- 1Department of Medical Microbiology and Nanobiomedical Engineering, Medical University of Białystok, Białystok, Poland
- 2Holy Cross Cancer Center, Kielce, Poland
- 3Institute of Health Science, Collegium Medicum, Jan Kochanowski University of Kielce, Kielce, Poland
- 42nd Department of Lung Diseases and Tuberculosis, Medical University of Bialystok, Bialystok, Poland
- 5Department of Chemistry and Biochemistry, Brigham Young University, Provo, UT, United States
The purpose of the work was to investigate the impact of sodium chloride (NaCl) on the antimicrobial efficacy of ceragenins (CSAs) and antimicrobial peptides (AMPs) against bacterial and fungal pathogens associated with cystic fibrosis (CF) lung infections. CF-associated bacterial (Pseudomonas aeruginosa, Ochrobactrum spp., and Staphylococcus aureus), and fungal pathogens (Candida albicans, and Candida tropicalis) were used as target organisms for ceragenins (CSA-13 and CSA-131) and AMPs (LL-37 and omiganan). Susceptibility to the tested compounds was assessed using minimal inhibitory concentrations (MICs) and bactericidal concentrations (MBCs), as well as by colony counting assays in CF sputum samples supplemented with various concentrations of NaCl. Our results demonstrated that ceragenins exhibit potent antimicrobial activity in CF sputum regardless of the NaCl concentration when compared to LL-37 and omiganan. Given the broad-spectrum antimicrobial activity of ceragenins in the microenvironments mimicking the airways of CF patients, ceragenins might be promising agents in managing CF disease.
1 Introduction
Cystic fibrosis (CF) is an inherited disorder characterized by the malfunction of the CF transmembrane conductance regulator (CFTR) protein, which plays an essential role in maintaining the balance of salt and water in airway epithelial cells (Saint-Criq and Gray, 2017). Due to the loss or impairment of this function in CF, the passage of chloride and sodium ions across the cell membrane is impaired, producing thick, sticky mucus (Boucher, 2004). Compared to healthy individuals, CF sputum contains about 5% less water, as well as a higher concentration of several mucins, anionic polyelectrolytes such as DNA, actin, and increased concentrations of proteases, resulting in the presence of very viscous mucus in the airways (Bhat et al., 1996; Lapierre et al., 2017; McKelvey et al., 2020; Akkerman-Nijland et al., 2021). The accumulation of mucus and impaired mucosal clearance create a favorable environment for bacterial and fungal colonization, causing chronic and recurrent infections that significantly affect the quality of life and survival of CF patients (Bhagirath et al., 2016; Turcios, 2020).
Furthermore, Pseudomonas aeruginosa and other CF pathogens produce highly antibiotic-tolerant biofilms that significantly impair the treatment of CF patients (Pragman et al., 2016; Simonin et al., 2019). Up to 95% of CF individuals not treated with CFTR potentiators and modulators, struggle with respiratory failure due to chronic bacterial infections accompanied by airway inflammation (Lyczak et al., 2002; Schaupp et al., 2023).
Cationic antimicrobial peptides (AMPs) are components of the innate immune response and serve as the first line of defense against pathogens. Broad-spectrum antimicrobial activity and immunomodulatory properties make AMPs promising candidates for antimicrobial agents (Rossi et al., 2008; Mansour et al., 2014; Lei et al., 2019). For instance, cathelicidin LL-37 is an endogenous AMP released from hCAP-18 protein that is synthesized by a variety of cell types, including mucosal epithelial cells, and immune cells, (Majewski et al., 2018) while omiganan is a new synthetic cationic peptide, consisting of 12 amino acids (Javia et al., 2022).
However, AMPs have certain limitations, such as susceptibility to protease or a high salt concentration, and a high propensity to interact with various extracellular matrix components (ECM), compromising their antimicrobial activity and widespread use as antimicrobial agents (Ng et al., 2017; McKelvey et al., 2020). For example, the high ionic strength and decreased pH of the surface liquid in CF lungs, via disruption of hydrogen bonding patterns, are potent AMPs inhibitors (Anderson and Yu, 2005; Kandasamy and Larson, 2006). Likewise, their direct interactions with highly abundant CF sputum and negatively charged biopolymers, including DNA, F-actin (Tang et al., 2005), and mucins (Felgentreff et al., 2006), lead to the formation of large and elongated aggregates.
Synthetic ceragenins (CSAs) are non-peptide analogs of natural AMPs that retain their antimicrobial properties while addressing some drawbacks, leading to an extended half-life in body fluids and tissues (Leszczyńska et al., 2011; Pollard et al., 2012; Sinclair et al., 2012; Durnaś et al., 2017). In addition, ceragenins show reduced potential for resistance induction, likely due to multiple mechanisms of action, including permeabilization of the microbial cell membranes and induction of reactive oxygen species. Therefore, ceragenins possess a broad spectrum of microorganisms, including multidrug-resistant (MDR) strains growing in planktonic and biofilm forms (Chmielewska et al., 2020; Paprocka et al., 2022; Tokajuk et al., 2022). Ceragenins have also demonstrated immunomodulatory and antiviral effects, making them promising candidates for versatile therapeutic applications (Howell et al., 2009; Bucki et al., 2015; Suprewicz et al., 2023).
The introduction of CFTR modulators has undoubtedly been a groundbreaking achievement in the management of CF, alleviating the underlying defect and substantially improving clinical outcomes, including reductions in infectious complications (Haq et al., 2022). However, continued research into new antimicrobial strategies is essential, as not all CF patients respond equally to CFTR modulators, and some continue to face persistent and difficult infections (McGarry et al., 2022). For instance, individuals with CFTR mutations, such as Phe508del, may not experience significant improvements in lung function or other symptoms, and infectious complications in the airways continue to pose a substantial challenge (Habib et al., 2019). Preventing the latter requires the prolonged use of antibiotics, which has led to the emergence of drug-resistant strains, underscoring the urgency for novel antimicrobial strategies. In addition, CFTR modulators may cause side effects, such as gastrointestinal and respiratory issues or headaches, impairing patients’ quality of life (Dagenais et al., 2020). Moreover, the cost of CFTR modulators may be a barrier to access for some patients or healthcare systems, thereby limiting this treatment to the world’s wealthiest nations (Zampoli et al., 2023). Finally, although short-term studies indicate that CFTR modulators improve lung function and quality of life, their long-term effects are still under investigation (Taylor-Cousar et al., 2023). Therefore, research and development of innovative antimicrobial strategies to ensure the health of individuals with CF is far from complete. This study evaluated the antimicrobial efficacy of ceragenins and AMPs against CF-associated pathogens in CF sputum samples alone and supplemented with NaCl excess.
2 Materials and methods
2.1 Bacterial strains
The following reference bacterial and fungal strains were tested in the study: Staphylococcus aureus Xen29, (Caliper Life Science Inc., Hopkinton, MA, USA), Pseudomonas aeruginosa ATCC 27853 (non-mucoid strain) (ATCC, Manassas, VA, USA), and Candida albicans ATCC 10231 (ATCC, Manassas, VA, USA). In addition, in experiments with artificially contaminated sputum, P. aeruginosa PAO1 DSM 19880 strain (mucoid strain) (DSMZ, DSMZ, Germany Germany) with inserted pMF230 plasmid encoding a beta-lactamase gene ensuring resistance to carbenicillin (Nivens et al., 2001) was used to differentiate it from P. aeruginosa (susceptible to carbenicillin) present in sputum sample collected from CF patient (see below). The pMF230 plasmid (Addgene, Watertown, MA, USA) was electroporated into P. aeruginosa PAO1 DSM 19880 using MicroPulser Electroporator (BioRad, Hercules, CA, USA) according to the procedure described by Choi et al. (2006), and transformants were further selected and maintained on LB agar plates with carbenicillin (400 μg/mL).
In addition, single clinical isolates of P. aeruginosa 4B (mucoid strain), Ochrobactrum spp. 10B, C. albicans 12B, and C. tropicalis 178 collected at the Department of Medical Microbiology and Nanobiomedical Engineering in Bialystok, Poland, were included in the study. S. aureus, P. aeruginosa, Ochrobactrum spp., and Candida strains were cultured and maintained on the recommended selective media purchased from Biomaxima (Lublin, Poland), i.e., Chapman, Cetrimide, and Sabouraud dextrose agar with chloramphenicol, respectively.
2.2 Compounds and experimental settings
Ceragenins were synthesized as described previously (Ding et al., 2002), whereas LL-37 and omiganan were commercially purchased from Lipopharm company (Gdańsk, Poland). The ceragenins and AMPs were dissolved in deionized water to ensure the absence of NaCl in the solution. The impact of NaCl concentrations on the antimicrobial activity of ceragenins and AMPs against the bacteria and fungi was analyzed using Mueller–Hinton (MH) broth, RPMI medium supplemented with D-(+)-glucose, and MOPS and deionized water. To that end, NaCl (Chempur, Piekary Śląskie, Poland) was suspended in these media to the final concentrations of 20 mM, 60 mM, 100 mM, 150 mM, and 300 mM. In addition, sputum samples from four cystic fibrosis patients, one positive for P. aeruginosa, one positive for S. aureus, the third positive for P. aeruginosa, Aspergillus and methicillin-resistant Staphylococcus aureus (MRSA) and the fourth positive for C. albicans, were included in the respective experiments in conditions mimicking CF lungs. For this purpose, sputum samples were diluted with deionized water to obtain 10 and 20% sputum solutions and used alone or supplemented with 150 mM or 300 mM NaCl.
2.3 Susceptibility testing
Microbial susceptibility testing was carried out using the serial microdilution method by current EUCAST (European Committee on Antimicrobial Susceptibility Testing) recommendations on 96-well microtiter plates with final volumes of 200 μL. For bacteria, minimum inhibitory concentrations (MICs) were determined in Mueller-Hinton broth (Sigma-Aldrich, Burlington, MA, USA), and minimum bactericidal concentrations (MBCs) were determined by placing 10 μL dilutions with no visible growth in the MIC test on the appropriate selective agar medium. For fungi, MIC was determined in 2xRPMI medium (Sigma-Aldrich, Burlington, MA, USA) supplemented with D-(+)-glucose (Sigma-Aldrich, Burlington, MA, USA) and MOPS (Sigma-Aldrich, Burlington, MA, USA) diluted twice with deionized water, while minimum fungicidal concentration (MFC) was determined by plating each sample (10 μL) on Sabouraud dextrose agar with the chloramphenicol. The final microbial concentration in the well was approximately 5 × 105 CFU (colony-forming units)/mL. MIC, MBC, and MFC values were determined after 24 h incubation.
2.4 Killing assay
A killing assay (colony counting assay) was performed to determine the bactericidal and fungicidal activity of LL-37, omiganan, CSA-13, and CSA-131 against selected clinical strains of P. aeruginosa and C. tropicalis. Briefly, individual colonies of bacteria and fungi were resuspended at ~108 CFU/mL and diluted to 105 CFU/mL in sterile deionized water. Tests were performed using the AMPs and ceragenins in the 1–100 μg/mL concentration range. After 60 min of incubation at 37°C, the plates were transferred to ice, and samples were serially diluted from 10 to 1,000 times. Then 10 μL aliquots of each dilution were placed on Luria-Bertani (LB) low-salt agar containing 400 μg/mL carbenicillin (Sigma-Aldrich, Burlington, MA, USA) or on Sabouraud dextrose agar with chloramphenicol and incubated overnight at 37°C to determine the number of visible colonies. The addition of carbenicillin to LB agar selected P. aeruginosa PAO1 (resistant to carbenicillin) and inhibited the growth of carbenicillin-susceptible to P. aeruginosa present in the sputum. The colony-forming units (CFU/mL) of each sample were determined by the dilution factor.
Furthermore, the above procedure was repeated using sterile deionized water with 150 mM and 300 mM NaCl as well as 10 and 20% sputum collected from CF patients alone and supplemented with 150 mM and 300 mM NaCl. Due to higher bacterial survival in the presence of 20% compared to 10% sputum, the 20% sputum was selected for further experiments. After incubating the sputum samples 2 μL of Sputasol (Oxoid, Basingstoke, Hampshire, UK) was added to liquefy them, followed by the pathogens counting on the selective agars.
2.5 Optical microscopy and DNA measurement
DNA concentration was quantified by absorbance at 260 nm using a NanoDrop One spectrophotometer (Thermo Fisher Scientific, Waltham, MA, USA). In our study of sputum samples from cystic fibrosis patients, YOYO-1 staining (Invitrogen, Carlsbad, CA) was used to visualize DNA at a final concentration of 1 μM. Additionally, F-actin was visualized using Rhodamine Phalloidin (Thermo Fisher Scientific, Waltham, MA, USA) at a final concentration of 0.15 μM. Fluorescence images were recorded using a Leica DMi8 microscope (Wetzlar, Germany).
2.6 Statistical analysis
All statistical analyses were conducted using Graph Pad Prism, version 8 (GraphPad Software, Inc., San Diego, CA). The data collected were reported as the mean ± standard deviation (SD) of three experiments. The significance of differences was determined using the two-tailed Student’s test and a p-value ≤0.05 was considered to be statistically significant. All results were compared to the control, which were samples treated only with CSAs: CSA-13 and CSA-131, and AMPs: LL-37 and omiganan for each inducible concentration.
3 Results
3.1 The antimicrobial activity of ceragenins is not affected by high NaCl concentration
The antimicrobial activity of CSA-13 and CSA-131 against all tested bacterial and fungal strains was not affected by increasing NaCl concentration (Table 1 and Table 2), since variation in the MIC and MBC values was limited to only one dilution. On the contrary, NaCl at a concentration of 300 mM elevated 8-fold MIC and ≥ 8-fold MBC values for LL-37 and P. aeruginosa PAO1 as well as 8-fold MIC and ≥ 16-fold MBC values for Ochrobactrum spp. 10B (Table 1). A similar increase in MIC/MBC values was also noted for omiganan and C. tropicalis 178 (Table 2), where the MIC value increased from 16 μg/mL in the absence of salt concentration to 64 μg/mL at 300 mM NaCl, and the MFC increased from 32 μg/mL to 128 μg/mL, respectively.
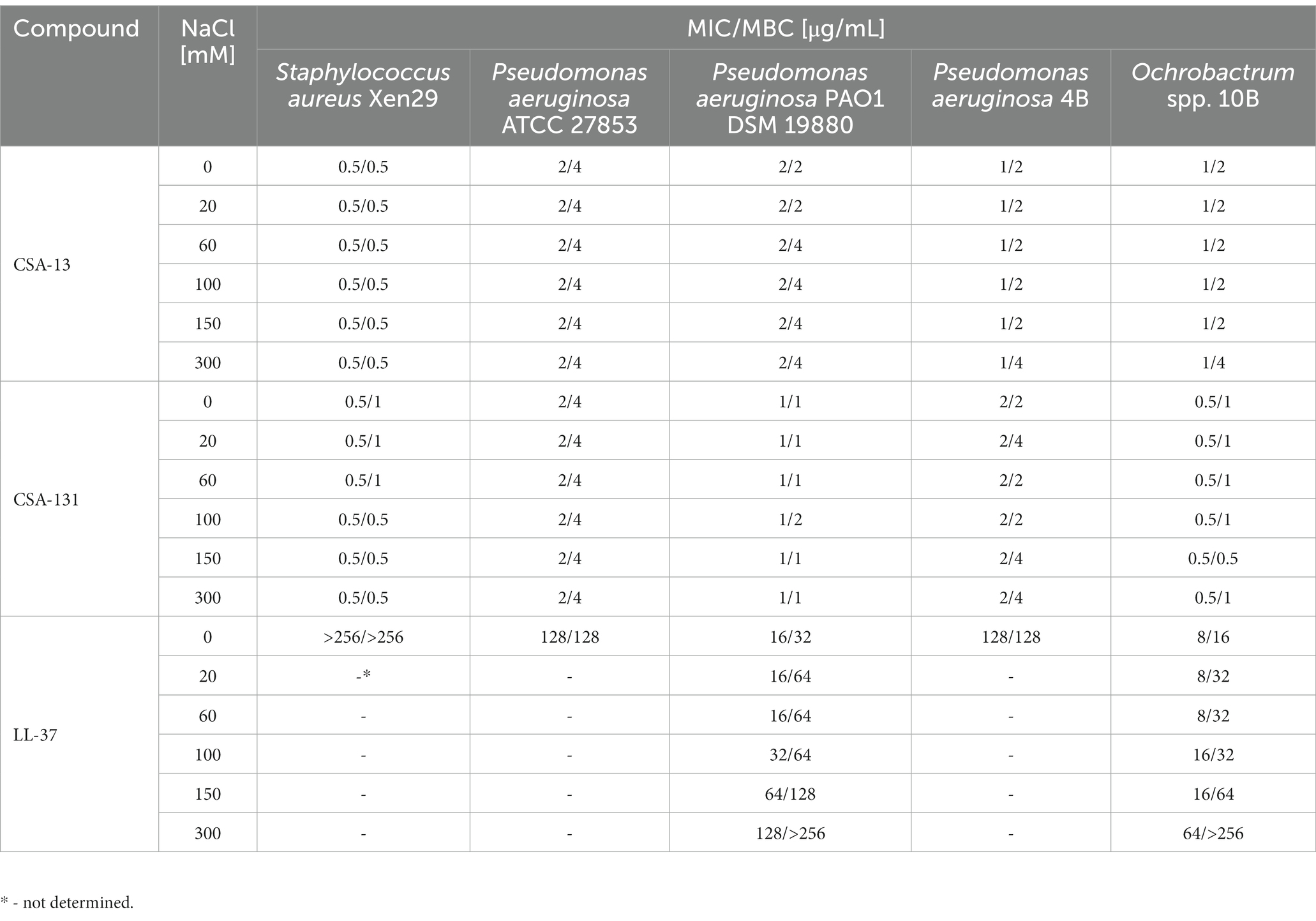
Table 1. Minimum inhibitory concentration (MIC) and minimum bactericidal concentration (MBC) values of CSA-13, CSA-131, and LL-37 against Staphylococcus aureus Xen29, and Pseudomonas aeruginosa ATCC 27853 and DSM 19880, and two clinical isolates – P. aeruginosa 4B and Ochrobactrum spp. 10B in the presence of NaCl concentration (0–300 mM).
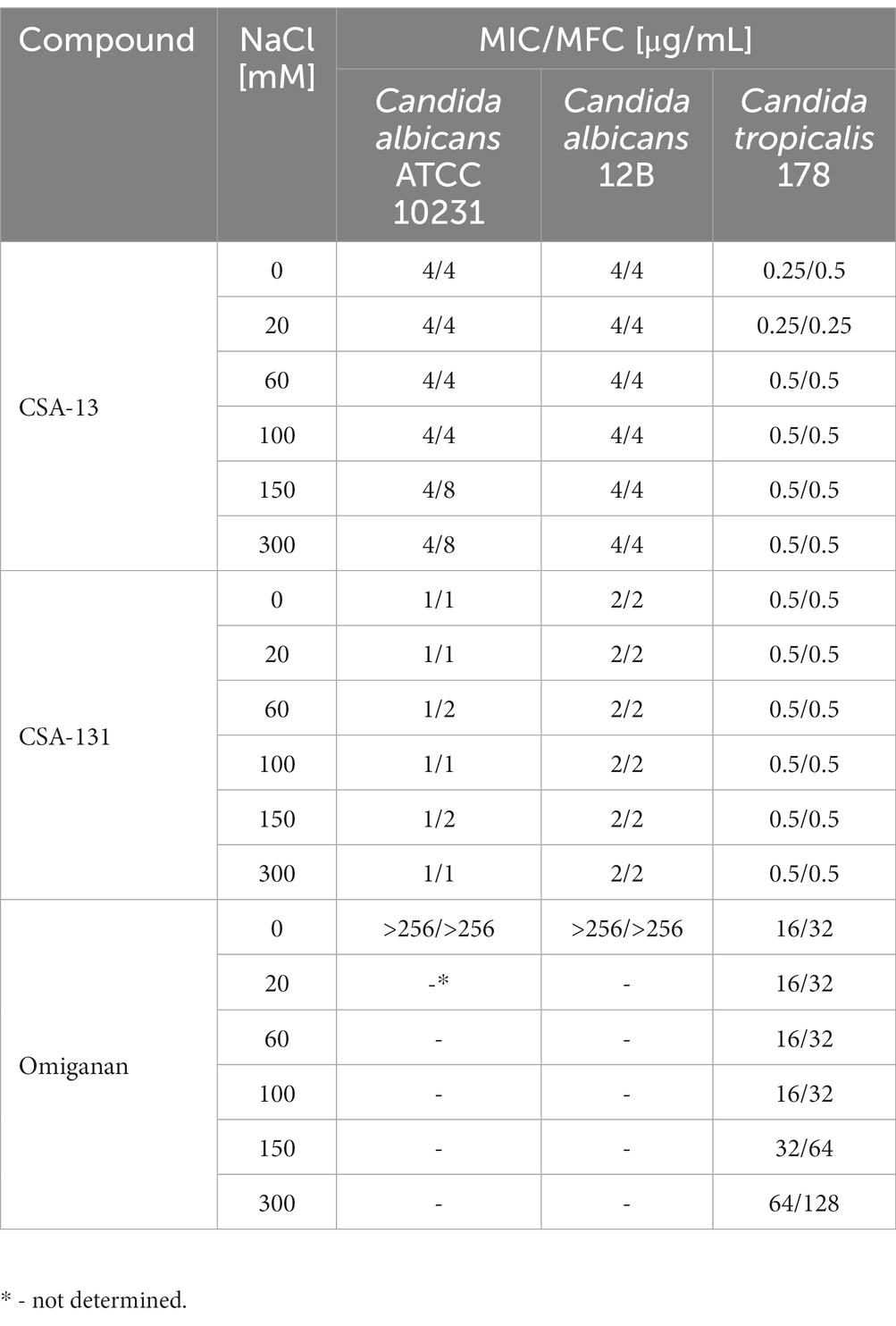
Table 2. Minimum inhibitory concentration (MIC) and minimum fungicidal concentration (MFC) values of CSA-13, CSA-131, and omiganan against Candida albicans ATCC 10231, and two clinical isolates – C. albicans 12B and C. tropicalis 178 in the presence of NaCl concentration (0–300 mM).
3.2 Ceragenins retain antimicrobial activity in CF sputum and NaCl excess
A killing assay was performed to evaluate the potential inhibitory effect on ceragenins and AMPs of CF sputum (diluted to 10 and 20%) alone and supplemented with 150 mM and 300 mM NaCl.
The highest NaCl concentration was obtained by combining 20% sputum (containing on average 65 mM NaCl; data not shown) and 300 mM NaCl, yielding an approximate concentration of 340 mM NaCl. As shown in Figure 1, 20% sputum from Patient A decreased the antimicrobial activity of CSA-131 against all P. aeruginosa. In contrast, in the presence of 10% sputum, the antibacterial activity of CSA-131 against P. aeruginosa 1,414 remained unchanged but decreased four-fold for both P. aeruginosa ATCC 27853 and P. aeruginosa 4B. Moreover, in 20% of sputum, the antibacterial activity of CSA-131 decreased four-fold across all strains (Figure 1). Nevertheless, this inhibitory effect was not augmented by 150 mM and 300 mM NaCl (Figures 2A,B). Similar results were obtained for CSA-13, CSA-131, and Candida tropicalis 178 (Figures 3A,B). On the contrary, the antimicrobial activity of LL-37 and omiganan was significantly attenuated in the presence of both sputum and NaCl (Figures 2F, 3F, 4C, 4F, 5C, 5F). Briefly, NaCl alone resulted in ≥2.5-fold increase of the killing dose (concentrations at which all pathogens are effectively eradicated) for LL-37, i.e., from 40 μg/mL to over 100 μg/mL, against P. aeruginosa PAO1 DSM19880 strain (Figure 2C), and ≥ 5-fold increase of the killing dose for omiganan, i.e., from 20 μg/mL to over 100 μg/mL, against C. tropicalis 178 (Figure 3C). Furthermore, in 20% sputum from Patient B, the killing doses against P. aeruginosa increased from 10 to 40 μg/mL for CSA-13, and from 5 to 20 μg/mL for CSA-131 (Figures 2D,E). Similarly, the killing doses against C. tropicalis increased from 20 to 100 μg/mL for CSA-13 and CSA-131 (Figures 3D,E). In Patient D’s 20% sputum solution, the killing doses of both P. aeruginosa and C. tropicalis were unchanged for CSA-13 and CSA-131 (5 μg/mL) (Figures 4A,B, 5A,B), while in 20% of the sputum of Patient C, the killing doses against the test strains increased (Figures 4D,E5D,E). In addition, the presence of 20% solutions of sputum samples from each patient increased the lethal doses from 40 to 100 μg/mL for LL-37 and from 20 to 100 μg/mL for omiganan for the tested strains (Figures 2F, 3F, 4C, 4F, 5C, 5F). It should be noted that omiganan was ineffective against C. tropicalis in the presence of 20% sputum (regardless of the patient sample) and 300 mM (Figures 3F, 5C, 5F), while the log (CFU) for LL-37 increase from ~4.8 to ~5.05 (Figures 2F, 4C, 4F).
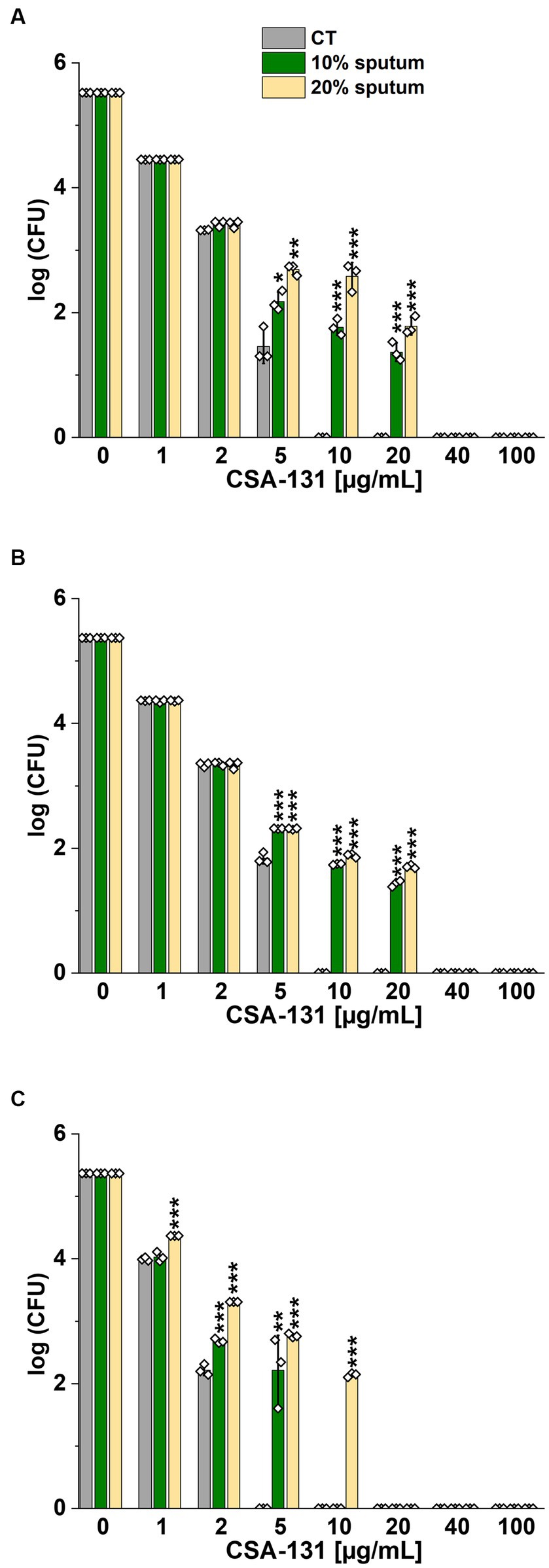
Figure 1. Bactericidal activity of CSA-131 against Pseudomonas aeruginosa ATCC 27853 (A) and P. aeruginosa PAO1 DSM 19880 (B) and clinical isolate P. aeruginosa 4B (C) in 10 and 20% solution of CF sputum collected from Patient A. Results show the mean ± SD, n = 3; * indicates statistical significance at p ≤ 0.05, ** p ≤ 0.01, and *** p ≤ 0.001 by Student’s t-test.
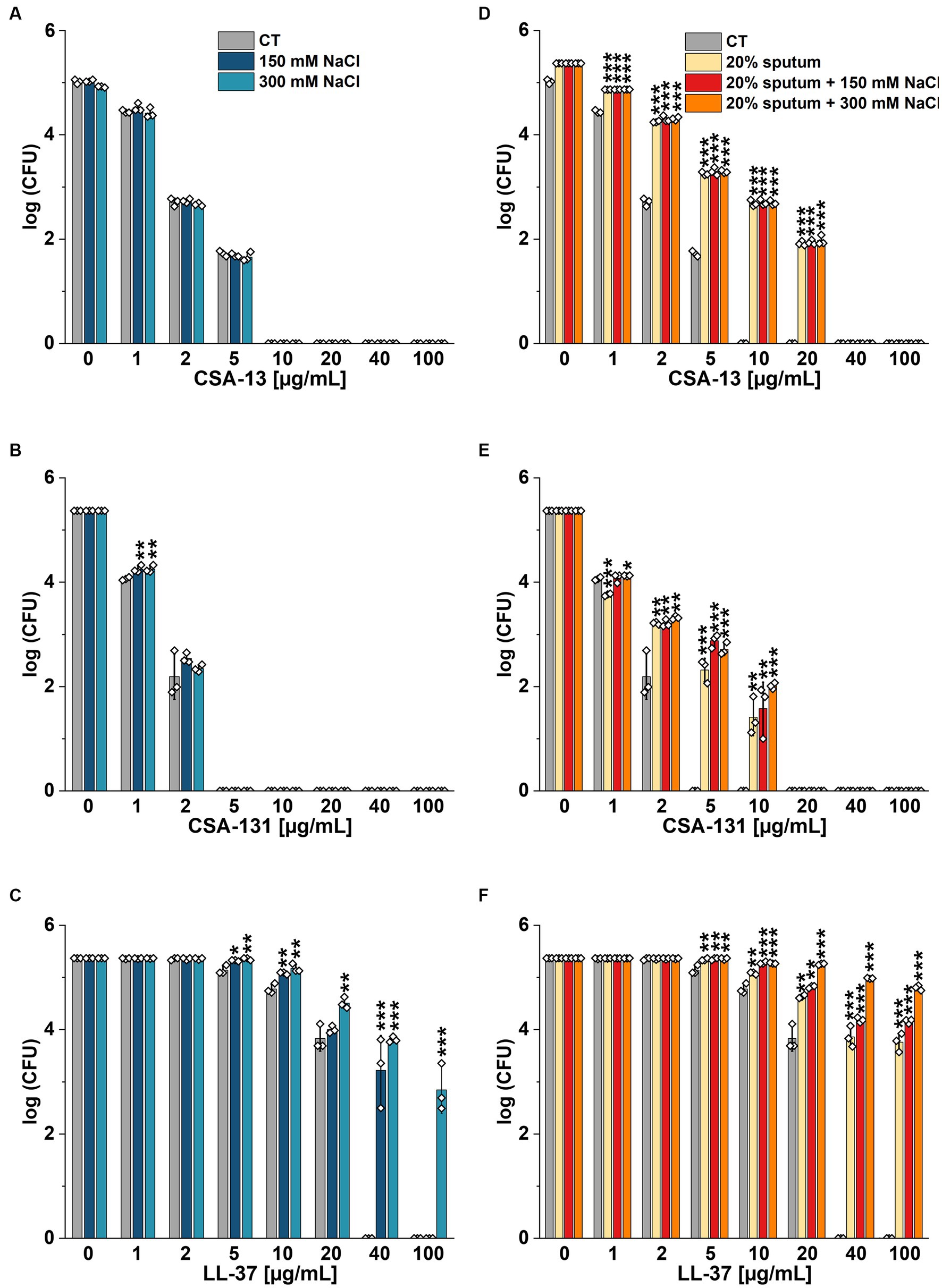
Figure 2. Bactericidal activity of CSA-13 (A,D), CSA-131 (B,E), and LL-37 (C,F) against Pseudomonas aeruginosa PAO1 DSM 19880 strain. Bacterial survival was evaluated in sterile deionized water (CT) (A–C), and in 20% solution of CF sputum collected from Patient B (D-F), without NaCl and with NaCl at a concentration of 150 mM and 300 mM. Results show the mean ± SD (n = 3). * indicates statistical significance at p ≤ 0.05, ** ≤0.01, and *** ≤0.001 by Student’s t-test.
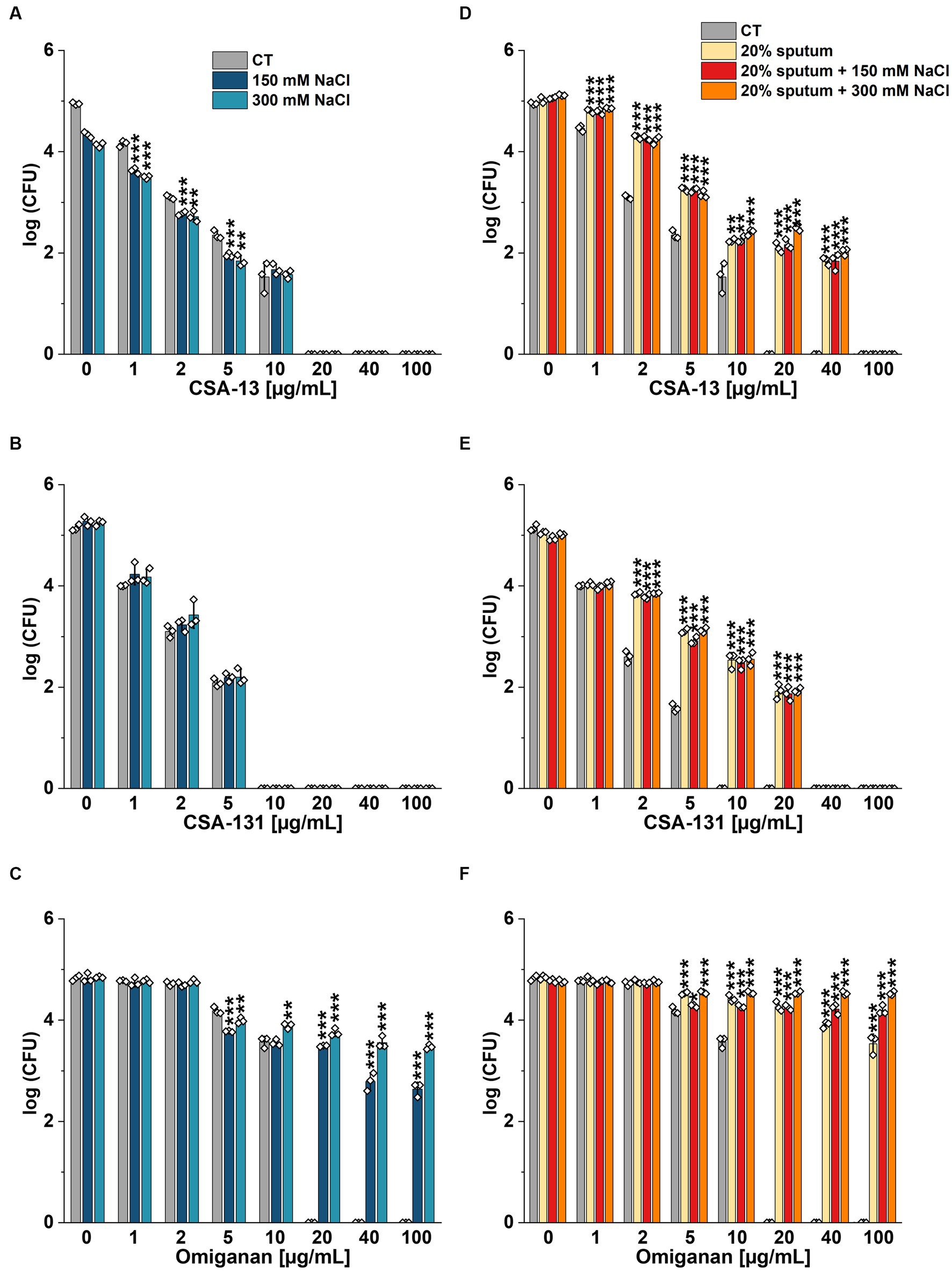
Figure 3. Fungicidal activity of CSA-13 (A,D), CSA-131 (B,E), and omiganan (C,F) against the clinical strain of Candida tropicalis 178. Fungal survival was evaluated in distilled water supplemented with NaCl (A–C), and in 20% solution of CF sputum collected from Patient B (D-F) alone and supplemented with NaCl at a concentration of 150 mM and 300 mM. Results show the mean ± SD (n = 3). * indicates statistical significance at p ≤ 0.05, ** ≤0.01, and *** ≤0.001 by Student’s t-test.
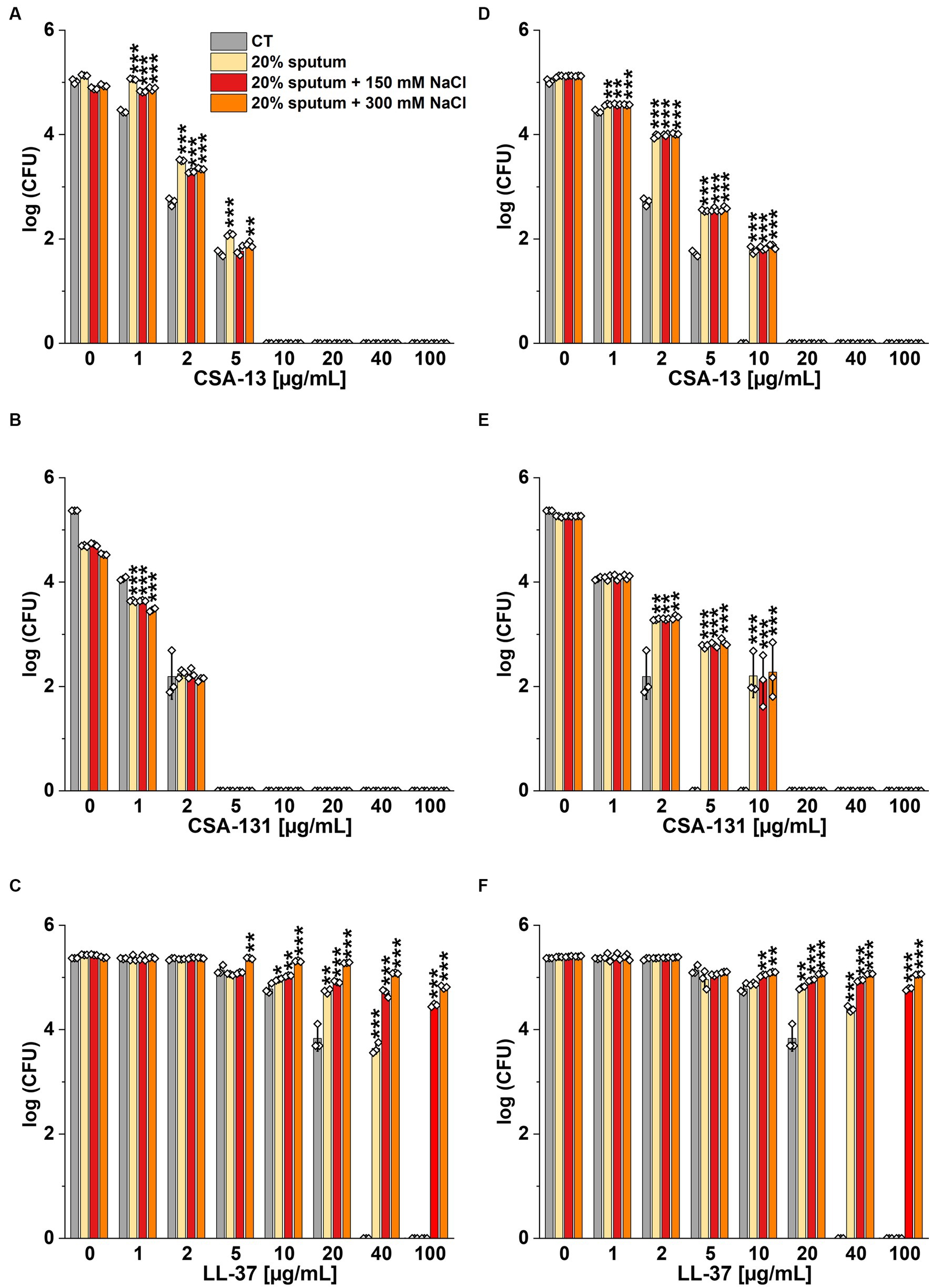
Figure 4. Bactericidal activity of CSA-13 (A,D), CSA-131 (B,E), and LL-37 (C,F) against Pseudomonas aeruginosa PAO1 DSM 19880 strain. Bacterial survival was evaluated in 20% solution of CF sputum collected from patient D (A-C), and from Patient C (D-F), without NaCl and with NaCl at a concentration of 150 mM and 300 mM. Results show the mean ± SD (n = 3). * indicates statistical significance at p ≤ 0.05, ** ≤0.01, and *** ≤0.001 by Student’s t-test.
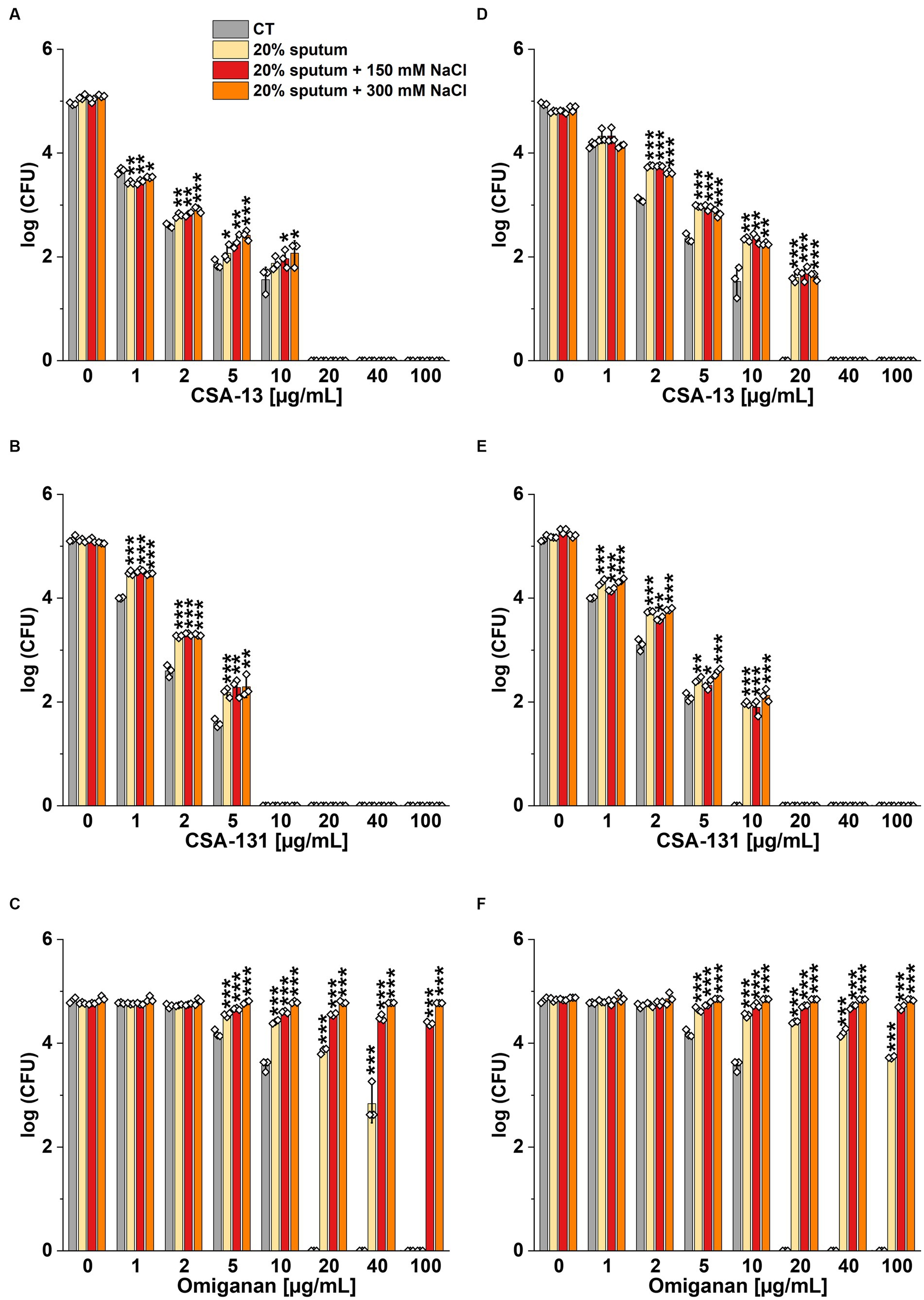
Figure 5. Fungicidal activity of CSA-13 (A,D), CSA-131 (B,E), and omiganan (C,F) against the clinical strain of Candida tropicalis 178. Fungal survival was evaluated in 20% solution of CF sputum from Patient D (A-C), and from Patient C (D-F), alone and supplemented with NaCl at a concentration of 150 mM and 300 mM. Results show the mean ± SD (n = 3). * indicates statistical significance at p ≤ 0.05, ** ≤0.01, and *** ≤0.001 by Student’s t-test.
3.3 CF sputum contains high concentrations of DNA
Among the sputum samples analyzed, the one containing P. aeruginosa, MRSA, and Aspergillus exhibited the highest DNA concentration, with a value of 6.66 ± 0.38 mg/mL. In contrast, lower DNA concentrations were observed in sputum samples containing only P. aeruginosa (3.86 ± 0.43 mg/mL), and the lowest DNA concentrations were found in samples with C. albicans (1 ± 0.01 mg/mL) and S. aureus (0.75 ± 0.21 mg/mL) (Figure 6B). The representative images show highly condensed bundles containing DNA and F-actin, which is characteristic of the sputum of CF patients (Figure 6A) (Sheils et al., 1996; Tomkiewicz et al., 1998; Tang et al., 2005).
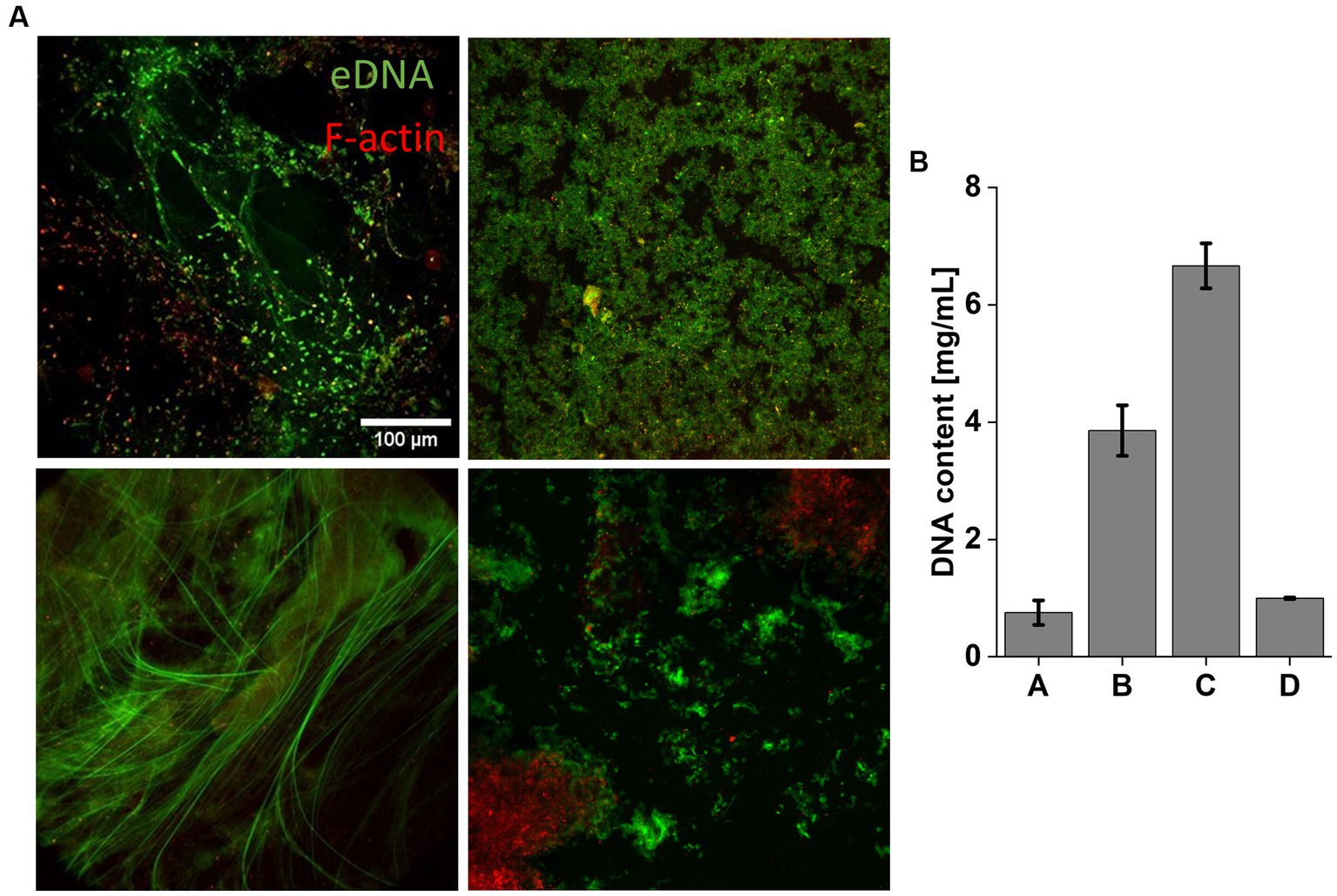
Figure 6. Representative microscopic images of sputum collected from CF patients. Top left image shows sputum from Patient A, top right image – Patient B, bottom left image – Patient C, bottom right image – Patient D. The DNA are shown green and F-actin in red. Scale bar 100 μm (A). (B) Mean DNA concentration in CF sputum. Results show the mean ± SD (n = 3).
4 Discussion
This study presents a comparative analysis of the antimicrobial efficacy of ceragenins and AMPs in the context of CF sputum and varying NaCl levels. The investigation aimed to reveal the potential of these agents as alternative therapeutic options for managing CF-associated infections, where conventional antibiotics frequently face limitations due to microbial resistance and the challenging CF microenvironment (López-Causapé et al., 2015).
Respiratory colonization and infection by P. aeruginosa are the leading causes of morbidity and mortality in CF patients. It is noteworthy that around 80% of patients are chronically colonized with P. aeruginosa by the age of 20 (Koch, 2002). Additionally, CF patients are commonly colonized by other bacterial and fungal pathogens, including methicillin-resistant Staphylococcus aureus, Mycobacterium abscessus complex, Burkholderia cepacia complex, Aspergillus fumigatus, and Candida species (Chotirmall et al., 2010; Bhagirath et al., 2016). Although Ochrobactrum infections are relatively uncommon in CF patients, this pathogen deserves attention due to its inherent resistance to antibiotics, the potential to cause chronic infections with clinical implications, and problems with identification (Carvalho Filho et al., 2018). Ochrobactrum spp. isolates have been found to exhibit high resistance to beta-lactam antibiotics, except carbapenems, due to the presence of an AmpC-like β-lactamase. Additionally, resistance to aminoglycosides and fluoroquinolones is an emerging issue that further complicates treatment efforts (Alonso et al., 2017; Yagel et al., 2020).
CF sputum is a complex milieu consisting of DNA, mucins, inflammatory cells, and various proteins (Morrison et al., 2019). It also contains varying levels of sodium chloride, a critical electrolyte. Studies have reported that CF sputum shows elevated levels of sodium chloride compared to this healthy individuals. In detail, the concentration of NaCl in the sputum of CF patients and healthy individuals is approximately 179.66 mM (10.5 g/L) and 126.62 mM (7.4 g/L), respectively. It is consistent with in vivo measurements in animal models of CFs in mice and in vitro in human bronchial epithelial models (Zabner et al., 1998; Jayaraman et al., 2001; Lapierre et al., 2017). Similarly, according to Joris et al., NaCl concentration in the airway surface fluid (ASF) of CF patients was 120–170 mM compared to 80 mM in subjects without cystic fibrosis (Joris et al., 1993). On the other hand, the average DNA concentration in CF sputum was found to be within the range of 0.2–20 mg/mL (White et al., 1985; Brandt et al., 1995; Lewenza, 2013; Sarkar, 2020), consistent with our findings.
The decreased antimicrobial activity of ceragenins against P. aeruginosa strains in the sputum may result from the presence of S. aureus, which was isolated from the sputum used in the study. Indeed, in the case of chronic CF infection, the number of microorganisms in the sputum of the respiratory tract reaches 107–109 CFU/mL, resulting from the inoculum effect, which substantially affects antimicrobial susceptibility testing results (Turner et al., 2015). Furthermore, while charge-based interactions can influence the antimicrobial activity of ceragenins in the presence of abundant in sputum linear polyelectrolytes, like actin or DNA, they are notably weaker compared to their impact on cationic AMPs, such as LL37, HB71, and WLBU2 (Bucki et al., 2007).
A widespread application of AMPs as antimicrobial agents is hindered by their sensitivity to several physicochemical factors frequently present in infected sites, such as a high salt concentration in the CF airway surface fluid (Goldman et al., 1997). For instance, in patients with cystic fibrosis, the activity of AMPs is significantly lower in the ASL than in normal ASL (Smith et al., 1996; Hiemstra, 2007).
Indeed, our results show that the antimicrobial activity of both LL-37 and omiganan against CF-associated pathogens is NaCl concentration-dependent. Similarly, Bals et al. and Tanaka et al. demonstrated that the antimicrobial activity of LL-37 diminishes with rising NaCl concentrations (Bals et al., 1998; Tanaka et al., 2000). Several studies have highlighted the limitations of AMPs in their antimicrobial activity. A high-salt environment has been found to impair the effectiveness of AMPs, as observed in the case of peptides like indolicidin, human beta-defensin-1, histidine-rich peptide P-113, gramicidins, magainins, and bactenecins (Goldman et al., 1997; Lee et al., 1997; Wu et al., 1999; Rothstein et al., 2001). In CF sputum, the presence of bacterial endotoxin lipopolysaccharide (LPS) is one of the factors leading to the inhibition of LL-37 antimicrobial activity (Bucki et al., 2007). Additionally, the secretion of two zinc-dependent metalloproteases, ZmpA and ZmpB, by Burkholderia cepacia can lead to the inactivation of AMPs, such as human beta-defensin-1 and LL-37 (Kooi and Sokol, 2009). These findings shed light on the challenges in utilizing AMPs as effective therapeutic agents in CF patients.
The structure of an AMP plays a vital role in determining its antimicrobial activity. It is believed that the helical, oligomeric conformation of LL-37 is crucial to the protein’s antimicrobial activity. Hence, the highest LL-37 antimicrobial activity is observed with maximum helix content, while intermediate and low activity corresponds to lower helix content and a disordered secondary structure. These findings suggest that the optimal antimicrobial activity of LL-37 necessitates an oligomeric α-helical structure before its interaction with the bacterial membrane (Johansson et al., 1998). Studies have demonstrated that increased NaCl concentration can induce conformational changes in LL-37. Specifically, at higher NaCl concentrations, LL-37 may undergo structural alterations and adopt a more random or disordered conformation resulting in decreased antimicrobial activity (Park et al., 2004; Huang et al., 2014).
In contrast, omiganan does not display a helical structure. However, the presence of positively charged sodium ions affects electrostatic interactions of this cationic AMP with microbial cell membranes (Mojsoska and Jenssen, 2015; Ghosh et al., 2021). Hence, in high-salt environments, the antimicrobial activity of omiganan may decrease, rendering it less effective against certain microorganisms. This dependence on salt concentration can affect its efficacy in physiological conditions where salt levels fluctuate, such as respiratory tract or skin infections (Brown, 2021).
On the other hand, the antimicrobial activity of ceragenins is independent of the concentration of NaCl, likely due to their unique structural properties. The smaller size of CSA-13 and CSA-131 than LL-37 and omiganan, along with distinct charge densities and lower positive charges, ensure their stability and resistance to changes in the ionic environment, including fluctuations in NaCl concentration and anionic polyelectrolytes, such as extracellular DNA (eDNA), F-actin, and mucin (Hashemi et al., 2018). In addition, the direct targeting of membrane lipids by ceragenins makes them less vulnerable to salt concentration compared to the highly charge-based AMPs mechanism of action (Epand et al., 2007).
Mucoid strains of P. aeruginosa that produce a thick alginate biofilm are typically more resistant to antimicrobials than non-mucoid strains due to the protective nature of the biofilm, which limits their penetration and, consequently, their efficacy (Nichols et al., 1989; Meluleni et al., 1995; Stewart, 1996; Hentzer et al., 2001). P. aeruginosa is well-known for its ability to develop antibiotic resistance, for example, through upregulation of genes encoding efflux pumps, which effectively remove antibiotics from the cell (Tomás et al., 2010). The favorable biocompatibility of CSA has been reported in previous work (Piktel et al., 2020; Paprocka et al., 2021; Suprewicz et al., 2023). For CSA-13 and CSA-131, the viability of human basal alveolar epithelial adenocarcinoma cells (A549) at a concentration of 10 μg/mL CSA-13 and CSA-131 was maintained at approximately 60 and 80%, respectively (Piktel et al., 2020; Paprocka et al., 2021; Suprewicz et al., 2023). Additionally, the problem of cytotoxicity can be effectively solved by the incorporation of Pluronic, a compound that increases cell viability and alleviates the hemolytic effects of CSAs while maintaining their antimicrobial activity (Leszczyńska et al., 2011; Paprocka et al., 2021).
Understanding the disparate modes of action and resistance to changes in salt concentration provides valuable insights into the therapeutic potential of ceragenins as effective antimicrobial agents, particularly in managing infections in complex biological environments, such as CF lungs. Further research in this area is critical to elucidate the intricacies of ceragenin activity and its implications for clinical applications. The intrinsic stability and resistance to changes in NaCl concentration make ceragenins promising candidates for combating infections in complex environments like the CF sputum (Bucki et al., 2007; Leszczyńska et al., 2011).
5 Conclusion
Our study provides a comprehensive comparative analysis of the efficacy of ceragenins and AMPs in the context of CF sputum and varying NaCl concentrations. We investigated the antimicrobial activity of CSA-13, CSA-131, LL-37, and omiganan against CF-associated pathogens, evaluating their performance under different conditions. Our findings revealed that ceragenins, specifically CSA-13 and CSA-131, exhibited remarkable antimicrobial efficacy, surpassing natural (LL-37) and synthetic (omiganan) AMPs. Notably, the antimicrobial activity of ceragenins remained consistent across a wide range of NaCl concentrations, underscoring their resistance to changes in ionic environments. In contrast, the antimicrobial activity of LL-37 and omiganan was reduced in high-salt environments, potentially compromising their performance in CF sputum, a condition where NaCl levels can vary. The structural properties of ceragenins and their lipid-based mode of action likely contribute to their stability and sustained antimicrobial activity, even under salt excess condition. These properties highlight the potential of ceragenins as promising candidates for combating CF-associated infections and overcoming limitations observed in natural and synthetic AMPs.
Scope statement
The study investigated the effect of sodium chloride (NaCl) on the antimicrobial efficacy of the cathelicidin LL-37, and their synthetic mimetic ceragenins (Ceragenins), which are considered as potential therapeutic agents in cystic fibrosis (CF) lung infections. The susceptibility of cystic fibrosis-associated bacterial pathogens (Pseudomonas aeruginosa, Ochrobactrum spp. and Staphylococcus aureus) and fungal pathogens (Candida albicans and C. tropicalis) to these compounds was determined using Minimum Inhibitory Concentrations (MIC) and Bactericidal Concentrations (MBC) tests in sputum samples collected from patients diagnosed with CF. Furthermore, a colony-counting assay was used to assess the effect of varying NaCl concentrations on tested agents’ antimicrobial activity. The findings reveal that ceragenins exhibit potent antimicrobial activity in CF sputum, regardless of the NaCl concentration when compared to LL-37 and omiganan. Given the broad-spectrum antimicrobial activity of ceragenins in microenvironments resembling the airways of CF patients, they represent promising agents for managing CF disease. This manuscript aligns with the journal’s scope as it contributes to the field of microbiology and infectious diseases. The investigation provides valuable insights into the development of effective antimicrobial strategies to combat CF-associated infections.
Data availability statement
The raw data supporting the conclusions of this article will be made available by the authors, without undue reservation.
Ethics statement
The studies involving humans were approved by Bioethics Committee of Medical University of Bialystok. The studies were conducted in accordance with the local legislation and institutional requirements. The participants provided their written informed consent to participate in this study.
Author contributions
KS: Conceptualization, Formal analysis, Writing – original draft, Data curation, Investigation, Methodology, Software, Writing – review & editing. ŁS: Formal analysis, Investigation, Methodology, Software, Writing – review & editing, Visualization. SC-D: Methodology, Software, Visualization, Writing – review & editing. SK: Methodology, Writing – review & editing, Investigation, Resources. SO: Methodology, Writing – review & editing, Software, Visualization. MZ: Software, Formal analysis, Investigation, Writing – original draft. ŁM: Formal analysis, Investigation, Data curation, Resources, Writing – review & editing. RM: Formal analysis, Investigation, Resources, Writing – review & editing, Supervision. TD: Formal analysis, Investigation, Writing – review & editing, Methodology, Validation. PS: Formal analysis, Investigation, Validation, Writing – review & editing, Supervision. KF: Formal analysis, Supervision, Validation, Writing – review & editing, Project administration, Visualization, Writing – original draft. RB: Formal analysis, Validation, Writing – original draft, Conceptualization, Resources.
Funding
The author(s) declare financial support was received for the research, authorship, and/or publication of this article. This work was supported by the National Science Center, Poland, under grant: UMO-2018/30/M/NZ6/00502 and the Medical University of Bialystok (SUB/1/DN/22/001/1122), Poland.
Conflict of interest
PS and RB are the consultants for N8 Medical, Inc. and report personal fees from N8.
The remaining authors declare that the research was conducted in the absence of any commercial or financial relationships that could be construed as a potential conflict of interest.
The author(s) declared that they were an editorial board member of Frontiers, at the time of submission. This had no impact on the peer review process and the final decision.
Publisher’s note
All claims expressed in this article are solely those of the authors and do not necessarily represent those of their affiliated organizations, or those of the publisher, the editors and the reviewers. Any product that may be evaluated in this article, or claim that may be made by its manufacturer, is not guaranteed or endorsed by the publisher.
References
Akkerman-Nijland, A. M., Akkerman, O. W., Grasmeijer, F., Hagedoorn, P., Frijlink, H. W., Rottier, B. L., et al. (2021). The pharmacokinetics of antibiotics in cystic fibrosis. Expert Opin. Drug Metab. Toxicol. 17, 53–68. doi: 10.1080/17425255.2021.1836157
Alonso, C. A., Kwabugge, Y. A., Anyanwu, M. U., Torres, C., and Chah, K. F. (2017). Diversity of Ochrobactrum species in food animals, antibiotic resistance phenotypes and polymorphisms in the bla OCH gene. FEMS Microbiol. Lett. 364:178. doi: 10.1093/femsle/fnx178
Anderson, R. C., and Yu, P.-L. (2005). Factors affecting the antimicrobial activity of ovine-derived cathelicidins against E. Coli 0157: H7. Int. J. Antimicrob. Agents 25, 205–210. doi: 10.1016/j.ijantimicag.2004.10.010
Bals, R., Wang, X., Zasloff, M., and Wilson, J. M. (1998). The peptide antibiotic LL-37/hCAP-18 is expressed in epithelia of the human lung where it has broad antimicrobial activity at the airway surface. Proc. Natl. Acad. Sci. 95, 9541–9546. doi: 10.1073/pnas.95.16.9541
Bhagirath, A. Y., Li, Y., Somayajula, D., Dadashi, M., Badr, S., and Duan, K. (2016). Cystic fibrosis lung environment and Pseudomonas aeruginosa infection. BMC Pulm. Med. 16:174. doi: 10.1186/s12890-016-0339-5
Bhat, P. G., Flanagan, D. R., and Donovan, M. D. (1996). Drug diffusion through cystic fibrotic mucus: steady-state permeation, rheologic properties, and glycoprotein morphology. J. Pharm. Sci. 85, 624–630. doi: 10.1021/js950381s
Boucher, R. (2004). New concepts of the pathogenesis of cystic fibrosis lung disease. Eur. Respir. J. 23, 146–158. doi: 10.1183/09031936.03.00057003
Brandt, T., Breitenstein, S., von der Hardt, H., and Tümmler, B. (1995). DNA concentration and length in sputum of patients with cystic fibrosis during inhalation with recombinant human DNase. Thorax 50, 880–882. doi: 10.1136/thx.50.8.880
Brown, R. B. (2021). Sodium toxicity in the nutritional epidemiology and nutritional immunology of COVID-19. Medicina 57:739. doi: 10.3390/medicina57080739
Bucki, R., Byfield, F. J., and Janmey, P. A. (2007). Release of the antimicrobial peptide LL-37 from DNA/F-actin bundles in cystic fibrosis sputum. Eur. Respir. J. 29, 624–632. doi: 10.1183/09031936.00080806
Bucki, R., Niemirowicz, K., Wnorowska, U., Byfield, F. J., Piktel, E., Wątek, M., et al. (2015). Bactericidal activity of ceragenin CSA-13 in cell culture and in an animal model of peritoneal infection. Antimicrob. Agents Chemother. 59, 6274–6282. doi: 10.1128/AAC.00653-15
Bucki, R., Sostarecz, A. G., Byfield, F. J., Savage, P. B., and Janmey, P. A. (2007). Resistance of the antibacterial agent ceragenin CSA-13 to inactivation by DNA or F-actin and its activity in cystic fibrosis sputum. J. Antimicrob. Chemother. 60, 535–545. doi: 10.1093/jac/dkm218
Carvalho Filho, É. B., Marson, F. A. L., and Levy, C. E. (2018). Challenges in the identification of Ochrobactrum anthropi in blood and sputum cultures of patients with cystic fibrosis. Rev. Epidemiol. E Controle Infecção. 8, 189–191. doi: 10.17058/reci.v1i2.9967
Chmielewska, S. J., Skłodowski, K., Piktel, E., Suprewicz, Ł., Fiedoruk, K., Daniluk, T., et al. (2020). NDM-1 Carbapenemase-producing Enterobacteriaceae are highly susceptible to Ceragenins CSA-13, CSA-44, and CSA-131. Infect. Drug Resist. 13, 3277–3294. doi: 10.2147/IDR.S261579
Choi, K.-H., Kumar, A., and Schweizer, H. P. (2006). A 10-min method for preparation of highly electrocompetent Pseudomonas aeruginosa cells: application for DNA fragment transfer between chromosomes and plasmid transformation. J. Microbiol. Methods 64, 391–397. doi: 10.1016/j.mimet.2005.06.001
Chotirmall, S. H., Greene, C. M., and McElvaney, N. G. (2010). Candida species in cystic fibrosis: a road less travelled. Med. Mycol. 48, S114–S124. doi: 10.3109/13693786.2010.503320
Dagenais, R. V., Su, V. C., and Quon, B. S. (2020). Real-world safety of CFTR modulators in the treatment of cystic fibrosis: a systematic review. J. Clin. Med. 10:23. doi: 10.3390/jcm10010023
Ding, B., Guan, Q., Walsh, J. P., Boswell, J. S., Winter, T. W., Winter, E. S., et al. (2002). Correlation of the antibacterial activities of cationic peptide antibiotics and cationic steroid antibiotics. J. Med. Chem. 45, 663–669. doi: 10.1021/jm0105070
Durnaś, B., Piktel, E., Wątek, M., Wollny, T., Góźdź, S., Smok-Kalwat, J., et al. (2017). Anaerobic bacteria growth in the presence of cathelicidin LL-37 and selected ceragenins delivered as magnetic nanoparticles cargo. BMC Microbiol. 17:167. doi: 10.1186/s12866-017-1075-6
Epand, R. F., Savage, P. B., and Epand, R. M. (2007). Bacterial lipid composition and the antimicrobial efficacy of cationic steroid compounds (Ceragenins). Biochim. Biophys. Acta 1768, 2500–2509. doi: 10.1016/j.bbamem.2007.05.023
Felgentreff, K., Beisswenger, C., Griese, M., Gulder, T., Bringmann, G., and Bals, R. (2006). The antimicrobial peptide cathelicidin interacts with airway mucus. Peptides 27, 3100–3106. doi: 10.1016/j.peptides.2006.07.018
Ghosh, S., Pandit, G., Debnath, S., Chatterjee, S., and Satpati, P. (2021). Effect of monovalent salt concentration and peptide secondary structure in peptide-micelle binding. RSC Adv. 11, 36836–36849. doi: 10.1039/D1RA06772A
Goldman, M. J., Anderson, G. M., Stolzenberg, E. D., Kari, U. P., Zasloff, M., and Wilson, J. M. (1997). Human β-defensin-1 is a salt-sensitive antibiotic in lung that is inactivated in cystic fibrosis. Cells 88, 553–560. doi: 10.1016/S0092-8674(00)81895-4
Habib, A.-R. R., Kajbafzadeh, M., Desai, S., Yang, C. L., Skolnik, K., and Quon, B. S. (2019). A systematic review of the clinical efficacy and safety of CFTR modulators in cystic fibrosis. Sci. Rep. 9:7234. doi: 10.1038/s41598-019-43652-2
Haq, I., Almulhem, M., Soars, S., Poulton, D., and Brodlie, M. (2022). Precision medicine based on CFTR genotype for people with cystic fibrosis. Pharmgenomics Pers. Med. 15, 91–104. doi: 10.2147/PGPM.S245603
Hashemi, M. M., Holden, B. S., and Savage, P. B. (2018). “Ceragenins as non-peptide mimics of endogenous antimicrobial peptides” in Fighting Antimicrobial Resistance. ed. A. Budimir (Zagreb, Croatia: IAPC Publishing), 139–169.
Hentzer, M., Teitzel, G. M., Balzer, G. J., Heydorn, A., Molin, S., Givskov, M., et al. (2001). Alginate overproduction affects Pseudomonas aeruginosa biofilm structure and function. J. Bacteriol. 183, 5395–5401. doi: 10.1128/JB.183.18.5395-5401.2001
Hiemstra, P. (2007). Antimicrobial peptides in the real world: implications for cystic fibrosis. Eur. Respir. J. 29, 617–618. doi: 10.1183/09031936.00017007
Howell, M. D., Streib, J. E., Kim, B. E., Lesley, L. J., Dunlap, A. P., Geng, D., et al. (2009). Ceragenins: a class of antiviral compounds to treat orthopox infections. J. Investig. Dermatol. 129, 2668–2675. doi: 10.1038/jid.2009.120
Huang, Y., He, L., Li, G., Zhai, N., Jiang, H., and Chen, Y. (2014). Role of helicity of α-helical antimicrobial peptides to improve specificity. Protein Cell 5, 631–642. doi: 10.1007/s13238-014-0061-0
Javia, A., Misra, A., and Thakkar, H. (2022). Liposomes encapsulating novel antimicrobial peptide Omiganan: characterization and its pharmacodynamic evaluation in atopic dermatitis and psoriasis mice model. Int. J. Pharm. 624:122045. doi: 10.1016/j.ijpharm.2022.122045
Jayaraman, S., Song, Y., Vetrivel, L., Shankar, L., and Verkman, A. (2001). Noninvasive in vivo fluorescence measurement of airway-surface liquid depth, salt concentration, and pH. J. Clin. Invest. 107, 317–324. doi: 10.1172/JCI11154
Johansson, J., Gudmundsson, G. H., MnE, R., Berndt, K. D., and Agerberth, B. (1998). Conformation-dependent antibacterial activity of the naturally occurring human peptide LL-37. J. Biol. Chem. 273, 3718–3724. doi: 10.1074/jbc.273.6.3718
Joris, L., Dab, I., and Quinton, P. M. (1993). Elemental composition of human airway surface fluid in healthy and diseased airways. Am. J. Respir. Crit. Care Med. 148, 1633–1637. doi: 10.1164/ajrccm/148.6_Pt_1.1633
Kandasamy, S. K., and Larson, R. G. (2006). Effect of salt on the interactions of antimicrobial peptides with zwitterionic lipid bilayers. Biochim. Biophys. Acta 1758, 1274–1284. doi: 10.1016/j.bbamem.2006.02.030
Koch, C. (2002). Early infection and progression of cystic fibrosis lung disease. Pediatr. Pulmonol. 34, 232–236. doi: 10.1002/ppul.10135
Kooi, C., and Sokol, P. A. (2009). Burkholderia cenocepacia zinc metalloproteases influence resistance to antimicrobial peptides. Microbiology 155, 2818–2825. doi: 10.1099/mic.0.028969-0
Lapierre, S. G., Phelippeau, M., Hakimi, C., Didier, Q., Reynaud-Gaubert, M., and Dubus, J.-C. (2017). Cystic fibrosis respiratory tract salt concentration: an exploratory cohort study. Medicine 96:e8423. doi: 10.1097/MD.0000000000008423
Lee, I. H., Cho, Y., and Lehrer, R. I. (1997). Effects of pH and salinity on the antimicrobial properties of clavanins. Infect. Immun. 65, 2898–2903. doi: 10.1128/iai.65.7.2898-2903.1997
Lei, J., Sun, L., Huang, S., Zhu, C., Li, P., He, J., et al. (2019). The antimicrobial peptides and their potential clinical applications. Am. J. Transl. Res. 11, 3919–3931.
Leszczyńska, K., Namiot, A., Cruz, K., Byfield, F., Won, E., and Mendez, G. (2011). Potential of ceragenin CSA-13 and its mixture with pluronic F-127 as treatment of topical bacterial infections. J. Appl. Microbiol. 110, 229–238. doi: 10.1111/j.1365-2672.2010.04874.x
Lewenza, S. (2013). Extracellular DNA-induced antimicrobial peptide resistance mechanisms in Pseudomonas aeruginosa. Front. Microbiol. 4:21. doi: 10.3389/fmicb.2013.00021
López-Causapé, C., Rojo-Molinero, E., Macia, M. D., and Oliver, A. (2015). The problems of antibiotic resistance in cystic fibrosis and solutions. Expert Rev. Respir. Med. 9, 73–88. doi: 10.1586/17476348.2015.995640
Lyczak, J. B., Cannon, C. L., and Pier, G. B. (2002). Lung infections associated with cystic fibrosis. Clin. Microbiol. Rev. 15, 194–222. doi: 10.1128/CMR.15.2.194-222.2002
Majewski, K., Kozłowska, E., Żelechowska, P., and Brzezińska-Błaszczyk, E. (2018). Serum concentrations of antimicrobial peptide cathelicidin LL-37 in patients with bacterial lung infections. Cent. Eur. J. Immunol. 43, 453–457. doi: 10.5114/ceji.2018.81355
Mansour, S. C., Pena, O. M., and Hancock, R. E. (2014). Host defense peptides: front-line immunomodulators. Trends Immunol. 35, 443–450. doi: 10.1016/j.it.2014.07.004
McGarry, M. E., Gibb, E. R., Oates, G. R., and Schechter, M. S. (2022). Left behind: the potential impact of CFTR modulators on racial and ethnic disparities in cystic fibrosis. Paediatr. Respir. Rev. 42, 35–42. doi: 10.1016/j.prrv.2021.12.001
McKelvey, M. C., Weldon, S., McAuley, D. F., Mall, M. A., and Taggart, C. C. (2020). Targeting proteases in cystic fibrosis lung disease. Paradigms, progress, and potential. Am. J. Respir. Crit. Care Med. 201, 141–147. doi: 10.1164/rccm.201906-1190PP
Meluleni, G. J., Grout, M., Evans, D. J., and Pier, G. B. (1995). Mucoid Pseudomonas aeruginosa growing in a biofilm in vitro are killed by opsonic antibodies to the mucoid exopolysaccharide capsule but not by antibodies produced during chronic lung infection in cystic fibrosis patients. J. Immunol. 155, 2029–2038. doi: 10.4049/jimmunol.155.4.2029
Mojsoska, B., and Jenssen, H. (2015). Peptides and peptidomimetics for antimicrobial drug design. Pharmaceuticals 8, 366–415. doi: 10.3390/ph8030366
Morrison, C. B., Markovetz, M. R., and Ehre, C. (2019). Mucus, mucins, and cystic fibrosis. Pediatr. Pulmonol. 54, S84–S96. doi: 10.1002/ppul.24530
Ng, S. M. S., Teo, S. W., Yong, Y. E., Ng, F. M., Lau, Q. Y., Jureen, R., et al. (2017). Preliminary investigations into developing all-D Omiganan for treating mupirocin-resistant MRSA skin infections. Chem. Biol. Drug Des. 90, 1155–1160. doi: 10.1111/cbdd.13035
Nichols, W. W., Evans, M. J., Slack, M. P., and Walmsley, H. L. (1989). The penetration of antibiotics into aggregates of mucoid and non-mucoid Pseudomonas aeruginosa. Microbiology 135, 1291–1303. doi: 10.1099/00221287-135-5-1291
Nivens, D. E., Ohman, D. E., Williams, J., and Franklin, M. J. (2001). Role of alginate and its O acetylation in formation of Pseudomonas aeruginosa microcolonies and biofilms. J. Bacteriol. 183, 1047–1057. doi: 10.1128/JB.183.3.1047-1057.2001
Paprocka, P., Durnaś, B., Mańkowska, A., Skłodowski, K., Król, G., Zakrzewska, M., et al. (2021). New β-lactam antibiotics and ceragenins–a study to assess their potential in treatment of infections caused by multidrug-resistant strains of Pseudomonas aeruginosa. Infect. Drug Resist. 14, 5681–5698. doi: 10.2147/IDR.S338827
Paprocka, P., Mańkowska, A., Skłodowski, K., Król, G., Wollny, T., Lesiak, A., et al. (2022). Bactericidal activity of Ceragenin in combination with ceftazidime, levofloxacin, co-Trimoxazole, and Colistin against the opportunistic pathogen Stenotrophomonas maltophilia. Pathogens 11:621. doi: 10.3390/pathogens11060621
Park, I. Y., Cho, J. H., Kim, K. S., Kim, Y.-B., Kim, M. S., and Kim, S. C. (2004). Helix stability confers salt resistance upon helical antimicrobial peptides. J. Biol. Chem. 279, 13896–13901. doi: 10.1074/jbc.M311418200
Piktel, E., Markiewicz, K. H., Wilczewska, A. Z., Daniluk, T., Chmielewska, S., Niemirowicz-Laskowska, K., et al. (2020). Quantification of synergistic effects of Ceragenin CSA-131 combined with iron oxide magnetic nanoparticles against cancer cells. Int. J. Nanomedicine 15, 4573–4589. doi: 10.2147/IJN.S255170
Pollard, J. E., Snarr, J., Chaudhary, V., Jennings, J. D., Shaw, H., Christiansen, B., et al. (2012). In vitro evaluation of the potential for resistance development to ceragenin CSA-13. J. Antimicrob. Chemother. 67, 2665–2672. doi: 10.1093/jac/dks276
Pragman, A. A., Berger, J. P., and Williams, B. J. (2016). Understanding persistent bacterial lung infections: clinical implications informed by the biology of the microbiota and biofilms. Clin. Pulm. Med. 23, 57–66. doi: 10.1097/CPM.0000000000000108
Rossi, L. M., Rangasamy, P., Zhang, J., Qiu, X. Q., and Wu, G. Y. (2008). Research advances in the development of peptide antibiotics. J. Pharm. Sci. 97, 1060–1070. doi: 10.1002/jps.21053
Rothstein, D. M., Spacciapoli, P., Tran, L. T., Xu, T., Roberts, F. D., Dalla Serra, M., et al. (2001). Anticandida activity is retained in P-113, a 12-amino-acid fragment of histatin 5. Antimicrob. Agents Chemother. 45, 1367–1373. doi: 10.1128/AAC.45.5.1367-1373.2001
Saint-Criq, V., and Gray, M. A. (2017). Role of CFTR in epithelial physiology. Cell. Mol. Life Sci. 74, 93–115. doi: 10.1007/s00018-016-2391-y
Sarkar, S. (2020). Release mechanisms and molecular interactions of Pseudomonas aeruginosa extracellular DNA. Appl. Microbiol. Biotechnol. 104, 6549–6564. doi: 10.1007/s00253-020-10687-9
Schaupp, L., Addante, A., Völler, M., Fentker, K., Kuppe, A., Bardua, M., et al. (2023). Longitudinal effects of elexacaftor/tezacaftor/ivacaftor on sputum viscoelastic properties, airway infection and inflammation in patients with cystic fibrosis. Eur. Respir. J. 62:2202153. doi: 10.1183/13993003.02153-2022
Sheils, C. A., Käs, J., Travassos, W., Allen, P. G., Janmey, P. A., Wohl, M. E., et al. (1996). Actin filaments mediate DNA fiber formation in chronic inflammatory airway disease. Am. J. Pathol. 148, 919–927.
Simonin, J., Bille, E., Crambert, G., Noel, S., Dreano, E., Edwards, A., et al. (2019). Author correction: airway surface liquid acidification initiates host defense abnormalities in cystic fibrosis. Sci. Rep. 9:17535. doi: 10.1038/s41598-019-54253-4
Sinclair, K., Pham, T., Farnsworth, R., Williams, D., Loc-Carrillo, C., Horne, L., et al. (2012). Development of a broad spectrum polymer-released antimicrobial coating for the prevention of resistant strain bacterial infections. J. Biomed. Mater. Res. A 100, 2732–2738. doi: 10.1002/jbm.a.34209
Smith, J. J., Travis, S. M., Greenberg, E. P., and Welsh, M. J. (1996). Cystic fibrosis airway epithelia fail to kill bacteria because of abnormal airway surface fluid. Cells 85, 229–236. doi: 10.1016/S0092-8674(00)81099-5
Stewart, P. S. (1996). Theoretical aspects of antibiotic diffusion into microbial biofilms. Antimicrob. Agents Chemother. 40, 2517–2522. doi: 10.1128/AAC.40.11.2517
Suprewicz, Ł., Szczepański, A., Lenart, M., Piktel, E., Fiedoruk, K., Barreto-Duran, E., et al. (2023). Ceragenins exhibit antiviral activity against SARS-CoV-2 by increasing the expression and release of type I interferons upon activation of the host’s immune response. Antivir. Res. 217:105676. doi: 10.1016/j.antiviral.2023.105676
Tanaka, D., Miyasaki, K., and Lehrer, R. (2000). Sensitivity of Actinobacillus actinomycetemcomitans and Capnocytophaga spp. to the bactericidal action of LL-37: a cathelicidin found in human leukocytes and epithelium. Oral Microbiol. Immunol. 15, 226–231. doi: 10.1034/j.1399-302x.2000.150403.x
Tang, J. X., Wen, Q., Bennett, A., Kim, B., Sheils, C. A., Bucki, R., et al. (2005). Anionic poly (amino acid) s dissolve F-actin and DNA bundles, enhance DNase activity, and reduce the viscosity of cystic fibrosis sputum. Am. J. Phys. Lung Cell. Mol. Phys. 289, L599–L605. doi: 10.1152/ajplung.00061.2005
Taylor-Cousar, J. L., Robinson, P. D., Shteinberg, M., and Downey, D. G. (2023). CFTR modulator therapy: transforming the landscape of clinical care in cystic fibrosis. Lancet 402, 1171–1184. doi: 10.1016/S0140-6736(23)01609-4
Tokajuk, J., Deptuła, P., Chmielewska, S. J., Skłodowski, K., Mierzejewska, Ż. A., Grądzka-Dahlke, M., et al. (2022). Ceragenin CSA-44 as a means to control the formation of the biofilm on the surface of tooth and composite fillings. Pathogens 11:491. doi: 10.3390/pathogens11050491
Tomás, M., Doumith, M., Warner, M., Turton, J. F., Beceiro, A., and Bou, G. (2010). Efflux pumps, Opr D porin, amp C β-lactamase, and multiresistance in Pseudomonas aeruginosa isolates from cystic fibrosis patients. Antimicrob. Agents Chemother. 54, 2219–2224. doi: 10.1128/AAC.00816-09
Tomkiewicz, RP, Kishioka, C, Freeman, J, and Rubin, BK. DNA and actin filament ultrastructure in cystic fibrosis sputum. Cilia, mucus, and mucociliary interactions New York: Dekker. (1998): 333–341.
Turcios, N. L. (2020). Cystic fibrosis lung disease: an overview. Respir. Care 65, 233–251. doi: 10.4187/respcare.06697
Turner, K. H., Wessel, A. K., Palmer, G. C., Murray, J. L., and Whiteley, M. (2015). Essential genome of Pseudomonas aeruginosa in cystic fibrosis sputum. Proc. Natl. Acad. Sci. 112, 4110–4115. doi: 10.1073/pnas.1419677112
White, R., Woodward, S., Leppert, M., O’Connell, P., Hoff, M., Herbst, J., et al. (1985). A closely linked genetic marker for cystic fibrosis. Nature 318, 382–384. doi: 10.1038/318382a0
Wu, M., Maier, E., Benz, R., and Hancock, R. E. (1999). Mechanism of interaction of different classes of cationic antimicrobial peptides with planar bilayers and with the cytoplasmic membrane of Escherichia coli. Biochemistry 38, 7235–7242. doi: 10.1021/bi9826299
Yagel, Y., Sestito, S., Motro, Y., Shnaiderman-Torban, A., Khalfin, B., Sagi, O., et al. (2020). Genomic characterization of antimicrobial resistance, virulence, and phylogeny of the genus Ochrobactrum. Antibiotics 9:177. doi: 10.3390/antibiotics9040177
Zabner, J., Smith, J. J., Karp, P. H., Widdicombe, J. H., and Welsh, M. J. (1998). Loss of CFTR chloride channels alters salt absorption by cystic fibrosis airway epithelia in vitro. Mol. Cell 2, 397–403. doi: 10.1016/S1097-2765(00)80284-1
Keywords: ceragenins, antimicrobial peptides, cystic fibrosis, sodium chloride, sputum scope statement
Citation: Skłodowski K, Suprewicz Ł, Chmielewska-Deptuła SJ, Kaliniak S, Okła S, Zakrzewska M, Minarowski Ł, Mróz R, Daniluk T, Savage PB, Fiedoruk K and Bucki R (2023) Ceragenins exhibit bactericidal properties that are independent of the ionic strength in the environment mimicking cystic fibrosis sputum. Front. Microbiol. 14:1290952. doi: 10.3389/fmicb.2023.1290952
Edited by:
Octavio Luiz Franco, Catholic University of Brasilia (UCB), BrazilReviewed by:
Giovanna Batoni, University of Pisa, ItalyLucia Lombardi, Imperial College London, United Kingdom
Copyright © 2023 Skłodowski, Suprewicz, Chmielewska-Deptuła, Kaliniak, Okła, Zakrzewska, Minarowski, Mróz, Daniluk, Savage, Fiedoruk and Bucki. This is an open-access article distributed under the terms of the Creative Commons Attribution License (CC BY). The use, distribution or reproduction in other forums is permitted, provided the original author(s) and the copyright owner(s) are credited and that the original publication in this journal is cited, in accordance with accepted academic practice. No use, distribution or reproduction is permitted which does not comply with these terms.
*Correspondence: Robert Bucki, YnVja2lyb2JlcnRAZ21haWwuY29t