- 1College of Agriculture, College of Life Sciences, Guizhou University, Guiyang, China
- 2International Jointed Institute of Plant Microbial Ecology and Resource Management in Guizhou University, Ministry of Agriculture, China Association of Agricultural Science Societies, Guiyang, China
- 3Guizhou-Europe Environmental Biotechnology and Agricultural Informatics Oversea Innovation Center in Guizhou University, Guizhou Provincial Science and Technology Department, Guiyang, China
- 4School of Ecology and Environment, Tibet University, Lhasa, China
- 5Microbiology Research Institute, Guangxi Agricultural Science Academy, Nanning, China
Aucuba japonica Thunb is an evergreen woody ornamental plant with significant economic and ecological values. It also produces aucubin, showing a variety of biological activities. It is widely planted in the southwest region of China, including karst landscape areas in Guizhou Province. In January 2022, a serious leaf blight disease was observed on the leaves of A. japonica in the outdoor gardens of Guizhou University, Guiyang, Guizhou, China. The causal agent was identified as Colletotrichum aenigma through amplification and sequencing of the internal transcribed spacer (ITS) region, translation of the chitin synthase (CHS) and actin (ACT) genes, and morphological characterizations. Koch’s postulates were confirmed by its pathogenicity on healthy leaves, including re-isolation and identification. To our knowledge, this is the first report of C. aenigma causing leaf blight on A. japonica worldwide. To identify pathogen characteristics that could be utilized for future disease management, the effects of temperature and light on mycelial growth, conidia production, and conidial germination, and the effects of humidity on conidial germination were studied. Optimal temperatures for mycelial growth of C. aenigma BY827 were 25–30°C, while 15°C and 35°C were favorable for conidia production. Concurrently, alternating 10-h light and 14-h dark, proved to be beneficial for mycelial growth and conidial germination. Additionally, conidial germination was enhanced at 90% humidity. In vitro screenings of ten chemical pesticides to assess their efficacy in suppressing C. aenigma representative strain BY827. Among them, difenoconazole showed the best inhibition rate, with an EC50 (concentration for 50% of maximal effect) value of 0.0148 μg/ml. Subsequently, field experiment results showed that difenoconazole had the highest control efficiency on A. japonica leaf blight (the decreasing rate of disease incidence and decreasing rate of disease index were 44.60 and 47.75%, respectively). Interestingly, we discovered that C. aenigma BY827 may develop resistance to mancozeb, which is not reported yet among Colletotrichum spp. strains. In conclusion, our study provided new insights into the causal agent of A. japonica leaf blight, and the effective fungicides evaluated provided an important basis and potential resource for the sustainable control of A. japonica leaf blight caused by C. aenigma in the field.
1. Introduction
In the plant world, Aucuba (Garryaceae) genus contains 11 evergreen woody species mainly distributed in East and Southeast Asia, including China, Korea, Japan, Myanmar, and Vietnam (Xiang and Boufford, 2005). Some species of this genus, such as Aucuba japonica Thunb. and A. chinensis Benth., are commonly used as traditional medicines (Kimura et al., 1997; Song et al., 2007). Meanwhile, other species have high economic values as they are usually planted as ornamental plants, for instance, A. chinensis Benth., A. obcordate (Rehder) Fu ex W.K. Hu and Soong., and A. japonica (Robertson, 2010). Consequently, the evergreen shrub A. japonica, renowned for its special variegated leaves often referred to as “spotted laurel,” has been widely introduced and cultivated as a garden plant worldwide, including in Europe and the United States (Ali and Kikuzawa, 2005). In addition, the wood of A. japonica can be used for handicrafts in the culture and entertainment industry (Huang et al., 2022).
To date, studies on A. japonica have mainly focused on geographic structure, systematic, physiology, and pharmacological effects (Ohi et al., 2003; Xue et al., 2009; WCSP, 2019). Although originally distributed in warm temperate and subtropical moist forests of East Asia, A. japonica can tolerate heavy shade, air pollution, poor soils, and other stresses like drought (Ali and Kikuzawa, 2005; Zhang et al., 2011). The branching of A. japonica is caused by flower bud formation, and previous studies suggested that flower bud abortion by females may reduce its sexual dimorphism in terms of clonal growth (Abe, 2002). Aucubin, extracted from the leaves of A. japonica, is widely reported for its significant antioxidant, anti-inflammatory, and neuroprotective properties (Kim et al., 2014; Wang et al., 2015; Yang et al., 2018). However, plant diseases, mainly caused by bacteria, fungi, and viruses, pose major threats to A. japonica growth. For instance, in 2019, an outbreak of a disease with southern blight symptoms was first reported on A. japonica, and the pathogenic fungus was identified as Athelia rolfsii (Sun et al., 2022). Similarly, from 2018 to 2021, anthracnose disease became a major disease in A. japonica, and the pathogenic fungus was confirmed to be Colletotrichum boninense (Liu et al., 2022). In addition, the aucuba ringspot virus (AuRV) caused A. japonica to show mild mosaic, vein banding, and yellow ringspot symptoms on the leaves, and the vector of AuRV is predicted to be aphids (Uke et al., 2021). Chemical control, using pesticides to suppress pests, is one of the most common and reliable approaches to managing ornamental plant diseases. By way of illustration, ten different insecticides were evaluated for their efficacies against the false oleander scale, Pseudaulacaspis cockerelli (Cooley) on A. japonica plants (Carson et al., 2021). However, little is known about active fungicide resources against particular fungal diseases on A. japonica. Commonly used fungicides for crops and horticulture include tebuconazole, mancozeb, difenoconazole, pentachloronitrobenzene, and others, which have achieved relatively good inhibitory effects (Homdork et al., 2000; Fan et al., 2016; Wang et al., 2021). Nevertheless, it has been proven that misuse or overuse of pesticides has caused many problems for the environment and the quality and safety of agricultural products (Smith et al., 2013; Yesica et al., 2020). Thus, it is important to screen chemical pesticides with high efficiency, low toxicity, and low residue to control the disease.
Among the Colletotrichum gloeosporioides species complex, C. aenigma is an agronomically important phytopathogenic fungal species causing anthracnose diseases in a variety of hosts, including cereals, legumes, vegetables, perennial crops, and tree fruits (Than et al., 2008; Damm et al., 2012; Guarnaccia et al., 2017). In general, infections caused by Colletotrichum spp. start with spore attachment to the host plant surface, followed by spore germination and the development of an appressorium that penetrates the cuticle of the plant (Moral et al., 2021). The majority of Colletotrichum spp. colonies exhibited similar texture and density characteristics, characterized by abundant aerial mycelium with regular margins. These colonies display a wide range of color variations, ranging from white and whitish to dark gray and pinkish-orange (Zheng et al., 2021). The occurrences of plant disease were influenced by environmental factors and management strategies, especially high temperature and humidity, which can lead to enhanced pathogenesis of phytopathogens (Ram et al., 1999; Zhang et al., 2012; Romero et al., 2022). Moreover, Estrada et al. (2000) reported that temperature and humidity variations could affect the pathogenicity, conidial germination, and appressoria formation of Colletotrichum spp., which can be used to control anthracnose in the field condition. For this reason, exploring the interactions between different factors such as humidity, temperature, photoperiod, and growth duration is critical for our understanding of the pathogenic mechanism and effective control of C. aenigma. The first report of C. aenigma causing anthracnose diseases on Pyrus pyrifolia was in Japan (Weir et al., 2012). Subsequently, C. aenigma was reported as a phytopathogen on a variety of hosts, such as Pyrus bretschneideri, Malus pumila, and Vitis vinifera (Yan et al., 2015; Fu et al., 2019; Lee et al., 2021), resulting in significant yield and quality losses in these plants. To our knowledge, C. aenigma has not yet been reported as a pathogen for A. japonica.
In general, C. aenigma poses a major threat to plants, causing dramatic losses in agriculture, forestry, and fruit production worldwide. The primary means of prevention and control of Colletotrichum spp. has been through the use of chemical fungicides in the last decades. However, the excessive use of these chemical fungicides may cause a series of problems over time, such as food safety issues due to pesticide residues (Abrol and Singh, 2003), off-target and side effects on the environment, human health, and other living organisms (Wilson and Tisdell, 2001; Williams et al., 2004; Schmitt-Jansen and Altenburger, 2005). Hence, it is significant to screen effective chemical fungicides to manage newly emerging diseases like leaf blight of A. japonica caused by C. aenigma. Therefore, the objectives of the present study were to: (1) isolate and identify the potential pathogens that caused serious anthracnose disease on A. japonica in Guiyang, Guizhou, China; (2) confirm the pathogenesis of the representative isolates; (3) characterize the biological characteristics of the pathogen referring to local karst landscape environmental factors; and (4) screening effective and sustainable fungicides for the management of the disease.
2. Materials and methods
2.1. Sample collection and fungal isolation
In January 2022, a serious leaf blight disease was observed on the leaves of A. japonica in the outdoor gardens of Guizhou University, Huaxi District, Guiyang City, Guizhou Province, China (26°44′58”N, 106°65′91″E). On the leaf surface of A. japonica, a burn-like black blight with wilting signs was frequently observed, leading to rapid and extensive foliage death. The disease affected an area of approximately 0.52 hectares, with disease incidence ranging from 60 to 70%, resulting in a mortality rate of 20 to 30% in plants. For fungal isolation, every two symptomatic leaves were collected from ten different plants using disposable gloves. The diseased leaves were first soaked in 75% v/v ethanol for 1 min, then in a 4% w/v sodium hypochlorite (NaClO) solution for 3 min, and finally washed thrice with sterile distilled water (dH2O). Subsequently, the leaves were placed on potato dextrose agar (PDA) medium and incubated at 25 ± 2°C for 7 days. The fungal colonies were purified by transferring single spores, pure cultures were obtained. Representative isolates were selected for further identification.
2.2. Morphological and molecular identification
The morphological characteristics of five representative isolates were examined under the microscope. Fungal conidia were observed and photographed using a Zeiss microscopic system (Carl Zeiss-Axioscope 5, Germany). The length and width of 60 randomly selected conidia were measured and recorded. For molecular identification and phylogeny analysis, the genomic DNA of the representative isolates was extracted from mycelia of seven-day-old cultures according to the manufacturer’s instructions (Biomiga Fungal DNA Extraction Kit, CA, United States). Subsequently, polymerase chain reaction (PCR) amplification of internal transcribed spacer (rDNA-ITS), a partial sequence of the chitin synthase (CHS) gene and actin (ACT) gene were performed using the following primers: ITS1 (5’-TCCGTAGGTGAACCTGCGG-3′) and ITS2 (5′-GCTGCGTTCTTCATCGATGC-3′; White et al., 1990), CHS-79F (5′-TGGGGCAAGGATGCTTGGAAGAAG-3′) and CHS-345R (5′-TGGAAGAACCATCTGTGAGAGTTG-3′; Carbone and Kohn, 1999), and ACT-512F (5′-ATGTGCAAGGCCGGTTTCGC-3′) and ACT-783R (5′-TACGAGTCCTTCTGGCCCAT-3′; Carbone and Kohn, 1999), respectively. The PCR amplifications were carried out in a Bio-Rad S1000 Thermal Cycler in a 25 μl reaction mixture containing 1 μl of DNA sample, 1 μl of each primer, 12.5 μl 2 × SanTaq PCR Mix (Sangon Biotech, Shanghai, China), and 9.5 μl double distilled water (ddH2O). The PCR conditions were as follows: initial denaturation at 94°C for 3 min, followed by 35 cycles of denaturation at 95°C for 30 s, annealing for 30 s at the corresponding temperatures (53°C for ITS, 58°C for CHS gene, and 61°C for ACT gene), extension at 72°C for 45 s, then a final extension for 10 min.
The DNA sequences obtained in this study were queried against other DNA sequences in the GenBank database in the National Center for Biotechnology Information (NCBI).1 Sequences of the sequenced DNA regions of strains have been deposited with GenBank. The phylogenetic tree was constructed by the neighbor-joining algorithms based on concatenated ITS region, CHS gene, and ACT gene sequences using MEGA 6.05 software (Tamura et al., 2013).
2.3. Pathogenicity test
To confirm Koch’s postulates, the pathogenicity of the representative strain C. aenigma BY827 was tested on healthy leaves of A. japonica. Firstly, inoculation was performed in a laminar flow workstation (Haier, Qingdao, China) using sterilized needles to perform pathogen inoculation on healthy A. japonica detached leaves (n = 9; Bajwa et al., 2010). Then, strain C. aenigma BY827 plugs (d = 5 mm) were inoculated onto the leaves, obtained from active growing C. aenigma BY827 colonies that were 7 days old. As a control, nine leaves were mock-inoculated with sterile non-inoculated PDA medium plugs. After inoculation, all leaves were incubated at 25 ± 2°C, with a 16 h: 8 h photoperiod, and 70 ± 2% relative humidity (RH). In parallel, the pathogenicity of the representative strain C. aenigma BY827 was verified on each of six leaves of three healthy 5-year-old A. japonica plants grown in the campus green space of Guizhou University, Guiyang, Guizhou, China. Each leaf was spray inoculated with 500 μL conidial suspension (1 × 105 conidia/mL) of C. aenigma BY827. Another three plants sprayed with equal amounts of sterile distilled water served as controls. The pure cultures of the pathogen were reisolated from diseased A. japonica leaves and confirmed through the molecular analysis mentioned above. The experiment was repeated three times.
2.4. The effect of temperature on mycelial growth, conidia production, and conidial germination rate
To study the mycelial growth and conidia production of C. aenigma BY827 at different temperatures, a 5 mm diameter mycelial plug was taken from the edge of a seven-day-old colony and placed in the center of a petri dish with PDA medium and then incubated at different temperatures. The petri dishes were then incubated at temperatures, ranging from 15°C to 35°C at 5°C intervals. Mycelial growth was recorded on the 5th day after inoculation. Three repetitions were conducted for each treatment, and the fungal colony’s diameters were measured by the criss-cross-method (Hu et al., 2022). Ten agar-mycelium plugs (5 mm diameter) were taken from the edge of the active growing colonies simultaneously and transferred into a centrifuge tube containing sterile water. The solution was vigorously vortexed and then filtered through a double-layer gauze. Afterwards, the solution was diluted, and a hemocytometer was used to count the number of conidia per square centimeter of the colony, which was calculated using the formula below. For the germination rate analysis, conidia from seven-day-old colonies were washed with sterile distilled water, and the conidia concentration was adjusted to 1 × 105 conidia/mL using a hemocytometer. Subsequently, 0.2 mL of conidial suspension was added to 0.2 mL potato dextrose broth (PDB). After 12 h, the germination rate was calculated by microscopy.
X: dilution fold, N: the number of conidia in five squares of the hemacytometer, n: the number of perforated agar-mycelium plugs, r: the inner diameter of the perforator (Deng et al., 2012; Cao et al., 2022).
2.5. The effect of photoperiod on mycelial growth, conidia production, and conidial germination rate
To assay the effects of photoperiod on C. aenigma BY827, inoculated growth medium (PDA for mycelial growth and conidia production; PDB for conidial germination rate) was placed in incubators at 25 ± 2°C with the following settings: (1) 6 h light and 18 h dark; (2) 10 h light and 14 h dark; (3) 14 h light and 10 h dark; (4) 18 h light and 6 h dark. Each treatment was performed in triplicates.
2.6. The effect of humidity on conidial germination rate
The experiment aimed to determine the effect of humidity on the conidial germination rate of C. aenigma BY827. A conidial suspension (1 × 105 conidia/mL) was smeared onto glass slides, dried with sterile air, and placed in a closed petri dish. The relative humidity was set to 60, 70, 80, and 90%, respectively. The plates were incubated for 24 h at 25 ± 2°C in the dark, and the conidial germination rate was determined by microscopic observation after 12 h. Each treatment was repeated three times.
2.7. In vitro antifungal activity of fungicides on mycelial growth
Antifungal activities of eight chemical fungicides and two biopesticides against a representative strain of C. aenigma BY827 were screened to explore sustainable control resources for managing the pathogen. The mycelial growth rate method was used (Xin et al., 2020). The employed fungicides were formulations of tebuconazole (43% suspension concentrate; SC), mancozeb (43% SC), difenoconazole (10% water-dispersible granule; WG), pentachloronitrobenzene (40% dust powder; DP), myclobutanil (12.5% emulsifiable concentrates; EC), dimethachlone (40% wettable powder; WP), hymexazol (70% WP), thiram (50% WP), carvacrol (5% aqueous solutions; AS), and kasugamycin (2% AS). They were purchased from a local distributor and diluted to various concentrations for further experiments (Table 1). Different fungicides were dissolved in organic solvents or water (myclobutanil was dissolved in acetone, and all other fungicides were dissolved in water). For those fungicides dissolved in acetone, a comparable final concentration of the indicated solvents was added into the PDA medium, which served as control plates (CK). Different concentrations of fungicides were mixed with PDA medium, and 10 mL was dispensed into sterile 9 cm diameter petri dishes. Pathogenic mycelial plugs of representative strain C. aenigma BY827 (5 mm in diameter) were inoculated into the center of PDA plates containing varying concentrations of gradient agents, and each treatment was repeated three times. The plates were then cultured at 25 ± 2°C under dark conditions. After 7 days, the colony diameter of each treatment was measured. The following equation was used to determine the inhibition efficiency of the fungicides, and EC50 (concentration for 50% of maximal effect) values were calculated.
R: relative inhibition rates, C: diameter of the fungus in control, T: diameter of the fungus in the treatment, F: diameter of fungus plugs (Luo et al., 2021).
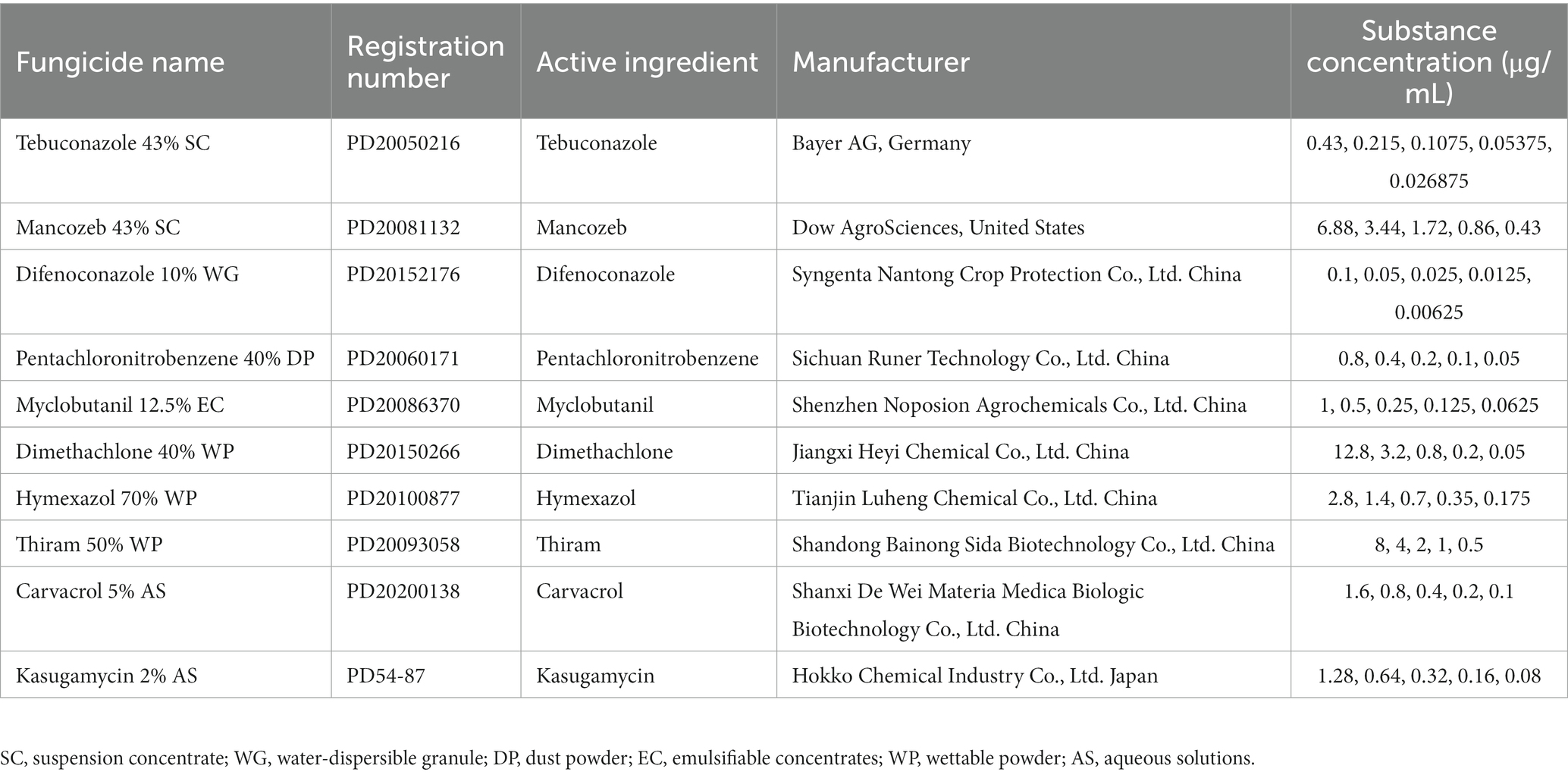
Table 1. Concentrations of substances used for fungicide sensitivity assays and their China pesticide registration numbers (http://www.chinapesticide.org.cn).
2.8. Field experiments
Four pesticides, namely difenoconazole, tebuconazole, pentachloronitrobenzene, and mancozeb, were selected to determine their effectiveness in controlling the leaf blight disease caused by C. aenigma in A. japonica. The experiments were carried out at the campus green space of Guizhou University, Guiyang, Guizhou, China, which was naturally infested with A. japonica leaf blight. The pesticides were formulated according to the recommended concentration for field use. To conduct the experiment tebuconazole (at a concentration of 2.87 μg/mL in sterile water containing 43% active pesticide ingredients), mancozeb (at a concentration of 10.75 μg/mL in sterile water containing 43% active pesticide ingredients), difenoconazole (at a concentration of 1.67 μg/mL in sterile water with 10% active pesticide ingredients), and pentachloronitrobenzene (at a concentration of 266.67 μg/mL in sterile water with 40% active pesticide ingredients) were used as the pesticide treatments, while sterile water was used as the control treatment. Each treatment was repeated three times. A pump sprayer was used to evenly spray the 5-year-old plants of A. japonica. The volume of sprayed liquid was 5 mL per plant. Leaf blight was investigated before and 14 days after spraying. A total of 100 plants were randomly selected in each treatment (6 m2). According to Fang (1998), the disease grading was based on the percentage of disease spots on leaves in the whole leaf area: Grade I, healthy, representative value was 0; Grade II, diseased area ≤ 25%, representative value was 1; Grade III, diseased area > 25 and ≤ 50%, representative value was 2; Grade IV, diseased area > 50 and ≤ 75%, representative value was 3; and Grade V, diseased area > 75%, representative value was 4. Meanwhile, the disease incidence, disease index, and control efficiency were calculated using the following formula (Henderson and Tilton, 1955; Sun and Song, 2002):
pt0: disease incidence in pesticide treatment plot before spraying, pt1: disease incidence in pesticide treatment plot after spraying.
CK0: disease index in control plot before spraying, CK1: disease index in control plot after spraying, PT0: disease index in pesticide treatment plot before spraying, PT1: disease index in pesticide treatment plot after spraying.
2.9. Statistical analysis
All percentage data were subjected to arcsine transformation and expressed as the mean ± SE (standard error of the mean). The statistical significance of the results was calculated using a one-way analysis of variance followed by Duncan’s new complex polar difference method at a significant level of p < 0.05 using IBM SPSS Statistics software version 25.0. The EC50 of different fungicides was calculated with a toxicity regression equation using the Data Processing System (DPS) v7.05 software (Pasche et al., 2004). Figures were created using GraphPad Prism (v8.0.2) software.
3. Results
3.1. Morphological characterization
A total of 31 Colletotrichum-like isolates were obtained from 20 diseased A. japonica leaves (Figure 1A). The mycelial growth rate ranged from 4.7 to 5.0 mm/day. Initially, the colonies appeared white, but as time progressed, the reverse side of the colonies gradually turned pale gray from the center. The colonies were velutinous to woolly and had a regular circular shape (Figures 1B,C). Five representative strains were selected from the obtained isolates for microscopic observation. Conidia were cylindrical, with one end slightly acute or broadly rounded, and ranged in size from 13.67 to 21.09 μm × 4.24 to 7.30 μm (n = 60; Figures 1D,E). Based on these morphological characteristics, the isolates matched the description of the genus Colletotrichum spp. (Weir et al., 2012).
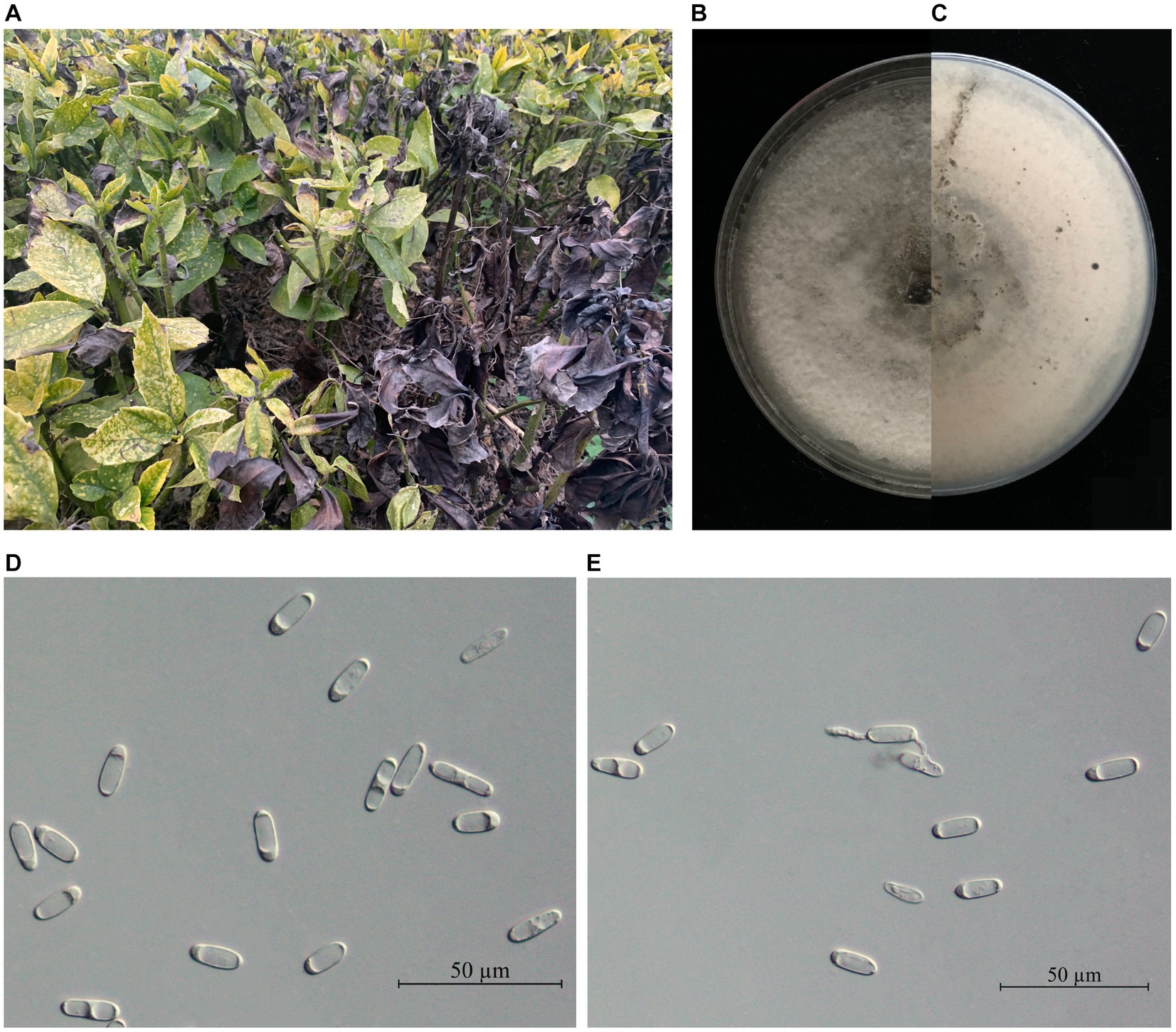
Figure 1. (A) Leaf blight disease of Aucuba japonica caused by Colletotrichum spp. (B) The front and (C) the reverse side of C. aenigma representative strain BY827 colonies were cultured on PDA for 7 days. (D,E) Conidia. Scale bars: D = 50 μm.
3.2. Molecular characterizations
Nucleotide sequences were submitted for BLAST analysis using the NCBI-BLAST program. BLAST searches of the sequenced fragments resulted in the best match to C. aenigma sequences (Table 2). The sequences of the DNA regions of strains BY825, BY826, BY827, BY828, and BY829 were deposited in GenBank and are included in the Supplementary Material (Table 2). In addition, a phylogenetic analysis of the 5 strains was conducted, and the phylogenetic tree was constructed based on the ITS region, CHS, and ACT genes sequences (Figure 2). The results confirmed that the strains BY825, BY826, BY827, BY828, and BY829 belong to the C. aenigma group when compared with sequences of the type strains.
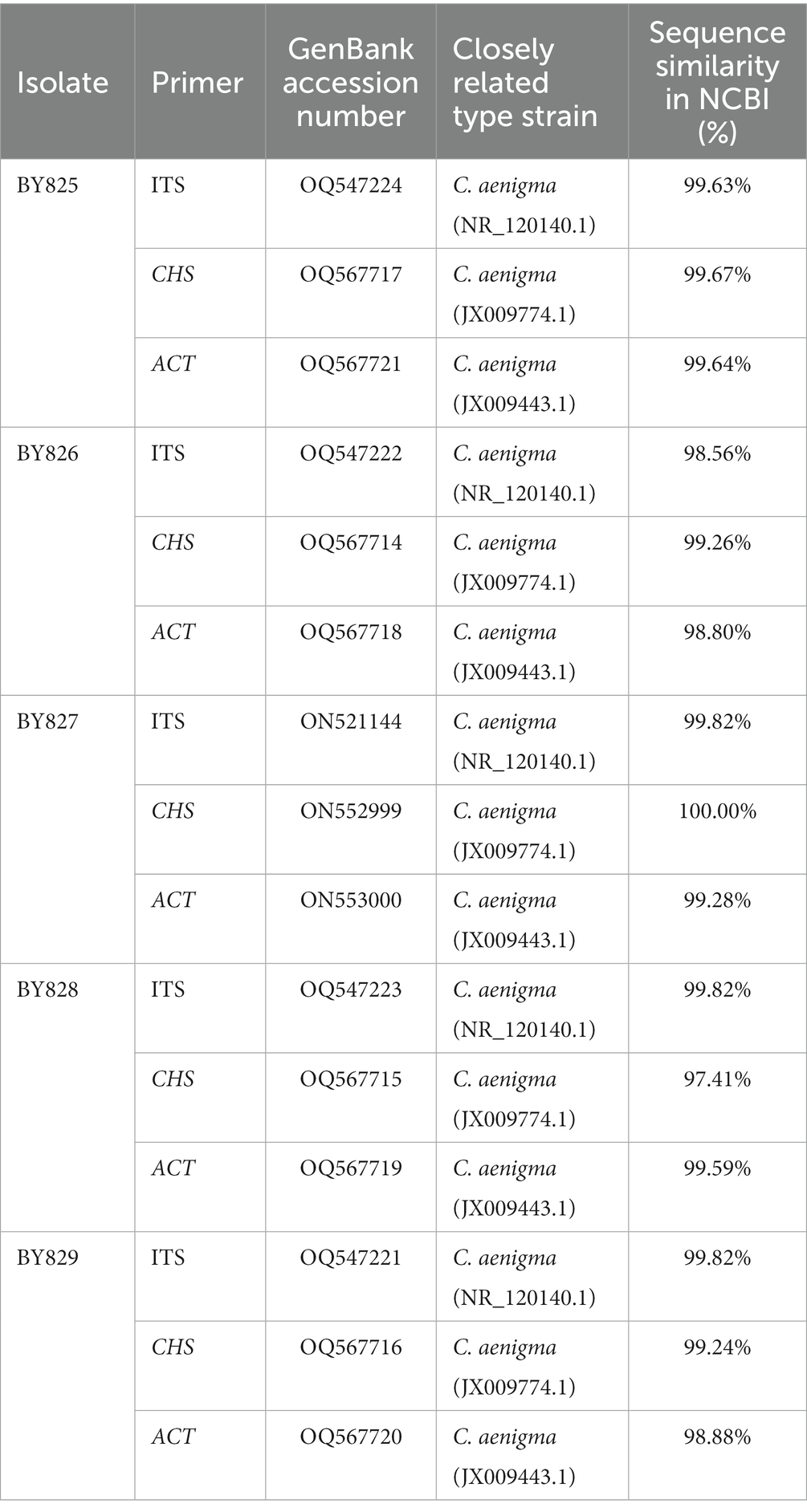
Table 2. Identification of isolates from Aucuba japonica leaves based on the comparison of ITS region, chitin synthase (CHS), and actin (ACT) sequences with the type strains in the database.
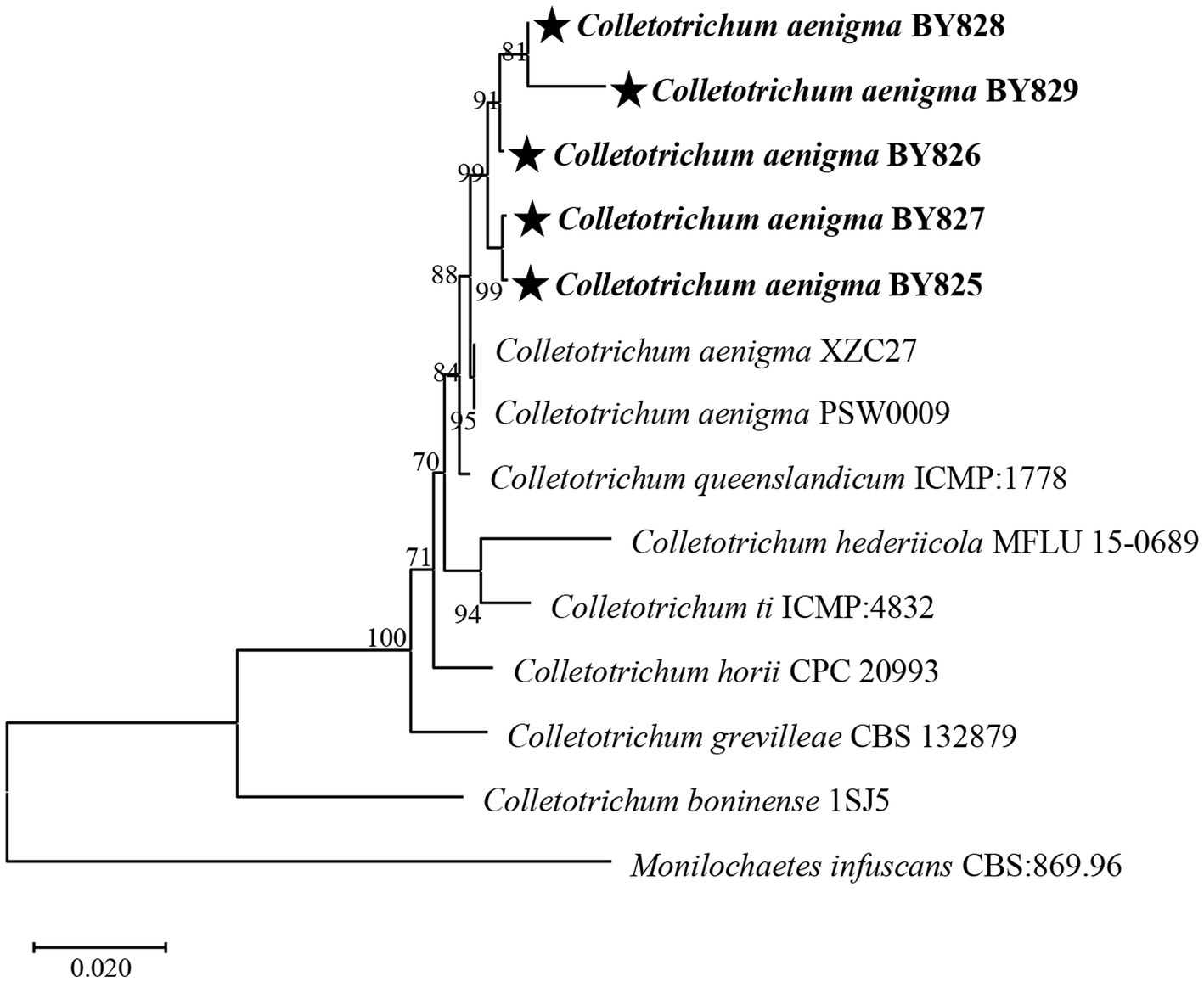
Figure 2. Neighbour-joining phylogenetic tree of concatenated sequences from the ITS region together with the chitin synthase (CHS), actin (ACT) genes of Colletotrichum aenigma BY825, C. aenigma BY826, C. aenigma BY827, C. aenigma BY828, and C. aenigma BY829 from this study and reference sequences of Colletotrichum spp. type materials. Monilochaetes infuscans CBS: 869.96 was used as the outgroup. Bootstrap values are provided next to the respective branches.
3.3. Pathogenicity test
Seven days after inoculation, typical disease symptoms (early stage of blight: black spots) were visible on all inoculated plants (Figure 3). In contrast, leaves in the control group did not show any disease symptoms. The pathogenicity test was repeated and confirmed three times. Pure cultures were re-isolated from diseased leaves and confirmed to be C. aenigma BY827 based on the morphological and molecular methods mentioned above (ITS region, CHS, and ACT sequences).
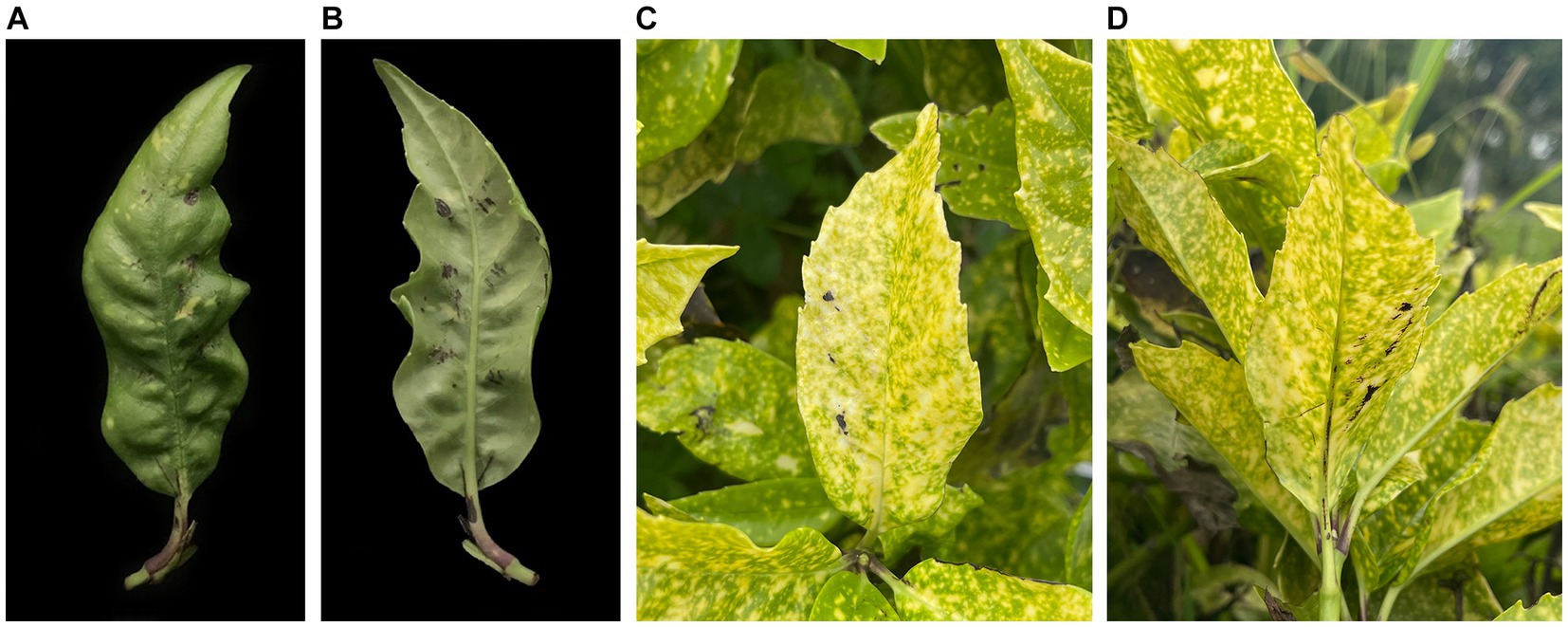
Figure 3. The leaf blight symptoms of Colletotrichum aenigma BY827 on Aucuba japonica detached leaves [(A) front side; (B) reverse side] and inoculated plants [(C) front side; (D) reverse side].
3.4. Effect of temperature
The experimental results indicated that the pathogen was capable of growing within a temperature range of 15–35°C. The highest mycelial growth rate of C. aenigma BY827 was observed at 25°C and 30°C (Figure 4A). However, neither mycelium nor conidia could grow at 10°C and 40°C. The number of conidia produced by the pathogen was significantly higher at 15°C and 35°C, as shown in Figure 4B. Furthermore, the results showed that the conidial germination rate at 15°C was significantly higher at 30°C (Figure 4C).
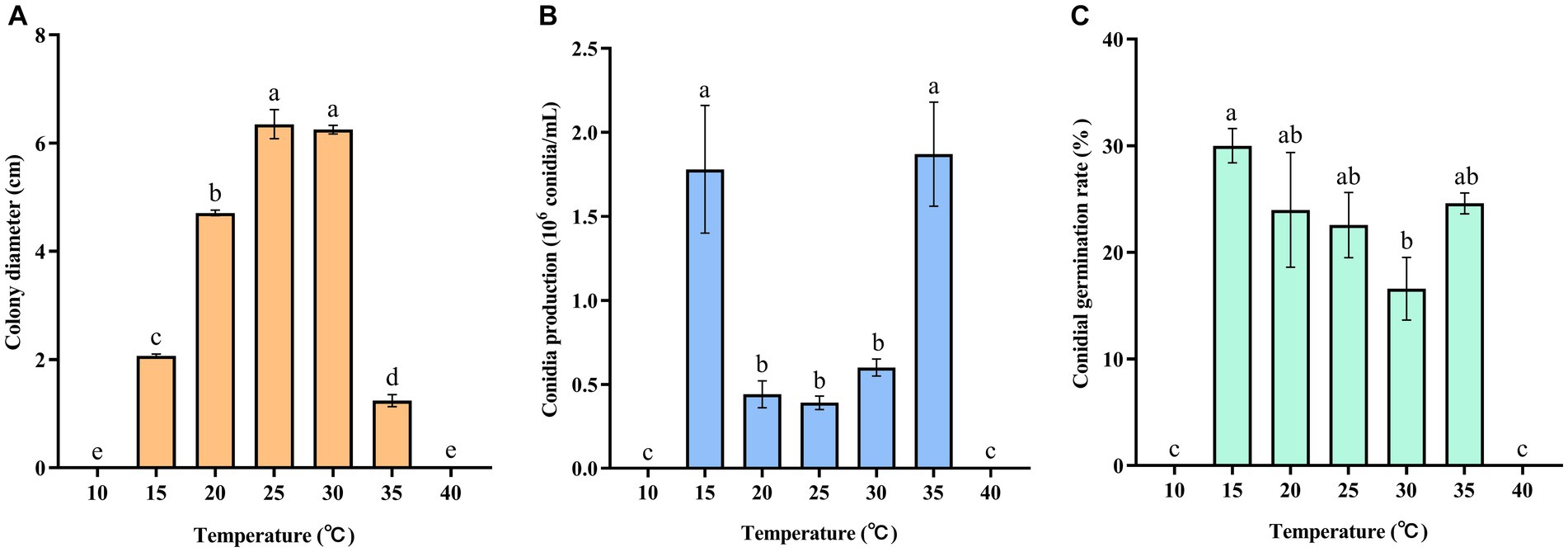
Figure 4. Effects of temperature on (A) mycelial growth, (B) conidia production, and (C) conidial germination rate of Colletotrichum aenigma BY827. Different lowercase letters indicate significant differences (p < 0.05). Data are mean ± SE.
3.5. Effect of photoperiod
The result indicated that 10-h/14-h alternating light increased the mycelial growth of C. aenigma BY827 (Figure 5A). There was no significant difference in conidia production under different photoperiods (Figure 5B). However, the alternation of 10 h light and 14 h dark, as well as 14 h light and 10 h dark, increased the conidial germination of C. aenigma BY827 (Figure 5C).
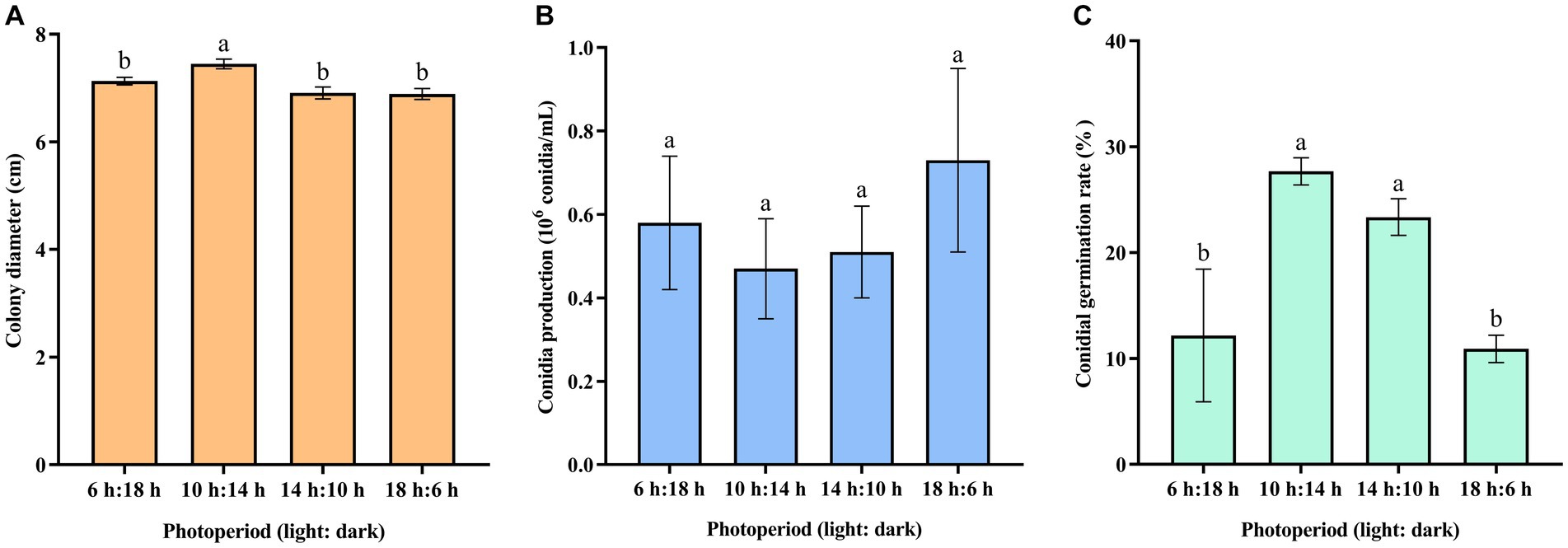
Figure 5. Effects of photoperiod on (A) mycelial growth, (B) conidia production, and (C) conidial germination rate of Colletotrichum aenigma BY827. Different lowercase letters indicate significant differences (p < 0.05). Data are mean ± SE.
3.6. Effect of humidity
The results showed that at 90% humidity, the conidial germination rate of C. aenigma BY827 was significantly higher than that of other humidifies (Figure 6).
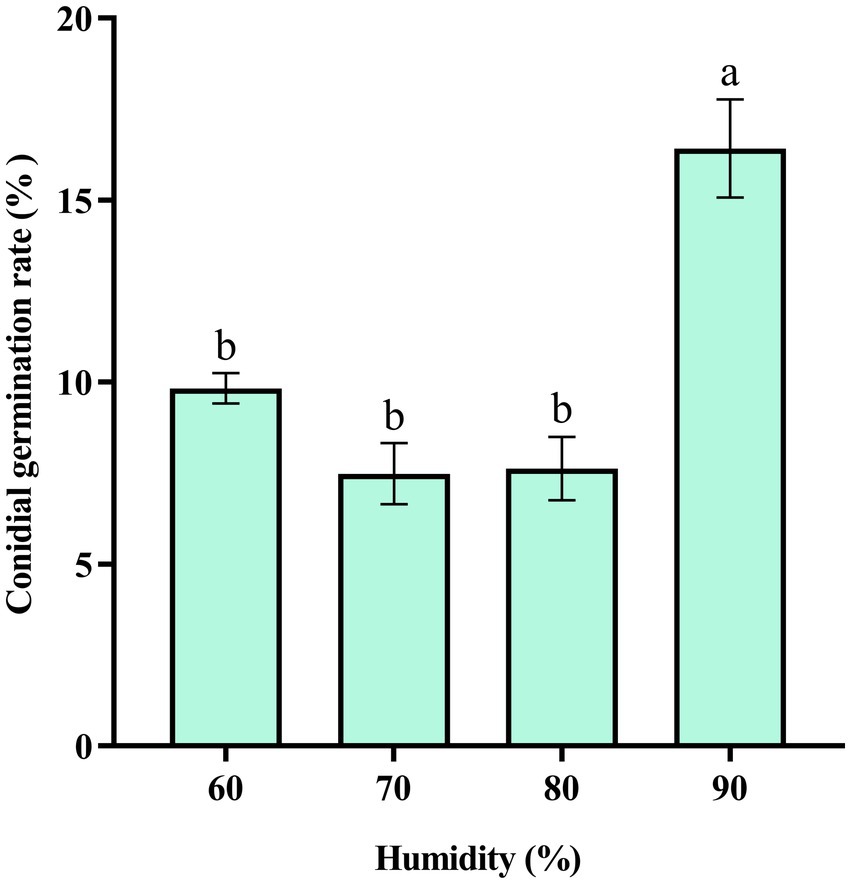
Figure 6. Effects of humidity on the conidial germination rate of Colletotrichum aenigma BY827. Different lowercase letters indicate significant differences (p < 0.05). Data are mean ± SE.
3.7. Fungicide assays
The sensitivities of the representative isolate C. aenigma BY827 to ten selected fungicides are presented in Table 3. Except for mancozeb, increasing concentrations of the fungicides inhibited the mycelial growth of C. aenigma BY827, as illustrated in Figure 7. Among the ten tested fungicides, the EC50 values of nine fungicides were less than 1 μg/mL. Difenoconazole showed the highest inhibition rate, with an EC50 value of 0.0148 μg/mL, followed by tebuconazole, pentachloronitrobenzene, myclobutanil, carvacrol, and kasugamycin, which also showed an effective inhibitory effect against C. aenigma BY827, with EC50 values of 0.0388 μg/mL, 0.1890 μg/mL, 0.1906 μg/mL, 0.2081 μg/mL, and 0.2996 μg/mL, respectively. In contrast, mancozeb did not show effective inhibitory effects against C. aenigma BY827, with EC50 values of 202.1461 μg/mL.
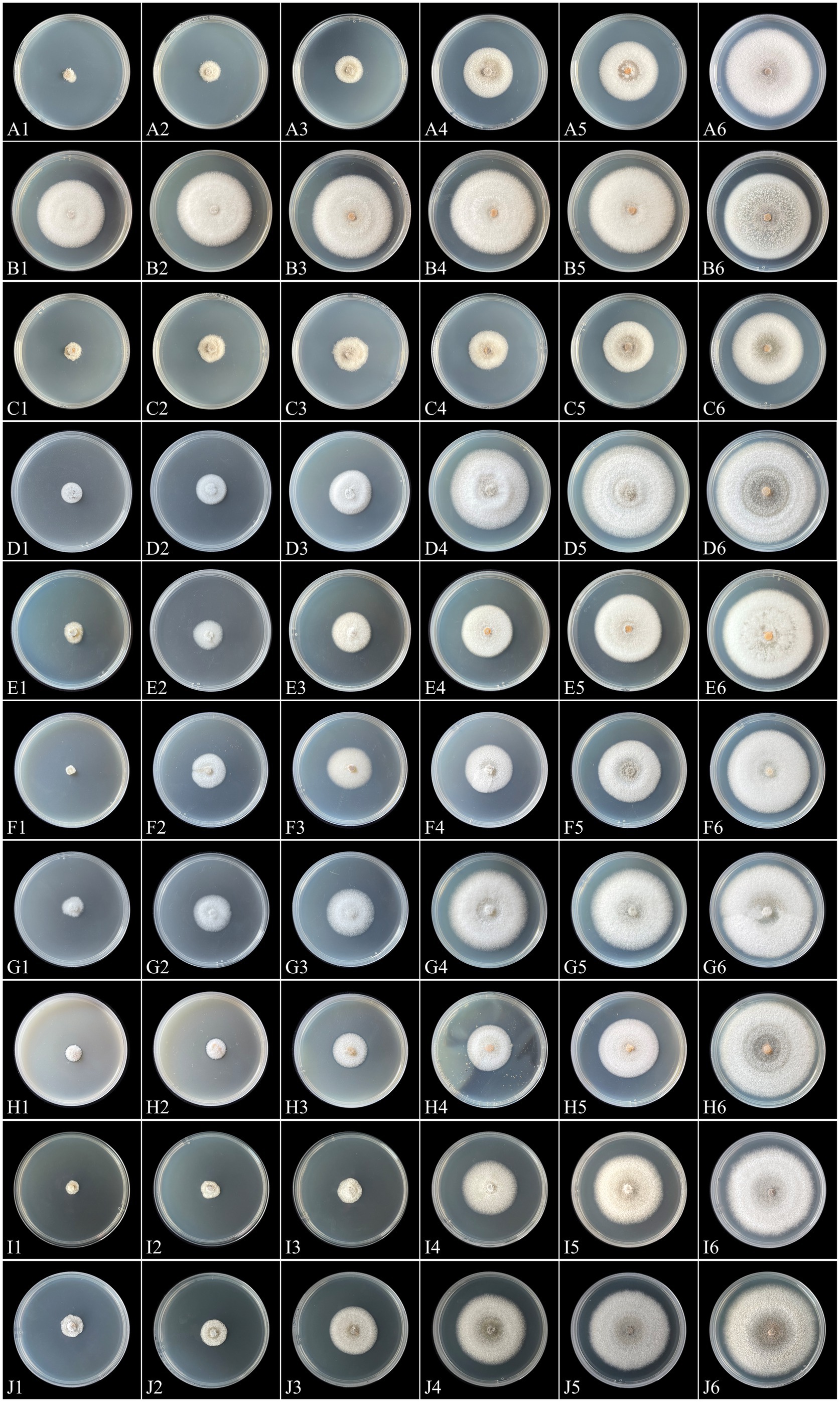
Figure 7. Mycelial growth of Colletotrichum aenigma BY827 on PDA plates incubated for 7 days in the absence (CK) or presence of different concentrations of tebuconazole (A1: 0.43 μg/mL, A2: 0.215 μg/mL, A3: 0.1075 μg/mL, A4: 0.05375 μg/mL, A5: 0.026875 μg/mL, A6: CK), mancozeb (B1: 6.88 μg/mL, B2: 3.44 μg/mL, B3: 1.72 μg/mL, B4: 0.86 μg/mL, B5: 0.43 μg/mL, B6: CK), difenoconazole (C1: 0.1 μg/mL, C2: 0.05 μg/mL, C3: 0.025 μg/mL, C4: 0.0125 μg/mL, C5: 0.00625 μg/mL, C6: CK), pentachloronitrobenzene (D1: 0.8 μg/mL, D2: 0.4 μg/mL, D3: 0.2 μg/mL, D4: 0.1 μg/mL, D5: 0.05 μg/mL, D6: CK), myclobutanil (E1: 1 μg/mL, E2: 0.5 μg/mL, E3: 0.25 μg/mL, E4: 0.125 μg/mL, E5: 0.0625 μg/mL, E6: CK), dimethachlone (F1: 12.8 μg/mL, F2: 3.2 μg/mL, F3: 0.8 μg/mL, F4: 0.2 μg/mL, F5: 0.05 μg/mL, F6: CK), hymexazol (G1: 2.8 μg/mL, G2: 1.4 μg/mL, G3: 0.7 μg/mL, G4: 0.35 μg/mL, G5: 0.175 μg/mL, G6: CK), thiram (H1: 8 μg/mL, H2: 4 μg/mL, H3: 2 μg/mL, H4: 1 μg/mL, H5: 0.5 μg/mL, H6: CK), carvacrol (I1: 1.6 μg/mL, I2: 0.8 μg/mL, I3: 0.4 μg/mL, I4: 0.2 μg/mL, I5: 0.1 μg/mL, I6: CK), and kasugamycin (J1: 1.28 μg/mL, J2: 0.64 μg/mL, J3: 0.32 μg/mL, J4: 0.16 μg/mL, J5: 0.08 μg/mL, J6: CK).
3.8. Field experiments
The results of the field experiment were presented in Table 4. Difenoconazole exhibited the highest control efficiency with a decreasing rate of disease incidence of 44.60% and decreasing rate of disease index of 47.75%, respectively, which were significantly higher than other treatments. Tebuconazole and pentachloronitrobenzene showed no differences in disease incidence and disease index. Moreover, mancozeb exhibited the lowest control efficiency with decreasing rate of disease incidence of 3.53% and decreasing rate of disease index of 3.77%, respectively, which were significantly lower than other treatments.
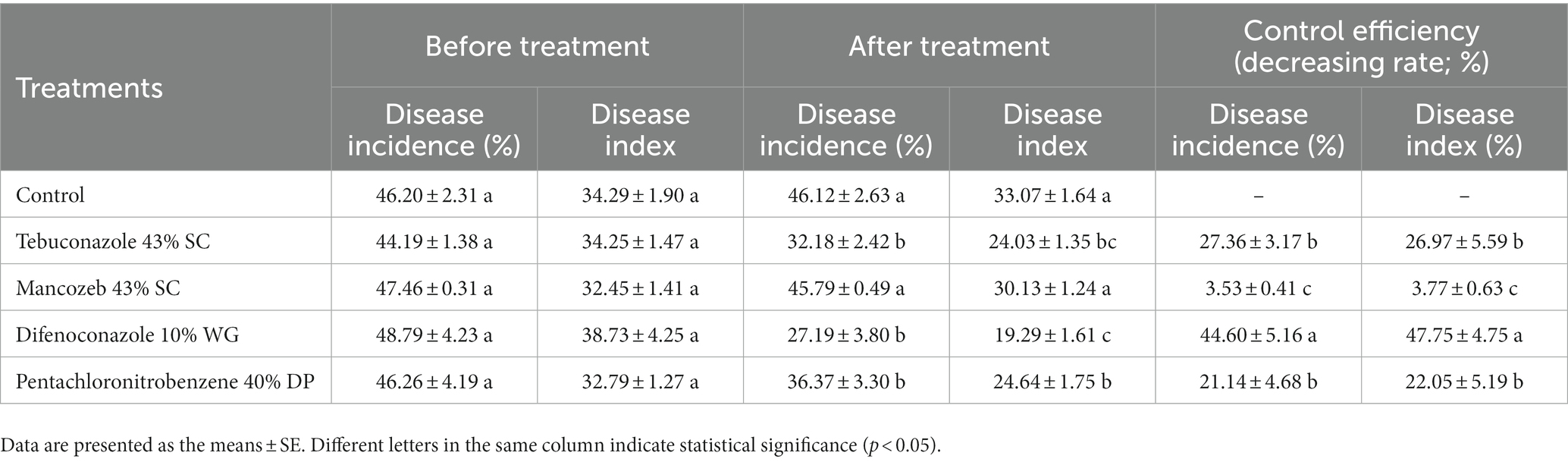
Table 4. Control effect of four fungicides on the leaf blight of Aucuba japonica in field experiments.
4. Discussion
Globally, Colletotrichum spp. are well-known for their high pathogenicity in causing anthracnose, a serious leaf blight disease that results in significant yield loss or quality reduction in various plants, including agricultural crops, fruit trees, and ornamental shrubs, across different ecosystems (Sharma et al., 2014). Previously, C. boninense was confirmed as the causative agent of anthracnose in A. japonica (Liu et al., 2022). In our study conducted in Guiyang, Guizhou, China, we isolated and identified C. aenigma as the causal agent of leaf blight disease in A. japonica. To our knowledge, this is the first report of C. aenigma causing leaf blight on A. japonica worldwide. This identification may help decision-makers to establish targeted control measures against the disease.
Understanding the biological characteristics of phytopathogens is crucial for the efficient control of related plant diseases. The results of the biological characteristics study showed that the optimum temperature for C. aenigma BY827 mycelial growth was 25–30°C. This was similar to other pathogenic Colletotrichum spp., such as C. truncatum and C. camelliae, optimum mycelial growth temperatures ranged from 25.0–29.3°C (Lu et al., 2018; Júnior et al., 2021). In addition, C. aenigma BY827 could not grow above 40°C condition, which is consistent with C. asianum T0408, another pathogenic strain caused mango anthracnose (Sun et al., 2016). The mycelial growth and conidial germination rate of C. aenigma BY827 were higher under the photoperiod of 10 h light and 14 h dark, which aligns with the local photoperiod conditions of Guiyang City, Guizhou Province, China (The climate data were compiled from the China Meteorological Data Network).2 Moreover, the conidial germination rate of C. aenigma BY827 increased when the relative humidity reached 90%, which is consistent with C. gloeosporioides I-2, also the causal agent of anthracnose disease on mango (Estrada et al., 2000).
Currently, chemical control is the most common and widely applied strategy for controlling plant diseases caused by Colletotrichum spp. (Yin et al., 2018). However, the long-term and extensive use of chemical fungicides resulted in various threats, including concerns about food safety, environmental pollution, and adverse effects on human health (Chen et al., 2021). Moreover, the development of resistance by pathogenic fungi is one of the main reasons for the resurgence of diseases, and residual issues of pesticides (Fair and Tor, 2014; Duan et al., 2020; Black et al., 2022; Tang et al., 2022). Therefore, it is necessary to screen effective pesticides for managing newly emerging diseases, such as leaf blight of A. japonica caused by C. aenigma. In our study, we conducted in vitro screening of ten different pesticides (eight chemical fungicides, and two biopesticides widely used to control pathogens like Colletotrichum spp.), and performed field trials of four fungicides (selected based on their effectiveness in the in vitro screening) against C. aenigma (Morsy and Elshahawy, 2016; Zhang et al., 2017; Fan et al., 2022). The results consistently showed that three pesticides, tebuconazole, difenoconazole, and pentachloronitrobenzene, significantly suppressed C. aenigma BY827 both in vitro and in vivo.
Furthermore, difenoconazole exhibited the highest inhibition rate, with an EC50 value of 0.0148 μg/ml, followed by tebuconazole and pentachloronitrobenzene. Similar results were observed for difenoconazole when used against its target pathogen Aspergillus fumigatus (Schoustra et al., 2019). Moreover, difenoconazole exhibited the highest control efficiency (47.75%), indicating potent antifungal activity against Colletotrichum spp. (Zhang et al., 2020; Peng et al., 2022). In contrast, mancozeb showed no potent inhibitory activity against C. aenigma BY827, with EC50 values of 202.1461 μg/mL. Mancozeb is a broad-spectrum fungicide that normally exhibits good inhibitory effects against fungi pathogens. For example, it significantly suppressed the tuber blight pathogen Pyricularia oryzae under both laboratory and field conditions (Kongcharoen et al., 2020). Until now, very few pathogens have been reported to be resistant to mancozeb (Tang et al., 2022). Torres-Calzada et al. (2015) reported that there was no mancozeb resistance in Colletotrichum truncatum isolates in Mexico. However, our study found that C. aenigma BY827 may have developed resistance to mancozeb, which was confirmed in field experiments. Our research indicated that mancozeb exhibited the lowest control efficiency (the decreasing rate of disease incidence and decreasing rate of disease index were 3.53 and 3.77%, respectively) and was significantly less effective than other fungicides. Furthermore, the intensive or overuse of mancozeb in such circumstances could increase the risks of environmental issues, especially in sensitive ecosystems like the karst landscapes.
In summary, our study provided new insights into the occurrence of A. japonica leaf blight disease in Guiyang, Guizhou, China, and identified C. aenigma as the new causal agent. Moreover, we evaluated the influences of crucial environmental factors for ornamental plant production, including temperature, photoperiod, as well as humidity, on the biological characteristics of representative strain C. aenigma BY827. Interestingly, we found that the biological characteristics of C. aenigma BY827 showed good adaptions to the local environmental conditions of Guiyang City. These could be taken into consideration for its future management. Furthermore, the results of in vitro fungicide screening assays and field experiments provided effective fungicide candidates, including difenoconazole, pentachloronitrobenzene, and tebuconazole for the control of A. japonica leaf blight caused by C. aenigma. Further studies could include the assessing of practical control efficiencies of these fungicides against C. aenigma on A. japonica plants in large-scale and in different application scenarios. In addition, monitoring mancozeb resistance among C. aenigma isolates, as well as possible cross-resistance between other pathogen populations, would provide valuable information on the evolution of fungicide resistance in unique ecosystems like karst landscapes in Guizhou, China. As a whole, the main findings from our study could contribute to developing future sustainable and effective management strategies to control A. japonica leaf blight disease caused by C. aenigma, especially in Guiyang, Guizhou, China.
Data availability statement
The datasets presented in this study can be found in online repositories. The names of the repository/repositories and accession number(s) can be found in the article/supplementary material.
Author contributions
XC and XH designed the experiments. RF, YjL, YB, JH, BY, XT, YxL, YC, ZY, MY, JS, and QP performed the experiments. RF, YjL, YB, MIG, and XC drafted the manuscript. RF, YB, YjL, and XH analyzed data. RF, XH, ZL, MIG, and XC conducted visualization and proofreading of the manuscript.
Funding
This study was supported by National Key Research and Development Program of China (2021YFE0107700), Science and Technology Base and Talent Project of Guangxi Province (Guike AA21196003), Guizhou Provincial Science and Technology Program (2019-1410; HZJD[2022]001; 2021-229), Outstanding Young Scientist Program of Guizhou Province (KY2021-026), Guizhou University Cultivation Project (2019-04; 2022-085; SYSKF2023-093), and Program for Introducing Talents to Chinese Universities (111 Program; D20023).
Acknowledgments
The authors gratefully acknowledge Shiweini Chen, Aiping Zhan, and Xi Wei for laboratory assistant work.
Conflict of interest
The authors declare that the research was conducted in the absence of any commercial or financial relationships that could be construed as a potential conflict of interest.
Publisher’s note
All claims expressed in this article are solely those of the authors and do not necessarily represent those of their affiliated organizations, or those of the publisher, the editors and the reviewers. Any product that may be evaluated in this article, or claim that may be made by its manufacturer, is not guaranteed or endorsed by the publisher.
Footnotes
References
Abe, T. (2002). Flower bud abortion influences clonal growth and sexual dimorphism in the understorey dioecious shrub Aucuba japonica (Cornaceae). Ann. Bot. 89, 675–681. doi: 10.1093/aob/mcf111
Abrol, D. P., and Singh, J. B. (2003). Relative efficacy of some insecticides against brinjal fruit and shoot borer, Leucinodes orbonalis Guen., and their impact on fruit yield. J. Asia Pac. Entomol. 6, 83–90. doi: 10.1016/S1226-8615(08)60172-7
Ali, M. S., and Kikuzawa, K. (2005). Shoot morphology of Aucuba japonica incurred by anisophylly: ecological implications. J. Plant Res. 118, 329–338. doi: 10.1007/s10265-005-0230-6
Bajwa, R., Mukhtar, I., and Mushtaq, S. (2010). New report of Alternaria alternata causing leaf spot of Aloe vera in Pakistan. Can. J. Plant Pathol. 32, 490–492. doi: 10.1080/07060661.2010.510644
Black, E., Weber, J., and Theelen, M. (2022). Biocide resistance in Acinetobacter baumannii: appraising the mechanisms. J. Hosp. Infect. 119:79. doi: 10.1016/j.jhin.2021.09.019
Cao, W. P., Zhen, W., Chen, D., Feng, S., and Song, J. (2022). Influence of plant growth regulators on sporulation and conidia characteristics of the entomopathogenic fungus Beauveria bassiana. Chin J Biolog Control. 38, 555–564. doi: 10.16409/j.cnki.2095-039x.2021.05.022
Carbone, I., and Kohn, L. M. (1999). A method for designing primer sets for speciation studies in filamentous ascomycetes. Mycologia. 91, 553–556. doi: 10.1080/00275514.1999.12061051
Carson, K. M. H., Carroll, E., Ibiyemi, O., William, B. B., Thurmond, A., Vinson, A., et al. (2021). Efficacy of foliar sprays of various products against false oleander scale on potted Aucuba japonica, 2019. Arthropod Manage. Tests. 46, 1–2. doi: 10.1093/amt/tsab123
Chen, X., Wicaksono, W. A., Berg, G., and Cernava, T. (2021). Bacterial communities in the plant phyllosphere harbour distinct responders to a broad-spectrum pesticide. Sci. Total Environ. 751:141799. doi: 10.1016/j.scitotenv.2020.141799
Damm, U., Cannon, P. F., Woudenberg, J. H., and Crous, P. W. (2012). The Colletotrichum acutatum species complex. Stud. Mycol. 73, 37–113. doi: 10.3114/sim0010
Deng, Y. Z., Qu, Z., He, Y., and Naqvi, N. I. (2012). Sorting nexin Snx41 is essential for conidiation and mediates glutathione-based antioxidant defense during invasive growth in Magnaporthe oryzae. Autophagy. 8, 1058–1070. doi: 10.4161/auto.20217
Duan, Y., Lu, F., Zhou, Z., Zhao, H., Zhang, J., Mao, Y., et al. (2020). Quinone outside inhibitors affect DON biosynthesis, mitochondrial structure and toxisome formation in fusarium graminearum. J. Hazard. Mater. 398:122908. doi: 10.1016/j.jhazmat.2020.122908
Estrada, A. B., Dodd, J. C., and Jeffries, P. (2000). Effect of humidity and temperature on conidial germination and appressorium development of two Philippine isolates of the mango anthracnose pathogen Colletotrichum gloeosporioides. Plant Pathol. 49, 608–618. doi: 10.1046/j.1365-3059.2000.00492.x
Fair, R. J., and Tor, Y. (2014). Antibiotics and bacterial resistance in the 21st century. Perspect. Med. Chem. 11, 25–64. doi: 10.4137/PMC.S14459
Fan, G., Shen, T., Jia, K., Xiao, X., Wu, Z., Gong, F., et al. (2022). Pentachloronitrobenzene reduces the proliferative capacity of zebrafish embryonic cardiomyocytes via oxidative stress. Toxics. 10:299. doi: 10.3390/toxics10060299
Fan, K., Wang, J., Fu, L., Li, X. J., Zhang, Y., Zhang, X. D., et al. (2016). Sensitivity of Botryosphaeria dothidea from apple to tebuconazole in China. Crop Prot. 87, 1–5. doi: 10.1016/j.cropro.2016.04.018
Fu, M., Crous, P. W., Bai, Q., Zhang, P. F., Xiang, J., Guo, Y. S., et al. (2019). Colletotrichum species associated with anthracnose of Pyrus spp. China. Persoonia. 42, 1–35. doi: 10.3767/persoonia.2019.42.01
Guarnaccia, V., Groenewald, J. Z., Polizzi, G., and Crous, P. W. (2017). High species diversity in Colletotrichum associated with citrus diseases in Europe. Persoonia. 39, 32–50. doi: 10.3767/persoonia.2017.39.02
Henderson, C. F., and Tilton, E. W. (1955). Tests with acaricides against the brown wheat mite. J. Econ. Entomol. 48, 157–161. doi: 10.1093/jee/48.2.157
Homdork, S., Fehrmann, H., and Beck, R. (2000). Effects of field application of tebuconazole on yield, yield components and the mycotoxin content of fusarium-infected wheat grain. J. Phytopathol. 148, 1–6. doi: 10.1111/j.1439-0434.2000.tb04617.x
Hu, J., Chang, R., Yuan, Y., Li, Z., and Wang, Y. (2022). Identification of key residues essential for the activation of plant immunity by subtilisin from Bacillus velezensis LJ02. Front. Microbiol. 13:869596. doi: 10.3389/fmicb.2022.869596
Huang, Y., Fan, L., Huang, J., Zhou, G., Chen, X., and Chen, J. (2022). Plastome phylogenomics of aucuba (Garryaceae). Front. Genet. 13:753719. doi: 10.3389/fgene.2022.753719
Júnior, M. B. D., Resende, M. L. V., Pozza, E. A., Machado, J. C., Resende, A. R. M., Cardoso, A. M. S., et al. (2021). Effect of temperature on Colletotrichum truncatum growth, and evaluation of its inoculum potential in soybean seed germination. Eur. J. Plant Pathol. 160, 1–6. doi: 10.1007/s10658-021-02293-w
Kim, Y. M., Sim, U. C., Shin, Y., and Kwon, Y. K. (2014). Aucubin promotes neurite outgrowth in neural stem cells and axonal regeneration in sciatic nerves. Experiment Neurobiol. 23, 238–245. doi: 10.5607/en.2014.23.3.238
Kimura, T., But, P. P. H., Guo, J. X., and Sung, C. K. (1997). International collation of traditional and folk medicine: Northeast Asia-part 1. World Sci. 2:238. doi: 10.1142/3041
Kongcharoen, N., Kaewsalong, N., and Dethoup, T. (2020). Efficacy of fungicides in controlling rice blast and dirty panicle diseases in Thailand. Sci. Rep. 10:16233. doi: 10.1038/s41598-020-73222-w
Lee, S. Y., Ten, L. N., Ryu, J. J., Kang, I. K., and Jung, H. Y. (2021). Colletotrichum aenigma associated with apple bitter rot on newly bred cv. RubyS apple. Res Plant Dis. 27, 70–75. doi: 10.5423/rpd.2021.27.2.70
Liu, Q., Wang, R., Song, Q., Wang, J., Shi, Y., Ding, W., et al. (2022). First report of Colletotrichum boninense causing anthracnose on Aucuba japonica in Guizhou Province of China. Plant Dis. 106:2755. doi: 10.1094/PDIS-12-21-2629-PDN
Lu, Q., Wang, Y., Li, N., Ni, D., Yang, Y., and Wang, X. (2018). Differences in the characteristics and pathogenicity of Colletotrichum camelliae and C. fructicola isolated from the tea plant [Camellia sinensis (L.) O. Kuntze]. Front. Microbiol. 9:3060. doi: 10.3389/fmicb.2018.03060
Luo, M., Chen, Y. M., He, J. L., Tang, X., Wu, X. D., and Xu, C. G. (2021). Identification of a new Talaromyces strain DYM25 isolated from the yap trench as a biocontrol agent against fusarium wilt of cucumber. Microbiol. Res. 251:126841. doi: 10.1016/j.micres.2021.126841
Moral, J., Agustí-Brisach, C., Raya, M. C., Jurado-Bello, J., López-Moral, A., Roca, L. F., et al. (2021). Diversity of Colletotrichum species associated with olive anthracnose worldwide. J Fungi (Basel, Switzerland). 7:741. doi: 10.3390/jof7090741
Morsy, A. A., and Elshahawy, I. E. (2016). Anthracnose of lucky bamboo Dracaena sanderiana caused by the fungus Colletotrichum dracaenophilum in Egypt. J. Adv. Res. 7, 327–335. doi: 10.1016/j.jare.2016.01.002
Ohi, T., Kajita, T., and Murata, J. (2003). Distinct geographic structure as evidenced by chloroplast DNA haplotypes and ploidy level in Japanese Aucuba (Aucubaceae). Am. J. Bot. 90, 1645–1652. doi: 10.3732/ajb.90.11.1645
Pasche, J. S., Wharam, C. M., and Gudmestad, N. C. (2004). Shift in sensitivity of Alternaria solani in response to QoI fungicides. Plant Dis. 88, 181–187. doi: 10.1094/PDIS.2004.88.2.181
Peng, K., Pan, Y., Tan, T., Zeng, X., Lin, M., Jiang, S., et al. (2022). Characterization and fungicide sensitivity of Colletotrichum godetiae causing sweet cherry fruit anthracnose in Guizhou, China. Front Microbiol. 13:923181. doi: 10.3389/fmicb.2022.923181
Ram, P. C., Singh, A. K., Singh, B. B., Singh, V. K., Singh, H. P., Setter, T. L., et al. (1999). Environmental characterization of floodwater in eastern India: relevance to submergence tolerance of lowland rice. Exp. Agric. 35, 141–152. doi: 10.1017/S0014479799002057
Robertson, P. S. I. (2010). On the flowering and fruiting of Aucuba japonica. Plant Ecol. Divers. 10, 386–387. doi: 10.1080/03746607009468727
Romero, F., Cazzato, S., Walder, F., Vogelgsang, S., Bender, S. F., and van der Heijden, M. G. A. (2022). Humidity and high temperature are important for predicting fungal disease outbreaks worldwide. New Phytol. 234, 1553–1556. doi: 10.1111/nph.17340
Schmitt-Jansen, M., and Altenburger, R. (2005). Toxic effects of isoproturon on periphyton communities-a microcosm study. Estuar. Coast. Shelf Sci. 62, 539–545. doi: 10.1016/j.ecss.2004.09.016
Schoustra, S. E., Debets, A. J. M., Rijs, A. J. M. M., Zhang, J., Snelders, E., Leendertse, P. C., et al. (2019). Environmental hotspots for azole resistance selection of Aspergillus fumigatus, the Netherlands. Emerg. Infect. Dis. 25, 1347–1353. doi: 10.3201/eid2507.181625
Sharma, K., Merrit, J. L., Palmateer, A., Goss, E., Smith, M., and Schubert, T. (2014). Isolation, characterization, and management of Colletotrichum spp. causing anthracnose on lucky bamboo (Dracaena sanderiana). Hort Scienc. 9, 453–459. doi: 10.21273/HORTSCI.49.4.453
Smith, K. M., Loh, E. H., Rostal, M. K., Zambrana-Torrelio, C. M., Mendiola, L., and Peter, D. (2013). Pathogens, pests, and economics: drivers of honey bee colony declines and losses. Eco Health. 10, 434–445. doi: 10.1007/s10393-013-0870-2
Song, L. K., Wei, Y., Jiang, S. Y., Zhao, M. X., and Wang, L. P. (2007). Research on aucuba omeinensis Fang the peculiar plant in Sichuan. Special wild economic animal and plant. Research 3:19-20+23. doi: 10.16720/j.cnki.tcyj.2007.03.003
Sun, R. H., Lu, G. L., Wang, F., Sun, X. T., Gao, L., Li, Y. Z., et al. (2022). First report of southern blight caused by Athelia rolfsii on spotted laurel (Aucuba japonica) in China. Plant Dis. 107:1949. doi: 10.1094/PDIS-09-22-2138-PDN
Sun, G. Y., and Song, Z. F. (2002). Experimental Techniques of Plant Pathology. Beijing: China Agriculture Press.
Sun, S., Tan, L. L., Pang, X. J., Wu, W. Q., Huang, D. Y., and Huang, X. L. (2016). Identification and biological characteristics of Colletotrichum asianum from post-harvest mango (Mangifera indica L.). Chin J Trop Crops. 37, 2392–2397. doi: 10.3969/j.issn.1000-2561.2016.12.023
Tamura, K., Stecher, G., Peterson, D., Filipski, A., and Kumar, S. (2013). MEGA6: molecular evolutionary genetics analysis version 6.0. Mol. Biol. Evol. 30, 2725–2729. doi: 10.1093/molbev/mst197
Tang, X., Yangjing, G., Zhuoma, G., Guo, X., Cao, P., Yi, B., et al. (2022). Biological characterization and in vitro fungicide screenings of a new causal agent of wheat fusarium head blight in Tibet, China. Front. Microbiol. 13:941734. doi: 10.3389/fmicb.2022.941734
Than, P. P., Prihastuti, H., Phoulivong, S., Taylor, P. W., and Hyde, K. D. (2008). Chilli anthracnose disease caused by Colletotrichum species. J. Zhejiang Univ. Sci. B. 9, 764–778. doi: 10.1631/jzus.B0860007
Torres-Calzada, C., Tapia-Tussell, R., Higuera-Ciapara, I., Martin-Mex, R., Nexticapan-Garcez, A., and Perez-Brito, D. (2015). Sensitivity of Colletotrichum truncatum to four fungicides and characterization of thiabendazole-resistant isolates. Plant Dis. 99, 1590–1595. doi: 10.1094/PDIS-11-14-1183-RE
Uke, A., Pinili, M. S., Natsuaki, K. T., and Geering, A. D. W. (2021). Complete genome sequence of aucuba ringspot virus. Arch. Virol. 166, 1227–1230. doi: 10.1007/s00705-021-04977-4
Wang, X., Peng, F., Cheng, C., Chen, L., Shi, X., Gao, X., et al. (2021). Synergistic antifungal activity of graphene oxide and fungicides against fusarium head blight in vitro and in vitro. Nanomaterials (Basel). 11:2393. doi: 10.3390/nano11092393
Wang, S. N., Xie, G. P., Qin, C. H., Chen, Y. R., Zhang, K. R., Li, X., et al. (2015). Aucubin prevents interleukin-1 beta induced inflammation and cartilage matrix degradation via inhibition of NF-κB signaling pathway in rat articular chondrocytes. Int. Immunopharmacol. 24, 408–415. doi: 10.1016/j.intimp.2014.12.029
WCSP. (2019). World Checklist of Selected Plant Families. Facilitated by the Royal Botanic Gardens, Kew. Published on the Internet; Available at: http://wcsp.science.kew.org/ (Accessed February 15, 2023).
Weir, B. S., Johnston, P. R., and Damm, U. (2012). The Colletotrichum gloeosporioides species complex. Stud. Mycol. 73, 115–180. doi: 10.3114/sim0011
White, T. J., Bruns, T., Lee, S. J. W. T., and Taylor, J. (1990). “Amplification and direct sequencing of fungal ribosomal RNA genes for phylogenetics.” In PCR Protocols: A Guide to Methods and Applications. eds. M. A. lnnis, D. H. Gelfand, J. Sninsky, and T. J. White. New York. 315–322. doi: 10.1016/B978-0-12-372180-8.50042-1
Williams, P., Whitfield, M., Biggs, J., Bray, S., Fox, G., and Nicolet, P. (2004). Comparative biodiversity of rivers, streams, ditches and ponds in an agricultural landscape in southern England. Biol. Conserv. 115, 329–341. doi: 10.1016/S0006-3207(03)00153-8
Wilson, C., and Tisdell, C. (2001). Why farmers continue to use pesticides despite environmental, health and sustainability costs. Ecol. Econ. 39, 449–462. doi: 10.1016/S0921-8009(01)00238-5
Xiang, Q. Y., and Boufford, D. E. (2005). Aucuba. Flora of China, 2nd. Beijing and St. Louis: Science Press, 222–226.
Xin, W., Mao, Y., Lu, F., Li, T., Wang, J., Duan, Y., et al. (2020). In vitro fungicidal activity and in planta control efficacy of coumoxystrobin against Magnaporthe oryzae. Pestic. Biochem. Physiol. 162, 78–85. doi: 10.1016/j.pestbp.2019.09.004
Xue, H. Y., Jin, L., Jin, L. J., Li, X. Y., and Zhang, P. (2009). Aucubin prevents loss of hippocampal neurons and regulates antioxidative activity in diabetic encephalopathy rats. Phytother. Res. 23, 980–986. doi: 10.1002/ptr.2734
Yan, J. Y., Jayawardena, M. M. R. S., Goonasekara, I. D., Wang, Y., Zheng, W., Liu, M., et al. (2015). Diverse species of Colletotrichum associated with grapevine anthracnose in China. Fungal Divers. 71, 233–246. doi: 10.1007/s13225-014-0310-9
Yang, Z., Wu, Q. Q., Xiao, Y., Duan, M. X., Liu, C., Yuan, Y., et al. (2018). Aucubin protects against myocardial infarction-induced cardiac remodeling via nNOS/NO-regulated oxidative stress. Oxidative Med. Cell. Longev. 2018:4327901. doi: 10.1155/2018/4327901
Yesica, P. C., José, C. R., Samuel, P., Hilda, V. S., Jacinto, B., Manuel, T., et al. (2020). Identification of thrips species and resistance of Frankliniella occidentalis (Thysanoptera: Thripidae) to malathion, spinosad, and bifenthrin in blackberry crops. Fla. Entomol. 102:738. doi: 10.1653/024.102.0411
Yin, Y. J., Chen, C. J., Guo, S. W., Li, K. M., Ma, Y. N., Sun, W. M., et al. (2018). The fight against Panax notoginseng root-rot disease using Zingiberaceae essential oils as potential weapons. Front. Plant Sci. 9:1346. doi: 10.3389/fpls.2018.0134
Zhang, C., Diao, Y., Wang, W., Hao, J., Imran, M., Duan, H., et al. (2017). Assessing the risk for resistance and elucidating the genetics of Colletotrichum truncatum that is only sensitive to some DMI fungicides. Front. Microbiol. 15:1779. doi: 10.3389/fmicb.2017.01779
Zhang, C. Y., Fang, Y. M., Ji, H. L., and Ma, C. T. (2011). Effects of shading on photosynthesis characteristics of Photinia × frasery and Aucuba japonica var. variegate. Chin. J. Appl. Ecol. 22, 1743–1749. doi: 10.13287/j.1001-9332.2011.0246
Zhang, L., Song, L., Xu, X., Zou, X., Duan, K., and Gao, Q. (2020). Characterization and fungicide sensitivity of Colletotrichum species causing strawberry anthracnose in eastern China. Plant Dis. 104, 1960–1968. doi: 10.1094/PDIS-10-19-2241-RE
Zhang, Y., Xu, L., Fan, X., Tan, J., Chen, W., and Xu, M. (2012). QTL mapping of resistance to gray leaf spot in maize. Theor. Appl. Genet. 125, 1797–1808. doi: 10.1007/s00122-012-1954-z
Keywords: Aucuba japonica, leaf blight, Colletotrichum aenigma, fungicides screening and resistance, agroecological disease
Citation: Fan R, Liu Y, Bin Y, Huang J, Yi B, Tang X, Li Y, Cai Y, Yang Z, Yang M, Song J, Pan Q, Liu Z, Ghani MI, Hu X and Chen X (2023) Identification of Colletotrichum aenigma as the new causal agent of leaf blight disease on Aucuba japonica Thunb., and screenings of effective fungicides for its sustainable management. Front. Microbiol. 14:1222844. doi: 10.3389/fmicb.2023.1222844
Edited by:
Stanislav Trdan, University of Ljubljana, SloveniaReviewed by:
Kandeeparoopan Prasannath, Eastern University, Sri LankaBeibei Ge, Chinese Academy of Agricultural Sciences, China
Liming Shi, Chinese Academy of Agricultural Sciences, China
Copyright © 2023 Fan, Liu, Bin, Huang, Yi, Tang, Li, Cai, Yang, Yang, Song, Pan, Liu, Ghani, Hu and Chen. This is an open-access article distributed under the terms of the Creative Commons Attribution License (CC BY). The use, distribution or reproduction in other forums is permitted, provided the original author(s) and the copyright owner(s) are credited and that the original publication in this journal is cited, in accordance with accepted academic practice. No use, distribution or reproduction is permitted which does not comply with these terms.
*Correspondence: Xiaoyulong Chen, Y2hlbnhpYW95dWxvbmdAc2luYS5jbg==; Xiaojing Hu, Z2RoeGp5eUAxNjMuY29t
†These authors have contributed equally to this work