Corrigendum: A long-awaited taxogenomic investigation of the family Halomonadaceae
- 1Department of Microbiology and Parasitology, Faculty of Pharmacy, University of Sevilla, Sevilla, Spain
- 2Departament of Microbiology and Ecology, University of Valencia, Valencia, Spain
- 3The University of Queensland, School of Chemistry and Molecular Biosciences, Australian Centre for Ecogenomics, St Lucia, QLD, Australia
- 4Research Group Environmental Ecology and Applied Microbiology, Department of Bioscience Engineering, University of Antwerp, Antwerp, Belgium
The family Halomonadaceae is the largest family composed of halophilic bacteria, with more than 160 species with validly published names as of July 2023. Several classifications to circumscribe this family are available in major resources, such as those provided by the List of Prokaryotic names with Standing in Nomenclature (LPSN), NCBI Taxonomy, Genome Taxonomy Database (GTDB), and Bergey’s Manual of Systematics of Archaea and Bacteria (BMSAB), with some degree of disagreement between them. Moreover, regardless of the classification adopted, the genus Halomonas is not phylogenetically consistent, likely because it has been used as a catch-all for newly described species within the family Halomonadaceae that could not be clearly accommodated in other Halomonadaceae genera. In the past decade, some taxonomic rearrangements have been conducted on the Halomonadaceae based on ribosomal and alternative single-copy housekeeping gene sequence analysis. High-throughput technologies have enabled access to the genome sequences of many type strains belonging to the family Halomonadaceae; however, genome-based studies specifically addressing its taxonomic status have not been performed to date. In this study, we accomplished the genome sequencing of 17 missing type strains of Halomonadaceae species that, together with other publicly available genome sequences, allowed us to re-evaluate the genetic relationship, phylogeny, and taxonomy of the species and genera within this family. The approach followed included the estimate of the Overall Genome Relatedness Indexes (OGRIs) such as the average amino acid identity (AAI), phylogenomic reconstructions using amino acid substitution matrices customized for the family Halomonadaceae, and the analysis of clade-specific signature genes. Based on our results, we conclude that the genus Halovibrio is obviously out of place within the family Halomonadaceae, and, on the other hand, we propose a division of the genus Halomonas into seven separate genera and the transfer of seven species from Halomonas to the genus Modicisalibacter, together with the emendation of the latter. Additionally, data from this study demonstrate the existence of various synonym species names in this family.
1. Introduction
First described in 1988 to accommodate the genera Halomonas and Deleya based on 16S rRNA oligonucleotide cataloging, the family Halomonadaceae (Franzmann et al., 1988) is the largest bacterial family harboring halophilic microorganisms. Since then, 13 new genera with validly published names and one non-validly published name have been added to the family, as stated in the List of Prokaryotic names with Standing in Nomenclature (LPSN) (Parte et al., 2020). As of July 2023, it comprises 168 species with validly published names (according to the LPSN) that share the following features: Gram-stain-negative, non–endospore-forming rods or coccoid cells, chemo-organotrophic, aerobic or facultatively anaerobic, catalase-positive and oxidase-variable, and halophilic or halotolerant bacteria (Ventosa et al., 2021b). In accordance with the LPSN, this family belongs to the order Oceanospirillales (Garrity et al., 2005b), class Gammaproteobacteria (Garrity et al., 2005a), and other taxonomic sources indicate that it is most closely related to three genera belonging to the family Oceanospirillaceae (Garrity et al., 2005c): Oceanospirillum, Marinospirillum, or Pseudospirillum. The most widely used prokaryotic classification resources have different taxonomic opinions on this family. According to Bergey’s Manual of Systematics of Archaea and Bacteria (BMSAB), the most recent description of the family Halomonadaceae comprises 12 genera with validly published names: Halomonas (type genus), Aidingimonas, Carnimonas, Chromohalobacter, Cobetia, Halotalea, Kushneria, Larsenimonas, Modicisalibacter, Pistricoccus, Salinicola, and Zymobacter (Ventosa et al., 2021b), as well as one genus with an effectively published name, “Phytohalomonas” (Liu et al., 2020). As of July 2023, LPSN and NCBI Taxonomy also assign these 13 genera to the Halomonadaceae and 2 additional genera: Halovibrio and Terasakiispira in LPSN (Parte et al., 2020) and Halovibrio and Salicola in NCBI Taxonomy (Schoch et al., 2020), while Genome Taxonomy Database (GTDB) (Parks et al., 2018) release 08-RS214 comprises the 13 BMSAB genera together with the genus Terasakiispira, the 3 Oceanospirillaceae genera listed above, and an unnamed genus (TA22) based on environmental sequence data. GTDB also proposes the division of the genus Halomonas into five distinct genera (denoted with alphabetical suffixes) for a total of 22 genera in this family. Hence, currently, there is some disagreement on the circumscription of this family, which warrants further investigation.
For the present study, we followed the taxonomy proposed by BMSAB, but irrespective of the classification adopted, most genera of the family Halomonadaceae are well-defined and conserved, with the notable exception of the type genus of the family, Halomonas. Halomonas is the 15th largest genus across the prokaryotes in terms of the number of species with validly published names (121 as of July 2023) according to the LPSN.1 The species of this genus seldom form a monophyletic group and are instead scattered amongst the other genera of the family Halomonadaceae (de la Haba et al., 2010, 2012, 2014; Ventosa et al., 2021a,b). The polyphyly of Halomonas probably stems from the use of this genus as a default parent taxon for newly described members of the family that did not fall in any of the well-defined genera. Members of this genus exhibit a high degree of heterogeneity, as can be evidenced by their broad DNA G + C range (51.4–74.3 mol%), the widest across the family (Ventosa et al., 2021a). These observations point to the necessity of dividing the genus Halomonas and reclassifying some of its species.
After its description, three studies have proposed amendments to the family Halomonadaceae, accompanied by the inclusion of new genera into this family (Dobson and Franzmann, 1996; Ben Ali Gam et al., 2007; Ntougias et al., 2007). Additionally, the type genus of the family, Halomonas, has been amended on one occasion to accommodate nine new species in the genus (Dobson and Franzmann, 1996). Initially, a number of characteristic signatures for the 16S rRNA gene sequence were defined for members of this family (Dobson et al., 1993). Nevertheless, as new members have been described, it has been necessary to readapt the 16S rRNA gene sequence signatures (Ben Ali Gam et al., 2007; de la Haba et al., 2014). As whole-genome sequencing has become easier and cheaper, the analysis of clade-specific signature genes has been proposed as a tool to supplement the use of 16S rRNA gene sequence signatures (Zheng et al., 2020).
Several taxonomic studies have been carried out on the Halomonadaceae, some of them based on the 16S rRNA gene sequence analysis (Dobson et al., 1993; Mellado et al., 1995; Dobson and Franzmann, 1996), and the most recent focused on alternative phylogenetic markers, such as the 23S rRNA gene (Arahal et al., 2002; de la Haba et al., 2010) and multilocus sequence analysis based on single-copy housekeeping genes (de la Haba et al., 2012). Those approaches allowed, at that time, to establish the phylogenetic relationships among the members of this family and to propose taxonomic rearrangements accordingly. More than 10 years later, the number of species within the Halomonadaceae has doubled, and many of the type strains have a publicly available genome sequence. To the best of our knowledge, no studies specifically addressing the taxonomic status of the family Halomonadaceae in the genomic era have been conducted.
Therefore, in this study, we re-evaluate the genetic relationship, phylogeny, and taxonomy of the species and genera within the present family Halomonadaceae, as delineated in BMSAB, with the primary focus on its type genus. For this, we considered various overall genome relatedness indexes (OGRIs) based on average nucleotide identity (ANI), digital DNA–DNA hybridization (dDDH), average amino acid identity (AAI), core-protein average amino acid identity (cAAI), and the core-genome and GTDB phylogenies together with a signature gene analysis. To resolve the polyphyly within the genus Halomonas, we propose a division of the genus into seven different genera and the reclassification of several Halomonas species into the genus Modicisalibacter. Furthermore, the present study demonstrated the need to exclude the genus Halovibrio from the family Halomonadaceae and the existence of nine synonym species across this family.
2. Materials and methods
2.1. Genome retrieval and sequencing of type strains
The databases scanned for genomic data were NCBI Assembly,2 NCBI Sequence Read Archive (SRA),3 JGI Genome Portal,4 ATCC Genome Portal,5 and Global Catalogue of Type Strain (Shi et al., 2021). A total of 147 genome sequences of distinct type strains of species of the family Halomonadaceae (as per the BMSAB resource) and the genera Halovibrio and Terasakiispira were available in those public databases at the beginning of this study. Since some databases searched contained redundant data, the final set of genomes used in this research was only retrieved from the NCBI Assembly and NCBI SRA databases. One out of those 147 available genomes was discarded due to a failed quality check according to GTDB (Parks et al., 2018), while another marked as contaminated and excluded from RefSeq was finally retained after the verification of its contamination and completeness in GTDB, giving a total number of 146 genome sequences (Supplementary Table 1). All except one of the downloaded genomic data were assembled either at contig, scaffold, or chromosome level. The remaining one was only available in the NCBI SRA database, and thus, only raw reads could be retrieved, which were processed as indicated below.
To make our study as comprehensive as possible, we sequenced the genomes of an additional 17 type strains of species of Halomonadaceae that were not publicly available when the analyses were designed and performed (Supplementary Table 1). Seven out of those type strains were sequenced and assembled by the Global Catalogue of Microorganisms (Shi et al., 2021) as part of the type strain sequencing project (Wu and Ma, 2019). The genomic DNA of the other 10 type strains, retrieved from culture collections, was extracted and purified as described elsewhere (Galisteo et al., 2022) and further sequenced by Novogene Europe (Cambridge, United Kingdom) on the Illumina NovaSeq PE150 platform after library preparation of genomic material using the Novogene NGS DNA Library Prep Set (Cat. No. PT004). Raw reads were quality trimmed and filtered with BBTools v.38.44 (Bushnell, 2020) and further assembled with SPAdes v3.15.2 (option “—isolate”) (Prjibelski et al., 2020).
For all the retrieved or obtained sequences, assembly quality, and basic statistics were estimated with QUAST v.2.3 (Gurevich et al., 2013). Genome sequence completeness and contamination were verified by using CheckM v.1.1.5 (Parks et al., 2015). Prodigal v.2.6.3 (Hyatt et al., 2010) was employed to extract the translated coding sequences from the assembled genomes, and Prokka v.1.14.6 (Seemann, 2014) was employed for the automated annotation. When more than one genome sequence was available for the same type strain, we selected the one with a better assessment of contig number, completeness, contamination, 16S rRNA gene sequence presence and length, and the presence of 20 essential amino acids coded by distinct tRNA-coding genes, resulting in a final set of 161 genomes of type strains of the family Halomonadaceae.
2.2. Pangenome and core-genome inference
The complete pangenome of the selected set of genome sequences was calculated with Anvi’o suite v.7.1 (Eren et al., 2021), using BLASTP+ (Camacho et al., 2009) as the amino acid sequence similarity search program. Specifically, the “anvi-pan-genome” script was used with default values except that the MCL inflation parameter was set to 5 (in order to increase the sensitivity of the Markov Cluster Algorithm) (van Dongen, 2008), and the minimum percent identity between two amino acid sequences was set to 40%. The pangenome database containing orthologous gene clusters was employed to extract the gene family presence/absence matrix (“anvi-compute-functional-enrichment-in-pan” script) as well as the translated single-copy core genes present in at least 90% of the analyzed genomes, denoted as “core90” (“anvi-get-sequences-for-gene-clusters” script).
The “core90” proteins were individually aligned with MUSCLE v.3.8.1551 (default parameters) (Edgar, 2004). Gappy columns (>50% gaps) from each alignment were removed and further pruned using the chi2 statistic to filter out the 20% least conserved columns, as implemented in the “alignment_pruner.pl” Perl script.6 Final alignments were concatenated whenever necessary using AMAS (Borowiec, 2016).
2.3. Estimation of amino acid substitution models
Model-based phylogenetic analyses of protein sequences strongly rely on amino acid substitution models, which are generally summarized in a 20-by-20 replacement matrix, designated as Q matrices. Since those matrices are computationally very expensive to estimate due to their high parametrization, they are not usually estimated during a phylogenetic analysis but selected from a pre-estimated set of Q matrices using model selection software (Minh et al., 2021).
With the aim of constructing very reliable phylogenies, in this study, we estimated empirical general time-reversible Q matrices customized for the family Halomonadaceae derived from multiple sequence alignments of selected gene sets. For that purpose, QMarker software (Minh et al., 2021), which follows a maximum-likelihood approach, was used. Two gene datasets were chosen to generate Halomonadaceae-specific Q matrices: the “core90” set and the “bac120” set (120 single-copy bacterial proteins used by GTDB taxonomy) (Parks et al., 2018).
2.4. Phylogenomic reconstructions and overall genome relatedness indexes
The trimmed and concatenated “core90” protein alignment was used to infer the maximum-likelihood phylogeny of the family Halomonadaceae with the IQ-TREE v.2.2.0 program (Minh et al., 2020), using the custom Q_core90 reversible matrix as the substitution model combined with profile mixture models C10–C60 (Quang et al., 2008). The best-fit model parameters (mixture models, amino acid frequencies, and rate heterogeneity across sites) were determined with ModelFinder (Kalyaanamoorthy et al., 2017) according to Bayesian Information Criterion (BIC) (Schwarz, 1978). Branch support was estimated via 1,000 ultrafast bootstrap approximations (Hoang et al., 2018). Normalization of taxonomic ranks at the genus level according to relative evolutionary divergence (RED) was carried out with PhyloRank.7
A second phylogenomic tree including type and non-type strains as well as metagenome-assembled genomes (MAGs) was constructed to assess the cluster stability when new genome sequences are added to the tree and to analyze the relationships between members of the family Halomonadaceae and other closely related genera of the order Oceanospirillales (i.e., Halospina, Marinospirillum, Oceanospirillum, and Pseudospirillum). This second tree was based on the “bac120” protein set retrieved from GTDB Release 202 data files using GTDB-Tk v. 1.7.0 (Chaumeil et al., 2019). However, unlike the original GTDB-Tk pipeline that randomly selected 42 columns per marker to reduce computational requirements, we obtained the full-length multiple sequence alignments for each protein, which were later trimmed and concatenated as explained above for the “core90” dataset. Phylogenetic inference was carried out as stated for the first tree, but the Q_bac120 reversible matrix was used instead. Arrangements and visualization of both trees were accomplished by using ARB v.7.0 (Westram et al., 2011).
Commonly used OGRIs for taxonomic purposes were pairwise calculated among the analyzed genome set. ANI metrics were estimated with PyANI v.0.2.10 (Pritchard et al., 2016) using the ANIb method (i.e., BLASTN+ to align 1,020-bp fragments of the input sequences) (Goris et al., 2007). The genome-to-genome distance calculator (GGDC) webserver was used to infer dDDH relatedness according to formula 2 (Meier-Kolthoff et al., 2013). AAI values were determined using the “aai.rb” script from the Enveomics collection (Rodriguez-R and Konstantinidis, 2016). Since horizontal gene transfer (HGT) events can distort the molecular clock of bacterial evolution (Novichkov et al., 2004) and can, thus, impact the pairwise AAI values (Zheng et al., 2020), we calculated an additional genome relatedness index denoted as cAAI, similar to AAI but based on the protein sequences of core orthologous gene families, which are less impacted by HGT. For that purpose, the previously defined “core90” protein dataset (unaligned and untrimmed) was selected to calculate cAAI values with the “aai.rb” script.
2.5. Representation of clade-specific signature genes
The gene family presence/absence matrix inferred from the pangenome was used to detect “signature genes,” which are defined as gene families exclusive to specific phylogenetic clades (phylogroups), that is, gene families present in all species of a clade and absent in all other species (Zheng et al., 2020). Several phylogroups were investigated, aimed at testing different taxonomic arrangements and splitting into the family Halomonadaceae. For each phylogroup, a representative species was chosen, and the remaining species under study were assigned to the phylogroup of the representative species with whom they shared a most recent common ancestor.
The signature gene UpSet plots were generated by gathering gene families with the same presence/absence pattern across the analyzed genomes, ruling out two types of trivial patterns: gene families present in a single genome (singleton genes) and gene families detected in all genomes (core genes). The patterns were then displayed in descending order of frequency (number of gene families). R packages “tidyverse” v.1.2.1 (Wickham et al., 2019) and “tidygenomes” v.0.1.3,8 as well as other R functions and scripts,9 were used for data processing and visualization of gene family presence/absence and signature genes.
3. Results and discussion
3.1. Core-genome phylogenomics suggest several rearrangements in the family Halomonadaceae
A genome-based analysis was carried out aimed at shedding light on the relationships among species and genera of the family Halomonadaceae. The pangenome of the 161 genome sequences, which included type strains of the species belonging to the family Halomonadaceae, consisted of 608,808 genes organized into 59,992 orthologous gene clusters. Only 55 single-copy core gene clusters were detected across all genomes, so a more relaxed set of 189 orthologous genes shared by at least 90% of the genomes (“core90”) encompassing a total of 23,924 residues after alignment and trimming was selected to calculate a Halomonadaceae-specific Q reversible matrix (Q_core90; Supplementary Table 2) and to infer a phylogenomic tree (Figure 1 and Supplementary Figure 1). The best-fit model selected by ModelFinder according to the BIC criterion to construct the tree was Q_core90 + C60 + F + R10. The resulting tree showed that, apart from the genus Halomonas, all the remaining genera within this family formed monophyletic groups. Noteworthy, the genera Terasakiispira and primarily Halovibrio were by far the most distantly related to the other genera within Halomonadaceae, suggesting that they might not belong to this family (see below).
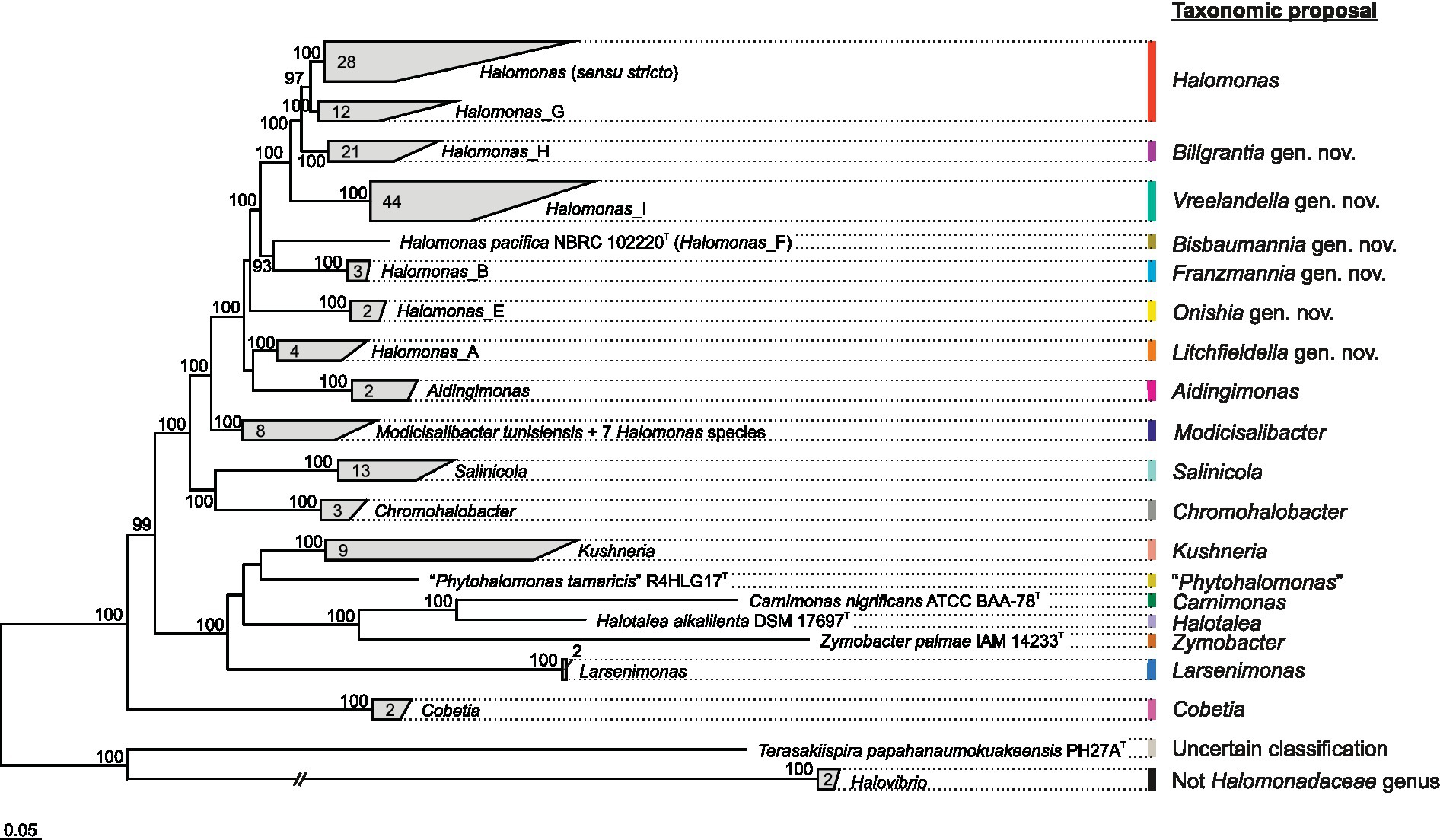
Figure 1. Clade collapsed maximum-likelihood phylogenomic tree based on the concatenation of the translated sequence of 189 single-copy genes shared by at least 90% of the members of the family Halomonadaceae under study (“core90” set). The genus Pistricoccus is missing because no representative genome sequences from type strains of this genus were recovered. The number of species comprised within each clade is displayed. Ultrafast bootstrap values ≥70% are shown above the branches. Bar, 0.05 changes per position.
Given the polyphyletic origin of the genus Halomonas and the heterogeneous characteristics of the species it harbors, we evaluated several proposals to split this genus into several genera with the following constraints: (i) the new proposed genera must be monophyletic; (ii) they must be supported by a 100% bootstrap value when possible; and (iii) they must fall within the relative evolutionary divergence (RED) interval defined for the rank of genus (Parks et al., 2018). The first attempt (proposal I) was to divide the genus Halomonas into eight phylogroups, five of them denoted according to GTDB taxonomy as Halomonas (sensu stricto), Halomonas_A, Halomonas_B, Halomonas_E, and Halomonas_F, and three newly proposed phylogroups designated as Halomonas_G, Halomonas_H, and Halomonas_I, as well as to transfer the species Halomonas coralii, Halomonas ilicicola, Halomonas muralis, Halomonas radicis, Halomonas xianhensis, and Halomonas zincidurans to the genus Modicisalibacter. Subsequent proposals were similar to this but included merging some of the previous phylogroups: proposal II = Halomonas (sensu stricto) + Halomonas_G; proposal III = Halomonas (sensu stricto) + Halomonas_G + Halomonas_H; proposal IV = Halomonas (sensu stricto) + Halomonas_G + Halomonas_H + Halomonas_I. It should be noted that proposal II involved the formation of a phylogroup (Halomonas + Halomonas_G) supported “only” by a 97% bootstrap, which does not meet the desired constraints indicated above but is high enough to consider this option of grouping. To select the best-fitting scenario for this genus reclassification among the above proposals, we performed further comparative genomic analyses.
3.2. Assessment on the basis of AAI and cAAI values does not provide sound clues to split the genus Halomonas
To evaluate the proposed phylogroups under the four scenarios, we plotted the all-vs-all AAI and cAAI results clustered by the phylogroup while distinguishing between intra-genus and inter-genus values (Figure 2). Theoretically, a well-delimited genus or phylogroup should not display any overlap between intra-clade and inter-clade AAI/cAAI values. As shown in Figure 2, all the currently existing genera within the family Halomonadaceae harboring more than a single species except Halomonas (sensu stricto) (i.e., Aidingimonas, Salinicola, Chromohalobacter, Kushneria, Larsenimonas, and Cobetia), as well as the new phylogroups shared by the four proposals (Halomonas_B, Halomonas_F, Halomonas_E, Halomonas_A, and the enlarged Modicisalibacter) showed minimal or no overlap between the inter- and intra-cluster AAI and cAAI values, confirming their clear separation and exclusivity as different genera within this family.
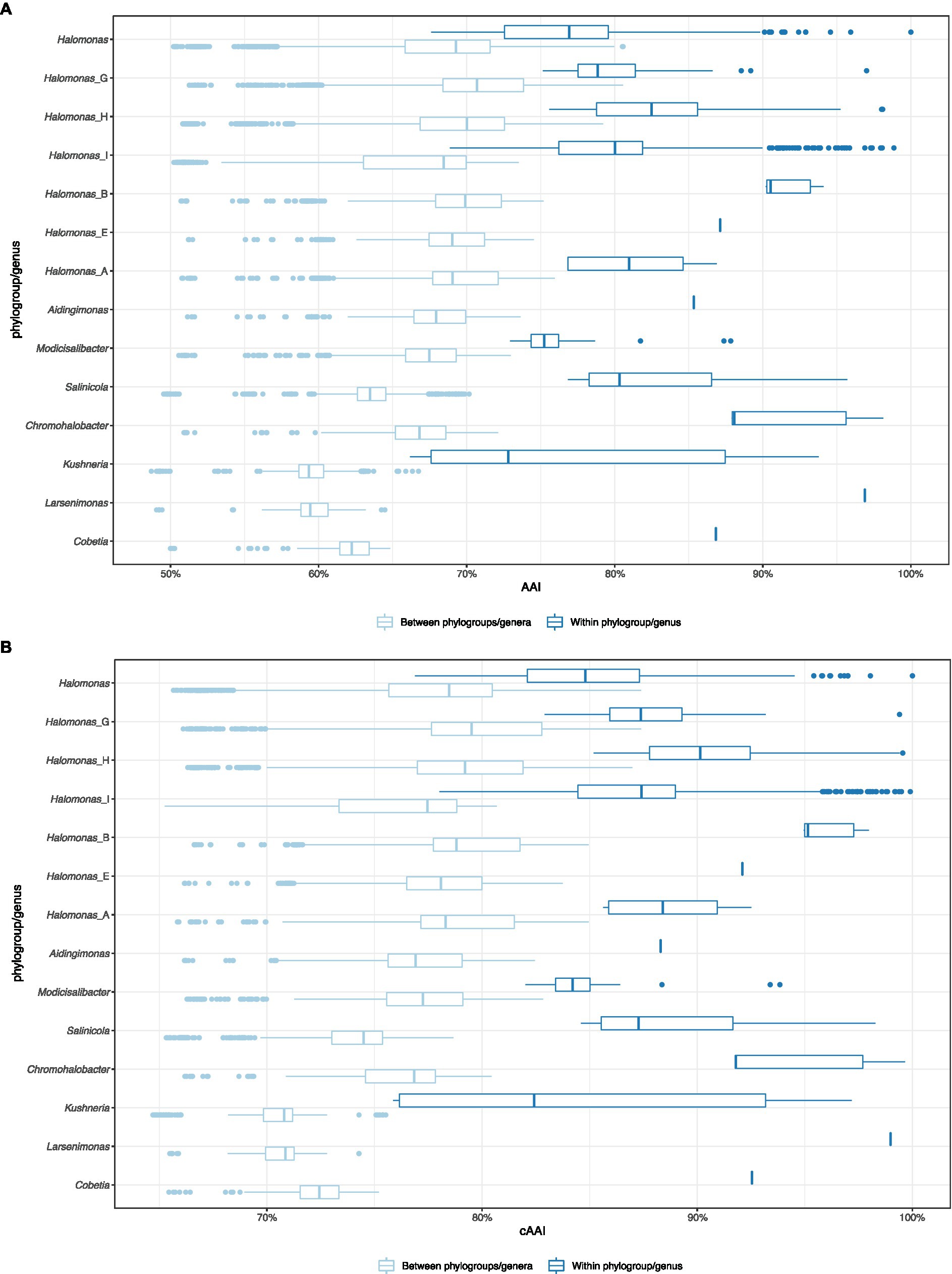
Figure 2. Box plot of the pairwise intra-clade and inter-clade AAI (A) and cAAI (B) values for the potential phylogroups/genera in the family Halomonadaceae, according to proposal I.
By contrast, the intra-group AAI and cAAI values largely overlapped with their inter-group counterparts for the phylogroups Halomonas, Halomonas_G, Halomonas_H, and Halomonas_I (proposal I), indicating their heterogeneity as well as the lack of clear differentiation among them. No significant overlap reduction was observed after the consecutive merger of these phylogroups (proposals II, III, and IV), although proposals II and III led to slightly decreased overlap (Supplementary Figures 2–4). Based on the above observations, we believe that AAI and cAAI data may be useful for the delineation of most of the genera of the family Halomonadaceae, but they are not reliable for fine phylogroup demarcation within the genus Halomonas. The lowest intra-clade AAI and cAAI values for the current genera and proposals I, II, and III were 66.2 and 75.9%, respectively, while the cutoffs for proposal IV were 65.5 and 73.2%, respectively. These threshold values might be useful for future genus circumscription within the family Halomonadaceae.
3.3. Signature genes support the inclusion of Halomonas_G into the genus Halomonas
Since AAI and cAAI values did not allow us to determine the best of the four proposals to split the genus Halomonas, we analyzed whether the currently existing genera and suggested phylogroups can be defined by clade-specific signature genes. For that purpose, we examined the gene family presence/absence matrix extracted from the pangenome of the family Halomonadaceae to detect gene families present in all genomes of a certain group but lacking in all other genomes. Signature genes may be the result of a shared evolutionary history within a phylogenetic clade, or they could be acquired by horizontal gene transfer reflecting common lifestyles, ecologies, and physiological properties (Zheng et al., 2020). Therefore, signature genes enable estimates of the evolutionary forces that shaped the cluster, and, thus, they might be of help to opt for one of the proposals over the others.
Except for Halomonas, all the current genera of the family Halomonadaceae according to the BMSAB classification containing more than a single species, together with Halomonas_B, Halomonas_E, Halomonas_A, the newly proposed phylogroups Halomonas_H and Halomonas_I, and the enlarged Modicisalibacter, were well supported by signature genes ranging from 5 to 548 (Figure 3 and Supplementary Figure 5). As can be expected, phylogroups/genera with a smaller number of species showed, generally, a larger number of signature genes; nevertheless, the impact of the clade size is limited, as can be evidenced from larger genera, such as Salinicola and Kushneria, displaying a relatively large number of signature genes. Considering that singleton genes (those present in a single genome) were omitted from the analysis, it was impossible to evaluate the signature genes harbored by phylogroups/genera containing only one species (i.e., Halomonas_F, “Phytohalomonas,” Carnimonas, Halotalea, and Zymobacter). Interestingly, no signature genes were identified for phylogroups Halomonas (sensu stricto) and Halomonas_G, which a priori dismisses proposal I. However, when merging these phylogroups (proposal II), a signature gene was detected (Figure 3). Although it is a single signature gene, it is conserved across a large number of genomes (40), comprising the phylogroups Halomonas and Halomonas_G and, therefore, it likely reflects a common evolutionary history of both phylogroups providing an important hint into the delineation of the genus Halomonas. Proposal III, which considers the merging of phylogroups Halomonas, Halomonas_G, and Halomonas_H, was also endorsed by a single signature gene (Supplementary Figure 5); however, proposal II is favored over proposal III due to the fact that phylogroup Halomonas_H is very well supported by 21 signature genes and, thus, it is preferable to keep it as a separate cluster. The last proposal, proposal IV, consisting of the fusion of phylogroups Halomonas, Halomonas_G, Halomonas_H, and Halomonas_I, can also be rejected because the combined clade did not show any signature genes (Supplementary Figure 5) and, in addition, phylogroup Halomonas_I was supported by five signature genes.
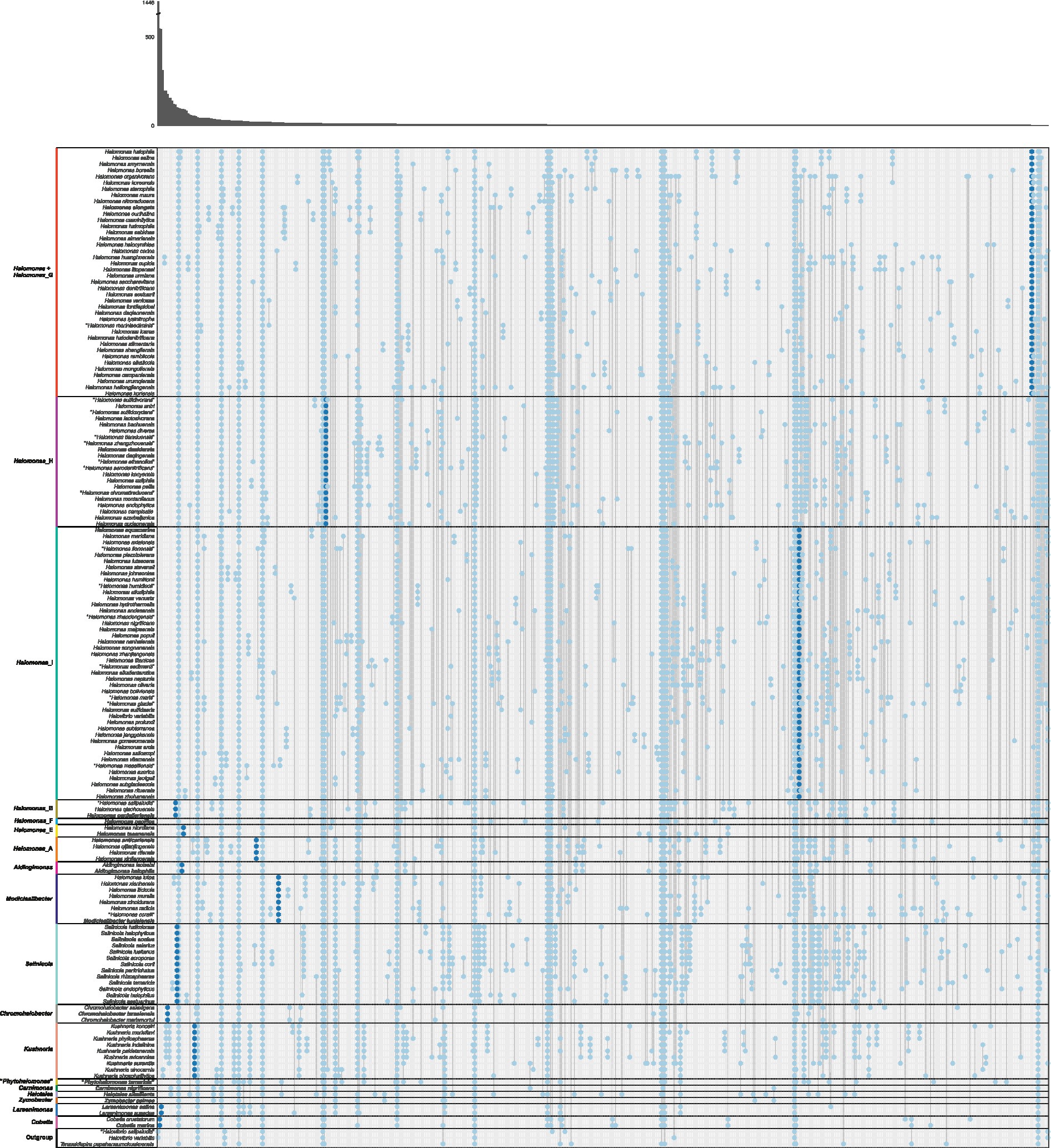
Figure 3. Gene family presence/absence patterns inferred from the pangenome of the family Halomonadaceae arranged according to proposal II. Each column represents a gene family pattern, where presence is indicated with a dot in the corresponding species. The absolute number of gene families that conform to each pattern is visualized in the marginal bar plot at the top. Separations between phylogroups/genera are indicated with horizontal black lines and the representative species of each phylogroup/genus is highlighted in bold. Genes that were present in all genomes of a clade and in none of the genomes outside of that clade, denoted as “signature genes,” are displayed in dark blue; other genes are shown in light blue. Patterns of presence in a single species or all species are not shown. The species Halovibrio salipaludis, Halovibrio variabilis, and Terasakiispira papahanaumokuakeensis were not considered members of the Halomonadaceae and were only used as an “outgroup”.
3.4. GTDB-based phylogeny is consistent with proposal II and delineates the family Halomonadaceae
Although signature gene analyses endorsed proposal II as the most reliable one to split the genus Halomonas, this proposal involves the creation of a cluster with “only” a 97% branch bootstrap, as stated above. While this support value cannot be regarded as too low, we attempted to establish a long-term and trustworthy classification of the family for which only 100% supported phylogroups are preferred. This concern raises questions about the suitability of proposal II, making it necessary to provide some additional evidence to accept or reject it.
Accordingly, an alternative family-specific Q time-reversible matrix (Q_bac120; Supplementary Table 3) and phylogenomic tree (Figure 4) were calculated on the basis of the “bac120” marker protein dataset used to infer the bacterial GTDB taxonomy (Parks et al., 2018), comprising a total of 32,354 aligned columns after trimming. These ubiquitous single-copy proteins have been identified as being suitable for phylogenetic inference (Parks et al., 2017) and may yield better resolved trees than those obtained by using the almost entire set of core proteins (“core90” set). In fact, some of the “core90” non-curated protein-coding genes might have been laterally transferred or undergone homologous recombination (Stott and Bobay, 2020) or might lack congruent phylogenetic signals or sufficient homology to make comparisons valid and conclusive (Wu et al., 2013; Capella-Gutierrez et al., 2014). In addition, genomes from non-type strains and MAGs were also included in this complementary reconstruction to evaluate tree topology preservation.

Figure 4. GTDB-derived maximum-likelihood phylogenomic tree based on the concatenation of 120 single-copy bacterial proteins (“bac120” set) showing the relationships among members of the family Halomonadaceae and related taxa. The type strain of the species Pistricoccus aurantiacus is missing because no genome sequences from this strain could be recovered. The number of species in each cluster is displayed inside wedges. Ultrafast bootstrap values ≥70% are shown above the branches. Bar, 0.05 changes per amino acid position.
The topology of the “bac120”-based tree (Figure 4) recovered the same phylogroups observed in the “core90”-based phylogeny (Figure 1). Although it is well-known that the selection of different phylogenetic markers can result in different topologies (Capella-Gutierrez et al., 2014; Ankenbrand and Keller, 2016; Tsai et al., 2019), in our analysis, the bias associated with marker choice did not affect the delineation of genera and phylogroups, demonstrating the stability of the obtained genome-based trees with independence of the protein set used for their inference. A good agreement between core-genome and “bac120” phylogenies has been previously demonstrated for the taxa of the Pseudomonadaceae (Lalucat et al., 2020), which is another family in the class Gammaproteobacteria. The inclusion of 82 additional GTDB representative genomes from non-type strains and MAGs of members of the family Halomonadaceae, as well as genomes from the closest genera of the order Oceanospirillales, did not alter the retrieved clades either (Figure 4). The most remarkable difference between our “core90” and “bac120” trees is the stronger support in the latter of the branch collapsing phylogroups Halomonas (sensu stricto) and Halomonas_G (corresponding to proposal II). Hence, this 100% bootstrap value recovered in the “bac120” tree after merging both phylogroups, together with the signature gene analysis, enables proposal II as the most appropriate to prune the genus Halomonas to the species comprising phylogroups Halomonas (sensu stricto) and Halomonas_G, while transferring the remaining species to six new genera and to the already described genus Modicisalibacter.
Concerning the taxonomic status of the family Halomonadaceae, the “bac120”-based phylogeny evidenced that all 12 genera currently affiliated to this family according to BMSAB, together with the non-validly published genus name “Phytohalomonas,” formed a monophyletic group of microorganisms (Figure 4), whose shared feature, which was at the same time differential to the closest related genera, was their halophily or halotolerance. The genus Halovibrio, also considered a member of the family Halomonadaceae by LPSN, was intimately related to the genus Halospina, belonging to the family Hahellaceae; 10,11 whereas the genus Terasakiispira, included into the Halomonadaceae by both LPSN and GTDB resources, was rather a taxon within the family Oceanospirillaceae given its closest relationship to the genera Marinospirillum, Oceanospirillum, and Pseudospirillum (Figure 4). GTDB taxonomy (release 08-RS214) has suggested the incorporation of these three genera into the family Halomonadaceae, even if they harbor marine (slight) halophiles in contrast to the majority of moderately halophilic bacteria characteristic of the family Halomonadaceae. Therefore, it becomes clear that the genus Halovibrio should be kept apart from the family Halomonadaceae, while further investigation including genomic, phylogenomic, and phenotypic comparisons is required to delineate the taxonomic affiliation of the genera Terasakiispira, Marinospirillum, Oceanospirillum, and Pseudospirillum at the family level.
3.5. Heterotypic synonyms revealed by ANI and dDDH values
Phylogenomic inference using both the “core90” and the “bac120” protein sets revealed the presence of some very closely related species pairs and even one set of three among the studied taxa that might be considered cases of heterotypic synonymy. To verify those hypotheses, ANI (Figure 5) and dDDH relatedness indexes were estimated between the genomes in question. It is widely accepted that cutoff values for species delineation based on these genomic indexes are 95–96% for ANI (Goris et al., 2007; Richter and Rossello-Mora, 2009; Chun and Rainey, 2014) and 70% for dDDH (Auch et al., 2010). Therefore, two or more strains can be considered to belong to the same species if both ANI values are ≥96% and dDDH values are ≥70%. According to this criterion, our results confirmed the existence of the following sets of heterotypic synonyms (ANI and dDDH values in parenthesis, respectively): Chromohalobacter israelensis – Chromohalobacter salexigens (98.1 and 83.6%); Halomonas alkaliphila – “Halomonas humidisoli” (97.7 and 80.5%); Halomonas antri – “Halomonas sulfidivorans” (97.7 and 80.2%); Halomonas aquamarina – Halomonas meridiana – Halomonas axialensis (96.5–97.5% and 71.2–79.1%); Halomonas halophila – Halomonas salina (100 and 100%); Halomonas icarae – “Halomonas marinisediminis” (97.4 and 79.2%); Halomonas neptunia – Halomonas alkaliantarctica (98.9 and 91.1%); Halomonas hamiltonii – Halomonas johnsoniae (97.3 and 76.8%); and Halomonas venusta – Halomonas hydrothermalis (96.8 and 73.0%).
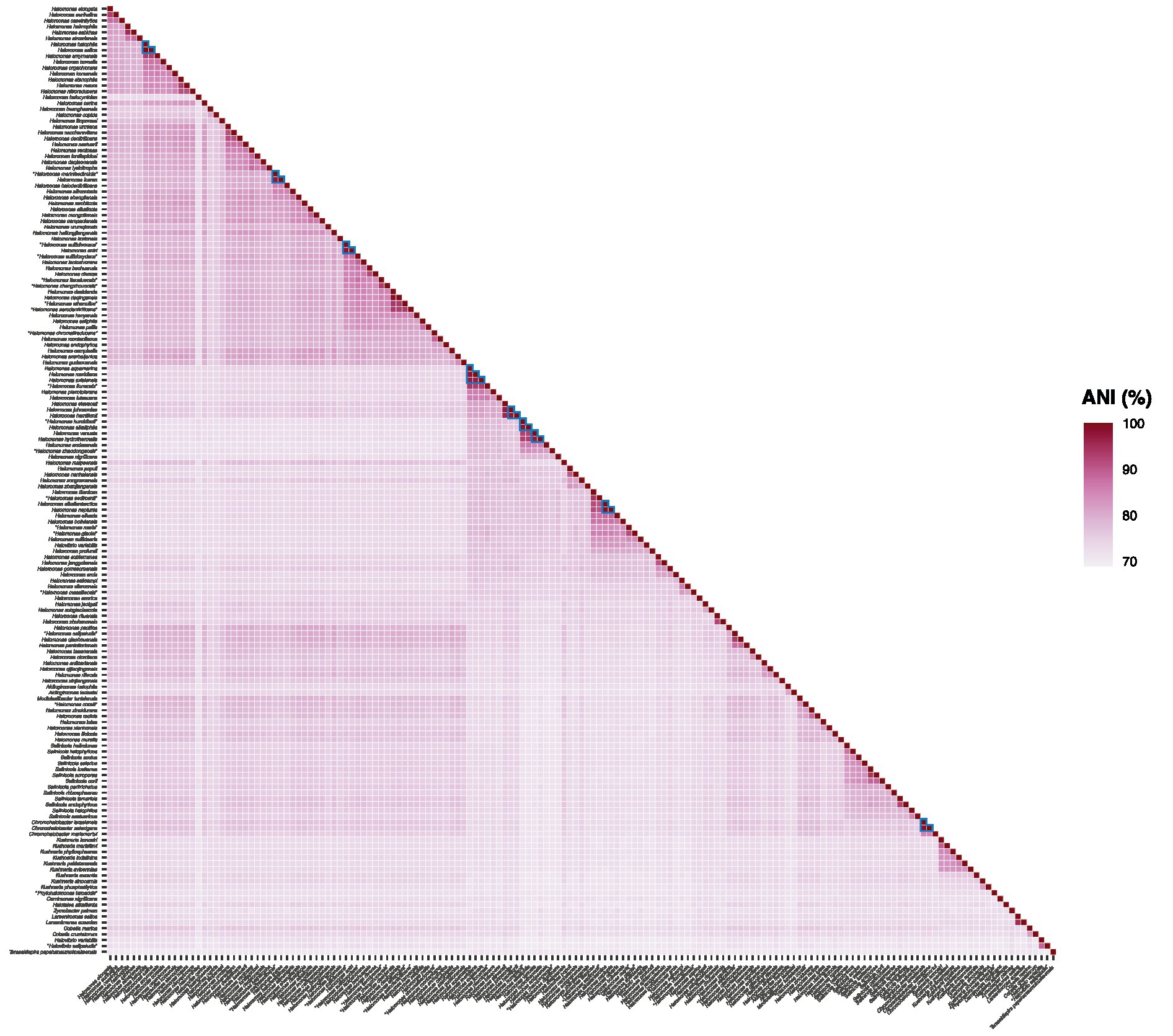
Figure 5. ANI values (%) from pairwise whole-genome comparisons between the type strains of members of the family Halomonadaceae. The ANI percentages are expressed as a heatmap in purple color. Values supporting the proposed synonym species names are surrounded by a blue triangle.
4. Taxonomic conclusions
The comparative genomic analyses (phylogenomics, OGRI, and signature genes) conducted among the type strains of the species of the family Halomonadaceae allow us to propose several taxonomic rearrangements within this family:
i. The genus Halomonas comprises the following species: H. elongata (type species), H. aestuarii, H. alimentaria, H. alkalicola, H. almeriensis, H. borealis, H. campaniensis, H. caseinilytica, H. cerina, H. cupida, H. daqiaonensis, H. denitrificans, H. eurihalina, H. fontilapidosi, H. halmophila, H. halocynthiae, H. halodenitrificans, H. halophila (synonym H. salina), H. heilongjiangensis, H. huangheensis, H. icarae (synonym “H. marinisediminis”), H. koreensis, H. korlensis, H. litopenaei, H. lysinitropha, H. maura, H. mongoliensis, H. nitroreducens, H. organivorans, H. ramblicola, H. sabkhae, H. saccharevitans, H. shengliensis, H. smyrnensis, H. stenophila, H. urmiana, H. urumqiensis, and H. ventosae.
ii. The following species are transferred to the genus Modicisalibacter: “Halomonas coralii,” Halomonas ilicicola, Halomonas lutea, Halomonas muralis, Halomonas radicis, Halomonas xianhensis, and Halomonas zincidurans, as “Modicisalibacter coralii,” Modicisalibacter ilicicola, Modicisalibacter luteus, Modicisalibacter muralis, Modicisalibacter radicis, Modicisalibacter xianhensis, and Modicisalibacter zincidurans, respectively. Therefore, it has been necessary to extend the description of the genus Modicisalibacter, as indicated below.
iii. The remaining analyzed species of the genus Halomonas are reclassified into new genera belonging to the family Halomonadaceae as follows, where the type species of each genus is highlighted in bold, according to the rule of priority of publication:
• Genus Vreelandella (corresponding to the phylogroup Halomonas_I), including the species H. alkaliphila (synonym “H. humidisoli”), H. andesensis, H. aquamarina (synonyms H. meridiana and H. axialensis), H. arcis, H. azerica, H. boliviensis, “H. glaciei,” H. gomseomensis, H. hamiltonii (synonym H. johnsoniae), H. janggokensis, H. jeotgali, “H. lionensis,” H. lutescens, H. malpeensis, “H. maris,” “H. massiliensis,” H. nanhaiensis, H. neptunia (synonym H. alkaliantarctica), H. nigrificans, H. olivaria, H. piezotolerans, H. populi, H. profundi, H. rituensis, H. salicampi, “H. sedimenti,” H. songnenensis, H. stevensii, H. subglaciescola, H. subterranea, H. sulfidaeris, H. titanicae, H. utahensis, H. venusta (synonym H. hydrothermalis), H. vilamensis, H. zhanjiangensis, “H. zhaodongensis,” and H. zhuhanensis.
• Genus Bisbaumannia (corresponding to the phylogroup Halomonas_F), including the single species H. pacifica.
• Genus Billgrantia (corresponding to the phylogroup Halomonas_H), including the species “H. aerodenitrificans,” H. antri (synonym “H. sulfidivorans”), H. azerbaijanica, H. bachuensis, H. campisalis, “H. chromatireducens,” H. desiderata (synonym H. daqingensis), H. diversa, H. endophytica, “H. ethanolica,” H. gudaonensis, H. kenyensis, H. lactosivorans, “H. montanilacus,” H. pellis, H. saliphila, “H. sulfidoxydans,” “H. tianxiuensis,” and “H. zhangzhouensis.”
• Genus Franzmannia (corresponding to the phylogroup Halomonas_B), including the species H. pantelleriensis, H. qiaohouensis, and “H. salipaludis.”
• Genus Litchfieldella (corresponding to the phylogroup Halomonas_A), including the species H. anticariensis, H. qijiaojingensis, H. rifensis, and H. xinjiangensis.
• Genus Onishia (corresponding to the phylogroup Halomonas_E), including the species H. niordiana and H. taeanensis.
i. The genus Halovibrio should be excluded from the family Halomonadaceae. According to GTBD, it should be transferred to the family Oleiphilaceae.
ii. The following species sets are considered heterotypic synonyms (the species name holding priority in the application of the International Code of Nomenclature of Prokaryotes (Oren et al., 2023) is listed in first place): Chromohalobacter israelensis – Chromohalobacter salexigens; Halomonas alkaliphila – “Halomonas humidisoli”; Halomonas antri – “Halomonas sufidivorans”; Halomonas aquamarina – Halomonas meridiana – Halomonas axialensis; Halomonas halophila – Halomonas salina; Halomonas hamiltonii – Halomonas johnsoniae; Halomonas icarae – “Halomonas marinisediminis”; Halomonas neptunia – Halomonas alkaliantarctica; and Halomonas venusta - Halomonas hydrothermalis.
iii. The species whose genome sequence was not publicly available or could not be sequenced when this study was accomplished, together with the new species described after the finalization of this research, should be further studied to determine their correct placement according to the taxonomy proposed here or if they may constitute new genera within the family Halomonadaceae.
Moreover, we strongly recommend the use of the clade-specific amino acid substitution matrices Q_core90 and Q_bac120, empirically calculated for the family Halomonadaceae, for future phylogenomic studies within this family instead of the pre-computed Q matrices generally selected by model selection software.
Description of Vreelandella gen. nov.
Vreelandella (Vree.land.el’la N.L. fem. dim. n. Vreelandella, named after Russell Vreeland, American scientist who described the genus Halomonas and studied halophilic microorganisms for over 40 years).
Cells are Gram-staining-negative rods, 0.3–1.9 × 0.5–6.0 μm in size, aerobic or facultatively anaerobic, and mostly motile. Endospores are not formed. Catalase-positive and oxidase-variable. Colonies are translucent, beige, black, cream, glistening-colored, ochre, orange, pale-pink, reddish-brown, white, or yellow pigmented. Slightly to moderately halophilic, growing at 0–27% (w/v) NaCl, with optimal growth at 0–15% (w/v) NaCl. Mesophilic or psychrotolerant, thriving at −5–60°C, showing optimal growth at 20–37°C. Alkaliphilic or alkalitolerant, growing at pH values in the range of 5.0–12.0, with optimal growth at pH 7.0–10.0. Chemo-organotrophic. Nitrate reduction is variable. The major respiratory quinones are Q9, Q8, and Q6. The major fatty acids are C16:0, C16:1ω6c/C16:1ω7c/iso-C15:0 2-OH, C18:1ω6c/C18:1ω7c, C19:0 cyclo ω8c, C12:0 3-OH, C17:0 cyclo, C10:0, iso-C16:1ω7c 2-OH, and C18:0. The major polar lipids are diphosphatidylglycerol, phosphatidylglycerol, and phosphatidylethanolamine.
The DNA G + C content ranges between 52.1 and 63.8 mol%.
The genus Vreelandella belongs to the family Halomonadaceae. The type species is Vreelandella aquamarina.
Description of Vreelandella aquamarina comb. nov.
Vreelandella aquamarina (a.qua.ma.ri’na. L. fem. n. aqua, water; L. masc. adj. marinus, of the sea, marine; N.L. fem. adj. aquamarina, pertaining to seawater).
Basonym: “Achromobacter aquamarinus” ZoBeAll and Upham 1944.
Homotypic synonyms: Halomonas aquamarina (ZoBell and Upham 1944) Dobson and Franzmann, 1996; Deleya aquamarina (ZoBell and Upham 1944) Akagawa and Yamasato 1989; Alcaligenes aquamarinus (ZoBell and Upham 1944) Hendrie et al. 1974 (Approved Lists 1980).
Cells are Gram-stain-negative rods, 0.6–1.9 × 1.0–4.5 μm in size, with rounded ends, occurring singly or in doublets, facultatively anaerobic, and motile by means of peritrichous or lateral flagella. Endospores are not formed. Catalase and oxidase are positive. Colonies are convex, smooth, circular, entire, whitish or cream-colored, and 2 mm in diameter after 2 days of incubation on Marine Agar 2,216 at 20°C. Slightly to moderately halophilic, growing at 0–30% (w/v) NaCl, with optimal growth at 0.5–10% (w/v) NaCl. Psychrotolerant, thriving at −1–47°C, showing optimal growth at 20–40°C. Alkalitolerant, growing at pH values in the range of 5.0–12.0, with optimal growth at pH 7.0–8.0. Chemo-organotrophic. Negative for Simmons’ citrate utilization, indole production, methyl red and Voges–Proskauer tests, lysine decarboxylase, and β-galactosidase. Aesculin, casein, gelatin, and DNA are not hydrolyzed. Variable for nitrate reduction to nitrite, H2S production, urease, hydrolysis of starch and Tween 80, ornithine decarboxylase, phosphatase, and phenylalanine deaminase. Produces acid but no gas from glucose. Does not ferment glycerol or xylose. Utilizes L-arabinose, D-fructose, D-glucose, maltose, sucrose, and fumarate as sole carbon and energy sources. The major respiratory quinone is Q9. The major fatty acids are C16:0, C18:1ω7c, C16:1ω7c/iso-C15:0 2-OH, C19:0 cyclo ω8c, and C12:0 3-OH. The major polar lipids are diphosphatidylglycerol, phosphatidylglycerol, and phosphatidylethanolamine.
The DNA G + C content is 56.7–57.0 mol%.
The type strain is 558T = ATCC 14400T = BCRC 12878T = CCUG 16157T = CECT 5000T = CGMCC 1.2324T = CIP 105454T = DSM 30161T = IAM 12550T = KCTC 22193T = LMD 73.17T = LMG 2853T = NCIMB 557T. The genome size of the type strain is 3.50 Mbp, and its DNA G + C content is 56.7 mol%. Isolated from seawater (Pacific Ocean).
Type strain genome sequence accession number: GCA_900110265.1.
Type strain 16S rRNA gene sequence accession number: AJ306888.
Halomonas meridiana and Halomonas axialensis should be regarded as heterotypic synonyms of Vreelandella aquamarina.
Description of Vreelandella venusta comb. nov.
Vreelandella venusta (ve.nus’ta. L. fem. adj. venusta, lovely, beautiful).
Basonym: Alcaligenes venustus Baumann et al. 1972 (Approved Lists 1980).
Homotypic synonyms: Halomonas venusta (Baumann et al. 1972) Dobson and Franzmann 1996; Deleya venusta (Baumann et al. 1972) Baumann et al. 1983.
Cells are Gram-stain-negative rods, 1.5 × 2.0–3.0 μm in size, occurring singly or in doublets, aerobic or facultatively anaerobic, and motile by means of peritrichous flagella. Accumulate β-polyhydroxybutyrate as an intracellular reserve product. Endospores are not formed. Catalase and oxidase are positive. Colonies are round, smooth, and yellow or cream-colored. Slightly to moderately halophilic, growing at 0–22% (w/v) NaCl, with optimal growth at 0.5–7% (w/v) NaCl. Psychrotolerant, thriving at 2–40°C, showing optimal growth at 30°C. Alkalitolerant, growing at pH values in the range of 5.0–12.0, with optimal growth at pH 7.0–8.0. Chemo-organotrophic. Positive for nitrate reduction to nitrite. Negative for indole production and methyl red and Voges–Proskauer tests. DNA is hydrolyzed, but alginate, casein, chitin, gelatin, starch, and Tween 80 are not. Variable for H2S production, lysine decarboxylase, ornithine decarboxylase, and phenylalanine deaminase. Acid production from D-glucose is variable, but negative from L-arabinose, D-fructose, D-galactose, lactose, maltose, D-mannose, sucrose, D-trehalose, D-xylose, or glycerol. Utilizes D-glucose, glycerol, acetate, butyrate, citrate, fumarate, DL-malate, propionate, L-glutamate, L-lysine, and L-proline as sole carbon (nitrogen) and energy sources. The major respiratory quinone is Q9. The major fatty acids are C16:0, C18:1ω7c, C16:1ω7c/iso-C15:0 2-OH, C19:0 cyclo ω8c, and C12:0 3-OH. The major polar lipids are diphosphatidylglycerol, phosphatidylglycerol, and phosphatidylethanolamine.
The DNA G + C content is 52.6–53.2 mol%.
The type strain is 86T = ATCC 27125T = CCUG 16063T = CIP 103201T = DSM 4743T = JCM 20634T = LMG 3445T = NBRC 102221T. The genome size of the type strain is 4.27 Mbp, and its DNA G + C content is 52.6 mol%. Isolated from seawater (off the coast of Oahu, Hawaii, United States).
Type strain genome sequence accession number: GCA_007989605.1.
Type strain 16S rRNA gene sequence accession number: AJ306894.
Halomonas hydrothermalis should be regarded as a heterotypic synonym of Vreelandella venusta.
Description of Vreelandella subglaciescola comb. nov.
Vreelandella subglaciescola (sub.gla.ci.es’co.la. L. prep. sub-, under, below; L. fem. n. glacies, ice; L. masc. n. suff. -cola, dweller; N.L. fem. n. subglaciescola, dwelling below the ice).
Basonym: Halomonas subglaciescola Franzmann et al. 1987.
The description is as given in the proposal of the basonym (Franzmann et al., 1987), with the following addition. The genome size of the type strain is 3.11 Mbp. The DNA G + C content is 60.8 mol%.
Isolated from saline water from Organic Lake, Antarctica.
The type strain is ACAM 12T = ATCC 43668T = CIP 104042T = DSM 4683T = IAM 14167T = JCM 21045T = LMG 8824T = NBRC 14766T = UQM 2926T.
Type strain genome sequence accession number: GCA_900142895.1.
Type strain 16S rRNA gene sequence accession number: AJ306892.
Description of Vreelandella neptunia comb. nov.
Vreelandella neptunia (nep.tu’ni.a. L. fem. adj. neptunia, Neptunian, pertaining to Neptunus, the Roman god of the sea).
Basonym: Halomonas neptunia Kaye et al. 2004.
Cells are Gram-stain-negative rods, 0.5–1.0 × 1.0–3.0 μm in size, occurring singly or in doublets, aerobic or facultatively anaerobic, and motile by means of peritrichous flagella. Produces exopolysaccharides and accumulates glycine-betaine but not β-polyhydroxybutyrate. Endospores are not formed. Oxidase-positive, but catalase-variable. Colonies are round, smooth, and cream-colored. Slightly to moderately halophilic, growing at 0.5–27% (w/v) NaCl, with optimal growth at 2–10% (w/v) NaCl. Psychrotolerant, thriving at −1–37°C, showing optimal growth at 30°C. Alkalitolerant, growing at pH values in the range of 5.0–12.0, with optimal growth at pH 7.0–9.0. Chemo-organotrophic. Positive for nitrate reduction to nitrite, but nitrite is not reduced. Negative for Simmons’ citrate utilization, H2S production, methyl red and Voges–Proskauer tests, lysine decarboxylase, ornithine decarboxylase, and phenylalanine deaminase. Able to synthesize α-glucosidase. Casein, DNA, gelatin, starch, and Tween 80 are not hydrolyzed. Variable for indole production. Acid production from D-galactose and D-glucose is positive, but negative from L-arabinose, D-fructose, lactose, maltose, D-mannose, sucrose, D-trehalose, D-xylose, or glycerol. Utilizes D-cellobiose, D-fructose, D-galactose, D-glucose, maltose, D-ribose, sucrose, D-trehalose, glycerol, and acetate as sole carbon and energy sources. The only respiratory quinone is Q9. The major fatty acids are C18:1ω7c, C16:0, C16:1ω7c, C19:0 cyclo ω8c, and C12:0 3-OH. The major polar lipids are phosphatidylglycerol, diphosphatidylglycerol, and phosphatidylethanolamine.
The DNA G + C content is 54.8–55.0 mol%.
The type strain is Eplume1T = ATCC BAA-805T = CCM 7107T = CECT 5815T = DSM 15720T. The genome size of the type strain is 4.93 Mbp, and its DNA G + C content is 55.0 mol%. Isolated from a deep-sea hydrothermal plume (NE Pacific Ocean, Juan de Fuca Ridge).
Type strain genome sequence accession numbers: GCA_030409295.1 and GCA_019903445.1.
Type strain 16S rRNA gene sequence accession number: AF212202.
Halomonas alkaliantarctica should be regarded as a heterotypic synonym of Vreelandella neptunia.
Description of Vreelandella sulfidaeris comb. nov.
Vreelandella sulfidaeris (sul.fid.ae’ris. N.L. neut. n. sulfidum, sulfide; L. neut. n. aes, ore; N.L. gen. n. sulfidaeris, from sulfide ore).
Basonym: Halomonas sulfidaeris Kaye et al. 2004.
The description is as given in the proposal of the basonym (Kaye et al., 2004), with the following addition. The genome size of the type strain is 4.48 Mbp. The DNA G + C content is 53.7 mol%.
Isolated from a deep-sea sulfide rock (NE Pacific Ocean, Juan de Fuca Ridge).
The type strain is Esulfide1T = ATCC BAA-803T = CCM 7108T = CECT 5817T = DSM 15722T.
Type strain genome sequence accession number: GCA_007182875.1.
Type strain 16S rRNA gene sequence accession number: AF212204.
Description of Vreelandella boliviensis comb. nov.
Vreelandella boliviensis (bo.li.vi.en’sis. N.L. fem. adj. boliviensis, from Bolivia, relating to the country where the bacteria were isolated).
Basonym: Halomonas boliviensis Quillaguamán et al. 2004.
The description is as given in the proposal of the basonym (Quillaguamán et al., 2004), with the following addition. The genome size of the type strain is 4.21 Mbp. The DNA G + C content is 54.7 mol%.
Isolated from the soil around a Bolivian hypersaline lake.
The type strain is LC1T = ATCC BAA-759T = DSM 15516T.
Type strain genome sequence accession numbers: GCA_000236035.1 and GCA_002265845.1.
Type strain 16S rRNA gene sequence accession number: AY245449.
Description of Vreelandella utahensis comb. nov.
Vreelandella utahensis (u.ta.hen’sis. N.L. fem. adj. utahensis, referring to Utah).
Basonym: Halomonas utahensis Sorokin and Tindall, 2006.
The description is as given in the proposal of the basonym (Sorokin and Tindall, 2006), with the following addition. The genome size of the type strain is 3.73 Mbp. The DNA G + C content is 55.8 mol%.
Isolated from surface water from the North Arm of Great Salt Lake (United States).
The type strain is isolate IIIT = ATCC 49240T = CECT 5286T = CIP 105504T = DSM 3051T = IAM 14440T = JCM 21223T = NBRC 102410T.
Type strain genome sequence accession number: GCA_007991175.1.
Type strain 16S rRNA gene sequence accession number: AJ306893.
Description of Vreelandella gomseomensis comb. nov.
Vreelandella gomseomensis (gom.se.om.en’sis. N.L. fem. adj. gomseomensis, referring to Gomseom in Anmyeondo, from where the first strains were isolated).
Basonym: Halomonas gomseomensis Kim et al. 2007.
The description is as given in the proposal of the basonym (Kim et al., 2007), with the following addition. The genome size of the type strain is 3.73 Mbp. The DNA G + C content is 59.8 mol%.
Isolated from saline water of the Gomseom solar saltern in Anmyeondo (Korea).
The type strain is M12T = CIP 109897T = DSM 18042T = KCTC 12662T.
Type strain genome sequence accession number: GCA_031451645.1.
Type strain 16S rRNA gene sequence accession number: AM229314.
Description of Vreelandella janggokensis comb. nov.
Vreelandella janggokensis (jang.gok.en’sis. N.L. fem. adj. janggokensis, referring to Janggok in Anmyeondo, from where the first strains were isolated).
Basonym: Halomonas janggokensis Kim et al. 2007.
The description is as given in the proposal of the basonym (Kim et al., 2007), with the following addition. The genome size of the type strain is 3.87 Mbp. The DNA G + C content is 57.3 mol%.
Isolated from saline water of the Janggok solar saltern in Anmyeondo (Korea).
The type strain is M24T = CIP 109896T = DSM 18043T = KCTC 12663T.
Type strain genome sequence accession number: GCA_031451615.1.
Type strain 16S rRNA gene sequence accession number: AM229315.
Description of Vreelandella arcis comb. nov.
Vreelandella arcis (ar’cis. L. gen. n. arcis, of a height, summit, or peak, referring to the isolation of the organism from a salt lake on the Qinghai-Tibet Plateau).
Basonym: Halomonas arcis Xu et al. 2007.
The description is as given in the proposal of the basonym (Xu et al., 2007), with the following addition. The genome size of the type strain is 4.14 Mbp. The DNA G + C content is 55.9 mol%.
Isolated from the water of a salt lake located in Altun Mountain on the Qinghai-Tibet Plateau (China).
The type strain is AJ282T = CGMCC 1.6494T = DSM 23549T = JCM 14607T = LMG 23978T.
Type strain genome sequence accession number: GCA_900103865.1.
Type strain 16S rRNA gene sequence accession number: EF144147.
Description of Vreelandella subterranea comb. nov.
Vreelandella subterranea (sub.ter.ra’ne.a. L. fem. adj. subterranea, underground, subterranean, referring to the isolation of the organism from the subterranean brines).
Basonym: Halomonas subterranea Xu et al. 2007.
The description is as given in the proposal of the basonym (Xu et al., 2007), with the following addition. The genome size of the type strain is 3.73 Mbp. The DNA G + C content is 58.0 mol%.
Isolated from water of a subterranean saline well at Si-Chuan Basin (China).
The type strain is ZG16T = CIP 109673T = CGMCC 1.6495T = DSM 23550T = JCM 14608T = LMG 23977T.
Type strain genome sequence accession number: GCA_900111305.1.
Type strain 16S rRNA gene sequence accession number: EF144148.
Description of Vreelandella alkaliphila comb. nov.
Vreelandella alkaliphila (al.ka.li’phi.la. N.L. neut. n. alkali, the ashes of saltwort [al-qaliy]; N.L. masc. adj. philus [from Gr. masc. adj. philos], friend, loving; N.L. fem. adj. alkaliphila, loving alkaline media).
Basonym: Halomonas alkaliphila Romano et al. 2007.
Cells are Gram-stain-negative rods, 0.3–0.7 × 0.5–2.6 μm in size, aerobic, and motile or non-motile. Produces exopolysaccharides and accumulates β-polyhydroxybutyrate, ectoine, and glycine-betaine. Catalase and oxidase are positive. Colonies are circular, wet, convex, and beige or cream-colored. Moderately halophilic, growing at 0–25% (w/v) NaCl, with optimal growth at 4–10% (w/v) NaCl. Mesophilic, thriving at 5–50°C, showing optimal growth at 30–37°C. Alkaliphilic or alkalitolerant, growing at pH values in the range of 5.0–12.0, with optimal growth at pH 7.5–9.0. Chemo-organotrophic. Positive for nitrate reduction to nitrite and tyrosine decomposition. Negative for H2S production. Hippurate and pectin are hydrolyzed, but casein, gelatin, phenylalanine, starch, Tween 80, and tyrosine are not. Variable for urease production. Utilizes D-glucose, D-galactose, D-fructose, sucrose, D-maltose, D-cellobiose, D-trehalose, glycerol, and acetate as sole carbon and energy sources. The major respiratory quinones are Q9, Q8, and Q6. The major fatty acids are C18:1ω6c/C18:1ω7c, C16:0, C16:1ω6c/C16:1ω7c, and C18:0. The major polar lipids are phosphatidylethanolamine, diphosphatidylglycerol, and phosphatidylglycerol.
The DNA G + C content is 52.5–52.6 mol%.
The type strain is 18bAGT = ATCC BAA-953T = DSM 16354T. The genome size of the type strain is 4.10 Mbp, and its DNA G + C content is 52.6 mol%. Isolated from a salt pool in Campania (Italy).
Type strain genome sequence accession number: GCA_016107625.1.
Type strain 16S rRNA gene sequence accession number: AJ640133.
Description of Vreelandella zhanjiangensis comb. nov.
Vreelandella zhanjiangensis (zhan.ji.ang.en’sis. N.L. fem. adj. zhanjiangensis, pertaining to Zhanjiang, a city in China near where the sample was collected).
Basonym: Halomonas zhanjiangensis Chen et al., 2009.
The description is as given in the proposal of the basonym (Chen et al., 2009), with the following addition. The genome size of the type strain is 4.06 Mbp. The DNA G + C content is 54.5 mol%.
Isolated from a sea urchin, Hemicentrotus pulcherrimus, South China Sea, tidal flat of Naozhou Island near Zhanjiang (China).
The type strain is JSM 078169T = CCTCC AB 208031T = DSM 21076T = KCTC 22279T.
Type strain genome sequence accession number: GCA_000377665.1.
Type strain 16S rRNA gene sequence accession number: FJ429198.
Description of Vreelandella stevensii comb. nov.
Vreelandella stevensii (ste.ven’si.i. N.L. gen. n. stevensii, of Stevens, named after Dr. David A. Stevens, a physician/epidemiologist who isolated and characterized the first strains).
Basonym: Halomonas stevensii Kim et al. 2010.
The description is as given in the proposal of the basonym (Kim K. K. et al., 2010), with the following addition. The genome size of the type strain is 3.69 Mbp. The DNA G + C content is 60.3 mol%.
Isolated from the blood of a renal care patient at California, San Jose, Santa Clara Valley Medical Center (United States).
The type strain is S18214T = DSM 21198T = KCTC 22148T.
Type strain genome sequence accession number: GCA_000275725.1.
Type strain 16S rRNA gene sequence accession number: AM941388.
Description of Vreelandella hamiltonii comb. nov.
Vreelandella hamiltonii (ha.mil.to’ni.i. N.L. gen. n. hamiltonii, of Hamilton, named after Dr. John R. Hamilton, a microbiologist who isolated and characterized the first strains).
Basonym: Halomonas hamiltonii Kim et al. 2010.
The description is as given in the proposal of the basonym (Kim K. K. et al., 2010), with the following addition. Cells are 0.7–1.0 × 1.5–4.0 μm in size and motile with lateral or lateral/polar flagella. Optimal growth occurs at sea-salt concentrations of 0–7.5%, w/v. Grown on cetrimide agar. Nitrate reduction to nitrite, the Voges–Proskauer test, and urease are variable. Utilization of adipate, D-galactose, malonate, L-lysine, DL-isoleucine, and L-valine as sole sources of carbon (nitrogen) and energy is variable. Acid production from L-arabinose, D-fucose, D-galactose, methyl α-D-glucoside, glycerol, D-mannitol, and melezitose is variable. Susceptibility to neomycin, penicillin G, and chloramphenicol is strain-dependent. The DNA G + C content is 60.1 mol%.
The type strain is W1025T = DSM 21196T = KCTC 22154T. The genome size of the type strain is 3.93 Mbp, and its DNA G + C content is 60.1 mol%. Isolated from the blood of a dialysis machine drain at California, San Jose, Santa Clara Valley Medical Center (USA).
Type strain genome sequence accession number: GCA_014651775.1.
Type strain 16S rRNA gene sequence accession number: AM941396.
Halomonas johnsoniae should be regarded as a heterotypic synonym of Vreelandella hamiltonii.
Description of Vreelandella andesensis comb. nov.
Vreelandella andesensis (an.de.sen’sis. N.L. fem. adj. andesensis, pertaining to the Andes).
Basonym: Halomonas andesensis Guzmán et al. 2010.
The description is as given in the proposal of the basonym (Guzmán et al., 2010), with the following addition. The genome size of the type strain is 3.91 Mbp. The DNA G + C content is 52.1 mol%.
Isolated from water from saline lake Laguna Colorada (22° 12’ S 67° 49’ W), 4,300 m above sea level (Bolivia).
The type strain is LC6T = CCUG 54844T = DSM 19434T = LMG 24243T.
Type strain genome sequence accession number: GCA_003989795.1.
Type strain 16S rRNA gene sequence accession number: EF622233.
Description of Vreelandella titanicae comb. nov.
Vreelandella titanicae (ti.tan’ic.ae. N.L. fem. n. titanica, the ship Titanic; N.L. gen. fem. n. titanicae, of or from the ship Titanic).
Basonym: Halomonas titanicae Mann et al. 2010.
The description is as given in the proposal of the basonym (Sánchez-Porro et al., 2010), with the following addition. The genome size of the type strain is 5.34 Mbp. The DNA G + C content is 54.6 mol%.
Isolated from the rusticles of the RMS Titanic wreck.
The type strain is BH1T = ATCC BAA-1257T = CECT 7585T = DSM 22872T = JCM 16411T = LMG 25388T.
Type strain genome sequence accession number: GCA_000336575.1.
Type strain 16S rRNA gene sequence accession number: FN433898.
Description of Vreelandella vilamensis comb. nov.
Vreelandella vilamensis (vi.la.men’sis. N.L. fem. adj. vilamensis, pertaining to Laguna Vilama, Jujuy, Argentina).
Basonym: Halomonas vilamensis Menes et al. 2011.
The description is as given in the proposal of the basonym (Menes et al., 2011), with the following addition. The genome size of the type strain is 3.47 Mbp. The DNA G + C content is 55.2 mol%.
Isolated from the sediment of hypersaline lake Laguna Vilama (22° 35’ S 66° 55’ W, 4,600 m above sea level) at Andean Puna desert, Jujuy (Argentina).
The type strain is SV325T = DSM 21020T = LMG 24332T.
Type strain genome sequence accession number: GCA_031451755.1.
Type strain 16S rRNA gene sequence accession number: EU557315.
Description of Vreelandella jeotgali comb. nov.
Vreelandella jeotgali (je.ot.ga’li. N.L. gen. n. jeotgali, of jeotgal, a traditional Korean fermented seafood).
Basonym: Halomonas jeotgali Kim et al. 2011.
The description is as given in the proposal of the basonym (Kim M.-S. et al., 2010), with the following addition. The genome size of the type strain is 2.85 Mbp. The DNA G + C content is 62.9 mol%.
Isolated from jeotgal, a traditional Korean fermented seafood.
The type strain is HwaT = JCM 15645T = KCTC 22487T.
Type strain genome sequence accession number: GCA_000334215.1.
Type strain 16S rRNA gene sequence accession number: EU909458.
Description of Vreelandella nanhaiensis comb. nov.
Vreelandella nanhaiensis (nan.hai.en’sis. N.L. fem. adj. nanhaiensis, pertaining to Nanhai, a sea in South China where the sample was collected).
Basonym: Halomonas nanhaiensis Long et al. 2013.
The description is as given in the proposal of the basonym (Long et al., 2013), with the following addition. The genome size of the type strain is 4.03 Mbp. The DNA G + C content is 54.4 mol%.
Isolated from a sample of marine sediment at a depth of 310 m (74°52′35” S 163°53′03″ E), South China Sea.
The type strain is YIM M 13059T = CCTCC AB 2012911T = DSM 25561T = JCM 18142T.
Type strain genome sequence accession number: GCA_003990185.1.
Type strain 16S rRNA gene sequence accession number: JX870002.
Description of Vreelandella olivaria comb. nov.
Vreelandella olivaria (o.li.va’ri.a. L. fem. adj. olivaria, of or belonging to olives, related to olive-processing effluents from where the type strain was isolated).
Basonym: Halomonas olivaria Amouric et al. 2014.
The description is as given in the proposal of the basonym (Amouric et al., 2014), with the following addition. The genome size of the type strain is 5.00 Mbp. The DNA G + C content is 55.3 mol%.
Isolated from salted olive-processing effluents from an evaporation pond (Morocco).
The type strain is TYRC17T = CCUG 53850 BT = DSM 19074T.
Type strain genome sequence accession number: GCA_004295565.1.
Type strain 16S rRNA gene sequence accession number: DQ645593.
Description of Vreelandella songnenensis comb. nov.
Vreelandella songnenensis (song.nen.en’sis. N.L. fem. adj. songnenensis, pertaining to Songnen Plain, north-east China, where the type strain was isolated).
Basonym: Halomonas songnenensis Jiang et al. 2014.
The description is as given in the proposal of the basonym (Jiang et al., 2014), with the following addition. The genome size of the type strain is 3.69 Mbp. The DNA G + C content is 59.1 mol%.
Isolated from saline and alkaline soil in an oilfield (46° 36′ 05.36” N 124° 55′ 00.36″ E), Songnen Plain (China).
The type strain is NEAU-ST10-39T = CGMCC 1.12152T = DSM 25870T.
Type strain genome sequence accession number: GCA_003002925.1.
Type strain 16S rRNA gene sequence accession number: JQ762289.
Description of Vreelandella salicampi comb. nov.
Vreelandella salicampi (sa.li.cam’pi. L. masc. n. sal, salt; L. masc. n. campus, field; N.L. gen. n. salicampi, of a salt field).
Basonym: Halomonas salicampi Lee et al. 2015.
The description is as given in the proposal of the basonym (Lee et al., 2015), with the following addition. The genome size of the type strain is 3.86 Mbp. The DNA G + C content is 56.2 mol%.
Isolated from a saltern soil at Gomso (Korea).
The type strain is BH103T = KACC 17609T = NBRC 109914T = NCAIM B 02528T.
Type strain genome sequence accession number: GCA_013415105.1.
Type strain 16S rRNA gene sequence accession number: KF963827.
Description of Vreelandella lutescens comb. nov.
Vreelandella lutescens (lu.tes’cens. L. part. adj. lutescens, becoming muddy, related to the muddy color of the mature colony).
Basonym: Halomonas lutescens Wang et al. 2016.
The description is as given in the proposal of the basonym (Wang et al., 2016), with the following addition. The genome size of the type strain is 3.70 Mbp. The DNA G + C content is 56.0 mol%.
Isolated from a sediment sample from Qinghai Lake (China).
The type strain is Q1UT = CGMCC 1.15122T = KCTC 42517T.
Type strain genome sequence accession number: GCA_014640815.1.
Type strain 16S rRNA gene sequence accession number: KP259554.
Description of Vreelandella nigrificans comb. nov.
Vreelandella nigrificans (nig.rif’i.cans. L. part. adj. nigrificans, making black).
Basonym: Halomonas nigrificans Oguntoyinbo et al. 2018.
The description is as given in the proposal of the basonym (Oguntoyinbo et al., 2018), with the following addition. The genome size of the type strain is 4.93 Mbp. The DNA G + C content is 52.8 mol%.
Isolated from cheese (Germany).
The type strain is MBT G8648T = DSM 105749T = LMG 29097T.
Type strain genome sequence accession number: GCA_002374315.1.
Type strain 16S rRNA gene sequence accession number: MG030686.
Description of Vreelandella malpeensis comb. nov.
Vreelandella malpeensis (mal.pe.en’sis. N.L. fem. adj. malpeensis, of or belonging to Malpe, a coastal town in Udupi City, Karnataka, India).
Basonym: Halomonas malpeensis Kämpfer et al. 2018.
The description is as given in the proposal of the basonym (Kämpfer et al., 2018), with the following addition. The genome size of the type strain is 3.61 Mbp. The DNA G + C content is 63.8 mol%.
Isolated from the rhizosphere of sand dune coastal plant, Coast of Malpe (India).
The type strain is YU-PRIM-29T = CCM 8737T = LMG 28855T.
Type strain Genome sequence accession number: GCA_020622355.1.
Type strain 16S rRNA gene sequence accession number: JQ730736.
Description of Vreelandella piezotolerans comb. nov.
Vreelandella piezotolerans (pie.zo.to’le.rans. Gr. v. piezô, to press; L. pres. part. tolerans, tolerating; N.L. part. adj. piezotolerans, pressure-tolerating).
Basonym: Halomonas piezotolerans Yan et al. 2020.
The description is as given in the proposal of the basonym (Yan et al., 2020), with the following addition. The genome size of the type strain is 3.95 Mbp. The DNA G + C content is 57.9 mol%.
Isolated from a deep-sea sediment sample of the New Britain Trench.
The type strain is NBT06E8T = KCTC 72680T = MCCC 1K04228T.
Type strain genome sequence accession numbers: GCA_012427705.1 and GCA_009660035.1.
Type strain 16S rRNA gene sequence accession number: MN435603.
Description of Vreelandella rituensis comb. nov.
Vreelandella rituensis (ri.tu.en’sis. N.L. fem. adj. rituensis, pertaining to Ritu, Tibet, where the type strain was isolated).
Basonym: Halomonas rituensis Gao et al. 2020.
The description is as given in the proposal of the basonym (Gao et al., 2020), with the following addition. The genome size of the type strain is 4.47 Mbp. The DNA G + C content is 57.2 mol%.
Isolated from a salt marsh sediment of a saline lake, Dongqian Lake, (33°31′ 51.06″N 80°14′13.64″E), Tibetan Plateau (China).
The type strain is TQ8ST = CICC 24572T = KCTC 62530T.
Type strain genome sequence accession number: GCA_003336665.1.
Type strain 16S rRNA gene sequence accession number: MH071181.
Description of Vreelandella zhuhanensis comb. nov.
Vreelandella zhuhanensis (zhu.han.en’sis. N.L. fem. adj. zhuhanensis, pertaining to Zhuhan marsh on the Tibetan Plateau, where the type strain was isolated).
Basonym: Halomonas zhuhanensis Gao et al. 2020.
The description is as given in the proposal of the basonym (Gao et al., 2020), with the following addition. The genome size of the type strain is 3.25 Mbp. The DNA G + C content is 57.1 mol%.
Isolated from a saline lake, Zhuhan Lake, (33°32′50.89″N 80°09′38.51″E), Tibetan Plateau (China).
The type strain is ZH2ST = CICC 24505T = KCTC 62531T.
Type strain genome sequence accession number: GCA_009793355.1.
Type strain 16S rRNA gene sequence accession number: MH071182.
Description of Vreelandella azerica comb. nov.
Vreelandella azerica (a.ze’ri.ca. N.L. fem. adj. azerica, pertaining to Azerbaijan, where the Urmia Lake is located and the type strain was isolated).
Basonym: Halomonas azerica Wenting et al. 2021.
The description is as given in the proposal of the basonym (Wenting et al., 2021), with the following addition. The genome size of the type strain is 3.42 Mbp. The DNA G + C content is 55.4 mol%.
Isolated from Urmia Lake (Iran).
The type strain is TBZ9T = KACC 21783T = LMG 25775T.
Type strain genome sequence accession number: GCA_013112225.1.
Type strain 16S rRNA gene sequence accession number: MN900573.
Description of Vreelandella profundi comb. nov.
Vreelandella profundi (pro.fun’di. L. gen. n. profundi, of the depth of the sea).
Basonym: Halomonas profundi Wang et al. 2022.
The description is as given in the proposal of the basonym (Wang et al., 2022), with the following addition. The genome size of the type strain is 3.60 Mbp. The DNA G + C content is 54.0 mol%.
Isolated from the deep-sea sediment of the Mariana Trench (11.12°N, 142.32°E).
The type strain is MT13T = KCTC 82923T = MCCC 1K06389T.
Type strain genome sequence accession number: GCA_019504685.1.
Type strain 16S rRNA gene sequence accession number: MZ411491.
Description of Vreelandella populi comb. nov.
Vreelandella populi (po’pu.li. L. gen. n. populi, of the genus Populus, referring to Populus euphratica).
Basonym: Halomonas populi Xu et al. 2021.
The description is as given in the proposal of the basonym (Xu et al., 2021), with the following addition. The genome size of the type strain is 3.80 Mbp. The DNA G + C content is 55.0 mol%.
Isolated from Populus euphratica in Ebinur Lake Wetland Nature Reserve (China).
The type strain is MCT = MCCC 1K03942T = JCM 33545T.
Type strain genome sequence accession number: GCA_003989825.1.
Type strain 16S rRNA gene sequence accession number: MK045667.
Description of Vreelandella glaciei sp. nov.
Vreelandella glaciei (gla.ci.e’i. L. gen. n. glaciei, meaning of the cold).
The description is as given in the original proposal of “Halomonas glaciei” (Reddy et al., 2003), with the following addition. The genome size of the type strain is 4.96 Mbp. The DNA G + C content is 54.4 mol%.
Isolated from the fast ice of Adelie Land, Antarctica.
The type strain is DD 39T = CGMCC 1.7263T = JCM 11692T = MTCC 4321T.
Type strain genome sequence accession number: GCA_013415125.1.
Type strain 16S rRNA gene sequence accession number: AJ431369.
Description of Vreelandella zhaodongensis sp. nov.
Vreelandella zhaodongensis (zhao.dong.en’sis. N.L. fem. adj. zhaodongensis, pertaining to Zhaodong City, North East of China, where the strain was isolated).
The description is as given in the original proposal of “Halomonas zhaodongensis” (Jiang et al., 2013), with the following addition. The genome size of the type strain is 3.72 Mbp. The DNA G + C content is 53.0 mol%.
Isolated from saline-alkaline soils in Zhaodong (China).
The type strain is NEAU-ST10-25T = CGMCC 1.12286T = DSM 25869T.
Type strain genome sequence accession number: GCA_013415115.1.
Type strain 16S rRNA gene sequence accession number: JQ762286.
Description of Vreelandella lionensis sp. nov.
Vreelandella lionensis (li.on.en’sis. N.L. fem. adj. lionensis, of or belonging to Golfe du Lion [Gulf of Lions], in reference to the origin of the type strain).
The description is as given in the original proposal of “Halomonas lionensis” (Gaboyer et al., 2014), with the following addition. The genome size of the type strain is 3.65 Mbp. The DNA G + C content is 55.9 mol%.
Isolated from the Mediterranean Sea sediment, Gulf of Lions (France).
The type strain is RHS90T = CIP 110370T = DSM 25632T = UBOCC 3186T.
Type strain genome sequence accession number: GCA_002087295.1.
Type strain 16S rRNA gene sequence accession number: HE661586.
Description of Vreelandella massiliensis sp. nov.
Vreelandella massiliensis (mas.si.li.en’sis. L. fem. adj. massiliensis, of Massilia, the old Roman name for Marseille, where the strain was isolated).
The description is as given in the original proposal of “Halomonas massiliensis” (Seck et al., 2016), with the following addition. The genome size of the type strain is 3.44 Mbp. The DNA G + C content is 58.4 mol%.
Isolated from the human gut (France).
The type strain is Marseille-P2426T = CSUR P2426T = DSM 103116T.
Type strain genome sequence accession number: GCA_900155385.1.
Type strain 16S rRNA gene sequence accession number: LT223576.
Description of Vreelandella maris sp. nov.
Vreelandella maris (ma’ris. L. gen. n. maris, of the sea).
The description is as given in the original proposal of “Halomonas maris” (Qiu et al., 2021a), with the following addition. The genome size of the type strain is 4.52 Mbp. The DNA G + C content is 54.4 mol%.
Isolated from the deep-sea sediment in the Southwest Indian Ocean (China).
The type strain is QX-1T = KCTC 82198T = MCCC 1A17875T = NBRC 114670T.
Type strain genome sequence accession number: GCA_013371085.1.
Type strain 16S rRNA gene sequence accession number: MT372903.
Description of Vreelandella sedimenti sp. nov.
Vreelandella sedimenti (se.di.men’ti. L. gen. n. sedimenti, of sediment, referring to the sediment of the Southwest Indian Ocean, where the type strain was isolated).
The description is as given in the original proposal of “Halomonas sedimenti” (Qiu et al., 2021b), with the following addition. The genome size of the type strain is 5.06 Mbp. The DNA G + C content is 54.3 mol%.
Isolated from the deep-sea sediment in the Southwest Indian Ocean (China).
The type strain is QX-2T = KCTC 82199T = MCCC 1A17876T.
Type strain genome sequence accession number: GCA_013416325.1.
Type strain 16S rRNA gene sequence accession number: MT372904.
Description of Bisbaumannia gen. nov.
Bisbaumannia (Bis.bau.mann’i.a. L. adv. bis, twice; N.L. fem. n. Bisbaumannia, referring to both microbiologist Linda Baumann and Paul Baumann, who first studied these microorganisms).
Cells are Gram-staining-negative straight rods, 0.8–1.1 × 1.5–3.0 μm in size, aerobic, and motile by means of peritrichous flagella. Endospores are not formed. Oxidase-positive. Colonies are convex with entire edges and cream-colored. Na+ is required for growth. Mesophilic. Chemo-organotrophic. Nitrate reduction is negative. The major respiratory quinone is Q9. The major fatty acids are C18:1ω7c, C19:0 cyclo ω8c, C16:0, and C16:1ω7c. The major polar lipids are phosphatidylglycerol, diphosphatidylglycerol, and phosphatidylethanolamine.
The DNA G + C content is 67.2 mol%.
The genus Bisbaumannia belongs to the family Halomonadaceae. The type species is Bisbaumannia pacifica.
Description of Bisbaumannia pacifica comb. nov.
Bisbaumannia pacifica (pa.ci’fi.ca. L. fem. adj. pacifica, peaceful, pertaining to the Pacific Ocean).
Basonym: Alcaligenes pacificus corrig. Baumann et al. 1972 (Approved Lists 1980).
Homotypic synomyms: Halomonas pacifica (Baumann et al. 1972) Dobson and Franzmann 1996; Deleya pacifica (Baumann et al. 1972) Baumann et al. 1983.
The description is as given in the original proposal of the basonym (Baumann et al., 1972), with the following addition. The genome size of the type strain is 3.85 Mbp. The DNA G + C content is 67.2 mol%.
Isolated from the seawater off the coast of Oahu (Hawaii, United States), Pacific Ocean.
The type strain is 62T = ATCC 27122T = CIP 103200T = DSM 4742T = JCM 20633T = LMG 3446T = NBRC 102220T = NCIMB 1977T.
Type strain genome sequence accession number: GCA_007989625.1.
Type strain 16S rRNA gene sequence accession number: AB681734.
Description of Billgrantia gen. nov.
Billgrantia (Bill.grant’i.a. N.L. fem. n. Billgrantia, named after the microbiologist William [Bill] D. Grant for his great contribution to the study of halophilic microorganisms).
Cells are Gram-staining-negative rods, 0.3–1.1 × 0.8–6.0 μm in size, aerobic or facultatively anaerobic, and mostly motile. Endospores are not formed. Catalase and oxidase are positive for most of the strains. Colonies are brown, cream, light beige, pinkish white, white, or yellow pigmented. Slightly to moderately halophilic, growing at 0–26% (w/v) NaCl, with optimal growth at 1–15% (w/v) NaCl. Mesophilic, thriving at 4–55°C, showing optimal growth at 25–42°C. Alkaliphilic or alkalitolerant, growing at pH values in the range of 5.0–12.0, with optimal growth at pH 7.0–10.0. Chemo-organotrophic. Nitrate reduction is mostly positive. The major respiratory quinones are Q9 and Q8. The major fatty acids are C18:1ω6c/C18:1ω7c, C16:0, C16:1ω6c/C16:1ω7c/iso-C15:0 2-OH, C19:0 cyclo ω8c, C12:0 3-OH, C16:1ω9c, and C17:1ω9c. The major polar lipids are diphosphatidylglycerol, phosphatidylglycerol, and phosphatidylethanolamine.
The DNA G + C content ranges between 62.1 and 67.5 mol%.
The genus Billgrantia belongs to the family Halomonadaceae. The type species is Billgrantia desiderata.
Description of Billgrantia desiderata comb. nov.
Billgrantia desiderata (de.si.de.ra’ta. L. fem. adj. desiderata, wished for, the strain wished for).
Basonym: Halomonas desiderata Berendes et al. 1997.
The description is as given in the original proposal of the basonym (Berendes et al., 1996), with the following addition. The DNA G + C content is 64.7–64.9 mol%.
The type strain is FB2T = CIP 105505T = DSM 9502T = LMG 19548T. The genome size of the type strain is 4.89 Mbp, and its DNA G + C content is 64.7 mol%. Isolated from a municipal sewage treatment plant in Göttingen (Germany).
Type strain genome sequence accession number: GCA_011742915.1.
Type strain 16S rRNA gene sequence accession number: X92417.
Description of Billgrantia campisalis comb. nov.
Billgrantia campisalis (cam.pi.sa’lis. L. masc. n. campus, field, plain; L. masc. n. sal, salt; N.L. gen. n. campisalis, of the plain of salt, of the salt plain).
Basonym: Halomonas campisalis Mormile et al. 2000.
The description is as given in the original proposal of the basonym (Mormile et al., 1999), with the following addition. The genome size of the type strain is 4.27 Mbp. The DNA G + C content is 66.3 mol%.
Isolated from a soil sample collected from a salt flat south of Alkali Lake, Washington State (USA).
The type strain is 4AT = ATCC 700597T = CIP 106639T = DSM 15413T.
Type strain genome sequence accession numbers: GCA_031451595.1 and GCA_022341425.1.
Type strain 16S rRNA gene sequence accession number: AF054286.
Description of Billgrantia gudaonensis comb. nov.
Billgrantia gudaonensis (gu.dao.nen’sis. N.L. fem. adj. gudaonensis, pertaining to Gudao, in the Shengli oilfield, PR China, where the type strain was isolated).
Basonym: Halomonas gudaonensis Wang et al. 2007.
The description is as given in the original proposal of the basonym (Wang et al., 2007), with the following addition. The genome size of the type strain is 4.17 Mbp. The DNA G + C content is 64.9 mol%.
Isolated from saline soil contaminated by crude oil, Gudao (China).
The type strain is SL014B-69T = CGMCC 1.6133T = DSM 23417T = LMG 23610T.
Type strain genome sequence accession number: GCA_900100195.1.
Type strain 16S rRNA gene sequence accession number: DQ421808.
Description of Billgrantia kenyensis comb. nov.
Billgrantia kenyensis (ke.ny.en’sis. N.L. fem. adj. kenyensis, Kenyan, of Kenya, the region of isolation).
Basonym: Halomonas kenyensis Boltyanskaya et al. 2008.
The description is as given in the original proposal of the basonym (Boltyanskaya et al., 2007), with the following addition. The genome size of the type strain is 4.42 Mbp. The DNA G + C content is 63.8 mol%.
Isolated from sediments from soda lakes (Kenya).
The type strain is AIR-2T = DSM 17331T = VKM B-2354T.
Type strain genome sequence accession number: GCA_013697085.1.
Type strain 16S rRNA gene sequence accession number: AY962237.
Description of Billgrantia saliphila comb. nov.
Billgrantia saliphila (sa.li’phi.la. L. masc. n. sal, salt; Gr. masc. adj. philos, loving; N.L. fem. adj. saliphila, salt-loving).
Basonym: Halomonas saliphila Gan et al. 2018.
The description is as given in the original proposal of the basonym (Gan et al., 2018), with the following addition. The genome size of the type strain is 4.34 Mbp. The DNA G + C content is 64.1 mol%.
Isolated from saline soil (China).
The type strain is LCB169T = CGMCC 1.15818T = KCTC 52618T.
Type strain genome sequence accession number: GCA_002930105.1.
Type strain 16S rRNA gene sequence accession number: KX008964.
Description of Billgrantia endophytica comb. nov.
Billgrantia endophytica (en.do.phy’ti.ca. Gr. pref. endo-, within; Gr. neut. n. phyton, plant; L. fem. adj. suff. -ica, adjectival suffix used with the sense of belonging to; N.L. fem. adj. endophytica, within plant, pertaining to the endophytic nature of the strain and its isolation from internal plant tissues).
Basonym: Halomonas endophytica Chen et al. 2018.
The description is as given in the original proposal of the basonym (Chen et al., 2018), with the following addition. The genome size of the type strain is 4.98 Mbp. The DNA G + C content is 62.1 mol%.
Isolated from liquid in the stems of Populus euphratica in Xinjiang (China).
The type strain is MC28T = KCTC 52999T = MCCC 1K03343T.
Type strain genome sequence accession number: GCA_002879615.1.
Type strain 16S rRNA gene sequence accession number: MF850257.
Description of Billgrantia montanilacus comb. nov.
Billgrantia montanilacus (mon.ta.ni.la’cus. L. masc. adj. montanus, a mountain; L. masc. n. lacus, lake; N.L. gen. n. montanilacus, of a mountain lake).
Basonym: Halomonas montanilacus Lu et al. 2020.
The description is as given in the original proposal of the basonym (Lu et al., 2020), with the following addition. The genome size of the type strain is 4.79 Mbp. The DNA G + C content is 62.9 mol%.
Isolated from hypersaline Lake Pengyanco on the Tibetan Plateau (China).
The type strain is PYC7WT = CICC 24506T = KCTC 62529T.
Type strain genome sequence accession number: GCA_003336675.1.
Type strain 16S rRNA gene sequence accession number: MH071180.
Description of Billgrantia lactosivorans comb. nov.
Billgrantia lactosivorans (lac.to.si.vo’rans. L. neut. adj. lactosum, lactose; L. pres. part. vorans, eating; N.L. part. adj. lactosivorans, eating lactose).
Basonym: Halomonas lactosivorans Ming et al. 2020.
The description is as given in the original proposal of the basonym (Ming et al., 2020), with the following addition. The genome size of the type strain is 4.36 Mbp. The DNA G + C content is 66.7 mol%.
Isolated from salt-lake sediment in Shanxi Province (China).
The type strain is CFH 90008T = DSM 103220T = KCTC 52281T.
Type strain genome sequence accession number: GCA_003254665.1.
Type strain 16S rRNA gene sequence accession number: KY039330.
Description of Billgrantia pellis comb. nov.
Billgrantia pellis (pel’lis. L. gen. n. pellis, of a hide, indicating the source of the type strain).
Basonym: Halomonas pellis Li et al. 2020.
The description is as given in the original proposal of the basonym (Li et al., 2020), with the following addition. The genome size of the type strain is 4.35 Mbp. The DNA G + C content is 63.6 mol%.
Isolated from wet salted hides (China).
The type strain is L5T = CGMCC 1.17335T = KCTC 72573T.
Type strain genome sequence accession number: GCA_008297955.1.
Type strain 16S rRNA gene sequence accession number: MN099429.
Description of Billgrantia azerbaijanica comb. nov.
Billgrantia azerbaijanica (a.zer.bai.ja’ni.ca. N.L. fem. adj. azerbaijanica, pertaining to Azerbaijan, a region in the north-west of Iran, where Urmia Lake is located and from which the type strain was isolated).
Basonym: Halomonas azerbaijanica Kazemi et al. 2021.
The description is as given in the original proposal of the basonym (Kazemi et al., 2021), with the following addition. The genome size of the type strain is 4.58 Mbp. The DNA G + C content is 67.5 mol%.
Isolated from water from Urmia Lake (Iran).
The type strain is TBZ202T = CECT 9693T = KCTC 62817T.
Type strain genome sequence accession number: GCA_004551485.1.
Type strain 16S rRNA gene sequence accession number: MK138622.
Description of Billgrantia diversa comb. nov.
Billgrantia diversa (di.ver’sa. L. fem. part. adj. diversa, different, distinct).
Basonym: Halomonas diversa Wang et al. 2021.
The description is as given in the original proposal of the basonym (Wang et al., 2021), with the following addition. The genome size of the type strain is 4.49 Mbp. The DNA G + C content is 62.9 mol%.
Isolated from the deep-sea sediment of the Pacific Ocean.
The type strain is D167-6-1T = KCTC 72441T = MCCC 1A13316T.
Type strain genome sequence accession number: GCA_014931605.1.
Type strain 16S rRNA gene sequence accession number: MW172430.
Description of Billgrantia bachuensis comb. nov.
Billgrantia bachuensis (ba.chu.en’sis. N.L. fem. adj. bachuensis, pertaining to Bachu, north-western China, where the strain was isolated).
Basonym: Halomonas bachuensis Xiao et al. 2021.
The description is as given in the original proposal of the basonym (Xiao et al., 2021), with the following addition. The genome size of the type strain is 4.70 Mbp. The DNA G + C content is 63.6 mol%.
Isolated from Gobi soil, Bachu (China).
The type strain is DX6T = CCTCC AB 2020094T = KCTC 82196T.
Type strain genome sequence accession number: GCA_011742165.1.
Type strain 16S rRNA gene sequence accession number: MT180568.
Description of Billgrantia antri comb. nov.
Billgrantia antri (an’tri. L. gen. n. antri, of a cave, referring to the location of the isolate).
Basonym: Halomonas antri So et al. 2022.
The description is as given in the original proposal of the basonym (So et al., 2022), with the following addition. Cells are Gram-stain-negative rods, 0.6–0.9 × 1.6–2.4 μm in size, strictly aerobic or facultatively anaerobic, and motile by means of one polar flagellum or peritrichous flagella. Catalase and oxidase activities are variable. Slightly to moderately halophilic, growing at 0–20% (w/v) NaCl, with optimal growth at 1–8% (w/v) NaCl. Mesophilic, thriving at 4–55°C, showing optimal growth at 25–40°C. Alkalitolerant, growing at pH values in the range of 6.0–10.0, with optimal growth at pH 7.0–8.0. Voges–Proskauer test is variable. Starch is hydrolyzed, but Tween 40 and Tween 60 are not. Hydrolysis of DNA and urea is variable. Esterase lipase (C8), lipase (C14), cystine arylamidase, α-glucosidase, and tryptophan deaminase activities are variable. Utilization of N-acetyl-glucosamine, potassium gluconate, adipate, and citrate is variable. The major respiratory quinone is Q9. The major fatty acids are C18:1ω6c/C18:1ω7c, C16:0, C16:1ω6c/C16:1ω7c, C19:0 cyclo ω8c, and C12:0 3-OH. The major polar lipids are diphosphatidylglycerol, phosphatidylglycerol, and phosphatidylethanolamine.
The DNA G + C content is 64.2–64.3 mol%.
The type strain is Y3S6T = KACC 21536T = NBRC 114315T = TBRC 15164T. The genome size of the type strain is 4.39 Mbp, and its DNA G + C content is 64.3 mol%. Isolated from surface seawater, Busan (Korea).
Type strain genome sequence accession number: GCA_019430905.1.
Type strain 16S rRNA gene sequence accession number: MN625868.
Description of Billgrantia chromatireducens sp. nov.
Billgrantia chromatireducens (chro.ma.ti.re.du’cens. N.L. masc. n. chromas, chromate; L. pres. part. reducens, converting to a different state; N.L. part. adj. chromatireducens, reducing chromate).
The description is as given in the original proposal of “Halomonas chromatireducens” (Shapovalova et al., 2009), with the following addition. The genome size of the type strain is 3.97 Mbp. The DNA G + C content is 62.8 mol%.
Isolated from soda salt marshes, Altai (Russia).
The type strain is AGD 8-3T = NCCB 100225T = VKM B-2497T.
Type strain genome sequence accession number: GCA_001545155.1.
Type strain 16S rRNA gene sequence accession number: EU447163.
Description of Billgrantia aerodenitrificans sp. nov.
Billgrantia aerodenitrificans (a.e.ro.de.ni.tri’fi.cans. Gr. masc. n. aêr, air; N.L. inf. v. denitrificare, to denitrify; N.L. part. adj. aerodenitrificans, denitrifying with or in air).
The description is as given in the original proposal of “Halomonas aerodenitrificans” (Wang and Shao, 2021), with the following addition. The genome size of the type strain is 5.08 Mbp. The DNA G + C content is 64.0 mol%.
Isolated from coastal water, 5 m depth, from the Taiwan Strait (China).
The type strain is CYD-9T = KCTC 72088T = MCCC 1A11058T.
Type strain genome sequence accession number: GCA_021404405.1.
Type strain 16S rRNA gene sequence accession number: MW205680.
Description of Billgrantia ethanolica sp. nov.
Billgrantia ethanolica (e.tha.no’li.ca. N.L. neut. n. ethanol, ethanol; L. fem. adj. suff. -ica, suffix used with various meanings; N.L. fem. adj. ethanolica, belonging to ethanol, in reference to the ability of the species to utilize ethanol as a substrate for growth).
The description is as given in the original proposal of “Halomonas ethanolica” (Wang and Shao, 2021), with the following addition. The genome size of the type strain is 4.57 Mbp. The DNA G + C content is 64.5 mol%.
Isolated from the sediment from shrimp culture pond, Zhangzhou (China).
The type strain is CYT3-1-1T = KCTC 72090T = MCCC 1A11081T.
Type strain genome sequence accession number: GCA_021404305.1.
Type strain 16S rRNA gene sequence accession number: MW205683.
Description of Billgrantia sulfidoxydans sp. nov.
Billgrantia sulfidoxydans (sul.fid.o’xy.dans. N.L. neut. n. sulfidum, sulfide; N.L. pres. part. oxydans, oxidizing; N.L. part. adj. sulfidoxydans, oxidizing sulfides).
The description is as given in the original proposal of “Halomonas sulfidoxydans” (Wang and Shao, 2021), with the following addition. The genome size of the type strain is 4.49 Mbp. The DNA G + C content is 66.0 mol%.
Isolated from surface sediments in the coastal sea at Taiwan Strait (China).
The type strain is CYN-1-2T = KCTC 72089T = MCCC 1A11059T.
Type strain genome sequence accession number: GCA_017868775.1.
Type strain 16S rRNA gene sequence accession number: MW205681.
Description of Billgrantia tianxiuensis sp. nov.
Billgrantia tianxiuensis (tian.xiu.en’sis. N.L. fem. adj. tianxiuensis, pertaining to the Tianxiu Hydrothermal Field, on the Northwest Indian Ridge, from where the type strain was isolated).
The description is as given in the original proposal of “Halomonas tianxiuensis” (Wang and Shao, 2021), with the following addition. The genome size of the type strain is 5.02 Mbp. The DNA G + C content is 63.9 mol%.
Isolated from sulfide from Tianxiu hydrothermal vents, 3,440 m depth, Northwest Indian Ocean.
The type strain is BC-M4-5T = KCTC 72092T = MCCC 1A14433T.
Type strain genome sequence accession number: GCA_009834345.1.
Type strain 16S rRNA gene sequence accession number: MW205685.
Description of Billgrantia zhangzhouensis sp. nov.
Billgrantia zhangzhouensis (zhang.zhou.en’sis. N.L. fem. adj. zhangzhouensis, of or pertaining to Zhangzhou, a city in Fujian, China, where the type strain was isolated).
The description is as given in the original proposal of “Halomonas zhangzhouensis” (Wang and Shao, 2021), with the following addition. The genome size of the type strain is 4.41 Mbp. The DNA G + C content is 63.3 mol%.
Isolated from the sediments from a shrimp culture pond in Zhangzhou (China).
The type strain is CXT3-11T = KCTC 72087T = MCCC 1A11036T.
Type strain genome sequence accession number: GCA_021404465.1.
Type strain 16S rRNA gene sequence accession number: MW205678.
Description of Franzmannia gen. nov.
Franzmannia (Franz.man’ni.a. N.L. fem. n. Franzmannia, in honor of Peter D. Franzmann, Australian microbiologist and polar researcher).
Cells are Gram-staining-negative rod-shaped or pleomorphic, 0.3–0.7 × 1.4–2.8 μm in size, aerobic, and motile or non-motile. Endospores are not formed. Catalase-variable and oxidase-positive. Colonies are cream, cream-pink, or light yellow pigmented. Moderately halophilic, growing at 0.5–20% (w/v) NaCl, with optimal growth at 2–10% (w/v) NaCl. Mesophilic, thriving at 10–45°C, showing optimal growth at 25–37°C. Alkalitolerant, growing at pH values in the range of 5.5–11.0, with optimal growth at pH 7.0–9.0. Chemo-organotrophic. Nitrate reduction is variable. The major respiratory quinone is Q9. The major fatty acids are C18:1ω6c/C18:1ω7c, C16:0, C19:0 cyclo ω8c, and C16:1ω6c/C16:1ω7c. The major polar lipids are phosphatidylglycerol, diphosphatidylglycerol, and phosphatidylethanolamine.
The DNA G + C content ranges between 63.8 and 64.5 mol%.
The genus Franzmannia belongs to the family Halomonadaceae. The type species is Franzmannia pantelleriensis.
Description of Franzmannia pantelleriensis comb. nov.
Franzmannia pantelleriensis (pan.tel.le.ri.en’sis. L. fem. adj. pantelleriensis, pertaining to Pantelleria Island [the place of isolation] in the south of Sicily, Italy).
Basonym: Halomonas pantelleriensis corrig Romano et al. 1997.
The description is as given in the proposal of the basonym (Romano et al., 1996), with the following addition. The genome size of the type strain is 4.40 Mbp. The DNA G + C content is 63.9 mol%.
Isolated from the hard sand of Venere Lake, Pantelleria Island (Italy).
The type strain is AAPT = ATCC 700273T = CIP 105506T = DSM 9661T = LMG 19550T.
Type strain genome sequence accession number: GCA_900102875.1.
Type strain 16S rRNA gene sequence accession number: X93493.
Description of Franzmannia qiaohouensis comb. nov.
Franzmannia qiaohouensis (qiao.hou.en’sis. N.L. fem. adj. qiaohouensis, pertaining to Qiaohou salt mine, south-west China, where the type strain was isolated).
Basonym: Halomonas qiaohouensis Wang et al. 2015.
The description is as given in the proposal of the basonym (Wang et al., 2014), with the following addition. The genome size of the type strain is 4.65 Mbp. The DNA G + C content is 64.5 mol%.
Isolated from the salt mine soil in southwest China.
The type strain is YIM QH88T = ACCC 60021T = CCTCC AB 2012965T = DSM 26770T.
Type strain genome sequence accession number: GCA_031451695.1.
Type strain 16S rRNA gene sequence accession number: KC237714.
Description of Franzmannia salipaludis sp. nov.
Franzmannia salipaludis (sa.li.pa.lu’dis. L. masc. n. sal, salt; L. fem. n. palus, swamp, marsh; N.L. gen. n. salipaludis, of a salt marsh).
The description is as given in the original proposal of “Halomonas salipaludis” (Xing et al., 2021), with the following addition. The genome size of the type strain is 5.48 Mbp. The DNA G + C content is 63.8 mol%.
Isolated from the saline-alkali wetland soil (China).
The type strain is WRN001T = ACCC 19974T = KCTC 52853T.
Type strain genome sequence accession number: GCA_002286975.1.
Type strain 16S rRNA gene sequence accession number: MF782428.
Description of Litchfieldella gen. nov.
Litchfieldella (Litch.field.el’la. N.L. fem. n. Litchfieldella, named after Dr. Carol D. Litchfield [1936–2012], in recognition of her many contributions to the study of halophilic microorganisms).
Cells are Gram-staining-negative rods, 0.4–1.0 × 1.2–4.4 μm in size, aerobic, and motile or non-motile. Endospores are not formed. Catalase-positive and oxidase-variable. Colonies are cream, yellow, brown-orange, or creamy-white pigmented. Moderately halophilic, growing at 0–23% (w/v) NaCl, with optimal growth at 5–13% (w/v) NaCl. Mesophilic, thriving at 15–50°C, showing optimal growth at 32–37°C. Alkalitolerant, growing at pH values in the range of 5.0–10.0, with optimal growth at pH 6.0–9.0. Chemo-organotrophic. Nitrate reduction is variable. The major respiratory quinone is Q9. The major fatty acids are C18:1ω7c, C16:0, C16:1ω7c/iso-C15:0 2-OH, and C19:0 cyclo ω8c.
The DNA G + C content ranges between 58.5 and 62.2 mol%.
The genus Litchfieldella belongs to the family Halomonadaceae. The type species is Litchfieldella anticariensis.
Description of Litchfieldella anticariensis comb. nov.
Litchfieldella anticariensis (an.ti.ca.ri.en’sis. L. fem. adj. anticariensis, pertaining to Antequera, originally the Roman city of Anticaria, in the province of Málaga, southern Spain, where the strains were isolated).
Basonym: Halomonas anticariensis Martínez-Cánovas et al. 2004.
The description is as given in the proposal of the basonym (Martínez-Cánovas et al., 2004), with the following addition. The genome size of the type strain is 5.07 Mbp. The DNA G + C content is 58.5 mol%.
Isolated from a soil sample from Fuente de Piedra, a saline-wetland wildfowl reserve in Málaga (Spain).
The type strain is FP35T = CECT 5854T = CIP 108499T = DSM 16096T = LMG 22089T.
Type strain genome sequence accession numbers: GCA_000409775.1 and GCA_000428505.1.
Type strain 16S rRNA gene sequence accession number: AY489405.
Description of Litchfieldella xinjiangensis comb. nov.
Litchfieldella xinjiangensis (xin.ji.ang.en’sis. N.L. fem. adj. xinjiangensis, pertaining to Xinjiang, a region of China, from where the type strain was isolated).
Basonym: Halomonas xinjiangensis Guan et al. 2010.
The description is as given previously (Guan et al., 2010; Navarro-Torre et al., 2020), with the following addition. The genome size of the type strain is 3.79 Mbp. The DNA G + C content is 60.7 mol%.
Isolated from a soil sample from Lop Nur salt lake (4° 23′ N 9° 18′ E, 778 m altitude) in Xinjiang Province, north-west China.
The type strain is TRM 0175T = CCTCC AB 208329T = KCTC 22608T.
Type strain genome sequence accession number: GCA_000759345.1.
Type strain 16S rRNA gene sequence accession number: EU822512.
Description of Litchfieldella rifensis comb. nov.
Litchfieldella rifensis (ri.fen’sis. N.L. fem. adj. rifensis, pertaining to the Rif Mountains in northern Morocco, where the strain was isolated).
Basonym: Halomonas rifensis Amjres et al. 2011.
The description is as given in the proposal of the basonym (Amjres et al., 2011), with the following addition. The genome size of the type strain is 4.83 Mbp. The DNA G + C content is 62.2 mol%.
Isolated from a solar saltern in the Rif Mountains (Morocco).
The type strain is HK31T = CECT 7698T = LMG 25695T.
Type strain genome sequence accession number: GCM10020179.
Type strain 16S rRNA gene sequence accession number: HM026177.
Description of Litchfieldella qijiaojingensis comb. nov.
Litchfieldella qijiaojingensis (qi.jiao’jing.en’sis. N.L. fem. adj. qijiaojingensis, pertaining to Qijiaojing Lake, Xinjiang Province, north-west China, where the sample from which the type strain was isolated and was collected).
Basonym: Halomonas qijiaojingensis Chen et al. 2012.
The description is as given in the proposal of the basonym (Chen et al., 2011), with the following addition. The genome size of the type strain is 4.77 Mbp. The DNA G + C content is 60.8 mol%.
Isolated from the shore sediment from a salt lake, Qijiaojing Lake (43°23′01” N 91°36′11″ E), Xinjiang province (China).
The type strain is YIM 93003T = CCTCC AB 208133T = DSM 22403T = KCTC 22228T.
Type strain genome sequence accession number: GCA_014651875.1.
Type strain 16S rRNA gene sequence accession number: HQ832735.
Description of Onishia gen. nov.
Onishia (O.ni’shi.a. N.L. fem. n. Onishia, named after Dr. Hiroshi Ōnishi [Japan], in recognition of his many contributions to the study of halophilic microorganisms).
Cells are Gram-staining-negative rods, 0.6–1.0 × 1.8–3.2 μm in size, aerobic or facultatively anaerobic, and motile. Endospores are not formed. Catalase and oxidase are positive. Colonies are cream pigmented. Moderately halophilic, growing at 1–25% (w/v) NaCl, with optimal growth at 10–15% (w/v) NaCl. Mesophilic, thriving at 4–45°C, showing optimal growth at 22–35°C. Alkalitolerant, growing at pH values in the range of 4.0–10.0, with optimal growth at pH 7.5–8.0. Chemo-organotrophic. Nitrate reduction is positive. The major respiratory quinones are Q9 and, according to the genome sequence, also Q8. The major fatty acids are C18:1ω6c/C18:1ω7c, C16:0, C16:1ω6c/C16:1ω7c/iso-C15:0 2-OH, C19:0 cyclo ω8c, and C12:0 3-OH. According to the genome sequence, the major polar lipids are phosphatidylglycerol, phosphatidylethanolamine, and diphosphatidylglycerol.
The DNA G + C content ranges between 61.1 and 62.3 mol%.
The genus Onishia belongs to the family Halomonadaceae. The type species is Onishia taeanensis.
Description of Onishia taeanensis comb. nov.
Onishia taeanensis (tae.an.en’sis. N.L. fem. adj. taeanensis, belonging to Taean, from where the organism was isolated).
Basonym: Halomonas taeanensis Lee et al. 2005.
The description is as given in the proposal of the basonym (Lee et al., 2005), with the following addition. The genome size of the type strain is 3.76 Mbp. The DNA G + C content is 62.3 mol%.
Isolated from the soil from a solar saltern (Korea).
The type strain is BH539T = CIP 109003T = DSM 16463T = KCTC 12284T.
Type strain genome sequence accession number: GCA_900100755.1.
Type strain 16S rRNA gene sequence accession number: AY671975.
Description of Onishia niordana comb. nov.
Onishia niordana (nior.di.a’na. N.L. fem. adj. niordana, pertaining to Njörd [Niord], Nordic god of marine coast).
Basonym: Halomonas niordiana Diéguez et al. 2020.
The description is as given in the proposal of the basonym (Diéguez et al., 2020), with the following addition. The genome size of the type strain is 3.68 Mbp. The DNA G + C content is 61.1 mol%.
Isolated from seawater (Norway).
The type strain is ATF 5.4T = CECT 9779T = LMG 31227T.
Type strain genome sequence accession number: GCA_004798965.1.
Type strain 16S rRNA gene sequence accession number: SDSD01000014.
Emended description of the genus Modicisalibacter Ben Ali Gam et al. 2007
Modicisalibacter (Mo.di.ci.sa.li.bac’ter. L. masc. adj. modicus, moderate, limited; L. masc. n. sal [gen. salis], salt; N.L. masc. n. bacter, a rod; N.L. masc. n. Modicisalibacter, a moderately halophilic rod).
Cells are Gram-staining-negative rods or short rods, 0.1–1.0 × 0.2–4.0 μm in size, aerobic, and mostly motile. Endospores are not formed. Catalase-positive and oxidase-variable. Colonies are cream, orange, pale/light orange, or yellow pigmented or colorless. Moderately halophilic, growing at 0–27.5% (w/v) NaCl, with optimal growth at 2.5–10% (w/v) NaCl. Mesophilic, thriving at 4–45°C, showing optimal growth at 25–37°C. Alkalitolerant, growing at pH values in the range of 5.0–10.0, with optimal growth at pH 6.5–8.5. Chemo-organotrophic. Nitrate reduction is positive. The major respiratory quinones are Q9 and Q8. The major fatty acids are C18:1ω6c/C18:1ω7c, C16:0, C16:1ω7c/iso-C15:0 2-OH, C19:0 cyclo ω8c, and C12:0 3-OH. The major polar lipids are diphosphatidylglycerol, phosphatidylglycerol, and phosphatidylethanolamine.
The DNA G + C content ranges between 59.1 and 67.4 mol%.
The genus Modicisalibacter belongs to the family Halomonadaceae. The type species is Modicisalibacter tunisiensis.
Description of Modicisalibacter muralis comb. nov.
Modicisalibacter muralis (mu.ra’lis. L. masc. adj. muralis, pertaining or belonging to walls).
Basonym: Halomonas muralis Heyrman et al. 2002.
The description is as given in the proposal of the basonym (Heyrman et al., 2002), with the following addition. The genome size of the type strain is 4.14 Mbp. The DNA G + C content is 61.9 mol%.
Isolated from microbial biofilms colonizing the walls and murals of the Saint-Catherine chapel, Castle Herberstein (Austria).
The type strain is R-5058T = CIP 108825T = DSM 14789T = LMG 20969T.
Type strain genome sequence accession number: GCA_900102945.1.
Type strain 16S rRNA gene sequence accession number: AJ320530.
Description of Modicisalibacter luteus comb. nov.
Modicisalibacter luteus (lu’te.us. L. masc. adj. luteus, orange-colored).
Basonym: Halomonas lutea Wang et al. 2008.
The description is as given in the proposal of the basonym (Wang et al., 2008), with the following addition. The genome size of the type strain is 4.53 Mbp. The DNA G + C content is 59.1 mol%.
Isolated from a salt lake, Xinjiang province (China).
The type strain is YIM 91125T = CCTCC AB 206093T = DSM 23508T = KCTC 12847T.
Type strain genome sequence accession number: GCA_000378505.1.
Type strain 16S rRNA gene sequence accession number: EF674852.
Description of Modicisalibacter ilicicola comb. nov.
Modicisalibacter ilicicola (i.li.ci’co.la. L. n. ilici, the Roman name of Elche, the city close to the solar salterns where the type strain was isolated; L. masc. n. suff. -cola, inhabitant, dweller; N.L. masc. n. ilicicola, inhabitant of Ilici).
Basonym: Halomonas ilicicola Arenas et al. 2009.
The description is as given in the proposal of the basonym (Arenas et al., 2009), with the following addition. The genome size of the type strain is 3.96 Mbp. The DNA G + C content is 63.2 mol%.
Isolated from saline water from a solar saltern, Santa Pola (38° 11′ 35” N 0° 35′ 45” W), Alicante (Spain).
The type strain is SP8T = CCM 7522T = CECT 7331T = DSM 19980T.
Type strain genome sequence accession number: GCA_900128925.1.
Type strain 16S rRNA gene sequence accession number: EU218533.
Description of Modicisalibacter xianhensis comb. nov.
Modicisalibacter xianhensis (xianh.en’sis. N.L. masc. adj. xianhensis, of or pertaining to Xianhe, Shandong Province, China, where the type strain was isolated).
Basonym: Halomonas xianhensis Zhao et al. 2012.
The description is as given in the proposal of the basonym (Zhao et al., 2012), with the following addition. The genome size of the type strain is 4.36 Mbp. The DNA G + C content is 61.3 mol%.
Isolated from saline soil contaminated with crude oil from Xianhe, Shandong Province (China).
The type strain is A-1T = CGMCC 1.6848T = JCM 14849T.
Type strain genome sequence accession number: GCA_900113605.1.
Type strain 16S rRNA gene sequence accession number: EF421176.
Description of Modicisalibacter zincidurans comb. nov.
Modicisalibacter zincidurans (zin.ci.du’rans. N.L. neut. n. zincum, zinc; L. pres. part. durans, enduring, being insensible; N.L. part. adj. zincidurans, zinc tolerating).
Basonym: Halomonas zincidurans Xu et al. 2013.
The description is as given previously (Xu et al., 2013; Navarro-Torre et al., 2020), with the following addition. The genome size of the type strain is 3.55 Mbp. The DNA G + C content is 64.4 mol%.
Isolated from deep-sea sediment from the South Atlantic Ocean.
The type strain is B6T = CGMCC 1.12450T = JCM 18472T.
Type strain genome sequence accession number: GCA_000731955.1.
Type strain 16S rRNA gene sequence accession number: JQ781698.
Description of Modicisalibacter radicis comb. nov.
Modicisalibacter radicis (ra’di.cis. L. gen. n. radicis, of a root).
Basonym: Halomonas radicis Navarro-Torre et al. 2020.
The description is as given in the proposal of the basonym (Navarro-Torre et al., 2020), with the following addition. The genome size of the type strain is 4.65 Mbp. The DNA G + C content is 64.9 mol%.
Isolated from Arthrocnemum macrostachyum growing in the Odiel marshes (Spain).
The type strain is EAR18T = CECT 9077T = LMG 29859T.
Type strain genome sequence accession number: GCA_900961225.1.
Type strain 16S rRNA gene sequence accession number: KU320882.
Description of Modicisalibacter coralii sp. nov.
Modicisalibacter coralii (co.ra’li.i. L. gen. n. coralii, from the coral Mussismilia braziliensis).
The description is as given in the original proposal of “Halomonas coralii” (Vidal et al., 2019), with the following addition. The genome size of the type strain is 4.44 Mbp. The DNA G + C content is 66.3 mol%.
Isolated from Mussismilia braziliensis (Brazil).
The type strain is 362.1T = CBAS 715T.
Type strain genome sequence accession number: GCA_004117855.1.
Type strain 16S rRNA gene sequence accession number: QWBW01000001.
Data availability statement
The datasets presented in this study can be found in online repositories. The names of the repository/repositories and accession number(s) can be found in Supplementary material.
Author contributions
RRH: Conceptualization, Formal analysis, Writing – original draft, Writing – review & editing. DRA: Conceptualization, Investigation, Writing – original draft, Writing – review & editing. CS-P: Conceptualization, Investigation, Writing – review & editing. MC: Formal analysis, Writing – review & editing. SW: Supervision, Writing – review & editing. PH: Supervision, Writing – review & editing. AV: Conceptualization, Writing – review & editing.
Funding
The author(s) declare financial support was received for the research, authorship, and/or publication of this article. This study was supported by grants PID2020-118136GB-I00 funded by MCIN/AEI/10.13039/501100011033, and from the Junta de Andalucía (P20_01066 and BIO-213), both with FEDER funds. RRH was a recipient of a short-stay grant (PRX21/00598) from the Spanish Ministry of Universities. MC and PH were funded by an Australian Research Council Discovery Project (grant number DP220100900).
Acknowledgments
The authors thank A. Oren (The Hebrew University of Jerusalem) for his help on the nomenclature of the new taxa and Brian Kemish (The University of Queensland, Australia) for his generous help and advice on the computing resources used for the presented data analyses.
Conflict of interest
The authors declare that the research was conducted in the absence of any commercial or financial relationships that could be construed as a potential conflict of interest.
The author(s) declared that they were an editorial board member of Frontiers, at the time of submission. This had no impact on the peer review process and the final decision.
Publisher’s note
All claims expressed in this article are solely those of the authors and do not necessarily represent those of their affiliated organizations, or those of the publisher, the editors and the reviewers. Any product that may be evaluated in this article, or claim that may be made by its manufacturer, is not guaranteed or endorsed by the publisher.
Supplementary material
The Supplementary material for this article can be found online at: https://www.frontiersin.org/articles/10.3389/fmicb.2023.1293707/full#supplementary-material
Footnotes
1. ^https://lpsn.dsmz.de/text/largest-genera
2. ^https://www.ncbi.nlm.nih.gov/assembly
3. ^https://www.ncbi.nlm.nih.gov/sra
4. ^https://genome.jgi.doe.gov/portal
6. ^https://github.com/novigit/davinciCode/blob/master/perl
7. ^https://github.com/dparks1134/PhyloRank
8. ^https://github.com/SWittouck/tidygenomes
9. ^https://github.com/SWittouck/lacto_genera
References
Amjres, H., Béjar, V., Quesada, E., Abrini, J., and Llamas, I. (2011). Halomonas rifensis sp. nov., an exopolysaccharide-producing, halophilic bacterium isolated from a solar saltern. Int. J. Syst. Evol. Microbiol. 61, 2600–2605. doi: 10.1099/ijs.0.027268-0
Amouric, A., Liebgott, P.-P., Joseph, M., Brochier-Armanet, C., and Lorquin, J. (2014). Halomonas olivaria sp. nov., a moderately halophilic bacterium isolated from olive-processing effluents. Int. J. Syst. Evol. Microbiol. 64, 46–54. doi: 10.1099/ijs.0.049007-0
Ankenbrand, M. J., and Keller, A. (2016). bcgTree: automatized phylogenetic tree building from bacterial core genomes. Genome 59, 783–791. doi: 10.1139/gen-2015-0175
Arahal, D. R., Ludwig, W., Schleifer, K. H., and Ventosa, A. (2002). Phylogeny of the family Halomonadaceae based on 23S and 16S rDNA sequence analyses. Int. J. Syst. Evol. Microbiol. 52, 241–249. doi: 10.1099/00207713-52-1-241
Arenas, M., Bañón, P. I., Copa-Patiño, J. L., Sánchez-Porro, C., Ventosa, A., and Soliveri, J. (2009). Halomonas ilicicola sp. nov., a moderately halophilic bacterium isolated from a saltern. Int. J. Syst. Evol. Microbiol. 59, 578–582. doi: 10.1099/ijs.0.003509-0
Auch, A. F., von Jan, M., Klenk, H.-P., and Göker, M. (2010). Digital DNA-DNA hybridization for microbial species delineation by means of genome-to-genome sequence comparison. Stand. Genomic Sci. 2, 117–134. doi: 10.4056/sigs.531120
Baumann, L., Baumann, P., Mandel, M., and Allen, R. D. (1972). Taxonomy of aerobic marine eubacteria. J. Bacteriol. 110, 402–429. doi: 10.1128/jb.110.1.402-429.1972
Ben Ali Gam, Z., Abdelkafi, S., Casalot, L., Tholozan, J. L., Oueslati, R., and Labat, M. (2007). Modicisalibacter tunisiensis gen. nov., sp. nov., an aerobic, moderately halophilic bacterium isolated from an oilfield-water injection sample, and emended description of the family Halomonadaceae Franzmann et al. 1989 emend Dobson and Franzmann 1996 emend. Ntougias et al. 2007. Int. J. Syst. Evol. Microbiol. 57, 2307–2313. doi: 10.1099/ijs.0.65088-0
Berendes, F., Gottschalk, G., Heine-Dobbernack, E., Moore, E. R. B., and Tindall, B. J. (1996). Halomonas desiderata sp. nov, a new alkaliphilic, halotolerant and denitrifying bacterium isolated from a municipal sewage works. Syst. Appl. Microbiol. 19, 158–167. doi: 10.1016/S0723-2020(96)80041-5
Boltyanskaya, Y. V., Kevbrin, V. V., Lysenko, A. M., Kolganova, T. V., Tourova, T. P., Osipov, G. A., et al. (2007). Halomonas mongoliensis sp. nov. and Halomonas kenyensis sp. nov., new haloalkaliphilic denitrifiers capable of N2O reduction, isolated from soda lakes. Microbiology (English translation of Mikrobiologiia) 76, 739–747. doi: 10.1134/S0026261707060148
Borowiec, M. L. (2016). AMAS: a fast tool for alignment manipulation and computing of summary statistics. PeerJ 4:e1660. doi: 10.7717/peerj.1660
Bushnell, B. (2020). BBMap short read aligner, and other bioinformatic tools. Available at: https://sourceforge.net/projects/bbmap. Last accessed: 2020.04.23.
Camacho, C., Coulouris, G., Avagyan, V., Ma, N., Papadopoulos, J., Bealer, K., et al. (2009). BLAST+: architecture and applications. BMC Bioinformatics 10, 1–9. doi: 10.1186/1471-2105-10-421
Capella-Gutierrez, S., Kauff, F., and Gabaldón, T. (2014). A phylogenomics approach for selecting robust sets of phylogenetic markers. Nucleic Acids Res. 42:e54. doi: 10.1093/nar/gku071
Chaumeil, P.-A., Mussig, A. J., Hugenholtz, P., and Parks, D. H. (2019). GTDB-Tk: a toolkit to classify genomes with the genome taxonomy database. Bioinformatics 36, 1925–1927. doi: 10.1093/bioinformatics/btz848
Chen, C., Anwar, N., Wu, C., Fu, G., Wang, R., Zhang, C., et al. (2018). Halomonas endophytica sp. nov., isolated from liquid in the stems of Populus euphratica. Int. J. Syst. Evol. Microbiol. 68, 1633–1638. doi: 10.1099/ijsem.0.002585
Chen, C., Shi, R., Liu, B.-B., Zhang, Y.-J., Sun, H.-Z., Li, C.-T., et al. (2011). Halomonas qijiaojingensis sp. nov. and Halomonas flava sp. nov., two moderately halophilic bacteria isolated from a salt lake. Antonie van Leeuwenhoek 100, 365–373. doi: 10.1007/s10482-011-9591-0
Chen, Y.-G., Zhang, Y.-Q., Huang, H.-Y., Klenk, H.-P., Tang, S.-K., Huang, K., et al. (2009). Halomonas zhanjiangensis sp. nov., a halophilic bacterium isolated from a sea urchin. Int. J. Syst. Evol. Microbiol. 59, 2888–2893. doi: 10.1099/ijs.0.010173-0
Chun, J., and Rainey, F. A. (2014). Integrating genomics into the taxonomy and systematics of the Bacteria and Archaea. Int. J. Syst. Evol. Microbiol. 64, 316–324. doi: 10.1099/ijs.0.054171-0
de la Haba, R. R., Arahal, D. R., Márquez, M. C., and Ventosa, A. (2010). Phylogenetic relationships within the family Halomonadaceae based on comparative 23S and 16S rRNA gene sequence analysis. Int. J. Syst. Evol. Microbiol. 60, 737–748. doi: 10.1099/ijs.0.013979-0
de la Haba, R. R., Arahal, D. R., Sánchez-Porro, C., and Ventosa, A. (2014). “The family Halomonadaceae” in The Prokaryotes – Gammaproteobacteria, eds. E. Rosenberg, E. F. Delong, S. Lory, E. Stackebrandt, and F. Thompson (Berlin Heidelberg: Springer-Verlag), 325–360. doi: 10.1007/978-3-642-38922-1_235
de la Haba, R. R., Carmen Márquez, M., Thane Papke, R., and Ventosa, A. (2012). Multilocus sequence analysis of the family Halomonadaceae. Int. J. Syst. Evol. Microbiol. 62, 520–538. doi: 10.1099/ijs.0.032938-0
Diéguez, A. L., Balboa, S., and Romalde, J. L. (2020). Halomonas borealis sp. nov. and Halomonas niordiana sp. nov., two new species isolated from seawater. Syst. Appl. Microbiol. 43:126040. doi: 10.1016/j.syapm.2019.126040
Dobson, S. J., and Franzmann, P. D. (1996). Unification of the genera Deleya (Baumann et al. 1983), Halomonas (Vreeland et al. 1980), and Halovibrio (Fendrich 1988) and the species Paracoccus halodenitrificans (Robinson and Gibbons 1952) into a single genus, Halomonas, and placement of the genus Zymobacter in the family Halomonadaceae. Int. J. Syst. Bacteriol. 46, 550–558. doi: 10.1099/00207713-46-2-550
Dobson, S. J., McMeekin, T. A., and Franzmann, P. D. (1993). Phylogenetic relationships between some members of the genera Deleya, Halomonas, and Halovibrio. Int. J. Syst. Bacteriol. 43, 665–673. doi: 10.1099/00207713-43-4-665
Edgar, R. C. (2004). MUSCLE: multiple sequence alignment with high accuracy and high throughput. Nucleic Acids Res. 32, 1792–1797. doi: 10.1093/nar/gkh340
Eren, A. M., Kiefl, E., Shaiber, A., Veseli, I., Miller, S. E., Schechter, M. S., et al. (2021). Community-led, integrated, reproducible multi-omics with anvi’o. Nat. Microbiol. 6, 3–6. doi: 10.1038/s41564-020-00834-3
Franzmann, P. D., Burton, H. R., and Mcmeekin, T. A. (1987). Halomonas subglaciescola, a new species of halotolerant bacteria isolated from Antarctica. Int. J. Syst. Bacteriol. 37, 27–34. doi: 10.1099/00207713-37-1-27
Franzmann, P. D., Wehmeyer, U., and Stackebrandt, E. (1988). Halomonadaceae fam. nov., a new family of the class Proteobacteria to accommodate the genera Halomonas and Deleya. Syst. Appl. Microbiol. 11, 16–19. doi: 10.1016/S0723-2020(88)80043-2
Gaboyer, F., Vandenabeele-Trambouze, O., Cao, J., Ciobanu, M.-C., Jebbar, M., Le Romancer, M., et al. (2014). Physiological features of Halomonas lionensis sp. nov., a novel bacterium isolated from a Mediterranean Sea sediment. Res. Microbiol. 165, 490–500. doi: 10.1016/j.resmic.2014.07.009
Galisteo, C., de la Haba, R. R., Sánchez-Porro, C., and Ventosa, A. (2022). Biotin pathway in novel Fodinibius salsisoli sp. nov., isolated from hypersaline soils and reclassification of the genus Aliifodinibius as Fodinibius. Front. Microbiol. 13:1101464. doi: 10.3389/fmicb.2022.1101464
Gan, L., Long, X., Zhang, H., Hou, Y., Tian, J., Zhang, Y., et al. (2018). Halomonas saliphila sp. nov., a moderately halophilic bacterium isolated from a saline soil. Int. J. Syst. Evol. Microbiol. 68, 1153–1159. doi: 10.1099/ijsem.0.002644
Gao, P., Lu, H., Xing, P., and Wu, Q. L. (2020). Halomonas rituensis sp. nov. and Halomonas zhuhanensis sp. nov., isolated from natural salt marsh sediment on the Tibetan plateau. Int. J. Syst. Evol. Microbiol. 70, 5217–5225. doi: 10.1099/ijsem.0.004395
Garrity, G. M., Bell, J. A., and Lilburn, T. (2005a). “Class III. Gammaproteobacteria class. nov.” in Bergey’s Manual of Systematic Bacteriology, eds. D. J. Brenner, N. R. Krieg, J. T. Staley, and G. M. Garrity (New York: Springer), 1.
Garrity, G. M., Bell, J. A., and Lilburn, T. (2005b). “Family I. Oceanospirillaceae fam. nov.” in Bergey’s Manual of Systematic Bacteriology. eds. D. J. Brenner, N. R. Krieg, J. T. Staley, and G. M. Garrity (New York: Springer), 271.
Garrity, G. M., Bell, J. A., and Lilburn, T. (2005c). “Order VIII. Oceanospirillales ord. nov.” in Bergey’s Manual of Systematic Bacteriology, eds. D. J. Brenner, N. R. Krieg, J. T. Staley, and G. M. Garrity (New York: Springer), 270. doi: 10.1007/0-387-28022-7_8
Goris, J., Konstantinidis, K. T., Klappenbach, J. A., Coenye, T., Vandamme, P., and Tiedje, J. M. (2007). DNA-DNA hybridization values and their relationship to whole-genome sequence similarities. Int. J. Syst. Evol. Microbiol. 57, 81–91. doi: 10.1099/ijs.0.64483-0
Guan, T.-W., Xiao, J., Zhao, K., Luo, X.-X., Zhang, X.-P., and Zhang, L.-L. (2010). Halomonas xinjiangensis sp. nov., a halotolerant bacterium isolated from a salt lake. Int. J. Syst. Evol. Microbiol. 60, 349–352. doi: 10.1099/ijs.0.011593-0
Gurevich, A., Saveliev, V., Vyahhi, N., and Tesler, G. (2013). QUAST: quality assessment tool for genome assemblies. Bioinformatics 29, 1072–1075. doi: 10.1093/bioinformatics/btt086
Guzmán, D., Quillaguamán, J., Muñoz, M., and Hatti-Kaul, R. (2010). Halomonas andesensis sp. nov., a moderate halophile isolated from the saline Lake Laguna Colorada in Bolivia. Int. J. Syst. Evol. Microbiol. 60, 749–753. doi: 10.1099/ijs.0.014522-0
Heyrman, J., Balcaen, A., De Vos, P., and Swings, J. (2002). Halomonas muralis sp. nov., isolated from microbial biofilms colonizing the walls and murals of the saint-Catherine chapel (castle Herberstein, Austria). Int. J. Syst. Evol. Microbiol. 52, 2049–2054. doi: 10.1099/00207713-52-6-2049
Hoang, D. T., Chernomor, O., von Haeseler, A., Minh, B. Q., and Vinh, L. S. (2018). UFBoot2: improving the ultrafast bootstrap approximation. Mol. Biol. Evol. 35, 518–522. doi: 10.1093/molbev/msx281
Hyatt, D., Chen, G. L., LoCascio, P. F., Land, M. L., Larimer, F. W., and Hauser, L. J. (2010). Prodigal: prokaryotic gene recognition and translation initiation site identification. BMC Bioinformatics 11:119. doi: 10.1186/1471-2105-11-119
Jiang, J., Pan, Y., Hu, S., Zhang, X., Hu, B., Huang, H., et al. (2014). Halomonas songnenensis sp. nov., a moderately halophilic bacterium isolated from saline and alkaline soils. Int. J. Syst. Evol. Microbiol. 64, 1662–1669. doi: 10.1099/ijs.0.056499-0
Jiang, J., Pan, Y., Meng, L., Hu, S., Zhang, X., Hu, B., et al. (2013). Halomonas zhaodongensis sp. nov., a slightly halophilic bacterium isolated from saline-alkaline soils in Zhaodong, China. Antonie van Leeuwenhoek 104, 685–694. doi: 10.1007/s10482-013-9976-3
Kalyaanamoorthy, S., Minh, B. Q., Wong, T. K. F., von Haeseler, A., and Jermiin, L. S. (2017). ModelFinder: fast model selection for accurate phylogenetic estimates. Nat. Methods 14, 587–589. doi: 10.1038/nmeth.4285
Kämpfer, P., Rekha, P. D., Busse, H.-J., Arun, A. B., Priyanka, P., and Glaeser, S. P. (2018). Halomonas malpeensis sp. nov., isolated from rhizosphere sand of a coastal sand dune plant. Int. J. Syst. Evol. Microbiol. 68, 1037–1046. doi: 10.1099/ijsem.0.002616
Kaye, J. Z., Márquez, M. C., Ventosa, A., and Baross, J. (2004). Halomonas neptunia sp. nov., Halomonas sulfidaeris sp. nov., Halomonas axialensis sp. nov. and Halomonas hydrothermalis sp. nov.: halophilic bacteria isolated from deep-sea hydrothermal-vent environments. Int. J. Syst. Evol. Microbiol. 54, 499–511. doi: 10.1099/ijs.0.02799-0
Kazemi, E., Tarhriz, V., Amoozegar, M. A., and Hejazi, M. S. (2021). Halomonas azerbaijanica sp. nov., a halophilic bacterium isolated from Urmia Lake after the 2015 drought. Int. J. Syst. Evol. Microbiol. 71:4578. doi: 10.1099/ijsem.0.004578
Kim, K. K., Jin, L., Yang, H. C., and Lee, S. T. (2007). Halomonas gomseomensis sp. nov., Halomonas janggokensis sp. nov., Halomonas salaria sp. nov. and Halomonas denitrificans sp. nov., moderately halophilic bacteria isolated from saline water. Int. J. Syst. Evol. Microbiol. 57, 675–681. doi: 10.1099/ijs.0.64767-0
Kim, K. K., Lee, K. C., Oh, H.-M., and Lee, J.-S. (2010). Halomonas stevensii sp. nov., Halomonas hamiltonii sp. nov. and Halomonas johnsoniae sp. nov., isolated from a renal care centre. Int. J. Syst. Evol. Microbiol. 60, 369–377. doi: 10.1099/ijs.0.004424-0
Kim, M.-S., Roh, S. W., and Bae, J.-W. (2010). Halomonas jeotgali sp. nov., a new moderate halophilic bacterium isolated from a traditional fermented seafood. J. Microbiol. 48, 404–410. doi: 10.1007/s12275-010-0032-y
Lalucat, J., Mulet, M., Gomila, M., and García-Valdés, E. (2020). Genomics in bacterial taxonomy: impact on the genus Pseudomonas. Genes (Basel) 11:139. doi: 10.3390/genes11020139
Lee, J.-C., Jeon, C. O., Lim, J.-M., Lee, S.-M., Lee, J.-M., Song, S.-M., et al. (2005). Halomonas taeanensis sp. nov., a novel moderately halophilic bacterium isolated from a solar saltern in Korea. Int. J. Syst. Evol. Microbiol. 55, 2027–2032. doi: 10.1099/ijs.0.63616-0
Lee, J.-C., Kim, Y.-S., Yun, B.-S., and Whang, K.-S. (2015). Halomonas salicampi sp. nov., a halotolerant and alkalitolerant bacterium isolated from a saltern soil. Int. J. Syst. Evol. Microbiol. 65, 4792–4799. doi: 10.1099/ijsem.0.000650
Li, X., Gan, L., Hu, M., Wang, S., Tian, Y., and Shi, B. (2020). Halomonas pellis sp. nov., a moderately halophilic bacterium isolated from wetsalted hides. Int. J. Syst. Evol. Microbiol. 70, 5417–5424. doi: 10.1099/ijsem.0.004426
Liu, L., Wang, S.-Y., He, C.-F., Zhang, X.-X., Chi, M., Liang, L.-X., et al. (2020). Phytohalomonas tamaricis gen. nov., sp. nov., an endophytic bacterium isolated from Tamarix ramosissima roots growing in Kumtag desert. Arch. Microbiol. 202, 143–151. doi: 10.1007/s00203-019-01724-x
Long, M.-R., Zhang, D.-F., Yang, X.-Y., Zhang, X.-M., Zhang, Y.-G., Zhang, Y.-M., et al. (2013). Halomonas nanhaiensis sp. nov., a halophilic bacterium isolated from a sediment sample from the South China Sea. Antonie van Leeuwenhoek 103, 997–1005. doi: 10.1007/s10482-013-9879-3
Lu, H., Xing, P., Zhai, L., Li, H., and Wu, Q. (2020). Halomonas montanilacus sp. nov., isolated from hypersaline Lake Pengyanco on the Tibetan plateau. Int. J. Syst. Evol. Microbiol. 70, 2859–2866. doi: 10.1099/ijsem.0.004109
Martínez-Cánovas, M. J., Béjar, V., Martínez-Checa, F., and Quesada, E. (2004). Halomonas anticariensis sp. nov., from Fuente de Piedra, a saline-wetland wildfowl reserve in Málaga, southern Spain. Int. J. Syst. Evol. Microbiol. 54, 1329–1332. doi: 10.1099/ijs.0.63108-0
Meier-Kolthoff, J. P., Auch, A. F., Klenk, H.-P., and Göker, M. (2013). Genome sequence-based species delimitation with confidence intervals and improved distance functions. BMC Bioinformatics 14:60. doi: 10.1186/1471-2105-14-60
Mellado, E., Moore, E. R., Nieto, J. J., and Ventosa, A. (1995). Phylogenetic inferences and taxonomic consequences of 16S ribosomal DNA sequence comparison of Chromohalobacter marismortui, Volcaniella eurihalina, and Deleya salina and reclassification of V. eurihalina as Halomonas eurihalina comb. nov. Int. J. Syst. Bacteriol. 45, 712–716. doi: 10.1099/00207713-45-4-712
Menes, R. J., Viera, C. E., Farías, M. E., and Seufferheld, M. J. (2011). Halomonas vilamensis sp. nov., isolated from high-altitude Andean lakes. Int. J. Syst. Evol. Microbiol. 61, 1211–1217. doi: 10.1099/ijs.0.023150-0
Ming, H., Ji, W.-L., Li, M., Zhao, Z.-L., Cheng, L.-J., Niu, M.-M., et al. (2020). Halomonas lactosivorans sp. nov., isolated from salt-lake sediment. Int. J. Syst. Evol. Microbiol. 70, 3504–3512. doi: 10.1099/ijsem.0.004209
Minh, B. Q., Dang, C. C., Vinh, L. S., and Lanfear, R. (2021). QMaker: fast and accurate method to estimate empirical models of protein evolution. Syst. Biol. 70, 1046–1060. doi: 10.1093/sysbio/syab010
Minh, B. Q., Schmidt, H. A., Chernomor, O., Schrempf, D., Woodhams, M. D., von Haeseler, A., et al. (2020). IQ-TREE 2: new models and efficient methods for phylogenetic inference in the genomic era. Mol. Biol. Evol. 37, 1530–1534. doi: 10.1093/molbev/msaa015
Mormile, M. R., Romine, M. F., Garcia, M. T., Ventosa, A., Bailey, T. J., and Peyton, B. M. (1999). Halomonas campisalis sp. nov., a denitrifying, moderately haloalkaliphilic bacterium. Syst. Appl. Microbiol. 22, 551–558. doi: 10.1016/S0723-2020(99)80008-3
Navarro-Torre, S., Carro, L., Rodríguez-Llorente, I. D., Pajuelo, E., Caviedes, M. Á., Igual, J. M., et al. (2020). Halomonas radicis sp. nov., isolated from Arthrocnemum macrostachyum growing in the Odiel marshes(Spain) and emended descriptions of Halomonas xinjiangensis and Halomonas zincidurans. Int. J. Syst. Evol. Microbiol. 70, 220–227. doi: 10.1099/ijsem.0.003742
Novichkov, P. S., Omelchenko, M. V., Gelfand, M. S., Mironov, A. A., Wolf, Y. I., and Koonin, E. V. (2004). Genome-wide molecular clock and horizontal gene transfer in bacterial evolution. J. Bacteriol. 186, 6575–6585. doi: 10.1128/JB.186.19.6575-6585.2004
Ntougias, S., Zervakis, G. I., Fasseas, C., and Fasseas, C. (2007). Halotalea alkalilenta gen. nov., sp. nov., a novel osmotolerant and alkalitolerant bacterium from alkaline olive mill wastes, and emended description of the family Halomonadaceae Franzmann et al. 1989, emend. Dobson and Franzmann 1996. Int. J. Syst. Evol. Microbiol. 57, 1975–1983. doi: 10.1099/ijs.0.65078-0
Oguntoyinbo, F. A., Cnockaert, M., Cho, G.-S., Kabisch, J., Neve, H., Bockelmann, W., et al. (2018). Halomonas nigrificans sp. nov., isolated from cheese. Int. J. Syst. Evol. Microbiol. 68, 371–376. doi: 10.1099/ijsem.0.002515
Oren, A., Arahal, D. R., Göker, M., Moore, E. R. B., Rossello-Mora, R., and Sutcliffe, I. C. (2023). International Code of Nomenclature of Prokaryotes. Prokaryotic Code (2022 Revision). Int. J. Syst. Evol. Microbiol. 73:005585. doi: 10.1099/ijsem.0.005585
Parks, D. H., Chuvochina, M., Waite, D. W., Rinke, C., Skarshewski, A., Chaumeil, P.-A., et al. (2018). A standardized bacterial taxonomy based on genome phylogeny substantially revises the tree of life. Nat. Biotechnol. 36, 996–1004. doi: 10.1038/nbt.4229
Parks, D. H., Imelfort, M., Skennerton, C. T., Hugenholtz, P., and Tyson, G. W. (2015). CheckM: assessing the quality of microbial genomes recovered from isolates, single cells, and metagenomes. Genome Res. 25, 1043–1055. doi: 10.1101/gr.186072.114
Parks, D. H., Rinke, C., Chuvochina, M., Chaumeil, P. A., Woodcroft, B. J., Evans, P. N., et al. (2017). Recovery of nearly 8,000 metagenome-assembled genomes substantially expands the tree of life. Nat. Microbiol. 2, 1533–1542. doi: 10.1038/s41564-017-0083-5
Parte, A. C., Sardà Carbasse, J., Meier-Kolthoff, J. P., Reimer, L. C., and Göker, M. (2020). List of prokaryotic names with standing in nomenclature (LPSN) moves to the DSMZ. Int. J. Syst. Evol. Microbiol. 70, 5607–5612. doi: 10.1099/ijsem.0.004332
Pritchard, L., Glover, R. H., Humphris, S., Elphinstone, J. G., and Toth, I. K. (2016). Genomics and taxonomy in diagnostics for food security: soft-rotting enterobacterial plant pathogens. Anal. Methods 8, 12–24. doi: 10.1039/C5AY02550H
Prjibelski, A., Antipov, D., Meleshko, D., Lapidus, A., and Korobeynikov, A. (2020). Using SPAdes de novo assembler. Curr. Protoc. Bioinformatics 70:e102. doi: 10.1002/cpbi.102
Qiu, X., Cao, X., Xu, G., Wu, H., and Tang, X. (2021a). Halomonas maris sp. nov., a moderately halophilic bacterium isolated from sediment in the southwest Indian Ocean. Arch. Microbiol. 203, 3279–3285. doi: 10.1007/s00203-021-02317-3
Qiu, X., Yu, L., Cao, X., Wu, H., Xu, G., and Tang, X. (2021b). Halomonas sedimenti sp. nov., a halotolerant bacterium isolated from deep-sea sediment of the southwest Indian Ocean. Curr. Microbiol. 78, 1662–1669. doi: 10.1007/s00284-021-02425-9
Quang, L. S., Gascuel, O., and Lartillot, N. (2008). Empirical profile mixture models for phylogenetic reconstruction. Bioinformatics 24, 2317–2323. doi: 10.1093/bioinformatics/btn445
Quillaguamán, J., Hatti-Kaul, R., Mattiasson, B., Alvarez, M. T., and Delgado, O. (2004). Halomonas boliviensis sp. nov., an alkalitolerant, moderate halophile isolated from soil around a Bolivian hypersaline lake. Int. J. Syst. Evol. Microbiol. 54, 721–725. doi: 10.1099/ijs.0.02800-0
Reddy, G. S. N., Raghavan, P. U. M., Sarita, N. B., Prakash, J. S. S., Nagesh, N., Delille, D., et al. (2003). Halomonas glaciei sp. nov. isolated from fast ice of Adelie Land Antarctica. Extremophiles 7, 55–61. doi: 10.1007/s00792-002-0295-2
Richter, M., and Rossello-Mora, R. (2009). Shifting the genomic gold standard for the prokaryotic species definition. Proc. Natl. Acad. Sci. U. S. A. 106, 19126–19131. doi: 10.1073/pnas.0906412106
Rodriguez-R, L. M., and Konstantinidis, K. T. (2016). The enveomics collection: a toolbox for specialized analyses of microbial genomes and metagenomes. PeerJ Prepr. 4:e1900v1. doi: 10.7287/peerj.preprints.1900v1
Romano, I., Nicolaus, B., Lama, L., Manca, M. C., and Gambacorta, A. (1996). Characterization of a haloalkalophilic strictly aerobic bacterium, isolated from Pantelleria island. Syst. Appl. Microbiol. 19, 326–333. doi: 10.1016/S0723-2020(96)80059-2
Sánchez-Porro, C., Kaur, B., Mann, H., and Ventosa, A. (2010). Halomonas titanicae sp. nov., a halophilic bacterium isolated from the RMS titanic. Int. J. Syst. Evol. Microbiol. 60, 2768–2774. doi: 10.1099/ijs.0.020628-0
Schoch, C. L., Ciufo, S., Domrachev, M., Hotton, C. L., Kannan, S., Khovanskaya, R., et al. (2020). NCBI taxonomy: a comprehensive update on curation, resources and tools. Database 2020, baaa062. doi: 10.1093/database/baaa062,
Schwarz, G. (1978). Estimating the dimension of a model. Ann. Stat. 6, 461–464. doi: 10.1214/aos/1176344136
Seck, E. H., Fournier, P.-E., Raoult, D., and Khelaifia, S. (2016). “Halomonas massiliensis” sp. nov., a new halotolerant bacterium isolated from the human gut. New Microb. New Infect. 14, 19–20. doi: 10.1016/j.nmni.2016.07.013
Seemann, T. (2014). Prokka: rapid prokaryotic genome annotation. Bioinformatics 30, 2068–2069. doi: 10.1093/bioinformatics/btu153
Shapovalova, A. A., Khijniak, T. V., Tourova, T. P., and Sorokin, D. Y. (2009). Halomonas chromatireducens sp. nov., a new denitrifying facultatively haloalkaliphilic bacterium from solonchak soil capable of aerobic chromate reduction. Microbiology (English translation of Mikrobiologiia) 78, 102–111. doi: 10.1134/S0026261709010135
Shi, W., Sun, Q., Fan, G., Hideaki, S., Moriya, O., Itoh, T., et al. (2021). gcType: a high-quality type strain genome database for microbial phylogenetic and functional research. Nucleic Acids Res. 49, D694–D705. doi: 10.1093/nar/gkaa957
So, Y., Chhetri, G., Kim, I., Kang, M., Kim, J., Lee, B., et al. (2022). Halomonas antri sp. nov., a carotenoid-producing bacterium isolated from surface seawater. Int. J. Syst. Evol. Microbiol. 72:5272. doi: 10.1099/ijsem.0.005272
Sorokin, D. Y., and Tindall, B. J. (2006). The status of the genus name Halovibrio Fendrich 1989 and the identity of the strains Pseudomonas halophila DSM 3050 and Halomonas variabilis DSM 3051. Request for an opinion. Int. J. Syst. Evol. Microbiol. 56, 487–489. doi: 10.1099/ijs.0.63965-0
Stott, C. M., and Bobay, L.-M. (2020). Impact of homologous recombination on core genome phylogenies. BMC Genomics 21:829. doi: 10.1186/s12864-020-07262-x
Tsai, M.-H., Liu, Y.-Y., Soo, V.-W., and Chen, C.-C. (2019). A new genome-to-genome comparison approach for large-scale revisiting of current microbial taxonomy. Microorganisms 7:161. doi: 10.3390/microorganisms7060161
van Dongen, S. (2008). Graph clustering via a discrete uncoupling process. SIAM J. Matrix Anal. Appl. 30, 121–141. doi: 10.1137/040608635
Ventosa, A., de la Haba, R. R., Arahal, D. R., and Sánchez-Porro, C. (2021a). “Halomonas” in Bergey’s Manual of Systematics of Archaea and Bacteria, ed. A. Oren (Hoboken, NJ: Wiley), 1–111. doi: 10.1002/9781118960608.gbm01190.pub2
Ventosa, A., de la Haba, R. R., Arahal, D. R., and Sánchez-Porro, C. (2021b). “Halomonadaceae” in Bergey’s Manual of Systematics of Archaea and Bacteria, ed. A. Oren (Hoboken, NJ: Wiley), 1–10. doi: 10.1002/9781118960608.fbm00228.pub2
Vidal, L. M. R., Gonçalves, A., Venas, T. M., Campeão, M. E., Calegario, G., Walter, J. M., et al. (2019). Halomonas coralii sp. nov. isolated from Mussismilia braziliensis. Curr. Microbiol. 76, 678–680. doi: 10.1007/s00284-019-01674-z
Wang, Y.-N., Cai, H., Yu, S.-L., Wang, Z.-Y., Liu, J., and Wu, X.-L. (2007). Halomonas gudaonensis sp. nov., isolated from a saline soil contaminated by crude oil. Int. J. Syst. Evol. Microbiol. 57, 911–915. doi: 10.1099/ijs.0.64826-0
Wang, L., Liu, X., Lai, Q., Gu, L., and Shao, Z. (2021). Halomonas diversa sp. nov., isolated from deep-sea sediment of the Pacific Ocean. Int. J. Syst. Evol. Microbiol. 71:4790. doi: 10.1099/ijsem.0.004790
Wang, L., and Shao, Z. (2021). Aerobic denitrification and heterotrophic sulfur oxidation in the genus Halomonas revealed by six novel species characterizations and genome-based analysis. Front. Microbiol. 12:652766. doi: 10.3389/fmicb.2021.652766
Wang, Y., Tang, S.-K., Lou, K., Mao, P.-H., Jin, X., Jiang, C.-L., et al. (2008). Halomonas lutea sp. nov., a moderately halophilic bacterium isolated from a salt lake. Int. J. Syst. Evol. Microbiol. 58, 2065–2069. doi: 10.1099/ijs.0.65436-0
Wang, F., Wan, J.-J., Zhang, X.-Y., Xin, Y., Sun, M.-L., Wang, P., et al. (2022). Halomonas profundi sp. nov., isolated from deep-sea sediment of the Mariana trench. Int. J. Syst. Evol. Microbiol. 72:5210. doi: 10.1099/ijsem.0.005210
Wang, T., Wei, X., Xin, Y., Zhuang, J., Shan, S., and Zhang, J. (2016). Halomonas lutescens sp. nov., a halophilic bacterium isolated from a lake sediment. Int. J. Syst. Evol. Microbiol. 66, 4697–4704. doi: 10.1099/ijsem.0.001413
Wang, Y.-X., Xiao, W., Dong, M.-H., Zhao, Q., Li, Z.-Y., Lai, Y.-H., et al. (2014). Halomonas qiaohouensis sp. nov., isolated from salt mine soil in southwest China. Antonie van Leeuwenhoek 106, 253–260. doi: 10.1007/s10482-014-0189-1
Wenting, R., Montazersaheb, S., Khan, S. A., Kim, H. M., Tarhriz, V., Hejazi, M. A., et al. (2021). Halomonas azerica sp. nov., isolated from Urmia Lake in Iran. Curr. Microbiol. 78, 3299–3306. doi: 10.1007/s00284-021-02482-0
Westram, R., Bader, K., Prüsse, E., Kumar, Y., Meier, H., Glöckner, F. O., et al. (2011). “ARB: a software environment for sequence data” in Handbook of Molecular Microbial Ecology I: Metagenomics and Complementary Approaches, ed. F. J. de Bruijn (Hoboken, NJ: Wiley-Blackwell), 399–406. doi: 10.1002/9781118010518.ch46
Wickham, H., Averick, M., Bryan, J., Chang, W., McGowan, L., François, R., et al. (2019). Welcome to the Tidyverse. J. Open Source Softw. 4:1686. doi: 10.21105/joss.01686
Wu, D., Jospin, G., and Eisen, J. A. (2013). Systematic identification of gene families for use as “markers” for phylogenetic and phylogeny-driven ecological studies of bacteria and archaea and their major subgroups. PLoS One 8:e77033. doi: 10.1371/journal.pone.0077033
Wu, L., and Ma, J. (2019). The global catalogue of microorganisms (GCM) 10K type strain sequencing project: providing services to taxonomists for standard genome sequencing and annotation. Int. J. Syst. Evol. Microbiol. 69, 895–898. doi: 10.1099/ijsem.0.003276
Xiao, Z., Shen, J., Wang, Z., Dong, F., and Zhao, J.-Y. (2021). Halomonas bachuensis sp. nov., isolated from Gobi soil. Curr. Microbiol. 78, 397–402. doi: 10.1007/s00284-020-02268-w
Xing, J., Gong, Q., Tang, L., Li, J., Fan, H., Wang, X., et al. (2021). Halomonas salipaludis sp. nov., isolated from a saline-alkali wetland soil. Arch. Microbiol. 203, 6033–6039. doi: 10.1007/s00203-021-02560-8
Xu, X.-W., Wu, Y.-H., Zhou, Z., Wang, C.-S., Zhou, Y.-G., Zhang, H.-B., et al. (2007). Halomonas saccharevitans sp. nov., Halomonas arcis sp. nov. and Halomonas subterranea sp. nov., halophilic bacteria isolated from hypersaline environments of China. Int. J. Syst. Evol. Microbiol. 57, 1619–1624. doi: 10.1099/ijs.0.65022-0
Xu, L., Xu, X.-W., Meng, F.-X., Huo, Y.-Y., Oren, A., Yang, J.-Y., et al. (2013). Halomonas zincidurans sp. nov., a heavy-metal-tolerant bacterium isolated from the deep-sea environment. Int. J. Syst. Evol. Microbiol. 63, 4230–4236. doi: 10.1099/ijs.0.051656-0
Xu, L., Ying, J.-J., Fang, Y.-C., Zhang, R., Hua, J., Wu, M., et al. (2021). Halomonas populi sp. nov. isolated from Populus euphratica. Arch. Microbiol. 204:86. doi: 10.1007/s00203-021-02704-w
Yan, F., Fang, J., Cao, J., Wei, Y., Liu, R., Wang, L., et al. (2020). Halomonas piezotolerans sp. nov., a multiple-stress-tolerant bacterium isolated from a deep-sea sediment sample of the New Britain Trench. Int. J. Syst. Evol. Microbiol. 70, 2560–2568. doi: 10.1099/ijsem.0.004069
Zhao, B., Wang, H., Mao, X., Li, R., Zhang, Y.-J., Tang, S., et al. (2012). Halomonas xianhensis sp. nov., a moderately halophilic bacterium isolated from a saline soil contaminated with crude oil. Int. J. Syst. Evol. Microbiol. 62, 173–178. doi: 10.1099/ijs.0.025627-0
Zheng, J., Wittouck, S., Salvetti, E., Franz, C. M. A. P., Harris, H. M. B., Mattarelli, P., et al. (2020). A taxonomic note on the genus Lactobacillus: description of 23 novel genera, emended description of the genus Lactobacillus Beijerinck 1901, and union of Lactobacillaceae and Leuconostocaceae. Int. J. Syst. Evol. Microbiol. 70, 2782–2858. doi: 10.1099/ijsem.0.004107
Keywords: phylogenomics, signature genes, halophiles, taxonomic reclassification, genus delineation
Citation: de la Haba RR, Arahal DR, Sánchez-Porro C, Chuvochina M, Wittouck S, Hugenholtz P and Ventosa A (2023) A long-awaited taxogenomic investigation of the family Halomonadaceae. Front. Microbiol. 14:1293707. doi: 10.3389/fmicb.2023.1293707
Edited by:
Ram Karan, University of Delhi, IndiaReviewed by:
Javier Pascual, Darwin Bioprospecting Excellence, SpainElena Plotnikova, Institute of Ecology and Genetics of Microorganisms (RAS), Russia
Copyright © 2023 de la Haba, Arahal, Sánchez-Porro, Chuvochina, Wittouck, Hugenholtz and Ventosa. This is an open-access article distributed under the terms of the Creative Commons Attribution License (CC BY). The use, distribution or reproduction in other forums is permitted, provided the original author(s) and the copyright owner(s) are credited and that the original publication in this journal is cited, in accordance with accepted academic practice. No use, distribution or reproduction is permitted which does not comply with these terms.
*Correspondence: Rafael R. de la Haba, cnJoQHVzLmVz; Antonio Ventosa, dmVudG9zYUB1cy5lcw==