- 1Department of Biology, Faculty of Science, University of Zagreb, Zagreb, Croatia
- 2Department of Biology, Josip Juraj Strossmayer University of Osijek, Osijek, Croatia
- 3Swiss Federal Research Institute WSL, Birmensdorf, Switzerland
- 4Faculty of Forestry and Wood Technology, University of Zagreb, Zagreb, Croatia
Introduction: Forest ecosystems are highly threatened by the simultaneous effects of climate change and invasive pathogens. Chestnut blight, caused by the invasive phytopathogenic fungus Cryphonectria parasitica, has caused severe damage to European chestnut groves and catastrophic dieback of American chestnut in North America. Within Europe, the impacts of the fungus are widely mitigated through biological control that utilizes the RNA mycovirus: Cryphonectria hypovirus 1 (CHV1). Viral infections, similarly to abiotic factors, can cause oxidative stress in their hosts leading to physiological attrition through stimulating ROS (reactive oxygen species) and NOx production.
Methods: To fully understand the interactions leading to the biocontrol of chestnut blight, it is vital to determine oxidative stress damage arising during CHV1 infection, especially considering that other abiotic factors, like long-term cultivation of model fungal strains, can also impact oxidative stress. Our study compared CHV1-infected C. parasitica isolates from two Croatian wild populations with CHV1-infected model strains (EP713, Euro7 and CR23) that have experienced long-term laboratory cultivation.
Results and Discussion: We determined the level of oxidative stress in the samples by measuring stress enzymes’ activity and oxidative stress biomarkers. Furthermore, for the wild populations, we studied the activity of fungal laccases, expression of the laccase gene lac1, and a possible effect of CHV1 intra-host diversity on the observed biochemical responses. Relative to the wild isolates, the long-term model strains had lower enzymatic activities of superoxide dismutase (SOD) and glutathione S-transferase (GST), and higher content of malondialdehyde (MDA) and total non-protein thiols. This indicated generally higher oxidative stress, likely arising from their decades-long history of subculturing and freeze–thaw cycles. When comparing the two wild populations, differences between them in stress resilience and levels of oxidative stress were also observed, as evident from the different MDA content. The intra-host genetic diversity of the CHV1 had no discernible effect on the stress levels of the virus-infected fungal cultures. Our research indicated that an important determinant modulating both lac1 expression and laccase enzyme activity is intrinsic to the fungus itself, possibly related to the vc type of the fungus, i.e., vegetative incompatibility genotype.
1. Introduction
The most important stress factors affecting contemporary forest ecosystems are not only climate change related, but also include pressure from invasive pathogenic species (Trumbore et al., 2015). Invasive phytopathogenic fungi have caused significant damage to several important tree species. The most well-known examples of such pathogens include Ophiostoma novo-ulmi (Brasier and Buck, 2001; Solla et al., 2008; Katanić, 2020), Hymenoscyphus fraxineus (Pautasso et al., 2013; Burokiene et al., 2015) and Cryphonectria parasitica (Anagnostakis, 1987; Rigling and Prospero, 2018). Each fungal pathogen has been responsible for the serious dieback of trees in Europe and North America, jeopardizing forest ecosystems. This is particularly notable for chestnut blight, caused by C. parasitica, which led to the functional extinction of the North American chestnut tree and severe damage to European chestnut groves.
Within Europe, chestnut blight is now mediated by natural biological control that utilises the RNA mycovirus Cryphonectria hypovirus 1 (CHV1) (MacDonald and Fulbright, 1991; Heiniger and Rigling, 1994). This biocontrol is highly successful, as it allows the trees to survive the fungal attack, has no secondary environmental impacts, and is self-sustaining due to chronic infections of the fungus and natural spread of the virus.
CHV1 infection undoubtedly exerts biotic stress on the host fungus which has been confirmed in model long-term laboratory strains (Allen et al., 2003; Kim et al., 2012; Nuskern et al., 2017), but understanding of the virus-induced stress in naturally occurring (wild) hypovirulent strains is scarce. Furthermore, most studies were performed using one or a limited number of laboratory model strains. Long-term laboratory culturing conditions have been shown in many systems to introduce artefacts that are not present in the natural environment, e.g., in vitro cell culture (Olsavsky et al., 2007; Kim et al., 2012; Li et al., 2014).
Reactive oxygen species (ROS) are generated either in low concentrations to engage in many important cell processes and signaling pathways, or pathologically where high ROS concentrations can cause significant cellular damage (da Silva Caetano et al., 2019; Almeida et al., 2020). The increase in ROS concentrations inside the cell and/or the reduction of the cell’s antioxidant capacity leads to the disruption of the redox control, resulting in oxidative stress (da Silva Caetano et al., 2019). Thus, controlling ROS levels is essential to maintain cellular homeostasis. This is ensured by the cellular antioxidant system which is comprised of enzymes such as superoxide dismutase (SOD), catalase (CAT), glutathione peroxidase (GPx), and non-enzymatic antioxidants including vitamin C, vitamin E, carotenoids, glutathione (GSH) and flavonoids (da Silva Caetano et al., 2019). Like plants and animals, fungi produce several antioxidative enzymes like CAT, SOD, GPx and glutathione reductase to counteract oxidative stress caused by ROS (Jamieson, 1998; Azevedo et al., 2007; Breitenbach et al., 2015), but alteration of their activity during virus infection is still understudied (Nuskern et al., 2017). As obligate intracellular parasites, viruses can interfere with the cell’s ROS homeostasis and modulate the host’s antioxidant defences, contributing to overall pathogenesis (Reshi et al., 2014). Viral infections usually stimulate ROS production in cells, which leads to increased levels of oxidative stress biomarkers and a change in the activities of antioxidant enzymes (Reshi et al., 2014; Camejo et al., 2016; da Silva Caetano et al., 2019; Almeida et al., 2020). Oxygen radicals and NOx, generated in excess during oxidative stress have been implicated in the generation of point mutations in a viral RNA genome and contribute to the high diversity of viral intra-host populations (Akaike et al., 2000; Akaike, 2001). However, research on viral intra-host genetic diversity is relatively scarce as often only human and plant-crop pathogens are explored (Lauring and Andino, 2010; Simmons et al., 2011; Dunham et al., 2014; Lauring, 2020; Feder et al., 2021; Leigh et al., 2021).
The pathosystem C. parasitica – CHV1 has long been used as a model for studying mycovirus biology, hypovirulence, virus-host interactions, fungal physiology, and biological disease control e.g. (Chen and Nuss, 1999; Peever et al., 2000; Kim et al., 2002; Hillman and Suzuki, 2004; Dawe and Nuss, 2013; Muñoz-Adalia et al., 2016). The early work on CHV1 cDNA cloning, sequence analysis and genomic organization was conducted only on the virus strain CHV1-EP713 (Shapira et al., 1991). Now considered “prototypic strain” it has been used in many studies of hypovirus effects on the host e.g. (Choi et al., 1992; Allen et al., 2003; Dawe et al., 2003; Wang et al., 2016). In addition to CHV1-EP713, CHV1-Euro7 has been studied in detail and established as a comparative prototypic strain (Chen and Nuss, 1999; Gobbin et al., 2003; Allen and Nuss, 2004).
Notably, these two CHV1 prototypic strains were isolated as early as the 1970s (Allemann et al., 1999; Chen and Nuss, 1999), and have been subsequently subculturing, stored freeze-dried or as glycerol stocks, and shipped to be shared between laboratories. These processes are unlikely to be benign to both, the virus and fungal host. In particular, the freeze/thaw cycles are likely a source of stress for the fungal host. In other species of fungi, long-term subculturing has been shown to lead to morphological heterogeneity, culture degeneration, physiological changes and reduced viability of the frozen stock (Ryan et al., 2002; Li et al., 2014; Jukic et al., 2017; Cabrera et al., 2020). Consequently, some conclusions about fungal physiology derived from the experiments on long-term C. parasitica model strains and prototypic hypovirus strains may not accurately reflect natural populations. For example, fungal enzymes such as laccases, considered a hallmark of pathogenic potential of C. parasitica, are important in many other biological processes including development of fungal structures, pigment synthesis, lignin decomposition and fungal/plant interactions (Baldrian, 2006) but previous studies have shown inconsistent results regarding changes in the laccase activity across different strains and experimental settings (Rigling et al., 1989; Rigling and Van Alfen, 1991; Allen et al., 2003; Gong et al., 2017; Nuskern et al., 2021). Most recently the intra-host populations of CHV1 from naturally infected Swiss and Croatian C. parasitica isolates were characterised (Leigh et al., 2021). These isolates offer an interesting opportunity to compare physiological differences between ‘wild’ cultures and ‘laboratory’ model strains of the C. parasitica – CHV1 system, in order to demonstrate the impacts of long-term manipulation.
The aims of this study were to (i) compare the oxidative stress parameters between naturally CHV1-infected C. parasitica isolates and CHV1-infected long-term laboratory model strains; (ii) determine the level of oxidative stress in two wild C. parasitica populations naturally infected with CHV1, by measuring stress enzymes activity and oxidative stress biomarkers; (iii) compare the enzyme activity of fungal laccases and the expression of the laccase gene lac1 in naturally infected populations; (iv) determine the possible effect of CHV1 intra-host diversity on the observed biochemical response of naturally infected fungus.
2. Materials and methods
2.1. Wild populations
2.1.1. Sample collection
To obtain samples naturally infected with CHV1, two wild Cryphonectria parasitica populations were sampled in July 2019 from two chestnut growing areas in Croatia, Ozalj (45.59°N, 15.46°E, elevation approx. 220–320 m) and Kast (45.70°N, 15.36°E, elevation approx. 540–640 m). The fungal populations’ diversity and CHV1 prevalence were previously determined and published (Ježić et al., 2021; Leigh et al., 2021). Fifty chestnut trees infected with C. parasitica, which causes bark wounds called cankers, were randomly chosen in each population and only one canker per tree was sampled. A bark plug was collected from each canker with a 2 mm bone marrow biopsy needle, which was sterilized after each sampling by dipping in 96% ethanol and flaming [(see Leigh et al., 2021) for full sampling methods].
2.1.2. Laboratory culturing
Bark plugs were surface sterilized by dipping them in 70% ethanol for several seconds, then drying on sterile filter paper, after which they were placed in φ 90 mm Petri plates containing 20 mL of potato dextrose agar (PDA) (Difco, BD, Franklin Lakes, NJ), followed by incubation in a growth chamber at 24°C and 70% humidity in the dark. After several days, most of the freshly grown mycelium expanding from bark samples was transferred to four new Petri plates with fresh PDA overlaid with cellophane. This was done to preserve most of the CHV1 population variability within each sample. After 10 days of growth, mycelia from all four Petri plates were stripped from the cellophane and the samples were combined into a single composite sample, transferred to previously weighted 2 mL tubes and lyophilized. All composite samples were used for RNA and DNA extraction (hypovirulence assessment and vic genotyping).
2.1.3. CHV1 detection
The virus was detected by RT-PCR using dsRNA as template. For dsRNA extraction, the Double-RNA Viral dsRNA extraction mini kit (iNtRON Biotechnology Inc., Seongnam, South Korea) was used. First-strand cDNA synthesis was conducted using the GoScript™ Reverse Transcription System (Promega, Madison, WI, USA) or High-Capacity cDNA Reverse Transcription Kit with RNase Inhibitor (Applied Biosystems by Thermo Fisher Scientific Waltham, MA, USA), with 10 μL of isolated dsRNA. The PCR reaction was performed as described by Allemann et al. (1999) and the obtained amplicons separated on 1% (wt/vol) agarose gel in 0.5× TBE buffer at 5 V/cm and visualized with GelStar DNA stain (Lonza, Basel, Switzerland).
2.1.4. Vegetative compatibility genotyping of Cryphonectria parasitica
Vegetative compatibility (vc) genotyping was done first by pairing all samples with tester strains of the most abundant vc types in Croatia – EU-1 and EU-2, as described in (Krstin et al., 2008). For all isolates which could not be unequivocally assigned to either of the aforementioned EU types, molecular vic genotyping was performed. DNA extractions were done with OmniPrep™ for Fungi extraction kit (G-Biosciences, Saint Louis, MO, USA), PCR reaction were performed as described in Mlinarec et al. (2018a) and the obtained amplicons separated on 1.5% (wt/vol) agarose gel in 0.5× TBE buffer at 5 V/cm and visualized with GelStar DNA stain (Lonza, Basel, Switzerland).
From each population, 10 CHV1-infected isolates were randomly chosen for subsequent genetic and biochemical analyses. Intra-host diversity of CHV1 was successfully determined by PacBio long-read HiFi sequencing for 10 isolates from the population Ozalj and nine from Kast, as published in Leigh et al. (2021). Those previously characterised isolates were further used in this study for biochemical analyses.
2.2. Model strains
Besides the C. parasitica samples from the wild populations, we used three well-characterized, long-term subculturing, CHV1-infected C. parasitica model strains (hereafter referred to as “model strains”) regenerated from stock cultures kept at −80°C in 22% glycerol. Those were the model strains harboring the prototypic CHV1 strains EP713 and Euro7, as well as isolate CR23 – a Croatian CHV1-infected field isolate of C. parasitica collected in the early 2000s, whose CHV1 genome has been fully sequenced (Krstin et al., 2008; Mlinarec et al., 2018b). Growth conditions followed those used for the field isolates from the wild populations (described in 2.1.2): small agar blocks of model strains tissue stocks stored at −80°C were placed in φ 60 mm Petri plates containing ca. 10 mL PDA, followed by incubation in a growth chamber at 24°C and 70% humidity in the dark. After several days of growth, model strains colonies were subcultured to four new φ 90 mm Petri plates containing 20 mL PDA overlaid with cellophane. After 10 days of growth, mycelia were stripped from the cellophane, combined into a single composite sample, transferred to previously weighted 2 mL tubes and lyophilized for further analysis.
2.3. Oxidative stress parameters of Cryphonectria parasitica
2.3.1. Preparation of protein extracts
Lyophilized samples were ground to a fine powder with φ 5 mm steel ball in TissueLyser II (Qiagen, Venlo, Netherlands) for 2 min at 30 Hz. From each ground sample, three replicates were prepared and weighed separately. To prepare the protein extracts for measurement of the level of lipid peroxidation and the activities of GST, SOD and LAC, 1750 μL of cold extraction buffer (100 mM potassium phosphate buffer, with 0.1 mM EDTA, pH 7.0) was added to the ground lyophilized tissue, mixed with a micro pestle and vortexed briefly. The homogenate was centrifuged at 20000× g for 20 min at 4°C. Supernatant was collected and protein concentration was determined according to Bradford (1976) using bovine serum albumin (Protein Standard, 2 mg/vial BSA, Sigma-Aldrich® Burlington, MA, United States) as a standard. The samples were aliquoted and stored at −20°C. All spectrophotometric measurements were done with a Multiscan Sky microplate spectrophotometer (Thermo Scientific™ ver 5.0) in 96-well plates (Nunc MaxiSorp™ flat-bottom, Thermo Scientific™).
2.3.2. Stress enzymes and laccase activity measurements
The activity of glutathione S-transferase (GST) was assayed spectrophotometrically as described in Bocchetti and Regoli (2006). The increase in absorbance at 340 nm was measured 10 times every 10 s, using 1-chloro-2,4-dinitrobenzene (CDNB) and reduced glutathione (GSH) as substrates. The reaction mixture was 100 mM potassium phosphate buffer (pH 6.4) with 1 mM CDNB. The reaction was done in plates by pipetting 277.5 μL of the reaction mixture, 7.5 μL of 100 mM GSH (final concentration was 2.5 mM) and 15 μL of the sample. GST activity was expressed in nanomoles of generated GS-DNB per minute per milligram of dry weight (DW) (ε = 9.6 mM−1 cm−1).
The activity of superoxide dismutase (SOD) was determined based on the inhibition of the photochemical reduction of nitroblue tetrazolium (NBT, Sigma-Aldrich) at 560 nm as described by Beauchamp and Fridovich (1971). The reaction mixture was 50 mM potassium phosphate buffer (pH 7.8) with 75 μM NBT and 0.1 mM EDTA. The reaction was done by pipetting 247.5 μL of the reaction mixture, 22.5 μL of 10.8 mM xanthine (final concentration was 0.81 mM), 10 μL of extraction buffer, 15 μL of 0.05 U/mL XOD (final concentration was 2.5 mU/mL) and 5 μL of the sample. The uninhibited reaction (max) contained 15 μL of extraction buffer instead of the sample. After mixing all reactants the plate was shaken for 5 min at low speed, and the absorbance was measured after 10 min. The inhibition of the reaction by SOD (%) was calculated as:
SOD activity was calculated from the calibration curve, obtained from % inhibition data plotted against log-SOD concentration, using bovine recombinant SOD (expressed in Escherichia coli, Sigma-Aldrich) as standard. One unit of SOD activity was defined as the amount of enzyme which caused 50% inhibition of NBT reduction. Results were expressed as enzyme units per milligram of dry weight (DW).
Laccase activity (LAC) was measured as an increase in absorbance at 468 nm due to 2,4-dimethoxyphenol DMOP oxidation to 3,3′,5,5′-tetramethoxydiphenylquinone (DMOPox, ε = 14.8 mM−1 cm−1) combining the methods described by Rigling et al. (1989) and Palmieri et al. (1997). The final reaction mixture consisted of 240 μL of citrate–phosphate buffer (0.1 M Na2HPO4 and 0.05 M citric acid, pH 3.4), 15 μL of 50 mM DMOP (prepared in the same citrate–phosphate buffer; final concentration 2.5 mM) and 45 μL of protein extract. The activity was expressed in nmol of the generated DMOPox per minute per mg of dry weight (DW).
2.3.3. Measurement of oxidative stress biomarkers
The content of total non-protein thiols (THIOLS) was determined using Ellman’s method (Ellman, 1959). Lyophilized ground tissue was homogenized with 1 mL 6.67% sulfosalicylic acid, incubated for 30 min on ice and centrifuged for 10 min at 13000× g at 4°C to precipitate proteins. Afterwards, supernatants (600 μL) were mixed with 150 μL of 5 mM 5,5′-dithiobis (2-nitrobenzoic acid), (DTNB; final concentration 1 mM), incubated for 15 min at room temperature, followed by measurement of absorbance at 412 nm. The non-protein thiol content was determined by the use of an extinction coefficient ε = 14.53 mM−1 cm−1 and expressed in nmol of the generated DTNB per mg of dry weight (DW).
Lipid peroxidation was determined by estimating the malondialdehyde (MDA) content using the thiobarbituric acid (TBA) method described by Heath and Packer (1968). First, to precipitate proteins, 500 μL of protein extracts were mixed with 100% (w/v) trichloroacetic acid (TCA) to a final concentration of 10% TCA, incubated for 30 min on ice and then centrifuged for 10 min at 13000× g at 4°C. 500 μL of supernatant was mixed with the same volume of MDA reagent, consisting of 0.5% (w/v) TBA in 10% (w/v) TCA resulting in a final concentration of TBA of 0.25% (w/v). The mixture was heated at 95°C for 30 min, after which the reaction was stopped in an ice bath. The cooled reaction mixture was centrifuged at 10000× g for 10 min at 4°C to remove any leftover precipitate. MDA content in nmol per mg of dry weight (DW) was calculated from the absorbance at 532 nm, corrected for unspecific turbidity by subtracting the absorbance at 600 nm, by using an extinction coefficient ε = 155 mM−1 cm−1.
2.4. Expression of the laccase gene lac1
2.4.1. RNA isolation and cDNA synthesis
Total RNA was extracted from lyophilized mycelia using the NucleoZOL reagent (Macherey-Nagel, Düren, Germany), following the manufacturer’s instructions. RNA concentration and purity were determined using a NanoPhotometer NP80 (Implant, München, Germany), while RNA integrity was verified by agarose gel electrophoresis and SYBR safe staining (Invitrogen, Carlsbad, CA, USA). The average RNA yield was around 1,500 ng/μL, while A260/280 ratio was approximately 1.9–2.0. Before cDNA synthesis, residual DNA in the obtained RNA solution was removed by RQ1 RNase-Free DNase (Promega, Madison, WI, USA). First-strand cDNA was synthesized from 3 μg of total RNA by the GoTaq® 2-Step RT-qPCR System (Promega, Madison, WI, USA) according to the manufacturer’s recommendation using a random hexamer mixture provided in the kit.
2.4.2. qPCR of lac1
Quantitative PCR (qPCR), using dye-based detection, was performed to analyse transcript levels of the lac1 gene. Gene encoding beta-tubulin was used as a reference gene for normalization. The oligonucleotide primers for laccase (lac1-FW: ATCAATCCGGCTAACACGAC; REV: TGTCATAGAATGGCCCACAA) and beta-tubulin (tubulin-FW: AGTGGATTCCCAACAACGTC; REV: CTTGAAGAGCTCCTGGATGG) were designed based on sequences in the GeneBank database (accession no. M73257 for lac1, accession no. AH014582 for tubulin) using Primer3 software. qPCR analysis was performed on StepOnePlus™ Real-Time PCR System with StepOnePlus™ Software v2.3 (Applied Biosystems, Waltham, MA, USA) and by using GoTaq® 2-Step RT-qPCR System (Promega, Madison, WI, USA), according to the manufacturer’s recommendation. For qPCR analysis, cDNA was diluted two-fold and measured in three technical replicates. Standard curves based on five points, corresponding to a twofold dilution series from selected cDNA served as standard and were used to compute the PCR efficiency of each primer pair. The average PCR efficiencies were 100% for the tubulin gene and 97.5% for the lac1 gene, while R2 values calculated for standard curves were 0.98 and 0.99 for the tubulin and lac1 genes, respectively. The specificity of the qPCR reaction was confirmed by melting curve analysis, and the relative expression of the lac1 gene was quantified by the comparative 2−ΔΔCt method (Pfaffl, 2001).
2.5. CHV1 intra-host population variability
For the same wild population samples used in this study, the genetic diversity of intra-host CHV1 populations was determined previously by Leigh et al. (2021). Briefly, Nei’s H was calculated to characterize CHV1 intra-host haplotype diversity (Nei and Tajima, 1980) and intra-host mutational diversity was measured using nucleotide diversity, i.e., π, estimated in SNPGenie (Nelson et al., 2015). In this research, we used those previously obtained viral variant diversity data to compare them with oxidative stress parameters of the same host isolates.
2.6. Data analysis of oxidative stress parameters
Statistical analyses were done with Statistica 13 (StatSoft Inc., Tulsa, OK, USA). Data of biochemical analysis are presented as mean ± standard error. Each data point on an individual level is the average of three replicates (n = 3). The normality of the data was tested by Kolmogorov–Smirnov and Lilliefors test of normality. The estimation of relationships between analyzed variables was done with Pearson’s correlation coefficient, at p < 0.05. To determine the differences between the population of wild isolates and model strains for measured physiological stress parameters t-test was used. Factorial ANOVA was used to determine which factors affect stress-related parameters between isolates from wild populations, followed by post hoc Tukey honest significant difference (HSD) test. For this, we analyzed the oxidative stress parameters by grouping the samples according to (1) their population of origin (Ozalj or Kast) or (2) vc type: EU-1 or EU-2 which are the two most abundant vc types in Croatia, or “EU-other” (comprised of all other samples represented by a single isolate of a particular vc type: e.g. EU3, EU5, EU13, EU15).
3. Results
3.1. Model strains vs. wild isolates
First, we analyzed the difference in the oxidative stress parameters between CHV1-infected long-term laboratory model strains – Euro7, EP713, CR23 (MODEL) and population isolates naturally infected with CHV1 – Kast and Ozalj (WILD). Those two groups differed significantly in all measured parameters, i.e., the activity of GST and SOD, content of non-protein thiols, and level of lipid peroxidation (Figure 1.). The enzymatic activities were significantly higher in wild populations [mean(GST) = 13.77 ± 0.79 nmol (GS-DNB) min−1 (mg DW)−1; mean(SOD) = 0.13 ± 0.01 U (mg DW)−1] than in model strains [mean(GST) = 5.80 ± 1.38 nmol (GS-DNB) min−1 (mg DW)−1; mean(SOD) = 0.07 ± 0.02 U (mg DW)−1]. On the other hand, the content of non-protein thiols and the level of lipid peroxidation were significantly higher in model strains [mean(THIOLS) = 5.41 ± 0.25 nmol (DTNB) (mg DW)−1; mean(MDA) = 0.11 ± 0.01 nmol (mg DW)−1] than in wild populations [mean(THIOLS) = 1.75 ± 0.14 nmol (DTNB) (mg DW)−1; mean(MDA) = 0.06 ± 0.00 nmol (mg DW)−1]. For model strains, there was a significant positive correlation between MDA content and SOD activity (0.695), the content of total non-protein thiols and SOD activity (0.622) and the content of MDA and total non-protein thiols (0.576), all at p < 0.05.
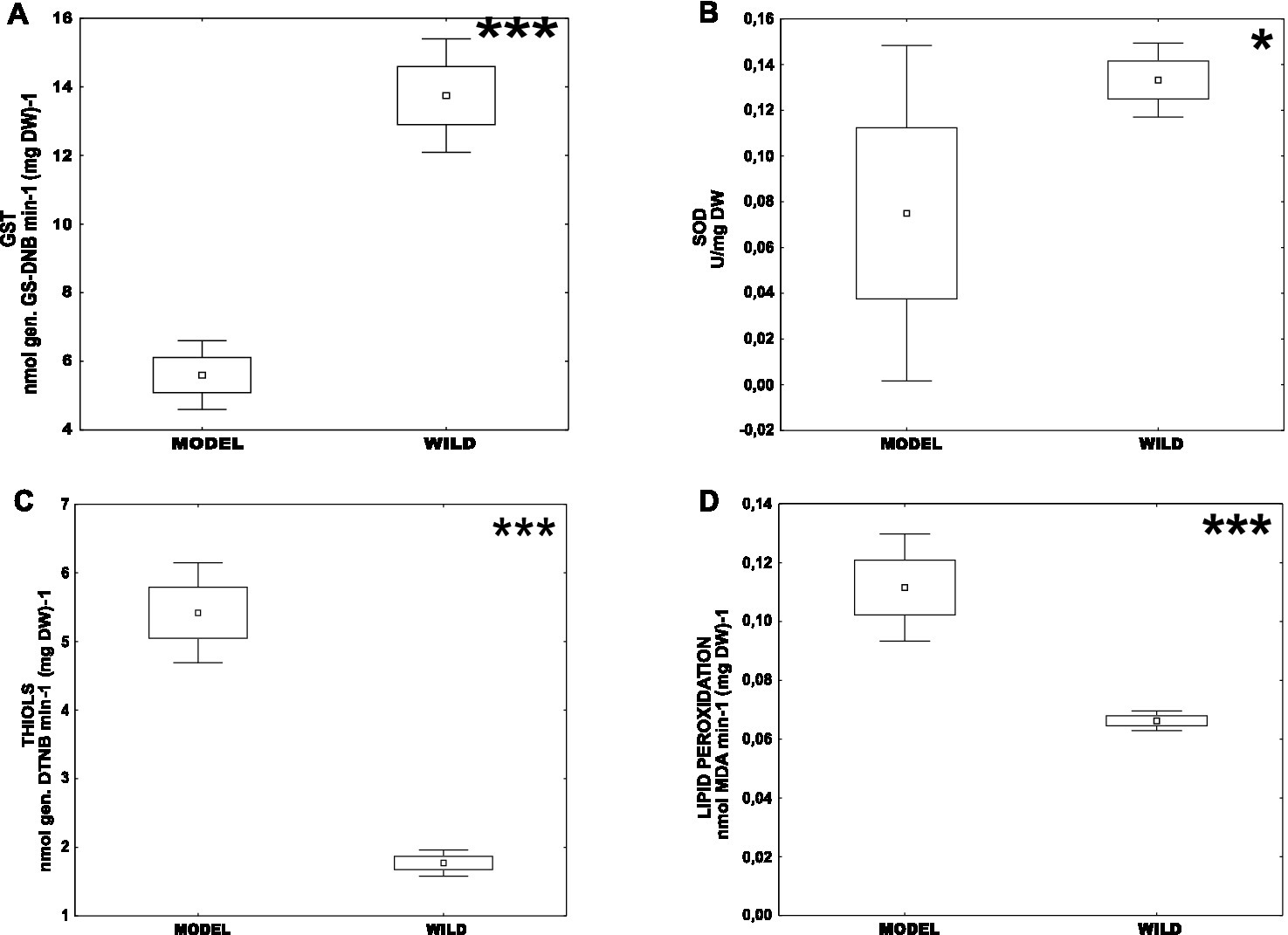
Figure 1. Difference of oxidative stress parameters between CHV1-infected long-term laboratory model strains (MODEL) – Euro7, EP713, CR23 and wild populations naturally infected with CHV1 – Kast and Ozalj (WILD) for: (A) activity of GST, (B) activity of SOD, (C) content of non-proteins thiols and (D) level of lipid peroxidation. Data are represented as mean, mean ± SE (box), mean ± 1.96 SE (Whiskers). Statistically significant differences are denoted by asterisks as follows: *p < 0.05; **p < 0.001; ***p < 0.0001.
3.2. Wild Cryphonectria parasitica populations
To test the effect of the CHV1 intra-host diversity on the host physiology we compared oxidative stress parameters of fungal isolates with parameters of viral genetic diversity (Nei’s H and π) for the CHV1 populations from the same isolates. We found no statistically significant correlation between Nei’s H and any of the tested parameters, while π slightly negatively correlated with SOD (−0.3619) and positively with lipid peroxidation (0.3823) at p < 0.05 (Supplementary Figures 1, 2). However, individual samples like KS 06_3, which had the highest genetic diversity of CHV1 also had the highest values for the activity of GST, the content of thiols and the level of lipid peroxidation. Otherwise, isolate KS 11_2 which had the lowest CHV1 diversity also had among the lowest values for analyzed oxidative stress parameters.
While the intra-population differences in oxidative stress parameters between samples were noticeable, ANOVA followed by post hoc Tukey HSD test indicated statistically significant differences between some samples in all parameters except for GST (Figure 2A), which might be explained by the large variability of the measured parameter in both populations.
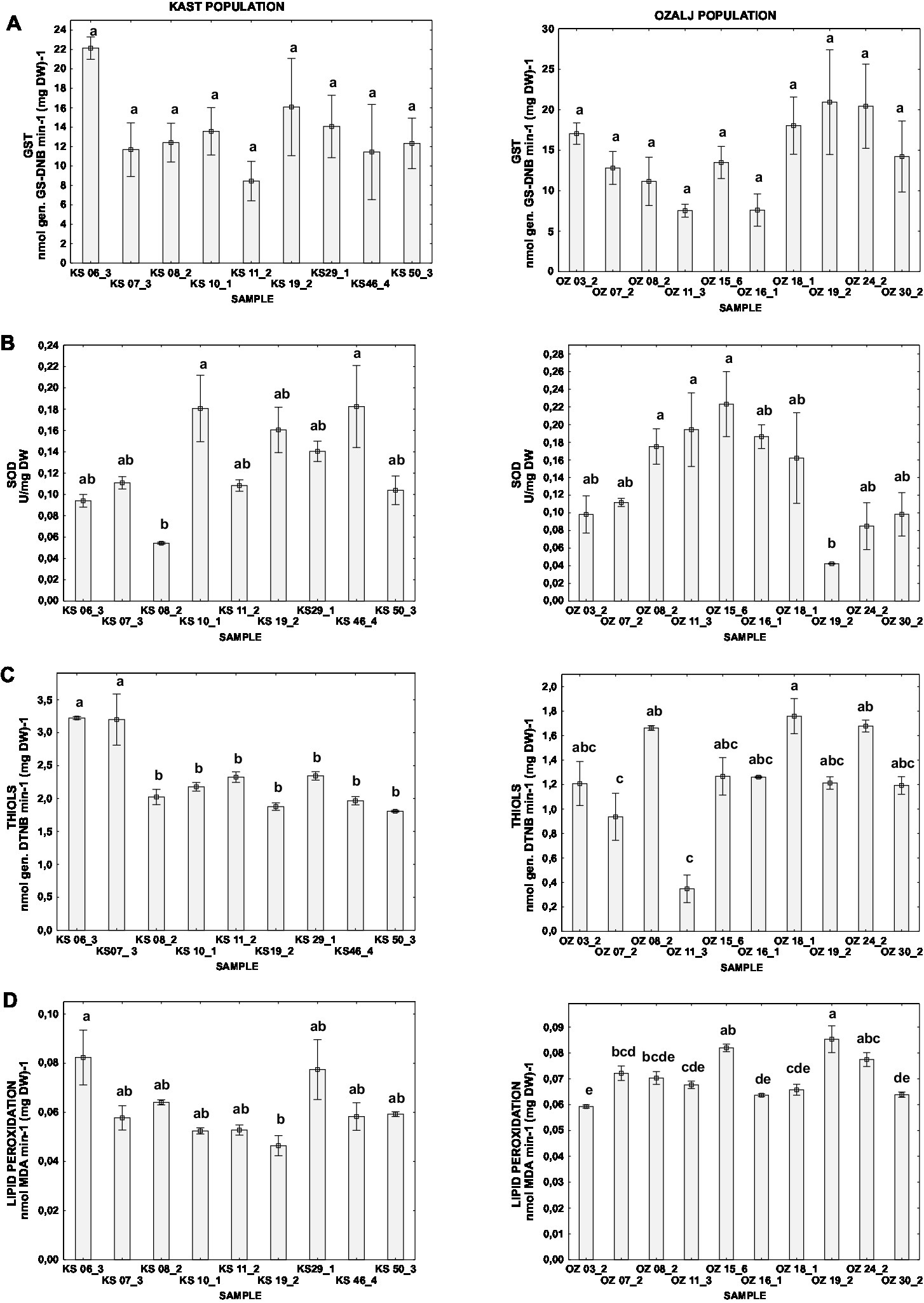
Figure 2. Difference of oxidative stress parameters for individual isolates of Kast (KS) and Ozalj (OZ) populations naturally infected with CHV1: (A) activity of GST, (B) activity of SOD, (C) content of non-proteins thiols, and (D) level of lipid peroxidation. Data are presented as mean ± SE. Letters denote the statistical difference between individual isolates for each population (Tukey HSD), respectively, at p < 0.05.
To test the possible effect of each isolates’ vc type or population of origin on the stress parameters factorial ANOVA was used. The activity of GST or SOD was not influenced by either of the two aforementioned factors (Table 1; Figures 3A,B). Surprisingly, total thiol content was affected by both studied factors (i.e., the population of origin and the vc type of the isolate) and lipid peroxidation level by isolates’ vc type. The population Kast had a higher content of thiols [mean(THIOLS) = 2.33 ± 0.10 nmol (DTNB) (mg DW)−1] and lower level of lipid peroxidation [mean(MDA) = 0.06 ± 0.00 nmol (mg DW)−1] in comparison to the population Ozalj [mean(THIOLS) = 1.25 ± 0.10 nmol (DTNB) (mg DW)−1; mean(MDA) = 0.07 ± 0.00 nmol (mg DW)−1] (Table 1; Figures 3C,D).
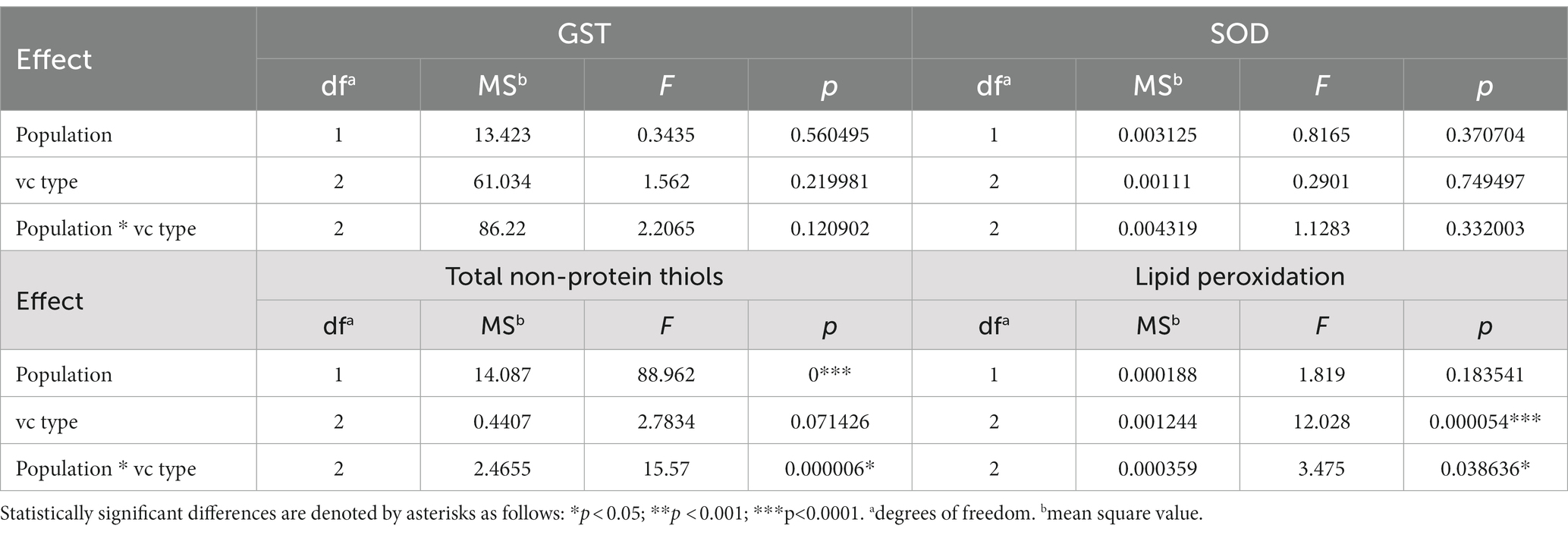
Table 1. Factorial ANOVA – Univariate test of significance for oxidative stress parameters between different groups of isolates based on their population of origin (Population), vc type (vc type), or the combination of both (Population * vc type).
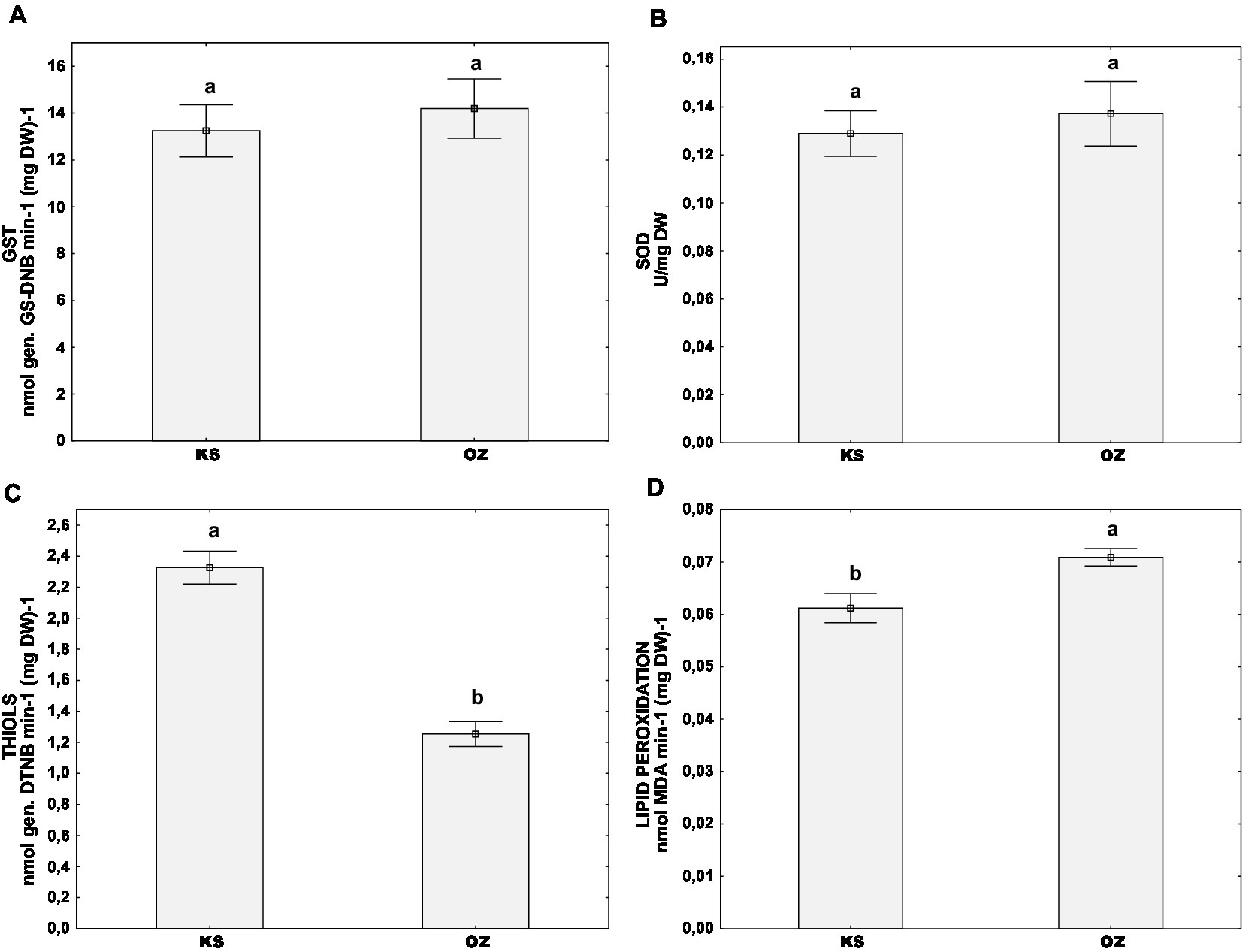
Figure 3. Difference of oxidative stress parameters between two wild populations naturally infected with CHV1 – Kast (KS) and Ozalj (OZ) for: (A) activity of GST, (B) activity of SOD, (C) content of non-proteins thiols and (D) level of lipid peroxidation. Data are represented as mean ± SE. Letters denote the statistical difference between individual isolates for each population (Tukey HSD), respectively, at p < 0.05.
Furthermore, we analyzed the oxidative stress parameters by grouping the samples according to their vc type: EU-1 or EU-2 which are the two most abundant vc types in Croatia, or “EU-other” (comprised of all other vc types, eg. EU-3, EU-5, EU-13 and EU-15). Post hoc Tukey HSD test did not indicate that the activities of measured stress-related enzymes GST and SOD were significantly different between the aforementioned vc type groups (Figures 4A,B). However, we noticed significant differences in the content of non-protein thiols (Figure 4C) and the level of lipid peroxidation (Figure 4D) between vc types. Isolates of the vc type EU-1 had the lowest content of thiols [mean(THIOLS) = 1.36 ± 0.16 nmol (DTNB) (mg DW)−1), but the highest level of lipid peroxidation (mean(MDA) = 0.08 ± 0.00 nmol (mg DW)−1], significantly different from both parameters in two other groups: EU-2 and “EU-other.” On the other hand, EU-2 and “EU-other” were similar in both the content of total thiols – for EU-2: [mean(THIOLS) = 1.77 ± 0.16 nmol (DTNB) (mg DW)−1], for “EU-other”: [mean(THIOLS) = 2.12 ± 0.15 nmol (DTNB) (mg DW)−1], and the level of lipid peroxidation – for EU-2: [mean(MDA) = 0.05 ± 0.00 nmol (mg DW)−1] and EU-other: [mean(MDA) = 0.06 ± 0.00 nmol (mg DW)−1].
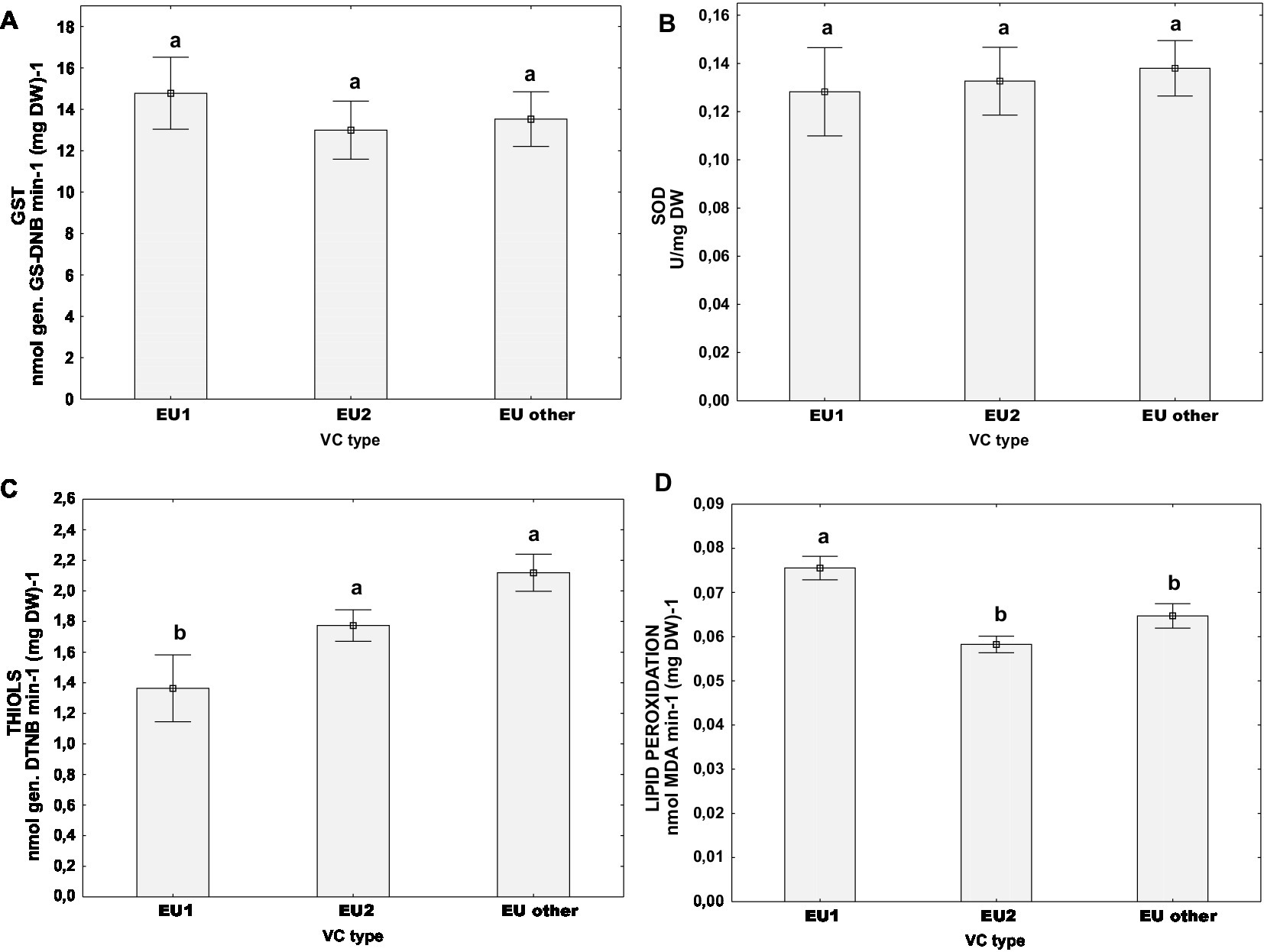
Figure 4. Difference of oxidative stress parameters for populations naturally infected with CHV1 grouped by vc types (EU-1, EU-2, “EU-other”): (A) activity of GST, (B) activity of SOD, (C) content of non-proteins thiols, and (D) level of lipid peroxidation. Data are represented as mean ± SE. Letters denote the statistical difference between vc type groups (Tukey HSD), at p < 0.05.
3.3. Laccase activity and lac1 gene expression
To assess the physiology of naturally CHV1-infected C. parasitica, we measured the activity of fungal laccases. In the population Kast, the mean value of laccase activity was 0.79 ± 0.05 nmol (DMOP) min−1 (mg DW)−1 (0.40–1.29 nmol (DMOP) min−1 (mg DW)−1), and in Ozalj the mean value was 1.06 ± 0.12 nmol (DMOP) min−1 (mg DW)−1 (0.29–2.73 nmol (DMOP) min−1 (mg DW)−1). The t-test showed no difference between populations. Similarly, the t-test did not indicate a significant difference in the relative expression of the lac1 between the two populations which ranged from 0.245 to 1.850 in Kast and from 0.027 to 2.425 in Ozalj.
Furthermore, we measured the activity of fungal laccases and the relative expression level of lac1 encoding extracellular laccase1 in naturally infected populations. Univariate tests of significance singled out the vc type of the fungus as a factor that has a statistically significant effect on both parameters. Thus, we further analyzed the laccase parameters by grouping the samples according to their vc type: EU-1, EU-2 and “EU-other,” as previously discussed. As shown in Figure 5. the relation between laccase activity and lac1 relative expression is opposite and inversely proportional between EU-1 and EU-2 vc types. If we analyse samples from both wild populations (Figure 5A), we see that the laccase activities were significantly higher in samples of EU-1 than of EU-2 vc type, while the lac1 relative gene expression was significantly lower for EU-1 vc type in comparison to EU-2. The trend is the same if we separate respective populations (Figures 5B,C), but the significance is lost for the Kast population (Figure 5B) due to the large data scatter.
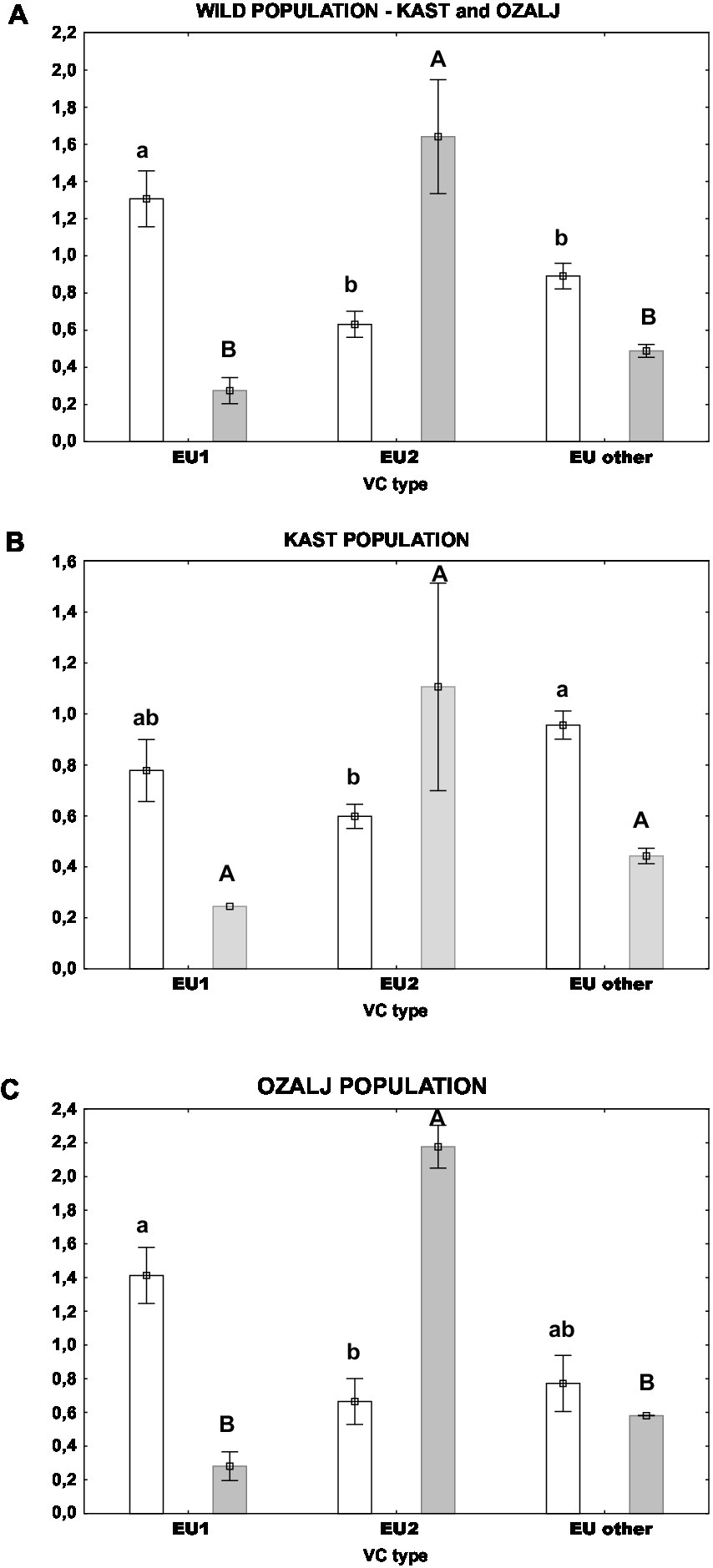
Figure 5. Laccase activity (white columns) and lac1 relative expression (grey columns) in isolates of different vc types: (A) both populations, (B) Kast population, (C) Ozalj population. Standard errors are indicated with error bars. Lower case letters denote statistical significance among samples for laccase activity; upper case letters denote statistical significance among samples for lac1 relative expression; Tukey HSD, all at p < 0.05.
4. Discussion
The present study broadens our knowledge of the physiology and stress response of a mycovirus-infected phytopathogenic fungus recently obtained from its natural habitat, versus physiological specificities of virus-infected long-term model strains. We have demonstrated clear differences in the physiology between wild populations and model strains; notably model strains had lower enzymatic activities of SOD and GST and higher content of MDA (i.e., level of lipid oxidation) and total non-protein thiols. These results indicate that the model strains are generally exposed to higher oxidative stress than the wild C. parasitica strains naturally infected with CHV1, i.e., high levels of lipid peroxidation induced by ROS (Birben et al., 2012) and the reduced SOD and GST activities suggest the depletion in cellular antioxidative enzymatic systems (Almeida et al., 2020). A similar correlation between the increase in MDA content and a decrease in SOD activity has been reported for Zika virus-infected cells, both in vitro and in vivo (Almeida et al., 2020). Additionally, a decrease in GST activity was observed in pathogenic yeast Trichosporon asahii exposed to other types of stressors like heavy metals (Ilyas et al., 2014). We have also observed the increase in the total non-protein thiols content, i.e., a gross estimate of GSH abundance (Noctor et al., 2011), whose secondary induction can compensate for the decrease in SOD activity under oxidative stress (Aquilano et al., 2014), further corroborating our observation that the model strains are under higher stress levels compared to wild populations.
Although virus infections undoubtedly lead to the increase of cellular ROS content (Reshi et al., 2014) and the CHV1 stress induction in C. parasitica has been reported previously (Nuskern et al., 2017), the high levels of oxidative stress observed in model strains cannot be assigned to CHV1 infection alone, since wild C. parasitica populations studied here were infected as well. A possible explanation of the observed phenomenon might be the ageing and deterioration of the model strains, since C. parasitica infected with prototypic CHV1 strains have been subculturing and exposed to freeze–thaw cycles for decades. In a study on the ageing of stationary cultures of the yeast Saccharomyces cerevisiae, the decrease in SOD activity accompanied by the oxidative damage of cellular proteins was observed, suggesting an increased exposure of macromolecules to ROS during ageing (Jakubowski et al., 2000). Similarly, in a filamentous fungus Aspergillus nidulans there was a correlation between fungal culture deterioration and cellular oxidative stress as well as a down-regulation of a SOD homolog in mitochondria of degenerated culture sectors (Li et al., 2014). Thus, thorough consideration of the physiological specificities of model laboratory strains is needed, especially when extrapolating the results to wild populations. Similar discrepancies have been observed previously when comparing the efficacy of horizontal CHV1 transmissions between different laboratory settings and natural conditions on chestnut trees (Deng et al., 2007; Brusini and Robin, 2013; Zhang and Nuss, 2016; Stauder et al., 2019).
While we have observed differences between oxidative stress levels of populations Ozalj and Kast, our work did not indicate that the CHV1 intra-host diversity had any effect on it (Leigh et al., 2021), but rather that these differences might be caused by some other factor, such as the accumulation of viral genome in the cytoplasm of the fungus, the genetic composition of the fungus or even the host tree (Dutech et al., 2010; Heller and Tudzynski, 2011; Ježić et al., 2014; Montibus et al., 2015; Ortiz-Urquiza and Keyhani, 2015; Poljak et al., 2017; Ježić et al., 2021).
It seems that the aforementioned differences in stress levels between C. parasitica strains from Ozalj and Kast can be associated with the genetic background of these populations. The higher stress resilience, or lower overall levels of oxidative stress in strains from Kast is evident by the lower level of lipid peroxidation observed in the strains from that population. Furthermore, the relatively higher content of non-protein thiols in this population might have a protective role against oxidative stress damage (Bai et al., 2003). Thus, when we analyzed isolates grouped by their vc type (EU-1, EU-2, EU-other), vc types EU-2 and “EU-other” had lower levels of lipid peroxidation and relatively higher content of non-protein thiols in comparison to EU-1. However, the prevalence of a particular vc type is significantly dependent on the population of origin – Kast population is mostly abundant with EU-2 and other vc types, while Ozalj contained more of the vc-type EU-1 (Supplementary Table 1), which might have contributed to the observed specificities of the stress level indicators.
The absolute values of laccase activity in samples of C. parasitica naturally infected with CHV1 measured in this study fell exclusively within the lower end of the range of values observed by Nuskern et al. (2021) in samples which have been infected with CHV1 by laboratory transmissions (Supplementary Table 2). It was possible to directly compare these two studies as the experimental setup was the same. This is important as the laccase activity is dependent of the experimental setup, e.g., the cultivation medium used, duration of cultivation and equipment used in experiment (Nuskern et al., 2021). This also applies to the studies that measured laccase mRNA accumulation – the level of accumulation was proven to be influenced by the culture medium, the age of the culture and exposure to the light (Choi et al., 1992). Importantly, lac1 expression was assessed almost exclusively on prototypic CHV1 strain EP713 and its isogenic virus-free fungal isolate EP155 (Rigling and Van Alfen, 1991; Choi et al., 1992; Larson et al., 1992; Rigling and van Alfen, 1993; Kazmierczak et al., 1996; Allen et al., 2003). This makes our study unique: to the best of our knowledge lac1 transcription levels have not been estimated in CHV 1-infected C. parasitica strains derived from the wild, but rather only from the aforementioned prototypic strains.
Our research indicated that the factor determining laccase physiology is intrinsic to the fungus itself, i.e., not related to CHV1 intra-host diversity nor the fungal population from which the samples have been obtained. Accordingly, we did demonstrate the dependence of both lac1 expression and laccase activity on the vegetative compatibility (vc) type of the fungus. As other filamentous Ascomycetes (Saupe, 2000), C. parasitica also possesses a heterokaryonic or vegetative compatibility (vc) system, that is a self–non-self-recognition system controlling the stability of anastomoses and heterokaryon formation. Vegetative compatibility is controlled by six known diallelic vic loci, defining 64 different vc types, also known as EU-types (Cortesi and Milgroom, 1998; Robin et al., 2000; Cortesi et al., 2001). It seems that laccase expression is regulated at a different pace in vc types EU-1 and EU-2: the expression levels of lac1 in EU-1 were lower, compared to the expression of lac1 in EU-2. The enzymatic activity of laccase was different as well between vc-types EU-1 and EU-2, but it was higher in EU-1 and lower in EU-2. Similarly, the lower lipid oxidation and higher concentration of non-protein thiols found in EU-2 compared to EU-1 suggest that the vc type (i.e., vic genotype) of the fungus might be an important physiological determinant. Since proteins with diverse domains are encoded by the vic loci (Choi et al., 2012; Dawe and Nuss, 2013; Zhang et al., 2014; Paoletti, 2016) they might be involved in several cellular processes beyond cell death/barrage formation, leading to the phenomenon observed. Specifically, the vc types EU-1 and EU-2 differ at locus vic2, which is responsible for the strongest incompatibility reaction (Cortesi et al., 2001), and that encodes for the patatin-like lipase and sec9 plasma membrane protein (Choi et al., 2012). In other fungal species, it was proposed that these protein analogs are involved in allorecognition, i.e., sec9 protein might bind to patatin-like phospholipase 1 and activate downstream metabolic response (Heller et al., 2018) which results in programmed cell death. Our results add to the slowly growing body of evidence (Leigh et al., 2021), that suggests that the aforementioned genes/proteins might be involved in another role beyond self/non-self recognition.
5. Conclusion
In our study, we observed lower enzymatic activities of SOD and GST and higher content of MDA and total non-protein thiols in model CHV1-infected C. parasitica isolates than in CHV1-infected isolates freshly obtained from wild populations. This indicates higher oxidative stress in the former, which can have serious implications if generalized inferences were to be drawn based solely on such model strains.
Furthermore, we observed significant differences between stress levels of the wild populations, underlying particular genetic distinctiveness of natural populations. While CHV1 intra-host diversity had no discernible effect, a particular vegetative incompatibility locus of the fungus host was shown to influence both lac1 expression and laccase enzyme activity.
Data availability statement
The original contributions presented in the study are included in the article/Supplementary material, further inquiries can be directed to the corresponding author.
Author contributions
MP and LN: physiology experiments, data analysis, and writing. KP: fungal culture preparation and physiology experiments. RV, ZK, and LK: expression analyses and writing. MĆ-P, DL, IP, and MI: writing. DR and MJ: experimental design, writing, and funding. All authors contributed to the article and approved the submitted version.
Funding
This research was funded by the Swiss Enlargement Contribution in the framework of the Croatian-Swiss Research Programme (project number IZHRZ0_180651), Croatian Science Foundation (grant number IP-2018-01-1295), and University of Zagreb financial support.
Conflict of interest
The authors declare that the research was conducted in the absence of any commercial or financial relationships that could be construed as a potential conflict of interest.
Publisher’s note
All claims expressed in this article are solely those of the authors and do not necessarily represent those of their affiliated organizations, or those of the publisher, the editors and the reviewers. Any product that may be evaluated in this article, or claim that may be made by its manufacturer, is not guaranteed or endorsed by the publisher.
Supplementary material
The Supplementary material for this article can be found online at: https://www.frontiersin.org/articles/10.3389/fmicb.2023.1192996/full#supplementary-material
References
Akaike, T. (2001). Role of free radicals in viral pathogenesis and mutation. Rev. Med. Virol. 11, 87–101. doi: 10.1002/rmv.303
Akaike, T., Fujii, S., Kato, A., Yoshitake, J., Miyamoto, Y., Sawa, T., et al. (2000). Viral mutation accelerated by nitric oxide production during infection in vivo. FASEB J. 14, 1447–1454. doi: 10.1096/fj.14.10.1447
Allemann, C., Hoegger, P. J., Heiniger, U., and Rigling, D. (1999). Genetic variation of Cryphonectria Hypoviruses (CHV1) in Europe, assessed using restriction fragment length polymorphism (RFLP) markers. Mol. Ecol. 8, 843–854. doi: 10.1046/j.1365-294X.1999.00639.x
Allen, T. D., Dawe, A. L., and Nuss, D. L. (2003). Use of CDNA microarrays to monitor transcriptional responses of the chestnut blight fungus Cryphonectria parasitica to infection by virulence-attenuating hypoviruses. Eucaryotic Cell 2, 1253–1265. doi: 10.1128/EC.2.6.1253
Allen, T. D., and Nuss, D. L. (2004). Specific and common alterations in host gene transcript accumulation following infection of the chestnut blight fungus by mild and severe hypoviruses. J. Virol. 78, 4145–4155. doi: 10.1128/jvi.78.8.4145-4155.2004
Almeida, L. T., Ferraz, A. C., da Silva Caetano, C. C., da Silva Menegatto, M. B., dos Santos Pereira, A. C., Andrade, R. L. S., et al. (2020). Zika virus induces oxidative stress and decreases antioxidant enzyme activities in vitro and in vivo. Virus Res. 286:198084. doi: 10.1016/j.virusres.2020.198084
Anagnostakis, S. L. (1987). Chestnut blight : the classical problem of an introduced pathogen. Mycologia 79, 23–37. doi: 10.1080/00275514.1987.12025367
Aquilano, K., Baldelli, S., and Ciriolo, M. R. (2014). Glutathione: new roles in redox signalling for an old antioxidant. Front. Pharmacol. 5, 1–12. doi: 10.3389/fphar.2014.00196
Azevedo, M. M., Carvalho, A., Pascoal, C., Rodrigues, F., and Cássio, F. (2007). Responses of antioxidant defenses to Cu and Zn stress in two aquatic fungi. Sci. Total. Environ. 377: 233–243. doi: 10.1016/j.scitotenv.2007.02.027
Bai, Z., Harvey, L. M., and McNeil, B. (2003). Oxidative stress in submerged cultures of Fungi. Crit. Rev. Biotechnol. 23, 267–302. doi: 10.1080/07388550390449294
Baldrian, P. (2006). Fungal Laccases – occurrence and properties. FEMS Microbiol. Rev. 30, 215–242. doi: 10.1111/j.1574-4976.2005.00010.x
Beauchamp, C., and Fridovich, I. (1971). Superoxide dismutase: improved assays and an assay applicable to acrylamide gels. Anal. Biochem. 44, 276–287. doi: 10.1016/0003-2697(71)90370-8
Birben, E., Sahiner, U. M., Sackesen, C., Erzurum, S., and Kalayci, O. (2012). Oxidative stress and antioxidant defense. WAO J. 5, 9–19. doi: 10.1097/WOX.0b013e3182439613
Bocchetti, R., and Regoli, F. (2006). Seasonal variability of oxidative biomarkers, lysosomal parameters, metallothioneins and peroxisomal enzymes in the Mediterranean mussel Mytilus galloprovincialis from Adriatic Sea. Chemosphere 65, 913–921. doi: 10.1016/j.chemosphere.2006.03.049
Bradford, M. M. (1976). A rapid and sensitive method for the quantitation of microgram quantities of protein utilizing the principle of protein-dye binding. Analyt. Bochem. 72, 248–254. doi: 10.1016/0003-2697(76)90527-3
Brasier, C. M., and Buck, K. W. (2001). Rapid evolutionary changes in a globally invading fungal pathogen (Dutch elm disease). Biol. Invasions 3, 223–233. doi: 10.1023/A:1015248819864
Breitenbach, M., Weber, M., Rinnerthaler, M., Karl, T., and Breitenbach-Koller, L. (2015). Oxidative stress in fungi: its function in signal transduction, interaction with plant hosts, and lignocellulose degradation. Biomolecules. 5, 318–342. doi: 10.3390/biom5020318
Brusini, J., and Robin, C. (2013). Mycovirus transmission revisited by in situ pairings of vegetatively incompatible isolates of Cryphonectria Parasitica. J. Virol. Methods 187, 435–442. doi: 10.1016/j.jviromet.2012.11.025
Burokiene, D., Prospero, S., Jung, E., Marciulyniene, D., Moosbrugger, K., Norkute, G., et al. (2015). Genetic population structure of the invasive ash dieback pathogen Hymenoscyphus fraxineus in its expanding range. Biol. Invasions 17, 2743–2756. doi: 10.1007/s10530-015-0911-6
Cabrera, E., Welch, L. C., Robinson, M. R., Sturgeon, C. M., Crow, M. M., and Segarra, V. A. (2020). Cryopreservation and the freeze-thaw stress response in yeast. Genes 11:835. doi: 10.3390/genes11080835
Camejo, D., Guzmán-Cedeño, Á., and Moreno, A. (2016). Reactive oxygen species, essential molecules, during plant-pathogen interactions. Plant Physiol. Biochem. 103, 10–23. doi: 10.1016/j.plaphy.2016.02.035
Chen, B., and Nuss, D. L. (1999). Infectious CDNA clone of hypovirus CHV1-Euro7: a comparative virology approach to investigate virus-mediated hypovirulence of the chestnut blight fungus Cryphonectria parasitica. J. Virol. 73, 985–992. doi: 10.1128/JVI.73.2.985-992.1999
Choi, G. H., Dawe, A. L., Churbanov, A., Smith, M. L., Milgroom, M. G., and Nuss, D. L. (2012). Molecular characterization of vegetative incompatibility genes that restrict hypovirus transmission in the chestnut blight fungus Cryphonectria parasitica. Genetics 190, 113–127. doi: 10.1534/genetics.111.13398
Choi, G. H., Larson, T. G., and Nuss, D. L. (1992). Molecular analysis of the laccase gene from the chestnut blight fungus and selective suppression of its expression in Na isogenic Hypovirulent strain. Mol. Plant Microbe Interact. 5, 119–128. doi: 10.1094/MPMI-5-119
Cortesi, P., McCulloch, C. E., Song, H., Lin, H., and Milgroom, M. G. (2001). Genetic control of horizontal virus transmission in the chestnut blight fungus, Cryphonectria parasitica. Genetics 159, 107–118. doi: 10.1093/genetics/159.1.107
Cortesi, P., and Milgroom, M. G. (1998). Genetics of vegetative incompatibility in Cryphonectria parasitica. Appl. Environ. Microbiol. 64, 2988–2994. doi: 10.1128/AEM.64.8.2988-2994.1998
da Silva Caetano, C. C., Camini, F. C., Almeida, L. T., Ferraz, A. C., da Silva, T. F., Lima, R. L. S., et al. (2019). Mayaro virus induction of oxidative stress is associated with liver pathology in a non-lethal mouse model. Sci. Rep. 9:15289. doi: 10.1038/s41598-019-51713-9
Dawe, A. L., McMains, V. C., Panglao, M., Kasahara, S., Chen, B., and Nuss, D. L. (2003). An ordered collection of expressed sequences from Cryphonectria parasitica and evidence of genomic microsynteny with Neurospora crassa and Magnaporthe grisea. Microbiology 149, 2373–2384. doi: 10.1099/mic.0.26371-0
Dawe, A. L., and Nuss, D. L. (2013). Hypovirus molecular biology: from Koch’s postulates to host self-recognition genes that restrict virus transmission. Adv. Virus Res. 86, 109–147. doi: 10.1016/B978-0-12-394315-6.00005-2
Deng, F., Allen, T. D., Hillman, B. I., and Nuss, D. L. (2007). Comparative analysis of alterations in host phenotype and transcript accumulation following hypovirus and mycoreovirus infections of the chestnut blight fungus Cryphonectria parasitica. Eukaryot. Cell 6, 1286–1298. doi: 10.1128/EC.00166-07
Dunham, J. P., Simmons, H. E., Holmes, E. C., and Stephenson, A. G. (2014). Analysis of viral (zucchini yellow mosaic virus) genetic diversity during systemic movement through a Cucurbita pepo vine. Virus Res. 191, 172–179. doi: 10.1016/j.virusres.2014.07.030
Dutech, C., Fabreguettes, O., Capdevielle, X., and Robin, C. (2010). Multiple introductions of divergent genetic lineages in an invasive fungal pathogen, Cryphonectria parasitica, in France. Heredity 105, 220–228. doi: 10.1038/hdy.2009.164
Ellman, G. L. (1959). Tissue sulfhydryl groups. Arch. Biochem. Biophys. 82, 70–77. doi: 10.1016/0003-9861(59)90090-6
Feder, A. F., Pennings, P. S., and Petrov, D. A. (2021). The clarifying role of time series data in the population genetics of HIV. PLoS Genet. 17:e1009050. doi: 10.1371/journal.pgen.1009050
Gobbin, D., Hoegger, P. J., Heiniger, U., and Rigling, D. (2003). Sequence variation and evolution of Cryphonectria hypovirus 1 (CHV-1) in Europe. Virus Res. 97, 39–46. doi: 10.1016/S0168-1702(03)00220-X
Gong, C., Liu, Y., Liu, S., Cheng, M., Zhang, Y., Wang, R., et al. (2017). Analysis of Clonostachys rosea-induced resistance to grey mould disease and identification of the key proteins induced in tomato fruit. Postharvest Biol. Technol. 123, 83–93. doi: 10.1016/j.postharvbio.2016.08.004
Heath, R. L., and Packer, L. (1968). Photoperoxidation in isolated chloroplasts. I-kinetics and stoichiometry of fatty acid peroxidation. Arch. Biochem. Biophys. 125, 189–198. doi: 10.1016/0003-9861(68)90654-1
Heiniger, U., and Rigling, D. (1994). Biological control of chestnut blight in Europe. Annu. Rev. Phytopathol. 32, 581–599. doi: 10.1146/annurev.py.32.090194.003053
Heller, J., Clave, C., Gladieux, P., and Glass, N. L. (2018). NLR surveillance of essential SEC-9 SNARE proteins induces programmed cell death upon allorecognition in filamentous Fungi. Proc. Natl. Acad. Sci. U. S. A. 115, E2292–E2301. doi: 10.1073/pnas.1719705115
Heller, J., and Tudzynski, P. (2011). Reactive oxygen species in phytopathogenic fungi: signaling, development, and disease. Annu. Rev. Phytopathol. 49, 369–390. doi: 10.1146/annurev-phyto-072910-095355
Hillman, B. I., and Suzuki, N. (2004). Viruses of the chestnut blight fungus, Cryphonectria parasitica. Adv. Virus Res. 63, 423–472. doi: 10.1016/S0065-3527(04)63007-7
Ilyas, S., Rehman, A., Varela, A. C., and Sheehan, D. (2014). Redox proteomics changes in the fungal pathogen Trichosporon asahii on arsenic exposure: identification of protein responses to metal-induced oxidative stress in an environmentally-sampled isolate. PLoS One 9, 24–26. doi: 10.1371/journal.pone.0102340
Jakubowski, W., Bilinski, T., and Bartosz, G. (2000). Oxidative stress during aging of stationary cultures of Saccharomyces Cerevisiae. Free Radic. Biol. Med. 28, 659–664. doi: 10.1016/S0891-5849(99)00266-X
Jamieson, D. J. (1998). Oxidative stress responses of the yeast Saccharomyces cerevisiae. Yeast. 14, 1511–1527. doi: 10.1002/(SICI)1097-0061(199812)14:16<1511::AID-YEA356>3.0.CO;2-S
Ježić, M., Krstin, L., Poljak, I., Liber, Z., Idžojtić, M., Jelić, M., et al. (2014). Castanea Sativa: genotype-dependent recovery from chestnut blight. Tree Genet. Genomes 10, 101–110. doi: 10.1007/s11295-013-0667-z
Ježić, M., Schwarz, J. M., Prospero, S., Sotirovski, K., Risteski, M., Ćurković-Perica, M., et al. (2021). Temporal and spatial genetic population structure of Cryphonectria parasitica and its associated hypovirus across an invasive range of chestnut blight in Europe. Phytopathology 111, 1327–1337. doi: 10.1094/PHYTO-09-20-0405-R
Jukic, E., Blatzer, M., Binder, U., Mayr, L., Lass-Flörl, C., and Lackner, M. (2017). Impact of morphological sectors on antifungal susceptibility testing and virulence studies. Antimicrob. Agents Chemother. 61, e00755–e00717. doi: 10.1128/AAC.00755-17
Katanić, Z., Krstin, L., Ježić, M., Ćaleta, B., Stančin, P., Zebec, M., et al. (2020). Identification and characterization of the causal agent of Dutch elm disease in Croatia. Eur. J. For. Res. 139, 805–815. doi: 10.1007/s10342-020-01286-4
Kazmierczak, P., Pfeiffer, P., Zhang, L., and Van Alfen, N. K. (1996). Transcriptional repression of specific host genes by the mycovirus Cryphonectria hypovirus 1. J. Virol. 70, 1137–1142. doi: 10.1128/jvi.70.2.1137-1142.1996
Kim, M. J., Choi, J. W., Park, S. M., Cha, B. J., Yang, M. S., and Kim, D. H. (2002). Characterization of a fungal protein kinase from Cryphonectria parasitica and its transcriptional Upregulation by Hypovirus. Mol. Microbiol. 45, 933–941. doi: 10.1046/j.1365-2958.2002.03079.x
Kim, J.-M., Park, J.-A., and Kim, D.-H. (2012). Comparative proteomic analysis of chestnut blight fungus, Cryphonectria parasitica, under tannic-acid-inducing and hypovirus-regulating conditions. Can. J. Microbiol. 58, 863–871. doi: 10.1139/w2012-065
Krstin, L., Novak-Agbaba, S., Rigling, D., Krajačić, M., and Ćurković-Perica, M. (2008). Chestnut blight fungus in Croatia: diversity of vegetative compatibility types, mating types and genetic variability of associated Cryphonectria Hypovirus 1. Plant Pathol. 57, 1086–1096. doi: 10.1111/j.1365-3059.2008.01905.x
Larson, T. G., Choi, G. H., and Nuss, D. L. (1992). Regulatory pathways governing modulation of fungal gene expression by a virulence-attenuating Mycovirus. EMBO J. 11, 4539–4548. doi: 10.1002/j.1460-2075.1992.tb05555.x
Lauring, A. S. (2020). Within-host viral diversity: a window into viral evolution. Annu. Rev. Virol. 7, 63–81. doi: 10.1146/annurev-virology-010320-061642
Lauring, A. S., and Andino, R. (2010). Quasispecies theory and the behavior of RNA viruses. PLoS Pathog. 6:e1001005. doi: 10.1371/journal.ppat.1001005
Leigh, D. M., Peranić, K., Prospero, S., Cornejo, C., Ćurković-Perica, M., Kupper, Q., et al. (2021). Long-read sequencing reveals the evolutionary drivers of intra-host diversity across natural RNA mycovirus infections. Virus Evolut. 7, 1–14. doi: 10.1093/ve/veab101
Li, L., Hu, X., Xia, Y., Xiao, G., Zheng, P., and Wang, C. (2014). Linkage of oxidative stress and mitochondrial dysfunctions to spontaneous culture degeneration in Aspergillus nidulans. Mol. Cell. Proteomics 13, 449–461. doi: 10.1074/mcp.M113.028480
MacDonald, W. L., and Fulbright, D. W. (1991). Biological control of chestnut blight: use and limitations of transmissible hypovirulence. Plant Dis. 75, 653–661. doi: 10.1094/pd-75-053
Mlinarec, J., Ježić, M., Ćosić, J., and Ćurković-Perica, M. (2018a). Multilocus PCR assay reveals high diversity of vegetative compatibility types in populations of Cryphonectria parasitica in Croatia. Plant Pathol. 67, 741–749. doi: 10.1111/ppa.12751
Mlinarec, J., Nuskern, L., Ježić, M., Rigling, D., and Ćurković-Perica, M. (2018b). Molecular evolution and invasion pattern of Cryphonectria hypovirus 1 in Europe: mutation rate, and selection pressure differ between genome domains. Virology 514, 156–164. doi: 10.1016/j.virol.2017.11.011
Montibus, M., Pinson-Gadais, L., Richard-Forget, F., Barreau, C., and Ponts, N. (2015). Coupling of transcriptional response to oxidative stress and secondary metabolism regulation in filamentous Fungi. Crit. Rev. Microbiol. 41, 295–308. doi: 10.3109/1040841X.2013.829416
Muñoz-Adalia, E. J., Fernández, M. M., and Diez, J. J. (2016). The use of mycoviruses in the control of forest diseases. Biocontrol Sci. Tech. 26, 577–604. doi: 10.1080/09583157.2015.1135877
Nei, M., and Tajima, F. (1980). DNA polymorphism detectable by restriction endonucleases. Genetics 97, 145–163. doi: 10.1093/genetics/97.1.145
Nelson, C. W., Moncla, L. H., and Hughes, A. L. (2015). SNPGenie: estimating evolutionary parameters to detect natural selection using pooled next-generation sequencing data. Bioinformatics 31, 3709–3711. doi: 10.1093/bioinformatics/btv449
Noctor, G., Queval, G., Mhamdi, A., Chaouch, S., and Foyer, C. H. (2011). Glutathione. Arabidopsis Book 9, 1–32. doi: 10.1199/tab.0142
Nuskern, L., Tkalec, M., Ježić, M., Katanić, Z., Krstin, L., and Ćurković-Perica, M. (2017). Cryphonectria hypovirus 1-induced changes of stress enzyme activity in transfected phytopathogenic fungus Cryphonectria parasitica. Microb. Ecol. 74, 302–311. doi: 10.1007/s00248-017-0945-7
Nuskern, L., Tkalec, M., Srezović, B., Ježić, M., Gačar, M., and Ćurković-Perica, M. (2021). Laccase activity in fungus Cryphonectria parasitica is affected by growth conditions and fungal – viral genotypic interactions. J. Fungi 7:958. doi: 10.3390/jof7110958
Olsavsky, K. M., Page, J. L., Johnson, M. C., Zarbl, H., Strom, S. C., and Omiecinski, C. J. (2007). Gene expression profiling and differentiation assessment in primary human hepatocyte cultures, established hepatoma cell lines, and human liver tissues. Toxicol. Appl. Pharmacol. 222, 42–56. doi: 10.1016/j.taap.2007.03.032
Ortiz-Urquiza, A., and Keyhani, N. O. (2015). Stress response signaling and virulence: insights from entomopathogenic fungi. Curr. Genet. 61, 239–249. doi: 10.1007/s00294-014-0439-9
Palmieri, G., Giardina, P., Scaloni, A., Capasso, A., Sannia, G., and Bianco, C. (1997). A novel white laccase from Pleurotus ostreatus. J. Biol. Chem. 272, 31301–31307. doi: 10.1074/jbc.272.50.31301
Paoletti, M. (2016). Vegetative incompatibility in fungi: from recognition to cell death, whatever does the trick. Fungal Biol. Rev. 30, 152–162. doi: 10.1016/j.fbr.2016.08.002
Pautasso, M., Aas, G., Queloz, V., and Holdenrieder, O. (2013). European ash (Fraxinus excelsior) dieback – a conservation biology challenge. Biol. Conserv. 158, 37–49. doi: 10.1016/j.biocon.2012.08.026
Peever, T. L., Liu, Y. C., Cortesi, P., and Milgroom, M. G. (2000). Variation in tolerance and virulence in the chestnut blight fungus-hypovirus interaction. Appl. Environ. Microbiol. 66, 4863–4869. doi: 10.1128/AEM.66.11.4863-4869.2000
Pfaffl, M. W. (2001). A new mathematical model for relative quantification in real-time RT–PCR. Nucleic Acids Res. 29, 2002–2007. doi: 10.1093/nar/29.9.e45
Poljak, I., Idžojtić, M., Šatović, Z., Ježić, M., Ćurković-Perica, M., Simovski, B., et al. (2017). Genetic diversity of the sweet chestnut (Castanea sativa mill.) in Central Europe and the western part of the Balkan Peninsula and evidence of marron genotype introgression into wild populations. Tree Genet. Genomes 13:18. doi: 10.1007/s11295-017-1107-2
Reshi, M. L., Su, Y. C., and Hong, J. R. (2014). RNA viruses: ROS-mediated cell death. Int. J. Cell Biol. 2014:467452, 1–16. doi: 10.1155/2014/467452
Rigling, D., Heiniger, U., and Hohl, H. R. (1989). Reduction of laccase activity in DsRNA-containing hypovirulent strains of Cryphonectria (Endothia) parasitica. Phytopathology 79, 219–223. doi: 10.1094/Phyto-79-219
Rigling, D., and Prospero, S. (2018). Cryphonectria parasitica, the causal agent of chestnut blight: invasion history, population biology and disease control. Mol. Plant Pathol. 19, 7–20. doi: 10.1111/mpp.12542
Rigling, D., and Van Alfen, N. K. (1991). Regulation of laccase biosynthesis in the plant-pathogenic fungus Cryphonectria parasitica by double-stranded RNA. J. Bacteriol. 173, 8000–8003. doi: 10.1128/jb.173.24.8000-8003.1991
Rigling, D., and van Alfen, N. K. (1993). Extra- and intracellular laccases of the chestnut blight fungus, Cryphonectria parasitica. Appl. Environ. Microbiol. 59, 3634–3639. doi: 10.1128/aem.59.11.3634-3639.1993
Robin, C., Anziani, C., and Cortesi, P. (2000). Relationship between biological control, incidence of hypovirulence, and diversity of vegetative compatibility types of Cryphonectria parasitica in France. Phytopathology 90, 730–737. doi: 10.1094/PHYTO.2000.90.7.730
Ryan, M. J., Bridge, P. D., Smith, D., and Jeffries, P. (2002). Phenotypic degeneration occurs during sector formation in Metarhizium anisopliae. J. Appl. Microbiol. 93, 163–168. doi: 10.1046/j.1365-2672.2002.01682.x
Saupe, S. J. (2000). Molecular genetics of heterokaryon incompatibility in filamentous ascomycetes. Microbiol. Mol. Biol. Rev. 64, 489–502. doi: 10.1128/MMBR.64.3.489-502.2000
Shapira, R., Choi, G. H., and Nuss, D. L. (1991). Virus-like genetic organization and expression strategy for a double-stranded RNA genetic element associated with biological control of chestnut blight. EMBO J. 10, 731–739. doi: 10.1002/j.1460-2075.1991.tb08004.x
Simmons, H. E., Holmes, E. C., and Stephenson, A. G. (2011). Rapid turnover of intra-host genetic diversity in zucchini yellow mosaic virus. Virus Res. 155, 389–396. doi: 10.1016/j.virusres.2010.11.007
Solla, A., Dacasa, M. C., Nasmith, C., Hubbes, M., and Gil, L. (2008). Analysis of Spanish populations of Ophiostoma ulmi and O. novo-ulmi using phenotypic characteristics and RAPD markers. Plant Pathol. 57, 33–44. doi: 10.1111/j.1365-3059.2007.01692.x
Stauder, C. M., Nuss, D. L., Zhang, D. X., Double, M. L., MacDonald, W. L., Metheny, A. M., et al. (2019). Enhanced hypovirus transmission by engineered super donor strains of the chestnut blight fungus, Cryphonectria parasitica, into a natural population of strains exhibiting diverse vegetative compatibility genotypes. Virology 528, 1–6. doi: 10.1016/j.virol.2018.12.007
Trumbore, S., Brando, P., and Hartmann, H. (2015). Forest health and global change. Science 349, 814–818. doi: 10.1126/science.aaa9092
Wang, J., Shi, L., He, X., Lu, L., Li, X., and Chen, B. (2016). Comparative Secretome analysis reveals perturbation of host secretion pathways by a hypovirus. Sci. Rep. 6:34308. doi: 10.1038/srep34308
Zhang, D.-X., and Nuss, D. L. (2016). Engineering super mycovirus donor strains of chestnut blight fungus by systematic disruption of multilocus vic genes. Proc. Natl. Acad. Sci. 113, 2062–2067. doi: 10.1073/pnas.1522219113
Keywords: culture deterioration, fungal laccases, fungal metabolism, long term sub-culturing, oxidative stress, virus-host interactions
Citation: Popović M, Nuskern L, Peranić K, Vuković R, Katanić Z, Krstin L, Ćurković-Perica M, Leigh DM, Poljak I, Idžojtić M, Rigling D and Ježić M (2023) Physiological variations in hypovirus-infected wild and model long-term laboratory strains of Cryphonectria parasitica. Front. Microbiol. 14:1192996. doi: 10.3389/fmicb.2023.1192996
Edited by:
F. Wayne Outten, University of South Carolina, United StatesReviewed by:
Leticia Botella, Mendel University in Brno, CzechiaLukasz Stepien, Institute of Plant Genetics, Polish Academy of Sciences, Poland
Copyright © 2023 Popović, Nuskern, Peranić, Vuković, Katanić, Krstin, Ćurković-Perica, Leigh, Poljak, Idžojtić, Rigling and Ježić. This is an open-access article distributed under the terms of the Creative Commons Attribution License (CC BY). The use, distribution or reproduction in other forums is permitted, provided the original author(s) and the copyright owner(s) are credited and that the original publication in this journal is cited, in accordance with accepted academic practice. No use, distribution or reproduction is permitted which does not comply with these terms.
*Correspondence: Marin Ježić, marin.jezic@biol.pmf.hr
†These authors have contributed equally to this work and share first authorship