- 1Innovation Center for Feeding and Utilization of Special Animals in Jilin Province, Research Center for Microbial Feed Engineering of Special Animals in Jilin Province, Institute of Special Animal and Plant Sciences, Chinese Academy of Agricultural Sciences, Changchun, China
- 2Jilin Agricultural Science and Technology University, Jilin City, China
- 3Key Laboratory of Agro-ecological Protection and Exploitation and Utilization of Animal and Plant Resources in Eastern Inner Mongolia, Chifeng University, Chifeng, China
- 4Hongjiu BioTech Co., Ltd., Tonghua, China
- 5Jilin Province Shuangyang Deer Industry Stock Breeding Co., Ltd., Changchun, China
American ginseng residue is an industrial by-product of ginseng saponin extraction, including polysaccharides and amino acids; however, it is often discarded into the natural environment, representing a waste of resources as well as an environmental issue. In this study, we examined the effects of adding American ginseng residue to the basal diet of sika deer. Twelve antler-bearing male sika deer were assigned randomly to groups fed a diet supplemented with 0% (CON), 1% (LGR), and 3% (HGR) American ginseng residue, respectively, (n = 4 per group) for 5 weeks. Supplementation with 3% American ginseng residue significantly increased antler production and feed utilization efficiency in antler-bearing sika deer (p < 0.05). There were no significant differences in serum biochemical indexes among the three groups, but serum immunoglobulin A and glutathione peroxidase levels were significantly increased in the LGR and HGR groups (p < 0.05). Supplementation with American ginseng residue affected rumen fermentation in sika deer, significantly increasing the rumen contents of acetic acid, propionic acid, and total volatile fatty acids, and decreasing rumen fluid pH (p < 0.05), but had no significant effect on microbial protein or ammoniacal nitrogen content. American ginseng residue also affected the rumen bacterial composition, with significant up-regulation of Bacteroidota abundance in the HGR group, significant increases in Fibrobacterota and Fibrobacter abundance in the LGR group, and a significant decrease in Oscillospiraceae_UCG-005. Supplementation with ginseng residue had no significant effect on volatile fatty acids in the feces of sika deer, but did affect the composition of fecal bacteria, with significant decreases in Desulfobacterota and Rikenellaceae_RC9_gut_group in the HGR group, and a significant increase in Ruminococcus in the LGR group (p < 0.05). In addition, the abundance of Paeniclostridium in the feces decreased linearly with increasing concentration of ginseng residue, with a significant difference among the groups (p < 0.05). This study comprehensively evaluated the effects of American ginseng residue as a potential feed additive on the production performance and gastrointestinal bacterial community in antler-bearing sika deer. The results indicated that ginseng residue was a suitable feed additive for improving production performance and health in sika deer.
1 Introduction
Ginseng residue is an industrial by-product resulting from the extraction of ginseng plants, such as American ginseng (Panax quinquefolius L.) and ginseng (Panax ginseng C. A. Mey.). These residues were previously disposed of as landfill, with adverse effects on the ecological environment. Given the efficacy and importance of ginsenosides in human health care products, many residues after ginsenoside extraction still contain active ingredients, such as polysaccharides and amino acids (Sun et al., 2022, 2023); however, extracting these would entail additional costs. Ginseng residue has previously been reported as a novel source of cellulose to provide energy to piglets, after microbial fermentation to break down lignocellulose (Xiao et al., 2022); however, this likely disrupted the functions of the active substances. We therefore considered that it would be possible to avoid wasting the functional ingredients of ginseng residue, such as active polysaccharides, oligopeptides, and sterols, by direct feeding, while simultaneously reducing environmental pressures.
Panax quinquefolius, P. ginseng, P. notoginseng, and P. japonicus are all well-known medicinal plants with excellent antioxidant and immunity-boosting effects (Li et al., 2017; Paik et al., 2023), which have different qualities in Chinese medicine and can be used for different diseases (Liu et al., 2020). Its main medicinal components are saponins and polysaccharides (Xiong et al., 2020; Qi et al., 2021). American ginseng is currently widely planted globally, with huge production, with the United States, Canada, China, and South Korea among the main production areas (Punja, 2011). The most commonly reported biological functions of American ginseng polysaccharides involve modulation of the inflammatory response and improvement of immune function. Previous studies showed that neutral polysaccharides in American ginseng significantly reduced expression levels of the pro-inflammatory cytokines interleukin (IL)-1β, IL-6, and tumor necrosis factor-α, and inhibited the massive recruitment of neutrophils in the neural thalamus of zebrafish, thus alleviating inflammation (Xie et al., 2023). Another oligopolysaccharide isolated from American ginseng, CVT-E002, acted on B-lymphocytes and stimulated the proliferation of splenocytes and the production of immunoglobulin (Ig) G in mice (Wang et al., 2001). Three acidic polysaccharides in American ginseng, PPQA2, PPQA4, and PPQA5, also showed immunostimulatory functions (Wang et al., 2015), while the polysaccharide WQP significantly ameliorated antibiotic-induced diarrhea in rats by inhibiting the mitogen-activated protein kinase inflammatory signaling pathway, decreasing inflammatory cytokine levels and the infiltration of inflammatory cells in the ileum and colon, and increasing short-chain fatty acids by increasing the abundance of beneficial bacteria, such as Lactobacillus and Bacteroides intestinalis, which promote restoration of the intestinal barrier (Ren et al., 2022). In addition to saponins and polysaccharides, protopanaxadiol in American ginseng significantly inhibited the growth of cancer cells by acting on signaling pathways such as TRAIL (Zhang et al., 2015). Some small molecule oligopeptides in American ginseng were shown to increase the activity of oxide-producing dismutase and glutathione peroxidase, reduce malondialdehyde content, and increase expression levels of nuclear respiratory factor 1 and mitochondrial transcription factor A in mice, thus enhancing the body’s scavenging function of reactive oxygen species and relieving fatigue (Li D. et al., 2018). These results indicate that saponin-extracted American ginseng residue retains medicinal value.
Sika deer are an important component of China’s livestock industry, providing humans with high-quality medicinal and edible products such as antlers, blood, and meat; notably however, nutritional research in sika deer is lacking (Bao et al., 2021; Zhou Z. et al., 2022; Takeda et al., 2023). The antler provide a traditional, valuable, and expensive medicinal product with a variety of effects, such as improving sexual performance in mice and boosting immunity (Zang et al., 2016; Xia et al., 2022). Antler production is thus one of the most important stages in the breeding of sika deer, with distinct economic impacts. Sika deer are sensitive and timid by nature and are thus susceptible to stress caused by frequent human interventions in captivity and the inability to change their living environment in response to changes in the natural environment (Zidon et al., 2009). During the antler-growth period, deer also need to cope with high energy and protein requirements, as well as adapting to rapid changes in hormone levels (Price and Allen, 2004; Bartos et al., 2009; Bao et al., 2021).
The effect of American ginseng residue in sika deer farming is unknown. We therefore comprehensively assessed the effect of American ginseng residue on the production performance, digestibility of nutrients, serum biochemical indexes, and gastrointestinal bacteria and bacterial fermentation in antler-bearing sika deer, with the aim of providing a reference for the reuse of waste and the development of novel feed additives.
2 Materials and methods
2.1 Animal ethics statement
All animal procedures were approved and authorized by the Animal Ethics Committee of the Institute of Special Animal and Plant Sciences, Chinese Academy of Agricultural Sciences (NO. ISAPSAEC-2021-59D).
2.2 Experimental material
American ginseng residue is a by-product of ethanol extraction of saponins. The ginseng residue used in these experiments was purchased from Jilin Hongjiu Bio-technology Co. (Tongliao, Jilin, China). The average polysaccharide content of this batch of residue was 31.61%, and no total ginsenosides were detected in the laboratory.
2.3 Experimental design and animal management
Twelve healthy 4-year-old male sika deer with similar body weights and antler-regeneration times were selected for this experiment. Each deer was housed in a separate enclosure and assigned randomly to one of three groups fed basal diets supplemented with 0, 1, and 3% American ginseng residue, respectively. The entire experiment lasted for 5 weeks, including a 1-week pre-experimental period to determine the maximum feed intake of the animals and acclimatize them to the diet, followed by a 4-week experimental period. Throughout the 5-week period, the animals were fed at 4 am and 5 pm each day with a total of 2.8 kg of diet (air-dried basis), during which time the animals had free access to water and the pens were cleaned regularly to ensure a clean environment. All the feed provided was consumed by the animals. The composition and nutrient content of the basal diet are shown in Table 1. The experiment was carried out in Shuangyang District, Changchun City, Jilin Province, China (longitude: 125.724, dimension: 43.539, temperature: 19–28°C, wind scale: <3).
2.4 Sample collection and measurement
Approximately 200 g of fresh feces were collected from the deer each day before morning feeding for 4 days prior to the end of the experiment. Hair and grit were removed from the collected feces and the feces were sprayed with 10% dilute hydrochloric acid for nitrogen fixation. The samples were then divided into two parts and processed immediately. One part was dried at 65°C to constant weight, pulverized, and passed through a 40-mesh sieve to produce air-dried samples to detect crude protein and so on (Yang L. et al., 2022), and the other was sterilized at 85°C for 2 h, dried at 65°C to constant weight, and sieved through an 18-mesh sieve for fiber determination.
On the last day of the experiment, the deer were anesthetized using an anesthesia gun (xylazine hydrochloride injection, 2 mL/100 kg) prior to the morning feeding, the antlers were cut off completely using a sterilized saw and weighed, and approximately 30 mL of blood was obtained. The blood was centrifuged (1,200 × g) and the serum was aspirated and stored at −80°C for later use. A special hose is inserted through the mouth to reach the rumen, and then the rumen fluid is extracted by means of negative pressure. Approximately 200 mL of rumen fluid was obtained from each deer via the rumen, of which the first 100 mL was discarded to avoid salivary contamination (Chang et al., 2022). Approximately 20 g of fresh feces were obtained from the rectum of the deer using sterile disposable gloves. The rumen fluid and feces were transferred quickly to liquid nitrogen and stored at −80°C for further analysis.
2.4.1 Digestibility of nutrients
Crude protein, ether extract, and dry matter were determined as described previously [Association of Official Analytical Chemists (AOAC), 2006] using a fully automated Kjeldahl nitrogen analyzer KDN-520 (Hangzhou Lvbo Instrument Co., Hangzhou, Zhejiang, China) and Soxhlet fat extraction B-811 (Büchi Labortechnik AG., Flawil, Switzerland). Neutral detergent fiber and acid detergent fiber (ADF) were determined using a fiber analyzer ANKOM A2000i (Ankom Technology Co., Macedon, NY, USA) according to the methods (Raffrenato and Van Amburgh, 2011; Barbosa et al., 2015). Acid-insoluble ash was used as an indicator and determined as described by the reference (Prawirodigdo et al., 2021). Apparent digestibility of a nutrient = 100 − (100 × A/A1 × B1/B), where A is the percentage of 2 mol/L hydrochloric acid-insoluble ash in the sample, A1 is the percentage of 2 mol/L hydrochloric acid-insoluble ash in the feces, B1 is the percentage of that nutrient in the feces, and B is the percentage of that nutrient in the sample.
2.4.2 Serum biochemical, antioxidant, and immunological indicators
The concentrations of serum triglycerides, total cholesterol, high-density lipoprotein cholesterol, low-density lipoprotein cholesterol, glucose, total protein, albumin, globulin, alkaline phosphatase, and aspartate aminotransferase were analyzed using commercial colorimetric kits (Nanjing Jiancheng Bioengineering Institute, Nanjing, Jiangsu, China) with a Beckman AU480 automatic biochemistry analyzer (Vitalab Selectra E, Spankeren, The Netherlands). Serum concentrations of IgA, IgM, and IgG were quantified using enzyme-linked immunoassay kits (MLBIO, Shanghai, China). Serum antioxidant levels of total superoxide dismutase, glutathione peroxidase, catalase, and total antioxidant capacity were measured using kits (Nanjing Jiancheng Bioengineering Institute, Nanjing, Jiangsu, China), in accordance with the manufacturer’s instructions.
2.4.3 Volatile fatty acids and rumen fermentation
Rumen fluid samples were centrifuged at 5000 × g for 10 min at 4°C, and the supernatant was added to 25% metaphosphoric acid solution containing an internal standard at a ratio of 5:1 (supernatant: internal standard), mixed well, frozen at −20°C overnight, centrifuged at 10,000 × g for 10 min at 4°C, and the supernatant was prepared. Fecal samples (30 mg) were placed in a glass tube and 900 μL of 0.5% phosphoric acid was added, mixed well, centrifuged at 14,000 × g for 10 min at 4°C. An equal amount of ethyl acetate was added to 800 μL of supernatant for extraction, mixed well, and centrifuged at 14,000 × g for 10 min at 4°C. The upper layer of the organic phase was mixed with 4-methylglutaric acid and prepared for use. The prepared samples were analyzed by mass spectrometry using an Agilent 7890A/5975C gas-mass spectrometer (Agilent Technologies Inc., Santa Clara, CA, USA). The chromatographic peak areas and retention times were extracted using MSD ChemStation software (Version B.08.00, Agilent Technologies Inc., Santa Clara, CA, USA). Standardized curves were plotted to calculate the contents of volatile fatty acids in the samples (Wang M. et al., 2016; Zhang et al., 2017).
The concentrations of ammoniacal nitrogen and microbial proteins in the rumen fluid were determined using appropriate kits (Nanjing Jiancheng Bioengineering Institute, Nanjing, Jiangsu, China) (Shahinian and Reinhold, 1971; Liu S. et al., 2023) and the pH of the rumen fluid was determined using a PHS-3C pH meter (Shanghai INESA Scientific Institute Co., Shanghai, China) (Kong et al., 2020).
2.4.4 16S rRNA amplicon sequencing
DNA was extracted from the samples using the CTAB method (Minas et al., 2011), tested for purity and concentration, and then diluted to 1 ng/μL using sterile water and used as a template for polymerase chain reaction (PCR) amplification using the following primers: 338 F (5′-ACTCCTACGGGGAGGCAGCA-3′) and 806 R (5′-GGACTACHVGGGTWTCTAAT-3′). PCR products were purified by magnetic beads, and detected by electrophoresis using a 2% agarose gel after mixing in equal amounts according to the PCR product concentration. A TruSeq DNA PCR-Free sample preparation kit (Illumina Inc., San Diego, CA, USA) was used for library construction, and the constructed libraries were quantified by Qubit and Q-PCR, qualified, and analyzed by NovaSeq6000 (Illumina Inc.) for on-line sequencing (Li et al., 2015; Zhang et al., 2023).
2.5 Data analysis
The Shannon index, Simpson index, abundance-based coverage estimator index, Chao1 index, and UniFrac distance were calculated using Qiime software (Version 1.9.1). Principal coordinates analysis (PCoA) plots were plotted using R software (Version 2.15.3). PCoA analysis was performed using the WGCNA, stats, and ggplot2 packages in R. Linear discriminant analysis effect size (LEfSe) was performed using the LEfSe package with a default setting of linear discriminant analysis (LDA) score of 3. Adonis analysis was performed using the adonis function in the R vegan package.
Data were analyzed using SPSS software (IBM SPSS Statistics 26; IBM-SPSS Inc., Chicago, IL, USA). The Shapiro–Wilk test was used to determine if the data conformed to a normal distribution, the Kruskal–Wallis test was used to determine non-conformity, and the Bonferroni method was used for multiple comparisons if p < 0.05. Normally distributed data were analyzed using parametric tests and the F-test was used to determine variance alignment. One-way ANOVA was used and post hoc multiple comparisons were made using Tukey’s test if variance alignment was met, and the t-test was used if variance alignment was not met. Values were expressed as mean ± standard error, with a significance value of p < 0.05.
3 Results
3.1 Effect of American ginseng residue on antler production and apparent digestibility of nutrients in sika deer
The addition of American ginseng residue to the diet significantly increased antler production by sika deer during the antler-bearing period (Table 2), with significantly greater production in the HGR group compared with the CON group (p < 0.05). Antler production was also increased in the LGR group, but the difference was not significant. The apparent digestibility of the ADF was significantly increased in the LGR and HGR groups compared with the CON group (p < 0.05), but there was no significant difference in the apparent digestibility of other nutrients among the groups.
3.2 Serum biochemical indexes in the three groups of sika deer
The addition of American ginseng residue to the diet had no significant effect on any of the measured serum biochemical indexes (Table 3), and no effect on glycolipid metabolism, protein metabolism, or enzymes related to liver and kidney metabolism (p > 0.05). Serum globulin levels tended to increase linearly with increasing ginseng residue concentration, but the difference was not significant (p < 0.1).
3.3 Effects of American ginseng residue on antioxidants and immune status of sika deer
Supplementation with American ginseng residue improved the immune and antioxidant statuses of sika deer during the antler-bearing period (Table 4). Serum IgA levels increased significantly (p < 0.05) and linearly in the LGR and HGR groups compared with the CON group. In addition, catalase and glutathione peroxidase activities increased significantly (p < 0.05) in the HGR group and glutathione peroxidase activity increased significantly (p < 0.05) in the LGR group compared with the CON group, but there were no significant differences in the other indexes among the three groups.
3.4 Supplementation of American ginseng residue altered rumen fermentation and bacterial communities in sika deer
We compared the rumen fermentation parameters among the three groups of sika deer (Table 5), and found that the acetate content was significantly higher in the LGR group compared with the CON group, and the propionate content was significantly higher in the LGR and HGR groups (p < 0.05). In addition, the total volatile fatty acid content was also significantly higher in the LGR group than in the CON group. The ammonia nitrogen and microbial protein contents of the rumen fluid were similar in all three groups of sika deer (p > 0.05), but the rumen fluid pH was significantly lower in the HGR group compared with the CON group (p < 0.05).
Illumina Nova sequencing, construction of PCR-free libraries, followed by paired-end sequencing. After splicing the reads, an average of 85,245 tags were measured per sample, and an average of 84,552 valid data were obtained after quality control, with the amount of valid data for quality control amounting to 65,081, and the effective quality control rate of 76.42%. The sequences were clustered into operational taxonomic units (OTUs) with 97% concordance. A total of 4,869 OTUs were obtained, and 3,289 OTUs were filtered after removing data for Archaea, unknown, and no blast hits. Among these, 1,928 OTUs were common to all three groups of Figure 1C. The sequences of the OTUs were then annotated for species using the Silva138 database. At the phylum level, Bacillota and Bacteroidota were the dominant rumen bacteria in sika deer, accounting for 47.5 and 35.5% of the total bacterial abundance, respectively (Figure 1A). At the genus level, Prevotella, Ruminococcus, Rikenellaceae_RC9_gut_group, Pseudomonas, Christensenellaceae_R-7_group, and Succiniclasticum were the dominant genera (Figure 1B), accounting for about half of all genera. We plotted a curve by randomly selecting the amount of sequencing data for the sample and its corresponding number of species to obtain the dilution curve of the sample (Figure 1D); the curve gradually flattened out and the sequencing data were reasonable.
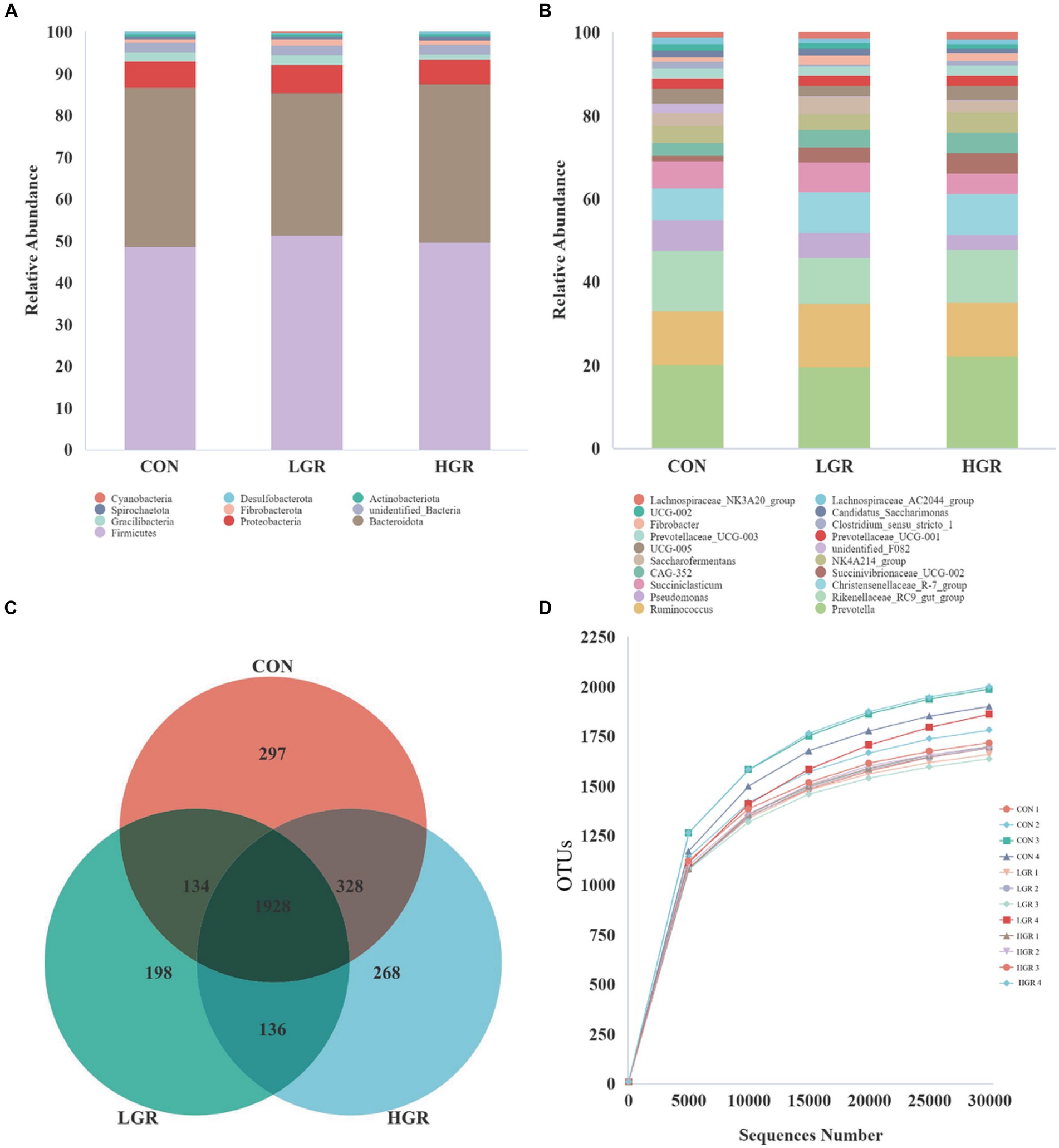
Figure 1. Abundances of top 10 bacteria at phylum level (A) and top 20 at genus level (B) in the rumen. Venn diagrams (C) and dilution curves (D) of rumen bacteria.
We investigated the effect of American ginseng residue on the abundance and diversity of rumen bacterial communities by comparing the alpha diversities among the three groups. There were no significant differences (p > 0.05) in the Shannon index, Simpson index, Chao1 index, and ACE index of the rumen bacteria (Figure 2). Based on the Bray–Curtis, binary-Jaccard, weighted UniFrac, and unweighted UniFrac distances, PCoA (Figure 3) and adonis analysis (Table 6) showed that the bacterial compositions of the CON and LGR groups were significantly separate (binary-Jaccard distances: p < 0.05, [CON vs. LGR]). We further compared bacteria with significantly different abundances at the phylum and genus levels. At the phylum level, Bacteroidota were significantly more abundant in the HGR group than in the LGR group (Figure 4A) and Fibrobacterota were significantly more abundant in the LGR group compared with the CON group (Figure 4B). At the genus level, Oscillospiraceae_UCG-005 were significantly less abundant in the LGR group (Figure 4C) and Fibrobacter were significantly more abundant in the LGR group compared with the CON group (Figure 4D). LDA showed that 11 bacterial species differed significantly from phylum level to genus level in the rumen fluid of the three groups (Figure 5A), Fibrobacteria, Fibrobacterales, and Fibrobacteraceae were significantly enriched under the same branch of the developmental tree in the LGR group, and Aeromonadales and Succinivibrionaceae were enriched in the HGR group under the same branch, while Oscillospirales__UCG-010 and Bacteroidales in the CON group were enriched on different branches (Figure 5B).
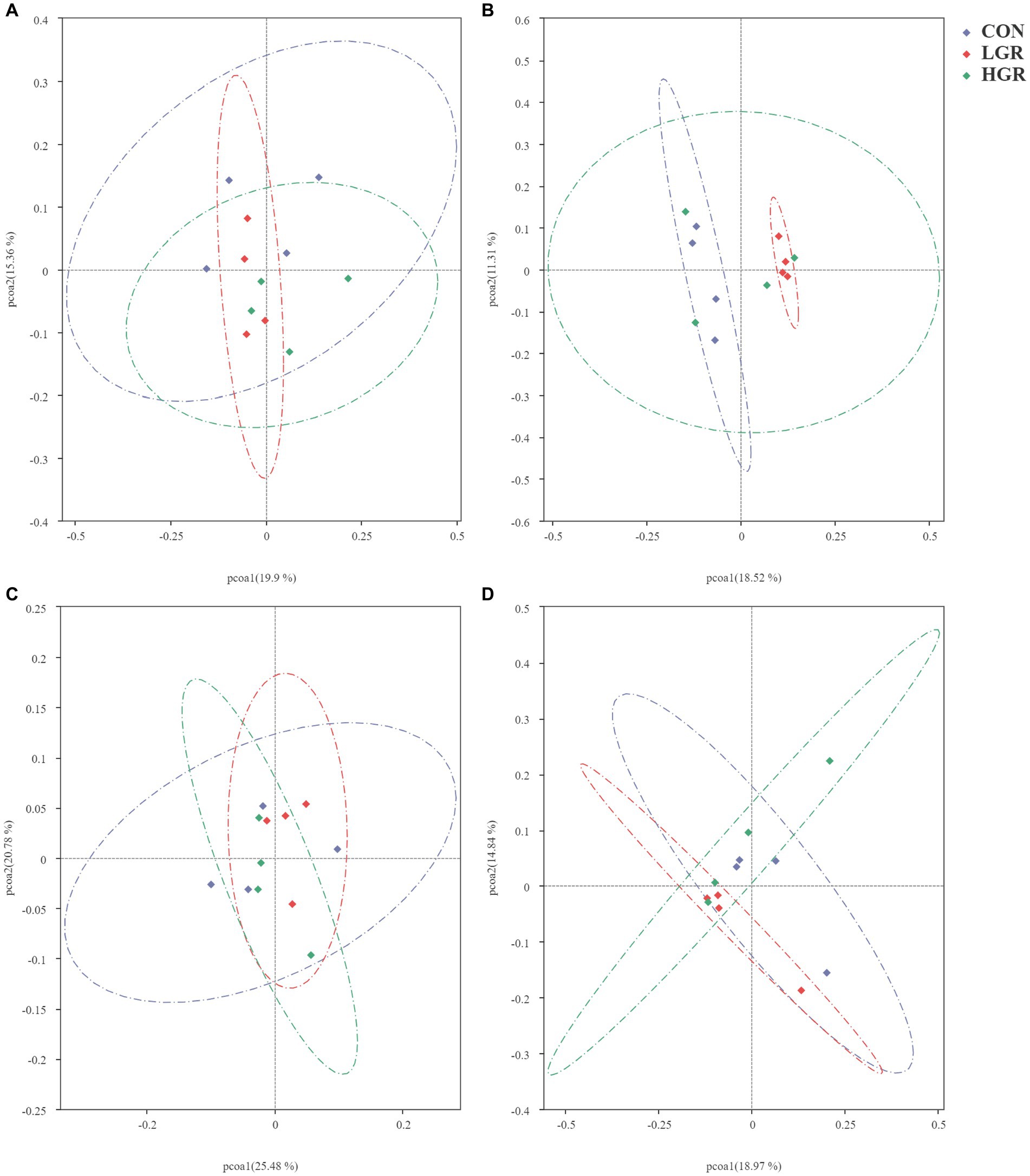
Figure 3. Principal co-ordinates analysis of bacterial communities in the rumen of sika deer based on Bray–Curtis distance (A), binary-Jaccard distances (B), weighted-UniFrac distance (C), and unweighted-UniFrac distance (D).
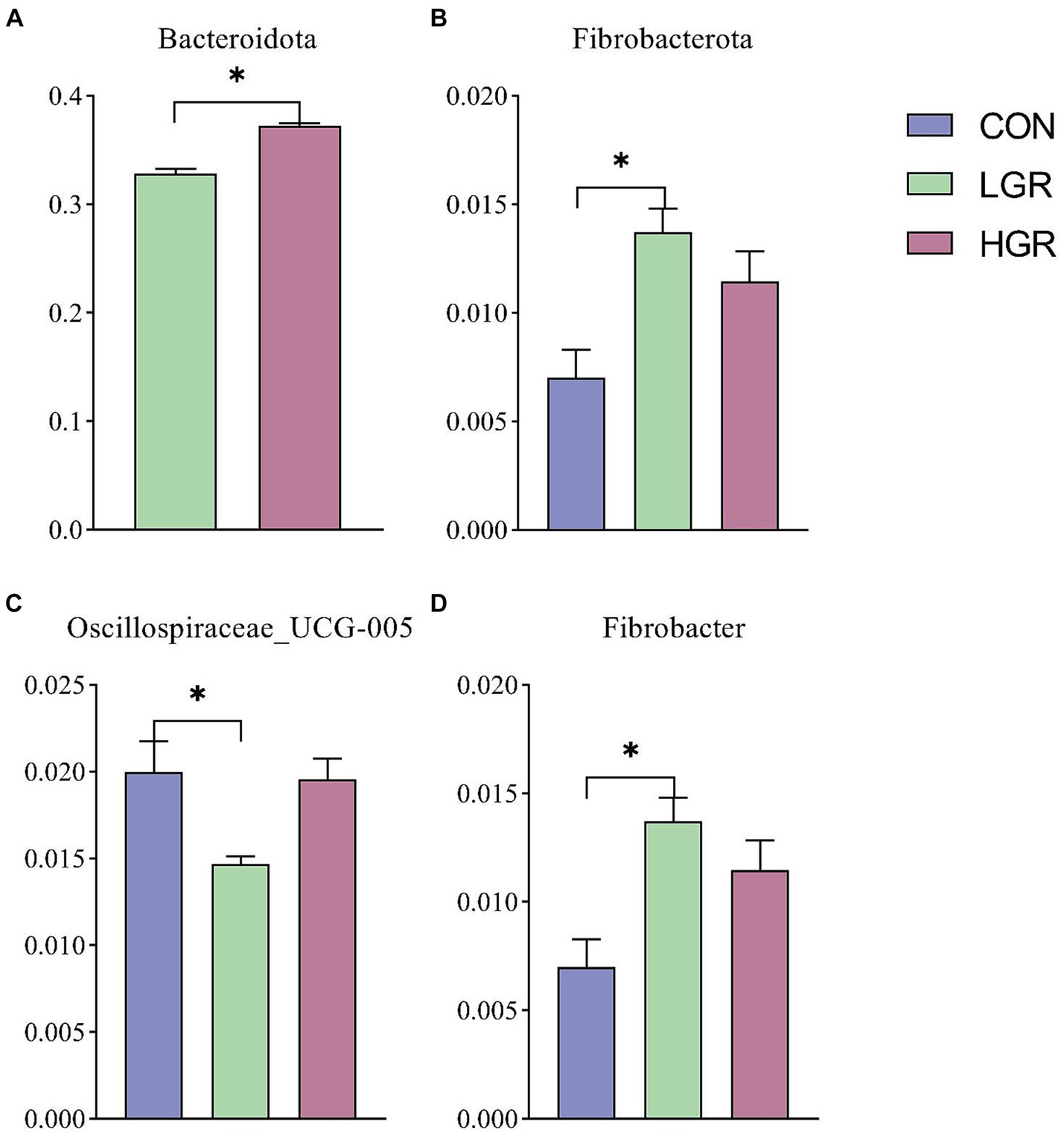
Figure 4. Differences in rumen bacteria at phylum level by t-test (A) and ANOVA (B). Differences in rumen bacteria at genus level (C,D). *p < 0.05.
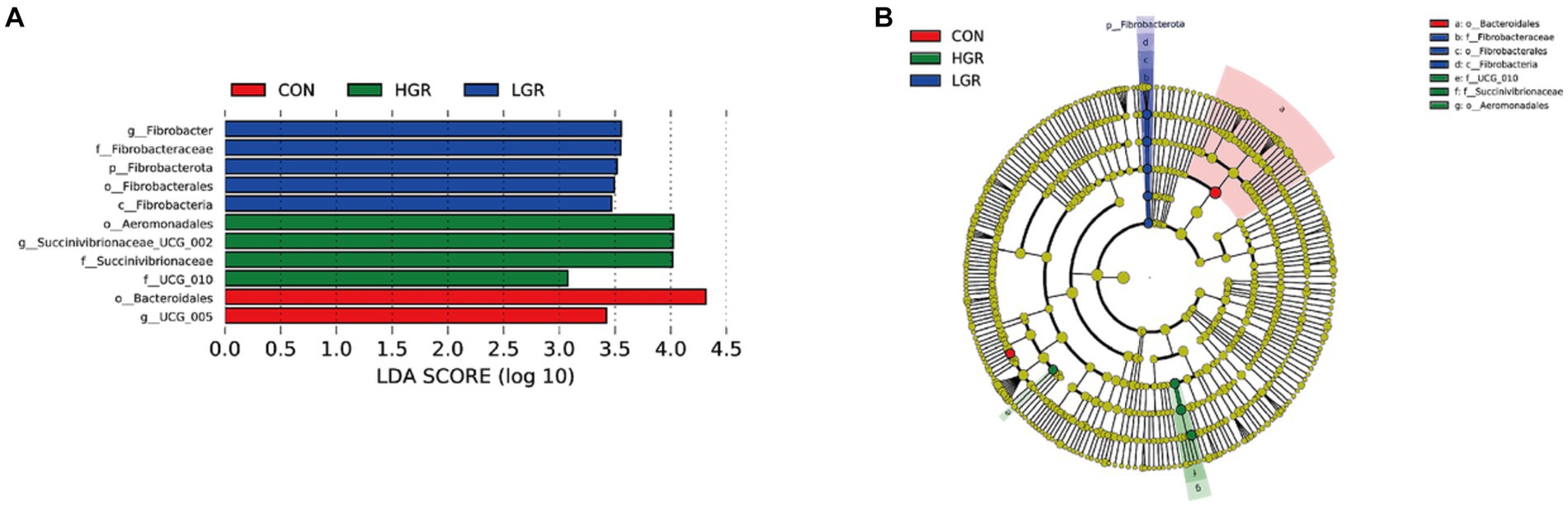
Figure 5. Linear discriminant analysis (LDA) effect size (LEfSe) analysis of rumen bacteria in sika deer. (A) LDA score of rumen microbiota (score > 3 significant). (B) Cladogram of LEfSe showing nodes of significant bacteria on evolutionary branches. Yellow nodes, no difference; other colored nodes, significant differences.
3.5 Effect of American ginseng residue on fecal volatile fatty acids and bacterial communities in sika deer
Fecal volatile fatty acids can reflect bacterial fermentation in the intestine. The feces contents of acetate, propionate, isobutyrate, butyrate, isovalerate, valerate, caproate, and total volatile fatty acids were similar in the three groups of deer (p > 0.05) (Table 7).
The fecal samples were sequenced and an average of 73,449 tags were measured for each sample. 73,020 data were obtained on average after quality control, and the amount of valid data for quality control amounted to 55,270, and the effective rate of quality control was 75.25%. A total of 5,981 OTUs were obtained after clustering with 97% concordance, and 4,794 OTUs were obtained after screening, of which 2,058 OTUs were common to all three groups (Figure 6C). The dominant bacteria in feces at the phylum level were Bacillota and Bacteroidota, which accounted for 48.6 and 29.2% of the total abundance, respectively (Figure 6A). The dominant bacteria at the genus level were Oscillospiraceae_UCG-005 (16.1%), Treponema (5.7%), Rikenellaceae_RC9_gut_group (5.7%), and Bacteroides (4.3%) (Figure 6B). The dilution curve gradually flattened out (Figure 6D) and the data were reasonable.
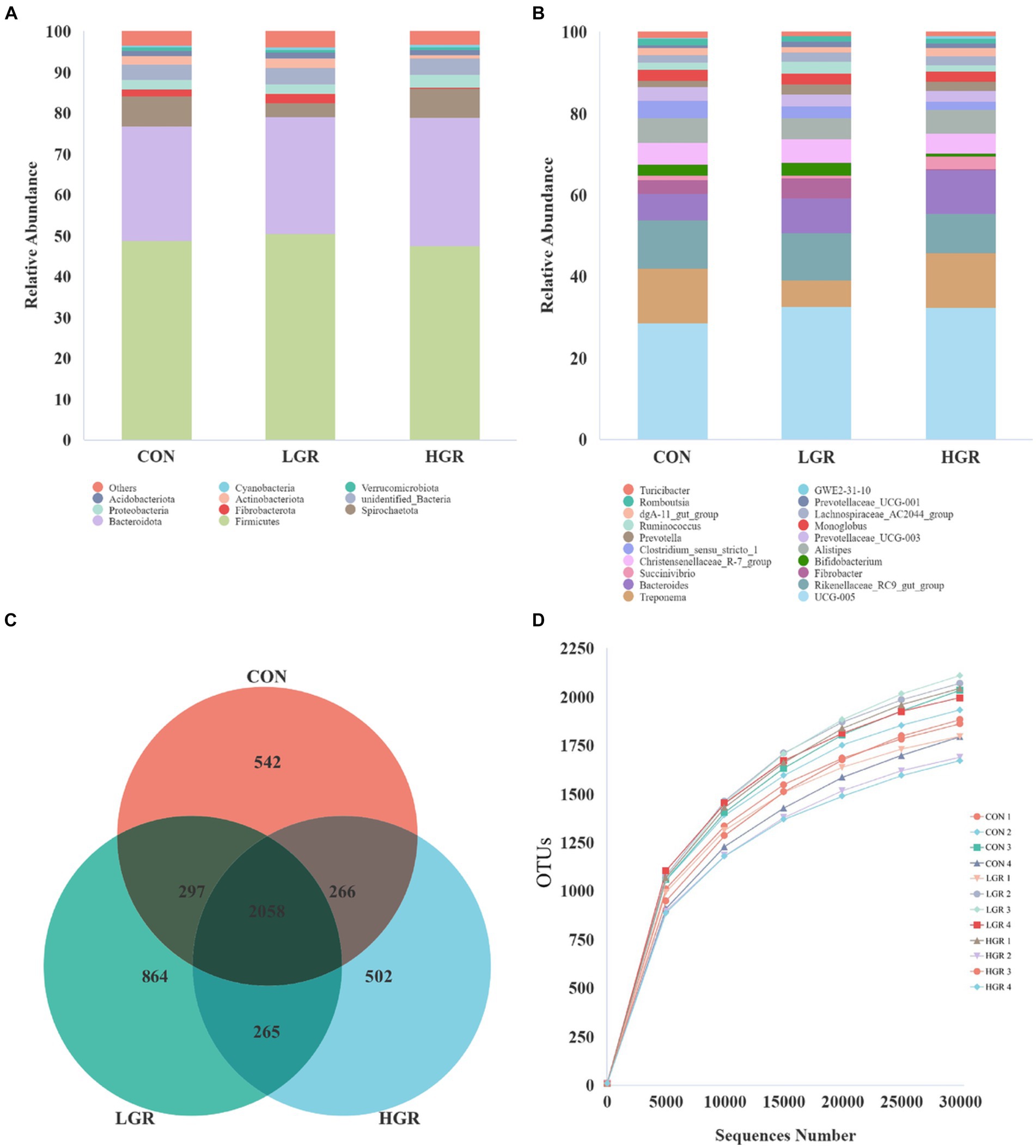
Figure 6. Abundance of top 10 bacteria in feces at phylum level (A) and top 20 at genus level (B). Venn diagrams (C) and dilution curves (D) of fecal bacteria.
The fecal alpha diversity results were similar for all three groups (p > 0.05) (Figure 7).
The results of PCoA (Figure 8) and adonis analysis (Table 8) showed that the fecal bacterial composition differed significantly between the CON and HGR groups, especially in terms of binary-Jaccard and unweighted-UniFrac distances (p < 0.05). Further analysis of the differential bacteria in the feces of the three groups (Figure 9) showed a significant decrease in Desulfobacterota abundance at the phylum level in the HGR group compared with the CON group (p < 0.05). At the genus level, the abundance of Rikenellaceae_RC9_gut_group was highly significantly decreased in the HGR group compared with the CON group (p < 0.01), and the abundance of Ruminococcus was highly significantly increased in the HGR group compared with the CON and HGR groups (p < 0.01), while the abundance of Paeniclostridium declined linearly in line with increasing addition of ginseng residue, with significant differences among the groups (p < 0.05). LDA (Figure 10A) showed significant enrichment from the phylum to the genus level for 19 bacterial species in the three groups, with Fibrobacterota providing the greatest contribution to the evolutionary tree (Figure 10B) in the LGR group. Fibrobacterota, Fibrobacteria, Fibrobacterales, Fibrobacteraceae, and Fibrobacter were enriched in the same branch, and Actinobacteriota, Actinobacteria, Bifidobacteriales, Bifidobacteriaceae, and Bifidobacterium were also equally enriched in the same branch, in addition to homology between Rikenellaceae and Rikenellaceae_RC9_gut_group in the CON group.
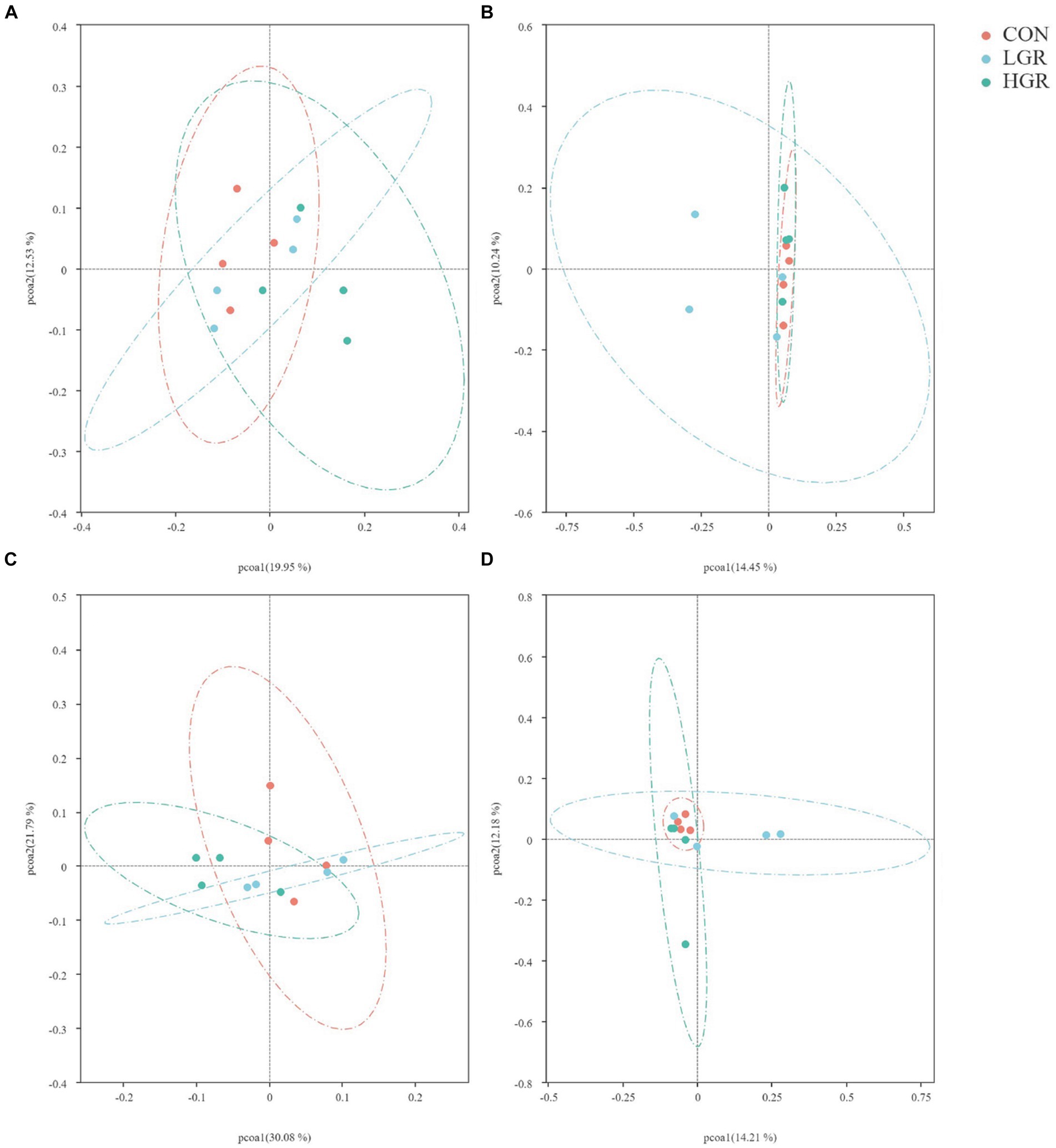
Figure 8. Principal co-ordinates analysis of bacterial communities in the feces of sika deer based on Bray–Curtis distance (A), binary-Jaccard distances (B), weighted-UniFrac distance (C), and unweighted-UniFrac distance (D).
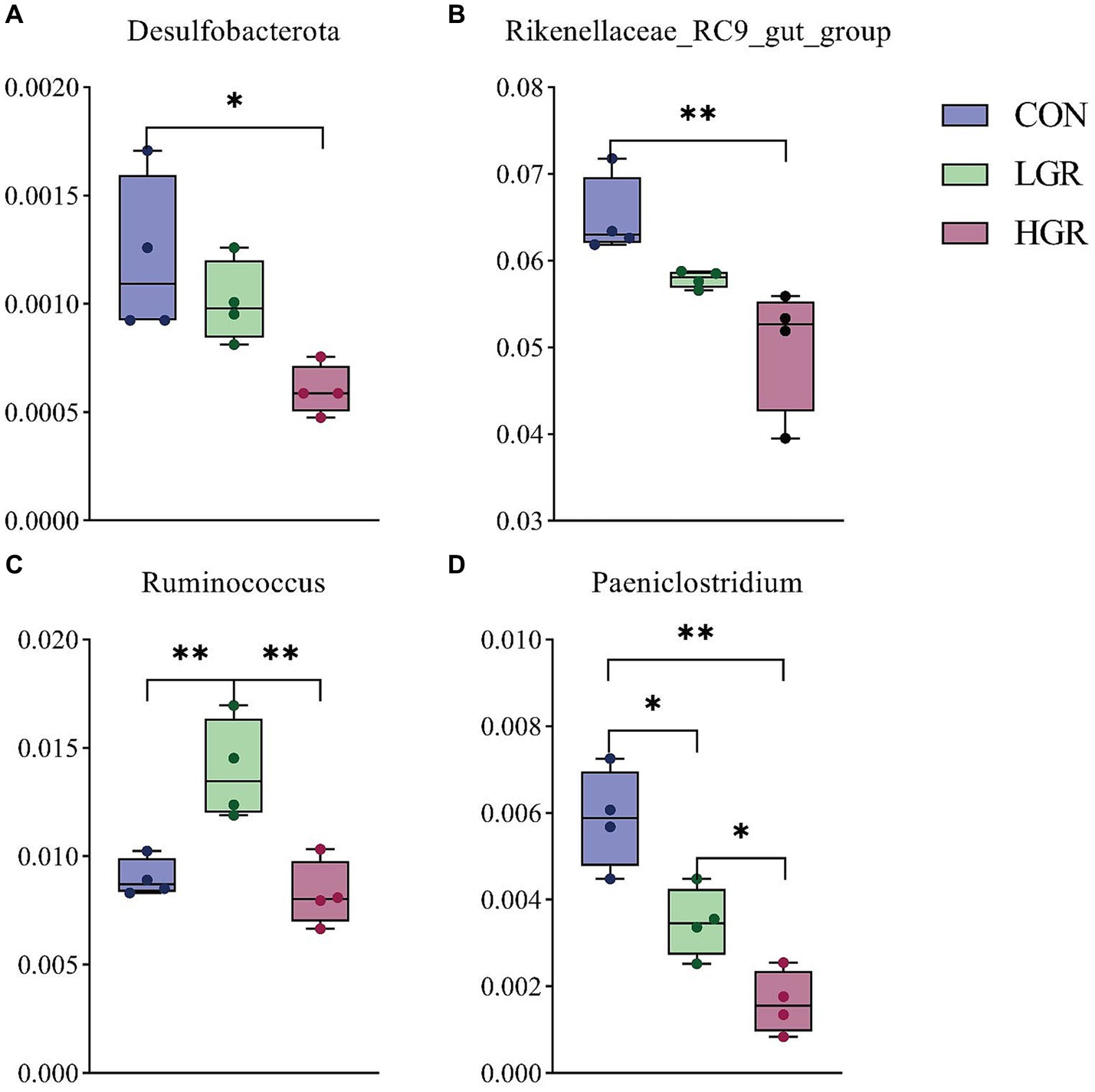
Figure 9. Differences in feces bacteria at phylum level (A) and genus level (B–D). *p < 0.05; **p < 0.01.
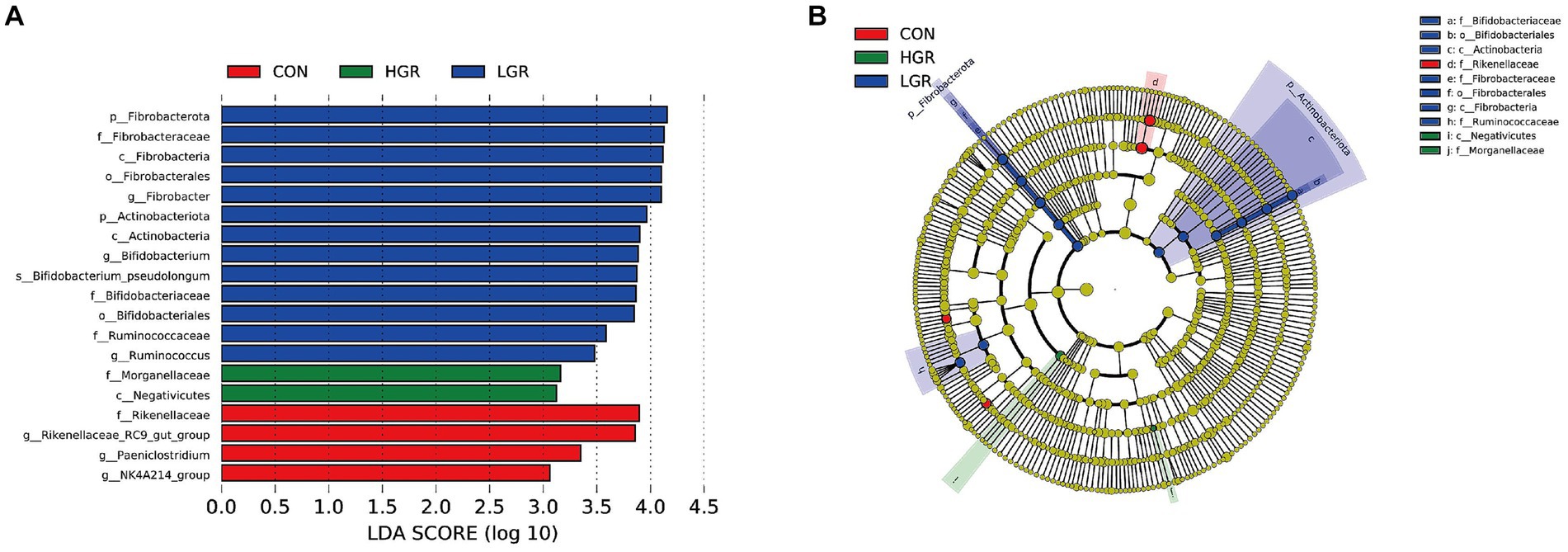
Figure 10. Linear discriminant analysis (LDA) effect size (LEfSe) analysis of fecal bacteria in three groups of sika deer. (A) LDA score of fecal microbiota (score > 3 significant). (B) Cladogram of LEfSe showing nodes of significant bacteria on evolutionary branches. Yellow nodes, no significant difference; other colored nodes, significant differences.
4 Discussion
American ginseng and sika deer are specific agricultural and animal husbandry industries, respectively, in Northeast China (Li et al., 2014), and the application of American ginseng by-products in sika deer breeding can thus maximize local advantages with mutual benefits. However, evidence for the role of American ginseng residue in the production of antler-bearing sika deer is currently lacking. Previous studies showed that animals experienced stress during specific physiological phases due to environmental changes or rapid changes in their own hormone levels, potentially leading to gastrointestinal tract damage, redox imbalance, and microflora dysbiosis, and ultimately affecting the performance and health of the animal (Campbell et al., 2013; Gresse et al., 2017; Deng et al., 2020). The current results showed that dietary supplementation with American ginseng residue significantly increased antler production as a direct result of improved utilization of cellulose in the feed, possibly related to the amelioration of gut damage and restoration of function. Previous studies found that American ginseng polysaccharides effectively treated intestinal mucosal injuries by up-regulating the ratio of villus height to crypt depth, increasing the number of cuprocytes and the expression of tight junction proteins (Zhou et al., 2021; Ren et al., 2022). In a mouse model of dextran sodium sulfate-induced enteritis, American ginseng extract reduced ileal inflammation and edema, increased villus length to alleviate diarrhea symptoms, and was effective in both prophylactic and therapeutic treatments (Jin et al., 2008; Wang C. Z. et al., 2016). Differences in serum activities and metabolite contents of various enzymes among the three subgroups in the present study demonstrated further the effects of ginseng residue in sika deer. Serum levels of various enzymes and transport products related to hepatic and renal metabolism were unaffected by the residue, indicating that the drug components in the residue did not affect hepatic and renal functions. Notably however, diet supplementation with American ginseng residue significantly enhanced the activities of catalase and glutathione peroxidase, which play key antioxidant roles in animals. Catalase is found widely in living organisms, and its main role is to scavenge the strongly cytotoxic hydrogen peroxide produced by the body’s metabolism (Alfonso-Prieto et al., 2009). Glutathione peroxidase exerts its antioxidant function by catalyzing the reduction of hydrogen peroxide by glutathione to achieve the scavenging of oxygen free radicals, and the oxidized glutathione generated can be reduced by other enzymes to play a role in the glutathione cycle (Arthur, 2000). In addition, serum IgA levels were significantly increased in the LGR and HGR groups, consistent with previous reports that American ginseng polysaccharides and other components exerted various effects to achieve immune-enhancing effects, such as activation of macrophages and enhanced phagocytosis (Yu et al., 2014), promotion of lymphocyte proliferation to increase Ig expression (Yu et al., 2017), and activation and inhibition of inflammatory responses (Jin et al., 2008; Yang S. et al., 2022). It mainly acts through binding to Toll-like receptors 2 ad 4 (Yin et al., 2019), to achieve the activation and recruitment of IL receptor-related kinases and phosphorylation of various immune-response-related protein kinases, ultimately resulting in immunomodulatory effects (Hoshino et al., 1999; Liu et al., 2017). In addition, American ginseng polysaccharides bind to complement receptor CR3, C-type lectin receptor, and scavenger receptor to regulate the immune response (Loh et al., 2017; Prado et al., 2020; Su et al., 2020). Polysaccharides and other active ingredients in ginseng dregs thus have good antioxidant and immunomodulatory effects that may improve the utilization of nutrients and the overall health of sika deer.
Ruminant diets contain a large proportion of crude feed components that are difficult to digest, and they thus rely on microorganisms in the rumen to ferment and degrade the cellulose and hemicellulose, which can produce large amounts of short-chain fatty acids (Wang et al., 2018; Liang et al., 2022), which then enter the animal’s body via the gastrointestinal tract epithelial cells and serve as an important source of energy. The composition of rumen microorganisms thus has an important impact on the animal (Baldwin and Connor, 2017). The current results showed that Bacillota and Bacteroidota dominated the rumen in sika deer at the phylum level, while Prevotella, Ruminococcus, and Rikenellaceae_RC9_gut_group were the dominant bacteria at the genus level, in agreement with previous studies of rumen bacteria in sika deer (Li et al., 2013, 2015; Si et al., 2021). The results of β-diversity analysis indicated that dietary supplementation with American ginseng residue resulted in significant differences in rumen bacterial composition between the LGR and CON groups, with significant differences at the phylum and genus levels for Fibrobacterota, Oscillospiraceae_UCG-005, and Fibrobacter. Fibrobacterota is an important bacterial phylum that degrades cellulose in the gastrointestinal tract of herbivores and provides an important physiological basis for the adaptation of animals to harsh dietary conditions (Ransom-Jones et al., 2012; Jewell et al., 2013). In addition to being present in ruminants, this group of bacteria is also widely present in the intestinal tracts of termites, camels, and other animals that rely heavily on cellulose-based diets (König et al., 2013; Gharechahi et al., 2022; Zhao et al., 2023). Fibrobacter, a genus of Fibrobacterota, has likewise been reported as an important cellulose-degrading bacterium (Béra-Maillet et al., 2004). The current results thus explain the significantly higher apparent digestibility of ADF in the LGR group compared with the CON group, as well as the differentially increased levels of acetic acid and propionic acid in the rumen fluid in the LGR and HGR groups. In the same way, red ginseng polysaccharides have a positive effect on the production of short-chain fatty acids in the cattle rumen (Ju et al., 2024). Oscillospiraceae_UCG-005 is also a class of cellulose-degrading bacteria in the rumen (Li et al., 2021), but is also thought to play a role in the catabolism and utilization of dietary lipids, with significant increases in abundance, especially in animals exposed to high-fat diets (Liu Y. et al., 2023; Wu Z. L. et al., 2023). This is consistent with the results of a decrease in apparent digestibility of ether extracts in the three groups of deer, although the difference was not significant. In addition, deer in the HGR group showed a significant decrease in rumen fluid pH, which may imply an effect on the homeostasis of the rumen internal environment. However, it remained within the healthy range according to previous reports (Zhou X. et al., 2022), and the decrease in rumen fluid pH within the appropriate range was more favorable for the transport of short-chain fatty acids (Yan et al., 2014). Although American ginseng residue reduced the abundance of some beneficial bacteria in the rumen of sika deer, the overall effect on rumen function was positive.
Unlike the rumen microbiome, gut microbes are essential for the survival of all animals. Gut microbes are involved in the digestion and absorption of nutrients in the host (Zhao et al., 2019), influence host growth and development (Sommer and Bäckhed, 2013), participate in the onset and progression of disease (Hsu and Schnabl, 2023), can even affect the host’s mood and sleep (Li Y. et al., 2018). Microorganisms interact with the host via mediators, including metabolites such as short-chain fatty acids, bile acids, and endogenous cannabinoids (de Vos et al., 2022). The dominant bacteria at the phylum level in the intestine in sika deer were Bacillota and Bacteroidota, and the most abundant genera were Oscillospiraceae_UCG-005, followed by Treponema and Rikenellaceae_RC9_gut_group, with clear inconsistency between the dominant bacteria in the intestines and rumen, in agreement with previous reports (Wu Y. et al., 2023). Diversity analysis and adonis analysis showed that the composition of gut bacteria in the HGR group was significantly different from the other two groups, specifically in terms of Desulfobacterota at the phylum level, and Rikenellaceae_RC9_gut_group, Ruminococcus, and Paeniclostridium at the genus level. Previous studies demonstrated that Desulfobacterota were mainly concentrated in the hindgut of animals and were significantly enriched in a wide range of diet-induced intestinal inflammation conditions in mice (Panah et al., 2023; Ruan et al., 2023). They have also been suggested to be a marker of intestinal barrier damage (Rao et al., 2021), largely due to the fact that Desulfobacterota lipopolysaccharides are severe inflammatory stimulants for the host (Huang et al., 2021). Rikenellaceae_RC9_gut_group is a beneficial bacterium in the gastrointestinal tract of animals, involved in the fermentation of cellulose and capable of producing short-chain fatty acids (Xi et al., 2023). Ruminococcus is a common bacterium in the feces of ruminants, mainly colonizing the jejunum and ileum of the intestinal lumen (Guerra et al., 2022; Zhang et al., 2022), and is involved in the degradation of starch and other complex polysaccharides (Rangarajan et al., 2022). Ruminococcus has also been well-documented as an inducer of active inflammation in animals (Hall et al., 2017; Henke et al., 2021), and has been shown to play a role in the maintenance of homeostasis by acting as a key symbiotic component of the intestinal ecosystem (La Reau and Suen, 2018; Crost et al., 2023; Juge, 2023). Paeniclostridium is a pathogenic bacterium (DeCandia et al., 2023) that induces inflammation in the intestinal tract and in various tissues in animals (Nyaoke et al., 2020; Gryaznova et al., 2021), via its ability to produce cytotoxic endotoxins (Watts et al., 2019; Li et al., 2022). Consistent with our results, these studies demonstrated that polysaccharide components contained in American ginseng and red ginseng can effectively regulate the bacterial composition of the intestinal tract of animal animals, significantly increase the abundance of beneficial bacteria and reduce the level of pathogenic bacteria, which is beneficial to the improvement of diarrhea in animals (Sha et al., 2023; Song et al., 2023; Min et al., 2024). We also detected Fibrobacterota in the intestine, and although its abundance did not differ not significantly among the groups, LEfSe analysis revealed that Fibrobacterota and its subordinate multiple bacteria, from the class to the genus level, were significantly enriched, with the highest contribution in the LGR group. This may facilitate the digestion of cellulose in the hindgut, and also suggests that, despite differences in microbial composition between the rumen and intestine (Zou et al., 2020), like communication between the upper and lower intestinal tracts, the effects of changes in the dominant bacteria in the rumen are transmitted to the intestinal tract (Steele et al., 2016); however, it is not known whether this microbial migration and crosstalk can occur in the reverse direction.
5 Conclusion
Overall, dietary supplementation with American ginseng residue increased the apparent digestibility of nutrients, improved the immune and antioxidant statuses, and promoted antler production in sika deer during the antler-bearing period, as well as positively regulating the gastrointestinal flora and bacterial fermentation. These results suggest that American ginseng residue may be a suitable feed additive for the production of sika deer.
Data availability statement
The datasets presented in this study can be found in online repositories. The names of the repository/repositories and accession number(s) can be found at: NCBI – https://www.ncbi.nlm.nih.gov/bioproject/?term=PRJNA1078378.
Author contributions
YW: Data curation, Writing – original draft, Writing – review & editing. SZ: Data curation, Formal analysis, Writing – review & editing. PZ: Conceptualization, Funding acquisition, Writing – review & editing. HL: Supervision, Writing – review & editing. ZQ: Investigation, Writing – review & editing. WH: Supervision, Writing – review & editing. WY: Visualization, Writing – review & editing. TF: Resources, Writing – review & editing. XZ: Resources, Writing – review & editing. JS: Resources, Writing – review & editing. KW: Conceptualization, Funding acquisition, Resources, Supervision, Writing – review & editing.
Funding
The author(s) declare financial support was received for the research, authorship, and/or publication of this article. This work was supported by the Central Public-interest Scientific Institution Basal Research Fund (no.1610342023014) and the earmarked fund of JARS-SIKA DEER (no. 202300801).
Conflict of interest
TF and XZ were employed by Hongjiu BioTech Co., Ltd. JS was employed by Jilin Province Shuangyang Deer Industry Stock Breeding Co., Ltd.
The remaining authors declare that the research was conducted in the absence of any commercial or financial relationships that could be construed as a potential conflict of interest.
Publisher’s note
All claims expressed in this article are solely those of the authors and do not necessarily represent those of their affiliated organizations, or those of the publisher, the editors and the reviewers. Any product that may be evaluated in this article, or claim that may be made by its manufacturer, is not guaranteed or endorsed by the publisher.
References
Alfonso-Prieto, M., Biarnés, X., Vidossich, P., and Rovira, C. (2009). The molecular mechanism of the catalase reaction. J. Am. Chem. Soc. 131, 11751–11761. doi: 10.1021/ja9018572
Arthur, J. R. (2000). The glutathione peroxidases. Cell. Mol. Life Sci. 57, 1825–1835. doi: 10.1007/pl00000664
Association of Official Analytical Chemists (AOAC). (2006). Official methods of analysis, 18th Edn. AOAC: Gaithersburg, MD.
Baldwin, R. L., and Connor, E. E. (2017). Rumen function and development. Vet. Clin. North Am. Food Anim. Pract. 33, 427–439. doi: 10.1016/j.cvfa.2017.06.001
Bao, K., Wang, X., Wang, K., Li, G., and Liu, H. (2021). Energy and protein requirements for the maintenance of growing male sika deer (cervus nippon). Front. Vet. Sci. 8:745426. doi: 10.3389/fvets.2021.745426
Barbosa, M. M., Detmann, E., Rocha, G. C., Franco, M. O., and Filho, S. C. V. (2015). Evaluation of laboratory procedures to quantify the neutral detergent fiber content in forage, concentrate, and ruminant feces. J. AOAC Int. 98, 883–889. doi: 10.5740/jaoacint.14-156
Bartos, L., Schams, D., and Bubenik, G. A. (2009). Testosterone, but not IGF-1, LH, prolactin or cortisol, may serve as antler-stimulating hormone in red deer stags (Cervus elaphus). Bone 44, 691–698. doi: 10.1016/j.bone.2008.12.004
Béra-Maillet, C., Ribot, Y., and Forano, E. (2004). Fiber-degrading systems of different strains of the genus Fibrobacter. Appl. Environ. Microbiol. 70, 2172–2179. doi: 10.1128/AEM.70.4.2172-2179.2004
Campbell, J. M., Crenshaw, J. D., and Polo, J. (2013). The biological stress of early weaned piglets. J. Anim. Sci. Biotechnol. 4:19. doi: 10.1186/2049-1891-4-19
Chang, M., Wang, F., Ma, F., Jin, Y., and Sun, P. (2022). Supplementation with galacto-oligosaccharides in early life persistently facilitates the microbial colonization of the rumen and promotes growth of preweaning Holstein dairy calves. Anim Nutr. 10, 223–233. doi: 10.1016/j.aninu.2022.04.009
Crost, E. H., Coletto, E., Bell, A., and Juge, N. (2023). Ruminococcus gnavus: friend or foe for human health. FEMS Microbiol. Rev. 47:fuad014. doi: 10.1093/femsre/fuad014
de Vos, W. M., Tilg, H., van Hul, M., and Cani, P. D. (2022). Gut microbiome and health: mechanistic insights. Gut 71, 1020–1032. doi: 10.1136/gutjnl-2021-326789
DeCandia, A. L., Adeduro, L., Thacher, P., Crosier, A., Marinari, P., Bortner, R., et al. (2023). Gut bacterial composition shows sex-specific shifts during breeding season in ex situ managed black-footed ferrets. J. Hered. :esad065. doi: 10.1093/jhered/esad065
Deng, Q., Tan, X., Wang, H., Wang, Q., Huang, P., Li, Y., et al. (2020). Changes in cecal morphology, cell proliferation, antioxidant enzyme, volatile fatty acids, lipopolysaccharide, and cytokines in piglets during the postweaning period. J. Anim. Sci. 98:skaa046. doi: 10.1093/jas/skaa046
Gharechahi, J., Sarikhan, S., Han, J. L., Ding, X. Z., and Salekdeh, G. H. (2022). Functional and phylogenetic analyses of camel rumen microbiota associated with different lignocellulosic substrates. NPJ Biofilms Microbiomes 8:46. doi: 10.1038/s41522-022-00309-9
Gresse, R., Chaucheyras-Durand, F., Fleury, M. A., van de Wiele, T., Forano, E., and Blanquet-Diot, S. (2017). Gut microbiota dysbiosis in postweaning piglets: understanding the keys to health. Trends Microbiol. 25, 851–873. doi: 10.1016/j.tim.2017.05.004
Gryaznova, M. V., Syromyatnikov, M. Y., Dvoretskaya, Y. D., Solodskikh, S. A., Klimov, N. T., Mikhalev, V. I., et al. (2021). Microbiota of cow's milk with udder pathologies. Microorganisms 9:1974. doi: 10.3390/microorganisms9091974
Guerra, V., Tiago, I., Aires, A., Coelho, C., Nunes, J., Martins, L. O., et al. (2022). The gastrointestinal microbiome of browsing goats (Capra hircus). PLoS One 17:e0276262. doi: 10.1371/journal.pone.0276262
Hall, A. B., Yassour, M., Sauk, J., Garner, A., Jiang, X., Arthur, T., et al. (2017). A novel Ruminococcus gnavus clade enriched in inflammatory bowel disease patients. Genome Med. 9:103. doi: 10.1186/s13073-017-0490-5
Henke, M. T., Brown, E. M., Cassilly, C. D., Vlamakis, H., Xavier, R. J., and Clardy, J. (2021). Capsular polysaccharide correlates with immune response to the human gut microbe Ruminococcus gnavus. Proc. Natl. Acad. Sci. USA 118:e2007595118. doi: 10.1073/pnas.2007595118
Hoshino, K., Takeuchi, O., Kawai, T., Sanjo, H., Ogawa, T., Takeda, Y., et al. (1999). Cutting edge: toll-like receptor 4 (TLR4)-deficient mice are hyporesponsive to lipopolysaccharide: evidence for TLR4 as the Lps gene product. J. Immunol. 162, 3749–3752. doi: 10.4049/jimmunol.162.7.3749
Hsu, C. L., and Schnabl, B. (2023). The gut-liver axis and gut microbiota in health and liver disease. Nat. Rev. Microbiol. 21, 719–733. doi: 10.1038/s41579-023-00904-3
Huang, Y., Wang, Z., Ma, H., Ji, S., Chen, Z., Cui, Z., et al. (2021). Dysbiosis and implication of the gut microbiota in diabetic retinopathy. Front. Cell. Infect. Microbiol. 11:646348. doi: 10.3389/fcimb.2021.646348
Jewell, K. A., Scott, J. J., Adams, S. M., and Suen, G. (2013). A phylogenetic analysis of the phylum Fibrobacteres. Syst. Appl. Microbiol. 36, 376–382. doi: 10.1016/j.syapm.2013.04.002
Jin, Y., Kotakadi, V. S., Ying, L., Hofseth, A. B., Cui, X., Wood, P. A., et al. (2008). American ginseng suppresses inflammation and DNA damage associated with mouse colitis. Carcinogenesis 29, 2351–2359. doi: 10.1093/carcin/bgn211
Ju, M. S., Jo, Y. H., Kim, Y. R., Ghassemi Nejad, J., Lee, J. G., and Lee, H. G. (2024). Supplementation of complex natural feed additive containing (C. militaris, probiotics and red ginseng by-product) on rumen-fermentation, growth performance and carcass characteristics in Korean native steers. Front. Vet. Sci. 10:1300518. doi: 10.3389/fvets.2023.1300518
Juge, N. (2023). Microbe profile: Ruminococcus gnavus: the yin and yang of human gut symbionts. Microbiology 169:001383. doi: 10.1099/mic.0.001383
Kong, F., Gao, Y., Tang, M., Fu, T., Diao, Q., Bi, Y., et al. (2020). Effects of dietary rumen-protected Lys levels on rumen fermentation and bacterial community composition in Holstein heifers. Appl. Microbiol. Biotechnol. 104, 6623–6634. doi: 10.1007/s00253-020-10684-y
König, H., Li, L., and Fröhlich, J. (2013). The cellulolytic system of the termite gut. Appl. Microbiol. Biotechnol. 97, 7943–7962. doi: 10.1007/s00253-013-5119-z
La Reau, A. J., and Suen, G. (2018). The Ruminococci: key symbionts of the gut ecosystem. J. Microbiol. 56, 199–208. doi: 10.1007/s12275-018-8024-4
Li, Y., Hao, Y., Fan, F., and Zhang, B. (2018). The role of microbiome in insomnia, circadian disturbance and depression. Front. Psych. 9:669. doi: 10.3389/fpsyt.2018.00669
Li, X., He, L., Luo, J., Zheng, Y., Zhou, Y., Li, D., et al. (2022). Paeniclostridium sordellii hemorrhagic toxin targets TMPRSS2 to induce colonic epithelial lesions. Nat. Commun. 13:4331. doi: 10.1038/s41467-022-31994-x
Li, Z. P., Liu, H. L., Li, G. Y., Bao, K., Wang, K. Y., Xu, C., et al. (2013). Molecular diversity of rumen bacterial communities from tannin-rich and fiber-rich forage fed domestic sika deer (Cervus nippon) in China. BMC Microbiol. 13:151. doi: 10.1186/1471-2180-13-151
Li, F., Lv, C., Li, Q., Wang, J., Song, D., Liu, P., et al. (2017). Chemical and bioactive comparison of flowers of Panax ginseng Meyer, Panax quinquefolius L., and Panax notoginseng Burk. J. Ginseng Res. 41, 487–495. doi: 10.1016/j.jgr.2016.08.008
Li, H., Ma, L., Li, Z., Yin, J., Tan, B., Chen, J., et al. (2021). Pvolution of the gut microbiota and its fermentation characteristics of ningxiang pigs at the young stage. Animals 11:638. doi: 10.3390/ani11030638
Li, D., Ren, J. W., Zhang, T., Liu, R., Wu, L., Du, Q., et al. (2018). Anti-fatigue effects of small-molecule oligopeptides isolated from Panax quinquefolium L. in mice. Food Funct. 9, 4266–4273. doi: 10.1039/c7fo01658a
Li, Z., Wright, A.-D. G., Liu, H., Fan, Z., Yang, F., Zhang, Z., et al. (2015). Response of the rumen microbiota of sika deer (Cervus nippon) fed different concentrations of tannin rich plants. PLoS One 10:e0123481. doi: 10.1371/journal.pone.0123481
Li, C., Yan, Z., Zhang, L., and Li, Y. (2014). Research and implementation of good agricultural practice for traditional Chinese medicinal materials in Jilin Province, China. J. Ginseng Res. 38, 227–232. doi: 10.1016/j.jgr.2014.05.007
Liang, J., Fang, W., Chang, J., Zhang, G., Ma, W., Nabi, M., et al. (2022). Long-term rumen microorganism fermentation of corn Stover in vitro for volatile fatty acid production. Bioresour. Technol. 358:127447. doi: 10.1016/j.biortech.2022.127447
Liu, Y., Hu, J., Li, M. M., and Zhao, G. (2023). Effects of taurine on rumen fermentation, nutrient digestion, rumen bacterial community and metabolomics and nitrogen metabolism in beef steers. J. Sci. Food Agric. 103, 3414–3426. doi: 10.1002/jsfa.12474
Liu, H., Lu, X., Hu, Y., and Fan, X. (2020). Chemical constituents of Panax ginseng and Panax notoginseng explain why they differ in therapeutic efficacy. Pharmacol. Res. 161:105263. doi: 10.1016/j.phrs.2020.105263
Liu, S., Wei, Z., Deng, M., Xian, Z., Liu, D., Liu, G., et al. (2023). Effect of a high-starch or a high-fat diet on the milk performance, apparent nutrient digestibility, hindgut fermentation parameters and microbiota of lactating cows. Animals 13:2508. doi: 10.3390/ani13152508
Liu, T., Zhang, L., Joo, D., and Sun, S. C. (2017). NF-κB signaling in inflammation. Signal Transduct. Target. Ther. 2:17023. doi: 10.1038/sigtrans.2017.23
Loh, S. H., Park, J. Y., Cho, E. H., Nah, S. Y., and Kang, Y. S. (2017). Animal lectins: potential receptors for ginseng polysaccharides. J. Ginseng Res. 41, 1–9. doi: 10.1016/j.jgr.2015.12.006
Min, S. J., Kim, H., Yambe, N., and Shin, M. S. (2024). Ameliorative effects of Korean-red-ginseng-derived polysaccharide on antibiotic-associated diarrhea. Polymers 16:231. doi: 10.3390/polym16020231
Minas, K., McEwan, N. R., Newbold, C. J., and Scott, K. P. (2011). Optimization of a high-throughput CTAB-based protocol for the extraction of qPCR-grade DNA from rumen fluid, plant and bacterial pure cultures. FEMS Microbiol. Lett. 325, 162–169. doi: 10.1111/j.1574-6968.2011.02424.x
Nyaoke, A. C., Navarro, M. A., Fresneda, K., Diab, S. S., Moore, J., Lyras, D., et al. (2020). Paeniclostridium (Clostridium) sordellii-associated enterocolitis in 7 horses. J. Vet. Diagn. Invest. 32, 239–245. doi: 10.1177/1040638720903738
Paik, S., Song, G. Y., and Jo, E. K. (2023). Ginsenosides for therapeutically targeting inflammation through modulation of oxidative stress. Int. Immunopharmacol. 121:110461. doi: 10.1016/j.intimp.2023.110461
Panah, F. M., Nielsen, K. D., Simpson, G. L., Schönherz, A., Schramm, A., Lauridsen, C., et al. (2023). A westernized diet changed the colonic bacterial composition and metabolite concentration in a dextran sulfate sodium pig model for ulcerative colitis. Front. Microbiol. 14:1018242. doi: 10.3389/fmicb.2023.1018242
Prado, S. B. R., Beukema, M., Jermendi, E., Schols, H. A., de Vos, P., and Fabi, J. P. (2020). Pectin interaction with immune receptors is modulated by ripening process in papayas. Sci. Rep. 10:1690. doi: 10.1038/s41598-020-58311-0
Prawirodigdo, S., Gannon, N. J., Leury, B. J., and Dunshea, F. R. (2021). Acid-insoluble ash is a better indigestible marker than chromic oxide to measure apparent total tract digestibility in pigs. Anim Nutr. 7, 64–71. doi: 10.1016/j.aninu.2020.07.003
Price, J., and Allen, S. (2004). Exploring the mechanisms regulating regeneration of deer antlers. Philos. Trans. R. Soc. Lond. Ser. B Biol. Sci. 359, 809–822. doi: 10.1098/rstb.2004.1471
Punja, Z. K. (2011). American ginseng: research developments, opportunities, and challenges. J. Ginseng Res. 35, 368–374. doi: 10.5142/jgr.2011.35.3.368
Qi, H., Zhang, Z., Liu, J., Chen, Z., Huang, Q., Li, J., et al. (2021). Comparisons of isolation methods, structural features, and bioactivities of the polysaccharides from three common panax species: a review of recent progress. Molecules 26:4997. doi: 10.3390/molecules26164997
Raffrenato, E., and Van Amburgh, M. E. (2011). Technical note: improved methodology for analyses of acid detergent fiber and acid detergent lignin. J. Dairy Sci. 94, 3613–3617. doi: 10.3168/jds.2010-3701
Rangarajan, A. A., Chia, H. E., Azaldegui, C. A., Olszewski, M. H., Pereira, G. V., Koropatkin, N. M., et al. (2022). Ruminococcus bromii enables the growth of proximal Bacteroides thetaiotaomicron by releasing glucose during starch degradation. Microbiology 168:1180. doi: 10.1099/mic.0.001180
Ransom-Jones, E., Jones, D. L., McCarthy, A. J., and McDonald, J. E. (2012). The Fibrobacteres: an important phylum of cellulose-degrading bacteria. Microb. Ecol. 63, 267–281. doi: 10.1007/s00248-011-9998-1
Rao, J., Xie, R., Lin, L., Jiang, J., Du, L., Zeng, X., et al. (2021). Fecal microbiota transplantation ameliorates gut microbiota imbalance and intestinal barrier damage in rats with stress-induced depressive-like behavior. Eur. J. Neurosci. 53, 3598–3611. doi: 10.1111/ejn.15192
Ren, D. D., Li, S. S., Lin, H. M., Xia, Y. S., Li, Z. M., Bo, P. P., et al. (2022). Panax quinquefolius polysaccharides ameliorate antibiotic-associated diarrhoea induced by lincomycin hydrochloride in rats via the MAPK signaling pathways. J Immunol Res 2022, 1–19. doi: 10.1155/2022/4126273
Ruan, S., Gao, X., Li, B., and Tian, J. (2023). The synergic effects and mechanism of KGM-DMY complex in the prevention of obesity and enhancement of fatigue resistance in mice. Food Funct. 14, 2607–2620. doi: 10.1039/d2fo03677k
Sha, J., Liu, Z., Yu, H., Huo, X., Wang, J., Duan, Y., et al. (2023). Dietary supplementation with American ginseng dietary fiber ameliorates intestinal mucosal barrier injury in immunosuppressed mice. Food Biosci. 56:103237. doi: 10.1016/j.fbio.2023.103237
Shahinian, A. H., and Reinhold, J. G. (1971). Application of the phenol-hypochlorite reaction to measurement of ammonia concentrations in Kjeldahl digests of serum and various tissues. Clin. Chem. 17, 1077–1080. doi: 10.1093/clinchem/17.11.1077
Si, H., Liu, H., Nan, W., Li, G., Li, Z., and Lou, Y. (2021). Effects of arginine supplementation on serum metabolites and the rumen bacterial community of sika deer (Cervus nippon). Front. Vet. Sci. 8:630686. doi: 10.3389/fvets.2021.630686
Sommer, F., and Bäckhed, F. (2013). The gut microbiota--masters of host development and physiology. Nat. Rev. Microbiol. 11, 227–238. doi: 10.1038/nrmicro2974
Song, H., Lee, J., Yi, S., Kim, W. H., Kim, Y., Namgoong, B., et al. (2023). Red ginseng dietary Fiber shows prebiotic potential by modulating gut microbiota in dogs. Microbiol. Spectr. 11:e0094923. doi: 10.1128/spectrum.00949-23
Steele, M. A., Penner, G. B., Chaucheyras-Durand, F., and Guan, L. L. (2016). Development and physiology of the rumen and the lower gut: targets for improving gut health. J. Dairy Sci. 99, 4955–4966. doi: 10.3168/jds.2015-10351
Su, C. H., Lu, M. K., Lu, T. J., Lai, M. N., and Ng, L. T. (2020). A (1→6)-branched (1→4)-β-d-glucan from Grifola frondosa inhibits lipopolysaccharide-induced cytokine production in RAW264.7 macrophages by binding to TLR2 rather than Dectin-1 or CR3 receptors. J. Nat. Prod. 83, 231–242. doi: 10.1021/acs.jnatprod.9b00584
Sun, J., Zhong, X., Sun, D., Cao, X., Yao, F., Shi, L., et al. (2022). Structural characterization of polysaccharides recovered from extraction residue of ginseng root saponins and its fruit nutrition preservation performance. Front. Nutr. 9:934927. doi: 10.3389/fnut.2022.934927
Sun, J., Zhong, X., Sun, D., Xu, L., Shi, L., Sui, J., et al. (2023). Anti-aging effects of polysaccharides from ginseng extract residues in Caenorhabditis elegans. Int. J. Biol. Macromol. 225, 1072–1084. doi: 10.1016/j.ijbiomac.2022.11.168
Takeda, S., Ahhmed, A. M., Sogawa, K., Mouri, S., Kaneko, S., Sakata, R., et al. (2023). Antioxidant activity of venison subjected to in vitro cooking and gastrointestinal digestion and isolation of its 2,2-diphenyl-1-picrylhydrazyl radical scavenging peptides. Anim. Sci. J. 94:e13870. doi: 10.1111/asj.13870
Wang, M., Guilbert, L. J., Ling, L., Li, J., Wu, Y., Xu, S., et al. (2001). Immunomodulating activity of CVT-E002, a proprietary extract from north American ginseng (Panax quinquefolium). J. Pharm. Pharmacol. 53, 1515–1523. doi: 10.1211/0022357011777882
Wang, M., Wang, R., Janssen, P. H., Zhang, X. M., Sun, X. Z., Pacheco, D., et al. (2016). Sampling procedure for the measurement of dissolved hydrogen and volatile fatty acids in the rumen of dairy cows. J. Anim. Sci. 94, 1159–1169. doi: 10.2527/jas.2015-9658
Wang, L., Yao, Y., Sang, W., Yang, X., and Ren, G. (2015). Structural features and immunostimulating effects of three acidic polysaccharides isolated from Panax quinquefolius. Int. J. Biol. Macromol. 80, 77–86. doi: 10.1016/j.ijbiomac.2015.06.007
Wang, C. Z., Yu, C., Wen, X. D., Chen, L., Zhang, C. F., Calway, T., et al. (2016). American ginseng attenuates colitis-associated colon carcinogenesis in mice: impact on gut microbiota and metabolomics. Cancer Prev. Res. (Phila.) 9, 803–811. doi: 10.1158/1940-6207.CAPR-15-0372
Wang, S., Zhang, G., Zhang, P., Ma, X., Li, F., Zhang, H., et al. (2018). Rumen fluid fermentation for enhancement of hydrolysis and acidification of grass clipping. J. Environ. Manag. 220, 142–148. doi: 10.1016/j.jenvman.2018.05.027
Watts, T. D., Vidor, C. J., Awad, M. M., Lyras, D., Rood, J. I., and Adams, V. (2019). pCP13, a representative of a new family of conjugative toxin plasmids in Clostridium perfringens. Plasmid 102, 37–45. doi: 10.1016/j.plasmid.2019.02.002
Wu, Z. L., Yang, X., Zhang, J., Wang, W., Liu, D., Hou, B., et al. (2023). Effects of forage type on the rumen microbiota, growth performance, carcass traits, and meat quality in fattening goats. Front. Vet. Sci. 10:1147685. doi: 10.3389/fvets.2023.1147685
Wu, Y., Zhu, Y., Guo, X., Wang, X., Yuan, W., Ma, C., et al. (2023). Methionine supplementation affects fecal bacterial community and production performance in sika deer (Cervus nippon). Animals (Basel). 13:2606. doi: 10.3390/ani13162606
Xi, L., Wen, X., Jia, T., Han, J., Qin, X., Zhang, Y., et al. (2023). Comparative study of the gut microbiota in three captive Rhinopithecus species. BMC Genomics 24:398. doi: 10.1186/s12864-023-09440-z
Xia, P., Liu, D., Jiao, Y., Wang, Z., Chen, X., Zheng, S., et al. (2022). Health effects of peptides extracted from deer antler. Nutrients 14:4183. doi: 10.3390/nu14194183
Xiao, D., Shao, H., Huo, Y., Agung Nugroho, W., Ifeoluwa Ogunniran, B., Fan, W., et al. (2022). Reclamation of ginseng residues using two-stage fermentation and evaluation of their beneficial effects as dietary feed supplements for piglets. Waste Manag. 154, 293–302. doi: 10.1016/j.wasman.2022.10.020
Xie, L., Yan, H., Han, L., Cui, L., Hussain, H., Feng, Q., et al. (2023). Structural characterization and anti-inflammatory activity of neutral polysaccharides from American ginseng. Int. J. Biol. Macromol. 248:125586. doi: 10.1016/j.ijbiomac.2023.125586
Xiong, H., Zhang, A. H., Zhao, Q. Q., Yan, G. L., Sun, H., and Wang, X. J. (2020). Discovery of quality-marker ingredients of Panax quinquefolius driven by high-throughput chinmedomics approach. Phytomedicine 74:152928. doi: 10.1016/j.phymed.2019.152928
Yan, L., Zhang, B., and Shen, Z. (2014). Dietary modulation of the expression of genes involved in short-chain fatty acid absorption in the rumen epithelium is related to short-chain fatty acid concentration and pH in the rumen of goats. J. Dairy Sci. 97, 5668–5675. doi: 10.3168/jds.2013-7807
Yang, S., Li, F., Lu, S., Ren, L., Bian, S., Liu, M., et al. (2022). Ginseng root extract attenuates inflammation by inhibiting the MAPK/NF-κB signaling pathway and activating autophagy and p62-Nrf2-Keap1 signaling in vitro and in vivo. J. Ethnopharmacol. 283:114739. doi: 10.1016/j.jep.2021.114739
Yang, L., Zhang, L., Zhang, P., Zhou, Y., Huang, X., Yan, Q., et al. (2022). Alterations in nutrient digestibility and performance of heat-stressed dairy cows by dietary L-theanine supplementation. Anim. Nutr. 11, 350–358. doi: 10.1016/j.aninu.2022.08.002
Yin, M., Zhang, Y., and Li, H. (2019). Advances in research on immunoregulation of macrophages by plant polysaccharides. Front. Immunol. 10:145. doi: 10.3389/fimmu.2019.00145
Yu, X. H., Liu, Y., Wu, X. L., Liu, L. Z., Fu, W., and Song, D. D. (2017). Isolation, purification, characterization and immunostimulatory activity of polysaccharides derived from American ginseng. Carbohydr. Polym. 156, 9–18. doi: 10.1016/j.carbpol.2016.08.092
Yu, X., Yang, X., Cui, B., Wang, L., and Ren, G. (2014). Antioxidant and immunoregulatory activity of alkali-extractable polysaccharides from north American ginseng. Int. J. Biol. Macromol. 65, 357–361. doi: 10.1016/j.ijbiomac.2014.01.046
Zang, Z. J., Tang, H. F., Tuo, Y., Xing, W. J., Ji, S. Y., Gao, Y., et al. (2016). Effects of velvet antler polypeptide on sexual behavior and testosterone synthesis in aging male mice. Asian J. Androl. 18, 613–619. doi: 10.4103/1008-682X.166435
Zhang, H., Lang, X., Zhang, Y., and Wang, C. (2022). Distribution of bacteria in different regions of the small intestine with Zanthoxylum bungeanum essential oil supplement in small-tailed Han sheep. Front. Microbiol. 13:1062077. doi: 10.3389/fmicb.2022.1062077
Zhang, Z., Li, Z., Wu, X., Zhang, C. F., Calway, T., He, T. C., et al. (2015). TRAIL pathway is associated with inhibition of colon cancer by protopanaxadiol. J. Pharmacol. Sci. 127, 83–91. doi: 10.1016/j.jphs.2014.11.003
Zhang, J., Shi, H., Wang, Y., Li, S., Cao, Z., Ji, S., et al. (2017). Effect of dietary forage to concentrate ratios on dynamic profile changes and interactions of ruminal microbiota and metabolites in Holstein heifers. Front. Microbiol. 8:2206. doi: 10.3389/fmicb.2017.02206
Zhang, J., Yang, Y., Lei, X., Wang, Y., Li, Y., Yang, Z., et al. (2023). Active dry yeast supplementation benefits ruminal fermentation, bacterial community, blood immunoglobulins, and growth performance in young dairy goats, but not for intermittent supplementation. Anim. Nutr. 13, 289–301. doi: 10.1016/j.aninu.2023.02.001
Zhao, F., Yang, L., Zhang, T., Zhuang, D., Wu, Q., Yu, J., et al. (2023). Gut microbiome signatures of extreme environment adaption in Tibetan pig. NPJ Biofilms Microbiomes 9:27. doi: 10.1038/s41522-023-00395-3
Zhao, J., Zhang, X., Liu, H., Brown, M. A., and Qiao, S. (2019). Dietary protein and gut microbiota composition and function. Curr. Protein Pept. Sci. 20, 145–154. doi: 10.2174/1389203719666180514145437
Zhou, R., He, D., Xie, J., Zhou, Q., Zeng, H., Li, H., et al. (2021). The synergistic effects of polysaccharides and ginsenosides from American ginseng (Panax quinquefolius L.) ameliorating cyclophosphamide-induced intestinal immune disorders and gut barrier dysfunctions based on microbiome-metabolomics analysis. Front. Immunol. 12:665901. doi: 10.3389/fimmu.2021.665901
Zhou, Z., Wang, T., Jia, Y., Wang, T., Luo, E., Zhong, J., et al. (2022). Transcriptional sequencing analysis reveals the potential use of deer antler for "tonifying the kidney and strengthening bone". J. Orthop. Surg. Res. 17:419. doi: 10.1186/s13018-022-03308-w
Zhou, X., Zhang, N., Zhang, J., Gu, Q., Dong, C., Lin, B., et al. (2022). Microbiome and fermentation parameters in the rumen of dairy buffalo in response to ingestion associated with a diet supplemented with cysteamine and hemp seed oil. J. Anim. Physiol. Anim. Nutr. (Berl) 106, 471–484. doi: 10.1111/jpn.13616
Zidon, R., Saltz, D., Shore, L. S., and Motro, U. (2009). Behavioral changes, stress, and survival following reintroduction of Persian fallow deer from two breeding facilities. Conserv. Biol. 23, 1026–1035. doi: 10.1111/j.1523-1739.2008.01163.x
Keywords: American ginseng residue, antioxidant, gastrointestinal bacteria, rumen fermentation, sika deer
Citation: Wu Y, Zhao S, Zheng P, Liu H, Qu Z, Hou W, Yuan W, Feng T, Zhan X, Shen J and Wang K (2024) Beneficial effects of American ginseng (Panax quinquefolius L.) extract residue as a feed additive on production, health status, and gastrointestinal bacteria in sika deer (Cervus nippon). Front. Microbiol. 15:1344905. doi: 10.3389/fmicb.2024.1344905
Edited by:
Li Wang, Guangdong Academy of Agricultural Sciences (GDAAS), ChinaReviewed by:
Tarique Hussain, Nuclear Institute for Agriculture and Biology, PakistanAlberto Torres, Cobb Vantress, Inc., United States
George Grant, University of Aberdeen, United Kingdom
Copyright © 2024 Wu, Zhao, Zheng, Liu, Qu, Hou, Yuan, Feng, Zhan, Shen and Wang. This is an open-access article distributed under the terms of the Creative Commons Attribution License (CC BY). The use, distribution or reproduction in other forums is permitted, provided the original author(s) and the copyright owner(s) are credited and that the original publication in this journal is cited, in accordance with accepted academic practice. No use, distribution or reproduction is permitted which does not comply with these terms.
*Correspondence: Kaiying Wang, dGNzd2t5QDEyNi5jb20=
†These authors have contributed equally to this work