- 1The First School of Clinical Medicine, Gannan Medical University, Ganzhou, Jiangxi, China
- 2Laboratory Medicine, First Affiliated Hospital of Gannan Medical University, Ganzhou, Jiangxi, China
Fungi are eukaryotic microorganisms found in nature, which can invade the human body and cause tissue damage, inflammatory reactions, organ dysfunctions, and diseases. These diseases can severely damage the patient’s body systems and functions, leading to a range of clinical symptoms that can be life-threatening. As the incidence of invasive fungal infections has progressively increased in the recent years, a wealth of evidence has confirmed the “double-edged sword” role of fungal extracellular vesicles (EVs) in intercellular communication and pathogen-host interactions. Fungal EVs act as mediators of cellular communication, affecting fungal-host cell interactions, delivering virulence factors, and promoting infection. Fungal EVs can also have an induced protective effect, affecting fungal growth and stimulating adaptive immune responses. By integrating recent studies, we discuss the role of EVs in fungi, providing strong theoretical support for the early prevention and treatment of invasive fungal infections. Finally, we highlight the feasibility of using fungal EVs as drug carriers and in vaccine development.
1. Introduction
Fungi are a class of eukaryotic microorganisms with a membrane-enclosed nucleus and additional organelles. Fungi exist in nature in a parasitic or saprophytic form and are capable of asexual or sexual reproduction (Sun et al., 2020). Among the millions of documented fungal species, approximately 300 are known to cause human diseases (Casadevall, 2022). In recent years, with the application of large doses of antibiotics, hormones, and immunosuppressants, an increasing number of fungi have invaded the human organism, where they have grown and multiplied in tissues, organs, or in the blood. In addition to their main infectious diseases (also known as invasive fungal diseases, IFD; Lamoth et al., 2022), they can cause inflammatory reactions and tissue damage. National and international epidemiological studies have shown that the overall incidence of IFD has been progressively increasing each year (Rayens et al., 2022). Nevertheless, the outcome of these fungal infections has often been overlooked. For example, the World Health Organization does not have any program on fungal infections and most public health facilities rarely conduct fungal surveillance procedures. In addition, most physicians handling fungal infections have no formal training in medical mycology. Moreover, severe fungal infections remain the leading cause of HIV or AIDS-related deaths worldwide (Limper et al., 2017; Tan et al., 2020).
Extracellular vesicles (EVs) are secreted by the vast majority of the cells, having an irreducible phospholipid bilayer structure. Due to the lack of reliable specific markers of their subcellular origin, the International Society for Extracellular Vesicles (ISEV) recommends their description in experimental systems to be based on specific physicochemical properties of EVs, such as their size, biochemical composition, and conditions describing their origin (Théry et al., 2018). Based on their size, they are classified as small EVs (sEVs; Liu et al., 2022), medium EVs, and/or large EVs (Gabrielli et al., 2022). EVs that are classified according to their biogenesis as originating from cells undergoing apoptosis are called apoptotic vesicles (with a diameter of 50–1,000 nm). EVs released from living cells include microvesicles (with a diameter of 50–1,000 nm) and exosomes (30–150 nm). Because of their overlapping sizes, surface markers, and the lack of proteins restricted to specific populations, the generic term “extracellular vesicles (EVs)” will be used in this review, in accordance with the latest ISEV guidelines.
2. Biogenesis of fungal EVs
Exosome formation (through the traditional Golgi pathway) begins with the formation of intracellular luminal vesicles (iLVs) by membranous organelles (e.g., endoplasmic reticulum, Golgi apparatus, and others), followed by the fusion of iLVs into multivesicular bodies (MVBs). Then, MVBs form vesicles of various sizes in an outgrowth manner. Finally, MVBs fuse with the cell membrane to release the vesicles into the extracellular space (Shao et al., 2018; Figure 1). On the other hand, microvesicles originate from the direct outward budding of the plasma membrane, generating a population of EVs with heterogeneous sizes. Apoptotic vesicles are also produced from the cell surface; however, they are only released by dying cells during their fragmentation. Recently, research advances have shown that specific fungal EVs are secreted via unconventional secretory pathways, including the Golgi reorganization and accumulation protein (GRASP) pathway, autophagy, the intracellular vesicle clusters (IVC) pathway, and endocytosis. GRASP maintains the Golgi structure and flows between different cisterns with Golgi-fused vesicles. Autophagosomes with IVC are not associated with the traditional secretory pathway. Endocytosis is accomplished through the sequential recruitment at endocytic sites of the proteins driving cargo sorting, membrane invagination, and vesicle release (De Toledo et al., 2019; Rizzo et al., 2020a). The mechanism by which fungal EVs cross the cell wall and are released into the extracellular environment is still unknown. Currently, there are three main working hypotheses to explain this process. According to the first hypothesis, the accumulation of EVs between the cell membrane and the cell wall generates a directional swelling pressure that forces EVs to cross the cell wall toward the extracellular space. In this case, the size of the EVs would be determined by the size of the wall pore and/or the thickness of the cell wall. According to the second hypothesis, cell wall remodeling provides access to the extracellular environment, through the action of degradative and synthetic enzymes associated with EVs. The third hypothesis postulates that protein channels (or structural cables) can direct EVs into the extracellular environment. Proteomics data suggest that some fungal EVs contain microtubulin or actin, which are components of structural cables (Brown et al., 2015).
3. Composition of fungal EVs
Initially, EVs were thought to be “garbage bags” for cells to remove debris or specific macromolecules (Jiang et al., 2022). Nevertheless, an increasing number of studies have demonstrated that fungal EVs contain a variety of bioactive substances, mainly proteins, nucleic acids, lipids, and carbohydrates. These molecules bind to the surface of the bilayer on the inner side of the vesicle, protecting these molecules from any external interference. With a better understanding of fungal EVs, the role and mechanisms of their carrier components in the physiological regulation of the organism and in disease development have been elucidated in detail. In this section, we outline general information on the composition of fungal EVs.
3.1. Proteins
The proteins in fungal EVs mainly include cytoplasmic and membrane-associated proteins. They also include various types of enzymes, which can increase intercellular communication, by secreting proteins to the surrounding environment. From recent studies, it became established that the plasma membrane proteins Sur7 and EVp1, the actin-binding protein Apb1, and the cell wall mannosylated protein are present in Candida albicans EVs (Nimrichter et al., 2016; Dawson et al., 2020). Proteomic analysis from different fungal EVs revealed the presence of virulence factors and a large number of proteins involved in biofilm formation, pathogenicity, and host-cell interactions (Sun et al., 2020; Zarnowski et al., 2021; Kulig et al., 2022). Proteins involved in vesicle biogenesis, or in the regulation of its size, production, and cargo, were also identified in a mouse model of aflatoxin endophthalmitis (Gandhi et al., 2022a). In recent years, the exploration of fungal virulence-associated proteins has become a hot topic of research. Cryptococcus EVs include heat shock protein (HSP70, etc.), hospholipase B, urease, and laccase (Marina et al., 2020; Park et al., 2020; Parreira et al., 2021; Jung et al., 2022). Analysis of Histoplasma capsulatum vesicles showed the presence of catalase B and superoxide dismutase precursors in these vesicles (Albuquerque et al., 2008). Proteins with antifungal effects, such as histone G and aspergillin, were found in EVs released by Aspergillus fumigatus-triggered polymorphonuclear granulocytes, which inhibited mycelial extension and affected fungal growth (Shopova et al., 2020). Recent studies have established that proteins from Penicillium marneffei and Paracoccidioides brasiliensis-derived vesicles are classified into six categories, based on their functions. These functions include protein/amino acid metabolism, stress/immune response, signal transduction/vesicle formation, carbohydrate/lipid metabolism, cellular organization/biogenesis, and others (Vallejo et al., 2012a; Yang et al., 2020).
3.2. Nucleic acids
Many studies have shown that fungal-derived EVs contain nucleic acids, including RNA (ribonucleic acid) and DNA (deoxyribonucleic acid), which have many different functions. A variety of RNA molecules are present in fungal EVs, including ncRNA, mRNA, snoRNA, snRNA, tRNA, microRNA, miRNA, and asRNA (De Toledo et al., 2019; Munhoz da Rocha et al., 2020). Less extensive research has been conducted on their DNA content, which remains to be explored in depth. EVs mediate nucleic acid export, participate in fungal intercellular communication, and in other cellular processes. Cryptococcus neoformans, Paraccidioides brasiliensis, Candida albicans, and EVs from Saccharomyces cerevisiae could export RNA molecules with different properties and biological functions, including interstrain virulence transfer in Cryptococcus (Peres da Silva et al., 2015; Marina et al., 2020). Cellular small RNA levels differed before and after treatment with the antifungal drug caspofungin. This observation provided useful material for the regulation of gene expression and intrinsic multidrug resistance mechanisms in Candida auris. Differences in virulence among distinct fungal isolates have been related to the RNA composition and content of the respective EVs. Moreover, RNAs from Candida auris EVs were shown to play a role in drug-induced stress signaling and in cell wall maintenance (Peres da Silva et al., 2019; Munhoz da Rocha et al., 2021; Zamith-Miranda et al., 2021; Amatuzzi et al., 2022). In addition, bioinformatic analysis of small RNAs carried by Malassezia EVs showed that these RNAs were not dependent on the biogenesis pathway of the RNAi (Rayner et al., 2017). Several studies have shown that EVs have a different RNA composition from their originating fungal cells. For example, one of the most enriched transcripts in EV exhibits low levels of expression in cells (CNAG_06651 amidohydrolase). there are 73 different ncRNA sequences in Histoplasma capsulatum and EVs of strain G186AR (Peres da Silva et al., 2018; Alves et al., 2019). These results reveal the specificity of nucleic acid transport in fungal vesicles.
3.3. Lipids
Lipids are important components of fungal EVs. The biogenesis of Aspergillus fumigatus EVs includes the formation of vesicles at the cell membrane level (Rizzo et al., 2020b). There is evidence that the lipid composition of fungal EVs also differs from that of its originating cell. For example, comparing the lipid composition of EVs from Candida albicans and Candida auris to the respective whole-cell composition indicates an enrichment of conserved hexosylceramide (HexCer, a lipid substance) in EVs of 67- and 109-fold, respectively (Zamith-Miranda et al., 2021). Therefore, the lipid composition could be a key factor to elucidating the EV biogenesis mechanism. Lipid components of fungal vesicles have the potential to modulate the interaction of fungi with their hosts. It has been shown that fatty acid and triglyceride-rich vesicles extracted from Paraccidioides brasiliensis induced a strong granulomatous response in mice (Alves et al., 1987). In Cryptococcus neoformans EVs, phosphatidylcholine (PC), phosphatidylethanolamine (PE), and sphingomyelin (SM) were the most differentially expressed lipids among the lipidomics associated with Cryptococcus infection. The deletion of the sterol biosynthetic gene Erg6 induced changes in the lipid and protein content of Cryptococcus neoformans EVs, suggesting a role for sterols in their formation (Oliveira et al., 2020; Zhang et al., 2021). In addition, the lipid profiles of EVs cultured in different media were clearly different. Moreover, differences in lipid composition caused by growth conditions may affect virulence and immune potential (Cleare et al., 2020).
3.4. Other compounds
Fungal EVs contain a variety of biologically active substances, including cytochromes, carbohydrates, and other compounds. Cryptococcus neoformans produces vesicles containing podoconjugates (the main virulence factor of Cryptococcus neoformans) which are composed of two polysaccharides, glucuronosyl xyloglucan (GXM) and galactomannan (GalXM; LaRocque-de-Freitas et al., 2018; Marina et al., 2020). The carbohydrate metabolites found in Histoplasma capsulatum EVs are also potentially interesting. These compounds have a different glucose content and function in Histoplasma capsulatum EVs cultured in different media sources, possibly due to increased synthesis of glucose in fungal cells or differences in the EV biogenesis mechanism (Cleare et al., 2020). EVs also act as vehicles for the transmission of pathogens, including prions, protein infection factors, and others. Prions from Saccharomyces cerevisiae sup35 could be vertically and horizontally transferred between yeast cells, causing transmissible spongiform encephalopathy (TSE; Kabani and Melki, 2016; Liu et al., 2017).
4. Biological functions of EVs
EVs are secreted by almost all cells and can be extracted from a wide range of tissue and cell culture supernatants. They can also be isolated from various body fluids, including urine, saliva, synovial fluid, bile, plasma, amniotic fluid, breast milk, semen, and ascites (Yáñez-Mó et al., 2015). EVs from several pathogenic fungi play a “double-edged sword” role in the regulation of the immune system (Figure 2). On the one hand, EVs have an important role in fungal infection and transmission, acting as mediators of cellular communication, to influence the interaction between fungal and host cells (e.g., macrophages, dendritic cells, and others), and to spread virulence factors into the host (Table 1). On the other hand, EVs have specific characteristics that induce protection, such as increased survival rates in Cryptococcus-infected Galleria mellonella (Reis et al., 2021), indicating their immunological potential (Table 2).
4.1. Immunological potential of EVs in the course of fungal infections
Fungal EVs affect the morphogenesis and biofilm formation capacity of Candida albicans, stimulating adaptive immune responses. It has been shown that Candida albicans EVs could in vitro modulate yeast to mycelial differentiation, thereby inhibiting biofilm formation and attenuating virulence. Candida albicans EVs treated for 24 h lost their ability to penetrate agar, reduced fungal load in invertebrate models of Candida albicans infection, and were nontoxic when inoculated into Galleria mellonella (Honorato et al., 2022). In addition, both the presence and absence of Candida albicans genes could have qualitative and quantitative effects on EV size, composition, and immunostimulatory phenotype. Genes involved in phospholipid synthesis could affect not only multiple secretory phenotypes, but also the release of EVs carrying microbial cargo. Factors affecting lipid synthesis are important aspects for future studies of secreted virulence factors (Wolf et al., 2015). The characteristics of Candida albicans filamentous cell-secreted EVs (HEV) were compared with yeast EVs (YEV). Interesting differences were observed in the proteomic analyses of the biogenesis and function of mycelial EVs. These were shown to differ from yeast EVs and could be used to discover novel diagnostic markers and targets for the treatment of Candida albicans infections (Martínez-López et al., 2022). To understand the pathogenesis properties of Candida in endophthalmitis, and to validate potential diagnostic and prognostic markers, EVs associated with transport, cell adhesion, membrane fusion, and cytoskeletal organization of differentially expressed proteins (such as aquaporin-5, CD5 antigen-like protein, Gasdermin and vesicle-associated proteins) were studied in a mouse model of Candida albicans and Aspergillus flavus endophthalmitis (Gandhi et al., 2022a,b). Under an atopic dermatitis (AD) setting, Candida albicans promoted altered glycosylation patterns in keratinocyte-derived EVs, to interact with inhibitory Siglecs on antigen-presenting cells. Strategies that target this pathway to enhance antifungal responses and limit pathogen transmission may provide new therapeutic options for AD cutaneous candidiasis (Kobiela et al., 2022). In Candida albicans EVs, the plasma membrane proteins Sur7 and EVp1 could be fungal equivalents of four transmembrane protein tags for EV studies (Dawson et al., 2020). The new antifungal drug turbinmicin disrupts the transport of EVs during biofilm growth and thus exhibits antifungal effects (Zhao et al., 2021). Candida auris EVs induced the activation of phagocytes and increased phagocytosis, thereby regulating host cell defense mechanisms (Zamith-Miranda et al., 2021; Chan et al., 2022). Saccharomyces cerevisiae EVs activated receptors on immune cells and could be used as a novel vaccine material for immune cell maturation (Higuchi et al., 2023).
Cryptococcus neoformans mutants lacking the gene coding for steryl glucosidase (Δsgl1), enriched in sterol glucosidase-catalyzed steryl glucoside (SGs), and containing polysaccharide glucuronide oxyglycans (GXM) extracellular vesicles could delay the acute lethality of Galleria mellonella infection to Cryptococcus neoformans. This observation demonstrated the potential use of Δsgl1 EVs as a vaccination strategy for Cryptococcus neoformans (Colombo et al., 2019; Rizzo et al., 2021). A vesicular peptide (isoleucine-proline-isoleucine, Ile-Pro-Ile) from EVs produced by Cryptococcus gattii improved the survival of Galleria mellonella infected by Cryptococcus (Reis et al., 2021). Immunization with EVs had a positive impact on mice infected with Paracoccidioides brasiliensis. Specifically, it induced the mobilization of activated T lymphocytes and natural killer T cells to the infected lungs, improved the production of pro-inflammatory cytokines and histopathological features, and reduced the fungal load (Baltazar et al., 2021). Sporothrix brasiliensis EVs co-cultured with macrophages could enhance the fungicidal activity (assessed through the phagocytic index) of the macrophages (Campos et al., 2021). Aspergillus EVs enhanced phagocytosis and macrophage cytotoxicity, triggering the release of a unique set of antifungal EVs (afEVs) by polymorphonuclear granulocytes (PMNs). Infection with differentiated PLB-985 neutrophil-derived extracellular vesicles limited the growth of Aspergillus fumigatus hyphae, which also demonstrated antifungal effects (Rafiq et al., 2022). As an immunological tool, pre-exposing mice to EVs from Aspergillus fumigatus induced the production of a fungal antigen-specific IgG-rich serum, reduced alveolar infiltration by neutrophils, prevented extensive lung tissue damage, and improved phagocytosis and fungal clearance (Souza et al., 2022). EVs released by Aspergillus flavus enhanced the phagocytosis and cytotoxicity of macrophages. In addition, proteins from these vesicles could produce marker molecules that provided information about the physiological and disease status induced by the fungal infection. These marker molecules could serve as novel prognostic targets for fungal fundus disease (Brauer et al., 2020; Gandhi et al., 2022a).
4.2. Pro-inflammatory effects of fungal extracellular vesicles
Candida albicans EVs can mediate the host inflammatory response. Short exposure of macrophages to EVs resulted in the internalization of these vesicles and in the production of nitric oxide (NO), IL-12, TGF-β, and IL-10. Similarly, dendritic cells treated with EVs produced IL-12, IL-10, and TNF-α (Honorato et al., 2022). Significantly elevated levels of IL-6, IL-1β, TNF-α, and IFN-γ were observed in EVs released from mice with Candida albicans endophthalmitis (Gandhi et al., 2022b). In addition, Candida albicans EVs activated the L-arginine/NO pathway to reduce the intracellular levels of reactive oxygen species (ROS) and apoptosis. This outcome was achieved by increasing NO levels and upregulating the expression of the NO dioxygenase gene YHB1, thereby promoting the growth of Candida albicans. During host cell infection, Candida albicans EVs synergistically enhanced the pathogenesis and destructive effects of the fungus on host cells, as well as in the RAW264.7, HOK, TR146, and HGEC cell lines (Wei et al., 2023). EVs originating from Candida glabrata, Candida parapsilosis, and Candida tropicalis induced pro-inflammatory responses in human immune cells and stimulated the secretion of TNF-α and IL-8 (Karkowska-Kuleta et al., 2020; Kulig et al., 2022). Moreover, Candida auris EVs enhanced yeast cell proliferation in macrophages, and Saccharomyces cerevisiae EVs were shown to transport pathogenic prions (Zamith-Miranda et al., 2021; Higuchi et al., 2023).
The EVs secreted by Cryptococcus neoformans could disseminate virulence factors, including heat shock protein (Parreira et al., 2021), phospholipase B (Jung et al., 2022), urease (Marina et al., 2020), laccase (Park et al., 2020), and polysaccharides (Jung et al., 2022). These EVs could also induce an immune response on macrophages, to escape intracellular restriction (Zhang et al., 2021). Cryptococcus gattii promoted intracellular multiplication in macrophages, by secreting EVs and disseminating virulence factors (such as melanin, laccase, and podoconjugates) into the host (Goulart et al., 2010; De Sousa et al., 2022). Malassezia released EVs that induced IL-4 and TNF-α reactions in peripheral blood mononuclear cells (PBMCs; Gehrmann et al., 2011). These could also promote IL-6 production, by participating in NF-κB-dependent signaling pathways (Zhang et al., 2019; Vallhov et al., 2020). Histoplasma capsulatum EVs contain several virulence-related proteins, such as peroxidase (C0NND7) and alkaline phosphatase (C0NBI7; Cleare et al., 2020). Attenuated Paracoccidioides brasiliensis EVs induced in vitro expression of fungal virulence traits and enhanced infection in mice. In addition, EVs from virulent Paracoccidioides brasiliensis stimulated RAW 264.7 cells and bone marrow-derived macrophages to express high levels of inflammatory mediators, specifically TNF-α, IL-6, MCP-1 and NO (da Silva et al., 2016; Octaviano et al., 2022). Incubation of Penicillium marneffei EVs with macrophages resulted in elevated expression levels of ROS, NO, and several inflammatory factors, including IL-1β, IL-6, IL-10, and TNF-α. Proteomic studies have shown that they contain a number of virulence factors, including HSP, MP1p, and peroxidase (Yang et al., 2020). EVs isolated from Sporothrix brasiliensis stimulated the production of IL-12 and IL-6 by macrophages and increased the phagocytic index and fungal load in DCs (dendritic cells). They also increased the levels of immunogenic components and proteins in EVs, which, according to proteomics data, could be related to their virulence (Ikeda et al., 2018; Campos et al., 2021). Aspergillus fumigatus EVs mediated the β-glucan-stimulated IL-1α secretion by neutrophils (Ratitong et al., 2021). Aspergillus flavus EVs induced the production of inflammatory mediators, such as NO, TNF-α, IL-6, and IL-1β, by macrophages (Brauer et al., 2020).
5. Interaction between fungal EVs and host cells and their immune regulation
5.1. Yeast and yeast-like bacteria
5.1.1. Candida albicans
Candida albicans is a commensal microorganism that colonizes a high percentage of the skin and mucosal surfaces of healthy individuals. However, when the immune defenses of an individual are disrupted, this fungus can cause invasive diseases and bloodstream infections. These infections can threaten the health and lives of immunocompromised patients (Andriantsitohaina and Papon, 2020). Numerous studies have shown that Candida albicans EVs could mediate host immune and inflammatory responses, being also associated with drug resistance in this fungus. A brief exposure of macrophages to EVs resulted in the internalization of these vesicles and in the production of NO, IL-12, TGF-β, and IL-10. Similarly, dendritic cells treated with EVs produced IL-12, IL-10, and TNF-α. In addition, Candida albicans EVs induced the upregulation of CD86 and MHC-II (Vargas et al., 2015), indicating their potential role in modulating the innate immune response to the fungus.
In addition, Candida albicans EVs contain proteins, lipids, and RNAs with immunogenic and pathogenic properties. The Candida albicans EV plasma membrane proteins Sur7 and EVp1 can be fungal equivalents of four transmembrane protein tags that can be exploited for the study of mammalian EVs (Dawson et al., 2020). The gene encoding phosphatidylserine synthase (CHO1) in Candida albicans is involved in phospholipid biosynthesis. In comparison with wild-type strains, the cho1Δ/Δ mutant strains produced EVs with different PC and protein contents, which could not activate the NF-κB signaling pathway in bone marrow-derived macrophages (Wolf et al., 2015). In addition, fungal cell walls allowed rapid changes in cell volume and the passage of large liposomal particles, such as EVs. Cell wall remodeling is a normal adaptive response of fungi to environmental changes and was shown to enhance fungal adaptation to these microenvironments (Childers et al., 2020).
Candida albicans EVs not only play an important role in modulating the host inflammatory response, but can also affect its drug resistance. EVs released during biofilm growth produced an adherent extracellular matrix, which provided the fungus with a protective layer against antifungal drugs (Taff et al., 2012; Zarnowski et al., 2021). Candida albicans EVs could regulate fungal virulence and biofilm formation (Honorato et al., 2022; Kulig et al., 2022). Reduced levels of EVs and matrix polysaccharides in ESCRT-deficient mutants greatly increased susceptibility to the antifungal drug fluconazole. Moreover, the addition of Candida albicans biofilm EVs reversed biofilm matrix accumulation and drug susceptibility in ESCRT mutants. As such, Candida albicans biofilm EVs could play a key role in substrate production and biofilm drug resistance (Zarnowski et al., 2018). These findings provide new ideas and directions for the development of novel antifungal drugs. A recently developed antifungal drug, turbinmicin, disrupted EV transport during biofilm growth and impaired the subsequent assembly of the biofilm matrix, thereby reducing fungal resistance and exhibiting a broad-spectrum antifungal activity (Zhao et al., 2021).
5.1.2. Non-Candida albicans
Although Candida albicans is the most common species of the Candida genus causing human infections, the global epidemiology of candidiasis is currently changing. As such, we collectively refer to other Candida genera as non-Candida Albicans (NCA) genera (Giacobbe et al., 2020). EVs from Candida glabrata, Candida tropicalis, and Candida parapsilosis induced pro-inflammatory responses in the THP-1 human monocyte cell line, stimulating the secretion of TNF-α and IL-8, and being involved in fungal infection and transport of virulence factors (Karkowska-Kuleta et al., 2020; Kulig et al., 2022). The prevalence of Candida glabrata, the second most common cause of invasive candidiasis, is currently increasing; however, the mechanisms by which it survives to the host’s antimicrobial and immune response remain unclear. Recent studies have demonstrated that phosphatidylinositol 3-kinase (PI3K), which is mainly involved in vesicle-mediated translocation and autophagy, is an important factor in the regulation of host immune responses, intracellular survival, and virulence in Candida glabrata (Rai et al., 2012, 2015). Candida auris, known as a “super fungus,” is prone to nosocomial outbreaks and has shown multiple drug resistance. Candida auris EVs regulated host cellular defense mechanisms, affecting adhesion to epithelial cells, intracellular cytotoxicity by macrophages, and the activation of dendritic cells (Zamith-Miranda et al., 2021). Application of the first-line antifungal agent caspofungin could increase the production levels of EVs, and also alter the types and amounts of their RNA and protein contents, which induced resistance in Candida auris (Chan et al., 2022).
Saccharomyces cerevisiae is a unicellular eukaryotic organism with many advantages for experimentation, such as rapid growth rates, a clear background, and easy biochemistry and molecular biology manipulation. As such, it is widely used as a natural carrier of gene therapy drugs and immune substances. Notably, the first genetically engineered vaccine, a recombinant hepatitis B vaccine, was produced using Saccharomyces cerevisiae (Yang et al., 2021). Recently, it was found that EVs from Saccharomyces cerevisiae could be used as a novel vaccine material (Higuchi et al., 2023). EVs also transport pathogenic prions and are vertically and horizontally transferred between yeast cells. Moreover, the number, size, morphology, and proteomic profile of yeast EVs were altered in strains with selective knockouts of ESCRT components. These observations suggest an important role of EVs in intercellular communication (Liu et al., 2017; Zhao et al., 2019). The EV biogenesis requires both conventional Golgi-derived pathways and unconventional secretory pathways, demonstrating the complexity of fungal cell biology (Oliveira et al., 2010). The yeast Eps15-like proteins Pan1p and Ent1p/2p synergistically regulated the interaction between endocytic vesicles and actin cables (Yoshida et al., 2021).
5.1.3. Cryptococcus spp.
Cryptococcus is a deep fungus which, despite being exclusively aerobic, is a serious threat to human life and health. It is capable of infecting both immunocompromised and immunocompetent individuals (Ellis et al., 2019; Baddley et al., 2021). The main cryptococci causing human infections are Cryptococcus neoformans and Cryptococcus gattii. Central nervous system cryptococcosis is the most common form of cryptococcal infection and has a serious prognosis with high morbidity and mortality rates (Alves Soares et al., 2019; Xu et al., 2020).
5.1.3.1. Cryptococcus neoformans
Cryptococcus neoformans is a parthenogenic intracellular pathogen that is most notably characterized by a surface envelope of polysaccharide pods. These structures are composed of two main polysaccharides, glucuronoxylated mannans (GXM) and galactosylated mannans (GalXM; LaRocque-de-Freitas et al., 2018). Numerous studies have shown that EVs secreted by Cryptococcus neoformans could induce immune responses (Zhang et al., 2021) and disseminate virulence factors into the host, including superoxide dismutase, phospholipase B, urease, and laccase (Cox et al., 2000). During the phenotypic characterization of Cryptococcus mutants lacking expression of the eukaryotic gene NOP16, this gene was found to be required for EVs production. The lack of NOP16 gene expression resulted in, not only reduced EV numbers, but also different small molecule compositions (Castelli et al., 2022). The galactose lectin Galectin-3 (Gal-3) protein regulates host innate and adaptive immunity. Increasing Gal-3 levels during infection could inhibit fungal growth by inducing vesicle rupture and disrupting the delivery of virulence factors (Almeida et al., 2017).
EVs released from Cryptococcus neoformans-activated macrophages mediated pro-inflammatory signals, both in vivo and in vitro. Moreover, they might also be involved in immune response regulation, by transferring antigens to receptor cells or by activating immune-related pathways (Zhang et al., 2021). The interaction of these EVs with alveolar macrophages is essential for the control of infection. The ingestion of nascent Cryptococcus leads to its intracellular replication and to the appearance of polysaccharide podocyte-containing vesicles in the macrophage cytoplasm. This phagocytosis process involves permeabilization of the lysosomal membrane and secretion of intracellular podocyte polysaccharides into the vesicles (Steenbergen et al., 2001; Tucker and Casadevall, 2002). Cryptococcus neoformans have a unique intracellular pathogenic mechanism. It involves cytoplasmic accumulation of polysaccharide-containing vesicles and their intracellular replication, leading to the formation of phagosomes. Alvarez et al. first reported the phenomenon of “phagosome extrusion,” in which nascent Cryptococcus was shown to leave macrophages under conditions of phagosome maturation, actin depolymerization, and homotrimeric phagosome fusion. As Lei demonstrated, cryptococcal pathogens could escape the intracellular confinement of mammalian macrophages to continue their spread and proliferation (Alvarez and Casadevall, 2006).
Liposomes containing amphotericin B could penetrate the fungal cell wall, which has deformable viscoelasticity to deliver amphotericin B to the cell membrane. This observation implies that the cell wall allows for a transmural vesicle flow. It was also shown that Cryptococcus neoformans EVs were able to cross the cell wall and deliver soluble membrane-bound effectors and other molecules to the extracellular space (Walker et al., 2018). The accumulation of sterol glucosides (SGs) has an effect on the glucuronide glucan (GXM) in fungal pod membranes. Cryptococcus neoformans mutants lacking the gene coding for sterol glucosidase (Δsgl1) were shown to be nonpathogenic. The deletion of Erg6, which is involved in fungal ergosterol biosynthesis, also affected Cryptococcus neoformans virulence and failed to induce protection in a vaccination model. GXM-containing EVs enriched in SGs delayed the acute lethality of Galleria mellonella against Cryptococcus neoformans infection (Colombo et al., 2019). A well-characterized vesicle transport adapter, the AP-3 complex, was shown to selectively transport the Arf1-GTPase and the Yck3 kinase to the vesicle membrane. These processes resulted in vesicle/lysosomal membrane permeabilization and the molecular triggering of cell death (Stolp et al., 2022).
5.1.3.2. Cryptococcus gattii
Cryptococcus gattii can be a causative agent of disease in immunocompetent individuals. Likewise, it can multiply within macrophages, in a process associated with intracellular replication and cytoplasmic accumulation of polysaccharide-containing vesicles (Goulart et al., 2010). Cryptococcus EVs have multiple biological roles related to the transport of various important virulence molecules, such as melanin, laccase, podoconjugates, and others (De Sousa et al., 2022). Genes coding for proteins involved in vesicle formation and transport may be associated with virulence. Representative difference analysis (RDA) was used to identify Cryptococcus neoformans and Cryptococcus gattii genes differentially expressed during growth in rat intraperitoneal macrophages. Upregulated genes in Cryptococcus gattii were found to be associated with cell growth, aerobic respiration, and protein binding (Goulart et al., 2010).
There is a unique “division of labor” mechanism in the pathogen population, in which specific cells adopt a dormant behavior, in order to support the growth of neighboring cells. Bielska et al. demonstrated that a pathogenic “division of labor” process could be triggered at large cellular distances and mediated by the release of EVs by the fungus (Bielska et al., 2018). Fungal EV molecules are characterized by induced protection. EVs obtained from Cryptococcus gattii were analyzed, in which a vesicular peptide (isoleucine-proline-isoleucine, Ile-Pro-Ile) improved the survival of Galleria mellonella infected by Cryptococcus gattii or Cryptococcus neoformans (Reis et al., 2021). It is suggested that the proteins in EVs produced by Cryptococcus gattii have an immunological potential. Not only the Cryptococcus Zip3 gene, a homolog of Saccharomyces cerevisiae Atx2p, affects the secretion of Cryptococcus EVs and GXM, but also the deletion of Zip3 leads to impaired virulence of Cryptococcus gattii (Garcia et al., 2020).
5.1.4. Malassezia
Malassezia is a lipophilic fungus that parasitizes the skin surface of humans and other mammals (White et al., 2014). It has been recently reported that the development of specific lipid-deficient skin diseases, such as atopic eczema (AE), is related to Malassezia spp. Moreover, EVs released by Malassezia can also play a role in the sensitization and maintenance of AE-related inflammation (Johansson et al., 2018). Meanwhile, Malassezia EVs can release EVs carrying antigens, small RNAs, and allergens, which induce IL-4 and TNF-α responses in PBMCs (Gehrmann et al., 2011). It can also be internalized into keratin-forming cells and promote IL-6 production, by participating in NF-κB-dependent signaling pathways (Zhang et al., 2019; Vallhov et al., 2020). Bioinformatic analysis of vesicular small RNAs indicates that these RNAs are likely not dependent on the RNAi biogenesis pathway (Rayner et al., 2017). According to a recent report, Malassezia globosa inhibited Staphylococcus aureus biofilm formation, through the ability to secrete the MgSAP1 aspartyl protease (Ianiri et al., 2018). Whether Malassezia EVs are involved in beneficial outcomes for the host is a subject for further research.
5.2. Temperature biphasic fungi
5.2.1. Histoplasma capsulatum
Histoplasma capsulatum is a common temperature biphasic fungus, which can produce different morphological features under different temperature conditions. It can also cause widely distributed granulomatous diseases. Histoplasma capsulatum produces EVs carrying lipids, proteins, nucleic acids, and polysaccharides of remarkable biological significance (Albuquerque et al., 2008; Rodrigues et al., 2008; Reis et al., 2019). Histoplasma capsulatum EVs contain multiple virulence-related proteins, such as peroxidase (C0NND7) and alkaline phosphatase (C0NBI7; Cleare et al., 2020). In comparison with EVs from Candida albicans, Cryptococcus neoformans, and Saccharomyces cerevisiae, the RNA content and class of Histoplasma capsulatum EVs are different (Alves et al., 2019). Monoclonal antibodies (mAb) could differentially regulate the loading and release of EVs by Histoplasma capsulatum in both concentration-dependent and independent manners, leading to different effects on host immune effector mechanisms (Matos Baltazar et al., 2016; Baltazar et al., 2018).
5.2.2. Paracoccidioides brasiliensis
Paracoccidioides brasiliensis could invade the mucous membranes, skin, and lungs, and cause chronic pyogenic granulomatosis. EVs from Paracoccidioides brasiliensis contain proteins, lipids, and RNAs. These molecules could induce the production of pro-inflammatory mediators by mouse macrophages (in a dose-dependent manner) and also modulate the innate immune response (da Silva et al., 2016). Protein characterization of EVs from the pathogenic stage of the Paracoccidioides brasiliensis yeast revealed multiple protein functions. These functions were mostly related to protein and carbohydrate metabolism, stress response, signaling pathways, cell division, transport, and additional potentially unclear functions (Vallejo et al., 2012a). Differences in virulence among fungal isolates could be related to their distinct EV-RNA content. These EVs might modulate the transcriptome of dendritic cells (Peres da Silva et al., 2019). Consistently, Vallejo observed differences in sterol composition and also between EVs and whole cells (Vallejo et al., 2012b).
Gal-3 affects the destruction and internalization of Paracoccidioides brasiliensis EVs by macrophages. It is also beneficial to mammalian hosts, by promoting host defense during infection (Hatanaka et al., 2019). In contrast, attenuated Paracoccidioides brasiliensis EVs induced in vitro expression of fungal virulence traits and enhanced infection in mice (Octaviano et al., 2022). The proteins from Paracoccidioides brasiliensis EVs could alter cell wall composition and affect fungal-host cell interactions. They could also release immunoreactive compounds into the extracellular environment, thereby affecting fungal pathogenesis. Therefore, the biosynthesis of EVs is a potential target for the development of novel antifungal drugs and vaccines (Nimrichter et al., 2016).
5.2.3. Penicillium marneffei
Penicillium marneffei is an opportunistic fungus that invades and proliferates within macrophages. It frequently causes disseminated infections in patients with AIDS. Incubation of EVs with macrophages leads to increased expression levels of ROS, NO, and several inflammatory factors, including IL-1β, IL-6, IL-10, and TNF-α. A study on the protein composition of EVs from Penicillium marneffei identified a total of 394 species, which were shown to be mostly involved in protein/amino acid metabolism and signal transduction. The proteins from these EVs were mainly localized in the cytoplasm, ribosomes, and nucleus. Most of the proteins were closely related to other members in the protein–protein interaction network of TM-derived EVs (Yang et al., 2020).
5.2.4. Sporothrix
Sporothrix can spread through the bloodstream and cause nodules and abscesses in the skin throughout the body. It has been established that ascomycetes, such as Sporothrix schenckii, Candida albicans, Candida parapsilosis, and Saccharomyces cerevisiae can produce EVs (Albuquerque et al., 2008). In addition, fungal EVs can facilitate fungal spread, by delivering essential proteins, lipids, nucleic acids, and some immunologically active components. EVs from Sporothrix brasiliensis have more immunologically active components, in comparison with those from Sporothrix schenckii, which could explain the greater virulence of Sporothrix brasiliensis (Ikeda and Ferreira, 2021). Consistently, macrophages at different concentrations showed increased fungicidal activity (as assessed by their phagocytic index) when co-cultured with Sporothrix brasiliensis EVs. Moreover, the levels of IL-12 and IL-6 levels were dose-dependently increased during infection. Molecules that are important in the immune response, such as MHC class II and immunoglobulin CD86, were overexpressed in Sporothrix brasiliensis-infected mice (Campos et al., 2021). These molecules showed that EVs could play a protective role during infection with Sporothrix brasiliensis. In addition, EVs isolated from Sporothrix brasiliensis induced an increase in the phagocytic index and fungal load in DCs, which, according to proteomics, could be related to the presence of virulence-associated immunogenic components and proteins in EVs (Ikeda et al., 2018).
5.3. Aspergillus
5.3.1. Aspergillus fumigatus
Aspergillus fumigatus is a conditionally pathogenic fungus that is widely present in the natural environment. When inhaled by humans, it can cause Aspergillus allergy-related diseases. Rizzo et al. first characterized fungal EVs in a protoplast model consisting of Aspergillus fumigatus cells without the cell wall. Protoplast EVs, which contain bioactive components, such as lipids, glycans, and proteins, could be a promising model for functional studies of fungal EVs (Rizzo et al., 2020b). UV irradiation of Aspergillus fumigatus EVs resulted in reduced colony formation levels, as well as in the upregulation of the MPKC stress response gene. These observations identified a role for EVs in fungal intraspecific communication. Thus, understanding the mechanism of EVs-fungal interaction can be useful for antifungal therapy (Bitencourt et al., 2022).
Aspergillus fumigatus EVs mediated the β-glucan stimulation of IL-1α secretion by neutrophils (Ratitong et al., 2021). Recently, it has been reported that Aspergillus fumigatus triggered the release of a unique set of antifungal EVs (afEVs) by PMNs. A proteomic analysis has shown that afEVs were rich in bioactive antifungal proteins that stimulated the production of inflammatory mediators and could lead to fungal clearance (Shopova et al., 2020). Multiple immunizations with EVs from Aspergillus fumigatus reduced neutrophil infiltration into the lungs, increased fungal clearance in the airways, and reduced lung injury. These effects were also related to a synergistic effect in the treatment of pulmonary aspergillosis infection with amphotericin B. This observation suggests that EVs can induce the activation of the host immune system and generate a protective response against Aspergillus fumigatus infection (Souza et al., 2022).
5.3.2. Aspergillus flavus
Aspergillus flavus is the second most common cause of aspergillosis worldwide. EVs from Aspergillus flavus could in vitro induce the production of inflammatory mediators, such as NO, TNF-α, IL-6, and IL-1β by macrophages, enhancing phagocytosis and cytotoxicity by these cells. Pre-stimulation with Aspergillus flavus EVs in wax borer larvae triggered an immune response and facilitated fungal clearance. This observation indicates that Aspergillus flavus EVs are biologically active and can stimulate the innate immune system (Brauer et al., 2020). EVs released from aflatoxin-infected retinal pigment epithelium (RPE) cells could also activate immune signaling pathways and might contribute to the pathogenesis of endophthalmitis, for which proteins in EVs may serve as clinical diagnostic and prognostic biomarkers (Gandhi and Joseph, 2022; Gandhi et al., 2022a).
6. Prospect and outlook
6.1. Drug carriers
Fungal-derived vesicles provide numerous outstanding advantages for the delivery of biological drugs. Specifically, they show unparalleled superiority as drug carriers, enhancing the solubility of insoluble molecules and their bioavailability, reducing the dose, and providing efficient drug targeting. In addition, bioengineered EVs offer advantages as drug delivery carriers: they have excellent hematological stability and biocompatibility, low toxicity and immunogenicity, as well as a long-distance and targeted transport capability, being also able to cross biological barriers (Figure 3).
Encapsulation of griseofulvin in deformable membrane vesicles (DMVs) for dermal administration has high drug permeability and skin retention, which can overcome its low bioavailability for oral administration. However, this approach also has many side effects and requires a long duration of the treatment (Indian patent application 208/DEL/2009; Aggarwal and Goindi, 2012). Multimolecular liposome/anionosomes containing clotrimazole (the vesicle gel system) were prepared by lipid hydrolysis to provide sustained and controlled release of appropriate drugs for local vaginal therapy (Ning et al., 2005). In addition, an effective ocular nano-vesicle carrier, which provides prolonged and controlled ocular delivery of clotrimazole (CLT) to the carrier system, has also been developed (Basha et al., 2013). Moreover, a novel nanosystem vesicle loaded with itraconazole enhanced corneal permeation and antimycotic activity, to combat Candida albicans infections (Elmeshad and Mohsen, 2016).
6.2. Vaccines
Although EV studies are becoming widespread in different biomedical fields, in mycology, this area of research is still in its infancy. Fungal resistance faced in the antibiotic era has created an urgent need for developing more vaccine types against fungal diseases, which are estimated to affect more than 1 billion people worldwide, with significant mortality (Bongomin et al., 2017; Rizzo et al., 2020a). Fungal EV vaccines have the following advantages: first, their high production yield, due to the rapid replication rates of fungal cells and their ability to secrete EVs in large quantities. Second, their multifunctionality indicates the possibility of modifying multiple antigens. Third, the low complexity of their composition. Fourth, their high stability.
The presence of known protective antigens on the surface of EVs suggests their potential for vaccine development. For example, Cryptococcus neoformans EVs contain proteins from the Mp88, Cda, and Gox families, which have immunomodulatory effects. Moreover, EVs obtained from Cryptococcus neoformans mutant strains produced a strong antibody response in mice, greatly prolonging the survival time of animals infected with Cryptococcus neoformans (Rizzo et al., 2021). In the last few years, substantial progress has been made in the host-fungal relationship. Recurrent vulvovaginal candidiasis (RVVC) is characterized by recurrent annual episodes. The virosomal vaccine (PEV7) and the immunotherapeutic vaccine (NDV-3A) can be used to prevent RVVC (de Bernardis et al., 2012; Gil-Bona et al., 2015). Through a comparative proteomic study of Candida albicans and its soluble secretory proteins, EVs from Candida albicans were identified as a new vaccine source by Ana et al. Interestingly, the Bgl2 protein found in vesicles and supernatants without EVs had the potential to prevent systemic candidiasis in a mouse model (Edwards et al., 2018).
7. Conclusion
The study of fungal EVs has been neglected for many years, due to the thick and hard properties of the cell walls of fungi. However, according to recent findings, fungal EVs are important mediators of intercellular communication, allowing the functional transfer of bioactive molecules, which include lipids, proteins, and nucleic acids. Fungal EVs provide access to nutrients, toxin delivery, and cell adhesion. They also promote biofilm formation, to evade host defense systems. More importantly, fungal EVs characterize the induction of protection in fungal infections and have a remarkable potential for clinical diagnosis, treatment, and prevention. EVs represent a new generation of drug delivery systems, which offer high delivery capacity and efficiency, intrinsic targeting properties, and low immunogenicity. In recent years, a large amount of research has been directed toward developing safe and efficient bioengineered EVs (Figure 4).
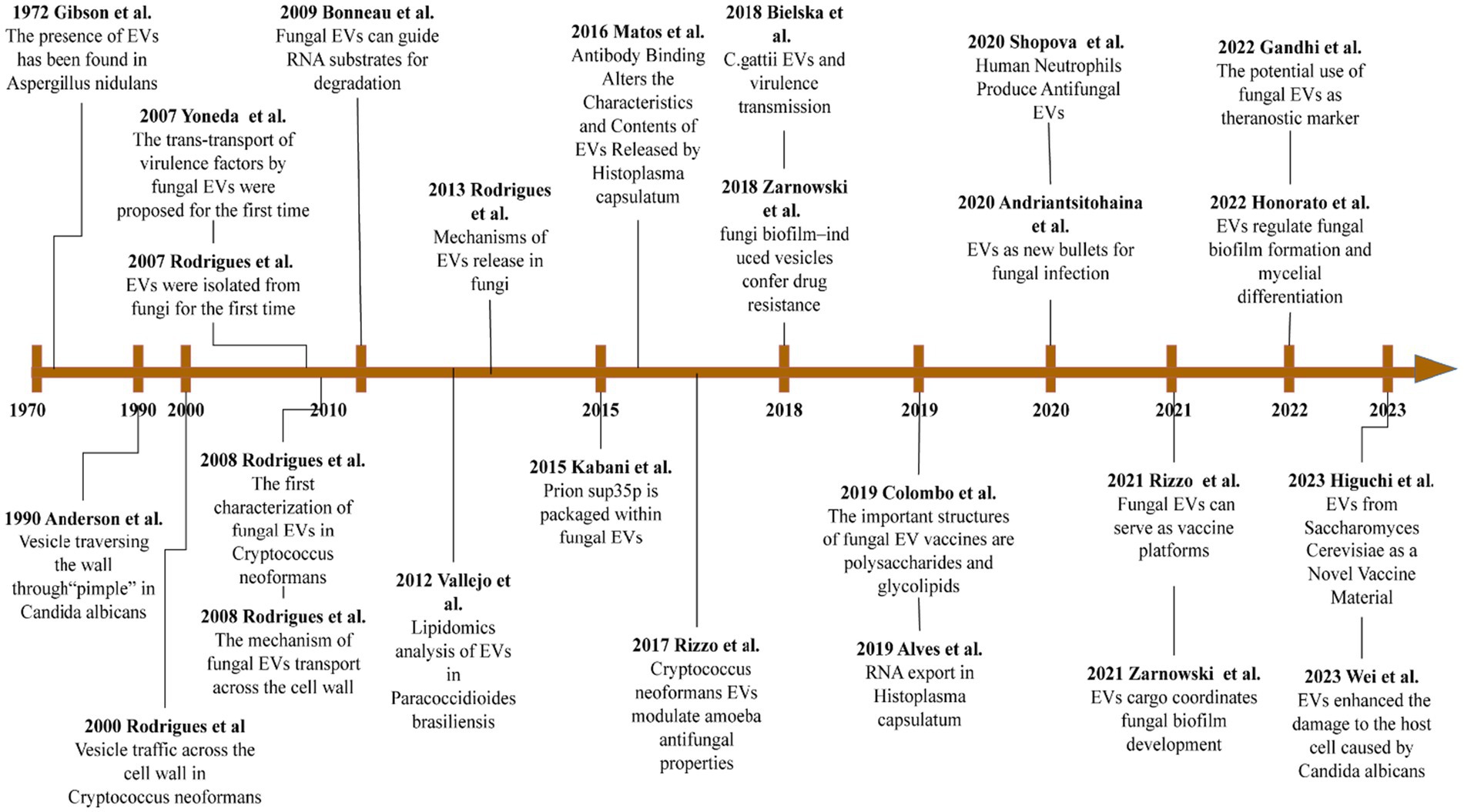
Figure 4. Important advances in the study of fungi EVs. This image references the image32760680 and updates on it.
However, there are still important limitations in the application of EVs as vaccines, such as: how to isolate fungal EVs with high efficiency and standardization, their scalable production, and storage of standard procedures. Due to our insufficient understanding of the mechanisms of EVs production, these are still urgent issues to be solved. In recent years, there has been an increase in vesicle production utilizing genetic engineering modifications (e.g., gene mutations, pathway modifications, and knockout of specific genes). Additional limitations to overcome include reducing the virulence of EVs and exploring their complex delivery mechanisms for precise delivery of antigens, as different pathogens express different virulence factors. In the near future, we need to develop vesicle materials with both improved safety and biodegradability. We also need to continuously optimize the vesicle preparation process. Such optimization will contribute to solving the limitations of their low encapsulation capacity, poor physical stability, low drug loading efficiency, and high cost of lipid-like vesicle production. We anticipate that, after continuous efforts, fungal EVs will provide a new direction for the development of its medical utilization.
Author contributions
YL wrote the first draft alone. All authors contributed to the article and approved the submitted version.
Conflict of interest
The authors declare that the research was conducted in the absence of any commercial or financial relationships that could be construed as a potential conflict of interest.
Publisher’s note
All claims expressed in this article are solely those of the authors and do not necessarily represent those of their affiliated organizations, or those of the publisher, the editors and the reviewers. Any product that may be evaluated in this article, or claim that may be made by its manufacturer, is not guaranteed or endorsed by the publisher.
References
Aggarwal, N., and Goindi, S. (2012). Preparation and evaluation of antifungal efficacy of griseofulvin loaded deformable membrane vesicles in optimized guinea pig model of Microsporum canis—Dermatophytosis. Int. J. Pharm. 437, 277–287. doi: 10.1016/j.ijpharm.2012.08.015
Albuquerque, P. C., Nakayasu, E. S., Rodrigues, M. L., Frases, S., Casadevall, A., Zancope-Oliveira, R. M., et al. (2008). Vesicular transport in Histoplasma capsulatum: an effective mechanism for trans-cell wall transfer of proteins and lipids in ascomycetes. Cell. Microbiol. 10, 1695–1710. doi: 10.1111/j.1462-5822.2008.01160.x
Almeida, F., Wolf, J. M., da Silva, T. A., DeLeon-Rodriguez, C. M., Rezende, C. P., Pessoni, A. M., et al. (2017). Galectin-3 impacts Cryptococcus neoformans infection through direct antifungal effects. Nat. Commun. 8:1968. doi: 10.1038/s41467-017-02126-7
Alvarez, M., and Casadevall, A. (2006). Phagosome extrusion and host-cell survival after Cryptococcus neoformans phagocytosis by macrophages. Curr. Biol. 16, 2161–2165. doi: 10.1016/j.cub.2006.09.061
Alves, L. M., Figueiredo, F., Filho, S. L. B., Tincani, I., and Silva, C. L. (1987). The role of fractions from Paracoccidioides brasiliensis in the genesis of inflammatory response. Mycopathologia 97, 3–7. doi: 10.1007/BF00437324
Alves, L. R., Peres da Silva, R., Sanchez, D. A., Zamith-Miranda, D., Rodrigues, M. L., Goldenberg, S., et al. (2019). Extracellular Vesicle-Mediated RNA Release inHistoplasma capsulatum. mSphere 4. doi: 10.1128/mSphere.00176-19
Alves Soares, E., Lazera, M. D. S., Wanke, B., Faria Ferreira, M., Carvalhaes de Oliveira, R. V., Oliveira, A. G., et al. (2019). Mortality by cryptococcosis in Brazil from 2000 to 2012: a descriptive epidemiological study. PLoS Negl. Trop. Dis. 13:e0007569. doi: 10.1371/journal.pntd.0007569
Amatuzzi, R. F., Zamith-Miranda, D., Munhoz da Rocha, I. F., Lucena, A. C. R., de Toledo Martins, S., Streit, R., et al. (2022). Caspofungin affects extracellular vesicle production and cargo in Candida auris. JoF 8:990. doi: 10.3390/jof8100990
Andriantsitohaina, R., and Papon, N. (2020). Extracellular vesicles: new bullets to fight fungal infections. Trends Cell Biol. 30, 589–590. doi: 10.1016/j.tcb.2020.05.008
Baddley, J. W., Chen, S. C., Huisingh, C., Benedict, K., DeBess, E. E., Galanis, E., et al. (2021). MSG07: an international cohort study comparing epidemiology and outcomes of patients With cryptococcus neoformans orcryptococcus gattiiInfections. Clin. Infect. Dis. 73, 1133–1141. doi: 10.1093/cid/ciab268
Baltazar, L. M., Ribeiro, G. F., Freitas, G. J., Queiroz-Junior, C. M., Fagundes, C. T., Chaves-Olórtegui, C., et al. (2021). Protective response in experimental Paracoccidioidomycosis elicited by extracellular vesicles containing antigens of Paracoccidioides brasiliensis. Cells 10:1813. doi: 10.3390/cells10071813
Baltazar, L. M., Zamith-Miranda, D., Burnet, M. C., Choi, H., Nimrichter, L., Nakayasu, E. S., et al. (2018). Concentration-dependent protein loading of extracellular vesicles released by Histoplasma capsulatum after antibody treatment and its modulatory action upon macrophages. Sci. Rep. 8:8065. doi: 10.1038/s41598-018-25665-5
Basha, M., Abd el-Alim, S. H., Shamma, R. N., and Awad, G. E. A. (2013). Design and optimization of surfactant-based nanovesicles for ocular delivery of Clotrimazole. J. Liposome Res. 23, 203–210. doi: 10.3109/08982104.2013.788025
Bielska, E., Sisquella, M. A., Aldeieg, M., Birch, C., O’Donoghue, E. J., and May, R. C. (2018). Pathogen-derived extracellular vesicles mediate virulence in the fatal human pathogen Cryptococcus gattii. Nat. Commun. 9:1556. doi: 10.1038/s41467-018-03991-6
Bitencourt, T. A., Hatanaka, O., Pessoni, A. M., Freitas, M. S., Trentin, G., Santos, P., et al. (2022). Fungal extracellular vesicles are involved in Intraspecies intracellular communication. MBio 13:e0327221. doi: 10.1128/mbio.03272-21
Bongomin, F., Gago, S., Oladele, R. O., and Denning, D. (2017). Global and multi-National Prevalence of fungal diseases—estimate precision. J Fungi 3:57. doi: 10.3390/jof3040057
Brauer, V. S., Pessoni, A. M., Bitencourt, T. A., de Paula, R. G., de Oliveira Rocha, L., Goldman, G. H., et al. (2020). Extracellular vesicles from aspergillus flavus induce M1 polarization in vitro. mSphere 5:20. doi: 10.1128/mSphere.00190-20
Brown, L., Wolf, J. M., Prados-Rosales, R., and Casadevall, A. (2015). Through the wall: extracellular vesicles in gram-positive bacteria, mycobacteria and fungi. Nat. Rev. Microbiol. 13, 620–630. doi: 10.1038/nrmicro3480
Campos, R. M. S., Jannuzzi, G. P., Ikeda, M., de Almeida, S. R., and Ferreira, K. S. (2021). Extracellular vesicles from Sporothrix brasiliensis yeast cells increases fungicidal activity in macrophages. Mycopathologia 186, 807–818. doi: 10.1007/s11046-021-00585-7
Casadevall, A. (2022). Immunity to invasive fungal diseases. Annu. Rev. Immunol. 40, 121–141. doi: 10.1146/annurev-immunol-101220-034306
Castelli, R. F., Pereira, A., Honorato, L., Valdez, A., de Oliveira, H. C., Bazioli, J. M., et al. (2022). Extracellular vesicle formation in Cryptococcus deuterogattii impacts fungal virulence and requires the NOP16 gene. Infect. Immun. 90:e0023222. doi: 10.1128/iai.00232-22
Chan, W., Chow, F. W., Tsang, C. C., Liu, X., Yao, W., Chan, T. T., et al. (2022). Induction of amphotericin B resistance in susceptible Candida auris by extracellular vesicles. Emerg Microbes Infect 11, 1900–1909. doi: 10.1080/22221751.2022.2098058
Childers, D. S., Avelar, G. M., Bain, J. M., Larcombe, D. E., Pradhan, A., Budge, S., et al. (2020). Impact of the environment upon the Candida albicans Cell Wall and resultant effects upon immune surveillance. Curr. Top. Microbiol. Immunol. 425, 297–330. doi: 10.1007/82_2019_182
Cleare, L. G., Zamith, D., Heyman, H. M., Couvillion, S. P., Nimrichter, L., Rodrigues, M. L., et al. (2020). Media matters! Alterations in the loading and release ofHistoplasma capsulatumextracellular vesicles in response to different nutritional milieus. Cell. Microbiol. 22:e13217. doi: 10.1111/cmi.13217
Colombo, A. C., Rella, A., Normile, T., Joffe, L. S., Tavares, P. M., Araújo, G. R., et al. (2019). Cryptococcus neoformans Glucuronoxylomannan and Sterylglucoside are required for host protection in an animal vaccination model. MBio 10:18. doi: 10.1128/mBio.02909-18
Cox, G. M., Mukherjee, J., Cole, G. T., Casadevall, A., and Perfect, J. R. (2000). Urease as a virulence factor in experimental Cryptococcosis. Infect. Immun. 68, 443–448. doi: 10.1128/IAI.68.2.443-448.2000
da Silva, T. A., Roque-Barreira, M. C., Casadevall, A., and Almeida, F. (2016). Extracellular vesicles from Paracoccidioides brasiliensis induced M1 polarization in vitro. Sci. Rep. 6:35867. doi: 10.1038/srep35867
Dawson, C. S., Garcia-Ceron, D., Rajapaksha, H., Faou, P., Bleackley, M. R., and Anderson, M. A. (2020). Protein markers for Candida albicans EVs include claudin-like Sur7 family proteins. J Extracell Vesicles 9:1750810. doi: 10.1080/20013078.2020.1750810
de Bernardis, F., Amacker, M., Arancia, S., Sandini, S., Gremion, C., Zurbriggen, R., et al. (2012). A virosomal vaccine against candidal vaginitis: immunogenicity, efficacy and safety profile in animal models. Vaccine 30, 4490–4498. doi: 10.1016/j.vaccine.2012.04.069
De Sousa, H. R., De Oliveira, G. P., Frazão, S. O., KCM, G., Rosa, C. P., Garcez, E. M., et al. (2022). Faster Cryptococcus Melanization increases virulence in experimental and human Cryptococcosis. J Fungi 8:393. doi: 10.3390/jof8040393
De Toledo, M. S., Szwarc, P., Goldenberg, S., and Alves, L. R. (2019). Extracellular vesicles in Fungi: composition and functions. Curr. Top. Microbiol. Immunol. 422, 45–59. doi: 10.1007/82_2018_141
Edwards, J. E., Schwartz, M. M., Schmidt, C. S., Sobel, J. D., Nyirjesy, P., Schodel, F., et al. (2018). A fungal immunotherapeutic vaccine (NDV-3A) for treatment of recurrent vulvovaginal candidiasis—a phase 2 randomized, double-blind, placebo-controlled trial. Clin. Infect. Dis. 66, 1928–1936. doi: 10.1093/cid/ciy185
Ellis, J., Bangdiwala, A. S., Cresswell, F. V., Rhein, J., Nuwagira, E., Ssebambulidde, K., et al. (2019). The changing epidemiology of HIV-associated adult meningitis, Uganda 2015-2017. Open Forum Infect. Dis. 6:ofz419. doi: 10.1093/ofid/ofz419
Elmeshad, A. N., and Mohsen, A. M. (2016). Enhanced corneal permeation and antimycotic activity of itraconazole against Candida albicans via a novel nanosystem vesicle. Drug Deliv. 23, 2115–2123. doi: 10.3109/10717544.2014.942811
Gabrielli, M., Prada, I., Joshi, P., Falcicchia, C., D’Arrigo, G., Rutigliano, G., et al. (2022). Microglial large extracellular vesicles propagate early synaptic dysfunction in Alzheimer’s disease. Brain 145, 2849–2868. doi: 10.1093/brain/awac083
Gandhi, J., and Joseph, J. (2022). Proteomic profiling of extracellular vesicles derived from ARPE-19 cells challenged with aspergillus flavus and Candida albicans: application in fungal endophthalmitis. Pathog Dis 80:ftac042. doi: 10.1093/femspd/ftac042
Gandhi, J., Naik, M. N., Mishra, D. K., and Joseph, J. (2022a). Proteomic profiling of aspergillus flavus endophthalmitis derived extracellular vesicles in an in-vivo murine model. Med. Mycol. 60:myac064. doi: 10.1093/mmy/myac064
Gandhi, J., Sushma, M. V., Rengan, A. K., Naik, M. N., Mishra, D. K., Boyinpally, S. R., et al. (2022b). Proteomic profiling of exosomes in a mouse model of Candida albicans endophthalmitis. Exp. Cell Res. 417:113222. doi: 10.1016/j.yexcr.2022.113222
Garcia, A. W. A., Kinskovski, U. P., Diehl, C., Reuwsaat, J. C. V., Motta de Souza, H., Pinto, H. B., et al. (2020). Participation of Zip3, a ZIP domain-containing protein, in stress response and virulence in Cryptococcus gattii. Fungal Genet. Biol. 144:103438. doi: 10.1016/j.fgb.2020.103438
Gehrmann, U., Qazi, K. R., Johansson, C., Hultenby, K., Karlsson, M., Lundeberg, L., et al. (2011). Nanovesicles from Malassezia sympodialis and host exosomes induce cytokine responses – novel mechanisms for host-microbe interactions in atopic eczema. PLoS One 6:e21480. doi: 10.1371/journal.pone.0021480
Giacobbe, D. R., Maraolo, A. E., Simeon, V., Magnè, F., Pace, M. C., Gentile, I., et al. (2020). Changes in the relative prevalence of candidaemia due to non-albicans Candida species in adult in-patients: a systematic review, meta-analysis and meta-regression. Mycoses 63, 334–342. doi: 10.1111/myc.13054
Gil-Bona, A., Llama-Palacios, A., Parra, C. M., Vivanco, F., Nombela, C., Monteoliva, L., et al. (2015). Proteomics unravels extracellular vesicles as carriers of classical cytoplasmic proteins in Candida albicans. J. Proteome Res. 14, 142–153. doi: 10.1021/pr5007944
Goulart, L., Silva, R. E., Chiapello, L., Silveira, C., Crestani, J., Masih, D., et al. (2010). Cryptococcus neoformansandCryptococcus gattiigenes preferentially expressed during rat macrophage infection. Med. Mycol. 48, 932–941. doi: 10.3109/13693781003677494
Hatanaka, O., Rezende, C. P., Moreno, P., Freitas Fernandes, F., Oliveira Brito, P. K. M., Martinez, R., et al. (2019). Galectin-3 inhibits Paracoccidioides brasiliensis growth and impacts Paracoccidioidomycosis through multiple mechanisms. mSphere 4:e00209. doi: 10.1128/mSphere.00209-19
Higuchi, A., Morishita, M., Nagata, R., Maruoka, K., Katsumi, H., and Yamamoto, A. (2023). Functional characterization of extracellular vesicles from Baker’s yeast Saccharomyces Cerevisiae as a novel vaccine material for immune cell maturation. J. Pharm. Sci. 112, 525–534. doi: 10.1016/j.xphs.2022.08.032
Honorato, L., de Araujo, J. F. D., Ellis, C. C., Piffer, A. C., Pereira, Y., Frases, S., et al. (2022). Extracellular vesicles regulate biofilm formation and yeast-to-hypha differentiation in Candida albicans. MBio 13:e0030122. doi: 10.1128/mbio.00301-22
Ianiri, G., Heitman, J., and Scheynius, A. (2018). The skin commensal yeast Malassezia globosa thwarts bacterial biofilms to benefit the host. J. Invest. Dermatol. 138, 1026–1029. doi: 10.1016/j.jid.2018.01.008
Ikeda, M., de Almeida, J. R. F., Jannuzzi, G. P., Cronemberger-Andrade, A., Torrecilhas, A. C. T., Moretti, N. S., et al. (2018). Extracellular vesicles from Sporothrix brasiliensis are an important virulence factor that induce an increase in fungal burden in experimental Sporotrichosis. Front. Microbiol. 9:2286. doi: 10.3389/fmicb.2018.02286
Ikeda, M. A. K., and Ferreira, K. S. (2021). Extracellular vesicles from Sporothrix yeast cells. Curr. Top. Microbiol. Immunol. 432, 35–44. doi: 10.1007/978-3-030-83391-6_4
Jiang, Y., Wang, F., Wang, K., Zhong, Y., Wei, X., Wang, Q., et al. (2022). Engineered exosomes: a promising drug delivery strategy for brain diseases. Curr. Med. Chem. 29, 3111–3124. doi: 10.2174/0929867328666210902142015
Johansson, H. J., Vallhov, H., Holm, T., Gehrmann, U., Andersson, A., Johansson, C., et al. (2018). Extracellular nanovesicles released from the commensal yeast Malassezia sympodialis are enriched in allergens and interact with cells in human skin. Sci. Rep. 8:9182. doi: 10.1038/s41598-018-27451-9
Jung, E. H., Park, Y. D., Dragotakes, Q., Ramirez, L. S., Smith, D. Q., Reis, F. C. G., et al. (2022). Cryptococcus neoformans releases proteins during intracellular residence that affect the outcome of the fungal-macrophage interaction. Microlife 3:uqac015. doi: 10.1093/femsml/uqac015
Kabani, M., and Melki, R. (2016). More than just trash bins? Potential roles for extracellular vesicles in the vertical and horizontal transmission of yeast prions. Curr. Genet. 62, 265–270. doi: 10.1007/s00294-015-0534-6
Karkowska-Kuleta, J., Kulig, K., Karnas, E., Zuba-Surma, E., Woznicka, O., Pyza, E., et al. (2020). Characteristics of extracellular vesicles released by the pathogenic yeast-like Fungi Candida glabrata, Candida parapsilosis and Candida tropicalis. Cells 9:1722. doi: 10.3390/cells9071722
Kobiela, A., Frackowiak, J. E., Biernacka, A., Hovhannisyan, L., Bogucka, A. E., Panek, K., et al. (2022). Exposure of keratinocytes to Candida Albicans in the context of atopic milieu induces changes in the surface glycosylation pattern of small extracellular vesicles to enhance their propensity to interact with inhibitory Siglec receptors. Front. Immunol. 13:884530. doi: 10.3389/fimmu.2022.884530
Kulig, K., Karnas, E., Woznicka, O., Kuleta, P., Zuba-Surma, E., Pyza, E., et al. (2022). Insight into the properties and Immunoregulatory effect of extracellular vesicles produced by Candida glabrata, Candida parapsilosis, and Candida tropicalis biofilms. Front. Cell. Infect. Microbiol. 12:879237. doi: 10.3389/fcimb.2022.879237
Lamoth, F., Lewis, R. E., and Kontoyiannis, D. P. (2022). Investigational antifungal agents for invasive mycoses: a clinical perspective. Clin. Infect. Dis. 75, 534–544. doi: 10.1093/cid/ciab1070
LaRocque-de-Freitas, I. F., Rocha, J. D. B., Nunes, M. P., Oliveira, P. A. V., Nascimento, D. O., Freire-de-Lima, L., et al. (2018). Involvement of the capsular GalXM-induced IL-17 cytokine in the control of Cryptococcus neoformans infection. Sci. Rep. 8:16378. doi: 10.1038/s41598-018-34649-4
Limper, A. H., Adenis, A., Le, T., et al. (2017). Fungal infections in HIV/AIDS. Lancet Infect. Dis. 17, e334–e343. doi: 10.1016/S1473-3099(17)30303-1
Liu, S., Hossinger, A., Göbbels, S., and Vorberg, I. M. (2017). Prions on the run: how extracellular vesicles serve as delivery vehicles for self-templating protein aggregates. Prion 11, 98–112. doi: 10.1080/19336896.2017.1306162
Liu, X., Tan, S., Liu, H., Jiang, J., Wang, X., Li, L., et al. (2022). Hepatocyte-derived MASP1-enriched small extracellular vesicles activate HSCs to promote liver fibrosis. Hepatology 77, 1181–1197. doi: 10.1002/hep.32662
Marina, C. L., Bürgel, P. H., Agostinho, D. P., Zamith-Miranda, D., Las-Casas, L. O., Tavares, A. H., et al. (2020). Nutritional conditions Modulate C. neoformans extracellular Vesicles’ capacity to elicit host immune response. Microorganisms 8:1815. doi: 10.3390/microorganisms8111815
Martínez-López, R., Hernáez, M. L., Redondo, E., Calvo, G., Radau, S., Pardo, M., et al. (2022). Candida albicans hyphal extracellular vesicles are different from yeast ones, carrying an active proteasome complex and showing a different role in host immune response. Microbiol Spectr 10:e0069822. doi: 10.1128/spectrum.00698-22
Matos Baltazar, L., Nakayasu, E. S., Sobreira, T. J., Choi, H., Casadevall, A., Nimrichter, L., et al. (2016). Antibody binding alters the characteristics and contents of extracellular vesicles released by Histoplasma capsulatum. mSphere 1:e00085. doi: 10.1128/mSphere.00085-15
Munhoz da Rocha, I. F., Amatuzzi, R. F., Lucena, A. C. R., Faoro, H., and Alves, L. R. (2020). Cross-kingdom extracellular vesicles EV-RNA communication as a mechanism for host–pathogen interaction. Front. Cell. Infect. Microbiol. 10:593160. doi: 10.3389/fcimb.2020.593160
Munhoz da Rocha, I. F., Martins, S. T., Amatuzzi, R. F., Zamith-Miranda, D., Nosanchuk, J. D., Rodrigues, M. L., et al. (2021). Cellular and extracellular vesicle RNA analysis in the global threat FungusCandida auris. Microbiol Spectr 9:e0153821. doi: 10.1128/Spectrum.01538-21
Nimrichter, L., de Souza, M. M., del Poeta, M., Nosanchuk, J. D., Joffe, L., Tavares, P. M., et al. (2016). Extracellular vesicle-associated transitory Cell Wall components and their impact on the interaction of Fungi with host cells. Front. Microbiol. 7:1034. doi: 10.3389/fmicb.2016.01034
Ning, M., Guo, Y., Pan, H., Chen, X., and Gu, Z. (2005). Preparation, in vitro and in vivo evaluation of liposomal/niosomal gel delivery systems for clotrimazole. Drug Dev. Ind. Pharm. 31, 375–383. doi: 10.1081/DDC-54315
Octaviano, C. E., Abrantes, N. E., and Puccia, R. (2022). Extracellular vesicles from Paracoccidioides brasiliensis can induce the expression of fungal virulence traits in vitro and enhance infection in mice. Front. Cell. Infect. Microbiol. 12:834653. doi: 10.3389/fcimb.2022.834653
Oliveira, D. L., Nakayasu, E. S., Joffe, L. S., Guimarães, A. J., Sobreira, T. J. P., Nosanchuk, J. D., et al. (2010). Characterization of yeast extracellular vesicles: evidence for the participation of different pathways of cellular traffic in vesicle biogenesis. PLoS One 5:e11113. doi: 10.1371/journal.pone.0011113
Oliveira, F. F. M., Paes, H. C., Peconick, L. D. F., Fonseca, F. L., Marina, C. L. F., Bocca, A. L., et al. (2020). Erg6 affects membrane composition and virulence of the human fungal pathogen Cryptococcus neoformans. Fungal Genet. Biol. 140:103368. doi: 10.1016/j.fgb.2020.103368
Park, Y. D., Chen, S. H., Camacho, E., Casadevall, A., and Williamson, P. R. (2020). Role of the ESCRT pathway in laccase trafficking and virulence of Cryptococcus neoformans. Infect. Immun. 88. doi: 10.1128/IAI.00954-19
Parreira, V., Santos, L. G. C., Rodrigues, M. L., and Passetti, F. (2021). ExVe: the knowledge base of orthologous proteins identified in fungal extracellular vesicles. Comput. Struct. Biotechnol. J. 19, 2286–2296. doi: 10.1016/j.csbj.2021.04.031
Peres da Silva, R., Longo, L. G. V., Cunha, J., Sobreira, T. J. P., Rodrigues, M. L., Faoro, H., et al. (2019). Comparison of the RNA content of extracellular vesicles derived from Paracoccidioides brasiliensis and Paracoccidioides lutzii. Cells 8. doi: 10.3390/cells8070765
Peres da Silva, R., Martins, S. T., Rizzo, J., dos Reis, F. C. G., Joffe, L. S., Vainstein, M., et al. (2018). Golgi reassembly and stacking protein (GRASP) participates in vesicle-mediated RNA export in Cryptococcus Neoformans. Genes 9:400. doi: 10.3390/genes9080400
Peres da Silva, R., Puccia, R., Rodrigues, M. L., Oliveira, D. L., Joffe, L. S., César, G. V., et al. (2015). Extracellular vesicle-mediated export of fungal RNA. Sci. Rep. 5:7763. doi: 10.1038/srep07763
Rafiq, M., Rivieccio, F., Zimmermann, A. K., Visser, C., Bruch, A., Krüger, T., et al. (2022). PLB-985 neutrophil-like cells as a model to study Aspergillus fumigatus pathogenesis. mSphere 7:e0094021. doi: 10.1128/msphere.00940-21
Rai, M. N., Balusu, S., Gorityala, N., Dandu, L., and Kaur, R. (2012). Functional genomic analysis of Candida glabrata-macrophage interaction: role of chromatin remodeling in virulence. PLoS Pathog. 8:e1002863. doi: 10.1371/journal.ppat.1002863
Rai, M. N., Sharma, V., Balusu, S., and Kaur, R. (2015). An essential role for phosphatidylinositol 3-kinase in the inhibition of phagosomal maturation, intracellular survival and virulence in Candida glabrata. Cell. Microbiol. 17, 269–287. doi: 10.1111/cmi.12364
Ratitong, B., Marshall, M., and Pearlman, E. (2021). β-Glucan-stimulated neutrophil secretion of IL-1α is independent of GSDMD and mediated through extracellular vesicles. Cell Rep. 35:109139. doi: 10.1016/j.celrep.2021.109139
Rayens, E., Norris, K. A., and Cordero, J. F. (2022). Mortality trends in risk conditions and invasive mycotic disease in the United States, 1999-2018. Clin. Infect. Dis. 74, 309–318. doi: 10.1093/cid/ciab336
Rayner, S., Bruhn, S., Vallhov, H., Andersson, A., Billmyre, R. B., and Scheynius, A. (2017). Identification of small RNAs in extracellular vesicles from the commensal yeast Malassezia sympodialis. Sci. Rep. 7:39742. doi: 10.1038/srep39742
Reis, F. C. G., Borges, B. S., Jozefowicz, L. J., Sena, B. A. G., Garcia, A. W. A., Medeiros, L. C., et al. (2019). A novel protocol for the isolation of fungal extracellular vesicles reveals the participation of a putative scramblase in polysaccharide export and capsule construction in Cryptococcus gattii. mSphere 4. doi: 10.1128/mSphere.00080-19
Reis, F. C. G., Costa, J. H., Honorato, L., Nimrichter, L., Fill, T. P., and Rodrigues, M. L. (2021). Small molecule analysis of extracellular vesicles produced by Cryptococcus gattii: identification of a tripeptide controlling Cryptococcal infection in an invertebrate host model. Front. Immunol. 12:654574. doi: 10.3389/fimmu.2021.654574
Rizzo, J., Chaze, T., Miranda, K., Roberson, R. W., Gorgette, O., Nimrichter, L., et al. (2020b). Characterization of extracellular vesicles produced by Aspergillus fumigatus protoplasts. mSphere 5:20. doi: 10.1128/mSphere.00476-20
Rizzo, J., Rodrigues, M. L., and Janbon, G. (2020a). Extracellular vesicles in Fungi: past, present, and future perspectives. Front. Cell. Infect. Microbiol. 10:346. doi: 10.3389/fcimb.2020.00346
Rizzo, J., Wong, S. S. W., Gazi, A. D., Moyrand, F., Chaze, T., Commere, P. H., et al. (2021). Cryptococcus extracellular vesicles properties and their use as vaccine platforms. J Extracell Vesicles 10:e12129. doi: 10.1002/jev2.12129
Rodrigues, M. L., Nimrichter, L., Oliveira, D. L., Nosanchuk, J. D., and Casadevall, A. (2008). Vesicular trans-Cell Wall transport in Fungi: a mechanism for the delivery of virulence-associated macromolecules? Lipid Insights 2, 27–40. doi: 10.4137/lpi.s1000
Shao, H., Im, H., Castro, C. M., Breakefield, X., Weissleder, R., and Lee, H. (2018). New Technologies for Analysis of extracellular vesicles. Chem. Rev. 118, 1917–1950. doi: 10.1021/acs.chemrev.7b00534
Shopova, I. A., Belyaev, I., Dasari, P., Jahreis, S., Stroe, M. C., Cseresnyés, Z., et al. (2020). Human neutrophils produce antifungal extracellular vesicles against Aspergillus fumigatus. MBio 11:20. doi: 10.1128/mBio.00596-20
Souza, J., Gurgel, I. L. S., Malacco, N. L. S. O., Martins, F. R. B., Queiroz-Junior, C. M., Teixeira, M. M., et al. (2022). Pre-exposure with extracellular vesicles from Aspergillus fumigatus attenuates inflammatory response and enhances fungal clearance in a murine model pulmonary aspergillosis. Front. Cell. Infect. Microbiol. 12:898619. doi: 10.3389/fcimb.2022.898619
Steenbergen, J. N., Shuman, H. A., and Casadevall, A. (2001). Cryptococcus neoformans interactions with amoebae suggest an explanation for its virulence and intracellular pathogenic strategy in macrophages. Proc. Natl. Acad. Sci. U. S. A. 98, 15245–15250. doi: 10.1073/pnas.261418798
Stolp, Z. D., Kulkarni, M., Liu, Y., Zhu, C., Jalisi, A., Lin, S., et al. (2022). Yeast cell death pathway requiring AP-3 vesicle trafficking leads to vacuole/lysosome membrane permeabilization. Cell Rep. 39:110647. doi: 10.1016/j.celrep.2022.110647
Sun, S., Hoy, M. J., and Heitman, J. (2020). Fungal pathogens. Curr. Biol. 30, R1163–R1169. doi: 10.1016/j.cub.2020.07.032
Taff, H. T., Nett, J. E., Zarnowski, R., Ross, K. M., Sanchez, H., Cain, M. T., et al. (2012). A Candida biofilm-induced pathway for matrix glucan delivery: implications for drug resistance. PLoS Pathog. 8:e1002848. doi: 10.1371/journal.ppat.1002848
Tan, B. H., Chakrabarti, A., Patel, A., Chua, M. M. M., Sun, P. L., Liu, Z., et al. (2020). Clinicians’ challenges in managing patients with invasive fungal diseases in seven Asian countries: an Asia fungal working group (AFWG) survey. Int. J. Infect. Dis. 95, 471–480. doi: 10.1016/j.ijid.2020.01.007
Théry, C., Witwer, K. W., Aikawa, E., Alcaraz, M. J., Anderson, J. D., Andriantsitohaina, R., et al. (2018). Minimal information for studies of extracellular vesicles 2018 (MISEV2018): a position statement of the International Society for Extracellular Vesicles and update of the MISEV2014 guidelines. J Extracell Vesicl 7:1535750. doi: 10.1080/20013078.2018.1535750
Tucker, S. C., and Casadevall, A. (2002). Replication of Cryptococcus neoformans in macrophages is accompanied by phagosomal permeabilization and accumulation of vesicles containing polysaccharide in the cytoplasm. Proc. Natl. Acad. Sci. U. S. A. 99, 3165–3170. doi: 10.1073/pnas.052702799
Vallejo, M. C., Nakayasu, E. S., Longo, L. V., Ganiko, L., Lopes, F. G., Matsuo, A. L., et al. (2012b). Lipidomic analysis of extracellular vesicles from the pathogenic phase of Paracoccidioides brasiliensis. PLoS One 7:e39463. doi: 10.1371/journal.pone.0039463
Vallejo, M. C., Nakayasu, E. S., Matsuo, A. L., Sobreira, T. J. P., Longo, L. V. G., Ganiko, L., et al. (2012a). Vesicle and vesicle-free extracellular proteome of Paracoccidioides brasiliensis: comparative analysis with other pathogenic fungi. J. Proteome Res. 11, 1676–1685. doi: 10.1021/pr200872s
Vallhov, H., Johansson, C., Veerman, R. E., and Scheynius, A. (2020). Extracellular vesicles released from the skin commensal yeast Malassezia sympodialis activate human primary keratinocytes. Front. Cell. Infect. Microbiol. 10:6. doi: 10.3389/fcimb.2020.00006
Vargas, G., Rocha, J. D., Oliveira, D. L., Albuquerque, P. C., Frases, S., Santos, S. S., et al. (2015). Compositional and immunobiological analyses of extracellular vesicles released Bycandida albicans. Cell. Microbiol. 17, 389–407. doi: 10.1111/cmi.12374
Walker, L., Sood, P., Lenardon, M. D., Milne, G., Olson, J., Jensen, G., et al. (2018). The viscoelastic properties of the fungal Cell Wall allow traffic of AmBisome as intact liposome vesicles. MBio 9:17. doi: 10.1128/mBio.02383-17
Wei, Y., Wang, Z., Liu, Y., Liao, B., Zong, Y., Shi, Y., et al. (2023). Extracellular vesicles of Candida albicans regulate its own growth through the L-arginine/nitric oxide pathway. Appl. Microbiol. Biotechnol. 107, 355–367. doi: 10.1007/s00253-022-12300-7
White, T. C., Findley, K., Dawson, T. L., Scheynius, A., Boekhout, T., Cuomo, C. A., et al. (2014). Fungi on the skin: dermatophytes and Malassezia. Cold Spring Harb. Perspect. Med. 4:9802. doi: 10.1101/cshperspect.a019802
Wolf, J. M., Espadas, J., Luque-Garcia, J., Reynolds, T., and Casadevall, A. (2015). Lipid biosynthetic genes affect Candida albicans extracellular vesicle morphology, cargo, and Immunostimulatory properties. Eukaryot. Cell 14, 745–754. doi: 10.1128/EC.00054-15
Xu, J., Neal, L. M., Ganguly, A., Kolbe, J. L., Hargarten, J. C., Elsegeiny, W., et al. (2020). Chemokine receptor CXCR3 is required for lethal brain pathology but not pathogen clearance during cryptococcal meningoencephalitis. Sci. Adv. 6:eaba2502. doi: 10.1126/sciadv.aba2502
Yáñez-Mó, M., Siljander, P. R., Andreu, Z., Zavec, A. B., Borràs, F. E., Buzas, E. I., et al. (2015). Biological properties of extracellular vesicles and their physiological functions. J Extracell Vesicles 4:27066. doi: 10.3402/jev.v4.27066
Yang, Q., Liu, Y., Jiang, Z., Xu, M., Yao, S., Li, C., et al. (2021). Multi-residue method for the detection of 40 β-lactam-antibiotics in vaccines by LC-MS/MS. Anal. Biochem. 631:114299. doi: 10.1016/j.ab.2021.114299
Yang, B., Wang, J., Jiang, H., Lin, H., Ou, Z., Ullah, A., et al. (2020). Extracellular vesicles derived from Talaromyces marneffei yeasts mediate inflammatory response in macrophage cells by bioactive protein components. Front. Microbiol. 11:603183. doi: 10.3389/fmicb.2020.603183
Yoshida, N., Ogura, I., Nagano, M., Ando, T., Toshima, J. Y., and Toshima, J. (2021). Cooperative regulation of endocytic vesicle transport by yeast Eps15-like protein Pan1p and epsins. J. Biol. Chem. 297:101254. doi: 10.1016/j.jbc.2021.101254
Zamith-Miranda, D., Heyman, H. M., Couvillion, S. P., Cordero, R. J. B., Rodrigues, M. L., Nimrichter, L., et al. (2021). Comparative molecular and Immunoregulatory analysis of extracellular vesicles from Candida albicans and Candida auris. mSystems 6:e0082221. doi: 10.1128/mSystems.00822-21
Zarnowski, R., Noll, A., Chevrette, M. G., Sanchez, H., Jones, R., Anhalt, H., et al. (2021). Coordination of fungal biofilm development by extracellular vesicle cargo. Nat. Commun. 12:6235. doi: 10.1038/s41467-021-26525-z
Zarnowski, R., Sanchez, H., Covelli, A. S., Dominguez, E., Jaromin, A., Bernhardt, J., et al. (2018). Candida albicans biofilm–induced vesicles confer drug resistance through matrix biogenesis. PLoS Biol. 16:e2006872. doi: 10.1371/journal.pbio.2006872
Zhang, Y. J., Han, Y., Sun, Y. Z., Jiang, H. H., Liu, M., Qi, R. Q., et al. (2019). Extracellular vesicles derived from Malassezia furfur stimulate IL-6 production in keratinocytes as demonstrated in in vitro and in vivo models. J. Dermatol. Sci. 93, 168–175. doi: 10.1016/j.jdermsci.2019.03.001
Zhang, L., Zhang, K., Li, H., Coelho, C., de Souza Gonçalves, D., Fu, M. S., et al. (2021). Cryptococcus neoformans-infected macrophages release Proinflammatory extracellular vesicles: insight into their components by multi-omics. MBio 12:21. doi: 10.1128/mBio.00279-21
Zhao, K., Bleackley, M., Chisanga, D., Gangoda, L., Fonseka, P., Liem, M., et al. (2019). Extracellular vesicles secreted by Saccharomyces cerevisiae are involved in cell wall remodelling. Commun Biol 2:305. doi: 10.1038/s42003-019-0538-8
Keywords: fungus, fungal infection, extracellular vesicles, fungal-host interaction, immunomodulation, drug carrier, vaccine
Citation: Lai Y, Jiang B, Hou F, Huang X, Ling B, Lu H, Zhong T and Huang J (2023) The emerging role of extracellular vesicles in fungi: a double-edged sword. Front. Microbiol. 14:1216895. doi: 10.3389/fmicb.2023.1216895
Edited by:
Andrea Masotti, Bambino Gesù Children’s Hospital (IRCCS), ItalyReviewed by:
Lysangela Ronalte Alves, Oswaldo Cruz Foundation, BrazilFarzam Vaziri, University of California, Davis, United States
Copyright © 2023 Lai, Jiang, Hou, Huang, Ling, Lu, Zhong and Huang. This is an open-access article distributed under the terms of the Creative Commons Attribution License (CC BY). The use, distribution or reproduction in other forums is permitted, provided the original author(s) and the copyright owner(s) are credited and that the original publication in this journal is cited, in accordance with accepted academic practice. No use, distribution or reproduction is permitted which does not comply with these terms.
*Correspondence: Tianyu Zhong, zhongtianyu@gmail.com; Junyun Huang, huangjunyun@gmu.edu.cn