- 1Research Group Pathogenesis of Bacterial Infections, TWINCORE, Centre for Experimental and Clinical Infection Research, a Joint Venture Between the Hannover Medical School and the Helmholtz Centre for Infection Research, Hannover, Germany
- 2Institute of Medical Microbiology and Hospital Epidemiology, Hannover Medical School, Hannover, Germany
Surface proteins of Gram-positive pathogens are key determinants of virulence that substantially shape host-microbe interactions. Specifically, these proteins mediate host invasion and pathogen transmission, drive the acquisition of heme-iron from hemoproteins, and subvert innate and adaptive immune cell responses to push bacterial survival and pathogenesis in a hostile environment. Herein, we briefly review and highlight the multi-facetted roles of cell wall-anchored proteins of multidrug-resistant Staphylococcus aureus, a common etiological agent of purulent skin and soft tissue infections as well as severe systemic diseases in humans. In particular, we focus on the functional diversity of staphylococcal surface proteins and discuss their impact on the variety of clinical manifestations of S. aureus infections. We also describe mechanistic and underlying principles of staphylococcal surface protein-mediated immune evasion and coupled strategies S. aureus utilizes to paralyze patrolling neutrophils, macrophages, and other immune cells. Ultimately, we provide a systematic overview of novel therapeutic concepts and anti-infective strategies that aim at neutralizing S. aureus surface proteins or sortases, the molecular catalysts of protein anchoring in Gram-positive bacteria.
Introduction
Staphylococcus aureus is a notorious pathogen that causes fatal diseases in the human population (Lowy, 1998; Lee et al., 2018). This microbe is a leading causative agent of skin and soft tissue infections (SSTIs), pneumonia, endocarditis, septic arthritis, osteomyelitis, bacteremia, and sepsis (Lowy, 1998; Kuehnert et al., 2006; Klevens et al., 2007). Of note, a global survey indicates that this pathogen accounts for more than 1 million deaths annually (GBD 2019 Antimicrobial Resistance Collaborators, 2022), an alarming death count which undoubtedly correlates with multidrug resistance (Chambers and Deleo, 2009; Lee et al., 2018), genetic flexibility and adaptive evolution (Chambers and Deleo, 2009; Malachowa and DeLeo, 2010; Smith et al., 2022; Howden et al., 2023), as well as refined immuno-evasive maneuvers this microbe evolved to overcome host immunity (Spaan et al., 2013; Thammavongsa et al., 2015a). Specifically, S. aureus secretes an extraordinary repertoire of virulence factors into the environment in order to establish acute and persistent infections in mammalian hosts (Foster, 2005; Thammavongsa et al., 2015a). Examples involve pore-forming and cytolytic toxins, superantigens, and multiple immuno-modulatory exoenzymes, which harbor an N-terminal signal peptide required for a Sec-machinery-dependent translocation across the cytoplasmic membrane (Foster, 2005; Spaan et al., 2013; Thammavongsa et al., 2015a; Tam and Torres, 2019). Moreover, S. aureus expresses up to 24 signal peptide-bearing and pathogenicity-associated cell surface proteins that are characterized by diverse functional domains and flexible host ligand binding properties, as well as by a short C-terminal sorting sequence (Foster, 2019; Schneewind and Missiakas, 2019). This sequence, typically an LPXTG motif (Schneewind et al., 1992; Schneewind and Missiakas, 2019), is sensed and cleaved by sortase A (SrtA), a type II membrane protein and transpeptidase that catalyzes anchoring of cell surface proteins to the peptidoglycan of S. aureus and other Gram-positive bacteria (Mazmanian et al., 1999; Ton-That et al., 1999; Schneewind and Missiakas, 2019). Remarkably, S. aureus srtA mutants largely fail to colonize the host and are strongly attenuated in animal models of infectious disease (Mazmanian et al., 2000; Jonsson et al., 2003; Schaffer et al., 2006; Bubeck Wardenburg et al., 2007; Weidenmaier et al., 2008; Cheng et al., 2009; Chen et al., 2014; Misawa et al., 2015), a striking phenotype that inspired the staphylococcal research community to examine the individual roles of cell surface-displayed proteins at the host-microbe interface.
Herein, we briefly summarize the multi-facetted and sometimes redundant functions of cell surface proteins during local and invasive S. aureus infections. We also discuss how these proteins affect staphylococcal immune evasion and interaction with professional and non-professional phagocytes. Ultimately, we highlight the potential role of staphylococcal surface proteins in the design of vaccines, unique anti-infective agents, and novel therapeutic intervention strategies.
Role of staphylococcal surface proteins during colonization and establishment of skin and soft tissue infections
S. aureus is a very frequent cause of SSTIs which include cellulitis, inflamed hair follicles (folliculitis), furuncles and deep-seated abscesses, and surgical site infections (Lowy, 1998; Lee et al., 2018). During the establishment of these infections, cell surface proteins play a substantial role and largely contribute to initial adhesion and invasion of host cells (Foster et al., 2014; Lee et al., 2018; Foster, 2019). For example, several staphylococcal surface proteins including clumping factor B (ClfB), fibronectin-binding protein B (FnBPB), and iron-regulated surface determinant protein A (IsdA) mediate binding to human loricrin (Clarke et al., 2009; Mulcahy et al., 2012; da Costa et al., 2022), the most abundant protein of the cornified cell envelope and terminally differentiated corneocytes (Candi et al., 2005). Thus, it is not unexpected that some surface proteins influence staphylococcal colonization of the nasal cavity which is the natural niche of S. aureus (Figure 1; O’Brien et al., 2002; Clarke et al., 2006; Wertheim et al., 2008; Mulcahy et al., 2012; Weidenmaier et al., 2012; Sun et al., 2018). Specifically, ClfB- and IsdA-mediated binding to loricrin has been shown to affect interaction with squamous nasal epithelial cells thereby facilitating stable colonization of rodent or human nares (Clarke et al., 2006, 2009; Wertheim et al., 2008; Mulcahy et al., 2012). This process is further strengthened by IsdA-mediated interaction with involucrin and cytokeratin-10 as well as other staphylococcal surface proteins such as serine aspartate repeat containing protein D (SdrD) and S. aureus surface protein G (SasG) which also confer attachment to desquamated epithelial cells (Clarke et al., 2009; Corrigan et al., 2009; Askarian et al., 2016; Mills et al., 2022). Nonetheless, colonization and initial binding to upper skin layers not necessarily correlate with establishment of purulent infections of the skin. Albeit colonization of the host is generally accepted to be a risk factor for acquiring local and invasive staphylococcal diseases (von Eiff et al., 2001; Wertheim et al., 2005), establishment of these infections often requires skin lesions, wounds, or other medical conditions that favor pathogen entry (Cheng et al., 2011; Tong et al., 2015). For example, patients with atopic dermatitis, a chronic inflammatory skin disease associated with an IgE-mediated allergic response (Bieber, 2008; Werfel, 2009), are at elevated risk to be colonized with S. aureus and therefore often suffer from local infections of the skin (Geoghegan et al., 2018; Ogonowska et al., 2020). During atopic dermatitis, ClfB and particularly fibronectin-binding proteins (FnBPs) not only mediate binding to skin cells but also react with IgE antibodies thereby triggering specific inflammatory and allergic immune responses (Cho et al., 2001; Reginald et al., 2011; Fleury et al., 2017; Farag et al., 2022). In that regard, we further note that ClfB contributes to SSTIs and early stages of abscess formation in experimental skin infection models (Figure 1; Lacey et al., 2019). Mice subcutaneously infected with a clfB mutant of the S. aureus MRSA isolate USA300 developed smaller skin lesions over the course of the infection as compared to animals infected with the parental strain (Lacey et al., 2019). This phenomenon is associated with loricrin, which was found to be a component of the abscess wall and major host factor required for the development of skin lesions in mammals (Lacey et al., 2019). Likewise, bacterial mutants lacking FnBPs exhibited attenuated virulence in skin abscess models (Kwiecinski et al., 2014), probably also as a result of impaired host cell invasion and altered interaction with loricrin or extracellular matrix components (Fowler et al., 2000; Edwards et al., 2011; da Costa et al., 2022). Reduced bacterial loads in these models may further be explained by FnBPB-mediated neutralization of histones (Pietrocola et al., 2019), an antimicrobial component of neutrophil extracellular traps (NETs) which are formed in response to S. aureus during infection of the skin or other body parts (Brinkmann et al., 2004; Yipp et al., 2012; von Kockritz-Blickwede and Winstel, 2022). With this in mind, it is also worth noting that various other S. aureus-derived surface proteins assist in protecting staphylococci against professional phagocytes thereby essentially contributing to the development of abscesses and SSTIs (Foster, 2019; Schneewind and Missiakas, 2019). In particular, staphylococcal protein A (SpA) is a chief factor required for proper abscess formation in the skin as staphylococcal mutants lacking this determinant display virulence defects and reduced abscess volume in experimental murine models of skin infection (Patel et al., 1987; Kwiecinski et al., 2014). Moreover, clumping factor A (ClfA) has been linked to skin infections inasmuch as subcutaneous abscesses from rabbits infected with clfA-deficient staphylococci differed in size and had only weak evidence of vasculitis and thrombosis when compared to lesions formed by the parental S. aureus isolate (Malachowa et al., 2016). Thus, SpA and ClfA influence the pathogenesis of skin abscesses and associated SSTIs, presumably due to their anti-phagocytic properties which are known to promote staphylococcal evasion from neutrophil-mediated killing (Dossett et al., 1969; Higgins et al., 2006). However, protein A was also found to affect infections of the skin by modulating inflammatory signaling cascades and cell death modalities in neutrophils and epithelial cells, highlighting the multi-facetted functions of staphylococcal cell surface proteins during establishment of SSTIs (Classen et al., 2011; Soong et al., 2012; Gonzalez et al., 2019; Ledo et al., 2020). Lastly, we note that not all cell surface proteins impacting SSTIs are part of the staphylococcal core genome. Specific MRSA clones with the sequence type ST239, for instance, carry a large ΦSPβ-like prophage in their genome that encodes a unique cell wall-anchored protein termed SasX (Li et al., 2012). Of note, mutant bacteria lacking sasX failed to colonize the nares of mice and were attenuated during experimental skin infection, a pioneering observation that has been linked to MRSA spread in China and other Asian countries (Li et al., 2012; Liu et al., 2015). Together, this comprehensive work underscores the relevance and importance of cell surface-displayed proteins during S. aureus colonization of host tissues and infections of the skin.
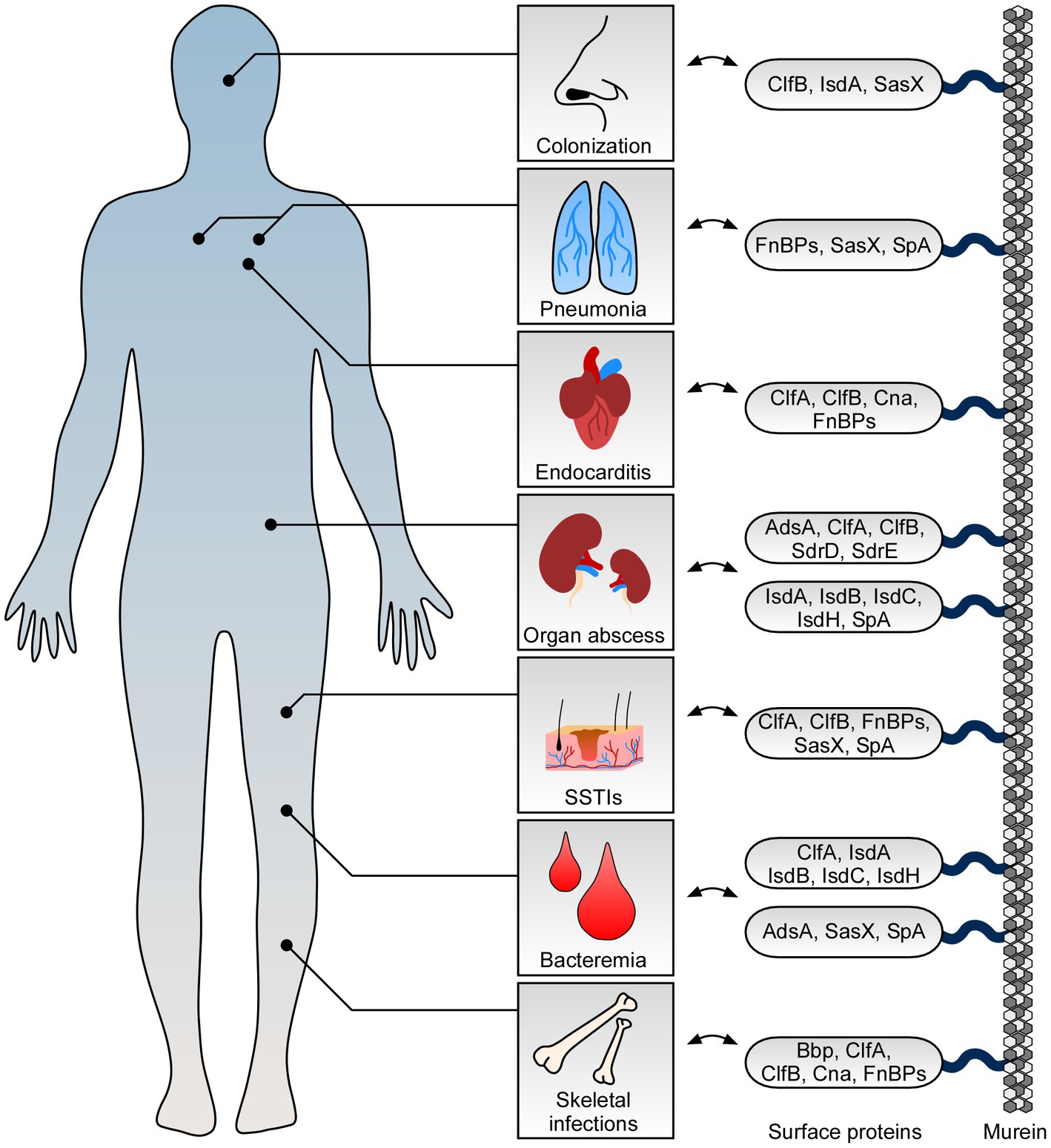
Figure 1. Role of staphylococcal surface proteins at the host-microbe interface. Staphylococcus aureus deploys surface proteins to promote interaction with mammalian hosts. While some surface proteins affect S. aureus nasal colonization, others contribute to skin and soft tissue infections (SSTIs) and fatal invasive diseases. Key surface proteins including adenosine synthase A (AdsA), bone sialoprotein (Bbp), clumping factor A and B (ClfA and ClfB), collagen adhesin (Cna), fibronectin-binding proteins (FnBPs), iron-regulated surface determinant proteins (IsdABCH), S. aureus surface protein X (SasX), serine aspartate repeat containing proteins D and E (SdrD and SdrE), and staphylococcal protein A (SpA) along with their proposed function during colonization and infection of human hosts are highlighted.
Impact of cell surface proteins on Staphylococcus aureus bacteremia and intra-organ abscess formation
S. aureus is also a frequently encountered agent of invasive and life-threatening diseases (Lowy, 1998; Lee et al., 2018). Bacteremia, for example, is a serious medical condition associated with high morbidity and mortality rates that often occurs upon staphylococcal entry into the bloodstream (Thomer et al., 2016; Bai et al., 2022). But how does S. aureus manage to survive within this hostile environment? Earlier studies and particularly transcriptional profiling of S. aureus in human blood identified multiple staphylococcal virulence determinants that became highly expressed under bacteremia-mimicking conditions (Malachowa et al., 2011). Among these factors are secreted toxins and various cell surface proteins including IsdA, IsdB, and IsdC, all elements of the iron-regulated surface determinant system (Isd; Figure 1; Malachowa et al., 2011). This system is required for iron and heme uptake in staphylococci and thus helps S. aureus to overcome iron limitation in the host (Mazmanian et al., 2003; Hammer and Skaar, 2011). Accordingly, S. aureus mutants lacking IsdA, IsdB, IsdC, as well as IsdH exhibit decreased survival in blood and virulence defects in mouse models of bloodstream infection (Cheng et al., 2009; Visai et al., 2009; Kim et al., 2010b). This also holds true for staphylococcal variants that fail to express protein A, SasX, or adenosine synthase A (AdsA; Palmqvist et al., 2002; Thammavongsa et al., 2009; Li et al., 2012; Falugi et al., 2013). While protein A and SasX predominantly aid in preventing phagocytic clearance of S. aureus by either capturing immunoglobulins or promoting intercellular aggregation (Forsgren and Sjoquist, 1966; Dossett et al., 1969; Forsgren and Quie, 1974; Li et al., 2012), AdsA along with its 5′–3′-nucleotidase activity dampens neutrophil responses and coupled killing of S. aureus during acute bloodstream infection by converting host-derived adenosine monophosphate into immuno-suppressive adenosine (Thammavongsa et al., 2009). Nevertheless, entry and survival of S. aureus in blood causes organism-wide dissemination and formation of new replicative niches that often manifest as abscesses (Thomer et al., 2016). Establishment of these lesions can occur in almost all organs and requires, inter alia, the activity of specific cell surface proteins (Cheng et al., 2011; Thomer et al., 2016). For example, elements of the Isd machinery, ClfA and ClfB, as well as protein A significantly contribute to intra-organ abscess formation and priming of persistent infections (Cheng et al., 2009). Moreover, lack of SdrD, a cell wall-anchored protein that is only prevalent in approximately 60% of all S. aureus isolates (Sabat et al., 2006), dramatically lowered abscess formation and bacterial loads in organ tissues in murine models of systemic infection (Cheng et al., 2009; Askarian et al., 2017). Likewise, genetic ablation of adsA decreased the staphylococcal burden in renal tissues following intravenous challenge and concurrently ameliorated overall disease outcomes in mice (Thammavongsa et al., 2009). However, this phenomenon not only correlated with the failure of adsA-deficient staphylococci to synthesize adenosine during the initial phase of a bloodstream infection. Previous work showed that AdsA, together with the help of a secreted nuclease, converts NETs and host-derived DNA molecules into phagocyte-eliminating deoxyadenosine and deoxyguanosine, two purine effector-deoxyribonucleosides that promote killing of immune cells by targeting the purine salvage pathway-apoptosis axis (Thammavongsa et al., 2013; Winstel et al., 2018, 2019; Tantawy et al., 2022). Following this strategy, phagocyte entry into purulent cavities of deep-seated abscesses is efficiently suppressed thereby enhancing staphylococcal survival and establishment of persistent infections in organ tissues (Thammavongsa et al., 2013; Winstel et al., 2019). Thus, staphylococcal surface proteins essentially contribute to S. aureus bloodstream infection and intra-organ abscess development.
Contribution of staphylococcal surface proteins to skeletal infections, endocarditis, and pneumonia
Not all of the aforementioned cell surface proteins exclusively affect abscess formation upon bloodstream infection and staphylococcal dissemination in the host (Figure 1; Foster, 2019). ClfA and protein A, for instance, play a key role during septic arthritis (Josefsson et al., 2001; Palmqvist et al., 2002), a dangerous joint disease which is characterized by fever, joint pain and swelling, as well as redness of affected body regions (Shirtliff and Mader, 2002; Mathews et al., 2010). Development of septic arthritis is also linked to the expression of staphylococcal collagen adhesin (Cna), a protein and member of the MSCRAMM (microbial surface component recognizing adhesive matrix molecule) family that mediates binding to collagen and cartilage (Patti et al., 1994; Xu et al., 2004). Moreover, fibrinogen-and fibronectin-binding proteins (i.e., ClfA, ClfB, FnBPA, and FnBPB) promote bacterial aggregation in human synovial fluid, a biofilm-like state that protects S. aureus from antibiotics and phagocytes within the joint cavity (Dastgheyb et al., 2015). In this regard, we further note that some of these proteins impact staphylococcal skeletal infections and chronic bone diseases (i.e., osteomyelitis; Gimza and Cassat, 2021; Masters et al., 2022). For example, S. aureus Cna and bone sialoprotein (Bbp), another MSCRAMM that facilitates adhesion to fibrinogen (Vazquez et al., 2011), confer binding to the bone matrix and thus contribute to the pathogenesis of osteomyelitis (Ryden et al., 1989; Elasri et al., 2002; Campoccia et al., 2009; Persson et al., 2009). Likewise, protein A is a major modulator of this disease as binding of SpA to osteoblasts prevents cellular proliferation and stimulates apoptotic cell death in bone-synthesizing cells (Claro et al., 2011; Widaa et al., 2012). Development of osteomyelitis and establishment of replicative niches in the bone environment is further promoted by FnBPs (Ahmed et al., 2001), crucial S. aureus surface proteins that also impact non-osseous and fatal staphylococcal diseases of the heart (Foster, 2019). More specifically, FnBPs along with fibrinogen-and collagen-binding proteins of S. aureus influence the pathogenesis of infective endocarditis (Kuypers and Proctor, 1989; Moreillon et al., 1995; Hienz et al., 1996; Entenza et al., 2000; Que et al., 2005; Claes et al., 2017), a serious and life-threatening disease affecting the endocardial surface of the heart (Holland et al., 2016). Mechanistically, these proteins promote attachment of S. aureus to vessel walls, thrombi, and traumatized or inflamed heart tissues (Kuypers and Proctor, 1989; Moreillon et al., 1995; Entenza et al., 2000; Que et al., 2005; Claes et al., 2017). At later stages, FnBPA together with other virulence factors trigger staphylococcal invasion of the valve endothelium thereby aiding in the establishment of novel proliferative sites that provoke tissue destruction, cardiac abscess formation, and organ failure (Hamill et al., 1986; Que et al., 2005; Holland et al., 2016). Not surprisingly perhaps that FnBPs have a similar role during acute lower respiratory tract infection (pneumonia) as these factors confer binding to and uptake of S. aureus into airway epithelial cells (Figure 1; McElroy et al., 2002; Mongodin et al., 2002). Yet, failure to enter host cells due to missing expression of FnBPs may also boost staphylococcal pathogenicity as demonstrated in a rat model of experimental pneumonia (McElroy et al., 2002). Presumably, intracellular replication and persistence is favored by specific S. aureus isolates and might help to better adapt to the inflamed lung environment. This is also exemplified by the persistent lifestyle of staphylococcal small colony variants (SCVs), an auxotrophic and hard-to-treat subpopulation of S. aureus that often emerges during airway infections and in patients with cystic fibrosis (Proctor et al., 2006; Kahl et al., 2016). SCVs particularly aim at infiltrating host cells by upregulating FnBPs and other cell surface proteins to establish a protective niche that shields the microbe from neutrophils and alveolar macrophages (Vaudaux et al., 2002; Kahl et al., 2005; Mitchell et al., 2008; Tuchscherr et al., 2010, 2011; Kriegeskorte et al., 2014). Since SCVs as well as wildtype S. aureus often co-infect the lung together with other pathogens (McCullers, 2014; Oliva and Terrier, 2021), we finally appreciate that staphylococcal surface proteins may even impact outcomes of polymicrobial infections. Most notably, recent advances suggest that S. aureus IsdA manipulates the Janus kinase-signal transducer and activator of transcription (JAK–STAT) signaling cascade thereby accelerating proliferation of severe acute respiratory syndrome coronavirus type 2 (SARS-CoV-2) in epithelial cells (Goncheva et al., 2023). Moreover, protein A was found to protect Pseudomonas aeruginosa from neutrophil-mediated killing and altered the capacity of this microbe to form biofilms (Armbruster et al., 2016). This mechanism involves binding of protein A to cell surface structures of P. aeruginosa and the release of SpA from the staphylococcal cell wall, an earlier described phenomenon that may even be linked to binding of protein A to tumor necrosis factor receptor 1 (TNFR1) on lung epithelial cells thereby shaping staphylococcal pneumonia (Gomez et al., 2004; Becker et al., 2014; Armbruster et al., 2016). Overall, these compelling studies highlight the variable functions of cell surface proteins during S. aureus bone and joint infections, endocarditis, and pneumonia.
Targeting cell surface proteins and sortase A to improve Staphylococcus aureus infection outcomes
Due to their near-essential role during S. aureus pathogenesis and colonization of the host, cell surface proteins represent attractive targets for the development of new prophylactic and anti-infective intervention strategies. Earlier studies demonstrated that vaccination of laboratory animals with staphylococcal cell surface proteins together with passive immunization approaches confer protective effects against S. aureus disease (Table 1). For example, IsdA-or IsdB-based immunization of mice and interference with heme-iron uptake attenuated the adaptive properties and virulence potential of staphylococci in multiple in vivo models (Table 1; Clarke et al., 2006; Kuklin et al., 2006; Brown et al., 2009; Kim et al., 2010b; Bennett et al., 2019a,b). Likewise, immunization of mice with SpAKKAA, a non-toxigenic protein A-based vaccine (Kim et al., 2010a), or safety-improved variants thereof abolished staphylococcal pathogenicity in murine and guinea pig models of bloodstream infection, and even promoted decolonization of rodent nares (Table 1; Kim et al., 2010a, 2015; Sun et al., 2018; Shi et al., 2021). Moreover, SpA-targeting monoclonal antibodies (mAbs) and derived humanized variants displayed therapeutic activity in abscess mouse models and concurrently offered protection against bacteremia and neonatal sepsis (Table 1; Kim et al., 2012; Thammavongsa et al., 2015b; Chen et al., 2019, 2020, 2022). These effects correlated with the antibody-mediated neutralization of the immunoglobulin Fcγ-binding and B-cell receptor crosslinking properties of SpA and enhanced opsonophagocytic killing of staphylococci in mouse or human blood (Kim et al., 2012; Thammavongsa et al., 2015b; Chen et al., 2019, 2020, 2022). Accelerated killing of S. aureus in host blood paired with ameliorated outcomes of septic arthritis or bacteremia was also observed in passively immunized animals that received ClfA-or SraP (serine-rich adhesin for platelets)-targeting antibodies (Josefsson et al., 2001; Tkaczyk et al., 2016; Yang et al., 2016; Zhou et al., 2021). Administration of an antiserum raised against staphylococcal AdsA further aided in rescuing mice from fatal bloodstream infection and peritonitis, presumably as a result of enhanced killing of staphylococci by circulating neutrophils that can no longer be suppressed by pathogen-derived adenosine (Zhang et al., 2017b). Accordingly, cell surface proteins and their immunogenic potential have often been exploited to formulate an effective vaccine against S. aureus (Clegg et al., 2021). Examples involve a recombinant, protein A-and IsdB-N2-containing five-antigen S. aureus vaccine (rFSAV) as well as SA4Ag, a multicomponent vaccine composed of capsular polysaccharide conjugates and recombinant forms of ClfA and the staphylococcal manganese transporter C (MntC; Creech et al., 2017; Frenck et al., 2017; Zeng et al., 2020). However, various clinical trials ended in failure due to adverse effects or limited efficacy in diseased patients (Clegg et al., 2021), thereby asking for improved vaccination strategies that may encompass probiotic-based immunization (Pan et al., 2023), advanced antibody engineering (Chen et al., 2020, 2022), or usage of live-attenuated vaccine platforms (Cabral et al., 2017; Moscoso et al., 2018). Alternatively, chemical interference with the transpeptidase activity of sortase A may also help to limit S. aureus colonization and severity of staphylococcal disease. In fact, previous work demonstrated that small molecule inhibitor-based blockade of sortase A can reduce S. aureus virulence in different animal model of infectious disease (Table 1). Computational drug engineering, for instance, identified 3,6-disubstituted triazolothiadiazole as a potent inhibitor of sortase A that improved infection outcomes of lethal S. aureus bacteremia (Table 1; Zhang et al., 2014). Further, compound library screening helped to isolate several natural products with sortase A-blocking and anti-infective properties (Table 1; Song et al., 2022a,b). Some of these agents even potentiated the efficacy of cell wall biosynthesis-targeting antibiotics during experimental pneumonia, presumably aiding in the design of poly-therapeutic approaches that may also encompass usage of allantodapsone, a prototype pan-inhibitor of staphylococcal adhesion to extracellular matrix proteins (Prencipe et al., 2022), to combat complicated MRSA infections in the future (Table 1; Song et al., 2022a,b; Wang et al., 2022b).
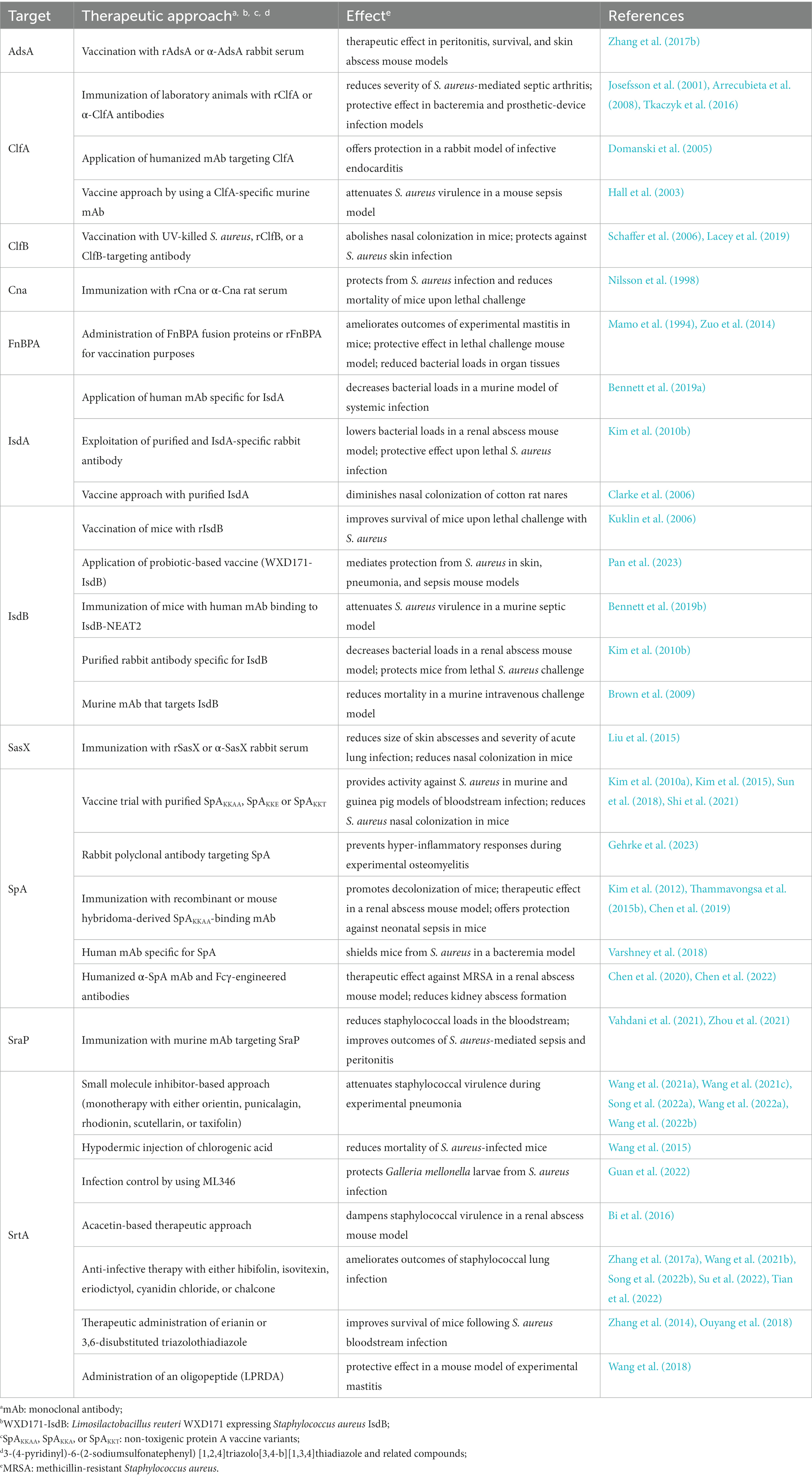
Table 1. Selected mono-therapeutic approaches to attenuate Staphylococcus aureus pathogenicity in vivo.
Concluding remarks
Cell surface proteins are key determinants of S. aureus virulence that largely affect host adaptation and immune evasion (Foster et al., 2014; Schneewind and Missiakas, 2019). Undoubtedly, many of these elements modulate host-microbe interaction and essentially contribute to the diverse clinical syndromes S. aureus may trigger in mammals (Foster et al., 2014; Schneewind and Missiakas, 2019). Staphylococcal surface proteins may even shape local outbreaks and emergence of new hyper-virulent clones (Li et al., 2012), as well as host tropism as exemplified by the biofilm-associated protein (Bap) which is prevalently expressed in S. aureus strains that provoke mastitis in animals (Valle et al., 2020). Notwithstanding, the antigenic variation, diversity, and functional multiplicity of cell surface proteins have hampered attempts to exploit these structures for the development of preventive therapeutics. Although active or passive immunization of laboratory animals conferred protective effects, neutralization of surface proteins may not necessarily represent a suitable approach to prevent staphylococcal infectious diseases in humans. Yet, experimental vaccines and antibody-based immunotherapies that seek to inactivate surface proteins in staphylococci may help to optimize future vaccine trials in diseased individuals. Concomitantly, resolving crystal structures of surface protein-antibody complexes, as recently implemented with ClfA and the mAb tefibazumab (Ganesh et al., 2016), along with an in-depth investigation of non-protective immune imprinting, a phenomenon that correlates with therapeutic failure of IsdB-based immunization trials (Tsai et al., 2022), could assist in the exploitation of S. aureus surface proteins for the reformulation of an effective vaccine candidate or fabrication of unique prophylactic tools that foster decolonization of high-risk patients. Ultimately, the discovery of new host ligands of non-excessively studied surface proteins such as the plasmin-sensitive surface protein (Pls), an MRSA-specific cell envelope-displayed glycoprotein (Savolainen et al., 2001; Josefsson et al., 2005; Bleiziffer et al., 2017), may also aid in the design of additional anti-infective strategies and further fuels our knowledge of staphylococcal infection dynamics.
Author contributions
NS and VW performed the literature review and wrote the manuscript. All authors substantially contributed to the article and approved the submitted version.
Funding
Work in the VW Laboratory is supported by the German Research Foundation (award WI4582/2-1 to VW; project number 449712894) and the Else Kröner-Fresenius-Stiftung (award 2021_EKEA.16 to VW).
Acknowledgments
We thank all laboratory members for helpful discussion. We apologize to authors whose work was either not referenced or not discussed. We are grateful for support from the German Research Foundation (project grant WI4582/2-1 to VW, project number 449712894) and from Else Kröner-Fresenius-Stiftung (award 2021_EKEA.16 to VW). NS was supported by the Hannover Biomedical Research School (HBRS) and the Center for Infection Biology (ZIB).
Conflict of interest
The authors declare that the research was conducted in the absence of any commercial or financial relationships that could be construed as a potential conflict of interest.
Publisher’s note
All claims expressed in this article are solely those of the authors and do not necessarily represent those of their affiliated organizations, or those of the publisher, the editors and the reviewers. Any product that may be evaluated in this article, or claim that may be made by its manufacturer, is not guaranteed or endorsed by the publisher.
References
Ahmed, S., Meghji, S., Williams, R. J., Henderson, B., Brock, J. H., and Nair, S. P. (2001). Staphylococcus aureus fibronectin binding proteins are essential for internalization by osteoblasts but do not account for differences in intracellular levels of bacteria. Infect. Immun. 69, 2872–2877. doi: 10.1128/IAI.69.5.2872-2877.2001
Armbruster, C. R., Wolter, D. J., Mishra, M., Hayden, H. S., Radey, M. C., Merrihew, G., et al. (2016). Staphylococcus aureus protein a mediates interspecies interactions at the cell surface of Pseudomonas aeruginosa. mBio 7:e00538–16. doi: 10.1128/mBio.00538-16
Arrecubieta, C., Matsunaga, I., Asai, T., Naka, Y., Deng, M. C., and Lowy, F. D. (2008). Vaccination with clumping factor a and fibronectin binding protein a to prevent Staphylococcus aureus infection of an aortic patch in mice. J. Infect. Dis. 198, 571–575. doi: 10.1086/590210
Askarian, F., Ajayi, C., Hanssen, A. M., van Sorge, N. M., Pettersen, I., Diep, D. B., et al. (2016). The interaction between Staphylococcus aureus SdrD and desmoglein 1 is important for adhesion to host cells. Sci. Rep. 6:22134. doi: 10.1038/srep22134
Askarian, F., Uchiyama, S., Valderrama, J. A., Ajayi, C., Sollid, J. U. E., van Sorge, N. M., et al. (2017). Serine-aspartate repeat protein D increases Staphylococcus aureus virulence and survival in blood. Infect. Immun. 85:e00559–16. doi: 10.1128/IAI.00559-16
Bai, A. D., Lo, C. K. L., Komorowski, A. S., Suresh, M., Guo, K., Garg, A., et al. (2022). Staphylococcus aureus bacteraemia mortality: a systematic review and meta-analysis. Clin. Microbiol. Infect. 28, 1076–1084. doi: 10.1016/j.cmi.2022.03.015
Becker, S., Frankel, M. B., Schneewind, O., and Missiakas, D. (2014). Release of protein a from the cell wall of Staphylococcus aureus. Proc. Natl. Acad. Sci. U. S. A. 111, 1574–1579. doi: 10.1073/pnas.1317181111
Bennett, M. R., Bombardi, R. G., Kose, N., Parrish, E. H., Nagel, M. B., Petit, R. A., et al. (2019a). Human mAbs to Staphylococcus aureus IsdA provide protection through both Heme-blocking and fc-mediated mechanisms. J. Infect. Dis. 219, 1264–1273. doi: 10.1093/infdis/jiy635
Bennett, M. R., Dong, J., Bombardi, R. G., Soto, C., Parrington, H. M., Nargi, R. S., et al. (2019b). Human V(H)1-69 gene-encoded human monoclonal antibodies against Staphylococcus aureus IsdB use at least three distinct modes of binding to inhibit bacterial growth and pathogenesis. mBio 10:e02473–19. doi: 10.1128/mBio.02473-19
Bi, C., Dong, X., Zhong, X., Cai, H., Wang, D., and Wang, L. (2016). Acacetin protects mice from Staphylococcus aureus bloodstream infection by inhibiting the activity of Sortase a. Molecules 21:1285. doi: 10.3390/molecules21101285
Bleiziffer, I., Eikmeier, J., Pohlentz, G., McAulay, K., Xia, G., Hussain, M., et al. (2017). The plasmin-sensitive protein Pls in methicillin-resistant Staphylococcus aureus (MRSA) is a glycoprotein. PLoS Pathog. 13:e1006110. doi: 10.1371/journal.ppat.1006110
Brinkmann, V., Reichard, U., Goosmann, C., Fauler, B., Uhlemann, Y., Weiss, D. S., et al. (2004). Neutrophil extracellular traps kill bacteria. Science 303, 1532–1535. doi: 10.1126/science.1092385
Brown, M., Kowalski, R., Zorman, J., Wang, X. M., Towne, V., Zhao, Q., et al. (2009). Selection and characterization of murine monoclonal antibodies to Staphylococcus aureus iron-regulated surface determinant B with functional activity in vitro and in vivo. Clin. Vaccine Immunol. 16, 1095–1104. doi: 10.1128/CVI.00085-09
Bubeck Wardenburg, J., Patel, R. J., and Schneewind, O. (2007). Surface proteins and exotoxins are required for the pathogenesis of Staphylococcus aureus pneumonia. Infect. Immun. 75, 1040–1044. doi: 10.1128/IAI.01313-06
Cabral, M. P., Garcia, P., Beceiro, A., Rumbo, C., Perez, A., Moscoso, M., et al. (2017). Design of live attenuated bacterial vaccines based on D-glutamate auxotrophy. Nat. Commun. 8:15480. doi: 10.1038/ncomms15480
Campoccia, D., Speziale, P., Ravaioli, S., Cangini, I., Rindi, S., Pirini, V., et al. (2009). The presence of both bone sialoprotein-binding protein gene and collagen adhesin gene as a typical virulence trait of the major epidemic cluster in isolates from orthopedic implant infections. Biomaterials 30, 6621–6628. doi: 10.1016/j.biomaterials.2009.08.032
Candi, E., Schmidt, R., and Melino, G. (2005). The cornified envelope: a model of cell death in the skin. Nat. Rev. Mol. Cell Biol. 6, 328–340. doi: 10.1038/nrm1619
Chambers, H. F., and Deleo, F. R. (2009). Waves of resistance: Staphylococcus aureus in the antibiotic era. Nat. Rev. Microbiol. 7, 629–641. doi: 10.1038/nrmicro2200
Chen, F., Liu, B., Wang, D., Wang, L., Deng, X., Bi, C., et al. (2014). Role of sortase a in the pathogenesis of Staphylococcus aureus-induced mastitis in mice. FEMS Microbiol. Lett. 351, 95–103. doi: 10.1111/1574-6968.12354
Chen, X., Schneewind, O., and Missiakas, D. (2022). Engineered human antibodies for the opsonization and killing of Staphylococcus aureus. Proc. Natl. Acad. Sci. U. S. A. 119:e2114478119. doi: 10.1073/pnas.2114478119
Chen, X., Shi, M., Tong, X., Kim, H. K., Wang, L. X., Schneewind, O., et al. (2020). Glycosylation-dependent opsonophagocytic activity of staphylococcal protein a antibodies. Proc. Natl. Acad. Sci. U. S. A. 117, 22992–23000. doi: 10.1073/pnas.2003621117
Chen, X., Sun, Y., Missiakas, D., and Schneewind, O. (2019). Staphylococcus aureus decolonization of mice with monoclonal antibody neutralizing protein a. J. Infect. Dis. 219, 884–888. doi: 10.1093/infdis/jiy597
Cheng, A. G., DeDent, A. C., Schneewind, O., and Missiakas, D. (2011). A play in four acts: Staphylococcus aureus abscess formation. Trends Microbiol. 19, 225–232. doi: 10.1016/j.tim.2011.01.007
Cheng, A. G., Kim, H. K., Burts, M. L., Krausz, T., Schneewind, O., and Missiakas, D. M. (2009). Genetic requirements for Staphylococcus aureus abscess formation and persistence in host tissues. FASEB J. 23, 3393–3404. doi: 10.1096/fj.09-135467
Cho, S. H., Strickland, I., Boguniewicz, M., and Leung, D. Y. (2001). Fibronectin and fibrinogen contribute to the enhanced binding of Staphylococcus aureus to atopic skin. J. Allergy Clin. Immunol. 108, 269–274. doi: 10.1067/mai.2001.117455
Claes, J., Liesenborghs, L., Peetermans, M., Veloso, T. R., Missiakas, D., Schneewind, O., et al. (2017). Clumping factor a, von Willebrand factor-binding protein and von Willebrand factor anchor Staphylococcus aureus to the vessel wall. J. Thromb. Haemost. 15, 1009–1019. doi: 10.1111/jth.13653
Clarke, S. R., Andre, G., Walsh, E. J., Dufrene, Y. F., Foster, T. J., and Foster, S. J. (2009). Iron-regulated surface determinant protein a mediates adhesion of Staphylococcus aureus to human corneocyte envelope proteins. Infect. Immun. 77, 2408–2416. doi: 10.1128/IAI.01304-08
Clarke, S. R., Brummell, K. J., Horsburgh, M. J., McDowell, P. W., Mohamad, S. A., Stapleton, M. R., et al. (2006). Identification of in vivo-expressed antigens of Staphylococcus aureus and their use in vaccinations for protection against nasal carriage. J. Infect. Dis. 193, 1098–1108. doi: 10.1086/501471
Claro, T., Widaa, A., O'Seaghdha, M., Miajlovic, H., Foster, T. J., O'Brien, F. J., et al. (2011). Staphylococcus aureus protein a binds to osteoblasts and triggers signals that weaken bone in osteomyelitis. PLoS One 6:e18748. doi: 10.1371/journal.pone.0018748
Classen, A., Kalali, B. N., Schnopp, C., Andres, C., Aguilar-Pimentel, J. A., Ring, J., et al. (2011). TNF receptor I on human keratinocytes is a binding partner for staphylococcal protein a resulting in the activation of NF kappa B, AP-1, and downstream gene transcription. Exp. Dermatol. 20, 48–52. doi: 10.1111/j.1600-0625.2010.01174.x
Clegg, J., Soldaini, E., McLoughlin, R. M., Rittenhouse, S., Bagnoli, F., and Phogat, S. (2021). Staphylococcus aureus vaccine research and development: the past, present and future including novel therapeutic strategies. Front. Immunol. 12:705360. doi: 10.3389/fimmu.2021.705360
Corrigan, R. M., Miajlovic, H., and Foster, T. J. (2009). Surface proteins that promote adherence of Staphylococcus aureus to human desquamated nasal epithelial cells. BMC Microbiol. 9:22. doi: 10.1186/1471-2180-9-22
Creech, C. B., Frenck, R. W. Jr., Sheldon, E. A., Seiden, D. J., Kankam, M. K., Zito, E. T., et al. (2017). Safety, tolerability, and immunogenicity of a single dose 4-antigen or 3-antigen Staphylococcus aureus vaccine in healthy older adults: results of a randomised trial. Vaccine 35, 385–394. doi: 10.1016/j.vaccine.2016.11.032
da Costa, T. M., Viljoen, A., Towell, A. M., Dufrene, Y. F., and Geoghegan, J. A. (2022). Fibronectin binding protein B binds to loricrin and promotes corneocyte adhesion by Staphylococcus aureus. Nat. Commun. 13:2517. doi: 10.1038/s41467-022-30271-1
Dastgheyb, S., Parvizi, J., Shapiro, I. M., Hickok, N. J., and Otto, M. (2015). Effect of biofilms on recalcitrance of staphylococcal joint infection to antibiotic treatment. J. Infect. Dis. 211, 641–650. doi: 10.1093/infdis/jiu514
Domanski, P. J., Patel, P. R., Bayer, A. S., Zhang, L., Hall, A. E., Syribeys, P. J., et al. (2005). Characterization of a humanized monoclonal antibody recognizing clumping factor a expressed by Staphylococcus aureus. Infect. Immun. 73, 5229–5232. doi: 10.1128/IAI.73.8.5229-5232.2005
Dossett, J. H., Kronvall, G., Williams, R. C. Jr., and Quie, P. G. (1969). Antiphagocytic effects of staphylococfcal protein a. J. Immunol. 103, 1405–1410.
Edwards, A. M., Potter, U., Meenan, N. A., Potts, J. R., and Massey, R. C. (2011). Staphylococcus aureus keratinocyte invasion is dependent upon multiple high-affinity fibronectin-binding repeats within FnBPA. PLoS One 6:e18899. doi: 10.1371/journal.pone.0018899
Elasri, M. O., Thomas, J. R., Skinner, R. A., Blevins, J. S., Beenken, K. E., Nelson, C. L., et al. (2002). Staphylococcus aureus collagen adhesin contributes to the pathogenesis of osteomyelitis. Bone 30, 275–280. doi: 10.1016/s8756-3282(01)00632-9
Entenza, J. M., Foster, T. J., Ni Eidhin, D., Vaudaux, P., Francioli, P., and Moreillon, P. (2000). Contribution of clumping factor B to pathogenesis of experimental endocarditis due to Staphylococcus aureus. Infect. Immun. 68, 5443–5446. doi: 10.1128/IAI.68.9.5443-5446.2000
Falugi, F., Kim, H. K., Missiakas, D. M., and Schneewind, O. (2013). Role of protein a in the evasion of host adaptive immune responses by Staphylococcus aureus. mBio 4, e00575–e00513. doi: 10.1128/mBio.00575-13
Farag, A. K., Roesner, L. M., Wieschowski, S., Heratizadeh, A., Eiz-Vesper, B., Kwok, W. W., et al. (2022). Specific T cells targeting Staphylococcus aureus fibronectin-binding protein 1 induce a type 2/type 1 inflammatory response in sensitized atopic dermatitis patients. Allergy 77, 1245–1253. doi: 10.1111/all.15120
Fleury, O. M., McAleer, M. A., Feuillie, C., Formosa-Dague, C., Sansevere, E., Bennett, D. E., et al. (2017). Clumping factor B promotes adherence of Staphylococcus aureus to Corneocytes in atopic dermatitis. Infect. Immun. 85:e00994–16. doi: 10.1128/IAI.00994-16
Forsgren, A., and Quie, P. G. (1974). Effects of staphylococcal protein a on heat labile opsonins. J. Immunol. 112, 1177–1180.
Forsgren, A., and Sjoquist, J. (1966). "protein a" from S. aureus. I. Pseudo-immune reaction with human gamma-globulin. J. Immunol. 97, 822–827.
Foster, T. J. (2005). Immune evasion by staphylococci. Nat. Rev. Microbiol. 3, 948–958. doi: 10.1038/nrmicro1289
Foster, T. J. (2019). Surface proteins of Staphylococcus aureus. Microbiol. Spectr. 7. doi: 10.1128/microbiolspec.GPP3-0046-2018
Foster, T. J., Geoghegan, J. A., Ganesh, V. K., and Hook, M. (2014). Adhesion, invasion and evasion: the many functions of the surface proteins of Staphylococcus aureus. Nat. Rev. Microbiol. 12, 49–62. doi: 10.1038/nrmicro3161
Fowler, T., Wann, E. R., Joh, D., Johansson, S., Foster, T. J., and Hook, M. (2000). Cellular invasion by Staphylococcus aureus involves a fibronectin bridge between the bacterial fibronectin-binding MSCRAMMs and host cell beta1 integrins. Eur. J. Cell Biol. 79, 672–679. doi: 10.1078/0171-9335-00104
Frenck, R. W. Jr., Creech, C. B., Sheldon, E. A., Seiden, D. J., Kankam, M. K., Baber, J., et al. (2017). Safety, tolerability, and immunogenicity of a 4-antigen Staphylococcus aureus vaccine (SA4Ag): results from a first-in-human randomised, placebo-controlled phase 1/2 study. Vaccine 35, 375–384. doi: 10.1016/j.vaccine.2016.11.010
Ganesh, V. K., Liang, X., Geoghegan, J. A., Cohen, A. L. V., Venugopalan, N., Foster, T. J., et al. (2016). Lessons from the crystal structure of the S. aureus surface protein clumping factor a in complex with Tefibazumab, an inhibiting monoclonal antibody. EBioMedicine 13, 328–338. doi: 10.1016/j.ebiom.2016.09.027
GBD 2019 Antimicrobial Resistance Collaborators (2022). Global mortality associated with 33 bacterial pathogens in 2019: a systematic analysis for the global burden of disease study 2019. Lancet 400, 2221–2248. doi: 10.1016/S0140-6736(22)02185-7
Gehrke, A. E., Mendoza-Bertelli, A., Ledo, C., Gonzalez, C. D., Noto Llana, M., Blanco, C., et al. (2023). Neutralization of Staphylococcus aureus protein a prevents exacerbated osteoclast activity and bone loss during osteomyelitis. Antimicrob. Agents Chemother. 67:e0114022. doi: 10.1128/aac.01140-22
Geoghegan, J. A., Irvine, A. D., and Foster, T. J. (2018). Staphylococcus aureus and atopic dermatitis: a complex and evolving relationship. Trends Microbiol. 26, 484–497. doi: 10.1016/j.tim.2017.11.008
Gimza, B. D., and Cassat, J. E. (2021). Mechanisms of antibiotic failure during Staphylococcus aureus osteomyelitis. Front. Immunol. 12:638085. doi: 10.3389/fimmu.2021.638085
Gomez, M. I., Lee, A., Reddy, B., Muir, A., Soong, G., Pitt, A., et al. (2004). Staphylococcus aureus protein a induces airway epithelial inflammatory responses by activating TNFR1. Nat. Med. 10, 842–848. doi: 10.1038/nm1079
Goncheva, M. I., Gibson, R. M., Shouldice, A. C., Dikeakos, J. D., and Heinrichs, D. E. (2023). The Staphylococcus aureus protein IsdA increases SARS CoV-2 replication by modulating JAK-STAT signaling. iScience 26:105975. doi: 10.1016/j.isci.2023.105975
Gonzalez, C. D., Ledo, C., Cela, E., Stella, I., Xu, C., Ojeda, D. S., et al. (2019). The good side of inflammation: Staphylococcus aureus proteins SpA and Sbi contribute to proper abscess formation and wound healing during skin and soft tissue infections. Biochim. Biophys. Acta Mol. basis Dis. 1865, 2657–2670. doi: 10.1016/j.bbadis.2019.07.004
Guan, X. N., Zhang, T., Yang, T., Dong, Z., Yang, S., Lan, L., et al. (2022). Covalent sortase a inhibitor ML346 prevents Staphylococcus aureus infection of galleria mellonella. RSC Med. Chem. 13, 138–149. doi: 10.1039/d1md00316j
Hall, A. E., Domanski, P. J., Patel, P. R., Vernachio, J. H., Syribeys, P. J., Gorovits, E. L., et al. (2003). Characterization of a protective monoclonal antibody recognizing Staphylococcus aureus MSCRAMM protein clumping factor a. Infect. Immun. 71, 6864–6870. doi: 10.1128/IAI.71.12.6864-6870.2003
Hamill, R. J., Vann, J. M., and Proctor, R. A. (1986). Phagocytosis of Staphylococcus aureus by cultured bovine aortic endothelial cells: model for postadherence events in endovascular infections. Infect. Immun. 54, 833–836. doi: 10.1128/iai.54.3.833-836.1986
Hammer, N. D., and Skaar, E. P. (2011). Molecular mechanisms of Staphylococcus aureus iron acquisition. Annu. Rev. Microbiol. 65, 129–147. doi: 10.1146/annurev-micro-090110-102851
Hienz, S. A., Schennings, T., Heimdahl, A., and Flock, J. I. (1996). Collagen binding of Staphylococcus aureus is a virulence factor in experimental endocarditis. J. Infect. Dis. 174, 83–88. doi: 10.1093/infdis/174.1.83
Higgins, J., Loughman, A., van Kessel, K. P., van Strijp, J. A., and Foster, T. J. (2006). Clumping factor a of Staphylococcus aureus inhibits phagocytosis by human polymorphonuclear leucocytes. FEMS Microbiol. Lett. 258, 290–296. doi: 10.1111/j.1574-6968.2006.00229.x
Holland, T. L., Baddour, L. M., Bayer, A. S., Hoen, B., Miro, J. M., and Fowler, V. G. Jr. (2016). Infective endocarditis. Nat Rev Dis Primers 2:16059. doi: 10.1038/nrdp.2016.59
Howden, B. P., Giulieri, S. G., Wong Fok Lung, T., Baines, S. L., Sharkey, L. K., Lee, J. Y. H., et al. (2023). Staphylococcus aureus host interactions and adaptation. Nat. Rev. Microbiol., 1–16. doi: 10.1038/s41579-023-00852-y
Jonsson, I. M., Mazmanian, S. K., Schneewind, O., Bremell, T., and Tarkowski, A. (2003). The role of Staphylococcus aureus sortase a and sortase B in murine arthritis. Microbes Infect. 5, 775–780. doi: 10.1016/s1286-4579(03)00143-6
Josefsson, E., Hartford, O., O'Brien, L., Patti, J. M., and Foster, T. (2001). Protection against experimental Staphylococcus aureus arthritis by vaccination with clumping factor a, a novel virulence determinant. J. Infect. Dis. 184, 1572–1580. doi: 10.1086/324430
Josefsson, E., Juuti, K., Bokarewa, M., and Kuusela, P. (2005). The surface protein Pls of methicillin-resistant Staphylococcus aureus is a virulence factor in septic arthritis. Infect. Immun. 73, 2812–2817. doi: 10.1128/IAI.73.5.2812-2817.2005
Kahl, B. C., Becker, K., and Loffler, B. (2016). Clinical significance and pathogenesis of staphylococcal small Colony variants in persistent infections. Clin. Microbiol. Rev. 29, 401–427. doi: 10.1128/CMR.00069-15
Kahl, B. C., Belling, G., Becker, P., Chatterjee, I., Wardecki, K., Hilgert, K., et al. (2005). Thymidine-dependent Staphylococcus aureus small-colony variants are associated with extensive alterations in regulator and virulence gene expression profiles. Infect. Immun. 73, 4119–4126. doi: 10.1128/IAI.73.7.4119-4126.2005
Kim, H. K., Cheng, A. G., Kim, H. Y., Missiakas, D. M., and Schneewind, O. (2010a). Nontoxigenic protein a vaccine for methicillin-resistant Staphylococcus aureus infections in mice. J. Exp. Med. 207, 1863–1870. doi: 10.1084/jem.20092514
Kim, H. K., DeDent, A., Cheng, A. G., McAdow, M., Bagnoli, F., Missiakas, D. M., et al. (2010b). IsdA and IsdB antibodies protect mice against Staphylococcus aureus abscess formation and lethal challenge. Vaccine 28, 6382–6392. doi: 10.1016/j.vaccine.2010.02.097
Kim, H. K., Emolo, C., DeDent, A. C., Falugi, F., Missiakas, D. M., and Schneewind, O. (2012). Protein A-specific monoclonal antibodies and prevention of Staphylococcus aureus disease in mice. Infect. Immun. 80, 3460–3470. doi: 10.1128/IAI.00230-12
Kim, H. K., Falugi, F., Thomer, L., Missiakas, D. M., and Schneewind, O. (2015). Protein a suppresses immune responses during Staphylococcus aureus bloodstream infection in guinea pigs. mBio 6:e02369–14. doi: 10.1128/mBio.02369-14
Klevens, R. M., Morrison, M. A., Nadle, J., Petit, S., Gershman, K., Ray, S., et al. (2007). Invasive methicillin-resistant Staphylococcus aureus infections in the United States. JAMA 298, 1763–1771. doi: 10.1001/jama.298.15.1763
Kriegeskorte, A., Block, D., Drescher, M., Windmuller, N., Mellmann, A., Baum, C., et al. (2014). Inactivation of thyA in Staphylococcus aureus attenuates virulence and has a strong impact on metabolism and virulence gene expression. mBio 5, e01447–e01414. doi: 10.1128/mBio.01447-14
Kuehnert, M. J., Kruszon-Moran, D., Hill, H. A., McQuillan, G., McAllister, S. K., Fosheim, G., et al. (2006). Prevalence of Staphylococcus aureus nasal colonization in the United States, 2001-2002. J. Infect. Dis. 193, 172–179. doi: 10.1086/499632
Kuklin, N. A., Clark, D. J., Secore, S., Cook, J., Cope, L. D., McNeely, T., et al. (2006). A novel Staphylococcus aureus vaccine: iron surface determinant B induces rapid antibody responses in rhesus macaques and specific increased survival in a murine S. aureus sepsis model. Infect. Immun. 74, 2215–2223. doi: 10.1128/IAI.74.4.2215-2223.2006
Kuypers, J. M., and Proctor, R. A. (1989). Reduced adherence to traumatized rat heart valves by a low-fibronectin-binding mutant of Staphylococcus aureus. Infect. Immun. 57, 2306–2312. doi: 10.1128/iai.57.8.2306-2312.1989
Kwiecinski, J., Jin, T., and Josefsson, E. (2014). Surface proteins of Staphylococcus aureus play an important role in experimental skin infection. APMIS 122, 1240–1250. doi: 10.1111/apm.12295
Lacey, K. A., Mulcahy, M. E., Towell, A. M., Geoghegan, J. A., and McLoughlin, R. M. (2019). Clumping factor B is an important virulence factor during Staphylococcus aureus skin infection and a promising vaccine target. PLoS Pathog. 15:e1007713. doi: 10.1371/journal.ppat.1007713
Ledo, C., Gonzalez, C. D., Garofalo, A., Sabbione, F., Keitelman, I. A., Giai, C., et al. (2020). Protein a modulates neutrophil and keratinocyte signaling and survival in response to Staphylococcus aureus. Front. Immunol. 11:524180. doi: 10.3389/fimmu.2020.524180
Lee, A. S., de Lencastre, H., Garau, J., Kluytmans, J., Malhotra-Kumar, S., Peschel, A., et al. (2018). Methicillin-resistant Staphylococcus aureus. Nat. Rev. Dis. Primers. 4:18033. doi: 10.1038/nrdp.2018.33
Li, M., Du, X., Villaruz, A. E., Diep, B. A., Wang, D., Song, Y., et al. (2012). MRSA epidemic linked to a quickly spreading colonization and virulence determinant. Nat. Med. 18, 816–819. doi: 10.1038/nm.2692
Liu, Q., Du, X., Hong, X., Li, T., Zheng, B., He, L., et al. (2015). Targeting surface protein SasX by active and passive vaccination to reduce Staphylococcus aureus colonization and infection. Infect. Immun. 83, 2168–2174. doi: 10.1128/iai.02951-14
Lowy, F. D. (1998). Staphylococcus aureus infections. N. Engl. J. Med. 339, 520–532. doi: 10.1056/NEJM199808203390806
Malachowa, N., and DeLeo, F. R. (2010). Mobile genetic elements of Staphylococcus aureus. Cell. Mol. Life Sci. 67, 3057–3071. doi: 10.1007/s00018-010-0389-4
Malachowa, N., Kobayashi, S. D., Porter, A. R., Braughton, K. R., Scott, D. P., Gardner, D. J., et al. (2016). Contribution of Staphylococcus aureus coagulases and clumping factor a to abscess formation in a rabbit Model of skin and soft tissue infection. PLoS One 11:e0158293. doi: 10.1371/journal.pone.0158293
Malachowa, N., Whitney, A. R., Kobayashi, S. D., Sturdevant, D. E., Kennedy, A. D., Braughton, K. R., et al. (2011). Global changes in Staphylococcus aureus gene expression in human blood. PLoS One 6:e18617. doi: 10.1371/journal.pone.0018617
Mamo, W., Jonsson, P., Flock, J. I., Lindberg, M., Müller, H. P., Wadström, T., et al. (1994). Vaccination against Staphylococcus aureus mastitis: immunological response of mice vaccinated with fibronectin-binding protein (FnBP-A) to challenge with S. aureus. Vaccine 12, 988–992. doi: 10.1016/0264-410x(94)90333-6
Masters, E. A., Ricciardi, B. F., Bentley, K. L. M., Moriarty, T. F., Schwarz, E. M., and Muthukrishnan, G. (2022). Skeletal infections: microbial pathogenesis, immunity and clinical management. Nat. Rev. Microbiol. 20, 385–400. doi: 10.1038/s41579-022-00686-0
Mathews, C. J., Weston, V. C., Jones, A., Field, M., and Coakley, G. (2010). Bacterial septic arthritis in adults. Lancet 375, 846–855. doi: 10.1016/S0140-6736(09)61595-6
Mazmanian, S. K., Liu, G., Jensen, E. R., Lenoy, E., and Schneewind, O. (2000). Staphylococcus aureus sortase mutants defective in the display of surface proteins and in the pathogenesis of animal infections. Proc. Natl. Acad. Sci. U. S. A. 97, 5510–5515. doi: 10.1073/pnas.080520697
Mazmanian, S. K., Liu, G., Ton-That, H., and Schneewind, O. (1999). Staphylococcus aureus sortase, an enzyme that anchors surface proteins to the cell wall. Science 285, 760–763. doi: 10.1126/science.285.5428.760
Mazmanian, S. K., Skaar, E. P., Gaspar, A. H., Humayun, M., Gornicki, P., Jelenska, J., et al. (2003). Passage of heme-iron across the envelope of Staphylococcus aureus. Science 299, 906–909. doi: 10.1126/science.1081147
McCullers, J. A. (2014). The co-pathogenesis of influenza viruses with bacteria in the lung. Nat. Rev. Microbiol. 12, 252–262. doi: 10.1038/nrmicro3231
McElroy, M. C., Cain, D. J., Tyrrell, C., Foster, T. J., and Haslett, C. (2002). Increased virulence of a fibronectin-binding protein mutant of Staphylococcus aureus in a rat model of pneumonia. Infect. Immun. 70, 3865–3873. doi: 10.1128/IAI.70.7.3865-3873.2002
Mills, K. B., Roy, P., Kwiecinski, J. M., Fey, P. D., and Horswill, A. R. (2022). Staphylococcal Corneocyte adhesion: assay optimization and roles of Aap and SasG Adhesins in the establishment of healthy skin colonization. Microbiol. Spectr. 10:e0246922. doi: 10.1128/spectrum.02469-22
Misawa, Y., Kelley, K. A., Wang, X., Wang, L., Park, W. B., Birtel, J., et al. (2015). Staphylococcus aureus colonization of the mouse gastrointestinal tract is modulated by wall Teichoic acid, capsule, and surface proteins. PLoS Pathog. 11:e1005061. doi: 10.1371/journal.ppat.1005061
Mitchell, G., Lamontagne, C. A., Brouillette, E., Grondin, G., Talbot, B. G., Grandbois, M., et al. (2008). Staphylococcus aureus SigB activity promotes a strong fibronectin-bacterium interaction which may sustain host tissue colonization by small-colony variants isolated from cystic fibrosis patients. Mol. Microbiol. 70, 1540–1555. doi: 10.1111/j.1365-2958.2008.06511.x
Mongodin, E., Bajolet, O., Cutrona, J., Bonnet, N., Dupuit, F., Puchelle, E., et al. (2002). Fibronectin-binding proteins of Staphylococcus aureus are involved in adherence to human airway epithelium. Infect. Immun. 70, 620–630. doi: 10.1128/IAI.70.2.620-630.2002
Moreillon, P., Entenza, J. M., Francioli, P., McDevitt, D., Foster, T. J., Francois, P., et al. (1995). Role of Staphylococcus aureus coagulase and clumping factor in pathogenesis of experimental endocarditis. Infect. Immun. 63, 4738–4743. doi: 10.1128/iai.63.12.4738-4743.1995
Moscoso, M., Garcia, P., Cabral, M. P., Rumbo, C., and Bou, G. (2018). A D-alanine auxotrophic live vaccine is effective against lethal infection caused by Staphylococcus aureus. Virulence 9, 604–620. doi: 10.1080/21505594.2017.1417723
Mulcahy, M. E., Geoghegan, J. A., Monk, I. R., O'Keeffe, K. M., Walsh, E. J., Foster, T. J., et al. (2012). Nasal colonisation by Staphylococcus aureus depends upon clumping factor B binding to the squamous epithelial cell envelope protein loricrin. PLoS Pathog. 8:e1003092. doi: 10.1371/journal.ppat.1003092
Nilsson, I. M., Patti, J. M., Bremell, T., Höök, M., and Tarkowski, A. (1998). Vaccination with a recombinant fragment of collagen adhesin provides protection against Staphylococcus aureus-mediated septic death. J. Clin. Invest. 101, 2640–2649. doi: 10.1172/jci1823
O'Brien, L. M., Walsh, E. J., Massey, R. C., Peacock, S. J., and Foster, T. J. (2002). Staphylococcus aureus clumping factor B (ClfB) promotes adherence to human type I cytokeratin 10: implications for nasal colonization. Cell. Microbiol. 4, 759–770. doi: 10.1046/j.1462-5822.2002.00231.x
Ogonowska, P., Gilaberte, Y., Baranska-Rybak, W., and Nakonieczna, J. (2020). Colonization with Staphylococcus aureus in atopic dermatitis patients: attempts to reveal the unknown. Front. Microbiol. 11:567090. doi: 10.3389/fmicb.2020.567090
Oliva, J., and Terrier, O. (2021). Viral and bacterial co-infections in the lungs: dangerous liaisons. Viruses 13:1725. doi: 10.3390/v13091725
Ouyang, P., He, X., Yuan, Z. W., Yin, Z. Q., Fu, H., Lin, J., et al. (2018). Erianin against Staphylococcus aureus infection via inhibiting Sortase a. Toxins (Basel) 10:385. doi: 10.3390/toxins10100385
Palmqvist, N., Foster, T., Tarkowski, A., and Josefsson, E. (2002). Protein a is a virulence factor in Staphylococcus aureus arthritis and septic death. Microb. Pathog. 33, 239–249. doi: 10.1006/mpat.2002.0533
Pan, N., Liu, Y., Zhang, H., Xu, Y., Bao, X., Sheng, S., et al. (2023). Oral vaccination with engineered probiotic Limosilactobacillus reuteri has protective effects against localized and systemic Staphylococcus aureus infection. Microbiol. Spectr. 11:e0367322. doi: 10.1128/spectrum.03673-22
Patel, A. H., Nowlan, P., Weavers, E. D., and Foster, T. (1987). Virulence of protein A-deficient and alpha-toxin-deficient mutants of Staphylococcus aureus isolated by allele replacement. Infect. Immun. 55, 3103–3110. doi: 10.1128/iai.55.12.3103-3110.1987
Patti, J. M., Bremell, T., Krajewska-Pietrasik, D., Abdelnour, A., Tarkowski, A., Ryden, C., et al. (1994). The Staphylococcus aureus collagen adhesin is a virulence determinant in experimental septic arthritis. Infect. Immun. 62, 152–161. doi: 10.1128/iai.62.1.152-161.1994
Persson, L., Johansson, C., and Ryden, C. (2009). Antibodies to Staphylococcus aureus bone sialoprotein-binding protein indicate infectious osteomyelitis. Clin. Vaccine Immunol. 16, 949–952. doi: 10.1128/CVI.00442-08
Pietrocola, G., Nobile, G., Alfeo, M. J., Foster, T. J., Geoghegan, J. A., De Filippis, V., et al. (2019). Fibronectin-binding protein B (FnBPB) from Staphylococcus aureus protects against the antimicrobial activity of histones. J. Biol. Chem. 294, 3588–3602. doi: 10.1074/jbc.RA118.005707
Prencipe, F., Alsibaee, A., Khaddem, Z., Norton, P., Towell, A. M., Ali, A. F. M., et al. (2022). Allantodapsone is a Pan-inhibitor of Staphylococcus aureus adhesion to fibrinogen, Loricrin, and cytokeratin 10. Microbiol. Spectr. 10:e0117521. doi: 10.1128/spectrum.01175-21
Proctor, R. A., von Eiff, C., Kahl, B. C., Becker, K., McNamara, P., Herrmann, M., et al. (2006). Small colony variants: a pathogenic form of bacteria that facilitates persistent and recurrent infections. Nat. Rev. Microbiol. 4, 295–305. doi: 10.1038/nrmicro1384
Que, Y. A., Haefliger, J. A., Piroth, L., Francois, P., Widmer, E., Entenza, J. M., et al. (2005). Fibrinogen and fibronectin binding cooperate for valve infection and invasion in Staphylococcus aureus experimental endocarditis. J. Exp. Med. 201, 1627–1635. doi: 10.1084/jem.20050125
Reginald, K., Westritschnig, K., Linhart, B., Focke-Tejkl, M., Jahn-Schmid, B., Eckl-Dorna, J., et al. (2011). Staphylococcus aureus fibronectin-binding protein specifically binds IgE from patients with atopic dermatitis and requires antigen presentation for cellular immune responses. J. Allergy Clin. Immunol. 128, 82–91.e88. doi: 10.1016/j.jaci.2011.02.034
Ryden, C., Yacoub, A. I., Maxe, I., Heinegard, D., Oldberg, A., Franzen, A., et al. (1989). Specific binding of bone sialoprotein to Staphylococcus aureus isolated from patients with osteomyelitis. Eur. J. Biochem. 184, 331–336. doi: 10.1111/j.1432-1033.1989.tb15023.x
Sabat, A., Melles, D. C., Martirosian, G., Grundmann, H., van Belkum, A., and Hryniewicz, W. (2006). Distribution of the serine-aspartate repeat protein-encoding sdr genes among nasal-carriage and invasive Staphylococcus aureus strains. J. Clin. Microbiol. 44, 1135–1138. doi: 10.1128/JCM.44.3.1135-1138.2006
Savolainen, K., Paulin, L., Westerlund-Wikstrom, B., Foster, T. J., Korhonen, T. K., and Kuusela, P. (2001). Expression of pls, a gene closely associated with the mecA gene of methicillin-resistant Staphylococcus aureus, prevents bacterial adhesion in vitro. Infect. Immun. 69, 3013–3020. doi: 10.1128/IAI.69.5.3013-3020.2001
Schaffer, A. C., Solinga, R. M., Cocchiaro, J., Portoles, M., Kiser, K. B., Risley, A., et al. (2006). Immunization with Staphylococcus aureus clumping factor B, a major determinant in nasal carriage, reduces nasal colonization in a murine model. Infect. Immun. 74, 2145–2153. doi: 10.1128/IAI.74.4.2145-2153.2006
Schneewind, O., and Missiakas, D. (2019). Sortases, surface proteins, and their roles in Staphylococcus aureus disease and vaccine development. Microbiol. Spectr. 7:PSIB-0004-2018. doi: 10.1128/microbiolspec.PSIB-0004-2018
Schneewind, O., Model, P., and Fischetti, V. A. (1992). Sorting of protein a to the staphylococcal cell wall. Cells 70, 267–281. doi: 10.1016/0092-8674(92)90101-h
Shi, M., Chen, X., Sun, Y., Kim, H. K., Schneewind, O., and Missiakas, D. (2021). A protein a based Staphylococcus aureus vaccine with improved safety. Vaccine 39, 3907–3915. doi: 10.1016/j.vaccine.2021.05.072
Shirtliff, M. E., and Mader, J. T. (2002). Acute septic arthritis. Clin. Microbiol. Rev. 15, 527–544. doi: 10.1128/CMR.15.4.527-544.2002
Smith, J. T., Eckhardt, E. M., Hansel, N. B., Rahmani Eliato, T., Martin, I. W., and Andam, C. P. (2022). Genome evolution of invasive methicillin-resistant Staphylococcus aureus in the Americas. Microbiol. Spectr. 10:e0020122. doi: 10.1128/spectrum.00201-22
Song, W., Wang, L., Jin, M., Guo, X., Wang, X., Guan, J., et al. (2022a). Punicalagin, an inhibitor of Sortase a, is a promising therapeutic drug to combat methicillin-resistant Staphylococcus aureus infections. Antimicrob. Agents Chemother. 66:e0022422. doi: 10.1128/aac.00224-22
Song, W., Wang, L., Zhao, Y., Lanzi, G., Wang, X., Zhang, C., et al. (2022b). Hibifolin, a natural Sortase a inhibitor, attenuates the pathogenicity of Staphylococcus aureus and enhances the antibacterial activity of Cefotaxime. Microbiol Spectr 10:e0095022. doi: 10.1128/spectrum.00950-22
Soong, G., Chun, J., Parker, D., and Prince, A. (2012). Staphylococcus aureus activation of caspase 1/calpain signaling mediates invasion through human keratinocytes. J. Infect. Dis. 205, 1571–1579. doi: 10.1093/infdis/jis244
Spaan, A. N., Surewaard, B. G., Nijland, R., and van Strijp, J. A. (2013). Neutrophils versus Staphylococcus aureus: a biological tug of war. Annu. Rev. Microbiol. 67, 629–650. doi: 10.1146/annurev-micro-092412-155746
Su, X., Yu, H., Wang, X., Zhang, C., Wang, H., Kong, X., et al. (2022). Cyanidin chloride protects mice from methicillin-resistant Staphylococcus aureus-induced pneumonia by targeting Sortase a. Virulence 13, 1434–1445. doi: 10.1080/21505594.2022.2112831
Sun, Y., Emolo, C., Holtfreter, S., Wiles, S., Kreiswirth, B., Missiakas, D., et al. (2018). Staphylococcal protein a contributes to persistent colonization of mice with Staphylococcus aureus. J. Bacteriol. 200:e00735–17. doi: 10.1128/JB.00735-17
Tam, K., and Torres, V. J. (2019). Staphylococcus aureus secreted toxins and extracellular enzymes. Microbiol. Spectr. 7:GPP3-0039-2018. doi: 10.1128/microbiolspec.GPP3-0039-2018
Tantawy, E., Schwermann, N., Ostermeier, T., Garbe, A., Bahre, H., Vital, M., et al. (2022). Staphylococcus aureus multiplexes death-effector Deoxyribonucleosides to neutralize phagocytes. Front. Immunol. 13:847171. doi: 10.3389/fimmu.2022.847171
Thammavongsa, V., Kern, J. W., Missiakas, D. M., and Schneewind, O. (2009). Staphylococcus aureus synthesizes adenosine to escape host immune responses. J. Exp. Med. 206, 2417–2427. doi: 10.1084/jem.20090097
Thammavongsa, V., Kim, H. K., Missiakas, D., and Schneewind, O. (2015a). Staphylococcal manipulation of host immune responses. Nat. Rev. Microbiol. 13, 529–543. doi: 10.1038/nrmicro3521
Thammavongsa, V., Missiakas, D. M., and Schneewind, O. (2013). Staphylococcus aureus degrades neutrophil extracellular traps to promote immune cell death. Science 342, 863–866. doi: 10.1126/science.1242255
Thammavongsa, V., Rauch, S., Kim, H. K., Missiakas, D. M., and Schneewind, O. (2015b). Protein A-neutralizing monoclonal antibody protects neonatal mice against Staphylococcus aureus. Vaccine 33, 523–526. doi: 10.1016/j.vaccine.2014.11.051
Thomer, L., Schneewind, O., and Missiakas, D. (2016). Pathogenesis of Staphylococcus aureus bloodstream infections. Annu. Rev. Pathol. 11, 343–364. doi: 10.1146/annurev-pathol-012615-044351
Tian, L., Wu, X., Yu, H., Yang, F., Sun, J., Zhou, T., et al. (2022). Isovitexin protects mice from methicillin-resistant Staphylococcus aureus-induced pneumonia by targeting Sortase a. J. Microbiol. Biotechnol. 32, 1284–1291. doi: 10.4014/jmb.2206.06007
Tkaczyk, C., Hamilton, M. M., Sadowska, A., Shi, Y., Chang, C. S., Chowdhury, P., et al. (2016). Targeting alpha toxin and ClfA with a multimechanistic monoclonal-antibody-based approach for prophylaxis of serious Staphylococcus aureus disease. mBio 7:e00528–16. doi: 10.1128/mBio.00528-16
Tong, S. Y., Davis, J. S., Eichenberger, E., Holland, T. L., and Fowler, V. G. Jr. (2015). Staphylococcus aureus infections: epidemiology, pathophysiology, clinical manifestations, and management. Clin. Microbiol. Rev. 28, 603–661. doi: 10.1128/CMR.00134-14
Ton-That, H., Liu, G., Mazmanian, S. K., Faull, K. F., and Schneewind, O. (1999). Purification and characterization of sortase, the transpeptidase that cleaves surface proteins of Staphylococcus aureus at the LPXTG motif. Proc. Natl. Acad. Sci. U. S. A. 96, 12424–12429. doi: 10.1073/pnas.96.22.12424
Tsai, C. M., Caldera, J. R., Hajam, I. A., Chiang, A. W. T., Tsai, C. H., Li, H., et al. (2022). Non-protective immune imprint underlies failure of Staphylococcus aureus IsdB vaccine. Cell Host Microbe 30, 1163–1172 e1166. doi: 10.1016/j.chom.2022.06.006
Tuchscherr, L., Heitmann, V., Hussain, M., Viemann, D., Roth, J., von Eiff, C., et al. (2010). Staphylococcus aureus small-colony variants are adapted phenotypes for intracellular persistence. J. Infect. Dis. 202, 1031–1040. doi: 10.1086/656047
Tuchscherr, L., Medina, E., Hussain, M., Volker, W., Heitmann, V., Niemann, S., et al. (2011). Staphylococcus aureus phenotype switching: an effective bacterial strategy to escape host immune response and establish a chronic infection. EMBO Mol. Med. 3, 129–141. doi: 10.1002/emmm.201000115
Vahdani, Y., Faraji, N., Haghighat, S., Yazdi, M. H., and Mahdavi, M. (2021). Molecular cloning and immunogenicity evaluation of IsdE protein of methicillin resistant Staphylococcus aureus as vaccine candidates. Microb. Pathog. 157:104953. doi: 10.1016/j.micpath.2021.104953
Valle, J., Fang, X., and Lasa, I. (2020). Revisiting bap multidomain protein: more than sticking Bacteria together. Front. Microbiol. 11:613581. doi: 10.3389/fmicb.2020.613581
Varshney, A. K., Kuzmicheva, G. A., Lin, J., Sunley, K. M., Bowling, R. A. Jr., Kwan, T. Y., et al. (2018). A natural human monoclonal antibody targeting Staphylococcus protein a protects against Staphylococcus aureus bacteremia. PLoS One 13:e0190537. doi: 10.1371/journal.pone.0190537
Vaudaux, P., Francois, P., Bisognano, C., Kelley, W. L., Lew, D. P., Schrenzel, J., et al. (2002). Increased expression of clumping factor and fibronectin-binding proteins by hemB mutants of Staphylococcus aureus expressing small colony variant phenotypes. Infect. Immun. 70, 5428–5437. doi: 10.1128/IAI.70.10.5428-5437.2002
Vazquez, V., Liang, X., Horndahl, J. K., Ganesh, V. K., Smeds, E., Foster, T. J., et al. (2011). Fibrinogen is a ligand for the Staphylococcus aureus microbial surface components recognizing adhesive matrix molecules (MSCRAMM) bone sialoprotein-binding protein (Bbp). J. Biol. Chem. 286, 29797–29805. doi: 10.1074/jbc.M110.214981
Visai, L., Yanagisawa, N., Josefsson, E., Tarkowski, A., Pezzali, I., Rooijakkers, S. H. M., et al. (2009). Immune evasion by Staphylococcus aureus conferred by iron-regulated surface determinant protein IsdH. Microbiology (Reading) 155, 667–679. doi: 10.1099/mic.0.025684-0
von Eiff, C., Becker, K., Machka, K., Stammer, H., and Peters, G. (2001). Nasal carriage as a source of Staphylococcus aureus bacteremia study group. N Engl. J. Med. 344, 11–16. doi: 10.1056/NEJM200101043440102
von Kockritz-Blickwede, M., and Winstel, V. (2022). Molecular prerequisites for neutrophil extracellular trap formation and evasion mechanisms of Staphylococcus aureus. Front. Immunol. 13:836278. doi: 10.3389/fimmu.2022.836278
Wang, L., Bi, C., Cai, H., Liu, B., Zhong, X., Deng, X., et al. (2015). The therapeutic effect of chlorogenic acid against Staphylococcus aureus infection through sortase a inhibition. Front. Microbiol. 6:1031. doi: 10.3389/fmicb.2015.01031
Wang, L., Jing, S., Qu, H., Wang, K., Jin, Y., Ding, Y., et al. (2021a). Orientin mediates protection against MRSA-induced pneumonia by inhibiting Sortase a. Virulence 12, 2149–2161. doi: 10.1080/21505594.2021.1962138
Wang, L., Li, Q., Li, J., Jing, S., Jin, Y., Yang, L., et al. (2021b). Eriodictyol as a potential candidate inhibitor of Sortase a protects mice from methicillin-resistant Staphylococcus aureus-induced pneumonia. Front. Microbiol. 12:635710. doi: 10.3389/fmicb.2021.635710
Wang, J., Li, H., Pan, J., Dong, J., Zhou, X., Niu, X., et al. (2018). Oligopeptide targeting Sortase a as potential anti-infective therapy for Staphylococcus aureus. Front. Microbiol. 9:245. doi: 10.3389/fmicb.2018.00245
Wang, X., Luan, Y., Hou, J., Jiang, T., Zhao, Y., Song, W., et al. (2022a). The protection effect of rhodionin against methicillin-resistant Staphylococcus aureus-induced pneumonia through sortase a inhibition. World J. Microbiol. Biotechnol. 39:18. doi: 10.1007/s11274-022-03457-4
Wang, L., Wang, G., Qu, H., Wang, K., Jing, S., Guan, S., et al. (2021c). Taxifolin, an inhibitor of Sortase a, interferes with the adhesion of methicillin-resistant staphylococcal aureus. Front. Microbiol. 12:686864. doi: 10.3389/fmicb.2021.686864
Wang, X., Wei, L., Wang, L., Chen, X., Kong, X., Luan, Y., et al. (2022b). Scutellarin potentiates vancomycin against lethal pneumonia caused by methicillin-resistant Staphylococcus aureus through dual inhibition of sortase a and caseinolytic peptidase P. Biochem. Pharmacol. 199:114982. doi: 10.1016/j.bcp.2022.114982
Weidenmaier, C., Goerke, C., and Wolz, C. (2012). Staphylococcus aureus determinants for nasal colonization. Trends Microbiol. 20, 243–250. doi: 10.1016/j.tim.2012.03.004
Weidenmaier, C., Kokai-Kun, J. F., Kulauzovic, E., Kohler, T., Thumm, G., Stoll, H., et al. (2008). Differential roles of sortase-anchored surface proteins and wall teichoic acid in Staphylococcus aureus nasal colonization. Int. J. Med. Microbiol. 298, 505–513. doi: 10.1016/j.ijmm.2007.11.006
Werfel, T. (2009). The role of leukocytes, keratinocytes, and allergen-specific IgE in the development of atopic dermatitis. J. Invest. Dermatol. 129, 1878–1891. doi: 10.1038/jid.2009.71
Wertheim, H. F., Melles, D. C., Vos, M. C., van Leeuwen, W., van Belkum, A., Verbrugh, H. A., et al. (2005). The role of nasal carriage in Staphylococcus aureus infections. Lancet Infect. Dis. 5, 751–762. doi: 10.1016/S1473-3099(05)70295-4
Wertheim, H. F., Walsh, E., Choudhurry, R., Melles, D. C., Boelens, H. A., Miajlovic, H., et al. (2008). Key role for clumping factor B in Staphylococcus aureus nasal colonization of humans. PLoS Med. 5:e17. doi: 10.1371/journal.pmed.0050017
Widaa, A., Claro, T., Foster, T. J., O'Brien, F. J., and Kerrigan, S. W. (2012). Staphylococcus aureus protein a plays a critical role in mediating bone destruction and bone loss in osteomyelitis. PLoS One 7:e40586. doi: 10.1371/journal.pone.0040586
Winstel, V., Missiakas, D., and Schneewind, O. (2018). Staphylococcus aureus targets the purine salvage pathway to kill phagocytes. Proc. Natl. Acad. Sci. U. S. A. 115, 6846–6851. doi: 10.1073/pnas.1805622115
Winstel, V., Schneewind, O., and Missiakas, D. (2019). Staphylococcus aureus exploits the host apoptotic pathway to persist during infection. mBio 10, e02270–e02219. doi: 10.1128/mBio.02270-19
Xu, Y., Rivas, J. M., Brown, E. L., Liang, X., and Hook, M. (2004). Virulence potential of the staphylococcal adhesin CNA in experimental arthritis is determined by its affinity for collagen. J. Infect. Dis. 189, 2323–2333. doi: 10.1086/420851
Yang, Y., Qian, M., Yi, S., Liu, S., Li, B., Yu, R., et al. (2016). Monoclonal antibody targeting Staphylococcus aureus surface protein a (SasA) protect against Staphylococcus aureus Sepsis and peritonitis in mice. PLoS One 11:e0149460. doi: 10.1371/journal.pone.0149460
Yipp, B. G., Petri, B., Salina, D., Jenne, C. N., Scott, B. N., Zbytnuik, L. D., et al. (2012). Infection-induced NETosis is a dynamic process involving neutrophil multitasking in vivo. Nat. Med. 18, 1386–1393. doi: 10.1038/nm.2847
Zeng, H., Yang, F., Feng, Q., Zhang, J., Gu, J., Jing, H., et al. (2020). Rapid and broad immune efficacy of a recombinant five-antigen vaccine against Staphylococcus Aureus infection in animal models. Vaccines (Basel) 8:134. doi: 10.3390/vaccines8010134
Zhang, B. Z., Cai, J., Yu, B., Xiong, L., Lin, Q., Yang, X. Y., et al. (2017b). Immunotherapy targeting adenosine synthase a decreases severity of Staphylococcus aureus infection in mouse Model. J. Infect. Dis. 216, 245–253. doi: 10.1093/infdis/jix290
Zhang, J., Liu, H., Zhu, K., Gong, S., Dramsi, S., Wang, Y. T., et al. (2014). Antiinfective therapy with a small molecule inhibitor of Staphylococcus aureus sortase. Proc. Natl. Acad. Sci. U. S. A. 111, 13517–13522. doi: 10.1073/pnas.1408601111
Zhang, B., Teng, Z., Li, X., Lu, G., Deng, X., Niu, X., et al. (2017a). Chalcone attenuates Staphylococcus aureus virulence by targeting Sortase a and alpha-Hemolysin. Front. Microbiol. 8:1715. doi: 10.3389/fmicb.2017.01715
Zhou, T. T., Yue, Y., Zheng, F., Liang, X. D., Cao, Q. X., Wang, Y. W., et al. (2021). Monoclonal antibody against l-lectin module of SraP blocks adhesion and protects mice against Staphylococcus aureus challenge. J. Microbiol. Immunol. Infect. 54, 420–428. doi: 10.1016/j.jmii.2019.08.019
Keywords: Staphylococcus aureus, surface proteins, sortase A, abscess, vaccine
Citation: Schwermann N and Winstel V (2023) Functional diversity of staphylococcal surface proteins at the host-microbe interface. Front. Microbiol. 14:1196957. doi: 10.3389/fmicb.2023.1196957
Edited by:
Axel Cloeckaert, Institut National de recherche pour l’agriculture, l’alimentation et l’environnement (INRAE), FranceReviewed by:
Timothy J Foster, Trinity College Dublin, IrelandPietro Speziale, University of Pavia, Italy
Silke Niemann, University Hospital Münster, Germany
Copyright © 2023 Schwermann and Winstel. This is an open-access article distributed under the terms of the Creative Commons Attribution License (CC BY). The use, distribution or reproduction in other forums is permitted, provided the original author(s) and the copyright owner(s) are credited and that the original publication in this journal is cited, in accordance with accepted academic practice. No use, distribution or reproduction is permitted which does not comply with these terms.
*Correspondence: Volker Winstel, d2luc3RlbC52b2xrZXJAbWgtaGFubm92ZXIuZGU=