- 1Research Center of Harmful Algae and Marine Biology, Key Laboratory of Eutrophication and Red Tide Prevention of Guangdong Higher Education Institutes, College of Life Science and Technology, Jinan University, Guangzhou, China
- 2School of Life Sciences, South China Normal University, Guangzhou, China
- 3Hubei Water Resources Research Institute, Hubei Water Resources and Hydropower Science and Technology Information Center, Wuhan, China
- 4Hubei Institute of Product Quality Supervision and Inspection, Wuhan, China
Lead (Pb) is a hazardous pollutant in water environments that can cause significant damage to aquatic animals and humans. In this study, crucian carp (Carassius auratus) were exposed to waterborne Pb for 96 h; then, histopathological analysis, quantitative qPCR analysis, and 16S high-throughput sequencing were performed to explore the effects of Pb on intestinal bioaccumulation, structural damage, oxidative stress, immune response, and microbiota imbalance of C. auratus. After Pb exposure, the intestinal morphology was obviously damaged, including significantly increasing the thickness of the intestinal wall and the number of goblet cells and reducing the depth of intestinal crypts. Pb exposure reduced the mRNA expressions of Claudin-7 and villin-1 while significantly elevated the level of GST, GSH, CAT, IL-8, IL-10, IL-1, and TNF-α. Furthermore, 16S rRNA analysis showed that the Shannon and Simpson indices decreased at 48 h after Pb exposure, and the abundance of pathogenic bacteria (Erysipelotrichaceae, Weeksellaceae, and Vibrionaceae) increased after Pb exposure. In addition, the correlation network analysis found that Proteobacteria were negatively correlated with Firmicutes and positively correlated with Bacteroidetes. Functional prediction analysis of bacteria speculated that the change in intestinal microbiota led to the PPAR signaling pathway and peroxisome function of the intestine of crucian carp was increased, while the immune system and membrane transport function were decreased. Finally, canonical correlation analysis (CCA) found that there were correlations between the intestinal microbiota, morphology, antioxidant factors, and immune factors of crucian carp after Pb exposure. Taken together, our results demonstrated that intestinal flora dysbiosis, morphological disruption, oxidative stress, and immune injury are involved in the toxic damage of Pb exposure to the intestinal structure and function of crucian carp. Meanwhile, Pb exposure rapidly increased the abundance of pathogenic bacteria, leading to intestinal disorders, further aggravating the damage of Pb to intestinal structure and function. These findings provide us a basis for the link between gut microbiome changes and heavy metal toxicity, and gut microbiota can be used as biomarkers for the evaluation of heavy metal pollution in future.
1. Introduction
With the development of the economy and the increase in population, the problem of environmental pollution is becoming more and more serious (Yu Y. Y. et al., 2021). Aquatic system, as an important part of the biosphere, has been seriously threatened by heavy metal pollution in water in the past few decades and has become a worldwide environmental problem (Shi et al., 2020; Yin et al., 2020; Rout et al., 2022a; Chen et al., 2023). In the past 5 years, the accumulation of heavy metals has been found in many water areas of China, such as Yangtze, Yellow, and Pearl Rivers (Xie et al., 2022; Chen et al., 2023; Liang et al., 2023). Heavy metal pollutants in the water environment were not only toxic to aquatic products but may also be absorbed by fish and passed up the food chain to humans through bioaccumulation (Francesco et al., 2014; Mario et al., 2019; Liu et al., 2020). As the major toxic heavy metals in the environment, Lead (Pb) is a key pollutant in environmental monitoring and contamination control, which has high toxicity to humans and organisms in the environment and has extensive pollution in the atmosphere, soil, and water (Chen et al., 2016; Behera et al., 2020, 2023; Shi et al., 2020; Parida et al., 2022). In 2015, Qi et al. found that the Pb content in the Fen River reached 21.2 mg/kg, and Huang et al. found that the Pb content in river soil caused by pollution in the Yunnan mining area reached 3,030.0 mg/kg in 2016 (Qi et al., 2015; Huang et al., 2016). Pb exposure has great toxic effects on the physiological, behavioral, and biochemical functions of animals. Previous studies have shown that Pb causes the accumulation of heavy metals, histopathology, and neurotoxicity in fish (Lee et al., 2019; Shahjahan et al., 2022). Lead accumulation can also cause oxidative stress in aquatic organisms and damage the antioxidant function of the body (Guo et al., 2021; Kumar et al., 2021). Therefore, several antioxidant markers, such as antioxidant enzymes (GST and CAT) and glutathione (GSH), are often used to evaluate the toxic effects of Pb-induced oxidative stress. However, with the development of toxicological research, the continued development of more reliable biomarkers to provide a more comprehensive monitoring and evaluation of pollution levels is warranted.
Intestinal microbiota is an intricate and unique ecosystem that is symbiotic with the host and is closely related to the host's antioxidant and immunity, as well as survival, metabolism, and behavior (Premysl et al., 2011; Daga et al., 2013; Wang et al., 2019; Meijerink et al., 2020; Zhang et al., 2020). Abnormal intestinal flora can affect host physiological activities, such as metabolic, inflammatory, and immune diseases, even increase host susceptibility to pathogens (Qi et al., 2019; Liu et al., 2020; Yu Z. W. et al., 2021). Stable intestinal microbiota structure is of great help to maintain the growth and development of the intestine of fish. On the other hand, foreign substances can interfere with the normal growth of the gut microbiota structure. For example, external low-temperature environment can improve the relative abundance of vibrio in the intestine, reduce the relative abundance of other probiotics, and change the structure of intestinal microbiota, leading to the occurrence of fish diseases (LeaMaster et al., 1997). Because the intestinal microbiota is a sensitive plastic factor responding to environmental changes, intestinal microbes have been used as a new biomarker for toxicity assessment in mammals (Candela et al., 2012; Li et al., 2021). Moreover, in recent years, the correlation between intestinal microbiota and heavy metal pollution has attracted attention (Duan et al., 2021). Therefore, understanding the toxic effect of waterborne Pb on fish intestinal microbiota, researching the correlation between microbes and the structure and function of the intestine under Pb pollution, has important research value for the development of fish intestinal microbiota as a biomarker for the assessment of aquatic environment pollution.
Fish, as a high tropic level in aquatic ecosystems, are widely present in aquatic environments around the world (Oost et al., 2003; Wang et al., 2021; Carlos et al., 2022; Kristine et al., 2022; Souza et al., 2022). Because of the accumulation of large amount of water pollutants through the food chain and bioaccumulation, fish are often considered as the suitable bioindicators of contaminated aquatic ecosystem (Yin et al., 2020; Mario et al., 2022). Crucian carp, belonging to the carp family, omnivorous, is one of the most common fish in freshwater environments (Dong and Li, 1994; Radke and Kahl, 2002; Li T. et al., 2015). Crucian carp is widely distributed in Eurasia, with excellent growth characteristics, good taste, and wide applicability in aquaculture systems and is an important commercial aquaculture fish in the world, and the annual global aquaculture production is millions of tons (FAO; Food and Agriculture Organization of the United Nations) (Rhee et al., 2014; Liu et al., 2017). As an energy carrier from lower to higher nutrient levels, the Pb accumulation of crucian carp would raise the risk of fish consumption (Oost et al., 2003). Thus, it is necessary to explore the toxicity of Pb to crucian carp to evaluate its hazards to aquatic organisms. In this study, crucian carp were exposed to simulated ponds with added lead contamination sources. We used inductively coupled plasma mass spectrometry (ICP-MS), histopathological symptom observation, and quantitative qPCR analysis to investigate the bioaccumulation levels of lead in the fish intestine, intestinal structural damage, and the expression levels of immune and antioxidant factors. In addition, the dynamics of the fish intestinal microbiota were detected by using 16s high-throughput sequencing technology to provide a detailed analysis report on the response of crucian carp to Pb stress. This will help to further understand the relationship between the intestinal structure, function, and microbial community of crucian carp under lead pollution and provide theoretical reference and experimental basis for intestinal microbes as a new water pollution toxicity biomarker.
2. Materials and methods
2.1. Fish maintenance and Pb exposure treatment
In this study, juvenile crucian carp (weight 13.4 ± 0.5 g, length 10.5 ± 0.3 cm) were provided by an aquaculture farm in Huadu, Guangzhou, China. The experiment was carried out at the aquaculture base of South China Normal University. Before the Pb exposure experiment, acclimation was performed as usual for 2 weeks (Liu et al., 2021). During the domestication period, the death rate of the experimental fish was <2% and had no clinical signs of disease.
Acute toxicity test and half lethal concentration (LC50) test for 96 h of Pb(NO3)2 were carried out according to the previous method (Pirsaheb et al., 2019). The 96-h LC50 of Pb for crucian carp was 216.62 mg/L. The safe concentration (SC) was calculated by multiplying the LC50 values with an application factor of 0.1 (Liu et al., 2020).
Sixty healthy crucian carp were randomly selected and cultured in three 1 m3 aquariums, 20 in each aquarium. Then, Pb(NO3)2 was added to make the Pb concentration in the culture water reach SC (21.66 mg/L) and maintained during the experiment. The posterior intestine of a fish was taken from each aquarium at 0 h (J 0h), 6 h (J 6h), 48 h (J 48h), and 96 h (J 96h), after Pb(NO3)2 exposure (n = 3). Fish were anesthetized with 0.05% tricaine methane sulfonate (MS-222; Aladdin, China) before sampling. A part of the posterior intestine of each fish was stored in Bouin's solution (Servicebio, China), and the other part of the posterior intestine of each fish was stored at −80°C after quick freezing in liquid nitrogen.
2.2. Pb content detection
The intestine from four groups (each group, n = 3) were collected, homogenized, and moved to the microwave digestion apparatus (Qiyao, China) for digestion (Shirin et al., 2015). Pb standard solution (Merck, Darmstadt, Germany) was injected into the inductively coupled plasma mass spectrometry (ICP-MS; Agilent, USA), to make the standard curve. Then, samples after microwave digestion were injected into the ICP-MS. According to the standard curve, the Pb content in the posterior intestine was obtained by referring to the previous methods (Li et al., 2020; Liu et al., 2020).
2.3. Intestinal histopathological examination
The intestine of crucian carp was fixed with Bouin's solution, embedded in paraffin, sectioned at 4 μm thickness, and stained with hematoxylin and eosin (HE). Finally, the intestinal tissue was observed under a polarized light microscope (Leica, Germany) (Tan et al., 2018).
2.4. qRT-PCR analysis
The total RNA from all samples was extracted by using TRIzol reagent (Vazyme, China), according to the manufacturer's instructions (Fu et al., 2019), and its quality and concentration were detected by electrophoresis in 1% agarose gel and NanoDrop 2000 spectrophotometer (NanoDrop Technologies, USA) (Fu et al., 2018). Then, using PrimeScript RT Reagent Kit (Takara, China) to synthesize cDNA and using the real-time PCR system (Bio-Rad, USA) to perform qRT-PCR (Huang et al., 2018). β-actin gene of crucian carp (GenBank accession NO. AB039726.2) was used as an internal control for the normalization of gene expression. Primers of genes (β-actin, Claudin-7, villin-1, GST, GSH, IL-1, IL-8, IL-10, and TNF-α) for qRT-PCR were designed by using Primer Premier 5 (Premier, Canada). The primer sequences are presented in Table 1.
2.5. DNA extraction and 16s rRNA gene amplification sequence analysis
All samples were sent to Guangzhou JiRui Gene Technology Co. Ltd. (China) for extraction of DNA and PCR amplification by the Illumina MiSeq Sequencing platform. PCR was performed from V3–V4 variable regions of 16S rRNA to taxonomically identify and analyze the bacteria (Liu et al., 2020; Dixit et al., 2021).
2.6. Statistical analysis
Unless otherwise stated, all experiments were performed with triplicate samples (n = 3), and the data are presented as means ± standard deviation. Before statistical analysis, the data tested for normality (Shapiro–Wilk) and homogeneity of variance (Levene's test) were assessed, and the assumptions were met. Differences in the expression levels of genes related to intestinal structure and function, intestinal structure-related indicators (intestinal wall thickness, crypt depth, and goblet cell number), relative abundance of intestinal microbiota, and functional proportion of intestinal microbiota were obtained by one-way ANOVA test. Graphs of Pb concentration and qPCR analysis were generated using GraphPad Prism 9, a number of intestinal goblet cells, intestinal wall thickness, and crypt depth were calculated using Image J, and the results were presented as means ± standard deviation (n = 3). Intestinal microbial operational taxonomic unit (OUT) classification statistics were performed using UPARSE. Alpha diversity analysis was performed using Mothur 1.30.2. Microbial functional prediction analysis was performed using the Kyoto Encyclopedia of Genes and Genomes database (KEGG, http://eggnog.embl.de/). Venn diagram analysis, network relationship analysis, and canonical correlation analysis (CCA) of microbiota were performed using the R software package (VennDiagram, Hmisc, and Vegan package). Statistical significance was set at P < 0.05 (*), and very significance was set at P < 0.01 (**) for all tests.
3. Results
3.1. Pb accumulation and histological analysis
Compared with the J 0 h group, the accumulation of Pb in the intestine was significantly increased (P < 0.05) in all the other groups, and the accumulation level of Pb was the highest (194.68 mg/kg) in the J 96 h group (Figure 1).
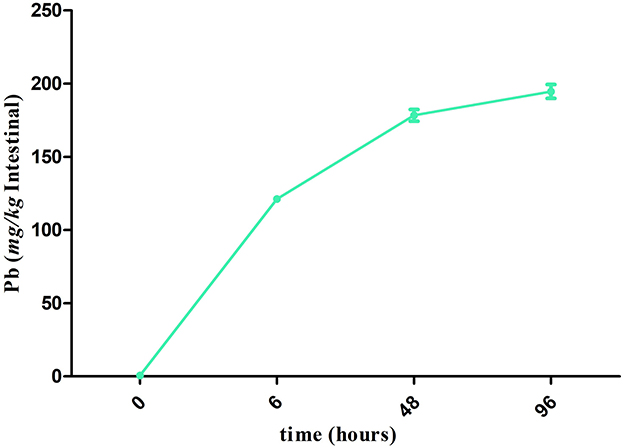
Figure 1. Accumulation of Pb in the crucian carp after Pb exposure during 96 h. The points in the line graphs represent the means ± SD of Pb content in the intestine of crucian carp (n =3).
The intestinal morphological changes are presented in Figure 2A. Compared with the J 0 h group, intestinal wall thickening, intestinal villi shortening, goblet cells increasing, and slight phagocytosis of leukocytes were observed in the 6, 48, and 96 h groups (Figures 2B–D). Specifically, after treated with Pb, the relative intestinal wall thickness was increased significantly and reached the highest level at 48 h (up to 1.54-fold, P < 0.01). After Pb exposure, the relative depth of intestinal crypts decreased and reached the lowest level at 96 h (down to 0.25-fold, P < 0.01) after Pb exposure, while the goblet cells' number of intestine increased and reached the highest level at 48 h (up to 4.24-fold, P < 0.01).
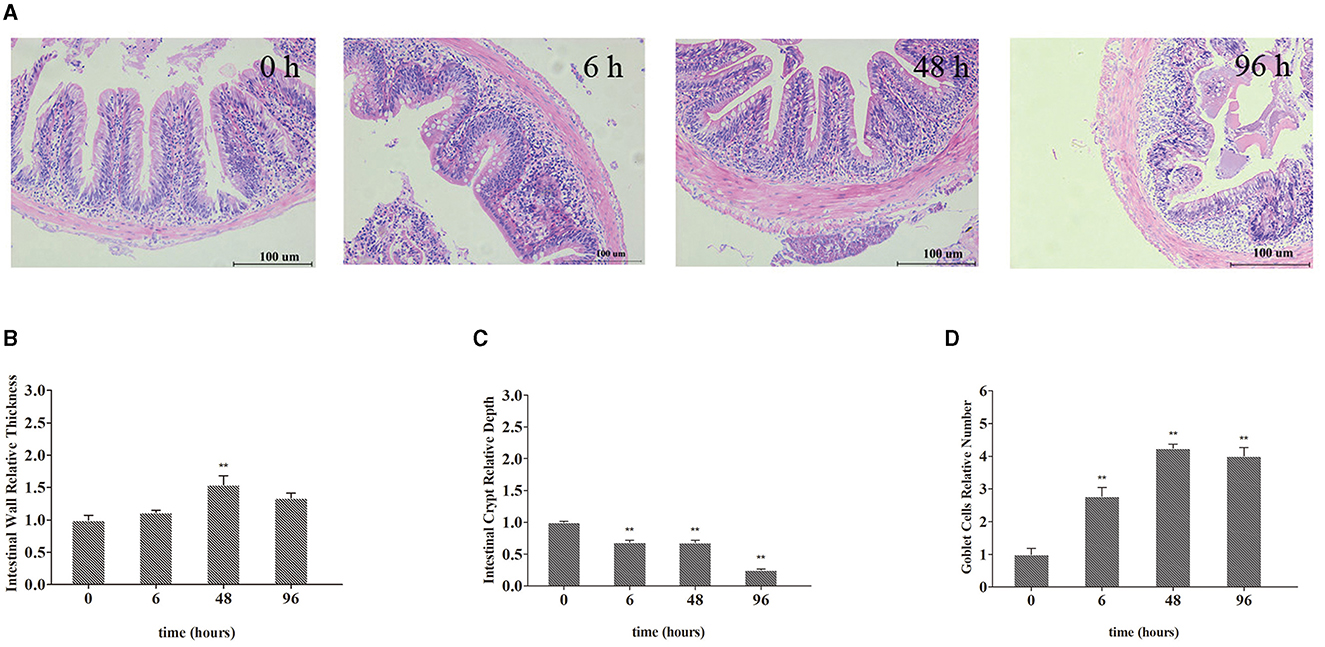
Figure 2. Representative micrographs of intestines from crucian carp. (A) Intestinal wall relative thickness. (B) Intestine crypt relative depth. (C) Goblet cells' relative number (D). Leukocyte infiltration occurs in the area indicated by the arrow. The bar plot denotes means ± SD of three replications, and the asterisk represents a statistically significant difference (*0.01 < P < 0.05 and **P < 0.01).
3.2. The expression of structure, antioxidant, and immune factor-related genes in the intestines
As shown in Figure 3, the expression of villin-1 and Claudin-7 in the intestine decreased significantly and reached the lowest level at 6 h (down to 0.60-fold, P < 0.01, and 0.62-fold, P < 0.01, respectively; Figures 3A, B) and returned to the normal level at 96 h, after Pb exposure. In Figures 3C, E, the expression of IL-1β and IL-10 in the intestine significantly increased to the highest level at 6 h (up to 6.48-fold, P < 0.01, and 2.31-fold, P < 0.01, respectively), after Pb exposure and gradually recovered later. In Figures 3D, F, the expression of IL-8 and TNF-α in the intestine significantly increased to the highest level at 48 h (up to 3.34-fold, P < 0.01, and 4.59-fold, P < 0.01, respectively) and gradually recovered at 96 h. As shown in Figures 3G, H, the expression of CAT and GST in the intestine significantly increased to the highest level at 6 h (up to 9.24-fold, p < 0.01, 2.18-fold, p < 0.01, respectively), after Pb exposure and gradually recovered later. In Figure 3I, the expression of GSH in the intestine significantly increased to the highest level at 48 h (up to 8.91-fold, P < 0.01) and gradually recovered at 96 h.
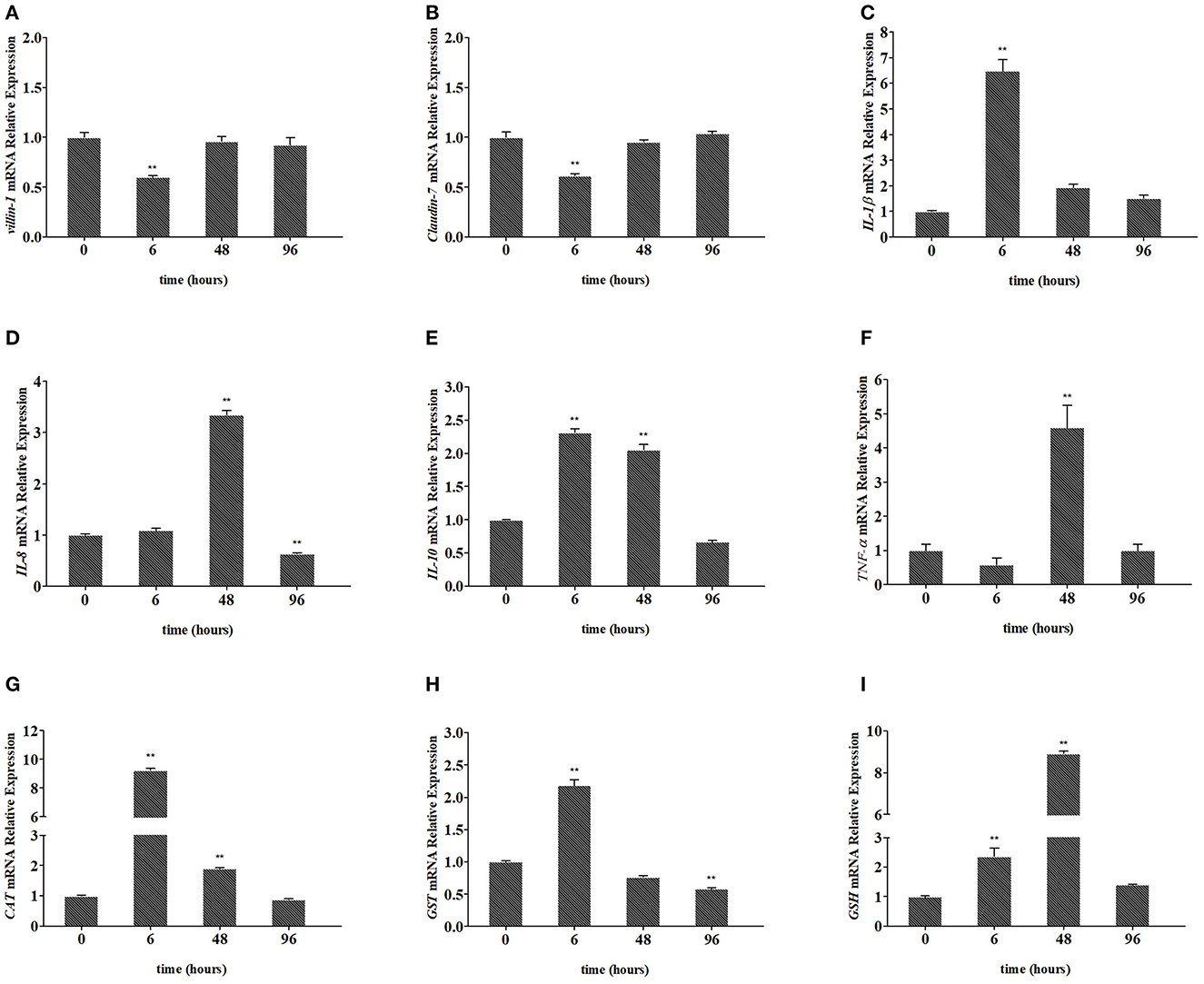
Figure 3. Relative expression of villin-1 (A), Claudin-7 (B), IL-1β (C), IL-8 (D), IL-10 (E), TNF-α (F), CAT (G), GST (H), and GSH (I) in the intestines of crucian carp. The mRNA levels of each gene were normalized to that of β-actin. The bar plot denotes means ± SD of three replications, and the asterisk represents a statistically significant difference (*0.01 < P < 0.05 and **P < 0.01).
3.3. Microbiota signature of the intestine of crucian carp
A total of 792,426 clean reads were obtained from the four groups, with valid sequences ranging from 21,391 to 152,300. Cluster analysis of the crucian carp's intestinal microbiota was divided into 592 OTUs, excluding monad sequences. There were 389 OTUs in the J 0 h group, 327 OTUs in the J 6 h group, 284 OTUs in the J 48 h group, and 453 OTUs in the J 96 h group, and 307 OTUs coexisted in the four groups (Supplementary Figure S1). The rarefaction curves of each sample tend to be saturated (Supplementary Figure S2). The good coverage (more than 99%) of sequencing and alpha diversity of microbiota are shown in Table 2. The trend of ACE and Chao1 was increased at 96 h (Supplementary Figures S3A, B), while Shannon and Simpson indices were decreased at 48 h after Pb exposure (Supplementary Figures S3C, D).
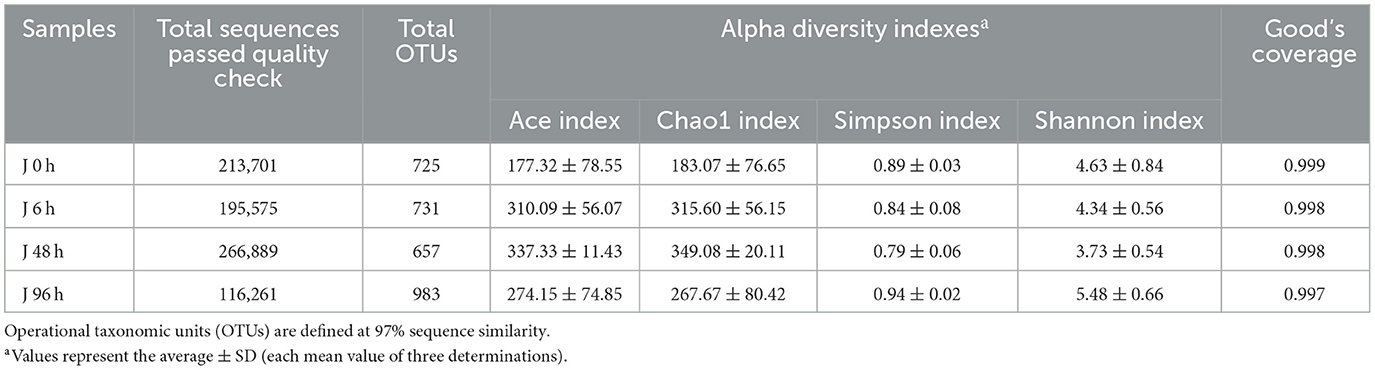
Table 2. Alpha diversity (diversity and richness) indexes as calculated by MOTHUR software (ver. 1.30.0).
3.4. Composition analysis of intestinal microbiota
At the phylum level, 19 bacterial phyla were identified in the intestine of crucian carp, and the relative abundance of (More than 5% in at least one sample) Proteobacteria, Cyanobacteria, Firmicutes, and Bacteroidetes were 22.80–45.95%, 2.46–10.82%, 16.56–34.08%, and 10.05–47.78%, respectively. The relative abundance of Firmicutes (34.08%), Proteobacteria (45.95%), Bacteroidete (47.78%), and Bacteroidete (31.77%) were the highest in the J 0 h group, J 6 h group, J 48 h group, and J 96 h group, respectively (Supplementary Figure S4). At the family level, 29 bacteria were classified by the community barplot analysis (Figure 4A), among which four main bacteria were selected for further analysis and they were Erysipelotrichaceae, Weeksellaceae, Vibrionaceae, and Flavobacteriaceae. The relative abundance of Erysipelotrichaceae was significantly decreased to the lowest level in the J 48 h group (down to 0.52-fold, P < 0.01; Figure 4B), while the abundance of Vibrionaceae, Weeksellaceae, and Flavobacteriaceae was significantly increased to the highest level in the J 6 h group (up to 2.29-fold, P < 0.01), J 48 h group (up to 353.88-fold, P < 0.01), and J 96 h group (up to 3.50-fold, P < 0.01), respectively (Figures 4C–E).
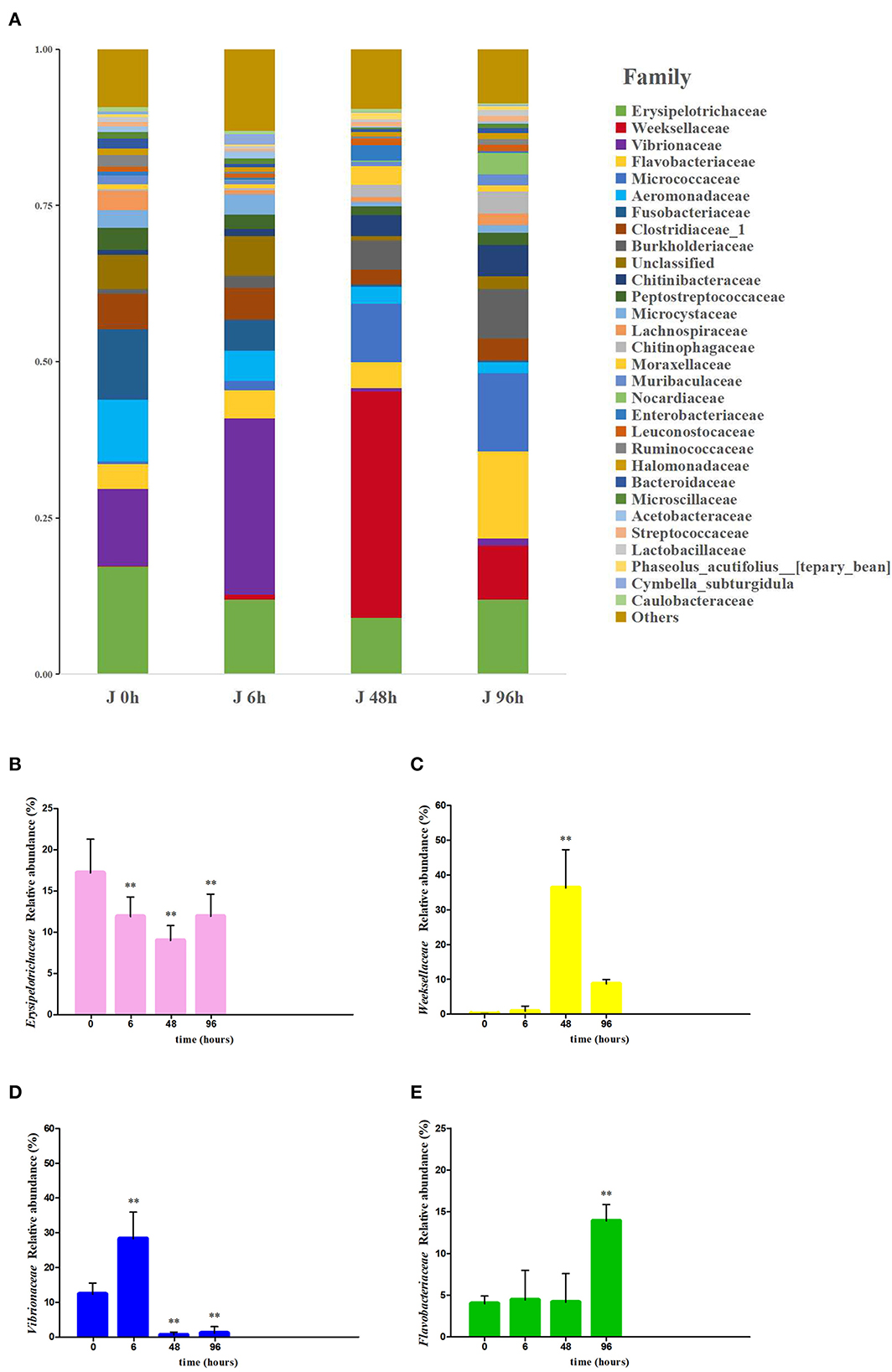
Figure 4. Effects of Pb exposure on the composition and relative abundance of microbiota crucian carp's intestines at the family level (A). The relative abundance of Erysipelotrichaceae (B), Weeksellaceae (C), Vibrionaceae (D), and Flavobacteriaceae (E) was measured. The asterisk represents a statistically significant difference (*0.01 < P < 0.05 and **P < 0.01).
3.5. Analysis of significantly different intestinal microbiota deviation values
Metastats analysis was used to screen the bacteria with significant differences in abundance among each group of crucial carp intestine, and the Integrated Biomarker Response (IBR) index and deviation index of the main difference bacteria at 0 h, 6 h, 48 h, and 96 h were calculated (Figure 5). Compared with 0 h, the IBR indexes for 6 h, 48 h, and 96 h were 4.80, 16.07, and 31.11, respectively; the deviation values of Aeromonas, Bacteroides, and Lachnospiraceae_NK4A136_group were decreased at 6 h, the deviation values of Aeromonas, Clostridium_sensu_stricto_1, and Microcystis_PCC-7914 were decreased at 48 h, and the deviation values of Aeromonas were decreased at 96 h. Instead, the deviation values of Chryseobacterium and Paenarthrobacter were increased at 6 h. The deviation values of Chryseobacterium and Paucibacter were increased at 48 h, and the deviation values of Deefgea, Paenarthrobacter, Paucibacter, and Sediminibacterium were increased at 96 h.
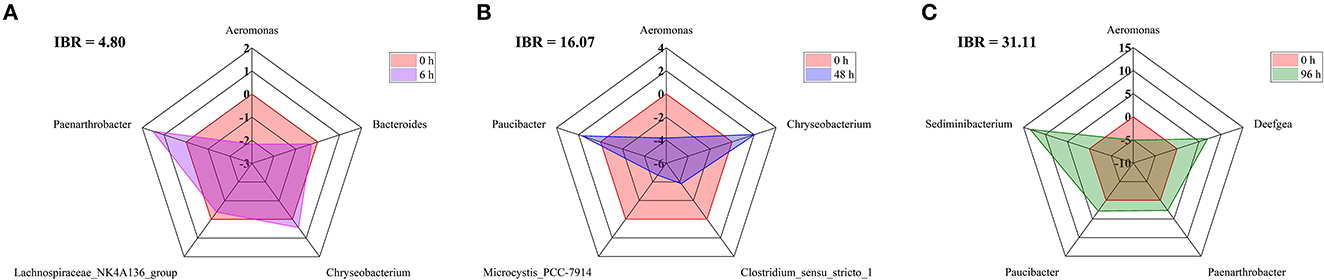
Figure 5. IBR index, Biomarker bias index, and associated star plots of the main difference bacteria at the genus level between 0 h, 6 h (A), 48 h (B), and 96 h (C) in the intestine of crucian carp.
3.6. Intestinal microbiota network analysis
According to the correlation network analysis of the abundance of OTUs, most OTUs of Proteobacteria were negatively correlated with Firmicutes, while most OTUs of Proteobacteria were positively correlated with Bacteroidetes (Figure 6). The most abundant taxa were OTU5 and OTU1 (Chryseobacterium soldanellicola). Among them, OTU1 (belonging to Weeksellaceae) was positively correlated with OTU10 (belonging to Chitinibacteraceae), OTU27 (belonging to Chitinibacteraceae), and OTU264 (belonging to Flavobacteriaceae). Meanwhile, OTU5 (belonging to Vibrionaceae) was positively correlated with OTU9 (belonging to Flavobacteriaceae), OTU157 (belonging to Burkholderiaceae), and OTU146 (belonging to Burkholderiaceae).
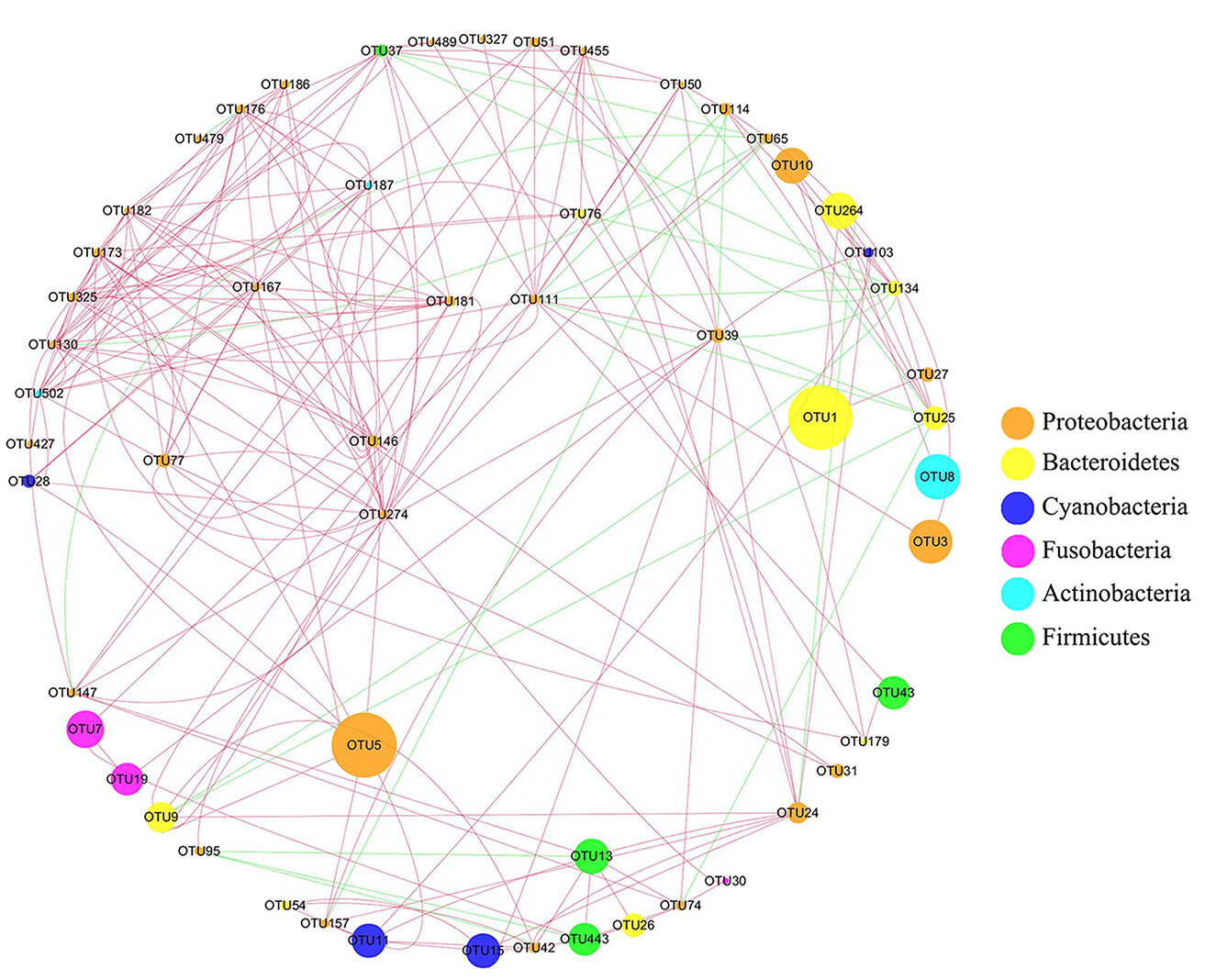
Figure 6. Network analysis of intestinal microbiota in crucian carp after Pb exposure. The circular nodes represent the species taxonomy of OTUs, and the size represents the abundance of OTUs. The lines indicate correlation, red indicates positive correlation, and green indicates negative correlation.
3.7. PICRUST analysis of intestinal microbiota in crucian carp
Based on the pathway comparison analysis of Kyoto Encyclopedia of Genes and Genomes (KEGG), the influence of intestinal microbiota change on the intestinal function of crucian carp were predicted. Level 1 functions were divided into six categories, among which the metabolism was the most abundant (Figure 7A). At the level 2, membrane transport and the immune system function were significantly changed after Pb exposure (Supplementary Table S1). Similarly, the peroxisome and PPAR signaling pathway function were significantly changed at level 3 (Supplementary Table S2). Functional changes in membrane transport, immune system, peroxisome, and PPAR signaling pathways are shown in Figures 7B–E, respectively. After Pb exposure, the relative abundance of peroxisome and PPAR signaling pathway functions in the intestine increased significantly, while membrane transport and immune system decreased significantly. The relative abundance levels of membrane transport function and immune system function were the lowest in the J 48 h group (down to 0.71-fold, P < 0.01) and J 6 h group (down to 0.79-fold, P < 0.05), respectively. Conversely, the relative abundance levels of peroxisome function and PPAR signaling pathway function were highest in the J 48 h group (up to 1.78-fold, P < 0.01) and J 96 h group (up to 1.65-fold, P < 0.01), respectively.
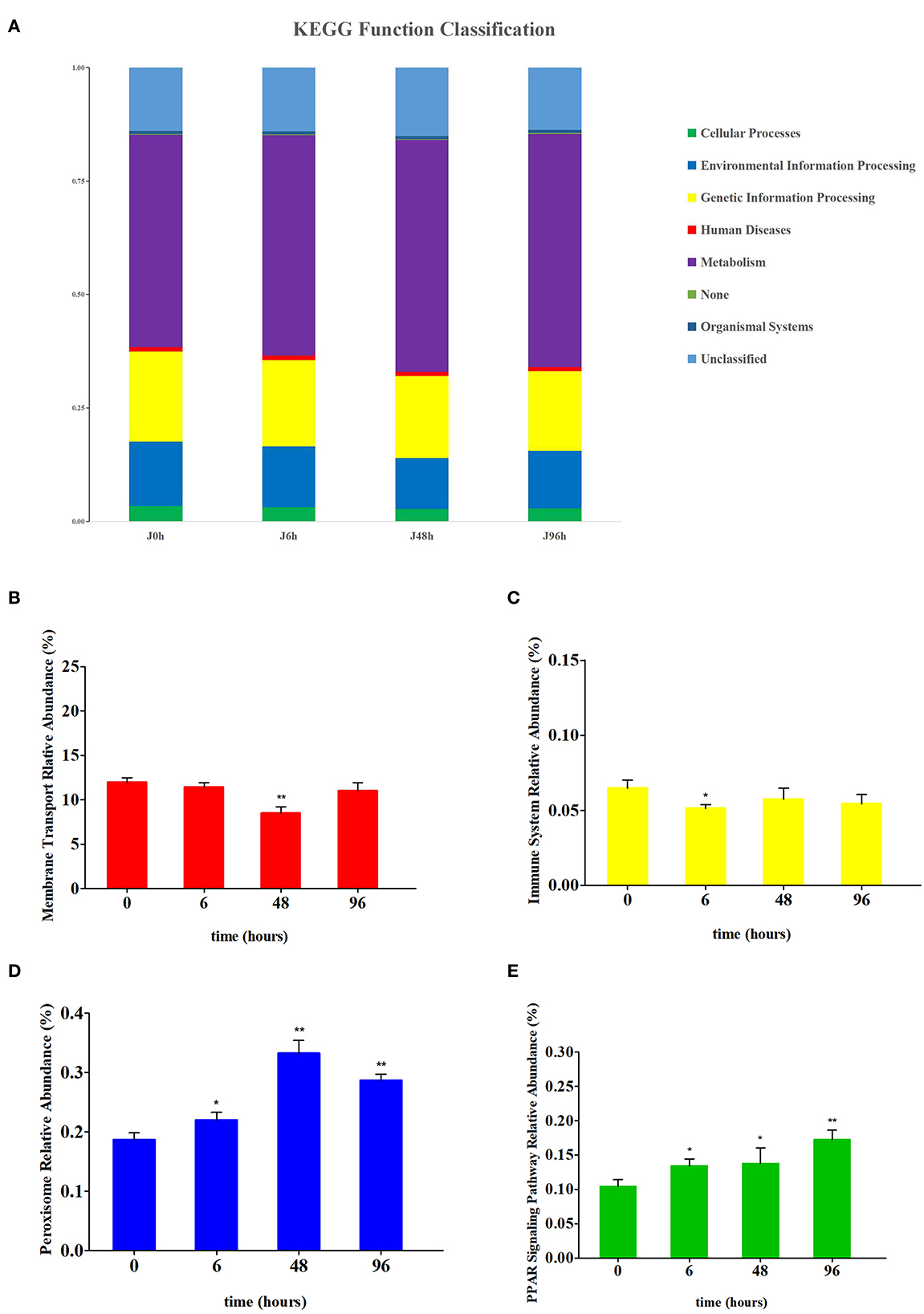
Figure 7. Comparison of the relative abundance of PICRUSt-generated function profile of crucian carp gut in each group. (A) The KEGG function classification at level 1 of each group. (B) Relative abundance changes of membrane transport in the intestines of crucian carp. (C) Relative abundance changes of the immune system in the intestines of crucian carp. (D) Relative abundance changes of peroxisome in the intestines of crucian carp. (E) Relative abundance changes of PPAR signaling pathway in the intestines of crucian carp. Asterisk represents a statistically significant difference (*0.01 < P < 0.05 and **P < 0.01).
3.8. CCA analysis of intestinal microbial community, Pb content, and intestinal physicochemical factors
CCA analysis showed that the microbial community was closely related to intestinal Pb content, antioxidant factors (CAT, GST, and GSH), and immune factors (IL-10, IL-8, and TNF- a; Figure 8). After Pb exposure, the intestinal microbiota structure in the J 0 h and J 6 h groups showed high similarity and short distance between each other. The changes in the intestinal microbial community in the J 48 h and J 96 h groups were particular, which with large distances from the J 0 h group. Among them, the intestinal microbial changes in the J 48 h group were positively correlated with goblet cell number, intestinal wall, GSH, IL-8, and TNF-a, while the intestinal microbial structure in the J 96 h group was positively correlated with Pb content.
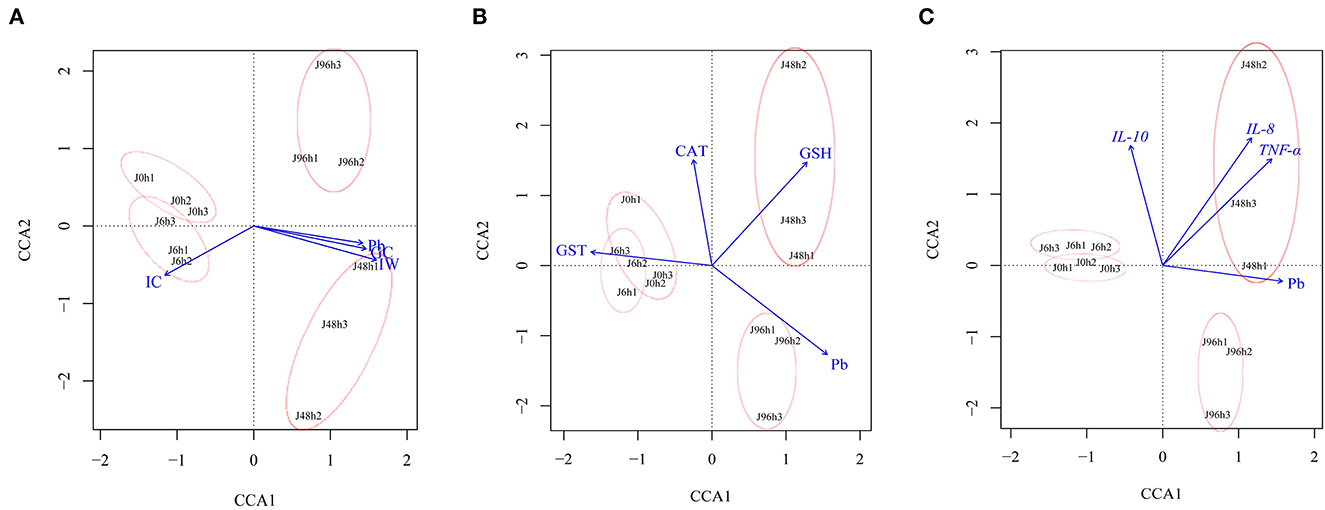
Figure 8. Canonical correlation analysis (CCA) between intestinal microbial community structure of crucian carp and (A) intestinal structure (IC, Intestine crypt relative depth; GC, Goblet cells relative number; IW, Intestinal wall relative thickness), (B) antioxidant factor (CAT, GST, GSH), (C) immune factors (IL-8, IL-10, TNF-α), and Pb content.
4. Discussion
Pb is widely present in the atmosphere, soil, and water and is a major pollutant in environmental monitoring and pollution control, with high toxicity to environmental organisms (Malik and Ahmad, 2002; Cruz et al., 2006; Melanie et al., 2015; Behera et al., 2021; Rout et al., 2022b). It can change the body function by breaking the intestinal wall barrier, destroying the intestinal structure, and changing the expression levels of some important genes in fish (Xia et al., 2018; Shi et al., 2020). Therefore, it is necessary to establish an effective toxicological evaluation of Pb. In this study, the effects of Pb on the morphological structure, antioxidant capacity, and immune capacity of the intestine of crucian carp under the SC were analyzed. It was found that Pb was enriched in the intestine of crucian carp and changed the morphological structure, antioxidant factors, and immune factors of the intestine. To further understand the effect of Pb on the intestinal microbial community of crucian carp, we used high-throughput sequencing technology to detect the composition of the intestinal microbial community of crucian carp. We found that there were significant correlations between the changes in the intestinal microbial community and intestinal structure indicators, antioxidant factors, and immune factors, indicating that the fish intestinal microbiota can be used as an important reference index for water pollution evaluation.
Intestine is an important organ for fish digestion, metabolism, endocrine regulation, and immunity (Buddington et al., 1997). Due to its communication with the external water environment, the intestine is one of the first organs to be affected by environmental pollutants, which is also one of the main organs for pollutants to accumulate (Yin et al., 2020). Therefore, the state of the intestine is often used as a good indicator for evaluating water quality and is a suitable model for studying the effects of environmental pollution (Ted et al., 2003; Karami et al., 2012; Li et al., 2014). Previous studies have shown that Pb accumulation can induce oxidative stress in the intestine of aquatic organisms (Duan et al., 2021). CAT, GST, and GSH play important roles in protecting tissues from oxidative stress. In multiple research studies, it was found that CAT plays an important role in the oxidative stress pathway against heavy metals, GST is a key factor in protecting organisms from oxidative stress, and GSH is involved in metal detoxification in animals (Narayanan et al., 2018; Yin et al., 2020). Therefore, CAT, GST, and GSH are generally considered as biomarkers of oxidative stress induced by exogenous exposure to aquatic organisms (Elia et al., 2003; Yin et al., 2020). In this study, the expression levels of CAT, GST, and GSH genes in the intestinal tissue increased, which further indicated that oxidative stress occurred in the intestine after Pb exposure. In this study, Duan's research suggested that Pb exposure will cause the occurrence of intestinal inflammation in fish, which was confirmed by the mRNA expression changes in immune-related genes (TNF-α, IL-1β, IL-8, and IL-10) (Duan et al., 2021). The occurrence of inflammation will lead to histopathological changes in the fish gut, such as enlarged intestinal wall, increased goblet cell numbers, and leukocyte infiltration (Liu et al., 2020). In this research, we observed intestinal histopathological changes in crucian carp, including enlargement of the intestinal wall, reduction of intestinal crypt length, increase in the number of goblet cells, and infiltration of leukocytes, which further promote the deepening of inflammation (Lofgren et al., 2002; Qiao et al., 2019; Liu et al., 2020).
Intestinal microbiota is an intricate and unique microbial community in the body, which is closely related to the body's metabolism, immunity, and behavior (Premysl et al., 2011; Wang et al., 2019; Zhang et al., 2020). Decreased microbial diversity and altered composition are considered to be the indicators for evaluating the occurrence of intestinal diseases (Xiong et al., 2015; Liu et al., 2020). This study found that Pb exposure reduced the intestinal microbiota diversity of crucian carp. Decreased microbial diversity may cause disorders such as intestinal microbial community and immune function, causing oxidative stress and leading to the occurrence of diseases (Goyette et al., 2007; Ganz et al., 2011; Kuno et al., 2016; Passos and Moraes, 2017). Therefore, the pathological response of lead exposure in the intestine of crucian carp may be related to the decrease in microbial diversity.
Waterborne lead significantly alters intestinal microbiota composition and abundance of fish. At the phylum level, Proteobacteria and Bacteroidetes became the most abundant bacteria in the intestine after Pb exposure implying that the stability of intestinal microbiota was influenced by Pb, which might affect the immune function, antioxidant function, and induce inflammation of crucian carp (Supplementary Figure S4). Feris and Kasemodel's research studies reported that gram-negative bacteria Proteobacteria are resistant to heavy metals, so there is no significant change in Pb-polluted areas (Feris et al., 2003; Kasemodel et al., 2019). Combined with previous research, Proteobacteria have a better heavy metal tolerance, so in the early stage, it becomes the dominant bacteria in the intestine after Pb stress (Hall and Meyer, 1998; Ancion et al., 2010). Bacteroidetes, the abundance of which increased significantly in the later stages of Pb exposure, are closely related to the induction of intestinal diseases (Zitomersky et al., 2013). Meanwhile, the increased abundance of Bacteroidetes can promote the occurrence of intestinal inflammation, which was further confirmed by the expression levels of immune genes in this study (Marchesi et al., 2016). Interestingly, intestinal microbiota network analysis found that the most abundant bacteria, OTU1 (belonging to Bacteroidetes), were positively correlated with OTU10 and OTU27 (belonging to Proteobacteria; Figure 6). These results imply that the increase in the abundance of Proteobacteria in the early stage may promote the increase in the abundance of Bacteroides in the later stage, after Pb exposure. On the contrary, Firmicutes, the dominant gram-positive bacteria present in the intestine of crucian carp, were significantly decreased after Pb exposure. Bacteria exhibited differential responses to Pb exposure, possibly because the cell membranes of gram-positive bacteria had highly electronegative chemicals that could bind heavy metal ions and destroy the function of bacterial membranes (Hyyryläinen et al., 2000; Huang et al., 2002; Lemire et al., 2013).
The family Vibrionaceae, belonging to the phylum Proteobacteria, which is a major pathogen that can cause severe economic loss in fish farming, was significantly increased in abundance under the influence of Pb (Sonia et al., 2015)., belonging to the phylum Bacteroidetes, which has been reported as a main fish pathogen in multiple salmon farms, widely recognized as one of the main sources of disease and economic loss of aquaculture (Nicolas et al., 2008). More than that, members of Bacteroides and Weeksellaceae have been found to promote inflammation and disrupt the formation of intestinal villi in fish (Liu et al., 2020). In this study, the changes in intestinal morphology may be related to the upregulation of the abundance of Weeksellaceae. On the contrary, Erysipelotrichaceae, which belongs to the phylum Tenericutes, had decreased after Pb exposure. Previous studies have found that the decline in the expression of Erysipelotrichaceae is significantly related to intestinal irritable bowel syndrome (Yang et al., 2016). Hence, the increase in the expression of Weeksellaceae, Vibrionaceae, and Flavobacteriaceae and the decrease in the expression of Erysipelotrichaceae might provide opportunities for the disease in the intestine of fish (Figure 4).
After Pb exposure, the deviated abundance value of the genus Aeromonas was reduced (Figure 5). Previous studies suggested that Aeromonas was resistant to heavy metals, such as Cd, Cu, and Cr (Kim et al., 2014). However, the deviation value of Aeromonas, in this study, was reduced, possibly because Aeromonas did not have Pb resistance. Conversely, the dominant levels of Sediminibacterium and Chryseobacterium increased significantly after Pb exposure. Sediminibacterium belongs to Bacteroidetes, and Chryseobacterium belongs to Flavobacteria, both are common pathogenic bacteria that cause inflammation in the intestines of fish and lead to fish death (Qu and Yuan, 2008; Satcolu et al., 2021). In addition, with the accumulation of Pb exposure time, the IBR index of the major bacteria at the genus level of the intestine of crucian carp increased, which reflects a gradual increase in the impact on the main bacteria in the intestine with the accumulation of Pb, further proved that the intestinal microbial community disorder of crucian carp caused by Pb was difficult to recover (Sanchez et al., 2013).
It was found that alterations in the intestinal microbiota would affect the intestinal structure and function of fish (Geovanny and Jose, 2010; Navarrete et al., 2013; Gao et al., 2017). As expected, the results of canonical correlation analysis indicated that changes in intestinal structure indices, as well as increased expression of immune factors in fish, were closely related to the changes in intestinal microbial communities (Figure 8). Surprisingly, this study also found that there is a correlation between antioxidant factors and intestinal microbiota, possibly because the imbalance of the intestinal microenvironment can promote intestinal oxidative stress. Crucian carp is an omnivorous fish and contains microorganisms in its diet (Li et al., 2013). Previous studies have also found that microorganisms can improve the physiological functions of crucian carp (Li Y. N. et al., 2015). Thus, the changes in the structure and function of the intestine may be closely related to intestinal microorganisms. The results of our study indicated that changes in intestinal microbiota will cause oxidative stress and affect the structure and immune function of the intestine. This was also confirmed by the functional changes (membrane transport, immune system, and peroxisome) of PICRUST analysis (Figure 7). In summary, when Pb pollution in the water environment causes structural changes and imbalances in the intestinal microbiota of crucian carp, the structure and function of the crucian carp will be affected.
5. Conclusion
In this study, we report the effects of Pb pollution on the intestine of crucian carp based on bioaccumulation, histopathology, gene expression, and high-throughput analysis for a comprehensive toxicological assessment of lead pollution in the aquatic environment. The results of this study indicate that waterborne Pb exposure cause structural damage, oxidative stress, and immune responses in the intestine of crucian carp. It is worth mentioning that after lead exposure, the changes in the abovementioned intestinal indicators may be closely related to intestinal microbiota. We found that the intestinal microbiota are sensitive to Pb pollution and altered the composition of the microbiota, increasing the abundance of pathogenic bacteria at an early stage, leading to intestinal disorders, and further aggravating the effect of Pb on the structure and function of the damaged intestine. In conclusion, our study provides the first report of comprehensively toxicological effects of Pb pollution on crucian carp and finds the relationship between the gut microbiota, morphology, antioxidant factors, and immune factors of crucian carp. The results of this study provide a basis for the link between gut microbiome changes and heavy metal toxicity, and gut microbiota can be used as biomarkers for the evaluation of heavy metal pollution.
Data availability statement
The datasets presented in this study can be found in online repositories. The names of the repository/repositories and accession number(s) can be found below: NCBI- PRJNA984177.
Ethics statement
All animal experimental procedures were carried out in accordance with the Regulations for Animal Experimentation of South China Normal University (SCNU-SLS-2020-001), and the animal facility was based on the National Institutes of Health guide for the care and use of Laboratory Animals (NIH Publications No. 8023). The study was conducted in accordance with the local legislation and institutional requirements.
Author contributions
HL: writing—reviewing and editing, investigation, and visualization. HZ: investigation and writing—reviewing and editing. QY, SZ, and FZ: writing—reviewing and editing and resources. XT: writing—reviewing and editing. SF: conceptualization, writing—reviewing and editing, investigation, and resources. All authors contributed to the article and approved the submitted version.
Funding
This project was supported by the Guangzhou Science and Technology Program Key projects (201804020058), Monitoring project of groundwater and important lakes along the main stream of the Yangtze River in Hubei Province (420000-2022-218-006-003), and the Natural Science Foundation of Guangdong Province, China (2020A1515111121 and 2022A1515011033).
Conflict of interest
The authors declare that the research was conducted in the absence of any commercial or financial relationships that could be construed as a potential conflict of interest.
Publisher's note
All claims expressed in this article are solely those of the authors and do not necessarily represent those of their affiliated organizations, or those of the publisher, the editors and the reviewers. Any product that may be evaluated in this article, or claim that may be made by its manufacturer, is not guaranteed or endorsed by the publisher.
Supplementary material
The Supplementary Material for this article can be found online at: https://www.frontiersin.org/articles/10.3389/fmicb.2023.1239323/full#supplementary-material
References
Ancion, P. Y., Lear, G., and Lewis, G. D. (2010). Three common metal contaminants of urban runoff (Zn, Cu and Pb) accumulate in freshwater biofilm and modify embedded bacterial communities. Environ. Pollut. 158, 2738–2745. doi: 10.1016/j.envpol.2010.04.013
Behera, B. K., Chakraborty, H. J., Patra, B., Rout, A. K., Dehury, B., Das, B. K., et al. (2020). Metagenomic analysis reveals bacterial and fungal diversity and their bioremediation potential from sediments of river Ganga and Yamuna in India. Front. Microbiol. 11, 556136. doi: 10.3389/fmicb.2020.556136
Behera, B. K., Dehury, B., Rout, A. K., Patra, B., Mantri, N., Chakraborty, H. J., et al. (2021). Metagenomics study in aquatic resource management: recent trends, applied methodologies and future needs. Gene Rep. 25, 101372. doi: 10.1016/j.genrep.2021.101372
Behera, B. K., Patra, B., Chakraborty, H. J., Rout, A. K., Dixit, S., Rai, A., et al. (2023). Bacteriophages diversity in India's major river Ganga: a repository to regulate pathogenic bacteria in the aquatic environment. Environ. Sci. Pollut. R. 30, 34101–34114. doi: 10.1007/s11356-022-24637-7
Buddington, R. K., Krogdahl, A., and Bakke, M. A. M. (1997). The intestines of carnivorous fish: structure and functions and the relations with diet. Acta Physiol. Scand. Suppl. 638, 67–80.
Candela, M., Biagi, E., Maccaferri, S., Turroni, S., and Brigidi, P. (2012). (2012). Intestinal microbiota is a plastic factor responding to environmental changes. Trends Microbiol. 20, 385–391. doi: 10.1016/j.tim.2012.05.003
Carlos, A. G. J., Christos, C. I., and Martin, J. G. (2022). Competitive dominance and broad environmental tolerance favor invasive success of Nile tilapia. Hydrobiologia 849, 1161–1176. doi: 10.1007/s10750-021-04778-5
Chen, M., Boyle, E. A., Switzer, A. D., and Gouramanis, C. (2016). A century long sedimentary record of anthropogenic lead (Pb), Pb isotopes and other trace metals in Singapore. Environ. Pollut. 213, 446–459. doi: 10.1016/j.envpol.2016.02.040
Chen, Z., Xu, J., Yang, F., Hou, Z., Ren, K., Yu, L., et al. (2023). Comprehensive monitoring and ecological risk assessment of heavy metals in soil and surface water of Chishui River basin in upper reaches of the Yangtze River. Water 15, 2069. doi: 10.3390/w15112069
Cruz, G. M., Celis, R., Hermosín, M. C., Koskinen, W. C., Nater, E. A., Cornejo, J., et al. (2006). Heavy metal adsorption by montmorillonites modified with natural organic cations. Soil Sci. Soc. Am. J. 70, 215–221. doi: 10.2136/sssaj2005.0131
Daga, P., Feijoo, G., Moreira, M. T., Costas, D., Villanueva, A. G., Lema, J. M., et al. (2013). Bioencapsulated probiotics increased survival, growth and improved gut flora of turbot (Psetta maxima) larvae. Aquacult. Int. 21, 337–345. doi: 10.1007/s10499-012-9556-y
Dixit, S., Gaur, M., Subudhi, E., Sahoo, R. K., Dey, S., Mahapatra, L. D., et al. (2021). Bacterial diversity and cazyme potential revealed in pandanus rich thermal spring cluster of India: a non-cultivable 16S rRNA sequencing approach. Front. Microbiol. 12, 760573. doi: 10.3389/fmicb.2021.760573
Dong, S., and Li, D. (1994). Comparative studies on the feeding capacity of silver carp and bighead carp. Chin. J. Oceanol. Limnol. 12, 185–190. doi: 10.1007/BF02850518
Duan, Y., Wang, Y., Huang, J., Li, H., Dong, H., Zhang, J., et al. (2021). Toxic effects of cadmium and lead exposure on intestinal histology, oxidative stress response, and microbial community of Pacific white shrimp Litopenaeus vannamei. Mar. Pollut. Bull. 167, 112220. doi: 10.1016/j.marpolbul.2021.112220
Elia, A. C., Galarini, R., Taticchi, M. I., Dorr, A. J. M., and Mantilacci, L. (2003). Antioxidant responses and bioaccumulation in Ictalurus melas under mercury exposure. Ecotoxicol. Environ. Saf. 55, 162–167. doi: 10.1016/S0147-6513(02)00123-9
Feris, K., Ramsey, P., Frazar, C., Moore, J. N., Gannon, J. E., Holben, W. E., et al. (2003). Differences in hyporheic-zone microbial community structure along a heavy-metal contamination gradient. Appl. Environ. Microb. 69, 5563–73. doi: 10.1128/AEM.69.9.5563-5573.2003
Francesco, F., Giuseppe, P., Katia, T., Vincenzo, F., Giuseppe, G., Francesca, A., et al. (2014). Bioaccumulation of heavy metals in blood and tissue of striped mullet in two Italian lakes. J. Aquat. Anim. Health 26, 278–284. doi: 10.1080/08997659.2014.938872
Fu, S., Ding, M., Liang, Q., Yang, Y., Chen, M., Wei, X., et al. (2019). The key differentially expressed genes and proteins related to immune response in the spleen of pufferfish (Takifugu obscurus) infected by Aeromonas hydrophila. Fish Shellfish Immunol. 91, 1–11. doi: 10.1016/j.fsi.2019.05.016
Fu, S., Ding, M., Yang, Y., Kong, J., Li, Y., Guo, Z., et al. (2018). Molecular cloning, characterization and expression analysis of caspase-6 in puffer fish (Takifugu obscurus). Aquaculture 490, 311–320. doi: 10.1016/j.aquaculture.2018.03.008
Ganz, M., Csak, T., Nath, B., and Szabo, G. (2011). Lipopolysaccharide induces and activates the Nalp3 inflammasome in the liver. World J. Gastroenterol. 17, 4772–4778. doi: 10.3748/wjg.v17.i43.4772
Gao, X., Xie, Q., Liu, L., Kong, P., Sheng, J., Xiang, H., et al. (2017). Metabolic adaptation to the aqueous leaf extract of Moringa oleifera Lam.-supplemented diet is related to the modulation of gut microbiota in mice. Appl. Microbiol. Biotechnol. 101, 5115–5130. doi: 10.1007/s00253-017-8233-5
Geovanny, D. G., and Jose, L. B. (2010). A review on the interactions between gut microbiota and innate immunity of fish. Fems. Immunol. Med. Mic. 52, 145–154. doi: 10.1111/j.1574-695X.2007.00343.x
Goyette, P., Labbé, C., Trinh, T. T., Xavier, R. J., and Rioux, J. D. (2007). Molecular pathogenesis of inflammatory bowel disease: genotypes, phenotypes and personalized medicine. Ann. Med. 39, 177–199. doi: 10.1080/07853890701197615
Guo, J., Pu, Y., Zhong, L., Wang, K., Duan, X., Chen, D., et al. (2021). Lead impaired immune function and tissue integrity in yellow catfish (Peltobargus fulvidraco) by mediating oxidative stress, inflammatory response and apoptosis. Ecotox. Environ. Safe. 226, 112857. doi: 10.1016/j.ecoenv.2021.112857
Hall, R. O., and Meyer, J. L. (1998). The trophic significance of bacteria in a detritus-based stream food web. Ecology 79, 1995–2012. doi: 10.1890/0012-9658(1998)079[1995:TTSOBI]2.0.CO;2
Huang, C., Wu, P., Jiang, W. D., Liu, Y., Zeng, Y. Y., Jiang, J., et al. (2018). Deoxynivalenol decreased the growth performance and impaired intestinal physical barrier in juvenile grass carp (Ctenopharyngodon idella). Fish Shellfish Immun. 80, 376–391. doi: 10.1016/j.fsi.2018.06.013
Huang, Q. R., Danek, T., Cheng, X. F., Dong, T., Qi, W. F., Zou, L. L., et al. (2016). Distribution and pollution characteristics analysis of heavy metals in surface sediment in Bi river. IOP Conf. Series 44, 052065. doi: 10.1088/1755-1315/44/5/052065
Huang, W., Zhang, Z., Han, X., Wang, J., Tang, J., Dong, S., et al. (2002). Concentration-dependent behavior of nisin interaction with supported bilayer lipid membrane. Biophys. Chem. 99, 271–279. doi: 10.1016/S0301-4622(02)00227-2
Hyyryläinen, H. L., Marika, V., Joanne, T., Hongyan, W., Matti, S., Colin, R. H., et al. (2000). Membrane transport structure function and biogenesis: D-alanine substitution of teichoic acids as a modulator of protein folding and stability at the cytoplasmic membrane-cell wall interface of Bacillus subtilis. J. Biol. Chem. 275, 26696–26703. doi: 10.1016/S0021-9258(19)61432-8
Karami, A., Christianus, A., Ishak, Z., Shamsuddin, Z. H., Masoumian, M., and Courtenay, S. C. (2012). Use of intestinal Pseudomonas aeruginosa in fish to detect the environmental pollutant benzo[a]pyrene. J. Hazard. Mater. 215–216, 108–114. doi: 10.1016/j.jhazmat.2012.02.038
Kasemodel, M. C., Sakamoto, I. K., Varesche, M. B. A., and Rodrigues, V. G. S. (2019). Potentially toxic metal contamination and microbial community analysis in an abandoned Pb and Zn mining waste deposit. Sci. Total Environ. 675, 367–379. doi: 10.1016/j.scitotenv.2019.04.223
Kim, D. C., You, M. J., Kim, B. S., Kim, W. I., Yi, S. W., Gee, W. S., et al. (2014). Antibiotic and heavy-metal resistance in motile Aeromonas strains isolated from fish. Afr. J. Microbiol. Res. 8, 1793–1797. doi: 10.5897/AJMR2013.6339
Kristine, C. V. B., Jason, D. E., Daniel, C. D., James, M., Iain, M. S., Hayden, T. S., et al. (2022). A global, historical database of tuna, billfish, and saury larval distributions. Sci. Data 9, 423. doi: 10.1038/s41597-022-01528-7
Kumar, E. K., Midhun, S. J., Vysakh, A., and James, T. J. (2021). Antagonistic effects of dietary Moringa oleifera on hemato-biochemical and oxidative stress of lead nitrate intoxicated Nile tilapia, Oreochromis niloticus. Aquac. Res. 52, 6164–6178. doi: 10.1111/are.15478
Kuno, T., Hirayama-Kurogi, M., Ito, S., and Ohtsuki, S. (2016). Effect of intestinal flora on protein expression of drug-metabolizing enzymes and transporters in the liver and kidney of germ-free and antibiotics-treated mice. Mol. Pharm. 13, 2691–2701. doi: 10.1021/acs.molpharmaceut.6b00259
LeaMaster, B., Walsh, W. A., Brock, J. A., and Fujioka, R. S. (1997). Cold stress-induced changes in the aerobic heterotrophic gastrointestinal tract bacterial flora of red hybrid tilapia. J. Fish Biol. 55, 770–780. doi: 10.1111/j.1095-8649.1997.tb01971.x
Lee, J. W., Choi, H., Hwang, U. K., Kang, J. C., Yue, J. K., Kwang, I. K., et al. (2019). Toxic effects of lead exposure on bioaccumulation, oxidative stress, neurotoxicity, and immune responses in fish: a review. Environ. Toxicol. Phar. 68, 101–108. doi: 10.1016/j.etap.2019.03.010
Lemire, J. A., Harrison, J. J., and Turner, R. J. (2013). Antimicrobial activity of metals: mechanisms, molecular targets and applications. Nat. Rev. Microbiol. 11, 371–384. doi: 10.1038/nrmicro3028
Li, L., Xie, P., Lei, H. H., and Zhang, X. Z. (2013). Renal accumulation and effects of intraperitoneal injection of extracted microcystins in omnivorous crucian carp (Carassius auratus). Toxicon 70, 62–69. doi: 10.1016/j.toxicon.2013.03.022
Li, T., Long, M., Gatesoupe, F. J., Zhang, Q. Q., Li, A. H., Gong, X. N., et al. (2015). Comparative analysis of the intestinal bacterial communities in different species of carp by pyrosequencing. Microb. Ecol. 69, 25–36. doi: 10.1007/s00248-014-0480-8
Li, W., Liu, B., Liu, Z., Yin, Y., Xu, G., Han, M., et al. (2021). Effect of dietary histamine on intestinal morphology, inflammatory status, and gut microbiota in yellow catfish (Pelteobagrus fulvidraco). Fish Shellfish Immun. 117, 95–103. doi: 10.1016/j.fsi.2021.07.017
Li, Y. H., Cha, C., Lv, X. J., Liu, J., He, J., Pang, Q., et al. (2020). Association between 10 urinary heavy metal exposure and attention deficit hyperactivity disorder for children. Environ. Sci. Pollut. Res. 27, 31233–31242. doi: 10.1007/s11356-020-09421-9
Li, Y. N., Zhu, H. Y., Kun, W. U., and Lian, J. Y. (2015). Application of a probiotics complex on the culture of Crucian Carp. Hebei Fisheries 2, 9–12. doi: 10.3969/j.issn.1004-6755.2015.02.004
Li, Z. H., Li, P., and Shi, Z. C. (2014). Molecular responses in digestive tract of juvenile common carp after chronic exposure to sublethal tributyltin. Ecotox. Environ. Saf. 109, 10–14. doi: 10.1016/j.ecoenv.2014.07.031
Liang, R. Z., Gu, Y. G., Li, H. S., Han, Y. J., Niu, J., Su, H., et al. (2023). Multi-index assessment of heavy metal contamination in surface sediments of the Pearl River estuary intertidal zone. Mar. Pollut. Bull. 186, 114445. doi: 10.1016/j.marpolbul.2022.114445
Liu, H., Fu, B., Pang, M., Feng, X., Yu, X., Tong, J., et al. (2017). A high-density genetic linkage map and QTL fine mapping for body weight in crucian carp (Carassius auratus) using 2b-RAD sequencing. G3 7, 2473–2487. doi: 10.1534/g3.117.041376
Liu, H. S., Fu, S. L., Zhang, S. S., Ding, M. M., and Wang, A. L. (2020). Lead induces structural damage, microbiota dysbiosis and cell apoptosis in the intestine of juvenile bighead carp (Hypophthalmichthys nobilis). Aquaculture 528, 735573. doi: 10.1016/j.aquaculture.2020.735573
Liu, H. S., Zhang, S. S., Qiu, M., Wang, A. L., Ye, J. M., Fu, S. L., et al. (2021). Garlic (Allium sativum) and Fu-ling (Poria cocos) mitigate lead toxicity by improving antioxidant defense mechanisms and chelating ability in the liver of grass carp (Ctenopharyngodon idella). Ecotoxicology 30, 885–898. doi: 10.1007/s10646-021-02405-6
Lofgren, M., Fagher, K., Wede, O. K., and Arner, A. (2002). Decreased shortening velocity and altered myosin isoforms in guinea-pig hypertrophic intestinal smooth muscle. J. Physiol. 544, 707–714. doi: 10.1113/jphysiol.2002.027060
Malik, A., and Ahmad, M. (2002). Seasonal variation in bacterial flora of the wastewater and soil in the vicinity of industrial area. Environ. Monit. Assess. 73, 263–273. doi: 10.1023/A:1013186218093
Marchesi, J. R., Adams, D. H., Fava, F., Hermes, G. D. A., Hirschfield, G. M., Hold, G., et al. (2016). The gut microbiota and host health: a new clinical frontier. Gut 65, 1–10. doi: 10.1136/gutjnl-2015-309990
Mario, A. B., Caterina, F., Miguel, B., Donají, J. G., and César, A. I. Z. (2022). Ecotoxicological perspectives of microplastic pollution in amphibians. J. Toxicol. Environ. Health B 8, 405–421. doi: 10.1080/10937404.2022.2140372
Mario, A. B., Lillà, L., and Caterina, F. (2019). Multidisciplinary haematology as prognostic device in environmental and xenobiotic stress-induced response in fish. Sci. Total Environ. 670, 1170–1183. doi: 10.1016/j.scitotenv.2019.03.275
Meijerink, N., Kers, J. G., Velkers, F. C., Haarlem, D. A., Lamot, D. M., Oliveira, J. E., et al. (2020). Early life inoculation with adult-derived microbiota accelerates maturation of intestinal microbiota and enhances NK cell activation in broiler chickens. Front. Vet. Sci. 2020, 7. doi: 10.3389/fvets.2020.584561
Melanie, D. L. R. A., Johannys, J. C., Marixa, M. R., Karlo, M. L., and Juan, C. M. W. (2015). Bacteria as potential indicators of heavy metal contamination in a tropical mangrove and the implications on environmental and human health. J. Trop. Life. Science 5, 110–116. doi: 10.11594/jtls.05.03.01
Narayanan, G., Baskaralingam, V., Ravichandran, R., Sekar, V., and Caterina, F. (2018). Bioaccumulation, cytotoxicity and oxidative stress of the acute exposure selenium in Oreochromis mossambicus. Ecotox. Environ. Saf. 162, 147–159. doi: 10.1016/j.ecoenv.2018.06.070
Navarrete, P., Fuentes, P., De la Fuente, L., Barros, L., Magne, F., Opazo, R., et al. (2013). Short-term effects of dietary soybean meal and lactic acid bacteria on the intestinal morphology and microbiota of Atlantic salmon (Salmo salar). Aquacult. Nutr. 19, 827–836. doi: 10.1111/anu.12047
Nicolas, P., Mondot, S., Achaz, G., Catherine, B., Bernardet, J. F., Duchaud, E., et al. (2008). Population structure of the fish-pathogenic bacterium Flavobacterium psychrophilum. Appl. Environ. Microb. 74, 3702–3709. doi: 10.1128/AEM.00244-08
Oost, R. V. D., Beyer, J., and Vermeulen, N. P. E. (2003). Fish bioaccumulation and biomarkers in environmental risk assessment: a review. Environ. Toxicol. Phar. 13, 57–149. doi: 10.1016/S1382-6689(02)00126-6
Parida, P. K., Behera, B. K., Dehury, B., Rout, A. K., Sarkar, D. J., Rai, A., et al. (2022). Community structure and function of microbiomes in polluted stretches of river Yamuna in New Delhi, India, using shotgun metagenomics. Environ. Sci. Pollut. R. 29, 71311–71325. doi: 10.1007/s11356-022-20766-1
Passos, M. D. C. F., and Moraes, F. J. P. (2017). Intestinal microbiota in digestive diseases. Arq. Gastroenterol. 54, 3. doi: 10.1590/s0004-2803.201700000-31
Pirsaheb, M., Azadi, N. A., Miglietta, M. L., Sayadi, M. H., Blahova, J., Fathi, M., et al. (2019). Toxicological effects of transition metal-doped titanium dioxide nanoparticles on goldfish (Carassius auratus) and common carp (Cyprinus carpio). Chemosphere 215, 904–915. doi: 10.1016/j.chemosphere.2018.10.111
Premysl, B., Emmanuel, D., Josh, C., Wendy, J., Jun, L., Jennifer, J., et al. (2011). The intestinal microbiota affects central levels of brain-derived neurotropic factor and behavior in mice. Gastroenterology 141, 599–609. doi: 10.1053/j.gastro.2011.04.052
Qi, X., Tu, X., Huang, A., Huang, A. G., Wang, G. X., Ling, F., et al. (2019). Immunosuppression-induced alterations in fish gut microbiota may increase the susceptibility to pathogens. Fish Shellfish Immun. 88, 540–545. doi: 10.1016/j.fsi.2019.03.035
Qi, Y., Ma, J., and Fan, G. S. (2015). Assessment of heavy metals contamination in fen river channels. Fresenius Environ. Bull. 24, 1990–1995.
Qiao, R., Sheng, C., Lu, Y., Zhang, Y., Ren, H., Lemos, B., et al. (2019). Microplastics induce intestinal inflammation, oxidative stress, and disorders of metabolome and microbiome in zebrafish. Sci. Total. Environ. 662, 246–253. doi: 10.1016/j.scitotenv.2019.01.245
Qu, J. H., and Yuan, H. L. (2008). Sediminibacterium salmoneum gen. nov. sp nov. a member of the phylum Bacteroidetes isolated from sediment of a eutrophic reservoir. Int. J. Syst. Evol. Micr. 58, 2191–2194. doi: 10.1099/ijs.0.65514-0
Radke, R. J., and Kahl, U. (2002). Effects of a filter-feeding fish [silver carp, Hypophthalmichthys molitrix (Val.)] on phyto- and zooplankton in a mesotrophic reservoir: results from an enclosure experiment. Freshwater Biol. 47, 2337–2344. doi: 10.1046/j.1365-2427.2002.00993.x
Rhee, J. S., Jeong, C. B., Kim, D. H., Kim, I. C., Lee, Y. S., Lee, C., et al. (2014). Immune gene discovery in the crucian carp Carassius auratus. Fish Shellfish Immun. 1, 240–251. doi: 10.1016/j.fsi.2013.11.009
Rout, A. K., Dehury, B., Parida, P. K., Sarkar, D. J., Behera, B., Das, B. K., et al. (2022a). Taxonomic profling and functional gene annotation of microbial communities in sediment of river Ganga at Kanpur, India: insights from whole-genome metagenomics study. Environ. Sci. Pollut. R. 29, 82309–82323. doi: 10.1007/s11356-022-21644-6
Rout, A. K., Dixit, S., Dey, S., Parida, P. K., Bhattacharya, M., Pradhan, S. K., et al. (2022b). “Role of modern biotechnology in the era of river water pollution,” in River Health and Ecology in South Asia, eds B. C. Patra, P. K. Shit, G. S. Bhunia, and M. Bhattacharya (Cham: Springer), 63–79. doi: 10.1007/978-3-030-83553-8_4
Sanchez, W., Burgeot, T., and Porcher, J. M. (2013). A novel “Integrated Biomarker Response” calculation based on reference deviation concept. Environ. Sci., Pollut. R. 20, 2721–2725. doi: 10.1007/s11356-012-1359-1
Satcolu, I. B., Duman, M., and Altun, S. (2021). Genome analysis and antimicrobial resistance characteristics of Chryseobacterium aquaticum isolated from farmed salmonids. Aquaculture 535, 736364. doi: 10.1016/j.aquaculture.2021.736364
Shahjahan, M., Taslima, K., Rahman, M. S., Al-Emran, M., Alam, S. I., and Faggio, C. (2022). Effects of heavy metals on fish physiology - a review. Chemosphere 300, 134519. doi: 10.1016/j.chemosphere.2022.134519
Shi, L. X., Wang, N. H., Hu, X. D., Yin, D. C., Wu, C. X., Liang, H. F., et al. (2020). Acute toxic effects of lead (Pb2+) exposure to rare minnow (Gobiocypris rarus) revealed by histopathological examination and transcriptome analysis. Environ. Toxicol. Phar. 78, 103385. doi: 10.1016/j.etap.2020.103385
Shirin, A., Mohammad, R. H., Zahra, M., and Shahram, S. (2015). Lead, cadmium, arsenic and mercury in canned tuna fish marketed in Tehran, Iran. Food Addit. Contam. B 8, 93–98. doi: 10.1080/19393210.2014.993430
Sonia, A. S. R., Gomez-Gil, B., Lozano-Olvera, R., Betancourt-Lozano, M., and Morales, C. M. S. (2015). Field and experimental evidence of Vibrio parahaemolyticus as the causative agent of acute hepatopancreatic necrosis disease of cultured shrimp (Litopenaeus vannamei) in northwestern Mexico. Appl. Environ. Microbiol. 81, 1689–1699. doi: 10.1128/AEM.03610-14
Souza, A. T., Argillier, C., Blabolil, P., Ded, V., Jaric, I., Monteoliva, A. P., et al. (2022). Empirical evidence on the effects of climate on the viability of common carp (Cyprinus carpio) populations in European lakes. Biol. Invasions 24, 1213–1227. doi: 10.1007/s10530-021-02710-5
Tan, X. H., Sun, Z. Z., Zhou, C. P., Huang, Z., Tan, L. J., Xun, P. W., et al. (2018). Effects of dietary dandelion extract on intestinal morphology, antioxidant status, immune function and physical barrier function of juvenile golden pompano Trachinotus ovatus. Fish Shellfish Immun. 73, 197–206. doi: 10.1016/j.fsi.2017.12.020
Ted, C. R., Jeffrey, R. J., and Miles, M. C. (2003). Cyprinid fishes as samplers of benthic diatom communities in freshwater streams of varying water quality. Can. J. Fish. Aquat. Sci. 60, 117–125. doi: 10.1139/f03-004
Wang, W., Cho, H. S., Kim, K., Park, K., and Oh, J. E. (2021).Tissue-specific distribution and bioaccumulation of cyclic and linear siloxanes in South Korean crucian carp (Carassius carassius). Environ. Pollut. 288, 1177899. doi: 10.1016/j.envpol.2021.117789
Wang, X., Shen, M., Zhou, J., and Jin, Y. (2019). Chlorpyrifos disturbs hepatic metabolism associated with oxidative stress and gut microbiota dysbiosis in adult zebrafish. Comp. Biochem. Physiol. C: Pharmacol. Toxicol. Endocrinol. 216, 19–28. doi: 10.1016/j.cbpc.2018.11.010
Xia, J., Lu, L., Jin, C., Wang, S., Zhou, J., Ni, Y., et al. (2018). Effects of short term lead exposure on gut microbiota and hepatic metabolism in adult zebrafish. Comp. Biochem. Physiol. C: Pharmacol. Toxicol. Endocrinol. 209, 1–8. doi: 10.1016/j.cbpc.2018.03.007
Xie, F., Yu, M., Yuan, Q., Meng, Y., Qie, Y., Shang, Z., et al. (2022). Spatial distribution, pollution assessment, and source identification of heavy metals in the Yellow River. J. Hazard. Mater. 436, 129309. doi: 10.1016/j.jhazmat.2022.129309
Xiong, J. B., Wang, K., Wu, J. F., Qiuqian, L. L., Yang, K. J., Qian, Y. X., et al. (2015). Changes in intestinal bacterial communities are closely associated with shrimp disease severity. Appl. Microbiol. Biotechnol. 99, 6911–6919. doi: 10.1007/s00253-015-6632-z
Yang, L. L., Wu, B. B., and Liang, Y. (2016). Effects of plant fermentation extract on intestinal microbiota in patients with irritable bowel syndrome. World Chin. J. Dig. 24, 2982. doi: 10.11569/wcjd.v24.i19.2982
Yin, J. J., Wang, L., Li, S., Chen, F. F., Abeer, M. H., Zhang, X. Z., et al. (2020). Accumulation and depuration of dissolved hexavalent chromium and effects on the antioxidant response in bighead carp (Aristichthys nobilis). Environ. Toxicol. Phar. 80, 103465. doi: 10.1016/j.etap.2020.103465
Yu, Y. Y., Tong, B., Liu, Y., Liu, H., and Yu, H. (2021). Bioaccumulation, histopathological and apoptotic effects of waterborne cadmium in the intestine of crucian carp Carassius auratus gibelio. Aquacult. Rep. 20, 100669. doi: 10.1016/j.aqrep.2021.100669
Yu, Z. W., Xie, Y., Huang, Z. C., Yang, K., Wang, Z. G., Hu, H. L., et al. (2021). Study of the therapeutic effect of raw and processed Vladimiriae Radix on ulcerative colitis based on intestinal flora, metabolomics and tissue distribution analysis. Phytomedicine 85, 153538. doi: 10.1016/j.phymed.2021.153538
Zhang, L., Gui, S., Wang, J., Chen, Q., Zeng, J., Liu, A., et al. (2020). Oral administration of green tea polyphenols (TP) improves ileal injury and intestinal flora disorder in mice with Salmonella typhimurium infection via resisting inflammation, enhancing antioxidant action and preserving tight junction. J. Funct. Foods 64, 103654. doi: 10.1016/j.jff.2019.103654
Zitomersky, N. L., Atkinson, B. J., Franklin, S. W., Mitchell, P. D., Snapper, S. B., Comstock, L. E., et al. (2013). Characterization of adherent Bacteroidales from intestinal biopsies of children and young adults with inflammatory bowel disease. PLoS ONE 8, e63686. doi: 10.1371/journal.pone.0063686
Keywords: Pb, Carassius auratus, oxidative stress, immune response, intestinal microbiota
Citation: Liu H, Zhang H, Yu Q, Zhang S, Tu X, Zhuang F and Fu S (2023) Lead induced structural and functional damage and microbiota dysbiosis in the intestine of crucian carp (Carassius auratus). Front. Microbiol. 14:1239323. doi: 10.3389/fmicb.2023.1239323
Received: 13 June 2023; Accepted: 16 August 2023;
Published: 04 September 2023.
Edited by:
Wei Zhu, Chinese Academy of Sciences, ChinaReviewed by:
Ajaya Kumar Rout, Rani Lakshmi Bai Central Agricultural University, IndiaMario Alberto Burgos-Aceves, Autonomous University of San Luis Potosí, Mexico
Mohamed Hamed, Al Azhar University, Egypt
Copyright © 2023 Liu, Zhang, Yu, Zhang, Tu, Zhuang and Fu. This is an open-access article distributed under the terms of the Creative Commons Attribution License (CC BY). The use, distribution or reproduction in other forums is permitted, provided the original author(s) and the copyright owner(s) are credited and that the original publication in this journal is cited, in accordance with accepted academic practice. No use, distribution or reproduction is permitted which does not comply with these terms.
*Correspondence: Fenghong Zhuang, 375952739@qq.com; Shengli Fu, fushengli@m.scnu.edu.cn