- 1Instituto de Ciências Biológicas, Universidade Federal do Pará, Belém, Brazil
- 2Núcleo de Pesquisas em Oncologia, Universidade Federal do Pará, Belém, Brazil
- 3Hospital Universitário João de Barros Barreto, Universidade Federal do Pará, Belém, Brazil
Cervical cancer ranks among the most prevalent cancers globally with high-risk human papillomaviruses implicated in nearly 99% of cases. However, hidden players such as changes in the microbiota are now being examined as potential markers in the progression of this disease. Researchers suggest that changes in the vaginal microbiota might correlate with cervical cancer. This review provides a comprehensive look at the microbiota changes linked with the advancement of cervical cancer. It also scrutinizes the databases from past studies on the microbiota during healthy and cancerous stages, drawing connections between prior findings concerning the role of the microbiota in the progression of cervical cancer. Preliminary findings identify Fusobacterium spp., Peptostreptococcus spp., Campylobacter spp., and Haemophilus spp., as potential biomarkers for cervical cancer progression. Alloscardovia spp., Eubacterium spp., and Mycoplasma spp. were identified as potential biomarkers for HPVs (+), while Methylobacterium spp. may be indicative of HPV (−). However, the study’s limitations, including potential biases and methodological constraints, underscore the need for further research to validate these findings and delve deeper into the microbiota’s role in HPV development. Despite these limitations, the review provides valuable insights into microbiota trends during cervical cancer progression, offering direction for future research. The review summarizes key findings from previous studies on microbiota during healthy and cancerous stages, as well as other conditions such as CIN, SIL, HPV (+), and HPV (−), indicating a promising area for further investigation. The consistent presence of HPV across all reported cervical abnormalities, along with the identification of distinct bacterial genera between cancerous and control samples, suggests a potential link that merits further exploration. In conclusion, a more profound understanding of the microbial landscape could elucidate the pathogenesis of cervical diseases and inform future strategies for diagnosis, prevention, and treatment.
1 Introduction
Cervical cancer (CAN) is a significant health concern for women worldwide, ranking as one of the most common cancers (Arbyn et al., 2020; WHO, 2020; Aobchey et al., 2022; Wang et al., 2022). As per the World Health Organization (WHO), in 2018, approximately 570,000 women were diagnosed with cervical cancer globally, resulting in roughly 311,000 fatalities (WHO, 2020; Wickramasinghe et al., 2021; Zhang et al., 2022). Furthermore, the WHO projected in 2020 that the annual incidence of new cases of this disease could rise from 570,000 to 700,000 between 2018 and 2030 (WHO, 2020).
The prevalence of this disease is notably higher in low-to middle-income countries, nonetheless, it affects women globally. The number of deaths in low and middle-income countries accounted for an estimated 90% of the 311,000 global fatalities. Consequently, age-standardized incidence rates fluctuate from 75 per 100,000 women in the highest-risk countries to fewer than 10 per 100,000 women in the lowest-risk countries (Bray et al., 2018; WHO, 2020). This disparity underscores the urgency to address cervical cancer, particularly in regions with higher risk factors.
Human papillomavirus (HPV) infection is pivotal in the development of cervical cancer, with nearly 99% of cases associated with high-risk HPV strains (WHO, 2020; Jiang and Wang, 2022). However, other contributing factors such as tobacco use, immunosuppression, malnutrition, and low socioeconomic status are also implicated (ACCP, 2004; Ghebre et al., 2017; Zhang et al., 2020). Persistent infection with various types of HPV is acknowledged as a contributing factor in the progression of cervical intraepithelial neoplasia (CIN) and invasive cervical cancer (ICC). Nevertheless, the complete involvement of HPV in the entire tumorigenic process remains a topic of ongoing debate due to insufficient data (Muñoz, 2000; Castellsagué, 2008; Wheeler, 2013; Kori and Arga, 2018; So et al., 2020; Kang et al., 2021).
Recent literature posits an intriguing hypothesis: microorganisms may play a significant role in malignancies. This theory suggests that there could be unexplored mechanisms during infections where these microscopic entities take a leading role (Parkin, 2006; Godoy-Vitorino et al., 2018).
This perspective necessitates a broader understanding of the microbial world and its potential influence on disease processes. The interplay between HPV and other microorganisms could add another layer of complexity to the etiology of cervical cancer, warranting further investigation. This new viewpoint not only challenges our current knowledge but also paves the way for innovative research directions in cervical cancer pathogenesis.
The detection of microbial diversity, first accomplished in 1677 by Van Leeuwenhoek through microscopic observation, has evolved significantly over time (Wei et al., 2021). In cervical cancer diagnostics, the Papanicolaou smear, a microscopic biopsy image analysis, has traditionally been the primary modality (Long et al., 2017; Kori and Arga, 2018). However, its reliability is debatable due to its dependence on human interpretation (Long et al., 2017). Despite several alternate cervical cancer screening methods proposed over the years such as cytological testing alone, standalone hrHPV testing, and cytological + hrHPV combination testing (co-testing) (Curry et al., 2018; Kim et al., 2018; Terasawa et al., 2022), the 5 years survival rate remains a dismal 66% (Long et al., 2017; Basic et al., 2021; Qu et al., 2021; Hou et al., 2022).
Treatment strategies for cervical cancer, such as surgical resection, radiotherapy, and chemotherapy, are frequently challenged by tumor metastasis and recurrence, complicating disease management (Mallmann and Mallmann, 2016; Vordermark, 2016; Koh et al., 2019; Li et al., 2021). Further, patients often suffer from side effects related to these treatments. This highlights a significant problem: the urgent need for novel, reliable diagnostic methods for cervical cancer that can improve early detection and thereby enhance survival rates (Zhu et al., 2016; Long et al., 2017; Liu et al., 2018; Koh et al., 2019; Han et al., 2021).
The exploration of microbial diversity has been significantly enhanced by advancements in culture technologies. However, due to inherent challenges associated with laboratory culturing procedures, our understanding is not yet exhaustive (Wei et al., 2021). In response to this, techniques centered around molecular sample analysis have emerged within the field of omics, paving the way for a more detailed investigation of microbial diversity (Wei et al., 2021).
The advent of laboratory automation has facilitated the deployment of high-throughput-omics technologies. These sophisticated methodologies enable an in-depth characterization of samples collected from both patients and healthy individuals, thereby expanding our knowledge of microbial ecosystems. One such transformative innovation is next-generation sequencing (NGS). This technique has unlocked the potential to delineate the intricate complexity of microbial communities and human microbiota, providing valuable insights into the influence of the microbiome on human health and disease pathologies (Peterson et al., 2009).
Among the various omic approaches, metagenomics stands out for its ability to divulge specific information about the genomes and genes within a microbial community. It serves as an essential first step in microbiome studies (Marchesi and Ravel, 2015; Aguiar-Pulido et al., 2016). The primary goal of metagenomics is to determine the taxonomic profile of a microbial community, typically involving NGS post-DNA extraction from samples, followed by assembly or mapping to a reference database, and subsequent annotation (Marchesi and Ravel, 2015; Aguiar-Pulido et al., 2016). This method has become particularly prevalent in investigating the microbial composition within the vaginal environment.
The female genital tract serves as a critical ecological niche for human microbiota (Gao et al., 2013), housing Lactobacillus species that contribute to metabolic processes, immunological responses, and overall gynecological health (Kang et al., 2021). Known for probiotic benefits, Lactobacillus species help combat vaginal dysbiosis (Machado et al., 2022; Pacha-Herrera et al., 2022; Rodríguez-Arias et al., 2022). Detailed insights follow in this review’s upcoming sections. There is an emerging body of literature suggesting that alterations in the vaginal microbiota may be linked to cervical cancer (Klein et al., 2020b; Norenhag et al., 2020; So et al., 2020; Tango et al., 2020; Kang et al., 2021; Sims et al., 2021; Wu et al., 2021; Zhou et al., 2021). Furthermore, numerous studies propose that the vaginal microbiota could play a crucial role in defending women against infections such as HPV, vulvovaginal candidiasis, and other sexually transmitted diseases (Liu et al., 2013; Lewis et al., 2017; Arroyo Mühr et al., 2021; Kang et al., 2021). As such, the cervical microbiota could potentially serve as a biomarker for assessing the risk of cancer progression (Mitra et al., 2016a,b; Curty et al., 2019; Arroyo Mühr et al., 2021).
The exploration of the human microbiome has been an exciting journey, with techniques evolving from 16S sequencing (Audirac-Chalifour et al., 2016; Dareng et al., 2016; di Paola et al., 2017; Klein et al., 2020b; Norenhag et al., 2020; So et al., 2020; Tango et al., 2020; Sims et al., 2021; Wu et al., 2021; Zhou et al., 2021), PCR (Norenhag et al., 2020), and microarray (Borgdorff et al., 2014; Norenhag et al., 2020) to cutting-edge methods like RNA-seq (Kori and Arga, 2018; Klein et al., 2020a; Chang et al., 2021) and Whole Genome Shotgun (WGS) (Klein et al., 2020a; Wei et al., 2021). A significant milestone in this journey was the commencement of the Human Microbiome Project (HMP) in 2008, which aimed to map the microbial landscape across various body parts, including the lower genital tract of healthy individuals (Castanheira et al., 2021). From this wealth of research, a startling revelation has emerged: approximately 20% of all fatal cancers are microbially induced (Godoy-Vitorino et al., 2018). Moreover, numerous studies have drawn significant correlations between alterations in the microbiome and cancer phenotypes (Elinav et al., 2019; Poore et al., 2020; Banavar et al., 2021). This underlines the potential of the microbiota as a treasure trove of biomarkers that could revolutionize clinical diagnostics and disease management.
This review is an ambitious endeavor to chart the intricate relationship between the microbiota and cervical cancer progression. We delve into the diverse universe of microorganisms implicated in cervicovaginal dysbiosis, providing an authoritative synthesis of prior research on both CONTROL (healthy) samples and CAN stage. Our goal is to offer an updated perspective on the role of microbiota in cervical cancer progression, thereby filling a crucial gap in the existing literature.
While our analysis provides a comprehensive overview based on the data available at the time of our research, it’s crucial to recognize the fluidity and rapid evolution of scientific knowledge. As such, newer developments may not have been captured. This underscores the need for ongoing research in this field. Therefore, we strongly advocate for broader studies using metagenomics and metatranscriptomics, as these techniques hold immense promise in untangling the intricate role of microbiota in cervical cancer progression. By deepening our understanding of this critical issue, we can pave the way for innovative therapeutic interventions, heralding a new era in women’s health management.
2 Cervical cancer and HPV
According to the World Health Organization (WHO, 2020), the principal instigator of cervical pre-cancer and squamous cervical cancer is the asymptomatic, persistent or chronic infection with one or more high-risk HPV types. While over 100 HPV types have been identified, only a fraction are associated with cervical cancer. Indeed, two specific types, HPV 16 and 18, are implicated in approximately 70% of all reported cervical cancer cases (Pappa et al., 2018; Cohen et al., 2019; Lin et al., 2019; WHO, 2020). Other high-risk HPV types, such as 31, 33, 45, and 58, are less frequently linked to cervical cancer, with prevalence varying by geographic location. Additionally, low-risk HPV types 6 and 11, although not contributing to cervical cancer, are responsible for most genital warts or condylomas (WHO, 2014).
The role of genetic variation in cervical cancer has been underscored by genome-wide association studies (GWAS). Lin et al. (2019), reported that cervical cancer harbors genetic variations across multiple susceptibility loci (Bahrami et al., 2018; Lin et al., 2019). The viral oncoproteins E6 and E7 appear to play a pivotal role in HPV-infected cervical cancers. Integration of the viral genome into the host DNA results in the upregulation of E6 and E7, leading to the deregulation of key proteins within cellular signaling pathways, including the inhibition of two vital tumor suppressor proteins, p53 and pRb (Oyervides-Muñoz et al., 2018; Lin et al., 2019). The combined effect of E6 and E7 viral proteins triggers the process of immortalization in HPV-infected cells. This precedes the malignant metamorphosis of these cells (Da Silva et al., 2021).
Furthermore, Lau et al. (2015) revealed that DNA tumor virus oncogenes, including E7, can bind to and suppress the cGAS-STING DNA-sensing pathway (Lau et al., 2015; Lin et al., 2019). However, it’s worth noting that not all integrations necessarily rely on the expression of the E6 and E7 oncogenes (Groves and Coleman, 2015; Lin et al., 2019). In addition to these findings, several reports have identified driver mutations in cervical cancer, such as PIK3CA (phosphatidylinositide 3-kinases catalytic subunit α), a central protein in the PI3K pathway, KRAS (Kirsten rat sarcoma viral oncogene homolog), and EGFR (epidermal growth factor receptor) (Lin et al., 2019).
3 Vaginal microbiota
The vaginal microbiota is a critical component of women’s health (Wu et al., 2021). This complex ecosystem, which operates in harmony with the host, provides protective mechanisms against dysbiosis and infection (Klein et al., 2020a). The function of the vaginal mucosa as a barrier against pathogens is facilitated by the interaction of epithelial cells, the immune system, and various microorganisms (Borgdorff et al., 2016; Taddei et al., 2018; Castanheira et al., 2021).
Dominating this ecosystem are Lactobacillus species, which play a significant role in maintaining vaginal health. By producing lactic acid, these bacteria sustain a low pH environment in the cervicovaginal setting, thereby preventing the colonization of harmful opportunistic pathogens, preserving the cervical epithelial barrier, and impeding mucin degradation (Amabebe and Anumba, 2018; Klein et al., 2020a; Norenhag et al., 2020; Salinas et al., 2020; So et al., 2020; Kang et al., 2021; Wu et al., 2021).
However, the composition of the vaginal microbiota is not static. It can be influenced by numerous factors such as genetics, diet, lifestyle, hygiene practices, ethnicity, reproductive age, infections, male factor, usage of antibiotics and contraceptives, sexual activity, physiological status, pregnancy and estrogen levels (Mitra et al., 2016b; Kwasniewski et al., 2018; Wu et al., 2021; Zhou et al., 2021; Baud et al., 2023).
Thanks to new molecular techniques, over 50 microbial species have been identified within the vaginal microbiota, with Lactobacillus spp. being the most prevalent (Norenhag et al., 2020; Wu et al., 2021). Among them, L. crispatus, L. gasseri, L. inners, and L. jensenii are the most commonly found (Wu et al., 2021).
Further research about the vaginal microbiota in healthy women from different ethnic groups (White, Black, Hispanic and Asian) by Ravel et al. (2011) led to the classification of vaginal bacterial communities into five distinct “community state types” (CST). In this classification, Lactobacillus species dominated groups I, II, III, and V. Group IV, on the other hand, was characterized by a diverse set of anaerobic bacteria, including bacteria like Prevotella spp., Streptococcus spp., Dialister spp., Fannyhessea spp. (previously known as Atopobium), Gardnerella spp., Megasphaera spp., Peptoniphilus spp., Sneathia spp., Eggerthella spp., Aerococcus spp., Finegoldia spp., and Mobiluncus spp. These findings were consistent with previous research employing 16S rRNA genes (Srinivasan and Fredricks, 2008; Zhou et al., 2010).
Despite the diversity in bacterial species, a commonality across all CST groups was the presence of lactic acid-producing bacteria, suggesting a conserved function throughout these communities.
4 Vaginal microbiota and cervical cancer
The human body is a dynamic ecosystem for a myriad of microbes, collectively known as the microbiome. This microbiome plays a pivotal role in maintaining normal bodily functions, including immune modulation and overall protection (Wei et al., 2021; Zhou et al., 2021). Over time, evidence has emerged highlighting the connection between the microbiome, inflammation, and the development and progression of cancer. According to Zhou et al. (2021) and Wei et al. (2021), disturbances in microbial homeostasis can trigger a cascade of immune responses. Chronic inflammation, a byproduct of such disruptions, is a known carcinogenic factor, heightening the host’s susceptibility to cancer (Zhou et al., 2021).
Given the profound implications of the microbiome on health and disease, specifically cervical cancer, advanced research is warranted. The advent of laboratory automation and high-throughput technologies has revolutionized our understanding of microbiome diversity and its potential impacts (Wei et al., 2021). There is mounting scientific evidence pointing towards a correlation between microbiota and cervical cancer (Castanheira et al., 2021; Kang et al., 2021; Wei et al., 2021; Wu et al., 2021; Zhou et al., 2021).
Cervicitis, or inflammation of the cervix, can stem from various conditions, including microbial infections. Chronic cervicitis has been linked to the development of cervical cancer. Pelvic inflammatory disease (PID) in women is typically triggered by ascending bacterial infections from the cervix to the uterus and fallopian tubes. Bacterial vaginosis (BV), a condition characterized by dysbiosis of cervicovaginal bacteria, is also associated with cervicitis. Notably, the microenvironment fostered by BV is reported to facilitate persistent HPV infection, a known precursor to cervical cancer (Castanheira et al., 2021; Zhou et al., 2021).
Various microorganisms, including Fusobacterium spp., Mycoplasma genitalium, Chlamydia trachomatis, Sneathia spp., Anaerococcus spp., Peptostreptococcus spp., Gardnerella spp., Prevotella spp., Fannyhessea spp., Streptococcus spp., Dialister spp., Megasphaera spp., Peptoniphilus spp., Finegoldia spp., Mobiluncus spp. and Lactobacillus iners have been implicated in the onset of cervical cancer. Interestingly, L. iners is found more frequently in infected women compared to their healthy counterparts. Table 1 provides a detailed overview of these microorganisms and their association with cervical cancer.
As illustrated in Table 1, microorganisms such as Fusobacterium spp., Sneathia spp., Anaerococcus spp., Peptostreptococcus spp., Gardnerella spp., Prevotella spp., Dialister spp., and Megasphaera spp. have been identified as biological markers for cervical cancer (CAN), high-grade squamous intraepithelial lesions (HSIL), and cervical intraepithelial neoplasia (CIN). Conversely, Lactobacillus crispatus, Lactobacillus gasseri, and Lactobacillus jensenii are associated with a decreased risk of infections, including HPV, CIN, and CAN. For an organized overview of this information, please refer to Table 2. This table presents a clear view of the microorganisms found at different disease stages in the vagina. These stages include squamous intraepithelial lesions (SIL)—further divided into low-grade (LSIL) and high-grade (HSIL), cervical intraepithelial neoplasia (CIN), invasive cervical cancer (ICC) or cervical cancer (CAN), and other infections such as pelvic inflammatory disease (PID), HPV, and bacterial vaginitis (BV). Additionally, a column has been included to indicate the microorganisms found in healthy controls (HC). Figure 1 provides a visual representation of the microorganisms present during vaginal inflammation, offering insight into the microbial landscape under these conditions.
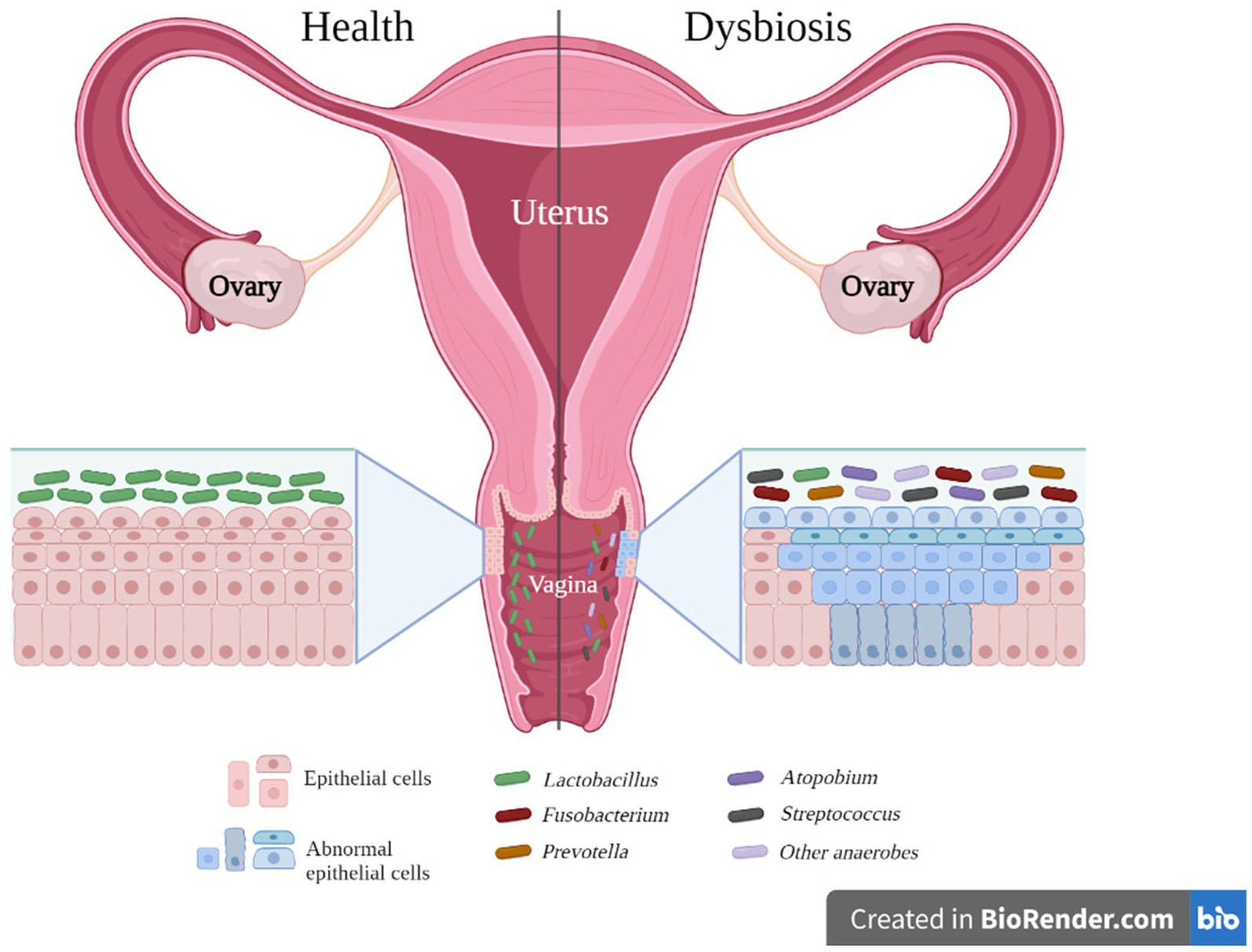
Figure 1. Vaginal microorganisms in a healthy vagina versus a dysbiosis stage in the vagina. Source of image: Authors and adapted from Zhou et al. (2021). The figure reveals the transformation that the vaginal microbiota undergoes between healthy and dysbiotic conditions. In a healthy state, the environment is primarily characterized by the presence of Lactobacillus species. On the other hand, in a state of dysbiosis, the environment is largely dominated by a variety of other bacteria, including Prevotella spp., the bacterium now known as Fannyhessea spp. (formerly Atopobium spp.), Streptococcus spp., and other anaerobic bacteria.
5 Microbial markers and cervical cancer
Advancements in microbiome research have unveiled new avenues for understanding the root causes of various diseases, including cancer. With the advent of high-throughput technologies such as genomics, transcriptomics, metagenomics, and metatranscriptomics, researchers can now generate an enormous amount of data (Wei et al., 2021). When it comes to cervical cancer, this vast repository of data is meticulously scrutinized to identify potential biomarkers that could transform its diagnosis and prognosis (Norenhag et al., 2020; Han et al., 2021; Kang et al., 2021; Wei et al., 2021; Zhou et al., 2021).
Researchers propose that certain microorganisms serve as beneficial diagnostic markers for cervical cancer or as indicators of infection severity. From the host’s perspective, diverse types of biomarkers (prognostic, predictive, and diagnostic) are being explored to enhance the management of cervical cancer.
High-throughput technologies have paved the way for a deeper exploration of the complex relationship between microbiota and cancer. The capacity to examine the entire microbiome and its intricate micro-ecosystems has led to the identification of specific microbial entities as predictive markers of cancer (Wei et al., 2021). At present, research is primarily centered around four main areas: characterizing microbial diversity and composition, conducting microbial functional analyses, predicting biomarkers, and investigating potential therapeutic applications. However, these areas are still nascent and need to be solidified in clinical practice (Wei et al., 2021).
To fully unravel the correlation between the microbiome and cancer, the consistent use of high-throughput methodologies is deemed necessary. Various studies conducted on the microbiota associated with cervical cancer or cervical disease have reiterated the urgent need for reliable biomarkers to improve the diagnosis of cervical cancer or prevent it. There is a pressing need to devise novel diagnostic strategies incorporating microbiological markers for early detection of cervical cancer in patients (Kang et al., 2021; Sims et al., 2021; Wei et al., 2021; Wu et al., 2021; Zhou et al., 2021).
Several microorganisms, including Fusobacterium spp., Sneathia spp. (S. amnii), Anaerococcus spp., Peptostreptococcus spp., Gardnerella spp. (G. vaginalis), Prevotella spp., Dialister spp., Fannyhessea spp. (F. vaginae), Streptococcus spp., Megasphaera spp., L. crispatus, and L. gasseri (Kori and Arga, 2018; Klein et al., 2020b; Tango et al., 2020), have been suggested as microbiological markers for cervical cancer. The profound implications of these findings for the future of cancer diagnostics underscore the importance of continued research in this promising field.
6 Data exploration
In an effort to gain deeper insights from the literature, a rigorous process of search was carried out. This process targeted papers that provided accessible information in the NCBI databases. Initially, six potential articles were identified, each providing specific details on vaginal microbiota and cervical health conditions, as illustrated in Table 3. However, upon further exploration for raw data within the NCBI, only four of these articles—marked with asterisk—offered the required information.
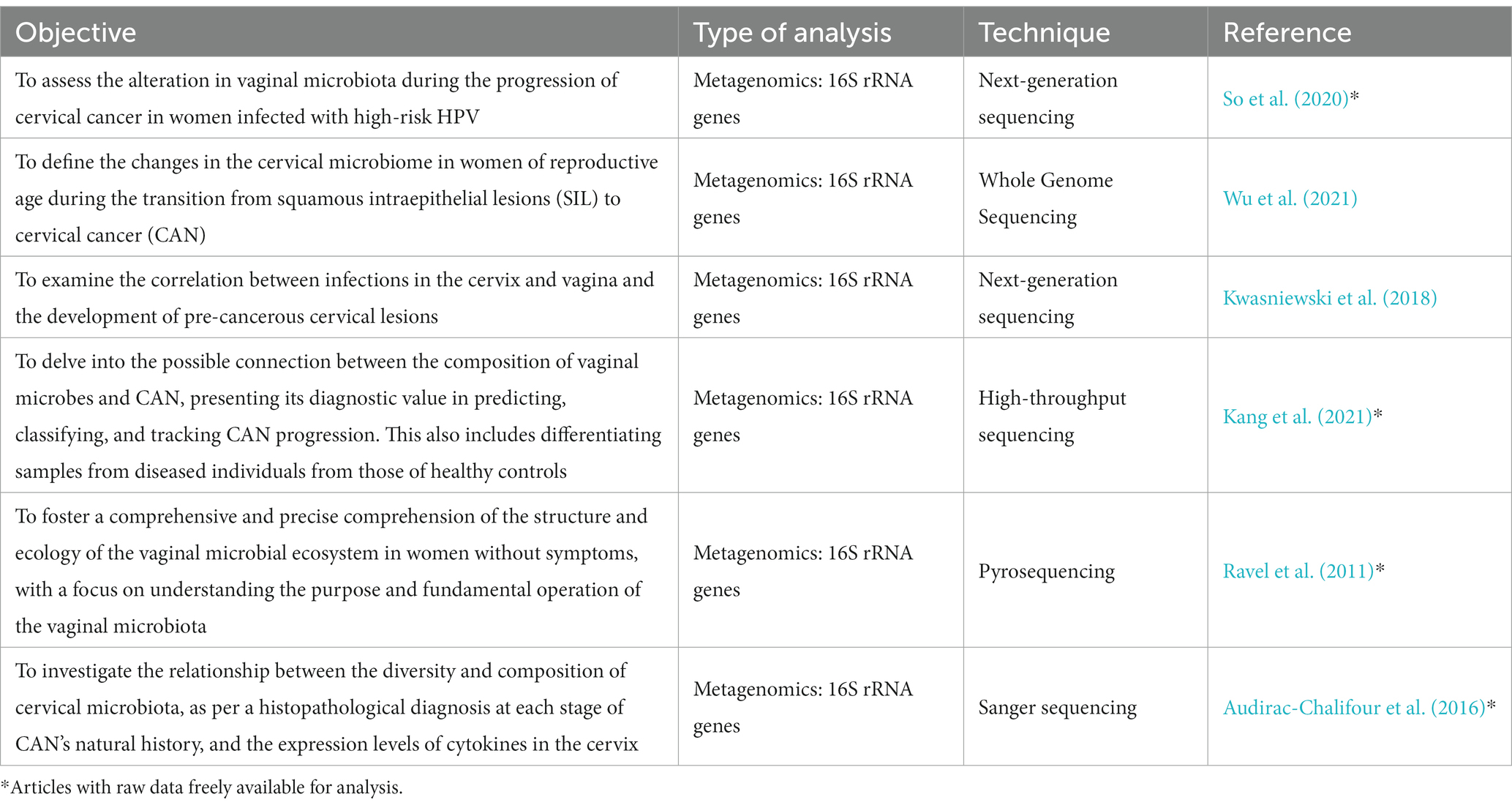
Table 3. Potential articles containing information on vaginal microbiota and cervical health conditions.
Despite the heterogeneity inherent in each database, we undertook a data exploration process to confirm if earlier published data (Ravel et al., 2011; Audirac-Chalifour et al., 2016) aligns with recent publications (So et al., 2020; Kang et al., 2021). It is worth noting that such analyses invariably encounter limitations rooted in the data source, standardization of metadata information, and the procedures employed for sequencing results, among other factors. Nevertheless, our investigation focused on deciphering microbiota patterns across various cervical health conditions.
Regarding the fourth study under scrutiny (Kang et al., 2021), the absence of raw data within the manuscript necessitated a comprehensive reanalysis of the samples provided, guided solely by accession numbers for sequences in the NCBI database. This re-evaluation was executed employing the QIIME-2022.8 pipeline, strictly adhering to the author’s guidelines delineated within their paper. Denoising was performed utilizing DADA2 (Divisive Amplicon Denoising Algorithm 2) (Callahan et al., 2016), and despite Kang et al. (2021) usage of the Silva v138 database, we elected to use the Silva (16S/18S rRNA) (Quast et al., 2013; Yilmaz et al., 2014) database v132. Similar to the authors, the sequences among the reanalyzed samples were rarefied to a sequencing depth of 6,919 reads.
Subsequent to the successful acquisition of data from each of the four papers, the next step was to distill this data, as showed in Table 4. All data abundances were normalized to values ranging from 0 to 1. We successfully compiled a total of 496 samples, encapsulating information pertaining to the type of cervical health condition (CAN, CIN, SIL, and control), the respective study reported, and HPV presence (restricted to CAN and Control samples). The statistical analysis and graphic representation were executed using the R 4.2.1 version.
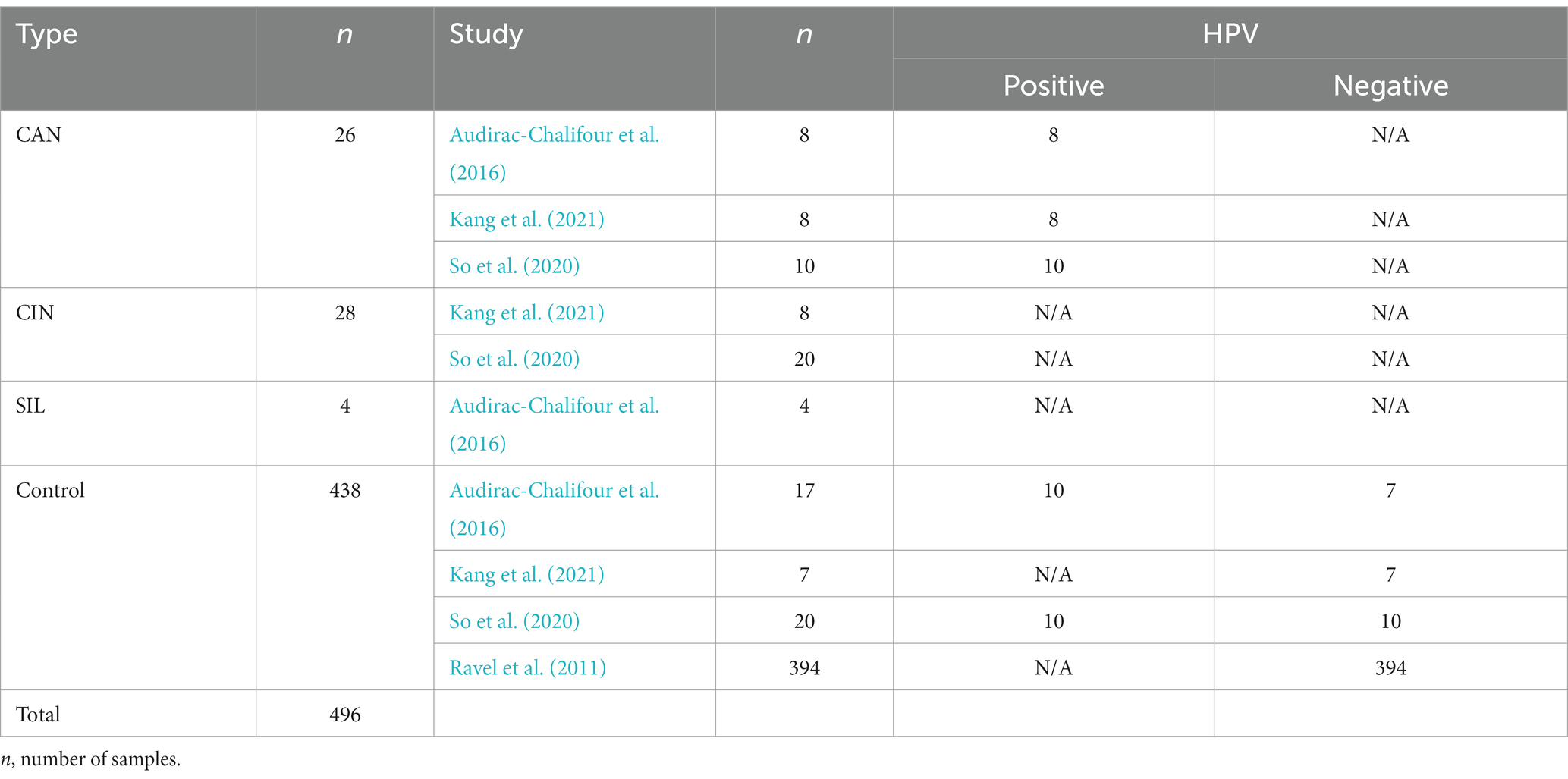
Table 4. Data derived from databases on NCBI with accessible raw information (Ravel et al., 2011; Audirac-Chalifour et al., 2016; So et al., 2020; Kang et al., 2021).
It is important to highlight that our analysis was conducted within certain constraints. The availability of raw data posed a significant limitation, necessitating the reanalysis of samples in specific instances. Additionally, the low number of articles utilized for our analysis, owing to our commitment to use only freely accessible information, may have affected the comprehensiveness of our study. Despite these challenges, we remained committed to conducting a meticulous and robust exploration of the available data.
According to the previous Table 1, where is presented the microorganisms proposed as microbiological markers in cervical cancer or cervix inflammation, the common bacteria genera found in each one of the four articles that were also mentioned in this table (as potential biomarkers) were: Fusobaterium spp., Sneathia spp., Streptococcus spp., Gardnerella spp., Dialister spp., Megasphaera spp., Peptostreptococcus spp., Peptoniphilus spp., Prevotella spp., Anaerococcus spp., and Lactobacillus spp.
Our findings, as outlined in Table 4, consistently demonstrate the presence of HPV in all documented cases of cervical abnormalities (CAN). To gain a deeper understanding of the bacterial profile associated with CAN, we employed a Venn diagram, as illustrated in Figure 2A. This visualization not only highlights the commonly identified bacteria in CAN cases, but also those observed in control samples. In the context of CAN, our analysis revealed 17 frequently reported bacterial genera: Fusobacterium spp., Sneathia spp., Streptococcus spp., Gardnerella spp., Dialister spp., Megasphaera spp., Peptostreptococcus spp., Peptoniphilus spp., Staphylococcus spp., Campylobacter spp., Parvimonas spp., Prevotella spp., Haemophilus spp., Porphyromonas spp., Anaerococcus spp., Lactobacillus spp., Ureaplasma spp.
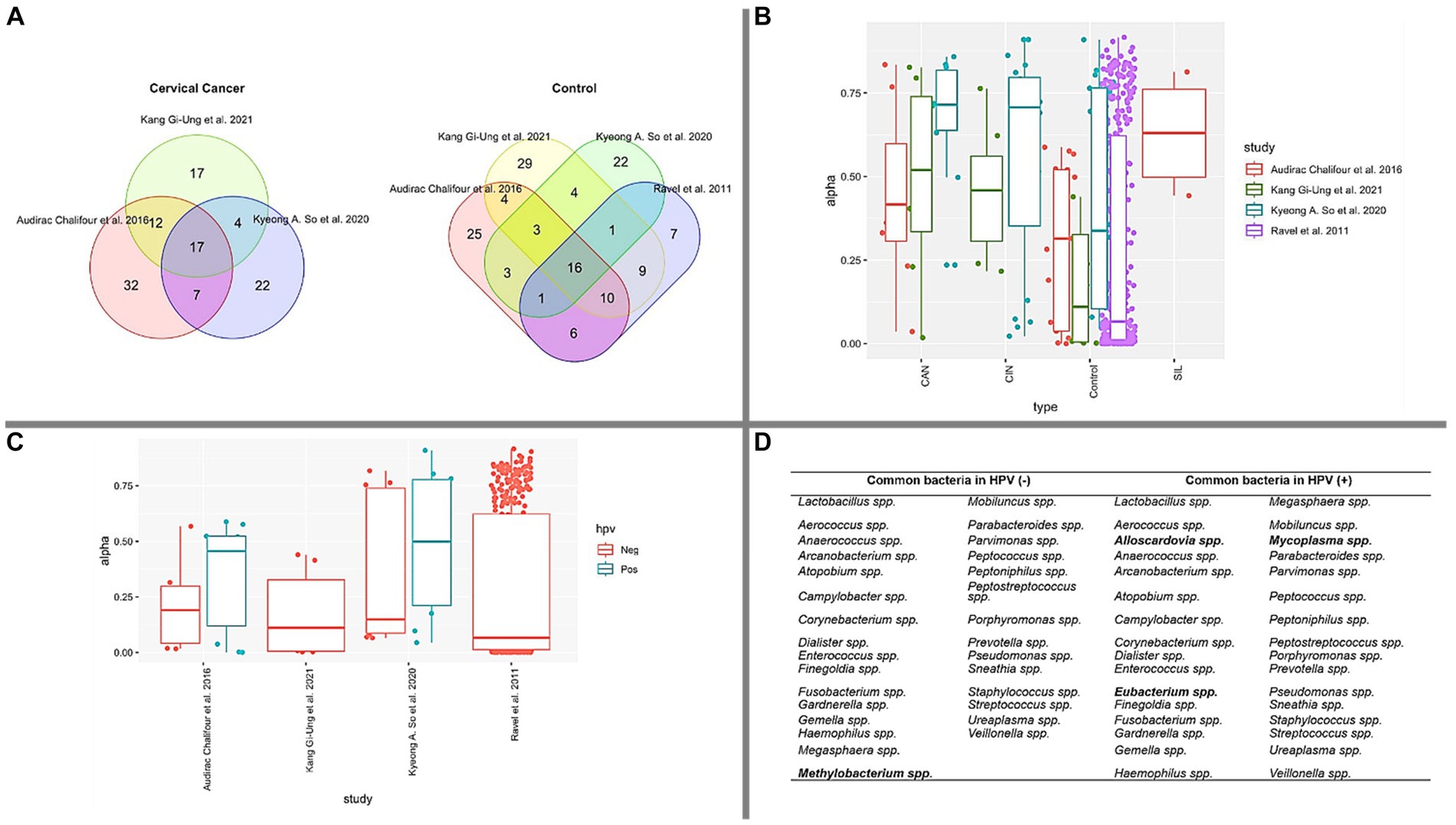
Figure 2. Bacterial composition and diversity. (A) The Venn diagram depicts the overlap of common bacterial types identified in CAN and CONTROL groups, based on the 50 most abundant bacteria in each study. (B) Simpson’s index measures genera diversity across various cervical health conditions. Notably, CONTROL samples exhibit lower diversity compared to CAN, CIN, and SIL conditions. The Kruskal-Wallis test yielded a p-value <0.05, signifying significant differences in diversity between CONTROL vs. CAN and CONTROL vs. CIN. (C) A box plot illustrating the prevalence of HPV infection in control samples across the studied papers. (D) Identification of common bacterial types in HPV (−) and HPV (+) groups in each paper, with unique bacteria within each group highlighted in bold.
To further elucidate the bacterial landscape, an additional Venn diagram was constructed to identify common bacterial genera in control samples from the studies examined (Figure 2A). We observed that 16 genera were common in these samples: Sneathia spp., Streptococcus spp., Gardnerella spp., Dialister spp., Megasphaera spp., Peptoniphilus spp., Staphylococcus spp., Parvimonas spp., Prevotella spp., Porphyromonas spp., Anaerococcus spp., Lactobacillus spp., Ureaplasma spp., Aerococcus spp., Finegoldia spp., Enterococcus spp.
In reference to Table 1, which proposes certain microorganisms as potential microbiological markers for cervical cancer or cervix inflammation, we noted that the following genera were shared between our four selected articles and those suggested as potential biomarkers: Fusobacterium spp., Sneathia spp., Streptococcus spp., Gardnerella spp., Dialister spp., Megasphaera spp., Peptostreptococcus spp., Peptoniphilus spp., Prevotella spp., Anaerococcus spp., and Lactobacillus spp. This overlap may indicate a significant link between these bacterial genera and cervical health disorders.
Simpson’s diversity index, a standard tool for determining alpha diversity, gauges the prevalence of dominant species and inversely correlates with species diversity (Sagar and Sharma, 2012). As illustrated in Figure 2B, our analyses calculated this alpha diversity. The data suggests that as the cervical health condition transitions from Control to SIL, CIN, and CAN, there is an observable increase in microbiota, corroborating previous literature (Klein et al., 2020a; Norenhag et al., 2020; So et al., 2020; Tango et al., 2020; Kang et al., 2021; Sims et al., 2021; Wu et al., 2021; Zhou et al., 2021). Figure 2C presents a similar pattern for HPV (−) samples, where bacterial diversity is comparatively lower than HPV (+) samples. The median value for boxes representing HPV (−) samples is less than 0.25, contrasting with those representing HPV (+) samples. Furthermore, we compiled a list of the top 50 bacterial genera present in HPV (+) and HPV (−) samples across the four studied papers. As depicted in Figure 2D, these bacteria are displayed accordingly.
It is crucial to emphasize that our exploration of the data did not follow the strict guidelines of a meta-analysis or systematic review. This absence of a structured approach may introduce a potential bias in our findings, as we might have overlooked certain studies or emphasized others disproportionately. Also, because we focused on information that’s freely available, there may be some limits to the scope and depth of our analysis. Despite these limitations, we see our work as an initial step in understanding the overall trends in microbiota composition during cervical cancer progression. Our findings should be interpreted with caution, considering the potential biases and methodological constraints. However, we believe our research provides valuable insights that can pave the way for future, more thorough investigations in this crucial area.
6.1 Microbiota and HPV
Based on the distinctive microorganisms identified solely in HPV (+) and HPV (−) samples (Figure 2D), existing literature has associated Methylobacterium spp. as a predominant bacteria in the ovary (Amabebe and Anumba, 2020) and ovarian cancer (Peric et al., 2019). Our data, as presented in Figure 2D, identified Methylobacterium spp. within the HPV (−) groups. Notably, this microorganism emerged as the sole differential entity when compared to HPV (+) samples. However, given the inherent limitations of a review paper, such as data heterogeneity, further research is required to substantiate these findings.
Contrarily, Alloscardovia spp., Eubacterium spp., and Mycoplasma spp. were exclusively detected in HPV (+) samples. Previous reports have also documented Alloscardovia spp. in HPV (+) samples (Gao et al., 2013), and a 2019 case study associated this microorganism with preterm premature rupture of membranes (PPROM) (Cardona-Benavides et al., 2019). Eubacterium spp., meanwhile, has been reported more frequently in HPV (+) patients than in HPV (−) patients (Carrillo-Ng et al., 2021) and is also associated with BV cases (Fredricks et al., 2005; Srinivasan and Fredricks, 2008). Moreover, Mycoplasma spp. has been found to be prevalent among women with HPV (+) (Klein et al., 2020b), with its abundance noted to increase in women with cervical lesions (Pacha-Herrera et al., 2022). Mycoplasma spp. has also been implicated as a potential cause of persistent HPV infection (Zhou et al., 2021) and has been identified in BV infections (Ferris et al., 2004; Verhelst et al., 2004; Kwasniewski et al., 2018).
Our review suggests that Alloscardovia spp., Eubacterium spp., and Mycoplasma spp. could potentially serve as biomarkers for HPV (+), while Methylobacterium spp. might be a marker for HPV (−). However, it’s important to note that due to the limitations inherent in this review and the data evaluated, these observations remain tentative. There is a clear need for continued research to further explore the role of the microbiota in the development of HPV, as this could provide valuable insights that may aid in the fight against this condition.
6.2 Microbiota and cervical cancer progression
Based on the findings outlined in Section 6, Table 5 encapsulates the shared bacteria identified in the intersection of the Venn diagram depicted in Figure 2A. This table effectively illustrates the genera of bacteria that appear to be prevalent as cervical cancer progresses, as well as in healthy controls.
Focusing first on the CONTROL samples, Fusobacterium spp., Peptostreptococcus spp., Campylobacter spp., and Haemophilus spp. were conspicuously absent from the bacterial genera identified. Drawing from existing literature, Fusobacterium spp. has been exclusively reported in CAN or CIN samples, which may account for the numerous propositions of Fusobacterium spp. as a potential marker of CAN (Audirac-Chalifour et al., 2016; Norenhag et al., 2020; So et al., 2020; Castanheira et al., 2021; Sims et al., 2021; Wu et al., 2021; Zhou et al., 2021). The potential of Fusobacterium spp. as an oncogenic entity and a promoter of dysplasia development has also been deliberated (Norenhag et al., 2020). Additional characteristics of this microorganism are detailed in Table 1. Thus, Table 5 in alignment with the literature, indicates that Fusobacterium spp. is only present in CIN, SIL, and CAN samples.
Peptostreptococcus spp., a bacterial genus not detected in control samples (refer to Table 5), is associated with cervical conditions such as cervical intraepithelial neoplasia (CIN) and cervical cancer (CAN) (Mitra et al., 2016b; So et al., 2020). This bacterium also plays a role in female genital tract infections like bacterial vaginosis and pelvic inflammatory disease (So et al., 2020) and is considered a distinctive marker for the CAN group (Wu et al., 2021).
Campylobacter spp., identified as a CAN marker (Wu et al., 2021), was first reported in CIN cases in 2018 (Zhang et al., 2018). This review’s analysis revealed the presence of this bacterium exclusively in CAN samples (Table 5).
Haemophilus spp. is another bacterial genus absent in control samples. It is believed to contribute to trichomoniasis as a colonizing microorganism (Kwasniewski et al., 2018) and has been reported only in CAN samples (So et al., 2020), explaining its absence in the control group (Table 5).
Three bacterial genera—Finegoldia spp., Enterococcus spp., and Aerococcus spp.—were found solely in control samples, contrasting with CAN samples (Table 5). Finegoldia’s spp. exclusive presence in control samples might be due to its higher abundance compared to other conditions, as analyses focused on the top 50 most abundant bacteria from each studied paper. More information about Finegoldia spp. can be found in Table 1.
Enterococcus spp., commonly found in healthy samples and associated with HPV clearance (Verhelst et al., 2004; Zhou et al., 2007; Ravel et al., 2011; Borgdorff et al., 2014; di Paola et al., 2017), aligns with the results observed in Table 5.
Aerococcus spp., another genus found exclusively in control samples, corroborates previous literature (Verhelst et al., 2004; Zhou et al., 2007; Srinivasan and Fredricks, 2008; Ravel et al., 2011). Additional information about Aerococcus spp. is available in Table 1.
Sneathia spp., Streptococcus spp., Gardnerella spp., Dialister spp., Megasphaera spp., Peptoniphilus spp., Staphylococcus spp., Parvimonas spp., Prevotella spp., Porphyromonas spp., Anaerococcus spp., Lactobacillus spp., and Ureaplasma spp. were found in both CAN and control samples.
Sneathia spp. has been reported in CIN samples (So et al., 2020), bacterial vaginosis (BV) cases (Srinivasan and Fredricks, 2008; Liu et al., 2013; Borgdorff et al., 2014; Klein et al., 2020a; Zhou et al., 2021), squamous intraepithelial lesion (SIL) samples (Audirac-Chalifour et al., 2016; Wu et al., 2021), HPV infections (Audirac-Chalifour et al., 2016; Dareng et al., 2016; di Paola et al., 2017; Castanheira et al., 2021; Zhou et al., 2021) and as a biomarker of cervical neoplasia (Godoy-Vitorino et al., 2018). However, it is also a common member of the vaginal community (Verhelst et al., 2004; Verstraelen et al., 2004; Kang et al., 2021), suggesting it should not be considered a biomarker. More information about Sneathia spp. is provided in Table 1.
Streptococcus spp. has been observed in CAN samples (So et al., 2020; Tango et al., 2020; Wu et al., 2021), cervical disease cases (So et al., 2020), and CIN patients (Tango et al., 2020; Arroyo Mühr et al., 2021). Still, it was also identified as part of the vaginal composition (Verhelst et al., 2004; Zhou et al., 2007; Srinivasan and Fredricks, 2008; Gao et al., 2013; Audirac-Chalifour et al., 2016; Arroyo Mühr et al., 2021; Kang et al., 2021; Wu et al., 2021; Zhou et al., 2021) and associated with HPV clearance (di Paola et al., 2017), which aligns with our findings that this microorganism appears in both groups. Further information about Streptococcus can be found in Table 1.
Gardnerella spp., a bacterium identified in both disease and control samples, is classified as an anaerobic carcinogen (Zhou et al., 2021). It has been found in cervical intraepithelial neoplasia (CIN) samples (Mitra et al., 2016b; So et al., 2020; Sims et al., 2021), bacterial vaginosis (BV) cases (Borgdorff et al., 2016; Mitra et al., 2016b; Amabebe and Anumba, 2018; Klein et al., 2020a; Castanheira et al., 2021), HPV persistent infections (Norenhag et al., 2020), and control samples (Audirac-Chalifour et al., 2016; Tango et al., 2020). This aligns with our paper analysis results. Additional information about Gardnerella can be found in Table 1.
Dialister spp., another genus present in both groups (cervical cancer and control), is an opportunistic pathogen influenced by Lactobacillus spp. (Wu et al., 2021). It has been reported as a marker genus in cervical cancer (So et al., 2020; Sims et al., 2021; Wu et al., 2021), and found in CIN samples (So et al., 2020), BV (van de Wijgert et al., 2014; di Paola et al., 2017; Amabebe and Anumba, 2020), and HPV cases (Gao et al., 2013; Audirac-Chalifour et al., 2016; Dareng et al., 2016). However, it’s also been observed in control samples (Ravel et al., 2011; So et al., 2020), which concurs with our findings. More details about Dialister spp. are provided in Table 1.
Megasphaera spp., found in both disease and normal samples, has been reported in squamous intraepithelial lesion (SIL) and cervical cancer samples (Wu et al., 2021), CIN cases (Mitra et al., 2016b; Sims et al., 2021), BV patients (Amabebe and Anumba, 2018; Klein et al., 2020a; Castanheira et al., 2021) and normal samples (Ravel et al., 2011; Arroyo Mühr et al., 2021). Table 1 provides more details on this bacterium.
Peptoniphilus spp., another common bacterium, has been reported as a cervical cancer marker (So et al., 2020; Wu et al., 2021), found in BV samples (Fredricks et al., 2005; Srinivasan and Fredricks, 2008; van de Wijgert et al., 2014), HPV infections (Shannon et al., 2017; Kang et al., 2021), and control samples (Srinivasan and Fredricks, 2008; Ravel et al., 2011). Further details about Peptoniphilus spp. can be found in Table 1.
Staphylococcus spp., although common in control samples (Tango et al., 2020), has also been observed in conditions such as cervical cancer (Tango et al., 2020; Arroyo Mühr et al., 2021), aerobic vaginitis (di Paola et al., 2017), and SIL (Klein et al., 2020a; Arroyo Mühr et al., 2021; Sims et al., 2021; Wu et al., 2021).
Parvimonas spp. is another bacterium observed in both cervical cancer and control samples. It has been reported in control samples (Shannon et al., 2017), cervical diseases (Godoy-Vitorino et al., 2018; So et al., 2020), and BV patients (van de Wijgert et al., 2014).
Prevotella spp. has been found in control samples (Lewis et al., 2017; Zhou et al., 2021) and other conditions like HPV infections (Norenhag et al., 2020), CIN (Mitra et al., 2016b; Godoy-Vitorino et al., 2018; Tango et al., 2020; Sims et al., 2021), CAN (Wu et al., 2021), and BV (di Paola et al., 2017; Amabebe and Anumba, 2018; Kwasniewski et al., 2018; Castanheira et al., 2021). This aligns with the results summarized in Table 5 and detailed in Table 1.
Porphyromonas spp., also present in both disease and control cases (Table 5), has been observed in CAN (Sims et al., 2019, 2021; Wu et al., 2021), BV samples (Fredricks et al., 2005; Srinivasan and Fredricks, 2008; van de Wijgert et al., 2014), and control cases (Verhelst et al., 2004; Srinivasan and Fredricks, 2008).
Anaerococcus spp. has been reported in control samples (Verhelst et al., 2004; Zhou et al., 2007; Srinivasan and Fredricks, 2008; Arroyo Mühr et al., 2021), and diseases such as CAN (Wu et al., 2021), SIL (Mitra et al., 2015, 2016b), and CIN cases (Mitra et al., 2016b; Godoy-Vitorino et al., 2018).
Lactobacillus spp., another common bacterium (Table 5), is seen in various conditions depending on the species—either as a disease marker or a health biomarker in the vaginal composition. For instance, it has been reported in conditions like CAN (Castanheira et al., 2021), CIN (Mitra et al., 2016b; Norenhag et al., 2020; Sims et al., 2021), SIL (Norenhag et al., 2020), HPV clearance (Mitra et al., 2016b; Norenhag et al., 2020), cervical dysplasia (Norenhag et al., 2020), and control samples (Mitra et al., 2016b; Amabebe and Anumba, 2018; Klein et al., 2020a; Norenhag et al., 2020; Castanheira et al., 2021; Sims et al., 2021; Zhou et al., 2021). More information about different Lactobacillus species (L. jensenii, L. gasseri, L. crispatus, L. inners) can be found in Table 1.
Lastly, Ureaplasma spp. has been reported in both CAN (Tango et al., 2020) and control samples (Verhelst et al., 2004; Srinivasan and Fredricks, 2008; Wu et al., 2021), as well as in CIN (Tango et al., 2020), and BV patients (Fredricks et al., 2005; van de Wijgert et al., 2014; Amabebe and Anumba, 2018; Klein et al., 2020a).
The exclusive presence of specific microorganisms in cervical adenocarcinoma cases such as Fusobacterium spp., Peptostreptococcus spp., Campylobacter spp., and Haemophilus spp., underscores their potential significance in the pathology of this disease. These unique bacteria could play important roles in the onset and progression of CAN, and their further investigation may provide valuable insights for the development of new diagnostic markers or therapeutic strategies. Understanding the specific functions and influences of these bacteria in CAN is a crucial step toward improving our ability to prevent and treat this form of cervical cancer.
7 Discussion
The evolution of sequencing methodologies has paved the way for revolutionary advancements in our understanding of microbiomes and associated diseases, including cervical cancer. This progression can be traced through the various technological tools utilized in the quest for comprehension.
In this context, innovative technologies like NextSeq500 (by Illumina) have been employed for parallel DNA and RNA sequencing to comprehensively detect detectable and actively transcribed DNA and RNA microbes in cervical specimens. The results from such studies suggest that the choice of approach (RNA-Seq, DNA-Seq) can influence the number of transcripts obtained. The focus of current research endeavors is to maximize sequence retention in order to amass a wealth of data that could prove beneficial for multiple investigations reliant on database information.
These technological advancements present an exciting opportunity to delve deeper into the intricacies of cervical cancer and its relationship with the microbiota. The wealth of information that these technologies can provide will undoubtedly fuel further research, and potentially lead to breakthroughs in diagnostic and therapeutic strategies.
The analyses conducted herein strongly advocate for continued biomarker exploration and the need for up-to-date data to inform the development of new strategies to combat cervical cancer.
Our review provides a comprehensive overview of the microbiota associated with the progression of cervical cancer and enumerates several microorganisms implicated in cervicovaginal dysbiosis.
Furthermore, we outline the principal discoveries of past research related to the microbiota present during the CONTROL (healthy) and CAN stages, as well as other conditions such as CIN, SIL, HPV (+), and HPV (−). This analysis allowed us to identify promising bacteria frequently reported as biomarkers, suggesting that biomarker identification is a compelling field with potential for numerous research projects.
A consistent presence of HPV was demonstrated in all reported cases of cervical abnormalities. We have identified noteworthy bacterial genera that differ between both CAN and control samples through our investigation. The intersection of these identified bacteria with those suggested as microbiological indicators for cervical health issues in existing research implies a potential connection that needs additional exploration. This understanding of the microbial landscape may provide valuable insights into the pathogenesis of cervical diseases and potentially guide future diagnostic and prevention strategies and treatment plans.
The advent of sequencing techniques has shed new light on our understanding of microbial biomarkers. The rise of this technology holds the promise of facilitating more in-depth studies examining the relationship between cancer and the microbiome. Nevertheless, there is a pressing need for additional research and the standardization of methods for metadata acquisition. This will enhance the scalability of results, ultimately aiming to positively impact the health and wellness of women worldwide.
Author contributions
WF: Conceptualization, Investigation, Methodology, Visualization, Writing – original draft, Writing – review & editing. PA: Conceptualization, Project administration, Supervision, Visualization, Writing – review & editing. LV: Visualization, Writing – review & editing. FM: Investigation, Methodology, Project administration, Supervision, Visualization, Writing – review & editing.
Funding
The author(s) declare financial support was received for the research, authorship, and/or publication of this article. This work was supported by the Universidade Federal do Pará, Coordenação de Aperfeiçoamento de Pessoal de Nível Superior (CAPES).
Acknowledgments
The authors would like to thank CAPES (Coordenação de Aperfeiçoamento de Pessoal de Nível Superior) for providing a doctoral scholarship to the author W. M. Fong Amaris. In addition, we would also like to thank the CCAD (Centro de computação de Alto Desempenho da Universidade Federal do Pará - High Performance Computer Center) for their support in developing this research.
Conflict of interest
The authors declare that the research was conducted in the absence of any commercial or financial relationships that could be construed as a potential conflict of interest.
Publisher’s note
All claims expressed in this article are solely those of the authors and do not necessarily represent those of their affiliated organizations, or those of the publisher, the editors and the reviewers. Any product that may be evaluated in this article, or claim that may be made by its manufacturer, is not guaranteed or endorsed by the publisher.
References
ACCP (2004). Cervical cancer prevention FACT SHEET risk factors for cervical cancer: evidence to date. J. Natl. Cancer Inst. 96, 1866–1869. doi: 10.1093/jnci/dji001
Aguiar-Pulido, V., Huang, W., Suarez-Ulloa, V., Cickovski, T., Mathee, K., and Narasimhan, G. (2016). Approaches for microbiome analysis. Evol. Bioinform. 12, 5–16. doi: 10.4137/EBO.S36436
Amabebe, E., and Anumba, D. O. C. (2018). The vaginal microenvironment: the physiologic role of Lactobacilli. Front. Med. 5:181. doi: 10.3389/fmed.2018.00181
Amabebe, E., and Anumba, D. O. C. (2020). Female gut and genital tract microbiota-induced crosstalk and differential effects of short-chain fatty acids on immune sequelae. Front. Immunol. 11, 2184–2115. doi: 10.3389/fimmu.2020.02184
Aobchey, P., Utama, K., Niamsup, H., and Sangthong, P. (2022). Gene expression analysis of RCC1, VAV2, RPA3, and SRPK1 for human cervical cancer biomarkers. Gene Rep. 26:101445. doi: 10.1016/j.genrep.2021.101445
Arbyn, M., Weiderpass, E., Bruni, L., de Sanjosé, S., Saraiya, M., Ferlay, J., et al. (2020). Estimates of incidence and mortality of cervical cancer in 2018: a worldwide analysis. Lancet Glob. Health 8, e191–e203. doi: 10.1016/S2214-109X(19)30482-6
Arroyo Mühr, L. S., Dillner, J., Ure, A. E., Sundström, K., and Hultin, E. (2021). Comparison of DNA and RNA sequencing of total nucleic acids from human cervix for metagenomics. Sci. Rep. 11:18852. doi: 10.1038/s41598-021-98452-4
Audirac-Chalifour, A., Torres-Poveda, K., Bahena-Román, M., Téllez-Sosa, J., Martínez-Barnetche, J., Corina-Ceballos, B., et al. (2016). Cervical microbiome and cytokine profile at various stages of cervical cancer: a pilot study. PLoS One 11:E0153274. doi: 10.1371/journal.pone.0153274
Bahrami, A., Hasanzadeh, M., Shahidsales, S., Farazestanian, M., Hassanian, S. M., Moetamani Ahmadi, M., et al. (2018). Genetic susceptibility in cervical cancer: from bench to bedside. J. Cell. Physiol. 233, 1929–1939. doi: 10.1002/jcp.26019
Banavar, G., Ogundijo, O., Toma, R., Rajagopal, S., Lim, Y. K., Tang, K., et al. (2021). The salivary metatranscriptome as an accurate diagnostic indicator of oral cancer. npj Genom. Med. 6:105. doi: 10.1038/s41525-021-00257-x
Basic, V., Zhang, B., Domert, J., Pellas, U., and Tot, T. (2021). Integrative meta analysis of gene expression profiles identifies FEN1 and ENDOU as potential diagnostic biomarkers for cervical squamous cell carcinoma. Oncol. Lett. 22, 1–18. doi: 10.3892/ol.2021.13101
Baud, A., Hillion, K. H., Plainvert, C., Tessier, V., Tazi, A., Mandelbrot, L., et al. (2023). Microbial diversity in the vaginal microbiota and its link to pregnancy outcomes. Sci. Rep. 13:9061. doi: 10.1038/s41598-023-36126-z
Borgdorff, H., Gautam, R., Armstrong, S. D., Xia, D., Ndayisaba, G. F., van Teijlingen, N. H., et al. (2016). Cervicovaginal microbiome dysbiosis is associated with proteome changes related to alterations of the cervicovaginal mucosal barrier. Mucosal Immunol. 9, 621–633. doi: 10.1038/mi.2015.86
Borgdorff, H., Tsivtsivadze, E., Verhelst, R., Marzorati, M., Jurriaans, S., Ndayisaba, G. F., et al. (2014). Lactobacillus-dominated cervicovaginal microbiota associated with reduced HIV/STI prevalence and genital HIV viral load in African women. ISME J. 8, 1781–1793. doi: 10.1038/ismej.2014.26
Bray, F., Ferlay, J., Soerjomataram, I., Siegel, R. L., Torre, L. A., and Jemal, A. (2018). Global cancer statistics 2018: GLOBOCAN estimates of incidence and mortality worldwide for 36 cancers in 185 countries. CA Cancer J Clin. 68, 394–424. doi: 10.3322/caac.21492
Callahan, B. J., McMurdie, P. J., Rosen, M. J., Han, A. W., Johnson, A. J. A., and Holmes, S. P. (2016). DADA2: high-resolution sample inference from Illumina amplicon data. Nat. Methods 13, 581–583. doi: 10.1038/nmeth.3869
Cardona-Benavides, I., Puertas-Prieto, A., Pinilla-Martín, F. J., Navarro-Marí, J. M., and Gutiérrez-Fernández, J. (2019). Alloscardovia omnicolens emerging presence in premature rupture of membranes. New Microbiol. 42, 237–239.
Carrillo-Ng, H., Becerra-Goicochea, L., Tarazona-Castro, Y., Pinillos-Vilca, L., del Valle, L. J., Aguilar-Luis, M. A., et al. (2021). Variations in cervico-vaginal microbiota among HPV-positive and HPV-negative asymptomatic women in Peru. BMC. Res. Notes 14:4. doi: 10.1186/s13104-020-05422-6
Castanheira, C. P., Sallas, M. L., Nunes, R. A. L., Lorenzi, N. P. C., and Termini, L. (2021). Microbiome and cervical cancer. Pathobiology 88, 187–197. doi: 10.1159/000511477
Castellsagué, X. (2008). Natural history and epidemiology of HPV infection and cervical cancer. Gynecol. Oncol. 110, S4–S7. doi: 10.1016/j.ygyno.2008.07.045
Chang, Y. S., Hsu, M. H., Tu, S. J., Yen, J. C., Lee, Y. T., Fang, H. Y., et al. (2021). Metatranscriptomic analysis of human lung metagenomes from patients with lung cancer. Genes 12:1458. doi: 10.3390/genes12091458
Cohen, P. A., Jhingran, A., Oaknin, A., and Denny, L. (2019). Cervical cancer. Lancet 393, 169–182. doi: 10.1016/S0140-6736(18)32470-X
Curry, S. J., Krist, A. H., Owens, D. K., Barry, M. J., Caughey, A. B., Davidson, K. W., et al. (2018). Screening for cervical cancer us preventive services task force recommendation statement. JAMA 320, 674–686. doi: 10.1001/jama.2018.10897
Curty, G., de Carvalho, P. S., and Soares, M. A. (2019). The role of the cervicovaginal microbiome on the genesis and as a biomarker of premalignant cervical intraepithelial neoplasia and invasive cervical cancer. Int. J. Mol. Sci. 21:222. doi: 10.3390/ijms21010222
Da Silva, M. L. R., De Albuquerque, B. H. D. R., De Medeiros Fernandes, T. A. A., De Almeida, V. D., Cobucci, R. N. D. O., Bezerra, F. L., et al. (2021). The role of HPV-induced epigenetic changes in cervical carcinogenesis (review). Biomed. Rep. 15:60. doi: 10.3892/br.2021.1436
Dareng, E. O., Ma, B., Famooto, A. O., Akarolo-Anthony, S. N., Offiong, R. A., Olaniyan, O., et al. (2016). Prevalent high-risk HPV infection and vaginal microbiota in Nigerian women. Epidemiol. Infect. 144, 123–137. doi: 10.1017/S0950268815000965
di Paola, M., Sani, C., Clemente, A. M., Iossa, A., Perissi, E., Castronovo, G., et al. (2017). Characterization of cervico-vaginal microbiota in women developing persistent high-risk human papillomavirus infection. Sci. Rep. 7:10200. doi: 10.1038/s41598-017-09842-6
Elinav, E., Garrett, W. S., Trinchieri, G., and Wargo, J. (2019). The cancer microbiome. Nat. Rev. Cancer 19, 371–376. doi: 10.1038/s41568-019-0155-3
Ferris, M. J., Masztal, A., Aldridge, K. E., Fortenberry, D., Fidel, P. L., and Martin, D. H. (2004). Association of Atopobium vaginae, a recently described metronidazole resistant anaerobe, with bacterial vaginosis. BMC Infect. Dis. 4:5. doi: 10.1186/1471-2334-4-5
Fredricks, D. N., Fiedler, T. L., and Marrazzo, J. M. (2005). Molecular identification of bacteria associated with bacterial vaginosis. N. Engl. J. Med. 353, 1899–1911. doi: 10.1056/NEJMoa043802
Gao, W., Weng, J., Gao, Y., and Chen, X. (2013). Comparison of the vaginal microbiota diversity of women with and without human papillomavirus infection: a cross-sectional study. BMC Infect. Dis. 13:271. doi: 10.1186/1471-2334-13-271
Ghebre, R. G., Grover, S., Xu, M. J., Chuang, L. T., and Simonds, H. (2017). Cervical cancer control in HIV-infected women: past, present and future. Gynecol. Oncol. Rep. 21, 101–108. doi: 10.1016/j.gore.2017.07.009
Godoy-Vitorino, F., Romaguera, J., Zhao, C., Vargas-Robles, D., Ortiz-Morales, G., Vázquez-Sánchez, F., et al. (2018). Cervicovaginal fungi and bacteria associated with cervical intraepithelial neoplasia and high-risk human papillomavirus infections in a Hispanic population. Front. Microbiol. 9:2533. doi: 10.3389/fmicb.2018.02533
Groves, I. J., and Coleman, N. (2015). Pathogenesis of human papillomavirus-associated mucosal disease. J. Pathol. 235, 527–538. doi: 10.1002/path.4496
Han, H. Y., Mou, J. T., Jiang, W. P., Zhai, X. M., and Deng, K. (2021). Five candidate biomarkers associated with the diagnosis and prognosis of cervical cancer. Biosci. Rep. 41:BSR20204394,. doi: 10.1042/BSR20204394
Hou, S., Zhang, X., and Yang, J. (2022). Long non-coding RNA ABHD11-AS1 facilitates the progression of cervical cancer by competitively binding to miR-330–5p and upregulating MARK2. Exp. Cell Res. 410:112929. doi: 10.1016/j.yexcr.2021.112929
Jiang, L., and Wang, X. (2022). The miR-133b/brefeldin A-inhibited guanine nucleotide-exchange protein 1 (ARFGEF1) axis represses proliferation, invasion, and migration in cervical cancer cells. Bioengineered 13, 3323–3332. doi: 10.1080/21655979.2022.2027063
Kang, G. U., Jung, D. R., Lee, Y. H., Jeon, S. Y., Han, H. S., Chong, G. O., et al. (2021). Potential association between vaginal microbiota and cervical carcinogenesis in Korean women: a cohort study. Microorganisms 9:294. doi: 10.3390/microorganisms9020294
Kim, J. J., Burger, E. A., Regan, C., and Sy, S. (2018). Screening for cervical cancer in primary care a decision analysis for the us preventive services task force. JAMA 320, 706–714. doi: 10.1001/jama.2017.19872
Klein, C., Kahesa, C., Mwaiselage, J., West, J. T., Wood, C., and Angeletti, P. C. (2020a). How the cervical microbiota contributes to cervical cancer risk in sub-Saharan Africa. Front. Cell. Infect. Microbiol. 10, 23–11. doi: 10.3389/fcimb.2020.00023
Klein, C., Samwel, K., Kahesa, C., Mwaiselage, J., West, J. T., Wood, C., et al. (2020b). Mycoplasma co-infection is associated with cervical cancer risk. Cancers 12:1093. doi: 10.3390/cancers12051093
Koh, W. J., Abu-Rustum, N. R., Bean, S., Bradley, K., Campos, S. M., Cho, K. R., et al. (2019). Cervical cancer, version 3.2019. J. Natl. Compr. Canc. Netw. 17, 64–84. doi: 10.6004/jnccn.2019.0001
Kori, M., and Arga, K. Y. (2018). Potential biomarkers and therapeutic targets in cervical cancer: insights from the meta-analysis of transcriptomics data within network biomedicine perspective. PLoS One 13:e0200717. doi: 10.1371/journal.pone.0200717
Kwasniewski, W., Wolun-Cholewa, M., Kotarski, J., Warchol, W., Kuzma, D., Kwasniewska, A., et al. (2018). Microbiota dysbiosis is associated with HPV-induced cervical carcinogenesis. Oncol. Lett. 16, 7035–7047. doi: 10.3892/ol.2018.9509
Lau, A., Gray, E. E., Brunette, R. L., and Stetson, D. B. (2015). DNA tumor virus oncogenes antagonize the cGAS-STING DNA-sensing pathway. Science 350, 568–571. doi: 10.1126/science.aab3291
Lewis, F. M. T., Bernstein, K. T., and Aral, S. O. (2017). Vaginal microbiome and its relationship to behavior, sexual health, and sexually transmitted diseases. Obstet. Gynecol. 129, 643–654. doi: 10.1097/AOG.0000000000001932
Li, Z., Chen, J., Zhao, S., Li, Y., Zhou, J., Liang, J., et al. (2021). Discovery and validation of novel biomarkers for detection of cervical cancer. Cancer Med. 10, 2063–2074. doi: 10.1002/cam4.3799
Lin, M., Ye, M., Zhou, J., Wang, Z. P., and Zhu, X. (2019). Recent advances on the molecular mechanism of cervical carcinogenesis based on systems biology technologies. Comput. Struct. Biotechnol. J. 17, 241–250. doi: 10.1016/j.csbj.2019.02.001
Liu, M., Jia, J., Wang, X., Liu, Y., Wang, C., and Fan, R. (2018). Long non-coding RNA HOTAIR promotes cervical cancer progression through regulating BCL2 via targeting miR-143-3p. Cancer Biol. Ther. 19, 391–399. doi: 10.1080/15384047.2018.1423921
Liu, M. B., Xu, S. R., He, Y., Deng, G. H., Sheng, H. F., Huang, X. M., et al. (2013). Diverse vaginal microbiomes in reproductive-age women with vulvovaginal candidiasis. PLoS One 8:e81857. doi: 10.1371/journal.pone.0081857
Long, N. P., Jung, K. H., Yoon, S. J., Anh, N. H., Nghi, T. D., Kang, Y. P., et al. (2017). Systematic assessment of cervical cancer initiation and progression uncovers genetic panels for deep learning-based early diagnosis and proposes novel diagnostic and prognostic biomarkers. Oncotarget 8, 109436–109456. doi: 10.18632/oncotarget.22689
Machado, A., Foschi, C., and Marangoni, A. (2022). Editorial: vaginal dysbiosis and biofilms. Front. Cell. Infect. Microbiol. 12:976057. doi: 10.3389/fcimb.2022.976057
Mallmann, P., and Mallmann, C. (2016). Neoadjuvant and adjuvant chemotherapy of cervical cancer. Oncol. Res. Treat. 39, 522–524. doi: 10.1159/000449023
Marchesi, J. R., and Ravel, J. (2015). The vocabulary of microbiome research: a proposal. Microbiome 3:31. doi: 10.1186/s40168-015-0094-5
Mitra, A., MacIntyre, D. A., Lee, Y. S., Smith, A., Marchesi, J. R., Lehne, B., et al. (2015). Cervical intraepithelial neoplasia disease progression is associated with increased vaginal microbiome diversity. Sci. Rep. 5:16865. doi: 10.1038/srep16865
Mitra, A., MacIntyre, D., Lee, Y., Smith, A., Marchesi, J., Lehne, B., et al. (2016a). Characterisation of the vaginal microbiome in cervical intraepithelial neoplasia. Lancet 387:S75. doi: 10.1016/S0140-6736(16)00462-1
Mitra, A., MacIntyre, D. A., Marchesi, J. R., Lee, Y. S., Bennett, P. R., and Kyrgiou, M. (2016b). The vaginal microbiota, human papillomavirus infection and cervical intraepithelial neoplasia: what do we know and where are we going next? Microbiome 4:58. doi: 10.1186/s40168-016-0203-0
Muñoz, N. (2000). Human papillomavirus and cancer: the epidemiological evidence. J. Clin. Virol. 19, 1–5. doi: 10.1016/S1386-6532(00)00125-6
Norenhag, J., Du, J., Olovsson, M., Verstraelen, H., Engstrand, L., and Brusselaers, N. (2020). The vaginal microbiota, human papillomavirus and cervical dysplasia: a systematic review and network meta-analysis. BJOG 127, 171–180. doi: 10.1111/1471-0528.15854
Oyervides-Muñoz, M. A., Pérez-Maya, A. A., Rodríguez-Gutiérrez, H. F., Gómez-Macias, G. S., Fajardo-Ramírez, O. R., Treviño, V., et al. (2018). Understanding the HPV integration and its progression to cervical cancer. Infect. Genet. Evol. 61, 134–144. doi: 10.1016/j.meegid.2018.03.003
Pacha-Herrera, D., Erazo-Garcia, M. P., Cueva, D. F., Orellana, M., Borja-Serrano, P., Arboleda, C., et al. (2022). Clustering analysis of the multi-microbial consortium by Lactobacillus species against vaginal dysbiosis among Ecuadorian women. Front. Cell. Infect. Microbiol. 12:863208. doi: 10.3389/fcimb.2022.863208
Pappa, K. I., Kontostathi, G., Lygirou, V., Zoidakis, J., and Anagnou, N. P. (2018). Novel structural approaches concerning HPV proteins: insight into targeted therapies for cervical cancer (review). Oncol. Rep. 39, 1547–1554. doi: 10.3892/or.2018.6257
Parkin, D. M. (2006). The global health burden of infection-associated cancers in the year 2002. Int. J. Cancer 118, 3030–3044. doi: 10.1002/ijc.21731
Peric, A., Weiss, J., Vulliemoz, N., Baud, D., and Stojanov, M. (2019). Bacterial colonization of the female upper genital tract. Int. J. Mol. Sci. 20:3405. doi: 10.3390/ijms20143405
Peterson, J., Garges, S., Giovanni, M., McInnes, P., Wang, L., Schloss, J. A., et al. (2009). The NIH human microbiome project. Genome Res. 19, 2317–2323. doi: 10.1101/gr.096651.109
Plisko, O., Zodzika, J., Jermakova, I., Pcolkina, K., Prusakevica, A., Liepniece-Karele, I., et al. (2021). Aerobic vaginitis—underestimated risk factor for cervical intraepithelial neoplasia. Diagnostics 11:97. doi: 10.3390/diagnostics11010097
Poore, G. D., Kopylova, E., Zhu, Q., Carpenter, C., Fraraccio, S., Wandro, S., et al. (2020). Microbiome analyses of blood and tissues suggest cancer diagnostic approach. Nature 579, 567–574. doi: 10.1038/s41586-020-2095-1
Qu, X., Shi, Z., Guo, J., Guo, C., Qiu, J., and Hua, K. (2021). Identification of a novel six-gene signature with potential prognostic and therapeutic value in cervical cancer. Cancer Med. 10, 6881–6896. doi: 10.1002/cam4.4054
Quast, C., Pruesse, E., Yilmaz, P., Gerken, J., Schweer, T., Yarza, P., et al. (2013). The SILVA ribosomal RNA gene database project: improved data processing and web-based tools. Nucleic Acids Res. 41, D590–D596.
Ravel, J., Gajer, P., Abdo, Z., Schneider, G. M., Koenig, S. S. K., McCulle, S. L., et al. (2011). Vaginal microbiome of reproductive-age women. Proc. Natl. Acad. Sci. U. S. A. 108, 4680–4687. doi: 10.1073/pnas.1002611107
Rodríguez-Arias, R. J., Guachi-Álvarez, B. O., Montalvo-Vivero, D. E., and Machado, A. (2022). Lactobacilli displacement and Candida albicans inhibition on initial adhesion assays: a probiotic analysis. BMC. Res. Notes 15:239. doi: 10.1186/s13104-022-06114-z
Sagar, R., and Sharma, G. P. (2012). Measurement of alpha diversity using Simpson index (1/λ): the jeopardy. Environ. Skept. Crit. 1, 23–24.
Salinas, A. M., Osorio, V. G., Pacha-Herrera, D., Vivanco, J. S., Trueba, A. F., and Machado, A. (2020). Vaginal microbiota evaluation and prevalence of key pathogens in Ecuadorian women: an epidemiologic analysis. Sci. Rep. 10:18358. doi: 10.1038/s41598-020-74655-z
Shannon, B., Yi, T. J., Perusini, S., Gajer, P., Ma, B., Humphrys, M. S., et al. (2017). Association of HPV infection and clearance with cervicovaginal immunology and the vaginal microbiota. Mucosal Immunol. 10, 1310–1319. doi: 10.1038/mi.2016.129
Sims, T. T., Colbert, L. E., and Klopp, A. H. (2021). The role of the cervicovaginal and gut microbiome in cervical intraepithelial neoplasia and cervical cancer. J. Immunother. Precis. Oncol. 4, 72–78. doi: 10.36401/JIPO-20-17
Sims, T. T., Colbert, L. E., Zheng, J., Delgado Medrano, A. Y., Hoffman, K. L., Ramondetta, L., et al. (2019). Gut microbial diversity and genus-level differences identified in cervical cancer patients versus healthy controls. Gynecol. Oncol. 155, 237–244. doi: 10.1016/j.ygyno.2019.09.002
So, K. A., Yang, E. J., Kim, N. R., Hong, S. R., Lee, J. H., Hwang, C. S., et al. (2020). Changes of vaginal microbiota during cervical carcinogenesis in women with human papillomavirus infection. PLoS One 15:e0238705. doi: 10.1371/journal.pone.0238705
Srinivasan, S., and Fredricks, D. N. (2008). The human vaginal bacterial biota and bacterial vaginosis. Interdiscip. Perspect. Infect. Dis. 2008:750479. doi: 10.1155/2008/750479
Taddei, C. R., Cortez, R. V., Mattar, R., Torloni, M. R., and Daher, S. (2018). Microbiome in normal and pathological pregnancies: a literature overview. Am. J. Reprod. Immunol. 80:e12993. doi: 10.1111/aji.12993
Tango, C. N., Seo, S. S., Kwon, M., Lee, D. O., Chang, H. K., and Kim, M. K. (2020). Taxonomic and functional differences in cervical microbiome associated with cervical cancer development. Sci. Rep. 10:9720. doi: 10.1038/s41598-020-66607-4
Terasawa, T., Hosono, S., Sasaki, S., Hoshi, K., Hamashima, Y., Katayama, T., et al. (2022). Comparative accuracy of cervical cancer screening strategies in healthy asymptomatic women: a systematic review and network meta-analysis. Sci. Rep. 12:94. doi: 10.1038/s41598-021-04201-y
van de Wijgert, J. H. H. M., Borgdorff, H., Verhelst, R., Crucitti, T., Francis, S., Verstraelen, H., et al. (2014). The vaginal microbiota: what have we learned after a decade of molecular characterization? PLoS One 9:e105998. doi: 10.1371/journal.pone.0105998
Verhelst, R., Verstraelen, H., Claeys, G., Verschraegen, G., Delanghe, J., van Simaey, L., et al. (2004). Cloning of 16S rRNA genes amplified from normal and disturbed vaginal microflora suggests a strong association between Atopobium vaginae, Gardnerella vaginalis and bacterial vaginosis. BMC Microbiol. 4:16. doi: 10.1186/1471-2180-4-16
Verstraelen, H., Verhelst, R., Claeys, G., Temmerman, M., and Vaneechoutte, M. (2004). Culture-independent analysis of vaginal microflora: the unrecognized association of Atopobium vaginae with bacterial vaginosis. Am. J. Obstet. Gynecol. 191, 1130–1132. doi: 10.1016/j.ajog.2004.04.013
Vordermark, D. (2016). Radiotherapy of cervical cancer. Oncol. Res. Treat. 39, 516–520. doi: 10.1159/000448902
Wang, R., Zhang, Y., and Shan, F. (2022). PD-L1: can it be a biomarker for the prognosis or a promising therapeutic target in cervical cancer? Int. Immunopharmacol. 103:108484. doi: 10.1016/j.intimp.2021.108484
Wei, L. Q., Cheong, I. H., Yang, G. H., Li, X. G., Kozlakidis, Z., Ding, L., et al. (2021). The application of high-throughput technologies for the study of microbiome and cancer. Front. Genet. 12:699793. doi: 10.3389/fgene.2021.699793
Wheeler, C. M. (2013). The natural history of cervical human papillomavirus infections and cervical cancer: gaps in knowledge and future horizons. Obstet. Gynecol. Clin. N. Am. 40, 165–176. doi: 10.1016/j.ogc.2013.02.004
WHO (2014). Comprehensive cervical cancer control: a guide to essential practice WHO Library Cataloguing-in-Publication Data.
WHO (2020). Global strategy to accelerate the elimination of cervical cancer as a public health problem and its associated goals and targets for the period 2020–2030 World Health Organization.
Wickramasinghe, R. N., Goonawardhana, N. D. S., Premaratne, S. P., and Perera, P. P. R. (2021). Quantitative real-time PCR as a novel detection method for micro-RNAs expressed by cervical cancer tissue: a review. J. Biosci. Med. 9, 100–115. doi: 10.4236/jbm.2021.99009
Wu, S., Ding, X., Kong, Y., Acharya, S., Wu, H., Huang, C., et al. (2021). The feature of cervical microbiota associated with the progression of cervical cancer among reproductive females. Gynecol. Oncol. 163, 348–357. doi: 10.1016/j.ygyno.2021.08.016
Yilmaz, P., Parfrey, L. W., Yarza, P., Gerken, J., Pruesse, E., Quast, C., et al. (2014). The SILVA and “all-species living tree project (LTP)” taxonomic frameworks. Nucleic Acids Res. 42, D643–D648. doi: 10.1093/nar/gkt1209
Zhang, C., Liu, Y., Gao, W., Pan, Y., Gao, Y., Shen, J., et al. (2018). The direct and indirect association of cervical microbiota with the risk of cervical intraepithelial neoplasia. Cancer Med. 7, 2172–2179. doi: 10.1002/cam4.1471
Zhang, S., Xu, H., Zhang, L., and Qiao, Y. (2020). Cervical cancer: epidemiology, risk factors and screening. Chin. J. Cancer Res. 32, 720–728. doi: 10.21147/j.issn.1000-9604.2020.06.05
Zhang, P., Zhao, F., Jia, K., and Liu, X. (2022). The LOXL1 antisense RNA 1 (LOXL1-AS1)/microRNA-423-5p (miR-423-5p)/ectodermal-neural cortex 1 (ENC1) axis promotes cervical cancer through the mitogen-activated protein kinase (MEK)/extracellular signal-regulated kinase (ERK) pathway. Bioengineered 13, 2567–2584. doi: 10.1080/21655979.2021.2018975
Zhou, X., Brown, C. J., Abdo, Z., Davis, C. C., Hansmann, M. A., Joyce, P., et al. (2007). Differences in the composition of vaginal microbial communities found in healthy Caucasian and black women. ISME J. 1, 121–133. doi: 10.1038/ismej.2007.12
Zhou, X., Hansmann, M. A., Davis, C. C., Suzuki, H., Brown, C. J., Schütte, U., et al. (2010). The vaginal bacterial communities of Japanese women resemble those of women in other racial groups. FEMS Immunol. Med. Microbiol. 58, 169–181. doi: 10.1111/j.1574-695X.2009.00618.x
Zhou, Z. W., Long, H. Z., Cheng, Y., Luo, H. Y., Wen, D. D., and Gao, L. C. (2021). From microbiome to inflammation: the key drivers of cervical cancer. Front. Microbiol. 12:767931. doi: 10.3389/fmicb.2021.767931
Keywords: microbiota changes, cervical cancer, progression, biomarkers, vaginal microbiota
Citation: Fong Amaris WM, Assumpção PPd, Valadares LJ and Moreira FC (2024) Microbiota changes: the unseen players in cervical cancer progression. Front. Microbiol. 15:1352778. doi: 10.3389/fmicb.2024.1352778
Edited by:
Svetlana Khaiboullina, University of Nevada, Reno, United StatesReviewed by:
Izabela Korona-Glowniak, Medical University of Lublin, PolandAntónio Machado, Universidad San Francisco de Quito, Ecuador
Copyright © 2024 Fong Amaris, Assumpção, Valadares and Moreira. This is an open-access article distributed under the terms of the Creative Commons Attribution License (CC BY). The use, distribution or reproduction in other forums is permitted, provided the original author(s) and the copyright owner(s) are credited and that the original publication in this journal is cited, in accordance with accepted academic practice. No use, distribution or reproduction is permitted which does not comply with these terms.
*Correspondence: W. M. Fong Amaris, wendy.amaris@icb.ufpa.br; Fabiano Cordeiro Moreira, fcmoreira@ufpa.br