.biocontrol.2008.07.010
Doan, T. T., Henry-des-Tureaux, T., Rumpel, C., Janeau, J.-L., and Jouquet, P. (2015). Impact of compost, vermicompost and biochar on soil fertility, maize yield and soil erosion in Northern Vietnam: a three year mesocosm experiment. Sci. Total Environ. 514, 147–154. doi: 10.1016/j.scitotenv.2015.02.005
Elad, Y., Cytryn, E., Harel, Y. M., Lew, B., and Graber, E. R. (2011). The biochar effect: plant resistance to biotic stresses. Phytopathol. Mediterr. 50, 335–349.
Estefan, G. (2013). “Methods of soil, plant, and water analysis: a manual for the West Asia and North Africa region,” in International Center for Agricultural Research in the Dry Areas (ICARDA).
Freeman, S., Minz, D., Kolesnik, I., Barbul, O., Zveibil, A., Maymon, M., et al. (2004). Trichoderma biocontrol of Colletotrichum acutatum and Botrytis cinerea and survival in strawberry. Eur. J. Plant Pathol. 110, 361–370. doi: 10.1023/B:EJPP.0000021057.93305.d9
Graber, E., Frenkel, O., Jaiswal, A., and Elad, Y. (2014). How may biochar influence severity of diseases caused by soilborne pathogens? Carbon Manag. 5, 169–183. doi: 10.1080/17583004.2014.913360
Graber, E. R., Meller Harel, Y., Kolton, M., Cytryn, E., Silber, A., Rav David, D., et al. (2010). Biochar impact on development and productivity of pepper and tomato grown in fertigated soilless media. Plant Soil. 337, 481–496. doi: 10.1007/s11104-010-0544-6
Hu, L., Cao, L., and Zhang, R. (2014). Bacterial and fungal taxon changes in soil microbial community composition induced by short-term biochar amendment in red oxidized loam soil. World J. Microbiol. Biotechnol. 30, 1085–1092. doi: 10.1007/s11274-013-1528-5
Hugh, R., and Leifson, E. (1953). The taxonomic significance of fermentative versus oxidative metabolism of carbohydrates by various gram negative bacteria. J. Bacteriol. 66, 24–26. doi: 10.1128/jb.66.1.24-26.1953
Hussain, F., and Abid, M. (2011). Pest and diseases of chilli crop in Pakistan: a review. Int. J. Biol. Biotech. 8, 325–332.
Kaur, C., and Kapoor, H. C. (2002). Anti-oxidant activity and total phenolic content of some Asian vegetables. Int. J. Food Sci. Technol. 37, 153–161. doi: 10.1046/j.1365-2621.2002.00552.x
Kaur, H., Salh, P., and Singh, B. (2017). Role of defense enzymes and phenolics in resistance of wheat crop (Triticum aestivum L.) towards aphid complex. J. Plant Inter. 12, 304–311. doi: 10.1080/17429145.2017.1353653
Khaledi, N., and Taheri, P. (2016). Biocontrol mechanisms of Trichoderma harzianum against soybean charcoal rot caused by Macrophomina phaseolina. J. Plant Protect. Res. 56, 1–11. doi: 10.1515/jppr-2016-0004
Khomari, S., Golshan-Doust, S., Seyed-Sharifi, R., and Davari, M. (2018). Improvement of soybean seedling growth under salinity stress by biopriming of high-vigour seeds with salt-tolerant isolate of Trichoderma harzianum. N Z J. Crop. Hortic. Sci. 46, 117–132. doi: 10.1080/01140671.2017.1352520
Kim, S. G., Hur, O.-S., Ro, N.-Y., Ko, H.-C., Rhee, J.-H., Sung, J. S., et al. (2016). Evaluation of resistance to Ralstonia solanacearum in tomato genetic resources at seedling stage. Plant Pathol. J. 32:58. doi: 10.5423/PPJ.NT.06.2015.0121
Kloss, S., Zehetner, F., Wimmer, B., Buecker, J., Rempt, F., Soja, G., et al. (2014). Biochar application to temperate soils: effects on soil fertility and crop growth under greenhouse conditions. J. Plant Nutr. Soil Sci. 177, 3–15. doi: 10.1002/jpln.201200282
Kongkiattikajorn, J., and Thepa, S. (2007). Increased tomato yields by heat treatment for controlling Ralstonia solanacearum in soil. Agric. Nat. Resour. 41, 219–224.
Lalay, G., Ullah, S., and Ahmed, I. (2022). Physiological and biochemical responses of Brassica napus L. to drought-induced stress by the application of biochar and Plant Growth Promoting Rhizobacteria. Microsc. Res. Techn. 85, 1267–1281. doi: 10.1002/jemt.23993
Larena, I., De Cal, A., and Melgarejo, P. (2010). Enhancing the adhesion of Epicoccum nigrum conidia to peach surfaces and its relationship to the biocontrol of brown rot caused by Monilinia laxa. J. Appl. Microbiol. 109, 583–593. doi: 10.1111/j.1365-2672.2010.04681.x
Lehmann, J., Rillig, M. C., Thies, J., Masiello, C. A., Hockaday, W. C., Crowley, D., et al. (2011). Biochar effects on soil biota–a review. Soil Biol. Biochem. 43, 1812–1836. doi: 10.1016/j.soilbio.2011.04.022
Muter, O., Grantina-Ievina, L., Makarenkova, G., Vecstaudza, D., Strikauska, S., Selga, T., et al. (2017). Effect of biochar and Trichoderma application on fungal diversity and growth of Zea mays in a sandy loam soil. Environ. Exper. Biol. 15, 289–296. doi: 10.22364/eeb.15.30
Nigussie, A., Kissi, E., Misganaw, M., and Ambaw, G. (2012). Effect of biochar application on soil properties and nutrient uptake of lettuces (Lactuca sativa) grown in chromium polluted soils. Am. Euras. J. Agric. Environ. Sci. 12, 369–376.
Oskiera, M., Szczech, M., Stepowska, A., Smolińska, U., and Bartoszewski, G. (2017). Monitoring of Trichoderma species in agricultural soil in response to application of biopreparations. Biol. Control. 113, 65–72. doi: 10.1016/j.biocontrol.2017.07.005
Ozdal, T., Capanoglu, E., and Altay, F. (2013). A review on protein–phenolic interactions and associated changes. Food Res. Int. 51, 954–970. doi: 10.1016/j.foodres.2013.02.009
Patel, A., Khare, P., and Patra, D. D. (2017). “Biochar mitigates salinity stress in plants,” in Plant Adaptation Strategies in Changing Environment, eds. V. Shukla, S. Kumar, N. Kumar (Singapore: Springer). doi: 10.1007/978-981-10-6744-0_6
Rajendiran, R., Jegadeeshkumar, D., Sureshkumar, B., and Nisha, T. (2010). In vitro assessment of antagonistic activity of Trichoderma viride against post harvest pathogens. J. Agric. Technol. 6, 31–35.
Rasool, M., Akhter, A., Soja, G., and Haider, M. S. (2021). Role of biochar, compost and plant growth promoting rhizobacteria in the management of tomato early blight disease. Sci. Rep. 11:6092. doi: 10.1038/s41598-021-85633-4
Saha, P., Singh, J., Bhanushree, N., Harisha, S. M., and Tomar, B. S. (2023). “Eggplant (Solanum melongena L.) nutritional and health promoting phytochemicals,” in Compendium of Crop Genome Designing for Nutraceuticals (Singapore: Springer Nature Singapore), 1463–1493. doi: 10.1007/978-981-19-4169-6_53
Savary, S., Ficke, A., Aubertot, J.-N., and Hollier, C. (2012). Crop losses due to diseases and their implications for global food production losses and food security. Food Secur. 4, 519–537. doi: 10.1007/s12571-012-0200-5
Savary, S., Willocquet, L., Pethybridge, S. J., Esker, P., McRoberts, N., Nelson, A., et al. (2019). The global burden of pathogens and pests on major food crops. Nat. Ecol. Evol. 3, 430–439. doi: 10.1038/s41559-018-0793-y
Schmitz, J., Hahn, M., and Brühl, C. A. (2014). Agrochemicals in field margins–An experimental field study to assess the impacts of pesticides and fertilizers on a natural plant community. Agric. Ecosyst. Environ. 193, 60–69. doi: 10.1016/j.agee.2014.04.025
Semida, W. M., Beheiry, H. R., Sétamou, M., Simpson, C. R., Abd El-Mageed, T. A., Rady, M. M., et al. (2019). Biochar implications for sustainable agriculture and environment: a review. South Afr. J. Botany. 127, 333–347. doi: 10.1016/j.sajb.2019.11.015
Sharma, M., and Kaushik, P. (2021). Biochemical composition of eggplant fruits: a review. Appl. Sci. 11:7078. doi: 10.3390/app11157078
Shoaib, A., Awan, Z. A., and Khan, K. A. (2019). Intervention of antagonistic bacteria as a potential inducer of disease resistance in tomato to mitigate early blight. Sci. Hortic. 252, 20–28. doi: 10.1016/j.scienta.2019.02.073
Singleton, V. L., and Rossi, J. A. (1965). Colorimetry of total phenolics with phosphomolybdic-phosphotungstic acid reagents. Am. J. Enol. Vitic. 16, 144–158. doi: 10.5344/ajev.1965.16.3.144
Širić, I., Alhag, S. K., Al-Shuraym, L. A., Mioč, B., DrŽaić, V., Abou Fayssal, S., et al. (2023). Combined Use of TiO2 nanoparticles and biochar produced from moss (Leucobryum glaucum (Hedw.) Ångstr) biomass for Chinese Spinach (Amaranthus dubius L.) cultivation under saline stress. Horticulturae 9:1056. doi: 10.3390/horticulturae9091056
Širić, I., Eid, E. M., Taher, M. A., El-Morsy, M. H., Osman, H. E., Kumar, P., et al. (2022). Combined use of spent mushroom substrate biochar and PGPR improves growth, yield, and biochemical response of cauliflower (Brassica oleracea var. botrytis): a preliminary study on greenhouse cultivation. Horticulturae 8:830. doi: 10.3390/horticulturae8090830
Steiner, C., Glaser, B., Geraldes Teixeira, W., Lehmann, J., Blum, W. E., Zech, W., et al. (2008). Nitrogen retention and plant uptake on a highly weathered central Amazonian Ferralsol amended with compost and charcoal. J. Plant Nutr. Soil Sci. 171, 893–899. doi: 10.1002/jpln.200625199
Sunesson, A., Vaes, W., Nilsson, C., Blomquist, G., Andersson, B., Carlson, R., et al. (1995). Identification of volatile metabolites from five fungal species cultivated on two media. Appl. Environ. Microbiol. 61, 2911–2918. doi: 10.1128/aem.61.8.2911-2918.1995
Tao, S., Wu, Z., He, X., Ye, B. C., and Li, C. (2018). Characterization of biochar prepared from cotton stalks as efficient inoculum carriers for Bacillus subtilis SL-13. Bioresources 13, 1773–1786. doi: 10.15376/biores.13.1.1773-1786
Wang, F., Wang, X., and Song, N. (2021). Biochar and vermicompost improve the soil properties and the yield and quality of cucumber (Cucumis sativus L.) grown in plastic shed soil continuously cropped for different years. Agric. Ecosyst. Environ. 315:107425. doi: 10.1016/j.agee.2021.107425
Winstead, N. (1952). Inoculation techniques for evaluating resistance to Pseudomonas solanacearum. Phytopathology 42, 623–634.
Keywords: carbon sequestration, plant protection, bacterial wilt, Trichoderma harzianum, soil amendment, organic agriculture
Citation: Ahmad CA, Akhter A, Haider MS, Abbas MT, Hashem A, Avila-Quezada GD and Abd_Allah EF (2024) Demonstration of the synergistic effect of biochar and Trichoderma harzianum on the development of Ralstonia solanacearum in eggplant. Front. Microbiol. 15:1360703. doi: 10.3389/fmicb.2024.1360703
Received: 04 January 2024; Accepted: 09 April 2024;
Published: 25 April 2024.
Edited by:
Ravinder Kumar, Indian Agricultural Research Institute (ICAR), IndiaReviewed by:
Triwidodo Arwiyanto, Gadjah Mada University, IndonesiaSami Abou Fayssal, University of Forestry, Sofia, Bulgaria
Copyright © 2024 Ahmad, Akhter, Haider, Abbas, Hashem, Avila-Quezada and Abd_Allah. This is an open-access article distributed under the terms of the Creative Commons Attribution License (CC BY). The use, distribution or reproduction in other forums is permitted, provided the original author(s) and the copyright owner(s) are credited and that the original publication in this journal is cited, in accordance with accepted academic practice. No use, distribution or reproduction is permitted which does not comply with these terms.
*Correspondence: Adnan Akhter, adnanakhter.iags@pu.edu.pk