- 1College of Animal Science, Guizhou University, Guiyang, China
- 2Key Laboratory of Animal Genetics, Breeding and Reproduction in the Plateau Mountainous Region, Ministry of Education, Guizhou University, Guiyang, Guizhou, China
The epiphytic microbiota source on plants plays a crucial role in the production of high-quality silage. To gain a better understanding of its contribution, the microbiota of alfalfa (M1C0), corn (M0C1) and the resulting mixture (M1C1) was applied in alfalfa-corn mixed silage production system. M1C0 decreased ammonia-N levels in terms of total nitrogen (57.59–118.23 g/kg TN) and pH (3.59–4.40) values (p < 0.01), which increased lactic acid (33.73–61.89 g/kg DM) content (p < 0.01). Consequently, this resulted in higher residual water-soluble carbohydrate (29.13–41.76 g/kg DM) and crude protein (152.54–167.91 g/kg DM) contents, as well as lower NDF (427.27 g/kg DM) and ADF (269.53 g/kg DM) contents in the silage compared to M1C1- and M0C1-treated samples. Moreover, M1C0 silage showed significantly higher bacterial alpha diversity indices (p < 0.05), including the number of observed species and Chao1 and Shannon diversity indices, at the later stages of ensiling. Lactobacillus, Kosakonia and Enterobacter were the dominant bacterial species in silages, with a relative abundance of >80%. However, the abundance of Lactobacillus amylovorus in M0C1- and M1C1-treated silage increased (p < 0.01) in the late stages of ensiling. These findings confirmed that the epiphytic microbiota source exerts competitive effects during anaerobic storage of alfalfa-corn mixed silage.
1 Introduction
Ensiling is a well-established and low-cost method for preserving fresh forage with high biological potential (Pakarinen et al., 2011). In the natural ensiling process, the epiphytic bacteria converting water soluble carbohydrates (WSC) in forage under anaerobic conditions, thereby producing organic acids (mainly lactic acid), which creates an acid environment to inhibits undesirable microorganisms (Dong et al., 2022), allowing the nutritional properties of forage to be preserved for a long time, this acidification inhibits the growth of spoilage microorganisms (Xu et al., 2017), thus allowing for long-term preservation of forage nutritional characteristics. Silage has become increasingly important worldwide due to its resilience against climatic variations. More recently, the role of the epiphytic microbiota source found on forage surface in silage fermentation has emerged as an important research hotspot.
The epiphytic microbiota source is a critical factor affecting silage quality. Previous studies have shown that inoculating epiphytic lactic acid bacteria (LAB) isolated from forage is more effective in promoting ensiling than using commercial bacterial fermentation starters (Yahaya et al., 2004). Wang et al. (2020) reported changes in the microbial community and fermentation quality of oat forage silage when using epiphytic microbiota source from maize and sorghum. Guo et al. (2015) reported that the napier grass silage showed lactic acid-type fermentation rather than acetic acid-type as usual. It is important to explore the dynamic changes occurring in the epiphytic microbiota source during ensiling (Xu et al., 2017). The composition of epiphytic microbiota source on fresh forage surface is highly heterogenous (Dong et al., 2022), being primarily composed of LAB, yeasts, and molds, and it varies considerably among different types of forage. The contribution of the epiphytic microbiota source to silage fermentation quality also varies. High-temperature-sterilized forage affect lactic acid, acetic acid, ammonia nitrogen and 2,3-butanediol content, while microbial origin only affect acetic acid content (Mogodiniyai Kasmaei et al., 2017). In this context, mixed silage has been proposed as an alternative to preserve nutrients and improve silage quality compared to conventional silage (Larsen et al., 2017), as the interactions among fermenting microorganisms are more complex.
Ensiling involves a variety of microorganisms and driven by the epiphytic microbiota source initially found on harvested grass. LAB is often associated with high-quality silage fermentation, whereas other microorganisms such as intestinal bacteria, clostridia, yeasts, and molds are undesirable. The majority of the bacteria involved in lactic acid fermentation of silage belong to the genera Lactobacillus, Pedicoccus, Weissella and Leuconostoc (Ni et al., 2018). Pahlow et al. (2003) reported that yeast, Bacillus, and Panicus compete with LAB for the utilization of water-soluble carbohydrates (WSC) in the fermentation substrate, leading to LAB growth inhibition. As silage fermentation progresses, the total relative abundance of Levilactobacillus, Lactococcus and Weissella decreases, whereas that of Bacillus and Panicus increases (Guan et al., 2018). The increase in the relative abundance of Bacillus indicates that silage becomes more susceptible to decay when exposed to air (Ávila and Carvalho, 2020). It is known that yeasts compete with LAB for sugars and lactic acid as energy sources during ensiling, which results in higher dry matter loss in silage (Carvalho et al., 2014). Furthermore, understanding the dynamics occurring in the epiphytic microbiota source during mixed silage fermentation is particularly challenging. Certain techniques have been used to obtain sterile substrates for silage production, among which are included γ-ray radiation (Heron et al., 1986), autoclaving (Graham et al., 1985) and heating (121°C, 20 min; Mogodiniyai Kasmaei et al., 2014). Heating and autoclaving can damage enzyme activity and the physical structure of raw materials, thus resulting in poor-quality silage, while γ-ray irradiation would not destroy the enzyme activity and the physical structure of raw materials (Ding et al., 2013). Wang et al. (2020) reported that γ-ray irradiation is the least likely method to cause change in the chemical composition of forage grass compared to heat or chemical sterilization. γ-ray irradiation to successfully isolate the contribution of epiphytic microbiota and chemical properties in alfalfa silage quality, avoiding changes in chemical composition during sterilization (Yang et al., 2019). Nevertheless, the current knowledge on the dynamics within the epiphytic microbiota from different forage sources during mixed silage fermentation is still scant. As these re-search technologies developed, we used γ-ray irradiation to irradiate alfalfa or corn to elucidate the adaptation process of epiphytic microbiota in alfalfa-corn mixed silage.
Therefore, the present study aimed to analyze fermentation quality and microbial succession patterns in gamma-irradiated alfalfa-corn mixed silage, and evaluate dynamic changes in the epiphytic microbiota source. Based on the findings discussed herein, it can be suggested that epiphytic microbiota source. From single-source or mixed-source forage has a positive effect on fermentation quality in mixed silage.
2 Materials and methods
2.1 Silage production
Alfalfa (Medicago sativa L, Gannong No. 9, peak flowering stage) and corn (Zea mays L, JinFuYu66 YNP64YNW02,wax ripening stage) were manually harvested in 10-cm above the soil in Jianggu Town, Zhenyuan City, Qiandongnan Miao and Dong Autonomous Prefecture, Guizhou Province China (N27.17°, E108.54°) in August 5, 2022, and immediately chopped into approximately 2 ~ 3 cm sections using a chopper. Approximately 500 g of alfalfa or corn was packed into polyethylene plastic bags (35 cm × 50 cm) and sealed in a vacuum sealer. Half of the bags containing alfalfa or corn were subjected to irradiation using a 60Co source at an absorbed dose of 25 kGy for 2 h to eliminate epiphytic microorganisms (Dong et al., 2019).
All bags were divided into the following treatments: (1) M1C1: non-irradiated alfalfa (50%) and non-irradiated maize (50%); (2) M1C0: non-irradiated alfalfa (50%) and irradiated corn (50%); (3) M0C1: irradiated alfalfa (50%) and non-irradiated corn (50%). The preparation of the aforementioned treatments was conducted under sterile conditions. A total of 54 samples were prepared (3 treatments × 6 storage periods × 3 replicates), and three bags from each treatment were opened at room temperature (>25°C) after 1, 3, 5, 7, 15, and 30 days of ensiling. Samples were collected for the analysis of chemical composition, fermentation characteristics, microbial populations, and bacterial community composition.
2.2 Determination of chemical composition of prepared mixed silages
Approximately 150 g of the sample obtained from each sample bag was weighed and dried at 65°C until a constant weight was achieved over a period of 72 h to determine dry matter (DM) content. The samples were then ground using a pulverizer and then passed through a 0.20-mm sieve prior to chemical composition analysis. Crude protein (CP) was determined according to the method described by AOAC (1990) using an automatic Kjeldahl nitrogen analyzer. The contents of neutral detergent fibers (NDF) and acid detergent fibers (ADF) were determined using the washing method as proposed by Van Soest et al. (1991) and referred to the GB/T 20806-2006 analytical standard. The content of water soluble carbohydrates (WSC) was determined using anthrone-sulfuric acid colorimetry. The buffering capacity was measured by electro-metric titration using a Model PHS-3E pH meter (Shanghai Precision & Scientific Instrument Co., Ltd.; Playne and McDonald, 1966).
We determine pH or other variables by shaking 20 g of the sample obtained from each sample bag was mixed with 180 mL of sterile distilled water for 30 min at 150 rpm on a shaker at 37°C and mixing the sample with sterile distilled water for 1 min using a juicer, then filtered through four layers of gauze. The obtained filtrate was centrifuged at 4,500 ×g for 15 min at 4°C, and the pH of the filtrate was measured using a pH meter (Model PHS-3E pH meter, Shanghai Precision & Scientific Instrument Co., Ltd.). Furthermore, the ammonia nitrogen (ammonia-N) content was determined using the phenol-sodium hypochlorite colorimetric method. Lactic acid (LA), acetic acid (AA), propionic acid (PA), and butyric acid (BA) were analyzed in approximately 5 mL of the supernatant by high performance liquid chromatography (HPLC; Li et al., 2018).
2.3 Microbial enumeration in prepared mixed silages
LAB, yeasts, molds and coliform bacteria were enumeration by plate culture method (Cai et al., 1999). LAB were enumerated on MRS agar plates (BEIJING AOBOXING BIO-TECH Co., Ltd., Beijing, China) incubated anaerobically at 37°C for 48 ~ 72 h. Yeasts and molds were enumerated on Bengal red agar plates (BEIJING AOBOXING BIO-TECH Co., Ltd., Beijing, China) after 72 h of incubation at 28°C. Coliform bacteria were enumerated on Eosin Methylene Blue Agar Plate (BEIJING AOBOXING BIO-TECH Co., Ltd., Beijing, China) after 18 ~ 24 h of incubation at 37°C.
2.4 Evaluation of bacterial community composition by full-length 16S rRNA gene sequencing
Total genomic DNA of each sample was extracted using the CTAB method. The purified DNA samples were diluted to a concentration of 1 ng/μL using sterile water. Specific primers with barcodes 27F (AGAGTTTGATCCTGGCTCAG) and 1492R (GNTACCTTGTTACGACTT) were used for conducting full-length 16S ribosomal RNA (rRNA) gene sequencing. PCR products with the same concentration were mixed, and the mixture was subjected to 2% agarose gel electrophoresis. The target bands were recovered using a gel recovery kit (Qiagen). The SMRT Bell library was constructed by ligating sequencing linkers to both ends of the amplified DNA fragment using a DNA binder, and purification selection was performed using AMpure PB magnetic beads. The constructed library was quantified using a Qubit fluorometer and then sequenced on the PacBio platform. Functional prediction, principal coordinate analysis (PCoA) and alpha diversity of the microbial community of silages were analyzed using the NovoMagic platform (Novogene Bio Technology Co., Ltd., Beijing, China). Raw sequencing data of bacterial community in silages prepared in this study have been submitted to the GenBank NCBI database under the accession number PRJNA973813.
2.5 Statistical analysis
Data were initially processed in Excel 2010 and then analyzed using SPSS software version 26.0 (IBM Corp., Armonk. NY, United States). Duncan’s test was performed to analyze changes in chemical composition, microbial population, and bacterial community indices during forage fermentation for silage production, and multiple comparisons were performed. Spearman correlation analysis was conducted to explore the relationship between fermentation parameters and bacterial community composition. Statistical significance was considered when p values were < 0.05.
3 Results and discussion
3.1 Chemical composition of raw materials of single-source silage
Table 1 depicts the chemical composition and microbial composition of fresh and sterilized raw materials before ensiling. Fresh alfalfa was harvested at the peak flowering stage with DM content of 256.96 g/kg, which was below the recommended DM range of 300–400 g/kg fresh matter (FM; Yuan et al., 2017). WSC content (>50.00 g/kg DM) is a crucial factors to affect the speed and extent of silage fermentation (Ni et al., 2018). WSC content in fresh alfalfa was 58.71 g/kg FM, which was sufficient to ensure the smooth progress of silage fermentation. In addition, NDF, ADF and buffering capacity in fresh alfalfa were 438.60 g/kg DM, 323.40 g/kg DM and 478.00 mEq/kg DM, respectively. Corn was harvested at the wax ripening stage exhibited DM, WSC, NDF, ADF and buffering capacity of 257.54 g/kg FM, 125.93 g/kg DM, 528.20 g/kg DM, 338.20 g/kg DM and 231.00 mEq/kg DM, respectively. No significant differences were observed in DM, WSC, NDF and ADF contents in fresh alfalfa-corn or γ-ray irradiation mixed silage and γ-ray irradiation alfalfa-corn mixed silage, thus indicating that γ-ray irradiation in forage silage did not affect the chemical composition with alfalfa and corn, which is in accordance with the study of Yang et al. (2019). A study by Oliveira et al. (2017) reported that a LAB load >5.0 log10 cfu/g FM led to improved silage quality. The initial counts of yeasts and coliform bacteria in fresh alfalfa were 5.47 log10 cfu/g FM and 6.09 log10 cfu/g FM, respectively, and whereas in fresh corn they were 6.31 log10 cfu/g FM and 5.83 log10 cfu/g FM, respectively. After 25 kGy of γ-ray irradiation for 2 h, no LAB, yeasts, or coliform bacteria were detected in alfalfa-corn mixed silage, thus indicating the efficacy of γ-ray irradiation for forage sterilization.
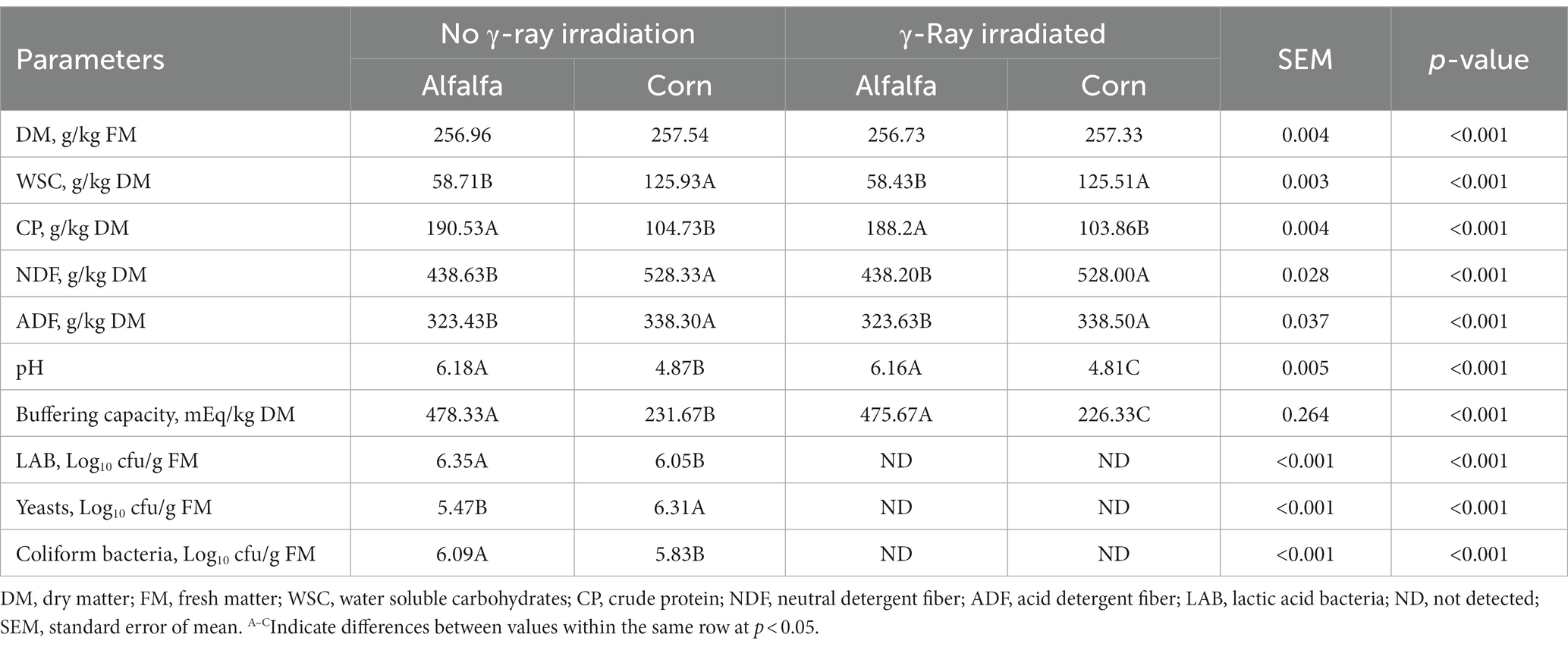
Table 1. Chemical composition and microbial population composition of fresh and sterilized alfalfa and corn before ensiling.
3.2 Chemical composition of alfalfa-corn mixed silage
Table 2 depicts an overview of the chemical composition of alfalfa-corn mixed silage during ensiling. Alfalfa-corn mixed silage during silage time, treatment and their interactions significantly affect WSC, CP, pH, ammonia-N, lactic acid, acetic acid, propionic acid (p < 0.05), but had no effect on DM, NDF and ADF (p > 0.05). Changes in DM content during ensiling were considered indicators for evaluating high-quality silage, and an indication the amount of water required for microbial growth. The nutrient content in raw material for silage, especially the dry matter content, is a key factor to affect the quality of silage fermentation (Xu D. M. et al., 2018). Throughout the storage period, the DM content in M1C1-, M1C0- and M0C1-treated samples showed an upward trend, with the highest DM content in observed in M1C0-treated samples. Moreover, a decrease in WSC content in all treatments (p < 0.001). However, both NDF and ADF contents showed initially an upward trend and then decreased. After 30 days of ensiling, the ADF content in M1C1- and M0C1-treated samples was significantly higher compared to M1C0-treated samples (p < 0.001), likely due to the consumption of digestible nutrients by microorganisms.
The pH of silage is an important indicator reflecting the utilization rate of WSC by microorganisms. McDonald et al. (1991) reported that the pH of high-quality silage should be below 4.2. Throughout the storage period, the pH of M1C1-, M1C0- and M0C1-treated silage showed a downward trend (p < 0.001). In particular, the pH of M1C0- and M0C1-treated silage was significantly lower than that of M1C1-treated silage (p < 0.001), which was consistent with the findings of McDonald et al. (1991). Moreover, the pH decrease of silage primarily occurred during the initial 15 days of ensiling, and did not significantly change as the storage period progressed, which was consistent with the findings of Ni et al. (2017a).
During the silage process, crude protein degradation is unavoidable, and some true protein is converted to non-protein-N (especially ammonia-N) under the action of plant enzymes and microorganisms, thereby reducing the utilization of feed protein by ruminants and increasing nitrogen emissions in animal production (He et al., 2020). Crude protein was significantly reduced during the fermentation process (p < 0.001). Ammonia-N is an important indicator reflecting protein hydrolysis in silage. Meanwhile, ammonia-N is produced by the activity of fermentative coliform bacteria or plant proteases (Ogunade et al., 2018). In the present study, the proportion of ammonia-N to total-N increased linearly in the initial 15 days of ensiling (p < 0.001) and then stabilized in M1C0-treated silages. However, the proportion of ammonia-N to total-N in each treatment decreased after 30 days of ensiling. M1C0-treated improves microbial fermentation and protein preservation in silage and reduces ammonia nitrogen production. In addition, M1C0-treated silage exhibited the lowest the ammonia-N content during ensiling, which indicated the lowest rate of protein degradation.
The decline in silage pH during fermentation is mainly caused by the production of organic acid. As shown in Table 1, lactic acid and acetic acid were the dominant organic acids during ensiling. Within 30 days of ensiling, the lactic acid content significantly increased in sterilized silage (p < 0.05). Compared to M1C0-treated silage, the lactic acid content in M1C1- and M0C1-treated silage was significantly lower (p < 0.05). However, the lactic acid content in M0C1-treated silage was lower than that in M1C0-treated silage (p < 0.05) but higher than that in M1C1-treated silage. These observations revealed that lactic acid production was primarily attributed to the epiphytic microbiota associated with alfalfa. Compared to M1C0- and M0C1-treated silage, the lactic acid content was lower in M1C1-treated silage, thus indicating the antagonistic effects established during fermentation by the epiphytic microbiota from the two forage grasses. Compared to M0C1-treated silage, the epiphytic microbiota from alfalfa in M1C0-treated silage had a promoting effect on lactic acid fermentation, whereas the epiphytic microbiota from corn had an inhibitory effect.
Furthermore, the acetic acid content gradually increased during ensiling (p < 0.001). According to Pahlow et al. (2003), under low DM content and high buffering capacity, lactic acid and propionic acid produced are converted into acetic acid. The propionic acid content (p < 0.001) gradually increased after 30 days of ensiling, except for M1C0-treated silage. The increase in acetic acid and propionic acid contents with prolonged ensiling time is consistent with previous studies (Jahanzad et al., 2016).
Finally, butyric acid was not detected in silages, which could be attributed to the production of large amounts of lactic acid and acetic acid during the initial 30 days of ensiling, thus preventing coliform bacteria from fermenting sugars into butyric acid or converting lactic acid into butyric acid (Kung et al., 2018).
3.3 Microbial population composition of alfalfa-corn mixed silage
Silage fermentation is initiated and regulated by microorganisms. As shown in Table 3, the microbial composition of the evaluated silages was examined. Ensiling led to an increase LAB counts in silages (p < 0.001). In particularly, LAB counts in M1C1- and M1C0-treated silage decreased during the initial 7 days of ensiling and then increased after 15 days of ensiling. Kung et al. (2018) reported that yeasts and coliform bacteria competed with LAB for WSC as fermentation substrates during ensiling. The counts of yeasts, coliform bacteria, and molds in all samples exceeded the detection level (<2.0 log10 cfu/g FM). After 30 days of ensiling, the population of yeasts, coliform bacteria, and molds in M1C1- and M0C1-treated silage was higher than that in M1C0-treated silage (p < 0.001). This suggests that the M1C0 treatment effectively inhibited the growth of yeasts, coliform bacteria, and molds, thus creating a favorable condition for the proliferation of LAB.
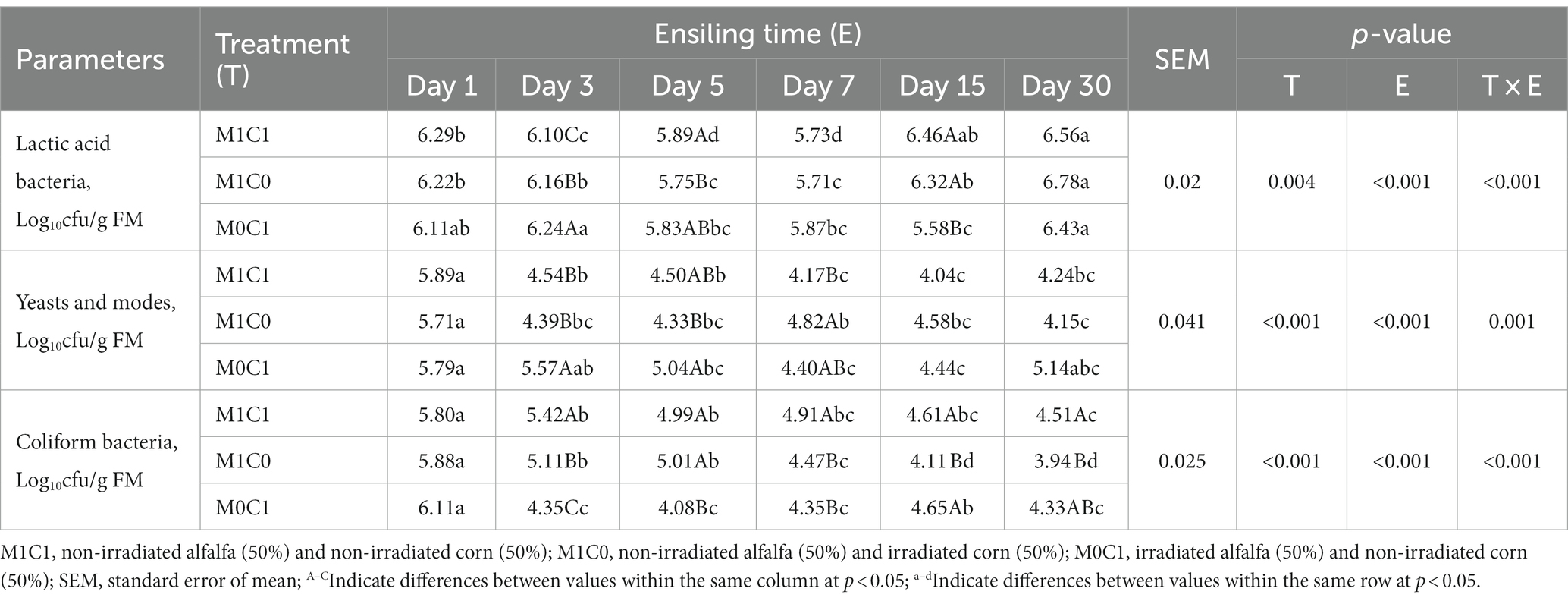
Table 3. Effects of different treatments on lactic acid bacteria, fungi, coliform bacteria count counts in alfalfa-corn mixed silage during ensiling.
3.4 Alpha diversity in the bacterial community of alfalfa-corn mixed silage
Table 4 depicts the results of alpha diversity analysis of the bacterial community in silage samples. Less than 99% of Good’s coverage index indicated that the depth of high-throughput se-quencing was satisfactory for the microbial compositions in silage samples and was enough for credible analysis. It is well known that beneficial bacteria contribute rapidly dominate the microbiota to improve silage quality in the middle and late stages (Ren et al., 2019). The Shannon diversity index varied significantly during ensiling, ranged from 1.14 to 4.34 (p < 0.001). Decreased in bacterial diversity and richness in M1C1-treated silage during the early stage of ensiling, which is the same finding by Xu Z. S. et al. (2018). The highest Shannon index (3.64–4.21) was observed during the initial 15 days in M1C1-treated silage. Comparisons between untreated soybean silage and pre-silage have shown that pre-silage showed higher bacterial diversity (Ni et al., 2017b). This could be due to the relatively high pH value of M1C1-treated silage, which was not sufficiently low to inhibit microbial growth. The Chao1 index was used to measure species richness in the microbial community of silages, which was found increased and then decreased in M1C1-treated during ensiling (p < 0.05). Compared to M1C0-treated, the decrease in Chao1 index in M0C1 silage was greater (p < 0.05).
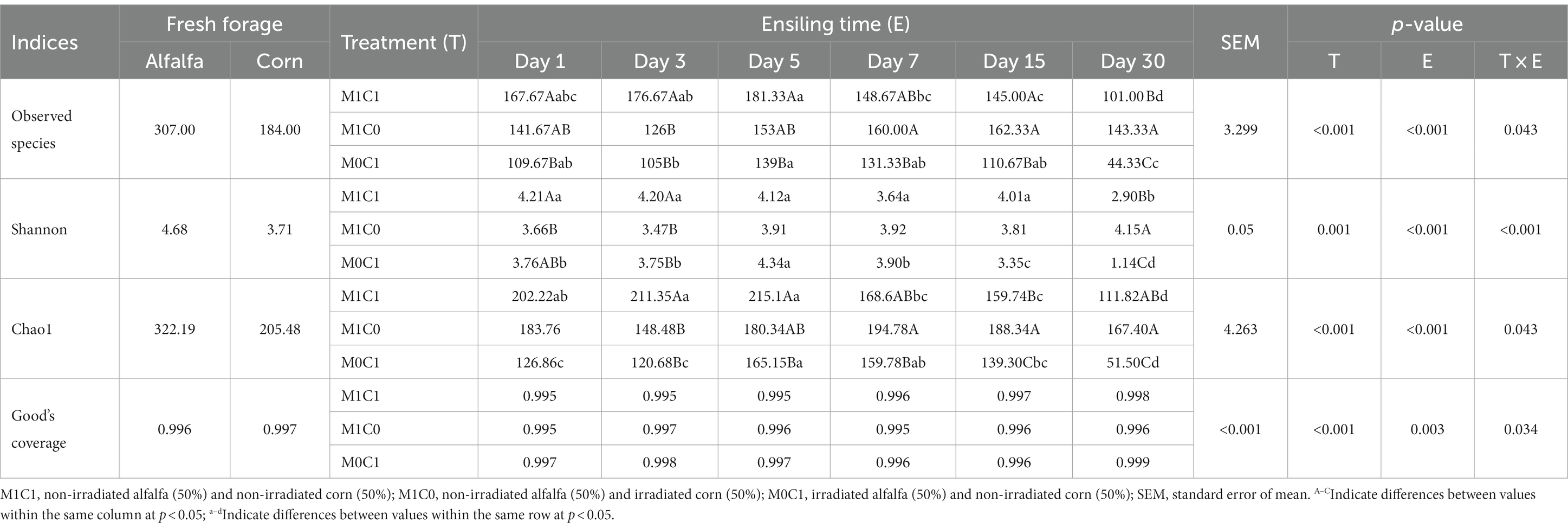
Table 4. Effects of different treatments on bacterial alpha-diversity indices in alfalfa-corn mixed silage during ensiling.
The difference of the bacterial community composition was markedly described in the principal co-ordinates analysis (PCoA) plot. As shown in Figure 1, PCoA analysis revealed that principal component (PC) 1 and principal component (PC) 2 explained 51.52 and 25.19% of the difference in bacterial community composition, respectively. M1C1-, M1C0-and M0C1-treated silages clustered separately, which showed that γ-ray irradiation altered the bacterial community structure of silage.
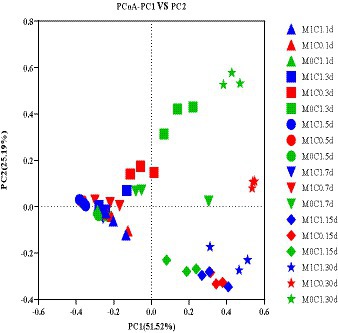
Figure 1. Principal coordinate analysis (PCoA) of the bacterial community structure of alfalfa-corn mixed silage. M1C1, non-irradiated alfalfa (50%) and non-irradiated maize (50%); M1C0, non-irradiated alfalfa (50%) and irradiated corn (50%); M0C1, irradiated alfalfa (50%) and non-irradiated corn (50%).
Yang et al. (2019) evaluated the addition of Lactobacillus plantarum to untreated and sterilized alfalfa silage, and showed that gamma irradiation did not affect the bacterial community composition. Ni et al. (2017b) reported that, with prolonged ensiling, non-LAB species of the epiphytic microbiota were inhibited or inactivated in pre-ensiled Italian ryegrass and crop corn. In the present study, the bacterial communities of M1C1- and M0C1-treated silages were similar, unlike M1C0-treated silage. Taken together, these results showed that γ-ray irradiation had significant impact on the composition of surface epiphytic microbiota in alfalfa-corn mixed silage.
3.5 Bacterial community composition of alfalfa-corn mixed silage
Parvin et al. (2010) described several microbial communities and succession patterns in different silages, which contributed to a better understanding of the complex ensiling process by evaluating microbial community composition (Xu D. M. et al., 2018). Changes in bacterial community composition of alfalfa-corn mixed silage at the phylum level are shown in Figure 2A. At the phylum level, Firmicutes followed by Proteobacteria dominated in fresh alfalfa and corn, with a higher relative abundance of Firmicutes in corn.
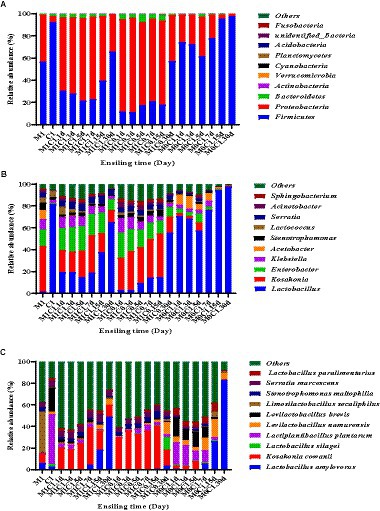
Figure 2. Effects of different treatments on the relative abundance of the first ten phyla (A), genus (B), and species (C) in alfalfa-corn mixed silage. M1C1, non-irradiated alfalfa (50%) and non-irradiated corn (50%); M1C0, non-irradiated alfalfa (50%) and irradiated corn (50%); M0C1, irradiated alfalfa (50%) and non-irradiated corn (50%).
At the genus level (Figure 2B), the relative abundance of Lactobacillus increased with prolonged ensiling time, whereas the relative abundance of Enterobacter and Kosakonia gradually decreased. Interestingly, the relative abundance of Lactobacillus in M1C0-treated increased linearly (p < 0.01). Enterobacter and Kosakonia were found in M1C1- and M1C0-treated silages. It may be that Kosakonia has a strong acid resistance, which remains to be verified. In the early stages of ensiling, certain acid-producing cocci conduct lactic acid fermentation. In contrast, in the late stages of ensiling, LAB with strong acid-resistant ability plays an important role in reducing the pH of silage (Cai et al., 1998).
At the species level (Figure 2C), Lactobacillus plantarum played a crucial role in promoting fermentation during the early stages of ensiling, produced lactic acid and decreased pH to inhibit the growth of undesirable microorganisms and prevents further decomposition of sugars and proteins in silage (Yan et al., 2019). The relative higher abundance of Lactobacillus plantarum in fresh corn can explain its abundance in M0C1 silage. In M0C1 silage, the relative abundance of L. plantarum gradually decreased from day 1 to day 30 of ensiling. Lactobacillus brevis is an obligatory heterofermentative LAB species which produces high acetic acid contents, which can improve silage aerobic stability and prevent deterioration caused by undesirable microorganisms (Guo et al., 2017). Lactobacillus brevis was found in all samples of M1C1-, M1C0- and M0C1-treated, which explained the high acetic acid contents. Compared to M0C1-treated, Kosakonia cowanii was observed in high relative abundance in M1C1- and M1C0-treated. As shown in Figure 3, pH value was negatively correlated with Kosakonia cowanii counts (p < 0.01), thus indicating that Kosakonia cowanii had strong acid resistance ability. However, further studies are required to elucidate whether Kosakonia cowanii has acid-producing properties.
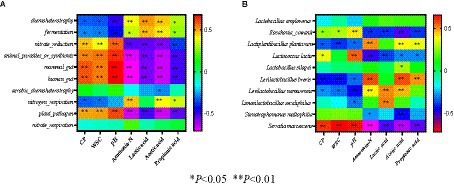
Figure 3. (A) Spearman correlation between microbiota dominant bacteria and silage fermentation parameters, (B) Spearman correlation between functional predictions and silage fermentation parameters.
3.6 Correlation analysis and function prediction analysis of silage quality parameters and bacterial community composition of alfalfa-corn mixed silage
The function prediction analysis of the bacterial community of silage is shown in Figure 4. The main functions of the bacterial community identified during ensiling of alfalfa-corn mixed silage were chemical heterotrophic, fermentation, and nitrate reduction. Chemical heterotrophic and fermentation increased gradually in the late stages of ensiling, with greater changes in M0C1-treated silages compared to M1C1- and M1C0-treated. This increase in chemical heterotrophy and fermentation may be attributed to the degradation of starch by Lactobacillus amylovorus during the late stages of ensiling, leading to the production of WSC. Zhang et al. (2018) reported that with the increase in chemoheterotrophy and fermentation, most bacteria utilize carbon and energy for oxidizing organic components for carbon fixation. Moreover, Li et al. (2021) reported that a correlation between the continuous production of ammonia-N and nitrogen respiration, nitrite ammonification and nitrite respiration. Higher rates of nitrogen respiration, nitrite ammonification and nitrite respiration were detected in all silage samples, which explained the continuous generation of ammonia-N.
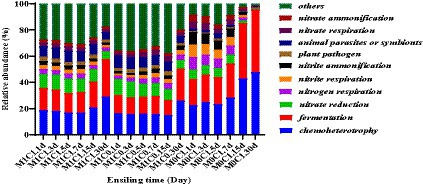
Figure 4. Function prediction of the bacterial community of mixed silage during silage fermentation.
Figure 3 depicts Spearman correlation coefficients between dominant bacteria /function prediction of microbiota and silage fermentation parameters. The WSC content was negatively correlated with Lactobacillus plantarum in silage samples (p < 0.01), indicating that Lactobacillus plantarum utilizes WSC as a fermentation substrate (Chen et al., 2020). This could explain the decrease in WSC. It is well known that at the late stages of ensiling, Lactobacillus plays an important role in increasing the lactic acid content and reducing pH value (Cai et al., 1998). The pH value was negatively (p < 0.01) correlated with the abundance of Lactobacillus species (including Lactobacillus plantarum, Lactobacillus brevis, Lactobacillus namurensis and Lactobacillus secaliphilus), but positively (p < 0.01) correlated with Kosakonia cowanii and Serratia marcescens. Ammonia-N production was positively correlated with the abundance of Lactobacillus brevis, Lactobacillus plantarum and Lactobacillus namurensis, indicating that chemoheterotrophy and fermentation continued slowly, thus allowing for the consumption of WSC and CP during ensiling, which are in agreement with the results reported by Li et al. (2022). Finally, the results revealed that the accumulation of lactic acid was positively correlated with the presence of Lactobacillus namurensis and Lactobacillus secaliphilus (p < 0.01).
4 Conclusion
This study highlights the antagonistic effects of the epiphytic microbiota source from alfalfa and corn during the fermentation process of mixed silage. The epiphytic microbiota source from alfalfa promoted lactic acid fermentation, while epiphytic microbiota source from corn inhibited acetic acid fermentation and had a positive impact on propionic acid fermentation. Therefore, understanding the role of the epiphytic microbiota source in mixed silage fermentation quality and the related dynamic changes occurring among microorganisms is crucial. The findings of the present work contribute to the knowledge base of mixed silage production.
Data availability statement
Raw sequencing data of bacterial community in silages prepared in this study have been submitted to the GenBank NCBI database under the accession number PRJNA97381.
Author contributions
XT and CL: data curation, formal analysis, visualization, writing – original draft, and writing – review and editing. XH, CheC, and DX: investigation. ChaC: conceptualization, methodology, validation, writing – review and editing, supervision, funding acquisition, project administration, and funding acquisition. All authors contributed to the article and approved the submitted version.
Conflict of interest
The authors declare that the research was conducted in the absence of any commercial or financial relationships that could be construed as a potential conflict of interest.
Publisher’s note
All claims expressed in this article are solely those of the authors and do not necessarily represent those of their affiliated organizations, or those of the publisher, the editors and the reviewers. Any product that may be evaluated in this article, or claim that may be made by its manufacturer, is not guaranteed or endorsed by the publisher.
References
AOAC , (1990). Official Methods of Analysis. Association of Official Analytical Chemists, Arlington, VA.
Ávila, C. L. S., and Carvalho, B. F. (2020). Silage fermentation-updates focusing on the performance of micro-organisms. J. Appl. 128, 966–984. doi: 10.1111/jam.14450
Cai, Y. M., Benno, Y., Ogawa, M., and Kumai, S. (1999). Effect of applying lactic acid bacteria isolated from forage crops on fermentation characteristics and aerobic deterioration of silage. J. Dairy Sci. 82, 520–526. doi: 10.3168/jds.S0022-0302(99)75263-X
Cai, Y. M., Benno, Y., Ogawa, M., Ohmomo, S., and Nakase, T. (1998). Influence of lactobacillus spp. from an inoculant and of weissella and leuconostoc spp. from forage crops on silage fermentation. Appl. Environ. Microbiol. 64, 2982–2987. doi: 10.1128/AEM.64.8.2982-2987.1998
Carvalho, B. F., Ávila, C. L. S., Pinto, J. C., Neri, J., and Schwan, R. F. (2014). Microbiological and chemical profile of sugar cane silage fermentation inoculated with wild strains of lactic acid bacteria. Anim. Feed Sci. Technol. 195, 1–13. doi: 10.1016/j.anifeedsci.2014.04.003
Chen, L. Y., Qu, H., Bai, S. Q., Yan, L. J., You, M. H., Gou, W. L., et al. (2020). Effect of wet sea buckthorn pomace utilized as an additive on silage fermentation profile and bacterial community composition of alfalfa. Bioresour. Technol. 314:123773. doi: 10.1016/j.biortech.2020.123773
Ding, W. R., Long, R. J., and Guo, X. S. (2013). Effects of plant enzyme inactivation or sterilization on lipolysis and proteolysis in alfalfa silage. J. Dairy Sci. 96, 2536–2543. doi: 10.3168/jds.2012-6438
Dong, Z. H., Li, J. F., Chen, L., Yuan, X. J., and Shao, T. (2019). Comparison of nitrogen transformation dynamics in non-irradiated and irradiated alfalfa and red clover during ensiling. Asian Australas. J. Anim. Sci. 32, 1521–1527. doi: 10.5713/ajas.18.0695
Dong, Z. H., Li, J. F., Wang, S. R., Zhao, J., Don, D., and Shao, T. (2022). Gamma-ray irradiation and microbiota transplantation to separate the effects of chemical and microbial diurnal variations on the fermentation characteristics and bacterial community of Napier grass silage. J. Sci. Food Agric 102, 4322–4332. doi: 10.1002/JSFA.11784
Graham, H., Åman, P., Theander, O., Kolankaya, N., and Stewart, C. S. (1985). Influence of heat sterilization and ammoniation on straw composition and degradation by pure cultures of cellulolytic rumen bacteria. Animal Feed Sci. Technol. 12, 195–203. doi: 10.1016/0377-8401(85)90013-6
Guan, H., Yan, Y. H., Li, X. L., Li, X. M., Shuai, Y., Feng, G. Y., et al. (2018). Microbial communities and natural fermentation of corn silages prepared with farm bunker-silo in Southwest China. Bioresour. Technol. 265, 282–290. doi: 10.1016/j.biortech.2018.06.018
Guo, G., Yuan, X. J., Wen, A. Y., Liu, Q., Zhang, S. L., and Shao, T. (2015). Silage fermentation characteristics of Napier grass harvested at various times on a sunny day. Crop Sci. 55, 458–464. doi: 10.2135/cropsci2014.03.0172
Guo, T. T., Zhang, L., Xin, Y. P., Xu, Z. S., He, H. Y., and Kong, J. (2017). Oxygen-inducible conversion of lactate to acetate in heterofermentative Lactobacillus brevis ATCC 367. Appl. Environ. Microbiol. 83:e01659-17. doi: 10.1128/AEM.01659-17
He, L. W., Lv, H. J., Xing, Y. Q., Chen, X. Y., and Zhang, Q. (2020). Intrinsic tannins affect ensiling characteristics and proteolysis of Neolamarckia cadamba leaf silage by largely altering bacterial community. Bioresour. Technol. 311:123496. doi: 10.1016/j.biortech.2020.123496
Heron, S. J. E., Edwards, R. A., and McDonald, P. (1986). Changes in the nitrogenous compo-nents of gamma-irradiated and inoculated ensiled ryegrass. J. Sci. Food Agric. 37, 979–985. doi: 10.1002/jsfa.2740371005
Jahanzad, E., Sadeghpour, A., Hashemi, M., Keshavarz Afshar, R., Hosseini, M. B., and Barker, A. V. (2016). Silage fermentation profile, chemical composition and economic evaluation of millet and soya bean grown in monocultures and as intercrops. Grass Forage Sci. 71, 584–594. doi: 10.1111/gfs.12216
Kung, L. M., Shaver, R. D., Grant, R. J., and Schmidt, R. J. (2018). Silage review: interpretation of chemical, microbial, and organoleptic components of silages. J. Dairy Sci. 101, 4020–4033. doi: 10.3168/jds.2017-13909
Larsen, S. U., Hjort-Gregersen, K., Vazifehkhoran, A. H., and Triolo, J. M. (2017). Co-ensiling of straw with sugar beet leaves increases the methane yield from straw. Bioresour. Technol. 245, 106–115. doi: 10.1016/j.biortech.2017.08.117
Li, Z., Gu, J. Y., Ding, J., Ren, N. Q., and Xing, D. F. (2021). Molecular mechanism of ethanol-H2 co-production fermentation in anaerobic acidogenesis: challenges and perspectives. Biotechnol. Adv. 46:107679. doi: 10.1016/J.BIOTECHADV.2020.107679
Li, P., Zhang, Y., Gou, W. L., Cheng, Q. M., Bai, S. Q., and Cai, Y. M. (2018). Silage fermentation and bacterial community of bur clover, annual ryegrass and their mixtures prepared with microbial inoculant and chemical additive. Anim. Feed Sci. Technol. 247, 285–293. doi: 10.1016/j.anifeedsci.2018.11.009
Li, P., Zhao, W. J., Yan, L. J., Chen, L. Y., Chen, Y. L., Gou, W. L., et al. (2022). Inclusion of abandoned rhubarb stalk enhanced anaerobic fermentation of alfalfa on the Qinghai Tibetan plateau. Bioresour. Technol. 347:126347. doi: 10.1016/j.biortech.2021.126347
McDonald, P., Hernderson, N., and Heron, S., (1991). The biochemistry of silage. Chalcombe Publications, USA.
Mogodiniyai Kasmaei, K., Dicksved, J., Spörndly, R., and Udén, P. (2017). Separating the effects of forage source and field microbiota on silage fermentation quality and aerobic stability. J. Br. Grassl. Soc. 72, 281–289. doi: 10.1111/gfs.12238
Mogodiniyai Kasmaei, K., Spörndly, R., and Udén, P. (2014). A sterilization technique with applications to silage research and inoculant evaluation. Grass Forage Sci. 69, 724–728. doi: 10.1111/gfs.12100
Ni, K. K., Minh, T. T., Tu, T. T. M., Tsuruta, T., Pang, H. L., and Nishino, N. (2017b). Comparative microbiota assessment of wilted Italian ryegrass, whole crop corn, and wilted alfalfa silage using denaturing gradient gel electrophoresis and next-generation sequencing. Appl. Microbiol. Biotechnol. 101, 1385–1394. doi: 10.1007/s00253-016-7900-2
Ni, K. K., Wang, F. F., Zhu, B. G., Yang, J. X., Zhou, G. A., Pan, Y., et al. (2017a). Effects of lactic acid bacteria and molasses additives on the microbial community and fermentation quality of soybean silage. Bioresour. Technol. 238, 706–715. doi: 10.1016/j.biortech.2017.04.055
Ni, K. K., Zhao, J. Y., Zhu, B. G., Su, R. N., Pan, Y., Ma, J. K., et al. (2018). Assessing the fermentation quality and microbial community of the mixed silage of forage soybean with crop corn or sorghum. Bioresour. Technol. 265, 563–567. doi: 10.1016/j.biortech.2018.05.097
Ogunade, I. M., Jiang, Y., Cervantes, A. A. P., Kim, D. H., Oliveira, A. S., Vyas, D., et al. (2018). Bacterial diversity and composition of alfalfa silage as analyzed by Illumina MiSeq sequencing: effects of Escherichia coli O157:H7 and silage additives. J. Dairy Sci. 101, 2048–2059. doi: 10.3168/jds.2017-12876
Oliveira, A. S., Weinberg, Z. G., Ogunade, I. M., Cervantes, A. A. P., Arriola, K. G., Jiang, Y., et al. (2017). Meta-analysis of effects of inoculation with homofermentative and facultative heterofermentative lactic acid bacteria on silage fermentation, aerobic stability, and the performance of dairy cows. J. Dairy Sci. 100, 4587–4603. doi: 10.3168/jds.2016-11815
Pahlow, G., Muck, R.E., Driehuis, F., Elferink, S.J.W.H.O., and Spoelstra, S.F., (2003). Microbiology of Ensiling. Silage Science and Technology. Madison, WI, USA.
Pakarinen, A., Maijala, P., Jaakkola, S., Stoddard, F. L., Kymäläinen, M., and Viikari, L. (2011). Evaluation of preservation methods for improving biogas production and enzymatic conversion yields of annual crops. Biotechnol. Biofuels 4:20. doi: 10.1186/1754-6834-4-20
Parvin, S., Wang, C., Li, Y., and Nishino, N. (2010). Effects of inoculation with lactic acid bacteria on the bacterial communities of Italian ryegrass, whole crop maize, Guinea grass and Rhodes grass silages. Anim. Feed Sci. Technol. 160, 160, 160–166. doi: 10.1016/j.anifeedsci.2010.07.010
Playne, M. J., and McDonald, P. (1966). The buffering constituents of herbage and of silage. J. Sci. Food Agric. 17, 264–268. doi: 10.1002/jsfa.2740170609
Ren, F. Y., He, R. C., Zhou, X. K., Gu, Q. C., Xia, Z. S., Liang, M. Z., et al. (2019). Dynamic changes in fermentation profiles and bacterial community composition during sugarcane top silage fermentation: a preliminary study. Bioresour. Technol. 285:121315. doi: 10.1016/j.biortech.2019.121315
Van Soest, P. J., Robertson, J. B., and Lewis, B. A. (1991). Methods for dietary fiber, neutral detergent fiber, and non-starch-polysaccharides in relation to animal nutrition. J. Dairy Sci. 74, 3583–3597. doi: 10.3168/jds.S0022-0302(91)78551-2
Wang, S. R., Zhao, J., Dong, Z. H., Li, J. F., Kaka, N. A., and Shao, T. (2020). Sequencing and microbiota transplantation to determine the role of microbiota on the fermentation type of oat silage. Bioresour. Technol. 309:123371. doi: 10.1016/j.biortech.2020.123371
Xu, D. M., Ding, W. R., Ke, W. C., Li, F. H., Zhang, P., and Guo, X. S. (2018). Modulation of metabolome and bacterial community in whole crop corn silage by inoculating homofermentative lactobacillus plantarum and heterofermentative Lactobacillus buchneri. Front. Microbiol. 9:3299. doi: 10.3389/fmicb.2018.03299
Xu, Z. S., He, H. Y., Zhang, S. S., and Kong, J. (2017). Effects of inoculants lactobacillus brevis and Lactobacillus parafarraginis on the fermentation characteristics and microbial communities of corn Stover silage. Sci. Rep. 7:13614. doi: 10.1038/s41598-017-14052-1
Xu, Z. S., Zhang, S. S., Zhang, R. L., Li, S. X., and Kong, J. (2018). The changes of dominant lactic acid bacteria and their metabolites during corn Stover ensiling. J. Appl. 125, 675–685. doi: 10.1111/jam.13914
Yahaya, M. S., Goto, M., Yimiti, W., Smerjai, B., and Kawamoto, Y. (2004). Evaluation of fermentation quality of a tropical and temperate forage crops ensiled with additives of fermented juice of epiphytic lactic acid bacteria (FJLB). Asian Aust. J. Animal Sci. 17, 942–946. doi: 10.5713/ajas.2004.942
Yan, Y. H., Li, X. M., Guan, H., Huang, L. K., Ma, X., Peng, Y., et al. (2019). Microbial community and fermentation characteristic of Italian ryegrass silage prepared with corn Stover and lactic acid bacteria. Bioresour. Technol. 279, 166–173. doi: 10.1016/j.biortech.2019.01.107
Yang, L. L., Yuan, X. J., Li, J. F., Dong, Z. H., and Shao, T. (2019). Dynamics of microbial community and fermentation quality during ensiling of sterile and nonsterile alfalfa with or without Lactobacillus plantarum inoculant. Bioresour. Technol. 275, 280–287. doi: 10.1016/j.biortech.2018.12.067
Yuan, X., Wen, A., Dong, Z., Desta, S. T., and Shao, T. (2017). Effects of formic acid and potassium diformate on the fermentation quality, chemical composition and aerobic stability of alfalfa silage. Grass Forage Sci. 72, 833–839. doi: 10.1111/gfs.12296
Keywords: epiphytic microbiota source, alfalfa, corn, fermentation profile, microbial diversity
Citation: Tang X, Liao C, Huang X, Chen C, Xu D and Chen C (2024) Epiphytic microbiota source stimulates the fermentation profile and bacterial community of alfalfa-corn mixed silage. Front. Microbiol. 14:1247254. doi: 10.3389/fmicb.2023.1247254
Edited by:
Yanhong Yan, Sichuan Agricultural University, ChinaReviewed by:
Jingrong Cheng, Guangdong Academy of Agricultural Sciences, ChinaMuhammad Tahir, Chinese Academy of Sciences (CAS), China
Hao Guan, Southwest Minzu University, China
Yanbing Li, Heilongjiang Bayi Agricultural University, China
Copyright © 2024 Tang, Liao, Huang, Chen, Xu and Chen. This is an open-access article distributed under the terms of the Creative Commons Attribution License (CC BY). The use, distribution or reproduction in other forums is permitted, provided the original author(s) and the copyright owner(s) are credited and that the original publication in this journal is cited, in accordance with accepted academic practice. No use, distribution or reproduction is permitted which does not comply with these terms.
*Correspondence: Chao Chen, gzgyxgc3855218@163.com
†These authors have contributed equally to this work and share first authorship