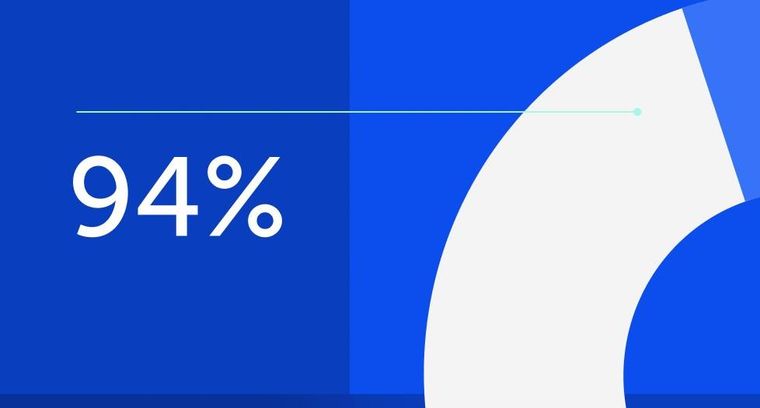
94% of researchers rate our articles as excellent or good
Learn more about the work of our research integrity team to safeguard the quality of each article we publish.
Find out more
REVIEW article
Front. Microbiol., 15 December 2023
Sec. Virology
Volume 14 - 2023 | https://doi.org/10.3389/fmicb.2023.1283361
This article is part of the Research TopicCharacteristics and Prevention of the Emerging Avian Influenza A Viruses in Birds and MammalsView all 8 articles
Avian influenza causes substantial economic loss in the poultry industry and potentially threatens human health. Over recent years, the highly pathogenic avian influenza A/H5N1 virus has led to devastating losses in poultry flocks and wild birds. At the same time, the number of mammalian species identified to be infected with A/H5N1 is increasing, with recent outbreaks in domestic cats, including household individuals, evidenced in July 2023 in Poland, ultimately creating opportunities for the virus to adapt better to mammalian hosts, including humans. Overall, between 2003 and 2023, over 10 outbreaks in felids have been documented globally, and in six of them, feed based on raw chicken was suspected as a potential source of A/H5N1, fuelling a debate on threats posed by A/H5N1 and methods to decrease the associated risks. This article debates that technology allowing the production of slaughter-free meat, including poultry, from cell and tissue cultures could be considered as a part of a mitigation strategy to decrease the overall burden and threat of adaptation of avian influenza viruses to human hosts. By shifting poultry production to the cultured meat industry, the frequency of A/H5N1 outbreaks in farmed birds may be decreased, leading to a reduced risk of virus acquisition by wild and domesticated mammals that have direct contact with birds or eat raw poultry and have close contact with human (including domestic cats), ultimately minimizing the potential of A/H5N1 to adapt better to mammalian host, including humans. This adds to the list of other benefits of cultured meat that are also reviewed in this paper, including decreased antibiotic use, risk of microbial contamination and parasite transmission, and environmental and ethical advantages over conventional slaughtered meat. In conclusion, further development and implementation of this technology, also in the context of poultry production, is strongly advocated. Although cultured poultry is unlikely to replace the conventional process in the near future due to challenges with scaling up the production and meeting the continuously increased demand for poultry meat, it may still decrease the pressures and threats related to the transmission of highly pathogenic avian influenza in selected world regions.
In July 2023, the United States Department of Agriculture approved chicken meat made by two companies using animal cell culture technology for human consumption (The Good Food Institute, 2023). This decision followed an earlier pre-marketing assessment by the United States Food and Drug Administration, which included an evaluation of the production process and quality of the final product (FDA, 2022, 2023). Cultured meat (or cultivated meat, clean meat, slaughter-free meat, in vitro meat, lab-grown meat, or cellular agriculture) refers to the product obtained using emerging technologies that integrate the laboratory methods of in vitro cell culture with tissue engineering to produce animal muscle under a controlled environment (Choi et al., 2021). The starting point of this process is a collection of tissue through animal biopsy, isolation of stem cells (myosatellites and others, depending on the product, e.g., embryonic stem cells, adipose-derived stem cells, fibroblasts, and endothelial stem cells), their proliferation and differentiation, followed by tissue structuration using different techniques, depending on the type of produced meat (Post, 2012; Bodiou et al., 2020; Kang et al., 2021; Reiss et al., 2021; Handral et al., 2022).
Historically, the idea of cultured meat dates back to 1931 when Winston Churchill published an essay entitled “Fifty Years Hence” in Strand Magazine, republished in 1932 by Popular Mechanics, in which he envisioned the future and stated: “We shall escape the absurdity of growing a whole chicken in order to eat the breast or wing, by growing these parts separately under a suitable medium” (Churchill, 1931). However, it took eight decades to obtain the first meat for human consumption, a beef burger, using an in vitro cell culture technique in 2013. Since then, the technology has received substantial interest from the scientific community, private investors, as well as some national stakeholders, e.g., in China, which included the development of cultured meat in their recent 5-years agriculture plan covering the period of 2021–2025 (FAO, 2022). The first regulatory agency to approve cultured meat, i.e., cell-cultured chicken, was the Singapore Food Agency in December 2020 (Singapore Food Agency, 2020). However, considering that Singapore is not a major market for chicken (USDA, 2023b), this decision could be regarded as symbolic and important from a marketing point of view. On the contrary, the authorization of similar products in the United States represents a historical milestone in the food industry if the U.S. market is the largest chicken production globally, with approximately 21 million metric tons of broiler meat obtained in 2022 alone (USDA, 2023b). By the end of 2023, cultured meat is not authorized for consumption in the European Union, but it is known that the safety and quality of products obtained using such technology will be evaluated by the European Food Safety Authority acting upon Regulation 2015/2283 of the European Parliament and of the Council of 25 November 2015 on novel foods (European Food Safety Authority, European Centre for Disease Prevention and Control, European Union Reference Laboratory for Avian Influenza et al., 2023).
In the present paper, cultured meat is discussed in the context of the benefits of its introduction, with a particular focus on the potential mitigation of emerging threats posed by avian influenza viruses. The increasing number of A/H5N1 transmissions to different mammalian species increases the risk of better viral adaptation to a human host (Imai et al., 2013; Leguia et al., 2023; Venkatesan, 2023; WHO, 2023c). A recent outbreak of A/H5N1 infections in domestic cats, documented in 2023 in Poland (ECDC, 2023a), represents another reminder of these risks, mitigation of which justifies consideration of multifaceted measures, which also includes changes to conventional poultry production that are offered by the cultured meat technology. As indicated, cultured meat, in addition to its ethical and environmental advantages, which are also reviewed in the present article, may also be superior from the public health perspective and decrease the risk of zoonoses, including those related to A/H5N1, although there are some hurdles that this technology is yet to overcome.
There are numerous benefits that can arise from the introduction of cultured meat, including cultured poultry. Firstly, it does not require the use of slaughtered animals and, therefore, is ethically superior, especially if one considers that the number of animals slaughtered for meat is increasing (Figure 1). In 2021 alone, 73.8 billion chickens, 1.4 billion pigs, 617 million sheep, 603 turkeys, 501 million goats, and 332 million cattle were slaughtered for meat (Our World in Data, 2023b). The global poultry meat production output amounted to nearly 138 million tonnes in 2021 (Figure 1), with the highest share of China (17.2%), United States (16.8%), Brazil (11.0%), Russia (3.3%), Indonesia (2.8%), India (2.7%), Mexico (2.7%), Poland (1.8%), Japan (1.8%), and Argentina (1.7%) (FAOSTAT, 2023).
Figure 1. (A) Number of chickens slaughtered annually for meat in different world regions in 1981, 2001, and 2021. (B) Number of different animals slaughtered globally for meat in 2021. Prepared based on data from the Food and Agriculture Organization of the United Nations collected by Our World in Data (Our World in Data, 2023b). (C) The poultry meat production outputs in different world regions with the share of main producers in particular continent (FAOSTAT, 2023).
Secondly, cultured meat can also offer a number of environmental advantages, including a significant reduction in land use, water withdrawal, energy consumption, and greenhouse emissions (Tuomisto and de Mattos, 2011; Lynch and Pierrehumbert, 2019). For example, the recent analysis based on data collected from over 15 companies and research institutes active in the production and supply chain of cultured meat demonstrated the particular benefits in this regard could be achieved when producing beef and pork with a carbon footprint reduced by over 95 and 9% compared to the global average from conventional production in 2018, respectively (Sinke et al., 2023). In the case of chicken production, the carbon footprint between conventional production and cultured meat does not differ, but the latter is postulated to significantly decrease land use and acidification (Sinke et al., 2023). Using renewable energy sources and optimizing culture medium composition may further reduce environmental impacts (Rzymski et al., 2021; Tuomisto et al., 2022).
Thirdly, the production of cultured meat is a more efficient way of turning crops into meat, regardless of the type of conventional meat it was compared to, including chicken, in case of which an estimated feed conversion rate was two-fold lower (Sinke et al., 2023).
Fourth, the production span of cultured meat, depending on the type of meat, is 2–8 weeks (shorter for chicken, longer for beef) (Bellani et al., 2020; Swartz, 2021) as opposed to 2 months to produce chicken, 6 months for swine, and 2 years for cattle (USDA, 1996, 2018). This makes production more predictable and stable, which is important from the food safety perspective, particularly if one considers the predicted climate change impacts on the food system (Miraglia et al., 2009; Nelson et al., 2014; Sharma et al., 2022).
Fifth, cultured meat has some advantages regarding public health (Halabowski and Rzymski, 2020; Rzymski et al., 2021). These include (i) a decrease in antibiotic use and the contribution of meat production to antibiotic resistance, which is recognized as one of the major human health threats (Antimicrobial Resistance Collaborators, 2022), (ii) better control over bacterial and fungal contamination of the final product, (iii) elimination of macroparasite presence, e.g., Taenia sp., Trichinella spp., in meat, and (iv) unpredictable emergence and spread of animal viruses, such as African Swine Fever virus or avian influenza viruses that can cause substantial economic damage (Rzymski et al., 2021; Ruiz-Saenz et al., 2022; Farahat et al., 2023). In addition, avian influenza is also a potential threat to human health. As stated in the guidance of the European Center for Disease Prevention and Control (ECDC) released in July 2023: “Transmission to humans cannot be excluded when avian influenza is circulating in wild birds and mammals, and people are directly exposed without wearing protective equipment” (ECDC, 2023b). Over the last 3 years, avian influenza has caused large outbreaks in wild birds and poultry in Europe. In June 2023, the A/H5N1 outbreak in domestic cats was documented in Poland (ECDC, 2023a; General Veterinary Inspectorate, 2023a,b,c). This is particularly concerning since it allows the virus to adapt to mammals, including humans, through reassortment between avian and mammalian influenza viruses (Marschall and Hartmann, 2008). Therefore, any technology that would offer to produce meat but decrease the risk of circulation of avian influenza viruses in poultry and its further spread to mammals, including humans, is highly needed, as discussed in the subsequent sections. One should note that the risk of viral infections, including infections with avian influenza viruses, is not non-existent in the industrial production of cultured poultry. However, it is significantly limited due to no contact with wild birds, sanitation measures, automatization of the process, and the ability to test cells deposited in the master-cell banks for avian pathogens to ensure no introduction of avian influenza viruses (Rzymski et al., 2021; Pasitka et al., 2022). Contrary to this, it is virtually impossible to test every farmed bird individual for avian influenza. The pre-market assessment of cultured poultry meat authorized in the United States revealed that the tested products were free of influenza A (as well as B) viruses (FDA, 2022).
In conclusion, cultured meat, including cultured poultry, is ethically superior, while its introduction to diet is not associated with excluding animal-derived products. It also provides substantial environmental benefits, which, in the case of chicken, particularly involve decreased land use and acidification impacts. Production of cultured poultry is more predictable and less prone to uncontrolled environmental factors, which is important from a food security standpoint. There are also premises that it also offers substantial benefits for public health, including decreased promotion of antibiotic resistance and risk of zoonoses.
Avian influenza viruses type A (Orthomyxoviridae family) naturally circulate in various wild birds and can infect poultry (Shriner and Root, 2020; Rafique et al., 2023). These viruses are classified into different subtypes based on hemagglutinin (H) and neuraminidase (N) genes, with 16 H (H1-H16) and 9 N (N1-N9) subtypes of avian influenza identified in birds. Based on clinical significance for poultry, the avian influenza viruses can be classified into low pathogenic and highly pathogenic (HPAI) (Spackman, 2020). The infection with the former is usually mild or asymptomatic, while the latter is characterized by severe disease and high mortality rates (Verhagen et al., 2011). However, when H5 and H7 avian influenza subtypes infect poultry, they may mutate into highly pathogenic forms (Horimoto et al., 1995; Alexander, 2000; Banks et al., 2001; Alexander and Brown, 2009; Monne et al., 2014; Shriner and Root, 2020). With time, the circulation of HPAI has become more frequent, leading to substantial economic loss in the poultry industry. For example, from 1959 to 1995, the emergence of HPAI was documented on 15 occasions with limited losses, but between 1996 and 2008, four outbreaks of HPAI affected millions of birds (Alexander and Brown, 2009). The most recent HPAI outbreak involves A/H5N1, the virus isolated for the first time in 1996 from a sick goose during an outbreak in Guangdong province, China (Xu et al., 1999), which over the years had diverged into distinct lineages and expanded into various reservoir hosts (Sonnberg et al., 2013). In the 2021–2022 epidemic season, a total of 2,467 outbreaks in poultry were identified in Europe alone, with 48 million culled birds. In addition, 187 events of HPAI A/H5N1 were detected in captive birds and 3,573 in wild birds (European Food Safety Authority, European Centre for Disease Prevention and Control, European Union Reference Laboratory for Avian Influenza et al., 2022). The A/H5N1 epidemic continues to cause significant impacts also in both Americas, resulting in 40 million animal losses in the United States in 2022 alone, with economic costs estimated in the range of 2.5–3.0 billion USD (Farahat et al., 2023). The situation continues to evolve in 2023 in various world parts (European Food Safety Authority, European Centre for Disease Prevention and Control, European Union Reference Laboratory for Avian Influenza et al., 2023). One should note that when an A/H5N1 outbreak occurs in poultry, the affected flock is subjected to depopulation, often requiring the culling of healthy birds to prevent further viral spread. This further undermines the ethics of poultry production and highlights that A/H5N1 leads to substantial waste generation, loss of resources, and protein wastage, making conventional poultry production less sustainable (WOAH, 2021).
In general, avian influenza viruses reveal a strong preference for using α2,3-linked sialic acid as a receptor, which dominates in birds. They usually exhibit low infectivity in mammals due to the prevalence of α2,6-linked sialic acid in the respiratory tract (Couceiro et al., 1993; Kimble et al., 2010). In order to become infective for mammals, including humans, several amino acid changes, mostly in the hemagglutinin protein, must occur. They may result from spontaneous mutations, to which influenza viruses are highly prone since their RNA polymerases lack proofreading activity (Boivin et al., 2010). The other process involves the reassortation of genome segments between distinct influenza stains in coinfected cells (Taylor et al., 2023). The first A/H5N1 outbreak virus in humans was documented in Hong Kong in 1997 when 18 individuals were infected by direct contact with chickens, of whom six died (Shortridge et al., 1998). As of 31 May 2023, 876 human infections with A/H5N1 were reported to the World Health Organization between 2003 and 2023, with a high mortality rate of 52% (WHO, 2023a). Only five cases were noted during the recent A/H5N1 epidemic in Europe (2021–2023), with no deaths reported (WHO, 2023a). Moreover, two of these cases that occurred in poultry workers in Spain are suspected not to represent real viral infections but rather arise from environmental contamination of nasal mucosa (Aznar et al., 2023). At the same time, a rising number of terrestrial and aquatic mammalian species have been identified to be infected with this virus, resulting in mortality and causing concern about the potential threat to public health. These species mostly include wild animals or those kept in captivity, e.g., in zoos (Keawcharoen et al., 2004; Kaplan and Webby, 2013). No transmission between these animals and humans has been evidenced in the past. However, recent investigations documented A/H5N1 transmission in minks farmed in 2022 in Spain (Agüero et al., 2023). It is also suspected that it may spread between seals in coastal New England in 2022 (Puryear et al., 2022).
In summary, A/H5N1 is a significant threat to food supply and security, requires culling a high number of birds, including healthy individuals, only to contain outbreaks, leads to substantial resources and economic losses, and continues to create a risk to human health. These risks are especially concerning given the increased outbreaks of A/H5N1 in mammals, including those with close contact with humans, such as domestic cats (WHO, 2023c).
The first cases of A/H5N1 infections in felids were noted in 2003, and within the last two decades, nearly 10 species belonging to the Felidae family have been affected, including wild and domestic cats (Table 1). These findings demonstrate that A/H5N1 can eventually evolve into genotypes posing a threat to mammals, and this risk cannot be neglected, given the devastating outcomes such infections could have on human health (Abbasi, 2023). The risk of the introduction of A/H5N1 to the human population is particularly high when companion animals, such as cats, are affected because of close contact and the tendency of cat owners to increase caregiver burden when a cat becomes ill (Spitznagel et al., 2023). However, A/H5N1 infections in companion animals such as dogs and domestic cats have so far been very sporadic (Songserm et al., 2006a,b).
Recently, an outbreak of A/H5N1 in domestic cats has been identified in different parts of Poland (WHO, 2023b). The first A/H5N1 cases in cats were confirmed by the General Veterinary Inspectorate in Poland on 26 June 2023, with new cases evidenced in subsequent days – the involved cats included household and free-ranging individuals, which died after experiencing fever, dyspnea, and neurological symptoms (General Veterinary Inspectorate, 2023a,b,c). These symptoms are in line with previous A/H5N1 infections documented in felids (Keawcharoen et al., 2004; Marschall and Hartmann, 2008; Thiry et al., 2009; Harder and Vahlenkamp, 2010). On 30 July 2023, the first genomic sequence of A/H5N1 isolated from the oro-pharyngeal swab of a dead cat was deposited in GISAID (ID: EPI_ISL_17949824). Genomic analysis of the virus sequences obtained from other dead cats from various locations in Poland indicated that they all belonged to the H5 clade 2.3.4.4b and were highly related to each other, suggesting a common origin (e.g., ID: EPI_ISL_17951056, EPI_ISL_17951055, EPI_ISL_17951054, EPI_ISL_17951053, EPI_ISL_17951052, EPI_ISL_17951051, and EPI_ISL_17950995) (WHO, 2023b). In addition, the isolates revealed two mutations in the gene encoding PB2 protein, i.e., E672K and K526R, that have been previously recognized to expand the host range of A/H5N1 and adapt it better to replication in mammalian cells (Long et al., 2013; Song et al., 2014; Kupferschmidt, 2023). The phylogenetic trees generated for each genome segment of HPAI H5N1 viruses from cats and avian species in Poland and H5 sequences collected in different European countries are available in the work by Domańska-Blicharz et al. (2023). Their topology indicates that the HPAI H5N1 viruses collected from the cats belonged to the CH (H5N1_A/Eurasian_Wigeon/Netherlands/3/2022-like) genotype (Domańska-Blicharz et al., 2023). According to the World Health Organization, as of 11 July 2023, 47 samples from 46 cats and one captive caracal have been tested for A/H5N1, of which 29 (61.7%) were positive. At least 23 of the cats were indoor individuals, with 18 having access to a balcony, backyard, or terrace and 5 having no access to the outside environment. As of 30 June 2023, 11 infected cats were reported to die, and 14 were euthanized. As noted, this outbreak is the first to report a high number of infected cats over a wide geographical area within a country (WHO, 2023b). By mid-July 2023, samples obtained from 33 domestic cats and one caracal were confirmed to be A/H5N1-positive (General Veterinary Inspectorate, 2023d) One should also note that a real morbidity and mortality was likely higher due to underreporting.
It has been recognized for a long time that an outbreak of A/H5N1 infections in domestic cats, particularly household, may create the opportunity for a virus to adapt to a mammalian host and potentially spread in humans (Songserm et al., 2006b; Marschall and Hartmann, 2008). This is particularly a threat if cases of feline A/H5N1 infection coincide with increased human influenza cases, which in the temperate zone are noted between autumn and early spring (Marschall and Hartmann, 2008; Tamerius et al., 2011). Although the route of infection of A/H5N1 in cats in this particular outbreak remains to be elucidated, it is certainly plausible that infections with avian influenza viruses in domestic cats may occur through the consumption of bird meat, including raw chicken, which is often offered to them by owners (Thiry et al., 2009). This hypothesis is supported by (i) the genomic similarities of A/H5N1 isolated from different cats, (ii) numerous cases of avian disease over a short period of time, which occurred in domestic cats, including indoor individuals, from different locations in Poland, often hundreds of kilometers apart, (iii) the fact that infections occurred during the breeding season for wild birds, which are then characterized by limited migration, and (iv) similarity of viral sequences to those of H5 clade 2.3.4.4b viruses causing an outbreak in poultry in Poland (although they were also similar to those circulating in wild birds; WHO, 2023b). In line with this, the World Organization for Animal Health/Food and Agriculture Organization Network of Expertise on Animal Influenza stated that a direct role from infected wild birds is unlikely as a common source of A/H5N1 in this particular case since not all infected cats had outdoor access, while the wide geographical distribution of infections in cats excludes cat-to-cat transmission as a primary mode of spread (WOAH/FAO, 2023).
The investigation of five frozen meat samples provided by caregivers of cats infected by A/H5N1 in Poland confirmed high levels of viral RNA in a sample of chicken meat purchased fresh and intended for human consumption and which was consumed by the household, A/H5N1-infected cat prior to the emergence of symptoms (Rabalski et al., 2023). Considering that viral sequences isolated from different cat-derived samples were nearly identical, although infected animals inhabited different geographical locations in the country, it is most plausible that they shared the same infection source (Rabalski et al., 2023). Notably, the cat-derived sequences were also highly similar to sequences of A/H5N1 isolated from chicken meat (bearing E627K and K526R mutations). In addition, an A/H5N1 virus isolated from the chicken meat demonstrated the ability to infect both canine and feline cells in vitro and was found to infect human airway epithelia in cell culture and induce cytopathic alterations (Rabalski et al., 2023).
Importantly, feed based on raw chicken was already suspected as a source of A/H5N1 infection in other outbreaks in felids (Table 1), and such a route of transmission in Felis catus, has also been evidenced experimentally (Kuiken et al., 2004; Rimmelzwaan et al., 2006). Repeatedly reported cases of A/H5N1 infection in domestic cats from different parts of the world, including cases in which feed based on raw chicken or contact with farmed birds was the most probable source of the virus, should be regarded as a warning sign and motivate the development of solutions that could mitigate future transmissions and reduce the risk of A/H5N1 adaptation to a human host through an intermediate host, such as domestic cat or other company animals that come in close contact with humans. To this end, the subsequent section discusses cultured poultry meat technology as one of many potential measures of mitigation strategy worth being considered.
The present situation of the A/H5N1 epidemic, increasing economic loss, and potential health threats indicate the urgent need to implement various mitigation strategies, including active surveillance, biosecurity measures, culling of affected poultry, and vaccination (Bertran et al., 2018; Hill et al., 2018). Nevertheless, due to the increasing number of poultry farmed in different world regions, the potential reservoir of A/H5N1 to emerge in poultry and spread to other birds and animals is expanding. Between 2000 and 2021, the number of chickens slaughtered globally for meat increased by over 33 billion and nearly doubled in Europe (Figure 1). Poultry meat production is expected to increase at a 1.9% annual rate (Alkhtib et al., 2023; Our World in Data, 2023a). Therefore, this clearly creates the need to find alternative approaches to meet the demand and decrease various risks arising from poultry production, including those related to A/H5N1.
This is also important if one considers that the HPAI viruses were demonstrated experimentally to survive up to several days on raw poultry meat at varying temperatures (Dai et al., 2022). There are some reports on the presence of such viruses in duck and chicken meat (Wu et al., 2020; Rabalski et al., 2023). This ability increases the risk of their transmission and infection, e.g., during contact with meat products and their further processing. Moreover, in some flocks, A/H5N1 infections may not be accompanied by increased mortality or clinical signs of disease and ultimately remain undetected (Gobbo et al., 2022). In addition, the HPAI viruses, including A/H5N1, can also survive on tissues and feathers of bird carcasses for some time in infective form, creating the risk of transmission when handling culled individuals due to avian influenza outbreaks on farms (Yamamoto et al., 2017). There are some reports from developed countries on human infections with A/H5N1 in individuals involved in culling birds in response to viral outbreaks (Adlhoch et al., 2023). Last but not least, biosecurity measures are often not effective enough in controlling avian influenza even in developed countries as highlighted by nearly 2,500 outbreaks of A/H5N1 reported in European poultry between 2021 and 2022, resulting in the culling of nearly 48 million domestic birds in 37 countries (European Food Safety Authority, European Centre for Disease Prevention and Control, European Union Reference Laboratory for Avian Influenza et al., 2022). All in all, there is an urgent necessity to introduce changes to poultry meat production to limit the economic loss and decrease health risks associated with avian influenza. Although, at present, cultured meat does not offer a viable solution to these issues, it may potentially decrease the avian influenza threats in the future if the technology undergoes further development, scaling up, and introduction to different markets.
With the recent United States Department of Agriculture approval of cultured poultry produced by two companies, more producers are expected to apply for evaluation in the United States (FDA, 2022, 2023). In late 2020, cell-cultured chicken products (containing 70% cultured chicken cells hybridized with plant proteins) were also authorized in Singapore (Singapore Food Agency, 2020). As of September 2023, no other country authorized such products, but there has been substantial interest and investments in their development in Israel, Netherlands, United Kingdom, China, South Korea, Japan, France, and Spain (Food Industry Executive, 2023). In May 2023, the European Food Safety Authority reported that it is ready to pursue a scientific assessment of cultured meat products (European Food Safety Authority, 2023).
Their introduction may provide various benefits, as already discussed in the previous section of this article, and which also encompass health issues (Table 2). It will likely decrease foodborne illnesses, which is substantial for poultry and accounts for the highest number of food-related hospitalizations in the United States (Chai et al., 2017). However, it should also be regarded as a chance to limit the economic losses and health threats associated with A/H5N1 circulation. Although all these benefits could be achieved by switching to plant-based diets (Rzymski et al., 2021; Gibbs and Cappuccio, 2022; Pieczyńska and Rzymski, 2022; Carey et al., 2023), they cannot be regarded as the ultimate solution. A relevant percentage of the human population is unwilling to exclude or limit meat from their diet while developing plant-based alternatives that sufficiently mimic the organoleptic and nutritional parameters of livestock products is challenging, and there are additional barriers related to cost and allergenicity issues (Graça et al., 2015; Verhoeckx et al., 2016). Cultured meat offers an alternative that does not force a consumer to abandon the products he/she is attached to. At the same, the production of cultured poultry does not involve continuous animal farming and decreases the risk of A/H5N1 mutation and cross-species infections. Furthermore, the production of cultured poultry allows one to produce raw meat for companion animals, including cats, which are obligatory carnivores, should not be fed plant-based products, and are frequently given raw meat, including poultry, by their caregivers. Using cultured meat in the diet of household cats creates a much lower risk of avian influenza transmission and, in addition, may decrease the ecological footprint of these animals, which was calculated to constitute 25% of the environmental impact of meat consumption in the United States (Okin, 2017).
Table 2. The main advantages and challenges of poultry obtained with cultured meat technology as compared to conventional meat production.
This said, one should note that cultured meat production is also met with several challenges (Table 2). To be fully ethically superior, the production of cultured meat cannot involve animal serum (e.g., fetal bovine serum), which is classically used in in vitro cell cultures to support their growth. Instead, non-animal substitutes should be employed. The efforts to develop and introduce them are being pursued, with some animal-origin-free media already commercialized, but it is yet to be shown whether they perform similarly to animal serum in culturing cells for meat production while being economically affordable (Benjaminson et al., 2002; Gstraunthaler, 2003; Piletz et al., 2018; Rzymski et al., 2021; Yamanaka et al., 2023). Nevertheless, there has apparently been progress in producing cultured chicken meat without animal serum. As long as the first products of this type authorized in Singapore in 2020 were produced using small quantities of fetal bovine serum, in early 2023, the approval for cultured chicken meat produced using serum-free media was granted. According to the documents submitted by the manufacturer to the Food and Drug Administration, cultured meat of Gallus gallus can be produced with and without serum media (FDA, 2022), although the company states to phase out the use of bovine serum albumin with recombinant forms of albumin protein (Food Navigator USA, 2022).
Moreover, a significant obstacle in introducing cultured poultry obtained with cultured meat technology may be its price, although it can only be projected at this point. According to one analysis, the wholesale cost of cell-cultured meat obtained using large-scale production was optimistically estimated at as high as 63 USD per kg (Garrison et al., 2022). However, CE Delft’s TEA study has projected that reducing the costs of growth factors and limiting the use of recombinant proteins (e.g., albumin, insulin, transferrin) could reduce the production costs to 15 USD per kg, still exceeding those of conventional products (Vergeer et al., 2021). Further reductions in the requirement for payback time, production run time, and volume of cultivated cells could eventually put down the costs to approx. 6 USD per kg (Vergeer et al., 2021), below the price of chicken filets on the US market and the majority of European ones (Numbeo, 2023). However, when these targets could be reached remains unknown. In order to fulfill consumers’ expectations and gain acceptance, cultured poultry must also match (or be superior to) the taste, structure, texture, flavor, nutritional quality, and overall appearance of the conventional meat counterpart (Ye et al., 2022). The difficulty of reaching these goals is likely higher for some meat types, e.g., beef steaks or pork chops (Fraeye et al., 2020). Whether cell-based poultry meat can be comparable to its conventional counterpart requires comparative consumer studies, preferentially blinded, the results of which are not available at the moment. According to the data presented to the U.S. Food and Drug Administration over the course of the first pre-marketing assessment, compared to standard counterparts, serum-free cultured chicken revealed lower levels of total fat, saturated fat, monounsaturated and polyunsaturated fatty acids, and sodium, similar contents of moisture, total amino acids, niacin (B3), magnesium and manganese, but higher levels of cholesterol, pantothenic acid (B5), pyridoxine (B6), vitamin A, calcium, iron, potassium, phosphorus, selenium, and zinc (FDA, 2022). Although some of these differences could be perceived as a nutritional advantage, further studies are needed to understand their effect on the general consumer’s reception of the product. Importantly, serum-free chicken contained higher levels of lead and cadmium compared to conventional ground chicken (FDA, 2022). It is also important to decrease the carbon footprint of cultured chicken production, which was recently shown to be similar to that of conventional products (Sinke et al., 2023). Therefore, it is critical to ensure that the production of cultured meat will rely on renewable energy sources, a factor that may likely also increase the public acceptance of such products. Consumer acceptance itself is also a challenge as cultured meat may appear unnatural, especially in more conservative groups, with lower openness to novelty and high skepticism over scientific achievements (Bryant and Barnett, 2018; Sikora and Rzymski, 2023). It is essential to ensure the appropriate communication of aims, advantages, and shortcomings of cultured meat to provide balanced and fair views to the potential consumers; otherwise, it may be subject to similar opposition as in the case of genetically modified foods (Sikora and Rzymski, 2021). As recently shown, both proponents and opponents of the cultured meat concept raise concerns over safety issues (Sikora and Rzymski, 2023). Last but not least, scaling the production to the level of having a substantial contribution to the global production of poultry meat remains challenging if one considers that poultry meat production in 2021 amounted to 137,979,970.00 tonnes, representing a 93.5% increase compared to 2001 (Figure 1). Some cultured meat facilities in the United States are planned to potentially produce approximately 13,607 tonnes of cultured meat (30 million lbs) a year (UPSIDE Foods, 2023), which would constitute only 0.05% of the poultry meat produced in this country in 2021 (FAOSTAT, 2023). Moreover, switching to cultured poultry technology would require scaling up the production and delivery of various reagents, i.e., culture media (which is the leading cost driver) and growth factors (O’Neill et al., 2022). As calculated, up to 50 L of culture media is required to produce 1 kg of cultured meat (Post, 2014). Therefore, despite the potential benefits that cultured poultry could deliver, it can be expected that unless novel circumstances arise or significant developments in scaling up the manufacturing are made, it may, for a long time, play only an accessory role in the global production output. Despite this, it may still decrease the pressures and threats of transmitting highly pathogenic avian influenza in some world regions.
The spread of the HPAI A/H5N1 virus in poultry leads to significant economic loss and concerns over the emergence of avian influenza in humans. It leads to a continuous need to pursue various mitigation strategies and seek novel solutions to the problem. This paper postulates that introducing cultured poultry meat is ethically superior, has environmental advantages, provides various public health benefits, and could also be regarded as a potential opportunity to mitigate the impacts and threats of avian influenza. By shifting poultry production to the cultured meat industry, the frequency of A/H5N1 outbreaks in farmed birds may decrease, leading to a reduced risk of virus acquisition by wild and domesticated mammals (including company animals, such as cats and dogs) that have direct contact with birds or eat raw poultry, ultimately minimizing the potential of A/H5N1 to adapt better to mammalian host, including human. This strongly advocates the further development and implementation of this technology in the context of poultry meat, even though, at present, it may be challenging to scale it up to the level competitive to conventional production.
PR: Conceptualization, Investigation, Methodology, Project administration, Visualization, Writing – original draft, Writing – review & editing.
The author(s) declare that no financial support was received for the research, authorship, and/or publication of this article.
The author declares that the research was conducted in the absence of any commercial or financial relationships that could be construed as a potential conflict of interest.
All claims expressed in this article are solely those of the authors and do not necessarily represent those of their affiliated organizations, or those of the publisher, the editors and the reviewers. Any product that may be evaluated in this article, or claim that may be made by its manufacturer, is not guaranteed or endorsed by the publisher.
Abbasi, J. (2023). Bird flu has begun to spread in mammals-here’s what’s important to know. JAMA 329, 619–621. doi: 10.1001/jama.2023.1317
Adlhoch, C., Fusaro, A., Gonzales, J. L., Kuiken, T., Marangon, S., Mirinaviciute, G., et al. (2023). Avian influenza overview December 2022 – march 2023. EFSA J. 21:e07917. doi: 10.2903/j.efsa.2023.7917
Agüero, M., Monne, I., Sánchez, A., Zecchin, B., Fusaro, A., Ruano, M. J., et al. (2023). Highly pathogenic avian influenza a(H5N1) virus infection in farmed minks, Spain, October 2022. Euro Surveill. 28:1. doi: 10.2807/1560-7917.ES.2023.28.3.2300001
Alexander, D. J. (2000). A review of avian influenza in different bird species. Vet. Microbiol. 74, 3–13. doi: 10.1016/S0378-1135(00)00160-7
Alexander, D. J., and Brown, I. H. (2009). History of highly pathogenic avian influenza. Rev. Sci. Tech. 28, 19–38. doi: 10.20506/rst.28.1.1856
Alkhtib, A., Wilson, P., Bedford, M. R., O’Neill, H., Nell, M., and Burton, E. (2023). Can the broiler industry rely on results of existing life cycle assessment and environmental assessments studies to inform broilers’ nutritional strategies? Poult. Sci. 102:102667. doi: 10.1016/j.psj.2023.102667
Antimicrobial Resistance Collaborators (2022). Global burden of bacterial antimicrobial resistance in 2019: a systematic analysis. Lancet 399, 629–655. doi: 10.1016/S0140-6736(21)02724-0
Aznar, E., Casas, I., González Praetorius, A., Ruano Ramos, M. J., Pozo, F., Sierra Moros, M. J., et al. (2023). Influenza a(H5N1) detection in two asymptomatic poultry farm workers in Spain, September to October 2022: suspected environmental contamination. Euro Surveill. 28:107. doi: 10.2807/1560-7917.ES.2023.28.8.2300107
Banks, J., Speidel, E. S., Moore, E., Plowright, L., Piccirillo, A., Capua, I., et al. (2001). Changes in the haemagglutinin and the neuraminidase genes prior to the emergence of highly pathogenic H7N1 avian influenza viruses in Italy. Arch. Virol. 146, 963–973. doi: 10.1007/s007050170128
Bellani, C. F., Ajeian, J., Duffy, L., Miotto, M., Groenewegen, L., and Connon, C. J. (2020). Scale-up technologies for the manufacture of adherent cells. Front. Nutr. 7:575146. doi: 10.3389/fnut.2020.575146
Benjaminson, M. A., Gilchriest, J. A., and Lorenz, M. (2002). In vitro edible muscle protein production system (MPPS): stage 1, fish. Acta Astronaut. 51, 879–889. doi: 10.1016/S0094-5765(02)00033-4
Bertran, K., Clark, A., and Swayne, D. E. (2018). Mitigation strategies to reduce the generation and transmission of airborne highly pathogenic avian influenza virus particles during processing of infected poultry. Int. J. Hyg. Environ. Health 221, 893–900. doi: 10.1016/j.ijheh.2018.05.013
Bodiou, V., Moutsatsou, P., and Post, M. J. (2020). Microcarriers for upscaling cultured meat production. Front. Nutr. 7:10. doi: 10.3389/fnut.2020.00010
Boivin, S., Cusack, S., Ruigrok, R. W. H., and Hart, D. J. (2010). Influenza a virus polymerase: structural insights into replication and host adaptation mechanisms. J. Biol. Chem. 285, 28411–28417. doi: 10.1074/jbc.R110.117531
Briand, F.-X., Souchaud, F., Pierre, I., Beven, V., Hirchaud, E., Hérault, F., et al. (2023). Highly pathogenic avian influenza a(H5N1) clade 2.3.4.4b virus in domestic cat, France, 2022. Emerg. Infect. Dis. 29, 1696–1698. doi: 10.3201/eid2908.230188
Bryant, C., and Barnett, J. (2018). Consumer acceptance of cultured meat: a systematic review. Meat Sci. 143, 8–17. doi: 10.1016/j.meatsci.2018.04.008
Carey, C. N., Paquette, M., Sahye-Pudaruth, S., Dadvar, A., Dinh, D., Khodabandehlou, K., et al. (2023). The environmental sustainability of plant-based dietary patterns: a scoping review. J. Nutr. 153, 857–869. doi: 10.1016/j.tjnut.2023.02.001
Chai, S. J., Cole, D., Nisler, A., and Mahon, B. E. (2017). Poultry: the most common food in outbreaks with known pathogens, United States, 1998–2012. Epidemiol. Infect. 145, 316–325. doi: 10.1017/S0950268816002375
Choi, K.-H., Yoon, J. W., Kim, M., Lee, H. J., Jeong, J., Ryu, M., et al. (2021). Muscle stem cell isolation and in vitro culture for meat production: a methodological review. Compr. Rev. Food Sci. Food Saf. 20, 429–457. doi: 10.1111/1541-4337.12661
Couceiro, J. N. S. S., Paulson, J. C., and Baum, L. G. (1993). Influenza virus strains selectively recognize sialyloligosaccharides on human respiratory epithelium; the role of the host cell in selection of hemagglutinin receptor specificity. Virus Res. 29, 155–165. doi: 10.1016/0168-1702(93)90056-S
Dai, M., Yan, N., Huang, Y., Zhao, L., and Liao, M. (2022). Survivability of highly pathogenic avian influenza virus on raw chicken meat in different environmental conditions. Lancet Microbe 3:e92. doi: 10.1016/S2666-5247(21)00333-5
Della Prevenzione Sanitaria DG. (2023). Ministero della Salute. Available at: https://www.anmvioggi.it/images/Nota_DGSAF_copy.pdf (Accessed August 8, 2023).
Desvaux, S., Marx, N., Ong, S., Gaidet, N., Hunt, M., Manuguerra, J. C., et al. (2009). Highly pathogenic avian influenza virus (H5N1) outbreak in captive wild birds and cats, Cambodia. Emerg. Infect. Dis. 15, 475–478. doi: 10.3201/eid1503.081410
Domańska-Blicharz, K., Świętoń, E., Świątalska, A., Monne, I., Fusaro, A., Tarasiuk, K., et al. (2023). Outbreak of highly pathogenic avian influenza a(H5N1) clade 2.3.4.4b virus in cats, Poland, June to July 2023. Euro Surveill. 28:366. doi: 10.2807/1560-7917.es.2023.28.31.2300366
ECDC. (2023a). Communicable disease threats report. Available at: https://www.ecdc.europa.eu/sites/default/files/documents/communicable-disease-threats-report-week-26-2023.pdf (Accessed July 3, 2023).
ECDC. (2023b). Enhanced surveillance of severe avian influenza virus infections in hospital settings in the EU/EEA. European Centre for Disease Prevention and Control. Available at: https://www.ecdc.europa.eu/en/publications-data/enhanced-surveillance-severe-avian-influenza-virus-infections-hospital-settings (Accessed June 27, 2023).
European Food Safety Authority. (2023). The safety of cell culture-derived food – Ready for scientific evaluation. European food safety authority. Available at: https://www.efsa.europa.eu/en/news/safety-cell-culture-derived-food-ready-scientific-evaluation (Accessed June 27, 2023).
European Food Safety Authority, European Centre for Disease Prevention and Control, European Union Reference Laboratory for Avian InfluenzaAdlhoch, C., Fusaro, A., Gonzales, J. L., Kuiken, T., Marangon, S., et al. (2022). Avian influenza overview June - September 2022. EFSA J. 20:e07597. doi: 10.2903/j.efsa.2022.7597
European Food Safety Authority, European Centre for Disease Prevention and Control, European Union Reference Laboratory for Avian InfluenzaAdlhoch, C., Fusaro, A., Gonzales, J. L., Kuiken, T., Mirinaviciute, G., et al. (2023). Avian influenza overview march - April 2023. EFSA J. 21:e08039. doi: 10.2903/j.efsa.2023.8039
FAO. (2022). “14th five-year” National Agricultural Product Origin Place Market System Development Plan. Available at: https://www.fao.org/faolex/results/details/es/c/LEX-FAOC208248. (Accessed July 1, 2023).
FAOSTAT. (2023). FAOSTAT. https://www.faostat.fao.org. (Accessed March 20, 2016).
Farahat, R. A., Khan, S. H., Rabaan, A. A., and Al-Tawfiq, J. A. (2023). The resurgence of avian influenza and human infection: a brief outlook. New Microbes New Infect. 53:101122. doi: 10.1016/j.nmni.2023.101122
FDA. (2022). FDA completes first pre-market consultation for human food made using animal cell culture technology. U.S. Food and Drug Administration. Available at: https://www.fda.gov/food/cfsan-constituent-updates/fda-completes-first-pre-market-consultation-human-food-made-using-animal-cell-culture-technology (Accessed June 26, 2023).
FDA. (2023). FDA completes second pre-market consultation for human food made using animal cell culture technology. U.S. Food and Drug Administration. Available at: https://www.fda.gov/food/cfsan-constituent-updates/fda-completes-second-pre-market-consultation-human-food-made-using-animal-cell-culture-technology (Accessed June 26, 2023).
Food Industry Executive. (2023). US Winning the Cultured Meat Race, With 60% of Global Investment. Available at: https://foodindustryexecutive.com/2023/01/us-winning-the-cultured-meat-race-with-60-of-global-investment/ (Accessed September 26, 2023).
Food Navigator USA. (2022). ‘A watershed moment in the history of food…’ cultivated meat edges closer to commercialization in the US as UPSIDE foods gets FDA green light. foodnavigator-usa.com. Available at: https://www.foodnavigator-usa.com/Article/2022/11/17/A-watershed-moment-in-the-history-of-food-Cultivated-meat-edges-closer-to-commercialization-in-the-US-as-UPSIDE-Foods-gets-FDA-green-light (Accessed September 26, 2023).
Fraeye, I., Kratka, M., Vandenburgh, H., and Thorrez, L. (2020). Sensorial and nutritional aspects of cultured meat in comparison to traditional meat: much to be inferred. Front. Nutr. 7:35. doi: 10.3389/fnut.2020.00035
Garrison, G. L., Biermacher, J. T., and Brorsen, B. W. (2022). How much will large-scale production of cell-cultured meat cost? J. Agric. Food Res. 10:100358. doi: 10.1016/j.jafr.2022.100358
General Veterinary Inspectorate. (2023a). Komunikat III GLW w sprawie choroby kotów. Available at: https://www.wetgiw.gov.pl/main/komunikaty/Komunikat-III-GLW-w-sprawie-choroby-kotow/idn:2283 (Accessed July 1, 2023).
General Veterinary Inspectorate. (2023b). Komunikat IV GLW w sprawie choroby kotów. Available at: https://www.wetgiw.gov.pl/main/komunikaty/Komunikat-IV-GLW-w-sprawie-choroby-kotow/idn:2287 (Accessed July 1, 2023).
General Veterinary Inspectorate. (2023c). Komunikat V GLW w sprawie choroby kotów. Available at: https://www.wetgiw.gov.pl/main/komunikaty/Komunikat-V-GLW-w-sprawie-choroby-kotow/idn:2290 (Accessed July 1, 2023).
General Veterinary Inspectorate. (2023d). Komunikat VII GLW w sprawie choroby kotów. Available at: https://www.wetgiw.gov.pl/main/komunikaty/Komunikat-VII-GLW-w-sprawie-choroby-kotow/idn:2302 (Accessed August 8, 2023).
Gibbs, J., and Cappuccio, F. P. (2022). Plant-based dietary patterns for human and planetary health. Nutrients 14:1614. doi: 10.3390/nu14081614
Gobbo, F., Zanardello, C., Bottinelli, M., Budai, J., Bruno, F., De Nardi, R., et al. (2022). Silent infection of highly pathogenic avian influenza virus (H5N1) clade 2.3.4.4b in a commercial chicken broiler flock in Italy. Viruses 14:1600. doi: 10.3390/v14081600
Graça, J., Calheiros, M. M., and Oliveira, A. (2015). Attached to meat? (un)willingness and intentions to adopt a more plant-based diet. Appetite 95, 113–125. doi: 10.1016/j.appet.2015.06.024
Gstraunthaler, G. (2003). Alternatives to the use of fetal bovine serum: serum-free cell culture. ALTEX 20, 275–281. doi: 10.14573/altex.2003.4.257
Halabowski, D., and Rzymski, P. (2020). Taking a lesson from the COVID-19 pandemic: Preventing the future outbreaks of viral zoonoses through a multi-faceted approach. Sci. Total Environ 757:143723. doi: 10.1016/j.scitotenv.2020.143723
Handral, H., Hua Tay, S., Wan Chan, W., and Choudhury, D. (2022). 3D printing of cultured meat products. Crit. Rev. Food Sci. Nutr. 62, 272–281. doi: 10.1080/10408398.2020.1815172
Harder, T. C., and Vahlenkamp, T. W. (2010). Influenza virus infections in dogs and cats. Vet. Immunol. Immunopathol. 134, 54–60. doi: 10.1016/j.vetimm.2009.10.009
Harvey, J. A., Mullinax, J. M., Runge, M. C., and Prosser, D. J. (2023). The changing dynamics of highly pathogenic avian influenza H5N1: next steps for management & science in North America. Biol. Conserv. 282:110041. doi: 10.1016/j.biocon.2023.110041
He, S., Shi, J., Qi, X., Huang, G., Chen, H., and Lu, C. (2015). Lethal infection by a novel reassortant H5N1 avian influenza a virus in a zoo-housed tiger. Microbes Infect. 17, 54–61. doi: 10.1016/j.micinf.2014.10.004
Hill, E. M., House, T., Dhingra, M. S., Kalpravidh, W., Morzaria, S., Osmani, M. G., et al. (2018). The impact of surveillance and control on highly pathogenic avian influenza outbreaks in poultry in Dhaka division, Bangladesh. PLoS Comput. Biol. 14:e1006439. doi: 10.1371/journal.pcbi.1006439
Horimoto, T., Rivera, E., Pearson, J., Senne, D., Krauss, S., Kawaoka, Y., et al. (1995). Origin and molecular changes associated with emergence of a highly pathogenic H5N2 influenza virus in Mexico. Virology 213, 223–230. doi: 10.1006/viro.1995.1562
Hu, T., Zhao, H., Zhang, Y., Zhang, W., Kong, Q., Zhang, Z., et al. (2016). Fatal influenza a (H5N1) virus infection in zoo-housed tigers in Yunnan Province. China. Sci. Rep. 6:5845. doi: 10.1038/srep25845
Imai, M., Herfst, S., Sorrell, E. M., Schrauwen, E. J. A., Linster, M., De Graaf, M., et al. (2013). Transmission of influenza a/H5N1 viruses in mammals. Virus Res. 178, 15–20. doi: 10.1016/j.virusres.2013.07.017
Jaeeun, L. (2023). Another cat with bird flu confirmed in Seoul. Asia News Network. Available at: https://asianews.network/another-cat-with-bird-flu-confirmed-in-seoul/ (Accessed August 8, 2023).
Kang, D.-H., Louis, F., Liu, H., Shimoda, H., Nishiyama, Y., Nozawa, H., et al. (2021). Engineered whole cut meat-like tissue by the assembly of cell fibers using tendon-gel integrated bioprinting. Nat. Commun. 12:5059. doi: 10.1038/s41467-021-25236-9
Kaplan, B. S., and Webby, R. J. (2013). The avian and mammalian host range of highly pathogenic avian H5N1 influenza. Virus Res. 178, 3–11. doi: 10.1016/j.virusres.2013.09.004
Keawcharoen, J., Oraveerakul, K., Kuiken, T., Fouchier, R. A. M., Amonsin, A., Payungporn, S., et al. (2004). Avian influenza H5N1 in tigers and leopards. Emerg. Infect. Dis. 10, 2189–2191. doi: 10.3201/eid1012.040759
Kimble, B., Nieto, G. R., and Perez, D. R. (2010). Characterization of influenza virus sialic acid receptors in minor poultry species. Virol. J. 7:365. doi: 10.1186/1743-422X-7-365
Korea Times. (2023). Highly pathogenic avian influenza virus confirmed in cat food. The Korea times. Available at: https://www.koreatimes.co.kr/www/nation/2023/08/113_356361.html (Accessed August 8, 2023).
Kuiken, T., Rimmelzwaan, G., van Riel, D., van Amerongen, G., Baars, M., Fouchier, R., et al. (2004). Avian H5N1 influenza in cats. Science 306:241. doi: 10.1126/science.1102287
Kupferschmidt, K. (2023). From bad to worse: how avian flu must change to trigger a human pandemic. Science 380, 28–29. doi: 10.1126/science.adi1013
Leguia, M., Garcia-Glaessner, A., Muñoz-Saavedra, B., Juarez, D., Barrera, P., Calvo-Mac, C., et al. (2023). Highly pathogenic avian influenza a (H5N1) in marine mammals and seabirds in Peru. Nat. Commun. 14, 1–11. doi: 10.1038/s41467-023-41182-0
Leschnik, M., Weikel, J., Möstl, K., Revilla-Fernández, S., Wodak, E., Bagó, Z., et al. (2007). Subclinical infection with avian influenza a H5N1 virus in cats. Emerg. Infect. Dis. 13, 243–247. doi: 10.3201/eid1302.060608
Long, J. S., Howard, W. A., Núñez, A., Moncorgé, O., Lycett, S., Banks, J., et al. (2013). The effect of the PB2 mutation 627K on highly pathogenic H5N1 avian influenza virus is dependent on the virus lineage. J. Virol. 87, 9983–9996. doi: 10.1128/JVI.01399-13
Lynch, J., and Pierrehumbert, R. (2019). Climate impacts of cultured meat and beef cattle. Front Sustain Food Syst 3:5. doi: 10.3389/fsufs.2019.00005
Marschall, J., and Hartmann, K. (2008). Avian influenza a H5N1 infections in cats. J. Feline Med. Surg. 10, 359–365. doi: 10.1016/j.jfms.2008.03.005
Miraglia, M., Marvin, H. J. P., Kleter, G. A., Battilani, P., Brera, C., Coni, E., et al. (2009). Climate change and food safety: an emerging issue with special focus on Europe. Food Chem. Toxicol. 47, 1009–1021. doi: 10.1016/j.fct.2009.02.005
Monne, I., Fusaro, A., Nelson, M. I., Bonfanti, L., Mulatti, P., Hughes, J., et al. (2014). Emergence of a highly pathogenic avian influenza virus from a low-pathogenic progenitor. J. Virol. 88, 4375–4388. doi: 10.1128/JVI.03181-13
Nelson, G. C., Valin, H., Sands, R. D., Havlík, P., Ahammad, H., Deryng, D., et al. (2014). Climate change effects on agriculture: economic responses to biophysical shocks. Proc. Natl. Acad. Sci. U. S. A. 111, 3274–3279. doi: 10.1073/pnas.1222465110
Numbeo. (2023). Price rankings by country of chicken fillets (1kg) (markets). Available at: https://www.numbeo.com/cost-of-living/country_price_rankings?itemId=19 ([Accessed November 4, 2023).
O’Neill, E. N., Ansel, J. C., Kwong, G. A., Plastino, M. E., Nelson, J., Baar, K., et al. (2022). Spent media analysis suggests cultivated meat media will require species and cell type optimization. Npj Sci. Food 6, 1–10. doi: 10.1038/s41538-022-00157-z
Okin, G. S. (2017). Environmental impacts of food consumption by dogs and cats. PLoS One 12:e0181301. doi: 10.1371/journal.pone.0181301
Our World in Data. (2023a). Global meat consumption. Our world in data. Available at: https://ourworldindata.org/grapher/global-meat-projections-to-2050 (Accessed June 27, 2023).
Our World in Data. (2023b). Yearly number of animals slaughtered for meat. Our world in data. Available at: https://ourworldindata.org/grapher/animals-slaughtered-for-meat (Accessed June 27, 2023).
Pasitka, L., Cohen, M., Ehrlich, A., Gildor, B., Reuveni, E., Ayyash, M., et al. (2022). Spontaneous immortalization of chicken fibroblasts generates stable, high-yield cell lines for serum-free production of cultured meat. Nat. Food 4, 35–50. doi: 10.1038/s43016-022-00658-w
Pieczyńska, K., and Rzymski, P. (2022). Health benefits of vegetarian and Mediterranean diets: narrative review. Pol. J. Food Nutr. Sci. 72, 327–346. doi: 10.31883/pjfns/156067
Piletz, J. E., Drivon, J., Eisenga, J., Buck, W., Yen, S., McLin, M., et al. (2018). Human cells grown with or without substitutes for fetal bovine serum. Cell Med. 10:5514. doi: 10.1177/2155179018755140
Post, M. J. (2012). Cultured meat from stem cells: challenges and prospects. Meat Sci. 92, 297–301. doi: 10.1016/j.meatsci.2012.04.008
Post, M. J. (2014). An alternative animal protein source: cultured beef. Ann. N. Y. Acad. Sci. 1328, 29–33. doi: 10.1111/nyas.12569
Puryear, W., Sawatzki, K., Hill, N. J., Foss, A. D., Stone, J. J., Doughty, L., et al. (2022). Outbreak of highly pathogenic avian influenza H5N1 in New England seals. bioRxiv 2022:1155. doi: 10.1101/2022.07.29.501155
Rabalski, L., Milewska, A., Pohlmann, A., Gackowska, K., Lepionka, T., Szczepaniak, K., et al. (2023). Emergence and potential transmission route of avian influenza a (H5N1) virus in domestic cats in Poland, June 2023. Euro Surveill. 28:390. doi: 10.2807/1560-7917.es.2023.28.31.2300390
Rafique, S., Rashid, F., Mushtaq, S., Ali, A., Li, M., Luo, S., et al. (2023). Global review of the H5N8 avian influenza virus subtype. Front. Microbiol. 14:1200681. doi: 10.3389/fmicb.2023.1200681
Reiss, J., Robertson, S., and Suzuki, M. (2021). Cell sources for cultivated meat: applications and considerations throughout the production workflow. Int. J. Mol. Sci. 22:7513. doi: 10.3390/ijms22147513
Rimmelzwaan, G. F., van Riel, D., Baars, M., Bestebroer, T. M., van Amerongen, G., Fouchier, R. A. M., et al. (2006). Influenza a virus (H5N1) infection in cats causes systemic disease with potential novel routes of virus spread within and between hosts. Am. J. Pathol. 168, 176–183. doi: 10.2353/ajpath.2006.050466
Ruiz-Saenz, J., Diaz, A., Bonilla-Aldana, D. K., Rodríguez-Morales, A. J., Martinez-Gutierrez, M., and Aguilar, P. V. (2022). African swine fever virus: a re-emerging threat to the swine industry and food security in the Americas. Front. Microbiol. 13:1011891. doi: 10.3389/fmicb.2022.1011891
Rzymski, P., Kulus, M., Jankowski, M., Dompe, C., Bryl, R., Petitte, J. N., et al. (2021). COVID-19 pandemic is a call to search for alternative protein sources as food and feed: a review of possibilities. Nutrients 13:150. doi: 10.3390/nu13010150
Sharma, R. K., Kumar, S., Vatta, K., Bheemanahalli, R., Dhillon, J., and Reddy, K. N. (2022). Impact of recent climate change on corn, rice, and wheat in southeastern USA. Sci. Rep. 12:16928. doi: 10.1038/s41598-022-21454-3
Shortridge, K. F., Zhou, N. N., Guan, Y., Gao, P., Ito, T., Kawaoka, Y., et al. (1998). Characterization of avian H5N1 influenza viruses from poultry in Hong Kong. Virology 252, 331–342. doi: 10.1006/viro.1998.9488
Shriner, S. A., and Root, J. J. (2020). A review of avian influenza a virus associations in synanthropic birds. Viruses 12:1209. doi: 10.3390/v12111209
Sikora, D., and Rzymski, P. (2021). “Public acceptance of GM foods: a global perspective (1999–2019)” in Policy issues in genetically modified crops. eds. P. Singh, A. Borthakur, A. A. Singh, A. Kumar, and K. K. Singh (San Diego, CA: Elsevier), 293–315.
Sikora, D., and Rzymski, P. (2023). The heat about cultured meat in Poland: a cross-sectional acceptance study. Nutrients 15:4649. doi: 10.3390/nu15214649
Singapore Food Agency. (2020). SFA. Available at: https://www.sfa.gov.sg/food-information/risk-at-a-glance/safety-of-alternative-protein (Accessed June 28, 2023).
Sinke, P., Swartz, E., Sanctorum, H., van der Giesen, C., and Odegard, I. (2023). Ex-ante life cycle assessment of commercial-scale cultivated meat production in 2030. Int. J. Life Cycle Assess. 28, 234–254. doi: 10.1007/s11367-022-02128-8
Song, W., Wang, P., Mok, B. W.-Y., Lau, S.-Y., Huang, X., Wu, W.-L., et al. (2014). The K526R substitution in viral protein PB2 enhances the effects of E627K on influenza virus replication. Nat. Commun. 5:5509. doi: 10.1038/ncomms6509
Songserm, T., Amonsin, A., Jam-on, R., Sae-Heng, N., Meemak, N., Pariyothorn, N., et al. (2006a). Avian influenza H5N1 in naturally infected domestic cat. Emerg. Infect. Dis. 12, 681–683. doi: 10.3201/eid1204.051396
Songserm, T., Amonsin, A., Jam-on, R., Sae-Heng, N., Pariyothorn, N., Payungporn, S., et al. (2006b). Fatal avian influenza A H5N1 in a dog. Emerg. Infect. Dis. 12, 1744–1747. doi: 10.3201/eid1211.060542
Sonnberg, S., Webby, R. J., and Webster, R. G. (2013). Natural history of highly pathogenic avian influenza H5N1. Virus Res. 178, 63–77. doi: 10.1016/j.virusres.2013.05.009
Spitznagel, M. B., Gober, M. W., and Patrick, K. (2023). Caregiver burden in cat owners: a cross-sectional observational study. J. Feline Med. Surg. 25:1098612X2211458. doi: 10.1177/1098612X221145835
Starick, E., Beer, M., Hoffmann, B., Staubach, C., Werner, O., Globig, A., et al. (2008). Phylogenetic analyses of highly pathogenic avian influenza virus isolates from Germany in 2006 and 2007 suggest at least three separate introductions of H5N1 virus. Vet. Microbiol. 128, 243–252. doi: 10.1016/j.vetmic.2007.10.012
Swartz, E. (2021). The science of cultivated meat. The Good Food Institute. Available at: https://gfi.org/science/the-science-of-cultivated-meat/ (Accessed June 27, 2023).
Tamerius, J., Nelson, M. I., Zhou, S. Z., Viboud, C., Miller, M. A., and Alonso, W. J. (2011). Global influenza seasonality: reconciling patterns across temperate and tropical regions. Environ. Health Perspect. 119, 439–445. doi: 10.1289/ehp.1002383
Taylor, K. Y., Agu, I., José, I., Mäntynen, S., Campbell, A. J., Mattson, C., et al. (2023). Influenza a virus reassortment is strain dependent. PLoS Pathog. 19:e1011155. doi: 10.1371/journal.ppat.1011155
Thanawongnuwech, R., Amonsin, A., Tantilertcharoen, R., Damrongwatanapokin, S., Theamboonlers, A., Payungporn, S., et al. (2005). Probable tiger-to-tiger transmission of avian influenza H5N1. Emerg. Infect. Dis. 11, 699–701. doi: 10.3201/eid1105.050007
The Good Food Institute. (2023). GOOD meat and UPSIDE foods approved to sell cultivated chicken following landmark USDA action. The good food institute. Available at: https://gfi.org/press/good-meat-and-upside-foods-approved-to-sell-cultivated-chicken-following-landmark-usda-action/ (Accessed June 28, 2023).
Thiry, E., Addie, D., Belák, S., Boucraut-Baralon, C., Egberink, H., Frymus, T., et al. (2009). H5N1 avian influenza in cats. ABCD guidelines on prevention and management. J. Feline Med. Surg. 11, 615–618. doi: 10.1016/j.jfms.2009.05.011
Tuomisto, H. L., Allan, S. J., and Ellis, M. J. (2022). Prospective life cycle assessment of a bioprocess design for cultured meat production in hollow fiber bioreactors. Sci. Total Environ. 851:158051. doi: 10.1016/j.scitotenv.2022.158051
Tuomisto, H. L., and de Mattos, M. J. T. (2011). Environmental impacts of cultured meat production. Environ. Sci. Technol. 45, 6117–6123. doi: 10.1021/es200130u
UPSIDE Foods. (2023). UPSIDE Foods. Available at: https://upsidefoods.com/blog/reshaping-the-future-of-food-production-upside-foods-announces-cultivated-meat-production-plant-in-chicagoland (Accessed September 26, 2023).
USDA. (1996). United States standards for grades of slaughter cattle. Available at: https://www.ams.usda.gov/sites/default/files/media/Slaughter_Cattle_Standard1.pdf (Accessed June 27, 2023).
USDA. (2018). United States classes, standards, and grades for poultry. Available at: https://www.ams.usda.gov/sites/default/files/media/PoultryStandard.pdf (Accessed June 27, 2023).
USDA. (2023a). 2022–2023 detections of highly pathogenic avian influenza in mammals. Available at: https://www.aphis.usda.gov/aphis/ourfocus/animalhealth/animal-disease-information/avian/avian-influenza/hpai-2022/2022-hpai-mammals (Accessed August 8, 2023).
USDA. (2023b). Livestock and poultry: World markets and trade. USDA Foreign Agricultural Service. Available at: https://www.fas.usda.gov/data/livestock-and-poultry-world-markets-and-trade (Accessed June 27, 2023).
Venkatesan, P. (2023). Avian influenza spillover into mammals. Lancet Microbe 4:e492. doi: 10.1016/S2666-5247(23)00173-8
Vergeer, R., Sinke, P., and Odegard, I. (2021). TEA of cultivated meat. Future projections of different scenarios – corrigendum. Available at: https://cedelft.eu/wp-content/uploads/sites/2/2021/02/CE_Delft_190254_TEA_of_Cultivated_Meat_FINAL_corrigendum.pdf (Accessed November 4, 2023).
Verhagen, J. H., Munster, V. J., and Fouchier, R. A. M. (2011). “Ecology and evolution of avian influenza viruses” in Genetics and Evolution of Infectious Disease. ed. M. Tibayrenc (Amsterdam, Netherlands: Elsevier), 729–749.
Verhoeckx, K., Broekman, H., Knulst, A., and Houben, G. (2016). Allergenicity assessment strategy for novel food proteins and protein sources. Regul. Toxicol. Pharmacol. 79, 118–124. doi: 10.1016/j.yrtph.2016.03.016
WHO. (2023a). Cumulative number of confirmed human cases for avian influenza a(H5N1) reported to WHO, 2003–2023, 31 May 2023. Available at: https://www.who.int/publications/m/item/cumulative-number-of-confirmed-human-cases-for-avian-influenza-a(h5n1)-reported-to-who--2003-2023--31-may-2023 (Accessed June 27, 2023).
WHO. (2023b). Influenza a(H5N1) in cats – Poland. Available at: https://www.who.int/emergencies/disease-outbreak-news/item/2023-DON476 (Accessed August 8, 2023).
WHO. (2023c). Ongoing avian influenza outbreaks in animals pose risk to humans. Available at: https://www.who.int/news/item/12-07-2023-ongoing-avian-influenza-outbreaks-in-animals-pose-risk-to-humans (Accessed August 8, 2023).
WOAH. (2021). Avian influenza. WOAH - world organisation for animal health. Available at: https://www.woah.org/en/disease/avian-influenza/ (Accessed August 8, 2023).
WOAH/FAO. (2023). OFFLU statement on infections with avian influenza a(H5N1) virus in cats in Poland. Offlu. Available at: https://www.offlu.org/index.php/2023/06/28/offlu-statement-on-infections-with-avian-influenza-ah5n1-virus-in-cats-in-poland/ (Accessed July 6, 2023).
Wu, L., Mitake, H., Kiso, M., Ito, M., Iwatsuki-Hirimoto, K., Yamayoshi, S., et al. (2020). Characterization of H7N9 avian influenza viruses isolated from duck meat products. Transbound. Emerg. Dis. 67, 792–798. doi: 10.1111/tbed.13398
Xu, X., Subbarao, K., Cox, N. J., and Guo, Y. (1999). Genetic characterization of the pathogenic influenza a/goose/Guangdong/1/96 (H5N1) virus: similarity of its hemagglutinin gene to those of H5N1 viruses from the 1997 outbreaks in Hong Kong. Virology 261, 15–19. doi: 10.1006/viro.1999.9820
Yamamoto, Y., Nakamura, K., and Mase, M. (2017). Survival of highly pathogenic avian influenza H5N1 virus in tissues derived from experimentally infected chickens. Appl. Environ. Microbiol. 83:17. doi: 10.1128/aem.00604-17
Yamanaka, K., Haraguchi, Y., Takahashi, H., Kawashima, I., and Shimizu, T. (2023). Development of serum-free and grain-derived-nutrient-free medium using microalga-derived nutrients and mammalian cell-secreted growth factors for sustainable cultured meat production. Sci. Rep. 13, 1–13. doi: 10.1038/s41598-023-27629-w
Ye, Y., Zhou, J., Guan, X., and Sun, X. (2022). Commercialization of cultured meat products: current status, challenges, and strategic prospects. Future Foods 6:100177. doi: 10.1016/j.fufo.2022.100177
Keywords: avian influenza, A/H5N1, zoonotic diseases, poultry industry, cultured meat, food safety, Felis catus
Citation: Rzymski P (2023) Avian influenza outbreaks in domestic cats: another reason to consider slaughter-free cell-cultured poultry? Front. Microbiol. 14:1283361. doi: 10.3389/fmicb.2023.1283361
Received: 25 August 2023; Accepted: 24 November 2023;
Published: 15 December 2023.
Edited by:
Kaicheng Wang, China Animal Health and Epidemiology Center, ChinaReviewed by:
Mohammad Enamul Hoque Kayesh, Patuakhali Science and Technology University, BangladeshCopyright © 2023 Rzymski. This is an open-access article distributed under the terms of the Creative Commons Attribution License (CC BY). The use, distribution or reproduction in other forums is permitted, provided the original author(s) and the copyright owner(s) are credited and that the original publication in this journal is cited, in accordance with accepted academic practice. No use, distribution or reproduction is permitted which does not comply with these terms.
*Correspondence: Piotr Rzymski, cnp5bXNraXBpb3RyQHVtcC5lZHUucGw=
Disclaimer: All claims expressed in this article are solely those of the authors and do not necessarily represent those of their affiliated organizations, or those of the publisher, the editors and the reviewers. Any product that may be evaluated in this article or claim that may be made by its manufacturer is not guaranteed or endorsed by the publisher.
Research integrity at Frontiers
Learn more about the work of our research integrity team to safeguard the quality of each article we publish.