- 1Department of Genetics, Ecology, and Evolution, Federal University of Minas Gerais, Belo Horizonte, Brazil
- 2Department of Clinical Analysis and Toxicology, Federal University of Minas Gerais Belo Horizonte, Minas Gerais, Brazil
- 3Department of Microbiology, Federal University of Minas Gerais, Belo Horizonte, Minas Gerais, Brazil
- 4Department of General Pathology, Federal University of Minas Gerais, Belo Horizonte, Minas Gerais, Brazil
- 5Department of Dermatology, School of Medicine and Public Health, University of Wisconsin-Madison, Madison, WI, United States
- 6Department of Biochemistry and Immunology, Federal University of Minas Gerais, Belo Horizonte, Minas Gerais, Brazil
- 7School of Biosciences and Veterinary Medicine, University of Camerino, Matelica, Italy
- 8STLO, INRAE, Institut Agro, Rennes, France
Introduction and objective: p62 is a human multifunctional adaptor protein involved in key cellular processes such as tissue homeostasis, inflammation, and cancer. It acts as a negative regulator of inflammasome complexes. It may thus be considered a good candidate for therapeutic use in inflammatory bowel diseases (IBD), such as colitis. Probiotics, including recombinant probiotic strains producing or delivering therapeutic biomolecules to the host mucosal surfaces, could help prevent and mitigate chronic intestinal inflammation. The objective of the present study was to combine the intrinsic immunomodulatory properties of the probiotic Lactococcus lactis NCDO2118 with its ability to deliver health-promoting molecules to enhance its protective and preventive effects in the context of ulcerative colitis (UC).
Material and methods: This study was realized in vivo in which mice were supplemented with the recombinant strain. The intestinal barrier function was analyzed by monitoring permeability, secretory IgA total levels, mucin expression, and tight junction genes. Its integrity was evaluated by histological analyses. Regarding inflammation, colonic cytokine levels, myeloperoxidase (MPO), and expression of key genes were monitored. The intestinal microbiota composition was investigated using 16S rRNA Gene Sequencing.
Results and discussion: No protective effect of L. lactis NCDO2118 pExu:p62 was observed regarding mice clinical parameters compared to the L. lactis NCDO2118 pExu: empty. However, the recombinant strain, expressing p62, increased the goblet cell counts, upregulated Muc2 gene expression in the colon, and downregulated pro-inflammatory cytokines Tnf and Ifng when compared to L. lactis NCDO2118 pExu: empty and inflamed groups. This recombinant strain also decreased colonic MPO activity. No difference in the intestinal microbiota was observed between all treatments. Altogether, our results show that recombinant L. lactis NCDO2118 delivering p62 protein protected the intestinal mucosa and mitigated inflammatory damages caused by dextran sodium sulfate (DSS). We thus suggest that p62 may constitute part of a therapeutic approach targeting inflammation.
1 Introduction
Inflammatory bowel diseases (IBD) constitute chronic or recurrent inflammatory ailments of the gastrointestinal tract (GIT). They constitute a growing public health challenge worldwide (Ng et al., 2017) and are divided into two types: Ulcerative Colitis (UC) and Crohn’s Disease (CD; Abraham and Cho, 2009; Glassner et al., 2020). Genetics and environmental factors such as antibiotic usage and the type of diet may alter immune responses and intestinal microbiota composition and participate in IBD etiology (Glassner et al., 2020).
UC treatments include topical or systemic 5-aminosalicylic acids (5-ASA), Azathioprine and 6-mercaptopurine immunomodulators, corticoids, and cyclosporine (Derikx et al., 2016). However, these current therapies have limited efficacy for many patients, leading to surgical intervention (Frei et al., 2012; Derikx et al., 2016). In this context, due to their anti-inflammatory and immunomodulatory properties, probiotic microorganisms have been considered a promising therapeutic tool for reducing IBD progression (Santos Rocha et al., 2014; Wang et al., 2018). Strong evidence of efficacy was proven for a probiotic product, VSL#3, in a meta-analysis of clinical trials (Sniffen et al., 2018). Wang et al. (2018) demonstrated that the administration of this probiotic cocktail of eight strains (e.g., Lactobacillus acidophilus, Lactobacillus delbrueckii subsp. bulgaricus, Lacticaseibacillus casei, Lactiplantibacillus plantarum, Streptococcus thermophilus, Bifidobacterium breve, Bifidobacterium longum subsp. infantis and Bifidobacterium longum subsp. longum) was able to reduce the tumor in azoxymethane/dextran sulfate sodium (AOM/DSS) induced mice ulcerative colitis-associated carcinogenesis model and to decrease the TNFα and IL6 levels in the colon tissue.
Probiotics are live microorganisms that benefit the host when ingested adequately (Hill et al., 2014). The beneficial effects of these microorganisms are attributed to their ability to regulate the intestinal microbiota, increase short-chain fatty acids (SCFA) production (e.g., acetate, propionate, and butyrate), inhibit the proliferation of pathogenic bacteria, modulate the systemic and local immune responses, and to reinforce epithelial barrier via stimulation of tight junctions proteins expression and of mucus-producing goblet cells (Jonkers et al., 2012; Bellavia et al., 2013; Derikx et al., 2016; Jakubczyk et al., 2020).
Many bacterial strains have been used as probiotics to mitigate colitis. This includes Lacticaseibacillus rhamnosus GG, Escherichia coli Nissle 1917 (Kruis et al., 2004; Zocco et al., 2006; Derikx et al., 2016), Lactobacillus delbrueckii (Santos Rocha et al., 2014), and Lactococcus lactis (Luerce et al., 2014; Wong et al., 2017). According to Plaza-Díaz et al. (2017), Lacticaseibacillus casei strain Shirota could modulate the immune system, increasing secretion of interleukin 4 (IL-4) production while reducing that of interferon-gamma (IFNγ), in cultured human dendritic cells. It further restored the normal stimulatory capacity of cells by reducing the expression of Toll-like receptors 2 (TLR2) and 4 (TLR4). The ability to induce immunomodulatory cytokines in vitro was indeed shown to correlate with that to mitigate colitis in vivo (Foligne et al., 2007).
In addition to wild-type probiotics, recombinant probiotic bacteria, aiming to express and deliver bioactive molecules with anti-inflammatory properties, is also being explored as a promising therapeutic approach in intestinal inflammatory conditions. These bioactive molecules include the surface layer protein B (SlpB) of Propionibacterium freudenreichii CIRM-BIA 129 (Belo et al., 2021), the 65 kDa heat shock protein (Hsp65) of Mycobacterium leprae (Gomes-Santos et al., 2017; Barroso et al., 2021), the antimicrobial pancreatitis-associated protein I (PAP; Carvalho et al., 2017) and the human cathelicidin (hCAP18; Noguès et al., 2022), among others.
One of the bioactive molecules that reportedly have immunomodulatory properties is the p62 protein, which is also named sequestosome 1 (SQSTM1), a multifactor molecule that is involved in many processes, including proliferation, apoptosis, inflammation, autophagy, and immune response (Pankiv et al., 2007; Komatsu et al., 2012; Vincent et al., 2020). This protein participates in physiological pathways related to different human diseases, such as neurodegenerative (Geetha et al., 2012), metabolic (insulin resistance and obesity; Long et al., 2017), osteoporosis (Krela-Kaźmierczak et al., 2016), IBD (Moscat and Diaz-Meco, 2012; Sabbieti et al., 2015; Vincent et al., 2020) and cancer (Venanzi et al., 2013, 2019). Ortiz-Masiá et al. (2014) observed increased levels of p62 in epithelial cells of IBD patients’ damaged mucosa and in mice treated with DSS, when compared to non-damaged mucosa. The authors suggested impaired epithelial autophagy is associated with human and murine colon injury. p62 protein is a well-known autophagy adaptor. It plays a role in selective autophagy activation. This essential scaffold can bind a variety of partner proteins and participate in biological signaling, innate immunity, apoptosis, and inflammatory response (Kim et al., 2019). Indeed, impaired autophagy promotes IBD, and defective autophagic flux with elevated p62 was observed in IBD tissues. Besides, p62 constitutes not only a cargo receptor for autophagy, but also a central signaling hub, linking several important pro- and anti-inflammatory pathways (Hennig et al., 2021). As an example, it restricts inflammasome signaling. Indeed, ablation of p62 expression restricts mitophagy and enhances NLRP3 inflammasome activation. Furthermore, p62 supports the autophagy-dependent degradation of ubiquitinated inflammasome proteins, such as NLRP3, ASC, and AIM2.
The anti-inflammatory property of recombinant p62 protein was proposed by Cecarini et al. (2020) in a mouse model of Alzheimer’s disease. The authors demonstrated that consumption of the recombinant lactic acid bacterium L. lactis MG1363 carrying the pExu vector harboring the p62 encoding gene (pExu:p62) improved memory, modulated the ubiquitin-proteasome system, and reduced oxidative process and neural inflammation in mice.
We have previously shown the anti-inflammatory properties of another L. lactis strain, NCDO2118, during the remission period of chemically induced colitis (Luerce et al., 2014). The objective of the present study was to combine the intrinsic immunomodulatory properties of L. lactis NCDO2118 with its ability to deliver p62 to enhance its protective and preventive effects in the context of UC.
2 Materials and methods
2.1 Bacteria culture conditions
Lactococcus lactis NCDO2118 strain was provided by (The National Collection of Dairy Organisms, now NCFB (q.v.) National Collection of Food Bacteria, originally at Shenfield, Berkshire, United Kingdom). This strain was transformed, either by the empty pExu plasmid or by the pExu:p62 plasmid containing a eukaryotic expression cassette encoding the human p62 protein (pExu:p62), which was reported previously (Mancha-Agresti et al., 2017; Cecarini et al., 2020). Recombinant strains (L. lactis pExu: empty and L. lactis pExu:p62) were grown overnight without agitation at 30°C on M17 broth (Difco), supplemented with glucose 0.5% and erythromycin (125 μg/mL). Bacteria were centrifuged (3,000 x g, 10 min, RT), and the pellets were resuspended in 0.1 M Phosphate Buffered Saline (PBS). Doses were prepared daily (1×109 CFU/mL) from fresh cultures.
2.2 Animals
Six weeks old C57BL/6 mice (male) were obtained from the Bioterism Center (CEBIO) at the Federal University of Minas Gerais (UFMG, Belo Horizonte, Brazil) together. Then, they were randomly split into six experimental groups (n = six animals per group). Before starting the experiment, all mice were weighed, and the body weight was similar between the groups (16–17 g). Mice were housed in polycarbonate-ventilated cages (3 per cage) under controlled room conditions: 12 h-light/dark cycles, temperature (25 ± 2°C). They had access to standard chow and drinking water ad libitum. The experiment design was approved by the Local Animal Experimentation Ethics Committee (CEUA-UFMG, Protocol n° 178/2022), and all procedures were conducted according to the Brazilian Society of Sciences in Laboratory Animals (SBCAL) (n.d.).
2.3 Experimental design
Mice were randomly split into three non-inflamed groups: Negative control (NC), L. lactis pExu: empty (pExu), L. lactis pExu:p62 (P62), and three inflamed groups: Inflamed DSS 2% (DSS), L. lactis pExu: empty (DSS-pExu) and L. lactis pExu:p62 (DSS-P62). During the experimental period, NC and DSS groups received 300 μL of phosphate-buffered saline (PBS, 0.1 M) solution daily by gavage for five consecutive days. In the same period, the other groups received daily treatments (300 μL) with L. lactis NCDO2118 (pExu: empty or pExu:p62; 109 CFU/animal/day). After 10 days of intermission, DSS, DSS-pExu, and DSS-P62 groups were inflamed by the addition of DSS 2% in the drinking water (MP Biomedicals, Lot: S6132, Cat: 160110) provided ad libitum for 7 days. NC, pExu, and P62 groups received sterile water in the same period. Liquid and chow intake were measured during all experiment periods. On the 22nd day, mice were anesthetized by a single intraperitoneal injection (16 mg/kg of xylazine and 80 mg/kg of ketamine, Syntec, Tamboré, Brazil; i.p) for blood collection via an axillary vein. Afterward, the animals were euthanized by cervical dislocation, and liver, spleen, and colon tissues were harvested and stored immediately at −80°C (Figure 1).
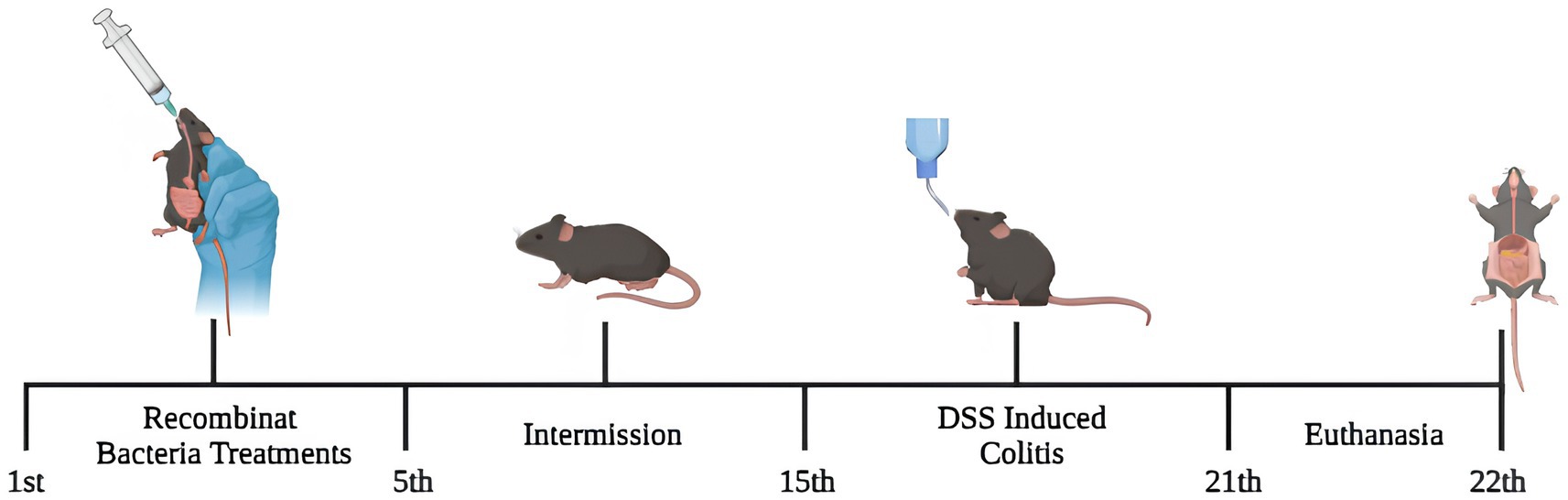
Figure 1. The experimental design. For 5 days, mice received treatments via gavage with recombinant Lactococcus lactis (pExu:empty or pExu:p62). After 10 days of intermission, mice were continuously inflamed by DSS 2% in drinking water and euthanized on the 22nd.
2.4 Intestinal permeability
Intestinal permeability was analyzed by determination of radioactivity in the blood. For that, 4 h before the procedures of euthanasia, all mice received, via gavage, 100 μL containing 18.5 MBq of Diethylenetriaminepentaacetic acid (DPTA) labeled with 99mtechnetium (99mTc-DTPA) to evaluate the impact of recombinant p62 protein on the epithelial barrier (Carvalho et al., 2021). After this period, mice were anesthetized, and 300 μL of blood was collected. According to the manufacturer’s instructions, the radioactivity was measured by a gamma radiation counter (Wizard, PerkinElmer). Data was expressed in dose percentage (%) of 99mTc-DTPA/g, according to the equation below:
cpm = count per minute.
2.5 Myeloperoxidase enzyme activity
Neutrophils’ recruitment to intestinal mucosa was evaluated by measuring the MPO enzymatic activity in colonic extracts (Barroso et al., 2021). Briefly, colon tissue was homogenized with PBS 0.1 M and centrifuged at 3,000 x g for 10 min at 4°C. Afterward, pellets hypotonic lysis was performed with a buffer containing 0.5% hexadecyltrimethylammonium bromide (Sigma-Aldrich) with three freeze–thaw cycles in liquid nitrogen. After centrifugation (3,000 x g, 15 min, 4°C), the supernatant was collected for colorimetric assay using 1.6 mM 3,3,5,5′-Tetramethylbenzidine (Sigma-Aldrich) as a chromogenic enzyme substrate. The absorbance was measured at 450 nm (MPO) on a microplate spectrophotometer (Bio-Rad 450 model, Bio-Rad Laboratories). The result was expressed as MPO arbitrary units/mg of tissue.
2.6 Secretory IgA dosage in the intestinal fluid
Secretory IgA total levels were analyzed by enzyme-linked immunosorbent assay (ELISA), according to Martins et al. (2009). Small intestine content was removed, weighed and intestinal secretion was washed out using 2 mL of 0.1 M PBS (pH 7.2) supplemented with protease inhibitor (1 μM Aprotinin; 25 μM Leupeptin; 1 μM Pepstatin; 1 mM Phenylmethylsulfonyl fluoride-PMSF). Samples were centrifuged at 2000 rpm for 30 min at 4°C; the supernatant was stored at −80°C until immunoglobulin dosage. The ELISA assay was performed in Nunc-Immuno MaxiSorp microtiter plates using goat anti-mouse IgA antibody (M-8769, Sigma, St. Louis, United States) in coat buffer (1 M Na2CO3; 0.1 M NaHCO3; pH 9.6) for 18 h at 4°C, washed (0.1 M PBS + 0.05% Tween 20), and blocked (1% albumin in PBS-Tween 20). Afterward, pre-diluted intestinal fluids (1:1000 in 0.1 M PBS-Tween 20) were incubated for 1 h. The plates were then washed, and a biotin-conjugated anti-mouse IgA antibody (dilution 1:1000, A4789, Sigma-Aldrich, St. Louis, MO, United States) was added and incubated for 1 h. Finally, 100 μL/well of OPD (1 mg/mL) and 0.04% H2O2 substrates were added and set for 10 min. The reaction was stopped with 20 μL/well of 1 M H2SO4 (Sigma-Aldrich, St. Louis, MO, United States). The assay reaction absorbance was measured at 492 nm using a microplate reader (Bio-Rad Laboratories, Hercules, CA, United States). Results were expressed in intestinal content’s sIgA concentration (μg/mL).
2.7 Cytokine concentration
Samples (100 mg) of colon tissue were weighed and homogenized in 500 ul of cytokine extraction solution (2 μL of Aprotinin (Sigma Aldrich), 500 mg of Bovine serum albumin (BSA), 4.40 mg of Benzethonium chloride (Sigma Aldrich), 2.34 g of Sodium Chloride (NaCl), 100 μL of DMSO, 37.2 mg of EDTA, 1.7 mg of PMSF in 100 mL of 0.1 M PBS plus 50 μL of Tween 20) using the Precellys tissue homogenizer and then samples were frozen at −80°C. IL10, IL6, and TGFβ cytokines concentrations were analyzed by enzyme-linked immunosorbent assay (Elisa), according to fabricant recommendation (DuoSet® Elisa Development System).
2.8 Histological analysis
After euthanasia, the liver, spleen, and entire colon were removed, and colon length was measured. For histological analysis, the liver, spleen, and colon were washed with PBS 0.1 M, and the proximal portion of the colon was rolled up. After, the tissues were immersed in a 10% buffered formaldehyde solution (Labsynth, São Paulo, Brazil) for fixation. Tissues were embedded in paraffin, and 4 μm thick slices of samples were placed on a glass slide and stained with hematoxylin and eosin (HE) or periodic acid–Schiff (PAS). The intestinal histological inflammation score was determined as described by Erben et al. (2014). Ten colon slide images from each animal were captured by a BX41 optical microscope (Olympus, Tokyo, Japan) for morphometric examination, and the crypt depth was measured. These analyses were performed using ImageJ 1.51j.8 software (NIH, Bethesda, MD, United States). The spleen and liver histological scores were analyzed according to Cesta (2006) and Liu et al. (2022), respectively (Supplementary Figure S1).
2.9 Mice colon relative gene expression analysis
Mice colon slices were collected during the euthanasia procedures and stored at −80°C. According to the recommended protocol, total RNA isolation of the colon was carried out using Pure Link™ RNA Mini Kit (Invitrogen). The samples were analyzed by NanoDrop 2000 spectrophotometer to verify the total RNA concentration and quality (Thermo Scientific; 260/280 ratio: 2.0–2.2). Also, RNA integrity was verified in 1.5% agarose gel. Residual genomic DNA was removed using the Turbo DNA-free™ Kit (Invitrogen). Total RNA was used to make 1,000 ng of complementary DNA (cDNA) by High-Capacity cDNA Reverse Transcription kit (Applied Biosystems™, ThermoFisher), according to recommended instructions. Quantitative PCR was carried out with the PowerUp™ SYBR® Green Master Mix (ThermoFisher) on the ABI PRISM 7900HT Sequence Detection System (Applied Biosystems™) under the following steps: 95°C for 10 min and 40 cycles of 95°C for 15 s, and 60°C for 1 min. Glyceraldehyde dehydrogenase (Gapdh) and β-actin (Actb) were used as housekeeping genes. Mucin 2 (Muc2), Zonulin (Hp), and Occluding (Ocln) were used as intestinal barrier markers. Also, Interleukins 1β (Il1b), 17A (Il17a), Tumor necrosis factor (Tnf), Interferon-gamma (Ifng), and Transforming growth factor beta 1 (Tgfb1) were used as inflammatory cytokines (Table 1). Gene expression results were analyzed following the 2−ΔΔCT method.
2.10 DNA sequencing
Mice stools were collected during the 21°day of the experimental period and stored at - 80°C for future analyses. For that, animals were separated, and the collection was done according to the natural physiology of each animal. The DNA from mice stool was extracted according to Yu and Morrison (2004) with modifications. Samples were sent to Neoprospectra Microbiome Technologies-Brazil, and they processed the next steps. The primer sequence used in this study was 341F (CCTACGGGRSGCAGCAG) and 806R (GGACTACHVGGGTWTCTAAT). The libraries were prepared using the TruSeq Library Prep kit (Illumina, United States) for sequencing bacterial amplicons using oligonucleotides 341F and 806R specific for the V3/V4 region of the 16S rRNA (Caporaso et al., 2012). Libraries were sequenced on the MiSeq system (Illumina, United States). Paired reads of 500 cycles were performed using the V3x600 sequencing kit (Illumina, United States) with 100,000 times per sample coverage.
2.11 Bioinformatic analysis
The Bioinformatics analysis for taxonomic classification was performed as follows. Quality filters were applied to fastq files, including the removal of truncated and low-quality readings (Phred score < 20) using the Trimmomatic tool (Bolger et al., 2014). Then, sense and antisense paired reads were merged into contigs. The sequences were subjected to singleton and chimera removal, and subsequently, the sequences were grouped into Taxonomic Operational Units (OTUs) using Uchime v. 4.2.40 and Vsearch v. 2.22.1 (Edgar et al., 2011; Rognes et al., 2016) and assigned taxonomically, considering a 97% similarity against sequences from the SILVA database (Quast et al., 2013). The Shannon index was employed to estimate alpha diversity using the RStudio packages Vegan, Fossil, and Microbiome. Shannon (H′) index is expressed as H′ = −Σni/n ln (ni/n), where ni represents the number of individuals in taxon i, and n is the total number of individuals. This index, serving as a measure of heterogeneity, considers species richness and evenness. The evenness of species diversity was computed using the Pielou formula: H′/H′max, where H′ is the Shannon index, and H′max is the maximum potential diversity of the number of species (S) within the community, determined by H′max = ln S (Shannon, 1948). Regarding beta diversity, PCoA analyses were conducted using the Bray–Curtis distance matrix to investigate group differences. The relative abundance of OTU across the groups was determined using STAMP software (Parks et al., 2014).
2.12 Statistical analysis
The results are presented as the mean ± standard error (SE). The Kolmogorov–Smirnov test was performed to determine the normality of distribution. Parametric data were evaluated by one-way ANOVA followed by the Tukey post-test. The normal distribution of OTU data was validated using the Shapiro–Wilk Test. A one-way ANOVA followed by the Tukey post-hoc test was applied to assess the variance in mean values across various group comparisons. In the case of multivariate data, distinctions among groups were identified using ANOSIM. Statistical significance was established with a value of p below 0.05. Data were analyzed using GraphPad Prism 8.0 software (GraphPad Software, San Diego, California, United States), RStudio v 4.2.1, and STAMP v. 2.1.3.
3 Results
3.1 p62 protein attenuates colon length shortening
Food intake and liquid consumption were analyzed daily. There was no statistically significant difference regarding food and liquid consumption among the groups during the experimental period (p > 0.05; Figures 2A,B). In addition, no alteration was observed in the body weight among the groups (p > 0.05; Figure 2C). Moderate DSS-induced colitis triggered colon length shortening. Indeed, mice in the DSS group presented a shorter colon length (5.83 ± 0.33 cm) when compared to the control (NC; 7.68 ± 0.28; p < 0.05; Figure 2D). L. lactis NCDO2118 pExu:empty consumption did not mitigate this shortening, as the colon length was similar in the DSS-pExu (6.37 ± 0.20) and the DSS groups. However, consumption of L. lactis NCDO2118 pExu:p62 attenuated the colon length shortening (6.97 ± 0.29) when compared to the DSS group (5.83 ± 0.33; p < 0.05).
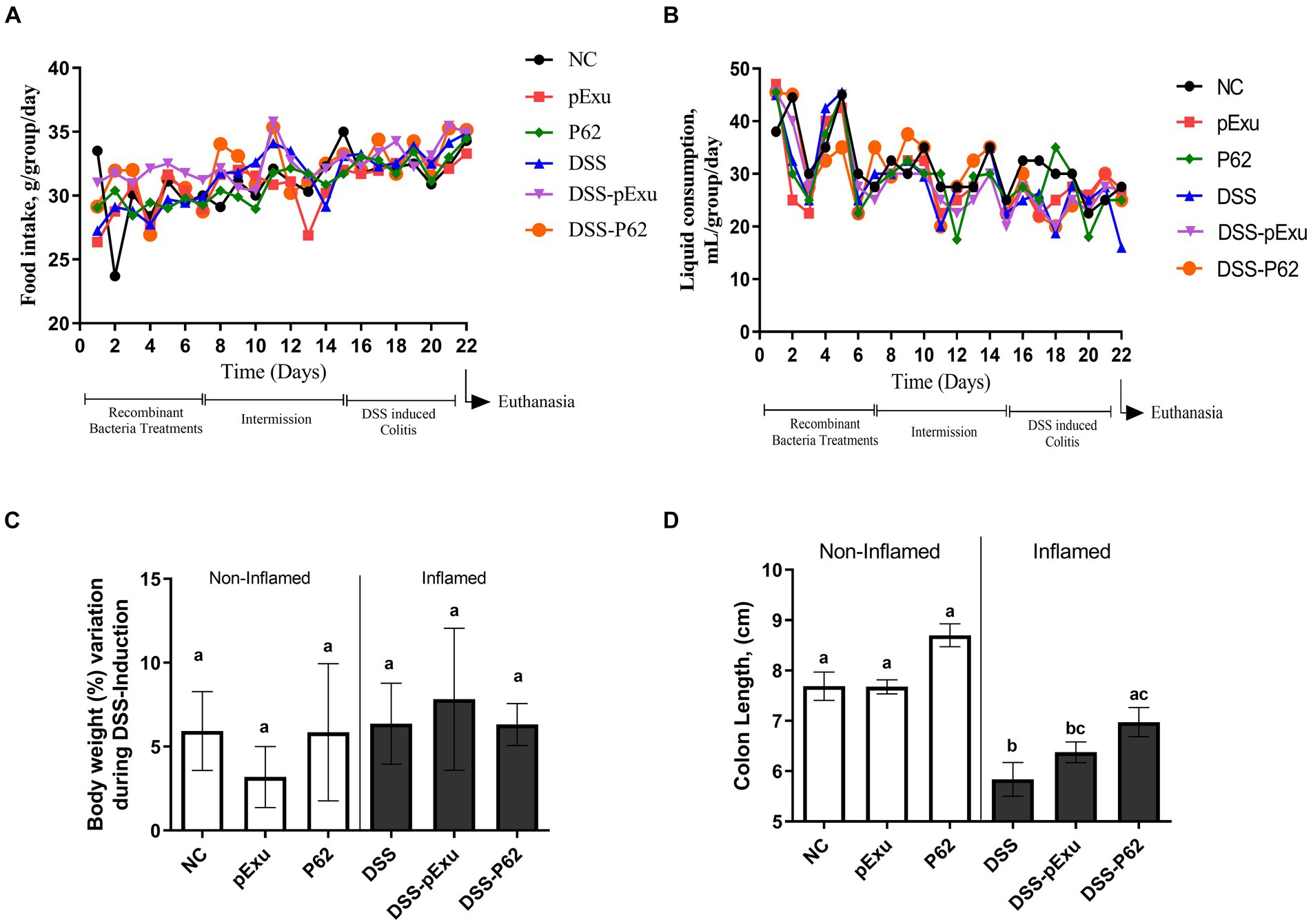
Figure 2. Effect of p62 protein on mice clinical parameters and colon length. Analysis of food (A) and liquid intake (B), body weight loss (C), and colon length (D) in mice inflamed with DSS 2%. Means ± SE followed by different lowercase letters indicate a statistically significant difference (p < 0.05) assessed by one-way ANOVA to multiple comparisons among treatments, followed by Tukey’s test.
3.2 p62 Protein expression does not improve L. lactis ability to prevent colon mucosa damage
Histological analysis showed that mice inflamed with DSS presented higher histopathological scores (Figure 3B) and lower crypt depth compared to the NC group (p = 0.003; Figure 3C) than healthy control mice. These alterations were mainly characterized by inflammatory cell infiltration into the lamina propria and damage to muscle extension. Both inflamed groups consuming L. lactis NCDO2118 pExu:empty or L. lactis NCDO2118 pExu:p62 (DSS-pExu and DSS-P62) have ameliorated the histopathological scores and crypt depth when compared to DSS group. However, no difference was observed between the two groups receiving L. lactis strains (Figures 3A–C). Regarding the liver and spleen, no significant histopathological alterations were observed in these organs among the groups (p > 0.05; Supplementary Figure S1).
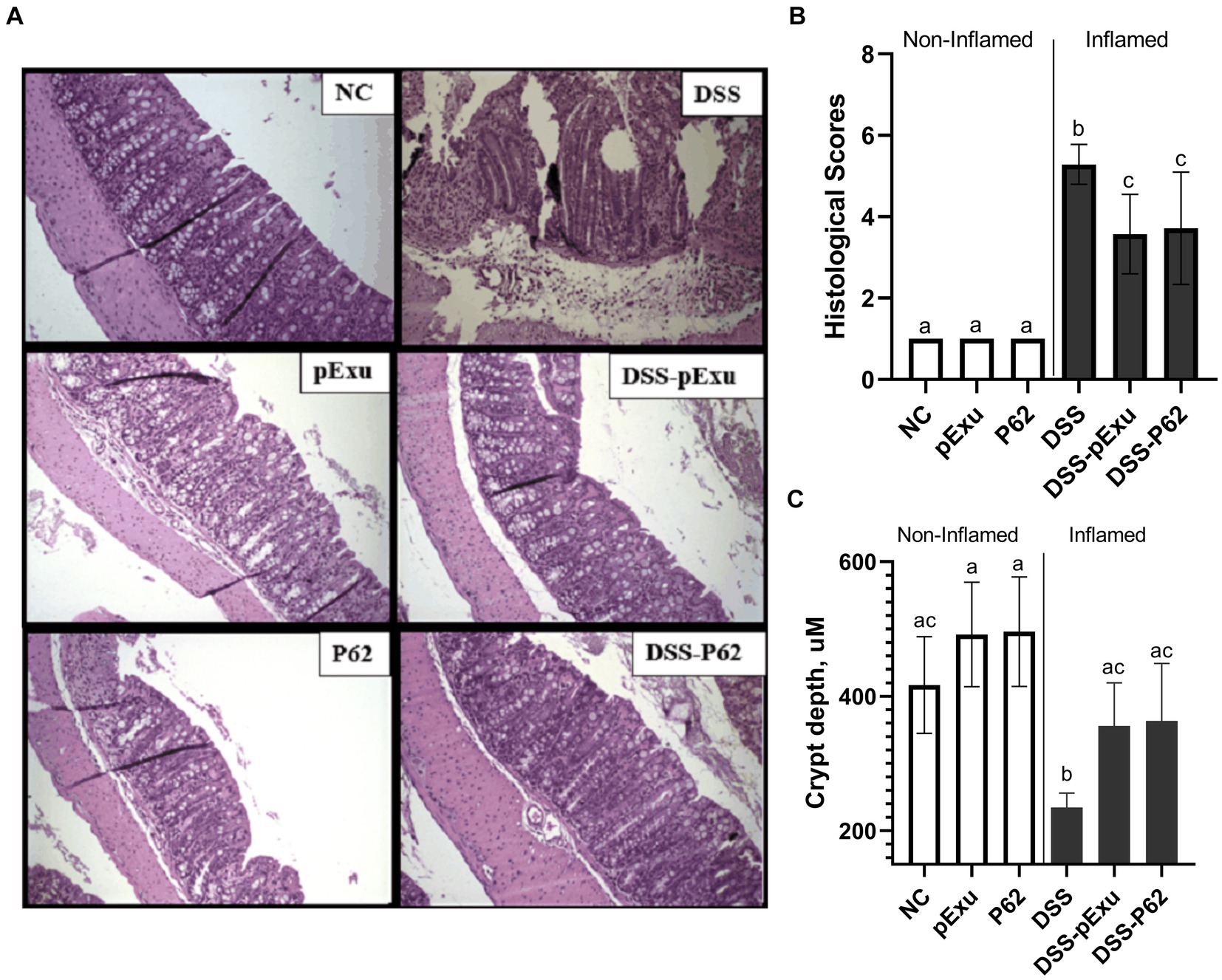
Figure 3. p62 protein Does not improve intestinal mucosa damage induced by DSS 2%. (A) Colon mucosa histopathology (B) histopathological score; and (C) crypt depth. Means ± SE followed by different lowercase letters indicate a statistically significant difference (p < 0.05) assessed by one-way ANOVA to multiple comparisons among treatments, followed by Tukey’s test.
3.3 p62 protein increased goblet cells number and Muc2 gene expression
The protective effect of L. lactis NCDO2118 pExu:p62 toward the epithelial barrier was evaluated by monitoring intestinal permeability, goblet cell count, and expression of genes encoding mucin 2 and tight junction proteins.
DSS-induced mice colitis group (DSS) exhibited a significant increase in intestinal permeability (0.8064 ± 0.44% ID/g; 84.40 average of goblet cells) and a decrease in goblet cells number when compared to the control group (0.235 ± 0.04 %ID/g; 151.5 average of goblet cells; p = 0.003; Figures 4A,B). Consumption of L. lactis NCDO2118 pExu:empty (0.437 ± 0.07% ID/g; p = 0.014) and of L. lactis NCDO2118 pExu:p62 both reduced the intestinal permeability (0.300 ± 0.08% ID/g; p = 0.0004) when compared to DSS group (Figure 4A). An increase was observed in the goblet cell count (118.3 average of goblet cells) when comparing the DSS-P62 group with other inflamed groups (Figures 4B,C).
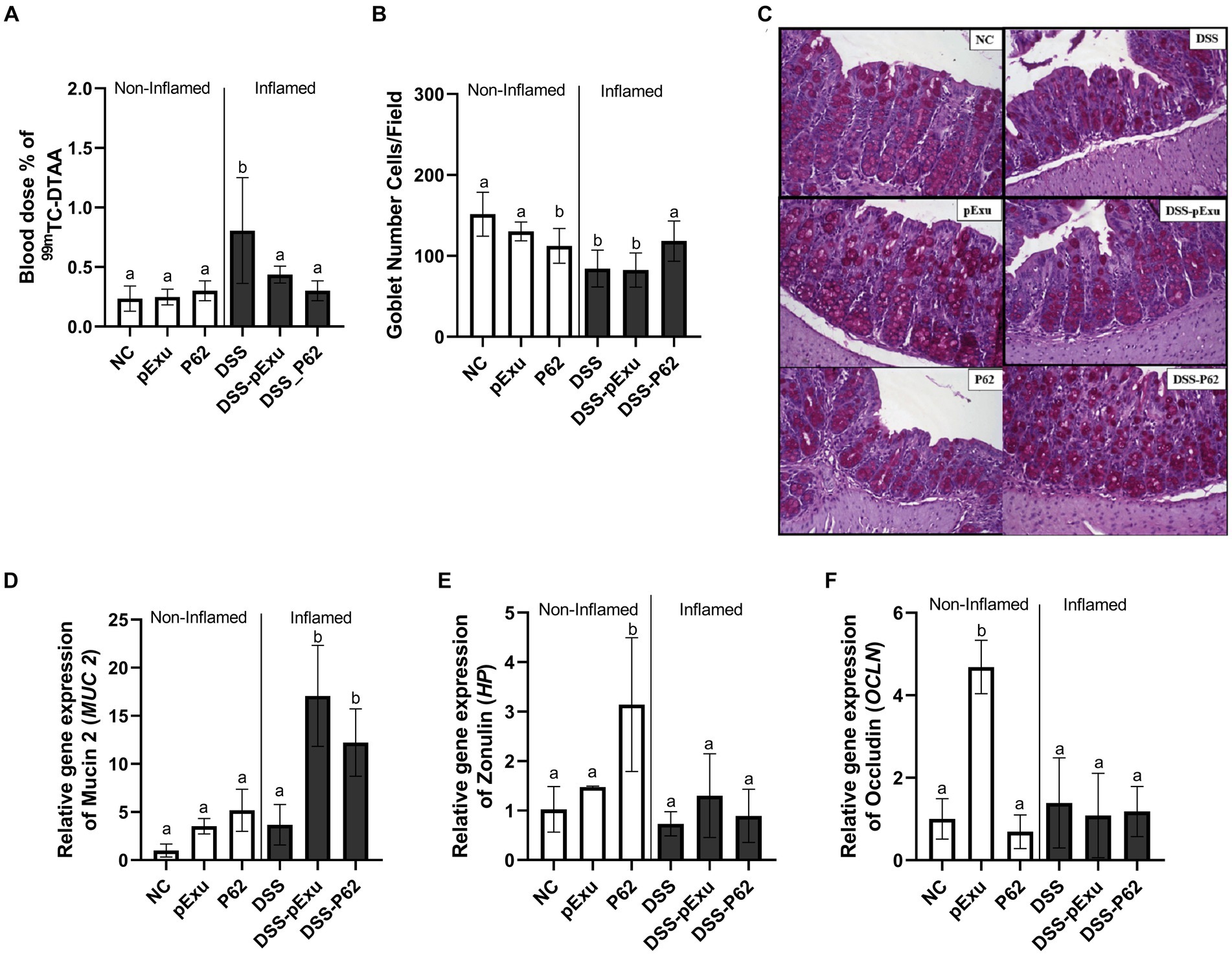
Figure 4. Protective effect of recombinant p62 protein on epithelial barrier. Intestinal permeability (A); The number of goblet cells/field (B); Representative photomicrographs from colon section stained with Periodic Acid-Schiff (PAS) (C); and level of mRNA transcript levels of mucin 2 (Muc2) (D), Zonulin (Hp) (E), Occludin (Ocln) (F). Means ± SE followed by different lowercase letters indicate a statistically significant difference (p < 0.05) assessed by one-way ANOVA followed by Tukey’s test.
Gene expression of markers associated with the epithelial barrier was also evaluated. For the non-inflamed and DSS group, no statistical differences were observed in the Muc2 (1.00 ± 0.27 vs. 3.68 ± 0.93) gene expression (p > 0.05; Figure 4D). However, upregulation of Hp and Ocln mRNA transcripts was observed for non-inflamed P62 and pExu groups, respectively, compared to other groups (Figures 4E,F). High transcript levels of Muc2 gene expression for the inflamed groups DSS-pExu (17.08 ± 1.71, p < 0.0001) and DSS-P62 (12.21 ± 1.56, p = 0.0009) were observed compared to the NC (1.00 ± 0.27) and DSS (3.68 ± 0.93) groups (Figure 4D).
3.4 p62 protein reduced inflammatory infiltration and modulated Tnf and Ifng gene expression
Inflammatory infiltration by neutrophils in the colon was assessed by monitoring the MPO enzyme activity. High levels of MPO enzyme activity were observed in the DSS (0.0248 ± 0.05; p = 0.0077) and DSS-pExu (0.2672 ± 0.04; p = 0.0027) groups when compared to the NC group (0.0630 ± 0.01; Figure 5A). In contrast to the other inflamed groups, consumption of L. lactis NCDO2118 pExu:p62 prevented this increase. Indeed, the activity in the DSS-P62 group (0.1322 ± 0.04) was lower than in the DSS-pExu group and close to that in the control NC group (p > 0.05).
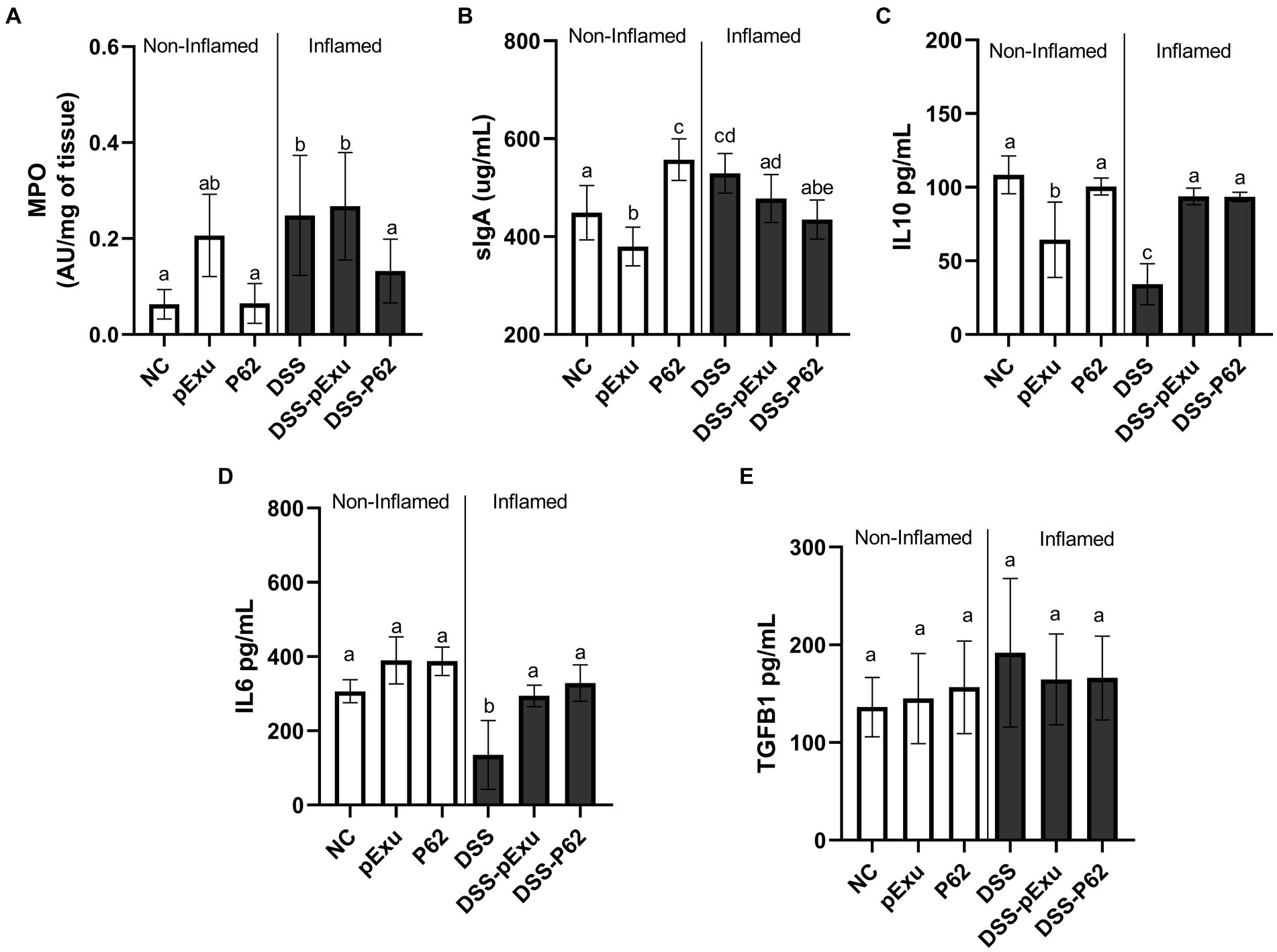
Figure 5. Effect of recombinant p62 protein on inflammatory markers. (A) Myeloperoxidase activity; (B) sIgA levels and total protein pg./mL of IL10 (C), IL6 (D), and TGFβ (E). Means ± SE followed by different lowercase letters indicate a statistically significant difference (p < 0.05) assessed by one-way ANOVA to multiple comparisons among treatments, followed by Tukey’s test.
Regarding sIgA levels in the intestinal fluid, a high concentration of this immunoglobulin was measured in the inflamed group (DSS; 529.4 ± 15.28 μg/mL), compared to the NC group (448.82 ± 20.98 μg/mL; p = 0.019; Figure 5B). Also, compared to the NC group, sIgA secretion was lower in the pExu group (379.6 ± 13.06 μg/mL; p = 0.041) and higher in the P62 (557.2 ± 16.06 μg/mL; p = 0.0007) groups. In the context of colitis, the increase in sIgA level was prevented by the consumption of L. lactis NCDO2118 pExu:p62 (DSS-P62; 434.7 ± 15.18 μg/mL), yet not by consumption of L. lactis NCDO2118 pExu:empty (Figure 5B).
The concentrations of pro-inflammatory (IL6 and TGFβ) and anti-inflammatory (IL10) cytokines were determined to evaluate the immunoregulatory effects of recombinant L. strains in the context of DSS-induced colitis in mice.
IL10 (34.18 pg./mL) and IL6 (135 pg./mL) cytokine concentrations were lower in the DSS group compared to the NC group (108 and 306 pg./mL, p < 0.05; Figures 5C,D). Recombinant L. lactis strains (DSS-pExu and DSS-P62 groups) increased the IL10 (93.73 vs. 93.44 pg./mL) and IL6 (294.2 vs. 328.6 pg./mL) cytokines concentration, compared to the DSS group (34.10 and 135 pg./mL, respectively; p < 0.05). There was no significant difference among groups regarding TGFβ cytokine concentration (Figure 5E). However, when using transcriptomics, it was observed that Tgfb1 mRNA levels were lower in the non-inflamed P62 group, as well as in DSS-pExu and DSS-P62 groups, when compared to the colitis DSS group (Figure 5E; p < 0.05).
The expression of pro-inflammatory cytokines genes (Tnf, Ifng, Il17a, Tgfb1, and Il1b) was also monitored further to evaluate the immunoregulatory effects of recombinant L. lactis strains.
The DSS treatment triggered upregulation of Tnf (3.29 ± 0.63), Ifng (12.41 ± 1.32), and Il17a (9.97 ± 0.93) gene expression when compared to the control NC group (1.00 ± 0.11; Figures 6A–C). There was no significant difference between the DSS-pExu and DSS-P62 groups regarding the Il17a and Tgfb1 mRNA transcript levels (Figures 6D,E). However, genes were downregulated in the DSS-P62 (p < 0.05; Figures 6A,B). When compared to the DSS group, gene expression of Tnf (0.42 ± 0.14), Ifng (11.06 ± 0.34 vs. 2.08 ± 0.41) and Tgfb1 (1.23 ± 0.04 vs. 0.12 ± 0.17) was downregulated, and that of Il1b (4.75 ± 0.89) was upregulated in the DSS-P62 group (p < 0.05; Figures 6A–E).
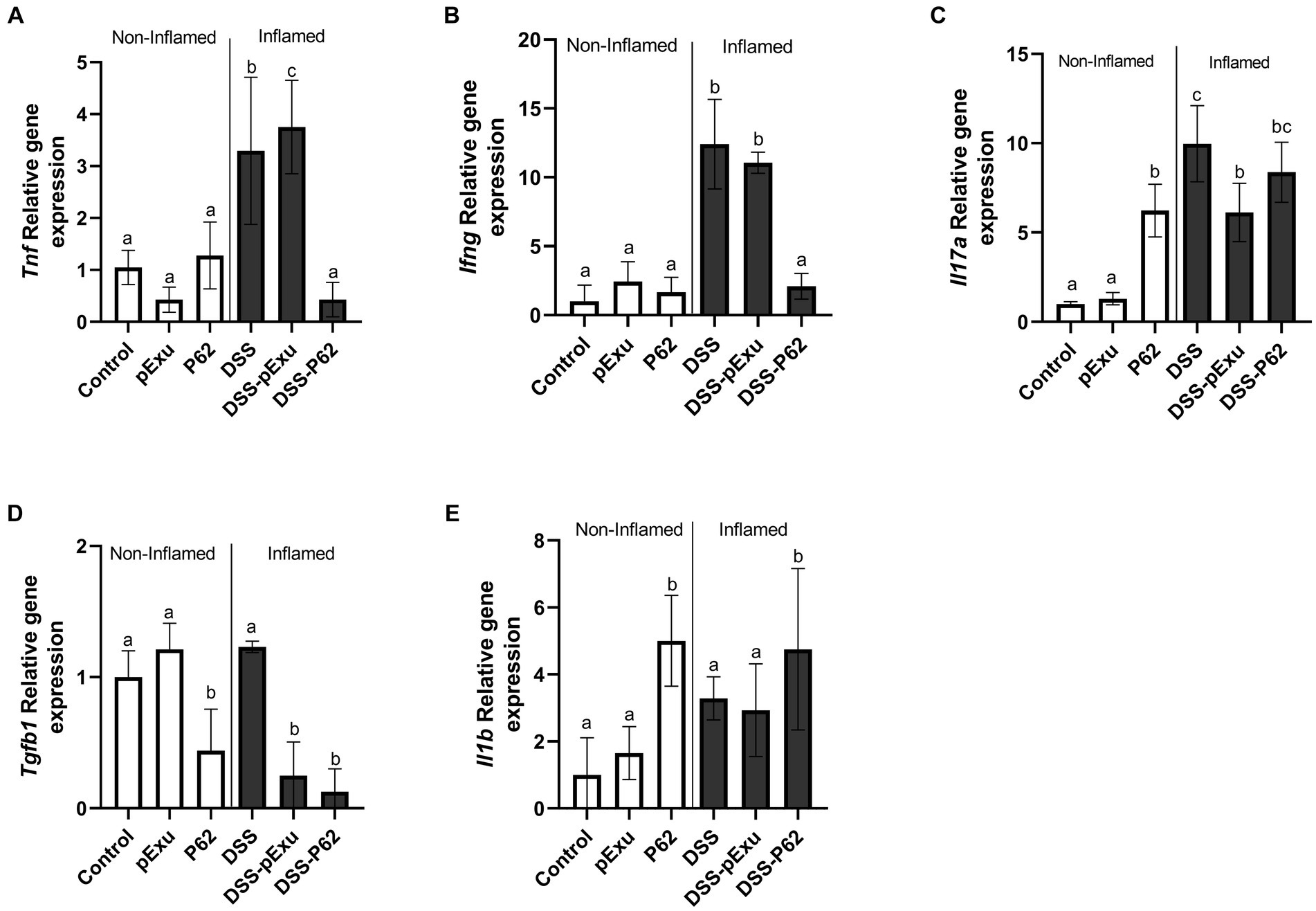
Figure 6. Effect of recombinant p62 protein on inflammatory markers. (A) Tnf, (B) Ifng, (C) Il17a, (D) Tgfb1, and (E) Il1b. Means ± SE followed by different lowercase letters indicate a statistically significant difference (p < 0.05) assessed by one-way ANOVA to multiple comparisons among treatments, followed by Tukey’s test.
3.5 p62 protein did not modulate the intestinal microbiota
Intestinal microbiota biodiversity was evaluated in this study. No difference was observed between the groups regarding alpha-diversity (ANOVA, p = 0.11) and beta-diversity (ANOSIM, p = 0.090). The proportion of Actinobacteriota and Firmicutes phyla population was decreased in the DSS group when compared to the negative control, while the treatment of mice receiving DSS with L. lactis harboring pExu empty vector restored the population of Firmicutes (p = 0,00146). At the genus level, the population of Anaerotruncus, Colidextribacter, Enterorhabdus, Eubacterium (Brachy group), Lachnoclostridium, UCG-001 (Lachnospiracea) and Tuzzerella were found decreased in the DSS control group, when compared to healthy mice. The administration of L. lactis harboring pExu empty vector significantly increased all these Genera, except for UCG-001 (Lachnospiracea). The treatment with L. lactis harboring p62 did not affect the mice’s gut microbiota composition (Figure 7).
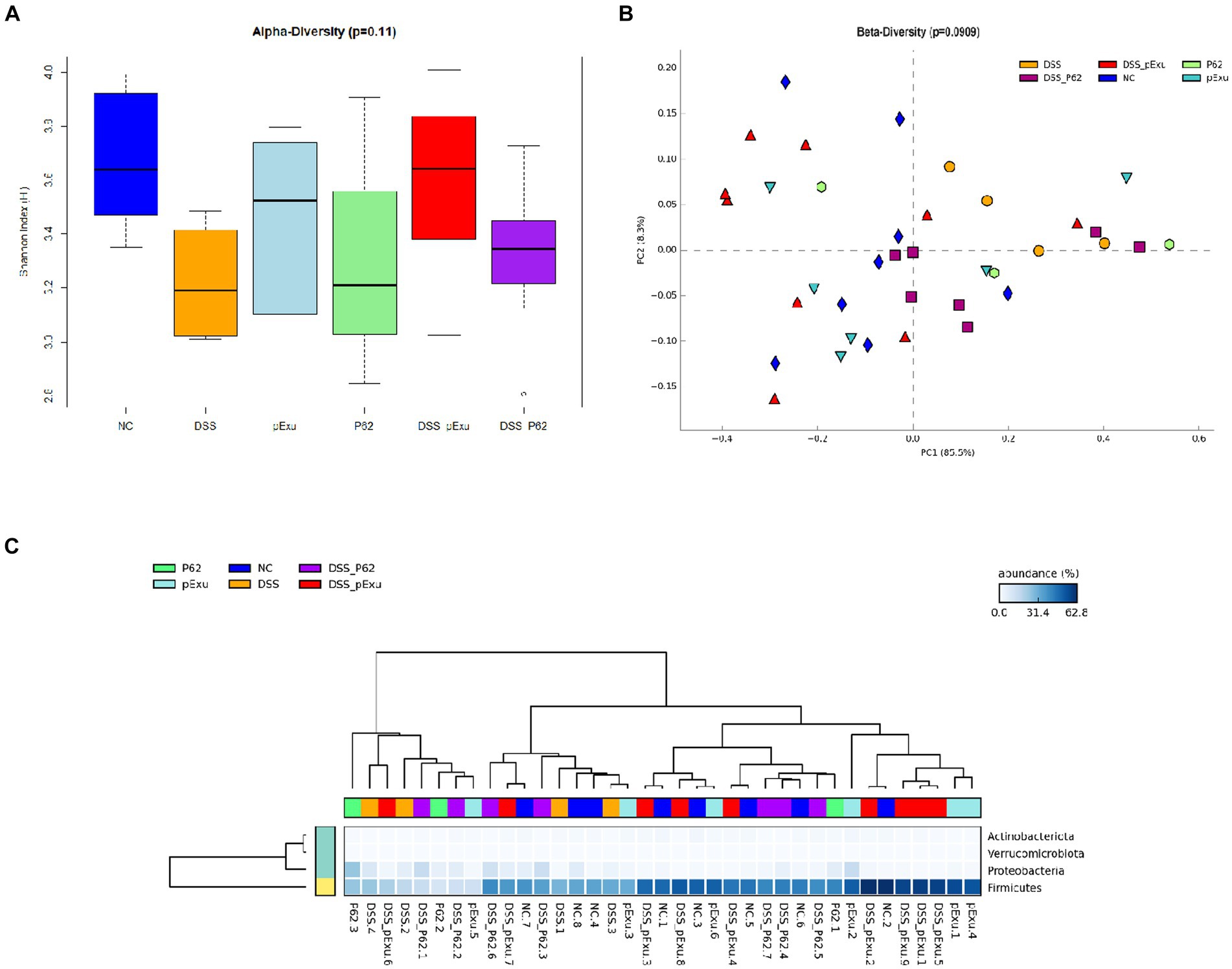
Figure 7. Effect of recombinant p62 protein on intestinal microbiota. (A) Shannon index, (B) Principal component analysis (PCA), and (C) Heatmap of bacterial taxon relative abundance. Data in the graph represent the proportion of reads assigned to a given phylum-level taxon. The dendrogram represents a hierarchical clustering based on the Unweighted Pair Group Method using the Arithmetic averages method.
4 Discussion
IBD constitutes a growing concern and encompasses diseases that affect people worldwide, with a higher occurrence in industrialized countries. IBD occurrence has been increasing with increasing consumption of fast food, yet decreasing consumption of fibers, with alteration of the immune system, gut microbiota, and intestinal permeability (Durchschein et al., 2016; Glassner et al., 2020). According to Kaplan (2015), the data might be underestimated due to people’s limited access to information, diagnostics, and adequate treatments.
Probiotics, prebiotics, symbiotics, paraprobiotics, and postbiotics constitute promising therapeutic alternatives to ameliorate IBD (Martyniak et al., 2021). Selected strains of probiotic bacteria have already been demonstrated to modulate the immune response, intestinal barrier, and permeability in vivo (Bellavia et al., 2013; Batista et al., 2020; Jakubczyk et al., 2020; Wei et al., 2022). Among them, Lactococcus lactis is of great interest for probiotic applications. Indeed, this lactic acid bacterium has a long history of safe use in producing fermented foods. According to its Generally Recognized as Safe (GRAS) status under United States Food and Drug Administration (FDA) regulations, its applications can be regarded. L. lactis strain NCDO2118, notably, revealed immunomodulatory properties in vitro in Caco-2 human intestinal epithelial cells (Luerce et al., 2014), as well as protective properties in vivo in a chronic colitis mouse model (Alves et al., 2023). L. lactis can be used as a tool to deliver therapeutic molecules aiming at the modulation of the immune system. Indeed, numerous studies have examined in vivo the potential of L. lactis as a delivery vector of heterologous proteins for vaccination purposes (Levit et al., 2022) or for treating IBD and mucositis (Carvalho et al., 2017).
The objective of the present study was to combine the intrinsic immunomodulatory properties of L. lactis NCDO2118 with its ability to deliver health-promoting molecules to enhance its protective and preventive effects in the context of UC. Several studies already evidenced the interest in using this strain to deliver in vivo therapeutic proteins in gut inflammation. It was shown that NCDO2118 expressing mycobacterial Hsp65 reduced intestinal inflammation and fibrosis in the TNBS-induced chronic colitis model, a preclinical, experimental model of Crohn’s disease (da Cunha et al., 2020). However, only the recombinant strain expressing Hsp65 was tested in vivo, and its benefits, compared with the wild-type strain, have not been assessed. Another study used a DSS-induced IBD mouse model to compare the effects of the NCDO2118 wild-type strain and a recombinant strain producing 15-lipoxygenase-1 (Carvalho, 2016). Finally, cloning of the Growth Differentiation Factor 11(GDF11) protein in this strain enhanced its anti-inflammatory against mucositis compared to the wild-type strain (Américo et al., 2023). However, this work was carried out in a different inflammation context, i.e., mucositis, an inflammation of the mucous membranes of patients undergoing chemotherapy.
Recently, Alves et al. (2023) explored the protective effects of the wild-type strain NCDO2118 in a DSS-induced colitis mouse model. Interestingly, the effects of administration of L. lactis NCDO2118 pExu: empty observed here on clinical parameters, secretory IgA, permeability, and production of IL10 and IL6, among others, are in agreement with those reported by the above authors when using the NCDO2118 wild-type strain.
One major difference with our study is the partial preservation of weight loss in the presence of the NCDO2118 wild-type strain (Alves et al., 2023). However, it is essential to note that this difference may be attributed to different experimental models of inflammation used between the two studies. Notably, while Alves et al. (2023) induced inflammation and treated mice with bacteria during the same time over 7 consecutive days, our experimental model included a pretreatment of mice with bacterial formulations for 5 consecutive days, followed by 10 days without treatment, and then by a period of 7 days of DSS exposure to induce inflammation. Our experimental protocol was designed to evaluate the preventive effects of recombinant L. lactis strains in the context of UC, while the protocol used by Alves et al. (2023) was conducted to test the curative effects of the wild-type NCDO2118 as a treatment in UC.
We noted, however, that administration of NCDO2118 pExu:empty induced the expression of Ocln and decreased the production of sIgA and IL10 in healthy animals. These results were similar to those reported by De Jesus et al. (2022), and Do Carmo et al. (2020). Whether this is due to the plasmid or the properties of the strain remains unknown. These results confirm, nevertheless, the protective effect of strain NCDO2118 in another moderate DSS-induced colitis mice model and indicate that the pExu: empty plasmid does not appear to interfere with its health properties. The administration of L. lactis NCDO2118 pExu:p62 ameliorated colon length, MPO activity, and secretory IgA levels. The number of goblet cells, notably, was higher than that found in the other groups of DSS-treated mice and similar to that in healthy mice. Goblet cells are key players in protecting intestinal mucosa (Pelaseyed et al., 2014). Their preservation might be one of the mechanisms associated with controlling colitis development by probiotics (Alves et al., 2023).
Our results suggest that the protective effects of NCDO2118 pExu:p62 are mediated via goblet cell preservation. Maintenance of this key component of the gut barrier opens promising perspectives. Indeed, patients with intestinal inflammation exhibit alterations in the structure and biosynthesis of mucin due to reduced expression of mucin genes or goblet cells number (Strous and Dekker, 2008; Bankole et al., 2021; Wang et al., 2021). Autophagy was shown to prevent intestinal inflammation and alleviate colitis in mice treated with 2,4,6-trinitrobenzene sulfonic acid (TNBS; Macias-Ceja et al., 2017). Tiwari et al. (2021) observed that autophagy was required when mucin biosynthesis was higher in goblet cells during the endoplasmic reticulum stress and apoptosis induction. According to them, autophagy is essential for goblet cells to survive during high mucin biosynthesis, thereby regulating cellular homeostasis. The importance of autophagy was also reported by Huang et al. (2018) during intestinal endoplasmic reticulum stress. p62 protein is present in all kinds of cells and is involved in cell autophagy and immune system regulation. In the autophagy pathway, kelch like ECH associated protein 1 and nuclear factor erythroid 2 related factor 2 (Keap1 Nrf2) pathway is activated due to a dysregulation as an inflammation associated with human diseases such as IBD, for example (Moscat and Diaz-Meco, 2012; Sabbieti et al., 2015). The induction of Nrf2 can bind to ubiquitinated protein, leading to the degradation of autophagosomes (Jain et al., 2010; Ichimura et al., 2013; Wong et al., 2017; Bartolini et al., 2018).
The analysis of inflammatory parameters further revealed that L. lactis NCDO2118 pExu:p62 also prevented the DSS-mediated induction of the expression of Tnf and Ifng genes, coding for pro-inflammatory cytokines. In line with this, Cecarini et al. (2020) demonstrated that the neuroprotective effect of recombinant L. lactis MG1363 pExu:p62 can be associated with its ability to modulate the TNF and IFNγ pro-inflammatory cytokines. According to Nakase et al. (2022), these cytokines are significant mediators of colitis progression and are highly secreted in the gut mucosa of UC patients. The over-expression of these pro-inflammatory cytokines is associated with increased intestinal epithelial cell death, leading to increased intestinal barrier permeability and aggravating inflammation. The genome (Oliveira et al., 2014) and proteomic (Da Silva et al., 2019) analysis was already reported for wild-type L. lactis NCDO2118. However, the effects of this strain on the healthy or unhealthy intestinal microbiota were never mentioned. Our work demonstrated no side effects of recombinant L. lactis NCDO2118 pExu:empty or pExu:p62 on the microbiota diversity and composition. We conclude, thus, that the absence of microbiota modification could have been caused by low-dose DSS induction colitis, which leaded to moderate inflammation. According to Park et al. (2021), microbiota alteration depends on the dose and duration of exposure. This study a protective effect of the p62 protein delivered by L. lactis NCDO2118 against mice with moderate DSS-induced colitis was observed and characterized. Indeed, while both strains of L. lactis, with or without the p62 coding insert, mitigated the histopathological score, the crypt depth, and the permeability, only L. lactis with the p62 coding insert, restored goblet cells, MPO activity, sIgA, as well as Tnf and Ifng expression. Research efforts will be necessary to elucidate better the autophagy pathway and immunological mechanisms of the p62 protein in colitis prevention.
Our study has some limitations that should be improved in future works. First, new doses of DSS and new IBD induction protocols (acute versus chronic colitis) should be tested to evaluate better the impact of L. lactis expressing p62 on these models, using different approaches for the treatments and prevention. Moreover, one should evaluate the expansion of immune cells (regulatory T cells, dendritic cells, macrophages) via flow cytometry to evaluate the impact of p62 on the host’s local and systemic immune response. Furthermore, more studies should be carried out to evaluate the beneficial effect of p62 on the intestinal microbiota.
Despite these limitations, our results demonstrated that L. lactis NCDO2118 harboring a plasmid encoding p62 protected the intestinal mucosa in moderate DSS-induced colitis by increasing goblet cells and mucin production. It further downregulated the pro-inflammatory cytokines Tnf and Ifng gene expression. This protective effect was more efficient than the same strain devoid of p62-encoding sequence. This suggests that local delivery of p62 by an immunomodulatory probiotic may constitute an alternative therapy to mitigate a moderate UC. This opens new avenues for developing biotherapies to potentiate the existing treatment of inflammatory diseases. Indeed, immunomodulatory probiotics, in conjunction with local delivery of bioactive proteins to the colitis site, may prolong therapy-induced remissions, as was evidenced for mixtures of probiotic bacteria (Sniffen et al., 2018).
Data availability statement
The data present in the study are deposited in the National Center for Biotechnology Information (NCBI), Bioproject: PRJNA1043697 (https://www.ncbi.nlm.nih.gov/sra/PRJNA1043697) repository, accession numbers: SAMN38350495, SAMN38350496, SAMN38350497, SAMN38350498, SAMN38350499, SAMN38350500, SAMN38350501, SAMN38350502, SAMN38350503, SAMN38350504, SAMN38350505, SAMN38350506, SAMN38350507, SAMN38350508, SAMN38350509, SAMN38350510, SAMN38350511, SAMN38350512, SAMN38350513, SAMN38350514, SAMN38350515, SAMN38350516, SAMN38350517, SAMN38350518, SAMN38350519, SAMN38350520, SAMN38350521, SAMN38350522, SAMN38350523, SAMN38350524, SAMN38350525, SAMN38350526, SAMN38350527, SAMN38350528, SAMN38350529, SAMN38350530, SAMN38350531.
Ethics statement
The animal study was approved by Local Animal Experimental Ethics Committee of the Federal University of Minas Gerais (CEUA-UFMG) (Protocol number 178/2022). The study was conducted in accordance with the local legislation and institutional requirements.
Author contributions
JL: Conceptualization, Data curation, Formal analysis, Funding acquisition, Methodology, Writing – original draft, Writing – review & editing. AF: Conceptualization, Methodology, Writing – review & editing. FB: Conceptualization, Methodology, Writing – review & editing. LJ: Conceptualization, Data curation, Formal analysis, Methodology, Writing – review & editing. OV: Methodology, Writing – review & editing. LQ: Methodology, Writing – review & editing. MA: Methodology, Writing – review & editing. GC: Methodology, Writing – review & editing. RG: Methodology, Writing – review & editing. JD: Methodology, Writing – review & editing. TSi: Conceptualization, Writing – review & editing. KV: Formal analysis, Methodology, Writing – review & editing. SF: Methodology, Writing – review & editing. RS: Formal analysis, Methodology, Writing – review & editing. FM: Writing – review & editing. EF: Formal analysis, Methodology, Writing – review & editing. TSa: Writing – review & editing. AB: Writing – review & editing. MO: Formal analysis, Writing – review & editing. AF: Writing – review & editing. RC: Methodology, Writing – review & editing. FV: Writing – review & editing. YL: Writing – review & editing. GJ: Writing – review & editing. EG: Writing – review & editing. VA: Funding–acquisition, Supervision, Writing – review & editing.
Funding
The author(s) declare financial support was received for the research and authorship for of this article funded by Conselho Nacional de Desenvolvimento Científico e Tecnológico (CNPq), grant number 152400/2022–2 and the publication funded by L’Institut National de Recherche en Agriculture, Alimentation et Environnement (INRAе); UMR, Science et Technologie du Lait et des Œufs, (STLO), France.
Acknowledgments
The authors would like to acknowledge the Pró-Reitoria de Pesquisa—Universidade Federal de Minas Gerais, Conselho Nacional de Desenvolvimento Científico e Tecnológico (CNPq), Coordenação de Aperfeiçoamento de Pessoal de Nível Superior (CAPES), Fundação de Amparo à Pesquisa do Estado de Minas Gerais (FAPEMIG) and L’Institut National de Recherche en Agriculture, Alimentation et Environnement (INRAе); UMR, Science et Technologie du Lait et des Œufs, (STLO), France for the financial support.
Conflict of interest
The authors declare that the research was conducted in the absence of any commercial or financial relationships that could be construed as a potential conflict of interest.
The author(s) declared that they were an editorial board member of Frontiers, at the time of submission. This had no impact on the peer review process and the final decision.
Publisher’s note
All claims expressed in this article are solely those of the authors and do not necessarily represent those of their affiliated organizations, or those of the publisher, the editors and the reviewers. Any product that may be evaluated in this article, or claim that may be made by its manufacturer, is not guaranteed or endorsed by the publisher.
References
Abraham, C., and Cho, J. H. (2009). Inflammatory bowel disease. N. Engl. J. Med. 361, 2066–2078. doi: 10.1056/nejmra0804647
Alves, J. L., Lemos, L., Rodrigues, N. M., Pereira, V. B., Barros, P. A. V., Canesso, M. C. C., et al. (2023). Immunomodulatory effects of different strains of Lactococcus lactis in DSS-induced colitis. Braz. J. Microbiol. 54, 1203–1215. doi: 10.1007/s42770-023-00928-0
Américo, M. F., Freitas, A. S., da Silva, T. F., de Jesus, L. C. L., Barroso, F. A. L., Campos, G. M., et al. (2023). Growth differentiation factor 11 delivered by dairy Lactococcus lactis strains modulates inflammation and prevents mucosal damage in a mice model of intestinal mucositis. Front. Microbiol. 14:1157544. doi: 10.3389/fmicb.2023.1157544
Bankole, E., Read, E., Curtis, M. A., Neves, J. F., and Garnett, J. A. (2021). The relationship between mucins and ulcerative colitis: A systematic review. J. Clin. Med. 10:1935. doi: 10.3390/jcm10091935
Barroso, F. A. L., de Jesus, L. C. L., de Castro, C. P., Batista, V. L., Ferreira, Ê., Fernandes, R. S., et al. (2021). Intake of Lactobacillus delbrueckii (pExu: hsp65) prevents the inflammation and the disorganization of the intestinal mucosa in a mouse model of mucositis. Microorganisms 9, 1–27. doi: 10.3390/microorganisms9010107
Bartolini, D., Dallaglio, K., Torquato, P., Piroddi, M., and Galli, F. (2018). Nrf2-p62 autophagy pathway and its response to oxidative stress in hepatocellular carcinoma. Transl. Res. 193, 54–71. doi: 10.1016/j.trsl.2017.11.007
Batista, V. L., da Silva, T. F., de Jesus, L. C. L., Coelho-Rocha, N. D., Barroso, F. A. L., Tavares, L. M., et al. (2020). Probiotics, prebiotics, Synbiotics, and Paraprobiotics as a therapeutic alternative for intestinal mucositis. Front. Microbiol. 11:544490. doi: 10.3389/fmicb.2020.544490
Bellavia, M., Tomasello, G., Romeo, M., Damiani, P., Lo Monte, A. I., Lozio, L., et al. (2013). Gut microbiota imbalance and chaperoning system malfunction are central to ulcerative colitis pathogenesis and can be counteracted with specifically designed probiotics: a working hypothesis. Med. Microbiol. Immunol. 202, 393–406. doi: 10.1007/S00430-013-0305-2
Belo, G. A., Cordeiro, B. F., Oliveira, E. R., Braga, M. P., da Silva, S. H., Costa, B. G., et al. (2021). SlpB protein enhances the probiotic potential of L. lactis NCDO 2118 in colitis mice model. Front. Pharmacol. 12:755825. doi: 10.3389/fphar.2021.755825
Bolger, A. M., Lohse, M., and Usadel, B. (2014). Trimmomatic: a flexible trimmer for Illumina sequence data. Bioinformatics 30, 2114–2120. doi: 10.1093/BIOINFORMATICS/BTU170
Brazilian Society of Sciences in Laboratory Animals (SBCAL). (n.d.) Sociedade Brasileira de Ciência em Animais de Laboratório (sbcal.org.br). (Accessed November 25, 2023).
Caporaso, J. G., Lauber, C. L., Walters, W. A., Berg-Lyons, D., Huntley, J., Fierer, N., et al. (2012). Ultra-high-throughput microbial community analysis on the Illumina HiSeq and MiSeq platforms. ISME J. 6, 1621–1624. doi: 10.1038/ismej.2012.8
Carvalho, R. (2016). Oral administration of Lactococcus lactis expressing recombinant 15-lipoxygenase-1 (15 LOX-1) modulates chemically induced colitis in mice. Med Res Arch 4. doi: 10.18103/mra.v4i7.612
Carvalho, P. L. A., Andrade, M. E. R., Trindade, L. M., Leocádio, P. C. L., Alvarez-Leite, J. I., dos Reis, D. C., et al. (2021). Prophylactic and therapeutic supplementation using fructo-oligosaccharide improves the intestinal homeostasis after mucositis induced by 5- fluorouracil. Biomed. Pharmacother. 133:111012. doi: 10.1016/j.biopha.2020.111012
Carvalho, R. D., Breyner, N., Menezes-Garcia, Z., Rodrigues, N. M., Lemos, L., Maioli, T. U., et al. (2017). Secretion of biologically active pancreatitis-associated protein I (PAP) by genetically modified dairy Lactococcus lactis NZ9000 in the prevention of intestinal mucositis. Microb. Cell Factories 16:27. doi: 10.1186/s12934-017-0624-x
Cecarini, V., Bonfili, L., Gogoi, O., Lawrence, S., Venanzi, F. M., Azevedo, V., et al. (2020). Neuroprotective effects of p62(SQSTM1)-engineered lactic acid bacteria in Alzheimer’s disease: a pre-clinical study. Aging (Albany NY) 12:15995. doi: 10.18632/aging.103900
Cesta, M. F. (2006). Normal structure, function, and histology of the spleen. Toxicol. Pathol. 34, 455–465. doi: 10.1080/01926230600867743
da Cunha, V. P., Preisser, T. M., Santana, M. P., Machado, D. C. C., Pereira, V. B., and Miyoshi, A. (2020). Mycobacterial Hsp65 antigen delivered by invasive Lactococcus lactis reduces intestinal inflammation and fibrosis in TNBS-induced chronic colitis model. Sci. Rep. 10:20123. doi: 10.1038/S41598-020-77276-8
Da Silva, W. M., Oliveira, L. C., Soares, S. C., Sousa, C. S., Tavares, G. C., Resende, C. P., et al. (2019). Quantitative proteomic analysis of the response of probiotic putative Lactococcus lactis NCDO 2118 strain to different oxygen availability under temperature variation. Front. Microbiol. 10:436709. doi: 10.3389/fmicb.2019
De Jesus, L. C. L., Sousa, T. J., Coeslho-Rocha, N. D., Profeta, R., Barroso, F. A. L., Drumond, M. M., et al. (2022). Safety evaluation of Lactobacillus delbrueckii subsp. Lactis CIDCA 133: a health-promoting Bacteria. Probiotics and Antimicrobial Proteins. 14, 816–829. doi: 10.1007/s12602-021-09826-z
Derikx, L. A. A. P., Dieleman, L. A., and Hoentjen, F. (2016). Probiotics and prebiotics in ulcerative colitis. Best Pract. Res. Clin. Gastroenterol. 30, 55–71. doi: 10.1016/j.bpg.2016.02.005
Do Carmo, F. L. R., Rabah, H., Cordeiro, B. F., Silva, S. H., Pessoa, R. M., Fernandes, S. O. A., et al. (2020). Probiotic Propionibacterium freudenreichii requires SlpB protein to mitigate mucositis induced by chemotherapy. Oncotarget 10, 7198–7219. doi: 10.18632/oncotarget.27319
Durchschein, F., Petritsch, W., and Hammer, H. F. (2016). Diet therapy for inflammatory bowel diseases: the established and the new. World J. Gastroenterol. 22, 2179–2194. doi: 10.3748/wjg.v22.i7.2179
Edgar, R. C., Haas, B. J., Clemente, J. C., Quince, C., and Knight, R. (2011). UCHIME improves sensitivity and speed of chimera detection. Bioinformatics 27, 2194–2200. doi: 10.1093/bioinformatics/btr381
Erben, U., Loddenkemper, C., Doerfel, K., Spieckermann, S., Haller, D., Heimesaat, M. M., et al. (2014). A guide to histomorphological evaluation of intestinal inflammation in mouse models. Int. J. Clin. Exp. Pathol. 7:4557.
Foligne, B., Nutten, S., Grangette, C., Dennin, V., Goudercourt, D., Poiret, S., et al. (2007). Correlation between in vitro and in vivo immunomodulatory properties of lactic acid bacteria. World J. Gastroenterol. 13, 236–243. doi: 10.3748/wjg.v13.i2.236
Frei, P., Biedermann, L., Manser, C. N., Wilk, M., Manz, M., Vavricka, S. R., et al. (2012). Topical therapies in inflammatory bowel disease. Digestion 86, 36–44. doi: 10.1159/000341947
Geetha, T., Vishwaprakash, N., Sycheva, M., and Babu, J. R. (2012). Sequestosome 1/p62: across diseases. Biomarkers 17, 99–103. doi: 10.3109/1354750X.2011.653986
Giulietti, A., Overbergh, L., Valckx, D., Decallonne, B., Bouillon, R., and Mathieu, C. (2001). An overview of real-time quantitative PCR: applications to quantify cytokine gene expression. Methods 25, 386–401. doi: 10.1006/meth.2001.1261
Glassner, K. L., Abraham, B. P., and Quigley, E. M. M. (2020). The microbiome and inflammatory bowel disease. J. Allergy Clin. Immunol. 145, 16–27. doi: 10.1016/j.jaci.2019.11.003
Gomes-Santos, A. C., de Oliveira, R. P., Moreira, T. G., Castro-Junior, A. B., Horta, B. C., Lemos, L., et al. (2017). Hsp65-producing Lactococcus lactis prevents inflammatory intestinal disease in mice by IL-10- and TLR2-dependent pathways. Front. Immunol. 8:30. doi: 10.3389/fimmu.2017.00030
Hennig, P., Fenini, G., Di Filippo, M., Karakaya, T., and Beer, H.-D. (2021). The Pathways Underlying the Multiple Roles of p62 in Inflammation and Cancer. Biomedicines. 9:707. doi: 10.3390/biomedicines9070707
Hill, C., Guarner, F., Reid, G., Gibson, G. R., Merenstein, D. J., Pot, B., et al. (2014). The international scientific Association for Probiotics and Prebiotics consensus statement on the scope and appropriate use of the term probiotic. Nat. Rev. Gastroenterol. Hepatol. 11, 506–514. doi: 10.1038/nrgastro.2014.66
Huang, Y., Feng, Y., Wang, Y., Wang, P., Wang, F., and Ren, H. (2018). Severe burn-induced intestinal epithelial barrier dysfunction is associated with endoplasmic reticulum stress and autophagy in mice. Front. Physiol. 9:339329. doi: 10.3389/fphys.2018.00441
Ichimura, Y., Waguri, S., Sou, Y., Kageyama, S., Hasegawa, J., Ishimura, R., et al. (2013). Phosphorylation of p62 activates the Keap1-Nrf2 pathway during selective autophagy. Mol. Cell 51, 618–631. doi: 10.1016/j.molcel.2013.08.003
Jain, A., Lamark, T., Sjøttem, E., Larsen, K. B., Awuh, J. A., Øvervatn, A., et al. (2010). p62/SQSTM1 is a target gene for transcription factor NRF2 and creates a positive feedback loop by inducing antioxidant response element-driven gene transcription. J. Biol. Chem. 285, 22576–22591. doi: 10.1074/jbc.m110.118976
Jakubczyk, D., Leszczyńska, K., and Górska, S. (2020). The effectiveness of probiotics in the treatment of inflammatory bowel disease (IBD)-A critical review. Nutrients 12:1973. doi: 10.3390/nu12071973
Jonkers, D., Penders, J., Masclee, A., and Pierik, M. (2012). Probiotics in the management of inflammatory bowel disease: a systematic review of intervention studies in adult patients. Drugs 72, 803–823. doi: 10.2165/11632710-000000000-00000
Kaplan, G. G. (2015). The global burden of IBD: from 2015 to 2025. Nat. Rev. Gastroenterol. Hepatol. 12, 720–727. doi: 10.1038/nrgastro.2015.150
Kim, S., Eun, H. S., and Eun-Kyeong, J. (2019). Roles of autophagy-related genes in the pathogenesis of inflammatory bowel disease. Cells 8:077. doi: 10.3390/cells8010077
Komatsu, M., Kageyama, S., and Ichimura, Y. (2012). p62/SQSTM1/A170: physiology and pathology. Pharmacol. Res. 66, 457–462. doi: 10.1016/j.phrs.2012.07.004
Krela-Kaźmierczak, I., Szymczak, A., Łykowska-Szuber, L., Eder, P., and Linke, K. (2016). Osteoporosis in gastrointestinal diseases. Adv. Clin. Exp. Med. 25, 185–190. doi: 10.17219/acem/33746
Kruis, W., Frič, P., Pokrotnieks, J., Lukáš, M., Fixa, B., Kaščák, M., et al. (2004). Maintaining remission of ulcerative colitis with the probiotic Escherichia coli Nissle 1917 is as effective as with standard mesalazine. Gut 53, 1617–1623. doi: 10.1136/gut.2003.037747
Levit, R., Cortes-Perez, N. G., De Moreno De Leblanc, A., Loiseau, J., Aucouturier, A., Langella, P., et al. (2022). Use of genetically modified lactic acid bacteria and bifidobacteria as live delivery vectors for human and animal health. Gut Microbes 14:821. doi: 10.1080/19490976.2022.2110821
Liu, F., Wei, L., Leow, W. Q., Liu, S. H., Ren, Y. Y., Wang, X. X., et al. (2022). Developing a new qFIBS model assessing histological features in pediatric patients with non-alcoholic steatohepatitis. Front Med (Lausanne) 9:925357. doi: 10.3389/fmed.2022.925357
Long, M., Li, X., Li, L., Dodson, M., Zhang, D. D., and Zheng, H. (2017). Multifunctional p62 effects underlie diverse metabolic diseases. Trends Endocrinol. Metab. 28, 818–830. doi: 10.1016/j.tem.2017.09.001
Luerce, T. D., Gomes-Santos, A. C., Rocha, C. S., Moreira, T. G., Cruz, D. N., Lemos, L., et al. (2014). Anti-inflammatory effects of Lactococcus lactis NCDO 2118 during the remission period of chemically induced colitis. Gut Pathog 6:33. doi: 10.1186/1757-4749-6-33
Machain-Williams, C., Reagan, K., Wang, T., Zeidner, N. S., and Blair, C. D. (2013). Immunization with Culex tarsalis mosquito salivary gland extract modulates West Nile virus infection and disease in mice. Viral Immunol. 26, 84–92. doi: 10.1089/vim.2012.0051
Macias-Ceja, D. C., Cosín-Roger, J., Ortiz-Masiá, D., Salvador, P., Hernández, C., Esplugues, J. V., et al. (2017). Stimulation of autophagy prevents intestinal mucosal inflammation and ameliorates murine colitis. Br. J. Pharmacol. 174:2501. doi: 10.1111/bph.13860
Mancha-Agresti, P., Drumond, M. M., Carmo, F. L. R., Santos, M. M., Santos, J. S., Venanzi, F., et al. (2017). A new broad range plasmid for DNA delivery in eukaryotic cells using lactic acid Bacteria: in vitro and in vivo assays. Mol Ther Methods Clin Dev 4, 83–91. doi: 10.1016/J.omtm.2016.12.005
Martins, R. A., Cunha, M. R., Neves, A. P., Martins, M., Teixeira-Veríssimo, M., and Teixeira, A. M. (2009). Effects of aerobic conditioning on salivary IgA and plasma IgA, IgG and IgM in older men and women. Int. J. Sports Med. 30, 906–912. doi: 10.1055/S-0029-1237389
Martyniak, A., Medyńska-Przęczek, A., Wędrychowicz, A., Skoczeń, S., and Tomasik, P. J. (2021). Prebiotics, probiotics, Synbiotics, Paraprobiotics and Postbiotic compounds in IBD. Biomol. Ther. 11:1903. doi: 10.3390/biom11121903
Moscat, J., and Diaz-Meco, M. T. (2012). p62: a versatile multitasker takes on cancer. Trends Biochem. Sci. 37, 230–236. doi: 10.1016/J.tibs.2012.02.008
Nakase, H., Sato, N., Mizuno, N., and Ikawa, Y. (2022). The influence of cytokines on the complex pathology of ulcerative colitis. Autoimmun. Rev. 21:103017. doi: 10.1016/j.autrev.2021.103017
Ng, S. C., Shi, H. Y., Hamidi, N., Underwood, F. E., Tang, W., Benchimol, E. I., et al. (2017). Worldwide incidence and prevalence of inflammatory bowel disease in the 21st century: a systematic review of population-based studies. Lancet 390, 2769–2778. doi: 10.1016/S0140-6736(17)32448-0
Noguès, E. B., Kropp, C., Bétemps, L., de Sousa, C., Chain, F., Auger, S., et al. (2022). Lactococcus lactis engineered to deliver hCAP18 cDNA alleviates DNBS-induced colitis in C57BL/6 mice by promoting IL17A and IL10 cytokine expression. Sci. Rep. 12, 1–12. doi: 10.1038/s41598-022-19455-3
Oliveira, L. C., Saraiva, T. D. L., Soares, S. C., Ramos, R. T. J., Sá, P. H. C. G., Carneiro, A. R., et al. (2014). Genome sequence of Lactococcus lactis subsp. lactis NCDO 2118, a GABA-producing strain. Genome Announc. 2, 980–994. doi: 10.1128/genomea.00980-14
Ortiz-Masiá, D., Cosín-Roger, J., Calatayud, S., Hernández, C., Alós, R., Hinojosa, J., et al. (2014). Hypoxic macrophages impair autophagy in epithelial cells through Wnt1: relevance in IBD. Mucosal/immunology. 7, 929–938. doi: 10.1038/mi.2013.108
Pankiv, S., Clausen, T. H., Lamark, T., Brech, A., Bruun, J. A., Outzen, H., et al. (2007). p62/SQSTM1 binds directly to Atg8/LC3 to facilitate degradation of ubiquitinated protein aggregates by autophagy. J. Biol. Chem. 282, 24131–24145. doi: 10.1074/jbc.m702824200
Park, H., Yeo, S., Kang, S., and Huh, C. S. (2021). Longitudinal microbiome analysis in a dextran sulfate sodium-induced colitis mouse model. Microorganisms. 9:370. doi: 10.3390/microorganisms9020370
Parks, D. H., Tyson, G. W., Hugenholtz, P., and Beiko, R. G. (2014). STAMP: statistical analysis of taxonomic and functional profles. Bioinformatics (Oxford, England) 30, 3123–3124. doi: 10.1093/bioinformatics/btu494
Pelaseyed, T., Bergström, J. H., Gustafsson, J. K., Ermund, A., Birchenough, G. M. H., Schütte, A., et al. (2014). The mucus and mucins of the goblet cells and enterocytes provide the first defense line of the gastrointestinal tract and interact with the immune system. Immunol. Rev. 260, 8–20. doi: 10.1111/imr.12182
Plaza-Díaz, J., Ruiz-Ojeda, F. J., Vilchez-Padial, L. M., and Gil, A. (2017). Evidence of the anti-inflammatory effects of probiotics and Synbiotics in intestinal chronic diseases. Nutrients 9:555. doi: 10.3390/nu9060555
Quast, C., Pruesse, E., Yilmaz, P., Gerken, J., Schweer, T., Yarza, P., et al. (2013). The SILVA ribosomal RNA gene database project: improved data processing and web-based tools. Nucleic Acids Res. 41, D590–D596. doi: 10.1093/NAR/GKS1219
Rognes, T., Flouri, T., Nichols, B., Quince, C., and Mahé, F. (2016). VSEARCH: A versatile open source tool for metagenomics. PeerJ 2016:e2584. doi: 10.7717/peerj.2584
Sabbieti, M. G., Agas, D., Capitani, M., Marchetti, L., Concetti, A., Vullo, C., et al. (2015). Plasmid DNA-coding p62 as a bone effective anti-inflammatory/anabolic agent. Oncotarget 6:3590. doi: 10.18632/oncotarget.2884
Santos Rocha, C., Gomes-Santos, A. C., Garcias Moreira, T., De Azevedo, M., Diniz Luerce, T., Mariadassou, M., et al. (2014). Local and systemic immune mechanisms underlying the anti-colitis effects of the dairy bacterium Lactobacillus delbrueckii. PLoS One 9:e85923. doi: 10.1371/journal.pone.0085923
Shannon, C. E. (1948). A mathematical theory of communication. Bell Syst. Tech. J. 27, 379–423. doi: 10.1002/j.1538-7305.1948.tb01338.x
Sniffen, J. C., McFarland, L. V., Evans, C. T., and Goldstein, E. J. C. (2018). Choosing an appropriate probiotic product for your patient: an evidence-based practical guide. PLoS One 13:e0209205. doi: 10.1371/journal.pone.0209205
Song, M.-K., Park, M.-Y., and Sung, M.-K. (2013). 5-fluorouracil-induced changes of intestinal integrity biomarkers in BALB/C mice. J. Cancer Prev. 18, 322–329. doi: 10.15430/JCP.2013.18.4.322
Strous, G. J., and Dekker, J. (2008). Mucin-type glycoproteins. Crit. Rev. Biochem. Mol. Biol. 27, 57–92. doi: 10.3109/10409239209082559
Tiwari, S., Begum, S., Moreau, F., Gorman, H., and Chadee, K. (2021). Autophagy is required during high MUC2 mucin biosynthesis in colonic goblet cells to contend metabolic stress. Am. J. Physiol. Gastrointest. Liver Physiol. 321, G489–G499. doi: 10.1152/ajpgi.00221.2021
Venanzi, F. M., Gabai, V., Mariotti, F., Magi, G. E., Vullo, C., Sufianov, A. A., et al. (2019). p62-DNA-encoding plasmid reverts tumor grade, changes tumor stroma, and enhances anticancer immunity. Aging 11:10711. doi: 10.18632/aging.102486
Venanzi, F., Shifrin, V., Sherman, M. Y., Gabai, V., Kisilev, O., Komissarov, A., et al. (2013). Broad-spectrum anti-tumor and anti-metastatic DNA vaccine based on p62-encoding vector. Oncotarget 4, 1829–1835. doi: 10.18632/oncotarget.1397
Vincent, G., Novak, E. A., Siow, V. S., Cunningham, K. E., Griffith, B. D., Comerford, T. E., et al. (2020). Nix-mediated Mitophagy modulates mitochondrial damage during intestinal inflammation. Antioxid. Redox Signal. 33:1. doi: 10.1089/ars.2018.7702
Volynets, V., Rings, A., Bárdos, G., Ostaff, M. J., Wehkamp, J., and Bischoff, S. C. (2016). Intestinal barrier analysis by assessment of mucins, tight junctions, and α-defensins in healthy C57BL/6J and BALB/cJ mice. Tissue Barriers 4:e1208468. doi: 10.1080/21688370.2016.1208468
Wang, C.-S.-E., Li, W.-B., Wang, H.-Y., Ma, Y.-M., Zhao, X.-H., Yang, H., et al. (2018). VSL#3 can prevent ulcerative colitis-associated carcinogenesis in mice. World J. Gastroenterol. 24, 4254–4262. doi: 10.3748/wjg.v24.i37.4254
Wang, R., Moniruzzaman, M., Wong, K. Y., Wiid, P., Harding, A., Giri, R., et al. (2021). Gut microbiota shape the inflammatory response in mice with an epithelial defect. Gut Microbes 13, 1–18. doi: 10.1080/19490976.2021.1887720
Wei, L., Duan, L., Zhao, H., and Tai, J. (2022). Role of a mixed probiotic product, VSL#3, in the prevention and treatment of colorectal cancer. Eur. J. Pharmacol. 930:175152. doi: 10.1016/j.ejphar.2022.175152
Wong, C. C. M., Zhang, L., Wu, W. K. K., Shen, J., Chan, R. L. Y., Lu, L., et al. (2017). Cathelicidin-encoding Lactococcus lactis promotes mucosal repair in murine experimental colitis. J. Gastroenterol. Hepatol. 32, 609–619. doi: 10.1111/jgh.13499
Yu, Z., and Morrison, M. (2004). Improved extraction of PCR-quality community DNA from digesta and fecal samples. BioTechniques 36, 808–812. doi: 10.2144/04365ST04
Keywords: inflammatory bowel disease, probiotic bacteria, recombinant protein, immunomodulation, epithelial barrier, gut microbiota
Citation: Laguna JG, Freitas AS, Barroso FAL, De Jesus LCL, De Vasconcelos OAGG, Quaresma LS, Américo MF, Campos GM, Glória RA, Dutra JCF, Da Silva TF, Vital KD, Fernandes SO, Souza RO, dos Santos Martins FS, Ferreira E, Santos TM, Birbrair A, De Oliveira MFA, Faria AMC, Carvalho RDO, Venanzi FM, Le Loir Y, Jan G, Guédon É and Azevedo VAC (2024) Recombinant probiotic Lactococcus lactis delivering P62 mitigates moderate colitis in mice. Front. Microbiol. 15:1309160. doi: 10.3389/fmicb.2024.1309160
Edited by:
Irfan A. Rather, Yeungnam University, Republic of KoreaReviewed by:
Saurabh Kumar, Kerry Inc., United StatesXinran Xu, Niagara bottling LLC, United States
Neeraja Recharla, University of Texas Medical Branch at Galveston, United States
Copyright © 2024 Laguna, Freitas, Barroso, De Jesus, De Vasconcelos, Quaresma, Américo, Campos, Glória, Dutra, Da Silva, Vital, Fernandes, Souza, Martins, Ferreira, Santos, Birbrair, De Oliveira, Faria, Carvalho, Venanzi, Le Loir, Jan, Guédon and Azevedo. This is an open-access article distributed under the terms of the Creative Commons Attribution License (CC BY). The use, distribution or reproduction in other forums is permitted, provided the original author(s) and the copyright owner(s) are credited and that the original publication in this journal is cited, in accordance with accepted academic practice. No use, distribution or reproduction is permitted which does not comply with these terms.
*Correspondence: Vasco Ariston de Carvalho Azevedo, dmFzY29hcmlzdG9uQGdtYWlsLmNvbQ==