- 1School of Life Sciences, Henan University, Kaifeng, China
- 2Department of Blood Transfusion, Henan Provincial People’s Hospital, Zhengzhou, Henan, China
Background: Emerging evidence reveals that SARS-CoV-2 possesses the capability to disrupt the gastrointestinal (GI) homeostasis, resulting in the long-term symptoms such as loss of appetite, diarrhea, gastroesophageal reflux, and nausea. In the current review, we summarized recent reports regarding the long-term effects of COVID-19 (long COVID) on the gastrointestine.
Objective: To provide a narrative review of abundant clinical evidence regarding the development and management of long-term GI symptoms in COVID-19 patients.
Results: Long-term persistent digestive symptoms are exhibited in a majority of long-COVID patients. SARS-CoV-2 infection of intestinal epithelial cells, cytokine storm, gut dysbiosis, therapeutic drugs, psychological factors and exacerbation of primary underlying diseases lead to long-term GI symptoms in COVID-19 patients. Interventions like probiotics, prebiotics, fecal microbiota transplantation, and antibiotics are proved to be beneficial in preserving intestinal microecological homeostasis and alleviating GI symptoms.
Conclusion: Timely diagnosis and treatment of GI symptoms in long-COVID patients hold great significance as they may contribute to the mitigation of severe conditions and ultimately lead to the improvement of outcomes of the patients.
1 Introduction
Coronavirus disease 2019 (COVID-19) caused by severe acute respiratory syndrome coronavirus 2 (SARS-CoV-2), is primarily recognized as an acute respiratory infectious disease (Zhu et al., 2020). SARS-CoV-2 has unique virological characteristics, associated with the rapid spread of SARS-CoV-2, which brings new challenges for the prevention and control of the long term outcomes of the epidemic (Hakim et al., 2021). Despite it remains challenging to determine exact number of individuals infected with COVID-19 over the last three or more years, evaluating the population affected by long-COVID is relatively easier to assess (Baroni et al., 2023). Long-COVID is defined as post-COVID-19 symptoms or post-acute sequelae of COVID-19 that involve illnesses associated with both direct and indirect impacts of SARS-CoV-2 infection (Levine, 2022). The World Health Organization defines “post-COVID-19 symptoms” as those of individuals with probable or confirmed SARS-CoV-2 infection who continue to have symptoms 3 months after infection, persisting for at least 2 months, and with no other obvious cause. The US Centers for Disease Control and Prevention uses a definition of persistent symptoms or health problems 4 weeks after infection, while the UK uses a 12-week time standard (Mansell et al., 2022; Soriano et al., 2022). In September 2022, leading data assessed that approximately 17 million people in Europe have been suffered from long-COVID and potentially millions may have to endure its effects for several consecutive years (Baroni et al., 2023). Three years after the onset of irresistible COVID-19, we have characterized major of its features, but we are still exploring about the potential prognosis and long-term outcomes of COVID-19 (Wang Z. et al., 2022).
Patients who have afflicted with COVID-19 for a prolonged duration predominantly experience persistent appearance of general influenza, including fever, cough, myalgia, and fatigue along with digestive manifestations such as abdominal pain, vomiting, anorexia, nausea, diarrhea, and elevated transaminase (Ren et al., 2021). Huang et al. (2020) also reported instances of “atypical” COVID-19 patients presenting with digestive symptoms as either their primary or sole manifestation (Chen N. et al., 2020). In addition, it has been reported that patients exhibiting digestive symptoms experience prolonged hospitalization and a less favorable prognosis compared with patients without gastrointestinal (GI) symptoms (Pan et al., 2020). Therefore, it is critical to investigate characteristics and underlying mechanisms of digestive system in long-COVID patients. In this review, we highlighted the prognosis and mechanism of intestinal symptoms with long-COVID more than 3 years since the outbreak onset, providing valuable and comprehensive evidence on the enduring effects of COVID-19. These endeavors aimed at monitoring the long-term outcomes of COVID-19 are critical for better understanding these clinical digestive manifestations and thus alleviating their consequences effectively.
2 Long-term GI effects of long-COVID
The spike protein of SARS-CoV-2 targets and attacks GI cells by specifically binding with the angiotensin-converting enzyme 2 receptor (ACE2) present on the surface of intestinal epithelial cells (Liang et al., 2020). Huang et al. (2020) revealed that 76% of hospitalized patients experienced at least one symptom that continues at least 6 months after infection of SARS-CoV-2, with intestinal manifestations being among those reported (Zhou et al., 2017; Cheung et al., 2020; Ong et al., 2020; Xiao et al., 2020; Joshee et al., 2022). Liang et al. (2020) identified the expression of ACE2 in various tissues showing that the small intestine bears the highest localization of ACE2, rendering IECs are more susceptible to SARS-CoV-2 infection (Carfì et al., 2020). SARS-CoV-2 is detected in feces of nearly half (48.1%) of COVID-19 patients (Cheung et al., 2020). A total of 70.3% of the patients with respiratory samples negative are notably stool samples positive for SARS-CoV-2 (Dhar and Mohanty, 2020; Pan et al., 2020; Xiao et al., 2020). The persistent existence of the SARS-CoV-2 genome in feces suggests that the virus continues to interact with the GI cells and cause prolonged clinical symptoms over time, with diarrhea being the primary manifestation (Zhou et al., 2017; Cheung et al., 2020; Ong et al., 2020; Xiao et al., 2020). Researchers detected the level of fecal SARS-CoV-2 RNA in mild-to-moderate COVID-19 patients 10 months after diagnosis and found that viral RNA shedding in feces was positively correlated with GI adverse conditions in patients with long COVID (Natarajan et al., 2022). A number of additional studies showed a similar result (Zollner et al., 2022; Upadhyay et al., 2023), suggesting there is an important biological significance for the continuous presence of SARS-CoV-2 in feces.
Zonulin (pre-Haptoglobin 2) is the precursor of haptoglobin (Hp)-2, whose uncleaved form detected in human serum is considered as a biomarker of increased gut permeability (Fasano, 2011). It has been reported that SARS-CoV-2 spike stimulates the expression of zonulin, leading to the increase of gut permeability (Llorens et al., 2021). Moreover, it was found that levels of zonulin in patients dying of severe COVID-19 are higher than those in patients recovered from the disease (Giron et al., 2021; Palomino-Kobayashi et al., 2022). These data suggest that zonulin might be associated with poor outcomes in COVID-19.
The majority of acute COVID-19-associated GI manifestations are mild and self-limiting and include anorexia, acute diarrhea, nausea, vomiting and abdominal pain/discomfort (Han et al., 2020; Pan et al., 2020). However, Weng et al. (2021) followed up 117 patients after Covid-19 for 90 days and found that patients with long COVID exhibit loss of appetite (24%), nausea (18%), acid reflux (18%), diarrhea (15%), and abdominal distension (14%) with < 10% of patients reporting belching, vomiting, and bloody stools. GI symptoms in long COVID are partially related to psycho-psychological factors, such as stress, anxiety, and depression, after recovery from COVID-19 (Freedberg and Chang, 2022). GI symptoms may not be evident during or prior of infection, but they can arise after a certain period or even manifest months after initial infection. Patients who have developed severe pneumonia followed by diminished blood oxygen saturation values are at higher risk of developing GI sequelae. This phenomenon could be attributed to the presence of multi-organ dysfunction syndrome that can exhibit in severe COVID-19 infection, particularly under the condition of septic shock (Luo et al., 2020; Wan et al., 2020). Notably, it is reported that GI manifestations during hospitalization for severe COVID-19 can lead to malnutrition, and this has been associated with raised mortality rates in patients (Zhang P. et al., 2021; Afrisham et al., 2023). Moreover, supplemental nutrition plays a pivotal role not only during hospitalization but also in alleviating GI sequelae effectively.
3 Mechanisms of long-term GI injury caused by long-COVID
3.1 SARS-CoV-2 infection of IECs
Intestinal organoid culture plays an important role in researches bridging the cell culture and in vivo animal work for disease models. SARS-CoV-2 is highly infectious in primates (including humans, rhesus monkeys, and crab eating monkeys), but has low transmission in wild-type mice, which greatly limits the research of SARS-CoV-2 in animals. SARS-CoV-2 is mainly thought to infect the lungs. In order to determine that SARS-CoV-2 can infect and replicate in the intestine, Zhou et al. (2020) established intestinal organoids from humans, a “mini-gut” cultured in a dish, and demonstrated that the virus can infect and replicate in intestinal cells of human intestinal organoids (Lamers et al., 2020), supporting the notion that the gut could be another crucial target organ for SARS-CoV-2. Moreover, researchers found that intestinal cells are susceptible to SARS-CoV and SARS-CoV-2 infection using human small intestinal organoids (hSIOs), and demonstrated that the intestinal epithelium supports SARS-CoV-2 replication (Lamers et al., 2020). Han et al. (2021) used intestinal organoids as a disease model for studying SARS-CoV-2 infection and screened inhibitors of SARS-CoV-2, providing valuable resources for drug screening and identifying candidates for COVID-19 therapeutics, arguing that intestinal organoids can indeed serve as an experimental model for coronavirus infection and therapeutic studies.
Multiple reports suggesting that viral nucleic acids are detected in anal/rectal swabs and stool samples from patients with mild and severe coronavirus pneumonia (Chen Y. et al., 2020; Liang et al., 2020; Lin et al., 2020; Wu et al., 2020; Xiao et al., 2020; Xu et al., 2020; Young et al., 2020), and the typical particles of SARS-CoV-2 are observed in IECs (Qian et al., 2021), indicating that SARS-CoV-2 actively replicates in the intestine. There are also studies suggesting that the presence of SARS-CoV-2 viral RNAs in GI tissue may be related to more severe cases (El Hajra Martínez et al., 2020). Massive infiltration of plasma cells and lymphocytes is observed in the lamina propria of the stomach, duodenum, and rectum, accompanied by the SARS-CoV-2 shell throughout the GI lumen, indicating that virus infection has triggered the inflammatory response in intestine (Liang et al., 2020; Xiao et al., 2020). Plasma VEGF level is markedly increased in patients with GI symptoms and positively correlated with intestinal inflammation. Mechanistically, Zeng et al. (2022) found that the spike of SARS-CoV-2 contributes to VEGF production in the duodenum of mice through activating the Ras-Raf-MEK-ERK pathway in IECs, but not in endothelium, and leading to an increase of permeability and inflammation. Moreover, epithelial-enteric neuronal crosstalk plays a vital role in SARS-CoV-2-induced inflammation. SARS-CoV-2 infection in enterocyte results in endoplasmic reticulum (ER) stress and the release of damage-associated molecular patterns (DAMPs) that induces the expression and release of vasoactive intestinal peptides (VIP) by enteric neurons (EN), hence disrupts gut electrolyte homeostasis. These findings highlight the role of epithelial-enteric neuronal crosstalk in COVID-19-related GI symptoms (Balasubramaniam et al., 2023). In addition, overexpression of interleukin (IL)-1β, IL-6 and TNF-α are also important in SARS-CoV-2-induced inflammation (Hoffmann et al., 2020; Villapol, 2020; Triana et al., 2021), supporting the notion that virus infection has triggered the inflammatory response in intestine.
Angiotensin-converting enzyme 2, as the main receptor for SARS-CoV-2 invasion, predominantly has higher expression and activity in the intestine. Therefore, the high expression of ACE2 in the gut is the key factor for SARS-CoV-2 to enter into host cells and induce GI symptoms (Du et al., 2020; Xiao et al., 2020; Zou et al., 2020). Structural biology and biochemical analysis reveal that SARS-CoV-2 is highly homologous to SARS-CoV, both belonging to β coronaviruses. The S protein of SARS-CoV-2 shares similarities with that in SARS-CoV, and the RBD structure of the SARS-CoV-2 S protein enhances its binding affinity to ACE2 on IECs. Proteins such as transmembrane serine protease 2 (TMPRSS2), endoprotease (Furin) and cathepsin L (CTSL) activate the S protein of SARS-CoV-2 to facilitate the fusion of SARS-CoV-2 and IECs membranes, thereby enhancing virus infection and leading to GI symptoms (D’Amico et al., 2020; Hoffmann et al., 2020; Lu et al., 2020; Wang M. Y. et al., 2020; Zhong et al., 2020). It can be inferred that SARS-CoV-2 adheres to ACE2-expressing cells and enters into IECs through receptor recognition, protease cleavage activation and membrane fusion, resulting in infiltration of plasma cells and lymphocytes and GI interstitial edema (Figure 1). The infected intestinal cells are thus damaged, leading to long-term malabsorption and intestinal inflammation.
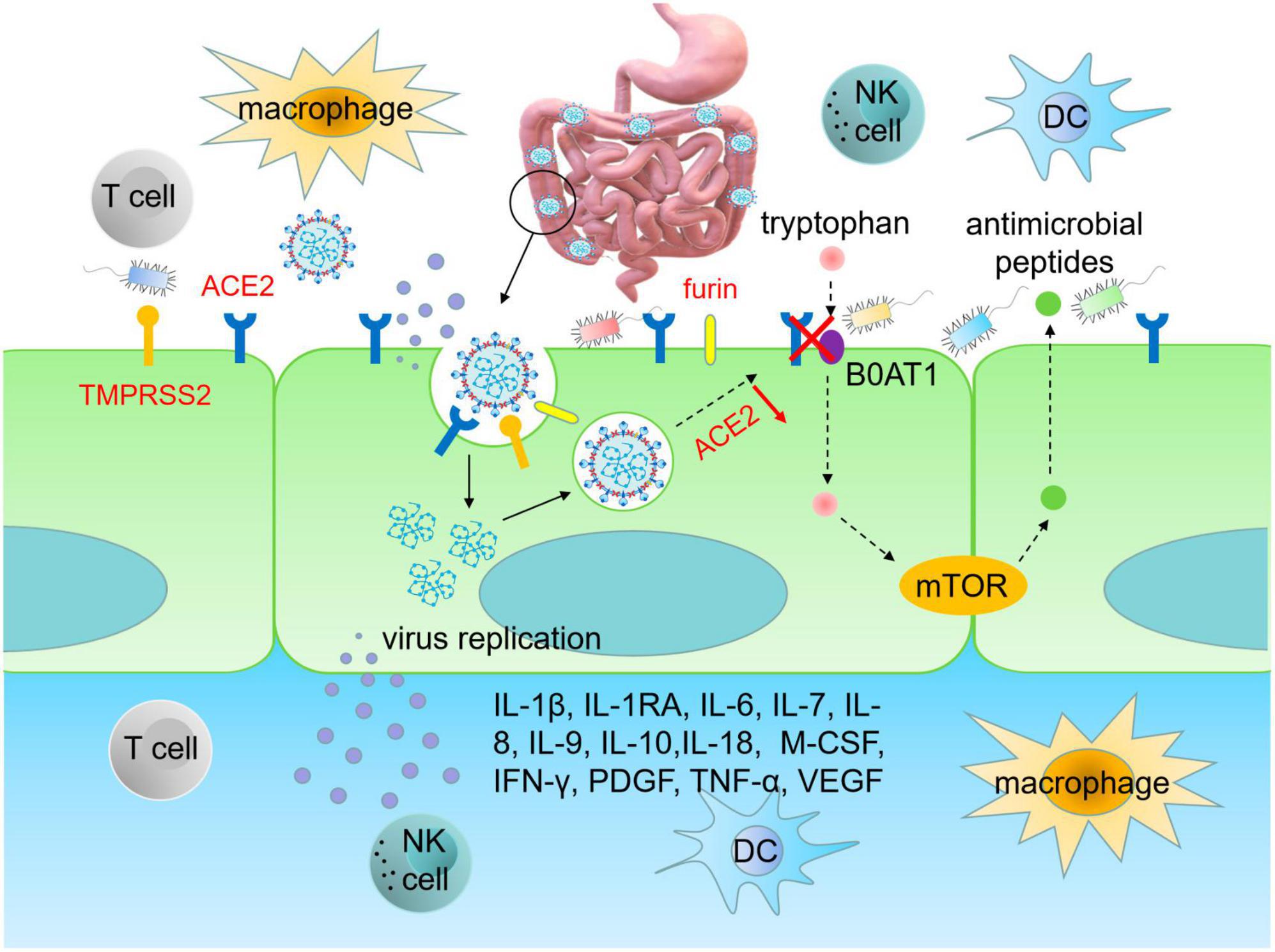
Figure 1. SARS-CoV-2 infects intestinal epithelial cells. The RBD region of S protein enhances the ability of SARS-CoV-2 to bind to ACE2 on intestinal cells. TMPRSS2 and furin promote RBD binding to ACE2 in IECs, thereby enhancing viral infection and causing GI damage. Upon infecting IECs, SARS-CoV-2 disrupts immune system, leading to an upsurge of pro-inflammatory cytokines, such as IL-1β, IL-1RA, IL-6, IL-7, IL-8, IL-9, IL-10, IL-18, M-CSF, IFN-γ, PDGF, TNF-α, and VEGF, which triggers cytokine storms. Infection of IECs by SARS-CoV-2 also leads to gut dysbiosis. The homeostasis of gut microbiota is related to the amino acid transport function of ACE2, which binds to the neutral amino acid transporter (B0AT1). Tryptophan, a neutral amino acid, regulates the expression of antimicrobial peptides, which maintain the flora of small intestine and large intestine in a stable state. RBD, receptor binding domain; ACE2, angiotensin converting enzyme 2; TMPRSS2, transmembrane serine protease 2; DC, dendritic cell; M-CSF, macrophage colony-stimulating factor; PDGF, platelet derived growth factor; VEGF, vascular endothelial growth factor.
3.2 Cytokine storm
Cytokine storm refers to the uncontrolled and excessive release of inflammatory factors due to immune dysregulation after stimulation. After SARS-CoV-2 infection, the immune system is disordered, resulting in cytokine storm (Chen N. et al., 2020). During conditions like COVID-19, homeostasis of intestinal microbiota is essential to maintenance of balanced immune reactions preventing an array of excessively detrimental inflammatory responses. The microbe-associated molecular patterns (MAMPs) of gram-negative bacteria activate inflammatory cells in COVID-19 conditions. Plasma levels of LPS produced by gram-negative bacteria are positively correlated with the severity of intestinal permeability in SARS-CoV-2 infection. The small intestine contains a significant amount of lymphoid tissues and numerous activated immune cells (Wang et al., 2023). Researchers have found the association of LPS with activated T cell and increased pro-inflammatory responses in the intestine (Clerbaux et al., 2022). Intestinal dysbiosis in some COVID-19 patients may contribute to the translocation of LPS from intestine into the portal circulation, hence stimulating the Kupffer cells in the liver and leading to the activation of NF-κB signaling and release of IFN-β and TNF-α (Li et al., 2015; Zuo et al., 2020b). When LPS enters into the systemic circulation, aforementioned response leads to an elevated hepatic and systemic inflammation (Kawaratani et al., 2013). Moreover, researchers found that low dose of LPS circulates in the plasma of patients with intestine dysbiosis and endotoxemia may act as a predictive cofactor in accelerating the severity of cytokine storm in COVID-19 patients (Szeto et al., 2008; Vignesh et al., 2020). In addition, IECs infected with SARS-CoV-2 release massive inflammatory mediators and chemokines, leading to the accumulation of neutrophils and further promoting the inflammatory response (Chen N. et al., 2020). When SARS-CoV-2 infects IECs, cytokines are excessively released, leading to long-term GI symptoms including predominantly diarrhea. According to Huang et al. (2020), levels of IL-1β, IL-1RA, IL-6, IL-7, IL-8, IL-9, IL-10, macrophage colony-stimulating factor (M-CSF), IFN-γ, platelet-derived growth factor (PDGF), TNF-α and vascular endothelial growth factor (VEGF) in COVID-19 patients were much higher as compared to healthy individuals, indicating that cytokine storm is being associated with the occurrence of extrapulmonary multiple organ dysfunction during the progression of COVID-19 (Effenberger et al., 2020; Ojetti et al., 2020).
Tao et al. (2021) followed 173 COVID-19 patients after post recovery and discharge and found that 52.3% of patients exhibit a mucosal immune response primarily driven by IgA, accompanied by increased serum creatinine, deteriorating proteinuria, and elevated levels of pro-inflammatory cytokines, notably IL-18 (Zhang P. et al., 2021). Plasma levels of IL-2, IL-7, IL-10, granulocytic stimulator, IP-10, human monocyte chemotactic protein 1, and human macrophage inflammatory protein 1α in ICU patients with COVID-19 are higher than those in mild patients, indicating strong correlation between cytokine storm and disease severity (Ma et al., 2020; Neurath, 2020; Au et al., 2021; Kumar et al., 2021). Virus-induced cytokine storm leads to rapid deterioration in COVID-19 patients with GI diseases, so immune-mediated cytokine storm plays a crucial role in the progression and development of COVID-19 (Figure 1).
3.3 Gut dysbiosis during long-COVID exposure
Researchers analyzed the composition of gut microbiota in feces from COVID-19 patients in acute, convalescent and post-discharge periods, and found that while the abundance of gut commensals in COVID-19 patients gradually increases as the patient showed clinical improvement and recovery, the profusion of flora remains significantly lower than that of healthy subjects at the three time points (Table 1). Even after the clearance of SARS-CoV-2 (confirmed by throat swabs) and resolution of respiratory symptoms, commensal depletion and gut dysbiosis persist and contribute to long-term (up to 30 days after clearance of SARS-CoV-2) GI complications (Zuo et al., 2020b; Yeoh et al., 2021). The baseline abundance of Clostridium ramosum, Coprobacillus, and Clostridium hathewayi is positively correlated with the severity of COVID-19 while disease severity inversely correlates with abundance of Faecalibacterium prausnitzii, Eubacterium rectale and Bifidobacteria, which possesses anti-inflammatory properties (Figure 2). Bacteroides dorei, Bacteroides thetaiotaomicron, Bacteroides massiliensis, and Bacteroides ovatus, which downregulate expression of ACE2 in murine intestine, are negatively correlated with SARS-CoV-2 load in fecal samples from COVID-19 patients during hospitalization. However, the abundance of Granulicatella and Rothia mucilaginosa in oral and intestinal samples of COVID-19 patients shows a positive correlation with the presence of SARS-CoV-2 (Zuo et al., 2020b; Gou et al., 2021; Wu et al., 2021; Yeoh et al., 2021). Tao et al. (2021) tracked and monitored 173 discharged COVID-19 patients, and observed that the level of virus-specific IgA antibody in serum of relapsed patients increases, while the diversity of gut microbiota decreases, but the abundance of conditional pathogens such as Streptococcus is amplified (Zhang P. et al., 2021). In addition, SARS-CoV-2 leads to dysbiosis of multiple microbiota. The abundance of bacteria, such as Coprobacillus, Clostridium ramosum, Clostridium hathewayi, Erysipelotrichaceae, Actinomyces, Enterobacteriaceae, Parabacteroides, Alistipes_indistinctus, Fusobacterium, Streptococcus, Morganella, Neisseria, Burkholderia, Desulfovibrionaceae, Granulicatella, and Rothia mucilaginosa is positively correlated with disease severity in COVID-19 patients (Table 2). Whereas the abundance of Bifidobacterium, Dorea, Bacteroides, Anaerostipes, Lachnospiraceae, Roseburia, Alistipes_onderdonkii, Faecalibacterium, Blautia, Ruminococcus, Coprococcus, Eggerthella, Akkermansia, and Eubacterium rectale is negatively correlated with disease severity in COVID-19 patients (Table 2).
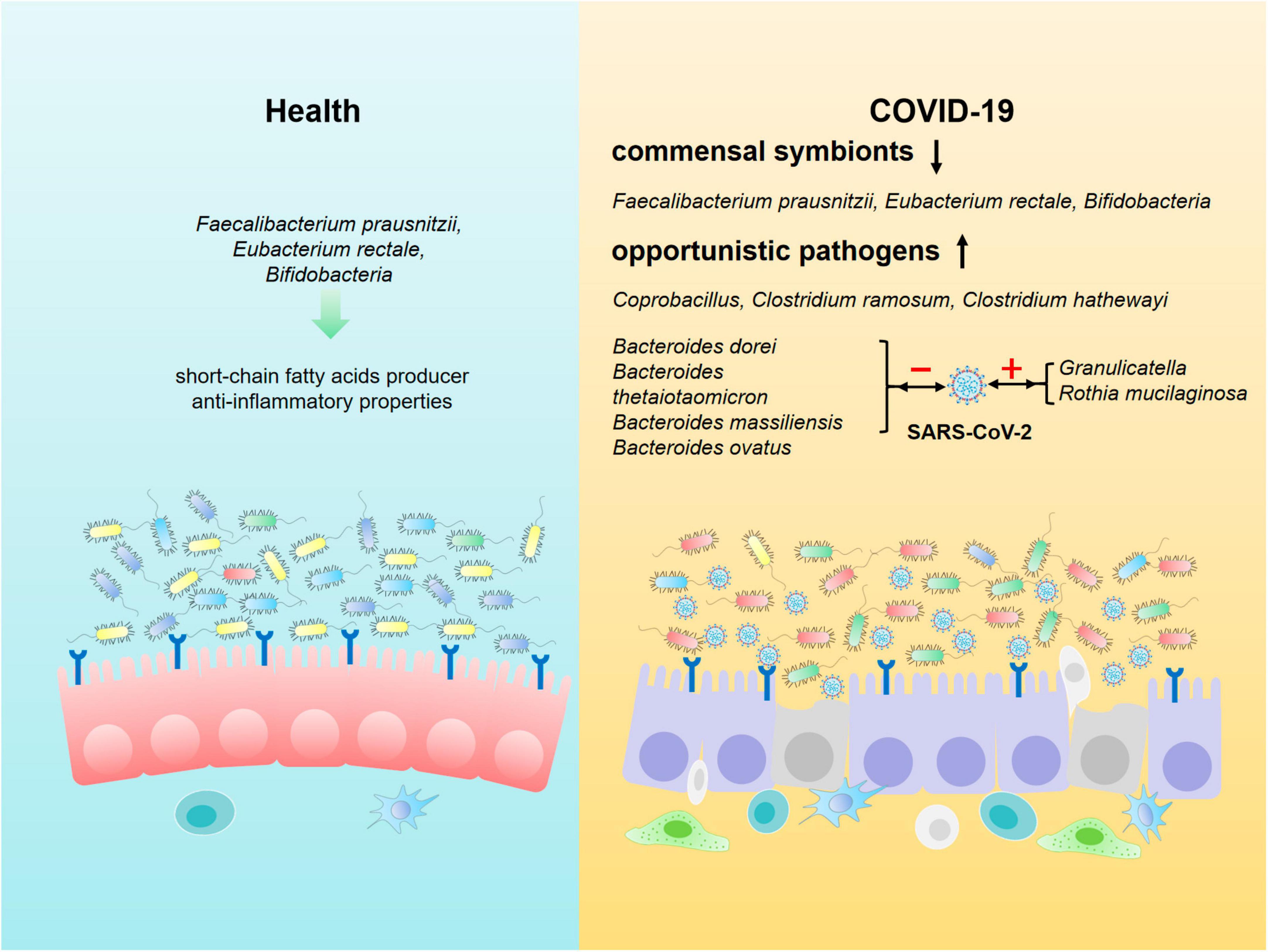
Figure 2. Composition of intestinal microbiota in healthy individuals and COVID-19 patients. Beneficial commensal bacteria such as Faecalibacterium prausnitzii, Eubacterium rectale, Bifidobacteria are dominant in the intestine of healthy population. However, the commensal symbionts are depleted, and opportunistic pathogens such as Coprobacillus, Clostridium ramosum and Clostridium hathewayi are markedly enriched in gut of COVID-19 patients. In addition, the composition of intestinal microbiota is closely associated with SARS-CoV-2 load in COVID-19 patients. Bacteroides dorei, Bacteroides thetaiotaomicron, Bacteroides massiliensis and Bacteroides ovatus are negatively correlated with the viral load, whereas the abundance of Granulicatella and Rothia mucilaginosa in oral and intestine of COVID-19 patients shows a positive correlation with SARS-CoV-2 load.
The homeostasis of gut microbiota is associated with the amino acid transport function of ACE2, which binds to the neutral amino acid transporter protein (B0AT1) on the luminal surface of IECs. Tryptophan, a neutral amino acid, is a key regulator of gut microbiota and inflammatory response. Tryptophan regulates the expression of antimicrobial peptides through the B0AT1-mTOR pathway to maintain the homeostasis of small intestinal and colonic flora (Hashimoto et al., 2012). After SARS-CoV-2 infects intestinal cells, IECs become dysfunctional, with some even necrotic and shedding, resulting in a reduction in the quantity of ACE2 (Trottein and Sokol, 2020; Verdecchia et al., 2020; Guo et al., 2021; Rajput et al., 2021). In addition, SARS-CoV-2 also co-internalizes with ACE2 through endocytosis, leading to a decreased expression of ACE2 and impaired transport of neutral amino acids, such as tryptophan, and inhibits the expression of antibacterial peptides, which contributes to gut dysbiosis and heightens vulnerability to intestinal inflammation (Figure 2). There is evidence showing that gut microbiota imbalance is related to changes in gut-lung axis (Dang and Marsland, 2019), gut-liver axis (Tripathi et al., 2018; Manzoor et al., 2022), and gut-brain axis (Mitrea et al., 2022) homeostasis in COVID-19 patients. Transmission electron microscopy analysis showed that the existence of SARS-CoV-2 particles is on the surface and inside of intestinal bacteria, suggesting that SARS-CoV-2 infection with human gut bacteria may be another mechanism leading to ecological imbalance in COVID-19 patients (Clerbaux et al., 2022). Moreover, SARS-CoV-2 infection in IECs triggers an immune response leading to gut dysbiosis, characterized by reduced levels of short-chain fatty acid produced by anti-inflammatory bacteria and an increase in the abundance of opportunistic pathogens, such as Enterobacteriaceae (Gu et al., 2020; Gaibani et al., 2021; Ren et al., 2021; Xu et al., 2021). Hence it is crucial to thoroughly assess the gut dysbiosis in long-COVID patients with diarrhea. If necessary, fecal metagenomic sequencing should be employed to identify novel therapeutic targets for the treatment of long-term GI pathogenesis by analyzing the characteristics of gut microbiota changes in COVID-19 patients.
3.4 Drug toxicity
Numerous anti-COVID-19 drugs have been undergone clinical trials. A combination of two or three drugs were often used for the treatment of COVID-19, which increased the risk of GI injury. It was reported that acute diarrhea was associated with the use of drugs such as oseltamivir and arbidol in 55.2% of COVID-19 patients (Ren et al., 2021). Furthermore, a study revealed that remdesivir, while used to combat COVID-19, may have adverse effects such as nausea, elevated ALT, and constipation (Panda et al., 2020), indicating that drug toxicity plays a critical role in the occurrence of GI injury among COVID-19 patients.
Moreover, antibiotics, non-steroidal anti-inflammatory drugs (NSAIDs) and corticosteroids are frequently employed in clinical settings to treat tissue hypoxia and cells injury caused by respiratory infection, including COVID-19. It was observed that many patients experience symptoms including nausea, abdominal pain and acute diarrhea following such treatment (Tian et al., 2020). In a study involving 1,099 patients with COVID-19, intravenous antibiotics were used in 57.5% of the patients, and among these patients 3.8% experienced acute diarrhea as an adverse effect (Guan and Zhong, 2020). By analysis of stool samples from 96 COVID-19 patients, Bernard-Raichon et al. (2022) concluded that opportunistic pathogens with antibiotic resistance massively proliferate and contribute to the aggravation of gut dysbiosis. Without administration of antibiotics, COVID-19 patients showed an enrichment of abundance in Ruminococcus gnavus, Ruminococcus torques and Bacteroides dorei and a reduction of Bifidobacterium adolescentis, Faecalibacterium prausnitzii and Eubacterium rectale. However, COVID-19 with antibiotic treatment was primarily related to an increase of Parabacteroides, Sutterella wadsworthensis and Bacteroides caccae and a decrease in Adlercreutzia equolifaciens, Dorea formicigenerans and Clostridium leptum. Administration of antibiotics during hospitalization was associated with the severity of COVID-19 (Yeoh et al., 2021). Therefore, digestive symptoms in COVID-19 patients could also be caused by the administration of anti-viral drugs, antibiotics, NSAIDs and corticosteroids. To mitigate long-term GI injury these drugs should be accompanied by protective agents as a combinational therapy.
3.5 Psychological effects of long-COVID
COVID-19 is a rapidly evolving infectious disease characterized by swift transmission, high pathogenicity, elevated mortality and lacks specific drugs for treatment. Consequently, most patients experience negative emotions including panic and anxiety. Stress-induced brain interactions stimulate GI tract and lead to abnormalities in digestive, sensory, and immune functions, resulting in GI symptoms such as abdominal pain and anorexia (Heinen et al., 2022; Taylor, 2022; Bassey et al., 2023). COVID-19 patients experience a wide range of physical and psychological symptoms, confirming that psychological factors can significantly slow down gastric emptying rates, that can ultimately lead to dysplasia (Aiyegbusi et al., 2021). A survey on the mental health and sleep status of COVID-19 patients found that compared with the control, the incidence of anxiety and sleep disorders are significantly higher in COVID-19 patients (Aiyegbusi et al., 2021), and the long-term GI symptoms in these patients has increased substantially (Anaya et al., 2021). Several studies suggest a causal impact of psychosocial stress on increased permeability of the intestine, possibly by corticotropin-releasing hormone-induced mast cell activation and reduced blood flow to the intestine under stimulation (Söderholm et al., 2002; Keita et al., 2010; Vicario et al., 2010). This suggests that psychological factors play a critical role in the development of GI symptoms in COVID-19 patients.
On the other hand, increased permeability of the intestine and abnormal influx of antigens from food and bacteria disrupts systemic immune homeostasis, which in turn harms brain function and structure (Genedi et al., 2019). Patients with structural and functional abnormalities in GI barrier, such as in colitis ulcerosa and Crohn’s disease, display a more frequently psychiatric comorbidity (Faresjö et al., 2007; Nicholl et al., 2008). Thus, GI symptoms mutually affect the outcome of psychological disorders.
3.6 Exacerbation of primary diseases
Patients with underlying diseases, such as chronic obstructive pulmonary disease, hypertension, coronary heart disease, diabetes, cerebrovascular disease, viral hepatitis B, and cancer are more likely to develop severe pneumonia and have poor prognosis from COVID-19 (Chen N. et al., 2020; Guan and Zhong, 2020). Patients with inflammatory bowel disease (IBD), including ulcerative colitis (UC) and Crohn’s disease (CD) are at high risk of developing COVID-19 complications, while long-term use of corticosteroids and immunosuppressants in IBD patients also elevates the risk of opportunistic SARS-CoV-2 infection (Bezzio et al., 2020). Moreover, IBD patients are more prone to stress and anxiety, which can lead to long-term GI symptoms (Figure 3).
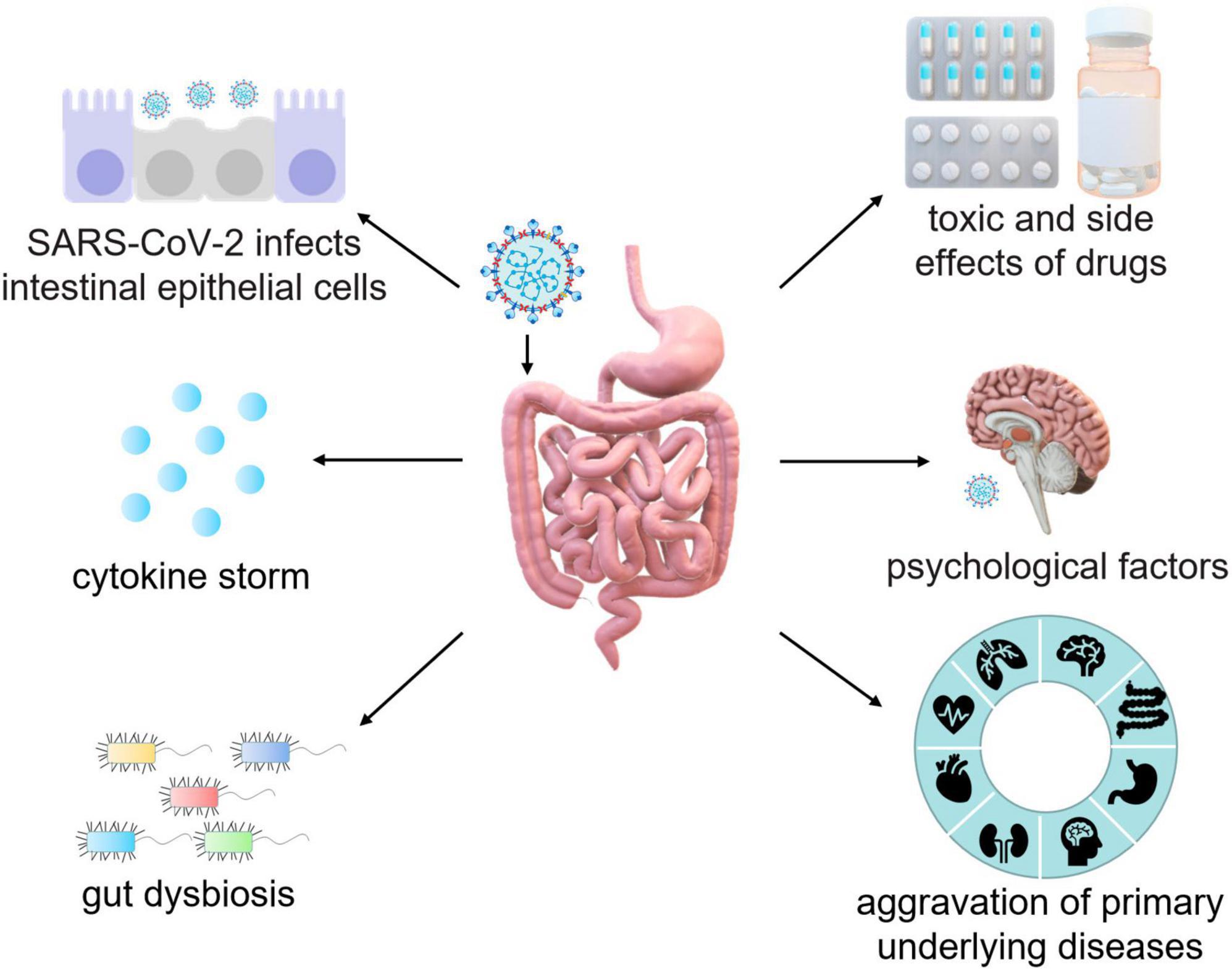
Figure 3. Schematic mechanisms of intestinal injury caused by SARS-CoV-2. The pathogenesis of intestinal injury in COVID-19 patients is mediated by the interaction between SARS-CoV-2 and ACE2, which leads to the destruction of epithelial cells and subsequent intestinal inflammation. In addition, of cytokine storm, gut dysbiosis, psychosocial factors, the aggravation of primary diseases, and potential side effects of antiviral drugs and antibiotics should also be considered during the treatment of GI injury in COVID-19 patients.
4 Preventive measures for long-term GI injury induced by SARS-CoV-2
4.1 Probiotics alleviate SARS-CoV-2-induced long-term GI injury
Probiotics are considered as an effective intervention for reducing morbidity and mortality in severe COVID-19 patients (Finlay et al., 2021). Despite of the continuous emergence of novel SARS-CoV-2 variant strains, the most potent prevention and control measure remains the widespread vaccination of the population with protective vaccines (Lynn et al., 2022). Studies have shown that gut microbiota of pre-vaccinated individuals can influence the immune balance within the host, consequently impacting the vaccine efficacy and immunogenicity (Pulendran, 2019; de Jong et al., 2020). It was reported that probiotics could improve the vaccine immunogenicity, and increase the ratio of seroprotection and seroconversion in adults vaccinated with influenza vaccine (Lei et al., 2017). Thus, the effectiveness and immune response of the vaccine in vulnerable populations can be improved by increasing the abundance of probiotics. Lactobacilli in the intestine of COVID-19 patients are beneficial to the maintenance of gastrointestinal homeostasis and alleviation of inflammatory illnesses (Sun et al., 2018; Huang et al., 2020). Wang M. et al. (2020) applied Lactobacillus plantarum to express SARS-CoV-2 S protein and showed that the protein could be expressed on the surface of Lactobacillus plantarum and bears a high antigenicity for stimulating the generation of specific monoclonal and polyclonal antibody against SARS-Cov-2-S-protein. Thus, Lactobacillus plantarum recombined with the gene expressing SARS-CoV-2 S protein could be developed as an ideal oral vaccine for SARS-CoV-2 infection (Wang M. et al., 2020). In addition, probiotics downregulate the proportion of pro-inflammatory and anti-inflammatory cytokines, which is of great benefit to the alleviation of cytokine storms in patients with COVID-19 (Aziz et al., 2020; Li Q. et al., 2021). In a short, due to the role of probiotics in the regulation of innate immunity, preventive intake of probiotics (formulations) might be helpful to promote protective antiviral response and inhibit harmful excessive inflammatory response of host (Tomkinson et al., 2023).
Judicious use of probiotics and prebiotics can safeguard the intestinal barrier and reduce intestinal permeability, and hence alleviate intestinal symptoms in COVID-19 patients by regulating immune homeostasis and inflammatory response (Trompette et al., 2018; Mak et al., 2020; Hu et al., 2021). Emerging studies have shown that probiotics can increase the number and activity of T cells, which directly contribute to the immune response induced by T cells and enhances immune functions (Dhar and Mohanty, 2020). Furthermore, probiotics also enhance microbiome diversity and fortify the integrity of intestinal barrier functions, thereby effectively preventing microbial translocation (Mack et al., 2003; Reiff and Kelly, 2010; Wei et al., 2018; Suez et al., 2019; Badgeley et al., 2021). VSL#3, a mixture of 8 probiotics, has been safely and effectively used worldwide for decades. It is proven that VSL#3 alleviates enteritis and reinforces intestinal mucous barrier by improving the gut microecology (Mimura et al., 2004; Sartor, 2006; Tankou et al., 2018). Administration with VSL#3 is able help to improve the biodiversity of microbiota in patients, reduce fungal colonization, and increase the abundance of Lactobacillus and Bifidobacterium, thereby could diminish the severity of COVID-19-induced GI symptoms (Kühbacher et al., 2006; Chitapanarux et al., 2010). A comprehensive meta-analysis evaluated the clinical efficacy of probiotics in preventing treatment-related diarrhea (Wei et al., 2018), and showed that combined capsules containing diverse strains of Bifidobacterium and Lactobacillus were beneficial in reducing the incidence of acute diarrhea (Osterlund et al., 2007; Wada et al., 2010). Moreover, d’Ettorre et al. (2020) treated COVID-19 patients with a combination of Streptococcus thermophilus DSM 32345, L. acidophilus DSM 32241, L. helveticus DSM 32242, Lacticaseibacillus paracasei DSM 32243, L. plantarum DSM 32244, LeviL. brevis DSM 27961, B. lactis DSM 32246, and B. lactis DSM 32247 for 7 days and found that patients given probiotics showed a reduction in GI symptoms as compared to those treated with the placebo. Gutiérrez-Castrellón et al. (2022) performed a randomized clinical trial involving 150 outpatients with COVID-19 treated with L. plantarum KABP022, KABP023 and KAPB033, and Pediococcus acidilactici KABP021 for 30 days and found that acute diarrhea remission rates are 53% and 28% in probiotic and placebo treatment groups, respectively. Similarly, Tang et al. (2021) applied probiotics to eliminate COVID-19 transmission in exposed household contacts involving 1,132 COVID-19 patients treated with L. rhamnosus GG or placebo for 28 days and beneficial effects were obtained. Collectively, current clinical evidence highlights that probiotics help to improve the immunity in COVID-19 patients by inhibiting pathogens colonization in the intestine, hence alleviating the disease severity (Olaimat et al., 2020).
Healthy dietary helps to maintain the homeostasis of gut microbiota in COVID-19 patients. Multiple studies showed that inflammatory response in COVID-19-positive patients is related to dietary styles (Ebrahimzadeh et al., 2022; Majidi et al., 2022; Moludi et al., 2022). Supplements of fruits, nuts, olive oil, vegetables, and whole grains, promote the abundance of probiotics and are negatively related to inflammation status in COVID-19 patients (Majidi et al., 2021; Hajipour et al., 2022). Moreover, vitamins D and A, selenium, flavonoids, zinc, and unsaturated fatty acids can alleviate inflammatory response in patients with COVID-19 via inhibiting the activation of nuclear factor kappa-B (NF-κB), hence reducing the production of pro-inflammatory cytokines, such as IL-6 and TNF-α. However, foods with higher glycemic load, carbohydrates, saturated fatty acids, and processed foods are found to disrupt the homeostasis of gut microbiota and are positively related to inflammation conditions in COVID-19 patients (Faghfouri et al., 2020, 2021; Zabetakis et al., 2020). In addition, appropriate intake of specific dietary fiber (Zhao et al., 2018), promote the growth of beneficial bacteria in the intestine, which may reduce the risk of SARS-CoV-2 infection (Kalantar-Zadeh et al., 2020; Merino et al., 2021). Fermentable dietary fiber (such as inulin) is fermented by gut microbiota to produce short-chain fatty acids (SCAFs), such as butyric acid, which alleviate the excessive inflammatory response caused by leukocytes in the lung, and enhance the immunoregulatory function of CD8 + T cells (Trompette et al., 2018). Antunes et al. (2019) administrated the acetate, butyrate, or propionate in the drinking water into mice infected with respiratory syncytial virus (RSV), and found that acetate exerts an anti-viral effect by binding with G protein coupled receptor 43 (GPR43). It suggests that increased abundance of probiotics could boost anti-viral ability and reduce long term GI symptoms in COVID-19 patients through preventive and therapeutic strategies.
4.2 FMT maintains intestinal flora homeostasis and relieves long-term GI symptoms caused by SARS-CoV-2
Fecal microbiota transplantation (FMT) is a therapeutic intervention to restructure the intestinal microbes of patients by transplanting the bacteria of healthy subjects into patient’s intestine (Xu et al., 2016; Cheng et al., 2018; Juul et al., 2018). Eiseman et al. (1958) treated pseudomembranous enteritis induced by antibiotics through FMT therapy, and obtained a notable improvement in patient condition, demonstrating the efficacy of FMT in treating Clostridium difficile infection and in the alleviation of intestinal inflammation (Hui et al., 2019). A significant proportion of COVID-19 patients bear gut dysbiosis, which indicates a close relationship between intestinal flora imbalance and SARS-CoV-2 (Cao et al., 2021). FMT treatment in severe COVID-19 patients, rapidly alleviates COVID-19 symptoms and cures Clostridium difficile infection (CDI) (Ianiro et al., 2020a; Biliński et al., 2022). Furthermore, Liu et al. (2021) observed that FMT improves the recovery of COVID-19 patients, alleviates residual gastrointestinal symptoms, and promotes the recovery of normal intestinal microbiota. Lastly, a retrospective study of 86 patients with CDI and COVID-19 showed that combination of antibiotics and FMT promotes the alleviation of abdominal pain and reduces relapse of CDI, and decreases levels of inflammation cytokines as compared to those treated with antibiotics alone (Boicean et al., 2022). Taken together, these studies suggest that FMT facilitates the relief of gastrointestinal symptoms, reduces intestinal inflammation, and promotes the recovery of COVID-19 patients.
Study in the germ-free SD rat model showed that the colonization of intestinal flora affects the expression of intestinal Ace2, Lcn2, and Nlrc5, and regulates systemic inflammatory responses, which impacts the susceptibility of IECs to SARS-CoV-2, indicating that normal gut microbiota may play a role in mitigating SARS-CoV-2 infectivity and resultant injury in gastrointestine (Yang et al., 2020). Therefore, selection of healthy subjects for FMT donor may prevent potential side effects transmitted from donor’s stool in gastrointestinal therapy (Ianiro et al., 2020b).
4.3 Rational medication for Long-COVID-19
COVID-19 patients may experience long term digestive symptoms due to the higher intake of antiviral drugs, antibiotics, NSAIDs and corticosteroids, with antibiotics being particularly associated with antibiotic-induced acute diarrhea (Ng T. M. et al., 2022). Despite the international community emphasis on reasonable antibacterial intervention for COVID-19 patients, clinical practice often deviates from this principle, resulting in an upsurge risk of developing antibiotic-induced acute diarrhea. Antibacterial treatment should be reserved for cases with clear evidence of bacterial infection (phlegm, procalcitonin, leukopenia and neutropenia) (Shchikota et al., 2021). Traditional Chinese medicine (TCM) has been reported to reduce the risk of mild and severe cases of COVID-19 developing to critical stages and significantly shorten the course of disease and improve overall clinical effectiveness (Liu et al., 2020). A large number of clinical trials tested the administration of TCM to treat COVID-19 and showed that some herbs could regulate the immune response, limit SARS-CoV-2 infection, and protect organs from viral infection-induced damage (Li et al., 2022). For example, Qiao et al. (2021) found that application of Qingfei Paidu decoction, Lianhua Qingwen Capsule, and Xuanfei Baidu formula could relieve the symptoms and reduce the production of pro-inflammatory cytokines in COVID-19 patients. Moreover, Li et al. reported that Lianhua Qingwen Capsule and Pudilan Xiaoyan Oral Liquid inhibit the expression of inflammatory factors, such as TNF-α, IL-6, CCL2/MCP-1, and CCL10/IP-10 (Deng et al., 2020; Runfeng et al., 2020). Polysaccharides are a kind of substances with active pharmacological activity and widely found in TCM. Polysaccharides play a crucial role in immunomodulatory, anti-fibrotic and antiviral functions. It was reported that polysaccharides alleviate the symptoms of COVID-19 by targeting the regulatory axis of transforming growth factor-β/Smad2/3 and DANCER/AUF-1/FOXO3 (Chen R. R. et al., 2020; Chen N. et al., 2020). In addition, polysaccharides that regulate homeostasis of intestinal flora for a long time by promoting the growth of probiotics (Xu et al., 2017), These findings suggest that TCM herbs and their active substances may be used in the prevention and treatment of COVID-19. Rational use of antibiotic or combination with TCM may be an effective way to reduce antibiotic related acute diarrhea in COVID-19.
4.4 Psychotherapy for overcoming post COVID-19 symptoms
COVID-19 patients often experienced concomitant psychological disorders like anxiety. Unfortunately, clinical focus tends to minimize physical symptoms, neglecting the accompanying mental and psychological problems that can contribute to the exacerbation of COVID-19 condition. The psychological state of COVID-19 patients has a significant impact on the development of the disease as an excessive psychological stress can lead to increased activation of GI tract, which increases the risk of dyspepsia and intestinal inflammation (Everitt et al., 2019). Psychotherapy served as a significant approach to enhance the quality of life and alleviate stress could shorten the course of COVID-19. In the realm of clinical care, medical staff should prioritize psychological care to ensure comprehensive wellbeing of patient.
5 Challenges and opportunities for long-COVID-19 treatment
Currently, there are multiple types of vaccine against SARS-CoV-2 for the prevention of the virus. However, there is no specific vaccination or authorized drug developed to directly treat COVID-19 (Majumder and Minko, 2021; Gasmi et al., 2023). The pathogenesis of long-term intestinal injury in COVID-19 patients is well-understood. Interaction between SARS-CoV-2 and ACE2 destroys epithelial cells leading to intestinal inflammation. Furthermore, during the treatment of COVID-19 patients it is critically needed to pay more attention toward underlying symptoms such as cytokine storm, gut dysbiosis, psychosocial factors, the aggravation of primary diseases, side effects of antiviral drugs and antibiotics (Figure 3). Therefore, clinicians must maintain heightened vigilance regarding digestive symptoms in COVID-19 patients. The expectation is for medical professionals to delve further into the comprehension of long-term symptoms in COVID-19 patients and better comprehend the pathogenesis of the disease, so as to provide more targeted treatment while effectively reducing the spread of the virus.
6 Conclusion
The pathogenesis of long-COVID is undoubtedly multifactorial. Even after the acute effects of COVID-19 subside, vigilant attention is required for an extended period to monitor the poor prognosis and sequelae of long-COVID. This review aims to comprehensively summarize and enhance the understanding of long-term GI injury caused by long-COVID, thereby improving the efficacy of clinical diagnosis and treatment, and paves a way for new therapeutic strategies and targets for GI injury.
Author contributions
K-YH: Writing—original draft, Writing—review & editing. X-YL: Writing—original draft, Writing—review & editing. LZ: Writing—original draft. D-HW: Writing—original draft. J-QL: Writing—original draft. L-YL: Writing—original draft. UEL: Writing—original draft. C-YC: Writing—original draft. Y-PJ: Conceptualization, Funding acquisition, Writing—original draft, Writing—review & editing. Z-XX: Conceptualization, Funding acquisition, Writing—review & editing.
Funding
The author(s) declare financial support was received for the research, authorship, and/or publication of this article. This work was supported by the National Natural Science Foundation of China (Nos. 82020108024 and 82200596), the National Key Research and Development Program of China (2023YFE0109800), the China Postdoctoral Science Foundation (No. 2022M721014), and the Scientific and Technological Research Project of Henan Province (No. 232102311034).
Conflict of interest
The authors declare that the research was conducted in the absence of any commercial or financial relationships that could be construed as a potential conflict of interest.
Publisher’s note
All claims expressed in this article are solely those of the authors and do not necessarily represent those of their affiliated organizations, or those of the publisher, the editors and the reviewers. Any product that may be evaluated in this article, or claim that may be made by its manufacturer, is not guaranteed or endorsed by the publisher.
References
Afrisham, R., Jadidi, Y., Davoudi, M., Moayedi, K., Soliemanifar, O., Eleni Xirouchaki, C., et al. (2023). Gastrointestinal, liver, pancreas, oral and psychological long-term symptoms of COVID-19 after recovery: a review. Mini Rev. Med. Chem. 23, 852–868. doi: 10.2174/1389557523666221116154907
Aiyegbusi, O. L., Hughes, S. E., Turner, G., Rivera, S. C., Mcmullan, C., Chandan, J. S., et al. (2021). Symptoms, complications and management of long COVID: a review. J. R. Soc. Med. 114, 428–442.
Al Bataineh, M. T., Henschel, A., Mousa, M., Daou, M., Waasia, F., Kannout, H., et al. (2021). Gut microbiota interplay With COVID-19 reveals links to host lipid metabolism among middle eastern populations. Front. Microbiol. 12:761067. doi: 10.3389/fmicb.2021.761067
Albrich, W. C., Ghosh, T. S., Ahearn-Ford, S., Mikaeloff, F., Lunjani, N., Forde, B., et al. (2022). A high-risk gut microbiota configuration associates with fatal hyperinflammatory immune and metabolic responses to SARS-CoV-2. Gut Microbes 14:2073131. doi: 10.1080/19490976.2022.2073131
Amarsy, R., Trystram, D., Cambau, E., Monteil, C., Fournier, S., Oliary, J., et al. (2022). Surging bloodstream infections and antimicrobial resistance during the first wave of COVID-19: a study in a large multihospital institution in the Paris region. Int. J. Infect. Dis. 114, 90–96. doi: 10.1016/j.ijid.2021.10.034
Amin-Chowdhury, Z., Aiano, F., Mensah, A., Sheppard, C. L., Litt, D., Fry, N. K., et al. (2021). Impact of the coronavirus disease 2019 (COVID-19) pandemic on invasive pneumococcal disease and risk of pneumococcal coinfection with severe acute respiratory syndrome coronavirus 2 (SARS-CoV-2): prospective national cohort study, England. Clin. Infect. Dis. 72, e65–e75. doi: 10.1093/cid/ciaa1728
Anaya, J. M., Rojas, M., Salinas, M. L., Rodríguez, Y., Roa, G., Lozano, M., et al. (2021). Post-COVID syndrome. A case series and comprehensive review. Autoimmun. Rev. 20:102947.
Antunes, K. H., Fachi, J. L., De Paula, R., Da Silva, E. F., Pral, L. P., Dos Santos, A., et al. (2019). Microbiota-derived acetate protects against respiratory syncytial virus infection through a GPR43-type 1 interferon response. Nat. Commun. 10:3273. doi: 10.1038/s41467-019-11152-6
Au, L., Fendler, A., Shepherd, S. T. C., Rzeniewicz, K., Cerrone, M., Byrne, F., et al. (2021). Cytokine release syndrome in a patient with colorectal cancer after vaccination with BNT162b2. Nat. Med. 27, 1362–1366. doi: 10.1038/s41591-021-01387-6
Aziz, M., Fatima, R., and Assaly, R. (2020). Elevated interleukin-6 and severe COVID-19: a meta-analysis. J. Med. Virol. 92, 2283–2285.
Badgeley, A., Anwar, H., Modi, K., Murphy, P., and Lakshmikuttyamma, A. (2021). Effect of probiotics and gut microbiota on anti-cancer drugs: mechanistic perspectives. Biochim. Biophys. Acta Rev. Cancer 1875:188494. doi: 10.1016/j.bbcan.2020.188494
Balasubramaniam, A., Tedbury, P. R., Mwangi, S. M., Liu, Y., Li, G., Merlin, D., et al. (2023). SARS-CoV-2 induces epithelial-enteric neuronal crosstalk stimulating VIP release. Biomolecules 13:207. doi: 10.3390/biom13020207
Baroni, C., Potito, J., Perticone, M. E., Orausclio, P., and Luna, C. M. (2023). How does long-COVID impact prognosis and the long-term sequelae? Viruses 15:1173.
Bassey, E. E., Gupta, A., Kapoor, A., and Bansal, A. (2023). COVID-19 and poverty in South America: the mental health implications. Int. J. Ment. Health Addict. 21, 2954–2960. doi: 10.1007/s11469-022-00765-6
Bernard-Raichon, L., Venzon, M., Klein, J., Axelrad, J. E., Zhang, C., Sullivan, A. P., et al. (2022). Gut microbiome dysbiosis in antibiotic-treated COVID-19 patients is associated with microbial translocation and bacteremia. Nat. Commun. 13:5926. doi: 10.1038/s41467-022-33395-6
Bezzio, C., Saibeni, S., Variola, A., Allocca, M., Massari, A., Gerardi, V., et al. (2020). Outcomes of COVID-19 in 79 patients with IBD in Italy: an IG-IBD study. Gut 69, 1213–1217. doi: 10.1136/gutjnl-2020-321411
Biliński, J., Winter, K., Jasiński, M., Szczȩś, A., Bilinska, N., Mullish, B. H., et al. (2022). Rapid resolution of COVID-19 after faecal microbiota transplantation. Gut 71, 230–232.
Boicean, A., Neamtu, B., Birsan, S., Batar, F., Tanasescu, C., Dura, H., et al. (2022). Fecal microbiota transplantation in patients co-infected with SARS-CoV2 and Clostridioides difficile. Biomedicines 11:7.
Cao, J., Wang, C., Zhang, Y., Lei, G., Xu, K., Zhao, N., et al. (2021). Integrated gut virome and bacteriome dynamics in COVID-19 patients. Gut Microbes 13, 1–21.
Carfì, A., Bernabei, R., and Landi, F. (2020). Persistent symptoms in patients after acute COVID-19. JAMA 324, 603–605.
Chen, N., Zhou, M., Dong, X., Qu, J., Gong, F., Han, Y., et al. (2020). Epidemiological and clinical characteristics of 99 cases of 2019 novel coronavirus pneumonia in Wuhan, China: a descriptive study. Lancet 395, 507–513. doi: 10.1016/S0140-6736(20)30211-7
Chen, R. R., Li, Y. J., Chen, J. J., and Lu, C. L. (2020). A review for natural polysaccharides with anti-pulmonary fibrosis properties, which may benefit to patients infected by 2019-nCoV. Carbohydr. Polym. 247:116740. doi: 10.1016/j.carbpol.2020.116740
Chen, X., Han, W., Wang, G., and Zhao, X. (2020). Application prospect of polysaccharides in the development of anti-novel coronavirus drugs and vaccines. Int. J. Biol. Macromol. 164, 331–343. doi: 10.1016/j.ijbiomac.2020.07.106
Chen, Y., Chen, L., Deng, Q., Zhang, G., Wu, K., Ni, L., et al. (2020). The presence of SARS-CoV-2 RNA in the feces of COVID-19 patients. J. Med. Virol. 92, 833–840.
Cheng, S., Ma, X., Geng, S., Jiang, X., Li, Y., Hu, L., et al. (2018). Fecal microbiota transplantation beneficially regulates intestinal mucosal autophagy and alleviates gut barrier injury. mSystems 3:e00137–18.
Cheung, K. S., Hung, I. F. N., Chan, P. P. Y., Lung, K. C., Tso, E., Liu, R., et al. (2020). Gastrointestinal manifestations of SARS-CoV-2 infection and virus load in fecal samples from a Hong Kong cohort: systematic review and meta-analysis. Gastroenterology 159, 81–95. doi: 10.1053/j.gastro.2020.03.065
Chitapanarux, I., Chitapanarux, T., Traisathit, P., Kudumpee, S., Tharavichitkul, E., and Lorvidhaya, V. (2010). Randomized controlled trial of live Lactobacillus acidophilus plus Bifidobacterium bifidum in prophylaxis of diarrhea during radiotherapy in cervical cancer patients. Radiat. Oncol. 5:31. doi: 10.1186/1748-717X-5-31
Clerbaux, L. A., Fillipovska, J., Muñoz, A., Petrillo, M., Coecke, S., Amorim, M. J., et al. (2022). Mechanisms leading to gut dysbiosis in COVID-19: current evidence and uncertainties based on adverse outcome pathways. J. Clin. Med. 11:5400. doi: 10.3390/jcm11185400
Cortes, G. M., Marcialis, M. A., Bardanzellu, F., Corrias, A., Fanos, V., and Mussap, M. (2022). Inflammatory bowel disease and COVID-19: how microbiomics and metabolomics depict two sides of the same coin. Front. Microbiol. 13:856165. doi: 10.3389/fmicb.2022.856165
D’Amico, F., Baumgart, D. C., Danese, S., and Peyrin-Biroulet, L. (2020). Diarrhea during COVID-19 infection: pathogenesis, epidemiology, prevention, and management. Clin. Gastroenterol. Hepatol. 18, 1663–1672.
d’Ettorre, G., Ceccarelli, G., Marazzato, M., Campagna, G., Pinacchio, C., Alessandri, F., et al. (2020). Challenges in the management of SARS-CoV2 infection: the role of oral bacteriotherapy as complementary therapeutic strategy to avoid the progression of COVID-19. Front. Med. (Lausanne) 7:389. doi: 10.3389/fmed.2020.00389
Dang, A. T., and Marsland, B. J. (2019). Microbes, metabolites, and the gut-lung axis. Mucosal Immunol. 12, 843–850.
de Jong, S. E., Olin, A., and Pulendran, B. (2020). The impact of the microbiome on immunity to vaccination in humans. Cell Host Microbe 28, 169–179.
De Maio, F., Ianiro, G., Coppola, G., Santopaolo, F., Abbate, V., Bianco, D. M., et al. (2021). Improved gut microbiota features after the resolution of SARS-CoV-2 infection. Gut Pathog. 13:62. doi: 10.1186/s13099-021-00459-9
Deng, W., Xu, Y., Kong, Q., Xue, J., Yu, P., Liu, J., et al. (2020). Therapeutic efficacy of Pudilan Xiaoyan Oral Liquid (PDL) for COVID-19 in vitro and in vivo. Signal Transduct. Target. Ther. 5:66. doi: 10.1038/s41392-020-0176-0
Dhar, D., and Mohanty, A. (2020). Gut microbiota and Covid-19- possible link and implications. Virus Res. 285:198018.
Doubravská, L., Htoutou Sedláková, M., Fišerová, K., Pudová, V., Urbánek, K., Petrželová, J., et al. (2022). Bacterial resistance to antibiotics and clonal spread in COVID-19-positive patients on a tertiary hospital intensive care unit, Czech Republic. Antibiotics (Basel) 11:783. doi: 10.3390/antibiotics11060783
Du, M., Cai, G., Chen, F., Christiani, D. C., Zhang, Z., and Wang, M. (2020). Multiomics evaluation of gastrointestinal and other clinical characteristics of COVID-19. Gastroenterology 158, 2298–2301.e2297. doi: 10.1053/j.gastro.2020.03.045
Ebrahimzadeh, A., Taghizadeh, M., and Milajerdi, A. (2022). Major dietary patterns in relation to disease severity, symptoms, and inflammatory markers in patients recovered from COVID-19. Front. Nutr. 9:929384. doi: 10.3389/fnut.2022.929384
Effenberger, M., Grabherr, F., Mayr, L., Schwaerzler, J., Nairz, M., Seifert, M., et al. (2020). Faecal calprotectin indicates intestinal inflammation in COVID-19. Gut 69, 1543–1544.
Eiseman, B., Silen, W., Bascom, G. S., and Kauvar, A. J. (1958). Fecal enema as an adjunct in the treatment of pseudomembranous enterocolitis. Surgery 44, 854–859.
El Hajra Martínez, I., Relea Pérez, L., and Calvo Moya, M. (2020). Presence of SARS-coronavirus-2 in the ileal mucosa: another evidence for infection of GI tract by this virus. Gastroenterology 159, 1624–1625. doi: 10.1053/j.gastro.2020.05.101
Everitt, H. A., Landau, S., O’reilly, G., Sibelli, A., Hughes, S., Windgassen, S., et al. (2019). Cognitive behavioural therapy for irritable bowel syndrome: 24-month follow-up of participants in the ACTIB randomised trial. Lancet Gastroenterol. Hepatol. 4, 863–872. doi: 10.1016/S2468-1253(19)30243-2
Faghfouri, A. H., Baradaran, B., Khabbazi, A., Khaje Bishak, Y., Zarezadeh, M., Tavakoli-Rouzbehani, O. M., et al. (2021). Profiling inflammatory cytokines following zinc supplementation: a systematic review and meta-analysis of controlled trials. Br. J. Nutr. 126, 1441–1450.
Faghfouri, A. H., Zarrin, R., Maleki, V., Payahoo, L., and Khajebishak, Y. (2020). A comprehensive mechanistic review insight into the effects of micronutrients on toll-like receptors functions. Pharmacol. Res. 152:104619. doi: 10.1016/j.phrs.2019.104619
Faresjö, A., Grodzinsky, E., Johansson, S., Wallander, M. A., Timpka, T., and Akerlind, I. (2007). Psychosocial factors at work and in every day life are associated with irritable bowel syndrome. Eur. J. Epidemiol. 22, 473–480. doi: 10.1007/s10654-007-9133-2
Farsi, Y., Tahvildari, A., Arbabi, M., Vazife, F., Sechi, L. A., Shahidi Bonjar, A. H., et al. (2022). Diagnostic, prognostic, and therapeutic roles of gut microbiota in COVID-19: a comprehensive systematic review. Front. Cell. Infect. Microbiol. 12:804644. doi: 10.3389/fcimb.2022.804644
Fasano, A. (2011). Zonulin and its regulation of intestinal barrier function: the biological door to inflammation, autoimmunity, and cancer. Physiol. Rev. 91, 151–175. doi: 10.1152/physrev.00003.2008
Finlay, B. B., Amato, K. R., Azad, M., Blaser, M. J., Bosch, T. C. G., Chu, H., et al. (2021). The hygiene hypothesis, the COVID pandemic, and consequences for the human microbiome. Proc. Natl. Acad. Sci. U.S.A. 118: e2010217118.
Freedberg, D. E., and Chang, L. (2022). Gastrointestinal symptoms in COVID-19: the long and the short of it. Curr. Opin. Gastroenterol. 38, 555–561. doi: 10.1097/MOG.0000000000000876
Gaibani, P., D’amico, F., Bartoletti, M., Lombardo, D., Rampelli, S., Fornaro, G., et al. (2021). The gut microbiota of critically Ill patients with COVID-19. Front. Cell. Infect. Microbiol. 11:670424. doi: 10.3389/fcimb.2021.670424
Gasmi, A., Noor, S., Menzel, A., Khanyk, N., Semenova, Y., Lysiuk, R., et al. (2023). Potential drugs in COVID-19 management. Curr. Med. Chem. doi: 10.2174/0929867331666230717154101
Genedi, M., Janmaat, I. E., Haarman, B., and Sommer, I. E. C. (2019). Dysregulation of the gut-brain axis in schizophrenia and bipolar disorder: probiotic supplementation as a supportive treatment in psychiatric disorders. Curr. Opin. Psychiatry 32, 185–195. doi: 10.1097/YCO.0000000000000499
Giron, L. B., Dweep, H., Yin, X., Wang, H., Damra, M., Goldman, A. R., et al. (2021). Plasma markers of disrupted gut permeability in severe COVID-19 patients. Front. Immunol. 12:686240. doi: 10.3389/fimmu.2021.686240
Gou, W., Fu, Y., Yue, L., Chen, G. D., Cai, X., Shuai, M., et al. (2021). Gut microbiota, inflammation, and molecular signatures of host response to infection. J. Genet. Genomics 48, 792–802.
Gu, S., Chen, Y., Wu, Z., Chen, Y., Gao, H., Lv, L., et al. (2020). Alterations of the gut microbiota in patients with coronavirus disease 2019 or H1N1 influenza. Clin. Infect. Dis. 71, 2669–2678.
Guan, W. J., and Zhong, N. S. (2020). Clinical characteristics of Covid-19 in China. reply. N. Engl. J. Med. 382, 1861–1862.
Guo, Y., Wang, B., Gao, H., Gao, L., Hua, R., and Xu, J. D. (2021). ACE2 in the gut: the center of the 2019-nCoV infected pathology. Front. Mol. Biosci. 8:708336. doi: 10.3389/fmolb.2021.708336
Gutiérrez-Castrellón, P., Gandara-Martí, T., Abreu, Y. a. a. T., Nieto-Rufino, C. D., López-Orduña, E., Jiménez-Escobar, I., et al. (2022). Probiotic improves symptomatic and viral clearance in Covid19 outpatients: a randomized, quadruple-blinded, placebo-controlled trial. Gut Microbes 14:2018899. doi: 10.1080/19490976.2021.2018899
Hajipour, A., Afsharfar, M., Jonoush, M., Ahmadzadeh, M., Gholamalizadeh, M., Hassanpour Ardekanizadeh, N., et al. (2022). The effects of dietary fiber on common complications in critically ill patients; with a special focus on viral infections; a systematic reveiw. Immun. Inflamm. Dis. 10:e613. doi: 10.1002/iid3.613
Hakim, A., Hasan, M. M., Hasan, M., Lokman, S. M., Azim, K. F., Raihan, T., et al. (2021). Major insights in dynamics of host response to SARS-CoV-2: impacts and challenges. Front. Microbiol. 12:637554. doi: 10.3389/fmicb.2021.637554
Han, C., Duan, C., Zhang, S., Spiegel, B., Shi, H., Wang, W., et al. (2020). Digestive symptoms in COVID-19 patients with mild disease severity: clinical presentation, stool viral RNA testing, and outcomes. Am. J. Gastroenterol. 115, 916–923. doi: 10.14309/ajg.0000000000000664
Han, Y., Duan, X., Yang, L., Nilsson-Payant, B. E., Wang, P., Duan, F., et al. (2021). Identification of SARS-CoV-2 inhibitors using lung and colonic organoids. Nature 589, 270–275. doi: 10.1038/s41586-020-2901-9
Hashimoto, T., Perlot, T., Rehman, A., Trichereau, J., Ishiguro, H., Paolino, M., et al. (2012). ACE2 links amino acid malnutrition to microbial ecology and intestinal inflammation. Nature 487, 477–481.
Heinen, A., Varghese, S., Krayem, A., and Molodynski, A. (2022). Understanding health anxiety in the COVID-19 pandemic. Int. J. Soc. Psychiatry 68, 1756–1763.
Hoffmann, M., Kleine-Weber, H., Schroeder, S., Krüger, N., Herrler, T., Erichsen, S., et al. (2020). SARS-CoV-2 cell entry depends on ACE2 and TMPRSS2 and is blocked by a clinically proven protease inhibitor. Cell 181, 271–280.e278.
Howell, M. C., Green, R., Mcgill, A. R., Dutta, R., Mohapatra, S., and Mohapatra, S. S. (2021). SARS-CoV-2-induced gut microbiome dysbiosis: implications for colorectal cancer. Cancers (Basel) 13:2676. doi: 10.3390/cancers13112676
Hu, J., Zhang, L., Lin, W., Tang, W., Chan, F. K. L., and Ng, S. C. (2021). Review article: probiotics, prebiotics and dietary approaches during COVID-19 pandemic. Trends Food Sci. Technol. 108, 187–196.
Huang, C., Wang, Y., Li, X., Ren, L., Zhao, J., Hu, Y., et al. (2020). Clinical features of patients infected with 2019 novel coronavirus in Wuhan, China. Lancet 395, 497–506.
Hughes, S., Troise, O., Donaldson, H., Mughal, N., and Moore, L. S. P. (2020). Bacterial and fungal coinfection among hospitalized patients with COVID-19: a retrospective cohort study in a UK secondary-care setting. Clin. Microbiol. Infect. 26, 1395–1399. doi: 10.1016/j.cmi.2020.06.025
Hui, W., Li, T., Liu, W., Zhou, C., and Gao, F. (2019). Fecal microbiota transplantation for treatment of recurrent C. difficile infection: An updated randomized controlled trial meta-analysis. PLoS One 14:e0210016. doi: 10.1371/journal.pone.0210016
Ianiro, G., Bibbó, S., Masucci, L., Quaranta, G., Porcari, S., Settanni, C. R., et al. (2020a). Maintaining standard volumes, efficacy and safety, of fecal microbiota transplantation for C. difficile infection during the COVID-19 pandemic: A prospective cohort study. Dig. Liver Dis. 52, 1390–1395.
Ianiro, G., Mullish, B. H., Kelly, C. R., Kassam, Z., Kuijper, E. J., Ng, S. C., et al. (2020b). Reorganisation of faecal microbiota transplant services during the COVID-19 pandemic. Gut 69, 1555–1563.
Joshee, S., Vatti, N., and Chang, C. (2022). Long-term effects of COVID-19. Mayo Clin. Proc. 97, 579–599.
Juul, F. E., Garborg, K., Bretthauer, M., Skudal, H., Øines, M. N., Wiig, H., et al. (2018). Fecal microbiota transplantation for primary Clostridium difficile infection. N. Engl. J. Med. 378, 2535–2536.
Kalantar-Zadeh, K., Ward, S. A., Kalantar-Zadeh, K., and El-Omar, E. M. (2020). Considering the effects of microbiome and diet on SARS-CoV-2 infection: nanotechnology roles. ACS Nano 14, 5179–5182.
Kawaratani, H., Tsujimoto, T., Douhara, A., Takaya, H., Moriya, K., Namisaki, T., et al. (2013). The effect of inflammatory cytokines in alcoholic liver disease. Mediators Inflamm. 2013:495156.
Keita, A. V., Söderholm, J. D., and Ericson, A. C. (2010). Stress-induced barrier disruption of rat follicle-associated epithelium involves corticotropin-releasing hormone, acetylcholine, substance P, and mast cells. Neurogastroenterol. Motil. 22, 770–778, e221–e222. doi: 10.1111/j.1365-2982.2010.01471.x
Khan, M., Mathew, B. J., Gupta, P., Garg, G., Khadanga, S., Vyas, A. K., et al. (2021). Gut dysbiosis and IL-21 response in patients with severe COVID-19. Microorganisms 9:1292. doi: 10.3390/microorganisms9061292
Kühbacher, T., Ott, S. J., Helwig, U., Mimura, T., Rizzello, F., Kleessen, B., et al. (2006). Bacterial and fungal microbiota in relation to probiotic therapy (VSL#3) in pouchitis. Gut 55, 833–841. doi: 10.1136/gut.2005.078303
Kumar, S., De Souza, R., Nadkar, M., Guleria, R., Trikha, A., Joshi, S. R., et al. (2021). A two-arm, randomized, controlled, multi-centric, open-label phase-2 study to evaluate the efficacy and safety of Itolizumab in moderate to severe ARDS patients due to COVID-19. Expert Opin. Biol. Ther. 21, 675–686. doi: 10.1080/14712598.2021.1905794
Lamers, M. M., Beumer, J., Van Der Vaart, J., Knoops, K., Puschhof, J., Breugem, T. I., et al. (2020). SARS-CoV-2 productively infects human gut enterocytes. Science 369, 50–54.
Lei, W. T., Shih, P. C., Liu, S. J., Lin, C. Y., and Yeh, T. L. (2017). Effect of probiotics and prebiotics on immune response to influenza vaccination in adults: a systematic review and meta-analysis of randomized controlled trials. Nutrients 9:1175. doi: 10.3390/nu9111175
Li, L., Wu, Y., Wang, J., Yan, H., Lu, J., Wang, Y., et al. (2022). Potential treatment of COVID-19 with traditional Chinese medicine: what herbs can help win the battle with SARS-CoV-2? Engineering (Beijing) 19, 139–152. doi: 10.1016/j.eng.2021.08.020
Li, Q., Cheng, F., Xu, Q., Su, Y., Cai, X., Zeng, F., et al. (2021). The role of probiotics in coronavirus disease-19 infection in Wuhan: a retrospective study of 311 severe patients. Int. Immunopharmacol. 95:107531. doi: 10.1016/j.intimp.2021.107531
Li, S., Yang, S., Zhou, Y., Disoma, C., Dong, Z., Du, A., et al. (2021). Microbiome profiling using shotgun metagenomic sequencing identified unique microorganisms in COVID-19 patients with altered gut microbiota. Front. Microbiol. 12:712081. doi: 10.3389/fmicb.2021.712081
Li, Y., Zeng, Z., Li, Y., Huang, W., Zhou, M., Zhang, X., et al. (2015). Angiotensin-converting enzyme inhibition attenuates lipopolysaccharide-induced lung injury by regulating the balance between angiotensin-converting enzyme and angiotensin-converting enzyme 2 and inhibiting mitogen-activated protein kinase activation. Shock 43, 395–404. doi: 10.1097/SHK.0000000000000302
Liang, W., Feng, Z., Rao, S., Xiao, C., Xue, X., Lin, Z., et al. (2020). Diarrhoea may be underestimated: a missing link in 2019 novel coronavirus. Gut 69, 1141–1143.
Lin, L., Jiang, X., Zhang, Z., Huang, S., Zhang, Z., Fang, Z., et al. (2020). Gastrointestinal symptoms of 95 cases with SARS-CoV-2 infection. Gut 69, 997–1001.
Liu, F., Ye, S., Zhu, X., He, X., Wang, S., Li, Y., et al. (2021). Gastrointestinal disturbance and effect of fecal microbiota transplantation in discharged COVID-19 patients. J. Med. Case Rep. 15:60.
Liu, Q., Mak, J. W. Y., Su, Q., Yeoh, Y. K., Lui, G. C., Ng, S. S. S., et al. (2022). Gut microbiota dynamics in a prospective cohort of patients with post-acute COVID-19 syndrome. Gut 71, 544–552. doi: 10.1136/gutjnl-2021-325989
Liu, Y., Yang, Y., Zhang, C., Huang, F., Wang, F., Yuan, J., et al. (2020). Clinical and biochemical indexes from 2019-nCoV infected patients linked to viral loads and lung injury. Sci. China Life Sci. 63, 364–374. doi: 10.1007/s11427-020-1643-8
Llorens, S., Nava, E., Muñoz-López, M., Sánchez-Larsen, Á., and Segura, T. (2021). Neurological symptoms of COVID-19: the zonulin hypothesis. Front. Immunol. 12:665300. doi: 10.3389/fimmu.2021.665300
Lu, R., Zhao, X., Li, J., Niu, P., Yang, B., Wu, H., et al. (2020). Genomic characterisation and epidemiology of 2019 novel coronavirus: implications for virus origins and receptor binding. Lancet 395, 565–574. doi: 10.1016/S0140-6736(20)30251-8
Luo, Y., Xue, Y., Mao, L., Yuan, X., Lin, Q., Tang, G., et al. (2020). Prealbumin as a predictor of prognosis in patients with coronavirus disease 2019. Front. Med. (Lausanne) 7:374. doi: 10.3389/fmed.2020.00374
Lynn, D. J., Benson, S. C., Lynn, M. A., and Pulendran, B. (2022). Modulation of immune responses to vaccination by the microbiota: implications and potential mechanisms. Nat. Rev. Immunol. 22, 33–46.
Ma, C., Cong, Y., and Zhang, H. (2020). COVID-19 and the digestive system. Am. J. Gastroenterol. 115, 1003–1006.
Mack, D. R., Ahrne, S., Hyde, L., Wei, S., and Hollingsworth, M. A. (2003). Extracellular MUC3 mucin secretion follows adherence of Lactobacillus strains to intestinal epithelial cells in vitro. Gut 52, 827–833. doi: 10.1136/gut.52.6.827
Majidi, N., Bahadori, E., Shekari, S., Gholamalizadeh, M., Tajadod, S., Ajami, M., et al. (2022). Effects of supplementation with low-dose group B vitamins on clinical and biochemical parameters in critically ill patients with COVID-19: a randomized clinical trial. Expert Rev. Anti Infect. Ther. 1–7. doi: 10.1080/14787210.2022.2125867
Majidi, N., Rabbani, F., Gholami, S., Gholamalizadeh, M., Bourbour, F., Rastgoo, S., et al. (2021). The effect of vitamin C on pathological parameters and survival duration of critically Ill coronavirus disease 2019 patients: a randomized clinical trial. Front. Immunol. 12:717816. doi: 10.3389/fimmu.2021.717816
Majumder, J., and Minko, T. (2021). Recent developments on therapeutic and diagnostic approaches for COVID-19. AAPS J. 23:14.
Mak, J. W. Y., Chan, F. K. L., and Ng, S. C. (2020). Probiotics and COVID-19: one size does not fit all. Lancet Gastroenterol. Hepatol. 5, 644–645.
Mansell, V., Hall Dykgraaf, S., Kidd, M., and Goodyear-Smith, F. (2022). Long COVID and older people. Lancet Healthy Longev. 3, e849–e854.
Manzoor, R., Ahmed, W., Afify, N., Memon, M., Yasin, M., Memon, H., et al. (2022). Trust your gut: the association of gut microbiota and liver disease. Microorganisms 10:1045. doi: 10.3390/microorganisms10051045
Marotz, C., Belda-Ferre, P., Ali, F., Das, P., Huang, S., Cantrell, K., et al. (2021). SARS-CoV-2 detection status associates with bacterial community composition in patients and the hospital environment. Microbiome 9:132. doi: 10.1186/s40168-021-01083-0
Marsico, C., Capretti, M. G., Aceti, A., Vocale, C., Carfagnini, F., Serra, C., et al. (2022). Severe neonatal COVID-19: challenges in management and therapeutic approach. J. Med. Virol. 94, 1701–1706.
Merino, J., Joshi, A. D., Nguyen, L. H., Leeming, E. R., Mazidi, M., Drew, D. A., et al. (2021). Diet quality and risk and severity of COVID-19: a prospective cohort study. Gut 70, 2096–2104.
Mimura, T., Rizzello, F., Helwig, U., Poggioli, G., Schreiber, S., Talbot, I. C., et al. (2004). Once daily high dose probiotic therapy (VSL#3) for maintaining remission in recurrent or refractory pouchitis. Gut 53, 108–114. doi: 10.1136/gut.53.1.108
Mitrea, L., Nemeş, S. A., Szabo, K., Teleky, B. E., and Vodnar, D. C. (2022). Guts imbalance imbalances the brain: a review of gut microbiota association with neurological and psychiatric disorders. Front. Med. (Lausanne) 9:813204. doi: 10.3389/fmed.2022.813204
Moludi, J., Qaisar, S. A., Alizadeh, M., Jafari Vayghan, H., Naemi, M., Rahimi, A., et al. (2022). The relationship between Dietary Inflammatory Index and disease severity and inflammatory status: a case-control study of COVID-19 patients. Br. J. Nutr. 127, 773–781.
Nair, G. B., and Niederman, M. S. (2021). Updates on community acquired pneumonia management in the ICU. Pharmacol. Ther. 217:107663.
Najmi, N., Megantara, I., Andriani, L., Goenawan, H., and Lesmana, R. (2022). Importance of gut microbiome regulation for the prevention and recovery process after SARS-CoV-2 respiratory viral infection (Review). Biomed. Rep. 16:25. doi: 10.3892/br.2022.1508
Natarajan, A., Zlitni, S., Brooks, E. F., Vance, S. E., Dahlen, A., Hedlin, H., et al. (2022). Gastrointestinal symptoms and fecal shedding of SARS-CoV-2 RNA suggest prolonged gastrointestinal infection. Med 3, 371–387.e379. doi: 10.1016/j.medj.2022.04.001
Newsome, R. C., Gauthier, J., Hernandez, M. C., Abraham, G. E., Robinson, T. O., Williams, H. B., et al. (2021). The gut microbiome of COVID-19 recovered patients returns to uninfected status in a minority-dominated United States cohort. Gut Microbes 13, 1–15. doi: 10.1080/19490976.2021.1926840
Ng, S. C., Peng, Y., Zhang, L., Mok, C. K., Zhao, S., Li, A., et al. (2022). Gut microbiota composition is associated with SARS-CoV-2 vaccine immunogenicity and adverse events. Gut 71, 1106–1116. doi: 10.1136/gutjnl-2021-326563
Ng, T. M., Ong, S. W. X., Loo, A. Y. X., Tan, S. H., Tay, H. L., Yap, M. Y., et al. (2022). Antibiotic therapy in the treatment of COVID-19 pneumonia: who and when? Antibiotics (Basel) 11:184.
Nicholl, B. I., Halder, S. L., Macfarlane, G. J., Thompson, D. G., O’brien, S., Musleh, M., et al. (2008). Psychosocial risk markers for new onset irritable bowel syndrome–results of a large prospective population-based study. Pain 137, 147–155. doi: 10.1016/j.pain.2007.08.029
Ojetti, V., Saviano, A., Covino, M., Acampora, N., Troiani, E., and Franceschi, F. (2020). COVID-19 and intestinal inflammation: role of fecal calprotectin. Dig. Liver Dis. 52, 1231–1233.
Olaimat, A. N., Aolymat, I., Al-Holy, M., Ayyash, M., Abu Ghoush, M., Al-Nabulsi, A. A., et al. (2020). The potential application of probiotics and prebiotics for the prevention and treatment of COVID-19. NPJ Sci. Food 4:17.
Ong, J., Young, B. E., and Ong, S. (2020). COVID-19 in gastroenterology: a clinical perspective. Gut 69, 1144–1145.
Osterlund, P., Ruotsalainen, T., Korpela, R., Saxelin, M., Ollus, A., Valta, P., et al. (2007). Lactobacillus supplementation for diarrhoea related to chemotherapy of colorectal cancer: a randomised study. Br. J. Cancer 97, 1028–1034. doi: 10.1038/sj.bjc.6603990
Palomino-Kobayashi, L. A., Ymaña, B., Ruiz, J., Mayanga-Herrera, A., Ugarte-Gil, M. F., and Pons, M. J. (2022). Zonulin, a marker of gut permeability, is associated with mortality in a cohort of hospitalised peruvian COVID-19 patients. Front. Cell. Infect. Microbiol. 12:1000291. doi: 10.3389/fcimb.2022.1000291
Pan, L., Mu, M., Yang, P., Sun, Y., Wang, R., Yan, J., et al. (2020). Clinical characteristics of COVID-19 patients with digestive symptoms in Hubei, China: a descriptive, cross-sectional, multicenter study. Am. J. Gastroenterol. 115, 766–773. doi: 10.14309/ajg.0000000000000620
Panda, P. K., Bandyopadhyay, A., Singh, B. C., Moirangthem, B., Chikara, G., Saha, S., et al. (2020). Safety and efficacy of antiviral combination therapy in symptomatic patients of Covid-19 infection - a randomised controlled trial (SEV-COVID Trial): a structured summary of a study protocol for a randomized controlled trial. Trials 21:866. doi: 10.1186/s13063-020-04774-5
Parker, A. M., Jackson, N., Awasthi, S., Kim, H., Alwan, T., Wyllie, A. L., et al. (2022). Association of upper respiratory Streptococcus pneumoniae colonization with SARS-CoV-2 infection among adults. Clin. Infect. Dis. ciac907.
Prasad, R., Patton, M. J., Floyd, J. L., Fortmann, S., Dupont, M., Harbour, A., et al. (2022). Plasma microbiome in COVID-19 subjects: an indicator of gut barrier defects and dysbiosis. Int. J. Mol. Sci. 23:9141.
Qian, Q., Fan, L., Liu, W., Li, J., Yue, J., Wang, M., et al. (2021). Direct evidence of active SARS-CoV-2 replication in the intestine. Clin. Infect. Dis. 73, 361–366. doi: 10.1093/cid/ciaa925
Qiao, L., Huang, W., Zhang, X., Guo, H., Wang, D., Feng, Q., et al. (2021). Evaluation of the immunomodulatory effects of anti-COVID-19 TCM formulae by multiple virus-related pathways. Signal Transduct. Target. Ther. 6:50. doi: 10.1038/s41392-021-00475-w
Rahaman, M. M., Sarkar, M. M. H., Rahman, M. S., Islam, M. R., Islam, I., Saha, O., et al. (2022). Genomic characterization of the dominating Beta, V2 variant carrying vaccinated (Oxford-AstraZeneca) and nonvaccinated COVID-19 patient samples in Bangladesh: a metagenomics and whole-genome approach. J. Med. Virol. 94, 1670–1688. doi: 10.1002/jmv.27537
Rajput, S., Paliwal, D., Naithani, M., Kothari, A., Meena, K., and Rana, S. (2021). COVID-19 and gut microbiota: a potential connection. Indian J. Clin. Biochem. 36, 266–277.
Reiff, C., and Kelly, D. (2010). Inflammatory bowel disease, gut bacteria and probiotic therapy. Int. J. Med. Microbiol. 300, 25–33.
Ren, Z., Wang, H., Cui, G., Lu, H., Wang, L., Luo, H., et al. (2021). Alterations in the human oral and gut microbiomes and lipidomics in COVID-19. Gut 70, 1253–1265. doi: 10.1136/gutjnl-2020-323826
Romani, L., Del Chierico, F., Macari, G., Pane, S., Ristori, M. V., Guarrasi, V., et al. (2022). The relationship between pediatric gut microbiota and SARS-CoV-2 infection. Front. Cell. Infect. Microbiol. 12:908492. doi: 10.3389/fcimb.2022.908492
Runfeng, L., Yunlong, H., Jicheng, H., Weiqi, P., Qinhai, M., Yongxia, S., et al. (2020). Lianhuaqingwen exerts anti-viral and anti-inflammatory activity against novel coronavirus (SARS-CoV-2). Pharmacol. Res. 156:104761.
Sartor, R. B. (2006). Mechanisms of disease: pathogenesis of Crohn’s disease and ulcerative colitis. Nat. Clin. Pract. Gastroenterol. Hepatol. 3, 390–407.
Schult, D., Reitmeier, S., Koyumdzhieva, P., Lahmer, T., Middelhoff, M., Erber, J., et al. (2022). Gut bacterial dysbiosis and instability is associated with the onset of complications and mortality in COVID-19. Gut Microbes 14, 2031840. doi: 10.1080/19490976.2022.2031840
Seibert, B., Cáceres, C. J., Cardenas-Garcia, S., Carnaccini, S., Geiger, G., Rajao, D. S., et al. (2021). Mild and severe SARS-CoV-2 infection induces respiratory and intestinal microbiome changes in the K18-hACE2 transgenic mouse model. Microbiol. Spectr. 9:e0053621. doi: 10.1128/Spectrum.00536-21
Sencio, V., Machelart, A., Robil, C., Benech, N., Hoffmann, E., Galbert, C., et al. (2022). Alteration of the gut microbiota following SARS-CoV-2 infection correlates with disease severity in hamsters. Gut Microbes 14:2018900. doi: 10.1080/19490976.2021.2018900
Shchikota, A. M., Pogonchenkova, I. V., Turova, E. A., Starodubova, A. V., and Nosova, N. V. (2021). [COVID-19-associated diarrhea]. Vopr. Pitan. 90, 18–30.
Söderholm, J. D., Yang, P. C., Ceponis, P., Vohra, A., Riddell, R., Sherman, P. M., et al. (2002). Chronic stress induces mast cell-dependent bacterial adherence and initiates mucosal inflammation in rat intestine. Gastroenterology 123, 1099–1108. doi: 10.1053/gast.2002.36019
Soriano, J. B., Murthy, S., Marshall, J. C., Relan, P., and Diaz, J. V. (2022). A clinical case definition of post-COVID-19 condition by a Delphi consensus. Lancet Infect. Dis. 22, e102–e107.
Suez, J., Zmora, N., Segal, E., and Elinav, E. (2019). The pros, cons, and many unknowns of probiotics. Nat. Med. 25, 716–729. doi: 10.1038/s41591-019-0439-x
Sun, Y., Qian, J., Xu, X., Tang, Y., Xu, W., Yang, W., et al. (2018). Dendritic cell-targeted recombinant Lactobacilli induce DC activation and elicit specific immune responses against G57 genotype of avian H9N2 influenza virus infection. Vet. Microbiol. 223, 9–20. doi: 10.1016/j.vetmic.2018.07.009
Sun, Z., Song, Z. G., Liu, C., Tan, S., Lin, S., Zhu, J., et al. (2022). Gut microbiome alterations and gut barrier dysfunction are associated with host immune homeostasis in COVID-19 patients. BMC Med. 20:24. doi: 10.1186/s12916-021-02212-0
Szeto, C. C., Kwan, B. C., Chow, K. M., Lai, K. B., Chung, K. Y., Leung, C. B., et al. (2008). Endotoxemia is related to systemic inflammation and atherosclerosis in peritoneal dialysis patients. Clin. J. Am. Soc. Nephrol. 3, 431–436.
Tang, H., Bohannon, L., Lew, M., Jensen, D., Jung, S. H., Zhao, A., et al. (2021). Randomised, double-blind, placebo-controlled trial of Probiotics To Eliminate COVID-19 Transmission in Exposed Household Contacts (PROTECT-EHC): a clinical trial protocol. BMJ Open 11:e047069. doi: 10.1136/bmjopen-2020-047069
Tang, L., Gu, S., Gong, Y., Li, B., Lu, H., Li, Q., et al. (2020). Clinical significance of the correlation between changes in the major intestinal bacteria species and COVID-19 severity. Engineering (Beijing) 6, 1178–1184. doi: 10.1016/j.eng.2020.05.013
Tankou, S. K., Regev, K., Healy, B. C., Cox, L. M., Tjon, E., Kivisakk, P., et al. (2018). Investigation of probiotics in multiple sclerosis. Mult. Scler. 24, 58–63.
Tao, W., Wang, X., Zhang, G., Guo, M., Ma, H., Zhao, D., et al. (2021). Re-detectable positive SARS-CoV-2 RNA tests in patients who recovered from COVID-19 with intestinal infection. Protein Cell 12, 230–235. doi: 10.1007/s13238-020-00778-8
Tian, Y., Rong, L., Nian, W., and He, Y. (2020). Review article: gastrointestinal features in COVID-19 and the possibility of faecal transmission. Aliment. Pharmacol. Ther. 51, 843–851.
Tian, Y., Sun, K. Y., Meng, T. Q., Ye, Z., Guo, S. M., Li, Z. M., et al. (2021). Gut microbiota may not be fully restored in recovered COVID-19 patients after 3-month recovery. Front. Nutr. 8:638825.
Tomkinson, S., Triscott, C., Schenk, E., and Foey, A. (2023). The potential of probiotics as ingestible adjuvants and immune modulators for antiviral immunity and management of SARS-CoV-2 infection and COVID-19. Pathogens 12:928. doi: 10.3390/pathogens12070928
Triana, S., Metz-Zumaran, C., Ramirez, C., Kee, C., Doldan, P., Shahraz, M., et al. (2021). Single-cell analyses reveal SARS-CoV-2 interference with intrinsic immune response in the human gut. Mol. Syst. Biol. 17:e10232. doi: 10.15252/msb.202110232
Tripathi, A., Debelius, J., Brenner, D. A., Karin, M., Loomba, R., Schnabl, B., et al. (2018). The gut-liver axis and the intersection with the microbiome. Nat. Rev. Gastroenterol. Hepatol. 15, 397–411.
Trompette, A., Gollwitzer, E. S., Pattaroni, C., Lopez-Mejia, I. C., Riva, E., Pernot, J., et al. (2018). Dietary fiber confers protection against flu by shaping Ly6c(-) patrolling monocyte hematopoiesis and CD8(+) T cell metabolism. Immunity 48, 992–1005.e1008. doi: 10.1016/j.immuni.2018.04.022
Trottein, F., and Sokol, H. (2020). Potential causes and consequences of gastrointestinal disorders during a SARS-CoV-2 infection. Cell Rep. 32:107915.
Upadhyay, V., Suryawanshi, R. K., Tasoff, P., Mccavitt-Malvido, M., Kumar, R. G., Murray, V. W., et al. (2023). Mild SARS-CoV-2 infection results in long-lasting microbiota instability. mBio 14:e0088923.
Venzon, M., and Cadwell, K. (2022). COVID-19 and the forgotten organ: prolonged changes to the metabolic output of the gut microbiome. Gastroenterology 162, 394–396. doi: 10.1053/j.gastro.2021.11.017
Verdecchia, P., Cavallini, C., Spanevello, A., and Angeli, F. (2020). The pivotal link between ACE2 deficiency and SARS-CoV-2 infection. Eur. J. Intern. Med. 76, 14–20.
Vestad, B., Ueland, T., Lerum, T. V., Dahl, T. B., Holm, K., Barratt-Due, A., et al. (2022). Respiratory dysfunction three months after severe COVID-19 is associated with gut microbiota alterations. J. Intern. Med. 291, 801–812. doi: 10.1111/joim.13458
Vicario, M., Guilarte, M., Alonso, C., Yang, P., Martínez, C., Ramos, L., et al. (2010). Chronological assessment of mast cell-mediated gut dysfunction and mucosal inflammation in a rat model of chronic psychosocial stress. Brain Behav. Immun. 24, 1166–1175. doi: 10.1016/j.bbi.2010.06.002
Vignesh, R., Swathirajan, C. R., Tun, Z. H., Rameshkumar, M. R., Solomon, S. S., and Balakrishnan, P. (2020). Could perturbation of gut microbiota possibly exacerbate the severity of COVID-19 via cytokine storm? Front. Immunol. 11:607734. doi: 10.3389/fimmu.2020.607734
Villapol, S. (2020). Gastrointestinal symptoms associated with COVID-19: impact on the gut microbiome. Transl. Res. 226, 57–69.
Vodnar, D. C., Mitrea, L., Teleky, B. E., Szabo, K., Cãlinoiu, L. F., Nemeş, S. A., et al. (2020). Coronavirus disease (COVID-19) caused by (SARS-CoV-2) infections: a real challenge for human gut microbiota. Front. Cell. Infect. Microbiol. 10:575559. doi: 10.3389/fcimb.2020.575559
Wada, M., Nagata, S., Saito, M., Shimizu, T., Yamashiro, Y., Matsuki, T., et al. (2010). Effects of the enteral administration of Bifidobacterium breve on patients undergoing chemotherapy for pediatric malignancies. Support. Care Cancer 18, 751–759. doi: 10.1007/s00520-009-0711-6
Wan, Y., Li, J., Shen, L., Zou, Y., Hou, L., Zhu, L., et al. (2020). Enteric involvement in hospitalised patients with COVID-19 outside Wuhan. Lancet Gastroenterol. Hepatol. 5, 534–535. doi: 10.1016/S2468-1253(20)30118-7
Wang, M. Y., Zhao, R., Gao, L. J., Gao, X. F., Wang, D. P., and Cao, J. M. (2020). SARS-CoV-2: structure, biology, and structure-based therapeutics development. Front. Cell. Infect. Microbiol. 10:587269. doi: 10.3389/fcimb.2020.587269
Wang, M., Fu, T., Hao, J., Li, L., Tian, M., Jin, N., et al. (2020). A recombinant Lactobacillus plantarum strain expressing the spike protein of SARS-CoV-2. Int. J. Biol. Macromol. 160, 736–740. doi: 10.1016/j.ijbiomac.2020.05.239
Wang, S., Liu, B., Huang, J., He, H., Zhou, L., He, Y., et al. (2023). Succinate and mitochondrial DNA trigger atopic march from atopic dermatitis to intestinal inflammation. J. Allergy Clin. Immunol. 151, 1050–1066.e1057. doi: 10.1016/j.jaci.2022.11.026
Wang, Y., Wu, G., Zhao, L., and Wang, W. (2022). Nutritional modulation of gut microbiota alleviates severe gastrointestinal symptoms in a patient with post-acute COVID-19 syndrome. mBio 13:e0380121. doi: 10.1128/mbio.03801-21
Wang, Z., Yang, L., and Song, X. Q. (2022). Oral GS-441524 derivatives: next-generation inhibitors of SARS-CoV-2 RNA-dependent RNA polymerase. Front. Immunol. 13:1015355. doi: 10.3389/fimmu.2022.1015355
Wei, D., Heus, P., Van De Wetering, F. T., Van Tienhoven, G., Verleye, L., and Scholten, R. J. (2018). Probiotics for the prevention or treatment of chemotherapy- or radiotherapy-related diarrhoea in people with cancer. Cochrane Database Syst. Rev. 8:Cd008831.
Weng, J., Li, Y., Li, J., Shen, L., Zhu, L., Liang, Y., et al. (2021). Gastrointestinal sequelae 90 days after discharge for COVID-19. Lancet Gastroenterol. Hepatol. 6, 344–346.
Wolff, L., Martiny, D., Deyi, V. Y. M., Maillart, E., Clevenbergh, P., and Dauby, N. (2021). COVID-19-associated Fusobacterium nucleatum Bacteremia, Belgium. Emerg. Infect. Dis. 27, 975–977. doi: 10.3201/eid2703.202284
Wu, Y., Cheng, X., Jiang, G., Tang, H., Ming, S., Tang, L., et al. (2021). Altered oral and gut microbiota and its association with SARS-CoV-2 viral load in COVID-19 patients during hospitalization. NPJ Biofilms Microbiomes 7:61.
Wu, Y., Guo, C., Tang, L., Hong, Z., Zhou, J., Dong, X., et al. (2020). Prolonged presence of SARS-CoV-2 viral RNA in faecal samples. Lancet Gastroenterol. Hepatol. 5, 434–435.
Xiao, F., Tang, M., Zheng, X., Liu, Y., Li, X., and Shan, H. (2020). Evidence for gastrointestinal infection of SARS-CoV-2. Gastroenterology 158, 1831–1833.e1833.
Xu, J., Chen, H. B., and Li, S. L. (2017). Understanding the molecular mechanisms of the interplay between herbal medicines and gut microbiota. Med. Res. Rev. 37, 1140–1185.
Xu, L., Zhang, T., Cui, B., He, Z., Xiang, J., Long, C., et al. (2016). Clinical efficacy maintains patients’ positive attitudes toward fecal microbiota transplantation. Medicine 95:e4055.
Xu, R., Lu, R., Zhang, T., Wu, Q., Cai, W., Han, X., et al. (2021). Temporal association between human upper respiratory and gut bacterial microbiomes during the course of COVID-19 in adults. Commun. Biol. 4:240. doi: 10.1038/s42003-021-01796-w
Xu, X., Zhang, W., Guo, M., Xiao, C., Fu, Z., Yu, S., et al. (2022). Integrated analysis of gut microbiome and host immune responses in COVID-19. Front. Med. 16:263–275. doi: 10.1007/s11684-022-0921-6
Xu, Y., Li, X., Zhu, B., Liang, H., Fang, C., Gong, Y., et al. (2020). Characteristics of pediatric SARS-CoV-2 infection and potential evidence for persistent fecal viral shedding. Nat. Med. 26, 502–505. doi: 10.1038/s41591-020-0817-4
Yang, T., Chakraborty, S., Saha, P., Mell, B., Cheng, X., Yeo, J. Y., et al. (2020). Gnotobiotic rats reveal that gut microbiota regulates colonic mRNA of Ace2, the receptor for SARS-CoV-2 Infectivity. Hypertension 76, e1–e3.
Yeoh, Y. K., Zuo, T., Lui, G. C., Zhang, F., Liu, Q., Li, A. Y., et al. (2021). Gut microbiota composition reflects disease severity and dysfunctional immune responses in patients with COVID-19. Gut 70, 698–706.
Young, B. E., Ong, S. W. X., Kalimuddin, S., Low, J. G., Tan, S. Y., Loh, J., et al. (2020). Epidemiologic features and clinical course of patients infected with SARS-CoV-2 in Singapore. JAMA 323, 1488–1494.
Zabetakis, I., Lordan, R., Norton, C., and Tsoupras, A. (2020). COVID-19: the inflammation link and the role of nutrition in potential mitigation. Nutrients 12:1466. doi: 10.3390/nu12051466
Zeng, F. M., Li, Y. W., Deng, Z. H., He, J. Z., Li, W., Wang, L., et al. (2022). SARS-CoV-2 spike spurs intestinal inflammation via VEGF production in enterocytes. EMBO Mol. Med. 14:e14844. doi: 10.15252/emmm.202114844
Zhang, F., Wan, Y., Zuo, T., Yeoh, Y. K., Liu, Q., Zhang, L., et al. (2022). Prolonged impairment of short-chain fatty acid and L-isoleucine biosynthesis in gut microbiome in patients with COVID-19. Gastroenterology 162, 548–561.e544. doi: 10.1053/j.gastro.2021.10.013
Zhang, P., He, Z., Yu, G., Peng, D., Feng, Y., Ling, J., et al. (2021). The modified NUTRIC score can be used for nutritional risk assessment as well as prognosis prediction in critically ill COVID-19 patients. Clin. Nutr. 40, 534–541. doi: 10.1016/j.clnu.2020.05.051
Zhang, Z., Zhang, G., Guo, M., Tao, W., Liu, X., Wei, H., et al. (2021). The potential role of an aberrant mucosal immune response to SARS-CoV-2 in the pathogenesis of IgA nephropathy. Pathogens 10:881. doi: 10.3390/pathogens10070881
Zhao, L., Zhang, F., Ding, X., Wu, G., Lam, Y. Y., Wang, X., et al. (2018). Gut bacteria selectively promoted by dietary fibers alleviate type 2 diabetes. Science 359, 1151–1156. doi: 10.1126/science.aao5774
Zhong, H., Wang, Y., Shi, Z., Zhang, L., Ren, H., He, W., et al. (2021). Characterization of respiratory microbial dysbiosis in hospitalized COVID-19 patients. Cell Discov. 7:23.
Zhong, P., Xu, J., Yang, D., Shen, Y., Wang, L., Feng, Y., et al. (2020). COVID-19-associated gastrointestinal and liver injury: clinical features and potential mechanisms. Signal Transduct. Target. Ther. 5:256. doi: 10.1038/s41392-020-00373-7
Zhou, J., Li, C., Liu, X., Chiu, M. C., Zhao, X., Wang, D., et al. (2020). Infection of bat and human intestinal organoids by SARS-CoV-2. Nat. Med. 26, 1077–1083.
Zhou, J., Li, C., Zhao, G., Chu, H., Wang, D., Yan, H. H., et al. (2017). Human intestinal tract serves as an alternative infection route for Middle East respiratory syndrome coronavirus. Sci. Adv. 3:eaao4966. doi: 10.1126/sciadv.aao4966
Zhu, N., Zhang, D., Wang, W., Li, X., Yang, B., Song, J., et al. (2020). A novel coronavirus from patients with pneumonia in China, 2019. N. Engl. J. Med. 382, 727–733.
Zollner, A., Koch, R., Jukic, A., Pfister, A., Meyer, M., Rössler, A., et al. (2022). Postacute COVID-19 is characterized by gut viral antigen persistence in inflammatory bowel diseases. Gastroenterology 163, 495–506.e498. doi: 10.1053/j.gastro.2022.04.037
Zou, X., Chen, K., Zou, J., Han, P., Hao, J., and Han, Z. (2020). Single-cell RNA-seq data analysis on the receptor ACE2 expression reveals the potential risk of different human organs vulnerable to 2019-nCoV infection. Front. Med. 14:185–192. doi: 10.1007/s11684-020-0754-0
Zuo, T., Liu, Q., Zhang, F., Lui, G. C., Tso, E. Y., Yeoh, Y. K., et al. (2021). Depicting SARS-CoV-2 faecal viral activity in association with gut microbiota composition in patients with COVID-19. Gut 70, 276–284. doi: 10.1136/gutjnl-2020-322294
Zuo, T., Zhang, F., Lui, G. C. Y., Yeoh, Y. K., Li, A. Y. L., Zhan, H., et al. (2020b). Alterations in gut microbiota of patients with COVID-19 during time of hospitalization. Gastroenterology 159, 944–955.e948.
Keywords: long-term effects of COVID-19 (long-COVID), SARS-CoV-2, gastrointestinal symptoms, prognosis, therapeutics, gut microbiota, immune responses
Citation: He K-Y, Lei X-Y, Zhang L, Wu D-H, Li J-Q, Lu L-Y, Laila UE, Cui C-Y, Xu Z-X and Jian Y-P (2023) Development and management of gastrointestinal symptoms in long-term COVID-19. Front. Microbiol. 14:1278479. doi: 10.3389/fmicb.2023.1278479
Received: 16 August 2023; Accepted: 20 November 2023;
Published: 14 December 2023.
Edited by:
Jaffar Al-Tawfiq, Johns Hopkins Aramco Healthcare (JHAH), Saudi ArabiaReviewed by:
François Trottein, Centre National de la Recherche Scientifique (CNRS), FranceSonia Villapol, Houston Methodist Research Institute, United States
Copyright © 2023 He, Lei, Zhang, Wu, Li, Lu, Laila, Cui, Xu and Jian. This is an open-access article distributed under the terms of the Creative Commons Attribution License (CC BY). The use, distribution or reproduction in other forums is permitted, provided the original author(s) and the copyright owner(s) are credited and that the original publication in this journal is cited, in accordance with accepted academic practice. No use, distribution or reproduction is permitted which does not comply with these terms.
*Correspondence: Yong-Ping Jian, eW9uZ3BpbmdqaWFuMTIzQDE2My5jb20=; Zhi-Xiang Xu, emhpeGlhbmd4dTA4QGdtYWlsLmNvbQ==
†These authors have contributed equally to this work