- 1National Key Laboratory of Agricultural Microbiology, Hubei Hongshan Laboratory, Huazhong Agricultural University, Wuhan, Hubei, China
- 2College of Veterinary Medicine, Huazhong Agricultural University, Wuhan, Hubei, China
- 3Key Laboratory of Preventive Veterinary Medicine in Hubei Province, The Cooperative Innovation Center for Sustainable Pig Production, Wuhan, Hubei, China
Although the bacterial composition of boar ejaculate has been extensively studied, the bacterial composition of extended boar semen is often overlooked, despite the potential risks these microorganisms may pose to the long-term preservation of extended boar semen at 15–17°C. In this study, we characterized the bacterial community composition of extended semen and discovered that Pseudomonas spp. was the dominant flora. The dominant strains were further isolated and identified as a potential new species in the Pseudomonas fluorescens group and named GXZC strain, which had adverse effects on sperm quality and was better adapted to growth at 17°C. Antimicrobial susceptibility testing showed that the GXZC strain was resistant to all commonly used veterinary antibiotics. Whole-genome sequencing (WGS) and genome annotation revealed the large genetic structure and function [7,253,751 base pairs and 6,790 coding sequences (CDSs)]. Comparative genomic analysis with the closest type strains showed that the GXZC strain predicted more diversity of intrinsic and acquired resistance genes to multi-antimicrobial agents. Taken together, our study highlights a problem associated with the long-term storage of extended boar semen caused by a P. fluorescens group strain with unique biological characteristics. It is essential to develop a new antibacterial solution for the long-term preservation of boar semen.
Introduction
Artificial insemination (AI) is widely used in global pig production to facilitate improvements in fertility, genetics, labor, and herd health (Knox, 2016). In pig AI, more than 99% of boar semen is stored in a nutrient-rich liquid state at 15–20°C (Johnson et al., 2000; Pezo et al., 2019). Long-term extenders are now widely used in commercial extended semen to meet the rapid development of AI and increase the flexibility of semen use, based on their ability to preserve sperm for 7–12 days after collection (Karageorgiou et al., 2016). However, the boar semen collection process is not aseptic, and freshly collected boar ejaculate often contains bacterial contamination which sources animal (e.g., feces, hair, and human) and non-animal (e.g., water, feed, and air) (Althouse et al., 2000). The bacterial composition of boar ejaculate is complex (Althouse et al., 2000, 2008; Althouse and Lu, 2005; Godia et al., 2020; Zhang et al., 2020). Some bacterial strains isolated from boar semen have been shown to reduce sperm quality (motility, plasma membrane integrity, acrosome, etc.), such as Enterobacteriaceae family (Luis Ubeda et al., 2013), Clostridium perfringens (Sepulveda et al., 2013), Pseudomonas aeruginosa (Sepulveda et al., 2016), Enterobacter cloacae (Prieto-Martinez et al., 2014), Staphylococcus aureus (Li et al., 2017), Proteus mirabilis (Gao et al., 2018), and Proteus vulgaris (Delgado-Bermudez et al., 2020). Previous studies have reported bacterial thresholds between × 103 and × 107 CFU/ml before adverse effects on sperm quality or fertility become apparent (Auroux et al., 1991; Diemer et al., 1996; Bussalleu et al., 2011; Sepulveda et al., 2013, 2014; Prieto-Martinez et al., 2014; Pinart et al., 2017; Delgado-Bermudez et al., 2020).
Antibiotics are commonly added to commercial extenders as the primary approach to control bacteria (Althouse et al., 2000). According to national regulatory requirements (Union, 1992; CSAMR, 2019), antibacterial substances, as essential components of commercial extenders, are strictly regulated by the requirements of local laws or regulations. Antibiotics commonly used in semen diluents include the β-lactams (penicillins, cephalosporins), aminoglycosides (gentamicin, streptomycin, and amikacin), macrolides (tylosin, spectinomycin), and lincosamides (lincomycin) (Santos and Silva, 2020). Aminoglycosides are the most commonly used antibiotics in boar semen (Mazurova and Vinter, 1991; Bryla and Trzcinska, 2015; Waberski et al., 2019).
Recently, the demand for long-term semen preservation has increased in the efficient and competitive pig industry in China. However, long-term storage in nutrient-rich container with antimicrobial agents facilitates the development of drug resistance in bacteria. The potential risks associated with bacterial contamination in long-term storage of extended boar semen cannot be ignored. Therefore, this study aimed to characterize the composition of the extended boar semen microbiome, isolate the predominant microorganisms, and explore their potential risks.
Materials and methods
Sampling processing
Three healthy and sexually mature (1–2 years of age) boars were randomly selected from the artificial insemination (AI) station of Yangxiang Farming Co., Ltd., which is a floor building with air filtration, temperature, humidity, and wind speed-controlled automatically. Boars were farmed in the same condition. The brief procedures are presented in Figure 1.
The collection and processing of ejaculates were conducted according to the minimum bacterial contamination protocol (Althouse et al., 2000). Each ejaculate was filtered through gauze to remove the gel. In total, 10 ml of ejaculate is diluted in sterile Beltsville thawing solution (BTS) to the concentration of 2.5 × 107 spermatozoa/ml (Pursel and Johnson, 1975) and seems as antibiotic-free group (detailed descriptions in the Supplementary material). Each of the remaining ejaculates was processed with the commercial manufacturing requirements of the AI station. In brief, the ejaculates diluted in Zenolong® (Beikang, Taizhou, China; with gentamicin added), to the same sperm concentration, and cooled to 17°C. Then, they were packaged in 60 ml plastic bags (IMV, Shanghai, China). Three of packaged extended semen doses of each boar were stored at 17°C for 12 days. After 0, 3, 6, 9, and 12 days of fluid storage at 17°C, the extended semen total sperm motility (TSM, including progressive motility and non-progressive motility) was measured using a computer-assisted semen analysis (CASA) system (HTR-IVOS II, Hamilton Thorne Research, Beverly, MA, United States; software settings are presented in Supplementary Table S1), and composition of bacterial community was characterized by 16S rRNA high-throughput sequencing. Considering the low bacterial concentrations which were not 16S rRNA sequenced for analysis at the early stages of stored extended semen, samples collected each time were shaken (37°C, 180 rpm) for 24 h to get the high-concentration bacterial communities and seem as group II. Previously unprocessed samples were seemed as group I.
DNA extraction and 16s rRNA high-throughput sequencing
The MagBeads Fast DNATM Kit for Soil (MP Biomedicals, Santa Ana, CA, United States) was used to extract total genomic DNA from groups I and II. The concentration was determined using a fluorometer (Qubit Fluorometer, Invitrogen), and <0.3 ng/μl did not meet the detection requirements. The samples were discarded.
PCR amplification of the bacterial 16S rRNA gene V3-V4 region was performed using the forward primer 338F and reverse primer 806R (Chen et al., 2017; Hu et al., 2018). The PCR products were purified using the QIAquick PCR Purification Kit (Qiagen, China), followed by end-repair mix and incubation at 20°C for 30 min. The end-repaired DNA was, then, purified, followed by A-tail mix and incubation at 37°C for 30 min. The purified adenylated 3′-end DNA was combined with adapter and ligation mix, and the ligation reaction was incubated at 16°C for 12–16 h. Adapter-ligated DNA was selected by running a 2.5% agarose gel for approximately 2.5 to 3 h to recover the target fragments. The gel is purified using QIAquick Gel Extraction Kit (Qiagen, China). The final library was quantified using two methods: determining the average molecular length using the Agilent 2100 Bioanalyzer instrument and quantifying the library by real-time quantitative PCR (qPCR) (TaqMan Probe). The raw reads were filtered to remove adaptors and low-quality and ambiguous bases. The paired-end reads were, then, merged to the tags using the Fast Length Adjustment of SHort reads (FLASH) (version 1.2.11, http://ccb.jhu.edu/software/FLASH/) (Magoc and Salzberg, 2011). These tags were clustered into operational taxonomic units (OTUs) at a 97% cutoff value using UPARSE software (version 7.0.1090, http://drive5.com/uparse/) (Edgar, 2013). Chimera sequences were detected by comparing the Gold database using UCHIME (version 4.2.40, http://drive5.com/uchime/uchime) (Edgar et al., 2011). The OTU representative sequences were, then, taxonomically classified with a minimum confidence threshold of 0.6 using the Ribosomal Database Project (RDP) Classifier (version 2.2, http://rdp.cme.msu.edu/) and trained on the Greengenes database (version 201305) using QIIME (version 1.8, http://qiime.sourceforge.net/) (Caporaso et al., 2010). The OTU abundance statistics table of each sample was obtained by comparing all tags with the OTUs using the USEARCH global method (version 7.0.1090, http://www.drive5.com/usearch) (Edgar, 2010). Bar graphs of different classification levels were plotted using the R package (version 3.4.1).
Bacterial isolation and characterization
The samples stored for the last day of group I were inoculated on TSA and stored at 17°C and 37°C, respectively, for 36 h. Five colonies with visually distinguishable colony morphologies were picked from the 17°C and 37°C samples. These colonies were designated as GXZC-number. The single primary colony was continuously purified for three passages. The resulting colonies were subjected to 16S DNA sequencing (Tsingke, Wuhan, China), and the sequences were identified using the BLAST (blastn) tool on the National Center for Biotechnology Information (NCBI) website (https://www.ncbi.nlm.nih.gov/).
The growth of the isolated strains was analyzed in flat-bottomed 100-well microtiter plates by measuring the optical density at 600 nm (OD600) every 30 min using the Bioscreen C system (Labsystems Oy, Helsinki, Finland) with shaking (180 rpm) at 17°C, 27°C, and 37°C.
Antimicrobial susceptibility testing was performed according to the Clinical Laboratory Standards Institute (CLSI) guidelines using the Kirby–Bauer agar diffusion or microdilution method (CLSI, 2022). Standard strains of Escherichia coli ATCC 25922 and Pseudomonas aeruginosa ATCC 27853 were used for quality control. Susceptibility testing was conducted against ampicillin, ceftiofur, cefquinome, gentamicin, neomycin, kanamycin, spectinomycin, sulfisoxazole, trimethoprim–sulfamethoxazole, doxycycline, enrofloxacin, chloramphenicol, florfenicol, tilmicosin, tiamulin, and colistin (according to the CLSI guidelines, the microdilution method is acceptable, and disk diffusion methods should not be performed for colistin). These antibiotics are commonly used as veterinary drugs (CSAMR, 2019). CLSI (2018, 2022) and European Committee on Antimicrobial Susceptibility Testing EUCAST (2022) criteria only provide minimum inhibitory concentration (MIC) for gentamicin, sulfisoxazole, doxycycline, and chloramphenicol. For the remaining 12 antibiotics, zone diameter and MIC breakpoints were not provided, and thus, the results are presented as zone diameter or MIC (colistin) values.
Assessment of the effect of isolated strains on boar semen
The isolated strains were cultured in tryptic soy broth (TSB) (BD, Spark MD, USA) at 27°C or 37°C for 16 h in a shaking bath to assess the pathogenicity of the isolated bacteria on boar sperm. Then, strains were inoculated into the extended semen. The commercial extended semen doses (Yangxiang, Guigang, China), with a concentration of 2.5 × 107 spermatozoa/ml, were collected from the healthy boar and divided into seven aliquots of 8 ml each. One aliquot was used as a control (non-infected samples), while the others were infected with isolated strain at the following initial bacterial concentrations (day 0): 2 × 102, 2 × 104, and 2 × 106 CFU/ml. Non-infected and infected samples were stored in sealed tubes at 17°C for 12 days. TSM was measured using the CASA system (HTR-IVOS II, Hamilton Thorne Research, Beverly, MA, United States) at 0, 2, 4, 6, 8,10, and 12 days. Simultaneously, bacterial growth was evaluated at each infectious dose and time point using plate culture with tryptic soy agar (TSA) (BD, Spark MD, United States).
Whole-genome sequencing, annotation, and gene prediction
The genome was sequenced using a combination of Illumina NovaSeq6000 (Illumina, San Diego, CA, USA) and Nanopore PromethION sequencing platforms (Oxford Nanopore Technologies, Oxford, UK). The original image data were converted into sequence data by base calling, resulting in raw reads that were saved as a FASTQ file with read sequences and quality information. After removing low-quality data using quality information statistics, the reads were assembled into a contig to generate a complete genome with seamless chromosomes and plasmids using CAUN (version 1.6) and the Hierarchical Genome Assembly Process (HGAP) (Chin et al., 2013; Koren et al., 2017). Finally, the PacBio assembly results were corrected using Illumine reads.
Glimmer (version 3.02, http://ccb.jhu.edu/software/glimmer/index.shtml) and GeneMarkS (version 4.3, http://topaz.gatech.edu/GeneMark) were used for CDS prediction (Besemer et al., 2001; Delcher et al., 2007). tRNA-scan-SE (version 2.0, http://trna.ucsc.edu/software/) was used for tRNA prediction (Chan and Lowe, 2019). Barrnap (https://github.com/tseemann/barrnap) was used for rRNA prediction.
The predicted CDSs were annotated from NCBI non-redundant (NR) (ftp://ftp.ncbi.nlm.nih.gov/blast/db/), Swiss-Prot (https://web.expasy.org/docs/swiss-prot_guideline.html), Protein families (Pfam) (http://pfam.xfam.org/), Gene Ontology (GO) (http://geneontology.org/), Clusters of Orthologous Groups (COG) (https://www.ncbi.nlm.nih.gov/research/cog/), and Kyoto Encyclopedia of Genes and Genomes (KEGG) (https://www.genome.jp/kegg/) databases using Blast2go (version 2.5, https://www.blast2go.com/), Diamond (version 0.8.35, https://github.com/bbuchfink/diamond), and HMMER (version 3.1, http://www.hmmer.org/) sequence alignment tools. Each set of query proteins was aligned with the databases, and the annotations of best-matched subjects (e-value <10−5) were obtained for gene annotation.
Genome-based species identification
To carry out subsequent comparative genomic analyses, we performed species identification based on whole-genome sequence using the online Type (Strain) Genome Server (TYGS) (https://tygs.dsmz.de/) (Meier-Kolthoff and Goeker, 2019) and JSpeciesWS (version3.9.8, https://jspecies.ribohost.com/jspeciesws/#analyse) (Richter et al., 2016).
Antibiotic resistance genes and comparative genomics analysis
Antibiotic resistance genes were annotated and identified using the online Resistance Gene Identifier (RGI) (version 6.0.1, https://card.mcmaster.ca/analyze/rgi) (select criteria: “Perfect, Strict and Loose hits,” Exclude nudge, High quality/coverage) (Alcock et al., 2022). The ResFinder software (version 4.1, https://cge.food.dtu.dk/services/ResFinder-4.1/) program (identity ≥ 80%, coverage ≥ 60%) within ResFinder data was used to identify the acquired antimicrobial resistance genes. Based on the average nucleotide identity (ANI) data and the phylogenetic tree, the closest homologs to the isolated strain were selected for resistance gene prediction and comparative analysis.
Data analysis
The data were analyzed using GraphPad Prism 8.0 (San Diego, CA, USA). The data from three independent experiments were presented as means and standard deviations (SD). TSM was compared by Duncan's multiple range tests using one-way analysis of variance (ANOVA) when the F-value was significant (p < 0.05). The correlation among the above-mentioned methods was evaluated by linear regression analysis.
Data availability
Whole-genome sequencing data of the P. GXZC strain were submitted to the NCBI (Pseudomonas sp. GXZC, assembly accession: GCF_026967615.1). The BioProject number for the complete genome sequence was PRJNA909457.
Result
Pseudomonas spp. was the dominant genus in the later stages of semen storage
After 6 months of investigation in a group with approximately 10,000 boars from three clusters of AI stations distributed throughout China, we observed a high percentage of Pseudomonas isolates and flora composition during the late storage stage (storage time > 9 days) of extended boar semen (data not shown). The three randomly selected boars were consistent with the previous background investigation. The extended semen of three boars also showed similar results, with a rapid decrease in total sperm motility (TSM) (Supplementary Figure S1) and an increased proportion of Pseudomonas spp. with increasing storage time (Figure 2).
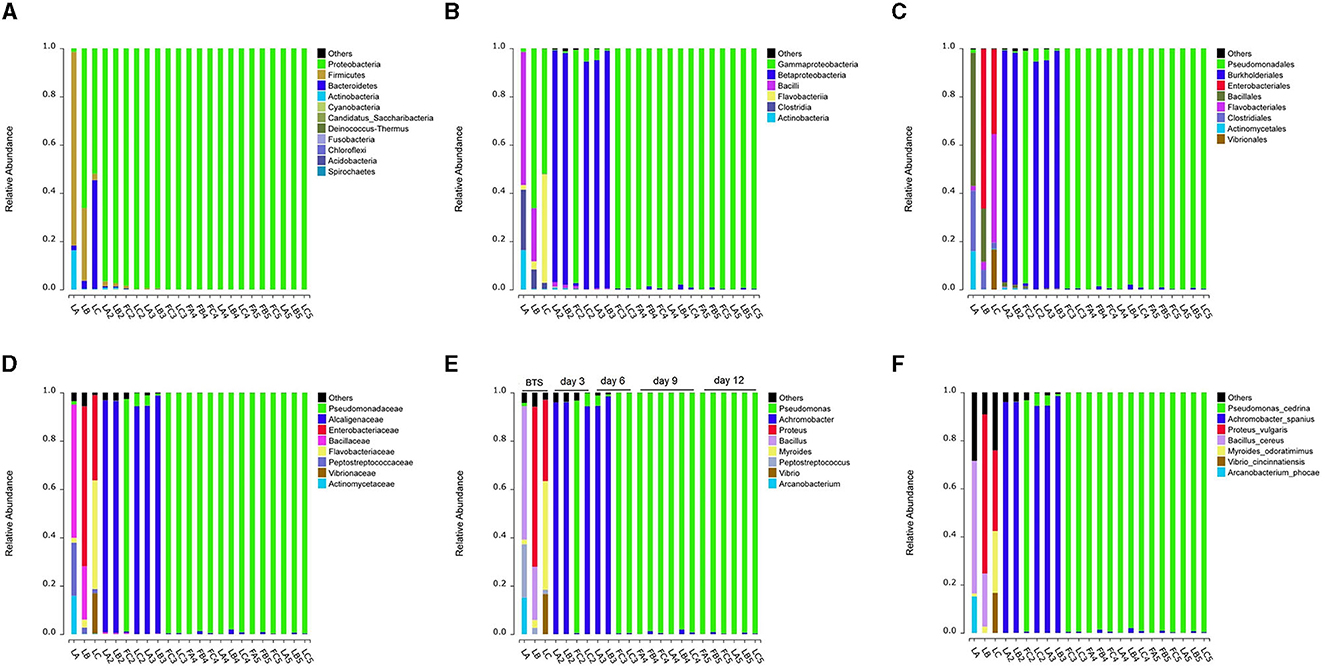
Figure 2. Variations in bacterial community composition of extended semen stored for days. (A–E) Shifts in bacterial taxonomic compositions at several taxonomic levels, including phylum (A), class (B), order (C), family (D), genus (E), and species (F). “LA, LB, and LC” are samples that the ejaculate was diluted in sterile BTS. All remaining are samples that the ejaculate was diluted in Zenolong®. “FC2, FC3, FA4, FB4, FC4, FA5, FB5, and FC5” are Group I in which the samples were stored at 17°C for the right time (3, 6, 9, or 12 days). Moreover, “LA, LB, LC, LA2, LB2, LC2, LA3, LB3, LC3, LA4, LB4, LC4, LA5, LB5, and LC5” are Group II in which the samples were stored in an incubator under shaking condition with 180 rpm at 37°C for 24 h after having 17°C storages. A, B, and C represent three boars, respectively; numbers 2, 3, 4, and 5 represent 3, 6, 9, and 12 days, where the extended semen was stored. “F” and “L” represent groups I and II, respectively.
Due to the initially low bacterial levels present in semen during the early stage of semen storage, 12 samples contained <0.3 ng/μl of genomic DNA and were discarded (Supplementary Table S2). To better comprehend the diversity of bacterial composition, the collected semen was incubated at a temperature of 37°C to facilitate rapid bacterial growth. By utilizing 16S rRNA high-throughput sequencing, we were able to partially elucidate the bacterial population, particularly in the extenders without antibiotics (BTS extender), which can be notably more complex. In conclusion, the community diversity decreased after the addition of antibiotics and after 12-day storage at 17°C (Supplementary Figure S2). In the later stages of storage (9 and 12 days), all samples were dominated by Pseudomonas spp. (relative abundance: 99.05%−99.86%), followed by Achromobacter spp. (relative abundance: 0.06%−1.95%) (Figure 2E, Supplementary Table S3).
P. GXZC strain identified as a strain of Pseudomonas fluorescens group with adaptation to 17°C temperature and multidrug resistance
All colony morphologies of samples inoculated on TSA were visually consistent at the same temperature. However, colony morphologies were different between 17°C and 37°C. Sequence alignment exhibited that the isolates stored at 17°C were all Pseudomonas spp. The sequences shared 100% identity, designated as P. GXZC strain. Similarly, the isolates stored at 37°C were all Achromobacter spp., and the sequences shared 100% identity, designated as A. GXZC strain. No other bacteria were isolated. However, it could not identify the groups or species using only 16S rRNA sequence comparisons. Multilocus sequence typing (MLST) was performed to identify the groups and species of Pseudomonas spp. using combined 16S rRNA, gyrB, rpoD, and rpoB sequences (Ait Tayeb et al., 2005; Mulet et al., 2009; Edgar, 2010), and Achromobacter spp. were identified based on 16S rRNA and recA sequences (Gomila et al., 2014). The sequencing primers are shown in Supplementary Table S4. P. GXYX was identified as P. azotoformans (with a threshold of 97% similarity for the species) within P. fluorescens group (Mulet et al., 2010), and A. GXYX was identified as A. xylosoxidans (with a threshold of 98% similarity for the species) (Gomila et al., 2014).
P. GXZC and A. GXZC had significantly different proliferation curves at 17°C, 27°C, and 37°C, respectively (Figure 3). At 17°C, P. GXZC proliferated more actively than A. GXZC. The strain entered the log phase from 2 to 8 h and then the stationary phase from 28 to 34 h, inoculating three 10-fold ratio gradient concentrations. Conversely, A. GXZC proliferation entered the log phase at the later stages of the experiment and did not enter the stationary phase until the end time. However, at 37°C, P. GXZC could not proliferate, while A. GXZC proliferation entered the log phase from 2 to 8 h and the stationary phase from 24 to 30 h. Although both isolated strains proliferated actively at 27°C, P. GXZC exhibited faster proliferation than A. GXZC, with an earlier point of entering the log and stationary phases.
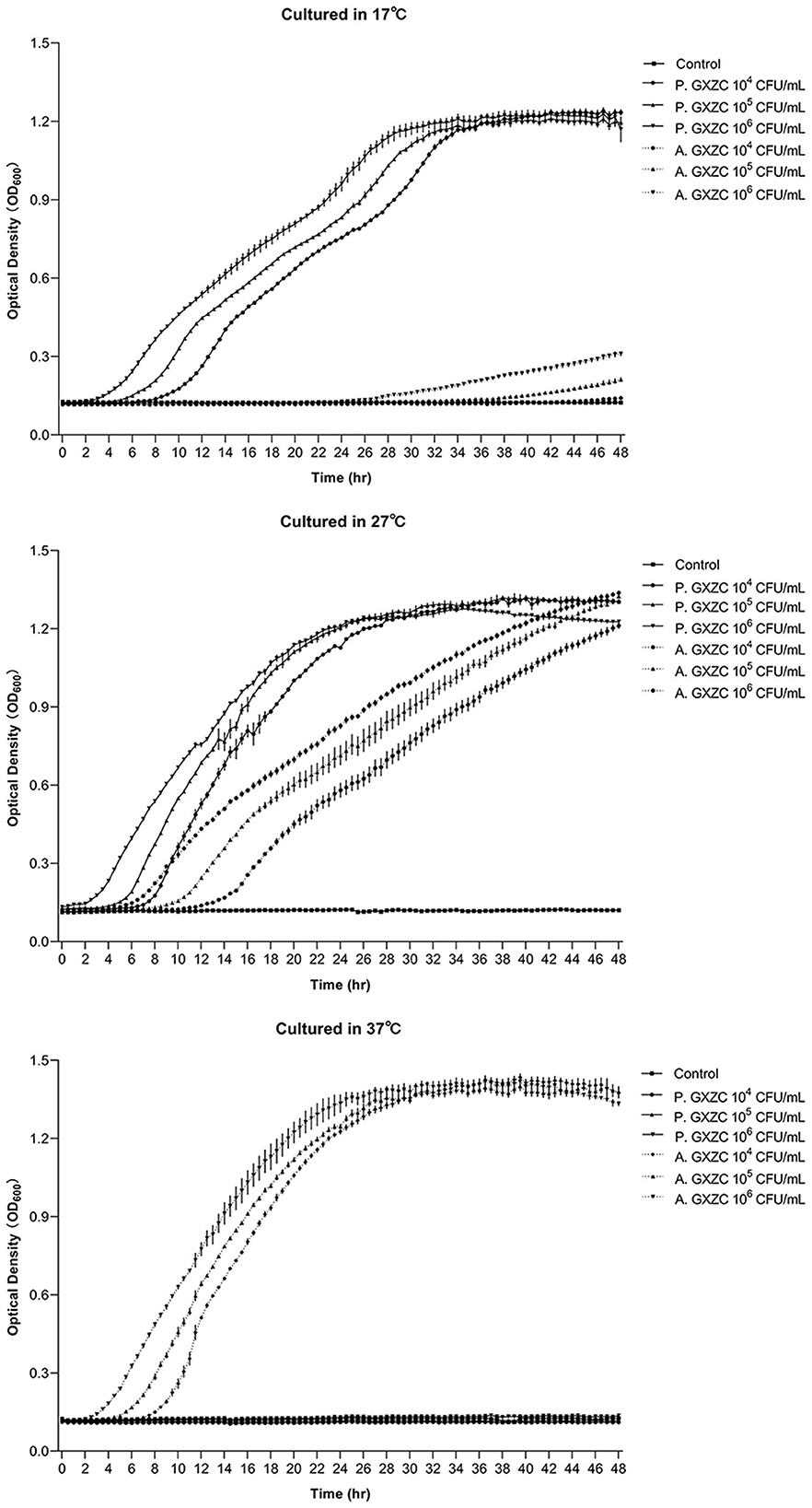
Figure 3. Bacterial proliferation curve at different temperature. The proliferation curve of P. GXZC and A. GXZC with inoculation of three 10-fold ratio gradient concentrations at 17°C, 27°C, and 37°C, respectively. Data are shown as mean ± SD. n = 3.
Antimicrobial susceptibility testing showed that P. GXZC strain exhibited resistance to gentamicin, sulfisoxazole, doxycycline, and chloramphenicol (Table 1). For the 12 antibiotics lacking zone diameter or MIC breakpoints, only zone diameter or MIC values are provided (referring to the criteria of P. aeruginosa, P. GXZC strain could identify resistance to colistin).
The P. GXZC strain harmed sperm vitality in extended semen storage
A study has confirmed a positive correlation between pregnancy rate and semen motility (Lucca et al., 2021). Herein, the effect of P. GXZC strain on the vitality of sperm was determined. The growth dynamics of the P. GXZC and A. GXZC strains are shown in Figure 4 and are notably different during the 12-day storage period at 17°C. Consistent with the assessment results above, P. GXZC proliferates actively in extended semen at 17°C (Figure 4A). All groups inoculated with different concentration of P. GXZC strain reach the stationary phase at approximately 108 CFU/ml in liquid extended semen. The proliferation of the P. GXZC strain adversely affected sperm vitality in the later stages of extended semen storage. On day 6, the tube containing 2 × 106 CFU/ml or 2 × 104 CFU/ml of P. GXZC differed significantly from the negative control. On day 12, all treatments differed from the control. It seems that only when the bacteria proliferate to a certain concentration (> 106 CFU/ml), they show significant negative effects on sperm vitality. In contrast, A. GXZC proliferation was extremely slow in liquid extended semen at 17°C. Moreover, there were no differences (Figure 4B) in the TSM between the treatments and the negative control over the entire time.
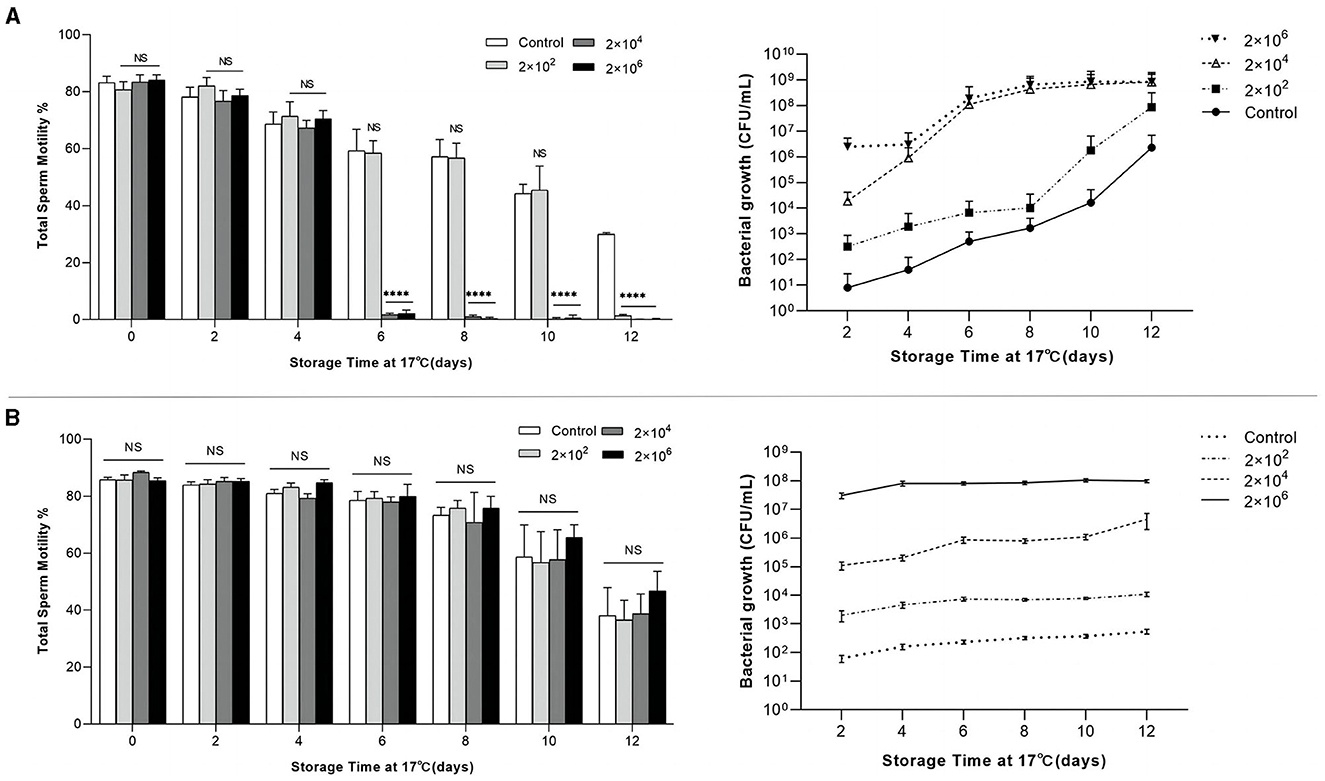
Figure 4. Relation of total sperm motility and bacterial growth in extended semen. Total sperm motility of extended semen incubated with different infectious concentrations (2 × 102, 2 × 104, and 2 × 106 CFU/ml) over 12 days of storage at 17°C, and bacterial growth plotted by colonies counted directly after incubating the TSA plates at 28°C for 24 h. (A) Incubated with P. GXYX strain. (B) Incubated with A. GXYX strain. Data are shown as mean ± SD. n = 3. “NS”: no significant; ****p < 0.0001.
Genomic characteristics of P. GXZC strain
The general characteristics of the P. GXZC strain genome are shown in Table 2, which consisted of 7,253,751 base pairs with an average G + C content of 60.23%. The genome contained approximately 6,790 predicted CDSs and 73 tRNA and 20 rRNA genes (8, 5S; 6, 16S; and 6, 23S) (Figure 5). The CDS numbers allocated to the different databases are presented in Table 2.
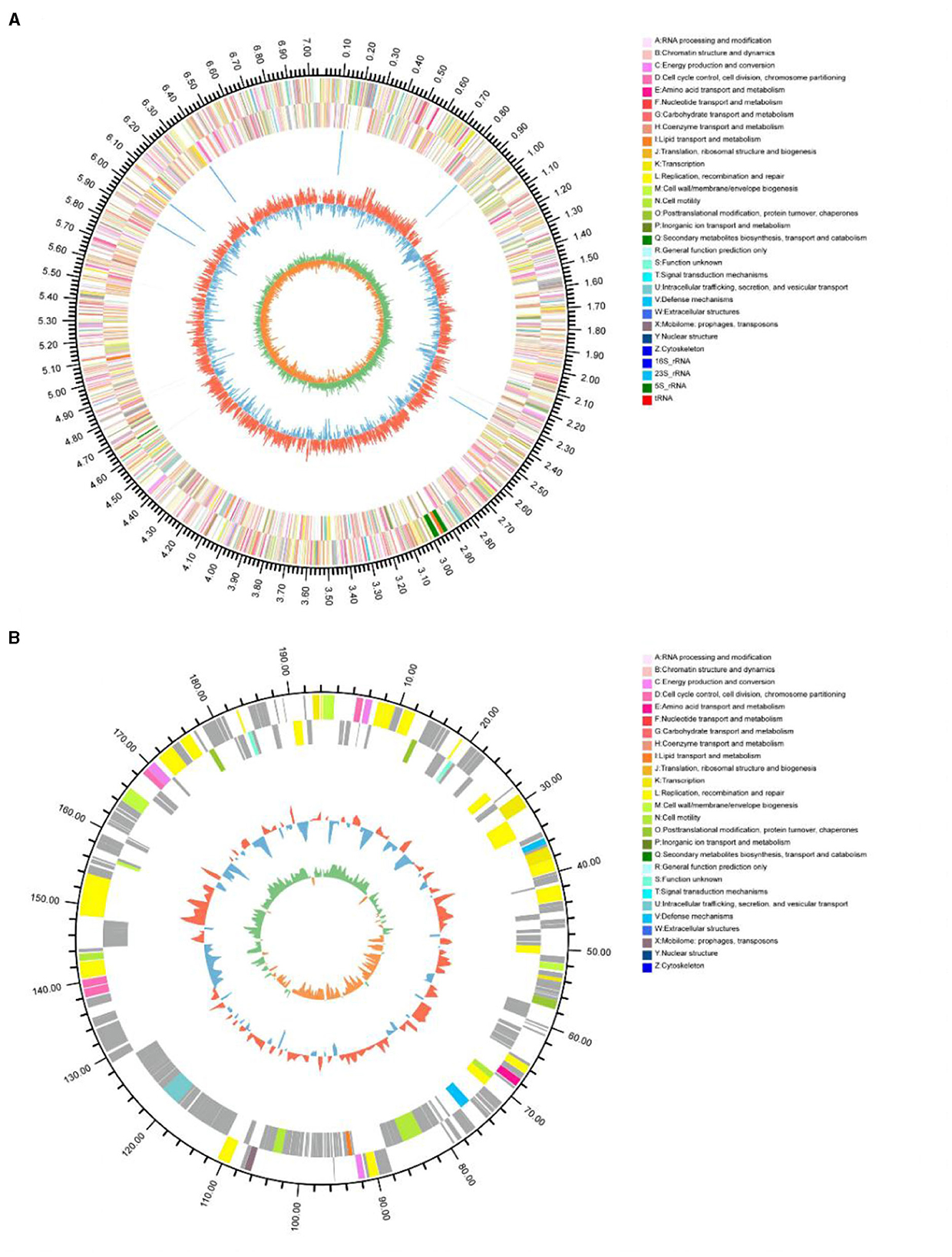
Figure 5. Circular representation of the P. GXZC genome structure. (A) Circular representation of chromosome; (B) Circular representation of plasmid. The outermost circle of the diagram is the genome size identification; the second and third circles are CDS on positive and negative strands, and different colors indicate the functional classification of different COGs of CDS; the fourth circle is rRNA, tRNA; the fifth circle is GC content, the outer red part indicates that the GC content of the region is higher than the (average GC content of the whole genome, and the higher the peak indicates the larger the difference with the average GC content The innermost circle is the GC-Skew value, the specific algorithm is G-C/G+C.
Species determination of P. GXZC based on whole-genome sequencing
P. GXZC strain does not belong to any species in the Type (Strain) Genome Server (TYGS) database and is potentially a new species in P. fluorescens group. The closest type strain genomes are Pseudomonas canadensis Feb-92 strain (assembly accession: GCF_026967615.1) and Pseudomonas simiae CCUG 50988 (assembly accession: GCF_900111895.1) (Figure 6).
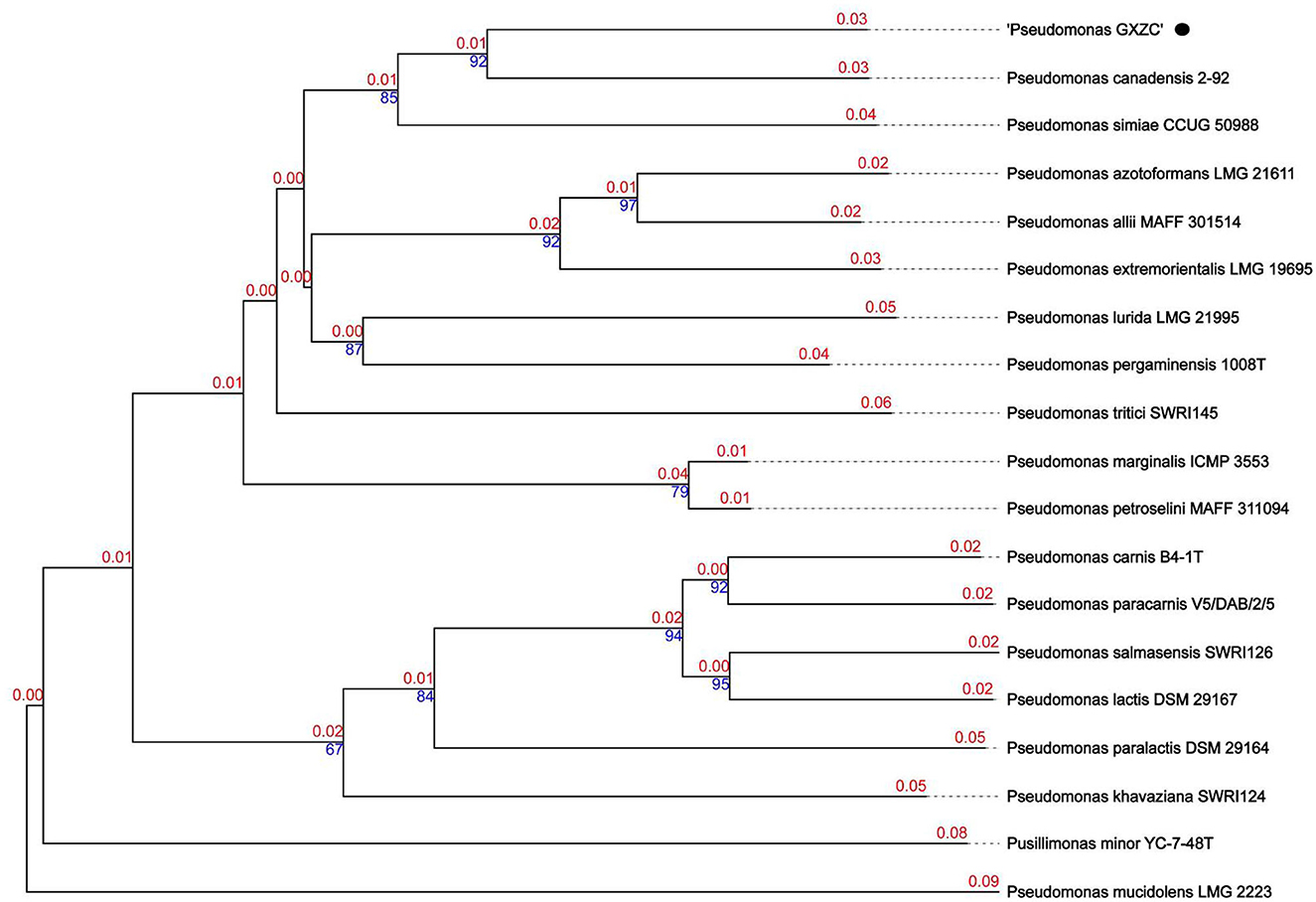
Figure 6. Phylogenetic relationships of P. GXZC whole genome was conducted in Type (Strain) Genome Server (TYGS) (date: 02 March 2023).
The best-matching type strain is P. canadensis Feb-92 (assembly accession: GCF_026967615.1, type strain), with an average nucleotide identity based on BLAST+ (ANIb) data of 92.4% using the JSpeciesWS (Supplementary Table S5). We selected the four best-matching type strains with ANIb > 90%, namely, P. canadensis Feb-92 (assembly accession: GCF_000503215.1), P. canadensis PA-6-2A (assembly accession: GCF_021605905.1), P. simiae CCUG 50988 (assembly accession: GCF_900111895.1), and P. simiae PCL1751 (assembly accession: GCF_000934565.1), to conduct a comparative analysis with P. GXZC.
Comparative analysis of antibiotic resistance genes (ARGs)
Based on the phylogenetic tree and ANIb, the four Pseudomonas strains conducted a comparative analysis with P. GXZC. ARGs of all strains were predicted and categorized by drug classes using online RGI 6.0.1. All strains were predicted diverse and large drug-resistant genes (Table 3). However, the GXZC strain has more ARG hits than other comparison strains with the following drug classes: aminoglycoside antibiotic (with perfect or strict criteria only), tetracycline antibiotic, fluoroquinolone antibiotic, macrolide antibiotic, penam, cephalosporin, disinfecting agents and antiseptics, sulfonamide antibiotic, nitroimidazole antibiotic, and lincosamide antibiotic. Except for penam, all drug classes are widely used in pig farms.
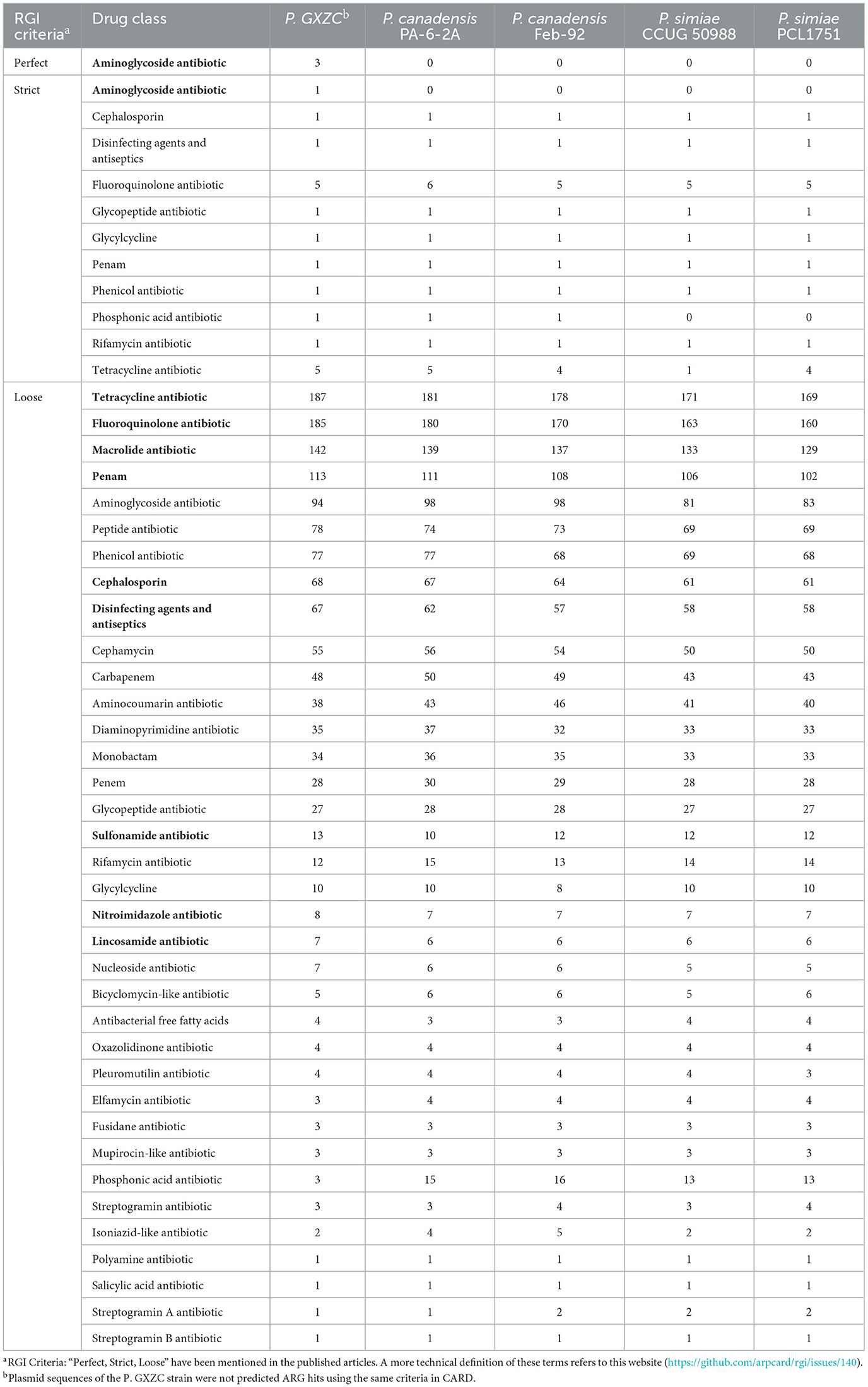
Table 3. Summary of predicted ARGs categorized by drug classes using Resistance Gene Identifier (RGI).
Antimicrobial resistance island of P. GXZC
The study identified nine acquired AMR genes conferring resistance to three drug classes of antibiotics in the chromosome of the GXZC strain using ResFinder 4.1 (Table 4). Four other close-type strains were unidentified. P. GXZC strain accepted nine acquired AMR genes conferring resistance to aminoglycoside antibiotics. Aminoglycosides are the most commonly used antibacterial additives in extended boar semen.
Using IslandViewer 4 with the IslandPath-DIMOB method (Bertelli et al., 2017), we identified 15 gene islands (GI) in the chromosome. One acquired AMR is located in one GI. The other eight acquired AMR genes were clustered in another 81.6-kb GI. This GI contains three types of mobile genetic elements, two integrons, three IS elements, and two transposons (Figure 7). This antimicrobial resistance island structure represents the potential for rapidly acquiring resistance markers under antimicrobial pressure. Alignment with MAUVE (version 1.1.3) revealed that similar GI structures are absent at the homologous location of the other two best-matching type strains (Figure 7).
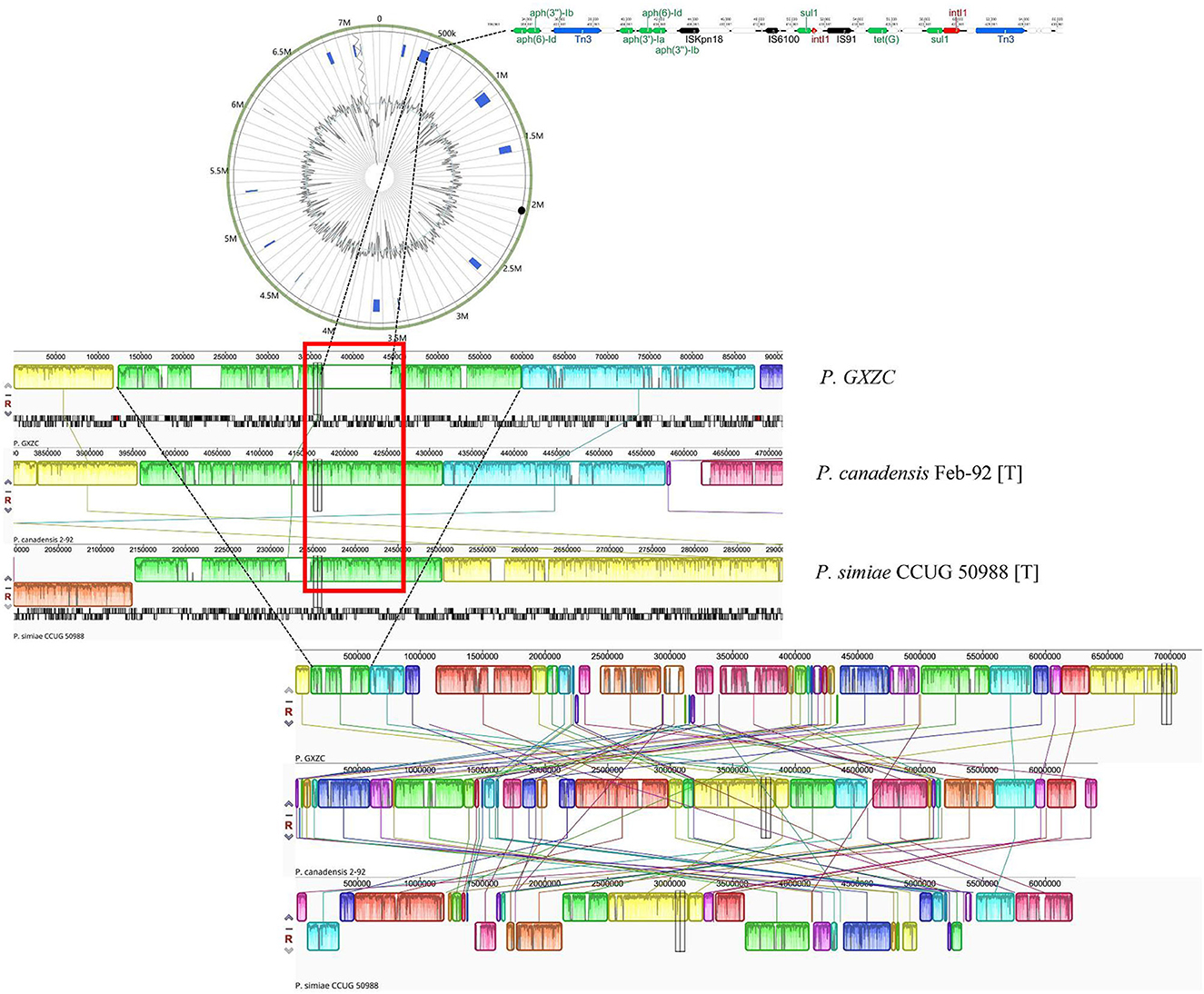
Figure 7. Antimicrobial resistance island of P. GXZC strain. Whole-genome alignment of P. GXZC, with two types of strains, P. canadensis Feb-92 and P. simiae CCUG 50988, is conducted using MAUVE. At the homologous site (in the red box), P. GXZC strain has an approximately 81kb insertion which is identified as an antimicrobial resistance island.
Discussion
Our study reviewed previous experiments on the assessment of semen quality during long-term preservation (Huo et al., 2002; Dube et al., 2004; Bussalleu et al., 2017; Shaoyong et al., 2019; Li et al., 2022), and we found that sperm motility analysis is the most widely used and effective method. In commercial extended semen production, TSM analysis performed by CASA is essential for quality control (Amann and Waberski, 2014). To simplify the evaluation of our experiments and facilitate statistical analysis, TSM analysis was exclusively used in our research.
Although various factors contribute to semen deterioration in a commercial extender containing antibiotics during extended storage, microbial contamination is a significant risk factor. High-throughput sequencing revealed dynamic bacterial proliferation during extended semen storage. Pseudomonas spp. was observed to gradually become the dominant flora in 12-day long-term semen storage. The result appears to be relative to an environment of 17°C and antibiotic supplements.
Pseudomonas is one of the most complex bacterial genus and comprises the largest number of gram-negative bacteria species (Gomila et al., 2015). Many isolations initially identified as “P. fluorescens species” are now reclassified as “P. fluorescens species complex” (Scales et al., 2014) or P. fluorescens intrageneric groups based on MLST (Mulet et al., 2010). MLST is a rapid genotyping method, but it has low resolution and is insufficient for species typing for P. fluorescens group to perform comparative genomic analysis. With the phylogenetic tree and ANIb database on WGS (Richter et al., 2016), P. GXZC strain was identified as a potential new species in Pseudomonas fluorescens group eventually. WGS is a gold standard method to identify Pseudomonas species (Tohya et al., 2022).
Most members of the P. fluorescens species complex (from the environment) optimum growth temperature are <30 °C, and their growth decreases as temperature rises above 30°C (Buchon et al., 2000; Zhang et al., 2019). This species complex is the most frequently reported “psychrotrophic bacteria” in cold-stored raw fluid milk and deteriorates stored milk (Craven and Macauley, 1993; Shah, 1994; Wiedmann et al., 2000; Dogan and Boor, 2003; Gunasekera et al., 2003; Du et al., 2022). It was the primary isolated strain among all contaminants in some studies (Dogan and Boor, 2003; de Oliveira et al., 2015; Du et al., 2022). The storage environment of the extended boar semen appears to be similar to that of raw fluid milk. In our study, P. GXZC actively proliferated at 17°C and 27°C but did not proliferate at 37°C and entered a viable-but-not-culturable (VBNC) state (Bunker et al., 2004). This may be one of the main reasons for P. GXZC to be the dominant flora in the 12-day storage of extended semen experiment.
We first confirmed that the isolated strain of P. fluorescens had a negative effect on sperm vitality when the bacteria proliferated to a certain concentration (> 106 CFU/ml). The negative effect of P. fluorescens on sperm motility is concentration-dependent. The characteristic may be related to sperm damage caused by bacterial outer membrane vesicles (OMVs) or lipopolysaccharide (LPS) (He et al., 2017; Gao et al., 2018) and the reduction in sperm protein phosphorylation levels (Sepulveda et al., 2016).
Pasteurization (70–80°C) can treat refrigerated raw milk, but for extended semen, antibiotics are one of the few options to inhibit bacterial proliferation in extended semen. However, antimicrobial susceptibility testing demonstrated that P. GXZC developed a high level of resistance to almost all commonly used veterinary antibiotics, including colistin, a the last resort for combating multidrug-resistant Gram-negative bacteria (MDR-GNB) (Singhal et al., 2022).
As a member of the species complex, P. GXZC strain has 6,790 predicted CDSs and diverse mechanisms of drug resistance. Numerous resistant phenotypes in this complex were related to intrinsic, adaptive, and acquired antimicrobial resistance mechanisms (Silverio et al., 2022). Whole-genome sequencing has revealed that the P. GXZC strain has more drug-resistant genes than closely related strains (identified based on WGS), which may be related to antibiotic use in extended boar semen or treating boars. Although previous studies have reported that transferable resistance mechanisms are rare in this complex, we confirmed horizontal gene transfer (HGT) of antimicrobial resistance in P. GXZC (Silverio et al., 2022). The mechanism of antibiotic resistance includes reduced permeability to antibiotic, antibiotic efflux, antibiotic inactivation, and antibiotic target alteration (Chopra and Roberts, 2001; Zeng and Jin, 2003; Xiao and Hu, 2012; Ashenafi et al., 2014; Chung et al., 2015; Kapoor et al., 2017). The resistance island with eight clustered resistance genes and various mobile genetic elements may indicate that these strains acquire resistance genes more rapidly than previously assumed. Our results suggest that adding antibiotics seems difficult to resolve the multidrug-resistant P. fluorescens strains and is an unsustainable approach to microbial control in extended semen.
P. fluorescens species complex are ubiquitous microorganisms in the environment (Scales et al., 2014). Although several previous studies have identified the presence of P. fluorescens in boar semen, the risks it poses in boar semen storage have not been taken seriously. This may be attributed to the past practice of storing extended semen for a short period, during which bacteria did not have sufficient time to proliferate and cause significant damage to sperm. However, with the growing adoption of commercial long-term extended semen which semen storage time shift from shorter storage durations to longer periods, the adverse effects of bacterial proliferation on extended semen storage should be given more attention. The biological characteristics exhibited by P. GXZC, as explored in this study, may pose a challenge for long-term semen storage. Several studies have attempted boar semen storage at 5°C in the absence of antibiotics (Paschoal et al., 2020; Jakel et al., 2021), which seem to similarly consider the impact of these cold-adapted proliferating or psychrotrophic bacteria.
Conclusion
Our study identified a potential new species GXZC strain in P. fluorescens group as the dominant flora in extended boar semen. This strain exhibited adverse effects on sperm quality. The strain can better adapt to growth at a moderately low temperature (17°C) and has multidrug resistance. Whole-genome sequencing and comparative genomic analysis revealed that the strain developed devise intrinsic resistance and horizontal resistance gene transfer. The unregulated proliferation of multidrug-resistant P. fluorescens within commercial extenders presents potential risks to the long-term preservation of extended boar semen at 17°C and can impact the success of artificial insemination (AI) procedures. In AI facilities, heightened attention should be given to the prevalence of this type of bacterial contamination, and more effective antimicrobial and hygienic management strategies should be developed to control it.
Data availability statement
The datasets presented in this study can be found in online repositories. The names of the repository/repositories and accession number(s) can be found in the article/Supplementary material.
Ethics statement
The animal study was approved by Laboratory Animal Monitoring Committee of Huazhong Agricultural University. The study was conducted in accordance with the local legislation and institutional requirements.
Author contributions
ZX: Conceptualization, Data curation, Investigation, Methodology, Writing—original draft, Writing—review and editing. ZH: Writing—review and editing, Data curation. XinL: Writing—review and editing. DG: Writing—review and editing. LW: Writing—review and editing. SL: Data curation, Investigation, Writing—review and editing. JZ: Data curation, Investigation, Writing—review and editing. XiaL: Funding acquisition, Supervision, Writing—review and editing. PQ: Conceptualization, Writing—review and editing.
Funding
This study was supported by grants from the Yingzi Tech and Huazhong Agricultural University Intelligent Research Institute of Food Health [Nos. IRIFH202302 and IRIFH202209] and the Fundamental Research Funds for the Central Universities [2662016PY004].
Conflict of interest
The authors declare that the research was caonducted in the absence of any commercial or financial relationships that could be construed as a potential conflict of interest.
Publisher's note
All claims expressed in this article are solely those of the authors and do not necessarily represent those of their affiliated organizations, or those of the publisher, the editors and the reviewers. Any product that may be evaluated in this article, or claim that may be made by its manufacturer, is not guaranteed or endorsed by the publisher.
Supplementary material
The Supplementary Material for this article can be found online at: https://www.frontiersin.org/articles/10.3389/fmicb.2023.1279630/full#supplementary-material
References
Ait Tayeb, L., Ageron, E., Grimont, F., and Grimont, P. A. D. (2005). Molecular phylogeny of the genus pseudomonas based on rpob sequences and application for the identification of isolates. Res. Microbiol. 156, 763–773 doi: 10.1016/j.resmic.2005.02.009
Alcock, B. P., Huynh, W., Chalil, R., Smith, K. W., Raphenya, A. R., Wlodarski, M. A., et al. (2022). Card 2023: expanded curation, support for machine learning, and resistome prediction at the comprehensive antibiotic resistance database. Nucleic. Acids. Res. 51, D690–D699. doi: 10.1093/nar/gkac920
Althouse, G., and Lu, K. (2005). Bacteriospermia in extended porcine semen. Theriogenology 63, 573–584. doi: 10.1016/j.theriogenology.2004.09.031
Althouse, G. C., Kuster, C. E., Clark, S. G., and Weisiger, R. M. (2000). Field investigations of bacterial contaminants and their effects on extended porcine semen. Theriogenology 53, 1167–1176. doi: 10.1016/S0093-691X(00)00261-2
Althouse, G. C., Pierdon, M. S., and Lu, K. G. (2008). Thermotemporal dynamics of contaminant bacteria and antimicrobials in extended porcine semen. Theriogenology 70, 1317–1323. doi: 10.1016/j.theriogenology.2008.07.010
Amann, R. P., and Waberski, D. (2014). Computer-assisted sperm analysis (casa): capabilities and potential developments. Theriogenology 81, 5–17. doi: 10.1016/j.theriogenology.2013.09.004
Ashenafi, M., Ammosova, T., Nekhai, S., and Byrnes, W. M. (2014). Purification and characterization of aminoglycoside phosphotransferase aph(6)-id, a streptomycin-inactivating enzyme. Mol. Cell. Biochem. 387, 207–216. doi: 10.1007/s11010-013-1886-1
Auroux, M., Jacques, L., Mathieu, D., and Auer, J. (1991). Is the sperm bacterial ratio a determining factor in impairment of sperm motility—an in vitro study in man with escherichia-coli. Int. J. Androl. 14, 264–270. doi: 10.1111/j.1365-2605.1991.tb01091.x
Bertelli, C., Laird, M. R., Williams, K. P., Lau, B. Y., Hoad, G., Winsor, G. L., et al. (2017). Islandviewer 4: expanded prediction of genomic islands for larger-scale datasets. Nucleic Acids Res. 45, W30–W35. doi: 10.1093/nar/gkx343
Besemer, J., Lomsadze, A., and Borodovsky, M. (2001). Genemarks: a self-training method for prediction of gene starts in microbial genomes. Implications for finding sequence motifs in regulatory regions. Nucleic. Acids. Res. 29, 2607–2618. doi: 10.1093/nar/29.12.2607
Bryla, M., and Trzcinska, M. (2015). Quality and fertilizing capacity of boar spermatozoa during liquid storage in extender supplemented with different antibiotics. Anim. Reprod. Sci. 163, 157–163. doi: 10.1016/j.anireprosci.2015.11.005
Buchon, L., Laurent, P., Gounot, A., and Guespin-Michel, J. (2000). Temperature dependence of extracellular enzymes production by psychrotrophic and psychrophilic bacteria. Biotechnol. Lett. 22, 1577–1581. doi: 10.1023/A:1005641119076
Bunker, S. T., Bates, T. C., and Oliver, J. D. (2004). Effects of temperature on detection of plasmid or chromosomally encoded gfp- and lux-labeled pseudomonas fluorescens in soil. Environ. Biosafety Res. 3, 83–90. doi: 10.1051/ebr:2004008
Bussalleu, E., Sancho, S., Briz, M. D., Yeste, M., and Bonet, S. (2017). Do antimicrobial peptides pr-39, pmap-36 and pmap-37 have any effect on bacterial growth and quality of liquid-stored boar semen? Theriogenology 89, 235–243. doi: 10.1016/j.theriogenology.2016.11.017
Bussalleu, E., Yeste, M., Sepúlveda, L., Torner, E., Pinart, E., and Bonet, S. (2011). Effects of different concentrations of enterotoxigenic and verotoxigenic e. Coli on boar sperm quality. Anim. Reprod. Sci. 127, 176–182. doi: 10.1016/j.anireprosci.2011.07.018
Caporaso, J. G., Kuczynski, J., Stombaugh, J., Bittinger, K., Bushman, F. D., Costello, E. K., et al. (2010). Qiime allows analysis of high-throughput community sequencing data. Nat. Methods. 7, 335–336. doi: 10.1038/nmeth.f.303
Chan, P. P., and Lowe, T. M. (2019). Trnascan-se: searching for trna genes in genomic sequences. Meth. Mol. Biol. (Clifton, N.J.). 1962, 1–14. doi: 10.1007/978-1-4939-9173-0_1
Chen, C., Song, X., Wei, W., Zhong, H., Dai, J., Lan, Z., et al. (2017). The microbiota continuum along the female reproductive tract and its relation to uterine-related diseases. Nat. Commun. 8, 875. doi: 10.1038/s41467-017-00901-0
Chin, C., Alexander, D. H., Marks, P., Klammer, A. A., Drake, J., Heiner, C., et al. (2013). Nonhybrid, finished microbial genome assemblies from long-read smrt sequencing data. Nat. Methods. 10, 563. doi: 10.1038/nmeth.2474
Chopra, I., and Roberts, M. (2001). Tetracycline antibiotics: mode of action, applications, molecular biology, and epidemiology of bacterial resistance. Microbiol. Mol. Biol. Rev. Mmbr. 65, 232–260. doi: 10.1128/MMBR.65.2.232-260.2001
Chung, H., Kim, K., Hong, S. S., Hong, S. G., Lee, K., and Chong, Y. (2015). The sul1 gene in stenotrophomonas maltophilia with high-level resistance to trimethoprim/sulfamethoxazole. Ann. Lab. Med. 35, 246–249. doi: 10.3343/alm.2015.35.2.246
CLSI (2018). Performance Standards for Antimicrobial Disk and Dilution Susceptibility Tests for Bacteria Isolated from Animals [(5th ed.), CLSI VET01ed., Vol.] Wayne, PA: Clinical and Laboratory Standards Institute.
CLSI (2022). Performance Standards for antimicrobial susceptibility testing ((30th ed.), CLSI supplement M100ed., Vol.). Wayne, PA, USA: Clinical and Laboratory Standards Institute. (Reprinted.
Craven, H. M., and Macauley, B. J. (1993). Microorganisms in pasteurised milk after refrigerated storage. I. Identification of types. Aust. J. Dairy Technol. 47, 38–45
CSAMR (2019). National food safety standard maximum residue limits of veterinary drugs in foods (GB 31650-2019 ed., Vol.). Beijing, China: China State Administration For Marketregulation.
de Oliveira, G. B., Favarin, L., Luchese, R. H., and McIntosh, D. (2015). Psychrotrophic bacteria in milk: how much do we really know? Braz. J. Microbiol. 46, 313–321. doi: 10.1590/S1517-838246220130963
Delcher, A. L., Bratke, K. A., Powers, E. C., and Salzberg, S. L. (2007). Identifying bacterial genes and endosymbiont dna with glimmer. Bioinformatics 23, 673–679. doi: 10.1093/bioinformatics/btm009
Delgado-Bermudez, A., Bonet, S., Yeste, M., and Pinart, E. (2020). Long-term storage of boar seminal doses contaminated with proteus vulgaris: a dose-dependent effect on sperm motility and sperm-bacteria interaction. Anim. Reprod. Sci. 216, 6349. doi: 10.1016/j.anireprosci.2020.106349
Diemer, T., Weidner, W., Michelmann, H., Schiefer, H., Rovan, E., and Mayer, F. (1996). Influence of escherichia coli on motility parameters of human spermatozoa in vitro. Int. J. Androl. 19, 271–277. doi: 10.1111/j.1365-2605.1996.tb00475.x
Dogan, B., and Boor, K. (2003). Genetic diversity and spoilage potentials among pseudomonas spp. Isolated from fluid milk products and dairy processing plants. Appl. Environ. Microbiol. 69, 130–138. doi: 10.1128/AEM.69.1.130-138.2003
Du, B., Meng, L., Liu, H., Zheng, N., Zhang, Y., Zhao, S., et al. (2022). Diversity and proteolytic activity of pseudomonas species isolated from raw cow milk samples across china. Sci. Total Environ. 838. doi: 10.1016/j.scitotenv.2022.156382
Dube, C., Beaulieu, M., Reyes-Moreno, C., Guillemette, C., and Bailey, J. (2004). Boar sperm storage capacity of bts and androhep plus: viability, motility, capacitation, and tyrosine phosphorylation. Theriogenology 62, 874–886. doi: 10.1016/j.theriogenology.2003.12.006
Edgar, R. C. (2010). Search and clustering orders of magnitude faster than blast. Bioinformatics 26, 2460–2461. doi: 10.1093/bioinformatics/btq461
Edgar, R. C. (2013). Uparse: highly accurate otu sequences from microbial amplicon reads. Nat. Methods. 10, 996. doi: 10.1038/nmeth.2604
Edgar, R. C., Haas, B. J., Clemente, J. C., Quince, C., and Knight, R. (2011). Uchime improves sensitivity and speed of chimera detection. Bioinformatics 27, 2194–2200. doi: 10.1093/bioinformatics/btr381
EUCAST (2022). Breakpoints tables for interpretation of mics and zone diameter (Version 12.0ed., Vol.). Sweden: European Committee on Antimicrobial Susceptibility Testing.
Gao, H., Gao, Y., Yang, C., Dong, D., Yang, J., Peng, G., et al. (2018). Influence of outer membrane vesicles of proteus mirabilis isolated from boar semen on sperm function. Vet. Microbiol. 224, 34–42. doi: 10.1016/j.vetmic.2018.08.017
Godia, M., Ramayo-Caldas, Y., Zingaretti, L. M., Darwich, L., Lopez, S., Rodriguez-Gil, J. E., et al. (2020). A pilot rna-seq study in 40 pietrain ejaculates to characterize the porcine sperm microbiome. Theriogenology 157, 525–533. doi: 10.1016/j.theriogenology.2020.08.001
Gomila, M., Pena, A., Mulet, M., Lalucat, J., and Garcia-Valdes, E. (2015). Phylogenomics and systematics in pseudomonas. Front. Microbiol. 6, 214. doi: 10.3389/fmicb.2015.00214
Gomila, M., Prince-Manzano, C., Svensson-Stadler, L., Busquets, A., Erhard, M., Martinez, D. L., et al. (2014). Genotypic and phenotypic applications for the differentiation and species-level identification of achromobacter for clinical diagnoses. PLoS ONE. 9, doi: 10.1371/journal.pone.0114356
Gunasekera, T., Dorsch, M., Slade, M., and Veal, D. (2003). Specific detection of pseudomonas spp. In milk by fluorescence in situ hybridization using ribosomal rna directed probes. J. Appl. Microbiol. 94, 936–945. doi: 10.1046/j.1365-2672.2003.01930.x
He, B., Guo, H., Gong, Y., and Zhao, R. (2017). Lipopolysaccharide-induced mitochondrial dysfunction in boar sperm is mediated by activation of oxidative phosphorylation. Theriogenology 87, 1–8. doi: 10.1016/j.theriogenology.2016.07.030
Hu, J., Ma, L., Nie, Y., Chen, J., Zheng, W., Wang, X., et al. (2018). A microbiota-derived bacteriocin targets the host to confer diarrhea resistance in early-weaned piglets. Cell Host Microbe. 24, 817–832. doi: 10.1016/j.chom.2018.11.006
Huo, L., Ma, X., and Yang, Z. (2002). Assessment of sperm viability, mitochondrial activity, capacitation and acrosome intactness in extended boar semen during long-term storage. Theriogenology 58, 1349–1360. doi: 10.1016/S0093-691X(02)00953-6
Jakel, H., Scheinpflug, K., Muhldorfer, K., Gianluppi, R., Lucca, M. S., Mellagi, A., et al. (2021). In vitro performance and in vivo fertility of antibiotic-free preserved boar semen stored at 5 degrees c. J. Anim. Sci. Biotechnol. 12, 9. doi: 10.1186/s40104-020-00530-6
Johnson, L., Weitze, K., Fiser, P., and Maxwell, W. (2000). Storage of boar semen. Anim. Reprod. Sci. 64, 133–134. doi: 10.1016/S0378-4320(00)00200-1
Kapoor, G., Saigal, S., and Elongavan, A. (2017). Action and resistance mechanisms of antibiotics: a guide for clinicians. J. Anaesthesiol. Clin. Pharmacol. 33, 300–305. doi: 10.4103/joacp.JOACP_349_15
Karageorgiou, M. A., Tsousis, G., Boscos, C. M., Tzika, E. D., Tassis, P. D., and Tsakmakidis, I. A. (2016). A comparative study of boar semen extenders with different proposed preservation times and their effect on semen quality and fertility. Acta Vet. Brno. 85, 23–31. doi: 10.2754/avb201685010023
Knox, R. V. (2016). Artificial insemination in pigs today. Theriogenology 85, 83–93. doi: 10.1016/j.theriogenology.2015.07.009
Koren, S., Walenz, B. P., Berlin, K., Miller, J. R., Bergman, N. H., and Phillippy, A. M. (2017). Canu: scalable and accurate long-read assembly via adaptive k-mer weighting and repeat separation. Genome Res. 27, 722–736. doi: 10.1101/gr.215087.116
Li, D., Zhang, W., Tian, X., He, Y., Xiao, Z., Zhao, X., et al. (2022). Hydroxytyrosol effectively improves the quality of pig sperm at 17 degrees c. Theriogenology 177, 172–182. doi: 10.1016/j.theriogenology.2021.10.018
Li, Y., Fu, J., Li, P., Yang, Q., Wang, L., Xie, W., et al. (2017). Effects of staphylococcus aureus on motility and protein phosphorylation modification of boar sperm during storage in vitro. Acta Veterinaria Et Zootechnica Sinica. 48, 1665–1673
Lucca, M. S., Gianluppi, R. D. F., Goncalves Mellagi, A. P., Bortolozzo, F. P., Wentz, I., and Ulguim, R. D. R. (2021). Effects of the classification of boars according to progressive sperm motility and the extender type on the reproductive performance of a single fixed-time insemination. Theriogenology 161, 120–125. doi: 10.1016/j.theriogenology.2020.11.018
Luis Ubeda, J., Ausejo, R., Dahmani, Y., Falceto, M. V., Usan, A., Malo, C., et al. (2013). Adverse effects of members of the enterobacteriaceae family on boar sperm quality. Theriogenology 80, 565–570. doi: 10.1016/j.theriogenology.2013.05.022
Magoc, T., and Salzberg, S. L. (2011). Flash: fast length adjustment of short reads to improve genome assemblies. Bioinformatics 27, 2957–2963. doi: 10.1093/bioinformatics/btr507
Mazurova, J., and Vinter, P. (1991). The effects of some antibiotics on microorganisms contaminating boar ejaculates. Vet. Med. 36, 213–223
Meier-Kolthoff, J. P., and Goeker, M. (2019). Tygs is an automated high-throughput platform for state-of-the-art genome-based taxonomy. Nat. Commun. 10, 3. doi: 10.1038/s41467-019-10210-3
Mulet, M., Bennasar, A., Lalucat, J., and García-Valdés, E. (2009). An rpod-based pcr procedure for the identification of pseudomonas species and for their detection in environmental samples. Mol. Cell. Probes. 23, 140–147. doi: 10.1016/j.mcp.2009.02.001
Mulet, M., Lalucat, J., and García-Valdés, E. (2010). Dna sequence-based analysis of the pseudomonas species. Environ. Microbiol. 12, 1513–1530. doi: 10.1111/j.1462-2920.2010.02181.x
Paschoal, A. F. L., Luther, A., Jaekel, H., Scheinpflug, K., Muehldorfer, K., Bortolozzo, F. P., et al. (2020). Determination of a cooling-rate frame for antibiotic-free preservation of boar semen at 5 degrees c. PLoS ONE. 15, e0234339. doi: 10.1371/journal.pone.0234339
Pezo, F., Romero, F., Zambrano, F., and Segundo Sanchez, R. (2019). Preservation of boar semen: an update. Reprod. Domest. Anim. 54, 423–434. doi: 10.1111/rda.13389
Pinart, E., Domenech, E., Bussalleu, E., Yeste, M., and Bonet, S. (2017). A comparative study of the effects of escherichia coli and clostridium perfringens upon boar semen preserved in liquid storage. Anim. Reprod. Sci. 177, 65–78. doi: 10.1016/j.anireprosci.2016.12.007
Prieto-Martinez, N., Bussalleu, E., Garcia-Bonavila, E., Bonet, S., and Yeste, M. (2014). Effects of enterobacter cloacae on boar sperm quality during liquid storage at 17 degrees c. Anim. Reprod. Sci. 148, 72–82. doi: 10.1016/j.anireprosci.2014.05.008
Pursel, V., and Johnson, L. (1975). Freezing of boar spermatozoa - fertilizing-capacity with concentrated semen and a new thawing procedure. J. Anim. Sci. 40, 99–102. doi: 10.2527/jas1975.40199x
Richter, M., Rossello-Mora, R., Gloeckner, F. O., and Peplies, J. (2016). Jspeciesws: a web server for prokaryotic species circumscription based on pairwise genome comparison. Bioinformatics 32, 929–931. doi: 10.1093/bioinformatics/btv681
Santos, C. S., and Silva, A. R. (2020). Current and alternative trends in antibacterial agents used in mammalian semen technology. Anim. Reprod. 17, 111. doi: 10.21451/1984-3143-AR2019-0111
Scales, B. S., Dickson, R. P., LiPuma, J. J., and Huffnagle, G. B. (2014). Microbiology, genomics, and clinical significance of the pseudomonas fluorescens species complex, an unappreciated colonizer of humans. Clin. Microbiol. Rev. 27, 927–948. doi: 10.1128/CMR.00044-14
Sepulveda, L., Bussalleu, E., Yeste, M., and Bonet, S. (2014). Effects of different concentrations of pseudomonas aeruginosa on boar sperm quality. Anim. Reprod. Sci. 150, 96–106. doi: 10.1016/j.anireprosci.2014.09.001
Sepulveda, L., Bussalleu, E., Yeste, M., and Bonet, S. (2016). Effect of pseudomonas aeruginosa on sperm capacitation and protein phosphorylation of boar spermatozoa. Theriogenology 85, 1421–1431. doi: 10.1016/j.theriogenology.2015.12.025
Sepulveda, L., Bussalleu, E., Yeste, M., Torner, E., and Bonet, S. (2013). How do different concentrations of clostridium perfringens affect the quality of extended boar spermatozoa? Anim. Reprod. Sci. 140, 83–91. doi: 10.1016/j.anireprosci.2013.04.013
Shaoyong, W., Li, Q., Ren, Z., Xiao, J., Diao, Z., Yang, G., et al. (2019). Effects of kojic acid on boar sperm quality and anti-bacterial activity during liquid preservation at 17 c. Theriogenology 140, 124–135. doi: 10.1016/j.theriogenology.2019.08.020
Silverio, M. P., Kraychete, G. B., Rosado, A. S., and Bonelli, R. R. (2022). Pseudomonas fluorescens complex and its intrinsic, adaptive, and acquired antimicrobial resistance mechanisms in pristine and human-impacted sites. Antibiotics-Basel. 11, 985. doi: 10.3390/antibiotics11080985
Singhal, L., Singh, C., and Gautam, V. (2022). “7.06 – colistin,” in ed. T. Kenakin. Cambridge, MA: Elsevier. 123–135.
Tohya, M., Teramoto, K., Watanabe, S., Hishinuma, T., Shimojima, M., Ogawa, M., et al. (2022). Whole-genome sequencing-based re-identification of pseudomonas putida/fluorescens clinical isolates identified by biochemical bacterial identification systems. Microbiol. Spectr. 10, 21. doi: 10.1128/spectrum.02491-21
Union, E. (1992). Council directive 92/65/eec laying down animal health requirements governing trade in and imports into the community of animals, semen, ova and embryos not subject to animal health requirements laid down in specific community rules referred to in annex a (i) to directive 90/425/eec. Bruxels: Official Journal of the European Communities, L254–L268.
Waberski, D., Luther, A., Gruenther, B., Jaekel, H., Henning, H., Vogel, C., et al. (2019). Sperm function in vitro and fertility after antibiotic-free, hypothermic storage of liquid preserved boar semen. Sci. Rep. 9. doi: 10.1038/s41598-019-51319-1
Wiedmann, M., Weilmeier, D., Dineen, S., Ralyea, R., and Boor, K. (2000). Molecular and phenotypic characterization of pseudomonas spp. isolated from milk. Appl. Environ. Microbiol. 66, 2085–2095. doi: 10.1128/AEM.66.5.2085-2095.2000
Xiao, Y., and Hu, Y. (2012). The major aminoglycoside-modifying enzyme aac(3)-ii found in escherichia coli determines a significant disparity in its resistance to gentamicin and amikacin in china. Microbial. Drug Res. (Larchmont, N.Y.). 18, 42–46. doi: 10.1089/mdr.2010.0190
Zeng, L., and Jin, S. (2003). Aph(3′)-iib, a gene encoding an aminoglycoside-modifying enzyme, is under the positive control of surrogate regulator hpaa. Antimicrob. Agents Chemother. 47, 3867–3876 doi: 10.1128/AAC.47.12.3867-3876.2003
Zhang, C., Bijl, E., Svensson, B., and Hettinga, K. (2019). The extracellular protease aprx from pseudomonas and its spoilage potential for uht milk: a review. Compr. Rev. Food. Sci. Food Saf. 18, 834–852. doi: 10.1111/1541-4337.12452
Keywords: boar semen preservation, Pseudomonas fluorescens, microbial resistance, whole-genome sequencing, antibiotic resistance island
Citation: Xiong Z, Hong Z, Li X, Gao D, Wang L, Liu S, Zhao J, Li X and Qian P (2023) The multidrug-resistant Pseudomonas fluorescens strain: a hidden threat in boar semen preservation. Front. Microbiol. 14:1279630. doi: 10.3389/fmicb.2023.1279630
Received: 18 August 2023; Accepted: 18 September 2023;
Published: 06 October 2023.
Edited by:
Vijay Soni, NewYork-Presbyterian, United StatesReviewed by:
Tushar Dhanani, Florida Agricultural and Mechanical University, United StatesBiplab Singha, University of Massachusetts Medical School, United States
Copyright © 2023 Xiong, Hong, Li, Gao, Wang, Liu, Zhao, Li and Qian. This is an open-access article distributed under the terms of the Creative Commons Attribution License (CC BY). The use, distribution or reproduction in other forums is permitted, provided the original author(s) and the copyright owner(s) are credited and that the original publication in this journal is cited, in accordance with accepted academic practice. No use, distribution or reproduction is permitted which does not comply with these terms.
*Correspondence: Ping Qian, cWlhbnBAbWFpbC5oemF1LmVkdS5jbg==