- 1Department of Microbiology, University of Dhaka, Dhaka, Bangladesh
- 2Department of Pharmacy, Faculty of Allied Health Sciences, Daffodil International University, Dhaka, Bangladesh
- 3Department of Microbiology, Jashore University of Science and Technology, Jessore, Bangladesh
- 4Stamford University Bangladesh, Dhaka, Bangladesh
- 5Department of Pharmacy, State University, Dhaka, Bangladesh
- 6Department of Biology, Bahir Dar University, Bahir Dar, Ethiopia
- 7Department of Botany and Microbiology, College of Sciences, King Saud University, Riyadh, Saudi Arabia
- 8Department of Food Science, Faculty of Agricultural and Food Sciences, Laval University, Quebec City, QC, Canada
- 9Department of Chemistry and Biochemistry, Faculty of Medicine and Pharmacy, Ibn Zohr University, Laayoune, Morocco
Wastewater discharged from hospitals is a recognized contributor to the dissemination of antibiotic-resistant bacteria and their associated genetic traits into the environment. This study focused on the analysis of β-lactamase-producing pathogenic bacteria within untreated biomedical wastewater originating from various hospitals in Dhaka City, Bangladesh, as well as in silico evaluation and structural activity relationship mentioned antibiotics were evaluated. In silico drug design techniques were applied to identify the relationship with how the functional group impacts the binding energy. Out of the 184 isolates obtained from well-established hospital sewage discharge points in Dhaka, 89 were identified as β-lactamase positive. These bacteria were subjected to antimicrobial susceptibility testing using the VITEK-2 assay, and their profiles of extended-spectrum beta-lactamase (ESBL) production were determined through molecular methodologies. Among the β-lactamase-positive isolates, considerable resistance was observed, particularly against ampicillin, Ceftriaxone, Cefuroxime, and Meropenem. The predominant resistant species included Escherichia coli, Acinetobacter baumannii, Pseudomonas aeruginosa, and Enterobacter cloacae. The study identified the prevalence of ESBL-producing genes, with blaNDM-1 being the most prevalent, followed by blaOXA-1, blaSHV, blaCTX-M, and blaKPC. None of the isolates carried the blaTEM gene. In addition to characterizing these bacteria, the research explored ways to enhance the binding energy of four existing antibiotics as new inhibitors through computational studies. The findings revealed significant improvements in binding energy. Specifically, Meropenem initially exhibited a binding energy of −7.5 kcal/mol, notably increasing to −8.3 kcal/mol after modification. With an initial binding energy was only −7.9 kcal/mol, Ampicillin experienced an enhancement, reaching −8.0 kcal/mol post-modification. Similarly, Ceftriaxone, with an initial binding energy of −8.2 kcal/mol, increased to −8.5 kcal/mol following structural adjustments. Finally, Cefuroxime, initially registering a binding energy of −7.1 kcal/mol, substantially increased to −8.9 kcal/mol after modification. This finding establishes a foundation for future investigations in the development of modified antibiotics to address the issue of antibiotic resistance. It presents prospective remedies for the persistent problem of antibiotic-resistant bacteria in healthcare and the environment.
1 Introduction
In densely populated urban areas like Dhaka City, the management of biomedical effluents poses a serious challenge due to the vast quantities of waste generated by healthcare facilities. Hospital wastewater generally contains a high concentration of biochemical oxygen demand (BOD), chemical oxygen demand (COD), total organic carbon (TOC), ammonia nitrogen, organic nitrogen, nitrites, nitrates, total phosphorus, as well as total solids (Emmanuel et al., 2005; Akin, 2016; Young and Lipták, 2018). In addition, it also acts as a reservoir for a significant concentration of pathogens (bacteria, viruses, protozoa, and fungi), antibiotic-resistance genes (ARGs), and antibiotic-resistant bacteria (Weiner et al., 2016). It has been observed that hospital effluents discharged into the sewer system, typically without pre-treatment, can act as a potential source of antimicrobial residues and antibiotic-resistant bacteria for the wastewater treatment plant (WWTP) (Deguenon et al., 2022). In healthcare settings, the emergence and spread of pathogenic bacteria that produce β-lactamases have become a severe issue because they can cause resistance to commonly used β-lactam antibiotics like penicillin. Multidrug-resistant ESKAPE bacteria and Escherichia coli (E. coli) cause cephalosporin-resistant infections. These infections are the majority of life-threatening bacterial infections among critically ill and immunocompromised patients worldwide. The ESKAPE bacteria include Enterococcus spp., Staphylococcus aureus, Klebsiella pneumoniae, Acinetobacter baumannii, Pseudomonas aeruginosa, and Enterobacter spp. Therefore, the current study was primarily done to identify the occurrence and characterize the pathogenic bacteria producing β-lactamase isolated from the biomedical, along with E. coli (Weiner et al., 2016). The most remarkable resistance mechanism among Gram-negative bacteria to the selective pressure of antibiotics is caused by β-lactamase enzymes. The genes that confer resistance can be transferred either vertically (to bacterial offspring) or horizontally (among different bacterial species) (Adekunle, 2012; Baquero et al., 2021). ESBL enzymes can be classified into around 300 subtypes, and among them, genes encoding blaCTX, blaTEM, blaOXA, and blaSHV are the most common types (Korzeniewska and Harnisz, 2013). The carbapenemase family of β-lactamases (a distinct subgroup) is one of the most threatening enzymes responsible for the resistance of the aforementioned strains. Several different forms of genes encode carbapenemase found in Enterobacteriaceae, including blaKPC and blaNDM-1 (El Salabi et al., 2013).
These enzymes have been found in numerous Enterobacteria species that have been isolated in hospitals worldwide. E. coli and Klebsiella pneumoniae were the first organisms to contain plasmids that encoded the blaNDM-1 gene; nevertheless, it has been demonstrated that these plasmids may be transferred to other species, aiding in the spread of the blaNDM-1 gene to other species as well. The prevalence of β-lactamase enzymes has steadily risen, leading to the reduced efficacy of many commonly prescribed antibiotics (Bush, 2010; Dortet et al., 2014). This phenomenon seriously affects public health, as it limits the treatment options available to medical professionals and increases the risk of infections becoming untreatable. Identifying the presence and understanding of the characteristics of pathogenic bacteria producing β-lactamase in hospital wastewater is of utmost importance to develop effective strategies for combating antibiotic resistance and safeguarding public health (Muteeb et al., 2022a; Irfan et al., 2023).
Moreover, the transmission of multidrug-resistant bacteria has become a severe threat in Bangladesh, as antibiotics can be easily purchased without a prescription from a registered physician (Islam, 2021). As hospital effluents can harbor different pathogens, they can act as reservoirs for superbugs. This study aimed to identify and characterize pathogenic bacteria producing β-lactamase in biomedical wastewater samples from Dhaka City hospitals. Additionally, we employed computational methods to enhance the antibacterial effectiveness of four commonly encountered antibiotics: Meropenem (100% resistance), Ampicillin (80–93% resistance), Ceftriaxone (73–100% resistance), and Cefuroxime (80–95% resistance). These modifications were designed to inhibit the growth of E. coli bacteria and reduce the likelihood of antibiotic resistance development in this pathogen.
Since these antibiotics are almost unable to fight against bacteria. Our work utilizes in silico approaches to evaluate the effects of functional groups on binding affinity, in light of the inadequate efficacy of Meropenem, Ampicillin, Ceftriaxone, and Cefuroxime against bacteria. β-lactamase-producing bacteria in biomedical wastewater often develop resistance to these antibiotics. Therefore, it is necessary to investigate improved formulations of these antibiotics to improve their effectiveness against bacteria. Our goal is to analyze the structural and chemical factors that affect binding interactions. This analysis will help us identify ways to enhance the therapeutic properties of these antibiotics. By accomplishing so, we can tackle the problem of bacterial resistance and improve their effectiveness in healthcare and environmental settings.
2 Materials and methods
2.1 Sample collection
A total of 300 sewage samples were collected from the wastewater disposal units of both public and private hospitals in Dhaka City between December 2022 and March 2023. The samples were acquired on weekdays in the morning, from 8: 00 AM to 11: 00 AM, and stored in 5 mL sterile Falcon tubes, each labeled according to its location. During transportation to the laboratory, the samples were kept in an icebox and processed within 2 h upon arrival.
2.2 Growth in nutrient agar
Samples obtained from hospitals were streaked onto nutrient agar medium (HiMedia) containing 2 and 4 μg/mL of Meropenem (Opsonin Pharmaceuticals, Bangladesh). Subsequently, they were incubated at 37°C for 24–48 h under aerobic conditions. Colonies that grew under meropenem selection were sub-cultured on nutrient agar and reserved for later stages of the study.
2.3 Identification of organisms using VITEK 2
For identification through the VITEK 2 platform, single colonies were streaked onto nutrient agar plates and incubated at 37°C overnight. One to three pure and distinct colonies were selected from each agar plate and suspended in normal saline to prepare an inoculum with an absorbency of approximately 0.5 McFarland standard before use in the assay. Bacterial strains were identified using the VITEK 2 GN ID card. As a positive control, Enterobacter hormaechei (ATCC-700323) was employed.
2.4 Antibiotic susceptibility test
The antibiotic susceptibility profile of the respective isolates was determined for 19 antimicrobial agents following CLSI guidelines and the manufacturer’s recommendations. The VITEK 2 system with VITEK 2 Cards (AST N280) was utilized for this analysis. Enterbacter hormaechei strain LBM 93.03.067 (ATCC-700323), susceptible to all drugs, served as the quality control for the system. The panel (bioMérieux) of 19 tested antibiotics included amikacin, amoxicillin/clavulanic acid, ampicillin, cefepime, cefoperazone/sulbactam, ceftazidime, Ceftriaxone, Cefuroxime, Cefuroxime Axetil, ciprofloxacin, co-trimoxazole, colistin, ertapenem, gentamicin, imipenem, Meropenem, nalidixic acid, nitrofurantoin, piperacillin, and tigecycline. The isolates were categorized as resistant, intermediate, or susceptible based on the minimum inhibitory concentration of the antibiotics, following CLSI guidelines. Bacterial strains displaying resistance to three or more antibiotic classes were classified as multidrug-resistant. These all antibiotic were taken from Opsonin pharmaceuticals, Bangladesh.
2.5 Characterization of ESBL and carbapenemase genes
All the confirmed β-lactamase-positive isolates were screened for the presence of molecular determinants of ESBL and carbapenem resistance, including the blaCTX, blaTEM, blaOXA1, blaSHV, blaNDM-1, and blaKPC families. The screening was conducted using a thermal cycler following a published protocol, with minor optimizations made for the primer annealing temperature. The optimal annealing temperature for each primer (Macrogen inc) was determined through a temperature gradient test ranging from 55 to 65°C, as detailed in Table 1.
2.6 Ligand preparation and optimization
The initial structures of Meropenem, Ampicillin, Ceftriaxone, and Cefuroxime were obtained from the PubChem database. Subsequently, we introduced modifications by adding a benzene ring and a -COOH group, as illustrated in Figure 1, using the ChemDraw application. These altered ligand structures were then imported into Gaussian 16 software. For optimization, we employed the hybrid B3LYP exchange-correlation functional in conjunction with the 6-311G(d) basis set, as implemented in Gaussian 16. Semi-core pseudo-potentials were also considered in density-functional theory (DFT) method (Obot et al., 2015; Ribeiro et al., 2015). Finally, the structures were saved in pdb format for subsequent molecular docking analysis.
2.7 Protein preparation and molecular docking studies
Molecular docking analysis is one of the essential method to predict the binding energy of drug protein complexes (Muteeb et al., 2022b). So, in this investigation, we applied molecular docking with our modified ligand and targeted E. coli bacterial protein (PDB ID: 1BTL) (Figure 2). Prior to experiment of molecular docking, the protein was acquired in PDB format from the RCSB Protein Data Bank and prepared using Discovery Studio. Subsequently, molecular docking analysis was conducted using PyRx, with grid centre points set at X = −11.873884, Y = −24.3376, Z = 19.0138, and box dimensions specified as X = 29.7641, Y = 27.76183, Z = 28.766. Finally, BIOVIA Discovery Studio Visualizer and ChimeraX were instrumental in visualizing the active amino acid residues (Dallakyan and Olson, 2015; Ahmad et al., 2022).
3 Result analysis
3.1 Bacterial screening
A total of 184 isolated bacterial colonies were obtained under meropenem selection. These isolates were further sub-cultured on nutrient agar medium and identified using VITEK-2.0 system. Of the 184 isolates, 48.4% (n = 89) were found to be beta-lactamase positive. The most prominent species identified in hospital effluents throughout the study period were Escherichia coli (42.7%, n = 38), Acinetobacter baumanii (25.9%, n = 23), Pseudomonas aeruginosa (25.9%, n = 23) and Enterobacter cloacae (5.6%, n = 5) (Figure 3).
3.2 Antibiogram of Acinetobacter baumannii
In the case of A. baumannii, the highest resistance level was observed against Meropenem, closely followed by ampicillin (with an 87% resistance rate). Notably, all of the isolated strains of this bacterial species exhibited multidrug resistance, as they displayed resistance to antibiotics from three distinct classes (Figure 4).
3.3 Antibiogram of Pseudomonas aeruginosa
Meropenem-resistant Pseudomonas aeruginosa strains exhibited increased resistance to three distinct antibiotic classes. Among these, the most pronounced resistance was observed against ampicillin, with a resistance rate of 94% (as shown in Figure 5). The resistance patterns against other antibiotics were as follows: Ceftriaxone (80%), Cefuroxime (80%), Nalidixic acid (53%), and Cefuroxime axetil (80%).
3.4 Antibiogram of Enterobacter cloacae
Among the five tested Enterobacter cloacae isolates, 100% were resistant to Ceftriaxone, Meropenem, and Cefuroxime Axetil, 80% to ampicillin, 60% to Cefuroxime and nalidixic acid, 40% to co-trimoxazole and nitrofurantoin and 20% to ciprofloxacin and amoxiclav. Multidrug resistance (MDR) was detected in all isolates (100%) as shown in Figure 6.
3.5 Characterization of ESBL and carbapenemase genes
Among all isolates that tested positive for β-lactamase, an examination was conducted to determine the presence of molecular determinants of ESBL genes. Out of the 89 isolates, the two most predominant carbapenemase genes were blaNDM-1 (100%), followed by blaOXA (41.2%). Additionally, other β-lactamase genes, such as asblaSHV (5.9%), blaCTX-M (5.9%) and blaKPC (5.9%), were also detected. Notably, none of the isolates harbored the blaTEM β-lactamase gene.
The NDM producers were predominantly found in members of both Enterobacteriaceae, such as E. coli and Enterobacter cloacae, and non-Enterobacteriaceae families, including Pseudomonas aeruginosa and Acinetobacter baumannii. Furthermore, KPC producers were mainly identified in Acinetobacter baumannii, while the OXA gene was predominantly present in Pseudomonas aeruginosa. In contrast, the distribution of CTX and SHV was less widespread among the aforementioned species (Figure 7).
The gel electrophoresis result shows a molecular weight marker (Gene Ruler 100 bp DNA ladder) in lane L, with the positive control (PC) showing a clear band. Lanes 1 to 16 and 18 exhibit 220 bp PCR product bands, while the negative control (NC-) in lane NC shows no such band (Figure 8).
In Figure 9, lane L contains a molecular weight marker (Gene Ruler 100 bp DNA ladder). Notably, lanes 12, 17, and 21 display distinct 893 bp PCR products.
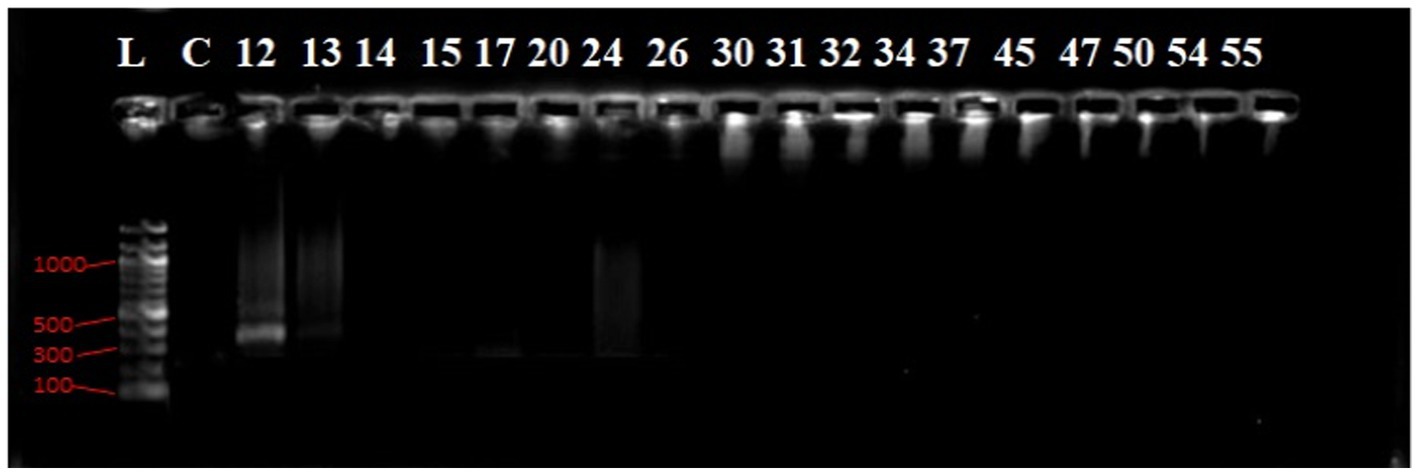
Figure 9. Agarose gel electrophoresis image of the PCR products of blaCTX-M GeneL lane, molecular weight marker; Gene Ruler 100 bp DNA ladder, lanes numbered 12 shows (300 bp) bands of PCR products while lane NC show negative control.
In Figure 10, Lane 10 and 13 reveal 764 bp PCR product bands, while lane NC- represents the negative control.
The gel electropherogram shows a molecular weight marker (Gene Ruler 100 bp DNA ladder) in lane L. The positive control (PC) and PCR products in lanes 15, 17, 20, 24, 26, and 32 exhibit 564 bp bands (Figure 11).
3.6 Molecular docking against Escherichia coli
The molecular docking investigation aimed to determine drug-protein complexes’ binding affinity and active site. In this study, Meropenem (100%), Ampicillin (80–93%), Ceftriaxone (73–100%), and Cefuroxime (80–95%) exhibited the highest levels of resistance against E. coli. Consequently, these four established drugs underwent structural modifications and molecular docking experiments.
The results revealed notable changes in binding energy. Specifically, Meropenem exhibited a binding energy of −7.5 kcal/mol, which increased significantly to −8.3 kcal/mol after modification. Ampicillin showed a binding energy of −7.9 kcal/mol, which improved to −8.0 kcal/mol post-modification. Similarly, Ceftriaxone had an initial binding energy of −8.2 kcal/mol, which rose to −8.5 kcal/mol following structural modification. Lastly, Cefuroxime initially had a binding energy of −7.1 kcal/mol, which substantially increased to −8.9 kcal/mol after modification (Table 2).
These findings underscore the potential for structural enhancements to boost the binding affinity of these antibiotics, thereby offering promising strategies to combat antibiotic resistance effectively.
3.7 Active residue analysis and binding pocket
We conducted an analysis of the active residues within the binding pocket to unveil the potential modes and specific amino acids involved in the formation of drug- protein complex complexes. In Figure 12, it becomes evident that the primary Meropenem featured only five active amino acid residues. However, upon the addition of functional groups, the number of active amino acid residues increased significantly. This augmentation in active amino acid residues led to the establishment of robust bonds between E. coli and Meropenem derivatives.
This observation highlights the dynamic nature of drug-protein interactions, particularly the impact of functional group additions on the binding characteristics. The increased active amino acid residues likely contribute to enhanced binding affinity, which could have implications for the efficacy and specificity of Meropenem derivatives in combating E. coli infections. These findings underscore the importance of the addition of functional group for improved the binding affinity.
Our investigation revealed that the original ampicillin encompassed just six active amino acid residues inside its binding region. However, when introducing a benzene ring in derivatives 02, the count of active amino acid residues detected a substantial rise, reaching a total of 11. Consequently, the incorporation of the benzene ring increased the antibiotic’s affinity for its target. Interestingly, the addition of other functional groups in derivatives 03 resulted in a decrease in the number of active residues, reducing it to five active sites (as shown in Figure 13).
This observation underscores the dynamic nature of ampicillin and its derivatives in interacting with their target proteins. The changes in active amino acid residues upon structural modifications can have important implications for their binding affinity and, consequently, their effectiveness as antibiotics.
In the case of Ceftriaxone and its derivatives, the primary Ceftriaxone molecule exhibits only seven active amino acid residues. However, upon adding a benzene ring and a COOH functional group, the active site expands to encompass 10 residues in the case of derivatives 02 and 09, as illustrated in Figure 14.
In the context of Cefuroxime and its derivatives, there’s a notable observation regarding active amino acid residues. In the case of the primary Cefuroxime molecule, it exhibits a total of 11 active amino acid residues. Interestingly, when we examine derivatives 02, they also demonstrate the same number of active amino acid residues as the primary ligand, which is 11. However, for derivatives 03, there is a slight reduction in the active site, with 10 amino acid residues being involved (as shown in Figure 15).
4 Discussion
According to the literature, hospital effluents have been identified as a possible repository for high concentrations of antimicrobials and microorganisms carrying antimicrobial resistance determinants (Chagas et al., 2011). As the -harboring ESBL can hydrolyze a wide variety of β-lactam antibiotics, including penicillin and cephalosporins, they pose significant hurdles in clinical settings, making the increased incidence of these bacteria a worrying global health concern. According to the findings of this study, there is a high burden of ESBL-producing bacteria in hospital effluents, underscoring the crucial role that healthcare facilities play in the spread of antibiotic resistance. Hospital, may act as hub for the emergence and dissemination of these resistant pathogens, given the high prevalence of ESBL-positive isolates in this environment. Moreover, hospital effluents may contain ESBL pathogens for several reasons, including poor disposal of patient waste that contains antibiotic residues, ineffective wastewater treatment methods, and the transfer of resistant bacteria from patients to the hospital environment. During our study, E. coli was found to be the most common β-lactamase lactamase-producing species in hospital wastewater.
All four bacterial species displayed the highest levels of resistance to MMeropenem (100%), ampicillin (80–93%), Ceftriaxone (73–100%), and Cefuroxime (80–95%), according to the AMR results analysis. Resistance patterns varied amongst isolates; however, E. coli isolates showed the highest levels of resistance, and this finding correlated with previous reports (Rabbani et al., 2017). Furthermore, it was discovered that E. coli has developed resistance to the antibiotic colistin, which is used as a last resort antibiotic. In previous research, urban sludge samples from Dhaka, Bangladesh, were shown to have colistin-resistant E. coli (Islam et al., 2017). On the contrary, considering these four bacterial species, tigecycline, gentamicin, imipenem, ertapenem, and cefotaxime/sulbactam remained the most effective antibiotics as the isolated strains were susceptible to these antibiotics. Increased levels of Enterobacteriaceae-producing carbapenemase have been seen in recent years in both clinical and community settings (Nordmann et al., 2009). While blaNDM-1 was previously exclusively found in E. coli and Klebsiella pneumoniae, current investigations have shown it in various organisms, which is concerning (Walsh et al., 2011). The studies carried out in China and Singapore have similar findings regarding intestinal bacteria having high levels of blaNDM-1in clinical samples (Wang et al., 2013).
Both Enterobacteriaceae (Escherichia coli, Enterobacter cloacae) and Non- Enterobacteriaceae (Pseudomonas aeruginosa, Acinetobacter baumannii) were shown to have blaNDM-1 in our investigation. Till now, the Enterobacteriaceae group and Pseudomonas aeruginosa have been the primary source of blaKPC in clinical settings. However, blaKPC gene was absent in these mentioned bacterial species of our study. In contrast, blaKPC was present only in Acinetobacter baumannii species. The occurrence of blaKPC producing isolates from environment are increasing day by day and they have been recovered from various sources.
Furthermore, this investigation conducted in silico experiments on four highly resistant antibiotics: Meropenem, Ampicillin, Ceftriaxone, and Cefuroxime, with the aim of enhancing their efficacy and ultimately reducing the likelihood of antibacterial resistance. The findings revealed that initially, the binding affinity was significantly lower in each case. However, upon the addition of functional groups, the binding energy gradually increased. In most instances, the benzene ring played a pivotal role in augmenting the binding energy, although the COOH group was also introduced into these four antibiotics.
These results suggest promising strategies for structural modifications to bolster the effectiveness of these antibiotics, thereby offering potential solutions in the ongoing battle against antibiotic resistance. By improving the binding affinity and energy, these modified antibiotics may exhibit enhanced antimicrobial properties, reducing the risk of bacterial resistance development. This in silico approach opens up new avenues for research into combating antibiotic-resistant pathogens and underscores the importance of innovative methods in the field of antibiotic development.
5 Conclusion
In summary, this study was conducted to determine the occurrence and characterization of β-Lactamase-producing Bacteria in Biomedical Wastewater and modified Meropenem, Ampicillin, Ceftriaxone, and Cefuroxime to enhance efficacy of mentioned antibiotics using in silico studies. It is noted that the alarming presence and attributes of pathogenic β-lactamase-producing bacteria in biomedical wastewater samples collected from hospitals in Dhaka City. The detection of these bacteria, along with their antibiotic resistance profiles, underscores a significant public health concern. These pathogens have the potential to disseminate antibiotic resistance genes within the environment, thus compromising the efficacy of β-lactam antibiotics.
These findings emphasize the critical need for robust wastewater management strategies and stringent infection control measures within healthcare facilities to curb the spread of these resistant pathogens.
Furthermore, we also performed computational investigation and improved the efficacy of these mentioned antibiotics so that possible new antibacterial agent could be developed. Besides, the policymakers and healthcare administrators should prioritize the implementation of rigorous infection control policies and the pre-treatment of effluents before releasing them into the environment. This concerted approach is essential to combat the emergence and transmission of multidrug-resistant and ESBL-producing bacteria. In essence, this research underscores the significance of proactive measures in safeguarding public health and combating antibiotic resistance, particularly in densely populated urban areas like Dhaka City, where the management of biomedical effluents presents a significant challenge.
Data availability statement
The original contributions presented in the study are included in the article/supplementary material, further inquiries can be directed to the corresponding authors.
Author contributions
SJM: Conceptualization, Investigation, Writing – original draft, Data curation, Methodology. SA: Conceptualization, Investigation, Writing – original draft, Methodology, Validation. SAJ: Methodology, Writing – original draft, Data curation, Validation. ATS: Writing – original draft, Data curation, Writing – review & editing. KB: Data curation, Project administration, Resources, Validation, Writing – review & editing. MY: Conceptualization, Formal analysis, Supervision, Resources, Writing – review & editing. CRA: Formal analysis, Project administration, Writing – original draft. BS: Investigation, Conceptualization, Writing – original draft, Writing – review & editing, Funding acquisition. TMD: Investigation, Methodology, Writing – review & editing. H-AN: Supervision, Project administration, Funding acquisition. MB: Investigation, Data curation, and Project administration.
Funding
The author(s) declare financial support was received for the research, authorship, and/or publication of this article. This work is financially supported by the Researchers Supporting Project number (RSP2024R197), King Saud University, Riyadh, Saudi Arabia.
Conflict of interest
The authors declare that the research was conducted in the absence of any commercial or financial relationships that could be construed as a potential conflict of interest.
Publisher’s note
All claims expressed in this article are solely those of the authors and do not necessarily represent those of their affiliated organizations, or those of the publisher, the editors and the reviewers. Any product that may be evaluated in this article, or claim that may be made by its manufacturer, is not guaranteed or endorsed by the publisher.
References
Adekunle, O. O. (2012). Mechanisms of antimicrobial resistance in bacteria, general approach. Int. J. Phar. Med. Biol. Sci. 1, 166–187.
Ahmad, R., Rosandy, A. R., Sahidin, I., Ab Ghani, N. S., Noor, N. M., and Baharum, S. N. (2022). Bioassay analysis and molecular docking study revealed the potential medicinal activities of active compounds Polygonumins B, C and D from Polygonum minus (Persicaria minor). Plan. Theory 12:59. doi: 10.3390/plants12010059
Akin, B. S. (2016). Contaminant properties of hospital clinical laboratory wastewater: a physiochemical and microbiological assessment. J. Environ. Prot. 7, 635–642. doi: 10.4236/jep.2016.75057
Baquero, F., Martinez, J. L., Lanza, V. F., Rodríguez-Beltrán, J., Galán, J. C., San Millán, A., et al. (2021). Evolutionary pathways and trajectories in antibiotic resistance. Clin. Microbiol. Rev. 34:e00050-19. doi: 10.1128/CMR.00050-19
Birkett, C. I., Ludlam, H. A., Woodford, N., Brown, D. F., Brown, N. M., Roberts, M. T., et al. (2007). Real-time TaqMan PCR for rapid detection and typing of genes encoding CTX-M extended-spectrum β-lactamases. J. Med. Microbiol. 56, 52–55. doi: 10.1099/jmm.0.46909-0
Bratu, S., Mooty, M., Nichani, S., Landman, D., Gullans, C., Pettinato, B., et al. (2005). Emergence of KPC-possessing Klebsiella pneumoniae in Brooklyn, New York: epidemiology and recommendations for detection. Antimicrob. Agents Chemother. 49, 3018–3020. doi: 10.1128/AAC.49.7.3018-3020.2005
Bush, K. (2010). Alarming β-lactamase-mediated resistance in multidrug-resistant Enterobacteriaceae. Curr. Opin. Microbiol. 13, 558–564. doi: 10.1016/j.mib.2010.09.006
Chagas, T. P. G., Seki, L., Cury, J., Oliveira, J., Dávila, A., Silva, D., et al. (2011). Multiresistance, beta-lactamase-encoding genes and bacterial diversity in hospital wastewater in Rio de Janeiro, Brazil. J. Appl. Microbiol. 111, 572–581. doi: 10.1111/j.1365-2672.2011.05072.x
Dallakyan, S., and Olson, A. J. (2015). Small-molecule library screening by docking with PyRx. Methods Mol. Biol., 243–250. doi: 10.1007/978-1-4939-2269-7_19
Deguenon, E., Dougnon, V., Houssou, V. M. C., Gbotche, E., Ahoyo, R. A., Fabiyi, K., et al. (2022). Hospital effluents as sources of antibiotics residues, resistant bacteria and heavy metals in Benin. SN App. Sci. 4:206. doi: 10.1007/s42452-022-05095-9
Dortet, L., Poirel, L., and Nordmann, P. (2014). Worldwide dissemination of the NDM-type carbapenemases in gram-negative bacteria. Biomed. Res. Int. 2014, 1–12. doi: 10.1155/2014/249856
El Salabi, A., Walsh, T. R., and Chouchani, C. (2013). Extended spectrum β-lactamases, carbapenemases and mobile genetic elements responsible for antibiotics resistance in gram-negative bacteria. Crit. Rev. Microbiol. 39, 113–122. doi: 10.3109/1040841X.2012.691870
Emmanuel, E., Perrodin, Y., Keck, G., Blanchard, J.-M., and Vermande, P. (2005). Ecotoxicological risk assessment of hospital wastewater: a proposed framework for raw effluents discharging into urban sewer network. J. Hazard. Mater. 117, 1–11. doi: 10.1016/j.jhazmat.2004.08.032
Haller, L., Chen, H., Ng, C., Le, T. H., Koh, T. H., Barkham, T., et al. (2018). Occurrence and characteristics of extended-spectrum β-lactamase-and carbapenemase-producing bacteria from hospital effluents in Singapore. Sci. Total Environ. 615, 1119–1125. doi: 10.1016/j.scitotenv.2017.09.217
Irfan, M., Almotiri, A., and AlZeyadi, Z. A. (2023). Antimicrobial resistance and β-lactamase production in clinically significant gram-negative Bacteria isolated from hospital and municipal wastewater. Antibiotics 12:653. doi: 10.3390/antibiotics12040653
Islam, M. M. (2021). Bacterial resistance to antibiotics: access, excess, and awareness in Bangladesh. Expert Rev. Anti-Infect. Ther. 19, 973–981. doi: 10.1080/14787210.2021.1865804
Islam, A., Rahman, Z., Monira, S., Rahman, M. A., Camilli, A., George, C. M., et al. (2017). Colistin resistant Escherichia coli carrying mcr-1 in urban sludge samples: Dhaka, Bangladesh. Gut Pathogens 9, 1–3. doi: 10.1186/s13099-017-0227-4
Korzeniewska, E., and Harnisz, M. (2013). Beta-lactamase-producing Enterobacteriaceae in hospital effluents. J. Environ. Manag. 123, 1–7. doi: 10.1016/j.jenvman.2013.03.024
Muteeb, G., Alsultan, A., Farhan, M., and Aatif, M. (2022a). Risedronate and methotrexate are high-affinity inhibitors of New Delhi Metallo-β-Lactamase-1 (NDM-1): a drug repurposing approach. Molecules 27:1283. doi: 10.3390/molecules27041283
Muteeb, G., Rehman, M. T., AlAjmi, M. F., Aatif, M., Farhan, M., and Shafi, S. (2022b). Identification of a potential inhibitor (MCULE-8777613195-0-12) of New Delhi Metallo-β-Lactamase-1 (NDM-1) using in silico and in vitro approaches. Molecules 27:5930. doi: 10.3390/molecules27185930
Nordmann, P., Cuzon, G., and Naas, T. (2009). The real threat of Klebsiella pneumoniae carbapenemase-producing bacteria. Lancet Infect. Dis. 9, 228–236. doi: 10.1016/S1473-3099(09)70054-4
Obot, I., Macdonald, D., and Gasem, Z. J. C. S. (2015). Density functional theory (DFT) as a powerful tool for designing new organic corrosion inhibitors. Part 1: an overview. Corr. Sci. 99, 1–30. doi: 10.1016/j.corsci.2015.01.037
Ogutu, J. O., Zhang, Q., Huang, Y., Yan, H., Su, L., Gao, B., et al. (2015). Development of a multiplex PCR system and its application in detection of blaSHV, bla TEM, bla CTX-M-1, bla CTX-M-9 and bla OXA-1 group genes in clinical Klebsiella pneumoniae and Escherichia coli strains. J. Antibiot. 68, 725–733. doi: 10.1038/ja.2015.68
Perez, F., Hujer, A. M., Hujer, K. M., Decker, B. K., Rather, P. N., and Bonomo, R. A. (2007). Global challenge of multidrug-resistant Acinetobacter baumannii. Antimicrob. Agents Chemother. 51, 3471–3484. doi: 10.1128/AAC.01464-06
Rabbani, M., Howlader, M. Z. H., and Kabir, Y. (2017). Detection of multidrug resistant (MDR) bacteria in untreated waste water disposals of hospitals in Dhaka City, Bangladesh. J. Glob. Antimicrob. Resist. 10, 120–125. doi: 10.1016/j.jgar.2017.04.009
Ribeiro, R., De Lazaro, S., Pianaro, S. J. J. O. M., and Materials, M. (2015). Density functional theory applied to magnetic materials: Mn3O4 at different hybrid functionals. J. Magnet. Magnetic Mater. 391, 166–171. doi: 10.1016/j.jmmm.2015.04.091
Soltan, D. M., Sabbaghi, A., Molla, A. H., Rastegar, L. A., Eshraghian, M., Fallah, M. J., et al. (2013). Prevalence of ampc and shv α-lactamases in clinical isolates of Escherichia coli from Tehran hospitals. Jundishapur J Microbiol. 6, 176–180. doi: 10.5812/jjm.5043
Walsh, T. R., Weeks, J., Livermore, D. M., and Toleman, M. A. (2011). Dissemination of NDM-1 positive bacteria in the New Delhi environment and its implications for human health: an environmental point prevalence study. Lancet Infect. Dis. 11, 355–362. doi: 10.1016/S1473-3099(11)70059-7
Wang, X., Liu, W., Zou, D., Li, X., Wei, X., Shang, W., et al. (2013). High rate of New Delhi Metallo-β-lactamase 1–producing bacterial infection in China. Clin. Infect. Dis. 56, 161–162. doi: 10.1093/cid/cis782
Weiner, L. M., Webb, A. K., Limbago, B., Dudeck, M. A., Patel, J., Kallen, A. J., et al. (2016). Antimicrobial-resistant pathogens associated with healthcare-associated infections: summary of data reported to the National Healthcare Safety Network at the Centers for Disease Control and Prevention, 2011–2014. Infect. Control Hosp. Epidemiol. 37, 1288–1301. doi: 10.1017/ice.2016.174
Keywords: hospital effluents, antibiotic-resistant, β-lactamase diversity, molecular docking, binding affinity, ESBL-producing genes
Citation: Mannan SJ, Akash S, Jahin SA, Saqif AT, Begum K, Yasmin M, Ahsan CR, Sitotaw B, Dawoud TM, Nafidi H-A and Bourhia M (2024) Occurrence and characterization of β-lactamase-producing bacteria in biomedical wastewater and in silico enhancement of antibiotic efficacy. Front. Microbiol. 14:1292597. doi: 10.3389/fmicb.2023.1292597
Edited by:
Ana B. Lanham, University of Lisbon, PortugalReviewed by:
Pawan Kumar Kanaujia, Mahayogi Gorakhnath University, IndiaMohammad Aatif, King Faisal University, Saudi Arabia
Hussein Al-Dahmoshi, University of Babylon, Iraq
Copyright © 2024 Mannan, Akash, Jahin, Saqif, Begum, Yasmin, Ahsan, Sitotaw, Dawoud, Nafidi and Bourhia. This is an open-access article distributed under the terms of the Creative Commons Attribution License (CC BY). The use, distribution or reproduction in other forums is permitted, provided the original author(s) and the copyright owner(s) are credited and that the original publication in this journal is cited, in accordance with accepted academic practice. No use, distribution or reproduction is permitted which does not comply with these terms.
*Correspondence: Baye Sitotaw, bWVyc2hhYmF5ZUBnbWFpbC5jb20=; Shopnil Akash, c2hvcG5pbC5waEBnbWFpbC5jb20=