- Department of Plant and Environmental Protection Sciences, University of Hawaiʻi at Mānoa, Honolulu, HI, United States
Xanthomonas and Stenotrophomonas are closely related genera in the family Lysobacteraceae. In our previous study of aroid-associated bacterial strains, most strains isolated from anthurium and other aroids were reclassified as X. phaseoli and other Xanthomonas species. However, two strains isolated from Spathiphyllum and Colocasia were phylogenetically distant from other strains in the Xanthomonas clade and two strains isolated from Anthurium clustered within the Stenotrophomonas clade. Phylogenetic trees based on 16S rRNA and nine housekeeping genes placed the former strains with the type strain of X. sacchari from sugarcane and the latter strains with the type strain of S. bentonitica from bentonite. In pairwise comparisons with type strains, the overall genomic relatedness indices required delineation of new species; digital DNA–DNA hybridization and average nucleotide identity values were lower than 70 and 95%, respectively. Hence, three new species are proposed: S. aracearum sp. nov. and S. oahuensis sp. nov. for two strains from anthurium and X. hawaiiensis sp. nov. for the strains from spathiphyllum and colocasia, respectively. The genome size of X. hawaiiensis sp. nov. is ~4.88 Mbp and higher than S. aracearum sp. nov. (4.33 Mbp) and S. oahuensis sp. nov. (4.68 Mbp). Gene content analysis revealed 425 and 576 core genes present in 40 xanthomonads and 25 stenotrophomonads, respectively. The average number of unique genes in Stenotrophomonas spp. was higher than in Xanthomonas spp., implying higher genetic diversity in Stenotrophomonas.
1 Introduction
Genera Xanthomonas (Dowson, 1939) and Stenotrophomonas (Palleroni and Bradbury, 1993) are the groups of gram-negative and aerobic bacteria belonging to the family Lysobacteraceae (syn. Xanthomonadaceae) of Lysobacterales (syn. Xanthomonadales) order of Gammaproteobacteria class in the phylum Proteobacteria (Kumar et al., 2019; Parte et al., 2020; Bansal et al., 2021a,b; Bansal et al., 2023). Stenotrophomonas and Xanthomonas are phylogenetically closely related genera along with Xylella and Pseudoxanthomonas in Lysobacteraceae (Bansal et al., 2021b; Bansal et al., 2023). Before the current generic name, Xanthomonas was described as Bacterium in 1921 and later reclassified into the genus Phytomonas in 1923 (Doidge, 1921; Bergey et al., 1923; Dowson, 1939). The taxonomy of the first reported xanthomonad from pepper and tomato was changed several times, as described below. The pathogen was originally classified as B. vesicatorium and then X. vesicatoria; subsequently, it was given the trinomial pathovar vesicatoria first under X. campestris (Young et al., 1978) and later under X. axonopodis (Vauterin et al., 1995) and finally reclassified as a separate species, X. euvesicatoria (Jones et al., 2004; Constantin et al., 2016). Interestingly, before designating the genus Stenotrophomonas, the first stenotrophomonad isolated from pleural fluid of a hospitalized patient had been referred to as X. maltophilia; initially identified within the Bacterium genus, it was subsequently reclassified under Pseudomonas for a decade (Hugh and Ryschenkow, 1961; Swings et al., 1983; Palleroni and Bradbury, 1993). In 1993, due to distinct phylogenetic lineage from the other phytopathogens in Xanthomonas, X. maltophilia was replaced by S. maltophilia (Palleroni and Bradbury, 1993). At the time of writing this manuscript, there are 36 and 17 validly published species of Xanthomonas and Stenotrophomonas, respectively, as well as some other invalid species shown in quotation marks (““) throughout the rest of this article in the List of Prokaryotic names with Standing in Nomenclature (LPSN, last accessed on December 2022) (Parte et al., 2020).
Most Xanthomonas species are pathogenic to more than 400 different monocot and dicot plants, including economically important crops and ornamentals. Additionally, some Xanthomonas strains are non-pathogenic and associated with plants (Bradbury, 1984; Leyns et al., 1984; Vauterin et al., 2000; Ryan et al., 2011; Vandroemme et al., 2013; Parte et al., 2020; Timilsina et al., 2020; Mafakheri et al., 2022). On monocotyledonous hosts, X. oryzae pv. oryzae and X. oryzae pv. oryzicola are listed on the USDA Select Agent list, causing severe diseases of rice (Oryza sativa). In addition, X. albilineans causes leaf scorch of sugarcane (Saccharum officinarum), and X. vasicola is a causal agent of banana wilt (Ryan et al., 2011). Whereas X. maliensis, “X. sontii,” and “X. indica” were reported associated with the rice phytobiome (Triplett et al., 2015; Bansal et al., 2021a; Rana et al., 2022), X. sacchari was associated with sugarcane, causing rice sheath rot disease (Ivayani et al., 2023). Constantin et al. (2016) reclassified bacterial strains isolated from Anthurium, Dieffenbachia, and other ornamental Araceae plants into the species and/or pathovars, X. phaseoli including two pathovars dieffenbachiae and syngonii, X. citri pv. aracearum and X. euvesicatoria. The Araceae strains of X. phaseoli pv. dieffenbachiae, X. phaseoli pv. syngonii, and X. citri pv. aracearum were pathogenic on their original hosts, but X. euvesicatoria strains isolated from Philodendron caused weak symptoms and lacked host specificity on tested Aracea hosts (Constantin et al., 2017).
As for Stenotrophomonas spp., they are ubiquitous environmental bacteria isolated from various sources. The type species, S. maltophilia, is an opportunistic pathogen on humans infecting through clinical materials and equipment, and “S. sepilia” was isolated from a nosocomial patient’s blood specimen (Hugh and Ryschenkow, 1961; Al-Anazi and Al-Jasser, 2014; Gautam et al., 2021). In addition to the clinical species, Stenotrophomonas species are also from various sources, such as S. humi and S. terrae from soil, S. daejeonensis and S. geniculata from water, S. pavanii, S. rhizophila, and “S. cyclobalanopsidis” associated with plants, “S. pennii” and “S. muris” from animals, S. lactitubi and S. indicatrix from surfaces in contact with food, and S. acidaminiphila and S. chelatiphaga from sludges (Assih et al., 2002; Wolf, 2002; Heylen et al., 2007; Kaparullina et al., 2009; Lee et al., 2011; Ramos et al., 2011a; Weber et al., 2018; Bian et al., 2020; Gilroy et al., 2021; Afrizal et al., 2022). Notably, S. maltophilia was also encountered frequently in aquatic and plant-associated environments, and S. rhizophila strains isolated from the rhizosphere and geocaulosphere were separated from S. maltophilia based on 16S rDNA analysis and DNA–DNA hybridization data (Berg et al., 1996; Denton and Kerr, 1998; Minkwitz and Berg, 2001; Wolf, 2002).
Among monocot plants, the Araceae family includes the most economically important ornamental plants in Hawaii, especially the genus Anthurium. During the 1980s to 1990s, the anthurium industry was seriously damaged due to X. phaseoli pv. dieffenbachiae outbreaks (formerly called X. axonopodis pv. dieffenbachiae) (Alvarez et al., 2006; Constantin et al., 2016). Hundreds of bacterial strains were isolated from various plant genera in Araceae worldwide, including the strains collected during the outbreaks in Hawaii, and stored in the Pacific Bacterial Collection at the University of Hawaii at Manoa.1 In our previous five-gene multilocus sequence analysis (MLSA) of Lysobacteraceae strains isolated from the Araceae family, a strain from Spathiphyllum and another strain from Colocasia clustered within the Xanthomonas clade but formed a distinct monophyletic lineage, while two strains from Anthurium grouped with the Stenotrophomonas clade instead of the Xanthomonas clade (Chuang, 2023). Moreover, these two stenotrophomonads were distinct from the former two xanthomonads based on the utilizations of N-acetyl-D-galactosamine (GalNAc) and D-serine, and the inability to oxidize D-galactose, glycerol, pectin, and sucrose based on Biolog GEN III microplate assays (Chuang, 2023).
Hence, we sequenced the whole genomes of the former strains isolated from Araceae, which are potential novel species, comparing them with the genomes of Xanthomonas spp. and Stenotrophomonas spp. type strains. Based on the nine-gene MLSA, overall genomic relatedness index (OGRI) values, and pan-core genomic analyses, strains A6251T from Spathiphyllum and A2111 from Colocasia are described as new species X. hawaiiensis sp. nov., strain A5588T from Anthurium is described as S. aracearum sp. nov., and strain A5586T from Anthurium is described as S. oahuensis sp. nov.
2 Materials and methods
2.1 Bacterial DNA isolation and genome sequencing
Bacteria were streaked out from the culture stock and grew on 2, 3, 5-triphenyltetrazolium chloride (TZC) agar medium (dextrose 5 gL−1, peptone 10 gL−1, 0.001% sterilized TZC, and agar 18 gL−1) at 28°C for 2 days. Bacterial genomic DNA was isolated from pure culture using QIAGEN Genomic-tip 100/G, following the manufacturer’s instruction (QIAGEN, Valencia, CA, USA). The Seqwell plexWell LP384 Library Preparation Kit and Native Barcoding Kit 24 V14 (SQK-NBD112.24) were used for barcode-indexed whole genome sequencing with Illumina NovaSeq system (Illumina San Diego, CA, USA) and Oxford Nanopore MinIoN Mk1C device (Oxford Nanopore Technologies, ONT, Oxford, UK), respectively. ONT long reads were base called and demultiplexed using basecaller and barcoder of GUPPY v6.3.2 on MANA, a high-performance computing cluster at the University of Hawaii at Manoa.
2.2 Hybrid genome assembly and genome annotation
The hybrid assembler, Unicycler v0.4.8 plugged in the web-server of BV-BRC 3.26.4,2 was employed by uploading paired-end (2 × 150 bp) Illumina short-read, high-accuracy basecalled ONT long reads for de novo genome assemblies (Wattam et al., 2017; Wick et al., 2017; Klair et al., 2022; Olson et al., 2023). In brief, Unicycler carried out SPAdes (v3.13.0) to assemble the Illumina short reads, and then, miniasm, minimap2 (v2.17), and Racon (v1.4.13) were run for long-read plus contig assembly, long-read bridging, and contig polishing, respectively. Alternatively, the genome of the strain (A2111) with a lower coverage of short reads was assembled by performing Flye v2.9.1 and genome assembly pipeline in the web server of BV-BRC 3.26.4 (see text footnote 2). Moreover, the genomic completeness and contamination were assessed by implementing the CheckM algorithm (Parks et al., 2015). The genome annotations were performed using Prokaryotic Genome Annotation Pipeline (PGAP v4.10) on NCBI (Tatusova et al., 2016) and Rapid Annotation using Subsystem Technology (RAST v2.0) web server (Aziz et al., 2008) as well.
2.3 Phylogenetic analyses
The partial 16S rRNA gene sequences of new species strains were amplified using primer set P16S-F1 (5’-AGACTCCTACGGGAGGCAGCA-3′) and P16S-R1 (5’-TTGACGTCATCCCC ACCTTCC-3′) by end-point PCR (Larrea-Sarmiento et al., 2019). Each 25 μL of PCR reaction mix contained 5 μL of 5X Q5 buffer, 5 μL of GC enhance, 2.5 μL of 5 μM primer F and R, 0.5 μL of 2.5 mM dNTPs, 0.5 μL of Q5 polymerase, 1 μL of gDNA, and 6.5 μL of nuclease-free water. The PCR reaction was run as follows: 10 s at 98°C; 35 cycles of 10 s denaturing at 98°C, 30 s of annealing at 58°C, and 30 s extending at 72°C; and 2 min at 72°C for final extension in a T100 Thermal Cycler (BIO-RAD Lab. Inc., Hercules, CA, USA). The sizes of PCR products were checked by running agarose gel electrophoresis, purified using Exo (exonuclease I)-SAP (shrimp alkaline phosphatase) method (GE Healthcare, Little Chalfont, UK) following the manufacturer’s instruction, and sent for Sanger sequencing service at the GENEWIZ company (South Plainfield, NJ, USA). The sequences were double checked with the assembled genomes.
For the phylogenetic analysis of 16S rRNA gene, the full-length sequences of 65 Stenotrophomonas and Xanthomonas species type strains were retrieved from their whole genome sequences and downloaded from GenBank on NCBI (Supplementary Table 1). The multiple alignment was performed using Geneious Prime 2021.2.2.3 The module of finding the best DNA/Protein model for the multiple alignment data was conducted, and the maximum likelihood (ML) phylogenetic tree was built using MEGA X (Kumar et al., 2018). The consistency of the phylogenetic tree was assessed by computing 1,000 bootstrapping analyses.
Additionally, the precise MLSA was performed to reveal the phylogenetic relations between the novel species and other Stenotrophomonas and Xanthomonas species. Nine housekeeping genes (atpD, dnaA, dnaK, gltA, gyrB, nuoD, ppsA, rpoH, and uvrB) used from the previous studies (Ramos et al., 2011b; Vasileuskaya-Schulz et al., 2011; Chuang, 2023) were retrieved from downloaded genomes. The sequences of the nine housekeeping genes were aligned with free end gaps algorithm separately. After trimming the both sequence ends of each gene, nine gene sequences were concatenated in alphabetic order using Geneious Prime for further analyzing. The ML phylogenetic tree was formed using MEGA X following the process as detailed above. The phylogenetic trees with bootstrapping analyses were created using web-based tool Interactive Tree Of Life (iTOL v6)4 (Letunic and Bork, 2021).
2.4 Genome similarity
To define new species, the pairwise comparisons of overall genomic relatedness indices (OGRIs) among the genomes of new species strains and other type strains of Stenotrophomonas and Xanthomonas species retrieved from NCBI database were calculated. The pairwise ANI and AP (alignment percentage) values were calculated using CLC Genomics Workbench 22.0.2 (CLC Bio-QIAGEN, Arahus, Denmark). Due to the inclusion of some incomplete genomes, OrthoANI (Average Nucleotide Identity by Orthology), which only considered the orthologous fragment pairs, was additionally calculated by performing Orthologous Average Nucleotide Identity tool (OAT) (Lee et al., 2016). Moreover, the pairwise dDDH values and the differences in G + C content (mol%) were inferred by estimating precise distance from whole genome sequences using the Genome-Genome Distance Calculator (GGDC) v3.0 on Type Strain Genome Server (TYGS) web server5 (Meier-Kolthoff et al., 2013, 2022).
2.5 Pan-genome analysis
Whole genome sequences of the new species and closely phylogenetically related species in each genus were used for pan-genome and core-genome analyses. Prokka v1.14.6 (Seemann, 2014) was used to re-annotate representative genomes, and the output gff files were used as input files for the Roary v3.13.0 pipeline (Page et al., 2015). For Roary, core and accessory genes were assessed with 80% minimum BLASTp identity, and multi-FASTA alignment of the core genome was generated using highly accurate PRANK, which is a probabilistic multiple alignment program (Löytynoja, 2014; Page et al., 2015). The number of core and unique genes among species of each genus was assessed from the Roary output and was used for the flower plots by computing R script in RStudio (R Core Team, 2022). A core gene phylogenetic tree was established using an ML tree inference tool Randomized Axelerated Maximum Likelihood – Next Generation (RAxML -NG) v0.8.0 (Kozlov et al., 2019), which combine the strengths of RAxML (Stamatakis, 2014) and Exascale Maximum Likelihood (ExaML) (Kozlov et al., 2015). The DNA substitution model, General Time Reversible (GTR) + GAMMA (G), was performed and ran separately with core genomes of type species of Xanthomonas spp. and Stenotrophomonas spp., with 1,000 bootstrap replicates. The core genome phylogenetic tree was displayed using a web-based tool Interactive Tree Of Life (iTOL v6, see text footnote 4) (Letunic and Bork, 2021). The Roary matrix with the presence and absence of core and accessory genes was combined with the core genome ML tree, and the results were visualized by conducting roary_plots.py (Page et al., 2015).
2.6 Antibiotic sensitivity assay
Antibiotic sensitivity assays were performed using disc diffusion methods described by Klair et al. (2022). Single colonies were picked from the pure culture plates of four new species strings and incubated in 10 mL of Luria-Bertani (LB) broth at 28°C with shaking at 200 rpm for 16 h. Light absorbance at 600 nm (OD600) of bacterial inoculum was adjusted to the value ~1.0, and 100 μL of inoculum was spread evenly on nutrient agar (NA, CRITERION™, Hardy Diagnostics). Seven antibiotics with different concentrations of bacitracin (50 mg/mL), chloramphenicol (50 mg/mL), gentamicin (50 mg/mL), kanamycin (50 mg/mL), penicillin (50 mg/mL), tetracycline (40 mg/mL), and polymyxin B sulfate (50 mg/mL) were tested. One Petri dish was divided into four zones, and three discs impregnated with each antibiotic solution and one disc soaked with sterile distilled water as control were placed in the center of each zone. Inhibition zones were observed and measured after incubating the plates at 28°C for 24 h.
3 Results
3.1 Genome assembly and annotation
The high-quality genomes of the strains A6251T, A5588T, and A5586T were assembled using Unicycler v0.4.8, whereas strain A2111 had a better de novo assembly using another hybrid genome assembler, Flye v2.9.1 (Table 1). The genome sizes of new species strains from anthurium, A5588T and A5586T, are 4.33 Mbp and 4.68 Mbp with 66.44 mol% and 65.3 mol% of GC content, respectively. In comparison, the GC content was higher in the other two strains, i.e., A6251T (4.88 Mbp) from spathiphyllum and A2111 (4.87 Mbp) from colocasia, with 68.93 mol% and 68.88 mol% GC content, respectively (Table 1). Based on the annotation of NCBI-PGAPservice, the average CDS number of the four strains was 4,016. The strain A5588T has the lowest CDS number, whereas the strain A5586T has the highest number (Table 1). The CheckM completeness estimates were 99.9% in A6251T and A2111 and 100% in A5588T and A5586T (Table 1). Although the CDS numbers estimated by RAST web server were slightly different from PGAP annotation, the strain A5588T had the lowest CDS number which correlated with its genome size (data not shown). By contrast, the coverage of subsystem features presented in A5588T was the highest and in A5586T was the lowest (Figure 1A). Four strains comprised 23 out of the total 27 subsystem feature categories including virulence, stress response, membrane transport, DNA, and protein metabolism (Figure 1B). Notably, only strains A6251T and A2111 contained proteins in the iron acquisition and metabolism subsystem but not strains A5588T and A5586T (Figure 1B). Stenotrophomonas maltophilia was reported to use two putative iron acquisition systems for the mediation of siderophores and heme as iron starvation (Kalidasan et al., 2018), implying that strains A5588T and A5586T from anthurium were different from the opportunistic human pathogen.
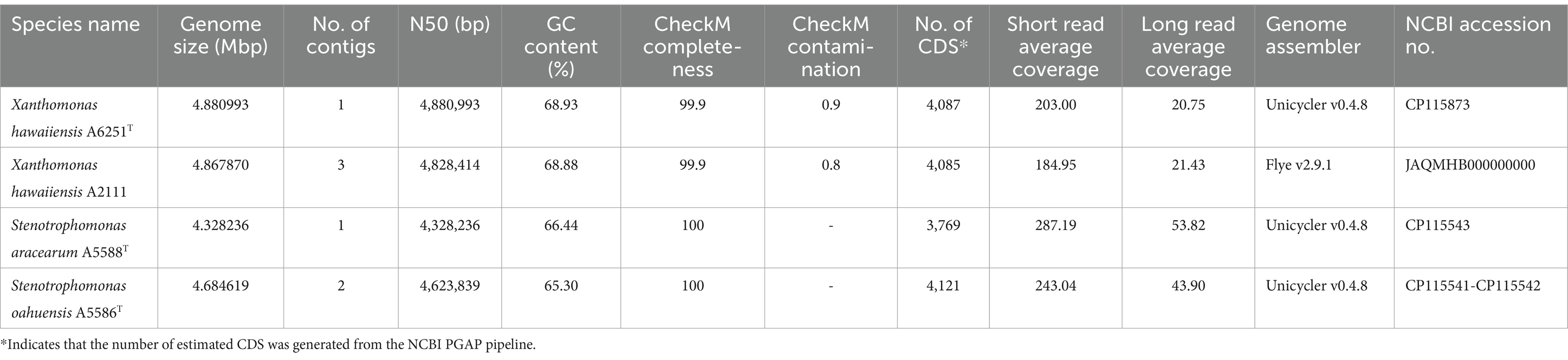
Table 1. Genome features of Xanthomonas hawaiiensis sp. nov., Stenotrophomonas aracearum sp. nov., and S. oahuensis sp. nov. in this study.
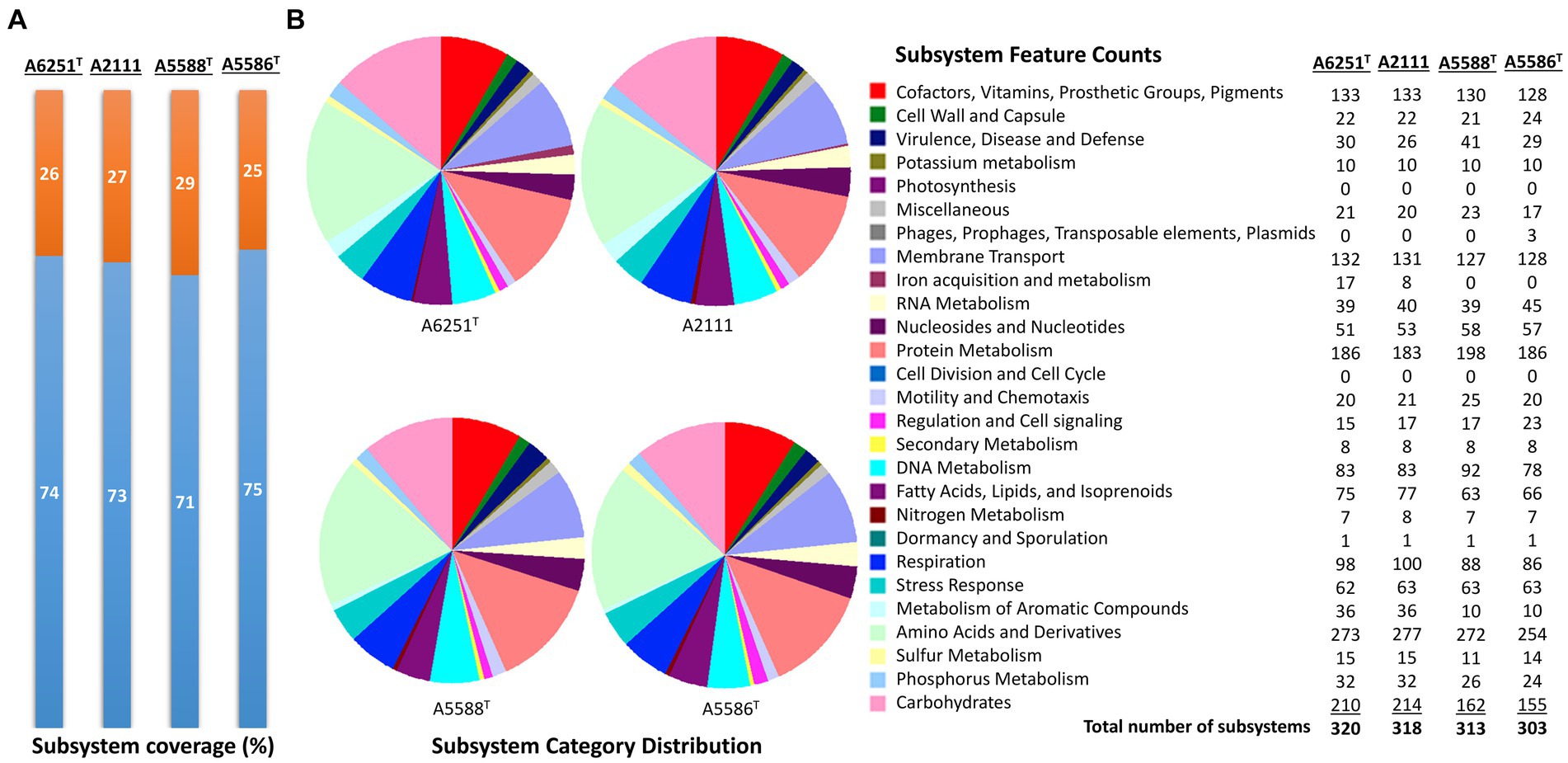
Figure 1. Subsystem annotation summary of new species strains, A6251T, A2111, A5588T, and A5586T by conducting RAST web server. (A) The percentages of protein-coding genes present (orange portions) or absent (blue portions) in the RAST subsystem. (B) The pie chart and the number of subsystem features in total 27 categories found in four genomes of aroid strains.
3.2 Phylogenetic analyses
The partial sequences of 16S rRNA gene were amplified using primer set P16S-F1 and P16S-R1 and deposited in the NCBI GenBank database under accession numbers OP962219 (A6251T), OP962220 (A2111), OP964727 (A5586T), and OP964728 (A5588T). The 16S rRNA gene sequences were retrieved from the whole genomes of new species strains, and 38 type strains of Xanthomonas species and 23 type strains of Stenotrophomonas species published in the NCBI database (Supplementary Table 1). The sequences of the nearly entire 16S rRNA gene ranging from 1,415 bp (S. bentonitica LMG 29893T) to 1,421 bp (S. chelatiphaga DSM 21508T) were analyzed for phylogenetic relationships. The 16S rRNA gene sequences of A6251T and A2111 were identical with X. sacchari CFBP 4641T and only one base was different from “X. sontii” PPL1T. A5588T and A5586T were closely related to each other and S. bentonitica LMG 29893T and showed higher similarity values of 16S rRNA ranging from 99.6 to 99.8%. In the maximum likelihood (ML) phylogenetic tree, 16S rRNA gene sequences depicted better resolution within Stenotrophomonas species than Xanthomonas species because of very poor species discrimination, which was higher than the 98.7% cutoff of 16S similarity (Figure 2).
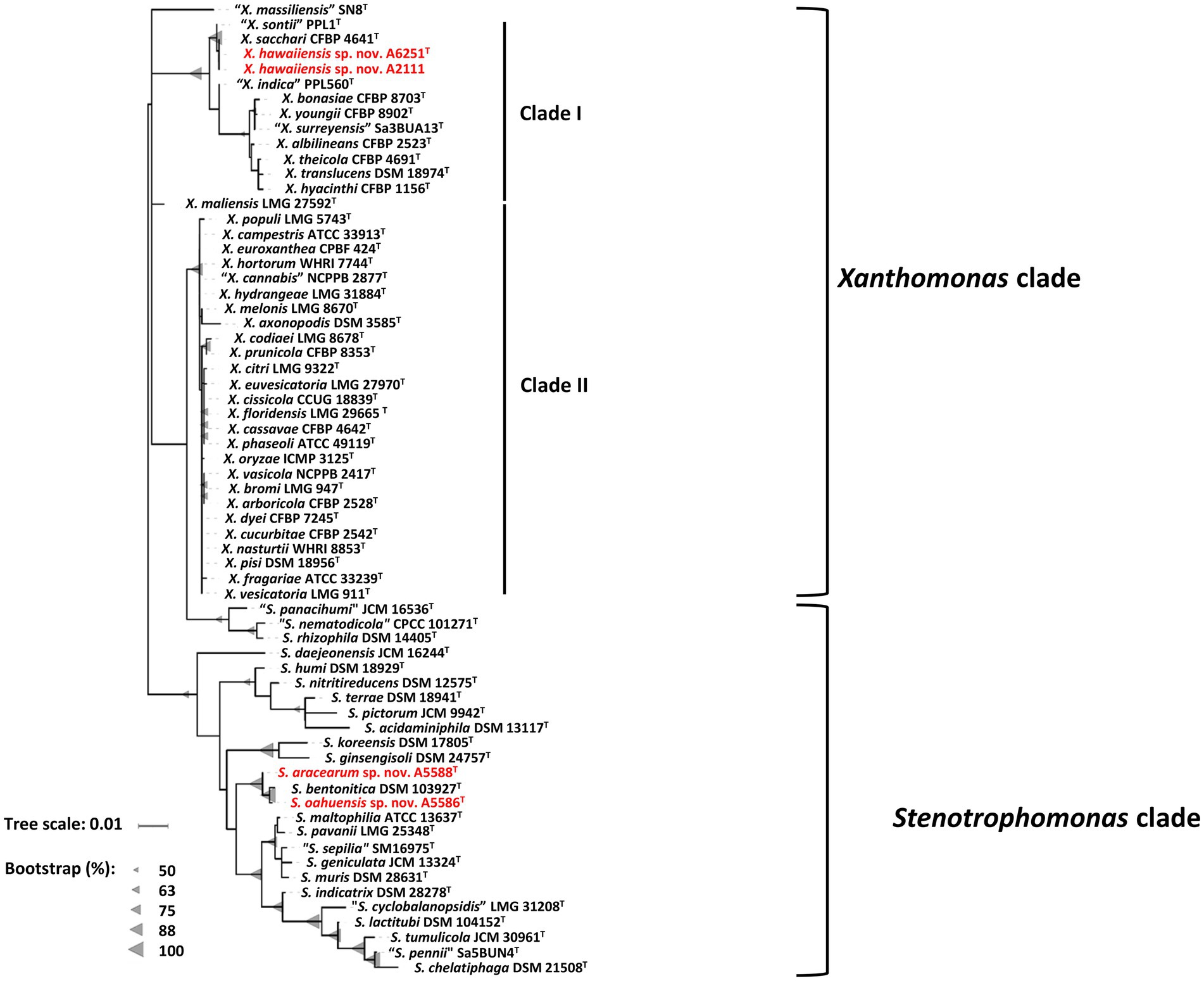
Figure 2. Maximum Likelihood phylogenetic tree based on almost full-length 16S rRNA gene sequences among three new species strains and type strains of Xanthomonas and Stenotrophomonas species. The tree scale bar indicates the number of nucleotide substitutions per sequence position. The range of gray triangles represents the degree of bootstrapping values.
For more detailed phylogenetic analysis, nine housekeeping genes (atpD, dnaA, dnaK, gltA, gyrB, nuoD, ppsA, rpoH, and uvrB) were selected and retrieved from whole genomes of formerly mentioned type strains of Xanthomonas and Stenotrophomonas species. Total length of concatenated sequence with nine genes in alphabetic order was approximately 14.3 Kb, which contained the maximum ~2,443 bp of gyrB gene and the minimum ~879 bp of uvrB gene sequences. The similarity of the concatenated gene sequences of two strains A6251T and A2111 was 99.3%; strain A5588T and strain A5586T showed 89.8% similarity. Based on nine housekeeping genes, the ML tree indicated that two major phylogenetic clades, Clade I and Clade II, were present within the Xanthomonas clade with high bootstrapping value support (Figure 3). Similar Clade I and II phylogenetic groupings were reported in the previous studies (Koebnik et al., 2021; Mafakheri et al., 2022; Rana et al., 2022). The strains A6251T and A2111 formed a monoclade clustering with X. sacchari, X. indica, X. sontii, and X. albilineans in Clade I, which also include X. surreyensis, X. bonasiae, X. traslucens, X. hyacinthi, X. theicola, and X. youngii (Figure 3). Stenotrophomonas bentonitica consistently clustered with strains A5588T and A5586T with strong bootstrapping value. Stenotrophomonas rhizophila and S. nematodicola formed a clade closely related to the A5588T-A5586T-S. bentonitica clade (Figure 3); however, no grouping was formed in the 16S rRNA phylogenetic tree (Figure 2).
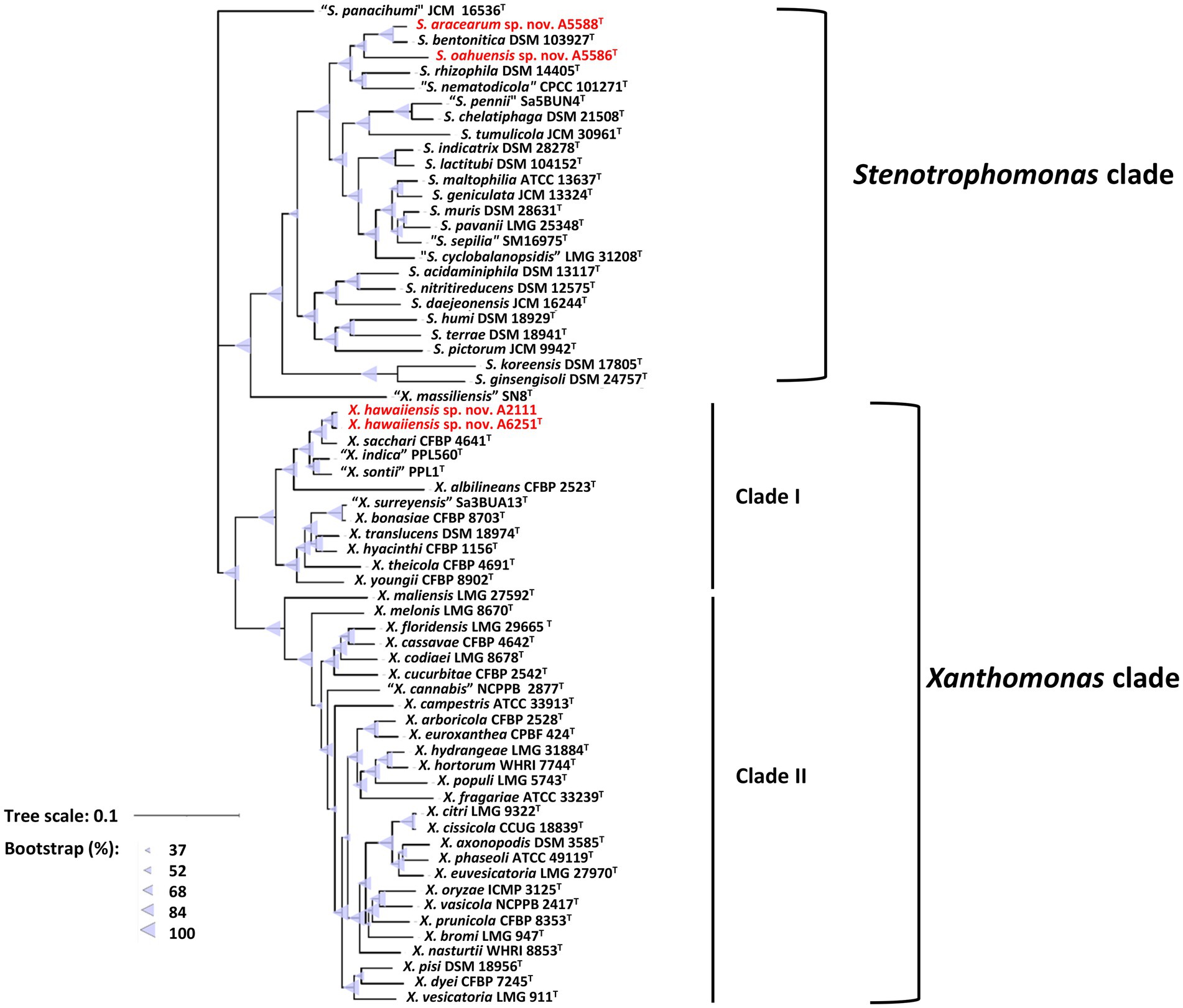
Figure 3. Maximum Likelihood phylogenetic tree based on concatenated sequence set of nine housekeeping genes, atpD, dnaA, dnaK, gltA, gyrB, nuoD, ppsA, rpoH, and uvrB of Xanthomonas and Stenotrophomonas species type strains. The scale bar represents the nucleotide substitutions per site. The range of purple triangles indicates the degree of bootstrapping support.
3.3 Overall genomic relatedness indices
To examine the accurate taxonomic classification, the overall genomic relatedness indices (OGRIs) including the values of ANI and dDDH of A6251T from spathiphyllum and A2111 from colocasia were analyzed with other type strains of Xanthomonas species. Meanwhile, A5588T and A5586T strains were compared with other type strains in Stenotrophomonas. The general cutoff values of ANI and dDDH for species delineation are lower than 95–96 and 70%, respectively (Goris et al., 2007; Richter and Rosselló-Móra, 2009; Meier-Kolthoff et al., 2013). Strains A6251T and A2111 shared 98.4% ANI and 85.2% dDDH with each other, which indicated that two strains belong to the same species. Based on the pairwise comparisons of the other Xanthomonas spp. reference genomes with either A6251T or A2111, the ANI and dDDH values were 83.4–94.9% and 22.3–59.3%, respectively, which strongly signified that A6251T and A2111 are distinguished from the others and should be considered a novel lineage (Table 2). Despite that X. sacchari CFBP 4641T shared slightly higher OrthoANI values (95.04, 95.1) with A6251T and A2111, other OGRIs supported the assignment as a new species (Table 2). The estimations of ANI and dDDH of anthurium strains, A5588T and A5586T, were 86.4 and 28.2%, respectively. Both strains shared ANI and dDDH values lower than 90% (83.4–86.8%) and 30% (20.7–29.9%) with other type strains of Stenotrophomonas spp., respectively, except for that A5588T and S. bentonitica LMG 29893T shared 94.7% of ANI and 56.4% of dDDH sequence identities (Table 3). In addition to ANI and dDDH values, other OGRIs including AP, OrthoANI, and G + C differences supported that A5588T and A5586T are two novel species (Table 3). To combine the phylogenetic analyses and evidence of OGRIs, three novel species were proposed, i.e., X. hawaiiensis sp. nov. strains A6251T and A2111; S. aracearum sp. nov. strain A5588T; and, S. oahuensis sp. nov. strain A5586T.
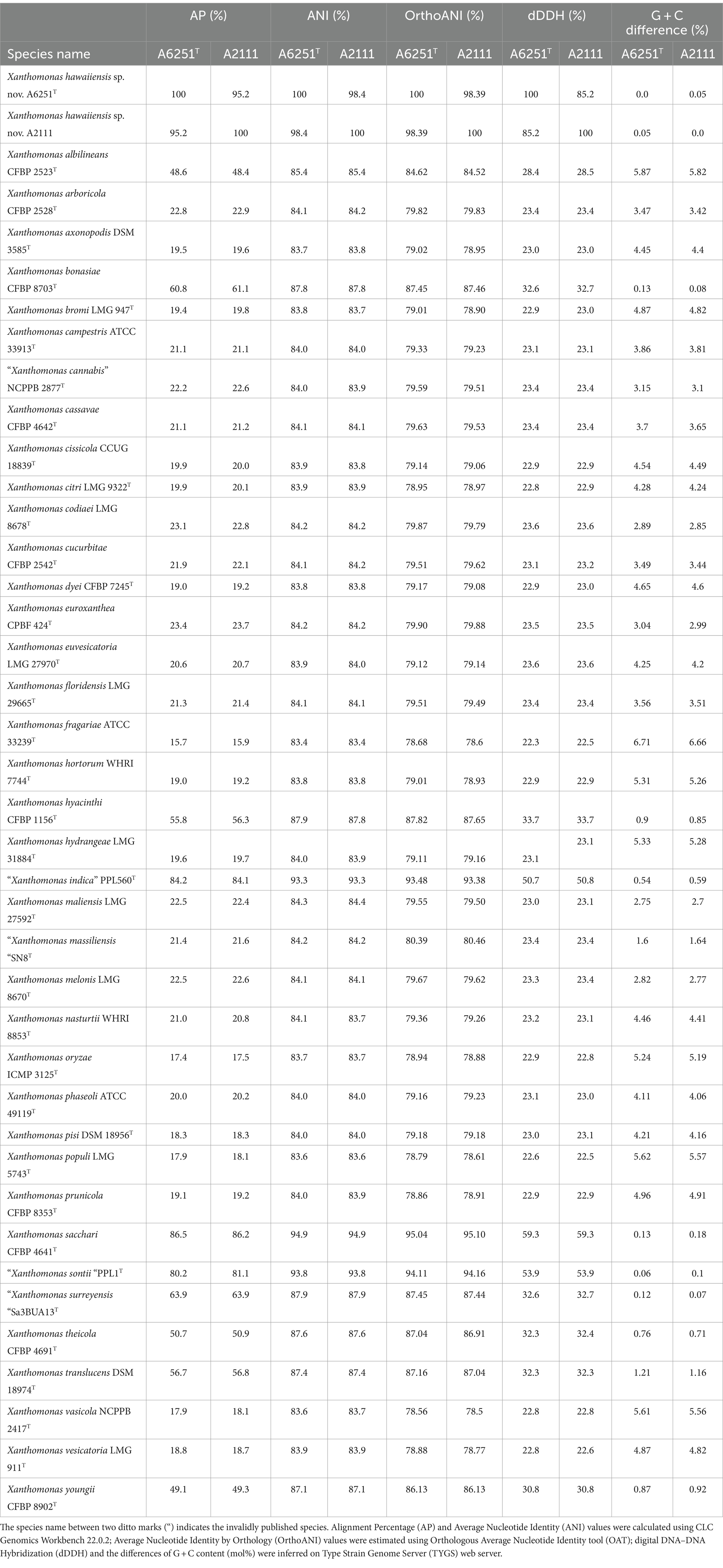
Table 2. Overall genomic relatedness indices (OGRIs) comparison of new species, Xanthomonas hawaiienesis sp. nov., strains with other type strains of Xanthomonas species.
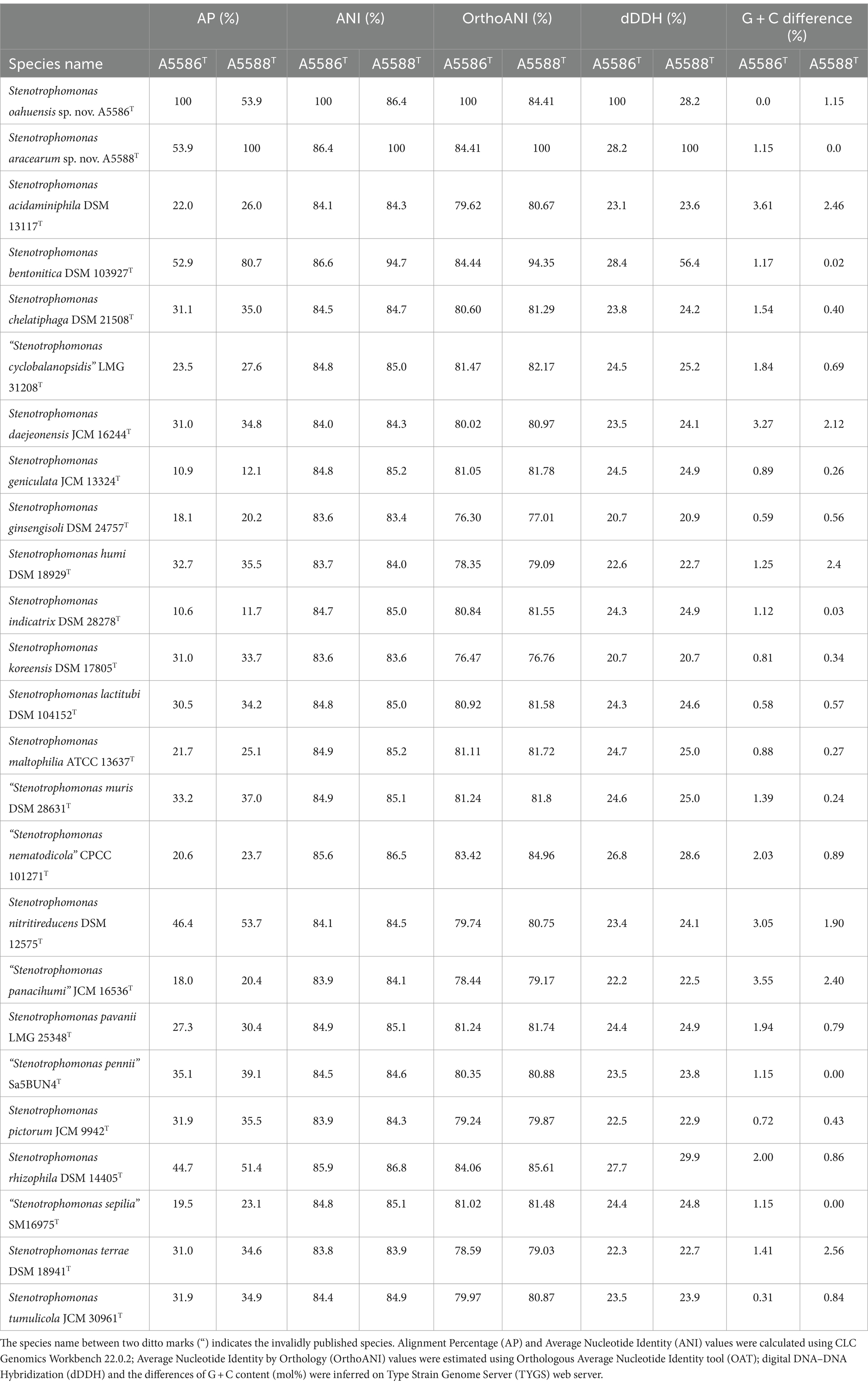
Table 3. Overall genomic relatedness indices (OGRIs) comparison of new species, Stenotrophomonas oahuensis sp. nov. and S. aracearum sp. nov. within other species in the genus.
3.4 Pan- and core-genomic analyses
Among 40 reference genomes of Xanthomonas spp. including X. hawaiiensis sp. nov. strains A6251T and A2111, 425 core orthologous genes (99% ≤ strains ≤100%) and 28,285 cloud genes (0% ≤ strains <15%) were found (Figure 4A). The lowest two numbers of unique genes present in A6251T and A2111 were 87 and 103, respectively, and follow X. sacchari CFBP 4641T which had 171 unique genes, as shown in the Figure 4B. The number of exclusive hypothetical protein encoded genes was comparatively lower in strains A6251T and A2111, whereas 50 common hypothetical proteins existed in all type strains of Xanthomonas spp. (Figure 4C). Based on the phylogenetic tree constituted with 425 core genes, the closest relative of X. hawaiiensis sp. nov. was X. sacchari CFBP 4641T, which successively clustered with “X. sontii” PPL1T and “X. indica” CFBP 9039T in Xanthomonas clade I species (Figure 5). The groupings were concordant with the previously described MLSA tree (Figure 3). The 34,713 gene clusters estimated in the Roary matrix revealed that the genomes of xanthomonads were highly diversified (Figure 5).
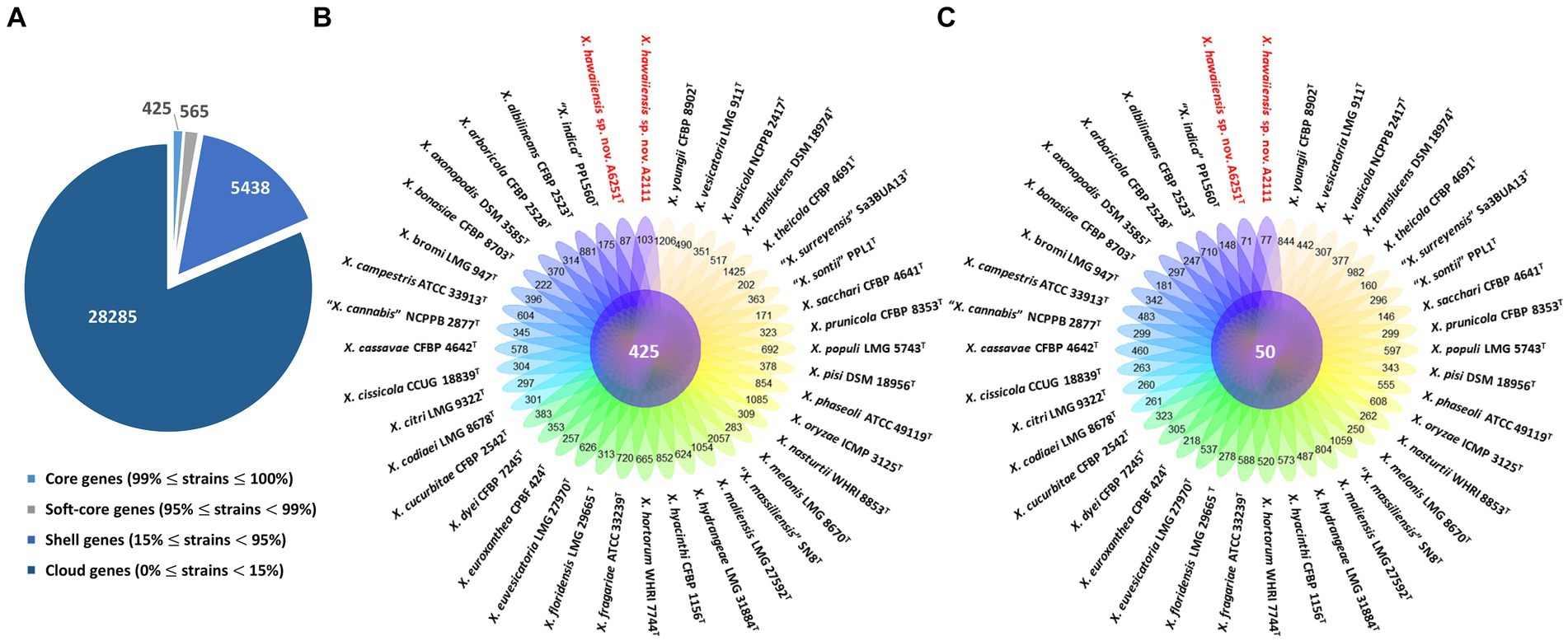
Figure 4. Pan-genome analyses of Xanthomonas hawaiienses sp. nov. (A6251T and A2111) with other type strains of Xanthomonas species. (A) Numbers of core, soft-core, shell, and cloud genes within 40 genomes of type strains of Xanthomonas species. (B) Floral plot showing the number of core orthologous genes in the center and the number of unique genes on each petal. (C) The number of common hypothetical protein encoding genes in the center of floral plot and the number of unique hypothetical protein encoding genes of each Xanthomonas strain on each petal.
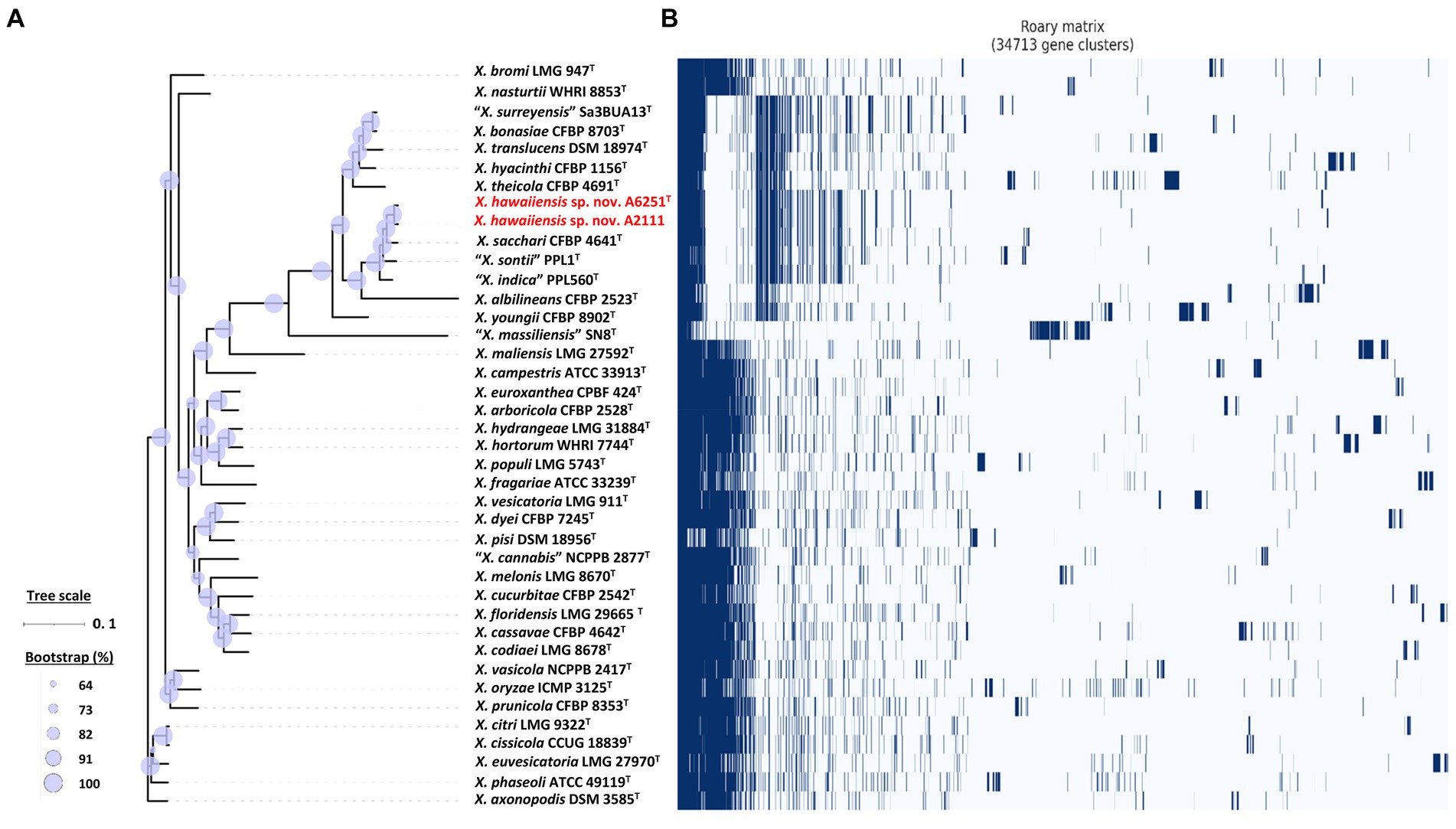
Figure 5. Core- and pan-genome analyses of 40 Xanthomonas species including new species strains. (A) Core genome-based ML phylogenetic tree of Xanthomonas hawaiienses sp. nov. (A6251T and A2111) with other type strains of Xanthomonas species. Tree scale bar represents the nucleotide substitutions per site. The range of purple circles indicates the percentage of bootstrapping confidence. (B) Pan genome-based Roary matrix of the presence and absence of genes among 40 coordinated Xanthomonas species. Dark blue blocks represent genes and pale blue blocks are missing genes in the genomes.
On the other hand, the pan genome size of 20 Stenotrophomonas spp. type strains, including S. aracearum sp. nov. (A5588T) and S. oahuensis sp. nov. (A5586T), was 31,069 with 576 core genes (Figures 6A, 7). The genome of the strain A5588T contained 396 unique genes, 317 of which were hypothetical protein encoding genes; whereas, a high number of hypothetical protein encoding genes (1,242 genes) were harbored in the genome of the strain A5586T, possessing total 1,526 unique genes (Figures 6B–C). As presented in the 9-gene ML tree (Figure 3), A5588T and S. bentonitica DSM 103927T were closely clustered together and grouped with A5586T, which was a sister group of the clade formed with S. rhizophila DSM 14405T and “S. nematodicola” CPCC 101271T (Figure 7). The average number of unique genes with unknown functions was higher in 25 Stenotrophomonas spp. than 40 Xanthomonas spp. (806 > 538), implying higher genetic diversity within stenotrophomonads, which warrants further investigations on Stenotrophomonas species.
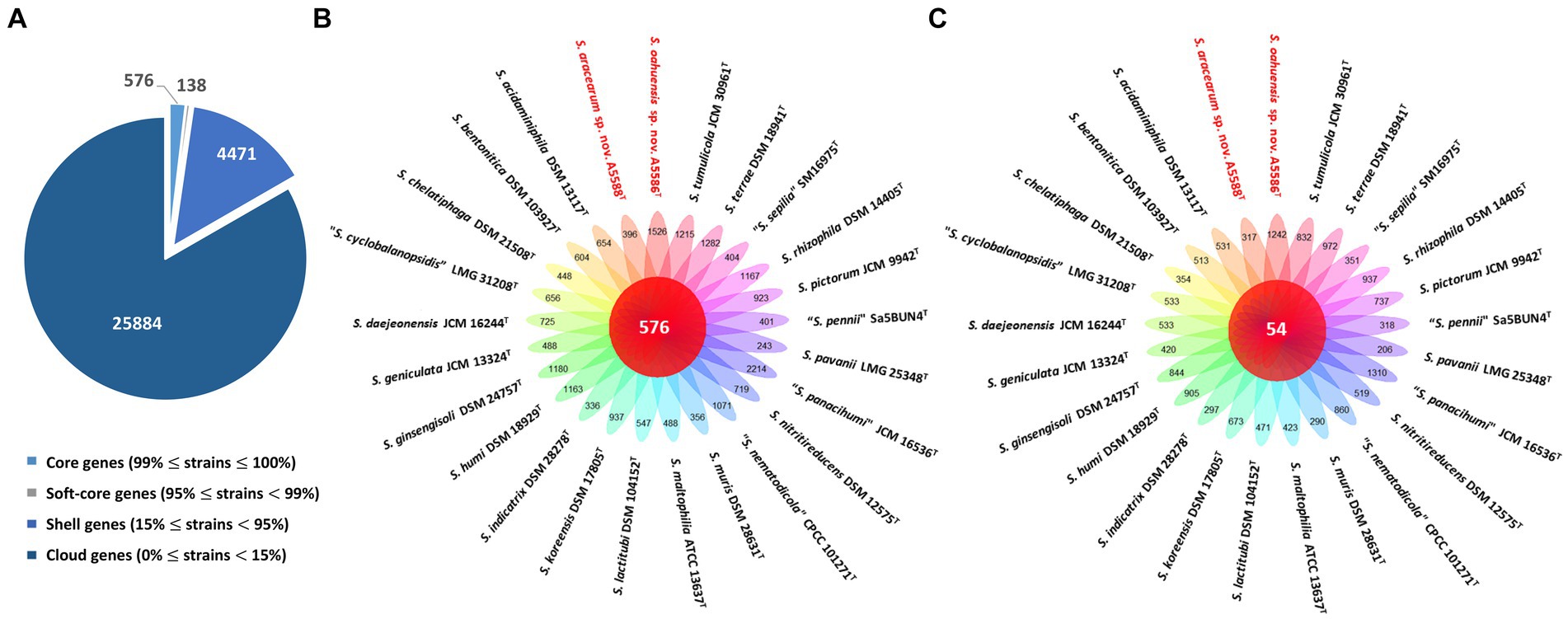
Figure 6. Pan-genome analyses of Stenotrophomonas aracearum sp. nov. (A5588T) and S. oahuensis sp. nov. (A5586T) with other type strains of Stenotrophomonas species. (A) Numbers of core, soft-core, shell, and cloud genes within 25 genomes of type strains of Stenotrophomonas species. (B) Floral plot showing the number of core orthologous genes in the center and the number of unique genes on each petal. (C) The number of common hypothetical protein encoding genes in the center of floral plot and the number of unique hypothetical protein encoding genes of each Stenotrophomonas species on each petal.
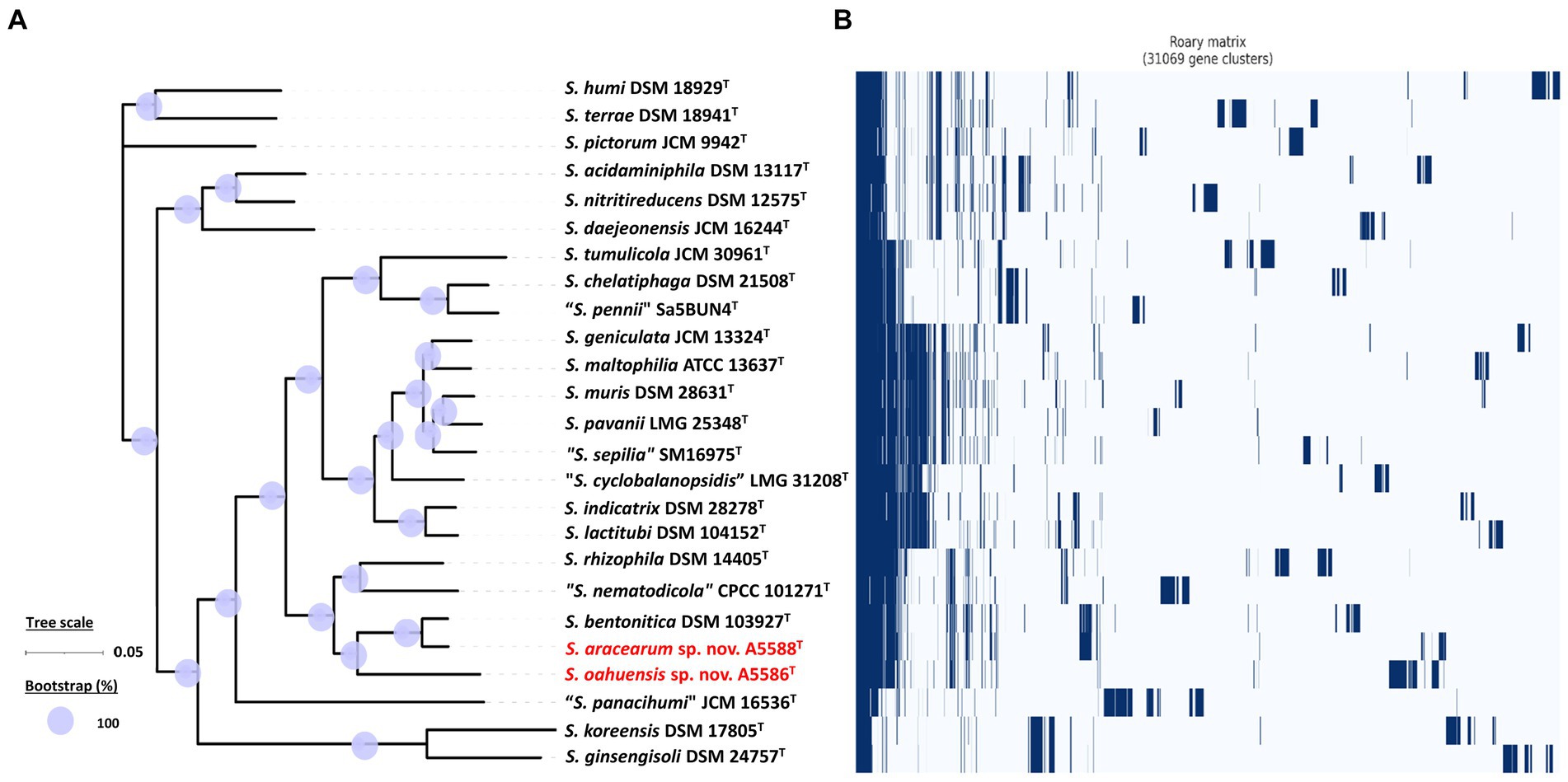
Figure 7. Core- and pan-genome analyses of 25 Stenotrophomonas species including two new species type strains. (A) Core genome-based ML phylogenetic tree of S. aracearum sp. nov. (A5588T) and S. oahuensis sp. nov. (A5586T) along with other type strains of Stenotrophomonas species. Tree scale bar represents the substitutions per nucleotide position. The purple circles represent 100% bootstrapping support. (B) Pan genome-based Roary matrix of the presence and absence of genes among all coordinated Stenotrophomonas species. Dark blue blocks indicate genes present and pale blue blocks indicate genes absent in the genomes.
3.5 Antibiotic sensitivity assays
The inhibition zones with seven tested antibiotics, namely, bacitracin (50 mg/mL), chloramphenicol (50 mg/mL), gentamicin (50 mg/mL), kanamycin (50 mg/mL), penicillin (50 mg/mL), tetracycline (40 mg/mL), and polymyxin B sulfate (50 mg/mL), indicated various degrees of sensitivity of four new species strains. Strains A6251T, A2111, and A5586T were sensitive to all tested antibiotics, whereas strain A5588T was sensitive to all tested antibiotics except penicillin (Supplementary Table 2). Strains A6251T and A2111, belonging to the same new species, displayed similar results, except for the tolerance to polymyxin B sulfate. Notably, A5586T displayed a very small inhibition zone (0.1 cm in radius) surrounding the discs of bacitracin on the NA plate after incubating at 28°C for 24 h (Supplementary Table 2).
4 Descriptions of new species
4.1 Xanthomonas hawaiiensis sp. nov. (ha.waii.en′sis. N.L. fem. adj. hawaiiensis, of or belonging to Hawaii, a state of the United States, referring to the geographical origin of the new species)
Colonies of the type strain A6251T are yellow (Honey, Hex code #FFC30B), circular shape, mucoid consistency, smooth surface, convex relief with entire margins, and 0.3–0.6 (avg. 0.45) mm in diameter on yeast dextrose calcium carbonate (YDC) medium plate after incubating at 28°C for 2 days. Cells are gram-negative and able to utilize dextrin, D-maltose, D-trehalose, D-cellobiose, gentiobiose, sucrose, D-turanose, α-D-lactose, D-melibiose, Β-methyl-D-glucoside, D-salicin, N-acetyl-D-glucosamine, α-D-glucose, D-mannose, D-fructose, D-galactose, L-fucose, 1% NaCl, 1% sodium lactate, glycerol, gelatin, L-glutamic acid, lincomycin, pectin, quinic acid, vancomycin, tetrazolium violet, tetrazolium blue, citric acid, bromo-succinic acid, lithium chloride, Tween 40, and acetic Acid. In contrast, cells are unable to oxidize stachyose, D-raffinose, N-acetyl-β-D-mannosamine, N-acetyl-D-galactosamine, N-acetyl-neuraminic acid, 8% NaCl, inosine, fusidic acid, D-sorbitol, D-mannitol, D-arabitol, myo-inositol, D-aspartic acid, minocycline, L-arginine, L-histidine, L-pyroglutamic acid, guanidine HCl, D-gluconic acid, mucic acid, D-saccharic acid, p-hydroxy-phenylacetic acid, D-lactic acid methyl ester, α-keto-glutaric acid, D-malic acid, γ-aminobutryric acid, α-hydroxybutyric acid, α-ketobutyric acid, formic acid, sodium butyrate, and sodium bromate. Some utilization of carbon resources and chemical components showed borderline results or inconsistency between two strains after growing cell suspension in GEN III Microplate (Biolog Inc., Hayward, CA, USA) at 28°C for 24 h.
X. hawaiiensis sp. nov. is sensitive to seven tested antibiotics, including bacitracin (50 mg/mL), chloramphenicol (50 mg/mL), gentamicin (50 mg/mL), kanamycin (50 mg/mL), penicillin (50 mg/mL), tetracycline (40 mg/mL), and polymyxin B sulfate (50 mg/mL). The genome size of type strain A6251T is 4.88 Mbp with 68.93 mol% of DNA G + C content.
The type strain A6251T = D-93T = ICMP 25022T = LMG 33200T was isolated from Spathiphyllum (Araceae family) in 1985 in Hawaii, USA. Another strain A2111 = D-194 = ICMP 25023 = LMG 33199 was isolated from Colocasia (Araceae family) in 1986 in Hawaii, USA.
4.2 Stenotrophomonas aracearum sp. nov. (a.ra.ce.a’rum. N.L. gen. fem. pl. n. aracearum, representative of plants belonging to the Araceae family)
Colonies of S. aracearum strain A5588T are dark yellow (Mustard, Hex code #E8B828), irregular shape, butyrous consistency, smooth surface, raised relief with entire margins, and 0.4–0.5 mm (average 0.45) in diameter on YDC medium plates after incubation at 28°C for 2 days. Cells are gram-negative and able to utilize D-maltose, D-cellobiose, gentiobiose, N-acetyl-D-glucosamine, N-acetyl-D-galactosamine, α-D-glucose, D-mannose, 1% sodium lactate, D-serine, troleandomycin, rifamycin SV, gelatin, lincomycin, guanidine HCl, vancomycin, tetrazolium violet, tetrazolium Blue, α-ketoglutaric acid, L-malic acid, bromo-succinic acid, acetic acid, and aztreonam. Cells grow under pH 6 and 1% NaCl but neither at pH 5 nor 8% NaCl. In the contrary, cells are unable to oxidize sucrose, D-turanose, stachyose, D-raffinose, α-D-lactose, D-melibiose, Β-methyl-D-glucoside, N-acetyl-β-D-mannosamine, N-acetyl-neuraminic acid, D-galactose, 3-methyl-glucose, inosine, fusidic acid, D-sorbitol, D-mannitol, D-arabitol, myo-inositol, glycerol, D-glucose-6-PO4, D-aspartic acid, D-serine, minocycline, L-arginine, L-aspartic acid, L- glutamic acid, L-histidine, L-pyroglutamic acid, L-serine, pectin, D-galacturonic acid, D-gluconic acid, mucic acid, quinic acid, D-saccharic acid, p-hydroxy-phenylacetic acid, D-lactic acid methyl ester, L-lactic acid, citric acid, D-malic acid, nalidixic acid, potassium tellurite, γ-aminobutryric acid, α-hydroxybutyric acid, α-ketobutyric acid, β-hydroxy-D, L-butyric acid, acetoacetic acid, formic acid, sodium butyrate, and sodium bromate. Some utilization of carbon sources and chemical components, such as dextrin and glucuronamide showed faded positive results after growing A5588T cell suspension in GEN III Microplate (Biolog Inc., Hayward, CA, USA) at 28°C for 24 h.
S. aracearum sp. nov. was sensitive to six tested antibiotics, namely, bacitracin (50 mg/mL), chloramphenicol (50 mg/mL), gentamicin (50 mg/mL), kanamycin (50 mg/mL), tetracycline (40 mg/mL), and polymyxin B sulfate (50 mg/mL) but resistant to penicillin (50 mg/mL) on NA plates. The genome size of type strain A5588T is 4.33 Mbp with 66.44 mol% of DNA G + C content.
The type strain A5588T = D-61-1LT = ICMP 25025T = LMG 33202T was isolated from Anthurium (Araceae family) in 1985 in Hawaii, USA.
4.3 Stenotrophomonas oahuensis sp. nov. (o.a.hu.en’sis. N.L. fem. adj. oahuensis, of or belonging to the island of Oahu in Hawaii, referring to the geographical origin of the new species)
Colonies of the S. oahuensis strain A5586T are dark yellow (Butterscotch, Hex code #FABD02), circular shape, butyrous consistency, smooth surface, flat relief with undulate margins, and 0.4–0.7 mm (average 0.55) in diameter on YDC medium plates after incubation at 28°C for 2 days. Cells are gram-negative and able to utilize dextrin, D-maltose, D-trehalose, D-cellobiose, gentiobiose, Β-methyl-D-glucoside, D-salicin, N-acetyl-D-glucosamine, α-D-glucose, D-mannose, 1% sodium lactate, gelatin, glycyl-L-proline, lincomycin, guanidine HCl, vancomycin, tetrazolium violet, tetrazolium blue, citric acid, α-ketoglutaric acid, L-malic acid, bromo-succinic acid, lithium chloride, propionic acid, acetic acid, and aztreonam. Cells grow under the conditions of pH 6, 1% NaCl, or 4% NaCl but cells survive neither pH 5 nor 8% NaCl solution. In contrast, cells are unable to oxidize sucrose, stachyose, D-raffinose, N-acetyl-β-D-mannosamine, N-acetyl-neuraminic acid, D-galactose, 3-methyl-glucose, inosine, D-fucose, L-fucose, L-rhamnose, inosine, fusidic acid, D-sorbitol, D-mannitol, D-arabitol, myo-inositol, glycerol, D-glucose-6-PO4, D-aspartic acid, D-serine, rifamycin SV, minocycline, L-arginine, L-aspartic acid, L- glutamic acid, L-histidine, L-pyroglutamic acid, L-serine, pectin, D-galacturonic acid, D-gluconic acid, D-glucuronic acid, mucic acid, quinic acid, D-saccharic acid, p-hydroxy-phenylacetic acid, D-lactic acid methyl ester, L-lactic acid, D-malic acid, nalidixic acid, potassium tellurite, γ-amino-butryric acid, α-hydroxy-butyric acid, β-hydroxy-D, L-butyric acid, α-keto-butyric acid, acetoacetic acid, formic acid, and sodium bromate. Some utilization of carbon resources and chemical components, such as D-turanose and sodium butyrate, showed faded positive results after growing A5586T cell suspension in GEN III Microplate (Biolog Inc., Hayward, CA, USA) at 28°C for 24 h.
S. oahuensis sp. nov. was sensitive to seven tested antibiotics, namely, bacitracin (50 mg/mL), chloramphenicol (50 mg/mL), gentamicin (50 mg/mL), kanamycin (50 mg/mL), penicillin (50 mg/mL), tetracycline (40 mg/mL), and polymyxin B sulfate (50 mg/mL). The genome size of type strain A5586T is 4.68 Mbp, which includes a chromosome (4.62 Mbp) and a plasmid (60.78 Kbp). The DNA G + C content of the type strain is 65.3 mol%.
The type strain A5586T = D-31T = ICMP 25024T = LMG 33201T was isolated from Anthurium (Araceae family) in 1981 in Hawaii, USA.
5 Discussion
The genera Xanthomonas and Stenotrophomonas are phylogenetically and evolutionarily linked and are also found frequently together in several niches, including environmental reservoirs (plants and soil) and biofilters used for waste gas treatment of animal-rendering plants (Lipski and Altendorf, 1997; Finkmann et al., 2000; Ryan et al., 2009). Although more studies are focused on phyto- and human-pathogenic species, the versatility of Xanthomonas and Stenotrophomonas spp. has the potential to be applied to many different fields and needs to be explored further.
The well-known industrial biopolymer, which is also a food additive, is xanthan gum produced by X. campestris and other Xanthomonas species (Margaritis and Zajic, 1978; Kennedy and Bradshaw, 1984; Gumus et al., 2010). Production of other bioactive secondary metabolites from xanthomonads include the siderophore xanthoferrin, which acts as a bioproduction agent under low iron conditions (Pandey et al., 2017), and the pigment xanthomonadin, analogs of which have antioxidant potential (Madden et al., 2019). The subsystem features of iron acquisition and metabolism based on RAST annotation webserver (Figure 1B) suggest that X. hawaiiensis sp. nov. strains, A6251T and A2111, are capable of surviving inside the hosts (Expert et al., 1996). The xss gene cluster encodes proteins including XssABCDE (Xanthomonas siderophore synthesis) and XsuA (Xanthomonas siderophore utilization), which are homologous to PvsABCDE (Vibrioferrin biosynthesis) and PsuA (Vibrioferrin receptor) (Pandey and Sonti, 2010; Pandey et al., 2017). The xss gene loci involved in biosynthesis, uptake, and export of xanthoferrin is found in both X. hawaiiensis sp. nov. strains A6251T and A2111. In addition, the xanC gene, which encodes an acyl carrier protein and is essential for yellow xanthomonadin pigment biosystem (Cao et al., 2018), is harbored in the genomes of A6251T and A2111.
The increasing number of studies on non-pathogenic xanthomonads isolated from rice, banana, citrus, walnut, and so on suggests that they have the potential for biocontrol and bioprotection against the causal agent of their host plants (Fernandes et al., 2021; Bansal et al., 2021a,b; Rana et al., 2022). For example, X. sontii strain R1 (formerly misclassified as X. sacchari) isolated from rice seed was reported to have an antagonistic ability against Burkholderia glumae, which caused rice panicle blight disease (Xie et al., 2003; Ham et al., 2011; Fang et al., 2015). In addition, Xanthomonas sp. from ryegrass, which was phylogenetically closely related to X. translucens, showed bioprotection activities against broad tested fungal pathogens (Li et al., 2020). In the previous studies (Leite et al., 1994; Lee et al., 2020), the gene cluster involved in type III secretion system (T3SS) formation was amplified to identify the non-pathogenicity and pathogenicity strains of X. campestris. While deciphering the genomes of X. hawaiiensis sp. nov. strains, the T3SS gene cluster was missing in both genomes of A6251T and A2111 (Chuang, 2023). The absence of T3SS was also observed in non-pathogenic strains of X. campestris (Lee et al., 2020), X. sacchari NCPPB 4393 and R1 strains (Studholme et al., 2011; Fang et al., 2015), and considerably commensal X. arboricola CFBP 6771 (Cesbron et al., 2015; Merda et al., 2017). Furthermore, based on 16S rRNA and nine housekeeping genes, the ML trees (Figures 2, 3) revealed that X. hawaiiensis sp. nov. strains A6251T and A2111 were placed in Clade I along with X. sacchari and X. traslucens, which have potential biocontrol and bioprotective agents, as previously described. Hence, the genomic constituents of A6251T and A2111 strains not only suggest that X. hawaiiensis sp. nov. strains isolated from Araceae should be commensal but also provide insight into the potential biocontrol capabilities of X. hawaiiensis sp. nov.
Recent research has begun to unravel the potential for biotechnological applications and biological control of stenotrophomonads. In agriculture, for example, Stenotrophomonas strains are known for promoting plant growth, protecting plants against biotic and abiotic stresses, and serving as biocontrol agents for plant diseases (Zhang and Yuen, 1999; Wolf, 2002; Messiha et al., 2007; Alavi et al., 2013; Berg and Martinez, 2015). As bioremediators and phytoremediators, Stenotrophomonas strains are capable of metabolizing and degrading a broad range of organic compounds, such as benzene and toluene, and tolerating antibiotics and heavy metals, such as mercury and silver (Binks et al., 1995; Alonso et al., 2000; Lee et al., 2002; Pages et al., 2008). Although S. aracearum sp. nov. A5588T strain and S. oahuensis sp. nov. A5586T strain showed no subsystem features of iron acquisition and metabolism, the higher number of RNA metabolism in A5586T strain and protein metabolism in A5588T strain (Figure 1B) might shed light on some unique metabolic activities in these new Stenotrophomonas species. Interestingly, the high number of unique genes and hypothetical protein encoding genes unraveled from detailed genomic contents of the novel species, especially in S. oahuensis sp. nov., imply that novel or useful enzymatic properties and metabolic capabilities of Xanthomonas and Stenotrophomonas spp. from different environmental sources are worth exploring for biocontrol and bioprotection purposes. Preliminary data from pathogenicity tests on anthurium indicated that strains A5588T and A5586T from anthurium are non-pathogenic stenotrophomonads due to lack of symptom development on their original host. In this study, we proposes three new species, namely, X. hawaiiensis sp. nov., S. aracearum sp. nov., and S. oahuensis sp. nov., isolated from Araceae and provides high quality whole genome sequences for further studies relative to their pathogenicity on Araceae host plants and other possible bioactivities.
Data availability statement
The datasets presented in this study can be found in online repositories. The names of the repository/repositories and accession number(s) can be found at: https://www.ncbi.nlm.nih.gov/genbank/, CP115541-CP115542; https://www.ncbi.nlm.nih.gov/genbank/, CP115543; https://www.ncbi.nlm.nih.gov/genbank/, CP115873.
Author contributions
S-CC: Data curation, Formal analysis, Investigation, Methodology, Software, Validation, Visualization, Writing – original draft, Writing – review & editing. SD: Methodology, Project administration, Supervision, Writing – review & editing. AA: Funding acquisition, Methodology, Resources, Writing – review & editing. MA: Conceptualization, Funding acquisition, Investigation, Project administration, Resources, Supervision, Validation, Visualization, Writing – review & editing.
Funding
The author(s) declare financial support was received for the research, authorship, and/or publication of this article. This research was funded by the USDA National Institute of Food and Agriculture, Hatch project 9038H, managed by the College of Tropical Agriculture and Human Resources. This study was also supported by the USDA-ARS Agreement No. 58-2040-9-011, System Approaches to Improve Production and Quality of Specialty Crops Grown in the U.S. Pacific Basin; sub-project: Genome Informed Next Generation Detection Protocols for Pests and Pathogens of Specialty Crops in Hawaii. The strains were stored and maintained by the National Science Foundation funded project (NSF-CSBR Grant No. DBI-1561663). The bioinformatics analyses were supported by NIGMS of the National Institutes of Health under award number P20GM125508.
Conflict of interest
The authors declare that the research was conducted in the absence of any commercial or financial relationships that could be construed as a potential conflict of interest.
The author(s) declared that they were an editorial board member of Frontiers, at the time of submission. This had no impact on the peer review process and the final decision.
Publisher’s note
All claims expressed in this article are solely those of the authors and do not necessarily represent those of their affiliated organizations, or those of the publisher, the editors and the reviewers. Any product that may be evaluated in this article, or claim that may be made by its manufacturer, is not guaranteed or endorsed by the publisher.
Supplementary material
The Supplementary material for this article can be found online at: https://www.frontiersin.org/articles/10.3389/fmicb.2024.1356025/full#supplementary-material
Footnotes
References
Afrizal, A., Jennings, S. A. V., Hitch, T. C. A., Riedel, T., Basic, M., Panyot, A., et al. (2022). Enhanced cultured diversity of the mouse gut microbiota enables custom-made synthetic communities. Cell Host Microbe 30, 1630–45.e25. doi: 10.1016/j.chom.2022.09.011
Al-Anazi, K. A., and Al-Jasser, A. M. (2014). Infections caused by Stenotrophomonas maltophilia in recipients of hematopoietic stem cell transplantation. Front. Oncol. 4:232. doi: 10.3389/fonc.2014.00232
Alavi, P., Starcher, M. R., Zachow, C., Müller, H., and Berg, G. (2013). Root-microbe systems: the effect and mode of interaction of stress protecting agent (SPA) Stenotrophomonas rhizophila DSM14405T. Front. Plant Sci. 4:4. doi: 10.3389/fpls.2013.00141
Alonso, A., Sanchez, P., and Martinez, J. L. (2000). Stenotrophomonas maltophilia D457R contains a cluster of genes from gram-positive bacteria involved in antibiotic and heavy metal resistance. Antimicrob. Agents Chemother. 44, 1778–1782. doi: 10.1128/AAC.44.7.1778-1782.2000
Alvarez, A. M., Toves, P. J., and Vowell, T. S. (2006). Bacterial blight of anthuriums: Hawaii's experience with a global disease. APSnet Features. doi: 10.1094/APSnetFeature-2006-0206
Assih, E. A., Ouattara, A. S., Thierry, S., Cayol, J.-L., Labat, M., and Macarie, H. (2002). Stenotrophomonas acidaminiphila sp. nov., a strictly aerobic bacterium isolated from an upflow anaerobic sludge blanket (UASB) reactor. Int. J. Syst. Evol. Microbiol. 52, 559–568. doi: 10.1099/00207713-52-2-559
Aziz, R. K., Bartels, D., Best, A. A., DeJongh, M., Disz, T., Edwards, R. A., et al. (2008). The RAST server: rapid annotations using subsystems technology. BMC Genomics 9:75. doi: 10.1186/1471-2164-9-75
Bansal, K., Kaur, A., Midha, S., Kumar, S., Korpole, S., and Patil, P. B. (2021a). Xanthomonas sontii sp. nov., a non-pathogenic bacterium isolated from healthy basmati rice (Oryza sativa) seeds from India. Antonie Van Leeuwenhoek 114, 1935–1947. doi: 10.1007/s10482-021-01652-1
Bansal, K., Kumar, S., Kaur, A., Singh, A., and Patil, P. B. (2021b). Deep phylo-taxono genomics reveals Xylella as a variant lineage of plant associated Xanthomonas and supports their taxonomic reunification along with Stenotrophomonas and Pseudoxanthomonas. Genomics 113, 3989–4003. doi: 10.1016/j.ygeno.2021.09.021
Bansal, K., Kumar, S., Singh, A., Chaudhary, A., and Patil, P. B. (2023). Redefining the taxonomic boundaries of genus Xanthomonas. Taxonomy 3, 452–465. doi: 10.3390/taxonomy3040026
Berg, G., Marten, P., and Ballin, G. (1996). Stenotrophomonas maltophilia in the rhizosphere of oilseed rape — occurrence, characterization and interaction with phytopathogenic fungi. Microbiol. Res. 151, 19–27. doi: 10.1016/S0944-5013(96)80051-6
Berg, G., and Martinez, J. L. (2015). Friends or foes: can we make a distinction between beneficial and harmful strains of the Stenotrophomonas maltophilia complex? Front. Microbiol. 6:241. doi: 10.3389/fmicb.2015.00241
Bergey, DH, Harrison, FC, Breed, RS, Hammer, BW, and Huntoon, FM. Bergey’s manual of determinative bacteriology. 1st Baltimore: The Williams & Wilkins Company. (1923).
Bian, D.-r., Xue, H., Piao, C.-g., and Li, Y. (2020). Stenotrophomonas cyclobalanopsidis sp. nov., isolated from the leaf spot disease of Cyclobalanopsis patelliformis. Antonie Van Leeuwenhoek 113, 1447–1454. doi: 10.1007/s10482-020-01453-y
Binks, P. R., Nicklin, S., and Bruce, N. C. (1995). Degradation of hexahydro-1,3,5-trinitro-1,3,5-triazine (RDX) by Stenotrophomonas maltophilia PB1. Appl. Environ. Microbiol. 61, 1318–1322. doi: 10.1128/aem.61.4.1318-1322.1995
Bradbury, JF. Bergey’s manual of systematic bacteriology. Baltimore: Williams & Wilkins Co.; (1984), p. 199–211.
Cao, X. Q., Wang, J. Y., Zhou, L., Chen, B., Jin, Y., and He, Y. W. (2018). Biosynthesis of the yellow xanthomonadin pigments involves an ATP-dependent 3-hydroxybenzoic acid: acyl carrier protein ligase and an unusual type II polyketide synthase pathway. Mol. Microbiol. 110, 16–32. doi: 10.1111/mmi.14064
Cesbron, S., Briand, M., Essakhi, S., Gironde, S., Boureau, T., Manceau, C., et al. (2015). Comparative genomics of pathogenic and nonpathogenic strains of Xanthomonas arboricola unveil molecular and evolutionary events linked to pathoadaptation. Front. Plant Sci. 6:1126. doi: 10.3389/fpls.2015.01126
Chuang, S-C. (2023). Genomic analyses and pathogenicity determinants of xanthomonads associated with Araceae and Araliaceae. PhD’s dissertation. Honolulu: University of Hawai‘I at Mānoa.
Constantin, E. C., Cleenwerck, I., Maes, M., Baeyen, S., Van Malderghem, C., De Vos, P., et al. (2016). Genetic characterization of strains named as Xanthomonas axonopodis pv. dieffenbachiae leads to a taxonomic revision of the X. axonopodis species complex. Plant Pathol. 65, 792–806. doi: 10.1111/ppa.12461
Constantin, E. C., Haegeman, A., Van Vaerenbergh, J., Baeyen, S., Van Malderghem, C., Maes, M., et al. (2017). Pathogenicity and virulence gene content of Xanthomonas strains infecting Araceae, formerly known as Xanthomonas axonopodis pv. dieffenbachiae. Plant Pathol. 66, 1539–1554. doi: 10.1111/ppa.12694
Denton, M., and Kerr, K. G. (1998). Microbiological and clinical aspects of infection associated with Stenotrophomonas maltophilia. Clin. Microbiol. Rev. 11, 57–80. doi: 10.1128/CMR.11.1.57
Doidge, E. M. (1921). A tomato canker. Ann. Appl. Biol. 7, 407–430. doi: 10.1111/j.1744-7348.1921.tb05528.x
Dowson, W. J. (1939). On the systematic position and generic names of the gram negative bacterial plant pathogens. Zentralblatt fur Bakteriologie, Parasitenkunde, Infektionskrankheiten und Hygiene 100, 177–193.
Expert, D., Enard, C., and Masclaux, C. (1996). The role of iron in plant host-pathogen interactions. Trends Microbiol. 4, 232–237. doi: 10.1016/0966-842X(96)10038-X
Fang, Y., Lin, H., Wu, L., Ren, D., Ye, W., Dong, G., et al. (2015). Genome sequence of Xanthomonas sacchari R1, a biocontrol bacterium isolated from the rice seed. J. Biotechnol. 206, 77–78. doi: 10.1016/j.jbiotec.2015.04.014
Fernandes, C., Albuquerque, P., Mariz-Ponte, N., Cruz, L., and Tavares, F. (2021). Comprehensive diversity assessment of walnut-associated xanthomonads reveal the occurrence of distinct Xanthomonas arboricola lineages and of a new species (Xanthomonas euroxanthea) within the same tree. Plant Pathol. 70, 943–958. doi: 10.1111/ppa.13355
Finkmann, W., Altendorf, K., Stackebrandt, E., and Lipski, A. (2000). Characterization of N2O-producing Xanthomonas-like isolates from biofilters as Stenotrophomonas nitritireducens sp nov., Luteimonas mephitis gen. Nov., sp nov and Pseudoxanthomonas broegbernensis gen. Nov., sp nov. Int. J. Syst. Evol. Microbiol. 50, 273–282. doi: 10.1099/00207713-50-1-273
Gautam, V., Patil, P. P., Bansal, K., Kumar, S., Kaur, A., Singh, A., et al. (2021). Description of Stenotrophomonas sepilia sp. nov., isolated from blood culture of a hospitalized patient as a new member of Stenotrophomonas maltophilia complex. New Microbes New Infect. 43:100920. doi: 10.1016/j.nmni.2021.100920
Gilroy, R., Ravi, A., Getino, M., Pursley, I., Horton, D. L., Alikhan, N.-F., et al. (2021). Extensive microbial diversity within the chicken gut microbiome revealed by metagenomics and culture. PeerJ 9:e10941. doi: 10.7717/peerj.10941
Goris, J., Konstantinidis, K. T., Klappenbach, J. A., Coenye, T., Vandamme, P., and Tiedje, J. M. (2007). DNA-DNA hybridization values and their relationship to whole-genome sequence similarities. Int. J. Syst. Evol. Microbiol. 57, 81–91. doi: 10.1099/ijs.0.64483-0
Gumus, T., Sukru Demirci, A., Mirik, M., Arici, M., and Aysan, Y. (2010). Xanthan gum production of Xanthomonas spp. isolated from different plants. Food Sci. Biotechnol. 19, 201–206. doi: 10.1007/s10068-010-0027-9
Ham, J. H., Melanson, R. A., and Rush, M. C. (2011). Burkholderia glumae: next major pathogen of rice? Mol. Plant Pathol. 12, 329–339. doi: 10.1111/j.1364-3703.2010.00676.x
Heylen, K., Vanparys, B., Peirsegaele, F., Lebbe, L., and De Vos, P. (2007). Stenotrophomonas terrae sp. nov. and Stenotrophomonas humi sp. nov., two nitrate-reducing bacteria isolated from soil. Int. J. Syst. Evol. Microbiol. 57, 2056–2061. doi: 10.1099/ijs.0.65044-0
Hugh, R., and Ryschenkow, E. (1961). Pseudomonas maltophilia, an alcaligenes-like species. J. Gen. Microbiol. 26, 123–132. doi: 10.1099/00221287-26-1-123
Ivayani, I., Widiastuti, A. N. I., Suryanti, S., Suharjo, R., and Priyatmojo, A. (2023). Short communication: first report of Xanthomonas sacchari causing rice sheath rot disease in Lampung, Indonesia. Biodiversitas 23, 6463–6470. doi: 10.13057/biodiv/d231245
Jones, J. B., Lacy, G. H., Bouzar, H., Stall, R. E., and Schaad, N. W. (2004). Reclassification of the xanthomonads associated with bacterial spot disease of tomato and oepper. Syst. Appl. Microbiol. 27, 755–762. doi: 10.1078/0723202042369884
Kalidasan, V., Azman, A., Joseph, N., Kumar, S., Hamat, R. A., and Neela, V. K. (2018). Putative iron acquisition systems in Stenotrophomonas maltophilia. Molecules 23:2048. doi: 10.3390/molecules23082048
Kaparullina, E., Doronina, N., Chistyakova, T., and Trotsenko, Y. (2009). Stenotrophomonas chelatiphaga sp. nov., a new aerobic EDTA-degrading bacterium. Syst. Appl. Microbiol. 32, 157–162. doi: 10.1016/j.syapm.2008.12.003
Kennedy, J. F., and Bradshaw, I. J. (1984). Production, properties and applications of xanthan. Prog. Ind. Microbiol. 19, 319–371.
Klair, D, Arizala, D, Dobhal, S, Boluk, G, Alvarez, AM, and Arif, M. Pectobacterium colocasium sp. nov. isolated from taro (Colocasia esculenta). Cold Spring Harbor: Cold Spring Harbor Laboratory Press; (2022).
Koebnik, R., Burokiene, D., Bragard, C., Chang, C., Saux, M. F.-L., Kölliker, R., et al. (2021). The complete genome sequence of Xanthomonas theicola, the causal agent of canker on tea plants, reveals novel secretion systems in clade-1 xanthomonads. Phytopathology 111, 611–616. doi: 10.1094/PHYTO-07-20-0273-SC
Kozlov, A. M., Aberer, A. J., and Stamatakis, A. (2015). ExaML version 3: a tool for phylogenomic analyses on supercomputers. Bioinformatics 31, 2577–2579. doi: 10.1093/bioinformatics/btv184
Kozlov, A. M., Darriba, D., Flouri, T., Morel, B., and Stamatakis, A. (2019). RAxML-NG: a fast, scalable and user-friendly tool for maximum likelihood phylogenetic inference. Bioinformatics 35, 4453–4455. doi: 10.1093/bioinformatics/btz305
Kumar, S., Bansal, K., Patil, P. P., and Patil, P. B. (2019). Phylogenomics insights into order and families of Lysobacterales. Access Microbiol. 1:e000015. doi: 10.1099/acmi.0.000015
Kumar, S., Stecher, G., Li, M., Knyaz, C., and Tamura, K. (2018). MEGA X: molecular evolutionary genetics analysis across computing platforms. Mol. Biol. Evol. 35, 1547–1549. doi: 10.1093/molbev/msy096
Larrea-Sarmiento, A., Alvarez, A. M., Stack, J. P., and Arif, M. (2019). Synergetic effect of non-complementary 5′ AT-rich sequences on the development of a multiplex TaqMan real-time PCR for specific and robust detection of Clavibacter michiganensis and C. michiganensis subsp. nebraskensis. PLoS One 14:e0218530. doi: 10.1371/journal.pone.0218530
Lee, E. Y., Jun, Y. S., Cho, K.-S., and Ryu, H. W. (2002). Degradation characteristics of toluene, benzene, ethylbenzene, and xylene by Stenotrophomonas maltophilia T3-c. J. Air Waste Manag. Assoc. 52, 400–406. doi: 10.1080/10473289.2002.10470796
Lee, I., Ouk Kim, Y., Park, S.-C., and Chun, J. (2016). OrthoANI: an improved algorithm and software for calculating average nucleotide identity. Int. J. Syst. Evol. Microbiol. 66, 1100–1103. doi: 10.1099/ijsem.0.000760
Lee, M., Woo, S.-G., Chae, M., Shin, M.-C., Jung, H.-M., and Ten, L. N. (2011). Stenotrophomonas daejeonensis sp. nov., isolated from sewage. Int. J. Syst. Evol. Microbiol. 61, 598–604. doi: 10.1099/ijs.0.017780-0
Lee, Y.-A., Yang, P.-Y., and Huang, S.-C. (2020). Characterization, phylogeny, and genome analyses of nonpathogenic Xanthomonas campestris strains isolated from Brassica seeds. Phytopathology 110, 981–988. doi: 10.1094/PHYTO-08-19-0319-R
Leite, R. P. Jr., Minsavage, G. V., Bonas, U., and Stall, R. E. (1994). Detection and identification of phytopathogenic Xanthomonas strains by amplification of DNA sequences related to the hrp genes of Xanthomonas campestris pv. Vesicatoria. Appl. Environ. Microbiol. 60, 1068–1077. doi: 10.1128/aem.60.4.1068-1077.1994
Letunic, I., and Bork, P. (2021). Interactive tree of life (iTOL) v5: an online tool for phylogenetic tree display and annotation. Nucleic Acids Res. 49, W293–W296. doi: 10.1093/nar/gkab301
Leyns, F., De Cleene, M., Swings, J. G., and De Ley, J. (1984). The host range of the genus Xanthomonas. Bot. Rev. 50, 308–356. doi: 10.1007/BF02862635
Li, T., Mann, R., Sawbridge, T., Kaur, J., Auer, D., and Spangenberg, G. (2020). Novel Xanthomonas species from the perennial ryegrass seed microbiome – assessing the bioprotection activity of non-pathogenic relatives of pathogens. Front. Microbiol. 11:1991. doi: 10.3389/fmicb.2020.01991
Lipski, A., and Altendorf, K. (1997). Identification of heterotrophic bacteria isolated from ammonia-supplied experimental biofilters. Syst. Appl. Microbiol. 20, 448–457. doi: 10.1016/S0723-2020(97)80014-8
Löytynoja, A. (2014). Phylogeny-aware alignment with PRANK. Methods Mol. Biol. 1079, 155–170. doi: 10.1007/978-1-62703-646-7_10
Madden, K. S., Jokhoo, H. R. E., Conradi, F. D., Knowles, J. P., Mullineaux, C. W., and Whiting, A. (2019). Using nature’s polyenes as templates: studies of synthetic xanthomonadin analogues and realising their potential as antioxidants. Org. Biomol. Chem. 17, 3752–3759. doi: 10.1039/C9OB00275H
Mafakheri, H., Taghavi, S. M., Zarei, S., Portier, P., Dimkić, I., Koebnik, R., et al. (2022). Xanthomonas bonasiae sp. nov. and Xanthomonas youngii sp. nov., isolated from crown gall tissues. Int. J. Syst. Evol. Microbiol. 72:005418. doi: 10.1099/ijsem.0.005418
Margaritis, A., and Zajic, J. E. (1978). Mixing, mass transfer, and scale-up of polysaccharide fermentations. Biotechnol. Bioeng. 20, 939–1001. doi: 10.1002/bit.260200702
Meier-Kolthoff, J. P., Auch, A. F., Klenk, H.-P., and Goeker, M. (2013). Genome sequence-based species delimitation with confidence intervals and improved distance functions. BMC Bioinformatics 14:60. doi: 10.1186/1471-2105-14-60
Meier-Kolthoff, J. P., Carbasse, J. S., Peinado-Olarte, R. L., and Göker, M. (2022). TYGS and LPSN: a database tandem for fast and reliable genome-based classification and nomenclature of prokaryotes. Nucleic Acids Res. 50, D801–D807. doi: 10.1093/nar/gkab902
Merda, D., Briand, M., Bosis, E., Rousseau, C., Portier, P., Barret, M., et al. (2017). Ancestral acquisitions, gene flow and multiple evolutionary trajectories of the type three secretion system and effectors in Xanthomonas plant pathogens. Mol. Ecol. 26, 5939–5952. doi: 10.1111/mec.14343
Messiha, N. A. S., van Diepeningen, A. D., Farag, N. S., Abdallah, S. A., Janse, J. D., and van Bruggen, A. H. C. (2007). Stenotrophomonas maltophilia: a new potential biocontrol agent of Ralstonia solanacearum, causal agent of potato brown rot. Eur. J. Plant Pathol. 118, 211–225. doi: 10.1007/s10658-007-9136-6
Minkwitz, A., and Berg, G. (2001). Comparison of antifungal activities and 16S ribosomal DNA sequences of clinical and environmental isolates of Stenotrophomonas maltophilia. J. Clin. Microbiol. 39, 139–145. doi: 10.1128/JCM.39.1.139-145.2001
Olson, R. D., Assaf, R., Brettin, T., Conrad, N., Cucinell, C., Davis, J. J., et al. (2023). Introducing the bacterial and viral bioinformatics resource center (BV-BRC): a resource combining PATRIC, IRD and ViPR. Nucleic Acids Res. 51, D678–D689. doi: 10.1093/nar/gkac1003
Page, A. J., Cummins, C. A., Hunt, M., Wong, V. K., Reuter, S., Holden, M. T. G., et al. (2015). Roary: rapid large-scale prokaryote pan genome analysis. Bioinformatics 31, 3691–3693. doi: 10.1093/bioinformatics/btv421
Pages, D., Rose, J., Conrod, S., Cuine, S., Carrier, P., Heulin, T., et al. (2008). Heavy metal tolerance in Stenotrophomonas maltophilia. PLoS One 3:e1539. doi: 10.1371/journal.pone.0001539
Palleroni, N. J., and Bradbury, J. F. (1993). Stenotrophomonas, a new bacterial genus for Xanthomonas maltophilia (Hugh 1980) Swings et al. 1983. Int. J. Syst. Bacteriol. 43, 606–609. doi: 10.1099/00207713-43-3-606
Pandey, S. S., Patnana, P. K., Rai, R., and Chatterjee, S. (2017). Xanthoferrin, the α-hydroxycarboxylate-type siderophore of Xanthomonas campestris pv. campestris, is required for optimum virulence and growth inside cabbage. Mol. Plant Pathol. 18, 949–962. doi: 10.1111/mpp.12451
Pandey, A., and Sonti, R. V. (2010). Role of the FeoB protein and siderophore in promoting virulence of Xanthomonas oryzae pv. oryzae on rice. J. Bacteriol. 192, 3187–3203. doi: 10.1128/JB.01558-09
Parks, D. H., Imelfort, M., Skennerton, C. T., Hugenholtz, P., and Tyson, G. W. (2015). CheckM: assessing the quality of microbial genomes recovered from isolates, single cells, and metagenomes. Genome Res. 25, 1043–1055. doi: 10.1101/gr.186072.114
Parte, A. C., Sardà Carbasse, J., Meier-Kolthoff, J. P., Reimer, L. C., and Göker, M. (2020). List of prokaryotic names with standing in nomenclature (LPSN) moves to the DSMZ. Int. J. Syst. Evol. Microbiol. 70, 5607–5612. doi: 10.1099/ijsem.0.004332
R Core Team (2022). R: A language and environment for statistical computing. R Foundation for Statistical Computing: Vienna, Austria.
Ramos, P. L., Moreira-Filho, C. A., Van Trappen, S., Swings, J., De Vos, P., Barbosa, H. R., et al. (2011a). An MLSA-based online scheme for the rapid identification of Stenotrophomonas isolates. Mem. Inst. Oswaldo Cruz 106, 394–399. doi: 10.1590/S0074-02762011000400003
Ramos, P. L., Van Trappen, S., Thompson, F. L., Rocha, R. C. S., Barbosa, H. R., De Vos, P., et al. (2011b). Screening for endophytic nitrogen-fixing bacteria in Brazilian sugar cane varieties used in organic farming and description of Stenotrophomonas pavanii sp. nov. Int. J. Syst. Evol. Microbiol. 61, 926–931. doi: 10.1099/ijs.0.019372-0
Rana, R., Madhavan, V. N., Saroha, T., Bansal, K., Kaur, A., Sonti, R. V., et al. (2022). Xanthomonas indica sp. nov., a novel member of non-pathogenic Xanthomonas community from healthy rice seeds. Curr. Microbiol. 79:304. doi: 10.1007/s00284-022-03001-5
Richter, M., and Rosselló-Móra, R. (2009). Shifting the genomic gold standard for the prokaryotic species definition. Proc. Natl. Acad. Sci. 106, 19126–19123. doi: 10.1073/pnas.0906412106
Ryan, R. P., Monchy, S., Cardinale, M., Taghavi, S., Crossman, L., Avison, M. B., et al. (2009). The versatility and adaptation of bacteria from the genus Stenotrophomonas. Nat. Rev. Microbiol. 7, 514–525. doi: 10.1038/nrmicro2163
Ryan, R. P., Vorhölter, F.-J., Potnis, N., Jones, J. B., Van Sluys, M.-A., Bogdanove, A. J., et al. (2011). Pathogenomics of Xanthomonas: understanding bacterium–plant interactions. Nat. Rev. Microbiol. 9, 344–355. doi: 10.1038/nrmicro2558
Seemann, T. (2014). Prokka: rapid prokaryotic genome annotation. Bioinformatics 30, 2068–2069. doi: 10.1093/bioinformatics/btu153
Stamatakis, A. (2014). RAxML version 8: a tool for phylogenetic analysis and post-analysis of large phylogenies. Bioinformatics 30, 1312–1313. doi: 10.1093/bioinformatics/btu033
Studholme, D. J., Wasukira, A., Paszkiewicz, K., Aritua, V., Thwaites, R., Smith, J., et al. (2011). Draft genome sequences of Xanthomonas sacchari and two banana-associated Xanthomonads reveal insights into the Xanthomonas group 1 clade. Genes 2, 1050–1065. doi: 10.3390/genes2041050
Swings, J., De Vos, P., Van den Mooter, M., and De Ley, J. (1983). Transfer of Pseudomonas maltophilia Hugh 1981 to the genus Xanthomonas as Xanthomonas maltophilia (Hugh 1981) comb. nov. Int. J. Syst. Bacteriol. 33, 409–413. doi: 10.1099/00207713-33-2-409
Tatusova, T., DiCuccio, M., Badretdin, A., Chetvernin, V., Nawrocki, E. P., Zaslavsky, L., et al. (2016). NCBI prokaryotic genome annotation pipeline. Nucleic Acids Res. 44, 6614–6624. doi: 10.1093/nar/gkw569
Timilsina, S., Potnis, N., Newberry, E. A., Liyanapathiranage, P., Iruegas-Bocardo, F., White, F. F., et al. (2020). Xanthomonas diversity, virulence and plant-pathogen interactions. Nat. Rev. Microbiol. 18, 415–427. doi: 10.1038/s41579-020-0361-8
Triplett, L. R., Verdier, V., Campillo, T., Van Malderghem, C., Cleenwerck, I., Maes, M., et al. (2015). Characterization of a novel clade of Xanthomonas isolated from rice leaves in Mali and proposal of Xanthomonas maliensis sp. nov. Antonie Van Leeuwenhoek 107, 869–881. doi: 10.1007/s10482-015-0379-5
Vandroemme, J., Cottyn, B., Pothier, J. F., Pflüger, V., Duffy, B., and Maes, M. (2013). Xanthomonas arboricola pv. fragariae: what's in a name? Plant Pathol. 62, 1123–1131. doi: 10.1111/ppa.12028
Vasileuskaya-Schulz, Z., Kaiser, S., Maier, T., Kostrzewa, M., and Jonas, D. (2011). Delineation of Stenotrophomonas spp. by multi-locus sequence analysis and MALDI-TOF mass spectrometry. Syst. Appl. Microbiol. 34, 35–39. doi: 10.1016/j.syapm.2010.11.011
Vauterin, L., Hoste, B., Kersters, K., and Swings, J. (1995). Reclassification of Xanthomonas. Int. J. Syst. Bacteriol. 45, 472–489. doi: 10.1099/00207713-45-3-472
Vauterin, L., Rademaker, J., and Swings, J. (2000). Synopsis on the taxonomy of the genus Xanthomonas. Phytopathology 90, 677–682. doi: 10.1094/PHYTO.2000.90.7.677
Wattam, A. R., Davis, J. J., Assaf, R., Boisvert, S., Brettin, T., Bun, C., et al. (2017). Improvements to PATRIC, the all-bacterial bioinformatics database and analysis resource center. Nucleic Acids Res. 45, D535–D542. doi: 10.1093/nar/gkw1017
Weber, M., Schünemann, W., Fuß, J., Kämpfer, P., and Lipski, A. (2018). Stenotrophomonas lactitubi sp. nov. and Stenotrophomonas indicatrix sp. nov., isolated from surfaces with food contact. Int. J. Syst. Evol. Microbiol. 68, 1830–1838. doi: 10.1099/ijsem.0.002732
Wick, R. R., Judd, L. M., Gorrie, C. L., and Holt, K. E. (2017). Unicycler: resolving bacterial genome assemblies from short and long sequencing reads. PLoS Comput. Biol. 13:e1005595. doi: 10.1371/journal.pcbi.1005595
Wolf, A. (2002). Stenotrophomonas rhizophila sp. nov., a novel plant-associated bacterium with antifungal properties. Int. J. Syst. Evol. Microbiol. 52, 1937–1944. doi: 10.1099/ijs.0.02135-0
Xie, G.-L., Soad, A., Swings, J., and Mew, T. W. (2003). Diversity of gram negative bacteria antagonistic against major pathogens of rice from rice seed in the tropic environment. J. Zhejiang Univ. Sci. A 4, 463–468. doi: 10.1631/jzus.2003.0463
Young, J. M., Dye, D. W., Bradbury, J. F., Panagopoulos, C. G., and Robbs, C. F. (1978). A proposed nomenclature and classification for plant pathogenic bacteria. New Zealand J. Agricu. Res. 21, 153–177.
Keywords: Araceae, MLSA, pan-genome, Xanthomonas hawaiiensis sp. nov., Stenotrophomonas aracearum sp. nov., Stenotrophomonas oahuensis sp. nov.
Citation: Chuang S-C, Dobhal S, Alvarez AM and Arif M (2024) Three new species, Xanthomonas hawaiiensis sp. nov., Stenotrophomonas aracearum sp. nov., and Stenotrophomonas oahuensis sp. nov., isolated from the Araceae family. Front. Microbiol. 15:1356025. doi: 10.3389/fmicb.2024.1356025
Edited by:
Kanika Bansal, Institute of Microbial Technology (CSIR), IndiaReviewed by:
Sanjeet Kumar, Illumina, Inc., IndiaVishnu Udayakumaran Nair Sunitha Kumary, EpiCypher, Inc., United States
Copyright © 2024 Chuang, Dobhal, Alvarez and Arif. This is an open-access article distributed under the terms of the Creative Commons Attribution License (CC BY). The use, distribution or reproduction in other forums is permitted, provided the original author(s) and the copyright owner(s) are credited and that the original publication in this journal is cited, in accordance with accepted academic practice. No use, distribution or reproduction is permitted which does not comply with these terms.
*Correspondence: Mohammad Arif, YXJpZkBoYXdhaWkuZWR1