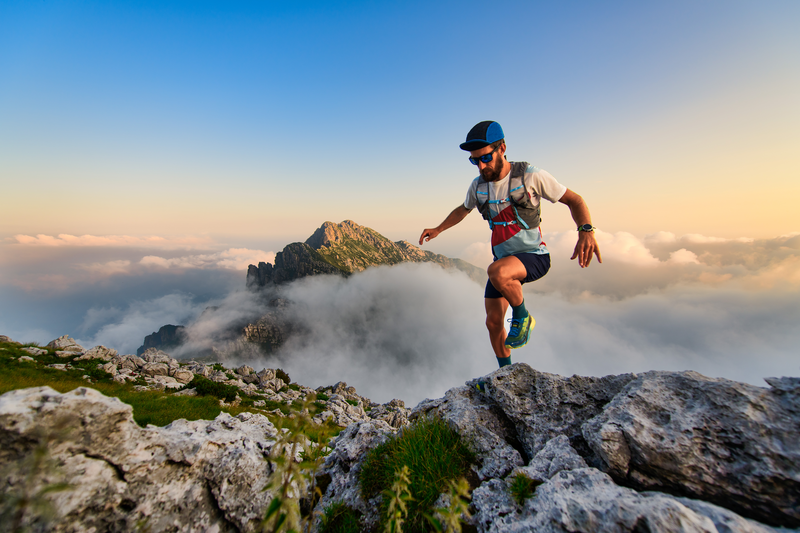
94% of researchers rate our articles as excellent or good
Learn more about the work of our research integrity team to safeguard the quality of each article we publish.
Find out more
ORIGINAL RESEARCH article
Front. Microbiol. , 07 March 2024
Sec. Microbial Physiology and Metabolism
Volume 15 - 2024 | https://doi.org/10.3389/fmicb.2024.1354140
The genus Paracoccidioides includes Paracoccidioides lutzii and the Paracoccidioides brasiliensis complex, which comprises four phylogenetic species. A key feature distinguishing planktonic growth from biofilm is the presence of a 3D extracellular matrix (ECM). Therefore, in this study, we analyzed biofilm formation in different species of Paracoccidioides yeast phase, characterized the structural elements of the matrix of P. brasiliensis (Pb18), P. lutzii (Pl01 and 8334) and P. restrepiensis (339 and 192) and evaluated the expression of glucan genes, according to the stage of biofilm evolution for P. brasiliensis. The strains were cultivated in planktonic and biofilm form for 24–144 h. The fungi biomass and metabolic activity were determined by crystal violet and tetrazolium salt reduction (XTT) tests and colony-forming unit (CFU) by plating. The biofilm structure was designed using scanning electron microscopy and confocal laser scanning microscopy techniques. The extracellular matrix of P. brasiliensis and P. lutzii biofilms was extracted by sonication, and polysaccharides, proteins, and extracellular DNA (eDNA) were quantified. The RNA was extracted with the Trizol® reagent and quantified; then, the cDNA was synthesized to analyze the enolase expression, 14-3-3, FKS1, AGS1, GEL3, and KRE6 genes by real-time PCR. All strains of Paracoccidioides studied form a biofilm with more significant metabolic activity and biomass values in 144 h. The extracellular matrix of P. brasiliensis and P. lutzii had a higher content of polysaccharides in their composition, followed by proteins and eDNA in smaller quantities. The P. brasiliensis biofilm kinetics of formation showed greater expression of genes related to glucan's synthesis and its delivery to the external environment in addition adhesins during the biofilm's adhesion, initiation, and maturation. The GEL3 and enolase genes increased in expression within 24 h and during the biofilm maturation period, there was an increase in 14-3-3, AGS1, and FKS1. Furthermore, at 144 h, there was a decrease in KRE6 expression and an increase in GEL3. This study highlights the potential for biofilm formation for three species of Paracoccidioides and the main components of the extracellular matrix that can contribute to a better understanding of biofilm organization.
Paracoccidioidomycosis (PCM) is a systemic mycosis that assumes clinical importance due to increased prevalence and mortality rates. PCM was included on the World Health Organization (WHO) Fungal Priority Pathogens List due to their endemicity in the Americas, high morbidity, and potential for sequelae that negatively impact the quality of life (WHO, 2022). The genus Paracoccidioides includes Paracoccidioides lutzii and the Paracoccidioides brasiliensis complex, which comprises four phylogenetic species, including Paracoccidioides brasiliensis (S1a and S1b), Paracoccidioides americana (PS2), Paracoccidioides restrepiensis (PS3), and Paracoccidioides venezuelensis (PS4) (Turissini et al., 2017; Teixeira et al., 2020). PCM affects the human lungs and can progress to systemic granulomatous disease with tegumentary and visceral involvement (de Oliveira et al., 2015; Hahn et al., 2022).
The pathogenicity of fungi in humans depends on various virulence factors. Dimorphism, cell wall composition, production of metalloproteins, adhesins, melanin, and indirectly the formation of biofilm has been reported as virulence factors of the genus Paracoccidioides (Mendes-Giannini et al., 2008; Sardi et al., 2015; Scorzoni et al., 2015; Camacho and Niño-Vega, 2017; Santos et al., 2020). The presence and content of α-glucans in the cell wall of yeast forms of P. brasiliensis was related to their virulence (San-Blas and Niño-Vega, 2008). Proteins such as 14-3-3 and enolase act as adhesins, playing an important role during Paracoccidioides spp. infection. A previous study showed a correlation between the expression of adhesins and virulence since the blockade of 14-3-3 and enolase adhesins resulted in reduced adhesion of Paracoccidioides spp. to lung cells of the A549 lineage (de Oliveira et al., 2015). Additionally, when adhesins were blocked, the survival rate was higher in mice (C57BL/6) and Galleria mellonella larvae (de Oliveira et al., 2015).
The biofilm formation by P. brasiliensis was first reported by Sardi et al. (2015) in abiotic surfaces (polystyrene plate) under hypoxic conditions. Also, the fungal agglomeration resembling biofilm was observed when interacting with epithelial and macrophage cells, an aspect that can be considered similar to that found in granulomas, which consists of a nodular arrangement in a hypoxic environment, containing many types of cells, as epithelioid and Langhans cells, and also yeasts (Fortes et al., 2011; Grahl et al., 2012). The gene expression at mature biofilm of P. brasiliensis demonstrates higher expression of gp43 and GAPDH known to be adhesins in P. brasiliensis, as well as up-regulation of aspartyl proteinase gene that can contribute to invasion and tissue destruction as described for Candida albicans (Vicentini et al., 1997; Hanna et al., 2000; Barbosa et al., 2006; Ramage et al., 2012; Sardi et al., 2015).
Posteriorly, Cattana et al. (2017) reported the presence of a dense network of Paracoccidioides yeast cells held by a dense ECM, observed by scanning electron microscope, forming a biofilm at a vascular prosthesis in a patient that's suffered intestinal infarct and acute ischemia in the right lower limb. Therefore, this prosthesis was removed for analysis, being this the first description of in vivo biofilm formation of P. brasiliensis.
The ECM can trigger higher resistance to antimicrobials, mainly due to the difficulty of their diffusion through the matrix, making the treatment of infections related to the biofilm challenging (Chandra et al., 2001; Mitchell et al., 2015; Silva et al., 2017; Oshiro et al., 2019).
The “matrixome” is the inventory of currently known biomolecules (polysaccharides, nucleic acids, proteins, lipids, and lipoproteins), their molecular, structural, and functional diversity associated with biofilm assembly, and their physicochemical and virulence attributes. The biofilm properties are related to surface adhesion, spatial and chemical heterogeneity, synergistic/competitive polymicrobial interactions, antimicrobial recalcitrance, and biofilm virulence (Karygianni et al., 2020).
The ECM corresponds to 70–95% of the organic matter of the biofilm mass, of heterogeneous and complex composition composed of four classes of macromolecular: proteins, carbohydrates, lipids, and nucleic acid (Flemming et al., 2000; Mitchell et al., 2016; Bamford et al., 2020). Studies on the matrix have reported that it is assembled in the extracellular environment, from the incorporation of a variety of products from the community to create a unique structure (Sutherland, 2001; Garny et al., 2010; Mitchell et al., 2015; Desmond et al., 2018; Zarnowski et al., 2018).
The composition of the Paracoccidioides extracellular matrix has not yet been characterized. Polysaccharides are essential in most biofilm matrices (Flemming et al., 2007), representing ~25% of their dry weight (Zarnowski et al., 2014). Alpha-mannan and β-1,6 glucan comprise 85 and 14%, respectively, of C. albicans' matrix carbohydrates, with β-glucan secretion in its biofilms regulated by gene expression in response to quorum sensing (Mitchell et al., 2015) and the increased expression of β-glucans in the matrix implies the sequestration of antifungal agents, reducing the susceptibility of C. albicans biofilms (Nett et al., 2010a; Zarnowski et al., 2018). Several glucan and mannan synthase and modification genes in C. albicans were important for producing the extracellular matrix components (Nett et al., 2010a,b; Taff et al., 2012; Mitchell et al., 2015).
Another constituent of the biofilm matrix is extracellular deoxyribonucleic acid (eDNA) found in the extracellular medium. The mechanism by which eDNA is secreted into the biofilm microenvironment is not fully understood. For bacteria, some mechanisms have been proposed, such as cell lysis and secretion via vesicles (Allesen-Holm et al., 2006) and for C. albicans and Aspergillus fumigatus (Rajendran et al., 2014, 2016), maybe cell lysis is involved in this process. In addition, the accumulation of toxic metabolites and the scarcity of nutrients resulting from limited diffusion by the matrix favors the expression of enzymes that remodel the cell wall, releasing nutrients to the microbial community, such as chitinases, which, when degrading chitin of the cell wall, cause DNA leakage to the outside environment (Rajendran et al., 2014). A previous study reports that chitin expression in the mature biofilm is increased and that chitinase inhibition alters the growth and stability of A. fumigatus and C. albicans biofilms (Rajendran et al., 2014).
Thus, in this study, we intended to analyze biofilm formation in different species of Paracoccidioides, characterize the structural elements of the matrix of Paracoccidioides spp., and describe the differential expression of genes related to glucan's synthesis and its delivery to the external environment and adhesins during the adhesion, initiation, and maturation of the P. brasiliensis biofilm. These factors may be necessary to understand biofilm formation better, giving insights into the determination of targets to be genetically modified to elucidate its role and, in the future, contribute to the development of anti-biofilm therapies.
The strains P. brasiliensis (Pb18), P. lutzii (Pl01 and 8334), and P. restrepiensis (339 and 192) were used in the yeast phase, obtained from the Mycology Laboratory of the Department of Clinical Analysis of the Faculty of Pharmaceutical Sciences, Campus of Araraquara, UNESP. The strains were cultivated in solid Fava-Netto medium at 37°C for 5 days (Restrepo et al., 2015).
The Paracoccidioides spp. strains were cultured in Fava-Netto medium for 5 days at 37°C. We followed the biofilm growth condition described by Oliveira et al. (2020). Briefly, a suspension containing 1 × 106 cells/mL in Brain Heart Infusion (BHI) medium supplemented with 1% of glucose at a higher viability condition, >90%, evaluated with Trypan blue assay, was plated on a polystyrene 24-well microtiter plate, adding 1 mL of inoculum. The plates were incubated at 37°C without agitation for 24, 48, 96, 144, and 168 h (two plates each time), changing the medium every 24 h. Concomitantly to the planktonic growth conditions, the same initial inoculum was seeded in 200 mL of BHI plus 1% glucose at the same final concentration and incubated at 37°C under the agitation of 150 rpm and at the periods of 24, 48, 96, 144, and 168 h. An aliquot of 48 mL was taken for RNA extraction.
The quantification of the total biomass of biofilms was determined based on the ability of crystal violet to penetrate the cell wall of microorganisms and remain retained in the cytoplasm. After the growth of biofilms in microplates (96 wells), the supernatant from each well was aspirated and washed twice with PBS. Two hundred microliter of methanol was added per well, which was maintained for 15 min for fixation and then aspirated and dried for 45 min. After 200 μL of 0.1% crystal violet (CV) for 20 min was added. After washing with distilled water to remove excess, the CV was solubilized with 200 μL of 33% acetic acid. After 10 min, the absorbance was read at 570 nm in a microplate reader (Sherry et al., 2014).
The kinetic of monospecies biofilm formation for P. brasiliensis, P. lutzii, and P. restrepiensis was obtained using the tetrazolium salt reduction test—XTT [2,3-Bis-(2-Methoxy-4-Nitro-5-Sulfophenyl)-2H-Tetrazolium-5-Carboxanilide], The reduction of XTT is used to measure the cellular viability of fungal biofilms by evaluating the metabolic activity, which promotes an intracellular reduction of salt forming a compound called formazan, which can be quantified by the color change (orange). A solution of XTT salt (0.1 mg/mL in PBS) and menadione (1 mM in ethanol; Sigma Aldrich, São Paulo, SP, Brazil) was prepared for these assays. A 100 μL aliquot of menadione XTT was then added to both prewashed biofilms and controls. The plates were incubated in the dark for 3 h at 37°C, and the colorimetric change was measured in a microplate reader at 492 nm. Wells containing culture medium/biofilms/XTT/menadione (positive control) and wells containing culture medium/XTT/menadione (negative control) were included (Martinez and Casadevall, 2007; Silva et al., 2010). The measurement is carried out at defined time intervals, providing data for constructing a kinetic curve of biofilm formation.
After biofilm formation, at different incubation times (0, 24, 48, 72, 96, 120, 144, and 168 h), the wells were washed thrice with PBS (pH 7.2–7.4) to remove non-adherent cells. Then, 100 μL of BHI broth was added to each well; biofilms were scraped off, and the well of plate contents were individually transferred to microtubes containing 900 μL of BHI broth. Biofilms were mechanically disrupted by vigorous agitation for 30 s on a vortex, serially diluted, and plated on BHI agar supplemented with 1% glucose, 5% P. brasiliensis culture filtrate, and 4% fetal bovine serum to obtain cell counts of Paracoccidioides spp. in monospecies biofilms.
In this step, only the strains of P. brasiliensis (Pb18) and P. lutzii (Pl01) were employed due to the clinical importance and incidence of these species. After the growth of biofilms in 24-well plates, the supernatant from each well was aspirated, and the biofilms were washed three times with PBS (pH 7.2–7.4). The first labeling was performed with fluorescein isothiocyanate (FITC—Fluorescein Isothiocyanate) showing the fungal cell, adding 500 μL of FITC (100 μg/mL, Invitrogen, Life Technologies, Brazil) per well for 30 min at room temperature. After incubation, washing was performed twice with PBS, and 500 μL of SYPRO Ruby (Invitrogen, Molecular Probes, Eugene, OR) was added for 20 min, evidencing the protein content of the biofilm matrix. After washing twice with PBS (pH 7.2–7.4), 500 μL of HOECHST (10 mM, Invitrogen, Life Technologies, USA) was added for 30 min to mark the nucleic content. After incubation, the biofilms were washed with PBS, and 1 mL of PBS (pH 7.2–7.4) was added for analysis. Analyzes were performed using a laser scanning confocal microscope (Carls Zeiss LSM 800, Germany). Each field of view was imaged using the 405 nm laser to capture HOECHST with an emission range of up to 450 nm, 488 nm for FITC detection with an emission range of up to 540 nm, and 280 nm for SYPRO Ruby with a range of emission up to 450 nm. Images were acquired using Image J 1.51p software.
Biofilms of P. brasiliensis and P. lutzii monospecies were subjected to matrix extraction by sonication with modifications, as previously described by Martins et al. (2010). Briefly, after biofilm formation in 24-well plates, biofilms were collected and transferred to conical tubes, resuspended in 10 mL of sterile water, and vortexed for 1 min. They were then sonicated for 20 min, followed by a 2-min vortex step, and centrifuged at 1,500 × g for 20 min. The supernatant was recovered, and then the analyses were performed.
The total biofilm and matrix polysaccharide content was determined following the protocol described by DuBois et al. (1956). After extracting the extracellular matrix, 0.5 mL of the extract was used, and 12.5 μL of a phenol solution (80%) was added, followed by 1.25 mL of concentrated sulfuric acid and mixed. The samples were left to rest for 10 min and incubated in a water bath at 30°C for 20 min, with the absorption measured at 485 nm in a spectrophotometer. The polysaccharide content was calculated using glucose to obtain the standard curve (0–200 μg/mL).
Protein content was quantified using Bradford's (1976) method. Briefly, after matrix extraction, 5 μL of samples were placed in 250 μL of Bradford reagent (BioRad) and incubated in the dark at room temperature for 10 min. After incubation, the reading was taken at 595 nm in the spectrophotometer. Protein content was calculated using bovine serum albumin (BSA) for the standard curve.
The eDNA was extracted as previously described (Martins et al., 2010) with some modifications. Briefly, phenol:chloroform: isoamyl alcohol (25:24:1, v/v) was added to the planktonic cell, biofilm, and extracellular matrix samples, vortexed and centrifuged at 1,732 × g for 20 min (Sorvall X1R, Thermo Scientific, West Chester, PA, USA). The supernatant was transferred to another tube, and ice-cold isopropanol (1:1) was added for DNA precipitation, followed by incubation for 1 h on ice. After centrifugation at 10,000 × g; 15 min at 4°C, the pellet was washed with ice-cold 70% ethanol, air-dried, and resuspended in 50 μL Tris-HCl EDTA buffer (TE). The eDNA concentration was measured by determining the absorbance at 260 nm in a spectrophotometer.
After incubation of pre-determined cultivation periods to biofilm condition, the supernatant was removed, followed by gentle washing with PBS to remove non-adherent cells. The same was collected after adding 750 μL of Trizol® reagent (Ambion Life Technology) per plate and with a mechanical detachment with a rubber scrapper. To planktonic condition, the aliquots were centrifuged at 3,000 rpm for 5 min, the supernatant was discarded, and the cell pellets were resuspended with three volumes of Trizol® reagent. The RNA extraction was performed as described by the manufacturer's instructions with some modifications, including cell disruption with glass beads, vigorous vortexing, and intercalating with ice baths. The total RNAs were quantified by spectrophotometry and their integrity was assessed by 0.8% agarose gel electrophoresis. Aliquots of extracted RNA were stored at −80°C until the use.
At first, genomic DNA from 1 μg of total RNA was removed with DNAse (Thermo Fisher Scientific) according to the manufacturer's instructions. Secondly, cDNA was synthesized using RevertAIDTM H Minus Reverse Transcriptase (Fermentas). The relative expression levels of enolase, 14-3-3, FKS1 (1,3-beta-glucan synthase), AGS1 (1,3-alpha-glucan synthase), GEL3 (1,3-beta glucanosyltransferase) and KRE6 (glycosyl hydrolase) genes were analyzed. ACT1 (actin-1) and L34 (ribosomal 60S subunit) were compared to the use as endogenous control genes, and the most adequate used the analysis (Goes et al., 2014; de Curcio, 2018). Real-time polymerase chain reactions (qRT-PCR) were performed in 20 μL reaction volumes, containing 1 × QuantiNOVA SYBR Green (Qiagen) master mix, 0.1 μL of Rox, cDNA templates (following four 10-fold serial dilutions: 10 ng, 1 ng, 100 pg, and 10 pg), 0.7 μmol of each primer pairs (Table 1) to generate a standard curve for each pair of primers. Control reactions were performed without template DNA, and cDNA was synthesized without the DNAse treatment. The real-time PCR amplification conditions were as follows: 95°C for 1 min for enzyme activation, a pre-denaturation step at 95°C for 1 min, followed by 40 cycles of 95°C for 5 s and 60°C for 32 s at Applied Biosystems 7500 cycler (Applied Biosystems). All melting curves were analyzed to confirm a single product that was also evaluated by electrophoresis. The efficiency (E) of the qPCR amplification was calculated using the slope value derived from the standard curve through the formula E = (10−1/slope – 1) × 100. Data were analyzed using the 2−ΔΔCt method, where the Ct is the threshold cycle (Livak and Schmittgen, 2001).
Graphs and statistical analysis were performed using GraphPad Prism, version 5.0 (GraphPad Software, Inc., San Diego, CA). Results were presented as mean ± standard deviation and compared by analysis of variance (ANOVA) followed by the Bonferroni test. Statistical significance was considered when p < 0.05.
P. brasiliensis (Pb18), P. lutzii (01, 8334), and P. restrepiensis (339, 192) were able to form a biofilm, with large amounts of biomass production (Figure 1) increasing over time. According to the statistical analyses performed between the strains, there was a significant difference in biofilm formation at 120, 144, and 168 h for the Pb18 strain when compared with the Pl01, Pl8334, Pr339, and Pr192 strains, showing significant p < 0.05, p < 0.01, and p < 0.001, respectively. The maturation of the biofilms occurred in 144 h of incubation, observing a significant increase of the biomass in this time when compared to the times of 24, 48, 72, and 96 h (p < 0.001) and the decrease in the time of 168 h, which can be explained as the dispersion phase of biofilms.
Figure 1. Quantification of the total biomass of P. brasiliensis (Pb18), P. lutzii (Pl01/8334), and P. restrepiensis (339/192) biofilms. Quantification of biomass of different biofilm P. brasiliensis strains by crystal violet methodology. Optical density reading at 570 nm of Pb18, Pl01, Pr339, Pr192, Pl8334 biomass. The results were expressed as the mean and standard error of the mean, and the experiments were carried out in six replicates and on three different occasions (n = 18). Two-way ANOVA. ***p < 0.001.
Paracoccidioides is a slow-growing and demanding fungus that was initially assessed at 24 h. Previous studies indicated that biofilm formation before this time was nearly negligible. In the period between 24 and 120 h, this activity was significantly increased for all analyzed strains. At 144 h, the biomass and metabolic activity reached the maximum value for all strains, and there was a significant difference (p < 0.001) between them, except for strains Pr339 and Pb8334 (p > 0.05). After 144 h, readings were decreased, except for strain Pr192, where no changes were observed (Figure 2). After 168 h, the biofilms can enter the dispersion phase.
Figure 2. Quantification of metabolic activity of biofilms formed by P. brasiliensis (Pb18), P. lutzii (Pl01/8334), and P. restrepiensis (339/192) determined by XTT. Optical density reading at 492 nm. The results were expressed as the mean and standard error of the mean, and the experiments were carried out in six replicates and on three different occasions. Two-way ANOVA analysis. **p < 0.01 and ***p < 0.001.
In this step, only P. brasiliensis (Pb18) and P. lutzii (Pl01) strains were used due to the clinical importance of these species and their incidence in Brazil and other countries. The images obtained by confocal microscopy show the overlap of the three dyes and indicate the orthogonal section of the biofilm in 3D. The biofilms gradually increased their thickness over the analyzed times, up to the total maturation time (144 h). A cluster of yeast-like forms was observed, indicating the formation of microcolonies and an increase in thickness for both species as the incubation period progressed. Two points per well of biofilms were evaluated, and the images were edited in ImageJ 1.51p software and ZEN Support Software 4.1.
The protein content was more evident in the mature biofilm of P. brasiliensis, but in P. lutzii, the red coloration occurred more intensely from 96 h of biofilm formation (Figures 3, 4). Microcolonies of different sizes are observed, spaced on the surface and with little protein content in the matrix. At 96 h, the spacing between microcolonies is reduced compared to 24 h and is comparable to 144 h (Figure 3). This decrease in space may be due to the multiplication of cells in the microcolonies and the production of extracellular matrix components that promote their growth. However, from 96 to 144 h, there is an increase in the abundance of protein content in the extracellular matrix, which may have contributed to the increase in the thickness of these biofilms.
Figure 3. Representative confocal microscopy images of P. brasiliensis (Pb18) biofilms obtained by confocal microscopy (CLSM). Biofilm of P. brasiliensis observed in confocal microscopy (24–144 h). The nucleic content was marked with Hoechst (blue), the fungal cell walls with FITC (green), and the protein content with SYPRO Ruby (red) and merge-overlapping. The clusters characteristic of biofilms of this species (microcolonies) are observed.
Figure 4. Representative confocal microscopy images of P. lutzii (Pl01) biofilms obtained by confocal microscopy (CLSM). Biofilm of P. lutzii observed in confocal microscopy (24–144 h). The nucleic content was marked with Hoechst (blue), the fungal cell walls with FITC (green), the protein content with SYPRO Ruby (red), and merge-overlapping. The clusters characteristic of biofilms of this species (microcolonies) are observed.
P. lutzii builds microcolonies much larger than those of P. brasiliensis. P. lutzii (Pl01) biofilm has a smaller thickness than that observed for P. brasiliensis (Pb18), and the increase over time was also less pronounced, as observed in Figure 4. The same was observed when the biomass was quantified by crystal violet (Figure 1). Furthermore, the distribution and size of microcolonies are quite different between the two strains, which may influence how these species respond to therapeutic interventions.
Quantitative data of polysaccharides, proteins, and eDNA from biofilms of P. brasiliensis (Pb18) and P. lutzii (Pl01) are shown in Figure 5. In the first 24 h, P. lutzii (Pl01) biofilm produced more polysaccharides (p < 0.01) than P. brasiliensis (Pb18) biofilm, as shown in Figure 5A. In the period of 96 h, there was no significant difference in the production of polysaccharides by Pb18 or Pl01 (p > 0.05). In 144 h, Pl01 produced more polysaccharides than Pb18 (p < 0.01).
Figure 5. Results of polysaccharides, proteins, and extracellular DNA quantity of P. brasiliensis (Pb18) and P. lutzii (Pl01) biofilms. (A) Amount of total polysaccharides measured by the Dubois method. (B) Amount of total proteins measured by the Bradford method, and (C) the amount of DNA evaluated by the phenol:chloroform: isoamyl alcohol method. The polysaccharide content was calculated using glucose for the standard curve (0–200 μg/mL) and bovine serum albumin protein (BSA). Data expressed as mean and standard deviation performed in duplicate on three different occasions using two-way ANOVA analyses. **p < 0.01 and ***p < 0.001.
P. brasiliensis (Pb18) showed higher protein production at 24 h, with a slight decline at 96 h and, at 144 h, comparable to the 24 h period (p < 0.01). P. lutzii (Pl01) did not show a significant increase in the period from 24 to 96 h (p > 0.05), but a significant increase in protein production at 144 h compared to the production at 24 and 96 h (p < 0.001) (Figure 5B). This data agrees with the confocal microscopy results (Figure 4).
P. brasiliensis (Pb18) showed significant eDNA production during the biofilm maturation (p < 0.01). P. lutzii (Pl01) produced eDNA in the first 24 h and a slight increase at 144 h (Figure 5C).
Among the evaluated components, polysaccharides are responsible for ~96.9 and 96.2% of the matrix of P. brasiliensis and P. lutzii, respectively, corresponding to the highest amount concerning the other components. Proteins are responsible for 2.7 and 3.5% of the matrix of P. brasiliensis and P. lutzii, respectively. Another key component of the matrix is eDNA. In the biofilm matrix of P. brasiliensis, a small amount of eDNA of approximately 0.3% was verified, and 0.4% for P. lutzii. When comparing the two species of Paracoccidioides, concerning the components of the extracellular matrix of the biofilm, we did not observe a significant difference in the values.
The mean ratio value of A260/280 for all RNA samples was 2.1–2.2, reflecting high purity (>1.8 to A260/280 nm), and the integrity of RNA was verified by electrophoresis (data not shown). Real-time PCR standardization was performed to determine the primer concentration as well as the efficiency of amplification. We calculated the slope for each primer pair and found values near the ideal, −3.2, efficiencies ranging from 90.99 to 105.1%, and an R2 value of 0.99, meeting the requirement for qRT-PCR (Table 2). Primers were tested for a non-specific product by amplicon separation on agarose gel electrophoresis and size confirmation (ranging from 118 to 193 bp according to the gene) at the end of a qPCR run as well as the analysis of melting curves that show only one peak for all samples indicating the amplification of a single product (Supplementary Figures S1A, B). The best endogenous control was the L34 gene, which showed lower expression variation between the samples and was used for the qRT-PCR analysis.
Table 2. Oligonucleotides used in qPCR and its respective amplification efficiencies, correlation coefficients (R2)‡, and slope values.
Quantitative PCR was performed to analyze the expression of some genes involved in P. brasiliensis adhesion and the synthesis and maintenance of glucans to assess whether they would be altered during the biofilm condition. During the early phase of biofilm formation (24 h), the genes GEL3 and enolase were up-regulated, with an increased expression of 3.4- and 3.1-fold compared to the planktonic cells. Posteriorly, at 48 h of biofilm maturation, GEL3, enolase, and 14-3-3 had their expression increased 2-, 3-, and 1.6-fold, respectively. At the intermediate time of biofilm maturation (96 h), we observed the most significant increases in several genes, such as FKS1 (3.4-fold), AGS1 (1.9-fold), enolase (5.4-fold), and 14-3-3 (2.6-fold). Finally, at 144 h, considered the stage of mature biofilm, a decreased expression of KRE6 (2.7-fold) and increased expression of GEL3 (1.8-fold) was found (Figure 6). The results of qRT-PCR are summarized in Figure 7.
Figure 6. Temporal analysis of expression of genes related to synthesis and maintenance of cell wall components and adhesins. Differential expression of genes in biofilm condition when compared to the planktonic growth at 24, 48, 96, and 144 h. Genes were evaluated by qRT-PCR. Conditions were compared using two-way ANOVA followed by a Bonferroni post-test. ***p < 0.0001; **p < 0.01; *p < 0.05. FKS1, 1,3-β-glucan synthase; AGS1, 1,3-α-glucan synthase; KRE6, glycosyl hydrolase required for 1,6-β-glucan synthesis; GEL3, 1,3-β-glucanosyltransferase, enolase and 14-3-3 genes.
Figure 7. Summary of gene modulation during the formation and maturation stages of P. brasiliensis biofilm. In red, genes are significantly more expressed, and in green, with reduced expression. 1,3-β glucan is highlighted in purple, and α-glucan in green.
In this work, we analyze biofilm formation in different species of Paracoccidioides. The ideal time for the biofilm maturation of most studied strains was 144 h, a result compatible with those described by Sardi et al. (2015) for P.brasiliensis biofilm. The new classification concerning the genus Paracoccidioides includes five species (Carrero et al., 2008; Hrycyk et al., 2018), but as can be seen in the crystal violet and XTT assays, the studied strains of three different species have behavior similar in terms of ability to form a biofilm (biomass and metabolic activity).
According to the literature, biofilms share several common characteristics, such as the production of extracellular polymeric substances. The composition of the extracellular matrix varies according to the microorganism and can be affected by the environment and changes in growth conditions, such as the amount of nutrients, temperature, and pH. Zarnowski et al. (2014) reported that the C. albicans biofilm matrix comprises 55% protein, 25% carbohydrates, 15% lipids, and 5% nucleic acid. For Aspergillus fumigatus, the biofilm matrix contains ~40% proteins, 43% polysaccharides, and at least 8% lipids (Reichhardt et al., 2015). For C. albicans, eDNA was reported to contribute to biofilms' structural integrity and role in antifungal resistance (Martins et al., 2010; Sapaar et al., 2014).
The matrix is self-produced in the extracellular environment, from incorporating diverse community products to creating a unique structure (Mitchell et al., 2015). The exact composition of the ECM of P. brasiliensis biofilm and the regulatory mechanisms of biofilm formation are still unclear. Here, variations in the amount of polysaccharides, proteins, and extracellular DNA (eDNA) were demonstrated after ECM extraction of P. brasiliensis and P. lutzii biofilms. Increased polysaccharide production at different stages of the in vitro biofilm (24, 96, and 144 h) was observed, and the same with total proteins and eDNA. Differences between the two species of Paracoccidioides were detected in biofilm growth.
Studies carried out with Candida have shown an alternation in the amount of polysaccharides and proteins in the biofilm matrix of different species of this genus (Silva et al., 2009). Generally, C. parapsilosis biofilm matrices had large amounts of carbohydrates and relatively smaller amounts of proteins. In contrast, biofilm matrices of C. tropicalis strains showed lower concentrations of proteins and carbohydrates, and C. glabrata biofilm matrices had relatively higher amounts of protein and carbohydrates than other species (Silva et al., 2009).
Polysaccharides are key constituents of the ECM of fungal biofilms. The polysaccharide 1,3-β-glucan is present in Candida albicans biofilm, associated with biofilm protection against antifungal agents (Taff et al., 2012). Additionally, they act as “scaffolds” for connecting other biomolecules, playing an essential role in the maintenance and function of biofilms (Sheppard and Howell, 2016). Therefore, the present study evaluated the genes' expression in controlling matrix delivery and enzymes related to carbohydrate production and modification to be incorporated into the ECM.
The 1,3-β-glucan synthase (Fks1) is required for 1,3-β-glucan production and biofilm matrix development in fungi and can also mention enzymes involved in its modification and delivery and/or excretion to the ECM matrix, as the 1,3-β-glucanosyltransferase (Gel3) (Douglas et al., 1997; Nett et al., 2007; Massey et al., 2023). The FKS1 gene of P. brasiliensis showed an increased expression throughout the maturation of biofilm, which was more expressive at 96 h (intermediate phase), with a 3.4-fold increase when compared to the planktonic culture, suggesting that this polysaccharide can be a component of the ECM of P. brasiliensis biofilm that coincides with the maturation phase with the most significant thickening of ECM.
Evidence suggests that Gel3, a glucan elongation protein, has 1,3-β-glucanosyltransferase activity, acting at the cross-linking of cell wall components in fungi, and therefore associated with cell wall morphogenesis (Popolo and Vai, 1999; Mouyna et al., 2000). In A. fumigatus, AfGel1p catalyzes the 1,3-β-glucanosyltransferase reaction in vitro in two steps: (1) a glycosidic bond of a 1,3-β-glucan donor is cleaved, and the reduced moiety is released, (2) then the new reduced end is transferred to a non-reducing end of a 1,3-β-glucan acceptor chair. Therefore, forming a new bond between the 1,3-β-glucan donor and acceptor results in the 1,3-β-glucan elongation at branch points of other glucans and also creates anchorage points for mannoproteins, chitin, and galactomannan. Thus, 1,3-β-glucanosyltransferase acts similarly to glycosidic hydrolases in the first step but as a carbohydrate in the second, being preferable to a water molecule (Mouyna et al., 2005; Ragni et al., 2007).
Furthermore, we have α-glucan as the main polysaccharide (95%) located in the outermost layer of the yeast form of P. brasiliensis; its presence is correlated with virulence, possibly due to the masking of β-glucan, which leads to a decrease in the recognition of the immune system (Souza et al., 2019). Among the genes involved in the α-glucan synthesis to P. brasiliensis, we have the AGS1, in which we observed a 1.9-fold increase in expression at 96 h of biofilm growth, and perhaps this polysaccharide can be part of the ECM component produced by the biofilm of this pathogen. In Aspergillus fumigatus, the presence of α-glucan in the ECM has already been described (Beauvais et al., 2007). The biofilm present in the aspergilloma produces a thicker layer of ECM containing α-glucan, which is absent in biofilms formed in invasive aspergillosis (Fontaine et al., 2010; Loussert et al., 2010).
Gel(s) are proteins homologous to glycophospholipid anchoring (Gas) proteins, pH-regulated (Phr), and essential to the development of pseudohyphae protein (Epd), which are present in different fungal species belonging to the GH72 (glycosyl hydrolase 72) family cluster (Saporito-Irwin et al., 1995; Mühlschlegel and Fonzi, 1997; Nakazawa et al., 1998; Mouyna et al., 2000; Ragni et al., 2007). The GEL3 expression in P. brasiliensis, as well as the amount of the respective protein, is increased in the mycelial phase, suffering a drastic reduction after 24 h when the temperature changes from 22 to 36°C, reaching low levels in the yeast phase, that corroborates with the predominance of 1,3-β glucan in the mycelium phase of this fungus (da Silva Castro et al., 2009). Additionally, P. brasiliensis GEL3 can partially rescue the GAS1 mutation in S. cerevisiae (da Silva Castro et al., 2009). The respective mutation leads to reduced growth phenotypes, morphological alterations, sensitivity to calcofluor, and increased cell wall permeability (Popolo and Vai, 1999; Vai et al., 2000). In the present study, we observed that GEL3 is more expressed at all evaluated times of biofilm maturation, mainly at 24, 48, and 144 h.
Gas1 (Saccharomyces cerevisiae), Phr1 and Phr2 (C. albicans), Gel1 and Gel2 (A. fumigatus) act actively in the biosynthesis and morphogenesis through the correct incorporation of glucan molecules in the cell wall being also related to the virulence of C. albicans and A. fumigatus in a murine model of infection (Ghannoum et al., 1995; Mouyna et al., 2005). PHR1 expression is increased in the biofilm vs. planktonic culture, and its deletion reduces the glucan in C. albicans (Murillo et al., 2005; Nett et al., 2009). In contrast, another study showed that the overexpression of PHR2, but not PHR1, increased biofilm occupancy but not adherence or biomass (Cabral et al., 2014). Such differences can reflect variations related to the medium composition and pH used for biofilm formation and the implication of these enzymes in this process (Popolo et al., 2017).
In the present study, the expression of GEL3 is increased. On the contrary, we have a greater expression of FKS1 and a decrease in the expression of GEL3 at 96 h, suggesting that in P. brasiliensis there is a feedback signaling for the fungus to produce additional 1,3-β glucan during biofilm growth when production occurs of enzymes necessary for its modification and delivery to the ECM is reduced. Previously, Taff et al. (2012) correlated the expression level of enzymes related to 1,3 β-glucan synthesis and glucan modification to their delivery to ECM and maturation. Mutants for glucan-modifying enzymes, which have reduced glucan delivery to the extracellular space, signal the fungus to undergo increased 1,3-β glucan synthesis.
In addition to glucan synthesis, Kre6 is a glycosyl hydrolase associated with 1,6-β-glucan synthesis, and its deletion in C. albicans did not impact biofilm formation (Kurita et al., 2011). In P. brasiliensis the KRE6 behavior was not like GEL3. KRE6 has a slight increase at 24 h, but at later times of biofilm maturation (48, 96, and 144 h), its expression was lower when compared to planktonic cells, being more evident at 144 h, possibly this polysaccharide is also present in lower amounts in the ECM to this microorganism. In yeast cells of P. brasiliensis we have 95% of glucan and 5% of β-glucan in both anomeric forms, predominantly 1,3-β-glucan and too little of 1,6-β-glucan (Kanetsuna et al., 1972).
Other genes evaluated in this study refer to adhesins. Adhesion is the first step to biofilm formation and pathogenesis, so this process underlies many consequences for fungal lifestyle, commensalism, and infection. Adhesion to several substrates can occur through different interactions, such as hydrophobic interactions, electrostatic attraction, and specific ligands called adhesins (Chaffin et al., 1998; Chandra et al., 2001; Jeng et al., 2005; Ramage et al., 2005).
Adhesins on the fungal cell surface are often identified as downstream effectors in biofilm proteomic and transcriptomics studies. Once the biofilm is formed, there is a progression from one adhesin to another, and we have limited knowledge regarding the consequences of its expression changes. A related question would be the role of external signaling: how does a cell know it is “stuck” to a substrate or a neighboring cell? In this sense, we have the quorum-sensing acting, but once immobilized, the cells act differently from planktonic cells, and the adhesion itself must be a determinant for this (Lipke, 2018).
In our qRT-PCR analysis, we evaluated the expression of two well-described adhesins for P. brasiliensis, enolase and 14-3-3 (Donofrio et al., 2009; Marcos et al., 2012, 2016; da Silva et al., 2013; de Oliveira et al., 2015). Enolase (ENO) is an enzyme of the glycolytic pathway considered also known to be a moonlighting protein in P. brasiliensis (Marcos et al., 2014) as well as for other pathogens (Fox and Smulian, 2001; Jong et al., 2003). Previously, Sardi et al. (2015) evaluated the expression of some adhesins and hydrolytic enzymes in mature biofilm condition (144 h) of P. brasiliensis, under low oxygen tension and culturing the fungi in mFUM medium (Modified Fluid Universal Medium) and observed 1.5-fold increase to enolase expression. Here, we included initial and intermediate times of biofilm formation and BHI as a medium and observed a considerable increase of expression at 24, 48, and 96 h, and to 144 h, this increase was not so expressive (1.8-fold).
These data may suggest that enolase is more important in the initial phases (probably in adhesion) of P. brasiliensis biofilm formation or changes in the metabolic process required by biofilm formation. The increase in the assimilation of carbohydrates via glycolysis with the production of a pool of pyruvate and amino acids is necessary for the increase in biomass that requires greater energy availability. Once the phase is reached, there is a decrease in the expression of related genes, resulting in a decrease in metabolic activity or stationary phase of the biofilm, as demonstrated in C. albicans (Yeater et al., 2007).
C. albicans is capable of secreting enolase, which is detected in the ECM of the biofilm (Silva et al., 2014); it can be re-adsorbed on the cell surface through ALS3 interaction, acting as a “bridge” for the interaction of the latter with host proteins (plasminogen and kininogen) (Karkowska-Kuleta et al., 2021) and also support for polymicrobial interaction in biofilms (Bartnicka et al., 2019).
Widely distributed among eukaryotes, 14-3-3 proteins are highly conserved and ubiquitous, with subunits of approximately 30 kDa, showing multiple isoforms. In the present study, we observed an increased expression of 14-3-3 at initial and intermediate times. This higher expression can probably contribute to changing the expression of other factors related to fungal adhesion with consequent ECM increase and biofilm formation; once biofilm is consolidated, its expression is reduced.
Several studies demonstrate that 14-3-3 proteins act as regulators of multiple physiological processes, signal transduction, primary metabolism, cell cycle regulation, protein trafficking, and reactions to abiotic and biotic stress; due to its lack of catalytic activity, many of its functions occur through the binding to other proteins (Shi et al., 2019). In P. brasiliensis, 14-3-3 seems involved in fungal dimorphism (Marcos et al., 2016). Previously, Carlton (2018) demonstrated that the isolate with low expression of 14-3-3 has more significant metabolic activity during biofilm growth, unlike the planktonic form, suggesting that the biofilm growth condition presents an altered state of metabolism (Borriello et al., 2006; Nguyen et al., 2011). Sardi et al. (2024) showed that the isolate silenced for 14-3-3 presents a reduced expression of other genes in planktonic or biofilm growth conditions, such as enolase, gp43, and GAPDH, as well as produces biofilm with a lower amount of ECM. Then, 14-3-3 is known to have multiple functions and can also act in the gene expression modulation of virulence factors and adhesin and influence the biogenesis of ECM directly or indirectly.
In conclusion, this study highlights the potential for biofilm formation by three species of Paracoccidioides and the main components of the extracellular matrix that may contribute to pathogenicity, mainly through its roles in promoting antifungal drug tolerance and immune evasion. The pathway related to the synthesis and delivery of polysaccharides, constituents of the extracellular matrix, considered the key to differentiating biofilm and planktonic growth conditions, is temporally modulated along the biofilm kinetics of formation in P. brasiliensis, and has a greater expression of important multifunctional proteins mainly involved in the adhesion at early stages and biofilm initiation. In the future, there is a need to study the composition of the extracellular matrix of the P. brasiliensis biofilm and isolates with low expression of the elucidated targets to deepen the knowledge about their fundamental importance and whether they can be considered attractive for the development of new therapies targeting their inhibition to decrease biofilm formation or even as biofilm markers.
The original contributions presented in the study are included in the article/Supplementary material, further inquiries can be directed to the corresponding author.
LO: Formal analysis, Investigation, Software, Writing—original draft. CM: Data curation, Formal analysis, Investigation, Software, Validation, Writing—original draft, Writing—review & editing. AC: Conceptualization, Visualization, Writing—review & editing. KM-A: Conceptualization, Supervision, Writing—review & editing. RP: Conceptualization, Supervision, Visualization, Writing—original draft. AF-A: Data curation, Methodology, Supervision, Visualization, Writing—review & editing. MM-G: Conceptualization, Data curation, Funding acquisition, Methodology, Project administration, Resources, Supervision, Visualization, Writing—original draft, Writing—review & editing.
The author(s) declare that financial support was received for the research, authorship, and/or publication of this article. This study was supported by the Fundação de Amparo à Pesquisa do Estado de São Paulo (FAPESP Grant Nos. 2016/17048-4, 2017/06658-9, and 2018/15877-9), the Coordenação de Aperfeiçoamento de Pessoal de Nível Superior–Brasil (PROEX-CAPES), the Conselho Nacional de Desenvolvimento Científico e Tecnológico (CNPq Grants Nos. 431455/2018-0, 310524/2018-0, and 164217/2020-7), and the Programa de Apoio ao Desenvolvimento Científico da Faculdade de Ciências Farmacêuticas da UNESP PADC-FCF-UNESP.
The authors declare that the research was conducted in the absence of any commercial or financial relationships that could be construed as a potential conflict of interest.
The author(s) declared that they were an editorial board member of Frontiers, at the time of submission. This had no impact on the peer review process and the final decision.
All claims expressed in this article are solely those of the authors and do not necessarily represent those of their affiliated organizations, or those of the publisher, the editors and the reviewers. Any product that may be evaluated in this article, or claim that may be made by its manufacturer, is not guaranteed or endorsed by the publisher.
The Supplementary Material for this article can be found online at: https://www.frontiersin.org/articles/10.3389/fmicb.2024.1354140/full#supplementary-material
Supplementary Figure S1. Specific amplification at the end of the qPCR run. (A) Melt curve analysis of each primer pair showing a single peak. (B) Agarose electrophoresis gel of amplicons demonstrating a single band with correspondent size according to the gene. MW, molecular weight.
Allesen-Holm, M., Barken, K. B., Yang, L., Klausen, M., Webb, J. S., Kjelleberg, S., et al. (2006). A characterization of DNA release in Pseudomonas aeruginosa cultures and biofilms. Mol. Microbiol. 59, 1114–1128. doi: 10.1111/j.1365-2958.2005.05008.x
Bamford, N. C., Le Mauff, F., Van Loon, J. C., Ostapska, H., Snarr, B. D., Zhang, Y., et al. (2020). Structural and biochemical characterization of the exopolysaccharide deacetylase Agd3 required for Aspergillus fumigatus biofilm formation. Nat. Commun. 11, 1–13. doi: 10.1038/s41467-020-16144-5
Barbosa, M. S., Báo, S. N., Andreotti, P. F., de Faria, F. P., Felipe, M. S. S., dos Santos Feitosa, L., et al. (2006). Glyceraldehyde-3-phosphate dehydrogenase of Paracoccidioides brasiliensis is a cell surface protein involved in fungal adhesion to extracellular matrix proteins and interaction with cells. Infect. Immun. 74, 382–389. doi: 10.1128/IAI.74.1.382-389.2006
Bartnicka, D., Karkowska-Kuleta, J., Zawrotniak, M., Satała, D., Michalik, K., Zielinska, G., et al. (2019). Adhesive protein-mediated cross-talk between Candida albicans and Porphyromonas gingivalis in dual species biofilm protects the anaerobic bacterium in unfavorable oxic environment. Sci. Rep. 9:4376. doi: 10.1038/s41598-019-40771-8
Beauvais, A., Schmidt, C., Guadagnini, S., Roux, P., Perret, E., Henry, C., et al. (2007). An extracellular matrix glues together the aerial-grown hyphae of Aspergillus fumigatus. Cell. Microbiol. 9, 1588–1600. doi: 10.1111/j.1462-5822.2007.00895.x
Borriello, G., Richards, L., Ehrlich, G. D., and Stewart, P. S. (2006). Arginine or nitrate enhances antibiotic susceptibility of Pseudomonas aeruginosa in biofilms. Antimicrob. Agents Chemother. 50, 382–384. doi: 10.1128/AAC.50.1.382-384.2006
Bradford, M. (1976). A rapid and sensitive method for the quantitation of microgram quantities of protein utilizing the principle of protein-dye binding. Anal. Biochem. 72, 248–254. doi: 10.1016/0003-2697(76)90527-3
Cabral, V., Znaidi, S., Walker, L. A., Martin-Yken, H., Dague, E., Legrand, M., et al. (2014). Targeted changes of the cell wall proteome influence Candida albicans ability to form single- and multi-strain biofilms. PLoS Pathog. 10:e1004542. doi: 10.1371/journal.ppat.1004542
Camacho, E., and Niño-Vega, G. A. (2017). Paracoccidioidesspp.: virulence factors and immune-evasion strategies. Mediators Inflamm. 2017, 1–19. doi: 10.1155/2017/5313691
Carlton, J. D. (2018). Importância da proteína 14-3-3 de Paracoccidioides brasiliensis na formação de biofilmes e caracterização funcional do isolado selvagem e silenciado de 14-3-3. Faculdade de Ciências Farmacêuticas de Araraquara - UNESP, Brazil.
Carrero, L. L., Niño-Vega, G., Teixeira, M. M., Carvalho, M. J. A., Soares, C. M. A., Pereira, M., et al. (2008). New Paracoccidioides brasiliensis isolate reveals unexpected genomic variability in this human pathogen. Fung. Genet. Biol. 45, 605–612. doi: 10.1016/j.fgb.2008.02.002
Cattana, M. E., Tracogna, M. F., Marques, I., Rojas, F., Fernández, M., de los Ángeles Sosa, M., et al. (2017). In vivo paracoccidioides sp. biofilm on vascular prosthesis. Mycopathologia 182, 747–749. doi: 10.1007/s11046-017-0126-8
Chaffin, W. L., López-Ribot, J. L., Casanova, M., Gozalbo, D., and Martínez, J. P. (1998). Cell wall and secreted proteins of Candida albicans: identification, function, and expression. Microbiol. Mol. Biol. Rev. 62, 130–180. doi: 10.1128/MMBR.62.1.130-180.1998
Chandra, J., Kuhn, D. M., Mukherjee, P. K., Hoyer, L. L., McCormick, T., and Ghannoum, M. A. (2001). Biofilm formation by the fungal pathogen Candida albicans: development, architecture, and drug resistance. J. Bacteriol. 183, 5385–5394. doi: 10.1128/JB.183.18.5385-5394.2001
da Silva Castro, N., de Castro, K. P., Orlandi, I., Feitosa, L. S, Rosa e Silva, L. K., Vainstein, M., et al. (2009). Characterization and functional analysis of the β-1,3-glucanosyltransferase 3 of the human pathogenic fungus Paracoccidioides brasiliensis. FEMS Yeast Res. 9, 103–114. doi: 10.1111/j.1567-1364.2008.00463.x
da Silva, J. F., de Oliveira, H. C., Marcos, C. M., da Silva, R. A. M., da Costa, T. A., Calich, V. L. G., et al. (2013). Paracoccidoides brasiliensis 30 kDa adhesin: identification as a 14-3-3 protein, cloning and subcellular localization in infection models. PLoS ONE 8:e62533. doi: 10.1371/journal.pone.0062533
de Curcio, J. S. (2018). Caracterização e análise da expressão de microRNAs-like de Paracoccidioides brasiliensis. Available online at: https://repositorio.unb.br/handle/10482/35211 (accessed January 01, 2023).
de Oliveira, H. C., da Silva, J. F., Scorzoni, L., Marcos, C. M., Rossi, S. A., de Paula e Silva, A. C., et al. (2015). Importance of adhesins in virulence of Paracoccidioides spp. Front. Microbiol. 6:431. doi: 10.3389/fmicb.2015.00431
Desmond, P., Best, J. P., Morgenroth, E., and Derlon, N. (2018). Linking composition of extracellular polymeric substances (EPS) to the physical structure and hydraulic resistance of membrane biofilms. Water Res. 132, 211–221. doi: 10.1016/j.watres.2017.12.058
Donofrio, F. C., Calil, A. C. A., Miranda, E. T., Almeida, A. M. F., Benard, G., Soares, C. P., et al. (2009). Enolase from Paracoccidioides brasiliensis: isolation and identification as a fibronectin-binding protein. J. Med. Microbiol. 58 (Pt 6), 706–713. doi: 10.1099/jmm.0.003830-0
Douglas, C. M., D'Ippolito, J. A., Shei, G. J., Meinz, M., Onishi, J., Marrinan, J. A., et al. (1997). Identification of the FKS1 gene of Candida albicans as the essential target of 1,3-beta-D-glucan synthase inhibitors. Antimicrob. Agents Chemother. 41, 2471–2479. doi: 10.1128/AAC.41.11.2471
DuBois, M., Gilles, K. A., Hamilton, J. K., Rebers, P. A., and Smith, F. (1956). Colorimetric method for determination of sugars and related substances. Anal. Chem. 28, 350–356. doi: 10.1021/ac60111a017
Flemming, H.-C., Neu, T. R., and Wozniak, D. J. (2007). The EPS matrix: the “house of biofilm cells”. J. Bacteriol. 189, 7945–7947. doi: 10.1128/JB.00858-07
Flemming, H.-C., Wingender, J., Mayer, C., Körstgens, V., and Borchard, W. (2000). “Cohesiveness in biofilm matrix polymers,” in Community Structure and Co-operation in Biofilms, eds D. G. Allison, P. Gilbert, H. M. Lappin-Scott, and M. Wilson (Cambridge: Cambridge University Press), 87–106.
Fontaine, T., Beauvais, A., Loussert, C., Thevenard, B., Fulgsang, C. C., Ohno, N., et al. (2010). Cell wall alpha1-3glucans induce the aggregation of germinating conidia of Aspergillus fumigatus. Fung. Genet. Biol. 47, 707–712. doi: 10.1016/j.fgb.2010.04.006
Fortes, M. R. P., Miot, H. A., Kurokawa, C. S., Marques, M. E. A., and Marques, S. A. (2011). Immunology of paracoccidioidomycosis. An. Bras. Dermatol. 86, 516–524. doi: 10.1590/S0365-05962011000300014
Fox, D., and Smulian, A. G. (2001). Plasminogen-binding activity of enolase in the opportunistic pathogen Pneumocystis carinii. Med. Mycol. 39, 495–507. doi: 10.1080/mmy.39.6.495.507
Garny, K., Neu, T. R., Horn, H., Volke, F., and Manz, B. (2010). Combined application of 13C NMR spectroscopy and confocal laser scanning microscopy—Investigation on biofilm structure and physico-chemical properties. Chem. Eng. Sci. 65, 4691–4700. doi: 10.1016/j.ces.2010.05.013
Ghannoum, M. A., Spellberg, B., Saporito-Irwin, S. M., and Fonzi, W. A. (1995). Reduced virulence of Candida albicans PHR1 mutants. Infect. Immun. 63, 4528–4530. doi: 10.1128/iai.63.11.4528-4530.1995
Goes, T., Bailão, E. F. L. C., Correa, C. R., Bozzi, A., Santos, L. I., Gomes, D. A., et al. (2014). New developments of RNAi in Paracoccidioides brasiliensis: prospects for high-throughput, genome-wide, functional genomics. PLoS Negl. Trop. Dis. 8:e3173. doi: 10.1371/journal.pntd.0003173
Grahl, N., Shepardson, K. M., Chung, D., and Cramer, R. A. (2012). Hypoxia and fungal pathogenesis: to air or not to air? Eukaryot. Cell 11, 560–570. doi: 10.1128/EC.00031-12
Hahn, R. C., Hagen, F., Mendes, R. P., Burger, E., Nery, A. F., Siqueira, N. P., et al. (2022). Paracoccidioidomycosis: current status and future trends. Clin. Microbiol. Rev. 35:e0023321. doi: 10.1128/cmr.00233-21
Hanna, S. A., Monteiro da Silva, J. L., and Mendes-Giannini, M. J. S. (2000). Adherence and intracellular parasitism of Paracoccidioides brasiliensis in Vero cells. Microbes Infect. 2, 877–884. doi: 10.1016/S1286-4579(00)00390-7
Hrycyk, M. F., Garcia Garces, H., Bosco, S., de, M. G., de Oliveira, S. L., Marques, S. A., et al. (2018). Ecology of Paracoccidioides brasiliensis, P. lutzii and related species: infection in armadillos, soil occurrence and mycological aspects. Med. Mycol. 56, 950–962. doi: 10.1093/mmy/myx142
Jeng, H. W., Holmes, A. R., and Cannon, R. D. (2005). Characterization of two Candida albicans surface mannoprotein adhesins that bind immobilized saliva components. Med. Mycol. 43, 209–217. doi: 10.1080/13693780410001731637
Jong, A. Y., Chen, S. H. M., Stins, M. F., Kim, K. S., Tuan, T.-L., and Huang, S.-H. (2003). Binding of Candida albicans enolase to plasmin(ogen) results in enhanced invasion of human brain microvascular endothelial cells. J. Med. Microbiol. 52 (Pt 8), 615–622. doi: 10.1099/jmm.0.05060-0
Kanetsuna, F., Carbonell, L. M., Azuma, I., and Yamamura, Y. (1972). Biochemical studies on the thermal dimorphism of Paracoccidioides brasiliensis. J. Bacteriol. 110, 208–218. doi: 10.1128/jb.110.1.208-218.1972
Karkowska-Kuleta, J., Wronowska, E., Satala, D., Zawrotniak, M., Bras, G., Kozik, A., et al. (2021). Als3-mediated attachment of enolase on the surface of Candida albicans cells regulates their interactions with host proteins. Cell. Microbiol. 23:e13297. doi: 10.1111/cmi.13297
Karygianni, L., Ren, Z., Koo, H., and Thurnheer, T. (2020). Biofilm matrixome: extracellular components in structured microbial communities. Trends Microbiol. 28, 668–681. doi: 10.1016/j.tim.2020.03.016
Kurita, T., Noda, Y., Takagi, T., Osumi, M., and Yoda, K. (2011). Kre6 protein essential for yeast cell wall beta-1,6-glucan synthesis accumulates at sites of polarized growth. J. Biol. Chem. 286, 7429–7438. doi: 10.1074/jbc.M110.174060
Lipke, P. (2018). What we do not know about fungal cell adhesion molecules. J. Fungi 4:59. doi: 10.3390/jof4020059
Livak, K. J., and Schmittgen, T. D. (2001). Analysis of relative gene expression data using real-time quantitative PCR and the 2(-Delta Delta C(T)) method. Methods 25, 402–408. doi: 10.1006/meth.2001.1262
Loussert, C., Schmitt, C., Prevost, M.-C., Balloy, V., Fadel, E., Philippe, B., et al. (2010). In vivo biofilm composition of Aspergillus fumigatus. Cell. Microbiol. 12, 405–410. doi: 10.1111/j.1462-5822.2009.01409.x
Marcos, C. M., de Fátima da Silva, J., de Oliveira, H. C., Moraes da Silva, R. A., Mendes-Giannini, M. J. S., and Fusco-Almeida, A. M. (2012). Surface-expressed enolase contributes to the adhesion of Paracoccidioides brasiliensis to host cells. FEMS Yeast Res. 12, 557–570. doi: 10.1111/j.1567-1364.2012.00806.x
Marcos, C. M., de Oliveira, H. C., da Silva, J. F., Assato, P. A., Fusco-Almeida, A. M., and Mendes-Giannini, M. J. S. (2014). The multifaceted roles of metabolic enzymes in the Paracoccidioides species complex. Front. Microbiol. 5:719. doi: 10.3389/fmicb.2014.00719
Marcos, C. M., Silva, J. F., de Oliveira, H. C., Assato, P. A., Singulani, J. L., Lopez, A. M., et al. (2016). Decreased expression of 14-3-3 in Paracoccidioides brasiliensis confirms its involvement in fungal pathogenesis. Virulence 7, 72–84. doi: 10.1080/21505594.2015.1122166
Martinez, L. R., and Casadevall, A. (2007). Cryptococcus neoformans biofilm formation depends on surface support and carbon source and reduces fungal cell susceptibility to heat, cold, and UV light. Appl. Environ. Microbiol. 73, 4592–4601. doi: 10.1128/AEM.02506-06
Martins, M., Uppuluri, P., Thomas, D. P., Cleary, I. A., Henriques, M., Lopez-Ribot, J. L., et al. (2010). Presence of extracellular DNA in the Candida albicans biofilm matrix and its contribution to biofilms. Mycopathologia 169, 323–331. doi: 10.1007/s11046-009-9264-y
Massey, J., Zarnowski, R., and Andes, D. (2023). Role of the extracellular matrix in Candida biofilm antifungal resistance. FEMS Microbiol. Rev. 47:fuad059. doi: 10.1093/femsre/fuad059
Mendes-Giannini, M. J. S., Monteiro da Silva, J. L., de Fátima da Silva, J., Donofrio, F. C., Miranda, E. T., Andreotti, P. F., et al. (2008). Interactions of Paracoccidioides brasiliensis with host cells: recent advances. Mycopathologia 165, 237–248. doi: 10.1007/s11046-007-9074-z
Mitchell, K. F., Zarnowski, R., and Andes, D. R. (2016). The extracellular matrix of fungal biofilms. Adv. Exp. Med. Biol. 931, 21–35. doi: 10.1007/5584_2016_6
Mitchell, K. F., Zarnowski, R., Sanchez, H., Edward, J. A., Reinicke, E. L., Nett, J. E., et al. (2015). Community participation in biofilm matrix assembly and function. Proc. Natl. Acad. Sci. U. S. A. 112, 4092–4097. doi: 10.1073/pnas.1421437112
Mouyna, I., Fontaine, T., Vai, M., Monod, M., Fonzi, W. A., Diaquin, M., et al. (2000). Glycosylphosphatidylinositol-anchored glucanosyltransferases play an active role in the biosynthesis of the fungal cell wall. J. Biol. Chem. 275, 14882–14889. doi: 10.1074/jbc.275.20.14882
Mouyna, I.sabelle, Morelle, W., Vai, M., Monod, M., Léchenne, B., Fontaine, T., Beauvais, A., et al. (2005). Deletion of GEL2 encoding for a beta(1-3)glucanosyltransferase affects morphogenesis and virulence in Aspergillus fumigatus: GELdeletions affectA. Fumigatus morphogenesis and virulence. Mol. Microbiol. 56, 1675–1688. doi: 10.1111/j.1365-2958.2005.04654.x
Mühlschlegel, F. A., and Fonzi, W. A. (1997). PHR2 of Candida albicans encodes a functional homolog of the pH-regulated gene PHR1 with an inverted pattern of pH-dependent expression. Mol. Cell. Biol. 17, 5960–5967. doi: 10.1128/MCB.17.10.5960
Murillo, L. A., Newport, G., Lan, C.-Y., Habelitz, S., Dungan, J., and Agabian, N. M. (2005). Genome-wide transcription profiling of the early phase of biofilm formation by Candida albicans. Eukaryot. Cell 4, 1562–1573. doi: 10.1128/EC.4.9.1562-1573.2005
Nakazawa, T., Horiuchi, H., Ohta, A., and Takagi, M. (1998). Isolation and characterization of EPD1, an essential gene for pseudohyphal growth of a dimorphic yeast, Candida maltosa. J. Bacteriol. 180, 2079–2086. doi: 10.1128/JB.180.8.2079-2086.1998
Navarro, M. V., de Barros, Y. N., Segura, W. D., Chaves, A. F. A., Jannuzzi, G. P., Ferreira, K. S., et al. (2021). The role of dimorphism regulating histidine kinase (Drk1) in the pathogenic fungus Paracoccidioides brasiliensis cell wall. J. Fungi 7:1014. doi: 10.3390/jof7121014
Nett, J., Lincoln, L., Marchillo, K., Massey, R., Holoyda, K., Hoff, B., et al. (2007). Putative role of beta-1,3 glucans in Candida albicans biofilm resistance. Antimicrob. Agents Chemother. 51, 510–520. doi: 10.1128/AAC.01056-06
Nett, J. E., Crawford, K., Marchillo, K., and Andes, D. R. (2010a). Role of Fks1p and matrix glucan in Candida albicans biofilm resistance to an echinocandin, pyrimidine, and polyene. Antimicrob. Agents Chemother. 54, 3505–3508. doi: 10.1128/AAC.00227-10
Nett, J. E., Lepak, A. J., Marchillo, K., and Andes, D. R. (2009). Time course global gene expression analysis of an in vivo Candida biofilm. J. Infect. Dis. 200, 307–313. doi: 10.1086/599838
Nett, J. E., Marchillo, K., Spiegel, C. A., and Andes, D. R. (2010b). Development and validation of an in vivo Candida albicans biofilm denture model. Infect. Immun. 78, 3650–3659. doi: 10.1128/IAI.00480-10
Nguyen, D., Joshi-Datar, A., Lepine, F., Bauerle, E., Olakanmi, O., Beer, K., et al. (2011). Active starvation responses mediate antibiotic tolerance in biofilms and nutrient-limited bacteria. Science 334, 982–986. doi: 10.1126/science.1211037
Oliveira, L. T., Medina-Alarcón, K. P., Singulani, J., de, L., Fregonezi, N. F., Pires, R. H., et al. (2020). Dynamics of mono-and dual-species biofilm formation and interactions between Paracoccidioides brasiliensis and Candida albicans. Front. Microbiol. 11:551256. doi: 10.3389/fmicb.2020.551256
Oshiro, K. G. N., Rodrigues, G., Monges, B. E. D., Cardoso, M. H., and Franco, O. L. (2019). Bioactive peptides against fungal biofilms. Front. Microbiol. 10:e02169. doi: 10.3389/fmicb.2019.02169
Popolo, L., Degani, G., Camilloni, C., and Fonzi, W. (2017). The PHR family: the role of extracellular transglycosylases in shaping candida albicans cells. J. Fungi 3:59. doi: 10.3390/jof3040059
Popolo, L., and Vai, M. (1999). The Gas1 glycoprotein, a putative wall polymer cross-linker. Biochim. Biophys. Acta 1426, 385–400. doi: 10.1016/S0304-4165(98)00138-X
Ragni, E., Fontaine, T., Gissi, C., Latgè, J. P., and Popolo, L. (2007). The Gas family of proteins of Saccharomyces cerevisiae: characterization and evolutionary analysis. Yeast 24, 297–308. doi: 10.1002/yea.1473
Rajendran, R., Sherry, L., Lappin, D. F., Nile, C. J., Smith, K., Williams, C., et al. (2014). Extracellular DNA release confers heterogeneity in Candida albicans biofilm formation. BMC Microbiol. 14:303. doi: 10.1186/s12866-014-0303-6
Rajendran, R., Sherry, L., Nile, C. J., Sherriff, A., Johnson, E. M., Hanson, M. F., et al. (2016). Biofilm formation is a risk factor for mortality in patients with Candida albicans bloodstream infection—Scotland, 2012–2013. Clin. Microbiol. Infect. 22, 87–93. doi: 10.1016/j.cmi.2015.09.018
Ramage, G., Coco, B., Sherry, L., Bagg, J., and Lappin, D. F. (2012). In vitro Candida albicans biofilm induced proteinase activity and SAP8 expression correlates with in vivo denture stomatitis severity. Mycopathologia 174, 11–19. doi: 10.1007/s11046-012-9522-2
Ramage, G., Saville, S. P., Thomas, D. P., and López-Ribot, J. L. (2005). Candida biofilms: an update. Eukaryot. Cell 4, 633–638. doi: 10.1128/EC.4.4.633-638.2005
Reichhardt, C., Ferreira, J. A. G., Joubert, L.-M., Clemons, K. V., Stevens, D. A., and Cegelski, L. (2015). Analysis of the Aspergillus fumigatus biofilm extracellular matrix by solid-state nuclear magnetic resonance spectroscopy. Eukaryot. Cell 14, 1064–1072. doi: 10.1128/EC.00050-15
Restrepo, A., Cano, L. E., and Gonzalez, Á. (2015). The power of the small: the example of Paracoccidioides brasiliensis conidia. Rev. Inst. Med. Trop. São Paulo 5, 5–10. doi: 10.1590/S0036-46652015000700003
San-Blas, G., and Niño-Vega, G. (2008). Paracoccidioides brasiliensis: chemical and molecular tools for research on cell walls, antifungals, diagnosis, taxonomy. Mycopathologia 165, 183–195. doi: 10.1007/s11046-007-9040-9
Santos, L. A., Grisolia, J. C., Burger, E., de Araujo Paula, F. B., Dias, A. L. T., and Malaquias, L. C. C. (2020). Virulence factors of Paracoccidioides brasiliensis as therapeutic targets: a review. Antonie Van Leeuwenhoek 113, 593–604. doi: 10.1007/s10482-019-01382-5
Sapaar, B., Nur, A., Hirota, K., Yumoto, H., Murakami, K., Amoh, T., et al. (2014). Effects of extracellular DNA from Candida albicans and pneumonia-related pathogens on Candida biofilm formation and hyphal transformation. J. Appl. Microbiol. 116, 1531–1542. doi: 10.1111/jam.12483
Saporito-Irwin, S. M., Birse, C. E., Sypherd, P. S., and Fonzi, W. A. (1995). PHR1, a pH-regulated gene of Candida albicans, is required for morphogenesis. Mol. Cell. Biol. 15, 601–613. doi: 10.1128/MCB.15.2.601
Sardi, J. C. O., Derissi Braz Carlton, J., Marcos, C. M., Fusco Almeida, A. M., and Mendes Giannini, M. J. S. (2024). Unveiling the functional significance of the 14.3.3 protein: a key player in Paracoccidioides brasiliensis biofilm formation. Microb. Pathog. 188:106537. doi: 10.1016/j.micpath.2024.106537
Sardi, J. C. O., Pitangui, N. S., Voltan, A. R., Braz, J. D., Machado, M. P., Fusco Almeida, A. M., et al. (2015). In vitro Paracoccidioides brasiliensisbiofilm and gene expression of adhesins and hydrolytic enzymes. Virulence 6, 642–651. doi: 10.1080/21505594.2015.1031437
Scorzoni, L., de Paula e Silva, A. C. A., Singulani, J. L., Leite, F. S., de Oliveira, H. C., Moraes da Silva, R. A., et al. (2015). Comparison of virulence betweenParacoccidioides brasiliensisandParacoccidioides lutziiusingGalleria mellonellaas a host model. Virulence 6, 766–776. doi: 10.1080/21505594.2015.1085277
Sheppard, D. C., and Howell, P. L. (2016). Biofilm exopolysaccharides of pathogenic fungi: Lessons from bacteria. J. Biol. Chem. 291, 12529–12537. doi: 10.1074/jbc.R116.720995
Sherry, L., Rajendran, R., Lappin, D. F., Borghi, E., Perdoni, F., Falleni, M., et al. (2014). Biofilms formed by Candida albicans bloodstream isolates display phenotypic and transcriptional heterogeneity that are associated with resistance and pathogenicity. BMC Microbiol. 14:182. doi: 10.1186/1471-2180-14-182
Shi, L., Ren, A., Zhu, J., Yu, H., Jiang, A., Zheng, H., et al. (2019). 14-3-3 proteins: a window for a deeper understanding of fungal metabolism and development. World J. Microbiol. Biotechnol. 35, 24. doi: 10.1007/s11274-019-2597-x
Silva, R. C., Padovan, A. C. B., Pimenta, D. C., Ferreira, R. C., da Silva, C. V., and Briones, M. R. S. (2014). Extracellular enolase of Candida albicans is involved in colonization of mammalian intestinal epithelium. Front. Cell. Infect. Microbiol. 4:66. doi: 10.3389/fcimb.2014.00066
Silva, S., Henriques, M., Martins, A., Oliveira, R., Williams, D., and Azeredo, J. (2009). Biofilms of non-Candida albicans Candida species: quantification, structure and matrix composition. Med. Mycol. 47, 681–689. doi: 10.3109/13693780802549594
Silva, S., Henriques, M., Oliveira, R., Williams, D., and Azeredo, J. (2010). In vitro biofilm activity of non-Candida albicans Candida species. Curr. Microbiol. 61, 534–540. doi: 10.1007/s00284-010-9649-7
Silva, S., Rodrigues, C., Araújo, D., Rodrigues, M., and Henriques, M. (2017). Candida species biofilms' antifungal resistance. J. Fungi 3:8. doi: 10.3390/jof3010008
Souza, A. C. O., Favali, C., Soares, N. C., Tavares, N. M., Jerônimo, M. S., Veloso Junior, P. H., et al. (2019). New role of P. brasiliensis α-glucan: differentiation of non-conventional dendritic cells. Front. Microbiol. 10:2445. doi: 10.3389/fmicb.2019.02445
Sutherland, I. (2001). The biofilm matrix – an immobilized but dynamic microbial environment. Trends Microbiol. 9, 222–227. doi: 10.1016/S0966-842X(01)02012-1
Taff, H. T., Nett, J. E., Zarnowski, R., Ross, K. M., Sanchez, H., Cain, M. T., et al. (2012). A Candida biofilm-induced pathway for matrix glucan delivery: implications for drug resistance. PLoS Pathog. 8:e1002848. doi: 10.1371/journal.ppat.1002848
Teixeira, M. M., Cattana, M. E., Matute, D. R., Muñoz, J. F., Arechavala, A., Isbell, K., et al. (2020). Genomic diversity of the human pathogen Paracoccidioides across the South American continent. Fung. Genet. Biol. 140:103395. doi: 10.1016/j.fgb.2020.103395
Turissini, D. A., Gomez, O. M., Teixeira, M. M., McEwen, J. G., and Matute, D. R. (2017). Species boundaries in the human pathogen Paracoccidioides. Fung. Genet. Biol. 106, 9–25. doi: 10.1016/j.fgb.2017.05.007
Vai, M., Brambilla, L., Orlandi, I., Rota, N., Ranzi, B. M., Alberghina, L., et al. (2000). Improved secretion of native human insulin-like growth factor 1 from gas1 mutant Saccharomyces cerevisiae cells. Appl. Environ. Microbiol. 66, 5477–5479. doi: 10.1128/AEM.66.12.5477-5479.2000
Vicentini, A. P., Moraes, J. Z., Gesztesi, J. L., Franco, M. F., de Souza, W., and Lopes, J. D. (1997). Laminin-binding epitope on gp43 from Paracoccidioides brasiliensis is recognized by a monoclonal antibody raised against Staphylococcus aureus laminin receptor. J. Med. Vet. Mycol. 35:851. doi: 10.1080/02681219780000851
WHO (2022). WHO Fungal Priority Pathogens List to Guide Research, Development and Public health action. Available online at: https://www.who.int/publications/i/item/9789240060241 (accessed May 07, 2023).
Yeater, K. M., Chandra, J., Cheng, G., Mukherjee, P. K., Zhao, X., Rodriguez-Zas, S. L., et al. (2007). Temporal analysis of Candida albicans gene expression during biofilm development. Microbiology 153 (Pt 8), 2373–2385. doi: 10.1099/mic.0.2007/006163-0
Zarnowski, R., Sanchez, H., Covelli, A. S., Dominguez, E., Jaromin, A., Bernhardt, J., et al. (2018). Candida albicans biofilm–induced vesicles confer drug resistance through matrix biogenesis. PLoS Biol. 16:e2006872. doi: 10.1371/journal.pbio.2006872
Keywords: Paracoccidioides spp., biofilm, extracellular matrix, qRT-PCR, glucans, adhesins
Citation: Oliveira LT, Marcos CM, Cabral AKLF, Medina-Alarcón KP, Pires RH, Fusco-Almeida AM and Mendes-Giannini MJS (2024) Paracoccidioides spp.: the structural characterization of extracellular matrix, expression of glucan synthesis and associated genes and adhesins during biofilm formation. Front. Microbiol. 15:1354140. doi: 10.3389/fmicb.2024.1354140
Received: 11 January 2024; Accepted: 19 February 2024;
Published: 07 March 2024.
Edited by:
Fernando Rodrigues, University of Minho, PortugalReviewed by:
Rosana Puccia, Federal University of São Paulo, BrazilCopyright © 2024 Oliveira, Marcos, Cabral, Medina-Alarcón, Pires, Fusco-Almeida and Mendes-Giannini. This is an open-access article distributed under the terms of the Creative Commons Attribution License (CC BY). The use, distribution or reproduction in other forums is permitted, provided the original author(s) and the copyright owner(s) are credited and that the original publication in this journal is cited, in accordance with accepted academic practice. No use, distribution or reproduction is permitted which does not comply with these terms.
*Correspondence: Maria José Soares Mendes-Giannini, bWFyaWEuZ2lhbm5pbmlAdW5lc3AuYnI=
Disclaimer: All claims expressed in this article are solely those of the authors and do not necessarily represent those of their affiliated organizations, or those of the publisher, the editors and the reviewers. Any product that may be evaluated in this article or claim that may be made by its manufacturer is not guaranteed or endorsed by the publisher.
Research integrity at Frontiers
Learn more about the work of our research integrity team to safeguard the quality of each article we publish.