- 1College of Animal Science and Technology, Shihezi University, Shihezi, China
- 2College of Life Sciences, Shihezi University, Shihezi, China
- 3Xinjiang Tianshan Junken Animal Husbandry Co., Ltd.,Shihezi, China
Introduction: Weaning stress seriously affects the welfare of calves and causes huge economic losses to the cattle breeding industry. Probiotics play an important role in improving animal growth performance, enhancing immune function, and improving gut microbiota. The newly isolated strains of Lactobacillus reuteri L81 and Lactobacillus johnsonii L29 have shown potential as probiotics. Here, we studied the probiotic properties of these two strains on weaned calves.
Methods: Forty calves were randomly assigned to four groups before weaning, with 10 calves in each group, control group (Ctrl group), L. reuteri L81 supplementation group (2 g per day per calf), L. johnsonii L29 supplementation group (2 g per day per calf), L. reuteri L81 and L. johnsonii L29 composite group (2 g per day per calf), and the effects of Lactobacillus reuteri L81 and Lactobacillus johnsonii L29 supplementation on growth performance, immune status, antioxidant capacity, and intestinal barrier function of weaned calves were evaluated.
Results: The results showed that probiotics supplementation increased the average daily weight gain of calves after weaning, reduced weaning diarrhea index (p < 0.05), and increased serum IgA, IgM, and IgG levels (p < 0.05). L. reuteri L81 supplementation significantly decreased IL-6, increased IL-10 and superoxide dismutase (SOD) levels at 21 d after weaning (p < 0.05). Moreover, probiotics supplementation significantly decreased serum endotoxin (ET), diamine oxidase (DAO), and D-lactic acid (D-LA) levels at different time points (p < 0.05). In addition, supplementation with L. reuteri L81 significantly reduced the crypt depth and increased the ratio of villus height to crypt depth (p < 0.05) in the ileum, increased gene expression of tight junction protein ZO-1, Claudin-1 and Occludin in jejunum and ileum mucosa, reduced the gene expression of INF- γ in ileum mucosa and IL-8 in jejunum mucosa, and increased the abundance of beneficial bacteria, including Bifidobacterium, Lactobacillus, Oscillospira, etc.
Discussion: verall, these results showed that the two strains isolated from cattle feces after low concentration fecal microbiota transplantation improved the growth performance, immune performance, antioxidant capacity, and intestinal barrier function of weaned calves, indicating their potential as supplements to alleviate weaning diarrhea in calves.
1. Introduction
Weaning is one of the most stressful periods in calf growth owing to the underdevelopment of the gastrointestinal system, incomplete intestinal microecosystem, low immune and antioxidant capacity, and weak digestion and absorption capacity of calves during the early weaning stage (Clark et al., 2016). Weaning stress, such as diarrhea, growth retardation, and even death, can occur as a result of dietary and psychological changes, which can seriously affect the welfare of calves and cause huge economic losses to the cattle breeding industry (Sweeney et al., 2010; Pempek et al., 2019). Antibiotics are widely used in animal husbandry to treat or prevent diarrhea and promote livestock growth (Brown et al., 2017); however, long-term antibiotics use can destroy the normal community structure of intestinal microorganisms and increase the presence of intestinal drug-resistant bacteria (Laxminarayan et al., 2013; Brand et al., 2017). Additionally, drug residues can pose potential problems, such as food safety issues, and threaten human health. Therefore, it is important to identify novel antibiotic substitutes capable of preventing or alleviating weaning stress in calves.
In 2001, the Food and Agriculture Organization of the United Nations and the World Health Organization (FAO/WHO) definition of probiotics -- “live microorganisms which when administered in adequate amounts confer a health benefit on the host” (Hill et al., 2014). Studies have shown that supplementation with probiotics plays an important role in improving animal production performance, reducing the incidence of diarrhea, enhancing immune function and antioxidant capacity, and improving intestinal microbial flora (Kelsey and Colpoys, 2018). Recently, there has been increasing research on effective antibiotics substitutes owing to health concerns associated with antibiotics use, and probiotics have been used as antibiotic substitutes in several countries. Lactobacillus plays a good role in improving intestinal epithelial barrier function, maintaining mucosal integrity, competing with pathogenic bacterial, and ameliorating intestinal mucosal damage (Wu et al., 2020). Our previous studies have shown that low-concentration fecal microbiota transplantation (LFMT) reduces the diarrhea incidence of weaned calves with Xinjiang yaks as the donor of fecal microbiota transplantation and Chinese Holstein calves as the recipient of fecal microbiota transplantation, and Lactobacillus was enriched in the intestine (Li et al., 2022). To develop more effective probiotics to alleviate weaning diarrhea in calves, we screened Lactobacillus in the feces of weaned calves after LFMT treatment in a previous study, and identified two potential probiotics for alleviating weaning diarrhea in calves, Lactobacillus reuteri L81 and Lactobacillus johnsonii L29.
L. reuteri and L. johnsonii have successfully been evaluated in humans and animals. L. reuteri probiotic preparations can improve gut function and enhance immunity, thereby promoting human health, enhancing pig growth, improving feed utilization efficiency, preventing diarrhea, and regulating the immune system in pigs (Hou et al., 2015). L. reuteri probiotics can replace antibiotics to promote the growth of weaned piglets and improve their intestinal health (Hong, 2016). L. johnsonii has been shown to prevent salmonella infection in weaned piglets, maintain metabolic homeostasis, enhance intestinal health in piglets (He et al., 2019), and regulate intestinal microbiota in mice. Based on these findings, the aim of this study was to examine the effects of these two strains on growth performance, diarrhea incidence, serum metabolites, immune performance, antioxidant capacity, and intestinal barrier function in early weaned calves. It is anticipated that the findings of this study would provide important evidence for implementing probiotic interventions and improving intestinal health in early weaned calves.
2. Materials and methods
2.1. Ethics statement
Animal experiment was conducted in accordance with the guidelines of the animal welfare and research institutions, and all procedures were approved by the Bioethics Committee of Shihezi University (License No.: A2021-32).
2.2. Animal management and diet
Forty Chinese Holstein calves of similar weight and birth date were randomly divided into four groups, with 10 calves in each group. To avoid cross-infection, all calves were placed on Calf Island. At 7 and 18 o’clock daily, the calves were fed twice with two equal volumes of milk in plastic buckets. At 50–56 d, the amount of milk consumed was 5 L/d. At 57–60 d, the calves were fed once daily, with the amount of milk reduced to 1 L/d, after weaning at 61 d, the feed amount was decreased to 0. The milk was produced on the same farm and pasteurized at 60°C before use. Starter concentrate was provided by Xinjiang Urumqi Zhengda Feed Co., Ltd. (Urumqi, China). All calves can drink water, take starter and Arrhenatherum elatius freely after weaning. The nutritional compositions of the diets are listed in Supplementary Table S1.
2.3. Experimental design, sample collection, and analysis
At 50–60 d of age, group 1 was fed normally, without any treatment, so it was the control group (Ctrl group). Group 2 was given L. reuteri L81 every day (2 g per day per calf, R group). Group 3 was given L. johnsonii L29 every day (2 g per day per calf, J group). Group 4 was given L. reuteri L81 and L. johnsonii L29 compound bacteria (2 g per day per calf, R + J group). The concentration of Lactobacillus used in the study was adjusted to 1 × 108 CFU/g. To ensure that Lactobacillus was fully consumed by the calves, freeze-dried Lactobacillus powder was dissolved in milk for morning feeding. This study was conducted at the Zhenxing Farm of the Tianshan Military Reclamation Company in Shihezi, Xinjiang, China, from May to July 2022.
2.4. Growth performance, diarrhea rate and diarrhea index
The body weight of each calf was measured before morning feeding on the day of weaning (T0), and at 7 days (T1), 14 days (T2), and 21 days (T3) after weaning. Feed intake was recorded daily, and the average daily gain (ADG), average daily feed intake (ADFI), and feed conversion rate (F/G) were calculated.
Fecal samples from the calves were scored according to the diarrhea index scoring method. The diarrhea index was scored as follows: normal feces (solid), 0 points; wet feces (semi-solid),1 point; mild diarrhea (pasty feces), 2 points; and severe diarrhea (watery stool), 3 points. The number of days and heads of calves with diarrhea during the trial period were recorded to determine the diarrhea index and diarrhea rate statistics (Mcguirk, 2008). The following formulas were used to calculate the diarrhea rate and index for each group of calves,
Diarrhea rate (%) = number of calves with diarrhea per group during the trial period × diarrhea days / (trial days × number of calves per group) × 100%.
2.5. Sample collection
Blood samples were collected from the jugular veins of six calves in each group before morning feeding at 7 (T1), 14 (T2), and 21 d (T3) after weaning in a 10 mL vacuum blood collection tube without anticoagulant. The blood samples were centrifugated at 3000 × g for 15 min, and the supernatant was stored in a 2 mL tube at −20°C until further analysis.
Calves were weaned at 60 d of age. At 21 d after weaning, five calves from the control (Ctrl group) and L. reuteri supplementation groups (R group) were anesthetized via intramuscular injection of 4% pentobarbital sodium solution at a dose of 40 mg/kg body weight and euthanized by jugular venous bleeding. The abdominal cavity was quickly opened, and the contents of the duodenum, jejunum, and ileum were collected and divided them into 2 mL and 10 mL sterile enzyme free tubes. The contents of the tubes were snap-frozen in liquid nitrogen. After flushing out the contents of the intestinal canal with phosphate buffer solution (pH 7.4), the intestinal mucosa was carefully cleaned of fatty tissues and stored in a 2 mL sterile enzyme free EP tube for rapid freezing. Intestinal contents and intestinal mucosa were transported to the laboratory in a frozen tube and stored at −80°C until further analysis.
In addition, the middle segments of duodenum, jejunum and ileum were selected. After the contents of the intestinal tube were flushed out with phosphate buffer solution with pH = 7.4, they were quickly placed in 4% paraformaldehyde fixative at 4°C for 48 h for tissue sectioning.
2.6. Determination of biochemical indicators
Serum total protein (TP), albumin (ALB), glucose (GLU), triglyceride (TG), and blood urea nitrogen (BUN) levels were determined using a biochemical detection kit from Nanjing Jiancheng Biotechnology Research Institute, according to the manufacturer’s instructions.
2.7. Antioxidant indicators, immune indicators, cytokines and intestinal permeability indicators in serum
Superoxide dismutase (SOD) and malondialdehyde (MDA) levels were determined by spectrophotometry using corresponding kits (Nanjing Jiancheng Biotechnology Research Institute, Nanjing, China), according to the manufacturer’s instructions.
IgA, IgG, IgM, IL-2, IL-6, IL-10, TNF- α, endotoxin (ET), diamine oxidase (DAO), and D-lactic acid (D-LA) contents were determined using ELISA kits (Shanghai Enzymes Biotechnology Co., Ltd.), according to the manufacturer’s instructions.
2.8. Intestinal tissue morphology
Each intestinal segment was sectioned by haematoxylin eosin (HE) staining method described by Hu J. et al. (2018) for intestinal histomorphology measurement. The main steps include fixation, dehydration, immersing the tissue block in xylene until completely transparent, placing the transparent tissue in paraffin, embedding, slicing, 37°C water bath development, drying in a 37°C oven, dewaxing, ethanol gradient elution, hematoxylin staining, eosin staining, ethanol gradient dehydration, sealing, and air drying. And its morphology and structure were observed at 10× under an optical microscope. The villus height and recess depth of small intestine were measured, and the ratio of villus height to recess depth was calculated.
2.9. Detection of intestinal tight junction protein and cytokine gene expression
The frozen duodenum, jejunum and ileum mucosa were taken out and placed in a autoclaved mortar, and the liquid nitrogen was added to grind and homogenize. Then, an appropriate amount of powder samples were taken, and total RNA was extracted from the tissue mucosa with TRIzol reagent. The total RNA was reverse transcribed and cDNA was synthesized according to the instructions of the reverse transcription kit. Primer 3 was used for primer design, and the primers were detailed in Supplementary Table S2. The primers were synthesized by Shanghai Shenggong Biotechnology Co., Ltd. The mRNA expression levels of intestinal tight junction proteins Claudin-1, Occuludin and ZO-1, intestinal mucosal cytokines TLR4, INF-γ, TGF-β1, IL-8 and IL-2 were detected by real-time fluorescent quantitative PCR with specific primers.
2.10. Determination of enzyme activity in the small intestine
After the calves were slaughtered, the intestinal contents were taken and placed in a clean centrifuge tube. They were transported to the laboratory and their pH value were immediately measured using a pH meter.
0.5 g of duodenal, jejunal, and ileal contents were diluted with physiological saline at a ratio of 1:9, followed by homogenization in an ice bath and centrifugation at 4500 × g for 20 min to obtain supernatant for enzyme activity assay. Amylase, trypsin, lipase, lactase, and Na-K-ATPase activities were determined using the respective ELISA kits (Shanghai Enzymes Biotechnology Co., Ltd.), according to the manufacturer’s instructions.
2.11. DNA extraction, PCR amplification of 16SrRNA, and sequencing
DNA extraction, genome library construction, and intestinal microbial sequencing were performed by Shanghai Parsenor Biotechnology Co. Ltd. Briefly, total DNA was extracted from the intestinal contents, and the V3-V4 hypervariable region of bacterial 16S rRNA was amplified using the universal primers 338F (5′-ACTCCTACGGGGAGCA-3′) and 806R (5′- GGACTACHVGGGTWTCTAAT-3′). After purification, all amplified products were sequenced using the Illumina MiSeq platform. The PCR amplification reaction system contained 5 μL of buffer (5 ×), 0.25 μL of fast pfu DNA polymerase (5 U/μL), 2 μL (2.5 mM) of dNTPs, 1 μL (10 uM) forward primer and 1 μL reverse primer, 1 μL of DNA template, and 14.75 μL of ddH2O. The PCR conditions were as follows: initial denaturation at 98°C for 5 min, followed by 25 cycles at 98°C for 30 s, annealing at 53°C for 30 s, and extension at 72°C for 45 s, and final extension at 72°C of 5 min. PCR amplicons were purified using Vazyme VAHTSTM DNA clean beads (Vazyme, Nanjing, China) and quantified using a Quant-iT PicoGreen dsDNA Assay Kit (Invitrogen, Carlsbad, CA, USA). After the individual quantification step, amplicons were pooled in equal amounts, and paired-end sequencing (2 × 250 bp) was performed by Shanghai Personal Biotechnology Co., Ltd. (Shanghai, China) on the Illumina MiSeq platform using the MiSeq Reagent Kit v3.
Bioinformatics analysis of the intestinal bacteria was conducted using QIIME2 2019.4, with slight modifications based on the official manual. Microbial α-diversity was evaluated using Shannon, Simpson, Chao1, and Coverage indices, while β-diversity was analyzed using nonmetric multidimensional scale. Shared and unique ASVs between samples or groups were visualized using the “VennDiam” function in R software. LEfSe analysis was used to compare the relative abundance of the intestinal microbiota between the control and L. reuteri L81 supplemented groups at the phylum and genus levels.
2.12. Statistics and analysis
One-way analysis of variance was used to analyze multiple sets of data, and the results were expressed as mean and standard deviation. Calculate the gene expression data of the sample using the 2-ΔΔCt method. The differences of intestinal villus height, crypt depth and the ratio of villus height to crypt depth, intestinal tight junction protein and cytokine gene expression were performed using t-tests. The data were expressed by mean value and standard error, means were considered statistically significant at p < 0.05. All statistical analyses were performed using SPSS 26.0 software (SPSS, Inc., Chicago, IL, United States).
3. Results
3.1. Effects of Lactobacillus reuteri L81 and Lactobacillus johnsonii L29 on growth performance, diarrhea incidence, and diarrhea index of weaned calves
There was no significant difference (p > 0.05) in the initial weight of the calves among the experimental groups (Figure 1). In contrast, calves in the R + J group had significantly higher ADG at T0–T1, while those in the R and R + J groups had significantly higher ADG at T1–T2 and T2–T3 compared with the Ctrl group (p < 0.05). However, there was no significant difference in ADG between the J and Ctrl groups, and no significant difference between R, J and R + J groups. Additionally, there was no significant difference in ADFI between the experimental groups at all time points. Moreover, F/G ratio was significantly lower in the R + J group than in the Ctrl group at T1–T2 (p < 0.05); however, there was no significant difference between the groups at T0–T1 and T2–T3.
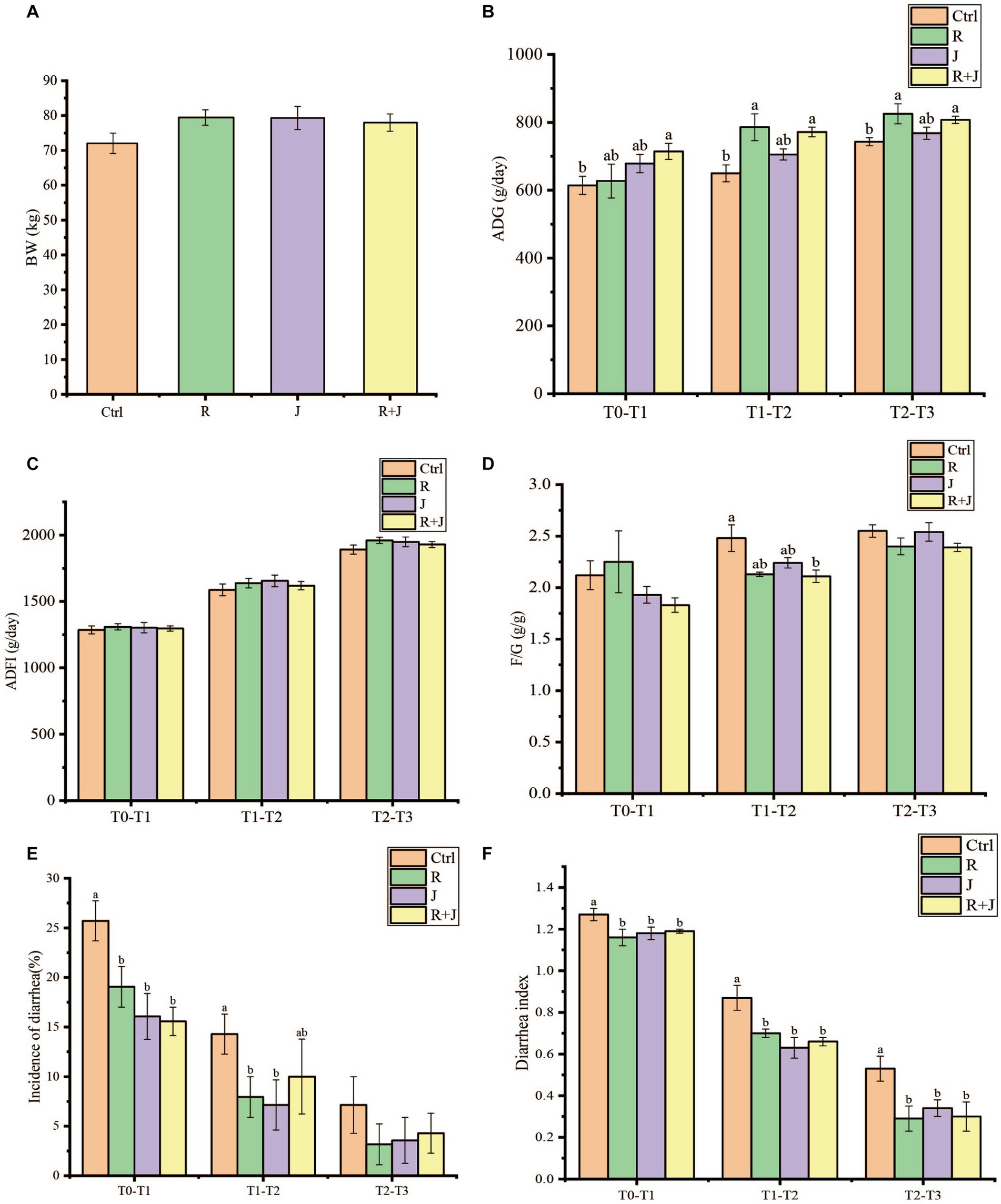
Figure 1. Effect of L. reuteri L81 and L. johnsonii L29 on growth performance, diarrhea incidence and diarrhea index of weaned calves. (A) Initial calf weight (BW). (B) Average daily weight gain (ADG). (C) Average daily food intake (ADFI). (D) Material weight ratio (F/G). (E) Incidence of calves diarrhea. (F) Calves diarrhea index. At the same time point, if there was no letter or the same letter was marked, the difference was not significant (p > 0.05), and different lower case letters indicate significant difference (p < 0.05). The same below.
Calf diarrhea incidence was significantly higher in the Ctrl group than in the R, J, and R + J groups at T0–T1 and T2–T3 (p < 0.05) (Figure 1); however, there was no significant difference between the lactobacilli supplementation groups. Similarly, diarrhea incidence was significantly lower in the R and J groups at T1–T2 (p < 0.05); however, there was no significant difference in diarrhea incidence between the R + J and Ctrl groups at the time point. Additionally, average fecal score was significantly higher in the Ctrl group than in the other treatment groups at all time points. However, there was no significant difference in average fecal score between the probiotics supplementation groups, indicating that the selected lactic acid bacteria are capable of alleviating and preventing calf diarrhea.
3.2. Effects of Lactobacillus reuteri L81 and Lactobacillus johnsonii L29 on serum biochemical indicators
Serum TP level was significantly higher (p < 0.05) in the R, J, and R + J groups than in the Ctrl group at T3 (Table 1); however, there was no significant difference (p > 0.05) in serum TP level between the probiotics supplementation groups. Similarly, serum GLB level was significantly higher (p < 0.05) in the R and R + J groups than in the Ctrl group at T3. Additionally, serum BUN level was significantly higher in the R group than in the Ctrl group at T1 and T2; however, there was no significant difference in serum BUM levels between the probiotics and Ctrl groups at T3. Moreover, there was no significant difference in GLU levels between the R and Ctrl groups. Interestingly, serum GLU level was significantly higher in the J group than in the Ctrl group at T2, and significantly higher in the R + J group than in the R and J groups at T3.
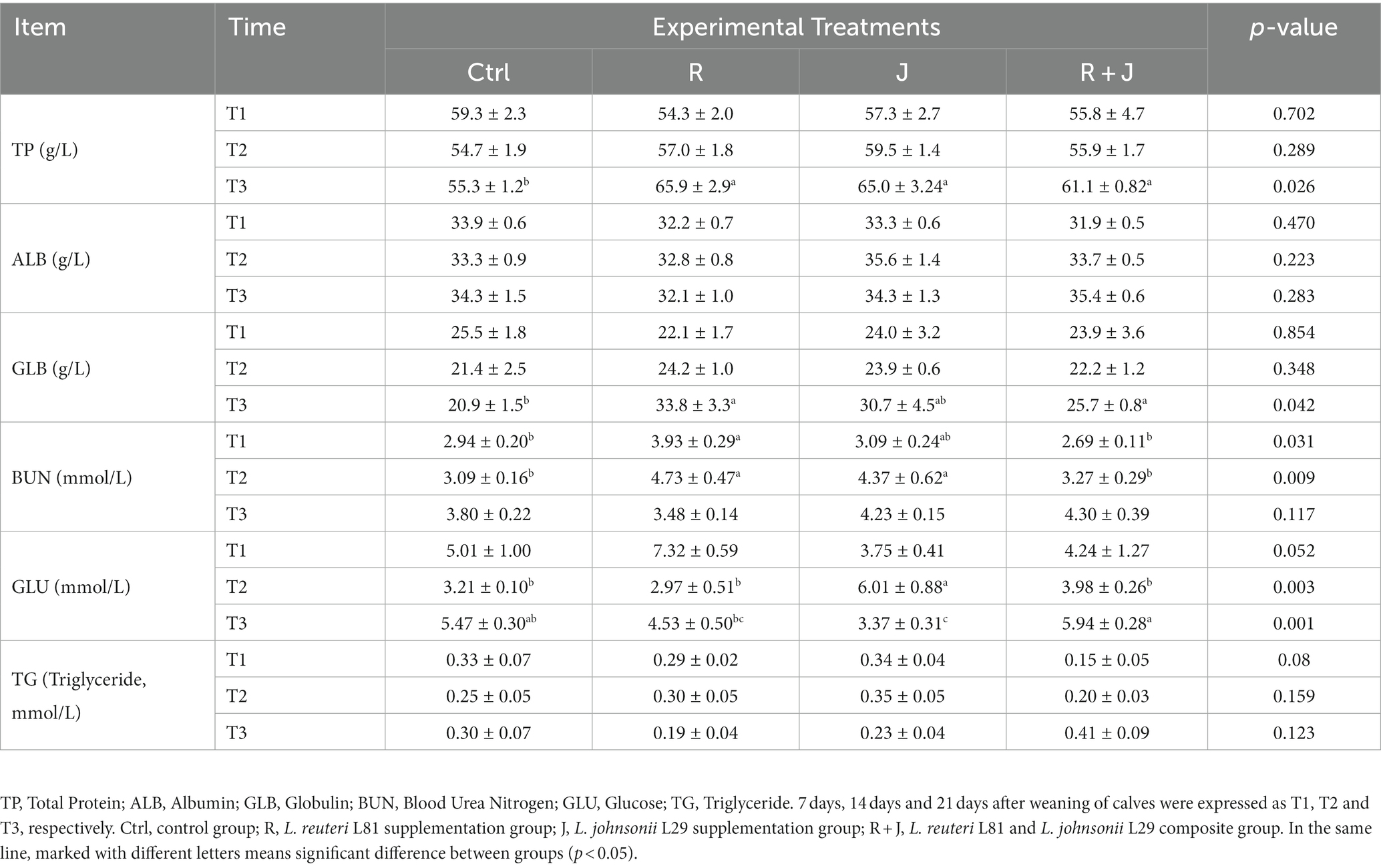
Table 1. Effect of L. reuteri L81 and L. johnsonii L29 on serum biochemical indexes of weaned calves.
3.3. Effects of Lactobacillus reuteri L81 and Lactobacillus johnsonii L29 on immune indicators, antioxidant indicators, cytokines, and intestinal permeability indicators
To evaluate the role of L. reuteri L81 and L. johnsonii L29 in the immune system, the serum immunoglobulins levels of the weaned calves were measured after probiotics supplementation (Table 2). Compared with the Ctrl group, serum IgA and IgM levels were significantly higher (p < 0.05) in the Lactobacillus-supplemented group at T1, T2, and T3. Specifically, calves in the J group had significantly higher serum IgM levels than those in the other groups at T1 and T2; however, there was no significant difference in IgM levels among the Lactobacillus supplementation groups at T3. Serum IgG level was significantly higher in the R group than in the Ctrl group at T2 and T3. Additionally, calves in the J group had significantly higher serum IgG levels than those in the other groups at T1. However, there was no significant difference (p > 0.05) in serum TNF-α level between the Ctrl and probiotic groups at T1, T2, and T3. Serum IL-10 concentration was significantly higher in the R group than in the Ctrl group at T1 and T3. Similarly, serum IL-10 concentration was significantly higher (p < 0.05) in the J group than in other groups at T2. Although there was no significant difference in serum IL-6 concentration between the groups at T1 and T2, the R group had significant lower serum IL-6 concentration at T3.
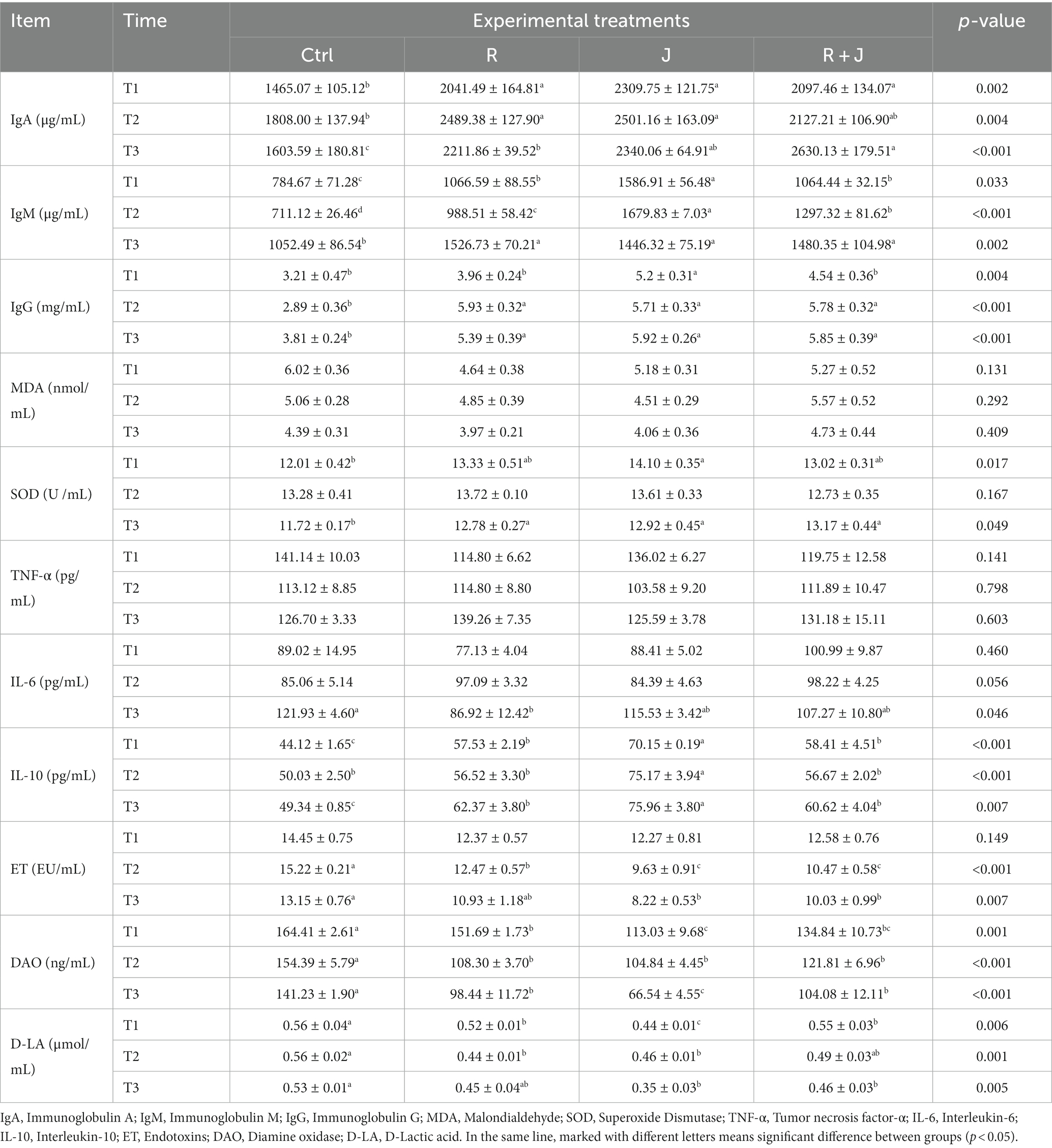
Table 2. Effect of L. reuteri L81 and L. johnsonii L29 on immune indexes, antioxidant indexes, biochemical indexes of intestinal barrier function in serum of weaned calves.
Calves in the J group had significantly higher serum SOD concentration than those in the Ctrl group at T1 (p < 0.05); however, there was no significant difference in serum SOD level between the J, R, and R + J groups at the time point. Calves in the probiotic groups (R, J, and R + J) had significantly higher (p < 0.05) serum SOD concentrations than those in the Ctrl group at T3.
Furthermore, the effects of the probiotics on intestinal barrier function in weaned calves were examined by determination the serum levels of intestinal permeability indicators (Table 2). There was no significant difference in serum ET levels between the Lactobacillus supplementation groups (R, J, and R + J) and the Ctrl group at T1; however, serum DAO and D-LA levels were significantly lower in the Lactobacillus supplementation groups than in the Ctrl group (p < 0.05). Calves in the Lactobacillus supplementation groups had significantly lower serum ET and DAO levels than those in the Ctrl group at T2; additionally, serum D-LA level was significant lower in the R and J groups than in the Ctrl group at T2 (p < 0.05). At T3, serum ET and D-LA levels were significantly lower in the J and R + J groups than in the Ctrl group (p < 0.05), meanwhile there was no significant difference between the Ctrl and R groups. Lactobacillus supplementation significantly decreased serum DAO levels compared with the Ctrl group, with the lowest level observed in the J group.
3.4. Effect of Lactobacillus reuteri L81 on intestinal morphology of weaned calves
The effect of L. reuteri L81 on the intestinal tissue morphology of weaned calves is shown in Table 3. The results showed that the villus height and the ratio of villus height to crypt depth of each small intestinal segment in the R group of Holstein weaned calves were higher than those in the Ctrl group, and the crypt depth was lower than that in the Ctrl group. The crypt depth of ileal in the R group was significantly reduced compared to the Ctrl group, and the villus height / crypt depth was significantly increased (p < 0.05).
3.5. Effects of Lactobacillus reuteri L81 on the expression of intestinal tight junction protein and cytokines related genes in weaned calves
It can be seen from Figure 2 that, compared with the Ctrl group, the gene expression of tight junction protein in duodenum of group R has no significant change. The gene related expression of tight junction protein Claudin-1, Occludin and ZO-1 in jejunum was significantly increased (p < 0.05). The relative expression of Claudin-1 gene in the ileum increased significantly (p < 0.05). There was no significant difference in the relative expression of TLR4 and IL-2 genes between the R group and the Ctrl group (p > 0.05). The expression of TGF-β1 in the jejunum and ileum of group R was significantly increased, and the expression of IL-8 in the jejunum and INF-γ in the ileum were significantly reduced (p < 0.05).
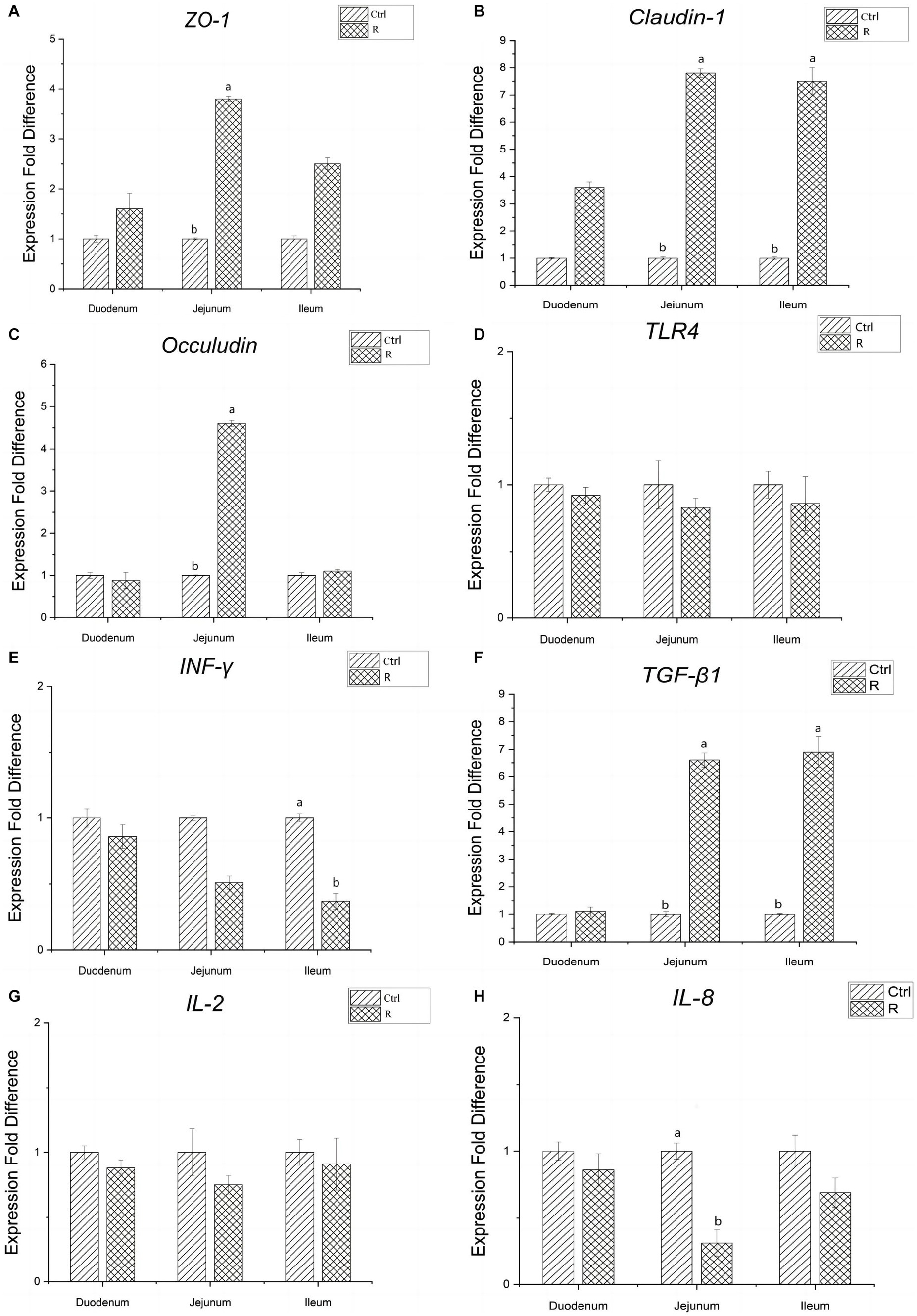
Figure 2. Effects of L. reuteri L81 on the expression of intestinal tight junction protein and cytokines related genes in weaned calves. (A) Relative gene expression of ZO-1. (B) Relative gene expression of Claudin-1. (C) Relative gene expression of Occludin. (D) Relative gene expression of TLR4. (E) Relative gene expression of INF-γ. (F) Relative gene expression of TGF-β1. (G) Relative gene expression of IL-2. (H) Relative gene expression of IL-8.
3.6. Effect of Lactobacillus reuteri L81 on pH value and enzyme activity in small intestine
The effects of L. reuteri L81 on intestinal content pH and enzyme activity in the duodenum, jejunum, and ileum were shown in Figure 3. It can be seen from Figure 3A that supplementation of L. reuteri L81 before weaning had no significant effect on intestinal pH after weaning. However, Na+-K+-ATPase activity in the duodenum, jejunum, and ileum was significantly higher in the R group than in the Ctrl group (p < 0.05). Compared with the Ctrl group, the supplementation of L. reuteri L81 had no significant effect on the activities of protease, amylase, lipase and lactase.
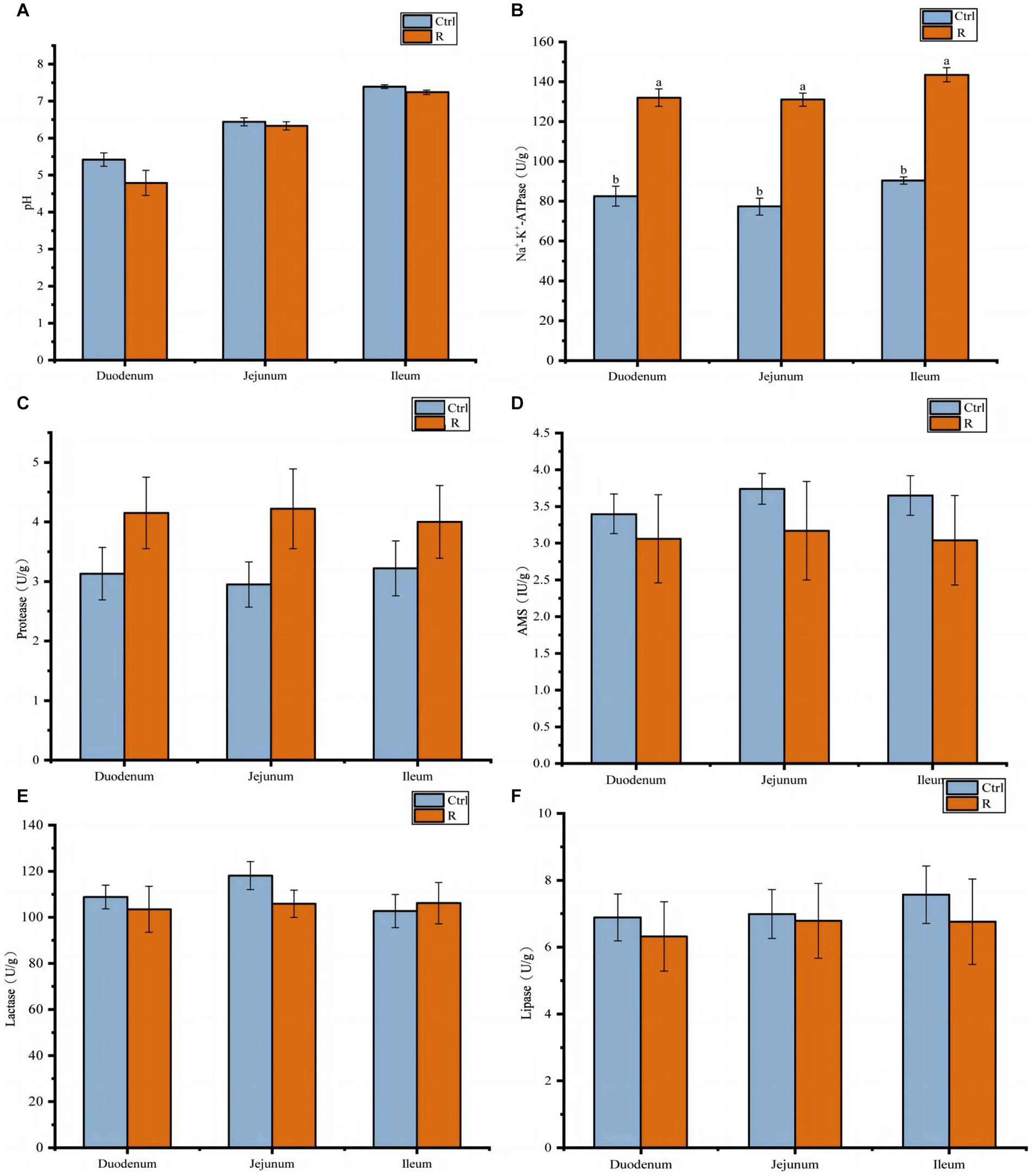
Figure 3. Effect of L. reuteri L81 on pH value and digestive enzyme activities of intestinal contents in weaned calves. (A) pH value. (B) Na+-K+-ATPase. (C) Protease. (D) AMS. (E) Lactase. (F) Lipase.
3.7. Quality evaluation of sequencing data of intestinal microbial communities
Sequencing of the V3–V4 regions of the 16S rRNA gene in DNA samples generated a total of 2,211,341 raw reads. After double-ended quality control, splicing, noise removal, chimerism removal, and singleton removal of raw reads, 1,308,705 valid sequences were obtained for subsequent analysis. The fungal ITS1-2 region was sequenced and 2,512,684 valid sequences were obtained for subsequent analysis. The sparse curves of all samples were nearly flat, indicating that the sequencing amount of each sample was sufficient to cover most of the bacterial and fungal communities and that the sequencing results reflected the composition and diversity of the bacterial and fungal communities in the samples (Figure 4). To assess the commonality and uniqueness of microorganisms in different intestinal segments, the number of ASVs common to different intestinal segments among the groups was analyzed using a Venn diagram. In the Ctrl and R groups, there were 123 ASVs for bacteria and 44 ASVs for fungi in the different intestinal segments, and each group of samples had unique ASVs. The number of unique ASVs in the ileum was higher than those in the duodenum and jejunum. Compared with the Ctrl group, a higher number of unique ASVs were observed in the jejunum and ileum of calves in the R group.
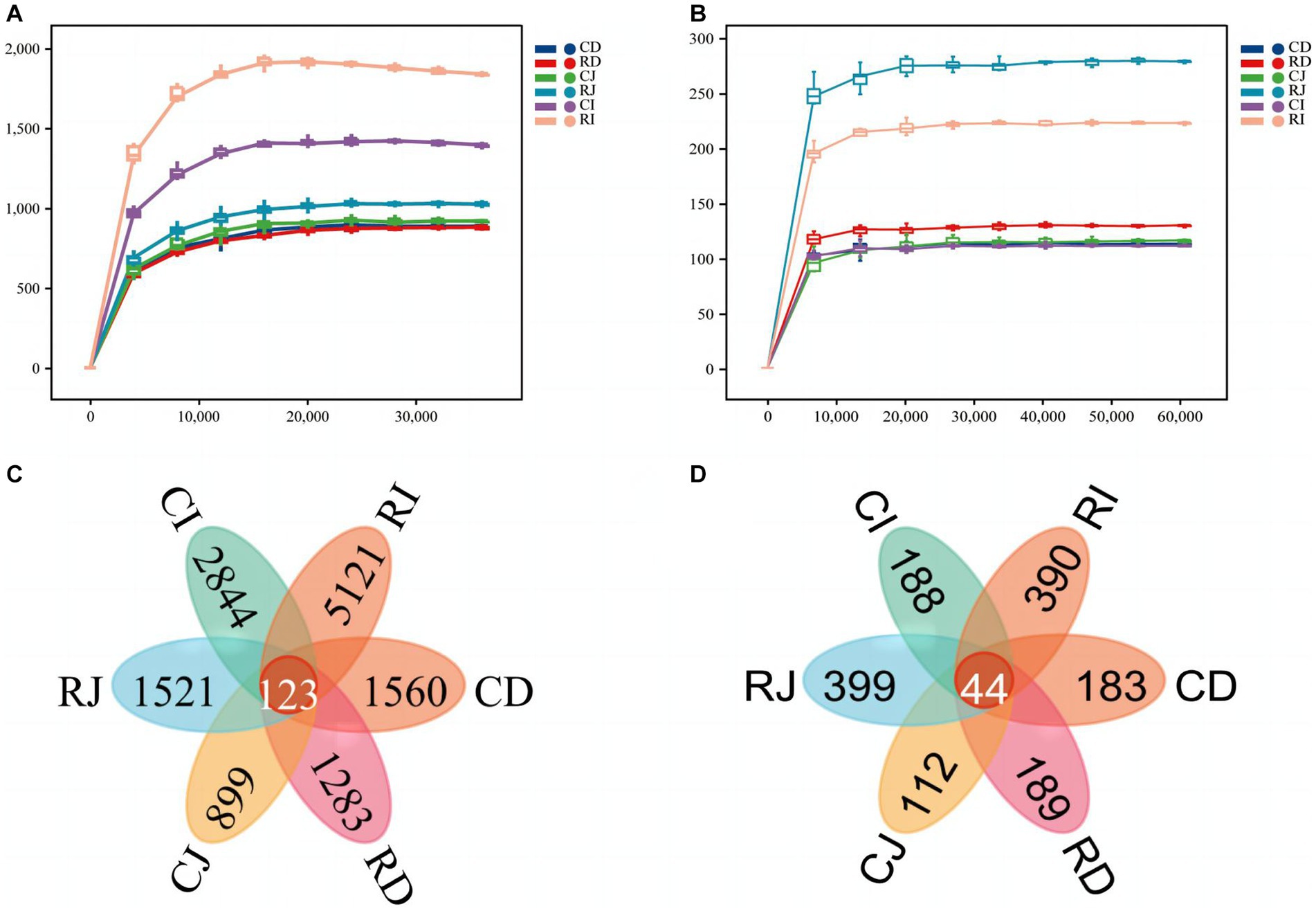
Figure 4. Bacterial and fungal rarefaction curve and venn diagrams of ASVs in different intestinal segments of different sample groups. (A) Bacterial rarefaction curve based on Chao index. (B) Fungal rarefaction curve based on Chao index. The X-axis is the sequencing depth, and the Y-axis is the median value and boxplot of Chao index calculated for 10 times. (C) Venn diagram of bacteria ASVs. (D) Fungus ASVs Venn diagram. CD, ctrl group duodenum; CJ, ctrl group jejunum; CI, ctrl group ileum; RD, L. reuteri L81 group duodenum; RJ, L. reuteri L81 group jejunum; RI, L. reuteri L81 group ileum.
3.8. Effect of Lactobacillus reuteri L81 on the diversity of intestinal microbiota in weaned calves
Furthermore, the effect of L. reuteri L81 supplementation on duodenal, jejunal, and ileal microbiota diversity were examined (Figure 5). The Good’s coverage index of each group of samples was above 0.99, indicating that the sequencing data sufficiently reflected the composition and distribution of the intestinal microbiota. Compared with the control group, the Chao1 and Shannon indices of bacteria and fungi in the different intestinal segments showed an upward trend in the R group; however, there was no significant difference in Simpson index between the groups.
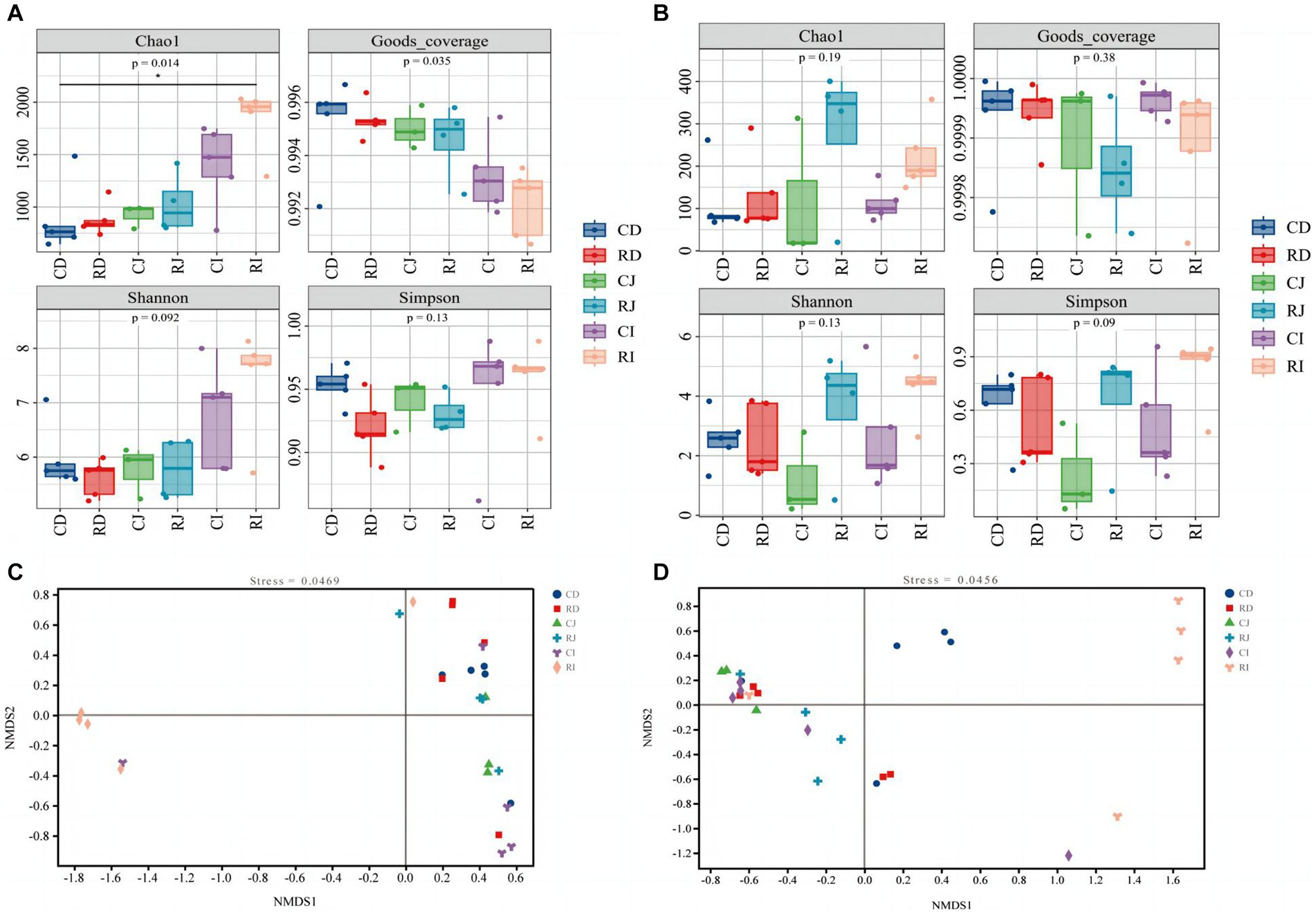
Figure 5. Effect of L. reuteri L81 on diversity of intestinal microbiota in weaned calves. (A) Bacterial α diversity index. (B) Fungal α diversity index. (C) NMDS diagram of bacteria. (D) NMDS diagram of fungal. 0.01 < p < 0.05 in the figure indicates significant difference, marked with *; p < 0.01 indicates extremely significant difference, marked with **. CD, ctrl group duodenum; CJ, ctrl group jejunum; CI, ctrl group ileum; RD, L. reuteri L81 group duodenum; RJ, L. reuteri L81 group jejunum; RI, L. reuteri L81 group ileum.
A non-metric multidimensional scale (NMDS) analysis of the composition of microbes in the duodenum, jejunum, and ileum of weaned calves was performed based on Bray Curtis distance (Figure 5). Intestinal microbiota in the same intestinal tract formed distinct clusters among individuals; however, there were individual differences between the groups, with the R group showing a significant separation of the ileal microbiota from the duodenal and jejunal microbiota.
3.9. Effect of Lactobacillus reuteri L81 on the structure of intestinal microbiota in weaned calves
At the phylum level, the dominant bacterial microbiota in the duodenum, jejunum, and ileum were Firmicutes, followed by Actinobacteria and Bacteroides. The dominant bacteria in the duodenum and jejunum were Firmicutes and Actinobacteria, while those in the ileum were Firmicutes and Bacteroides (Figure 6). The relative abundance of Bacteroidetes was significantly higher in the ileum than in the duodenum and jejunum. Compared with the Ctrl group, L. reuteri L81 supplementation significantly decreased the relative abundance of Proteobacteria in the ileum of the calves. Ascomycota was the dominant fungal phylum in the duodenum, jejunum, and ileum, followed by Basidiomycota and Mucoromycota. Ascomycota fluctuated between groups, and the relative abundance of Ascomycota in the ileum of the R group was significantly lower than that in the other experimental groups.
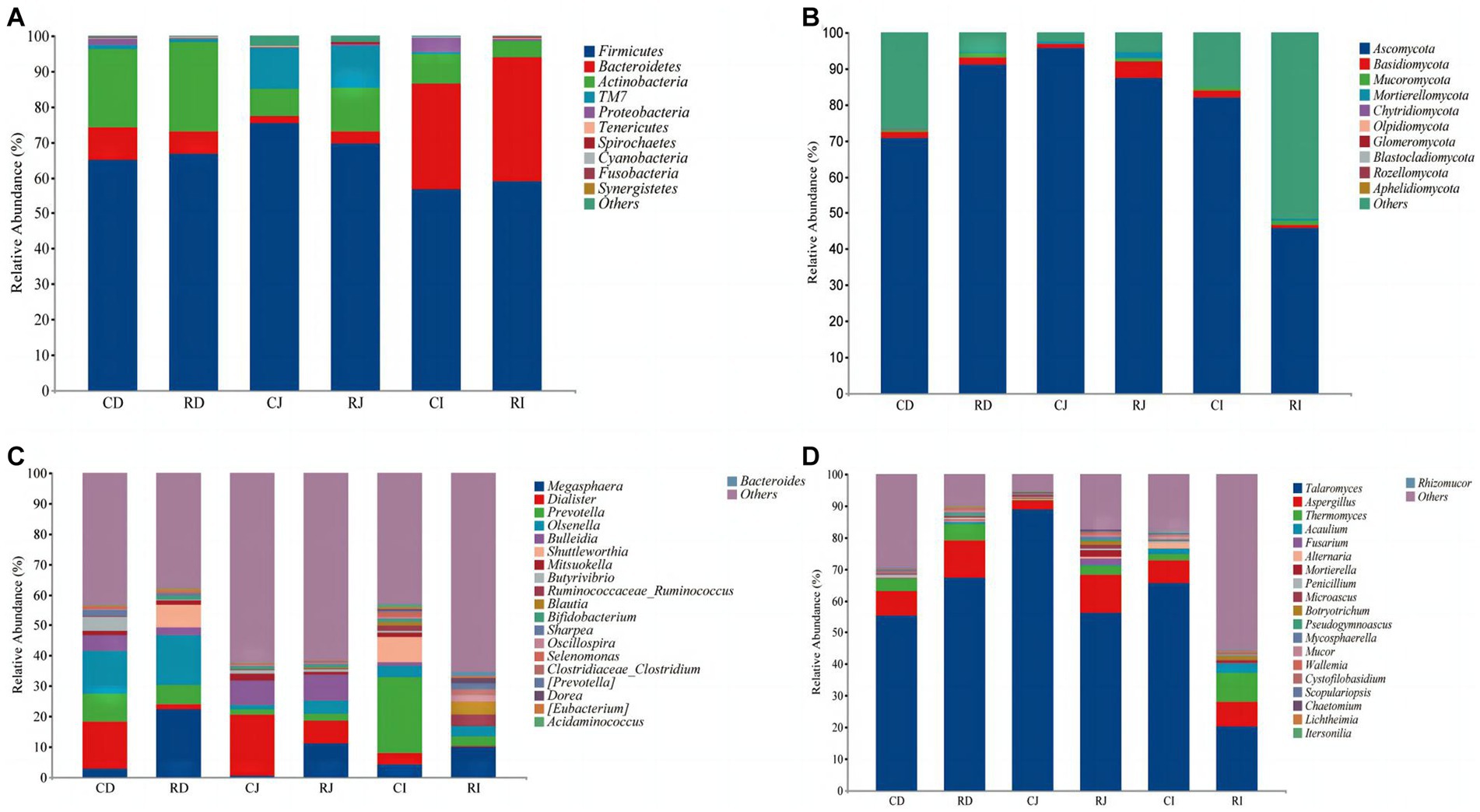
Figure 6. Effects of L. reuteri L81 on the intestinal microbiota composition of weaned calves at the level of phylum and genus. (A) The microbiota composition of bacteria at the phylum level. (B) The microbiota composition of fungal at the phylum level. (C) The microbiota composition of bacteria at the genus level. (D) The microbiota composition of fungal at the genus level. CD, ctrl group duodenum; CJ, ctrl group jejunum; CI, ctrl group ileum; RD, L. reuteri L81 group duodenum; RJ, L. reuteri L81 group jejunum; RI, L. reuteri L81 group ileum.
At the genus level, Megaspaera, Dialister, Prevotella, Olsenella, and Bulleidia were the predominant bacteria in the duodenum and jejunum. Megaspaera, Prevotella, and Dialister were the dominant genera in the ileum, followed by Ruminococcaceae (Ruminococcus) and Blautia. However, L. reuteri L81 supplementation increased the relative abundances of Ruminoccaceae_Ruminococcus, Blautia, and Oscillospira in the ileum and decreased the relative abundance of Dialister in the duodenum, jejunum, and ileum. Talaromyces and Aspergillus were the dominant fungal genera in the duodenum, jejunum, and ileum, followed by Thermomyces.
LEfSe analysis was performed to further identify microbial compositional differences in intestinal segments between the Ctrl and R groups (Figure 7). L. reuteri L81 supplementation enriched Bifidobacteriales, Lactobacillus, Mobilucus and Corynebacterium in the duodenum; Thermomyces in the jejunum; and Blautia, Collinsella, Oscillospira, Roseburia, Coprobacillus, Odoribacter, Lactobacillus, Thermogenes, Lophotrichus, and Botryotrruchum in the ileum.
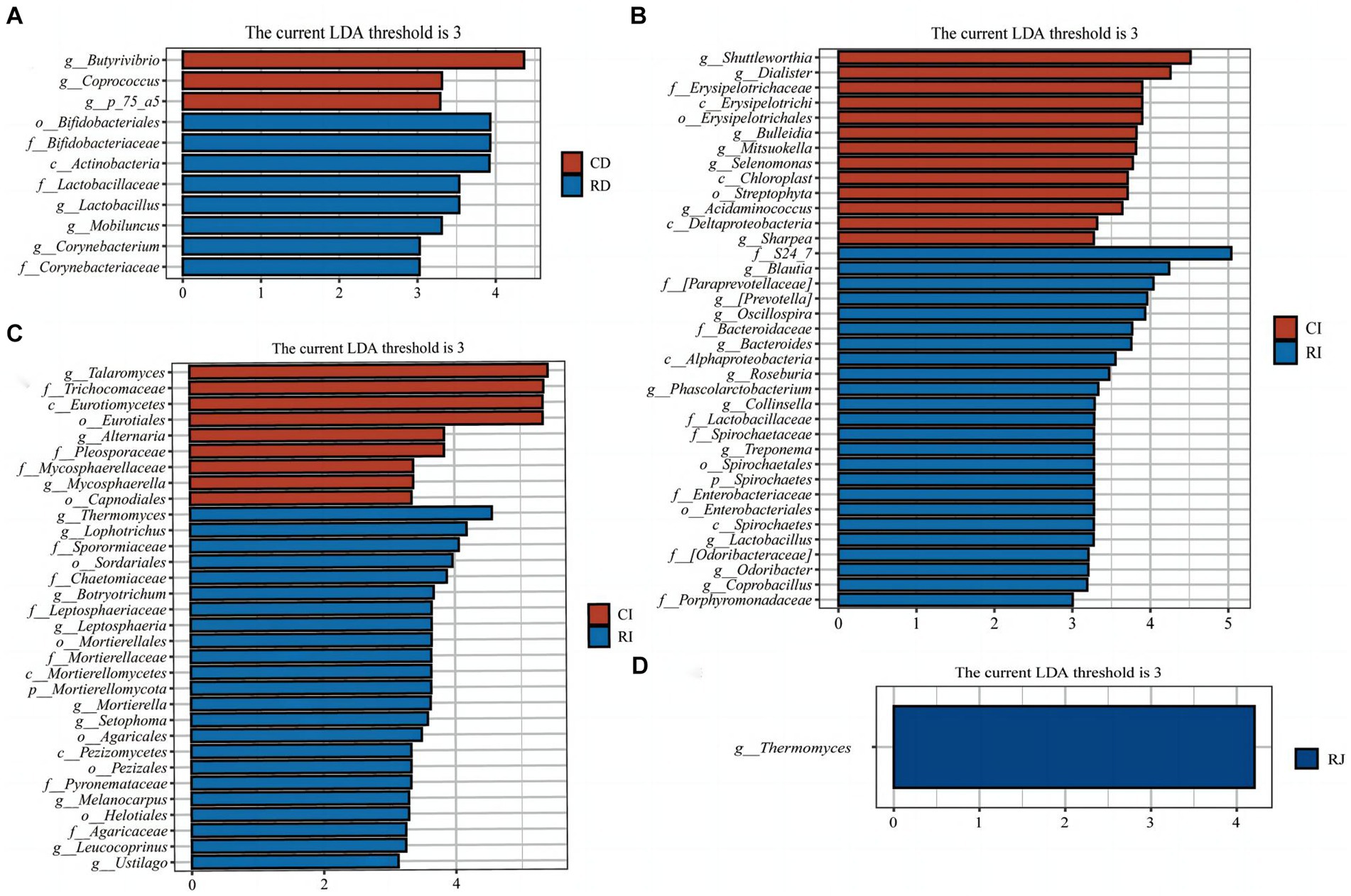
Figure 7. LEfSe analysis of intestinal microbiota in weaned calves. (A) Histograms of LEfSe analysis of duodenal bacteria in the Ctrl group and L. reuteri L81 group. (B) Histograms of LEfSe analysis of ileal bacteria in the Ctrl group and L. reuteri L81 group. (C) Histogram of LEfSe analysis of ileal fungi in the Ctrl group and L. reuteri L81 group. (D) Histogram of LEfSe analysis of jejunum fungi in the Ctrl group and L. reuteri L81 group. The ordinate of the histogram is the classification group with significant difference between groups, and the abscissa is the bar chart showing the logarithmic score of LDA of each classification group. The longer the length is, the more significant the difference of taxon is. The color of the bar chart indicates the sample group with the highest abundance corresponding to the taxon.
4. Discussion
During weaning, calves are prone to stress, which can affect their health and growth performance, and lead to stunted growth and development. Probiotics play a positive role in promoting animal health, improving host immunity, combating pathogenic bacteria, regulating intestinal microecology, and improving enhancing intestinal epithelial barrier function (Aoudia et al., 2016; David et al., 2016; Kelsey and Colpoys, 2018).
4.1. Growth performance, diarrhea incidence, and diarrhea index
In the present study, supplementation with L. reuteri L81and L. reuteri L81 + L. johnsonii L29 improved ADG in wean calves compared with the Ctrl group at T1–T2 and T2–T3. Similarly, L. reuteri supplementation has been shown to significantly improve the ADG of piglets (Wang et al., 2020); in contrast, some studies have shown that the addition of L. reuteri or the combination of L. reuteri and other probiotics had no significant effect on ADG in weaned piglets (Dell’Anno et al., 2021), which could be attributed to differences in probiotic strains, supplementation amount, or combinations. Additionally, supplementation with L. reuteri L81 + L. johnsonii L29 significantly reduced the F/G ratio at T1–T2. Previous studies have shown that the supplementation of L. reuteri or probiotics before weaning can improve the growth of calves.
Early intervention with probiotics has been reported to prevent calf diarrhea, reduce calf mortality, and improve calf health by promoting gastrointestinal health and maintaining intestinal barrier integrity in pre-weaning calves (Liu et al., 2015). A previous study showed that L. reuteri and L. johnsonii exhibited potent antibacterial activity against pathogens that commonly cause bovine diarrhea (Fan et al., 2021). L. reuteri has a strong ability to adhere to the intestinal mucosa, which can improve the distribution of intestinal microbiota and inhibit colonization by harmful bacteria (Deng et al., 2020). Moreover, L. johnsonii supplementation has been shown to improve intestinal environment, immunity, and disease resistance in piglets (Xin et al., 2019). In the present study, supplementation with L. reuteri L81and L. johnsonii L29 reduced diarrhea incidence at T0–T1 and T1–T2 after weaning, which was consistent with previous findings. Although there was no significant difference in diarrhea rate between the groups at T2–T3, Lactobacillus supplementation significantly reduced the diarrhea index of calves. Overall, these results indicated that the probiotics were effective in preventing weaning diarrhea in calves when administered individually or in combinations.
4.2. Serum biochemical indicators
ALB has several physiological functions, such as maintaining normal colloidal osmotic pressure in blood vessels, transporting various metabolic substances, and regulating transported substances. GLB is primarily involved in immune regulation, while BUN is a product of protein metabolism and an important indicator of renal function. In the present study, serum TP and GLB levels were significantly higher in the R group than in the Ctrl group at T3, indicating that L. reuteri L81 improved immune performance in weaned calves. Similarly, calves in the R group had significantly higher BUN levels than those in the Ctrl group at T1 and T2, indicating that amino acid metabolism was elevated in weaned calves and that accumulated nitrogen could not be discharged in a timely manner (Lu et al., 2021; Han et al., 2022). GLU is the main indicator of glucose metabolism in the body and is a direct energy source for the body’s activities. In the present study, L. reuteri L81 and L. johnsonii L29 supplementation had different effects on serum GLU levels, indicating that different microecological preparations have different effects on energy and lipid metabolism in weaned calves.
4.3. Immune function, antioxidant capacity, and intestinal permeability
Serum IgG, IgM, and IgA levels reflect the functional state of humoral immunity (Kotnik and Zaletel, 2011; Panahi et al., 2020; Tan et al., 2021). Some studies have shown that L. johnsonii supplementation can improve serum IgG levels in sows (Wang et al., 2014), restore serum IgA and IgG levels in elderly mice with protein energy malnutrition (PEM), and contribute to the recovery of the immune system in immunocompromised hosts (Kaburagi et al., 2007). Additionally, L. johnsonii BS15 supplementation can prevent subclinical necrotizing enteritis (SNE) in broilers by improving immune related blood parameters and enhancing intestinal immunity (Wang et al., 2018). Consistent with previous findings, L. johnsonii L29 supplementation significantly increased serum IgG, IgM, and IgA levels in weaned calves in the present study, indicating that L. johnsonii L29 has a promoting effect on enhancing the immunity of weaned calves. Similarly, some studies have shown that L. reuteri can significantly increase serum IgG and IgA levels and enhance immune response in mice. In the present study, L. reuteri L81 supplementation significantly increased serum IgG and IgA levels at T2 and T3 compared with the Ctrl group. Moreover, supplementation with L. reuteri L81 and L. johnsonii L29 mixture significantly improved serum IgG, IgM, and IgA levels in calves at T3, indicating that supplementation with L. reuteri L81, L. johnsonii L29, or a combination of both can alleviate weaning-induced diarrhea by increasing calf immunoglobulin levels and enhancing immunity against viruses and bacteria.
SOD activity indirectly reflects the ability of the body to scavenge free oxygen radicals. Studies have shown that L. reuteri supplementation can significantly improve SOD activity in piglets (Yang et al., 2019). Similarly, oral administration of L. reuteri significantly increased SOD activity and decreased MDA levels in the intestinal tissue of mice with necrotizing enterocolitis (Tang et al., 2019). In the present study, probiotics supplementation significantly increased SOD activity at T1 and T3 compared with the Ctrl group, which was consistent with previous findings, indicating that probiotics can increase the activity of antioxidant enzymes in calves.
Weaning stress can damage the immune system and alter the production of inflammatory cytokines (Kim et al., 2012; Gurung et al., 2021), making the body extremely susceptible to infection by various pathogens. Studies have shown that some L. reuteri strains (including GMNL-263) can reduce the production of proinflammatory cytokines, such as TNF-α and IL-6, in mice fed a high fat diet (Hsieh et al., 2016). Additionally, L. reuteri significantly suppressed sodium dextran sulfate-induced expression of TNF-α (Hou et al., 2018). Consistent with previous findings, L. reuteri L81 supplementation significantly reduced serum IL-6 level at T3 compared with the Ctrl group in the present study. IL-10 is an anti-inflammatory cytokine that inhibits the expression of several pro-inflammatory cytokines and cell surface antigens, and improves immunopathology (Couper et al., 2008). In the present study, L. johnsonii L29 supplementation significantly increased serum level of IL-10 compared with the Ctrl group. Additionally, supplementation with L. reuteri L81 and a mixture of L. johnsonii L29 and L. reuteri L81 significantly increased serum IL-10 levels, which was consistent with previous findings that probiotics can bind to intestinal mucosal epithelial cells via toll-like receptors and mediate cytokine secretion to regulate the expression of immune T cells and the production of IL-10 to enhance the body’s immunity (Carolina et al., 2019). L. reuteri L81 and L. johnsonii L29 have been speculated to alleviate inflammatory reaction in the body, improve intestinal mucosal barrier function, and reduce the occurrence of diarrhea by stimulating the anti-inflammatory cytokine IL-10.
Factors, such as stress and autoimmune system dysfunction, have been shown to damage mucosal barrier function and alter intestinal wall permeability (Wang et al., 2016). ET, D-LA, and DAO can enter blood circulation via the damaged intestinal mucosa, making them important markers for evaluating intestinal mucosal barrier function (Kuang et al., 2020; He et al., 2022). In the present study, L. johnsonii L29 supplementation inhibited the leakage of D-LA and DAO into the peripheral blood and suppressed serum ET levels compared with the Ctrl group, indicating that L. johnsonii L29 had a protective effect on intestinal mucosal integrity. Similarly, L. reuteri L81 supplementation significantly reduced serum DAO levels, and serum concentration of D-LA at T1 and T2. L. reuteri has been speculated to alleviate weaning diarrhea by reducing intestinal mucosal damage. Additionally, L. reuteri L81supplementation significantly reduced serum levels of ET, indicating that L. reuteri can inhibit the growth of harmful intestinal bacteria and reduce the production of intestinal toxins. Similarly, supplementation with a mixture of L. reuteri L81 and L. johnsonii L29 decreased the serum levels of ET, D-LA, and DAO in weaned calves at different time points compared with the Ctrl group, indicating that the probiotics reduced intestinal mucosal permeability, improved mucosal barrier function, and reduced the leakage of endotoxins into blood circulation, thereby relieving weaning diarrhea in calves. In our research, we found that compared with L. johnsonii L29, under the same nutritional and cultural conditions, L. reuteri L81 has faster growth rate, shorter passage and preparation cycle, and lower economic and time costs for large-scale cultivation. Considering economic cost factors, it is more suitable for the preparation and production of probiotic formulations and is convenient for later promotion and application. Therefore, in the subsequent experiments, just L. reuteri L81 was studied.
4.4. Intestinal morphology
The villus height, crypt depth, and the ratio of villus height to crypt depth are major indicators in evaluating the integrity and digestive ability of the small intestine. When calves are weaned, due to the imperfect digestive system of calves and the change of dietary structure, it is easy to lead to the contraction of intestinal villi, the decrease of digestion and absorption enzyme activity of calves. We found that the supplementation of L. reuteri L81 increased the height of small intestinal villi, reduced the depth of crypts, and also had a positive effect on increasing the ratio between the two. The small intestinal villi mainly absorb amino acids, glucose, inorganic salts and other substances from the epithelial cells of the intestinal mucosa to provide nutrition for the body. The ratio of villus height to crypt depth can comprehensively reflect the digestion and absorption of the intestine. The larger the ratio, the more epithelial cells in the small intestine, the larger the absorption area of the small intestine, and the higher the absorption and utilization of nutrients. The results showed that the L.reuteri L81 increased the average daily gain of calves after weaning. It was speculated that this was related to the increase of villus height and the ratio of villus height / crypt depth, which was similar to the results of previous studies (Wang et al., 2020). In short, L. reuteri L81 enhances the integrity of intestinal tissue morphology in weaned calves, thereby promoting nutrient absorption and weight gain.
4.5. Expression of intestinal tight junction protein and cytokines related genes
There is a group of protein complexes composed of transmembrane proteins such as ZO-1, Occludin and Claudins between intestinal epithelial cells, which play a key regulatory role in maintaining intestinal barrier function and preventing intestinal mucosal injury (Günzel and Yu, 2013; Kuo et al., 2021). Bacteria and toxins can enter other tissues or organs through the damaged intestinal epithelial cell tight junction protein, thus causing bacterial and toxin translocation and many diseases. It has been reported that the supplementation of probiotics can enhance the intestinal physical barrier function by increasing the expression of intestinal epithelial tight junction protein. For example, Lactobacillus rhamnosus GG can protect the intestinal mucosa of rats from PTG induced damage by preventing the decrease of the expression of intercellular connexin (Orlando et al., 2018). Lactobacillus reuteri LR1 isolated from the feces of weaned piglets can increase the protein content of tight junction protein, ZO-1 and Occludin in IPEC-1 cells infected by ETEC K88 (Yi et al., 2018). In this study, it was found that the gene expression of ZO-1, Claudin-1 and Occludin in jejunum mucosa and Claudin-1 in the ileum were significantly increased after weaning of Holstein calves supplemented with L. reuteri L81 before weaning, which was consistent with the results of previous studies. It shows that L. reuteri L81 plays an important role in enhancing intestinal barrier function and maintaining intestinal health. In addition, we also found that L. reuteri L81 significantly enhanced the gene expression of TGF-β1 in jejunum and ileum mucosa, and significantly reduced the gene expression of IL-8 in jejunum and INF-γ in ileum, indicating that this strain can regulate the immune performance of calves after weaning by enhancing the expression of anti-inflammatory factors and inhibiting the expression of pro-inflammatory factors, and reduce the intestinal mucosal damage caused by pathogenic bacteria, thereby alleviating the occurrence of weaning diarrhea in calves.
4.6. Enzyme activity in small intestine
The activity of digestive enzymes in the digestive tract is an important indicator of digestive function in animals. Na+/K+-ATPase is a transmembrane protein that plays an important role in maintaining Na+ −dependent transport vectors, such as sodium glucose co-transporter 1 (SGLT1)-mediated glucose transport (Manoharan et al., 2018). A previous study showed that children with ulcerative colitis with severe proctitis have significantly lower Na+/K+-ATPase activity than those with remission stage ulcerative colitis or with normal rectal mucosa, which may lead to impaired sodium transport-induced diarrhea (Ejderhamn et al., 1989). Additionally, B. amyloliquefaciens supplementation significantly increased intestinal Na+/K+-ATPase activity in piglets (Hu S. et al., 2018). Consistent with the previous findings, L. reuteri supplementation significantly increased the activity of Na+/K+-ATPase in the duodenum, jejunum, and ileum of weaned calves.
4.7. Regulation of intestinal microbiota
The intestinal microbiota plays crucial roles in the development, maturation, and homeostasis of the mucosal immune system (Hou et al., 2022), and an imbalance in the intestinal microbiota is associated with several diseases that cause inflammation of the intestinal tissue (Malmuthuge et al., 2015). Maintaining normal intestinal microbiota structure is necessary to maintain a healthy gut. There is increasing evidence that early intervention of intestinal microbiota during critical periods may be a promising method for improving intestinal microbial colonization (Xiang et al., 2020). Supplementation with probiotics improves early intestinal health and reduces intestinal diseases in calves (Aidy et al., 2013; Gensollen et al., 2016). This study conducted 16S rRNA and ITS gene amplicon sequencing on the small intestine contents of Ctrl and R groups of calves on the 21st day after weaning to analyze the effect of L. reuteri L81 on the composition and diversity of intestine microbiota in weaned calves.
Intestinal microbial diversity is related to the age, disease status and growth rate of calves. For example, intestinal microbial diversity was significantly lower in calves with diarrhea than in healthy calves (Oikonomou et al., 2013). Previous studies have shown that the supplementation of probiotics to calf diets can alter intestinal bacterial diversity and colony composition in the gastrointestinal tract (Wen et al., 2022). An abundant intestinal microbiota can resist pathogenic bacteria invasion; moreover, the higher the microbiota diversity the better is the nutritional status of the intestinal tract. In the present study, L. reuteri L81 supplementation increased the number of unique ASVs compared with the Ctrl group, with an increase in Chao1 and Shannon indices of intestinal microbiota, indicating that Lactobacillus enhanced the abundance and diversity of intestinal microbiota and improved disease resistance. Some studies have shown relatively low microbial diversity in the small intestine, similar microbial composition and diversity in the ileum and hindgut of Aohan Fine Wool Sheep, and significantly lower intestinal microbial diversity and abundance in the foregut than in the hindgut (Ma et al., 2022). In the present study, the number of unique ASVs and Chao1 and Shannon indices was significantly higher in the ileum than in the duodenum and jejunum, indicating a higher bacterial diversity and abundance in the ileum than in the duodenum and jejunum, which was consistent with previous findings (Myer et al., 2016; Freetly et al., 2020). However, there was no significant difference in Simpson index among the groups, indicating that L. reuteri L81 supplementation did not significantly affect bacterial uniformity in each intestinal segment. Additionally, NMDS analysis showed significant separation of microbial composition in the ileum, duodenum, and jejunum of calves with supplemented L. reuteri L81, indicating a certain difference in microbial community composition between the ileum and other intestinal regions, which is consistent with the research results of Chinese Mongolian sheep. NMDS analysis showed that there was a significant separation in microorganism composition in the ileum, duodenum, and jejunum of calves fed Lactobacillus-supplemented diet, indicating that there was a certain difference in the composition of microbial communities in the ileum and other intestinal regions, which was consistent with findings in Chinese Mongolian sheep (Zeng et al., 2017).
High-throughput sequencing showed that Firmicutes, Bacteroides, and Actinobacteria were the dominant bacteria in the small intestine of weaned calves. Firmicutes can participate in energy absorption in the intestine (Tap et al., 2010), and Firmicutes have been shown to be the dominant flora in the small intestine of ruminant and monogastric animals (Costea et al., 2017; Liu et al., 2019). The small intestinal microbial community is mainly composed of rapidly growing facultative anaerobic bacteria that can tolerate the combined effects of bile acids and antibiotics and effectively compete with the host and other bacteria for simple carbohydrates. Bile acids secreted by the proximal bile duct can inhibit the growth of Bacteroides spp. (Donaldson et al., 2016), which was confirmed by the relatively low abundance of Bacteroidetes in the duodenum and jejunum of calves in the present study, regardless of whether L. reuteri L81 was added or not.
At the genus level, there were similarities and differences in the dominant bacterial genera in various segments of the small intestine, which may be related to the physiological function of each intestinal segment. Specifically, L. reuteri L81 supplementation decreased the relative abundance of Dialister in the various segments of the small intestine. Among the human intestinal microbiota, Dialister has one of the lowest average abundances of core bacteria, but its role in the microbiota is poorly understood. However, the low abundance of Dialister has been shown to be related to Crohn’s disease (Joossens et al., 2011). L. acidophilus NCFM strain and cellobiose symbiotic bacteria supplementation for three weeks increased the relative abundance of Bifidobacterium, Collinsella, and Eubacterium, and decreased the relative abundance of Dialister, which is similar to the results of the present study. Bifidobacteria can improve the intestinal epithelial barrier function in animals by inhibiting the secretion of pro-inflammatory factors and improving the expression of intestinal tight junction proteins (Ling et al., 2016; Dong et al., 2022). An in-depth study of Lactobacillus showed that this bacterium could maintain the intestinal epithelial barrier function by inhibiting intestinal epithelial cell apoptosis, upregulating the expression of intestinal tight junction proteins, and improving the integrity of the intestinal mucosa (Hu S. et al., 2018; Zhou et al., 2022). Oscillospira is a genus of bacteria capable of producing short-chain fatty acids, such as butyric acid, which has positive effects in certain specific diseases and is considered a candidate for the next generation of probiotics (Yang et al., 2021). Several factors, including probiotics and prebiotics, promote the enrichment of Oscillospira spp. In the present study, L. reuteri L81 supplementation enriched Bifidobacterium, Lactobacillus, and Oscillospira in the duodenum, jejunum, and ileum, indicating that L. reuteri L81 can improve the intestinal barrier function of calves by promoting the relative abundance of probiotics, thereby alleviating weaning diarrhea. Several studies have shown that Collinsella and Bifidobacterium can modify host bile acids and regulate the virulence of intestinal pathogens (Elisha et al., 2017). Bacteroides and Odoribacter play important roles in maintaining the balance between intestinal microbiota and intestinal barrier function (Li et al., 2012). The enrichment of these bacteria in the ileum plays a positive role in maintaining the intestinal health in weaned calves. Additionally, L. reuteri L81 supplementation enriched fungi in the jejunum and ileum, indicating that the addition of this bacterium also had an impact on the composition of the fungal. For example, the Thermomycetes was enriched in the jejunum and ileum of the L. reuteri L81 group. Research has shown that Thermomyces is an excellent hemicellulose degrading bacterium that secretes xylanase (Lu et al., 2022). Increased serum triglyceride concentration and metabolic biomarkers in mice, including the deposition of hepatic lipids, weight gain, correlate with increased abundance of the fungal Thermomyces and decreased Saccharomyces (Mims et al., 2021). Research suggests that intestinal fungi are related to host health and disease; however, the role of fungi in intestinal health requires further study.
5. Conclusion
In our previous study, fecal microbial transplantation was carried out with Xinjiang yak as donor and Holstein calf as recipient. In this research, two strains of Lactobacillus (L. reuteri L81and L. johnsonii L29) isolated from the feces of Holstein calves after low concentration fecal microbiota transplantation had a positive effect on the growth performance, immune and antioxidant capacity in weaned calves, and alleviated weaning diarrhea by reducing intestinal permeability. Supplementation of L. reuteri L81 before weaning significantly reduced the ileal crypt depth and increased the ratio of villus height to crypt depth of ileal after weaning. It significantly up-regulated gene expression of tight junction proteins ZO-1, Claudin-1, Occludin and cytokine TGF-β1 in jejunum mucosa, and increased gene expression of Claudin-1 and TGF-β1 in the ileum mucosa, reduced gene expression of pro-inflammatory factor IL-8 in the jejunal mucosa and INF- γ in the ileal mucosa. L. reuteri L81 also significantly increased the Na+- K+- ATPase activity in the small intestine of weaned calves. In addition, it promotes the enrichment of beneficial bacteria Bifidobacterium, Lactobacillus, and Oscillospira in the small intestine. To sum up, L. reuteri L81 can effectively improve the intestinal tissue morphology of calves after weaning, enhance the intestinal epithelial barrier function, alleviate stress reactions and intestinal damage caused by early weaning, and help enhance the resistance of calves to weaning diarrhea.
Data availability statement
The datasets presented in this study can be found in online repositories. The names of the repository/repositories and accession number(s) can be found in the article/Supplementary material.
Ethics statement
The animal study was approved by Bioethics Committee of Shihezi University. The study was conducted in accordance with the local legislation and institutional requirements.
Author contributions
WZ and YL designed the study. RL contributed to managing animals and sample collection. XL and YL contributed to the writing of the manuscript and the preparation of the first draft. CC, CN, YW, and JN have contributed to the comprehensive revision and editing of the manuscript. All authors contributed to the article and approved the submitted version.
Funding
This work was supported by the Key Scientific and Technological Research Project in Key Fields of Xinjiang Production and Construction Corps (No. 2018AB041) and the Major Science and Technology Project of Xinjiang Production and Construction Corps (No. 2021AA004).
Acknowledgments
The authors thank all members of Laboratory who have contributed comments and ideas. At the same time, we would like to thank Editage (www.editage.cn) for English language editing.
Conflict of interest
RL was employed by Xinjiang Tianshan Junken Animal Husbandry Co., Ltd.
The remaining authors declare that the research was conducted in the absence of any commercial or financial relationships that could be construed as a potential conflict of interest.
Publisher’s note
All claims expressed in this article are solely those of the authors and do not necessarily represent those of their affiliated organizations, or those of the publisher, the editors and the reviewers. Any product that may be evaluated in this article, or claim that may be made by its manufacturer, is not guaranteed or endorsed by the publisher.
Supplementary material
The Supplementary material for this article can be found online at: https://www.frontiersin.org/articles/10.3389/fmicb.2023.1249628/full#supplementary-material
References
Aidy, S. E., Hooiveld, G., Tremaroli, V., Bäckhed, F., and Kleerebezem, M. (2013). The gut microbiota and mucosal homeostasis: colonized at birth or at adulthood, does it matter? Gut Microbes 4, 118–124. doi: 10.4161/gmic.23362
Aoudia, N., Rieu, A., Briandet, R., Deschamps, J., Chluba, J., Jego, G., et al. (2016). Biofilms of Lactobacillus plantarum and Lactobacillus fermentum: effect on stress responses, antagonistic effects on pathogen growth and immunomodulatory properties. Food Microbiol. 53, 51–59. doi: 10.1016/j.fm.2015.04.009
Brand, P., Gobeli, S., and Perreten, V. (2017). Pathotyping and antibiotic resistance of porcine enterovirulent Escherichia coli strains from Switzerland (2014-2015). Schweiz. Arch. Tierheilkd. 159, 373–380. doi: 10.17236/sat00120
Brown, K., Uwiera, R., Kalmokoff, M. L., Brooks, S., and Inglis, G. D. (2017). Antimicrobial growth promoter use in livestock: a requirement to understand their modes of action to develop effective alternatives. Int. J. Antimicrob. Agents 49, 12–24. doi: 10.1016/j.ijantimicag.2016.08.006
Carolina, M. G., Silvia, I. C., María, J., Lemme, D., Eva, V., and Gabriela, P. (2019). Beneficial effects of probiotic consumption on the immune system. Ann. Nutr. Metab. 74, 115–124. doi: 10.1159/000496426
Clark, E. O., Deller, A. N., Harrelson, P., and Harrelson, F. W. (2016). Effects of two-stage weaning duration on beef cattle growth and vocalizations. J. Anim. Sci. 95:58. doi: 10.2527/ssasas2017.0118
Costea, P. I., Hildebrand, F., Arumugam, M., Bäckhed, F., Blaser, M. J., Bushman, F. D., et al. (2017). Enterotypes in the landscape of gut microbial community composition. Nat. Microbiol. 3, 8–16. doi: 10.1038/s41564-017-0072-8
Couper, K. N., Blount, D. G., and Riley, E. M. (2008). IL-10: the master regulator of immunity to infection. J. Immunol. 180, 5771–5777. doi: 10.4049/jimmunol.180.9.5771
David, R. C., Patricia, R. M., Abelardo, M., Miguel, G., De, L., and Nuria, S. (2016). Intestinal short chain fatty acids and their link with diet and human health. Front. Microbiol. 7:185. doi: 10.3389/fmicb.2016.00185
Dell’Anno, M., Callegari, M. L., Reggi, S., Caprarulo, V., Giromini, C., Spalletta, A., et al. (2021). Lactobacillus plantarum and Lactobacillus reuteri as functional feed additives to prevent diarrhoea in weaned piglets. Animals 11:1766. doi: 10.3390/ani11061766
Deng, Z., Dai, T., Zhang, W., Zhu, J., Luo, X. A.-O., Fu, D., et al. (2020). Glyceraldehyde-3-phosphate dehydrogenase increases the adhesion of Lactobacillus reuteri to host mucin to enhance probiotic effects. Int. J. Mol. Sci. 21:9756. doi: 10.3390/ijms21249756
Donaldson, G. P., Lee, S. M., and Mazmanian, S. K. (2016). Gut biogeography of the bacterial microbiota. Nat. Rev. Microbiol. 14, 20–32. doi: 10.1038/nrmicro3552
Dong, J., Ping, L., Cao, T., Sun, L., Liu, D., Wang, S., et al. (2022). Immunomodulatory effects of the Bifidobacterium longum BL-10 on lipopolysaccharide-induced intestinal mucosal immune injury. Front. Immunol. 13:947755. doi: 10.3389/fimmu.2022.947755
Ejderhamn, J., Finkel, Y., and Strandvik, B. (1989). Na, K-ATPase activity in rectal mucosa of children with ulcerative colitis and Crohn's disease. Scand. J. Gastroenterol. 24, 1121–1125. doi: 10.3109/00365528909089265
Elisha, I. L., Botha, F. S., McGaw, L. J., and Eloff, J. N. (2017). The antibacterial activity of extracts of nine plant species with good activity against Escherichia coli against five other bacteria and cytotoxicity of extracts. BMC Complement. Altern. Med. 17:133. doi: 10.1186/s12906-017-1645-z
Fan, P., Kim, M., Liu, G., Zhai, Y., Liu, T., Driver, J. D., et al. (2021). The gut microbiota of newborn calves and influence of potential probiotics on reducing diarrheic disease by inhibition ofpathogen colonization. Front. Microbiol. 12:772863. doi: 10.3389/fmicb.2021.772863
Freetly, H. C., Aaron, D., Lindholm-Perry, A. K., Thallman, R. M., Keele, J. W., Foote, A. P., et al. (2020). Digestive tract microbiota of beef cattle that differed in feed efficiency. J. Anim. Sci. 98:skaa 008. doi: 10.1093/jas/skaa008
Gensollen, T., Iyer, S. S., Kasper, D. L., and Blumberg, R. S. (2016). How colonization by microbiota in early life shapes the immune system. Science 352, 539–544. doi: 10.1126/science.aad9378
Günzel, D., and Yu, A. S. L. (2013). Claudins and the modulation of tight junction permeability. Physiol. Rev. 93, 525–569. doi: 10.1152/physrev.00019.2012
Gurung, R., Abrahamsen, F. W., Mullenix, K. K., Abdela, W., McElhenney, W., and Gurung, N. (2021). Evaluation of KemTRACE (R) chromium on animal performance and immune system of weaned beef calves. J. Anim. Sci. 99, 11–12. doi: 10.1093/jas/skab096.019
Han, L., Azad, M. A. K., Huang, P., Wang, W., Zhang, W., Blachier, F., et al. (2022). Maternal supplementation with different probiotic mixture from late pregnancy to day 21 postpartum: consequences for litter size, plasma and colostrum parameters, and fecal microbiota and metabolites in sows. Front. Vet. Sci. 9:726276. doi: 10.3389/fvets.2022.726276
He, L., Wang, C., Simujide, H., Aricha, H., Zhang, J., Liu, B., et al. (2022). Effect of early pathogenic escherichia coli infection on the intestinal barrier and immune function in newborn calves. Front. Cell. Infect. Microbiol. 12:818276. doi: 10.3389/fcimb.2022.818276
He, T., Zhu, Y., Yu, J., Xia, B., Liu, X., Yang, G., et al. (2019). Lactobacillus johnsonii L531 reduces pathogen load and helps maintain short-chain fatty acid levels in the intestines of pigs challenged with salmonella enterica infantis. Vet. Microbiol. 230, 187–194. doi: 10.1016/j.vetmic.2019.02.003
Hill, C., Guarner, F., Reid, G., Gibson, G. R., Merenstein, D. J., Pot, B., et al. (2014). The international scientific association for probiotics and prebiotics consensus statement on the scope and appropriate use of the term probiotic. Nat. Rev. Gastroenterol. Hepatol. 11, 506–514. doi: 10.1038/nrgastro.2014.66
Hong, W. (2016). Effect of Lactobacillus on growth performance and mucosal immunity in weaned piglets. Master's thesis. Guangzhou (Guangdong Province): South China Agricultural University.
Hou, K., Wu, Z., Chen, X., Wang, J., Zhang, D., Xiao, C., et al. (2022). Microbiota in health and diseases. Signal Transduct. Target. Ther. 7:135. doi: 10.1038/s41392-022-00974-4
Hou, Q., Ye, L., Liu, H., Huang, L., Yang, Q., Turner, J. R., et al. (2018). Lactobacillus accelerates ISCs regeneration to protect the integrity of intestinal mucosa through activation of STAT3 signaling pathway induced by LPLs secretion of IL-22. Cell Death Differ. 25, 1657–1670. doi: 10.1038/s41418-018-0070-2
Hou, C., Zeng, X., Yang, F., Liu, H., and Qiao, S. (2015). Study and use of the probiotic Lactobacillus reuteri in pigs: a review. J. Anim. Sci. Biotechnol. 6, 14–33. doi: 10.1186/s40104-015-0014-3
Hsieh, F., Lan, C. E., Huang, T., Chen, K., Chai, C., Chen, W., et al. (2016). Heat-killed and live Lactobacillus reuteri GMNL-263 exhibit similar effects on improving metabolic functions in high-fat diet-induced obese rats. Food Funct. 7, 2374–2388. doi: 10.1039/c5fo01396h
Hu, S., Cao, X., Wu, Y., Mei, X., Xu, H., Wang, Y., et al. (2018). Effects of probiotic Bacillus as an alternative of antibiotics on digestive enzymes activity and intestinal integrity of piglets. Front. Microbiol. 9:2427. doi: 10.3389/fmicb.2018.02427
Hu, J., Chen, L., Zheng, W., Shi, M., Liu, L., Xie, C., et al. (2018). Lactobacillus frumenti facilitates intestinal epithelial barrier function maintenance in early-weaned piglets. Front. Microbiol. 9:897. doi: 10.3389/fmicb.2018.00897
Joossens, M., Huys, G., Cnockaert, M., Preter, V. D., and Vermeire, S. (2011). Dysbiosis of the faecal microbiota in patients with Crohn's disease and their unaffected relatives. Gut 60, 631–637. doi: 10.1136/gut.2010.223263
Kaburagi, T., Yamano, T., Fukushima, Y., Yoshino, H., Mito, N., and Sato, K. (2007). Effect of Lactobacillus johnsonii La1 on immune function and serum albumin in aged and malnourished aged mice. Nutrition 23, 342–350. doi: 10.1016/j.nut.2007.02.001
Kelsey, A. J., and Colpoys, J. D. (2018). Effects of dietary probiotics on beef cattle performance and stress. J. Vet. Behav. 27, 8–14. doi: 10.1016/j.jveb.2018.05.010
Kim, M. H., Yun, C. H., Lee, C. H., and Ha, J. K. (2012). The effects of fermented soybean meal on immunophysiological and stress-related parameters in Holstein calves after weaning. J. Dairy Sci. 95, 5203–5212. doi: 10.3168/jds.2012-5317
Kotnik, V., and Zaletel, J. (2011). Complement in diabetic nephropathy. Mol. Immunol. 48, 1719–1720. doi: 10.1016/j.molimm.2011.06.391
Kuang, J. H., Huang, Y. Y., Hu, J. S., Yu, J. J., Zhou, Q. Y., and Liu, D. M. (2020). Exopolysaccharides from Bacillus amyloliquefaciens DMBA-K4 ameliorate dextran sodium sulfate-induced colitis via gut microbiota modulation. J. Funct. Foods 75:104212. doi: 10.1016/j.jff.2020.104212
Kuo, W., Zuo, L., Odenwald, M. A., Madha, S., Singh, G., Gurniak, C. B., et al. (2021). The tight junction protein zo-1 is dispensable for barrier function but critical for effective mucosal repair. Gastroenterology 161, 1924–1939. doi: 10.1053/j.gastro.2021.08.047
Laxminarayan, R., Duse, A., Wattal, C., Zaidi, A. K. M., Wertheim, H. F. L., Sumpradit, N., et al. (2013). Antibiotic resistance-the need for global solutions. Lancet Infect. Dis. 13, 1057–1098. doi: 10.1016/S1473-3099(13)70318-9
Li, R. W., Connor, E. E., Li, C., Vi, R. L. B., and Sparks, M. E. (2012). Characterization of the rumen microbiota of pre-ruminant calves using metagenomic tools. Environ. Microbiol. 14, 129–139. doi: 10.1111/j.1462-2920.2011.02543.x
Li, Y., Li, X., Wu, Y., and Zhang, W. (2022). Effects of fecal microbiota transplantation from yaks on weaning diarrhea, fecal microbiota composition, microbial network structure and functional pathways in Chinese Holstein calves. Front. Microbiol. 13:898505. doi: 10.3389/fmicb.2022.898505
Ling, X., Linglong, P., Weixia, D., and Hong, W. (2016). Protective effects of Bifidobacterium on intestinal barrier function in LPS-induced enterocyte barrier injury of Caco-2 monolayers and in a rat NEC model. PLoS One 11:e0161635. doi: 10.1371/journal.pone.0161635
Liu, H., Roos, S., Jonsson, H., Ahl, D., Dicksved, J., Lindberg, J. E., et al. (2015). Effects of Lactobacillus johnsonii and Lactobacillus reuteri on gut barrier function and heat shock proteins in intestinal porcine epithelial cells. Physiol. Rep. 3:e12355. doi: 10.14814/phy2.12355
Liu, J., Taft, D. H., Maldonado-Gomez, M. X., Johnson, D., Treiber, M. L., Lemay, D. G., et al. (2019). The fecal resistome of dairy cattle is associated with diet during nursing. Nat. Commun. 10:4406. doi: 10.1038/s41467-019-12111-x
Lu, J., Wang, J., Gao, Q., Liu, Z., Chen, Z., Huang, Q., et al. (2021). Effects of different probiotics on growth performance, serum biochemical indexes and fecal microflora of weaned goats. Chin. J. Anim. Nutr. 33, 2752–2764. doi: 10.3969/j.issn.1006-267x.2021.05.034
Lu, X., Yang, Y., Hong, C., Zhu, W., Yao, Y., Zhu, F., et al. (2022). Optimization of vegetable waste composting and the exploration of microbial mechanisms related to fungal communities during composting. J. Environ. Manag. 319:115694. doi: 10.1016/j.jenvman.2022.115694
Ma, Y., Deng, X., Yang, X., Wang, J., Li, T., Hua, G., et al. (2022). Characteristics of bacterial microbiota in different intestinal segments of Aohan fine-wool sheep. Front. Microbiol. 13:874536. doi: 10.3389/fmicb.2022.874536
Malmuthuge, N., Griebel, P. J., and Guan, L. L. (2015). The gut microbiome and its potential role in the development and function of newborn calf gastrointestinal tract. Front. Vet. Sci. 2:36. doi: 10.3389/fvets.2015.00036
Manoharan, P., Sundaram, S., Singh, S., and Sundaram, U. (2018). Inducible nitric oxide regulates brush border membrane na-glucose co-transport, but not na:h exchange via p38 map kinase in intestinal epithelial cells. Cells 7:111. doi: 10.3390/cells7080111
Mcguirk, S. M. (2008). Disease management of dairy calves and heifers. Vet. Clin. North Am. Food Anim. Pract. 24, 139–153. doi: 10.1016/j.cvfa.2007.10.003
Mims, T. S., Abdallah, Q. A., Stewart, J. D., Watts, S. P., White, C. T., Rousselle, T. V., et al. (2021). The gut mycobiome of healthy mice is shaped by the environment and correlates with metabolic outcomes in response to diet. Commun. Biol. 4:281. doi: 10.1038/s42003-021-01820-z
Myer, P. R., Wells, J. E., Smith, T., Kuehn, L. A., and Freetly, H. C. (2016). Analysis of the gut bacterial communities in beef cattle and their association with feed intake, growth, and efficiency. J. Anim. Sci. 95, 3215–3224. doi: 10.2527/jas.2016.1059
Oikonomou, G., Teixeira, A. G., Foditsch, C., Bicalho, M. L., Machado, V. S., Bicalho, R. C., et al. (2013). Fecal microbial diversity in pre-weaned dairy calves as described by pyrosequencing of metagenomic 16S rDNA. Associations of faecalibacterium species with health and growth. PLoS One 8:e63157. doi: 10.1371/journal.pone.0063157
Orlando, A., Linsalata, M., Bianco, G., Notarnicola, M., D’Attoma, B., Scavo, M. P., et al. (2018). Lactobacillus rhamnosus GG protects the epithelial barrier of wistar rats from the pepsin-trypsin-digested gliadin (PTG)-induced enteropathy. Nutrients 10:1698. doi: 10.3390/nu10111698
Panahi, Z., Kia, V., Moghiman, M., Doroud, D., and Paryan, M. (2020). A fast and straightforward method for the purification of anti-immunoglobulin G (IgG) for coombs wright assay. J. Med. Microbiol. Infect. Dis. 8, 155–160. doi: 10.29252/JOMMID.8.4.155
Pempek, J. A., Watkins, L. R., Bruner, C. E., and Habing, G. (2019). A multisite, randomized field trial to evaluate the influence of lactoferrin on the morbidity and mortality of dairy calves with diarrhea. J. Dairy Sci. 102, 9259–9267. doi: 10.3168/jds.2019-16476
Sweeney, B. C., Rushen, J., Weary, D. M., and Passillé, A. M. D. (2010). Duration of weaning, starter intake, and weight gain of dairy calves fed large amounts of milk. J. Dairy Sci. 93, 148–152. doi: 10.3168/jds.2009-2427
Tan, J., Cho, H., Pholcharee, T., Pereira, L. S., Doumbo, S., Doumtabe, D., et al. (2021). Functional human IgA targets a conserved site on malaria sporozoites. Sci. Transl. Med. 13:eabg2344. doi: 10.1126/scitranslmed.abg2344
Tang, J., Guo, C., and Gong, F. (2019). Protective effect of Lactobacillus reuteri against oxidative stress in neonatal mice with necrotizing enterocolitis. Nan Fang Yi Ke Da Xue Xue Bao 39, 1221–1226. doi: 10.12122/j.issn.1673-4254.2019.10.14
Tap, J., Mondot, S., Levenez, F., Pelletier, E., Caron, C., Furet, J. P., et al. (2010). Towards the human intestinal microbiota phylogenetic core. Environ. Microbiol. 11, 2574–2584. doi: 10.1111/j.1462-2920.2009.01982.x
Wang, H., Ni, X., Xiaodan, Q., Lei, L., Xin, J., Luo, M., et al. (2018). Probiotic Lactobacillus johnsonii BS15 improves blood parameters related to immunity in broilers experimentally infected with subclinical necrotic enteritis. Front. Microbiol. 9:49. doi: 10.3389/fmicb.2018.00049
Wang, M., Wu, H., Lu, L., Jiang, L., and Yu, Q. (2020). Lactobacillus reuteri promotes intestinal development and regulates mucosal immune function in newborn piglets. Front. Vet. Sci. 7:42. doi: 10.3389/fvets.2020.00042
Wang, L., Zhang, J., Guo, Z., Kwok, L., Ma, C., Zhang, W., et al. (2014). Effect of oral consumption of probiotic Lactobacillus planatarum P-8 on fecal microbiota, SIgA, SCFAs, and TBAs of adults of different ages. Nutrition 30, 776–783.e1. doi: 10.1016/j.nut.2013.11.018
Wang, S., Zhao, S., Fang, J., Ma, D., Fu, H., Li, Z., et al. (2016). Role of stress and intestinal barrier dysfunction in inflammatory bowel disease. World Chin. J. Digestol. 24, 3248–3254. doi: 10.11569/wcjd.v24.i21.3248
Wen, J., Zhao, W., Li, J., Hu, C., Zou, X., and Dong, X. (2022). Dietary supplementation of chitosan oligosaccharide-clostridium butyricum synbiotic relieved early-weaned stress by improving intestinal health on pigeon squabs (columba livia). Front. Immunol. 13:926162. doi: 10.3389/fimmu.2022.926162
Wu, H., Xie, S., Miao, J., Li, Y., Wang, Z., Wang, M., et al. (2020). Lactobacillus reuteri maintains intestinal epithelial regeneration and repairs damaged intestinal mucosa. Gut Microbes 11, 997–1014. doi: 10.1080/19490976.2020.1734423
Xiang, Q., Wu, X., Pan, Y., and Wang, L. (2020). Early intervention using fecal microbiota transplantation combined with probioticsinfluence the growth performance, diarrhea, and intestinal barrier function of piglets. Appl. Sci. 10:568. doi: 10.3390/app10020568
Xin, J., Chai, Z., Zhang, C., Zhang, Q., Zhu, Y., Cao, H., et al. (2019). Comparing the microbial community in four stomach of dairy cattle, yellow cattle and three yak herds in Qinghai-Tibetan plateau. Front. Microbiol. 10:1547. doi: 10.3389/fmicb.2019.01547
Yang, J., Li, Y., Wen, Z., Liu, W., Meng, L., and Huang, H. (2021). Oscillospira-a candidate for the next-generation probiotics. Gut Microbes 13:1987783. doi: 10.1080/19490976.2021.1987783
Yang, J., Wang, C., Liu, L., and Zhang, M. (2019). Lactobacillus reuteri KT260178 supplementation reduced morbidity of piglets through its targeted colonization, improvement of cecal microbiota profile, and immune functions. Probiotics Antimicrob. Proteins 12, 194–203. doi: 10.1007/s12602-019-9514-3
Yi, H., Wang, L., Xiong, Y., Wang, Z., Qiu, Y., Wen, X., et al. (2018). Lactobacillus reuteri LR1 improved expression of genes of tight junction proteins via the MLCK pathway in IPEC-1 cells during infection with Enterotoxigenic Escherichia coli K88. Mediat. Inflamm. 2018:6434910. doi: 10.1155/2018/6434910
Zeng, Y., Zeng, D., Ni, X., Zhu, H., Jian, P., Zhou, Y., et al. (2017). Microbial community compositions in the gastrointestinal tract of chinese mongolian sheep using Illumina MiSeq sequencing revealed high microbial diversity. AMB Express 7:75. doi: 10.1186/s13568-017-0378-1
Keywords: weaned calf, Lactobacillus , growth performance, immune capacity, intestinal barrier function
Citation: Li Y, Li X, Nie C, Wu Y, Luo R, Chen C, Niu J and Zhang W (2023) Effects of two strains of Lactobacillus isolated from the feces of calves after fecal microbiota transplantation on growth performance, immune capacity, and intestinal barrier function of weaned calves. Front. Microbiol. 14:1249628. doi: 10.3389/fmicb.2023.1249628
Edited by:
Renpeng Du, Heilongjiang University, ChinaReviewed by:
Yu Ping, University of California, Irvine, United StatesJana Koscova, University of Veterinary Medicine and Pharmacy in Košice, Slovakia
Copyright © 2023 Li, Li, Nie, Wu, Luo, Chen, Niu and Zhang. This is an open-access article distributed under the terms of the Creative Commons Attribution License (CC BY). The use, distribution or reproduction in other forums is permitted, provided the original author(s) and the copyright owner(s) are credited and that the original publication in this journal is cited, in accordance with accepted academic practice. No use, distribution or reproduction is permitted which does not comply with these terms.
*Correspondence: Wenju Zhang, emhhbmd3ajEwMjJAc2luYS5jb20=
†These authors have contributed equally to this work and share first authorship