- 1Ministry of Education Key Laboratory of Cell Activities and Stress Adaptations, School of Life Sciences, Lanzhou University, Lanzhou, China
- 2Key Laboratory of Extreme Environmental Microbial Resources and Engineering, Lanzhou, China
- 3Key Laboratory of Desert and Desertification, Northwest Institute of Eco-Environment and Resources, Chinese Academy of Sciences, Lanzhou, China
A rich diversity of radiation-resistant (Rr) and desiccation-resistant (Dr) bacteria has been found in arid habitats of the world. Evidence from scientific research has linked their origin to reactive oxygen species (ROS) intermediates. Rr and Dr. bacteria of arid regions have the potential to regulate imbalance radicals and evade a higher dose of radiation and oxidation than bacterial species of non-arid regions. Photochemical-activated ROS in Rr bacteria is run through photo-induction of electron transfer. A hypothetical model of the biogeochemical cycle based on solar radiation and desiccation. These selective stresses generate oxidative radicals for a short span with strong reactivity and toxic effects. Desert-inhibiting Rr bacteria efficiently evade ROS toxicity with an evolved antioxidant system and other defensive pathways. The imbalanced radicals in physiological disorders, cancer, and lung diseases could be neutralized by a self-sustaining evolved Rr bacteria antioxidant system. The direct link of evolved antioxidant system with intermediate ROS and indirect influence of radiation and desiccation provide useful insight into richness, ecological diversity, and origin of Rr bacteria capabilities. The distinguishing features of Rr bacteria in deserts present a fertile research area with promising applications in the pharmaceutical industry, genetic engineering, biological therapy, biological transformation, bioremediation, industrial biotechnology, and astrobiology.
1 Introduction
Radiation-resistant (Rr) and desiccation-resistant (Dr) bacteria can survive with exposure to radiation and desiccation cycles in natural habitats. Their resistance capabilities were confirmed during laboratory experimentation (Singh and Gabani, 2011; Paulino-Lima et al., 2013), while the radiosensitive bacterial cells immediately reduced in number with radiation exposure (Sghaier, 2011). Based on the wavelength, electromagnetic radiation comprises ionizing radiation (IR) and non-IR. The wavelength of IR falls below 100 nm and includes X-rays and gamma rays and is extremely hazardous because it produces ions in the cell. Other IR includes alpha and beta particles with undetectable wavelengths and high penetrating ability (Griffiths, 2020; Chaudhary and Kumar, 2023). Non-IR does not result in the formation of ions but includes ultraviolet radiation (UVR), whose wavelengths fall between 100 and 400 nm (Mba et al., 2012; Leszczynski, 2014). These Rr bacteria of desiccated soil evolved in response to photo-irradiation and can survive with exposure to radiation environments. Due to selective stresses that prevail in deserts, they are regarded as a unique environment for studying ecological diversity and function potentiality of Rr bacteria. In a desiccated environment, the photochemical production of ROS interlinks defensive pathways against radiation and desiccation, and bacterial communities can survive multiple stress conditions.
Desiccation is a typical feature of the desert’s environment and an important factor that helps microbial cells evolve with resistant features. Microbial communities of deserts have resistant deoxyribonucleic acid (DNA), efficient proteomic systems, richness in metabolites, enzymes, and dissimilar pigmentation (Castillo and Smith, 2017; Orellana et al., 2020; Kanekar and Kanekar, 2022). Desiccated regions such as the Taklamakan Desert, Sonoran Desert, Sahara Desert, and Atacama Desert are extensively studied for their rich bacterial diversity with extreme resistance to radiation, desiccation, and oxidation (Sajjad et al., 2017; Belov et al., 2018; Guesmi et al., 2021; Liu et al., 2022). Radiation and desiccation are the main ecological factors affecting the microbial diversity of the desert ecosystem. However, researchers endeavor to reveal the actual factor affecting the evolved abilities of desert-borne bacteria. Scientists contemplate that the radiation tolerance found in bacterial species of desiccated regions is unusual. This is because the intensity of radiation survived by bacteria inhabiting deserts does not even reach the surface of the Earth. In particular, D. radiodurans exhibits an ability to resist radiation that is four times greater in magnitude, with no loss in cell numbers, than the highest recorded radiation on earth (Battista, 1997). Rr bacteria of the desert environment, especially D. radiodurans, resist 15,000 Gy of gamma, which is explained by its ability to scavenge ROS (Daly, 2006). ROS plays an intermediate role in interlinking resistance mechanisms against radiation and desiccation.
The evolved abilities of Rr bacteria have wide applications in applied sciences. Rr bacteria utilize the imbalanced charge radicals for their energy generation and consumption. As the redox cycle of Rr bacteria evolved and operated with the intensity of radiation, it might be with desiccation. These bacteria managed intracellular induction of electrons by their counter-response to these environmental stresses. The by-products of radiations and desiccation of bacterial cells in the form of charge radicals and their counter-response help in the evolved abilities (Daly, 2006). Rr bacteria also produce distinct pigmentation with absorbance and reflection of incoming radiation. These pigments are important for scavenging radicals produced by radiation and desiccation. Rr bacteria have an efficient antioxidant system, highlighting their importance in chemotherapy and curing lung diseases, the main obstacle of which is overcoming imbalanced radicals. Rr bacteria efficiently balance elevated radicals that are induced by radiation, desiccation, or any other stress factor (Latifi et al., 2009; Yoboue et al., 2014; Fagliarone et al., 2017; Han et al., 2020). Rr bacteria resist osmotic and acid stress temperature variation and have a pool of enzymes, making them a suitable candidate in fermentation biotechnology. In addition, Rr bacteria exhibit tolerance to heavy metals, playing a crucial role in the bioremediation of pollutants and the biodegradation of radioactive and radionucleotide waste (Luo et al., 2014; Beblo-Vranesevic et al., 2017; Chen et al., 2021; Nayak et al., 2021; Kumar et al., 2022). Furthermore, Rr bacteria produce various extremolytes, and their potential sunscreen ability is already commercialized and effectively utilized by skincare industries (Mendes-Silva et al., 2020).
Therefore, it is evident that the interaction of Rr and Dr. bacteria with various stresses is interesting to study further. Thus, the current review summarized the most recent knowledge about the origin, abundance, and diversity of Rr bacteria in response to radiation and desiccation. This article also covers the following topics: (i) desert environmental traits, (ii) a common antioxidant system by which Rr bacteria sustain in radiation and desiccated environment, and (iii) the potential application of Rr bacteria in the field of biotechnology, bioremediation, biological therapy, fermentation, energy, and pharmaceutical industry.
2 Environmental traits of deserts and their influence
2.1 ROS is elicited through radiation and desiccation
Desiccated soil exposed to photoradiation generates ROS species in the desert environment. ROS is also produced inside a cell in response to radiation and desiccation. These selective environmental stresses influence essential components and cellular processes of microbial cells directly or indirectly through ROS derivatives. These ROS are reactive, damage biological molecules with strong affinity, and interfere with cellular processes. ROS interferes with the genome, nucleotides, mitochondrial DNA, proteins, lipids, and the redox cycle of the target cell (Krisko and Radman, 2010; Daly, 2012; Imlay, 2013, 2015; Schieber and Chandel, 2014). Despite their toxicity on cellular contents, a high abundance of microbial communities have evolved ROS scavenging mechanisms with the ability to resist desiccation, radiation, and their derivatives. It is postulated that similar mechanisms work in both cell corrosion and cell protection through ROS intermediates (Figure 1).
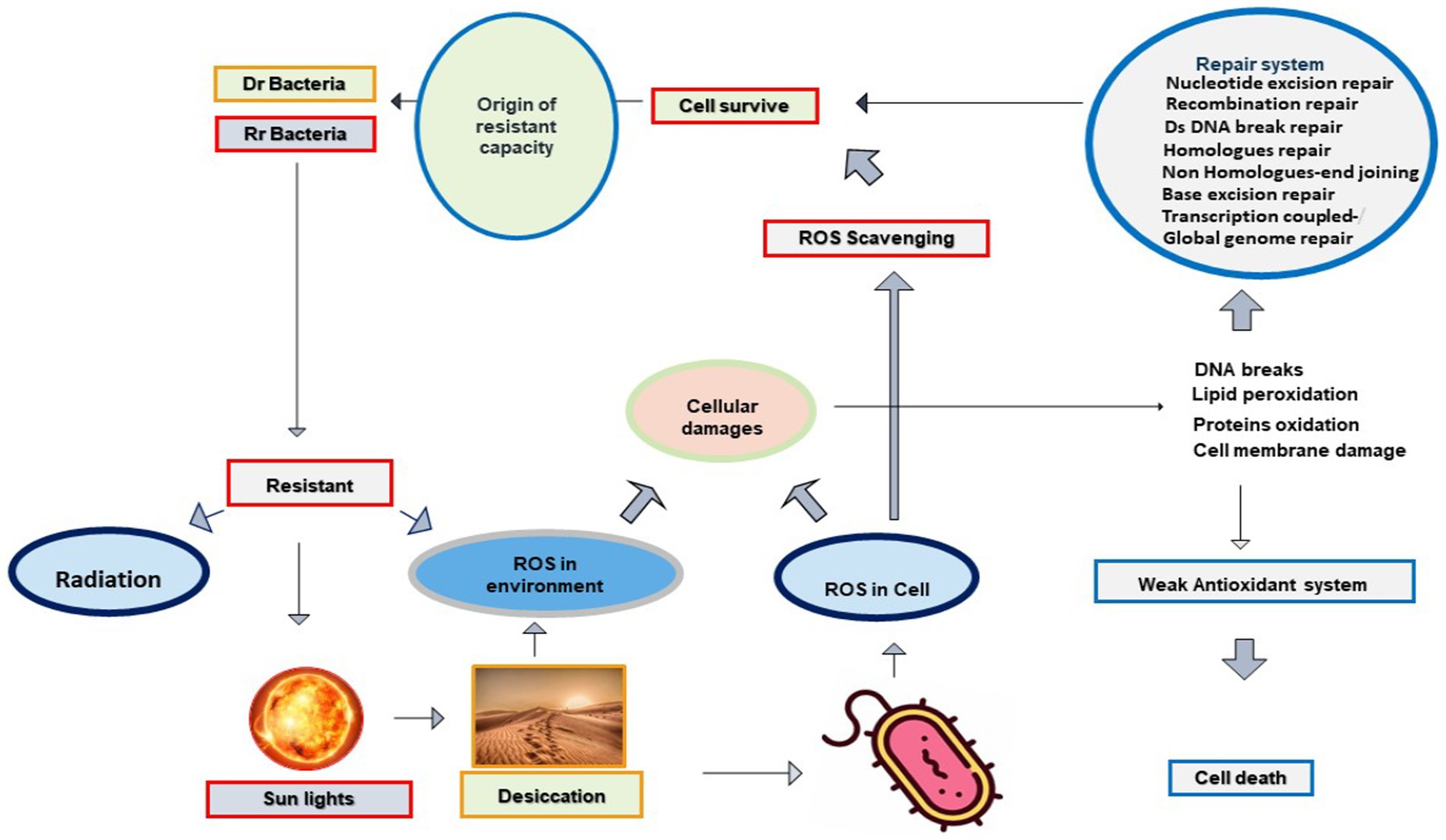
Figure 1. Radiation and desiccation initiate the origin of Rr and Dr. capabilities in deserts through the antioxidant system and repair mechanisms: Radiation and desiccation triggered the production of ROS species in bacterial cells. Due to their strong reactivity, ROS causes DNA damage, protein oxidation, and lipid peroxidation. Bacterial cells having no proper antioxidant system lose their viability and cells marked with evolved antioxidant systems survive with exposure to radiation, desiccation, and oxidation.
2.2 Photochemical redox reactions
ROS are produced in the cell and are also found in the surrounding desert environment. These ROS drive the abundance, diversity, and origin of the Rr and Dr. bacteria. ROS are generated by solar radiation and desiccation. Normally, photodesiccated soils accumulate peroxides and superoxide at higher levels than non-desert soils. A study highlighted evidence of superoxide (O2¯) and hydroxyl (-OH) production in desert soil (Georgiou et al., 2015). Nitrogen oxide (NOx) is released from the desiccated soil of the Mojave Desert at rates comparable to wet soil if the soil is irradiated by solar radiation. This shows the importance of radiation and desiccated soil in regulating ROS and reactive nitrogen species (RNS) (Austin and Vivanco, 2006; McCalley and Sparks, 2009). However, due to the short lifespan of reactive species and the lack of suitable techniques for their detection in the soil, these photochemical oxidative reactions and their associated mechanisms are not fully understood (Ito et al., 1985; Schneider et al., 2014; Halliwell and Gutteridge, 2015).
3 Hypothetical models of Rr bacteria origin in deserts
3.1 A naturally occurring desiccation
The most accepted and main driving force for the origin of Rr bacteria is desiccation. Rr bacteria are naturally resistant to desiccation. It is known that desiccation and radiation share a common target in a bacterial cell by causing the disintegration of the DNA molecules (Singh, 2018), while in response, Dr. and Rr bacteria also effectively recover the lesions of DNA caused by either desiccation or radiation. The similar target of radiation and desiccation in the cell and the similar mechanism of the cell to evade damaging effects in both conditions give a clear understanding that the desiccated environment of deserts is one of the important aspects of the rich diversity of Rr bacteria (Rainey et al., 2005). The hypothesis that Rr Bacterial community is a consequence of desiccation was previously studied. It was shown that resistance to a high radiation dose and desiccation is regulated through ROS scavenging system and efficient DNA repair mechanisms. It was shown that such mechanisms compensate for desiccation and also evolved to resist radiation (Makarova et al., 2001; Cox and Battista, 2005; Yu et al., 2015). To correlate Rr bacteria with desiccation, a phylogenetically diversified Rr bacteria belonging to Bacteroidetes, Proteobacteria, Deinococcus-Thermus, Firmicutes, and Actinobacteria were studied from the Taklimakan Desert (Yu et al., 2015). Interestingly, all Rr bacteria have the potentiality of carotenoid-like molecules, an efficient scavenger of ROS and reactive nitrogen species (RNS) (Asker et al., 2012). These ecologically distributed Rr bacteria and their associated ROS scavengers possess higher activities against desiccation and oxidation. Research on the desiccated environment of Taklimakan supports the hypothesis that the resistant phenotype is a consequence of the evolution of the ROS scavenging system, which defends cells against oxidative damage caused by desiccation (Yu et al., 2015).
3.2 A naturally occurring radiation
The Rr bacteria at desiccated habitat balance radicals generated by radiation at the atomic level. This mechanism is based on the availability of free electrons with ROS species and their immediate balancing. These ROS species and their unstable revolving electron are hit by incoming radiation. These electrons are excited after they gain energy from the incoming photon; these electrons circulate freely in the cytoplasm and damage cellular structures, commonly DNA, lipids, and proteins. Due to available free electrons, ROS species are very reactive and damage biological and cellular structures (Atri et al., 2022). To balance charge particles caused by radiation, these ROS species have to lose or gain more charge ions either to build a new molecule or convert the toxic to a less toxic form. In simple words, this redox cycle is imbalanced by photon–electron interaction through ROS intermediates and balanced again by an antioxidant system of Rr bacteria. Due to this evolved feature, Rr bacteria are more resistant than radiosensitive cells (Tiquia-Arashiro and Rodrigues, 2016).
Photosynthetic cells also have a photo-induced electron cycle at the atomic level. The electrons derive excitation as soon as the incoming photons collide with the electrons in the antenna complex of the photosystem. These excited electrons are consumed in photophosphorylation (Kramer and Evans, 2011; Maróti et al., 2020). Photon energy from electromagnetic radiation transforms visible light into electrical energy and chemical energy (Chandra et al., 2018). Bacteria inhibiting in desiccated soil are naturally pigmented in response to radiation. Biologists assume that the Rr bacterial community of deserts drives this useful phenomenon at the cellular level in response to radiation. The radiation (photon–electrons) interaction or ionic interaction mediates the flow of charge radicals in the cell. Thus, it is concluded that radiation is a source of energy for physiological function and also the transformation of energy in the cell from one form to another (Shukla and Subba Rao, 2017), while a considerable amount of radiation converts to heat, as an answer is why the Rr bacterial community evades from a dose higher than the natural radiation.
3.3 Temperature, salinity, and Rr bacteria
The extreme Rr bacteria and rich diversity of desert-borne bacteria also have temperature dependency. At freezing temperature, the ROS produced due to radiation is restricted to diffuse, whereas, at room temperature, the ROS produced freely moves and disintegrates DNA molecules higher than ROS at freezing conditions. The gamma radiation resistance of D. radiodurans and isolated strains from the extreme cold desert of Antarctica Dry Valley was compared under varying temperatures. D. radiodurans withstand a high dose of gamma radiation at −79°C when frozen on dry ice compared to room temperature (Dartnell et al., 2010). The IR resistance of microbes is also studied with correlation to salinity. There is no correlation between high Rr and Dr. bacteria with salt concentration (Shukla et al., 2007).
4 Geographical distribution of Rr bacteria
Rr bacterial communities are ecologically distributed in hot and cold deserts (Chanal et al., 2006; Shukla et al., 2007; Azua-Bustos et al., 2012; Musilova et al., 2015; Shirsalimian et al., 2018; Guesmi et al., 2022) Deinococcus thermus strains LD4 and LD5 were isolated from the Lut Desert of Iran (the hottest place on earth) and are resistant to a dose of >15 KGy of gamma radiation and > 600 j/m2 of UV-C (Mohseni et al., 2014). Their extreme radiation resistance is explained by desiccation and temperature fluctuation during the day and night. Similarly, Hymenobacter xinjiangensis X2-1gT has been reported from the Xinjiang Desert, China, which can resist 8 KGy of gamma radiation from a 60Co source at a dose rate of 10 Gy min−1 (Zhang Q. et al., 2007). Desertibacter roseus strain 2622T has been reported from the Xinjiang Taklamakan Desert of China, which can survive at 10 KGy of gamma radiation at a dose rate of 300 Gy min −1 at room temperature (Liu et al., 2011). These species are ecologically dispersed, and the genus Deinococcus is widely found in the Deserts. For instance, 60 strains of this genus were isolated from the irradiated arid soil of the Sonoran Desert, including 9 strains identified for the first time (Rainey et al., 2005). In total, 14 Rr strains, such as species of Bacillus cereus/thuringiensis of phylum Firmicutes, have been reported from the Atacama Desert. These strains showed 300 j/m2 of UV-C tolerance except for Bacillus strain S3.300–2, which showed a 10% survival rate even at the higher dose of 318 j/m2 (Paulino-Lima et al., 2013). The details of Rr bacteria and their potential to resist radiation are described in Table 1. Due to harsh ecological elements prevailing in deserts and most commonly fluctuations of chemical and physical weathering, Rr strains have evolved to produce antiviral, antibacterial, and antifungal metabolites. As a result of rapid emerging resistance against the preexisting antibiotics, the search for new metabolites obtained from such extreme habitats is crucial to contain human and animal diseases (Gabani and Singh, 2013; Nayak et al., 2021). Rr bacteria are not only resistant to oxidation and radiation but survive with fluctuation of temperature and are categorized into mesophile, thermophile, and hyperthermophile subgroups (Ranawat and Rawat, 2017; Li et al., 2020; Marszalkowski et al., 2021). These bacteria are found in less non-arid habitats than in arid ones, as plenty of photochemical production of ROS in the desert ecosystem facilitates their origin, diversity, and abundance (Rainey et al., 2005). It is therefore concluded that Rr bacteria is found in soil either irradiated or desiccated and eventually grows by following a cycle of oxidative regulation (Schulze-Makuch et al., 2017).
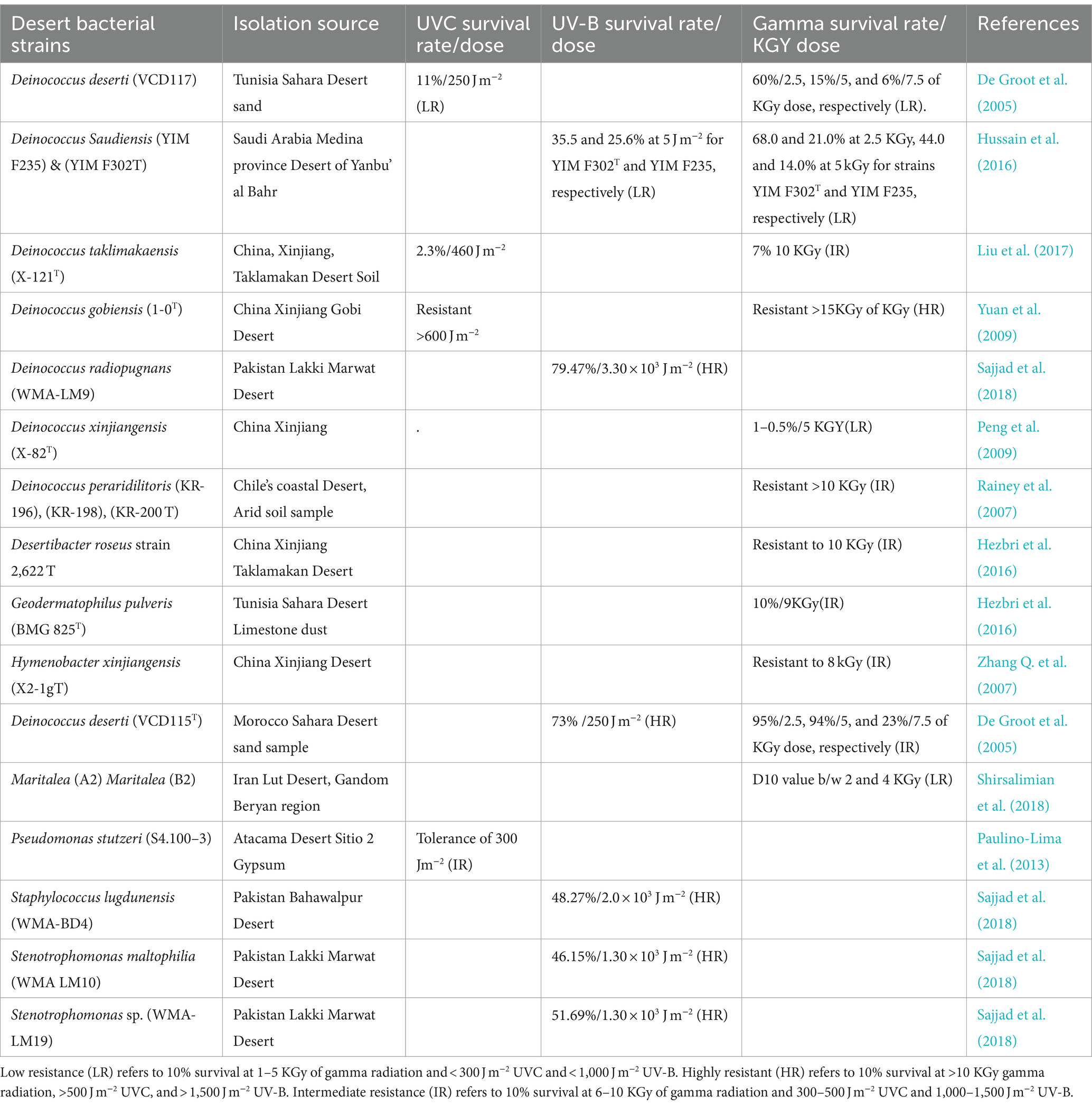
Table 1. Rr bacteria and their resistance capabilities: Rr bacteria isolated from various desiccated soil across the world.
5 Mechanism for ionizing radiation resistance
5.1 Copy number of DNA and nucleoid organization
IR resistance in bacteria has focused on explaining how cells cope with DNA and protein damage and detoxify ROS (Villa et al., 2021; Rai and Dutta, 2024). DNA and protein damage are believed to have the most significant impact on cell survival. Increased DNA copy number has been positively correlated to increased IR resistance in E. coli (Krasin and Hutchinson, 1977) and Saccharomyces cerevisiae (Mortimer, 1958). Increased numbers of DNA copies provide enough genetic information that DNA repair systems can use to correct the damages caused by IR. Recombination, a process that bacteria frequently use to repair DNA double-strand breaks (DSBs), requires more than one copy of the DNA being repaired (Cox and Battista, 2005). The nucleoid of IR-resistant bacteria, including Rubrobacter radiotolerans and Deinococcus spp., appears to be more condensed than the genome of IR-sensitive species such as Thermus aquaticus and E. coli (Zimmerman and Battista, 2005). The condensation prevents DNA fragment diffusion and limits the post-irradiation activity of intracellular DNases, thus facilitating cell survival.
5.2 Enzymatic and non-enzymatic processes and proteins
The oxidative stress caused by IR is prevented through enzymatic processes. The activity of the following proteins protecting against oxidative stress (peroxidases, catalases, and superoxide dismutases) relates to ROS accessibility and may be a contributive factor for IR resistance (Wang and Schellhorn, 1995; Tian et al., 2004). As for non-enzymatic processes, antioxidant molecules, including carotenoid pigments and intracellular salts, are involved in protection against oxidative stress. Novel proteins in D. radiodurans have been linked to IR resistance. Five novel protein genes are highly expressed and encoded proteins of unknown function in D. radiodurans following exposure to IR, such as DdrA, DdrB, DdrC, DdrD, and PprA. These proteins mediate Rec A-independent processes related to IR (Harris et al., 2004; Tanaka et al., 2004).
5.3 Radiation resistance mechanism against UV radiation
DNA damage caused by UV radiation is dependent on wavelength. UV-A (320 to 400 nm) induced only indirect damage to DNA, proteins, and lipids through ROS species intermediates. UV-B radiation (280 to 320 nm) and UV-C (100 to 280 nm) cause direct and indirect damage. The most common products formed by UV-B irradiation are cyclobutane pyrimidine dimers (CPD) (Mitchell and Karentz, 1993). Rr bacteria have numerous repair mechanisms in response to damage caused by UVR. These mechanisms are divided into photoreactivation and dark repair (DR). There are a total of three different dark repair mechanisms (i) nucleotide excision repair, (ii) error-prone repair, and (iii) postreplication recombinational repair (Fernández Zenoff et al., 2006).
6 Potentiality and diversity in applications of Rr bacteria
The Rr bacteria are under consideration in different domains (Figure 2). The extraordinary capabilities of Rr bacteria are defined by known features such as radiation resistance, regulation of ROS, antioxidation, DNA repair mechanism, efficient proteomic system, enzymes, and metabolites such as carotenoid pigments. These features of Rr bacteria are very effective in natural conditions. These natural traits of Rr bacteria can be manipulated and cloned by modern biotechnological approaches. Rr bacteria could be utilized for their importance in the pharmaceutical industry, lung disorders, chemotherapy, bioremediation, fermentation, radioactive waste management, and biodegradation of biomass to yield valued-added compounds (Kumar et al., 2010; Guan et al., 2017; Nayak et al., 2021; He et al., 2022).
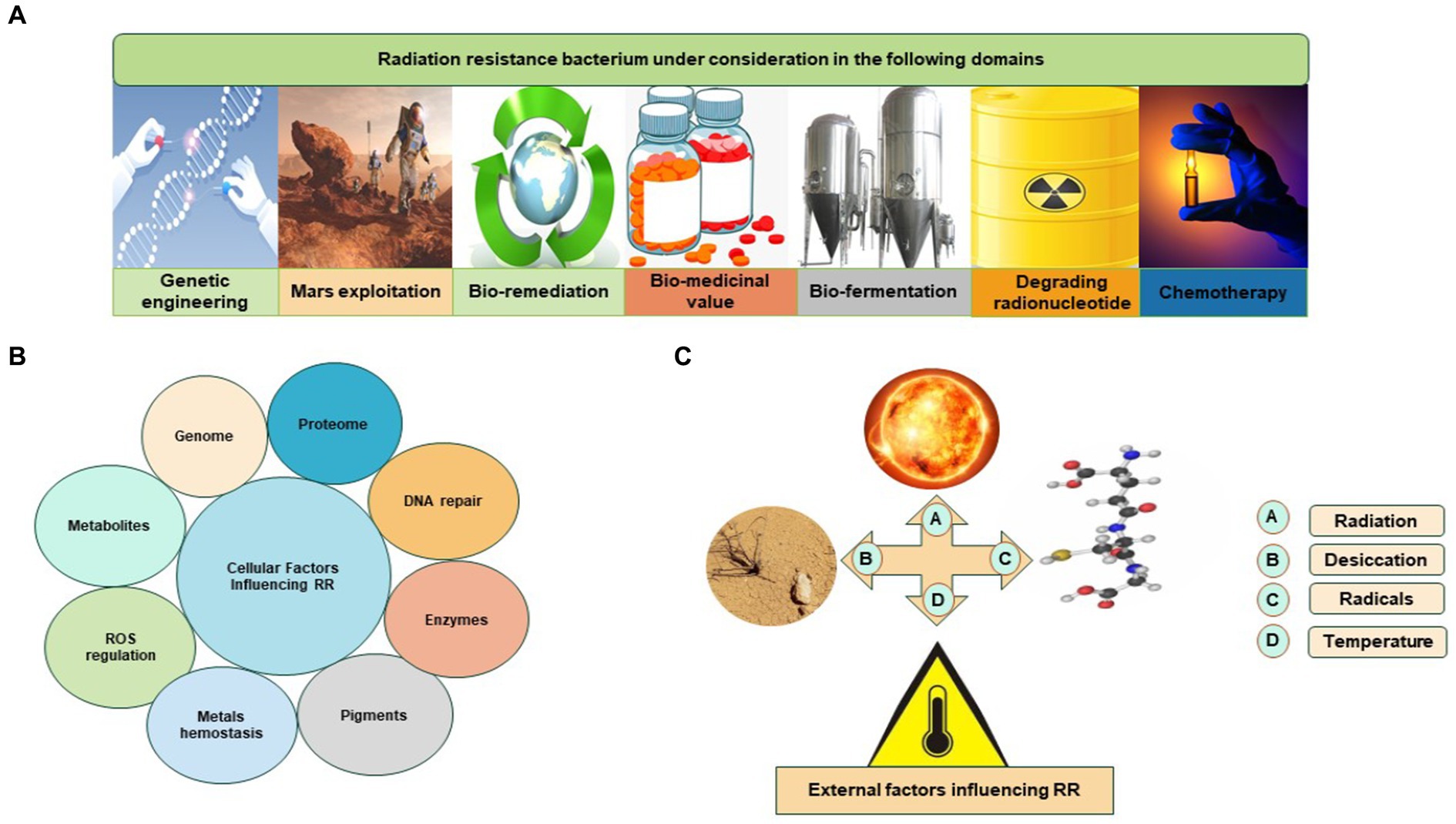
Figure 2. Rr bacteria is under different considerations: (A) Rr bacterium in various sectors; (B) cellular factors influencing Rr bacteria; (C) external factors influencing Rr bacteria.
6.1 Antioxidant system of Rr bacteria
The antioxidant system of Rr bacteria efficiently protects cells from internal and external stresses. The antioxidant system of Rr bacteria has both enzymatic and non-enzymatic pathways. The homeostasis of metal ions (Peana et al., 2018) further strengthens the antioxidant system of bacteria. Metal ions such as Cu2+, Mn2+, and Zn2+ are important to balance free radicals in bacterial cells exposed to UV-B radiation (Santos et al., 2013). Rr bacteria regulate intracellular Cu2+, Mn2+, and Zn2+. These ions reduce peroxide stress by blocking the Fenton and Haber–Weiss reactions (Bagwell et al., 2008; Daly et al., 2010). The cellular metabolism of Rr bacteria is least affected by environmental stresses with evolved antioxidant systems (McLean and McLean, 2010; Etemadifar et al., 2016). Rr bacteria with efficient antioxidant systems are less, vulnerable to protein oxidation, lipid peroxidation, and nucleic acid breaks with radiation, desiccation, and oxidation than radiosensitive bacteria (Sajjad et al., 2018).
6.2 Non-enzymatic antioxidants and their applications
Carotenoids are non-enzymatic antioxidants (Krinsky and Johnson, 2005; Bing Tian et al., 2007). Carotenoids are natural pigments, tetra-terpenoids including C40 hydrocarbon backbones (Carotenes) and their oxygenated derivatives xanthophylls. Carotenoids have a major role in radioresistance due to their efficient ROS scavenging ability (Yang, 2021). These ROS include hydrogen peroxide (H2O2) singlet molecular oxygen (1O2), -OH, O2¯, and RNS, such as 2,2-diphenyl-1-picrylhydrazyl (DPPH•) and nitric oxide (NO) (Tian et al., 2009). Microorganisms and plants manufacture carotenoids and have wide applications (Figure 3). D. radiodurans and other bacterial species synthesize different types of carotenoids (Krinsky and Johnson, 2005; Asker et al., 2012). The 13 genes of D. radiodurans are involved in the biosynthesis of red color carotenoids (Saito, 2011). However, bacteria produce carotenoids of different colors. The pink, yellow, and red color appearance of Rr isolates from the Taklamakan Desert is due to the production of carotenoid-like molecules (Yu et al., 2015). A unique carotenoid deinoxanthin efficiently scavenges O2¯and H2O2. Deionxanthin prevents protein oxidation at lower concentrations and has potent scavenging ability than lycopene and alpha-carotene (Zhang L. et al., 2007). These pigments and metabolites are responsible for the photoprotection of cells against UV light in deserts (Yu et al., 2015). Carotenoid pigments are significant to human health. They are efficient quenchers of reactive O2 and are linked to several diseases in humans, such as life-threatening cancer and other chronic disorders. The epidemiological data on carotenoid supplementation showed that ROS-mediated disorders are largely controlled by their consumption in a normal diet. Carotenoids regulate the balance of free radicals in a cell and are potential supplements for aging control, cancer prevention, and other beneficial aspects of human health (Burda, 2014; Rivera-Madrid et al., 2020).
6.3 Enzymatic antioxidants and curative measures of the lungs
Rr bacteria produce various enzymes, such as catalase (CAT) and superoxide dismutase (SOD), and some species also possess oxidases. These enzymes scavenge free radicals and ions. ROS detoxification is vital for the survival of the irradiated cell. Therefore, bacterial cells evolved to yield various enzymes to maintain the balance of ROS production and their removal. These enzymes are the first line of defense to protect microbial cells from radiation. D. radiodurans enzymatic antioxidants CAT and SOD are involved in the chemical conversion of ROS to less toxic substances (Markillie et al., 1999; Makarova et al., 2007).
Rr bacteria evolved with such efficient enzymatic pathways could, fortunately, serve as a supplement in the injured lung tissue. These enzymes can potentially detoxify harmful radicals caused by smoking or any other inhaled chemical inducer. A balance of oxidant and the antioxidant system is important in the cellular hemostasis of normal lung tissue, but physiological disturbance occurs when the antioxidant system fails to neutralize a high amount of ROS. These lung complications include chronic obstructive pulmonary disease (COPD) and asthma (Leibel and Post, 2016). Cigarette smoking causes nearly 90% of all lung cancer deaths and 80% of COPD, such as emphysema and chronic bronchitis. An innovative approach involving these enzymes is crucial to overcome lung disorders caused and worsened by a high amount of ROS. A competent Rr bacteria evolved with an enzymatic antioxidant system is important to control ROS toxicity in lung disorders, which might overcome radicals and prevent their entry into blood through air sacs and other body tissue. ROS induces oxidative damage to DNA, carbohydrates, lipids, and proteins, initiating an array of downstream processes promoting the progression of COPD in affected lungs. The genetic ability of Rr bacteria against oxidative damage could be engineered as probiotics supplementing to treat smoking lung disorders.
6.4 Pharmaceutical products
The extremolytes of Rr bacteria include mycosporine-like amino acids (MAAs), systonemin, bacterioruberin, ectoine, sphaerophorin, melanin, pannarin, and polyhydroxyalkanoates (PHA). These extremolytes reduce photodamage associated with UV radiation. The enhanced potential sunscreen ability of MAA is already commercialized and effectively utilized for skin care industries (De la Coba et al., 2009; Slaninova et al., 2018; He et al., 2022). Extremolytes such as asterina, palythine, palythene, and palythinol indicate anticancer and skin care products (Llewellyn and Airs, 2010). Scytonemin may act as an anti-inflammatory and antiproliferative drug to offer a novel pharmacophore for protein kinase inhibitors. Scytonemin has been shown as a competitive ATP inhibitor of Polo-like kinases (PLKs) (Murugan et al., 2011). PLKs have been the cancer target for many years because PLKs control many oncogenes. Previous studies have shown that scytonemin has a major role in hyperproliferative disorder because of its ability to inhibit PLK1 (Stevenson et al., 2002). Another research showed PLK1 inhibition mediated by scytonemin-induced apoptosis of cancer cell types such as osteosarcoma (Strebhardt and Ullrich, 2006; de Cárcer et al., 2007; Duan et al., 2010), suggesting that scytonemin is a promising anticancer agent. Similarly, ectoine also has therapeutic potential; for example, the human kidney keratinocytes irradiated with UV-A were effectively treated with ectoine. Ectoine has also been studied for skincare applications against desiccation, water loss, and UV damage. Due to water loss, ectoine is a prophylactic agent for dry skin (Buenger and Driller, 2004). Ectoine was also observed to prevent the damage associated with bacterial lipopolysaccharide (Buommino et al., 2005). Rubrobacter radiotolerans produce another compound, bacterioruberin, which prevents cancer and repairs the DNA breaks caused by IR in human cells.
6.5 Rr bacteria impact on cancer control
Oxidative stress is linked with cancer, a physiological disorder. Rr bacteria scavenge imbalanced radicals and potentially prevent protein oxidation and DNA damage. D. radiodurans could be a model bacteria for cancer control because of their resistance to extreme radiation levels, desiccation, and other oxidative stress conditions (Slade and Radman, 2011; Krisko and Radman, 2013; Qi et al., 2020). High levels of ROS promote disease pathological conditions. These ROS stimulate the apoptosis of cancer cells and are efficiently utilized as a chemotherapeutic tool (Li et al., 2011; Redza-Dutordoir and Averill-Bates, 2016). Recently, a study highlighted the role of crude secondary metabolite extract (CSME) of D. radiodurans in triple-negative human breast carcinoma MDA-MB-231 cells. CSME-induced ROS production encourages nuclear membrane alterations with apoptotic destruction of MDA-MB-231 cells. D. radiodurans CSME upregulates apoptotic marker expression in breast cancer chemotherapy (Maqbool et al., 2020). Further study is required to explore the role of CSME as a bioactive compound for cancer control on a molecular level.
6.6 Fermentation of organic waste
The Rr microbes that are utilized for the production of different useful compounds, for example, use the recombinant D. radiodurans for the fermentation of organic waste, particularly lignocellulosic biomass (Jiang et al., 2017) to produce value-added compounds. Using its extremophilic properties and resistance to many stresses, like heavy metal resistance, pollutants resistance, osmotic and acid stress, temperature, and the ability to resist DNA-damaging agents of this host will help in survival in fermentation along with a high expression of the desired pathway and high production. The engineering of this host for the pretreatment of lignocellulosic biomass can be coupled with physicochemical pre-treatments such as radiation and acid–alkali under different conditions (temperature, suspension pH, and nutrient availability).
6.7 Efficient expression system of Rr bacteria
Rr bacteria could also be utilized as an expression system to clone desired products. The deep genome study of D. radiodurans has provided insight into the genetic makeup for tolerating multiple stress conditions. The presence of different pathways and some unique genomics ability to uptake genetic material from outside has made this bacterium suitable for use in a different application through genetic engineering (Makarova et al., 2001). The introduction of novel bioremediation capabilities into D. radiodurans has been successfully achieved. Chromosomal integration and vector-based expression of foreign genes in trans have proved effective (Misra et al., 2012). Using such multiresistant microbes will help to overcome the limitations of the enzymatic processes, such as the high costs of the pure enzyme, the fragile nature of the enzyme, very narrow reaction conditions, the low half-life of the enzyme, and the need for exogenous cofactors. Instead of using regulators and genes from D. radiodurans (Pan et al., 2009), introducing the whole pathway in D. radiodurans can help increase the desired product’s expression in extreme conditions. Due to the remarkable DNA repair mechanism, engineering this host will help to design a process where other microbes are unable to survive due to the presence of DNA-damaging agents.
6.8 Energy conversion by Rr bacteria
Rr bacteria have distinctive morphological pigmentation. Rr bacteria abundantly display red, yellow, pink, orange, or simultaneous contrast such as red-orange or yellow-green characteristics colors. These features and colors are inclined from one trait to another (Kreusch and Duarte, 2021; Liu et al., 2022). Most of the reflected colors match the wavelength specified for the respective range of the visible light spectrum. This raises an interesting scientific query to investigate whether color rich diversity of the arid habitats evolved through the influence of different wavelengths of electromagnetic radiation (Figure 4). The characteristic color of bacteria is due to the reflected spectrum of radiation, which cannot be absorbed by a cell. Meanwhile, the absorbed radiation is a driving force for cellular processes, energy generation, and consumption from raised ROS species. A considerable amount of radiation also dissipates as thermal energy in the form of heat (Castillo et al., 2015; Makhneva et al., 2020). Bacteriochlorophylls (B) Chls and carotenoids are bound non-covalently to specific apoproteins, as the main light-harvesting and energy-transforming pigments of photosynthetic organisms. B Chls are vital components of the photochemical reaction centers and account for most of the antenna pigments in anoxygenic photosynthetic bacteria. Due to electromagnetic exposure, the excitation reaching the photochemical reaction centers of B Chls at the lowest singlet excited state causes initial charge separation. Thus, the conversion of light energy into electrochemical energy is initiated and ultimately provides a driving force for all essential processes in photosynthetic and heterotrophic organisms (Renger, 2007).
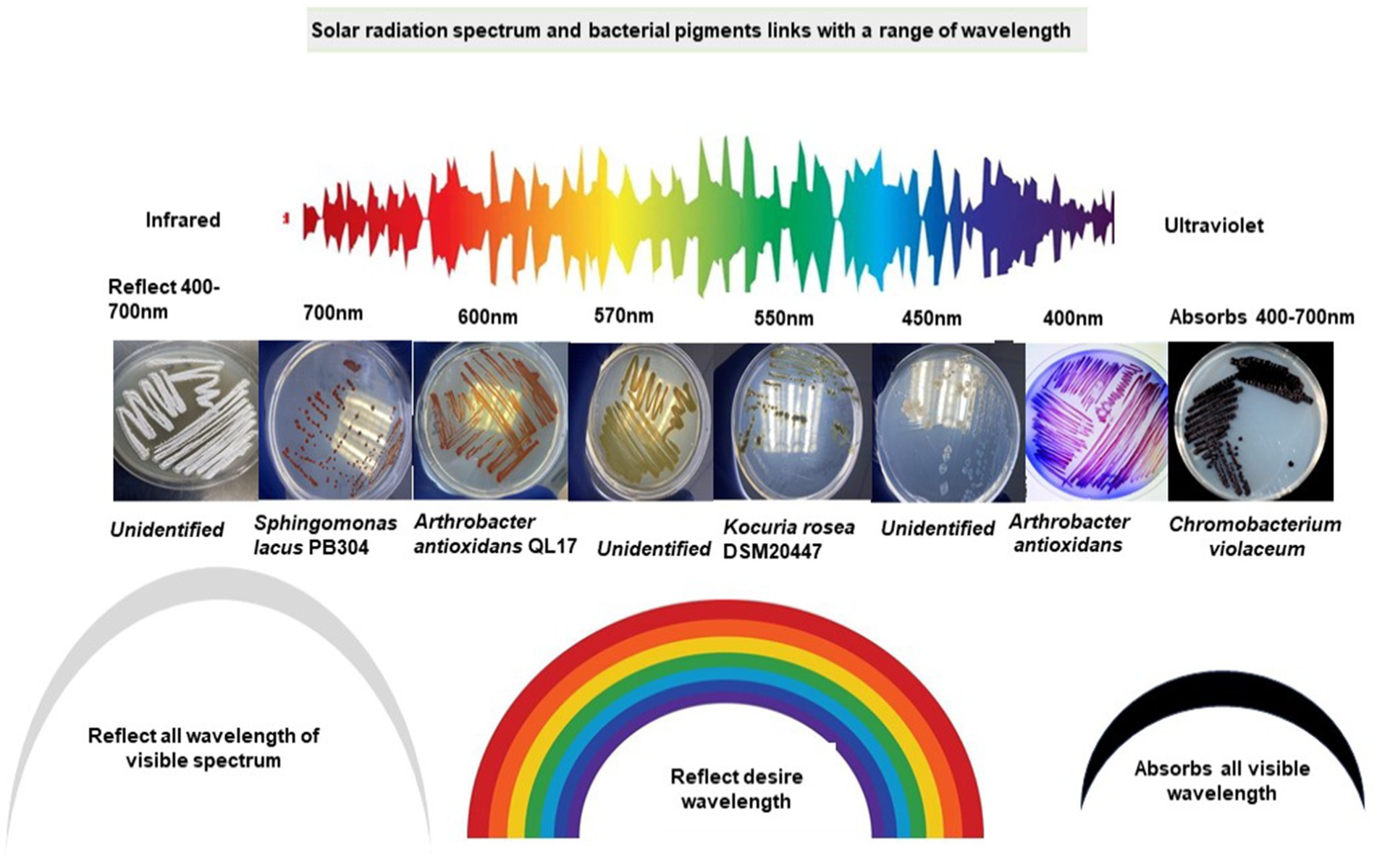
Figure 4. Visible spectrum of solar radiation and characteristics of bacteria pigments with a range of wavelengths.
6.9 Radioactive waste management through Rr bacteria
The world is concerned about discharging radioactive waste from anthropogenic sources before it is converted to less hazardous or fully non-hazardous form. Recently, alarming news about Fukushima tritium discharge in the marine ecosystem was circulated. This raised responsiveness about the risk to aquatic life and the food chain it disturbs. Reconsidering and global management of both natural and anthropogenic reservoirs of tritium discharge in the ocean and its impact on aquatic life is necessary. The insight of earlier studies has revealed the effects of the tritium on natural biota and the potential risk to human health it poses through the food chain (Galeriu et al., 2008; Melintescu and Galeriu, 2011; Zhao et al., 2021). Tritiated water has 99% of tritium, rapidly reaching the ocean through precipitation, river runoff, and evaporation (Liger et al., 2018; Oms et al., 2019). Three main reactors are located on the shore of Canada’s Great Lakes with a maximum level of tritium (8.4 Bq L − 1) greater than twofold as offshore waters (3.5 Bq L − 1). Non-nuclearized coastal rivers in France have a tritium concentration of 3 to 4 Bq L − 1, but in Rhone River, this concentration varied from 2.50 to 12.85 Bq L − 1 with a mean of 6.31 Bq L − 1, and this is because of high nuclear reactors (Jean-Baptiste et al., 2018). The prevalence of tritium in oceans and other contaminated sites is important to conserve aquatic biota and to reduce its potential risk to human health. This would not been possible without innovative ways. Tritium releases negative charge beta particles from radioactive decay and causes cellular damage if inhaled or ingested (Roch-Lefèvre et al., 2018). Humans acquire a considerable amount of organically bound tritium from aquatic biota, mainly through fishes that rely on zooplankton and phytoplankton for their food cycle. The evolved features of bacterial cells that receive and transform harmful charge radicals into a thermal spectrum are fascinating. This could be utilized to balance charge radicals produced through radioactive decay by introducing its consortium into radioactive sites. Rr bacteria could transform radioactive contents into a non-hazardous form or possibly energy exchange (Figure 5). Another approach is E. coli, and Saccharomyces cerevisiae are genetic engineering platforms of biotechnological applications. However, due to the specific ability to grow and express novel engineered functions in recent years, research has begun using D. radiodurans in biotechnologies (Basu, 2022) and bioremediation (Manobala et al., 2019). Toxic waste management is achieved by successful gene transfer, and expression is reported in D. radiodurans for bioremediation of nuclear radioactive and heavy metal-polluted environments (Shukla et al., 2017; Khan et al., 2021). Using D. radiodurans regulators in yeast and bacteria enhances its activity, and production is successful in fermentation. These IR-resistant bacteria have achieved their target for radioactive waste degradation and could be utilized for radioactive-contaminated sites (Pan et al., 2009; Ma et al., 2011).
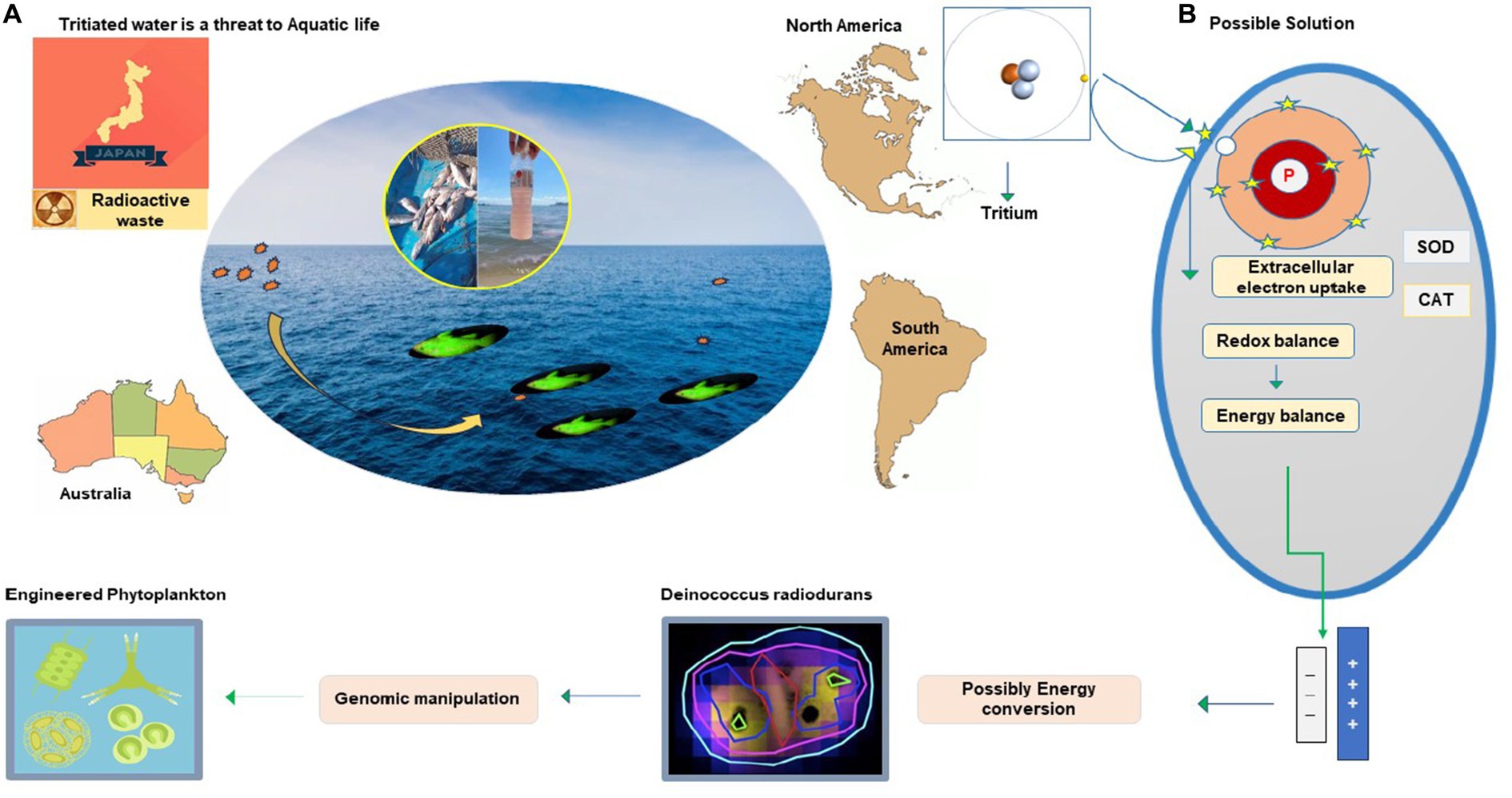
Figure 5. Radioactive waste degradation at the contaminated site by utilizing the antioxidant potential of Rr bacteria.
7 Gaps and future recommendations
ROS regulation and common defensive pathways in Rr bacteria help to understand the origin of resistance against radiation, desiccation, and oxidation. Rr- and Dr-resistant bacteria found in deserts are important for understanding ROS-associated toxicities in human pathological and physiological conditions. The features of the antioxidant system found in the Rr bacterium can be inserted in human disorders, which are known by a disturbance in the regulation of the redox cycle. Rr bacterium regulates a high level of ROS due to radiation exposure. The sensitivity of human cancer cells to radiation underscores the knowledge of the fact that if bacteria can resist radiation, could human cells also evade doses capable of causing damage? Due to its radio-tolerant nature, the Rr bacterium also opens opportunities for degrading radioactive waste. Rr bacterium is a possible solution to generate useful energy in response to incoming photon flux and a platform for producing novel antimicrobial agents and useful metabolites of commercial significance.
7.1 Strength of the review
This review article has mainly emphasized the origin, applications, and geographical distribution of Rr bacteria in extreme desert environments. Their by-products, such as enzymes and non-enzymatic antioxidants, were linked to disease pathological conditions. Desert environmental traits such as the photochemical production of ROS in desiccated soil and their impact on radiation resistance have been highlighted. The review article also summarized Rr bacteria from different geographical locations, and data were collected on their survival potential in varying intensities of electromagnetic radiation. Furthermore, the importance of Rr bacteria was evaluated in several sectors, of which microbial biotechnology, bioremediations, fermentation, the pharmaceutical industry, and biological therapy are mainly described.
7.2 Limitation of the review
Extensive research is further required to evaluate the intermediate role of ROS, desiccation, and radiation in influencing the abundance, diversity, and evolved capabilities of Rr bacteria. An antioxidant system of Rr bacteria must be carefully studied to investigate its role in cancer therapy, lung disorders, and the importance of bioremediation of radioactive waste.
8 Conclusion
The deserts of the world are rich in Rr and Dr. bacteria. Deserts are mostly desiccated and irradiated. These ecological factors are evidence of the rich diversity of radio-tolerant bacteria. The higher resistance against radiation, desiccation, and oxidation is more evident in the link between ROS species. ROS plays a central role in the origin of a common defensive system, such as an antioxidant system of bacteria against radiation and desiccation. Deserts are home to ROS due to photodesiccated soil. These ROS are evident in the adaptation of bacterial communities in deserts. The antioxidant system regulates cellular stresses by scavenging free radicals among the bacterial community, which inhibits them in deserts. A rise of ROS in the cell damages cellular components and breaks DNA molecules, causing protein oxidation, lipid peroxidation, and abnormality in metabolism. ROS species are mediums that link the toxicity of desiccation to radiation. ROS induction through these stresses also activates counter-responses, which include DNA repair enzymes, an efficient proteomic system, distinct pigmentation, and metabolic and anti-scavenging pathways that reduce toxicity to cellular contents. The similar targets of radiation and desiccation through ROS and the common protection mechanism of Rr bacteria to combat these stresses explained their coevolution. The Rr bacteria in deserts tend to possess more enzymes and extremolytes of biotechnological importance. Due to their resistant nature, Rr bacteria could be efficiently utilized as an expression system in gene cloning. E. coli and Saccharomyces cerevisiae are platforms of choice for genetic engineering and biotechnological applications. However, in recent years, due to the specific ability to grow and express novel engineered functions, research has begun using Rr bacteria in biotechnologies and bioremediation. Rr bacterial species appear to have a distinct pigmented appearance. Most bacterial species bear blue, red, green, and yellow appearances, and researchers have studied them as reliable sources of natural colorants. Furthermore, more microbial extracts of these species are tested for their antioxidant ability in biomedical research (Sajjad et al., 2020). These bacterial species absorb incoming radiations, dissipate a certain amount in the form of heat, and remain transformed into photoelectric, electronic, and chemical based on their consumption and cellular needs. Rr bacteria is worth exploring due to its importance in pharmaceutical companies, radioactive site decontamination, industrial economic interest, genetic engineering, and biotechnological products.
Author contributions
AK: Conceptualization, Visualization, Writing – original draft. GL: Formal analysis, Validation, Writing – review & editing. GZ: Conceptualization, Funding acquisition, Supervision, Validation, Writing – review & editing. XL: Formal analysis, Funding acquisition, Supervision, Validation, Visualization, Writing – review & editing.
Funding
The author(s) declare that financial support was received for the research, authorship, and/or publication of this article. This study was supported by the Third Xinjiang Scientific Expedition Program (2022xjkk1200), the West Light Foundation of the Chinese Academy of Sciences (xbzg-zdsys-202105), and Gansu Province Talent Project in 2024.
Conflict of interest
The authors declare that the research was conducted in the absence of any commercial or financial relationships that could be constructed as a potential conflict of interest.
Publisher’s note
All claims expressed in this article are solely those of the authors and do not necessarily represent those of their affiliated organizations, or those of the publisher, the editors and the reviewers. Any product that may be evaluated in this article, or claim that may be made by its manufacturer, is not guaranteed or endorsed by the publisher.
References
Asker, D., Awad, T. S., Beppu, T., and Ueda, K. (2012). Isolation, characterization, and diversity of novel radiotolerant carotenoid-producing bacteria. Microb. Caroten. Bact. Microalgae Methods Protoc. 892, 21–60. doi: 10.1007/978-1-61779-879-5_3
Atri, D., Kamenetskiy, M., May, M., Kalra, A., Castelblanco, A., and Quiñones-Camacho, A. (2022). Estimating the potential of ionizing radiation-induced radiolysis for microbial metabolism in terrestrial planets with rarefied atmospheres arXiv preprint arXiv:2207.14675.
Austin, A. T., and Vivanco, L. (2006). Plant litter decomposition in a semi-arid ecosystem controlled by photodegradation. Nature 442, 555–558. doi: 10.1038/nature05038
Azua-Bustos, A., Urrejola, C., and Vicuña, R. (2012). Life at the dry edge: microorganisms of the Atacama Desert. FEBS Lett. 586, 2939–2945. doi: 10.1016/j.febslet.2012.07.025
Bagwell, C., Milliken, C., Ghoshroy, S., and Blom, D. (2008). Intracellular copper accumulation enhances the growth of Kineococcus radiotolerans during chronic irradiation. Appl. Environ. Microbiol. 74, 1376–1384. doi: 10.1128/AEM.02175-07
Basu, B. (2022). The radiophiles of Deinococcaceae family: resourceful microbes for innovative biotechnological applications. Curr. Res. Microb. Sci. 3:100153. doi: 10.1016/j.crmicr.2022.100153
Battista, J. R. (1997). Against all odds: the survival strategies of Deinococcus radiodurans. Ann. Rev. Microbiol. 51, 203–224. doi: 10.1146/annurev.micro.51.1.203
Beblo-Vranesevic, K., Galinski, E. A., Rachel, R., Huber, H., and Rettberg, P. (2017). Influence of osmotic stress on desiccation and irradiation tolerance of (hyper)-thermophilic microorganisms. Arch. Microbiol. 199, 17–28. doi: 10.1007/s00203-016-1269-6
Belov, A. A., Cheptsov, V. S., and Vorobyova, E. A. (2018). Soil bacterial communities of Sahara and Gibson deserts: physiological and taxonomical characteristics. AIMS Microbiol. 4, 685–710. doi: 10.3934/microbiol.2018.4.685
Buenger, J., and Driller, H. (2004). Ectoin: an effective natural substance to prevent UVA-induced premature photoaging. Skin Pharmacol. Appl. Ski. Physiol. 17, 232–237. doi: 10.1159/000080216
Buommino, E., Schiraldi, C., Baroni, A., Paoletti, I., Lamberti, M., De Rosa, M., et al. (2005). Ectoine from halophilic microorganisms induces the expression of hsp70 and hsp70B′ in human keratinocytes modulating the proinflammatory response. Cell Stress Chaperones 10, 197–203. doi: 10.1379/CSC-101R.1
Burda, K. (2014). Potential role of carotenoids as antioksidants in human healihand disease. Nutrients 6, 466–488. doi: 10.3390/nu6020466
Castillo, H., Schoderbek, D., Dulal, S., Escobar, G., Wood, J., Nelson, R., et al. (2015). Stress induction in the bacteria Shewanella oneidensis and Deinococcus radiodurans in response to below-background ionizing radiation. Int. J. Radiat. Biol. 91, 749–756. doi: 10.3109/09553002.2015.1062571
Castillo, H., and Smith, G. B. (2017). Below-background ionizing radiation as an environmental cue for bacteria. Front. Microbiol. 8:177. doi: 10.3389/fmicb.2017.00177
Chanal, A., Chapon, V., Benzerara, K., Barakat, M., Christen, R., Achouak, W., et al. (2006). The desert of Tataouine: an extreme environment that hosts a wide diversity of microorganisms and radiotolerant bacteria. Environ. Microbiol. 8, 514–525. doi: 10.1111/j.1462-2920.2005.00921.x
Chandra, R., Venkata Mohan, S., Roberto, P.-S., Ritmann, B. E., and Cornejo, R. A. S. (2018). Biophotovoltaics: Conversion of light energy to bioelectricity through photosynthetic microbial fuel cell technology. Microbial Fuel Cell: A Bioelectrochemical System that Converts Waste to Watts, 373–387. doi: 10.1007/978-3-319-66793-5_19
Chaudhary, N., and Kumar, G. (2023). Mutagenic radiations: X-rays, ionizing particles, and ultraviolet. Biotechnologies and genetics in plant mutation breeding: 1: mutagenesis and crop improvement.
Chen, A., Hernandez-Vargas, J., Han, R., Cortazar-Martinez, O., Gonzalez, N., Patel, S., et al. (2021). Small RNAs as a new platform for tuning the biosynthesis of silver nanoparticles for enhanced material and functional properties. ACS Appl. Mater. Interfaces 13, 36769–36783. doi: 10.1021/acsami.1c07400
Cox, M. M., and Battista, J. R. (2005). Deinococcus radiodurans—the consummate survivor. Nat. Rev. Microbiol. 3, 882–892. doi: 10.1038/nrmicro1264
Daly, M. J. (2006). Modulating radiation resistance: insights based on defenses against reactive oxygen species in the radioresistant bacterium Deinococcus radiodurans. Clin. Lab. Med. 26, 491–504. doi: 10.1016/j.cll.2006.03.009
Daly, M. J. (2012). Death by protein damage in irradiated cells. DNA Repair 11, 12–21. doi: 10.1016/j.dnarep.2011.10.024
Daly, M. J., Gaidamakova, E. K., Matrosova, V. Y., Kiang, J. G., Fukumoto, R., Lee, D.-Y., et al. (2010). Small-molecule antioxidant proteome-shields in Deinococcus radiodurans. PLoS One 5:e12570. doi: 10.1371/journal.pone.0012570
Dartnell, L. R., Hunter, S. J., Lovell, K. V., Coates, A. J., and Ward, J. M. (2010). Low-temperature ionizing radiation resistance of Deinococcus radiodurans and Antarctic Dry Valley bacteria. Astrobiology 10, 717–732. doi: 10.1089/ast.2009.0439
de Cárcer, G., Perez de Castro, I., and Malumbres, M. (2007). Targeting cell cycle kinases for cancer therapy. Curr. Med. Chem. 14, 969–985. doi: 10.2174/092986707780362925
De Groot, A., Chapon, V., Servant, P., Christen, R., Saux, M. F.-L., Sommer, S., et al. (2005). Deinococcus deserti sp. nov., a gamma-radiation-tolerant bacterium isolated from the Sahara Desert. Int. J. Syst. Evol. Microbiol. 55, 2441–2446. doi: 10.1099/ijs.0.63717-0
De la Coba, F., Aguilera, J., De Galvez, M., Alvarez, M., Gallego, E., Figueroa, F., et al. (2009). Prevention of the ultraviolet effects on clinical and histopathological changes, as well as the heat shock protein-70 expression in mouse skin by topical application of algal UV-absorbing compounds. J. Dermatol. Sci. 55, 161–169. doi: 10.1016/j.jdermsci.2009.06.004
Duan, Z., Ji, D., Weinstein, E. J., Liu, X., Susa, M., Choy, E., et al. (2010). Lentiviral shRNA screen of human kinases identifies PLK1 as a potential therapeutic target for osteosarcoma. Cancer Lett. 293, 220–229. doi: 10.1016/j.canlet.2010.01.014
Etemadifar, Z., Gholami, M., and Derikvand, P. (2016). UV-resistant bacteria with multiple-stress tolerance isolated from desert areas in Iran. Geomicrobiol J. 33, 1–7. doi: 10.1080/01490451.2015.1063025
Fagliarone, C., Mosca, C., Ubaldi, I., Verseux, C., Baqué, M., Wilmotte, A., et al. (2017). Avoidance of protein oxidation correlates with the desiccation and radiation resistance of hot and cold desert strains of the cyanobacterium Chroococcidiopsis. Extremophiles 21, 981–991. doi: 10.1007/s00792-017-0957-8
Fernández Zenoff, V., Siñeriz, F., and Farias, M. E. (2006). Diverse responses to UV-B radiation and repair mechanisms of bacteria isolated from high-altitude aquatic environments. Appl. Environ. Microbiol. 72, 7857–7863. doi: 10.1128/AEM.01333-06
Gabani, P., and Singh, O. V. (2013). Radiation-resistant extremophiles and their potential in biotechnology and therapeutics. Appl. Microbiol. Biotechnol. 97, 993–1004. doi: 10.1007/s00253-012-4642-7
Galeriu, D., Davis, P., Raskob, W., and Melintescu, A. (2008). Recent progresses in tritium radioecology and dosimetry. Fusion Sci. Technol. 54, 237–242. doi: 10.13182/FST08-A1803
Georgiou, C. D., Sun, H. J., McKay, C. P., Grintzalis, K., Papapostolou, I., Zisimopoulos, D., et al. (2015). Evidence for photochemical production of reactive oxygen species in desert soils. Nat. Commun. 6:7100. doi: 10.1038/ncomms8100
Guan, N., Li, J., Shin, H.-D., Du, G., Chen, J., and Liu, L. (2017). Microbial response to environmental stresses: from fundamental mechanisms to practical applications. Appl. Microbiol. Biotechnol. 101, 3991–4008. doi: 10.1007/s00253-017-8264-y
Guesmi, S., Najjari, A., Pujic, P., Ghedira, K., Ouertani, R., Jabberi, M., et al. (2022). Roots of the xerophyte Panicum turgidum host a cohort of ionizing-radiation-resistant biotechnologically-valuable bacteria. Saudi J. Biolog. Sci. 29, 1260–1268. doi: 10.1016/j.sjbs.2021.09.020
Guesmi, S., Pujic, P., Nouioui, I., Dubost, A., Najjari, A., Ghedira, K., et al. (2021). Ionizing-radiation-resistant Kocuria rhizophila PT10 isolated from the Tunisian Sahara xerophyte Panicum turgidum: Polyphasic characterization and proteogenomic arsenal. Genomics 113, 317–330. doi: 10.1016/j.ygeno.2020.11.029
Halliwell, B., and Gutteridge, J. M. (2015). Free radicals in biology and medicine. Oxford: Oxford University Press.
Han, R., Fang, J., Jiang, J., Gaidamakova, E. K., Tkavc, R., Daly, M. J., et al. (2020). Signal recognition particle RNA contributes to oxidative stress response in Deinococcus radiodurans by modulating catalase localization. Front. Microbiol. 11:613571. doi: 10.3389/fmicb.2020.613571
Harris, D. R., Tanaka, M., Saveliev, S. V., Jolivet, E., Earl, A. M., Cox, M. M., et al. (2004). Preserving genome integrity: the DdrA protein of Deinococcus radiodurans R1. PLoS Biol. 2:e304. doi: 10.1371/journal.pbio.0020304
He, X., Xue, J., Shi, L., Kong, Y., Zhan, Q., Sun, Y., et al. (2022). Recent antioxidative nanomaterials toward wound dressing and disease treatment via ROS scavenging. Mater. Today Nano 17:100149. doi: 10.1016/j.mtnano.2021.100149
Hezbri, K., Ghodhbane-Gtari, F., Montero-Calasanz, M. D. C., Nouioui, I., Rohde, M., Spröer, C., et al. (2016). Geodermatophilus pulveris sp. nov., a gamma-radiation-resistant actinobacterium isolated from the Sahara desert. Int. J. Syst. Evol. Microbiol. 66, 3828–3834. doi: 10.1099/ijsem.0.001272
Hussain, F., Khan, I. U., Habib, N., Xian, W.-D., Hozzein, W. N., Zhang, Z.-D., et al. (2016). Deinococcus saudiensis sp. nov., isolated from desert. Int. J. Syst. Evol. Microbiol. 66, 5106–5111. doi: 10.1099/ijsem.0.001479
Imlay, J. A. (2013). The molecular mechanisms and physiological consequences of oxidative stress: lessons from a model bacterium. Nat. Rev. Microbiol. 11, 443–454. doi: 10.1038/nrmicro3032
Imlay, J. A. (2015). Diagnosing oxidative stress in bacteria: not as easy as you might think. Curr. Opin. Microbiol. 24, 124–131. doi: 10.1016/j.mib.2015.01.004
Ito, T., Kato, M., Toi, K., Shirakawa, T., Ikemoto, I., and Tokuda, T. (1985). Oxygen species adsorbed on ultraviolet-irradiated magnesium oxide. J. Chem. Soc. Faraday Trans. Phys. Chem. Condens. Phases 81, 2835–2844. doi: 10.1039/f19858102835
Jean-Baptiste, P., Fontugne, M., Fourré, E., Marang, L., Antonelli, C., Charmasson, S., et al. (2018). Tritium and radiocarbon levels in the Rhône river delta and along the French Mediterranean coastline. J. Environ. Radioact. 187, 53–64.
Jiang, L.-L., Zhou, J.-J., Quan, C.-S., and Xiu, Z.-L. (2017). Advances in industrial microbiome based on microbial consortium for biorefinery. Bioresour. Bioprocess. 4, 1–10. doi: 10.1186/s40643-017-0141-0
Kanekar, P. P., and Kanekar, S. P. (2022). Psychrophilic, Psychrotrophic, and Psychrotolerant microorganisms diversity and biotechnology of Extremophilic microorganisms from India. Divers. Biotechnol. Extremop. Microorgan. India Microorgan. Sustain., 215–249. doi: 10.1007/978-981-19-1573-4_7
Khan, A., Naeem, M., Bilal, M., Khan, A., Subhan, F., Ikram, M., et al. (2021). Assessing the physico-chemical parameters and some metals of underground water and associated soil in the arid and semiarid regions of Tank District, Khyber Pakhtunkhwa, Pakistan. Environ. Monit. Assess. 193:610. doi: 10.1007/s10661-021-09370-x
Kramer, D. M., and Evans, J. R. (2011). The importance of energy balance in improving photosynthetic productivity. Plant Physiol. 155, 70–78. doi: 10.1104/pp.110.166652
Krasin, F., and Hutchinson, F. (1977). Repair of DNA double-strand breaks in Escherichia coli, which requires recA function and the presence of a duplicate genome. J. Mol. Biol. 116, 81–98. doi: 10.1016/0022-2836(77)90120-6
Kreusch, M. G., and Duarte, R. T. D. (2021). Photoprotective compounds and radioresistance in pigmented and non-pigmented yeasts. Appl. Microbiol. Biotechnol. 105, 3521–3532. doi: 10.1007/s00253-021-11271-5
Krinsky, N. I., and Johnson, E. J. (2005). Carotenoid actions and their relation to health and disease. Mol. Asp. Med. 26, 459–516. doi: 10.1016/j.mam.2005.10.001
Krisko, A., and Radman, M. (2010). Protein damage and death by radiation in Escherichia coli and Deinococcus radiodurans. Proc. Natl. Acad. Sci. U.S.A. 107, 14373–14377. doi: 10.1073/pnas.1009312107
Krisko, A., and Radman, M. (2013). Biology of extreme radiation resistance: the way of Deinococcus radiodurans. Cold Spring Harb. Perspect. Biol. 5:a012765. doi: 10.1101/cshperspect.a012765
Kumar, V., Kumari, A., Pandey, M., and Sharma, M. (2022). Molecular mechanism of radio-resistance and heavy metal tolerance adaptation in microbes. Microb. Extremoz. B978, 275–293. doi: 10.1016/B978-0-12-822945-3.00003-8
Kumar, R., Patel, D. D., Bansal, D. D., Mishra, S., Mohammed, A., Arora, R., et al. (2010). Extremophiles: sustainable resource of natural compounds-extremolytes. Sustain. Biotechnol. Sourc. Renew. Ener. 3295, 279–294. doi: 10.1007/978-90-481-3295-9_15
Latifi, A., Ruiz, M., and Zhang, C.-C. (2009). Oxidative stress in cyanobacteria. FEMS Microbiol. Rev. 33, 258–278. doi: 10.1111/j.1574-6976.2008.00134.x
Leibel, S., and Post, M. (2016). Endogenous and exogenous stem/progenitor cells in the lung and their role in the pathogenesis and treatment of pediatric lung disease. Front. Pediatr. 4:36. doi: 10.3389/fped.2016.00036
Leszczynski, D. (2014). Radiation proteomics: a brief overview. Proteomics 14, 481–488. doi: 10.1002/pmic.201300390
Li, J., Gao, R., Chen, Y., Xue, D., Han, J., Wang, J., et al. (2020). Isolation and identification of Microvirga thermotolerans HR1, a novel thermo-tolerant bacterium, and comparative genomics among Microvirga species. Microorganisms 8:101. doi: 10.3390/microorganisms8010101
Li, Z.-Y., Yang, Y., Ming, M., and Liu, B. (2011). Mitochondrial ROS generation for regulation of autophagic pathways in cancer. Biochem. Biophys. Res. Commun. 414, 5–8. doi: 10.1016/j.bbrc.2011.09.046
Liger, K., Grisolia, C., Cristescu, I., Moreno, C., Malard, V., Coombs, D., et al. (2018). Overview of the TRANSAT (TRANSversal Actions for Tritium) project. Fusion Eng. Des. 136, 168–172.
Liu, Y., Chen, T., Li, J., Wu, M., Liu, G., Zhang, W., et al. (2022). High proportions of radiation-resistant strains in Culturable bacteria from the Taklimakan Desert. Biology 11:501. doi: 10.3390/biology11040501
Liu, M., Dai, J., Liu, Y., Cai, F., Wang, Y., Rahman, E., et al. (2011). Desertibacter roseus gen. Nov., sp. nov., a gamma radiation-resistant bacterium in the family Rhodospirillaceae, isolated from desert sand. Int. J. Syst. Evol. Microbiol. 61, 1109–1113. doi: 10.1099/ijs.0.021246-0
Liu, Z., Kim, M. C., Wang, L., Zhu, G., Zhang, Y., Huang, Y., et al. (2017). Deinococcus taklimakanensis sp. nov., isolated from desert soil. Int. J. Syst. Evol. Microbiol. 67, 4311–4316. doi: 10.1099/ijsem.0.002168
Llewellyn, C. A., and Airs, R. L. (2010). Distribution and abundance of MAAs in 33 species of microalgae across 13 classes. Mar. Drugs 8, 1273–1291. doi: 10.3390/md8041273
Luo, X., Zeng, X.-C., He, Z., Lu, X., Yuan, J., Shi, J., et al. (2014). Isolation and characterization of a radiation-resistant bacterium from Taklamakan Desert showing potent ability to accumulate Lead (II) and considerable potential for bioremediation of radioactive wastes. Ecotoxicology 23, 1915–1921. doi: 10.1007/s10646-014-1325-4
Ma, R., Zhang, Y., Hong, H., Lu, W., Lin, M., Chen, M., et al. (2011). Improved osmotic tolerance and ethanol production of ethanologenic Escherichia coli by IrrE, a global regulator of radiation-resistance of Deinococcus radiodurans. Curr. Microbiol. 62, 659–664. doi: 10.1007/s00284-010-9759-2
Makarova, K. S., Aravind, L., Wolf, Y. I., Tatusov, R. L., Minton, K. W., Koonin, E. V., et al. (2001). Genome of the extremely radiation-resistant bacterium Deinococcus radiodurans viewed from the perspective of comparative genomics. Microbiol. Mol. Biol. Rev. 65, 44–79. doi: 10.1128/MMBR.65.1.44-79.2001
Makarova, K. S., Omelchenko, M. V., Gaidamakova, E. K., Matrosova, V. Y., Vasilenko, A., Zhai, M., et al. (2007). Deinococcus geothermalis: the pool of extreme radiation resistance genes shrinks. PLoS One 2:e955. doi: 10.1371/journal.pone.0000955
Makhneva, Z., Ashikhmin, A., Bolshakov, M., and Moskalenko, A. (2020). Carotenoids are probably involved in singlet oxygen generation in the membranes of purple photosynthetic bacteria under light irradiation. Microbiology 89, 164–173. doi: 10.1134/S0026261720010099
Manobala, T., Shukla, S. K., Subba Rao, T., and Dharmendira Kumar, M. (2019). A new uranium bioremediation approach using radio-tolerant Deinococcus radiodurans biofilm. J. Biosci. 44, 1–9. doi: 10.1007/s12038-019-9942-y
Maqbool, I., Sudharsan, M., Kanimozhi, G., Alrashood, S. T., Khan, H. A., and Prasad, N. R. (2020). Crude cell-free extract from Deinococcus radiodurans exhibit anticancer activity by inducing apoptosis in triple-negative breast cancer cells. Front. Cell Dev. Biol. 8:707. doi: 10.3389/fcell.2020.00707
Markillie, L. M., Varnum, S. M., Hradecky, P., and Wong, K.-K. (1999). Targeted mutagenesis by duplication insertion in the radioresistant bacterium Deinococcus radiodurans: radiation sensitivities of catalase (katA) and superoxide dismutase (sodA) mutants. J. Bacteriol. 181, 666–669. doi: 10.1128/JB.181.2.666-669.1999
Maróti, P., Kovács, I. A., Kis, M., Smart, J. L., and Iglói, F. (2020). Correlated clusters of closed reaction centers during induction of intact cells of photosynthetic bacteria. Sci. Rep. 10:14012. doi: 10.1038/s41598-020-70966-3
Marszalkowski, M., Werner, A., Feltens, R., Helmecke, D., Gößringer, M., Westhof, E., et al. (2021). Comparative study on tertiary contacts and folding of RNase P RNAs from a psychrophilic, a mesophilic/radiation-resistant, and a thermophilic bacterium. RNA 27, 1204–1219. doi: 10.1261/rna.078735.121
Mba, C., Afza, R., and Shu, Q. (2012). Mutagenic radiations: X-rays, ionizing particles and ultraviolet plant mutation breeding and biotechnology. Wallingford UK: CABI.
McCalley, C. K., and Sparks, J. P. (2009). Abiotic gas formation drives nitrogen loss from a desert ecosystem. Science 326, 837–840. doi: 10.1126/science.1178984
McLean, R., and McLean, M. (2010). Microbial survival mechanisms and the interplanetary transfer of life through space. J. Cosmol. 7, 1802–1820.
Melintescu, A., and Galeriu, D. (2011). Dynamic model for tritium transfer in an aquatic food chain. Radiat. Environ. Biophys. 50, 459–473. doi: 10.1007/s00411-011-0362-0
Mendes-Silva, T. D. C. D., da Silva Andrade, R. F., Ootani, M. A., Mendes, P. V. D., Da Silva, M. R. F., Souza, K. S., et al. (2020). Biotechnological potential of carotenoids produced by extremophilic microorganisms and application prospects for the cosmetics industry. Adv. Microbiol. 10, 397–410. doi: 10.4236/aim.2020.108029
Misra, C. S., Appukuttan, D., Kantamreddi, V. S. S., Rao, A. S., and Apte, S. K. (2012). Recombinant D. radiodurans cells for bioremediation of heavy metals from acidic/neutral aqueous wastes. Bioengineered 3, 44–48. doi: 10.4161/bbug.3.1.18878
Mitchell, D. L., and Karentz, D. (1993). The induction and repair of DNA photodamage in the environment. Environ. UV Photobiol., 345–377. doi: 10.1007/978-1-4899-2406-3_12
Mohseni, M., Abbaszadeh, J., and Nasrollahi Omran, A. (2014). Radiation resistant of native Deinococcus spp. isolated from the lout desert of Iran “the hottest place on earth”. Int. J. Environ. Sci. Technol. 11, 1939–1946. doi: 10.1007/s13762-014-0643-7
Mortimer, R. K. (1958). Radiobiological and genetic studies on a polyploid series (haploid to hexaploid) of Saccharomyces cerevisiae. Radiat. Res. 9, 312–326. doi: 10.2307/3570795
Murugan, R. N., Park, J.-E., Kim, E.-H., Shin, S. Y., Cheong, C., Lee, K. S., et al. (2011). Plk1-targeted small molecule inhibitors: molecular basis for their potency and specificity. Mol. Cells 32, 209–220. doi: 10.1007/s10059-011-0126-3
Musilova, M., Wright, G., Ward, J. M., and Dartnell, L. R. (2015). Isolation of radiation-resistant bacteria from Mars analog Antarctic dry valleys by preselection, and the correlation between radiation and desiccation resistance. Astrobiology 15, 1076–1090. doi: 10.1089/ast.2014.1278
Nayak, T., Sengupta, I., and Dhal, P. K. (2021). A new era of radiation resistance bacteria in bioremediation and production of bioactive compounds with therapeutic potential and other aspects: An in-perspective review. J. Environ. Radioact. 237:106696. doi: 10.1016/j.jenvrad.2021.106696
Oms, P. E., Du Bois, P. B., Dumas, F., Lazure, P., Morillon, M., Voiseux, C., et al. (2019). Inventory and distribution of tritium in the oceans in 2016. Sci. Total Environ. 656, 1289–1303.
Orellana, G., Gómez-Silva, B., Urrutia, M., and Galetović, A. (2020). UV-A irradiation increases scytonemin biosynthesis in cyanobacteria inhabiting halites at Salar Grande, Atacama Desert. Microorganisms 8:1690. doi: 10.3390/microorganisms8111690
Pan, J., Wang, J., Zhou, Z., Yan, Y., Zhang, W., Lu, W., et al. (2009). IrrE, a global regulator of extreme radiation resistance in Deinococcus radiodurans, enhances salt tolerance in Escherichia coli and Brassica napus. PLoS One 4:e4422. doi: 10.1371/journal.pone.0004422
Paulino-Lima, I. G., Azua-Bustos, A., Vicuña, R., González-Silva, C., Salas, L., Teixeira, L., et al. (2013). Isolation of UVC-tolerant bacteria from the hyperarid Atacama Desert, Chile. Microb. Ecol. 65, 325–335. doi: 10.1007/s00248-012-0121-z
Peana, M., Chasapis, C., Simula, G., Medici, S., and Zoroddu, M. (2018). A model for manganese interaction with Deinococcus radiodurans proteome network involved in ROS response and defense. J. Trace Elem. Med. Biol. 50, 465–473. doi: 10.1016/j.jtemb.2018.02.001
Peng, F., Zhang, L., Luo, X., Dai, J., An, H., Tang, Y., et al. (2009). Deinococcus xinjiangensis sp. nov., isolated from desert soil. Int. J. Syst. Evol. Microbiol. 59, 709–713. doi: 10.1099/ijs.0.004564-0
Qi, H.-Z., Wang, W.-Z., He, J.-Y., Ma, Y., Xiao, F.-Z., and He, S.-Y. (2020). Antioxidative system of Deinococcus radiodurans. Res. Microbiol. 171, 45–54. doi: 10.1016/j.resmic.2019.11.002
Rai, S. N., and Dutta, T. (2024). A novel ionizing radiation-induced small RNA, DrsS, promotes the detoxification of reactive oxygen species in Deinococcus radiodurans. Appl. Environ. Microbiol. 90, e01538–e01523. doi: 10.1128/aem.01538-23
Rainey, F. A., Ferreira, M., Nobre, M. F., Ray, K., Bagaley, D., Earl, A. M., et al. (2007). Deinococcus peraridilitoris sp. nov., isolated from a coastal desert. Int. J. Syst. Evol. Microbiol. 57, 1408–1412. doi: 10.1099/ijs.0.64956-0
Rainey, F. A., Ray, K., Ferreira, M., Gatz, B. Z., Nobre, M. F., Bagaley, D., et al. (2005). Extensive diversity of ionizing-radiation-resistant bacteria recovered from Sonoran Desert soil and description of nine new species of the genus Deinococcus obtained from a single soil sample. Appl. Environ. Microbiol. 71, 5225–5235. doi: 10.1128/AEM.71.9.5225-5235.2005
Ranawat, P., and Rawat, S. (2017). Radiation resistance in thermophiles: mechanisms and applications. World J. Microbiol. Biotechnol. 33, 1–22. doi: 10.1007/s11274-017-2279-5
Redza-Dutordoir, M., and Averill-Bates, D. (2016). Biochim Biophys Acta-Mol. Cell Res. 1863, 2977–2992.
Renger, G. (ed.). (2007). Primary processes of photosynthesis: Principles and apparatus. (Vol. 8). Royal Society of Chemistry.
Rivera-Madrid, R., Carballo-Uicab, V. M., Cárdenas-Conejo, Y., Aguilar-Espinosa, M., and Siva, R. (2020). Overview of carotenoids and beneficial effects on human health. Carotenoids Proper. Process. Appl., 1–40.
Roch-Lefèvre, S., Grégoire, E., Martin-Bodiot, C., Flegal, M., Fréneau, A., Blimkie, M., et al. (2018). Cytogenetic damage analysis in mice chronically exposed to low-dose internal tritium beta-particle radiation. Oncotarget 9, 27397–27411. doi: 10.18632/oncotarget.25282
Saito, T. (2011). The red pigments contained in the radioresistant bacteria and the radioresistant mechanism of these bacteria.
Sajjad, W., Ahmad, M., Khan, S., Ilyas, S., Hasan, F., Celik, C., et al. (2017). Radio-protective and antioxidative activities of astaxanthin from newly isolated radio-resistant bacterium Deinococcus sp. strain WMA-LM9. Ann. Microbiol. 67, 443–455. doi: 10.1007/s13213-017-1269-z
Sajjad, W., Din, G., Rafiq, M., Iqbal, A., Khan, S., Zada, S., et al. (2020). Pigment production by cold-adapted bacteria and fungi: colorful tale of cryosphere with wide range applications. Extremophiles 24, 447–473. doi: 10.1007/s00792-020-01180-2
Sajjad, W., Khan, S., Ahmad, M., Rafiq, M., Badshah, M., Zada, S., et al. (2018). Effects of ultra-violet radiation on cellular proteins and lipids of radioresistant bacteria isolated from desert soil. Folia Biologica (Kraków) 66, 41–52. doi: 10.3409/fb_66-1.05
Santos, A. L., Gomes, N. C., Henriques, I., Almeida, A., Correia, A., and Cunha, A. (2013). Role of transition metals in UV-B-induced damage to Bacteria. Photochem. Photobiol. 89, 640–648. doi: 10.1111/php.12049
Schieber, M., and Chandel, N. S. (2014). ROS function in redox signaling and oxidative stress. Curr. Biol. 24, R453–R462. doi: 10.1016/j.cub.2014.03.034
Schneider, J., Matsuoka, M., Takeuchi, M., Zhang, J., Horiuchi, Y., Anpo, M., et al. (2014). Understanding TiO2 photocatalysis: mechanisms and materials. Chem. Rev. 114, 9919–9986. doi: 10.1021/cr5001892
Schulze-Makuch, D., Airo, A., and Schirmack, J. (2017). The adaptability of life on earth and the diversity of planetary habitats. Front. Microbiol. 8:2011. doi: 10.3389/fmicb.2017.02011
Sghaier, H. (2011). DNA repair: lessons from the evolution of ionizing- radiation-resistant prokaryotes – fact and theory. Select. Top. DNA Repair, 145–156. doi: 10.5772/22312
Shirsalimian, M., Akhavan Sepahy, A., Amoozegar, M., Kalantar, S., and Dabbagh, R. (2018). Isolation of two radiation resistant and desiccation tolerant bacteria, Modestobacter sp. A2 and maritalea sp. B9, from gandom beryan hill in the lut desert of Iran. Microbiology 87, 363–371. doi: 10.1134/S0026261718030104
Shukla, M., Chaturvedi, R., Tamhane, D., Vyas, P., Archana, G., Apte, S., et al. (2007). Multiple-stress tolerance of ionizing radiation-resistant bacterial isolates obtained from various habitats: correlation between stresses. Curr. Microbiol. 54, 142–148. doi: 10.1007/s00284-006-0311-3
Shukla, A., Parmar, P., and Saraf, M. (2017). Radiation, radionuclides and bacteria: An in-perspective review. J. Environ. Radioact. 180, 27–35. doi: 10.1016/j.jenvrad.2017.09.013
Shukla, S. K., and Subba Rao, T. (2017). The first recorded incidence of Deinococcus radiodurans R1 biofilm formation and its implications in heavy metals bioremediation. BioRxiv 234–781.
Singh, H. (2018). Desiccation and radiation stress tolerance in cyanobacteria. J. Basic Microbiol. 58, 813–826. doi: 10.1002/jobm.201800216
Singh, O. V., and Gabani, P. (2011). Extremophiles: radiation resistance microbial reserves and therapeutic implications. J. Appl. Microbiol. 110, 851–861. doi: 10.1111/j.1365-2672.2011.04971.x
Slade, D., and Radman, M. (2011). Oxidative stress resistance in Deinococcus radiodurans. Microbiol. Mol. Biol. Rev. 75, 133–191. doi: 10.1128/MMBR.00015-10
Slaninova, E., Sedlacek, P., Mravec, F., Mullerova, L., Samek, O., Koller, M., et al. (2018). Light scattering on PHA granules protects bacterial cells against the harmful effects of UV radiation. Appl. Microbiol. Biotechnol. 102, 1923–1931. doi: 10.1007/s00253-018-8760-8
Stevenson, C. S., Capper, E. A., Roshak, A. K., Marquez, B., Eichman, C., Jackson, J. R., et al. (2002). The identification and characterization of the marine natural product scytonemin as a novel antiproliferative pharmacophore. J. Pharmacol. Exp. Ther. 303, 858–866. doi: 10.1124/jpet.102.036350
Strebhardt, K., and Ullrich, A. (2006). Targeting polo-like kinase 1 for cancer therapy. Nat. Rev. Cancer 6, 321–330. doi: 10.1038/nrc1841
Tanaka, M., Earl, A. M., Howell, H. A., Park, M.-J., Eisen, J. A., Peterson, S. N., et al. (2004). Analysis of Deinococcus radiodurans's transcriptional response to ionizing radiation and desiccation reveals novel proteins that contribute to extreme radioresistance. Genetics 168, 21–33. doi: 10.1534/genetics.104.029249
Tian, B., Sun, Z., Shen, S., Wang, H., Jiao, J., Wang, L., et al. (2009). Effects of carotenoids from Deinococcus radiodurans on protein oxidation. Lett. Appl. Microbiol. 49, 689–694. doi: 10.1111/j.1472-765X.2009.02727.x
Tian, B., Wu, Y., Sheng, D., Zheng, Z., Gao, G., and Hua, Y. (2004). Chemiluminescence assay for reactive oxygen species scavenging activities and inhibition on oxidative damage of DNA in Deinococcus radiodurans. Lumin. J. Biol. Chem. Lumin. 19, 78–84. doi: 10.1002/bio.761
Tian, B., Xu, Z., Sun, Z., Lin, J., and Hua, Y. (2007). Evaluation of the antioxidant effects of carotenoids from Deinococcus radiodurans through targeted mutagenesis, chemiluminescence, and DNA damage analyses. Biochimica et Biophysica Acta (BBA)-General Subjects 1770, 902–911. doi: 10.1016/j.bbagen.2007.01.016
Tiquia-Arashiro, S., and Rodrigues, D. F. (2016). Extremophiles: applications in nanotechnology. Springer.
Villa, J. K., Han, R., Tsai, C.-H., Chen, A., Sweet, P., Franco, G., et al. (2021). A small RNA regulates pprM, a modulator of pleiotropic proteins promoting DNA repair, in Deinococcus radiodurans under ionizing radiation. Sci. Rep. 11:12949. doi: 10.1038/s41598-021-91335-8
Wang, P., and Schellhorn, H. E. (1995). Induction of resistance to hydrogen peroxide and radiation in Deinococcus radiodurans. Can. J. Microbiol. 41, 170–176. doi: 10.1139/m95-023
Yang, Q. (2021). Crucial roles of carotenoids as bacterial endogenous defense system for bacterial radioresistance of Deinococcus radiodurans. BioRxiv 2021:811.
Yoboue, E. D., Mougeolle, A., Kaiser, L., Averet, N., Rigoulet, M., and Devin, A. (2014). The role of mitochondrial biogenesis and ROS in the control of energy supply in proliferating cells. Biochimica et Biophysica Acta (BBA)-Bioenergetics 1837, 1093–1098. doi: 10.1016/j.bbabio.2014.02.023
Yu, L. Z. H., Luo, X. S., Liu, M., and Huang, Q. (2015). Diversity of ionizing radiation-resistant bacteria obtained from the Taklimakan Desert. J. Basic Microbiol. 55, 135–140. doi: 10.1002/jobm.201300390
Yuan, M., Zhang, W., Dai, S., Wu, J., Wang, Y., Tao, T., et al. (2009). Deinococcus gobiensis sp. nov., an extremely radiation-resistant bacterium. Int. J. Syst. Evol. Microbiol. 59, 1513–1517. doi: 10.1099/ijs.0.004523-0
Zhang, Q., Liu, C., Tang, Y., Zhou, G., Shen, P., Fang, C., et al. (2007). Hymenobacter xinjiangensis sp. nov., a radiation-resistant bacterium isolated from the desert of Xinjiang, China. Int. J. Syst. Evol. Microbiol. 57, 1752–1756. doi: 10.1099/ijs.0.65033-0
Zhang, L., Yang, Q., Luo, X., Fang, C., Zhang, Q., and Tang, Y. (2007). Knockout of crtB or crtI gene blocks the carotenoid biosynthetic pathway in Deinococcus radiodurans R1 and influences its resistance to oxidative DNA-damaging agents due to change of free radicals scavenging ability. Arch. Microbiol. 188, 411–419. doi: 10.1007/s00203-007-0262-5
Zhao, C., Wang, G., Zhang, M., Wang, G., de With, G., Bezhenar, R., et al. (2021). Transport and dispersion of tritium from the radioactive water of the Fukushima Daiichi nuclear plant. Mar. Pollut. Bull. 169:112515. doi: 10.1016/j.marpolbul.2021.112515
Keywords: desert, radiation, ROS species, antioxidant, environmental stresses, applications
Citation: Khan A, Liu G, Zhang G and Li X (2024) Radiation-resistant bacteria in desiccated soil and their potentiality in applied sciences. Front. Microbiol. 15:1348758. doi: 10.3389/fmicb.2024.1348758
Edited by:
Philippe M. Oger, UMR5240 Microbiologie, Adaptation et Pathogenie (MAP), FranceReviewed by:
Xuedong Luo, Hashing Agricultural University, ChinaRunhua Han, University of Manitoba, Canada
Copyright © 2024 Khan, Liu, Zhang and Li. This is an open-access article distributed under the terms of the Creative Commons Attribution License (CC BY). The use, distribution or reproduction in other forums is permitted, provided the original author(s) and the copyright owner(s) are credited and that the original publication in this journal is cited, in accordance with accepted academic practice. No use, distribution or reproduction is permitted which does not comply with these terms.
*Correspondence: Gaosen Zhang, gaosenzhang@hotmail.com; Xiangkai Li, xkli@lzu.edu.cn