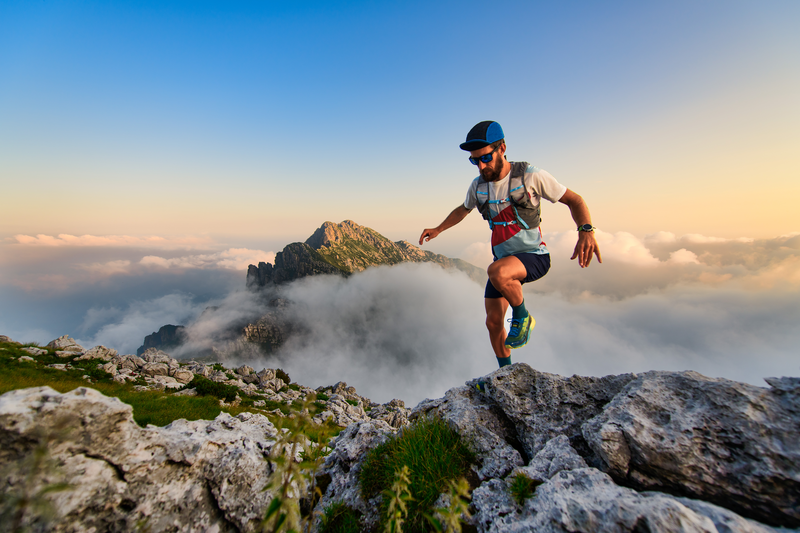
94% of researchers rate our articles as excellent or good
Learn more about the work of our research integrity team to safeguard the quality of each article we publish.
Find out more
ORIGINAL RESEARCH article
Front. Microbiol. , 05 January 2024
Sec. Virology
Volume 14 - 2023 | https://doi.org/10.3389/fmicb.2023.1325473
This article is part of the Research Topic Transmission and Infection of Arboviruses View all 14 articles
Introduction: Culicoides biting midges (Diptera: Ceratopogonidae) are vectors of arboviral pathogens that primarily affect livestock represented by Schmallenberg virus (SBV), epizootic hemorrhagic disease virus (EHDV) and bluetongue virus (BTV). In Kenya, studies examining the bionomic features of Culicoides including species diversity, blood-feeding habits, and association with viruses are limited.
Methods: Adult Culicoides were surveyed using CDC light traps in two semi-arid ecologies, Baringo and Kajiado counties, in Kenya. Blood-fed specimens were analysed through polymerase chain reaction (PCR) and sequencing of cytochrome oxidase subunit 1 (cox1) barcoding region. Culicoides pools were screened for virus infection by generic RT-PCR and next-generation sequencing (NGS).
Results: Analysis of blood-fed specimens confirmed that midges had fed on cattle, goats, sheep, zebra, and birds. Cox1 barcoding of the sampled specimens revealed the presence of known vectors of BTV and epizootic hemorrhagic disease virus (EHDV) including species in the Imicola group (Culicoides imicola) and Schultzei group (C. enderleni, C. kingi, and C. chultzei). Culicoides leucostictus and a cryptic species distantly related to the Imicola group were also identified. Screening of generated pools (11,006 individuals assigned to 333 pools) by generic RT-PCR revealed presence of seven phylogenetically distinct viruses grouping in the genera Goukovirus, Pacuvirus and Orthobunyavirus. The viruses showed an overall minimum infection rate (MIR) of 7.0% (66/333, 95% confidence interval (CI) 5.5-8.9). In addition, full coding sequences of two new iflaviruses, tentatively named Oloisinyai_1 and Oloisinyai_2, were generated by next-generation sequencing (NGS) from individual homogenate of Culicoides pool.
Conclusion: The results indicate a high genetic diversity of viruses in Kenyan biting midges. Further insights into host-vector-virus interactions as well as investigations on the potential clinical significance of the detected viruses are warranted.
Culicoides or biting midges (Diptera: Ceratopogonidae) are tiny insects measuring about 1–3 mm in length, commonly identified by unique wing pigmentation and macrotrichia pattern (Mathieu et al., 2012; Kirk-Spriggs and Sinclair, 2017; Borkent and Dominiak, 2020). They have a global distribution with species important for veterinary and public health mainly grouped into the genus Culicoides Latreille (Diptera: Ceratopogonidae) comprising about 1,340 species. The Afrotropical region is home to diverse Culicoides species, with over 120 species described in southern Africa alone (Garros et al., 2019; Borkent and Dominiak, 2020). Examples of medically important Culicoides species include invasive species of the Culicoides imicola complex (C. imicola, C. brevitarsis and C. bolitinos) and Schultzei group (C. enderleni, C. kingi, and C. schultzei) (Bakhoum et al., 2013; Leta et al., 2019).
Both sexes of Culicoides feed on plants as a primary energy source, but females also blood-feed on vertebrates such as mammals, birds and lizards to acquire additional protein to fertilize their eggs (Martínez-de la Puente et al., 2015; Borkent and Dominiak, 2020). Some Culicoides species rely on insect haemolymph as a protein source (Lassen et al., 2012). Further, there is a record of C. anophelis and C. nubeculosus feeding on engorged mosquitoes (Kremer et al., 1974; Ma et al., 2013). Like other insects, Culicoides harbour insect-specific viruses of the genera Iflavirus (Langat et al., 2021). Iflaviruses are arthropod-infecting viruses which are not known to be pathogenic to animals and have been largely identified in arthropods of the orders Lepidoptera and Hemiptera (Carrillo-Tripp et al., 2014).
The hematophagous feeding tendency ranks Culicoides among arthropods of veterinary and public health importance as they transmit parasites, protozoa and arboviruses that can cause severe disease, such as Schmallenberg virus (SBV) (family Peribunyaviridae, genus orthobunyavirus) and Bluetongue virus (BTV) (family Reoviridae, genus orbivirus) (Elbers et al., 2008; Santiago-Alarcon et al., 2012; Elbers et al., 2013; Sick et al., 2019; Žiegytė et al., 2021). Schmallenberg virus is grouped into the simbu serogroup together with Akabane virus (AKV) and Shuni virus (SHUV) and predominantly infects domestic ruminants causing fever, diarrhoea, and serious fetal malformation in gestating cattle and sheep (Saeed et al., 2001). Other viruses in the simbu serogroup include Oropouche virus (OROV) which has been associated with febrile illness in humans and is common in the neotropics (Romero-Alvarez and Escobar, 2018; Gaillet et al., 2021). On the other hand, viruses of the genus Orbivirus such as epizootic hemorrhagic disease virus (EHDV) and BTV are known to cause haemorrhagic disease and high morbidity in livestock leading to movement bans and strict trade restrictions which cause huge economic losses (Elbers et al., 2008; Rivera et al., 2021). Outbreaks of Culicoides-borne viruses have been common in Europe, for instance SBV in Germany, Italy, Netherlands, UK and Belgium (Hoffmann et al., 2012,; Elbers et al., 2013; Stokes et al., 2018; Ferrara et al., 2023a,b), but there are also reports of EHDV in Canada and North-eastern United States (Stallknecht et al., 2015; Allen et al., 2019). In Africa, BTV is endemic in South Africa, Morocco and Algeria (Youssef et al., 2015; Grewar, 2016; Moustafa et al., 2016; Durr et al., 2017).
The epizootics of Culicoides-borne viruses demonstrate the important role of neglected insect groups in veterinary health as outbreaks often mirror distribution of vectors (Purse et al., 2004). While the development and host biting rate of vectors such as C. enderleni and C. imicola are associated with warm temperatures, colder temperatures distinctly enable sustained EHDV and BTV transmission in an episystem (Grimaud et al., 2019; Mayo et al., 2020; Ferrara et al., 2023a,b). Another factor facilitating sustained virus transmission is the availability of vertebrate hosts that support large insect populations and may also serve as reservoir and maintenance hosts (Kuno et al., 2017). To understand the transmission dynamics of Culicoides-borne viruses, it is important to identify blood-meal sources of the vectors and abundant vector species. However, apart from reports highlighting active circulation of BTV serotypes in Kenya, studies on Culicoides-borne viruses are generally scarce in Africa (Toye et al., 2013; Onyango et al., 2015; Langat et al., 2021). In this study, we sought to identify Culicoides-borne viruses in two arid ecosystems, Baringo and Kajiado counties in Kenya using generic RT-PCR assays and next-generation sequencing (NGS). We further examined the vertebrate blood-meal sources of Culicoides in this area and identified abundant species by molecular barcoding.
Adult Culicoides were collected between August 2019 and July 2020 in Baringo and Kajiado counties at the end of rainy season and coinciding with peak abundance (Diarra et al., 2014). The study spanned 4 sites, Ntepes, Sandai, Logumgum, and Kapkuikui, in Baringo County, and 2 sites in Kajiado county, namely Soweto and Oloisinyai (Figure 1). The 2 study areas have similar semi-arid ecologies. Baringo had mean daily temperatures of 21.4°C, mean daily rainfall of 3.0 mm and a mean relative humidity of 66.8% during the sample collection periods. In Kajiado, the mean daily temperature was 23.7°C, mean daily rainfall was 0.3 mm and mean relative humidity 66.0% during sampling period. Using GIS-coordinates, the weather variable data were retrived from https://power.larc.nasa.gov/, and mean daily temperature and humidity in Kajiado documented using Thermochron iButton (Sunnyvale, CA) attached to each trap. The sparse human population in both areas rely on nomadic pastoralism as the major economic activity. A typical family has large herds of cattle, goat and/or sheep which they move from one place to another in search of water and pasture. The areas also have conservancies haboring wild animals including Kiborgoch community conservancy in Baringo and Olkirimatian conservancy in Kajiado. Some of the common wild animals in the conservancies include zebra, impala antelope and diverse bird species, including ostriches.
Figure 1. Map showing specimen collection sites in two semi-arid counties Kajiado and Baringo, in Kenya during entomological surveillance activities conducted between August 2019 and July 2020. The map was generated in QGIS 2.12 with shape files provided by Natural Earth (http://www.naturalearthdata.com/) and Africa Open data (https://africaopendata.org/dataset/kenya-counties-shapefile) (QGIS Development Team, 2019).
Centers for Disease Control (CDC) light traps model 512 (John W. Hock Company, Gainesville, United States) were used to catch adult Culicoides as part of a larger arbovirus surveillance project. Eight CDC light traps were baited with yeast-produced CO2 (Laguna-Aguilar et al., 2012), and deployed overnight from 18:00 h to 06:00 h for 3 consecutive nights at each sampling site. The traps were deployed outdoors with an inter-trap distance of about 25 m geo-referenced positions near farms/animal shelters (in the vicinity of human-settlement) as well as inside wild animal conservancies. After retrieval, the samples were initially taken to a temporary field laboratory. Biting midges were anesthetized with triethylamine and cryopreserved in liquid nitrogen for transportation to the laboratory at icipe in Nairobi and stored at −80°C until further analysis. In the laboratory, the Culicoides were sorted from other insects on a pre-chilled ice pack under a dissecting microscope (Stemi 2000-C microscope, Zeiss, Germany) (Glick, 1990; Bakhoum et al., 2018). Unfed Culicoides were pooled in groups of 2 to 50 according to collection date and sampling site. Engorged (blood-fed) specimen were analyzed individually.
The collected Culicoides were homogenized for 30 s in 1.5 mL microcentrifuge tubes containing 2.0 mm zirconia beads and DPBS (Dulbeccos phosphate-buffered saline, pH 7.4) using a Mini-Beadbeater-16 (Biospec, Bartlesville, OK, United States). For each pool, 1 mL of DPBS was used, whereas for individual engorged specimens the volume was reduced to 500 μL. The homogenate was centrifuged for 10 min in a bench top centrifuge (Eppendorf, United States) at 2500 revolutions per minute (rpm) at 4°C. After phase separation, the supernatant was used for virus screening and isolation. The pellet of individual engorged samples were preserved for DNA analyzes inclusive of species identification and blood-meal source determination.
DNA was extracted from the homogenate pellets of individual engorged Culicoides using DNeasy Blood and Tissue Kit (Qiagen, Hilden, Germany) following manufacturer’s recommendations and stored at −20°C until further use. Culicoides species molecular identification involved polymerase chain reaction (PCR) and Sanger sequencing of a 710 bp region of the cytochrome oxidase subunit 1 (cox1) gene as previously described (Folmer et al., 1994). To identify different blood meal host sources a different cox1 fragment was targeted using vertebrate specific primers (Reeves et al., 2018). ExoSAP-IT (USB Corporation, Cleveland, OH, United States) was used to remove unincorporated dNTPs and PCR primers from the amplicons before submitting purified amplicons for Sanger sequencing (Microsynth Seqlab GmbH, Göttingen, Germany). Sequences were edited in Geneious prime and used to query Barcode of Life (BOLD) and GenBank databases (Altschul et al., 1990; Ratnasingham and Hebert, 2007).
Viral RNA was extracted from 140 μL of the homogenate supernatants using the Viral RNA Mini Kit (Qiagen, Hilden, Germany) as described by the manufacturer. Double-stranded cDNA was synthesized using random hexamer primers (Endoh et al., 2005) and High Capacity cDNA Reverse Transcription (RT) kit (Life Technologies, CA, United States) following the manufacturers’ protocol. Pan-PCR assays targeting the RNA-dependent RNA polymerase (RdRp) gene of peribunyaviruses and phenuiviruses were utilized to screen for viral infections as described earlier (Hermanns et al., 2023). The resulting PCR products were examined on 1.5% agarose gels stained with ethidium bromide and amplicons of the correct size were purified for Sanger sequencing (Macrogen, Amsterdam, Netherlands) using ExoSAP-IT (USB Corporation, Cleveland, OH, United States).
Virus-positive samples were inoculated onto semi-confluent monolayers of mammalian Vero E6 (Ceropithecus aethiops) and insect C6/36 (Aedes albopictus) and KC (Drosophila melanogaster) cell lines, as described previously (Junglen et al., 2009). Briefly, Vero E6 cells were maintained in Dulbecco’s modified Eagle’s medium (DMEM)supplemented with 5% fetal calf serum (FCS) and 1% l-glutamine. C6/36 and KC cells were maintained in Leibovitz’s L-15 medium (L-15) supplemented with 5% FCS. Vero E6 cells were maintained in a 5% CO2 incubator at 37°C while C6/36 and KC cells were maintained at 28°C incubator without CO2. The cells were observed regularly for up to 7 days for occurrence of cytopathic effects (CPE). Samples were passaged on fresh cells 8 days after inoculation and a 75 μL aliqout of cell culture supernatant was taken from each sample. The blind passage was repeated 3 times and aliquots from cell culture supernatants from each passage were tested for viral replication.
Sequencing libraries were constructed using the KAPA HyperPlus kit (Roche Diagnostics, Rotkreuz, Switzerland) following the manufacturers’ instructions. Sequencing was performed on the Illumina MiSeq platform (Illumina, United States) as described (Marklewitz et al., 2019). Low-quality reads and adaptor sequences were removed from paired-end reads using BBDuk (filterk = 27, trimk = 30; http://jgi.doe.gov/data-and-tools/bb-tools/). After quality control, the cleaned reads were de novo assembled using Spades v3.11 implemented in Geneious Prime (Kearse et al., 2012). The resulting contigs were queried against the NCBI reference sequence database using BLASTn search.
Sequences were analyzed using Geneious prime (Kearse et al., 2012). Obtained sequences were compared to publicly available sequences in BOLD and GenBank (Altschul et al., 1990; Ratnasingham and Hebert, 2007). Sequences were aligned to related sequences using MAFFT as implemented in Geneious prime (Kearse et al., 2012). Maximum likelihood (ML) phylogenetic analyzes were executed using PhyML v. 2.2.4 in Geneious prime with each of the aligned cox1 nucleotide datasets. Nodal support was assessed by 1,000 bootstrap replicates using standard parameters (Guindon et al., 2010). Flavivirus and iflavirus genome organization were predicted using interProScan executed in Geneious prime (Kearse et al., 2012; Jones et al., 2014).
Metadata including Culicoides trap catches, sampling area, GPS coordinates, collection date, trapping method and abdominal status were captured in Microsoft Excel (2020) and relative abundance of Culicoides in sampling areas analyzed in R version 4.1.2 using funrar package (R Core Team, 2016). Minimum infection rate (MIR) was determined using PooledInfRate (https://github.com/CDCgov/PooledInfRate) under the assumption that there was at least one infected specimen in every positive Culicoides pool (Ségard et al., 2018). The relative abundance of Culicoides was estimated binomially and evaluated by Chi square tests at 95% confidence intervals in R version 4.1.2 (R Core Team, 2016).
A total of 11,006 Culicoides were collected from the six sampling sites in the two counties using CDC light traps (Table 1). More Culicoides were collected from Baringo County (55.9%, n = 6,149) than from Kajiado County (44.1%, n = 4,857) (Table 1). Overall, the majority of Culicoides specimens were collected in Oloisinyai, Kajiado County (42.0%; n = 4,622). In Baringo County, Logumgum (23.8%, n = 2,620) and Kaptombes (13.3%, n = 1,462) yielded the highest numbers of Culicoides. Seventy-four individuals (0.7% of the total collection) were engorged (blood-fed) specimens.
Seventy-four blood-fed specimens mainly originating from Baringo (93.2%, n = 69) and only few from Kajiado county (6.8%, n = 5) were identified to species by PCR. Most prevalent species were C. imicola (45.9%, n = 34) followed by C. enderleni (37.8%, n = 28). Other species identified in the blood-fed cohorts included C. kingi (6.8%, n = 5), C. leucostictus (1.4%, n = 1) and C. schultzei (1.4%, n = 1). Five samples could not be identified to species level by cox1 gene analyzes. The sequences of these 5 samples showed maximum nucleotide similarity of 85–86% to C. brevitarsis and C. imicola, and grouped in a distinct monophyletic clade in distant relationship to the Imicola group (Figure 2A). Among the collected blood-fed specimen, the highest species richness was observed in Kaptombes, Baringo County. Only one species (C. enderleni) was found in Sandai and Ntepes in Baringo County. Culicoides enderleni was common at all sampling sites, while C. schultzei was identified in Oloisinyai, Kajiado County, only.
Figure 2. Phylogenetic relationship of identified Culicoides species and their blood-feeding patterns. (A) Maximum likelihood phylogenetic analysis based on the Cox1 (nt 710 bp) gene inferred with PhyML v. 2.2.4 using the GTR substitution model. Bootstrap values of 70% and above are shown. (B) Alluvial diagram showing Culicoidies species blood-meal sources. The diagram was visualized in RAWGraphs (Mauri et al., 2017). UN: Unknown blood-meal source (identification was not successful).
In total, blood-meal sources were successfully identified from 63 Culicoides specimens (85.1%, 63/74; Table 2). Five different vertebrate blood-meal hosts were identified including livestock such as cattle (Bos taurus), goat (Capra hircus) and sheep (Ovis aries), and wild animals exemplified by zebra (Equus burchelli boehmi) and birds (Crinifer piscator) (Table 2; Figure 2B). Culicoides imicola showed the most diverse blood-meal sources having fed on cattle, goat and sheep followed by C. enderleni which had fed on cattle and goat. The other species C. kingi and C. schultzei were found to have fed on cattle only while C. leucostictus blood meals were from birds. Analysis of sequence chromatograms did not show evidence for mixed blood-meal sources. No human blood-meal source was identified, despite the trapping conducted in the vicinity of human-settlements.
All collected 11,006 Culicoides specimens were tested in pools (n = 333, ≤50 Culicoides/pool) for infections with phenui- and peribunyaviruses. Iflaviruses were detected during analysis of NGS data from Culicoides homogenates. An overall minimum infection rate (MIR) of 7.0% (66/333, 95% confidence interval (CI) 5.5–8.9) was found (Table 3). Highest MIR were registered in Sandai (MIR = 47.0, 95% CI 18.3–107.8) and Ntepes (MIR = 11.8, 95% CI 5.4–22.2), both in Baringo County (Table 3).
Two potential Goukovirus species were detected in 56 samples (MIR of 5.7, 95% CI 4.3–7.3, 56/333). The viral sequences were detected at all sampling sites in Baringo and Kajiado counties. Detections were made in C. enderleni that had fed on cattle and in C. imicola with a blood-meal from a goat (Figure 3A). The 2 potential Goukovirus species showed 98–100% and 89–100% nucleotide identities among each at the RdRp gene of 521–233 nucleotides in length. Maximum pairwise amino acid identities of 57 to 71% were found to Gouleako virus described in mosquitoes from Cote d’Ivoire (Supplementary Figure S1) (Marklewitz et al., 2011). The phylogenetic tree revealed that the 56 viruses characterized in this study clustered within a monophyletic clade sister to Gouleako goukovirus.
Figure 3. Maximum likelihood RNA-dependent RNA polymerase (RdRp) gene phylogenies depicting the genetic relatedness of (A) goukoviruses and samples sequenced in the present study; (B) peribunyaviruses and members of the Peribunyaviridae family; (C) iflaviruses and members of the Iflaviridae family. The phylogenetic trees were inferred with PhyML v. 2.2.4. *: Samples with mixed infection; ‡: sequence not deposited to GeneBank due to short length.
Eight RdRp gene sequences that fell within the family Peribunyaviridae were identified (Figure 3B; MIR of 0.7%; 95% CI 0.2–1.4, 8/333). BLAST analysis showed that the sequence of the sample MC1 from Sandai, Baringo county, shared 90% amino acid identity to Bahig virus from the Tete serogroup that was isolated from birds in Egypt (Shchetinin et al., 2015). Sample KC97 from Oloisinyai, Kajiado was distantly related to the clade comprising Akhtuba and Khurdun viruses isolated from birds in Russia (Figure 3B) (Al’kovskhovskiĭ et al., 2013). Another sample MC18 was distantly related to Pacui virus (genus Pacuvirus) detected in Brazilian rodents (Rodrigues et al., 2014). The remaining samples from Baringo and Kajiado counties clustered together forming a unique monophyletic clade. A distance matrix of pairwise similarity scores of detected viruses and representative peribunyaviruses is shown in Figure S2. There was no peribunyavirus detection in blood-fed specimens.
Seventeen Culicoides homogenate pools positive by Pan-PCR assay were randomly selected and analyzed by NGS. Analysis of NGS data from revealed full coding sequences of two novel iflaviruses (tentatively named Oloisinyai_1 and Oloisinyai_2) in sample KC102 collected from Oloisinyai (Kajiado County) with overall MIR of 0.2% (95% CI 0.0–0.6, 2/17). Comparison of the two sequences against the GenBank database using BLASTx showed 80–82% amino acid similarity to Lymantria dispar iflavirus 1 isolated in the US (Carrillo-Tripp et al., 2014) (Figure 3C). The detected viral sequences had 85% nucleotide similarity to each other. Genome annotation of Oloisinyai_1 and Oloisinyai_2 revealed a single-stranded positive sense RNA [ssRNA (+)] genome and a genomic organization that includes a capsid protein at N-terminal end and non-structural proteins (helicase and RNA-dependent RNA polymerase) at the C-terminal end (Figure 4). Attempts to isolate the detected phenuiviruses, peribunyaviruses and iflaviruses in KC, C6/36, and Vero E6 cell lines were not successful.
Figure 4. Genome organization of detected Oloisinyai_1 and Oloisinyai_2 iflaviruses. Colored sections highlight regions of functional significance. (A) Oloisinyai_1 virus; (B) Oloisinyai_2 virus.
Culicoides are vectors of several arboviral pathogens of veterinary and public health importance (Elbers et al., 2008; Romero-Alvarez and Escobar, 2018; Sick et al., 2019; Gaillet et al., 2021). Similar to earlier association of Culicoides abundance with warm temperatures, the high Culicoides collection in Baringo and Kajiado counties can be attributed to the favorable weather conditions observed in study sites (Grimaud et al., 2019; Mayo et al., 2020). Although the present study involved barcoding of a limited number of blood-fed Culicoides (n = 74), it revealed the presence of established/known vectors including members of Imicola group (C. imicola) and Schultzei group (C. enderleni, C. kingi, and C. schultzei) (Bakhoum et al., 2013). The rarely encountered species C. leucostictus and a cryptic Culicoides sp. distantly related to the Imicola group species (C. imicola and C. brevitarsis) were also identified (Table 2; Figure 2A). Culicoides imicola was the most abundant blood-fed Culicoides. This finding is similar to an earlier survey in Senegal showing higher collections of C. imicola in traps set near farms/animal shelters suggesting that animal shelters provide dung important for successful breeding of the vector (Acevedo et al., 2010; Diarra et al., 2014, 2015). Culicoides imicola is a known vector of BTV and its presence in Kenya provides an important avenue for the virus transmission (Sick et al., 2019). It is noteworthy that there was no record of blood-fed C. imicola in Kajiado County. However, the presence of C. imicola in the region cannot be excluded since only blood-fed Culicoides species were barcoded. Culicoides schultzei group species identified in the present study are known vectors of EHDV and BTV (Mellor et al., 1984; Bakhoum et al., 2016) and have been previously described in Kenya (Walker and Boreham, 1976; Cornet and Brunhes, 1994). Their presence highlight the risk of the virus’ transmission and is notable in light of reports of high BTV and EHDV prevalence in western part of the country (Toye et al., 2013). Considering the relatively low numbers of Culicoides samples that were barcoded, it is possible that more species and haplotypes could be identified with analysis of larger number of samples. Additional Culicoides species characterization would provide valuable information on vector species composition in the region.
Culicoides have diverse blood-meal sources. Therefore, information about their blood-feeding pattern is important in elucidating vector-host interactions and Culicoides-borne virus eco-epidemiology. Overall, our data confirmed five blood-meal hosts including cattle, goats, sheep, zebras, and birds in agreement with previous studies which reported diverse hosts for Culicoides species sampled in Romania (Tomazatos et al., 2020). All Culicoides species demonstrated mammophilic blood-feeding habits except C. leucostictus which had fed on birds (Table 2; Figure 2B). While ornithophilic tendencies in C. leucostictus has been reported before, there is little information on the role of the species in virus transmission (Scheffer et al., 2012). At each sampling site, there appeared to be a correlation between available vertebrate hosts and the blood-meal source detected. For example, at Kaptombes there is a wildlife conservancy inhabited by zebra explaining their detection in Culicoides blood-meals. The finding that Culicoides were feeding on livestock hosts such as cattle, goat and sheep confirm opportunistic feeding habits, underscoring the role of large animal herds serving as blood-meal sources for vectors (Table 2; Figure 2B). Although hosts availability seemed to influence the observed blood-feeding pattern, it is interesting that human blood-meals were not detected, an indication of potential impact on veterinary rather than human health by Culicoides-associated viruses in these ecological settings. A blood-meal source could not be established for 14.9% (n = 11) of the blood-fed Culicoides (Table 2). The blood-meal analysis involved nested PCR using 3 primer pair combinations of which one, targeting a shorter cox1 gene of 244 bp, is ideal for detecting degraded host DNA (Reeves et al., 2018). Therefore, blood-meal source identification failure can be attributed to highly degraded host DNA due to advanced digestion in the insect gut. Nevertheless, the study shows that Culicoides species in the two counties take diverse blood-meal sources and that the vertebrate hosts can potentially act as reservoirs of Culicoides-borne viruses. However, the observed blood-feeding pattern should be interpreted with caution due to the small sample size. A wider study focused on blood-fed Culicoides would provide greater insight into Culicoides feeding patterns and vector-host interactions.
Virus screening assays revealed the presence of seven potential virus species belonging to the genera Goukovirus, Pacuvirus, Orthobunyavirus and Iflavirus (Table 3). The family Phenuiviridae represents both medically important viruses such as Rift Valley fever virus and the recently discovered sandfly-borne phleboviruses in Baringo County, as well as insect specific viruses like the gouleako virus, prototype of the genus Goukovirus (Marklewitz et al., 2011; Tchouassi et al., 2019; Marklewitz et al., 2020). Since the discovery of Gouleako virus, several further mosquito-associated goukoviruses have been described (Lefkowitz et al., 2018). However, information associating Culicoides with goukoviruses transmission is scarce. The data presented here expand the list of viruses in the genus, describing detection of uncharacterised goukoviruses identified in Culicoides. These viruses had a MIR of 5.7% (95% CI 4.3–7.3, 56/333) and were widely distributed, occurring at all sampling sites (Table 3). Further, the viruses were detected in established vectors of EHDV and BTV (C. enderleni and C. imicola) that had fed on cattle and goat.
One detected vial sequence (MC1) clustered with the pathogenic Simbu group including SBV, AKV, and OROV (Saeed et al., 2001; Romero-Alvarez and Escobar, 2018; Gaillet et al., 2021). Further, sample KC97 was related to Akhtuba and Khurdun viruses isolated from birds in Russia (Al’kovskhovskiĭ et al., 2013; Rodrigues et al., 2014). These virus detections are not surprising as the genus is probably the most medically relevant one within the family Peribunyaiviridae and comprises the majority of Culicoides-borne viruses (Lefkowitz et al., 2018). Orthobunyaviruses are known to have a wide vertebrate host range inclusive of humans, livestock and birds. In the present study, there was no detection of orthobunyaviruses in Culicoides that had fed on livestock and birds, however, this cannot be ruled out due to the small sample size. Unfortunately, attempts to generate more sequence information of the detected peribunyaviruses failed, as well as virus isolation attempts were not successful. Hence, further studies including genome sequencing and analyzes as well as phenotypic studies with virus isolates in cell culture are needed for further classification and assessment.
In the present study, seventeen samples were analyzed by NGS revealing two insect-specific viruses of genus Iflaviruses were detected. The low diversity of detected viruses in relation to a similar study could be attributed to the few representative samples that were analyzed by NGS (Langat et al., 2021). The identified iflaviruses showed 80–82% amino acid similarity to Lymantria dispar iflavirus 1 (LdIV1) isolated in the US (Carrillo-Tripp et al., 2014), suggesting that the identified iflaviruses represent novel species in the genus as stipulated in the Executive Committee (EC) 51, 2019 report (Lefkowitz et al., 2018). Apart from recent report of iflaviruses in Culicoides in Kenya, reports of Culicoides iflaviruses infection in the country remains scarce (Langat et al., 2021).
This study has provided evidence that established Culicoides vectors (C. imicola, C. enderleni, C. kingi, and C. schultzei) of BTV and EHDV were feeding mainly on livestock host in Kenya. Blood-meal analyzes revealed opportunistic feeding of Culicoides species on livestock and wildlife and the potential for spillover of viruses of veterinary importance. Expanded studies on vector-host-virus interactions, inclusive of Culicoides blood-meal source determination, virus genome characterization and seroprevalence studies are needed to establish the clinical and modulatory impacts of Culicoides-borne viruses.
The datasets presented in this study can be found in online repositories. The names of the repository/repositories and accession number(s) can be found in the article/supplementary material.
The animal study was approved by Kenya Medical Research Institute Scientific and Ethics Review Unit (KEMRI-SERU). The study was conducted in accordance with the local legislation and institutional requirements.
EO: Conceptualization, Data curation, Formal analysis, Investigation, Methodology, Software, Validation, Visualization, Writing – original draft, Writing – review & editing. AB: Conceptualization, Resources, Supervision, Writing – review & editing. IS: Writing – review & editing, Formal analysis, Investigation. CG: Formal analysis, Investigation, Writing – review & editing. JO: Writing – review & editing, Formal analysis, Investigation. DOm: Formal analysis, Investigation, Writing – review & editing. DOn: Formal analysis, Investigation, Writing – review & editing. RS: Conceptualization, Funding acquisition, Resources, Supervision, Writing – review & editing. BT: Conceptualization, Funding acquisition, Resources, Supervision, Writing – review & editing. SJ: Conceptualization, Formal analysis, Funding acquisition, Methodology, Resources, Supervision, Validation, Writing – review & editing. DT: Conceptualization, Formal analysis, Funding acquisition, Methodology, Resources, Supervision, Validation, Writing – review & editing.
The author(s) declare financial support was received for the research, authorship, and/or publication of this article. The work was funded by the Deutsche Forschungsgemeinschaft (JU 2857/9-1 / and -2 to SJ). Edwin Ogola was supported by a German Academic Exchange Service (DAAD) through the icipe ARPPIS-DAAD scholarship and a UP postgraduate bursary. DT received support from the Norad-funded project Combatting Arthropod Pests for better Health, Food and Climate Resilience (CAP-Africa; project number RAF-3058 KEN-18/0005). We also gratefully acknowledge the financial support and technical support of ICIPE core donors: Swiss Agency for Development and Cooperation (SDC), Switzerland; Swedish International Development Cooperation Agency (Sida), Sweden; Australian Centre for International Agricultural Research (ACIAR), Federal Democratic Republic of Ethiopia and the Government of the Republic of Kenya. The views expressed herein do not necessarily reflect the official opinion of the donors. The funders had no role in study design, data collection and analysis, decision to publish, or preparation of the manuscript.
We are grateful for the technical support of Christian Hieke and Verena Hyde of Institute of Virology, Charité Universitätsmedizin Berlin, and Gibert Rotich of icipe’s ML-EID laboratory.
The authors declare that the research was conducted in the absence of any commercial or financial relationships that could be construed as a potential conflict of interest.
The author(s) declared that they were an editorial board member of Frontiers, at the time of submission. This had no impact on the peer review process and the final decision.
All claims expressed in this article are solely those of the authors and do not necessarily represent those of their affiliated organizations, or those of the publisher, the editors and the reviewers. Any product that may be evaluated in this article, or claim that may be made by its manufacturer, is not guaranteed or endorsed by the publisher.
The Supplementary material for this article can be found online at: https://www.frontiersin.org/articles/10.3389/fmicb.2023.1325473/full#supplementary-material
SUPPLEMENTARY FIGURE 1 | Goukoviruses distance matrix. Distance matrix of the RdRp gene showing representative viruses of genus Goukovirus and samples sequenced in the present study. Light grey to dark shades highlights amino acid distances.
SUPPLEMENTARY FIGURE 2 | Peribunyaviruses distance matrix. Distance matrix of the RdRp gene showing representative viruses and samples sequenced in the present study (bold). Light grey to dark shades indicates nucleotide distances.
Acevedo, P., Ruiz-Fons, F., Estrada, R., Márquez, A. L., Miranda, M. A., Gortázar, C., et al. (2010). A broad assessment of factors determining Culicoides imicola abundance: modelling the present and forecasting its future in climate change scenarios. PLoS One 5:e14236. doi: 10.1371/JOURNAL.PONE.0014236
Al’kovskhovskiĭ, S. V., Shchetinin, A. M., Lvov, D. K., Shchelkanov, M. I., Deriabin, P. G., L’vov, D. N., et al. (2013). The Khurdun virus (KHURV): a new representative of the orthobunyavirus (Bunyaviridae). Vopr. Virusol. 58, 10–13.
Allen, S. E., Rothenburger, J. L., Jardine, C. M., Ambagala, A., Hooper-McGrevy, K., Colucci, N., et al. (2019). Epizootic hemorrhagic disease in white-tailed deer, Canada. Emerg. Infect. Dis. 25, 832–834. doi: 10.3201/eid2504.180743
Altschul, S. F., Gish, W., Miller, W., Myers, E. W., and Lipman, D. J. (1990). Basic local alignment search tool. J. Mol. Biol. 215, 403–410. doi: 10.1016/S0022-2836(05)80360-2
Bakhoum, M. T., Fall, M., Fall, A. G., Bellis, G. A., Gottlieb, Y., Labuschagne, K., et al. (2013). First record of Culicoides oxystoma Kieffer and diversity of species within the Schultzei group of Culicoides Latreille (Diptera: Ceratopogonidae) biting midges in Senegal. PLoS One 8:e84316. doi: 10.1371/journal.pone.0084316
Bakhoum, M. T., Fall, M., Seck, M. T., Gardes, L., Fall, A. G., Diop, M., et al. (2016). Foraging range of arthropods with veterinary interest: new insights for Afrotropical Culicoides biting midges (Diptera: Ceratopogonidae) using the ring method. Acta Trop. 157, 59–67. doi: 10.1016/J.ACTATROPICA.2016.01.023
Bakhoum, M. T., Sarr, M., Fall, A. G., Huber, K., Fall, M., Sembène, M., et al. (2018). DNA barcoding and molecular identification of field-collected Culicoides larvae in the Niayes area of Senegal. Parasit. Vectors 11:615. doi: 10.1186/s13071-018-3176-y
Borkent, A., and Dominiak, P. (2020). Catalog of the biting midges of the world (Diptera: Ceratopogonidae). Zootaxa 4787, 1–377. doi: 10.11646/zootaxa.4787.1.1
Carrillo-Tripp, J., Krueger, E. N., Harrison, R. L., Toth, A. L., Miller, W. A., and Bonning, B. C. (2014). Lymantria dispar iflavirus 1 (LdIV1), a new model to study iflaviral persistence in lepidopterans. J. Gen. Virol. 95, 2285–2296. doi: 10.1099/vir.0.067710-0
Cornet, M., and Brunhes, J. (1994). Révision des espèces de Culicoides apparentés à C. schultzei (Enderlein, 1908) dans la région afrotropicales (Diptera, Ceratopogonidae). Bull. Soc. entomol. Fr. 99, 149–164. doi: 10.3406/BSEF.1994.17053
Diarra, M., Fall, M., Fall, A. G., Diop, A., Seck, M. T., Garros, C., et al. (2014). Seasonal dynamics of Culicoides (Diptera: Ceratopogonidae) biting midges, potential vectors of African horse sickness and bluetongue viruses in the Niayes area of Senegal. Parasit. Vectors 7:147. doi: 10.1186/1756-3305-7-147/FIGURES/4
Diarra, M., Fall, M., Lancelot, R., Diop, A., Fall, A. G., Dicko, A., et al. (2015). Modelling the abundances of two major Culicoides (Diptera: Ceratopogonidae) species in the Niayes area of Senegal. PLoS One 10:e0131021. doi: 10.1371/JOURNAL.PONE.0131021
Durr, P. A., Graham, K., and van Klinken, R. D. (2017). Sellers’ revisited: a big data reassessment of historical outbreaks of bluetongue and African horse sickness due to the long-distance wind dispersion of Culicoides midges. Front. Vet. Sci. 4:98. doi: 10.3389/fvets.2017.00098
Elbers, A. R., Backx, A., Meroc, E., Gerbier, G., Staubach, C., Hendrickx, G., et al. (2008). Field observations during the bluetongue serotype 8 epidemic in 2006. Detection of first outbreaks and clinical signs in sheep and cattle in Belgium, France and the Netherlands. Prev. Vet. Med. 87, 21–30. doi: 10.1016/j.prevetmed.2008.06.004
Elbers, A. R., Meiswinkel, R., van Weezep, E., van Oldruitenborgh-Oosterbaan, M. M. S., and Kooi, E. A. (2013). Schmallenberg virus in Culicoides spp. biting midges, the Netherlands, 2011. Emerg. Infect. Dis. 19, 106–109. doi: 10.3201/eid1901.121054
Endoh, D., Mizutani, T., Kirisawa, R., Maki, Y., Saito, H., Kon, Y., et al. (2005). Species-independent detection of RNA virus by representational difference analysis using non-ribosomal hexanucleotides for reverse transcription. Nucleic Acids Res. 33:e65. doi: 10.1093/nar/gni064
Ferrara, G., Improda, E., Piscopo, F., Esposito, R., Iovane, G., Pagnini, U., et al. (2023b). Bluetongue virus seroprevalence and risk factor analysis in cattle and water buffalo in southern Italy (Campania region). Vet. Res. Commun. 1–6. doi: 10.1007/s11259-023-10215-w
Ferrara, G., Wernike, K., Iovane, G., Pagnini, U., and Montagnaro, S. (2023a). First evidence of Schmallenberg virus infection in southern Italy. BMC Vet. Res. 19:95. doi: 10.1186/s12917-023-03666-5
Folmer, O., Black, M., Hoeh, W., Lutz, R., and Vrijenhoek, R. (1994). DNA primers for amplification of mitochondrial cytochrome C oxidase subunit I from diverse metazoan invertebrates. Mol. Mar. Biol. Biotechnol. 5, 294–299. doi: 10.1371/journal.pone.0013102
Gaillet, M., Pichard, C., Restrepo, J., Lavergne, A., Perez, L., Enfissi, A., et al. (2021). Outbreak of Oropouche virus in French Guiana. Emerg. Infect. Dis. 27, 2711–2714. doi: 10.3201/eid2710.204760
Garros, C., Labuschagne, K., Dommergues, L., Balenghien, T., Muñoz, F., Bakhoum, M. T., et al. (2019). Culicoides Latreille in the sun: faunistic inventory of Culicoides species (Diptera: Ceratopogonidae) in Mayotte (Comoros archipelago, Indian Ocean). Parasit. Vectors 12:135. doi: 10.1186/s13071-019-3379-x
Glick, J. I. (1990). Culicoides biting midges (Diptera: Ceratopogonidae) of Kenya. J. Med. Entomol. 27, 85–195. doi: 10.1093/jmedent/27.2.85
Grewar, J. D. (2016). The economic impact of bluetongue and other orbiviruses in sub-Saharan Africa, with special reference to southern Africa. Vet. Ital. 52, 375–381. doi: 10.12834/VetIt.503.2427.3
Grimaud, Y., Guis, H., Chiroleu, F., Boucher, F., Tran, A., Rakotoarivony, I., et al. (2019). Modelling temporal dynamics of Culicoides Latreille (Diptera: Ceratopogonidae) populations on Reunion Island (Indian Ocean), vectors of viruses of veterinary importance. Parasit. Vectors 12, 562–517. doi: 10.1186/s13071-019-3812-1
Guindon, S., Dufayard, J. F., Lefort, V., Anisimova, M., Hordijk, W., and Gascuel, O. (2010). New algorithms and methods to estimate maximum-likelihood phylogenies: assessing the performance of PhyML 3.0. Syst. Biol. 59, 307–321. doi: 10.1093/sysbio/syq010
Hermanns, K., Marklewitz, M., Zirkel, F., Kopp, A., Kramer-Schadt, S., and Junglen, S. (2023). Mosquito community composition shapes virus prevalence patterns along anthropogenic disturbance gradients. elife 12:e66550. doi: 10.7554/eLife.66550
Hoffmann, B., Scheuch, M., Höper, D., Jungblut, R., Holsteg, M., Schirrmeier, H., et al. (2012). Novel orthobunyavirus in cattle, Europe, 2011. Emerg. Infect. Dis. 18, 469–472. doi: 10.3201/eid1803.111905
Jones, P., Binns, D., Chang, H. Y., Fraser, M., Li, W., McAnulla, C., et al. (2014). InterProScan 5: genome-scale protein function classification. Bioinformatics 30, 1236–1240. doi: 10.1093/BIOINFORMATICS/BTU031
Junglen, S., Kopp, A., Kurth, A., Pauli, G., Ellerbrok, H., and Leendertz, F. H. (2009). A new flavivirus and a new vector: characterization of a novel flavivirus isolated from Uranotaenia mosquitoes from a tropical rain Forest. J. Virol. 83, 4462–4468. doi: 10.1128/JVI.00014-09
Kearse, M., Moir, R., Wilson, A., Stones-Havas, S., Cheung, M., Sturrock, S., et al. (2012). Geneious basic: an integrated and extendable desktop software platform for the organization and analysis of sequence data. Bioinformatics 28, 1647–1649. doi: 10.1093/bioinformatics/bts199
Kirk-Spriggs, A. H., and Sinclair, B. J. (2017). Manual of Afrotropical Diptera, volume 2: Nematocera & Lower Brachycera | NHBS Academic & Professional Books. SANBI Publishing. Coimbatore
Kremer, M., Molet, B., Rebholtz-hirtzel, C., and Delecolle, J. C. (1974). Culicoides nubeculosus (Ceratopogonidae) feeding on engorged Aedes aegypti under laboratory conditions. Mosq. News 34, 471–472.
Kuno, G., Mackenzie, J. S., Junglen, S., Hubálek, Z., Plyusnin, A., and Gubler, D. J. (2017). Vertebrate reservoirs of arboviruses: myth, synonym of amplifier, or reality? Viruses 9, 1–28. doi: 10.3390/v9070185
Laguna-Aguilar, M., Alvarado-Moreno, M. S., Sánchez-Rodríguez, O. S., Ramírez-Jiménez, R., Zárate-Nahón, E. A., Sánchez-Casas, R. M., et al. (2012). Field evaluation of a novel trap baited with carbon dioxide produced by yeast for the collection of female Aedes aegypti mosquitoes in Mexico. Southwest. Entomol. 37, 495–504. doi: 10.3958/059.037.0407
Langat, K. S., Eyase, F., Bulimo, W., Lutomiah, J., Oyola, S. O., Imbuga, M., et al. (2021). Profiling of RNA viruses in biting midges (Ceratopogonidae) and related Diptera from Kenya using metagenomics and metabarcoding analysis. mSphere 6, e00551–e00521. doi: 10.1128/mSphere.00551-21
Lassen, S. B., Nielsen, S. A., and Kristensen, M. (2012). Identity and diversity of blood meal hosts of biting midges in Denmark. Parasit. Vectors 5:143. doi: 10.1186/1756-3305-5-143
Lefkowitz, E. J., Dempsey, D. M., Hendrickson, R. C., Orton, R. J., Siddell, S. G., and Smith, D. B. (2018). Virus taxonomy: the database of the international committee on taxonomy of viruses (ICTV). Nucleic Acids Res. 46, D708–D717. doi: 10.1093/nar/gkx932
Leta, S., Fetene, E., Mulatu, T., Amenu, K., Jaleta, M. B., Beyene, T. J., et al. (2019). Updating the global occurrence of Culicoides imicola, a vector for emerging viral diseases. Sci. Data 6, 185–188. doi: 10.1038/s41597-019-0197-0
Ma, Y., Xu, J., Yang, Z., Wang, X., Lin, Z., Zhao, W., et al. (2013). A video clip of the biting midge Culicoides anophelis ingesting blood from an engorged Anopheles mosquito in Hainan, China. Parasit. Vectors 6:326. doi: 10.1186/1756-3305-6-326/TABLES/1
Marklewitz, M., Dutari, L. C., Paraskevopoulou, S., Page, R. A., Loaiza, J. R., and Junglen, S. (2019). Diverse novel phleboviruses in sandflies from the Panama Canal area, Central Panama. J. Gen. Virol. 100, 938–949. doi: 10.1099/jgv.0.001260
Marklewitz, M., Handrick, S., Grasse, W., Kurth, A., Lukashev, A., Drosten, C., et al. (2011). Gouleako virus isolated from west African mosquitoes constitutes a proposed novel genus in the family Bunyaviridae. J. Virol. 85, 9227–9234. doi: 10.1128/jvi.00230-11
Marklewitz, M., Tchouassi, D. P., Hieke, C., Heyde, V., Torto, B., Sang, R., et al. (2020). Insights into the evolutionary origin of Mediterranean sandfly fever viruses. mSphere 5, e00598–e00520. doi: 10.1128/mSphere.00598-20
Martínez-de la Puente, J., Figuerola, J., and Soriguer, R. (2015). Fur or feather? Feeding preferences of species of Culicoides biting midges in Europe. Trends Parasitol. 31, 16–22. doi: 10.1016/j.pt.2014.11.002
Mathieu, B., Cêtre-Sossah, C., Garros, C., Chavernac, D., Balenghien, T., Carpenter, S., et al. (2012). Development and validation of IIKC: an interactive identification key for Culicoides (Diptera: Ceratopogonidae) females from the Western Palaearctic region. Parasit Vectors 5, 1–11. doi: 10.1186/1756-3305-5-137
Mauri, M., Elli, T., Caviglia, G., Uboldi, G., and Azzi, M. (2017). RAWGraphs: a visualisation platform to create open outputs. Proc. ACM Int. Conf. Part F131371 28, 1–5. doi: 10.1145/3125571.3125585
Mayo, C., McDermott, E., Kopanke, J., Stenglein, M., Lee, J., Mathiason, C., et al. (2020). Ecological dynamics impacting bluetongue virus transmission in North America. Front. Vet. Sci 7:186. doi: 10.3389/fvets.2020.00186
Mellor, P. S., Osborne, R., and Jennings, D. M. (1984). Isolation of bluetongue and related viruses from Culicoides spp. in the Sudan. Epidemiol. Infect. 93, 621–628. doi: 10.1017/S0022172400065190
Moustafa, K., Pam, D. L., and Meriem, H. B. (2016). Sero-epidemiology of bluetongue in Algerian ruminants. Afr. J. Biotechnol. 15, 868–871. doi: 10.5897/ajb2016.15343
Onyango, M. G., Michuki, G. N., Ogugo, M., Venter, G. J., Miranda, M. A., Elissa, N., et al. (2015). Delineation of the population genetic structure of Culicoides imicola in east and South Africa. Parasit. Vectors 8:660. doi: 10.1186/s13071-015-1277-4
Purse, B. V., Baylis, M., Tatem, A. J., Rogers, D. J., Mellor, P. S., Van Ham, M., et al. (2004). Predicting the risk of bluetongue through time: climate models of temporal patterns of outbreaks in Israel. Rev. Sci. Tech. 23, 761–775. doi: 10.20506/rst.23.3.1515
QGIS Development Team, (2019). Welcome to the QGIS project. Available at: https://qgis.org/en/site/ (accessed June 11, 2019).
R Core Team, (2016). R: A language and environment for statistical computing. Vienna, Austria: R Foundation for Statistical Computing.
Ratnasingham, S., and Hebert, P. D. N. (2007). BOLD: the barcode of life data system (http://www.barcodinglife.org). Mol. Ecol. Notes 7:355-64. doi: 10.1111/J.1471-8286.2007.01678.X
Reeves, L. E., Gillett-Kaufman, J. L., Kawahara, A. Y., and Kaufman, P. E. (2018). Barcoding blood meals: new vertebrate-specific primer sets for assigning taxonomic identities to host DNA from mosquito blood meals. PLoS Negl. Trop. Dis. 12:e0006767. doi: 10.1371/journal.pntd.0006767
Rivera, N. A., Varga, C., Ruder, M. G., Dorak, S. J., Roca, A. L., Novakofski, J. E., et al. (2021). Bluetongue and epizootic Hemorrhagic disease in the United States of America at the wildlife-livestock Interface. Pathogens 10:915. doi: 10.3390/pathogens10080915
Rodrigues, D. S. G., Medeiros, D. B. D. A., Rodrigues, S. G., Martins, L. C., de Lima, C. P. S., de Oliveira, L. F., et al. (2014). Pacui virus, Rio Preto da Eva virus, and Tapirape virus, three distinct viruses within the family Bunyaviridae. Genome Announcents 2, e00923–e00914. doi: 10.1128/genomeA.00923-14
Romero-Alvarez, D., and Escobar, L. E. (2018). Oropouche fever, an emergent disease from the Americas. Microbes Infect. 20, 135–146. doi: 10.1016/j.micinf.2017.11.013
Saeed, M. F., Li, L., Wang, H., Weaver, S. C., and Barrett, A. D. T. (2001). Phylogeny of the Simbu serogroup of the genus bunyavirus. J. Gen. Virol. 82, 2173–2181. doi: 10.1099/0022-1317-82-9-2173
Santiago-Alarcon, D., Havelka, P., Schaefer, H. M., and Segelbacher, G. (2012). Bloodmeal analysis reveals avian plasmodium infections and broad host preferences of culicoides (diptera: Ceratopogonidae) vectors. PLoS One 7:e31098. doi: 10.1371/journal.pone.0031098
Scheffer, E. G., Venter, G. J., Labuschagne, K., Page, P. C., Mullens, B. A., MacLachlan, N. J., et al. (2012). Comparison of two trapping methods for Culicoides biting midges and determination of African horse sickness virus prevalence in midge populations at Onderstepoort, South Africa. Vet. Parasitol. 185, 265–273. doi: 10.1016/J.VETPAR.2011.09.037
Ségard, A., Gardès, L., Jacquier, E., Grillet, C., Mathieu, B., Rakotoarivony, I., et al. (2018). Schmallenberg virus in Culicoides Latreille (Diptera: Ceratopogonidae) populations in France during 2011-2012 outbreak. Transbound Emerg. Dis. 65, e94–e103. doi: 10.1111/tbed.12686
Shchetinin, A. M., Lvov, D. K., Deriabin, P. G., Botikov, A. G., Gitelman, A. K., Kuhn, J. H., et al. (2015). Genetic and phylogenetic characterization of Tataguine and Witwatersrand viruses and other Orthobunyaviruses of the anopheles a, Capim, Guamá, Koongol, Mapputta, Tete, and Turlock serogroups. Viruses 7, 5987–6008. doi: 10.3390/V7112918
Sick, F., Beer, M., Kampen, H., and Wernike, K. (2019). Culicoides biting midges-underestimated vectors for arboviruses of public health and veterinary importance. Viruses 11:376. doi: 10.3390/v11040376.MDPIAG
Stallknecht, D. E., Allison, A. B., Park, A. W., Phillips, J. E., Goekjian, V. H., Nettles, V. F., et al. (2015). Apparent increase of reported hemorrhagic disease in the midwestern and northeastern USA. J. Wildl. Dis. 51, 348–361. doi: 10.7589/2013-12-330
Stokes, J. E., Tarlinton, R. E., Lovatt, F., Baylis, M., Carson, A., and Duncan, J. S. (2018). Survey to determine the farm-level impact of Schmallenberg virus during the 2016-2017 United Kingdom lambing season. Vet. Rec. 183:690. doi: 10.1136/vr.104866
Tchouassi, D. P., Marklewitz, M., Chepkorir, E., Zirkel, F., Agha, S. B., Tigoi, C. C., et al. (2019). Sand fly-associated Phlebovirus with evidence of neutralizing antibodies in humans, Kenya. Emerg. Infect. Dis. 25, 681–690. doi: 10.3201/eid2504.180750
Tomazatos, A., Jöst, H., Schulze, J., Spînu, M., Schmidt-Chanasit, J., Cadar, D., et al. (2020). Blood-meal analysis of Culicoides (Diptera: Ceratopogonidae) reveals a broad host range and new species records for Romania. Parasit. Vectors 13:79. doi: 10.1186/s13071-020-3938-1
Toye, P. G., Batten, C. A., Kiara, H., Henstock, M. R., Edwards, L., Thumbi, S., et al. (2013). Bluetongue and epizootic haemorrhagic disease virus in local breeds of cattle in Kenya. Res. Vet. Sci. 94, 769–773. doi: 10.1016/j.rvsc.2012.11.001
Walker, A. R., and Boreham, P. F. L. (1976). Blood feeding of Culicoides (Diptera, Ceratopogonidae) in Kenya in relation to the epidemiology of bluetongue and ephemeral fever. Bull. Entomol. Res. 66, 181–188. doi: 10.1017/S000748530000660X
Youssef, L., Mounir, K., Mohamed, B., El Harrak, M., and Fihri, O. F. (2015). Spatial and seasonal distribution of Culicoides species in Morocco in relation to the transmission of bluetongue viruses. Br. J. Virol. 2, 88–95. doi: 10.17582/journal.bjv/2015.2.6.88.95
Keywords: arbovirus surveillance, vertebrate hosts, next generation sequencing, Culicoides biting midges, Goukovirus, Pacuvirus, Orthobunyavirus, Iflavirus
Citation: Ogola EO, Bastos ADS, Slothouwer I, Getugi C, Osalla J, Omoga DCA, Ondifu DO, Sang R, Torto B, Junglen S and Tchouassi DP (2024) Viral diversity and blood-feeding patterns of Afrotropical Culicoides biting midges (Diptera: Ceratopogonidae). Front. Microbiol. 14:1325473. doi: 10.3389/fmicb.2023.1325473
Received: 21 October 2023; Accepted: 13 December 2023;
Published: 05 January 2024.
Edited by:
Ke Liu, Chinese Academy of Agricultural Sciences, ChinaReviewed by:
Devojit Kumar Sarma, ICMR-National Institute for Research in Environmental Health, IndiaCopyright © 2024 Ogola, Bastos, Slothouwer, Getugi, Osalla, Omoga, Ondifu, Sang, Torto, Junglen and Tchouassi. This is an open-access article distributed under the terms of the Creative Commons Attribution License (CC BY). The use, distribution or reproduction in other forums is permitted, provided the original author(s) and the copyright owner(s) are credited and that the original publication in this journal is cited, in accordance with accepted academic practice. No use, distribution or reproduction is permitted which does not comply with these terms.
*Correspondence: Sandra Junglen, c2FuZHJhLmp1bmdsZW5AY2hhcml0ZS5kZQ==; David P. Tchouassi, ZHRjaG91YXNzaUBpY2lwZS5vcmc=
†These authors have contributed equally to this work
Disclaimer: All claims expressed in this article are solely those of the authors and do not necessarily represent those of their affiliated organizations, or those of the publisher, the editors and the reviewers. Any product that may be evaluated in this article or claim that may be made by its manufacturer is not guaranteed or endorsed by the publisher.
Research integrity at Frontiers
Learn more about the work of our research integrity team to safeguard the quality of each article we publish.