- 1Zhejiang Provincial People's Hospital Affiliated to Hangzhou Medical College, Hangzhou Medical College, Hangzhou, China
- 2The Marine Biomedical Research Institute, Guangdong Medical University, Zhanjiang, China
- 3Department of Biological and Chemical Sciences, New York Institute of Technology—Manhattan Campus, New York, NY, United States
- 4Department of Clinical Medicine, Medicine and Technology, School of Zunyi Medical University, Zunyi, China
- 5Guangdong Provincial Key Laboratory of Systems Biology and Synthetic Biology for Urogenital Tumors, Shenzhen Key Laboratory of Genitourinary Tumor, Department of Urology, The First Affiliated Hospital of Shenzhen University, Shenzhen Second People's Hospital(Shenzhen Institute of Translational Medicine), Shenzhen, China
- 6Department of Gastroenterology, Zibo Central Hospital, Zibo, China
- 7Department of Clinical Laboratory, Institute of Translational Medicine, The First Affiliated Hospital of Shenzhen University, Shenzhen Second People’s Hospital, Shenzhen, China
Viruses and tumors are two pathologies that negatively impact human health, but what occurs when a virus encounters a tumor? A global consensus among cancer patients suggests that surgical resection, chemotherapy, radiotherapy, and other methods are the primary means to combat cancer. However, with the innovation and development of biomedical technology, tumor biotherapy (immunotherapy, molecular targeted therapy, gene therapy, oncolytic virus therapy, etc.) has emerged as an alternative treatment for malignant tumors. Oncolytic viruses possess numerous anti-tumor properties, such as directly lysing tumor cells, activating anti-tumor immune responses, and improving the tumor microenvironment. Compared to traditional immunotherapy, oncolytic virus therapy offers advantages including high killing efficiency, precise targeting, and minimal side effects. Although oncolytic virus (OV) therapy was introduced as a novel approach to tumor treatment in the 19th century, its efficacy was suboptimal, limiting its widespread application. However, since the U.S. Food and Drug Administration (FDA) approved the first OV therapy drug, T-VEC, in 2015, interest in OV has grown significantly. In recent years, oncolytic virus therapy has shown increasingly promising application prospects and has become a major research focus in the field of cancer treatment. This article reviews the development, classification, and research progress of oncolytic viruses, as well as their mechanisms of action, therapeutic methods, and routes of administration.
1. Introduction
In recent years, significant advances in cancer genomics and the application of various therapeutic interventions, such as chemotherapy, radiation therapy, and immunotherapy, have led to remarkable improvements in the prognosis of cancer patients. However, these treatments still have substantial limitations, including (1) serious side effects associated with chemotherapy and radiation therapy, (2) development of drug resistance in tumor cells, and (3) suboptimal efficacy of immunotherapy in severe and highly immunosuppressed diseases (Chiocca and Rabkin, 2014; Zhu et al., 2022; Tripodi et al., 2023). Therefore, the development of a novel therapeutic approach is necessary, which should avoid adverse effects on normal tissue cells while exhibiting high specificity for tumor cells. Oncolytic viruses (OVs) are natural or genetically modified drugs that possess a characteristic of elevated sensitivity to innate antiviral signaling and dependence on tumor signaling pathways, allowing for targeted infection and intracellular proliferation within tumor cells, triggering both innate and adaptive immune responses in the host, ultimately resulting in tumor cell death (Chiocca and Rabkin, 2014; Ghasemi Darestani et al., 2023; Malhotra and Kim, 2023). This property renders oncolytic viruses a promising therapeutic approach for cancer treatment. Simultaneously, the ruptured tumor cells can release their progeny OVs to continue infecting the remaining tumor cells, playing a role in continuously killing tumor cells (Russell et al., 2012; Lawler et al., 2017). After all the tumor cells are infected and cleared by the virus, the virus generally breaks the immune system’s tolerance and triggers an immune response, which results in its elimination (Russell and Barber, 2018). Therefore, as an emerging cancer treatment approach, OVs treatment has significant research prospects and is of great research importance.
With the development of genetic engineering, several OVs treatment has been applied, such as the marketing of Rigvir (Doniņa et al., 2015; Alberts et al., 2018) was approved in Latvia, H101 (Liang, 2018) in China, T-VEC (Wall and Baldwin-Medsker, 2017) in the United States, and the Japanese herpes simplex virus Deltyact (Teserparturev/G47 ∆; Frampton, 2022). However, these viruses have not been particularly widely used after being marketed due to the different structures and biological characteristics of oncolytic viruses. Their production processes and quality control are quite diverse. At present, the understanding of their biological mechanism of action and the quality of oncolytic viruses used for treatment are not comprehensive, which poses great challenges for the research and application of oncolytic viruses in cancer treatment. Moreover, there is currently a lack of comprehensive reviews on clinical trials of most oncolytic viruses in the research field. Therefore, our study aims to fill this gap.
In this review, we will focus on the diversity of OVs, the potential harm to the human body, the high efficiency of killing tumor cells, and their therapeutic methods, and clarify the great role of OVs in the treatment of tumor cells. By searching the PubMed database and ClinicalTrials.gov, we collected many articles and data about OVs in clinical studies and summarized the treatment methods of most OVs and the efficacy of combination therapy.
2. Development, species, and research progress of oncolytic viruses
2.1. The development of oncolytic viruses
In 1896, a case of leukemia was reported in which the white blood cells of a woman infected with the influenza virus decreased dramatically (Tian et al., 2022). The phenomenon that cancer remission following influenza infection made people realize that viruses and tumors, as diseases that are difficult to cure, may interact with each other. After that, cases of tumor reduction or disappearance following virus infection were reported one after another. In 1912, an Italian doctor discovered that a cervical cancer patient’s tumor began to recede after a rabies vaccination (Das et al., 2021). Since then, scientists conducted clinical trials using various wild-type viruses to treat tumors from the 1950s to the 1980s. However, due to limited medical conditions, technology, and understanding of viruses and tumor mechanisms, viruses did not revolutionize tumor treatment, and the development of oncolytic viruses was slow (Moore, 1952; Southam and Moore, 1952; Asada, 1974). With advancements in modern genetic engineering and research on viral genes, designing and manipulating viruses using genetic engineering has emerged as a promising approach for OVs. In 1991, the first OV was used to inhibit the growth of glioma in mice (Ezzeddine et al., 1991), and the development of OVs began to advance rapidly. In 1996, research proved that the adenovirus with an E1B55K gene mutation could selectively replicate in p53 defective tumor cells (Bischoff et al., 1996), and then the genetically modified adenovirus ONYX-015 entered phase I clinical trials (Rothmann et al., 1998). In 2004, RIGVIR was approved for melanoma treatment in Latvia, followed by H101 in China in 2005, and T-VEC in the United States and Europe in 2015 (Bilsland et al., 2016; Kaufman et al., 2022; Zhang et al., 2022), marking the maturity of oncolytic virus technology and recognition of the efficacy of oncolytic virus. In 2017, Cell reported that the combination of oncolytic virus T-VEC and PD-1 anti-tumor drug Keytruda was used to treat melanoma (Ribas et al., 2017), setting off an upsurge of research on oncolytic virus combined therapy. In 2021, Deltyact (Teserpatricev/G47 ∆) in Japan was approved for listing (Zeng et al., 2021). Until now, numerous genetically modified OVs have undergone various clinical trials and achieved notable results (Macedo et al., 2020), making oncolytic virus one of the promising tumor immunotherapy methods in clinics.
2.2. Introduction and development of some oncolytic viruses
Current research shows that the size of oncolytic viruses is mostly distributed between 20 and 200 nm. Selective replication in tumor cells is a critical characteristic of oncolytic viruses for tumor immunotherapy. The replication ability of OV depends on the viral infection ability, the characteristics of tumor cells, and the host resistance (Sostoa et al., 2020). Not all viruses are suitable for use as oncolytic viruses, and their selection depends on factors such as the potential pathogenicity, immunogenicity, and genes encoding the therapeutic ability of the virus (Russell et al., 2012). Several oncolytic viruses are currently in use, including adenovirus (Ad; Gállego Pérez-Larraya et al., 2022; Nisar et al., 2022; Yngve et al., 2022), herpes simplex virus (HSV; Kawamura et al., 2022; Scanlan et al., 2022; Shayan et al., 2022), vaccinia virus (VV; Zhou et al., 2020; Yang Z. et al., 2021), reovirus (Seyed-Khorrami et al., 2021; Yang C. et al., 2021), poliovirus (Ghajar-Rahimi et al., 2022; Wei et al., 2022), coxsackie virus (CV; Lu et al., 2020; Sarwar et al., 2021), Newcastle disease virus (NDV; Chan et al., 2021), vesicular stomatitis virus (VSV; Sarwar et al., 2021), myxoma virus (Villa et al., 2022), and some enteroviruses (Xu et al., 2021; Kolyasnikova et al., 2023).
Nonetheless, the ability to selectively infect certain tumors may also limit the virus’s applicability to specific tumor types. Su et al. found a way to overcome this limitation by employing the survivin promoter as a regulatory element to direct the adenovirus toward cancer cells (Su et al., 2022). Additionally, they reduced the expression of Elb-55kD to augment the virus’s tumor selectivity, allowing for adenovirus replication in various tumor cells while minimizing excessive viral replication in normal cells. Consequently, the modified adenovirus demonstrated a broad-spectrum anti-cancer effect.
2.2.1. Adenovirus
Adenovirus (Ad) is a type of double-stranded DNA virus. Ads are the primary focus of oncolytic virus research. Oncolytic adenovirus can infect almost all types of cells and is easy to prepare and purify. However, it also has the disadvantage that Ad in the body is easy to cause induction of autoimmunity to reject the Ad. Back in 2005, China approved the first OV, an Ad called H101 (Liang, 2012; Wei et al., 2018), for the treatment of squamous cell carcinoma of the head and neck (SCCNH; Garber, 2006). Ads are used to treat several types of cancer, including melanoma (García et al., 2019; Milenova et al., 2021), lung cancer (Watanabe et al., 2006), colorectal cancer (Kuhn et al., 2008), prostate adenocarcinoma (PRAD ; Chen et al., 2001; DeWeese et al., 2001; Ramesh et al., 2006; Small et al., 2006; Huang et al., 2008; Burke et al., 2012; Rojas-Martínez et al., 2013; Packiam et al., 2018; Oronsky et al., 2022), malignant glioma (MG; Chiocca et al., 2004; Lang et al., 2018; Kieran et al., 2019; Carpenter et al., 2021), ovarian cancer (OC; Kimball et al., 2010; Kim et al., 2013; Vassilev et al., 2015), head and neck cancer (HNC; Chang et al., 2009; Nakajima et al., 2009), and solid tumors (Lin et al., 2007; Li et al., 2009; Nemunaitis et al., 2010; Nokisalmi et al., 2010; Pesonen et al., 2012; Machiels et al., 2019).
Adenovirus is commonly modified by four methods: the first method involves modifying the gene sequence in the E1 region. For example, the E1b55K of the virus does not prevent the apoptosis pathway in normal cells, but it does in tumor cells (Cheng et al., 2015). The second method involves using tumor-specific promoters to replace the necessary promoters in Ad, such as the promoters of telomerase reverse transcriptase, to regulate E1A gene expression in cancer cells with high levels of telomerase reverse transcriptase (Tazawa et al., 2020). The third method is to modify the Ad capsid protein to make the virus bind more selectively to tumor cells (Wang et al., 2016; Franke et al., 2023). Furthermore, inserting genes to express pro-apoptotic proteins can enhance the ability of the virus to kill tumor cells (Cerullo et al., 2010). Some of the oncolytic adenoviruses under study are listed in Table 1.
2.2.2. Herpes simplex virus
Herpes virus is a group of DNA viruses enclosed by a cellular membrane. It exhibits strong replication ability and is commonly used in genetic engineering. Herpesvirus has numerous advantages, including a wide range of infected hosts, high infection efficiency, large genome capacity, clear gene and protein functions, and low pathogenicity. Research on HSV-1 in the herpesvirus group has shown that it has a positive effect on the treatment of tumor cells. HSV has demonstrated a significant effect on melanoma (Senzer et al., 2009; Kaufman et al., 2010; Andtbacka et al., 2015; Johnson et al., 2015; Killock, 2015; Puzanov et al., 2016; Chesney et al., 2018a,b; Andtbacka et al., 2019; Yun et al., 2022), breast cancer (BC; Teshigahara et al., 2004; Gholami et al., 2014; Fan et al., 2021; Parmar et al., 2022), HNC (Mace et al., 2008; Harrington et al., 2010), glioblastoma (Papanastassiou et al., 2002; Kanai et al., 2011; Patel et al., 2016; Omar et al., 2021), PRAD (Wang et al., 2019), peritoneal cancer (Nakano et al., 2005), colorectal cancer (CC; Kemeny et al., 2006; Geevarghese et al., 2010), pancreatic cancer (PAAD; Nakao et al., 2011; Kelly et al., 2016; Hirooka et al., 2018), solid tumor (Zhang et al., 2021), and other tumor cells. Currently, there are several strategies for HSV virus transformation, including deleting UL39 and other genes required for replication in nondividing cells and deleting the neural virulence gene ICP34.5 and immune escape-related gene ICP47 (Campadelli-Fiume et al., 2011). For example, in 2015, the T-VEC virus became the first OV approved by the FDA for the treatment of malignant melanoma (Johnson et al., 2015). The T-VEC deleted ICP34.5 and ICP47 genes and inserted foreign genes that can express immune-activating protein granulocyte-macrophage colony-stimulating factor (Harrington et al., 2015). The protein encoded by the ICP34.5 gene is critical for the infection of healthy cells. Therefore, deleting the ICP34.5 gene is a strategy to improve the virus’s tumor selectivity (Russell and Barber, 2018; Jin et al., 2021; Tian et al., 2022). However, deleting the ICP34.5 gene may make the virus more susceptible to clearance by immune cells (Yin et al., 2017). To avoid this, researchers have deleted the ICP47 gene to prevent the virus from being cleared by immune cells (Jin et al., 2021). And Japan’s Deltyact (testerpaturev/G47 Δ) also deleted these two genes and mutated the ICP6 gene (Frampton, 2022). The second strategy involves improving the targeting of the virus to tumor cells. For example, the glycoprotein of the virus can be modified to enhance the targeting of the virus to tumor cells (Menotti et al., 2008). In addition, the specific recognition receptor can also be constructed into the gene sequence of the virus to build a virus mutant that recognizes and expresses tumor cell-related receptors (Menotti et al., 2006; Grandi et al., 2010). The third strategy involves inserting immune stimulator-related genes to enhance local immune toxicity, such as GM-CSF, PD1/PD-L1, IL-2, etc. Table 2 shows some of the HSVs currently under study.
2.2.3. Vaccinia virus
Vaccinia virus is a giant double-stranded DNA virus that comes in two forms. It is a vaccine against smallpox infection with detailed human safety data. And have multiple mechanisms of action that target human and rodent tumors, making the virus more effective. For example, the Pexa-VEC contains a deletion of viral thymidine kinase gene (Chaurasiya et al., 2021). The advantage of utilizing VV to treat tumors is that it replicates quickly. The genome does not integrate itself into the host cell, and the genome is large enough to insert whole segments of foreign genes. VV is effective in targeting hepatocellular carcinoma (HCC) (Park et al., 2008; Heo et al., 2013), melanoma (Hwang et al., 2011), CC (Park et al., 2015), HNC (Mell et al., 2017), peritoneal cancer (Lauer et al., 2018), OC (Mori et al., 2019), BC (Beguin et al., 2020), glioblastoma (Breton et al., 2010), and some other tumors (Cripe et al., 2015; Downs-Canner et al., 2016; Peng et al., 2018). At present, the first modification strategy for the vaccinia virus is to delete the genes necessary for virus replication in normal cells, such as thymidine kinase (Parato et al., 2012), and vaccinia growth factor (McCart et al., 2001), so that the virus can only replicate in tumor cells. The second strategy is to insert genes encoding specific tumor-associated antigens or tumor-specific antigens to increase specific T-cell-mediated immune responses. In addition, immunogenicity is further enhanced by arming the virus or modifying the viral vector with immune-stimulating molecules such as interleukins and colony-stimulating factors (Guo et al., 2019). Some of the VV under-studying can be seen in Table 3.
2.2.4. Newcastle disease virus
Newcastle disease virus is an RNA virus that causes Newcastle disease in birds but has mild symptoms in humans. It is a typical example of oncolytic viruses. Recent studies have shown that NDV combined with radiotherapy and combined point inhibitors has been shown to improve the clearance rate of melanoma in mice (Vijayakumar et al., 2019). Similarly, NDV infects melanoma (Lazar et al., 2010), HNC (Prince et al., 2005), glioma (Freeman et al., 2006), solid tumor (Pecora et al., 2002), and other tumors (Yaacov et al., 2008). NDV can increase its number in tumor cells by using the B cell lymphoma overexpressed by tumor cells (Mansour et al., 2011). NDV is mainly used for immune regulation, such as by introducing IL-2 (Zamarin et al., 2009) or reverse genetics (Vigil et al., 2007) to improve the efficacy of tumor lysis. One example shows that the L289A mutation of the F gene has increased cytotoxicity, which can be used to treat HCC patients with improved safety and effectively (Altomonte et al., 2010). Some of the NDVs under-studying can be seen in Table 4.
2.2.5. Reovirus
A naturally occurring double-stranded RNA virus, reovirus normally infects the respiratory and intestinal systems of mammals. The oncolytic ability of reovirus is associated with the good replication and dissolution of these cells found in various cancer cell lines. The virus works against a variety of cancers, including BC, SCCNH, melanoma (Galanis et al., 2012; Mahalingam et al., 2017), multiple myeloma, PAAD, CC (Goel et al., 2020; Chaurasiya et al., 2021), glioma (Forsyth et al., 2008; Kicielinski et al., 2014), and lung cancer. Reovirus likes to replicate in cells with dysregulated growth factor signaling cascade (Shmulevitz et al., 2005). In tumor cells lacking anti-virus PKR signal transduction defects, tumor lysis can occur directly. At the same time, cytotoxicity mediated by NK cells and T cells is a determining factor for anti-tumor efficacy (Rajani et al., 2016). Transformed cells that activate the Ras signaling pathway are tolerant to reovirus by enhancing viral membrane detachment, increasing particle infectivity, and viral apoptosis release (Marcato et al., 2007). The reovirus situation can be seen in Table 5.
2.2.6. Some other viruses
In 2004, Latvia launched Rigvir (Doniņa et al., 2015), a naturally occurring virus with oncolytic features, which is used to treat melanoma, CC, PAAD, BDC, renal carcinoma, PRAD, lung cancer, uterine cancer, lymphoid sarcoma, gastrointestinal cancer (GC), and other cancers (Alberts et al., 2016; Brokane et al., 2019). A case study involving the use of Rigvir therapy demonstrated the therapeutic value of this treatment in advanced melanoma. The patient, a 1972-born female, was diagnosed with malignant melanoma in the lumbar region. Following surgical and chemotherapy interventions, the tumor showed a poor response and was associated with severe systemic side effects (Alberts et al., 2016). In February 2013, the patient began receiving Rigvir treatment as the sole therapy. After treatment, the size of the inguinal lymph nodes was reduced by half, and the patient’s condition remained stable until the time of publication in 2016. These findings highlight the significant therapeutic potential of Rigvir in late-stage melanoma (Alberts et al., 2016).
Coxsackie virus (CV) is an enterovirus that infects people with symptoms such as fever, cough, and cold. Research by British scientists has shown that CV has the potential to target, infect, and destroy cancer cells in patients with BDC (Annels et al., 2019). CV is also used against many types of cancer (Bradley et al., 2014; Li et al., 2022), including melanoma, BDC, lung cancer, and PRAD. It can enter tumor cells through cells via intercellular adhesion molecule 1 (CD54), decal accelerating factor (CD55), or connectors that may be overexpressed in multiple myeloma, melanoma, breast cancer, and other tumor cells (Shafren et al., 2004; Au et al., 2007; Guo et al., 2014).
Poliovirus is a tiny RNA enterovirus that invades nerve cells, and humans are the only host for the virus. Poliovirus can be used as a new cancer treatment tool (Brown et al., 2014). The poliovirus solely relies on the CD155 receptor to enter the host cell and CD155 is abundant in malignant cells (Gromeier et al., 2000). This CD155 receptor may damage the response of anti-tumor immune cells and be overexpressed by tumor cells (Carlsten et al., 2009). Poliovirus is used to treat glioblastoma, BDC, melanoma, and other symptoms. In 2018, PVSRIPO, a treatment developed at Duke University, significantly improved long-term survival in patients with recurrent glioma. However, some studies have shown the cytotoxicity of poliovirus (Goetz et al., 2010; Brown and Gromeier, 2015).
In 2014, the research group Yan found that the natural oncolytic virus M1 can selectively infect a variety of human cancers with ZAP deficiency (Lin et al., 2014), including Hepatocellular carcinoma (HCC), BDC, and melanoma, and it has no toxic side effects on normal cells (Zhang et al., 2016; Cai and Yan, 2021).
There are also relevant studies on other oncolytic viruses, such as measles virus (Hasegawa et al., 2006; Galanis et al., 2010; Zhang et al., 2012; Aref et al., 2016; Dispenzieri et al., 2017; Packiriswamy et al., 2020), H1 Parvovirus (Geletneky et al., 2017; Hajda et al., 2017), myxoma virus (Cornejo et al., 2020), Seneca Valley virus (Rudin et al., 2011; Burke et al., 2015; Burke, 2016; Schenk et al., 2020), and VSV (Tober et al., 2014; Schreiber et al., 2019), to prove the development of these OVs in fighting tumors.
Table 6 shows some of the viruses currently under study.
2.3. Clinical research status of oncolytic virus
By visiting Clinicaltrials.gov and searching for the keyword “oncolytic,” 186 clinical study registries were identified, 147 of which had detailed clinical data. The remaining clinical trial registrations were rejected for further analysis because their status was withdrawn, terminated, unknown, or suspended and because the articles did not conform to the contents of this review. Further analysis of 147 clinical study registries was performed, and multiple variables were assessed, including clinical trial phase, number of patients, route of administration, and indications of application. A complete list of clinical study registries is in Tables 1-6.
2.3.1. Research stage
A total of 7,776 cancer patients were enrolled and completed 147 clinical trials. Most clinical studies were in Phase I (n = 72), Phase I/II (n = 34), Phase II (n = 36), and Phase III (n = 5; Figure 1). This shows that OVs research is currently in its early stages and has great potential for development.
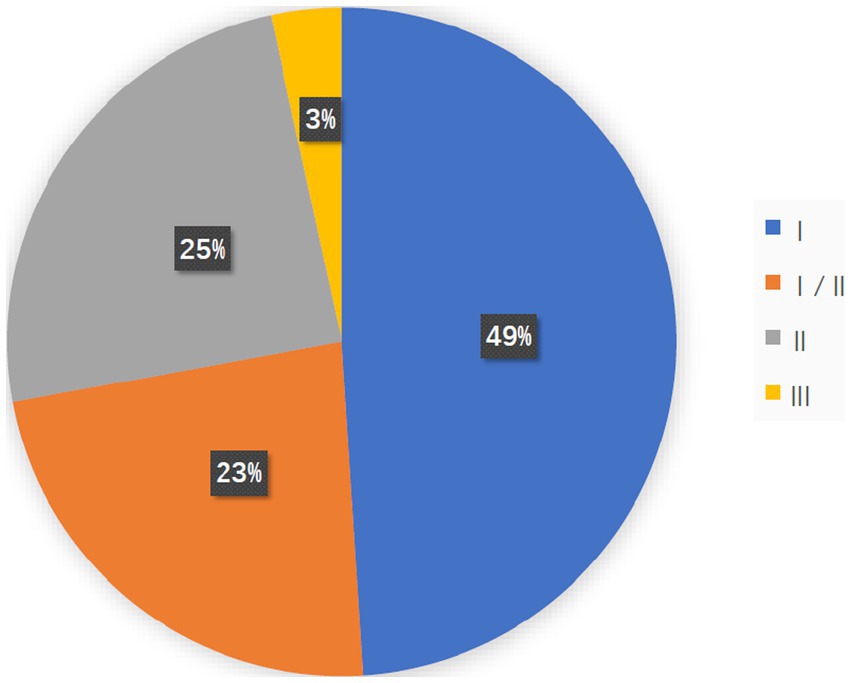
Figure 1. The pie chart shows the study stage of 147 clinical studies, most of which were in Phase I (n = 72, 48.9%), Phase I/II (n = 34, 23.1%), and Phase II (N = 36, 24.5%) and Phase III (n = 5, 3.40%).
2.3.2. Route of administration and indications of application
A collation of clinical study registries found that 73 trials (49.7%) involved intratumor injection, and 43 (29.3%) involved intravenous injection. There were 5 (3.4%) experiments of both intratumor injection and intravenous injection, eight involving Intraperitoneal administration, five involving intravesical injections, four involving Convection-enhanced delivery (CED), two involving intraperitoneal injections, one involving intra-arterial injection, and six without explanation of how to conduct the administration (Figure 2A).
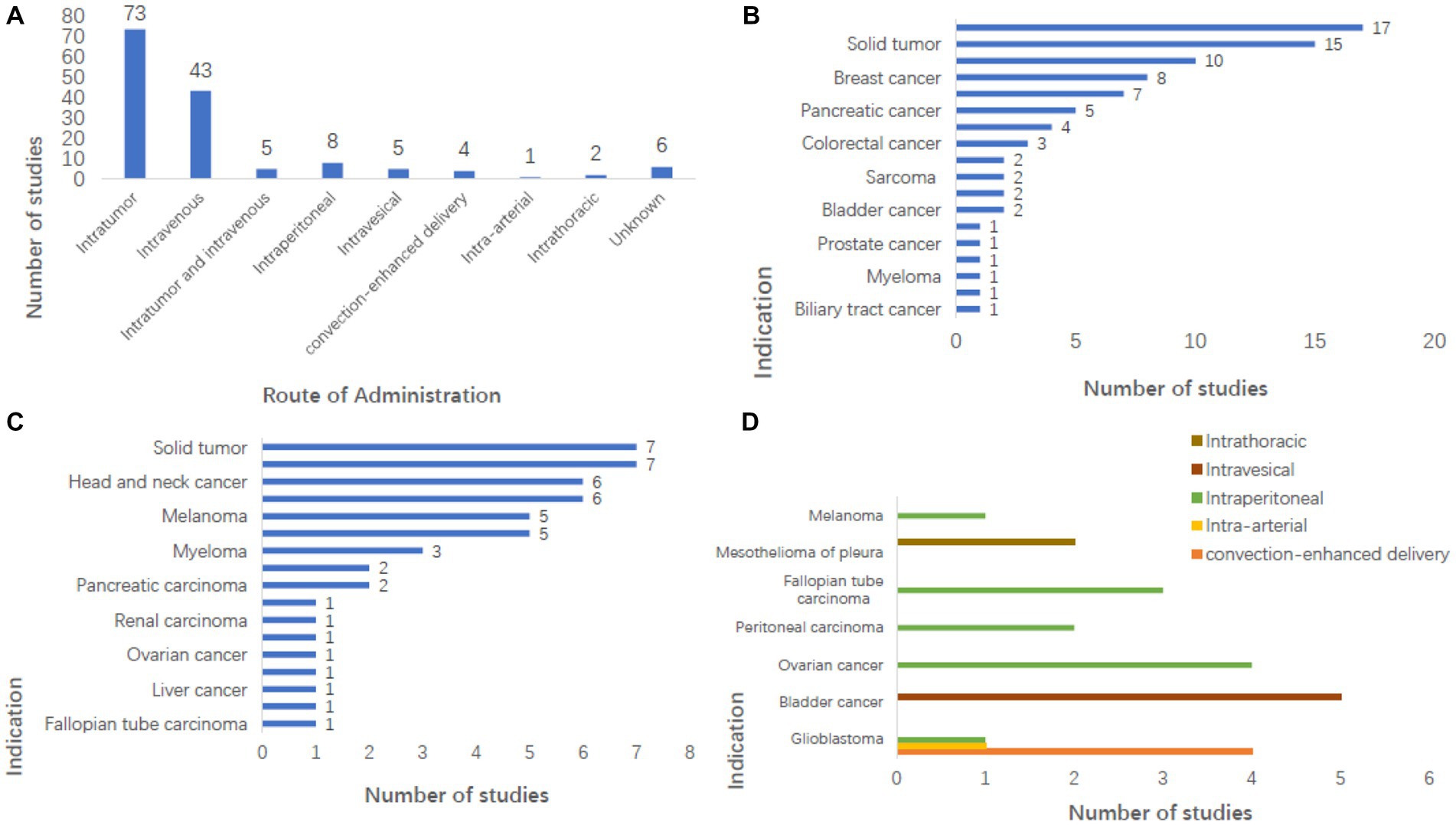
Figure 2. Analysis of administration pattern and indications in clinical study registry. (A) Intratumoral injection (n = 73) and intravenous injections (n = 43) were the main administrative methods in the study, and the number of other administrative methods is shown in the figure. (B) Shows the number of studies on tumors where intratumoral injection can be used, among which melanoma has the largest number of studies (n = 17, 20.5%), which are mostly used in benign solid tumors but also some malignant tumors. (C) Shows the number of studies of tumors that can be injected intravenously, the majority of which are malignant solid tumors. (D) Showing tumors that can be treated by administration other than intratumoral and intravenous injections, it can be seen that glioblastoma is a tumor that can be treated by virtually every administration method.
Tumor site administration is also referred to as an intratumoral injection. Currently, intratumoral virus injection, which allows the virus to expand in tumor cells, is the preferred method for most OVs in clinical and development stages. However, intratumoral injection becomes very difficult once tumor cells develop total metastasis due to the need to accurately locate tumor cells and the difficulty of monitoring and controlling the therapeutic effect. Although OVs will not migrate to the location of the metastatic tumor, they will activate the host’s anti-tumor response to control the metastatic tumor (Twumasi-Boateng et al., 2018; Hinterberger et al., 2021). The intratumoral injection is used for melanoma, glioblastoma, BC, liver cancer, PAAD, HNC, CC, BDC, and other benign solid tumors (Figure 2B). A total of 4,411 patients received intratumoral injections, including over 1,300 in the melanoma study. At the same time, many articles have studied the effect of intratumoral injection (Li et al., 2008; Nakao et al., 2011; Morris et al., 2013; Streby et al., 2017; Hirooka et al., 2018).
Intravenous injections avoid the need to look for specific tumor cells and allow for a single treatment option for the patient, something that intratumoral drugs cannot. Intravenous injection can accurately deliver the drug to a location that the local injection cannot reach. However, drugs given intravenously need to be minimal or harmless to normal cells in the body, and some neutralizing substances in the blood may neutralize the virus, and the immune system’s response may eliminate the virus too quickly to be effective in treating tumor cells. In addition, the combination of the virus with certain components in the blood limits the delivery route of the virus and makes treatment less effective (Kaufman and Bommareddy, 2019). It may even produce adverse reactions of varying degrees (Imperiale, 2008). And Intravenous injection is one of the main research directions at present (Laurie et al., 2006; Lorence et al., 2007; White et al., 2008; Comins et al., 2010; Breitbach et al., 2011a; Chakrabarty et al., 2013; Park et al., 2015; Garcia-Carbonero et al., 2017; Mell et al., 2017; Streby et al., 2019; Beguin et al., 2021).
Several oncolytic viruses are being tested intravenously, including REOLYSIN®(Pelareorep; Adair et al., 2012; Galanis et al., 2012), Enadenotucirev (ColoAdl; Garcia-Carbonero et al., 2017; Marino et al., 2017; Machiels et al., 2019), HSV1716 (Streby et al., 2019), JX594 (Breitbach et al., 2011b), MV-NIS (Dispenzieri et al., 2017), NDV-HUJ (Freeman et al., 2006), PV701 (Hotte et al., 2007), RT3D (White et al., 2008; Karapanagiotou et al., 2012), vvDD (Downs-Canner et al., 2016), and TG6002 (Beguin et al., 2021). Other OVs such as Seneca Valley virus and Coxsackievirus A21, have also been studied to show the feasibility and safety of intravenous administration (Kennedy et al., 2022). Intravenous injection can be used for lung cancer, HNC, CC, gynecological tumor, and other malignant tumors (Figure 2C). The number of patients who received intravenous injections was 2,198, accounting for 28% of the total.
Intra-arterial injection, in which the virus is selectively transported throughout the body, and because of the limited volume of the blood and target organs, can avoid the reaction of the antibodies in intravenous injection with the virus, which can reduce the effectiveness of the virus. Experiments on the treatment of OVs with intrahepatic artery administration have been conducted (Reid et al., 2002; Fong et al., 2009). Quantum-enhanced delivery can be used for the treatment of glioblastoma. Intravesical injection, intraperitoneal injection, and intrathoracic injection are all used for tumors in the bladder, abdominal cavity, and thoracic cavity (Figure 2D).
Although the effect of intratumoral injection of OVs is good, intravenous and arterial administration also has a certain effect. The elimination of OVs by antibodies in the body, the destruction of viruses by complements, and the clearance of viruses by organs and tissues such as the liver, spleen, and kidney will affect the effect of OVs in treating tumors (Kolb et al., 2015; Roy et al., 2020). Therefore, many studies have attempted to use carrier tools to improve the problem of how to avoid being attacked by the immune system after intravenous administration, such as using liposomes (Iscaro et al., 2022), nanoparticles (Fusciello et al., 2019), immunoliposomes (Li et al., 2016), mesenchymal stem cells (Naji et al., 2019; Franco-Luzon et al., 2020), and other carrier tools.
Mesenchymal stem cells have great advantages as the carrier tool of OVs in systematic administration because they can be isolated from a variety of tissues, have low immunogenicity, and possess chemotaxis to solid tumors (Volarevic et al., 2018). Mesenchymal stem cells can be used as the cell carrier of OVs for multiple injections and as the replication site of OVs to generate more progeny viruses and promote the tumor-killing effect of OVs. In the latest research, mesenchymal stem cells have the characteristics of chemotaxis to solid tumors (Najar et al., 2016; Karagiannis et al., 2017; Vangala et al., 2019; Kostadinova and Mourdjeva, 2020). At the same time, some studies have shown that cytokines secreted by tumor cells are one of the key reasons for inducing mesenchymal stem cells to chemotaxis to tumor cells (Lejmi et al., 2015; Pavon et al., 2018), and which may promote the occurrence of anti-tumor immunity (Morales-Molina et al., 2018).
Although mesenchymal stem cells carrying oncolytic viruses have shown potential efficacy in preclinical studies, but their effectiveness in clinical trials is still being evaluated. They can also promote the occurrence of tumor cells, inhibit apoptosis of tumor cells, and promote tumor metastasis through a variety of mechanisms (Naji et al., 2019; Atiya et al., 2020; Kostadinova and Mourdjeva, 2020). There are some controversies about the delivery of oncolytic virus by mesenchymal stem cells, but in general, the destructive effect on tumors may be greater than the promoting effect.
2.4. Combination therapy with oncolytic virus
Oncolytic viruss can enhance the exposure of tumor antigens, regulate the tumor microenvironment, activate immune cells, and so on through a variety of ways to induce the anti-tumor immune response of the whole-body system to kill tumor cells. The clinical efficacy of first-generation OVs (weakly effective wild-type or naturally occurring variants) was limited, while the new generation of genetically modified OVs has achieved tumor eradication in clinical studies (Bilsland et al., 2016; Russell and Barber, 2018; Jin et al., 2021; Tian et al., 2022). However, current research trends favor the use of combination therapies because monotherapy with OVs faces challenges such as T cell loss due to tumor heterogeneity and the immunosuppressive microenvironment and the difficulty of expression of inhibitory molecules in tumor microenvironment (Zou et al., 2023). Clinical studies have confirmed that the combination of OVs with chemotherapy and the combination of OVs with immunotherapy have achieved remarkable therapeutic effects, surpassing the efficacy of chemotherapy or immunotherapy alone (Tian et al., 2022; Zou et al., 2023). A tumor is not a large number of isolated cancer cells but a complex tissue composed of many different types of cells that perform heterogeneous interactions with each other. This is one reason why a single drug cannot cure cancer completely. Of the 147 clinical registries, 82 (55.8%) were combined studies. Among them, 41% were combined with immune checkpoint inhibitors, and 31% were combined with chemotherapy (Figure 3A). The tumors that can be tested include most solid tumors of the brain, chest, abdomen, and genitals (Figure 3B), which can be treated in combination with other conventional tumor treatment methods. Therefore, OVs can exert greater benefits, promote and increase therapeutic efficacy, and give the oncolytic virus more attention in the international clinical oncology community (Roulstone et al., 2015; Husseini et al., 2017).
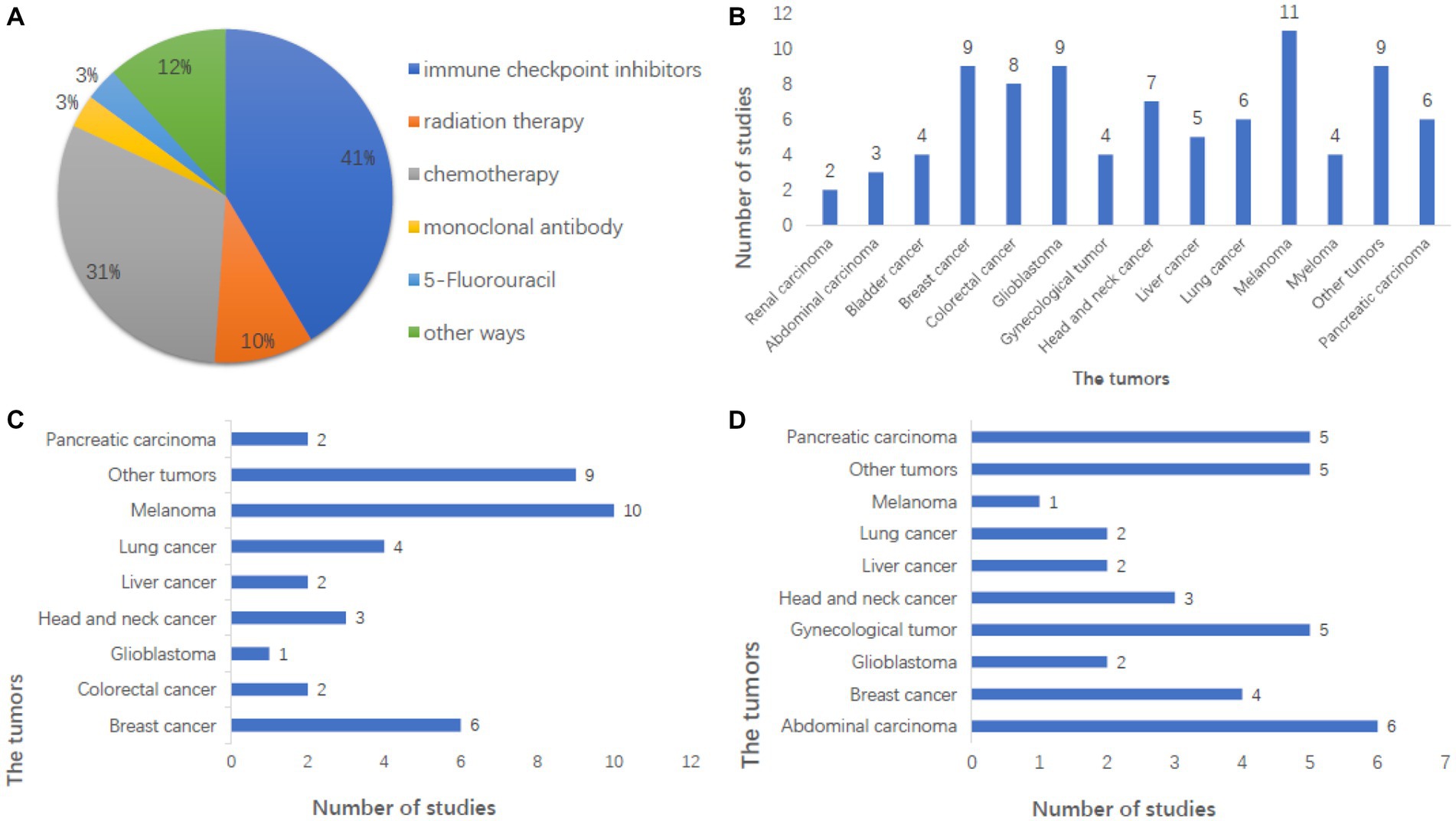
Figure 3. Analysis of combination therapy in the registry of clinical studies. (A) Combination therapy in the study was mainly immune checkpoint inhibitors and chemotherapy, and the other combination therapy methods were also studied in A certain number of studies. (B) This shows the number of studies on tumors that can be treated with combination therapy, with melanoma being the largest. And breast cancer and glioblastoma also have a large number of studies, including the majority of solid tumors. (C) Showed the number of studies on tumors treated with immune checkpoint inhibitors combined with OVs, with melanoma being the largest, accounting for 25.6%. (D) This shows the number of studies on tumors treated by chemotherapy combined with OVs, with the largest number being abdominal tumors, followed by brain tumors.
The efficacy of immune checkpoint inhibitors has been widely recognized for their efficacy in cancer treatment, but the response rate is not high, and patients with programmed cell death protein 1 (PD-1) resistance make tumor treatment more difficult. Studies have shown that OVs combined with checkpoint inhibitors can significantly improve the objective response rate of various solid tumors, induce a large number of immune cells to infiltrate tumors, and change the tumor microenvironment (Chon et al., 2019), thus enhancing the anti-tumor activity of immunotherapy and the survival rate of human infected with tumor cells. It is an ideal immune platform to enhance immune responses and tumor types in patients with poor response to immune checkpoint suppression, inducing auto proliferation and antitumor activity through a variety of mechanisms. Melanoma was the most tested tumor in combination with immune checkpoint inhibitors, followed by breast cancer (Figure 3C). In 2017, the efficacy of T-VEC combined with PD-1 antibody in the treatment of melanoma was significantly improved (Drescher et al., 2019). The combination of PD-1 inhibitors and oncolytic viruses seems to be the darling of the new age for treating cancer cells, and it is showing signs of potential in clinical trials (Havunen et al., 2021).
Chemotherapy combined with OVs is the most common combination. Chemotherapy is one of the main therapeutic methods that induce DNA damage by inhibiting DNA synthesis, mitosis, and cell division of cancer cells with intracellular toxins and other chemicals. Tumors treated by chemotherapy combined with OVs include celiac tumors, breast cancer, etc. (Figure 3D). Not only does Pelareorep have research reports on the treatment of combined chemotherapy (Mahalingam et al., 2018, 2020) that show that combined chemotherapy is effective, but also other types of oncolytic viruses have research reports that combined chemotherapy is safe (Karapanagiotou et al., 2012; Siurala et al., 2015). Radiotherapy combined with OVs therapy is also a common combination, and some studies show the safety of combined radiotherapy (Markert et al., 2014).
Surgical treatment can generally cure early tumors, but once the tumor metastases, surgery is very difficult, and the tumor cannot be completely cleared. Radiation and chemotherapy have limited effects, serious damage to normal cells and tissues, and great side effects. The overall effectiveness of antibody therapy is not high, and drugs are difficult to break through the barrier and tumor microenvironment. In comparison, OV therapy is safe and reliable, more effective than other immunotherapies, has a simple preparation method, low price, and wide adaptability, and can be used for a variety of cancers. The opportunity for OVs to combine drugs in cancer is huge. It can alter the tumor microenvironment and induce immune cells to infiltrate tumor cells, and various undiscovered anti-tumor properties are being revealed through experiments.
3. The process of treating a tumor with an oncolytic virus
3.1. Mechanism of action of oncolytic viruses
The oncolytic virus represents a promising immunotherapeutic agent in current cancer therapy. Its anti-tumor activity is mediated by a variety of mechanisms that impact the immune system in tumors.
Natural oncolytic viruses exhibit poor controllability, weak killing ability toward tumor cells, and are easily cleared by the body’s immune system. In contrast, the genetically modified oncolytic virus can be highly expressed in specific areas of the body to avoid triggering the systemic immune response, thereby prolonging the virus’s action time and enhancing its ability to kill tumor cells.
Tumor cells in the tumor suppressor gene are inactivated or missing after their antiviral infection ability is abated to allow OVs to multiply and ultimately destroy tumor cells in the body. When tumor cells are infected by the virus, they break and die, and then new virus particles are released, further infecting the surrounding tumor cells. This is a kind of antitumor mechanism similar to the chain reaction (Figure 4). OVs mainly eliminate tumor cells through the host’s immune system. That is, OVs not only kill tumor cells on their own but also stimulate the body’s immune system to enhance the effect of treating tumor cells (Kelly and Russell, 2007). This can be applied both systematically and locally and is much better than traditional treatments for cancer cells. OVs fight tumor cells in four ways: oncolysis, antitumor immunity, transgenic expression, and collapse of blood vessels supplying tumor cells (Gujar et al., 2019).
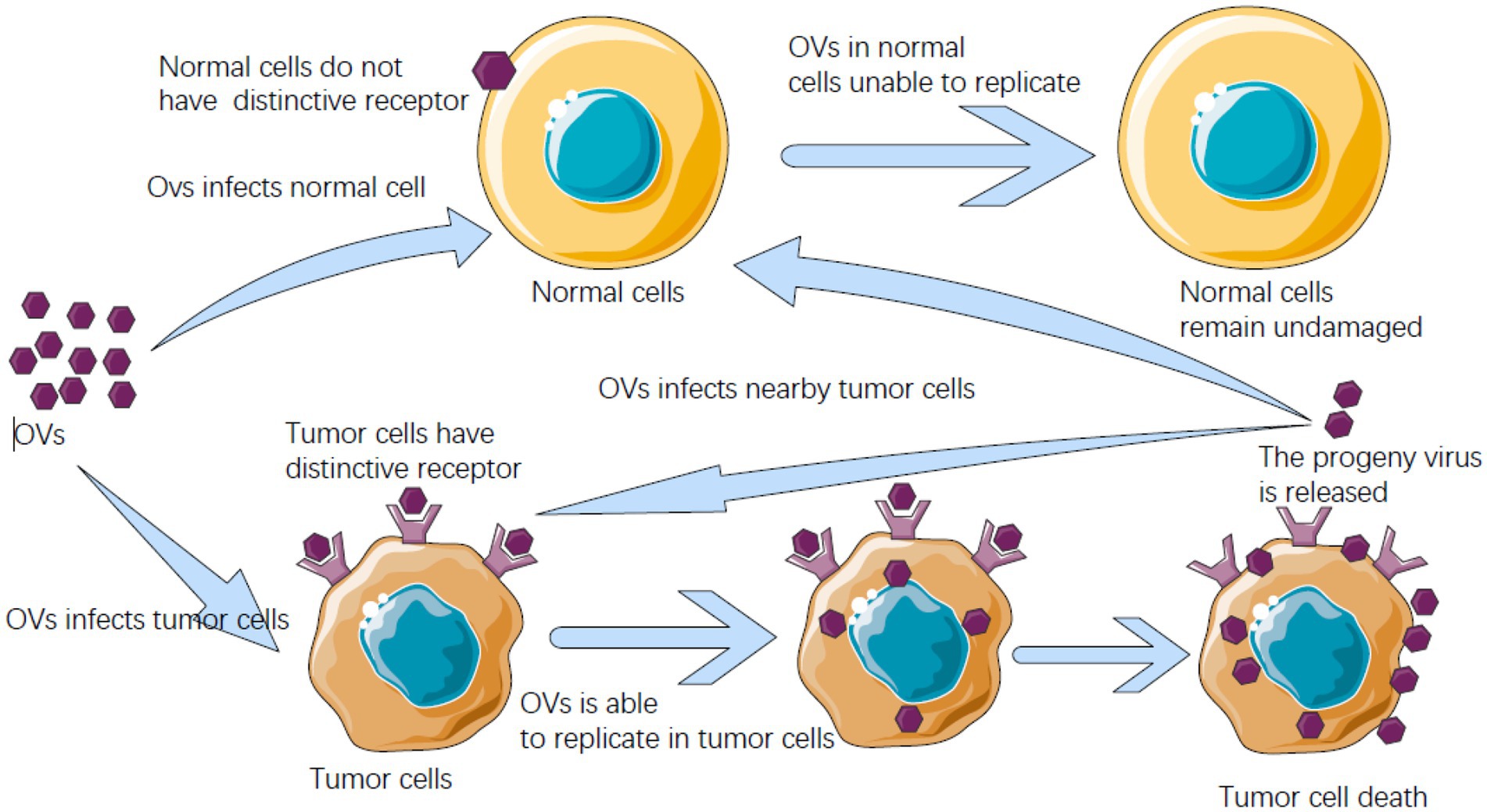
Figure 4. Oncolytic virus (OV) after entering the host can identify receptors on cells, meet with receptors unique to cancer cells can enter the cancer cells, and can breed will kill cancer cells in cancer cells, and cancer cells burst into offspring OVs are released, and can infect nearby cancer cells and kill, and in the normal cells of the human body does not have this kind of peculiar receptor, OVs cannot get into normal cells to multiply.
The mechanism of action of OVs can be roughly divided into two parts, one is the direct tumor-killing mechanism mediated by a virus, and the other is the anti-tumor immune response mechanism activated by the body to kill tumor cells.
3.2. Oncolytic viruses enter tumor cells
Oncolytic refers to the ability to dissolve tumor cells. To enhance the effectiveness of OVs in targeting tumor cells, research, and development are focused on tumor-specific targeting. Currently, many OVs can mutate to target the surface proteins abnormally expressed by cancer cells, weakening the antiviral ability of tumor cells and providing OVs with an opportunity to enter the tumor cells.
For example, adenoviruses can be designed to target the unique receptors expressed by tumor cells (DeWeese et al., 2001), or they can be inserted into the promoters in tumor cells to utilize the signal pathways to enter tumor cells directly. HSV may enter tumor cells through herpesvirus entry mediator or Nectin (Yu et al., 2005), which are overexpressed in cancer cells, especially melanoma, or it can be designed to bind integrins highly expressed on tumor cells (You et al., 2001). Vaccinia virus can replicate in some cancer cells that overexpress epidermal growth factor receptors by enhancing the induction of RAS signal (Parato et al., 2012). NDV can increase its number in tumor cells by using the B cell lymphoma overexpressed by tumor cells (Mansour et al., 2011). In normal healthy cells, reovirus can enter the cells and start to produce viral RNA, thus activating the double-translated RNA-dependent protein kinase pathway, which is an immune mode that can inhibit protein translation and prevent virus transmission (Bischoff and Samuel, 1989). However, the double-translated RNA-dependent protein kinase pathway will not be activated in cancer cells, causing the virus to infect tumor cells and eventually leading to the splitting of cancer cells (Strong et al., 1998).
At the same time, many OVs can rapidly replicate by using the defect of the antiviral mechanism of tumor cells in type I interferon signal (Stojdl et al., 2000; Wilden et al., 2009). The measles virus can use the surface receptor CD46, a factor that prevents cells from being destroyed and is overexpressed by cancer cells through the complement pathway of the immune system (Dorig et al., 1993; Anderson et al., 2004), or it can be designed to recognize carcinoembryonic antigen and directly enter tumor cells (Hammond et al., 2001).
3.3. Tumor-killing mechanism mediated by an oncolytic virus
Here are some ways in which OVs can kill tumors.
Firstly, through virus-mediated cytotoxic killing, OVs use the energy and raw materials of tumor cells as a breeding site for their proliferation. The virus can inhibit the growth of tumor cells and use the cell as a factory for replication and lysis of tumor cells. After the release of the progeny virus, it continues to infect neighboring tumor cells, creating a cycle that continues until the immune response weakens the replication of the virus or the tumor cells in the host are depleted (Hamid et al., 2017). Because the antiviral response of tumor cells has certain defects, oncolytic viruses will preferentially replicate in tumor cells, while in normal cells, viruses can hardly replicate due to the presence of interferon and related factors. Additionally, some viruses can produce substances with certain cytotoxicity and oncolytic activity. For example, proteins encoded by the E3 region of Ad can directly mediate the lysis of tumor cells (Suzuki et al., 2002).
Secondly, OVs can indirectly kill tumors by destroying the vascular system of tumor cells. Tumor growth depends on the nutrients provided by the tumor vascular system, so the destruction of the tumor vascular system can effectively inhibit tumor growth (Figure 5A). Angiogenesis is one of the markers of malignant tumor cells, and research shows that oncolytic viruses can specifically infect and destroy tumor vascular endothelial cells and stromal cells, as well as destroy tumor blood vessels by promoting the production of endostatin and angiostatin (Hutzen et al., 2014). For example, the oncolytic vaccinia virus has been proven to inhibit the formation of vascular endothelial cells of tumor cells, thereby damaging the tumor vascular system and inhibiting the formation of new blood vessels. This reduces the blood flow to supply tumor cells and ultimately limits the growth and development of tumors (Goradel et al., 2017; Hashemi Goradel et al., 2018). VSV can directly infect and damage tumor blood vessels in the body without affecting normal blood vessels (Breitbach et al., 2011b).
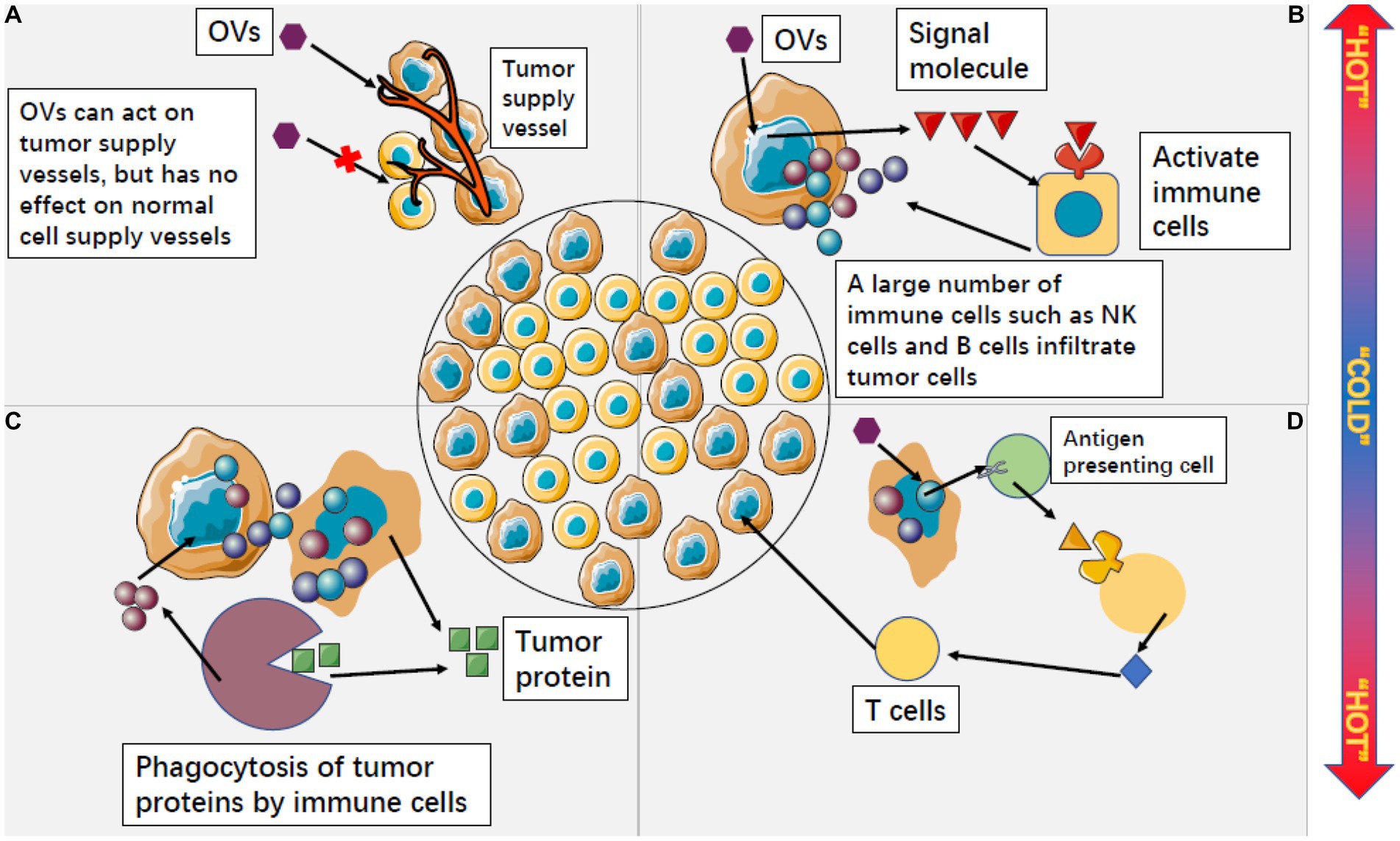
Figure 5. Oncolytic viruses can change the microenvironment of tumor cells from “cold” to “hot.” (A) OVS can specifically attack the supply vessels of tumor cells, making tumor cells have no nutritional supply to kill tumor cells, while OVS have no response to the supply vessels of normal cells. (B) After OVs act on tumor cells, tumor cells can release a large number of signal molecules to activate non-specific immune cells outside tumor cells. A large number of activated immune cells infiltrate tumor cells and accelerate the process of killing tumor cells. (C) Cleaved tumor cells will release a large amount of tumor protein, but non-specific immune cells can use this tumor protein to continuously play an immune role and form a long-term anti-tumor effect. (D) OV activates specific antigens in tumor cells, which can be expressed by antigen-presenting cells and induce the killing effect of T cells.
3.4. Mechanism of anti-tumor immune response induced by oncolytic viruses
The tumor-bearing host cannot make an effective immune response to prevent tumor growth. The inhibition state of the tumor microenvironment is due to the inactivation of cytotoxic T lymphocytes (Jiang and Shapiro, 2014) or active inhibitory T cells (Postow et al., 2018), leading to tumor immune escape.
Oncolytic viruss have a strong stimulation effect on the immune cells in the existing tumor tissues and can greatly change the tumor microenvironment, changing the tumor from cold to hot. By doing so, OVs can convert the immunosuppressive tumor microenvironment into an immunostimulatory one, thereby promoting anti-tumor immune responses (Chaurasiya et al., 2018; Sivanandam et al., 2019). Tumor cells infected with OVs can express some signaling molecules that induce immune cells outside the tumor to infiltrate the tumor in large numbers (Figure 5B; Ribas et al., 2017). Activated nonspecific immune cells kill and engulf other tumor cells, and lysed tumor cells release large amounts of tumor proteins that can be engulfed by innate immune cells and produce long-term adaptive immune responses against tumor-specific antigens (Figure 5C; Del Papa et al., 2019; Chouljenko et al., 2020; Kdimati et al., 2021). Some tumor-specific antigens can also be expressed by antigen-presenting cells, inducing T cells to attack uninfected tumor cells. This process is similar to a vaccine, but it occurs within tumor tissues and ultimately induces the killing effect of CD8 T cells, effectively preventing tumor recurrence and metastasis (Figure 5D; Macleod et al., 2014; Eissa et al., 2021; Yamashita et al., 2021).
Type I interferon can not only promote the immune response of clearing a virus but also reduce cell proliferation and activate apoptosis-promoting protein p53 (Takaoka et al., 2003). Therefore, OVs are more specific for tumor cell environment or an environment with limited type I interferon response.
The anti-tumor effect of the oncolytic virus seems to have immune memory. Some experiments have shown that the efficacy of OV injected into the mice immunized with OV in advance is better than that of the mice immunized without OV (Zhu et al., 2007). At the same time, some studies have shown that repeated intratumoral injection of OV can prolong its anti-tumor effect and induce lasting anti-tumor memory (Ghonime et al., 2018). It is possible that vaccines that have been injected as OV, such as the measles virus, may also act on anti-tumor (Qi et al., 2018; Leb-Reichl et al., 2021), but may also inhibit the anti-tumor effect (Laksono et al., 2018; Rudak et al., 2021).
Although OVs infection can trigger anti-tumor immunity, the immune system can also inhibit the virus, leading to reduced efficacy. OVs may cause the human body to acquire live viruses with acute toxicity and, in some cases, may cause latent infections and chronic diseases. This pathogenicity largely depends on the type of virus, virulence, immune destructiveness, and the host’s immune response. At present, no serious adverse events have been reported in the clinical trials, but the follow-up of the experimental patients is incomplete. Most OVs currently use toxicity reduction to prevent acute and chronic poisoning (Mejias-Perez et al., 2018; Nakatake et al., 2019).
As a pathogen, the pathogenicity of the oncolytic virus cannot be ignored. Some viruses may damage normal tissues during tumor lysis, so the biological safety of oncolytic virus therapy deserves further research, especially for people with low immunity. After chemotherapy, radiotherapy, and other traditional treatment schemes for cancer patients, bone marrow is usually damaged, and the body cannot produce a normal number of red blood cells, white blood cells, and platelets. When the body does not have enough white blood cells, oncolytic virus therapy is fatal (Perkins, 2002). Although some oncolytic viruses are not toxic, the body does not have enough immunity to resist the oncolytic virus, which will lead to toxic reactions. T-VEC guidelines clearly state that people with low immunity or who are pregnant should avoid using T-VECs. At present, the potential safety problems and long-term adverse events of the oncolytic virus are still unclear (Li et al., 2020).
4. Problems and challenges of oncolytic virus therapy for tumors
Although OVs can respond well to tumor cells, they still face several challenges. One challenge is how OV manipulates the host’s immune system to promote anti-tumor immune responses while limiting antiviral immune responses and virus clearance with minimal damage (Tan et al., 2020; Wu et al., 2021). Systemic drug administration has higher value and application prospects and is expected to cure metastatic tumors or blood tumors (Zhang et al., 2020; Tang et al., 2021; Xie et al., 2021). However, the therapeutic effect of systemic drug delivery is not ideal, and researchers need to spend a lot of energy to limit the antiviral immune response of systemic drug delivery. Using cell vectors to deliver oncolytic viruses to tumors without damaging surrounding tissues is one strategy to overcome this challenge.
Another huge challenge is to select the right patients to use OVs for clinical treatment. Tumor patients receiving OV treatment may have already undergone multiple conventional tumor treatments, which may have damaged their immune systems. One of the mechanisms underlying the efficacy of oncolytic viruses is their ability to trigger immune responses in cancer patients (Chaurasiya et al., 2021). Therefore, future research can focus on how to assess the immune system function of tumor patients and determine whether they should receive oncolytic virus therapy based on these evaluations. And selecting appropriate biomarkers may effectively improve the treatment of OVs.
As a live virus, OV can activate the immune system of the body. How to inhibit antiviral immunity and enhance anti-tumor immunity is a new challenge in the treatment process. Moreover, current research shows that OV needs to be combined with some tumor therapies to achieve the maximum therapeutic effect. In the future, the application value of monotherapy remains to be explored.
5. Conclusion and prospects
Although OVs therapy has made great progress, there are many obstacles in the process of treatment. With the continuous development of science and technology, as well as people’s in-depth research on the mechanism of action of oncolytic virus, the mode of administration, and the pathogenesis of tumors, the use of an oncolytic virus, combined with other therapies to treat tumors and other methods provide a new direction for targeted treatment of tumors. Oncolytic viruses will become the main force in the treatment of tumors in the future.
Author contributions
XL, XZ, and TT conceived the work. XZ, CF, ZX, MC, and ZL wrote and drafted the manuscript. XZ, ZX, TT, and XL discussed and edited the manuscript. All authors contributed to the article and approved the submitted version.
Funding
This work was supported partly by The Wu Jieping Medical Foundation Clinical Research Special Grant—Application of High-Throughput Rapid Detection Technology for Pathogenic Pathogens (No. 320.6750.2021-06-29).
Conflict of interest
The authors declare that the research was conducted in the absence of any commercial or financial relationships that could be construed as a potential conflict of interest.
Publisher’s note
All claims expressed in this article are solely those of the authors and do not necessarily represent those of their affiliated organizations, or those of the publisher, the editors and the reviewers. Any product that may be evaluated in this article, or claim that may be made by its manufacturer, is not guaranteed or endorsed by the publisher.
References
Abate-Daga, D., Andreu, N., Camacho-Sanchez, J., Alemany, R., Herance, R., Millan, O., et al. (2011). Oncolytic adenoviruses armed with thymidine kinase can be traced by PET imaging and show potent antitumoural effects by ganciclovir dosing. PLoS One 6:e26142. doi: 10.1371/journal.pone.0026142
Adair, R. A., Roulstone, V., Scott, K. J., Morgan, R., Nuovo, G. J., Fuller, M., et al. (2012). Cell carriage, delivery, and selective replication of an oncolytic virus in tumor in patients. Sci. Transl. Med. 4:138ra77. doi: 10.1126/scitranslmed.3003578
Alberts, P., Olmane, E., Brokane, L., Krastiņa, Z., Romanovska, M., Kupčs, K., et al. (2016). Long-term treatment with the oncolytic ECHO-7 virus Rigvir of a melanoma stage IV M1c patient, a small cell lung cancer stage IIIA patient, and a histiocytic sarcoma stage IV patient-three case reports. APMIS 124, 896–904. doi: 10.1111/apm.12576
Alberts, P., Tilgase, A., Rasa, A., Bandere, K., and Venskus, D. (2018). The advent of oncolytic virotherapy in oncology: the Rigvir(R) story. Eur. J. Pharmacol. 837, 117–126. doi: 10.1016/j.ejphar.2018.08.042
Altomonte, J., Marozin, S., Schmid, R. M., and Ebert, O. (2010). Engineered Newcastle disease virus as an improved oncolytic agent against hepatocellular carcinoma. Mol. Ther. 18, 275–284. doi: 10.1038/mt.2009.231
Anderson, B. D., Nakamura, T., Russell, S. J., and Peng, K. W. (2004). High CD46 receptor density determines preferential killing of tumor cells by oncolytic measles virus. Cancer Res. 64, 4919–4926. doi: 10.1158/0008-5472.CAN-04-0884
Andtbacka, R. H. I., Amatruda, T., Nemunaitis, J., Zager, J. S., Walker, J., Chesney, J. A., et al. (2019). Biodistribution, shedding, and transmissibility of the oncolytic virus talimogene laherparepvec in patients with melanoma. EBioMedicine 47, 89–97. doi: 10.1016/j.ebiom.2019.07.066
Andtbacka, R. H., Kaufman, H. L., Collichio, F., Amatruda, T., Senzer, N., Chesney, J., et al. (2015). Talimogene Laherparepvec improves durable response rate in patients with advanced melanoma. J. Clin. Oncol. 33, 2780–2788. doi: 10.1200/JCO.2014.58.3377
Annels, N. E., Mansfield, D., Arif, M., Ballesteros-Merino, C., Simpson, G. R., Denyer, M., et al. (2019). Phase I trial of an ICAM-1-targeted immunotherapeutic-Coxsackievirus A21 (CVA21) as an oncolytic agent against non muscle-invasive bladder Cancer. Clin. Cancer Res. 25, 5818–5831. doi: 10.1158/1078-0432.CCR-18-4022
Aref, S., Bailey, K., and Fielding, A. (2016). Measles to the rescue: a review of oncolytic measles virus. Viruses 8:294. doi: 10.3390/v8100294
Asada, T. (1974). Treatment of human cancer with mumps virus. Cancer 34, 1907–1928. doi: 10.1002/1097-0142(197412)34:6<1907::AID-CNCR2820340609>3.0.CO;2-4
Atiya, H., Frisbie, L., Pressimone, C., and Coffman, L. (2020). Mesenchymal stem cells in the tumor microenvironment. Adv. Exp. Med. Biol. 1234, 31–42. doi: 10.1007/978-3-030-37184-5_3
Au, G. G., Lincz, L. F., Enno, A., and Shafren, D. R. (2007). Oncolytic Coxsackievirus A21 as a novel therapy for multiple myeloma. Br. J. Haematol. 137, 133–141. doi: 10.1111/j.1365-2141.2007.06550.x
Beguin, J., Foloppe, J., Maurey, C., Accart, N., Aubertin, G., Grellier, B., et al. (2020). Preclinical evaluation of the oncolytic vaccinia virus TG6002 by translational research on canine breast Cancer. Mol. Ther. Oncolytics 19, 57–66. doi: 10.1016/j.omto.2020.08.020
Beguin, J., Gantzer, M., Farine, I., Foloppe, J., Klonjkowski, B., Maurey, C., et al. (2021). Safety, biodistribution and viral shedding of oncolytic vaccinia virus TG6002 administered intravenously in healthy beagle dogs. Sci. Rep. 11:2209. doi: 10.1038/s41598-021-81831-2
Bilsland, A. E., Spiliopoulou, P., and Evans, T. R. (2016). Virotherapy: cancer gene therapy at last? F1000Res 5:F1000. doi: 10.12688/f1000research.8211.1
Bischoff, J. R., Kirn, D. H., Williams, A., Heise, C., Horn, S., Muna, M., et al. (1996). An adenovirus mutant that replicates selectively in p53-deficient human tumor cells. Science 274, 373–376. doi: 10.1126/science.274.5286.373
Bischoff, J. R., and Samuel, C. E. (1989). Mechanism of interferon action. Activation of the human P1/eIF-2 alpha protein kinase by individual reovirus s-class mRNAs: s1 mRNA is a potent activator relative to s4 mRNA. Virology 172, 106–115. doi: 10.1016/0042-6822(89)90112-8
Bradley, S., Jakes, A. D., Harrington, K., Pandha, H., Melcher, A., and Errington-Mais, F. (2014). Applications of coxsackievirus A21 in oncology. Oncolytic Virother 3, 47–55. doi: 10.2147/OV.S56322
Bramante, S., Koski, A., Kipar, A., Diaconu, I., Liikanen, I., Hemminki, O., et al. (2014). Serotype chimeric oncolytic adenovirus coding for GM-CSF for treatment of sarcoma in rodents and humans. Int J Cancer 135, 720–730. doi: 10.1002/ijc.28696
Breitbach, C. J., Burke, J., Jonker, D., Stephenson, J., Haas, A. R., Chow, L. Q., et al. (2011a). Intravenous delivery of a multi-mechanistic cancer-targeted oncolytic poxvirus in humans. Nature 477, 99–102. doi: 10.1038/nature10358
Breitbach, C. J., De Silva, N. S., Falls, T. J., Aladl, U., Evgin, L., Paterson, J., et al. (2011b). Targeting tumor vasculature with an oncolytic virus. Mol. Ther. 19, 886–894. doi: 10.1038/mt.2011.26
Breton, E., Goetz, C., Kintz, J., Accart, N., Aubertin, G., Grellier, B., et al. (2010). In vivo preclinical low-field MRI monitoring of tumor growth following a suicide-gene therapy in an orthotopic mice model of human glioblastoma. C. R. Biol. 333, 220–225. doi: 10.1016/j.crvi.2009.12.012
Brokane, L., Jaunalksne, I., Tilgase, A., Olmane, E., Petroška, D., Rasa, A., et al. (2019). Combination treatment with nivolumab and Rigvir of a progressive stage IIC skin melanoma patient. Clin. Case Rep. 7, 1191–1196. doi: 10.1002/ccr3.2182
Brown, M. C., Dobrikova, E. Y., Dobrikov, M. I., Walton, R. W., Gemberling, S. L., Nair, S. K., et al. (2014). Oncolytic polio virotherapy of cancer. Cancer 120, 3277–3286. doi: 10.1002/cncr.28862
Brown, M. C., and Gromeier, M. (2015). Cytotoxic and immunogenic mechanisms of recombinant oncolytic poliovirus. Curr. Opin. Virol. 13, 81–85. doi: 10.1016/j.coviro.2015.05.007
Burke, M. J. (2016). Oncolytic Seneca Valley virus: past perspectives and future directions. Oncolytic Virother 5, 81–89. doi: 10.2147/OV.S96915
Burke, M. J., Ahern, C., Weigel, B. J., Poirier, J. T., Rudin, C. M., Chen, Y., et al. (2015). Phase I trial of Seneca Valley virus (NTX-010) in children with relapsed/refractory solid tumors: a report of the Children's oncology group. Pediatr. Blood Cancer 62, 743–750. doi: 10.1002/pbc.25269
Burke, J. M., Lamm, D. L., Meng, M. V., Nemunaitis, J. J., Stephenson, J. J., Arseneau, J. C., et al. (2012). A first in human phase 1 study of CG0070, a GM-CSF expressing oncolytic adenovirus, for the treatment of nonmuscle invasive bladder cancer. J. Urol. 188, 2391–2397. doi: 10.1016/j.juro.2012.07.097
Cai, J., and Yan, G. (2021). The identification and development of a novel oncolytic virus: alphavirus M1. Hum. Gene Ther. 32, 138–149. doi: 10.1089/hum.2020.271
Campadelli-Fiume, G., De Giovanni, C., Gatta, V., Nanni, P., Lollini, P. L., and Menotti, L. (2011). Rethinking herpes simplex virus: the way to oncolytic agents. Rev. Med. Virol. 21, 213–226. doi: 10.1002/rmv.691
Carlsten, M., Norell, H., Bryceson, Y. T., Poschke, I., Schedvins, K., Ljunggren, H. G., et al. (2009). Primary human tumor cells expressing CD155 impair tumor targeting by down-regulating DNAM-1 on NK cells. J. Immunol. 183, 4921–4930. doi: 10.4049/jimmunol.0901226
Carpenter, A. B., Carpenter, A. M., Aiken, R., and Hanft, S. (2021). Oncolytic virus in gliomas: a review of human clinical investigations. Ann. Oncol. 32, 968–982. doi: 10.1016/j.annonc.2021.03.197
Cerullo, V., Pesonen, S., Diaconu, I., Escutenaire, S., Arstila, P. T., Ugolini, M., et al. (2010). Oncolytic adenovirus coding for granulocyte macrophage colony-stimulating factor induces antitumoral immunity in cancer patients. Cancer Res. 70, 4297–4309. doi: 10.1158/0008-5472.CAN-09-3567
Chakrabarty, R., Tran, H., Boulay, I., Moran, T., Parenteau, A., Tavca, R., et al. (2013). Bio-distribution study of Reolysin(R) (pelareorep) through a single intravenous infusion in Sprague-Dawley rats. Investig. New Drugs 31, 1476–1486. doi: 10.1007/s10637-013-0033-x
Chan, L. C., Kalyanasundram, J., Leong, S. W., Masarudin, M. J., Veerakumarasivam, A., Yusoff, K., et al. (2021). Persistent Newcastle disease virus infection in bladder cancer cells is associated with putative pro-survival and anti-viral transcriptomic changes. BMC Cancer 21:625. doi: 10.1186/s12885-021-08345-y
Chang, J., Zhao, X., Wu, X., Guo, Y., Guo, H., Cao, J., et al. (2009). A phase I study of KH901, a conditionally replicating granulocyte-macrophage colony-stimulating factor: armed oncolytic adenovirus for the treatment of head and neck cancers. Cancer Biol. Ther. 8, 676–682. doi: 10.4161/cbt.8.8.7913
Chaurasiya, S., Chen, N. G., and Fong, Y. (2018). Oncolytic viruses and immunity. Curr. Opin. Immunol. 51, 83–90. doi: 10.1016/j.coi.2018.03.008
Chaurasiya, S., Fong, Y., and Warner, S. G. (2021). Oncolytic Virotherapy for Cancer: clinical experience. Biomedicine 9:419. doi: 10.3390/biomedicines9040419
Chen, Y., DeWeese, T., Dilley, J., Zhang, Y., Li, Y., Ramesh, N., et al. (2001). CV706, a prostate cancer-specific adenovirus variant, in combination with radiotherapy produces synergistic antitumor efficacy without increasing toxicity. Cancer Res. 61, 5453–5460.
Cheng, P. H., Wechman, S. L., McMasters, K. M., and Zhou, H. S. (2015). Oncolytic replication of E1b-deleted adenoviruses. Viruses 7, 5767–5779. doi: 10.3390/v7112905
Chesney, J., Awasthi, S., Curti, B., Hutchins, L., Linette, G., Triozzi, P., et al. (2018b). Phase IIIb safety results from an expanded-access protocol of talimogene laherparepvec for patients with unresected, stage IIIB-IVM1c melanoma. Melanoma Res. 28, 44–51. doi: 10.1097/CMR.0000000000000399
Chesney, J., Puzanov, I., Collichio, F., Singh, P., Milhem, M. M., Glaspy, J., et al. (2018a). Randomized, open-label phase II study evaluating the efficacy and safety of Talimogene Laherparepvec in combination with Ipilimumab versus Ipilimumab alone in patients with advanced, Unresectable Melanoma. J. Clin. Oncol. 36, 1658–1667. doi: 10.1200/JCO.2017.73.7379
Chiocca, E. A., Abbed, K. M., Tatter, S., Louis, D. N., Hochberg, F. H., Barker, F., et al. (2004). A phase I open-label, dose-escalation, multi-institutional trial of injection with an E1B-attenuated adenovirus, ONYX-015, into the peritumoral region of recurrent malignant gliomas, in the adjuvant setting. Mol. Ther. 10, 958–966. doi: 10.1016/j.ymthe.2004.07.021
Chiocca, E. A., and Rabkin, S. D. (2014). Oncolytic viruses and their application to cancer immunotherapy. Cancer Immunol. Res. 2, 295–300. doi: 10.1158/2326-6066.CIR-14-0015
Chon, H. J., Lee, W. S., Yang, H., Kong, S. J., Lee, N. K., Moon, E. S., et al. (2019). Tumor microenvironment remodeling by Intratumoral oncolytic vaccinia virus enhances the efficacy of immune-checkpoint blockade. Clin. Cancer Res. 25, 1612–1623. doi: 10.1158/1078-0432.CCR-18-1932
Chouljenko, D. V., Ding, J., Lee, I. F., Murad, Y. M., Bu, X., Liu, G., et al. (2020). Induction of durable antitumor response by a novel oncolytic herpesvirus expressing multiple immunomodulatory transgenes. Biomedicine 8:484. doi: 10.3390/biomedicines8110484
Comins, C., Spicer, J., Protheroe, A., Roulstone, V., Twigger, K., White, C. M., et al. (2010). REO-10: a phase I study of intravenous reovirus and docetaxel in patients with advanced cancer. Clin. Cancer Res. 16, 5564–5572. doi: 10.1158/1078-0432.CCR-10-1233
Cornejo, Y., Li, M., Dellinger, T. H., Mooney, R., Rahman, M. M., Mcfadden, G., et al. (2020). NSCs are permissive to oncolytic Myxoma virus and provide a delivery method for targeted ovarian cancer therapy. Oncotarget 11, 4693–4698. doi: 10.18632/oncotarget.27845
Cripe, T. P., Ngo, M. C., Geller, J. I., Louis, C. U., Currier, M. A., Racadio, J. M., et al. (2015). Phase 1 study of intratumoral Pexa-Vec (JX-594), an oncolytic and immunotherapeutic vaccinia virus, in pediatric cancer patients. Mol. Ther. 23, 602–608. doi: 10.1038/mt.2014.243
Das, R., Langou, S., Le, T. T., Prasad, P., Lin, F., and Nguyen, T. D. (2021). Electrical stimulation for immune modulation in Cancer treatments. Front. Bioeng. Biotechnol. 9:795300. doi: 10.3389/fbioe.2021.795300
Del Papa, J., Petryk, J., Bell, J. C., and Parks, R. J. (2019). An oncolytic adenovirus vector expressing p14 FAST protein induces widespread syncytium formation and reduces tumor growth rate in vivo. Mol. Ther. Oncolytics 14, 107–120. doi: 10.1016/j.omto.2019.05.001
DeWeese, T. L., van der Poel, H., Li, S., Mikhak, B., Drew, R., Goemann, M., et al. (2001). A phase I trial of CV706, a replication-competent, PSA selective oncolytic adenovirus, for the treatment of locally recurrent prostate cancer following radiation therapy. Cancer Res. 61, 7464–7472.
Dilley, J., Reddy, S., Ko, D., Nguyen, N., Rojas, G., Working, P., et al. (2005). Oncolytic adenovirus CG7870 in combination with radiation demonstrates synergistic enhancements of antitumor efficacy without loss of specificity. Cancer Gene Ther 12, 715–722. doi: 10.1038/sj.cgt.7700835
Dispenzieri, A., Tong, C., LaPlant, B., Lacy, M. Q., Laumann, K., Dingli, D., et al. (2017). Phase I trial of systemic administration of Edmonston strain of measles virus genetically engineered to express the sodium iodide symporter in patients with recurrent or refractory multiple myeloma. Leukemia 31, 2791–2798. doi: 10.1038/leu.2017.120
Dong, W., van Ginkel, J. W., Au, K. Y., Alemany, R., Meulenberg, J. J., and van Beusechem, V. W. (2014). ORCA-010, a novel potency-enhanced oncolytic adenovirus, exerts strong antitumor activity in preclinical models. Hum Gene Ther 25, 897–904. doi: 10.1089/hum.2013.229
Doniņa, S., Strēle, I., Proboka, G., Auziņš, J., Alberts, P., Jonsson, B., et al. (2015). Adapted ECHO-7 virus Rigvir immunotherapy (oncolytic virotherapy) prolongs survival in melanoma patients after surgical excision of the tumour in a retrospective study. Melanoma Res. 25, 421–426. doi: 10.1097/CMR.0000000000000180
Dorig, R. E., Marcil, A., Chopra, A., and Richardson, C. D. (1993). The human CD46 molecule is a receptor for measles virus (Edmonston strain). Cells 75, 295–305. doi: 10.1016/0092-8674(93)80071-L
Downs-Canner, S., Guo, Z. S., Ravindranathan, R., Breitbach, C. J., O'Malley, M. E., Jones, H. L., et al. (2016). Phase 1 study of intravenous oncolytic poxvirus (vvDD) in patients with advanced solid cancers. Mol. Ther. 24, 1492–1501. doi: 10.1038/mt.2016.101
Drescher, C., Drexler, K., Hilber, H., Schmidt, B., and Haferkamp, S. (2019). Regression of mucosal melanoma following intralesional talimogene laherparepvec (T-VEC) injection in combination with immunotherapy. J. Dtsch. Dermatol. Ges. 17, 321–323. doi: 10.1111/ddg.13763_g
Eissa, I. R., Mukoyama, N., Abdelmoneim, M., Naoe, Y., Matsumura, S., Bustos-Villalobos, I., et al. (2021). Oncolytic herpes simplex virus HF10 (canerpaturev) promotes accumulation of CD8(+) PD-1(−) tumor-infiltrating T cells in PD-L1-enriched tumor microenvironment. Int. J. Cancer 149, 214–227. doi: 10.1002/ijc.33550
Eriksson, E., Milenova, I., Wenthe, J., Stahle, M., Leja-Jarblad, J., Ullenhag, G., et al. (2017). Shaping the Tumor Stroma and Sparking Immune Activation by CD40 and 4-1BB Signaling Induced by an Armed Oncolytic Virus. Clin Cancer Res 23, 5846–5857. doi: 10.1158/1078-0432.CCR-17-0285
Ezzeddine, Z. D., Martuza, R. L., Platika, D., Short, M. P., Malick, A., Choi, B., et al. (1991). Selective killing of glioma cells in culture and in vivo by retrovirus transfer of the herpes simplex virus thymidine kinase gene. New Biol. 3, 608–614.
Fan, J., Jiang, H., Cheng, L., Ma, B., and Liu, R. (2021). Oncolytic herpes simplex virus and temozolomide synergistically inhibit breast cancer cell tumorigenesis in vitro and in vivo. Oncol. Lett. 21:99. doi: 10.3892/ol.2020.12360
Fong, Y., Kim, T., Bhargava, A., Brown, K., Brody, L., Covey, A., et al. (2009). A herpes oncolytic virus can be delivered via the vasculature to produce biologic changes in human colorectal cancer. Mol. Ther. 17, 389–394. doi: 10.1038/mt.2008.240
Forsyth, P., Roldan, G., George, D., Wallace, C., Palmer, C. A., Morris, D., et al. (2008). A phase I trial of intratumoral administration of reovirus in patients with histologically confirmed recurrent malignant gliomas. Mol. Ther. 16, 627–632. doi: 10.1038/sj.mt.6300403
Frampton, J. E. (2022). Teserpaturev/G47Delta: First Approval. BioDrugs 36, 667–672. doi: 10.1007/s40259-022-00553-7
Franco-Luzon, L., Gonzalez-Murillo, A., Alcantara-Sanchez, C., García-García, L., Tabasi, M., Huertas, A. L., et al. (2020). Systemic oncolytic adenovirus delivered in mesenchymal carrier cells modulate tumor infiltrating immune cells and tumor microenvironment in mice with neuroblastoma. Oncotarget 11, 347–361. doi: 10.18632/oncotarget.27401
Franke, A. C., Hardet, R., Prager, L., Bentler, M., Demeules, M., John-Neek, P., et al. (2023). Capsid-modified adeno-associated virus vectors as novel vaccine platform for cancer immunotherapy. Mol. Ther. Methods Clin. Dev. 29, 238–253. doi: 10.1016/j.omtm.2023.03.010
Freeman, A. I., Zakay-Rones, Z., Gomori, J. M., Linetsky, E., Rasooly, L., Greenbaum, E., et al. (2006). Phase I/II trial of intravenous NDV-HUJ oncolytic virus in recurrent glioblastoma multiforme. Mol. Ther. 13, 221–228. doi: 10.1016/j.ymthe.2005.08.016
Fujita, K., Kato, T., Hatano, K., Kawashima, A., Ujike, T., Uemura, M., et al. (2020). Intratumoral and s.c. injection of inactivated hemagglutinating virus of Japan envelope (GEN0101) in metastatic castration-resistant prostate cancer. Cancer Sci 111, 1692–1698. doi: 10.1111/cas.14366
Fusciello, M., Fontana, F., Tahtinen, S., Capasso, C., Feola, S., Martins, B., et al. (2019). Artificially cloaked viral nanovaccine for cancer immunotherapy. Nat. Commun. 10:5747. doi: 10.1038/s41467-019-13744-8
Galanis, E., Hartmann, L. C., Cliby, W. A., Long, H. J., Peethambaram, P. P., Barrette, B. A., et al. (2010). Phase I trial of intraperitoneal administration of an oncolytic measles virus strain engineered to express carcinoembryonic antigen for recurrent ovarian cancer. Cancer Res. 70, 875–882. doi: 10.1158/0008-5472.CAN-09-2762
Galanis, E., Markovic, S. N., Suman, V. J., Nuovo, G. J., Vile, R. G., Kottke, T. J., et al. (2012). Phase II trial of intravenous administration of Reolysin((R)) (Reovirus Serotype-3-Dearing strain) in patients with metastatic melanoma. Mol. Ther. 20, 1998–2003. doi: 10.1038/mt.2012.146
Gállego Pérez-Larraya, J., Garcia-Moure, M., Labiano, S., Patiño-García, A., Dobbs, J., Gonzalez-Huarriz, M., et al. (2022). Oncolytic DNX-2401 virus for pediatric diffuse intrinsic pontine glioma. N. Engl. J. Med. 386, 2471–2481. doi: 10.1056/NEJMoa2202028
Garber, K. (2006). China approves world's first oncolytic virus therapy for cancer treatment. J. Natl. Cancer Inst. 98, 298–300. doi: 10.1093/jnci/djj111
García, M., Moreno, R., Gil-Martin, M., Cascallò, M., de Olza, M. O., Cuadra, C., et al. (2019). A phase 1 trial of oncolytic adenovirus ICOVIR-5 administered intravenously to cutaneous and uveal melanoma patients. Hum. Gene Ther. 30, 352–364. doi: 10.1089/hum.2018.107
Garcia-Carbonero, R., Salazar, R., Duran, I., Osman-Garcia, I., Paz-Ares, L., Bozada, J. M., et al. (2017). Phase 1 study of intravenous administration of the chimeric adenovirus enadenotucirev in patients undergoing primary tumor resection. J. Immunother. Cancer 5:71. doi: 10.1186/s40425-017-0277-7
Geevarghese, S. K., Geller, D. A., de Haan, H. A., Hörer, M., Knoll, A. E., Mescheder, A., et al. (2010). Phase I/II study of oncolytic herpes simplex virus NV1020 in patients with extensively pretreated refractory colorectal cancer metastatic to the liver. Hum. Gene Ther. 21, 1119–1128. doi: 10.1089/hum.2010.020
Geletneky, K., Hajda, J., Angelova, A. L., Leuchs, B., Capper, D., Bartsch, A. J., et al. (2017). Oncolytic H-1 parvovirus shows safety and signs of immunogenic activity in a first phase I/IIa glioblastoma trial. Mol. Ther. 25, 2620–2634. doi: 10.1016/j.ymthe.2017.08.016
Ghajar-Rahimi, G., Kang, K. D., Totsch, S. K., Gary, S., Rocco, A., Blitz, S., et al. (2022). Clinical advances in oncolytic virotherapy for pediatric brain tumors. Pharmacol. Ther. 239:108193. doi: 10.1016/j.pharmthera.2022.108193
Ghasemi Darestani, N., Gilmanova, A. I., al-Gazally, M. E., Zekiy, A. O., Ansari, M. J., Zabibah, R. S., et al. (2023). Mesenchymal stem cell-released oncolytic virus: an innovative strategy for cancer treatment. Cell Commun. Signal 21:43. doi: 10.1186/s12964-022-01012-0
Gholami, S., Chen, C. H., Gao, S., Lou, E., Fujisawa, S., Carson, J., et al. (2014). Role of MAPK in oncolytic herpes viral therapy in triple-negative breast cancer. Cancer Gene Ther. 21, 283–289. doi: 10.1038/cgt.2014.28
Ghonime, M. G., Jackson, J., Shah, A., Roth, J., Li, M., Saunders, U., et al. (2018). Chimeric HCMV/HSV-1 and Deltagamma(1)34.5 oncolytic herpes simplex virus elicit immune mediated antigliomal effect and antitumor memory. Transl. Oncol. 11, 86–93. doi: 10.1016/j.tranon.2017.10.005
Goel, S., Ocean, A. J., Parakrama, R. Y., Ghalib, M. H., Chaudhary, I., Shah, U., et al. (2020). Elucidation of Pelareorep pharmacodynamics in a phase I trial in patients with KRAS-mutated colorectal Cancer. Mol. Cancer Ther. 19, 1148–1156. doi: 10.1158/1535-7163.MCT-19-1117
Goetz, C., Everson, R. G., Zhang, L. C., and Gromeier, M. (2010). MAPK signal-integrating kinase controls cap-independent translation and cell type-specific cytotoxicity of an oncolytic poliovirus. Mol. Ther. 18, 1937–1946. doi: 10.1038/mt.2010.145
Goradel, N. H., Asghari, M. H., Moloudizargari, M., Negahdari, B., Haghi-Aminjan, H., and Abdollahi, M. (2017). Melatonin as an angiogenesis inhibitor to combat cancer: mechanistic evidence. Toxicol. Appl. Pharmacol. 335, 56–63. doi: 10.1016/j.taap.2017.09.022
Grandi, P., Fernandez, J., Szentirmai, O., Carter, R., Gianni, D., Sena-Esteves, M., et al. (2010). Targeting HSV-1 virions for specific binding to epidermal growth factor receptor-vIII-bearing tumor cells. Cancer Gene Ther. 17, 655–663. doi: 10.1038/cgt.2010.22
Gromeier, M., Solecki, D., Patel, D. D., and Wimmer, E. (2000). Expression of the human poliovirus receptor/CD155 gene during development of the central nervous system: implications for the pathogenesis of poliomyelitis. Virology 273, 248–257. doi: 10.1006/viro.2000.0418
Gujar, S., Bell, J., and Diallo, J. S. (2019). SnapShot: Cancer immunotherapy with oncolytic viruses. Cells 176:1240. doi: 10.1016/j.cell.2019.01.051
Guo, P., Huang, J., Wang, L., Jia, D., Yang, J., Dillon, D. A., et al. (2014). ICAM-1 as a molecular target for triple negative breast cancer. Proc. Natl. Acad. Sci. U. S. A. 111, 14710–14715. doi: 10.1073/pnas.1408556111
Guo, Z. S., Lu, B., Guo, Z., Giehl, E., Feist, M., Dai, E., et al. (2019). Vaccinia virus-mediated cancer immunotherapy: cancer vaccines and oncolytics. J. Immunother. Cancer 7:6. doi: 10.1186/s40425-018-0495-7
Haines, B. B., Denslow, A., Grzesik, P., Lee, J. S., Farkaly, T., Hewett, J., et al. (2021). ONCR-177, an Oncolytic HSV-1 Designed to Potently Activate Systemic Antitumor Immunity. Cancer Immunol Res 9, 291–308. doi: 10.1158/2326-6066.CIR-20-0609
Hajda, J., Lehmann, M., Krebs, O., Kieser, M., Geletneky, K., Jäger, D., et al. (2017). A non-controlled, single arm, open label, phase II study of intravenous and intratumoral administration of ParvOryx in patients with metastatic, inoperable pancreatic cancer: ParvOryx02 protocol. BMC Cancer 17:576. doi: 10.1186/s12885-017-3604-y
Hamid, O., Hoffner, B., Gasal, E., Hong, J., and Carvajal, R. D. (2017). Oncolytic immunotherapy: unlocking the potential of viruses to help target cancer. Cancer Immunol. Immunother. 66, 1249–1264. doi: 10.1007/s00262-017-2025-8
Hammond, A. L., Plemper, R. K., Zhang, J., Schneider, U., Russell, S. J., and Cattaneo, R. (2001). Single-chain antibody displayed on a recombinant measles virus confers entry through the tumor-associated carcinoembryonic antigen. J. Virol. 75, 2087–2096. doi: 10.1128/JVI.75.5.2087-2096.2001
Harrington, K. J., Hingorani, M., Tanay, M. A., Hickey, J., Bhide, S. A., Clarke, P. M., et al. (2010). Phase I/II study of oncolytic HSV GM-CSF in combination with radiotherapy and cisplatin in untreated stage III/IV squamous cell cancer of the head and neck. Clin. Cancer Res. 16, 4005–4015. doi: 10.1158/1078-0432.CCR-10-0196
Harrington, K. J., Puzanov, I., Hecht, J. R., Hodi, F. S., Szabo, Z., Murugappan, S., et al. (2015). Clinical development of talimogene laherparepvec (T-VEC): a modified herpes simplex virus type-1-derived oncolytic immunotherapy. Expert. Rev. Anticancer. Ther. 15, 1389–1403. doi: 10.1586/14737140.2015.1115725
Hasegawa, K., Pham, L., O'Connor, M. K., Federspiel, M. J., Russell, S. J., and Peng, K. W. (2006). Dual therapy of ovarian cancer using measles viruses expressing carcinoembryonic antigen and sodium iodide symporter. Clin. Cancer Res. 12, 1868–1875. doi: 10.1158/1078-0432.CCR-05-1803
Hashemi Goradel, N., Ghiyami-Hour, F., Jahangiri, S., Negahdari, B., Sahebkar, A., Masoudifar, A., et al. (2018). Nanoparticles as new tools for inhibition of cancer angiogenesis. J. Cell. Physiol. 233, 2902–2910. doi: 10.1002/jcp.26029
Havunen, R., Kalliokoski, R., Siurala, M., Sorsa, S., Santos, J. M., Cervera-Carrascon, V., et al. (2021). Cytokine-coding oncolytic adenovirus TILT-123 is safe, selective, and effective as a single agent and in combination with immune checkpoint inhibitor anti-PD-1. Cells 10:246. doi: 10.3390/cells10020246
Heise, C., Sampson-Johannes, A., Williams, A., McCormick, F., Von Hoff, D. D., and Kirn, D. H. (1997). ONYX-015, an E1B gene-attenuated adenovirus, causes tumor-specific cytolysis and antitumoral efficacy that can be augmented by standard chemotherapeutic agents. Nat Med 3, 639–645. doi: 10.1038/nm0697-639
Hemminki, O., Parviainen, S., Juhila, J., Turkki, R., Linder, N., Lundin, J., et al. (2015). Immunological data from cancer patients treated with Ad5/3-E2F-Delta24-GMCSF suggests utility for tumor immunotherapy. Oncotarget 6, 4467–4481. doi: 10.18632/oncotarget.2901
Heo, J., Reid, T., Ruo, L., Rose, S., Bloomston, M., Cho, M., et al. (2013). Randomized dose-finding clinical trial of oncolytic immunotherapeutic vaccinia JX-594 in liver cancer. Nat. Med. 19, 329–336. doi: 10.1038/nm.3089
Hinterberger, M., Giessel, R., Fiore, G., Graebnitz, F., Bathke, B., Wennier, S., et al. (2021). Intratumoral virotherapy with 4-1BBL armed modified vaccinia Ankara eradicates solid tumors and promotes protective immune memory. J. Immunother. Cancer 9:e001586. doi: 10.1136/jitc-2020-001586
Hirooka, Y., Kasuya, H., Ishikawa, T., Kawashima, H., Ohno, E., Villalobos, I. B., et al. (2018). A phase I clinical trial of EUS-guided intratumoral injection of the oncolytic virus, HF10 for unresectable locally advanced pancreatic cancer. BMC Cancer 18:596. doi: 10.1186/s12885-018-4453-z
Hotte, S. J., Lorence, R. M., Hirte, H. W., Polawski, S. R., Bamat, M. K., O'Neil, J. D., et al. (2007). An optimized clinical regimen for the oncolytic virus PV701. Clin. Cancer Res. 13, 977–985. doi: 10.1158/1078-0432.CCR-06-1817
Huang, P., Watanabe, M., Kaku, H., Kashiwakura, Y., Chen, J., Saika, T., et al. (2008). Direct and distant antitumor effects of a telomerase-selective oncolytic adenoviral agent, OBP-301, in a mouse prostate cancer model. Cancer Gene Ther. 15, 315–322. doi: 10.1038/cgt.2008.3
Husseini, F., Delord, J. P., Fournel-Federico, C., Guitton, J., Erbs, P., Homerin, M., et al. (2017). Vectorized gene therapy of liver tumors: proof-of-concept of TG4023 (MVA-FCU1) in combination with flucytosine. Ann. Oncol. 28, 169–174. doi: 10.1093/annonc/mdw440
Hutzen, B., Bid, H. K., Houghton, P. J., Pierson, C. R., Powell, K., Bratasz, A., et al. (2014). Treatment of medulloblastoma with oncolytic measles viruses expressing the angiogenesis inhibitors endostatin and angiostatin. BMC Cancer 14:206. doi: 10.1186/1471-2407-14-206
Hwang, T. H., Moon, A., Burke, J., Ribas, A., Stephenson, J., Breitbach, C. J., et al. (2011). A mechanistic proof-of-concept clinical trial with JX-594, a targeted multi-mechanistic oncolytic poxvirus, in patients with metastatic melanoma. Mol. Ther. 19, 1913–1922. doi: 10.1038/mt.2011.132
Imperiale, M. J. (2008). Keeping adenovirus away from the liver. Cell Host Microbe 3, 119–120. doi: 10.1016/j.chom.2008.02.007
Iscaro, A., Jones, C., Forbes, N., Mughal, A., Howard, F. N., Janabi, H. A., et al. (2022). Targeting circulating monocytes with CCL2-loaded liposomes armed with an oncolytic adenovirus. Nanomedicine 40:102506. doi: 10.1016/j.nano.2021.102506
Jiang, X., and Shapiro, D. J. (2014). The immune system and inflammation in breast cancer. Mol. Cell. Endocrinol. 382, 673–682. doi: 10.1016/j.mce.2013.06.003
Jin, K. T., Tao, X. H., Fan, Y. B., and Wang, S. B. (2021). Crosstalk between oncolytic viruses and autophagy in cancer therapy. Biomed. Pharmacother. 134:110932. doi: 10.1016/j.biopha.2020.110932
Johnson, D. B., Puzanov, I., and Kelley, M. C. (2015). Talimogene laherparepvec (T-VEC) for the treatment of advanced melanoma. Immunotherapy 7, 611–619. doi: 10.2217/imt.15.35
Kanai, R., Wakimoto, H., Martuza, R. L., and Rabkin, S. D. (2011). A novel oncolytic herpes simplex virus that synergizes with phosphoinositide 3-kinase/Akt pathway inhibitors to target glioblastoma stem cells. Clin. Cancer Res. 17, 3686–3696. doi: 10.1158/1078-0432.CCR-10-3142
Kaneda, Y. (2010). A non-replicating oncolytic vector as a novel therapeutic tool against cancer. BMB Rep 43, 773–780. doi: 10.5483/BMBRep.2010.43.12.773
Kanerva, A., Nokisalmi, P., Diaconu, I., Koski, A., Cerullo, V., Liikanen, I., et al. (2013). Antiviral and antitumor T-cell immunity in patients treated with GM-CSF-coding oncolytic adenovirus. Clin Cancer Res 19, 2734–2744. doi: 10.1158/1078-0432.CCR-12-2546
Karagiannis, K., Proklou, A., Tsitoura, E., Lasithiotaki, I., Kalpadaki, C., Moraitaki, D., et al. (2017). Impaired mRNA expression of the migration related chemokine receptor CXCR4 in mesenchymal stem cells of COPD patients. Int. J. Inflamm. 2017:6089425. doi: 10.1155/2017/6089425
Karapanagiotou, E. M., Roulstone, V., Twigger, K., Ball, M., Tanay, M., Nutting, C., et al. (2012). Phase I/II trial of carboplatin and paclitaxel chemotherapy in combination with intravenous oncolytic reovirus in patients with advanced malignancies. Clin. Cancer Res. 18, 2080–2089. doi: 10.1158/1078-0432.CCR-11-2181
Kaufman, H. L., and Bommareddy, P. K. (2019). Two roads for oncolytic immunotherapy development. J. Immunother. Cancer 7:26. doi: 10.1186/s40425-019-0515-2
Kaufman, H. L., Kim, D. W., DeRaffele, G., Mitcham, J., Coffin, R. S., and Kim-Schulze, S. (2010). Local and distant immunity induced by intralesional vaccination with an oncolytic herpes virus encoding GM-CSF in patients with stage IIIc and IV melanoma. Ann. Surg. Oncol. 17, 718–730. doi: 10.1245/s10434-009-0809-6
Kaufman, H. L., Shalhout, S. Z., and Iodice, G. (2022). Talimogene Laherparepvec: moving from first-in-class to best-in-class. Front. Mol. Biosci. 9:834841. doi: 10.3389/fmolb.2022.834841
Kawamura, Y., Hua, L., Gurtner, A., Wong, E., Kiyokawa, J., Shah, N., et al. (2022). Histone deacetylase inhibitors enhance oncolytic herpes simplex virus therapy for malignant meningioma. Biomed. Pharmacother. 155:113843. doi: 10.1016/j.biopha.2022.113843
Kdimati, S., Mullins, C. S., and Linnebacher, M. (2021). Cancer-cell-derived IgG and its potential role in tumor development. Int. J. Mol. Sci. 22:11597. doi: 10.3390/ijms222111597
Kelly, E., and Russell, S. J. (2007). History of oncolytic viruses: genesis to genetic engineering. Mol. Ther. 15, 651–659. doi: 10.1038/sj.mt.6300108
Kelly, K. J., Wong, J., Gönen, M., Allen, P., Brennan, M., Coit, D., et al. (2016). Human trial of a genetically modified herpes simplex virus for rapid detection of positive peritoneal cytology in the staging of pancreatic Cancer. EBioMedicine 7, 94–99. doi: 10.1016/j.ebiom.2016.03.043
Kemeny, N., Brown, K., Covey, A., Kim, T., Bhargava, A., Brody, L., et al. (2006). Phase I, open-label, dose-escalating study of a genetically engineered herpes simplex virus, NV1020, in subjects with metastatic colorectal carcinoma to the liver. Hum. Gene Ther. 17, 1214–1224. doi: 10.1089/hum.2006.17.1214
Kennedy, E. M., Denslow, A., Hewett, J., Kong, L., De Almeida, A., Bryant, J. D., et al. (2022). Development of intravenously administered synthetic RNA virus immunotherapy for the treatment of cancer. Nat. Commun. 13:5907. doi: 10.1038/s41467-022-33599-w
Kicielinski, K. P., Chiocca, E. A., Yu, J. S., Gill, G. M., Coffey, M., and Markert, J. M. (2014). Phase 1 clinical trial of intratumoral reovirus infusion for the treatment of recurrent malignant gliomas in adults. Mol. Ther. 22, 1056–1062. doi: 10.1038/mt.2014.21
Kieran, M. W., Goumnerova, L., Manley, P., Chi, S. N., Marcus, K. J., Manzanera, A. G., et al. (2019). Phase I study of gene-mediated cytotoxic immunotherapy with AdV-tk as adjuvant to surgery and radiation for pediatric malignant glioma and recurrent ependymoma. Neuro-Oncology 21, 537–546. doi: 10.1093/neuonc/noy202
Killock, D. (2015). Skin cancer: T-VEC oncolytic viral therapy shows promise in melanoma. Nat. Rev. Clin. Oncol. 12:438. doi: 10.1038/nrclinonc.2015.106
Kim, K. H., Dmitriev, I. P., Saddekni, S., Kashentseva, E. A., Harris, R. D., Aurigemma, R., et al. (2013). A phase I clinical trial of Ad5/3-Delta24, a novel serotype-chimeric, infectivity-enhanced, conditionally-replicative adenovirus (CRAd), in patients with recurrent ovarian cancer. Gynecol. Oncol. 130, 518–524. doi: 10.1016/j.ygyno.2013.06.003
Kimball, K. J., Preuss, M. A., Barnes, M. N., Wang, M., Siegal, G. P., Wan, W., et al. (2010). A phase I study of a tropism-modified conditionally replicative adenovirus for recurrent malignant gynecologic diseases. Clin. Cancer Res. 16, 5277–5287. doi: 10.1158/1078-0432.CCR-10-0791
Kirn, D., Hermiston, T., and McCormick, F. (1998). ONYX-015: clinical data are encouraging. Nat Med 4, 1341–1342. doi: 10.1038/3902
Kiyohara, E., Tanemura, A., Nishioka, M., Yamada, M., Tanaka, A., Yokomi, A., et al. (2020). Intratumoral injection of hemagglutinating virus of Japan-envelope vector yielded an antitumor effect for advanced melanoma: a phase I/IIa clinical study. Cancer Immunol Immunother 69, 1131–1140. doi: 10.1007/s00262-020-02509-8
Kolb, E. A., Sampson, V., Stabley, D., Walter, A., Sol-Church, K., Cripe, T., et al. (2015). A phase I trial and viral clearance study of reovirus (Reolysin) in children with relapsed or refractory extra-cranial solid tumors: a Children's oncology group phase I consortium report. Pediatr. Blood Cancer 62, 751–758. doi: 10.1002/pbc.25464
Kolyasnikova, N. M., Pestov, N. B., Sanchez-Pimentel, J. M., Barlev, N. A., and Ishmukhametov, A. A. (2023). Anti-Cancer Virotherapy in Russia: lessons from the past, current challenges and prospects for the future. Curr. Pharm. Biotechnol. 24, 266–278. doi: 10.2174/1389201023666220516121813
Kostadinova, M., and Mourdjeva, M. (2020). Potential of mesenchymal stem cells in anti-Cancer therapies. Curr. Stem Cell Res. Ther. 15, 482–491. doi: 10.2174/1574888X15666200310171547
Kuhn, I., Harden, P., Bauzon, M., Chartier, C., Nye, J., Thorne, S., et al. (2008). Directed evolution generates a novel oncolytic virus for the treatment of colon cancer. PLoS One 3:e2409. doi: 10.1371/journal.pone.0002409
Laksono, B. M., Grosserichter-Wagener, C., de Vries, R. D., Langeveld, S. A. G., Brem, M. D., van Dongen, J. J. M., et al. (2018). In vitro measles virus infection of human lymphocyte subsets demonstrates high susceptibility and permissiveness of both naive and memory B cells. J. Virol. 92, e00131–e00218. doi: 10.1128/JVI.00131-18
Lang, F. F., Conrad, C., Gomez-Manzano, C., Yung, W. K. A., Sawaya, R., Weinberg, J. S., et al. (2018). Phase I study of DNX-2401 (Delta-24-RGD) oncolytic adenovirus: replication and immunotherapeutic effects in recurrent malignant glioma. J. Clin. Oncol. 36, 1419–1427. doi: 10.1200/JCO.2017.75.8219
Lauer, U. M., Schell, M., Beil, J., Berchtold, S., Koppenhöfer, U., Glatzle, J., et al. (2018). Phase I study of oncolytic vaccinia virus GL-ONC1 in patients with peritoneal Carcinomatosis. Clin. Cancer Res. 24, 4388–4398. doi: 10.1158/1078-0432.CCR-18-0244
Laurie, S. A., Bell, J. C., Atkins, H. L., Roach, J., Bamat, M. K., O'Neil, J. D., et al. (2006). A phase 1 clinical study of intravenous administration of PV701, an oncolytic virus, using two-step desensitization. Clin. Cancer Res. 12, 2555–2562. doi: 10.1158/1078-0432.CCR-05-2038
Lawler, S. E., Speranza, M. C., Cho, C. F., and Chiocca, E. A. (2017). Oncolytic viruses in Cancer treatment: a review. JAMA Oncol. 3, 841–849. doi: 10.1001/jamaoncol.2016.2064
Lazar, I., Yaacov, B., Shiloach, T., Eliahoo, E., Kadouri, L., Lotem, M., et al. (2010). The oncolytic activity of Newcastle disease virus NDV-HUJ on chemoresistant primary melanoma cells is dependent on the proapoptotic activity of the inhibitor of apoptosis protein Livin. J. Virol. 84, 639–646. doi: 10.1128/JVI.00401-09
Leb-Reichl, V. M., Kienzl, M., Kaufmann, A., Stoecklinger, A., Tockner, B., Kitzmueller, S., et al. (2021). Leveraging immune memory against measles virus as an antitumor strategy in a preclinical model of aggressive squamous cell carcinoma. J. Immunother. Cancer 9:e002170. doi: 10.1136/jitc-2020-002170
Lejmi, E., Perriraz, N., Clement, S., Baertschiger, R., Christofilopoulos, P., Meier, R., et al. (2015). Inflammatory chemokines MIP-1delta and MIP-3alpha are involved in the migration of multipotent mesenchymal stromal cells induced by hepatoma cells. Stem Cells Dev. 24, 1223–1235. doi: 10.1089/scd.2014.0176
Li, H. L., Li, S., Shao, J. Y., Cao, Y., Jiang, W. Q., Liu, R. Y., et al. (2008). Pharmacokinetic and pharmacodynamic study of intratumoral injection of an adenovirus encoding endostatin in patients with advanced tumors. Gene Ther. 15, 247–256. doi: 10.1038/sj.gt.3303038
Li, Z. L., Liang, X., Li, H. C., Wang, Z. M., and Chong, T. (2016). Dendritic cells serve as a "Trojan horse" for oncolytic adenovirus delivery in the treatment of mouse prostate cancer. Acta Pharmacol. Sin. 37, 1121–1128. doi: 10.1038/aps.2016.59
Li, L., Liu, S., Han, D., Tang, B., and Ma, J. (2020). Delivery and biosafety of oncolytic Virotherapy. Front. Oncol. 10:475. doi: 10.3389/fonc.2020.00475
Li, J. L., Liu, H. L., Zhang, X. R., Xu, J. P., Hu, W. K., Liang, M., et al. (2009). A phase I trial of intratumoral administration of recombinant oncolytic adenovirus overexpressing HSP70 in advanced solid tumor patients. Gene Ther. 16, 376–382. doi: 10.1038/gt.2008.179
Li, K., Zhao, Y., Hu, X., Jiao, J., Wang, W., and Yao, H. (2022). Advances in the clinical development of oncolytic viruses. Am. J. Transl. Res. 14, 4192–4206.
Liang, M. (2012). Clinical development of oncolytic viruses in China. Curr. Pharm. Biotechnol. 13, 1852–1857. doi: 10.2174/138920112800958760
Liang, M. (2018). Oncorine, the world first oncolytic virus medicine and its update in China. Curr. Cancer Drug Targets 18, 171–176. doi: 10.2174/1568009618666171129221503
Lin, X., Huang, H., Li, S., Li, H., Li, Y., Cao, Y., et al. (2007). A phase I clinical trial of an adenovirus-mediated endostatin gene (E10A) in patients with solid tumors. Cancer Biol. Ther. 6, 648–653. doi: 10.4161/cbt.6.5.4004
Lin, Y., Zhang, H., Liang, J., Li, K., Zhu, W., Fu, L., et al. (2014). Identification and characterization of alphavirus M1 as a selective oncolytic virus targeting ZAP-defective human cancers. Proc. Natl. Acad. Sci. U. S. A. 111, E4504–E4512. doi: 10.1073/pnas.1408759111
Lorence, R. M., Roberts, M. S., O'Neil, J. D., Groene, W. S., Miller, J. A., Mueller, S. N., et al. (2007). Phase 1 clinical experience using intravenous administration of PV701, an oncolytic Newcastle disease virus. Curr. Cancer Drug Targets 7, 157–167. doi: 10.2174/156800907780058853
Lu, D., Huang, Y., Kong, Y., Tao, T., and Zhu, X. (2020). Gut microecology: why our microbes could be key to our health. Biomed. Pharmacother. 131:110784. doi: 10.1016/j.biopha.2020.110784
Mace, A. T., Ganly, I., Soutar, D. S., and Brown, S. M. (2008). Potential for efficacy of the oncolytic herpes simplex virus 1716 in patients with oral squamous cell carcinoma. Head Neck 30, 1045–1051. doi: 10.1002/hed.20840
Macedo, N., Miller, D. M., Haq, R., and Kaufman, H. L. (2020). Clinical landscape of oncolytic virus research in 2020. J. Immunother. Cancer 8:1486. doi: 10.1136/jitc-2020-001486
Machiels, J. P., Salazar, R., Rottey, S., Duran, I., Dirix, L., Geboes, K., et al. (2019). A phase 1 dose escalation study of the oncolytic adenovirus enadenotucirev, administered intravenously to patients with epithelial solid tumors (EVOLVE). J. Immunother. Cancer 7:20. doi: 10.1186/s40425-019-0510-7
Macleod, B. L., Bedoui, S., Hor, J. L., Mueller, S. N., Russell, T. A., Hollett, N. A., et al. (2014). Distinct APC subtypes drive spatially segregated CD4+ and CD8+ T-cell effector activity during skin infection with HSV-1. PLoS Pathog. 10:e1004303. doi: 10.1371/journal.ppat.1004303
Mahalingam, D., Fountzilas, C., Moseley, J., Noronha, N., Tran, H., Chakrabarty, R., et al. (2017). A phase II study of REOLYSIN((R)) (pelareorep) in combination with carboplatin and paclitaxel for patients with advanced malignant melanoma. Cancer Chemother. Pharmacol. 79, 697–703. doi: 10.1007/s00280-017-3260-6
Mahalingam, D., Goel, S., Aparo, S., Patel Arora, S., Noronha, N., Tran, H., et al. (2018). A phase II study of Pelareorep (REOLYSIN((R))) in combination with gemcitabine for patients with advanced pancreatic adenocarcinoma. Cancers 10:160. doi: 10.3390/cancers10060160
Mahalingam, D., Wilkinson, G. A., Eng, K. H., Fields, P., Raber, P., Moseley, J. L., et al. (2020). Pembrolizumab in combination with the oncolytic virus Pelareorep and chemotherapy in patients with advanced pancreatic adenocarcinoma: a phase Ib study. Clin. Cancer Res. 26, 71–81. doi: 10.1158/1078-0432.CCR-19-2078
Malhotra, J., and Kim, E. S. (2023). Oncolytic viruses and Cancer immunotherapy. Curr. Oncol. Rep. 25, 19–28. doi: 10.1007/s11912-022-01341-w
Mansour, M., Palese, P., and Zamarin, D. (2011). Oncolytic specificity of Newcastle disease virus is mediated by selectivity for apoptosis-resistant cells. J. Virol. 85, 6015–6023. doi: 10.1128/JVI.01537-10
Marcato, P., Shmulevitz, M., Pan, D., Stoltz, D., and Lee, P. W. (2007). Ras transformation mediates reovirus oncolysis by enhancing virus uncoating, particle infectivity, and apoptosis-dependent release. Mol. Ther. 15, 1522–1530. doi: 10.1038/sj.mt.6300179
Marino, N., Illingworth, S., Kodialbail, P., Patel, A., Calderon, H., Lear, R., et al. (2017). Development of a versatile oncolytic virus platform for local intra-tumoural expression of therapeutic transgenes. PLoS One 12:e0177810. doi: 10.1371/journal.pone.0177810
Markert, J. M., Razdan, S. N., Kuo, H. C., Cantor, A., Knoll, A., Karrasch, M., et al. (2014). A phase 1 trial of oncolytic HSV-1, G207, given in combination with radiation for recurrent GBM demonstrates safety and radiographic responses. Mol. Ther. 22, 1048–1055. doi: 10.1038/mt.2014.22
McCart, J. A., Ward, J. M., Lee, J., Hu, Y., Alexander, H. R., Libutti, S. K., et al. (2001). Systemic cancer therapy with a tumor-selective vaccinia virus mutant lacking thymidine kinase and vaccinia growth factor genes. Cancer Res. 61, 8751–8757.
Mejias-Perez, E., Carreno-Fuentes, L., and Esteban, M. (2018). Development of a safe and effective vaccinia virus oncolytic vector WR-Delta4 with a set of gene deletions on several viral pathways. Mol. Ther. Oncolytics 8, 27–40. doi: 10.1016/j.omto.2017.12.002
Mell, L. K., Brumund, K. T., Daniels, G. A., Advani, S. J., Zakeri, K., Wright, M. E., et al. (2017). Phase I trial of intravenous oncolytic vaccinia virus (GL-ONC1) with cisplatin and radiotherapy in patients with Locoregionally advanced head and neck carcinoma. Clin. Cancer Res. 23, 5696–5702. doi: 10.1158/1078-0432.CCR-16-3232
Menotti, L., Cerretani, A., and Campadelli-Fiume, G. (2006). A herpes simplex virus recombinant that exhibits a single-chain antibody to HER2/neu enters cells through the mammary tumor receptor, independently of the gD receptors. J. Virol. 80, 5531–5539. doi: 10.1128/JVI.02725-05
Menotti, L., Cerretani, A., Hengel, H., and Campadelli-Fiume, G. (2008). Construction of a fully retargeted herpes simplex virus 1 recombinant capable of entering cells solely via human epidermal growth factor receptor 2. J. Virol. 82, 10153–10161. doi: 10.1128/JVI.01133-08
Milenova, I., Lopez Gonzalez, M., Quixabeira, D. C. A., Santos, J. M., Cervera-Carrascon, V., Dong, W., et al. (2021). Oncolytic adenovirus ORCA-010 activates Proinflammatory myeloid cells and facilitates T cell recruitment and activation by PD-1 blockade in melanoma. Hum. Gene Ther. 32, 178–191. doi: 10.1089/hum.2020.277
Moore, A. E. (1952). Viruses with oncolytic properties and their adaptation to tumors. Ann. N. Y. Acad. Sci. 54, 945–952. doi: 10.1111/j.1749-6632.1952.tb39969.x
Morales-Molina, A., Gambera, S., Cejalvo, T., Moreno, R., Rodríguez-Milla, M. Á., Perisé-Barrios, A. J., et al. (2018). Antitumor virotherapy using syngeneic or allogeneic mesenchymal stem cell carriers induces systemic immune response and intratumoral leukocyte infiltration in mice. Cancer Immunol. Immunother. 67, 1589–1602. doi: 10.1007/s00262-018-2220-2
Mori, K. M., Giuliano, P. D., Lopez, K. L., King, M. M., Bohart, R., and Goldstein, B. H. (2019). Pronounced clinical response following the oncolytic vaccinia virus GL-ONC1 and chemotherapy in a heavily pretreated ovarian cancer patient. Anti-Cancer Drugs 30, 1064–1066. doi: 10.1097/CAD.0000000000000836
Morris, D. G., Feng, X., DiFrancesco, L. M., Fonseca, K., Forsyth, P. A., Paterson, A. H., et al. (2013). REO-001: a phase I trial of percutaneous intralesional administration of reovirus type 3 Dearing (Reolysin(R)) in patients with advanced solid tumors. Investig. New Drugs 31, 696–706. doi: 10.1007/s10637-012-9865-z
Najar, M., Raicevic, G., Fayyad-Kazan, H., Bron, D., Toungouz, M., and Lagneaux, L. (2016). Mesenchymal stromal cells and immunomodulation: a gathering of regulatory immune cells. Cytotherapy 18, 160–171. doi: 10.1016/j.jcyt.2015.10.011
Naji, A., Eitoku, M., Favier, B., Deschaseaux, F., Rouas-Freiss, N., and Suganuma, N. (2019). Biological functions of mesenchymal stem cells and clinical implications. Cell. Mol. Life Sci. 76, 3323–3348. doi: 10.1007/s00018-019-03125-1
Nakajima, O., Ichimaru, D., Urata, Y., Fujiwara, T., Horibe, T., Kohno, M., et al. (2009). Use of telomelysin (OBP-301) in mouse xenografts of human head and neck cancer. Oncol. Rep. 22, 1039–1043. doi: 10.3892/or_00000533
Nakano, K., Todo, T., Zhao, G., Yamaguchi, K., Kuroki, S., Cohen, J. B., et al. (2005). Enhanced efficacy of conditionally replicating herpes simplex virus (G207) combined with 5-fluorouracil and surgical resection in peritoneal cancer dissemination models. J. Gene Med. 7, 638–648. doi: 10.1002/jgm.700
Nakao, A., Kasuya, H., Sahin, T. T., Nomura, N., Kanzaki, A., Misawa, M., et al. (2011). A phase I dose-escalation clinical trial of intraoperative direct intratumoral injection of HF10 oncolytic virus in non-resectable patients with advanced pancreatic cancer. Cancer Gene Ther. 18, 167–175. doi: 10.1038/cgt.2010.65
Nakatake, M., Kurosaki, H., Kuwano, N., et al. (2019). Partial deletion of glycoprotein B5R enhances vaccinia virus neutralization escape while preserving oncolytic function. Mol. Ther. Oncolytics 14, 159–171. doi: 10.1016/j.omto.2019.05.003
Nemunaitis, J., Tong, A. W., Nemunaitis, M., Senzer, N., Phadke, A. P., Bedell, C., et al. (2010). A phase I study of telomerase-specific replication competent oncolytic adenovirus (telomelysin) for various solid tumors. Mol. Ther. 18, 429–434. doi: 10.1038/mt.2009.262
Nisar, M., Paracha, R. Z., Gul, A., Arshad, I., Ejaz, S., Murad, D., et al. (2022). Interaction analysis of adenovirus L5 protein with pancreatic Cancer cell surface receptor to analyze its affinity for oncolytic virus therapy. Front. Oncol. 12:832277. doi: 10.3389/fonc.2022.832277
Nokisalmi, P., Pesonen, S., Escutenaire, S., Särkioja, M., Raki, M., Cerullo, V., et al. (2010). Oncolytic adenovirus ICOVIR-7 in patients with advanced and refractory solid tumors. Clin. Cancer Res. 16, 3035–3043. doi: 10.1158/1078-0432.CCR-09-3167
Omar, N. B., Bentley, R. T., Crossman, D. K., Foote, J. B., Koehler, J. W., Markert, J. M., et al. (2021). Safety and interim survival data after intracranial administration of M032, a genetically engineered oncolytic HSV-1 expressing IL-12, in pet dogs with sporadic gliomas. Neurosurg. Focus. 50:E5. doi: 10.3171/2020.11.FOCUS20844
Oronsky, B., Gastman, B., Conley, A. P., Reid, C., Caroen, S., and Reid, T. (2022). Oncolytic adenoviruses: the cold war against Cancer finally turns hot. Cancer 14:4701. doi: 10.3390/cancers14194701
Packiam, V. T., Lamm, D. L., Barocas, D. A., Trainer, A., Fand, B., Davis, R. L. III, et al. (2018). An open label, single-arm, phase II multicenter study of the safety and efficacy of CG0070 oncolytic vector regimen in patients with BCG-unresponsive non-muscle-invasive bladder cancer: interim results. Urol. Oncol. 36, 440–447. doi: 10.1016/j.urolonc.2017.07.005
Packiriswamy, N., Upreti, D., Zhou, Y., Khan, R., Miller, A., Diaz, R. M., et al. (2020). Oncolytic measles virus therapy enhances tumor antigen-specific T-cell responses in patients with multiple myeloma. Leukemia 34, 3310–3322. doi: 10.1038/s41375-020-0828-7
Papanastassiou, V., Rampling, R., Fraser, M., Petty, R., Hadley, D., Nicoll, J., et al. (2002). The potential for efficacy of the modified (ICP 34.5(−)) herpes simplex virus HSV1716 following intratumoural injection into human malignant glioma: a proof of principle study. Gene Ther. 9, 398–406. doi: 10.1038/sj.gt.3301664
Parato, K. A., Breitbach, C. J., Le Boeuf, F., Wang, J., Storbeck, C., Ilkow, C., et al. (2012). The oncolytic poxvirus JX-594 selectively replicates in and destroys cancer cells driven by genetic pathways commonly activated in cancers. Mol. Ther. 20, 749–758. doi: 10.1038/mt.2011.276
Park, S. H., Breitbach, C. J., Lee, J., Park, J. O., Lim, H. Y., Kang, W. K., et al. (2015). Phase 1b trial of biweekly intravenous Pexa-Vec (JX-594), an oncolytic and immunotherapeutic vaccinia virus in colorectal Cancer. Mol. Ther. 23, 1532–1540. doi: 10.1038/mt.2015.109
Park, B. H., Hwang, T., Liu, T. C., Sze, D. Y., Kim, J. S., Kwon, H. C., et al. (2008). Use of a targeted oncolytic poxvirus, JX-594, in patients with refractory primary or metastatic liver cancer: a phase I trial. Lancet Oncol. 9, 533–542. doi: 10.1016/S1470-2045(08)70107-4
Parmar, H. S., Nayak, A., Kataria, S., Tripathi, V., Jaiswal, P., Gavel, P. K., et al. (2022). Restructuring the ONYX-015 adenovirus by using spike protein genes from SARS-CoV-2 and MERS-CoV: possible implications in breast cancer treatment. Med. Hypotheses 159:110750. doi: 10.1016/j.mehy.2021.110750
Pascual-Pasto, G., Bazan-Peregrino, M., Olaciregui, N. G., Restrepo-Perdomo, C. A., Mato-Berciano, A., Ottaviani, D., et al. (2019). Therapeutic targeting of the RB1 pathway in retinoblastoma with the oncolytic adenovirus VCN-01. Sci Transl Med 11. doi: 10.1126/scitranslmed.aat9321
Patel, D. M., Foreman, P. M., Nabors, L. B., Riley, K. O., Gillespie, G. Y., and Markert, J. M. (2016). Design of a Phase I Clinical Trial to evaluate M032, a genetically engineered HSV-1 expressing IL-12, in patients with recurrent/progressive glioblastoma Multiforme, anaplastic astrocytoma, or Gliosarcoma. Hum. Gene. Ther. Clin. Dev. 27, 69–78. doi: 10.1089/humc.2016.031
Pavon, L. F., Sibov, T. T., de Souza, A. V., SMF, M., Cabral, F. R., de Souza, J. G., et al. (2018). Tropism of mesenchymal stem cell toward CD133(+) stem cell of glioblastoma in vitro and promote tumor proliferation in vivo. Stem Cell Res Ther 9:310. doi: 10.1186/s13287-018-1049-0
Pecora, A. L., Rizvi, N., Cohen, G. I., Meropol, N. J., Sterman, D., Marshall, J. L., et al. (2002). Phase I trial of intravenous administration of PV701, an oncolytic virus, in patients with advanced solid cancers. J. Clin. Oncol. 20, 2251–2266. doi: 10.1200/JCO.2002.08.042
Peng, J., Wang, S., Fan, W., Li, S., Wu, Y., Mou, X., et al. (2018). Synergistic suppression effect on tumor growth of acute myeloid leukemia by combining cytarabine with an engineered oncolytic vaccinia virus. Onco. Targets. Ther. 11, 6887–6900. doi: 10.2147/OTT.S172037
Perkins, D. (2002). Targeting apoptosis in neurological disease using the herpes simplex virus. J. Cell. Mol. Med. 6, 341–356. doi: 10.1111/j.1582-4934.2002.tb00513.x
Pesonen, S., Diaconu, I., Cerullo, V., Escutenaire, S., Raki, M., Kangasniemi, L., et al. (2012). Integrin targeted oncolytic adenoviruses Ad5-D24-RGD and Ad5-RGD-D24-GMCSF for treatment of patients with advanced chemotherapy refractory solid tumors. Int. J. Cancer 130, 1937–1947. doi: 10.1002/ijc.26216
Postow, M. A., Sidlow, R., and Hellmann, M. D. (2018). Immune-related adverse events associated with immune checkpoint blockade. N. Engl. J. Med. 378, 158–168. doi: 10.1056/NEJMra1703481
Prince, H. M., Regester, G., Gates, P., Jablonskis, L., Seymour, J. F., Lillie, K., et al. (2005). A phase Ib clinical trial of PV701, a milk-derived protein extract, for the prevention and treatment of oral mucositis in patients undergoing high-dose BEAM chemotherapy. Biol. Blood Marrow Transplant. 11, 512–520. doi: 10.1016/j.bbmt.2005.04.001
Puzanov, I., Milhem, M. M., Minor, D., Hamid, O., Li, A., Chen, L., et al. (2016). Talimogene Laherparepvec in combination with Ipilimumab in previously untreated, Unresectable stage IIIB-IV melanoma. J. Clin. Oncol. 34, 2619–2626. doi: 10.1200/JCO.2016.67.1529
Qi, Y., Xing, K., Zhang, L., Zhao, F., Yao, M., Hu, A., et al. (2018). Protective immunity elicited by measles vaccine exerts anti-tumor effects on measles virus hemagglutinin gene-modified cancer cells in a mouse model. J. Cancer Res. Clin. Oncol. 144, 1945–1957. doi: 10.1007/s00432-018-2720-7
Rajani, K., Parrish, C., Kottke, T., Thompson, J., Zaidi, S., Ilett, L., et al. (2016). Combination therapy with Reovirus and anti-PD-1 blockade controls tumor growth through innate and adaptive immune responses. Mol. Ther. 24, 166–174. doi: 10.1038/mt.2015.156
Ramesh, N., Ge, Y., Ennist, D. L., Zhu, M., Mina, M., Ganesh, S., et al. (2006). CG0070, a conditionally replicating granulocyte macrophage colony-stimulating factor--armed oncolytic adenovirus for the treatment of bladder cancer. Clin. Cancer Res. 12, 305–313. doi: 10.1158/1078-0432.CCR-05-1059
Reid, T., Galanis, E., Abbruzzese, J., Sze, D., Wein, L. M., Andrews, J., et al. (2002). Hepatic arterial infusion of a replication-selective oncolytic adenovirus (dl1520): phase II viral, immunologic, and clinical endpoints. Cancer Res. 62, 6070–6079.
Ribas, A., Dummer, R., Puzanov, I., VanderWalde, A., Andtbacka, R. H. I., Michielin, O., et al. (2017). Oncolytic Virotherapy promotes Intratumoral T cell infiltration and improves anti-PD-1 immunotherapy. Cells 170, 1109–1119.e10. doi: 10.1016/j.cell.2017.08.027
Rincon, E., Cejalvo, T., Kanojia, D., Alfranca, A., Rodriguez-Milla, M. A., Gil Hoyos, R. A., et al. (2017). Mesenchymal stem cell carriers enhance antitumor efficacy of oncolytic adenoviruses in an immunocompetent mouse model. Oncotarget 8, 45415–45431. doi: 10.18632/oncotarget.17557
Rodriguez-Garcia, A., Gimenez-Alejandre, M., Rojas, J. J., Moreno, R., Bazan-Peregrino, M., Cascallo, M., et al. (2015). Safety and efficacy of VCN-01, an oncolytic adenovirus combining fiber HSG-binding domain replacement with RGD and hyaluronidase expression. Clin Cancer Res 21, 1406–1418. doi: 10.1158/1078-0432.CCR-14-2213
Rojas, J. J., Cascallo, M., Guedan, S., Gros, A., Martinez-Quintanilla, J., Hemminki, A., et al. (2009). A modified E2F-1 promoter improves the efficacy to toxicity ratio of oncolytic adenoviruses. Gene Ther 16, 1441–1451. doi: 10.1038/gt.2009.103
Rojas-Martínez, A., Manzanera, A. G., Sukin, S. W., Esteban-María, J., González-Guerrero, J. F., Gomez-Guerra, L., et al. (2013). Intraprostatic distribution and long-term follow-up after AdV-tk immunotherapy as neoadjuvant to surgery in patients with prostate cancer. Cancer Gene Ther. 20, 642–649. doi: 10.1038/cgt.2013.56
Rothmann, T., Hengstermann, A., Whitaker, N. J., and Scheffner, M. (1998). Zur Hausen H. replication of ONYX-015, a potential anticancer adenovirus, is independent of p53 status in tumor cells. J. Virol. 72, 9470–9478. doi: 10.1128/JVI.72.12.9470-9478.1998
Roulstone, V., Khan, K., Pandha, H. S., Rudman, S., Coffey, M., Gill, G. M., et al. (2015). Phase I trial of cyclophosphamide as an immune modulator for optimizing oncolytic reovirus delivery to solid tumors. Clin. Cancer Res. 21, 1305–1312. doi: 10.1158/1078-0432.CCR-14-1770
Roy, D. G., Bell, J. C., and Bourgeois-Daigneault, M. C. (2020). Magnetic targeting of oncolytic VSV-based therapies improves infection of tumor cells in the presence of virus-specific neutralizing antibodies in vitro. Biochem. Biophys. Res. Commun. 526, 641–646. doi: 10.1016/j.bbrc.2020.03.135
Rudak, P. T., Yao, T., Richardson, C. D., and Haeryfar, S. M. M. (2021). Measles virus infects and programs MAIT cells for apoptosis. J. Infect. Dis. 223, 667–672. doi: 10.1093/infdis/jiaa407
Rudin, C. M., Poirier, J. T., Senzer, N. N., Stephenson, J., Loesch, D., Burroughs, K. D., et al. (2011). Phase I clinical study of Seneca Valley virus (SVV-001), a replication-competent picornavirus, in advanced solid tumors with neuroendocrine features. Clin. Cancer Res. 17, 888–895. doi: 10.1158/1078-0432.CCR-10-1706
Russell, S. J., and Barber, G. N. (2018). Oncolytic viruses as antigen-agnostic Cancer vaccines. Cancer Cell 33, 599–605. doi: 10.1016/j.ccell.2018.03.011
Russell, S. J., Peng, K. W., and Bell, J. C. (2012). Oncolytic virotherapy. Nat. Biotechnol. 30, 658–670. doi: 10.1038/nbt.2287
Sarwar, A., Hashim, L., Faisal, M. S., Haider, M. Z., Ahmed, Z., Ahmed, T. F., et al. (2021). Advances in viral oncolytics for treatment of multiple myeloma—a focused review. Expert. Rev. Hematol. 14, 1071–1083. doi: 10.1080/17474086.2021.1972802
Scanlan, H., Coffman, Z., Bettencourt, J., Shipley, T., and Bramblett, D. E. (2022). Herpes simplex virus 1 as an oncolytic viral therapy for refractory cancers. Front. Oncol. 12:940019. doi: 10.3389/fonc.2022.940019
Schenk, E. L., Mandrekar, S. J., Dy, G. K., Aubry, M. C., Tan, A. D., Dakhil, S. R., et al. (2020). A randomized double-blind phase II study of the Seneca Valley virus (NTX-010) versus placebo for patients with extensive-stage SCLC (ES SCLC) who were stable or responding after at least four cycles of platinum-based chemotherapy: north central Cancer treatment group (Alliance) N0923 study. J. Thorac. Oncol. 15, 110–119. doi: 10.1016/j.jtho.2019.09.083
Schreiber, L. M., Urbiola, C., Das, K., Spiesschaert, B., Kimpel, J., Heinemann, F., et al. (2019). The lytic activity of VSV-GP treatment dominates the therapeutic effects in a syngeneic model of lung cancer. Br. J. Cancer 121, 647–658. doi: 10.1038/s41416-019-0574-7
Senzer, N. N., Kaufman, H. L., Amatruda, T., Nemunaitis, M., Reid, T., Daniels, G., et al. (2009). Phase II clinical trial of a granulocyte-macrophage colony-stimulating factor-encoding, second-generation oncolytic herpesvirus in patients with unresectable metastatic melanoma. J. Clin. Oncol. 27, 5763–5771. doi: 10.1200/JCO.2009.24.3675
Seyed-Khorrami, S. M., Soleimanjahi, H., Soudi, S., and Habibian, A. (2021). MSCs loaded with oncolytic reovirus: migration and in vivo virus delivery potential for evaluating anti-cancer effect in tumor-bearing C57BL/6 mice. Cancer Cell Int. 21:244. doi: 10.1186/s12935-021-01848-5
Shafren, D. R., Au, G. G., Nguyen, T., Newcombe, N. G., Haley, E. S., Beagley, L., et al. (2004). Systemic therapy of malignant human melanoma tumors by a common cold-producing enterovirus, coxsackievirus a21. Clin. Cancer Res. 10, 53–60. doi: 10.1158/1078-0432.ccr-0690-3
Shayan, S., Arashkia, A., Bahramali, G., Abdoli, A., Nosrati, M. S. S., and Azadmanesh, K. (2022). Cell type-specific response of colon cancer tumor cell lines to oncolytic HSV-1 virotherapy in hypoxia. Cancer Cell Int. 22:164. doi: 10.1186/s12935-022-02564-4
Shen, F. B., Chang, J. H., Yang, C., Li, J., Guo, Y., Yi, B., et al. (2007a). Tumor-selective replication, cytotoxicity and GM-CSF production of oncolytic recombinant adenovirus in KH901 injection. Sichuan Da Xue Xue Bao Yi Xue Ban 38, 31–34.
Shen, F. B., Yang, C., Lei, N., Ju, Q., Guo, Y., Yi, B., et al. (2007b). Effects of KH901, a tumor-specific oncolytic recombinant adenovirus, on antitumor and expressing GM-CSF in xenograft tumor models. Sichuan Da Xue Xue Bao Yi Xue Ban 38, 386–390.
Shirakawa, Y., Tazawa, H., Tanabe, S., Kanaya, N., Noma, K., Koujima, T., et al. (2021). Phase I dose-escalation study of endoscopic intratumoral injection of OBP-301 (Telomelysin) with radiotherapy in oesophageal cancer patients unfit for standard treatments. Eur J Cancer 153, 98–108. doi: 10.1016/j.ejca.2021.04.043
Shmulevitz, M., Marcato, P., and Lee, P. W. (2005). Unshackling the links between reovirus oncolysis, Ras signaling, translational control and cancer. Oncogene 24, 7720–7728. doi: 10.1038/sj.onc.1209041
Siurala, M., Bramante, S., Vassilev, L., Hirvinen, M., Parviainen, S., Tähtinen, S., et al. (2015). Oncolytic adenovirus and doxorubicin-based chemotherapy results in synergistic antitumor activity against soft-tissue sarcoma. Int. J. Cancer 136, 945–954. doi: 10.1002/ijc.29048
Sivanandam, V., LaRocca, C. J., Chen, N. G., Fong, Y., and Warner, S. G. (2019). Oncolytic viruses and immune checkpoint inhibition: the best of both worlds. Mol. Ther. Oncolytics 13, 93–106. doi: 10.1016/j.omto.2019.04.003
Small, E. J., Carducci, M. A., Burke, J. M., Rodriguez, R., Fong, L., van Ummersen, L., et al. (2006). A phase I trial of intravenous CG7870, a replication-selective, prostate-specific antigen-targeted oncolytic adenovirus, for the treatment of hormone-refractory, metastatic prostate cancer. Mol. Ther. 14, 107–117. doi: 10.1016/j.ymthe.2006.02.011
Sostoa, J., Dutoit, V., and Migliorini, D. (2020). Oncolytic viruses as a platform for the treatment of malignant brain tumors. Int. J. Mol. Sci. 21:7449. doi: 10.3390/ijms21207449
Southam, C. M., and Moore, A. E. (1952). Clinical studies of viruses as antineoplastic agents with particular reference to Egypt 101 virus. Cancer 5, 1025–1034. doi: 10.1002/1097-0142(195209)5:5<1025::AID-CNCR2820050518>3.0.CO;2-Q
Stojdl, D. F., Lichty, B., Knowles, S., Marius, R., Atkins, H., Sonenberg, N., et al. (2000). Exploiting tumor-specific defects in the interferon pathway with a previously unknown oncolytic virus. Nat. Med. 6, 821–825. doi: 10.1038/77558
Streby, K. A., Currier, M. A., Triplet, M., Ott, K., Dishman, D. J., Vaughan, M. R., et al. (2019). First-in-human intravenous Seprehvir in young Cancer patients: a phase 1 clinical trial. Mol. Ther. 27, 1930–1938. doi: 10.1016/j.ymthe.2019.08.020
Streby, K. A., Geller, J. I., Currier, M. A., Warren, P. S., Racadio, J. M., Towbin, A. J., et al. (2017). Intratumoral injection of HSV1716, an oncolytic herpes virus, is safe and shows evidence of immune response and viral replication in young Cancer patients. Clin. Cancer Res. 23, 3566–3574. doi: 10.1158/1078-0432.CCR-16-2900
Strong, J. E., Coffey, M. C., Tang, D., Sabinin, P., and Lee, P. W. (1998). The molecular basis of viral oncolysis: usurpation of the Ras signaling pathway by reovirus. EMBO J. 17, 3351–3362. doi: 10.1093/emboj/17.12.3351
Su, Y., Li, J., Ji, W., Wang, G., Fang, L., Zhang, Q., et al. (2022). Triple-serotype chimeric oncolytic adenovirus exerts multiple synergistic mechanisms against solid tumors. J. Immunother. Cancer 10:10. doi: 10.1136/jitc-2022-004691
Suzuki, K., Alemany, R., Yamamoto, M., and Curiel, D. T. (2002). The presence of the adenovirus E3 region improves the oncolytic potency of conditionally replicative adenoviruses. Clin. Cancer Res. 8, 3348–3359.
Takaoka, A., Hayakawa, S., Yanai, H., Stoiber, D., Negishi, H., Kikuchi, H., et al. (2003). Integration of interferon-alpha/beta signalling to p53 responses in tumour suppression and antiviral defence. Nature 424, 516–523. doi: 10.1038/nature01850
Tan, S., Li, D., and Zhu, X. (2020). Cancer immunotherapy: pros, cons and beyond. Biomed. Pharmacother. 124:109821. doi: 10.1016/j.biopha.2020.109821
Tang, Z., Xu, Z., Zhu, X., and Zhang, J. (2021). New insights into molecules and pathways of cancer metabolism and therapeutic implications. Cancer Commun. 41, 16–36. doi: 10.1002/cac2.12112
Tazawa, H., Hasei, J., Yano, S., Kagawa, S., Ozaki, T., and Fujiwara, T. (2020). Bone and soft-tissue sarcoma: a new target for telomerase-specific oncolytic Virotherapy. Cancers 12:478. doi: 10.3390/cancers12020478
Teshigahara, O., Goshima, F., Takao, K., Kohno, S. I., Kimata, H., Nakao, A., et al. (2004). Oncolytic viral therapy for breast cancer with herpes simplex virus type 1 mutant HF 10. J. Surg. Oncol. 85, 42–47. doi: 10.1002/jso.20005
Tian, Y., Xie, D., and Yang, L. (2022). Engineering strategies to enhance oncolytic viruses in cancer immunotherapy. Signal Transduct. Target. Ther. 7:117. doi: 10.1038/s41392-022-00951-x
Tober, R., Banki, Z., Egerer, L., Behmüller, S., Kreppel, F., Greczmiel, U., et al. (2014). VSV-GP: a potent viral vaccine vector that boosts the immune response upon repeated applications. J. Virol. 88, 4897–4907. doi: 10.1128/JVI.03276-13
Tripodi, L., Sasso, E., Feola, S., Vitale, M., Coluccino, L., Leoni, G., et al. (2023). Systems biology approaches for the improvement of oncolytic virus-based immunotherapies. Cancers 15, 1297. doi: 10.3390/cancers15041297
Twumasi-Boateng, K., Pettigrew, J. L., Kwok, Y. Y. E., Bell, J. C., and Nelson, B. H. (2018). Oncolytic viruses as engineering platforms for combination immunotherapy. Nat. Rev. Cancer 18, 419–432. doi: 10.1038/s41568-018-0009-4
Vangala, G., Imhoff, F. M., Squires, C. M. L., Cridge, A. G., and Baird, S. K. (2019). Mesenchymal stem cell homing towards cancer cells is increased by enzyme activity of cathepsin D. Exp. Cell Res. 383:111494. doi: 10.1016/j.yexcr.2019.07.007
Vassilev, L., Ranki, T., Joensuu, T., Jäger, E., Karbach, J., Wahle, C., et al. (2015). Repeated intratumoral administration of ONCOS-102 leads to systemic antitumor CD8(+) T-cell response and robust cellular and transcriptional immune activation at tumor site in a patient with ovarian cancer. Onco. Targets. Ther. 4:e1017702. doi: 10.1080/2162402X.2015.1017702
Vigil, A., Park, M. S., Martinez, O., Chua, M. A., Xiao, S., Cros, J. F., et al. (2007). Use of reverse genetics to enhance the oncolytic properties of Newcastle disease virus. Cancer Res. 67, 8285–8292. doi: 10.1158/0008-5472.CAN-07-1025
Vijayakumar, G., Palese, P., and Goff, P. H. (2019). Oncolytic Newcastle disease virus expressing a checkpoint inhibitor as a radioenhancing agent for murine melanoma. EBioMedicine 49, 96–105. doi: 10.1016/j.ebiom.2019.10.032
Villa, N. Y., Rahman, M. M., Mamola, J., Sharik, M. E., de Matos, A. L., Kilbourne, J., et al. (2022). Transplantation of autologous bone marrow pre-loaded ex vivo with oncolytic myxoma virus is efficacious against drug-resistant Vk*MYC mouse myeloma. Oncotarget 13, 490–504. doi: 10.18632/oncotarget.28205
Volarevic, V., Markovic, B. S., Gazdic, M., Volarevic, A., Jovicic, N., Arsenijevic, N., et al. (2018). Ethical and safety issues of stem cell-based therapy. Int. J. Med. Sci. 15, 36–45. doi: 10.7150/ijms.21666
Wall, L. M., and Baldwin-Medsker, A. (2017). Safe and effective standards of care: supporting the administration of T-VEC for patients with advanced melanoma in the outpatient oncology setting. Clin. J. Oncol. Nurs. 21, E260–E266. doi: 10.1188/17.CJON.E260-E266
Wang, L., Ning, J., Wakimoto, H., Wu, S., Wu, C. L., Humphrey, M. R., et al. (2019). Oncolytic herpes simplex virus and PI3K inhibitor BKM120 synergize to promote killing of prostate Cancer stem-like cells. Mol. Ther. Oncolytics 13, 58–66. doi: 10.1016/j.omto.2019.03.008
Wang, Z., Yu, B., Wang, B., Yan, J., Feng, X., Wang, Z., et al. (2016). A novel capsid-modified oncolytic recombinant adenovirus type 5 for tumor-targeting gene therapy by intravenous route. Oncotarget 7, 47287–47301. doi: 10.18632/oncotarget.10075
Watanabe, T., Hioki, M., Fujiwara, T., Nishizaki, M., Kagawa, S., Taki, M., et al. (2006). Histone deacetylase inhibitor FR901228 enhances the antitumor effect of telomerase-specific replication-selective adenoviral agent OBP-301 in human lung cancer cells. Exp. Cell Res. 312, 256–265. doi: 10.1016/j.yexcr.2005.10.026
Wei, C., Li, M., Li, X., Lyu, J., and Zhu, X. (2022). Phase separation: "the master key" to deciphering the physiological and pathological functions of cells. Adv Biol 6:e2200006. doi: 10.1002/adbi.202200006
Wei, D., Xu, J., Liu, X. Y., Chen, Z. N., and Bian, H. (2018). Fighting Cancer with viruses: oncolytic virus therapy in China. Hum. Gene Ther. 29, 151–159. doi: 10.1089/hum.2017.212
Wenthe, J., Naseri, S., Hellstrom, A. C., Wiklund, H. J., Eriksson, E., and Loskog, A. (2020). Immunostimulatory oncolytic virotherapy for multiple myeloma targeting 4-1BB and/or CD40. Cancer Gene Ther 27, 948–959. doi: 10.1038/s41417-020-0176-9
Wenthe, J., Naseri, S., Labani-Motlagh, A., Enblad, G., Wikstrom, K. I., Eriksson, E., et al. (2021). Boosting CAR T-cell responses in lymphoma by simultaneous targeting of CD40/4-1BB using oncolytic viral gene therapy. Cancer Immunol Immunother 70, 2851–2865. doi: 10.1007/s00262-021-02895-7
White, C. L., Twigger, K. R., Vidal, L., De Bono, J. S., Coffey, M., Heinemann, L., et al. (2008). Characterization of the adaptive and innate immune response to intravenous oncolytic reovirus (Dearing type 3) during a phase I clinical trial. Gene Ther. 15, 911–920. doi: 10.1038/gt.2008.21
Wilden, H., Fournier, P., Zawatzky, R., and Schirrmacher, V. (2009). Expression of RIG-I, IRF3, IFN-beta and IRF7 determines resistance or susceptibility of cells to infection by Newcastle disease virus. Int. J. Oncol. 34, 971–982. doi: 10.3892/ijo_00000223
Wu, Z., Li, S., and Zhu, X. (2021). The mechanism of stimulating and mobilizing the immune system enhancing the anti-tumor immunity. Front. Immunol. 12:682435. doi: 10.3389/fimmu.2021.682435
Xie, S., Wu, Z., Qi, Y., Wu, B., and Zhu, X. (2021). The metastasizing mechanisms of lung cancer: recent advances and therapeutic challenges. Biomed. Pharmacother. 138:111450. doi: 10.1016/j.biopha.2021.111450
Xu, J. Y., Liu, M. T., Tao, T., Zhu, X., and Fei, F. Q. (2021). The role of gut microbiota in tumorigenesis and treatment. Biomed. Pharmacother. 138:111444. doi: 10.1016/j.biopha.2021.111444
Yaacov, B., Eliahoo, E., Lazar, I., Ben-Shlomo, M., Greenbaum, I., Panet, A., et al. (2008). Selective oncolytic effect of an attenuated Newcastle disease virus (NDV-HUJ) in lung tumors. Cancer Gene Ther. 15, 795–807. doi: 10.1038/cgt.2008.31
Yamashita, M., Tasaki, M., Murakami, R., Arai, Y., Nakamura, T., and Nakao, S. (2021). Oncolytic vaccinia virus induces a novel phenotype of CD8(+) effector T cells characterized by high ICOS expression. Mol. Ther. Oncolytics 20, 422–432. doi: 10.1016/j.omto.2021.01.016
Yang, Z., Gray, M., and Winter, L. (2021). Why do poxviruses still matter? Cell Biosci. 11:96. doi: 10.1186/s13578-021-00610-8
Yang, C., Hua, N., Xie, S., Wu, Y., Zhu, L., Wang, S., et al. (2021). Oncolytic viruses as a promising therapeutic strategy for hematological malignancies. Biomed. Pharmacother. 139:111573. doi: 10.1016/j.biopha.2021.111573
Yin, J., Markert, J. M., and Leavenworth, J. W. (2017). Modulation of the Intratumoral immune landscape by oncolytic herpes simplex virus Virotherapy. Front. Oncol. 7:136. doi: 10.3389/fonc.2017.00136
Yngve, E., Gao, M., and Yu, D. (2022). A qPCR-based method for quantification of replication competent adenovirus (RCA) in conditionally replicating oncolytic adenoviruses. Methods Mol. Biol. 2521, 249–258. doi: 10.1007/978-1-0716-2441-8_13
You, Z., Fischer, D. C., Tong, X., Hasenburg, A., Aguilar-Cordova, E., and Kieback, D. G. (2001). Coxsackievirus-adenovirus receptor expression in ovarian cancer cell lines is associated with increased adenovirus transduction efficiency and transgene expression. Cancer Gene Ther. 8, 168–175. doi: 10.1038/sj.cgt.7700284
Yu, Z., Chan, M. K., O-Charoenrat, P., Eisenberg, D. P., Shah, J. P., Singh, B., et al. (2005). Enhanced nectin-1 expression and herpes oncolytic sensitivity in highly migratory and invasive carcinoma. Clin. Cancer Res. 11, 4889–4897. doi: 10.1158/1078-0432.CCR-05-0309
Yun, C. O., Hong, J., and Yoon, A. R. (2022). Current clinical landscape of oncolytic viruses as novel cancer immunotherapeutic and recent preclinical advancements. Front. Immunol. 13:953410. doi: 10.3389/fimmu.2022.953410
Zamarin, D., Vigil, A., Kelly, K., Garcia-Sastre, A., and Fong, Y. (2009). Genetically engineered Newcastle disease virus for malignant melanoma therapy. Gene Ther. 16, 796–804. doi: 10.1038/gt.2009.14
Zeng, J., Li, X., Sander, M., Zhang, H., Yan, G., and Lin, Y. (2021). Oncolytic Viro-immunotherapy: an emerging option in the treatment of gliomas. Front. Immunol. 12:721830. doi: 10.3389/fimmu.2021.721830
Zhang, M., Eshraghian, E. A., Jammal, O. A., Zhang, Z., and Zhu, X. (2020). CRISPR technology: the engine that drives cancer therapy. Biomed. Pharmacother. 133:111007. doi: 10.1016/j.biopha.2020.111007
Zhang, B., Huang, J., Tang, J., Hu, S., Luo, S., Luo, Z., et al. (2021). Intratumoral OH2, an oncolytic herpes simplex virus 2, in patients with advanced solid tumors: a multicenter, phase I/II clinical trial. J. Immunother. Cancer 9:e002224. doi: 10.1136/jitc-2020-002224
Zhang, H., Lin, Y., Li, K., Liang, J., Xiao, X., Cai, J., et al. (2016). Naturally existing oncolytic virus M1 is nonpathogenic for the nonhuman Primates after multiple rounds of repeated intravenous injections. Hum. Gene Ther. 27, 700–711. doi: 10.1089/hum.2016.038
Zhang, S. C., Wang, W. L., Cai, W. S., Jiang, K. L., and Yuan, Z. W. (2012). Engineered measles virus Edmonston strain used as a novel oncolytic viral system against human hepatoblastoma. BMC Cancer 12:427. doi: 10.1186/1471-2407-12-427
Zhang, J., Zhang, Q., Liu, Z., Wang, J., Shi, F., Su, J., et al. (2022). Efficacy and safety of recombinant human adenovirus type 5 (H101) in persistent, recurrent, or metastatic gynecologic malignancies: a retrospective study. Front. Oncol. 12:877155. doi: 10.3389/fonc.2022.877155
Zhou, Y., Kong, Y., Fan, W., Tao, T., Xiao, Q., Li, N., et al. (2020). Principles of RNA methylation and their implications for biology and medicine. Biomed. Pharmacother. 131:110731. doi: 10.1016/j.biopha.2020.110731
Zhu, Z., McGray, A. J. R., Jiang, W., Lu, B., Kalinski, P., and Guo, Z. S. (2022). Improving cancer immunotherapy by rationally combining oncolytic virus with modulators targeting key signaling pathways. Mol. Cancer 21:196. doi: 10.1186/s12943-022-01664-z
Zhu, H., Su, Y., Zhou, S., Xiao, W., Ling, W., Hu, B., et al. (2007). Immune analysis on mtHSV mediated tumor therapy in HSV-1 seropositive mice. Cancer Biol. Ther. 6, 724–731. doi: 10.4161/cbt.6.5.3953
Keywords: anti-tumor immune response, oncolytic virus, tumor cells, PD-1, adenovirus, herpes simplex virus
Citation: Zhu X, Fan C, Xiong Z, Chen M, Li Z, Tao T and Liu X (2023) Development and application of oncolytic viruses as the nemesis of tumor cells. Front. Microbiol. 14:1188526. doi: 10.3389/fmicb.2023.1188526
Edited by:
Tejabhiram Yadavalli, University of Illinois Chicago, United StatesReviewed by:
Pankaj Sharma, University of Illinois Chicago, United StatesMadavaraju Krishnaraju, Northwestern University, United States
Copyright © 2023 Zhu, Fan, Xiong, Chen, Li, Tao and Liu. This is an open-access article distributed under the terms of the Creative Commons Attribution License (CC BY). The use, distribution or reproduction in other forums is permitted, provided the original author(s) and the copyright owner(s) are credited and that the original publication in this journal is cited, in accordance with accepted academic practice. No use, distribution or reproduction is permitted which does not comply with these terms.
*Correspondence: Xiuqing Liu, liuxiuqing86@163.com; Tao Tao, tao_tao79@163.com
†These authors have contributed equally to this work