- 1Monoclonal Antibody Discovery Laboratory, Fondazione Toscana Life Sciences, Siena, Italy
- 2Department of Biotechnology, Chemistry and Pharmacy, University of Siena, Siena, Italy
- 3Fondazione Biotecnopolo di Siena, Siena, Italy
Neisseria gonorrhoeae (gonococcus) is an obligate human pathogen and the etiological agent of the sexually transmitted disease gonorrhea. The rapid rise in gonococcal resistance to all currently available antimicrobials has become a significant public health burden and the need to develop novel therapeutic and prophylactic tools is now a global priority. While high-throughput screening methods allowed rapid discovery of extremely potent monoclonal antibodies (mAbs) against viral pathogens, the field of bacteriology suffers from the lack of assays that allow efficient screening of large panels of samples. To address this point, we developed luminescence-based (L-ABA) and resazurin-based (R-ABA) antibody bactericidal assays that measure N. gonorrhoeae metabolic activity as a proxy of bacterial viability. Both L-ABA and R-ABA are applicable on the large scale for the rapid identification of bactericidal antibodies and were validated by conventional methods. Implementation of these approaches will be instrumental to the development of new medications and vaccines against N. gonorrhoeae and other bacterial pathogens to support the fight against antimicrobial resistance.
Introduction
Gonorrhea is a sexually transmitted disease with an estimated incidence of 86.9 million cases globally per annum (Kirkcaldy et al., 2019). It is caused by the host-adapted human pathogen N. gonorrhoeae (Quillin and Seifert, 2018), which is listed as a high priority pathogen for research into novel treatments by the World Health Organization (WHO) because of its ability to quickly develop resistance to antibiotics (Unemo and Shafer, 2011; Suay-García and Pérez-Gracia, 2018). Recently emerged gonococcus strains showed resistance to most currently available antibiotics including ceftriaxone which represents the last remaining option for first-line treatment (Unemo and Shafer, 2014; Unemo et al., 2016). Despite efforts over many decades, no vaccine or specific treatment have yet been successfully developed to counter gonococcal infections and novel strategies need to be implemented to achieve this goal (Russell et al., 2019). Human monoclonal antibodies (mAbs) have shown great potential in the fight against infectious diseases, especially against viral pathogens. Indeed, mAbs are extremely effective and specific toward their targets, and nowadays can be developed faster than any other drug. The coronavirus disease 2019 (COVID-19) pandemic case has highlighted their great potential and the possibility to bring mAbs from bench to bedside in less than 6 months (Kelley, 2020). This milestone was achieved thanks to the unprecedented technological and methodological advancement of the last two decades in the field of B cell analyses and mAb screening. Indeed, this scenario has permitted to interrogate the human antibody repertoire and to quickly screen hundreds of thousands of antibodies to select the optimal candidates for clinical development. Conversely, the field of mAb screening and selection against bacterial pathogens is hampered by the lack of efficient screening assays. The serum bactericidal assay (SBA), conventionally used to evaluate the functional activity of sera or mAbs in vitro, is a long and lab-intensive method that relies on counting the number of colonies of survived bacteria on agar plates (Figure 1C; Mountzouros and Howell, 2000). While this approach has been extensively used as an in vitro surrogate assay to evaluate the immunogenicity of bacterial vaccines against cholera (Jang et al., 2016) and meningococcal disease (Balmer and Borrow, 2004), it is not suitable for high-throughput mAb screening. To respond to this unmet need, we herein report the development of two high-throughput (HT) assays named luminescence-based (L-ABA) and resazurin-based (R-ABA) antibody bactericidal assays (Figures 1A,B), for rapid screening and identification of antibodies against gonococcus and AMR bacteria.
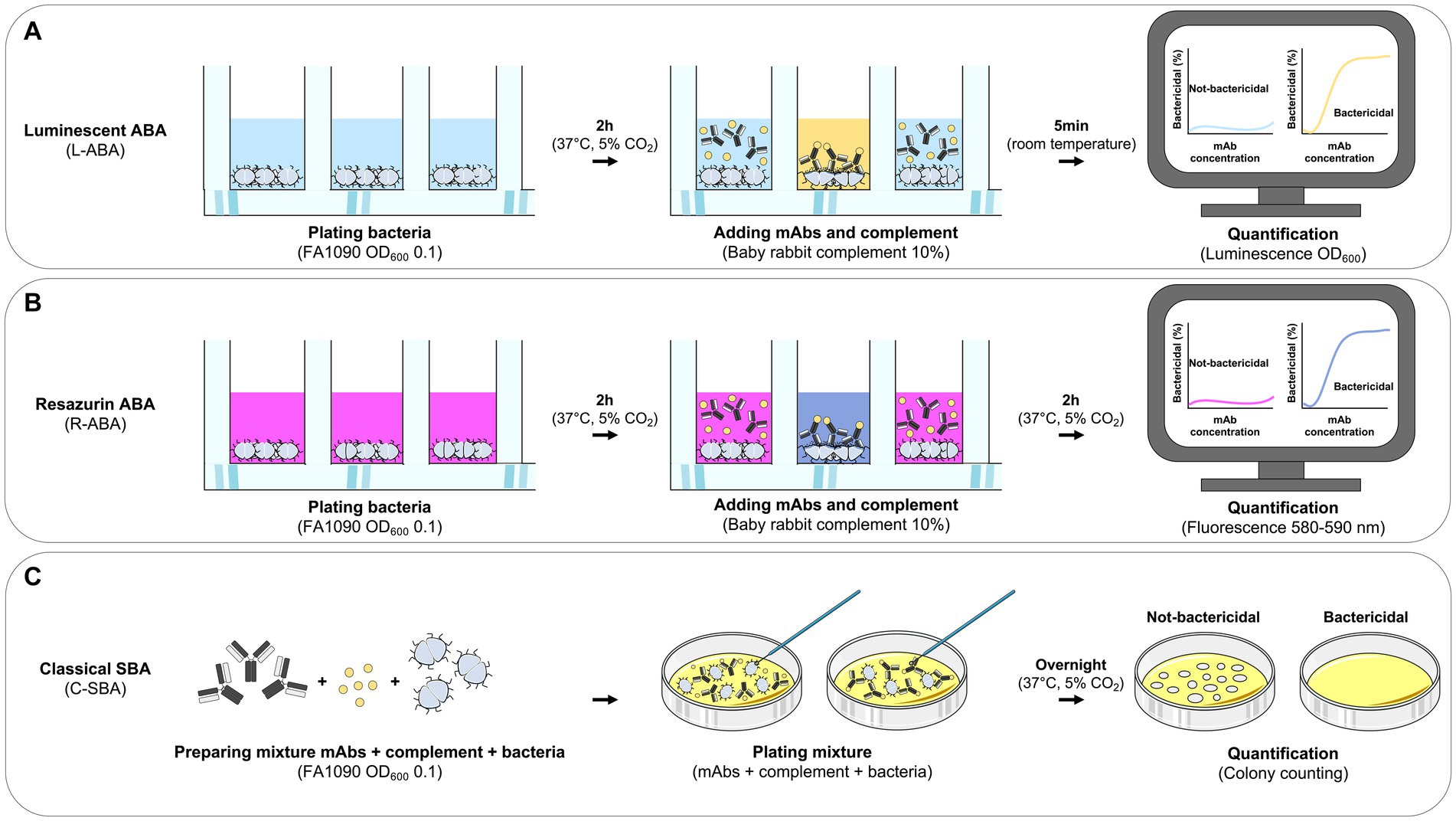
Figure 1. Workflow for monoclonal antibody based high-throughput serum bactericidal assays. (A–C) Schematic representation of the workflows implemented for the L-ABA (A), R-ABA (B), and C-SBA (C).
Materials and methods
Bacterial strain and preparation
Neisseria gonorrhoeae strain FA1090 (ATCC 700825), was used in this study. Fresh cultures of bacteria were prepared from frozen stocks by streaking onto gonococcal agar (GCA) consisting of gonococcal (GC) agar base supplemented with 1% v/v IsoVitaleX (BD Biosciences, Franklin Lakes, NJ, United States). On the following day, bacteria were grown in GC liquid medium at 37°C, 5% CO2 starting from an optical density at 600 nanometers (OD600) 0.1 until mid-log phase cultures, i.e., OD600 0.5.
Source of complement
Baby rabbit complement (BRC; Cedarlane) was used as a source of complement for serum sensitivity and for all ABA assays. For optimized ABA assays, BRC was diluted to 10% v/v for use as a complement source, which is within the recommended range of 10–20% v/v for gonococcal bactericidal assays (McQuillen et al., 1994). For negative controls with heat-inactivated complement (hiBRC), BRC was heated at 56°C for 1 h.
Complement toxicity assay
Neisseria gonorrhoeae was grown to mid-log phase (OD600 = 0.5) as described above. Bacterial cultures were then directly diluted in sterile Dulbecco’s phosphate-buffered saline (DPBS) supplemented with 2% fetal bovine serum (FBS; Hyclone) and 0.1% glucose (from now on named as ABA buffer). To assess the optimal OD600, different dilutions were used. Specifically, starting from cultures at OD600 0.5, bacteria were diluted 1:5, 1:10, and 1:100 to obtain final OD600 0.1, 0.01, and 0.001, respectively. Reactions were performed by independently seeding 10 μL of diluted bacteria (ABA buffer) in the presence of 5, 10, 20, or 40% v/v of BRC (or hiBRC in negative controls) in a final volume of 50 μL. Reactions were incubated for 1 or 2 h at 37°C, 5% CO2 in sterile round bottom 96-well plates. After the incubation, plates were centrifuged for 8 min at 4,500 × g. The supernatant was discarded to remove dead or lysed bacteria and the pellets were re-suspended in 30 μL of DPBS. This suspension was transferred into an opaque white 96-well flat bottom plate (Optiplate 96, Perkin Elmer) and 30 μL of BacTiter-Glo reagent (Promega, United States) were added to each well. The reaction was incubated for 5 min. After incubation, the luminescence signal was read using a Varioskan™ LUX multimode microplate reader (Thermo Fisher Scientific, Waltham, MA, United States).
L-ABA assay with purified mAbs
Mid-log phase bacteria (OD600 0.5) were diluted 1:10 in ABA buffer to prepare a 5x working stock. Reactions were performed by seeding 10 μL of 5x bacterial stock with serial dilutions of the purified mAb 2C7 (or a negative control) in ABA buffer and in the presence of 10% v/v BRC (or hiBRC in negative controls) in a final volume of 50 μL for each well. Reactions were incubated for 2 h at 37°C, 5% CO2 in sterile round bottom 96-well plates. After the incubation, plates were centrifuged for 8 min at 4,500 × g. The supernatant was discarded to remove dead or lysed bacteria and the pellets were re-suspended in 30 μL of DPBS. This suspension was transferred into an opaque white 96-well flat bottom plate (Optiplate 96, Perkin Elmer) and 30 μL of BacTiter-Glo reagent (Promega, United States) were added to each well. The reaction was incubated for 5 min before the luminescence signal was read using a Varioskan™ LUX multimode microplate reader (Thermo Fisher Scientific, Waltham, MA, United States). Acquired data were analyzed using GraphPad Prism version 9.4.1 by fitting the data to a sigmoidal dose–response curve to calculate the IC50.
R-ABA assay with purified mAbs
Neisseria gonorrhoeae was grown to mid-log phase (OD600 0.5) as described above. Assay plates were then prepared as described above for the L-ABA, with the only difference that black 96-well plates (Culturplate 96, Perkin Elmer) were used, instead of the round bottom ones. Reactions were incubated for 2 h at 37°C, 5% CO2. Then, 10 μL of 0.025% resazurin solution in sterile distilled water were added to each well. The reactions were then further incubated for 2 h at 37°C, 5% CO2. At the end of the incubation, fluorescence signals were measured by a Varioskan™ LUX multimode microplate reader (Thermo Fisher, Waltham, MA, United States) using 560 nm for excitation and 590 nm for emission. After the measurement, the assay plate was kept at 37°C overnight to allow complete conversion of resazurin to resorufin in the wells containing live bacteria. On the following day, the plate was observed by eye and pictures were taken.
C-SBA assay with purified mAbs
Neisseria gonorrhoeae was grown to mid-log phase (OD600 0.5) as described above. Assay plates were then prepared as described above for the L-ABA, using round bottom 96-well plates. Reactions were incubated for 2 h at 37°C, 5% CO2. Then, samples were diluted five times 1:10 in ABA buffer and plated onto solid GC agar plates. Plates were incubated overnight at 37°C, 5% CO2 and the following day colonies were counted. To measure the IC50 values, CFU counts were normalized by assigning the value 100% to negative control samples treated with only complement, but not mAb 2C7.
Transcriptionally active PCR for 2C7 transfection
To obtain PCR fragments that could be directly used to transfect cells and express mAb 2C7, TAP-PCR reactions of the heavy/light chain fragments were performed in replicates, as previously described (Andreano et al., 2021). Briefly, the appropriate vectors of heavy and light chains, carrying the variable region of the 2C7 mAb, were used as templates. Primer sequences: TAP-Fwd: TTAGGCACCCCAGGCTTTAC; TAP-Rev: AGATGGTTCTTTCCGCCTCA. Reaction was performed using 0.25 μL of Q5 polymerase (NEB), 5 μL of GC Enhancer (NEB), 5 μL of 5X buffer,10 mM dNTPs, 0.125 μL of 100 μM forward/reverse primers, and 3 μL of template (i.e., vectors carrying heavy and light chains) using the following PCR program: 98°C/2′, 35 cycles composed of 98°C/10″, 61°C/20″, 72°C/1′, and 72°C/5′. TAP-PCR products were purified and quantified by Nanodrop and used for transient transfection in the Expi293F cell line following manufacturing instructions (Thermo Fisher Scientific).
96-well/plate small-scale transfection
Expi293F cells (Thermo Fisher Scientific) were handled as recommended by the manufacturer. Briefly, on the day before the transfection, cells were counted and adjusted at a concentration of 2.5 × 106 cells/mL in Expi293 transfection medium. The day after, cells were counted, centrifuged at 310 × g for 8 min and then resuspended to achieve a concentration of 2.5 × 106 cells/mL. 0.8 mL/well of this cell suspension were then added into Deep Well 96-well plates (Eppendorf) and kept at 37°C, 8% CO2, in a plate shaker set at 1,000 rpm until cell transfection. Small scale transfection was then carried out according to the manufacturer protocol with the layout described below. Cells in rows A–D were transfected with 300 ng of heavy chain TAP product + 600 ng of light chain TAP product to obtain 2C7-containing supernatants (positive controls). Cells in rows E–H were transfected with lipofectamine-Optimem only, as negative controls (mock transfection of Expi293F cells). After transfection, cells were incubated shaking at 1,000 rpm for 6 days and then centrifuged to collect the supernatants that were used in the HT-ABA assays.
L-ABA for high-throughput screening of mAb supernatants
Up to 96 different supernatants can be assayed within each plate, using the same setup described above. To evaluate the applicability of this assay for bactericidal screenings, a round-bottom 96-well plate was prepared as follows:
- Rows A–D: each well contained 10 μL supernatant from Expi293F cells transfected with 2C7 (positive control, 1:5 dilution), 5 μL BRC (10% v/v), 10 μL of bacteria (OD600 0.05), and 25 μL of ABA buffer to reach a final volume of 50 μL.
- Rows E–H: each well contained 10 μL supernatant of mock Expi293F cells (negative control, 1:5 dilution), 5 μL BRC (10% v/v), 10 μL of bacteria (OD600 0.05), and 25 μL of ABA buffer to reach a final volume of 50 μL.
After 2 h incubation, the plates were centrifuged and then processed as described above. Luminescence data were then analyzed to calculate the Z′-factor, to evaluate the reliability of the established assay for primary mAb screenings.
R-ABA for high-throughput screening of mAb supernatants
The resazurin test plate was set-up as described above. After 2 h incubation, 10 μL/well of resazurin solution were added and the samples further incubated for 2 h before fluorescence measurement.
Results
Development of the luminescent antibody bactericidal assay
Accurate selection of the right source of complement to be used in bactericidal assays is crucial to correctly evaluate and interpret the functionality of antibacterial mAbs (Ercoli et al., 2015; Findlow et al., 2019). Optimization of the amount of complement that can be used with a specific pathogen is equally important to ensure lack of toxicity and low non-specific killing of bacteria by complement alone. Baby rabbit complement (BRC) has been extensively used to evaluate the bactericidal potency of sera against N. gonorrhoeae and N. meningitidis (Maslanka et al., 1997; Borrow et al., 2005; Frasch et al., 2009). In addition, when compared to human complement, BRC is more easily available in large batches and subject to standardization (Findlow et al., 2019). For all the above reasons, we decided to develop the L-ABA using BRC as the source of complement. The first steps consisted in optimizing the number of bacteria, the amount of BRC and incubation time to avoid complement toxicity and non-specific killing (NSK). Therefore, we incubated three different amounts of N. gonorrhoeae FA1090 (OD600 0.1, 0.01, and 0.001) with four different percentages of BRC (5, 10, 20, and 40%), for 1 or 2 h at 37°C (Figure 2). Bacterial viability was then evaluated by using the BacTiter Glo reagent as previously reported (Rossi et al., 2020). This reagent measures the amount of ATP in the reaction which correlates with the number of viable microbial cells. Heat inactivated (hi) BRC was used as the control to evaluate the NSK induced by active BRC. Our data showed that 20 and 40% BRC caused the highest percentage of NSK in almost all tested conditions. Conversely, 5 and 10% of BRC, with the exception of 2 h OD600 0.001 condition, showed the least NSK. As for the number of bacteria, OD600 0.1 and 0.01 resulted in the highest relative luminescence unit (RLU) values which are needed to correctly discriminate live from dead bacteria. Finally, both incubation times (1 and 2 h) gave similar results, with the 2 h condition resulting in higher RLUs at all ODs tested. Based on our data, four parameters were selected (10% BRC, 1 and 2 h incubation, and OD600 0.1 and 0.01) to evaluate the bactericidal efficacy of an anti-N. gonorrhoeae monoclonal antibody. In particular, the published anti-N. gonorrhoeae mAb 2C7, directed against the gonococcal lipooligosaccharide and functional against the strain FA1090 used here, was used to determine the optimal settings for this assay (Figure 3; Gulati et al., 2019). The antibody 2C7 showed complement-dependent killing of N. gonorrhoeae in all the tested conditions. However, OD600 0.01 and 2 h incubation resulted in the lowest half-maximal inhibitory concentration (IC50) value, suggesting higher assay sensitivity which is a desired feature to study the bactericidal activity of anti-N. gonorrhoeae mAbs in high throughput.
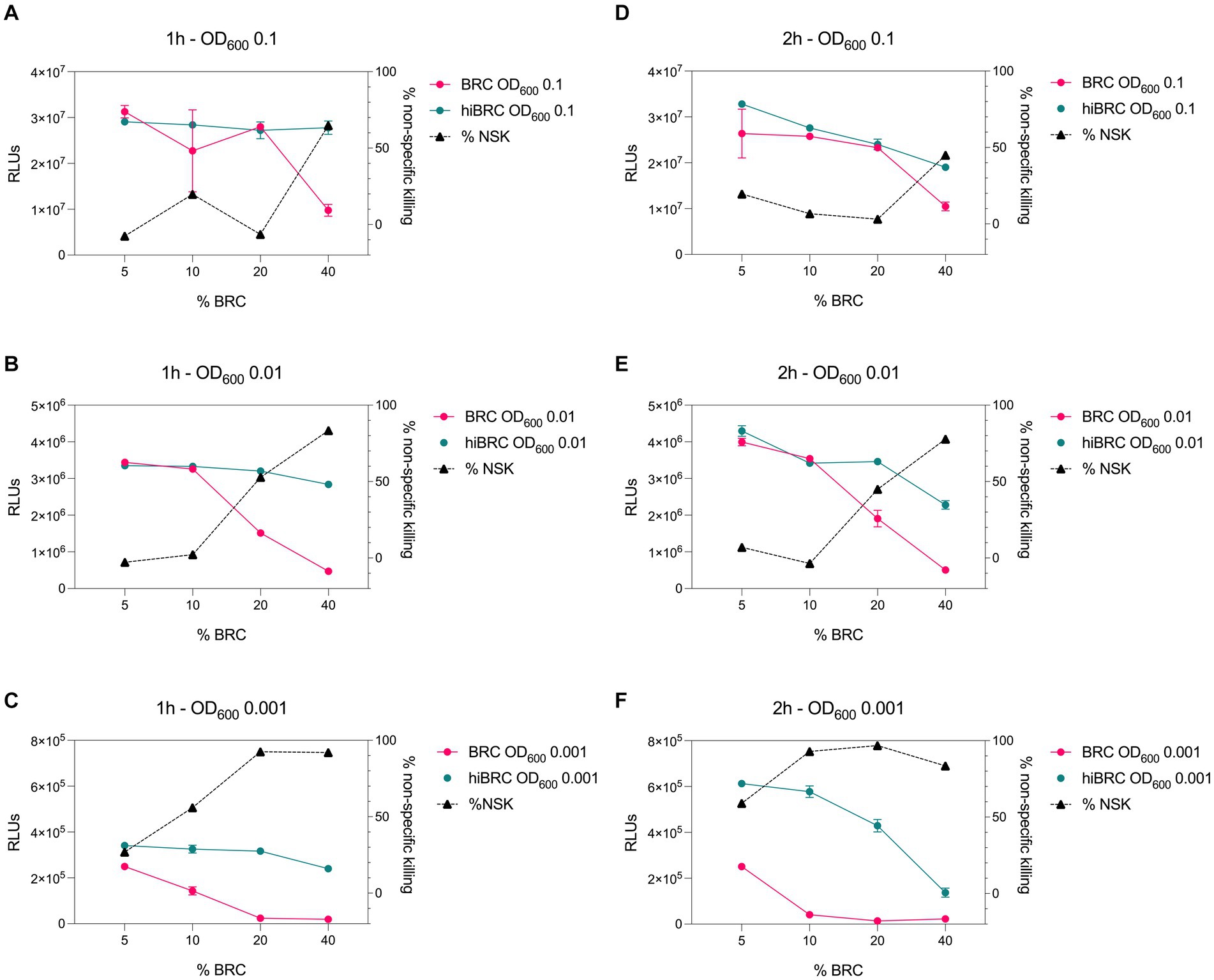
Figure 2. Evaluation of different conditions for L-ABA implementation. (A–C) Graphs show the viability and non-specific killing (NSK) of bacteria tested at OD600 0.1 (A), 0.01 (B), and 0.001 (C) incubated with different amounts of BRC or hi-BRC (40, 20, 10, and 5%) for 1 h. (D–F) Graphs show the viability and non-specific killing of bacteria tested at OD600 0.1 (D), 0.01 (E), and 0.001 (F) incubated with different amounts of BRC or hi-BRC (40, 20, 10, and 5%) for 2 h.
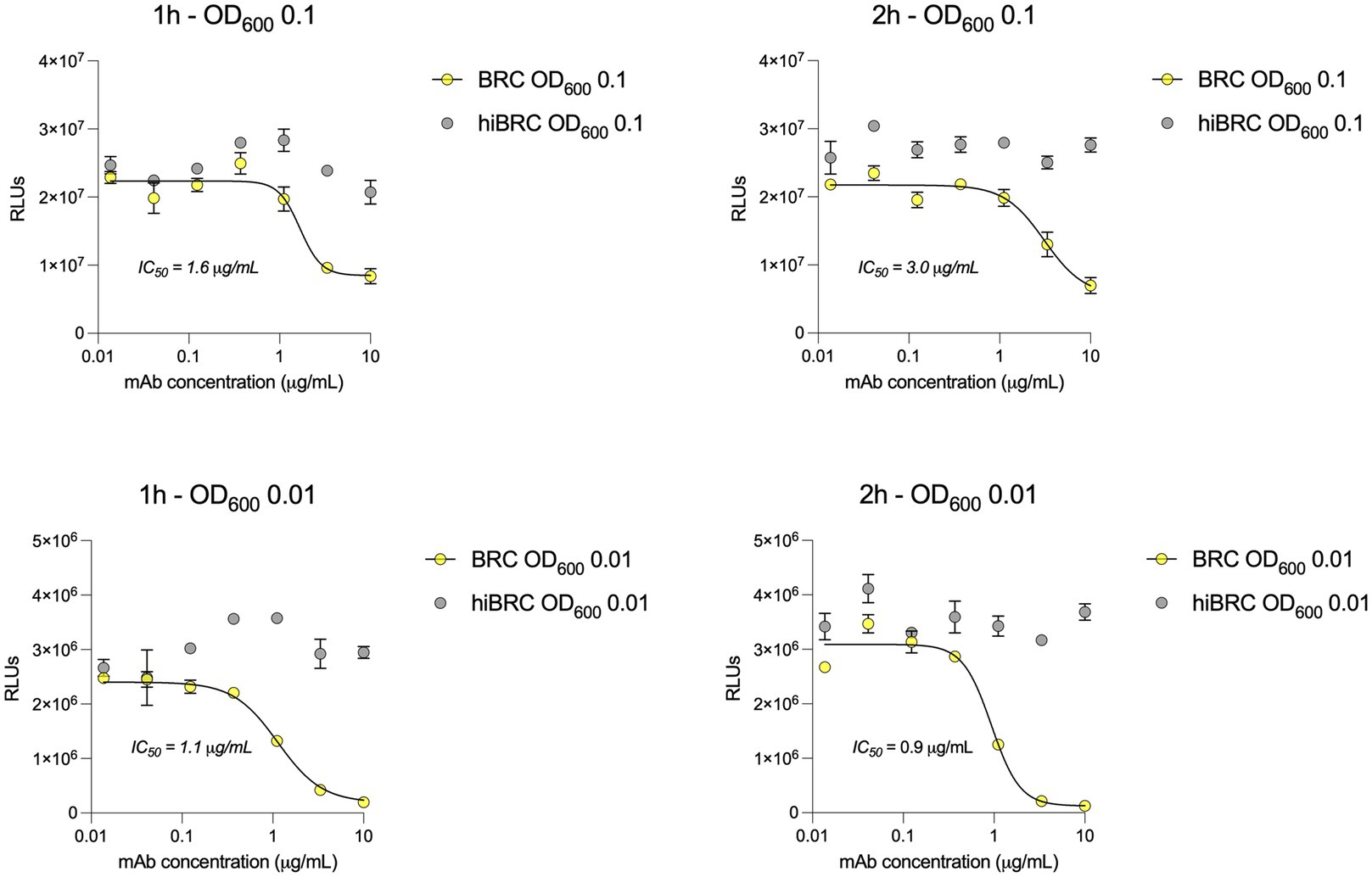
Figure 3. 2C7 bactericidal activity evaluated by L-SBA. (A,B) Graphs show the bactericidal curves of 2C7 after 1 h incubation with 10% BRC or hiBRC at OD600 0.1 (A) and 0.01 (B). (C,D) Graphs show the bactericidal curves of 2C7 after 2 h incubation with 10% BRC or hiBRC at OD600 0.1 (C) and 0.01 (D). The IC50 values obtained with each condition are reported on the graphs.
Correlation between L-ABA, R-ABA, and C-SBA
The experimental conditions described above (OD600 0.01, 10% BRC, 2 h incubation) allowed to quantitatively evaluate the potency of the anti-gonococcal mAb 2C7 in terms of reduction of the ATP levels in the sample. To validate our assay, we compared the 2C7 bactericidal activity observed in L-ABA with a classical serum bactericidal assay (C-SBA), and a third approach that we named resazurin-based ABA (R-ABA). C-SBA relies on colony forming unit (CFU) counting and is conventionally used to evaluate serum activity. Differently, R-ABA is based on the reduction of the resazurin blue dye to a pink, fluorescent resorufin product by live bacteria. The amount of resorufin produced, and consequent fluorescent signal, in the assay is proportional to the number of live bacteria and to their metabolic activity. When we compared the IC50 obtained with the three assays, we observed almost identical values for L-ABA and R-ABA (0.25 and 0.23 μg/mL, respectively), while a slightly lower IC50 was observed for the C-SBA (0.05 μg/mL; Figures 4A–C). A possible explanation for the IC50 difference observed with these approaches is that compromised, but still alive bacteria are still detected with the L-or R-ABA assays as they do not require overnight incubation times. On the other hand, damaged bacteria could be unable to form visible colonies in the solid plates required for the C-SBA assay, leading to an overestimation of the mAb bactericidal potency with this latter assay. Furthermore, L-and R-SBA assays are run entirely in a liquid medium, while the C-SBA is run on solid plates, which could limit molecule diffusion and therefore stabilize mAb-antigen interactions. Despite these differences, the similarities among L-ABA, R-ABA, and C-SBA are also demonstrated by a strong correlation among the three assays (r = 0.99, p < 0.001 L-ABA vs. R-ABA and r = 0.83 p < 0.0039 L-ABA/R-ABA vs. C-SBA; Figures 4D–F).
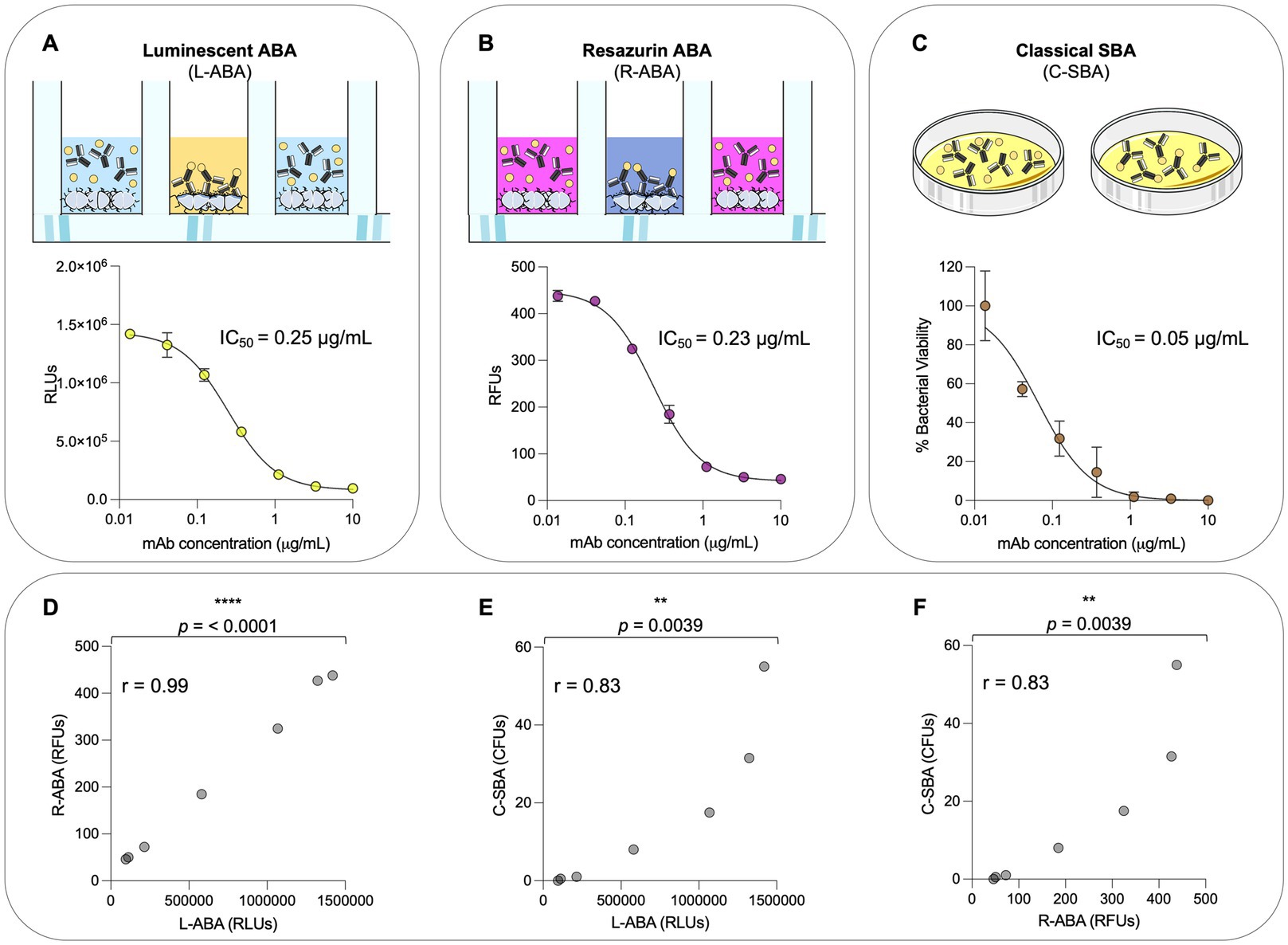
Figure 4. Comparison between L-ABA, R-ABA, and C-SBA assays. (A–C) Bactericidal curves of 2C7 mAb starting from 10 μg/mL to 4 ng/mL (three-step serial dilutions) against Neisseria gonorrhoeae FA1090 tested by L-SBA (A), R-SBA (B), and C-SBA (C). The IC50 values are reported on each graph. (D–F) Two-tailed Pearson coefficient correlation was performed between L-ABA and R-SBA (D), L-ABA and C-SBA (E), and R-ABA and C-SBA (F). The r squared values (r) are indicated on each graph. A nonparametric Mann–Whitney t test was used to evaluate statistical significances between groups. Two-tailed value of p significances are shown as **p < 0.01 and ****p < 0.0001.
Implementation of high-throughput L-ABA and R-ABA
In high-throughput screenings, robust and sensitive assays are required to avoid false negative results and maximize the number of positive hits. This is particularly true in the case of primary screening, where hundreds or thousands of samples must be tested without the possibility to perform technical replicates. One of the most used parameters to assess the robustness and reliability of a primary screening assay is the Z factor, which is defined by two metrics: the mean (μ) and the standard deviation (σ) of both the positive (p) and negative (n) controls. The Z factor value can range from 0 to 1.0, denoting, respectively, a non-useful assay displaying overlap between the positive and negative controls, and an excellent, ideal assay. Values between 0.5 and 1.0 denote an assay of excellent quality, in which positive and negative controls are well separated (Zhang et al., 1999). Below, we report the formula used to calculate the Z factor:
To mimic high-throughput mAb screening, small-scale 2C7 mAb expression in deep 96-well plates was obtained. This approach allowed to rapidly produce 48 independently expressed positive control replicates (2C7-containing Expi293F supernatants diluted 1:5) and 48 negative control replicates (mock transfections diluted 1:5; Figure 5A). The supernatants were used to implement high-throughput versions of our luminescence (HT-L-ABA) and fluorescence (HT-R-ABA) assays which aimed to assess in parallel all samples from a 96-well plate. The same experimental conditions previously described for the luminescence and fluorescence-based assays were applied to HT-L-ABA and HT-R-ABA. For both assays, all 48 wells containing 2C7 resulted in positive hits. Conversely, none of the mock transfection supernatants gave a false positive result. The experimental conditions adopted allowed us to reach a Z factor of 0.52 with HT-L-ABA and 0.68 with HT-R-ABA (Figures 5B,C), indicating high robustness and specificity for both assays.
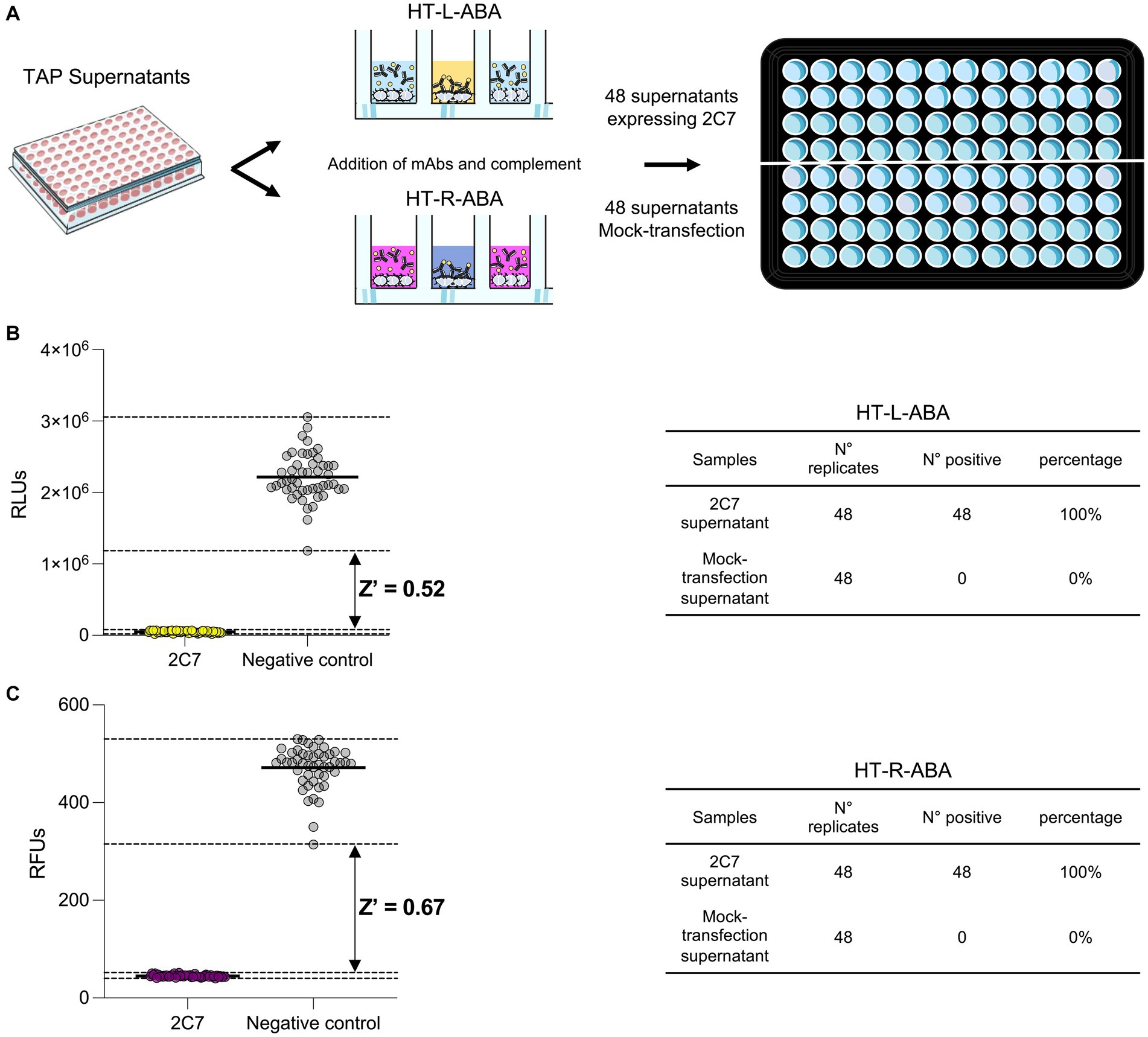
Figure 5. High-throughput assays for mAb screening. (A) Schematic representation of the two high-throughput assays (HT-L-ABA and HT-R-ABA) used to evaluate 2C7 bactericidal activity in a 96-well plate. (B) The graph shows the HT-L-ABA screening. The table describes number of replicates, positive hits, and percentage of positive hits for 2C7 supernatants and mock-transfected supernatants. (C) The graph shows the HT-R-ABA screening. The table describes number of replicates, positive hits, and percentage of positive hits for 2C7 supernatants and mock-transfected supernatants. Black lines represent the mean values, while black dotted lines display the maximum and minimum RLU values for both positive (2C7) and negative controls. The Z factor was calculated for both assays and is reported on the graphs.
Discussion
The global spread of multidrug-resistant gonorrhea has spurred efforts to develop safe and effective therapeutics and vaccines against this disease. Monoclonal antibodies have shown great potential as therapeutics and tools for antigen discovery and vaccine development against viral pathogens, while their use in the bacterial field is still limited. New assays are needed to implement high-throughput strategies to screen and identify highly bactericidal antibodies. In this paper, we described the development of two new assays named L-ABA (high-throughput version HT-L-ABA) and R-ABA (high-throughput version HT-R-ABA). Differently from conventional assays, like the C-SBA, the methods presented in this work offer multiple advantages by eliminating the labor-intensive steps of plating hundreds or thousands of samples and counting individual CFU (2–4 vs. >24 h), decreasing hands-on time by laboratory personnel and increasing the capacity to screen large sets of samples in a single day, thereby accelerating data acquisition and analyses. In addition, these approaches could also be applied to other N. gonorrhoeae strains, for rapid testing of new drugs, as well as other Gram-negative bacteria. Finally, the resazurin-based assay is an affordable method for mAb (or other drugs) screening, being resazurin not expensive, and results can be easily visualized by the naked eye. All these advantages can expedite the development of new therapeutic solutions and vaccines to fight N. gonorrhoeae and AMR.
Data availability statement
The raw data supporting the conclusions of this article will be made available by the authors, without undue reservation.
Author contributions
SS, MT, EA, and RR conceived the study and wrote the manuscript. SS and MT designed the experiments. SS, MT, and VA conducted the experiments and analyzed the data. SS, MT, VA, CS, EA, and RR reviewed the manuscript. All authors contributed to the article and approved the submitted version.
Funding
This work received funding by the European Research Council (ERC) advanced grant agreement number 787552 (vAMRes).
Conflict of interest
The authors declare that the research was conducted in the absence of any commercial or financial relationships that could be construed as a potential conflict of interest.
Publisher’s note
All claims expressed in this article are solely those of the authors and do not necessarily represent those of their affiliated organizations, or those of the publisher, the editors and the reviewers. Any product that may be evaluated in this article, or claim that may be made by its manufacturer, is not guaranteed or endorsed by the publisher.
References
Andreano, E., Nicastri, E., Paciello, I., Pileri, P., Manganaro, N., Piccini, G., et al. (2021). Extremely potent human monoclonal antibodies from COVID-19 convalescent patients. Cells 184, 1821–1835.e16. doi: 10.1016/j.cell.2021.02.035
Balmer, P., and Borrow, R. (2004). Serologic correlates of protection for evaluating the response to meningococcal vaccines. Expert Rev. Vaccines 3, 77–87. doi: 10.1586/14760584.3.1.77
Borrow, R., Balmer, P., and Miller, E. (2005). Meningococcal surrogates of protection—serum bactericidal antibody activity. Vaccine 23, 2222–2227. doi: 10.1016/j.vaccine.2005.01.051
Ercoli, G., Baddal, B., Alessandra, G., Marchi, S., Petracca, R., Aricò, B., et al. (2015). Development of a serological assay to predict antibody bactericidal activity against non-typeable Haemophilus influenzae. BMC Microbiol. 15:87. doi: 10.1186/s12866-015-0420-x
Findlow, J., Balmer, P., and Borrow, R. (2019). A review of complement sources used in serum bactericidal assays for evaluating immune responses to meningococcal ACWY conjugate vaccines. Hum Vaccin Immunother 15, 2491–2500. doi: 10.1080/21645515.2019.1593082
Frasch, C. E., Borrow, R., and Donnelly, J. (2009). Bactericidal antibody is the immunologic surrogate of protection against meningococcal disease. Vaccine 27, B112–B116. doi: 10.1016/j.vaccine.2009.04.065
Gulati, S., Beurskens, F. J., de Kreuk, B. J., Roza, M., Zheng, B., Deoliveira, R. B., et al. (2019). Complement alone drives efficacy of a chimeric antigonococcal monoclonal antibody. PLoS Biol. 17:e3000323. doi: 10.1371/journal.pbio.3000323
Jang, M. S., Sahastrabuddhe, S., Yun, C. H., Han, S. H., and Yang, J. S. (2016). Serum bactericidal assay for the evaluation of typhoid vaccine using a semi-automated colony-counting method. Microb. Pathog. 97, 19–26. doi: 10.1016/j.micpath.2016.05.013
Kelley, B. (2020). Developing therapeutic monoclonal antibodies at pandemic pace. Nat. Biotechnol. 38, 540–545. doi: 10.1038/s41587-020-0512-5
Kirkcaldy, R. D., Weston, E., Segurado, A. C., and Hughes, G. (2019). Epidemiology of gonorrhoea: a global perspective. Sex. Health 16, 401–411. doi: 10.1071/SH19061
Maslanka, S. E., Gheesling, L. L., Libutti, D. E., Donaldson, K. B., Harakeh, H. S., Dykes, J. K., et al. (1997). Standardization and a multilaboratory comparison of Neisseria meningitidis serogroup a and C serum bactericidal assays. The multilaboratory study group. Clin. Diagn. Lab. Immunol. 4, 156–167. doi: 10.1128/cdli.4.2.156-167.1997
McQuillen, D. P., Gulati, S., and Rice, P. A. (1994). Complement-mediated bacterial killing assays. Methods Enzymol. 236, 137–147. doi: 10.1016/0076-6879(94)36013-8
Mountzouros, K. T., and Howell, A. P. (2000). Detection of complement-mediated antibody-dependent bactericidal activity in a fluorescence-based serum bactericidal assay for group B Neisseria meningitidis. J. Clin. Microbiol. 38, 2878–2884. doi: 10.1128/JCM.38.8.2878-2884.2000
Quillin, S. J., and Seifert, H. S. (2018). Neisseria gonorrhoeae host adaptation and pathogenesis. Nat. Rev. Microbiol. 16, 226–240. doi: 10.1038/nrmicro.2017.169
Rossi, O., Molesti, E., Saul, A., Giannelli, C., Micoli, F., and Necchi, F. (2020). Intra-laboratory evaluation of luminescence based high-throughput serum bactericidal assay (L-SBA) to determine bactericidal activity of human sera against Shigella. High Throughput 9, 1–12. doi: 10.3390/ht9020014
Russell, M. W., Jerse, A. E., and Gray-Owen, S. D. (2019). Progress toward a gonococcal vaccine: the way forward. Front. Immunol. 10:2417. doi: 10.3389/fimmu.2019.02417
Suay-García, B., and Pérez-Gracia, M. T. (2018). Future prospects for Neisseria gonorrhoeae treatment. Antibiotics 7:49. doi: 10.3390/antibiotics7020049
Unemo, M., Golparian, D., Sánchez-Busó, L., Grad, Y., Jacobsson, S., Ohnishi, M., et al. (2016). The novel 2016 WHO Neisseria gonorrhoeae reference strains for global quality assurance of laboratory investigations: phenotypic, genetic and reference genome characterization. J. Antimicrob. Chemother. 71, 3096–3108. doi: 10.1093/jac/dkw288
Unemo, M., and Shafer, W. M. (2011). Antibiotic resistance in Neisseria gonorrhoeae: origin, evolution, and lessons learned for the future. Ann. N. Y. Acad. Sci. 1230, E19–E28. doi: 10.1111/j.1749-6632.2011.06215.x
Unemo, M., and Shafer, W. M. (2014). Antimicrobial resistance in Neisseria gonorrhoeae in the 21st century: past, evolution, and future. Clin. Microbiol. Rev. 27, 587–613. doi: 10.1128/CMR.00010-14
Keywords: monoclonal antibodies, Neisseria gonorrhoeae, bacteria, antimicrobial resistance, therapeutics, high-throughput screening
Citation: Stazzoni S, Troisi M, Abbiento V, Sala C, Andreano E and Rappuoli R (2023) High-throughput bactericidal assays for monoclonal antibody screening against antimicrobial resistant Neisseria gonorrhoeae. Front. Microbiol. 14:1243427. doi: 10.3389/fmicb.2023.1243427
Edited by:
Daniel Golparian, Örebro University, SwedenReviewed by:
Tania Rivera-Hernandez, National Council of Science and Technology (CONACYT), MexicoMariaelena Caboni, Holobiome, United States
Copyright © 2023 Stazzoni, Troisi, Abbiento, Sala, Andreano and Rappuoli. This is an open-access article distributed under the terms of the Creative Commons Attribution License (CC BY). The use, distribution or reproduction in other forums is permitted, provided the original author(s) and the copyright owner(s) are credited and that the original publication in this journal is cited, in accordance with accepted academic practice. No use, distribution or reproduction is permitted which does not comply with these terms.
*Correspondence: Rino Rappuoli, cmluby5yYXBwdW9saUBiaW90ZWNub3BvbG8uaXQ=
†These authors have contributed equally to this work