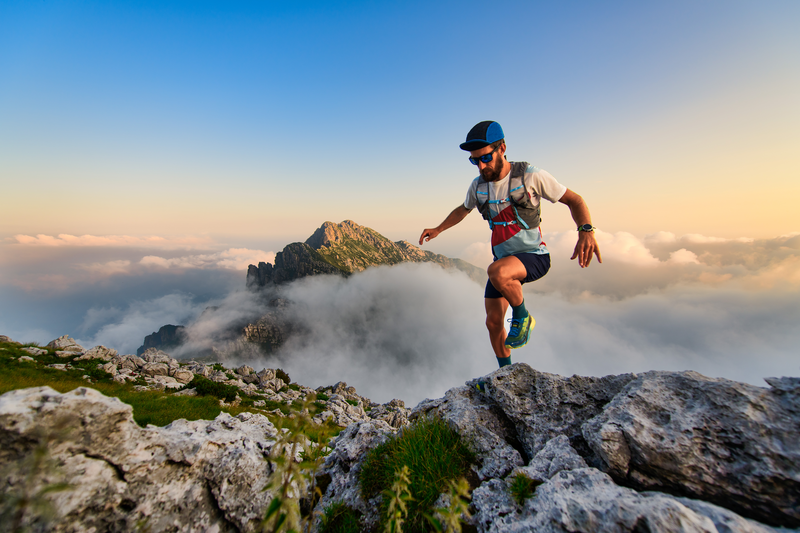
94% of researchers rate our articles as excellent or good
Learn more about the work of our research integrity team to safeguard the quality of each article we publish.
Find out more
ORIGINAL RESEARCH article
Front. Microbiol. , 17 August 2023
Sec. Antimicrobials, Resistance and Chemotherapy
Volume 14 - 2023 | https://doi.org/10.3389/fmicb.2023.1239538
This article is part of the Research Topic Insights in Antimicrobials, Resistance & Chemotherapy: 2022 View all 17 articles
Introduction: The increase in clinical Enterobacteriaceae with dual carbapenemase has become a serious healthcare concern. It is essential to characterize the transferability and potential dissemination of blaKPC−2- and blaNDM−1-coharboring carbapenem-resistant Citrobacter freundii (CRCF).
Methods: Four blaKPC−2- and blaNDM−1-coharboring CRCF strains were collected from our surveillance of the prevalence of carbapenem-resistant Enterobacteriaceae. The isolates were assessed using species identification, antimicrobial susceptibility testing, conjugation assays, whole-genome sequencing, plasmid stability, and fitness costs. Clonality, genome, plasmidome, and phylogeny were analyzed to reveal potential dissemination.
Results: Three ST523 blaKPC−2- and blaNDM−1-coharboring CRCF strains, collected from the same hospital within 1 month, exhibited high homology (both identity and coverage >99%), implying clonal dissemination and a small-scale outbreak. Moreover, the blaKPC−2 and blaNDM−1 genes were coharbored on an IncR plasmid, probably generated by a blaKPC−2-harboring plasmid acquiring blaNDM−1, in these three strains. Importantly, the IncR plasmid may form a transferable hybrid plasmid, mediated by IS6100 via transposition, with another IncFII plasmid included in the same C. freundii strain. Furthermore, the blaKPC−2 and blaNDM−1 of the fourth CRCF strain are located on two different non-transferable plasmids lacking complete transfer elements. Additionally, throughout the course of the 10-day continuous passage, the genetic surroundings of blaNDM−1 in four CRCF strains were gradually excised from their plasmids after the 8th day, whereas they maintained 100% retention for blaKPC−2. Genome and plasmidome analyses revealed that blaKPC−2- or blaNDM−1-harboring C. freundii were divergent, and these plasmids have high homology to plasmids of other Enterobacteriaceae.
Conclusion: Clonal dissemination of ST523 blaKPC−2- and blaNDM−1-coharboring CRCF strains was detected, and we first reported blaKPC−2 and blaNDM−1 concomitantly located on one plasmid, which could be transferred with mediation by IS6100 via transposition. Continued surveillance should urgently be implemented.
Citrobacter freundii, a Gram-negative and facultative anaerobic bacillus, is an opportunistic pathogen and causes diverse infections, including those of the urinary tract, respiratory tract, and bloodstream (Liu et al., 2018). Carbapenemase-producing C. freundii (CPCF), carrying blaKPC encoding a class A serine β-lactamase KPC or blaNDM encoding a class B metallo-β-lactamase NDM, has been increasingly reported in recent years and resulted in a small-scale, sporadic outbreak (Hammerum et al., 2016; Bartsch et al., 2017; Jimenez et al., 2017; Bitar et al., 2019; Babiker et al., 2020). Both KPC-2 and NDM-1, the subclasses of KPC and NDM, respectively, show a broad spectrum of hydrolytic activity against penicillins, cephalosporins, and carbapenems. The former can also hydrolyze monobactams and be inhibited by most β-lactamase inhibitors, such as avibactam and relebactam. By comparison, the latter cannot hydrolyze monobactams and is unresponsive to the above β-lactamase inhibitors (Bush, 2013; Bush and Bradford, 2019). Importantly, blaKPC−2- and blaNDM−1-coproducing CPCF strains have been recovered from patient specimens and clinical waste in China, leading to limited options for antibacterial treatment (Feng et al., 2015; Wu et al., 2016; Ouyang et al., 2018; Li et al., 2022). Nevertheless, each of those studies only described a single CRCF strain carrying blaKPC−2 and blaNDM−1 on two separate plasmids.
Herein, we identified four blaKPC−2- and blaNDM−1-coharboring CRCF strains isolated from urine specimens and renal perfusion fluid of four patients in China. We first identified blaKPC−2 and blaNDM−1 concomitantly located on one IncR plasmid of three CRCF strains and also compared their genetic environments in conjunction with data available on NCBI, revealing its potential dissemination mechanism.
Four blaKPC−2- and blaNDM−1-coharboring CRCF strains were collected during our surveillance of the prevalence of carbapenem-resistant Enterobacteriaceae isolates. Species identification was determined using matrix-assisted laser desorption ionization–time of flight mass spectrometry (MALDI-TOF MS) (Bruker Daltonik, Bremen, Germany), as described previously (Lu et al., 2021).
In vitro susceptibility tests were performed using N335 susceptibility cards and the Vitek-2 system (bioMérieux, France), including amikacin, minocycline, doxycycline, ceftazidime, cefepime, piperacillin/tazobactam, aztreonam, levofloxacin, ciprofloxacin, and sulfamethoxazole/trimethoprim. The minimal inhibitory concentrations (MICs) of imipenem, meropenem, tigecycline, colistin, ceftazidime–avibactam, and aztreonam–avibactam were determined using the microdilution broth method (bio-KONT, Ltd. China) with E. coli ATCC 25922 as the quality control strain, as we described previously (Zhang et al., 2023). The breakpoint of tigecycline was defined by the U.S. Food and Drug Administration (FDA) (Marchaim et al., 2014). The results of other antimicrobial agents were interpreted following the standards of the Clinical Laboratory Standards Institute (CLSI, 2021) (2021). The production of carbapenemases was determined using the modified carbapenem inactivation method (mCIM) and the EDTA-modified carbapenem inactivation method (eCIM), as recommended by the CLSI 2021 (2021).
As we described previously (Zhang et al., 2023), WGS was performed using the Illumina HiSeq 2500 platform and the nanopore sequencing method on MinION flow cells (for CPCF strains CF2075, CF2084, CF2085, and CF26019 and the transconjugant E. coli J53_K-N, respectively). Raw reads were filtered to remove the low-quality sequences and adaptors using skewer (Jiang et al., 2014) and Porechop (https://github.com/rrwick/Porechop), respectively. De novo assembly was performed via the SPAdes Genome Assembler v3.13.1 (Prjibelski et al., 2020) and Unicycler (Wick et al., 2017). Gene prediction for CRCF genomes, including four from this study and three retrieved from the NCBI genome database, was performed using Prokka 1.12 (Seemann, 2014). Insertion sequences were identified using the ISfinder database (Siguier et al., 2006). The antimicrobial resistance genes, multilocus sequence types (MLSTs), and plasmid replicon were analyzed via the CGE server (https://cge.food.dtu.dk/services/). Single-nucleotide polymorphisms (SNPs) were determined using Snippy (https://github.com/tseemann/snippy). Homology analysis was performed using BLASTn, FastANI, and SNP. Linear alignments of blaKPC−2- and blaNDM−1-bearing structures were generated using genoPlotR and gggenes in R-4.1.2. The transferability of blaKPC−2 and blaNDM−1-carrying plasmids in four CRCF strains was evaluated using oriTfinder (Li et al., 2018).
Plasmid conjugation experiments were conducted for four CRCF strains, as described previously (Zhang et al., 2023). Azide-resistant E. coli J53 and amikacin-resistant K. pneumoniae KP54 were used as the recipient strains. In brief, the four CRCF strains and recipient KP54 were adjusted to a McFarland standard of 0.5 and mixed at a ratio of 1:3, and a 0.1-milliliter aliquot of mixture was transferred into LB broth without antibiotics. After an 18-h incubation at 37°C, 200-ml cultures were streaked onto China blue agar (CBA, addition of rosolic acid as the pH indicator) plates containing both amikacin (16 mg/L) and meropenem (1 mg/L) to screen the blaKPC−2− or blaNDM−1-carrying transconjugants. Similarly, KP54_CF2075K-N and recipient E. coli J53 were mixed, and transconjugants carrying blaKPC−2 or blaNDM−1 were also selected on CBA plates containing both azide (150 mg/L) and meropenem (1 mg/L). The above transconjugants were confirmed by antimicrobial susceptibility testing (AST), polymerase chain reaction (PCR), and pulsed-field gel electrophoresis (PFGE), respectively (Zhang et al., 2023).
The plasmid stability and fitness cost were assessed as previously described but with slight modifications (Gao et al., 2020). The stability of CF2075, CF2084, CF2085, CF26019, and the transconjugants was evaluated by a passage experiment. In brief, the aforementioned strains were grown in LB broth and transferred at a 24-h interval for 10 consecutive days (approximately 200 generations), at a 1:1000 dilution, into fresh LB broth. The cultures at the 2nd, 4th, 6th, 8th, and 10th days were serially diluted and streaked onto the antibiotic-free LB agar. Approximately 50 colonies were randomly selected to identify the retention of blaKPC−2 and blaNDM−1 using PCR. All the above experiments were conducted in triplicate on different days.
Fitness cost was evaluated by growth curves. In brief, CF2085, CF2085ΔNDM (obtained in the above passage assay), CF26019, CF26019ΔNDM, the recipient KP54, and its transconjugants were cultured and shaken at 200 rpm overnight at 37°C in 10 mL LB broth. The overnight cultures were diluted and incubated at 37°C for 25 h to measure the optical density values (OD600). The experiment was repeated two times. The growth curves were estimated using Tukey's multiple comparison tests with a one-way analysis of variance (ANOVA).
To better unravel the plasmidome of blaKPC−2- or blaNDM−1-harboring plasmids in C. freundii, we searched the RefSeq database on NCBI and obtained the intact plasmids harboring blaKPC−2 (42) or blaNDM−1 (21) in C. freundii worldwide as of 20 November 2022. Blasting was performed with BLASTn and illustrated with the R ggplot2 package.
A phylogenetic tree from relevant plasmids was built using PhyML (Guindon et al., 2010), under model GTR running 1,000 bootstrap replicates, from the alignment results generated by mafft (Kuraku et al., 2013). The maximum-likelihood phylogenetic tree of blaKPC−2- and blaNDM−1-coharboring CRCF strains in this study with three complete genomes was built using RaxML (Stamatakis, 2014), running 1,000 bootstrap replicates under model GTR-G, from the alignment generated by SNPs and filtered to remove recombination using Gubbins v2.4.1 (Croucher et al., 2015). Visualization was performed using iTOL (https://itol.embl.de).
Data analyses were performed using GraphPad Prism 8.2.1. One-way analysis of variance was used for assessing significant differences, with a P-value of < 0.05 being considered statistically significant.
From 11 September 2020 to 12 October 2020, three blaKPC−2- and blaNDM−1-coharboring CRCF strains (CF2075, CF2084, and CF2085) were recovered from urine specimens of three inpatients with congenital hydronephrosis post-operation or urinary infection (aged 8 months to 9 years) at the same urological ward of a tertiary hospital in Henan, China (Table 1). The CRCF strain CF26019 was isolated from the renal perfusion fluid of Patient 4 with chronic kidney disease at another tertiary hospital in Beijing, China, after kidney transplantation (Table 1). The four patients all finally recovered from urological diseases.
Multilocus sequence typing (MLST) revealed that all CRCF strains, CF2075, CF2084, and CF2085, belonged to ST523. The strains CF2084 and CF2085 had >99% coverage and identity to CF2075 by BLASTn and FastANI, >99% vice versa. Further analysis revealed that there were only 6 and 18 single-nucleotide polymorphisms (SNPs) between CF2084 and CF2075 and CF2085 and CF2075, respectively. Moreover, Xbal and S1-PFGE also revealed that CF2075, CF2084, and CF2085 had identical bands (Supplementary Figures S1A, B). Overall, PFGE and WGS both indicated that there was a nosocomial outbreak of ST523 CRCF strains through clonal dissemination.
The CRCF strains CF2075, CF2084, CF2085, and CF26019 showed resistance to imipenem, meropenem, ceftazidime, ceftazidime–avibactam, aztreonam, piperacillin/tazobactam, and cefepime but were susceptible to amikacin, tigecycline, colistin, and aztreonam/avibactam for limited treatment options (Table 2). WGS revealed they all carried blaKPC−2 and blaNDM−1, in line with resistance phenotypes. Moreover, CF26019 also harbored aac(3)-IIa and aac(6′)-Ib-cr, resulting in resistance to tobramycin.
Table 2. Carbapenemase genes located on plasmids and MICs (mg/L) of CF2075, CF2084, CF2085, CF26019, and the transconjugantsa.
Due to the high homology and clonal spread of the strains of CF2075, CF2084, and CF2085, only CF2075 was chosen for further analysis in detail. WGS and S1-PFGE revealed that CF2075 consisted of a chromosome (5,013,357 bp and 52% GC content) and two plasmids designated as pCF2075-1 (46,049 bp and 56% GC content; identical plasmids pCF2084-1 and pCF2085-1 were identified in CF2084 and CF2085, respectively) and pCF2075-2 (81,274 bp and 53% GC content; identical plasmids pCF2084-2 and pCF2085-2 in CF2084 and CF2085, respectively), classified as IncR and IncFII incompatibility groups, respectively (Supplementary Figures S1A, B). Interestingly, pCF2075-1 concomitantly carried blaKPC−2 and blaNDM−1, and the genetic surroundings of the two genes were also contiguous. The blaKPC−2-harboring genetic environment was Tn1721-klcA-korC-ΔISKpn6-blaKPC−2-ISKpn27-Tn3 (13,760 bp), which is particularly prevalent in China (Figure 1A). It is likely that the blaNDM−1-harboring genetic context was ISCR1-dsbD-trpF-ble-blaNDM−1-ΔISAba125-Δsul1 (5,250 bp). The blaNDM−1 gene flanked by ISCR1 was rarely reported before (Figure 1A). No resistance gene was detected in pCF2075-2.
Figure 1. Origin and formation of pCF2075-1. (A) Linear sequence alignment analysis on plasmid pA1732-KPC (MT810373.1), pCF2075-1, pM218A-NDM-1 gene cluster (KX687851.1), and pB72B (CP069803.1). (B) Proposed formation mechanism of pCF2075-1 as follows. Step 1: pA1732-KPC evolves to generate a cointegrate by losing insignificant segments (arc dotted line) via similar recombination or transposition. Step 2: ISCR1, IS5075, and Tn3 mediate the production of transposable elements (TEs), and the cointegrate is integrated into the hybrid plasmid pCF2075-1 by these TEs.
To our knowledge, we have first reported the co-existence of blaKPC−2 and blaNDM−1 located on one plasmid (pCF2075-1) in C. freundii. The plasmid pCF2075-1, an IncR plasmid, exhibited low coverage (< 82.0%) compared to these plasmids on NCBI. Therefore, pCF2075-1 is a previously unreported fusion plasmid. To decipher the forming mechanism of pCF2075-1, its structure was analyzed in detail. It consisted of pA1732-KPC (63.5%, MT810373.1), the pM218A-NDM-1 gene cluster (11.4%, KX687851.1), and pB72B (22.4%, CP069803.1). The pA1732-KPC was contained in K. pneumoniae A1732 and harbored the same replicon IncR and blaKPC−2-harboring genetic context as pCF2075. Consequently, pA1732-KPC could be considered the backbone of pCF2075-1. However, the genetic environment of blaKPC−2 of pCF2075-1 did not harbor IRL-2 (Tang et al., 2022), which could embed blaKPC−2 into transposon Tn1721 for transfer. This transposon is non-transferable by carrying blaKPC−2. The pM218A-NDM-1 in Escherichia coli harbored blaNDM−1, flanked by ISCR1 of a class 1 Integron. ISCR1 can generate a transposable element (TE) mediating the transfer of blaNDM−1. It implied that the blaNDM−1-harboring TE was integrated into the backbone of pCF2075-1 to generate the blaKPC−2− and blaNDM−1-co-carrying cointegrate (Figure 1B). Furthermore, the other two convergences of blaKPC−2 and blaNDM−1 in the K. pneumoniae strain CHS5 chromosome (CP110688.1) and the K. michiganensis strain K254 plasmid pK254-KPC_NDM (OM938013.1) were retrieved from NCBI (Figure 2A). The blaKPC−2-harboring genetic contexts of the CHS5 chromosome and pK254-KPC_NDM were similar to Tn6296, which originated from the Tn1722-based transposon inserted by the core blaKPC−2 region. Notably, the blaNDM−1-harboring genetic contexts of both the CHS5 chromosome and pK254-KPC_NDM were contained in a class 1 Integron, in which the core blaNDM−1 region was flanked by ISCR1. Collectively, a propensity for the co-existence of blaKPC−2 and blaNDM−1 was overwhelmingly dominated by the ISCR1-mediating transfer of blaNDM−1 and the Tn1721/Tn6296-based-transposon-mediating transfer of blaKPC−2.
Figure 2. Linear alignment of blaKPC−2- or blaNDM−1-bearing plasmid. (A) Linear sequence analysis of blaKPC−2- and blaNDM−1-bearing chromosome and plasmid on CHS5 chromosome (CP110688.1), pCF2075-1, and pK254-KPC_NDM (OM938013.1). (B) Linear comparison of pCF2085-1 between CF2085 and CF2085ΔNDM. CF2085ΔNDM was derived from excision of blaNDM−1 core structure for CF2085 in conjugation. (C) Linear alignment of pCF26019-1 structure on CF26019 and CF26019ΔNDM. CF26019ΔNDM was derived from excision of blaNDM−1 core structure for CF26019 in conjugation.
In addition, CF26019 belonged to ST118 and contained a chromosome (5,177,371bp and 52% GC content) and four plasmids. Unlike CF2075, blaNDM−1 and blaKPC−2 of CF26019 were separately located on plasmid pCF26019-1 (44,169 bp and 56% GC content) and pCF26019-3 (35,420 bp and 57% GC content), belonging to IncFII/IncFIB and IncP6 incompatibility groups, respectively. Furthermore, pCF26019-1 showed a maximum query coverage of 73% with a nucleotide identity of 100% to the plasmids p205880-NDM of K. pneumoniae MH909345.1 and pK218-NDM of C. portucalensis OL988824.1. The genetic surrounding of blaNDM−1 on pCF26019-1 was IS5-ΔISEhe3-groL-groS-cutA-dsbD-trpF-ble-blaNDM−1-ΔISAba125(8,208 bp), which is a common genetic surrounding observed in blaNDM−1-harboring Enterobacteriaceae strains. The pCF26019-3 exhibited 91% coverage and 100% identity to eight plasmids on NCBI, including CF121SC21 plasmid1 (LT992437.1) of C. freundii and plasmid pA1705-KPC (MH909348.1) of K. pneumoniae A1705. The genetic context of blaKPC−2 on pCF26019-3 was ΔISEc33-Tn3-ISApu1-hp-ISApu2-ISKpn27-ΔblaTEM − 1-blaKPC−2-ΔISKpn6-korC-klcA (13,066 bp), which commonly exists on different plasmids in Enterobacteriaceae (MN539620.1, MN477223.1, and MH909348.1) and Pseudomonas species (CP040685.1).
The transferability of blaKPC−2 and blaNDM−1 was evaluated by conjugation assays. The results revealed that blaKPC−2 and blaNDM−1 of CF2075, CF2084, and CF2085 could be concomitantly transferred to the recipient K. pneumoniae KP54, a clinical strain isolated from urine samples, and the transconjugants were designated as KP54_CF2075K-N, KP54_CF2084K-N, and KP54_CF2085K-N, respectively (Supplementary Figure S1B). Further conjugation experiments revealed that blaKPC−2 and blaNDM−1 of KP54_CF2075K-N could also be simultaneously conjugated into recipient E. coli J53, designated as E. coli J53_K-N (Supplementary Figure S1C). The above transconjugants were verified by resistance phenotype using PCR and PFGE. However, no transconjugant was obtained after co-culturing CF26019 with KP54, even though we repeated the conjugation assay dozens of times.
Unexpectedly, S1-PFGE showed that all the transconjugants, KP54_CF2075K-N, KP54_CF2084K-N, and KP54_CF2085K-N, contained a novel fusion plasmid co-carrying blaKPC−2 and blaNDM−1, named pCfr_tK-N, which is different from any plasmids of CF2075, CF2084, and CF2085 in size. However, the size of the pCfr_tK-N is almost equal to the sum of the size of pCF2075-1 and pCF2075-2; that is to say, pCfr_tK-N was probably generated by the recombination of pCF2075-1 and pCF2075-2. To unravel the forming mechanism of pCfr_tK-N, WGS was performed. Sequence analysis revealed that pCfr_tK-N was indeed the recombination result of pCF2075-1 and pCF2075-2 (Figures 3A, B), and the junctions of these two plasmids were two insertion sequence IS6100s. Of note, two 8-base-pair direct repeat (DR, ATGCTCAG) sequences were adjacent to the left inverted repeat sequence (IRL) of one IS6100 and the right inverted repeat sequence (IRR) of another IS6100, which implied that the recombination was mediated by IS6100 (Figure 3C). The plasmid stability of four CRCF strains and transconjugants was evaluated by passage experiment (Supplementary Figure S1E). Excisions of blaNDM−1 strains were obtained from CF2085 and CF26019 on the 8th day, named CF2085ΔNDM and CF26019ΔNDM, respectively. However, S1-PFGE and the sequence analysis showed that blaNDM−1-harboring genetic surroundings were excised from the plasmids of CF2085 and CF26019, which were inconsistent with the loss of blaNDM−1 usually caused by the removal of plasmids harboring blaNDM−1 in most cases (Figure 2B and Supplementary Figure S1B). A similar phenomenon was also observed in CF2075 and CF2084 on the 10th day of the passage experiment. However, the transconjugants (KP54_CF2075K-N, KP54_CF2084K-N, KP54_CF2085K-N, and E. coli J53_K-N) maintained 100% retention during the process of 10-day continuous passage, showing that the hybrid plasmids harboring blaKPC−2 and blaNDM−1 could be stably inherited. In summary, blaKPC−2- and blaNDM−1-coharboring CRCF strains and transconjugants can retain the stable inheritance of blaKPC−2- and blaNDM−1-co-carrying plasmids (>90% retention in the 10-day passage) that might slow the clearance of resistance genes and facilitate clonal dissemination.
Figure 3. Emergence and recombination mechanism of hybrid plasmid pCfr_tK-N generated during conjugation. (A) Linear sequence comparison of hybrid plasmid pCfr_tK-N with pCF2075-1 and pCF2075-2. (B) Circular alignment analysis of pCF2075-1, pCF2075-2, and hybrid plasmid pCfr_tK-N. Alignments were generated using the BLAST Ring Image Generator (BRIG). (C) Proposed model for the formation of the fusion plasmid pCfr_tK-N mediated by IS6100 transposition.
Growth rates were measured to assess the impact of the acquisition of blaKPC−2- and blaNDM−1-coharboring plasmids on biological fitness cost. A significant growth difference (P < 0.0001) was shown between CF2085 and CF2085ΔNDM, KP54 and KP54_CF2075K-N, and E. coli J53 and E. coli J53_K-N, whereas no significant difference (P > 0.5) was observed in the growth rates between CF26019 and CF26019ΔNDM. Taken together, the blaKPC−2- and blaNDM−1-coharboring plasmids exhibited punishment of fitness in CF2075, CF2084, CF2085, and their transconjugants.
A total of 46 blaKPC−2-harboring plasmids contained in C. freundii strains, including 42 retrieved from NCBI and 4 in this study, were analyzed via phylogenetic tree (Figure 4). These 42 C. freundii strains were recovered from urine, sediment, rectal swab, and blood and also from wastewater in the hospital environment and were gathered in Spain, the Czech Republic, China, and USA. Heterogeneous STs were identified in blaKPC−2-harboring C. freundii, including ST8, ST18, ST22, ST65, ST118, ST257, ST259, ST523, and ST632. The C. freundii strains belonging to the same STs might carry both closely related plasmids (such as pCF2075-1, pCF2084-1, and pCF2085-1 or CP037739.1 and CP054297.1), largely implying a clonal spread, and distantly related ones (CP011608.1 and CP011656.1). Plasmid incompatibility groups revealed that the above closely related plasmids had the same replicon, including IncP6, IncR, and IncN. Furthermore, blaKPC−2-harboring plasmids showed ≤ 52% and ≤ 59% coverage compared with the blaKPC−2- and blaNDM−1-co-carrying plasmids pCF2075-1 and pK254-KPC_NDM, respectively. Notably, most blaKPC−2-harboring plasmids (43/46, 93.5%) exhibited ≥82.0% coverage at 100% identity to the plasmids contained in non-C. freundii Enterobacteriaceae. Similarly, blaKPC−2-harboring plasmids (34/46, 74.0%) also exhibited ≥82.0% coverage to the plasmids of C. freundii.
Figure 4. Plasmidome analysis of blaKPC−2-bearing plasmid in C. freundii. (A) blaKPC−2 genetic surrounding was analyzed in blaKPC−2-bearing plasmid of C. freundii strains, including 42 strains from NCBI and 4 from this study. (B) The maximum-likelihood phylogenetic tree was built by PhyML from 46 complete plasmids' sequence alignment generated by mafft. The tree was visualized and annotated using Interactive Tree Of Life (iTOL, https://itol.embl.de). Class—different types of blaKPC−2 genetic surrounding on the left. Alignment—alignment to pCF2075-1 and pK254-KPC_NDM. Coverage—coverage to plasmids in non-Citrobacter sp., Enterobacteriaceae, and Citrobacter sp.
Four blaNDM−1-harboring plasmids in this study and twenty-one on NCBI were comprehensively analyzed (Figure 5). Their STs also showed diversity, including ST18, ST19, ST88, ST98, ST116, ST252, ST257, ST396, and ST523. Incompatibility groups mainly focus on IncX3 (10/25, 40.0%), IncC (5/25, 20.0%), and IncR (4/25, 16.0%), while identical replicons showed an obvious cluster (MK101346.1 and MH995506.1; CP097106.1 and CP055250.1; and pCF2075-1, p-CF2084-1, and pCF2085-1). However, a similar core blaNDM−1 region was not always contained in a cluster. The blaNDM−1-harboring plasmids were isolated from clinical species and environments in line with blaKPC−2-harboring plasmids and were mainly from China (16/25, 64.0%) since 2010. In contrast with pCF2075-1 and pK254-KPC_NDM, all blaNDM−1-harboring plasmids exhibited ≤ 55% coverage. To better evaluate the origin of blaNDM−1-harboring plasmids in C. freundii, these plasmids were compared with those on NCBI. The results revealed that 72.0% (18/25) plasmids in C. freundii and 92.0% (23/25) plasmids in other Enterobacteriaceae shared >80% of their length with the aforementioned blaNDM−1-containing plasmids, respectively.
Figure 5. Plasmidome analysis of blaNDM−1-bearing plasmid in C. freundii. (A) blaNDM−1 genetic surrounding was analyzed in blaNDM−1-bearing plasmid of C. freundii strains, including 21 strains from NCBI and 4 from this study. (B) The maximum-likelihood phylogenetic tree was built by PhyML from 25 complete plasmids' sequence alignment generated by mafft. The Interactive Tree Of Life (https://itol.embl.de) was used for visualization. Class—different types of blaNDM−1 genetic surrounding on the left. Alignment—alignment to pCF2075-1 and pK254-KPC_NDM. Coverage—coverage to plasmids in non-Citrobacter sp., Enterobacteriaceae, and Citrobacter sp.
To decipher the underlying mechanism in forming blaKPC−2- and blaNDM−1-coharboring CRCF strains, seven blaKPC−2- and blaNDM−1-coharboring CRCF strains having complete genomes, including three reported previously (Wu et al., 2016; Ouyang et al., 2018; Li et al., 2022) and four in this study, were analyzed (Figure 6). All were recovered in China after 2013 and could be divided into three clusters based on genome (cluster 1: CF26019; cluster 2: P10159 and WCHCF65; cluster 3: CF2075, CF2084, CF2085, and SCLZS47). STs showed an extensive distribution, including ST88, ST116, ST118, ST252, and ST523.
Figure 6. Maximum-likelihood phylogenetic tree of seven blaKPC−2- and blaNDM−1-coharboring CRCF strains was built by RaxML. The tree is annotated based on ST, Inc_pKPC (incompatibility group of blaKPC−2-bearing plasmids), Inc_pNDM (incompatibility group of blaNDM−1-bearing plasmids), transferability, species, year, geography, and similarity among blaKPC−2-bearing plasmids (blue heatmap) and blaNDM−1-bearing plasmids (purple heatmap) in C. freundii. The similarity is defined as the coverage of homology regions for query plasmid (row-wise) and subject plasmid (column-wise).
The blaKPC−2-harboring plasmids in both P10159 and SCLZS47 were identical, although not grouped into the same cluster, and CF2075, CF2084, and CF2085 also contained the same plasmids. Therefore, four different blaKPC−2-harboring plasmids were identified in the seven CRCF strains. These different plasmids, other than the type of pCF2075-1, have a high coverage of similar sequence (bi-directional ≥90%) in Enterobacteriaceae. For blaNDM−1-harboring plasmids, three different plasmid types were identified. It is likely that the coverage of similar sequences among these plasmids was also distantly related (4.0–16.0%). Only the type of pP10159-1 (MF072961.1) had similar plasmids (bi-directional coverage and identity ≥99.9%) reported before in Enterobacteriaceae, and others were novel ones. Furthermore, P10159 and SCLZS47 were isolated from Chongqing and Sichuan in China, which are geographically close, and had identical blaKPC−2- and blaNDM−1-harboring plasmids, but the chromosomes of P10159 had 81.0% coverage of similar sequence at 95.94% to SCLZS47 and 80.0% the other way around, hinting at a horizontal gene transfer (HGT) by plasmids.
CRCF coharboring blaKPC−2 and blaNDM−1 was first reported in 2015 (Feng et al., 2015) and has emerged continually in recent years, especially in China (Feng et al., 2015; Wu et al., 2016; Ouyang et al., 2018; Li et al., 2022). However, comprehensive analyses for the CRCF strains were lacking, and the potential dissemination mechanism has remained unclear.
In the current study, four blaKPC−2- and blaNDM−1-coharboring CRCF strains were identified. Three of them (CF2075, CF2084, and CF2085), recovered from urine samples within 1 month in the same ward, indicated a clonal outbreak according to PFGE, WGS, and phylogenetic tree analyses. WGS revealed a blaKPC−2- and blaNDM−1-coharboring plasmid in these three CRCF strains, which was first reported here. The clonal outbreak of coharboring dual carbapenemase genes will pose a severe threat to public health. Only the plasmid pK254-KPC_NDM (OM938013.1) in K. michiganensis and the chromosome (CP110688.1) CHS5 in K. pneumoniae are coharboring blaKPC−2 and blaNDM−1, in line with the data on NCBI. The blaNDM−1-carrying genetic surroundings of the above plasmid and chromosome were highly identical, facilitating the generation of TE and further spread. However, the blaKPC−2-carrying genetic surroundings of them were Tn1721/Tn6296-based transposons, which were usually generated in an ancestor transposon by inserting another transposon, and lacked an intact inverted repeat sequence (IR) at both sides of the core blaKPC−2 region, resulting in difficult transfer. Taken together, it is reasonable to hypothesize that a blaKPC−2- and blaNDM−1-coharboring plasmid or chromosome might be derived from blaKPC−2-harboring plasmid or chromosome progenitors that acquired blaNDM−1 by HGT, such as TUs and transposons. AST revealed that aztreonam–avibactam was probably an option for blaKPC−2- and blaNDM−1-coharboring CRCF strains.
To evaluate the transferability of blaKPC−2 and blaNDM−1, a series of transconjugants were obtained by conjugation assays and showed consistency of resistance phenotype and genotype. However, S1-PFGE showed that pCF2075-1 was not transferred alone to the recipient, and further analysis revealed that during conjugation, the fusion plasmid pCfr_tK-N was generated and could be transferred to the recipient strains KP54 and E. coli J53, respectively. Sequence analysis indicated that pCfr_tK-N was a recombinant of pCF2075-1 and pCF2075-2 mediated by IS6100, and this intermolecular transposition was first reported. Similar recombination, mediated by IS26, ISKpn14, ISKpn74, and IS903B, was also reported in conjugations among different resistant or virulent plasmids in recent years, which could accelerate the dissemination of resistance and virulence genes (Wang et al., 2022a,b; Yang et al., 2022). The pCF2075-1 being transferred by generating recombinants might be explained by the fact that the self-transferrable plasmids usually contain four modules: origin of transfer site oriT, relaxase gene, gene encoding type IV coupling protein (T4CP), and gene cluster for bacterial type IV secretion system (T4SS). Putative transferability analysis revealed that pCF2075-1 only contained oriT and relaxase gene, which prevented it from being transferred alone. However, pCF2075-2 contained all four complete modules. Therefore, pCF2075-1 is required to be integrated into pCF2075-2 to be transferrable. Furthermore, the stability and fitness cost of the CRCF strains and transconjugants were evaluated. These exhibited higher retention for blaKPC−2 and blaNDM−1 (>90.0% after 10 days) but required biological cost.
Plasmidome is extremely essential for understanding its origin and taking measures to prevent its propagation. In total, 46 blaKPC−2-harboring plasmids and 25 blaNDM−1-harboring plasmids retrieved from NCBI were systematically analyzed. Analysis revealed that C. freundii strains with blaKPC−2- or blaNDM−1-harboring plasmids shared the same STs, such as ST18 and ST257, that might generate more blaKPC−2- and blaNDM−1-coharboring CRCF strains in future. Importantly, most blaKPC−2- or blaNDM−1-harboring plasmids in C. freundii had high homology to the plasmids of other Enterobacteriaceae, hinting at the high transferability of blaKPC−2- or blaNDM−1-harboring plasmids. Moreover, the types of core blaKPC−2 and blaNDM−1 regions were relatively conserved, although blaKPC−2 or blaNDM−1 genetic surroundings exhibited multiple types by different ISs, indicating that ISs play a significant role in driving resistance genes' transfer.
We next comprehensively elaborated on the evolutionary relationships of seven blaKPC−2- and blaNDM−1-coharboring CRCF strains. These were grouped into three clusters. Notably, P10159 and SCLZS47 had the same blaKPC−2- and blaNDM−1-harboring plasmids, although they did not belong to the same cluster. The discordance of homology of blaKPC−2- or blaNDM−1-harboring plasmids to the genome evolution indicated that these have a strong ability to transfer and adapt to different hosts. As mentioned above, blaKPC−2- or blaNDM−1-harboring plasmids in C. freundii belong to diverse STs. Therefore, the formation of blaKPC−2- and blaNDM−1-coharboring CRCF strains probably occurs in two ways: blaKPC−2-harboring C. freundii acquires blaNDM−1-harboring plasmids, or blaNDM−1-harboring C. freundii acquires blaKPC−2-harboring plasmids.
In conclusion, we identified four blaKPC−2- and blaNDM−1-coharboring CRCF strains and reported, for the first time, blaKPC−2 and blaNDM−1 concomitantly located on one plasmid. Notably, the plasmid was integrated into another plasmid to generate an uncommon fusion plasmid, mediated by IS6100 via transposition, which could be transferred into a different genus in Enterobacteriaceae. Genome and plasmidome analyses revealed that blaKPC−2- or blaNDM−1-harboring C. freundii were divergent, and these plasmids have high homology to plasmids of other Enterobacteriaceae.
The datasets presented in this study can be found in online repositories. The names of the repository/repositories and accession number(s) can be found below: https://www.ncbi.nlm.nih.gov/, BioProject: PRJNA937448.
FZ and ZiyL performed the experiments. FZ, ZiyL, and XL analyzed the data. FZ and BL wrote the main manuscript text and prepared Figures 1–6 and Supplementary Figure S1. ZiyL, XL, YH, YW, JZ, YZ, YF, ZicL, XY, ZhiL, and CL prepared Tables 1, 2. All authors reviewed the manuscript. All authors contributed to the article and approved the submitted version.
This study was supported by the Chinese Academy of Medical Sciences (CAMS) Innovation Fund for Medical Sciences (Grant Number CIFMS 2021-I2M-1-030).
The authors declare that the research was conducted in the absence of any commercial or financial relationships that could be construed as a potential conflict of interest.
All claims expressed in this article are solely those of the authors and do not necessarily represent those of their affiliated organizations, or those of the publisher, the editors and the reviewers. Any product that may be evaluated in this article, or claim that may be made by its manufacturer, is not guaranteed or endorsed by the publisher.
The Supplementary Material for this article can be found online at: https://www.frontiersin.org/articles/10.3389/fmicb.2023.1239538/full#supplementary-material
Supplementary Figure S1. PFGE, the stability and growth curves of CF2075, CF2084, CF2085, CF26019, and the transconjugants. (A) Xbal-PFGE of CF2075, CF2084, CF2085, CF26019, and the transconjugants. (B, C) S1-PFGE of these strains. Red arrows indicate transferable hybrid plasmid pCfr_tK-N. (D) PCR for blaKPC−2 and blaNDM−1 and excision sequences of CF2085, CF2085ΔNDM, CF26019, and CF26019ΔNDM. Green arrows indicate the remains of pCF2085-1 and pCF26019-1 excising of blaNDM−1-bearing surrounding in CF2085ΔNDM and CF26019ΔNDM, respectively. (E) The stability of these strains throughout the course of the 10-day continuous passage. (F) Growth curves of these strains within 25 h.
Babiker, A., Evans, D. R., Griffith, M. P., McElheny, C. L., Hassan, M., Clarke, L. G., et al. (2020). Clinical and genomic epidemiology of carbapenem-nonsusceptible citrobacter spp. at a tertiary health care center over 2 decades. J. Clin. Microbiol. 58, e00275–20. doi: 10.1128/JCM.00275-20
Bartsch, S. M., McKinnell, J. A., Mueller, L. E., Miller, L. G., Gohil, S. K., Huang, S. S., et al. (2017). Potential economic burden of carbapenem-resistant Enterobacteriaceae (CRE) in the United States. Clin. Microbiol. Infect. 23, 48 e49–48. doi: 10.1016/j.cmi.2016.09.003
Bitar, I., Caltagirone, M., Villa, L., Mattioni Marchetti, V., Nucleo, E., Sarti, M., et al. (2019). Interplay among IncA and bla(KPC)-Carrying Plasmids in Citrobacter freundii. Antimicrob. Agents Chemother. 63, e02609–18. doi: 10.1128/AAC.02609-18
Bush, K. (2013). Proliferation and significance of clinically relevant beta-lactamases. Ann. N. Y. Acad. Sci. 1277, 84–90. doi: 10.1111/nyas.12023
Bush, K., and Bradford, P. A. (2019). Interplay between beta-lactamases and new beta-lactamase inhibitors. Nat. Rev. Microbiol. 17, 295–306. doi: 10.1038/s41579-019-0159-8
CLSI (2021). Performance Standards for Antimicrobial Susceptibility Testing. 31st ed. CLSI supplement M100. Wayne, PA: Clinical and Laboratory Standards Institute.
Croucher, N. J., Page, A. J., Connor, T. R., Delaney, A. J., Keane, J. A., Bentley, S. D., et al. (2015). Rapid phylogenetic analysis of large samples of recombinant bacterial whole genome sequences using Gubbins. Nucleic. Acids. Res. 43, e15. doi: 10.1093/nar/gku1196
Feng, J., Qiu, Y., Yin, Z., Chen, W., Yang, H., Yang, W., et al. (2015). Coexistence of a novel KPC-2-encoding MDR plasmid and an NDM-1-encoding pNDM-HN380-like plasmid in a clinical isolate of Citrobacter freundii. J. Antimicrob. Chemother. 70, 2987–2991. doi: 10.1093/jac/dkv232
Gao, H., Liu, Y., Wang, R., Wang, Q., Jin, L., and Wang, H. (2020). The transferability and evolution of NDM-1 and KPC-2 co-producing Klebsiella pneumoniae from clinical settings. EBioMedicine 51, 102599. doi: 10.1016/j.ebiom.2019.102599
Guindon, S., Dufayard, J. F., Lefort, V., Anisimova, M., Hordijk, W., and Gascuel, O. (2010). New algorithms and methods to estimate maximum-likelihood phylogenies: assessing the performance of PhyML 3.0. Syst. Biol. 59, 307–321. doi: 10.1093/sysbio/syq010
Hammerum, A. M., Hansen, F., Nielsen, H. L., Jakobsen, L., Stegger, M., Andersen, P. S., et al. (2016). Use of WGS data for investigation of a long-term NDM-1-producing Citrobacter freundii outbreak and secondary in vivo spread of blaNDM-1 to Escherichia coli, Klebsiella pneumoniae and Klebsiella oxytoca. J. Antimicrob. Chemother. 71, 3117–3124. doi: 10.1093/jac/dkw289
Jiang, H., Lei, R., Ding, S. W., and Zhu, S. (2014). Skewer: a fast and accurate adapter trimmer for next-generation sequencing paired-end reads. BMC Bioinform. 15, 182. doi: 10.1186/1471-2105-15-182
Jimenez, A., Castro, J. G., Munoz-Price, L. S., de Pascale, D., Shimose, L., Mustapha, M. M., et al. (2017). Outbreak of klebsiella pneumoniae carbapenemase-producing citrobacter freundii at a tertiary acute care facility in Miami, Florida. Infect. Control Hosp. Epidemiol. 38, 320–326. doi: 10.1017/ice.2016.273
Kuraku, S., Zmasek, C. M., Nishimura, O., and Katoh, K. (2013). aLeaves facilitates on-demand exploration of metazoan gene family trees on MAFFT sequence alignment server with enhanced interactivity. Nucleic Acids Res. 41, W22–W28. doi: 10.1093/nar/gkt389
Li, X., Xie, Y., Liu, M., Tai, C., Sun, J., Deng, Z., et al. (2018). oriTfinder: a web-based tool for the identification of origin of transfers in DNA sequences of bacterial mobile genetic elements. Nucleic. Acids. Res. 46, W229–W234. doi: 10.1093/nar/gky352
Li, Y., Fang, C., Qiu, Y., Dai, X., and Zhang, L. (2022). Genomic characterization of a carbapenem-resistant Citrobacter freundii cocarrying bla(KPC-2) and bla(NDM-1). J. Glob. Antimicrob. Resist. 29, 289–292. doi: 10.1016/j.jgar.2022.04.014
Liu, L. H., Wang, N. Y., Wu, A. Y., Lin, C. C., Lee, C. M., and Liu, C. P. (2018). Citrobacter freundii bacteremia: Risk factors of mortality and prevalence of resistance genes. J. Microbiol. Immunol. Infect. 51, 565–572. doi: 10.1016/j.jmii.2016.08.016
Lu, B., Yang, J., Gao, C., Li, D., Cui, Y., Huang, L., et al. (2021). Listeriosis cases and genetic diversity of Their L. monocytogenes isolates in China, 2008-2019. Front. Cell Infect. Microbiol. 11, 608352. doi: 10.3389/fcimb.2021.608352
Marchaim, D., Pogue, J. M., Tzuman, O., Hayakawa, K., Lephart, P. R., Salimnia, H., et al. (2014). Major variation in MICs of tigecycline in Gram-negative bacilli as a function of testing method. J. Clin. Microbiol. 52, 1617–1621. doi: 10.1128/JCM.00001-14
Ouyang, J., Sun, F., Zhou, D., Feng, J., Zhan, Z., Xiong, Z., et al. (2018). Comparative genomics of five different resistance plasmids coexisting in a clinical multi-drug resistant Citrobacter freundii isolate. Infect. Drug Resist. 11, 1447–1460. doi: 10.2147/IDR.S165818
Prjibelski, A., Antipov, D., Meleshko, D., Lapidus, A., and Korobeynikov, A. (2020). Using SPAdes De Novo assembler. Curr. Protoc. Bioinformatics 70, e102. doi: 10.1002/cpbi.102
Seemann, T. (2014). Prokka: rapid prokaryotic genome annotation. Bioinformatics 30, 2068–2069. doi: 10.1093/bioinformatics/btu153
Siguier, P., Perochon, J., Lestrade, L., Mahillon, J., and Chandler, M. (2006). ISfinder: the reference centre for bacterial insertion sequences. Nucleic. Acids. Res. 34, D32–36. doi: 10.1093/nar/gkj014
Stamatakis, A. (2014). RAxML version 8: a tool for phylogenetic analysis and post-analysis of large phylogenies. Bioinformatics 30, 1312–1313. doi: 10.1093/bioinformatics/btu033
Tang, Y., Li, G., Shen, P., Zhang, Y., and Jiang, X. (2022). Replicative transposition contributes to the evolution and dissemination of KPC-2-producing plasmid in Enterobacterales. Emerg. Microbes Infect. 11, 113–122. doi: 10.1080/22221751.2021.2013105
Wang, X., Tang, B., Liu, G., Wang, M., Sun, J., Tan, R., et al. (2022a). Transmission of nonconjugative virulence or resistance plasmids mediated by a self-transferable IncN3 plasmid from carbapenem-resistant klebsiella pneumoniae. Microbiol. Spectr. 10, e0136422. doi: 10.1128/spectrum.01364-22
Wang, X., Wang, Y., Jiang, X., Gong, X., Wang, Y., and Shen, Z. (2022b). Co-transfer of mcr-8 with bla(NDM-1) or tmexCD1-toprJ1 by plasmid hybridisation. Int. J. Antimicrob. Agents 60, 106619. doi: 10.1016/j.ijantimicag.2022.106619
Wick, R. R., Judd, L. M., Gorrie, C. L., and Holt, K. E. (2017). Unicycler: Resolving bacterial genome assemblies from short and long sequencing reads. PLoS Comput. Biol. 13, e1005595. doi: 10.1371/journal.pcbi.1005595
Wu, W., Espedido, B., Feng, Y., and Zong, Z. (2016). Citrobacter freundii carrying blaKPC-2 and blaNDM-1: characterization by whole genome sequencing. Sci. Rep. 6, 30670. doi: 10.1038/srep30670
Yang, X., Xie, M., Xu, Q., Ye, L., Yang, C., Dong, N., et al. (2022). Transmission of pLVPK-like virulence plasmid in Klebsiella pneumoniae mediated by an Incl1 conjugative helper plasmid. iScience 25, 104428. doi: 10.1016/j.isci.2022.104428
Zhang, F., Li, Z., Liu, X., Luo, G., Wu, Y., Li, C., et al. (2023). Molecular characteristics of an NDM-4 and OXA-181 Co-producing K51-ST16 carbapenem-resistant klebsiella pneumoniae: study of its potential dissemination mediated by conjugative plasmids and insertion sequences. Antimicrob. Agents Chemother. 67, e0135422. doi: 10.1128/aac.01354-22
Keywords: carbapenem-resistant Citrobacter freundii, KPC-2- and NDM-1-coproducing CRCF, hybrid plasmid, transposition recombination, IS6100
Citation: Zhang F, Li Z, Liu X, Hu Y, Zhao J, Zhang Y, Fan Y, Lei Z, Yang X, Li Z, Li C, Wu Y and Lu B (2023) Carbapenem-resistant Citrobacter freundii harboring blaKPC−2 and blaNDM−1: a study on their transferability and potential dissemination via generating a transferrable hybrid plasmid mediated by IS6100. Front. Microbiol. 14:1239538. doi: 10.3389/fmicb.2023.1239538
Received: 13 June 2023; Accepted: 31 July 2023;
Published: 17 August 2023.
Edited by:
Mona I. Shaaban, Mansoura Universiy, EgyptReviewed by:
Yanan Wang, Henan Agricultural University, ChinaCopyright © 2023 Zhang, Li, Liu, Hu, Zhao, Zhang, Fan, Lei, Yang, Li, Li, Wu and Lu. This is an open-access article distributed under the terms of the Creative Commons Attribution License (CC BY). The use, distribution or reproduction in other forums is permitted, provided the original author(s) and the copyright owner(s) are credited and that the original publication in this journal is cited, in accordance with accepted academic practice. No use, distribution or reproduction is permitted which does not comply with these terms.
*Correspondence: Binghuai Lu, enMyNTA0MUAxMjYuY29t
Disclaimer: All claims expressed in this article are solely those of the authors and do not necessarily represent those of their affiliated organizations, or those of the publisher, the editors and the reviewers. Any product that may be evaluated in this article or claim that may be made by its manufacturer is not guaranteed or endorsed by the publisher.
Research integrity at Frontiers
Learn more about the work of our research integrity team to safeguard the quality of each article we publish.