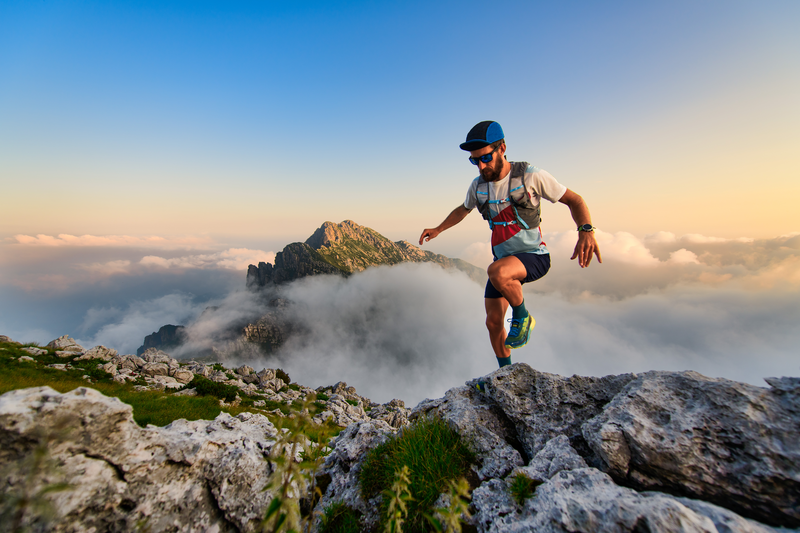
94% of researchers rate our articles as excellent or good
Learn more about the work of our research integrity team to safeguard the quality of each article we publish.
Find out more
MINI REVIEW article
Front. Microbiol. , 24 July 2023
Sec. Extreme Microbiology
Volume 14 - 2023 | https://doi.org/10.3389/fmicb.2023.1197823
This article is part of the Research Topic 55th Anniversary of Ivan Barnes: Microbial Communities of Serpentinite-Hosted Ecosystems View all 10 articles
Serpentinite-hosted systems are amongst the most challenging environments for life on Earth. Serpentinization, a geochemical alteration of exposed ultramafic rock, produces hydrothermal fluids enriched in abiotically derived hydrogen (H2), methane (CH4), and small organic molecules. The hyperalkaline pH of these fluids poses a great challenge for metabolic energy and nutrient acquisition, curbing the cellular membrane potential and limiting electron acceptor, carbon, and phosphorous availability. Nevertheless, serpentinization supports the growth of diverse microbial communities whose metabolic make-up might shed light on the beginning of life on Earth and potentially elsewhere. Here, we outline current hypotheses on metabolic energy production, carbon fixation, and nutrient acquisition in serpentinizing environments. A taxonomic survey is performed for each important metabolic function, highlighting potential key players such as H2 and CH4 cycling Serpentinimonas, Hydrogenophaga, Methanobacteriales, Methanosarcinales, and novel candidate phyla. Methodological biases of the available data and future approaches are discussed.
The beginnings of life remain one of the most outstanding scientific issues and have been dubbed the “black hole at the heart of biology” (Lane, 2015). One of the central requirements for living systems is a continuous physicochemical disequilibrium driving biological activity (Russell et al., 1988). In marine alkaline hydrothermal systems, strong electrochemical gradients develop between the ultrabasic hydrothermal fluid rising from the deep subsurface, and the seawater. These gradients are maintained across the porous hydrothermal chimney wall, which can be compared to an osmotic membrane. It is hypothesized that this rudimentary proton motive force has driven chimney nanopores to develop into protocells at the emergence of life (Russell et al., 2010; Sojo et al., 2016). Modern ecosystems at hydrothermal vents might thus provide a glimpse into very early microbial life forms.
Most alkaline hydrothermal systems are formed in environments where mantle rocks have been tectonically uplifted and exposed, either above sea level or on the seafloor. Contact with water initiates serpentinization, a geochemical alteration of the ultramafic rock. This process yields large amounts of hydrogen (H2) and constitutes one of the most important sources of H2 on Earth (Reaction 1) (Truche et al., 2020).
The oxidation of ferrous iron in olivine or pyroxene to ferric iron and magnetite by water creates reducing conditions. Catalyzed by minerals, this facilitates abiotic reactions of the produced H2 with mantle-derived carbon dioxide (CO2) or carbon monoxide (CO) (McCollom and Seewald, 2001). In Sabatier (Reaction 2) and Fischer-Tropsch (Reaction 3) type processes, methane (CH4) and small organic molecules [Cn H(2n + 2)] are enriched in the hydrothermal fluid (Barbier et al., 2020):
The serpentinization reaction produces hydrothermal fluids with pH values commonly surpassing 12. In these ultrabasic conditions, the carbonate equilibrium is permanently shifted from CO2 to carbonate species, removing most dissolved inorganic carbon (DIC) from the environment. Upon reaching the rock surface, much of the carbonate precipitates with fluid-derived calcium. Over time, calcium carbonate amalgamates with brucite and forms the chimneys or travertines typical for serpentinizing environments (Barnes et al., 1978; Früh-Green et al., 2004; McCollom and Seewald, 2013) (Figure 1A).
Figure 1. The biogeochemical environment of marine serpentinite-hosted systems. (A) Production of compounds of interest in microbial metabolism. Reactions are color-coded with solid arrows showing abiotic processes, empty arrows showing biotic processes, and striped arrows indicating external supply. The metabolic potential of shown compounds as an energy source, electron acceptor, carbon source, or other nutrient source is indicated with different shapes. Note that compound concentrations, external supply, temperature, and pH are strongly site dependent. In continental serpentinizing systems, travertines form instead of chimneys. (B) Redox potential of available electron acceptors along a gradient from the oxic surface to the anoxic interior of the chimney wall. Adapted from Boyd et al. (2014).
The products of serpentinization can support chemosynthetic microbial ecosystems growing independently from sunlight. Such serpentinite-hosted ecosystems are found in marine and continental environments, with hydrothermal fluids originating from marine, meteoric and/or groundwater sources.
Most marine serpentinizing ecosystems are located along rather slow-spreading mid-ocean ridges, where continuous tectonic activity facilitates frequent exposure of ultramafic rock (Schrenk et al., 2013; Albers et al., 2021). The most prominent example is the Lost City hydrothermal field near the Mid-Atlantic ridge (e.g., Kelley et al., 2005). A similar system, the Old City hydrothermal field, has recently been discovered along the Southwest Indian ridge (Lecoeuvre et al., 2021). Several other marine sites host mixed-type ecosystems that feature characteristics of both alkaline and acidic hydrothermal vents, such as the Rainbow (Flores et al., 2011), Logatchev (Perner et al., 2007), Ashadze (Fabri et al., 2011) and Kairei fields (Nakamura et al., 2009).
The most well-studied continental systems include the Samail ophiolite in Oman (Rempfert et al., 2017; Kraus et al., 2021), the Tablelands (Bay of Islands) ophiolite in Newfoundland (Brazelton et al., 2013), the Cabeço de Vide aquifer in Portugal (Tiago and Veríssimo, 2013), the Leka ophiolite complex in Norway (Daae et al., 2013), the Italian Gruppo di Voltri (Quéméneur et al., 2015), the Zambales ophiolite in the Philippines (Woycheese et al., 2015), The Cedars (Suzuki et al., 2013; Kohl et al., 2016) and Coast Range (Twing et al., 2017) ophiolites on the West Coast of the United States, the Chimaera (Tekirova) ophiolite in Turkey (Neubeck et al., 2017), the tropical Santa Elena ophiolite in Costa Rica (Crespo-Medina et al., 2017), the Del Puerto ophiolite in California (Blank et al., 2009) and the Troodos ophiolite in Cyprus (Rizoulis et al., 2016).
Terrestrial and marine serpentinizing systems are likely to differ in environmental variables such as salinity and the compounds available for microbial metabolism, which might influence the ecosystem’s overall functioning. An exciting transition site between terrestrial and marine serpentinite-hosted ecosystems is the Prony Bay Hydrothermal field located on the Southern Coast of New Caledonia, South Pacific (Launay and Fontes, 1985; Monnin et al., 2014). Prony Bay features several venting sites along a gradient from land to sea with a maximum depth of 50 m. The Prony Bay springs are fed by meteoric water, implying a strong salinity gradient between the hydrothermal fluid and ambient seawater (Monnin et al., 2014; Postec et al., 2015). Inversely, the Ney Springs system in Northern California features marine-type hydrothermal fluids in a continental context (Trutschel et al., 2022). Prony Bay is geochemically and microbiologically reflective of both ophiolitic and deep marine sites (Quéméneur et al., 2014; Postec et al., 2015; Frouin et al., 2018, 2022; Trutschel et al., 2022).
Serpentinite-hosted ecosystems are subjected to very challenging environmental conditions. The elevated pH poses a fundamental energetic problem on the cellular level, as it inverts the transmembrane pH gradient that typically drives all cellular processes. Furthermore, the high pH also reduces the bioavailability of electron acceptors, carbon, and other macronutrients (McCollom and Seewald, 2013; Schrenk et al., 2013). The microbial adaptations to these challenges are essential to understand life in a serpentinization context.
The central challenge for life in hyperalkaline conditions revolves around maintaining intracellular pH homeostasis and, more critically, conserving an electrochemical proton gradient across the cell membrane, which is the main driving force of the cell. The so-called proton motive force has two components: A transmembrane pH gradient (ΔpH), which is usually alkaline inside the cell relative to the outside, and a transmembrane electrical potential (Δψ), which is negative as long as the inner membrane surface is negatively charged. Maintaining a circum-neutral intracellular pH is crucial to ensure the stability of nucleic acids and proteins. In hyperalkaline conditions, however, the ΔpH is inverted and very low due to H+ limitation outside of the cell, as the concentration of H+ decreases by 10−4 at pH 11 compared to pH 7. This reduces the proton motive force and jeopardizes pH homeostasis (Krulwich et al., 2011 and references therein). Most alkaliphiles employ mechanisms increasing the efficiency of H+ uptake while maintaining a high Δψ, which is also essential for pH homeostasis. This is achieved via K+/H+ and Na+/H+ antiporters with high H+ affinity, such as the Mrp complex in alkaliphilic Bacillus spp. (Ito et al., 2017). Those antiporters contribute to creating a transmembrane Na+ gradient, generating a sodium motive force that requires specialized sodium-F1F0-ATP synthases or Na+-dependent respiratory complexes (Krulwich et al., 2011; Kuhns et al., 2020). Based on varying Na+ concentrations, it is likely that these mechanisms differ between terrestrial and marine serpentinization-influenced site microorganisms. In low salt environments, alkaliphiles might excrete Na+ via V1V0-ATPases to maintain a sodium motive force (Suzuki et al., 2014; Ohlsson et al., 2019). Other alkaliphiles such as Serpentinimonas spp. isolated from The Cedars maintain a proton motive force using specialized H+ binding F type ATPases (Hicks et al., 2010; Suzuki et al., 2014).
The membrane potential generated via H+ or Na+ translocation depends on the redox potential of electron donors and acceptors. Serpentinization yields a range of reduced compounds that can serve as metabolic energy sources, most importantly H2 and CH4 (Figure 1A) (Boyd et al., 2014). The relative concentration of these gases varies significantly between sites (Etiope et al., 2011; Monnin et al., 2014), rendering generalized statements on a primary source of electrons provided by serpentinization difficult. While methanotrophs are more easily detected than hydrogenotrophs in many serpentinite-hosted environments (e.g., Brazelton et al., 2006; Kraus et al., 2021) (Table 1), the energetic potential of H2 oxidation greatly surpasses the oxidation potential of CH4. The detection of hydrogenotrophs may be limited by the methodological approach, as the metabolic potential to oxidize H2 cannot be predicted from 16S rRNA sequences (Brazelton et al., 2012, 2022). Accordingly, metagenomic surveys show that many organisms in all types of serpentinite-hosted systems feature [FeFe]- and [NiFe]-hydrogenases (Brazelton et al., 2012; Mei et al., 2016; Kraus et al., 2021; Lecoeuvre et al., 2021; Frouin et al., 2022) (Table 1).
Table 1. Taxonomic survey for critical metabolic functions in all types of serpentinite-hosted environments, specifying the methodological approach including metagenome-assembled genomes (MAGs) and single-cell amplified genomes (SAGs).
Another potential electron source in serpentinizing systems is constituted by compounds not directly created by serpentinization such as reduced sulfur species (Sabuda et al., 2020; Trutschel et al., 2022) and CO (Brazelton et al., 2012; Morrill et al., 2014; Fones et al., 2019) (Table 1). While the oxidation potential of CO is very low, the ability to use this energy source may provide a valuable ecological advantage. In surface exposed serpentinization-influenced waters, light constitutes an additional energy source used by cyanobacterial phototrophs (e.g., Kamran et al., 2020) (Table 1).
While serpentinization provides an abundance of electron donors, the availability of terminal electron acceptors is limited, especially in terrestrial serpentinizing systems, and mainly derived from the ambient environment (Figure 1A). Oxygen represents a very potent electron acceptor on the chimney or travertine surface. With increasing proximity to the reduced hydrothermal endmember, however, the availability of oxygen or alternative electron acceptors sharply decreases. The microbial community near the oxic-anoxic interphase may use nitrate (Frouin et al., 2022), even though data on nitrate reduction is scarce. In addition, organisms from the Troodos and The Cedars ophiolites have been shown to reduce metals such as iron or magnetite (Rizoulis et al., 2016; Rowe et al., 2017) (Table 1). Towards the anoxic interior of the hydrothermal carbonate chimneys or in deep ophiolite groundwaters, sulfate reduction is a dominant metabolic strategy in all types of serpentinite-hosted systems (Brazelton et al., 2006; Tiago and Veríssimo, 2013; Postec et al., 2015; Glombitza et al., 2021) (Table 1 and Figure 1B).
To deal with electron acceptor limitation, many microbes also perform fermentation of sugars, simple organic acids and amino acids, including Stickland type reactions (Barker, 1981; Postec et al., 2021). Therefore, a large proportion of the anaerobic serpentinite-hosted community may not feature a bona fide electron transport chain with cytochromes or quinones (Table 1). Genomic analysis suggests that many serpentinite-hosted fermenters conserve energy by substrate-level phosphorylation (e.g., in glycolysis), or via bifurcative-confurcative [FeFe] H2-producing hydrogenases, which balance the reducing equivalents NADH and ferredoxin produced by fermentation (Westphal et al., 2018). This is often associated with the Rnf complex, a respiratory enzyme that catalyzes the oxidation of reduced ferredoxin to the reduction of NAD+. The negative free energy change of this reaction is used to generate a transmembrane H+ or Na+ gradient (Westphal et al., 2018). This system can be considered a primitive respiratory mechanism where the terminal electron acceptor is H+ (Buckel and Thauer, 2018).
Next to maintaining energy-yielding reactions, microorganisms in serpentinite-hosted environments must cope with severe nutrient limitation resulting from the decreased solubility of essential macronutrients at high pH (McCollom and Seewald, 2013; Schrenk et al., 2013). Especially relevant for the metabolic functioning of the community are the carbon, nitrogen, and phosphorous sources for primary production (Figure 1A).
One of the most significant issues regarding primary production in serpentinite-hosted environments is the absence of DIC, which precipitates as calcium carbonate in hyperalkaline conditions. While calcium carbonate is mostly insoluble and thus unavailable as a carbon source, it has nevertheless been shown to support the growth of some serpentinite-hosted microorganisms. This might be the result of local redissolution into bicarbonate catalyzed by the carbonic anhydrase (Suzuki et al., 2014; Fones et al., 2019; Bird et al., 2021) (Table 1). Alternatively, small organic molecules may serve as primary source of carbon. These include organic acids such as formate and acetate produced in Fischer-Tropsch and Sabatier-type reactions (Barbier et al., 2020; Fones et al., 2021) or via acetogenesis and fermentation (Kohl et al., 2016; Suzuki et al., 2017), as well as amino acids such as glycine produced in Strecker synthesis (Ménez et al., 2018; Nobu et al., 2022) (Figure 1A). The abiotic origin of those organic carbon sources tackles the definition of heterotrophy, which normally refers to the consumption of organic compounds derived from organic sources (Schönheit et al., 2016).
While bicarbonate, formate, acetate, and glycine have been shown to support the growth of microorganisms associated with serpentinization, their metabolic route remains hypothetical. Transferred across the cell membrane via specialized transporters, formate can be oxidized to CO2 via the formate dehydrogenase in the pH-neutral cytoplasm (Brazelton et al., 2022). Likewise, bicarbonate can be reduced to CO2 via the carbonic anhydrase (Suzuki et al., 2014; Bird et al., 2021). The produced CO2 is subsequently introduced to different carbon fixation pathways yielding acetyl-CoA. In serpentinite-hosted environments, the Wood-Ljungdahl pathway, reverse tricarboxylic acid cycle, and Calvin-Benson-Bassham cycle have been confirmed (Seyler et al., 2020). Based on a recent study expanding the phylogenetic range of most carbon fixation pathways, the 3-hydroxypropionate bi-cycle, dicarboxylate/4-hydroxybutyrate cycle, and 3-hydroxypropionate/4-hydroxybutyrate cycle might also be employed (Garritano et al., 2022). Contrary to formate and bicarbonate, glycine can be directly transformed into acetyl-phosphate and subsequently acetyl-coA via the lesser known reductive glycine pathway (Sánchez-Andrea et al., 2020). Genes encoding the glycine reductase are found in metagenomes from Lost City, The Cedars, and the Japanese Hakuba Happo hot springs (Brazelton et al., 2022; Nobu et al., 2022). Also acetate can be directly transformed into acetyl-phosphate and acetyl-CoA, rendering its metabolic route less complex (Rose et al., 1954).
Serpentinization also decreases the solubility of other macronutrients essential for microbial growth. Inorganic phosphorous is severely limited in serpentinizing environments because it is scavenged by the mineral brucite (Schrenk et al., 2013). A metagenomic survey revealed the high occurrence of genes involved in phosphonate catabolism in serpentinizing sites, suggesting that the microbial community might use phosphonates as an alternative phosphorous source (Frouin et al., 2022). The catabolism of methylphosphonate, the most commonly available phosphonate species in marine environments, may additionally contribute to the global carbon and energy budget in these ecosystems by releasing CH4 (Frouin et al., 2022). On the contrary, the availability of nitrogen in serpentinizing environments remains controversial. While some authors suggest that concentrations are low (Schrenk et al., 2013), others propose that N2 and nitrate are readily available to the serpentinite-hosted community (Lang et al., 2013; Rempfert et al., 2023). Potential nitrogen limitation may be alleviated by the fixation of N2 derived from the endmember fluids or ambient seawater (Morrill et al., 2013; Monnin et al., 2014) (Figure 1A). While a recent study found the associated genetic marker nifH in 10 different serpentinite-hosted systems, its overall abundance was low (Frouin et al., 2022).
The biochemical characteristics of serpentinite-hosted ecosystems reinforce the presumed link between serpentinization and the beginnings of life. Serpentinization is an ancient process which likely occurred on early Earth (Russell et al., 2010). The abiotic production of organic acids associated with serpentinization is for instance supported by isotopic signatures (McCollom and Seewald, 2013). Likewise, amino acids such as glycine may be formed abiotically, which is especially interesting in prebiotic chemistry (Aubrey et al., 2009; Ménez et al., 2018). The metabolic use of these compounds is linked to very deep-branching functions, such as the reductive tricarboxylic acid cycle and the Wood-Ljungdahl pathway, which are likely the most ancient carbon fixation pathways on Earth (Sumi and Harada, 2021). Another primordial function preserved in serpentinizing environments is CO oxidation. CO is not only one of the most ancient energy sources exploited in metabolism but is also suggested to have played a key role in several critical prebiotic reactions (King and Weber, 2007). It may thus constitute a direct link between abiotic and biotic chemistry.
The specific metabolic challenges posed by serpentinization suggest the presence of specialized taxonomic groups playing an important role in the trophic network. It might be possible that such “core” taxa are relevant in a wide variety of serpentinizing environments, even though the overall community structure can vary significantly in space and time (Suzuki et al., 2013; Postec et al., 2015; Fones et al., 2019; Brazelton et al., 2022; Trutschel et al., 2022).
The community of H2 oxidizers appears to be dominated by Gammaproteobacteria (Table 1). This includes a major proportion of Serpentinimonas (formerly grouped under Betaproteobacteria). Serpentinimonas is one of the taxa most commonly associated with serpentinization, and represented by some of the few available isolates from serpentinite-hosted ecosystems (Suzuki et al., 2014; Bird et al., 2021). So far, all of those isolated strains originate from The Cedars. Still, 16S rRNA analysis confirms the presence of Serpentinimonas and its sister genus Hydrogenophaga in other terrestrial systems, as well as in Prony Bay and Lost City (e.g., Brazelton et al., 2012; Quéméneur et al., 2015; Woycheese et al., 2015; Frouin et al., 2018) (Table 1).
While aerobic methane oxidation is mostly performed by the bacterial Methylococcales (Brazelton et al., 2017; Kraus et al., 2021), anaerobic methanotrophy and methanogenesis feature exclusively archaea (Table 1). Hydrogenotrophic methanogens belonging to Methanobacteriales are often detected in serpentinite-hosted terrestrial ecosystems (Woycheese et al., 2015; Brazelton et al., 2017; Kraus et al., 2021; Quéméneur et al., 2021, 2023; Mei et al., 2022). In addition, there is a subgroup of Methanosarcinales which is probably endemic to serpentinizing environments and includes two distinct phylotypes (e.g., Schrenk et al., 2004; Brazelton et al., 2010; Suzuki et al., 2013). Next to their systems of origin, The Cedars Methanosarcinales (TCMS) and Lost City Methanosarcinales (LCMS) have been observed in Prony Bay (Frouin et al., 2018) and Old City (Lecoeuvre et al., 2021) (Table 1). However, attempts to culture them have been unsuccessful so far.
The fermenting community seems almost entirely dominated by Clostridia (Table 1), of which several novel species have been isolated from Prony Bay (Ben Aissa et al., 2014, 2015; Mei et al., 2014; Bes et al., 2015; Postec et al., 2021).
Other energy yielding metabolic strategies including CO oxidation, sulfur oxidation and sulfate reduction are performed by a broader diversity of taxonomic groups (Table 1). Notably, the community of sulfate reducers includes Desulfovibrionales and Dethiobacter species, which can be very abundant in serpentinizing environments (Brazelton et al., 2012; Suzuki et al., 2013; Tiago and Veríssimo, 2013; Postec et al., 2015; Woycheese et al., 2015; Mei et al., 2016) (Table 1).
Regarding carbon uptake, it might be especially interesting to further investigate certain candidate phyla that occur in various serpentinizing systems. For example, Ca. Bipolaricaulota can use bicarbonate and formate (Brazelton et al., 2022) and plays a role in acetogenesis (Colman et al., 2022). Likewise, Ca. NPL-UPA2 grows on bicarbonate (Brazelton et al., 2022) and performs acetogenesis via the Wood-Ljungdahl pathway (Suzuki et al., 2018) (Table 1). The Wood-Ljungdahl pathway is also employed by Ca. Hakubanella thermoalkaliphilus, a novel Actinobacteriota from the Hakuba Happo hot springs serpentinizing system (Merino et al., 2020). Finally, Ca. Lithacetigena was recently shown to perform glycine reduction (Nobu et al., 2022). These candidate phyla might play an important role in the trophic chain by supplying fixed carbon to the community.
While several critical metabolic strategies and taxonomic groups could be identified, the scope of their distribution across serpentinizing ecosystems remains unclear due to methodological biases and shortcomings. Firstly, continental sites have been studied much more extensively than marine ones (Table 1). Our understanding of the latter is almost entirely based on the famous Lost City (Table 1) and most recently Old City (Lecoeuvre et al., 2021), which limits the generalization of findings on marine serpentinizing systems and reduces the meaningfulness of comparison with continental ones. This issue emphasizes the interest of the shallow marine transition field of Prony Bay. Its common characteristics with continental and marine sites may help establish the core metabolic properties of serpentinite-hosted ecosystems. In addition, the study of such shallow fields is facilitated by their geographical accessibility.
Another factor introducing bias is the methodological approach. Most studies rely on metabarcoding and metagenomic techniques (Table 1), which are strongly dependent on the scope of available reference databases. Moreover, the presence of a functional gene does not necessarily signify its activity. However, confirmation of gene expression is rare, notably due to technical difficulties in obtaining quality metatranscriptomes from such environments (Table 1). In addition, there is a lack of experimental evidence complementing bioinformatic hypotheses. Studies attempting to bridge this gap include activity measurements using 13C and 14C labeled substates in microcosms (Brazelton et al., 2011, 2017; Morrill et al., 2014; Fones et al., 2021), as well as isolation of Serpentinimonas and Clostridia species from cultures (e.g., Suzuki et al., 2014; Postec et al., 2021) (Table 1). Their small number is probably also associated with technical difficulties, including the cultivation of recalcitrant microorganisms such as obligate anaerobic chemolithoautotrophs. While combined omics approaches can provide valuable results, critical metabolic groups will eventually need to be cultivated to confirm their functional role in the ecosystem. This may be facilitated by implementing more sophisticated culture platforms to mimic the conditions associated with serpentinization in the laboratory.
Serpentinite-hosted environments are inhabited by microbial communities that cope with energetic challenges and severe nutrient limitation. It can be assumed that a significant proportion of those microorganisms yield energy from H2 oxidation with electron acceptors derived from external sources or CO2 degassed from mantle rocks. Calcium carbonate can serve as inorganic carbon source, and formate, acetate and glycine as organic carbon sources for primary production. Bicarbonate, formate, and glycine may be fixed via different carbon fixation pathways such as the Wood-Ljungdahl pathway, the reverse tricarboxylic acid and Calvin-Benson-Bassham cycles and the reductive glycine pathway. Moreover, the microbial communities might cope with nitrogen and phosphorous limitation by fixing N2 and breaking down phosphonates. The analysis of functional genes suggests that taxa such as Gammaproteobacteria, Desulfovibrionales, Clostridia and several candidate phyla play a crucial role in the trophic network and that the genera Serpentinimonas, Hydrogenophaga, and Methanobacterium as well as uncultivated Methanosarcinales, are characteristic for serpentinizing environments. However, the scope of research on serpentinite-hosted ecosystems needs to be broadened by including a greater diversity of marine and shallow transition sites. In addition, experimental evidence is needed to confirm the metabolic activity of hypothesized key players. A technological advancement of the methodological approach might not only contribute to the understanding of present serpentinite-hosted ecosystems, but also provide insights into the beginning of life on Earth and potentially elsewhere.
GE, AP, and RP: conceptualization. AL and MQ: validation. RP: investigation and writing of original draft. AL, MQ, AP, GE, and RP: review and edit of original draft. GE and AP: supervision. GE: project administration. All authors contributed to the article and approved the submitted version.
This project was financially supported by the ANR MICROPRONY (N°19-CE02-0020-02), the French Institute of Research for Development (IRD), and a Ph.D. fellowship granted to RP by the Aix-Marseille University’s Doctoral School “Sciences de l’Environnement” (ED 251).
The authors thank all project partners, including MICROPRONY.
The authors declare that the research was conducted in the absence of any commercial or financial relationships that could be construed as a potential conflict of interest.
All claims expressed in this article are solely those of the authors and do not necessarily represent those of their affiliated organizations, or those of the publisher, the editors and the reviewers. Any product that may be evaluated in this article, or claim that may be made by its manufacturer, is not guaranteed or endorsed by the publisher.
Albers, E., Bach, W., Pérez-Gussinyé, M., McCammon, C., and Frederichs, T. (2021). Serpentinization-driven H2 production from continental break-up to mid-ocean ridge spreading: unexpected high rates at the West Iberia margin. Front. Earth Sci. 9:673063. doi: 10.3389/feart.2021.673063
Aubrey, A. D., Cleaves, H. J., and Bada, J. L. (2009). The role of submarine hydrothermal systems in the synthesis of amino acids. Orig. Life Evol. Biosph. 39, 91–108. doi: 10.1007/s11084-008-9153-2
Barbier, S., Huang, F., Andreani, M., Tao, R., Hao, J., Eleish, A., et al. (2020). A review of H2, CH4, and hydrocarbon formation in experimental serpentinization using network analysis. Front. Earth Sci. 8:209. doi: 10.3389/feart.2020.00209
Barker, H. A. (1981). Amino acid degradation by anaerobic bacteria. Annu. Rev. Biochem. 50, 23–40. doi: 10.1146/annurev.bi.50.070181.000323
Barnes, I., O’Neil, J. R., and Trescases, J. J. (1978). Present day serpentinization in New Caledonia, Oman and Yugoslavia. Geochim. Cosmochim. Acta 42, 144–145. doi: 10.1016/0016-7037(78)90225-9
Ben Aissa, F., Postec, A., Erauso, G., Payri, C., Pelletier, B., Hamdi, M., et al. (2015). Characterization of Alkaliphilus hydrothermalis sp. nov., a novel alkaliphilic anaerobic bacterium, isolated from a carbonaceous chimney of the Prony hydrothermal field, New Caledonia. Extremophiles 19, 183–188. doi: 10.1007/s00792-014-0697-y
Ben Aissa, F., Postec, A., Erauso, G., Payri, C., Pelletier, B., Hamdi, M., et al. (2014). Vallitalea pronyensis sp. nov., isolated from a marine alkaline hydrothermal chimney. Int. J. Syst. Evol. Microbiol. 64, 1160–1165. doi: 10.1099/ijs.0.055756-0
Bes, M., Merrouch, M., Joseph, M., Quéméneur, M., Payri, C., Pelletier, B., et al. (2015). Acetoanaerobium pronyense sp. nov., an anaerobic alkaliphilic bacterium isolated from a carbonate chimney of the Prony hydrothermal field (New Caledonia). Int. J. Syst. Evol. Microbiol. 65, 2574–2580. doi: 10.1099/ijs.0.000307
Bird, L. J., Kuenen, J. G., Osburn, M. R., Tomioka, N., Ishii, S., Barr, C., et al. (2021). Serpentinimonas gen. Nov., Serpentinimonas raichei sp. nov., Serpentinimonas barnesii sp. nov. and Serpentinimonas maccroryi sp. nov., hyperalkaliphilic and facultative autotrophic bacteria isolated from terrestrial serpentinizing springs. Int. J. Syst. Evol. Microbiol. 71:004945. doi: 10.1099/ijsem.0.004945
Blank, J. G., Green, S. J., Blake, D., Valley, J. W., Kita, N. T., Treiman, A., et al. (2009). An alkaline spring system within the Del Puerto ophiolite (California, USA): a Mars analog site. Planet. Space Sci. 57, 533–540. doi: 10.1016/j.pss.2008.11.018
Boyd, E. S., Schut, G. J., Peters, J. W., and Adams, M. W. W. (2014). Hydrogen metabolism and the evolution of biological respiration: two separate families of enzymes that oxidize hydrogen and also produce it arose through convergent evolution. Microbe Mag. 9, 361–367. doi: 10.1128/microbe.9.361.1
Brazelton, W. J., Ludwig, K. A., Sogin, M. L., Andreishcheva, E. N., Kelley, D. S., Shen, C.-C., et al. (2010). Archaea and bacteria with surprising microdiversity show shifts in dominance over 1,000-year time scales in hydrothermal chimneys. Proc. Natl. Acad. Sci. USA 107, 1612–1617. doi: 10.1073/pnas.0905369107
Brazelton, W. J., McGonigle, J. M., Motamedi, S., Pendleton, H. L., Twing, K. I., Miller, B. C., et al. (2022). Metabolic strategies shared by basement residents of the Lost City hydrothermal field. Appl. Environ. Microbiol. 88:e0092922. doi: 10.1128/aem.00929-22
Brazelton, W. J., Mehta, M. P., Kelley, D. S., and Baross, J. A. (2011). Physiological differentiation within a single-species biofilm fueled by serpentinization. mBio 2, e00127–e00111. doi: 10.1128/mBio.00127-11
Brazelton, W. J., Morrill, P. L., Szponar, N., and Schrenk, M. O. (2013). Bacterial communities associated with subsurface geochemical processes in continental serpentinite springs. Appl. Environ. Microbiol. 79, 3906–3916. doi: 10.1128/AEM.00330-13
Brazelton, W. J., Nelson, B., and Schrenk, M. O. (2012). Metagenomic evidence for H2 oxidation and H2 production by serpentinite-hosted subsurface microbial communities. Front. Microbiol. 2:268. doi: 10.3389/fmicb.2011.00268
Brazelton, W. J., Schrenk, M. O., Kelley, D. S., and Baross, J. A. (2006). Methane- and sulfur-metabolizing microbial communities dominate the Lost City hydrothermal field ecosystem. Appl. Environ. Microbiol. 72, 6257–6270. doi: 10.1128/AEM.00574-06
Brazelton, W. J., Thornton, C. N., Hyer, A., Twing, K. I., Longino, A. A., Lang, S. Q., et al. (2017). Metagenomic identification of active methanogens and methanotrophs in serpentinite springs of the Voltri massif, Italy. PeerJ 5:e2945. doi: 10.7717/peerj.2945
Buckel, W., and Thauer, R. K. (2018). Flavin-based electron bifurcation, ferredoxin, flavodoxin, and anaerobic respiration with protons (Ech) or NAD+ (Rnf) as electron acceptors: a historical review. Front. Microbiol. 9:401. doi: 10.3389/fmicb.2018.00401
Colman, D. R., Kraus, E. A., Thieringer, P. H., Rempfert, K., Templeton, A. S., Spear, J. R., et al. (2022). Deep-branching acetogens in serpentinized subsurface fluids of Oman. Proc. Natl. Acad. Sci. USA 119:e2206845119. doi: 10.1073/pnas.2206845119
Crespo-Medina, M., Twing, K. I., Sánchez-Murillo, R., Brazelton, W. J., McCollom, T. M., and Schrenk, M. O. (2017). Methane dynamics in a tropical serpentinizing environment: the Santa Elena ophiolite, Costa Rica. Front. Microbiol. 8:916. doi: 10.3389/fmicb.2017.00916
Daae, F. L., Økland, I., Dahle, H., Jørgensen, S. L., Thorseth, I. H., and Pedersen, R. B. (2013). Microbial life associated with low-temperature alteration of ultramafic rocks in the Leka ophiolite complex. Geobiology 11, 318–339. doi: 10.1111/gbi.12035
Etiope, G., Schoell, M., and Hosgörmez, H. (2011). Abiotic methane flux from the Chimaera seep and Tekirova ophiolites (Turkey): understanding gas exhalation from low temperature serpentinization and implications for Mars. Earth Planet. Sci. Lett. 310, 96–104. doi: 10.1016/j.epsl.2011.08.001
Fabri, M.-C., Bargain, A., Briand, P., Gebruk, A., Fouquet, Y., Morineaux, M., et al. (2011). The hydrothermal vent community of a new deep-sea field, Ashadze-1, 12°58′N on the mid-Atlantic ridge. J. Mar. Biol. Assoc. U. K. 91, 1–13. doi: 10.1017/S0025315410000731
Flores, G. E., Campbell, J. H., Kirshtein, J. D., Meneghin, J., Podar, M., Steinberg, J. I., et al. (2011). Microbial community structure of hydrothermal deposits from geochemically different vent fields along the mid-Atlantic ridge: microbial communities of hydrothermal vent deposits. Environ. Microbiol. 13, 2158–2171. doi: 10.1111/j.1462-2920.2011.02463.x
Fones, E. M., Colman, D. R., Kraus, E. A., Nothaft, D. B., Poudel, S., Rempfert, K. R., et al. (2019). Physiological adaptations to serpentinization in the Samail ophiolite, Oman. ISME J. 13, 1750–1762. doi: 10.1038/s41396-019-0391-2
Fones, E. M., Colman, D. R., Kraus, E. A., Stepanauskas, R., Templeton, A. S., Spear, J. R., et al. (2021). Diversification of methanogens into hyperalkaline serpentinizing environments through adaptations to minimize oxidant limitation. ISME J. 15, 1121–1135. doi: 10.1038/s41396-020-00838-1
Frouin, E., Bes, M., Ollivier, B., Quéméneur, M., Postec, A., Debroas, D., et al. (2018). Diversity of rare and abundant prokaryotic phylotypes in the Prony hydrothermal field and comparison with other serpentinite-hosted ecosystems. Front. Microbiol. 9:102. doi: 10.3389/fmicb.2018.00102
Frouin, E., Lecoeuvre, A., Armougom, F., Schrenk, M. O., and Erauso, G. (2022). Comparative metagenomics highlight a widespread pathway involved in catabolism of phosphonates in marine and terrestrial serpentinizing. Ecosystems 7:e0032822. doi: 10.1128/msystems.00328-22
Früh-Green, G. L., Connolly, J. A. D., Plas, A., Kelley, D. S., and Grobéty, B. (2004). “Serpentinization of oceanic peridotites: implications for geochemical cycles and biological activity” in Geophysical monograph series. eds. W. S. D. Wilcock, E. F. DeLong, D. S. Kelley, J. A. Baross, and S. Craig Cary (Washington, D C: American Geophysical Union), 119–136. doi: 10.1029/144GM08
Garritano, A. N., Song, W., and Thomas, T. (2022). Carbon fixation pathways across the bacterial and archaeal tree of life. PNAS Nexus 1:pgac226. doi: 10.1093/pnasnexus/pgac226
Glombitza, C., Putman, L. I., Rempfert, K. R., Kubo, M. D., Schrenk, M. O., Templeton, A. S., et al. (2021). Active microbial sulfate reduction in fluids of serpentinizing peridotites of the continental subsurface. Commun. Earth Environ. 2:84. doi: 10.1038/s43247-021-00157-z
Hicks, D. B., Liu, J., Fujisawa, M., and Krulwich, T. A. (2010). F1F0-ATP synthases of alkaliphilic bacteria: lessons from their adaptations. Biochim. Biophys. Acta BBA – Bioenerg. 1797, 1362–1377. doi: 10.1016/j.bbabio.2010.02.028
Ito, M., Morino, M., and Krulwich, T. A. (2017). Mrp antiporters have important roles in diverse bacteria and archaea. Front. Microbiol. 8:2325. doi: 10.3389/fmicb.2017.02325
Kamran, A., Sauter, K., Reimer, A., Wacker, T., Reitner, J., and Hoppert, M. (2020). Cyanobacterial mats in calcite-precipitating serpentinite-hosted alkaline springs of the Voltri Massif, Italy. Microorganisms 9:62. doi: 10.3390/microorganisms9010062
Kelley, D. S., Karson, J. A., Früh-Green, G. L., Yoerger, D. R., Shank, T. M., Butterfield, D. A., et al. (2005). A serpentinite-hosted ecosystem: the Lost City hydrothermal field. Science 307, 1428–1434. doi: 10.1126/science.1102556
King, G. M., and Weber, C. F. (2007). Distribution, diversity and ecology of aerobic CO-oxidizing bacteria. Nat. Rev. Microbiol. 5, 107–118. doi: 10.1038/nrmicro1595
Kohl, L., Cumming, E., Cox, A., Rietze, A., Morrissey, L., Lang, S. Q., et al. (2016). Exploring the metabolic potential of microbial communities in ultra-basic, reducing springs at the cedars, CA, USA: experimental evidence of microbial methanogenesis and heterotrophic acetogenesis: methanogenesis at the cedars. J. Geophys. Res. Biogeosci. 121, 1203–1220. doi: 10.1002/2015JG003233
Kraus, E. A., Nothaft, D., Stamps, B. W., Rempfert, K. R., Ellison, E. T., Matter, J. M., et al. (2021). Molecular evidence for an active microbial methane cycle in subsurface serpentinite-hosted groundwaters in the Samail ophiolite, Oman. Appl. Environ. Microbiol. 87, e02068–e02020. doi: 10.1128/AEM.02068-20
Krulwich, T. A., Sachs, G., and Padan, E. (2011). Molecular aspects of bacterial pH sensing and homeostasis. Nat. Rev. Microbiol. 9, 330–343. doi: 10.1038/nrmicro2549
Kuhns, M., Trifunović, D., Huber, H., and Müller, V. (2020). The Rnf complex is a Na+ coupled respiratory enzyme in a fermenting bacterium, Thermotoga maritima. Commun. Biol. 3:431. doi: 10.1038/s42003-020-01158-y
Lane, N. (2015). The vital question: energy, evolution, and the origins of complex life. New York City: WW Norton & Company.
Lang, S. Q., Früh-Green, G. L., Bernasconi, S. M., and Butterfield, D. A. (2013). Sources of organic nitrogen at the serpentinite-hosted Lost City hydrothermal field. Geobiology 11, 154–169. doi: 10.1111/gbi.12026
Launay, J., and Fontes, J.-C. (1985). Les sources thermales de Prony (Nouvelle Calédonie) et leurs précipités chimiques, exemple de formation de brucite primaire. Geol. Fr. 1, 83–100.
Lecoeuvre, A., Ménez, B., Cannat, M., Chavagnac, V., and Gérard, E. (2021). Microbial ecology of the newly discovered serpentinite-hosted Old City hydrothermal field (southwest Indian ridge). ISME J. 15, 818–832. doi: 10.1038/s41396-020-00816-7
McCollom, T. M., and Seewald, J. S. (2001). A reassessment of the potential for reduction of dissolved CO 2 to hydrocarbons during serpentinization of olivine. Geochim. Cosmochim. Acta 65, 3769–3778. doi: 10.1016/S0016-7037(01)00655-X
McCollom, T. M., and Seewald, J. S. (2013). Serpentinites, hydrogen, and life. Elements 9, 129–134. doi: 10.2113/gselements.9.2.129
Mei, N., Postec, A., Bartoli, M., Vandecasteele, C., Wils, L., Gil, L., et al. (2022). Methanobacterium alkalithermotolerans sp. nov., a novel alkaliphilic and hydrogen-utilizing methanogen isolated from an alkaline geothermal spring (La Crouen, New Caledonia). Int. J. Syst. Evol. Microbiol. 72. doi: 10.1099/ijsem.0.005554
Mei, N., Postec, A., Monnin, C., Pelletier, B., Payri, C. E., Ménez, B., et al. (2016). Metagenomic and PCR-based diversity surveys of [FeFe]-hydrogenases combined with isolation of alkaliphilic hydrogen-producing bacteria from the serpentinite-hosted Prony hydrothermal field, New Caledonia. Front. Microbiol. 7:1301. doi: 10.3389/fmicb.2016.01301
Mei, N., Zergane, N., Postec, A., Erauso, G., Ollier, A., Payri, C., et al. (2014). Fermentative hydrogen production by a new alkaliphilic Clostridium sp. (strain PROH2) isolated from a shallow submarine hydrothermal chimney in Prony Bay, New Caledonia. Int. J. Hydrog. Energy 39, 19465–19473. doi: 10.1016/j.ijhydene.2014.09.111
Ménez, B., Pisapia, C., Andreani, M., Jamme, F., Vanbellingen, Q. P., Brunelle, A., et al. (2018). Abiotic synthesis of amino acids in the recesses of the oceanic lithosphere. Nature 564, 59–63. doi: 10.1038/s41586-018-0684-z
Merino, N., Kawai, M., Boyd, E. S., Colman, D. R., McGlynn, S. E., Nealson, K. H., et al. (2020). Single-cell genomics of novel actinobacteria with the Wood-Ljungdahl pathway discovered in a serpentinizing system. Front. Microbiol. 11:1031. doi: 10.3389/fmicb.2020.01031
Monnin, C., Chavagnac, V., Boulart, C., Ménez, B., Gérard, M., Gérard, E., et al. (2014). Fluid chemistry of the low temperature hyperalkaline hydrothermal system of Prony Bay (New Caledonia). Biogeosciences 11, 5687–5706. doi: 10.5194/bg-11-5687-2014
Morrill, P. L., Brazelton, W. J., Kohl, L., Rietze, A., Miles, S. M., Kavanagh, H., et al. (2014). Investigations of potential microbial methanogenic and carbon monoxide utilization pathways in ultra-basic reducing springs associated with present-day continental serpentinization: the tablelands, NL, CAN. Front. Microbiol. 5:613. doi: 10.3389/fmicb.2014.00613
Morrill, P. L., Kuenen, J. G., Johnson, O. J., Suzuki, S., Rietze, A., Sessions, A. L., et al. (2013). Geochemistry and geobiology of a present-day serpentinization site in California: the cedars. Geochim. Cosmochim. Acta 109, 222–240. doi: 10.1016/j.gca.2013.01.043
Nakamura, K., Morishita, T., Bach, W., Klein, F., Hara, K., Okino, K., et al. (2009). Serpentinized troctolites exposed near the Kairei hydrothermal field, central Indian ridge: insights into the origin of the Kairei hydrothermal fluid supporting a unique microbial ecosystem. Earth Planet. Sci. Lett. 280, 128–136. doi: 10.1016/j.epsl.2009.01.024
Neubeck, A., Sun, L., Müller, B., Ivarsson, M., Hosgörmez, H., Özcan, D., et al. (2017). Microbial community structure in a serpentine-hosted abiotic gas seepage at the Chimaera ophiolite, Turkey. Appl. Environ. Microbiol. 83, e03430–e03416. doi: 10.1128/AEM.03430-16
Nobu, M. K., Nakai, R., Tamazawa, S., Mori, H., Toyoda, A., Ijiri, A., et al. (2022). Unique H2-utilizing lithotrophy in serpentinite-hosted systems. ISME J. 17, 95–104. doi: 10.1038/s41396-022-01197-9
Ohlsson, J. I., Osvatic, J. T., Becraft, E. D., and Swingley, W. D. (2019). Microbial community in hyperalkaline steel slag-fill emulates serpentinizing springs. Diversity 11:103. doi: 10.3390/d11070103
Perner, M., Kuever, J., Seifert, R., Pape, T., Koschinsky, A., Schmidt, K., et al. (2007). The influence of ultramafic rocks on microbial communities at the Logatchev hydrothermal field, located 15°N on the mid-Atlantic ridge: influence of ultramafic rocks on microbial communities. FEMS Microbiol. Ecol. 61, 97–109. doi: 10.1111/j.1574-6941.2007.00325.x
Postec, A., Quéméneur, M., Bes, M., Mei, N., Benaïssa, F., Payri, C., et al. (2015). Microbial diversity in a submarine carbonate edifice from the serpentinizing hydrothermal system of the Prony Bay (New Caledonia) over a 6-year period. Front. Microbiol. 6:857. doi: 10.3389/fmicb.2015.00857
Postec, A., Quéméneur, M., Lecoeuvre, A., Chabert, N., Joseph, M., and Erauso, G. (2021). Alkaliphilus serpentinus sp. nov. and Alkaliphilus pronyensis sp. nov., two novel anaerobic alkaliphilic species isolated from the serpentinite-hosted Prony Bay hydrothermal field (New Caledonia). Syst. Appl. Microbiol. 44:126175. doi: 10.1016/j.syapm.2020.126175
Quéméneur, M., Bes, M., Postec, A., Mei, N., Hamelin, J., Monnin, C., et al. (2014). Spatial distribution of microbial communities in the shallow submarine alkaline hydrothermal field of the Prony Bay, New Caledonia: microbial communities of Prony hydrothermal field. Environ. Microbiol. Rep. 6, 665–674. doi: 10.1111/1758-2229.12184
Quéméneur, M., Mei, N., Monnin, C., Postec, A., Guasco, S., Jeanpert,, et al. (2023). Microbial taxa related to natural hydrogen and methane emissions in serpentinite-hosted hyperalkaline springs of New Caledonia. Front. Microbiol. 14:1196516. doi: 10.3389/fmicb.2023.1196516
Quéméneur, M., Mei, N., Monnin, C., Postec, A., Wils, L., Bartoli, M., et al. (2021). Procaryotic diversity and hydrogenotrophic methanogenesis in an alkaline spring (La Crouen, New Caledonia). Microorganisms 9:1360. doi: 10.3390/microorganisms9071360
Quéméneur, M., Palvadeau, A., Postec, A., Monnin, C., Chavagnac, V., Ollivier, B., et al. (2015). Endolithic microbial communities in carbonate precipitates from serpentinite-hosted hyperalkaline springs of the Voltri massif (Ligurian Alps, northern Italy). Environ. Sci. Pollut. Res. 22, 13613–13624. doi: 10.1007/s11356-015-4113-7
Rempfert, K. R., Miller, H. M., Bompard, N., Nothaft, D., Matter, J. M., Kelemen, P., et al. (2017). Geological and geochemical controls on subsurface microbial life in the Samail ophiolite, Oman. Front. Microbiol. 8:56. doi: 10.3389/fmicb.2017.00056
Rempfert, K. R., Nothaft, D. B., Kraus, E. A., Asamoto, C. K., Evans, R. D., Spear, J. R., et al. (2023). Subsurface biogeochemical cycling of nitrogen in the actively serpentinizing Samail ophiolite, Oman. Front. Microbiol. 14:1139633. doi: 10.3389/fmicb.2023.1139633
Rizoulis, A., Milodowski, A. E., Morris, K., and Lloyd, J. R. (2016). Bacterial diversity in the hyperalkaline allas springs (Cyprus), a natural analogue for cementitious radioactive waste repository. Geomicrobiol J. 33, 73–84. doi: 10.1080/01490451.2014.961107
Rose, I. A., Grunberg-Manago, M., Korey, S. R., and Ochoa, S. (1954). Enzymatic phosphorylation of acetate. J. Biol. Chem. 211, 737–756. doi: 10.1016/S0021-9258(18)71161-7
Rowe, A. R., Yoshimura, M., LaRowe, D. E., Bird, L. J., Amend, J. P., Hashimoto, K., et al. (2017). In situ electrochemical enrichment and isolation of a magnetite-reducing bacterium from a high pH serpentinizing spring. Environ. Microbiol. 19, 2272–2285. doi: 10.1111/1462-2920.13723
Russell, M. J., Hall, A. J., Cairns-Smith, A. G., and Braterman, P. S. (1988). Submarine hot springs and the origin of life. Nature 336:117. doi: 10.1038/336117a0
Russell, M. J., Hall, A. J., and Martin, W. (2010). Serpentinization as a source of energy at the origin of life: serpentinization and the emergence of life. Geobiology 8, 355–371. doi: 10.1111/j.1472-4669.2010.00249.x
Sabuda, M. C., Brazelton, W. J., Putman, L. I., McCollom, T. M., Hoehler, T. M., Kubo, M. D. Y., et al. (2020). A dynamic microbial sulfur cycle in a serpentinizing continental ophiolite. Environ. Microbiol. 22, 2329–2345. doi: 10.1111/1462-2920.15006
Sánchez-Andrea, I., Guedes, I. A., Hornung, B., Boeren, S., Lawson, C. E., Sousa, D. Z., et al. (2020). The reductive glycine pathway allows autotrophic growth of Desulfovibrio desulfuricans. Nat. Commun. 11:5090. doi: 10.1038/s41467-020-18906-7
Schönheit, P., Buckel, W., and Martin, W. F. (2016). On the origin of heterotrophy. Trends Microbiol. 24, 12–25. doi: 10.1016/j.tim.2015.10.003
Schrenk, M. O., Brazelton, W. J., and Lang, S. Q. (2013). Serpentinization, carbon, and deep life. Rev. Mineral. Geochem. 75, 575–606. doi: 10.2138/rmg.2013.75.18
Schrenk, M. O., Kelley, D. S., Bolton, S. A., and Baross, J. A. (2004). Low archaeal diversity linked to subseafloor geochemical processes at the Lost City hydrothermal field, mid-Atlantic ridge. Environ. Microbiol. 6, 1086–1095. doi: 10.1111/j.1462-2920.2004.00650.x
Seyler, L. M., Brazelton, W. J., McLean, C., Putman, L. I., Hyer, A., Kubo, M. D. Y., et al. (2020). Carbon assimilation strategies in ultrabasic groundwater: clues from the integrated study of a serpentinization-influenced aquifer. mSystems 5, e00607–e00619. doi: 10.1128/mSystems.00607-19
Sojo, V., Herschy, B., Whicher, A., Camprubí, E., and Lane, N. (2016). The origin of life in alkaline hydrothermal vents. Astrobiology 16, 181–197. doi: 10.1089/ast.2015.1406
Sumi, T., and Harada, K. (2021). Kinetics of the ancestral carbon metabolism pathways in deep-branching bacteria and archaea. Commun. Chem. 4:149. doi: 10.1038/s42004-021-00585-0
Suzuki, S., Ishii, S., Hoshino, T., Rietze, A., Tenney, A., Morrill, P. L., et al. (2017). Unusual metabolic diversity of hyperalkaliphilic microbial communities associated with subterranean serpentinization at the cedars. ISME J. 11, 2584–2598. doi: 10.1038/ismej.2017.111
Suzuki, S., Ishii, S., Wu, A., Cheung, A., Tenney, A., Wanger, G., et al. (2013). Microbial diversity in the cedars, an ultrabasic, ultrareducing, and low salinity serpentinizing ecosystem. Proc. Natl. Acad. Sci. USA 110, 15336–15341. doi: 10.1073/pnas.1302426110
Suzuki, S., Kuenen, J. G., Schipper, K., van der Velde, S., Ishii, S., Wu, A., et al. (2014). Physiological and genomic features of highly alkaliphilic hydrogen-utilizing betaproteobacteria from a continental serpentinizing site. Nat. Commun. 5:3900. doi: 10.1038/ncomms4900
Suzuki, S., Nealson, K. H., and Ishii, S. (2018). Genomic and in-situ transcriptomic characterization of the candida Monninte phylum NPL-UPL2 from highly alkaline highly reducing serpentinized groundwater. Front. Microbiol. 9:3141. doi: 10.3389/fmicb.2018.03141
Tiago, I., and Veríssimo, A. (2013). Microbial and functional diversity of a subterrestrial high pH groundwater associated to serpentinization: microbial and functional diversity of subsurface aquifer. Environ. Microbiol. 15, 1687–1706. doi: 10.1111/1462-2920.12034
Truche, L., McCollom, T. M., and Martinez, I. (2020). Hydrogen and abiotic hydrocarbons: molecules that change the world. Elements 16, 13–18. doi: 10.2138/gselements.16.1.13
Trutschel, L. R., Chadwick, G. L., Kruger, B., Blank, J. G., Brazelton, W. J., Dart, E. R., et al. (2022). Investigation of microbial metabolisms in an extremely high pH marine-like terrestrial serpentinizing system: Ney Springs. Sci. Total Environ. 836:155492. doi: 10.1016/j.scitotenv.2022.155492
Twing, K. I., Brazelton, W. J., Kubo, M. D. Y., Hyer, A. J., Cardace, D., Hoehler, T. M., et al. (2017). Serpentinization-influenced groundwater harbors extremely low diversity microbial communities adapted to high pH. Front. Microbiol. 8:308. doi: 10.3389/fmicb.2017.00308
Westphal, L., Wiechmann, A., Baker, J., Minton, N. P., and Müller, V. (2018). The Rnf complex is an energy-coupled transhydrogenase essential to reversibly link cellular NADH and ferredoxin pools in the acetogen Acetobacterium woodii. J. Bacteriol. 200:e00357. doi: 10.1128/JB.00357-18
Woycheese, K. M., Meyer-Dombard, D. R., Cardace, D., Argayosa, A. M., and Arcilla, C. A. (2015). Out of the dark: transitional subsurface-to-surface microbial diversity in a terrestrial serpentinizing seep (Manleluag, Pangasinan, the Philippines). Front. Microbiol. 6:44. doi: 10.3389/fmicb.2015.00044
Keywords: serpentinization, alkaline hydrothermal system, alkaliphile, hydrogenotroph, lithotroph, submarine alkaline vent theory, origin of life
Citation: Popall RM, Postec A, Lecoeuvre A, Quéméneur M and Erauso G (2023) Metabolic challenges and key players in serpentinite-hosted microbial ecosystems. Front. Microbiol. 14:1197823. doi: 10.3389/fmicb.2023.1197823
Received: 31 March 2023; Accepted: 29 June 2023;
Published: 24 July 2023.
Edited by:
William J. Brazelton, The University of Utah, United StatesReviewed by:
Daniel Colman, Montana State University, United StatesCopyright © 2023 Popall, Postec, Lecoeuvre, Quéméneur and Erauso. This is an open-access article distributed under the terms of the Creative Commons Attribution License (CC BY). The use, distribution or reproduction in other forums is permitted, provided the original author(s) and the copyright owner(s) are credited and that the original publication in this journal is cited, in accordance with accepted academic practice. No use, distribution or reproduction is permitted which does not comply with these terms.
*Correspondence: Gaël Erauso, Z2FlbC5lcmF1c29AbWlvLm9zdXB5dGhlYXMuZnI=
Disclaimer: All claims expressed in this article are solely those of the authors and do not necessarily represent those of their affiliated organizations, or those of the publisher, the editors and the reviewers. Any product that may be evaluated in this article or claim that may be made by its manufacturer is not guaranteed or endorsed by the publisher.
Research integrity at Frontiers
Learn more about the work of our research integrity team to safeguard the quality of each article we publish.