- 1School of Karst Science/State Engineering Technology Institute for Karst Desertification Control, Guizhou Normal University, Guiyang, China
- 2Department of Zoology, College of Science, King Saud University, Riyadh, Saudi Arabia
Different utilization patterns can alter the C, N, P cycles and their ecological stoichiometry characteristics in grassland soils. However, the effects of different utilization patterns on soil microbial biomass, microbial entropy and soil-microorganism stoichiometry imbalance of artificial grassland are not clear. So this study was took different utilization patterns of artificial grassland [i.e., grazing grassland (GG), mowing grassland (MG), enclosed grassland (EG)] as the research object to investigate responses of soil microbial biomass, microbial entropy and soil-microorganism stoichiometry imbalance to different utilization patterns in the karst rocky desertification control area. We found that the contents of microbial biomass carbon (MBC) and microbial biomass nitrogen (MBN) were highest in GG, and the content of microbial biomass phosphorus (MBP) was highest in EG. Soil microbial biomass entropy carbon (qMBC) and soil microbial biomass entropy nitrogen (qMBN) of GG and MG were higher than those of EG, but soil microbial biomass entropy phosphorus (qMBP) was opposite. C:N stoichiometry imbalance (C:Nimb) was EG > GG > MG, C:P stoichiometry imbalance (C:Pimb) was EG > MG > GG, N:P stoichiometry imbalance (N:Pimb) was MG > EG > GG. MBN was significantly positive correlated with C:Nimb and C:Pimb, MBC was significantly negative correlated with C:Pimb, MBP was significantly negative correlated with N:Pimb. The redundancy analysis (RDA) results showed that N:Pimb (p = 0.014), C:Nimb (p = 0.014), and C:P in the soil (C:Psoil, p = 0.028) had the most significant effect on microbial entropy. EG had a significant effect on soil microbial biomass and microbial entropy. The results of this study can directly or indirectly reflect the grassland soil quality under different utilization patterns in the karst rocky desertification area, which has a certain reference value for the degraded ecosystem restoration.
Introduction
Soil has the most diverse microbial community on the planet (Hartmann and Six, 2023), and soil microorganisms directly drive key functions in ecosystems, playing an important role in soil organic matter decomposition, nutrient cycling, C storage, maintaining soil ecosystem productivity, and determining soil fertility, crop yield, and stress tolerance (Bahram et al., 2018; Zhao et al., 2019; Sardar et al., 2021a,b). Soil microorganisms release inorganic nutrients available to plants by decomposing soil organic matter, modify nutrient availability through oxidation, reduction, dissolution and chelation processes, and store nutrients in microbial residues (Marschner and Rengel, 2007; Hu et al., 2014), ultimately driving global nutrient cycling processes (Falkowski et al., 2008). The biomass of soil microorganisms refers to the total biomass with a volume of less than 5.0 × 103 μm3 in the soil, mainly referring to some living parts of soil organic matter such as bacteria, fungi, algae and protozoa, which is an important parameter to characterize the material cycle and energy flow in the soil ecosystem (Rogers and Tate, 2001). Meanwhile, the amount of microbial biomass can also reflect mineralization capacity of the soil and indicate the vitality of the soil (Li et al., 2018). The contents of soil organic carbon (SOC), total nitrogen (TN) and total phosphorus (TP) change little in a short time, but soil microorganisms are very sensitive to environmental changes, and their biomass stoichiometry changes with climate, biota, land use and vegetation succession. Therefore, MBC, MBN, and MBP have the characteristics of sensitivity and fast turnover rate, which are more suitable for reflecting soil fertility changes and assessing soil quality (Nair and Ngouajio, 2012; Song et al., 2022; Zhang et al., 2023). The stoichiometry characteristics of soil C, N, and P significantly influence the community structure, microbial biomass, assimilation rates and metabolic activities of soil microorganisms (Mooshammer et al., 2014; Zechmeister-Boltenstern et al., 2015; Zhou and Wang, 2016). Therefore, clarifying the stoichiometric relationship between soil and soil microorganisms is of great importance to reveal the mechanism of soil nutrient balance (Zhang et al., 2019).
Soil microbial entropy is the proportion of soil microbial biomass C, N, and P to the SOC, TN, and TP content, and is mainly used to reflect the microbial biomass that can be supported by a unit of resources (Heuck et al., 2015). Soil microbial entropy is mainly influenced by the quality and content of soil organic matter. It reflects the dynamic change of soil ecosystem by reflecting the change of soil nutrient and its use efficiency, which is a sensitive index to characterize the change of soil quality (Ji et al., 2020), and has the function of indicating the accumulation and change of soil nutrient (Rogers and Tate, 2001). The results of a study by Zhou and Wang (2016) on MBC, MBN, and MBP in 6 grassland ecosystems and 13 forest ecosystems showed that MBC, MBN, and MBP all increased significantly with the secondary succession process. The results of a study by Wu et al. (2019) on soil microbial biomass and soil microbial entropy in desert grassland showed that soil microbial biomass and soil microbial entropy will decrease with the aggravation of land degradation. Although both succession processes and the degree of degradation have different effects on soil microbial entropy, the effect of difference use patterns on soil microbial entropy in grasslands has not yet been investigated.
Soil and microbial stoichiometry imbalances, which can measure the difference between the chemical composition of microorganisms and resource, the smaller the value indicates that the higher the quality of the resource, the higher the efficiency of microbial growth, which can help to clarify the dynamic balance of nutrients between the soil and microorganisms (Mooshammer et al., 2014; Zhou and Wang, 2016). The ecological stoichiometry of soil microbial C:N:P can enhance the understanding of soil microbial ecological processes and mechanisms (Wu et al., 2019). Studies have shown that soil microbial entropy changes with the change in soil stoichiometry (Anderson and Domsch, 1990; Ding et al., 1992) and decreases with increasing the stoichiometry imbalance of soil C:N:P (Zhou and Wang, 2016). Therefore, it is of great importance to study the changes of ecological stoichiometry, microbial entropy and soil-microorganism stoichiometry imbalance under different utilization patterns to maintain ecosystem stability and sustainable development.
The karst in southwest China is an ecological security barrier area in China, with a very fragile ecological environment, and its ecological protection and economic development have received much attention from the international community (He et al., 2023). The fragile ecological environment of the region, combined with a long history of traditional agriculture such as maize and potatoes, has resulted in severe soil erosion, shallow soil layers and prominent karst desertification problems (Xiong et al., 2016). Since the end of the last century, the research results on the rocky desertification control show that returning farmland to grassland and developing herbivorous animal husbandry in the rocky desertification areas are important measures for repairing the damaged ecological environment, and are of great significance for promoting ecological reconstruction and economic development (Xiong et al., 2002). Differences in grassland use will inevitably lead to differences in nutrient ratios and elemental balances of C, N, and P in grassland soils (Song et al., 2023). At present, grazing and mowing are the main utilization patterns of karst artificial grassland, and the different utilization patterns may alter the nutrient cycling relationship between grassland soil and microorganisms formed by long-term evolution (Pei et al., 2021), thus having a profound impact on the function of grassland ecosystem. However, the ecological stoichiometry studies of soil and microorganisms in the karst area have mostly focused on forests or different land uses (Song et al., 2019; Chen et al., 2020; Lu et al., 2022; Lei et al., 2023; Wen et al., 2023), while studies on the response of soil microbial biomass, microbial entropy and soil-microorganism stoichiometry imbalance to different utilization patterns in the artificial grassland of karst area are clearly lacking. Therefore, it is urgent to clarify the characteristics of soil microbial biomass, microbial entropy and soil-microorganism stoichiometry imbalance and reveal the nutrient balance and limiting factors of soil and microbial under different grassland utilization patterns in the karst desertification area.
For this reason, we hypothesized that soil microbial biomass, microbial entropy and soil-microorganism stoichiometry imbalance would have different response characteristics to different utilization patterns in the artificial grassland of karst area. In order to solve the above conjecture, this study was took different utilization patterns of artificial grassland (i.e., GG, MG, EG) as the research object to investigate responses of soil microbial biomass, microbial entropy and soil-microorganism stoichiometry imbalance to different utilization patterns in the karst desertification control area, so as to provide theoretical support for sustainable utilization of artificial grassland in ecologically fragile areas of China and even the world.
Materials and methods
Study area
The study area is located in Salaxi Town, Qixingguan District, Bijie City, Guizhou Province, China (105°02′01′′–105°08′09′′E, 27°11′36′′–27°16′51′′N), which is a typical karst plateau-mountain desertification control area. The rocky desertification area is 55.931 km2, accounting for 64.93% of the demonstration area. The climate type belongs to the subtropical monsoon climate, with annual rainfall of about 1,000 mm, mostly concentrated in July to September. The mean temperature in July (hottest month) is 21.2°C, the mean temperature in January (coldest month) is 2.3°C, the frost-free period is about 245 days, and the average annual sunshine hours are about 1,360 h. Groundwater resources are difficult to exploit and extract, and the main sources of water for production and domestic use are springs and surface water, which is abundant in summer but severely depleted in the dry season. The vegetation is dominated by subtropical deciduous broad-leaved and evergreen broad-leaved mixed forest, accounting for about 10% of the entire area. The soil is mainly yellow soil and calcareous, formed by the weathering of carbonate rock parent material. In order to restore the ecological environment of rocky desertification caused by population pressure, we have established soil and water conservation forests, economic forests and artificial grasslands with green vegetation that has both ecological and economic benefits. The main crops are maize and potatoes, which are grown for long periods of the year, with severe soil erosion and shallow soil layers. The basic chemical properties of the soil are as follows: SOC content is ranged from 17.37 to 22.45 mg/kg, TN content is ranged from 1.74 to 2.19 mg/kg, TP content is ranged from 0.53 to 1.26 mg/kg.
Plot setting
In April 2012, the research group established artificial grassland in the study area. The main planting method was mixed seeding, mainly with Lolium perenne + Dactylis glomerata + Trifolium repens, and the seed ratio was 2:2:1. After the grassland establishment, free grazing has been the main use, and the carrying capacity was 600 m2 per sheep unit. To reveal the difference in soil microbial biomass, microbial entropy and soil-microorganism stoichiometry imbalance under different utilization patterns, GG and MG were set up in August 2019, and EG was used as a control treatment. The area of grazing and mowing plots was about 3,000 m2, and the enclosure plot was about 100m2. Three replications were set up for three treatments, and the distance between the boundaries of each plot was more than 50 cm. The average number of grazing animals in each plot was 5 (in line with the local grazing situation), and the grazing animals were Guizhou semi-fine wool sheep about 1 year old. Except for extreme weather conditions, the grazing period was about 300 days per year. The stubble height of the mowed grassland was approximately 5 cm, and the mowing was carried out according to the normal grass phenological period or the mowing height. The EG was not used for any purpose.
Sampling method
In mid-August 2021, 15 sampling points were set up evenly in each sample plot using the “S” shaped multi-point sampling method. After removing the litter layer from the soil surface, soil samples were taken from the surface layer (0–10 cm) using soil auger. To reduce spatial heterogeneity, soil samples from 15 sampling points were mixed into one sample, and a total of 9 soil samples were obtained. Impurities were removed from the soil samples, and the samples were divided into two parts. One part of the soil samples was naturally air dried indoors, and then passed through a 2 mm sieve for the determination of soil C, N, and P contents. The other part was placed in dry ice at −78.5°C and brought back to the laboratory for the determination of microbial C, N, and P contents.
Samples determination
The potassium dichromate-external heating method was used to determine soil organic carbon (SOC), the sulfuric acid catalyst digestion-Kjeldahl method was used to determine total nitrogen (TN), the concentrated sulfuric acid digestion-Mo–Sb colorimetric method was used to determine total phosphorus (TP) (Bao, 2000). TN and TP were determined by the continuous flow analyzer (SYSTEA, FLOWSYS, Italy), and TP was determined by the ultraviolet spectrophotometer (Specord 200 PLUS, Analytik, Germany). The chloroform fumigation-K2SO4 leaching method was used to determine soil microbial biomass carbon (MBC), microbial biomass nitrogen (MBN), the chloroform fumigation-NaHCO3 extraction-Pi assay-additional Pi correction method was used to determine soil microbial biomass phosphorus (MBP) (Zhang et al., 2012; Wu et al., 2019).
Data analysis
The ecological stoichiometric ratio of C, N, and P of soil and microorganism was expressed in mass ratios (Lin, 2010; Wu et al., 2019; Zhang et al., 2023).
Soil microbial entropy C (qMBC) = MBC/SOC × 100%.
Soil microbial entropy N (qMBN) = MBN/TN × 100%.
Soil microbial biomass entropy P (qMBP) = MBP/TP × 100%.
C:N stoichiometry imbalance (C:Nimb) = C:Nsoil/C:Nmic.
C:P stoichiometry imbalance (C:Pimb) = C:Psoil/C:Pmic.
N:P stoichiometry imbalance (N:Pimb) = N:Psoil/N:Pmic (Wu et al., 2019; Zhang et al., 2023).
Data were analyzed using One-way ANOVA, multiple comparisons of least significant difference and Person correlation analysis in SPSS 22.0, and plotted using Origin 2018. Redundancy analysis (RDA) was performed in Canoco 5.0.
Results
Soil microbial biomass C, N, P and its ecological stoichiometry
As shown in Table 1, different utilization of grassland can have different effects on MBC, MBN, and MBP. The variation range of MBC content was from 270.89 to 350.24 mg/kg, and GG and MG were 22.66% and 14.17% higher than EG, respectively. The MBN content ranged from 81.53 to 142.46 mg/kg. The MBP content ranged from 7.50 to 18.82 mg/kg, and it was significantly lower in GG and MG than in EG with values of 48.24% and 137.74%, respectively. The C:Nmic and C:Pmic were highest in MG, C:Nmic was lowest in GG, C:Pmic and N:Pmic were lowest in EG, and C:Pmic was highest in GG.

Table 1. Soil microbial biomass C, N, P and its ecological stoichiometry of grassland under different utilization modes.
Soil microbial entropy and stoichiometry imbalance
As shown in Table 2, anthropogenic utilization practices significantly affected qMBC and qMBN but not qMBP compared natural closure. The qMBC was significantly higher in GG and MG than in EG, but the values between GG and MG were very close. The qMBN was significantly higher in GG than that in MG and EG, and there were significant differences among the three treatments. There was no significant difference in qMBP among the three treatments. The C:Nimb in EG was significantly higher than that in GG and MG, and there were significant differences among the three treatments. The C:Pimb was significantly higher in EG than that in GG and MG. The N:Pimb in MG was significantly higher than that in GG, but not significantly different from that in EG.

Table 2. Soil microbial entropy and stoichiometry imbalance of grassland under different utilization modes.
Correlation analysis of soil microbial biomass and soil-microorganism stoichiometry imbalance
It can be seen from Figure 1 that there was a significant negative correlation between MBC and C:Pimb at the 0.01 level. MBN was positively correlated with C:Nimb and C:Pimb at 0.01 level. MBP was negatively correlated with N:Pimb at 0.001 level. C:Nimb was positively correlated with C:Pimb at 0.05 level.
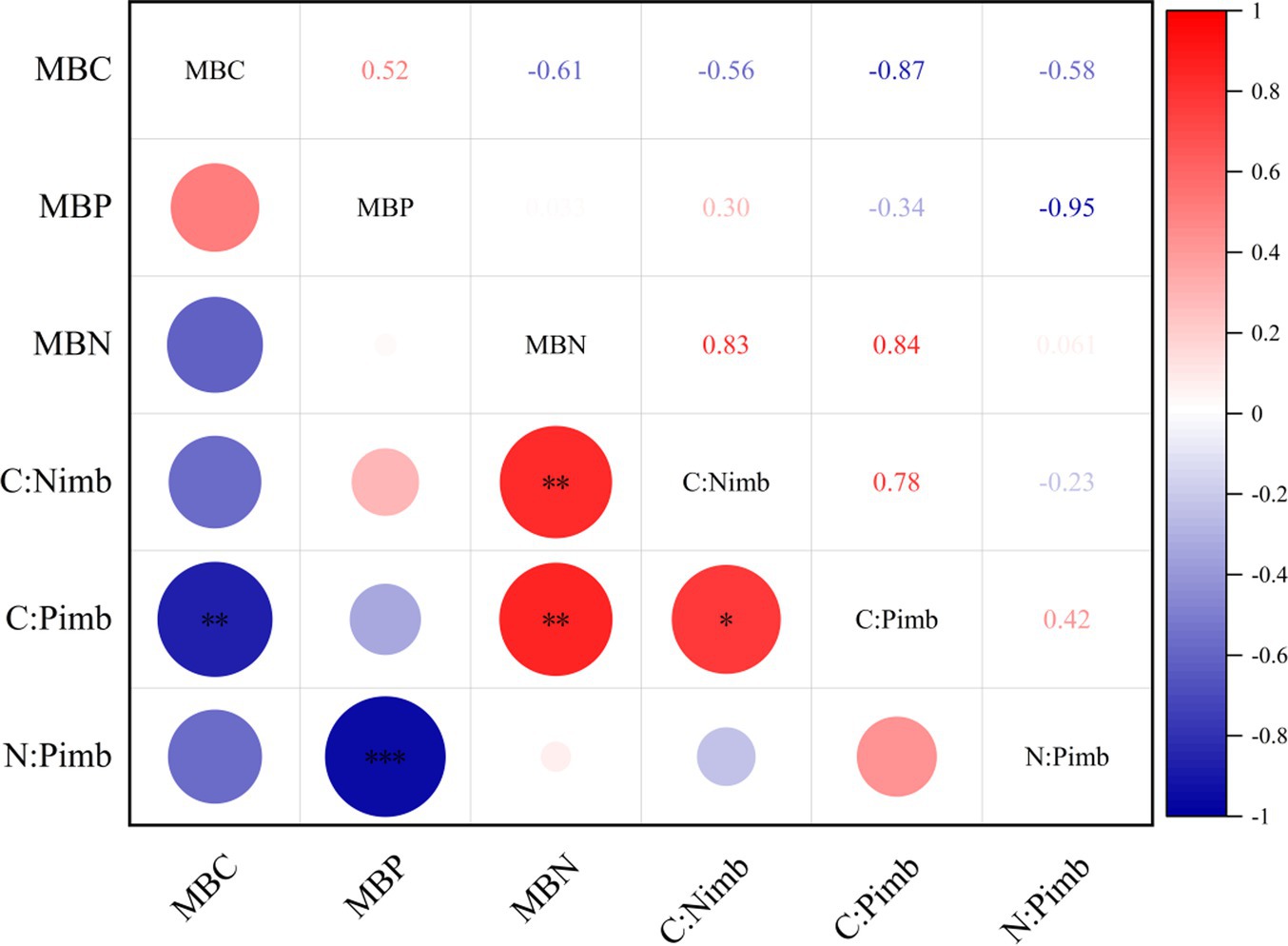
Figure 1. Correlation analysis between microbial biomass and soil-microorganism stoichiometric imbalance. *p <= 0.05, **p <= 0.01, ***p <= 0.001.
Relationship between soil microbial entropy and ecological stoichiometry of soil-microorganism C:N:P
The results of the RDA analysis (Figure 2) showed that the first axis (F = 2.8, p = 0.008) and all axes (F = 14.29, p = 0.002) were statistically significantly correlated. qMBC, qMBN, and qMBP explained 73.72% and 26.02% of the variation in soil microbial entropy in the first and second axes, respectively. The results showed that the first two axes could well reflect the relationship between soil microbial entropy and soil stoichiometry imbalance, and that was mainly determined by the first axis. Among them, N:Pimb (p = 0.014), C:Nimb (p = 0.014) and C:Psoil (p = 0.028) had the most significant effect on microbial entropy. qMBC was positively correlated with C:Nmic, C:Psoil, N:Psoil, C:Pmic and N:Pmic, but negatively correlated with C:Nimb and N:Pimb. qMBN was positively correlated with C:Nimb and N:Pmic, but negatively correlated with N:Pimb, C:Nmic, C:Psoil, N:Psoil, C:Pmic. qMBP was positively correlated with N:Pimb, C:Nmic, C:Psoil, and negatively correlated with N:Psoil, C:Pmic, N:Pmic.
Discussion
Effects of different utilization patterns on MBC, MBN, and MBP in grassland
Grassland has extremely important ecological and production functions (Bai and Cotrufo, 2022), and soil microbial biomass is an important indicator of soil development and nutrient cycling (Bastida et al., 2021). In this study, MBC, MBN, and MBP contents were different with different grassland utilization. The MBC and MBN content in GG was the highest, which may be related to that grazing livestock intensifies disturbed soil environment, promotes the accumulation of soil C and N, and improves soil organic matter and biological activity, which is consistent with the research results of Li et al. (2023). However, some studies suggest that increasing grazing intensity significantly reduces the MBN levels (Song et al., 2008). As the grazing intensity in this study was moderate, the MBN content was not affected by the grazing disturbance, but played a facilitating role, which also showed that moderate grazing will increase MBN. In this study, the MBP content in EG was significantly higher than that in GG and MG, which was closely related to the vegetation change, livestock influence, soil nutrients loss, and the litter return in the process of grassland utilization. In the process of grazing and mowing, soil nutrients, vegetation types and microbial activities will change, and the influence of artificial grazing and trampling may be the reason for the decrease in soil microbial biomass, which is consistent with the research results of Wu et al. (2019) in desert grassland of Ningxia, but inconsistent with the research of Chen (2018), who believed that enclosure measures increased P demand, and decreased soil available P content, ultimately reducing the P content of microbial biomass. The reason for the opposite results may be related to the enclosure time and environmental factors. This study was conducted in the subtropical zone of China, while Chen’s (2018) study was conducted in Inner Mongolia of northern China. The results also showed that soil microorganisms under different grassland use patterns would show different microbial activity and material metabolism ability, thus showing different MBC, MBN, MBP content, and ultimately affect the development trend of plant and soil environment.
Effects of different utilization patterns on soil microbial entropy and soil-microorganism stoichiometry imbalance in grassland
Soil microbial entropy can reflect the microbial biomass that can be supported by a unit of soil resources, reflect differences in soil nutrients and utilization efficiency, and reveal differences in soil fertility (Anderson, 2003). In general, the soil microbial entropy is greater, soil nutrient accumulation is greater, and soil nutrient loss is greater (Sparling, 1992). In this study, the changes in qMBC, qMBN, and qMBP were different in different utilization modes. The microbial activity in the soil was greatly activated by the artificial application of GG and MG, and the qMBC was greatly increased, which was significantly higher than that of EG. Relatively, qMBN was the highest in GG and the lowest in MG, indicating that N storage increased in GG and decreased in MG. There was no significant difference in qMBP among the three treatments, but EG was the highest, indicating that its P reserve was the highest, while GG was the lowest. A previous studies found that the qMBC, qMBN, and qMBP of 84% of the ecological succession series in 19 ecological succession processes increased with the ecological succession process, indicating that the variation in soil microbial entropy can largely reflect the evolution of soil quality (Zhou and Wang, 2016). In this study, qMBN was relatively high, while qMBC and qMBP were relatively low under different grassland use patterns, indicating that C and P were relatively poor in this study area. In order to maintain C, P and other nutrients needed for plant growth, it is necessary to increase the proportion of microbial biomass in C and P to maintain a higher material metabolism capacity. With the mowing of grassland vegetation and the feeding of livestock, the grazing grassland and the mowing grassland require more C, N, P and other nutrients to accelerate the activity of SOC, which promotes the faster rate of conversion of SOC into microbial biomass, and thus the microbial entropy increases. We also found that artificial utilization measures can improve qMBC, but qMBN and qMBP need to be analyzed according to specific conditions.
Studies have shown that the higher the soil-microorganism stoichiometry imbalance value, the worse the soil quality, and the lower the growth utilization efficiency of microorganisms (Zhou and Wang, 2016). In the study, the stoichiometry imbalance of C:Nimb, C:Pimb and N:Pimb in soil-microorganism was different under different utilization patterns. EG had the highest mean value of stoichiometric imbalance, indicating that the soil quality was poor and the growth and utilization efficiency of microorganisms was lower, while MG had the lowest mean value of stoichiometry imbalance, indicating that the soil quality was good and the growth and utilization efficiency of microorganisms was higher.
Coupling relationship between soil microbial biomass and soil-microorganism stoichiometry imbalance
MBC, MBN, and MBP were closely related to C:Pimb, C:Pimb and N:Pimb under different grassland use patterns. MBC and MBP were positively correlated, indicating that MBC and MBP had the same response to grassland utilization, and were strongly influenced by each other. The main reason may be that MBC and MBP are derived from the direct transformation of soil organic matter, and the transformation rate and decomposition amount of SOC and TP affect MBC and MBP. MBC and MBN were negatively correlated, which is inconsistent with the research results of northwestern Loess Plateau and Zoige sandy grassland in western Sichuan (Zhou and Wang, 2016; Zhong et al., 2017), which may be mainly related to the influence of grazing livestock, fertilization and other factors on grassland soil. The MBC was negatively correlated with C:Pimb, that MBN was significantly positively correlated with C:Nimb and C:Pimb, and the MBP was significantly negatively correlated with N:Pimb. It can be seen that the decrease or increase of soil MBC would cause the change of MBC:MBN, MBC:MBP, MBN:MBP, and further lead to the increase of soil and microorganism stoichiometry imbalance. Our results are consistent with those of Wu et al. (2019) and Zhou and Wang (2016), that microbes can adapt to changes in soil-microorganism stoichiometry imbalance by adjusting their own biomass.
Effect of soil-microorganism C:N:P stoichiometry on soil microbial entropy
The soil-microorganism stoichiometry imbalance can reflect the adaptation of microorganisms to soil nutrient fluctuations and the relationship between the two in the synergistic regulation of ecosystem nutrient dynamics balance (Mooshammer et al., 2014; Wu et al., 2019). In this study, the effects of the microbial ecological stoichiometry and soil-microorganism stoichiometry imbalance on soil microbial entropy were different under different utilization patterns. The qMBC was positively correlated with C:Nmic, C:Psoil, N:Psoil, C:Pmic and N:Pmic, and negatively correlated with C:Nimb and N:Pimb, which was mainly due to the coordinated supply of soil C, N, and P nutrients required for the growth and metabolism of soil microorganisms (Zhou and Wang, 2015), which is consistent with the findings of Xu et al. (2014) and Zhou and Wang (2015). The qMBN was positively correlated with C:Nimb and N:Pmic, but negatively correlated with N:Pimb, C:Nmic, C:Psoil, N:Psoil, C:Pmic. The qMBP was positively correlated with N:Pimb, C:Nmic, C:Psoil, but negatively correlated with N:Psoil, C:Pmic, N:Pmic, which was consistent with the findings of Xu et al. (2014) and Zhang et al. (2023). The results showed that the balance among microbial element utilization had a certain influence on the cycling of C, N, and P in the ecosystem (Zhang et al., 2023). In this study, under relatively high soil C:Nsoil and relatively low C:Psoil of EG, the microbial growth will be limited by N, resulting in the decrease of qMBC compared with GG and MG. Therefore, the decrease of microbial entropy in EG compared with GG and MG in this study may be related to the limitation of soil N, which is consistent with previous studies (Wu et al., 2019; Zhang et al., 2023). In general, the variation characteristics of soil microbial biomass, microbial entropy and stoichiometry imbalance can directly or indirectly reflect the soil quality of grassland under different utilization patterns, and provide scientific reference for sustainable management of degraded ecosystem grassland in the karst desertification area.
Conclusion
This study was investigated soil microbial biomass, microbial entropy and soil-microorganism stoichiometry imbalance of artificial grassland under different utilization patterns in the karst control area. We came to the following conclusion: Soil microbes showed different microbial activity and ability to metabolize materials under different grassland use patterns with different MBC, MBN, MBP content. The content of MBC and MBN was highest in GG, and the MBP content was highest in EG. GG and MG treatments strongly activated soil microbial activity, and significantly increased qMBC, which was significantly higher than that in EG, indicating that artificial utilization measures can improve qMBC. The mean value of soil-microorganism stoichiometry imbalance in EG was the highest, while that in MG was the lowest. The decrease or increase of MBC content would lead to the change of MBC:MBN, MBC:MBP, MBN:MBP, and finally lead to the increase of soil and microbial stoichiometry imbalance. N:Pimb (p = 0.014), C:Nimb (p = 0.014) and C:Psoil (p = 0.028) had the most significant effect on microbial entropy. The results of this study may directly or indirectly reflect the status of grassland soil quality under different utilization patterns in the karst desertification area, and have some reference value for the study of degraded ecosystem restoration.
Data availability statement
The datasets presented in this study can be found in online repositories. The names of the repository/repositories and accession number(s) can be found at: https://doi.org/10.6084/m9.figshare.24129651.
Author contributions
YC: Conceptualization, Data curation, Funding acquisition, Investigation, Resources, Visualization, Writing – original draft, Writing – review & editing, Formal analysis, Methodology, Project administration, Software, Supervision, Validation. SS: Conceptualization, Data curation, Validation, Visualization, Writing – original draft, Formal analysis, Funding acquisition, Project administration, Resources. KX: Conceptualization, Formal analysis, Funding acquisition, Investigation, Supervision, Writing – original draft. GA: Data curation, Formal analysis, Funding acquisition, Visualization, Writing – original draft, Writing – review & editing. JF: Supervision, Validation.
Funding
The author(s) declare financial support was received for the research, authorship, and/or publication of this article. This research was funded by the Oversea Expertise Introduction Program for Discipline Innovation of China (No. D17016); The Guiding Fund Project of Government’s Science and Technology (No. Qian Ke He Zhong Yin Di [2023]005); Research supporting project (RSP-2023/95, King Saud University, Riyadh, Saudi Arabia); Academic Talent Plan of Guizhou Normal University (No. Qian Shi Xin Miao [2022]B31; [2021]B15); Natural Science Research Project of Education Department of Guizhou Province [Qianjiaohe KY Zi (2022) 157].
Conflict of interest
The authors declare that the research was conducted in the absence of any commercial or financial relationships that could be construed as a potential conflict of interest.
Publisher’s note
All claims expressed in this article are solely those of the authors and do not necessarily represent those of their affiliated organizations, or those of the publisher, the editors and the reviewers. Any product that may be evaluated in this article, or claim that may be made by its manufacturer, is not guaranteed or endorsed by the publisher.
References
Anderson, T. H. (2003). Microbial eco-physiological indicators to asses soil quality. Agric. Ecosyst. Environ. 98, 285–293. doi: 10.1016/S0167-8809(03)00088-4
Anderson, T. H., and Domsch, K. H. (1990). Application of eco-physiological quotients (qCO2 and qD) on microbial biomasses from soils of different cropping histories. Soil Biol. Biochem. 22, 251–255. doi: 10.1016/0038-0717(90)90094-G
Bahram, M., Hildebrand, F., Forslund, S. K., Anderson, J. L., Soudzilovskaia, N. A., Bodegom, P. M., et al. (2018). Structure and function of the global topsoil microbiome. Nature 560, 233–237. doi: 10.1038/s41586-018-0386-6
Bai, Y. F., and Cotrufo, M. F. (2022). Grassland soil carbon sequestration: current understanding, challenges, and solutions. Science 377, 603–608. doi: 10.1126/science.abo2380
Bastida, F., Eldridge, D. J., García, C., Kenny Png, G., Bardgett, R. D., and Delgado-Baquerizo, M. (2021). Soil microbial diversity-biomass relationships are driven by soil carbon content across global biomes. ISME J. 15, 2081–2091. doi: 10.1038/s41396-021-00906-0
Chen, H. (2018). “Impact of water status on soil microbial biomass and community structure in typical grasslands of Inner Mongolia” in PhD Thesis (Beijing: Agricultural University)
Chen, H. J., Peng, W. X., Du, H., Song, T. Q., Zeng, F. P., and Wang, F. (2020). Effect of different grain for green approaches on soil bacterial community in a karst region. Front. Microbiol. 11:577242. doi: 10.3389/fmicb.2020.577242
Ding, M. M., Yi, W. M., Liao, L. Y., Martens, R., and Insam, H. (1992). Effect of afforestation on microbial biomass and activity in soils of tropical China. Soil Biol. Biochem. 24, 865–872. doi: 10.1016/0038-0717(92)90007-K
Falkowski, P. G., Fenchel, T., and Delong, E. F. (2008). The microbial engines that drive Earth’s biogeochemical cycles. Science 320, 1034–1039. doi: 10.1126/science.1153213
Hartmann, M., and Six, J. (2023). Soil structure and microbiome functions in agroecosystems. Nat. Rev. Earth Environ. 4, 4–18. doi: 10.1038/s43017-022-00366-w
He, S. Y., Xiong, K. N., Song, S. Z., Chi, Y. K., Fang, J. Z., and He, C. (2023). Research progress of grassland ecosystem structure and stability and inspiration for improving its service capacity in the karst desertification control. Plants 12:770. doi: 10.3390/plants12040770
Heuck, C., Weig, A., and Spohn, M. (2015). Soil microbial biomass C:N:P stoichiometry and microbial use of organic phosphorus. Soil Biol. Biochem. 85, 119–129. doi: 10.1016/j.soilbio.2015.02.029
Hu, C. J., Guo, L., and Liu, G. H. (2014). Soil microbial community structure under different vegetation restoration patterns in the loess hilly area. Acta Ecol. Sin. 34, 2986–2995. doi: 10.5846/stxb201306081455
Ji, J. J., Zheng, W., Yang, Z. J., Wang, Q. C., Xiong, D. C., Xu, C., et al. (2020). Effects of subtropical forest conversion on soil microbial respiration and its entropy. Acta Ecol. Sin. 40, 800–807. doi: 10.5846/stxb201810142226
Lei, J. J., Cao, Y. X., Wang, J., Chen, Y. Z., Peng, Y. Y., Shao, Q. W., et al. (2023). Soil nutrients, enzyme activities, and microbial communities along a chronosequence of Chinese fir plantations in subtropical China. Plants 12:1931. doi: 10.3390/plants12101931
Li, S. Y., Cui, Y. X., Sun, Z. J., Liu, H. X., and Ye, H. W. (2023). Effect of grazing exclusion on soil organic carbon and stoichiometry characteristics of soil microbial biomass in sagebrush desert. Acta Pratacu. Sin. 32, 58–70. doi: 10.11686/cyxb2022267
Li, L. J., Zhu-Barker, X., Ye, R. Z., Doane, T. A., and Horwath, W. R. (2018). Soil microbial biomass size and soil carbon influence the priming effect from carbon inputs depending on nitrogen availability. Soil Biol. Biochem. 119, 41–49. doi: 10.1016/j.soilbio.2018.01.003
Lin, X. G. (2010). Principles and methods of soil microbiology research. Beijing, Higher Education Press.
Lu, M., Liu, K., Zhang, L., Zeng, F., Song, T., Peng, W., et al. (2022). Stoichiometric variation in soil carbon, nitrogen, and phosphorus following cropland conversion to forest in Southwest China. Forests 13:1155. doi: 10.3390/f13081155
Marschner, P., and Rengel, Z. (2007). Nutrient cycling in terrestrial ecosystem. Springer, Berlin, 159–182
Mooshammer, M., Wanek, W., Zechmeister-Boltenstern, S., and Richter, A. (2014). Stoichiometric imbalances between terrestrial decomposer communities and their resources: mechanisms and implications of microbial adaptations to their resources. Front. Microbiol. 5:22. doi: 10.3389/fmicb.2014.00022
Nair, A., and Ngouajio, M. (2012). Soil microbial biomass, functional microbial diversity, and nematode community structure as affected by cover crops and compost in an organic vegetable production system. Appl. Soil Ecol. 58, 45–55. doi: 10.1016/j.apsoil.2012.03.008
Pei, G. T., Sun, J. F., He, T. X., and Hu, B. Q. (2021). Effects of long-term human disturbances on soil microbial diversity and community structure in a karst grassland ecosystem of northwestern Guangxi, China. Chin. J. Plant Ecol. 45, 74–84. doi: 10.1038/ismej.2011.32
Rogers, B. F., and Tate, R. L. (2001). Temporal analysis of the soil microbial community along a top sequence in pineland soils. Soil Biol. Biochem. 33, 1389–1401. doi: 10.1016/S0038-0717(01)00044-X
Sardar, M. F., Zhu, C. X., Geng, B., Ahmad, H. R., Song, T. T., and Li, H. N. (2021b). The fate of antibiotic resistance genes in cow manure composting: shaped by temperature-controlled composting stages. Bioresour. Technol. 320:124403. doi: 10.1016/j.biortech.2020.124403
Sardar, M. F., Zhu, C. X., Geng, B., Huang, Y. L., Abbasi, B., Zhang, Z. G., et al. (2021a). Enhanced control of sulfonamide resistance genes and host bacteria during thermophilic aerobic composting of cow manure. Environ. Pollut. 275:116587. doi: 10.1016/j.envpol.2021.116587
Song, J. F., Han, G. D., Zhang, G., and Wu, C. Y. (2008). The effects of grazing intensity on soil microorganisms and soil microbial biomass in meadow steppe. J. Inner Mongolia Normal Univ. 130, 237–240.
Song, M., Peng, W. X., Du, H., and Xu, Q. G. (2019). Responses of soil and microbial C:N:P stoichiometry to vegetation succession in a karst region of Southwest China. Forests 10:755. doi: 10.3390/f10090755
Song, S. Z., Wang, X. L., He, C., and Chi, Y. K. (2023). Effects of utilization methods on C, N, P rate and enzyme activity of artificial grassland in karst desertification area. Agronomy 13:1368. doi: 10.3390/agronomy13051368
Song, S. Z., Xiong, K. N., Chi, Y. K., He, C., Fang, J. Z., and He, S. Y. (2022). Effect of cultivated pastures on soil bacterial communities in the karst rocky desertification area. Front. Microbiol. 13:922989. doi: 10.3389/fmicb.2022.922989
Sparling, G. P. (1992). Ratio of microbial biomass carbon to soil organic carbon as a sensitive indicator of changes in soil organic matter. Soil Res. 30, 195–207. doi: 10.1071/SR9920195
Wen, L., Li, D., Xiao, X., and Tang, H. M. (2023). Alterations in soil microbial phospholipid fatty acid profile with soil depth following cropland conversion in karst region, Southwest China. Environ. Sci. Pollut. Res. 30, 1502–1519. doi: 10.1007/s11356-022-22178-7
Wu, X. Z., Liu, B. R., Yan, X., Liu, R. T., and An, H. (2019). Response of soil microbial biomass and microbial entropy to desertification in desert grassland. Chin. J. Appl. Ecol. 30, 2691–2698. doi: 10.13287/j.1001-9332.201908.009
Xiong, K. N., Li, P., Zhou, Z. F., An, Y. L., Lv, T., and Lan, A. J. (2002). Remote sensing of karst rocky desertification: a typical GIS study—taking Guizhou province as an example. Beijing, Beijing Geological Press.
Xiong, K. N., Zhu, D. Y., Peng, T., Yu, L. F., Xue, J. H., and Li, P. (2016). Study on ecological industry technology and demonstration for karst rocky desertification control of the karst plateau-gorge. Acta Ecol. Sin. 36, 7109–7113. doi: 10.5846/stxb201610172104
Xu, X. F., Schimel, J. P., Thornton, P. E., Song, X., Yuan, F. M., and Goswami, S. (2014). Substrate and environmental controls on microbial assimilation of soil organic carbon: a framework for earth system models. Ecol. Lett. 17, 547–555. doi: 10.1111/ele.12254
Zechmeister-Boltenstern, S., Keiblinger, K. M., Mooshammer, M., Peñuelas, J., Richter, A., Sardans, J., et al. (2015). The application of ecological stoichiometry to plant-microbial-soil organic matter transformations. Ecol. Monogr. 85, 133–155. doi: 10.1890/14-0777.1
Zhang, J. Y., Ai, Z. M., Liang, C. T., Wang, G. L., Liu, G. B., and Xue, S. (2019). How microbes cope with short-term N addition in a Pinus tabuliformis forest-ecological stoichiometry. Geoderma 337, 630–640. doi: 10.1016/j.geoderma.2018.10.017
Zhang, G. H., Niu, J., Yi, L., Sun, B. Y., Li, J. M., and Xiao, H. (2023). Ecological stoichiometry of soil and microbial biomass carbon, nitrogen and phosphorus in tea plantations with different ages. Chin. J. Appl. Ecol. 34, 969–976. doi: 10.13287/j.1001-9332.202304.015
Zhang, L. Q., Peng, W. X., Song, T. Q., Zou, D. S., Zeng, F. P., Song, M., et al. (2012). Spatial heterogeneity of soil microbial biomass carbon, nitrogen, and phosphorus in sloping farmland in a karst region on the Yunnan-Guizhou plateau. Acta Ecol. Sin. 32, 2056–2065. doi: 10.5846/stxb201108171204
Zhao, Z. B., He, J. Z., Geisen, S., Han, L. L., Wang, J. T., Shen, J. P., et al. (2019). Protist communities are more sensitive to nitrogen fertilization than other microorganisms in diverse agricultural soils. Microbiome 7:33. doi: 10.1186/s40168-019-0647-0
Zhong, B., Sun, G., Chen, D. M., and Zhang, N. N. (2017). Effects of different restoration measures on soil microbial biomass carbon and nitrogen and soil enzymes in the process of restoration of the desertified grassland in Zoige. Ecol. Environ. Sci. 26, 392–399. doi: 10.16258/j.cnki.1674-5906.2017.03.005
Zhou, Z. H., and Wang, C. K. (2015). Soil resources and climate jointly drive variations in microbial biomass carbon and nitrogen in China’s forest ecosystems. Biogeosciences 12, 6751–6760. doi: 10.5194/bg-12-6751-2015
Keywords: karst desertification, artificial grassland, soil microbial biomass, microbial entropy, stoichiometry imbalance
Citation: Chi Y, Song S, Xiong K, Albasher G and Fang J (2023) Responses of soil microbial biomass, microbial entropy and soil-microorganism stoichiometry imbalance to different utilization patterns in the artificial grassland of karst desertification area. Front. Microbiol. 14:1293353. doi: 10.3389/fmicb.2023.1293353
Edited by:
Marika Pellegrini, University of L’Aquila, ItalyReviewed by:
Asit Mandal, Indian Institute of Soil Science (ICAR), IndiaDebasis Mitra, National Rice Research Institute (ICAR), India
Copyright © 2023 Chi, Song, Xiong, Albasher and Fang. This is an open-access article distributed under the terms of the Creative Commons Attribution License (CC BY). The use, distribution or reproduction in other forums is permitted, provided the original author(s) and the copyright owner(s) are credited and that the original publication in this journal is cited, in accordance with accepted academic practice. No use, distribution or reproduction is permitted which does not comply with these terms.
*Correspondence: Kangning Xiong, eGlvbmdrbkBnem51LmVkdS5jbg==
†These authors have contributed equally to this work and share first authorship