- 1Key Laboratory of Veterinary Pharmaceutical Development, Ministry of Agricultural and Rural Affairs, Lanzhou Institute of Husbandry and Pharmaceutical Sciences of Chinese Academy of Agricultural Sciences, Lanzhou, China
- 2Department of Thoracic Surgery, Gansu Province Hospital, Lanzhou, China
- 3College of Food Science and Engineering, Gansu Agricultural University, Lanzhou, Gansu, China
Inflammatory bowel disease (IBD) is a serious global public health concern. Although the pathogenesis of the disease is currently unknown, it has been reported to be associated with both intestinal microbiota and inflammatory mediators. There is evidence suggesting that the feces of the Plateau pika is useful for treating gastrointestinal injuries and pain. Although fecal microbiota transplantation is highly efficacious intervention for IBD prevention, however, potential the transfer of pathogenic microbes or toxic substances is potentially hazardous. Fortunately, micropore filtering of the donor feces can minimize the risk of bacterial infection allowing retention of the therapeutic effects of the residual bacteriophages. Here, we demonstrated that Plateau pika feces not only alleviated the IBD symptoms but also promoted optimal structure and composition of the intestinal microbiota. Additionally, Plateau pika feces transfer also enhanced phenotypic features, such as, body-weight, disease activity index, and histological scores. In conclusion, Plateau pika feces was found to protect mice against colitis induced by dextran sodium sulfate by reducing inflammation and regulating microbial dysbiosis. These findings suggest the potential of Plateau pika feces as an alternative therapy for IBD.
Introduction
Inflammatory bowel disease (IBD) is a progressive immune-associated disease, characterized by persistent remission and relapse, that increases the risk of colorectal cancer (Cleynen et al., 2016). The incidence of the disease is currently increasing, and it is particularly common in recently industrialized countries (Gecse and Vermeire, 2018; Xiao et al., 2019). The risk factors of IBD include dysregulation of the mucosal immune response against bacteria-derived antigens, leading to the increased production of pro-inflammatory cytokines (Olén et al., 2020). Considering the crucial relevance of intestinal microbiota in keeping pathogens at bay and in maintaining overall health, there is growing research interest in supporting and enhancing the healthy microbial ecosystem to achieve better physical health (Jess et al., 2012). Moreover, these interventions may also assist in relieving the clinical manifestations of ulcerative colitis (UC) and Crohn’s disease (CD). The small lagomorph the Plateau pika (Ochotona curzoniae) from the alpine meadows of the Tibetan Plateau is a keystone species as it is food for predators, and provides shelter for small nesting tiny birds in its burrows (Olén et al., 2020).
Despite its overall protective actions against harmful circumstances such as pathogen infection, inflammation often involves pathways that aggravate pathogenic processes, thereby increasing the vulnerability of the host to further attacks (Ng et al., 2017). Many cytokines, such as, TNF-α, IL-6, and IL-1 are targets of the NF-кB pathway (Nadeem et al., 2020). While inhibitor proteins (IBs) typically restrict the activity of NF-кB in the cytoplasm, after translocation of the protein to the nucleus NF-кB promotes a series of complex phosphorylation and degradation events leading to the expression of downstream target genes and subsequent pro-inflammatory signaling (Kuipers et al., 2015). The NF-кB axis is reported to be closely associated with the pathogenesis of both UC and CD in humans and animals (Odenwald and Turner, 2017; Schirmer et al., 2019).
The interaction between intestinal microbiota and pro-inflammatory factors in UC is complex (Xavier and Podolsky, 2007). Prior investigations have revealed that the alleviation of UC symptoms occurs via an intricate balance between beneficial bacteria, host genetic factors, and common environmental stimuli. The recently introduced technique of fecal microbiota transplantation (FMT) has been shown to effectively enhance the structural regulation of the intestinal microbiota, thus relieving IBD and persistent gastrointestinal dysbiosis (Roda et al., 2016; Na and Moon, 2019). Nevertheless, the precise mechanism where FMT treatment relieves UC symptoms remains undetermined, and the majority of published information to date has been from case reports and allied research.
Previous studies have shown a reduced risk of UC when Plateau pika feces, host genetic factors, and common environmental stresses interact in a balanced manner (Paramsothy et al., 2019). Here, we explored the role of Plateau pika FMT in relieving IBD in a mouse model of colitis.
Materials and methods
Animals
All protocols involving the C57BL/6 J mice followed the Care and Use of Laboratory Animals (Gansu Province Animal Care Committee, Lanzhou, China) guidelines, and the study received ethical approval from the Lanzhou Institute of Husbandry and Pharmaceutical Sciences, CAAS. The mice were housed in an environment with constant temperature (20–24°C) and humidity and a 12-h / 12-h light/ dark cycle. Mice received food and water ad libitum.
The animal protocol is presented in Figure 1. Briefly, mice(n = 10/ group) were randomly assigned to one of three groups: (i) control; (ii) dextran sodium sulfate (DSS); (iii) FMT. For the first 7 days, all mice, apart from the controls received drinking water containing 3% DSS solution thus establishing the UC model. Subsequently, we collected, accumulated, gently homogenized, and froze the cecal contents of 10 Plateau pikas in 10% sterile glycerol. To generate a working FMT solution, the frozen fecal material was thawed and diluted to 0.05 g/ml with sterile saline, followed by homogenization, centrifugation (5,000 g 30 min, 4°C), and subsequent filtration of the supernatant using a 0.45 μm PES filter (Minisart® High Flow Syringe Filter, Sartorius™, Germany; Slack et al., 2022). The mice received FMT via a gavage of 200 μl of the supernatant from the Plateau pika fecal samples once a day over 2 weeks. Meanwhile, the control and DSS mice received a gavage of 200 μl of 0.9% saline solution. To establish the colitis mouse model, we supplemented sterile water with 3% DSS for 7 days, with 7 additional days of water without DSS. the pika feces supernatant was administered via gavage to the FMT mice, while control mice were given 0.9% saline solution. Daily parameters, including body-weight, changes in the consistency of the feces, and the presence of blood in the feces were monitored. To analyze the intestinal microbiota using 16S rRNA gene sequencing, feces were collected from the mice on day 14, and colonic tissue was harvested from the mice following euthanasia (Figure 1). DAI was scored as earlier described (Supplementary Table S1; Murthy et al., 1993; Camuesco et al., 2004).
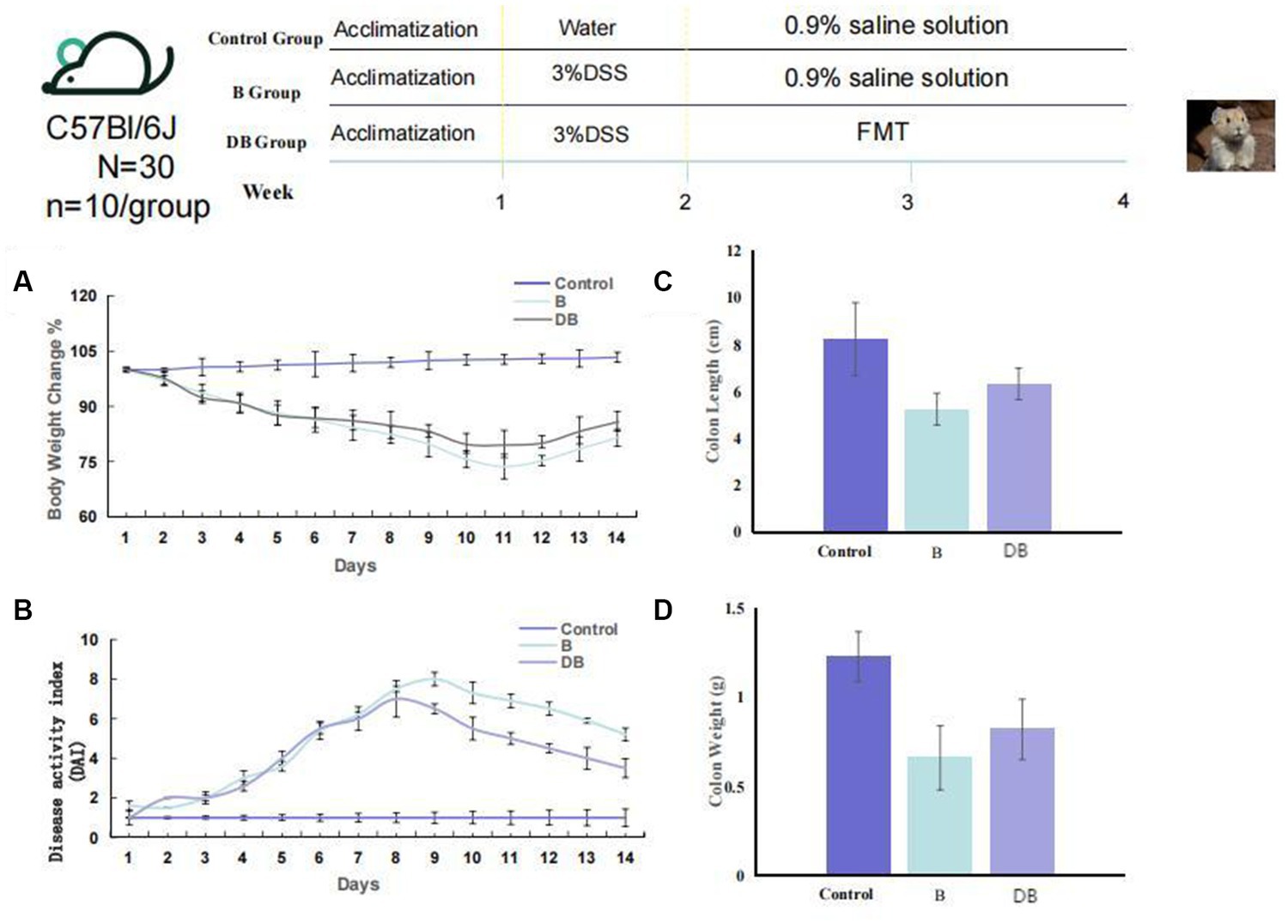
Figure 1. FMT reduced colonic damage of the colitis mouse model. Experimental design for FMT treatment on DSS-induced colitis in mice. (A) Weight loss (n = 10). (B) DAI (n = 6). (C) Colon length, and (D) Colon weight. Statistics were calculated with a two-tailed Student’s t test. *, p < 0.01; **, p, 0.01 (C,D). Data are presented as the mean 6 SEM.
After completion of the treatment, all animals were first anesthetized with sodium pentobarbital (5%), and then sacrificed by cervical dislocation immediately before dissection. Their colons opened, and the feces were gently moved using forceps. The distal colon (about 30 mm) together with additional intestinal regions were used for histological analysis using hematoxylin–eosin (HE) staining, respectively. We also collected colon samples for proinflammatory cytokine evaluation.
Histopathological analysis and HE staining
Mouse colons were fixed in 10% buffered formalin, before paraffin embedding, sectioning into 5 μm sections, HE staining, and examination under light microscopy.
Assessment of colonic oxidative stress (OS) parameter
Colonic parameters, namely, malondialdehyde (MDA) levels, total antioxidant capacity (T-AOC), catalase (CAT), and superoxide dismutase (SOD) activities, were measured using the appropriate kits (Nanjing Jiancheng Bioengineering Institute, China).
16S rRNA gene amplicon sequencing
We next determined the composition of the intestinal microbial communities in the distal ileal mucosa and intestinal lumen using 16S rRNA gene (V3-region) amplicon sequencing using NextSeq with v2 MID, 300-cycle, paired-end chemistry on a NextSeq (Illumina United States) platform. Total DNA was isolated using a Bead-Beat® Micro AX Gravity Kit (A&A Biotechnology, Poland), according to the provided directions. A library was generated, as reported previously (Slack et al., 2022). The mean amplicon sequencing depth per sample was 35,840 reads (minimum 9,286; maximum 63,584 reads).
Statistical analysis
Data were analyzed using GraphPad Prism and SPSS. p < 0.05 was set as the significance threshold. Data are presented as mean ± standard deviation (SD) and compared using one-way ANOVA and Duncan’s test.
Results
FMT-mediated regulation of mouse colitis development and clinicopathological manifestations
Among other consequences, DSS-triggered colitis causes the formation of ulcers in the intestinal epithelium, resulting in disruption of the inner mucosal layer, and promoting the invasion of acute and inflammatory immune cells (Das et al., 2020; Bell et al., 2022). Typical biomarkers of IBD are body weight, disease activity index (DAI) scoring, and the length/colon weight ratio (Ni et al., 2017; Chen et al., 2021). These variables were, therefore, used in the present study to evaluate the FMT-mediated regulation of colitis development and progression in vivo. It was found that all three variables were significantly reduced in DSS-treated mice (p < 0.01) compared with the controls (Figure 1). Significant weight gain was observed after FMT administration, which was equivalent to the weight of the control mice (p < 0.05). FMT-treated mice also showed substantially reduced DAI scores. Together, these results are consistent with previous findings (Weingarden and Vaughn, 2017), and indicated that treatment with FMT could successfully prevent DSS-driven IDB.
Plateau pika feces mitigated the pathological manifestations of DSS-induced experimental UC
We developed a DSS-induced mouse model of UC using 14-week-old C57BL/6 J mice to elucidate the influences of Plateau pika microbiota transplantation on UC. Relative to the control mice, the mice receiving Plateau pika feces microbiota transplantation showed significant improvements in their colons (Figure 1). The changes in body weight of the FMT-treated mice were also moderate. It was also found that the FMT treatment strongly prevented weight loss, relative to the control mice (p = 0.038; Figure 1A). Additionally, the DAI scores of the FMT-treated mice were substantially reduced, in comparison with the controls (p = 0.027; Figure 1B).
FMT-mediated regulation of histopathological changes in the colon
Representative images of the colon histology from each group of mice are shown in Figure 2. As expected, control mice showed normal colon histomorphology (Figure 2). In contrast, the DSS-treated mice showed thickening of the intestinal walls, submucosal edema, mucosal invasion, cytoplasmic mucin depletion, and ulceration of the colon (Figure 2). These characteristic symptoms of UC were significantly reduced after FMT administration (Figure 3). These results are consistent with those of Newman et al. (2017).
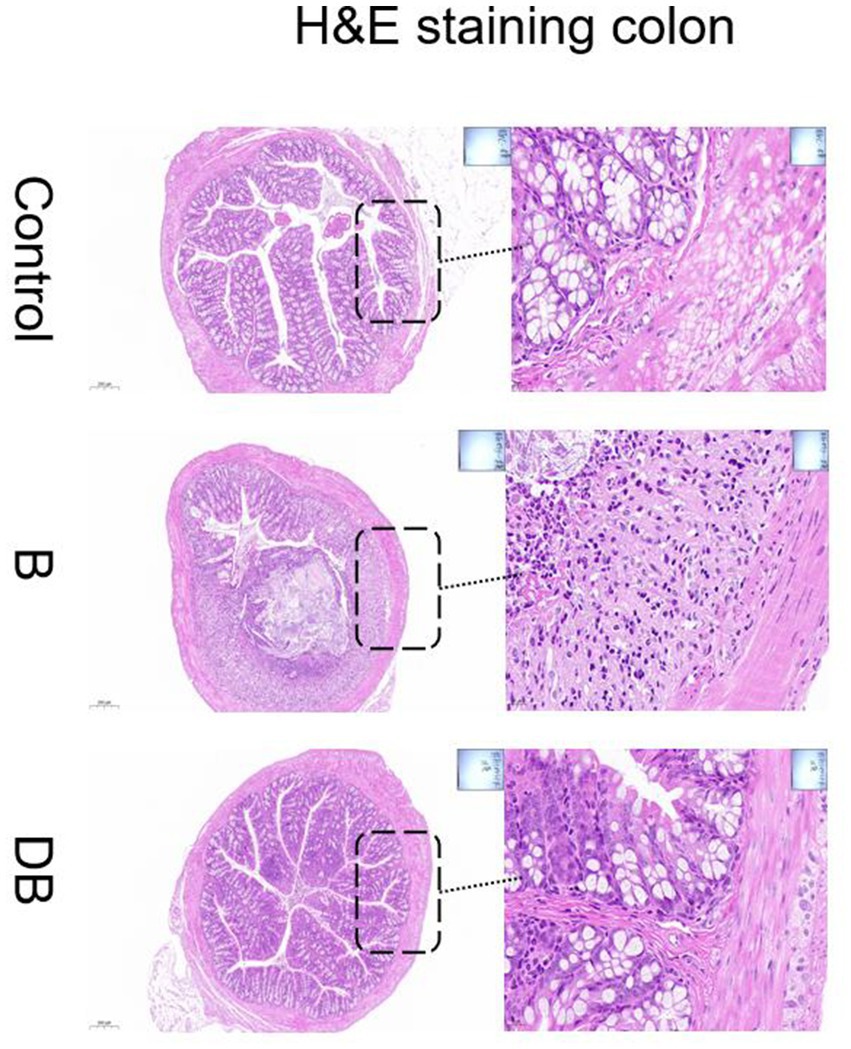
Figure 2. Effects of FMT intervention on the mouse colon histological changes (Representative hematoxylin and eosin staining) (Control) Control group, (B) B group, (DB) DB group.
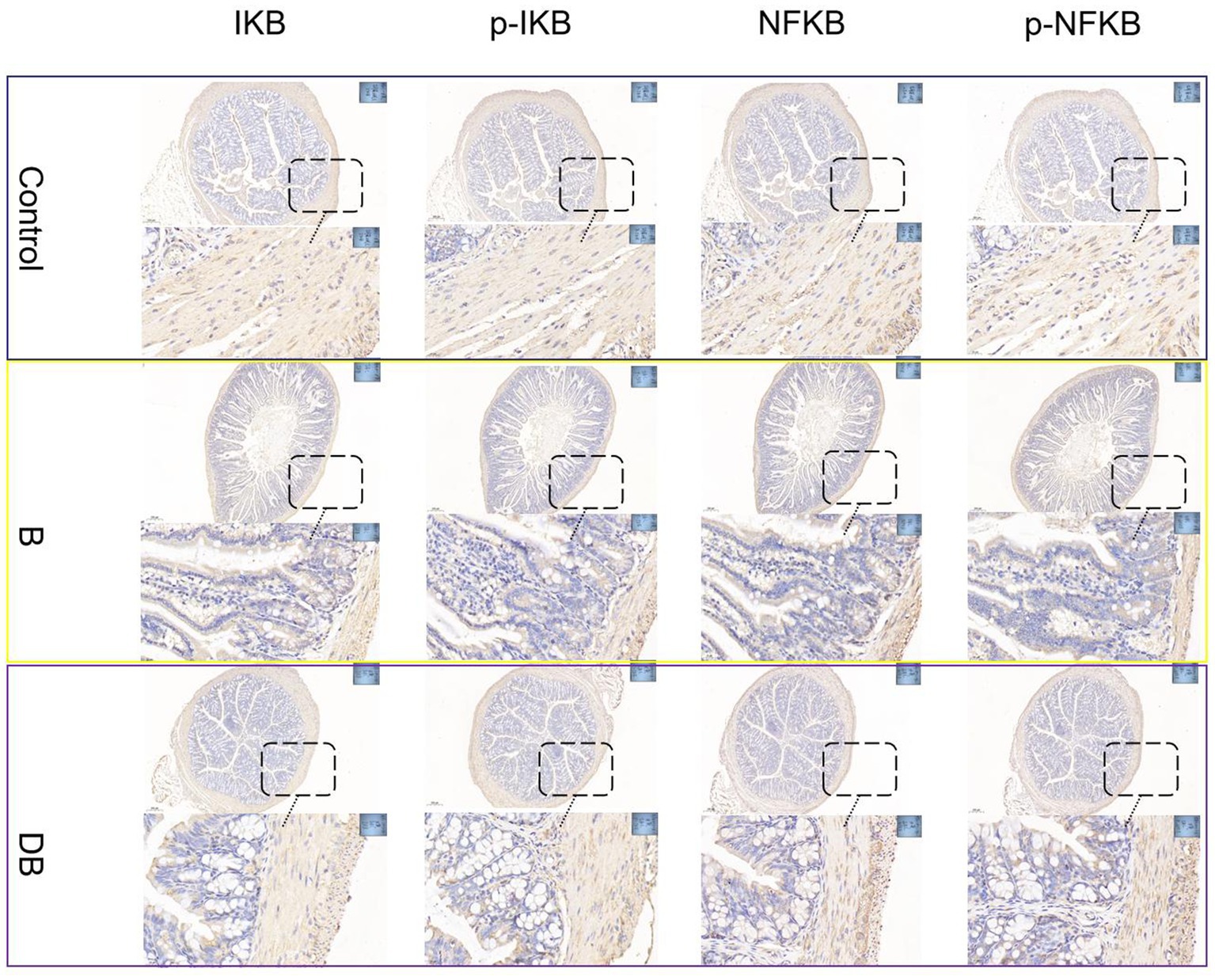
Figure 3. FMT modulated DSS-induced NF-κB activation in the colonic tissue. NF-κB protein levels in the colonic tissue were analyzed by immunohistochemical.
FMT-mediated regulation of oxidative status in colonic tissue
The present as well as prior UC studies demonstrated a link between intestinal OS and disease (Figure 4). Reactive oxygen species (ROS) are typically produced when proinflammatory factors activate phagocytes (Sun et al., 2017). Hence, any intervention that enhances the cellular antioxidant capacity can potentially prevent the development and severity of UC (Paramsothy et al., 2017; Mishra et al., 2022). Markedly reduced levels of CAT, SOD, and T-AOC together with significantly raised MDA levels were observed in DSS mice relative to the controls. CAT requires the splitting of the superoxide anion to catalyze O2 reduction to H2O2, as well as H2O2 conversion to water (Ortiz-Rivera et al., 2017), while T-AOC modulates body function and the susceptibility to certain diseases (Özçam et al., 2019). The MDA content is a well-established indicator of OS (Özçam et al., 2022). This evidence suggests that the colon undergoes OS during UC. Here, it was found that after FMT treatment, the CAT, SOD, and T-AOC levels rose significantly (p < 0.05) while those of MDA declined sharply (p < 0.01), thus indicating that FMT treatment could successfully reduce OS.
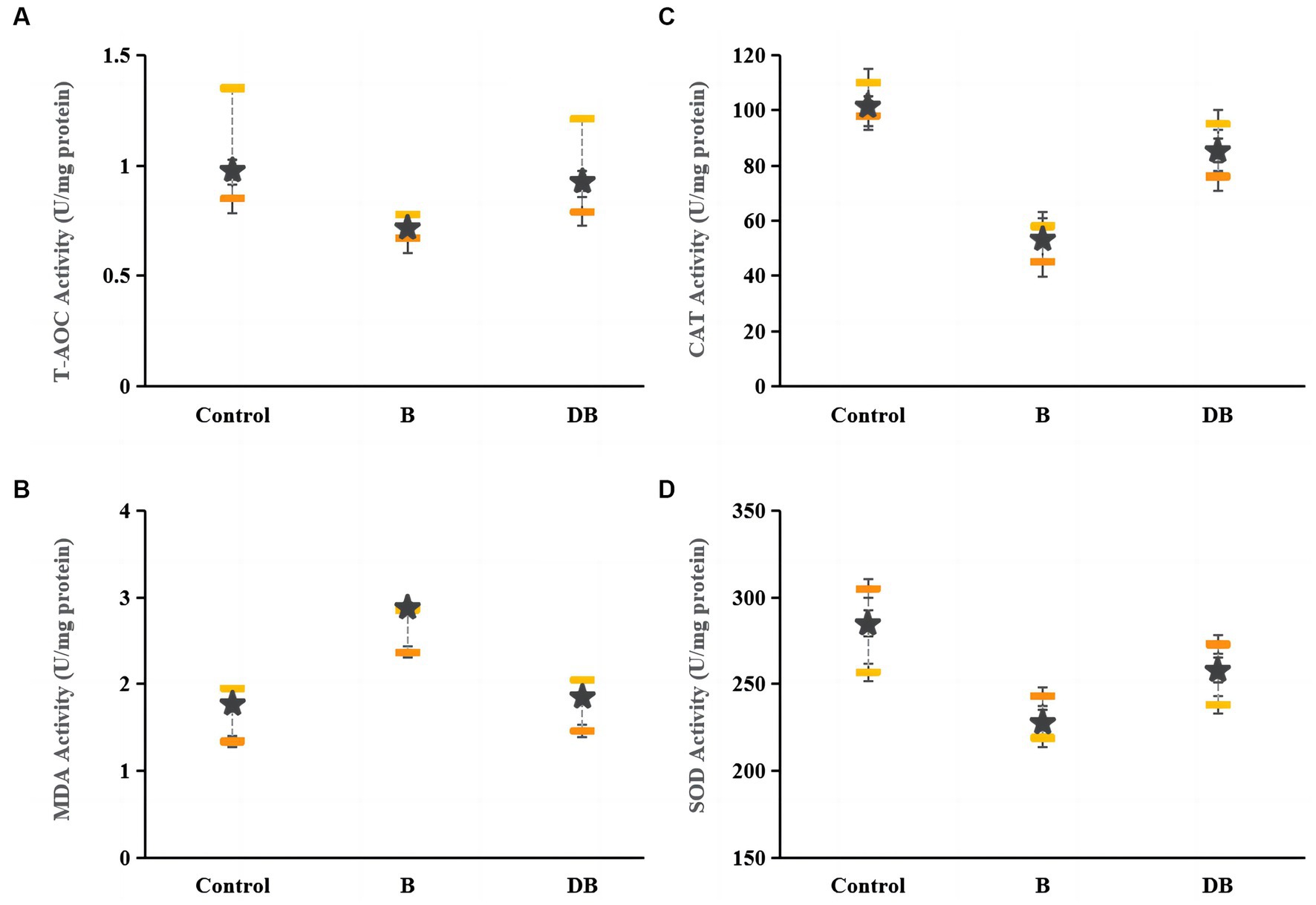
Figure 4. Effects of FMT intervention on colonic oxidative stress parameters in DSS-induced colitis mouse. (A) T-AOC, (B) MDA, (C) CAT, and (D) SOD. Data are presented as mean ± SD. ##p < 0.01 and #p < 0.05 vs. the control group. **p < 0.01 and *p < 0.05 vs. the B model group.
Plateau pika feces modulated the composition of the intestinal microbiota in a DSS-induced mouse model of UC
Colitis is closely linked to modifications in the composition and organization of the intestinal microbiota (Sartor and Wu, 2017). We used 16S rRNA sequencing of fecal samples collected after 2 weeks of DSS treatment to explore the significance of Plateau pika feces for regulating intestinal microbiota in a DSS-driven mouse model of colitis. The rarefaction analysis showed that the bacterial composition was quite diverse (Zhu et al., 2018). The intestinal microbiota of all three groups differed in their species compositions (Figure 5). The Simpson index was used to evaluate the alpha diversity at the species level in samples from all three groups collected on day 14. This showed that the Simpson index of FMT-treated mice differed markedly from that of the control mice (Figure 5). Treatment with Plateau pika feces increased the composition and variety of the microbiota throughout the chronic inflammatory phase, which, in turn, accelerated healing. Measurement of beta diversity using principal coordinate analysis (P-CoA) with the Bray Curtis distance metric revealed two distinct clusters for the FMT and control mice (Figure 5), further indicative of significantly altered microbial compositions, even in species diversity, in the FMT-treated mice by day 14. Our overall findings revealed that FMT treatment strongly regulated the intestinal microbiota throughout the chronic and recuperation stages of intestinal inflammation. Moreover, FMT-treated mice exhibited unusual microbial compositions throughout recovery from intestinal inflammation, namely, the presence of Dubosiella and Lactobacillus, while Ileibacterium, unclassified_f_Lachnospiraceae, Bifidobacterium, Turicibacter, Lachnospiraceae_NK4A136_group, and Candidatus_Saccharimonas were more prevalent on day 14.
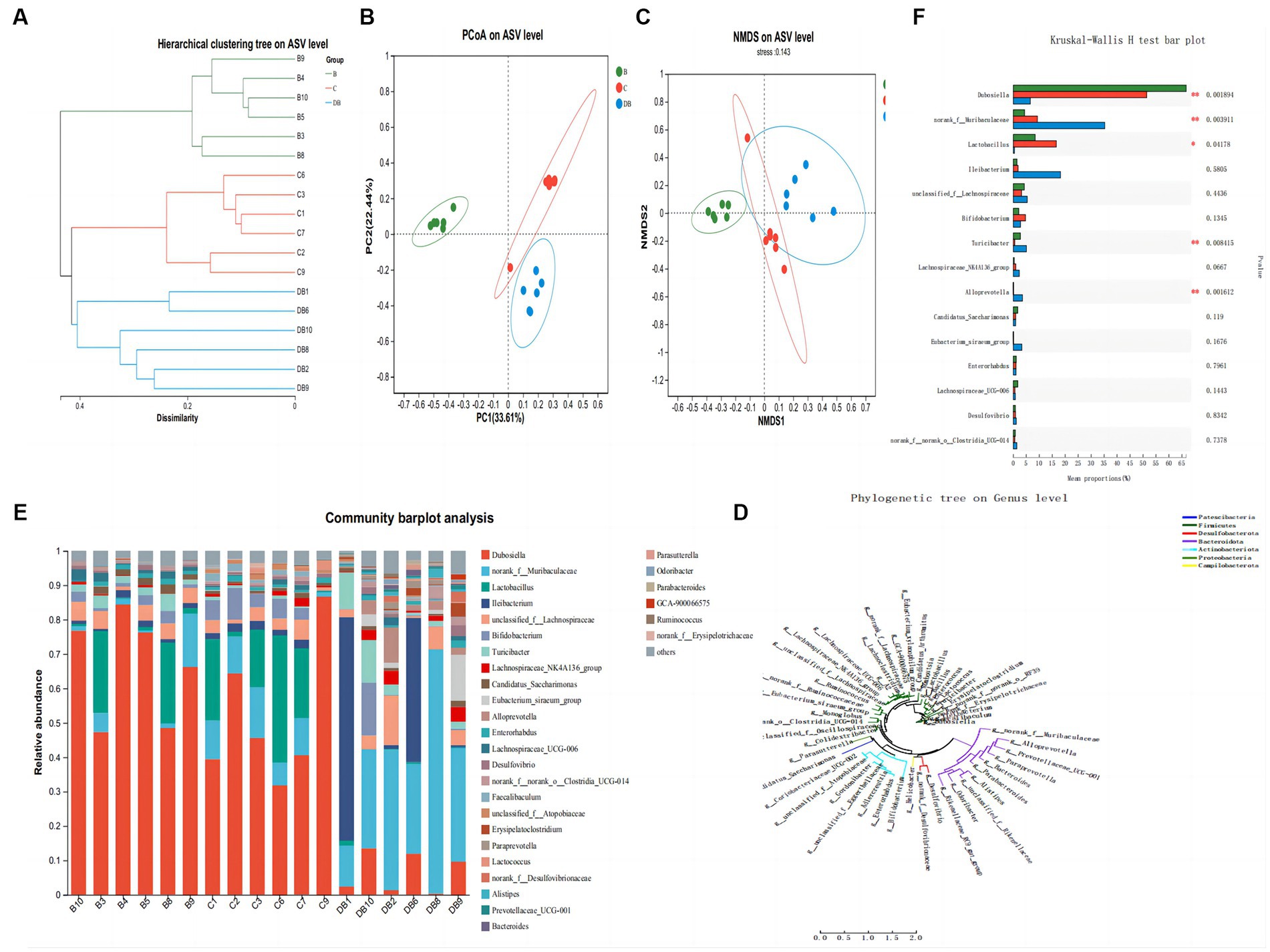
Figure 5. Influences of FMT administration on gut microbiota β-diversity. (A) Hierarchical clustering tree of weighted UniFrac distance, and (B) Principal coordinates analysis (PCoA) of ASV level. (C) NMDS of ASV level. (D) Phylogenetic tree on genus level. (E) Community barplot analysis. (F) Kruskal-Wallis H test bar plot. Data are presented as mean ± SD. ##p < 0.01 and #p < 0.05 vs. the control group. **p < 0.01 and *p < 0.05 vs. the DSS model group.
Discussion
The aim of this investigation was to determine the overall effects of Plateau pika feces on recovery from intestinal inflammation in a DSS-induced mouse model of colitis. The relief of UC symptoms may have resulted from the restoration of the intestinal structure, function, and micro-environment. The findings of this study provide important information on alternative treatment methods for colonic inflammation employing functional probiotics and the use of Plateau pika feces in IBD.
We demonstrated that Plateau pika feces alleviated the symptoms of DSS-triggered colitis, shown in reduced colon length, weight-loss, DAI scores, mucosal loss, and the invasion of inflammatory cells, as documented in previous publications (Park et al., 2018; Wang et al., 2020, 2021; Jeong et al., 2022).
Importantly, the Plateau pika feces cohort outperformed single-strain mice in terms of minimizing body-weight fluctuations, DAI, and colonic histological scores (Kühl et al., 2015; Gasaly et al., 2021). This may be because, after entry into the gut, bacterial strains may directly diminish inflammation by modulating the total microbial network, apart from their interaction with mucosal epithelial cells (Liang et al., 2021). We also confirmed that on day 8 of the development of colonic inflammation and on day 14 of healing in colitis mice, Plateau pika feces effectively rescued micro-ecological dysregulation. Based upon reports, altered microbiota may prevent colitis progression. The alpha and beta diversities revealed that the structural composition of the FMT mice intestinal microbiota differed significantly from that of the controls (Sun et al., 2020; Wang et al., 2021). The single strain-based intestinal microbiota recovery mostly occurred on day 14, which made it less efficacious than the Plateau pika feces. Metabolites that enter hosts are quickly absorbed into the intestine. They also reach the small intestinal epithelial cells, whereby their utilization is further augmented.
The intestinal microbiota is modulated directly by metabolites as they include bacteriocins with significant antibacterial activity (Salminen et al., 2021). The logical conclusion is that following absorption into the gastrointestinal tract, metabolites may potentially exhibit indirect anti-inflammatory activities through additional regulation of microbial communities.
Considering such significant alterations of the microbiota in FMT-treated colitis, we consider that treatments with Plateau pika feces have great potential in treating UC. Consequently, RFCV discovered 11 unique bacterial taxa. Given that only three taxa, namely, Lactobacillaceae Lactobacillus, Dubosiella Firmicutes, and Rikenellaceae Rikenellaceae were strongly influenced by the single strains suggests that single strains are less beneficial for enhancing the intestinal microbiota. The Plateau pika feces are particularly controlled by the other eight taxa. it was found that six taxa, namely, Muribaculaceae Muribaculaceae, Bacteroidaceae Bacteroides, Oscillospirales UCG.005, Lachnospiraceae Ruminococcus, Tissierellales Anaerovoracaceae, and Clostridia Clostridia, were abundant in the FMT-treated group, relative to controls. Muribaculaceae is known for its effect in lowering inflammation, inhibiting dangerous microorganisms, and fostering anticancer immunity (Setoyama et al., 2003). Bacteroides are potent candidates for next-generation probiotics due to their unique ability to generate short-chain fatty acids and sphingolipids that support intestinal barrier integrity, as well as modulation of the immune response, which, in turn, repairs intestinal damage (Brown et al., 2019; Wang et al., 2022). The other four species produce butyrate, with Oscillospirales UCG.005 and L. ruminococcus showing a relatively low abundance in patients with UC and CRC patients (Mancabelli et al., 2017). The remaining two Clostridia taxa enhance the proliferation and differentiation of regulatory T cells to alleviate IBD and allergic diarrhea (Atarashi et al., 2013). Conversely, here, DSS treatment strongly increased the relative abundances of Parasutterella Burkholderiales, and Bacteroidales Tannerellaceae in mice, consistent with the conclusions of prior publications (Silvestri et al., 2020).
IBD patients have increased numbers of P. burkholderiales, which is correlated with persistent colonic inflammation (Chen et al., 2018; Zhao et al., 2021). Importantly, there are no significant variations in the relative abundances of the eight taxa in the metabolite intervention experiment between the mix and control groups, indicating that mixed metabolites cannot fully control the colonic microbiota. As a result, we hypothesized that metabolite delivery would influence microbial ecology in the small intestine. The relative abundance of Acidiferrobacterales Acidiferrobacteraceae, Sphingomonadaceae Sphingosinicella, and Reyranellaceae Reyranella was markedly reduced in the controls, confirming the supposition that metabolites act by balancing the small intestine microenvironment.
Despite widespread acknowledgement that IBD is correlated with alterations in the composition and metabolism of the intestinal microbiota, there have been no reports of a causal association between dysbiosis and IBD in humans (Ni et al., 2017). There is very limited research on the association between intestinal microbiota and the IBD phenotype. Here, our RDA provided strong evidence for an association between microbial and colitis phenotypic data in the probiotic FMT group, whereby core species-level microbial data provided essential phenotypic data, such as disease severity and colonic pathology. Co-occurrence networks to established to investigate the relationship between the phenotypic data and the microbiological markers. In FMT-treated mice, we clearly identified the presence of B. bacteroides, M. muribaculaceae, B. tannerellaceae, and P. burkholderiales, as well as a stronger and broader interaction network on day 8. Since many microbial markers in the metabolite intervention experiments were stand-alone taxonomic units and not symbiotic communities, they obviously did not affect phenotypic parameters. In all the interventions, M. muribaculaceae was found to be pivotal in the co-occurrence networks, indicating its importance in the interplay between the general microbiota and disease phenotypes. Collectively, our findings and those of other published work demonstrated that Plateau pika feces might effectively reduce intestinal inflammation and restore function in a colitis animal model.
Conclusion
Here, we demonstrated that Plateau pika FMT relieved the intestinal inflammatory symptoms associated with colitis by altering the structure and composition of the intestinal microbiota. Our findings demonstrated a strong link between the intestinal microbiota and the colitis phenotype. These findings in an animal model highlight the potential use of Plateau pika FMT in the maintenance of intestinal health and may contribute toward the development of clinically applicable Plateau pika FMT-based interventions for IBD and other intestinal inflammatory diseases.
Limitations of our research
The current investigation has several limitations. Firstly, we did not employ metagenomics for the evaluation of bacterial community composition. This will be done in future investigations. Secondly, to identify suitable potential therapeutic targets, the associated intestinal microbiota- and inflammation-related pathways require further examination. Lastly, additional metabolomics research is warranted to clarify the methods of the purification and identification of vital components that operate via the mixed-metabolite intervention.
Importance
IBD is a chronic, nonspecific inflammatory disease. It is characterized by ulceration and erosion of the colonic mucosa and can also cause a wide variety of other lesions that frequently affect the entire colon. In this study, we investigated the efficacy of Plateau pika feces administration in reducing gut inflammation and the restoration of gut microecology restoration. The results showed that Plateau pika feces significantly reduced inflammation and accelerated recovery when compared with the control group. These findings support the use of Plateau pika feces as an alternative therapeutic approach for IBD.
Data availability statement
The data presented in the study are deposited in the NIH repository, accession number SUB13763520.
Ethics statement
The animal study was approved by Lanzhou Institute of Husbandry and Pharmaceutical Sciences, CAAS. The study was conducted in accordance with the local legislation and institutional requirements.
Author contributions
XD, PD, and YW designed the experiment and acquired grants. YY, BC, WS, and MF performed the experiments. YY, YL, and XL collected and analyzed the data. YY drafted and edited the manuscript. All authors contributed to the article and approved the submitted version.
Funding
This work was supported by Gansu Provincial Youth Science Foundation Project (grant no. 22JR5RA043), The Central Level Scientific Research Institutes for Basic R & D Special Fund (no. 1610322023010), Gansu Provincial Science and Technology Plan Project (grant no. 22JR5RA039), and National Key R&D Plan (grant no. 2021YFF0702405).
Conflict of interest
The authors declare that the research was conducted in the absence of any commercial or financial relationships that could be construed as a potential conflict of interest.
Publisher’s note
All claims expressed in this article are solely those of the authors and do not necessarily represent those of their affiliated organizations, or those of the publisher, the editors and the reviewers. Any product that may be evaluated in this article, or claim that may be made by its manufacturer, is not guaranteed or endorsed by the publisher.
Supplementary material
The Supplementary material for this article can be found online at: https://www.frontiersin.org/articles/10.3389/fmicb.2023.1228778/full#supplementary-material
References
Atarashi, K., Tanoue, T., Oshima, K., Suda, W., Nagano, Y., Nishikawa, H., et al. (2013). Treg induction by a rationally selected mixture of Clostridia strains from the human microbiota. Nature 500, 232–236. doi: 10.1038/nature12331
Bell, H. N., Rebernick, R. J., Goyert, J., Singhal, R., Kuljanin, M., Kerk, S. A., et al. (2022). Reuterin in the healthy gut microbiome suppresses colorectal cancer growth through altering redox balance. Cancer Cell 40, 185–200.e6. doi: 10.1016/j.ccell.2021.12.001
Brown, E. M., Ke, X., Hitchcock, D., Jeanfavre, S., Avila-Pacheco, J., Nakata, T., et al. (2019). Bacteroides-derived sphingolipids are critical for maintaining intestinal homeostasis and symbiosis. Cell Host Microbe 25, 668–680.e7. doi: 10.1016/j.chom.2019.04.002
Camuesco, D., Comalada, M., Rodríguez-Cabezas, M. E., Nieto, A., Lorente, M. D., Concha, A., et al. (2004). The intestinal anti-inflammatory effect of quercitrin is associated with an inhibition in iNOS expression. Br. J. Pharmacol. 143, 908–918. doi: 10.1038/sj.bjp.0705941
Chen, Y., Cui, W., Li, X., and Yang, H. (2021). Interaction between commensal bacteria, immune response and the intestinal barrier in inflflammatory bowel disease. Front. Immunol. 12:761981. doi: 10.3389/fifimmu.2021.761981
Chen, Y.-J., Wu, H., Wu, S.-D., Lu, N., Wang, Y.-T., Liu, H.-N., et al. (2018). Parasutterella, in association with irritable bowel syndrome and intestinal chronic inflflammation. J. Gastroenterol. Hepatol. 33, 1844–1852. doi: 10.1111/jgh.14281
Cleynen, I., Boucher, G., Jostins, L., Schumm, L. P., Zeissig, S., Ahmad, T., et al. (2016). Inherited determinants of Crohn’s disease and ulcerative colitis phenotypes: a genetic association study. Lancet 387, 156–167. doi: 10.1016/S0140-6736(15)00465-1
Das, N. K., Schwartz, A. J., Barthel, G., Inohara, N., Liu, Q., Sankar, A., et al. (2020). Microbial metabolite signaling is required for systemic iron homeostasis. Cell Metab. 31, 115–130.e6. doi: 10.1016/j.cmet.2019.10.005
Gasaly, N., de Vos, P., and Hermoso, M. A. (2021). Impact of bacterial metabolites on gut barrier function and host immunity: a focus on bacterial metabolism and its relevance for intestinal inflflammation. Front. Immunol. 12:658354. doi: 10.3389/fifimmu.2021.658354
Gecse, K. B., and Vermeire, S. (2018). Differential diagnosis of inflflammatory bowel disease: imitations and complications. Lancet Gastroenterol. Hepatol. 3, 644–653. doi: 10.1016/S2468-1253(18)30159-6
Jeong, Y., Kim, D. H., and Lee, K. (2022). Homeostasis effects of fermented Maillard reaction products by Lactobacillus gasseri 4M13 in dextran sulfate sodium-induced colitis mice. J. Sci. Food Agric. 102, 434–444. doi: 10.1002/jsfa.11374
Jess, T., Rungoe, C., and Peyrin–Biroulet, L. (2012). Risk of colorectal cancer in patients with ulcerative colitis: a meta-analysis of population-based cohort studies. Clin. Gastroenterol. Hepatol. 10, 639–645. doi: 10.1016/j.cgh.2012.01.010
Kühl, A. A., Erben, U., Kredel, L. I., and Siegmund, B. (2015). Diversity of intestinal macrophages in inflflammatory bowel diseases. Front. Immunol. 6:613. doi: 10.3389/fifimmu.2015.00613
Kuipers, E. J., Grady, W. M., Lieberman, D., Seufferlein, T., Sung, J. J., Boelens, P. G., et al. (2015). Colorectal cancer. Nat. Rev. Dis. Primers. 1:15065. doi: 10.1038/nrdp.2015.65
Liang, Y., Liu, M., Pu, J., Zhu, Z., Gao, Z., Zhou, Q., et al. (2021). Probiotics and their metabolites ameliorate inflflammatory bowel disease: a critical review. Infect. Microbes. Dis. 3, 4–13. doi: 10.1097/IM9.0000000000000046
Mancabelli, L., Milani, C., Lugli, G. A., Turroni, F., Cocconi, D., van Sinderen, D., et al. (2017). Identifification of universal gut microbial biomarkers of common human intestinal diseases by meta-analysis. FEMS Microbiol. Ecol. 93:fifix153. doi: 10.1093/femsec/fifix153
Mishra, J., Stubbs, M., Kuang, L., Vara, N., Kumar, P., and Kumar, N. (2022). Inflflammatory bowel disease therapeutics: a focus on probiotic engineering. Med. Inf. 2022:9621668. doi: 10.1155/2022/9621668
Murthy, S. N., Cooper, H. S., Shim, H., Shah, R. S., Ibrahim, S. A., and Sedergran, D. J. (1993). Treatment of dextran sulfate sodium-induced murine colitis by intracolonic cyclosporin. Dig. Dis. Sci. 38, 1722–1734. doi: 10.1007/BF01303184
Na, S.-Y., and Moon, W. (2019). Perspectives on current and novel treatments for inflflammatory bowel disease. Gut Liver 13, 604–616. doi: 10.5009/gnl19019
Nadeem, M. S., Kumar, V., Al-Abbasi, F. A., Kamal, M. A., and Anwar, F. (2020). Risk of colorectal cancer in inflflammatory bowel diseases. Semin. Cancer Biol. 64, 51–60. doi: 10.1016/j.semcancer.2019.05.001
Newman, K. M., Rank, K. M., Vaughn, B. P., and Khoruts, A. (2017). Treatment of recurrent Clostridium difficile infection using fecal microbiota transplantation in patients with inflammatory bowel disease. Gut Microbes 8, 303–309. doi: 10.1080/19490976.2017.1279377
Ng, S. C., Shi, H. Y., Hamidi, N., Underwood, F. E., Tang, W., Benchimol, E. I., et al. (2017). Worldwide incidence and prevalence of inflflammatory bowel disease in the 21st century: a systematic review of population-based studies. Lancet 390, 2769–2778. doi: 10.1016/S0140-6736(17)32448-0
Ni, J., Wu, G. D., Albenberg, L., and Tomov, V. T. (2017). Gut microbiota and IBD: causation or correlation? Nat. Rev. Gastroenterol. Hepatol. 14, 573–584. doi: 10.1038/nrgastro.2017.88
Odenwald, M. A., and Turner, J. R. (2017). The intestinal epithelial barrier: a therapeutic target? Nat. Rev. Gastroenterol. Hepatol. 14, 9–21. doi: 10.1038/nrgastro.2016.169
Olén, O., Erichsen, R., Sachs, M. C., Pedersen, L., Halfvarson, J., Askling, J., et al. (2020). Colorectal cancer in ulcerative colitis: a Scandinavian population-based cohort study. Lancet 395, 123–131. doi: 10.1016/S0140-6736(19)32545-0
Ortiz-Rivera, Y., Sánchez-Vega, R., Gutiérrez-Méndez, N., León-Félix, J., AcostaMuñiz, C., and Sepulveda, D. R. (2017). Production of reuterin in a fermented milk product by Lactobacillus reuteri: inhibition of pathogens, spoilage microorganisms, and lactic acid bacteria. J. Dairy Sci. 100, 4258–4268. doi: 10.3168/jds.2016-11534
Özçam, M., Oh, J.-H., Tocmo, R., Acharya, D., Zhang, S., Astmann, T. J., et al. (2022). A secondary metabolite drives intraspecies antagonism in a gut symbiont that is inhibited by cell-wall acetylation. Cell Host Microbe 30, 824–835.e6. doi: 10.1016/j.chom.2022.03.033
Özçam, M., Tocmo, R., Oh, J.-H., Afrazi, A., Mezrich, J. D., Roos, S., et al. (2019). Gut symbionts Lactobacillus reuteri R2lc and 2010 encode a polyketide synthase cluster that activates the mammalian arylhydrocarbon receptor. Appl. Environ. Microbiol. 85, e01661–e01618. doi: 10.1128/AEM.01661-18
Paramsothy, S., Kamm, M. A., Kaakoush, N. O., Walsh, A. J., van den Bogaerde, J., Samuel, D., et al. (2017). Multidonor intensive faecal microbiota transplantation for active ulcerative colitis: a randomised placebo-controlled trial. Lancet 389, 1218–1228. doi: 10.1016/S0140-6736(17)30182-4
Paramsothy, S., Nielsen, S., Kamm, M. A., Deshpande, N. P., Faith, J. J., Clemente, J. C., et al. (2019). Specific Bacteria and metabolites associated with response to fecal microbiota transplantation in patients with ulcerative colitis. Gastroenterology 156, 1440–1454.e2. doi: 10.1053/j.gastro.2018.12.001
Park, J.-S., Choi, J. W., Jhun, J., Kwon, J. Y., Lee, B.-I., Yang, C. W., et al. (2018). Lactobacillus acidophilus improves intestinal inflflammation in an acute colitis mouse model by regulation of Th17 and Treg cell balance and fifibrosis development. J. Med. Food 21, 215–224. doi: 10.1089/jmf.2017.3990
Roda, G., Jharap, B., Neeraj, N., and Colombel, J.-F. (2016). Loss of response to antiTNFs: defifinition, epidemiology, and management. Clin. Transl. Gastroenterol. 7:e135. doi: 10.1038/ctg.2015.63
Salminen, S., Collado, M. C., Endo, A., Hill, C., Lebeer, S., Quigley, E. M. M., et al. (2021). The international Scientifific Association of Probiotics and Prebiotics (ISAPP) consensus statement on the defifinition and scope of postbiotics. Nat. Rev. Gastroenterol. Hepatol. 18, 649–667. doi: 10.1038/s41575-021-00440-6
Sartor, R. B., and Wu, G. D. (2017). Roles for intestinal bacteria, viruses, and fungi in pathogenesis of inflflammatory bowel diseases and therapeutic approaches. Gastroenterology 152, 327–339.e4. doi: 10.1053/j.gastro.2016.10.012
Schirmer, M., Garner, A., Vlamakis, H., and Xavier, R. J. (2019). Microbial genes and pathways in inflflammatory bowel disease. Nat. Rev. Microbiol. 17, 497–511. doi: 10.1038/s41579-019-0213-6
Setoyama, H., Imaoka, A., Ishikawa, H., and Umesaki, Y. (2003). Prevention of gut inflflammation by Bififidobacterium in dextran sulfate-treated gnotobiotic mice associated with Bacteroides strains isolated from ulcerative colitis patients. Microbes Infect. 5, 115–122. doi: 10.1016/S1286-4579(02)00080-1
Silvestri, C., Pagano, E., Lacroix, S., Venneri, T., Cristiano, C., Calignano, A., et al. (2020). Fish oil, cannabidiol and the gut microbiota: an investigation in a murine model of colitis. Front. Pharmacol. 11:585096. doi: 10.3389/fphar.2020.585096
Slack, R. J., Macdonald, S. J. F., Roper, J. A., Jenkins, R. G., and Hatley, R. J. D. (2022). Emerging therapeutic opportunities for integrin inhibitors. Nat. Rev. Drug Discov. 21, 60–78. doi: 10.1038/s41573-021-00284-4
Sun, M., Liu, Y., Song, Y., Gao, Y., Zhao, F., Luo, Y., et al. (2020). The ameliorative effect of Lactobacillus plantarum-12 on DSS-induced murine colitis. Food Funct. 11, 5205–5222. doi: 10.1039/d0fo00007h
Sun, M., Wu, W., Liu, Z., and Cong, Y. (2017). Microbiota metabolite short chain fatty acids, GPCR, and inflflammatory bowel diseases. J. Gastroenterol. 52, 1–8. doi: 10.1007/s00535-016-1242-9
Wang, G., Huang, S., Cai, S., Yu, H., Wang, Y., Zeng, X., et al. (2020). Lactobacillusreuteri ameliorates intestinal inflflammation and modulates gut microbiota and metabolic disorders in dextran sulfate sodium-induced colitis in mice. Nutrients 12:2298. doi: 10.3390/nu12082298
Wang, N., Wang, S., Xu, B., Liu, F., Huo, G., and Li, B. (2021). Alleviation effects of Bififi-dobacterium animalis subsp. lactis XLTG11 on dextran sulfate sodiuminduced colitis in mice. Microorganisms 9:2093. doi: 10.3390/microorganisms9102093
Wang, C., Xiao, Y., Yu, L., Tian, F., Zhao, J., Zhang, H., et al. (2022). Protective effects of different Bacteroides vulgatus strains against lipopolysaccharide-induced acute intestinal injury, and their underlying functional genes. J. Adv. Res. 36, 27–37. doi: 10.1016/j.jare.2021.06.012
Weingarden, A. R., and Vaughn, B. P. (2017). Intestinal microbiota, fecal microbiota transplantation, and inflammatory bowel disease. Gut Microbes 8, 238–252. doi: 10.1080/19490976.2017.1290757
Xavier, R. J., and Podolsky, D. K. (2007). Unravelling the pathogenesis of inflammatory bowel disease. Nature 448, 427–434. doi: 10.1038/nature06005
Xiao, P., Zhang, H., Zhang, Y., Zheng, M., Liu, R., Zhao, Y., et al. (2019). Phosphatase Shp2 exacerbates intestinal inflflammation by disrupting macrophage responsiveness to interleukin-10. J. Exp. Med. 216, 337–349. doi: 10.1084/jem.20181198
Zhao, L., Tian, W., Qu, W., Li, T., Zhu, J., and Ma, H. (2021). Protease hydrolysates ameliorates inflflammation and intestinal flflora imbalance in DSS-induced colitis mice. J. Food Qual. 2021, 1–11. doi: 10.1155/2021/5536148
Keywords: inflammatory bowel disease, Plateau pika feces, fecal microbiota transplantation, gut microbiota, colitis murine model
Citation: Yang Y, Cui B, Lv Y, Lu X, Shen W, Feng M, Ding X, Dong P and Wang Y (2023) Plateau pika fecal microbiota transplantation ameliorates inflammatory bowel disease manifestations in a mouse model of colitis. Front. Microbiol. 14:1228778. doi: 10.3389/fmicb.2023.1228778
Edited by:
Xiaodan Huang, Lanzhou University, ChinaReviewed by:
Na Zhao, Chinese Academy of Sciences (CAS), ChinaJing Yang, National Institute for Communicable Disease Control and Prevention (China CDC), China
Copyright © 2023 Yang, Cui, Lv, Lu, Shen, Feng, Ding, Dong and Wang. This is an open-access article distributed under the terms of the Creative Commons Attribution License (CC BY). The use, distribution or reproduction in other forums is permitted, provided the original author(s) and the copyright owner(s) are credited and that the original publication in this journal is cited, in accordance with accepted academic practice. No use, distribution or reproduction is permitted which does not comply with these terms.
*Correspondence: Xuezhi Ding, dingxuezhi@caas.cn; Pengcheng Dong, dongpengceng@caas.cn; Yu Wang, wangyu02@caas.cn
†These authors have contributed equally to this work