- Department of Neurology and Neuroscience Center, The First Hospital of Jilin University, Changchun, China
Background: It has been suggested in several observational studies that migraines are associated with the gut microbiota. It remains unclear, however, how the gut microbiota and migraines are causally related.
Methods: We performed a bidirectional two-sample mendelian randomization study. Genome-wide association study (GWAS) summary statistics for the gut microbiota were obtained from the MiBioGen consortium (n = 18,340) and the Dutch Microbiota Project (n = 7,738). Pooled GWAS data for plasma metabolites were obtained from four different human metabolomics studies. GWAS summary data for migraine (cases = 48,975; controls = 450,381) were sourced from the International Headache Genetics Consortium. We used inverse-variance weighting as the primary analysis. Multiple sensitivity analyses were performed to ensure the robustness of the estimated results. We also conducted reverse mendelian randomization when a causal relationship between exposure and migraine was found.
Results: LachnospiraceaeUCG001 (OR = 1.12, 95% CI: 1.05–1.20) was a risk factor for migraine. Blautia (OR = 0.93, 95% CI: 0.88–0.99), Eubacterium (nodatum group; OR = 0.94, 95% CI: 0.90–0.98), and Bacteroides fragilis (OR = 0.97, 95% CI: 0.94–1.00) may have a suggestive association with a lower migraine risk. Functional pathways of methionine synthesis (OR = 0.89, 95% CI: 0.83–0.95) associated with microbiota abundance and plasma hydrocinnamate (OR = 0.85, 95% CI: 0.73–1.00), which are downstream metabolites of Blautia and Bacteroides fragilis, respectively, may also be associated with lower migraine risk. No causal association between migraine and the gut microbiota or metabolites was found in reverse mendelian randomization analysis. Both significant horizontal pleiotropy and significant heterogeneity were not clearly identified.
Conclusion: This Mendelian randomization analysis showed that LachnospiraceaeUCG001 was associated with an increased risk of migraine, while some bacteria in the gut microbiota may reduce migraine risk. These findings provide a reference for a deeper comprehension of the role of the gut–brain axis in migraine as well as possible targets for treatment interventions.
Introduction
Recurrent migraine attacks are a debilitating neurological disease that is the second leading cause of disability (GBD 2016 Disease and Injury Incidence and Prevalence Collaborators, 2017; Dodick, 2018). It is thought to affect over 1 billion individuals globally and imposes a substantial economic burden on individuals and society (Ashina et al., 2021). To date, the causes and pathology of migraines are still poorly understood. Evidence has indicated that background genetic and environmental factors influence the onset and progression of migraine (Goadsby et al., 2017). Genetic studies have suggested that approximately half of the individual risk variations for migraine are heritable, underscoring the genetic contribution to the etiology of migraine (Piane et al., 2007).
In addition to genetics and environment, the microbiota–gut–brain axis has been hypothesized as a key mechanism through which gut microbiota might trigger migraine attacks (Arzani et al., 2020). The microbiota–gut–brain axis is currently a subject of intense interest. Research focuses on studying the relationship between gut microbiota and hosts and describes the flow of information between the brain and gut microbiota (Cryan et al., 2019; Socała et al., 2021). Imbalances in the gut microbiota abundance may affect the release of neurotransmitters and inflammatory mediators, such as glutamate, calcitonin gene-related peptide, and interleukin (IL)-1β, which might increase the risk of migraine (Arzani et al., 2020; Crawford et al., 2022). Therefore, balancing the abundance of gut microbiota, such as by probiotic supplementation, is thought to be a promising and intriguing therapeutic target (Arzani et al., 2020).
Several observational studies have found notable differences in the makeup and quantity of certain resident microorganisms in people with migraine compared to those without, including increased amounts of Alcaligenes, Clostridium coccoides, and Eggerthella lenta and a decreased proportion of Faecalibacterium prausnitzii and Bifidobacterium adolescentis (Chen et al., 2019; Georgescu et al., 2019; Kopchak et al., 2022; Kopchak and Hrytsenko, 2022). Additionally, it was shown that the frequency and intensity of migraine attacks were negatively associated with the abundance of Alcaligenes; Eggerthella lenta levels were positively correlated with visual analog scores in people with migraine (Kopchak et al., 2022; Kopchak and Hrytsenko, 2022). The categories in the oral microbiome of people with migraine and people without differed in a study using 16S rRNA gene sequencing (Jiang et al., 2021). The frequency and severity of migraine attacks were improved by multiple probiotic formulations according to observational research including 1,020 people with migraine (Straube et al., 2018). However, the conclusions of several published studies have been contradictory (De Roos et al., 2017; Chen et al., 2019; Martami et al., 2019). The results of a meta-analysis, which included only three randomized controlled studies, suggested that probiotic supplementation may not significantly improve migraine frequency or severity (Parohan et al., 2022).
In observational study, confounding variables including age, environmental circumstances, and way of life could easily bias the connection between the gut microbiota and migraine (Rinninella et al., 2019). At the same time, considering that most observational studies are designed as case–control studies, it is difficult to judge the chronological order of exposure factors and disease outcomes or to clarify causal relationships between them (Davey Smith and Hemani, 2014). Randomized controlled trials serve a dual purpose by not only exploring causality but also providing valuable insights into the efficacy of treatments and prognosis. Greater benefits to public health will result from investigating the associations between the gut microbiota and migraine, which will help with primary migraine prevention.
Mendelian randomization (MR) using genetic variants of exposure as instrumental variables (IVs) is a novel approach investigating exposure factors and illness outcomes (Bowden et al., 2019). There is reduced confounding bias in MR investigations because genetic variations are allocated at random and appear before diseases do (Lin et al., 2021). In two-sample MR analysis, exposure and outcome data are derived from two independent genome-wide association studies (GWAS) to avoid bias caused by samples from the same population (Xu et al., 2021). In the present study, we applied a two-sample MR design to evaluate the association between gut microbiota and migraine, according to the STROBEMR guidelines (Skrivankova et al., 2021).
Methods
Assumptions for MR and study design
The IVs in the MR analysis must meet the following three assumptions (Davey Smith and Hemani, 2014): (1) IVs that are closely related to the gut microbiota, (2) IVs that are independent of confounding factors (affecting gut microbiota and migraine), and (3) IVs that affect migraine only through the gut microbiota and not through other factors. Figure 1 presents a flowchart of the MR analyses conducted in this study.
Genome-wide association study data
GWAS summary statistics for the gut microbiota were obtained from the MiBioGen consortium (Kurilshikov et al., 2021; Consortium M, 2022). This study included 24 cohorts with a total of 18,340 individuals, making it the largest GWAS meta-analysis of gut microbiota to date, with 13,266 participants of European ancestry. Notably, given the substantial representation of participants with European ancestry, this dataset is suitable for MR analysis within European populations. The microbial composition of the participants was analyzed. To ensure that host genetic variation correlated with the abundance of bacterial taxa, the mean abundance level of bacterial taxa had to be greater than 1%. This decision was based on the proportion of taxa present in the samples and microbial quantitative trait loci mapping analysis. Direct taxonomic binning was used to classify the taxa. The bacterial taxa included in this study included phylum (n = 9), class (n = 16), order (n = 20), family (n = 32) and genus (n = 119), respectively. Fifteen bacterial taxa (unidentified family or genus) without specific species names were omitted from the investigation. Another GWAS summary dataset for gut microbial taxa was obtained from the Dutch Microbiota Project, which included 7,738 individuals of European ancestry (Lopera-Maya et al., 2022). A total of 207 microbial taxa (5 phyla, 10 classes, 13 orders, 26 families, 48 genera, 105 species) and 205 functional pathways were included in this study.
Given the role of gut metabolites as a bridge between the microbiota and host, microbes may influence the activity and function of the host nervous system by altering metabolite levels. We included data from four human metabolomic GWAS conducted in individuals of European ancestry (Shin et al., 2014; Kettunen et al., 2016; Hemani et al., 2018; Richardson et al., 2022). One study, which included 7,824 participants, analyzed 452 plasma metabolites, and another study, which included 24,925 participants, analyzed 123 metabolites. The two remaining studies investigated 249 metabolites and included 115,078 and 115,082 participants each. First, plasma metabolites associated with migraine were identified. Next, the Human Metabolome Database (Wishart et al., 2022) and gutMGene (Cheng et al., 2020) were used to identify whether the plasma metabolites identified in the first step were associated with the gut microbiota.
The summary-level GWAS data for migraine were obtained from a meta-analysis of the GWAS of participants of European ancestry from the International Headache Genetics Consortium (IHGC). The acquisition of the data was approved through a direct application and material transfer agreement. This study was conducted by Hautakangas et al. (Hautakangas et al., 2022) and included data from five studies. A total of 102,048 migraine patients and 771,257 controls were included. In the present study, the data used were from 48,975 people with migraine and 540,381 control individuals from four studies (23andMe was not included because of the strict participant privacy protections in this cohort). Migraine cases were identified based on self-reported data or the International Classification of Headache Disorders. The cases included in this analysis were adjusted for sex, age, and ancestry. The original article has the rest of the information, such as demographic information (Hautakangas et al., 2022). Details of the cohorts included in both GWAS studies are available (Kurilshikov et al., 2021; Hautakangas et al., 2022). There was a limited amount of sample overlap between two GWAS data included in this study (<2%). Moreover, two-sample Mendelian analysis can be applied to large datasets with overlapping samples (Minelli et al., 2021). Detailed GWAS information is presented in Supplementary Table S1.
Instrumental variables selection
Screening criteria for the IVs included the following. A significance level of a single-nucleotide polymorphism (SNP) of p < 1.0 × 10−5 was considered a candidate IV. This threshold aligns with similar criteria used in published studies (Sanna et al., 2019). To ensure the independence of each IV, linkage disequilibrium was set at R2 < 0.001 and a clumping distance of 10,000 kb, using the European-based 1,000 Genome Projects in the R package. Minor allele frequencies ≤0.01 may represent rare variants, and SNPs with minor allele frequencies ≤0.01 are controversial in genetic association analyses, so these were excluded. F-statistics were calculated according to the calculation formula in the previous literature (Pierce et al., 2011). Weak IVs with F-statistics less than 10 were excluded to prevent violation of the first assumption of MR. Palindromic SNPs with intermediate allele frequencies were also removed. Finally, the PhenoScanner1 (PhenoScanner, n.d.), a platform capable of querying information related to genotypes and phenotypes, was employed. Additional checks to exclude potential associations between the included IVs and currently recognized risk factors for migraine, such as tobacco use, caffeine consumption, diastolic blood pressure, systolic blood pressure, vitamin D, depression, and anxiety, were conducted (Guo et al., 2020; Yuan et al., 2022; Zheng et al., 2023). If present, SNPs significantly associated with these potential confounders at genome-wide significance (p < 1.0 × 10−5) were removed.
Statistical analysis
Three different methods were used for the bidirectional two-sample MR analysis: random-effect inverse-variance weighting (IVW), MR-Egger, and weighted median (Burgess et al., 2013; Bowden et al., 2015, 2016). IVW is a meta-analysis of Wald ratios for each SNP. Without horizontal pleiotropy, the IVW results are unbiased and serve as the primary outcome of the analysis. When horizontal pleiotropy is present, the MR-egger method can be applied, but it requires that the pleiotropy effect be independent of the variant–exposure association. The weighted median was used to calculate the median effect of SNPs and allowed for invalid tools, provided that the proportion of invalid IVs was less than 50% in the MR analysis. Cochran’s Q test was used to assess heterogeneity. It was assumed that there was no significant heterogeneity among the included IVs when the p-value of the Q test was greater than 0.05. The intercept term of the MR-Egger regression can be used to determine whether there is horizontal pleiotropy. If the intercept is not statistically different from zero, no significant horizontal pleiotropy is suggested (Bowden et al., 2015). The MR-Pleiotropy RESidual Sum and Outlier (MR-PRESSO) analysis was employed to assess the presence of outliers and mitigate the effects of horizontal pleiotropy (Verbanck et al., 2018). Outliers that potentially influenced the results were systematically removed until the global test p-value exceeded 0.05 (Wootton et al., 2020). Power calculations for MR were performed using the mRnd website2 (Burgess, n.d.).
To avoid the possible interference of reverse causation in the causal inference between gut microbiota and migraine, reverse MR analysis should be considered. The present reverse MR analysis used migraine as the exposure and gut microbiota as the outcome. The methods and procedures used in the reverse Mendelian analysis were consistent with those used in the forward Mendelian analysis.
Association effects were described as odds ratios (OR) with 95% confidence intervals (CI). This result was considered robust when the estimated effects of the three methods of analysis were the same and at least the IVW method estimate was significant. An approach called false discovery rate (FDR) control was used to prevent bias caused by multiple testing. The q-value (corrected p-value) was calculated with an FDR of q-value <0.05. A suggestive association between the gut microbiota and migraine was considered when p < 0.05 but q ≥ 0.05.
R software was used to conduct all statistical analyses (Version 4.2.2; R Foundation for Statistical Computing, Vienna, Austria). The R package for MR analysis consisted of the TwoSampleMR (version 0.5.6), MR-PRESSO (version 1.0), and qvalue R packages (Storey and Tibshirani, 2003; Hemani et al., 2017; Verbanck et al., 2018).
Results
Gut microbiota (MiBioGen) and migraine
The selection standards for IVs ultimately resulted in the identification of 2,120 SNPs as IVs associated with 196 bacterial taxa (Supplementary Table S2). There was no bias driven by weak IVs in the MR analysis, as each of the included SNPs had an F-statistic greater than 10.
To determine whether any bacterial taxa were associated with migraine at the phylum, class, order, family, and genus levels, we first preprocessed the data using the IVW approach (Figure 2; Supplementary Table S3). We found that family Family XIII (OR = 0.90, 95% CI: 0.82–1.00, p = 0.048) and genus Eubacterium (nodatum group) (OR = 0.94, 95% CI: 0.90–0.98, p = 4.00 × 10−3) were associated with a lower risk of migraine, while genus Coprobacter (OR = 1.07, 95% CI: 1.00–1.14, p = 0.045), genera Hungatella (OR = 1.08, 95% CI: 1.00–1.15, p = 0.04), LachnospiraceaeUCG001 (OR = 1.12, 95% CI: 1.05–1.20, p = 3.65× 10−4), Marvinbryantia (OR = 1.13, 95% CI: 1.02–1.25, p = 0.02), Parabacteroides (OR = 1.14, 95% CI: 1.02–1.28, p = 0.02), and Roseburia (OR = 1.11, 95% CI: 1.02–1.21, p = 0.02) were linked to a higher risk of migraine (Figure 2; Supplementary Table S4). When FDR was considered, only the genus LachnospiraceaeUCG001 (q = 0.03) remained significantly associated with migraine. The remaining associations should only be considered suggestive (all q > 0.05). Regarding the sensitivity analysis, the estimated results of the other two analysis methods were in the same direction as those of the IVW method (Supplementary Table S4). Additionally, there was no strong reason to suspect horizontal pleiotropy based on the results of Cochran’s Q test, the MR-Egger regression intercept test, and the MR-PRESSO analysis (Supplementary Table S4). Thus, there was a causal relationship between the genus Lachnospiraceae UCG 001 and migraine.
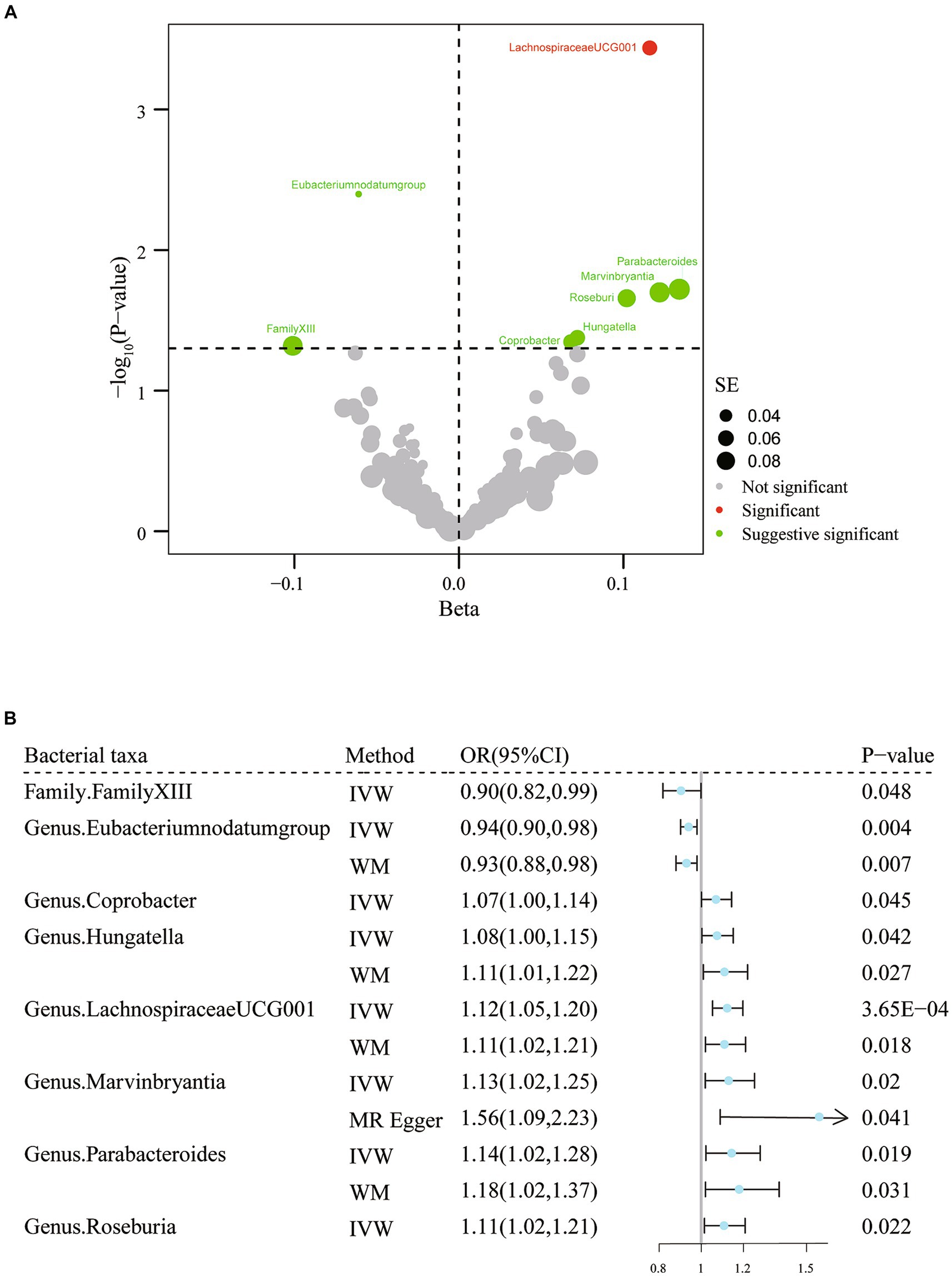
Figure 2. Results of IVW analysis of gut microbiota (MiBioGen) and migraine. (A) The volcano plot shows the association between 196 gut microbiota and migraine risk. The X-axis represents the β value and the Y-axis shows the logarithmic p-value in base 10. The red dots indicate the p-value <0.05 and false discovery rate < 0.05. Green dots indicate p-values <0.05 but the false discovery rate > 0.05. (B) Forest plot results for association estimates of gut microbiota and migraine. CI, confidence interval; IVW, inverse-variance weighted; WM, weighted median; OR, odds ratio; SE, standard error.
Gut microbiota (Dutch microbiota project) and migraine
In the preliminary exploration phase, we used the IVW approach to identify eight microbial taxa and three functional pathways that may be associated with migraine (Figure 3; Supplementary Table S5). There was no evidence of weak instrumental variables.
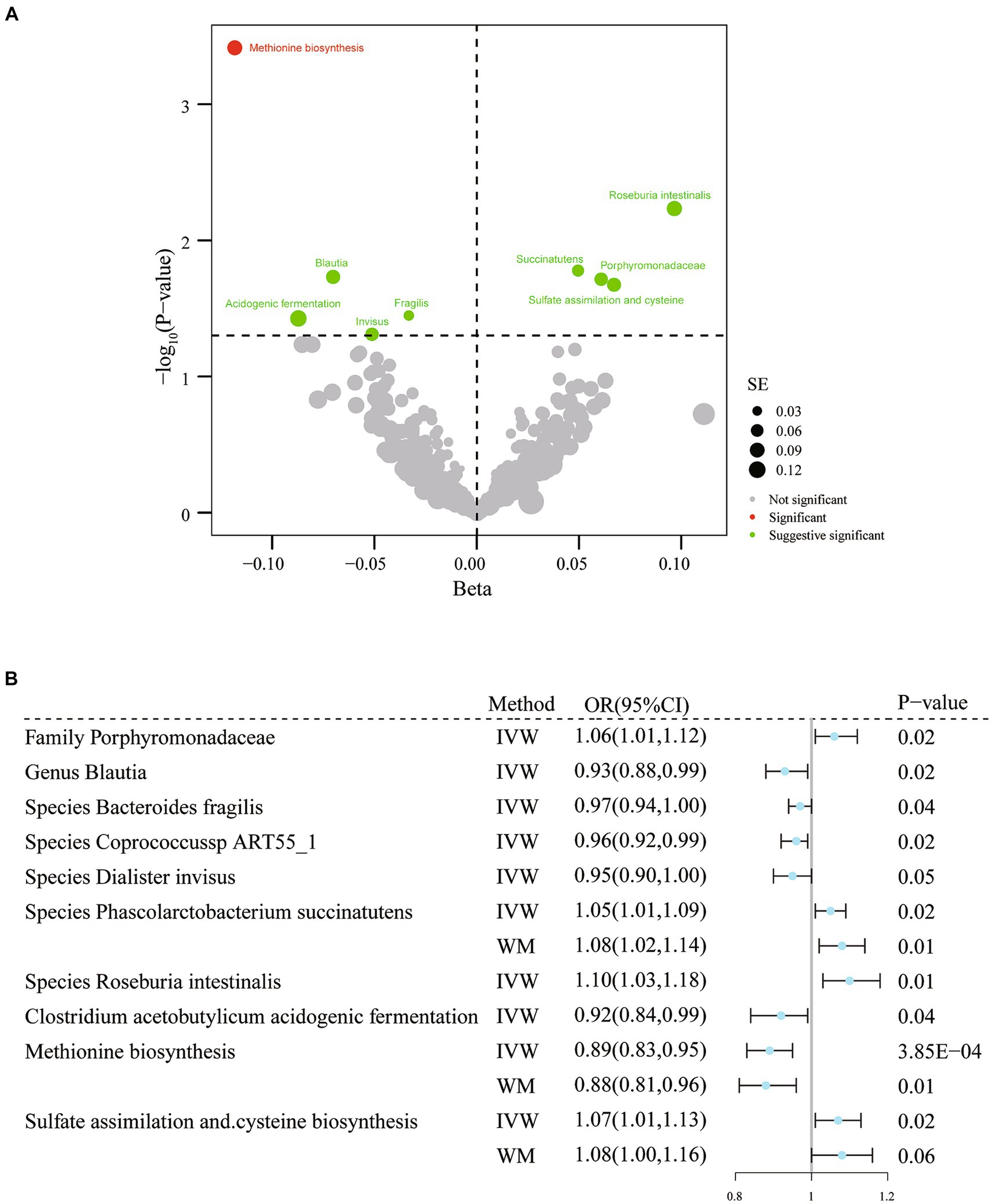
Figure 3. Results of IVW analysis of gut microbiota (Dutch Microbiota Project) and migraine. (A) The volcano plot shows the association between 207 gut microbiota and 205 functional pathways with migraine risk. The X-axis represents the β value and the Y-axis shows the logarithmic p-value in base 10. The red dots indicate the p-value <0.05 and false discovery rate < 0.05. Green dots indicate p-values <0.05 but the false discovery rate > 0.05. (B) Forest plot results for association estimation. CI, confidence interval; IVW, inverse-variance weighted; WM, weighted median; OR, odds ratio; MR, mendelian randomization; SE, standard error.
According to the results of IVW analysis, genus Blautia (OR = 0.93, 95% CI: 0.88–0.99, p = 0.02) and species Bacteroides fragilis (OR = 0.97, 95% CI: 0.94–1.00, p = 0.04), coprococcus sp_ART55_1 (OR = 0.96, 95% CI: 0.92–0.99, p = 0.02), and Dialister invisus (OR = 0.95, 95% CI: 0.90–1.00, p = 0.049) were linked to lower migraine risk. Furthermore, the family Porphyromonadaceae (OR = 1.06, 95% CI: 1.01–1.12, p = 0.02) and species Phascolarctobacterium succinatutens (OR = 1.05, 95% CI: 1.01–1.09 p = 0.02) and Roseburia intestinalis (OR = 1.10, 95% CI: 1.03–1.18, p = 5.84× 10−3) were associated with a higher risk of migraine (Figure 3; Supplementary Table S6). Additionally, three related functional pathways include Clostridium acetobutylicum acidogenic fermentation (OR = 0.92, 95% CI: 0.84–0.99, p = 0.04), methionine biosynthesis (OR = 0.89, 95% CI: 0.83–0.95, p = 3.85× 10−4), sulfate assimilation, and cysteine biosynthesis (OR = 1.07, 95% CI: 1.01–1.13, p = 0.02) were associated with migraine risk (Figure 3; Supplementary Table S6). However, when adjustment for multiplicity was considered, only the association between methionine synthesis (q = 0.01) and migraine remained significant; thus, the remaining associations should be considered suggestive (Supplementary Table S6). The association estimates were similar in the sensitivity analyses. No evidence of heterogeneity or horizontal pleiotropy was found (Supplementary Table S6).
Metabolites and migraine
Based on the pooled data from four human metabolome GWAS, 6 plasma metabolites were found to be associated with migraine (Supplementary Table S7). However, when adjustment for multiplicity was considered, these associations should be considered suggestive (all q > 0.05). A search of the gutMGene database revealed that only hydrocinnamate (OR = 0.85, 95% CI: 0.73–1.00, p = 0.046) was associated with gut microbial metabolism (Supplementary Table S8). The strength of the included instrumental variables was adequate. Heterogeneity or horizontal pleiotropy was not observed (Supplementary Table S8).
Reverse MR analysis
When migraine was included as an exposure, the screening threshold was established as 5 × 10−8 at the genome-wide level due to the availability of sufficient IVs. The details of the included IVs are provided in Supplementary Table S9. In the reverse MR analysis, there was no significant causal relationship between migraine and the gut microbiota or metabolites (Supplementary Table S10). No evidence of heterogeneity, horizontal pleiotropy, or outliers was found in most of the association analyses (Supplementary Table S10).
Discussion
The present study employed a two-sample MR design to explore the potential causal relationship between gut microbiota and migraines utilizing the summary statistics for migraine published by the IHGC and for gut microbiota from the MiBioGen or Dutch Microbiota project. According to our MR analysis, Lachnospiraceae UCG 001 increased the likelihood of migraine, while methionine biosynthesis pathways associated with the microbiota and hydrocinnamate were associated with a reduced migraine risk. Our study also revealed that multiple gut microbiota may be involved in the occurrence and development of migraine. For example, one family (family XIII), two genera (Blautia, Eubacterium (nodosa group)), and three species (Bacteroides fragilis, coprococcus sp_ART55_1, and Dialister invisus) of gut microbiota may have preventive properties against migraine. However, one family (Porphyromonadaceae), five genera (Coprobacter, Hungatella, Marvinbryantia, Parabacteroides, Roseburia) and two species (Phascolarctobacterium succinatutens, Roseburia intestinalis) of gut microbiota may increase the risk of migraine.
Some observational studies have reported a relationship between gut microbiota and migraine (Chen et al., 2019; Georgescu et al., 2019; Geisler et al., 2021; Kopchak et al., 2022; Kopchak and Hrytsenko, 2022; Liu et al., 2022; Miao et al., 2022; Bai et al., 2023; Yong et al., 2023). In the present study, the genus LachnospiraceaeUCG001, which had not previously been identified, was discovered to be a risk factor for migraine for the first time. The Lachnospiraceae family belongs to the phylum Firmicutes and comprises 58 genera and several unclassified strains, some of which generate butyrate along with various short-chain fatty acids (SCFAs) by hydrolyzing carbohydrates such as starch and sugar (Vacca et al., 2020). Some Lachnospiraceae species actively impair glucose metabolism, leading to inflammation (Vacca et al., 2020). The current study showed that LachnospiraceaeUCG001, at the genus level, might influence depressive symptoms or major depressive disorder in humans (Jiang et al., 2015; Zheng et al., 2016; Radjabzadeh et al., 2022), and it was also linked to anhedonia in mice at the species level (Yang et al., 2019). Overall, the available evidence has suggested that LachnospiraceaeUCG001 may affect health, such as in depression and multiple sclerosis. Thus, LachnospiraceaeUCG001 may adversely affect migraines. Information regarding the physiological role of LachnospiraceaeUCG001 in neurological disorders is limited and warrants further study.
Blautia is a genus of anaerobic bacteria with probiotic properties that is dominant in the gut microbiota (Maturana and Cárdenas, 2021). It has the potential to inhibit the colonization of pathogenic bacteria in the intestine and affects the composition of intestinal microbiota (Liu et al., 2021). Blautia can use glucose to produce acetic acid, thereby altering the intestinal environment. It may also be related to the metabolism of certain amino acids, such as methionine (Hosomi et al., 2022). Notably, functional pathway analysis of gut microbiota found that methionine synthesis was associated with reduced migraine risk. One of the derived metabolites of Blautia is S-adenosylmethionine. S-adenosylmethionine is important in health maintenance and a methyl donor for biological methylation. Recent studies have shown that Blautia can help prevent diabetes, obesity, and inflammation and is used to treat depression (Yang et al., 2020; Hosomi et al., 2022). In summary, accumulating evidence reveals that Blautia has beneficial effects on human health and can act as a probiotic (Figure 4). Eubacterium (nodatum group) may play a protective role against migraine, similar to the results of some observational studies (Chen et al., 2019; Mukherjee et al., 2020; Yong et al., 2023). Eubacterium can increase the levels of SCFAs, including acetic and butyric acids, and exert anti-inflammatory effects (Figure 4; Mukherjee et al., 2020). The role of Bacteroides fragilis in human health is controversial. It has demonstrated both an ability to suppress intestinal inflammation (IL-10) and an increased risk of colorectal cancer (Carrow et al., 2020). However, a search of the gutMGene database suggested that Bacteroides fragilis was associated with hydrocinnamate (Jain et al., 2019). Hydrocinnamate has potential antioxidant properties such as scavenging free radicals and activating antioxidant enzymes (Menni et al., 2020). Consistently, our study found a protective effect of genetically predicted higher plasma hydrocinnamate levels against migraine (Figure 4). Therefore, we suggest that Blautia and Eubacterium (nodatum group) at the genus level and Bacteroides fragilis at species level may be candidates for migraine treatment in the future. Currently, the literature lacks studies of the effect of Family XIII, coprococcus sp_ART55_1, and Dialister invisus on human health. Thus, it is difficult to judge their potential protective effect. Therefore, there is not enough confidence that these four microbial taxa have an a priori protective effect against migraine. One observational study reported a possible negative trend in the correlation between Prevotellaceae and migraine duration (Liu et al., 2022). Studies have reported a reduced abundance of Howardella at the species at the genus level in individuals with depression (iMSMS Consortium, 2022). However, the causal estimates of the relationship between Prevotellaceae, Eubacterium (rectale group), Howardella, and migraine showed inconsistent directions in the IVW and MR-Egger methods (Supplementary Table S3). Therefore, their causal association with migraines needs to be considered with caution in MR analyses.
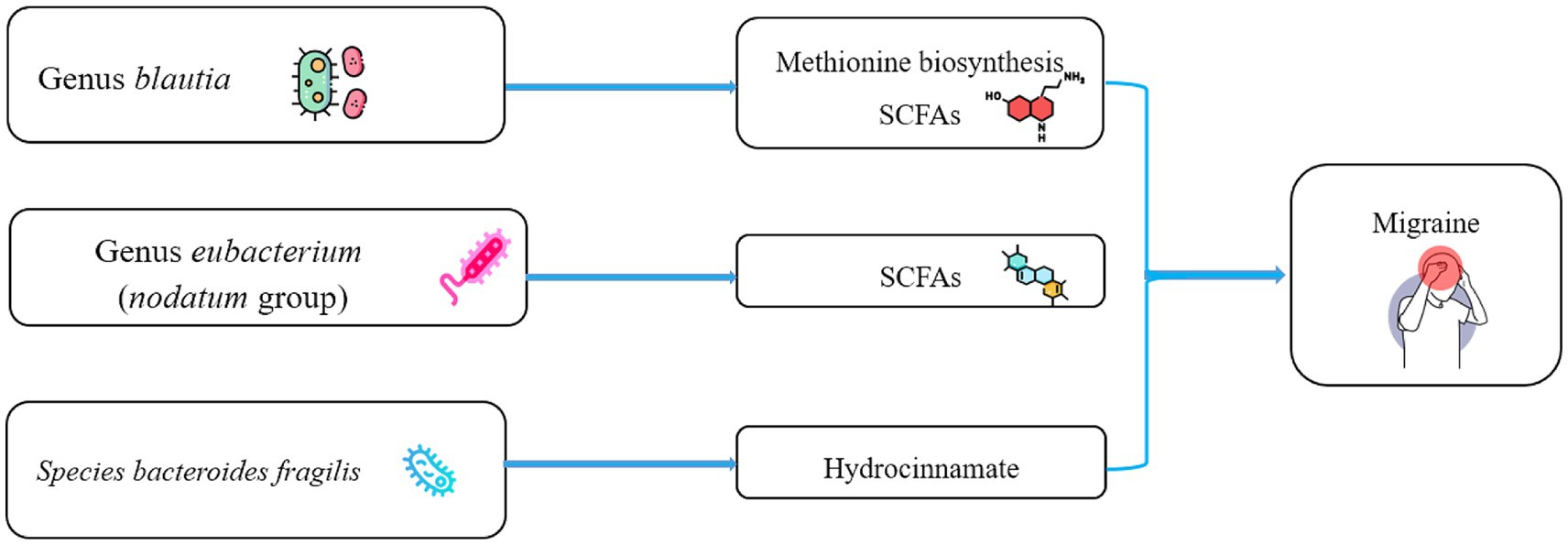
Figure 4. Schematic representation of the potential metabolites of the gut microbiota that influence migraine. SCFAs, short chain fatty acids.
Parabacteroides, Hungatella, Coprobacter, Marvinbryantia, and Roseburia are potentially positively related to the risk of migraines. In line with the present findings, the abundance of Parabacteroides was higher in patients with migraines than controls, and there was a possible positive correlation between Parabacteroides and Pittsburgh Sleep Quality Index score (Bai et al., 2023; Yong et al., 2023). Parabacteroides belongs to the phylum Bacteroidetes, a group of Gram-negative anaerobic bacteria that regularly colonize the human digestive system. Lipopolysaccharides and the metabolic end-product succinate are two ways that Parabacteroidetes cause inflammation (Ezeji et al., 2021). Observational studies have found a greater abundance of Hungatella in patients with chronic migraine compared to people without migraine (Yong et al., 2023). Studies have reported that the abundance of Hungatella was higher in patients with multiple sclerosis compared to controls and was positively associated with depressive symptoms (iMSMS Consortium, 2022; Radjabzadeh et al., 2022). Hungatella is a member of the family Clostridiaceae and the phylum Firmicutes. Hungatella produces the precursor molecule trimethylamine-N-oxide, which has been linked to neurological disorders like depression (Janeiro et al., 2018). Some studies have reported that the abundance of Coprobacter was higher in parents with neurosyphilis, general paresis, or sarcopenia, as well as kids suffering from autism spectrum disorder, compared with controls (Liu et al., 2019; Wang Y. et al., 2022; Wang G. et al., 2022). Coprobacter has a potential pathogenic role that may be related to the elevation of trimethylamine-N-oxide; further investigation should be done to better comprehend its function in the pathogenesis of migraine (Shirouchi et al., 2022).
In the reported observational studies, Marvinbryantia was positively correlated with vitamin D3, and Marvinbryantia abundance was inversely correlated with the likelihood of amyloid positivity (Zuo et al., 2019; Verhaar et al., 2021). This implies that Marvinbryantia is a beneficial genus. Marvinbryantia, which belongs to the phylum Firmicutes, is a cellulose-degrading bacterial genus. On the one hand, Marvinbryantia can produce acetate in the intestine, thereby promoting butyrate synthesis. In contrast, Gordon et al. found that Marvinbryantia can improve succinate production in vivo (Rey et al., 2010). By regulating IL-1β expression and reactive oxygen species generation, succinate has an effect on key inflammatory pathways in immune and non-immune cells (Lampropoulou et al., 2016). Roseburia is in the phylum Firmicutes and family Lachnospiraceae. Five well-characterized species of the Roseburia genus, including the Roseburia intestinalis, Roseburia hominis, Roseburia inulinivorans, Roseburia faecis, and Roseburia cecicola, all produce SCFAs (Nie et al., 2021). In addition, Roseburia can convey messages to colonic glia to trigger IL-22 release and influence 5-hydroxytryptamine levels and glial fibrillary acidic protein expression in colonic tissue (Peh et al., 2022). Therefore, Roseburia exert potential beneficial effects on the pathogenesis of neurological diseases like stroke, depression, and Parkinson’s disease (Nie et al., 2021; Peh et al., 2022). However, the present study revealed that Roseburia may increase migraine risk. One study found that Roseburia was less abundant at the genus level in people with migraine compared to those without (Yong et al., 2023). However, a mouse model of migraine did not reveal any significant differences (Nan et al., 2023). In this context, the relationship between Roseburia and migraines should be viewed cautiously and warrants further exploration.
As discussed previously, Eubacterium can increase levels of SCFAs and exert anti-inflammatory effects. The end-products of the metabolism of the human intestinal flora are SCFAs, which mainly contain acetic acid, propionic acid, and butyric acid. The SCFA-producing bacterial groups identified in this study as being associated with migraine were Blautia and Eubacterium (nodatum groups). Studies have reported that SCFAs can decrease microglial activation and pro-inflammatory cytokine secretion, such as mitogen-activated protein kinases, nuclear factor-κB, IL-1β, and TNF-α (Dalile et al., 2019; Silva et al., 2020). SCFAs affect inflammatory signaling pathways mainly by activating transmembrane G protein-coupled receptors, such as olfactory receptor 51E2, GPR109A, GPR41, and GPR42 (Dalile et al., 2019). For example, propionate and butyrate reduced the onset of pain in a nitroglycerine-induced mouse model of migraine and reduced the release of IL-1β and TNF-α in the ileum (Lanza et al., 2021). SCFAs regulate neurotransmitter and neurotrophic factor levels. For example, the neurotransmitters glutamate, glutamine, and γ-aminobutyric acid are altered by acetate in hypothalamus (Frost et al., 2014). Propionate and butyrate affect intracellular potassium levels and thus participate in the operation of cellular signaling systems (Oleskin and Shenderov, 2016). SCFA can also regulate tryptophan and tyrosine metabolism and affect serotonin and dopamine levels (Clarke et al., 2014). In a rat model of migraine known as the inflammatory soup model, it was found that bacteria associated with the production of SCFAs and 5-hydroxytryptamine were reduced, resulting in decreased levels of 5-hydroxytryptamine and tryptophan hydroxylase (Nan et al., 2023). Additionally, SCFAs, especially propionate and butyrate, have been found to inhibit the activity of histone deacetylases, thereby regulating the acetylation of histone lysine residues in nucleosomes and protecting nerve cells.
The findings of two randomized controlled trials suggested that supplementation with probiotics, specifically bifidobacterium, lactobacillus, and lactococcus strains, could potentially decrease the frequency of migraine. However, another randomized controlled trial did not support this claim (De Roos et al., 2017). Further, three studies did not find that probiotic supplementation could significantly improve intestinal permeability or reduce the secretion of inflammatory factors (Martami et al., 2019; Rinninella et al., 2019; Ghavami et al., 2021). However, the present study did not identify a potential causal relationship between Bifidobacterium, Lactobacillus, or Lactococcus and migraine.
This study has multiple strengths compared to previous studies. First, we complemented the gap in the species-level relationship between gut microbiota and migraine. Second, we identified three gut microbial taxa that serve as beneficial bacteria and show promise as candidate therapeutic targets for migraine treatment. Third, our findings revealed that a functional pathway for methionine synthesis associated with the gut microbiota is associated with a reduced risk of migraine. Fourth, we identified a plasma metabolite (hydrocinnamate) that may act as a hub between gut microbiota and migraine.
Nevertheless, this study had some limitations. First, to include more genetic variation in IVs, the significance level for gut microbiota selection of IVs was set at 1 × 10−5, rather than using the traditional GWAS significance threshold (p < 5 × 10−8). However, F-statistics >10 can rule out potential weak instrument bias, and FDR correction was used to limit the possibility of false positives. Second, some of the microbiota found to be associated with migraine were not successfully replicated in a different dataset. However, we provide possible directions for exploration that warrant further discussion regarding their true relationship with migraine. Third, the low statistical power of some association analyses should not be ignored. Fourth, since the sample size of the gut microbiota was relatively small, weak instrumental bias may have affected the reverse MR analysis; therefore, reverse causality could not be completely ruled out. Fifth, because the majority of the GWAS participants used as our gut microbiota data were of European heritage, our findings may not be generalizable to all populations. Further studies on the causal relationship between the gut microbiota and migraine in different global regions should be considered to obtain better generality. Sixth, given that the analysis used summary statistics, subgroup analyses according to migraine subtype and sex were not performed. Seventh, direct mechanistic studies supporting our findings are still lacking, and more studies are needed to gain more direct proof of any proposed connection.
Conclusion
This bidirectional two-sample MR study concluded that there is a causal relationship between Lachnospiraceae UCG 001 and migraine. However, further studies are required to clarify the underlying pathogenic mechanisms. Additionally, genus Blautia, genus Eubacterium (nodatum group), species Bacteroides fragilis, the methionine biosynthesis pathway related to gut bacteria, and hydrocinnamate were found to be protective factors against migraine. These findings provide potential targets for migraine intervention. Migraine and gut microbiota do not appear to be causally related; however, inverse MR analysis was unable to entirely rule out the effects of migraine on gut microbiota.
Data availability statement
The original contributions presented in the study are included in the article/Supplementary material, further inquiries can be directed to the corresponding author.
Author contributions
KQ: Conceptualization, Formal analysis, Methodology, Software, Writing – original draft. M-XL: Conceptualization, Methodology, Writing – original draft. LG: Formal analysis, Software, Writing – original draft. Z-TC: Visualization, Writing – original draft. J-JL: Investigation, Writing – original draft. RY: Investigation, Writing – original draft. MD: Data curation, Project administration, Supervision, Writing – review & editing.
Funding
The author(s) declare financial support was received for the research, authorship, and/or publication of this article. This study was funded by the Natural Science Foundation of Jilin Province of China (Grant No. 20200201606JC) and the National Natural Science Foundation of China (Grant No. 31872772) to MD supported this research.
Acknowledgments
The participants and researchers of the International Headache Genetics Consortium, MiBioGen consortium, and Dutch Microbiota Project are thanked by the authors. The members of the International Headache Genetics Consortium (full details in Supplementary Table S16). The authors thank all investigators who provided GWAS data on plasma metabolites. The authors also would like to thank Editage (www.editage.cn) for English language editing.
Conflict of interest
The authors declare that the research was conducted in the absence of any commercial or financial relationships that could be construed as a potential conflict of interest.
Publisher’s note
All claims expressed in this article are solely those of the authors and do not necessarily represent those of their affiliated organizations, or those of the publisher, the editors and the reviewers. Any product that may be evaluated in this article, or claim that may be made by its manufacturer, is not guaranteed or endorsed by the publisher.
Supplementary material
The Supplementary material for this article can be found online at: https://www.frontiersin.org/articles/10.3389/fmicb.2024.1325047/full#supplementary-material
Abbreviations
GWAS, genome-wide association study; MR, Mendelian randomization; IV, instrumental variable; IHGC, International Headache Genetics Consortium; SNP, single nucleotide polymorphism; IVW, inverse variance weighting; OR, odds ratio; SCFA, short-chain fatty acid; IL, interleukin; TMAO, tryptophan, serotonin, tyrosine, trimethylamine N-oxide; MR-PRESSO, MR-Pleiotropy RESidual Sum and Outlier; FDR, false discovery rate; CI, confidence interval.
Footnotes
References
Arzani, M., Jahromi, S. R., Ghorbani, Z., Vahabizad, F., Martelletti, P., Ghaemi, A., et al. (2020). Gut-brain Axis and migraine headache: a comprehensive review. J. Headache Pain 21:15. doi: 10.1186/s10194-020-1078-9
Ashina, M., Katsarava, Z., Do, T. P., Buse, D. C., Pozo-Rosich, P., Özge, A., et al. (2021). Migraine: epidemiology and systems of care. Lancet (London, England). 397, 1485–1495. doi: 10.1016/S0140-6736(20)32160-7
Bai, J., Shen, N., and Liu, Y. (2023). Associations between the gut microbiome and migraines in children aged 7-18 years: an analysis of the American gut project cohort. Pain Manag. Nurs. 24, 35–43. doi: 10.1016/j.pmn.2022.06.002
Bowden, J., Davey Smith, G., and Burgess, S. (2015). Mendelian randomization with invalid instruments: effect estimation and bias detection through Egger regression. Int. J. Epidemiol. 44, 512–525. doi: 10.1093/ije/dyv080
Bowden, J., Davey Smith, G., Haycock, P. C., and Burgess, S. (2016). Consistent estimation in Mendelian randomization with some invalid instruments using a weighted median estimator. Genet. Epidemiol. 40, 304–314. doi: 10.1002/gepi.21965
Bowden, J., Del Greco, M. F., Minelli, C., Zhao, Q., Lawlor, D. A., Sheehan, N. A., et al. (2019). Improving the accuracy of two-sample summary-data Mendelian randomization: moving beyond the NOME assumption. Int. J. Epidemiol. 48, 728–742. doi: 10.1093/ije/dyy258
Burgess . (n.d.). Online sample size and power calculator for Mendelian randomization with a binary outcome. Available at: http://cnsgenomics.com/shiny/mRnd.
Burgess, S., Butterworth, A., and Thompson, S. G. (2013). Mendelian randomization analysis with multiple genetic variants using summarized data. Genet. Epidemiol. 37, 658–665. doi: 10.1002/gepi.21758
Carrow, H. C., Batachari, L. E., and Chu, H. (2020). Strain diversity in the microbiome: lessons from Bacteroides fragilis. PLoS Pathog. 16:e1009056. doi: 10.1371/journal.ppat.1009056
iMSMS Consortium (2022). Gut microbiome of multiple sclerosis patients and paired household healthy controls reveal associations with disease risk and course. Cell 185, 3467–86.e16. doi: 10.1016/j.cell.2022.08.021
Chen, J., Wang, Q., Wang, A., and Lin, Z. (2019). Structural and functional characterization of the gut microbiota in elderly women with migraine. Front. Cell. Infect. Microbiol. 9:470. doi: 10.3389/fcimb.2019.00470
Cheng, L., Qi, C., Zhuang, H., Fu, T., and Zhang, X. (2020). gutMDisorder: a comprehensive database for dysbiosis of the gut microbiota in disorders and interventions. Nucleic Acids Res. 48, D554–D560. doi: 10.1093/nar/gkz843
Clarke, G., Stilling, R. M., Kennedy, P. J., Stanton, C., Cryan, J. F., and Dinan, T. G. (2014). Minireview: gut microbiota: the neglected endocrine organ. Mol. Endocrinol. 28, 1221–1238. doi: 10.1210/me.2014-1108
Consortium M . (2022). MiBioGen. Available at: https://mibiogen.gcc.rug.nl/.
Crawford, J., Liu, S., and Tao, F. (2022). Gut microbiota and migraine. Neurobiol. Pain 11:100090. doi: 10.1016/j.ynpai.2022.100090
Cryan, J. F., O’Riordan, K. J., Cowan, C. S. M., Sandhu, K. V., Bastiaanssen, T. F. S., Boehme, M., et al. (2019). The microbiota-gut-brain Axis. Physiol. Rev. 99, 1877–2013. doi: 10.1152/physrev.00018.2018
Dalile, B., Van Oudenhove, L., Vervliet, B., and Verbeke, K. (2019). The role of short-chain fatty acids in microbiota-gut-brain communication. Nat. Rev. Gastroenterol. Hepatol. 16, 461–478. doi: 10.1038/s41575-019-0157-3
Davey Smith, G., and Hemani, G. (2014). Mendelian randomization: genetic anchors for causal inference in epidemiological studies. Hum. Mol. Genet. 23, R89–R98. doi: 10.1093/hmg/ddu328
De Roos, N. M., van Hemert, S., Rovers, J. M. P., Smits, M. G., and Witteman, B. J. M. (2017). The effects of a multispecies probiotic on migraine and markers of intestinal permeability-results of a randomized placebo-controlled study. Eur. J. Clin. Nutr. 71, 1455–1462. doi: 10.1038/ejcn.2017.57
Dodick, D. W. (2018). Migraine. Lancet (London, England). 391, 1315–1330. doi: 10.1016/S0140-6736(18)30478-1
Ezeji, J. C., Sarikonda, D. K., Hopperton, A., Erkkila, H. L., Cohen, D. E., Martinez, S. P., et al. (2021). Parabacteroides distasonis: intriguing aerotolerant gut anaerobe with emerging antimicrobial resistance and pathogenic and probiotic roles in human health. Gut Microbes 13:1922241. doi: 10.1080/19490976.2021.1922241
Frost, G., Sleeth, M. L., Sahuri-Arisoylu, M., Lizarbe, B., Cerdan, S., Brody, L., et al. (2014). The short-chain fatty acid acetate reduces appetite via a central homeostatic mechanism. Nat. Commun. 5:3611. doi: 10.1038/ncomms4611
GBD 2016 Disease and Injury Incidence and Prevalence Collaborators (2017). Global, regional, and national incidence, prevalence, and years lived with disability for 328 diseases and injuries for 195 countries, 1990-2016: a systematic analysis for the global burden of disease study 2016. Lancet (London, England) 390, 1211–1259. doi: 10.1016/S0140-6736(17)32154-2
Geisler, C., Pankoke, J., Schlicht, K., Knappe, C., Rohmann, N., Hartmann, K., et al. (2021). BMI, alcohol consumption and gut microbiome species richness are related to structural and functional neurological abnormalities. Nutrients 13:743. doi: 10.3390/nu13113743
Georgescu, D., Iurciuc, M. S., Ionita, I., Dragan, S., Muntean, M., Ancusa, O. E., et al. (2019). Migraine without Aura and Subclinical atherosclerosis in Young females: is gut microbiota to blame? Medicina (Kaunas) 55:786. doi: 10.3390/medicina55120786
Ghavami, A., Khorvash, F., Heidari, Z., Khalesi, S., and Askari, G. (2021). Effect of synbiotic supplementation on migraine characteristics and inflammatory biomarkers in women with migraine: results of a randomized controlled trial. Pharmacol. Res. 169:105668. doi: 10.1016/j.phrs.2021.105668
Goadsby, P. J., Holland, P. R., Martins-Oliveira, M., Hoffmann, J., Schankin, C., and Akerman, S. (2017). Pathophysiology of migraine: a disorder of sensory processing. Physiol. Rev. 97, 553–622. doi: 10.1152/physrev.00034.2015
Guo, Y., Rist, P. M., Daghlas, I., Giulianini, F., Kurth, T., and Chasman, D. I. (2020). A genome-wide cross-phenotype meta-analysis of the association of blood pressure with migraine. Nat. Commun. 11:3368. doi: 10.1038/s41467-020-17002-0
Hautakangas, H., Winsvold, B. S., Ruotsalainen, S. E., Bjornsdottir, G., Harder, A. V. E., Kogelman, L. J. A., et al. (2022). Genome-wide analysis of 102,084 migraine cases identifies 123 risk loci and subtype-specific risk alleles. Nat. Genet. 54, 152–160. doi: 10.1038/s41588-021-00990-0
Hemani, G., Tilling, K., and Davey, S. G. (2017). Orienting the causal relationship between imprecisely measured traits using GWAS summary data. PLoS Genet. 13:e1007081. doi: 10.1371/journal.pgen.1007081
Hemani, G., Zheng, J., Elsworth, B., Wade, K. H., Haberland, V., Baird, D., et al. (2018). The MR-base platform supports systematic causal inference across the human phenome. eLife 7:7. doi: 10.7554/eLife.34408
Hosomi, K., Saito, M., Park, J., Murakami, H., Shibata, N., Ando, M., et al. (2022). Oral administration of Blautia wexlerae ameliorates obesity and type 2 diabetes via metabolic remodeling of the gut microbiota. Nat. Commun. 13:4477. doi: 10.1038/s41467-022-32015-7
Jain, A., Li, X. H., and Chen, W. N. (2019). An untargeted fecal and urine metabolomics analysis of the interplay between the gut microbiome, diet and human metabolism in Indian and Chinese adults. Sci. Rep. 9:9191. doi: 10.1038/s41598-019-45640-y
Janeiro, M. H., Ramírez, M. J., Milagro, F. I., Martínez, J. A., and Solas, M. (2018). Implication of trimethylamine N-oxide (TMAO) in disease: potential biomarker or new therapeutic target. Nutrients 10:1398. doi: 10.3390/nu10101398
Jiang, H., Ling, Z., Zhang, Y., Mao, H., Ma, Z., Yin, Y., et al. (2015). Altered fecal microbiota composition in patients with major depressive disorder. Brain Behav. Immun. 48, 186–194. doi: 10.1016/j.bbi.2015.03.016
Jiang, W., Wang, T., Liu, C., Deng, M., Ren, X., Wang, F., et al. (2021). A 16S rRNA gene sequencing based study of oral microbiota in migraine patients in China. Bioengineered 12, 2523–2533. doi: 10.1080/21655979.2021.1933840
Kettunen, J., Demirkan, A., Würtz, P., Draisma, H. H., Haller, T., Rawal, R., et al. (2016). Genome-wide study for circulating metabolites identifies 62 loci and reveals novel systemic effects of LPA. Nat. Commun. 7:11122. doi: 10.1038/ncomms11122
Kopchak, O., and Hrytsenko, O. (2022). Feature of gut microbiota in patients with migraine and healthy individuals. Georgian Med. News 327, 13–17.
Kopchak, O. O., Hrytsenko, O. Y., and Pulyk, O. R. (2022). Peculiarities of the gut microbiota in patients with migraine comparing to healthy individuals. Wiad. Lek. 75, 2218–2221. doi: 10.36740/WLek202209207
Kurilshikov, A., Medina-Gomez, C., Bacigalupe, R., Radjabzadeh, D., Wang, J., Demirkan, A., et al. (2021). Large-scale association analyses identify host factors influencing human gut microbiome composition. Nat. Genet. 53, 156–165. doi: 10.1038/s41588-020-00763-1
Lampropoulou, V., Sergushichev, A., Bambouskova, M., Nair, S., Vincent, E. E., Loginicheva, E., et al. (2016). Itaconate links inhibition of succinate dehydrogenase with macrophage metabolic remodeling and regulation of inflammation. Cell Metab. 24, 158–166. doi: 10.1016/j.cmet.2016.06.004
Lanza, M., Filippone, A., Ardizzone, A., Casili, G., Paterniti, I., Esposito, E., et al. (2021). SCFA treatment alleviates pathological signs of migraine and related intestinal alterations in a mouse model of NTG-induced migraine. Cells 10:756. doi: 10.3390/cells10102756
Lin, L., Zhang, R., Huang, H., Zhu, Y., Li, Y., Dong, X., et al. (2021). Mendelian randomization with refined instrumental variables from genetic score improves accuracy and reduces Bias. Front. Genet. 12:618829. doi: 10.3389/fgene.2021.618829
Liu, S., Li, E., Sun, Z., Fu, D., Duan, G., Jiang, M., et al. (2019). Altered gut microbiota and short chain fatty acids in Chinese children with autism spectrum disorder. Sci. Rep. 9:287. doi: 10.1038/s41598-018-36430-z
Liu, X., Mao, B., Gu, J., Wu, J., Cui, S., Wang, G., et al. (2021). Blautia-a new functional genus with potential probiotic properties? Gut Microbes 13, 1–23. doi: 10.1080/19490976.2021.1903826
Liu, J., Tang, W., Hou, L., Wang, J., Wang, R., Zhang, Y., et al. (2022). Alteration of gut microbiota in migraine patients with irritable bowel syndrome in a Chinese Han population. Front. Neurol. 13:899056. doi: 10.3389/fneur.2022.899056
Lopera-Maya, E. A., Kurilshikov, A., Van der Graaf, A., Hu, S., Andreu-Sánchez, S., Chen, L., et al. (2022). Effect of host genetics on the gut microbiome in 7,738 participants of the Dutch microbiome project. Nat. Genet. 54, 143–151. doi: 10.1038/s41588-021-00992-y
Martami, F., Togha, M., Seifishahpar, M., Ghorbani, Z., Ansari, H., Karimi, T., et al. (2019). The effects of a multispecies probiotic supplement on inflammatory markers and episodic and chronic migraine characteristics: a randomized double-blind controlled trial. Cephalalgia 39, 841–853. doi: 10.1177/0333102418820102
Maturana, J. L., and Cárdenas, J. P. (2021). Insights on the evolutionary genomics of the Blautia genus: potential new species and genetic content among lineages. Front. Microbiol. 12:660920. doi: 10.3389/fmicb.2021.660920
Menni, C., Zhu, J., Le Roy, C. I., Mompeo, O., Young, K., Rebholz, C. M., et al. (2020). Serum metabolites reflecting gut microbiome alpha diversity predict type 2 diabetes. Gut Microbes 11, 1632–1642. doi: 10.1080/19490976.2020.1778261
Miao, S., Tang, W., Li, H., Li, B., Yang, C., Xie, W., et al. (2022). Repeated inflammatory dural stimulation-induced cephalic allodynia causes alteration of gut microbial composition in rats. J. Headache Pain 23:71. doi: 10.1186/s10194-022-01441-9
Minelli, C., Del Greco, M. F., van der Plaat, D. A., Bowden, J., Sheehan, N. A., and Thompson, J. (2021). The use of two-sample methods for Mendelian randomization analyses on single large datasets. Int. J. Epidemiol. 50, 1651–1659. doi: 10.1093/ije/dyab084
Mukherjee, A., Lordan, C., Ross, R. P., and Cotter, P. D. (2020). Gut microbes from the phylogenetically diverse genus Eubacterium and their various contributions to gut health. Gut Microbes 12:1802866. doi: 10.1080/19490976.2020.1802866
Nan, N., Wang, Q., Li, M. J., Xu, Y. S., Guo, X. M., Xu, R., et al. (2023). The microbiota-dependent treatment of Wuzhuyu decoction for chronic migraine model rat associated with anxiety-depression like behavior. Oxidative Med. Cell. Longev. 2023, 1–20. doi: 10.1155/2023/2302653
Nie, K., Ma, K., Luo, W., Shen, Z., Yang, Z., Xiao, M., et al. (2021). Roseburia intestinalis: a beneficial gut organism from the discoveries in genus and species. Front. Cell. Infect. Microbiol. 11:757718. doi: 10.3389/fcimb.2021.757718
Oleskin, A. V., and Shenderov, B. A. (2016). Neuromodulatory effects and targets of the SCFAs and gasotransmitters produced by the human symbiotic microbiota. Microb. Ecol. Health Dis. 27:30971. doi: 10.3402/mehd.v27.30971
Parohan, M., Djalali, M., Sarraf, P., Yaghoubi, S., Seraj, A., Foroushani, A. R., et al. (2022). Effect of probiotic supplementation on migraine prophylaxis: a systematic review and meta-analysis of randomized controlled trials. Nutr. Neurosci. 25, 511–518. doi: 10.1080/1028415X.2020.1764292
Peh, A., O'Donnell, J. A., Broughton, B. R. S., and Marques, F. Z. (2022). Gut microbiota and their metabolites in stroke: a double-edged sword. Stroke 53, 1788–1801. doi: 10.1161/STROKEAHA.121.036800
PhenoScanner . (n.d.). A database of human genotype-phenotype associations. Available at: www.phenoscanner.medschl.cam.ac.uk.
Piane, M., Lulli, P., Farinelli, I., Simeoni, S., De Filippis, S., Patacchioli, F. R., et al. (2007). Genetics of migraine and pharmacogenomics: some considerations. J. Headache Pain 8, 334–339. doi: 10.1007/s10194-007-0427-2
Pierce, B. L., Ahsan, H., and Vanderweele, T. J. (2011). Power and instrument strength requirements for Mendelian randomization studies using multiple genetic variants. Int. J. Epidemiol. 40, 740–752. doi: 10.1093/ije/dyq151
Radjabzadeh, D., Bosch, J. A., Uitterlinden, A. G., Zwinderman, A. H., Ikram, M. A., Van Meurs, J. B. J., et al. (2022). Gut microbiome-wide association study of depressive symptoms. Nat. Commun. 13:7128. doi: 10.1038/s41467-022-34502-3
Rey, F. E., Faith, J. J., Bain, J., Muehlbauer, M. J., Stevens, R. D., Newgard, C. B., et al. (2010). Dissecting the in vivo metabolic potential of two human gut acetogens. J. Biol. Chem. 285, 22082–22090. doi: 10.1074/jbc.M110.117713
Richardson, T. G., Leyden, G. M., Wang, Q., Bell, J. A., Elsworth, B., Davey Smith, G., et al. (2022). Characterising metabolomic signatures of lipid-modifying therapies through drug target mendelian randomisation. PLoS Biol. 20:e3001547. doi: 10.1371/journal.pbio.3001547
Rinninella, E., Raoul, P., Cintoni, M., Franceschi, F., Miggiano, G. A. D., Gasbarrini, A., et al. (2019). What is the healthy gut microbiota composition? A changing ecosystem across age, environment, diet, and diseases. Microorganisms 7:14. doi: 10.3390/microorganisms7010014
Sanna, S., Van Zuydam, N. R., Mahajan, A., Kurilshikov, A., Vich Vila, A., Võsa, U., et al. (2019). Causal relationships among the gut microbiome, short-chain fatty acids and metabolic diseases. Nat. Genet. 51, 600–605. doi: 10.1038/s41588-019-0350-x
Shin, S. Y., Fauman, E. B., Petersen, A. K., Krumsiek, J., Santos, R., Huang, J., et al. (2014). An atlas of genetic influences on human blood metabolites. Nat. Genet. 46, 543–550. doi: 10.1038/ng.2982
Shirouchi, B., Fukuda, A., and Akasaka, T. (2022). Unlike Glycerophosphocholine or choline chloride, dietary phosphatidylcholine does not increase plasma trimethylamine-N-oxide levels in Sprague-Dawley rats. Meta 12:64. doi: 10.3390/metabo12010064
Silva, Y. P., Bernardi, A., and Frozza, R. L. (2020). The role of short-chain fatty acids from gut microbiota in gut-brain communication. Front. Endocrinol. 11:25. doi: 10.3389/fendo.2020.00025
Skrivankova, V. W., Richmond, R. C., Woolf, B. A. R., Yarmolinsky, J., Davies, N. M., Swanson, S. A., et al. (2021). Strengthening the reporting of observational studies in epidemiology using Mendelian randomization: the STROBE-MR statement. JAMA 326, 1614–1621. doi: 10.1001/jama.2021.18236
Socała, K., Doboszewska, U., Szopa, A., Serefko, A., Włodarczyk, M., Zielińska, A., et al. (2021). The role of microbiota-gut-brain axis in neuropsychiatric and neurological disorders. Pharmacol. Res. 172:105840. doi: 10.1016/j.phrs.2021.105840
Storey, J. D., and Tibshirani, R. (2003). Statistical significance for genomewide studies. Proc. Natl. Acad. Sci. USA 100, 9440–9445. doi: 10.1073/pnas.1530509100
Straube, A., Müller, H., Stiegelbauer, V., and Frauwallner, A. (2018). Migraine prophylaxis with a probiotic. Results of an uncontrolled observational study with 1,020 patients. MMW Fortschr. Med. 160, 16–21. doi: 10.1007/s15006-018-1052-5
Vacca, M., Celano, G., Calabrese, F. M., Portincasa, P., Gobbetti, M., and De Angelis, M. (2020). The controversial role of human gut Lachnospiraceae. Microorganisms 8:573. doi: 10.3390/microorganisms8040573
Verbanck, M., Chen, C. Y., Neale, B., and Do, R. (2018). Detection of widespread horizontal pleiotropy in causal relationships inferred from Mendelian randomization between complex traits and diseases. Nat. Genet. 50, 693–698. doi: 10.1038/s41588-018-0099-7
Verhaar, B. J. H., Hendriksen, H. M. A., de Leeuw, F. A., Doorduijn, A. S., van Leeuwenstijn, M., Teunissen, C. E., et al. (2021). Gut microbiota composition is related to AD pathology. Front. Immunol. 12:794519. doi: 10.3389/fimmu.2021.794519
Wang, Y., Zhang, Y., Lane, N. E., Wu, J., Yang, T., Li, J., et al. (2022). Population-based metagenomics analysis reveals altered gut microbiome in sarcopenia: data from the Xiangya sarcopenia study. J. Cachexia. Sarcopenia Muscle 13, 2340–2351. doi: 10.1002/jcsm.13037
Wang, G., Zou, D., Lu, X., Gu, X., Cheng, Y., Qi, T., et al. (2022). Gut microbiota alternation in disease progression of Neurosyphilis. Infect. Drug Resist. 15, 6603–6612. doi: 10.2147/IDR.S389155
Wishart, D. S., Guo, A., Oler, E., Wang, F., Anjum, A., Peters, H., et al. (2022). HMDB 5.0: the human metabolome database for 2022. Nucleic Acids Res. 50, D622–D631. doi: 10.1093/nar/gkab1062
Wootton, R. E., Richmond, R. C., Stuijfzand, B. G., Lawn, R. B., Sallis, H. M., Taylor, G. M. J., et al. (2020). Evidence for causal effects of lifetime smoking on risk for depression and schizophrenia: a Mendelian randomisation study. Psychol. Med. 50, 2435–2443. doi: 10.1017/S0033291719002678
Xu, Q., Ni, J. J., Han, B. X., Yan, S. S., Wei, X. T., Feng, G. J., et al. (2021). Causal relationship between gut microbiota and autoimmune diseases: a two-sample Mendelian randomization study. Front. Immunol. 12:746998. doi: 10.3389/fimmu.2021.746998
Yang, C., Fang, X., Zhan, G., Huang, N., Li, S., Bi, J., et al. (2019). Key role of gut microbiota in anhedonia-like phenotype in rodents with neuropathic pain. Transl. Psychiatry 9:57. doi: 10.1038/s41398-019-0379-8
Yang, J., Zheng, P., Li, Y., Wu, J., Tan, X., Zhou, J., et al. (2020). Landscapes of bacterial and metabolic signatures and their interaction in major depressive disorders. Sci. Adv. 6:8555. doi: 10.1126/sciadv.aba8555
Yong, D., Lee, H., Min, H. G., Kim, K., Oh, H. S., and Chu, M. K. (2023). Altered gut microbiota in individuals with episodic and chronic migraine. Sci. Rep. 13:626. doi: 10.1038/s41598-023-27586-4
Yuan, S., Daghlas, I., and Larsson, S. C. (2022). Alcohol, coffee consumption, and smoking in relation to migraine: a bidirectional Mendelian randomization study. Pain 163, e342–e348. doi: 10.1097/j.pain.0000000000002360
Zheng, H., Shi, Y. Z., Liang, J. T., Lu, L. L., and Chen, M. (2023). Modifiable factors for migraine prophylaxis: a mendelian randomization analysis. Front. Pharmacol. 14:1010996. doi: 10.3389/fphar.2023.1010996
Zheng, P., Zeng, B., Zhou, C., Liu, M., Fang, Z., Xu, X., et al. (2016). Gut microbiome remodeling induces depressive-like behaviors through a pathway mediated by the host's metabolism. Mol. Psychiatry 21, 786–796. doi: 10.1038/mp.2016.44
Keywords: migraine, gut microbiota, metabolite, gut-brain axis, causal inference, Mendelian randomization
Citation: Qu K, Li M-X, Gan L, Cui Z-T, Li J-J, Yang R and Dong M (2024) To analyze the relationship between gut microbiota, metabolites and migraine: a two-sample Mendelian randomization study. Front. Microbiol. 15:1325047. doi: 10.3389/fmicb.2024.1325047
Edited by:
Georgia Damoraki, National and Kapodistrian University of Athens, GreeceReviewed by:
Fang Liu, The 980th Hospital of the People’s Liberation Army Joint Service Support Force, ChinaHaibo Shi, South China University of Technology, China
Copyright © 2024 Qu, Li, Gan, Cui, Li, Yang and Dong. This is an open-access article distributed under the terms of the Creative Commons Attribution License (CC BY). The use, distribution or reproduction in other forums is permitted, provided the original author(s) and the copyright owner(s) are credited and that the original publication in this journal is cited, in accordance with accepted academic practice. No use, distribution or reproduction is permitted which does not comply with these terms.
*Correspondence: Ming Dong, ZG9uZ2dlQGpsdS5lZHUuY24=