- Department of Dermatology, Skin Research Institute of Peking University Shenzhen Hospital, Peking University Shenzhen Hospital, Shenzhen, China
Invasive fungal disease (IFD) poses a significant threat to immunocompromised patients and remains a global challenge due to limited treatment options, high mortality and morbidity rates, and the emergence of drug-resistant strains. Despite advancements in antifungal agents and diagnostic techniques, the lack of effective vaccines, standardized diagnostic tools, and efficient antifungal drugs contributes to the ongoing impact of invasive fungal infections (IFI). Recent studies have highlighted the presence of extracellular vesicles (EVs) released by fungi carrying various components such as enzymes, lipids, nucleic acids, and virulence proteins, which play roles in both physiological and pathological processes. These fungal EVs have been shown to interact with the host immune system during the development of fungal infections whereas their functional role and potential application in patients are not yet fully understood. This review summarizes the current understanding of the biologically relevant findings regarding EV in host-pathogen interaction, and aim to describe our knowledge of the roles of EV as diagnostic tools and vaccine vehicles, offering promising prospects for the treatment of IFI patients.
1. Introduction
Invasive fungal disease (IFD) is a severe and life-threatening condition caused by fungal infections. Recent reports indicate that fungal infections cause over 1.5 million deaths worldwide each year (GAFFI1), surpassing malaria (WHO2) and similar to tuberculosis (WHO3) in terms of mortality rates. This highlights the global significance of fungal infections, with an increasing number of high-risk groups being exposed to invasive fungal infections (IFIs), including individuals with hematologic malignancies and those affected by HIV/AIDS. Unfortunately, therapeutic options for IFD are severely limited by challenges such as incomplete drug efficacy, cytotoxicity, and the emergence of drug-resistant strains (Odds, 2003). This is further exacerbated by the growing population of immunocompromised patients, including individuals with HIV infection, leukemia, or cancer undergoing immunosuppressive therapy or chemotherapy (Mossad, 2018; Rawson et al., 2021). Given the global prevalence of fungal disease and the limitations in its treatment, it is essential to research the interaction between fungi and the host immune system during the development of fungal infections and to explore novel therapeutic strategies.
Extracellular vesicle (EV), including exosome, microvesicle, and apoptotic vesicle, is a lipid-bilayer-enclosed particle that is secreted by both prokaryotic and eukaryotic cells (Lotvall et al., 2014). Exosome is nanosized vesicle (30–100 nm) and its biogenesis encompasses a series of intricate steps including endosome formation, multivesicular bodies (MVBs) generation, and cargo sorting. The initiation of exosome biogenesis arises with the budding of an endosomal vesicle from the plasma membrane. There are three distinct mechanisms underlie the formation of endocytic vesicles including clathrin-mediated endocytosis (CME), caveolin-dependent endocytosis (CDE), and clathrin- and caveolin-independent endocytosis (Mayor et al., 2014). Fusion of endocytic vesicles results in the creation of intraluminal vesicles (ILVs) within early endosomes, a process governed by ESCRT-dependent and ESCRT-independent pathways that subsequently culminate in the formation of MVBs. Exosomes take shape within these ILVs, which originate within the framework of late endosomes, an essential facet of the endocytic apparatus. The sorting of cargoes into ILVs draws upon diverse organelles including the trans-Golgi network (TGN), endoplasmic reticulum (ER), and mitochondria. MVBs exhibit the capability to fuse with lysosomes, thereby undergoing lysosomal degradation (Kwon et al., 2016). Alternatively, MVBs undertake trafficking toward the plasma membrane, where membrane fusion facilitates the extracellular release of ILVs in the form of exosomes. Unlike exosomes, microvesicles (MVs) (100 nm to 1 μm) have a direct origin from the plasma membrane and are commonly categorized as ectosomes (Heijnen et al., 1999). The process governing MV formation initiates with the development of outward buds at specific membrane sites, followed by fission and eventual liberation of the vesicle into the extracellular milieu (Ratajczak et al., 2006). Molecular rearrangements within the plasma membrane transpire at the points of MV origination, leading to membrane budding. These rearrangements encompass alterations in lipid and protein composition as well as changes in Ca2+ levels. The perturbed Ca2+ levels trigger the recruitment and activation of calcium-dependent enzymes, such as scramblase and floppase, inducing subsequent modifications in the lipid composition of the plasma membrane. Notably, one of the principal attributes of MVs is the externalization of phosphatidylserine (PS) (Barteneva et al., 2013). The generation of microvesicles requires cytoskeleton components, such as actin and microtubules, along with molecular motors (kinesins and myosins), and fusion machinery (SNAREs and tethering factors), However, the precise route of MV formation is unclear.
EVs derived from fungi have generated increased attention and emerged as a promising field in recent years. Research has revealed that fungal EVs carry a range of components, including proteins, enzymes, lipids, nucleic acids, and carbohydrates, which enable them to facilitate biofilm formation, virulence factor transportation, and modulate of the host immune response (Rutter et al., 2022). Fungi release EVs that transport enzymes and metabolites involved in the infection process, thus being considered as virulence factors. Recent years have witnessed notable advancements in fungal EV research, unveiling their potential applications as immunomodulatory agents and therapeutic strategies that actively participate in biological processes, diagnosis, and therapy. However, the interaction between fungi and the host immune system remains poorly understood, and there is limited research investigating the potential clinical applications of fungal EVs. Therefore, it is imperative to summary the immune response triggered by fungal EVs in the host to facilitate their potential role in diagnosis and therapy.
2. Fungal EVs and virulence factors
2.1. Fungi infection
Fungal infections occur through two pathways: extrinsic and intrinsic. Extrinsic infections are mainly caused by environmental fungi, while intrinsic infections are promoted by the patient’s gut flora (Dimitriu et al., 2019; Podder et al., 2019). While infectivity, pathogenicity, and virulence are the three main variables that determine the outcome in patients exposed to fungi, the course of the infection is also influenced by fungal cell wall (Rodrigues et al., 2007). The fungal cell wall, consisting of β-1,3-glucan, chitin, chitosan, and glycosylated proteins, mediates the innate immune response against fungal infection. Targeting the fungal cell wall has emerged as a promising strategy for antifungal drugs due to its specificity for fungi and the ability to induce cell lysis. Studies have shown that Candida albicans β-glucan can invade uninfected monocytes, exacerbating immunodeficiency and increasing susceptibility to systemic fungal infection (Nisini et al., 2007). Similarly, caspofungin has been found to enhance the immune system’s ability to destroy mold hyphae mediated by human polymorphonuclear neutrophils (Lamaris et al., 2008). Conversely, Maligie and Selitrennikoff (2005) found that Cryptococcus neoformans develop resistance to caspofungin and cilofungin through a mechanism unrelated to β-1,3-glucan synthase resistance. The structure of fungal cell wall and virulence factors are summarized in Figure 1.
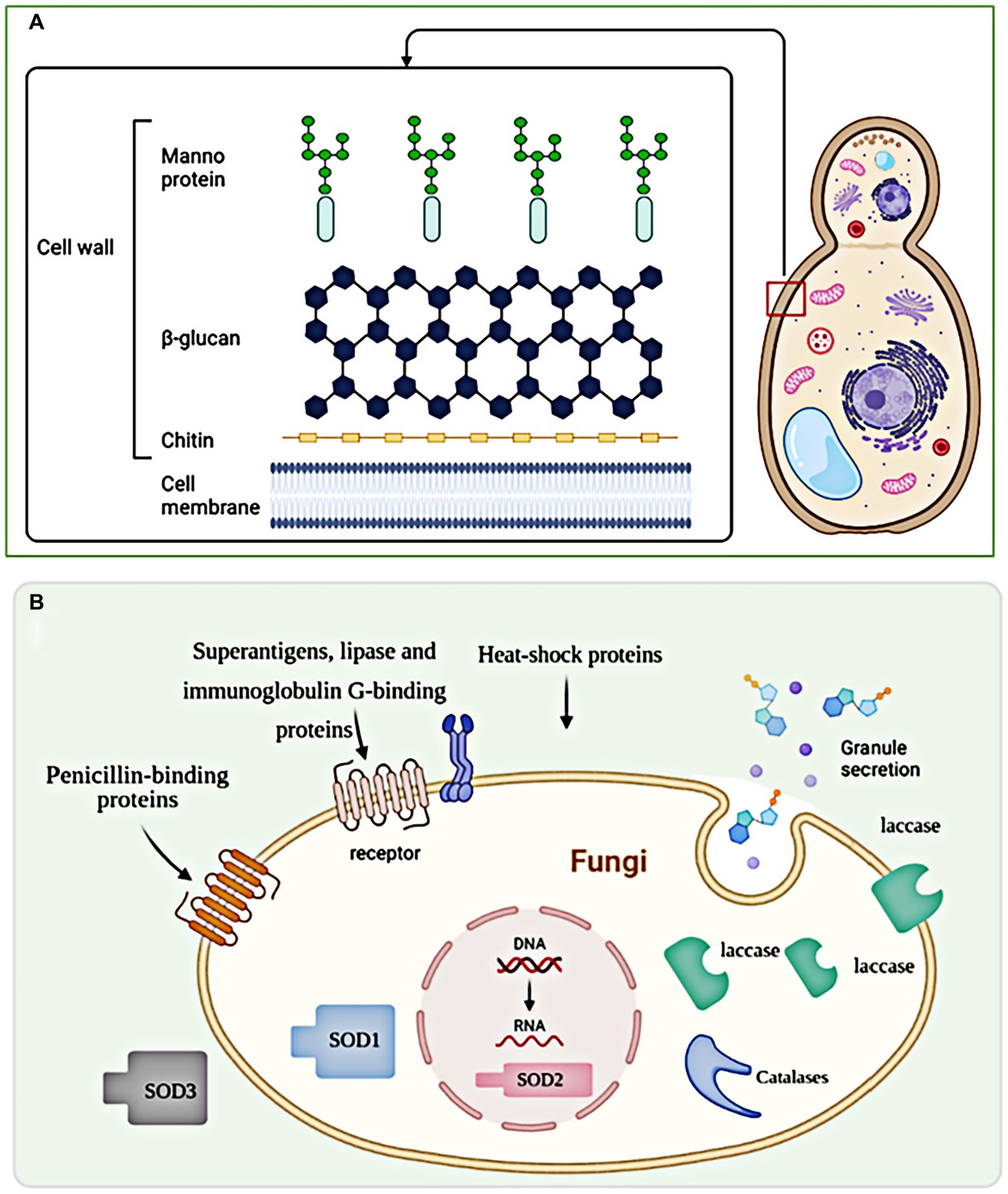
Figure 1. The structure of fungal cell wall and virulence factors. (A) The fungal cell wall is a complex structure composed of polysaccharides, proteins, and lipids that provide structural integrity and protection to fungal cells. (B) Exosomes, being larger vesicles, do not directly pass through the fungal cell wall but can indirectly influence it through the secretion of enzymes and other molecules involved in cell wall modification and remodeling.
Fungal pathogens have developed various strategies to evade immune recognition and cause systemic infections. Luo et al. demonstrated that Candida albicans, through sequence polymorphisms and differences in the expression levels of Gpm1 (phosphoglycerate mutase 1) and Pra1 (pH-regulated antigen 1), essential complement evasion proteins, increases its virulence by modulating immune fitness (Luo et al., 2015). Dasari et al. investigated the central immune evasion protein Aspf2 in Aspergillus fumigatus and found that it facilitates early infection stages by preventing host innate immune attacks and disrupting lung epithelial cell layers (Dasari et al., 2018). Aspergillus fumigatus also employs Dihydroxynaphthalene melanin (DHN-melanin) to inhibit acidification in the phagolysosome, interfering with the endocytic process and evading immune cell absorption (Thywissen et al., 2011; Akoumianaki et al., 2016). Furthermore, Taborda et al. discovered that the opsonic receptor CR3 plays a crucial role in antibody-mediated complement-independent phagocytosis of Cryptococcus neoformans, connecting the innate and adaptive immune systems (Taborda and Casadevall, 2002). These studies highlight the mechanisms by which fungal pathogens manipulate the immune response for their survival and dissemination.
Fungal pathogens employ various mechanisms to evade host defense and immune responses, including the detoxification of oxidative death mechanisms. Candida albicans, for example, utilizes the glyoxylate cycle to resist neutrophils and evade oxidative stress responses, enhancing its survival (Miramon et al., 2012). Despite their presence in the human microbiome, certain fungi can cause severe and fatal systemic infections, particularly in patients with haematologic malignancies like leukemia (Bhatt et al., 2011). Fungal dissemination into the bloodstream is a critical step in triggering invasive fungal infections (IFIs), leading to skin, bone, and central nervous system (CNS) involvement (Maphanga et al., 2020). Cryptococcus neoformans, by evading mucociliary clearance, enters deep alveolar spaces and subsequently targets the CNS after disseminating through the bloodstream (Rajasingham et al., 2017). The resistance of fungi to antifungal agents is also a concern, with specific mutations identified in Candida albicans that increase resistance without causing tissue damage or excessive inflammation. These mutations affect genes involved in cell signaling pathways, including those regulating pathogen recognition (Naseem et al., 2015; Wirnsberger et al., 2016; Xiao et al., 2016; Zhao et al., 2017).
2.2. Fungal EVs as virulence factors
The mechanisms of biogenesis and release of fungal EVs are not as well elucidated as in mammals. Genetic studies have implicated at least three different pathways in the release of EVs in fungi, including the conventional post-Golgi secretory pathway, ESCRT-mediated release of exosomes via MVBs, and an unconventional secretory pathway involving Golgi reassembly stacking proteins (GRASP). Fungal microvesicle biogenesis occurs via the direct outward budding and pinching of the plasma membrane releasing the nascent microvesicle into the extracellular space. Figure 2 summarizes the structure and generation of fungal EVs. Fungal EVs contain various native substances, including nucleic acids, pigments, proteins, lipids, carbohydrates, polysaccharides, and prions, which play roles in pathophysiological processes. Genetic and molecular evidence indicates similarities between fungal EVs and mammalian EVs (TerBush et al., 1996). Transmembrane proteins (annexins and tetraspanins), vesicle trafficking proteins (ESCRT proteins and Rab GTPases), cytoskeletal proteins, heat-shock proteins, metabolic enzymes, integrins, 14-3-3 proteins, and ribosomal subunits are all connected with mammalian EVs (Bonsergent et al., 2021). Similarly, fungal EVs were frequently abundant the same proteins involved in the plasma membrane, pathogenicity, stress responses, transport, signaling, and fundamental cellular metabolism (Zarnowski et al., 2021). Fungal EVs carry enzymes involved in metabolic pathway, such as amino acid biosynthesis and fatty acid metabolism, although their specific biological functions are yet to be fully understood (Rodrigues et al., 2007; Albuquerque et al., 2008; Vallejo et al., 2012b; Vargas et al., 2015). Comparable to EVs derived from tumors, fungal EV biogenesis and cargo loading involve the ESCRT-mediated MVB pathway and the conventional secretory pathway via the endoplasmic reticulum (ER)-Golgi Apparatus (GA)-exocyst-plasma membrane axis, with potential alternative pathways (Wenzel, 1995). Fungal EVs and mammalian EVs also show strong similarities in molecular content, such as both EVs transport several RNAs belonging to broad functional classes, including various functional classes of RNAs, such as messenger RNA (mRNA) (Lasser et al., 2017), which contribute to their biological functions. Table 1 summarizes early evidence and recent findings in the field of fungal EVs.
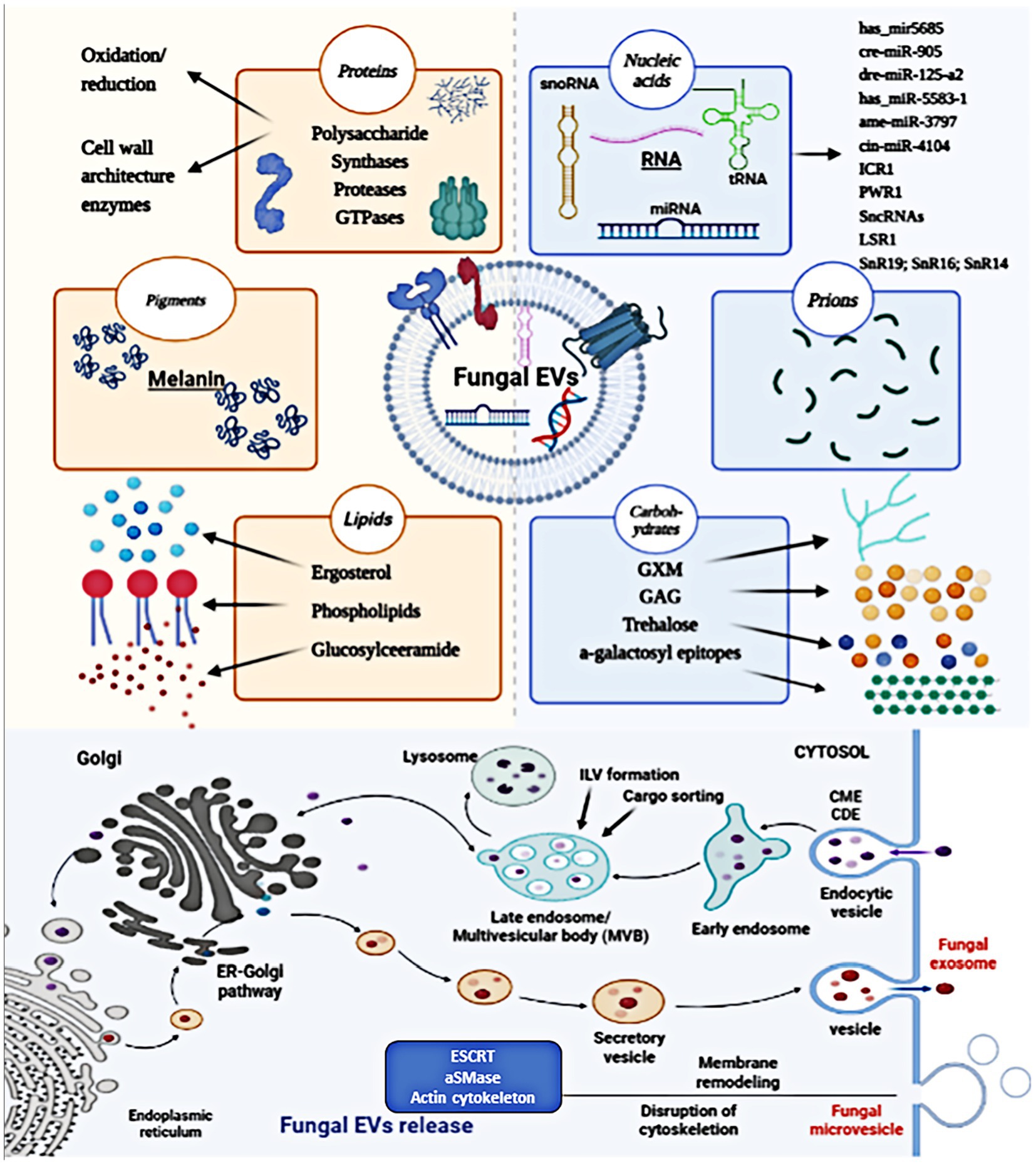
Figure 2. The structure and biogenesis of fungal EVs. Fungal EVs carry a variety of biologically native substances, such as nucleic acids, pigments, proteins, lipids, carbohydrates, polysaccharides, and prions, which are involved in pathophysiological processes. Fungal EVs biogenesis occurs in MVB endosomes, giving rise to secreted exosomes, and at the plasma membrane, resulting in the generation of MVs. Fungal microvesicle biogenesis occurs via the direct outward budding and pinching of the plasma membrane releasing the nascent microvesicle into the extracellular space.
Many virulence factors are transported from fungal cells via EVs (Schrettl et al., 2007; Silva et al., 2014; Bleackley et al., 2020; Garcia-Ceron et al., 2021), including those that lack signal peptides (Liu et al., 2020). Studies have focused on profiling EVs-associated RNAs in fungi and host cells, investigating their accumulation during infection and identifying potential target genes. RNA content in EVs has been found to contribute significantly to gene regulation (Yanez-Mo et al., 2015). In invasive fungi like Candida albicans, Aspergillus fumigatus, and Paracoccidioides brasiliensis, different intraspecific cells exhibit varied gene regulation induced by EVs. Goncalves et al. identified 10 up-regulated lncRNAs, including 1200007C13Rik, 4833418N02Rik, Gm12840, Gm15832, Gm20186, Gm38037, Gm45774, Gm4610, Mir22hg, and Mirt1, associated with biological processes during Candida albicans infection, particularly the response to injury (Goncalves et al., 2023). In addition, Rosenblad et al. discovered three novel ncRNA genes, namely nuclear RNase P RNA, RNase MRP RNA, and a potential snoRNA U14, originating from the ribosomal region of fungi, highlighting the important role of ncRNA genes in fungal EVs genetics (Rosenblad et al., 2022). The emergence of an increasing number of fungi suggests that the diversity of fungal species is generally underestimated. EVs derived from different fungal species exhibit different virulence characteristics when infecting hosts.
Fungal EVs are considered crucial for microorganisms-host interactions. Candida albicans, a major cause of lethal fungal infections in immunocompromised individuals, employs adhesins, invasins, and hydrolases as virulence factors to breach physical barriers and invade host tissues (Saville et al., 2003). EVs have been successfully isolated from various Candida spp., including Candida glabrata, Candida parapsilosis, and Candida tropicalis (Wellington et al., 2009; Gil-Bona et al., 2015; Karkowska-Kuleta et al., 2020). The endosomal sorting complex required for transport (ESCRT) pathway, specifically subunits Hse1 and Vps27, is involved in Candida albicans biofilm EV production. Mutants deficient in ESCRT subunits show decreased EVs production (Zarnowski et al., 2018). Dawson et al. performed the novo identification of EV protein for Candida albicans, which found that claudin-like Sur7 family (Pfam: PF06687) proteins Sur7 and Evp1 (orf19.671) had the correlation with the fungal infection (Dawson et al., 2020). Additionally, differences in cytokine release were observed among Candida species, with Candida glabrata producing the highest levels (Kulig et al., 2022). Moreover, Candida auris, a chronic infectious disease, can be resistant to antifungal drugs. EVs purified from Candida auris improved antifungal resistance to amphotericin B formulations (Chan et al., 2022). The biological phases of Candida albicans are yeast and hyphae. Hyphae can damage tissue by infiltrating mucosal epithelial cells, which eventually causes a blood infection and hyphal EVs derived from Candida albicans differ from yeast vesicles due to containing a greater number of virulence-related proteins despite both kinds of EVs showed immune reactively with human sera from invasive candidiasis patients (Martinez-Lopez et al., 2022).
In addition to Candida spp., Aspergillus fumigatus also secretes EVs, leading to alterations in fungal cell wall morphology and exerting antifungal effects. This indicates the involvement of Aspergillus fumigatus EVs in inhibiting mycelial growth within the fungal cytoplasm, possibly due to the presence of an antimicrobial protein (Shopova et al., 2020). Aspergillus fumigatus possesses membrane-delimited organelles involved in melanization and is recruited to the endosome through an unconventional secretion pathway, utilizing various factors. This pathway allows for the sequential concentration and utilization of substrates, galactomannan-anchor precursors, anti-reactive oxygen species (ROS), and P450 (CYP450) enzymes (Upadhyay et al., 2016). Additionally, the endosomal system facilitates the export of negatively charged macromolecules across the plasma membrane, which helps sequester toxic intermediates and minimizes the risk of damage to other cytoplasmic machinery (Upadhyay et al., 2016). Souza et al. purified EVs from Aspergillus fumigatus, showing that the presence of glucanosyltransferases (Gel1, Gel4, and Bgt1), Ecm33, and EglC among proteins involved in cell wall remodeling in Aspergillus fumigatus, which resulted in the elongation of the cell wall glucan chain and resulting in the maintenance and resistance of the cell wall, indicating that these proteins in the EVs participate of mechanisms of fungal virulence (Souza et al., 2019).
Rodrigues et al. found that approximately 76 different proteins have been detected in Cryptococcus neoformans EVs and implied that Cryptococcus neoformans secretes pathogenesis-related chemicals in an effective and widespread manner, and that EVs serve as “virulence bags” that transport a concentrated payload of fungi to host effector cells and tissues. However, there is minimal overlap and no analysis of their abundance or enrichment (Rodrigues et al., 2008b). Among these proteins, the immunogenic properties of three Cryptococcal proteins (Cda2, Fpd1, and MP88) have been previously confirmed (Levitz et al., 2001; Biondo et al., 2003). Recent studies have identified key virulence factors in Cryptococcus neoformans EVs, including superoxide dismutase, phospholipase B, urease, and glucuronoxylomannan (GXM), a high-molecular-weight polysaccharide. Specifically, GXM is produced intracellularly and transported through the cell wall within these secretory vesicles (Mariano Andrade et al., 2003; Monari et al., 2005; Yauch et al., 2006; Rodrigues et al., 2007; Monari et al., 2009). Cryptococcus EVs play a crucial role in the pathophysiology of fungal infection processes by acting as virulence factors by regulating prion transmission, virulence transfer, and antifungal drug resistance. Furthermore, applying an innovative protocol for the new isolation of Cryptococcus gattii EVs, Reis et al. studied Cryptococcus gattii and revealed the involvement of scrablase a phospholipid translocase, as a virulence factor in Cryptococcus gattii secretion (Reis et al., 2019). EVs have also been observed in fungal culture supernatants and body fluids of various human pathogenic fungi, including Histoplasma capsulatum (Albuquerque et al., 2008), Sporothrix brasiliensis (Ikeda et al., 2018), Sporothrix schenckii (Albuquerque et al., 2008), Malassezia sympodialis (Gehrmann et al., 2011), and Trichophyton interdigitale (Bitencourt et al., 2018). Zhao et al. demonstrated that EVs enriched with Saccharomyces cerevisiae Fks1 and Chs3 protected yeast cells from cell wall disruption, suggesting that EVs prevent the host immune system from killing fungal organisms through a potential role in intraspecific fungal communication (Zhao et al., 2019). Rayner et al. reported that Malassezia sympodialis EVs exhibited an RNAi-independent route for biogenesis, but no evidence was found linking small RNA expression in Malassezia sympodialis EVs to pH changes (Rayner et al., 2017). Therefore, the role of virulence factors of Malassezia sympodialis EVs in the host remains unclear. Additionally, Bitencourt et al. identified specific miRNA sequences in Paracoccidioides brasiliensis (has-mir5685, cre-MIR905, dre-MIR-125-a2, and has-mir-5583-1) and Saccharomyces cerevisiae (ame-mir-3797 and cin-mir-4104), suggesting their potential virulence significance in fungal EVs (Peres da Silva et al., 2015b).
3. The interaction of fungal EVs with the immune system
Fungi have developed effective immune evasion mechanisms to survive within the host environment, and long-term antigenic stimulation can significantly impact the modulation of the host immune response (Richie et al., 2009). Fungal pathogens possess the ability to evade human host immune responses by skillfully avoiding recognition and manipulating host responses, which may potentially trigger immune reactions against the fungi (Luo et al., 2015). Similarly, fungal EVs also have the capacity to modulate immunity activation, either positively or negatively, depending on the presence of fungal virulence factors (Oliveira et al., 2010a). Fungal EVs regulate the expression of co-stimulatory molecules in dendritic cells, macrophages polarization, and cytokine production in phagocytes. Figure 3 shows the immunoregulatory functions of fungal EVs. Table 2 summarizes the positive and negative regulation of activation of innate immunity by fungal EVs.
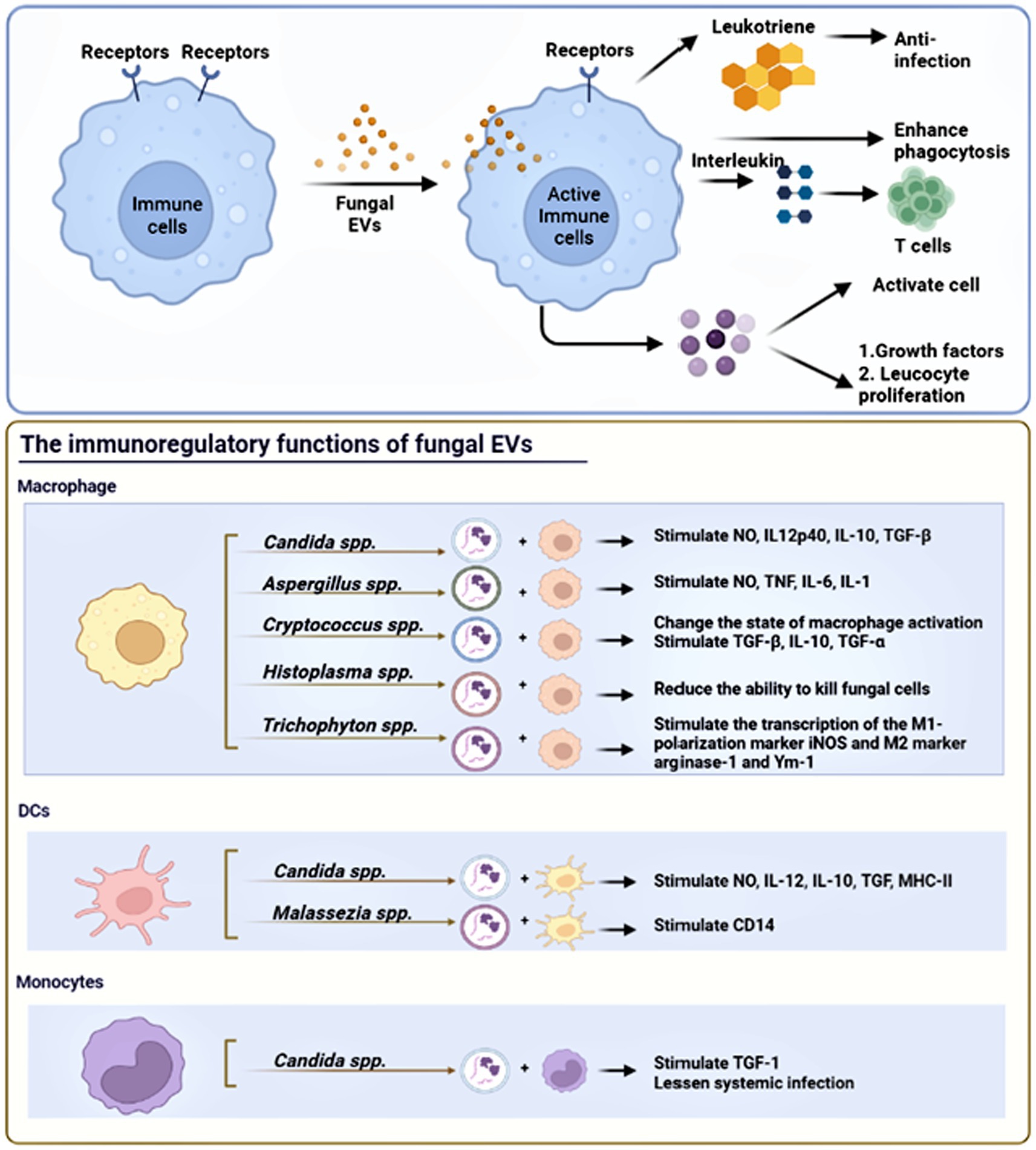
Figure 3. The immunoregulatory functions of fungal EVs. Fungal EVs can present antigen on their surface molecules directly to T cells, DCs, and monocytes. Fungal EVs could modulate positively or negatively the activation of innate immunity, which depend on the presence of fungal virulence factors and fungal species.
3.1. Fungal EVs positively modulate the activation of immunity
Candida albicans is more prone to cause diseases ranging from superficial mucosal to life-threatening systemic infections in patients with malignancy, leading to a variety of diseases, including mucosal infection, candidemia and disseminated candidiasis. Antimicrobial peptides and the complement system are two important, evolutionary conserved systems discovered as the first line of defense against bacteria in relation to the humoral response against fungi (Wenzel, 1995; Pfaller et al., 1998; Steele et al., 1999). Candida albicans EVs exhibit immunomodulatory effects that active the innate immune response. Co-stimulation of RAW 264.7 macrophages with EVs induces the production of NO, IL-12p40, IL-10, and TGF-β, similar to bone marrow-derived macrophages (BMDM) that produce NO, IL-12p40, TNF-α, and IL-10. In an in vivo experiment by Vargas et al., immunosuppressed mice vaccinated with EVs + Freund’s adjuvant (ADJ) showed higher levels of TNFα, IL-12p70, and IFN-γ in the blood. However, IL-12p70, TGFβ, IL-4, and IL-10 levels also increased without EVs + Freund’s adjuvant (ADJ) treatment (Vargas et al., 2020). Macrophages and DCs can internalize Candida spp. EVs, triggering a significant biological response characterized by increased NO, IL-12, IL-10, and TGF-β secretion, and MHC-II expression in DCs (Vargas et al., 2015). The morphological transition between yeast and hyphal forms is known to be linked to the virulence of Candida spp., affecting traits such as the ability to penetrate host tissues. Interactions on the cell surface and secreted proteins play crucial roles in the initial interaction between Candida spp. and the host (Hazen, 1989). Candida albicans EVs modulate the immune response by secreting TGF-1 transporter from human monocytes. It has been discovered that Candida-glucans activate the complement receptor 3 (CR3) on the plasma membrane of monocytes, leading to the production of TGF-1-transporting EVs. These EVs suppress the immune response in blood vessels, stimulate endothelial cells to produce TGF, and attenuate systemic infection (Mobius et al., 2002; Andriantsitohaina and Papon, 2020). Martinez-Lopez et al. observed that EVs secreted by hyphal cells (HEVs) of Candida albicans differ from yeast EVs (YEVs), with HEV exhibiting higher virulence and more pronounced effects on human immune cells (Martinez-Lopez et al., 2022). Oliveira et al. showed that low levels of Candida haemulonii var. EVs are not recognized by the traditional pathway of the oxidative burst produced by macrophages, which allow the transport of virulence factors via EVs that are not recognized by the host immune system and could act as regulators during infections caused by Candida haemulonii var. High EV concentrations and Candida haemulonii var., however, triggered microbicidal responses of macrophages (Oliveira et al., 2023). Therefore, EVs were shown to contribute to fungal virulence, and these vesicles may be a source of antigens that activate host immune responses and can be used to develop new therapeutic targets.
It has been reported that human neutrophils produce antifungal EVs against Aspergillus fumigatus (Shopova et al., 2020). The release of EVs by the pathogenic Aspergillus fumigatus was first demonstrated by Souza et al., showing that Aspergillus EVs can induce phagocytosis, production of pro-inflammatory mediators, and phagocyte recognition, all of which contribute to fungal elimination (Souza et al., 2019). Brauer et al. evaluated Aspergillus flavus EV production and immunomodulatory effects and observed that the presence of EVs induces the production of inflammatory mediators such as NO, TNF, IL-6, and IL-1 are produced by macrophages. Furthermore, Aspergillus flavus EVs regulate macrophage phagocytosis, killing, and M1 macrophage polarization in vitro (Brauer et al., 2020). Mesenchymal stem cells (MSCs) play a role in the fungal immune response. Studies found that EVs secreted by MSCs possess tissue repair capabilities, inhibit inflammation, improve immune response, and promote angiogenesis (Gatti et al., 2011). In a clinical model of allergic airway inflammation, Cruz et al. discovered that MSC-secreted EVs attenuated the Th2/Th17-mediated inflammatory response, thereby alleviating airway hyper-responsiveness induced by Aspergillus filaments (Cruz et al., 2015). This suggests that MSC-EV could be a valuable target in vaccine formulations. Freitas et al. evaluated the pro- and anti-inflammatory effects of Aspergillus fumigatus EVs in vitro, revealing EVs derived from Aspergillus fumigatus induced a partial proinflammatory response in macrophages by increasing the production of TNF-α and gene expression of induced nitric oxide synthase and adhesion molecules (Freitas et al., 2023).
In Cryptococcus spp., studies have shown that Cryptococcus neoformans EVs are more beneficial than detrimental to the host (Oliveira et al., 2010a). Oliveira et al. observed that Cryptococcus neoformans secretes EVs during interactions with phagocytes in vivo, which can directly modulate host-pathogen interactions and influence the outcome of Cryptococcus neoformans-macrophage interactions by regulating macrophage activation states (Oliveira et al., 2010a). Indeed, Cryptococcus neoformans EVs have been shown to stimulate macrophages in vitro, inducing the production anti-inflammatory cytokines such as TGF-β and IL-10 (Oliveira et al., 2010a). Additionally, it is crucial to investigate the role of Cryptococcus spp. EVs in inducing immunity, particularly in infected immunocompromised hosts, where innate immune cells develop a memory-like response to repeated exposure to Cryptococcus neoformans (Devi et al., 1991; Hole et al., 2019). The presence of functional T cells is undoubtedly essential for effective defense against Cryptococcus neoformans. Moreover, Oliveira et al. found that incubation of murine macrophage with Cryptococcus neoformans EV led to a significant elevation in extracellular levels of TNF-α, IL-10, and TGF-β, promoting localized fungal infection (Oliveira et al., 2010a).
It has been reported that various fungal species secrete EVs that modulate the immune response of patients. Bitencourt et al. were the first to report that Trichophyton interdigitale secretes EVs, which stimulate the transcription of the M1-polarization marker inducible nitric oxide synthase (iNOS) and inhibit the expression of the M2 markers arginase-1 and Ym-1. These EVs also induce the production of pro-inflammatory mediators by bone marrow-derived macrophages (BMDMs) and keratinocytes in a dose-dependent manner (Bitencourt et al., 2018). Gehrmann et al. discovered that EVs derived from DCs co-cultured with Malassezia sympodialis can carry Malassezia sympodialis antigens and stimulate cytokine release in autologous CD14 and CD34-depleted PBMCs from patients. Interestingly, they also found for the first time that Malassezia sympodialis EVs contain antigens and allergens that induce cytokine responses in CD14 and CD34-depleted PBMCs from patients (Gehrmann et al., 2011). To date, Paracoccidioides brasiliensis EVs have been shown to stimulate M1 Macrophages in vitro, leading to the production of pro-inflammatory cytokines and increased relative expression of the iNOS gene (da Silva et al., 2016).
3.2. Fungal EVs negatively modulate the activation of immunity
As mentioned above, EVs derived from fungal species contain a diverse range of virulence factors, regulators, and highly immunogenic components that may directly contribute to disease development (Vargas et al., 2020). Therefore, the presence of multiple virulence factors in fungal EVs suggests that their potential harm to the host cannot be disregarded entirely. However, only a limited number of studies have reported on fungal EVs negatively modulating the activation of host immunity, such as Sporothrix spp. and Histoplasma spp. (Baltazar et al., 2018; Ikeda et al., 2018). In an in vivo study, Ikeda et al. observed a significant increase in fungal load in animals infected with Sporothrix brasiliensis. They found that the amount of fungal growth in the tissue increased as the number of fungal EVs injected into the mice increased, suggesting that fungal EVs facilitate the growth of fungus in the host (Ikeda et al., 2018). Similarly, Baltazar et al. demonstrated that the determination of the pathogen’s intracellular fate depends on the signaling pathways activated when macrophages and Histoplasma capsulatum interact. Importantly, they investigated that Histoplasma capsulatum-EV-treated macrophages had a reduced ability to kill fungal cells, particularly phagocytes treated with 7B6-EV. The levels of glutathione peroxidase (C0NI23) in EVs were reduced due to 6B7mAb treatment. Therefore, yeast cells may be better equipped to handle peroxides produced by phagocytes because glutathione peroxidase is less abundant in 6B7-EV (Baltazar et al., 2018). Additionally, in the extensively studies case of the opportunistic fungal pathogen Cryptococcus neoformans, EVs contain the capsular antigen GXM, which has been shown to exert cytotoxic effects on macrophages directly through the Fas/FasL pathway and can suppress monocytes, neutrophils, and T lymphocytes (Diamond et al., 1972; Villena et al., 2008). Other evidence suggests that Cryptococcus neoformans EVs enhance brain infection by facilitating the crossing of the blood-brain barrier and modulating the host’s ability to fight against infection through cytokines induction, providing a remarkable example of cross-kingdom communication (Huang et al., 2012). Therefore, the functional role of fungal EVs in the host immune system is complex, and further molecular biology experiments are needed in the future to explore the main regulatory pathways, as well as key cytokines and proteins.
4. The application of fungal EVs
Although current multiple methods for diagnosing fungal infections are applied including culture, microscopic examination, molecular biology, histopathology, and serological tests (Ness et al., 1989), they cannot specifically detect invasive fungal diseases and accurately identify fungal species in an efficient and inexpensive manner. The development of novel diagnostic methods for fungi can benefit from investments in identifying fungal pathogens through EVs-based strategies, as they offer distinct advantages in disease biomarkers (Ahn et al., 2018). EVs have been found to carry reliable markers for the diagnosis of infectious diseases, including viral and misfolded proteins, enabling the development of sensitive tests that can identify the infection and monitor its progression. Infectious EVs are particularly useful in this regard due to their ability to detect both active and dormant intracellular infections (Kruh-Garcia et al., 2014). However, the absence of specific protein markers, such as those found in mammalian EVs, which have allowed for sophisticated isolation and analysis techniques, has hindered in-depth research into fungal EVs. For example, ESCRT proteins like Vps23 (Tsg101) and Bro1 (ALIX), involved in the biogenesis of fungal EVs, are not present in the cargo of fungal EVs. Tetraspanin homologs have been discovered in numerous fungi, including the model yeast Saccharomyces cerevisiae. Interestingly, 22 putative Candida albicans EVs protein markers were identified, including the claudin-like Sur7 family (Pfam: PF06687) proteins Evp1 (orf19.6741) and Sur7 (Dawson et al., 2020), providing evidence that fungal EVs can be useful tools for elucidating the role of EVs derived from fungi. Furthermore, Martinez-Lopez et al. discovered distinct differences between Candida albicans hyphal cells EVs (HEVs) and Candida albicans yeast EVs (YEVs), suggesting their relevance and potential use in developing new diagnostic markers and therapeutic targets for Candida albicans infection (Martinez-Lopez et al., 2022). Moreover, a broad-omics approach proposed by Zysset et al. could enhance our knowledge in the fields of fungal MV biology, biogenesis, composition, and immunomodulatory capabilities, and facilitating the discovery of new molecules with diagnostic biomarker potential (Zysset et al., 1988). Fungal EVs containing RNA could serve as potential diagnostic biomarkers due to their involvement in spliceosome machinery and pre-mRNA processing during fungal adaptation to different niches (e.g., cre-MIR905, has-mir5685, dre-MIR-125-a2, and has-mir5583-1) (Peres da Silva et al., 2015b). Interestingly, has-mir5685 was also found to be significantly decreased in breast cancer, while has-mir5583-1 has a strong correlation with nasopharyngeal carcinoma (Wen et al., 2019), indicating the possibility of fungal infection in tumor patients through RNA delivery and communication in EVs. However, most current molecular tests lack validity, exhibit cross-reactivity, and are limited to the detection of a few species. Therefore, the exploration of fungal EVs as diagnostic biomarkers is still in its early stages.
RNA-based methods, such as host-induced gene silencing and artificial vesicle-protected RNA antifungal strategies, have been proposed as EV-based approaches for preventing infectious diseases (Cai et al., 2018). Currently, only a limited number of vaccine candidates targeting vulvovaginal candidiasis are under development, and there are no licensed antifungal vaccines available (Levy et al., 1989; Odds, 2003). The development of antifungal vaccines focusing on the fungal cell wall presents attractive targets. Clinical trials are underway for a vaccine composed of the recombinant N terminus of Als3p, a surface protein expressed by Candida albicans that facilitates host entry. This vaccine has demonstrated effectiveness in treating oropharyngeal and vaginal candidiasis (Schmidt et al., 2012). Additionally, preclinical studies in rodents have shown promise for the major secreted aspartic protease (Sap2) as a vaccine against vaginal candidiasis (Vecchiarelli et al., 2012). Fungal EVs elicit robust immune responses in animals both in vitro and in vivo, suggesting their potential application as mycosis vaccines. The antifungal properties of Candida EVs have been extensively investigated, with several studies demonstrating their ability to inhibit the growth of other fungal strains. For instance, Vargas et al. discovered that EVs from Candida albicans exhibit a protective effect against candidiasis by stimulating macrophages and dendritic cells to produce cytokines and express costimulatory molecules (Vargas et al., 2020). Gandhi et al. revealed that EVs derived from fungal-infected Retinal Pigment Epithelial (RPE) cells may contribute to the pathogenesis of endophthalmitis and activate immune signaling pathways, indicating the potential of EVs as a cargo for treating fungal infections (Gandhi and Joseph, 2022). In an in vivo study, Souza et al. found that Aspergillus fumigatus EVs rescued 50% of mice with Aspergillus fumigatus-induced lethal fungal pneumonia, suggesting their potential use as immunizing agents (Souza et al., 2022). Shopova et al. discovered a previously unknown mechanism by which neutrophils combat Aspergillus fumigatus infection, holding significant clinical implications for the development of new antifungal therapies (Shopova et al., 2020). Specifically, the discovery that neutrophils release EVs carrying antifungal substances to target fungi highlights the diagnostic and therapeutic potential of these vehicles for fungal infections.
Furthermore, conducting additional research on the molecular mechanisms underlying this interaction has the potential to uncover novel targets for drug development. Ultimately, comprehending the intricate interplay between host defenses and fungal pathogens is critical for enhancing the outcomes of patients affected with invasive fungal infections (Shopova et al., 2020). Considering the substantial global burden of the disease on high-risk populations, the development of a vaccine against Cryptococcosis is an urgent priority. Wang et al. demonstrated that in immunocompromised animals, particularly those lacking CD4+ T cells, heat-killed fbp1 cells (HK-fbp1) from Cryptococcus neoformans can confer protection against a challenge by the virulent parental strain, suggesting its potential as a therapeutic agent for treating invasive Cryptococcus infection (Wang et al., 2023). Additionally, Specht et al. investigated four Cryptococcal proteins (GP-Cda1, GP-Cda2, GP-Cda3, and GP-Sod1) and their potential role as candidate vaccines, highlighting their ability to protect mice from a lethal Cryptococcal challenge (Specht et al., 2017). The clinical application of fungal EVs is summarized in Table 3.
5. Conclusion and perspective
Fungal infections are increasingly prevalent worldwide. However, unlike bacteria or viral infections, there is currently no available vaccine for fungal threats. In recent years, the incidence of fungal infections has risen, particularly among immunocompromised individuals such as organ transplant recipients, AIDS patients, and those undergoing chemotherapy. The identification of fungal EVs has provided a promising avenue for diagnosing and treating invasive fungal infections. Nonetheless, the field of fungal EV research is still in its early stages within mycology, despite the widespread use of EVs in various biomedical disciplines. The impact of fungal EVs on the host’s innate immunity can be either beneficial or detrimental, contingent upon the presence of fungal virulence factors. Interestingly, mounting evidence suggests that fungal EVs can serve as valuable tools for investigating the role of EVs derived from fungi. This review highlights the current progress and limitations in the study of fungal EVs, encompassing their potential clinical applications as diagnostic tools and therapeutic carriers. However, despite the growing interest and understanding of fungal EVs, their precise role in mediating the immune response between fungal cells and the host remains unknown. This includes elucidating the mechanisms by which fungal EVs enable fungal cells to evade immune surveillance and exploring the protective effects of fungal EVs in eliminating fungal pathogens. Furthermore, this review offers insights into the potential utility of fungal EVs in modulating host immune responses, improving antifungal drug delivery, and serving as a platform for vaccine development. It may also shed light on the unexplored therapeutic potential of fungal EVs in pathogenesis and their application in therapeutic interventions.
Author contributions
JL was the major contributor in designing and writing the manuscript. XH provided substantial advice in designing and revising the paper, supervised the study, and contributed to manuscript preparation. All authors contributed to the article and approved the submitted version.
Funding
This work was supported by grants from the Guangdong Basic and Applied Basic Research Foundation, Shenzhen Key Medical Discipline Construction Fund, Scientific Research Foundation of PEKING UNIVERSITY SHENZHEN HOSPITAL, Grant/Award Number: 2021A1515011558, SZXK040, and KYQD2021049.
Conflict of interest
The authors declare that the research was conducted in the absence of any commercial or financial relationships that could be construed as a potential conflict of interest.
Publisher’s note
All claims expressed in this article are solely those of the authors and do not necessarily represent those of their affiliated organizations, or those of the publisher, the editors and the reviewers. Any product that may be evaluated in this article, or claim that may be made by its manufacturer, is not guaranteed or endorsed by the publisher.
Footnotes
References
Ahn, S. Y., Park, W. S., Kim, Y. E., Sung, D. K., Sung, S. I., Ahn, J. Y., et al. (2018). Vascular endothelial growth factor mediates the therapeutic efficacy of mesenchymal stem cell-derived extracellular vesicles against neonatal hyperoxic lung injury. Exp. Mol. Med. 50, 1–12. doi: 10.1038/s12276-018-0160-8
Akoumianaki, T., Kyrmizi, I., Valsecchi, I., Gresnigt, M. S., Samonis, G., Drakos, E., et al. (2016). Aspergillus Cell Wall melanin blocks LC3-associated phagocytosis to promote pathogenicity. Cell Host Microbe 19, 79–90. doi: 10.1016/j.chom.2015.12.002
Albuquerque, P. C., Nakayasu, E. S., Rodrigues, M. L., Frases, S., Casadevall, A., Zancope-Oliveira, R. M., et al. (2008). Vesicular transport in Histoplasma capsulatum: an effective mechanism for trans-cell wall transfer of proteins and lipids in ascomycetes. Cell. Microbiol. 10, 1695–1710. doi: 10.1111/j.1462-5822.2008.01160.x
Alves, L. R., Peres da Silva, R., Sanchez, D. A., Zamith-Miranda, D., Rodrigues, M. L., Goldenberg, S., et al. (2019). Extracellular vesicle-mediated RNA release in Histoplasma capsulatum. mSphere 4:e00176-19. doi: 10.1128/mSphere.00176-19
Amatuzzi, R. F., Zamith-Miranda, D., Munhoz da Rocha, I. F., Lucena, A. C. R., de Toledo Martins, S., Streit, R., et al. (2022). Caspofungin affects extracellular vesicle production and cargo in Candida auris. J Fungi (Basel) 8:990. doi: 10.3390/jof8100990
Anderson, J., Mihalik, R., and Soll, D. R. (1990). Ultrastructure and antigenicity of the unique cell wall pimple of the Candida opaque phenotype. J. Bacteriol. 172, 224–235. doi: 10.1128/jb.172.1.224-235.1990
Andriantsitohaina, R., and Papon, N. (2020). Extracellular vesicles: new bullets to fight fungal infections. Trends Cell Biol. 30, 589–590. doi: 10.1016/j.tcb.2020.05.008
Baltazar, L. M., Ribeiro, G. F., Freitas, G. J., Queiroz-Junior, C. M., Fagundes, C. T., Chaves-Olortegui, C., et al. (2021). Protective response in experimental Paracoccidioidomycosis elicited by extracellular vesicles containing antigens of Paracoccidioides brasiliensis. Cells 10:1813. doi: 10.3390/cells10071813
Baltazar, L. M., Zamith-Miranda, D., Burnet, M. C., Choi, H., Nimrichter, L., Nakayasu, E. S., et al. (2018). Concentration-dependent protein loading of extracellular vesicles released by Histoplasma capsulatum after antibody treatment and its modulatory action upon macrophages. Sci. Rep. 8:8065. doi: 10.1038/s41598-018-25665-5
Barteneva, N. S., Fasler-Kan, E., Bernimoulin, M., Stern, J. N., Ponomarev, E. D., Duckett, L., et al. (2013). Circulating microparticles: square the circle. BMC Cell Biol. 14:23. doi: 10.1186/1471-2121-14-23
Bhatt, V. R., Viola, G. M., and Ferrajoli, A. (2011). Invasive fungal infections in acute leukemia. Ther. Adv. Hematol. 2, 231–247. doi: 10.1177/2040620711410098
Bielska, E., Sisquella, M. A., Aldeieg, M., Birch, C., O’Donoghue, E. J., and May, R. C. (2018). Pathogen-derived extracellular vesicles mediate virulence in the fatal human pathogen Cryptococcus gattii. Nat. Commun. 9:1556. doi: 10.1038/s41467-018-03991-6
Biondo, C., Beninati, C., Bombaci, M., Messina, L., Mancuso, G., Midiri, A., et al. (2003). Induction of T helper type 1 responses by a polysaccharide deacetylase from Cryptococcus neoformans. Infect. Immun. 71, 5412–5417. doi: 10.1128/IAI.71.9.5412-5417.2003
Bitencourt, T. A., Rezende, C. P., Quaresemin, N. R., Moreno, P., Hatanaka, O., Rossi, A., et al. (2018). Extracellular vesicles from the dermatophyte Trichophyton interdigitale modulate macrophage and keratinocyte functions. Front. Immunol. 9:2343. doi: 10.3389/fimmu.2018.02343
Bleackley, M. R., Samuel, M., Garcia-Ceron, D., McKenna, J. A., Lowe, R. G. T., Pathan, M., et al. (2020). Extracellular vesicles from the cotton pathogen Fusarium oxysporum f. sp. vasinfectum induce a phytotoxic response in plants. Front. Plant Sci. 10:1610. doi: 10.3389/fpls.2019.01610
Bonsergent, E., Grisard, E., Buchrieser, J., Schwartz, O., Thery, C., and Lavieu, G. (2021). Quantitative characterization of extracellular vesicle uptake and content delivery within mammalian cells. Nat. Commun. 12:1864. doi: 10.1038/s41467-021-22126-y
Brauer, V. S., Pessoni, A. M., Bitencourt, T. A., de Paula, R. G., de Oliveira Rocha, L., Goldman, G. H., et al. (2020). Extracellular vesicles from Aspergillus flavus induce M1 polarization in vitro. mSphere 5:e00190-20. doi: 10.1128/mSphere.00190-20
Cai, Q., Qiao, L., Wang, M., He, B., Lin, F. M., Palmquist, J., et al. (2018). Plants send small RNAs in extracellular vesicles to fungal pathogen to silence virulence genes. Science 360, 1126–1129. doi: 10.1126/science.aar4142
Castelli, R. F., Pereira, A., Honorato, L., Valdez, A., de Oliveira, H. C., Bazioli, J. M., et al. (2022). Extracellular vesicle formation in Cryptococcus deuterogattii impacts fungal virulence and requires the NOP16 gene. Infect. Immun. 90:e0023222. doi: 10.1128/iai.00232-22
Chan, W., Chow, F. W., Tsang, C. C., Liu, X., Yao, W., Chan, T. T., et al. (2022). Induction of amphotericin B resistance in susceptible Candida auris by extracellular vesicles. Emerg Microbes Infect 11, 1900–1909. doi: 10.1080/22221751.2022.2098058
Chigaleichik, A. G., Belova, L. A., Grishchenko, V. M., and Rylkin, S. S. (1977). Several properties of the extracellular vesicles of Candida tropicalis yeasts grown on n-alkanes. Mikrobiologiia 46, 467–471.
Cleare, L. G., Zamith, D., Heyman, H. M., Couvillion, S. P., Nimrichter, L., Rodrigues, M. L., et al. (2020). Media matters! Alterations in the loading and release of Histoplasma capsulatum extracellular vesicles in response to different nutritional milieus. Cell. Microbiol. 22:e13217. doi: 10.1111/cmi.13217
Colmenares, S. U., Buker, S. M., Buhler, M., Dlakic, M., and Moazed, D. (2007). Coupling of double-stranded RNA synthesis and siRNA generation in fission yeast RNAi. Mol. Cell 27, 449–461. doi: 10.1016/j.molcel.2007.07.007
Cruz, F. F., Borg, Z. D., Goodwin, M., Sokocevic, D., Wagner, D. E., Coffey, A., et al. (2015). Systemic administration of human bone marrow-derived mesenchymal stromal cell extracellular vesicles ameliorates Aspergillus hyphal extract-induced allergic airway inflammation in immunocompetent mice. Stem Cells Transl. Med. 4, 1302–1316. doi: 10.5966/sctm.2014-0280
da Silva, T. A., Roque-Barreira, M. C., Casadevall, A., and Almeida, F. (2016). Extracellular vesicles from Paracoccidioides brasiliensis induced M1 polarization in vitro. Sci. Rep. 6:35867. doi: 10.1038/srep35867
Dasari, P., Shopova, I. A., Stroe, M., Wartenberg, D., Martin-Dahse, H., Beyersdorf, N., et al. (2018). Aspf2 from Aspergillus fumigatus recruits human immune regulators for immune evasion and cell damage. Front. Immunol. 9:1635. doi: 10.3389/fimmu.2018.01635
Dawson, C. S., Garcia-Ceron, D., Rajapaksha, H., Faou, P., Bleackley, M. R., and Anderson, M. A. (2020). Protein markers for Candida albicans EVs include claudin-like Sur7 family proteins. J. Extracell. Vesicles 9:1750810. doi: 10.1080/20013078.2020.1750810
de Paula, R. G., Antoniêto, A. C. C., Nogueira, K. M. V., Ribeiro, L. F. C., Rocha, M. C., Malavazi, I., et al. (2019). Extracellular vesicles carry cellulases in the industrial fungus Trichoderma reesei. Biotechnol. Biofuels 12:146. doi: 10.1186/s13068-019-1487-7
Devi, S. J., Schneerson, R., Egan, W., Ulrich, T. J., Bryla, D., Robbins, J. B., et al. (1991). Cryptococcus neoformans serotype a glucuronoxylomannan-protein conjugate vaccines: synthesis, characterization, and immunogenicity. Infect. Immun. 59, 3700–3707. doi: 10.1128/iai.59.10.3700-3707.1991
Diamond, R. D., Root, R. K., and Bennett, J. E. (1972). Factors influencing killing of Cryptococcus neoformans by human leukocytes in vitro. J. Infect. Dis. 125, 367–376. doi: 10.1093/infdis/125.4.367
Dimitriu, P. A., Iker, B., Malik, K., Leung, H., Mohn, W. W., Hillebrand, G. G., et al. (2019). New insights into the intrinsic and extrinsic factors that shape the human skin microbiome. MBio 10:10. doi: 10.1128/mBio.00839-19
Eisenman, H. C., Frases, S., Nicola, A. M., Rodrigues, M. L., and Casadevall, A. (2009). Vesicle-associated melanization in Cryptococcus neoformans. Microbiology (Reading) 155, 3860–3867. doi: 10.1099/mic.0.032854-0
Freitas, M. S., Bitencourt, T. A., Rezende, C. P., Martins, N. S., Dourado, T. M. H., Tirapelli, C. R., et al. (2023). Aspergillus fumigatus extracellular vesicles display increased galleria mellonella survival but partial pro-inflammatory response by macrophages. J. Fungi (Basel) 9:9. doi: 10.3390/jof9050541
Gandhi, J., and Joseph, J. (2022). Proteomic profiling of extracellular vesicles derived from ARPE-19 cells challenged with aspergillus flavus and Candida albicans: application in fungal endophthalmitis. Pathog. Dis. 80:ftac042. doi: 10.1093/femspd/ftac042
Garcia-Ceron, D., Lowe, R. G. T., McKenna, J. A., Brain, L. M., Dawson, C. S., Clark, B., et al. (2021). Extracellular vesicles from fusarium graminearum contain protein effectors expressed during infection of corn. J Fungi (Basel) 7:7. doi: 10.3390/jof7110977
Gatti, S., Bruno, S., Deregibus, M. C., Sordi, A., Cantaluppi, V., Tetta, C., et al. (2011). Microvesicles derived from human adult mesenchymal stem cells protect against ischaemia-reperfusion-induced acute and chronic kidney injury. Nephrol. Dial. Transplant. 26, 1474–1483. doi: 10.1093/ndt/gfr015
Gehrmann, U., Qazi, K. R., Johansson, C., Hultenby, K., Karlsson, M., Lundeberg, L., et al. (2011). Nanovesicles from Malassezia sympodialis and host exosomes induce cytokine responses--novel mechanisms for host-microbe interactions in atopic eczema. PLoS One 6:e21480. doi: 10.1371/journal.pone.0021480
Gibson, R. K., and Peberdy, J. F. (1972). Fine structure of protoplasts of Aspergillus nidulans. J. Gen. Microbiol. 72, 529–538. doi: 10.1099/00221287-72-3-529
Gil-Bona, A., Llama-Palacios, A., Parra, C. M., Vivanco, F., Nombela, C., Monteoliva, L., et al. (2015). Proteomics unravels extracellular vesicles as carriers of classical cytoplasmic proteins in Candida albicans. J. Proteome Res. 14, 142–153. doi: 10.1021/pr5007944
Goncalves, G. F., de Faria Poloni, J., and Dorn, M. (2023). Transcriptomic analysis of Long non-coding RNA during Candida albicans infection. Genes (Basel) 14:251. doi: 10.3390/genes14020251
Hazen, K. C. (1989). Participation of yeast cell surface hydrophobicity in adherence of Candida albicans to human epithelial cells. Infect. Immun. 57, 1894–1900. doi: 10.1128/iai.57.7.1894-1900.1989
Heijnen, H. F., Schiel, A. E., Fijnheer, R., Geuze, H. J., and Sixma, J. J. (1999). Activated platelets release two types of membrane vesicles: microvesicles by surface shedding and exosomes derived from exocytosis of multivesicular bodies and alpha-granules. Blood 94, 3791–3799. doi: 10.1182/blood.V94.11.3791
Hole, C. R., Wager, C. M. L., Castro-Lopez, N., Campuzano, A., Cai, H., Wozniak, K. L., et al. (2019). Induction of memory-like dendritic cell responses in vivo. Nat. Commun. 10:2955. doi: 10.1038/s41467-019-10486-5
Honorato, L., de Araujo, J. F. D., Ellis, C. C., Piffer, A. C., Pereira, Y., Frases, S., et al. (2022). Extracellular vesicles regulate biofilm formation and yeast-to-hypha differentiation in Candida albicans. MBio 13:e0030122. doi: 10.1128/mbio.00301-22
Huang, S. H., Wu, C. H., Chang, Y. C., Kwon-Chung, K. J., Brown, R. J., and Jong, A. (2012). Cryptococcus neoformans-derived microvesicles enhance the pathogenesis of fungal brain infection. PLoS One 7:e48570. doi: 10.1371/journal.pone.0052576
Ikeda, M. A. K., de Almeida, J. R. F., Jannuzzi, G. P., Cronemberger-Andrade, A., Torrecilhas, A. C. T., Moretti, N. S., et al. (2018). Extracellular vesicles from Sporothrix brasiliensis are an important virulence factor that induce an increase in fungal burden in experimental Sporotrichosis. Front. Microbiol. 9:2286. doi: 10.3389/fmicb.2018.02286
Johansson, H. J., Vallhov, H., Holm, T., Gehrmann, U., Andersson, A., Johansson, C., et al. (2018). Extracellular nanovesicles released from the commensal yeast Malassezia sympodialis are enriched in allergens and interact with cells in human skin. Sci. Rep. 8:9182. doi: 10.1038/s41598-018-27451-9
Kabani, M., and Melki, R. (2015). Sup35p in its soluble and prion states is packaged inside extracellular vesicles. MBio 6:e01017-15. doi: 10.1128/mBio.01017-15
Kabani, M., Pilard, M., and Melki, R. (2020). Glucose availability dictates the export of the soluble and prion forms of Sup35p via periplasmic or extracellular vesicles. Mol. Microbiol. 114, 322–332. doi: 10.1111/mmi.14515
Karkowska-Kuleta, J., Kulig, K., Karnas, E., Zuba-Surma, E., Woznicka, O., Pyza, E., et al. (2020). Characteristics of extracellular vesicles released by the pathogenic yeast-like Fungi Candida glabrata, Candida parapsilosis and Candida tropicalis. Cells 9:1722. doi: 10.3390/cells9071722
Kruh-Garcia, N. A., Wolfe, L. M., Chaisson, L. H., Worodria, W. O., Nahid, P., Schorey, J. S., et al. (2014). Detection of Mycobacterium tuberculosis peptides in the exosomes of patients with active and latent M. tuberculosis infection using MRM-MS. PLoS One 9:e103811. doi: 10.1371/journal.pone.0103811
Kulig, K., Karnas, E., Woznicka, O., Kuleta, P., Zuba-Surma, E., Pyza, E., et al. (2022). Insight into the properties and Immunoregulatory effect of extracellular vesicles produced by Candida glabrata, Candida parapsilosis, and Candida tropicalis biofilms. Front. Cell. Infect. Microbiol. 12:879237. doi: 10.3389/fcimb.2022.879237
Kwon, S. H., Oh, S., Nacke, M., Mostov, K. E., and Lipschutz, J. H. (2016). Adaptor protein CD2AP and L-type lectin LMAN2 regulate exosome cargo protein trafficking through the Golgi complex. J. Biol. Chem. 291, 25462–25475. doi: 10.1074/jbc.M116.729202
Lamaris, G. A., Lewis, R. E., Chamilos, G., May, G. S., Safdar, A., Walsh, T. J., et al. (2008). Caspofungin-mediated beta-glucan unmasking and enhancement of human polymorphonuclear neutrophil activity against aspergillus and non-aspergillus hyphae. J. Infect. Dis. 198, 186–192. doi: 10.1086/589305
Las-Casas, L. O., Marina, C. L. F., de Castro, R. J. A., Coelho, L. C., Bao, S. N., de Hoog, G. S., et al. (2022). Pathogenicity and growth conditions modulate Fonsecaea extracellular Vesicles' ability to interact with macrophages. Front. Cell. Infect. Microbiol. 12:879018. doi: 10.3389/fcimb.2022.879018
Lasser, C., Shelke, G. V., Yeri, A., Kim, D. K., Crescitelli, R., Raimondo, S., et al. (2017). Two distinct extracellular RNA signatures released by a single cell type identified by microarray and next-generation sequencing. RNA Biol. 14, 58–72. doi: 10.1080/15476286.2016.1249092
Leone, F., Bellani, L., Muccifora, S., Giorgetti, L., Bongioanni, P., Simili, M., et al. (2018). Analysis of extracellular vesicles produced in the biofilm by the dimorphic yeast Pichia fermentans. J. Cell. Physiol. 233, 2759–2767. doi: 10.1002/jcp.25885
Levitz, S. M., Nong, S., Mansour, M. K., Huang, C., and Specht, C. A. (2001). Molecular characterization of a mannoprotein with homology to chitin deacetylases that stimulates T cell responses to Cryptococcus neoformans. Proc. Natl. Acad. Sci. U. S. A. 98, 10422–10427. doi: 10.1073/pnas.181331398
Levy, D. A., Bohbot, J. M., Catalan, F., Normier, G., Pinel, A. M., and Dussourd d'Hinterland, L. (1989). Phase II study of D.651, an oral vaccine designed to prevent recurrences of vulvovaginal candidiasis. Vaccine 7, 337–340. doi: 10.1016/0264-410X(89)90197-7
Liu, M., Bruni, G. O., Taylor, C. M., Zhang, Z., and Wang, P. (2018). Comparative genome-wide analysis of extracellular small RNAs from the mucormycosis pathogen Rhizopus delemar. Sci. Rep. 8:5243. doi: 10.1038/s41598-018-23611-z
Liu, J., Yang, J., Wang, R., Liu, L., Zhang, Y., Bao, H., et al. (2020). Comparative characterization of extracellular enzymes secreted by Phanerochaete chrysosporium during solid-state and submerged fermentation. Int. J. Biol. Macromol. 152, 288–294. doi: 10.1016/j.ijbiomac.2020.02.256
Lotvall, J., Hill, A. F., Hochberg, F., Buzas, E. I., Di Vizio, D., Gardiner, C., et al. (2014). Minimal experimental requirements for definition of extracellular vesicles and their functions: a position statement from the International Society for Extracellular Vesicles. J Extracell Vesicles 3:26913. doi: 10.3402/jev.v3.26913
Luo, S., Hipler, U. C., Munzberg, C., Skerka, C., and Zipfel, P. F. (2015). Sequence variations and protein expression levels of the two immune evasion proteins Gpm1 and Pra1 influence virulence of clinical Candida albicans isolates. PLoS One 10:e0113192. doi: 10.1371/journal.pone.0113192
Maligie, M. A., and Selitrennikoff, C. P. (2005). Cryptococcus neoformans resistance to echinocandins: (1,3)beta-glucan synthase activity is sensitive to echinocandins. Antimicrob. Agents Chemother. 49, 2851–2856. doi: 10.1128/AAC.49.7.2851-2856.2005
Maphanga, T. G., Birkhead, M., Munoz, J. F., Allam, M., Zulu, T. G., Cuomo, C. A., et al. (2020). Human Blastomycosis in South Africa caused by Blastomyces percursus and Blastomyces emzantsi sp. nov., 1967 to 2014. J. Clin. Microbiol. :58:e01661-19. doi: 10.1128/JCM.01661-19
Mariano Andrade, R., Monteiro Almeida, G., Alexandre DosReis, G., and Bento, C. A. M. (2003). Glucuronoxylomannan of Cryptococcus neoformans exacerbates in vitro yeast cell growth by interleukin 10-dependent inhibition of CD4+ T lymphocyte responses. Cell. Immunol. 222, 116–125. doi: 10.1016/S0008-8749(03)00116-3
Martinez-Lopez, R., Hernaez, M. L., Redondo, E., Calvo, G., Radau, S., Pardo, M., et al. (2022). Candida albicans hyphal extracellular vesicles are different from yeast ones, carrying an active proteasome complex and showing a different role in host immune response. Microbiol. Spectr. 10:e0069822. doi: 10.1128/spectrum.00698-22
Matos Baltazar, L., Nakayasu, E. S., Sobreira, T. J., Choi, H., Casadevall, A., Nimrichter, L., et al. (2016). Antibody binding alters the characteristics and contents of extracellular vesicles released by Histoplasma capsulatum. mSphere 1:1. doi: 10.1128/mSphere.00085-15
Mayor, S., Parton, R. G., and Donaldson, J. G. (2014). Clathrin-independent pathways of endocytosis. Cold Spring Harb. Perspect. Biol. 6:a016758. doi: 10.1101/cshperspect.a016758
Miramon, P., Dunker, C., Windecker, H., Bohovych, I. M., Brown, A. J., Kurzai, O., et al. (2012). Cellular responses of Candida albicans to phagocytosis and the extracellular activities of neutrophils are critical to counteract carbohydrate starvation, oxidative and nitrosative stress. PLoS One 7:e52850. doi: 10.1371/journal.pone.0052850
Mobius, W., Ohno-Iwashita, Y., van Donselaar, E. G., Oorschot, V. M., Shimada, Y., Fujimoto, T., et al. (2002). Immunoelectron microscopic localization of cholesterol using biotinylated and non-cytolytic perfringolysin O. J. Histochem. Cytochem. 50, 43–55. doi: 10.1177/002215540205000105
Monari, C., Bevilacqua, S., Piccioni, M., Pericolini, E., Perito, S., Calvitti, M., et al. (2009). A microbial polysaccharide reduces the severity of rheumatoid arthritis by influencing Th17 differentiation and proinflammatory cytokines production. J. Immunol. 183, 191–200. doi: 10.4049/jimmunol.0804144
Monari, C., Kozel, T. R., Bistoni, F., and Vecchiarelli, A. (2002). Modulation of C5aR expression on human neutrophils by encapsulated and acapsular Cryptococcus neoformans. Infect. Immun. 70, 3363–3370. doi: 10.1128/IAI.70.7.3363-3370.2002
Monari, C., Pericolini, E., Bistoni, G., Casadevall, A., Kozel, T. R., and Vecchiarelli, A. (2005). Cryptococcus neoformans capsular glucuronoxylomannan induces expression of fas ligand in macrophages. J. Immunol. 174, 3461–3468. doi: 10.4049/jimmunol.174.6.3461
Monari, C., Retini, C., Casadevall, A., Netski, D., Bistoni, F., Kozel, T. R., et al. (2003). Differences in outcome of the interaction between Cryptococcus neoformans glucuronoxylomannan and human monocytes and neutrophils. Eur. J. Immunol. 33, 1041–1051. doi: 10.1002/eji.200323388
Mossad, S. B. (2018). Management of Infections in solid organ transplant recipients. Infect. Dis. Clin. N. Am. 32, xiii–xvii. doi: 10.1016/j.idc.2018.06.001
Munhoz da Rocha, I. F., Martins, S. T., Amatuzzi, R. F., Zamith-Miranda, D., Nosanchuk, J. D., Rodrigues, M. L., et al. (2021). Cellular and extracellular vesicle RNA analysis in the global threat fungus Candida auris. Microbiol. Spectr. 9:e0153821. doi: 10.1128/Spectrum.01538-21
Naseem, S., Frank, D., Konopka, J. B., and Carpino, N. (2015). Protection from systemic Candida albicans infection by inactivation of the Sts phosphatases. Infect. Immun. 83, 637–645. doi: 10.1128/IAI.02789-14
Ness, M. J., Vaughan, W. P., and Woods, G. L. (1989). Candida antigen latex test for detection of invasive candidiasis in immunocompromised patients. J. Infect. Dis. 159, 495–502. doi: 10.1093/infdis/159.3.495
Nisini, R., Torosantucci, A., Romagnoli, G., Chiani, P., Donati, S., Gagliardi, M. C., et al. (2007). beta-glucan of Candida albicans cell wall causes the subversion of human monocyte differentiation into dendritic cells. J. Leukoc. Biol. 82, 1136–1142. doi: 10.1189/jlb.0307160
Octaviano, C. E., Abrantes, N. E., and Puccia, R. (2022). Extracellular vesicles from Paracoccidioides brasiliensis can induce the expression of fungal virulence traits in vitro and enhance infection in mice. Front. Cell. Infect. Microbiol. 12:834653. doi: 10.3389/fcimb.2022.834653
Odds, F. C. (2003). Reflections on the question: what does molecular mycology have to do with the clinician treating the patient? Med. Mycol. 41, 1–6. doi: 10.1080/714043892
Oliveira, B. T. M., Dourado, T. M. H., Santos, P. W. S., Bitencourt, T. A., Tirapelli, C. R., Colombo, A. L., et al. (2023). Extracellular vesicles from Candida haemulonii var. vulnera modulate macrophage oxidative burst. J. Fungi (Basel) 9:562. doi: 10.3390/jof9050562
Oliveira, D. L., Freire-de-Lima, C. G., Nosanchuk, J. D., Casadevall, A., Rodrigues, M. L., and Nimrichter, L. (2010a). Extracellular vesicles from Cryptococcus neoformans modulate macrophage functions. Infect. Immun. 78, 1601–1609. doi: 10.1128/IAI.01171-09
Oliveira, D. L., Nakayasu, E. S., Joffe, L. S., Guimarães, A. J., Sobreira, T. J., Nosanchuk, J. D., et al. (2010b). Characterization of yeast extracellular vesicles: evidence for the participation of different pathways of cellular traffic in vesicle biogenesis. PLoS One 5:e11113. doi: 10.1371/journal.pone.0011113
Panepinto, J., Komperda, K., Frases, S., Park, Y. D., Djordjevic, J. T., Casadevall, A., et al. (2009). Sec6-dependent sorting of fungal extracellular exosomes and laccase of Cryptococcus neoformans. Mol. Microbiol. 71, 1165–1176. doi: 10.1111/j.1365-2958.2008.06588.x
Peres da Silva, R., Heiss, C., Black, I., Azadi, P., Gerlach, J. Q., Travassos, L. R., et al. (2015a). Extracellular vesicles from Paracoccidioides pathogenic species transport polysaccharide and expose ligands for DC-SIGN receptors. Sci. Rep. 5:14213. doi: 10.1038/srep14213
Peres da Silva, R., Puccia, R., Rodrigues, M. L., Oliveira, D. L., Joffe, L. S., César, G. V., et al. (2015b). Extracellular vesicle-mediated export of fungal RNA. Sci. Rep. 5:7763. doi: 10.1038/srep07763
Peres da Silva, R., Martins, S. T., Rizzo, J., dos Reis, F. C. G., Joffe, L. S., Vainstein, M., et al. (2018). Golgi reassembly and stacking protein (GRASP) participates in vesicle-mediated RNA export in Cryptococcus Neoformans. Genes (Basel) 9:400. doi: 10.3390/genes9080400
Pfaller, M. A., Jones, R. N., Messer, S. A., Edmond, M. B., and Wenzel, R. P. (1998). National surveillance of nosocomial blood stream infection due to species of Candida other than Candida albicans: frequency of occurrence and antifungal susceptibility in the SCOPE program. SCOPE participant group. Surveillance and control of pathogens of epidemiologic. Diagn. Microbiol. Infect. Dis. 30, 121–129. doi: 10.1016/S0732-8893(97)00192-2
Podder, S., Saha, D., and Ghosh, T. C. (2019). Deciphering the intrinsic properties of fungal proteases in optimizing phytopathogenic interaction. Gene 711:143934. doi: 10.1016/j.gene.2019.06.024
Rajasingham, R., Smith, R. M., Park, B. J., Jarvis, J. N., Govender, N. P., Chiller, T. M., et al. (2017). Global burden of disease of HIV-associated cryptococcal meningitis: an updated analysis. Lancet Infect. Dis. 17, 873–881. doi: 10.1016/S1473-3099(17)30243-8
Ratajczak, J., Wysoczynski, M., Hayek, F., Janowska-Wieczorek, A., and Ratajczak, M. Z. (2006). Membrane-derived microvesicles: important and underappreciated mediators of cell-to-cell communication. Leukemia 20, 1487–1495. doi: 10.1038/sj.leu.2404296
Rawson, T. M., Wilson, R. C., and Holmes, A. (2021). Understanding the role of bacterial and fungal infection in COVID-19. Clin. Microbiol. Infect. 27, 9–11. doi: 10.1016/j.cmi.2020.09.025
Rayner, S., Bruhn, S., Vallhov, H., Andersson, A., Billmyre, R. B., and Scheynius, A. (2017). Identification of small RNAs in extracellular vesicles from the commensal yeast Malassezia sympodialis. Sci. Rep. 7:39742. doi: 10.1038/srep39742
Reis, F. C. G., Borges, B. S., Jozefowicz, L. J., Sena, B. A. G., Garcia, A. W. A., Medeiros, L. C., et al. (2019). A novel protocol for the isolation of fungal extracellular vesicles reveals the participation of a putative scramblase in polysaccharide export and capsule construction in Cryptococcus gattii. mSphere 4:4. doi: 10.1128/mSphere.00080-19
Richie, D. L., Hartl, L., Aimanianda, V., Winters, M. S., Fuller, K. K., Miley, M. D., et al. (2009). A role for the unfolded protein response (UPR) in virulence and antifungal susceptibility in Aspergillus fumigatus. PLoS Pathog. 5:e1000258. doi: 10.1371/journal.ppat.1000258
Rizzo, J., Albuquerque, P. C., Wolf, J. M., Nascimento, R., Pereira, M. D., Nosanchuk, J. D., et al. (2017). Analysis of multiple components involved in the interaction between Cryptococcus neoformans and Acanthamoeba castellanii. Fungal Biol. 121, 602–614. doi: 10.1016/j.funbio.2017.04.002
Rizzo, J., Chaze, T., Miranda, K., Roberson, R. W., Gorgette, O., Nimrichter, L., et al. (2020). Characterization of extracellular vesicles produced by Aspergillus fumigatus protoplasts. mSphere 5:5. doi: 10.1128/mSphere.00476-20
Rizzo, J., Oliveira, D. L., Joffe, L. S., Hu, G., Gazos-Lopes, F., Fonseca, F. L., et al. (2014). Role of the Apt1 protein in polysaccharide secretion by Cryptococcus neoformans. Eukaryot. Cell 13, 715–726. doi: 10.1128/EC.00273-13
Rizzo, J., Trottier, A., Moyrand, F., Coppee, J. Y., Maufrais, C., Zimbres, A. C. G., et al. (2023). Coregulation of extracellular vesicle production and fluconazole susceptibility in Cryptococcus neoformans. MBio :e0087023. doi: 10.1128/mbio.00870-23
Rizzo, J., Wong, S. S. W., Gazi, A. D., Moyrand, F., Chaze, T., Commere, P. H., et al. (2021). Cryptococcus extracellular vesicles properties and their use as vaccine platforms. J. Extracell. Vesicles 10:e12129. doi: 10.1002/jev2.12129
Rodrigues, M. L., Alvarez, M., Fonseca, F. L., and Casadevall, A. (2008a). Binding of the wheat germ lectin to Cryptococcus neoformans suggests an association of chitinlike structures with yeast budding and capsular glucuronoxylomannan. Eukaryot. Cell 7, 602–609. doi: 10.1128/EC.00307-07
Rodrigues, M. L., Nakayasu, E. S., Oliveira, D. L., Nimrichter, L., Nosanchuk, J. D., Almeida, I. C., et al. (2008b). Extracellular vesicles produced by Cryptococcus neoformans contain protein components associated with virulence. Eukaryot. Cell 7, 58–67. doi: 10.1128/EC.00370-07
Rodrigues, M. L., Nimrichter, L., Oliveira, D. L., Frases, S., Miranda, K., Zaragoza, O., et al. (2007). Vesicular polysaccharide export in Cryptococcus neoformans is a eukaryotic solution to the problem of fungal trans-cell wall transport. Eukaryot. Cell 6, 48–59. doi: 10.1128/EC.00318-06
Rosenblad, M. A., Larsson, E., Walker, A., Thongklang, N., Wurzbacher, C., and Nilsson, R. H. (2022). Evidence for further non-coding RNA genes in the fungal rDNA region. MycoKeys 90, 203–213. doi: 10.3897/mycokeys.90.84866
Rutter, B. D., Chu, T. T., Dallery, J. F., Zajt, K. K., O'Connell, R. J., and Innes, R. W. (2022). The development of extracellular vesicle markers for the fungal phytopathogen Colletotrichum higginsianum. J. Extracell. Vesicles 11:e12216. doi: 10.1002/jev2.12216
Saville, S. P., Lazzell, A. L., Monteagudo, C., and Lopez-Ribot, J. L. (2003). Engineered control of cell morphology in vivo reveals distinct roles for yeast and filamentous forms of Candida albicans during infection. Eukaryot. Cell 2, 1053–1060. doi: 10.1128/EC.2.5.1053-1060.2003
Schmidt, C. S., White, C. J., Ibrahim, A. S., Filler, S. G., Fu, Y., Yeaman, M. R., et al. (2012). NDV-3, a recombinant alum-adjuvanted vaccine for Candida and Staphylococcus aureus, is safe and immunogenic in healthy adults. Vaccine 30, 7594–7600. doi: 10.1016/j.vaccine.2012.10.038
Schrettl, M., Bignell, E., Kragl, C., Sabiha, Y., Loss, O., Eisendle, M., et al. (2007). Distinct roles for intra- and extracellular siderophores during Aspergillus fumigatus infection. PLoS Pathog. 3, 1195–1207. doi: 10.1371/journal.ppat.0030128
Shopova, I. A., Belyaev, I., Dasari, P., Jahreis, S., Stroe, M. C., Cseresnyés, Z., et al. (2020). Human neutrophils produce antifungal extracellular vesicles against Aspergillus fumigatus. MBio 11:11. doi: 10.1128/mBio.00596-20
Silva, B. M., Prados-Rosales, R., Espadas-Moreno, J., Wolf, J. M., Luque-Garcia, J. L., Goncalves, T., et al. (2014). Characterization of Alternaria infectoria extracellular vesicles. Med. Mycol. 52, 202–210. doi: 10.1093/mmy/myt003
Souza, J. A. M., Baltazar, L. M., Carregal, V. M., Gouveia-Eufrasio, L., de Oliveira, A. G., Dias, W. G., et al. (2019). Characterization of Aspergillus fumigatus extracellular vesicles and their effects on macrophages and neutrophils functions. Front. Microbiol. 10:2008. doi: 10.3389/fmicb.2019.02008
Souza, J. A. M., Gurgel, I., Malacco, N., Martins, F. R. B., Queiroz-Junior, C. M., Teixeira, M. M., et al. (2022). Pre-exposure with extracellular vesicles from Aspergillus fumigatus attenuates inflammatory response and enhances fungal clearance in a murine model pulmonary aspergillosis. Front. Cell. Infect. Microbiol. 12:898619. doi: 10.3389/fcimb.2022.898619
Specht, C. A., Lee, C. K., Huang, H., Hester, M. M., Liu, J., Luckie, B. A., et al. (2017). Vaccination with recombinant Cryptococcus proteins in glucan particles protects mice against Cryptococcosis in a manner dependent upon mouse strain and Cryptococcal species. MBio 8:8. doi: 10.1128/mBio.01872-17
Steele, C., Ratterree, M., and Fidel, P. L. Jr. (1999). Differential susceptibility of two species of macaques to experimental vaginal candidiasis. J. Infect. Dis. 180, 802–810. doi: 10.1086/314964
Taborda, C. P., and Casadevall, A. (2002). CR3 (CD11b/CD18) and CR4 (CD11c/CD18) are involved in complement-independent antibody-mediated phagocytosis of Cryptococcus neoformans. Immunity 16, 791–802. doi: 10.1016/S1074-7613(02)00328-X
Takeo, K., Uesaka, I., Uehira, K., and Nishiura, M. (1973). Fine structure of Cryptococcus neoformans grown in vitro as observed by freeze-etching. J. Bacteriol. 113, 1442–1448. doi: 10.1128/jb.113.3.1442-1448.1973
TerBush, D. R., Maurice, T., Roth, D., and Novick, P. (1996). The exocyst is a multiprotein complex required for exocytosis in Saccharomyces cerevisiae. EMBO J. 15, 6483–6494. doi: 10.1002/j.1460-2075.1996.tb01039.x
Thywissen, A., Heinekamp, T., Dahse, H. M., Schmaler-Ripcke, J., Nietzsche, S., Zipfel, P. F., et al. (2011). Conidial Dihydroxynaphthalene melanin of the human pathogenic fungus Aspergillus fumigatus interferes with the host endocytosis pathway. Front. Microbiol. 2:96. doi: 10.3389/fmicb.2011.00096
Upadhyay, S., Xu, X., Lowry, D., Jackson, J. C., Roberson, R. W., and Lin, X. (2016). Subcellular compartmentalization and trafficking of the biosynthetic machinery for fungal melanin. Cell Rep. 14, 2511–2518. doi: 10.1016/j.celrep.2016.02.059
Vallejo, M. C., Nakayasu, E. S., Longo, L. V., Ganiko, L., Lopes, F. G., Matsuo, A. L., et al. (2012a). Lipidomic analysis of extracellular vesicles from the pathogenic phase of Paracoccidioides brasiliensis. PLoS One 7:e39463. doi: 10.1371/journal.pone.0039463
Vallejo, M. C., Nakayasu, E. S., Matsuo, A. L., Sobreira, T. J., Longo, L. V., Ganiko, L., et al. (2012b). Vesicle and vesicle-free extracellular proteome of Paracoccidioides brasiliensis: comparative analysis with other pathogenic fungi. J. Proteome Res. 11, 1676–1685. doi: 10.1021/pr200872s
Vallhov, H., Johansson, C., Veerman, R. E., and Scheynius, A. (2020). Extracellular vesicles released from the skin commensal yeast Malassezia sympodialis activate human primary keratinocytes. Front. Cell. Infect. Microbiol. 10:6. doi: 10.3389/fcimb.2020.00006
Vargas, G., Honorato, L., Guimaraes, A. J., Rodrigues, M. L., Reis, F. C. G., Vale, A. M., et al. (2020). Protective effect of fungal extracellular vesicles against murine candidiasis. Cell. Microbiol. 22:e13238. doi: 10.1111/cmi.13238
Vargas, G., Rocha, J. D., Oliveira, D. L., Albuquerque, P. C., Frases, S., Santos, S. S., et al. (2015). Compositional and immunobiological analyses of extracellular vesicles released by Candida albicans. Cell. Microbiol. 17, 389–407. doi: 10.1111/cmi.12374
Vecchiarelli, A., Pericolini, E., Gabrielli, E., and Pietrella, D. (2012). New approaches in the development of a vaccine for mucosal candidiasis: progress and challenges. Front. Microbiol. 3:294. doi: 10.3389/fmicb.2012.00294
Vecchiarelli, A., Retini, C., Monari, C., Tascini, C., Bistoni, F., and Kozel, T. R. (1996). Purified capsular polysaccharide of Cryptococcus neoformans induces interleukin-10 secretion by human monocytes. Infect. Immun. 64, 2846–2849. doi: 10.1128/iai.64.7.2846-2849.1996
Vecchiarelli, A., Retini, C., Pietrella, D., Monari, C., and Kozel, T. R. (2000). T lymphocyte and monocyte interaction by CD40/CD40 ligand facilitates a lymphoproliferative response and killing of Cryptococcus neoformans in vitro. Eur. J. Immunol. 30, 1385–1393. doi: 10.1002/(SICI)1521-4141(200005)30:5<1385::AID-IMMU1385>3.0.CO;2-K
Villena, S. N., Pinheiro, R. O., Pinheiro, C. S., Nunes, M. P., Takiya, C. M., DosReis, G. A., et al. (2008). Capsular polysaccharides galactoxylomannan and glucuronoxylomannan from Cryptococcus neoformans induce macrophage apoptosis mediated by Fas ligand. Cell. Microbiol. 10, 1274–1285. doi: 10.1111/j.1462-5822.2008.01125.x
Wang, Y., Wang, K., Rivera, A., and Xue, C. (2023). Development of a heat-killed fbp1 mutant strain as a therapeutic agent to treat invasive Cryptococcus infection. Microbiol Spectr 11:e0495522. doi: 10.1128/spectrum.04955-22
Wellington, M., Dolan, K., and Krysan, D. J. (2009). Live Candida albicans suppresses production of reactive oxygen species in phagocytes. Infect. Immun. 77, 405–413. doi: 10.1128/IAI.00860-08
Wen, W., Mai, S. J., Lin, H. X., Zhang, M. Y., Huang, J. L., Hua, X., et al. (2019). Identification of two microRNA signatures in whole blood as novel biomarkers for diagnosis of nasopharyngeal carcinoma. J. Transl. Med. 17:186. doi: 10.1186/s12967-019-1923-2
Wenzel, R. P. (1995). Nosocomial candidemia: risk factors and attributable mortality. Clin. Infect. Dis. 20, 1531–1534. doi: 10.1093/clinids/20.6.1531
Winters, C. M., Hong-Brown, L. Q., and Chiang, H. L. (2020). Intracellular vesicle clusters are organelles that synthesize extracellular vesicle-associated cargo proteins in yeast. J. Biol. Chem. 295, 2650–2663. doi: 10.1074/jbc.RA119.008612
Wirnsberger, G., Zwolanek, F., Asaoka, T., Kozieradzki, I., Tortola, L., Wimmer, R. A., et al. (2016). Inhibition of CBLB protects from lethal Candida albicans sepsis. Nat. Med. 22, 915–923. doi: 10.1038/nm.4134
Wolf, J. M., Espadas, J., Luque-Garcia, J., Reynolds, T., and Casadevall, A. (2015). Lipid biosynthetic genes affect Candida albicans extracellular vesicle morphology, cargo, and Immunostimulatory properties. Eukaryot. Cell 14, 745–754. doi: 10.1128/EC.00054-15
Xiao, Y., Tang, J., Guo, H., Zhao, Y., Tang, R., Ouyang, S., et al. (2016). Targeting CBLB as a potential therapeutic approach for disseminated candidiasis. Nat. Med. 22, 906–914. doi: 10.1038/nm.4141
Yanez-Mo, M., Siljander, P. R., Andreu, Z., Zavec, A. B., Borras, F. E., Buzas, E. I., et al. (2015). Biological properties of extracellular vesicles and their physiological functions. J. Extracell. Vesicles 4:27066. doi: 10.3402/jev.v4.27066
Yang, B., Wang, J., Jiang, H., Lin, H., Ou, Z., Ullah, A., et al. (2020). Extracellular vesicles derived from Talaromyces marneffei yeasts mediate inflammatory response in macrophage cells by bioactive protein components. Front. Microbiol. 11:603183. doi: 10.3389/fmicb.2020.629894
Yauch, L. E., Lam, J. S., and Levitz, S. M. (2006). Direct inhibition of T-cell responses by the Cryptococcus capsular polysaccharide glucuronoxylomannan. PLoS Pathog. 2:e120. doi: 10.1371/journal.ppat.0020120
Zamith-Miranda, D., Heyman, H. M., Couvillion, S. P., Cordero, R. J. B., Rodrigues, M. L., Nimrichter, L., et al. (2021). Comparative molecular and Immunoregulatory analysis of extracellular vesicles from Candida albicans and Candida auris. mSystems 6:e0082221. doi: 10.1128/mSystems.00822-21
Zarnowski, R., Noll, A., Chevrette, M. G., Sanchez, H., Jones, R., Anhalt, H., et al. (2021). Coordination of fungal biofilm development by extracellular vesicle cargo. Nat. Commun. 12:6235. doi: 10.1038/s41467-021-26525-z
Zarnowski, R., Sanchez, H., Covelli, A. S., Dominguez, E., Jaromin, A., Bernhardt, J., et al. (2018). Candida albicans biofilm-induced vesicles confer drug resistance through matrix biogenesis. PLoS Biol. 16:e2006872. doi: 10.1371/journal.pbio.2006872
Zhao, K., Bleackley, M., Chisanga, D., Gangoda, L., Fonseka, P., Liem, M., et al. (2019). Extracellular vesicles secreted by Saccharomyces cerevisiae are involved in cell wall remodelling. Commun. Biol. 2:305. doi: 10.1038/s42003-019-0538-8
Zhao, X., Guo, Y., Jiang, C., Chang, Q., Zhang, S., Luo, T., et al. (2017). JNK1 negatively controls antifungal innate immunity by suppressing CD23 expression. Nat. Med. 23, 337–346. doi: 10.1038/nm.4260
Keywords: IFD, EVs, immune system, diagnosis, vaccine vehicles
Citation: Liu J and Hu X (2023) Fungal extracellular vesicle-mediated regulation: from virulence factor to clinical application. Front. Microbiol. 14:1205477. doi: 10.3389/fmicb.2023.1205477
Edited by:
R. Jayapradha, SASTRA University, IndiaReviewed by:
Luciana Pereira Ruas, Brazilian Biosciences National Laboratory (LNBio), BrazilMarcel I. Ramirez, Oswaldo Cruz Foundation, Brazil
Copyright © 2023 Liu and Hu. This is an open-access article distributed under the terms of the Creative Commons Attribution License (CC BY). The use, distribution or reproduction in other forums is permitted, provided the original author(s) and the copyright owner(s) are credited and that the original publication in this journal is cited, in accordance with accepted academic practice. No use, distribution or reproduction is permitted which does not comply with these terms.
*Correspondence: Xiaoping Hu, xiaoping7752@sohu.com