- 1Food Science and Nutrition Department, Veterinary Faculty, Regional Campus of International Excellence Campus Mare Nostrum, University of Murcia, Murcia, Spain
- 2Biomedical Research Institute of Murcia (IMIB-Arrixaca), Murcia, Spain
- 3Microbiology Service, Virgen de La Arrixaca University Hospital, Murcia, Spain
Maternal microbiota forms the first infant gut microbial inoculum, and perinatal factors (diet and use of antibiotics during pregnancy) and/or neonatal factors, like intra partum antibiotics, gestational age and mode of delivery, may influence microbial colonization. After birth, when the principal colonization occurs, the microbial diversity increases and converges toward a stable adult-like microbiota by the end of the first 3–5 years of life. However, during the early life, gut microbiota can be disrupted by other postnatal factors like mode of infant feeding, antibiotic usage, and various environmental factors generating a state of dysbiosis. Gut dysbiosis have been reported to increase the risk of necrotizing enterocolitis and some chronic diseases later in life, such as obesity, diabetes, cancer, allergies, and asthma. Therefore, understanding the impact of a correct maternal-to-infant microbial transfer and a good infant early colonization and maturation throughout life would reduce the risk of disease in early and late life. This paper reviews the published evidence on early-life gut microbiota development, as well as the different factors influencing its evolution before, at, and after birth, focusing on diet and nutrition during pregnancy and in the first months of life.
1. Introduction
The human body harbors trillions of microorganisms whose coordinated actions are believed to be important for human life. Such microbial cell populations reach their highest density in the gut compartment, where they collectively form a complex microbial community known as the gut microbiota, which develops over the first 3 years of life until it reaches its adult form (Milani et al., 2017). The gut microbiota is composed of a significant number of different bacteria, approximately 160 species per person per fecal sample, and this ecosystem plays an important role in human health (Rodríguez et al., 2015). Gut microbiota members may belong to any of the three domains of life, i.e., Archea, Bacteria, and Eucarya, and also includes viruses. The gut microbiota is dominated by two phyla, Bacteroidetes and Firmicutes, followed by Actinobacteria, Proteobacteria, Verrucomicrobia, Fusobacteria, and Cyanobacteria in lower proportions (González-Bermúdez et al., 2018). Bacteroidetes, which account for 90% of the gut microbiota, include bacteria belonging to the genus Bacteroides and Prevotella. Firmicutes is constituted by a large number of genera, the most important being Lactobacillus and Clostridium, and Proteobacteria contains the genera Enterobacteriaceae. The main genus belonging to the Actinobacteria phylum in the human intestine is Bifidobacterium (Sebastián-Domingo and Sánchez-Sánchez, 2018). These microorganisms are known to establish complex trophic relationships with each other and their human host, ranging from symbiosis to parasitism (Milani et al., 2017).
In general, during childhood, various behaviors of the infant such as skin-to-skin contact with the mother, the introduction of objects, as well as parts of the body in the mouth like hands and foots, especially during the stage of crawling, are actions that promote the exposure to microbes (Arrieta et al., 2014). In addition to these actions, there are some windows of opportunity for microbiota modulation, from pregnancy to childhood, where the microbiota is more affected by environmental factors.
Until now, it was believed that during the first year of life, the infant gastrointestinal microbiota should evolve from being almost sterile, as the “sterile uterus paradigm” proposes, to a complex and varied community, with its composition and structure subjected to great variability (Funkhouser and Bordenstein, 2013). However, recent studies suggest that infants host an initial microbiome from the mother through the amniotic fluid (DiGiulio, 2012; Wang et al., 2013; Collado et al., 2016; Rehbinder et al., 2018), umbilical cord blood (Jiménez et al., 2005; Wang et al., 2013) and fetal membranes (Jones et al., 2009) and receive a maternal microbe supplementation through birth and breastfeeding. As a result, for the process of transmission and when it takes place, there are currently different theories among the scientific community, but there is a certain consensus on the main factors that can influence the transmission and subsequent colonization of the intestinal microbiota of the infant, with maternal diet during gestation, use of antibiotics including intrapartum antibiotics and type of delivery being the three main influential prenatal factors (Biasucci et al., 2008; Huurre et al., 2008; Mueller et al., 2015a; Kuperman and Koren, 2016; Mirpuri, 2020; Taylor et al., 2023).
The gestation period is one of these critical windows and focusing on diet, maternal diet has been considered a key factor that could play an important role in the colonization process in several ways. One of these is because newborns are colonized with vaginal and rectal strains from the mother transiently during delivery; this maternal microbiota could be affected by the dietary pattern during pregnancy defining the maternal microbiota and therefore the colonization of the newborn (Jiménez et al., 2005).
For example, it has been shown that maternal diet and fetal immune development are related through changes in the mother’s gut microbiota (Kaplan et al., 2011; Li et al., 2014; Boutin and Finlay, 2016; Chu et al., 2016a; Mirpuri, 2020). Dietary changes during pregnancy may affect the microbial composition and diversity of the pregnant gut, as well as the production of short-chain fatty acids (SCFA). SCFA, propionate, acetate and butyrate, are the main metabolites formed by gut bacteria through the microbial fermentation of microbiota-accessible carbohydrates (MAC) and are the main source of energy for colon cells (Alsharairi, 2020a). Among many of their functions, these metabolites affect T lymphocytes and dendritic cells by binding to protein-coupled receptors and directly inhibiting histone deacetylases, which promotes the differentiation of T-helper cells (Th1, Th2; Stiemsma and Turvey, 2017; Panzer and Lynch, 2015). A diet low in MAC has been shown to reduce bacterial diversity and SCFA production, which may interfere with regulatory T-cell function and lead to inhibition of immunoglobulin A and G (IgA, IgG) production (Alsharairi, 2020a). Also, data from animal models suggests that gut microbiome transferred through the placenta produces several metabolites that act as important mediators in the development of fetal immunity (Gray et al., 2017). Therefore, understanding how the whole process of transmission, colonization, and microbial evolution takes place during the first months of neonatal life is crucial.
Another route by which maternal diet during pregnancy can lead to changes in the microbiota of the infant is through breast milk. Several authors have observed variations in breast milk microbiota and other important bioactive compounds due to the diet of the mother during pregnancy (review by Taylor et al., 2023). This microbiota, present in breast milk, will be another source of colonization during the infant’s early life and may therefore influence the colonization patterns and establishment of the infant’s gut microbiota.
To date, there are published studies that attempt to gather information on how the various factors mentioned above influence the gut microbiota of the infant (Funkhouser and Bordenstein, 2013; Milani et al., 2017; Chong et al., 2018; Selma-Royo et al., 2019). However, publications focusing on diet (García-Mantrana et al., 2016; Chu et al., 2016a; Maher et al., 2020; Mirpuri, 2020; Miko et al., 2022; Taylor et al., 2023) have not covered the prenatal and postnatal period together and have not considered other modulatory factors that play an important role in the colonization process and development of the infant gut microbiota. All these aspects have been included in this review.
The present review is intended as a synopsis summarizing the different research and articles that focus on all these factors, with emphasis on diet. A critical appraisal of other research has been made with the aim to put them in context in an orderly, precise, and analytical manner while pointing out the similarities and differences in the literature reviewed.
2. Methods
A literature search was performed using the electronic databases PubMed/Medline and Scopus with no date limits, for the description of the “theory of colonization in the uterus” in a timeline format, and with date limits (from January 1, 2000 to July 5, 2023) for the analysis of prenatal and postnatal factors that influence the intestinal microbiota of the infant. The most relevant published studies were identified in an independently way by the authors.
The keywords used were (alone or in combination): colonization, meconium, amniotic fluid, uterus, sterile uterus paradigm, intestinal microbiota, pregnancy, lactation, diet, perinatal/postnatal, factors, antibiotics, gestational age, delivery, geographical, siblings, lifestyle, probiotics, dietary pattern, breastmilk, infant formula, weaning, complementary feeding, vegetarian diet, high fat diet, ketogenic diet, gluten-free diet, and infant gut microbiota. The search included reviews/systematic reviews, meta-analyses, randomized controlled trials/experimental studies, and observational studies (case, cross-sectional, case–control, cohort reports) published in English.
3. Transmission, colonization and evolution of the infant gut microbiota
3.1. Prenatal transmission and colonization: “The sterile womb paradigm” vs. “in-utero colonization hypothesis”
Until recently, the in-utero environment has been considered sterile under normal conditions, and the colonization process was thought to begin at birth when the infant is exposed to the microbiota of the mother and the environment (Koleva et al., 2015). According to this concept, microbes are acquired both vertically (from the mother) and horizontally (from other humans or the environment) during and after the birth (Perez-Muñoz et al., 2017).
Since the studies of Escherich (1989), who first describe the meconium (the earliest stool from an infant) to be free of viable bacteria, the idea that term fetuses are sterile in utero has been widely accepted (Perez-Muñoz et al., 2017). A few years later, Tissier (1900) established that bacterial colonization of the newborn occurs when the newborn initiates transit through the birth channel via contamination by maternal vaginal and fecal bacteria. The process continues after delivery and progressively the intestinal lumen of the newborn becomes colonized by temporal microbiota. The abundance and variety suffer changes over time to culminate into a relatively stable microbial composition seen throughout adulthood (Jiménez et al., 2008). Most of the studies that established the sterile womb paradigm employed traditional culture-based methods and microscopy, which are still considered valid today despite these may fail to detect viable but non-cultivable microbes (Milani et al., 2017).
For more than a century, the hypothesis of Escherich was generally accepted even though other three additional independent studies conducted in 1927 (Burrage, 1927), 1934 (Hall, 1934), and 1936 (Snyder, 1936), suggesting some microbial activity in meconium which supposed a conflict between both ideas.
After the early studies on the meconium discussed above, further microbiological research on this topic ceased for a period of over 60 years (Koleva et al., 2015) until Jiménez et al. (2008). They reported 100% (n = 21) of meconium to be positive for bacteria by culture techniques and therefore rebut the non-sterility of infant meconium shown by earlier researchers. Two years later and using non-culture-based techniques, Mshvildadze et al. (2010) provided further evidence on the presence of bacteria in meconium obtained from neonates born at 22 to 32 weeks gestational age. These authors detected microbial DNA in 91% of samples and denaturing gradient gel electrophoresis (DGGE) profiling revealed an association between prematurity and reduced meconium microbial diversity.
There is now a multitude of recent studies employing next-generation DNA sequencing techniques that have challenged the traditional view of human microbiome acquisition. These studies propose that neither the fetus, the placenta, or the amniotic fluid are sterile, and that acquisition and colonization of the human gastrointestinal tract begins in utero, what is known as “in-utero colonization hypothesis” (Jiménez et al., 2008; Aagaard et al., 2014; Collado et al., 2016).
Madan et al. (2012), in a prospective longitudinal study, applied high throughput pyrosequencing of the hypervariable V6 region of the 16S rRNA gene to understand the gut microbial colonization in prematurity; they found that meconium of all subjects (n = 6) was not sterile, being Lactobacillus, Staphylococcus and Enterobacteriaceae the predominant bacterial genera. Gosalbes et al. (2013) characterized meconium microbiota in 20 term newborns from a Spanish birth cohort, to assess whether it contributes to the future microbiota of the infant’s gastrointestinal tract, and to evaluate how it relates to lifestyle variables and atopic-conditions. Their conclusion was that the microbial pattern differed from the microbiota of feces, vagina, and skin from adults but was similar to that of young infant feces. Another conclusion of this study was that meconium microbiota has an intrauterine origin, which is influenced by maternal factors and may have consequences for childhood health.
A few years later, Moles et al. (2013) aimed to characterize the evolution of gut microbiota during the first 3 weeks of life of 14 preterm neonates, including the meconium microbiota. The bacterial diversity and taxonomy were examined using culture-dependent and molecular techniques, including DGGE and Human Intestinal Tract Chip (HITChip) analysis of 16S rRNA amplicons. Both approaches showed that spontaneously released meconium of such neonates contains a specific microbiota that differs from those observed in early fecal samples (Rodríguez et al., 2015). The phylum Bacilli and Firmicutes were the main bacteria groups detected in meconium, while Proteobacteria phylum was more abundant in fecal samples. Another study, carried out by Hu et al. (2013), aimed to assess if the bacterial community of meconium is affected by maternal diabetes status. Samples were collected from 23 newborns stratified by maternal diabetes status and the microbiome was profiled using multi-barcode 16S rRNA sequencing followed by taxonomic assignment and diversity analysis. A diversified microbiota was found in all meconium samples, which was not affected by the mode of delivery, showing a lower species diversity, higher sample-to-sample variation, enrichment of Proteobacteria and reduction of Bacteroidetes compared to adult feces. The taxonomy analyses, among the meconium samples, suggested that the overall bacterial content in meconium significantly differed by maternal diabetes status. Specifically, in those samples of the diabetes group, the phyla Bacteroidetes and the genus Parabacteroides were enriched. Two years later, one study by Hansen et al. (2015), showed evidences of microbial presence in 66% (10 of 15) of meconium samples, from 15 healthy full-term vaginally-delivered infants. The taxa which predominated in meconium belonged to Bifidobacterium, Enterobacteriaceae, Enterococcaceae, and Bacteroides-Prevotella (Koleva et al., 2015).
Shi et al. (2018) used a metagenomic sequencing technique to characterize the meconium microbiome from a Chinese cohort of vaginally and C-section delivered infants (CSDI); the authors founded, in contrast to Hu et al. (2013) the meconium microbiome diversity was higher in vaginally delivered infants (VDI) than that in CSDI. Propionibacterium species were most abundant in the VDI, whereas the CSDI group had high levels of Bacillus licheniformis. Moreover, different modes of delivery affected the antibiotic resistance gene prevalence, what might influence the infant’s health later in life. In this sense, Dominguez-Bello et al. (2010) also studied the impact of birth mode on bacterial communities in a rectal swab obtained at birth. They also found that the meconium microbiota composition of full-term infants (FTI) was also influenced by the mode of delivery (Koleva et al., 2015).
Furthermore, Tapiainen et al. (2018) investigated if maternal factors during pregnancy, such as the environment, influence the microbiome of the first stool more than immediate perinatal factors. Regions of the bacterial 16S rRNA gene were sequenced to characterize the microbiome of the first-pass meconium samples (n = 212). With a relative abundance of 44 %, Firmicutes was the most abundant phyla, Proteobacteria, 28 %, and Bacteroidetes, 15 %. The diversity of microbiome was increased by the biodiversity of the home environment, whereas perinatal factors, such as the delivery mode or exposure to antimicrobials during the birth did not have any effect.
In summary, despite the fact that culture and molecular techniques in diverse publication have provided preliminary evidences for diverse groups of bacteria in meconium from both FTI and preterm infant, its origin remains unclear. Meconium microbial communities have low species diversity and high inter-individual variability, thus being very similar to early fecal microbiota (Hu et al., 2013; Moles et al., 2013). At the phylum level, meconium microbiota looks more closely to gut microbes of infants than of adults (Dominguez-Bello et al., 2010). Despite this similarity, when compared meconium with fecal samples at 3 and 12 months, meconium was found to be less abundant in Bacteroides and Bifidobacterium species and therefore more likely to be colonized with Escherichia-Shigella and Enterococcus (Bäckhed et al., 2015). Moreover, the high similarity between meconium and amniotic fluid microbes (Ardissone et al., 2014), and the fact that large quantities of amniotic fluid are swallowed by fetus in the last trimester of pregnancy (Gilbert and Brace, 1993), leads to the assumption that the meconium microbiota may have an intrauterine origin. As we reviewed in detail in the next section, these findings contradict the classic dogma whose main idea is that the newborn comes from a sterile environment and suggest that the establishment of intestinal microbiota is initiated in the prenatal gut (Koleva et al., 2015).
On the other hand, in two recently published papers (Dos Santos et al., 2021; Kennedy et al., 2021), the authors have compared the sequencing of meconium samples and negative controls (a swab exposed to operating theatre air during delivery, genomic prep reagents either exposed to PCR hood air during sample preparation or not exposed and PCR amplifications without added template DNA) traying to elucidate if meconium is sterile or not, and in both they observed no significant differences in the number of reads or taxonomic composition between negative controls and meconium, which again supports “the sterile womb paradigm.”
3.2. Origin of meconium microbiota and routes of transmission
Thanks to the new advances in sequencing and molecular technologies, it has been possible to study the microbiome of areas considered sterile. Some recent studies have provided evidences of the presence of microbes in placental tissue (Aagaard et al., 2014), amniotic fluid (DiGiulio, 2012; Wang et al., 2013), umbilical cord blood (Jiménez et al., 2005), fetal membranes (Jones et al., 2009) and meconium (Hu et al., 2013; Moles et al., 2013; Collado et al., 2016; Shi et al., 2018) the existence of functional pathways that allow a bacterial exposure with the fetus during the stage of pregnancy (Koleva et al., 2015).
While vaginal microbes associated with preterm birth can get access to the uterine environment through an ascending route, the mechanisms by which gut bacteria reach this human niche (placenta, amniotic fluid and umbilical cord) are not well understood.
There are still many doubts about what could be the mechanisms by which intestinal bacteria gain access to the uterine environment. It has been suggested that gut microorganisms after translocation of the gut epithelium, are able to travel to the placenta through the bloodstream. It is known that one of the main roles of the intestinal epithelial barrier is to prevent microbial entry into the circulatory system, but dendritic cells can actively penetrate the intestinal epithelium to the intestinal lumen where bacteria are present, elevate these live bacteria and transport them through the body as they migrate to the lymphoid organs (Rodríguez et al., 2015).
Furthermore, bacterial species that are normally found in the human oral cavity have also been isolated from the amniotic fluid and probably these bacteria, during periodontal infections, may have access to the bloodstream thanks to the inflammation of the gums. The main bacterial species, with an oral origin, found in amniotic fluid are Fusobacterium nucleatum, Streptococcus spp., Bergeyella spp., Porphyromonas gingivalis, Rothia dentocariosa, and Filfactor alocis (Funkhouser and Bordenstein, 2013).
To test whether maternal gut bacteria can be transferred to fetuses in utero, two pioneer studies investigated if oral administration of a genetically labelled Enterococcus faecium to pregnant mice resulted in its presence in amniotic fluid and meconium of term off-spring after sterile c-section (CS; Jiménez et al., 2005, 2008). In both studies, Enterococcus faecium genetically distinctive strain was detected by polymerase chain reaction in the intestinal lumen of pups delivered 1 day prematurely by CS. Also, E. faecium with the genetic label was cultured from amniotic fluid and meconium of pups from inoculated mothers. In contrast, it could not be detected in the samples obtained from a non-inoculated control group.
On the other hand, other studies have concluded that there is no evidence of a placenta, amniotic fluid and/or early contact with microbes (Lauder et al., 2016; Rehbinder et al., 2018; Panzer et al., 2023) and therefore, the in-utero colonization hypothesis continues to be the subject of debate (Selma-Royo et al., 2019). A review published by Perez-Muñoz et al. (2017) concluded that current scientific evidence does not support the existence of microorganisms in the fetal healthy environment.
With new molecular and sequencing techniques, it has been possible to overcome the limitation of culture-based methods, but it is true that the data that support the in-utero colonization hypothesis must be taken with extreme caution, because of particular methodological limitations. For example, the bacterial DNA detected may belong to non-viable microorganisms, that is, dead microorganisms which cannot be detected by culture-based techniques. In the case of studies carried out in placenta this consideration is particularly important because it plays a key role in the elimination of microbes and other components that may be ubiquitous in the blood (Perez-Muñoz et al., 2017; Panzer et al., 2023). Another important methodological issue is that the highly sensitive molecular techniques employed to study the low microbial biomass, may detect contaminating microbes and therefore produce false-positive results (Milani et al., 2017).
4. Microbial colonization and their evolution during the first months of life: how the establishment of the microbiota occurs?
It is from birth when the human microbial colonization process begins to a greater extent and continues to develop and modulate in species abundance for about 3 years, until the microbiota becomes adult-like. A great number of trials in both animal models and humans, suggests that this period constitutes a critical window for immunological and physiological development (Arrieta et al., 2015).
For example, the intestinal-barrier plays an important role in the defense against infections, and nutritional, endocrine, and immune functions. Increased permeability may cause beneficial effects but also may enhance the uptake of microorganisms and foreign antigens, leading to risk of development of infection, inflammation and systemic hypersensitivity (Łoniewska et al., 2020). At birth, intestine is highly permeable and then drops sharply after delivery, which leads to a process known as “gut closure” during the first year of life. It has been shown that the use of antibiotics and vaginal delivery have been associated with an increase in the intestinal permeability during this period (Łoniewska et al., 2020) and that Ruminococcus (torques group) might be one of the microorganisms most involved in controlling paracellular permeability (Kaczmarczyk et al., 2021).
The great advances in metagenomic technologies during the last 20 years have allowed us to know more accurately the composition of the intestinal microbiota since early infancy (Bäckhed et al., 2015; Hill et al., 2017; Murphy et al., 2017). Different genotype-based studies have provided definitive evidence of the transmission of specific microbes from mothers to the gut of their infants (Dominguez-Bello et al., 2010; Bäckhed et al., 2015; Asnicar et al., 2017). This concept of transmission between mother and infant is commonly known as vertical transmission or mother-newborn transmission (Figure 1). For example, Bäckhed et al. (2015), using metagenomic sequencing, compared the microbial species in 98 newborns and their mothers and found that 135 of 187 taxonomically annotated MetaOTUs present in vaginally delivered newborns also were found in their own mothers including important species such as Escherichia/Shigella, Bifidobacterium longum, Enterococcus faecalis, Bacteroides fragilis, B. thetaiotaomicron, and Bilophila wadsworthia, reinforcing the concept of vertical transference. Also, Dominguez-Bello et al. (2010) demonstrated that neonates born vaginally have a microbiota resembling the vaginal microbiome, enriched with Lactobacillus and Prevotella species, although other bacteria, such as the Enterobacteriaceae family, including Escherichia or Klebsiella, are also present (Selma-Royo et al., 2019). On the contrary, Dos Santos et al. (2023) conducted a longitudinal, prospective cohort study of 621 Canadian mother-newborn pairs and collected pre-delivery maternal vaginal swabs and infant stool samples at 10-days and 3-months of life. The aim was to evaluated the effect of maternal vaginal microbiome composition on the development of the infant stool microbiome. The authors concluded that maternal vaginal microbiome composition at delivery does not affect infant stool microbiome composition and development.
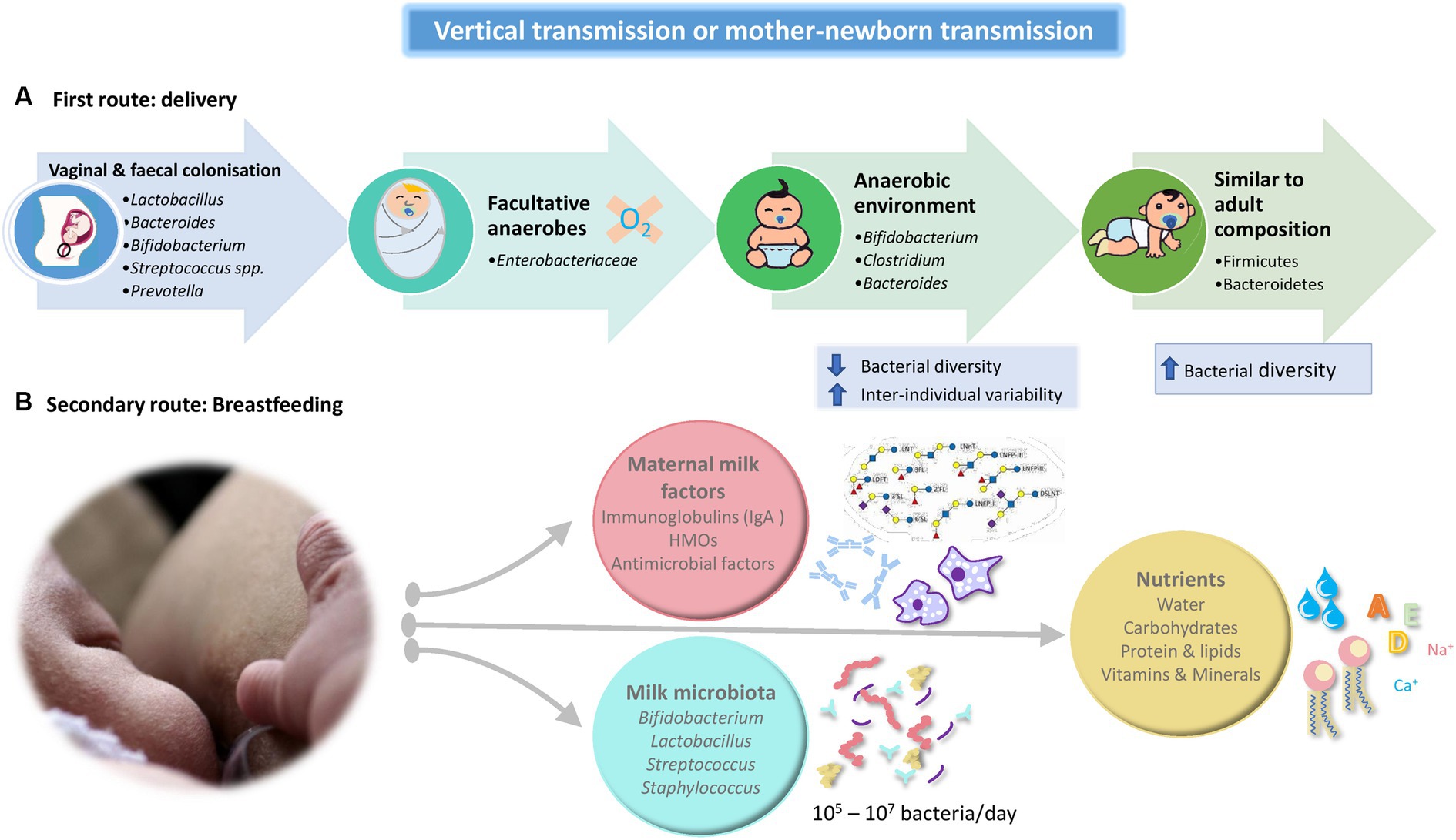
Figure 1. Schematic summary of the two main routes that comprise the vertical transmission or mother-newborn transmission. (A) Route linked to the type of delivery. (B) Route linked to breastfeeding. HMO: Human Milk Oligosaccharides.
During vaginal delivery, the infant gut becomes colonized by maternal vaginal and fecal bacteria (Lactobacillus, Prevotella of the Bacteroidetes phylum, Sneathia of the Fusobacteria phylum; Jiménez et al., 2008). First colonizers, facultative anaerobes such as members of the Enterobacteriacea family, create an anaerobic environment in the intestinal lumen which allows the growth of strict anaerobes as Bifidobacterium spp., Clostridium, and Bacteroides (Koleva et al., 2015; Rodríguez et al., 2015). The neonatal gut microbiota is characterized by low bacterial diversity and higher inter-individual variability as well as a relative dominance of the phyla Proteobacteria and Actinobacteria (mainly comprised of Bifidobacterium genus). The microbiota become more diverse with the emergence and dominance of Firmicutes and Bacteroidetes as time after birth increases, resembling the adult composition and diversity approximately between 2 and 5 years of age (Yatsunenko et al., 2012; Bäckhed et al., 2015; Rodríguez et al., 2015).
A secondary route of maternal microbial transmission is breastfeeding. Breast milk provides a mix of nutrients and pro microbial and antimicrobial agents, which satisfies the nutritional requirements of the infant and confers protection against pathogens through the transmission of maternal antibodies (IgA) and other antimicrobial factors (Arrieta et al., 2014; Milani et al., 2017). Maternal microbial transmission occurs in two different ways, indirectly, via maternal milk factors that affect bacterial growth and metabolism such as human milk oligosaccharides (HMO), secretory IgA, and anti-microbial factors and, directly, by the exposure of the neonate to the milk microbiota (van den Elsen et al., 2019).
Traditionally, through the use of culture-dependent techniques the presence of microbes in human milk has been confirmed. Most bacteria isolated from breast milk belong to Staphylococcus, Streptococcus, Lactobacillus, and Bifidobacterium spp., (Gomez-Gallego et al., 2016). It has been described that an infant can consume approximately 800 ml/day of milk, leading to ingest between 105 and 107 bacteria per day, with human milk constituting one of the main sources of bacteria to the breastfed infant gut (Fernández et al., 2013). However, the composition of breast milk microbiota is not stable over time. Various studies have been able to determine that there is an evolution throughout the period of breastfeeding. For example, Cabrera-Rubio et al. (2012) concluded that the colostrum microbiota has a higher diversity than mature milk, being dominated by Weissella, Leuconostoc (both lactic acid bacteria), Staphylococcus, Streptococcus, and Lactobacillus spp. One month later, the Staphylococcus level is dramatically reduced, and there are higher levels of Veillonella, Prevotella, Leptotrichia (typical inhabitants of the oral cavity), Lactobacillus, Streptococcus spp., and increasing levels of Bifidobacterium and Enterococcus spp. These techniques have confirmed the existence of a rich and diverse breast milk microbial community. Also, Ward et al. (2013) carried on a shotgun metagenomics analysis of 10 pooled human milk samples by total DNA sequencing and reported that Proteobacteria (65 %) and Firmicutes (34 %) are the predominant phyla and Pseudomonas spp. (61.1%), Staphylococcus spp. (33.4%), and Streptococcus spp. (0.5%) are the predominant genera.
Some reviews summarize that human milk microbiota could derive from colonization from mother’s skin and the infant’s oral cavity during suckling because breast milk flows back into the mammary ducts, which provides a route for bacteria found in their infant’s oral cavity to enter the mammary gland. But a number of studies also support the entero-mammary pathway hypothesis, wherein bacteria from the maternal gut may reach the mammary glands via dendritic cells and macrophages (Fernández et al., 2013; Gomez-Gallego et al., 2016; Milani et al., 2017; van den Elsen et al., 2019).
In addition to shaping the composition of the microbiota, the practice of early feeding affects the metabolism of the microbiota. The microbiomes of newborns and breastfed are enriched in genes necessary for the degradation of HMO. HMO are the third largest component of breast milk and are structurally complex sugars unique to human breast milk. They are not digestible, promote the proliferation and growth of specific microorganisms, including Bifidobacterium and Bacteroidetes, and the metabolism of these substrates resulted in the production of lactate and short-chain fatty acids (SCFA), which in turn increased the acidity of the surrounding environment, an important factor in preventing the invasion of pathogens. For all of that, HMO are considered a type of prebiotic and exert positive effects on health (Arrieta et al., 2014; Chong et al., 2018; van den Elsen et al., 2019).
Throughout the first year of life, gut bacterial diversity and richness continue to respond rapidly to changes in the infant diet. The first introduction of infants to solid foods occurs during weaning (complimentary feeding) when infants are exposed to a much larger array of non-digestible carbohydrates than those present in breast milk or formula. The presence of new substrates can significantly alter the gut microbiota, favoring the growth of polysaccharides fermenters such as Bacteroides, Clostridium, Ruminococcus, and Faecalibacterium (Arrieta et al., 2014; Vallès et al., 2014). During weaning the alpha diversity, concept that indicates how many different species could be detected in a microbial ecosystem, increases, resulting in the replacement of Proteobacteria and Actinobacteria by Firmicutes and Bacteroidetes phyla as the dominant members of the infant microbiota (Fallani et al., 2011; Bergström et al., 2014; Laursen et al., 2016; Milani et al., 2017; van den Elsen et al., 2019).
Additionally, the introduction of solid foods also changes the metabolic function of the gut bacteria as genes involved in the degradation of sugars from breast milk are less needed and utilized. Instead, the microbiota adapts to the available energy source, and functionally matures to be able to degrade complex sugars and starch found in solid food (Bäckhed et al., 2015). By the end of the first year of life, the composition of the infant gut microbiota is more similar to the microbiota of an adult; however, a typical adult microbial profile is not established until 2-3 years of age (Yatsunenko et al., 2012). The development of such condition reaches a climax status represented by the establishment of a homoeostasis among all its members. A wide range of factors can cause shifts in this microbiota balance, thereby disrupting the gut microbiota homoeostasis and causing a state of dysbiosis. There is a controversy on the exact meaning of dysbiosis, simply because of the lack of an accurate description of a “normal” or healthy microbiota. Dysbiosis is usually associated with harmful effects and may have long-term consequences leading to disorders or diseases. The process of development and maturation of the intestinal microbiota is a dynamic and non-random process, in which both positive and negative interactions take place between the main microbial taxa (Milani et al., 2017). Therefore, more research is needed to clarify what specific components of a solid food diet play the biggest role in the development of the infant gut microbiota and how it will affect the child’s health in the long term.
5. Main influential factors in the microbial colonization
Human microbial colonization represents the de novo assembly of a complex microbial community, a process that is influenced by a number of different factors (both intrinsic and extrinsic) such as mode of delivery, type of feeding, and antimicrobial treatments. Also, as previously discussed, the diet during the pregnancy period and during the first years of life has an influence in this development. The mother’s age, as well as environmental and life style, and family genetics have also been reported to impact the infant microbiota (González-Bermúdez et al., 2018). Various epidemiological studies have established a clear correlation between these factors that disrupt the gut microbiota during childhood, and immune and metabolic disorders later in life (Sevelsted et al., 2015; Milani et al., 2017). The following section highlights some perinatal, neonatal and postnatal factors that are thought to influence the development of infant gut microbiota (Figure 2).
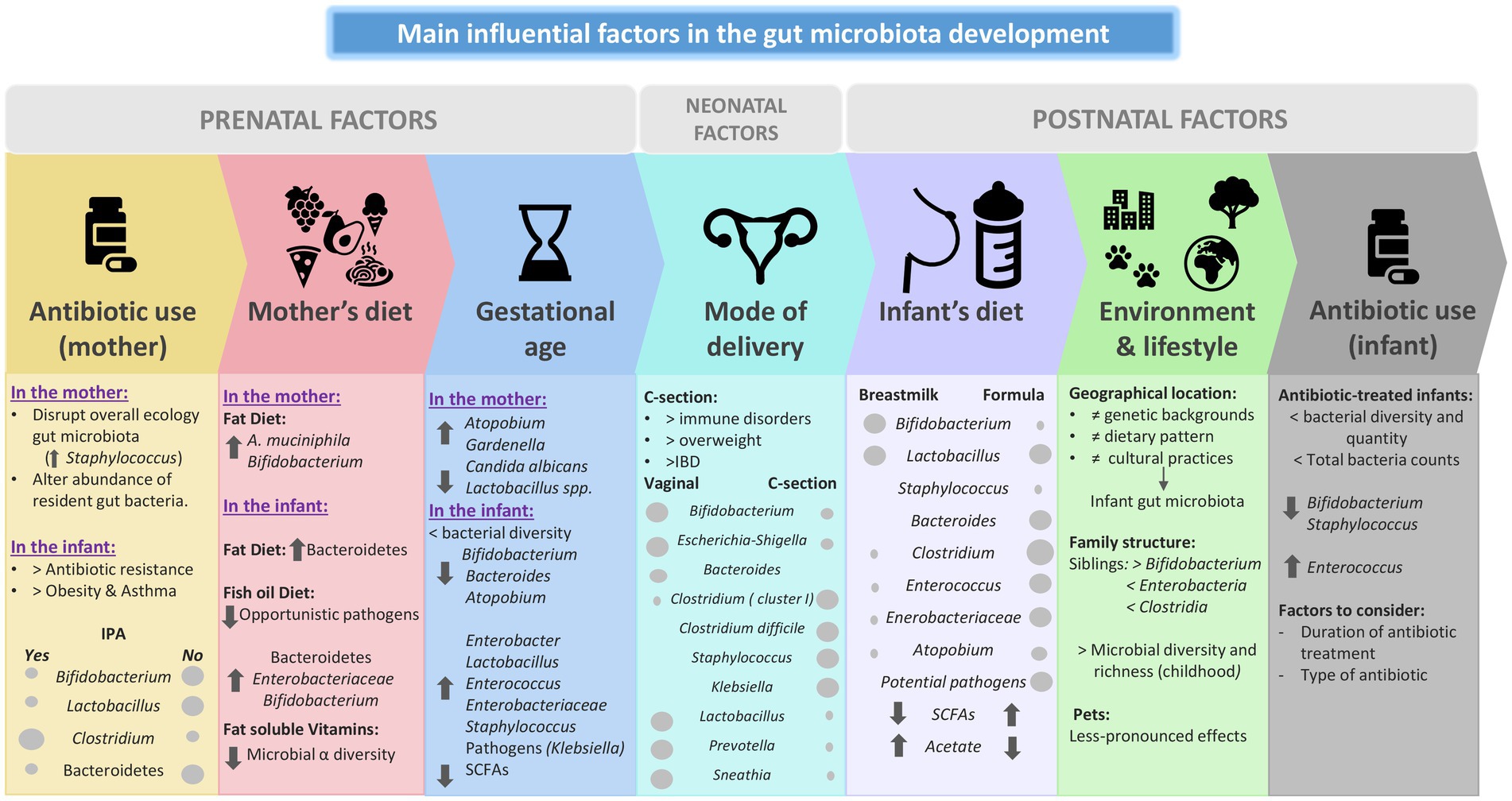
Figure 2. Main influential factors in the gut microbiota development. IPA: Intra partum antibiotic. SCFA: Short chain fatty acids. IBD: Infant bowel disease.
5.1. Prenatal and perinatal factors
Prenatal factors during pregnancy can affect the maternal gut microbiota, which in turn can affect the infant gut colonization and even influence the future development and behavior of the infant.
5.1.1. Antibiotics during pregnancy
Regarding the use of antibiotics, initial epidemiological evidence indicates that disrupting microbial exchange through the use of antibiotics in pregnancy may increase offspring risk of some chronic diseases later in life, such as childhood obesity and asthma (Mueller et al., 2015b). As many reviewed resume, inappropriate antibiotic usage may significantly disrupt the overall ecology of the gut microbiota, alter the abundance of resident gut bacteria, potentially lead the child toward certain diseases, and conferring antibiotic resistance in infancy (Arrieta et al., 2014; Kumbhare et al., 2019; Dierikx et al., 2020). In one of the previous studies carried out in mice, it was observed that prenatal antibiotics decrease the diversity and structure of the microbiota (Tormo-Badia et al., 2014).
Antibiotics treatment during pregnancy is widespread in Western countries, representing 80% of the medications prescribed at this stage. However, although antibiotic treatment can sometimes save lives, it can also have harmful consequences. The use of antibiotics in the prenatal period, has been associated with delayed colonization by some microbes especially Bifidobacterium and Lactobacillus species (Jauréguy et al., 2004; Westerbeek et al., 2006; Faa et al., 2013; Keski-Nisula et al., 2013). Also, a study carried out by Mueller et al. (2015a) found that children exposed to prenatal antibiotics in the second or third trimester had 84% higher risk of obesity compared with unexposed children.
Stokholm et al. (2014) reported an increase in vaginal colonization by species of Staphylococcus due to the use of antibiotics during pregnancy and also observed a mayor colonization by Escherichia coli at women treated at the third trimester of pregnancy. All these alterations may have effects on the early microbial colonization of the neonate. However, according to more recent studies (Kamal et al., 2019; Korpela, 2021), no significant differences in gut microbiota composition were found between infants exposed and unexposed to maternal antibiotic who born by C-section, but antimicrobial alters the gut microbiota in a significantly way in vaginally delivered infants.
During the perinatal period the main cause of antibiotic exposure is the use of intrapartum antibiotic prophylaxis (IPA) in over 30 % of total deliveries (Nogacka et al., 2018). Mothers are given IPA as standard treatment for prevention of vertical transmission of Group B Streptococcus to neonates. This standard of care treatment is an opportunity to examine how antibiotics can affect the infant microbiome (Kim et al., 2019). It has been reported in recent studies that IPA affects the development of later microbiota in the newborn (Jauréguy et al., 2004; Keski-Nisula et al., 2013; Aloisio et al., 2014; Arboleya et al., 2015; Azad et al., 2016; Gonzalez-Perez et al., 2016). As Korpela (2021), the vaginally delivered infant’s gut microbiota pattern, whose mothers were exposed to IPA, was represented by an increase in Clostridia, Enterobacteria, Streptococci and pathogens species, and a decrease in Bifidobacteria.
More specifically, in a longitudinal prospective birth cohort, the bacterial community in early life of infants exposed to IPA were different from that infant who were not exposure at 10 days and 6 weeks of age, and these differences disappeared at 12 weeks (Stearns et al., 2017). The authors observed that Actinobacteria had a delay pattern of colonization. Also, the time of exposure to treatment influences the pattern of colonization as in this study, there was a decrease of 7.2 % in the abundance of Bifidobacterium and a positive effect on the abundance of Clostridium for each hour of IPA administration during vaginal birth. Aloisio et al. (2016) observed significant differences in the microbial composition in newborns whose mothers received IPA during delivery, with increased level of Proteobacteria and a lower number of Actinobacteria and Bacteroidetes. A similar pattern and a higher abundance of Proteobacteria and Firmicutes was also observed by Nogacka et al. (2018). These findings underscore the fact that the consumption of antibiotics by mothers during pregnancy can affect the gut microbiome of the newborn, which in turn can affect the health and development of the infant. Therefore, this factor must be carefully observed and controlled.
5.1.2. Consumption of probiotics and supplements
Despite the existence of a wide range of research conducted on the use of probiotics during pregnancy and the influence on breast milk microbiota, the data on how probiotics affect the offspring’s gut microbiota are very limited. The consumption of probiotics during gestation had been related with a lower risk of atopic disease or asthma in infants (Lahtinen et al., 2009; Ismail et al., 2013).
The study carried out by Lahtinen et al. (2009) is the only research found to date that study the consumption of probiotic by the mother during gestation up to the time of delivery, and their effect in the offspring gut microbiota. The authors employed an exclusively prenatal supplementation strategy and observed an increase in the prevalence of B. longum among the infants (at 90 days of life) whose mothers consumed probiotics during late pregnancy and a trend towards a higher increased prevalence of B. breve. These results are important because these findings are in line with the entero-mammary hypothesis.
According to a recent systematic review conducted by Zaidi et al. (2021), consumption of probiotic during pregnancy and lactation was associated with the same beneficial bacteria colonization of the infant gut but with varying effects according to participant characteristics, type of supplement administered, and outcome measured. Also, this review concluded that nutritional supplementation with probiotic or vitamin D during pregnancy and lactation had very limited effects on the alpha and beta diversity of the breastmilk and infant gut microbiota (Zaidi et al., 2021). Regarding vitamin D supplementation, in the KOALA birth cohort (Talsness et al., 2017), the authors observed limited associations between maternal vitamin D supplementation, 25-hydroxyvitamin D concentration, and the infant gut microbiome. Just a negative correlation between levels of maternal vitamin D and Bifidobacterium spp. was also reported.
5.1.3. Diet and nutrition during pregnancy
As discussed above, the maternal intestinal flora is the major source of a healthy microbiota for the newborn, and the diet during pregnancy may modify the proportion of different groups, and therefore, the pattern of colonization of the newborn in utero and subsequently during delivery and early life. Moreover, the changes in the mother’s microbial composition can alter the abundance of genes that promote various metabolic processes during pregnancy (Gohir et al., 2015) also affecting the microbial composition of the newborn. Several previous studies, in addition to demonstrating a clear association between the diet and the intestinal microbiome, have also successfully revealed the important role of maternal dietary modulations, since they can influence changes in the infant’s intestinal microbiome (Table 1). Moreover, it has recently been reported that the maternal diet during pregnancy has a key impact on both the maternal and infant microbiota in a delivery mode-dependent manner (Chu et al., 2016b; Lundgren et al., 2018; Selma-Royo et al., 2021).
5.1.3.1. High fat diets
A high fat diet during pregnancy and its effects in offspring is one of the most studied patterns among researchers (Ma et al., 2014; Gohir et al., 2015; Chu et al., 2016b; Mann et al., 2018). Ma et al. (2014) concluded, in a primate model, that maternal consumption of high fat diet during pregnancy or post birth results in dysbiosis of the neonatal intestinal microbiome. They observed the dominance of Bacteroidetes and an increase in the abundance of Prevotella in the offspring’s gut (Ma et al., 2014). Chu et al. (2016b) carried out a study in humans and demonstrated that independently of the maternal body mass index, a high fat maternal diet reduced Bacteroides levels in the neonatal gut microbiome.
Some authors have focused their research on how intake or exposure to certain specific fats such as fish oil, polyunsaturated fatty acid (PUFA) and monounsaturated fatty acids (MUFA), or vitamins influence the microbiota. For example, Gibson et al. (2015) compared the intestinal microbiota among offspring of rats born to mothers fed either n-6 (PUFA), n-3 PUFA or control feed; the authors concluded that there is a general reduction in microbial richness due to maternal exposure to a diet rich in PUFA. It was also observed an increase in the level of Bacteroidetes in the gut microbiota of offspring born to mothers who had consumed a diet rich in fish oil and also a reduction of Enterobacteriaceae and Bifidobacteria spp. and an abundance of taxa of opportunistic pathogens like Bacteroides fragilis, Bilophila wadsworthia and Enterococcus faecium when compared to the control group (Gibson et al., 2015). However, Urwin et al. (2014) did not found significant effect on maternal or infant gut microbiota based on the consumption of salmon, rich in n-3 and n-6 PUFA. When the authors took into account the mode of feeding, they observed that formula-fed infants in the salmon group were associated with lower bacterial counts of the Atopobium cluster compared to controls, though this was not observed in breastfed infants (BFI).
Mandal et al. (2016) studied, in 60 women in the second trimester of pregnancy, the relationships between the intake of macro and micro nutrients from diet and their gut microbiota through the use of food frequency questionnaires and observed, after delivery, taxonomic differences. They concluded that some of the most important modulators of the mother’s gut microbiota are fats and fats soluble vitamins. Specifically, they observed that a higher dietary intake of fat-soluble vitamins, especially vitamin D, were associated with reduced microbial alpha diversity. In addition, vitamin D, mono unsaturated fat, cholesterol and retinol were associated with higher levels of Proteobacteria, a phylum known to be associated with multiple pathogens and to have pro-inflammatory properties. On the other hand, the authors observed that saturated fats, vitamin E and proteins were associated with a relative reduction in Proteobacteria levels (Mandal et al., 2016).
Newly, Selma-Royo et al. (2021), in a nested cross-sectional study (MAMI cohort) observed that intake of saturated (SFA) and MUFA, is associated with both maternal and neonatal microbiota at the time of birth, in a delivery mode-dependent manner. Members of Firmicutes in neonatal gut microbiota were positively associated with a high fat intake, more specifically SFA and MUFA, during pregnancy, and negatively associated with a high consumption of fiber, proteins from vegetable sources, and vitamins by the mother.
5.1.3.2. Very low-calorie ketogenic diet
The very low-calorie ketogenic diet (VLCKD) is a type of diet that is gaining much interest as a therapeutic approach to many diseases such as epilepsies (Lim et al., 2022; Zambrano et al., 2023). However, little is known about its effects during pregnancy on the mother’s or offspring’s gut microbiota and health. A few researchers have investigated the VLCKD, its possible influence on the gut microbiota of children and its therapeutic implications in asthma (Alsharairi, 2020b), obesity (Alsharairi, 2021) and inflammatory bowel diseases (Alsharairi, 2022) but not in pregnant women or infants.
The VLCKD is characterized by a low carbohydrate, moderate protein, and high fat diet (Lim et al., 2022). This low carbohydrate intake changes the energy source from glucose to ketone bodies (acetoacetate and β-hydroxybutyrate) allowing to achieve nutritional ketosis. The VLCKD includes sources of dietary fiber, fats (high in PUFA, moderate in MUFA and low in saturated fats) and plant-based protein, which may influence the development of SCFA-producing bacteria, and therefore, lead to an anti-inflammatory state. Furthermore, ketone bodies play an important role in regulating gut homeostasis and gene expression through epigenetic modifications similar to butyrate (Alsharairi, 2020b, 2022).
Despite all the potential benefits of the VLCKD, it would be premature to recommend this type of diet during pregnancy. Pregnancy is a period when nutritional requirements change according to the stage and where a shortage of macro- or micronutrients can trigger adverse health effects in both mother and offspring. At present, there is a paucity of high-quality clinical trials and prospective cohort studies to monitor changes in maternal gut microbiota composition during pregnancy and lactation following the VLCKD and their potential long-term effects on maternal and offspring health.
5.1.3.3. Vegetarian diet
Nowadays, plant-based and vegetarian eating pattern are very extended. This type of diet is related to lower risk of obesity, cardiovascular disease, cerebrovascular disease, diabetes mellitus, and chronic kidney disease in adults (Miko et al., 2022).
In relation to its role in the gut microbiota, the vegetarian diet, due to fiber content, could promote beneficial changes such as an increased synthesis of SCFA.
To date, there is limited information on how a vegetarian diet during gestation may affect the gut microbiota of the newborn. There are two studies that investigated the role of a vegetarian or fruit-rich diet during gestation on the gut microbiota of the infant and in both cases different results were observed. Lundgren et al. (2018) found a negative association between maternal diets high in fruits and vegetables and the beneficial microbe Bifidobacterium in vaginally born infants. On the other hand, Fan et al. (2021) studied the effects of high or low fruit and vegetable gestational intake on the infant gut microbiota and did not observe any change in the alpha diversity but the authors did observe a variation in the infant’s microbiome. Specifically, the counts of Propionibacteriales, Propionibacteriaceae, Cutibacterium, Tannerellaceae, Parabacteroides, and Lactococcus were higher in the microbiome of infants with high maternal vegetable and fruit consumption.
5.1.3.4. Probiotics food consumption
One of the nutritional recommendations for pregnant women is to increase the consumption of dairy products, including yogurt. Yogurt is a food produced by the bacterial fermentation of cow’s milk and it is considered a probiotic food since Lactobacillus bulgaricus and Streptococcus thermophilus are living in its preparation. Bisanz et al. (2015) assessed the influence of maternal consumption of probiotic yogurt with Lactobacillus rhamnosus GR-1 supplemented with Moringa plant on the infant microbiome and found an association between the maternal consumption of yogurt and an increase in the relative abundance of Bifidobacterium and a decrease in the relative abundance of Enterobacteriaceae.
Another food that is being consumed is Kefir. Kefir is a unique fermented dairy product that is produced by mixing lactic acid bacteria, acetic acid bacteria and yeast. Tunay and Kök (2022) investigated the possible effect of kefir consumption during gestation on the intestinal microbiota of the newborn and observed that had indeed transferred unique bacteria of kefir (Lactobacillus kefiranofaciens subsp. kefiranofaciens, Lentilactobacillus kefiri and Lentilactobacillus parakefiri) to the infant gut.
5.1.3.5. Sweetened consumption
The consumption of sweeteners is very popular among the population. Nowadays, there is a high concern, about the negative impact that high sugar intake has on health and maybe this is one of the reasons why the use of sweetener, such us aspartame, sucralose or acesulfame-K, it has become very popular. One of the main foods that include sweeteners in their list of ingredients are sweetened beverages.
Laforest-Lapointe et al. (2021) study if maternal consumption of artificially sweetened beverages during pregnancy is associated with modifications of infant gut bacterial community composition at 3-4 months and 1 year of live and identified 4 microbiome clusters. From immature (Cluster 1) to mature (Cluster 4) and two deviated from this trajectory (Clusters 2 and 3). Alterations in infant gut bacterial taxonomy structure at the community level were associated with maternal artificially sweetened beverages consumption. A reduction of several Bacteroides sp. in Cluster 2 was also observed. The authors concluded that regular intake of artificial sweetened beverages during gestation resulted in a higher BMI of 1 year old infants, suggesting that infant gut microbiota and their metabolites could be involved.
5.1.3.6. Alcohol consumption
Today there is no doubt about the negative effects of alcohol consumption during pregnancy, both for mothers and newborns. The effect of alcohol consumption during pregnancy was reviewed recently by Kumbhare et al. (2019), concluding that it is associated with various disorders such as hepatic diseases in the newborn and premature delivery.
Moreover, the initial intestinal colonization of the infant can be affected by such changes, making it more predisposed to infections and diseases later in life. However, how alcohol consumption during pregnancy affect infant gut microbiota are rarely investigated.
Wang et al. (2021) examined the relationship of maternal diet and alcohol consumption during pregnancy with the infant gut microbiota in Chinese mother-infant dyads. Alcohol intake leaded to a significantly lower abundance in Faecalibacterium genus compared with those without alcohol drinking. These bacteria have been shown an intestinal anti-inflammatory effect. Besides, Megamonas genus was abundant in infant exposed to alcohol consumption mothers. Nonalcoholic fatty liver disease, the most chronic liver disease in children and adolescents, is positively related to Megamonas in infant gut. However, it has been taken into account that more researches are needed to explore these relationships in a larger birth cohort.
In general, all these studies emphasize that maternal diet during pregnancy is a very influential factor in the development of the intestinal microbiota of both the mother and the newborn, and therefore, a key in the health of the infant during the first years of life.
5.1.4. Gestational Age
One of the most important perinatal factors in the establishment of the infant gut microbiota is the gestational age. The World Health Organization (WHO; UNICEF and WHO, 2010) defined preterm births as those occurring before 37 completed weeks of gestation and usually have very low birth weight. During the pregnancy period, microbial diversity within the vaginal microbiota decreases, while members of Lactobacillus species increase, potentially reinforcing their protective function. However, infections caused by bacteria, viruses, and fungi during the pregnancy period have been considered a cause of intrauterine growth restriction and preterm birth. Certain infectious agents can reach the amniotic fluid, establishing an intra-amniotic infection and initiating an inflammatory response at the maternal and fetal tissues, which promotes pre-labor rupture of membranes and preterm birth (Ruiz et al., 2016). Bacterial communities characterized by high levels of Atopobium, Gardnerella and Ureaplasma as well as lower levels of Lactobacillus spp. or a higher presence of Candida albicans have been found to be correlated with premature deliveries (Ruiz et al., 2016).
Preterm infants (PTI) have to overcome serious health challenges. In most cases, and depending on the degree of prematurity, PTI have a degree of immaturity in the digestive system and respiratory, immune and neurological problems. Because of that, hospitalization is necessary for the application of treatments such as intensive use of antibiotics and other medications, and/or even the use of artificial respiration and feeding such as sterile parenteral nutrition (Henderickx et al., 2019). Also, in most cases the births of premature infants are usually by CS and therefore they never come in contact with the mother’s vaginal microbiome. All these factors can interfere in the process of colonization and the correct development of the gut microbiota, resulting in an unusual establishment and a different or deviating composition with increased colonization by pathogenic microorganisms.
The pattern of colonization in PTI is characterized by a reduction in bacterial diversity (Rougé et al., 2010; Moles et al., 2013; Henderickx et al., 2019). Several authors have compared the gut microbial colonization in preterm and full-term infants (FTI) and reporting that PTI showed a reduced level of strict anaerobes such as Bifidobacterium, Bacteroides, and Atopobium, and high levels of facultative anaerobes like Enterobacter, Lactobacillus and Enterococcus. It was also observed an increased abundance of Enterobacteriaceae family and Staphylococcus, and a higher colonization by pathogens, such as Klebsiella (Mshvildadze et al., 2010; Rougé et al., 2010; Arboleya et al., 2012, 2015). Additionally, Hill et al. (2017) in the INFANTMET cohort (using 16S rRNA amplicon Illumina sequencing and bacteriological culture) observed that at the phylum level Proteobacteria and Firmicutes dominates PTI when compared to FTI. As a result of the alterations in the composition of the intestinal microbiota, differences have also been observed in the main microbial metabolites, the SCFA, whose concentration in feces is higher in FTI than in premature neonates (Arboleya et al., 2012).
As it has been mentioned previously, the infant’s microbiota is not only composed by bacteria, but also of non-pathogenic viruses or archaea. In this respect, Rao et al. (2021) studying the forces that shape the dynamics of microbiome assembly in preterm neonates, observed that there was an inverse correlation between bacterial and fungal loads in the infant gut. Specifically, the authors found that interactions between different kingdoms may influence assembly. For example, Candida albicans, a fungal species, inhibited multiple dominant genera of gut bacteria.
5.1.5. Mode of delivery
The mode of delivery has been recognized as an important driver of the early gut microbiota composition and have a major impact on the type of microbiota acquired during birth by the newborn. Several studies have shown that human neonatal microbiota across all body habitats (skin, oral, nasopharyngeal, and gut) is influenced by their mode of delivery (Penders et al., 2006; Biasucci et al., 2008; Dominguez-Bello et al., 2010). During the labor and immediately after birth, microbes from the mother and surrounding environment colonize the gastrointestinal tract of the infant leading to the development of a dense complex microbiota, being the first major exposure of the neonate to microbes (Munyaka et al., 2014; Korpela, 2021).Vaginal delivery infants (VDI) come into contact with the maternal vaginal and fecal microbiota, which results in neonatal gut colonization by vagina-associated microbes such as Lactobacillus, Escherichia, Bacteroides, Bifidobacterium, Streptococcus spp. and Prevotella (Penders et al., 2006; Dominguez-Bello et al., 2010; Fallani et al., 2010; Azad et al., 2013; Laursen et al., 2015). In contrast, children born by caesarean section (CS) are also exposed to their mother’s microbiota, but initial exposure is most likely to non-maternally derived environmental isolates from equipment, air, and other infants, with the nursing staff serving as vectors for transference (Staphylococcus, Corynebacterium, Propionibacterium; Biasucci et al., 2008; Dominguez-Bello et al., 2010; Munyaka et al., 2014).
According to the numbers provided by the WHO, in Spain more than 25 % of births are performed by CS and globally, the number of CS has grown from 12 % in the year 2000 to 21 % in 2015 over the world (Betran et al., 2016). The early colonization patterns of CSDI differ greatly from children born vaginally and being less diverse (Biasucci et al., 2008; Dominguez-Bello et al., 2010; Matamoros et al., 2013; Arrieta et al., 2014; Jakobsson et al., 2014). In particular, it was demonstrated that CSDI had minor amounts of Bifidobacteria (Dominguez-Bello et al., 2010; Azad et al., 2013), Escherichia -Shigella, and absence of Bacteroides (Fallani et al., 2010; Jakobsson et al., 2014) while were enriched with Clostridium (cluster I), Clostridium difficile, Staphylococcus species (Penders et al., 2006) and higher amounts of Klebsiella (Dogra et al., 2015). In contrast, VDI were characterized by Bifidobacteria (Hansen et al., 2015), predominantly B. longum and B. catenulatum species (Biasucci et al., 2008; Dogra et al., 2015) and vaginal-related microbes such as Lactobacillus, Prevotella, and Sneathia (Penders et al., 2006; Biasucci et al., 2008; Dominguez-Bello et al., 2010).
Also, Jakobsson et al. (2014) showed that the gut microbiota of CSDI at 24 months of age is less diverse than those delivered vaginally. The authors hypothesize that this drop in diversity may be due to delayed colonization of the gut by Bacteroidetes until 1 year of age. These differences between vaginally and CSDI gradually decrease, but remain more heterogeneous than VDI up to 12 months of life. In one recent research, focused on the long-term impact of delivery mode on gut microbiota development over the first 4 years of life, it has been found that Lachnospiraceae was dominant in CSDI while Parabacteroides was found to be more unique to VDI at one and 2 years of age; however, by the fourth year, Lachnospiraceae and other Clostridium spp. became more dominant in these VDI (Fouhy et al., 2019).
The differences observed in the microbiota between VDI and CSDI have been associated with the protective effect of vaginally delivered labor, particularly since it has been suggested that CS has long-term health implications. In fact, CS has been associated with an increased risk of immune disorders such as asthma (Ege et al., 2011; Roduit et al., 2009; Thavagnanam et al., 2008), allergy (Bager et al., 2008), type 1 diabetes (Cardwell et al., 2008), as well as an increased risk of overweight (Tun et al., 2018), a higher risk of development of inflammatory bowel disease (Bager et al., 2012) or an enhanced risk for developing celiac condition (Koletzko et al., 2018). Moreover, different modes of delivery affected the antibiotic resistance gene prevalence, that might influence the infant’s health later in life (Shi et al., 2018). The cause of this could be an inadequate development of the immune system since in several studies it has been observed that CSDI have a much lower level of Th1-related chemokines in their blood, which may be translated into less protection (Munyaka et al., 2014).
On the other hand, there are some authors who have not observed any association between CSDI and type 1 diabetes or the development of celiac disease (Sevelsted et al., 2015; Koletzko et al., 2018). Despite these studies, it can be concluded that the relevance of early gut microbiota in the maturation and development of the host’s immune system is supported by the finding that the mode of delivery influences the health status through adulthood, while the effects on gut microbiota composition decrease after the first year of life (Milani et al., 2017). The most accepted explanation for the association between mode of deliver and the development of diseases is the gut microbial dysbiosis, but it is necessary to carry out more research to adequately support this hypothesis.
5.2. Postnatal factors
Some of the most important postnatal factors, that may influence the gut microbiota composition of a growing neonate are the mode of infant feeding, the introduction to solid food, environment and lifestyle, the use of antibiotics and host genetics.
5.2.1. Infant feeding during the first months of life
5.2.1.1. Breast milk vs. infant formula
Mostly, breast milk (BM) is the first food to which the newborn is exposed, and it is during breastfeeding when one of the most important links between the mother and the newborn is formed. Although nowadays infant formulas (IF) are products increasingly similar to BM, large differences continue to be observed. This is because BM has a very complex composition mainly due to the presence of various growth factors and enzymes, and also this composition changes over time (colostrum, transitional milk and mature milk; Scholtens et al., 2009; Bezirtzoglou et al., 2011; Cabrera-Rubio et al., 2012; Guaraldi and Salvatori, 2012; Andreas et al., 2015). It has been reported, that BM and more specifically its bioactive compounds, such as HMO, probiotics polyamines, lactoferrin, nucleotides and whey proteins affect different biological processes, intervening in the optimal physical and intellectual development of the child, as well as in the reduction of the incidence of future diseases (Turfkruyer and Verhasselt, 2015; Oddy, 2017; Moossavi et al., 2018), as Kumbhare et al. (2019) and Porro et al. (2023) reviewed. For this reason, WHO recommends exclusive breastfeeding of infants up to 6 months due to the considerable potential to improve child health and wellbeing (UNICEF and WHO, 2010).
The differences in the gut microbial composition between the infants who has been fed with BM and those who have been fed with IF are well documented (O’Sullivan et al., 2015; van den Elsen et al., 2019) and Table 2 summarizes the main studies carried out to understand how diet and nutrition may have an influence on influent the infant gut microbiota during the first months of life.
The gut microbiome in formula fed infants exhibits higher diversity than that of BFI because the latter are exposed to different carbohydrates, bacteria, and nutrients, causing different microbial colonization patterns of the gut. In this context, different publications have reported that stools of BFI contain higher levels of Bifidobacterium species (phyla Actinobacteria), being the most prevalent B. breve, B. longum, B. dentium, B. infantis and B. pseudocatenulatum (Harmsen et al., 2000; Bäckhed et al., 2015). Also, BFI’s stools contain higher levels of Lactobacillus and lower levels of potential pathogens than those infants feeding with IF. Infants exclusively feed with IF were more often colonized by Staphylococci, Bacteroides, Clostridium species, Enterococci, Enterobacteria, and the genus Atopobium (Penders et al., 2006; Fallani et al., 2010; Bezirtzoglou et al., 2011; Guaraldi and Salvatori, 2012; Gritz and Bhandari, 2015; Azad et al., 2016; Martin et al., 2016).
As mentioned earlier, a different pattern of colonization also implies differences in microbiota metabolism and therefore SCFA levels (Porro et al., 2023). Exclusive breastfeeding has been associated with lower absolute concentrations of total SCFA, Acetate, Butyrate, Propionate, Valerate, Isobutyrate, and Isovalerate, and higher concentrations of lactate. Further, the relative proportion of acetate was higher with exclusive breastfeeding compared to infants feed with Infant formula (Bridgman et al., 2017).
5.2.1.2. Complementary feeding
Although it is well established that early infant feeding has a major influence on the establishment of the gut microbiota, very little is understood about how the introduction of first solid food and the gradually replace of the milk-base diet influences the infant gut microbiota.
The introduction of solid foods is an important dietary event during infancy that causes profound shifts in the gut microbial composition towards a more adult-like state (Penders, 2017; Homann et al., 2021; Schwab, 2022). One of the reasons why this profound change occurs may be due to the progressive decrease in the consumption of breast milk during the complementary feeding stage and consequently of its components (HMOs and bacteria). In addition, with the introduction of solid foods, the diversity and complexity of dietary carbohydrates increases, leading to an increase in the abundance of carbohydrate-degrading bacteria in the months after switching to solid foods (Schwab, 2022). All this leads to a transition process in which the fermentative capacity increases after weaning, and which takes several months to complete. This increase in fermentative capacity produces substantial changes not only in the intestinal microbiota, but also in its metabolites, SCFAs. Specifically, butyric acid is the SCFA with most dramatic higher levels after the introduction of solid foods together with bacteria that produce it.
As Laursen et al. (2017), a large number of longitudinal studies have showed a significant change in microbial composition around the stage of introduction to solid foods and cessation of milk-base diet.
Bergström et al. (2014) carried out a large Danish cohort (“SKOT study”) to study the fecal microbiota of 330 infants at 9, 18 and 36 months of age. The authors observed, during the period characterized by transition from breastfeeding/formula feeding to family diet, that a complementary feeding induces replacement of a microbiota characterized by Lactobacilli, Bifidobacteria, and Enterobacteriaceae towards a microbiota dominated by Clostridium spp. and Bacteroides spp. On the other hand, since the microbiota is influenced by the age at which the novel foods are introduced, its composition was also pronouncedly influenced by the time of cessation of breastfeeding.
Fallani et al. (2011) in a European cohort formed by 531 infants from five different countries, observed similar results and demonstrate that, regardless of differences in geographic location, antibiotic use, delivery mode and milk feeding practices, there are consistent changes in the microbial composition of infants. Specifically, was observed a decrease in Bifidobacteriaceae, Enterobacteriaceae and Clostridiaceae while increasing in Ruminococcaceae and Lachnospiraceae species, starting at 6 weeks up to 4 weeks after introduction of solid food.
These results are in agreement with those found by Thompson et al. (2015) who also observed an increase in the diversity of the intestinal microbiota of infants both in exclusively BFI and non-exclusively BFI (n = 10) after introduction of solid foods. The authors observed a higher diversity and species richness in non-exclusively BFI compared with exclusively BFI. On the other hand, the pattern of exclusively BFI gut microbiota showed increased proportions of Bifidobacterium and a decrease in abundance of Bacteroidetes and Clostridiales with respect to non- exclusively BFI.
Laursen et al. (2016) observed a decrease in saccharolytic bacteria, such as members of Bifidobacteria genus, and an increase in Lachnospiraceae, which are associated with breast milk. These changes were correlated with a higher protein intake. On the other hand, ingestion of fiber was demonstrated to be associated with higher levels of Prevotellacea. Interestingly, two species that are absent or present at very low levels during early infancy, Faecalibacterium prausnitzii and Akkermansia muciniphila, increase in abundance to adult levels at 12 months and 24 months, respectively, (Milani et al., 2017). Both species are being widely studied because they are considered to promote a correct state of health in adulthood. Interestingly, the microbiota composition in African and European infants is very similar until the introduction of solid foods, indicating the dominant role of diet over other variables in shaping the microbial composition of the gut in early life (van den Elsen et al., 2019).
More recently, Homann et al. (2021) characterized the infant gut microbiota of 24 healthy, full-term infants, belonging from two different countries and concluded that introducing a high variety of first foods may increase alpha diversity and stabilize the gut microbiome early in life.
5.2.2. Probiotic consumption in early life
Nowadays, the consumption of probiotics by infants during lactation and in the first years of life is very widespread as they are considered to have a collaborative role in solving different digestive problems. In this respect, Korpela et al. (2018) observed that probiotics had a strong overall impact on the infant gut microbiota composition, but the effect depended on the infant’s diet. Only breastfed infants showed the expected increase in Bifidobacteria and reduction in Proteobacteria and Clostridia. In the placebo group, both birth mode and antibiotic use were significantly associated with altered microbiota composition and function, particularly reduced Bifidobacterium abundance. In the probiotic group, the effects of antibiotics and birth mode were either completely eliminated or reduced. These indicate that it is possible to correct undesired changes in microbiota composition and function caused by antibiotic treatments or caesarean birth by supplementing infants with a probiotic mixture together with at least partial breastfeeding.
5.2.3. Environment and lifestyle
Geographical location has been described as a relevant environmental factor that may have an impact on human microbiota. Different ethnogeography populations have distinct genetic backgrounds, dietary patterns, and cultural practices.
For example, De Filippo et al. (2010) compared the fecal microbiota of children from a rural African village of Burkina Faso and that of European urban infants, residents in Florence, Italy (EU). The reason to select these two populations was that the diet of the children from Burkina Faso is characterized by the consumption of grains, legumes and high-fiber diet of vegetables with the absence of processed food and furthermore, it represents a society similar to that of early human colony at the Neolithic era. EU children have a western diet, which is characterized by a high consumption of animal fats, sugars and a greater caloric intake. Gut microbiota from Burkina Faso children were dominated by Bacteroidetes and showed a depletion in Firmicutes population. In addition, a significantly high levels of SCFA were found.
Subsequently, further studies have continued investigating and comparing the differences between developing vs. developed countries including a large cohort of pediatric and adult samples from the Amazonas of Venezuela, rural Malawi and urban United States areas. Bacteroides predominated in the North American samples, whereas Prevotella predominated in Malawian and Venezuelan samples (Yatsunenko et al., 2012). As well as, there were many more differences in the samples from USA than between Malawian and Venezuelan microbiota mainly in children older than 3 years of age.
Three years later, Lin et al. (2013) observed the same Prevotella-Bacteroides split when the authors compared American children living in wealthy neighborhoods with children living in a Bangladesh slum. Another research comparing southeaster African and northern European infants reported a different bacterial composition, containing higher abundance of the Prevotella-Bacteroides group and the Bifidobaterium genus in African children (Grzeskowiak et al., 2012a).
In Spain, Echarri et al. (2011) evaluate the microbiota of 40 breastfed FTI from two different Spanish locations, one in the northern Atlantic coast and the other in the south-east Mediterranean coast, at different times (fecal samples were collected at 8, 30 and 90 days of life). The authors showed a high inter-individual variability on the levels of the different microbial groups. However, despite this variability their results showed statistically significant higher levels of Bacteroides and Staphylococcus (at 8 days of age) and lower levels of Enterobacteriaceae in infants from the Mediterranean coast than in infants from northern Spain. Similar levels of Enterococcaceae, Clostridia XIVa and IV clusters, Atopobium, Bifidobacterium and Lactobacillus were found between both groups at 90 days of life. When sampling times were not taken into account, lower counts of Lactobacilli and higher counts of C. leptum group, as well as significantly higher levels of Bacteriodes and Staphylococcus were observed in infants from the south of the country compared to those from the north coast.
These differences have also been observed in comparative studies of colonization patterns between different countries belonging to the same continent. For example, it has been reported that infants from Northern areas from Europe have higher levels of Bifidobacterium spp. and some Clostridium spp. and Atopobium spp., while Southern European infants had a higher abundance of Eubacteria, Lactobacillus, and Bacteroides (Fallani et al., 2010). Also, other authors have showed significant differences between the gut microbiota of European infants from different countries (Grześkowiak et al., 2012b). For example, between German and Finnish infants, where German infants showed a higher abundance of the Bacteroides-Prevotella group and Akkermansia muciniphila while the proportion of Bifidobacterium spp. was higher in the Finnish infants (Grześkowiak et al., 2012b).
In addition to the geographical location, family structure and lifestyle may influence the model of colonization in infant gut microbiota and have also been describe as a relevant environmental factor (Rodríguez et al., 2015). Despite this, it is necessary to establish more decisive evidence about the effects of family structure, size, and birth order in the pattern of colonization (Fouhy et al., 2012).
Infants without siblings, who were recruited from the Child, Parent and Health: Lifestyle and Genetic Constitution (KOALA) Birth Cohort Study, had slightly lower numbers of Bifidobacteria, compared with infants with older siblings (Penders et al., 2006). These results agree with those reported by Adlerberth et al. (2007) in another research as part of the ALLERGYFLORA study. In this publication, infants with older siblings had lower proportions of Enterobacteria, other than Escherichia coli, as well as Clostridia, in the gut, but also a higher anaerobe/facultative anaerobe ratio. In a recent study performed with a Danish cohort, the presence of older siblings was shown to be associated with increased gut microbial diversity and richness during early childhood, while the presence of household pets had less-pronounced effects on the gut microbiota (Laursen et al., 2015).
Overall, geographical location (dietary patterns and lifestyle in a specific area and family structure (siblings) seems to affect gut microbiota colonization during early life, although more studies are needed to determined more accurately the factors that influence or contribute to a greater extent.
5.2.4. Antibiotics during early life
Antibiotic usage in early life can also disrupt the normal pattern of colonization, affecting the growth of otherwise dominant bacterial phyla in the human gut (Reyman et al., 2022). These alterations can remain for long periods of time, spanning months and even years with partial or complete recovery (Kumbhare et al., 2019) and leading to a greater susceptibility to numerous diseases later in life (Johnson et al., 2005; Penders et al., 2006; Fallani et al., 2010; Chu et al., 2015; Rasmussen et al., 2018) such as asthma (Kozyrskyj et al., 2007; Metsälä et al., 2015).
Yassour et al. (2016) carried out a longitudinal study to investigate changes in the gut microbiome from 39 infants, half of whom received, during the first 3 years of life, multiple courses of antibiotics. The microbiota of antibiotic-treated children was less diverse in terms of both bacterial species and strains, with some species often dominated by single strains. In addition, they observed short-term composition changes between consecutive samples from children treated with antibiotics.
Additionally, Korpela et al. (2016) observed in 2–7-year-old Finnish children (n = 142; sampled at two time points) that the use of macrolides is associated with changes in microbial composition, reducing diversity and metabolism of the microbiota that also proves to be durable. Specifically, after 1 year of administration of finishing antibiotics treatment, the authors observed the restoration in the levels of Bifidobacterium and Bacteroides and antibiotic resistance and also concluded that Penicillins imprints a weaker mark on the microbiota in comparison with macrolides (Korpela et al., 2016). Finally, Reyman et al. (2022) observed, in 147 infants exposed to antibiotics in their first week of life, a decreased abundance of Bifidobacterium spp. and increased abundance of Klebsiella and Enterococcus spp. compared to controls. Also, regarding the type of antibiotic employed, the authors observed that Amoxicillin + Cefotaxime show the largest effect on microbial composition and also in anti-microbial resistance gene profile. In contrast, Penicillin + Gentamicin had minor effects (Reyman et al., 2022).
Another factor to consider is the duration of antibiotic treatment. About this theme, one study has investigated how short (≤ 3 days) or long treatments (≥ 5 days) may influence gut microbiota of infants in early life. Zwittink et al. (2018) observed a depletion in infant gut Bifidobacterium levels after a short treatment with antibiotics until the third week of life. In long treatments, this depletion in Bifidobacterium remains decreased up to 6 weeks postpartum. In both type of treatments with antibiotics, Enterococcus became the dominant genus of the microbial community. These results are in line with other authors (Tanaka et al., 2009; Martin et al., 2016; Fouhy et al., 2019). Specifically, Martin et al. (2016) observed a slightly depletion in total bacterial counts and also decreased levels of Bifidobacterium and Staphylococcus in infants who received antibiotics.
All these results suggest that the first moths of life are indeed a critical window where the infant gut microbiota could be influenced by the use of antibiotics and linking these changes with later diseases. However, more research is necessary to understand the impact of antibiotic treatment and their long-term effects. Moreover, issues related with the increasing number of pathogenic strains and their antibiotic resistance should be considered because of both are growing due to the ever-increasing use of antibiotic.
6. Conclusion
Although there is no consensus about prenatal colonization through microbial transmission between mother and child, there does exist a broad agreement on the main factors that may influence the transmission and subsequent colonization of the infant’s intestinal microbiota. Prenatal factors such as consumption of antibiotics and diet during pregnancy can affect the maternal gut microbiota and their metabolites, as well as breast milk composition, which in turn can affect infant colonization and even influence offspring’s future development and health.
Focusing on the diet during pregnancy, and summarizing the different bibliographical sources consulted, while this review has outlined significant advances in our understanding of the role of diet during pregnancy in the development of infant gut microbiota, large gaps still exist. Despite all the studies carried out, both in humans and in animal models, with the aim of determining the role of the consumption of macro and micro-nutrients during pregnancy and how it affects the infant gut microbiota, the wide differences in methodology and in the results observed do not allow us to obtain a clear conclusion. However, as other authors have indicated, alcohol consumption should be totally restricted during the gestation period, not due to possible changes in the intestinal microbiota of the child, but due to its adverse effects on mother’s and offspring’s health.
On the other hand, as previously mentioned, pregnancy is a stage with specific nutritional needs which also change throughout the gestation period. Modifications in the diet can lead to the restriction of certain macro or micronutrients, thus leading to altered health states of both the mother and the offspring. For example, ketogenic diets that restrict the consumption of carbohydrates, the main source of glucose, have not been studied in pregnant women and its short- and long-term effects are unknown.
The first moths of life are also a critical window where the infant gut microbiota could be influenced by postnatal factors, such as the use of antibiotics, environment and lifestyle agents and of course the infant diet, being linked these changes with later diseases. Regarding feeding during the first years of life, accumulating evidence from human observational and animal studies suggests that diet during the first months of life, and more specifically breastfeeding may influence the offspring microbiota in a good way. The breastfed infants seem to present higher levels of Bifidobacterium species and lower levels of Bacteroides, Clostridium, Enterococcus, Enterobacteriaceae, Atopobium and potential pathogen species, comparing with formula fed infants. Regarding the introduction of first solid foods and the gradually replacement of milk-based diets, a large number of longitudinal studies have shown a significant change in microbial composition, characterized by dominance of complex carbohydrate-degrading bacteria although additional studies with larger sample size would be required.
Finally, we consider it necessary to take into account two issues. On the one hand, the procedure of development and maturation of the intestinal microbiota is a dynamic and non-random process, in which both positive and negative interactions occur between the main microbial taxa. On the other hand, it is not a single factor, but a wide range of factors acting together, that can cause changes in this balance, thus altering the homeostasis of the intestinal microbiota and causing the so-called state of dysbiosis. All this makes it difficult for the scientific community to reach a solid conclusion about which factors are really influencing the colonization pattern and evolution of the intestinal microbiota of the infant and its repercussions on future health, generating the need to carry out more studies that can help to know and understand in more detail these relationships, taking into account more than one as a whole and study their effects in the short and long term.
Author contributions
CM-G, MS-P, and GY-G: conceptualization and review and editing. CS-M: wrote the paper. All authors have read and agreed to the published version of the manuscript.
Funding
This research was supported by the Spanish Ministry of Science, Innovation and Universities (grant number PID2019-106693RB-100/AEI/10.13039/501100011033). CS-M was funded by a predoctoral fellowship (FPU MECD 15/05809) awarded by The Ministry of Education, Culture as part of the Government of Spain.
Conflict of interest
The authors declare that the research was conducted in the absence of any commercial or financial relationships that could be construed as a potential conflict of interest.
Publisher’s note
All claims expressed in this article are solely those of the authors and do not necessarily represent those of their affiliated organizations, or those of the publisher, the editors and the reviewers. Any product that may be evaluated in this article, or claim that may be made by its manufacturer, is not guaranteed or endorsed by the publisher.
References
Aagaard, K., Ma, J., Antony, K. M., Ganu, R., Petrosino, J., and Versalovic, J. (2014). The placenta harbors a unique microbiome. Sci. Transl. Med. 6:237ra65. doi: 10.1126/scitranslmed.3008599
Adlerberth, I., Strachan, D. P., Matricardi, P. M., Ahrné, S., Orfei, L., Åberg, N., et al. (2007). Gut microbiota and development of atopic eczema in 3 European birth cohorts. J. Allergy Clin. Immunol. 120, 343–350. doi: 10.1016/j.jaci.2007.05.018
Aloisio, I., Mazzola, G., Corvaglia, L. T., Tonti, G., Faldella, G., Biavati, B., et al. (2014). Influence of intrapartum antibiotic prophylaxis against group B Streptococcus on the early newborn gut composition and evaluation of the anti-Streptococcus activity of Bifidobacterium strains. Appl. Microbiol. Biotechnol. 98, 6051–6060. doi: 10.1007/s00253-014-5712-9
Aloisio, I., Quagliariello, A., De Fanti, S., Luiselli, D., De Filippo, C., Albanese, D., et al. (2016). Evaluation of the effects of intrapartum antibiotic prophylaxis on newborn intestinal microbiota using a sequencing approach targeted to multi hypervariable 16S rDNA regions. Appl. Microbiol. Biotechnol. 100, 5537–5546. doi: 10.1007/s00253-016-7410-2
Alsharairi, N. A. (2020a). The infant gut microbiota and risk of asthma: the effect of maternal nutrition during pregnancy and lactation. Microorganisms 8, 1–10. doi: 10.3390/microorganisms8081119
Alsharairi, N. A. (2020b). The role of short-chain fatty acids in the interplay between a very low-calorie ketogenic diet and the infant gut microbiota and its therapeutic implications for reducing asthma. Int. J. Mol. Sci. 21:9580. doi: 10.3390/ijms21249580
Alsharairi, N. A. (2021). The role of short-chain fatty acids in mediating very low-calorie ketogenic diet-infant gut microbiota relationships and its therapeutic potential in obesity. Nutrients 13:3702. doi: 10.3390/nu13113702
Alsharairi, N. A. (2022). The therapeutic role of short-chain fatty acids mediated very low-calorie ketogenic diet–gut microbiota relationships in Paediatric inflammatory bowel diseases. Nutrients 14:4113. doi: 10.3390/nu14194113
Andreas, N. J., Kampmann, B., and Mehring, L.-D. K. (2015). Human breast milk: a review on its composition and bioactivity. Early Hum. Dev. 91, 629–635. doi: 10.1016/j.earlhumdev.2015.08.013
Arboleya, S., Binetti, A., Salazar, N., Fernández, N., Solís, G., Hernández-Barranco, A., et al. (2012). Establishment and development of intestinal microbiota in preterm neonates. FEMS Microbiol. Ecol. 79, 763–772. doi: 10.1111/j.1574-6941.2011.01261.x
Arboleya, S., Sánchez, B., Milani, C., Duranti, S., Solís, G., Fernández, N., et al. (2015). Intestinal microbiota development in preterm neonates and effect of perinatal antibiotics. J. Pediatr. 166, 538–544. doi: 10.1016/j.jpeds.2014.09.041
Ardissone, AN, De La Cruz, DM, Davis-Richardson, AG, Rechcigl, KT, Li, N, Drew, JC, et al. Meconium microbiome analysis identifies bacteria correlated with premature birth. JH Weitkamp, editor. PLoS One. 2014;9:e90784, doi: 10.1371/journal.pone.0090784
Arrieta, M. C., Stiemsma, L. T., Amenyogbe, N., Brown, E., and Finlay, B. (2014). The intestinal microbiome in early life: health and disease. Front. Immunol. 5:427. doi: 10.3389/fimmu.2014.00427
Arrieta, M. C., Stiemsma, L. T., Dimitriu, P. A., Thorson, L., Russell, S., Yurist-Doutsch, S., et al. (2015). Early infancy microbial and metabolic alterations affect risk of childhood asthma. Sci. Transl. Med. 7:307ra152. doi: 10.1126/scitranslmed.aab2271
Asnicar, F., Manara, S., Zolfo, M., Truong, D. T., Scholz, M., Armanini, F., et al. (2017). Studying vertical microbiome transmission from mothers to infants by strain-level metagenomic profiling. mSystems 2:e00164-16. doi: 10.1128/mSystems.00164-16
Azad, M. B., Konya, T., Maughan, H., Guttman, D. S., Field, C. J., Chari, R. S., et al. (2013). Gut microbiota of healthy Canadian infants: profiles by mode of delivery and infant diet at 4 months. CMAJ 185, 385–394. doi: 10.1503/cmaj.121189
Azad, M. B., Konya, T., Persaud, R. R., Guttman, D. S., Chari, R. S., Field, C. J., et al. (2016). Impact of maternal intrapartum antibiotics, method of birth and breastfeeding on gut microbiota during the first year of life: a prospective cohort study. BJOG 123, 983–993. doi: 10.1111/1471-0528.13601
Bäckhed, F., Roswall, J., Peng, Y., Feng, Q., Jia, H., Kovatcheva-Datchary, P., et al. (2015). Dynamics and stabilization of the human gut microbiome during the first year of life. Cell Host Microbe 17, 690–703. doi: 10.1016/j.chom.2015.04.004
Bager, P., Simonsen, J., Nielsen, N. M., and Frisch, M. (2012). Cesarean section and offspring’s risk of inflammatory bowel disease: a national cohort study. Inflamm. Bowel Dis. 18, 857–862. doi: 10.1002/ibd.21805
Bager, P., Wohlfahrt, J., and Westergaard, T. (2008). Caesarean delivery and risk of atopy and allergic disesase: Meta-analyses. Clin. Exp. Allergy 38, 634–642. doi: 10.1111/j.1365-2222.2008.02939.x
Bergström, A., Skov, T. H., Bahl, M. I., Roager, H. M., Christensen, L. B., Ejlerskov, K. T., et al. (2014). Establishment of intestinal microbiota during early life: a longitudinal, explorative study of a large cohort of Danish infants. Appl. Environ. Microbiol. 80, 2889–2900. doi: 10.1128/AEM.00342-14
Betran, A. P., Torloni, M. R., Zhang, J. J., and Gülmezoglu, A. M. (2016). WHO statement on caesarean section rates. BJOG 123, 667–670. doi: 10.1111/1471-0528.13526
Bezirtzoglou, E., Tsiotsias, A., and Welling, G. W. (2011). Microbiota profile in feces of breast- and formula-fed newborns by using fluorescence in situ hybridization (FISH). Anaerobe 17, 478–482. doi: 10.1016/j.anaerobe.2011.03.009
Biasucci, G., Benenati, B., Morelli, L., Bessi, E., and Boehm, G. (2008). Cesarean delivery may affect the early biodiversity of intestinal bacteria. J. Nutr. 138, 1796S–1800S. doi: 10.1093/jn/138.9.1796S
Bisanz, J. E., Enos, M. K., PrayGod, G., Seney, S., Macklaim, J. M., Chilton, S., et al. (2015). Microbiota at multiple body sites during pregnancy in a rural tanzanian population and effects of Moringa-supplemented probiotic yogurt. Appl. Environ. Microbiol. 81, 4965–4975. doi: 10.1128/AEM.00780-15
Boutin, R. C. T., and Finlay, B. B. (2016). Microbiota-mediated immunomodulation and asthma: current and future perspectives. Curr. Treat. Options Allergy 3, 292–309. doi: 10.1007/s40521-016-0087-z
Bridgman, S. L., Azad, M. B., Field, C. J., Haqq, A. M., Becker, A. B., Mandhane, P. J., et al. (2017). Fecal short-chain fatty acid variations by breastfeeding status in infants at 4 months: differences in relative versus absolute concentrations. Front. Nutr. 4:11. doi: 10.3389/fnut.2017.00011
Cabrera-Rubio, R., Collado, M. C., Laitinen, K., Salminen, S., Isolauri, E., and Mira, A. (2012). The human milk microbiome changes over lactation and is shaped by maternal weight and mode of delivery. Am. J. Clin. Nutr. 96, 544–551. doi: 10.3945/ajcn.112.037382
Cardwell, C. R., Stene, L. C., Joner, G., Cinek, O., Svensson, J., Goldacre, M. J., et al. (2008). Caesarean section is associated with an increased risk of childhood-onset type 1 diabetes mellitus: a meta-analysis of observational studies. Diabetologia 51, 726–735. doi: 10.1007/s00125-008-0941-z
Chong, C. Y. L., Bloomfield, F. H., and O’Sullivan, J. M. (2018). Factors affecting gastrointestinal microbiome development in neonates. Nutrients 10:274. doi: 10.3390/nu10030274
Chu, D. M., Antony, K. M., Ma, J., Prince, A. L., Showalter, L., Moller, M., et al. (2016b). The early infant gut microbiome varies in association with a maternal high-fat diet. Genome Med. 8:77. doi: 10.1186/s13073-016-0330-z
Chu, D. M., Meyer, K. M., Prince, A. L., and Aagaard, K. M. (2016a). Impact of maternal nutrition in pregnancy and lactation on offspring gut microbial composition and function. Gut Microbes 7, 459–470. doi: 10.1080/19490976.2016.1241357
Chu, S, Yu, H, Chen, Y, Chen, Q, Wang, B, and Zhang, J. Periconceptional and gestational exposure to antibiotics and childhood asthma. P Latzin, editor. PLoS One 2015;10:e0140443, doi: 10.1371/journal.pone.0140443
Collado, M. C., Rautava, S., Aakko, J., Isolauri, E., and Salminen, S. (2016). Human gut colonisation may be initiated in utero by distinct microbial communities in the placenta and amniotic fluid. Sci. Rep. 6:23129. doi: 10.1038/srep23129
De Filippo, C., Cavalieri, D., Di Paola, M., Ramazzotti, M., Poullet, J. B., Massart, S., et al. (2010). Impact of diet in shaping gut microbiota revealed by a comparative study in children from Europe and rural Africa. Proc. Natl. Acad. Sci. U. S. A. 107, 14691–14696. doi: 10.1073/pnas.1005963107
Dierikx, T. H., Visser, D. H., Benninga, M. A., van Kaam, A. H. L. C., de Boer, N. K. H., de Vries, R., et al. (2020). The influence of prenatal and intrapartum antibiotics on intestinal microbiota colonisation in infants: a systematic review. J. Infect. 81, 190–204. doi: 10.1016/j.jinf.2020.05.002
DiGiulio, D. B. (2012). Diversity of microbes in amniotic fluid. Semin Fetal Neonatal. Med. 17, 2–11. doi: 10.1016/j.siny.2011.10.001
Dogra, S., Sakwinska, O., Soh, S. E., Ngom-Bru, C., Brück, W. M., Berger, B., et al. (2015). Dynamics of infant gut microbiota are influenced by delivery mode and gestational duration and are associated with subsequent adiposity. MBio 6, e02419–e02414. doi: 10.1128/mBio.02419-14
Dominguez-Bello, M. G., Costello, E. K., Contreras, M., Magris, M., Hidalgo, G., Fierer, N., et al. (2010). Delivery mode shapes the acquisition and structure of the initial microbiota across multiple body habitats in newborns. Proc. Natl. Acad. Sci. U. S. A. 107, 11971–11975. doi: 10.1073/pnas.1002601107
Dos Santos, S. J., Pakzad, Z., Albert, A. Y. K., Elwood, C. N., Grabowska, K., Links, M. G., et al. (2023). Maternal vaginal microbiome composition does not affect development of the infant gut microbiome in early life. Front. Cell. Infect. Microbiol. 13:1144254. doi: 10.3389/fcimb.2023.1144254
Dos Santos, S. J., Pakzad, Z., Elwood, C. N., Albert, A. Y. K., Gantt, S., Manges, A. R., et al. (2021). Early neonatal meconium does not have a demonstrable microbiota determined through use of robust negative controls with cpn 60-based microbiome profiling. Microbiol. Spectr. 9:e00067-21. doi: 10.1128/Spectrum.00067-21
Echarri, P. P., Graciá, C. M., Berruezo, G. R., Vives, I., Ballesta, M., Solís, G., et al. (2011). Assessment of intestinal microbiota of full-term breast-fed infants from two different geographical locations. Early Hum. Dev. 87, 511–513. doi: 10.1016/j.earlhumdev.2011.03.013
Ege, M. J., Mayer, M., Normand, A. C., Genuneit, J., Cookson, W. O. C. M., Braun-Fahrländer, C., et al. (2011). Exposure to environmental microorganisms and childhood asthma. N. Engl. J. Med. 364, 701–709. doi: 10.1056/NEJMoa1007302
Escherich, T. H. (1989). The intestinal bacteria of the neonate and breast-fed infant. Rev. Infect. Dis. 11, 352–356.
Faa, G., Gerosa, C., Fanni, D., Nemolato, S., Van Eyken, P., and Fanos, V. (2013). Factors influencing the development of a personal tailored microbiota in the neonate, with particular emphasis on antibiotic therapy. J. Matern. Fetal Neonatal Med. 26, 35–43. doi: 10.3109/14767058.2013.829700
Fallani, M., Amarri, S., Uusijarvi, A., Adam, R., Khanna, S., Aguilera, M., et al. (2011). Determinants of the human infant intestinal microbiota after the introduction of first complementary foods in infant samples from five European centres. Microbiology 157, 1385–1392. doi: 10.1099/mic.0.042143-0
Fallani, M., Young, D., Scott, J., Norin, E., Amarri, S., Adam, R., et al. (2010). Intestinal microbiota of 6-week-old infants across Europe: geographic influence beyond delivery mode, breast-feeding, and antibiotics. J. Pediatr. Gastroenterol. Nutr. 51, 77–84. doi: 10.1097/MPG.0b013e3181d1b11e
Fan, H. Y., Tung, Y. T., Yang, Y. C. S. H., Hsu, J. B., Lee, C. Y., Chang, T. H., et al. (2021). Maternal vegetable and fruit consumption during pregnancy and its effects on infant gut microbiome. Nutrients 13:1559. doi: 10.3390/nu13051559
Fernández, L., Langa, S., Martín, V., Maldonado, A., Jiménez, E., Martín, R., et al. (2013). The human milk microbiota: origin and potential roles in health and disease. Pharmacol. Res. 69, 1–10. doi: 10.1016/j.phrs.2012.09.001
Fouhy, F., Ross, R. P., Fitzgerald, G., Stanton, C., and Cotter, P. D. (2012). Composition of the early intestinal microbiota:knowledge, knowledge gaps and the use of high-throughput sequencing to address these gaps. Gut Microbes 3, 203–220. doi: 10.4161/gmic.20169
Fouhy, F., Watkins, C., Hill, C. J., O’Shea, C. A., Nagle, B., Dempsey, E. M., et al. (2019). Perinatal factors affect the gut microbiota up to four years after birth. Nat. Commun. 10:1517. doi: 10.1038/s41467-019-09252-4
Funkhouser, L. J., and Bordenstein, S. R. (2013). Mom knows best: the universality of maternal microbial transmission. PLoS Biol. 11:e1001631. doi: 10.1371/journal.pbio.1001631
García-Mantrana, I., Bertua, B., Martínez-Costa, C., and Collado, M. C. (2016). Perinatal nutrition: how to take care of the gut microbiota? Clin. Nutr. Exp. 6, 3–16. doi: 10.1016/j.yclnex.2016.02.002
Gibson, D. L., Gill, S. K., Brown, K., Tasnim, N., Ghosh, S., Innis, S., et al. (2015). Maternal exposure to fish oil primes offspring to harbor intestinal pathobionts associated with altered immune cell balance. Gut Microbes 6, 24–32. doi: 10.1080/19490976.2014.997610
Gilbert, W. M., and Brace, R. A. (1993). Amniotic fluid volume and normal flows to and from the amniotic cavity. Semin. Perinatol. 17, 150–157.
Gohir, W., Whelan, F. J., Surette, M. G., Moore, C., Schertzer, J. D., and Sloboda, D. M. (2015). Pregnancy-related changes in the maternal gut microbiota are dependent upon the mother’s periconceptional diet. Gut Microbes 6, 310–320. doi: 10.1080/19490976.2015.1086056
Gomez-Gallego, C., Garcia-Mantrana, I., Salminen, S., and Collado, M. C. (2016). The human milk microbiome and factors influencing its composition and activity. Semin. Fetal Neonatal Med. 21, 400–405. doi: 10.1016/j.siny.2016.05.003
González-Bermúdez, C. A., López-Nicolás, R., Peso-Echarri, P., Frontela-Saseta, C., and Martínez-Graciá, C. (2018). Effects of different thickening agents on infant gut microbiota. Food Funct. 9, 1768–1778. doi: 10.1039/C7FO01992K
Gonzalez-Perez, G., Hicks, A. L., Tekieli, T. M., Radens, C. M., Williams, B. L., and Lamousé-Smith, E. S. N. (2016). Maternal antibiotic treatment impacts development of the neonatal intestinal microbiome and antiviral immunity. J. Immunol. 196, 3768–3779. doi: 10.4049/jimmunol.1502322
Gosalbes, M. J., Llop, S., Vallès, Y., Moya, A., Ballester, F., and Francino, M. P. (2013). Meconium microbiota types dominated by lactic acid or enteric bacteria are differentially associated with maternal eczema and respiratory problems in infants. Clin. Exp. Allergy 43, 198–211. doi: 10.1111/cea.12063
Gray, L. E. K., O’Hely, M., Ranganathan, S., Sly, P. D., and Vuillermin, P. (2017). The maternal diet, gut bacteria, and bacterial metabolites during pregnancy influence offspring asthma. Front. Immunol. 8:365. doi: 10.3389/fimmu.2017.00365/full
Gritz, E. C., and Bhandari, V. (2015). The human neonatal gut microbiome: a brief review. Front. Pediatr. 3:17. doi: 10.3389/fped.2015.00017
Grzeskowiak, L., Collado, M. C., Mangani, C., Maleta, K., Laitinen, K., Ashorn, P., et al. (2012a). Distinct gut microbiota in southeastern African and northern European infants. J. Pediatr. Gastroenterol. Nutr. 54, 812–816. doi: 10.1097/MPG.0b013e318249039c
Grześkowiak, Ł., Grönlund, M. M., Beckmann, C., Salminen, S., von Berg, A., and Isolauri, E. (2012b). The impact of perinatal probiotic intervention on gut microbiota: double-blind placebo-controlled trials in Finland and Germany. Anaerobe 18, 7–13. doi: 10.1016/j.anaerobe.2011.09.006
Guaraldi, F., and Salvatori, G. (2012). Effect of breast and formula feeding on gut microbiota shaping in newborns. Front. Cell. Infect. Microbiol. 2:94. doi: 10.3389/fcimb.2012.00094
Hall, I. C. (1934). Bacterial Flora of first specimens of meconium passed by fifty new-born infants. Arch. Pediatr. Adolesc. Med. 47:1279. doi: 10.1001/archpedi.1934.01960130103007
Hansen, R, Scott, KP, Khan, S, Martin, JC, Berry, SH, Stevenson, M, et al. First-pass meconium samples from healthy term vaginally-delivered neonates: an analysis of the microbiota. TR Kollmann, editor. PLoS One. 2015;10:e0133320, doi: 10.1371/journal.pone.0133320
Harmsen, H. J. M., Wildeboer-Veloo, A. C. M., Raangs, G. C., Wagendorp, A. A., Klijn, N., Bindels, J. G., et al. (2000). Analysis of intestinal flora development in breast-fed and formula-fed infants by using molecular identification and detection methods. J. Pediatr. Gastroenterol. Nutr. 30, 61–67. doi: 10.1097/00005176-200001000-00019
Henderickx, J. G. E., Zwittink, R. D., Van Lingen, R. A., Knol, J., and Belzer, C. (2019). The preterm gut microbiota: an inconspicuous challenge in nutritional neonatal care. Front. Cell. Infect. Microbiol. 9:443877. doi: 10.3389/fcimb.2019.00085
Hill, C. J., Lynch, D. B., Murphy, K., Ulaszewska, M., Jeffery, I. B., O’Shea, C. A., et al. (2017). Evolution of gut microbiota composition from birth to 24 weeks in the INFANTMET cohort. Microbiome 5:4. doi: 10.1186/s40168-016-0213-y
Homann, C. M., Rossel, C. A. J., Dizzell, S., Bervoets, L., Simioni, J., Li, J., et al. (2021). Infants’ first solid foods: impact on gut microbiota development in two intercontinental cohorts. Nutrients 13:2639. doi: 10.3390/nu13082639
Hu, J., Nomura, Y., Bashir, A., Fernandez-Hernandez, H., Itzkowitz, S., Pei, Z., et al. (2013). Diversified microbiota of meconium is affected by maternal diabetes status. PLoS One 8:e78257. doi: 10.1371/journal.pone.0078257
Huurre, A., Kalliomäki, M., Rautava, S., Rinne, M., Salminen, S., and Isolauri, E. (2008). Mode of delivery - effects on gut microbiota and humoral immunity. Neonatology 93, 236–240. doi: 10.1159/000111102
Ismail, I. H., Licciardi, P. V., and Tang, M. L. K. (2013). Probiotic effects in allergic disease. J. Paediatr. Child Health 49, 709–715. doi: 10.1111/jpc.12175
Jakobsson, H. E., Abrahamsson, T. R., Jenmalm, M. C., Harris, K., Quince, C., Jernberg, C., et al. (2014). Decreased gut microbiota diversity, delayed Bacteroidetes colonisation and reduced Th1 responses in infants delivered by caesarean section. Gut 63, 559–566. doi: 10.1136/gutjnl-2012-303249
Jauréguy, F., Carton, M., Panel, P., Foucaud, P., Butel, M. J., and Doucet-Populaire, F. (2004). Effects of intrapartum penicillin prophylaxis on intestinal bacterial colonization in infants. J. Clin. Microbiol. 42, 5184–5188. doi: 10.1128/JCM.42.11.5184-5188.2004
Jiménez, E., Fernández, L., Marín, M. L., Martín, R., Odriozola, J. M., Nueno-Palop, C., et al. (2005). Isolation of commensal bacteria from umbilical cord blood of healthy neonates born by cesarean section. Curr. Microbiol. 51, 270–274. doi: 10.1007/s00284-005-0020-3
Jiménez, E., Marín, M. L., Martín, R., Odriozola, J. M., Olivares, M., Xaus, J., et al. (2008). Is meconium from healthy newborns actually sterile? Res. Microbiol. 159, 187–193. doi: 10.1016/j.resmic.2007.12.007
Johnson, C. C., Ownby, D. R., Alford, S. H., Havstad, S. L., Williams, L. K., Zoratti, E. M., et al. (2005). Antibiotic exposure in early infancy and risk for childhood atopy. J. Allergy Clin. Immunol. 115, 1218–1224. doi: 10.1016/j.jaci.2005.04.020
Jones, HE, Harris, KA, Azizia, M, Bank, L, Carpenter, B, Hartley, JC, et al. (2009). Differing prevalence and diversity of bacterial species in fetal membranes from very preterm and term labor. P Middleton, editor. PLoS One. 4:e8205. doi: 10.1371/journal.pone.0008205
Kaczmarczyk, M., Löber, U., Adamek, K., Węgrzyn, D., Skonieczna-Żydecka, K., Malinowski, D., et al. (2021). The gut microbiota is associated with the small intestinal paracellular permeability and the development of the immune system in healthy children during the first two years of life. J. Transl. Med. 19:177. doi: 10.1186/s12967-021-02839-w
Kamal, S. S., Hyldig, N., Krych, Ł., Greisen, G., Krogfelt, K. A., Zachariassen, G., et al. (2019). Impact of early exposure to cefuroxime on the composition of the gut microbiota in infants following cesarean delivery. J. Pediatr. 210, 99–105.e2. doi: 10.1016/j.jpeds.2019.03.001
Kaplan, J. L., Shi, H. N., and Walker, W. A. (2011). The role of microbes in developmental immunologic programming. Pediatr. Res. 69, 465–472. doi: 10.1203/PDR.0b013e318217638a
Kennedy, K. M., Gerlach, M. J., Adam, T., Heimesaat, M. M., Rossi, L., Surette, M. G., et al. (2021). Fetal meconium does not have a detectable microbiota before birth. Nat. Microbiol. 6, 865–873. doi: 10.1038/s41564-021-00904-0
Keski-Nisula, L., Kyynäräinen, H. R., Kärkkäinen, U., Karhukorpi, J., Heinonen, S., and Pekkanen, J. (2013). Maternal intrapartum antibiotics and decreased vertical transmission of Lactobacillus to neonates during birth. Acta Paediat. Int. J. Paediat. 102, 480–485. doi: 10.1111/apa.12186
Kim, H., Sitarik, A. R., Woodcroft, K., Johnson, C. C., and Zoratti, E. (2019). Birth mode, breastfeeding, pet exposure, and antibiotic use: associations with the gut microbiome and sensitization in children. Curr Allergy Asthma Rep 19:22. doi: 10.1007/s11882-019-0851-9
Koletzko, S., Lee, H. S., Beyerlein, A., Aronsson, C. A., Hummel, M., Liu, E., et al. (2018). Cesarean section on the risk of celiac disease in the offspring: the teddy study. J. Pediatr. Gastroenterol. Nutr. 66, 417–424. doi: 10.1097/MPG.0000000000001682
Koleva, P. T., Kim, J. S., Scott, J. A., and Kozyrskyj, A. L. (2015). Microbial programming of health and disease starts during fetal life. Birth Defects Res. C Embryo Today 105, 265–277. doi: 10.1002/bdrc.21117
Korpela, K. (2021). Impact of delivery mode on infant gut microbiota. Ann. Nutr. Metab. 77, 11–19. doi: 10.1159/000518498
Korpela, K., Salonen, A., Vepsäläinen, O., Suomalainen, M., Kolmeder, C., Varjosalo, M., et al. (2018). Probiotic supplementation restores normal microbiota composition and function in antibiotic-treated and in caesarean-born infants. Microbiome 6:182. doi: 10.1186/s40168-018-0567-4
Korpela, K., Salonen, A., Virta, L. J., Kekkonen, R. A., Forslund, K., Bork, P., et al. (2016). Intestinal microbiome is related to lifetime antibiotic use in Finnish pre-school children. Nat. Commun. 7:10410. doi: 10.1038/ncomms10410
Kozyrskyj, A. L., Ernst, P., and Becker, A. B. (2007). Increased risk of childhood asthma from antibiotic use in early life. Chest 131, 1753–1759. doi: 10.1378/chest.06-3008
Kumbhare, S. V., Patangia, D. V., Patil, R. H., Shouche, Y. S., and Patil, N. P. (2019). Factors influencing the gut microbiome in children: from infancy to childhood. J. Biosci. 44:49. doi: 10.1007/s12038-019-9860-z
Kuperman, A. A., and Koren, O. (2016). Antibiotic use during pregnancy: how bad is it? BMC Med. 14:91. doi: 10.1186/s12916-016-0636-0
Laforest-Lapointe, I., Becker, A. B., Mandhane, P. J., Turvey, S. E., Moraes, T. J., Sears, M. R., et al. (2021). Maternal consumption of artificially sweetened beverages during pregnancy is associated with infant gut microbiota and metabolic modifications and increased infant body mass index. Gut Microbes 13, 1–15. doi: 10.1080/19490976.2020.1857513
Lahtinen, S. J., Boyle, R. J., Kivivuori, S., Oppedisano, F., Smith, K. R., Robins-Browne, R., et al. (2009). Prenatal probiotic administration can influence Bifidobacterium microbiota development in infants at high risk of allergy. J. Allergy Clin. Immunol. 123, 499–501. doi: 10.1016/j.jaci.2008.11.034
Lauder, A. P., Roche, A. M., Sherrill-Mix, S., Bailey, A., Laughlin, A. L., Bittinger, K., et al. (2016). Comparison of placenta samples with contamination controls does not provide evidence for a distinct placenta microbiota. Microbiome 4:29. doi: 10.1186/s40168-016-0172-3
Laursen, M. F., Andersen, L. B. B., Michaelsen, K. F., Mølgaard, C., Trolle, E., Bahl, M. I., et al. (2016). Infant gut microbiota development is driven by transition to family foods independent of maternal obesity. mSphere 1:e00069-15. doi: 10.1128/mSphere.00069-15
Laursen, M. F., Bahl, M. I., Michaelsen, K. F., and Licht, T. R. (2017). First foods and gut microbes. Front. Microbiol. 8:356. doi: 10.3389/fmicb.2017.00356
Laursen, M. F., Zachariassen, G., Bahl, M. I., Bergström, A., Høst, A., Michaelsen, K. F., et al. (2015). Having older siblings is associated with gut microbiota development during early childhood. BMC Microbiol. 15:154. doi: 10.1186/s12866-015-0477-6
Li, M., Wang, M., and Donovan, S. M. (2014). Early development of the gut microbiome and immune-mediated childhood disorders. Semin. Reprod. Med. 32, 74–86. doi: 10.1055/s-0033-1361825
Lim, J. M., Letchumanan, V., Tan, L. T. H., Hong, K. W., Wong, S. H., Ab Mutalib, N. S., et al. (2022). Ketogenic diet: a dietary intervention via gut microbiome modulation for the treatment of neurological and nutritional disorders (a narrative review). Nutrients 14:3566. doi: 10.3390/nu14173566
Lin, A., Bik, E. M., Costello, E. K., Dethlefsen, L., Haque, R., Relman, D. A., et al. (2013). Distinct distal gut microbiome diversity and composition in healthy children from Bangladesh and the United States. PLoS One 8:e53838. doi: 10.1371/journal.pone.0053838
Łoniewska, B., Adamek, K., Węgrzyn, D., Kaczmarczyk, M., Skonieczna-żydecka, K., Clark, J., et al. (2020). Analysis of faecal zonulin and calprotectin concentrations in healthy children during the first two years of life. An observational prospective cohort study. J. Clin. Med. 9:777. doi: 10.3390/jcm9030777
Lundgren, S. N., Madan, J. C., Emond, J. A., Morrison, H. G., Christensen, B. C., Karagas, M. R., et al. (2018). Maternal diet during pregnancy is related with the infant stool microbiome in a delivery mode-dependent manner. Microbiome 6:109. doi: 10.1186/s40168-018-0490-8
Ma, J., Prince, A. L., Bader, D., Hu, M., Ganu, R., Baquero, K., et al. (2014). High-fat maternal diet during pregnancy persistently alters the offspring microbiome in a primate model. Nat. Commun. 5:3889. doi: 10.1038/ncomms4889
Madan, J. C., Salari, R. C., Saxena, D., Davidson, L., O’Toole, G. A., Moore, J. H., et al. (2012). Gut microbial colonisation in premature neonates predicts neonatal sepsis. Arch. Dis. Child. Fetal Neonatal Ed. 97, F456–F462. doi: 10.1136/fetalneonatal-2011-301373
Maher, S. E., O’Brien, E. C., Moore, R. L., Byrne, D. F., Geraghty, A. A., Saldova, R., et al. (2020). The association between the maternal diet and the maternal and infant gut microbiome: A systematic review. Br. J. Nutr. 129, 1–29. doi: 10.1017/S0007114520000847
Mandal, S., Godfrey, K. M., McDonald, D., Treuren, W. V., Bjørnholt, J. V., Midtvedt, T., et al. (2016). Fat and vitamin intakes during pregnancy have stronger relations with a proinflammatory maternal microbiota than does carbohydrate intake. Microbiome 4:55. doi: 10.1186/s40168-016-0200-3
Mann, P. E., Huynh, K., and Widmer, G. (2018). Maternal high fat diet and its consequence on the gut microbiome: a rat model. Gut Microbes 9, 143–154. doi: 10.1080/19490976.2017.1395122
Martin, R, Makino, H, Yavuz, AC, Ben-Amor, K, Roelofs, M, Ishikawa, E, et al. Early-life events, including mode of delivery and type of feeding, siblings and gender, shape the developing gut microbiota. JS Suchodolski, editor. PLoS One. 2016;11:e0158498, doi: 10.1371/journal.pone.0158498
Matamoros, S., Gras-Leguen, C., Le Vacon, F., Potel, G., and De La Cochetiere, M. F. (2013). Development of intestinal microbiota in infants and its impact on health. Trends Microbiol. 21, 167–173. doi: 10.1016/j.tim.2012.12.001
Metsälä, J., Lundqvist, A., Virta, L. J., Kaila, M., Gissler, M., and Virtanen, S. M. (2015). Prenatal and post-natal exposure to antibiotics and risk of asthma in childhood. Clin. Exp. Allergy 45, 137–145. doi: 10.1111/cea.12356
Miko, E., Csaszar, A., Bodis, J., and Kovacs, K. (2022). The maternal–fetal gut microbiota axis: physiological changes, dietary influence, and modulation possibilities. Life. 12:424. doi: 10.3390/life12030424
Milani, C., Duranti, S., Bottacini, F., Casey, E., Turroni, F., Mahony, J., et al. (2017). The first microbial colonizers of the human gut: composition, activities, and health implications of the infant gut microbiota. Microbiol. Mol. Biol. Rev. 81, e00036–e00017. doi: 10.1128/mmbr.00036-17
Mirpuri, J. (2020). Evidence for maternal diet-mediated effects on the offspring microbiome and immunity: implications for public health initiatives. Pediatr. Res. 89, 301–306. doi: 10.1038/s41390-020-01121-x
Moles, L., Gómez, M., Heilig, H., Bustos, G., Fuentes, S., de Vos, W., et al. (2013). Bacterial diversity in meconium of preterm neonates and evolution of their fecal microbiota during the first month of life. PLoS One 8:e66986. doi: 10.1371/journal.pone.0066986
Moossavi, S., Miliku, K., Sepehri, S., Khafipour, E., and Azad, M. B. (2018). The prebiotic and probiotic properties of human milk: implications for infant immune development and pediatric asthma. Front. Pediatr. 6:197. doi: 10.3389/fped.2018.00197
Mshvildadze, M., Neu, J., Shuster, J., Theriaque, D., Li, N., and Mai, V. (2010). Intestinal microbial ecology in premature infants assessed with non-culture-based techniques. J. Pediatr. 156, 20–25. doi: 10.1016/j.jpeds.2009.06.063
Mueller, N. T., Bakacs, E., Combellick, J., Grigoryan, Z., and Dominguez-Bello, M. G. (2015b). The infant microbiome development: mom matters. Trends Mol. Med. 21, 109–117. doi: 10.1016/j.molmed.2014.12.002
Mueller, N. T., Whyatt, R., Hoepner, L., Oberfield, S., Dominguez-Bello, M. G., Widen, E. M., et al. (2015a). Prenatal exposure to antibiotics, cesarean section and risk of childhood obesity. Int. J. Obes. 39, 665–670. doi: 10.1038/ijo.2014.180
Munyaka, P. M., Khafipour, E., and Ghia, J. E. (2014). External influence of early childhood establishment of gut microbiota and subsequent health implications. Front. Pediatr. 2:109. doi: 10.3389/fped.2014.00109
Murphy, K., Curley, D., O’Callaghan, T. F., O’Shea, C. A., Dempsey, E. M., O’Toole, P. W., et al. (2017). The composition of human milk and infant faecal microbiota over the first three months of life: a pilot study. Sci. Rep. 7:40597. doi: 10.1038/srep40597
Nogacka, A. M., Salazar, N., Arboleya, S., Suárez, M., Fernández, N., Solís, G., et al. (2018). Early microbiota, antibiotics and health. Cell. Mol. Life Sci. 75, 83–91. doi: 10.1007/s00018-017-2670-2
O’Sullivan, A., Farver, M., and Smilowitz, J. T. (2015). The influence of early infant-feeding practices on the intestinal microbiome and body composition in infants. Nutr. Metab. Insights 8, 1–9. doi: 10.4137/NMI.S29530
Oddy, W. H. (2017). Breastfeeding, childhood asthma, and allergic disease. Ann. Nutr. Metab. 70, 26–36. doi: 10.1159/000457920
Panzer, A. R., and Lynch, S. V. (2015). Influence and effect of the human microbiome in allergy and asthma. Curr. Opin. Rheumatol. 27, 373–380. doi: 10.1097/BOR.0000000000000191
Panzer, J. J., Romero, R., Greenberg, J. M., Winters, A. D., Galaz, J., Gomez-Lopez, N., et al. (2023). Is there a placental microbiota? A critical review and re-analysis of published placental microbiota datasets. BMC Microbiol. 23:76. doi: 10.1186/s12866-023-02764-6
Penders, J. (2017). “Chapter 5 early diet and the infant gut microbiome: how breastfeeding and solid foods shape the microbiome” in Microbiota in health and disease: From pregnancy to childhood eds. P. D. Brownw, E. Claassen and M. D. Cabana. (Nederland: Wageningen Academic Publishers), 105–118.
Penders, J., Thijs, C., Vink, C., Stelma, F. F., Snijders, B., Kummeling, I., et al. (2006). Factors influencing the composition of the intestinal microbiota in early infancy. Pediatrics 118, 511–521. doi: 10.1542/peds.2005-2824
Perez-Muñoz, M. E., Arrieta, M. C., Ramer-Tait, A. E., and Walter, J. (2017). A critical assessment of the “sterile womb” and “in utero colonization” hypotheses: implications for research on the pioneer infant microbiome. Microbiome 5:48. doi: 10.1186/s40168-017-0268-4
Porro, M., Kundrotaite, E., Mellor, D. D., and Munialo, C. D. (2023). A narrative review of the functional components of human breast milk and their potential to modulate the gut microbiome, the consideration of maternal and child characteristics, and confounders of breastfeeding, and their impact on risk of obesity later in life. Nutr. Rev. 81, 597–609. doi: 10.1093/nutrit/nuac072
Rao, C., Coyte, K. Z., Bainter, W., Geha, R. S., Martin, C. R., and Rakoff-Nahoum, S. (2021). Multi-kingdom ecological drivers of microbiota assembly in preterm infants. Nature 591, 633–638. doi: 10.1038/s41586-021-03241-8
Rasmussen, S. H., Shrestha, S., Bjerregaard, L. G., Ängquist, L. H., Baker, J. L., Jess, T., et al. (2018). Antibiotic exposure in early life and childhood overweight and obesity: a systematic review and meta-analysis. Diabetes Obes. Metab. 20, 1508–1514. doi: 10.1111/dom.13230
Rehbinder, E. M., Lødrup Carlsen, K. C., Staff, A. C., Angell, I. L., Landrø, L., Hilde, K., et al. (2018). Is amniotic fluid of women with uncomplicated term pregnancies free of bacteria? Am. J. Obstet. Gynecol. 219, 289.e1–289.e12. doi: 10.1016/j.ajog.2018.05.028
Reyman, M., van Houten, M. A., Watson, R. L., Chu, M. L. J. N., Arp, K., de Waal, W. J., et al. (2022). Effects of early-life antibiotics on the developing infant gut microbiome and resistome: a randomized trial. Nat. Commun. 13:893. doi: 10.1038/s41467-022-28525-z
Rodríguez, J. M., Murphy, K., Stanton, C., Ross, R. P., Kober, O. I., Juge, N., et al. (2015). The composition of the gut microbiota throughout life, with an emphasis on early life. Microb. Ecol. Health Dis. 26:26050. doi: 10.3402/mehd.v26.26050
Roduit, C., Scholtens, S., De Jongste, J. C., Wijga, A. H., Gerritsen, J., Postma, D. S., et al. (2009). Asthma at 8 years of age in children born by caesarean section. Thorax 64, 107–113. doi: 10.1136/thx.2008.100875
Rougé, C., Goldenberg, O., Ferraris, L., Berger, B., Rochat, F., Legrand, A., et al. (2010). Investigation of the intestinal microbiota in preterm infants using different methods. Anaerobe 16, 362–370. doi: 10.1016/j.anaerobe.2010.06.002
Ruiz, L., Moles, L., Gueimonde, M., and Rodriguez, J. M. (2016). Perinatal microbiomes’ influence on preterm birth and preterms’ health: influencing factors and modulation strategies. J. Pediatr. Gastroenterol. Nutr. 63, e193–e203. doi: 10.1097/MPG.0000000000001196
Scholtens, S., Wijga, A. H., Brunekreef, B., Kerkhof, M., Hoekstra, M. O., Gerritsen, J., et al. (2009). Breast feeding, parental allergy and asthma in children followed for 8 years. The PIAMA birth cohort study. Thorax 64, 604–609. doi: 10.1136/thx.2007.094938
Schwab, C. (2022). The development of human gut microbiota fermentation capacity during the first year of life. Microb. Biotechnol. 15, 2865–2874. doi: 10.1111/1751-7915.14165
Sebastián-Domingo, J. J., and Sánchez-Sánchez, C. (2018). From the intestinal flora to the microbiome. Rev. Esp. Enferm. Dig. 110, 51–56. doi: 10.17235/reed.2017.4947/2017
Selma-Royo, M., García-Mantrana, I., Calatayud, M., Parra-Llorca, A., Martínez-Costa, C., and Collado, M. C. (2021). Maternal diet during pregnancy and intestinal markers are associated with early gut microbiota. Eur. J. Nutr. 60, 1429–1442. doi: 10.1007/s00394-020-02337-7
Selma-Royo, M, Tarrazó, M, García-Mantrana, I, Gómez-Gallego, C, Salminen, S, and Collado, MC. (2019). Shaping microbiota during the first 1000 days of life. In: Advances in experimental medicine and biology. p. 3–24. Available at: http://www.ncbi.nlm.nih.gov/pubmed/30680645 [Accessed April 11, 2019].
Sevelsted, A., Stokholm, J., Bønnelykke, K., and Bisgaard, H. (2015). Cesarean section chronic immune disorders. Pediatrics 135, e92–e98. doi: 10.1542/peds.2014-0596
Shi, Y. C., Guo, H., Chen, J., Sun, G., Ren, R. R., Guo, M. Z., et al. (2018). Initial meconium microbiome in Chinese neonates delivered naturally or by cesarean section. Sci. Rep. 8:3255. doi: 10.1038/s41598-018-21657-7
Snyder, M. L. (1936). The bacterial flora of meconium specimenscollected from sixty-four infants within four hours after delivery. J. Pediatr. 9, 624–632. doi: 10.1016/S0022-3476(36)80069-9
Stearns, J. C., Simioni, J., Gunn, E., McDonald, H., Holloway, A. C., Thabane, L., et al. (2017). Intrapartum antibiotics for GBS prophylaxis alter colonization patterns in the early infant gut microbiome of low risk infants. Sci. Rep. 7:16527. doi: 10.1038/s41598-017-16606-9
Stiemsma, L. T., and Turvey, S. E. (2017). Asthma and the microbiome: defining the critical window in early life. Allergy, Asthma Clin. Immunol. 13:3. doi: 10.1186/s13223-016-0173-6
Stokholm, J., Schjørring, S., Eskildsen, C. E., Pedersen, L., Bischoff, A. L., Følsgaard, N., et al. (2014). Antibiotic use during pregnancy alters the commensal vaginal microbiota. Clin. Microbiol. Infect. 20, 629–635. doi: 10.1111/1469-0691.12411
Talsness, C. E., Penders, J., Jansen, E. H. J. M., Damoiseaux, J., Thijs, C., and Mommers, M. (2017). Influence of vitamin D on key bacterial taxa in infant microbiota in the KOALA birth cohort study. PLoS One 12:e0188011. doi: 10.1371/journal.pone.0188011
Tanaka, S., Kobayashi, T., Songjinda, P., Tateyama, A., Tsubouchi, M., Kiyohara, C., et al. (2009). Influence of antibiotic exposure in the early postnatal period on the development of intestinal microbiota. FEMS Immunol. Med. Microbiol. 56, 80–87. doi: 10.1111/j.1574-695X.2009.00553.x
Tapiainen, T., Paalanne, N., Tejesvi, M. V., Koivusaari, P., Korpela, K., Pokka, T., et al. (2018). Maternal influence on the fetal microbiome in a population-based study of the first-pass meconium. Pediatr. Res. 84, 371–379. doi: 10.1038/pr.2018.29
Taylor, R., Keane, D., Borrego, P., and Arcaro, K. (2023). Effect of maternal diet on maternal Milk and breastfed infant gut microbiomes: a scoping review. Nutrients 15:1420. doi: 10.3390/nu15061420
Thavagnanam, S., Fleming, J., Bromley, A., Shields, M. D., and Cardwell, C. R. (2008). A meta-analysis of the association between caesarean section and childhood asthma. Clin. Exp. Allergy 38, 629–633. doi: 10.1111/j.1365-2222.2007.02780.x
Thompson, A. L., Monteagudo-Mera, A., Cadenas, M. B., Lampl, M. L., and Azcarate-Peril, M. A. (2015). Milk- and solid-feeding practices and daycare attendance are associated with differences in bacterial diversity, predominant communities, and metabolic and immune function of the infant gut microbiome. Front. Cell. Infect. Microbiol. 5:3. doi: 10.3389/fcimb.2015.00003
Tissier, H. Recherches sur la flore intestinale normale et pathologique du nourrisson. Paris: G. Carré et C. Naud; 1900. 1–253 p.
Tormo-Badia, N., Håkansson, V. K., Molin, G., Ahrné, S., and Cilio, C. M. (2014). Antibiotic treatment of pregnant non-obese diabetic mice leads to altered gut microbiota and intestinal immunological changes in the offspring. Scand. J. Immunol. 80, 250–260. doi: 10.1111/sji.12205
Tun, H. M., Bridgman, S. L., Chari, R., Field, C. J., Guttman, D. S., Becker, A. B., et al. (2018). Roles of birth mode and infant gut microbiota in intergenerational transmission of overweight and obesity from mother to offspring. JAMA Pediatr. 172, 368–377. doi: 10.1001/jamapediatrics.2017.5535
Tunay, R. T., and Kök, T. T. (2022). Verticle transmission of unique bacterial strains from mother to infant via consuming natural kefir. Int. Dairy J. 126:105251. doi: 10.1016/j.idairyj.2021.105251
Turfkruyer, M., and Verhasselt, V. (2015). Breast milk and its impact on maturation of the neonatal immune system. Curr. Opin. Infect. Dis. 28, 199–206. doi: 10.1097/QCO.0000000000000165
UNICEF and WHO (2010). Vol. WHA55 A55/, World Health Organization. World Health Organization. p. 19 Indicators for assessing infant and young child feeding practices. Available at: http://apps.who.int/iris/bitstream/handle/10665/44306/9789241599290_eng.pdf?sequence=1%0Ahttp://whqlibdoc.who.int/publications/2008/9789241596664_eng.pdf%5Cnhttp://www.unicef.org/programme/breastfeeding/innocenti.htm%5Cnhttp://innocenti15.net/declaration [Accessed July 31, 2019].
Urwin, H. J., Miles, E. A., Noakes, P. S., Kremmyda, L. S., Vlachava, M., Diaper, N. D., et al. (2014). Effect of salmon consumption during pregnancy on maternal and infant faecal microbiota, secretory IgA and calprotectin. Br. J. Nutr. 111, 773–784. doi: 10.1017/S0007114513003097
Vallès, Y, Artacho, A, Pascual-García, A, Ferrús, ML, Gosalbes, MJ, Abellán, JJ, et al. Microbial succession in the gut: directional trends of taxonomic and functional change in a birth cohort of Spanish infants. DS Guttman, editor. PLoS Genet. 2014;10:e1004406, doi: 10.1371/journal.pgen.1004406
van den Elsen, L. W. J., Garssen, J., Burcelin, R., and Verhasselt, V. (2019). Shaping the gut microbiota by breastfeeding: the gateway to allergy prevention? Front. Pediatr. 7:47. doi: 10.3389/fped.2019.00047
Wang, X., Buhimschi, C. S., Temoin, S., Bhandari, V., Han, Y. W., and Buhimschi, I. A. (2013). Comparative microbial analysis of paired amniotic fluid and cord blood from pregnancies complicated by preterm birth and early-onset neonatal Sepsis. PLoS One 8:e56131. doi: 10.1371/journal.pone.0056131
Wang, Y, Xie, T, Wu, Y, Liu, Y, Zou, Z, and Bai, J. (2021). Impacts of Maternal Diet and Alcohol Consumption during Pregnancy on Maternal and Infant Gut Microbiota.
Ward, T. L., Hosid, S., Ioshikhes, I., and Altosaar, I. (2013). Human milk metagenome: a functional capacity analysis. BMC Microbiol. 13:116. doi: 10.1186/1471-2180-13-116
Westerbeek, E. A. M., van den Berg, A., Lafeber, H. N., Knol, J., Fetter, W. P. F., and van Elburg, R. M. (2006). The intestinal bacterial colonisation in preterm infants: a review of the literature. Clin. Nutr. 25, 361–368. doi: 10.1016/j.clnu.2006.03.002
Yassour, M., Vatanen, T., Siljander, H., Hämäläinen, A. M., Härkönen, T., Ryhänen, S. J., et al. (2016). Natural history of the infant gut microbiome and impact of antibiotic treatment on bacterial strain diversity and stability. Sci. Transl. Med. 8, 343ra81–343ra81. doi: 10.1126/scitranslmed.aad0917
Yatsunenko, T., Rey, F. E., Manary, M. J., Trehan, I., Dominguez-Bello, M. G., Contreras, M., et al. (2012). Human gut microbiome viewed across age and geography. Nature 486, 222–227. doi: 10.1038/nature11053
Zaidi, A. Z., Moore, S. E., and Okala, S. G. (2021). Impact of maternal nutritional supplementation during pregnancy and lactation on the infant gut or breastmilk microbiota: a systematic review. Nutrients 13:1137. doi: 10.3390/nu13041137
Zambrano, AK;, Zambrano, AK, Cadena-Ullauri, S, Guevara-Ramírez, P, Frias-Toral, E, Ruiz-Pozo, VA, et al. (2023). Citation: The Impact of a Very-Low-Calorie Ketogenic Diet in the Gut Microbiota Composition in Obesity.
Zwittink, R. D., Renes, I. B., van Lingen, R. A., van Zoeren-Grobben, D., Konstanti, P., Norbruis, O. F., et al. (2018). Association between duration of intravenous antibiotic administration and early-life microbiota development in late-preterm infants. Eur. J. Clin. Microbiol. Infect. Dis. 37, 475–483. doi: 10.1007/s10096-018-3193-y
Glossary
Keywords: gut microbiota, breast-feeding, diet, delivery mode, colonization, pregnancy, infant, newborn
Citation: Suárez-Martínez C, Santaella-Pascual M, Yagüe-Guirao G and Martínez-Graciá C (2023) Infant gut microbiota colonization: influence of prenatal and postnatal factors, focusing on diet. Front. Microbiol. 14:1236254. doi: 10.3389/fmicb.2023.1236254
Edited by:
Renqiang Yu, Women's Hospital of Jiangnan University, ChinaReviewed by:
Naser Alsharairi, Griffith University, AustraliaSimona Rolla, University of Turin, Italy
Beata Łoniewska, Pomeranian Medical University, Poland
Copyright © 2023 Suárez-Martínez, Santaella-Pascual, Yagüe-Guirao and Martínez-Graciá. This is an open-access article distributed under the terms of the Creative Commons Attribution License (CC BY). The use, distribution or reproduction in other forums is permitted, provided the original author(s) and the copyright owner(s) are credited and that the original publication in this journal is cited, in accordance with accepted academic practice. No use, distribution or reproduction is permitted which does not comply with these terms.
*Correspondence: Marina Santaella-Pascual, marinasp@um.es