- 1Key Laboratory of Dairy Science, Ministry of Education, College of Food Science, Northeast Agricultural University, Harbin, China
- 2Food Laboratory of Zhongyuan, Luohe, China
- 3School of Zhang Zhongjing Health Care and Food, Nanyang Institute of Technology, Nanyang, China
Powdered infant formula (PIF) is prone to Cronobacter sakazakii (C. sakazakii) contamination, which can result in infections that endanger the lives of newborns and infants. Slightly acidic electrolytic water (SAEW) has shown antibacterial effects on a variety of foodborne pathogens and has a wide applicability in the food industry. Here, the antibacterial activity of SAEW against C. sakazakii and its use as a disinfectant on contact surfaces with high infection transmission risk were investigated. The inactivation of SAEW on C. sakazakii was positively correlated to the SAEW concentration and treatment time. The antibacterial effect of SAEW was achieved by decreasing the intracellular adenosine triphosphate (ATP), K+, protein, and DNA contents of C. sakazakii, reducing the intracellular pH (pHin) and destroying the cell morphology, which led to inactivation of C. sakazakii ultimately. To test the applicability of this study, the results showed that approximately 103 CFU/cm2 of C. sakazakii were successfully inactivated on stainless steel and rubber surfaces after a 30 mg/L SAEW treatment for 20 s. These results indicate the antibacterial mechanism and potential application of SAEW against C. sakazakii, as well as a new strategy for the prevention and control of C. sakazakii on stainless steel and rubber surfaces.
1 Introduction
Cronobacter sakazakii (C. sakazakii) is a Gram-negative and non-spore-forming bacterium classified to the Enterobacteriaceae, which is an opportunistic foodborne pathogen. Neonatal meningitis, septicemia, and necrotizing enterocolitis are common illnesses of C. sakazakii infections. The death rate from C. sakazakii infections varies from 40 to 80% in the absence of effective treatment (Yan and Fanning, 2015; Phair et al., 2022).
Food contamination caused by C. sakazakii has received increasing attention because of its high osmotic pressure tolerance, good survival ability in low-moisture environments, and subsequent cross-contamination. Several foods and places have been reported to contain C. sakazakii contamination, powdered infant formula (PIF) in particular (Vojkovska et al., 2016; Lou et al., 2019). Gloves and stainless steel surfaces are the main external sources of food contamination by C. sakazakii (Lindsay et al., 2019). Therefore, the effective inhibition of C. sakazakii on the contact surfaces of dairy processing plants is very crucial. As of now, the food business has used peroxyacetic acid, organic acids, hydrogen peroxide, chlorine dioxide, and hydrogen peroxide as disinfectants (Sethi et al., 2020). Despite the fact that these disinfectants are effective, the food industry does not prioritize using them due to chemical management and environmental issues (Pacheappan et al., 2022).
As a new type of environmentally friendly antibacterial agent, slightly acidic electrolytic water (SAEW) has been widely used in food industry, agriculture and medical field (Roy et al., 2021). SAEW has the advantages of being less corrosive to equipment, less irritating to hands, and minimizing human health and safety issues in chemical disinfection (Zang et al., 2019). Electrolyzing diluted sodium chloride or hydrochloric acid solution is generally used as the raw material, from which SAEW can be generated using a special electrolytic device. By adjusting the devices, the pH value of SAEW can range from 5.0 to 6.5, and its oxidation–reduction potential (ORP) can be set to above 850 mV (Ye et al., 2017). The antimicrobial effect of SAEW is due to the interaction of its pH condition, redox potential and active chlorine (Li et al., 2023). SAEW can change the ultrastructure and permeability of Staphylococcus aureus and E. coli, leading to cell content leakage and inactivation (Ding et al., 2016; Kim et al., 2019). SAEW has the ability to effectively inhibit the growth of Listeria innocua on stainless steel tableware, rubber, and glass by limiting biofilm formation (Jeon et al., 2018). Spraying SAEW also can effectively reduce the number of Staphylococcus epidermidis in the air (Naka et al., 2020).
Although these investigations showed SAEW to have good antibacterial action against foodborne pathogens on surfaces that could potentially come into contact with food, few studies have reported the mechanism of action of SAEW against C. sakazakii. We hypothesize that SAEW can destroy the cell membrane of C. sakazakii, causing leakage of its intracellular contents and thus restraining the growth of C. sakazakii. As such, this work investigated the antibacterial effects of SAEW against C. sakazakii and evaluated the application of SAEW on rubber gloves and stainless steel surfaces. Our main objective is to offer a theoretical foundation for better SAEW use in the areas of food safety control, quality maintenance, and shelf-life extension.
2 Materials and methods
2.1 Activation and preservation of strains
The cryopreserved tube of C. sakazakii (ATCC 29544, American Type Culture Collection) stored at −80°C was removed and thawed. Then inoculated the C. sakazakii into sterile Luria-Bertani (LB) broth medium at 3% inoculum, cultured at 37°C for 8–12 h, and streaked on Trypticase Soy Agar (TSA) medium. The single colonies were inoculated into sterilized LB broth medium and incubated at 37°C for 24 h until the population reached 108 CFU/mL. This bacterial suspension was used in relevant tests. In addition, the bacterial solution and glycerin were transferred in a 3:1 ratio into a 1.5 mL cryo-storage tube, which was then stored at −80°C.
2.2 Preparation of SAEW
The preparation of SAEW followed the method of Hao et al. (2017). Sodium chloride solution of 0.1% and hydrochloric acid solution of 0.1% were added to the SAEW generator (KH SAEW-1500, Kanghui Water Treatment Equipment Co., Ltd., Shandong, China) to obtain SAEW. The pH and oxidation–reduction potential (ORP) were measured by a pH/ORP meter (Model ST2100, Ohaosi Instruments Changzhou Limited, China), and the available chlorine concentration (ACC) was determined using the iodometric method (Zeng et al., 2010). SAEW was replaced with deionized water in blank control test. Table 1 shows the pH, ORP, and ACC of the SAEW in this study.
2.3 Determination of the antibacterial efficacy of SAEW
The 2 mL of C. sakazakii suspension (108 CFU/mL) was treated with 4 mL of SAEW at different concentrations (0, 10, 15, 20, 25, and 30 mg/L) for 20 s, 40 s, and 60 s, respectively. According to Tao’s study, 1 mL of the mixture was transferred into a sterile tube containing 4 mL of neutralization buffer solution (0.85% NaCl solution and 0.5% sodium thiosulfate) to terminate the reaction (Tao et al., 2022). After 10 min, dilute the mixture to 105 CFU/mL with sterile normal saline. The 100 μL diluent was plated on TSA medium. The colonies were counted following a 37°C overnight incubation (DHP-9272, Yiheng Technology Co., Ltd., Shanghai).
2.4 Measurement of intracellular ATP concentrations
This experiment referred to the method of Sánchez et al. (2010), with minor modifications. The 2 mL of C. sakazakii suspension (108 CFU/mL) was treated with 4 mL of SAEW of different concentrations (0, 10, 20, and 30 mg/L) for 20 s, 40 s, or 60 s. Sterile normal saline was used as a control. The intracellular ATP concentrations were measured using an ATP assay kit (Biyuntian Biotechnology Co., Ltd., China). The supernatant was prepared by centrifuged at 12,000 × g for 5 min, and then stored the supernatant in ice box for later use.
2.5 Measurement of intracellular K+ concentrations
The K+ leakage was measured according to the study of Ding et al. (2016). The 2 mL of C. sakazakii suspension was treated with 4 mL of SAEW of different concentrations for 20 s, 40 s, or 60 s. Sterile normal saline was used as a control. The mixture was centrifuged at 8,000 × g for 10 min before being filtered through 0.22 μm filters (Pilot Experimental Equipment Co., Ltd., China). The filtrate was then used to measure the content of K+ by atomic absorption spectrophotometry (iCE3500, Saimer Fischer Technology Co., Ltd., China).
2.6 Measurement of intracellular protein concentrations
The protein leakage of the C. sakazakii cells was measured according to the Hao’s previous report (Hao et al., 2017). The 2 mL of cell suspension was treated with 4 mL of SAEW of different concentrations for 20 s, 40 s, or 60 s. The control was sterile normal saline. The samples were centrifuged at 8,000 × g for 10 min, and 1 mL of the supernatant was taken and 5 mL of the Coomassie brilliant blue solution was used to stain it (Solarbio Biotechnology Co., LTD., China). After staining for 5 min, the mixture was analyzed for protein leakage by ultraviolet–visible spectrophotometry (Qingdao Lubo Weiye Environmental Protection Technology Co., Ltd., China) at 595 nm.
2.7 Measurement of DNA fragmentation
The effect of DNA fragmentation caused by the SAEW on C. sakazakii was measured using agarose gel electrophoresis (AGE), according to previous report (Liu et al., 2011; Guo et al., 2019). The 2 mL of cell suspension was treated with 4 mL of SAEW of different concentrations for 20 s, 40 s, or 60 s. Sterile normal saline was used as a control. The genomic DNA was extracted using the bacterial genomic DNA extraction kit (Tiangen Biotech Co., Ltd., Beijing, China). The DNA samples were then added to the prepared agarose gelsand gel wells and stained with a fluorescent dye—ethidium bromide (EB). Finally, the electrophoresis was performed at 100 V for 30 min, from which the image was obtained from a gel imaging system (Tianchi Yicheng Biotechnology Co., LTD., Beijing, China).
2.8 Measurement of intracellular pH (pHin)
The changes in pHin were determined following a protocol described by Guo et al. (2020b). First, the C. sakazakii cell suspension was washed twice with a 50 mmol/L HEPES buffer solution before being mixed with a 3 μmol/L pHin fluorescent probe at 37°C for 20 min. The suspension was then washed continuously with a 50 mmol/L K3PO4 solution and resuspended again, to which a 10 μmol/L glucose solution was added, and the resulting suspension was cultured at 37°C for 30 min. Finally, the sample was washed twice with a neutral PBS buffer solution, and the bacteria were suspended again, then stored on ice for later use.
The C. sakazakii suspension treated as described above was taken and treated with 4 mL of SAEW of different concentrations for 20 s, 40 s, or 60 s. In addition, sterile normal saline was used as a control. The completed reaction samples were added to a 96-well opaque black plate, and the fluorescence intensities of the buffer solutions at various pHin values (3, 4, 5, 6, 7, 8, 9, 10) were measured using a microplate reader (Tecan Infinite 200 PRO, Putian Xinqiao Technology Co., Ltd., Beijing) at a wavelength of 520 nm for the emission and 490 nm for the excitation.
2.9 Transmission electron microscopy (TEM) analysis
The cellular morphology of C. sakazakii treated with different concentrations of SAEW (0, 15, and 30 mg/L) for 20 s, 40, or 60 s was observed by a transmission electron microscope (*/HT7800, Hitachi, Japan) following the previous report of Fei et al. (2019). The control was sterile normal saline. After treatments, the C. sakazakii cells were centrifuged at 8,000 × g for 10 min and then mixed with 2.5% glutaraldehyde at 4°C for 2 h. The cells were rinsed three times with 0.1 M phosphate buffer (pH = 7.2) for 15 min each before being fixed with 1% osmium tetraoxide for 120 min and rinsed three times more. The cells were treated with different concentrations of ethanol (50, 70, 90, 100%) and then treated with the mixture of 100% ethanol and 100% acetone. The samples were infiltrated with acetone and embedding solution, followed by embedding, sectioning and positive staining of the samples. Finally, observed under TEM.
2.10 Antibacterial activity of SAEW on stainless steel and rubber surfaces
The antibacterial activity of SAEW on stainless steel and rubber surfaces were analyzed following a previously reported protocol (Huang et al., 2006). The stainless steel and rubber had thicknesses of 0.05 mm and 0.5 mm (Harbin Hardware Market., China), respectively, and were cut into a sheet with dimensions of 25 (5 × 5) cm2. The squares were immersed in a C. sakazakii suspension (108 CFU/mL) for 5 min after sterilization and dried in a laminar flow cabinet for 20 min at room temperature (Jiasheng Together Manufacturing Co., LTD., China). Then the sterile cotton swabs were used to wipe the squares, and the swabs were thoroughly washed with 5 mL of sterile sodium chloride solution. Then the sterile cotton swabs were used to wiped the squares and washed the swabs adequately with 5 mL of sterile sodium chloride solution. The solution was properly diluted, plated on the TSA medium, and incubated overnight at 37°C. Finally, colony counting was performed to obtain the initial colony number on the surfaces.
The SAEW of different concentrations were sprayed on the surface of the above prepared squares with the bacterial solution, and treated for 20 s, 40 s and 60 s, respectively. The bacteria adhering to the stainless steel sheet squares and rubber sheet squares were removed with sterile cotton swabs, which were then thoroughly washed with the sterile sodium chloride solution. The diluted broth was plated on the TSA solid medium, and incubated overnight in an incubator at 37°C for colony counting.
2.11 Statistical analysis
Experiments were repeated in triplicate and data were analyzed using SPSS 19.0 software (SPSS, Chicago, IL). All data are expressed as the mean values ± Standard deviation (SD). The statistical differences at 5% significance level among means were determined by one-way analysis of variance (ANOVA).
3 Results and discussion
3.1 The antibacterial activity of SAEW against Cronobacter sakazakii
The antibacterial effect of SAEW with different concentrations against C. sakazakii was shown in Table 2. After the treatment of different concentrations of SAEW, the number of C. sakazakii significantly decreased. Furthermore, when the reaction time increased from 20 to 60 s, the total number of C. sakazakii significantly decreased (p < 0.05). The SAEW at a concentration of 30 mg/L inactivated about 108 CFU/mL of C. sakazakii strains within 60 s.
Previous research has found that SAEW can inhibit the growth of Rhizopus stolonifer colonies, and that the effect of inhibition increases with concentration (Li et al., 2021). The effect of acidic electrolyzed water can cause the decline of C. sakazakii colony on the surface of pear, melon and apple with values of 1.3, 1.7 and 1.8 log CFU/g, respectively, and there is no significant difference in the decline rate of each colony (Santo et al., 2016). In the present study, C. sakazakii significantly decreased with an increase in SAEW concentrations. In addition, due to its slightly acidic pH, SAEW does not corrode processing equipment or irritate producers’ hands as severely as strong acidic electrolyzed water (Abadias et al., 2008; Issa-Zacharia et al., 2010).
3.2 Changes in intracellular ATP content
The effects of different SAEW concentrations on the intracellular ATP content of C. sakazakii were determined, as shown in Figure 1. The SAEW concentration and time extension were found to be inversely related to the relative luminescence value, indicating that the intracellular ATP content of C. sakazakii showed a decreasing trend and that the content of intracellular ATP was influenced by both the SAEW concentration and action time. Similarly, the reduction of intracellular ATP also occurred during the SAEW against Pseudomonas fluoresces, E. coli, S. aureus, and Bacillus subtilis (Hao et al., 2017; Cai et al., 2019). In general, the intracellular ATP concentration of bacteria was stable but showed lower stability once the cell membrane was damaged (Kacprzyk et al., 2007). Therefore, it is presumed that the treatment of SAEW can damage the cell membrane of C. sakazakii, destroying the vitality of C. sakazakii.
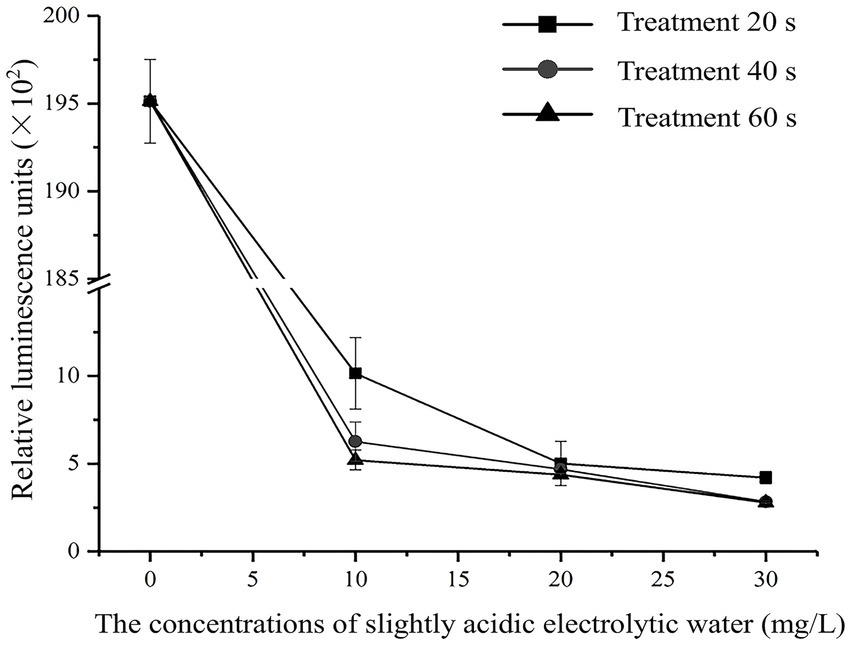
Figure 1. Effect of different concentration of SAEW in intracellular ATP content of C. sakazakii ATCC 29544.
3.3 Changes in the amount of K+ leakage
As shown in Figure 2, the effects of SAEW on K+ leakage at different concentrations and treatment times were analyzed. When treated with SAEW for 60 s, the K+ leakage of C. sakazakii increased with the concentration of SAEW, and the difference of leakage was significant (p < 0.05). Previous researches have explored the inhibitory effect of SAEW on B. subtilis and S. aureus, from which K+ leakage exhibited a positive correlation with the concentration and action time of SAEW (Tang et al., 2011; Ding et al., 2016). Zeng et al. (2010) reported the effect of SAEW (30.20 mg/L) on K+ leakage in S. aureus and E. coli, which results showed that K+ leakage in cells rapidly increased at the beginning of the SAEW treatment (approximately 1 min), but tended to slow down at the later stages. The large loss of K+ may be due to SAEW-induced denaturation of the K+ channel transmembrane protein (Hebert et al., 2005). The K+ leakage led to an imbalance of the ion channels and seriously damaged the physiological function of the bacteria, thus promoting bacterial death (Tao et al., 2022).
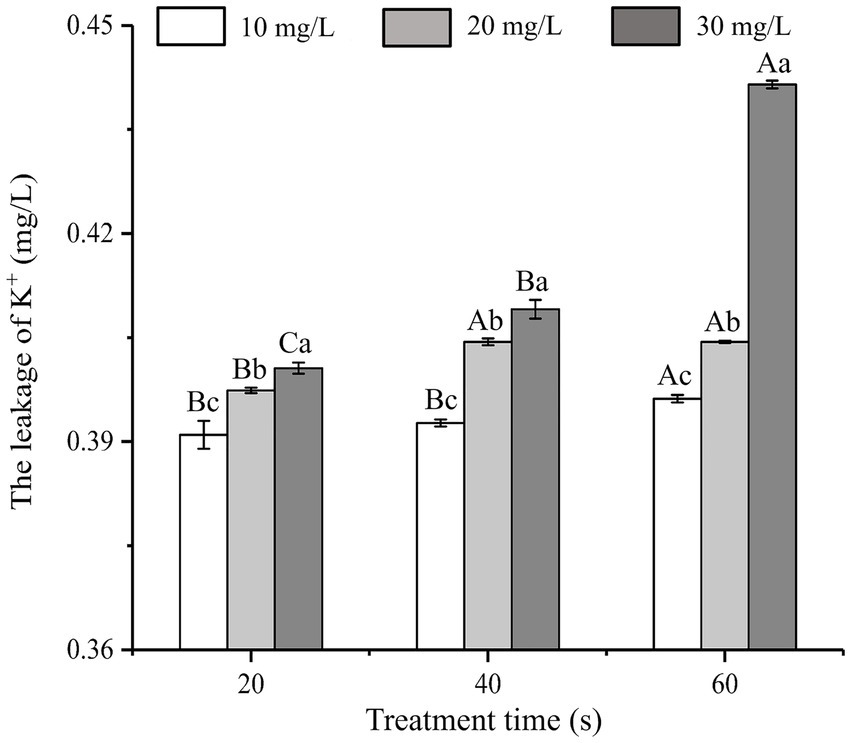
Figure 2. Effect of different concentration of SAEW in intracellular K+ content of C. sakazakii ATCC 29544.
3.4 Protein leakage
The influence of SAEW at different concentrations on protein leakage from C. sakazakii is shown in Figure 3. At the same treatment time, the protein leakage of C. sakazakii increased with the increase in SAEW concentration (p < 0.05). At identical concentration, the protein leakage showed a positive correlation with the action time of SAEW, indicating that the concentration of SAEW had a significant impact on the protein leakage of C. sakazakii. As the basic substance of bacterial life activities, excessive protein leakage can affect the normal metabolic function of C. sakazakii, leading to bacterial inactivation (Wang et al., 2015). Zeng also observed that SAEW caused protein leakage in S. aureus and E. coli (Zeng et al., 2010). Given that protein leakage is irreversible, higher protein leakage is expected at longer processing times. Therefore, SAEW can lead to the inactivation of C. sakazakii due to the leakage of bacterial protein.
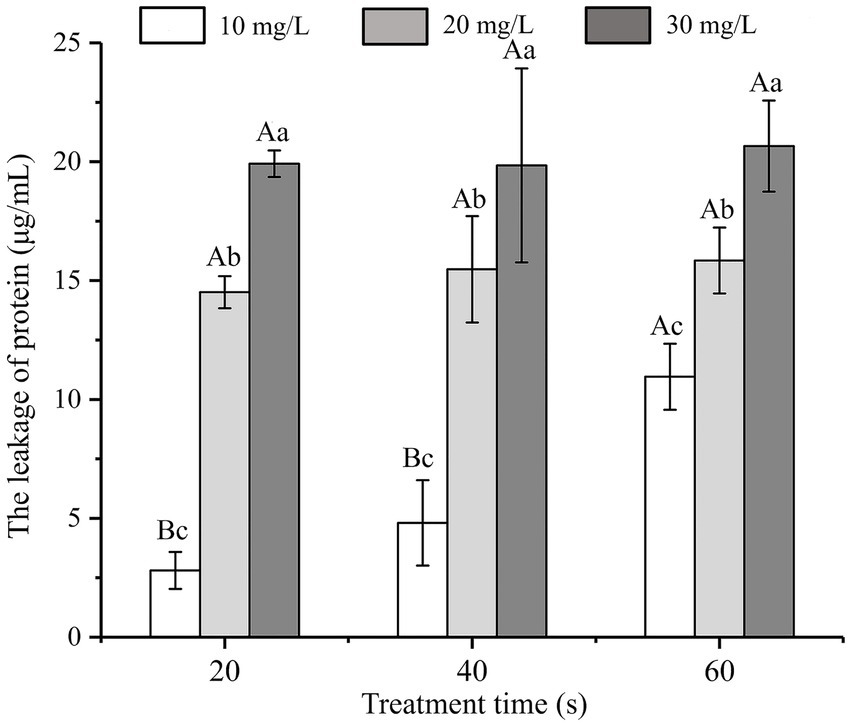
Figure 3. Effect of different concentration of SAEW in intracellular protein content of C. sakazakii ATCC 29544.
3.5 DNA cleavage analysis
The growth, development, and heredity of bacteria are inseparable from the control of DNA. DNA loss is very harmful to all cells (Cui et al., 2018). The effects of SAEW on the DNA of C. sakazakii are shown in Figure 4. The results showed a lighter and eventual disappearance of the strip color as the SAEW concentration increased. When the concentrations were the same, the color of the strips gradually became lighter as the action time increased, but the difference was not noticeable. The streaks of bacterial DNA were vague and imprecise at the SAEW concentration of 30 mg/L. The DNA bands of C. sakazakii disappeared after treatment with 30 mg/L SAEW for 60 s. The results demonstrated that the brightness of SAEW-treated bacterial DNA bands was lower than the control group, suggesting that DNA leakage occurred after the SAEW treatment in C. sakazakii. Other researchers used SAEW in S. aureus, E. coli, B. subtilis, and Listeria monocytogenes, from which SAEW reduced the intracellular DNA of these pathogens (Zeng et al., 2010; Hao et al., 2017). The decrease of DNA in bacterial cells, it is supposed that a result of SAEW damage toward bacterial cell membranes, resulting in macro-molecular nucleotide leakage, which combined with DNA in the groove mode and inhibited normal bacterial functions, ultimately leading to bacterial death (Wu et al., 2017).
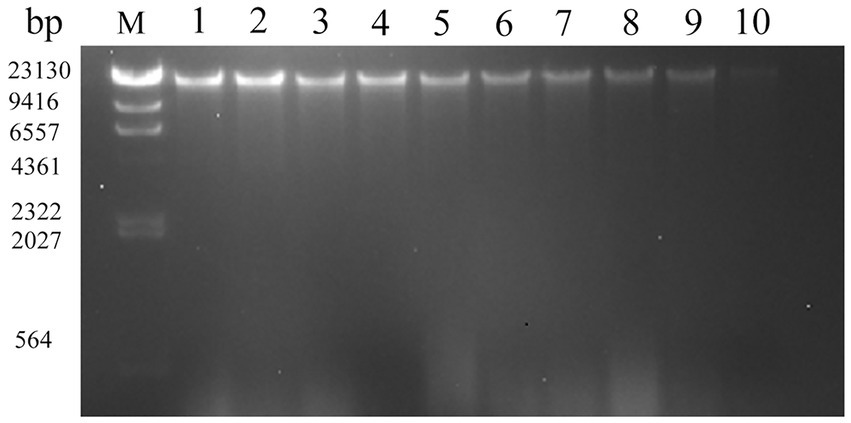
Figure 4. Effect of different concentration of SAEW in intracellular DNA content of C. sakazakii ATCC 29544. Lane M: marker; Lane 1: blank control; Lanes 2, 3, 4: the concentration of SAEW is 10 mg/L; Lanes 5, 6, 7: the concentration of SAEW is 20 mg/L; Lanes 8, 9, 10: the concentration of SAEW is 30 mg/L; Lanes 2, 5, 8: the action time of SAEW is 20 s; Lanes 3, 6, 9: the action time of SAEW is 40 s; Lanes 4, 7, 10: the action time of SAEW is 60 s.
3.6 Changes in intracellular pH (pHin)
SAEW can change the pHin gradient, which can increase the sensitivity of the cell membrane, thus promoting cell membrane destruction and cell inactivation (Hricova et al., 2008). The influence of SAEW on the pHin of C. sakazakii is shown in Figure 5. The pHin of bacteria decreased at longer action times at the same concentration (p < 0.05). The initial pHin of C. sakazakii control group was approximately 5.97 ± 0.19. According to Figure 5, the pHin values of C. sakazakii treated with SAEW were all lower than the normal range. The previous study also found that acidic electrolytic water could significantly lower the pHin of Pseudomonas fluorescens (Cai et al., 2019), which is consistent with this study. It is assumed that SAEW provided a slightly acidic environment around the bacteria to lower the pHin of C. sakazakii, thereby altering the permeability of the cell membrane and resulting in inactivation.
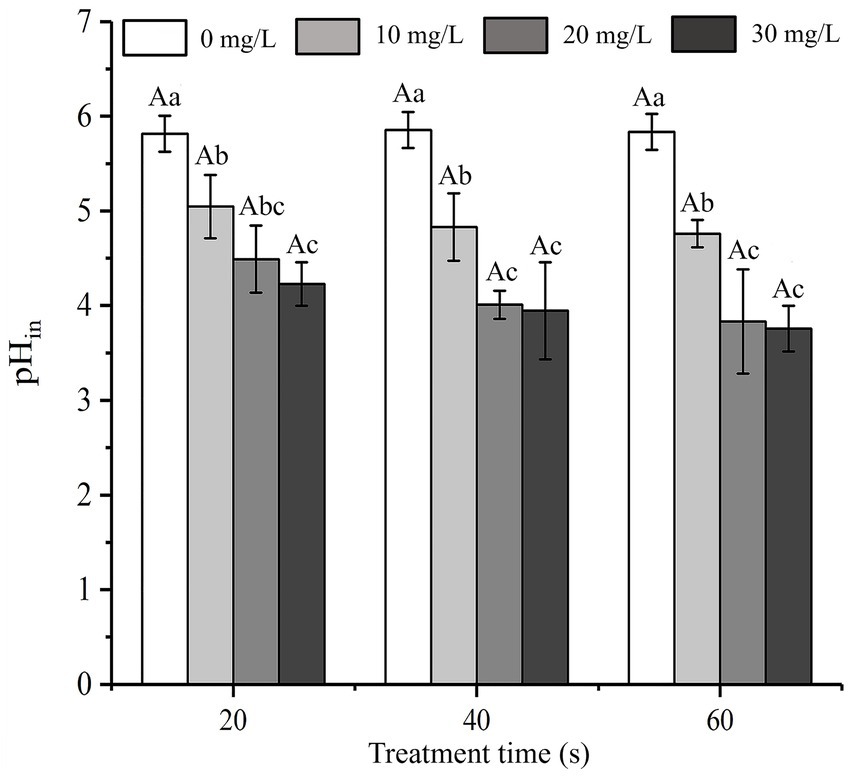
Figure 5. Effect of different concentration of SAEW on the intracellular pH of C. sakazakii ATCC 29544.
3.7 Changes in cell morphology
Transmission electron microscopy (TEM) was used to visually observe any changes in cell morphology and cytoplasm (Guo et al., 2020a). Figure 6 presents the TEM diagram of C. sakazakii treated with SAEW at different concentrations. The TEM image of C. sakazakii after treatment with 15 mg/L SAEW for 60 s was shown in Figure 6B, which exhibited cell wall and cell membrane dissolution. As a result, the cell contents showed an uneven distribution and even leaked and migrated to the edge. Figure 6C presents the TEM image of C. sakazakii after the 30 mg/L SAEW treatment for 60 s, wherein most of C. sakazakii showed internal voids and serious plasmolysis. Overall, a large amount of cell contents leaked, and the cells showed serious damage. After being treated with SAEW, C. sakazakii exhibited changes in its cell morphology, membrane damage, and uneven cell content distribution and leakage. Moreover, the study have reported that the antibacterial effect of SAEW can also destroy the integrity of cell membranes of Salmonella typhi, S. aureus, and Bacillus cereus, thereby resulting in cell content leakage and thus affecting cell function (Kim et al., 2019).
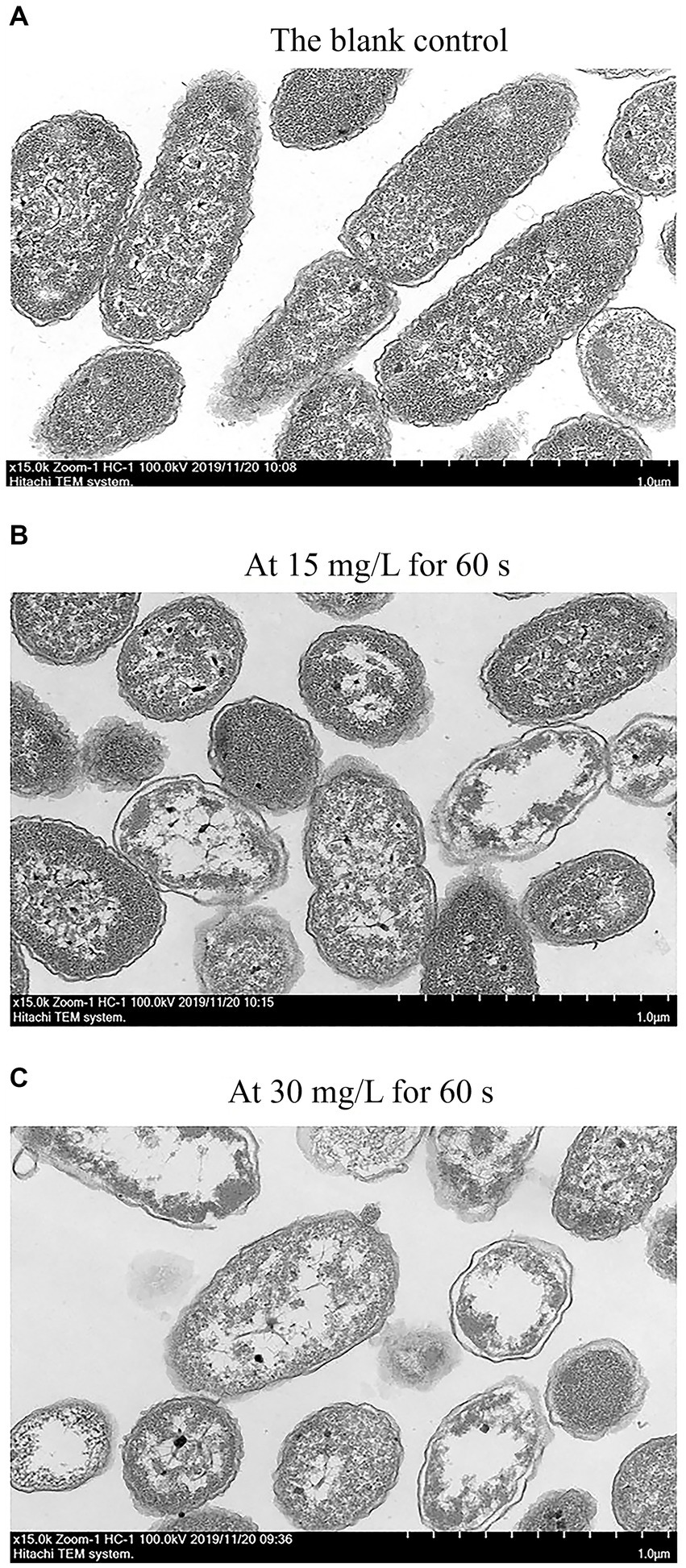
Figure 6. The TEM of C. sakazakii ATCC 29544 after treated with different concentration of SAEW. (A) The blank control. (B) At 15 mg/L for 60 s. (C) At 30 mg/L for 60 s.
3.8 Application of SAEW on the surfaces of stainless steel and rubber
The antibacterial effects of SAEW with different action times and concentrations against C. sakazakii inoculated on stainless steel and rubber surfaces are shown in Figures 7A,B, respectively. The initial inoculum amounts of C. sakazakii on the stainless steel and rubber surfaces were approximately 4.60 ± 0.05 log CFU/cm2 and 4.58 ± 0.06 log CFU/cm2, respectively. The number of C. sakazakii colonies inoculated on stainless steel and rubber decreased as SAEW concentration and action time increased. At a concentration of 30 mg/L and an action time of 60 s, almost all of the C. sakazakii colonies on the stainless steel and rubber surfaces were inactivated. The inactivation rates were 99.06 and 99.03%, respectively.
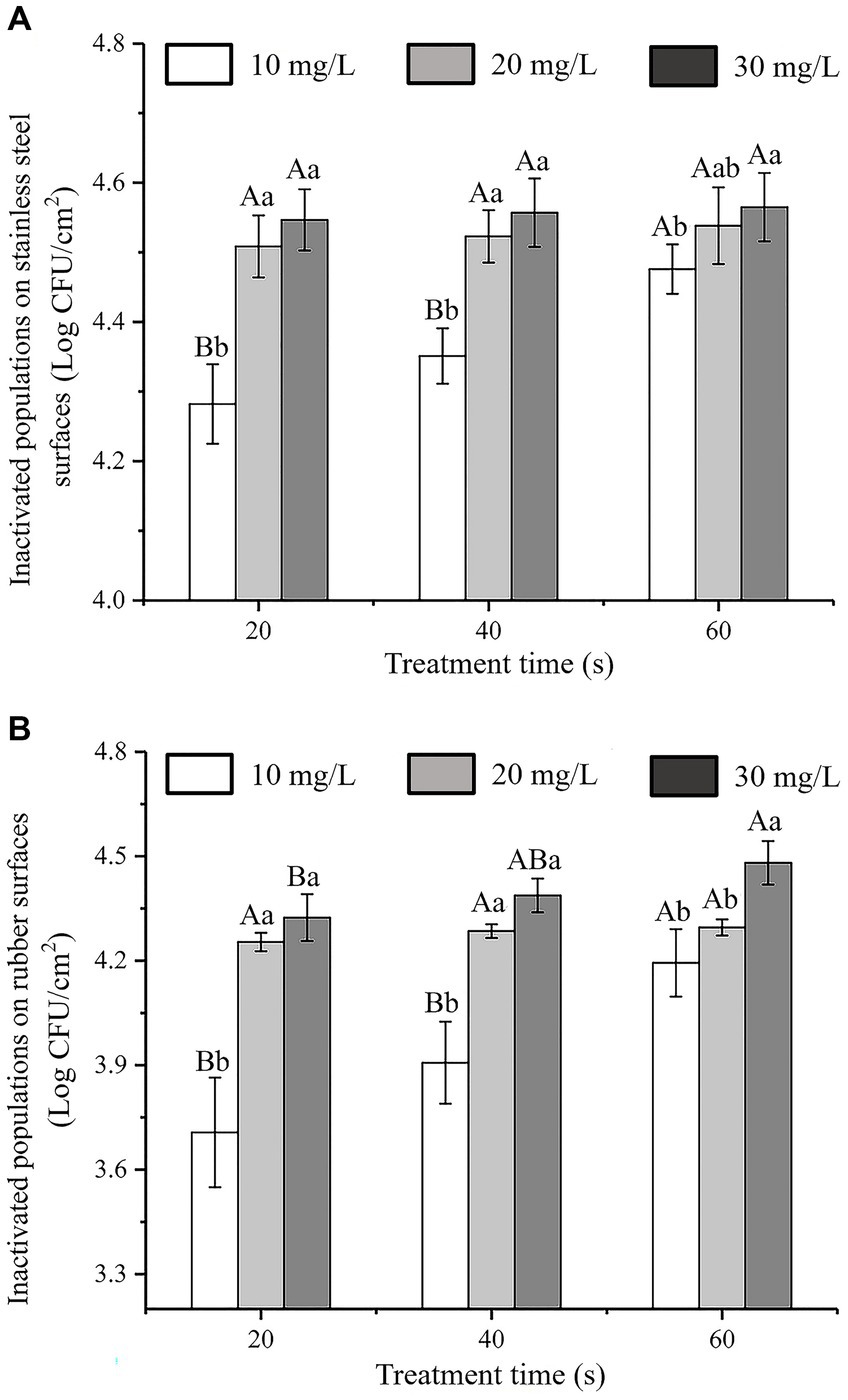
Figure 7. Antibacterial activity of SAEW against C. sakazakii ATCC 29544 inoculatedon to different surfaces. (A) Stainless steel surface. (B) Rubber surface.
According to Chauhan’s study on the inhibition of C. sakazakii by organic acids, it was found that SAEW had better inhibition of C. sakazakii by comparison, and compared with Chang’s study, SAEW had better inhibition of bacteria on C. sakazakii than Houttuynia cordata Thunb. crude extract (Chauhan et al., 2022; Chang et al., 2023). In addition, the experimental results show that SAEW with low ACC and near neutral pH can sterilize E. coli and Staphylococcus aureus in a short period of time, and will become a potential alternative to NaOCl solutions commonly used in the food industry (Issa-Zacharia et al., 2010).
Previous research on the antibacterial activity of SAEW to against other pathogenic bacteria on food contact surfaces have reported that spraying with SAEW for 0.5–2.0 min resulted in a reduction of E. coli, S. typhimurium, and S. aureus on stainless steel by 3.44–6.01, 4.51–5.64, and 4.68–5.38 log CFU/cm2, respectively (Ni et al., 2016). Similarly, L. monocytogenes inactivation on natural rubber latex gloves was reported after a 5 min treatment with acid electrolytic water (Huang et al., 2006). The results of our study were consistent with those of earlier investigations, which demonstrated that SAEW had an effective inhibitory effect on C. sakazakii on the surfaces of stainless steel and rubber gloves.
In general, chemical disinfectants are used in the food industry to inhibit C. sakazakii on equipment and material surfaces (Chang et al., 2021). However, the prolonged and excessive use of chemical disinfectants can result in bacterial drug dependence or resistance, thus lowering their effectiveness, such that their potential impact cannot be ignored (Mc Carlie et al., 2020; Notcovich et al., 2020). Lindsay et al. (2019) showed that rubber gloves and stainless-steel facilities used by the staff were the main sources of Cronobacter species contamination in the dairy plants. Therefore, it is necessary to control the growth of C. sakazakii on stainless-steel facilities and rubber gloves.
4 Conclusion
This study demonstrated that SAEW has effective antibacterial activity against C. sakazakii. At an SAEW concentration of 30 mg/L, C. sakazakii was completely inactivated after 60 s of treatment. Higher antibacterial efficacy was also observed at increased times and concentrations. The mechanism of SAEW against C. sakazakii was illustrated by damage to the cell membrane as well as changes in cell membrane permeability and cell morphology, causing the cell contents leakage, including its intracellular ATP, K+, protein, and DNA. As a result, the ion balance inside and outside the cell was disrupted, thus decreasing the pHin content, ultimately inactivating C. sakazakii. After treatment with 30 mg/L SAEW for 20 s, approximately 103 CFU/cm2 of C. sakazakii on stainless steel and rubber surfaces were inactivated. Overall, this study suggests that SAEW is a potential convenient disinfectant for use in the dairy industry to inhibit C. sakazakii on stainless steel and rubber surfaces.
Data availability statement
The original contributions presented in the study are included in the article/supplementary material, further inquiries can be directed to the corresponding authors.
Author contributions
LG: Conceptualization, Data curation, Investigation, Methodology, Writing – original draft. JH: Data curation, Investigation, Methodology, Software, Writing – original draft. YW: Data curation, Investigation, Writing – review & editing. YC: Methodology, Software, Writing – review & editing. WQ: Formal analysis, Writing – review & editing. CM: Software, Supervision, Writing – review & editing. PF: Methodology, Supervision, Writing – review & editing. YJ: Investigation, Supervision, Writing – review & editing.
Funding
The author(s) declare financial support was received for the research, authorship, and/or publication of this article. This research was supported by the Doctoral scientific research launch fund project of Nanyang Institute of Technology (NGBJ-2023-08), Interdisciplinary Sciences Project, Nanyang Institute of Technology (520079), and Natural Science Foundation of Guizhou Province (Qiankehe foundation-ZK [2022] General 008).
Conflict of interest
The authors declare that the research was conducted in the absence of any commercial or financial relationships that could be construed as a potential conflict of interest.
Publisher’s note
All claims expressed in this article are solely those of the authors and do not necessarily represent those of their affiliated organizations, or those of the publisher, the editors and the reviewers. Any product that may be evaluated in this article, or claim that may be made by its manufacturer, is not guaranteed or endorsed by the publisher.
References
Abadias, M., Usall, J., Oliveira, M., Alegre, I., and Viñas, I. (2008). Efficacy of neutral electrolyzed water (NEW) for reducing microbial contamination on minimally-processed vegetables. Int. J. Food Microbiol. 123, 151–158. doi: 10.1016/j.ijfoodmicro.2007.12.008
Cai, L.-L., Hu, H.-J., Lu, Q., Wang, H.-H., Xu, X.-L., Zhou, G.-H., et al. (2019). Morphophysiological responses of detached and adhered biofilms of Pseudomonas fluorescens to acidic electrolyzed water. Food Microbiol. 82, 89–98. doi: 10.1016/j.fm.2019.01.007
Chang, Y., Xia, S., Fei, P., Feng, H., Fan, F., Liu, Y., et al. (2023). Houttuynia cordata Thunb. crude extract inactivates Cronobacter sakazakii: antibacterial components, antibacterial mechanism, and application as a natural disinfectant. Food Control 145:109467. doi: 10.1016/j.foodcont.2022.109467
Chang, Y., Xing, M., Hu, X., Feng, H., Wang, Y., Guo, B., et al. (2021). Antibacterial activity of chrysanthemum buds crude extract against Cronobacter sakazakii and its application as a natural disinfectant. Food Microbiol. 11:632177. doi: 10.3389/fmicb.2020.632177
Chauhan, R., Kumari, S., Goel, G., and Azmi, W. (2022). Synergistic combination of malic acid with sodium hypochlorite impairs biofilm of Cronobacter sakazakii. Lwt 155:112902. doi: 10.1016/j.lwt.2021.112902
Cui, H., Zhang, C., Li, C., and Lin, L. (2018). Antimicrobial mechanism of clove oil on Listeria monocytogenes. Food Control 94, 140–146. doi: 10.1016/j.foodcont.2018.07.007
Ding, T., Xuan, X.-T., Li, J., Chen, S.-G., Liu, D.-H., Ye, X.-Q., et al. (2016). Disinfection efficacy and mechanism of slightly acidic electrolyzed water on Staphylococcus aureus in pure culture. Food Control 60, 505–510. doi: 10.1016/j.foodcont.2015.08.037
Fei, P., Xu, Y., Zhao, S., Gong, S., and Guo, L. (2019). Olive oil polyphenol extract inhibits vegetative cells of Bacillus cereus isolated from raw milk. J. Dairy Sci. 102, 3894–3902. doi: 10.3168/jds.2018-15184
Guo, L., Gong, S., Wang, Y., Sun, Q., Duo, K., and Fei, P. (2020a). Antibacterial activity of olive oil polyphenol extract against Salmonella typhimurium and Staphylococcus aureus: possible mechanisms. Foodborne Pathog. Dis. 17, 396–403. doi: 10.1089/fpd.2019.2713
Guo, L., Sun, Q., Gong, S., Bi, X., Jiang, W., Xue, W., et al. (2019). Antimicrobial activity and action approach of the olive oil polyphenol extract against Listeria monocytogenes. Front. Microbiol. 10:1586. doi: 10.3389/fmicb.2019.01586
Guo, L., Wang, Y., Bi, X., Duo, K., Sun, Q., Yun, X., et al. (2020b). Antimicrobial activity and mechanism of action of the Amaranthus tricolor crude extract against Staphylococcus aureus and potential application in cooked meat. Foods 9:359. doi: 10.3390/foods9030359
Hao, J., Wu, T., Li, H., and Liu, H. (2017). Differences of bactericidal efficacy on Escherichia coli, Staphylococcus aureus, and Bacillus subtilis of slightly and strongly acidic electrolyzed water. Food Bioprocess Technol. 10, 155–164. doi: 10.1007/s11947-016-1801-3
Hebert, S. C., Desir, G., Giebisch, G., and Wang, W. (2005). Molecular diversity and regulation of renal potassium channels. Physiol. Rev. 85, 319–371. doi: 10.1152/physrev.00051.2003
Hricova, D., Stephan, R., and Zweifel, C. (2008). Electrolyzed water and its application in the food industry. J Food Protect. 71, 1934–1947. doi: 10.4315/0362-028x-71.9.1934
Huang, Y.-R., Hsieh, H.-S., Lin, S.-Y., Lin, S.-J., Hung, Y.-C., and Hwang, D.-F. (2006). Application of electrolyzed oxidizing water on the reduction of bacterial contamination for seafood. Food Control 17, 987–993. doi: 10.1016/j.foodcont.2005.07.003
Issa-Zacharia, A., Kamitani, Y., Morita, K., and Iwasaki, K. (2010). Sanitization potency of slightly acidic electrolyzed water against pure cultures of Escherichia coli and Staphylococcus aureus, in comparison with that of other food sanitizers. Food Control 21, 740–745. doi: 10.1016/j.foodcont.2009.11.002
Jeon, H. R., Kwon, M. J., and Yoon, K. S. (2018). Control of Listeria innocua biofilms on food contact surfaces with slightly acidic electrolyzed water and the risk of biofilm cells transfer to duck meat. J Food Protect. 81, 582–592. doi: 10.4315/0362-028x.Jfp-17-373
Kacprzyk, L., Rydengard, V., Morgelin, M., Davoudi, M., Pasupuleti, M., Malmsten, M., et al. (2007). Antimicrobial activity of histidine-rich peptides is dependent on acidic conditions. BBA Biomembranes 1768, 2667–2680. doi: 10.1016/j.bbamem.2007.06.020
Kim, H.-J., Tango, C. N., Chelliah, R., and Oh, D.-H. (2019). Sanitization efficacy of slightly acidic electrolyzed water against pure cultures of Escherichia coli, Salmonella enterica, typhimurium, Staphylococcus aureus and Bacillus cereus spores, in comparison with different water hardness. Sci. Rep. 9, 4348–4314. doi: 10.1038/s41598-019-40846-6
Li, L., Mu, T. H., and Zhang, M. (2021). Contribution of ultrasound and slightly acid electrolytic water combination on inactivating Rhizopus stolonifer in sweet potato. Ultrason. Sonochem. 73:105528. doi: 10.1016/j.ultsonch.2021.105528
Li, Z., Wang, X., Li, X., Guo, S., Li, S., Chen, B., et al. (2023). Optimization of electrolysis process, storage conditions and sterilization effect of slightly acidic electrolytic water prepared by titanium suboxide electrode. J. Environ. Chem. Eng. 11:109679. doi: 10.1016/j.jece.2023.109679
Lindsay, D., Laing, S., Fouhy, K. I., Souhoka, L., Beaven, A. K., Soboleva, T. K., et al. (2019). Quantifying the uncertainty of transfer of Cronobacter spp. between fomites and floors and touch points in dairy processing plants. Food Microbiol. 84:103256. doi: 10.1016/j.fm.2019.103256
Liu, X.-L., Zhang, X.-J., Fu, Y.-J., Zu, Y.-G., Wu, N., Liang, L., et al. (2011). Cajanol inhibits the growth of Escherichia coli and Staphylococcus aureus by acting on membrane and DNA damage. Planta Med. 77, 158–163. doi: 10.1055/s-0030-1250146
Lou, X., Yu, H., Wang, X., Qi, J., Zhang, W., Wang, H., et al. (2019). Potential reservoirs and routes of Cronobacter transmission during cereal growing, processing and consumption. Food Microbiol. 79, 90–95. doi: 10.1016/j.fm.2018.12.004
Mc Carlie, S., Boucher, C. E., and Bragg, R. R. (2020). Molecular basis of bacterial disinfectant resistance. Drug Resist. Updat. 48:100672. doi: 10.1016/j.drup.2019.100672
Naka, A., Yakubo, M., Nakamura, K., and Kurahashi, M. (2020). Effectiveness of slightly acidic electrolyzed water on bacteria reduction: in vitro and spray evaluation. PeerJ 8:e8593. doi: 10.7717/peerj.8593
Ni, L., Zheng, W., Zhang, Q., Cao, W., and Li, B. (2016). Application of slightly acidic electrolyzed water for decontamination of stainless steel surfaces in animal transport vehicles. Prev. Vet. Med. 133, 42–51. doi: 10.1016/j.prevetmed.2016.09.010
Notcovich, S., Williamson, N., Flint, S., Yapura, J., Schukken, Y., and Heuer, C. (2020). Effect of bismuth subnitrate on in vitro growth of major mastitis pathogens. J. Dairy Sci. 103, 7249–7259. doi: 10.3168/jds.2019-17830
Pacheappan, G. D., Samsudin, N. I. P., and Hasan, H. (2022). The effects of different disinfectants and application conditions on microbial contaminants at dairy processing line. J. Food Process. Preserv. 46:e16172. doi: 10.1111/jfpp.16172
Phair, K., Pereira, S. G., Kealey, C., Fanning, S., and Brady, D. B. (2022). Insights into the mechanisms of Cronobacter sakazakii virulence. Microb. Pathog. 169:105643. doi: 10.1016/j.micpath.2022.105643
Roy, P. K., Mizan, M. F. R., Hossain, M. I., Han, N., Nahar, S., Ashrafudoulla, M., et al. (2021). Elimination of Vibrio parahaemolyticus biofilms on crab and shrimp surfaces using ultraviolet C irradiation coupled with sodium hypochlorite and slightly acidic electrolyzed water. Food Control 128:108179. doi: 10.1016/j.foodcont.2021.108179
Sánchez, E., García, S., and Heredia, N. (2010). Extracts of edible and medicinal plants damage membranes of Vibrio cholerae. Appl. Environ. Microbiol. 76, 6888–6894. doi: 10.1128/AEM.03052-09
Santo, D., Graça, A., Nunes, C., and Quintas, C. (2016). Survival and growth of Cronobacter sakazakii on fresh-cut fruit and the effect of UV-C illumination and electrolyzed water in the reduction of its population. Int. J. Food Microbiol. 231, 10–15. doi: 10.1016/j.ijfoodmicro.2016.04.023
Sethi, S., Nayak, S. L., Joshi, A., and Sharma, R. R. (2020). “Sanitizers for fresh-cut fruits and vegetables” in Fresh-cut fruits and vegetables. ed. M. W. Siddiqui (Cambridge, MA: Academic Press), 99–119.
Tang, W., Zeng, X., Zhao, Y., Ye, G., Gui, W., and Ni, Y. (2011). Disinfection effect and its mechanism of electrolyzed oxidizing water on spores of Bacillus subtilis var. niger. Food Sci. Biotechnol. 20, 889–895. doi: 10.1007/s10068-011-0123-5
Tao, H., Liao, Q., Xu, Y., and Wang, H.-L. (2022). Efficacy of slightly acidic electrolyzed water for inactivation of Cronobacter sakazakii and biofilm cells. J. Food Protect. 85, 511–517. doi: 10.4315/JFP-21-263
Vojkovska, H., Karpiskova, R., Orieskova, M., and Drahovska, H. (2016). Characterization of Cronobacter spp. isolated from food of plant origin and environmental samples collected from farms and from supermarkets in the Czech Republic. Int. J. Food Microbiol. 217, 130–136. doi: 10.1016/j.ijfoodmicro.2015.10.017
Wang, C., Chang, T., Yang, H., and Cui, M. (2015). Antibacterial mechanism of lactic acid on physiological and morphological properties of Salmonella enteritidis, Escherichia coli and Listeria monocytogenes. Food Control 47, 231–236. doi: 10.1016/j.foodcont.2014.06.034
Wu, Y., Bai, J., Zhong, K., Huang, Y., and Gao, H. (2017). A dual antibacterial mechanism involved in membrane disruption and DNA binding of 2R, 3R-dihydromyricetin from pine needles of Cedrus deodara against Staphylococcus aureus. Food Chem. 218, 463–470. doi: 10.1016/j.foodchem.2016.07.090
Yan, Q., and Fanning, S. (2015). Strategies for the identification and tracking of Cronobacter species: an opportunistic pathogen of concern to neonatal health. Front. Pediatr. 3:38. doi: 10.3389/fped.2015.00038
Ye, Z., Wang, S., Chen, T., Gao, W., Zhu, S., He, J., et al. (2017). Inactivation mechanism of Escherichia coli induced by slightly acidic electrolyzed water. Sci. Rep. 7:6279. doi: 10.1038/s41598-017-06716-9
Zang, Y. T., Bing, S., Li, Y. J., and Shu, D. Q. (2019). Application of slightly acidic electrolyzed water and ultraviolet light for Salmonella enteritidis decontamination of cell suspensions and surfaces of artificially inoculated plastic poultry transport coops and other facility surfaces. Poult. Sci. 98, 6445–6451. doi: 10.3382/ps/pez520
Keywords: slightly acidic electrolytic water, Cronobacter sakazakii, antibacterial mechanism, application, powdered infant formula
Citation: Guo L, Han J, Wang Y, Chang Y, Qu W, Man C, Fei P and Jiang Y (2024) Antibacterial action of slightly acidic electrolytic water against Cronobacter sakazakii and its application as a disinfectant on high-risk contact surfaces. Front. Microbiol. 15:1314362. doi: 10.3389/fmicb.2024.1314362
Edited by:
Beatrix Stessl, University of Veterinary Medicine Vienna, AustriaReviewed by:
Pantu Kumar Roy, Gyeongsang National University, Republic of KoreaBabak Pakbin, Technical University of Munich, Germany
Copyright © 2024 Guo, Han, Wang, Chang, Qu, Man, Fei and Jiang. This is an open-access article distributed under the terms of the Creative Commons Attribution License (CC BY). The use, distribution or reproduction in other forums is permitted, provided the original author(s) and the copyright owner(s) are credited and that the original publication in this journal is cited, in accordance with accepted academic practice. No use, distribution or reproduction is permitted which does not comply with these terms.
*Correspondence: Ling Guo, Z3VvbGluZ0BuZWF1LmVkdS5jbg==; Peng Fei, ZmVpcGVuZzAyMjBAMTI2LmNvbQ==; Yujun Jiang, eXVqdW5famlhbmdAMTYzLmNvbQ==