- College of Life Sciences, Northwest Normal University, Lanzhou, China
Morel mushroom (Morchella spp.) is a rare edible and medicinal fungus distributed worldwide. It is highly desired by the majority of consumers. Bacterial diseases have been commonly observed during artificial cultivation of Morchella sextelata. Bacterial pathogens spread rapidly and cause a wide range of infections, severely affecting the yield and quality of M. sextelata. In this study, two strains of bacterial pathogens, named M-B and M-5, were isolated, cultured, and purified from the tissues of the infected M. sextelata. Koch’s postulates were used to determine the pathogenicity of bacteria affecting M. sextelata, and the pathogens were identified through morphological observation, physiological and biochemical analyses, and 16S rRNA gene sequence analysis. Subsequently, the effect of temperature on the growth of pathogenic bacteria, the inhibitory effect of the bacteria on M. sextelata on plates, and the changes in mycelial morphology of M. sextelata mycelium were analyzed when M. sextelata mycelium was double-cultured with pathogenic bacteria on plates. The results revealed that M-B was Pseudomonas chlororaphis subsp. aureofaciens and M-5 was Bacillus subtilis. Strain M-B started to multiply at 10–15°C, and strain M-5 started at 15–20°C. On the plates, the pathogenic bacteria also produced significant inhibition of M. sextelata mycelium, and the observation of mycelial morphology under the scanning electron microscopy revealed that the inhibited mycelium underwent obvious drying and crumpling, and the healthy mycelium were more plump. Thus, this study clarified the pathogens, optimal growth environment, and characteristics of M. sextelata bacterial diseases, thereby providing valuable basic data for the disease prevention and control of Morchella production.
1. Introduction
Morels (Morchella spp.), a valuable class of fungi used for food and medicine, is the collective name of the fungi belong to the Morchella genus (Zhao, 2018; Du et al., 2020). Because of its uneven cap, the shape of this fungus resembles the belly of a sheep and hence named accordingly (Du et al., 2019; Sun et al., 2019). The fungus is rich in amino acids, adenosine, and other flavorful substances. Because of their crisp texture and unique flavor, these delicious mushrooms often serve as a perfect addition to any meal (Rotzoll et al., 2006; Wang et al., 2016). Morels are also rich in protein, lipids, fiber, vitamins, minerals, and other nutrients (Vieira et al., 2016; Tietel and Masaphy, 2018), and polysaccharides isolated from morels have good biological activities such as antioxidant, anti-inflammatory, antitumor, immunomodulatory, and regulation of intestinal flora (Chang and Wasser, 2012; Vieira Gomes et al., 2019; Goldman et al., 2021; Xu et al., 2022; Li et al., 2023). Other chemical constituents of morels such as phenolics, ascorbic acid, and carotenoids have attracted research interest because of their excellent biological activity, mainly its high antioxidant activity (Soobrattee et al., 2005; Leal et al., 2013; Tietel and Masaphy, 2018; Vieira Gomes et al., 2019). In traditional medicine, morels have been used as an antiseptic. The powder of the fruiting bodies is dumped into the wound to facilitate the rapid healing of wounds. They are also used to treat stomach pain (Mahmood et al., 2011).
Because of its high economic value, indoor cultivation of morel mushrooms has received considerable attention worldwide (Du et al., 2015). Outdoor cultivation of morel mushrooms was first performed in France and was reported by Roze (1882) in relation to Jerusalem artichokes (Liu et al., 2018). Successful indoor cultivation of morels was first reported in 1982 by Owwer (1982). This subsequently resulted in three successive patents for morel mushroom cultivation (US Patents 4594809, 4757640, and 4866878) (Du et al., 2012; Liu et al., 2018). However, this indoor morel cultivation method could not be applied on a large scale due to inconsistent harvesting yields. Based on the utilization of exogenous nutrients and successful domestication of easy-to-cultivate varieties since 2012, 16,466 ha of outdoor cultivation of morels has been achieved in mainland China during the 2021–2022 season (Tan et al., 2019; Shi et al., 2022). As morel cultivation is rapidly expanding, diseases are increasingly becoming the greatest threat to morel mushroom production (Tan et al., 2021a). Diseases affecting morel mushroom production are mainly caused by bacteria and fungi. More current studies have focused on fungal diseases, such as stipe rot disease caused by the Fusarium incarnatum–F. equiseti species complex (Guo et al., 2016) and by Purpureocillium lilacinum (Masaphy, 2022), cobweb disease caused by Hypomyces/Cladobotryum species (Lan et al., 2020), white mold disease caused by Paecilomyces penicillatus (He et al., 2018; Yu et al., 2022), and pileus rot disease caused by Diploöspora longispora (He et al., 2018; Liu et al., 2018; Sun et al., 2023). However, studies on bacterial diseases of morels are relatively scarce. At least two types of bacterial diseases were initially identified, namely soft rot and red-stipe diseases (Liu et al., 2019), but their specific causative pathogens have not yet been identified. Recent studied reported red-stipe disease in Morchella sextelata and identified several important genes, metabolites, and pathways through transcriptomic and metabolomic analyses of the infected M. sextelata. Based on the results, the possibility of F. nematophilum being the causative pathogen of red-stipe disease was ruled out (Yang et al., 2023).
In April 2022, a disease in the main area of morel production in the southern part of Gansu Province, China, severely affected M. sextelata production. It manifested as symptoms of red-stipe disease, stopping the growth of fruiting bodies. During the disease outbreak, the whole stipe was reddish brown, and then, the color gradually deepened and spread from the bottom. The whole fruit body turned red and released a foul odor. With the disease course, Morchella lost its vitality, stopped growing, and eventually rot. The disease can lead to a major reduction in yield or even extinction in a short period, thereby exerting a great impact on morel mushroom cultivation. In this study, field survey, sample collection, isolation, identification, and rewiring of suspected pathogenic bacteria were performed for the widespread occurrence of M. sextelata red-stipe disease in the main Morchella production area in the southern part of Gansu Province. The pathogenic strain causing red-stipe disease in M. sextelata was identified on the basis of Koch’s postulates as well as and biological characterization and 16S rRNA gene sequence analysis. The study thus provides basic data for the accurate identification of the causative agent and prevention and control of the bacterial disease affecting M. sextelata in the field.
2. Materials and methods
2.1. Collection of samples of infected M. sextelata and isolation of pathogenic bacteria
The infected M. sextelata fruiting bodies were collected from the Morchella cultivation base in Chengxian, Longnan City, Gansu Province. In total, 30 samples were collected, 20 diseased samples, and 10 non-diseased samples, packed in clean self-sealing bags, and stored in a 4°C refrigerator at the laboratory for pathogen isolation. The samples were first rinsed with tap water to remove the soil attached and then rinsed with sterile water. The samples were first immersed in 75% ethanol for approximately 10 s and then in 0.1% mercuric chloride for 10 s, and washed 3 times with sterile water. Then, the materials were cut into 1–2-mm tissue slices with sterile blades and inoculated on Luria–Bertani (LB) solid medium. The LB plates with the slices were incubated in a thermostatic electric incubator at 30°C for 24 h. The bacteria were isolated from the LB agar plates using the scratch method. Single colonies with different morphologies were picked for isolation and purification. A single colony was picked and incubated on the LB plate again by streaking for 24 h until a single colony was obtained. A part of the purified strain was used for subsequent experiments, and the remaining part was stored in 20% glycerol at −80°C for future use.
2.2. Pathogenicity test
Pathogenicity tests were conducted according to Koch’s postulates. Morchella sextelata was selected as the main cultivar of Morchella. The pathogenic strains to be tested were inoculated in LB liquid medium for activation and incubated at 30°C with 160 r/min oscillation. Overnight bacterial cultures (1 × 108 CFU/mL) grown in LB medium were injected into the ascocarps of morels by using a 1-mL sterile syringe. The experiment consisted of three treatment groups and three control groups. The first treatment group was only inoculated with M-B bacterial cultures, the second treatment group was only inoculated with M-5 bacterial cultures, the third treatment group was inoculated with both M-B and M-5 bacterial cultures, the fourth group was inoculated with sterilized M-B cultures as a killed bacterial control; the fifth group was inoculated with sterilized M-5 cultures as a killed bacterial control. The LB medium treatment was used as the blank control. Three replicates were established for each treatment group. In order to simulate field cultivation conditions in the laboratory, the inoculated M. sextelata was cultivated in a constant temperature incubation room at 17°C and 85% humidity. The disease incidence rate and symptoms were recorded. Two days after inoculation, some symptoms were observed on the surface. The pathogenic bacteria were reisolated from the infected M. sextelata fruiting bodies on LB plates, and the characteristics of the reisolated bacteria were compared with those of the original culture.
2.3. Identification of pathogens
The purified pathogenic bacteria were inoculated on LB plates and incubated at 30°C for 24 h. After observing the growth and morphological characteristics of the colonies, individual colonies were picked for microscopic examination. The isolates were stained using the Gram staining kit. The physiological and biochemical indicators of the isolated pathogenic bacteria isolated were determined by referring to the bacterial identification method presented in the Manual of Identification of Common Bacterial Systems (Dong and Cai, 2001). The physiological indicators included colony morphology, growth temperature, pseudocyanine production, fluorochrome production, utilization of carbon sources (glucose, mannitol, sucrose, trehalose, and fructose), and utilization of nitrogen sources (yeast powder, beef paste, ammonium sulfate, peptone, and sodium nitrate). The biochemical tests conducted included gelatin liquefaction, starch hydrolysis, H2S production, Voges–Proskauer test, methyl red test, and catalase and urease determination.
All strains isolated were subjected to phylogenetic analysis. DNA was extracted using the bacterial DNA extraction kit. The 16S rRNA gene fragment of the strain was amplified through PCR by using bacterial universal primers 27F (5′-AGAGTTTGATCATGGCTCAG-3′) and 1492R (5′-GGYTACCTTGTTACGACTT-3′). PCR reaction conditions were pre-denaturation at 95°C for 5 min, denaturation at 95°C for 1 min, annealing at 55°C for 30 s, extension at 72°C for 1 min, 34 cycles, extension at 72°C for 5 min amplification (Dou et al., 2018). Then, 1% agarose gel electrophoresis was performed to detect the PCR products. The PCR products were then selected and sent to Jinweizhi Biotechnology Co., Ltd., for sequencing. The sequences were compared with the known sequences in the NCBI database by using BLAST. The phylogenetic tree was constructed using the overlay method (neighbor joining) with MEGA 11.0 software and subjected to the bootstrap test (1,000). The outgroups were Azotobacter chroococcum and Aeribacillus pallidus, respectively.
Bacterial morphology was observed under scanning electron microscopy (SEM). The actively growing bacterial solution was centrifuged at 8,000 rpm for 3–5 min, and the supernatant was discarded and poured into 2.5% glutaraldehyde and fixed overnight. The samples were then washed 3 times with phosphate buffered saline (PBS), fixed in 1% osmium solution for 4–6 h, washed 3 times with phosphate buffered saline, then dehydrated once in 30, 50, 70, 85, and 95% ethanol, and treated twice with 100% ethanol for 15–20 min each. The samples are then subjected to critical-point drying and gold spraying before being ready for SEM (ULTRA Plus-type, Zeiss Company, Germany) (Xie et al., 2005).
2.4. Inhibition of growth and changes in mycelial morphology of M. sextelata by pathogenic bacteria on plates
The isolated pathogenic bacteria were inoculated on LB plates and incubated at 30°C for 24 h for activation. The laboratory-preserved M. sextelata strain ZW111 was inoculated on potato dextrose agar (PDA) plates and incubated at 20°C for 4 days for activation. The plate standoff method (Wang W. X. et al., 2014) was used to detect the inhibitory effect of the pathogenic bacteria. M. sextelata ZW111’s cake was placed in the center of the PDA plate. The antagonistic bacterial cake (diameter = 8 mm) was placed on three points at equal distance (2.5 cm) from the center of the M. sextelata. Three parallels were run for each treatment. The control group plate only contained M. sextelata ZW111. Two other B. subtilis preserved in this laboratory were selected for testing to preliminarily investigate whether all B. subtilis species inhibited M. sextelata growth or only the strain M-5, isolated in this study, inhibited M. sextelata. P. chlororaphis subsp. aureofaciens exhibits a strong antifungal activity (Raio et al., 2011; Zhang et al., 2021), and so, other strains were not tested. All plates were incubated at 25°C for 4 days in a constant temperature incubator. The pathogenic bacteria in the plates were observed for their inhibitory effect on M. sextelata, and observed morphological changes in the mycelium under SEM. The samples were taken from the mycelium of M. sextelata located in the marginal region of the inhibition zone, and the subsequent fixation, dehydration, drying and spraying gold treatments of the samples were referred to in 2.3.
2.5. Effect of temperature on the growth of pathogenic bacteria
The occurrence of bacterial diseases of M. sextelata during cultivation is closely related to the influence of temperature, especially high temperature and high humidity, we determined the effect of temperature on the growth of pathogenic bacteria to provide a better theoretical reference for preventing and controlling field diseases of morels. The prepared LB liquid medium was divided into test tubes, with each tube containing 10 mL LB medium. Then, 0.1 mL of bacterial solution (1 × 108 CFU/mL) was added to each of the test tubes. The inoculated tubes were placed on a shaker at 4, 10, 15, 20, 25, 28, 30, 35, 37, 40, and 42°C, 160 r/min and incubated with shaking for 24 h. Three replicates were established set for each group of temperature, and the culture medium without any inoculum was used as the control. After 24 h of incubation, the OD600nm values of the bacterial broth incubated at different temperatures were measured using a ultraviolet-visible spectrophotometer and recorded (Zhou, 2013).
2.6. Identification of cellulase production by pathogenic strains and determination of enzyme activity
The isolated pathogenic bacteria were inoculated in carboxymethyl cellulose (CMC) agar medium (0.5% CMC-Na, 0.1% NaNO3, 0.1% K2HPO4, 0.1% NaCl, 0.05% MgSO4, 0.05% yeast extract, 1.5% agar) and incubated at a constant temperature of 37°C. After incubation, the CMC agar plates were flooded with 0.2% Congo red for 30 min, and destained by washing twice with 1 mol/L NaCl solution for 20 min. Standard cellulase solution (MAKLIN Reagent Co.) was used as a positive control and inactivated enzyme solution as a negative control. The activity zones had observed against a red background after Congo red staining (Zhang et al., 2022).
The filter paper enzyme activity (FPase) of cellulase was measured through the amount of reducing sugars liberated during hydrolysis using filter paper as substrate by the DNS (3,5-dinitrosalicylic acid) method (Ghose, 1987; Xie et al., 2023). The strains to be tested were inoculated into cellulase production broth (1% CMC-Na, 1% peptone, 0.00075% FeSO4⋅7H2O, 0.00025% MnSO4⋅H2O, 0.0002% ZnSO4), incubated at 30°C for 3–4 days at 160 r/min, and centrifuged at 6,000 r/min for 10 min, and the supernatant was the crude enzyme solution. Cut the filter paper into 1 cm × 3 cm strips, roll them into small rolls and place them in a 5 mL test tube, add 1.75 mL of acetic acid-sodium acetate buffer (pH = 4.8) and 0.25 mL of enzyme solution, respectively. Place the tube in a water bath at 50°C for 1 h, then the reaction was terminated by 1 mL DNS. The color of the mixture was developed by incubating it for 10 min at 100°C. The inactivated crude enzyme solution was treated as above and used as a control. The absorbance was measured at 540 nm by using an ultraviolet-visible spectrophotometer. A standard curve was plotted using glucose. The equation of the standard curve is y = 0.5515 × +0.0206 and the correlation coefficient is R2 = 0.9996 (Supplementary Table 3). One unit (U) is defined as the amount of enzyme required to produce 1 μmol of glucose equivalent released per minute under the conditions described above (Li et al., 2020; Sarangthem et al., 2023).
2.7. Identification of chitinase production by pathogenic strains and determination of enzyme activity
The isolated pathogenic bacteria were inoculated on colloidal chitin agar medium [1% colloidal chitin, 0.5% NaCl, 0.05% MgSO4⋅7H2O, 0.07% K2HPO4, 0.03% KH2PO4, 1% (NH4)2SO4, 0.3% yeast extract powder, and 1.5% agar] and incubated at a constant temperature of 37°C. Colloidal chitin was prepared according to the method by Sandhya et al. (2004). After incubation, the plates were stained with 0.2% Congo red using the same method as in 2.6. Standard chitinase solution (MAKLIN Reagent Co.) was used as a positive control and inactivated enzyme solution as a negative control (Linda et al., 2018).
The chitinase activity of the strains was also determined by the DNS (3,5-dinitrosalicylic acid) method as in 2.6. The strains were inoculated into colloidal chitin broth (1% colloidal chitin, 0.05% MgSO4⋅7H2O, 1.2% urea, 0.03% KH2PO4, 0.5% NaCl, 0.07% K2HPO4, 0.3% yeast extract powder), the supernatant was the crude enzyme solution. Mix 0.5 ml crude enzyme solution with 0.5 ml colloid chitin and 0.5 ml PBS by volume. The reaction was carried out in a water bath at 37°C for 30 min, then the reaction was terminated by 1 mL DNS. The absorbance was measured at 540 nm. The inactivated crude enzyme solution was treated as above and used as a control. A standard curve was plotted using N-acetyl-D-glucosamine. The equation of the standard curve is y = 0.4063 × −0.0384 and the correlation coefficient is R2 = 0.9994 (Supplementary Table 4). One unit (U) is defined as the amount of enzyme required to catalyze the production of 1 μmol of N-acetyl-D-glucosamine from the substrate in 1 min under suitable conditions as one enzyme activity unit (U) (Du et al., 2021).
2.8. Statistical analysis
All experiments were repeated in triplicate. The data were processed using the SPASS statistical software to perform an ANOVA [least-significant difference (LSD) test and Duncan’s multiple range test, p < 0.05]. Origin software was used for statistical analysis and graph drawing.
3. Results
3.1. Collection of samples and culture properties of isolates from infected M. sextelata
The collected infected M. sextelata samples were reddish in color and had a foul odor (Figure 1A). We isolated two types of bacteria, named M-B and M-5, from the diseased M. sextelata, and we did not isolate these bacteria from the undiseased M. sextelata. Strain M-B was inoculated on LB solid medium and incubated at 30°C for 24 h. The colonies appeared orange, smooth, and opaque with a round, slightly elevated surface (Figure 1B). Strain M-5 was inoculated on LB solid medium and incubated at 30°C for 24 h. After incubation, the colonies appeared creamy white, subcircular, with irregular edges, rough and opaque, and a dry, wrinkled surface (Figure 1C).

Figure 1. Samples of infected M. sextelata collected (A); morphological characteristics of isolate colonies M-B (B) and M-5 (C).
3.2. Pathogenicity testing
The treated M. sextelata were placed in the incubation room at 17°C and 85% humidity. At 36 h after inoculation, reddish spots started to appear from the base of the stipe of M. sextelata in all bacterial solution treatment groups (Figure 2). After 60 h, the whole stipe began to turn red, and the red color of the stipe was the deepest in the mixed treatment group, and the M-5-treated M. sextelata started to produce a foul odor (Figure 2). At 84 h, the whole mushroom body in all bacterial solution treatment groups began to turn red and the red color continued to deepen (Figure 2). A total of 108 h later, the mushroom body began to turn reddish brown and soft rot (Figure 2). At 132 h, the whole body was dark red, wilted, and crumpled, accompanied by a large amount of unpleasant odor (Figure 2). Similar to field symptoms. The three control groups inoculated with sterilized M-B bacterial solution, sterilized M-5 bacterial solution, and LB blank medium showed slight browning over time, with no softening of the fruiting body and without symptoms of disease. Pathogenic bacteria were reisolated from these infected M. sextelata. These isolates exhibited similar morphologies on LB solid plates, satisfying Koch’s postulates.
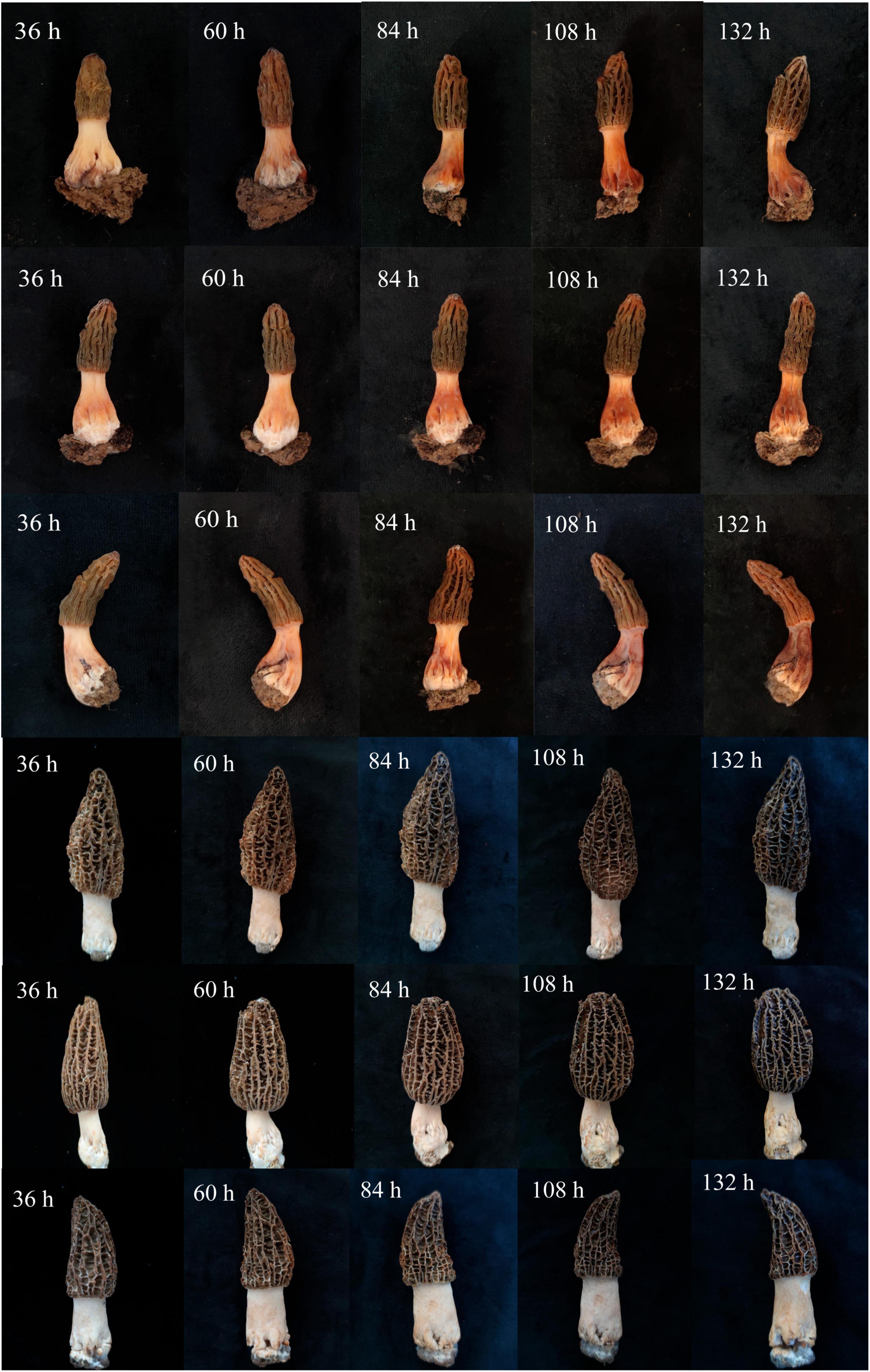
Figure 2. Pathogenicity test of isolates. The first row of M. sextelata was inoculated with only M-5 ramets, the second row of M. sextelata was inoculated with M-B alone, and the third row of M. sextelata was inoculated with both M-5 and M-B, the fourth row of control group was inoculated with sterilized M-B (CK1), the fifth row of control group was inoculated with sterilized M-5 (CK2), and the sixth row of control group was inoculated with LB blank medium (CK3).
3.3. Identification of pathogens
According to the results of Gram staining, strain M-5 (Supplementary Figure 1A) was stained purple and exhibited a rod-shaped body, with bluntly rounded ends and budding spores. It was tentatively determined to be Gram-positive. Morphological analysis through SEM (Supplementary Figure 1B) revealed that the bacterium was rod-shaped with no pods and a size of (0.6–0.7) μm × (2.3–1.4) μm. Its endospore was located in the center of the body or slightly deviated and did not expand after the bacterial cell became oval. Strain M-B (Supplementary Figure 1C) was stained red and exhibited a rod-shaped body. It was tentatively determined to be Gram-negative. SEM (Supplementary Figure 1D) revealed that the bacterium was rod-shaped, with a size of (0.4–0.5) μm × (1–1.3) μm.
Physiological and biochemical characterization of strains M-B and M-5 showed (Table 1) that both strains M-B and M-5 were able to utilize glucose, mannitol, sucrose, trehalose, fructose, yeast, beef extract, ammonium sulfate, peptone, sodium nitrate. Strain M-B was negative for starch hydrolysis, H2S production, methyl red test, positive for gelatin liquefaction, Voges–Proskauer test, catalase and urease reaction. Strain M-5 was negative for H2S production, methyl red test and urease reaction, and positive for gelatin liquefaction, catalase, Voges–Proskauer test and urease reaction. According to the physiological and biochemical tests, and combined with morphological characteristics, according to the Manual of Identification of Common Bacterial Systems, the strain M-B was preliminarily identified as Pseudomonas chlororaphis subsp. aureofaciens and strain M-5 was preliminarily identified as Bacillus subtilis.
After sequencing was completed, the sequences were compared with the known sequences in the NCBI database by using BLAST. The sequences with a high homology were downloaded and compared using MEGA 11.0 software to construct a phylogenetic tree by using the neighbor-joining method. The results revealed (Figure 3) that the isolated strains belonged to two main groups. M-5 and B. subtilis converged in the same species, and so, the bacterium was tentatively identified as B. subtilis. M-B and P. chlororaphis subsp. aureofaciens converged in the same species, and therefore, the bacterium was tentatively identified as P. chlororaphis subsp. aureofaciens.
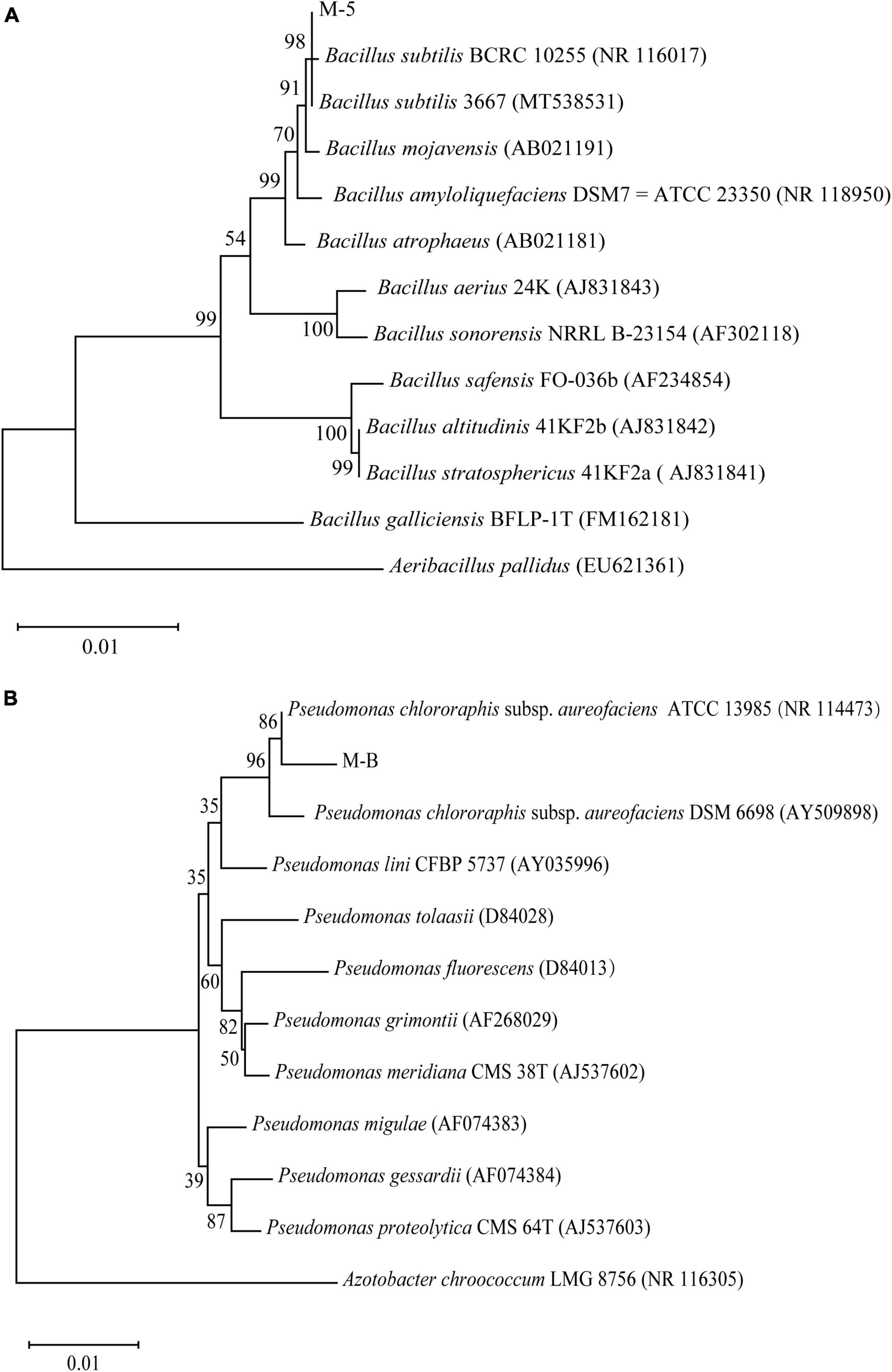
Figure 3. Phylogenetic analysis based on sequencing of the 16S ribosomal RNA gene. Bootstrap values (%) presented at the branches were calculated from 1,000 replications. Strain M-5 (A); strain M-B (B).
3.4. Inhibitory effect of pathogenic bacteria on M. sextelata on plates and changes in mycelial morphology of M. sextelata
The plate standoff results (Figure 4) revealed a clear inhibition circle between the pathogenic bacteria and M. sextelata. Strains M-B and M-5 severely inhibited mycelial growth in M. sextelata (Figures 4A, C). SEM showed that the mycelium of M. sextelata in the control group was healthy and full (Figure 4F), whereas that at the edge of the colonies of M. sextelata treated with the pathogenic strains exhibited an obvious distortion, surface depression, folding, drying, and rupture (Figures 4B, D). The results thus indicated that the pathogenic bacteria changed the morphology of the M. sextelata mycelium. The laboratory-preserved other strains of B. subtilis did not produce an inhibition circle around M. sextelata (Figures 4G, I), the M. sextelata mycelium covered the growth of the B. subtilis cake and the mycelium was full and strong. This indicated that the other B. subtilis strains had no inhibitory effect on the mycelial growth of M. sextelata on the plate. These results preliminarily indicate that perhaps not all B. subtilis strains inhibit M. sextelata growth. Only the B. subtilis strain M-5 isolated from the infected M. sextelata exhibited an inhibitory effect on M. sextelata. In the following research, we will continue our in-depth investigation on whether all B. subtilis will inhibit the growth of M. sextelata. The difference between different pathogenic and non-pathogenic strains among the same species also serves as a direction for subsequent research.
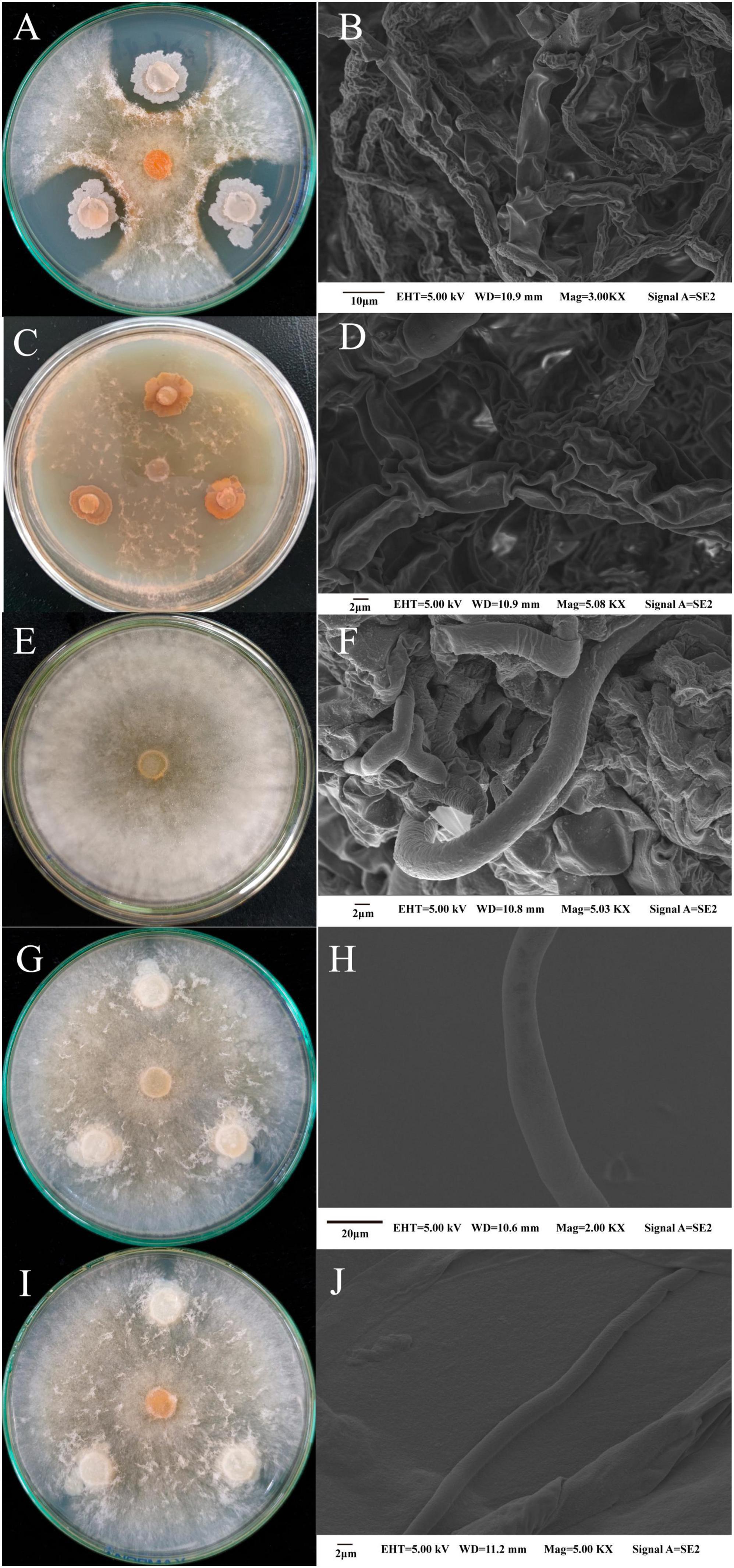
Figure 4. Changes in the mycelial morphology of M. sextelata were observed in the pathogenic bacteria and M. sextelata plate confrontation and through SEM: strain M-5 and M. sextelata confrontation (A) and M. sextelata mycelial morphology after confrontation (B); strain M-B and M. sextelata confrontation (C) and M. sextelata mycelial morphology after confrontation (D); control M. sextelata on the plate (E) and mycelial morphology observed through SEM (F). Two other B. subtilis strains cultured in standoff with M. sextelata ZW111, namely B. subtilis B626 (G) and mycelial morphology observed through SEM (H); B. subtilis B602 (I) and mycelial morphology observed through SEM (J).
3.5. Effect of temperature on the growth of pathogenic bacteria
The temperature effect results showed (Figure 5 and Supplementary Tables 1, 2) that the growth of strain M-B was inhibited at 4°C. When the temperature was increased, the OD600nm values were the maximum at 35°C, and the growth was inhibited when the temperature was higher than 40°C. Therefore, strain M-B did not grow at temperatures below 4°C or above 40°C. When the strain was incubated for 10 min in a water bath at 55°C and coated on the LB plate, no colonies were observed for 48 h, indicating that the lethal temperature for strain M-B was 55°C. The growth of strain M-5 was inhibited at 4°C, and the OD600nm values were the maximum at 25°C with an increase in temperature. After strain M-5 was incubated on LB plates at 110°C for 10 min, no colonies were observed for 48 h, indicating that the lethal temperature for strain M-5 was 110°C. Strain M-B started to multiply at 10–15°C, and strain M-5 started at 15–20°C. Therefore, the temperature can be controlled below 15°C to as the lower temperature could inhibit the growth of pathogenic bacteria during M. sextelata cultivation.
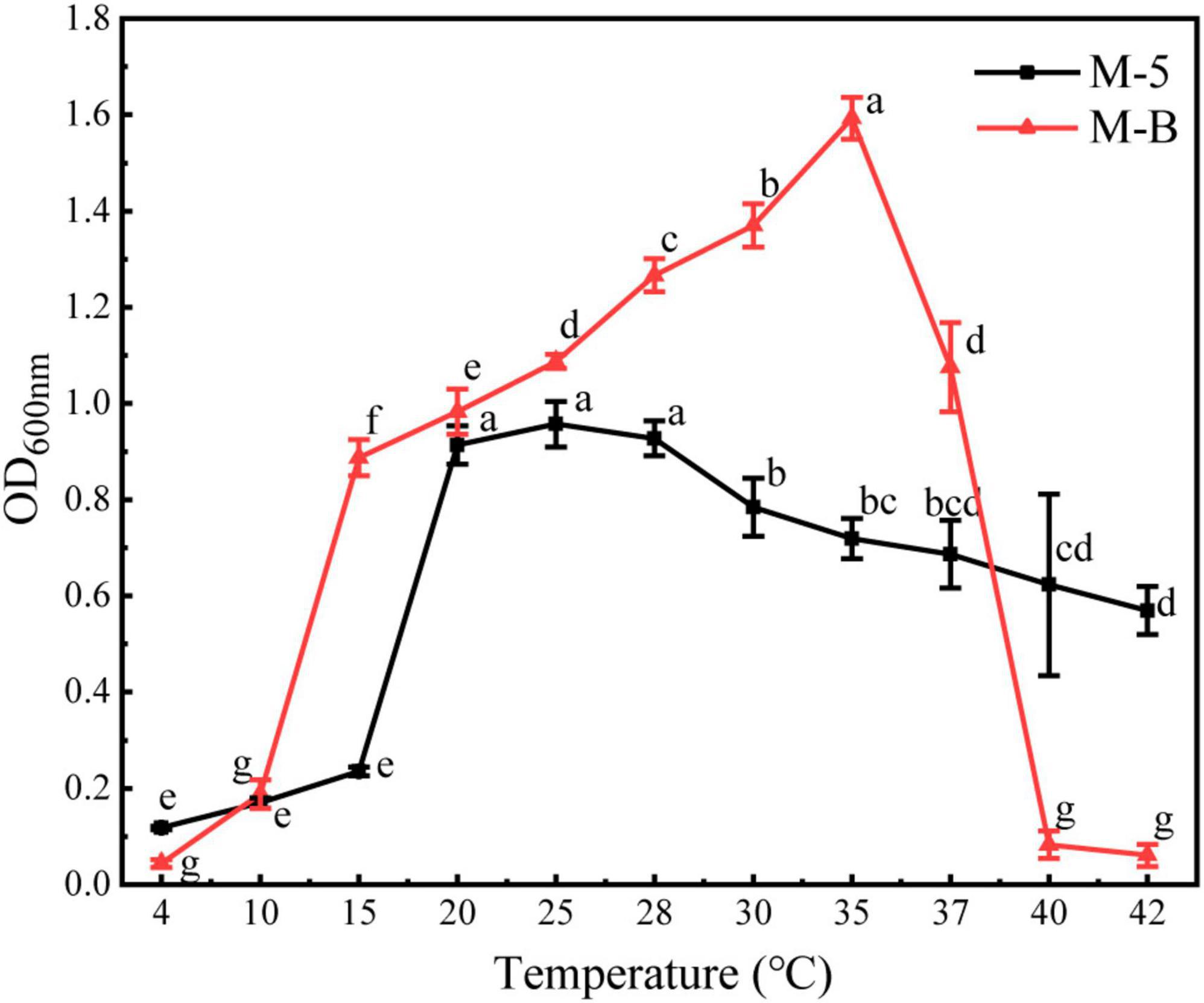
Figure 5. Effect of temperature on the growth of pathogenic bacteria. Different letters above values indicate significant differences (P < 0.05, Tukey’s test in ANOVA).
3.6. Identification of cellulase production by pathogenic strains and determination of enzyme activity
The results of cellulase secretion by two bacterial pathogens inoculated into cellulose medium by spot inoculation are shown in Figure 6. Congo red binds strongly to the large polysaccharide cellulose, which is hydrolyzed by the action of cellulase into smaller sugars that Congo red cannot bind, resulting in a hyaline ring around the colony. A clear circle can be seen around the edge of the colony of strain M-5, while no clear circle is evident around strain M-B, indicating that strain M-5 can secrete cellulase. Based on the enzyme activity data (Table 2 and Supplementary Table 5), the enzyme activity value of strain M-5 is much higher than that of strain M-B, indicating strong cellulase production ability. Strain M-5 has lower enzyme activity and weaker cellulase production ability.
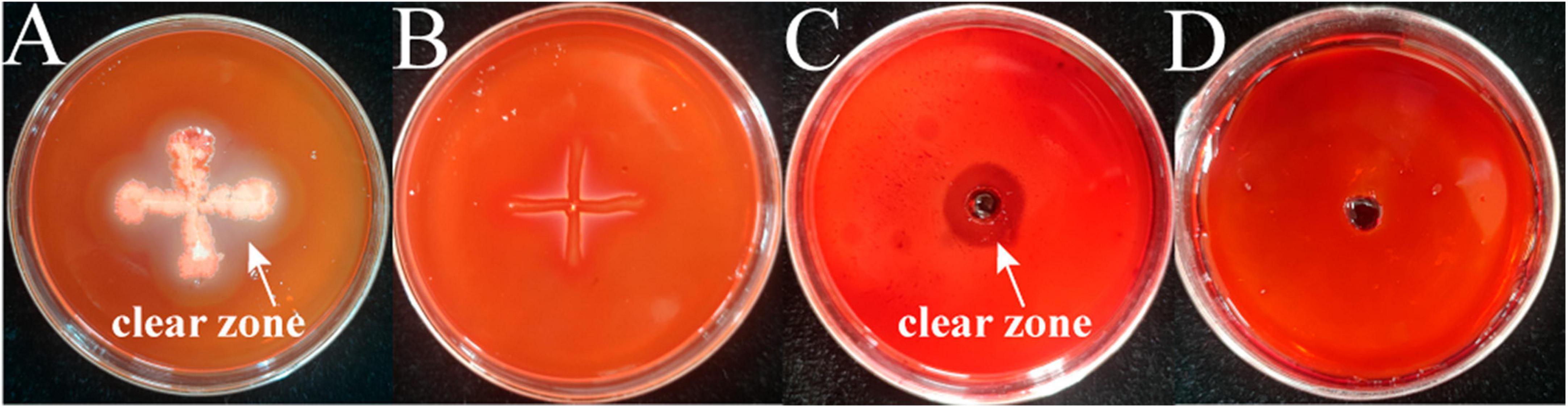
Figure 6. Identification of cellulase producing pathogenic strains. M-5 strain (A), M-B strain (B), cellulase solution positive control (C) and inactivated enzyme solution negative control (D).
3.7. Identification of chitinase production by pathogenic strains and determination of enzyme activity
The two bacterial pathogens were inoculated separately into the chitinase medium by spot inoculation and the results of chitinase secretion by both pathogens are shown in Figure 7, where clear circles can be seen at the edge of the colonies of both strains M-5 and M-B. This indicates that both strains can secrete chitinase. Under the action of chitinase, chitin can be hydrolyzed from white insoluble particles to soluble small molecules, resulting in a transparent ring around the colony of the bacterium to be tested. Combined with the enzyme activity data (Table 2 and Supplementary Table 6), both strain M-5 and strain M-B had strong chitinase production ability.
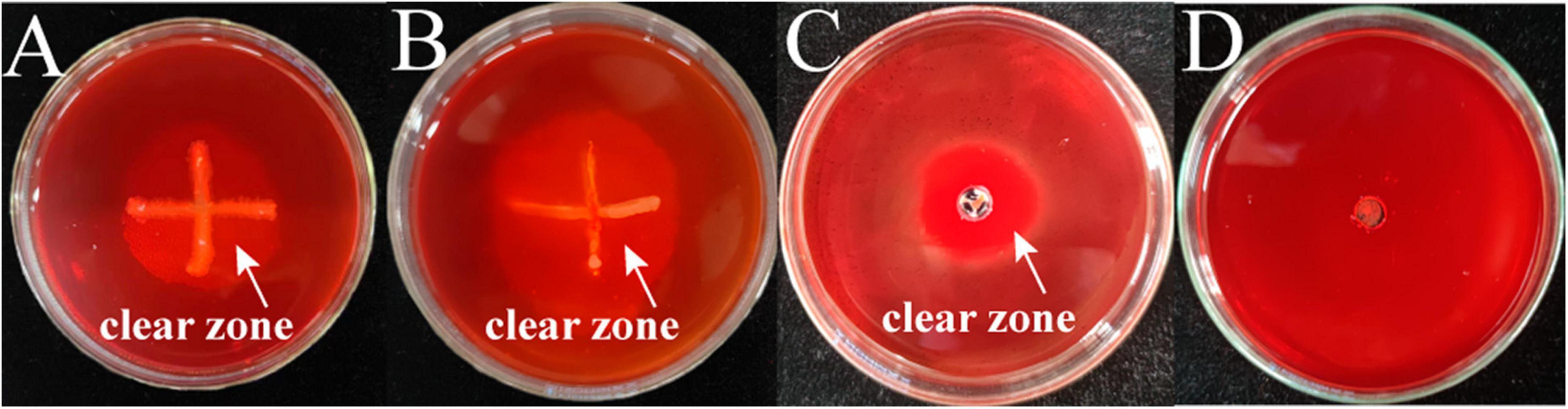
Figure 7. Identification of chitinase producing pathogenic strains. M-5 strain (A), M-B strain (B), chitinase solution positive control (C) and inactivated enzyme solution negative control (D).
4. Discussion
In recent years, Bacillus. spp and Pseudomonas. spp have been widely used as antagonists of pathogenic fungi (Haas and Défago, 2005; Lastochkina et al., 2019). In the present study, B. subtilis M-5 and P. chlororaphis subsp. aureofaciens M-B were identified as bacterial pathogens causing red-stipe disease of M. sextelata, a very unusual observation. However, in plate confrontation tests of other strains of B. subtilis with M. sextelata (Figures 4G, I), we found that not all B. subtilis strains inhibit M. sextelata growth. Only B. subtilis M-5, isolated from the fruiting bodies of infected morels, exerted an inhibitory effect on M. sextelata growth. P. chlororaphis subsp. aureofaciens exhibits inhibitory effects on fungi (Raio et al., 2011; Zhang et al., 2021). The inoculation of M. sextelata with P. chlororaphis subsp. aureofaciens possibly led to the inhibition of M. sextelata growth. Moreover, the occurrence of M. sextelata red-stipe disease may be related to the strong antifungal activity of P. chlororaphis subsp. aureofaciens.
Bacillus subtilis inhibits fungi mainly by producing numerous metabolites (Wang Z. et al., 2014; Kilani-Feki et al., 2016) including (i) bacteriocins such as chymotrypsin, polymyxin, and mycotoxin; (ii) proteases, amylases, lipases, chitinases, and other cell wall-degrading enzymes (Frändberg and Schnürer, 1994; Sadfi et al., 2001; Islam et al., 2012), which are among the more active enzymes (Islam et al., 2012; Zhang et al., 2014); and (iii) antibacterial substances such as small-molecule antimicrobial peptides, antimicrobial proteins, and polysaccharides (Xing et al., 2015). Chitin and β-l,3-glucan are enzymes crucial for fungal control, and B. subtilis produces chitinase and β-l,3-glucanase that degrade fungal cell walls and increase cell membrane permeability. Certain pathogenic bacteria have been shown to inhibit the growth of mycelium of morel mushrooms through antagonistic secretions (Tan et al., 2021b). In this experiment, we found that the isolated pathogenic strain M-5 could produce chitinase and cellulase, and had good enzyme-producing ability (Table 2). Antifungal proteins have a crucial role in the antagonistic effect of B. subtilis. These proteins can inhibit mycotoxin production, disrupt cell walls, deform the mycelium, cause abnormal spore germination, and inhibit the growth and reproduction of fungi (Rismondo and Schulz, 2021; Liu et al., 2022). A study investigating of the mechanism of inhibition of Colletotrichum gloeosporioides by antifungal proteins revealed that the mycelium structure after antifungal protein treatment was damaged, the cell wall was ruptured, the cytoplasmic structure was disturbed, and the contents of the cell flowed out (Liu et al., 2020; Oliveira Silva et al., 2022). Thus, antifungal proteins exert their antifungal effects by disrupting the cell wall structure and function. They also reduce the ergosterol content and affect the membrane structure and stability (Chen et al., 2023). In agreement with the present study results (Figure 4B), SEM observation of the M. sextelata mycelium after standoff culture with strain M-5 revealed that the mycelium structure was damaged, exhibiting dryness, wrinkling, and spillage of cell contents. This indicated that strain M-5 disrupted the cell wall of the M. sextelata mycelium. When the mycelial membrane integrity is disrupted, nucleic acids and proteins are released, leading to impaired cell function and loss of viability (Chen et al., 2023).
Pseudomonas chlororaphis is a common soil and plant root environment host bacterium that inhibits a wide range of pathogenic fungi (Raio and Puopolo, 2021). Production of several metabolites is essential for the inhibition of fungi by P. chlororaphis. (i) Antibiotics and antimicrobial compounds such as 2,4 diacetylphloroglucinol, hydrogen cyanide, pyrrolnitrin, pyoluteorin, and phenazines are among the most studied secondary metabolites produced by P. chlororaphis and many other Pseudomonas spp. (Arseneault and Filion, 2016; Raio and Puopolo, 2021). Phenazines have broad-spectrum antibacterial activity. These compounds are excreted as strongly diffused yellow-orange pigments during bacterial growth in vitro (Mavrodi et al., 2006). (ii) P. chlororaphis can also release volatile organic compounds (VOCs) that induce systemic resistance in plants (Ma et al., 2016). (iii) P. chlororaphis can produce siderophores, proteases, and lipases (Jain and Pandey, 2016). These hydrolases play a key role in the degradation of fungal cell walls and indirectly promote plant growth (Chin-A-Woeng et al., 2001). In this experiment, we found that the isolated pathogenic bacteria M-B had a strong ability to produce chitinase and a weak ability to produce cellulose (Table 2). VOCs produced by P. chlororaphis subsp. aureofaciens strain SPS-41 isolated from sweet potato roots exhibited strong antifungal activity and effectively inhibited mycelial growth and spore germination of Ceratocystis fimbriata, thereby causing massive cytoplasmic leakage, severely disrupting the cell membrane structure, and reducing the ergosterol content (Zhang et al., 2019, 2021). Consistent with the study results, SEM observation of the mycelial morphology of M. sextelata after confrontation with strain M-B (Figure 4D) revealed numerous cavities and dryness in the mycelium, thereby indicating loss of cell membrane integrity and release of cell contents. P. chlororaphis subsp. aureofaciens strain M71, isolated from tomato inter-rhizosphere, exhibited a strong inhibitory effect on Seiridium cardinale. Strain M71 inhibited the radial growth of fungal colonies in vitro by releasing phenazine compounds into the agar medium (Raio et al., 2011). In the standoff test, strain M-B also secreted a yellow-orange pigment around the colonies, indicating phenazine production.
The occurrence of bacterial diseases of M. sextelata during cultivation is closely related to the influence of temperature, especially high temperature and high humidity. The pathogenic bacteria are prone to attack the stalk part of M. sextelata ascocarp first. The infected mushroom body often produces an unpleasant fishy smell. Then, the ascocarp or the diseased part wilts, followed by reddening of the stalk, ceasing of growth, and softening of the mushroom body (Liu et al., 2019). In combination with previous studies, temperature is highly correlated with the occurrence of M. sextelata disease, and in this study, we found that temperature below 15°C could inhibit the growth of pathogenic bacteria. Therefore, to avoid the occurrence of bacterial diseases as much as possible, the temperature maintained during cultivation must be as low as possible so as to inhibit the growth and reproduction of pathogenic bacteria. To eliminate some pathogenic microorganisms remaining in the soil, the soil can also be disinfected at a high temperature after cultivation.
In this study, two strains, B. subtilis M-5 and P. chlororaphis subsp. aureofaciens M-B, were isolated from 20 samples of M. sextelata with red-stipe disease. The two pathogenic bacteria were identified using phylogenetic methods. This is the first study to investigate the pathogenicity, phylogenetic and morphological analysis, and biological properties of bacterial pathogens causing the M. sextelata red-stipe disease. Both strains exhibited strong antifungal activity in the pathogenicity test and plate confrontation test against M. sextelata. Both strains M-5 and M-B could produce chitinase and cellulase. B. subtilis M-5 and P. chlororaphis subsp. aureofaciens M-B were used here as pathogens causing bacterial diseases in M. sextelata. In this study, strains M-B and M-5 did cause red-stipe disease of M. sextelata, but what kind of relationship they have with M. sextelata at lower temperatures, whether it exists in M. sextelata or not, and whether it affects morel mushrooms in any other ways need to be further explored. In subsequent studies, we will continue to investigate the pathogenic mechanisms of B. subtilis and P. chlororaphis subsp. aureofaciens in M. sextelata bacterial diseases. We will also explore the differences between pathogenic and non-pathogenic strains and whether any factors are regulating the switch between them. The screening of antagonistic microorganisms for the M. sextelata red-stipe disease will also be a subsequent research direction.
Data availability statement
The datasets presented in this study can be found in online repositories. The names of the repository/repositories and accession number(s) can be found below: NCBI–OR053654 and OR053655.
Author contributions
XZ, SN, and KM designed the experiments. KM and JZ performed the experiments. KM, MS, and LL analyzed the data and processed the images. KM wrote the manuscript. XZ and SN performed the review and revision of the manuscript. All authors contributed to the article and approved the submitted version.
Funding
This study was supported by the National Natural Science Foundation of China (31770588).
Conflict of interest
The authors declare that the research was conducted in the absence of any commercial or financial relationships that could be construed as a potential conflict of interest.
Publisher’s note
All claims expressed in this article are solely those of the authors and do not necessarily represent those of their affiliated organizations, or those of the publisher, the editors and the reviewers. Any product that may be evaluated in this article, or claim that may be made by its manufacturer, is not guaranteed or endorsed by the publisher.
Supplementary material
The Supplementary Material for this article can be found online at: https://www.frontiersin.org/articles/10.3389/fmicb.2023.1231353/full#supplementary-material
References
Arseneault, T., and Filion, M. (2016). “Phenazine-producing Pseudomonas spp. as biocontrol agents of plant pathogens,” in Microbial Inoculants in Sustainable Agricultural Productivity, ed. D. P. Singh (New Delhi: Springer), 53–68. doi: 10.1007/978-81-322-2644-44
Chang, S. T., and Wasser, S. P. (2012). The role of culinary-medicinal mushrooms on human welfare with a pyramid model for human health. Int. J. Med. Mushrooms 14:95. doi: 10.1615/intjmedmushr.v14.i2.10
Chen, W., Wu, Z., and He, Y. (2023). Isolation, purification, and identification of antifungal protein produced by Bacillus subtilis SL-44 and anti-fungal resistance in apple. Environ. Sci. Pollut. Res. 30, 62080–62093.
Chin-A-Woeng, T. F., Thomas-Oates, J. E., Lugtenberg, B. J., and Bloemberg, G. V. (2001). Introduction of the phzH gene of Pseudomonas chlororaphis PCL1391 extends the range of biocontrol ability of phenazine-1-carboxylic acid-producing Pseudomonas spp. strains. Mol. Plant-Microbe Interact. 14, 1006–1015. doi: 10.1094/MPMI.2001.14.8.1006
Dong, X. Z., and Cai, M. Y. (2001). Manual for the systematic identification of common bacteria. Beijing: Science Press.
Dou, Y. N., Niu, S. Q., Dou, J. T., Zhao, D., Zhen, D. D., Zhou, X., et al. (2018). Screening, identification and preliminary investigation on the antagonistic activity of Bacillus sp. against apple tree rots. Microbiol. Bull. 45, 2684–2694.
Du, J., Duan, S., Miao, J., Zhai, M., and Cao, Y. (2021). Purification and characterization of chitinase from Paenibacillus sp. Biotechnol. Appl. Biochem. 68, 30–40. doi: 10.1002/bab.1889
Du, X. H., Wang, H., Sun, J., Xiong, L., and Yu, J. (2019). Hybridization, characterization and transferability of SSRs in the genus Morchella. Fungal Biol. 123, 528–538. doi: 10.1016/j.funbio.2019.05.005
Du, X. H., Wu, D., Kang, H., Wang, H., Xu, N., Li, T., et al. (2020). Heterothallism and potential hybridization events inferred for twenty-two yellow morel species. IMA Fungus 11, 1–19. doi: 10.1186/s43008-020-0027-1
Du, X. H., Zhao, Q., O’Donnell, K., Rooney, A. P., and Yang, Z. L. (2012). Multigene molecular phylogenetics reveals true morels (Morchella) are especially species-rich in China. Fungal Genet. Biol. 49, 455–469. doi: 10.1016/j.fgb.2012.03.006
Du, X. H., Zhao, Q., and Yang, Z. L. (2015). A review on research advances, issues, and perspectives of morels. Mycology 6, 78–85. doi: 10.1080/21501203.2015.1016561
Frändberg, E., and Schnürer, J. (1994). Evaluation of a chromogenic chito-oligosaccharide analogue, p-nitrophenyl−β−D-N, N’-diacetylchitobiose, for the measurement of the chitinolytic activity of bacteria. J. Appl. Bacteriol. 76, 259–263. doi: 10.1111/j.1365-2672.1994.tb01625.x
Ghose, T. K. (1987). Measurement of cellulase activities. Pure Appl. Chem. 59, 257–268. doi: 10.1351/pac198759020257
Goldman, G. H., Delneste, Y., and Papon, N. (2021). Fungal Polysaccharides Promote Protective Immunity. Trends Microbiol. 29, 379–381. doi: 10.1016/j.tim.2021.02.004
Guo, M. P., Chen, K., Wang, G. Z., and Bian, Y. B. (2016). First report of stipe rot disease on Morchella importuna caused by Fusarium incarnatum Fequiseti species complex in China. Plant Dis. 100, 2530–2530. doi: 10.1094/PDIS-05-16-0633-PDN
Haas, D., and Défago, G. (2005). Biological control of soil-borne pathogens by fluorescent pseudomonads. Nat. Rev. Microbiol. 3, 307–319. doi: 10.1038/nrmicro1129
He, P., Li, C., Cai, Y., Zhang, Y., Bian, Y., and Liu, W. (2018). First report of pileus rot disease on cultivated Morchella importuna caused by Diploöspora longispora in China. J. Gen. Plant Pathol. 84, 65–69. doi: 10.1007/s10327-017-0754-3
Islam, M. R., Jeong, Y. T., Lee, Y. S., and Song, C. H. (2012). Isolation and identification of antifungal compounds from Bacillus subtilis C9 inhibiting the growth of plant pathogenic fungi. Mycobiology 40, 59–65. doi: 10.5941/MYCO.2012.40.1.059
Jain, R., and Pandey, A. (2016). A phenazine-1-carboxylic acid producing polyextremophilic Pseudomonas chlororaphis (MCC2693) strain, isolated from mountain ecosystem, possesses biocontrol and plant growth promotion abilities. Microbiol. Res. 190, 63–71. doi: 10.1016/j.micres.2016.04.017
Kilani-Feki, O., Khedher, S. B., Dammak, M., Kamoun, A., Jabnoun-Khiareddine, H., Daami-Remadi, M., et al. (2016). Improvement of antifungal metabolites production by Bacillus subtilis V26 for biocontrol of tomato postharvest disease. Biol. Control. 95, 73–82. doi: 10.1016/j.biocontrol.2016.01.005
Lan, Y. F., Cong, Q. Q., Wang, Q. W., Tang, L. N., Li, X. M., Yu, Q. W., et al. (2020). First report of Cladobotryum protrusum causing cobweb disease on cultivated Morchella importuna. Plant Dis. 104, 977. doi: 10.1094/PDIS-08-19-1611-PDN
Lastochkina, O., Seifikalhor, M., Aliniaeifard, S., Baymiev, A., Pusenkova, L., Garipova, S., et al. (2019). Bacillus Spp.: Efficient biotic strategy to control postharvest diseases of fruits and vegetables. Plants. 8:97. doi: 10.3390/plants8040097
Leal, A. R., Barros, L., Barreira, J. C., Sousa, M. J., Martins, A., Santos-Buelga, C., et al. (2013). Portuguese wild mushrooms at the “pharma–nutrition” interface: Nutritional characterization and antioxidant properties. Food Res. Int. 50, 1–9. doi: 10.1016/j.foodres.2012.10.012
Li, Y., Chen, H., and Zhang, X. (2023). Cultivation, nutritional value, bioactive compounds of morels, and their health benefits: A systematic review. Front. Nutr. 10:1159029. doi: 10.3389/fnut.2023.1159029
Li, Z. F., Zhu, J., Li, T., D, G. F., W, T., L, T. G., et al. (2020). Screening, identification, and enzyme activity determination of cellulase producing bacteria from tobacco straw. Southw. Agric. J. 33, 645–650.
Linda, T. M., Siregar, S., Fitri, W. D., Martina, A., Lestari, W., and Roslim, D. I. (2018). Isolation and screening of culturable endophytic bacteria from leaf of rubber plant that produces of chitinase. J. Phys. Conf. Ser. 1116:052038.
Liu, Q., Ma, H., Zhang, Y., and Dong, C. (2018). Artificial cultivation of true morels: current state, issues and perspectives. Crit. Rev. Biotechnol. 38, 259–271. doi: 10.1080/07388551.2017.1333082
Liu, W., Cai, Y. L., He, P. X., Ma, X. L., and Bian, Y. B. (2019). Pest and disease occurrence patterns and prevention and control measures for cultivation of morel mushrooms. J. Edible Mushrooms 26, 128–134.
Liu, W., Yuan, L., and Wang, S. (2020). Recent progress in the discovery of antifungal agents targeting the cell wall. J. Med. Chem. 63, 12429–12459. doi: 10.1021/acs.jmedchem.0c00748
Liu, X., Jiang, X., Sun, H., Du, J., Luo, Y., Huang, J., et al. (2022). Evaluating the Mode of Antifungal Action of Heat-Stable Antifungal Factor (HSAF) in Neurospora crassa. J. Fungi. 8, 252. doi: 10.3390/jof8030252
Ma, Z., Hua, G. K. H., Ongena, M., and Höfte, M. (2016). Role of phenazines and cyclic lipopeptides produced by Pseudomonas sp. CMR12a in induced systemic resistance on rice and bean. Environ. Microbiol. Rep. 8, 896–904. doi: 10.1111/1758-2229.12454
Mahmood, A., Malik, R. N., Shinwari, Z. K., and Mahmood, A. Q. E. E. L. (2011). Ethnobotanical survey of plants from Neelum, Azad Jammu and Kashmir, Pakistan. Pak. J. Bot. 43, 105–110.
Masaphy, S. (2022). First report on Purpureocillium lilacinum infection of indoor-cultivated morel primordia. Agriculture 12:695. doi: 10.3390/agriculture12050695
Mavrodi, D. V., Blankenfeldt, W., and Thomashow, L. S. (2006). Phenazine compounds in fluorescent Pseudomonas spp. biosynthesis and regulation. Annu. Rev. Phytopathol. 44, 417–445. doi: 10.1146/annurev.phyto.44.013106.145710
Oliveira Silva, A. D., Aliyeva-Schnorr, L., Wirsel, S. G., and Deising, H. B. (2022). Fungal pathogenesis-related cell wall biogenesis, with emphasis on the maize anthracnose fungus Colletotrichum graminicola. Plants 11:849. doi: 10.3390/plants11070849
Owwer, R. (1982). Notes on the development of the morel ascocarp: Morchella esculenta. Mycologia 74, 142–144. doi: 10.1080/00275514.1982.12021480
Raio, A., and Puopolo, G. (2021). Pseudomonas chlororaphis metabolites as biocontrol promoters of plant health and improved crop yield. World J. Microbiol. Biotechnol. 37:99. doi: 10.1007/s11274-021-03063-w
Raio, A., Puopolo, G., Cimmino, A., Danti, R., Della Rocca, G., and Evidente, A. (2011). Biocontrol of cypress canker by the phenazine producer Pseudomonas chlororaphis subsp. aureofaciens strain M71. Biol. Control 58, 133–138. doi: 10.1016/j.biocontrol.2011.04.012
Rismondo, J., and Schulz, L. M. (2021). Not just transporters: Alternative functions of ABC transporters in Bacillus subtilis and Listeria monocytogenes. Microorganisms. 9:163. doi: 10.3390/microorganisms9010163
Rotzoll, N., Dunkel, A., and Hofmann, T. (2006). Quantitative studies, taste reconstitution, and omission experiments on the key taste compounds in morel mushrooms (Morchella deliciosa Fr.). J. Agric. Food Chem. 54, 2705–2711. doi: 10.1021/jf053131y
Roze, M. E. (1882). Adherence de la base d’appareils ascospores de Morchella sur Helianthus tuberosus. Bull. Soc. Bot. Fr. 191, 66–167.
Sadfi, N., Cherif, M., Fliss, I., Boudabbous, A., and Antoun, H. (2001). Evaluation of bacterial isolates from salty soils and Bacillus thuringiensis strains for the biocontrol of Fusarium dry rot of potato tubers. J. Plant Pathol. 83, 101–117.
Sandhya, C., Adapa, L. K., Nampoothiri, K. M., Binod, P., Szakacs, G., and Pandey, A. (2004). Extracellular chitinase production by Trichoderma harzianum in submerged fermentation. J. Basic Microbiol. 44, 49–58. doi: 10.1002/jobm.200310284
Sarangthem, I., Rajkumari, L., Ngashangva, N., Nandeibam, J., Yendrembam, R. B., and Mukherjee, P. K. (2023). Isolation and characterization of bacteria from natural hot spring and insights into the thermophilic cellulase production. Curr Microbiol. 80:64. doi: 10.1007/s00284-022-03168-x
Shi, X., Liu, D., He, X., Liu, W., and Yu, F. (2022). Epidemic identification of fungal diseases in Morchella cultivation across China. J. Fungi 8:1107. doi: 10.3390/jof8101107
Soobrattee, M. A., Neergheen, V. S., Luximon-Ramma, A., Aruoma, O. I., and Bahorun, T. (2005). Phenolics as potential antioxidant therapeutic agents: mechanism and actions. Mutat. Res. 579:200. doi: 10.1016/j.mrfmmm.2005.03.023
Sun, J. Z., Yu, S., Lu, Y. Z., Liu, H. W., and Liu, X. Z. (2023). Proposal of a new family Pseudodiploösporeaceae fam. nov. (Hypocreales) based on phylogeny of Diploöspora longispora and Paecilomyces penicillatus. Mycology 14, 60–73. doi: 10.1080/21501203.2022.2143919
Sun, Q. D., Zhang, J. P., Xie, Y. Y., and Yan, W. J. (2019). Advances in the research of nutrients, functional components and health functions of Sheep Tripe. Food Sci. 40, 323–328. doi: 10.7506/spkx1002-6630-20180411-135
Tan, H., Kohler, A., Miao, R., Liu, T., Zhang, Q., Zhang, B., et al. (2019). Multi-omic analyses of exogenous nutrient bag decomposition by the black morel Morchella importuna reveal sustained carbon acquisition and transferring. Environ. Microbiol. 21, 3909–3926. doi: 10.1111/1462-2920.14741
Tan, H., Liu, T., Yu, Y., Tang, J., Jiang, L., Martin, F. M., et al. (2021a). Morel production related to soil microbial diversity and evenness. Microbiol. Spectrum. 9:e0022921. doi: 10.1128/Spectrum.00229-21
Tan, H., Yu, Y., Tang, J., Liu, T., Miao, R., Huang, Z., et al. (2021b). Build your own mushroom soil: Microbiota succession and nutritional accumulation in semi-synthetic substratum drive the fructification of a soil-saprotrophic morel. Front. Microbiol. 12:656656. doi: 10.3389/fmicb.2021.656656
Tietel, Z., and Masaphy, S. (2018). True morels (Morchella)-nutritional and phytochemical composition, health benefits and flavor: A review. Crit. Rev. Food Sci. Nutr. 58, 1888–1901. doi: 10.1080/10408398.2017.1285269
Vieira, V., Fernandes, Â, Barros, L., Glamočlija, J., Ćirić, A., Stojković, D., et al. (2016). Wild Morchella conica Pers. from different origins: a comparative study of nutritional and bioactive properties. J. Sci. Food Agric. 96, 90–98. doi: 10.1002/jsfa.7063
Vieira Gomes, D. C., de Alencar, M. V. O. B., Dos Reis, A. C., de Lima, R. M. T., de Oliveira Santos, J. V., da Mata, A. M. O. F., et al. (2019). Antioxidant, anti-inflammatory and cytotoxic/antitumoral bioactives from the phylum Basidiomycota and their possible mechanisms of action. Biomed. Pharmacother. 112:108643. doi: 10.1016/j.biopha.2019.108643
Wang, W. K., Zhu, Y., Tang, Y., Lu, N., Song, J. L., Yuan, W. D., et al. (2016). Non-volatile taste components of different cultivated mushrooms at mycelia, primordium, and fruit body cultivation stages. Int. J. Food Prop. 19, 1938–1948. doi: 10.1080/10942912.2015.1089891
Wang, W. X., Xu, B. L., Xue, Y. Y., Chen, Z., and Liang, X. D. (2014). Identification of antagonistic bacteria against apple tree rots and determination of their inhibition effect. Chin. J. Ecol. Agric. 22, 1214–1221.
Wang, Z., Wang, Y., Zheng, L., Yang, X., Liu, H., and Guo, J. (2014). Isolation and characterization of an antifungal protein from Bacillus licheniformis HS10. Biochem. Biophys. Res. Commun. 454, 48–52. doi: 10.1016/j.bbrc.2014.10.031
Xie, H., Wu, B., Liu, G., and Li, X. (2023). Optimization of in situ cellulase production from Penicillium oxalicum P-07 under submerged fermentation conditions with different cellulose types. J. Environ. Chem. Eng. 11:110290. doi: 10.1016/j.jece.2023.110290
Xie, J. Y., Dong, G. J., and Liu, Z. Y. (2005). Microbial sample preparation for scanning electron microscopy. J. Electron Microsc 24:440. doi: 10.3969/j.issn.1000-6281.2005.04.191
Xing, Y., Wang, S., Fan, J., Oso, A. O., Kim, S. W., Xiao, D., et al. (2015). Effects of dietary supplementation with lysine-yielding Bacillus subtilis on gut morphology, cecal microflora, and intestinal immune response of Linwu ducks. J. Anim. Sci. 93, 3449–3457. doi: 10.2527/jas.2014-8090
Xu, Y., Tang, J., Wang, Y., He, X., Tan, H., Yu, Y., et al. (2022). Large-scale commercial cultivation of morels: Current state and perspectives. Appl. Microbiol. Biotechnol. 106, 4401–4412. doi: 10.1007/s00253-022-12012-y
Yang, C., Jiang, X., Ma, L., Xiao, D., Liu, X., Ying, Z., et al. (2023). Transcriptomic and Metabolomic Profiles Provide Insights into the Red-Stipe Symptom of Morel Fruiting Bodies. J. Fungi. 9:373. doi: 10.3390/jof9030373
Yu, Y., Liu, T., Liu, L., Chen, Y., Tang, J., Peng, W., et al. (2022). Application of the mushroom volatile 1-octen-3-ol to suppress a morel disease caused by Paecilomyces penicillatus. Appl. Microbiol. Biotechnol. 106, 4787–4799. doi: 10.1007/s00253-022-12038-2
Zhang, D. S., Xu, Y. X., S, S. M., and Zhou, W. (2022). Study on solid culture medium and inoculation and culture time for cellulase and lipase production by bacteria. J. Microbiol. 42, 44–53.
Zhang, X., Zgao, G., Li, D., Li, S., and Hong, Q. (2014). Identification and evaluation of strain B37 of Bacillus subtilis antagonistic to sapstain fungi on poplar wood. Sci. World J. 2014:149342. doi: 10.1155/2014/149342
Zhang, Y., Li, T., Liu, Y., Li, X., Zhang, C., Feng, Z., et al. (2019). Volatile organic compounds produced by Pseudomonas chlororaphis subsp. aureofaciens SPS-41 as biological fumigants to control Ceratocystis fimbriata in postharvest sweet potatoes. J. Agric. Food Chem. 67, 3702–3710. doi: 10.1021/acs.jafc.9b00289
Zhang, Y., Li, T., Xu, M., Guo, J., Zhang, C., Feng, Z., et al. (2021). Antifungal effect of volatile organic compounds produced by Pseudomonas chlororaphis subsp. aureofaciens SPS-41 on oxidative stress and mitochondrial dysfunction of Ceratocystis fimbriata. Pestic. Biochem. Physiol. 173:104777. doi: 10.1016/j.pestbp.2021.104777
Zhao, Q. (2018). Current situation, Prospect and suggestions for the development of China’s morel mushroom industry. Edible Med. Mushrooms 26, 148–151.
Keywords: Morchella sextelata, bacterial disease, isolation and identification, biological property, pathogenic bacteria
Citation: Zhu X, Ma K, Sun M, Zhang J, Liu L and Niu S (2023) Isolation and identification of pathogens of Morchella sextelata bacterial disease. Front. Microbiol. 14:1231353. doi: 10.3389/fmicb.2023.1231353
Received: 30 May 2023; Accepted: 17 October 2023;
Published: 06 November 2023.
Edited by:
Xi-Hui Du, Chongqing Normal University, ChinaReviewed by:
Pilar Junier, Université de Neuchâtel, SwitzerlandElsherbiny A. Elsherbiny, Mansoura University, Egypt
Copyright © 2023 Zhu, Ma, Sun, Zhang, Liu and Niu. This is an open-access article distributed under the terms of the Creative Commons Attribution License (CC BY). The use, distribution or reproduction in other forums is permitted, provided the original author(s) and the copyright owner(s) are credited and that the original publication in this journal is cited, in accordance with accepted academic practice. No use, distribution or reproduction is permitted which does not comply with these terms.
*Correspondence: Xuetai Zhu, zhuxuetai@nwnu.edu.cn
†These authors have contributed equally to this work