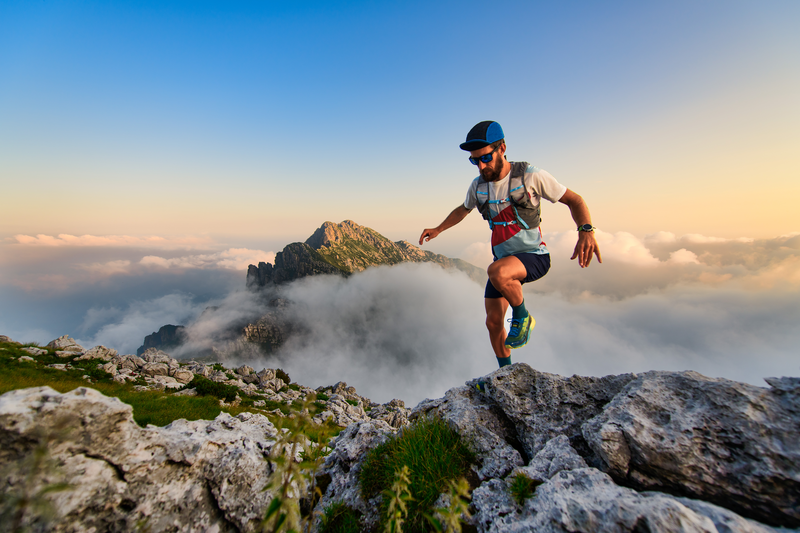
94% of researchers rate our articles as excellent or good
Learn more about the work of our research integrity team to safeguard the quality of each article we publish.
Find out more
OPINION article
Front. Microbiol. , 07 August 2023
Sec. Infectious Agents and Disease
Volume 14 - 2023 | https://doi.org/10.3389/fmicb.2023.1210747
This article is part of the Research Topic Reducing Healthcare-associated Infections through Antimicrobial Materials View all 6 articles
Central venous catheters (CVCs) play a clinically important role in various treatments, including venous pressure monitoring, the infusion of drugs, such as anti-cancer chemotherapy and antibiotics, parenteral nutrition, and blood transfusion (Saugel et al., 2017). However, compared with peripheral venous catheters, patients with indwelling CVCs are at increased risk of thrombosis, embolism, and infection (Ball and Singh, 2023). Catheter-related infections (CRIs) are thought to be associated with the formation of bacterial and fungal colonies on the catheter (Hanna et al., 2004). Microorganisms adhere to device surfaces, produce an extracellular polymeric matrix, and form biofilms. The biofilm-forming microorganisms then become a source of medical device-associated infection (Donlan, 2002). CRI is defined as a positive catheter tip culture and systemic inflammatory findings, while catheter-related bloodstream infection (CRBSI) is defined as CRI-criteria plus detection of the same organisms as in the catheter tip culture in peripheral blood cultures (Garner et al., 1988; Rockholt et al., 2023). CRBSIs have a reported mortality rate of 12%−40% depending on a variety of factors, such as comorbidities, the type of CVC used, and the type of pathogen, and are associated with longer hospital stays and increased medical costs (O'Grady et al., 2011; Böll et al., 2021; Ball and Singh, 2023).
Several preventive measures exist for CRIs, such as thorough hand hygiene, sterilization of the CVC site, and avoidance of catheter insertion at the femoral site. It is widely accepted that CVCs, which are foreign substances that can be a source of infection, should be removed as soon as possible. However, in clinical practice, situations arise in which long-term indwelling is required. Therefore, in such special situations, the Centers for Disease Control and Prevention (CDC) recommends the use of antimicrobial agents or antiseptic-impregnated catheters with comprehensive and adequate infection control measures, as shown Supplementary Figure S1 (O'Grady et al., 2011). Antimicrobial-impregnated catheters are a promising option for cases that require long-term CVC use (Böll et al., 2021). The purpose of this article was to summarize previous attempts to use antimicrobial/antiseptic impregnated catheters and provide a new perspective.
The control of CRI remains a major challenge to overcome; hence, a multifaceted attempt should be conducted. Certainly, antimicrobial-impregnated catheters have achieved some success to date, but no established clinical efficacy has been achieved with antiseptic substances (e.g., silver), which have been studied for many years (Viola et al., 2017). One such catheter is the chlorhexidine/silver sulfadiazine catheter, but most studies have found that its effect on reducing CRBSIs is not significant (Viola et al., 2017). Minocycline/rifampicin and miconazole/rifampicin catheters have also been shown to reduce the incidence of CRBSIs in several studies, where the incidence of antibiotic resistance, which remains a concern, was low (Viola et al., 2017; Reitzel et al., 2020; Böll et al., 2021). In clinical trials of patients with cancer, minocycline/rifampicin-impregnated catheters have also been shown to reduce the incidence of CRBSIs when used for longer than 2 months (Hanna et al., 2004). The combination of chlorhexidine with minocycline and rifampicin on catheters has been reported to have enhanced antimicrobial activity (Raad et al., 2012). Chlorhexidine acts on cell membranes, minocycline on protein synthesis, and rifampin on RNA synthesis. Thus, the mechanism of action of the combination of these three drugs may be additive. However, the confirmed enhancement of activity is only the result of in vitro studies, and the possibility of clinical application needs to be confirmed in clinical trials (Viola et al., 2017). As discussed above, a combined approach with different mechanisms of action may enhance antimicrobial activity and broaden the spectrum. Therefore, new approaches that are not antimicrobial agents or antiseptic substances may produce additional benefits.
From a different perspective, attempts have been made to inhibit iron, which is essential for the growth and proliferation of pathogenic microorganisms, as a strategy to increase antimicrobial activity (Schwarz et al., 2019; Scott et al., 2020). Specifically, the strategy is to use iron chelators in combination with antibacterial and antifungal agents. We hypothesize that the impregnation of catheters with antimicrobial agents and iron chelators would increase their antimicrobial and anti-biofilm activity and inhibit microbial colony and biofilm formation, resulting in reduced CRI incidence and longer catheter use, as shown in Supplementary Figure S2. The surface and lumen of the catheter are impregnated with antimicrobial and iron-chelating agents, which are assumed to inhibit bacterial and fungal colony and biofilm formation by exerting antimicrobial and iron-chelating activities on the bacteria and fungi that attach to the surface.
Because of its redox potential, iron is involved in many biochemical reactions. Iron is an essential element for all living organisms, including bacterial pathogens (Dev and Babitt, 2017). It also plays an important role as a cofactor in various intracellular pathways, such as DNA synthesis and repair, electron transfer, oxygen transport, and immune response (Scott et al., 2020). Iron levels in the human body are strictly regulated to protect against the toxic effects of free iron, which catalyzes the generation of reactive oxygen species (ROS), and the utilization of iron by invading microorganisms (Dixon and Stockwell, 2014). Through nutritional immunity, humans inhibit microbial growth by starving microorganisms of the metal ions they need (Hood and Skaar, 2012; Palmer and Skaar, 2016). Bacteria and fungi bind iron using high-affinity chelating compounds, called siderophores, as a strategy to acquire iron. Numerous types of siderophores have been reported. Siderophores are categorized into three main structural families—carboxylates, catecholates, and hydroxamates—with the catecholate siderophore, enterobactin, showing the highest affinity for iron, even higher than that for the host iron-binding protein, transferrin (Ellermann and Arthur, 2017). Iron chelators may be useful in the treatment of infectious diseases by limiting the availability of iron, thereby inhibiting the production of ROS, and by inhibiting microbial growth due to nutrient restriction (Lehmann et al., 2021; Paterson et al., 2022).
To date, the combinations of vancomycin and deferasirox against methicillin-resistant Staphylococcus aureus (Luo et al., 2014), doxycycline, and the iron chelator CP762 against Pseudomonas aeruginosa (Faure et al., 2021), and the triple combination of doxycycline, deferasirox, and thiostrepton (an antibiotic against gram-positive bacteria) against P. aeruginosa and Acinetobacter baumannii (Chan et al., 2020) have shown promising effects both in vitro and in animal models. For fungi, animal model experiments of combination therapy with liposomal amphotericin B (L-AMB), micafungin, and deferasirox against Aspergillus and Mucor (Ibrahim et al., 2011), animal experiments of combination therapy with L-AMB and deferasirox against Aspergillus fumigatus (Ibrahim et al., 2010), and in vitro experiments of combination therapy with AMB and deferoxamine against Cryptococcus spp. (Chayakulkeeree et al., 2020) have indicated that these combinations are useful. Despite promising experimental data for the combination of deferasirox and L-AMB for mucormycosis (Ibrahim et al., 2007, 2011; Schwarz et al., 2019), unexpectedly, a higher mortality rate at 90 days was seen with the combination than with L-AMB alone in a randomized controlled trial (Spellberg et al., 2012). Patients were enrolled at multiple centers with heterogeneous patient populations, resulting in an imbalance in underlying diseases and risk factors, with more patients in the deferasirox group having hematologic malignancies, neutropenia, and pulmonary infections. This imbalance in the patient's background may have influenced the patient's prognosis (Spellberg et al., 2012). Therefore, clinical trials are inconclusive regarding the efficacy of iron chelation assisted therapy.
Nevertheless, iron-chelation therapy still has potential for clinical applications. Different types of iron-chelating agents have been shown to have different antimicrobial activities. Of those tested, a water-soluble agent containing iron-selective copolymers (denying iron to bacterial infections; DIBI) was the most effective (Iron Binding Polymers | Fe Pharma, 2021; Lehmann et al., 2021). Recent studies have reported the promising finding that a combination of DIBI and antimicrobials may overcome drug-resistant bacteria and fungi. DIBI in combination with ciprofloxacin has been shown to be successful in treating ciprofloxacin-resistant A. baumannii-infected mice (Parquet et al., 2019). Moreover, the combination of DIBI and fluconazole was found to inhibit the growth of fluconazole-resistant Candida albicans in an in vitro study (Savage et al., 2018). These findings suggest that the appropriate selection of a type of iron chelator and its combination with an antimicrobial agent may be useful for dealing with a broad spectrum of infectious pathogens, and may overcome antibiotic resistance mechanisms.
One notable problem related to CVC implantation is biofilm formation. Here, we further explain the relationship between iron chelators and biofilm formation. It has already been mentioned that iron is essential for bacterial and fungal growth and proliferation, but it is also required for biofilm formation (Nazik et al., 2015; Coraça-Huber et al., 2018; Firoz et al., 2021). Microorganisms produce biofilms that protect them against antimicrobials, making them drug resistant (Høiby et al., 2010; Singh et al., 2010; Brauner et al., 2016; Thi et al., 2020). Hence, methods are needed to inhibit the formation of biofilms and attack the microorganisms that have formed within them. Iron-chelation therapy has been tested in P. aeruginosa to explore adjunctive therapy for chronic P. aeruginosa infection. Deferiprone, a synthetic iron chelator, has been shown to inhibit biofilm formation (Houshmandyar et al., 2021). Furthermore, the combination of iron chelators and antimicrobial agents has shown enhanced biofilm inhibitory activity; examples include combination therapy with the iron (VI) chelator N, N'-bis (2-hydroxybenzyl) ethylenediamine-N, N'-diacetic acid and colistin in an in vitro experiment (Mettrick et al., 2020), and with the iron chelators deferoxamine or deferasirox and tobramycin (Moreau-Marquis et al., 2009). Lactoferrin, a component of human secretions, also has iron-chelating activity (Singh, 2004), and ALX-109, an investigational agent containing lactoferrin and hypothiocyanite (a bactericidal agent), has shown activity against P. aeruginosa biofilms in combination with tobramycin and aztreonam (Moreau-Marquis et al., 2015). Deferasirox has shown biofilm inhibitory effects on the periodontal bacterium Prevotella intermedia (Moon et al., 2013), and lactoferrin treatment has significantly reduced proinflammatory cytokines production by gingival fibroblasts infected with P. intermedia. Moreover, an observational clinical trial of patients with periodontitis showed that treatment with lactoferrin reduced proinflammatory cytokines such as interleukin 6, edema, bleeding, pocket depth, and gingival and plaque indices in the crevicular fluid and improved clinical adhesion levels (Berlutti et al., 2011). Several studies were conducted on Staphylococcus spp. 1,2,3,4,6-Penta-O-galloyl-β-D-glucopyranose, a plant-derived ingredient with iron-chelating properties commonly used in Chinese medicine, has shown biofilm inhibitory effects on S. aureus (Lin et al., 2012). The combination of deferiprone and the heme analog gallium protoporphyrin in combination with gentamicin or ciprofloxacin increased the biofilm inhibitory effects in an S. aureus colony biofilm model (Richter et al., 2017). Additionally, for coagulase-negative staphylococci, deferiprone showed enhanced antibacterial activity in combination with clindamycin, gentamicin, or vancomycin by disrupting biofilms (Coraça-Huber et al., 2018). Regarding fungi, deferiprone inhibited biofilm formation by A. fumigatus, whereas deferoxamine had the opposite effect, and promoted biofilm formation. The result of the promotion of biofilm formation by deferoxamine may be due to A. fumigatus acquiring more iron chelator complexes through a siderophore-like mechanism (Nazik et al., 2015). In addition, deferoxamine is used by zygomycetes and A. fumigatus as a siderophore to promote bacterial growth (Boelaert et al., 1993). Thus, depending on the combination of the type of microorganism and iron chelator, the microorganism has a tolerance mechanism and takes advantage of iron starvation. However, iron chelators have a different point of action than do antibacterial and antifungal agents, and thus, may carry less risk of inducing drug resistance in microorganisms. This is a major advantage of combining drugs with different mechanisms of action.
The iron chelator CP762 in combination with tetracyclines against P. aeruginosa showed synergistic effects. Among the tetracyclines, doxycycline showed particularly high synergism (Faure et al., 2021). CP762 is a hexadentate hydroxypyridinone iron chelator that has high affinity and selectivity for iron and does not utilize many of the bacterial iron siderophore receptors, making it unlikely to donate iron to pathogenic microbes (Piyamongkol et al., 2005; Zhou et al., 2011). Tetracycline binds to the 30S bacterial ribosome via a magnesium bridge (White and Cantor, 1971; Pioletti et al., 2001), but iron can inhibit this mechanism by binding to the magnesium binding site (Faure et al., 2021). Sequestration of iron by iron chelators may minimize iron binding to tetracycline, which would facilitate its complexation with low-affinity ions such as magnesium, which is necessary for binding to the bacterial ribosome (Faure et al., 2021).
Most iron chelators examined in the past have been effective in inhibiting biofilm formation by bacteria and fungi, and their antimicrobial activity has been shown to be enhanced when used in combination with antimicrobial agents (Figure 1, Table 1). Therefore, we believe that the inclusion of iron chelators in antimicrobial- or antifungal-impregnated catheters could help overcome microbial resistance mechanisms and create CVCs with a lower risk of CRIs. Further research on iron chelators and antimicrobial-impregnated catheters, and confirmation of their effects on reducing the incidence of CRIs in clinical trials, are warranted.
Figure 1. Mechanisms of antimicrobial and anti-biofilm activity of iron chelators and enhancement of antimicrobial activity when used in combination with antimicrobial agents. (A) Iron chelators exert antimicrobial and anti-biofilm activity by depriving pathogenic microorganisms of iron, which is necessary for their growth and proliferation and for biofilm formation. (B) When used in combination with an antimicrobial agent (tetracycline), tetracycline acts on the bacterial 30S ribosome via magnesium, but iron competes with magnesium, so iron chelation reduces the amount of iron bound to tetracycline, thereby promoting binding to magnesium, resulting in enhanced antimicrobial activity. Figures created with BioRender (https://biorender.com/).
Table 1. Previous studies of antibacterial and anti-biofilm activity with antibacterial/antifungal agents and iron chelators.
Conceptualization, methodology, and writing—original draft: KI and HI. Resources and data curation: KI. Writing—review and editing and visualization: KI, HT, YM, and HI. Supervision: HT, YM, and HI. All authors contributed to the article and approved the submitted version.
The authors declare that the research was conducted in the absence of any commercial or financial relationships that could be construed as a potential conflict of interest.
All claims expressed in this article are solely those of the authors and do not necessarily represent those of their affiliated organizations, or those of the publisher, the editors and the reviewers. Any product that may be evaluated in this article, or claim that may be made by its manufacturer, is not guaranteed or endorsed by the publisher.
The Supplementary Material for this article can be found online at: https://www.frontiersin.org/articles/10.3389/fmicb.2023.1210747/full#supplementary-material
Ball, M., and Singh, A. (2023). “Care Of A Central Line,” in StatPearls (Treasure Island, FL: StatPearls Publishing). Available online at: http://www.ncbi.nlm.nih.gov/books/NBK564398/ (accessed April 22, 2023).
Berlutti, F., Pilloni, A., Pietropaoli, M., Polimeni, A., and Valenti, P. (2011). Lactoferrin and oral diseases: current status and perspective in periodontitis. Ann Stomatol (Roma) 2, 10–18.
Boelaert, J. R., de Locht, M., Van Cutsem, J., Kerrels, V., Cantinieaux, B., Verdonck, A., et al. (1993). Mucormycosis during deferoxamine therapy is a siderophore-mediated infection. In vitro and in vivo animal studies. J. Clin. Invest. 91, 1979–1986. doi: 10.1172/JCI116419
Böll, B., Schalk, E., Buchheidt, D., Hasenkamp, J., Kiehl, M., Kiderlen, T. R., et al. (2021). Central venous catheter-related infections in hematology and oncology: 2020 updated guidelines on diagnosis, management, and prevention by the Infectious Diseases Working Party (AGIHO) of the German Society of Hematology and Medical Oncology (DGHO). Ann. Hematol. 100, 239–259. doi: 10.1007/s00277-020-04286-x
Brauner, A., Fridman, O., Gefen, O., and Balaban, N. Q. (2016). Distinguishing between resistance, tolerance and persistence to antibiotic treatment. Nat. Rev. Microbiol. 14, 320–330. doi: 10.1038/nrmicro.2016.34
Chan, D. C. K., Guo, I., and Burrows, L. L. (2020). Forging new antibiotic combinations under iron-limiting conditions. Antimicrob. Agents Chemother. 64, e01909–e01919. doi: 10.1128/AAC.01909-19
Chayakulkeeree, M., Tangkoskul, T., Waywa, D., Tiengrim, S., Pati, N., and Thamlikitkul, V. (2020). Impact of iron chelators on growth and expression of iron-related genes of Cryptococcus species. J. Mycol. Med. 30, 100905. doi: 10.1016/j.mycmed.2019.100905
Coraça-Huber, D. C., Dichtl, S., Steixner, S., Nogler, M., and Weiss, G. (2018). Iron chelation destabilizes bacterial biofilms and potentiates the antimicrobial activity of antibiotics against coagulase-negative Staphylococci. Pathog. Dis. 76. doi: 10.1093/femspd/fty052
Dev, S., and Babitt, J. L. (2017). Overview of iron metabolism in health and disease. Hemodial. Int. 21, S6–S20. doi: 10.1111/hdi.12542
Dixon, S. J., and Stockwell, B. R. (2014). The role of iron and reactive oxygen species in cell death. Nat. Chem. Biol. 10, 9–17. doi: 10.1038/nchembio.1416
Donlan, R. M. (2002). Biofilms: microbial life on surfaces. Emerging Infect. Dis. 8, 881–890. doi: 10.3201/eid0809.020063
Ellermann, M., and Arthur, J. C. (2017). Siderophore-mediated iron acquisition and modulation of host-bacterial interactions. Free Radic. Biol. Med. 105, 68–78. doi: 10.1016/j.freeradbiomed.2016.10.489
Faure, M. E., Cilibrizzi, A., Abbate, V., Bruce, K. D., and Hider, R. C. (2021). Effect of iron chelation on anti-pseudomonal activity of doxycycline. Int. J. Antimicrob. Agents 58, 106438. doi: 10.1016/j.ijantimicag.2021.106438
Firoz, A., Haris, M., Hussain, K., Raza, M., Verma, D., Bouchama, M., et al. (2021). Can targeting iron help in combating chronic pseudomonas infection? A systematic review. Cureus 13, e13716. doi: 10.7759/cureus.13716
Garner, J. S., Jarvis, W. R., Emori, T. G., Horan, T. C., and Hughes, J. M. (1988). CDC definitions for nosocomial infections, 1988. Am. J. Infect. Control 16, 128–140. doi: 10.1016/0196-6553(88)90053-3
Hanna, H., Benjamin, R., Chatzinikolaou, I., Alakech, B., Richardson, D., Mansfield, P., et al. (2004). Long-term silicone central venous catheters impregnated with minocycline and rifampin decrease rates of catheter-related bloodstream infection in cancer patients: a prospective randomized clinical trial. J. Clin. Oncol. 22, 3163–3171. doi: 10.1200/JCO.2004.04.124
Høiby, N., Ciofu, O., and Bjarnsholt, T. (2010). Pseudomonas aeruginosa biofilms in cystic fibrosis. Future Microbiol. 5, 1663–1674. doi: 10.2217/fmb.10.125
Hood, M. I., and Skaar, E. P. (2012). Nutritional immunity: transition metals at the pathogen-host interface. Nat. Rev. Microbiol. 10, 525–537. doi: 10.1038/nrmicro2836
Houshmandyar, S., Eggleston, I. M., and Bolhuis, A. (2021). Biofilm-specific uptake of a 4-pyridone-based iron chelator by Pseudomonas aeruginosa. Biometals 34, 315–328. doi: 10.1007/s10534-020-00281-x
Ibrahim, A. S., Gebermariam, T., Fu, Y., Lin, L., Husseiny, M. I., French, S. W., et al. (2007). The iron chelator deferasirox protects mice from mucormycosis through iron starvation. J. Clin. Invest. 117, 2649–2657. doi: 10.1172/JCI32338
Ibrahim, A. S., Gebremariam, T., French, S. W., Edwards, J. E., and Spellberg, B. (2010). The iron chelator deferasirox enhances liposomal amphotericin B efficacy in treating murine invasive pulmonary aspergillosis. J. Antimicrob. Chemother. 65, 289–292. doi: 10.1093/jac/dkp426
Ibrahim, A. S., Gebremariam, T., Luo, G., Fu, Y., French, S. W., Edwards, J. E., et al. (2011). Combination therapy of murine mucormycosis or aspergillosis with iron chelation, polyenes, and echinocandins. Antimicrob. Agents Chemother. 55, 1768–1770. doi: 10.1128/AAC.01577-10
Iron Binding Polymers | Fe Pharma (2021). Available online at: https://fepharm.com/iron-binding-polymers/ (accessed April 22, 2023).
Lehmann, C., Aali, M., Zhou, J., and Holbein, B. (2021). Comparison of treatment effects of different iron chelators in experimental models of sepsis. Life (Basel) 11, 57. doi: 10.3390/life11010057
Lin, M.-H., Shu, J.-C., Huang, H.-Y., and Cheng, Y.-C. (2012). Involvement of iron in biofilm formation by Staphylococcus aureus. PLoS ONE 7, e34388. doi: 10.1371/journal.pone.0034388
Luo, G., Spellberg, B., Gebremariam, T., Lee, H., Xiong, Y. Q., French, S. W., et al. (2014). Combination therapy with iron chelation and vancomycin in treating murine staphylococcemia. Eur. J. Clin. Microbiol. Infect. Dis. 33, 845–851. doi: 10.1007/s10096-013-2023-5
Mettrick, K., Hassan, K., Lamont, I., and Reid, D. (2020). The Iron-chelator, N,N'-bis (2-hydroxybenzyl) ethylenediamine-N,N'-Diacetic acid is an effective colistin adjunct against clinical strains of biofilm-dwelling Pseudomonas aeruginosa. Antibiotics (Basel) 9, 144. doi: 10.3390/antibiotics9040144
Moon, J.-H., Kim, C., Lee, H.-S., Kim, S.-W., and Lee, J.-Y. (2013). Antibacterial and antibiofilm effects of iron chelators against Prevotella intermedia. J. Med. Microbiol. 62, 1307–1316. doi: 10.1099/jmm.0.053553-0
Moreau-Marquis, S., Coutermarsh, B., and Stanton, B. A. (2015). Combination of hypothiocyanite and lactoferrin (ALX-109) enhances the ability of tobramycin and aztreonam to eliminate Pseudomonas aeruginosa biofilms growing on cystic fibrosis airway epithelial cells. J. Antimicrob. Chemother. 70, 160–166. doi: 10.1093/jac/dku357
Moreau-Marquis, S., O'Toole, G. A., and Stanton, B. A. (2009). Tobramycin and FDA-approved iron chelators eliminate Pseudomonas aeruginosa biofilms on cystic fibrosis cells. Am. J. Respir. Cell Mol. Biol. 41, 305–313. doi: 10.1165/rcmb.2008-0299OC
Nazik, H., Penner, J. C., Ferreira, J. A., Haagensen, J. A. J., Cohen, K., Spormann, A. M., et al. (2015). Effects of iron chelators on the formation and development of aspergillus fumigatus biofilm. Antimicrob. Agents Chemother. 59, 6514–6520. doi: 10.1128/AAC.01684-15
O'Grady, N. P., Alexander, M., Burns, L. A., Dellinger, E. P., Garland, J., Heard, S. O., et al. (2011). Guidelines for the prevention of intravascular catheter-related infections. Am. J. Infect. Control 39, S1–34. doi: 10.1016/j.ajic.2011.01.003
Palmer, L. D., and Skaar, E. P. (2016). Transition metals and virulence in bacteria. Annu. Rev. Genet. 50, 67–91. doi: 10.1146/annurev-genet-120215-035146
Parquet, M. D. C., Savage, K. A., Allan, D. S., Ang, M. T. C., Chen, W., Logan, S. M., et al. (2019). Antibiotic-resistant acinetobacter baumannii is susceptible to the novel iron-sequestering anti-infective dibi in vitro and in experimental pneumonia in mice. Antimicrob. Agents Chemother. 63, e00855–e00819. doi: 10.1128/AAC.00855-19
Paterson, J. R., Beecroft, M. S., Mulla, R. S., Osman, D., Reeder, N. L., Caserta, J. A., et al. (2022). Insights into the antibacterial mechanism of action of chelating agents by selective deprivation of iron, manganese, and zinc. Appl. Environ. Microbiol. 88, e0164121. doi: 10.1128/AEM.01641-21
Pioletti, M., Schlünzen, F., Harms, J., Zarivach, R., Glühmann, M., Avila, H., et al. (2001). Crystal structures of complexes of the small ribosomal subunit with tetracycline, edeine and IF3. EMBO J. 20, 1829–1839. doi: 10.1093/emboj/20.8.1829
Piyamongkol, S., Zhou, T., Liu, Z. D., Khodr, H. H., and Hider, R. C. (2005). Design and characterisation of novel hexadentate 3-hydroxypyridin-4-one ligands. Tetrahedron Lett. 46, 1333–1336. doi: 10.1016/j.tetlet.2004.12.115
Raad, I., Mohamed, J. A., Reitzel, R. A., Jiang, Y., Raad, S., Al Shuaibi, M., et al. (2012). Improved antibiotic-impregnated catheters with extended-spectrum activity against resistant bacteria and fungi. Antimicrob. Agents Chemother. 56, 935–941. doi: 10.1128/AAC.05836-11
Reitzel, R. A., Rosenblatt, J., Gerges, B. Z., Jarjour, A., Fernández-Cruz, A., and Raad, I. I. (2020). The potential for developing new antimicrobial resistance from the use of medical devices containing chlorhexidine, minocycline, rifampicin and their combinations: a systematic review. JAC. Antimicrob. Resist. 2, dlaa002. doi: 10.1093/jacamr/dlaa002
Richter, K., Thomas, N., Zhang, G., Prestidge, C. A., Coenye, T., Wormald, P.-J., et al. (2017). Deferiprone and gallium-protoporphyrin have the capacity to potentiate the activity of antibiotics in staphylococcus aureus small colony variants. Front. Cell. Infect. Microbiol. 7, 280. doi: 10.3389/fcimb.2017.00280
Rockholt, M. M., Agrell, T., Thorarinsdottir, H., and Kander, T. (2023). Sustained low catheter related infection (CRI) incidence in an observational follow-up study of 9924 catheters using automated data scripts as quality assurance for central venous catheter (CVC) management. Infect. Prev. Pract. 5, 100273. doi: 10.1016/j.infpip.2023.100273
Saugel, B., Scheeren, T. W. L., and Teboul, J.-L. (2017). Ultrasound-guided central venous catheter placement: a structured review and recommendations for clinical practice. Crit. Care 21, 225. doi: 10.1186/s13054-017-1814-y
Savage, K. A., Parquet, M., del, C., Allan, D. S., Davidson, R. J., Holbein, B. E., et al. (2018). Iron restriction to clinical isolates of candida albicans by the novel chelator dibi inhibits growth and increases sensitivity to azoles in vitro and in vivo in a murine model of experimental vaginitis. Antimicrob. Agents Chemother. 62, e02576–e02517. doi: 10.1128/AAC.02576-17
Schwarz, P., Cornely, O. A., and Dannaoui, E. (2019). Antifungal combinations in Mucorales: a microbiological perspective. Mycoses 62, 746–760. doi: 10.1111/myc.12909
Scott, C. R., Holbein, B. E., and Lehmann, C. D. (2020). Iron should be restricted in acute infection. Front Biosci (Landmark Ed) 25, 673–682. doi: 10.2741/4827
Singh, P. K. (2004). Iron sequestration by human lactoferrin stimulates P. aeruginosa surface motility and blocks biofilm formation. Biometals 17, 267–270. doi: 10.1023/B:BIOM.0000027703.77456.27
Singh, R., Ray, P., Das, A., and Sharma, M. (2010). Penetration of antibiotics through Staphylococcus aureus and Staphylococcus epidermidis biofilms. J. Antimicrob. Chemother. 65, 1955–1958. doi: 10.1093/jac/dkq257
Spellberg, B., Ibrahim, A. S., Chin-Hong, P. V., Kontoyiannis, D. P., Morris, M. I., Perfect, J. R., et al. (2012). The deferasirox-ambisome therapy for mucormycosis (DEFEAT Mucor) study: a randomized, double-blinded, placebo-controlled trial. J. Antimicrob. Chemother. 67, 715–722. doi: 10.1093/jac/dkr375
Thi, M. T. T., Wibowo, D., and Rehm, B. H. A. (2020). Pseudomonas aeruginosa Biofilms. Int. J. Mol. Sci. 21, 8671. doi: 10.3390/ijms21228671
Viola, G. M., Rosenblatt, J., and Raad, I. I. (2017). Drug eluting antimicrobial vascular catheters: progress and promise. Adv. Drug Deliv. Rev. 112, 35–47. doi: 10.1016/j.addr.2016.07.011
White, J. P., and Cantor, C. R. (1971). Role of magnesium in the binding of tetracycline to Escherichia coli ribosomes. J. Mol. Biol. 58, 397–400. doi: 10.1016/0022-2836(71)90255-5
Keywords: central venous catheterization, catheter-related infections, catheter-related bacteremia, iron-chelating agents, biofilms
Citation: Itoh K, Tsutani H, Mitsuke Y and Iwasaki H (2023) Potential additional effects of iron chelators on antimicrobial-impregnated central venous catheters. Front. Microbiol. 14:1210747. doi: 10.3389/fmicb.2023.1210747
Received: 23 April 2023; Accepted: 27 July 2023;
Published: 07 August 2023.
Edited by:
Axel Cloeckaert, Institut national de recherche pour l'agriculture, l'alimentation et l'environnement (INRAE), FranceReviewed by:
Mostafa A. Elbahnasawy, Al-Azhar University, EgyptCopyright © 2023 Itoh, Tsutani, Mitsuke and Iwasaki. This is an open-access article distributed under the terms of the Creative Commons Attribution License (CC BY). The use, distribution or reproduction in other forums is permitted, provided the original author(s) and the copyright owner(s) are credited and that the original publication in this journal is cited, in accordance with accepted academic practice. No use, distribution or reproduction is permitted which does not comply with these terms.
*Correspondence: Kazuhiro Itoh, a2l0b2hAdS1mdWt1aS5hYy5qcA==
†ORCID: Kazuhiro Itoh orcid.org/0000000155747118
Disclaimer: All claims expressed in this article are solely those of the authors and do not necessarily represent those of their affiliated organizations, or those of the publisher, the editors and the reviewers. Any product that may be evaluated in this article or claim that may be made by its manufacturer is not guaranteed or endorsed by the publisher.
Research integrity at Frontiers
Learn more about the work of our research integrity team to safeguard the quality of each article we publish.