- 1Key Laboratory of Cultivation and Protection for Non-Wood Forest Trees, Central South University of Forestry and Technology, Changsha, China
- 2Key Laboratory of National Forestry and Grassland Administration on Control of Artificial Forest Diseases and Pests in South China, Central South University of Forestry and Technology, Changsha, China
- 3Hunan Provincial Key Laboratory for Control of Forest Diseases and Pests, Central South University of Forestry and Technology, Changsha, China
Camellia oleifera Abel, a woody oil plant, that is endemic to China. Tea oil, also referred to as “oriental olive oil,” is a superior quality plant-based cooking oil. The production of tea oil accounts for 8% of the total edible vegetable oil production in the country. Since 2022, the annual output value of C. oleifera industry has exceeded 100 billion yuan, making it one of the major economic contributors to China’s rural revitalization development strategy. In recent years, demand and production have grown in parallel. However, this has led to an increase in the incidence levels of pest and diseases. Pests and diseases significantly reduce the quality and yield of C. oleifera. C. oleifera diseases are mainly caused by pathogenic fungi. C. oleifera anthracnose, soft rot, leaf spot, coal stain, leaf gall disease, and root rot are the most important fungal diseases affecting the C. oleifera industry. However, the same disease may be caused by different pathogenic fungi. C. oleifera can be found in half of China and is found in several climatic zones. The geographical distribution of woody plant diseases is consistent with the distribution of the tree species and the ecology of the range, which also results in a highly complex distribution of fungal diseases of C. oleifera. The management of fungal diseases in C. oleifera is extremely challenging due to the variety of pathogenic fungal species, multiple routes of transmission, the lack of resistant plants, and the environmental safety of chemical measures. The optimal strategy for addressing fungal diseases in C. oleifera is to develop and apply an integrated disease management plan. This review provides a brief overview of the pathogenic species, pathogenesis, pathogenesis, geographical distribution, current management strategies, and potentially new methods of C. oleifera fungal diseases, to provide direction for the development of comprehensive management measures for C. oleifera fungal diseases in the future.
1 Introduction
Camellia oleifera Abel has been cultivated for more than 2,000 years in China, and the domestic cultivation area is the largest in the world (He and He, 2002). In addition, it is minor planting in Japan, South Korea, India, Southeast Asia, and certain Western countries (Aukkanimart et al., 2013; Suealek et al., 2018; He et al., 2021; Teixeira and Sousa, 2021). C. oleifera is a leading industry in China’s rural areas, promoting it enriching the people, and serving as a livelihood industry to combat poverty. Since 2022, the national C. oleifera planting area reached 467 million hectares, with a total output of tea oil of 1 million tons, and a total output value of the industry exceeding 1,160 billion yuan (The Central People’s Government of the People’s Republic of China, 2020; National Forestry and Grassland Administration, 2023). Tea oil boasts high nutritional value and an array of healthcare benefits (Hong et al., 2019; Du et al., 2020), including aging inhibiting, assistance to pregnant women during lactation and postpartum recovery (Zhang et al., 2019), antioxidant (Zhong et al., 2019), anti-cancer properties (Zong et al., 2016; Zheng et al., 2019), among others. In recent years, the Chinese government has strongly supported the expansion of the C. oleifera plantation area to foster the development of the advantageous C. oleifera industry (National Forestry and Grassland Administration, 2021; Central People’s Government of the People’s Republic of China, 2022). However, as the cultivation area of C. oleifera continues to increase, the occurrence of diverse diseases is increasing daily. During previous investigations, it was discovered that plant pathogenic fungi were the primary causes of diseases in C. oleifera. This causes the flower and fruit drop rates of C. oleifera to increase up to 70–80% in certain regions. The traditional diagnosis of diseases in C. oleifera often depends on the practitioner’s experience, with symptoms serving as the sole basis for deducing the disease type. However, the symptoms vary due to different pathogenic fungi in various environments, different stages of infection, and different plant tissues, resulting in confusion in the classification of C. oleifera disease. Researchers were not able to develop the fact that there is a wide range of pathogenic fungi causing C. oleifera disease and that the same disease can be caused by multiple pathogenic fungi until the advent of molecular tools (Zhu and He, 2023). The natural geographic distribution of woody plant diseases is closely linked to the distribution of tree species, pest biota, and ecological environment. C. oleifera is widely grown and can survive in complex environments, therefore, analyzing the distribution of fungal diseases in C. oleifera will aid in the implementation of integrated disease management strategies. Targeted and precise prevention and management of plant diseases is a hot topic of discussion in the academic community, which cannot be achieved without an in-depth investigation into the pathogenic mechanisms of pathogenic fungi. Emerging research findings on the causal fungi of C. oleifera fungal diseases are emerging, which providing a strong basis for new integrated management strategies. The majority of reviews pertaining to fungal diseases in C. oleifera merely provide a brief summary of the conditions under which they occur and the pathogenic fungal species involved, lacking detailed descriptions of their geographical distribution, pathogenic fungi, and the mechanisms of their pathogenicity. Furthermore, as more and more novel pathogenic fungi are identified, it is crucial to promptly summarize these details. The purpose of this review is to provide summary of the present condition of the primary fungal diseases affecting C. oleifera (pathogenic species, geographical distribution, pathogenic mechanisms). Also, discuss potential integrated management strategies for C. oleifera disease in the context of existing research.
2 Current occurrence of major fungal diseases of Camellia oleifera
Common fungal diseases of C. oleifera include anthracnose, soft rot, leaf spot, coal stain, leaf gall, and root rot. These diseases spread rapidly and can cause significant harm, often resulting in the widespread death of C. oleifera in severe cases. Rarely have white rot, scab disease, and algal spot disease of C. oleifera been reported, and systematic studies are lacking. Hence, solely the corresponding pathogenic fungal species are presented in this paper (Table 1).
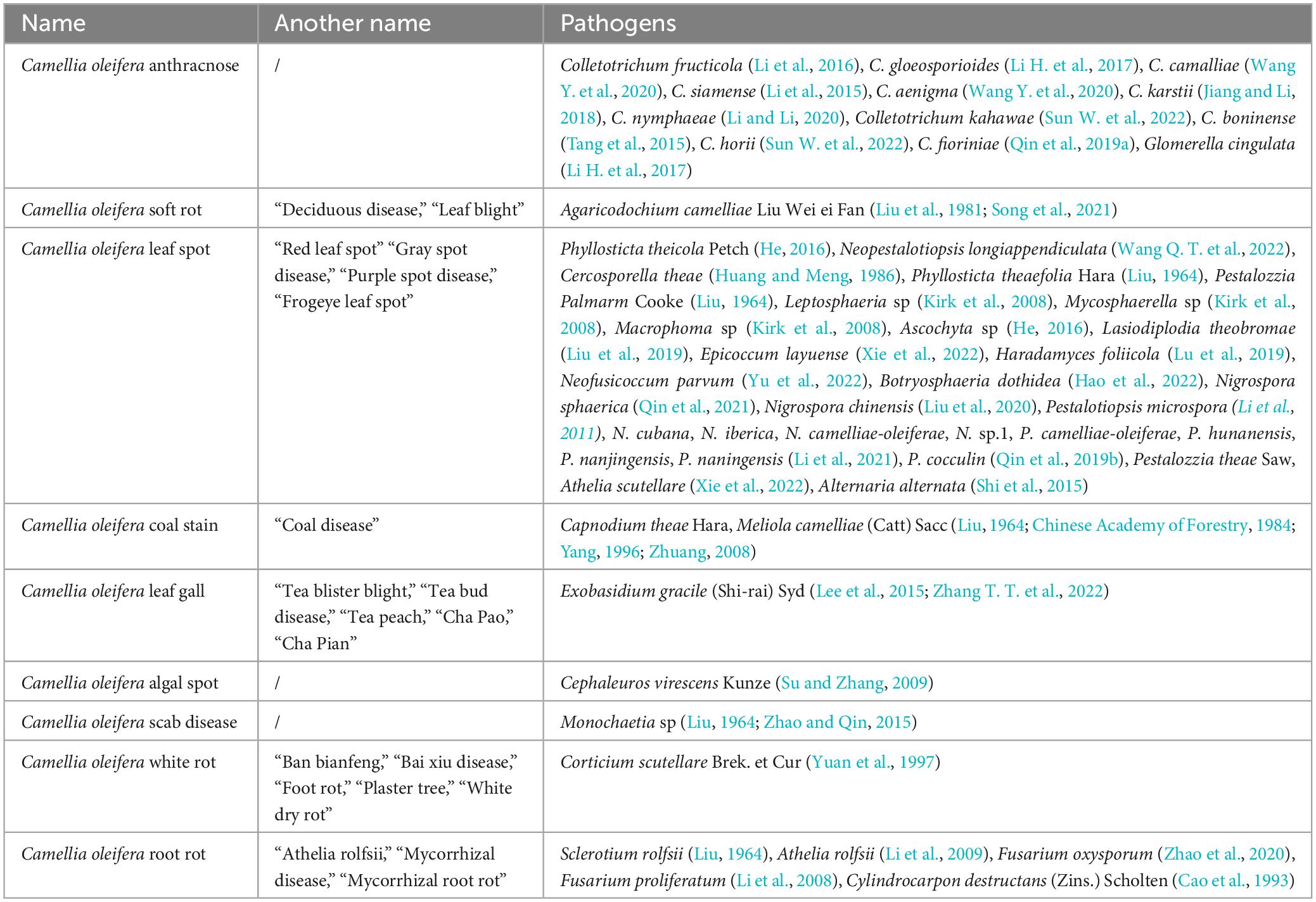
Table 1. Statistics on the names of essential fungal diseases and the types of pathogens in Camellia oleifera.
C. oleifera anthracnose has been the primary disease. It can cause severe fruit drop, fall buds, branch tip dieback, and even whole plant decline. Due to this disease, C. oleifera yields are frequently reduced by 10–30% in all provinces (districts), and by up to 40–80% in heavily affected regions (Zhou, 2023). The fungus of the genus Colletotrichum is the primary cause of C. oleifera anthracnose, with 12 identified species of Colletotrichum (Teleomorph: Glomerella cingulata) capable of infecting C. oleifera (Table 1). Colletotrichum fructicola is the predominant pathogenic fungus that cause anthracnose in C. oleifera, which is widely distributed across all C. oleifera growing areas in China (Li, 2018). Colletotrichum spp typically invades C. oleifera tissues in two forms, one by producing infestation pegs that can directly penetrate the cuticle of C. oleifera, and the other way is by producing bud tubes from conidia or invading stomata with their mycelium (Li, 2021). Colletotrichum spp as a typical semi-living trophic fungus with both living and dead trophic phases. During the live nutrient stage, the structural or functional integrity of the pathogenic fungal infestation is critical in determining it’s to successfully invade plant tissues. However, certain mutants can lose their pathogenicity due to conidia not properly germinating (Liang et al., 2021; Lee et al., 2022). In addition, the formation of the appressorium of plant pathogenic fungi and the strong turgor pressure within it determine the host penetration mediated by compression. Studies have shown that the deletion of autophagy-related proteins CfAtg8 and CfAtg9 leads to a reduction in the melanin layer of the appressorium of C. fructicola, accompanied by cytorrhysis and plasmolysis, ultimately affecting the pathogenicity of the pathogenic fungi (Zhang S. et al., 2022). Necrotrophic stage pathogenic fungi are more likely to interfere with plant immunity or induce plant cell death, which consequently fosters further infestation activity. If important PAMPs (es) or DAMPs (Damage associated molecular patterns) are absent, the causative agent cannot suppress the immune responses to ROS, callose, and hormone accumulation in plant tissues, leading to decreased virulence (Balmer et al., 2013; Sun et al., 2016). Anthracnose follows a distinct seasonal pattern, with the average daily temperature beginning to rise from early April, and the minimum dew-point temperature reaching approximately 24°C, moisture condenses on the leaves, and the pathogen begins to infect the young leaf and tip tissues (Figure 1A; Noriega Cantú et al., 2017). The peak was reached in August, and along with the phenology changes in C. oleifera, a large number of fruits were infected (Figure 1B). As temperatures and air humidity decrease at the end of October, the disease tends to slow down (Jing et al., 2009; Liu, 2012). The mycelia or conidia of the pathogen overwinter in the tissue of the spot, young fruit, or receptacle, serving as the origin of the initial infestation the following year (Liu, 2012; Wang et al., 2013).
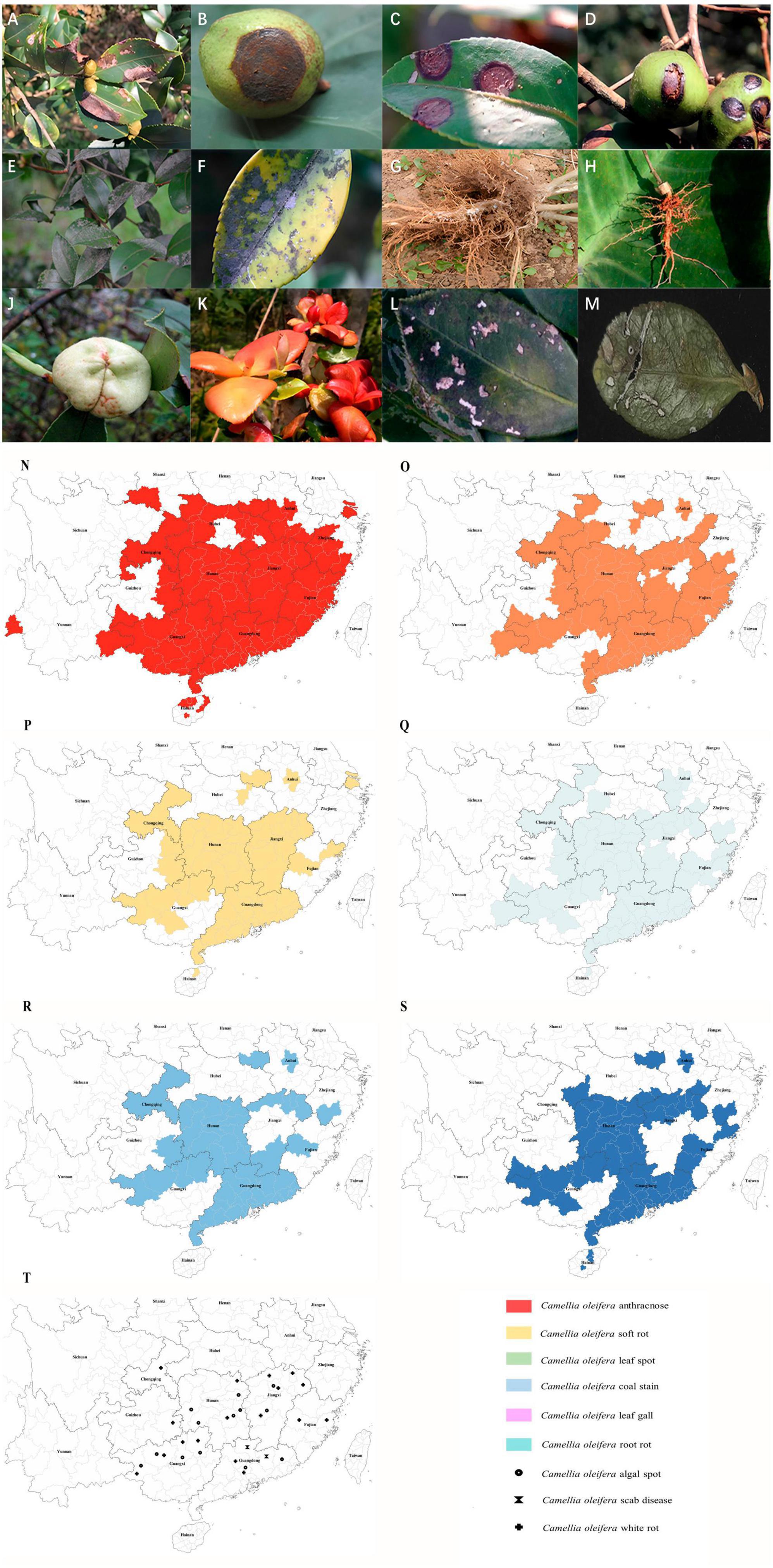
Figure 1. Images showing symptoms of major diseases of Camellia oleifera and geographical distribution of the main fungal diseases. (A) Anthracnose (leaf), (B) Anthracnose (fruit), (C) Soft rot (leaf), (D) Soft rot (fruit), (E,F) Coal stain (leaf), (G,H) Root rot, (J) Leaf gall (fruit), (K) Leaf gall (leaf), (L) Leaf spot (caused by Pestalotiopsis theae), (M) Leaf spot (caused by Neopestalotiopsis longiappendiculata), (N) Distribution of C. oleifera anthracnose, (O) Distribution of C. oleifera soft rot, (P) Distribution of C. oleifera leaf spot, (Q) Distribution of C. oleifera coal stain, (R) Distribution of C. oleifera leaf gall, (S) Distribution of C. oleifera root rot. (T) Distribution of C. oleifera algal spot, white rot and scab disease. Geographical distribution statistical criteria: the disease is mainly controlled or severely damaged in the area. See Supplementary material for reference.
In recent years, the occurrence of C. oleifera soft rot has been frequent and severe. In 1981, Agaricodochium camelliae Liu Wei ei Fan as the causal fungus responsible for causing C. oleifera soft rot (Liu et al., 1981). Later, the morphology of its conidiophores and conidia led to it also being referred to as Myrothecium camelliae (Liu Wei ei Fan) P. K. Chi, Wu ex Lin (Qi and Wei, 1983). In 2013, A. camelliae was included in the National List of Hazardous Pests in Forestry (National Forestry and Grassland Administration, 2013). A. camelliae differs from the majority of plant pathogenic fungi in that its invasion is directly achieved by sporodochium rather than conidia. During an infestation, sporodochium grows parallel brush-like mycelial tufts, invading the plant cells through the stomata or penetrating the epidermis. As the invasion process progresses, the sporodochium gradually shrinks (Wei and Qi, 1982). It mainly impacts the leaves and fruits of C. oleifera. In severe cases, up to 95% of the plants and 50% of the fruits can be affected by the disease (Figures 1C, D; Jiang, 2007; Wang Y. C. et al., 2020). The principal characteristic of the disease is the development of waterlogged state spots that are yellowish-brown or brownish-yellow, mushroom-like sporodochium. During the late period, the spots are scattered with earthy-yellow mushroom-like sporodochium, and the spots rot, causing the diseased fruit to split (Qi and Wei, 1983; Wei and Ma, 2010). The onset of C. oleifera soft rot is affected by the ambient temperature and humidity, with two peak development periods occurring from April to June and October to November each year, when temperatures average between 15–25°C. Temperatures that are too high or too low can hinder the progress of the disease (Lin et al., 1981; Wang Y. C. et al., 2020; Lu, 2021).
Capnodium theae Hara and Meliola camelliae (Catt) Sacc are recognized as the pathogenic fungi that cause C. oleifera coal stain. According to the nutritional mode, some scholars believe that bituminous coal pathogens can be classified into parasitic and saprophytic types (Yamamoto, 1955). As saprophytic type fungi, Ca. theae mainly derives nutrients from nectar secreted by insects. Spore germination and shoot tube elongation occur only in the presence of honeydew from insects or secretions of plants. Stimulation of germination and growth is not achieved through ordinary sugar water or nutrient solutions (Yamamoto, 1952). This type of fungus does not cause direct harm to plant tissues, instead, it covers the surface of branches and leaves, influencing photosynthesis and gas exchange, thus causing plant damage indirectly (Reynolds, 1999). Disease outbreaks caused by this pathogenic fungus are usually accompanied by severe insect infestations (Chomnunti et al., 2014; Khalkho et al., 2021). In contrast, M. camelliae, as a parasitic type, typically infects the extrafloral nectaries region of plant leaves and stems, drawing nutrients from plant tissues to fuel its growth (Mesquita et al., 2022). Severe cases of C. oleifera coal stain have been documented to cause a reduction in yields by 60–70%, which can persist for years to come, ultimately leading to no harvest (Chen, 1980). The key characteristics of C. oleifera coal stain are the presence of black sooty spots on branches and leaves during the initial stages, and black mycelium covering the entire leaf in the later stages (Figures 1E, F). The pathogenic fungi have a preference for cool, highly humid conditions, with the highest prevalence of the disease occurring during the months of March to May and September to November (Yu, 2019).
Camellia oleifera root rot can be subdivided into two categories (Figures 1G, H). Initially, it was believed that Sclerotium rolfsii (Teleomorph: Athelia rolfsii) was the causal agent of C. oleifera root rot (Table 1). The study discovered that S. rolfsii tends to infect the stems of plants close to the soil, but it can also damage various tissues, including shoots, buds, and petioles (Xu et al., 2008; Balamurugan et al., 2022; Thomas and Saravanakumar, 2023). Plant diseases caused by S. rolfsii are therefore also referred to as stem rot or blight. S. rolfsii can be found in soil or soil surface residues in the form of mycelia and sclerotia, utilizing two infection modes to fully colonize, including invading from damaged plant tissues and directly infecting the plant’s soft tissues (Bhuiyan et al., 2019). The pathogenic fungus produces the virulence factor oxalic acid, which inhibits the host’s defenses and causes stomatal opening, ultimately leading to increases transpiration rates and reduced biomass, resulting in wilting (Guimarães and Stotz, 2004; Kabbage et al., 2015). Infected plants of S. rolfsii produce white mycelial mats on the surface of the lesion, which are followed by the formation of globular nuclei either at the infection site or in the surrounding soil (Han et al., 2012; Amaradasa et al., 2020). C. oleifera is predominantly a seedling disease, and infection with S. rolfsii causes lesions to appear near the ground at the base of the stem or at the root base, which indicates a covering of white mycelium and dark-colored pycnidia. The affected seedlings grow poorly, their stem rots, their leaves turn yellow and wilt, and they can be easily pulled up (Huang and Xiao, 2015). Thus far, there have been no reports indicating that S. rolfsii causes harm to the buds, fruits, and other tissues of C. oleifera. In recent years, more Fusarium spp have been isolated and identified from diseased C. oleifera (Table 1). As weak parasites, they specialize in infesting plants that are poorly grown or have a lower resistant. Normally, they are harbored in the soil as saprophytic nutrients or spores that sprout and grow upon stimulated by plant root secretions (Ding et al., 2020). Fusarium spp tends to invade from the root tips of the plant, resulting in symptoms like yellowing of the leaves, reduced leaf size, and plant dwarfism. Some pathogenic fungi enter the vascular bundles and secrete pectinase and cellulase enzymes that degrade cells, and block the transport of water and nutrients. As a result, the plants exhibit symptoms of water loss and wilt (Garcia-Maceira et al., 2001). In addition to this, Fusarium spp secretes toxins, including monocotyledons and fusaric acid, that aid in the rapid colonization of disease-causing fungi by inhibiting protein synthesis, altering plant cell growth, mitochondrial activity, membrane permeability, or inducing programmed cell death in plant cells (Bouizgarne et al., 2006; Jiao et al., 2013). It’s worth noting that the toxin produced by Fusarium spp maintains a high degree of toxicity to humans and animals as well. Accidental ingestion of infected fruits or seeds may pose a life-threatening risk (Qiu et al., 2019). According to survey statistics, the incidence of C. oleifera root rot in seedlings is as high as 50%, and in severe cases, it can reach 30% or more in young stands (Zhao et al., 2020). Sclerotia spp is maintained at 15–25°C for 27–34 days to initiate germination, and higher soil moisture accelerates this process (Clarkson et al., 2004). Similarly, Fusarium spp demonstrated increased pathogenicity at around 25°C, with increased humidity accelerating the development of the disease (Marin et al., 1995; Lui and Kushalappa, 2002). The incidence of both types of root rot peaks during July to August (Li et al., 2008; He, 2016; Ma and Luo, 2019).
Camellia oleifera leaf gall drew attention in the 1940s (Yang, 1941). The pathogenic fungus causing the lesions is Exobasidium gracile (Shi-rai) Syd. Over 170 species of Exobasidium spp are plant pathogens, all of which share the common trait of infecting young, tender plant tissues, leading to the formation of leaf and flower galls (Figures 1J, K; Weille, 1960; Nickerson and Kloet, 1997). Currently only E. gracile is found in C. oleifera (Dong et al., 2019). After infecting the young flower and leaf buds of C. oleifera, the pathogen stimulates tissue proliferation and cell expansion, resulting in the formation of gall bodies (Liu et al., 2012; Huang et al., 2017). Once the flower buds proliferate, they do not produce fruit and seeds, resulting in reduced yields. The proliferation of leaves disrupts the usual process of photosynthesis, consequently leading to reduced yields. Documented that the average incidence of C. oleifera leaf swelling is around 39%, with a maximum of 80% or more causing serious damage (Qiu et al., 2011; Jia et al., 2017). Most Exobasidium spp produce both basidiospores and conidia, although both types of spores are capable of infection. However, conidia are more resilient, and the causal fungus often attaches itself to the tissue, surviving through winter or summer as the initial. The basidiospore is constrained by its structural specificity and serves primarily for dissemination (Ingram et al., 2019). The basidiospores are often present following the onset of disease, thus challenging the prediction of disease occurrence by detecting spores in the air. C. oleifera leaf gall often occurs in early spring, and a warm and humid environment is favorable for the disease. However, when the temperature exceeds 29°C, the growth of the pathogenic fungi is restricted, and the disease occurrence is slowed down (Ingram et al., 2019). Interestingly, C. oleifera leaf gall can reduce yields and affect the plant’s health. However, the galls are edible and have a fresh and sweet taste. In addition, it is rich in nutrients and has been found to have hypoglycemic and anticancer effects (Zhu et al., 2007a,b; Huang et al., 2018).
Camellia oleifera leaf spot generally refers to a disease that cause leaf damage and form “leaf spots,” including anthracnose and soft rot disease. Many different pathogenic fungi can cause these diseases (He, 2016). Leaf spots often lead to the destruction of over 50% of the infected leaves, resulting in serious economic losses (Lu et al., 2019; Qin et al., 2021; Hao et al., 2022; Yu et al., 2022). So far, we have identified 28 pathogenic fungi (excluding anthracnose and soft rot) that can cause C. oleifera leaf spot (Table 1). Pestalotiopsis spp and Neopestalotiopsis spp are the primary pathogenic fungi that cause C. oleifera leaf spot (Figures 1L, M). It is noteworthy that Neopestalotiopsis spp is a novel genus isolated from Pestalotiopsis spp, distinguished by analyses of systematics, sporulation structure, and conidial characteristics (Maharachchikumbura et al., 2014). Pestalotiopsis spp is a common pathogenic fungus, endophyte, and saprophyte. It is interesting to note that certain endophytic Pestalotiopsis spp can transform into a pathogenic phenotype, which can cause harm to the plant under specific conditions (Song, 2016). Conversely, when acting as an endophyte, it not only remains harmless but also produces a diverse array of compounds that assist the plant in resisting other pathogens (Rehman et al., 2022). Similar to other pathogenic fungi, the conidia of Pestalotiopsis spp have a crucial role in their pathogenicity, and any reduction in conidial numbers or functional deficiencies can significantly impact their ability to cause disease (Zhou et al., 2021; Yan et al., 2023). The pathogenic fungus overwinters in the form of mycelia or conidia in diseased leaf tissue and explodes in warm and humid conditions the following year, commonly in June and September, respectively (Liu et al., 2019; Lu et al., 2019). Some pathogenic fungi are more conducive to infestation during low temperatures and rainy weather (Kirk et al., 2008).
3 Geographical distribution of major fungal diseases of C. oleifera
Currently, there are 15 provinces (autonomous regions and municipalities directly under the central government) suitable for the cultivation of C. oleifera (National Forestry and Grassland Administration, 2023), primarily covering tropical monsoon, subtropical monsoon, and temperate monsoon climatic regions (Liu, 2019), all of which are characteristics by high temperature and rainy summer. It is highly conducive to the growth and spread of many pathogenic fungi. At present, C. oleifera anthracnose is currently the most serious disease, covering almost all the suitable areas for C. oleifera (Figure 1N). This is followed by soft rot, coal stain leaf gall, leaf spot, and root rot, which are also widespread (Figures 1O–S). There are only sporadic reports of white rot, scab disease, and algal spot (Figure 1T). C. oleifera leaf spot is difficult to distinguish because of the many different pathogenic fungi and the disease is likely to be confused with other foliar diseases. The actual extent of the real occurrence might be much larger than the areas depicted on the map, and we speculate that similar to anthracnose, it is present in all crucial production regions of C. oleifera in China. Due to global warming, the average temperature has increased, providing more opportunities for pathogens to thrive in the future (Roos et al., 2011). This means that more C. oleifera producing areas in China will be affected by more diseases in the future. C. oleifera plantation area in China is rapidly expanding, which also poses unprecedented challenges for disease management. According to the present statistical results, there are no significant geographical variations in the distribution of pathogenic fungi. Moreover, multiple pathogenic fungi can often be identified in C. oleifera stands, leading to the concurrence of several simultaneous diseases.
4 Management of fungal diseases in Camellia oleifera
Currently, the demand for tea oil and the associated quality requirements are on the rise in the international market, and there is also growing concern over domestic food safety. The development of the C. oleifera industry needs to maintain the excellent quality of tea oil while addressing the need to increase production significantly. To ensure the long-term health of C. oleifera forests, guarantee the high quality of tea oil, and prevent environmental pollution. It is necessary to establish a pollution-free management technology system that is founded on ecological management technology. Taking into account the entire ecosystem of the C. oleifera forest, we should establish an accurate monitoring and forecasting system, select and breed disease-resistant varieties, develop biological control techniques, and utilize chemical pesticides rationally to carry out integrated management. Both to management diseases in C. oleifera below the permissible economic threshold and to ensure the high and stable production of pollution-free tea oil. Currently, the management of C. oleifera diseases is primarily conducted through traditional chemical methods. The use of broad-spectrum fungicides increases both the cost of production and the risk of disease-causing fungal resistance. Although there are numerous methods for the prevention and control of plant diseases, each one possesses advantages, disadvantages, and limitations. Practice has demonstrated that no single control measure for any disease can entirely and efficiently address the issue of damage. Only by adapting measures to local conditions and implementing integrated disease management (IDM) can we achieve the best control effect by leveraging the strengths of various measures and addressing their shortcomings. However, the development of new methods and technologies for managing C. oleifera fungal disease is seriously lagging, which is not conducive to the sustainable development of the industry. In the context of C. oleifera fungal disease patterns and the pathogenic mechanisms of the causal fungi, we will discuss several potential management strategies.
4.1 Selection and breeding of disease-resistant varieties
Cultivating varieties that are resistant to diseases is considered the best way to manage plant diseases in the long term (de Resende et al., 2021). Crossbreeding and systematic selection are frequently employed in the selection of new C. oleifera varieties, while traditional selection methods, which are labor-intensive, time-consuming, and highly dependent on environmental conditions, have demonstrated limitations in the development of broad-spectrum disease-resistant varieties. Indeed, newly improved varieties are often unable to adapt to the frequent emergence of new virulent microspecies of pathogens. With the development of biotechnology and molecular biology, the use of molecular breeding can overcome the limitations imposed by traditional breeding. The technique of molecular marker-assisted selection (MAS) has found extensive application in variety selection. Molecular markers can improve the efficiency and precision of genetic analyses, particularly in cases where multiple resistance genes are present in a single variety (Paterson et al., 1991). The disease resistance (R) gene is characteristics by specific evolution, enables plants to recognize pathogen-specific Avr genes, initiate signal transduction to activate defense capabilities, and naturally become an ideal target. The causal fungi that cause C. oleifera disease typically complete the infestation through mechanical invasion, toxin production, or induction of cell death. However, R genes can prevent the invasion of pathogenic fungi by regulating the strength of structures such as the plant cell wall and callose in a relative manner (Bacete et al., 2020); Altering the target or direct detoxification of toxins secreted by pathogenic fungi (Wang et al., 2021); Scavenging excessively accumulated ROS while inhibiting cell death (Navarrete et al., 2022). R genes improve disease resistance in plants in various ways. Moreover, the proteins encoded by disease-resistance genes have much in common, which opens up the possibility of selecting broad-spectrum disease-resistant varieties. The mining of R genes within the C. oleifera genome is bound to significantly assist in the identification of C. oleifera varieties that possess broad-spectrum disease resistance. Naturally, the publication of genome-wide data for C. oleifera will make this task much easier (Lin et al., 2022). In addition to mining disease-resistance genes, another future task that needs to be completed is the construction of a mature C. oleifera tissue culture system. This will significantly shorten the selection cycle and minimize the impact on the environment. It has been reported that treating healing tissues with pathogenic fungal toxins can also induce plants to develop corresponding resistance under selection pressure (Hui et al., 2003). Furthermore, another method to obtain precisely resistant varieties is by utilizing tissue culture technology and gene editing technology to introduce genes encoding antimicrobial proteins into C. oleifera, resulting in the construction of disease-resistant transgenic plants. However, some legal, ethical, and experimental issues continue to hinder the commercial utilization of transgenic plants (Fritsche et al., 2018). However, few key disease resistance genes have been identified in C. oleifera, and there is a lack of a mature genetic transformation system. Currently, precise cultivation of plants with targeted resistance poses a challenge.
4.2 Establishing an accurate monitoring and forecasting system
Fungal diseases of C. oleifera can be said to be an important reason for the loss of C. oleifera yield. Therefore, accurate modeling and prediction of such diseases can facilitate advance understanding of the time and severity of disease outbreaks. The majority of pathogenic fungi, that cause C. oleifera diseases, are disseminated through the medium of spores. Pathogenic fungi produce a significant quantity of spores on affected tissues, including diseased branches, leaves, and fruits, which can then spread via air currents or animals or human activities, frequently leading to widespread disease outbreaks. As a result, the presence and variety of spores in the air are strongly linked to the incidence of diseases (Volz, 1997; Suffert et al., 2011; Mukherjee et al., 2021). The spore capture technology can collect and detect spores of pathogenic fungi in the air of a given area, and simultaneously integrate them with the phenological phase of C. oleifera and the corresponding meteorological factors. It can accurately predict and analyze the period and degree of occurrence of disease, as well as their spatial and temporal variations, in C. oleifera forest regions (Redondo et al., 2020; Galagedara et al., 2021; Miller et al., 2022). Initially, spore trapping techniques were frequently employed in conjunction with microscopic examination to identify and quantify spores, but the method entails a high degree of detection error, such that even seasoned professionals cannot ensure a high degree of accuracy. Moreover, the form and size of the spores of certain pathogenic fungi may vary over time. The qPCR technique is deemed to be the most dependable technique for measuring the relative levels of genes in various samples simultaneously, thanks to its high efficiency and sensitivity (Galli et al., 2015; Machado et al., 2015). Several studies have successfully combined spore-trapping techniques with qPCR to analyze the environmental spore densities of various plant pathogens (Dvořák et al., 2017; Quesada et al., 2018). It is noteworthy that a diverse array of fungal spores coexist in the air, encompassing non-pathogenic spores, and in addition, spore densities are lower in the air. Therefore, the specificity and sensitivity of qPCR protocols for the detection and quantification of C. oleifera pathogenic fungi spores are critical. The fungal ITS sequence is a suitable choice for the specificity requirement as it experiences minimal natural selection pressure during evolution and can accommodate greater variation. To achieve the best possible detection, the sensitivity can be optimized by optimizing primer sequences, DNA extraction techniques, and other factors. At present, there have been studies on high-precision detection technology for some C. oleifera diseases (Song, 2012), which provides support for improving the monitoring and prediction system for C. oleifera diseases. As a soil-borne disease, C. oleifera root rot is caused by a fungus that does not rely on conidia as its primary mode of transmission, making it unpredictable through the methods described above. Root rot is a so insidious disease, causing symptoms to go unnoticed in its early stages, and once the typical symptoms surface, they are often irreversible. Although the initial symptoms of root rot are not obvious, subtle changes in tissue metabolic pathways occur when plant tissues are attacked by the causal fungus. The NIR broad-spectrum method can rapidly identify this nuanced difference to diagnose the health status of the plant, making it effective for the early detection of plant root rot (Calamita et al., 2021). However, little research has been conducted on the differential changes in tissue metabolic pathways following infection by pathogenic fungi in C. oleifera, hindering the promotion and application of this technology in monitoring fungal diseases in this plant.
4.3 Use of biological control technology
Biological control is one of the practices of integrated plant disease management that uses biological agents to suppress the growth of pathogens, thereby preventing the development of plant disease (O’Brien, 2017; Kohl et al., 2019). These include the utilization of microbial antagonists like bacteria, fungi, viruses, or nematodes for disease suppression (Heydari and Pessarakli, 2010). Multiple studies have indicated that microorganisms isolated from the surface or inner part of plant tissues, as well as inter-root soil, can inhibit the growth of pathogenic bacteria, compete for colonization, or improve plant resistance (Dong et al., 2022; Kong et al., 2022; Sun Z. Z. et al., 2022; Zhou et al., 2022). Against Colletotrichum spp, a multitude of antagonistic microorganisms secrete extracellular enzymes, antibiotics, or volatile compounds that trigger mycelial disintegration, shrinkage, or abnormal conidial development of the pathogenic fungus, thus preventing its infestation (Bonatelli et al., 2016; Won et al., 2019). Furthermore, some antagonistic microorganisms can prevent disease by disrupting the cell wall structure of the parasitic mycelia (Alvindia, 2018). When selecting antagonistic microorganisms, it is important to consider the ability to colonize as well. The working principle of such antagonistic microorganisms is rapid dominance in plant tissues, access to more nutrients, and the simultaneous synthesis of more inhibitory substances. Moreover, possessing greater athleticism and the capability to ability to adapt to harsh environmental conditions is a necessary prerequisite for achieving success (Carvajal et al., 2002; Capdevila et al., 2004). Improving the plant’s resistance can better handle diverse disease-causing fungi. Undoubtedly, using beneficial microorganisms to indirectly improve the resistance of C. oleifera is an effective method in the practical setting of lacking highly resistant C. oleifera varieties. Trichoderma fungi and Bacillus bacteria are often applied for biological control. They can enhance plant growth by improving the soil environment or producing a range of secondary metabolites to stimulate the plant’s defense mechanism, thereby improving the plant’s resistance to diseases (Perelló and Bello, 2011; Miljakovic et al., 2020; Asad, 2022). In addition, the application of fungus-feeding nematodes has been found to effectively decrease the progression of soil-borne diseases (Hasna et al., 2008), and mycoviruses to control the incidence of sclerotinia blight (Jiang et al., 2013). The control techniques presented hold immense potential for managing fungal diseases of C. oleifera. The key to the effectiveness of microbial antagonists lies in obtaining high-quality microbial that have strong environmental adaptability and athletics, while maintaining good antibacterial activity. This results in an extremely high cost for the preparation and development of microbial agents. At the same time, the use of pharmaceuticals necessitates professional training. Therefore, high human and economic costs are one of the key constraints to the diffusion of microbial antagonists. In addition to microbial antagonists, plant-derived fungicides are also highly sought after by people. Many phytochemicals have the potential to suppress the progression of plant fungal diseases (Cho et al., 2007; Gahukar, 2018). Plant-derived fungicides have the characteristics of low toxicity to humans and can be rapidly degraded into non-toxic substances under natural conditions. The study discovered that aldehydes, phenols, alkaloids, and other substances derived from plants can impact the development of numerous plant pathogenic fungi, including Fusarium spp, Colletotrichum spp, and Sclerotinia spp (Cho et al., 2007). For example, cinnamaldehyde can inhibit the synthesis of chitin in fungal cell walls and inhibit the infection activity of F. solani (Wang J. et al., 2022); Berberine has the ability to suppress the growth of various pathogenic fungi, including C. capsici and Pyricularia oryzae Cav. by inhibiting the growth of fungal hyphae and the germination of spores (Singh et al., 2001; Kokkrua et al., 2019). Despite the fact that botanical fungicides have been used in production, their development has been hindered by factors such as unstable properties, susceptibility to degradation, and slow efficacy.
4.4 Reasonable use of chemical pesticides
In production practice, Fungicides such as carbendazim, Bordeaux mixture and thiophanate methyl are frequently employed to manage fungal diseases of C. oleifera. The indiscriminate use of these chemicals poses risks, including chemical residue transfer (Li Y. H. et al., 2017; Zhou et al., 2018) and severe plant toxicity (Gaikwad and Nimbalkar, 2005). They remain toxic to animals, animal embryos, and even humans (Fimognari et al., 1999; Ellis et al., 2010; Baurand et al., 2016). Apart from the safety issue, drug resistance is also a problem that cannot be disregarded. One of the key factors in the emergence of resistance is the reduction in protein affinity that is targeted by fungicides, resulting from a single target of drug action and a single gene mutation (e.g., carbendazim, thiophanate-methyl). Current chemical management methods, often in the disease-prone period of centralized application, and with a variety of agents used in combination, not only improve the efficiency of the use of chemical pesticides but also reduce the risk of drug resistance (Mueller et al., 2004). Fungicide resistance management is essential to slowing the emergence and spread of resistance for as long as possible while maintaining the necessary level of disease management. Developing a scientific pesticide application plan for the daily management of C. oleifera disease to reduce various risks is urgently needed in this regard. During the development of new chemical agents, the captivation of plant resistance using plant immune inducers can effectively achieve disease resistance (Caffi and Rossi, 2018). Immune inducers are mostly plant hormones or active small molecules produced during the interaction between pathogens and their hosts (Bektas and Eulgem, 2014), which do not induce resistance in disease-causing fungi as they do not significantly inhibit the pathogenic fungi themselves. They are also environmentally friendly with low toxicity to humans and animals, and can be used at low concentrations with a wide range of effects. The comprehensive and flexible application of plant immune inducers will facilitate the discovery of potential solutions to challenges related to food safety and agrochemicals (Guo et al., 2021). As a “vaccine” for plants, the development of drugs for immune inducers requires the search for corresponding “antigens,” which cannot be separated from the study of pathogenic mechanisms of pathogenic fungi. At present, the research on the pathogenic mechanism of pathogenic fungi in C. oleifera is still in its infancy, and the development of corresponding immune inducers is still very difficult.
5 Conclusion and outlook
This review provides a more detailed understanding of C. oleifera diseases by describing the species, geographic distribution, and pathogenesis of the major fungal diseases (Figure 2). Among the numerous fungal diseases of C. oleifera, anthracnose caused by Colletotrichum spp has the widest distribution range. Moreover, Colletotrichum spp possesses numerous hosts and exhibits robust destructive power, often leading to cross-infection of different plants in the same forest (Eaton et al., 2021; Sharma et al., 2022). Therefore, Camellia anthracnose is the key object of prevention in daily production. The causal fungi responsible for fungal diseases of C. oleifera are diverse, with multiple diseases co-occurring, but there is no evidence of significant geographic variation in them. Therefore, the management of C. oleifera fungal diseases in all regions needs to maintain a high level of attention to multiple diseases at the same time. A comprehensive examination of the pathogenesis of each disease can facilitate the development of more effective targeted prevention and management strategies. As an example, C. oleifera root rot, as a soil-borne disease, is greatly limited in its ability to spread by the soil environment, with the causal fungus tending to only attack poorly healthy and young C. oleifera plants. Therefore, by implementing effective fertilizer management strategies to maintain seedlings healthy, along with regular disinfecting the of soil in the seedbed, C. oleifera root rot can be effectively management.
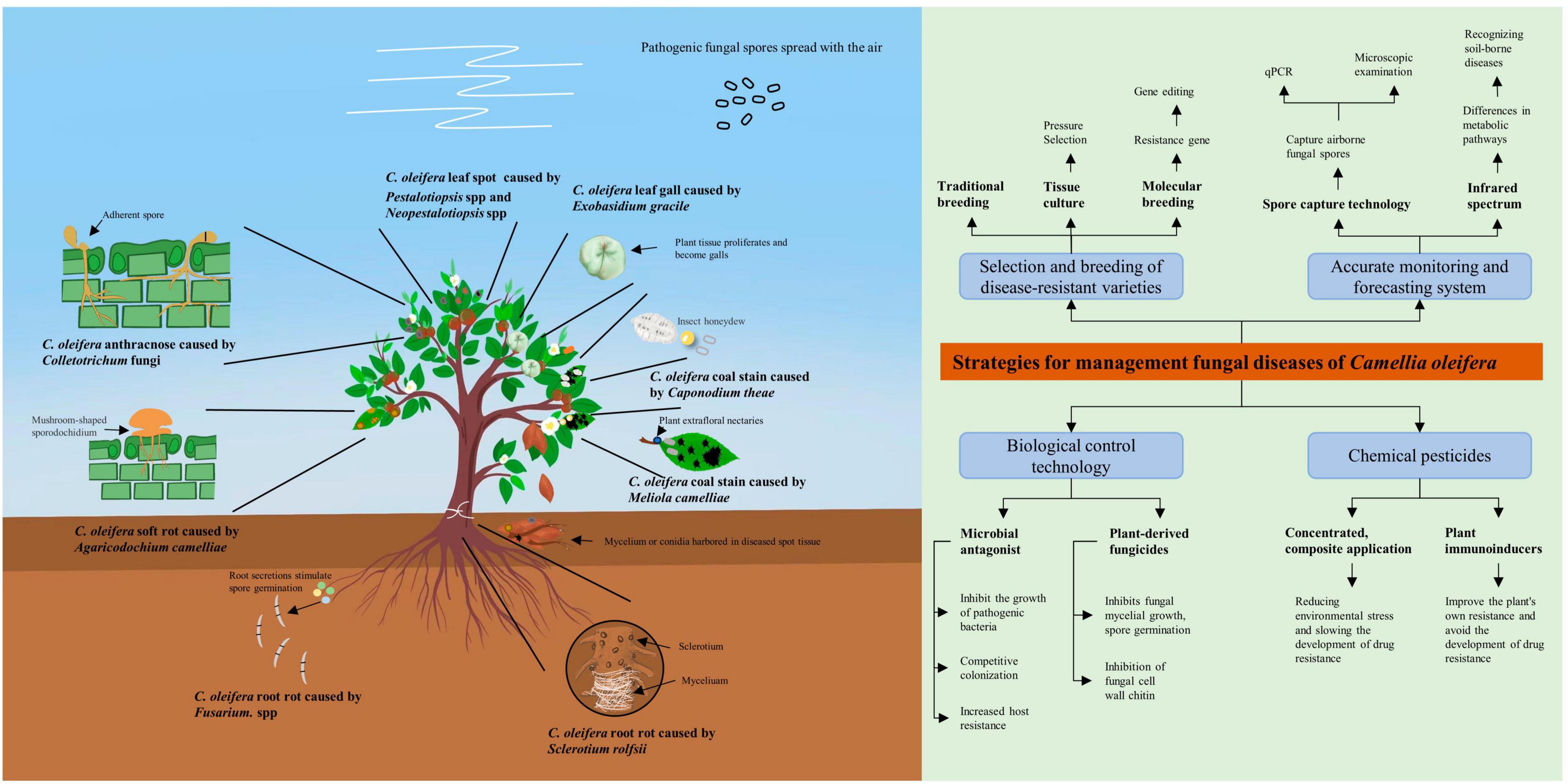
Figure 2. Schematic diagram of pathogenic bacteria infesting Camellia oleifera and integrated disease management strategies. This figure serves as an image summary outlining the basic elements of the literature review.
The management of fungal diseases in C. oleifera needs to combine a variety of measures. A single chemical management measure is often ineffective in suppressing the disease and plants are subjected to combined attacks by multiple pathogenic fungi (Yimer et al., 2018). The traditional methods of prevention and management have limitations. For example, an integrated management strategy that prioritizes selects of resistant varieties and combines them with rational chemical methods and cultivation measures can be more effective in minimizing losses caused by plant spotted wilt diseases (Culbreath et al., 2003). However, the present strategies for managing C. oleifera fungal disease remains relatively homogenous, and the advancement of new management strategies is inseparable from fundamental research on C. oleifera and the fungi responsible for causing the disease. Recent research should therefore focus on, but not be limited to, the following two areas. Firstly, the selection of resistant varieties is the best option to maximize the benefits of production. Not only is it environmentally friendly, but it’s also long-lasting. Therefore, it is worth identifying disease-resistance genes in C. oleifera. Secondly, a comprehensive understanding of the epidemiology and pathogenesis of various diseases, along with targeted early administration of drugs and the use of compound agents, would significantly reduce production costs and environmental hazards. This would also facilitate the establishment of accurate monitoring and prediction systems. Similarly, developing “specific drugs” for different diseases also requires a sufficient understanding of the pathogenic mechanisms of fungi. In addition, an in-depth understanding of the interactions between C. oleifera and pathogenic fungi will facilitate the development of highly effective targeting agents. For example, the development of plant immune inducers requires understanding the immune response mechanisms of C. oleifera in response to different pathogenic fungi, targeted stimulation of key gene regulation, and can effectively improve the overall disease resistance of C. oleifera without the risk of drug resistance. Furthermore, continuously screening high-quality microbial resources with the potential to develop microbial antagonists, establishing a database of plant-derived fungicide resources, and enhancing and optimizing extraction processes are directions of research that demand ongoing attention.
Author contributions
XC, GZ, and JL contributed to the conception of the manuscript. ZW, YH, AN, YX, and DZ contributed to the data analysis. All authors have read and agreed to the published version of the manuscript.
Funding
This research was funded by the National Natural Science Foundation of China (32271900) and the Hunan Provincial Innovation Foundation for Postgraduate (CX20230748).
Conflict of interest
The authors declare that the research was conducted in the absence of any commercial or financial relationships that could be construed as a potential conflict of interest.
Publisher’s note
All claims expressed in this article are solely those of the authors and do not necessarily represent those of their affiliated organizations, or those of the publisher, the editors and the reviewers. Any product that may be evaluated in this article, or claim that may be made by its manufacturer, is not guaranteed or endorsed by the publisher.
Supplementary material
The Supplementary Material for this article can be found online at: https://www.frontiersin.org/articles/10.3389/fmicb.2023.1215024/full#supplementary-material
References
Alvindia, D. G. (2018). The antagonistic action of Trichoderma harzianumstrain DGA01 against anthracnose-causing pathogen in mango cv. ‘Carabao’. Biocontrol Sci. Technol. 28, 591–602. doi: 10.1080/09583157.2018.1468998
Amaradasa, B. S., Turner, A., Lowman, S., and Mei, C. (2020). First report of southern blight caused by Sclerotium rolfsii in industrial hemp in southern virginia. Plant Dis. 104, 1563–1563. doi: 10.1094/pdis-10-19-2157-pdn
Asad, S. A. (2022). Mechanisms of action and biocontrol potential of Trichoderma against fungal plant diseases - A review. Ecol. Complexity 49:100978. doi: 10.1016/j.ecocom.2021.100978
Aukkanimart, R., Boonmars, T., Pinlaor, S., Tesana, S., Aunpromma, S., Booyarat, C., et al. (2013). Histopathological changes in tissues of Bithynia siamensis goniomphalos incubated in crude extracts of camellia seed and mangosteen pericarp. Korean J. Parasitol. 51, 537–544. doi: 10.3347/kjp.2013.51.5.537
Bacete, L., Melida, H., Lopez, G., Dabos, P., Tremousaygue, D., Denance, N., et al. (2020). Arabidopsis response regulator 6 (ARR6) modulates plant cell-wall composition and disease resistance. Mol. Plant Microbe Interact. 33, 767–780. doi: 10.1094/MPMI-12-19-0341-R
Balamurugan, A., Ashajyothi, M., Charishma, K., Prakash, G., and Kumar, A. (2022). Athelia rolfsii causing sprout and shoot rot of sugarcane in India. Aust. Plant Pathol. 51, 597–600. doi: 10.1007/s13313-022-00887-4
Balmer, D., de Papajewski, D. V., Planchamp, C., Glauser, G., and Mauch-Mani, B. (2013). Induced resistance in maize is based on organ-specific defence responses. Plant J. 74, 213–225. doi: 10.1111/tpj.12114
Baurand, P. E., Dallinger, R., Capelli, N., and de Vaufleury, A. (2016). Metallothionein gene expression in embryos of the terrestrial snail (Cantareus aspersus) exposed to cadmium and copper in the Bordeaux mixture. Environ. Sci. Pollut. Res. 23, 3068–3072. doi: 10.1007/s11356-015-5649-2
Bektas, Y., and Eulgem, T. (2014). Synthetic plant defense elicitors. Front. Plant Sci. 5:804. doi: 10.3389/fpls.2014.00804
Bhuiyan, S. A., Wickramasinghe, P., Mudge, S. R., Adhikari, P., and Magarey, R. C. (2019). Athelia rolfsii causes sett rots and germination failure in sugarcane (Saccharum hybrid): Pathogenicity and symptomatology. Australas. Plant Pathol. 48, 473–483. doi: 10.1007/s13313-019-00648-w
Bonatelli, M. L., Tsui, S., Marcon, J., Batista, B. D., Kitajima, E. W., Pereira, J. O., et al. (2016). Antagonistic activity of fungi from anthracnose lesions on paullinia cupana aganist Colletotrichum sp. J. Plant Pathol. 98, 197–205. doi: 10.4454/Jpp.V98i2.029
Bouizgarne, B., El-Maarouf-Bouteau, H., Frankart, C., Reboutier, D., Madiona, K., Pennarun, A. M., et al. (2006). Early physiological responses of Arabidopsis thaliana cells to fusaric acid: Toxic and signalling effects. New Phytol. 169, 209–218. doi: 10.1111/j.1469-8137.2005.01561.x
Caffi, T., and Rossi, V. (2018). Fungicide models are key components of multiple modelling approaches for decision-making in crop protection. Phytopathol. Mediterr. 57, 153–169. doi: 10.14601/Phytopathol_Mediterr-22471
Calamita, F., Imran, H. A., Vescovo, L., Mekhalfi, M. L., and La Porta, N. (2021). Early identification of root rot disease by using hyperspectral reflectance: The case of pathosystem Grapevine/Armillaria. Remote Sensing 13:2436 doi: 10.3390/rs13132436
Camacho Carvajal, M. M., Wijfjes, A. H., Mulders, I. H., Lugtenberg, B. J., and Bloemberg, G. V. (2002). Characterization of NADH dehydrogenases of Pseudomonas fluorescens WCS365 and their role in competitive root colonization. Mol. Plant Microbe Interact. 15, 662–671. doi: 10.1094/MPMI.2002.15.7.662
Cao, F. X., Wu, G. J., Tian, Z. R., Hu, Q. F., Zhang, C. X., and Jiang, G. B. (1993). Research on the pathogenesis of root rot of non-wood forest research. Non-wood Forest Res. S1, 323–326. doi: 10.14067/j.cnki.1003-8981.1993.s1.080
Capdevila, S., Martinez-Granero, F. M., Sanchez-Contreras, M., Rivilla, R., and Martin, M. (2004). Analysis of Pseudomonas fluorescens F113 genes implicated in flagellar filament synthesis and their role in competitive root colonization. Microbiology 150(Pt 11), 3889–3897. doi: 10.1099/mic.0.27362-0
Central People’s Government of the People’s Republic of China (2022). 2022 Central Document No. 1 [Online]. Bejing: Central People’s Government of the People’s Republic of China.
Chen, H. L. (1980). Preliminary report of a trial on the control of coal stain on oil tea by dried lego coating. J. Zhejiang For. Sci. Technol. 18, 2–4.
Chinese Academy of Forestry (1984). China Forest Diseases. Beijing: China Forestry Publishing House.
Cho, J. Y., Choi, G. J., Son, S. W., Jang, K. S., Lim, H. K., Lee, S. O., et al. (2007). Isolation and antifungal activity of lignans from Myristica fragrans against various plant pathogenic fungi. Pest Manage. Sci. 63, 935–940. doi: 10.1002/ps.1420
Chomnunti, P., Hongsanan, S., Aguirre-Hudson, B., Tian, Q., Persoh, D., Dhami, M. K., et al. (2014). The sooty moulds. Fungal Divers. 66, 1–36. doi: 10.1007/s13225-014-0278-5
Clarkson, J. P., Phelps, K., Whipps, J. M., Young, C. S., Smith, J. A., and Watling, M. (2004). Forecasting sclerotinia disease on lettuce: Toward developing a prediction model for carpogenic germination of sclerotia. Phytopathology 94, 268–279. doi: 10.1094/phyto.2004.94.3.268
Culbreath, A. K., Todd, J. W., and Brown, S. L. (2003). Epidemiology and management of tomato spotted wilt in peanut. Annu. Rev. Phytopathol. 41, 53–75. doi: 10.1146/annurev.phyto.41.052002.095522
de Resende, M. L. V., Pozza, E. A., Reichel, T., and Botelho, D. M. S. (2021). Strategies for coffee leaf rust management in organic crop systems. Agronomy-Basel 11:14. doi: 10.3390/agronomy11091865
Weille, A. G. (1960). Blister blight (Exobasidium vexans) in tea and its relationship with environmental conditions. Neth. J. Agric. Sci. 8, 183–210.
Khalkho, A., Nand Rai, A., and Bhardwaj, S. (2021). Capnodium variegatum – a new foliicolous species of sooty mould infecting Bauhinia Variegata L. from chhattisgarh, India. Plant Arch. 21, 218–225. doi: 10.51470/PLANTARCHIVES.2021.v21.no1.250
Ding, Y., Gardiner, D. M., Xiao, D., and Kazan, K. (2020). Regulators of nitric oxide signaling triggered by host perception in a plant pathogen. Proc. Natl. Acad. 117, 11147–11157. doi: 10.1073/pnas.1918977117
Dong, Y. J., Tang, B. B., He, M. M., Wang, L. L., Wu, K., Yang, S. X., et al. (2022). High concentrations of antagonistic bacterial strains from diseased sanqi ginseng rhizosphere suppressed Fusarium root rot. Eur. J. Plant Pathol. 163, 143–153. doi: 10.1007/s10658-022-02463-4
Dong, Z., Liu, W., Zhou, D., Li, P., Wang, T., Sun, K., et al. (2019). Bioactive exopolysaccharides reveal Camellia oleifera infected by the fungus exobasidium gracile could have a functional use. Molecules 24:2048. doi: 10.3390/molecules24112048
Du, M., Hu, L., Fang, X., and Zhang, J. (2020). Anti-hypertensive effects of blended Camellia oleifera Abel oil and eucommia extract on SHR mice. Open J. For. 10, 1–6. doi: 10.4236/ojf.2020.101001
Dvořák, M., Janoš, P., Botella, L., Rotková, G., and Zas, R. (2017). Spore dispersal patterns of Fusarium circinatum on an infested monterey pine forest in North-western Spain. Forests 8:432. doi: 10.3390/f8110432
Eaton, M. J., Edwards, S., Inocencio, H. A., Machado, F. J., Nuckles, E. M., Farman, M., et al. (2021). Diversity and cross-infection potential of Colletotrichum causing fruit rots in mixed-fruit orchards in kentucky. Plant Dis. 105, 1115–1128. doi: 10.1094/pdis-06-20-1273-re
Ellis, S. R., Hodson, M. E., and Wege, P. (2010). Determining the influence of rainfall patterns and carbendazim on the surface activity of the earthworm Lumbricus terrestris. Environ. Toxicol. Chem. 29, 1821–1827. doi: 10.1002/etc.219
Fimognari, C., Nusse, M., and Hrelia, P. (1999). Flow cytometric analysis of genetic damage, effect on cell cycle progression, and apoptosis by thiophanate-methyl in human lymphocytes. Environ. Mol. Mutagen. 33, 173–176.
Fritsche, S., Poovaiah, C., MacRae, E., and Thorlby, G. (2018). A new zealand perspective on the application and regulation of gene editing. Front. Plant Sci. 9:1323. doi: 10.3389/fpls.2018.01323
Gahukar, R. T. (2018). Management of pests and diseases of important tropical/subtropical medicinal and aromatic plants: A review. J. Appl. Res. Med. Aromat. Plants. 9, 1–18. doi: 10.1016/j.jarmap.2018.03.002
Gaikwad, A. P., and Nimbalkar, C. A. (2005). Phytotoxicity of copper fungicides to guava fruits. J. Environ. Biol. 26, 155–156.
Galagedara, N., Doyle, V., Price, P., Robertson, C. L., and Thomas-Sharma, S. (2021). Comparing the efficiency of spore traps to capture airborne inoculum of Cercospora spp. on soybean. Phytopathology 111, 29–29.
Galli, V., Borowski, J. M., Perin, E. C., Messias, R. D., Labonde, J., Pereira, I. D., et al. (2015). Validation of reference genes for accurate normalization of gene expression for real time-quantitative PCR in strawberry fruits using different cultivars and osmotic stresses. Gene 554, 205–214. doi: 10.1016/j.gene.2014.10.049
Garcia-Maceira, F. I., Di Pietro, A., Huertas-Gonzalez, M. D., Ruiz-Roldan, M. C., and Roncero, M. I. (2001). Molecular characterization of an endopolygalacturonase from Fusarium oxysporum expressed during early stages of infection. Appl. Environ. Microbiol. 67, 2191–2196. doi: 10.1128/AEM.67.5.2191-2196.2001
Guimarães, R. L., and Stotz, H. U. (2004). Oxalate production by sclerotinia sclerotiorum deregulates guard cells during infection. Plant Physiol. 136, 3703–3711. doi: 10.1104/pp.104.049650
Guo, S. X., He, F., Song, B. A., and Wu, J. (2021). Future direction of agrochemical development for plant disease in China. Food Energy Secur. 10:e293. doi: 10.1002/fes3.293
Han, K. S., Lee, S. C., Lee, J. S., Soh, J. W., and Kim, S. (2012). First report of sclerotium rot on cymbidium orchids caused by Sclerotium rolfsii in Korea. Mycobiology 40, 263–264. doi: 10.5941/MYCO.2012.40.4.263
Hao, Y., Liao, K., Guo, J., Jin, C., Guo, K., and Chen, M. (2022). First report of Botryosphaeria dothidea causing leaf spot of Camellia oleifera in China. Plant Dis. [Online ahead of print]. doi: 10.1094/PDIS-06-22-1452-PDN
Hasna, M. K., Lagerlöf, J., and Rämert, B. (2008). Effects of fungivorous nematodes on corky root disease of tomato grown in compost-amended soil. Acta Agric. Scand., Sect. B. 58, 145–153. doi: 10.1080/09064710701412767
He, F., and He, B. (2002). Cultural distribution and site classification for Camellia oleifera. Sci. Silvae Sin. 38, 64–72.
He, X. Y. (2016). The Illustrated Handbook of Diseases and Insects of Oil-tea Camellia. Beijing: Science Press.
He, Y.-C., Wu, M.-J., Lei, X.-L., Yang, J.-F., Gao, W., Soo, B. Y., et al. (2021). Gallotannins from nut shell extractives of Camellia oleifera. J. Korean Wood Sci. Technol. 49, 267–273.
Heydari, A., and Pessarakli, M. (2010). A review on biological control of fungal plant pathogens using microbial antagonists. J. Biol. Sci. 10, 273–290. doi: 10.3923/jbs.2010.273.290
Hong, C., Chang, C., Zhang, H., Jin, Q., Wu, G., and Wang, X. (2019). Identification and characterization of polyphenols in different varieties of Camellia oleifera seed cakes by UPLC-QTOF-MS. Food Res. Int. 126:108614. doi: 10.1016/j.foodres.2019.108614
Huang, C. W., and Xiao, C. Y. (2015). Characteristics of the occurrence and control trials of root rot of oil tea in Enshi. Hubei Plant Protect. 152, 9–10.
Huang, F. L., and Meng, M. Q. (1986). Preliminary report of purple spot disease of Camellia oleifena in Guangxi Province. For. Sci. Technol. 09, 29–30. doi: 10.13456/j.cnki.lykt.1986.09.017
Huang, R. J., Wang, Z. Q., Yang, Y., and Li, J. (2017). Observation on morphological traits and ultrastructure of abnormal leaf induced by Exobasidium gracile in Camellia oleifera. Acta Bot. Boreali-Occidentalia Sin. 37, 8–13.
Huang, Y. P., Huang, G., Liu, S. B., and Peng, X. L. (2018). Nutrient composition analysis of Camellia oleifera leaf swelling gall body tea buds and tea flakes. Jiangxi Agric. 145, 36–37. doi: 10.19394/j.cnki.issn1674-4179.2018.20.028
Hui, X. M., Yun, L. C., Bin, L. J., Tan, X. L., Tian, W. Z., and Tang, Z. S. (2003). Analysis of resistant spectrum to rice blast in transgenic rice lines introduced lysozyme gene from T4 Phage. Sci. Agric. Sin 2, 273–279.
Ingram, R. J., Ludwig, H. D., and Scherm, H. (2019). Epidemiology of Exobasidium leaf and fruit spot of rabbiteye blueberry: Pathogen overwintering, primary infection, and disease progression on leaves and fruit. Plant Dis. 103, 1293–1301. doi: 10.1094/PDIS-09-18-1534-RE
Jia, D. S., Mao, J. H., Chen, F., Mao, M. H., and Liao, Y. J. (2017). Occurrence and control of Camellia oleifera gall disease induced by Exobasidium gracile in highland areas. J. West China For. Sci. 46, 29–34. doi: 10.16473/j.cnki.xblykx1972.2017.05.006
Jiang, D. H., Fu, Y. P., Li, G. Q., and Ghabrial, S. A. (2013). Viruses of the Plant Pathogenic Fungus Sclerotinia Sclerotiorum. San Diego: Elsevier Academic Press Inc.
Jiang, S. Q., and Li, H. (2018). First report of leaf anthracnose caused by Colletotrichum karstii on tea-oil trees (Camellia oleifera) in China. Plant Dis. 102, 674–675. doi: 10.1094/PDIS-08-17-1195-PDN
Jiao, J., Zhou, B., Zhu, X., Gao, Z., and Liang, Y. (2013). Fusaric acid induction of programmed cell death modulated through nitric oxide signalling in tobacco suspension cells. Planta 238, 727–737. doi: 10.1007/s00425-013-1928-7
Jing, A., Zhou, G., and Li, H. (2009). Recent advances in tea-oil tree anthracnose research. Chin. For. Pathol. 28, 27–31.
Kabbage, M., Yarden, O., and Dickman, M. B. (2015). Pathogenic attributes of Sclerotinia sclerotiorum: Switching from a biotrophic to necrotrophic lifestyle. Plant Sci. 233, 53–60. doi: 10.1016/j.plantsci.2014.12.018
Kirk, P. M., Cannon, P. F., David, J. C., and Stalpers, J. A. (2008). Ainsworth and Bisby‘s Dictionary of the Fungi, 10th Edn. Wallingford: CAB international.
Kohl, J., Kolnaar, R., and Ravensberg, W. J. (2019). Mode of action of microbial biological control agents against plant diseases: Relevance beyond efficacy. Front. Plant Sci. 10:19. doi: 10.3389/fpls.2019.00845
Kokkrua, S., Ismail, S. I., Mazlan, N., and Dethoup, T. (2019). Efficacy of berberine in controlling foliar rice diseases. Eur. J. Plant Pathol. 156, 147–158. doi: 10.1007/s10658-019-01871-3
Kong, W. L., Chen, X., Sun, H., Sun, X. R., and Wu, X. Q. (2022). Identification of two fungal pathogens responsible for Liriodendron chinense x tulipifera black spot and screening of Trichoderma sp. for disease control. Plant Dis. 106, 2172–2181. doi: 10.1094/pdis-06-21-1266-re
Lee, C. K., Lee, S. H., Shin, H. D., Park, J. H., and Sieber, T. (2015). First report of Exobasidium gracile causing hypertrophied leaves of Camellia sasanquain South Korea. For. Pathol. 45, 258–261. doi: 10.1111/efp.12184
Lee, K. H., Gumilang, A., Fu, T., Kang, S. W., and Kim, K. S. (2022). The autophagy protein CsATG8 is involved in asexual development and virulence in the Pepper anthracnose fungus Colletotrichum scovillei. Mycobiology 50, 467–474. doi: 10.1080/12298093.2022.2148393
Li, H. (2018). Population Genetic Analyses of the Fungal Pathogen Colletotrichum on Tea-Oil Trees in China and Characterization of MAPK gene CfPMK1 in the Pathogen. Changsha: Central South University of Forestry and Technology.
Li, H., Li, Y., Jiang, S. Q., Liu, J. A., and Zhou, G. Y. (2017). Pathogen of oil-tea anthracnose caused by Colletotrichum spp. in Hunan Province. Sci. Silvae Sin. 53, 43–53. doi: 10.11707/j.1001-7488.20170806
Li, Y. H., Zhou, B. L., Qian, M. R., Wang, Q., and Zhang, H. (2017). Transfer assessment of carbendazim residues from rape flowers to apicultural products. J. Anal. Methods Chem. 2017:7. doi: 10.1155/2017/6075405
Li, H., Song, G. T., Zhou, G. Y., and Liu, J. A. (2009). Molecular detection of Athelia rolfsii of Camellia oleifera by PCR. Biotechnology 19, 40–41. doi: 10.16519/j.cnki.1004-311x.2009.05.030
Li, H., Zhou, G. Y., and He, M. J. (2008). Study on biological characteristics and molecular identification of pathogens causing root rot of Camellia oleifera. J. Southw. For. Univ. 4:56.
Li, H., Zhou, G. Y., Liu, J. A., and Xu, J. P. (2016). Population genetic analyses of the fungal pathogen Colletotrichum fructicola on tea-oil trees in China. PLoS One 11:e0156841. doi: 10.1371/journal.pone.0156841
Li, H., Zhou, G. Y., and Xu, J. P. (2015). Pathogen identification of a new anthracnose of Camellia oleiferain China based on multiple-gene sequences. Plant Protect. 41, 92–96.
Li, H., Zhou, G. Y., Zhang, H. Y., Song, G. T., and Liu, J. N. (2011). Study on isolated pathogen of leaf blight and screening antagonistic bacteria from healthy leaves of Camellia oleifera. Afr. J. Agric. Res. 6, 4560–4566. doi: 10.5897/AJAR09.099
Li, L., Yang, Q., and Li, H. (2021). Morphology, phylogeny, and pathogenicity of Pestalotioid species on Camellia oleifera in China. J. Fungi 7:1080. doi: 10.3390/jof7121080
Li, M. (2021). Studies on Leaf Cytology and Immunity of Colletotrichum fructicola-Camellia oleifera Interaction at Early Stage. Changsha: Central South University of Forestry and Technology.
Li, S. Z., and Li, H. (2020). First report of Colletotrichum nymphaeae causing anthracnose on Camellia oleifera in China. Plant Dis. 104, 1860–1861. doi: 10.1094/PDIS-09-19-2016-PDN
Liang, C., Zhang, B., Zhou, Y., Yin, H., An, B., Lin, D., et al. (2021). CgNPG1 as a novel pathogenic gene of Colletotrichum gloeosporioides from Hevea brasiliensis in mycelial growth, conidiation, and the invasive structures development. Front. Microbiol. 12:629387. doi: 10.3389/fmicb.2021.629387
Lin, P., Wang, K., Wang, Y., Hu, Z., Yan, C., Huang, H., et al. (2022). The genome of oil-Camellia and population genomics analysis provide insights into seed oil domestication. Genome Biol. 23, 14. doi: 10.1186/s13059-021-02599-2
Lin, X. J., Wu, G. J., and Wang, J. X. (1981). Study of the disease pattern of soft rot of Camellia oleifera. J. Central South Univ. For. Technol. 1, 78–84. doi: 10.14067/j.cnki.1673-923x.1981.01.007
Liu, H. L. (2019). Identification of the Anthraconse of Camellia oleifera Pathogen in Different Climate Zones and Cene Differential Expression. Changsha: Central South University of Forestry and Technology.
Liu, S. B., Li, Y., Long, H., and Zhou, T. T. (2012). Observation on morphological and structural characteristics of ovary gall and leaf gall induced by Exobasidium gracile in Camellia oleifera. Acta Bot. Boreali-Occidentalia Sin. 32, 1752–1757.
Liu, S. Q. (1964). Camellia oleifera disease survey report in Anhui province. J. Anhui Agric. Univ. 75–86. doi: 10.13610/j.cnki.1672-352x.1964.00.006
Liu, W. (2012). Studies on Etiology, Occurrence and Control of Camellia oleifera Anthracnose. Wuhan: Huazhong Agricultural University.
Liu, X. J., Wei, A. J., Fan, S. R., Yang, W. A., Li, H. Z., and Zhou, S. L. (1981). Studies on the pathogenic fungus of the soft-rot of tea-oil trees. Acta Microbiol. Sin. 2, 132–133.
Liu, X. Y., Yu, F. Y., Fu, D. Q., Yang, W. B., Jia, X. C., and Chen, L. Q. (2019). Identification and characterization of Camellia oleifera leaf spot disease pathogen. China Trop. Agric. 1, 38–42.
Liu, Y. J., Hu, F., Chen, L. S., and Xu, S. W. (2020). First report of Nigrospora sphaerica causing leaf blight on oil tea (Camellia oleifera) in China. Plant Dis. 104, 3252–3252. doi: 10.1094/pdis-03-20-0563-pdn
Lu, P. K., Hsieh, W. T., Pan, H. R., and Ou, J. H. (2019). First report of Haradamyces foliicola causing leaf blight on oil tea (Camellia oleifera) in Taiwan. Plant Dis. 103, 1027–1028. doi: 10.1094/pdis-07-18-1175-pdn
Lu, X. F. (2021). Survey on the occurrence of soft rot of oil tea and integrated control technology. Rural Sci. Technol. 12, 70–71. doi: 10.19345/j.cnki.1674-7909.2021.01.037
Lui, L. H., and Kushalappa, A. C. (2002). Response surface models to predict potato tuber infection by Fusarium sambucinum from duration of wetness and temperature, and dry rot lesion expansion from storage time and temperature. Int. J. Food Microbiol. 76, 19–25. doi: 10.1016/s0168-1605(01)00752-8
Ma, Y. L., and Luo, L. C. (2019). Isolation, detection and screening of control agents on pathogenic bacteria of Camellia oleifer root rot. J. Anhui Agric. Sci. 47, 154–156.
Machado, R. D., Christoff, A. P., Loss-Morais, G., Margis-Pinheiro, M., Margis, R., and Korbes, A. P. (2015). Comprehensive selection of reference genes for quantitative gene expression analysis during seed development in Brassica napus. Plant Cell Rep. 34, 1139–1149. doi: 10.1007/s00299-015-1773-1
Maharachchikumbura, S. S., Hyde, K. D., Groenewald, J. Z., Xu, J., and Crous, P. W. (2014). Pestalotiopsis revisited. Stud Mycol. 79, 121–186. doi: 10.1016/j.simyco.2014.09.005
Marin, S., Sanchis, V., and Magan, N. (1995). Water activity, temperature, and pH effects on growth of Fusarium moniliforme and Fusarium proliferatum isolates from maize. Can. J. Microbiol. 41, 1063–1070. doi: 10.1139/m95-149
Mesquita, N. L. S., Santos, A., de Oliveira, R. J. V., Soares, P. P. D., de Novaes, Q. S., and Bezerra, J. L. (2022). Sooty mould caused by Capnodium alfenasii on Azadirachta indica Key-words. J. Phytopathol. 170, 574–578. doi: 10.1111/jph.13120
Miljakovic, D., Marinkovic, J., and Balesevic-Tubic, S. (2020). The significance of Bacillus spp. in disease suppression and growth promotion of cield and vegetable crops. Microorganisms 8:1037 doi: 10.3390/microorganisms8071037
Miller, I. F., Jiranek, J., Brownell, M., Coffey, S., Gray, B., Stahl, M., et al. (2022). Predicting the effects of climate change on the cross-scale epidemiological dynamics of a fungal plant pathogen. Sci Rep. 12:14823. doi: 10.1038/s41598-022-18851-z
Mueller, D. S., Bradley, C. A., Grau, C. R., Gaska, J. M., Kurle, J. E., and Pedersen, W. L. (2004). Application of thiophanate-methyl at different host growth stages for management of sclerotinia stem rot in soybean. Crop Protect. 23, 983–988. doi: 10.1016/j.cropro.2004.02.013
Mukherjee, R., Gruszewski, H. A., Bilyeu, L. T., Schmale, D. G., and Boreyko, J. B. (2021). Synergistic dispersal of plant pathogen spores by jumping-droplet condensation and wind. Proc. Natl. Acad. Sci. U. S. A. 118:e2106938118. doi: 10.1073/pnas.2106938118
National Forestry and Grassland Administration (2013). National List of Dangerous Pests in Forestry. Available online at: http://www.forestry.gov.cn/portal/main/govfile/13/govfile_1983.htm (accessed January 12, 2013).
National Forestry and Grassland Administration (2021). Fourteen Five” Forestry and Grassland Protection and Development Plan Outline. Available online at: http://www.forestry.gov.cn/main/5461/20210819/091113145233764.html (accessed December 14, 2021).
National Forestry and Grassland Administration (2023). Three-Year Action Plan to Accelerate the Development of Oil Tea Industry (2023-2025). Available online at: http://www.forestry.gov.cn/main/6222/20230110/202930861565261.html (accessed December 22, 2022).
Navarrete, F., Gallei, M., Kornienko, A. E., Saado, I., Khan, M., Chia, K. S., et al. (2022). TOPLESS promotes plant immunity by repressing auxin signaling and is targeted by the fungal effector Naked1. Plant Commun. 3:100269. doi: 10.1016/j.xplc.2021.100269
Nickerson, N. L., and Vander Kloet, S. P. (1997). Exobasidium leaf spot of lowbush blueberry. Can. J. Plant Pathol. 19, 66–68. doi: 10.1080/07060669709500575
Noriega Cantú, D. H., Pereyda Hernández, J., and Garrido Ramírez, E. R. (2017). Efecto de factores climatológicos sobre la fluctuación de esporas en árboles de mango cv. Ataulfo en Guerrero, México. Rev. Mex. Fitopatol. 35, 227–241. doi: 10.18781/r.Mex.Fit.1610-1
O’Brien, P. A. (2017). Biological control of plant diseases. Austral. Plant Pathol. 46, 293–304. doi: 10.1007/s13313-017-0481-4
Paterson, A. H., Damon, S., Hewitt, J. D., Zamir, D., Rabinowitch, H. D., Lincoln, S. E., et al. (1991). Mendelian factors underlying quantitative traits in tomato: Comparison across species, generations, and environments. Genetics 127, 181–197. doi: 10.1093/genetics/127.1.181
Perelló, A. E., and Dal Bello, G. M. (2011). Suppression of tan spot and plant growth promotion of wheat by synthetic and biological inducers under field conditions. Ann. Appl. Biol. 158, 267–274. doi: 10.1111/j.1744-7348.2011.00460.x
Qi, Y. H., and Wei, A. J. (1983). Observation and study on the sporodochium receptacle of the pathogen of Camellia oleifera soft rot. J. South China Agric. Coll. 4, 16–22.
Qin, S. Z., Chen, X. Y. L., Zhou, X. H., Zhao, J., Baccelli, I., and Cernava, T. (2021). First report of Camellia oleifera leaf blight caused by Nigrospora chinensis. J. Plant Pathol. 103, 711–712. doi: 10.1007/s42161-021-00793-6
Qin, S. Z., Hong, Z. G., and Wang, Z. W. (2019a). Study on the identification of the pathogen of Colletotrichum fioriniae in Camellia oleifera in Guizhou province. Tea Fujian 41:3.
Qin, S. Z., Wang, J. W., and Zhang, Z. T. (2019b). Identification of the pathogen of oil tea leaf blight in Guizhou. South China Agric. 13, 134–135. doi: 10.19415/j.cnki.1673-890x.2019.26.067
Qiu, J., Xu, J., and Shi, J. (2019). Fusarium toxins in Chinese wheat since the 1980s. Toxins 11:248. doi: 10.3390/toxins11050248
Qiu, J. S., Yu, J. Y., Wu, Y. K., Zhu, X. E., Wang, J., Xu, J., et al. (2011). Preliminary report on the Exobasidium gracile(Shirai)Syd. of Camellia oleifera in Guizhou Province. Guizhou For. Sci. Technol. 39, 19–22.
Quesada, T., Hughes, J., Smith, K., Shin, K., James, P., and Smith, J. (2018). A low-cost spore trap allows collection and real-time PCR quantification of airborne Fusarium circinatum spores. Forests 9:586. doi: 10.3390/f9100586
Redondo, M. A., Berlin, A., Boberg, J., and Oliva, J. (2020). Vegetation type determines spore deposition within a forest-agricultural mosaic landscape. FEMS Microbiol. Ecol. 96:fiaa082. doi: 10.1093/femsec/fiaa082
Rehman, B., Khan, S. A., Hamayun, M., Iqbal, A., and Lee, I. J. (2022). Potent bioactivity of endophytic fungi isolated from Moringa oleifera leaves. Biomed. Res. Int. 2022:2461021. doi: 10.1155/2022/2461021
Reynolds, D. R. (1999). Capnodium citri: The sooty mold fungi comprising the taxon concept. Mycopathologia 148, 141–147. doi: 10.1023/a:1007170504903
Roos, J., Hopkins, R., Kvarnheden, A., and Dixelius, C. (2011). The impact of global warming on plant diseases and insect vectors in Sweden. Eur. J. Plant Pathol. 129, 9–19. doi: 10.1007/s10658-010-9692-z
Sharma, G., Maymon, M., Meshram, V., and Freeman, S. (2022). Characterization of Colletotrichum isolates from strawberry and other hosts with reference to cross-inoculation potential. Plants-Basel 11:16. doi: 10.3390/plants11182373
Shi, H. A., Fu, B. Z., Zhang, Z. L., Li, G. Y., and Wang, L. H. (2015). The study of two Camellia pathogens isolation, identification and biological characteristics. Hubei Agric. Sci. 54, 5908–5911. doi: 10.14088/j.cnki.issn0439-8114.2015.23.028
Singh, B., Srivastava, J. S., Khosa, R. L., and Singh, U. P. (2001). Individual and combined effects of berberine and santonin on spore germination of some fungi. Folia Microbiol. 46, 137–142. doi: 10.1007/bf02873592
Song, G. T. (2012). The Study on Detection and Biological Control to the Main Pathogens of Camellia oleifera. Changsha: Central South University of Forestry and Technology.
Song, L. S. (2016). Research on Taxonomy, Molecular Systematics and DNA Barcoding of Pestalotiopsis. Nanning: Guangxi University.
Song, Y. P., Wang, P., and Zhou, H. W. (2021). Detection and controlmeasures on Agaricodochium camellia. J. Jiujiang Univ. 36:56. doi: 10.19717/j.cnki.jjun.2021.02.001
Su, Q. L., and Zhang, L. F. (2009). Oil-tea Cultivation and Pest Control in China. Beijing: China Forestry Publishing House.
Suealek, N., Yokoyama, W. H., Rojpibulstit, P., Holt, R. R., and Hackman, R. M. (2018). Thai tea seed (Camellia oleifera) oil favorably affects plasma lipid responses in hamsters fed high-fat diets. Eur. J. Lipid Sci. Technol. 121:1800024. doi: 10.1002/ejlt.201800024
Suffert, F., Sache, I., and Lannou, C. (2011). Early stages of Septoria tritici blotch epidemics of winter wheat: Build-up, overseasoning, and release of primary inoculum. Plant Pathol. 60, 166–177. doi: 10.1111/j.1365-3059.2010.02369.x
Sun, W., Lei, T., Yuan, H., and Chen, S. (2022). First report of anthracnose caused by Colletotrichum kahawae and Colletotrichum horri on tea-oil tree in China. Plant Dis. 107:1944. doi: 10.1094/PDIS-06-22-1312-PDN
Sun, Z. Z., Liu, T. T., Liu, Z., Zeng, C. Z., and Liu, Z. X. (2022). Screening of antagonistic bacteria against the blue mold of citrus fruit from soil by a new parallel screening method without prior isolation of single strains. Biol. Control. 176:105066. doi: 10.1016/j.biocontrol.2022.105066
Sun, Y., Wang, Y., and Tian, C. (2016). bZIP transcription factor CgAP1 is essential for oxidative stress tolerance and full virulence of the poplar anthracnose fungus Colletotrichum gloeosporioides. Fungal Genet. Biol. 95, 58–66. doi: 10.1016/j.fgb.2016.08.006
Tang, Y. L., Zhou, G. Y., Li, H., Zhong, W. B., Long, H. E., and Wang, L. Y. (2015). Identification of a new anthracnose of Camellia oleifera based on multiple-gene phylogeny. Chin. J. Trop. Crops 36, 972–977.
Teixeira, A. M., and Sousa, C. (2021). A review on the biological activity of Camellia species. Molecules 26:2178 doi: 10.3390/molecules26082178
The Central People’s Government of the People’s Republic of China (2020). The Planting Area of Camellia oleifera in China Reaches 68 million mu. Available online at: http://www.gov.cn/xinwen/2020-11/17/content_5562082.htm (accessed November 17, 2020).
Thomas, A., and Saravanakumar, D. (2023). First report of Sclerotium rolfsii (Athelia rolfsii) causing basal rot in lettuce in Trinidad. J. Plant Pathol. 105, 611–611. doi: 10.1007/s42161-023-01319-y
Volz, P. A. (1997). Transmission of fungal spores in space and their conditions for survival: A review. Microbios 91, 145–151.
Wang, F., Li, X., Li, Y., Han, J., Chen, Y., Zeng, J., et al. (2021). Arabidopsis P4 ATPase-mediated cell detoxification confers resistance to Fusarium graminearum and Verticillium dahliae. Nat. Commun. 12:6426. doi: 10.1038/s41467-021-26727-5
Wang, J., Zhang, J., Ma, J., Liu, L., Li, J., Shen, T., et al. (2022). The major component of cinnamon oil as a natural substitute against Fusarium solani on Astragalus membranaceus. J. Appl. Microbiol. 132, 3125–3141. doi: 10.1111/jam.15458
Wang, Q. T., Cheng, Y. H., Zhuo, L., Wang, Y., Zhou, H., and Hou, C. L. (2022). Neopestalotiopsis longiappendiculata as the agent of grey blight disease of Camellia spp. J. Phytopathol. 170, 770–777. doi: 10.1111/jph.13139
Wang, Y., Chen, J. Y., Xu, X., Cheng, J., Zheng, L., Huang, J., et al. (2020). Identification and characterization of Colletotrichum species associated with anthracnose disease of Camellia oleifera in China. Plant Dis. 104, 474–482. doi: 10.1094/PDIS-11-18-1955-RE
Wang, Y. C., Yang, H., Deng, L. Q., and Yang, C. X. (2020). Survey on the occurrence of soft rot of oil tea and integrated control technology. Mod. Agric. Sci. Technol. 779, 145–153.
Wang, Y. X., Liu, W., Chen, J. Y., Liu, Y. J., and Huang, J. B. (2013). Overwintering sites, various stages and amount of Colletotrichum gloeosporioides on Camellia oleifera. Hubei For. Sci. Technol. 179, 32–46.
Wei, A. J., and Qi, Y. H. (1982). Preliminary studies on the mechanism of infestation by the causal agent of soft rot of Camellia oleifera. For. Sci. Technol. 3, 26–28. doi: 10.13456/j.cnki.lykt.1982.03.014
Wei, C. Y., and Ma, Y. L. (2010). Risk analysis and control technology of Myrothecium camelliae in China. J. Anhui Agric. Sci. 38, 18187–18189. doi: 10.13989/j.cnki.0517-6611.2010.32.163
Won, S. J., Kwon, J. H., Kim, D. H., and Ahn, Y. S. (2019). The effect of Bacillus licheniformis MH48 on control of foliar fungal diseases and growth promotion of Camellia oleifera seedlings in the coastal reclaimed land of Korea. Pathogens 8:6. doi: 10.3390/pathogens8010006
Xie, X., Zhang, S., Yu, Q., Li, X., Liu, Y., and Wang, L. (2022). First report of Epicoccum layuense causing leaf brown spot on Camellia oleifera in Hefei, China. Plant Dis. [Online ahead of print]. doi: 10.1094/PDIS-08-21-1726-PDN
Xu, Z., Gleason, M. L., Mueller, D. S., Esker, P. D., Bradley, C. A., Buck, J. W., et al. (2008). Overwintering of Sclerotium rolfsii and S. rolfsii var. delphinii in different latitudes of the United States. Plant Dis. 92, 719–724. doi: 10.1094/PDIS-92-5-0719
Yamamoto, W. (1952). The germination of spores of the sooty mould fungus, and the growth of their germ tubes, with special reference to the honeydews secreted by insects. Jpn. J. Phytopathol. 16, 49–53. doi: 10.3186/jjphytopath.16.49
Yamamoto, W. (1955). On the so-called host range of sooty mould fungi. Jpn. J. Phytopathol. 19, 97–103. doi: 10.3186/jjphytopath.19.97
Yan, X., Zhang, S., Yu, Z., Sun, L., Sohail, M. A., Ye, Z., et al. (2023). The MAP kinase PvMK1 regulates hyphal development, autophagy, and pathogenesis in the bayberry twig blight fungus Pestalotiopsis versicolor. J. Fungi 9:606 doi: 10.3390/jof9060606
Yimer, S. M., Ahmed, S., Fininsa, C., Tadesse, N., Hamwieh, A., and Cook, D. R. (2018). Distribution and factors influencing chickpea wilt and root rot epidemics in Ethiopia. Crop Protect. 106, 150–155. doi: 10.1016/j.cropro.2017.12.027
Yu, F. Y., Liu, X. Y., Jia, X. C., Fu, D. Q., Song, W. W., and Niu, X. Q. (2022). First report of Neofusicoccum parvum causing leaf spot of Camellia drupifera in China. J. Plant Pathol. 104, 431–431. doi: 10.1007/s42161-021-00992-1
Yu, Y. C. (2019). Occurrence and control of oil tea sooty mold in susong area. J. Agric. Catastrophol. 9, 17–18. doi: 10.19383/j.cnki.nyzhyj.2019.02.008
Yuan, S. L., Sao, L. P., and Li, C. D. (1997). Diseases of Trees and Shrubs in China. Beijing: China Science Publishing.
Zhang, J., Ying, Y., and Yao, X. (2019). Effects of turning frequency on the nutrients of Camellia oleifera shell co-compost with goat dung and evaluation of co-compost maturity. PLoS One 14:e0222841. doi: 10.1371/journal.pone.0222841
Zhang, S., Guo, Y., Li, S., Li, H., and Di Pietro, A. (2022). Histone acetyltransferase CfGcn5-mediated autophagy governs the pathogenicity of Colletotrichum fructicola. mBio 13:e01956-22. doi: 10.1128/mbio.01956-22.
Zhang, T. T., Cai, X. Y., Teng, L., Li, X., Zhong, N. F., and Liu, H. M. (2022). Molecular characterization of three novel mycoviruses in the plant pathogenic fungus Exobasidium. Virus Res. 307:198608. doi: 10.1016/j.virusres.2021.198608
Zhao, D. Y., and Qin, C. S. (2015). Original Color Ecological Atlas for Diagnosis and Control of Oil-Tea Pests and Diseases. Guangzhou: Guangdong Science and Technology Press.
Zhao, Z. X., Yan, W. R., Xiao, M., Xiao, D. B., and Lei, F. (2020). Molecular identification of pathogens causing root rot of Camellia oleifera in tropical. Mol. Plant Breed. 18, 6433–6440. doi: 10.13271/j.mpb.018.006433
Zheng, L. F., Chen, L., Li, J., Liang, L., Fan, Y. W., Qiu, L. Y., et al. (2019). Two kaempferol glycosides separated from Camellia oleifera meal by high-speed countercurrent chromatography and their possible application for antioxidation. J. Food Sci. 84, 2805–2811. doi: 10.1111/1750-3841.14765
Zhong, W. Q., Shen, J. L., Liao, X. D., Liu, X. L., Zhang, J., Zhou, C. H., et al. (2019). Camellia (Camellia oleifera Abel.) seed oil promotes milk fat and protein synthesis-related gene expression in bovine mammary epithelial cells. Food Sci. Nutr. 8, 419–427. doi: 10.1002/fsn3.1326
Zhou, G. Y. (2023). Green control techniques for oil tea diseases (I). For. Ecol. 808, 36–38. doi: 10.13552/j.cnki.lyyst.2023.01.014
Zhou, J. P., Xie, Y. Q., Liao, Y. H., Li, X. Y., Li, Y. M., Li, S. P., et al. (2022). Characterization of a Bacillus velezensis strain isolated from Bolbostemmatis rhizoma displaying strong antagonistic activities against a variety of rice pathogens. Front. Microbiol. 13:983781. doi: 10.3389/fmicb.2022.983781
Zhou, L., Jiang, Y. P., Lin, Q., Wang, X., Zhang, X. Z., Xu, J., et al. (2018). Residue transfer and risk assessment of carbendazim in tea. J. Sci. Food Agric. 98, 5329–5334. doi: 10.1002/jsfa.9072
Zhou, M., Li, Z., Liu, Y., Zhang, P., Hao, X., and Zhu, X. (2021). Transcription factors Pmr1 and Pmr2 cooperatively regulate melanin biosynthesis, conidia development and secondary metabolism in Pestalotiopsis microspora. J. Fungi 8:38. doi: 10.3390/jof8010038
Zhu, B. F., Peng, L., and Luo, L. F. (2007a). Study on health care functions of extract from fleshy fruit and fleshy leaf of Camellia oleifera Abel. J. Food Sci. Biotechnol. 26, 46–50.
Zhu, B. F., Peng, L., Luo, L. F., Liu, J. H., and He, Q. D. (2007b). The analysis of exploitive value and edible security in fleshy fruit and fleshy leaf of Camellia oleifera Abel. J. Food Sci. Biotechnol. 26, 1–6.
Zhu, H., and He, C. (2023). Identification and characterization of Colletotrichum Species causing tea-Oil Camellia (Camellia oleifera C.Abel) anthracnose in Hainan, China. Forests 14:1030. doi: 10.3390/f14051030
Keywords: Camellia oleifera, fungal pathogens, plant diseases, management strategies, geographical distribution
Citation: Chen X, He Y, Wang Z, Niu A, Xue Y, Zhou D, Zhou G and Liu J (2023) Research progress and management strategies of fungal diseases in Camellia oleifera. Front. Microbiol. 14:1215024. doi: 10.3389/fmicb.2023.1215024
Received: 01 May 2023; Accepted: 30 October 2023;
Published: 23 November 2023.
Edited by:
Jian-Wei Guo, Kunming University, ChinaReviewed by:
Abhay K. Pandey, Tea Research Association, North Bengal Regional R&D Centre, IndiaCopyright © 2023 Chen, He, Wang, Niu, Xue, Zhou, Zhou and Liu. This is an open-access article distributed under the terms of the Creative Commons Attribution License (CC BY). The use, distribution or reproduction in other forums is permitted, provided the original author(s) and the copyright owner(s) are credited and that the original publication in this journal is cited, in accordance with accepted academic practice. No use, distribution or reproduction is permitted which does not comply with these terms.
*Correspondence: Guoying Zhou, emd5aW5ncXFAMTYzLmNvbQ==; Junang Liu, a2pjOTYyMEAxNjMuY29t