- 1Academy of Agriculture and Forestry Sciences of Qinghai University, Qinghai Academy of Agriculture and Forestry Sciences, Xining, Qinghai, China
- 2State Key Laboratory of Plateau Ecology and Agriculture, Qinghai University, Xining, Qinghai, China
- 3College of Agriculture and Animal Husbandry, Qinghai University, Xining, Qinghai, China
Rhizobium-driven symbiotic nitrogen-fixation in legumes not only benefits the growth but also enhances the stress tolerance of plants. Isolating and characterizing efficient, drought-tolerant rhizobia is a central goal for improving crop yields in arid regions. Here, we phylogenetically and biochemically characterized a novel strain of Rhizobium (‘QHCD11’) sampled from the root nodules of faba beans growing in an arid agricultural area in Qinghai-Tibet. We further tested the drought tolerance of the strain as well as of ‘Qingcan 14’ faba bean seedlings inoculated with it. Biochemical characterization involved bromothymol blue (BTB) tests, carbon metabolic profiling (Biolog GENIII), DNA–DNA hybridization (dDDH) assays, average nucleotide identity (ANI) analyses, and 16S rRNA sequencing. The result indicated that strain ‘QHCD11’ likely belongs to the Rhizobium indicum species. Drought stress tolerance was assessed by exposure to polyethylene glycol (PEG-6000) at concentrations of 0, 10, 15, and 20%. Increasing concentrations of PEG-6000 tended to result in decreased growth of ‘QHCD11’, although the strain performed better at 20% PEG 6000 than at 15%. Inoculation of drought-stressed faba bean seedlings with strain ‘QHCD11’ improved root vitality, chlorophyll content, antioxidant enzyme activities, and plant height. We suggest that inoculation of faba beans with ‘QHCD11’ is an environmentally sound strategy for mitigating crop drought stress in arid and semi-arid regions. In addition, the results presents here will benefit future studies into faba bean-rhizobia symbioses under drought stress.
Introduction
Nitrogen (N) is arguably one of the most important in all of the essential plant nutrients. Globally, the nitrogen cycle transforms approximately 3 × 109 tons of gaseous N2 into plant- available forms annually. Importantly, approximately 60% of the total N conversion results from biochemical activities (Hardarson, 2003; Mabrouk and Belhadj, 2010). Rhizobium, a genus of Gram-negative soil bacteria, can develop symbiotic relationships with leguminous plants in the form of root nodules, they fix atmospheric nitrogen (Remigi et al., 2016; Stefan et al., 2018). As one of the most important contributors to total biological nitrogen fixation, Rhizobium-legume symbioses contribute more than 50% of the nitrogen available in agricultural soils (Herridge et al., 2008). Not only does this symbiotic relationship enhance nitrogen fixation capacity, but it also improves legume productivity and yield (Jida and Assefa, 2012; Laranjo et al., 2014; Alinia et al., 2022).
Rhizobium collected from the nodules and roots of legumes may be promising biofertilizers for use in improving crop growth, nodulation, nitrogen fixation, and resistance to stress and disease. Several studies indicate that naturally- occurring populations of rhizobia from certain geographic regions exhibit greater nodulation competency than many commercial strains (Gaci et al., 2021; Tuan Vo et al., 2021). The isolation and characterization of native rhizobial populations is likely to provide valuable biological resources for the enhancement of crop productivity (Humaira and Bano, 2011). Several studies have subjected native rhizobial populations isolated from legume crops to molecular identification through the use of genomic sequence-based digital DNA–DNA hybridization (dDDH), average nucleotide identity (ANI) analysis, and 16S rRNA sequencing (Deng et al., 2022; Zaw et al., 2022). It is clear from these studies that phylogenetic analysis is a stable and dependable method for the description and classification of species.
Unfavorable environmental conditions can compromise the legume-Rhizobium relationship, including drought and excessively high temperatures. Because of this, it is crucial to study legume-Rhizobium symbioses under such conditions. Studies suggest that host plants are often less tolerant of environmental stress than their microbial partners (Jebara et al., 2001; Mnasri et al., 2007; Vriezen et al., 2007). Inoculating seeds with Rhizobium has been shown to enhance the environmental stress tolerance of plants (Franzini et al., 2013; Zeenat et al., 2021). Such increased resistance likely results from a combination of decreased generation of reactive oxygen species (ROS), improvement of leaf water status, facilitation of water uptake, mitigation of ionic imbalance, and more efficient nutrient acquisition. Furthermore, utilizing highly efficient and stress-tolerant rhizobial inocula can further enhance the yield of legume crops in unfavorable environments (Peoples et al., 2009; Denton et al., 2017; Kanonge-Mafaune et al., 2018). Rhizobia appear to boost the stress resilience and growth of host plants through several mechanisms, including inducing the biosynthesis of osmolytes such as ectoines, polyamines, proline, and glycine betaine to guard cells from osmotic stress- and desiccation-related damage; promoting the activity of both enzymatic and non-enzymatic antioxidants; and increasing photosynthetic activity and biomass accumulation (Reina-Bueno et al., 2012; Wdowiak-Wróbel et al., 2013; Lunn et al., 2014; Ding, 2022). Taken together, it is clear that rhizobia can support plant health in unfavorable conditions. This ability may be crucial for enhancing legume cultivation on marginal land and in semi-arid and arid regions.
Qinghai is situated in northwestern China, in the northeastern section of the Qinghai-Tibet Plateau, and has an average altitude of more than 3,000 m. Seventy percent of the agricultural production of Qinghai occurs in semi-arid and arid regions (Li et al., 2018). Faba bean (Vicia faba L.; Fabaceae) has proven adaptable to the varied climates of this region, and is utilized as a grain, vegetable, and fodder crop. In addition, this leguminous crop enhances the sustainability of local cropping systems by contributing nitrogen to agricultural fields through biological fixation (Amede et al., 1999; Khan et al., 2007). At present, faba bean is the primary legume crop grown across the Qinghai-Tibet Plateau, and is a major contributor to economic and ecological development in the region. Unfortunately, faba bean is considerably less drought tolerant than other legumes such as chickpea, pea, and common bean (McDonald and Paulsen, 1997; Amede and Schubert, 2003; Sharma et al., 2017).
Here, we sought to determine the phylogenetic status and symbiotic efficacy of the Rhizobium strain ‘QHCD11’, which was isolated from an arid agricultural area in Qinghai-Tibet. We analyzed the morphological, physiological, biochemical, and genetic characteristics of Rhizobium strain ‘QHCD11’, as well as assessed its ability to colonize and enhance the drought resistance and growth of faba beans. The results of this study help to illuminate the complex relationship between rhizobia and legumes under stressful environmental conditions. We suggest that Rhizobium strain ‘QHCD11’ may be further developed into a useful inoculant for improving the drought tolerance of faba beans.
Materials and methods
Root nodule
The root nodule was obtained from an arid agriculture area in Haidong City, Minhe County, Qinghai Province, China (36.0607° N, 102.4520° E). The field soil pH was 8.1, with 16.65 g kg−1 organic matter (OM), 1.20 g kg−1 total N, 82 mg kg−1 alkaline N, 2.11 g kg−1 total phosphorus (P; P2O5), 24.6 mg kg−1 available P, 20.65 g kg−1 total potassium (K; K2O), 178 mg kg−1 available K, and 0.62 g kg−1 total salt. A single healthy plant at flowering stage was uprooted along with nodules and transported to the laboratory. The nodules was chose to isolate of red color (leghemoglobins).
Isolation of rhizobial cultures
Faba bean root nodules were isolated in accordance with the method of Vincent (1970). The Rhizobium isolate was grown on yeast mannitol agar (YMA) plates at 28°C. The pure culture was preserved in 10% (v/v) glycerol stock for subsequent analyses. All morphological, biochemical, and molecular studies were carried out as described previously (Li et al., 2021). The reference Rhizobium leguminosarum strain ‘ACCC15854’ was obtained from the Agriculture Culture Collection of China (ACCC; Beijing, China).
BTB test of Rhizobium ‘QHCD11’
To determine the alkali and acid production of the pure culture isolates, they were streaked onto fresh YMA plates (pH 6.8) containing 30 μg L−1 bromothymol blue (BTB) (Sharma et al., 2010). In a slight alteration of the original method, all plates were incubated for 3–5 d at 28°C, and the plates were evaluated each day. When acidic compounds are produced, the plate color changes from green to yellow, and when alkaline compounds are produced, the plate color changes from green to blue.
Biochemical evaluation of Rhizobium ‘QHCD11’
To evaluate the biochemical and metabolic profile of Rhizobium strain ‘QHCD11’, we studied the biochemical parameters associated with carbon metabolism. A Biolog GEN III MIDI system (BIOLOG, CA, United States) was used to test whether Rhizobium strain ‘QHCD11’ could utilize 95 different carbon sources, according to the manufacturer’s instructions. Briefly, the isolate was cultured for 24 h in YM broth. The cells were then purified twice by 3 min of centrifugation at 8000 rpm. Subsequently, the cells were resuspended in sterilized water and adjusted to 108 cells ml−1. Both experimental and control samples were prepared according to the manufacturer’s standard protocol. Positive (+) tests were indicated by a color change to purple, and a negative (−) tests were indicated by a lack of color change.
16S rRNA gene sequencing
To amplify the 16S rRNA gene cluster, the 16S-27F (5’-GTTTGATCM- TGGCTCAG-3′) and 16S-1492R (5’-TACGGYTACCTTGTTACGACTT-3′) primers were used, as described by Chelius and Triplett (2001). Each 25 μL PCR reaction mixture contained 3 μL of DNA template, 1 μL each of 16S rRNA reverse and forward primers, 12.5 μL of PCR mix, and 7.5 μL of double-distilled water (DDW). The Ezbiocloud database1 was used to search the partial 16S rRNA gene. A neighbor-joining (NJ) tree was constructed, using 1,000 bootstrap replications, in in MEGA 7.0. Kimura’s two-parameter model was utilized to determine distances (Kimura, 1980).
Genomic characteristics of Rhizobium ‘QHCD11’
After the removal of connector sequences, the sequencing data were assembled to construct contigs and scaffolds using A5-MiSeq and SPAdes. The assemblies were evaluated and compared, and base correction was performed, using Pilon. Subsequent to filtration, PGCGAP was utilized to assemble the high-quality paired-end reads (Liu et al., 2021). Genomic reannotation was performed with Prokka (Seemann, 2014). Using the draft genome, the RAST server was utilized to determine the G + C content (Brettin et al., 2015). Both the ANI and dDDH were utilized to determine the taxonomic status of the novel isolate. JSpecies WS2 was used to obtain ANI values and the Genome-to-Genome Distance Calculator (GGDC)3 was used to obtain dDDH values.
Drought tolerance of Rhizobium ‘QHCD11’
The drought tolerance of Rhizobium ‘QHCD11’ was assessed by culturing the isolate in YMA liquid culture medium supplemented with polyethylene glycol (PEG-6000) at 0, 10, 15%, or 20% (w/v). Each liquid culture vial was inoculated with 0.1 mL of standard bacterial suspension and each experiment was replicated three times. The tubes were incubated for 72 h at 28 ± 2°C in an orbital shaker at 160 rpm. Growth was assessed using a spectrophotometer to observe the optical densities at 600 nm (OD600nm), with PEG-free YMA liquid culture medium used as the blank. For comparison, the R. leguminosarum reference strain ‘ACCC15854’ was also tested.
Inoculation of faba beans with Rhizobium
Faba bean‘Qingcan 14’ seeds were provided by the Qinghai Academy of Agriculture and Forestry Science (Xining City, Qinghai Provence, China). The surfaces of healthy, uniform seeds were sterilized using 1% sodium hypochlorite and washed at least three times with sterilized water. The seeds were subsequently germinated in darkness at 28°C in a petri dish carrying wet filter paper. The stored strain QHCD11 with 10% glycerol was streaked onto Yeast Mannitol Agar (YMA) plates and incubated at 28°C for 3 to 5 days until good growth was observed. A standard curve describing the relationship between cell number and optical density at 600 nm (OD600nm), was developed for strain QHCD11 to enable the application of a standard CFU mL−1 across experiments. Moreover, population counts (CFU mL−1) were determined by dilution plating. One milliliter of rhizobial suspension (≈108 CFU mL−1) was used to inoculate each test tube containing 20 mL of YMB media. When the radicle reached 0.5–1.0 cm, seedlings were soaked in a Rhizobium standard suspension (108 CFU mL−1) for 30 min and then planted in sterile containers containing quartz sand. To the base of each 5-day-old seedling was added an additional 2 mL of inoculum (108 CFU mL−1). Each week, seedlings were provided with 20 mL of sterilized water and 20 mL of N-free McKnight’s nutrient solution. The experiment treatments consisted of four different treatments, no inoculation plants under well-watered conditions (NN-CK), no inoculation drought-stressed plants (NN-DS), inoculation plants under well-watered conditions (NA-CK), and inoculation plants under drought stress (NA-DS). Three replicates and three pots per replicate were designated for each treatment, making a total of 36 pots. All plants were grown in a greenhouse with natural daylight from 9 March to 9 Jun. The average day/night temperature was 26/20°C, air relative humidity was 70–80%. The plants were irrigated with distilled water every 1–2 days, and supplied with ½-strength Hoagland’s nutrient solution every 30 days (approximately 200 mL per pot). Drought stress was applied during faba bean flowerings stage and was subjected to withdrawing water for 3 days. Physiological index measurements were performed on plants after being subjected to drought stress for 3 days. As a positive control, additional seedlings were inoculated with the R. leguminosarum reference strain ‘ACCC15854’, while uninoculated seedlings were used as negative controls. All treatments were arranged in a randomized block design, and included uninoculated seedlings under well-watered conditions (NN-CK), uninoculated seedlings under drought conditions (NN-DS), inoculated seedlings under well-watered conditions (NA-CK), and inoculated seedlings under drought conditions (NA-DS). Each treatment consisted of three replicates, each replicate consisted of three pots, and a total of 36 pots were used for the experiment. All seedlings were cultivated in a climate-controlled chamber with 70–80% relative humidity and an average night/day temperature of 20/25°C. All morphological, physiological, and biochemical studies were carried out during the blooming stage. The tested indices included root mitochondrial respiration [triphenyltetrazolium chloride (TTC) method], superoxide dismutase (SOD) activity, proline (PRO) content, leaf relative water content (RWC), chlorophyll content (SPAD), root length, fresh root nodule weight, number of root nodules, and plant height. The root mitochondrial respiration (TTC), superoxide dismutase (SOD) and proline (PRO) content were determined using a TTC Assay Kit, a SOD Assay Kit and a PRO Assay Kit, respectively (Nanjing Jiancheng Bioengineering Institute, China). SPAD was evaluated with a SPAD- 502 meter (Konica Minolta, Japan). As described by Mayak et al. (2004), RWC (%) was calculated as [(FW-DW)/ (FTW-DW)] × 100, where FTW is the fully turgid weight, DW the dry weight, and FW is the fresh weight.
Statistical analysis
Statistically significant differences were determined using the standard error (SE) of at least three replicates with SPSS 17.0 (SPSS, IL, United States).
Results
Characterization of Rhizobium isolated from faba bean plants
The isolate was purified by repeated plate streaking, and cultured on YMA plates at 28°C for 3 d in darkness. Morphologically, the isolates were described as 3–5 mm diameter, mucilaginous, opaque, shiny white colonies with a wet surface, which produced copious amounts of sticky exopolysaccharides. Microscopically, the bacteria were Gram-negative, rot-shaped, and red in color, which matches the general description of Rhizobium. The YMA-BTB-based acid/alkali production test indicated that the isolate was producing acidic compounds (green to yellow plate color transition) in medium. These result suggested that the isolate was a species of Rhizobium.
Phylogenetic analysis and genomic characteristics of the Rhizobium isolate
The complete nucleotide sequence (1,477 bp) of the 16S rRNA gene was evaluated. An EzBioCloud sequence search revealed that strain ‘QHCD11’ shared 99.93% similarity were the Rhizobium type strains R. leguminosarum ‘USDA 2370’T, R. anhuiense ‘CCBAU 23252’T, R. laguerreae ‘FB206’T, R. ruizarguesonis ‘UMP1133’T, and R. indicum ‘JKLM12A2’T (Table 1). The constructed NJ phylogenetic tree indicated that Rhizobium ‘QHCD11’ formed a phyletic group with R. changzhiense ‘WYCCWR 11279’T (MH778807) (Figure 1). Our results were largely in agreement with previous studies suggesting that variation between Rhizobial strains may be more common at the species level, with diversity observed genus-wide.
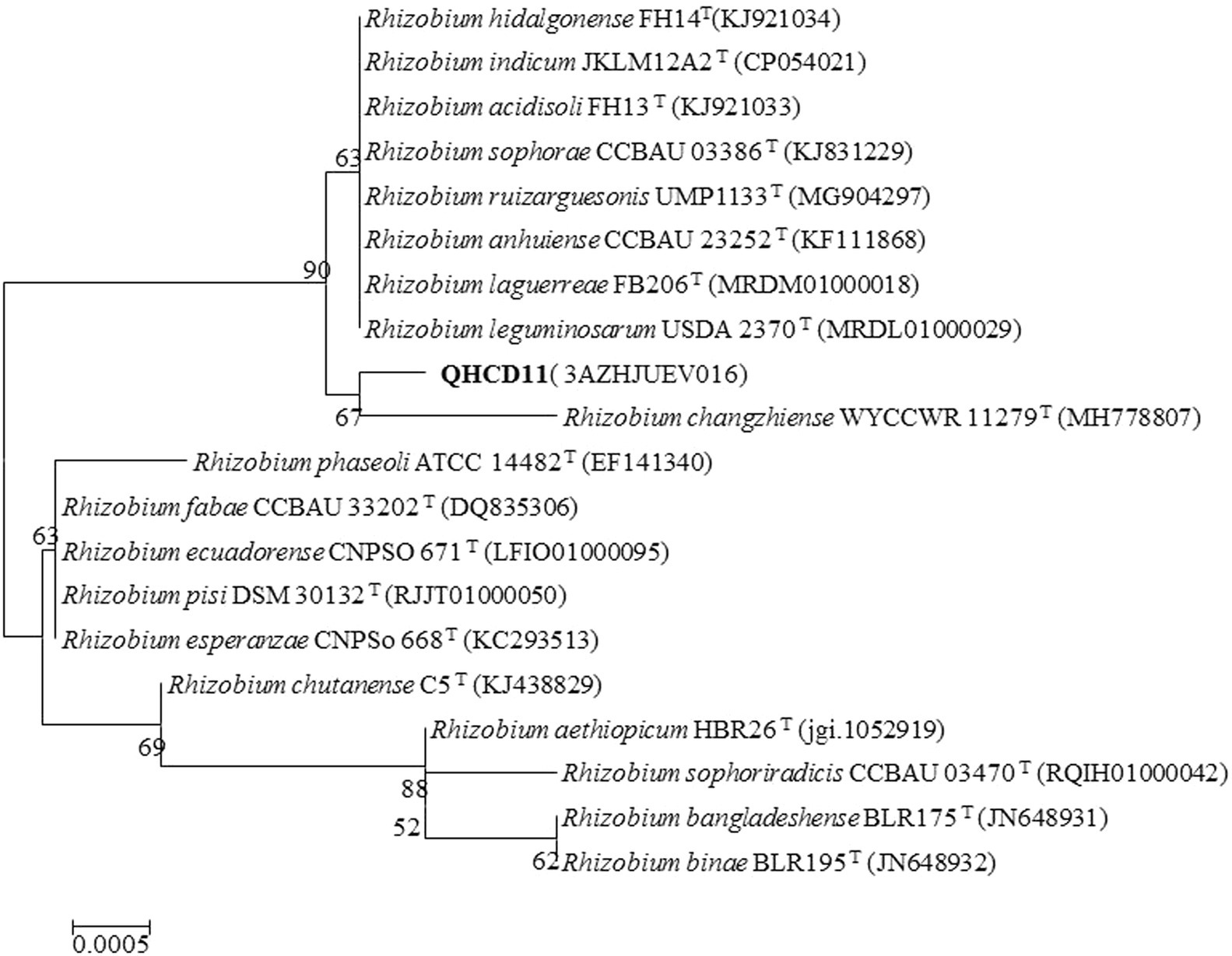
Figure 1. Neighbor-joining (NJ) phylogenetic analysis of Rhizobium ‘QHCD11’ near-full- length 16S rRNA gene sequences. Branch points show bootstrap values >50%. Scale = 0.0005 nucleotide substitutions per position.
The draft genome of Rhizobium ‘QHCD11’ was 7.50 Mbp in size and the G + C content was 60.9%. The ANI value of Rhizobium ‘QHCD11’ was 95.62%, which was the same as that of R. indicum ‘FA23’T (Table 2). To confirm the taxonomic status, dDDH values were also compared between strains (Tindall et al. 2010). The dDDH value of Rhizobium ‘QHCD11’ was 69.7%, indicating that it is a species of R. indicum ‘MCC 3961’T (Table 3). Furthermore, in the phylogenomic tree, strain ‘QHCD11’ clustered with R. indicum ‘MCC 3961’T (Figure 2).
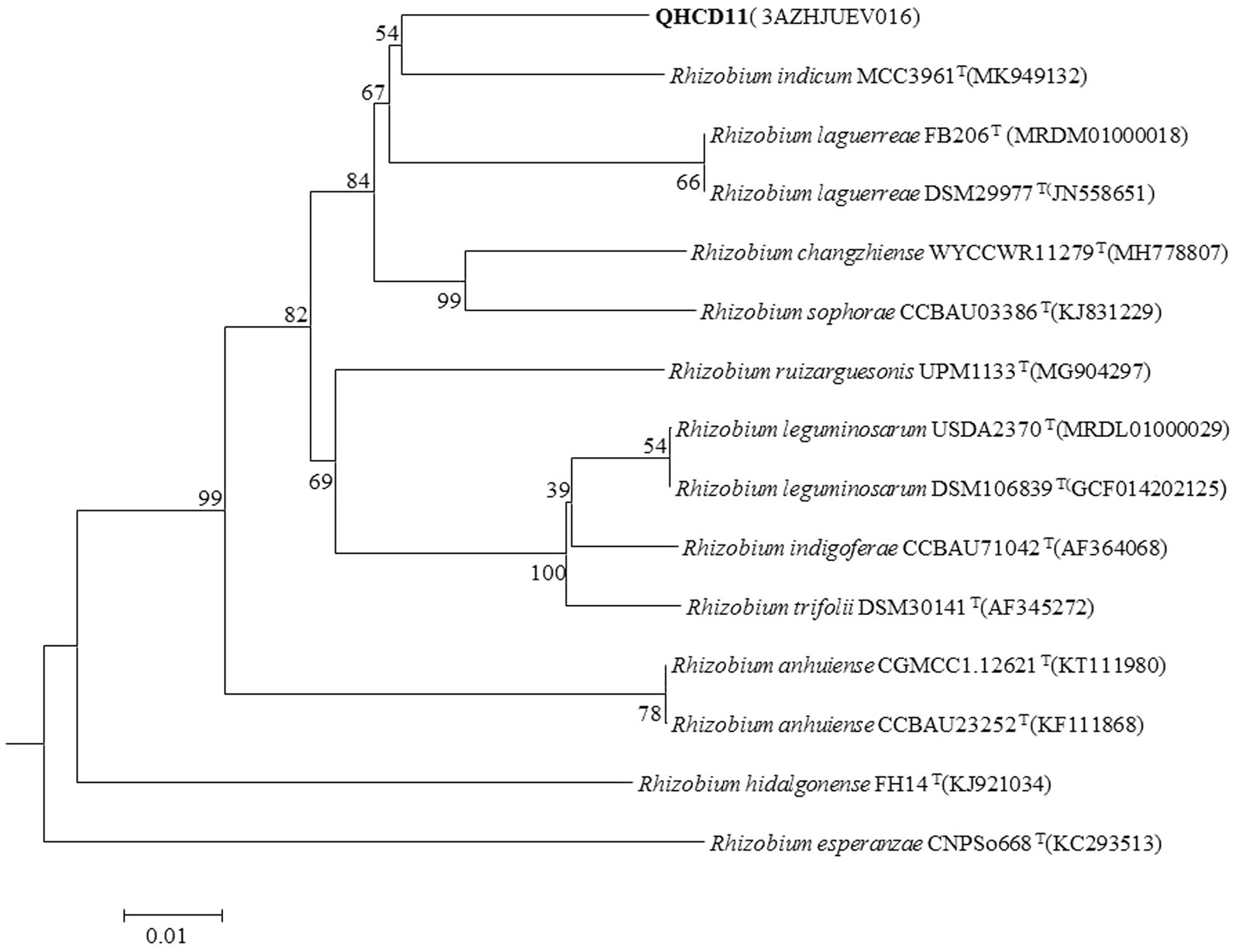
Figure 2. Neighbor-joining (NJ) phylogenetic analysis of Rhizobium ‘QHCD11’ whole genome sequence. Scale = 0.01 nucleotide substitutions per position. The outgroup was R. esperanzae ‘CNPSo668’T.
Chemotaxonomic characteristics of the Rhizobium isolate
We analyzed the fatty acid content of Rhizobium ‘QHCD11’ and found that the cells contain C16:0 (7.58%), C19:0 cyclo ω8c (6.18%), C18:0 (4.54%), C18:0 3OH (2.61%), 12:0 aldehyde (summed feature 2, 11.37%), and 16:1 ω7c/16:1 ω6c (summed feature 3, 1.14%) (Figure 3). Similar fatty acid profiles were observed in the Rhizobium reference strains.
Drought stress tolerance of the Rhizobium isolate
The drought tolerance of Rhizobium ‘QHCD11’ was evaluated by culturing the cells in YMA liquid culture media containing 0, 10, 15%, or 20% PEG-6000. The growth of the isolate, as determined spectrophotometrically based on OD600nm, was compared with R. leguminosarum reference strain ‘ACCC15854’ (Table 4). Increasing concentrations of PEG 6000 tended to result in decreased population size and optical density. Interestingly, Rhizobium ‘QHCD11’ performed better at 20% PEG 6000 than at 15% PEG 6000 (Figure 4), with the turbidity rising from 63.53 to 68.63%. Overall, Rhizobium ‘QHCD11’ was found to have superior drought tolerance. Research suggests that drought tolerant Rhizobium can improved the drought tolerance of host plants, so Rhizobium ‘QHCD11’ was selected for further studies of inoculation efficiency and growth improvement in faba bean under drought stress.
Inoculation on faba bean growth under drought stress
Inoculation of plants with beneficial microbes has been shown to ameliorate abiotic stress (Egamberdieva et al., 2016). Here, we studied the biochemical, physiological, and morphological effects of inoculation with Rhizobium ‘QHCD11’ on ‘Qingcan 14’ faba bean seedlings subjected to drought stress. The results were compared with an uninoculated negative control and a positive control inoculated with R. leguminosarum reference strain ‘ACCC15854’ (Table 5).
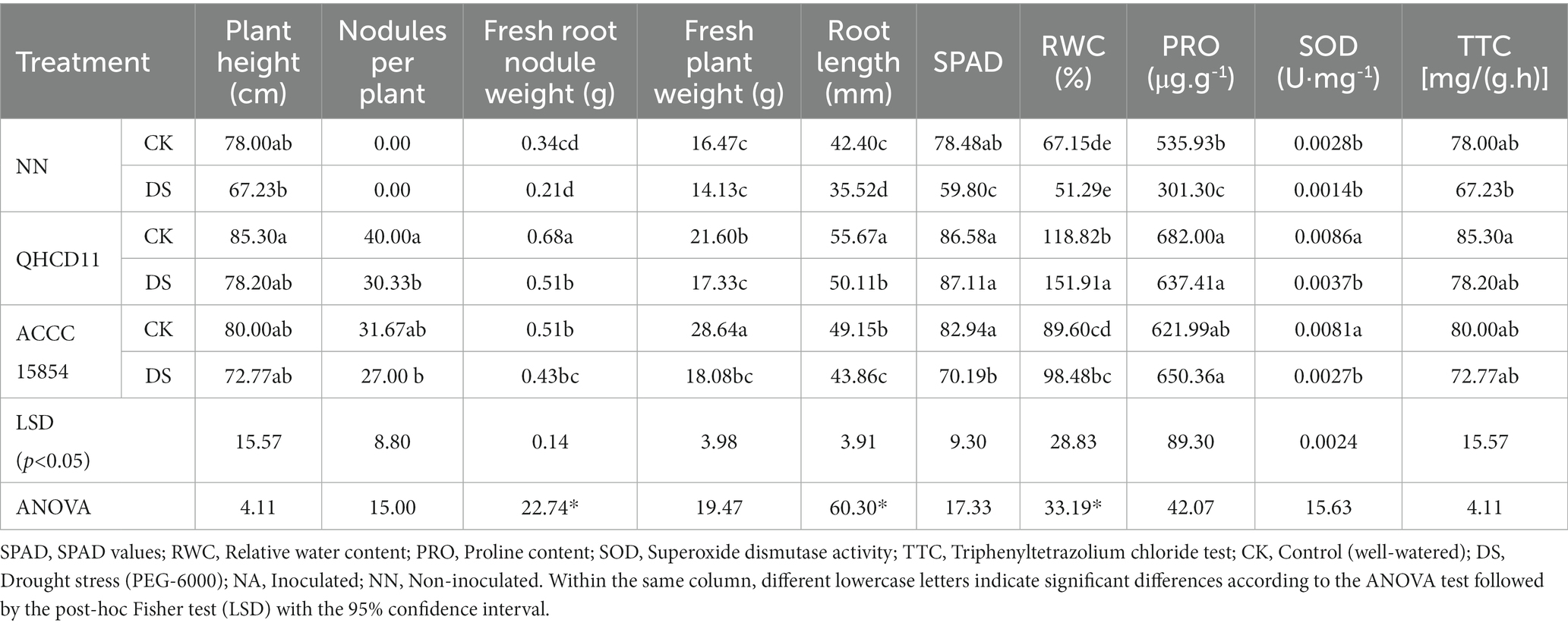
Table 5. Morphophysiological indices of inoculated and uninoculated faba bean seedlings under control and drought-stressed conditions.
Overall, inoculated faba beans (NA) exhibited substantially different characteristics than uninoculated faba beans (NN), with NA seedlings exhibiting improved drought resistance compared to NN seedlings. Unstressed inoculated seedlings exhibited the maximum for all tested parameters. Compared with matched controls, uninoculated faba bean seedlings were 13.8% shorter, had 59.52% fewer root nodules, and had 31.67% shorter roots (p ≤ 0.05). Unstressed Rhizobium ‘QHCD11’- inoculated seedlings were taller (85.3 cm) than unstressed uninoculated seedlings (78.0 cm). Drought-stressed Rhizobium ‘QHCD11’-inoculated seedlings had a greater number of root nodules (30.33) and a higher fresh root nodule weight (0.51 g) than uninoculated seedlings (11.33 and 0.21 g, respectively).
Physiological analyses revealed that drought stress not only hindered the biosynthesis of chlorophyll, but also accelerated the decomposition of existing chlorophyll. Rhizobium inoculation improved the SPAD value under both well-watered (+32.31%) and drought-stressed (+41.69%) conditions. Leaf RWC is an indicator of water status and reflects the balance between water supply and transpiration rate. Inoculation with Rhizobium ‘QHCD11’ significantly increased RWC by 81.05% under drought-stressed conditions and 48.05% under well-watered conditions.
Drought stress increased the proline content of faba bean seedlings, with Rhizobium ‘QHCD11’- inoculated seedlings exhibiting the greatest increase in proline. Compared to uninoculated seedlings, rhizobial inoculation resulted in a 38.14% increase in proline content under drought-stressed conditions. Furthermore, drought stress upregulated the activity of the enzymatic antioxidant SOD compared to well-watered conditions. Compared to un-inoculated seedlings, rhizobial inoculation increased SOD activity by 27.26% under well-watered conditions and a 33.76% increase in SOD activity under drought-stressed conditions. In water-limited environments, root health and vitality are predictors of survival (Zhang et al., 2022; Yu et al., 2023). The TTC test is an indicator of plant vitality broadly. Inoculated seedlings had significantly higher root vitality than uninoculated seedlings, especially Rhizobium ‘QHCD11’ -inoculated seedlings. Drought stress reduced root vitality, which was ameliorated with rhizobial inoculation. Increased root viability in inoculated plants is likely to have contributed to the increased drought tolerance of inoculated faba bean seedlings.
Discussion
Crop yield can be severely impacted by abiotic stress, such as high temperatures, alkalinity, high salt, and drought. Both naturally occurring and inoculated microbes have proven efficacious in allaying abiotic stress in crops and other plants. Specifically, Rhizobium inoculants enhance plant abiotic stress resistance by decreasing the generation of ROS, improving leaf water status, facilitating water uptake, and maintaining ionic balance (Thrall et al., 2008). Native rhizobia collected from targeted host plants and coexisting leguminous plants can serve as resources for the development of new and improved crop inocula. Here, we characterized a rhizobial isolate collected from the roots of a faba bean plant growing in the arid Haidong City region in Qinghai Province, China. The isolate, ‘QHCD11’, exhibited a fast growth rate, acid production, and a morphological appearance in line with other members of the genus Rhizobium.
The ‘QHCD11’ 16S rRNA sequence was 1,477 bp long, and the draft genome was 7.50 Mbp in size, with a G + C content of 60.9%. Analysis of sequence identity indicated that the strain shared 99.93% similarity with R. laguerreae ‘FB206’T, R. leguminosarum ‘USDA 2370’T, R. anhuiense ‘CCBAU 23252’T, R. ruizarguesonis ‘UMP1133’T, and R. indicum ‘JKLM12A2’T. NJ phylogenetic analysis revealed that ‘QHCD11’ formed a phyletic group with R. changzhiense ‘WYCCWR 11279’T (MH778807). Furthermore, phylogenomic analysis indicated that ‘QHCD11’ clustered with R. indicum ‘MCC3961’T. Our results were largely in agreement with previous studies suggesting that variation between Rhizobial strains may be more common at the species level, with diversity observed genus-wide. The ANI comparison value of strain ‘QHCD11’ to the closely-related R. indicum ‘FA23’T was 95.62%, which is greater than the ≥95% threshold to discriminate between different species of microbes (Chun et al., 2018). To confirm the taxonomic status, genome sequence-based DDH was also utilized. The DDH value was 69.7%, close to the DDH threshold value of 70% (Meier-Kolthoff et al., 2013), indicating that strain ‘QHCD11’ belonged to the R. indicum ‘MCC 3961’T species. Strain ‘QHCD11’ was found to contain a fatty acid array similar to Rhizobium reference strains, including18:1 ω7c (summed feature 8, 60.19%), 16:1 ω7c/16:1 ω6c (summed feature 3, 1.14%), 12:0 aldehyde (summed feature 2, 11.37%), C18:0 3OH (2.61%), C18:0 (4.54%), C19:0 cyclo ω8c (6.18%), and C16:0 (7.58%). These results clearly demarcate ‘QHCD11’ as a species of R. indicum (Richter and Rosselló-Móra, 2009). The Biolog GENIII bacterial identification system showed that strain ‘QHCD11’ was able to utilize several carbon sources, including tetrazolium blue, tetrazolium violet, acidum chinicum, glucuronamide, glucopyrone, L-glutamic acid, L-arginine, glycyl-L-proline, D-fructose-6-PO4, D-glucose-6-PO4, myo-phaseomannite, L-trehalose, D-galactose, N-Acetyl-D-galactosamine, N-Acetyl-β-D-mannosamine, D-meliodisaccharide, and D-honey trisaccharide. Based on these results, strain ‘QHCD11’ was preliminarily identified as belonging to the fast-growing genus Rhizobium.
Leguminous are important components of natural ecosystems and agricultural systems, and their microsymbionts of legumes have been intensively characterized (Han et al., 2010; Furseth et al., 2012; Zaw et al., 2021). Abiotic stresses are stress conditions to plants arising from the environment, it not only affected plants but also affect microbes. Many studies have shown that strains with drought tolerance can be screened out in arid environment, and inoculation of rhizobia can improve the growth of crops under abiotic stress conditions, increases crop yield and stress resistance (Enebe and Babalola, 2018; Bouhnik et al., 2022). Strain QHCD11 was identified by 16 s rRNA and whole genome sequencing as Rhizobium indicum species
Some researchers point out that inoculation of seeds with Rhizobium improved abiotic stress tolerance, it is attributed to produce light molecular weight organic solutes such as glycine betaine, proline, polyamines and ectoines, and these solutes protect the plant cells by stabilizing the structure and conformation of proteins as well as cell membranes from water stress, desiccation; increase photosynthetic and biomass productivity and also play role in the enhancement of the activity of various enzymatic and non-enzymatic antioxidants (Santos and Costa, 2002; Yurgel et al., 2013). In this study, we found that inoculation with strain ‘QHCD11’ initiates a wide response in various physiological activities like improves leaf water status, decreases the generation of reactive oxygen species, increase photosynthetic, enhance of the activity of various enzymatic antioxidants. Rhizobium inoculation improved the SPAD value under both drought-stressed and well-watered conditions. Furthermore, rhizobial inoculation can maintain leaf RWC by reducing dehydration-related cell damage, such as by increasing the proline content, as shown here. Enzymatic antioxidants like SOD alleviate oxidative stressed by converting ROS into O2 and water (Hasanuzzaman et al., 2012). Here, we found that inoculation with strain ‘QHCD11’ resulted in increased SOD activity, resulting in reduced oxidative stress and oxidative damage. And the same time, inoculated seedlings exhibited the best growth of all experimental groups under adequate water supply condition.
Conclusion
In the present study, we characterized a fast-growing Rhizobium isolate (‘QHCD11’) which can utilize a range of carbon sources. Based on phylogenetic, chemotaxonomic, genotypic, and phenotypic analyses, the ‘QHCD11’ strain putatively belongs to the R. indicum ‘MCC 3961’T species. Strain ‘QHCD11’ exhibited superior drought tolerance, and inoculation with this strain conferred drought tolerance and growth enhancement to faba bean seedlings. We suggest that Rhizobium strain ‘QHCD11’ may be further developed into a useful inoculant for improving the drought tolerance of faba beans and, potentially, other leguminous. These data help to illuminate the complex relationship between rhizobia and legumes under stressful environmental conditions.
Data availability statement
The original contributions presented in the study are included in the article/Supplementary materials, further inquiries can be directed to the corresponding author. The data presented in the study are deposited in the NCBI repository, accession number SUB13746821 Seq OR412372.
Author contributions
PL and TH designed experiments and wrote the manuscript. CT and JZ carried out experiments. CT and XW analyzed experimental results. YL analyzed sequencing data. All authors contributed to the article and approved the submitted version.
Funding
This work was supported by the Qinghai Provincial Department of Science and Technology Project (2020-ZJ-709).
Conflict of interest
The authors declare that the research was conducted in the absence of any commercial or financial relationships that could be construed as a potential conflict of interest.
Publisher’s note
All claims expressed in this article are solely those of the authors and do not necessarily represent those of their affiliated organizations, or those of the publisher, the editors and the reviewers. Any product that may be evaluated in this article, or claim that may be made by its manufacturer, is not guaranteed or endorsed by the publisher.
Abbreviations
ANI, Average nucleotide identity; dDDH, Digital DNA–DNA hybridization; NJ, Neighbor-joining; YMA, Yeast mannitol agar.
Footnotes
References
Ahmad, M., Zahir, Z. A., Nazli, F., Akram, F., Arshad, M., and Khalid, M. (2013). Effectiveness of halo-tolerant, auxin producing pseudomonas and rhizobium strains to improve osmotic stress tolerance in mung bean (Vigna radiata L.). Braz. J. Microbiol. 44, 1341–1348. doi: 10.1590/S1517-83822013000400045
Alinia, M., Kazemeini, S. A., Sepehri, M., and Dadkhodaie, A. (2022). Simultaneous application of rhizobium strain and melatonin improves the photosynthetic capacity and induces antioxidant defense system in common bean (Phaseolus vulgaris L.) under salinity stress. J. Plant Growth Regul. 41, 1367–1381. doi: 10.1007/s00344-021-10386-3
Amede, T., Kittlitz, E. V., and Schubert, S. (1999). Differential drought responses of faba bean (Vicia faba L.) inbred lines. J. Agron. Crop Sci. 183, 35–45. doi: 10.1046/j.1439-037x.1999.00310.x
Amede, T., and Schubert, S. (2003). Mechanisms of drought resistance in grain legumes I: osmotic adjustment. Ethiop. J. Sci. 26, 37–46. doi: 10.4314/sinet.v26i1.18198
Benidire, L., Lahrouni, M., Khalloufi, F. E., and Göttfert, M. (2017). Effects of Rhizobium leguminosarum inoculation on growth, nitrogen uptake and mineral assimilation in Vicia faba plants under salinity stress. J. Agric. Sci. Technol. 19, 889–901.
Bouhnik, O., Alami, S., Lamin, H., Lamrabet, M., Bennis, M., Ouajdi, M., et al. (2022). The fodder legume chamaecytisus albidus establishes functional symbiosis with different bradyrhizobial symbiovars in Morocco. Microb. Ecol. 84, 794–807. doi: 10.1007/s00248-021-01888-4
Brettin, T., Davis, J. J., Disz, T., Edwards, R. A., Gerdes, S., Olsen, G. J., et al. (2015). RASTtk: a modular and extensible implementation of the RAST algorithm for building custom annotation pipelines and annotating batches of genomes. Sci. Rep. 5, 8365–8370. doi: 10.1038/srep08365
Chelius, M. K., and Triplett, E. W. (2001). The diversity of archaea and bacteria in association with the roots of Zea mays L. Microb. Ecol. 41, 252–263. doi: 10.1007/s002480000087
Chun, J., Oren, A., Ventosa, A., Christensen, H., Arahal, D. R., da Costa, M. S., et al. (2018). Proposed minimal standards for the use of genome data for the taxonomy of prokaryotes. Int. J. Syst. Evol. Microbiol. 68, 461–466. doi: 10.1099/ijsem.0.002516
Deng, N., Huang, H. Q., Hu, Y. H., Wang, X., and Mo, K. L. (2022). Paenibacillus arenilitoris sp. nov., isolated from seashore sand and genome mining revealed the biosynthesis potential as antibiotic producer. Antonie Van Leeuwenhoek 115, 1307–1317. doi: 10.1007/s10482-022-01773-1
Denton, M. D., Phillips, L. A., Peoples, M. B., Pearce, D. J., Swan, A. D., Mele, P. M., et al. (2017). Legume inoculant application methods: effects on nodulation patterns, nitrogen fixation, crop growth and yield in narrow-leaf lupin and faba bean. Plant Soil 419, 25–39. doi: 10.1007/s11104-017-3317-7
Ding, W. L. (2022). The promotion of legume nodulation in plant-soil-microbe systems under phosphorus-limited conditions. Plant Soil 476, 251–262. doi: 10.1007/s11104-022-05564-5
Egamberdieva, D., Jabborova, D., and Berg, G. (2016). Synergistic interactions between Bradyrhizobium japonicum and the endophyte Stenotrophomonas rhizophila and their effects on growth, and nodulation of soybean under salt stress. Plant Soil 405, 35–45. doi: 10.1007/s11104-015-2661-8
Enebe, M. C., and Babalola, O. O. (2018). The influence of plant growth-promoting rhizobacteria in plant tolerance to abiotic stress: a survival strategy. Appl. Microbiol. Biotechnol. 102, 7821–7835. doi: 10.1007/s00253-018-9214-z
Franzini, V. I., Azcón, R., Méndes, F. L., and Aroca, R. (2013). Different interaction among glomus and rhizobium species on Phaseolus vulgaris and Zea mays plant growth, physiology and symbiotic development under moderate drought stress conditions. Plant Growth Regul. 70, 265–273. doi: 10.1007/s10725-013-9798-3
Furseth, B. J., Conley, S. P., and Ané, J. M. (2012). Soybean response to soil rhizobia and seed applied rhizobia inoculants in Wisconsin. Crop Sci. 52, 339–344. doi: 10.2135/cropsci2011.01.0041
Gaci, M., Benhizia, Y., Dems, M. A., Boukaous, L., Benguedouar, A., and Hynes, M. F. (2021). Phylogenetic diversity of indigenous rhizobium trapped from the natural habitat of Pisum sativum L. in eastern and Central Algeria. Plant Soil 461, 369–388. doi: 10.1007/s11104-020-04795-8
Han, T. X., Tian, C. F., Wang, E. T., and Chen, W. X. (2010). Associations among rhizobial chromosomal background, nod genes, and host plants based on the analysis of symbiosis of indigenous rhizobia and wild legumes native to Xinjiang. Microb. Ecol. 59, 311–323. doi: 10.1007/s00248-009-9577-x
Hardarson, G. (2003). Atkins C (2003) optimising biological N2 fixation by legumes in farming systems. Plant Soil 252, 41–54. doi: 10.1023/A:1024103818971
Hasanuzzaman, M., Hossain, M. A., da Silva, J. A. T., and Fujita, M. (2012). “Plant response and tolerance to abiotic oxidative stress: antioxidant defense is a key factor” in Crop stress and its management: Perspectives and strategies. eds. B. Venkateswarlu, A. K. Shanker, C. Shanker, and M. Maheswari (Berlin: Springer), 261–316.
Herridge, D. F., Peoples, M. B., and Boddey, R. M. (2008). Global inputs of biological nitrogen fixation in agricultural systems. Plant Soil 311, 1–18. doi: 10.1007/s11104-008-9668-3
Humaira, Y., and Bano, A. (2011). Isolation and characterization of phosphate solubilizing bacteria from rhizosphere soil of weeds of khewra salt range. Pak. J. Bot. 43, 1663–1668.
Hussain, A., Agrawal, N. K., and Leikanger, I. (2016). Action for Adaptation: Bringing climate change science to policy makers - a synthesis report of a conference held in Islamabad. Food Security 8, 285–289.
Khan, H. U. R., Link, W., Hocking, T. J., and Stoddard, F. L. (2007). Evaluation of physiological traits for improving drought tolerance in faba bean (Vicia faba L.). Plant Soil 292, 205–217. doi: 10.1007/s11104-007-9217-5
Jebara, M., Drevon, J. J., and Aouani, M. E. (2001). Effects of hydroponic culture system and NaCl on interactions between common bean lines and native rhizobia from Tunisian soils. Agronomie 21, 601–605. doi: 10.1051/agro:2001150
Jida, M., and Assefa, F. (2012). Phenotypic diversity and plant growth promoting characteristics of Mesorhizobium species isolated from chickpea (Cicer arietinum L.) growing areas of Ethiopia. African. J. Biotechnol. 11, 7483–7493. doi: 10.5897/AJMR11.400
Kanonge-Mafaune, G., Chiduwa, M. S., Chikwari, E., and Pisa, C. (2018). Evaluating the effect of increased rates of rhizobial inoculation on grain legume productivity. Symbiosis 75, 217–227. doi: 10.1007/s13199-018-0550-7
Kimura, M. (1980). A simple method for estimating evolutionary rates of base substitutions through comparative studies of nucleotide sequences. J. Mol. Evol. 16, 111–120. doi: 10.1007/BF01731581
Laranjo, M., Alexandre, A., and Oliveira, S. (2014). Legume growth promoting rhizobia: an overview on the Mesorhizobium genus. Microbiol. Res. 169, 2–17. doi: 10.1016/j.micres.2013.09.012
Li, P., Teng, C. C., Ding, B. J., Liu, Y. J., Hou, W. W., and He, T. (2021). A study on drought tolerance of rhizobia strains of faba bean (Vicia faba L.) isolated from drought regions in Qinghai plateau. Acta Agric. Univ. Jiangxiensis 43, 1241–1249. doi: 10.13836/j.jjau.2021133 (in Chinese)
Li, P., Zhang, Y. X., Wu, X. X., and Liu, Y. J. (2018). Drought stress impact on leaf proteome variations of faba bean(Vicia faba L.) in the Qinghai–Tibet plateau of China. 3 Biotech 8:110. doi: 10.1007/s13205-018-1088-3
Liu, H. L., Xin, B. Y., Zheng, J. S., and Zhong, H. (2021). Build a bioinformatic analysis platform and apply it to routine analysis of microbial genomics and comparative genomics. Protoc Exch. doi: 10.21203/rs.2.21224/v5
Lunn, J. E., Delorge, I., Figueroa, C. M., Dijck, P. V., and Stitt, M. (2014). Trehalose metabolism in plants. Plant J. 79, 544–567. doi: 10.1111/tpj.12509
Mabrouk, Y., and Belhadj, O. (2010). “The potential use of rhizobium–legume symbiosis for enhancing plant growth and management of plant diseases” in Microbes for legume improvement. eds. M. S. Khan, A. Zaidi, and J. Musarrat (Microbes for legume improvement, Wien, New York: Springer-Verlag), 1–25.
Mayak, S., Tirosh, T., and Glick, B. R. (2004). Plant growth-promoting bacteria that confer resistance to water stress in tomatoes and peppers. Plant Science 166, 525–530.
McDonald, G. K., and Paulsen, G. M. (1997). High temperature effects on photosynthesis and water relations of grain legumes. Plant Soil 196, 47–58. doi: 10.1023/A:1004249200050
Meier-Kolthoff, J. P., Auch, A. F., Klenk, H. P., and Göker, M. (2013). Genome sequence-based species delimitation with confidence intervals and improved distance functions. BMC Bioinformatics 14:60. doi: 10.1186/1471-2105-14-60
Mnasri, B., Mrabet, M., Laguerre, G., Aouani, M. E., and Mhamdi, R. (2007). Salt-tolerant rhizobia isolated from a Tunisian oasis that are highly effective for symbiotic N2-fixation with Phaseolus vulgaris constitute a novel biovar (bv. Mediterranense) of Sinorhizobium meliloti. Arch. Microbiol. 187, 79–85. doi: 10.1007/s00203-006-0173-x
Peoples, M. B., Brockwell, J., Herridge, D. F., Rochester, I. J., Alves, B. J. R., Urquiaga, S., et al. (2009). The contributions of nitrogen-fixing crop legumes to the productivity of agricultural systems. Symbiosis 48, 1–17. doi: 10.1007/BF03179980
Rahi, P., Giram, P., Chaudhari, D., DiCenzo, G. C., Kiran, S., Khullar, A., et al. (2020). Rhizobium indicum sp. nov., isolated from root nodules of pea (Pisum sativum) cultivated in the Indian trans-Himalayas. Syst. Appl. Microbiol. 43:126127. doi: 10.1016/j.syapm.2020.126127
Reina-Bueno, M., Argandoña, M., Nieto, J. J., Hidalgo-García, A., Iglesias Guerra, F., Delgado, M. J., et al. (2012). Role of trehalose in heat and desiccation tolerance in the soil bacterium Rhizobium etli. BMC Microbiol. 12:207. doi: 10.1186/1471-2180-12-207
Remigi, P., Zhu, J., Young, J. P. W., and Masson-Boivin, C. (2016). Symbiosis within symbiosis: evolving nitrogen-fixing legume symbionts. Trends Microbiol. 24, 63–75. doi: 10.1016/j.tim.2015.10.007
Richter and Rosselló-Móra (2009). Shifting the genomic gold standard for the prokaryotic species definition. PNAS 106, 19126–19131. doi: 10.1073/pnas.0906412106
Santos, H., and Costa, M. S. D. (2002). Compatible solutes of organisms that live in hot saline environments. Environ. Microbiol. 4, 501–509. doi: 10.1046/j.1462-2920.2002.00335.x
Seemann, T. (2014). Prokka: rapid prokaryotic genome annotation. Bioinformatics 30, 2068–2069. doi: 10.1093/bioinformatics/btu153
Sharma, A., Bandamaravuri, K. B., Sharma, A., and Arora, D. K. (2017). Phenotypic and molecular assessment of chickpea rhizobia from different chickpea cultivars of India. 3 Biotech 7, 7:327:327. doi: 10.1007/s13205-017-0952-x
Sharma, M. P., Srivastava, K., and Sharma, S. K. (2010). Biochemical characterization and metabolic diversity of soybean rhizobia isolated from Malwa region of Central India. Plant Soil Environ. 56, 375–383. doi: 10.17221/247/2009-PSE
Stefan, A., Van, C. J., Rosu, C. M., Stedela, C., Labroud, N. E., Flemetakise, E., et al. (2018). Genetic diversity and structure of rhizobium leguminosarum populations associated with clover plants are influenced by local environmental variables. Syst. Appl. Microbiol. 41, 251–259. doi: 10.1016/j.syapm.2018.01.007
Thrall, P. H., Bever, J. D., and Slattery, J. F. (2008). Rhizobial mediation of acacia adaptation to soil salinity: evidence of underlying trade-offs and tests offs and test of expected patterns. J. Ecol. 96, 746–755. doi: 10.1111/j.1365-2745.2008.01381.x
Tindall, B. J., Rosselló-Móra, R., Busse, H. J., Ludwig, W., and Kämpfer, P. (2010). Notes on the characterization of prokaryote strains for taxonomic purposes. Int J Syst Evol Microbiol 60, 249–266.
Tuan Vo, Q. A., Ballard, R. A., Barnett, S. J., and Franco, C. M. M. (2021). Isolation and characterisation of endophytic actinobacteria and their effect on the growth and nodulation of chickpea (Cicer arietinum). Plant Soil 466, 357–371. doi: 10.1007/s11104-021-05008-6
Vincent, J. M. (1970). In: International biological programme handbook: a manual for the practical study of root-nodule bacteria, Oxford Edinburgh: Blackwell Scientific Publications Oxford, 73–97.
Vriezen, J. A. C., de Bruijn, F. J., and Nusslein, K. (2007). Responses of rhizobia to desiccation in relation to osmotic stress, oxygen, and temperature. Appl. Environ. Microbiol. 73, 3451–3459. doi: 10.1128/AEM.02991-06
Wdowiak-Wróbel, S., Leszcz, A., and Małek, W. (2013). Salt tolerance in Astragalus cicer microsymbionts: the role of glycine betaine in osmoprotection. Curr. Microbiol. 66, 428–436. doi: 10.1007/s00284-012-0293-2
Yu, J. J., Fan, N. L., Hao, T., Bian, Y. J., Zhuang, L. L., Li, Q. G., et al. (2023). Ethionine-mitigation of drought stress associated with changes in root viability, antioxidant defense, osmotic adjustment, and endogenous hormones in tall fescue. Plant Growth Regul. 100, 119–132. doi: 10.1007/s10725-022-00944-4
Yurgel, S. N., Rice, J., Mulder, M., Kahn, M. L., Belova, V. S., and Roumiantseva, M. L. (2013). Truncated betB2-144 plays a critical role in Sinorhizobium meliloti Rm2011 osmoprotection and glycine-betaine catabolism. Eur. J. Soil Biol. 54, 48–55. doi: 10.1016/j.ejsobi.2012.10.004
Zaw, M., Rathjen, J. R., Zhou, Y., Ryder, M. H., and Denton, M. D. (2021). Symbiotic effectiveness, ecological adaptation and phylogenetic diversity of chickpea rhizobia isolated from a large-scale Australian soil collection. Plant Soil 469, 49–71. doi: 10.1007/s11104-021-05119-0
Zaw, M., Rathjen, J. R., Zhou, Y., Ryder, M. H., and Denton, M. D. (2022). Symbiotic effectiveness, ecological adaptation and phylogenetic diversity of chickpea rhizobia isolated from a large-scale Australian soil collection. Plant Soil 469, 49–71. doi: 10.1007/s11104-021-05119-0
Zeenat, M., Shahla, F., Basit, G., and Khalid, R. H. (2021). Inoculation of rhizobium alleviates salinity stress through modulation of growth characteristics, physiological and biochemical attributes, stomatal activities and antioxidant defence in Cicer arietinum L. J. Plant Growth Regul. 40, 2148–2163. doi: 10.1007/s00344-020-10267-1
Keywords: Rhizobium, phylogeny, biochemical characterization, drought tolerance, Vicia faba
Citation: Li P, Teng C, Zhang J, Liu Y, Wu X and He T (2023) Characterization of drought stress-mitigating Rhizobium from faba bean (Vicia faba L.) in the Chinese Qinghai-Tibet Plateau. Front. Microbiol. 14:1212996. doi: 10.3389/fmicb.2023.1212996
Edited by:
Asma Imran, National Institute for Biotechnology and Genetic Engineering, PakistanReviewed by:
Anandham Rangasamy, Tamil Nadu Agricultural University, IndiaMohsin Tariq, University of California, Berkeley, United States
Copyright © 2023 Li, Teng, Zhang, Liu, Wu and He. This is an open-access article distributed under the terms of the Creative Commons Attribution License (CC BY). The use, distribution or reproduction in other forums is permitted, provided the original author(s) and the copyright owner(s) are credited and that the original publication in this journal is cited, in accordance with accepted academic practice. No use, distribution or reproduction is permitted which does not comply with these terms.
*Correspondence: Tao He, aGV0YW94bkBhbGl5dW4uY29t