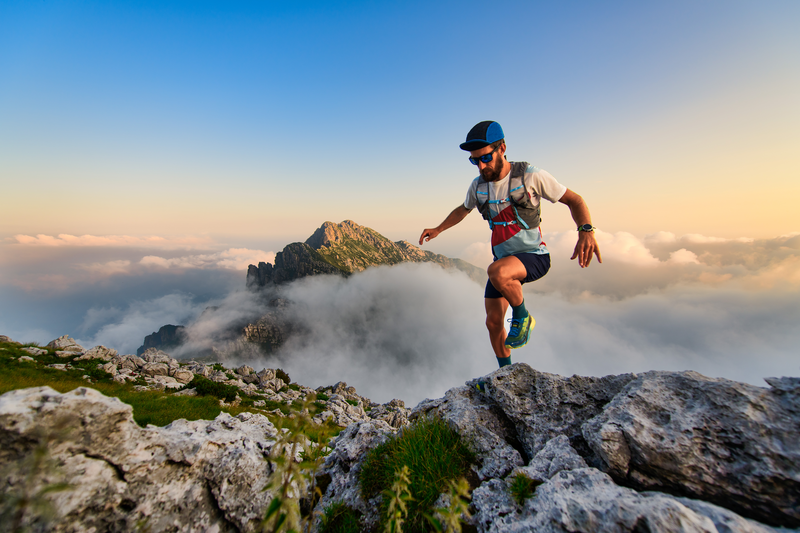
95% of researchers rate our articles as excellent or good
Learn more about the work of our research integrity team to safeguard the quality of each article we publish.
Find out more
ORIGINAL RESEARCH article
Front. Microbiol. , 22 September 2023
Sec. Antimicrobials, Resistance and Chemotherapy
Volume 14 - 2023 | https://doi.org/10.3389/fmicb.2023.1273397
This article is part of the Research Topic Systems Biology and Antimicrobial Drug Resistance View all 19 articles
Staphylococcus aureus (S. aureus) is an important zoonotic pathogen that causes a high incidence rate and mortality worldwide. This study investigated the prevalence of methicillin-resistant Staphylococcus aureus (MRSA) strains in a tertiary A hospital in Shanxi Province, China, in order to determine the major epidemic clones as well as their antibiotic resistance and virulence characteristics. A total of 212 S. aureus strains were collected in this hospital, and were subjected to antimicrobial susceptibility testing, detection of virulence genes, resistance genes, and efflux pump genes. Among them, 38 MRSA strains were further subjected to detection of biofilm genes, assessment of biofilm formation ability, MLST, spa typing, SCCmec typing, and phylogenetic analysis. The majority of S. aureus strains came from the neonatology department, with secretions and purulent fluid being the main source of samples. The strains showed high resistance to penicillin (98.11%), erythromycin (64.62%) and clindamycin (59.91%), while being sensitive to vancomycin and linezolid. The detection rates of efflux pump genes and resistance genes were high, and there was a significant correlation between resistance gene types and phenotypes, with mecA showing a close correlation with oxacillin. The detection rates of virulence genes and the toxin gene profiles of MSSA and MRSA strains showed significant differences. And the detection rate of biofilm genes in MRSA strains was relatively high, with 13.16% of MRSA strains showing strong biofilm formation ability. The most common epidemic clone of MRSA was ST59-SCCmecIV-t437, followed by ST59-SCCmecV-t437. The former had a higher detection rate of resistance genes and a stronger biofilm formation ability, while the latter had a higher positive rate for pvl gene and stronger pathogenicity, making it more likely to cause systemic infections. Phylogenetic analysis showed that all MRSA strains in this study clustered into three major branches, with distinct differences in antibiotic resistance and virulence characteristics among the branches. ST59-MRSA strains from different species showed consistency and inter-species transmission, but there were differences among ST59-MRSA strains from different geographical locations. In general, most MSSA and MRSA strains exhibited multidrug resistance and carried multiple resistance genes, virulence genes, and biofilm formation genes, warranting further research to elucidate the mechanisms of drug resistance and pathogenesis.
Staphylococcus aureus (S. aureus) is a widely distributed Gram-positive pathogenic bacterium that gets its name from the yellow grape-like colonies on culture media (Algammal et al., 2020b). It colonizes various parts of the human body, such as the nasal cavity, skin, throat, and digestive system, and is a major cause of endocarditis, bacteremia, osteomyelitis, and skin and soft tissue infections (Turner et al., 2019). The pathogenicity of S. aureus primarily stems from the production of exotoxins, including paton-valentine leukocidin (PVL), hemolysins, staphylococcal enterotoxins (SEs), adhesins, exfoliative toxins, etc., which enter the host’s body and cause disease through adhesion and invasion (Oliveira et al., 2018). SEs are the most common exotoxins and include sea-see, seg-sej, sel-seq, ser-set, selk-selq, selu-selx; they can disrupt intestinal functions and lead to food poisoning, often causing clinical symptoms of nausea, vomiting, abdominal pain, and diarrhea. PVL is mainly composed of two proteins encoded by the lukS-PV and lukF-PV genes, and it targets phagocytes, lymphocytes and natural killer cells, causing skin and soft tissue infections and severe necrotizing pneumonia (Bennett and Thomsen, 2020).
Due to the high pathogenicity of S. aureus, antibiotics have been widely used in clinical practice to treat S. aureus infections. However, just 2 years after the introduction of methicillin, methicillin-resistant Staphylococcus aureus (MRSA) emerged (Barber, 1961). Since then, MRSA strains have spread extensively in countries such as Europe and America, transitioning from hospitals to communities. MRSA has become an important pathogen causing both hospital-acquired and community-acquired infections and has evolved to some strains which develop resistance to virtually all clinically available antibiotics (Mlynarczyk-Bonikowska et al., 2022). MRSA is resistant to almost all β-lactam antibiotics, mainly due to the acquisition of a resistant genomic island called staphylococcal cassette chromosome (SCC) element carrying the mecA/mecC gene (SCCmec). The SCCmec element is a mobile genetic element that inserts into the chromosome of susceptible strains, resulting in the production of penicillin-binding protein (PBP2a/2c) and significantly reducing their affinity for β-lactam antibiotics, thus conferring resistance (Yamaguchi et al., 2020). Additionally, multidrug-resistant MRSA strains have acquired numerous resistance genes, which further enhance their resistance complexity and can be transmitted between different strains and species through mobile elements (Monaco et al., 2017).
As the understanding of the constantly changing epidemiology of MRSA increases, there is a need for rapid and reliable methods to characterize isolates to aid in investigating clone dissemination. The main methods for MRSA genotyping include pulsed field gel electrophoresis (PFGE), multilocus sequence typing (MLST), SCCmec typing, and spa typing. PFGE typing has high resolution, stable and reproducible results, and can effectively reflect the correlation between bacterial genotype and epidemiology. It is considered the “gold standard” for bacterial genotyping (He and Reed, 2020). MLST is based on the differences in seven housekeeping genes of S. aureus. This technique has high accuracy, good reproducibility, and allows typing results to be uploaded to public databases for data sharing and comparison, which is important for understanding strain epidemiological characteristics and genetic evolutionary relationships (Liu and Ji, 2020). SCCmec typing is based on the differences in mec gene complex and cassette chromosome recombinase (ccr) gene complex. It has been certified by the international working group on classification of SCC components and is divided into 14 types, SCCmec I - SCCmec XIV (Uehara, 2022). spa typing is a sequencing typing method based on the polymorphism of the X region sequence of spa gene, which encodes staphylococcal protein A. This method is fast, reproducible, and has high resolution, indicating both micro and macro variations, making it suitable for local and global epidemiological studies (Wang, 2020).
MRSA strains exhibit genetic diversity, and the main feature of their molecular epidemiology is the continuous emergence of regionally prevalent strains. The prevalence, drug resistance characteristics, virulence characteristics, and epidemic clones of MRSA vary in different regions and change over time. However, there were few reports on the molecular epidemiological characteristics of MRSA in Shanxi Province, China. Therefore, this study focused on 212 strains of S. aureus isolated from a tertiary A hospital in Shanxi Province. We investigated the colonization and departmental distribution of the strains, tested their resistance to commonly used clinical antibiotics, as well as the carriage rate of resistance genes, efflux pump genes and virulence genes. Molecular genetic methods such as SCCmec typing, MLST, spa typing, and whole-genome sequencing were used to genotype the MRSA isolates from different sources and perform comparative genomic analysis. This study provided initial insights into the prevalence characteristics, drug resistance, and genetic polymorphism of healthcare-associated MRSA in Shanxi Province, revealing the molecular features and evolutionary relationships of MRSA strains. It also provided a theoretical basis for the clinical treatment of MRSA infections and technical support for the tracking of healthcare-associated MRSA infections.
From May 2021 to October 2022, a total of 212 non-duplicate strains of S. aureus were collected and isolated from a tertiary A hospital in Shanxi Province. S. aureus isolates were recovered from inpatients and outpatients who had cough, fever, skin abscess, and other clinical symptoms related to infection. These strains included all the isolates of S. aureus obtained from patients during this period at this hospital. The identification of the strains was performed using matrix-assisted laser desorption/ionization time-of-flight mass spectrometry (MALDI-TOF/TOF) technology. The resistance to oxacillin was determined through antibiotic susceptibility testing, and the presence of the mecA gene was confirmed by PCR, identifying 38 strains as MRSA. This study was approved by the Ethics Committee of Fenyang College, Shanxi Medical University. Being a retrospective study, informed consent was not required.
All strains were subjected to antimicrobial susceptibility testing according to the methods recommended by the Clinical and Laboratory Standards Institute (CLSI, 2015). The VITEK2-compact automated antimicrobial susceptibility analyzer was used to determine the minimum inhibitory concentrations of the strains against 12 antibiotics, including penicllin (10 U), erythromycin (15 μg), clindamycin (2 μg), oxacillin (1 μg), sulfamethoxazole (25 μg), tetracycline (30 μg), gentamicin (10 μg), levofloxacin (5 μg), rifampicin (5 μg), nitrofurantoin (300 μg), vancomycin (30 μg), linezolid (30 μg). ATCC29213 was used as the quality control strain.
PCR was performed to detect the positive rates of various genes, including 8 efflux pump genes (Zhang Hang et al., 2019), 17 resistance genes (Merlino et al., 2002; Chen Cheng et al., 2020), 22 virulence genes (Brakstad et al., 1992; Chen Cheng et al., 2020), and 11 biofilm-related genes (Waryah et al., 2016). The primers and PCR programs for amplification of the relevant genes were performed according to the primer sequences and PCR procedures listed in Supplementary Table S1. After the reaction, 6 × DNA loading buffer was added to the PCR products, and 20 μL of the mixture was loaded onto a 1.5% agarose gel for electrophoresis at 110 V for 40 min. The gel was visualized and images were captured using a UV gel imaging system. The positive rates of each gene were calculated.
100 μL of the MRSA strains, stored at −80°C, were added to 10 mL of TSB medium and incubated at 37°C with shaking at 220 rpm for 12 h. Then, 100 μL of the cultured bacterial suspension was added to 10 mL of 0.5% TSBG medium and mixed well. Next, 200 μL of the bacterial suspension was added to each well of a 96-well plate, with each strain occupying 5 wells. The plate was then incubated at 37°C for 24 h. After incubation, the liquid and planktonic bacteria were discarded by adding 200 μL of PBS buffer to each well and washing it three times. The wells were then stained with 100 μL of crystal violet dye and incubated for 30 min. After removing the dye, each well was washed three times with 200 μL of PBS buffer. Finally, 200 μL of 95% ethanol was added to each well, and the optical density at 560 nm was measured within 30 min. TSBG medium served as the negative control, and the critical optical density value was defined as ODc (mean OD of the negative control plus three times its standard deviation). The ability to form biofilms was classified into four categories: OD ≤ ODc, no biofilm formation (−); ODc<OD ≤ 2ODc, weak biofilm formation (+); 2ODc<OD ≤ 4ODc, moderate biofilm formation (++); 4ODc<OD, strong biofilm formation (+++).
The genomes of a total of 38 MRSA strains were sequenced at the Beijing Genomics Institute (BGI, Shenzhen, China) using a PacBio Sequel II and DNBSEQ platform. The PacBio platform utilized four SMRT cells Zero-Mode Waveguide arrays for sequencing, and the resulting subreads set was generated. Subreads were repaired and the Canu program was employed for self-correction.
The assembled contigs were used for molecular typing, including MLST, spa-typing and SCCmec typing. These typings were predicted using the Center for Genomic Epidemiology website.1 For function annotation, the WGS data was annotated some databases, including VFDB (for virulence genes) (Chen et al., 2016), ARDB, CARD (for resistance genes) (Alcock et al., 2020) and COG (Galperin et al., 2015). Analyze the Core/Pan genes of all MRSA strains, as well as the COG functions of these genes. Cluster these strains based on Core/Pan genes, and construct the phylogenetic tree using the TreeBeST with the NJ method. Then 20 strains of ST59 MRSA strains were selected from NCBI, and these strains were compared with ST59 strains in this study to build a phylogenetic tree. The production and processing of images, such as heat maps, box plots, phylogenetic trees and so on, have been utilized on the chiplot online website.2
Statistical analyses were performed using Graphpad Pism 9.5 for Windows. Chi-squared test was used for rate analysis, and Pearson correlation test was used for consistency analysis. The p < 0.05 was considered to indicate statistical significance. *p ≤ 0.05; **p ≤ 0.01; ***p ≤ 0.001; ****p ≤ 0.0001.
A total of 38 out of the 212 identified S. aureus strains were confirmed to be MRSA (Table 1). These strains were sourced from various departments, with both methicillin-susceptible S. aureus (MSSA) and MRSA mainly originating from the neonatology department (31/174, 17.82%; 9/38, 23.68%). For MSSA, children (53/174, 30.46%), middle-aged individuals (59/174, 33.91%), and the elderly (54/174, 31.03%) were the predominant affected populations. On the other hand, MRSA primarily affected children (19/38, 50.00%) and the elderly (10/38, 26.32%). The main sources of the strains were secretions and purulent fluid, with 23 and 11 MRSA cases originating from secretions and purulent fluid, respectively. The remaining 4 cases were from sputum, throat swabs, vaginal swabs, and tissue samples. Statistical analysis showed significant differences in age and sample type, in addition to gender and department distribution. Detailed information on the MRSA strains can be found in Supplementary Table S2.
In this study, S. aureus exhibited high resistance rates to penicillin (208/212, 98.11%), erythromycin (137/212, 64.62%), and clindamycin (127/212, 59.91%). It showed sensitivity to vancomycin and linezolid. Oxacillin, sulfamethoxazole, tetracycline, gentamicin, levofloxacin, rifampicin, and nitrofurantoin demonstrated good activity against S. aureus, with resistance rates ranging from 0.5 to 17% (Figure 1A). Multiple drug resistance was observed in 57.47% of the MSSA strains and 81.58% of the MRSA strains. One MSSA strain exhibited resistance to all seven antibiotics tested, while 26/38 MRSA strains demonstrated resistance to four or five antibiotics (Figure 1B). Detection of efflux pump genes revealed high detection rates for all genes except qacA/B and smr, which were not detected in MRSA strains. The detection rates for norA, norB, norC, mepA, and mdeA genes in MRSA strains were 100%, slightly higher than their detection rates in MSSA strains (Figure 1C). The most common efflux pump gene profile in all strains was norA-norB-norC-sepA-mepA-mdeA (120/212, 56.60%). Detection of resistance genes showed that among the 37 oxacillin-resistant strains, 24 were mecA-positive, while one strain was sensitive to oxacillin but tested positive for the mecA gene. In all strains, quinolone-related genes gyrA and grlA (174/174, 100%; 32/38, 84.21%), and β-lactam-related gene blaZ (142/174, 81.61%; 31/38, 81.58%) exhibited the highest detection rates. But tet(O) and mecC were not detected in any of the strains (Figure 1D). The most common resistance gene profile in all strains was blaZ-ermC-gyrA-grlA (51/212, 24.06%). Consistency analysis of all efflux pump genes, resistance genes, and antibiotic detection results revealed a significant correlation between resistance genotypes and phenotypes, showing a positive correlation. When the Pearson correlation coefficient is greater than 0.4, it indicated a strong correlation. The closely related correlations found in this study were mecA and oxacillin (r = 0.7378), aacA-aphD and gentamicin (r = 0.6943), aacA-aphD and sulfamethoxazole (r = 0.598), ermB and gentamicin (r = 0.4772), ermB and sulfamethoxazole (r = 0.4127) (Figure 1E).
Figure 1. The drug resistance characteristics of S. aureus isolates. (A) The antibiotic susceptibility testing of all strains. PEN, penicillin; ERY, erythromycin; CLI, clindamycin; OXA, oxacillin; SXT, sulfamethoxazole; TET, tetracycline; GEN, gentamicin; LEV, levofloxacin; RFP, rifampicin; NIT, nitrofurantoin; VAN, vancomycin; LNZ, linezolid. (B) The number of antibiotic resistance of MSSA and MRSA. (C) Detection rate of efflux pump genes. (D) The detection rate of resistance genes. (E) Consistency between drug resistance genotypes and phenotypes. The size of the circle represents the correlation between the antibiotics and resistance genes, and the closer the correlation, the larger the circle. The p < 0.05 was considered to indicate statistical significance. *p ≤0.05; **p ≤0.01; ***p≤0.001; ****p≤0.0001.
In this study, all enterotoxin genes were detected, but the detection rates varied significantly among different strains. Among MSSA strains, seg had the highest detection rate (132/174, 75.86%), followed by sei (116/174, 66.67%), seln (110/174, 63.22%), selo (108/174, 62.07%), and selm (108/174, 62.07%). In MRSA strains, sea had the highest detection rate (32/38, 84.21%), followed by selq (21/38, 55.26%), selk (20/38, 52.63%), and seb (19/38, 50.00%). The detection rate of the pvl gene was relatively high, and the detection rate in MSSA strains (70/174, 40.23%) was higher than that in MRSA strains (9/38, 23.68%). The detection rates of toxic shock syndrome toxin (tst) gene and exfoliative toxin (eta) gene were maintained at 2 to 5.5%, while the exfoliative toxin (etb) gene was not detected in any strains (Figure 2A). The most common virulence gene profile in all strains was pvl-seg-sei-selm-seln-selo (43/212, 20.28%). The distribution of virulence gene numbers in MSSA strains ranged from 0 to 12, with 29.31% of strains harboring 6 virulence genes. Low-pathogenicity strains without virulence genes accounted for 6.32% of MSSA strains, while 1.72% of strains had 12 virulence genes and exhibited high pathogenicity. Considering the clinical information, all highly pathogenic strains were derived from the otolaryngology department. MRSA strains mainly had 4 to 6 or 10 virulence genes, generally demonstrating strong pathogenicity (Figure 2B). Figure 2C analyzed the virulence gene profiles of the 38 MRSA strains and showed a complex pattern, with a total of 22 types, among which sea-seb-selk-selq were the most common.
Figure 2. The virulence characteristics of S. aureus isolates. (A) The detection rate of virulence genes of all strains. (B) The number of virulence genes of MSSA and MRSA. The horizontal axis represents the number of strains, while the vertical axis represents the number of genes. (C) The upset plot of MRSA. The bar chart on the left represents the quantity of various toxic genes. The solid circles represent the intersection between data quantities, meaning that each vertical column of solid circles represents a specific toxic gene profile. The bar chart above represents the quantity of different toxic gene profiles. The p < 0.05 was considered to indicate statistical significance. **p ≤0.01; ****p≤0.0001.
The positive rates of biofilm-associated genes in the 38 MRSA strains were analyzed in Supplementary Figure S1A, and except for fib, which was not detected, the positive rates of the remaining genes were all above 80%. Among them, clfA, clfB, fnbA, icaA, icaB, icaC, and icaR were detected in all MRSA strains. The biofilm formation ability test results showed that, except for strains SA17 and SA35, all other strains were capable of biofilm formation (Supplementary Figure S1B). Most strains showed weak (17/38, 44.74%) or moderate (14/38, 36.84%) biofilm formation ability, while only 5 strains showed strong biofilm formation ability (Supplementary Figure S1C).
A total of 14 MLST types were identified among the 38 MRSA strains, with ST59 being the most prevalent type (19/38, 50.00%), followed by ST22 (3/38, 7.80%) and ST5 (3/38, 7.80%). SCCmec typing revealed the presence of three major types and five minor subtypes, including IVa (14/25, 56%), IVc (1/25, 4%), IVg (1/25, 4%), Vb (8/25, 32%), and XII (1/25, 4%). Additionally, 13 MRSA strains were detected to be mecA-negative and SCCmec element-negative, but still exhibited resistance to oxacillin. In terms of spa typing, except for strain SA27, the remaining 37 MRSA strains were classified into 15 types, with t437 being the most prevalent (17/37, 45.95%), followed by t441 (3/37, 8.11%) and t309 (3/37, 8.11%) (Supplementary Table S2). MLST typing, SCCmec typing, and spa typing showed a close relationship, with all ST22 types corresponding to t309, while ST59 types were predominantly associated with SCCmec-IV, V and spa t437 and t441. The most common epidemic clone in this study was ST59-SCCmec IV-t437 (8/38, 21.05%), followed by ST59-SCCmec V-t437 (6/38, 15.79%) and ST59-SCCmec IV-t441 (3/38, 7.89%). Analysis of the number of resistance genes and virulence genes in the major types showed that ST59 had a higher number of resistance genes but fewer virulence genes compared to ST5 and ST22. There was no significant difference in the number of resistance genes and virulence genes between SCCmec IV and V. The spa type t437 exhibited high levels of resistance genes and virulence genes, t441 had more resistance genes but very few virulence genes, and t309 showed the opposite pattern (Figure 3A). Different strains of different molecular types exhibited varying biofilm formation abilities, with ST59-MRSA strains showing higher biofilm formation abilities, and significant differences were observed between ST59-MRSA and ST5-MRSA strains (Figure 3B). Figure 3C analyzed the resistance gene profiles, virulence gene profiles, and biofilm formation abilities of the three major epidemic clones. ST59-SCCmec V-t437 had a high positive rate for the pvl gene, reaching 66.67%. The ser gene rate for the ST59-SCCmec IV-t437 type was relatively high at 37.5%, and this type of MRSA strain showed higher biofilm formation abilities.
Figure 3. The resistance and virulence characteristics of major typings. (A) The number of resistant and virulence genes in the main typing strains. The horizontal axis represents different subtypes, while the vertical axis represents the number of genes. The box plot represents the average, upper and lower quartiles of these gene numbers. (B) The biofilm formation ability in the main typing strains. The horizontal axis represents different subtypes, while the vertical axis represents OD value. The violin diagram shows the distribution and probability density of biofilm formation ability. (C) The gene heatmap of major epidemic typings. Dark blue indicates the presence of this gene, while light blue indicates the absence of this gene. The formation ability of biofilms varies from strong (+++) to weak (+). The p < 0.05 was considered to indicate statistical significance. *p ≤0.05; **p ≤0.01; ***p≤0.001; ****p≤0.0001.
Core-pan analysis of the 38 MRSA strains revealed a total of 2094 core genes and 0–66 dispensable genes distributed among the strains, with SA03, SA11, and SA26 having the highest number of dispensable genes (Figure 4A). COG functional annotation analysis was performed on the 2094 core genes and 309 dispensable genes, resulting in annotations to 23 and 19 categories, respectively. The core genes were involved in a wide range of functions, primarily in translation, ribosomal structure, and biogenesis (9.98%), followed by amino acid transport and metabolism (9.60%). The dispensable genes were primarily involved in mobilome: prophages, transposons (16.83%), and replication, recombination, and repair (15.86%) (Figure 4B). A heatmap was created based on the distribution of dispensable genes among different strains, which also showed clustering of the strains. The results revealed that these 38 strains mainly clustered into four major branches, with SA04 and SA07 forming a separate branch that was more distantly related to the other strains (Figure 4C).
Figure 4. The core and pan-genome analyses of 38 MRSA in this study. (A) The flower diagram of numbers of core genes and dispensable genes for all the strains. (B) The COG functional annotation of core genes and dispensable genes. (C) The heat map of dispensable genes. Top are Dispensable gene cluster and left are strain cluster. The similarities of gene are shown in the middle with different color represent different coverage by heat map.
According to the analysis of core-pan genes, a phylogenetic tree of the 38 strains was constructed (Figure 5). The results showed that these strains were closely related and mainly clustered into three major branches, clade I, clade II, and clade III. SA04 and SA07 formed a separate branch, clade I. Clade II mainly consisted of strains that were not classified in SCCmec typing, and these strains were resistant to oxacillin but did not contain the mecA gene. Additionally, the positive rate of the resistance gene blaZ, associated with β-lactam antibiotics, was higher than the average level, reaching 85.7% in clade II. Clade III mainly consisted of MRSA strains of ST59 genotype, which exhibited significant differences in resistance and virulence genes compared to clade II. In clade III, the vanRE gene had a high detection rate of 100%, while aph(3′)-IIIa, aad(6), and ant6-Ia had detection rates of 63.6%. In clade II, the detection rates of vanRE and ant6-Ia were 21.4 and 7.1% respectively, and aph(3′)-IIIa and aad(6) were not detected. The sulfonamide resistance genes dfrA and dfrG were not detected in clade III, while they had detection rates of 21.4% in clade II. The lukD/lukE gene, associated with leukocidin, had a high detection rate of 71.4% in clade II, while it was 4.5% in clade III. The lukF-PV/lukS-PV gene had similar detection rates in clade III and clade II, at 27.2 and 21.4%, respectively. Clade II and clade III had different profiles of common enterotoxin genes, which were seg-sei-selm-seln-selo-set and sea-seb-selk-selq-set, respectively.
Figure 5. The phylogenetic trees of 38 MRSA strains. Perform phylogenetic analysis on 38 MRSA strains and construct a phylogenetic tree. These strains were divided into three branches, namely green (clade I), blue (clade II), and red (clade III). The different colors of the circles represented the different subtypes of the strains. Blocks indicated the presence or absence of these genes, dark blue indicated the presence of these genes, and light blue indicated the absence of these genes.
In this study, ST59-MRSA was the most common clone type, and a phylogenetic tree of 19 ST59-MRSA strains was constructed based on core-pan genes (Figures 6A,B). The phylogenetic tree showed that these strains clustered into three branches, indicating diversity. Strains of the ST59-SCCmec V-t437 type were closely related and formed a single branch. Figures 6A,B showed that differences in departments and sample types did not have a significant impact on the phylogenetic relationship of strains. Strains from different departments exhibited similarities, while strains from the same department exhibited diversity. COG functional annotation was performed on the 19 ST59-MRSA strains, and they were annotated to 23 entries, showing some variation between the genomes, but primarily annotated to amino acid transport and metabolism and translation, ribosomal structure, and biogenesis (Figure 6C). To study the genetic evolutionary relationship of ST59-MRSA, 22 ST59-MRSA strains were selected from the NCBI database as reference sequences (Supplementary Table S3), and a phylogenetic tree was constructed with them and the 19 ST59-MRSA strains from this study (Figures 6D,E). Except for SA36, the remaining 18 MRSA strains from this study clustered into the same branch. SA36 had the closest relationship with strain SWF.27 from European human sources, both belonging to ST59-t172. Figure 6D showed that ST59-MRSA strains from humans, animals, and food sources were closely related and exhibited consistent relationships. Figure 6E showed that the phylogenetic relationship between strains was related to geographic location, with ST59-MRSA primarily originating from Asia and exhibiting distinct regional characteristics. ST59-MRSA strains from the Americas and Europe tended to be in different evolutionary branches compared to those from Asia.
Figure 6. The evolutionary analysis of ST59 typing. (A,B) The phylogenetic trees of ST59 MRSA strains in this study. Analyze the impact of differences in departments (A) and speciman types (B) on the genetic relationships of strains. (C) The COG functional annotation of ST59 MRSA strains in this study. (D,E) The phylogenetic trees of ST59 MRSA strains in this study and 20 reference strains. Analyze the impact of differences in sources (D) and continents (E) on the genetic evolution of strains.
MRSA is characterized by its wide spread, fast transmission, and complex resistance mechanisms, making the monitoring and treatment of MRSA an important topic of concern. According to the surveillance by the CHINET surveillance system, S. aureus has maintained a high detection rate, while the infection rate of MRSA decreased from 45 to 30% between 2013 and 2021. In some Asian countries, the hospital-acquired MRSA infection rates remain above 20%, with rates reaching as high as 70–80% in countries like South Korea and Vietnam (Chen and Huang, 2014). In our study, the MRSA infection rate was relatively low at 17.9%, below the national average. Children and elderly patients were the most common groups affected by MRSA infection, and there was a significant difference in MRSA infection rates based on age. Previous research has shown a decline in the outpatient visit rate for pyogenic skin and soft tissue infections in pediatrics, but S. aureus remains a major cause of invasive bacterial infections in children (Prochaska et al., 2023).
Antibiotic susceptibility testing results showed that most strains exhibited high resistance to penicillin, erythromycin, and clindamycin, while showing good antibacterial activity against vancomycin and linezolid, which is consistent with a multicenter longitudinal study of MRSA strains in China conducted by Wang B. et al. (2022). However, in this study, the resistance rates to tetracycline and gentamicin were 13.68 and 7.08%, respectively, significantly lower than the national average (42.8, 33.5%), which may be due to the lower usage rate of tetracycline and gentamicin in the Shanxi hospital. The multidrug resistance of S. aureus is not only present in clinical strains but also in retail water products in China. Monitoring of S. aureus in these products found that 90.6% of strains exhibited multidrug resistance, with high resistance against penicillin (88.2%), ampicillin (88.2%), and erythromycin (53.8%) (Rong et al., 2017). In this study, in addition to 81.58% of MRSA strains, 57.47% of MSSA strains also exhibited multidrug resistance, which was associated with the high detection rate of resistance genes and efflux pump genes. The resistance of beta-lactam antibiotics is mainly related to mecA and blaZ, with a high detection rate of blaZ in all strains. It encodes β-lactamase, which mediates the degradation of β-lactam antibiotics (Rocha et al., 2022). We also found 34.21% of MRSA strains showed resistance to oxacillin but were negative for the mecA and mecC genes. This may be due to the overproduction of β-lactamase or modifications in PBP genes caused by spontaneous amino acid substitutions in the transpeptidase domain (Hou et al., 2023). The detection rate of pvl gene in this type of MRSA was only 15.3%, which confirms that this type of MRSA generally does not contain the PVL locus that expresses leukocidin. Resistance to erythromycin is mainly determined by the resistance gene erm. Consistent with previous research, the highest positive rates of ermC (52.63%) and ermB (44.74%) were observed in MRSA strains, with ermC playing a dominant role in the mechanism of erythromycin resistance (Li et al., 2019). And also, erm can mediate the resistance of S. aureus to most macrolides, lincosamides, and streptogramins B (MLS-B), that is the ermA or ermC genes often determine the inducible resistance to MLS-B in S. aureus. The frequency of formation of constitutive variants from inducible ermA and ermC genes are usually high. The reasons behind constitutive variants include deletions, duplications, insertions, and, to a lesser extent, point mutations found in a region approximately 200 bp upstream of the 5′ end of the erm gene (Mlynarczyk-Bonikowska et al., 2022). But in this study, three point mutations (T222C, C224T, G299A) occurred in the ermB gene of SA05 and SA09 strains, resulting in ones synonymous mutation (Asn→Asn) and two missense mutations (Thr→Ile, Ser→Asn). However, these mutations do not affect the resistance of both strains to erythromycin and clindamycin. Pearson correlation analysis showed consistency between the resistance genotypes and phenotypes of S. aureus, and the use of conserved genes in S. aureus was able to successfully predict the resistance phenotype of strains. This suggests that a large hospital can use genomic data features to construct strain prediction models, which can be directly used to determine the drug resistance of strains and guide treatment in the absence of rapid susceptibility results (Wang S. et al., 2022). Therefore, the results of the study on the resistance genotypes and phenotypes of these S. aureus strains can serve as a medication guide for the treatment of S. aureus infections in this hospital and assist in the identification and treatment of multidrug-resistant bacteria in clinical practice.
In this study, all isolates contained at least one virulence gene, which plays an important role in colonization, invasion, and infection processes (Patel and Rawat, 2023). Enterotoxin is one of the main causes of foodborne illnesses caused by S. aureus. There were significant differences in the enterotoxin gene profiles between MSSA and MRSA strains. The classical enterotoxin genes sea (84.21%) and seb (50.00%) were more frequently detected in MRSA strains. In a study on subclinical mastitis in cows and water buffaloes, milk samples were collected, and MRSA isolates were tested for toxin genes, with the sea gene being the most prevalent (Algammal et al., 2020a). The sea gene is heat-stable and can maintain some activity under conditions of 28°C for 121 min. It is commonly detected in foodborne MRSA strains and has a significant association with outbreaks of food poisoning (Lv et al., 2021). The seb gene is usually highly expressed in ST59-MRSA, which contributes to widespread systemic infection, which may be the reason for the increased mortality rate of ST59 infection in China (Bae et al., 2021). In MSSA strains, the most common enterotoxin gene profile is seg-sei-selm-seln-selo, which belongs to the enterotoxin gene cluster (egc cluster). The egc cluster functions as a superantigen, activating T lymphocytes and antigen-presenting cells to produce a large amount of cytokines, which can directly cause toxicity to the cardiovascular system (Patel and Rawat, 2023). The egc cluster has become a major enterotoxin gene in S. aureus, with similar findings in oral S. aureus (Kwapisz et al., 2020) and foodborne S. aureus (Wu et al., 2019; Igbinosa et al., 2023). We found that the distribution of enterotoxin genes in this hospital is diverse, with different toxin gene distributions in different populations and disease types. Classical enterotoxin genes and egc clusters have become the main toxin genes in healthcare-associated and foodborne S. aureus. PVL is a two-component toxin composed of LukS and LukF proteins, which bind to C5aR and C5L2, forming channels on the cell membrane of white blood cells, leading to cell lysis and tissue necrosis, causing skin and soft tissue infections (Patel and Rawat, 2023). The detection rate of the pvl gene in this study was relatively high at 37.26%, higher than the detection rate of the pvl gene isolated from blood samples by Wang et al. (2021). PVL is commonly found in community-acquired methicillin-resistant Staphylococcus aureus (CA-MRSA) and has been considered a marker for CA-MRSA (Hou et al., 2023). Therefore, based on the detection rate of pvl and preliminary epidemiological investigations indicating that these strains in this study are mostly community-acquired infections, it suggests that CA-MRSA is not only spreading in the community but also increasing in prevalence in hospitals. In addition, the detection rate of pvl in MSSA strains (70/174, 40.23%) was higher than that in MRSA strains (9/38, 23.68%), which may be related to the source and number of samples. However, it also indicates that although MRSA has attracted attention due to its multidrug resistance, it is not necessarily more virulent than MSSA.
The clone types of S. aureus have geographical advantages, and ST59 is the predominant MRSA clone in China and even Asia (Huh and Chung, 2016). In this study, 50% of the MRSA isolates belonged to the ST59-MRSA. Systematic evolutionary analysis of ST59-MRSA showed consistency among strains isolated from humans, food, and animals, indicating close genetic relatedness. Additionally, the phylogenetic relationship among strains was associated with geographical location, with strains from different continents tending to diverge in evolution. In a study by Wang B. et al. (2022) in 2022, ST59-MRSA accounted for 27.1% of the clinical MRSA isolates in China. Wu et al. (2019) analyzed food-related MRSA in China in 2019 and found that 47.7% of the isolates belonged to ST59-MRSA, and it has been shown that food-related MRSA strains have a high degree of similarity to community-associated MRSA. The distribution of ST59-MRSA is geographically limited and mainly spreads within Asia. However, analysis of isolates from Europe showed that the resistance profiles of European ST59-t437 isolates were similar to those of Asian ST59-t437 isolates, but different from other MRSA isolates in Europe (Glasner et al., 2015).
In this study, the predominant epidemic clones were ST59-SCCmecIV-t437 and ST59-SCCmecV-t437, both belonging to clade III in the phylogenetic tree but relatively independent, forming two smaller branches. Both clones showed high rates of resistance and virulence gene detection, with resistance genes detected for all antibiotic classes except sulfonamides. The main enterotoxin genes in these clones were the classical enterotoxin genes sea and seb, and the ST59-SCCmecV-t437 clone had a high positive rate for the pvl gene, reaching 66.67%. These two clones belong to the Asian-Pacific clone and the Taiwan clone, respectively. Asian-Pacific clone isolates are mostly pvl-negative and colonize healthy children, while Taiwan clone carries the pvl gene and is generally isolated from critically ill patients. Due to the high expression of pvl and hla genes in Taiwan clone isolates, it has higher virulence and pathogenic potential compared to the Asian-Pacific clone (Chen et al., 2013). MLST is consistent with spa typing, and the ST59 typing is generally associated with t441 and t437. And then we found an ST59-t172 isolate, which is distantly related to other ST59-MRSA strains in this study but closely related to an ST59-t172 isolate from Finland in Europe. It is extremely rare elsewhere and mainly reported in Finland. The ST59-t172 strain mainly spreads among elderly patients and can cause outbreaks of S. aureus in environments with low MRSA incidence (Silvola et al., 2022).
This study investigated the colonization and distribution of S. aureus in a tertiary A hospital in Shanxi, China. It detected the antibiotic resistance, biofilm formation capability, as well as the carriage rates of resistance genes, virulence genes, and biofilm-related genes in the isolates. And then three molecular typing methods and whole-genome sequencing were used to study the molecular characteristics and genetic evolution of MRSA isolates from different sources. It was found that MRSA isolates displayed multidrug resistance, and the carriage of resistance genes and virulence genes exhibited diversity and complexity. The predominant clones were ST59-SCCmecIV-t437 and ST59-SCCmecV-t437, and ST59-MRSA was closely associated with various hosts, showing diverse patterns of transmission. This study elucidated the molecular characteristics and evolutionary relationship of MRSA isolates, which can contribute to the prevention and control of S. aureus infections.
The datasets presented in this study can be found in online repositories. The names of the repository/repositories and accession number(s) can be found at: https://www.ncbi.nlm.nih.gov/, PRJNA994481.
The studies involving humans were approved by Scientific Research Ethics Committee, Fenyang College of Shanxi Medical University. The studies were conducted in accordance with the local legislation and institutional requirements. Written informed consent for participation was not required from the participants or the participants’ legal guardians/next of kin in accordance with the national legislation and institutional requirements.
ZH: Conceptualization, Data curation, Investigation, Methodology, Visualization, Writing – original draft, Writing – review & editing. BX: Conceptualization, Data curation, Funding acquisition, Investigation, Methodology, Project administration, Supervision, Visualization, Writing – original draft, Writing – review & editing. LL: Conceptualization, Funding acquisition, Investigation, Project administration, Visualization, Writing – original draft, Writing – review & editing. RY: Writing – review & editing, Investigation, Methodology, Resources, Validation. JZ: Writing – review & editing, Investigation, Methodology, Resources, Validation. JY: Writing – review & editing, Data curation, Investigation, Methodology. PL: Writing – review & editing, Data curation, Investigation, Methodology. JW: Validation, Writing – review & editing, Resources, Software, Supervision.
The author(s) declare financial support was received for the research, authorship, and/or publication of this article. This study was supported by Fundamental Research Program of Shanxi Province (Grant nos. 20210302123397 and 202203021212351), Key R&D Projects of Introducing High-Level Scientific and Technological Talents in Lvliang City (Grant no. 2021RC-1-4), Project of Lvliang City Science and Technology Program (Grant no. 2020SHFZ29), Science and Technology Innovation Project of Colleges and Universities in Shanxi Province (Grant no. 2020L0749), Special Fund for Key Disciplines of Fenyang College of Shanxi Medical University (Grant no. 2022B14), National College Students’ Innovation and Entrepreneurship Training Program (Grant no. 20221569), and Projects of Innovation and Entrepreneurship Training Program for College Students of Fenyang College of Shanxi Medical University (Grant nos. FDC202209, FDC202214, and FDC202215).
The authors declare that the research was conducted in the absence of any commercial or financial relationships that could be construed as a potential conflict of interest.
All claims expressed in this article are solely those of the authors and do not necessarily represent those of their affiliated organizations, or those of the publisher, the editors and the reviewers. Any product that may be evaluated in this article, or claim that may be made by its manufacturer, is not guaranteed or endorsed by the publisher.
The Supplementary material for this article can be found online at: https://www.frontiersin.org/articles/10.3389/fmicb.2023.1273397/full#supplementary-material
Alcock, B. P., Raphenya, A. R., Lau, T. T. Y., Tsang, K. K., Bouchard, M., Edalatmand, A., et al. (2020). CARD 2020: antibiotic resistome surveillance with the comprehensive antibiotic resistance database. Nucleic Acids Res. 48, D517–D525. doi: 10.1093/nar/gkz935
Algammal, A. M., Enany, M. E., El-Tarabili, R. M., Ghobashy, M. O. I., and Helmy, Y. A. (2020a). Prevalence, antimicrobial resistance profiles, virulence and enterotoxins-determinant genes of MRSA isolated from subclinical bovine mastitis in Egypt. Pathogens 9:362. doi: 10.3390/pathogens9050362
Algammal, A. M., Hetta, H. F., Elkelish, A., Alkhalifah, D. H. H., Hozzein, W. N., Batiha, G. E., et al. (2020b). Methicillin-resistant Staphylococcus aureus (MRSA): one health perspective approach to the bacterium epidemiology, virulence factors, antibiotic-resistance, and zoonotic impact. Infect. Drug Resist. 13, 3255–3265. doi: 10.2147/IDR.S272733
Bae, J. S., Da, F., Liu, R., He, L., Lv, H., Fisher, E. L., et al. (2021). Contribution of staphylococcal enterotoxin B to Staphylococcus aureus systemic infection. J. Infect. Dis. 223, 1766–1775. doi: 10.1093/infdis/jiaa584
Barber, M. (1961). Methicillin-resistant staphylococci. J. Clin. Pathol. 14, 385–393. doi: 10.1136/jcp.14.4.385
Bennett, M. R., and Thomsen, I. P. (2020). Epidemiological and clinical evidence for the role of toxins in S. aureus human disease. Toxins 12:408. doi: 10.3390/toxins12060408
Brakstad, O. G., Aasbakk, K., and Maeland, J. A. (1992). Detection of Staphylococcus aureus by polymerase chain reaction amplification of the nuc gene. J. Clin. Microbiol. 30, 1654–1660. doi: 10.1128/jcm.30.7.1654-1660.1992
Chen Cheng, W. J., Tong, W., Jiawei, C., and Guiqin, W. (2020). Analysis of enterotoxin genes, resistance genes and molecular typing of MRSA isolated from dairy cow. Genomics Appl. Biol. 39, 1080–1088. doi: 10.13417/j.gab.039.001080
Chen, C. J., and Huang, Y. C. (2014). New epidemiology of Staphylococcus aureus infection in Asia. Clin. Microbiol. Infect. 20, 605–623. doi: 10.1111/1469-0691.12705
Chen, C. J., Unger, C., Hoffmann, W., Lindsay, J. A., Huang, Y. C., and Gotz, F. (2013). Characterization and comparison of 2 distinct epidemic community-associated methicillin-resistant Staphylococcus aureus clones of ST59 lineage. PLoS One 8:e63210. doi: 10.1371/journal.pone.0063210
Chen, L., Zheng, D., Liu, B., Yang, J., and Jin, Q. (2016). VFDB 2016: hierarchical and refined dataset for big data analysis--10 years on. Nucleic Acids Res. 44, D694–D697. doi: 10.1093/nar/gkv1239
CLSI (2015). Performance standards for antimicrobial susceptibility testing; twenty-fifth informational supplement. Wayne, PA: The Clinical and Laboratory Standards Institute.
Galperin, M. Y., Makarova, K. S., Wolf, Y. I., and Koonin, E. V. (2015). Expanded microbial genome coverage and improved protein family annotation in the COG database. Nucleic Acids Res. 43, D261–D269. doi: 10.1093/nar/gku1223
Glasner, C., Pluister, G., Westh, H., Arends, J. P., Empel, J., Giles, E., et al. (2015). Staphylococcus aureus spa type t437: identification of the most dominant community-associated clone from Asia across Europe. Clin. Microbiol. Infect. 21, 163.e1–163.e8. doi: 10.1016/j.cmi.2014.09.010
He, Y., and Reed, S. (2020). Pulsed-field gel electrophoresis typing of Staphylococcus aureus strains. Methods Mol. Biol. 2069, 79–88. doi: 10.1007/978-1-4939-9849-4_5
Hou, Z., Liu, L., Wei, J., and Xu, B. (2023). Progress in the prevalence, classification and drug resistance mechanisms of methicillin-resistant Staphylococcus aureus. Infect. Drug Resist. 16, 3271–3292. doi: 10.2147/IDR.S412308
Huh, K., and Chung, D. R. (2016). Changing epidemiology of community-associated methicillin-resistant Staphylococcus aureus in the Asia-Pacific region. Expert Rev. Anti Infect. Ther. 14, 1007–1022. doi: 10.1080/14787210.2016.1236684
Igbinosa, E. O., Beshiru, A., Igbinosa, I. H., Ogofure, A. G., Ekundayo, T. C., and Okoh, A. I. (2023). Prevalence, multiple antibiotic resistance and virulence profile of methicillin-resistant Staphylococcus aureus (MRSA) in retail poultry meat from Edo, Nigeria. Front. Cell. Infect. Microbiol. 13:1122059. doi: 10.3389/fcimb.2023.1122059
Kwapisz, E., Garbacz, K., Kosecka-Strojek, M., Schubert, J., Bania, J., and Miedzobrodzki, J. (2020). Presence of egc-positive major clones ST 45, 30 and 22 among methicillin-resistant and methicillin-susceptible oral Staphylococcus aureus strains. Sci. Rep. 10:18889. doi: 10.1038/s41598-020-76009-1
Li, X., Huang, T., Xu, K., Li, C., and Li, Y. (2019). Molecular characteristics and virulence gene profiles of Staphylococcus aureus isolates in Hainan, China. BMC Infect. Dis. 19:873. doi: 10.1186/s12879-019-4547-5
Liu, Y., and Ji, Y. (2020). Multilocus sequence typing of Staphylococcus aureus. Methods Mol. Biol. 2069, 95–102. doi: 10.1007/978-1-4939-9849-4_7
Lv, G., Jiang, R., Zhang, H., Wang, L., Li, L., Gao, W., et al. (2021). Molecular characteristics of Staphylococcus aureus from food samples and food poisoning outbreaks in Shijiazhuang, China. Front. Microbiol. 12:652276. doi: 10.3389/fmicb.2021.652276
Merlino, J., Watson, J., Rose, B., Beard-Pegler, M., Gottlieb, T., Bradbury, R., et al. (2002). Detection and expression of methicillin/oxacillin resistance in multidrug-resistant and non-multidrug-resistant Staphylococcus aureus in Central Sydney, Australia. J. Antimicrob. Chemother. 49, 793–801. doi: 10.1093/jac/dkf021
Mlynarczyk-Bonikowska, B., Kowalewski, C., Krolak-Ulinska, A., and Marusza, W. (2022). Molecular mechanisms of drug resistance in Staphylococcus aureus. Int. J. Mol. Sci. 23:8088. doi: 10.3390/ijms23158088
Monaco, M., Pimentel de Araujo, F., Cruciani, M., Coccia, E. M., and Pantosti, A. (2017). Worldwide epidemiology and antibiotic resistance of Staphylococcus aureus. Curr. Top. Microbiol. Immunol. 409, 21–56. doi: 10.1007/82_2016_3
Oliveira, D., Borges, A., and Simoes, M. (2018). Staphylococcus aureus toxins and their molecular activity in infectious diseases. Toxins 10:252. doi: 10.3390/toxins10060252
Patel, H., and Rawat, S. (2023). A genetic regulatory see-saw of biofilm and virulence in MRSA pathogenesis. Front. Microbiol. 14:1204428. doi: 10.3389/fmicb.2023.1204428
Prochaska, E. C., Xiao, S., Tamma, P. D., Sick-Samuels, A., Schumacher, C., Gadala, A., et al. (2023). Trends in pediatric community-onset Staphylococcus aureus antibiotic susceptibilities over a five-year period in a multihospital health system. Antimicrob. Steward. Healthc. Epidemiol. 3:e12. doi: 10.1017/ash.2022.370
Rocha, G. D., Nogueira, J. F., Gomes Dos Santos, M. V., Boaventura, J. A., Nunes Soares, R. A., de Simoni, J., et al. (2022). Impact of polymorphisms in blaZ, blaR1 and blaI genes and their relationship with beta-lactam resistance in S. aureus strains isolated from bovine mastitis. Microb. Pathog. 165:105453. doi: 10.1016/j.micpath.2022.105453
Rong, D., Wu, Q., Xu, M., Zhang, J., and Yu, S. (2017). Prevalence, virulence genes, antimicrobial susceptibility, and genetic diversity of Staphylococcus aureus from retail aquatic products in China. Front. Microbiol. 8:714. doi: 10.3389/fmicb.2017.00714
Silvola, J., Grondahl-Yli-Hannuksela, K., Hirvioja, T., Rantakokko-Jalava, K., Rintala, E., Auranen, K., et al. (2022). Whole genome sequencing reveals new links between spa t172/CC59 methicillin-resistant Staphylococcus aureus cases in low-endemicity region of Southwest Finland, 2007–2016. Sci. Rep. 12:21326. doi: 10.1038/s41598-022-25556-w
Turner, N. A., Sharma-Kuinkel, B. K., Maskarinec, S. A., Eichenberger, E. M., Shah, P. P., Carugati, M., et al. (2019). Methicillin-resistant Staphylococcus aureus: an overview of basic and clinical research. Nat. Rev. Microbiol. 17, 203–218. doi: 10.1038/s41579-018-0147-4
Uehara, Y. (2022). Current status of staphylococcal cassette chromosome mec (SCCmec). Antibiotics 11:86. doi: 10.3390/antibiotics11010086
Wang, X. (2020). Spa typing of Staphylococcus aureus isolates. Methods Mol. Biol. 2069, 89–94. doi: 10.1007/978-1-4939-9849-4_6
Wang, X., Lin, D., Huang, Z., Zhang, J., Xie, W., Liu, P., et al. (2021). Clonality, virulence genes, and antibiotic resistance of Staphylococcus aureus isolated from blood in Shandong, China. BMC Microbiol. 21:281. doi: 10.1186/s12866-021-02344-6
Wang, B., Xu, Y., Zhao, H., Wang, X., Rao, L., Guo, Y., et al. (2022). Methicillin-resistant Staphylococcus aureus in China: a multicentre longitudinal study and whole-genome sequencing. Emerg. Microbes Infect. 11, 532–542. doi: 10.1080/22221751.2022.2032373
Wang, S., Zhao, C., Yin, Y., Chen, F., Chen, H., and Wang, H. (2022). A practical approach for predicting antimicrobial phenotype resistance in Staphylococcus aureus through machine learning analysis of genome data. Front. Microbiol. 13:841289. doi: 10.3389/fmicb.2022.841289
Waryah, C. B., Gogoi-Tiwari, J., Wells, K., Eto, K. Y., Masoumi, E., Costantino, P., et al. (2016). Diversity of virulence factors associated with west Australian methicillin-sensitive Staphylococcus aureus isolates of human origin. Biomed. Res. Int. 2016:8651918. doi: 10.1155/2016/8651918
Wu, S., Huang, J., Zhang, F., Wu, Q., Zhang, J., Pang, R., et al. (2019). Prevalence and characterization of food-related methicillin-resistant Staphylococcus aureus (MRSA) in China. Front. Microbiol. 10:304. doi: 10.3389/fmicb.2019.00304
Yamaguchi, T., Ono, D., and Sato, A. (2020). Staphylococcal cassette chromosome mec (SCCmec) analysis of MRSA. Methods Mol. Biol. 2069, 59–78. doi: 10.1007/978-1-4939-9849-4_4
Zhang Hang, Y. F., Xinpu, L., Xurong, W., Jinyin, L., and Hongsheng, L. (2019). Study on relationship between antimicrobial resistance and efflux pump related genes of methicillin-resistant Staphylococcus aureus in dairy cow. Acta Vet. Zootech. Sin. 50, 2326–2332. doi: 10.11843/j.issn.0366-6964.2019.11.017
Keywords: MRSA, resistance genes, virulence genes, molecular typing, comparative genomics
Citation: Hou Z, Xu B, Liu L, Yan R, Zhang J, Yin J, Li P and Wei J (2023) Prevalence, drug resistance, molecular typing and comparative genomics analysis of MRSA strains from a tertiary A hospital in Shanxi Province, China. Front. Microbiol. 14:1273397. doi: 10.3389/fmicb.2023.1273397
Received: 06 August 2023; Accepted: 06 September 2023;
Published: 22 September 2023.
Edited by:
Vijay Soni, Weill Cornell Medical Center, NewYork-Presbyterian, United StatesReviewed by:
Arka Banerjee, Weill Cornell Medical Center, NewYork-Presbyterian, United StatesCopyright © 2023 Hou, Xu, Liu, Yan, Zhang, Yin, Li and Wei. This is an open-access article distributed under the terms of the Creative Commons Attribution License (CC BY). The use, distribution or reproduction in other forums is permitted, provided the original author(s) and the copyright owner(s) are credited and that the original publication in this journal is cited, in accordance with accepted academic practice. No use, distribution or reproduction is permitted which does not comply with these terms.
*Correspondence: Jianhong Wei, d2poNTEyM0AxNjMuY29t; Benjin Xu, YmowNzI2QHN4bXUuZWR1LmNu
†These authors have contributed equally to this work
Disclaimer: All claims expressed in this article are solely those of the authors and do not necessarily represent those of their affiliated organizations, or those of the publisher, the editors and the reviewers. Any product that may be evaluated in this article or claim that may be made by its manufacturer is not guaranteed or endorsed by the publisher.
Research integrity at Frontiers
Learn more about the work of our research integrity team to safeguard the quality of each article we publish.