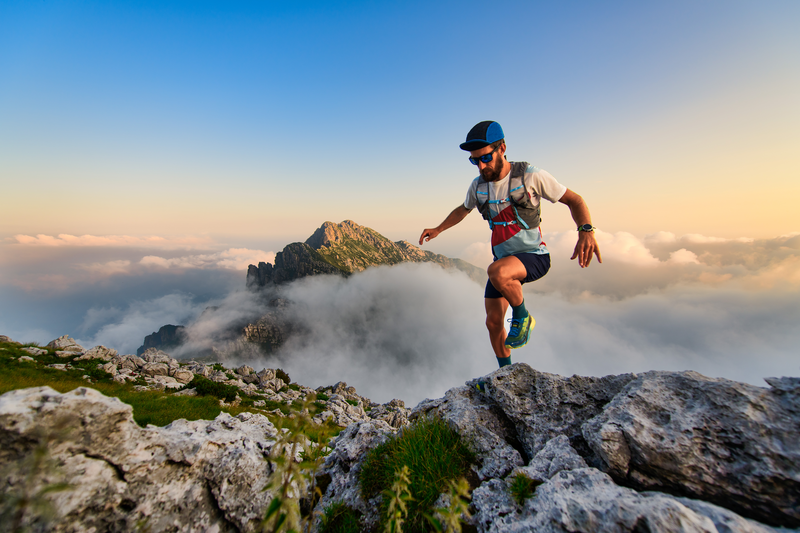
95% of researchers rate our articles as excellent or good
Learn more about the work of our research integrity team to safeguard the quality of each article we publish.
Find out more
ORIGINAL RESEARCH article
Front. Microbiol. , 11 January 2024
Sec. Ancient DNA and Forensic Microbiology
Volume 14 - 2023 | https://doi.org/10.3389/fmicb.2023.1326835
This article is part of the Research Topic Microbes and Cultural Heritage: From Biodiversity to Applications View all 4 articles
Kaihua paper is a type of precious hand-made paper in China that has been used throughout Chinese history. Due to its extraordinary whiteness and fine texture, it was adopted by the imperial palace in the reign of Emperor Kang Xi and Yong Zheng of the Qing Dynasty of China in the 17th and 18th century. It is stained by a special type of yellowish-brown spot after years of storage, which is called a “peachy spot.” The formation of such spots remains unclear, although complicated physicochemical processes or microbial activities might be involved. We performed nondestructive sampling and high-throughput sequencing on peachy spot surfaces, unstained areas, and air samples in the stack room to analysis the the bacterial and fungal communities, and performed prediction of functional genes of the bacterial communities. The results showed that peachy spot formation was mainly related to bacterial communities rather than fungal communities. Significantly more potential acid- producing, acidophilic or cellulase-producing bacteria, such as, Streptococcus, Staphylococcus, and Lysinibacillus, and pigment-producing bacteria, such as Methylobacterium and Rubrobacter, were identified in the peachy spot samples. Prediction of the functional genes of the bacterial community also suggested the production of acidic substance pigments. These findings provide new insights into the pigment formation mechanism in ancient paper and open an opportunity to develop new strategies to preserve the ancient paper documents.
Paper, as a carrier of human thought, art and history, is critical in human society. Paper relics, like all other cultural heritage materials, are nonrenewable and will degenerate over time. Thus, preserving paper relics is a cultural and technological challenge.
Microbial deterioration of paper materials has always been a focus in the preservation of paper relics (Cappitelli et al., 2010). Early studies on the biodegradation of cultural heritage have been carried out for approximately a century (Sterflinger et al., 2018), using mostly culture-dependent methods and microscopic observations (Mitchell and McNamara, 2010). Fungi, especially filamentous fungi, are considered the most important organisms in microbial hazards. Due to technical limitations, only culturable microbes, which represent only a small part of the total microbes, were studied (Amann et al., 1995). These culturable strains were probably not the dominant microbes that caused paper damage. In recent years, new methods have been applied to study the microbial community on various historical relics, including paper, parchment, storeroom objects, and photographic materials (Sclocchi et al., 2016; Migliore et al., 2017; Liu et al., 2018; Piñar et al., 2020; Branysova et al., 2022). Genomic analysis based on high-throughput sequencing is becoming a powerful approach to study the diversity and impact of microorganisms on historical objects (Kraková et al., 2018; Sterflinger et al., 2018; Beata, 2020).
Traditional Chinese handmade paper was made of plant fiber, following slightly different processes depending on the geographical area and historical period of production. The sources of plant fiber include bark, straw, and bamboo. Moreover, paper is often coated with gelatin or other substances to make it impermeable to ink (Area and Cheradame, 2011). These diverse organic and inorganic components in paper provide substrates for microbial growth and reproduction under favorable conditions, which in turn destroys the structure of the paper (Sterflinger, 2010). Therefore, different types of paper may favor different microorganisms that settle on its surface, resulting in different structures of microbial communities and ultimately different kinds of damage.
Kaihua paper is a unique type of ancient paper preserved to this day that is famous for its delicate and white and thin characteristics. It was one of the most valuable royal papers in the Qing Dynasty, especially during the reign of the three emperors, Kangxi, Yongzheng and Qianlong (1662–1795 A.D.). The plant fiber used to make Kaihua paper was from the family Thymelaeaceae. Ancient Kaihua paper commonly developed isolated or coalescent yellowish-brown spots, which are called “peachy spots.” Such spots affect the paper greatly and may lead to irreversible loss of historical content. Morphologically, peachy spots resemble the well-studied “foxing spots,” a common damage to paper cultural relics (Meynell and Newsam, 1978). Among the many hypotheses about foxing formation, metals and microorganisms are considered the two major causes (Choi, 2007). However, the role of microorganisms in the formation of “peachy spots” on Kaihua paper remains unknown.
In this study, culture-independent high-throughput sequencing was used to identify bacterial and fungal communities on Kaihua paper affected by peachy spots. The potential roles of bacterial and fungal groups in the formation of peachy spots were explored by correlation analysis and prediction of functional genes of the microbial community.
Three sets of ancient books made of Kaihua paper preserved in Fudan University Library were selected for this study (Figure 1). These books came from the reign of Emperor Kang Xi and Yong Zheng and Qian Long of the Qing Dynasty of China in the 18th century and have been kept in the same stack room for rare ancient books for a long time without being touched. The temperature of the stack room is kept at 16 ~ 20°C and the humidity at 50 ~ 60%. Although the books are in relatively good condition, there are obvious peachy spots on each set of books.
Figure 1. Representative images of the “peachy spots” of Kaihua paper from three sets of ancient books. (A) Zhu Zi Quan Shu dated approximately 1713–1717 A.D. (B) Zhu Pi Yu Zhi dated approximately 1713–1717 A.D. (C) Yu Zhuan Xing Li Jing Yi in Manchu dated approximately 1732–1738 A.D.
The sampling areas most suitable for the study were chosen and evaluated carefully, and the sampling protocol was designed to minimize impact to the paper. A sterilized polyester fiber swab (SwwipTM, Cleanmo, Shenzhen, China) was selected as the sampling tool (swab head size: 4.0 mm x 11.0 mm, total length: 70.0 mm). The swab head was fully moistened with 20 μL of sterilized wetting solution (0.15 M NaCl and 0.1% Tween-80, dissolved in ultrapure water) and used to gently wipe the preselected sampling position 20 times to collect the microorganisms on the paper surface. Then, the swab head was peeled off using sterile tweezers and immediately transferred into the PowerBead extraction tube in the MOBIO PowerSoil DNA Isolation Kit (Mo Bio, Carlsbad, CA, USA). For each set of books, three samples of peachy spots and one sample of unstained area of the inner page were collected.
To exclude interference from airborne microorganisms of the stack room at the time of sampling, the indoor air sedimentation sample were independently collected as the control. Three sterilized polyester fiber swabs were moistened as other sample swabs and allowed to stand on the sampling bench during the sampling, which lasted about 1 h. At the end of the sampling, the heads of three swabs were peeled off and transferred into a single extraction tube for DNA extraction and amplification process, following the same procedure as the other samples to keep consistency.
Samples were stored in a − 20°C freezer, and DNA extraction was performed within 12 h.
For DNA extraction, each sample was processed using the MOBIO PowerSoil DNA Isolation Kit following the manufacturer’s instructions with minor modifications. The C6 solution provided by the kit was replaced with sterile TE buffer to minimize DNA degradation.
Sequences of the 16S rDNA and ITS rDNA were amplified for bacteria and fungi, respectively, using primer sets of 515F (GTGCCAGCMGCCGCGGTAA)/806R (GGACTACHVGGGTWTCTAAT) for the V4 region of 16S rDNA (Kuczynski et al., 2011) and ITS5-1737F (GGAAGTAAAAGTCGTAACAAGG)/ITS2-2043R (GCTGCGTTCTTCATCGATGC) for region 1 of ITS rDNA (Bellemain et al., 2010). The amplification was conducted according to the following program: initial denaturation at 94°C for 5 min, 20 cycles of denaturation at 94°C for 45 s, annealing at 50°C for 30 s, and extension for 90 s at 72°C, final extension at 72°C for 10 min. Then the same amplification was performed once again to add the barcode sequences to the primers for sample distinction after sequencing.
The concentration, total amount and purity of the amplification products were determined, and then the qualified amplification products were sequenced on the Illumina MiSeq (300-bp paired-end reads) platform (Illumina Inc., San Diego, United States) by Novogene Bioinformatics Technology Co., Ltd. (Beijing, China).
The QIIME platform (Caporaso et al., 2010) was used to filter the acquired sequences to remove low quality (Q value less than 20), short length (shorter than 250 bp) and chimeric sequences. The sequences were split according to their taxonomy and assigned to operational taxonomic units (OTUs) at the 97% similarity level using MOTHUR1 (Schloss et al., 2009). The representative sequences of these OTUs were classified within the SILVA database release 119 for bacteria and UNITE for fungi (Abarenkov et al., 2010).
The QIIME platform was used to analyze the bacterial and fungal community structure and composition on the normalized OTU dataset. Rarefaction curves were created to assess sampling efficiency. Three alpha diversity indexes, Chao 1, PD value and Shannon index, were calculated to reflect the overall diversity and complexity of the microbial community.
Multivariate analyses on the OTU dataset were performed using the UniFrac unweighted metric in QIIME. Principal coordinates analysis (PCoA) was performed to show the differences between the sample sets. Species abundance clustering heatmaps were used to analyze the microbial composition structure.
Tax4Fun was implemented to predict the functional differences between unstained areas and peachy spots. Tax4Fun is an R package that links 16S rRNA gene sequences with the functional annotation of sequenced prokaryotic genomes, which is realized with a nearest-neighbor identification based on a minimum 16S rRNA sequence similarity (Aßhauer et al., 2015). It was used to predict the functional genes from the 16S rDNA information of “peachy spots” groups and samples from unstained area based on the Silva database, and the KEGG functional annotation abundance table was obtained. Then STAMP software (Parks et al., 2014) was used to screen for genes with significant differences in abundance between “peachy spots” samples and unstained samples. Only differences with p values less than 0.05 were reported.
All statistical analyses were conducted in the R environment (v3.4.3; http://www.r-project.org/), and all statistical tests were considered significant at p values less than 0.05. Two-way nested ANOSIM (analysis of similarity) was used to test for significant differences in microbial composition (Clarke and Warwick, 1994). Wilcoxon’s test was used to compare the relative abundance between the unstained areas and peachy spots.
Samples were taken from the surface of three sets of ancient books made of Kaihua paper: Zhu Zi Quan Shu, Zhu Pi Yu Zhi, and Yu Zhuan Xing Li Jing Yi in Manchu. Sequencing of the amplicon libraries of 16S rDNA and ITS rDNA yielded a total of 1,656,407 raw reads belonging to bacteria and fungi. After quality trimming and assigning reads to their respective samples, 789,811 high-quality 16S rDNA sequences and 810,781 high-quality ITS rDNA sequences were obtained for 13 samples, including 9 samples of peachy spots, 3 samples of unstained areas, and 1 stack room air sample (Table 1).
The rarefaction curves confirmed the efficiency of the sampling (Figures 2A, 3A). In the bacterial part, the alpha diversity index of the peachy spots was significantly higher than that of the unstained group (p < 0.05), and the alpha diversity indexes of the three groups of peachy spot samples were very close to each other, with no significant differences. The diversity of the bacterial community at the peachy spots was significantly higher than that of the unstained area of the same book (Figures 2B–D). However, no consistent difference was found in the diversity of the fungal community between peachy spots and unstained areas (Figures 3B–D). In addition, there was a significant within-group difference in the Chao1 index and PD value in the KTa group (Figures 3B,D), indicating that the fungal community structure varied greatly between spots in the same book. It seemed that peachy spots on Kaihua paper were more related to bacteria than fungi.
Figure 2. Rarefaction curves and alpha diversity index of bacterial communities of 9 “peachy spot” samples, 3 unstained samples and 1 air sample. (A) Rarefaction curves; (B) Chao1 index; (C) Shannon index; (D) PD value. The alpha diversity of the KT group (KTa, KTb and KTc) was significantly higher than that of the K group (p < 0.05).
Figure 3. Rarefaction curves and alpha diversity index of fungal communities of 9 “peachy spot” samples, 3 unstained samples and 1 air sample. (A) Rarefaction curves; (B) Chao1 index; (C) Shannon index; (D) PD value.
Species annotation was performed on all bacterial and fungal OTUs. Most identified bacteria belonged to the phyla Bacillota, Pseudomonadota, and Actinomycetota (Supplementary Table S1). All identified fungi belonged to 7 phyla. The phylum Ascomycota occurred with the highest abundance in all samples, followed by Basidiomycota and Zygomycota (Supplementary Table S2).
The top 35 most abundant genera of either bacteria or fungi were used for cluster analysis (Figure 4). The heatmap showed that the bacterial communities of high abundance were quite similar within peachy spot groups KTa, KTb, and KTc and were clearly different from the unstained group (K group) and the air deposition sample (control) (Figure 4A). Peachy spot groups had significantly higher proportions of Bacillota, such as Methylobacterium, Christensenellaceae, Streptococcus, Lysinibacillus, Staphylococcus and Lactobacillus, than the unstained group and air deposition sample (Figure 4A). In addition, Rubrobacter of Actinobacteria was also significantly aggregated in peachy spots, whereas the proportion of other genera of Actinobacteria and Pseudomonadota was relatively low (Figure 4A). Among the unstained paper samples, Paenibacillus and Bacillus were more prominent (Figure 4A). In the air deposition sample, there were significantly more Escherichia-Shigella (Figure 4A).
Figure 4. Heatmap of grouping clustering in bacterial communities (A) and fungal communities (B). Thirty-five genera with the highest proportion were used for this cluster analysis.
Furthermore, the clustering of the species abundance of fungi varied greatly. No significant similarity was found between different peachy spot groups or between peachy spot groups, the unstained paper group, and the air deposition sample (Figure 4B), indicating that fungi might not be the main cause of peachy spots. Aspergillus, which was frequently found on ancient books, aggregated in the air sample but not in the paper samples (Figure 4B). In addition, the proportions of Cercospora and Fusarium were also higher in the air deposition sample than in all paper samples (Figure 4B). In the unstained paper group, there were high abundances of Cladosporium, Neurospora and Talaromyces (Figure 4B). These genera include multiple species that are common plant endophytes.
To reveal the differences in community structure between peachy spot areas and unstained areas, we conducted PLS-DA to compare the composition of both bacteria and fungi in all samples. The results showed that the microbial community (both bacteria and fungi) on the paper surface could be completely separated from that of the indoor air of the stack room (Figure 5). The bacterial community of peachy spot samples could also be separated from the unstained paper samples (Figure 5A), whereas the fungal community of these two sets of samples overlapped (Figure 5B). Moreover, the bacterial community structures of the peachy spot groups were relatively close to each other, and the difference within each group was less than that of fungi (Figure 5). These results also suggested that the formation of peachy spots may be directly related to the bacterial community but not to the fungal community.
Figure 5. PLS-DA (partial least squares discrimination analysis) of bacterial communities (A) and fungal communities (B).
To elucidate the potential contribution of the bacteria to the formation of peachy spots, we defined the peachy spot samples in all books as the peachy spot group (KT) and the samples from unstained area of the inner page in all books as the unstained paper group (K). The R package Tax4Fun was used to predict functional genes in the bacterial community from the 16S rDNA data of the two groups. STAMP was used to screen for genes with significant differences in abundance between groups.
The abundance of some gene ortholog groups, such as mineral absorption, riboflavin metabolism, carotenoid biosynthesis, and lysine biosynthesis, was significantly higher in the peachy spot group than in the unstained paper group (Figure 6). This also suggested that the bacterial community at the peachy spots area might be associated with the accumulation of pigments and the increased production of acidic substances.
Figure 6. Predicted KEGG ortholog groups with significant differences (p < 0.05) in relative abundance between the “peachy spots” and unstained paper.
Ancient paper was made of many organic and inorganic compounds that can be used by bacteria and fungi. Traditionally, groups of fungi, including Penicillium, Aspergillus, Trichoderma, Chaetomium and Alternaria, were considered important for paper damage (Arai, 2000; Nitiu et al., 2020), as they are relatively easy to observe and culture. However, they may not necessarily be the key microbes that cause paper damage.
Most previous studies have also linked fungi to the cause of paper stains. Microscopic observation of the presence of mycelia has been used as a criterion to determine whether a stain is due to mold or mildew damage. The presence of UV fluorescence is used as a standard to define “foxing spots” (Meynell and Newsam, 1978; Koochakzaei and Alizadeh Gharetapeh, 2021), but fluorescence is also considered by other researchers as a factor in the formation of “foxing” spots (Bicchieri et al., 2001; Figueira et al., 2020). The peachy spots on the Kaihua paper resemble foxing spots by description, although in our previous tests, no UV fluorescence was observation at the spots (data not shown).
In this study, we used high-throughput sequencing technology to determine the bacterial and fungal communities on the surface of peachy spots and compared them with unstained surfaces as well as air samples from a stack room. The results showed that the abundance of Penicillium was not significantly higher in the position of peachy spots than that without it. The abundance of Aspergillus in the air deposition sample of the stack room was even higher than that of peachy spot samples. This implied that these typical filamentous fungi might have come from the stack room environment where the ancient books were stored but had not stably established on the surface of Kaihua paper and thus were not related to the formation of peachy spots. Cercospora and Fusarium were also found to be relatively abundant in the air deposition sample, both of which have multiple species that are pathogenic to plants (Daub and Ehrenshaft, 2000; Rampersad, 2020; Sinha et al., 2023). These fungi may come from other contaminated ancient books or old wooden bookshelves and furniture and pose a potential threat to paper (Şimşekli et al., 1999; Gravesen, 2000; Piñar et al., 2013). In addition, high abundances of Cladosporium, Neurospora and Talaromyces were found in the unstained paper samples, and multiple species of these genera belong to plant endophytes (Tian et al., 2009; Yamagiwa et al., 2011; Nitiu et al., 2020).
The results suggested that the formation of peachy spots on Kaihua paper might be more related to bacteria than fungi. The role of bacteria in the conservation and preservation of paper relics has long been neglected or underestimated. In recent years, more attention has been given to bacterial communities, where the bacterial genera Bacillus, Clostridium, Pseudomonas, Micrococcus, Actinomyces and Cellulophaga were often found to be related to paper damage (Kraková et al., 2012; Dunca et al., 2014; Lech, 2016; Szulc et al., 2018; Raeisnia et al., 2022; Pavlović et al., 2023). In this study, we found significantly more Actinomycetes of the genus Rubrobacter in peachy spot groups. Species of the genus Rubrobacter has previously isolated from deteriorated monuments (Piñar et al., 2013) and the carotenoid pigments they produce has been found to have a causal relationship with the rosy discoloration of ancient wall paintings (Imperi et al., 2007; Ettenauer et al., 2014). And Actinomycetes have been reported to possibly utilize insoluble matrix components and penetrate materials in parchment and canvas paintings and even increase the water solubility of the matrix for further utilization (Pavić et al., 2015; Lech, 2016).
Nevertheless, other bacteria commonly present on paper relics were not abundant (below 1% for Clostridium and Pseudomonas) or even absent (Micrococcus and Cellobacteria) in peachy spot samples. Instead, bacteria with acid-producing, acidophilic or cellulase-producing potential, including Lactobacillus, Streptococcus, Staphylococcus, and Lysinibacillus, and bacteria with pigment-producing potential, such as Methylobacterium (van Aken et al., 2004; Vadivukkarasi et al., 2018), were found to be highly abundant in peachy spots.
The result of functional gene prediction with Tax4fun supported the hypothesis regarding the role of bacteria in peachy spot formation. The abundance of predicted bacterial gene orthologs involved in the biosynthesis and metabolism of riboflavin, carotenoids and flavonoids was significantly higher in the peachy spot groups than in the unstained paper group. Some bacteria have the capability to produce organic acids and various enzymes to hydrolyze the paper components (Pavić et al., 2015), making the material easier to be colonized by more microbes, and the addition of pigment-producing bacteria may further help the stain formation. It is also possible that the chemical composition of peachy spot areas promoted the survival of certain bacteria and shaped the structure of the bacterial community.
The results obtained in the present study also showed that bacteria and fungi not only coexist in the microenvironment of the paper surface but also form relatively stable communities in each group. This may be because the paper itself continues to exert selective pressure on microorganisms. Studies of purple spots on ancient parchment hypothesized a succession model, starting from halophilic archaea, followed by halotolerant bacteria and actinomycetes, and then fungi (Migliore et al., 2019). Similarly, peachy spots on Kaihua paper may be the result of synergy and competition between different kinds of archaea, bacteria, and fungi. Their succession caused stains and the aging of Kaihua paper after a long time. Some archaea have been found to produce pink pigments in previous study (Mazzoli et al., 2018; Tichy et al., 2023). Although archaea were not investigated in this study, they could have played a role in the biodeterioration of Kaihua paper. The accumulation of bacteria with potential acid- and pigment-producing ability may be a relatively late phenomenon in the process of peachy spot formation. The results also remind us that without timely intervention, the bacteria at peachy spots will continue to destroy precious cultural relics.
Although studies based on DNA sequencing provide clues for the peachy spots, they may not tell the current microbial activities on the paper. Part of the detected DNA may be the remains of dead cells that have not been degraded completely. This gives us an opportunity to investigate the microbial community involved in the formation of peachy spots, but it also increases the difficulty of analyzing the process. One solution is to analyze RNA, which is much less stable than DNA. However, we found that the concentration of RNA recovered from the paper surface using noninvasive methods was too low to study. Due to the limitation of sample numbers and the lack of integrated analysis of multiple omics, the exact mechanism of peachy spot formation needs to be further studied.
In summary, through nondestructive sampling and high-throughput sequencing, it was found that the bacterial community on the peachy spots on Kaihua paper significantly differed from that on the unstained paper and the air in the stack room. No such difference was found in the fungal community. Bioinformatics analysis and functional gene prediction indicated that the formation of peachy spots may be mainly related to bacteria rather than fungi. The bacterial community may be mostly responsible for acidic substance and pigment accumulation in paper. The mechanisms of peachy spot formation are rather complex, and therefore, an increase in sample size and multiomics joint analysis will be useful to reveal the key microorganisms and their succession process that cause this phenomenon.
The datasets presented in this study can be found in online repositories. The names of the repository/repositories and accession number(s) can be found below: NCBI, PRJNA1031546, PRJNA1032408.
YZ: Data curation, Investigation, Visualization, Writing – original draft. YS: Data curation, Writing – review & editing. YH: Investigation, Methodology, Writing – original draft. JZ: Conceptualization, Funding acquisition, Resources, Supervision, Writing – review & editing.
The author(s) declare financial support was received for the research, authorship, and/or publication of this article. This work was financially supported by the National Key R&D Program of China (2019YFC1520400). The funding body had no role in the design of the study, the collection, analysis, and interpretation of data, or in writing the manuscript.
We gratefully thank the Fudan University Library and the Institute for Preservation and Conservation of Chinese Ancient Books of Fudan University for providing the ancient books for this study. We thank Academician Yuliang Yang (Institute for Preservation and Conservation of Chinese Ancient Books) and Guanghui Yang (Institute for Preservation and Conservation of Chinese Ancient Books) for their support and useful comments on research methods.
The authors declare that the research was conducted in the absence of any commercial or financial relationships that could be construed as a potential conflict of interest.
All claims expressed in this article are solely those of the authors and do not necessarily represent those of their affiliated organizations, or those of the publisher, the editors and the reviewers. Any product that may be evaluated in this article, or claim that may be made by its manufacturer, is not guaranteed or endorsed by the publisher.
The Supplementary material for this article can be found online at: https://www.frontiersin.org/articles/10.3389/fmicb.2023.1326835/full#supplementary-material
Abarenkov, K., Nilsson, R. H., Larsson, K. H., Alexander, I. J., Eberhardt, U., Erland, S., et al. (2010). The UNITE database for molecular identification of fungi--recent updates and future perspectives. New Phytol. 186, 281–285. doi: 10.1111/j.1469-8137.2009.03160.x
Amann, R. I., Ludwig, W., and Schleifer, K. H. (1995). Phylogenetic identification and in situ detection of individual microbial cells without cultivation. Microbiol. Rev. 59, 143–169. doi: 10.1128/mr.59.1.143-169.1995
Arai, H. (2000). Foxing caused by Fungi: twenty-five years of study. Int. Biodeterior. Biodegrad. 46, 181–188. doi: 10.1016/S0964-8305(00)00063-9
Area, M. C., and Cheradame, H. (2011). Paper aging and degradation: recent findings and research methods. Bioresources 6, 5307–5337. doi: 10.15376/biores.6.4.5307-5337
Aßhauer, K. P., Wemheuer, B., Daniel, R., and Meinicke, P. (2015). Tax4Fun: predicting functional profiles from metagenomic 16S rRNA data. Bioinformatics 31, 2882–2884. doi: 10.1093/bioinformatics/btv287
Beata, G. (2020). The use of-omics tools for assessing biodeterioration of cultural heritage: a review. J. Cult. Herit. 45, 351–361. doi: 10.1016/j.culher.2020.03.006
Bellemain, E., Carlsen, T., Brochmann, C., Coissac, E., Taberlet, P., and Kauserud, H. (2010). ITS as an environmental DNA barcode for fungi: an in silico approach reveals potential PCR biases. BMC Microbiol. 10:189. doi: 10.1186/1471-2180-10-189
Bicchieri, M., Pappalardo, G., Romano, F. P., Sementilli, F. M., and de Acutis, R. (2001). Characterization of foxing stains by chemical and spectrometric methods. Restaurator 22, 1–19. doi: 10.1515/REST.2001.1
Branysova, T., Demnerova, K., Durovic, M., and Stiborova, H. (2022). Microbial biodeterioration of cultural heritage and identification of the active agents over the last two decades. J. Cult. Herit. 55, 245–260. doi: 10.1016/j.culher.2022.03.013
Caporaso, J. G., Kuczynski, J., Stombaugh, J., Bittinger, K., Bushman, F. D., Costello, E. K., et al. (2010). QIIME allows analysis of high-throughput community sequencing data. Nat. Methods 7, 335–336. doi: 10.1038/nmeth.f.303
Cappitelli, F., Pasquariello, G., Tarsitani, G., and Sorlini, C. (2010). Scripta manent? Assessing microbial risk to paper heritage. Trends Microbiol. 18, 538–542. doi: 10.1016/j.tim.2010.09.004
Choi, S. (2007). Foxing on paper: a literature review. J. Am. Inst. Conserv. 46, 137–152. doi: 10.1179/019713607806112378
Clarke, K. R., and Warwick, R. M. (1994). Change in marine communities: An approach to statistical analysis and interpretation. Plymouth, UK: Plymouth Marine Laboratory, Natural Environment Research Council.
Daub, M. E., and Ehrenshaft, M. (2000). The photoactivated cercospora toxin cercosporin: contributions to plant disease and fundamental biology. Annu. Rev. Phytopathol. 38, 461–490. doi: 10.1146/annurev.phyto.38.1.461
Dunca, S. I., Tanase, C., Padurariu, C., Balaes, T., Ardelean, E., and Puica, N. M. (2014). Study of the contaminating microbiota of old paper supports. Eur. Sci. J. ESJ 10, 237–251.
Ettenauer, J. D., Jurado, V., Pinar, G., Miller, A. Z., Santner, M., Saiz-Jimenez, C., et al. (2014). Halophilic microorganisms are responsible for the rosy discolouration of saline environments in three historical buildings with mural paintings. PLoS One 9:e103844. doi: 10.1371/journal.pone.0103844
Figueira, F., Matos, M., Nunes, A., Afonso, M., Rocha, A. C., Campelo, J., et al. (2020). Considerations about foxing stains in three paper collections ranging from the 16th to the 20th century. Conservar Património 35, 45–57. doi: 10.1080/14786419.2016.1180600
Gravesen, S. (2000). Microbiology on indoor air ‘99--what is new and interesting? An overview of selected papers presented in Edinburgh, august, 1999. Indoor Air 10, 74–80. doi: 10.1034/j.1600-0668.2000.010002074.x
Imperi, F., Caneva, G., Cancellieri, L., Ricci, M. A., Sodo, A., and Visca, P. (2007). The bacterial aetiology of rosy discoloration of ancient wall paintings. Environ. Microbiol. 9, 2894–2902. doi: 10.1111/j.1462-2920.2007.01393.x
Koochakzaei, A., and Alizadeh Gharetapeh, S. (2021). Paper foxing stains on a historic manuscript from the early Qajar era: abiotic or biotic foxing? Heritage 4, 1366–1374. doi: 10.3390/heritage4030074
Kraková, L., Chovanová, K., Selim, S. A., Šimonovičová, A., Puškarová, A., Maková, A., et al. (2012). A multiphasic approach for investigation of the microbial diversity and its biodegradative abilities in historical paper and parchment documents. Int. Biodeterior. Biodegrad. 70, 117–125. doi: 10.1016/j.ibiod.2012.01.011
Kraková, L., Šoltys, K., Otlewska, A., Pietrzak, K., Purkrtová, S., Savická, D., et al. (2018). Comparison of methods for identification of microbial communities in book collections: culture-dependent (sequencing and MALDI-TOF MS) and culture-independent (Illumina MiSeq). Int. Biodeterior. Biodegrad. 131, 51–59. doi: 10.1016/j.ibiod.2017.02.015
Kuczynski, J., Stombaugh, J., Walters, W. A., González, A., Caporaso, J. G., and Knight, R. (2011). Using QIIME to analyze 16S rRNA gene sequences from microbial communities. Curr. Protoc. Bioinformatics Chapter Chapter 10:10.7.1. doi: 10.1002/0471250953.bi1007s36
Lech, T. (2016). Evaluation of a parchment document, the 13th century incorporation charter for the city of Krakow, Poland, for microbial hazards. Appl. Environ. Microbiol. 82, 2620–2631. doi: 10.1128/aem.03851-15
Liu, Z., Zhang, Y., Zhang, F., Hu, C., Liu, G., and Pan, J. (2018). Microbial community analyses of the deteriorated storeroom objects in the Tianjin museum using culture-independent and culture-dependent approaches. Front. Microbiol. 9, 311–312. doi: 10.3389/fmicb.2018.00802
Mazzoli, R., Giuffrida, M. G., and Pessione, E. (2018). Back to the past: “find the guilty bug—microorganisms involved in the biodeterioration of archeological and historical artifacts”. Applied Microbiology & Biotechnology. 102, 6393–6407. doi: 10.1007/s00253-018-9113-3
Meynell, G. G., and Newsam, R. J. (1978). Foxing, a fungal infection of paper. Nature 274, 466–468. doi: 10.1038/274466a0
Migliore, L., Perini, N., Mercuri, F., Orlanducci, S., Rubechini, A., and Thaller, M. C. (2019). Three ancient documents solve the jigsaw of the parchment purple spot deterioration and validate the microbial succession model. Sci. Rep. 9:1623. doi: 10.1038/s41598-018-37651-y
Migliore, L., Thaller, M. C., Vendittozzi, G., Mejia, A. Y., Mercuri, F., Orlanducci, S., et al. (2017). Purple spot damage dynamics investigated by an integrated approach on a 1244 a.D. parchment roll from the secret Vatican archive. Sci. Rep. 7, 9521–9512. doi: 10.1038/s41598-017-05398-7
Mitchell, R., and McNamara, C. J. (2010). Cultural heritage microbiology: Fundamental studies in conservation science. Washington, DC: ASM Press.
Nitiu, D. S., Mallo, A. C., and Saparrat, M. C. (2020). Fungal melanins that deteriorate paper cultural heritage: an overview. Mycologia 112, 859–870. doi: 10.1080/00275514.2020.1788846
Parks, D. H., Tyson, G. W., Philip, H., and adn Beiko, R. G. (2014). Stamp: statistical analysis of taxonomic and functional profiles. Bioinformatics 30, 3123–3124. doi: 10.1093/bioinformatics/btu494
Pavić, A., Ilić-Tomić, T., Pačevski, A., Nedeljković, T., Vasiljević, B., and Morić, I. (2015). Diversity and biodeteriorative potential of bacterial isolates from deteriorated modern combined-technique canvas painting. Int. Biodeterior. Biodegrad. 97, 40–50. doi: 10.1016/j.ibiod.2014.11.012
Pavlović, J., Puškárová, A., Planý, M., Farkas, Z., Rusková, M., Kvalová, K., et al. (2023). Colored stains: microbial survey of cellulose-based and lignin rich papers. Int. J. Biol. Macromol. 241:124456. doi: 10.1016/j.ijbiomac.2023.124456
Piñar, G., Piombino-Mascali, D., Maixner, F., Zink, A., and Sterflinger, K. (2013). Microbial survey of the mummies from the capuchin catacombs of Palermo, Italy: biodeterioration risk and contamination of the indoor air. FEMS Microbiol. Ecol. 86, 341–356. doi: 10.1111/1574-6941.12165
Piñar, G., Sclocchi, M. C., Pinzari, F., Colaizzi, P., Graf, A., Sebastiani, M. L., et al. (2020). The microbiome of Leonardo da Vinci’s drawings: a bio-archive of their history. Front. Microbiol. 11:593401. doi: 10.3389/fmicb.2020.593401
Raeisnia, N., Arefian, E., and Amoozegar, M. A. (2022). Microbial community of an 11th century manuscript by both culture-dependent and -independent approaches. Microbiology 91, 313–323. doi: 10.1134/S0026261722300117
Rampersad, S. N. (2020). Pathogenomics and management of fusarium diseases in plants. Pathogens. 9:340. doi: 10.3390/pathogens9050340
Schloss, P. D., Westcott, S. L., Ryabin, T., Hall, J. R., Hartmann, M., Hollister, E. B., et al. (2009). Introducing mothur: open-source, platform-independent, community-supported software for describing and comparing microbial communities. Appl. Environ. Microbiol. 75, 7537–7541. doi: 10.1128/aem.01541-09
Sclocchi, M. C., Kraková, L., Pinzari, F., Colaizzi, P., Bicchieri, M., Šaková, N., et al. (2016). Microbial life and death in a foxing stain: a suggested mechanism of photographic prints defacement. Microb. Ecol. 73, 815–826. doi: 10.1007/s00248-016-0913-7
Şimşekli, Y., Gücin, F., and Asan, A. (1999). Isolation and identification of indoor airborne fungal contaminants of food production facilities and warehouses in Bursa, Turkey. Aerobiologia 15, 225–231. doi: 10.1023/A:1007623831010
Sinha, S., Navathe, S., Singh, S., Gupta, D. K., Kharwar, R. N., and Chand, R. (2023). Genome sequencing and annotation of Cercospora sesami, a fungal pathogen causing leaf spot to Sesamum indicum. Three biotech 13:55. doi: 10.1007/s13205-023-03468-4
Sterflinger, K. (2010). Fungi: their role in deterioration of cultural heritage. Fungal Biol. Rev. 24, 47–55. doi: 10.1016/j.fbr.2010.03.003
Sterflinger, K., Little, B., Pinar, G., Pinzari, F., de los Rios, A., and Gu, J. D. (2018). Future directions and challenges in biodeterioration research on historic materials and cultural properties. Int. Biodeterior. Biodegrad. 129, 10–12. doi: 10.1016/j.ibiod.2017.12.007
Szulc, J., Otlewska, A., Ruman, T., Kubiak, K., Karbowska-Berent, J., Kozielec, T., et al. (2018). Analysis of paper foxing by newly available omics techniques. Int. Biodeterior. Biodegrad. 132, 157–165. doi: 10.1016/j.ibiod.2018.03.005
Tian, C., Beeson, W. T., Iavarone, A. T., Sun, J., Marletta, M. A., Cate, J. H., et al. (2009). Systems analysis of plant cell wall degradation by the model filamentous fungus Neurospora crassa. Proc. Natl. Acad. Sci. U. S. A. 106, 22157–22162. doi: 10.1073/pnas.0906810106
Tichy, J., Waldherr, M., Ortbauer, M., Graf, A., Sipek, B., Jembrih-Simbuerger, D., et al. (2023). Pretty in pink? Complementary strategies for analysing pink biofilms on historical buildings. Sci. Total Environ. 904:166737. doi: 10.1016/j.scitotenv.2023.166737
Vadivukkarasi, P., Jayashree, S., and Seshadri, S. (2018). Occurrence and ecological significance of Methylobacterium. Trop. Ecol. 59, 575–587.
van Aken, B., Peres, C. M., Doty, S. L., Yoon, J. M., and Schnoor, J. L. (2004). Methylobacterium populi sp. nov., a novel aerobic, pink-pigmented, facultatively methylotrophic, methane-utilizing bacterium isolated from poplar trees (Populus deltoides x nigra DN34). Int. J. Syst. Evol. Microbiol. 54, 1191–1196. doi: 10.1099/ijs.0.02796-0
Keywords: microbial community, traditional Chinese handmade paper, biodeterioration, Kaihua paper, functional prediction
Citation: Zhou Y, Shi Y, Huang Y and Zhong J (2024) Microbes on the “peachy spots” of ancient Kaihua paper: microbial community and functional analysis. Front. Microbiol. 14:1326835. doi: 10.3389/fmicb.2023.1326835
Received: 27 October 2023; Accepted: 26 December 2023;
Published: 11 January 2024.
Edited by:
Yingnan Bian, Enlight Medical, ChinaReviewed by:
Teresa Rinaldi, Sapienza University of Rome, ItalyCopyright © 2024 Zhou, Shi, Huang and Zhong. This is an open-access article distributed under the terms of the Creative Commons Attribution License (CC BY). The use, distribution or reproduction in other forums is permitted, provided the original author(s) and the copyright owner(s) are credited and that the original publication in this journal is cited, in accordance with accepted academic practice. No use, distribution or reproduction is permitted which does not comply with these terms.
*Correspondence: Jiang Zhong, anpob25nQGZ1ZGFuLmVkdS5jbg==
Disclaimer: All claims expressed in this article are solely those of the authors and do not necessarily represent those of their affiliated organizations, or those of the publisher, the editors and the reviewers. Any product that may be evaluated in this article or claim that may be made by its manufacturer is not guaranteed or endorsed by the publisher.
Research integrity at Frontiers
Learn more about the work of our research integrity team to safeguard the quality of each article we publish.