- 1School of Life Sciences and Agricultural Engineering, Nanyang Normal University, Nanyang, China
- 2Research Center of Henan Provincial Agricultural Biomass Resource Engineering and Technology, Nanyang Normal University, Nanyang, China
The genus Erythrobasidium is kind of species-scarce and undersampling basidiomycetes. Currently, only six species have been accepted into the genus and the diversity still remains incompletely understood. In this study, five Erythrobasidium strains were isolated in the surface of plant leaves collected from the Baotianman Nature Reserve, Henan Province, central China. Phylogenetic analyses of the small ribosomal subunit (SSU) rRNA gene, the internal transcribed spacer (ITS) region, the D1/D2 domain of the large subunit (LSU) rRNA gene, and the translation elongation factor 1-α (TEF1-α) gene coupled with morphological studies were employed to characterize and identify these isolates. As a result of these, two new species, namely E. turpiniae sp. nov. and E. nanyangense sp. nov., were delimited and proposed based on morphological and molecular evidence. A detailed description and illustration of both new species, as well as their differences with the close relatives in the genus are provided. An identification key for Erythrobasidium species is also provided. This study provides further insights into our understanding of Erythrobasidium species.
Introduction
The genus Erythrobasidium is a type of phyllosphere-inhabiting Basidiomycota. It was defined by Hamamoto et al. (1988) to accommodate Erythrobasidium hasegawae, the teleomorphic state of Rhodotorula hasegawae. The original species name, E. hasegawae, was soon after changed to E. hasegawianum to fit standard naming conventions (Hamamoto et al., 1991; Hamamoto, 2011). E. hasegawianum was initially placed in the Agaricomycotina, but it was later reclassified as a member of the Pucciniomycotina based on phylogenetic analyses of the small subunit (SSU) rRNA gene (Hamamoto and Nakase, 2000), the D1/D2 domain of the large subunit (LSU) rRNA gene (Fell et al., 2000; Hamamoto, 2011), and a seven-gene dataset consisting of SSU, D1/D2 LSU, ITS, RPB1, RPB2, TEF1-α, and CYTB (Wang et al., 2015a). Wang et al. (2015b) revised the genus Erythrobasidium based on a multi-gene phylogeny, and transferred two Sporobolomyces species, S. elongatum and S. yunnanensis, to this genus as new combinations. Recently, three new species, E. primogenitum, E. leptospermi, and E. proteacearum, have been proposed by Tan et al. (2021) in Index Fungorum and by Tan and Shivas (2022) in Index of Australian Fungi based only on the D1/D2 and ITS sequences. Worryingly, the representative cultures of these three species are not currently available in a reference culture collection. The genus is presently classified in the family Erythrobasidiaceae within the order Erythrobasidiales, class Cystobasidiomycetes, Pucciniomycotina (Denchev, 2009; Wang et al., 2015b).
Except the type strain of E. hasegawianum, all strains of Erythrobasidium species have been reported to isolate from leaf surfaces (Shivas et al., 1983; Bai et al., 2001; Hamamoto, 2011; Hamamoto et al., 2011; Zang et al., 2018; Li et al., 2020; Tan et al., 2021; Tan and Shivas, 2022) and are considered important phyllosphere-inhabiting yeasts (Hamamoto, 2011; Hamamoto et al., 2011; Wang et al., 2015b). E. hasegawianum, the type species of the genus, is known from its sexual morph, which is characterized by unicellular basidia without mating and the lack of teliospores (Hamamoto, 2011). The other two validly known species, E. elongatum and E. yunnanensis, have been reported to have asexual morphs that reproduce by polar budding and the formation of ballistoconidia (Shivas et al., 1983; Bai et al., 2001; Hamamoto, 2011; Hamamoto et al., 2011). Physiologically, Erythrobasidium species can utilize various carbon and nitrogen sources, but not methanol or myo-inositol. From a phenotypic perspective, there are no distinctive phenotypic traits that can reliably delineate the genus Erythrobasidium. Therefore, molecular phylogenetic analysis coupled with morphological studies is recommended for identification of Erythrobasidium species (Wang et al., 2015b).
Species of the genus Erythrobasidium are best known for their orange-red colonies and have been studied for a variety of applications. For example, Erythrobasidium species have the ability to produce carotenoids such as beta-carotene. The carotenoid-producing capability of Erythrobasidium yeasts has been of interest to the field of biotechnology to develop commodities such as pigments (Mannazzu et al., 2015). E. hasegawianum is often fermented to produce linalool and ocimene, two common perfume components. As a natural bioflavoring producer, E. hasegawianum has great potential for applications in beverage industry.
The surface of plant leaves, normally referred to as the phylloplane, is known to be an important habitats for microorganisms. Various yeast species in the phyllosphere of different plants have been reported by several authors (de Azeredo et al., 1998; Zang et al., 2018; Srisuk et al., 2019; Into et al., 2020a,b; Li et al., 2020) but a few species of Erythrobasidium in this particular habitat have been uncovered. Surveying phylloplane can help us gain a better understanding of the diversity, distribution, and ecology of Erythrobasidium and lead to the discovery of new fungal species that may have valuable functions.
The Baotianman Nature Reserve located in Henan Province, central China, measures 4,285 ha. With a forest coverage rate of 98%, it is classified as World Biosphere Reserve by the United Nations Educational, Scientific and Cultural Organization (UNESCO). The reserve represents a virgin forest with more than 2,000 species of higher plants. The local climate consists of cold, dry winters and fresh, rainy summers—typical of a transitional zone from a northern subtropical zone to a warm temperate (Hu et al., 2022). These weather patterns make Baotianman an excellent location for studying fungal diversity. During the survey, a number of phyllosphere-inhabiting yeasts were obtained, and some of them have been described as new species, namely B. ellipsoidea, B. foliicola, and B. pseudofoliicola, in earlier paper (Chai et al., 2023). Among these associates, five additional yeast strains could not be ascribed to any validly known species. The aim of this study was to identify these yeasts as two new species of the genus Erythrobasidium based on multi-locus phylogenetic analyses of SSU, ITS, LSU, and TEF1-α sequence data and morphological observations.
Materials and methods
Sample collection and yeast isolation
Leaf samples collected from Baotianman Nature Reserve (33°30′44″N, 111°55′47″E) were stored in sterile flasks and transported to the laboratory within 24 h. Yeast strains were isolated from leaf surfaces by the improved ballistospore-fall method as described in previous paper (Nakase and Takashima, 1993). Briefly, the leaf was cut into small pieces that were attached with a thin layer of petroleum jelly to the inner lid of a Petri dish containing yeast extract-malt extract (YM) agar (0.3% yeast extract, 0.3% malt extract, 0.5% peptone, 1% glucose, and 2% agar) with added 0.01% chloramphenicol, to avoid bacterial growth. Plates were then incubated at 25 °C and monitored daily by eye for presence of colonies, which were selected and purified by streaking them on separate YM agar plates. After purification, yeast strains were suspended in YM broth supplemented with 20% (v/v) glycerol and stored at −80 °C. Cultures of all obtained isolates were preserved in Microbiology Lab, Nanyang Normal University, Henan, China. All isolates used in this study and their origins are presented in Table 1.
Morphological and physiological characterization
Morphological and physiological characteristics of yeast strains were defined according to methods established by Kurtzman et al. (2011). Colony characteristics were observed and recorded on YM agar after 2 weeks of incubation at 25 °C. To investigate mycelium formation, colonies were transferred to corn meal (CM) agar (2% cornmeal infusion and 2% agar) slide cultures and incubated at 25 °C for 2 weeks. Sexual tests were conducted for individual strains and strain pairs on potato dextrose agar (PDA) (20% potato infusion, 2% glucose, and 1.5% agar), CM agar, and yeast carbon base plus 0.01% ammonium sulfate (YCBS) agar for 2 months and observed at weekly intervals (Hamamoto, 2011; Li et al., 2020). The inverted-plate method (do Carmo-Sousa and Phaff, 1962) was used to observe the ballistoconidium-forming activity of all yeasts after 2 weeks of incubation on CM agar at 20 °C. Glucose fermentation was carried out in a liquid medium using Durham fermentation tubes. Carbon and nitrogen source assimilation tests were conducted in a liquid medium and starved inoculum was used for the nitrogen test (Kurtzman et al., 2011). Cycloheximide resistance was performed in a liquid medium, while urea hydrolysis was conducted on agar slants. Acid production and the diazonium blue B (DBB) reactions were investigated using petri dishes with a solid medium (Kurtzman et al., 2011). Growth at different temperatures (15, 20, 25, 30, 35, and 37 °C) was determined by the amount of cultivation on YM agar. All experiments were carried out with three replicates. Cell morphology was examined with LEICA DM2500 cameras (LECIA, Wetzlar, Germany) and use LASV4.13 software. At least 50 representative measurements were randomly selected and measured to calculate the average size of the budding cells. All novel taxonomic descriptions and proposed names were deposited in the MycoBank database.1
DNA extraction, PCR amplification, and sequencing
The total genomic DNA was extracted from yeast strains using the Ezup Column Yeast Genomic DNA Purification Kit according to the manufacturer’s instructions (Sangon Biotech, China). Four nuclear loci, which include the SSU rRNA gene, the ITS region, the D1/D2 domain of the LSU rRNA gene, and TEF1-α gene were sequenced using NS1/NS8 (White et al., 1990), ITS1/ITS4 (White et al., 1990), NL1/NL4 (Kurtzman and Robnett, 1998), and EF1-526F/EF1-1567R (Rehner and Buckley, 2005) primers, respectively. The amplifications were performed in a 25 μL reaction-volume tube containing 9.5 μL of ddH2O, 12.5 μL of 2 × Taq PCR Master Mix with blue dye (Sangon Biotech, Shanghai, China), 1 μL of DNA template, and 1 μL of each primer. The following parameters were used to amplify the SSU, ITS, and D1/D2 regions: an initial denaturation step of 2 min at 95 °C, followed by 35 cycles of 30 s at 95 °C, 30 s at 51 °C, 40 s at 72 °C, and a final extension of 10 min at 72 °C (Toome et al., 2013). For TEF1-α, we used a touchdown PCR protocol as described (Wang et al., 2014). The PCR products were purified and sequenced at Sangon Biotech (shanghai) Co., Ltd (China) with the same primers. We determined the identity and accuracy of the newly-obtained sequences by comparing them to sequences in GenBank and assembled them using BioEdit 7.1.3.0 (Hall, 1999). All newly generated sequences were deposited in the GenBank database,2 and the accession numbers were listed in Table 2.
Phylogenetic analysis
A total of 21 taxa were included in the phylogenetic analyses in this study. Except for 20 sequences recognized in this study, the other sequences were obtained from previous studies (Wang et al., 2015b; Li et al., 2020) and GenBank (Table 2). Naohidea sebacea CBS 8477T was used as the outgroup. The phylogenetic relationships of the new Erythrobasidium species and their relatives were determined using a combined sequence dataset of four loci (SSU, ITS, LSU, and TEF1-α). Sequences of the individual loci were aligned with ClustalX v. 1.83 (Thompson et al., 1997) or MAFFT 7.110 (Katoh and Standley, 2013) using default settings. Phylosuit v. 1.2.2 (Zhang et al., 2020) was used to concatenate the aligned sequences of the different loci. Manual gap adjustments were performed to improve the alignment. Any ambiguously aligned regions were excluded before analysis.
Multi-locus phylogenetic analyses were carried out by using maximum likelihood (ML) and Bayesian inference (BI) methods. The ML was determined using 1,000 searches on RAxML v. 8.2.3 (Stamatakis, 2014). ML bootstrap values (BS) of the nodes were evaluated using 1,000 rapid bootstrap replicates under the GTRCAT model. For the BI approach, Modelfinder (Kalyaanamoorthy et al., 2017) was used to determine the appropriate substitution model that would best fit the DNA evolution for the combined dataset. MrBayes v. 3.2.7a (Ronquist et al., 2012) in the CIPRES Science Gateway version v. 3.3 was used to analyze the BI data. Best-fit evolution models were determined as GTR+I+G for the SSU, ITS, LSU, and TEF1-α partitions. Six simultaneous Markov chains were run for 50 million generations and trees were sampled every 1,000th generation. The first 25% of created sample trees were discarded as they represent the burn-in phase of analysis. The remaining trees were used to calculate Bayesian posterior probabilities (BPP) of the clades.
The resulting trees were viewed with FigTree v. 1.4.3 (Andrew, 2016) and processed with Adobe Illustrator CS5. Branches that received MLBS ≥ 50% and BPP ≥ 0.90 were considered significantly supported.
Results
Molecular phylogeny
During this study, five strains of two new Erythrobasidium species were discovered in the Baotianman Nature Reserve. To reveal the phylogenetic position of the new species, we performed phylogenetic analyses with combined SSU, ITS, LSU, and TEF1-α sequence data. The sequence dataset consisted of 3,875 characters including gaps (SSU, 1,763 characters; ITS, 569 characters; LSU, 616 characters; TEF1-α, 927 characters). Of these characters, 2,528 were constant, 604 were variable but parsimony-uninformative, and 743 were parsimony-informative. The topology of ML tree is consistent with that of BI tree, agreeing with previous studies (Wang et al., 2015b; Li et al., 2020). Therefore, only the tree inferred from the ML analysis is provided in Figure 1 with MLBS (≥ 50%) and BPP (≥ 0.90) labeled on branches. In our phylogenetic tree, five newly isolated strains were formed into two well supported separate groups (100% MLBS/1 BPP) in the Erythrobasidium clade, and were clearly distinct from other species of Erythrobasidium.
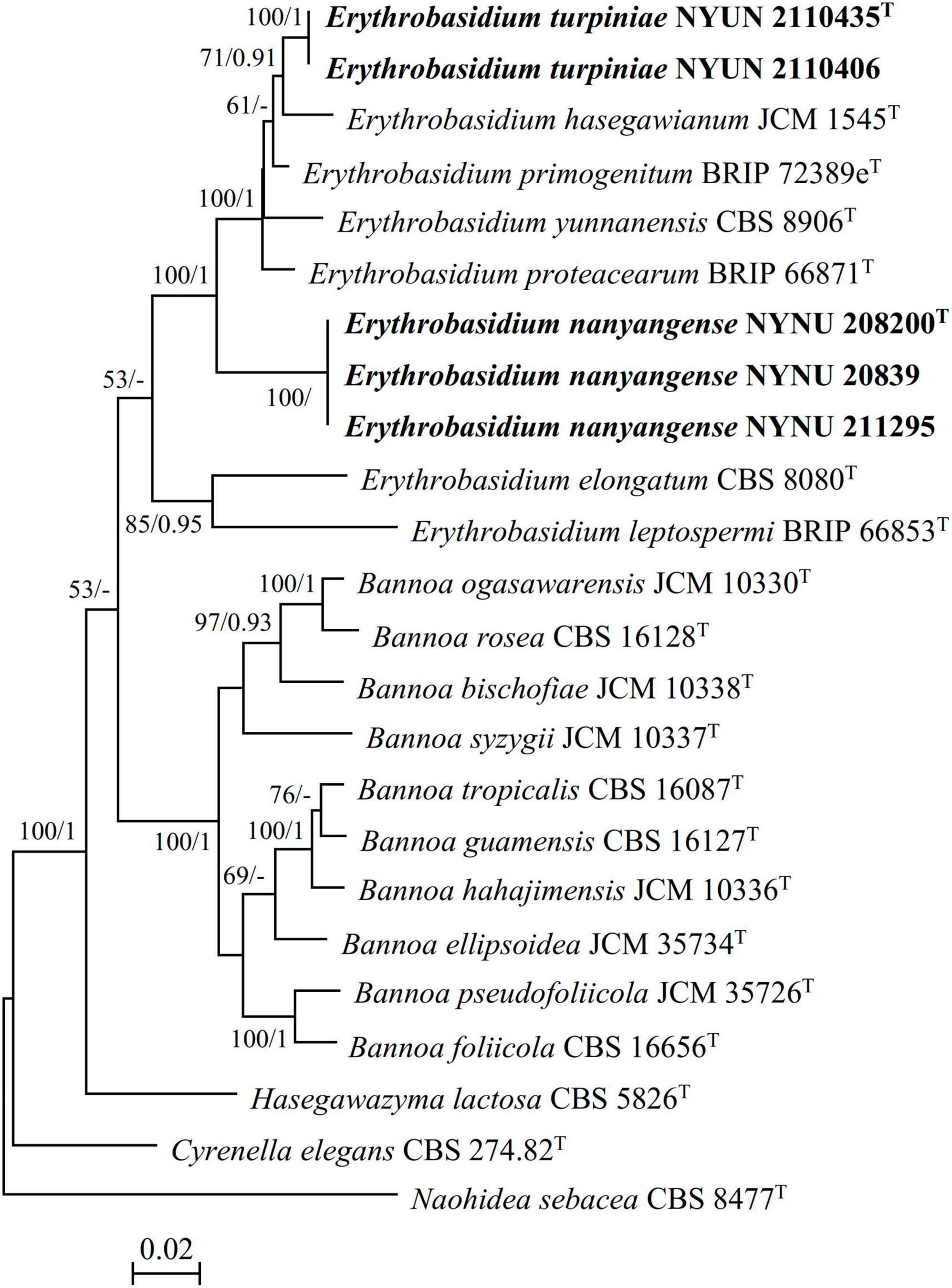
Figure 1. Maximum likelihood (ML) phylogram of the Erythrobasidium species based on combined SSU, ITS, LSU, and TEF1-α sequence data. Naohidea sebacea CBS 8477T was used as the outgroup. Branches are labeled with MLBS ≥ 50% and BPP ≥ 0.90, respectively. New strains described in this study are shown in bold.
Two strains NYNU 2110435 and NYNU 2110406 formed a well-supported clade that clustered with E. hasegawianum with moderate statistical support (71% MLBS/0.91 BPP) (Figure 1). The two strains of the NYUN 2110435 grope had identical sequences in both the D1/D2 domain and the ITS region, indicating they belong to the same species. This group differed from E. hasegawianum by four nt (∼0.7%) substitutions in the D1/D2 domain and by 9 nt (∼1.6) mismatches in the ITS region, respectively. In general, basidiomycetous yeast strains differing by two or more nucleotide substitutions in the D1/D2 domain or having nucleotide differences of 1-2% in the ITS region may represent different taxa (Fell et al., 2000; Vu et al., 2016). The differences in both the D1/D2 and ITS sequences have raised the possibility that the NYNU 2110435 group may represent a novel species distinct from E. hasegawianum. This hypothesis was supported by comparison of the partial TEF1-α gene sequences. While no difference among the partial TEF1-α gene sequences of the strains of the novel species, respectively, were detected; the novel species differed from E. hasegawianum by 40 nt (∼4.7 %) substitutions in this region. These findings confirm that the NYNU 2110435 group represents a novel species in the genus Erythrobasidium, for which the name E. turpiniae sp. nov. is proposed.
Three isolates NYNU 20839, NYNU 208200, and NYNU 211295 formed a distinct divergent lineage within Erythrobasidium (Figure 1). Three isolates of the NYNU 208200 grope shared 100% nucleotide identity based on the D1/D2 and ITS sequences, indicating that they are conspecific. BLASTn searches of the D1/D2 and ITS sequences indicated that this group was most closely related to E. elongatum, differing by 15 nt (∼2.6%) substitutions in the D1/D2 domain and 24 nt (∼4.3%) mismatches in the ITS region, respectively. Moreover, the partial TEF1-α gene sequences further confirm the novelty of this species, as they differed by 99 nt (∼11.6%) substitutions from E. elongatum. Hence, the NYNU 208200 group represents a novel Erythrobasidium species, for which the name E. nanyangense sp. nov. is proposed.
Taxonomy
Erythrobasidium turpiniae C.Y. Chai & F.L. Hui, sp. nov., Figure 2.
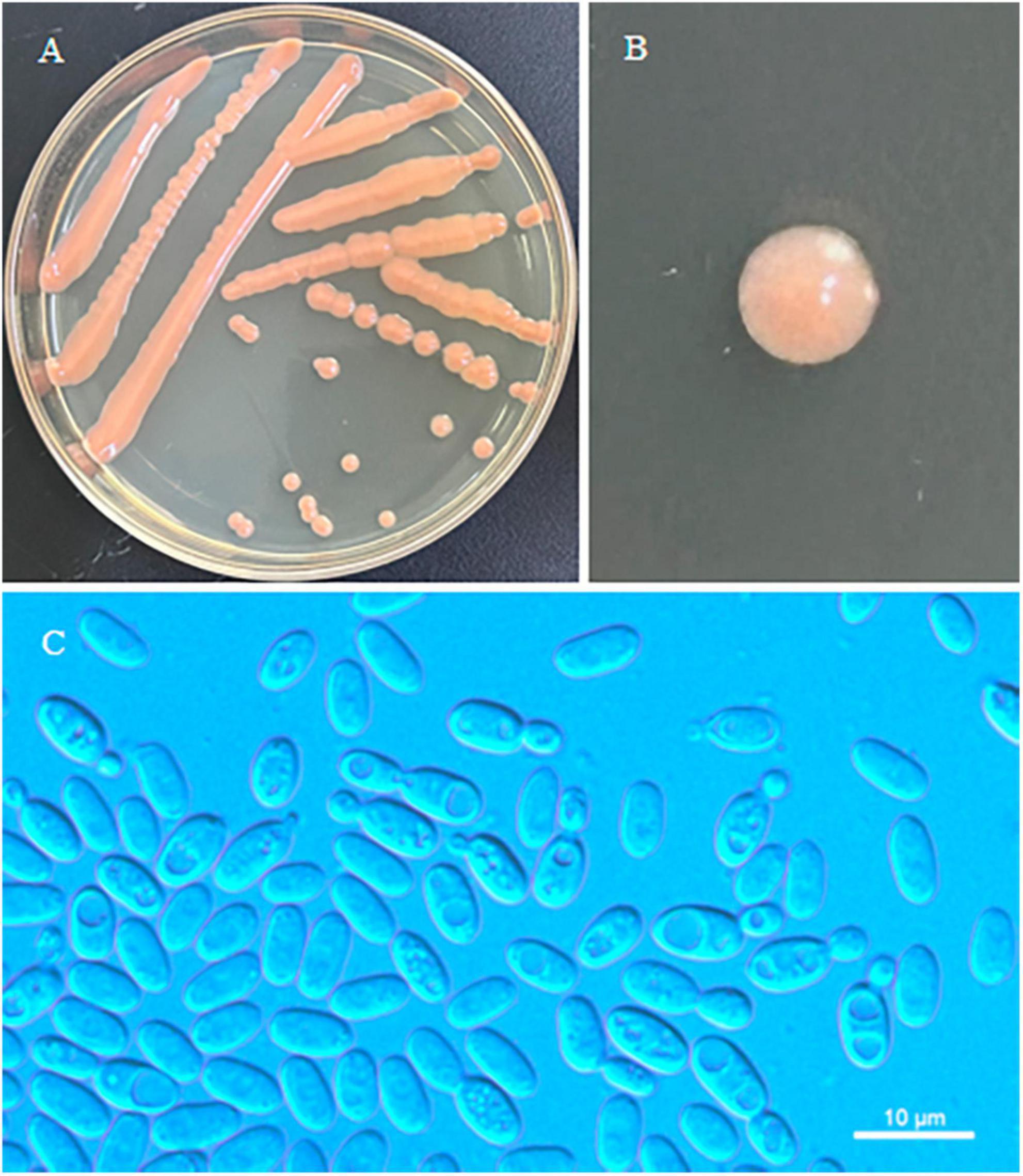
Figure 2. Morphological characteristics of Erythrobasidium turpiniae sp. nov (GDMCC 2.269, holotype). Culture (A), single colony (B) and budding cells (C) on YM agar after growth for 7 d at 25 °C. Scale bars = 10 μm.
MycoBank: MB 847948
Etymology: the specific epithet “turpiniae” refers to Turpinia, the plant genus, from which the type strain was isolated.
Typus: China, Henan Province, Neixiang County, Baotianman Nature Reserve, in phylloplane from leaf of Turpinia sp., October 2021, L. Zhang and H. Zhang, NYUN 2110435 (holotype GDMCC 2.269T preserved as a metabolically inactive state, culture ex-type JCM 35725).
Description: On YM agar, after 7 d at 25°C, the streak culture is orange, smooth, glistening and butyrous in texture. The margin is entire. On YM agar, after 7 d at 25 °C, cells are ellipsoidal and cylindrical, 2.8–4.9 μm × 5.3–7.7 μm and single, budding is polar. After 1 month at 25 °C, a ring and sediment are present. In Dalmau plate culture on corn meal agar, hyphae and pseudohyphae are not formed. Sexual structures are not observed for individual strains and strain pairs on PDA, CM agar and YCBS agar for 1 month. Ballistoconidia are not produced. Glucose fermentation is absent. Glucose, inulin, sucrose, galactose, lactose (weak), trehalose, maltose (weak), melezitose, cellobiose, salicin (weak), L-sorbose, L-rhamnose (weak), D-xylose, L-arabinose, D-arabinose (weak), 5-keto-D-gluconate (weak), glycerol, ribitol, D-mannitol, D-glucitol (weak), DL-lactate, succinate, citrate (weak), D-gluconate (weak), D-glucosamine, 2-keto-D-gluconate and D-glucuronate are assimilated as sole carbon sources. Raffinose, melibiose, methyl-α-D-glucoside, D-ribose, methanol, ethanol, erythritol, galactitol, myo-inositol and D-glucono-1,5-lactone are not assimilated. Nitrate, nitrite (weak), ethylamine (weak) and L-lysine are assimilated as sole nitrogen sources. Cadaverine is not assimilated. Maximum growth temperature is 30 °C. Growth in vitamin-free medium is negative. Starch-like substances are not produced. Urease activity is positive. Diazonium Blue B reaction is positive.
Additional strain examined: China, Henan Province, Neixiang County, Baotianman Nature Reserve, in phylloplane from undetermined leaf, October 2021, L. Zhang and H. zhang, 2110406.
GenBank accession numbers: holotype GDMCC 2.269T (SSU: OP221010, ITS: OM014199, D1/D2: OM014196, TEF1-α: OR785452); additional strain NYUN 2110406 (ITS: OQ130168, D1/D2: OQ130169, TEF1-α: OR785451).
Note: Physiologically, E. turpiniae sp. nov. can be differentiated from its closest relative E. hasegawianum (Hamamoto, 2011) by its ability to assimilate inulin, lactose, L-rhamnose, and D-gluconate, as well as its inability to assimilate D-ribose. In addition, E. turpiniae sp. nov. can grow at 30 °C while E. hasegawianum cannot (Table 3).
Erythrobasidium nanyangense C.Y. Chai & F.L. Hui, sp. nov., Figure 3.
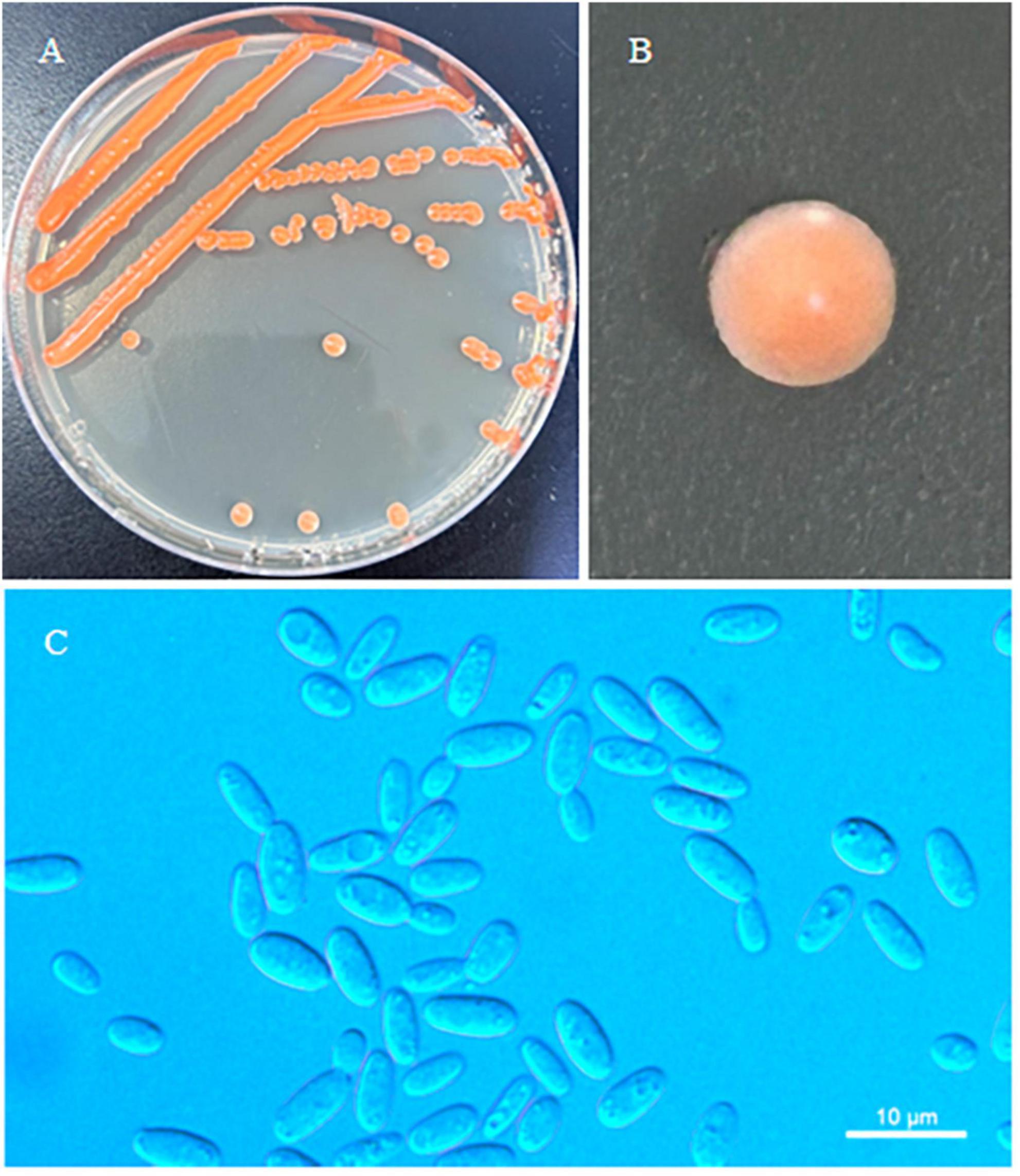
Figure 3. Morphological characteristics of Erythrobasidium nanyangense sp. nov (CICC 33505, holotype). Culture (A), single colony (B) and budding cells (C) on YM agar after growth for 7 d at 25 °C. Scale bars = 10 μm.
MycoBank: MB 847949
Etymology: the specific epithet “nanyangense” refers to the geographic origin of the type strain, Nanyang city, Henan Province.
Typus: China, Henan Province, Neixiang County, Baotianman Nature Reserve, in phylloplane from undetermined leaf, July 2016, L. Zhang and H. zhang, NYNU 208200 (holotype CICC 33505T preserved as a metabolically inactive state, culture ex-type CBS 16661).
Description: On YM agar, after 7 d at 25 °C, the streak culture is orange–red, smooth, glistening and butyrous in texture. The margin is entire. In YM broth, after 7 d at 25 °C, cells are ovoid or cylindrical, 2.5–3.7 μm × 4.8–7.8 μm and single, budding is polar. After 1 month at 25 °C, a ring and sediment are present. In Dalmau plate culture on corn meal agar, hyphae and pseudohyphae are not formed. Sexual structures are not observed for individual strains and strain pairs on PDA, CM agar and YCBS agar for 1 month. Ballistoconidia are not produced. Glucose fermentation is absent. Glucose, inulin, sucrose, galactose, trehalose, maltose, melezitose, methyl-α-D-glucoside, salicin, L-sorbose (weak), L-rhamnose (weak), D-xylose, L-arabinose, D-arabinose, 5-keto-D-gluconate, D-ribose (weak), glycerol, ribitol, galactitol, D-mannitol, D-glucitol, DL-lactate (weak), succinate, D-glucuronate and D-glucono-1,5-lactone are assimilated as sole carbon sources. Raffinose, melibiose, lactose, cellobiose, methanol, ethanol, erythritol, myo-inositol, citrate, D-gluconate, D-glucosamine and 2-keto-D-gluconate are not assimilated. Nitrate, nitrite, ethylamine (weak), L-lysine and cadaverine (weak) are assimilated as sole nitrogen sources. Maximum growth temperature is 30 °C. Growth in vitamin-free medium is negative. Starch-like substances are not produced. Urease activity is positive. Diazonium Blue B reaction is positive.
Additional strain examined: China, Henan Province, Neixiang County, Baotianman Nature Reserve, in phylloplane from Undetermined leaf, October 2021, L. Zhang and H. zhang, NYNU 20839 and NYUN 211295.
GenBank accession numbers: holotype CICC 33505T (ITS: MW362360, D1/D2: MW362359, TEF1-α: OP313688); additional strains NYNU 20839 (ITS: OQ130167, D1/D2: OQ130166, TEF1-α: OR785453) and NYUN 211295 (ITS: OQ130172, D1/D2: OQ130170, TEF1-α: OR785454).
Note: Physiologically, E. nanyangense sp. nov. can be differentiated from its closest relative, E. elongatum (Hamamoto et al., 2011), by its ability to assimilate inulin, galactose, methyl-α-D-glucoside, D-ribose, galactitol, D-glucuronate, nitrate, nitrite, ethylamine, L-lysine, and cadaverine, as well as its inability to assimilate cellobiose. In addition, E. nanyangense sp. nov. can grow at 30 °C while E. elongatum cannot (Table 3).
Key to species of Erythrobasidium
The four species now recognized in Erythrobasidium can be differentiated as indicated in the following key:
1. a. Methyl-α-D-glucoside is assimilated. .. .. .. .. .. .. .. .. .. .. .. E. nanyangense
b. Methyl-α-D-glucoside is not assimilated. .. .. .. .. .. .. .. .. .. .. .. .. .. .. .. .. .2
2. (1) a. Inulin is assimilated. .. .. .. .. .. .. .. .. .. .. .. .. .. .. .. .. .. .. . E. turpiniae
b. Inulin is not assimilated. .. .. .. .. .. .. .. .. .. .. .. .. .. .. .. .. .. .. .. .. . .3
3. (2) a. D-Glucuronate is assimilated. .. .. .. .. .. .. .. .. .. .. .. .. .. .E. hasegawianum
b. D-Glucuronate is not assimilated. .. .. .. .. .. .. .. .. .. .. .. .. .. .. .. .. .. .. . 4
4. (3) a. D-Glucitol is assimilated. .. .. .. .. .. .. .. .. .. .. .. .. .. .. .. . .E. elongatum
b. D-Glucitol is not assimilated. .. .. .. .. .. .. .. .. .. .. .. .. .. .. .. .E. yunnanensis
Discussion
Traditional methods of classification for Erythrobasidium species are based primarily on phenotypical features, such as colony morphology, cell shape, basidia formation, and details of physiological and biochemical characteristics etc. (Hamamoto, 2011). The classification based on these phenotypical features, however, was in many cases not consistent with the results obtained from phylogenetic analyses. For example, E. elongatum and E. yunnanensis, originally classified in the Sporobolomyces, are nested within the teleomorphic genus Erythrobasidium based on phylogenetic analyses (Bai et al., 2001; Hamamoto, 2011; Hamamoto et al., 2011; Wang et al., 2015a). As a result, these two species were then reassigned to the genus Erythrobasidium, according to the International Code of Nomenclature for Algae, Fungi, and Plants (McNeill et al., 2012). Therefore, a combination of phenotypical characteristics and phylogenetic analysis has been adopted as the standard method for concretely identifying Erythrobasidium species (Wang et al., 2015b).
In this study, we introduce E. turpiniae sp. nov. and E. nanyangense sp. nov as two new species of Erythrobasidium, and describe them in asexual morphs based on molecular analyses and morphological features. We found that E. turpiniae sp. nov. formed a basal clade related to E. hasegawianum (Figure 1). E. nanyangense sp. nov. clustered together with a separate clade within Erythrobasidium (Figure 1). We compared the sequences of the D1/D2 domain, ITS region, and TEF1-α gene of two new species with their closely related species. The differences in these regions were great enough to separate new Erythrobasidium strains into two species. The species shared high similarity in colony morphology, color, and individual cell shape, and they clearly differed from the closest known species in physiological and biochemical characteristics (Table 3). The combination of the morphological characteristics and molecular analyses recorded in our study strongly supports the recognition of two new Erythrobasidium species.
Members of the genus Erythrobasidium have not yet been sufficiently studied and the species diversity has long been underestimated. Up to now, only eight Erythrobasidium species, including E. turpiniae sp. nov. and E. nanyangense sp. nov. described in the present study, were found in nature. E. hasegawianum was the most widely distributed, occurring in China, USA, and South Africa (Hamamoto, 2011; Vu et al., 2016; Zang et al., 2018; Li et al., 2020). E. elongatum, E. leptospermi, E. primogenitum, E. proteacearum, E. nanyangense sp. nov., E. turpiniae sp. nov., and E. yunnanensis were scarce, occurring only in Australia or China (Bai et al., 2001; Hamamoto et al., 2011; Tan et al., 2021; Tan and Shivas, 2022). However, some unpublished strains of Erythrobasidium have also been isolated in different parts of the world; for example, Erythrobasidium sp. GY113362PS (LC272891) and Erythrobasidium sp. GY1131127PS (LC272890) have been obtained from Korea, Erythrobasidium sp. OTU655 (MK018684) and Erythrobasidium sp. LM681 (EF060964) from USA, Erythrobasidiales sp. DBP-2011 (KM527115) from Italy, and Erythrobasidium sp. UFMG-ABT330 (KM527115) and Erythrobasidium sp. BRT565 (OR430047) from Brazil. In addition, several environmental sequences of Erythrobasidium have also been reported from Brazil, from Finland (Pitkäranta et al., 2008), and from Germany (Renker et al., 2005; Neubert et al., 2006). Taken together, these suggests this genus could be broadly distributed and further large-scale studies are needed to explore the diversity and distribution of Erythrobasidium species worldwide.
The phylloplane is an important habitat for yeasts and these yeasts can alter nutrient availability for other microorganisms, antagonize pathogens, and stimulate plant defenses (Into et al., 2020a,b). As yeasts play crucial roles in nutrient cycling and symbiotic relationships with plants, discovering the diversity of yeast taxa helps in the functioning of ecosystems. Besides, protected areas like the Baotianman Nature Reserve are usually undisturbed, and conducting research in these areas has a high potential for discovering novel and endemic yeast species, consequently contributing to the expansion of yeast diversity.
Conclusion
Although Erythrobasidium is widely distributed in the world, the diversity of this genus has not been completely resolved. In this work, five phyllosphere-inhabiting yeast strains were identified as two new species, E. turpiniae sp. nov. and E. nanyangense sp. nov., based on morphological and molecular phylogenetic analysis, which provides us with further understanding of this genus diversity in China. In the future, we firmly believe that more and more species of the genus will be isolated from more plants around the world.
Data availability statement
The datasets presented in this study can be found in online repositories. The names of the repository/repositories and accession number(s) can be found in this article/supplementary material.
Author contributions
Y-FL: Investigation, Writing – original draft. C-YC: Investigation, Writing – review & editing. F-LH: Writing – review & editing.
Funding
The authors declare financial support was received for the research, authorship, and/or publication of this article. This research was funded by the National Natural Science Foundation of China (Project No. 31570021) and the State Key Laboratory of Motor Vehicle Biofuel Technology, Henan Tianguan Enterprise Group Co., Ltd., China (No. 2018001).
Acknowledgments
We are very grateful to our colleagues at the School of Life Sciences and Agricultural Engineering, Nanyang Normal University, including Dr. Lin Zhang and Dr. H. Zhang for providing specimens; to Ting Lei for help with phylogenetic analysis; and to Ran-Ran Jia and Wen-Ting Hu for help with morphological observations.
Conflict of interest
The authors declare that the research was conducted in the absence of any commercial or financial relationships that could be construed as a potential conflict of interest.
Publisher’s note
All claims expressed in this article are solely those of the authors and do not necessarily represent those of their affiliated organizations, or those of the publisher, the editors and the reviewers. Any product that may be evaluated in this article, or claim that may be made by its manufacturer, is not guaranteed or endorsed by the publisher.
Footnotes
References
Andrew, R. (2016). FigTree: Tree Figure Drawing Tool Version 1.4.3. Institute of Evolutionary Biology, United Kingdom. Edinburgh: University of Edinburgh Press.
Bai, F. Y., Takashima, M., Hamamoto, M., and Nakase, T. (2001). Sporobolomyces yunnanensis sp. nov., a Q-10(H2)-containing yeast species with a close phylogenetic relationship to Erythrobasidium hasegawianum. Int. J. Syst. Evol. Microbiol. 51, 231–235. doi: 10.1099/00207713-51-1-231
Chai, C. Y., Lei, T., Chu, X. Y., and Hui, F. L. (2023). Multi-gene phylogeny and taxonomy of the genus Bannoa with the addition of three new species from central China. Front. Microbiol. 14:1143156. doi: 10.3389/fmicb.2023
de Azeredo, L. A. I., Gomes, E. A. T., Mendonca-Hagler, L. C., and Hagler, A. N. (1998). Yeast communities associated with sugarcane in Campos. Rio de Janeiro, Brazil. Int. Microbiol. 1, 205–208.
Denchev, C. M. (2009). Validation of three names of families in the Pucciniomycotina. Mycol. Balcanica. 6, 87–88.
do Carmo-Sousa, L., and Phaff, H. J. (1962). An improved method for the detection of spore discharge in the Sporobolomycetaceae. J. Bacteriol. 83, 434–435. doi: 10.1128/jb.83.2.434-435.1962
Fell, J. W., Boekhout, T., Fonseca, A., Scorzetti, G., and StatzellTallman, A. (2000). Biodiversity and systematics of basidiomycetous yeasts as determined by large-subunit rDNA D1/D2 domain sequence analysis. Int. J. Syst. Evol. Microbiol. 50, 1351–1371. doi: 10.1099/00207713-50-3-1351
Hall, T. A. (1999). Bioedit: a user-friendly biological sequence alignment editor and analysis program for Windows 95/98/NT. Nucleic Acids Symp. Ser. 41, 95–98.
Hamamoto, M. (2011). “Erythrobasidium Hamamoto, Sugiyama & Komagata (1991),” in The Yeasts, a Taxonomic Study, 5 Edn, eds C. P. Kurtzman, J. W. Fell, and T. Boekhout (Amsterdam: Elsevier), 1433–11435. doi: 10.1016/B978-0-444-52149-1.00112-9
Hamamoto, M., Boekhout, T., and Takashi Nakase, T. (2011). “Sporobolomyces Kluyver and van Niel (1924),” in The Yeasts, a Taxonomic Study, 5 Edn, eds C. P. Kurtzman, J. W. Fell, and T. Boekhout (Amsterdam: Elsevier), 1929–1990. doi: 10.1016/B978-0-444-52149-1.00156-7
Hamamoto, M., and Nakase, T. (2000). Phylogenetic analysis of the ballistoconidium-forming yeast genus Sporobolomyces based on 18S rDNA sequences. Int. J. Syst. Evol. Microbiol. 50, 1373–1380. doi: 10.1099/00207713-50-3-1373
Hamamoto, M., Sugiyama, J., and Komagata, K. (1988). Transfer of Rhodotorula hasegawae to a new basidiomycetous genus Erythrobasidium as Erythrobasidium hasegawae comb. nov. J. Gen. Appl. Microbiol. 34, 279–287.
Hamamoto, M., Sugiyama, J., and Komagata, K. (1991). Nomenclature of the basidiomycetous yeast species Erythrobasidium hasegawae. J. Gen. Appl. Microbiol. 37, 131–132.
Hu, W. T., Chu, S. B., Li, Y., and Hui, F. L. (2022). Hyphopichia xiaguanensis f.a., sp. nov., an ascomycetous yeast species isolated from plant leaves. Int. J. Syst. Evol. Microbiol. 72:5398. doi: 10.1099/ijsem.0.005398
Into, P., Pontes, A., Sampaio, J. P., and Limtong, S. (2020a). Yeast diversity associated with the phylloplane of corn plants cultivated in Thailand. Microorganisms 8:80. doi: 10.3390/microorganisms8010080
Into, P., Khunnamwong, P., Jindamoragot, S., Am-In, S., Intanoo, W., and Limtong, S. (2020b). Yeast associated with rice phylloplane and their contribution to control of rice sheath blight disease. Microorganisms 8:362. doi: 10.3390/microorganisms8030362
Kalyaanamoorthy, S., Minh, B. Q., Wong, T. K. F., Von Haeseler, A., and Jermiin, L. S. (2017). Modelfinder: fast model selection for accurate phylogenetic estimates. Nat. Methods 14, 587–589. doi: 10.1038/nmeth.4285
Katoh, K., and Standley, D. M. (2013). MAFFT multiple sequence alignment software version 7: improvements in performance and usability. Mol. Biol. Evol. 30, 772–780. doi: 10.1093/molbev/mst010
Kurtzman, C. P., Fell, J. W., and Boekhout, T. (2011). “Methods for isolation, phenotypic characterization and maintenance of yeasts,” in The Yeasts, a Taxonomic Study, 5 Edn, eds C. P. Kurtzman, J. W. Fell, and T. Boekhout (Amsterdam: Elsevier), 87–110. doi: 10.1016/B978-0-444-52149-1.00007-0
Kurtzman, C. P., and Robnett, C. J. (1998). Identification and phylogeny of ascomycetous yeasts from analysis of nuclear large subunit (26S) ribosomal DNA partial sequences. Antonie van Leeuwenhoek 73, 331–371. doi: 10.1023/a:1001761008817
Li, A. H., Yuan, F. X., Groenewald, M., Bensch, K., Yurkov, A. M., Li, K., et al. (2020). Diversity and phylogeny of basidiomycetous yeasts from plant leaves and soil: proposal of two new orders, three new families, eight new genera and one hundred and seven new species. Stud. Mycol. 96, 17–140. doi: 10.1016/j.simyco.2020.01.002
Mannazzu, I., Landolfo, S., da Silva, T. L., and Buzzini, P. (2015). Red yeasts and carotenoid production: outlining a future for nonconventional yeasts of biotechnological interest. World J. Microbiol. Biotechnol. 31, 1665–1673. doi: 10.1007/s11274-015-1927-x
McNeill, J., Barrie, F., Buck, W., Demoulin, V., Greuter, W., Al, E., et al. (2012). International Code of Nomenclature for Algae, Fungi, and Plants (Melbourne Code). Regnum Vegetabile 154. Königstein: Koeltz Scientific Books.
Nakase, T., and Takashima, M. (1993). A simple procedure for the high frequency isolation of new taxa of ballistosporous yeasts living on the surfaces of plants. RIKEN Rev. 3, 33–34.
Neubert, K., Mendgen, K., Brinkmann, H., and Wirsel, S. G. (2006). Only a few fungal species dominate highly diverse mycofloras associated with the common reed. Appl. Environ. Microbiol. 72, 1118–1128. doi: 10.1128/AEM.72.2.1118-1128.2006
Pitkäranta, M., Meklin, T., Hyvärinen, A., Paulin, L., Auvinen, P., Nevalainen, A., et al. (2008). Analysis of fungal flora in indoor dust by ribosomal DNA sequence analysis, quantitative PCR, and culture. Appl. Environ. Microbiol. 74, 233–244. doi: 10.1128/AEM.00692-07
Rehner, S. A., and Buckley, E. A. (2005). Beauveria phylogeny inferred from nuclear ITS and EF1-α sequences: evidence for cryptic diversification and links to Cordyceps teleomorphs. Mycologia 97, 84–98. doi: 10.3852/mycologia.97.1.84
Renker, C., Otto, P., Schneider, K., Zimdars, B., Maraun, M., and Buscot, F. (2005). Oribatid mites as potential vectors for soil microfungi: study of mite-associated fungal species. Microb. Ecol. 50, 518–528. doi: 10.1007/s00248-005-5017-8
Ronquist, F., Teslenko, M., Mark, P., Avres, D. L., Darling, A., Höhna, S., et al. (2012). MrBayes3.2: efficient Bayesian phylogenetic inference and model choice, across a large model space. Syst. Biol. 61, 539–542. doi: 10.1093/sysbio/sys029
Shivas, R. G., and Rodrigues de Miranda, L. (1983). Two new species of the genus Sporobolomyces and a new Rhodotorula species from leaf surfaces. Antonie van Leeuwenhoek 49, 159–166.
Srisuk, N., Nutaratat, P., Surussawadee, J., and Limtong, S. (2019). Yeast communities in sugarcane phylloplane. Microbiology 88, 353–369. doi: 10.1134/S0026261719030135
Stamatakis, A. (2014). RAxML version 8: a tool for phylogenetic analyses and post analyses of large phylogenies. Bioinformatics 30, 1312–1313. doi: 10.1093/bioinformatics/btu033
Tan, Y. P., Bishop-Hurley, S. L., Marney, T. S., and Shivas, R. G. (2021). Nomenclatural novelties. Index Fungorum 503, 1–8.
Tan, Y. P., and Shivas, R. G. (2022). Nomenclatural novelties. Index Australian Fungi 1, 1–18. doi: 10.5281/zenodo.7250859
Thompson, J. D., Gibson, T. J., Plewniak, F., Jeanmougin, F., and Higgins, D. G. (1997). The CLUSTAL_X windows interface: flexible strategies for multiple sequence alignment aided by quality analysis tools. Nucleic Acids Res. 25, 4876–4882. doi: 10.1093/nar/25.24.4876
Toome, M., Roberson, R. W., and Aime, M. C. (2013). Meredithblackwellia eburnean gen. et sp. nov., Kriegeriaceae fam. nov. and Kriegeriales ord. nov. toward resolving higher-level classification in Microbotryomycetes. Mycologia 105, 486–495. doi: 10.3852/12-251
Vu, D., Groenewald, M., Szöke, S., Cardinali, G., Eberhardt, U., Stielow, B., et al. (2016). DNA barcoding analysis of more than 9 000 yeast isolates contributes to quantitative thresholds for yeast species and genera delimitation. Stud. Mycol. 85, 91–105. doi: 10.1016/j.simyco.2016.11.007
Wang, Q. M., Groenewald, M., Takashima, M., Theelen, B., Han, P. J., Liu, X. Z., et al. (2015a). Phylogeny of yeasts and related taxa within Pucciniomycotina determined from multigene sequence analyses. Stud. Mycol. 81, 27–53. doi: 10.1016/j.simyco.2015.08.002
Wang, Q. M., Yurkov, A. M., Göker, M., Lumbsch, H. T., Leavitt, S. D., Groenewald, M., et al. (2015b). Phylogenetic classification of yeasts and related taxa within Pucciniomycotina. Stud. Mycol. 81, 146–189. doi: 10.1016/j.simyco.2015.12.002
Wang, Q. M., Theelen, B., Groenewald, M., Bai, F. Y., and Boekhout, T. (2014). Moniliellomycetes and Malasseziomycetes, two new classes in Ustilaginomycotina. Persoonia 2014, 41–47. doi: 10.3767/003158514X682313
White, T. J., Bruns, T., Lee, S., and Taylor, J. (1990). “Amplification and direct sequencing of fungal ribosomal RNA genes for phylogenetics,” in PCR Protocols: a Guide to Methods and Applications, eds M. A. Innis, D. H. Gelfand, J. J. Sninsky, and T. J. White (San Diego, CA: Academic Press), 315–322. doi: 10.1016/B978-0-12-372180-8.50042-1
Zang, W., Liu, C. Y., Xie, G. F., Shen, C., Liu, X. Z., Zou, H. J., et al. (2018). Resources and species diversity of the phyllosphere yeasts from the Yandang Mountains in Zhejiang Province. Acta Ecol. Sin. 38, 3920–3930. doi: 10.5846/stxb201705040827
Keywords: Basidiomycota, Erythrobasidiaceae, phylogenetic analysis, orange-red yeast, leaf
Citation: Lu Y-F, Chai C-Y and Hui F-L (2024) Two new Erythrobasidium species inhabiting the phyllosphere discovered in the Baotianman Nature Reserve in China. Front. Microbiol. 15:1287984. doi: 10.3389/fmicb.2024.1287984
Received: 03 September 2023; Accepted: 22 January 2024;
Published: 06 February 2024.
Edited by:
George Tsiamis, University of Patras, GreeceReviewed by:
Nicolás D. Franco-Sierra, Vedas CII, ColombiaAbhay K. Pandey, North Bengal Regional R&D Center, India
Copyright © 2024 Lu, Chai and Hui. This is an open-access article distributed under the terms of the Creative Commons Attribution License (CC BY). The use, distribution or reproduction in other forums is permitted, provided the original author(s) and the copyright owner(s) are credited and that the original publication in this journal is cited, in accordance with accepted academic practice. No use, distribution or reproduction is permitted which does not comply with these terms.
*Correspondence: Feng-Li Hui, ZmVuZ2xpaHVpQHllYWgubmV0