- 1School of Water and Environment, Chang’an University, Xi’an, China
- 2Key Laboratory of Subsurface Hydrology and Ecological Effects in Arid Region, Ministry of Education, Chang’an University, Xi’an, China
- 3Power China Northwest Engineering Corporation Limited, Xi’an, Shaanxi, China
- 4Shaanxi Union Research Center of University and Enterprise for River and Lake Ecosystems Protection and Restoration, Xi’an, Shaanxi, China
The riparian zone is an important location of nitrogen removal in the terrestrial and aquatic ecosystems. Many studies have focused on the nitrogen removal efficiency and one or two nitrogen removal processes in the riparian zone, and less attention has been paid to the interaction of different nitrogen transformation processes and the impact of in situ environmental conditions. The molecular biotechnology, microcosm culture experiments and 15N stable isotope tracing techniques were used in this research at the riparian zone in Weinan section of the Wei River, to reveal the nitrogen removal mechanism of riparian zone with multi-layer lithologic structure. The results showed that the nitrogen removal rate in the riparian zone was 4.14–35.19 μmol·N·kg−1·h−1. Denitrification, dissimilatory reduction to ammonium (DNRA) and anaerobic ammonium oxidation (anammox) jointly achieved the natural attenuation process of nitrogen in the riparian zone, and denitrification was the dominant process (accounting for 59.6%). High dissolved organic nitrogen and nitrate ratio (DOC:NO3−) would promote denitrification, but when the NO3− content was less than 0.06 mg/kg, DNRA would occur in preference to denitrification. Furthermore, the abundances of functional genes (norB, nirS, nrfA) and anammox bacterial 16S rRNA gene showed similar distribution patterns with the corresponding nitrogen transformation rates. Sedimentary NOX−, Fe(II), dissolved organic carbon (DOC) and the nitrogen transformation functional microbial abundance were the main factors affecting nitrogen removal in the riparian zone. Fe (II) promoted NO3− attenuation through nitrate dependent ferrous oxidation process under microbial mediation, and DOC promotes NO3− attenuation through enhancing DNRA effect. The results of this study can be used for the management of the riparian zone and the prevention and control of global nitrogen pollution.
1. Introduction
As a basic component of organisms, nitrogen is also a necessary nutrient element for biological growth (Liu et al., 2019). According to statistics, with the growth of the world’s population and the intensification of agricultural production methods (Basu et al., 2022), the annual amount of nitrogen produced by human activities (about 210 Tg) is 3.6 times that of biological natural nitrogen fixation (Fowler et al., 2013; Zheng et al., 2021). A large amount of anthropogenic active nitrogen is input into terrestrial and marine river systems (Kuypers et al., 2018), which will lead to environmental problems such as eutrophication and acid rain worldwide (Lyu et al., 2021). Although a series of river protection policies have been implemented from local to international (Basu et al., 2022), the nitrate (NO3−) load in the river basin is still high and even increasing (Wurtsbaugh et al., 2019), which would affect water quality and environmental sustainability (Hu et al., 2023). At the same time, excessive NO3−/NO2− will interact with hemoglobin to generate methemoglobin, which will lose the ability to transport oxygen (Canfield et al., 2010) and seriously threaten human health. Therefore, the exploration of the biogeochemical processes and removal mechanisms of excess reactive nitrogen (especially NO3−) in rivers has become the focus of attention in the fields of hydrology and environmental ecology.
Although there have been a lot of comments on the research of nitrogen removal (Sharma and Ahlert, 1977; Bo and Tage, 2002; Lee et al., 2009; Deng et al., 2015; Ro et al., 2018; Sun et al., 2021a), the current understanding of the mechanism of nitrogen removal is far less than the understanding of its production mechanism (Zhao et al., 2021). Previous studies have found that denitrification and anaerobic ammonium oxidation (anammox) can permanently remove NO3−/NO2− in the system in the form of N2 under anaerobic or anoxic conditions (Mulder et al., 1995; Hamersley et al., 2009; Zheng et al., 2021). The dissimilatory nitrate reduction to ammonium (DNRA) reaction, as a further NO3− reduction process, also plays an important role in controlling nitrate conversion (Deng et al., 2015). Different from denitrification and anammox denitrification, DNRA cannot permanently remove nitrogen in the form of N2, but retains the converted nitrogen in the form of ammonia nitrogen (NH4+-N) in the system under anaerobic/anoxic conditions for biological utilization to promote the nitrogen cycle (Broman et al., 2021; Chen et al., 2022). For example, NH4+ can be oxidized to N2 by anammox bacteria (Hu et al., 2019), and can also be converted to NO3− by nitrification driven by ammonia-oxidizing archaea, bacteria and nitrite-oxidizing bacteria to provide substrates for denitrification (Wang et al., 2019).
Recent reports showed that denitrification and DNRA processes also occurred under aerobic conditions (Huang et al., 2020; Wen et al., 2020), and they were two microbial processes that compete for NO3− and dissolved organic carbon (DOC) (Jia et al., 2020), and the dominant position of the two processes was directly affected by environmental conditions. DNRA occurs preferentially at high carbon load (DOC: NO3− > 10) (Chen et al., 2022). The competition and symbiosis mechanism of different nitrogen transformation processes reflects the complexity of the nitrogen cycle process. In addition, some reports have shown that the nitrogen transformation process is controlled by microbial community structure, dissolved oxygen (DO), pH, DOC, iron and nitride content (Ding et al., 2017; Liu et al., 2017, 2022). Among them, DOC is considered to be a key factor limiting nitrogen conversion (Niswonger et al., 2017; Wang L. et al., 2022), and iron is considered to be an important element affecting the removal of nitrate, nitrite and ammonium (Lu et al., 2017; Jamieson et al., 2018). However, the dominant factors under different environmental conditions are different (Chen et al., 2023). Therefore, it is of great significance to fully reveal the mechanism of nitrogen removal and the corresponding environmental control to solve the nitrogen pollution in the basin.
As a transitional zone between river and terrestrial systems, the riparian zone of the river has a significant diversity of species and environmental conditions. It plays an important role in improving water quality and maintaining the health and sustainable development of watershed ecosystems (Zhao et al., 2019; Lyu et al., 2021). It is also considered to be a hot spot area for nitrogen buffering (Wang et al., 2019), which can prevent (remove) about 70% of nitrogen from entering the river (Mayer et al., 2007; Ding et al., 2017). The structural characteristics of sediments restrict the migration and transformation of solutes (Hou et al., 2017; Peralta-Maraver et al., 2018). For example, sediment particles with small particle size will reduce the solute exchange flux by 75% and hinder solute transport. Sediments with coarse particle size can promote solute transport and accelerate microbial reaction rate (Fetzer et al., 2017; Wang et al., 2020b). For the riparian zone with obvious heterogeneity of sediment structure, there are significant differences in nitrogen conversion rate. In addition, the fluctuation of river water and groundwater level controls the redox conditions and hydro-chemical indexes (such as pH, DOC, water content, etc.) of riparian sediments, which further affects the structure of microbial community and N-loss rate (Covatti and Grischek, 2021; Wang et al., 2021). Previous studies on nitrogen transformation in riparian zones showed that denitrification, anammox and DNRA processes were the main nitrogen removal pathways in riparian zones (Li J. et al., 2020; Ruan et al., 2020; Zhao et al., 2021). However, due to the high heterogeneity of riparian zones, the complexity of nitrogen transformation processes and the limitations of research methods and technologies, the contribution and limiting factors of these processes to nitrogen removal in riparian zones are still rarely reported.
In view of this background, this study aims to investigate the habitat conditions, N-loss process and distribution characteristics of related microbial communities under heterogeneous sedimentary structure of riparian zones by molecular biology technology, isotope tracer technology and microcosm control experiment. The purpose of this study is (1) to identify the nitrogen removal process under heterogeneous conditions in riparian zones, (2) to quantify the nitrogen removal rate of the riparian zone and the contribution of main nitrogen removal processes to nitrogen removal, and (3) to reveal the main controlling factors affecting the nitrogen removal in the riparian zone. This study can provide new insights into the nitrogen removal mechanism of riparian zones and help to more accurately assess the purification capacity of riparian zones.
2. Materials and methods
2.1. Study area and data collection
Located on the Loess Plateau in Northwest China, the Wei River is the largest tributary of the Yellow River, with a total length of 818 km, flowing through Gansu and Shaanxi provinces (Su et al., 2019). The Shaanxi section of the Wei River is 502 km long, which provides water for 60% of the population and 56% of the cultivated land in Shaanxi Province (He et al., 2020). The water flow in this section is from west to east, and the lithology of the riparian sediment gradually becomes finer from the upstream Baoji section (pebbles, gravels) to the downstream Weinan section (fine sand, silt), and the flow rate becomes slower. Affected by the input of excessive nitrogen fertilizer, inorganic nitrogen load (mainly NO3−) has become the main source of non-point source pollution in the Shaanxi section of the Wei River, and the nitrogen pollution problem in the downstream Weinan section is more serious (Wang et al., 2018; Shi et al., 2019).
The riparian zone of the Weinan section was selected as a typical heterogeneous profile and sampling points were set up (shown in Figure 1). According to the natural stratification of the riparian zone profile, the sediment samples of different layers were collected using a portable sediment sampler (Rigo Co., Ltd., Saitama, Japan) after disinfection with anhydrous alcohol. One group of samples (about 10 kg) was collected from each layer, and a total of 5 groups of samples were collected. The samples numbered from top to bottom were W1 (0–50 mm), W2 (50–150 mm), W3 (150–200 mm), W4 (200–300 mm), and W5 (300–350 mm). Among them, W1, W3 and W5 layers were mixed with black sludge. In order to avoid pollution during storage and transportation, the collected samples were packed into sterile self-sealing bags and sterile tube tubes (50 mL), respectively. The samples of sterile self-sealing bags were used for experiments and physical and chemical properties analysis, and were stored at −4°C. The samples of sterile tube tubes were used to determine microbial diversity and abundance, and were stored in an ultra-low temperature refrigerator at −80°C.
2.2. Analysis of sediment characteristics
As the sediment samples were collected, the pH value of the sediment was immediately measured using a pH400 portable soil pH meter (Spectrum Technologies, United States), the conductivity and total dissolved solids of the sediment were measured using a 2265FS conductivity meter (Spectrum Technologies, United States), and the water content (WC) and redox potential (ORP) of the sediment were measured using a soil multi-parameter tester (Delta-T WET-2, United States). In addition, some sediment samples were taken from each group to remove debris such as trunks, and then dried naturally and screened by a 2.0 mm filter. The treated samples were analyzed by laser particle size analyzer Mastersizer2000 (Malvern Instruments Ltd., United Kingdom), and the mineral structure of the samples was analyzed by SEM scanning electron microscopy (Thermo Fisher QUANTA 250PEG, United States) (Zhou et al., 2016). The dissolved organic carbon (DOC) in sediments was determined by total organic carbon analyzer (Shimadzu TOC-VCPH, Japan), and the dissolved inorganic carbon (DIC) was measured using a dissolved inorganic carbon analyzer (ApolloAS-C3, United States). The Fe(II) was determined by phenanthroline spectrophotometry (Zheng et al., 2020). After extraction with 2 mol L−1 KCl, the contents of total nitrogen (TN), ammonia nitrogen (NH4+-N), nitrate nitrogen (NO3−-N) and nitrite nitrogen (NO2−-N) in sediments were determined by UV-1800 ultraviolet spectrophotometer (Shimadzu, Kyoto, Japan), with detection limits of 0.015, 0.015, and 0.03 mg/kg, respectively. Dissolved organic nitrogen (DON) was determined by continuous flow analyzer (Skalar SAN++, Netherlands). The sample test was carried out in parallel for three times. The test results were expressed as the mean of three tests, and the error range was less than 5%.
2.3. DNA extraction and sequencing analysis
After thawing and mixing the sediments at each sampling point, 0.25 g was weighed, and then DNA was extracted in strict accordance with the instructions of PowerSoil ® DNA separation kit (Mo Bio Carlsbad, United States). The concentration and purity of DNA were detected by NanoDrop2000 spectrophotometer (Thermo Scientific, United States), and the qualified DNA samples were analyzed by fluorescence quantitative (qPCR). Sequencing was performed using the Illumina Miseq platform (Personal Biotechnology Company, Shanghai, China). The abundance of genes (nirS, norB, Amx-16S, nrfA) related to nitrogen removal processes (i.e., denitrification, anammox and DNRA) in sediment samples was determined by SYBR-Green dye using ABI7500 rapid real-time fluorescence quantitative PCR instrument (Applied Biosystems, Foster City, CA, United States). The Table 1 provides detailed information on primers, and three qPCR analyses were performed for each sample for error analysis.
2.4. Isotope tracer experiment
In order to quantitatively identify the nitrogen removal process in riparian zones, 15N isotope tracing experiments were carried out using isotope pairing technique (IPT) (Nielsen, 1992). The sediment and sterile water were mixed at a mass ratio of 1: 7 to make a uniform mud and filled with helium for 30 min, and then the mud was filled into a series of airtight serum bottles with syringes (Exetainer, Labco, United Kingdom). The bottles were placed in a dark environment for 24 h, and the remaining NO3−-N and NO2−-N in the bottles and DO in deionized water were consumed. 3 serum bottles were randomly selected after culture, 200 μL 50% ZnCl2 solution was added to inhibit microbial activity, and the remaining NO3−-N and NO2−-N concentrations in the bottles were extracted and determined. The remaining serum bottles were divided into 4 groups, numbered E1, E2, E3 and E4. The 100 μL helium-aerated isotope reagents were injected into the first three groups with syringes: E1 (15NH4+ standard solution with 99.6% 15N), E2 (15NH4++14NO3− standard solution, at a volume ratio of 1,1) and E3 (15NO3− standard solution with 99% 15N) (Zhang et al., 2020), and the final concentration of standard solution in each group was 100 μmol/L. Then, 200 μL of 50% ZnCl2 solution was injected into half of the bottles in each group as the initial sample, and 200 μL of 50% ZnCl2 solution was injected into the remaining serum bottles after 8 h of culture as the termination sample. The concentration of dissolved N2 (28N2, 29N2, 30N2) in the supernatant was determined by MAT253 stable isotope ratio mass spectrometer (Thermo Fisher Scientifie Inc., MA, United States) to calculate the denitrification and anammox rates.
The E4 was used to determine the DNRA reaction rate, and the injection solution was K15NO3 (99.0 atom%, final concentration approximately 100 μmol / L) aerated by helium, in addition, the starting and ending samples were filled with high-purity helium for aeration for 30 min to remove N2 (29N2, 30N2) produced by denitrification and anammox in the bottle (Deng et al., 2015). Then 200 μL HBrO was injected into the aerated serum bottle to completely oxidize 15NH4+ to 29N2 and 30N2, and the concentration of N2 and 15NH4+ in E4 was determined by mass spectrometer and ultraviolet spectrophotometer (Soonmo and Wayne, 2002; Hou et al., 2013; Yin et al., 2014), all samples needed three parallel experiments. Denitrification (Dtotal), anammox (Atotal) and dissimilatory ammonium reduction (RDNRA) rates (μmol·N·kg−1·h−1) were calculated from the following equations (Nils Risgaard-Petersen et al., 2003; Trimmer et al., 2006):
Where Q30 is the formation rate of 30N2 measured by mass spectrometer, FN is the proportion of 15NO3− in total NO3− after adding 15NO3−, Q29 is the formation rate of 29N2 measured by mass spectrometer, [15NH4+]1 is the concentration of 15NH4+ in the system at the initial time, [15NH4+]2 is the concentration of 15NH4+ μmol/L in the system after 8 h of culture, W is the sample mass kg, and T is the culture time h. Analysis of variance (ANOVA) was used to examine the spatial and temporal differences in denitrification, anammox and DNRA rates.
2.5. Microcosm static culture experiment
In order to further explore the role of Fe(II) and DOC in the N-loss process of riparian zone, batch static culture experiments were carried out in anaerobic glove box (COY-7150220, United States) by controlling variables. The samples were evenly mixed with each layer of sediment samples in riparian zone. Anaerobic deionized water (Ar/N2 for 20 min) was mixed with sediment samples at a ratio of 3: 1, and 12 g of the above homogeneous mixed slurry was taken into a 100 mL serum bottle (in triplicate). After standing, 80 mL of pure water was added, and then Ar/N2 was introduced for aeration for 20 min, and the bottle was filled with anaerobic deionized water. Cultured under anaerobic dark conditions for 2 days to remove the sample background to remove nitrate, nitrite and oxygen from the sample background. Different exogenous substances were added to each bottle (details in Supplementary Tables S1, S2), and anaerobic dark culture was performed for 31 days. The supernatant was collected regularly with a 1 mL syringe (interval of 24 h on the first 15 days, and interval of 48 h on the 16th day) to determine the concentration of Fe(II), nitrate nitrogen, nitrite nitrogen and ammonia nitrogen by the phenanthroline spectrophotometry and UV-1800 ultraviolet spectrophotometer (Shimadzu, Kyoto, Japan), all samples need three parallel experiments. After each sampling, it was sealed with a sealing film to prevent oxygen pollution.
2.6. Statistical analysis
In this study, the OriginPro 2022 and R language were used for graphic drawing and analysis. One-way analysis of variance (ANOVA) was used to test the differences in nitrogen conversion rate, microbial functional genes and sediment physical and chemical indicators (95% confidence interval). The SPSS software package (version; 25 IBM, United States) was used to estimate the relationship between different variables through pearson correlation and linear regression equation. The significance level of all statistical analyses was set as p < 0.05.
3. Results and discussion
3.1. Physicochemical properties and spatial distribution characteristics of nitrogen in riparian sediments
The lithology structure of the riparian zone in the study area is heterogeneous in the vertical direction (Table 2). The soil particle size ranged from 1.98 to 215.9 μm, mainly silt (4–63 μm), with clay (>63 μm), silt, and fine sand (<4 μm) contents accounting for 0–0.03%, 72.15–98.1%, and 1.86–27.85%, respectively. There were abundant minerals in the sediments of the riparian zone, mainly quartz (55.9–64.1%) and plagioclase (14.8–20.0%). Followed by calcite (6.0–8.6%), chlorite (4.7–6.6%), illite (3.4–5.4%), potassium feldspar (1.7–4.4%), dolomite (1.3–4.1%) and a small part of amphibole, which indicated that the parent rock of the sediment was completely weathered and the mineral maturity was high.
The soil particles of the riparian zone sediments had a high degree of polymerization, and micro-aggregates were observed in the sediment W1, W3, and W5 layers containing sludge, and there were obvious bridging substances between the soil particles (Supplementary Figure S1), which greatly hindered the uptake of organic matter by microorganisms, protected the ‘comprehensiveness’ of soil organic matter, and formed natural organic carbon. Therefore, the DOC content in this area was high (Table 2). In addition, the pH range of the sediment environment in the riparian zone was 7.77–8.02, which was weakly alkaline overall. Along the vertical direction of the riparian zone, conductivity (EC) and total dissolved solids (TDS) showed a decreasing trend, Fe(II) gradually increased, and oxidation reduction potential (ORP) gradually decreased, and the reducibility of the sedimentary environment in the riparian zone gradually increased.
As shown in Figure 2, the distribution of nitrogen content in different depths of sediments in the riparian zone of the Weinan section of the Wei River varied greatly, with the total nitrogen (TN) content ranged from 11.8 to 15.31 mg/kg, and the DON content ranged from 7.21 to 11.42 mg/kg, which was the main form of nitrogen. With the increase of sediment depth in the riparian zone, TN showed a downward trend, DON showed an increasing trend (Figure 2A), and dissolved organic nitrogen (DIN) content gradually decreased (Figure 2A), which confirmed that the riparian zone had a removal effect on inorganic nitrogen pollution.
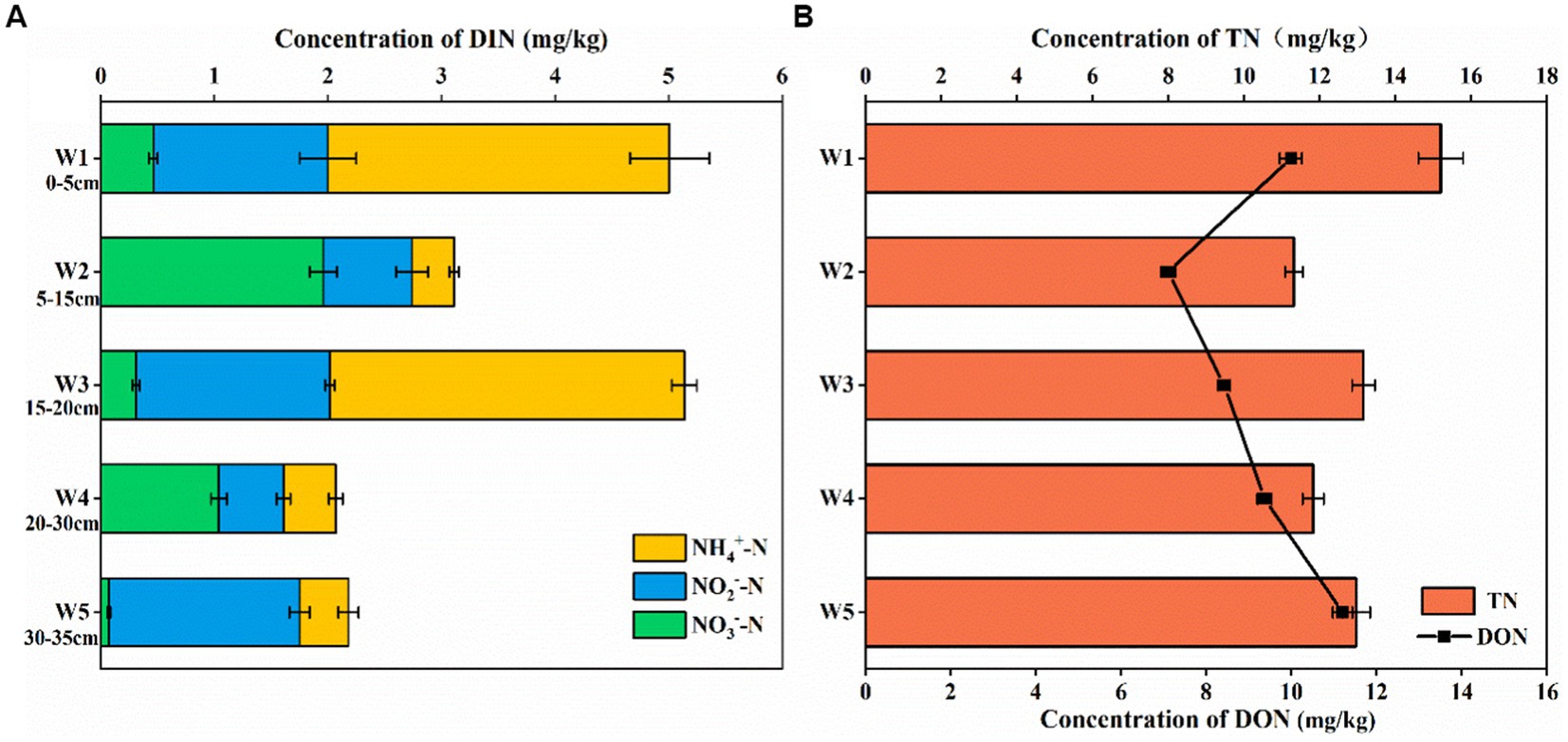
Figure 2. Vertical distribution of nitrogen in riparian sediments of Weinan section of Wei River [(A) The content of DIN, (B) The content of TN and DON, Error bars represent standard deviations].
It had been shown that the migration and transformation of nitrogen in the riparian zone is mainly completed by the geochemical biological effects of NH4+-N, NO3−-N and NO2−-N (nitrification, denitrification, anaerobic ammonium oxidation, etc.) (Zhao et al., 2021). In the riparian sediments of Weinan section of Wei River, the NO3−-N, NO2−-N and NH4+-N contents were 0.06–1.96 mg/kg, 0.03–1.71 mg/kg and 0.42–3.12 mg/kg, respectively. In layers W1, W3, and W5, which contained sludge, the DIN in the sediments mainly existed in the form of NO2−-N and NH4+-N, and the NO3−-N content was low and reached a minimum value of 0.06 mg/kg in the lowest layer W5, while in the sediment layers W2 and W4, the content of NO3−-N was significantly higher than that of NO2−-N and NH4+-N, which indicated that there was a complex nitrogen cycle in the sediments of the riparian zone in Weinan section.
3.2. Microbial community dynamics involved in nitrogen removal processes in riparian sediments
Metagenomics can determine the composition and function of microbial communities (Daims et al., 2015; Chen et al., 2022). In this study, genes associated with denitrification (nirS, norB), anammox (Amx-16S) and DNRA (nrfA) reactions were detected in the sediments of the riparian zone of the Weinan section of the Wei River by qPCR, which indicated that the riparian zone may remove nitrogen from the environment through denitrification, anammox and DNRA processes. Among them, denitrification combined with anammox removed NO3−-N ‘permanently’ from the riparian zone of the Wei River, while DNRA converted NO3−-N into inorganic nitrogen and retained it in the riparian zone for other organisms (Crowe et al., 2012; Deng et al., 2015). The Coverage index of each sample in this study was greater than 0.99, indicating that the determination results of sediment samples had high confidence (Supplementary Table S3). There were significant differences in the diversity and abundance of microbial communities involved in the nitrogen removal process along the sediment depth (Figure 3).
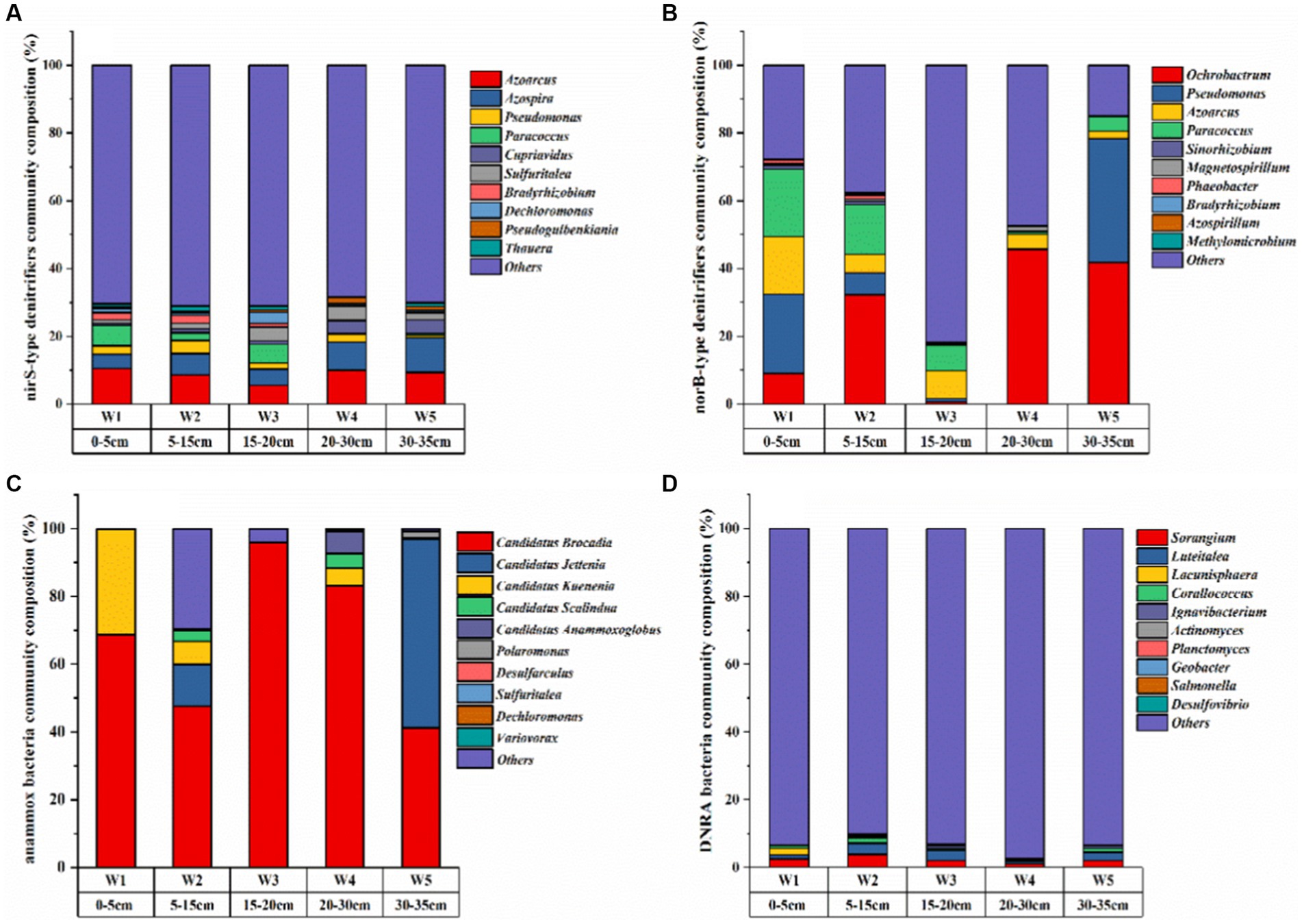
Figure 3. The community composition of nitrogen-transforming microorganisms in the riparian sediments of the Weinan section of the Wei River [top 10 species at the sample genus level: (A) nirS denitrifying bacteria; (B) norB denitrifying bacteria; (C) anammox bacteria; (D) DNRA bacteria].
The diversity of denitrifying bacteria community in the riparian zone of Weinan section was the highest, and Proteobacteria was the most important denitrifying bacteria (Figures 3A,B), which was the same as the results of other freshwater system sediments (Song et al., 2012; Dong et al., 2021). Aerobic denitrifying bacteria such as Azoarcus, Ochrobactrum, Pseudomonas, Paracoccus, and Azospira are the main dominant genera (Figures 3A,B). Which was attributed to the interaction between river water and groundwater. The transport of dissolved oxygen to sediments provided an oxidizing environment for microorganisms. The high redox potential in sediments also confirmed this view (Table 2). These aerobic microorganisms use oxygen/NO3−-N/NO2−-N as an electron acceptor for denitrification under aerobic conditions (Huang et al., 2021; Zhang et al., 2022).
In addition, the abundance of nirS gene was 2.01 × 104–6.92 × 105 copies g−1, and the abundance of norB gene was 1.74 × 105–3.68 × 105 copies g−1 (Figure 4). As a nitrite reductase marker gene nirS (Wang et al., 2019), the abundance of nirS gene was the same as the distribution of NO2−-N content (Figures 2A, 4A). With the increase of sediment depth, the abundance of nirS gene decreased first and then increased, and then decreased gradually, and decreased to the minimum in W5 layer (Figure 4A). As a marker gene of nitric oxide reductase (Liu et al., 2017), the abundance of norB gene was similar to the distribution of N2O content (Figure 4B and Supplementary Figure S2). The abundance of norB gene decreased sharply from W1 layer, and decreased to the minimum value in W2 layer and then increased gradually. It was shown that sediment denitrification capacity was positively correlated with the abundance of the nirS gene (Mosier and Francis, 2010). We also found that the spatial distribution pattern of nirS gene abundance and denitrification rate in the riparian zone of Weinan section was the same (Figures 4A, 5), which was higher in W1 and W3 layers and lower in other layers, which further indicated that the abundance of nirS gene could be approximated as an indicator of denitrification capacity in sediments.
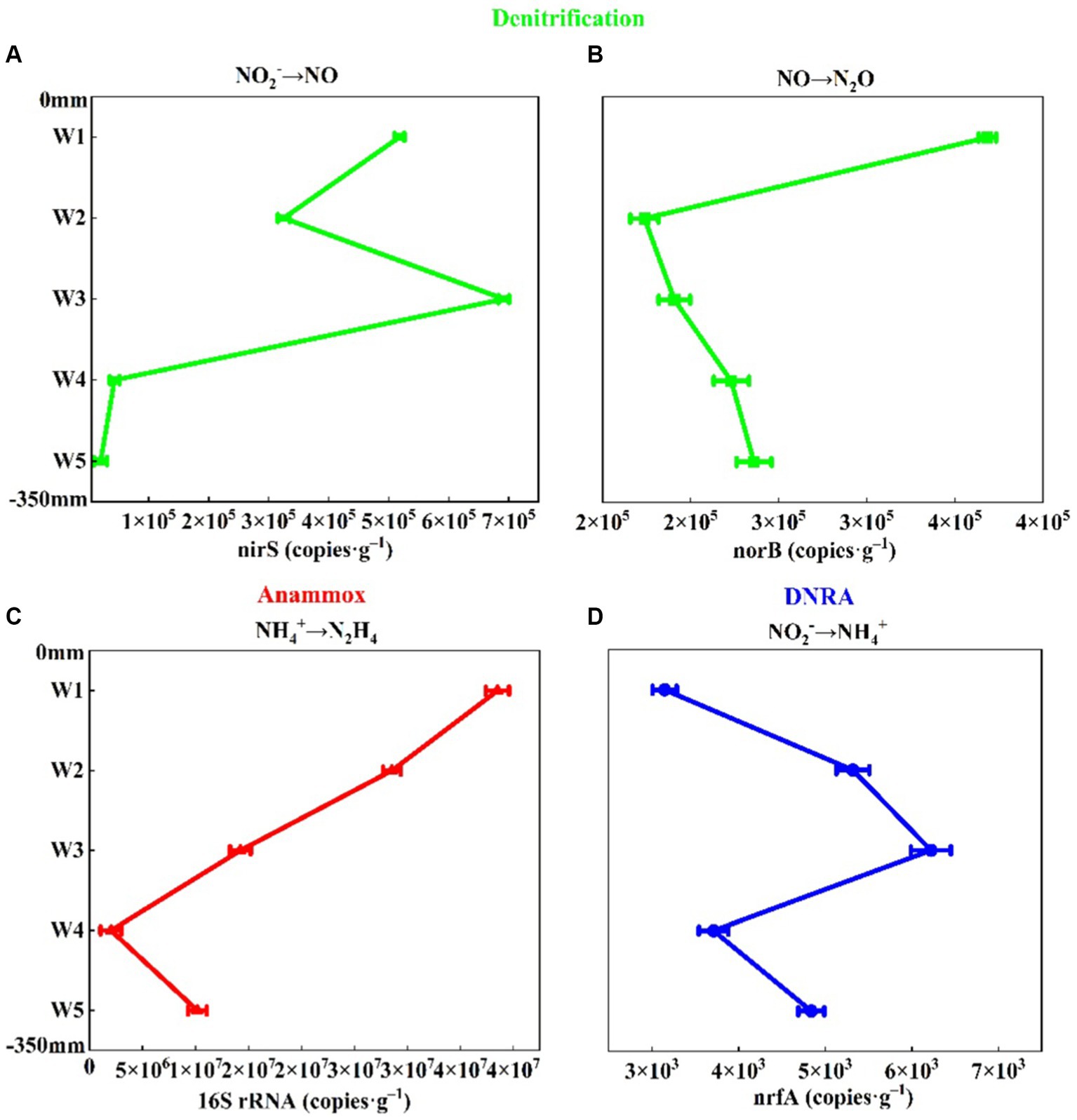
Figure 4. Abundance of genes for nitrogen removal in riparian sediments of Weinan section of Wei River [(A) nirS, (B) norB, (C) 16S rRNA, (D) nrfA, Error bars represent standard deviations].
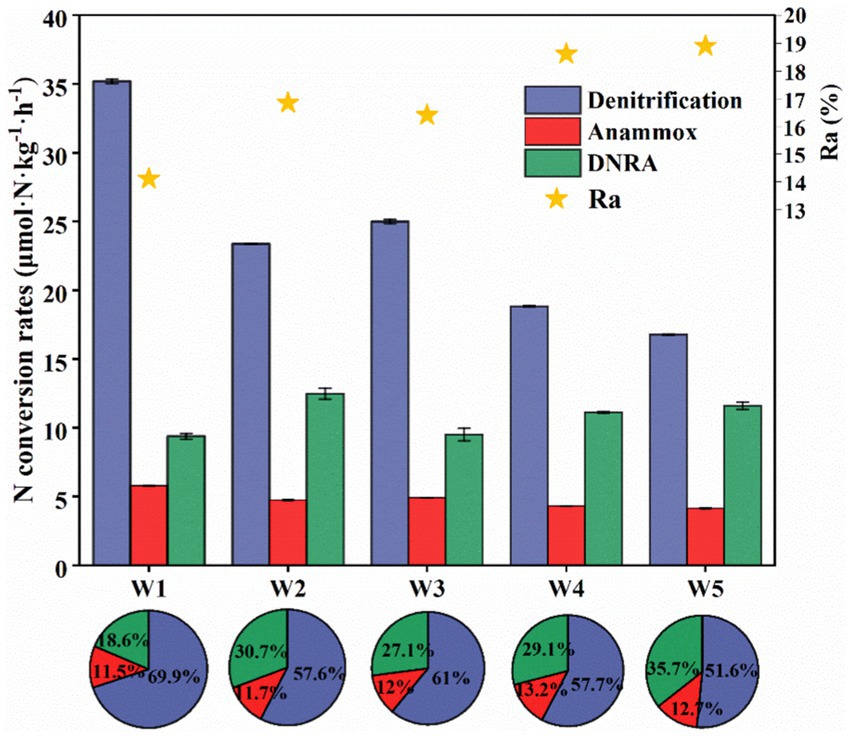
Figure 5. The spatial variation characteristics of denitrification, anammox and DRNA rates in the riparian zone of Weinan section of Weihe River (Ra is the N2 contribution of anammox rate in the N-loss process); the pie chart is the contribution of potential nitrogen removal rate, anammox rate and DNRA rate to the attenuation of NO3−-N or NO2−-N in different depth samples of riparian zone in the study area, Error bars represent standard deviations.
The dominant genera of anammox bacteria in the riparian zone of Weinan section of Wei River were Candidatus Brocadia, Candidatus Jettenia, Candidatus Kuenenia and Candidatus Scalindua (Figure 3C). Most of these dominant bacteria were autotrophic, which used inorganic carbon as a carbon source in the anaerobic environment, and were anaerobic ammonia-oxidizing bacteria commonly found in terrestrial ecosystems (Humbert et al., 2010). The lowest diversity and relatively homogeneous composition of anammox bacteria were found in the sludgy layers W1, W3 and W5 (Figure 3C), which could be attributed to the fact that the high DOC content in the sludgy layer inhibited the growth of anammox bacteria (Guven et al., 2005; Chamchoi et al., 2008). The abundance of anammox bacteria 16S rRNA gene was the highest in riparian sediments, which was two orders of magnitude higher than the abundance of denitrifying functional genes, ranging from 2.03 × 106 to 3.85 × 107 copies g−1 (Figure 4C), and gradually decreased along the sediment depth.
The dominant genera of DNRA bacteria in riparian zone were mainly Sorangium, Luteitalea, Lacunisphaera and Corallococcus (Figure 3D), which were similar to the reports of DNRA bacterial diversity in other rivers and lakes (Yuan et al., 2022; Zhao et al., 2022), and most of these microorganisms were heterotrophic (Wang et al., 2020a). Among them, Sorangium is an aerobic bacterium belonging to Myxococcales, which can use NO3−-N/NO2−-N to produce NO (Banerjee et al., 2018), and is considered to be a key microorganism for synergistic control of soil microbial composition. Luteitalea belongs to Acidobacteria, which are aerobic and chemoheterotrophic bacteria, and can use organic matter in the environment as a carbon source for life activities (Vieira et al., 2017; Qiao et al., 2020). Compared with other functional genes, the abundance of nrfA gene in DNRA bacteria was the lowest, ranging from 3147.43–6221.28 copies g−1 (Figure 4D). Meanwhile, along the depth direction of the sediment, the abundance of nrfA gene was completely consistent with the distribution of DOC content (Figure 4D and Table 2). DOC, as an important carbon source of DNRA, can promote DNRA reaction (Broman et al., 2021), which is an important factor in regulating DNRA in riparian zone.
Microorganisms are important mediators for nitrogen migration and transformation in riparian sediments (Kuypers et al., 2018; Liu et al., 2021). Our observations showed that heterogeneous riparian zones can provide microhabitats for denitrifying, anaerobic ammonia oxidation and DNRA bacteria. The differences in the diversity and abundance of these communities in sediment layers with different lithologic structures may affect the nitrogen removal process of the riparian zone.
3.3. Nitrogen removal mechanism and nitrogen removal capacity of riparian sediments
As shown in Figure 5, we calculated the denitrification rate (Dtotal), anammox rate (Atotal) and DNRA rate (RDNRA), which further confirmed that the natural attenuation process of nitrogen in the riparian zone of the study area was achieved by the combined action of these three processes. Unlike other regions where denitrification and anammox reactions are the main N-loss pathways in sediments (Choi et al., 2016; Liu et al., 2019; Zhang et al., 2020; Zheng et al., 2020), the contribution rate of anammox to nitrogen removal in the riparian zone of Weinan section of Wei River was extremely low (about 12.2%), and Atotal was 4.14–5.77 μmol·N·kg−1·h−1 (Figure 5), which was related to the unique properties of the riparian zone. Under the interaction of river water and groundwater, the sedimentary environment of the riparian zone was oxidizing and heterogeneous, while anammox bacteria were extremely sensitive and had extremely high requirements for environmental stability, using inorganic carbon (DIC) as a carbon source for anammox reactions in low or even anaerobic environments (Jin et al., 2012; Huang et al., 2022), and the content of DIC in the riparian zone was low (Table 2), thus the activity of anammox bacteria was suppressed. Even though the oxidation of the environment along the sediment depth direction became weaker, the abundance of anammox bacteria also decreased (Figure 4) and Atotal remained low. However, the anammox process contributed 14–19% of the N2 production (Figure 5), and its nitrogen removal capacity cannot be ignored.
In contrast, denitrification and DNRA played a major role in the nitrogen removal process in the riparian zone of Weinan section of Wei River, and their nitrogen removal contribution rates were about 59.6 and 28.2%, respectively. Among them, Dtotal was 17.76–35.19 μmol·N·kg−1·h−1, RDNRA was 9.37–12.47 μmol·N·kg−1·h−1 (Figure 5). DNRA and denitrification belong to the dissimilatory reduction process of NO3−-N, and both of them use NO3− as the electron acceptor with substrate competition (Yin et al., 2017; Wang et al., 2020b; Wang Z. et al., 2022). It is generally believed that denitrification requires less free energy than DNRA action in the same environment, and thus preferentially occurs in most cases (Kartal et al., 2007; Chen et al., 2015). Meanwhile, the community diversity and abundance of denitrifying bacteria in riparian zone sediments were much higher than that of DNRA bacteria (Figures 3, 4), thus denitrification dominated the N-loss process in riparian zones (Figure 5).
In addition, we also observed that both Dtotal and RDNRA showed significant spatial distribution characteristics. Along the sediment depth direction, Dtotal decreased first and then increased, and then gradually decreased (Figure 5), which was similar to the variation of denitrifying bacteria (especially nirS gene) abundance and its contribution rate to nitrogen removal (Figure 4A). The RDNRA fluctuated slightly, but with the increase of sediment depth, the contribution rate of DNRA to nitrogen removal increased significantly, and reached the maximum value of 35.7% in the bottom layer W5 (Figure 5), which we speculated might be related to the competitive mechanisms of denitrification and DNRA. Studies have shown that DOC: NO3− (electron donor: acceptor) is a key factor in regulating denitrification and DNRA (Tiedje et al., 1983; Rütting et al., 2011), but in the sediment of a single medium, it was not DOC: NO3− but resource concentration (NO3−) that determines the choice of DNRA and denitrification pathways, and when the content of NO3− becomes more limited, it is more conducive to DNRA (Vuono et al., 2019). Although the sediments in the riparian zone of the Weinan section were heterogeneous, the lithology structure of the bottom W5 layer was relatively simple, and the silt (accounting for about 98%) was the main lithology structure (Table 2). Meanwhile, the minimum NO3− content in the W5 layer was only 0.06 mg/kg, which might promote the NO3− to select the reduction path of DNRA and increase the contribution rate of DNRA in this layer.
Previous studies have shown that the abundance of functional genes is a good predictor of nitrogen transformation rate (Wang et al., 2017; Zhang et al., 2018). Interestingly, in this study, although the abundance of 16S rRNA gene of anammox bacteria was the highest and the abundance of nrfA gene of DNRA bacteria was the lowest, the anammox rate in riparian sediments was significantly lower than that of DNRA (Figure 5). Therefore, the abundance of microbial genes cannot systematically quantify the biogeochemical process of nitrogen. The nitrogen cycle in riparian zone is not only determined by microorganisms, but also related to the nature of riparian zone itself, environmental factors and specific nitrogen transformation process. This study shows that molecular biology techniques coupled with isotope tracing methods can better quantitatively reveal the N-loss process in the riparian zone.
To evaluate the nitrogen removal efficiency of the riparian zone of Weinan section of Wei River, we compared the nitrogen removal rate of sediments from different aquatic ecosystems in various regions of the world (Table 3). We found that the nitrogen removal rate of sediments in the Weinan section of the Wei River was 4.14–35.19 μmol·N·kg−1·h−1, which was higher than that of sediments in most regions, including wetlands, continental shelf and estuary sediments. This indicates that the riparian zone of Weinan section has a strong capacity to reduce inorganic nitrogen pollution. We also found that the nitrogen removal rate of riparian sediments in the study area was similar to that of estuarine turbidity maximum zone (TMZ) and wetland. In recent years, TMZ has been considered as an important place to remove reactive nitrogen from land to sea due to its large number of suspended particles that can easily adsorb reducing cations to consume oxygen, provide a growth environment for anaerobic microorganisms, and promote denitrification and anammox reactions (DeVries et al., 2012; Zheng et al., 2021), and wetland is considered to be a hot spot for nitrogen removal due to its high organic carbon and anaerobic environment prone to denitrification (Cheng et al., 2020). Therefore, the sediments in the riparian zone of the Weinan section share some common characteristics with TMZ and wetland, such as high organic matter content, low oxygen concentration and diverse microbial communities.
3.4. Effects of Fe (II) and DOC on N–loss process in riparian zone
Iron and DOC are important components in the riparian zone, and they can regulate the nitrogen transformation process in environmental media (Wu et al., 2021; Lu et al., 2022), which plays an important role in the natural attenuation of nitrogen (Yang et al., 2018; Lu et al., 2022). Large amounts of Fe(II) and DOC were present in the riparian zone of the Weinan section of the Wei River (Table 2), and we investigated the effects of Fe(II) and DOC on the process of natural attenuation of NO3−-N in the riparian zone through microcosmic incubation experiments.
We observed that compared with the in situ riparian zone sediments and the sediments supplemented with Fe(II) combined with sterilization, the riparian zone sediments supplemented with Fe(II) only had higher NO3−-N reduction rates (Figure 6A), which proved that there may be a microbial-driven nitrate-dependent ferrous oxidation process (NDFO) in the riparian zone, which can reduce NO3− to NO2−, N2O, and N2. Additionally, Supplementary Figure S3 showed that the riparian zone sediment consumed a large amount of DOC in the system after the addition of Fe(II), which further indicated that the microorganisms involved in the NDFO process were mainly chemoenergetic, inorganic allotrophic or parthenogenic and used DOC as a carbon source. In the first 10 days of incubation, the contents of NO3−-N and Fe(II) in the system showed a sharp decline, and the NO3−-N was completely consumed to the minimum (0.07 mg/L, Figure 6B and Supplementary Figure S4), while the contents of NO2−-N and NH4+-N showed a significant upward trend (Figure 6B). This result indicated that the NDFO reaction (Fe(II) + NO3− → Fe(III) + NO2−) was carried out in the riparian zone at this stage, which may be accompanied by denitrification (NO3− → NO2−), nitrogen mineralization (DON → NH4+) and DNRA reaction (NO3- → NH4+). During the 11–30 days of culture, the content of NO3−-N in the sediments remained at the minimum value, and the content of NO2−-N showed a significant downward trend, while the content of NH4+-N increased first and then decreased, and the content of Fe(II) decreased first and gradually increased after 20 days (Figure 6B and Supplementary Figure S4), indicating that NDFO (Fe(II) + NO2− → Fe (III) + N2), iron ammonia oxidation (Feammox, Fe(III) + NH4+ → Fe(II) + N2), and anammox (NO2− + NH4+ → N2) may exist at this stage. Thus, the Fe-N cycle exists in the riparian zone sediments and Fe(II) accelerates the attenuation of NO3−-N in the riparian zone sediments through the NDFO reaction driven by microorganisms, while the coupling of Feammox with the NDFO process achieved the recycling of Fe within the system.
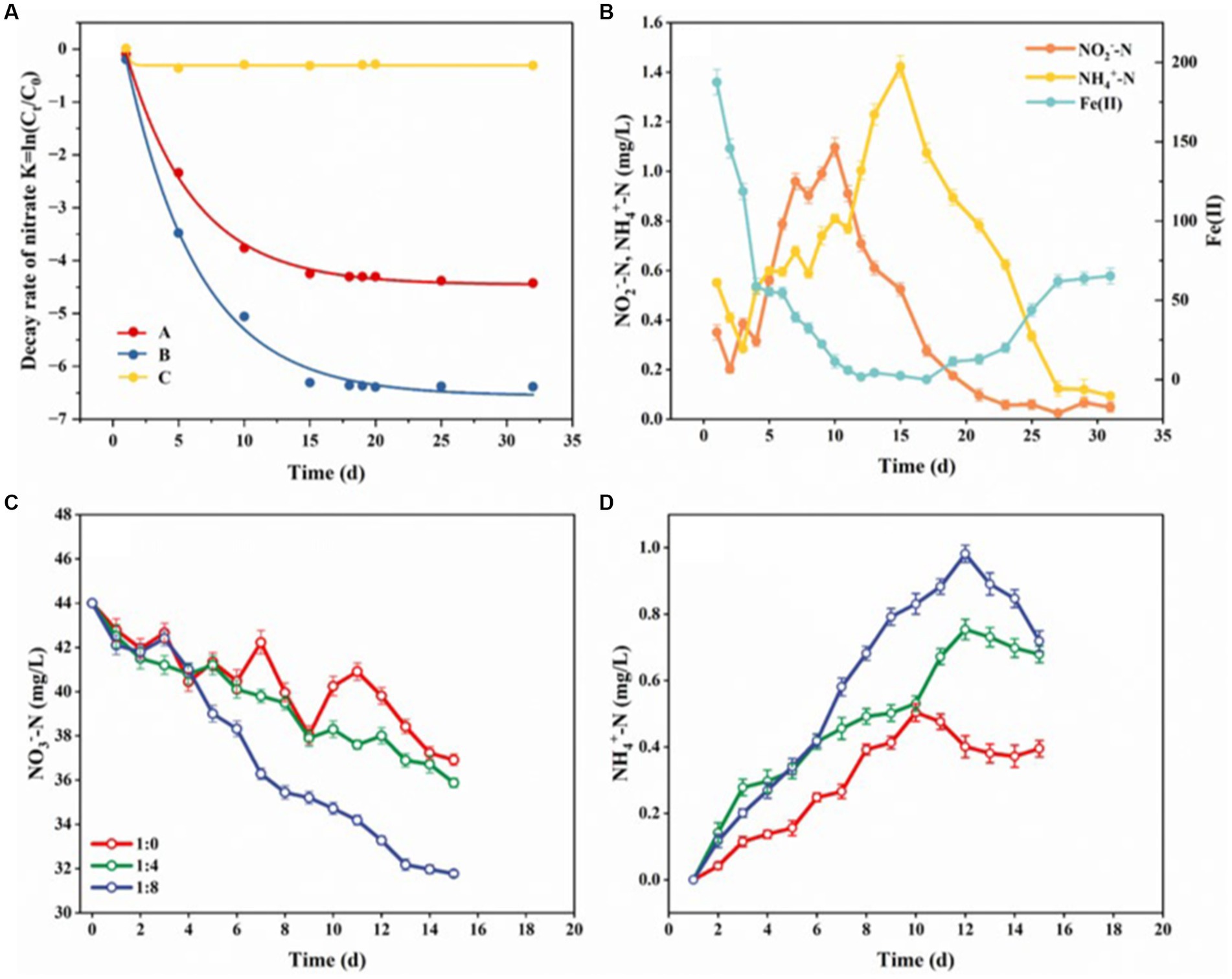
Figure 6. Patterns of NO3−-N decay rates and material changes in sediments in riparian zones under different exogenous conditions. (A,B) Show the effect of Fe(II) on sediment NO3−-N decay rates and the content of NO3−-N, Fe(II), NO2−-N, and NH4+-N. (C,D) Show the effect of DOC on NO3−-N and NH4+-N in sediments. Ct is the content of NO3−-N at t (d), C0 is the initial content of NO3−-N, (A) is the in situ riparian zone sediments, (B) is the sediment added with Fe(II), (C) is the sediment added with Fe(II) and sterilized, 1:0 indicates a DOC: NO3− of 0:1 sediment, 1:4 indicates a DOC: NO3− of 4:1 sediment, and 1:8 1:0 indicates a DOC: NO3− of 8:1 sediment. 1, Error bars represent standard deviations.
We also observed that under different carbon-nitrogen ratios (DOC: NO3−), the content of NO3−-N in the reaction system showed a downward trend (Figure 6C), while the content of NH4+ -N showed a trend of rapid increase and then gradual decrease (Figure 6D), and at the same time, the higher the value of DOC: NO3−, the more dramatic the changes in the NO3−-N and NH4+-N content (Figures 6C,D). In the pre-culture period (first 10 d), the NO3−-N content in the system decreased and the NH4+-N content increased, which might be attributed to the promotion of DNRA reaction by high carbon-nitrogen ratios. Numerous reports have shown that DOC is the substrate for DNRA and denitrification processes, denitrification process is a competitive advantage when the carbon-nitrogen ratios in the soil is lower than 2.5, but DNRA reaction occurs preferentially when the value of carbon-nitrogen ratios is higher than 4, and the sufficient DOC is beneficial to promote the activity and growth of DNRA bacteria (Tiedje et al., 1983; Wang et al., 2020a; Chen et al., 2022). In the later stage of culture (after 10 d), the content of NO3−-N and NH4+-N in the system decreased gradually, which may be due to the anammox. At this stage, DOC and NO3−-N in the system were consumed by denitrification and DNRA reaction, resulting in NO3−-N and NH4+-N, which provided sufficient substrate for anammox reaction and promoted the growth of anammox bacteria. Hence, in the riparian zone of Weinan section of Wei River, sufficient DOC increases the carbon-nitrogen ratios in the environment, which promotes the DNRA reaction and accelerates the attenuation of NO3−-N.
3.5. Correlation between environmental parameters, nitrogen transformation gene abundance and nitrogen removal rate
As the riparian zone is an active biogeochemical region with naturally developed physicochemical and microbiological gradients that are key drivers of the nitrogen cycle (Hou et al., 2017). We observed that Dtotal was significantly positively correlated with Atotal and negatively correlated with RDNRA (Supplementary Figures S5, S6), which suggested that denitrification and anammox process in the riparian zone had a mutually reinforcing relationship. Denitrification could provide electron acceptors (NO2−-N) for the anammox process, and the consumption of organic carbon by denitrifying bacteria could alleviate its inhibitory effect on anammox bacteria (Hou et al., 2015; Liu et al., 2019). On the other hand, denitrification and DNRA showed a competitive relationship, competing for the common electron donor DOC and electron acceptor NO3− in environmental media. Therefore, the synergistic and competitive relationship among denitrification, anammox and DNRA jointly promoted the nitrogen removal process in the riparian zone.
In the riparian zone of Weinan section of Wei River, DNRA and denitrification are the most active in the attenuation process of nitrate. For the denitrification process, TDS, EC, NH4+ and NO2− were the main environmental factors affecting Dtotal, which were significantly positively correlated with Dtotal (p < 0.05, Figure 7A). This conclusion was consistent with the results of Magalhaes et al. (2005) in the intertidal sediments of Douro estuary in Portugal and Liu et al. (2018) in the sediments of Yangtze River lakes, these substances directly or indirectly provide electron acceptor/donor or energy for denitrifying bacteria to promote denitrification. A large number of studies have shown that nitrate is the reaction substrate of denitrification and is proportional to the denitrification rate (Mulder et al., 1995; Yao et al., 2018; Broman et al., 2021). Interestingly, the correlation between NO3− content and Dtotal in this study was not strong and negatively correlated (Figure 7A), which may be related to the NO3− content and the abundance of denitrifying bacteria in the riparian zone. The electron affinity of NO3−-N and NO2−-N was different, and there was a competitive electron relationship (Xing et al., 2016). In the W1, W3, and W5 layers containing sludge, the contents of DOC, NH4+-N and NO2−-N were significantly higher (Table 2 and Figure 2A). Therefore, the electrons provided by a large amount of DOC may preferentially flow to NO2−-N for denitrification (nitrite reduction) or DNRA reaction. While in the W2 and W4 layers with higher NO3−-N content, the DOC content and denitrification function (nirS, norB) microbial abundance were lower (Table 2 and Figures 4A,B), and the denitrification process might be inhibited. Accordingly, Dtotal in the riparian zone was significantly positively correlated with DOC and NO2− contents, but not with NO3− contents in sediments.
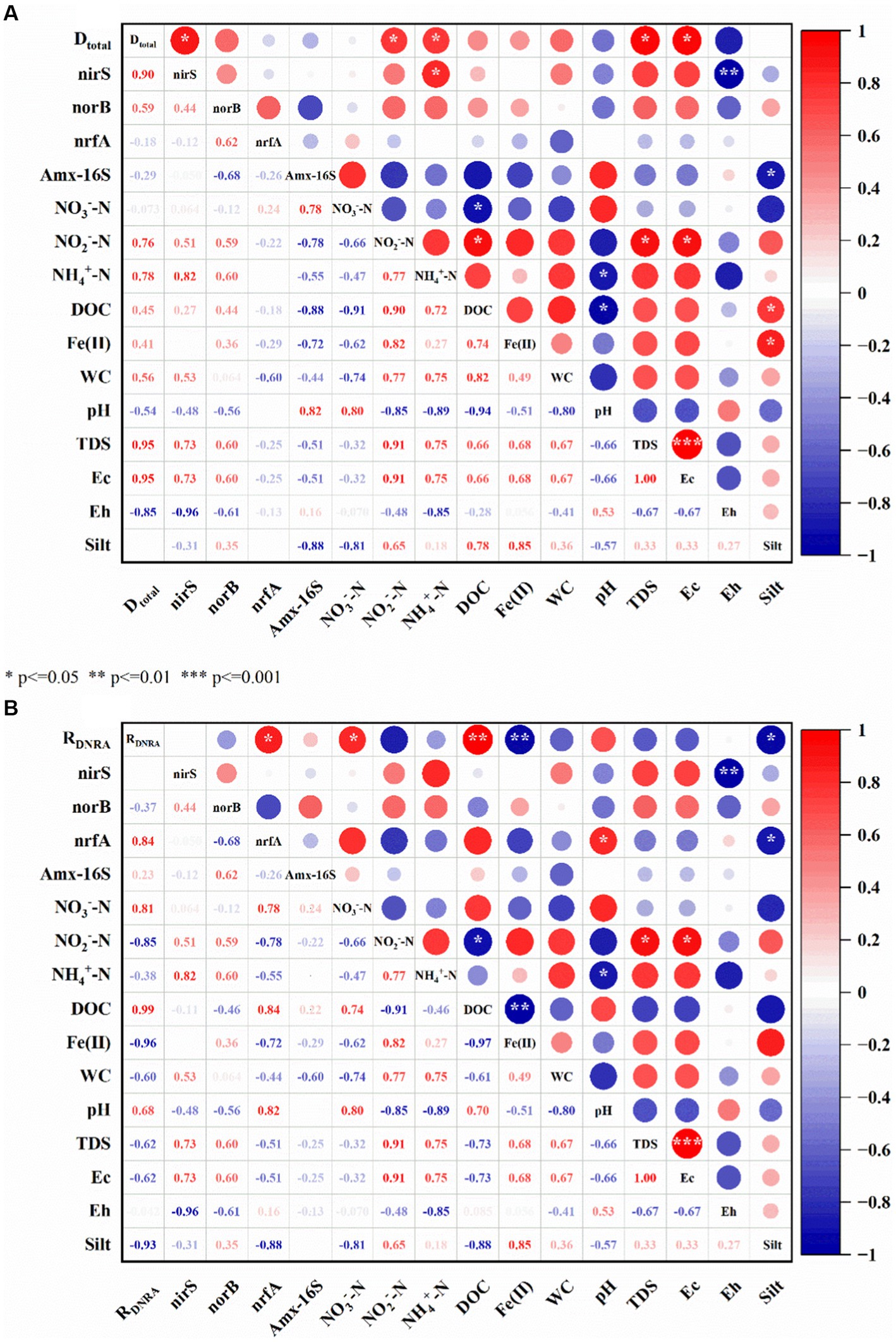
Figure 7. Analysis of driving factors of nitrogen removal rate. WC: water content, red represents a positive correlation, blue represents a negative correlation, the deeper the color, the stronger the correlation, the size of the circle is the correlation coefficient, *, **, *** represents the significant statistics at p < 0.05, 0.01, and 0.001, (A) is the driving factor affecting the denitrification rate, (B) is the driving factor affecting the DNRA rate.
For the DNRA process, NO3−, sediment lithology (Silt), DOC, Fe(II) and NO2− were the main environmental factors affecting RDNRA (p < 0.05, Figure 7B). Among them, NO3− and Fe(II) were significantly positively correlated with RDNRA, and the other environmental factors were significantly negatively correlated with RDNRA. Fetzer and Li et al. found that the microbial community of riparian sediments is very sensitive to lithologic structure, coarse particles promote microbial activity and accelerate material transport, and fine particles will block exchange channels, reduce microbial activity and hinder solute migration and transformation (Fetzer et al., 2017; Wang et al., 2020b). The lithology of the sediments in the riparian zone of the Weinan section of the Wei River was dominated by silt, followed by fine sand, and these fine particles might inhibit the DNRA reaction. Microcosmic experiments showed that there was a coupled Fe-N cycle in the riparian zone, and Fe(II) would promote the attenuation of NO3−-N through NDFO action, which reduced the reaction substrate of DNRA and inhibited the DNRA reaction. In contrast, NO3− and DOC provided electron acceptors and electron donors, respectively, for the DNRA reaction (Li Z et al., 2020; Chang et al., 2021), which facilitated the DNRA reaction.
In addition, Dtotal was significantly and positively correlated with the abundance of denitrification functional genes nirS and nroB, and RDNRA was also significantly and positively correlated with the abundance of DNRA functional gene nrfA. This conclusion is consistent with the research results of China’s Yangtze River estuary (Zheng et al., 2021), Northeast China Sea (Chang et al., 2021), and high-altitude rivers (Zhang et al., 2020). This further proves that the nitrogen removal process of riparian sediments is mediated by the redox reaction of different microorganisms. In addition, in the study of soil nitrogen cycle, it was found that the microorganisms involved in denitrification and DNRA processes usually lived in the same environment, and the optimal pH range was relatively close, denitrification was 6–8, and DNRA was 5–9. High pH will promote nitrate reduction through DNRA reaction and weaken the intensity of denitrification (Stevens et al., 1998; Fernandes et al., 2012). Interestingly, in this study, the pH of the sediment environment was 7.77–8.02, and the correlation analysis showed that pH was negatively correlated with Dtotal and denitrifying bacteria abundance, and significantly positively correlated with RDNRA and DNRA bacteria abundance (p < 0.05, Figure 7). It indicated that the pH of the sedimentary environment in the riparian zone was more suitable for the growth of DNRA bacteria.
In general, compared with the permanent submerged sediments, the riparian sediments in the Weinan section of the Wei River are in a dry-wet alternation state. The interaction between river water and groundwater causes the fluctuation of water level in the riparian zone, which changes the physical and chemical properties of the sediments, and then affects the microbial community structure and the distribution characteristics of inorganic nitrogen. The abundance of nitrogen-converting microorganisms, NOX−, Fe(II) and DOC jointly drove the N-loss process in the riparian zone.
4. Conclusion
This study investigated N-loss processes and the diversity and abundance of associated microbial communities in the heterogeneous riparian zone of the Weinan section of the Wei River. The results showed that the combined effects of denitrification (17.76–35.19 μmol·N·kg−1·h−1), DNRA (9.37–12.47 μmol·N·kg−1·h−1) and anammox (4.14–5.77 μmol·N·kg−1·h−1) achieved the natural attenuation of nitrogen in the riparian zone, and denitrification (accounting for 51.6–69.9%) was the dominant process. In addition, the riparian zone sediments harbor diverse and abundant microbial communities that mediate these processes. The abundance of functional genes and environmental factors (especially DOC and Fe(II)) are also the constraints of sediment nitrogen removal rate in riparian zone. These results suggest that riverbed sediments are a hot area for nitrogen removal in riparian zones, which is of great significance for strengthening the control of river nitrogen pollution. This study has important implications for understanding and managing the water quality and ecosystem health of inland rivers in arid and semi-arid regions.
Data availability statement
The datasets presented in this study can be found in online repositories. The names of the repository/repositories and accession number(s) can be found below: NCBI, PRJNA1002244.
Author contributions
YW, FYe, RL, FYa, and FG performed the material preparation, data collection, and analysis. FYe, YS, and LD wrote the first draft of the manuscript. FYe, YX, and YS wrote the revised manuscript. All authors contributed to the conception and design of the study, commented on previous versions of the manuscript, read, and approved the final manuscript.
Funding
This study was funded by the National Natural Science Foundation of China (Nos. 41877190 and 42277061), the Key Research and Development Program of Shaanxi (Nos. 2020ZDLSF06-04 and 2021ZDLSF05-05).
Conflict of interest
FYa, RL, and FG were employed by Power China Northwest Engineering Corporation Limited.
The remaining authors declare that the research was conducted in the absence of any commercial or financial relationships that could be construed as a potential conflict of interest.
Publisher’s note
All claims expressed in this article are solely those of the authors and do not necessarily represent those of their affiliated organizations, or those of the publisher, the editors and the reviewers. Any product that may be evaluated in this article, or claim that may be made by its manufacturer, is not guaranteed or endorsed by the publisher.
Supplementary material
The Supplementary material for this article can be found online at: https://www.frontiersin.org/articles/10.3389/fmicb.2023.1239055/full#supplementary-material
References
Banerjee, S., Schlaeppi, K., and van der Heijden, M. G. A. (2018). Keystone taxa as drivers of microbiome structure and functioning. Nat. Rev. Microbiol. 16, 567–576. doi: 10.1038/s41579-018-0024-1
Basu, N. B., Van Meter, K. J., Byrnes, D. K., Van Cappellen, P., Brouwer, R., Jacobsen, B. H., et al. (2022). Managing nitrogen legacies to accelerate water quality improvement. Nat. Geosci. 15, 97–105. doi: 10.1038/s41561-021-00889-9
Bo, T., and Tage, D. (2002). Production of N2 through anaerobic ammonium oxidation coupled to nitrate reduction in marine sediments. Appl. Environ. Microbiol. 68, 1312–1318. doi: 10.1128/AEM.68.3.1312-1318.2002
Braker, G., and Tiedje, J. M. (2003). Nitric oxide reductase (norB) genes from pure cultures and environmental samples. Appl. Environ. Microbiol. 69, 3476–3483. doi: 10.1128/AEM.69.6.3476-3483.2003
Broman, E., Zilius, M., Samuiloviene, A., Vybernaite-Lubiene, I., Politi, T., Klawonn, I., et al. (2021). Active DNRA and denitrification in oxic hypereutrophic waters. Water Res. 194:116954. doi: 10.1016/j.watres.2021.116954
Canfield, D. E., Glazer, A. N., and Falkowski, P. G. (2010). The evolution and future of earth’s nitrogen cycle. Science 330, 192–196. doi: 10.1126/science.1186120
Chamchoi, N., Nitisoravut, S., and Schmidt, J. E. (2008). Inactivation of ANAMMOX communities under concurrent operation of anaerobic ammonium oxidation (ANAMMOX) and denitrification. Bioresour. Technol. 99, 3331–3336. doi: 10.1016/j.biortech.2007.08.029
Chang, Y., Yin, G., Hou, L., Liu, M., Zheng, Y., Han, P., et al. (2021). Nitrogen removal processes coupled with nitrification in coastal sediments off the north East China Sea. J. Soils Sediments 21, 3289–3299. doi: 10.1007/s11368-021-02964-5
Chen, Z., Ding, W., Xu, Y., Müller, C., Rütting, T., Yu, H., et al. (2015). Importance of heterotrophic nitrification and dissimilatory nitrate reduction to ammonium in a cropland soil: evidences from a 15N tracing study to literature synthesis. Soil Biol. Biochem. 91, 65–75. doi: 10.1016/j.soilbio.2015.08.026
Chen, S., Gao, D., Zhang, J., Zheng, Y., Li, X., Dong, H., et al. (2023). Gross nitrogen transformations in intertidal sediments of the Yangtze estuary: distribution patterns and environmental controls. Geoderma 429:116233. doi: 10.1016/j.geoderma.2022.116233
Chen, Y., Su, X., Wan, Y., Lyu, H., Dong, W., Shi, Y., et al. (2022). Nitrogen biogeochemical reactions during bank filtration constrained by hydrogeochemical and isotopic evidence: a case study in a riverbank filtration site along the second Songhua River, NE China. Appl. Geochem. 140:105272. doi: 10.1016/j.apgeochem.2022.105272
Cheng, F. Y., Van Meter, K. J., Byrnes, D. K., and Basu, N. B. (2020). Maximizing US nitrate removal through wetland protection and restoration. Nature 588, 625–630. doi: 10.1038/s41586-020-03042-5
Choi, A., Cho, H., Kim, S.-H., Thamdrup, B., Lee, S., and Hyun, J.-H. (2016). Rates of N2 production and diversity and abundance of functional genes associated with denitrification and anaerobic ammonium oxidation in the sediment of the Amundsen Sea Polynya, Antarctica. Deep Sea Res. II Top. Stud. Oceanogr. 123, 113–125. doi: 10.1016/j.dsr2.2015.07.016
Covatti, G., and Grischek, T. (2021). Sources and behavior of ammonium during riverbank filtration. Water Res. 191:116788. doi: 10.1016/j.watres.2020.116788
Crowe, S. A., Canfield, D. E., Sundby, B., and Maranger, R. (2012). Anammox, denitrification and fixed-nitrogen removal in sediments from the lower St Lawrence Estuary. Biogeosciences 9, 4309–4321. doi: 10.5194/bg-9-4309-2012
Daims, H., Lebedeva, E. V., Pjevac, P., Han, P., Herbold, C., Albertsen, M., et al. (2015). Complete nitrification by Nitrospira bacteria. Nature 528, 504–509. doi: 10.1038/nature16461
Deng, F., Hou, L., Liu, M., Zheng, Y., Yin, G., Li, X., et al. (2015). Dissimilatory nitrate reduction processes and associated contribution to nitrogen removal in sediments of the Yangtze Estuary. J. Geophys. Res. Biogeosci. 120, 1521–1531. doi: 10.1002/2015jg003007
DeVries, T., Deutsch, C., Primeau, F., Chang, B., and Devol, A. (2012). Global rates of water-column denitrification derived from nitrogen gas measurements. Nat. Geosci. 5, 547–550. doi: 10.1038/ngeo1515
Ding, B., Li, Z., and Qin, Y. (2017). Nitrogen loss from anaerobic ammonium oxidation coupled to Iron(III) reduction in a riparian zone. Environ. Pollut. 231, 379–386. doi: 10.1016/j.envpol.2017.08.027
Dong, M., Kowalchuk, G. A., Liu, H., Xiong, W., Deng, X., Zhang, N., et al. (2021). Microbial community assembly in soil aggregates: a dynamic interplay of stochastic and deterministic processes. Appl. Soil Ecol. 163:103911. doi: 10.1016/j.apsoil.2021.103911
Fernandes, S. O., Bonin, P. C., Michotey, V. D., Garcia, N., and LokaBharathi, P. A. (2012). Nitrogen-limited mangrove ecosystems conserve N through dissimilatory nitrate reduction to ammonium. Sci. Rep. 2:419. doi: 10.1038/srep00419
Fetzer, J., Holzner, M., Plotze, M., and Furrer, G. (2017). Clogging of an Alpine streambed by silt-sized particles - insights from laboratory and field experiments. Water Res. 126, 60–69. doi: 10.1016/j.watres.2017.09.015
Fowler, D., Coyle, M., Skiba, U., Sutton, M. A., Cape, J. N., Reis, S., et al. (2013). The global nitrogen cycle in the twenty-first century. Philos. Trans. R. Soc. B Biol. Sci. 368:20130164. doi: 10.1098/rstb.2013.0164
Fozia, Z. Y., Hou, L., Zhang, Z., and Liu, M. (2020). Anaerobic ammonium oxidation (anammox) bacterial diversity, abundance, and activity in sediments of the Indus Estuary. Coastal and Shelf. Science 243:106925. doi: 10.1016/j.ecss.2020.106925
Guven, D., Dapena, A., Kartal, B., Schmid, M. C., Maas, B., van de Pas-Schoonen, K., et al. (2005). Propionate oxidation by and methanol inhibition of anaerobic ammonium-oxidizing bacteria. Appl. Environ. Microbiol. 71, 1066–1071. doi: 10.1128/AEM.71.2.1066-1071.2005
Hamersley, M. R., Woebken, D., Boehrer, B., Schultze, M., Lavik, G., and Kuypers, M. M. (2009). Water column anammox and denitrification in a temperate permanently stratified Lake (Lake Rassnitzer, Germany). Syst. Appl. Microbiol. 32, 571–582. doi: 10.1016/j.syapm.2009.07.009
He, Q. L., Zang, F. B., and Yang, M. Y. (2020). Source analysis and pollution evaluation of carbon, nitrogen and phosphorus in river sediments of the Weihe River in Shaanxi Province. J. Soil Water Conserv. :34. doi: 10.13870/j.cnki.stbcxb.2020.02.008
Hou, Z., Nelson, W. C., Stegen, J. C., Murray, C. J., Arntzen, E., Crump, A. R., et al. (2017). Geochemical and microbial community attributes in relation to hyporheic zone geological facies. Sci. Rep. 7:12006. doi: 10.1038/s41598-017-12275-w
Hou, L., Yin, G., Liu, M., Zhou, J., Zheng, Y., Gao, J., et al. (2015). Effects of sulfamethazine on denitrification and the associated N2O release in estuarine and coastal sediments. Environ. Sci. Technol. 49, 326–333. doi: 10.1021/es504433r
Hou, L., Zheng, Y., Liu, M., Gong, J., Zhang, X., Yin, G., et al. (2013). Anaerobic ammonium oxidation (anammox) bacterial diversity, abundance, and activity in marsh sediments of the Yangtze Estuary. J. Geophys. Res. Biogeosci. 118, 1237–1246. doi: 10.1002/jgrg.20108
Hu, J., Ouyang, W., and Yang, Z. (2023). Impacts of extreme climate on nitrogen loss in different forms and pollution risk with the copula model. J. Hydrol. 620:129412. doi: 10.1016/j.jhydrol.2023.129412
Hu, Z., Wessels, H. J. C. T., van Alen, T., Jetten, M. S. M., and Kartal, B. (2019). Nitric oxide-dependent anaerobic ammonium oxidation. Nat. Commun. 10:1244. doi: 10.1038/s41467-019-09268-w
Huang, D. Q., Fu, J. J., Li, Z. Y., Fan, N. S., and Jin, R. C. (2022). Inhibition of wastewater pollutants on the anammox process: a review. Sci. Total Environ. 803:150009. doi: 10.1016/j.scitotenv.2021.150009
Huang, C., Liu, Q., Li, Z.-L., Ma, X.-d., Hou, Y.-N., Ren, N.-Q., et al. (2021). Relationship between functional bacteria in a denitrification desulfurization system under autotrophic, heterotrophic, and mixotrophic conditions. Water Res. 188:116526. doi: 10.1016/j.watres.2020.116526
Huang, X., Weisener, C. G., Ni, J., He, B., Xie, D., and Li, Z. (2020). Nitrate assimilation, dissimilatory nitrate reduction to ammonium, and denitrification coexist in Pseudomonas putida Y-9 under aerobic conditions. Bioresour. Technol. 312:123597. doi: 10.1016/j.biortech.2020.123597
Humbert, S., Tarnawski, S., Fromin, N., Mallet, M.-P., Aragno, M., and Zopfi, J. (2010). Molecular detection of anammox bacteria in terrestrial ecosystems: distribution and diversity. ISME J. 4, 450–454. doi: 10.1038/ismej.2009.125
Jamieson, J., Prommer, H., Kaksonen, A. H., Sun, J., Siade, A. J., Yusov, A., et al. (2018). Identifying and quantifying the intermediate processes during nitrate-dependent Iron(II) oxidation. Environ. Sci. Technol. 52, 5771–5781. doi: 10.1021/acs.est.8b01122
Jia, M., Winkler, M. K. H., and Volcke, E. I. P. (2020). Elucidating the competition between heterotrophic denitrification and DNRA using the resource-ratio theory. Environ. Sci. Technol. 54, 13953–13962. doi: 10.1021/acs.est.0c01776
Jin, R.-C., Yang, G.-F., Yu, J.-J., and Zheng, P. (2012). The inhibition of the anammox process: a review. Chem. Eng. J. 197, 67–79. doi: 10.1016/j.cej.2012.05.014
Kartal, B., Kuypers, M. M. M., Lavik, G., Schalk, J., Op den Camp, H. J. M., Jetten, M. S. M., et al. (2007). Anammox bacteria disguised as denitrifiers: nitrate reduction to dinitrogen gas via nitrite and ammonium. Environ. Microbiol. 9, 635–642. doi: 10.1111/j.1462-2920.2006.01183.x
Kuypers, M. M. M., Marchant, H. K., and Kartal, B. (2018). The microbial nitrogen-cycling network. Nat. Rev. Microbiol. 16, 263–276. doi: 10.1038/nrmicro.2018.9
Lee, C.-g., Fletcher, T. D., and Sun, G. (2009). Nitrogen removal in constructed wetland systems. Eng. Life Sci. 9, 11–22. doi: 10.1002/elsc.200800049
Li, Z., Peng, Y., and Gao, H. (2020). Enhanced long-term advanced denitrogenation from nitrate wastewater by anammox consortia: dissimilatory nitrate reduction to ammonium (DNRA) coupling with anammox in an upflow biofilter reactor equipped with EDTA-2Na/Fe(II) ratio and pH control. Bioresour. Technol. 305:123083. doi: 10.1016/j.biortech.2020.123083
Li, J., Yu, S., and Qin, S. (2020). Removal capacities and environmental constrains of denitrification and anammox processes in eutrophic riverine sediments. Water Air Soil Pollut. 231:274. doi: 10.1007/s11270-020-04593-z
Lin, X. B., Liu, M., Hou, L. J., Gao, D. Z., Li, X. F., Lu, K. J., et al. (2017). Nitrogen losses in sediments of the East China Sea: spatiotemporal variations, controlling factors, and environmental implications. J. Geophys. Res. Biogeosci. 122, 2699–2715. doi: 10.1002/2017JG004036
Liu, C., Hou, L., Liu, M., Zheng, Y., Yin, G., Han, P., et al. (2019). Coupling of denitrification and anaerobic ammonium oxidation with nitrification in sediments of the Yangtze Estuary: importance and controlling factors. Estuar. Coast. Shelf Sci. 220, 64–72. doi: 10.1016/j.ecss.2019.02.043
Liu, X., Hu, S., Sun, R., Wu, Y., Qiao, Z., Wang, S., et al. (2021). Dissolved oxygen disturbs nitrate transformation by modifying microbial community, co-occurrence networks, and functional genes during aerobic-anoxic transition. Sci. Total Environ. 790:148245. doi: 10.1016/j.scitotenv.2021.148245
Liu, Y., Liu, C., Nelson, W. C., Shi, L., Xu, F., Liu, Y., et al. (2017). Effect of water chemistry and hydrodynamics on nitrogen transformation activity and microbial community functional potential in hyporheic zone sediment columns. Environ. Sci. Technol. 51, 4877–4886. doi: 10.1021/acs.est.6b05018
Liu, X., Sun, R., Hu, S., Zhong, Y., and Wu, Y. (2022). Aromatic compounds releases aroused by sediment resuspension alter nitrate transformation rates and pathways during aerobic-anoxic transition. J. Hazard. Mater. 424:127365. doi: 10.1016/j.jhazmat.2021.127365
Liu, W., Yao, L., Jiang, X., Guo, L., Cheng, X., and Liu, G. (2018). Sediment denitrification in Yangtze lakes is mainly influenced by environmental conditions but not biological communities. Sci. Total Environ. 616-617, 978–987. doi: 10.1016/j.scitotenv.2017.10.221
Lu, Y., Xu, L., Shu, W., Zhou, J., Chen, X., Xu, Y., et al. (2017). Microbial mediated iron redox cycling in Fe (hydr)oxides for nitrite removal. Bioresour. Technol. 224, 34–40. doi: 10.1016/j.biortech.2016.10.025
Lu, Y., Zhang, P., Liu, H., Bu, X., Li, Y., Wen, Z., et al. (2022). Effect of dam on iron species distribution and transformation in riparian zones. J. Hydrol. 610:127869. doi: 10.1016/j.jhydrol.2022.127869
Lyu, C., Li, X., Yuan, P., Song, Y., Gao, H., Liu, X., et al. (2021). Nitrogen retention effect of riparian zones in agricultural areas: a meta-analysis. J. Clean. Prod. 315:128143. doi: 10.1016/j.jclepro.2021.128143
Magalhaes, C. M., Joye, S. B., Moreira, R. M., Wiebe, W. J., and Bordalo, A. A. (2005). Effect of salinity and inorganic nitrogen concentrations on nitrification and denitrification rates in intertidal sediments and rocky biofilms of the Douro River estuary, Portugal. Water Res. 39, 1783–1794. doi: 10.1016/j.watres.2005.03.008
Mayer, P. M., Reynolds, S. K. Jr., McCutchen, M. D., and Canfield, T. J. (2007). Meta-analysis of nitrogen removal in riparian buffers. J. Environ. Qual. 36, 1172–1180. doi: 10.2134/jeq2006.0462
McPhillips, L. E., Groffman, P. M., Goodale, C. L., and Walter, M. T. (2015). Hydrologic and biogeochemical drivers of riparian denitrification in an agricultural watershed. Water Air Soil Pollut. 226:226. doi: 10.1007/s11270-015-2434-2
Mohan, S. B., Schmid, M., Jetten, M., and Cole, J. (2004). Detection and widespread distribution of the nrfA gene encoding nitrite reduction to ammonia, a short circuit in the biological nitrogen cycle that competes with denitrification. FEMS Microbiol. Ecol. 49, 433–443. doi: 10.1016/j.femsec.2004.04.012
Mosier, A. C., and Francis, C. A. (2010). Denitrifier abundance and activity across the San Francisco Bay estuary. Environ. Microbiol. Rep. 2, 667–676. doi: 10.1111/j.1758-2229.2010.00156.x
Mulder, A., van de Graaf, A. A., Robertson, L. A., and Kuenen, J. G. (1995). Anaerobic ammonium oxidation discovered in a denitrifying fluidized bed reactor. FEMS Microbiol. Ecol. 16, 177–184. doi: 10.1111/j.1574-6941.1995.tb00281
Nielsen, L. P. (1992). Denitrification in sediment determined from nitrogen isotope pairing. FEMS Microbiol. Lett. 86, 357–362. doi: 10.1111/j.1574-6968.1992.tb04828.x
Nils Risgaard-Petersen, L. P. N., Rysgaard, S., Dalsgaard, T., and Meyer, R. L. (2003). Application of the isotope pairing technique in sediments whereanammox and denitrification coexist. Limnol. Oceanogr. Methods 1, 63–73. doi: 10.4319/lom.2003.1.63
Niswonger, R., Naranjo, R., Smith, D., Constantz, J., Allander, K., Rosenberry, D., et al. (2017). Nutrient processes at the stream-lake interface for a channelized versus unmodified stream mouth. Water Resour. Res. 53, 237–256. doi: 10.1002/2016WR019538
Peralta-Maraver, I., Reiss, J., and Robertson, A. L. (2018). Interplay of hydrology, community ecology and pollutant attenuation in the hyporheic zone. Sci. Total Environ. 610-611, 267–275. doi: 10.1016/j.scitotenv.2017.08.036
Qiao, Z., Sun, R., Wu, Y., Hu, S., Liu, X., Chan, J., et al. (2020). Characteristics and metabolic pathway of the bacteria for heterotrophic nitrification and aerobic denitrification in aquatic ecosystems. Environ. Res. 191:110069. doi: 10.1016/j.envres.2020.110069
Ro, H. M., Kim, P. G., Park, J. S., Yun, S. I., and Han, J. (2018). Nitrogen removal through N cycling from sediments in a constructed coastal marsh as assessed by (15)N-isotope dilution. Mar. Pollut. Bull. 129, 275–283. doi: 10.1016/j.marpolbul.2018.02.037
Ruan, Y., Taherzadeh, M. J., Kong, D., Lu, H., Zhao, H., Xu, X., et al. (2020). Nitrogen removal performance and metabolic pathways analysis of a novel aerobic denitrifying halotolerant Pseudomonas balearica strain RAD-17. Microorganisms 8:72. doi: 10.3390/microorganisms8010072
Rütting, T., Boeckx, P., Müller, C., and Klemedtsson, L. (2011). Assessment of the importance of dissimilatory nitrate reduction to ammonium for the terrestrial nitrogen cycle. Biogeosciences 8, 1779–1791. doi: 10.5194/bg-8-1779-2011
Schmid, M. C., Risgaard-Petersen, N., van de Vossenberg, J., Kuypers, M. M. M., Lavik, G., Petersen, J., et al. (2007). Anaerobic ammonium-oxidizing bacteria in marine environments: widespread occurrence but low diversity. Environ. Microbiol. 9, 1476–1484. doi: 10.1111/j.1462-2920.2007.01266.x
Sharma, B., and Ahlert, R. C. (1977). Nitrification and nitrogen removal. Water Res. 11, 897–925. doi: 10.1016/0043-1354(77)90078-1
Shen, L. D., Liu, X., and Wu, H. S. (2018). Importance of anaerobic ammonium oxidation as a nitrogen removal pathway in freshwater marsh sediments. J. Appl. Microbiol. 125, 1423–1434. doi: 10.1111/jam.14042
Shi, P., Zhang, Y., Song, J., Li, P., Wang, Y., Zhang, X., et al. (2019). Response of nitrogen pollution in surface water to land use and social-economic factors in the Weihe River watershed, Northwest China. Sustain. Cities Soc. 50:101658. doi: 10.1016/j.scs.2019.101658
Song, H., Li, Z., Du, B., Wang, G., and Ding, Y. (2012). Bacterial communities in sediments of the shallow Lake Dongping in China. J. Appl. Microbiol. 112, 79–89. doi: 10.1111/j.1365-2672.2011.05187.x
Soonmo, A., and Wayne, S. G. (2002). Dissimilatory nitrate reduction to ammonium (DNRA) as a nitrogen link, versus denitrification as a sink in a shallow estuary (Laguna Madre/Baffin Bay, Texas). Mar. Ecol. Prog. Ser. 237, 41–50. doi: 10.3354/meps237041
Stevens, R. J., Laughlin, R. J., and Malone, J. P. (1998). Soil pH affects the processes reducing nitrate to nitrous oxide and di-nitrogen. Soil Biol. Biochem. 30, 1119–1126. doi: 10.1016/S0038-0717(97)00227-7
Su, P., Wang, X., Lin, Q., Peng, J., Song, J., Fu, J., et al. (2019). Variability in macroinvertebrate community structure and its response to ecological factors of the Weihe River Basin, China. Ecol. Eng. 140:105595. doi: 10.1016/j.ecoleng.2019.105595
Sun, L., Liang, X., Jin, M., Ma, B., Zhang, X., and Song, C. (2021a). Ammonium and nitrate sources and transformation mechanism in the quaternary sediments of Jianghan plain, China. Sci. Total Environ. 774:145131. doi: 10.1016/j.scitotenv.2021.145131
Sun, L., Wang, C., Yu, H., Liu, D., Houlton, B. Z., Wang, S., et al. (2021b). Biotic and abiotic controls on dinitrogen production in coastal sediments. Glob. Biogeochem. Cycles 35:35. doi: 10.1029/2021gb007069
Throback, I. N., Enwall, K., Jarvis, A., and Hallin, S. (2004). Reassessing PCR primers targeting nirS, nirK and nosZ genes for community surveys of denitrifying bacteria with DGGE. FEMS Microbiol. Ecol. 49, 401–417. doi: 10.1016/j.femsec.2004.04.011
Tiedje, J. M., Sexstone, A. J., Myrold, D. D., and Robinson, J. A. (1983). Denitrification: ecological niches, competition and survival. Antonie Van Leeuwenhoek 48, 569–583. doi: 10.1007/BF00399542
Trimmer, M., Risgaard-Petersen, N., Nicholls, J. C., and Engström, P. (2006). Direct measurement of anaerobic ammonium oxidation (anammox) and denitrification in intact sediment cores. Mar. Ecol. Prog. Ser. 326, 37–47. doi: 10.3354/meps326037
Vieira, S., Luckner, M., Wanner, G., and Overmann, J. (2017). Luteitalea pratensis gen. nov., sp. nov. a new member of subdivision 6 Acidobacteria isolated from temperate grassland soil. Int. J. Syst. Evol. Microbiol. 67, 1408–1414. doi: 10.1099/ijsem.0.001827
Vuono, D. C., Read Rw, J. H., Benjamin, W., and Sullivan, G. J. J. (2019). Resource limitation modulates the fate of dissimilated nitrogen in a dual-pathway Actinobacterium. Front. Microbiol. 10:3. doi: 10.3389/fmicb.2019.00003
Wang, Z., Ahmad, H. A., Teng, Z.-J., Sun, M., Ismail, S., Qiao, Z., et al. (2022). Widespread but overlooked DNRA process in a full-scale simultaneous partial nitrification, anammox, and denitrification plant. ACS ES&T Water 2, 1360–1369. doi: 10.1021/acsestwater.2c00088
Wang, H., Deng, N., Wu, D., Hu, S., and Kou, M. (2017). Long-term net transformation and quantitative molecular mechanisms of soil nitrogen during natural vegetation recovery of abandoned farmland on the Loess Plateau of China. Sci. Total Environ. 607-608, 152–159. doi: 10.1016/j.scitotenv.2017.07.014
Wang, S., Liu, C., Wang, X., Yuan, D., and Zhu, G. (2020a). Dissimilatory nitrate reduction to ammonium (DNRA) in traditional municipal wastewater treatment plants in China: widespread but low contribution. Water Res. 179:115877. doi: 10.1016/j.watres.2020.115877
Wang, S., Pi, Y., Song, Y., Jiang, Y., Zhou, L., Liu, W., et al. (2020b). Hotspot of dissimilatory nitrate reduction to ammonium (DNRA) process in freshwater sediments of riparian zones. Water Res. 173:115539. doi: 10.1016/j.watres.2020.115539
Wang, W., Song, J., Zhang, G., Liu, Q., Guo, W., Tang, B., et al. (2018). The influence of hyporheic upwelling fluxes on inorganic nitrogen concentrations in the pore water of the Weihe River. Ecol. Eng. 112, 105–115. doi: 10.1016/j.ecoleng.2017.12.012
Wang, L., Wang, Y., Li, Y., Wang, L., Zhu, J., Zhang, W., et al. (2022). Effect of water chemistry on nitrogen transformation, dissolved organic matter composition and microbial community structure in hyporheic zone sediment columns. Environ. Res. 215:114246. doi: 10.1016/j.envres.2022.114246
Wang, Z., Wang, W., Zhang, Z., Hou, X., Ma, Z., and Chen, B. (2021). River-groundwater interaction affected species composition and diversity perpendicular to a regulated river in an arid riparian zone. Glob. Ecol. Conserv. 27:e01595. doi: 10.1016/j.gecco.2021.e01595
Wang, S., Wang, W., Zhao, S., Wang, X., Hefting, M. M., Schwark, L., et al. (2019). Anammox and denitrification separately dominate microbial N-loss in water saturated and unsaturated soils horizons of riparian zones. Water Res. 162, 139–150. doi: 10.1016/j.watres.2019.06.052
Welsh, A., Chee-Sanford, J. C., Connor, L. M., Loffler, F. E., and Sanford, R. A. (2014). Refined NrfA phylogeny improves PCR-based nrfA gene detection. Appl. Environ. Microbiol. 80, 2110–2119. doi: 10.1128/AEM.03443-13
Wen, G., Wang, T., Huang, T.-L., Wan, Q.-Q., Cao, R.-H., Kou, L.-Q., et al. (2020). Nitrogen removal performance and nitrogen/carbon balance of oligotrophic aerobic denitrifiers. Huan Jing Ke Xue 41, 2339–2348. doi: 10.13227/j.hjkx.201911201
Wu, H., Hao, B., Zhou, Q., Xu, K., Cai, Y., and Liu, G. (2021). Contribution of various categories of environmental factors to sediment nitrogen-removal in a low C/N ratio river. Ecol. Eng. 159:106121. doi: 10.1016/j.ecoleng.2020.106121
Wurtsbaugh, W. A., Paerl, H. W., and Dodds, W. K. (2019). Nutrients, eutrophication and harmful algal blooms along the freshwater to marine continuum. WIREs Water 6:e1373. doi: 10.1002/wat2.1373
Xing, W., Li, D., Li, J., Hu, Q., and Deng, S. (2016). Nitrate removal and microbial analysis by combined micro-electrolysis and autotrophic denitrification. Bioresour. Technol. 211, 240–247. doi: 10.1016/j.biortech.2016.03.044
Yang, C., Zhang, Y.-K., Liu, Y., Yang, X., and Liu, C. (2018). Model-based analysis of the effects of dam-induced river water and groundwater interactions on hydro-biogeochemical transformation of redox sensitive contaminants in a hyporheic zone. Water Resour. Res. 54, 5973–5985. doi: 10.1029/2018WR023286
Yao, X., Zhang, L., Zhang, Y., Zhang, B., Zhao, Z., Zhang, Y., et al. (2018). Nitrogen fixation occurring in sediments: contribution to the nitrogen budget of Lake Taihu, China. J. Geophys. Res. 123, 2661–2674. doi: 10.1029/2018jg004466
Yin, G., Hou, L., Liu, M., Li, X., Zheng, Y., Gao, J., et al. (2017). DNRA in intertidal sediments of the Yangtze Estuary. J. Geophys. Res. Biogeosci. 122, 1988–1998. doi: 10.1002/2017JG003766
Yin, G., Hou, L., Liu, M., Liu, Z., and Gardner, W. S. (2014). A novel membrane inlet mass spectrometer method to measure (1)(5)NH4(4)(+) for isotope-enrichment experiments in aquatic ecosystems. Environ. Sci. Technol. 48, 9555–9562. doi: 10.1021/es501261s
Yuan, H., Jia, B., Zeng, Q., Zhou, Y., Wu, J., Wang, H., et al. (2022). Dissimilatory nitrate reduction to ammonium (DNRA) potentially facilitates the accumulation of phosphorus in lake water from sediment. Chemosphere 303:134664. doi: 10.1016/j.chemosphere.2022.134664
Zhang, H., Ma, B., Huang, T., Yang, W., Liu, X., and Niu, L. (2022). Nitrogen removal from low carbon/nitrogen polluted water is enhanced by a novel synthetic micro-ecosystem under aerobic conditions: novel insight into abundance of denitrification genes and community interactions. Bioresour. Technol. 351:127013. doi: 10.1016/j.biortech.2022.127013
Zhang, S., Qin, W., Bai, Y., Zhang, Z., Wang, J., Gao, H., et al. (2020). Linkages between anammox and denitrifying bacterial communities and nitrogen loss rates in high-elevation rivers. Limnol. Oceanogr. 66, 765–778. doi: 10.1002/lno.11641
Zhang, X., Zhang, Q., Yang, A., Hou, L., Zheng, Y., Zhai, W., et al. (2018). Incorporation of microbial functional traits in biogeochemistry models provides better estimations of benthic denitrification and anammox rates in coastal oceans. J. Geophys. Res. Biogeosci. 123, 3331–3352. doi: 10.1029/2018JG004682
Zhao, H., Huang, Y., You, S., Wu, Y., and Zheng, F. (2019). A framework for assessing the effects of afforestation and south-to-north water transfer on nitrogen and phosphorus uptake by plants in a critical riparian zone. Sci. Total Environ. 651, 942–952. doi: 10.1016/j.scitotenv.2018.09.226
Zhao, Y., Yuan, F., Li, S., Zhang, J., Li, Y., Shi, X., et al. (2022). Assembly mechanism and co-occurrence patterns of DNRA microbial communities and imprint of nitrate reduction in the Songhua River sediments of China’s largest old industrial base. J. Environ. Manag. 322:116091. doi: 10.1016/j.jenvman.2022.116091
Zhao, S., Zhang, B., Sun, X., and Yang, L. (2021). Hot spots and hot moments of nitrogen removal from hyporheic and riparian zones: a review. Sci. Total Environ. 762:144168. doi: 10.1016/j.scitotenv.2020.144168
Zheng, Y. L., Hou, L. J., Chen, F. Y., Zhou, J., Liu, M., Yin, G. Y., et al. (2020). Denitrifying anaerobic methane oxidation in intertidal marsh soils: occurrence and environmental significance. Geoderma 357:113943. doi: 10.1016/j.geoderma.2019.113943
Zheng, Y., Hou, L., Zhang, Z., Ge, J., Li, M., Yin, G., et al. (2021). Overlooked contribution of water column to nitrogen removal in estuarine turbidity maximum zone (TMZ). Sci. Total Environ. 788:147736. doi: 10.1016/j.scitotenv.2021.147736
Keywords: riparian zone, denitrification, nitrogen removal, stable isotope technology, functional genes
Citation: Ye F, Duan L, Sun Y, Yang F, Liu R, Gao F, Wang Y and Xu Y (2023) Nitrogen removal in freshwater sediments of riparian zone: N-loss pathways and environmental controls. Front. Microbiol. 14:1239055. doi: 10.3389/fmicb.2023.1239055
Edited by:
Yaoguo Wu, Northwestern Polytechnical University, ChinaReviewed by:
Qian Zhang, Chongqing University of Technology, ChinaXiaoxia Wang, Qingdao University, China
Lei Miao, Huazhong University of Science and Technology, China
Copyright © 2023 Ye, Duan, Sun, Yang, Liu, Gao, Wang and Xu. This is an open-access article distributed under the terms of the Creative Commons Attribution License (CC BY). The use, distribution or reproduction in other forums is permitted, provided the original author(s) and the copyright owner(s) are credited and that the original publication in this journal is cited, in accordance with accepted academic practice. No use, distribution or reproduction is permitted which does not comply with these terms.
*Correspondence: Yaqiao Sun, c3VueWFxaWFvQDEyNi5jb20=