- 1Liver Disease Department of Integrative Medicine, Ningbo No. 2 Hospital, Ningbo, Zhejiang, China
- 2Cixi Biomedical Research Institute, Wenzhou Medical University, Ningbo, Zhejiang, China
- 3Liver Diseases Institute, Ningbo No. 2 Hospital, Ningbo, Zhejiang, China
- 4Key Laboratory of Diagnosis and Treatment of Digestive System Tumors of Zhejiang Province, Ningbo, Zhejiang, China
Non-alcoholic fatty liver disease (NAFLD) is a prevalent chronic liver condition observed globally, with the potential to progress to non-alcoholic steatohepatitis (NASH), cirrhosis, and even hepatocellular carcinoma. Currently, the US Food and Drug Administration (FDA) has not approved any drugs for the treatment of NAFLD. NAFLD is characterized by histopathological abnormalities in the liver, such as lipid accumulation, steatosis, hepatic balloon degeneration, and inflammation. Dysbiosis of the gut microbiota and its metabolites significantly contribute to the initiation and advancement of NAFLD. Bacteroides, a potential probiotic, has shown strong potential in preventing the onset and progression of NAFLD. However, the precise mechanism by which Bacteroides treats NAFLD remains uncertain. In this review, we explore the current understanding of the role of Bacteroides and its metabolites in the treatment of NAFLD, focusing on their ability to reduce liver inflammation, mitigate hepatic steatosis, and enhance intestinal barrier function. Additionally, we summarize how Bacteroides alleviates pathological changes by restoring the metabolism, improving insulin resistance, regulating cytokines, and promoting tight-junctions. A deeper comprehension of the mechanisms through which Bacteroides is involved in the pathogenesis of NAFLD should aid the development of innovative drugs targeting NAFLD.
Introduction
With the global surge in obesity, there has been a parallel increase in diseases related to obesity, such as type 2 diabetes mellitus (T2DM), fatty liver disease, and cardiovascular disease (Badmus et al., 2022). Non-alcoholic fatty liver disease (NAFLD) is characterized by the excessive accumulation of intrahepatic fat, known as hepatic steatosis, in the absence of heavy alcohol abuse (Hrncir et al., 2021). NAFLD covers a spectrum of diseases, ranging from non-alcoholic hepatic steatosis to non-alcoholic steatohepatitis (NASH), and in severe cases, advanced cirrhosis and hepatocellular carcinoma (Kumar et al., 2020). The incidence and prevalence of NAFLD have surged globally in recent years, mirroring the rise in the obese population. In 1990–2019, NAFLD affected approximately 30% of the global population, with regional variations ranging from 25% in Western Europe to 44% in Latin America (Younossi et al., 2023). Mounting evidence suggests that NAFLD is closely associated with metabolic diseases and cardiovascular diseases, including obesity, T2DM, hypertriglyceridemia, and hypertension (Lonardo et al., 2018). With its escalating morbidity and mortality rates, NAFLD has emerged as a major chronic liver disease also in China, imposing a substantial economic burden on society and necessitating extensive further research to understand and tackle it (Chen H. et al., 2022).
Non-alcoholic fatty liver disease (NAFLD) is recognized as a multifactorial disease resulting from complex interactions between genetic and environmental factors, with its pathogenesis not yet fully understood (Bibbo et al., 2018). Initially, the “two-hit” theory was proposed to explain the development of NAFLD (Zhang et al., 2018). However, this theory has been deemed outdated by some researchers, who instead advocate for the “multiple-hit” theory, positing that NAFLD involves various mechanisms influenced by the host susceptibility, environment, genetics, and gut microbiota. The concept of the gut–liver axis has garnered increasing attention, with research highlighting the intricate relationship between the intestine and liver diseases (Paolella et al., 2014). A recent study linked the pathogenesis of NAFLD to alterations in the gut microbiota (Lee et al., 2021). This view was reinforced by Hoyles et al. (2018), who observed that the transplantation of gut microbiota from NAFLD patients into germ-free mice led to hepatic steatosis and the emergence of gut microbiota with similar characteristics to those found in NAFLD patients, suggesting that the gut microbiota play a pivotal role in the development of NAFLD.
Probiotics play a crucial role in maintaining or restoring the homeostasis of gut microbiota, representing a key factor in alleviating NAFLD and preserving the host’s overall health status (Kaufmann et al., 2023). Among the identified probiotics, Lactobacillus and Akkermansia have demonstrated the ability to mitigate liver damage, metabolic abnormalities in the host, and disruptions in the gut microbiota caused by a high-fat diet (HFD) (Cani and de Vos, 2017; Arellano-Garcia et al., 2023). However, the cultivation of these two species is challenging, and their therapeutic efficacy may be influenced by environmental factors. In contrast, Bacteroides, as potentially beneficial organisms, exhibit greater stability and provide energy to adjacent bacteria (Zafar and Saier, 2021). Consequently, Bacteroides has emerged as a promising probiotic (Tan et al., 2019a).
Bacteroides, the predominant genus in the Bacteroidetes phylum, comprise Gram-negative bacteria that exhibit a rod-shaped morphology. They are characterized by their anaerobic nature, resistance to bile, lack of motility, and inability to form spores (Fernandez-Julia et al., 2021; Béchon and Ghigo, 2022). Bacteroides display rapid growth in diverse complex media, with chopped meats being the primary medium for their growth and storage. Notably, Bacteroides colonies possess a distinct smooth and rounded morphology, exhibiting frosted surfaces that range in color from a dull white to light beige (Bacic and Smith, 2008). Bacteroides fragilis (B. fragilis), a prevalent member of the Bacteroides genus, is commonly found in the human intestinal tract. When cultivated on Bacteroides bile esculin medium, B. fragilis hydrolyzes esculin, resulting in the formation of characteristic black colonies (Sun et al., 2019). It is important to note that Bacteroides do not undergo cell division in the presence of oxygen. Therefore, the key consideration in culturing Bacteroides lies in maintaining an anaerobic atmosphere (Bacic and Smith, 2008). Due to their ability to tolerate oxygen, ease of manipulation on laboratory benches, and relatively short generation time, Bacteroides serve as an ideal microbiota for investigating bacteria–host interactions (Béchon and Ghigo, 2022).
In recent years, researchers have proposed new treatments for NAFLD, such as bariatric surgery, endoscopic therapy, glucagon-like peptide 1 (GLP-1) receptor agonists, statins, vitamin E and fecal microbiota transplantation (Smits et al., 2013; Nguyen and Varela, 2017; Hadi et al., 2018; Azemawah et al., 2019; Doumas et al., 2019; Lefere et al., 2021; Mandour et al., 2022; Geerts and Lefere, 2023; Lee and Kim, 2023; Igi et al., 2024). Compared to these treatments, Bacteroides, a single-strain probiotic supplementation, is not only safe but also regulates the gut microbiota and treats the root cause of NAFLD (Carpi et al., 2022). We summarize the advantages and disadvantages of these treatment modalities in Table 1. Thus, Bacteroides have received increasing attention as it is now believed they may play a critical role in regulating hepatic metabolic processes. However, no review has yet summarized the interactions between Bacteroides and NAFLD. Consequently, in the present review, we elucidate the potential mechanisms underlying the therapeutic effects of Bacteroides, a major gut microbiota, in the treatment of NAFLD. This review offers a fresh perspective for NAFLD management.
Reduced abundance of Bacteroides in NAFLD
Multiple studies have revealed the therapeutic impact of Bacteroides in metabolic disorders, including obesity and T2DM (Yoshida et al., 2021; Chen Q. et al., 2022; Li Y. et al., 2022). Subsequent investigations into the gut microbiota have demonstrated that augmenting the abundance of Bacteroides in the intestinal tract can effectively mitigate the spectrum of pathological manifestations associated with NAFLD (Li et al., 2019; Liu et al., 2019). Consequently, the correlation between Bacteroides and NAFLD has emerged as a focal point of scientific inquiry.
Several researchers have reported an inverse correlation between Bacteroides and indicators of body weight, such as the waistline and body mass index, as well as serum lipids levels, including low-density lipoprotein, total cholesterol, and triglycerides (Zeng et al., 2019; Romualdo et al., 2022; Lin et al., 2023). However, the results of current clinical research on changes in the abundance of Bacteroides in NAFLD patients are conflicting. One clinical study found that the abundance of Bacteroides increased as NAFLD pathology progressed, indicating a potential association between Bacteroides and NAFLD severity (Boursier et al., 2016). Conversely, a study on obese patients with NAFLD showed a significant reduction in Bacteroides abundance (Jin and Xu, 2023). In another study, compared to 28 healthy controls, 39 patients with biopsy-proven NAFLD had a prominently lower abundance of Bacteroides (Da Silva et al., 2018). Similar trends were observed in children with NAFLD, whereby one study demonstrated a decrease in Bacteroides abundance compared to in the controls (Del Chierico et al., 2017). Additionally, a recent study using a mouse model of NAFLD induced by a Western diet found an inverse correlation between Bacteroides abundance and the progression of NAFLD (Romualdo et al., 2022). Overall, these findings support the notion of a reduced abundance of Bacteroides in patients with NAFLD.
Therapeutic role of Bacteroides in NAFLD
In healthy adults, Bacteroides constitute 20–80% of the gut microbiota, playing a crucial role as the dominant flora in the gut (Tan et al., 2019a). As prominent commensals and mutualists in the human gut, Bacteroides species have adapted to the gut and established a solid association with the human host (Wexler and Goodman, 2017). Bacteroides spp. possess polysaccharide utilization loci that enable them to metabolize polysaccharides that are not easily absorbed in the intestine, providing energy to adjacent bacteria (Zafar and Saier, 2021). This process helps maintain the balance of the gut microbiota. Bacteroides have attracted significant attention from scientists due to their remarkable adaptive properties, particularly their potential benefits.
In recent years, various effective drugs, such as glucose-lowering drugs, antioxidants, lipid-lowering agents, and natural bile acid treatments, have been used to treat NAFLD (Mantovani and Dalbeni, 2021). Interestingly, gut microbiota analysis has shown an increase in Bacteroides abundance after treatment with Raw Bowl Tea polyphenols, atorvastatin, Ganoderma meroterpene derivative, and the prebiotic inulin for NAFLD (Liu et al., 2019; Qiao et al., 2020; Aoki et al., 2021; Li H. et al., 2022). For instance, atorvastatin was found to reduce lipid levels, improve HFD-induced hepatic steatosis, and increase Bacteroides abundance (Li H. et al., 2022). In fa/fa rats, the oral administration of Ganoderma meroterpene derivative was shown to reduce de novo lipogenesis, reverse non-alcoholic hepatic steatosis, and enhance Bacteroides abundance (Qiao et al., 2020). Furthermore, the knockout of sirtuin 2, which has a preventive role against NAFLD, was associated with a decreased abundance of Bacteroides and aggravation of NAFLD (Li et al., 2023). Bacteroides has also demonstrated potential for treating ethanol- or drug-induced liver damage in several studies (Sangineto et al., 2022; Wang H. et al., 2022). These findings suggest that Bacteroides might have a therapeutic role against NAFLD.
Effects of Bacteroides on the liver
Non-alcoholic fatty liver disease (NAFLD) is characterized by histopathologic abnormalities in the liver, including lipid deposition, steatosis, hepatic balloon degeneration, and inflammation, which can lead to liver fibrosis (Marchisello et al., 2019). Bacteroides species have shown excellent metabolic regulation. Recent studies strongly indicate that administering Bacteroides uniformis (B. uniformis), Bacteroides vulgatus (B. vulgatus), Bacteroides acidifaciens (B. acidifaciens) and Bacteroides thetaiotaomicron (B. thetaiotaomicron) can alleviate various metabolic disorders, such as insulin resistance, adiposity, and steatosis, which are the main manifestations of metabolic disorders in NAFLD mice (Gauffin Cano et al., 2012; Yang et al., 2017; Lee et al., 2021; Xu et al., 2023; Li et al., 2024). Importantly, Bacteroides has also been shown to reduce liver inflammation by enhancing the immune function and to contribute to the alleviation of liver fibrosis (Fernández-Murga and Sanz, 2016; López-Almela et al., 2021).
Effects of Bacteroides on the gut
Changes in gut microbiota and the gut barrier function can influence the development and progression of NAFLD, leading to elevated levels of endotoxin and increased permeability to bacterial endotoxins (Jin et al., 2016). However, numerous studies have demonstrated that Bacteroides can improve gut homeostasis by ameliorating gut microbiota disorders and regulating the tight-junction (TJ) proteins (El Kaoutari et al., 2013; Lopez-Siles et al., 2017; Nagpal et al., 2018; López-Almela et al., 2021). Moreover, Bacteroides influence the levels of various metabolites, including short-chain fatty acids (SCFAs), branched-chain amino acids (BCAAs), and taurine, that are key for the absorption of lipid-soluble foods, maintaining the integrity of the gut barrier to prevent the translocation of bacteria, and regulating glucolipid metabolism. These metabolites have emerged as crucial factors in attempts at regulation of the pathological processes of NAFLD (Ji et al., 2019; Yoshida et al., 2021; Xu et al., 2023; Zhou et al., 2023). Based on the available evidence, some Bacteroides strain may alleviate NAFLD. Additional details are provided in Table 2.
Underlying mechanisms of Bacteroides’ action in NAFLD
In recent years, there has been significant research on the role of Bacteroides in NAFLD. However, the precise mechanisms by which Bacteroides act remain unclear. NAFLD is strongly associated with hepatic steatosis, inflammation, and intestinal dysfunction. Existing evidence suggests that Bacteroides can prevent NAFLD by mitigating hepatic steatosis (Figure 1) and liver inflammation (Figure 2), and enhancing the intestinal barrier function (Figure 3).
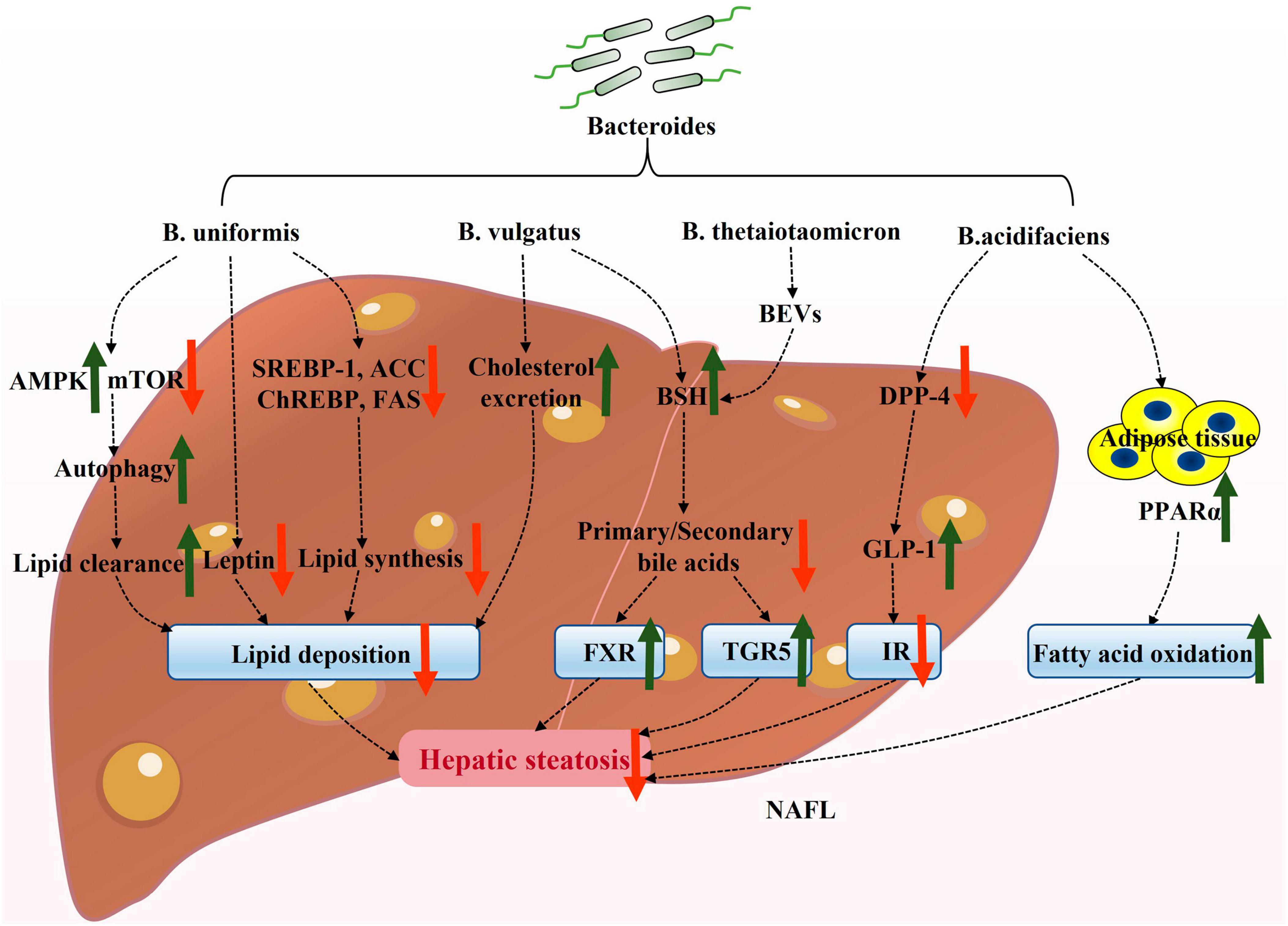
Figure 1. Potential mechanisms underlying the attenuation of hepatic steatosis by Bacteroides in NAFLD. Bacteroides and its active ingredient, bacterial extracellular vesicles (BEVs), alleviate hepatic steatosis and associated insulin resistance. AMPK, adenosine monophosphate-activated protein kinase; mTOR, mechanistic target of rapamycin; SREBP-1, sterol regulatory element-binding protein-1; ChREBP, carbohydrate response element-binding protein; ACC, acetyl-CoA carboxylase; FAS, fatty acid synthase; BEVs, bacterial extracellular vesicles; BSH, bile salt hydrolase; TGR5, takeda G protein-coupled receptor 5; FXR, farnesoid X receptor; DPP-4, dipeptidyl peptidase-4; GLP-1, glucagon-like peptide-1; IR, insulin resistance; PPARα, peroxisome proliferator-activated receptor α.
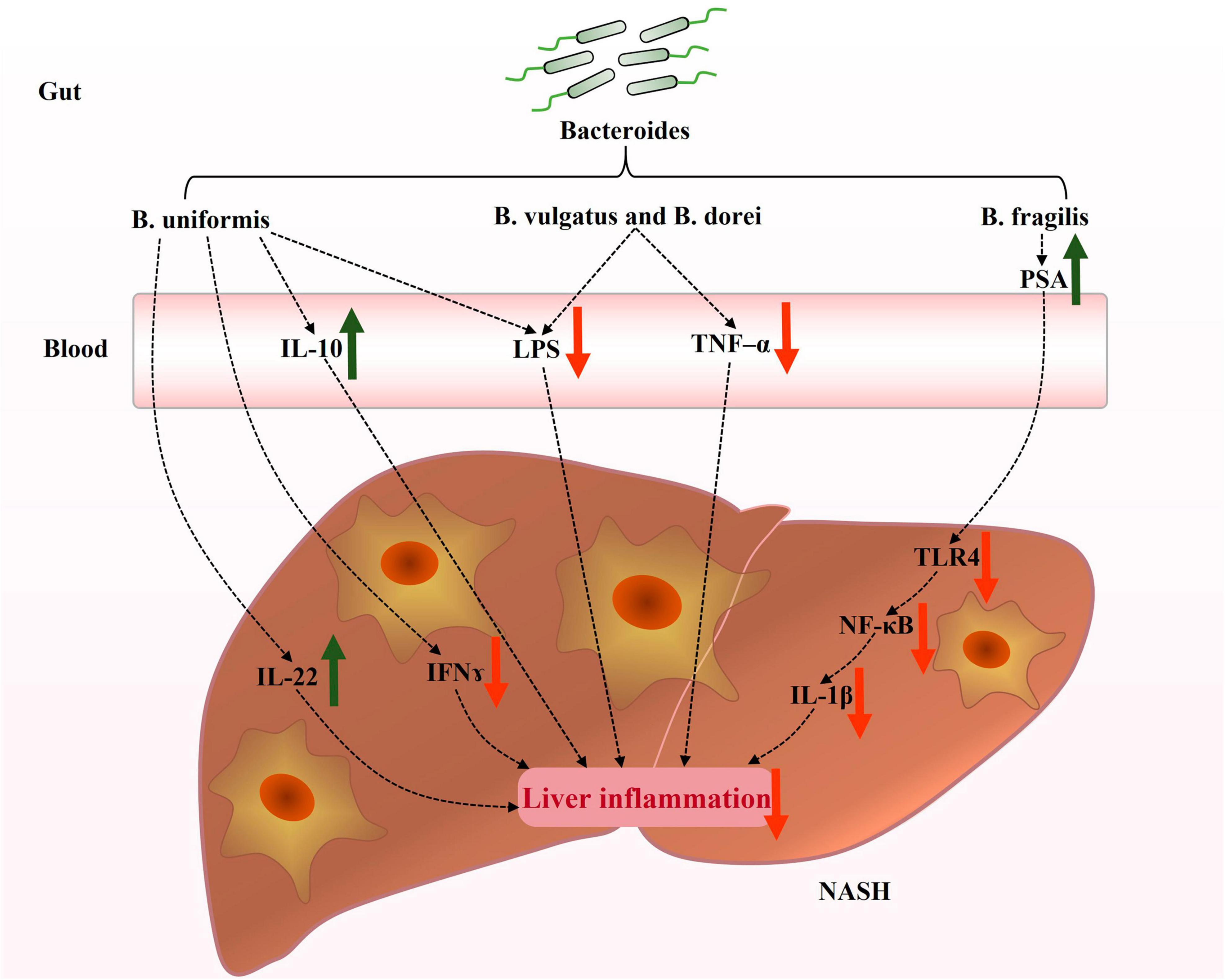
Figure 2. Potential mechanisms contributing to the prevention of hepatic inflammation by Bacteroides in NAFLD. Bacteroides regulated inflammation and immune response in the livers of NAFLD patients. The current focus on the mechanisms underlying the improvement of hepatic inflammation by Bacteroides primarily revolves around the production of inflammatory factors and LPS and TLR4 signaling. IL-22, interleukin-22; IFN-γ, interferon-γ; IL-10, interleukin-10; LPS, lipopolysaccharide; TNF-α, tumor necrosis factor-α; PSA, polysaccharide A; TLR4, toll-like receptor 4; NF-κB, nuclear factor-κB; IL-1β, interleukin-1β.
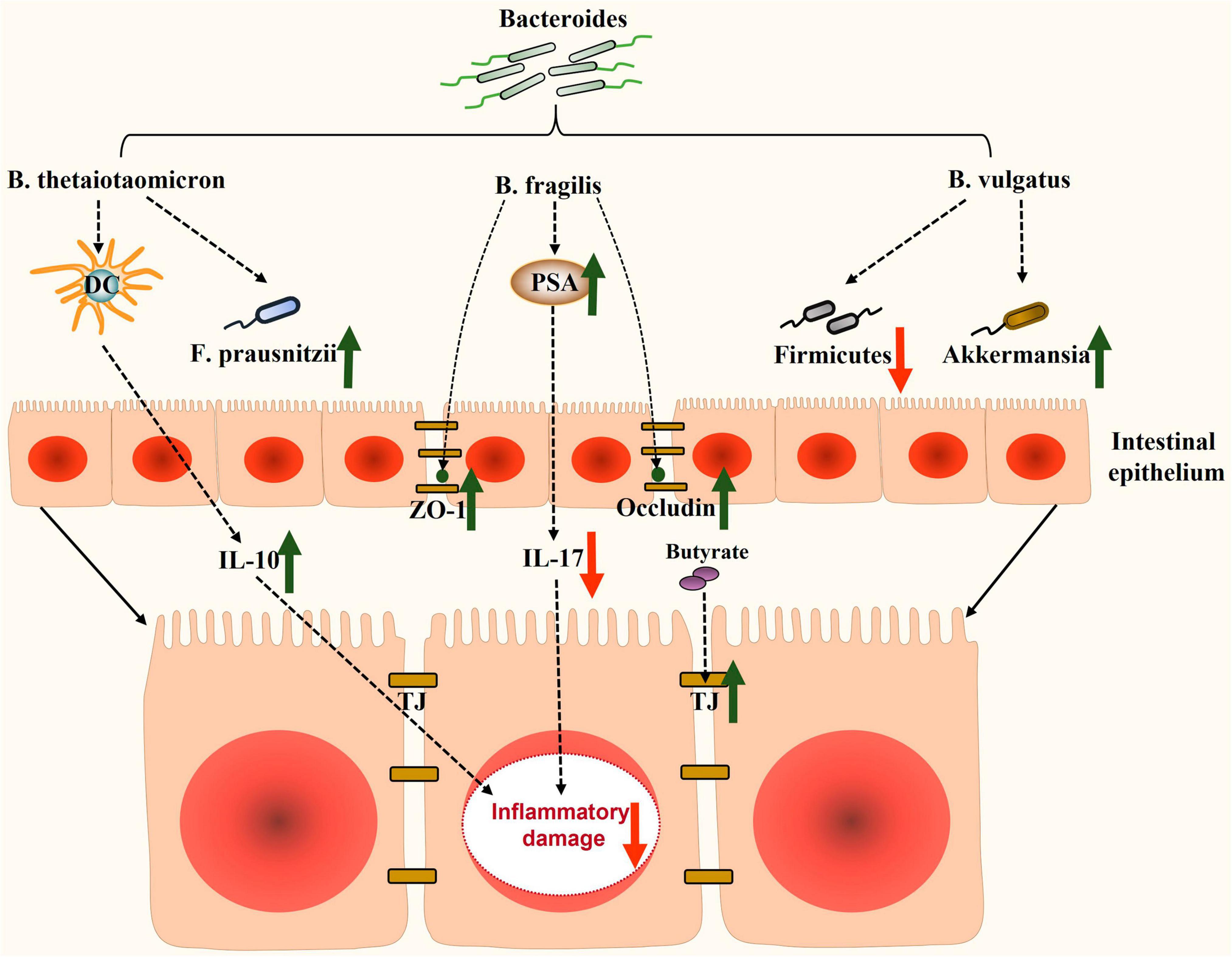
Figure 3. Potential mechanisms involved in enhancing the intestinal barrier function by Bacteroides in NAFLD. Bacteroides and its metabolites enhance the barrier function of the intestine through modulating intestinal inflammatory factors, improving the expression of tight-junction proteins, and restoring intestinal dysbiosis. DC, dendritic cells; F. prausnitzii, Faecalibacterium prausnitzii; IL-10, interleukin-10; ZO-1, zonula occludens-1; PSA, polysaccharide A; IL-17, interleukin 17; TJ, tight-junction.
Hepatic steatosis and Bacteroides
The excessive deposition of free fatty acids is a major contributor to the initiation of NAFLD. The excessive accumulation of free fatty acids in the liver promotes the progression from hepatic steatosis to NASH and cirrhosis through the generation of reactive oxygen species (Li et al., 2019). Interestingly, the transition from hepatic steatosis to inflammation is triggered by imbalances in the adipocytokines and lipotoxicity (Khan and Newsome, 2018). Therefore, effective treatment for NAFLD involves improving steatosis and its associated metabolic dysfunction.
Reducing lipid deposition
Liver lipid deposition is primarily caused by excessive fat production or impaired fatty acid oxidation (FAO) (Ipsen et al., 2018). De novo lipogenesis refers to the process of synthesizing saturated fatty acids from acetyl coenzyme A, derived from glycolysis (Ipsen et al., 2018). This process is regulated by multiple transcription factors, such as sterol regulatory element-binding protein-1 (SREBP-1) activated by insulin, and carbohydrate response element-binding protein (ChREBP) activated by glucose, which are critical regulators of hepatic lipogenesis (Sanders and Griffin, 2016). SREBP-1 and ChREBP also regulate the expression of hepatic lipid synthesis-related genes, including acetyl-CoA carboxylase (ACC), stearoyl-CoA desaturase 1 (SCD1), and fatty acid synthase (FAS) (Postic et al., 2007; Mu et al., 2020).
In obese ob/ob mice, the expressions of SREBP-1c and ChREBP were found to be significantly increased in the liver, and it was reported that reducing the levels of either factor could contribute to the attenuation of hepatic steatosis (Dentin et al., 2006). In a mouse model of HFD-induced NAFLD, supplementation with B. uniformis significantly downregulated the expression of hepatic lipogenesis-related proteins, such as SREBP-1, ChREBP, ACC, FAS, and leptin; thereby alleviating the progression of NAFLD (Lee et al., 2021). Another study also supported this point (López-Almela et al., 2021). In rats with metabolic dysfunction induced by HFD, the oral administration of B. vulgatus significantly reduced body weight gain, improved the lipid profile, promoted cholesterol excretion, and enhanced lipid homeostasis (Xu et al., 2023).
Bacteroides also appear to actively contribute to FAO. Peroxisome proliferator-activated receptor α (PPARα) is a key receptor involved in FAO, and its activation has been shown to reduce hepatic steatosis in obese ob/ob mice (Gao et al., 2015). Interestingly, research found that B. acidifaciens prevented obesity in mice by upregulating PPARα (Yang et al., 2017). Additionally, HFD-induced mice treated with B. uniformis showed an increased activation of adenosine monophosphate-activated protein kinase (AMPK) and a reduced expression of the mechanistic target of rapamycin (mTOR) (Lee et al., 2021). Yan et al. (2022) found that the activation of AMPK and the inactivation of mTOR initiated autophagy to clear hepatic lipids. Therefore, it was hypothesized that B. uniformis may induce autophagy through the AMPK/mTOR signaling pathway to regulate lipid homeostasis, thus promoting fat removal.
Bacteroides have been found to significantly modulate lipid metabolism in animal models (Lee et al., 2021; López-Almela et al., 2021; Li H. et al., 2022; Xu et al., 2023). These studies indicate that Bacteroides ultimately reduce the accumulation of fat in the liver via inhibiting SREBP-1 and ChREBP expression, as well as related adipogenic genes, and by promoting FAO-related pathways. This suggests that several Bacteroides spp., such as B. uniformis, B. vulgatus, and B. acidifaciens, play a critical role in reducing lipid deposition.
Improving insulin resistance
Insulin resistance is a key factor in the development of NAFLD. Impaired insulin signaling in adipose tissue leads to excessive fatty acids transport to the liver via dysregulated lipolysis, contributing to the development of NASH (Lomonaco et al., 2012; Lian et al., 2020). Insulin-dependent lipogenesis is another crucial mechanism in NAFLD (Smith et al., 2020). Studies have demonstrated the protective role of Bacteroides against insulin resistance related to steatosis and the accumulation of lipids in the liver. Some studies have shown that the beneficial effects of Bacteroides on insulin resistance were partly due to increased insulin levels in the body or the alleviation of metabolic endotoxemia (Rao et al., 2016; Lee et al., 2021).
In 2017, a study conducted by Yang et al. (2017) demonstrated that the oral administration of B. acidifaciens resulted in decreased blood glucose levels and increased insulin levels in mice fed a HFD. Subsequently, the authors further investigated the effect of B. acidifaciens in regulating insulin resistance. Importantly, the oral administration of B. acidifaciens or its culture supernatant led to a reduction in the levels of dipeptidyl peptidase-4 (DPP-4) in the ileum of the small intestine (Yang et al., 2017). DPP-4 is an enzyme known for degrading GLP-1. These findings suggest that B. acidifaciens may inhibit the activity of the DPP-4 enzyme, leading to the activation of GLP-1 and a subsequent improvement in glucose tolerance and insulin sensitivity. This evidence strongly suggests that B. acidifaciens may have the potential to alleviate hepatic steatosis by improving insulin resistance.
Regulating bile acid metabolism
Bacteroides spp. are prominent Gram-negative bacteria that produce bacterial extracellular vesicles (BEVs), a novel secretion system capable of transporting a wide range of chemically diverse cargoes, including lipids, membrane-embedded and associated proteins, peptidoglycan, and nucleic acids (Perez-Cruz et al., 2015; Rakoff-Nahoum et al., 2016; Cecil et al., 2019; McMillan and Kuehn, 2021). Studies have revealed that BEVs produced by B. thetaiotaomicron in the gastrointestinal tract were enriched with specific proteins and enzymes, such as dipeptidyl peptidases, bile salt hydrolase (BSH), and asparaginase, thus influencing the host cell’s biosynthetic pathways (Stentz et al., 2022). BSH activity is a gut microbial process that significantly impacts the host’s local (gastrointestinal) and systemic (hepatic) functions (Joyce et al., 2014b). Controlled experimental systems indicated that bacterial BSH activity had a profound effect on the host bile acid profiles, improving conditions like hypercholesterolemia and obesity, thus serving as a crucial mechanism by which microbiota regulate the host lipid metabolism (Joyce et al., 2014a).
Bacteroides vulgatus has been shown to exhibit BSH activity (Xu et al., 2023). BSH catalyzes the deconjugation of bile acids (BAs), liberating primary BAs and glycine/taurine moieties (Horáčková et al., 2018). Coincidentally, taurine, produced through deconjugation, has also been reported to play a role in physiological processes, such as antioxidant defense, immune regulation, and lipid homeostasis, thereby blunting the progression of NAFLD (Lambert et al., 2015; Kim et al., 2022). Subsequently, a portion of the primary BAs is affected by the gut microbiota through processes like dehydroxylation, dehydrogenation, and sulfation, resulting in the production of secondary BAs (Foley et al., 2019). An imbalance in the ratio of primary to secondary bile acids is a significant factor in accelerating NAFLD. The BSH enzyme produced from B. vulgatus restores this imbalance and regulates the host metabolism by activating farnesoid X receptor (FXR) and takeda G protein-coupled receptor 5 (TGR5) (Arab et al., 2017; Jiao et al., 2018; Pathak et al., 2018). As mentioned earlier, the ratio of primary to secondary bile acids is regulated by BSH. Therefore, we hypothesize that Bacteroides activate the expression of FXR and TGR5 by modulating the bile acids ratio through BSH activity, and by reducing hepatic steatosis.
Collectively, current evidence confirms the role of Bacteroides and its components in metabolic dysfunction associated with steatosis (Figure 1), which may partially explain the mechanism of action of Bacteroides in improving NAFLD.
Liver inflammation and Bacteroides
NAFLD is a manifestation of liver-related metabolic syndrome. Numerous studies have demonstrated there are alterations of the immune microenvironment during the development of NAFLD (Ilan, 2013; Huby and Gautier, 2022). The gut microbiota plays various roles in the intestinal barrier function, immunity, and metabolism by mediating different forms of host responses. The gut microbiota with its various metabolites influence immune homeostasis and liver metabolism, thereby impacting the progression of NAFLD (Kolodziejczyk et al., 2019; D’Alessandro et al., 2020). An association between low levels of Bacteroides and liver damage in alcoholic fatty liver disease has also been established (Ferrere et al., 2017). Further research on Bacteroides has revealed its essential role in regulating hepatic inflammation in NAFLD.
Regulating cytokines in the liver
Interferon-γ (IFN-γ) and tumor necrosis factor-α (TNF-α) are recognized as pro-inflammatory cytokines that play crucial roles in the pathophysiology of NAFLD. These factors recruit inflammatory cells and/or activate macrophages, leading to a hepatic inflammatory response (Tilg, 2010; Li et al., 2021). Importantly, one study found that mice treated with B. vulgatus and Bacteroides dorei (B. dorei) exhibited significantly reduced serum TNF-α levels (Yoshida et al., 2018). In obese mice, B. uniformis increased the expression of anti-inflammatory interleukin-22 (IL-22) and decreased the production of pro-inflammatory IFN associated with high-fat, high-sugar diet-induced liver damage, contributing to a greater balance of the hepatic immune status (López-Almela et al., 2021). Notably, IL-22 has also shown a protective response to liver tissue injury, attenuating the inflammatory response in NAFLD models, and also alleviating oxidative stress, endoplasmic reticulum stress, and apoptosis (Zai et al., 2021; Abdelnabi et al., 2022).
Furthermore, Bacteroides also exert a protective effect on the liver. The administration of B. uniformis increased interleukin-10 (IL-10) levels in mice fed a HFD (Gauffin Cano et al., 2012). Several studies have found an association between NAFLD severity and serum IL-10 levels reduction, suggesting the involvement of IL-10 in immune regulation in the liver (den Boer et al., 2006; Paredes-Turrubiarte et al., 2016). These findings suggest that Bacteroides modulate the hepatic cytokines related to immune regulation (such as TNF-α, IL-22, IFN-γ, and IL-10) and downregulate the hepatic inflammatory responses (Figure 2).
Inhibiting gut-derived LPS and TLR4 signaling
Lipopolysaccharide (LPS), a typical pathogen-associated molecular pattern, mediates inflammatory injury in hepatocytes and has been found to induce inflammatory liver injury through the downstream toll-like receptor 4 (TLR4) signaling pathway (Qi et al., 2020; An et al., 2022). LPS is a crucial factor in the induction of inflammatory responses in the liver and is expressed abundantly in patients with NASH (Carpino et al., 2020). Treatment with B. vulgatus and B. dorei has been reported to reduce circulating LPS levels via strengthening the gut barrier function (Yoshida et al., 2018). Additionally, B. uniformis reduces the expression of acyl carrier protein, a key component required for LPS biosynthesis (Wang et al., 2021b). Polysaccharide A (PSA), an immunogenic component of B. fragilis capsules, inhibited the TLR4/nuclear factor-κB (NF-κB) signaling pathway and suppressed the downstream inflammatory factor interleukin-1β (IL-1β) in a model of LPS-induced liver inflammation (Wang et al., 2021c). These findings suggest that the potential mechanism by which Bacteroides attenuate liver inflammation is by modulating LPS and TLR4 signaling in NAFLD (Figure 2).
In a safety assessment, B. uniformis reduced inducible nitric oxide synthase (iNOS) expression in the liver, which was increased in the livers of immunosuppressed mice (Fernández-Murga and Sanz, 2016). iNOS was demonstrated to be one of the key enzymes involved in producing nitric oxide (NO) from the amino acid L-arginine, while excessive NO production has been associated with the etiology of many liver diseases, such as liver fibrosis (Iwakiri, 2015). This finding indicated that Bacteroides might possess protective abilities against liver fibrosis. However, further studies are still needed to confirm Bacteroides’ effect on NAFLD progression to fibrosis and its exact functional mechanism.
Intestinal barrier function and Bacteroides
The gut–liver axis facilitates bidirectional communication between the biliary tract, portal vein, and systemic circulation. The intestinal barrier serves as the first line of defense against endotoxin-induced liver damage. Disruption of the intestinal barrier function, caused by factors such as intestinal barrier damage, inflammation, or microbial imbalances, can lead to increased intestinal permeability. This can allow endotoxins to translocate into the bloodstream and disseminate to the liver, thereby promoting the progression of NAFLD to NASH (Wigg et al., 2001; Mouries et al., 2019; Hrncir et al., 2021). Several theories support the protective effect of Bacteroides in maintaining the integrity of the intestinal barrier.
Promoting tight-junctions in the gut
The intestinal epithelial barrier function relies on the TJs of intestinal epithelial cells. These TJs consist of various tight-junction proteins, including zonula occludens-1 (ZO-1) and occludin, which play crucial roles in regulating intestinal permeability (Wigg et al., 2001). B. fragilis, for instance, promotes the expression of TJ proteins, such as ZO-1 and occludin (Wang et al., 2021a; Zhou et al., 2022). Bacteroides spp. also promote the fermentation of polysaccharides in the intestine, resulting in the production of SCFAs, like acetate, propionate, and butyrate (El Kaoutari et al., 2013). Butyrate, in particular, helps maintain the intestinal barrier function by providing essential energy to the epithelium and upregulating TJ protein expression (Donohoe et al., 2011; Nagpal et al., 2018). Additionally, butyrate exhibits anti-inflammatory, metabolic modulating, and antioxidant effects that contribute to the amelioration of NAFLD (Amiri et al., 2022).
Bacteroides also contribute to preservation of the mucus and microbial barrier of the gut. B. thetaiotaomicron, for example, maintains the function of the intestinal mucus barrier by inducing the expression of the mucin genes muc2 and muc4 and by increasing the number of goblet cells (Wrzosek et al., 2013). Moreover, Bacteroides play a role in constructing a biological barrier that protects the host from pathogens, like LPS-producing Proteobacteria (Wang L. et al., 2022).
Alleviating inflammatory damage in the gut
Bacteroides also play a significant role in maintaining gut immune homeostasis, including the regulation of IL-10 as well as T helper cell 17 (Th17). For instance, B. thetaiotaomicron promotes the response of mucosal dendritic cells and contributes to the expression of IL-10 levels (Durant et al., 2020; Fonseca et al., 2022). Research has shown that B. fragilis PSA suppressed the pro-inflammatory response, thus preventing colitis by inhibiting Th17 cells (Troy and Kasper, 2010). Th17 cells are known to secrete pro-inflammatory cytokines, such as interleukin 17 (IL-17), interleukin 21 (IL-21), and TNF-α (Chen and O’Shea, 2008). Interestingly, IL-17 significantly contributes to the major comorbidity of NAFLD, specifically early atherosclerosis, which was confirmed in a study involving obese NAFLD patients (Tarantino et al., 2014). Furthermore, studies have demonstrated that Bacteroides ovatus (B. ovatus) and B. fragilis alleviate LPS-related intestinal inflammation by restoring Treg/Th17 homeostasis or regulating cytokine production (Tan et al., 2019b). Additionally, SCFAs inhibited the NF-κB pathway and LPS-induced NOD-like receptor family pyrin domain containing 3 (NLRP3) activity, thereby reducing intestinal inflammatory injury (Feng et al., 2018).
Restoring the gut microbiota homeostasis
Long-term consumption of a HFD disrupts the balance of the gut microbiota, promoting the progression of NAFLD. Restoring gut microbiota homeostasis has been identified as a key target for NAFLD treatment, and various therapeutic modalities, such as fecal microbial transplantation and next-generation probiotics, have been proposed as treatments (Rotman and Sanyal, 2017; Campagnoli et al., 2022; Riezu-Boj et al., 2022; Maestri et al., 2023; Singh et al., 2023). Bacteroides play a crucial role in regulating gut microbiota homeostasis.
Firmicutes have been reported to be associated with the acquisition of more energy (Turnbaugh et al., 2006). An increase in Firmicutes was found to be one of the identified features of the gut microbiome in NAFLD model mice (Romualdo et al., 2022). B. vulgatus was found to decrease the levels of Firmicutes at the phylum level and reshape the imbalanced gut microbiota induced by HFD (Xu et al., 2023). In mice with obesity, the administration of B. uniformis effectively restored the gut microbiota induced by a high-fat, high-sugar diet (López-Almela et al., 2021). Another study showed that Bacteroides participated in the production of BCAAs in the colon (Diether and Willing, 2019), which promoted beneficial intestinal bacteria growth, further enhancing the protective effect of the intestinal barrier (Zhou et al., 2018).
In a study conducted in 2023, researchers discovered that Faecalibacterium prausnitzii (F. prausnitzii) exhibited a beneficial effect on liver inflammation and hepatic steatosis in NASH mice (Shin et al., 2023). It was observed that F. prausnitzii may engage in a mutually beneficial relationship with certain Bacteroides species. Heinken et al. (2014) demonstrated that the production of acetate by B. thetaiotaomicron facilitated the growth of F. prausnitzii. Furthermore, B. thetaiotaomicron is capable of fermenting pectin to release pectin derivatives that can be utilized by F. prausnitzii (Lopez-Siles et al., 2017). Interestingly, You et al. (2023) revealed that B. vulgatus restored the depletion of Akkermansia induced by a HFD. Akkermansia, known for its ability to improve metabolic disorders in humans, is negatively impacted by mucin deficiency (Van Herreweghen et al., 2018). The study by You et al. (2023) further demonstrated that a metabolite called N-acetyl-D-glucosamine (GlcNAc), induced by B. vulgatus, had a mucin-stimulating effect, effectively reversing the decline of Akkermansia in mice fed a HFD. Additionally, B. vulgatus serves as a provider of nutrients, supplying microbiota-accessible carbohydrates, such as mucin glycans, to maintain microbial homeostasis, particularly with Lactobacilli and Bifidobacteria, which play a crucial role in the mucus integrity, indirectly reinforcing the cooperative network with Akkermansia (You et al., 2023; Zhang et al., 2023). Therefore, it can be inferred that the therapeutic effects of Bacteroides in NAFLD are partially mediated by increasing the abundance of beneficial flora in the gut. In conclusion, Bacteroides contribute to the enhancement of the intestinal barrier function by modulating the epithelial, mucosal, and immune components, as well as the microbial ecosystem (Figure 3).
Conclusion and future perspectives
Recent findings have unveiled that gut microbiota dysbiosis serves as a risk factor that can exert an influence on and contribute to the pathogenesis of NAFLD. Based on the aforementioned in vivo studies, Bacteroides not only rectifies the dysbiosis of the gut microbiota but also mitigates NAFLD by modulating metabolic and inflammatory factors, while enhancing intestinal barrier functions. Consequently, Bacteroides exhibits the potential to be a novel therapeutic modality for NAFLD.
Bacteroides has been discovered to possess a remarkable ameliorating effect on NAFLD, thus paving the way for its prospective application in clinical settings. Reasonable assumptions regarding the clinical utilization of Bacteroides have been formulated based on animal experiments and clinical studies involving other probiotics. For the recommended clinical dosage of Bacteroides, we hypothesized that a daily dose ranging from 2 × 108 to 1 × 1010 colony forming units (CFU) (Silva-Sperb et al., 2019; Laue et al., 2023) is suitable for healthy adults. This dosage range is considered safe and effective. Similar to most probiotic delivery methods, Bacteroides can be administered through colony transplantation, oral formulations, injections, and enteric capsules. Among these modalities, we favor the use of enterosoluble capsules, as they not only protect the flora from gastric acid but also enhance portability, thereby improving patient adherence. In summary, Bacteroides is deemed beneficial, however, potential risks may arise, such as exacerbation of intestinal flora disorders leading to abdominal pain and diarrhea as well as allergic reactions triggered by sensitivities to specific ingredients.
There are still some questions that need to be addressed regarding the involvement of Bacteroides in the regulation of NAFLD. First, although the immune regulatory effects of Bacteroides in the liver have been demonstrated, the specific mechanisms by which Bacteroides and its active ingredients participate in immune status regulation in the liver have yet to be elucidated and require further exploration in future studies. Second, Bacteroides encompass numerous species, including B. uniformis, B. fragilis, B. vulgatus, B. thetaiotaomicron, and others mentioned in the text. These species play distinct roles in various aspects of NAFLD development, and it remains unclear whether their combined use would yield superior outcomes. The combination of probiotics is widely employed in clinical practice. Therefore, future experiments could employ germ-free mice to evaluate the effects of combinations of flora. Last, the current experimental findings are primarily based on in vitro studies or animal models, thus lacking substantial clinical evidence. By constructing relevant clinical cohorts, clinical studies combined with fecal microbiota transplantation could be conducted to validate the efficacy and safety of Bacteroides in human NAFLD treatment.
In summary, Bacteroides and its metabolism play a therapeutic role in NAFLD by ameliorating hepatic steatosis, insulin resistance, and dysbiosis. More clinical studies in the future will promote the clinical application of Bacteroides in NAFLD.
Author contributions
JuZ: Writing – original draft, Writing – review & editing. JiZ: Investigation, Writing – review & editing. ZH: Investigation, Writing – review & editing. HL: Conceptualization, Funding acquisition, Writing – review & editing.
Funding
The authors declare that financial support was received for the research, authorship, and/or publication of this article. This work was supported by the Zhejiang Provincial Natural Science Foundation of China under Grant No. LY23H290004, the Ningbo Natural Science Foundation, Project ID: 2022J242, the Major Special Science and Technology Project of Ningbo City (#2022Z128), National Administration of Traditional Chinese Medicine - Zhejiang Provincial Administration of Traditional Chinese Medicine Joint Construction Technology Plan (GZY-ZJ-KJ-23092), Zhejiang Province Traditional Chinese Medicine Science and Technology Plan (2022ZB332), and the Project of NINGBO Leading Medical and Health Discipline, Project Number: 2022Z01.
Acknowledgments
We thank Medjaden Inc. for scientific editing of this manuscript.
Conflict of interest
The authors declare that the research was conducted in the absence of any commercial or financial relationships that could be construed as a potential conflict of interest.
Publisher’s note
All claims expressed in this article are solely those of the authors and do not necessarily represent those of their affiliated organizations, or those of the publisher, the editors and the reviewers. Any product that may be evaluated in this article, or claim that may be made by its manufacturer, is not guaranteed or endorsed by the publisher.
Abbreviations
NAFLD, non-alcoholic fatty liver disease; NASH, non-alcoholic steatohepatitis; T2DM, type 2 diabetes mellitus; B. fragilis, Bacteroides fragilis; HFD, high-fat diet; GLP-1, glucagon-like peptide-1; B. uniformis, Bacteroides uniformis; B. vulgatus, Bacteroides vulgatus; B. acidifaciens, Bacteroides acidifaciens; B. thetaiotaomicron, Bacteroides thetaiotaomicron; TJ, tight-junction; SCFAs, short-chain fatty acids; BCAAs, branched-chain amino acids; FAO, fatty acid oxidation; SREBP-1, sterol regulatory element-binding protein-1; ChREBP, carbohydrate response element-binding protein; ACC, acetyl-CoA carboxylase; SCD1, stearoyl-CoA desaturase 1; FAS, fatty acid synthase; PPAR α , peroxisome proliferator-activated receptor α ; AMPK, adenosine monophosphate-activated protein kinase; mTOR, mechanistic target of rapamycin; DPP-4, dipeptidyl peptidase-4; BEVs, bacterial extracellular vesicles; BSH, bile salt hydrolase; BAs, bile acids; FXR, farnesoid X receptor; TGR5, takeda G protein-coupled receptor 5; IFN- γ , interferon- γ ; TNF- α , tumor necrosis factor- α ; B. dorei, Bacteroides dorei; IL-22, interleukin-22; IL-10, interleukin-10; LPS, lipopolysaccharide; TLR4, toll-like receptor 4; PSA, polysaccharide A; NF- κ B, nuclear factor- κ B; IL-1 β , interleukin-1 β ; iNOS, inducible nitric oxide synthase; NO, nitric oxide; ZO-1, zonula occludens-1; Th17, T helper cell 17; IL-17, interleukin 17; IL-21, interleukin 21; B. ovatus, Bacteroides ovatus; NLRP3, NOD-like receptor family pyrin domain containing 3; F. prausnitzii, Faecalibacterium prausnitzii; GlcNAc, N-acetyl -D-glucosamine; CFU, colony forming units.
References
Abdelnabi, M. N., Flores Molina, M., Soucy, G., Quoc-Huy Trinh, V., Bedard, N., Mazouz, S., et al. (2022). Sex-dependent hepatoprotective role of IL-22 receptor signaling in non-alcoholic fatty liver disease-related fibrosis. Cell Mol. Gastroenterol. Hepatol. 14, 1269–1294. doi: 10.1016/j.jcmgh.2022.08.001
Amiri, P., Arefhosseini, S., Bakhshimoghaddam, F., Jamshidi Gurvan, H., and Hosseini, S. A. (2022). Mechanistic insights into the pleiotropic effects of butyrate as a potential therapeutic agent on NAFLD management: a systematic review. Front. Nutr. 9:1037696. doi: 10.3389/fnut.2022.1037696
An, L., Wirth, U., Koch, D., Schirren, M., Drefs, M., Koliogiannis, D., et al. (2022). The role of gut-derived lipopolysaccharides and the intestinal barrier in fatty liver diseases. J. Gastrointest Surg. 26, 671–683. doi: 10.1007/s11605-021-05188-7
Aoki, R., Onuki, M., Hattori, K., Ito, M., Yamada, T., Kamikado, K., et al. (2021). Commensal microbe-derived acetate suppresses NAFLD/NASH development via hepatic FFAR2 signalling in mice. Microbiome 9:188. doi: 10.1186/s40168-021-01125-7
Arab, J. P., Karpen, S. J., Dawson, P. A., Arrese, M., and Trauner, M. (2017). Bile acids and nonalcoholic fatty liver disease: molecular insights and therapeutic perspectives. Hepatology 65, 350–362. doi: 10.1002/hep.28709
Arellano-Garcia, L., Trepiana, J., Martinez, J. A., Portillo, M. P., and Milton-Laskibar, I. (2023). Beneficial effects of viable and heat-inactivated Lactobacillus rhamnosus GG administration on oxidative stress and inflammation in diet-induced NAFLD in rats. Antioxidants 12:717. doi: 10.3390/antiox12030717
Azemawah, V., Movahed, M. R., Centuori, P., Penaflor, R., Riel, P. L., Situ, S., et al. (2019). State of the art comprehensive review of individual statins, their differences, pharmacology, and clinical implications. Cardiovasc. Drugs Ther. 33, 625–639. doi: 10.1007/s10557-019-06904-x
Bacic, M.K., and Smith, C.J. (2008). Laboratory maintenance and cultivation of bacteroides species. Curr. Protoc. Microbiol. Chapter 13, Unit 13C.11. doi: 10.1002/9780471729259.mc13c01s9
Badmus, O. O., Hillhouse, S. A., Anderson, C. D., Hinds, T. D., and Stec, D. E. (2022). Molecular mechanisms of metabolic associated fatty liver disease (MAFLD): functional analysis of lipid metabolism pathways. Clin. Sci. 136, 1347–1366. doi: 10.1042/CS20220572
Béchon, N., and Ghigo, J. M. (2022). Gut biofilms: Bacteroides as model symbionts to study biofilm formation by intestinal anaerobes. FEMS Microbiol. Rev. 46:fuab054. doi: 10.1093/femsre/fuab054
Bibbo, S., Ianiro, G., Dore, M. P., Simonelli, C., Newton, E. E., and Cammarota, G. (2018). Gut Microbiota as a driver of inflammation in nonalcoholic fatty liver disease. Mediators Inflamm. 2018:9321643. doi: 10.1155/2018/9321643
Boursier, J., Mueller, O., Barret, M., Machado, M., Fizanne, L., Araujo-Perez, F., et al. (2016). The severity of nonalcoholic fatty liver disease is associated with gut dysbiosis and shift in the metabolic function of the gut microbiota. Hepatology 63, 764–775. doi: 10.1002/hep.28356
Campagnoli, L. I. M., Marchesi, N., Vairetti, M., Pascale, A., Ferrigno, A., and Barbieri, A. (2022). Age-related NAFLD: the use of probiotics as a supportive therapeutic intervention. Cells 11:2827. doi: 10.3390/cells11182827
Cani, P. D., and de Vos, W. M. (2017). Next-generation beneficial microbes: the case of Akkermansia muciniphila. Front. Microbiol. 8:1765. doi: 10.3389/fmicb.2017.01765
Carpi, R. Z., Barbalho, S. M., Sloan, K. P., Laurindo, L. F., Gonzaga, H. F., Grippa, P. C., et al. (2022). The effects of probiotics, prebiotics and synbiotics in Non-Alcoholic Fat Liver Disease (NAFLD) and Non-Alcoholic Steatohepatitis (NASH): a systematic review. Int. J. Mol. Sci. 23:8805. doi: 10.3390/ijms23158805
Carpino, G., Del Ben, M., Pastori, D., Carnevale, R., Baratta, F., Overi, D., et al. (2020). Increased liver localization of lipopolysaccharides in human and experimental NAFLD. Hepatology 72, 470–485. doi: 10.1002/hep.31056
Cecil, J. D., Sirisaengtaksin, N., O’Brien-Simpson, N. M., and Krachler, A. M. (2019). Outer membrane vesicle-host cell interactions. Microbiol. Spectr. 7. doi: 10.1128/microbiolspec.PSIB-0001-2018
Chen, H., Zhan, Y., Zhang, J., Cheng, S., Zhou, Y., Chen, L., et al. (2022). The global, regional, and national burden and trends of NAFLD in 204 countries and territories: an analysis from global burden of disease 2019. JMIR Public Health Surveill. 8:e34809. doi: 10.2196/34809
Chen, Q., Wang, D., Gu, Y., Jiang, Z., and Zhou, Z. (2022). Tangeretin prevents obesity by modulating systemic inflammation, fat browning, and gut microbiota in high-fat diet-induced obese C57BL/6 mice. J. Nutr. Biochem. 101:108943. doi: 10.1016/j.jnutbio.2022.108943
Chen, Z., and O’Shea, J. J. (2008). Th17 cells: a new fate for differentiating helper T cells. Immunol. Res. 41, 87–102. doi: 10.1007/s12026-007-8014-9
Da Silva, H. E., Teterina, A., Comelli, E. M., Taibi, A., Arendt, B. M., Fischer, S. E., et al. (2018). Nonalcoholic fatty liver disease is associated with dysbiosis independent of body mass index and insulin resistance. Sci. Rep. 8:1466. doi: 10.1038/s41598-018-19753-9
D’Alessandro, G., Antonangeli, F., Marrocco, F., Porzia, A., Lauro, C., Santoni, A., et al. (2020). Gut microbiota alterations affect glioma growth and innate immune cells involved in tumor immunosurveillance in mice. Eur. J. Immunol. 50, 705–711. doi: 10.1002/eji.201948354
Del Chierico, F., Nobili, V., Vernocchi, P., Russo, A., De Stefanis, C., Gnani, D., et al. (2017). Gut microbiota profiling of pediatric nonalcoholic fatty liver disease and obese patients unveiled by an integrated meta-omics-based approach. Hepatology 65, 451–464. doi: 10.1002/hep.28572
den Boer, M. A., Voshol, P. J., Schröder-van, der Elst, J. P., Korsheninnikova, E., Ouwens, D. M., et al. (2006). Endogenous interleukin-10 protects against hepatic steatosis but does not improve insulin sensitivity during high-fat feeding in mice. Endocrinology 147, 4553–4558. doi: 10.1210/en.2006-0417
Dentin, R., Benhamed, F., Hainault, I., Fauveau, V., Foufelle, F., Dyck, J. R., et al. (2006). Liver-specific inhibition of ChREBP improves hepatic steatosis and insulin resistance in ob/ob mice. Diabetes 55, 2159–2170. doi: 10.2337/db06-0200
Diether, N. E., and Willing, B. P. (2019). Microbial fermentation of dietary protein: an important factor in diet–microbe–host interaction. Microorganisms 7:19. doi: 10.3390/microorganisms7010019
Donohoe, D. R., Garge, N., Zhang, X., Sun, W., O’Connell, T. M., Bunger, M. K., et al. (2011). The microbiome and butyrate regulate energy metabolism and autophagy in the mammalian colon. Cell Metab. 13, 517–526. doi: 10.1016/j.cmet.2011.02.018
Doumas, M., Imprialos, K., Dimakopoulou, A., Stavropoulos, K., Binas, A., and Athyros, V. G. (2019). The role of statins in the management of nonalcoholic fatty liver disease. Curr. Pharm. Des. 24, 4587–4592. doi: 10.2174/1381612825666190117114305
Durant, L., Stentz, R., Noble, A., Brooks, J., Gicheva, N., Reddi, D., et al. (2020). Bacteroides thetaiotaomicron-derived outer membrane vesicles promote regulatory dendritic cell responses in health but not in inflammatory bowel disease. Microbiome 8:88. doi: 10.1186/s40168-020-00868-z
El Kaoutari, A., Armougom, F., Gordon, J. I., Raoult, D., and Henrissat, B. (2013). The abundance and variety of carbohydrate-active enzymes in the human gut microbiota. Nat. Rev. Microbiol. 11, 497–504. doi: 10.1038/nrmicro3050
Feng, Y., Wang, Y., Wang, P., Huang, Y., and Wang, F. (2018). Short-chain fatty acids manifest stimulative and protective effects on intestinal barrier function through the inhibition of NLRP3 inflammasome and autophagy. Cell Physiol. Biochem. 49, 190–205. doi: 10.1159/000492853
Fernández-Murga, M. L., and Sanz, Y. (2016). Safety assessment of Bacteroides uniformis CECT 7771 isolated from stools of healthy breast-fed infants. PLoS One 11:e0145503. doi: 10.1371/journal.pone.0145503
Fernandez-Julia, P. J., Munoz-Munoz, J., and van Sinderen, D. (2021). A comprehensive review on the impact of β-glucan metabolism by Bacteroides and Bifidobacterium species as members of the gut microbiota. Int. J. Biol. Macromol. 181, 877–889. doi: 10.1016/j.ijbiomac.2021.04.069
Ferrere, G., Wrzosek, L., Cailleux, F., Turpin, W., Puchois, V., Spatz, M., et al. (2017). Fecal microbiota manipulation prevents dysbiosis and alcohol-induced liver injury in mice. J. Hepatol. 66, 806–815. doi: 10.1016/j.jhep.2016.11.008
Foley, M. H., O’Flaherty, S., Barrangou, R., and Theriot, C. M. (2019). Bile salt hydrolases: gatekeepers of bile acid metabolism and host-microbiome crosstalk in the gastrointestinal tract. PLoS Pathog. 15:e1007581. doi: 10.1371/journal.ppat.1007581
Fonseca, S., Carvalho, A. L., Miquel-Clopes, A., Jones, E. J., Juodeikis, R., Stentz, R., et al. (2022). Extracellular vesicles produced by the human gut commensal bacterium Bacteroides thetaiotaomicron elicit anti-inflammatory responses from innate immune cells. Front. Microbiol. 13:1050271. doi: 10.3389/fmicb.2022.1050271
Gao, Q., Jia, Y., Yang, G., Zhang, X., Boddu, P. C., Petersen, B., et al. (2015). PPARα-deficient ob/ob obese mice become more obese and manifest severe hepatic steatosis due to decreased fatty acid oxidation. Am. J. Pathol. 185, 1396–1408. doi: 10.1016/j.ajpath.2015.01.018
Gauffin Cano, P., Santacruz, A., and Moya, Á, and Sanz, Y. (2012). Bacteroides uniformis CECT 7771 ameliorates metabolic and immunological dysfunction in mice with high-fat-diet induced obesity. PLoS One 7:e41079. doi: 10.1371/journal.pone.0041079
Geerts, A., and Lefere, S. (2023). Bariatric surgery for non-alcoholic fatty liver disease: indications and post-operative management. Clin. Mol. Hepatol. 29(Suppl.), S276–S285. doi: 10.3350/cmh.2022.0373
Hadi, H., Vettor, R., and Rossato, M. (2018). Vitamin E as a treatment for nonalcoholic fatty liver disease: reality or myth? Antioxidants 7:12. doi: 10.3390/antiox7010012
Heinken, A., Khan, M. T., Paglia, G., Rodionov, D. A., Harmsen, H. J., and Thiele, I. (2014). Functional metabolic map of Faecalibacterium prausnitzii, a beneficial human gut microbe. J. Bacteriol. 196, 3289–3302. doi: 10.1128/jb.01780-14
Horáčková, Š, Plocková, M., and Demnerová, K. (2018). Importance of microbial defence systems to bile salts and mechanisms of serum cholesterol reduction. Biotechnol. Adv. 36, 682–690. doi: 10.1016/j.biotechadv.2017.12.005
Hoyles, L., Fernández-Real, J. M., Federici, M., Serino, M., Abbott, J., Charpentier, J., et al. (2018). Molecular phenomics and metagenomics of hepatic steatosis in non-diabetic obese women. Nat. Med. 24, 1070–1080. doi: 10.1038/s41591-018-0061-3
Hrncir, T., Hrncirova, L., Kverka, M., Hromadka, R., Machova, V., Trckova, E., et al. (2021). Gut microbiota and NAFLD: pathogenetic mechanisms, microbiota signatures, and therapeutic interventions. Microorganisms 9:957. doi: 10.3390/microorganisms9050957
Huby, T., and Gautier, E. L. (2022). Immune cell-mediated features of non-alcoholic steatohepatitis. Nat. Rev. Immunol. 22, 429–443. doi: 10.1038/s41577-021-00639-3
Igi, W. F., de Oliveira, V. L., Matar, A., and de Moura, D. T. H. (2024). Role of endoscopic duodenojejunal bypass liner in obesity management and glycemic control. Clin. Endosc. doi: 10.5946/ce.2023.217 Online ahead of print.
Ilan, Y. (2013). Immune therapy for nonalcoholic steatohepatitis: are we there yet? J. Clin. Gastroenterol. 47, 298–307. doi: 10.1097/MCG.0b013e31827873dc
Ipsen, D. H., Lykkesfeldt, J., and Tveden-Nyborg, P. (2018). Molecular mechanisms of hepatic lipid accumulation in non-alcoholic fatty liver disease. Cell Mol. Life Sci. 75, 3313–3327. doi: 10.1007/s00018-018-2860-6
Iwakiri, Y. (2015). Nitric oxide in liver fibrosis: the role of inducible nitric oxide synthase. Clin. Mol. Hepatol. 21, 319–325. doi: 10.3350/cmh.2015.21.4.319
Ji, Y., Yin, Y., Li, Z., and Zhang, W. (2019). Gut microbiota-derived components and metabolites in the progression of Non-Alcoholic Fatty Liver Disease (NAFLD). Nutrients 11:1712. doi: 10.3390/nu11081712
Jiao, N., Baker, S. S., Chapa-Rodriguez, A., Liu, W., Nugent, C. A., Tsompana, M., et al. (2018). Suppressed hepatic bile acid signalling despite elevated production of primary and secondary bile acids in NAFLD. Gut 67, 1881–1891. doi: 10.1136/gutjnl-2017-314307
Jin, C. J., Engstler, A. J., Sellmann, C., Ziegenhardt, D., Landmann, M., Kanuri, G., et al. (2016). Sodium butyrate protects mice from the development of the early signs of non-alcoholic fatty liver disease: role of melatonin and lipid peroxidation. Br. J. Nutr. 116, 1682–1693. doi: 10.1017/s0007114516004025
Jin, L. T., and Xu, M. Z. (2023). Characterization of gut dominant microbiota in obese patients with nonalcoholic fatty liver disease. Front. Cell Infect. Microbiol. 13:1113643. doi: 10.3389/fcimb.2023.1113643
Joyce, S. A., MacSharry, J., Casey, P. G., Kinsella, M., Murphy, E. F., Shanahan, F., et al. (2014a). Regulation of host weight gain and lipid metabolism by bacterial bile acid modification in the gut. Proc. Natl. Acad. Sci. U S A. 111, 7421–7426. doi: 10.1073/pnas.1323599111
Joyce, S. A., Shanahan, F., Hill, C., and Gahan, C. G. (2014b). Bacterial bile salt hydrolase in host metabolism: potential for influencing gastrointestinal microbe-host crosstalk. Gut Microbes 5, 669–674. doi: 10.4161/19490976.2014.969986
Kaufmann, B., Seyfried, N., Hartmann, D., and Hartmann, P. (2023). Probiotics, prebiotics, and synbiotics in nonalcoholic fatty liver disease and alcohol-associated liver disease. Am. J. Physiol. Gastrointest Liver Physiol. 325, G42–G61. doi: 10.1152/ajpgi.00017.2023
Khan, R. S., and Newsome, P. N. (2018). NAFLD in 2017: novel insights into mechanisms of disease progression. Nat. Rev. Gastroenterol. Hepatol. 15, 71–72. doi: 10.1038/nrgastro.2017.181
Kim, S. H., Seo, H., Kwon, D., Yuk, D. Y., and Jung, Y. S. (2022). Taurine ameliorates tunicamycin-induced liver injury by disrupting the vicious cycle between oxidative stress and endoplasmic reticulum stress. Life 12:354. doi: 10.3390/life12030354
Kolodziejczyk, A. A., Zheng, D., Shibolet, O., and Elinav, E. (2019). The role of the microbiome in NAFLD and NASH. EMBO Mol. Med. 11:e9302. doi: 10.15252/emmm.201809302
Kumar, R., Priyadarshi, R. N., and Anand, U. (2020). Non-alcoholic fatty liver disease: growing burden, adverse outcomes and associations. J. Clin. Transl. Hepatol. 8, 76–86. doi: 10.14218/jcth.2019.00051
Lambert, I. H., Kristensen, D. M., Holm, J. B., and Mortensen, O. H. (2015). Physiological role of taurine–from organism to organelle. Acta Physiol. 213, 191–212. doi: 10.1111/apha.12365
Laue, C., Papazova, E., Pannenbeckers, A., and Schrezenmeir, J. (2023). Effect of a probiotic and a synbiotic on body fat mass, body weight and traits of metabolic syndrome in individuals with abdominal overweight: a human, double-blind, randomised, controlled clinical study. Nutrients 15:3039. doi: 10.3390/nu15133039
Lee, H. A., and Kim, H. Y. (2023). Therapeutic mechanisms and clinical effects of glucagon-like peptide 1 receptor agonists in nonalcoholic fatty liver disease. Int. J. Mol. Sci. 24:9324. doi: 10.3390/ijms24119324
Lee, H. B., Do, M. H., Jhun, H., Ha, S. K., Song, H. S., Roh, S. W., et al. (2021). Amelioration of hepatic steatosis in mice through Bacteroides uniformis CBA7346-mediated regulation of high-fat diet-induced insulin resistance and lipogenesis. Nutrients 13:2989. doi: 10.3390/nu13092989
Lefere, S., Onghena, L., Vanlander, A., van Nieuwenhove, Y., Devisscher, L., and Geerts, A. (2021). Bariatric surgery and the liver—mechanisms, benefits, and risks. Obes. Rev. 22:e13294. doi: 10.1111/obr.13294
Li, H., Wang, S., Wang, S., Yu, H., Yu, W., Ma, X., et al. (2022). Atorvastatin inhibits high-fat diet-induced lipid metabolism disorders in rats by inhibiting Bacteroides reduction and improving metabolism. Drug Des. Devel. Ther. 16, 3805–3816. doi: 10.2147/dddt.s379335
Li, Y., Yang, Y., Wang, J., Cai, P., Li, M., Tang, X., et al. (2022). Bacteroides ovatus-mediated CD27(-) MAIT cell activation is associated with obesity-related T2D progression. Cell Mol. Immunol. 19, 791–804. doi: 10.1038/s41423-022-00871-4
Li, H., Wang, X. K., Tang, M., Lei, L., Li, J. R., Sun, H., et al. (2024). Bacteroides thetaiotaomicron ameliorates mouse hepatic steatosis through regulating gut microbial composition, gut-liver folate and unsaturated fatty acids metabolism. Gut Microbes 16:2304159. doi: 10.1080/19490976.2024.2304159
Li, J., Chen, Q., Yi, J., Lan, X., Lu, K., Du, X., et al. (2021). IFN-γ contributes to the hepatic inflammation in HFD-induced nonalcoholic steatohepatitis by STAT1β/TLR2 signaling pathway. Mol. Immunol. 134, 118–128. doi: 10.1016/j.molimm.2021.03.005
Li, X., Du, Y., Xue, C., Kang, X., Sun, C., Peng, H., et al. (2023). SIRT2 deficiency aggravates diet-induced nonalcoholic fatty liver disease through modulating gut microbiota and metabolites. Int. J. Mol. Sci. 24:8970. doi: 10.3390/ijms24108970
Li, X., Zeng, F., Huang, Y., and Liu, B. (2019). The positive effects of grifola frondosa heteropolysaccharide on NAFLD and regulation of the gut microbiota. Int. J. Mol. Sci. 20:5302. doi: 10.3390/ijms20215302
Lian, C. Y., Zhai, Z. Z., Li, Z. F., and Wang, L. (2020). High fat diet-triggered non-alcoholic fatty liver disease: a review of proposed mechanisms. Chem. Biol. Interact. 330:109199. doi: 10.1016/j.cbi.2020.109199
Lin, Y., Xu, Z., Yeoh, Y. K., Tun, H. M., Huang, W., Jiang, W., et al. (2023). Combing fecal microbial community data to identify consistent obesity-specific microbial signatures and shared metabolic pathways. iScience 26:106476. doi: 10.1016/j.isci.2023.106476
Liu, B., Zhang, J., Sun, P., Yi, R., Han, X., and Zhao, X. (2019). Raw bowl tea (Tuocha) polyphenol prevention of nonalcoholic fatty liver disease by regulating intestinal function in mice. Biomolecules 9:435. doi: 10.3390/biom9090435
Lomonaco, R., Ortiz-Lopez, C., Orsak, B., Webb, A., Hardies, J., Darland, C., et al. (2012). Effect of adipose tissue insulin resistance on metabolic parameters and liver histology in obese patients with nonalcoholic fatty liver disease. Hepatology 55, 1389–1397. doi: 10.1002/hep.25539
Lonardo, A., Nascimbeni, F., Mantovani, A., and Targher, G. (2018). Hypertension, diabetes, atherosclerosis and NASH: cause or consequence? J. Hepatol. 68, 335–352. doi: 10.1016/j.jhep.2017.09.021
López-Almela, I., Romaní-Pérez, M., Bullich-Vilarrubias, C., Benítez-Páez, A., Gómez Del, Pulgar, E. M., et al. (2021). Bacteroides uniformis combined with fiber amplifies metabolic and immune benefits in obese mice. Gut Microbes 13, 1–20. doi: 10.1080/19490976.2020.1865706
Lopez-Siles, M., Duncan, S. H., Garcia-Gil, L. J., and Martinez-Medina, M. (2017). Faecalibacterium prausnitzii: from microbiology to diagnostics and prognostics. ISME J. 11, 841–852. doi: 10.1038/ismej.2016.176
Maestri, M., Santopaolo, F., Pompili, M., Gasbarrini, A., and Ponziani, F. R. (2023). Gut microbiota modulation in patients with non-alcoholic fatty liver disease: effects of current treatments and future strategies. Front. Nutr. 10:1110536. doi: 10.3389/fnut.2023.1110536
Mandour, M. O., El-Hassan, M., Elkomi, R. M., and Oben, J. A. (2022). Non-alcoholic fatty liver disease: is surgery the best current option and can novel endoscopy play a role in the future? World J. Hepatol. 14, 1704–1717. doi: 10.4254/wjh.v14.i9.1704
Mantovani, A., and Dalbeni, A. (2021). Treatments for NAFLD: state of art. Int. J. Mol. Sci. 22:2350. doi: 10.3390/ijms22052350
Marchisello, S., Di Pino, A., Scicali, R., Urbano, F., Piro, S., Purrello, F., et al. (2019). Pathophysiological, molecular and therapeutic issues of nonalcoholic fatty liver disease: an overview. Int. J. Mol. Sci. 20:1948. doi: 10.3390/ijms20081948
McMillan, H. M., and Kuehn, M. J. (2021). The extracellular vesicle generation paradox: a bacterial point of view. EMBO J. 40:e108174. doi: 10.15252/embj.2021108174
Mouries, J., Brescia, P., Silvestri, A., Spadoni, I., Sorribas, M., Wiest, R., et al. (2019). Microbiota-driven gut vascular barrier disruption is a prerequisite for non-alcoholic steatohepatitis development. J. Hepatol. 71, 1216–1228. doi: 10.1016/j.jhep.2019.08.005
Mu, H., Zhou, Q., Yang, R., Zeng, J., Li, X., Zhang, R., et al. (2020). Naringin attenuates high fat diet induced non-alcoholic fatty liver disease and gut bacterial dysbiosis in mice. Front. Microbiol. 11:585066. doi: 10.3389/fmicb.2020.585066
Nagpal, R., Wang, S., Ahmadi, S., Hayes, J., Gagliano, J., Subashchandrabose, S., et al. (2018). Human-origin probiotic cocktail increases short-chain fatty acid production via modulation of mice and human gut microbiome. Sci. Rep. 8:12649. doi: 10.1038/s41598-018-30114-4
Nguyen, N. T., and Varela, J. E. (2017). Bariatric surgery for obesity and metabolic disorders: state of the art. Nat. Rev. Gastroenterol. Hepatol. 14, 160–169. doi: 10.1038/nrgastro.2016.170
Paolella, G., Mandato, C., Pierri, L., Poeta, M., Di Stasi, M., and Vajro, P. (2014). Gut-liver axis and probiotics: their role in non-alcoholic fatty liver disease. World J. Gastroenterol. 20, 15518–15531. doi: 10.3748/wjg.v20.i42.15518
Paredes-Turrubiarte, G., Gonzalez-Chavez, A., Perez-Tamayo, R., Salazar-Vazquez, B. Y., Hernandez, V. S., Garibay-Nieto, N., et al. (2016). Severity of non-alcoholic fatty liver disease is associated with high systemic levels of tumor necrosis factor alpha and low serum interleukin 10 in morbidly obese patients. Clin. Exp. Med. 16, 193–202. doi: 10.1007/s10238-015-0347-4
Pathak, P., Xie, C., Nichols, R. G., Ferrell, J. M., Boehme, S., Krausz, K. W., et al. (2018). Intestine farnesoid X receptor agonist and the gut microbiota activate G-protein bile acid receptor-1 signaling to improve metabolism. Hepatology 68, 1574–1588. doi: 10.1002/hep.29857
Perez-Cruz, C., Delgado, L., Lopez-Iglesias, C., and Mercade, E. (2015). Outer-inner membrane vesicles naturally secreted by gram-negative pathogenic bacteria. PLoS One 10:e0116896. doi: 10.1371/journal.pone.0116896
Postic, C., Dentin, R., Denechaud, P. D., and Girard, J. (2007). ChREBP, a transcriptional regulator of glucose and lipid metabolism. Annu. Rev. Nutr. 27, 179–192. doi: 10.1146/annurev.nutr.27.061406.093618
Qi, X., Yang, M., Stenberg, J., Dey, R., Fogwe, L., Alam, M. S., et al. (2020). Gut microbiota mediated molecular events and therapy in liver diseases. World J. Gastroenterol. 26, 7603–7618. doi: 10.3748/wjg.v26.i48.7603
Qiao, S., Bao, L., Wang, K., Sun, S., Liao, M., Liu, C., et al. (2020). Activation of a specific gut Bacteroides-folate-liver axis benefits for the alleviation of nonalcoholic hepatic steatosis. Cell Rep. 32:108005. doi: 10.1016/j.celrep.2020.108005
Rakoff-Nahoum, S., Foster, K. R., and Comstock, L. E. (2016). The evolution of cooperation within the gut microbiota. Nature 533, 255–259. doi: 10.1038/nature17626
Rao, A., Kosters, A., Mells, J. E., Zhang, W., Setchell, K. D., Amanso, A. M., et al. (2016). Inhibition of ileal bile acid uptake protects against nonalcoholic fatty liver disease in high-fat diet-fed mice. Sci. Transl. Med. 8:357ra122. doi: 10.1126/scitranslmed.aaf4823
Riezu-Boj, J. I., Barajas, M., Pérez-Sánchez, T., Pajares, M. J., Araña, M., Milagro, F. I., et al. (2022). Lactiplantibacillus plantarum DSM20174 attenuates the progression of non-alcoholic fatty liver disease by modulating gut microbiota, improving metabolic risk factors, and attenuating adipose inflammation. Nutrients 14:5212. doi: 10.3390/nu14245212
Romualdo, G. R., Valente, L. C., Sprocatti, A. C., Bacil, G. P., de Souza, I. P., Rodrigues, J., et al. (2022). Western diet-induced mouse model of non-alcoholic fatty liver disease associated with metabolic outcomes: features of gut microbiome-liver-adipose tissue axis. Nutrition 103-104:111836. doi: 10.1016/j.nut.2022.111836
Rotman, Y., and Sanyal, A. J. (2017). Current and upcoming pharmacotherapy for non-alcoholic fatty liver disease. Gut 66, 180–190. doi: 10.1136/gutjnl-2016-312431
Sanders, F. W., and Griffin, J. L. (2016). De novo lipogenesis in the liver in health and disease: more than just a shunting yard for glucose. Biol. Rev. Camb. Philos. Soc. 91, 452–468. doi: 10.1111/brv.12178
Sangineto, M., Grander, C., Grabherr, F., Mayr, L., Enrich, B., Schwärzler, J., et al. (2022). Recovery of Bacteroides thetaiotaomicron ameliorates hepatic steatosis in experimental alcohol-related liver disease. Gut Microbes 14:2089006. doi: 10.1080/19490976.2022.2089006
Shin, J. H., Lee, Y., Song, E. J., Lee, D., Jang, S. Y., Byeon, H. R., et al. (2023). Faecalibacterium prausnitzii prevents hepatic damage in a mouse model of NASH induced by a high-fructose high-fat diet. Front. Microbiol. 14:1123547. doi: 10.3389/fmicb.2023.1123547
Silva-Sperb, A. S., Moraes, H. A., de Moura, B. C., Alves, B. C., Bruch-Bertani, J. P., Azevedo, V. Z., et al. (2019). Effect of probiotic supplementation in nonalcoholic steatohepatitis patients: PROBILIVER TRIAL protocol. Trials 20:580. doi: 10.1186/s13063-019-3679-7
Singh, T. P., Kadyan, S., Devi, H., Park, G., and Nagpal, R. (2023). Gut microbiome as a therapeutic target for liver diseases. Life Sci. 322:121685. doi: 10.1016/j.lfs.2023.121685
Smith, G. I., Shankaran, M., Yoshino, M., Schweitzer, G. G., Chondronikola, M., Beals, J. W., et al. (2020). Insulin resistance drives hepatic de novo lipogenesis in nonalcoholic fatty liver disease. J. Clin. Invest. 130, 1453–1460. doi: 10.1172/jci134165
Smits, L. P., Bouter, K. E. C., de Vos, W. M., Borody, T. J., and Nieuwdorp, M. (2013). Therapeutic potential of fecal microbiota transplantation. Gastroenterology 145, 946–953. doi: 10.1053/j.gastro.2013.08.058
Stentz, R., Jones, E., Juodeikis, R., Wegmann, U., Guirro, M., Goldson, A. J., et al. (2022). The proteome of extracellular vesicles produced by the human gut bacteria Bacteroides thetaiotaomicron in vivo is influenced by environmental and host-derived factors. Appl. Environ. Microbiol. 88:e0053322. doi: 10.1128/aem.00533-22
Sun, F., Zhang, Q., Zhao, J., Zhang, H., Zhai, Q., and Chen, W. (2019). A potential species of next-generation probiotics? The dark and light sides of Bacteroides fragilis in health. Food Res. Int. 126:108590. doi: 10.1016/j.foodres.2019.108590
Tan, H., Zhai, Q., and Chen, W. (2019a). Investigations of Bacteroides spp. towards next-generation probiotics. Food Res. Int. 116, 637–644. doi: 10.1016/j.foodres.2018.08.088
Tan, H., Zhao, J., Zhang, H., Zhai, Q., and Chen, W. (2019b). Novel strains of Bacteroides fragilis and Bacteroides ovatus alleviate the LPS-induced inflammation in mice. Appl. Microbiol. Biotechnol. 103, 2353–2365. doi: 10.1007/s00253-019-09617-1
Tarantino, G., Costantini, S., Finelli, C., Capone, F., Guerriero, E., La Sala, N., et al. (2014). Is serum Interleukin-17 associated with early atherosclerosis in obese patients? J. Transl. Med. 12:214. doi: 10.1186/s12967-014-0214-1
Tilg, H. (2010). The role of cytokines in non-alcoholic fatty liver disease. Dig. Dis. 28, 179–185. doi: 10.1159/000282083
Troy, E. B., and Kasper, D. L. (2010). Beneficial effects of Bacteroides fragilis polysaccharides on the immune system. Front. Biosci. 15, 25–34. doi: 10.2741/3603
Turnbaugh, P. J., Ley, R. E., Mahowald, M. A., Magrini, V., Mardis, E. R., and Gordon, J. I. (2006). An obesity-associated gut microbiome with increased capacity for energy harvest. Nature 444, 1027–1031. doi: 10.1038/nature05414
Van Herreweghen, F., De Paepe, K., Roume, H., Kerckhof, F.M., and Van de Wiele, T. (2018). Mucin degradation niche as a driver of microbiome composition and Akkermansia muciniphila abundance in a dynamic gut model is donor independent. FEMS Microbiol. Ecol. 94. doi: 10.1093/femsec/fiy186
Wang, C., Li, S., Hong, K., Yu, L., Tian, F., Zhao, J., et al. (2021a). The roles of different Bacteroides fragilis strains in protecting against DSS-induced ulcerative colitis and related functional genes. Food Funct. 12, 8300–8313. doi: 10.1039/d1fo00875g
Wang, C., Zhao, J., Zhang, H., Lee, Y. K., Zhai, Q., and Chen, W. (2021b). Roles of intestinal bacteroides in human health and diseases. Crit. Rev. Food Sci. Nutr. 61, 3518–3536. doi: 10.1080/10408398.2020.1802695
Wang, X., Ye, C., Xun, T., Mo, L., Tong, Y., Ni, W., et al. (2021c). Bacteroides fragilis polysaccharide a ameliorates abnormal voriconazole metabolism accompanied with the inhibition of TLR4/NF-κB pathway. Front. Pharmacol. 12:663325. doi: 10.3389/fphar.2021.663325
Wang, H., Wang, Q., Yang, C., Guo, M., Cui, X., Jing, Z., et al. (2022). Bacteroides acidifaciens in the gut plays a protective role against CD95-mediated liver injury. Gut Microbes 14:2027853. doi: 10.1080/19490976.2022.2027853
Wang, L., Cao, Z. M., Zhang, L. L., Li, J. M., and Lv, W. L. (2022). The role of gut microbiota in some liver diseases: from an immunological perspective. Front. Immunol. 13:923599. doi: 10.3389/fimmu.2022.923599
Wexler, A. G., and Goodman, A. L. (2017). An insider’s perspective: Bacteroides as a window into the microbiome. Nat. Microbiol. 2:17026. doi: 10.1038/nmicrobiol.2017.26
Wigg, A. J., Roberts-Thomson, I. C., Dymock, R. B., McCarthy, P. J., Grose, R. H., and Cummins, A. G. (2001). The role of small intestinal bacterial overgrowth, intestinal permeability, endotoxaemia, and tumour necrosis factor alpha in the pathogenesis of non-alcoholic steatohepatitis. Gut 48, 206–211. doi: 10.1136/gut.48.2.206
Wrzosek, L., Miquel, S., Noordine, M. L., Bouet, S., Joncquel Chevalier-Curt, M., Robert, V., et al. (2013). Bacteroides thetaiotaomicron and Faecalibacterium prausnitzii influence the production of mucus glycans and the development of goblet cells in the colonic epithelium of a gnotobiotic model rodent. BMC Biol. 11:61. doi: 10.1186/1741-7007-11-61
Xu, M., Lan, R., Qiao, L., Lin, X., Hu, D., Zhang, S., et al. (2023). Bacteroides vulgatus ameliorates lipid metabolic disorders and modulates gut microbial composition in hyperlipidemic rats. Microbiol. Spectr. 11:e0251722. doi: 10.1128/spectrum.02517-22
Yan, L. S., Zhang, S. F., Luo, G., Cheng, B. C., Zhang, C., Wang, Y. W., et al. (2022). Schisandrin B mitigates hepatic steatosis and promotes fatty acid oxidation by inducing autophagy through AMPK/mTOR signaling pathway. Metabolism 131:155200. doi: 10.1016/j.metabol.2022.155200
Yang, J. Y., Lee, Y. S., Kim, Y., Lee, S. H., Ryu, S., Fukuda, S., et al. (2017). Gut commensal Bacteroides acidifaciens prevents obesity and improves insulin sensitivity in mice. Mucosal Immunol. 10, 104–116. doi: 10.1038/mi.2016.42
Yoshida, N., Emoto, T., Yamashita, T., Watanabe, H., Hayashi, T., Tabata, T., et al. (2018). Bacteroides vulgatus and Bacteroides dorei reduce gut microbial lipopolysaccharide production and inhibit atherosclerosis. Circulation 138, 2486–2498. doi: 10.1161/circulationaha.118.033714
Yoshida, N., Yamashita, T., Osone, T., Hosooka, T., Shinohara, M., Kitahama, S., et al. (2021). Bacteroides spp. promotes branched-chain amino acid catabolism in brown fat and inhibits obesity. iScience 24:103342. doi: 10.1016/j.isci.2021.103342
You, H. J., Si, J., Kim, J., Yoon, S., Cha, K. H., Yoon, H. S., et al. (2023). Bacteroides vulgatus SNUG 40005 restores Akkermansia depletion by metabolite modulation. Gastroenterology 164, 103–116. doi: 10.1053/j.gastro.2022.09.040
Younossi, Z. M., Golabi, P., Paik, J. M., Henry, A., Van Dongen, C., and Henry, L. (2023). The global epidemiology of nonalcoholic fatty liver disease (NAFLD) and nonalcoholic steatohepatitis (NASH): a systematic review. Hepatology 77, 1335–1347. doi: 10.1097/hep.0000000000000004
Zafar, H., and Saier, M. H. (2021). Gut Bacteroides species in health and disease. Gut Microbes 13, 1–20. doi: 10.1080/19490976.2020.1848158
Zai, W., Chen, W., Liu, H., and Ju, D. (2021). Therapeutic opportunities of IL-22 in non-alcoholic fatty liver disease: from molecular mechanisms to clinical applications. Biomedicines 9:1912. doi: 10.3390/biomedicines9121912
Zeng, Q., Li, D., He, Y., Li, Y., Yang, Z., Zhao, X., et al. (2019). Discrepant gut microbiota markers for the classification of obesity-related metabolic abnormalities. Sci. Rep. 9:13424. doi: 10.1038/s41598-019-49462-w
Zhang, X., Ji, X., Wang, Q., and Li, J. Z. (2018). New insight into inter-organ crosstalk contributing to the pathogenesis of non-alcoholic fatty liver disease (NAFLD). Protein Cell 9, 164–177. doi: 10.1007/s13238-017-0436-0
Zhang, B., Li, J., Fu, J., Shao, L., Yang, L., and Shi, J. (2023). Interaction between mucus layer and gut microbiota in non-alcoholic fatty liver disease: Soil and seeds. Chin. Med. J. (Engl) 136, 1390–1400. doi: 10.1097/cm9.0000000000002711
Zhou, C., Wang, Y., Li, C., Xie, Z., and Dai, L. (2023). Amelioration of colitis by a gut bacterial consortium producing anti-inflammatory secondary bile acids. Microbiol. Spectr. 11:e0333022. doi: 10.1128/spectrum.03330-22
Zhou, H., Yu, B., Gao, J., Htoo, J. K., and Chen, D. (2018). Regulation of intestinal health by branched-chain amino acids. Anim. Sci. J. 89, 3–11. doi: 10.1111/asj.12937
Keywords: Bacteroides, NAFLD, steatosis, liver inflammation, intestinal barrier
Citation: Zhang J, Zhou J, He Z and Li H (2024) Bacteroides and NAFLD: pathophysiology and therapy. Front. Microbiol. 15:1288856. doi: 10.3389/fmicb.2024.1288856
Received: 05 September 2023; Accepted: 07 March 2024;
Published: 20 March 2024.
Edited by:
Xiaozhen Jen Mou, Kent State University, United StatesReviewed by:
Jeehwan Oh, University of Wisconsin-Madison, United StatesSalvatore Pezzino, University of Catania, Italy
Copyright © 2024 Zhang, Zhou, He and Li. This is an open-access article distributed under the terms of the Creative Commons Attribution License (CC BY). The use, distribution or reproduction in other forums is permitted, provided the original author(s) and the copyright owner(s) are credited and that the original publication in this journal is cited, in accordance with accepted academic practice. No use, distribution or reproduction is permitted which does not comply with these terms.
*Correspondence: Hongshan Li, bGlob25nc2hhbl8xOTgyQDEyNi5jb20=
†These authors have contributed equally to this work and share first authorship