- 1Serviço de Doenças Infeciosas, Centro Hospitalar Universitário Lisboa Norte EPE, Lisbon, Portugal
- 2Clínica Universitária de Doenças Infeciosas, Faculdade de Medicina da Universidade de Lisboa, Lisbon, Portugal
- 3Instituto de Farmacologia e Neurociências, Faculdade de Medicina da Universidade de Lisboa, Lisbon, Portugal
- 4Laboratório de Microbiologia na Saúde Ambiental, Laboratório Associado TERRA, Instituto de Saúde Ambiental, Faculdade de Medicina da Universidade de Lisboa, Lisbon, Portugal
- 5Unidade Local do Programa de Prevenção e Controlo de Infeções e das Resistências aos Antimicrobianos, Centro Hospitalar Universitário Lisboa Norte EPE, Lisbon, Portugal
- 6Serviço de Patologia Clínica, Centro Hospitalar Universitário Lisboa Norte EPE, Lisbon, Portugal
- 7Instituto de Microbiologia, Faculdade de Medicina da Universidade de Lisboa, Lisbon, Portugal
- 8Egas Moniz Center for Interdisciplinary Research (CiiEM), Egas Moniz School of Health and Science, Monte da Caparica, Portugal
- 9Instituto de Medicina Preventiva e Saúde Pública, Faculdade de Medicina da Universidade de Lisboa, Lisbon, Portugal
Introduction: Extensively drug-resistant Pseudomonas aeruginosa (XDR-PA) is a growing concern due to its increasing incidence, limited therapeutic options, limited data on the optimal treatment, and high mortality rates. The study aimed to characterize the population, the outcome and the microbiological characteristics of XDR-PA identified in a Portuguese university hospital center.
Methods: All XDR-PA isolates between January 2019 and December 2021 were identified. XDR-PA was defined as resistance to piperacillin-tazobactam, third and fourth generation cephalosporins, carbapenems, aminoglycosides and fluoroquinolones. A retrospective analysis of the medical records was performed.
Results: One hundred seventy-eight individual episodes among 130 patients with XDR-PA detection were identified. The most common sources of infection were respiratory (32%) and urinary tracts (30%), although skin and soft tissue infections (18%) and primary bacteremia (14%) were also prevalent. Colonization was admitted in 64 cases. Several patients had risk factors for complicated infections, most notably immunosuppression, structural lung abnormalities, major surgery, hemodialysis or foreign intravascular or urinary devices. XDR-PA identification was more frequent in male patients with an average age of 64.3 ± 17.5 years. One non-susceptibility to colistin was reported. Only 12.4% were susceptible to aztreonam. Ceftazidime-avibactam (CZA) was susceptible in 71.5% of the tested isolates. Ceftolozane-tazobactam (C/T) was susceptible in 77.5% of the tested isolates. Antibiotic regimens with XDR-PA coverage were reserved for patients with declared infection, except to cystic fibrosis. The most frequently administered antibiotics were colistin (41 cases), CZA (39 cases), and C/T (16 cases). When combination therapy was used, CZA plus colistin was preferred. The global mortality rate among infected patients was 35.1%, significantly higher in those with hematologic malignancy (50.0%, p < 0.05), followed by the ones with bacteremia (44.4%, p < 0.05) and those medicated with colistin (39.0%, p < 0.05), especially the ones with respiratory infections (60.0%). Among patients treated with CZA or C/T, the mortality rate seemed to be lower.
Discussion: XDR-PA infections can be severe and difficult to treat, with a high mortality rate. Even though colistin seems to be a viable option, it is likely less safe and efficient than CZA and C/T. To the best of the authors’ knowledge, this is the first description of the clinical infection characteristics and treatment of XDR-PA in Portugal.
1 Introduction
Extensively drug resistant Pseudomonas aeruginosa (XDR-PA) is a growing concern due to its increasing incidence, limited therapeutic options, limited data on the optimal treatment, and high mortality rates. Being able to survive in many ecological settings, P. aeruginosa is highly flexible and can adapt to a wide array of environmental pressures. Having access to numerous metabolic pathways and a wide range of virulence and resistance factors thanks to its genetic plasticity, make it one of the most successful bacteria in Medical Microbiology (Behzadi et al., 2022a,b).
Indeed, although in the late 20th century the incidence of P. aeruginosa bloodstream infections was declining, by 2010 it had increased again to 6.5 per 10,000 (Werth et al., 2015). Moreover, an increase in the prevalence of multidrug resistant (MDR) and XDR-PA was evident, with XDR-PA prevalences ranging from 2.6 to 11.2% being described (Walkty et al., 2017; Sader et al., 2018a). Furthermore, according to the European Center for Disease Prevention and Control (ECDC), in 2021 most countries in Europe reported rates of resistance higher than 10% for the majority of the antimicrobial classes under surveillance and only two countries reported less than 5% resistance to carbapenems (European Centre for Disease Prevention and Control and World Health Organization, 2023). In Portugal, in 2021, 12.7% of all isolates were resistant to at least three classes. The highest resistance was seen to fluoroquinolones (18.1%) and piperacillin-tazobactam (16.4%). The most susceptible of the five surveilled classes was aminoglycosides (6.3% resistant) (European Centre for Disease Prevention and Control and World Health Organization, 2023). Moreover, carbapenem-resistant P. aeruginosa is of critical priority in the World Health Organization Priority Pathogens List (Behzadi et al., 2022b).
Even though P. aeruginosa is often a common cause of severe bloodstream infection, ventilator-associated pneumonia and other hospital-acquired infections (Bassetti et al., 2018b), XDR-PA carries an increased mortality. A meta-analysis by Matos et al. describes a mortality of 44.6% among patients infected with MDR P. aeruginosa versus 24.8% in those with non-MDR infections (de Matos et al., 2018). Recio et al. (2018) compared patients with bacteremia due XDR-PA versus susceptible strains and also found a difference in mortality (62.5% versus 30%).
Even though it is an opportunistic pathogen present in the environment, P. aeruginosa is seldom found in the microbiota of healthy humans (Silby et al., 2011; Estepa et al., 2014). Nevertheless, in patients at risk, such as those with a large exposure to the healthcare setting or with certain chronic illnesses (like cystic fibrosis), the colonization rate can reach up to 80% (Gómez-Zorrilla et al., 2014; Ciofu et al., 2015), while those submitted to antibiotic therapy have a higher risk of attaining MDR strains (Gómez-Zorrilla et al., 2014). It also has intrinsic resistance to many antimicrobial drugs and a high capacity of attaining resistance mutations and mobile genetic elements (Bassetti et al., 2018a,b; Horcajada et al., 2019). Although there are many factors contributing to the emergence of antimicrobial resistance, the misuse or overuse of antibiotics is paramount (Algammal et al., 2023). Indeed, despite the new antimicrobials or β-lactam inhibitors that have become commercially available in the last 5 years (Karvouniaris et al., 2023), the emergence of antimicrobial resistance remains one of the greatest threats to global health (Mendes et al., 2022).
Despite the existence of several articles depicting the molecular epidemiology and resistance mechanisms of P. aeruginosa, to the best of the authors’ knowledge there is a gap in the clinical characterization of these patients in Portugal, a country with one of the highest antimicrobial resistance rates in Europe (Pereira et al., 2013, 2015; Hernández-García et al., 2021a,b,c). The authors aim to characterize the population, the outcome and the microbiological characteristics of XDR-PA identified in a tertiary care university hospital center in Portugal.
2 Materials and methods
2.1 Study procedures
A cross-sectional and retrospective observational study was performed in a 1,000-bed tertiary care university hospital center in Lisbon, including inpatients and outpatients with XDR-PA detection between January 2019 and December 2021 (Figure 1). XDR-PA was defined as non-susceptibility to piperacillin-tazobactam, third and fourth generation cephalosporins, carbapenems, aminoglycosides and fluoroquinolones, which is included in the consensus defined by Magiorakos et al. (2012).
Electronic medical records were obtained and analyzed. The following population characteristics were collected: gender and age, risk factors such as presence of immunosuppressive status, invasive devices and certain chronic illnesses, site of infection or colonization, antimicrobial therapy performed and outcome. Colonization was defined as the positive identification of XDR-PA without clinical evidence of infection.
Statistical analysis was performed with MS Excel™ and IBM SPSS™ version 26. A descriptive analysis of the variables was performed. When comparing categorical variables, the Pearson’s Chi-square test was used. Applicability conditions were verified. The significance level was set at p < 0.05.
2.2 Bacterial identification and antimicrobial susceptibility testing
Bacterial identification and antimicrobial susceptibility testing were routinely performed at the hospital’s microbiology laboratory by automated systems (MicroScan WalkAway™ or Vitek™).
Susceptibility was tested by a panel of antibiotics: piperacillin-tazobactam, ceftazidime, cefepime, imipenem, meropenem, amikacin, gentamycin, and ciprofloxacin. When there were limited treatment options, ceftazidime-avibactam, ceftolozane-tazobactam, aztreonam, fosfomycin and colistin were also tested. The colistin susceptibility determination was been performed by microdilution. The clinical breakpoints were interpreted in accordance with European Committee on Antimicrobial Susceptibility Testing (EUCAST) Clinical breakpoints - breakpoints and guidance, available at the European Society of Clinical Microbiology and Infectious Diseases (ESCMID) website.1 The isolates were then categorized as susceptible and resistant by applying the breakpoints results.
When the production of metallo-beta lactamases was suspected, a GeneXpert™ assay was performed.
2.3 XDR-PA resistotyping
To better characterize the XDR-PA population, we performed a resistotype distribution analysis. Considering that all included XDR-PA isolates were by definition non-susceptible to piperacillin-tazobactam, third- and fourth generation cephalosporins, carbapenems, aminoglycosides and fluoroquinolones, the resistance patterns of those with available susceptibility results to colistin, ceftazidime-avibactam, ceftolozane-tazobactam, aztreonam, and fosfomycin were analyzed. Resistance pattern distribution (resistotyping) and MAR index of clinical XDR P. aeruginosa isolates were performed in accordance with previous studies (Behzadi et al., 2022b).
3 Results
Over the 3 years considered in this study (2019-2021) a total of 6,514 isolates of P. aeruginosa pertaining to 3,181 patients were identified. Considering XDR-PA, a total of 243 isolates pertaining to 178 episodes concerning 130 patients were found. The prevalence of XDR-PA identification among all P. aeruginosa isolates was 3.7%, corresponding to 4.1% of all patients with identification of this microorganism. A new episode was defined as a positive XDR-PA isolate when obtained more than 14 days after the last one, in the same patient. Of these 178 episodes, 114 (64.0%) were from infections and 64 (36.0%) were from colonizations. Moreover, 69 episodes (48 patients) happened in 2019, 61 (46 patients) occurred in 2020 and 48 (36 patients) in 2021. It is noteworthy that only six patients were co-infected with SARS-CoV-2, of which four of them died.
3.1 Clinical results
Patient demographics of the 130 patients in which was recovered an XDR-PA isolate are described in Table 1. The cohort was predominantly male (65.4%), with a mean age of 64.3 ± 17.5 years. The most frequent comorbidities described in patients with XDR-PA were diabetes mellitus, immunosuppressive therapy, the presence of a urinary tract device, malignancy and hemodialysis or renal transplant.
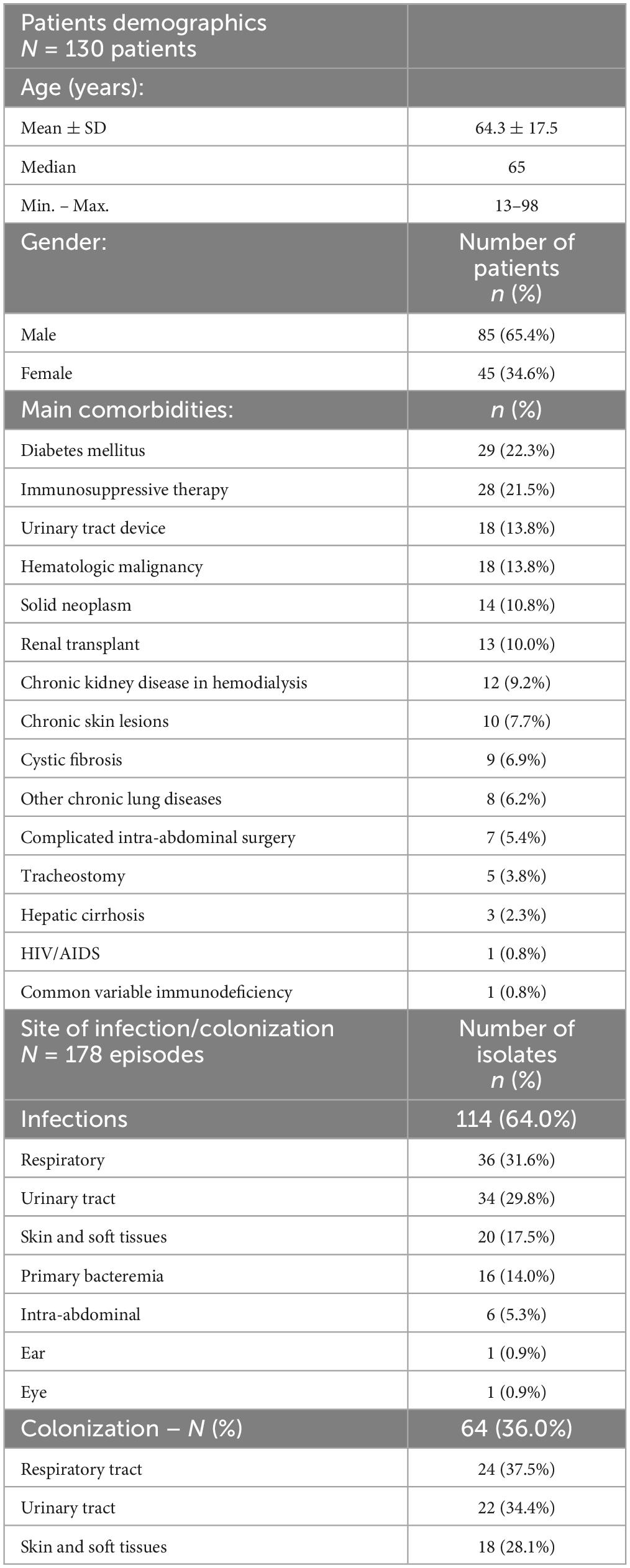
Table 1. Patient demographics of the 130 patients with XDR Pseudomonas aeruginosa (XDR-PA) and site of infection/colonization of the 178 XDR-PA episodes.
Extensively drug-resistant Pseudomonas aeruginosa (XDR-PA) was the etiology of infection in several organs and systems. In Table 1 there is a description of the main sources of infection. A total 114 infections were found, most frequently in the respiratory tract, urinary tract and skin and soft tissues. Sixteen (14.0%) cases presented with primary bacteremia. Of these, most were in immunosuppressed patients often with hematological malignancy. Data regarding mechanical ventilation or the presence of a central venous access was unavailable. It is noteworthy that 23.7% (27/114) of all infections presented with bacteremia. In 64 episodes XDR-PA identification was considered colonization, most frequently of the respiratory and urinary tracts.
When infected, patients were submitted to either monotherapy (52 episodes) with an active drug according to the antimicrobial susceptibility test, or to combined therapy (32 episodes). Whenever a patient was prescribed an active drug combined with another to which this agent was resistant (i.e., colistin plus meropenem), it was considered monotherapy. In 30 cases the information regarding appropriate antibiotic therapy was unavailable.
Table 2 describes the appropriate antimicrobials and combinations used and properly documented. Colistin was used in 41 episodes, ceftazidime-avibactam in 39 and ceftolozane-tazobactam in 16. Aztreonam was used in seven cases, but only once in monotherapy. Fosfomycin was used in seven cases but only in combination.
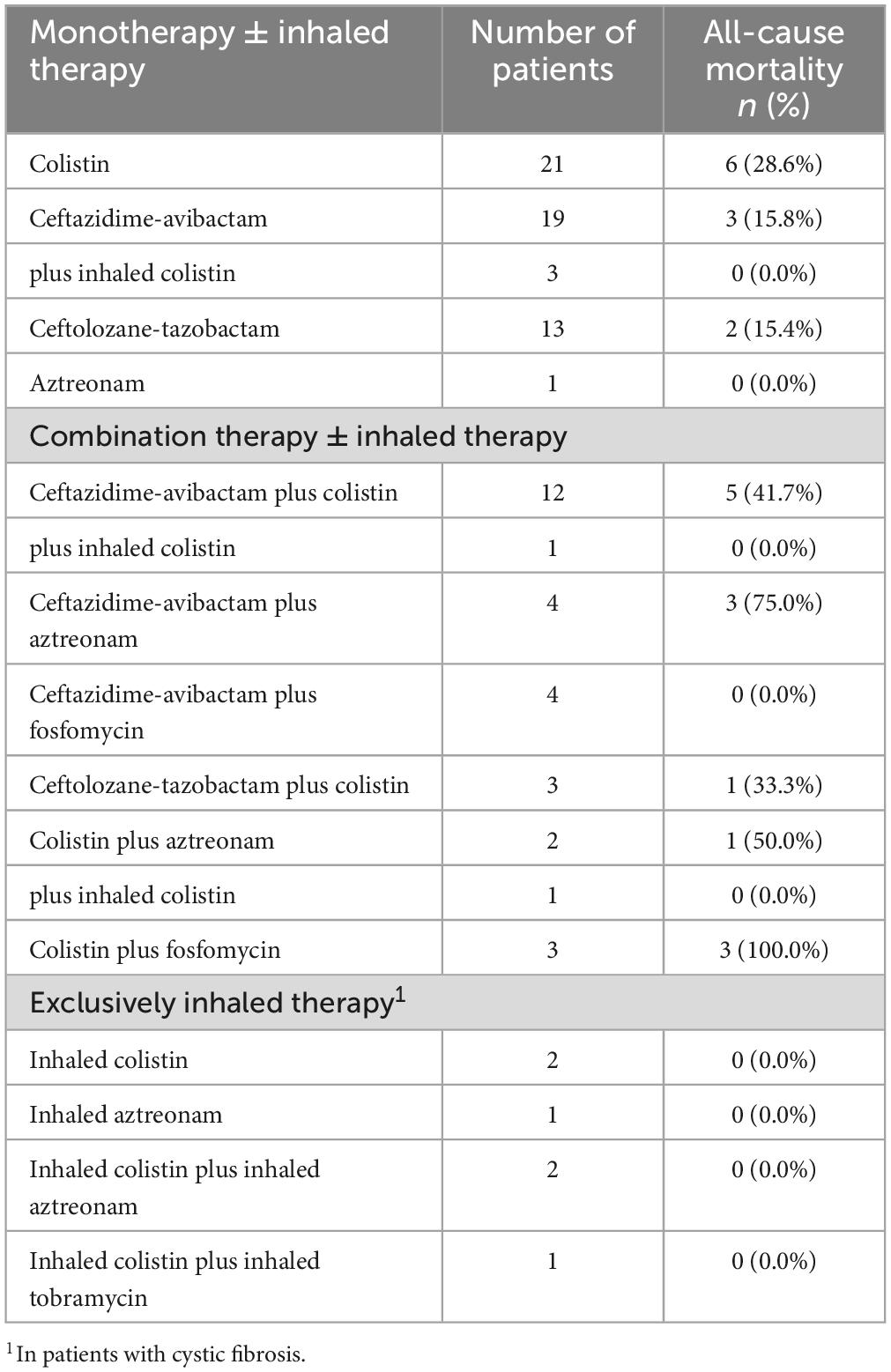
Table 2. Antimicrobial treatment to Pseudomonas aeruginosa infections and all-cause mortality by treatment.
Colonized patients were not submitted to antimicrobial therapy directed to the XDR-PA except in six episodes of cystic fibrosis that presented with respiratory colonization and who were under inhaled colistin (two cases), inhaled aztreonam (one case), both in combination (two cases) or inhaled colistin plus inhaled tobramycin (one case).
There were 40 deaths (35.1%) among infected patients (Table 3). The ones who were treated with combined therapy had a higher mortality (43.8%) than those that were treated with monotherapy (21.2%, p > 0.05). Adjusting for the antimicrobial used, the mortality rate was 39.0% (p > 0.05, 16 deaths) among colistin-treated patients, 28.2% (11 deaths) among ceftazidime-avibactam-treated patients and 18.8% (three deaths) among ceftolozane-tazobactam-treated patients. Considering only patients with respiratory disease, colistin therapy was associated with a mortality rate of 60.0% as opposed to 32.3% when excluding these patients. Comparison of mortality between patients treated with different antibiotics failed to yield statistically significant differences.
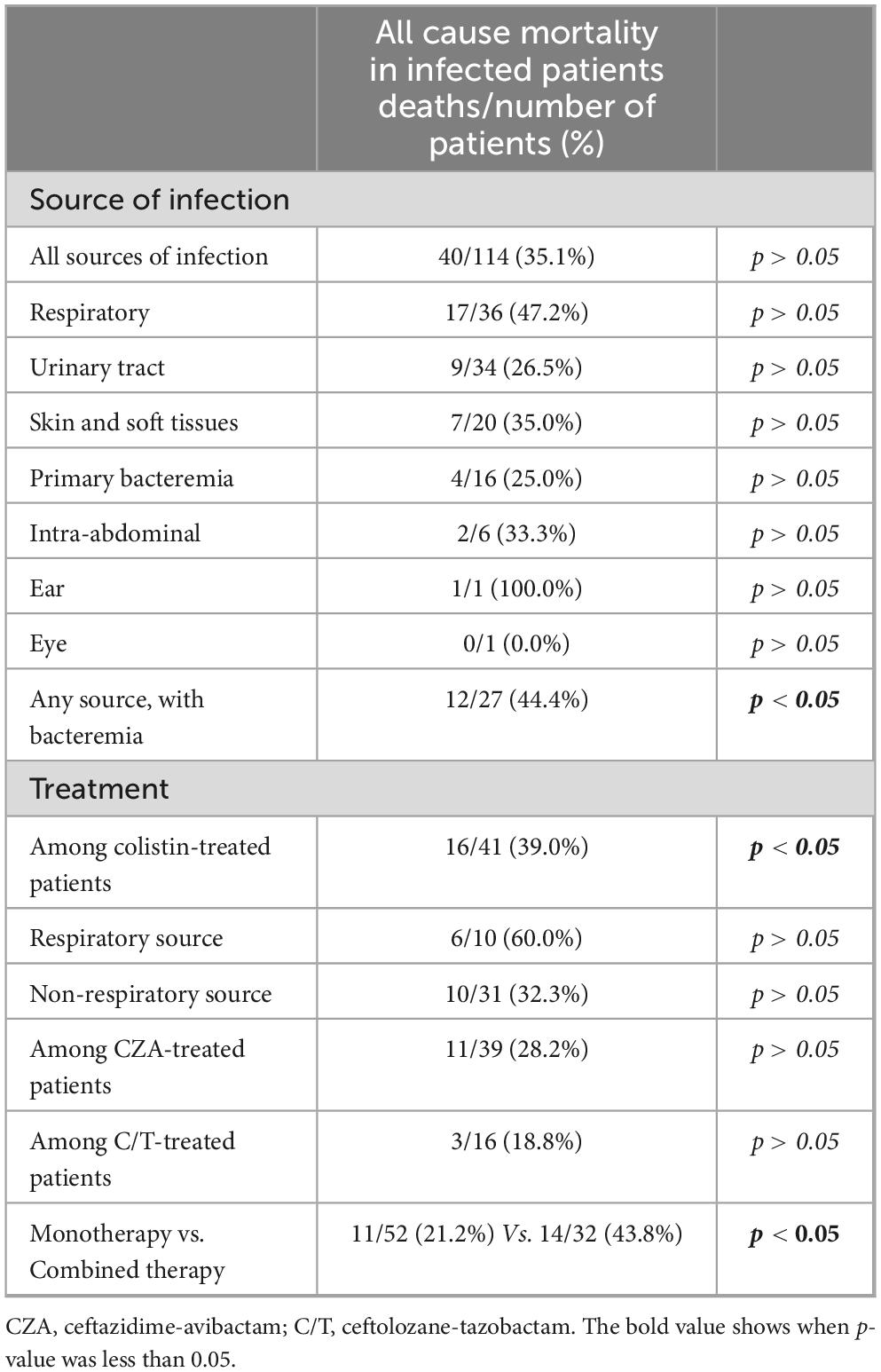
Table 3. Clinical outcome of infection considering source of infection and treatment including colistin, ceftazidime-avibactam or ceftolozane-tazobactam.
Regarding the source of infection (Figure 2), mortality was highest among patients with respiratory infections (47.2%), followed by those with skin and soft tissues infections (35.0%), intra-abdominal infections (33.3%), and urinary tract infections (26.5%). Among patients with positive blood cultures the mortality rate was 44.4% (p < 0.05), although only 25.0% when presenting with primary bacteremia. Considering all comorbidities, only hematologic malignancy was significantly associated with mortality (50.0% died, p < 0.05).
3.2 Microbiological results
Extensively drug-resistant Pseudomonas aeruginosa (XDR-PA) was identified in 243 microbiological examinations, corresponding to the 178 episodes. Antimicrobial susceptibility testing (AST) was performed in 216 (88.9%) isolates (Table 4). In the remaining, AST was not performed since it had already been done for another contemporary sample.
The only resistance found to colistin was in a 34-year-old patient with cystic fibrosis, and it remained susceptible exclusively to ceftazidime-avibactam and to ceftolozane-tazobactam novel combinations. Moreover, a 56-year-old patient with hematologic malignancy under chemotherapy presented with febrile neutropenia and primary bacteremia with a VIM-producing XDR-PA that was susceptible only to colistin. The patient was treated with colistin and fosfomycin and ultimately died.
Among all P. aeruginosa isolates, the yearly susceptibility rate to meropenem varied between 80 and 82%. Regarding XDR-PA isolates, when tested, most were susceptible to ceftazidime-avibactam and ceftolozane-tazobactam, while only 22.4% were susceptible to fosfomycin and 12.4% to aztreonam. Regarding the strains tested both for susceptibility to ceftazidime-avibactam and to ceftolozane-tazobactam, 63.2% were susceptible and 19.1% were resistant to both antibiotics. Of those resistant to ceftazidime-avibactam, 42.2% were susceptible to ceftolozane-tazobactam, while of those resistant to ceftolozane-tazobactam, 16.1% were susceptible to ceftazidime-avibactam. The related defaults for XDR in association with the used antibiotics and combinations are presented in Figure 3. Most of the strains tested for both colistin and ceftazidime-avibactam and colistin and ceftolozane-tazobactam were susceptible to both antimicrobials (70.9 and 76.6%, respectively). Two-antibiotic combinations with fosfomycin or aztreonam were less likely to be susceptible to both drugs, which is in accordance with the lower susceptibility rates found in those antimicrobials.
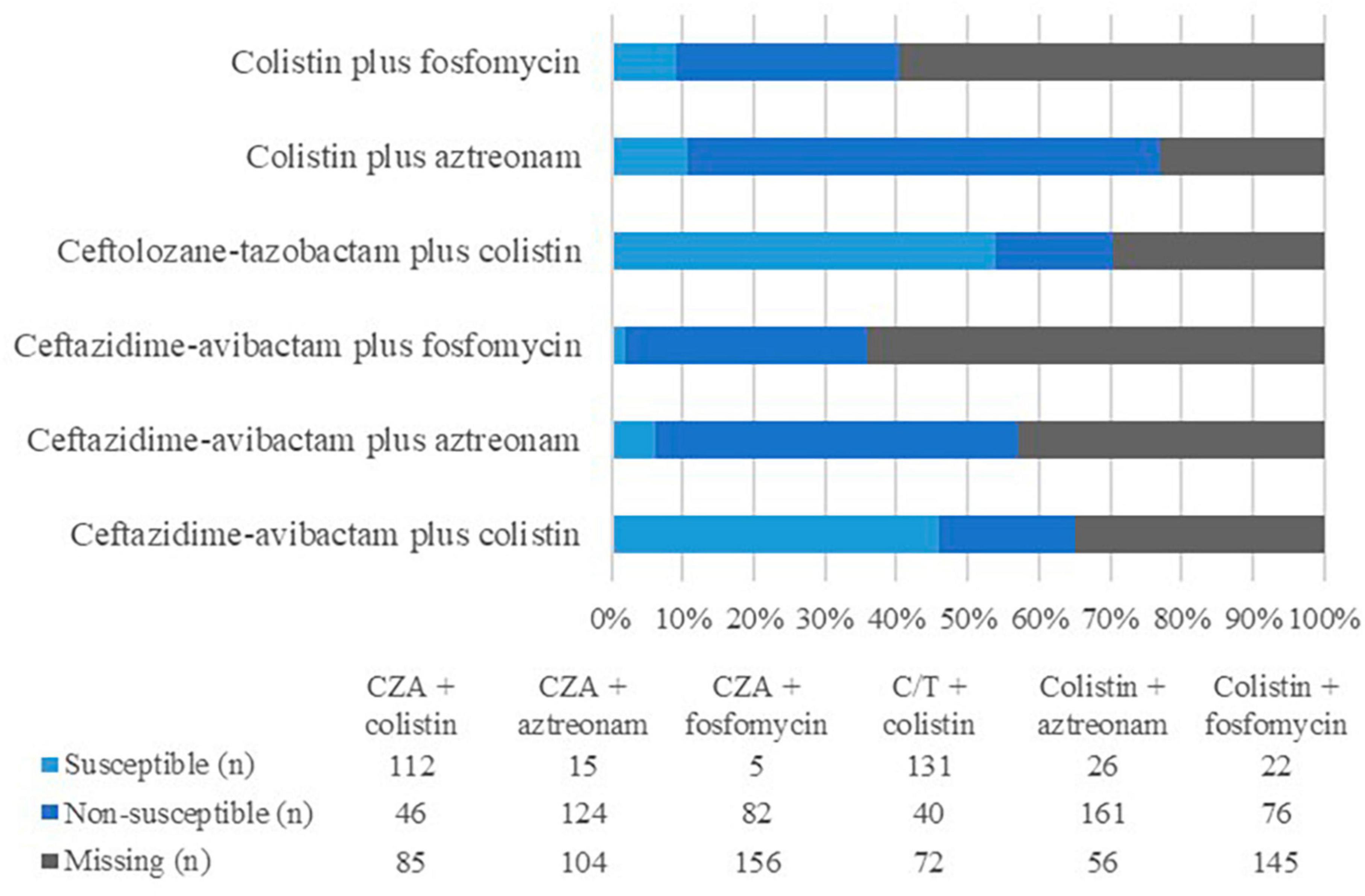
Figure 3. Antimicrobial susceptibilities of XDR-PA isolates (n = 243) to antimicrobial combinations used as treatment in the present study. CZA, ceftazidime-avibactam; C/T, ceftolozane-tazobactam.
3.3 XDR-PA resistotyping
Table 5 shows the resistance patterns of 65 clinical XDR-PA that were non-susceptible to piperacillin-tazobactam, third and fourth generation cephalosporins, carbapenems, aminoglycosides and fluoroquinolones and that, additionally had available AST results for colistin, ceftazidime-avibactam, ceftolozane-tazobactam, aztreonam and fosfomycin. Twelve different resistotypes were found.
The most common resistotype was the combined resistance to aztreonam and fosfomycin (n = 26, 40.0%), followed by ceftazidime-avibactam, aztreonam and fosfomycin (n = 13; 20.0%), and ceftazidime-avibactam, ceftolozane-tazobactam and aztreonam (n = 8; 12.3%). Resistance to at least two of the tested antibiotics occurred in 90.8%, while 43.1% were resistant to more than three antimicrobials.
4 Discussion
Extensively drug resistant Pseudomonas aeruginosa (XDR-PA) is a growing concern due to its increasing incidence, limited therapeutic options, limited data on the optimal treatment, and high mortality rates. Even though the criteria used to define XDR-PA did not match exactly those defined by Magiorakos et al. (2012), the prevalence reported in the present study is lower than the one displayed in 2017 by a large-scale Spanish multicenter study (17%) (Del Barrio-Tofinõ et al., 2019). Similarly, a trial including patients with ventilator-associated pneumonia in Spain, Greece and Italy showed a rate of 35.8% XDR isolates, mostly from Greece (Pérez et al., 2019). Other countries around the world also reported higher XDR-PA prevalence, such as 22.1% out of 447 P. aeruginosa isolates in Nepal (Mahto et al., 2021) or 15.5% out of 3248 isolates in Iran (Mirzaei et al., 2020). The prevalence reported in the present study is more similar to that described in Canada and in USA. McCracken et al. (2019) showed 4.5% XDR-PA among all 3864 Canadian isolates between 2007 and 2016, while Sader et al. (2017) depict 9.4% XDR-PA among 7452 American isolates from 2012 to 2015. Although there is a lack in data regarding the prevalence of XDR-PA in Portuguese isolates, ECDC reports a resistance rate to carbapenems of 14.1 and of 12.7% to any combination of at least three of the following antibiotics: piperacillin-tazobactam, ceftazidime, carbapenems, aminoglycosides and fluoroquinolones (Antimicrobial resistance surveillance in Europe 2023 - 2021 data. Stockholm: European Centre for Disease Prevention and Control and World Health Organization, 2023).
While P. aeruginosa is rarely found in the microbiota of healthy humans (Silby et al., 2011; Estepa et al., 2014), it can colonize up to 80% of patients with risk factors, such as a large exposure to the healthcare setting or certain chronic illnesses (such as cystic fibrosis, and solid or hematologic malignancies) (Gómez-Zorrilla et al., 2014; Ciofu et al., 2015). The presence of foreign devices, such as venous or urinary catheters, tracheostomy (especially in children), open abdominal surgery, diabetes, chronic hepatic disorder and end-stage renal disease also increase the risk for P. aeruginosa infection (Varaiya et al., 2008; Willmann et al., 2014; Miller et al., 2016; Bassetti et al., 2018a; Russell et al., 2019; Jean et al., 2020; Körpinar, 2021). Also prior antibiotic therapy (especially fluoroquinolones and carbapenems), so frequent in the healthcare setting and in patients with these comorbidities, is a major risk factor for attaining MDR strains (Falagas et al., 2006; Peña et al., 2012; Gómez-Zorrilla et al., 2014; Bassetti et al., 2018a; Jean et al., 2020). In fact, prior use of fluoroquinolones or carbapenems has been depicted as an independent risk factor for XDR-PA infections (Palavutitotai et al., 2018).
In P. aeruginosa, resistance mechanisms such as decreased permeability, expression of efflux pumps, target modifications and production of inactivating enzymes have all been described (Mesaros et al., 2007). In particular, production of extended-spectrum beta-lactamases and carbapenemases have been described, including metallo-beta-lactamases (Mesaros et al., 2007; Sacha et al., 2008; Ríos et al., 2018; Muddassir et al., 2021). Since these enzymes use zinc, which seems to be a valuable cofactor to hydrolyze beta-lactams, instead of serine in their active sites, they confer resistance to all beta-lactam antibiotics except aztreonam, while not being degraded by currently available beta-lactamase inhibitors (Sacha et al., 2008; Behzadi et al., 2020). In this study there was one VIM-producing XDR-PA that was susceptible only to colistin. Despite VIM not being able to degrade aztreonam, P. aeruginosa is capable of producing simultaneously additional inactivating enzymes, conferring resistance also to this drug (Mesaros et al., 2007; Ríos et al., 2018). So, the combination of aztreonam-avibactam (or ceftazidime-avibactam plus aztreonam if the former is not available) is pertinent as it combines the ability of avibactam to inactivate all serine-beta-lactamases, thus allowing aztreonam to be effective (Marshall et al., 2017; Mischnik et al., 2017). However, due to mechanisms of resistance to aztreonam independent of beta-lactamases, the activity of this combination has to be confirmed with synergy testing (Karakonstantis et al., 2020).
One drug that is often protected by the cross-resistance of other anti-pseudomonal antibiotics is colistin (Mesaros et al., 2007). And while pan-drug resistant isolates have been described, most often XDR-PA remains susceptible to colistin (Souli et al., 2008; Viedma et al., 2009; Giani et al., 2018). In this study all isolates but one were susceptible to colistin. However, while the prevalence of resistance to ceftazidime-avibactam and to ceftolozane-tazobactam appears to be overall low in Europe and the United States, the authors report approximately one quarter of non-susceptible isolates. This might be explained by the geographical variation of the predominance of different mechanisms of resistance (Flamm et al., 2014; Del Barrio-Tofiño et al., 2017; Giani et al., 2018; Livermore et al., 2018; Sader et al., 2018b; Evans et al., 2019), although when considering the cross-resistance of ceftolozane-tazobactam and ceftazidime-avibactam in this study, the authors believe the mechanism not to be predominantly related to carbapenemases. Regarding aztreonam and fosfomycin, the authors report high rates of non-susceptibility, as displayed by other centers and other Portuguese data (Sader et al., 2017; Safaei et al., 2017; Evans et al., 2019; Hernández-García et al., 2021a).
Regarding the analysis of the resistance patterns in XDR-PA isolates, it is not unexpected that the most frequent resistotype is resistance to both aztreonam and fosfomycin. It is noteworthy, however, that resistance patterns that included non-susceptibility to both aztreonam and ceftazidime-avibactam were frequent (40.0%, resistotypes V, VIII, X, and XII). Unfortunately, despite the knowledge that ceftazidime-avibactam resistance is emerging in K. pneumoniae in Portugal since its approval by the national regulatory authority in 2019 (Mendes et al., 2022, 2023), no data was available on synergy testing of ceftazidime-avibactam plus aztreonam or further study of resistance mechanisms in P. aeruginosa.
As XDR-PA is undoubtedly a growing threat with increasingly limited therapeutic options, it is essential to define new and innovative therapeutic strategies, either by developing new systemic drugs, new drug combinations, different methods of drug administration or alternative therapies (Mesaros et al., 2007; Marshall et al., 2017; Mischnik et al., 2017; Bassetti et al., 2018b; Horcajada et al., 2019; Ekkelenkamp et al., 2020; Gaurav et al., 2020; Yao et al., 2021). For example, the synergistic combination colistin-mefloquine has been suggested as a potential future therapeutic option against colistin-resistant strains due to its anti-biofilm activity (Behzadi et al., 2022a). Inhaled therapy has long since been used in cystic fibrosis and it can be a powerful adjunct in the treatment of XDR-PA infections. Since drug concentration in the lung is much higher with inhaled therapy than with the intravenous route, it can be effective even against in vitro resistant strains (Horcajada et al., 2019).
The authors report high mortality rates (35.1%), as observed in previous studies (Samonis et al., 2014; de Matos et al., 2018; Palavutitotai et al., 2018). Patients with hematologic malignancy have been described as being at an increased risk of a poor outcome (Samonis et al., 2014; Tofas et al., 2017; de Matos et al., 2018), perhaps due to their immunosuppressed status with frequent and recurrent infections and antimicrobial use. The authors report a 50.0% (p < 0.05) mortality among patients with hematologic malignancy.
Patients with bacteremia had a worse prognosis (mortality 44.4%, p < 0.05), similar to the 18.0% reported by Dantas et al. (2014) in their comparison between bacteremia due to susceptible and MDR strains. Having been established as an independent risk factor for mortality (Dantas et al., 2014), it surely is a marker for severe disease. Thus, it is interesting to note that in patients presenting with primary bacteremia the mortality was only 25.0%.
Pseudomonas aeruginosa is also a frequent cause of healthcare-associated respiratory and urinary tract infections (Quartin et al., 2013; Lamas Ferreiro et al., 2017; Jean et al., 2020), the most frequent sources of infection as reported in this study and consistent with the most frequent types of Healthcare Associated Infections, as reported by the ECDC (Suetens et al., 2023). Having been established that infections with resistant strains have a greater risk than susceptible strains (de Matos et al., 2018; Recio et al., 2018; Jean et al., 2020) it is not unexpected to find high mortality rates in this study. Nevertheless, 47.2% fatalities among patients with respiratory infections was higher than what had been previously described (Peña et al., 2013; Giaccari et al., 2021). The 26.5% mortality described among patients with urinary tract infections is similar to that described by Lamas Ferreiro et al. (2017).
Adjusting for the antimicrobial used, the authors report a higher mortality rate among colistin-treated patients (39.0%, p < 0.05). Although these results did not achieve statistical significance, it appears that the mortality among patients treated with ceftazidime-avibactam (28.2%) or ceftolozane-tazobactam (18.8%) was lower. Considering exclusively monotherapy, these results are consistent, with 28.6% of patients treated with colistin dying, against 15.8 and 15.4% of patients treated with ceftazidime-avibactam and ceftolozane-tazobactam, respectively. This seems paradoxical since colistin has been described as one of the most active antipseudomonal drugs (Del Barrio-Tofinõ et al., 2019; López Montesinos et al., 2021; Pinilla-Rello et al., 2021). The authors believe that this result might be related to both a better efficacy and safety profile of beta-lactams and a poorer penetration of colistin in the lung, which is supported by the 60.0% mortality when considering only patients with respiratory infection treated with colistin versus 32.3% when considering only other sources of infection. This findings support the last IDSA Guidance, where beta-lactams are put at the forefront of the treatment of MDR P. aeruginosa infections (Tamma et al., 2022). Patients that were submitted to combined directed therapy seemed to have a higher mortality rate than those treated with only one active drug. These results may be related to a possibly increased severity of disease among patients submitted to combined therapy.
The present study has several limitations. First, by using a narrower definition of XDR-PA than previously published, it is possible that other isolates were not considered. Therefore, comparisons with other studies must take this limitation into consideration. Second, several important variables, such as disease severity, exposure to venous catheterization or mechanical ventilation, recent or recurrent history of antibiotic therapy or healthcare exposure, and prior P. aeruginosa colonization status were most often not possible to ascertain.
The results of this study are relevant as they provide a first insight into the clinical outcome of Portuguese patients infected with XDR-PA, allowing for better care between relevant centers. However, in the future, it is essential to improve our understanding and to further characterize these patients, particularly in high-risk environments (such as focusing on Intensive Care Units and Hematology wards). Moreover, there is a crucial need for a current description of the molecular epidemiology and characterization of the resistance mechanisms of P. aeruginosa in Portugal. Furthermore, the integration of clinical data with molecular surveillance and the analysis of genetic resistance determinants may be relevant to improve the quality of care, allowing for a greater accent on research-based recommendations. Finally, with the potential impact of the COVID-19 pandemic on hospital epidemiology, it is paramount to evaluate this issue in the light of new therapeutic options.
5 Conclusion
In conclusion, patients infected with extensively drug resistant Pseudomonas aeruginosa are difficult to treat, with very limited therapeutic options. XDR-PA is a pathogen responsible for potentially severe disease that can have high mortality. Although the most frequent sources of infection were the respiratory and urinary sites, patients with bacteremia or hematologic malignancy had a higher risk. In this study, colistin was the most susceptible antibiotic in vitro. However, patients treated with ceftazidime-avibactam or ceftolozane-tazobactam appeared to have a better prognosis than those treated with colistin, especially considering those with respiratory infection. To the best of the authors’ knowledge, this is the first study to provide a clinical characterization of patients infected with XDR-PA strains in Portugal.
Data availability statement
The raw data supporting the conclusions of this article will be made available by the authors, without undue reservation.
Ethics statement
Ethical approval was not required for the study involving humans in accordance with the local legislation and institutional requirements. Written informed consent to participate in this study was not required from the participants or the participants’ legal guardians/next of kin in accordance with the national legislation and the institutional requirements.
Author contributions
DM: Conceptualization, Formal analysis, Methodology, Writing – original draft. SP: Conceptualization, Methodology, Writing – review & editing. CS: Writing – review & editing. AF: Writing – review & editing. JM: Writing – review & editing. ÁP: Writing – review & editing. CC: Supervision, Writing – review & editing.
Funding
The author(s) declare that no financial support was received for the research, authorship, and/or publication of this article.
Conflict of interest
JM and CC received research grants administered through university and honoraria for serving on the speaker’s bureaus of Pfizer and MSD that were not related to the present study.
The remaining authors declare that the research was conducted in the absence of any commercial or financial relationships that could be construed as a potential conflict of interest.
Publisher’s note
All claims expressed in this article are solely those of the authors and do not necessarily represent those of their affiliated organizations, or those of the publisher, the editors and the reviewers. Any product that may be evaluated in this article, or claim that may be made by its manufacturer, is not guaranteed or endorsed by the publisher.
Footnotes
References
Algammal, A., Hetta, H. F., Mabrok, M., and Behzadi, P. (2023). Editorial: emerging multidrug-resistant bacterial pathogens “superbugs”: a rising public health threat. Front. Microbiol. 14:1135614. doi: 10.3389/fmicb.2023.1135614
Bassetti, M., Vena, A., Russo, A., Croxatto, A., Calandra, T., and Guery, B. (2018b). Rational approach in the management of Pseudomonas aeruginosa infections. Curr. Opin. Infect. Dis. 31, 578–586. doi: 10.1097/QCO.0000000000000505
Bassetti, M., Vena, A., Croxatto, A., Righi, E., and Guery, B. (2018a). How to manage Pseudomonas aeruginosa infections. Drugs Context 7, 1–18. doi: 10.7573/dic.212527
Behzadi, P., Ambrosi, C., Scribano, D., Zanetti, S., Sarshar, M., Gajdács, M., et al. (2022a). Editorial: current perspectives on Pseudomonas aeruginosa: epidemiology, virulence and contemporary strategies to combat multidrug-resistant (MDR) pathogens. Front. Microbiol. 13:975616. doi: 10.3389/fmicb.2022.975616
Behzadi, P., Gajdács, M., Pallós, P., Ónodi, B., Stájer, A., Matusovits, D., et al. (2022b). Relationship between biofilm-formation, phenotypic virulence factors and antibiotic resistance in environmental Pseudomonas aeruginosa. Pathogens 11:1015.
Behzadi, P., García-Perdomo, H. A., Karpiński, T. M., and Issakhanian, L. (2020). Metallo-ß-lactamases: a review. Mol. Biol. Rep. 47, 6281–6294. doi: 10.1007/s11033-020-05651-9
Ciofu, O., Tolker-Nielsen, T., Jensen, P. Ø., Wang, H., and Høiby, N. (2015). Antimicrobial resistance, respiratory tract infections and role of biofilms in lung infections in cystic fibrosis patients. Adv. Drug Deliv. Rev. 85, 7–23. doi: 10.1016/j.addr.2014.11.017
Dantas, R. C., Ferreira, M. L., Gontijo-Filho, P. P., and Ribas, R. M. (2014). Pseudomonas aeruginosa bacteraemia: independent risk factors for mortality and impact of resistance on outcome. J. Med. Microbiol. 63, 1679–1687. doi: 10.1099/jmm.0.073262-0
de Matos, E. C. O., Andriolo, R. B., Rodrigues, Y. C., de Lima, P. D. L., Carneiro, I. C. D. R. S., and Lima, K. V. B. (2018). Mortality in patients with multidrug-resistant Pseudomonas aeruginosa infections: a meta-analysis. Rev. Soc. Bras. Med. Trop. 51, 415–420. doi: 10.1590/0037-8682-0506-2017
Del Barrio-Tofiño, E., López-Causapé, C., Cabot, G., Rivera, A., Benito, N., Segura, C., et al. (2017). Genomics and susceptibility profiles of extensively drug-resistant Pseudomonas aeruginosa isolates from Spain. Antimicrob. Agents Chemother. 61:e01589-17. doi: 10.1128/AAC.01589-17
Del Barrio-Tofinõ, E., Zamorano, L., Cortes-Lara, S., López-Causape, C., Sánchez-Diener, I., Cabot, G., et al. (2019). Spanish nationwide survey on Pseudomonas aeruginosa antimicrobial resistance mechanisms and epidemiology. J. Antimicrob. Chemother. 74, 1825–1835. doi: 10.1093/jac/dkz147
Ekkelenkamp, M. B., Tunney, M. M., Gilpin, D. F., Bernardini, F., Dale, G. E., Elborn, J. S., et al. (2020). Susceptibility of Pseudomonas aeruginosa recovered from cystic fibrosis patients to murepavadin and 13 comparator antibiotics. Antimicrob. Agents Chemother. 64:e01541-19.
Estepa, V., Rojo-Bezares, B., Torres, C., and Sáenz, Y. (2014). Faecal carriage of Pseudomonas aeruginosa in healthy humans: antimicrobial susceptibility and global genetic lineages. FEMS Microbiol. Ecol. 89, 15–19. doi: 10.1111/1574-6941.12301
European Centre for Disease Prevention and Control and World Health Organization (2023). Antimicrobial resistance surveillance in Europe 2023–2021 data. Stockholm: European Centre for Disease Prevention and Control and World Health Organization, doi: 10.2900/63495
Evans, S. R., Tran, T. T. T., Hujer, A. M., Hill, C. B., Hujer, K. M., Mediavilla, J. R., et al. (2019). Rapid molecular diagnostics to inform empiric use of ceftazidime/avibactam and ceftolozane/tazobactam against Pseudomonas aeruginosa: PRIMERS IV. Clin. Infect. Dis. 68, 1823–1830. doi: 10.1093/cid/ciy801
Falagas, M. E., Koletsi, P. K., Kopterides, P., and Michalopoulos, A. (2006). Risk factors for isolation of strains susceptible only to polymyxin among patients with Pseudomonas aeruginosa Bacteremia. Antimicrob. Agents Chemother. 50, 2541–2543. doi: 10.1128/AAC.00224-06
Flamm, R. K., Farrell, D. J., Sader, H. S., and Jones, R. N. (2014). Ceftazidime/avibactam activity tested against Gram-negative bacteria isolated from bloodstream, pneumonia, intra-abdominal and urinary tract infections in US medical centres (2012). J. Antimicrob. Chemother. 69, 1589–1598. doi: 10.1093/jac/dku025
Gaurav, A., Kothari, A., Omar, B. J., and Pathania, R. (2020). Assessment of polymyxin B–doxycycline in combination against Pseudomonas aeruginosa in vitro and in a mouse model of acute pneumonia. Int. J. Antimicrob. Agents 56:106022. doi: 10.1016/j.ijantimicag.2020.106022
Giaccari, L. G., Pace, M. C., Passavanti, M. B., Gargano, F., Aurilio, C., and Sansone, P. (2021). Ceftolozane/tazobactam for resistant drugs Pseudomonas aeruginosa respiratory infections: a systematic literature review of the real-world evidence. Life 11:474. doi: 10.3390/life11060474
Giani, T., Arena, F., Pollini, S., Di Pilato, V., D’Andrea, M. M., De Angelis, L. H., et al. (2018). Italian nationwide survey on Pseudomonas aeruginosa from invasive infections: activity of ceftolozane/tazobactam and comparators, and molecular epidemiology of carbapenemase producers. J. Antimicrob. Chemother. 73, 664–671. doi: 10.1093/jac/dkx453
Gómez-Zorrilla, S., Camoez, M., Tubau, F., Periche, E., Cañizares, R., Dominguez, M. A., et al. (2014). Antibiotic pressure is a major risk factor for rectal colonization by multidrug-resistant Pseudomonas aeruginosa in critically ill patients. Antimicrob. Agents Chemother. 58, 5863–5870. doi: 10.1128/AAC.03419-14
Hernández-García, M., Garciá-Castillo, M., Garciá-Fernández, S., Melo-Cristino, J., Pinto, M. F., Goncąlves, E., et al. (2021a). Distinct epidemiology and resistance mechanisms affecting ceftolozane/tazobactam in Pseudomonas aeruginosa isolates recovered from ICU patients in Spain and Portugal depicted by WGS. J. Antimicrob. Chemother. 76, 370–379. doi: 10.1093/jac/dkaa430
Hernández-García, M., Garciá-Fernández, S., Garciá-Castillo, M., Pássaro, L., Cantón, R., Melo-Cristino, J., et al. (2021b). In vitro characterization of Pseudomonas aeruginosa recovered in Portugal from low respiratory tract infections in ICU patients (STEP Study). FEMS Microbiol. Lett. 368:fnab099. doi: 10.1093/femsle/fnab099
Hernández-García, M., García-Castillo, M., García-Fernández, S., López-Mendoza, D., Díaz-Regañón, J., Romano, J., et al. (2021c). Presence of chromosomal crpp-like genes is not always associated with ciprofloxacin resistance in Pseudomonas aeruginosa clinical isolates recovered in ICU patients from Portugal and Spain. Microorganisms 9:388. doi: 10.3390/microorganisms9020388
Horcajada, J. P., Montero, M., Oliver, A., Sorlí, L., Luque, S., Gómez-Zorrilla, S., et al. (2019). Epidemiology and treatment of multidrug-resistant and extensively drug-resistant Pseudomonas aeruginosa infections. Clin. Microbiol. Rev. 32, 1–52. doi: 10.1128/CMR.00031-19
Jean, S. S., Chang, Y. C., Lin, W. C., Lee, W. S., Hsueh, P. R., and Hsu, C. W. (2020). Epidemiology, treatment, and prevention of nosocomial bacterial pneumonia. J. Clin. Med. 9:275. doi: 10.3390/jcm9010275
Karakonstantis, S., Kritsotakis, E. I., and Gikas, A. (2020). Treatment options for K. pneumoniae, P. aeruginosa and A. baumannii co-resistant to carbapenems, aminoglycosides, polymyxins and tigecycline: an approach based on the mechanisms of resistance to carbapenems. Infection 48, 835–851. doi: 10.1007/s15010-020-01520-6
Karvouniaris, M., Almyroudi, M. P., Abdul-Aziz, M. H., Blot, S., Paramythiotou, E., Tsigou, E., et al. (2023). Novel antimicrobial agents for gram-negative pathogens. Antibiotics 12:761. doi: 10.3390/antibiotics12040761
Körpinar, Ş (2021). A retrospective analysis of microbiologic profile of foot infections in patients with diabetic end-stage renal disease. Int. J. Low. Extrem. Wounds 20, 15–21. doi: 10.1177/1534734620958364
Lamas Ferreiro, J. L., Álvarez Otero, J., González Gónzalez, L., Novoa Lamazares, L., Arca Blanco, A., Bermúdez Sanjurjo, J. R., et al. (2017). Pseudomonas aeruginosa urinary tract infections in hospitalized patients: mortality and prognostic factors. PLoS One 12:e0178178. doi: 10.1371/journal.pone.0178178
Livermore, D. M., Meunier, D., Hopkins, K. L., Doumith, M., Hill, R., Pike, R., et al. (2018). Activity of ceftazidime/avibactam against problem Enterobacteriaceae and Pseudomonas aeruginosa in the UK, 2015-16. J. Antimicrob. Chemother. 73, 648–657. doi: 10.1093/jac/dkx438
López Montesinos, I., Gómez-Zorrilla, S., Palacios-Baena, Z. R., Prim, N., Echeverria-Esnal, D., Gracia, M. P., et al. (2021). Aminoglycoside or polymyxin monotherapy for treating complicated urinary tract infections caused by extensively drug-resistant Pseudomonas aeruginosa: a propensity score-adjusted and matched cohort study. Infect. Dis. Ther. 11, 335–350. doi: 10.1007/s40121-021-00570-z
Magiorakos, A. P., Srinivasan, A., Carey, R. B., Carmeli, Y., Falagas, M. E., Giske, C. G., et al. (2012). Multidrug-resistant, extensively drug-resistant and pandrug-resistant bacteria: an international expert proposal for interim standard definitions for acquired resistance. Clin. Microbiol. Infect. 18, 268–281. doi: 10.1111/j.1469-0691.2011.03570.x
Mahto, M., Shah, A., Show, K. L., Moses, F. L., and Stewart, A. G. (2021). Pseudomonas aeruginosa in Nepali hospitals: poor outcomes amid 10 years of increasing antimicrobial resistance. Public Health Action 11, 58–63. doi: 10.5588/pha.21.0048
Marshall, S., Hujer, A. M., Rojas, L. J., Papp-Wallace, K. M., Humphries, R. M., Spellberg, B., et al. (2017). Can ceftazidime-avibactam and aztreonam overcome β-lactam resistance conferred by metallo-β-lactamases in Enterobacteriaceae? Antimicrob. Agents Chemother. 61:e02243-16. doi: 10.1128/AAC.02243-16
McCracken, M. G., Adam, H. J., Blondeau, J. M., Walkty, A. J., Karlowsky, J. A., Hoban, D. J., et al. (2019). Characterization of carbapenem-resistant and XDR Pseudomonas aeruginosa in Canada: results of the CANWARD 2007-16 study. J. Antimicrob. Chemother. 74, iv32–iv38. doi: 10.1093/jac/dkz285
Mendes, G., Ramalho, J. F., Bruschy-Fonseca, A., Lito, L., Duarte, A., Melo-Cristino, J., et al. (2022). First description of ceftazidime/avibactam resistance in a ST13 KPC-70-producing Klebsiella pneumoniae strain from Portugal. Antibiotics 11:167. doi: 10.3390/antibiotics11020167
Mendes, G., Santos, M. L., Ramalho, J. F., Bruschy-Fonseca, A., Lito, L., Mendes-Pedro, D., et al. (2023). Genomic characterisation of a novel KPC-98-producing clinical Klebsiella pneumoniae strain conferring resistance to ceftazidime/avibactam. Int. J. Antimicrob. Agents 62:107013. doi: 10.1016/j.ijantimicag.2023.107013
Mesaros, N., Nordmann, P., Plésiat, P., Roussel-Delvallez, M., Van Eldere, J., Glupczynski, Y., et al. (2007). Pseudomonas aeruginosa: resistance and therapeutic options at the turn of the new millennium. Clin. Microbiol. Infect. 13, 560–578. doi: 10.1111/j.1469-0691.2007.01681.x
Miller, B., Popejoy, M. W., Hershberger, E., Steenbergen, J. N., and Alverdy, J. (2016). Characteristics and outcomes of complicated intra-abdominal infections involving Pseudomonas aeruginosa from a randomized, double-blind, phase 3 ceftolozane-tazobactam study. Antimicrob. Agents Chemother. 60, 4387–4390. doi: 10.1128/AAC.03074-15
Mirzaei, B., Bazgir, Z. N., Goli, H. R., Iranpour, F., Mohammadi, F., and Babaei, R. (2020). Prevalence of multi-drug resistant (MDR) and extensively drug-resistant (XDR) phenotypes of Pseudomonas aeruginosa and Acinetobacter baumannii isolated in clinical samples from Northeast of Iran. BMC Res. Notes 13:380. doi: 10.1186/s13104-020-05224-w
Mischnik, A., Baumert, P., Hamprecht, A., Rohde, A., Peter, S., Feihl, S., et al. (2017). Susceptibility to cephalosporin combinations and aztreonam/avibactam among third-generation cephalosporin-resistant Enterobacteriaceae recovered on hospital admission. Int. J. Antimicrob. Agents 49, 239–242. doi: 10.1016/j.ijantimicag.2016.10.013
Muddassir, M., Munir, S., Raza, A., Basirat, A., Ahmed, M., Farooq, U., et al. (2021). Epidemiology and high incidence of metallo-β-lactamase and AmpC-β-lactamases in nosocomial Pseudomonas aeruginosa. Iran. J. Basic Med. Sci. 24, 1373–1379. doi: 10.22038/ijbms.2021.57293.12748
Palavutitotai, N., Jitmuang, A., Tongsai, S., Kiratisin, P., and Angkasekwinai, N. (2018). Epidemiology and risk factors of extensively drug-resistant Pseudomonas aeruginosa infections. PLoS One 13:e0193431. doi: 10.1371/journal.pone.0193431
Peña, C., Gómez-Zorrilla, S., Oriol, I., Tubau, F., Dominguez, M. A., Pujol, M., et al. (2013). Impact of multidrug resistance on Pseudomonas aeruginosa ventilator-associated pneumonia outcome: predictors of early and crude mortality. Eur. J. Clin. Microbiol. Infect. Dis. 32, 413–420. doi: 10.1007/s10096-012-1758-8
Peña, C., Gómez-Zorrilla, S., Suarez, C., Dominguez, M. A., Tubau, F., Arch, O., et al. (2012). Extensively drug-resistant Pseudomonas aeruginosa: risk of bloodstream infection in hospitalized patients. Eur. J. Clin. Microbiol. Infect. Dis. 31, 2791–2797. doi: 10.1007/s10096-012-1629-3
Pereira, S. G., Marques, M., Pereira, J., and Cardoso, O. (2015). Multidrug and extensive drug resistance in Pseudomonas aeruginosa clinical isolates from a Portuguese central hospital: 10-year survey. Microb. Drug Resist. 21, 194–200. doi: 10.1089/mdr.2014.0137
Pereira, S. G., Reis, T., Mendez, I. P., and Cardoso, O. (2013). Prevalence and molecular epidemiology of imipenem-resistant Pseudomonas aeruginosa carrying metallo-beta-lactamases from two central hospitals in Portugal. Microb. Drug Resist. 19, 392–396. doi: 10.1089/mdr.2013.0029
Pérez, A., Gato, E., Pérez-Llarena, J., Fernández-Cuenca, F., Gude, M. J., Oviaño, M., et al. (2019). High incidence of MDR and XDR Pseudomonas aeruginosa isolates obtained from patients with ventilator-associated pneumonia in Greece, Italy and Spain as part of the MagicBullet clinical trial. J. Antimicrob. Chemother. 74, 1244–1252. doi: 10.1093/jac/dkz030
Pinilla-Rello, A., Huarte-Lacunza, R., Magallón-Martínez, A., Cazorla-Poderoso, L., Pereira-Blanco, O., Pérez-Moreno, M., et al. (2021). Utilization study in real clinical practice of ceftolozane/tazobactam vs aminoglycosides and/or colistin in the treatment of multirresistant or extremely resistant Pseudomonas aeruginosa. Rev. Esp. Quimioter. 34, 441–449. doi: 10.37201/req/006.2021
Quartin, A. A., Scerpella, E. G., Puttagunta, S., and Kett, D. H. (2013). A comparison of microbiology and demographics among patients with healthcare-associated, hospital-acquired, and ventilator-associated pneumonia: a retrospective analysis of 1184 patients from a large, international study. BMC Infect. Dis. 13:561. doi: 10.1186/1471-2334-13-561
Recio, R., Villa, J., Viedma, E., Orellana, M. Á, Lora-Tamayo, J., and Chaves, F. (2018). Bacteraemia due to extensively drug-resistant Pseudomonas aeruginosa sequence type 235 high-risk clone: facing the perfect storm. Int. J. Antimicrob. Agents 52, 172–179. doi: 10.1016/j.ijantimicag.2018.03.018
Ríos, P., Rocha, C., Castro, W., Vidal, M., Canal, E., Bernal, M., et al. (2018). Extensively drug-resistant (XDR) Pseudomonas aeruginosa identified in Lima, Peru co-expressing a VIM-2 metallo-β-lactamase, OXA-1 β-lactamase and GES-1 extended-spectrum β-lactamase. JMM Case Rep. 5:e005154. doi: 10.1099/jmmcr.0.005154
Russell, C. J., Simon, T. D., and Neely, M. N. (2019). Development of chronic Pseudomonas aeruginosa-positive respiratory cultures in children with tracheostomy. Lung 197, 811–817. doi: 10.1007/s00408-019-00285-6
Sacha, P., Wieczorek, P., Hauschild, T., Zórawski, M., Olszańska, D., and Tryniszewska, E. (2008). Metallo-β-lactamases of Pseudomonas aeruginosa–A novel mechanism resistance to β-lactam antibiotics. Folia Histochem. Cytobiol. 46, 137–142. doi: 10.2478/v10042-008-0020-9
Sader, H. S., Castanheira, M., Duncan, L. R., and Flamm, R. K. (2018a). Antimicrobial susceptibility of Enterobacteriaceae and Pseudomonas aeruginosa isolates from united states medical centers stratified by infection type: results from the international network for optimal resistance monitoring (INFORM) surveillance program. Diagn. Microbiol. Infect. Dis. 92, 69–74. doi: 10.1016/j.diagmicrobio.2018.04.012
Sader, H. S., Flamm, R. K., Carvalhaes, C. G., and Castanheira, M. (2018b). Antimicrobial susceptibility of Pseudomonas aeruginosa to ceftazidime-avibactam, ceftolozane-tazobactam, piperacillin-tazobactam, and meropenem stratified by U.S. census divisions: results from the 2017 INFORM program. Antimicrob. Agents Chemother. 62:e01587-18. doi: 10.1128/AAC.01587-18
Sader, H. S., Huband, M. D., Castanheira, M., and Flamm, R. K. (2017). Pseudomonas aeruginosa antimicrobial susceptibility results from four years (2012 to 2015) of the International Network for Optimal Resistance Monitoring Program in the United States. Antimicrob. Agents Chemother. 61:e02252-16. doi: 10.1128/AAC.02252-16
Safaei, H., Moghim, S., Isfahani, B., Fazeli, H., Poursina, F., Yadegari, S., et al. (2017). Distribution of the strains of multidrug-resistant, extensively drug-resistant, and pandrug-resistant Pseudomonas aeruginosa isolates from burn patients. Adv. Biomed. Res. 6:74. doi: 10.4103/abr.abr_239_16
Samonis, G., Vardakas, K. Z., Kofteridis, D. P., Dimopoulou, D., Andrianaki, A. M., Chatzinikolaou, I., et al. (2014). Characteristics, risk factors and outcomes of adult cancer patients with extensively drug-resistant Pseudomonas aeruginosa infections. Infection 42, 721–728. doi: 10.1007/s15010-014-0635-z
Silby, M. W., Winstanley, C., Godfrey, S. A. C., Levy, S. B., and Jackson, R. W. (2011). Pseudomonas genomes: diverse and adaptable. FEMS Microbiol. Rev. 35, 652–680. doi: 10.1111/j.1574-6976.2011.00269.x
Souli, M., Galani, I., and Giamarellou, H. (2008). Emergence of extensively drug-resistant and pandrug-resistant Gram-negative bacilli in Europe. Euro Surveill. 13:19045. doi: 10.2807/ese.13.47.19045-en
Suetens, C., Kärki, T., Plachouras, D., and European Centre for Disease Prevention and Control (2023). Point prevalence survey of healthcare-associated infections and antimicrobial use in European acute care hospitals: 2016-2017. Solna: European Centre for Disease Prevention and Control.
Tamma, P. D., Aitken, S. L., Bonomo, R. A., Mathers, A. J. van Duin, D., and Clancy, C. J. (2022). Infectious Diseases Society of America Antimicrobial-Resistant Treatment Guidance: Gram-Negative Bacterial Infections. Infectious Diseases Society of America 2023; Version 3.0. Available online at: https://www.idsociety.org/practice-guideline/amr-guidance/. (accessed November 8, 2023).
Tofas, P., Samarkos, M., Piperaki, E. T., Kosmidis, C., Triantafyllopoulou, I. D., Kotsopoulou, M., et al. (2017). Pseudomonas aeruginosa bacteraemia in patients with hematologic malignancies: risk factors, treatment and outcome. Diagn. Microbiol. Infect. Dis. 88, 335–341. doi: 10.1016/j.diagmicrobio.2017.05.003
Varaiya, A., Kulkarni, M., Bhalekar, P., and Dogra, J. (2008). Incidence of metallo-beta-lactamase-producing Pseudomonas aeruginosa in diabetes and cancer patients. Indian J. Pathol. Microbiol. 51, 200–203. doi: 10.4103/0377-4929.41683
Viedma, E., Juan, C., Acosta, J., Zamorano, L., Otero, J. R., Sanz, F., et al. (2009). Nosocomial spread of colistin-only-sensitive sequence type 235 Pseudomonas aeruginosa isolates producing the extended-spectrum β-lactamases GES-1 and GES-5 in Spain. Antimicrob. Agents Chemother. 53, 4930–4933. doi: 10.1128/AAC.00900-09
Walkty, A., Lagace-Wiens, P., Adam, H., Baxter, M., Karlowsky, J., Mulvey, M. R., et al. (2017). Antimicrobial susceptibility of 2906 Pseudomonas aeruginosa clinical isolates obtained from patients in Canadian hospitals over a period of 8 years: results of the Canadian Ward surveillance study (CANWARD), 2008–2015. Diagn. Microbiol. Infect. Dis. 87, 60–63. doi: 10.1016/j.diagmicrobio.2016.10.003
Werth, B. J., Carreno, J. J., and Reveles, K. R. (2015). Shifting trends in the incidence of Pseudomonas aeruginosa septicemia in hospitalized adults in the United States from 1996-2010. Am. J. Infect. Control 43, 465–468. doi: 10.1016/j.ajic.2015.01.028
Willmann, M., Klimek, A. M., Vogel, W., Liese, J., Marschal, M., Autenrieth, I. B., et al. (2014). Clinical and treatment-related risk factors for nosocomial colonisation with extensively drug-resistant Pseudomonas aeruginosa in a haematological patient population: a matched case control study. BMC Infect. Dis. 14:650. doi: 10.1186/s12879-014-0650-9
Keywords: extensively drug-resistant, Pseudomonas aeruginosa, antimicrobial resistance, difficult-to-treat infections, ceftazidime-avibactam, ceftolozane-tazobactam
Citation: Mendes Pedro D, Paulo SE, Santos CM, Fonseca AB, Melo Cristino J, Pereira ÁA and Caneiras C (2024) Extensively drug-resistant Pseudomonas aeruginosa: clinical features and treatment with ceftazidime/avibactam and ceftolozane/tazobactam in a tertiary care university hospital center in Portugal – A cross-sectional and retrospective observational study. Front. Microbiol. 15:1347521. doi: 10.3389/fmicb.2024.1347521
Received: 30 November 2023; Accepted: 15 January 2024;
Published: 13 February 2024.
Edited by:
Alessandra Oliva, Sapienza University of Rome, ItalyReviewed by:
Payam Behzadi, Islamic Azad University, ShahreQods, IranSamy Selim, Al Jouf University, Saudi Arabia
Copyright © 2024 Mendes Pedro, Paulo, Santos, Fonseca, Melo Cristino, Pereira and Caneiras. This is an open-access article distributed under the terms of the Creative Commons Attribution License (CC BY). The use, distribution or reproduction in other forums is permitted, provided the original author(s) and the copyright owner(s) are credited and that the original publication in this journal is cited, in accordance with accepted academic practice. No use, distribution or reproduction is permitted which does not comply with these terms.
*Correspondence: Diogo Mendes Pedro, ZGlvZ28ucGVkcm9AY2hsbi5taW4tc2F1ZGUucHQ=