- College of Wine and Horticulture, Ningxia University, Yinchuan, China
The substitution of chemical fertilizers with organic fertilizers is a viable strategy to enhance crop yield and soil quality. In this study, the aim was to investigate the changes in soil microorganisms, soil chemical properties, and growth of Chinese flowering cabbage under different fertilization treatments involving earthworms and cow manure. Compared with the control (100% chemical fertilizer), CE (30% reduction in chemical fertilizer + earthworms) and CFE (30% reduction in chemical fertilizer + cow dung + earthworms) treatments at soil pH 8.14 and 8.07, respectively, and CFC (30% reduction in chemical fertilizer + cow manure) and CFE treatments increased soil organic matter (SOM), total nitrogen (TN), available nitrogen (AN), and available potassium (AK) contents. Earthworms and cow manure promoted the abundance of Bacillus and reduced that of the pathogens Plectosphaerella and Gibberella. The mantle test revealed that pH was not correlated with the microbial community. Random forest analysis verified that AN, SOM, and TN were important factors that jointly influenced bacterial and fungal diversity. Overall, the synergistic effect of earthworms and cow manure increased soil fertility and microbial diversity, thereby promoting the growth and development of Chinese flowering cabbage. This study enhanced the understanding of how bioregulation affects the growth and soil quality of Chinese flowering cabbage, and thus provided a guidance for the optimization of fertilization strategies to maximize the yield and quality of Chinese flowering cabbage while reducing environmental risks.
1. Introduction
Increasing application of fertilizers has resulted in adverse consequences on soil and water quality, biodiversity, climate, and human health. These effects are more pronounced than the corresponding increases in crop yields (Kour et al., 2020). In many intensive farming regions, the farmers struggle to accurately determine fertilizer requirements for crops and prioritize high yields. This often results in excessive use of fertilizers that exceeds the actual needs of the crops (Zhu et al., 2019). This problem is primarily attributed to inadequate fertilizer management, leading to reduced fertilizer efficiency and environmental contamination. Agricultural systems face the formidable challenge of meeting the mounting food demand while minimizing the harmful environmental impacts associated with intensive use of fertilizers, particularly nitrogen (N) fertilizers (Steffen et al., 2015). Excessive N fertilizer application adversely affects soil physicochemical properties and disrupts microbial communities, causing imbalance in the soil ecosystem (Wu et al., 2020). Overuse of fertilizers reduces soil microbial biomass by reducing microbial abundance and diversity. Additionally, excessive fertilization weakens the stability of microbial community and diminishes the interactions among microbial taxa (Zhang et al., 2013). These findings highlight the negative impacts of excessive fertilization on sustainable soil productivity and microbial ecology; for example, long-term excessive application of synthetic N fertilizers is associated with the degradation of soil microbial communities. In a study, long-term application of inorganic fertilizers augmented total soil organic carbon levels and significantly increased maize yields (Belay et al., 2002). However, the excessive use of chemical fertilizers leads to nitrate accumulation in vegetable products, thereby compromising food safety. In recent years, organic fertilizers have garnered considerable attention as a sustainable alternative to mitigate N losses and restore soil fertility. Combining organic fertilizers with low dose of chemical fertilizer can help in alleviating the adverse effects of overuse of chemical fertilizers. Numerous studies have demonstrated that the partial substitution of chemical fertilizers with organic fertilizers is a promising strategy for enhancing soil fertility and crop yield. For example, replacement of chemical fertilizers with organic counterparts augments the effectiveness of soil N and phosphorus, ultimately increasing cabbage yield (Xiao et al., 2022). Application of compost along with organic fertilizers can enhance soil fertility, increase fungal abundance and diversity, and promote the abundance of favorable microbial taxa (Kamaa et al., 2011; Jiao et al., 2021).
Decline in the yield and quality of Chinese flowering cabbage, resulting from frequent succession and uncontrolled fertilizer application, can be attributed to reduced soil porosity and water content and microbial community imbalance caused by excessive fertilization. Earthworms, as crucial organisms in soil ecosystem, play a pivotal role in soil structure formation, nutrient cycling, and organic matter decomposition (Siebert et al., 2019). Their activity enhances soil porosity, water infiltration, and nutrient availability. Furthermore, earthworms facilitate the transportation and blending of organic material in soil, promoting the interaction between organic fertilizers and soil microbial communities (Wang et al., 2021). Studies have demonstrated that the activity of earthworms alters the abundance and proportions of bacteria, fungi, and actinomycetes, thereby altering the microbial community structure (Medina-Sauza et al., 2019). In addition, earthworm activity can influence soil pH and the availability of trace elements, thereby improving microbial activity and community structure. This, in turn, increases the abundance of beneficial soil microorganisms, enhances bacterial community diversity, and promotes microbially mediated organic matter cycling (Cai et al., 2020). Soil microbial communities serve as essential drivers of nutrient cycling and soil fertility and play a fundamental role in organic matter decomposition, N fixation, and nutrient conversion (Blouin et al., 2013). Therefore, understanding the impact of earthworms on soil quality and their interactions with organic fertilizers is crucial for implementing sustainable soil management practices.
Previous studies have primarily focused on the replacement of chemical fertilizers with organic fertilizers to reduce chemical fertilizer inputs and obtain high crop yield (Liu et al., 2015). In this study, the aim was to assess the interactions among soil chemical properties, microbial community structure, and yield and quality of Chinese flowering cabbage under various fertilizer treatments including chemical fertilizer, earthworms, and cow manure. The study aimed to assess whether the addition of earthworms, through bioregulation, can enhance the microbial characteristics of soil that is perennially successively cropped, ultimately improving the quality and yield of Chinese flowering cabbage. In this study, our primary goals included investigating the influence of various physicochemical and biological factors on the quality and yield of Chinese flowering cabbage under equal nitrogen inputs, examining the effects of earthworms and cow manure on the soil microbial community and their underlying mechanisms, and evaluating whether earthworms and cow manure demonstrate a synergistic effect in enhancing the yield and quality of Chinese flowering cabbage under reduced chemical fertilization.
2. Materials and methods
2.1. Site description and fertilization
The experiment was performed in the No. 2 glass greenhouse of the training base of Ningxia University, Yinchuan, Ningxia (38° 5032″ N, 106° 1322″ E). The test soil was the soil under continuous cropping for 9 years at the Lijun Town, Yongning County, Yinchuan, Ningxia. The physical and chemical properties of the soil were: soil pH = 8.21, total nitrogen (TN) = 0.22 g kg−1, available nitrogen (AN) = 1.43 mg kg−1, and soil organic matter (SOM) = 6.81 g kg−1. The experiment was performed in potting boxes measuring 0.41 m in length, 0.27 m in width, and 0.19 m in height. Each box contained 8 kg soil with 18 cm-deep layer. The experiment involved four treatments (Table 1).
Each treatment was performed with six replicates. The fertilizers consisted of urea (N 46%) for N, calcium superphosphate (P2O5 12%) for phosphorus, and potassium sulfate (K2O 50%) for potash. Chemical fertilizers (urea: 0.28 t/ha, calcium superphosphate: 0.13 t/ha, and potassium sulfate: 0.12 t/ha) were applied according to conventional fertilization methods. In the CFC and CFE treatments, the same nitrogen application rate was applied as in the CK treatment. The total nitrogen (TN) content of the cow manure was determined, and it was applied at the rate of 386 g/pot. The basic properties of cow manure were: water content 58%, organic carbon 163.35 g kg−1, and TN content 1.12 g kg−1. Cow manure and calcium superphosphate were used as a basal fertilizer, with 40% of urea and potassium sulfate applied as a basal fertilizer and remaining 60% as a follow-up fertilizer. Prior to the experiment, earthworms (Eisenia foetida) exhibiting high activity and relatively uniform size were carefully chosen. Earthworms were introduced before planting at a density of 60 g m−2, comprising approximately 23 red-seeded Aesop earthworms (each weighing 0.15 g). Except for the CE treatment, all treatments received the same amount of N. Chinese flowering cabbage was planted on July 10, 2022, by direct sowing at 6–7 kg/ha−2, Chinese flowering cabbage were interplanted after the first true leaf, harvested on August 26, 2022, and soil samples were sampled and collected.
2.2. Analysis of soil properties
After harvesting, plants, visible worms and insects, and stones were removed from the samples by multipoint sampling. Further, the samples were sieved through a 2 mm sieve. The soil samples were mixed, divided into multiple portions of 3–5 g each, quick-frozen in liquid nitrogen, and stored at −80°C for the determination of soil microorganisms. A portion of the soil was set aside for the determination of soil physicochemical indexes. Soil pH was determined using the potentiometric method (water:soil = 2.5:1) using a pH meter (FiveEasy Plus pH/mV, Mettler-Toledo (Schweiz) GmbH, Switzerland). Available nitrogen (AN) and TN were determined using the alkaline diffusion method and Kjeldahl digestion method, respectively. Soil available potassium (AK) and available phosphorus (AP) were extracted with CH3COONH4 solution (soil: CH3COONH4 solution = 1:10) and HCl-NH4F solution (soil: HCL-NH4F solution = 1:10), respectively. Soil AK was measured using a 6400A flame photometer (INESA, Shanghai, China), and soil AP was analyzed using a photometer at 660 nm. The soil organic matter (SOM) content was determined using oil bath method.
2.3. Soil DNA extraction and high-throughput sequencing
DNA was extracted using the E.Z.N.A. DNA Kit (Omega Bio-Tek, Norcross, GA, USA). The quality of the extracted DNA was verified using 1% agarose gel electrophoresis. DNA concentration and purity were assessed using NanoDrop 2000 spectrophotometer (Thermo Fisher Scientific). The target primer pairs were (5′-ACTCCTACG GGAGGCAGCAG-3′) and 806R (5′-GGACTACHVGGGTWTCT AAT-3′) for 16S rRNA gene in bacteria, and ITS1F (5′-CTTGG TCATTTAGAGGAAGTAA-3′) and ITS2R (5′-GCTGCGTTCTTCAT CGATGC-3′) in fungi (Zhang et al., 2020). The PCR products were separated using 2% agarose gel electrophoresis, purified with the AxyPrep DNA Gel Extraction Kit (Axygen Biosciences, Union City, CA, USA), and quantified using the QuantiFluor™-ST system (Promega, USA). Finally, bacterial and fungal amplicons were sequenced in pairs on an Illumina HiSeq sequencer. Duplicate reads were removed according to the UCHIME reference dataset, and the reads were sorted and clustered to operational taxonomic units (OTUs) using the USEARCH11-uparse algorithm with a default 97% similarity. A total of 674,096,280,450,803 bases of optimized sequences were obtained, with an average sequence length of 416 bp. All obtained raw sequence datasets have been uploaded to the NCBI Sequence Read Archive (SAR) with the accession number PRJNA1019528 (Supplementary Table S1).
2.4. Analysis of the quality and yield of Chinese flowering cabbage
In the harvested Chinese flowering cabbage, cabbage yield by measuring the fresh and dry weights of above- and below ground portions of cabbage. Nitrate levels were determined using the salicylic acid–sulfuric acid method. Vitamin C (VC) content was determined using 2,6-dichloroindol staining (Arya et al., 2000). The concentration of soluble sugars was determined using the anthrone sulfate method (Wang C. et al., 2020). The soluble protein content was determined using the Kormas Brilliant Blue method (Sedmak and Grossberg, 1977). To determine the nitrite levels, the vegetables samples were mixed with 0.4% p-aminobenzenesulphonic acid and 0.2% naphthylenediamine hydrochloride solution, followed by proper fixation, thorough shaking, and measurement of absorbance at 538 nm.
2.5. Statistical and bioinformatics analysis
Yield and quality of Chinese flowering cabbage were analyzed using one-way ANOVA in SPSS18.0 software. Estimated fungal and bacterial richness using Chao 1 indices, and the diversity index was estimated by the Shannon index. PCoA based on Bray–Curtis distance was conducted to examine the differences in microbial community composition among samples. Principal coordinate analysis (PCoA) was performed using R software (version 4.2.2). A permutation multivariate analysis of variance (PMANOVA) was performed to assess the effects of different fertilization treatments on soil microbial community structure. Species differences were analyzed using the Kruskal–Wallis rank sum test (Kruskal–Wallis h test). Circos mapping was conducted using Circos-0.67-67 software (Yan et al., 2020). Soil physicochemical factors and correlations between growth of Chinese flowering cabbage and soil microbial communities were assessed using mantle test in the Linket package of R software. In addition, to identify the main environmental factors affecting microbial diversity, random forest analysis was performed (Breiman, 2001), in which the relative importance of soil pH, SOM, TN, AN, AP, and AK was ranked. The random forest analysis was performed in the R statistical computing environment with the random forest package.
3. Results
3.1. Effect of different fertilizer treatments on chemical properties of soil
Inclusion of earthworms (CE) led to a reduction in soil pH, and the reduction in pH was significant when it was combined with cow manure (CFE) (p < 0.01). In contrast, partial replacement of fertilizer with cow manure (CFC) significantly increased soil pH (p < 0.05). The CE treatment decreased but both CFC and CFE treatments considerably increased SOM (Figure 1). The CFE treatment significantly changed soil AK compared with the other treatments. AP content in the CE and CFC treatments was different from that in the CFE treatment; however, AP content in the CK and CFE treatments was similar.
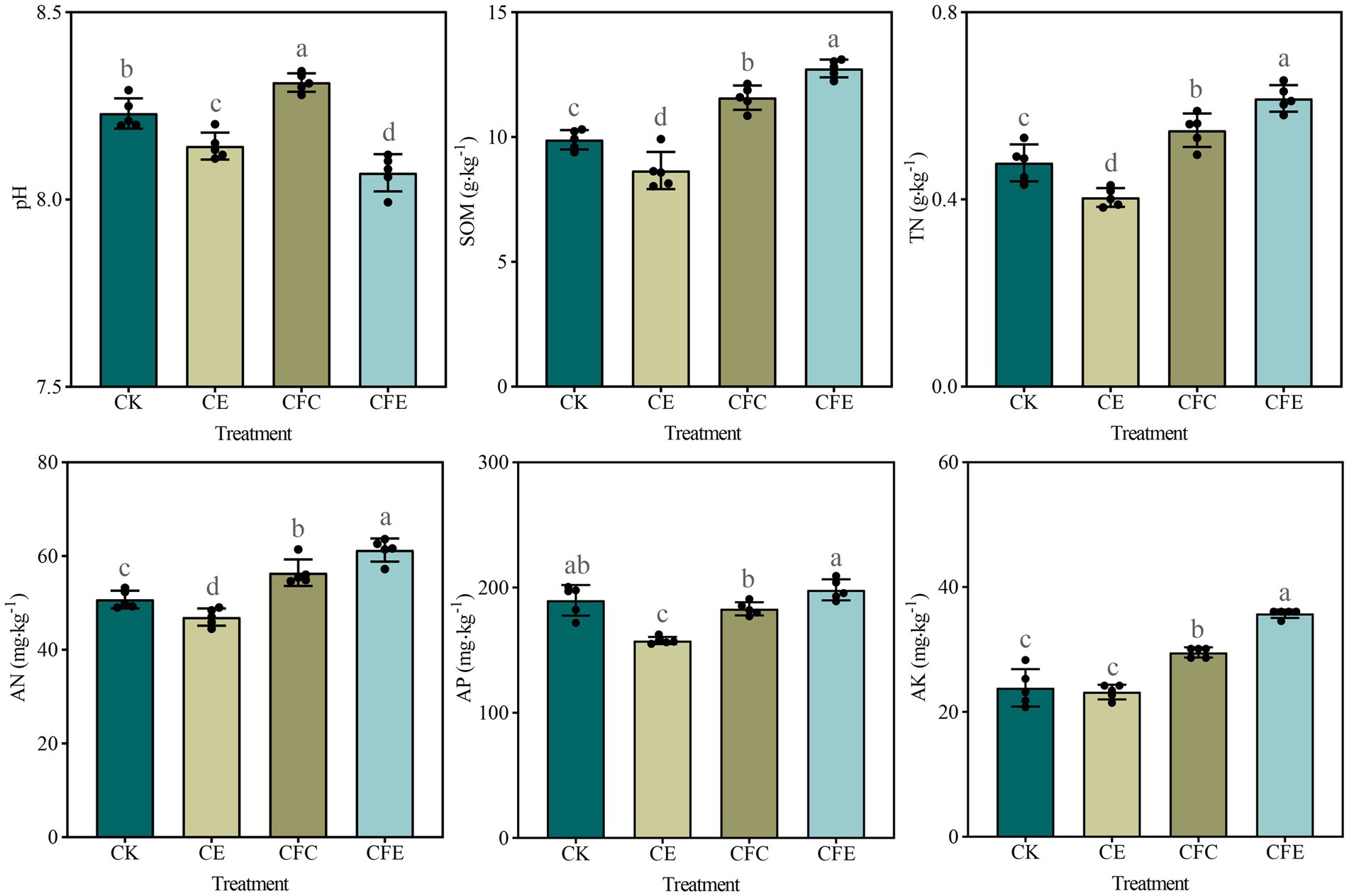
Figure 1. Soil physicochemical properties under different fertilizer treatments. CK: 100% chemical fertilizer; CE: 30% reduced chemical fertilizer + earthworms; CFC: 30% reduced chemical fertilizer + cow manure; CFE: 30% reduced chemical fertilizer + cow manure + earthworms. SOM: soil organic matter; TN: total nitrogen; AN: available nitrogen; AP: available phosphorus; AK: available potassium.
3.2. Yield and quality of Chinese flowering cabbage under different fertilizer treatments
The CFE treatment exhibited a significant increase in the yield of Chinese flowering cabbage, with above and below ground fresh weight of Chinese flowering cabbage exhibiting increase by 31.77 and 20.23%, respectively, compared with the CK treatment (Figure 2A). Furthermore, the CFE treatment showed significant differences in cabbage fresh weight compared to the CE and CFC treatment. In terms of aboveground dry weight, the CFE treatment resulted in 23.01% increase compared with the CK treatment, while CE and CFC treatment had no significant effect compared to CK (Figure 2B). Regarding the quality of Chinese flowering cabbage, the CFE treatment significantly enhanced soluble protein, soluble sugar, vitamin C, nitrate, and nitrite contents compared with the CK treatment (Figure 3). Similar trends were observed in the Chinese flowering cabbage stems, except for nitrite and nitrate.
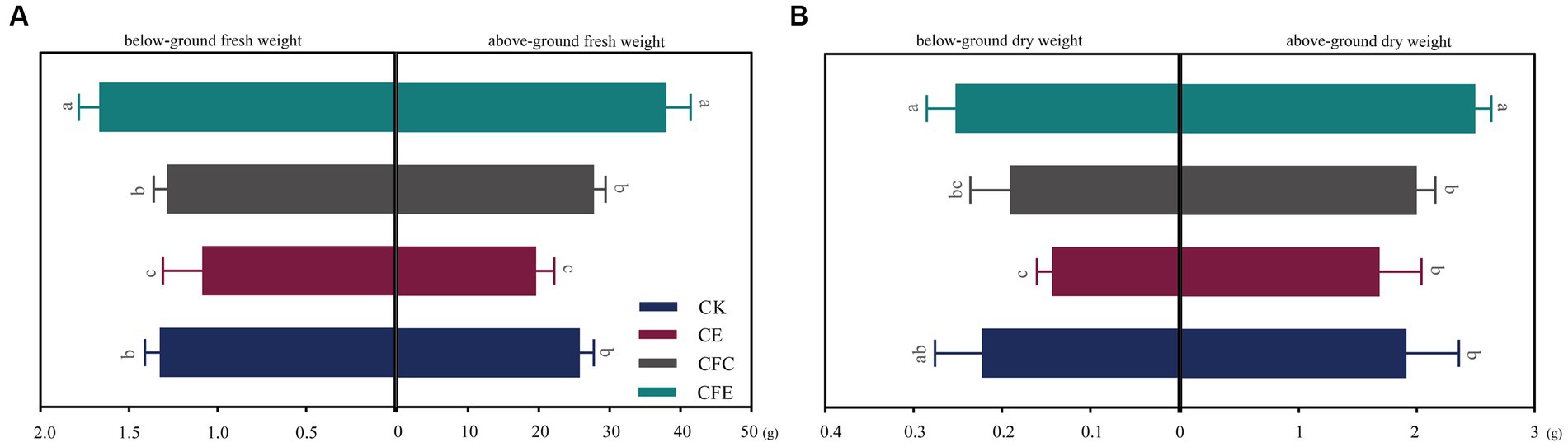
Figure 2. Chinese flowering cabbage yield under different treatments. Fresh weight of above- and belowground parts of Chinese flowering cabbage (A). Dry weight of above- and belowground parts of Chinese flowering cabbage (B). CK: 100% chemical fertilizer; CE: 30% reduced chemical fertilizer + earthworms; CFC: 30% reduced chemical fertilizer + cow manure; CFE: 30% reduced chemical fertilizer + cow manure + earthworms.
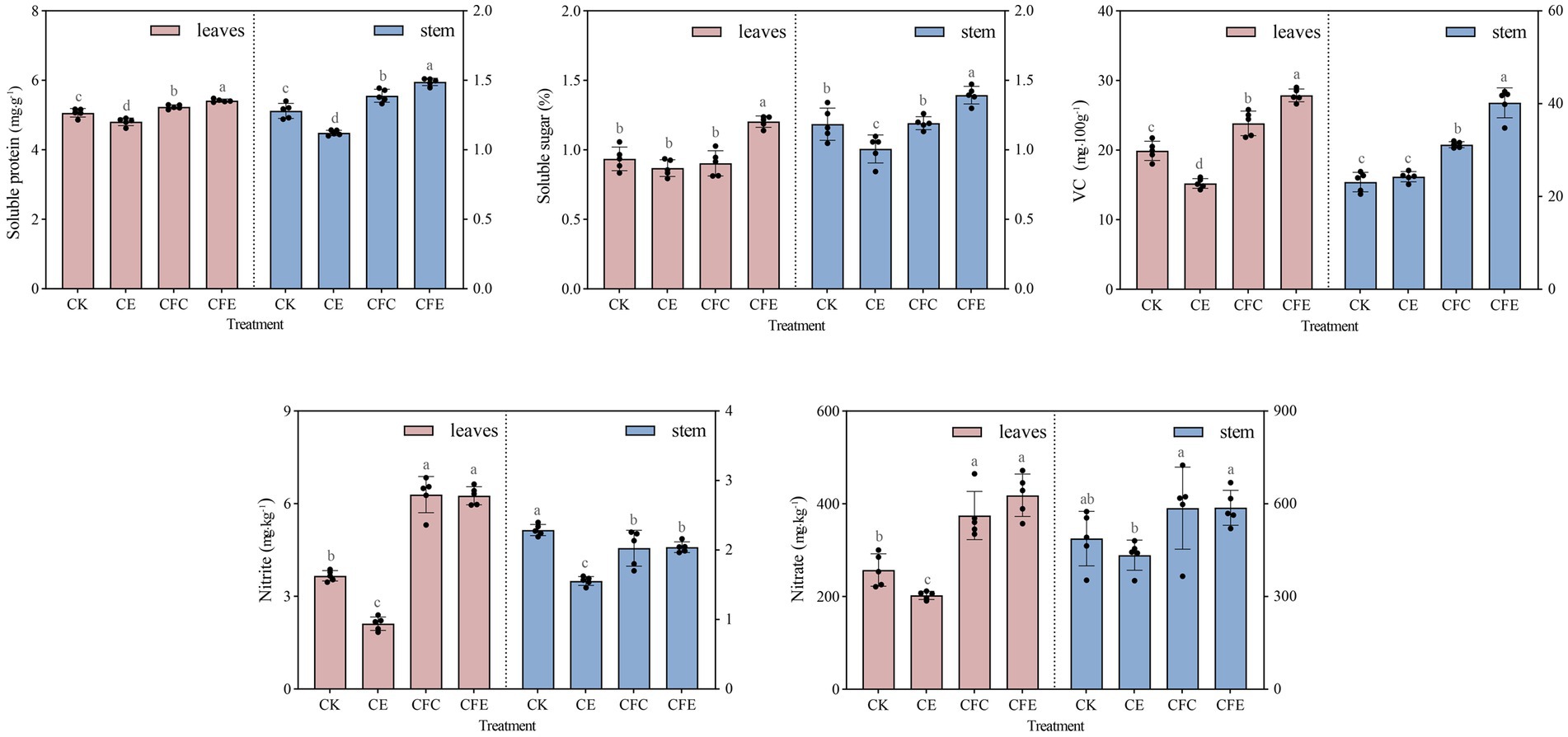
Figure 3. Chinese flowering cabbage quality under different treatments. CK: 100% chemical fertilizer; CE: 30% reduced chemical fertilizer + earthworms; CFC: 30% reduced chemical fertilizer + cow manure; CFE: 30% reduced chemical fertilizer + cow manure + earthworms.
3.3. Effect of different fertilizer treatments on the soil microbial community structure
The CFC and CFE treatments exhibited significant increases in both bacterial and fungal Chao 1 and Shannon indexes. However, no significant differences were observed in bacterial Chao 1 and Shannon indexes between the CE and CK treatments. The CE treatment increased fungal Chao 1 index. The bacterial Chao 1 index followed the order CFC < CFE < CE < CK, whereas the Shannon index followed the order CFC < CFE < CK < CE (Figure 4).
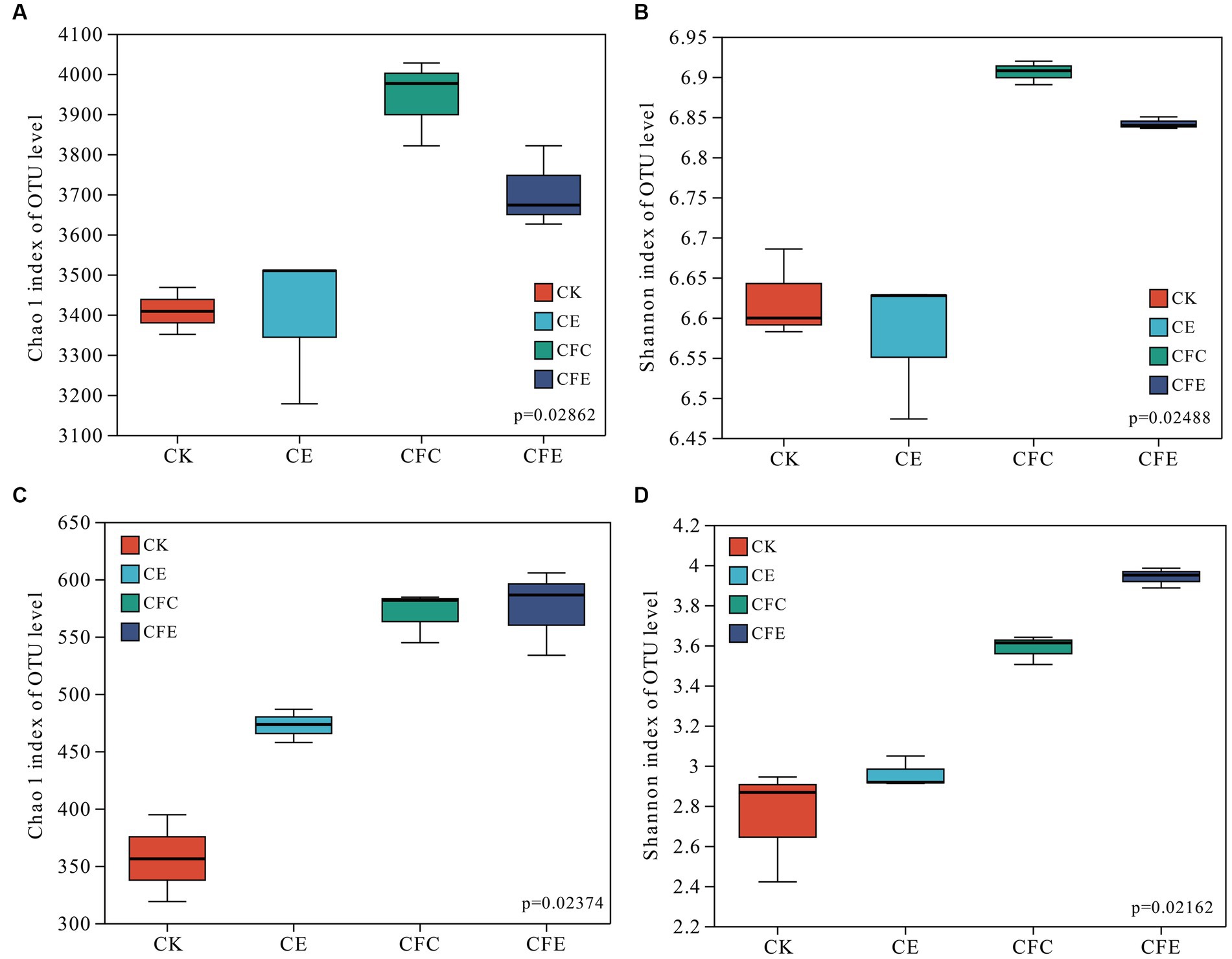
Figure 4. Fertilizer treatments on bacterial and fungal Chao 1 indices (A,C), Shannon index (B,D). CK: 100% chemical fertilizer; CE: 30% reduced chemical fertilizer + earthworms; CFC: 30% reduced chemical fertilizer + cow manure; CFE: 30% reduced chemical fertilizer + cow manure + earthworms.
3.4. Composition of soil microbial communities
Differences in soil bacterial diversity between the CFC and CFE treatments were not significant, whereas significant differences in bacterial diversity were observed between the CE, CFC, and CFE treatments compared with the CK treatment. Principal component 1 and principal component 2 accounted for 60.42 and 17.61% of the variance, respectively (Figure 5A). The treatments exhibited significant variations in fungal community composition, where principal component 1 and principal component 2 explained 59.07 and 17.42% of the variance, respectively (Figure 5B).
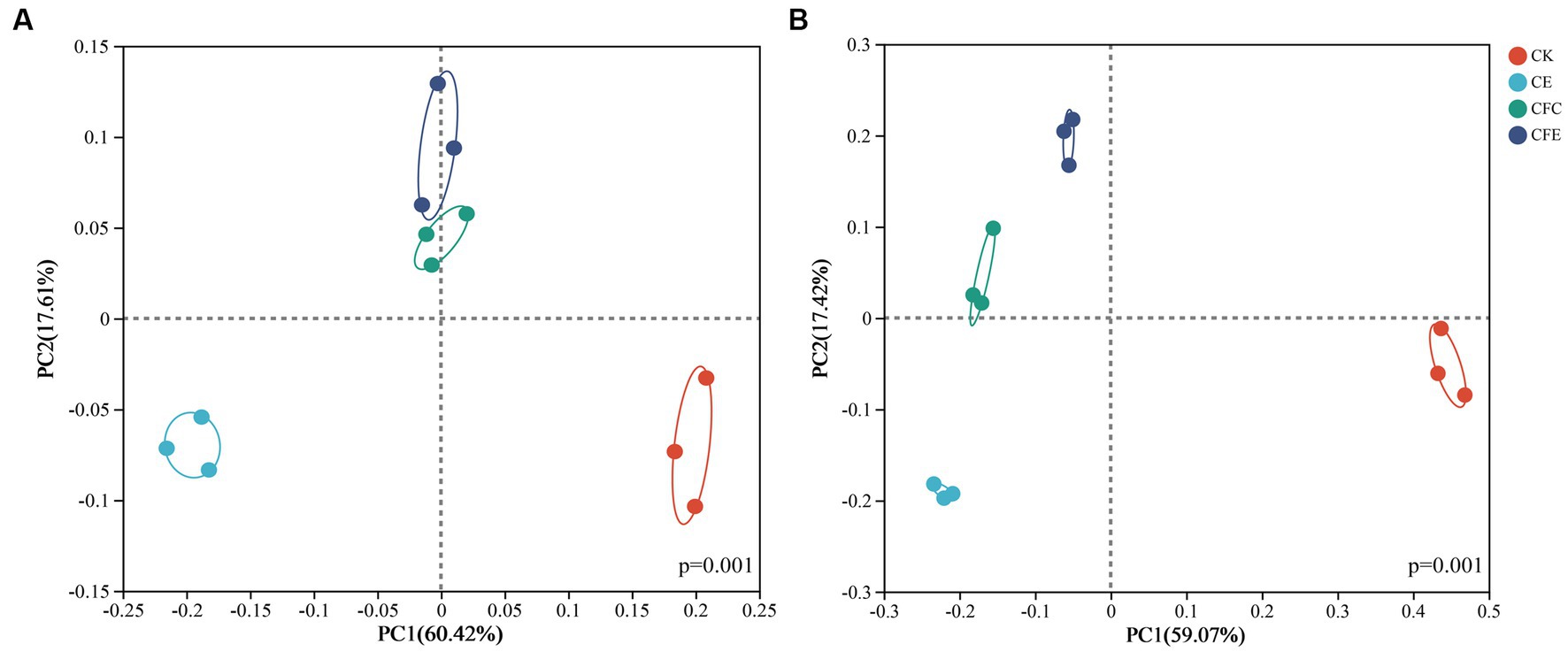
Figure 5. PCoA results of (A) bacterial (pseudo-F:8.21, p = 0.001, PERMANOVAR) and (B) fungal (pseudo-F:13.07, p = 0.001, PERMANOVAR) communities found with different fertilization types. CK: 100% chemical fertilizer; CE: 30% reduced chemical fertilizer + earthworms; CFC: 30% reduced chemical fertilizer + cow manure; CFE: 30% reduced chemical fertilizer + cow manure + earthworms.
The top-4 bacterial phyla identified in the four treatment groups were Proteobacteria, Actinobacteriota, Chloroflexi, and Firmicutes, representing 24.80, 19.71, 17.74, and 13.11% of the total sequences, respectively (Figure 6A). Moreover, Kruskal–Wallis h test demonstrated that the relative abundance of the Firmicutes clade was significantly higher in the CE, CFC, and CFE treatments compared with the CK treatment (Figure 6B; p < 0.05). Additionally, the relative abundance of the Firmicutes clade was higher under the CE and CFE treatments compared with the CFC treatment. Further analysis at the genus level revealed that the dominant genera were Bacillus, norank_f_JG30-KF-CM45, norank_f_norank_o_Actinomarinales, norank_f_norank_o_Vicinamibacterales, and Romboutsia (Figure 6C). The CFE treatment significantly increased the abundance of Bacillus.
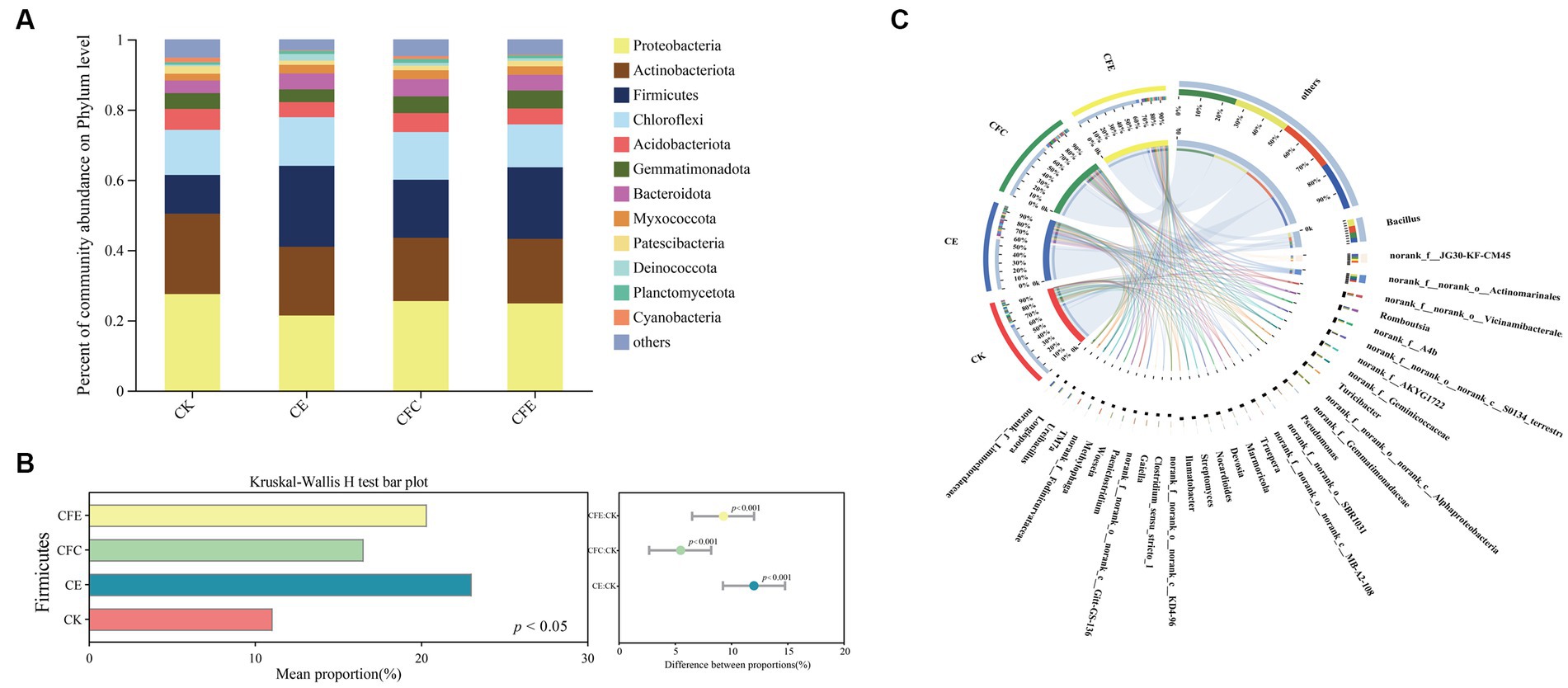
Figure 6. Relative abundance of bacterial taxa at the phylum level (A). The relative abundances of bacteria was tested by the Kruskal–Wallis H-test (B). Composition of bacterial community at the genus level (C). The data were visualized by circos. CK: 100% chemical fertilizer; CE: 30% reduced chemical fertilizer + earthworms; CFC: 30% reduced chemical fertilizer + cow manure; CFE: 30% reduced chemical fertilizer + cow manure + earthworms.
In terms of soil fungal community composition, the dominant fungal phylum was Ascomycota, comprising 62.86% of the total sequences. It was followed by Rozellomycota, unclassified_k_Fungi, Olpidiomycota, and Mortierellomycota (Figure 7A). The relative abundance of Ascomycota was higher under the CK treatment compared with the CE, CFC, and CFE treatments. Conversely, the relative abundance of Rozellomycota and unclassified_k_Fungi was higher under the CE and CFC treatments compared with the CFE treatment. Kruskal–Wallis h test indicated a significantly higher relative abundance of Rozellomycota under the CE, CFC, and CFE treatments compared with the CK treatment (Figure 7B; p < 0.05). At the genus level, the dominant fungal genera were unclassified_p_Rozellomycota, Plectosphaerella, unclassified_k_Fungi, unclassified_f_Chaetomiaceae, unclassified_c_Sordariomycetes, and Gibberella (Figure 7C).
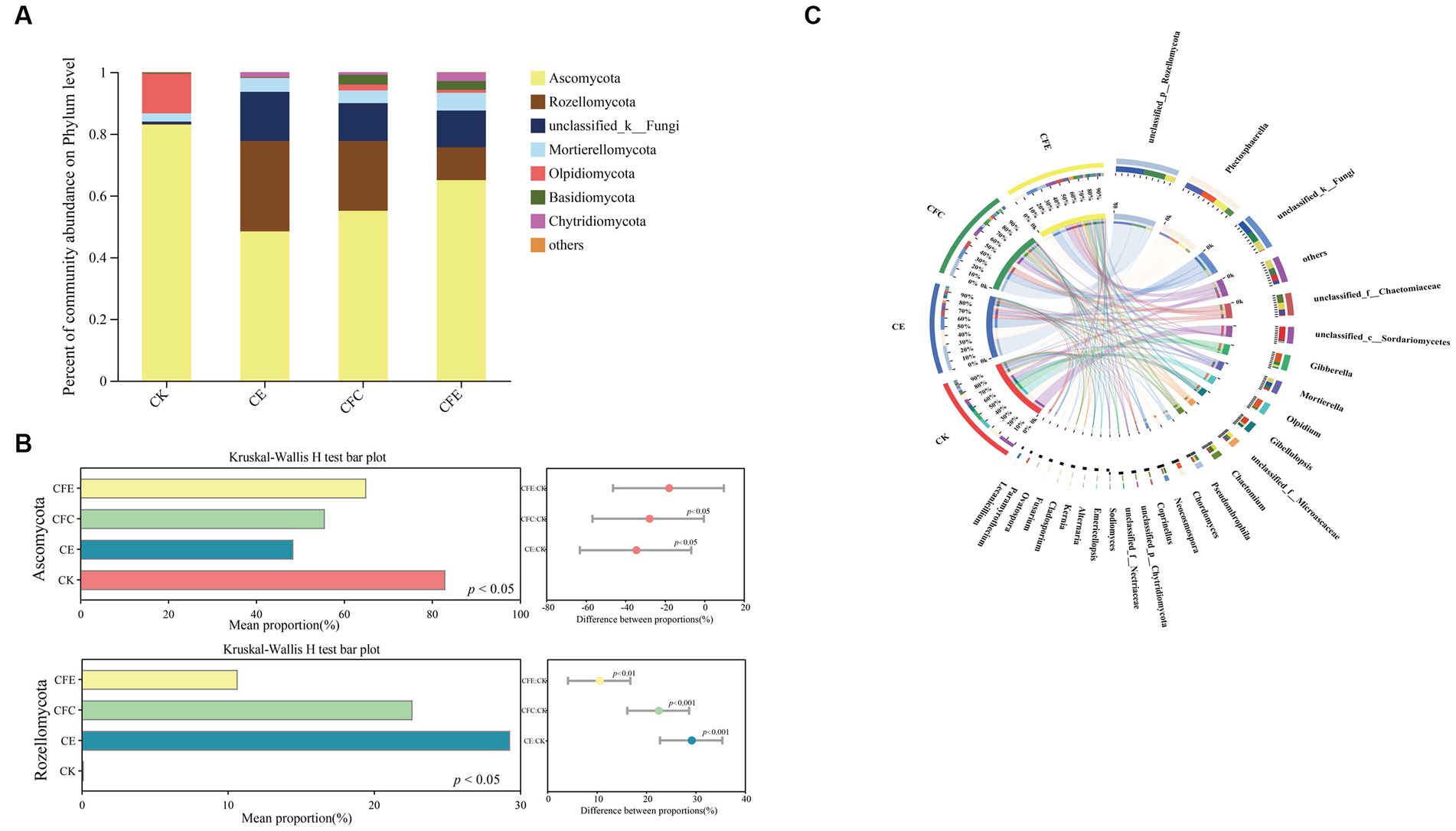
Figure 7. Relative abundance of fungal taxa at the phylum level (A). The relative abundances of bacteria was tested by the Kruskal–Wallis H-test (B). Composition of fungal community at the genus level (C). The data were visualized by circos. CK: 100% chemical fertilizer; CE: 30% reduced chemical fertilizer + earthworms; CFC: 30% reduced chemical fertilizer + cow manure; CFE: 30% reduced chemical fertilizer + cow manure + earthworms.
3.5. Interactions between soil physicochemistry, growth of Chinese flowering cabbage, and soil microbial communities
The relationship among soil bacterial and fungal communities, Chinese flowering cabbage growth, and soil physicochemical properties was analyzed using mantel test. The results revealed that the yield of Chinese flowering cabbage and soluble protein, nitrite, nitrate, VC, TN, AN, SOM, AP, and AK contents were significantly correlated with bacterial communities. TN, AN, SOM, AK, yield, and all qualities of Chinese flowering cabbage were highly significantly correlated with the fungal community but pH and AP did not (Figure 8). These results were verified using random forest analysis. Random forest analysis revealed the soil bacterial community diversity was affected by AN, followed by SOM, AK, TN, pH, and AP (Figure 9A), whereas the fungal community diversity was affected by SOM, followed by AK, AN, TN, pH, and AP (Figure 9B).
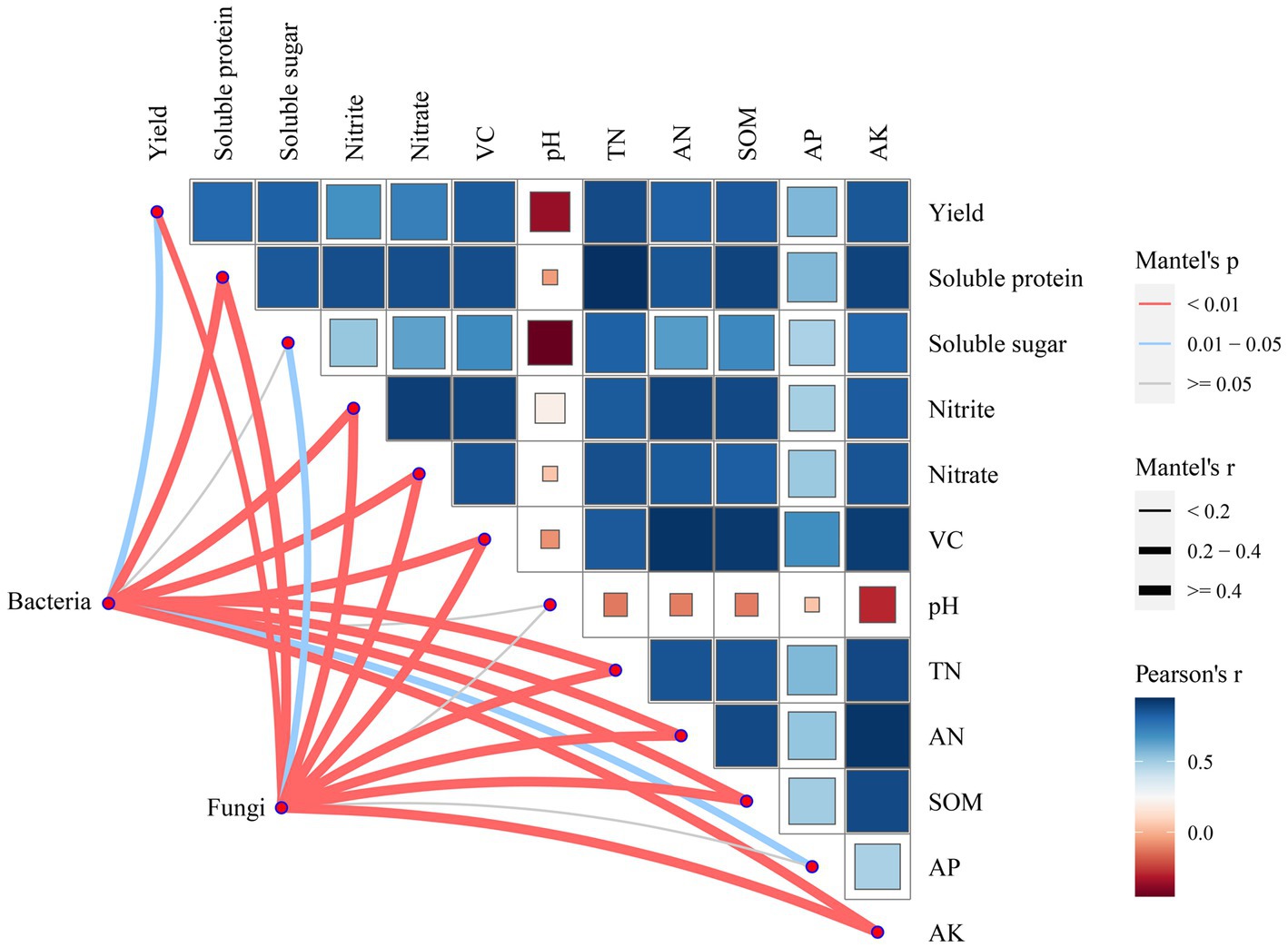
Figure 8. Chinese flowering cabbage growth, soil physicochemical and soil microbial interactions. CK: 100% chemical fertilizer; CE: 30% reduced chemical fertilizer + earthworms; CFC: 30% reduced chemical fertilizer + cow manure; CFE: 30% reduced chemical fertilizer + cow manure + earthworms.
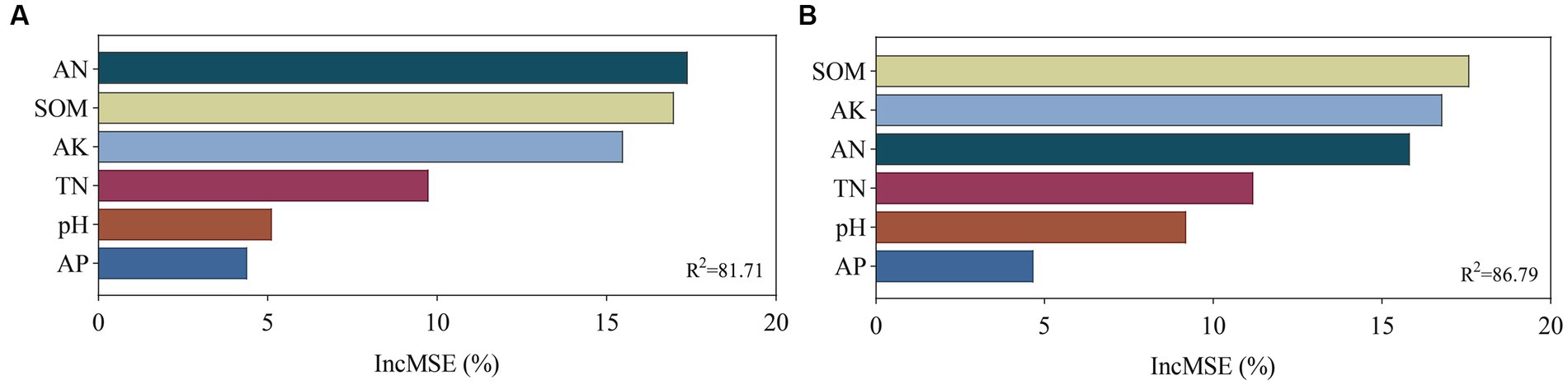
Figure 9. Main factors influencing microbial diversity under different treatments. This figure shows the stochastic forest importance (the percentage of increase in the mean variance error [MSE]) of soil chemical properties on the microbial diversity of bacteria (A) and fungi (B).
4. Discussion
The type of fertilizer significantly influenced both soil conditions and the growth of Chinese flowering cabbage. At equal N application levels, the CFC and CFE treatments exhibited higher yield than the CK treatment. This was mainly because the reduction in the amount of chemical fertilizer was based on the nutrient uptake characteristics of Chinese flowering cabbage and soil fertility, and nutrient requirements were balanced by increasing crop nutrients (van Wesenbeeck et al., 2021). An adequate supply of TN is essential as it provides the necessary N required by plants for promoting chlorophyll synthesis and photosynthesis (Zhao et al., 2003; Liu et al., 2017). Consequently, this leads to the development of greener leaves and higher photosynthetic efficiency, which ultimately promotes plant growth and nutrient accumulation and improves the quality of Chinese flowering cabbage.
The quality of Chinese flowering cabbage was significantly improved under the combined application of earthworms and cow manure (CFE). Cow manure provided abundant nutrients and provided additional nutritional support for plant growth. This, in turn, enhanced nutrient uptake and photosynthesis (Bansal and Kapoor, 2000; Arancon et al., 2008; Guo et al., 2016). Additionally, earthworms contributed to creating a more suitable growth environment for the plant by improving soil structure, aeration, and water retention capacity (Hallam and Hodson, 2020). The results indicated that the CE treatment could not exhibit the desired improvements in the yield and quality of Chinese flowering cabbage. This can be attributed to the severe soil compaction, resulting in reduced earthworm survival, as well as lower soil porosity and water content. The application of cow manure improved soil structure by increasing organic carbon, water-soluble starch, and carbon and N contents (Kacprzak et al., 2023). In addition, the inclusion of earthworms significantly reduced soil pH. This is consistent with previous studies reporting that earthworms regulate soil pH through N excretion and calcium secretion from their glands. Additionally, the organic acids excreted by earthworms and secreted by their epidermis contribute to the reduction in soil pH (Wang G. et al., 2020). Continuous cropping of Chinese flowering cabbage increases soil pH. Therefore, inclusion of earthworms acts as a buffer, maintaining soil pH (Brautigan et al., 2014). Earthworms play a crucial role in promoting soil aggregation through their burrowing and casting activities (Lavelle et al., 2020). In this study, the CFE treatment increased SOM and soil TN, AN, and AK. The stability of soil aggregates is essential for SOM dynamics and soil fertility and reflects the influence of soil biota and soil carbon and N dynamics (Bhattacharyya et al., 2022). This influence is partly attributed to the contribution of soil biota and microorganisms to carbon and nutrient transformation at the soil aggregate scale (Vogel et al., 2022).
Soil bacterial and fungal diversity and community structure are closely related to nutrient cycling, soil quality, and productivity. Different fertilizer treatments significantly affected the alpha diversity and community structure of soil bacteria and fungi. CFC and CFE treatments increased bacterial and fungal diversity, likely due to the positive effects of earthworms and cow manure on the bacterial and fungal activities. This is consistent with previous studies. The different fertilizer treatments in this study significantly enhanced soil nutrient content, and the vast majority of Ascomycetes, Actinobacteria, and Bacteroidota (which were previously reported to be eutrophic bacteria) became the dominant species. This is consistent with a previous study reporting that the spatial distribution of the bacteria was mainly driven by nutrients (Jankowski et al., 2014). Furthermore, the abundance of Firmicutes increased after inoculation with earthworms. Previous studies have indicated that Firmicutes, as a fast-growing phylum, thrives in environments rich in carbon substrates. The continuous digging and casting activities of earthworms contribute to carbon mineralization, which explains the observed increase in the abundance of Firmicutes after the inclusion of earthworms (Singh et al., 2016; He et al., 2020).
The Chao 1 and Shannon indexes were lower, and the abundance of Bacillus was higher in the CFE treatment than in the CFC treatment (Liu et al., 2021). The higher abundance of the Bacillus in the CFE treatment than in the other treatments can be attributed to the fact that this genus is mostly aerobic or partially anaerobic photosynthetic bacteria, and earthworms can form loose and porous vermicompost due to their own feeding and movement of the earthworm haptosphere (Przemieniecki et al., 2021). Norank_f_JG30-KF-CM45 was negatively correlated with TOC. This suggested that inoculation with earthworms can increase the decomposition rate of organic matter and improve the quality of organic fertilizer (Jiang et al., 2020). Norank_f_norank_o_Actinomarinales had the lowest abundance in the CK treatment. Actinomyces spp. promoted organic matter conversion, and the abundance of Actinomyces spp. increased after inoculation with earthworms and cow manure, which participated in soil nutrient cycling and improved soil fertility (Janvier et al., 2007). This confirmed that earthworms can enhance the beneficial effects of organic fertilizers on the abundance, activity, and community structure of soil microorganisms (Huang et al., 2014).
Among fungi, Ascomycota was the most abundant mycorrhizal fungal phylum in the CK treatment, with a decrease in abundance after inoculation with earthworms or addition of organic fertilizer. This is consistent with previous studies suggesting that the relative abundance of soil fungal phyla varies depending on cropping patterns (Wang et al., 2019). The significant abundance of Ascomycota may reflect the unique distribution pattern of fungi in agricultural soils, particularly where plant diversity is particularly low. The CFE treatments increased the abundance of Basidiomycota (Figure 7). A competitive relationship may exist between the ascomycetes and the Stramenopiles resulting in a decrease in the abundance of Ascomycota (Ye et al., 2020). Compared with the CK treatment, all other treatments significantly increased the abundance of Rozellomycota and unclassified_p_Rozellomycota. Interestingly, Rozellomycota is frequently detected in animal gut (Li et al., 2020). This suggested that earthworms or organic fertilizers may have a more pronounced positive effect on Rozellomycota compared with chemical fertilizers. At the fungal genus level, the CFE and CFC treatments reduced the abundance of Plectosphaerella and Gibberella, taxa that are known to be the major pathogens responsible for root and stem rot in many plant species (Farh et al., 2018). Inoculation with earthworms significantly increased the abundance of unclassified_k_Fungi and unclassified_f_Chaetomiaceae, which have cellulose-degrading capacity, and altered the community structure of soil fungi, which play a role in soil ecological cycle system (Song et al., 2020). Previous studies have associated Gibberella with severe decay of leaves, peduncles, and flowers in potted hyacinths and with cob rot disease (Erysipelas cob rot) in maize (Tomioka et al., 2008; Tian et al., 2021). The CFE treatment reduced the abundance of Gibberella. Various studies have reported that alterations in soil microbial communities are closely related to the inhibition of soilborne pathogenic fungi (Wang et al., 2017). Therefore, inoculation of earthworms and cow manure can inhibit the enrichment of these pathogenic fungi, thereby reducing the incidence of root rot and other diseases.
Soil microbial diversity and communities can significantly influence soil quality and the sustainability of soil ecosystems. Previous studies have reported that soil pH is an important factor in microbial community structure. However, in this study, this effect did not appear to be significant. Soil pH was not significantly correlated with bacterial and fungal communities and was negatively correlated with the yield and quality of Chinese flowering cabbage. This may be attributed to the fact that soil pH is alkaline because of the perennial continuous cropping of Chinese flowering cabbage; however, the optimum growth environment for bacteria is lower neutral, which resulted in no significant correlation between pH and bacterial community (Fernández-Calviño et al., 2011). Fungi are only slightly affected by soil pH as they have a strong ability to adapt to acidity (Nevarez et al., 2009; Rousk et al., 2010). Random forest analysis indicated that AN, SOM, AK, and TN were the main factors affecting bacterial and fungal diversities and indicated a significant positive correlation with the quality and yield of Chinese flowering cabbage. These physicochemical factors could partially explain the nutrient cycling and utilization by microorganisms in the ecosystem (Zhao et al., 2019). Numerous studies have indicated that more than 50% of the N required by crops is obtained from soil, whereas the remainder is derived from in-season fertilizer applications. In soil, soil organic N constitutes approximately 90% of the TN content (Xu et al., 2016). This is consistent with previous studies consistently demonstrating that the application of organic fertilizer significantly enhances soil organic N and SOM content, thereby promoting plant growth (Zhou et al., 2013). Abundant nutrients such as organic N and organic potassium in SOM provide a sustained and balanced supply of nutrients to crops (Han et al., 2021). These nutrients are gradually released into inorganic forms through microbial decomposition and mineralization, effectively meeting the nutrient requirements for crop growth and quality development (Tahat et al., 2020). Overall, different fertilization treatments altered the microbial diversity and community in the soil by regulating the soil properties, and the combined effect of earthworms and cow manure promoted the growth of Chinese flowering cabbage and optimized the soil structure.
5. Conclusion
This study revealed that the synergistic effect of earthworms and cow manure under reduced application of chemical fertilizer decreased soil pH; increased soil organic matter, total nitrogen, available nitrogen, and available potassium; and effectively improved soil chemical properties. This study indicated that the synergistic effect of earthworms and cow manure promoted the growth of Chinese flowering cabbage and increased microbial diversity and altered community structure. Earthworms and cow manure promoted the abundance of Bacillus and decreased the abundance of Plectosphaerella and Gibberella. This study provided a scientific basis for the establishment of environmentally friendly fertilization techniques to effectively promote sustainable agricultural development.
Data availability statement
The original contributions presented in the study are publicly available. This data can be found here: NCBI - PRJNA1019528.
Author contributions
FG: Writing – original draft, Writing – review & editing. LY: Methodology, Resources, Writing – review & editing. XM: Writing – review & editing. LX: Investigation, Writing – review & editing. ZS: Investigation, Writing – review & editing. YL: Investigation, Writing – review & editing.
Funding
The author(s) declare financial support was received for the research, authorship, and/or publication of this article. This research was funded by the National Key Research and Development Program (2021YFD1600300), Key Research and Development Projects in Ningxia Hui Autonomous Region (2021BBF02006), Key Research and Development Projects in Ningxia Hui Autonomous Region (2021BBF02019), and Ningxia Hui Autonomous Region Key Research and Development Program Project Talent Attraction Special (2021BEB04064).
Acknowledgments
We thank the reviewers for their suggestions and comments on the manuscript. We are particularly grateful to the editor and reviewers for their help in improving our manuscript.
Conflict of interest
The authors declare that the research was conducted in the absence of any commercial or financial relationships that could be construed as a potential conflict of interest.
Publisher’s note
All claims expressed in this article are solely those of the authors and do not necessarily represent those of their affiliated organizations, or those of the publisher, the editors and the reviewers. Any product that may be evaluated in this article, or claim that may be made by its manufacturer, is not guaranteed or endorsed by the publisher.
Supplementary material
The Supplementary material for this article can be found online at: https://www.frontiersin.org/articles/10.3389/fmicb.2023.1285464/full#supplementary-material
References
Arancon, N. Q., Edwards, C. A., Babenko, A., Cannon, J., Galvis, P., and Metzger, J. D. (2008). Influences of vermicomposts, produced by earthworms and microorganisms from cattle manure, food waste and paper waste, on the germination, growth and flowering of petunias in the greenhouse. Appl. Soil Ecol. 39, 91–99. doi: 10.1016/j.apsoil.2007.11.010
Arya, S. P., Mahajan, M., and Jain, P. (2000). Non-spectrophotometric methods for the determination of vitamin C. Anal. Chim. Acta 417, 1–14. doi: 10.1016/S0003-2670(00)00909-0
Bansal, S., and Kapoor, K. K. (2000). Vermicomposting of crop residues and cattle dung with Eisenia foetida. Bioresour. Technol. 73, 95–98. doi: 10.1016/S0960-8524(99)00173-X
Belay, A., Claassens, A., and Wehner, F. (2002). Effect of direct nitrogen and potassium and residual phosphorus fertilizers on soil chemical properties, microbial components and maize yield under long-term crop rotation. Biol. Fertil. Soils 35, 420–427. doi: 10.1007/s00374-002-0489-x
Bhattacharyya, S. S., Ros, G. H., Furtak, K., Iqbal, H. M. N., and Parra-Saldívar, R. (2022). Soil carbon sequestration – an interplay between soil microbial community and soil organic matter dynamics. Sci. Total Environ. 815:152928. doi: 10.1016/j.scitotenv.2022.152928
Blouin, M., Hodson, M. E., Delgado, E. A., Baker, G., Brussaard, L., Butt, K. R., et al. (2013). A review of earthworm impact on soil function and ecosystem services. Eur. J. Soil Sci. 64, 161–182. doi: 10.1111/ejss.12025
Brautigan, D. J., Rengasamy, P., and Chittleborough, D. J. (2014). Amelioration of alkaline phytotoxicity by lowering soil pH. Crop Pasture Sci. 65, 1278–1287. doi: 10.1071/CP13435
Cai, S. M., Wang, J. J., Lv, W. G., Xu, S. X., and Zhu, H. T. (2020). Nitrogen fertilization alters the effects of earthworms on soil physicochemical properties and bacterial community structure. Appl. Soil Ecol. 150:103478. doi: 10.1016/j.apsoil.2019.103478
Farh, M. E. A., Kim, Y. J., Kim, Y. J., and Yang, D. C. (2018). Cylindrocarpon destructans/Ilyonectria radicicola-species complex: causative agent of ginseng root-rot disease and rusty symptoms. J. Ginseng Res. 42, 9–15. doi: 10.1016/j.jgr.2017.01.004
Fernández-Calviño, D., Rousk, J., Brookes, P. C., and Bååth, E. (2011). Bacterial pH-optima for growth track soil pH, but are higher than expected at low pH. Soil Biol. Biochem. 43, 1569–1575. doi: 10.1016/j.soilbio.2011.04.007
Guo, L. Y., Wu, G. L., Li, Y., Li, C. H., Liu, W. J., Meng, J., et al. (2016). Effects of cattle manure compost combined with chemical fertilizer on topsoil organic matter, bulk density and earthworm activity in a wheat–maize rotation system in eastern China. Soil Tillage Res. 156, 140–147. doi: 10.1016/j.still.2015.10.010
Hallam, J., and Hodson, M. E. (2020). Impact of different earthworm ecotypes on water stable aggregates and soil water holding capacity. Biol. Fertil. Soils 56, 607–617. doi: 10.1007/s00374-020-01432-5
Han, J. Q., Dong, Y. Y., and Zhang, M. (2021). Chemical fertilizer reduction with organic fertilizer effectively improve soil fertility and microbial community from newly cultivated land in the loess plateau of China. Appl. Soil Ecol. 165:103966. doi: 10.1016/j.apsoil.2021.103966
He, Y., Lu, L., Peng, C., Li, H. L., Zhang, J., and Li, R. (2020). High-yield grass Pennisetum sinese Roxb plantation and organic manure alter bacterial and fungal communities structure in an ecological agriculture farm. AMB Express 10:86. doi: 10.1186/s13568-020-01018-2
Huang, K., Li, F. S., Wei, Y. F., Fu, X. Y., and Chen, X. M. (2014). Effects of earthworms on physicochemical properties and microbial profiles during vermicomposting of fresh fruit and vegetable wastes. Bioresour. Technol. 170, 45–52. doi: 10.1016/j.biortech.2014.07.058
Jankowski, K., Schindler, D. E., and Horner-Devine, M. C. (2014). Resource availability and spatial heterogeneity control bacterial community response to nutrient enrichment in lakes. PLoS One 9:e86991. doi: 10.1371/journal.pone.0086991
Janvier, C., Villeneuve, F., Alabouvette, C., Edel-Hermann, V., Mateille, T., and Steinberg, C. (2007). Soil health through soil disease suppression: which strategy from descriptors to indicators? Soil Biol. Biochem. 39, 1–23. doi: 10.1016/j.soilbio.2006.07.001
Jiang, J. S., Wang, Y., Guo, F. Q., Zhang, X. F., Dong, W., Zhang, X. D., et al. (2020). Composting pig manure and sawdust with urease inhibitor: succession of nitrogen functional genes and bacterial community. Environ. Sci. Pollut. Res. 27, 36160–36171. doi: 10.1007/s11356-020-09696-y
Jiao, S., Peng, Z. H., Qi, J. J., Gao, J. M., and Wei, G. H. (2021). Linking bacterial-fungal relationships to microbial diversity and soil nutrient cycling. mSystems 6:e01052-20. doi: 10.1128/msystems.01052-20
Kacprzak, M., Malińska, K., Grosser, A., Sobik-Szołtysek, J., Wystalska, K., Dróżdż, D., et al. (2023). Cycles of carbon, nitrogen and phosphorus in poultry manure management technologies – environmental aspects. Crit. Rev. Environ. Sci. Technol. 53, 914–938. doi: 10.1080/10643389.2022.2096983
Kamaa, M., Mburu, H., Blanchart, E., Chibole, L., Chotte, J. L., Kibunja, C., et al. (2011). Effects of organic and inorganic fertilization on soil bacterial and fungal microbial diversity in the Kabete long-term trial, Kenya. Biol. Fertil. Soils 47, 315–321. doi: 10.1007/s00374-011-0539-3
Kour, D., Rana, K. L., Yadav, A. N., Yadav, N., Kumar, M., Kumar, V., et al. (2020). Microbial biofertilizers: bioresources and eco-friendly technologies for agricultural and environmental sustainability. Biocatal. Agric. Biotechnol. 23:101487. doi: 10.1016/j.bcab.2019.101487
Lavelle, P., Spain, A., Fonte, S., Bedano, J. C., Blanchart, E., Galindo, V., et al. (2020). Soil aggregation, ecosystem engineers and the C cycle. Acta Oecol. 105:103561. doi: 10.1016/j.actao.2020.103561
Li, P. F., Liu, M., Ma, X. Y., Wu, M., Jiang, C. Y., Liu, K., et al. (2020). Responses of microbial communities to a gradient of pig manure amendment in red paddy soils. Sci. Total Environ. 705:135884. doi: 10.1016/j.scitotenv.2019.135884
Liu, G. Y., Du, Q. J., and Li, J. M. (2017). Interactive effects of nitrate-ammonium ratios and temperatures on growth, photosynthesis, and nitrogen metabolism of tomato seedlings. Sci. Hortic. 214, 41–50. doi: 10.1016/j.scienta.2016.09.006
Liu, H., Li, J., Li, X., Zheng, Y., Feng, S., and Jiang, G. (2015). Mitigating greenhouse gas emissions through replacement of chemical fertilizer with organic manure in a temperate farmland. Sci. Bul. 60, 598–606. doi: 10.1007/s11434-014-0679-6
Liu, J., Shu, A. P., Song, W. F., Shi, W. C., Li, M. C., and Zhang, W. X. (2021). Long-term organic fertilizer substitution increases rice yield by improving soil properties and regulating soil bacteria. Geoderma 404:115287. doi: 10.1016/j.geoderma.2021.115287
Medina-Sauza, R. M., Álvarez-Jiménez, M., Delhal, A., Reverchon, F., Blouin, M., Guerrero-Analco, J. A., et al. (2019). Earthworms building up soil microbiota, a review. Front. Environ. Sci. 7:81. doi: 10.3389/fenvs.2019.00081
Nevarez, L., Vasseur, V., Le Madec, A., Le Bras, M. A., Coroller, L., Leguérinel, I., et al. (2009). Physiological traits of Penicillium glabrum strain LCP 08.5568, a filamentous fungus isolated from bottled aromatised mineral water. Int. J. Food Microbiol. 130, 166–171. doi: 10.1016/j.ijfoodmicro.2009.01.013
Przemieniecki, S. W., Skwiercz, A., Damszel, M., Telesiński, A., Zapałowska, A., Sierota, Z., et al. (2021). Ecology, biology and enzymatic activity of the rhizosphere planted with Larix decidua seedlings after addition of vermicompost. Appl. Soil Ecol. 168:104101. doi: 10.1016/j.apsoil.2021.104101
Rousk, J., Bååth, E., Brookes, P. C., Lauber, C. L., Lozupone, C., Caporaso, J. G., et al. (2010). Soil bacterial and fungal communities across a pH gradient in an arable soil. ISME J. 4, 1340–1351. doi: 10.1038/ismej.2010.58
Sedmak, J. J., and Grossberg, S. E. (1977). A rapid, sensitive, and versatile assay for protein using Coomassie brilliant blue G250. Anal. Biochem. 79, 544–552. doi: 10.1016/0003-2697(77)90428-6
Siebert, J., Eisenhauer, N., Poll, C., Marhan, S., Bonkowski, M., Hines, J., et al. (2019). Earthworms modulate the effects of climate warming on the taxon richness of soil meso- and macrofauna in an agricultural system. Agric. Ecosyst. Environ. 278, 72–80. doi: 10.1016/j.agee.2019.03.004
Singh, S., Singh, J., and Vig, A. P. (2016). Earthworm as ecological engineers to change the physico-chemical properties of soil: soil vs vermicast. Ecol. Eng. 90, 1–5. doi: 10.1016/j.ecoleng.2016.01.072
Song, K., Sun, Y. F., Qin, Q., Sun, L. J., Zheng, X. Q., Terzaghi, W., et al. (2020). The effects of earthworms on fungal diversity and community structure in farmland soil with returned straw. Front. Microbiol. 11:594265. doi: 10.3389/fmicb.2020.594265
Steffen, W., Richardson, K., Rockstrom, J., Cornell, S., Fetzer, I., Bennett, E. M., et al. (2015). Planetary boundaries: guiding human development on a changing planet. Science 347:1259855. doi: 10.1126/science.1259855
Tahat, M. M., Alananbeh, K. M., Othman, Y. A., and Leskovar, D. I. (2020). Soil health and sustainable agriculture. Sustainability 12:4859. doi: 10.3390/su12124859
Tian, L. X., Ou, J. R., Sun, X., Miao, Y. J., Pei, J., Zhao, L., et al. (2021). The discovery of pivotal fungus and major determinant factor shaping soil microbial community composition associated with rot root of American ginseng. Plant Signal. Behav. 16:1952372. doi: 10.1080/15592324.2021.1952372
Tomioka, K., Hirooka, Y., Aoki, T., and Sato, T. (2008). Fusarium rot of hyacinth caused by Gibberella zeae (anamorph: fusarium graminearum). J. Gen. Plant Pathol. 74, 264–266. doi: 10.1007/s10327-008-0088-2
van Wesenbeeck, C. F. A., Keyzer, M. A., van Veen, W. C. M., and Qiu, H. (2021). Can China’s overuse of fertilizer be reduced without threatening food security and farm incomes? Agric. Syst. 190:103093. doi: 10.1016/j.agsy.2021.103093
Vogel, H. J., Balseiro-Romero, M., Kravchenko, A., Otten, W., Pot, V., Schlüter, S., et al. (2022). A holistic perspective on soil architecture is needed as a key to soil functions. Eur. J. Soil Sci. 73:e13152. doi: 10.1111/ejss.13152
Wang, L., Li, J., Yang, F., E, Y., Raza, W., Huang, Q., et al. (2017). Application of bioorganic fertilizer significantly increased apple yields and shaped bacterial community structure in orchard soil. Microb. Ecol. 73, 404–416. doi: 10.1007/s00248-016-0849-y
Wang, C., Lv, J., Coulter, J. A., Xie, J. M., Yu, J. H., and Li, J. (2020). Slow-release fertilizer improves the growth, quality, and nutrient utilization of wintering Chinese chives (Allium tuberosum Rottler ex Spreng.). Agronomy 10:381. doi: 10.3390/agronomy10030381
Wang, N., Wang, W. H., Jiang, Y. J., Dai, W., Li, P. F., Yao, D. D., et al. (2021). Variations in bacterial taxonomic profiles and potential functions in response to the gut transit of earthworms (Eisenia fetida) feeding on cow manure. Sci. Total Environ. 787:147392. doi: 10.1016/j.scitotenv.2021.147392
Wang, G., Wang, L., Ma, F., You, Y. Q., Wang, Y. J., and Yang, D. G. (2020). Integration of earthworms and arbuscular mycorrhizal fungi into phytoremediation of cadmium-contaminated soil by Solanum nigrum L. J. Hazard. Mater. 389:121873. doi: 10.1016/j.jhazmat.2019.121873
Wang, K. B., Zhang, Y. W., Tang, Z. S., Shangguan, Z. P., Chang, F., Jia, F. A., et al. (2019). Effects of grassland afforestation on structure and function of soil bacterial and fungal communities. Sci. Total Environ. 676, 396–406. doi: 10.1016/j.scitotenv.2019.04.259
Wu, L. N., Jiang, Y., Zhao, F. Y., He, X. F., Liu, H. F., and Yu, K. (2020). Increased organic fertilizer application and reduced chemical fertilizer application affect the soil properties and bacterial communities of grape rhizosphere soil. Sci. Rep. 10:9568. doi: 10.1038/s41598-020-66648-9
Xiao, X. M., Li, J., Lyu, J., Feng, Z., Zhang, G. B., Yang, H. X., et al. (2022). Chemical fertilizer reduction combined with bio-organic fertilizers increases cauliflower yield via regulation of soil biochemical properties and bacterial communities in Northwest China. Front. Microbiol. 13:922149. doi: 10.3389/fmicb.2022.922149
Xu, N., Tan, G. C., Wang, H. Y., and Gai, X. P. (2016). Effect of biochar additions to soil on nitrogen leaching, microbial biomass and bacterial community structure. Eur. J. Soil Biol. 74, 1–8. doi: 10.1016/j.ejsobi.2016.02.004
Yan, M., Chen, S., Huang, T., Li, B., Li, N., Liu, K., et al. (2020). Community compositions of phytoplankton and eukaryotes during the mixing periods of a drinking water reservoir: dynamics and interactions. Int. J. Environ. Res. Public Health 17:1128. doi: 10.3390/ijerph17041128
Ye, G. P., Lin, Y. X., Luo, J. F., Di, H. J., Lindsey, S., and Liu, D. Y. (2020). Responses of soil fungal diversity and community composition to long-term fertilization: field experiment in an acidic ultisol and literature synthesis. Appl. Soil Ecol. 145:103305. doi: 10.1016/j.apsoil.2019.06.008
Zhang, S. N., Wang, Y., Sun, L. T., Qiu, C., Ding, Y. Q., Gu, H. L., et al. (2020). Organic mulching positively regulates the soil microbial communities and ecosystem functions in tea plantation. BMC Microbiol. 20:103. doi: 10.1186/s12866-020-01794-8
Zhang, F. G., Zhu, Z., Yang, X. M., Ran, W., and Shen, Q. R. (2013). Trichoderma harzianum T-E5 significantly affects cucumber root exudates and fungal community in the cucumber rhizosphere. Appl. Soil Ecol. 72, 41–48. doi: 10.1016/j.apsoil.2013.05.016
Zhao, Z. B., He, J. Z., Geisen, S., Han, L. L., Wang, J. T., Shen, J. P., et al. (2019). Protist communities are more sensitive to nitrogen fertilization than other microorganisms in diverse agricultural soils. Microbiome 7:33. doi: 10.1186/s40168-019-0647-0
Zhao, D. L., Raja Reddy, K., Kakani, V. G., Read, J. J., and Carter, G. A. (2003). Corn (Zea mays L.) growth, leaf pigment concentration, photosynthesis and leaf hyperspectral reflectance properties as affected by nitrogen supply. Plant Soil 257, 205–218. doi: 10.1023/A:1026233732507
Zhou, Z. C., Gan, Z. T., Shangguan, Z. P., and Zhang, Z. P. (2013). Effects of long-term repeated mineral and organic fertilizer applications on soil organic carbon and total nitrogen in a semi-arid cropland. Eur. J. Agron. 45, 20–26. doi: 10.1016/j.eja.2012.11.002
Keywords: biodiversity, Chinese flowering cabbage, earthworm, environmental factors, fertilizer, yield
Citation: Gao F, Ye L, Mu X, Xu L, Shi Z and Luo Y (2023) Synergistic effects of earthworms and cow manure under reduced chemical fertilization modified microbial community structure to mitigate continuous cropping effects on Chinese flowering cabbage. Front. Microbiol. 14:1285464. doi: 10.3389/fmicb.2023.1285464
Edited by:
Ajar Nath Yadav, Eternal University, IndiaReviewed by:
Jiangbing Xu, Nanjing University of Information Science and Technology, ChinaXiaojing Li, Chinese Academy of Agricultural Sciences, China
Copyright © 2023 Gao, Ye, Mu, Xu, Shi and Luo. This is an open-access article distributed under the terms of the Creative Commons Attribution License (CC BY). The use, distribution or reproduction in other forums is permitted, provided the original author(s) and the copyright owner(s) are credited and that the original publication in this journal is cited, in accordance with accepted academic practice. No use, distribution or reproduction is permitted which does not comply with these terms.
*Correspondence: Lin Ye, eWVsaW4yMjExMTJAMTI2LmNvbQ==