- 1Key Laboratory of Agro-ecological Processes in Subtropical Region, Hunan Provincial Key Laboratory of Animal Nutrition Physiology and Metabolic Processes, Institute of Subtropical Agriculture, Chinese Academy of Sciences, Changsha, Hunan, China
- 2School of Biology and Food Engineering, Fuyang Normal University, Fuyang, Anhui, China
- 3College of Animal Science and Technology, Hunan Agricultural University, Changsha, Hunan, China
Introduction: Chinese medicinal herbs play important roles in anti-inflammatory, antioxidant, and antibacterial activities. However, the effects of Chinese herb ultrafine powder (CHUP) on laying hens still need to be elucidated. Therefore, this study aimed to evaluate the effects of dietary CHUP supplementation on jejunal morphology, physical barrier function, and microbiota in laying hens.
Methods: A total of 576 Xinyang black-feather laying hens (300 days old) were randomly assigned into eight groups, with eight replicates per group and nine hens per replicate. The hens were fed a basal diet (control group) and a basal diet supplemented with 0.5% Leonuri herba (LH group), 0.25% Ligustri lucidi fructus (LF group), 0.25% Taraxaci herba (TH group), 0.5% LH + 0.25% LF (LH-LF group), 0.5% LH + 0.25% TH (LH-TH group), 0.25% LF + 0.25% TH (LF-TH group), and 0.5% LH + 0.25% LF + 0.25% TH (LH-LF-TH group), respectively, for 120 days.
Results: The results showed that dietary LH-LF and LH-LF-TH supplementation increased (p < 0.05) the jejunal villus height to crypt depth ratio of laying hens. Dietary LF-TH supplementation up-regulated jejunal claudin-5 expression, while LH supplementation up-regulated jejunal claudin-1 expression and increased the jejunal abundances of potentially beneficial bacteria related to short-chain fatty acids and bacteriocins production, such as Blautia, Carnobacterium, Clostridiales, and Erysipelotrichales (p < 0.05). In addition, dietary LH supplementation enriched (p < 0.05) the tetracycline biosynthesis, butirosin/neomycin biosynthesis, and D-arginine/D-ornithine metabolism, whereas steroid biosynthesis and limonene/pinene degradation were enriched (p < 0.05) in the LH-LF and LH-LF-TH groups. Moreover, Spearman’s correlation analysis revealed the potential correlation between the abundance of the jejunal microbiota and jejunal morphology and the physical barrier function of laying hens.
Discussion: Collectively, these findings suggest that dietary CHUP supplementation could enhance the beneficial bacteria abundance, physical barrier function, and metabolic function associated with short-chain fatty acids and bacteriocins production. Moreover, combined supplementation of dietary CHUP showed better effects than the sole CHUP supplementation.
Introduction
Laying hens during the late laying phase are characterized by decreased laying performance, egg quality, and an imbalanced immune system (Ma et al., 2014). In addition, intestinal lipid metabolism, energy production, and antioxidant metabolism are also affected during the late laying phase of laying hens (Wang et al., 2019). It is well-known that gut microbiota plays important roles in overall host health (Shu et al., 2011). Intestinal microbiota not only regulates digestion and absorption of nutrients (Chang and Martinez-Guryn, 2019) but also influences immune homeostasis and chronic diseases (Gao et al., 2018). In addition, the intestinal microbiota of high-yield laying hens could increase the egg-laying rates of low-yield laying hens by modulating the relative abundance of intestinal microbiota (Wang et al., 2020). Previous studies also reported that the enhancement of intestinal mucosal barrier functions could increase egg production by enhancing the immunity of the intestinal tissue in laying hens (Nii, 2022). Thus, maintaining a healthy intestinal development is critical for improving the overall health of laying hens, as disorders of the intestinal microbiota have several adverse effects. Therefore, it is necessary to explore the effects of feed additives on laying hens that can improve laying performance and egg quality by maintaining intestinal barrier integrity and microbiota balance.
Feed additives are commonly used to improve animal health and productivity and inhibit toxins in the intestine to promote nutrient absorption (Ashour et al., 2020). Antibiotics, a type of traditional feed additives, are banned in most countries due to the major threat to public health caused by antibiotic resistance (Huang et al., 2018). In the recent few decades, various antibiotic alternatives, such as natural polyamines (Ma et al., 2020), amino acids (Dong et al., 2017), organic acids (Gong et al., 2021), vitamins (Li et al., 2022), probiotics (Abd El-Hack et al., 2020), and Chinese herbs and their extracts (Cheng et al., 2014) have been used to improve the production performance and product quality in poultry. These feed additives could improve animal health by transplantation of spermidine-altered microbiota (Ma et al., 2020), producing intestinal mucin (Cheng et al., 2014), and altering the selection pressures on the resident microbiota (Bedford and Cowieson, 2012). Meanwhile, Chinese herbs have shown various beneficial effects, such as anti-inflammation, antioxidant, and anti-bacterial properties (Han et al., 2019), which further influence the gut microbiota structure and microbiota-mediated bioconversion (Chen et al., 2016). In addition, Chinese herbs were also found to modulate intestinal barrier function and improve metabolic endotoxemia (Zhang et al., 2021). Moreover, Chinese herbs could promote the production of intestinal short-chain fatty acids (SCFAs) by increasing the abundance of Roseburia and Eubacterium (Zhang et al., 2019), and thereby showed ideal anti-inflammatory effects by increasing the abundance of anti-inflammatory associated gut bacteria (Wei et al., 2018). Therefore, Chinese herbs might have the potential to be served as a prophylactic or therapeutic way to affect gut barrier integrity and intestinal microbiota. However, to date, few studies have investigated the interplays between Chinese herbs and the intestinal microbiota of laying hens.
According to the medical experience from a traditional Chinese medical doctor and the theory of “sovereign, minister, assistant, and courier”, Leonuri herba (LH), Ligustri lucidi fructus (LF), and Taraxaci herba (TH) design appropriate dose to prepare Chinese herb ultrafine powder (CHUP). The LH is a sovereign medicine that activates the blood to regulate menstruation, heat-clearing, and detoxification, therefore, its dosage is larger than that of LF and TH. The LF showed better effects on the liver and kidney, while TH could effectively reduce swelling and disperse stagnation (Chinese Pharmacopoeia Commission, 2020). Moreover, LF and TH have been used together as minister medicine since one was a tonifying and replenishing medicine, and the other was a heat-clearing medicine. In recent years, ultrafine grinding technology has been applied to develop novel feed formulations. Compared with the traditional decoction pieces, due to the advantage of smaller particle size, CHUP showed improved dissolution of the active ingredients of drugs by increasing the damage rate of the plant cell walls, thereby improving the bioavailability of Chinese herbs in humans (Rao et al., 2019).
We hypothesized that supplementing Chinese herbs in laying hens diet during the late laying phase might positively stimulate the overall health of laying hens by influencing the intestinal morphology and altering the microbial community composition. Hence, to test these hypotheses, this study investigated the effects of dietary CHUP supplementation on the intestinal health of the laying hens, which might provide a feasible nutritional and low-cost dietary formulation approach to promote intestinal health and extend the laying period of laying hens.
Materials and methods
Experimental birds, diets, and management
A total of 576 healthy 300 days old Xinyang black-feather laying hens were randomly assigned into eight groups. Each group contained eight replicates with nine hens per replicate. After 7 days of the adaptation period, the birds in the control group were fed a basal diet, and the birds in the treatment groups were fed a basal diet supplemented with 0.5% Leonuri herba (LH group), 0.25% Ligustri lucidi fructus (LF group), 0.25% Taraxaci herba (TH group), 0.5% LH + 0.25% LF (LH-LF group), 0.5% LH + 0.25% TH (LH-TH group), 0.25% LF + 0.25% TH (LF-TH group), and 0.5% LH + 0.25% LF + 0.25% TH (LH-LF-TH group), respectively. The CHUP (particle size distribution, D95 < 48 μm) additives were provided by Kangxing Pharmaceutical Co., Ltd. (Henan, China). The experiment lasted 120 days. Three hens were housed per cage, kept in four-layer ladder cages, and had free access to feed and drinking water at all times. The birds were housed in a room with a 16 h/8 h light/dark cycle and fed twice daily at 07:30 and 16:00 h with their respective diets throughout the experimental period; water was available by a nipple-type drinker. The room temperature was maintained at approximately 25°C. According to the layer feeding standard (NY/T 33-2004), the basal diet was formulated based on the nutritional requirements during the late laying period (Table 1).
Sample collection
At the end of the experiment (days 120 of the trial), eight laying hens were selected for euthanization from each group. The middle sections of the jejunum (2 cm in length) were collected and then fixed in 10% neutral-buffered formalin for morphological analysis. Jejunal (middle position) contents were collected into sterile tubes, immediately frozen into liquid nitrogen, and stored at −80°C for intestinal microbiota analysis. The jejunal tissues were collected into RNase-free tubes, frozen into liquid nitrogen, and stored at −80°C for gene expression analysis.
Histomorphological observation
The fixed segments of the jejunal tissues were dehydrated, clarified, and embedded in paraffin. Slides were selected from the sections (approximately 5-μm thickness), stained with hematoxylin-eosin (HE), and finally sealed. The villus height (VH, from the tip of the villus to the villus-crypt junction) and crypt depth (CD, from the crypt opening to the base) of the jejunum were determined using an optical microscope (Nikon Eclipse CI, Tokyo, Japan) with image-processing software. The VH to CD ratio (VCR) was then calculated.
Gene expression analysis related to jejunal physical barrier function
The total RNA of the jejunal tissues was extracted using the AG RNAex Pro reagent (Accurate Biology, Changsha, China). The purity and concentration of the extracted total RNA were determined using a NanoDrop ND-2000 spectrophotometer (Thermo Fischer Scientific, Waltham, MA, US). The total RNA (1 μg) was reversely transcribed into cDNA using the PrimeScript RT Reagent Kit with gDNA Eraser (Accurate Biology) for quantitative PCR analysis. The real-time quantitative PCR (RT-qPCR) was performed on the Light Cycler® 480 II Real-Time PCR System (Roche, Basel, Switzerland). Primer sequences were synthesized by the Tsingke Biotechnology Co., Ltd. (Beijing, China) and used in this study (listed in Supplementary Table S1). The RT-qPCR was carried out in a 10-μL reaction system, consisting of 4.2 μl of cDNA template, 0.4 μl of each primer, and 5.0 μl SYBR® Green mix (AG, Changsha, China). The RT-qPCR conditions were as follows: an initial step at 95°C for 5 min, followed by 40 cycles of denaturation at 95°C for 5 s and annealing at 60°C for 30 s, and a final extension at 72°C for 30 s. The relative mRNA expression levels were calculated using β-actin as the internal control and the 2−ΔΔCt method (Rao et al., 2013).
Jejunal microbiota analysis
The microbial DNA from the individual jejunal contents (200 mg) was extracted using the QIAamp DNA Stool Mini Kit (Qiagen, Hilden, Germany) according to the manufacturer’s instructions. The V3–V4 regions of the 16S rRNA gene were amplified using PCR with primers 338F (5′-ACTCCTACGGGAGGCAGCA-3′) and 806R (5′-GGACTACHVGGGTWTCTAAT-3′). The PCR reaction conditions were as follows: an initial denaturation at 98°C for 2 min, followed by 25 cycles of denaturation at 95°C for 15 s and annealing at 55°C for 30 s, extension at 72°C for 30 s, and a final extension at 72°C for 5 min. PCR amplified products were recovered by 1.20% Agarose Gel Extraction Kit (Invitrogen, Carlsbad, CA, US) and quantified using the Quant-iT PicoGreen dsDNA Assay Kit (Invitrogen, Carlsbad, CA, US). Illumina MiSeq sequencing and bioinformatics analysis were performed by the Shanghai Personal Biotechnology Co., Ltd. (Shanghai, China) on the Illumina NovaSeqPE 250 platform (Illumina, San Diego, CA, US). Sequences were processed and taxonomy was assigned using the Quantitative Insights into Microbial Ecology 2 (QIIME2). Amplicon sequence variants (ASVs) were determined with DADA2 by denoising. The taxonomic annotation of ASVs was implemented using the Greengenes database. Alpha diversity indices (including Observed_species richness, Shannon index, Faith’s Phylogenetic Diversity, and Pielo’s Evenness index) were determined to evaluate the richness and evenness of microbial species. Beta diversity was evaluated by principal coordinate analysis (PCoA) based on the Jaccard distance. Taxonomic distributions at the phylum, family, and genus levels were statistically compared based on the results of taxonomic annotation among different treatment groups. Linear discriminant analysis (LDA) combined effect size (LEfSe) was performed to explore the biomarkers in the relative abundances of bacteria among different groups using Wilcoxon’s test. Spearman’s correlation analysis (R > 0.5, p < 0.05) among the top 50 phyla, families, and genera was conducted to determine the potential relationship between jejunal morphology, gene expression level, and microbial composition.
Statistical analysis
After the test data were initially organized by Excel, one-way ANOVA was performed using the SPSS (version 26.0) software, and Tukey’s post-hoc test was performed to compare the statistical significance among different treatment groups. Data are presented as means and standard error of mean. p value < 0.05 was considered for statistical significance.
Results
Effects of dietary Chinese herb ultrafine powder on jejunal morphology of laying hens
The effects of dietary CHUP supplementation on the jejunal morphology of laying hens are presented in Table 2 and Figure 1. The jejunal VH was lower (p < 0.05) in the TH and LH-TH groups compared with the control and other treatment groups (except the LF group). In addition, jejunal VH was higher (p < 0.05) in the LH-LF group compared with the other treatment groups (except the LH group). The jejunal VCR was higher (p < 0.05) in the LH-LF and LH-LF-TF groups compared with the LH-TH group. However, dietary CHUP supplementation did not affect (p > 0.05) the jejunal CD of laying hens (Table 2). Moreover, there were no visible differences in the jejunal morphology of laying hens (Figure 1).
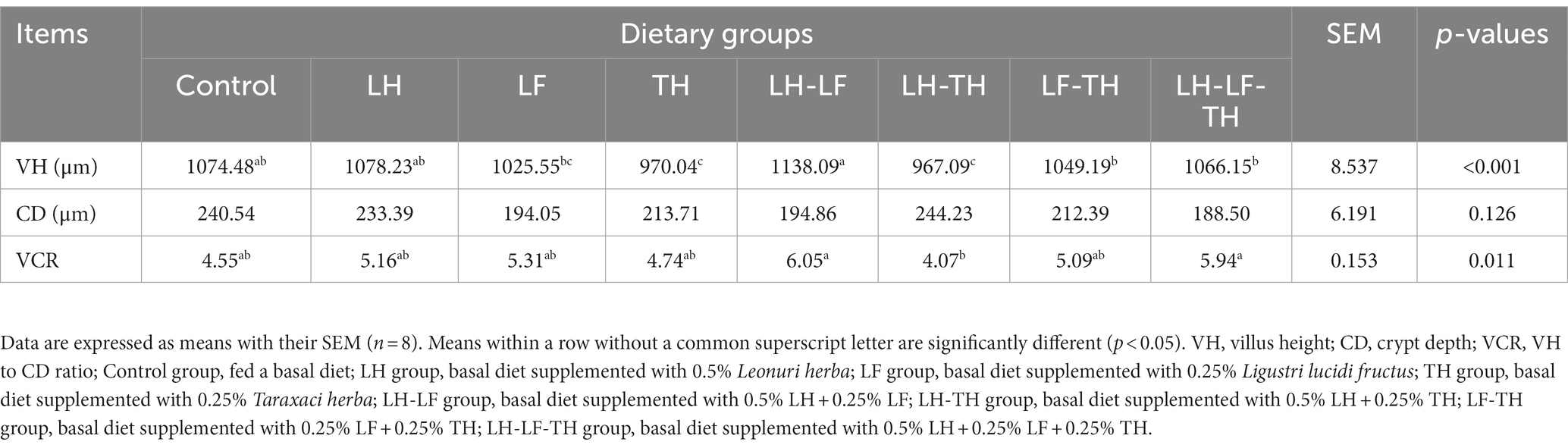
Table 2. Effects of dietary Chinese herb ultrafine powder supplementation on jejunal morphology of laying hens.
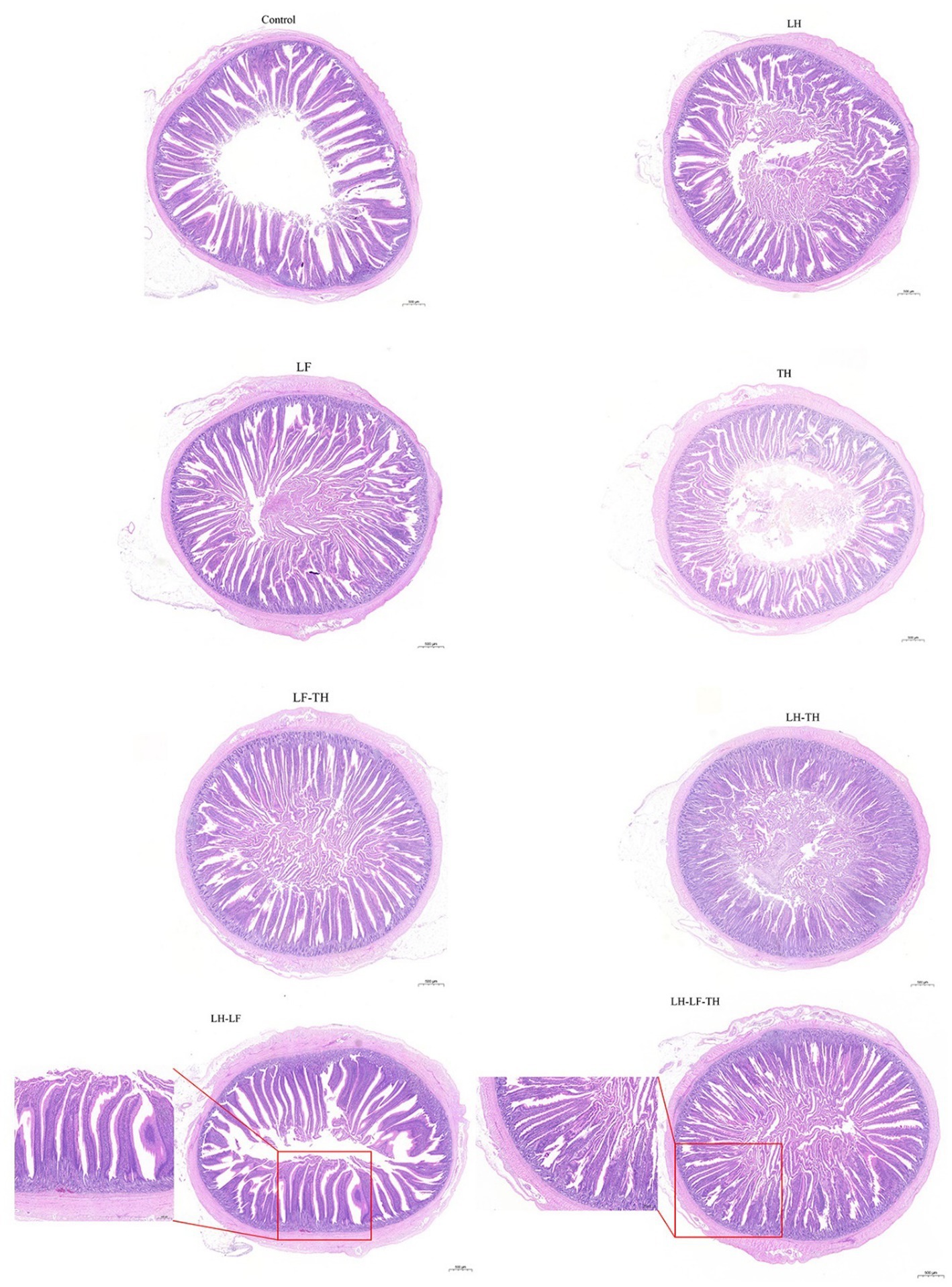
Figure 1. Effects of dietary Chinese herb ultrafine powder supplementation on jejunal morphology of laying hens (HE staining). Scale bars, 500 μm (magnification 20×). Control group, fed a basal diet; LH group, basal diet supplemented with 0.5% Leonuri herba; LF group, basal diet supplemented with 0.25% Ligustri lucidi fructus; TH group, basal diet supplemented with 0.25% Taraxaci herba; LH-LF group, basal diet supplemented with 0.5% LH + 0.25% LF; LH-TH group, basal diet supplemented with 0.5% LH + 0.25% TH; LF-TH group, basal diet supplemented with 0.25% LF + 0.25% TH; LH-LF-TH group, basal diet supplemented with 0.5% LH + 0.25% LF + 0.25% TH.
Effects of dietary Chinese herb ultrafine powder on gene expressions related to jejunal physical barrier functions in laying hens
The gene expressions related to the jejunal physical barrier function of different treatment groups are shown in Figure 2. Dietary LH-TH and LF-TH supplementation down-regulated (p < 0.05) the jejunal claudin-1 expression compared with the LH group. In addition, dietary LF-TH supplementation up-regulated (p < 0.05) the jejunal claudin-5 expression compared with the other groups. Moreover, jejunal occludin expression was down-regulated (p < 0.05) in all treatment groups (except the LH group) compared with the control group. However, no significant differences (p > 0.05) were observed in mucin-2 (MUC-2) and zonula occludens-1 (ZO-1) expressions in the jejunum of laying hens in response to dietary CHUP supplementation.
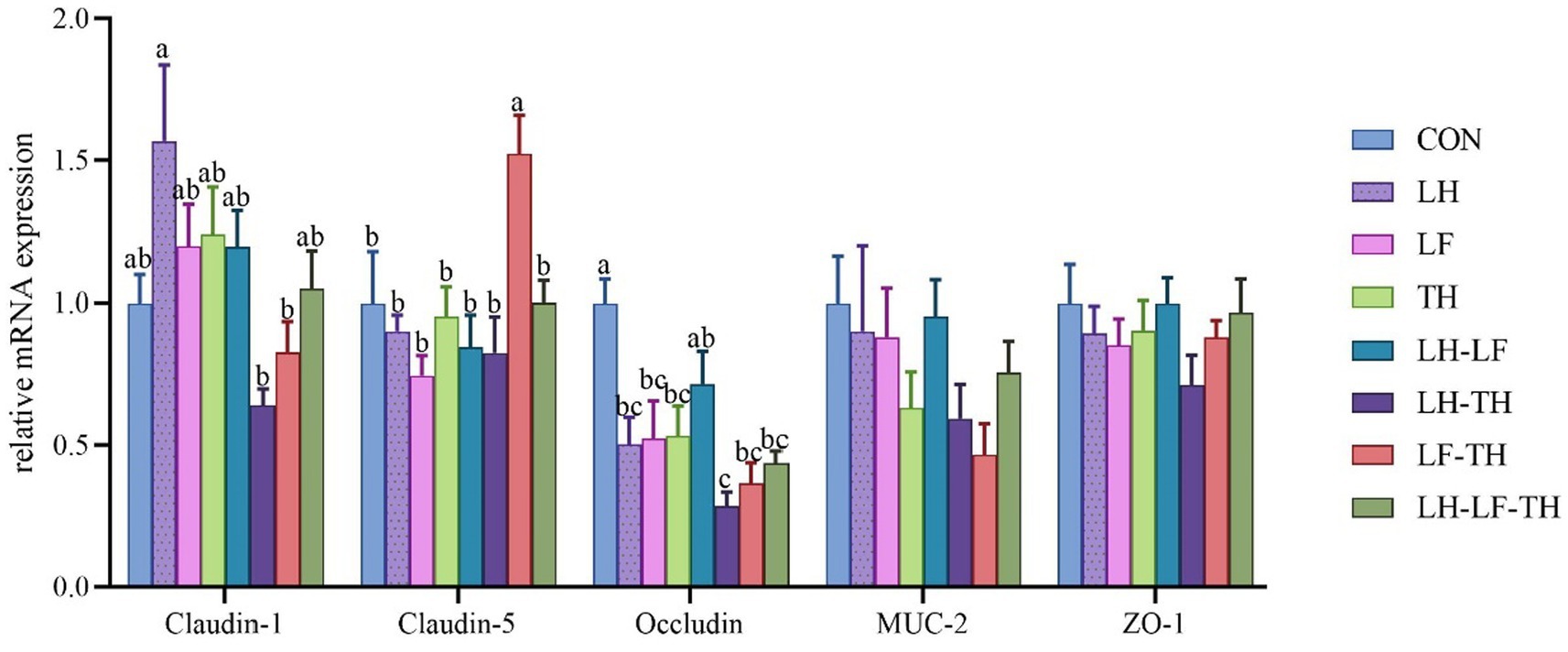
Figure 2. Effects of dietary Chinese herb ultrafine powder supplementation on physical barrier function-related gene expressions in the jejunum of laying hens (n = 8). a–c Different superscript letters in the histogram indicate significant differences among different groups (p < 0.05). MUC-2, mucin-2; ZO-1, zonula occludens-1. CON, control group, fed a basal diet; LH group, basal diet supplemented with 0.5% Leonuri herba; LF group, basal diet supplemented with 0.25% Ligustri lucidi fructus; TH group, basal diet supplemented with 0.25% Taraxaci herba; LH-LF group, basal diet supplemented with 0.5% LH + 0.25% LF; LH-TH group, basal diet supplemented with 0.5% LH + 0.25% TH; LF-TH group, basal diet supplemented with 0.25% LF + 0.25% TH; LH-LF-TH group, basal diet supplemented with 0.5% LH + 0.25% LF + 0.25% TH.
Effects of dietary Chinese herb ultrafine powder on jejunal microbiota profile in laying hens
A total of 7,988,670 valid sequences were obtained from 64 samples of the jejunal contents in laying hens using the DADA2 plugin. Alpha diversity analysis was conducted to evaluate the diversity and structure of the jejunal microbiota communities in different dietary treatments. As shown in Figure 3, no significant differences (p > 0.05) were observed between the treatment and control groups; however, there were distinct separations (p < 0.05) between the LH and LF-TH groups in Observed_species, Faith’s PD, Pielou_e, and Simpson indices. As shown in Figure 4, the PCoA analysis revealed that there were significant separations between the control and LH-LF, LH-TH, or LH-LF-TH groups.
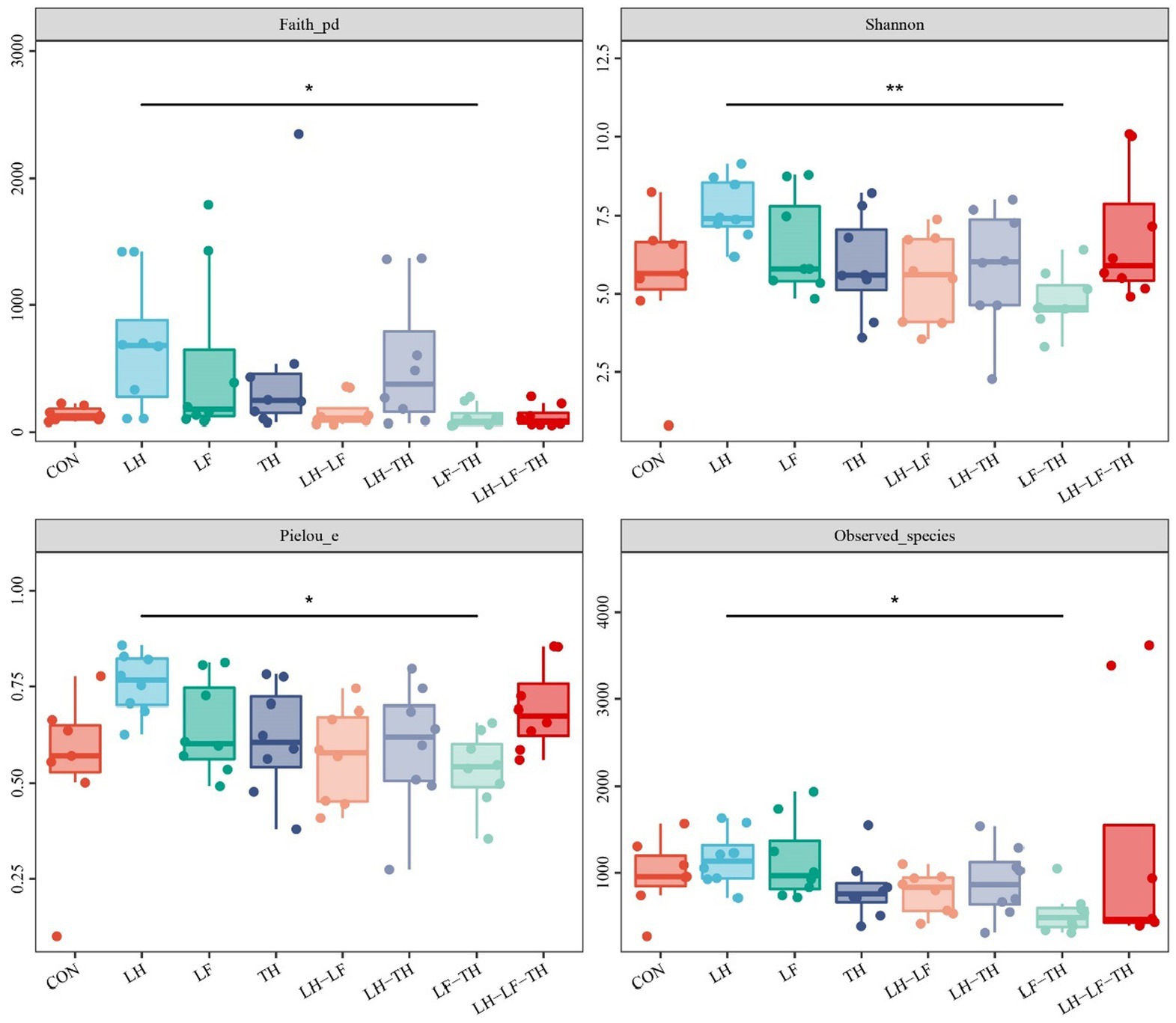
Figure 3. Effects of dietary Chinese herb ultrafine powder supplementation on alpha diversity indices of jejunal microbiota of laying hens (n = 7–8). *p < 0.05, **p < 0.01. CON, control group, fed a basal diet; LH group, basal diet supplemented with 0.5% Leonuri herba; LF group, basal diet supplemented with 0.25% Ligustri lucidi fructus; TH group, basal diet supplemented with 0.25% Taraxaci herba; LH-LF group, basal diet supplemented with 0.5% LH + 0.25% LF; LH-TH group, basal diet supplemented with 0.5% LH + 0.25% TH; LF-TH group, basal diet supplemented with 0.25% LF + 0.25% TH; LH-LF-TH group, basal diet supplemented with 0.5% LH + 0.25% LF + 0.25% TH.
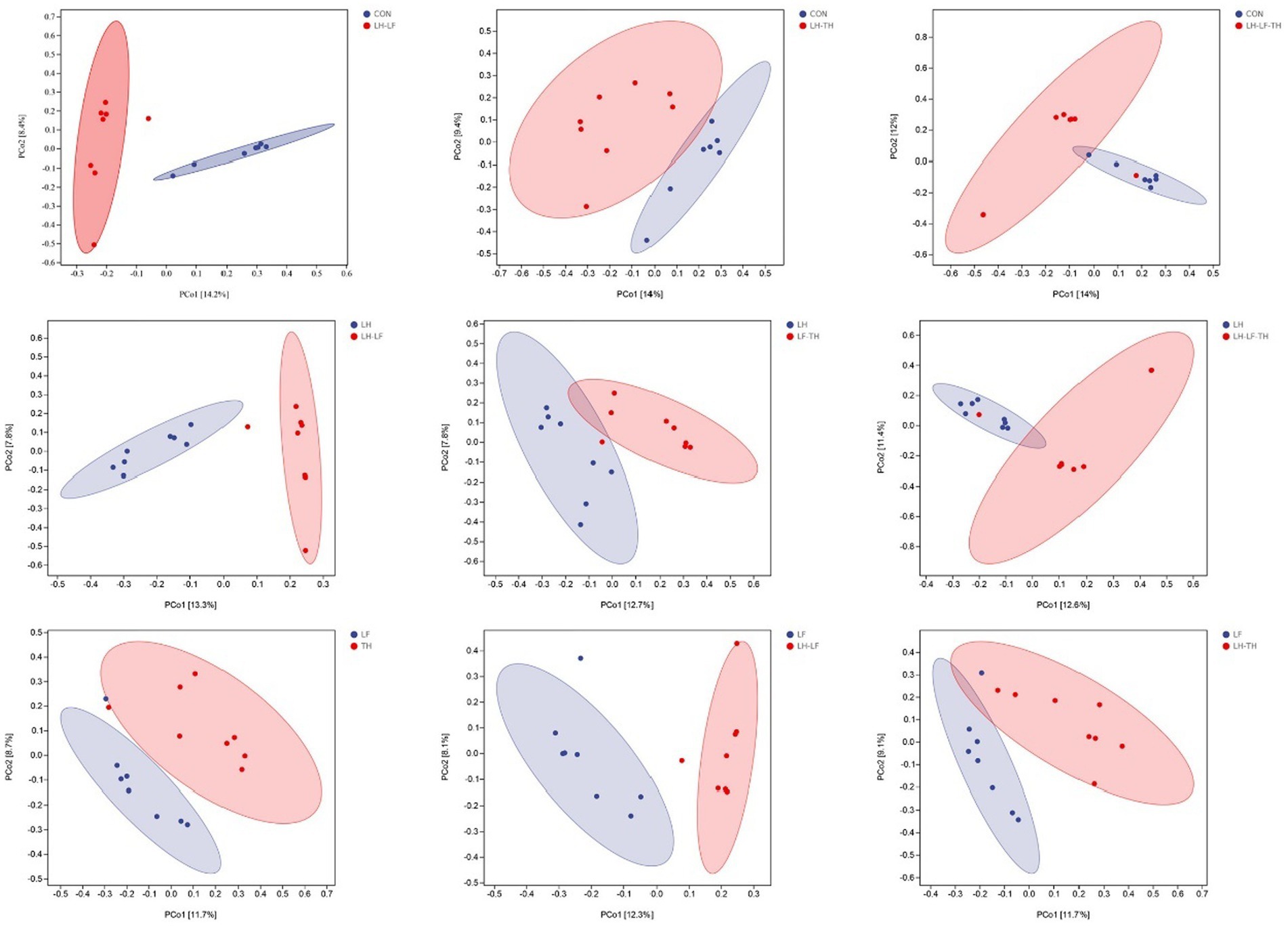
Figure 4. Effects of dietary Chinese herb ultrafine powder supplementation on β-diversity of jejunal microbiota of laying hens (n = 7–8). Principal coordinate analysis (PCoA) of microbiota based on Jaccard distances. CON, control group, fed a basal diet; LH group, basal diet supplemented with 0.5% Leonuri herba; LF group, basal diet supplemented with 0.25% Ligustri lucidi fructus; TH group, basal diet supplemented with 0.25% Taraxaci herba; LH-LF group, basal diet supplemented with 0.5% LH + 0.25% LF; LH-TH group, basal diet supplemented with 0.5% LH + 0.25% TH; LF-TH group, basal diet supplemented with 0.25% LF + 0.25% TH; LH-LF-TH group, basal diet supplemented with 0.5% LH + 0.25% LF + 0.25% TH.
The jejunal microbiota composition was analyzed at the phylum (Figure 5), family (Figure 6), and genus (Figure 7) levels. At the phylum level (Figure 5A), Firmicutes (59.07%) and Proteobacteria (18.41%) were the most dominant phyla, followed by Actinobacteria (10.14%), Bacteroidetes (8.79%), Tenericutes (0.25%), Verrucomicrobia (0.23%), and others (3.12%). The relative abundances of Cyanobacteria and Chloroflexi were higher (p < 0.05) in the LH-LF-TH group compared with the other groups (Figure 5B). At the family level (Figure 6A), Lactobacillaceae (33.97%) and Enterococcaceae (6.70%) were the most dominant families, followed by Helicobacteraceae (5.45%), Peptostreptococcaceae (4.51%), Sphingomonadaceae (4.38%), Ruminococcaceae (3.97%), and others (41.01%). The relative abundance of Enterococcaceae was higher (p < 0.05) in the LH-LF and LF-TH groups, as well as Lachnospiraceae in the LH group, when compared with the control group. However, the abundance of Lachnospiraceae in the TH, LH-LF, LH-TH, and LH-LF-TH groups was lower (p < 0.05) compared with the control group (Figure 6B). At the genus level (Figure 7A), Lactobacillus (33.92%) and Enterococcus (6.69%) were the two most dominant phyla, followed by Helicobacter (5.45%), Sphingomonas (4.30%), Bacteroides (2.74%), Aeriscardovia (2.49%), and others (44.41%). The relative abundances of Enterococcus in the LH-LF and LF-TH groups, Aeriscardovia in the LH-LF group, Chelativorans and Halomonas in the LH-LF-TH group, and Blautia in the LH and LF groups were higher (p < 0.05) compared with the control group. The relative abundance of Faecalibacterium in the LH-LF group was lower (p < 0.05) compared with the control and LF groups, whereas Ruminococcus was lower (p < 0.05) in the LH-LF and LH-LF-TH groups compared with the control group (Figure 7B).
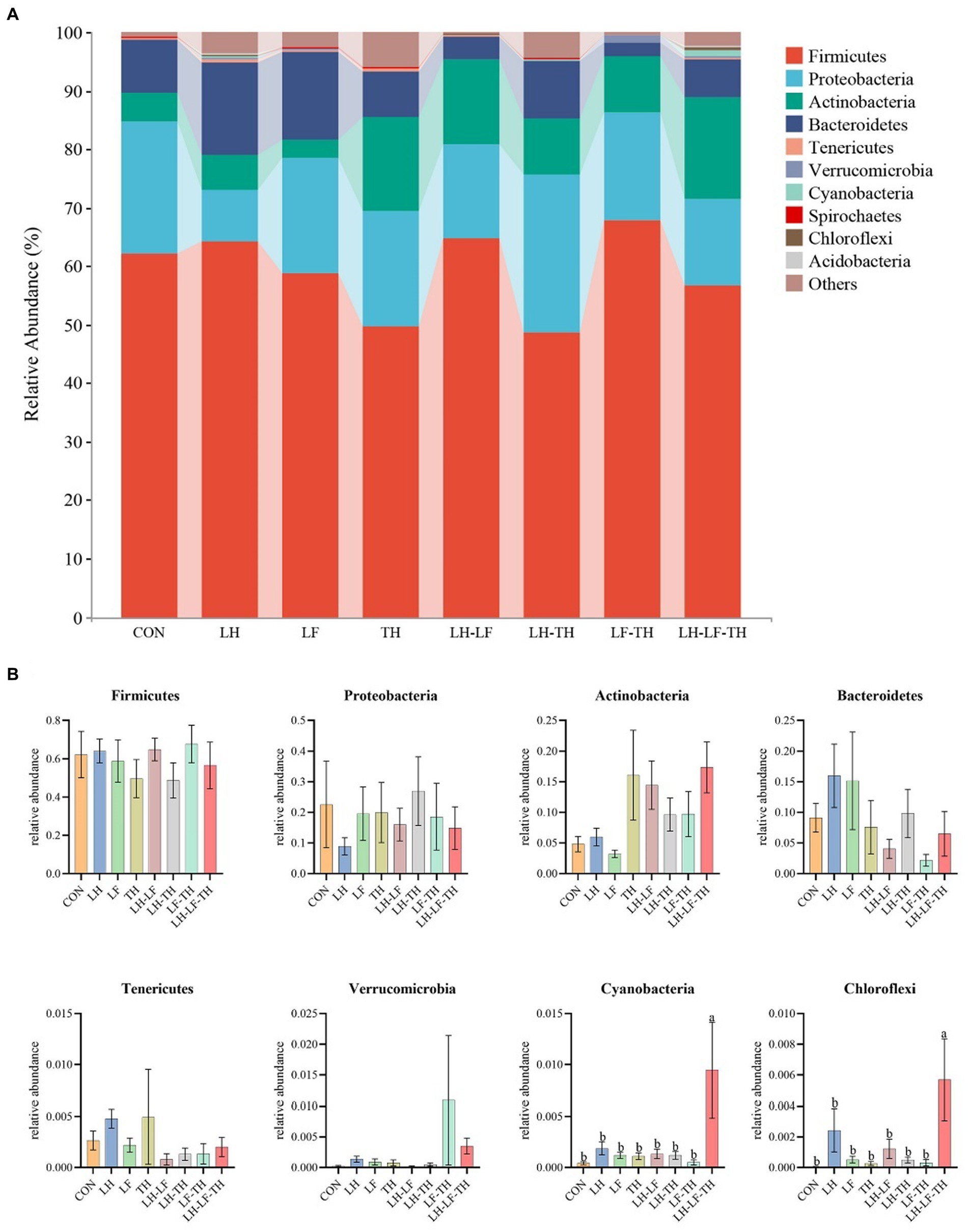
Figure 5. Effects of dietary Chinese herb ultrafine powder supplementation on microbial community composition in the jejunum of laying hens at the phylum level (n = 7–8). (A) Taxonomic composition at the phylum level, and (B) taxonomic differences among different groups. a,b Different superscript letters in the histogram indicate significant differences among different groups (p < 0.05). CON, control group, fed a basal diet; LH group, basal diet supplemented with 0.5% Leonuri herba; LF group, basal diet supplemented with 0.25% Ligustri lucidi fructus; TH group, basal diet supplemented with 0.25% Taraxaci herba; LH-LF group, basal diet supplemented with 0.5% LH + 0.25% LF; LH-TH group, basal diet supplemented with 0.5% LH + 0.25% TH; LF-TH group, basal diet supplemented with 0.25% LF + 0.25% TH; LH-LF-TH group, basal diet supplemented with 0.5% LH + 0.25% LF + 0.25% TH.
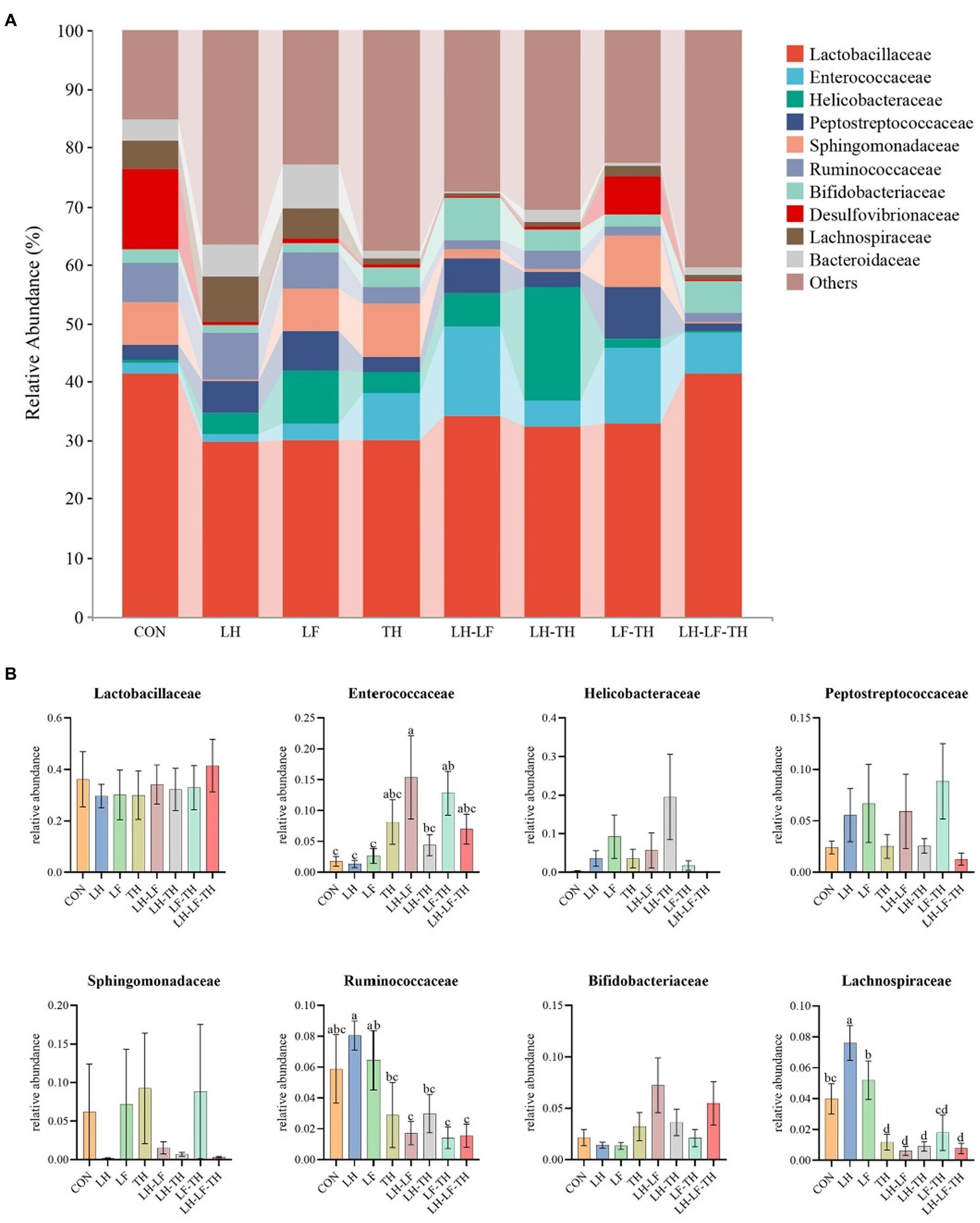
Figure 6. Effects of dietary Chinese herb ultrafine powder supplementation on microbial community composition in the jejunum of laying hens at the family level (n = 7–8). (A) Taxonomic composition at the family level, and (B) taxonomic differences among different groups. a–d Different superscript letters in the histogram indicate significant differences among different groups (p < 0.05). CON, control group, fed a basal diet; LH group, basal diet supplemented with 0.5% Leonuri herba; LF group, basal diet supplemented with 0.25% Ligustri lucidi fructus; TH group, basal diet supplemented with 0.25% Taraxaci herba; LH-LF group, basal diet supplemented with 0.5% LH + 0.25% LF; LH-TH group, basal diet supplemented with 0.5% LH + 0.25% TH; LF-TH group, basal diet supplemented with 0.25% LF + 0.25% TH; LH-LF-TH group, basal diet supplemented with 0.5% LH + 0.25% LF + 0.25% TH.
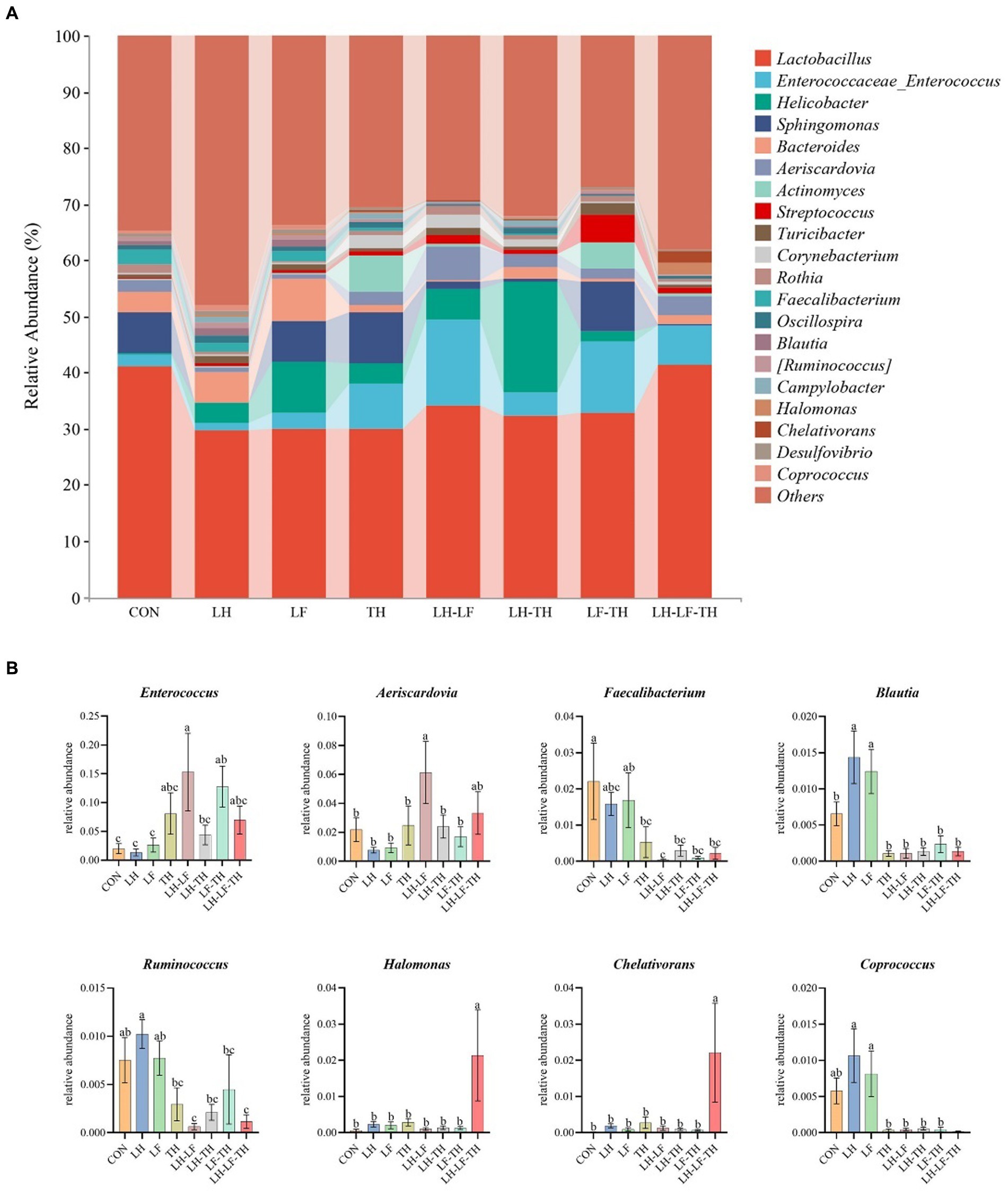
Figure 7. Effects of dietary Chinese herb ultrafine powder supplementation on microbial community composition in the jejunum of laying hens at the genus levels (n = 7–8). (A) Taxonomic composition at the genus levels, and (B) taxonomic differences among different groups. a–c Different superscript letters in the histogram indicate significant differences among different groups (p < 0.05). CON, control group, fed a basal diet; LH group, basal diet supplemented with 0.5% Leonuri herba; LF group, basal diet supplemented with 0.25% Ligustri lucidi fructus; TH group, basal diet supplemented with 0.25% Taraxaci herba; LH-LF group, basal diet supplemented with 0.5% LH + 0.25% LF; LH-TH group, basal diet supplemented with 0.5% LH + 0.25% TH; LF-TH group, basal diet supplemented with 0.25% LF + 0.25% TH; LH-LF-TH group, basal diet supplemented with 0.5% LH + 0.25% LF + 0.25% TH.
The LEfSe analysis (Figures 8A,B) revealed that 32 bacterial biomarkers were enriched (LDA threshold > 2) in the jejunum of the LH group, including (LDA > 4) Clostridiales, Clostridia, and Lachnospiraceae. The abundances of Springobacteriaceae, Sphingobacterium, Methylopila, Luteimonsa, and Erythrobacteraceae were enriched in the LH-LF group. In the LH-LF-TH group, 12 bacterial biomarkers were enriched (LDA > 2) in the jejunum of laying hens, including (LDA > 4) Rhizobiales, Phyllobacteriaceae, and Chelativorans. Moreover, 19 bacterial biomarkers were enriched (LDA > 2) in the jejunum of the LH-TH group, including (LDA > 4) Camphylobacerales, Epsilonproteobacteria, Helicobacter, and Helicobacteraceae. However, only Eubacteriaceae was enriched (LDA > 2) in the control group.
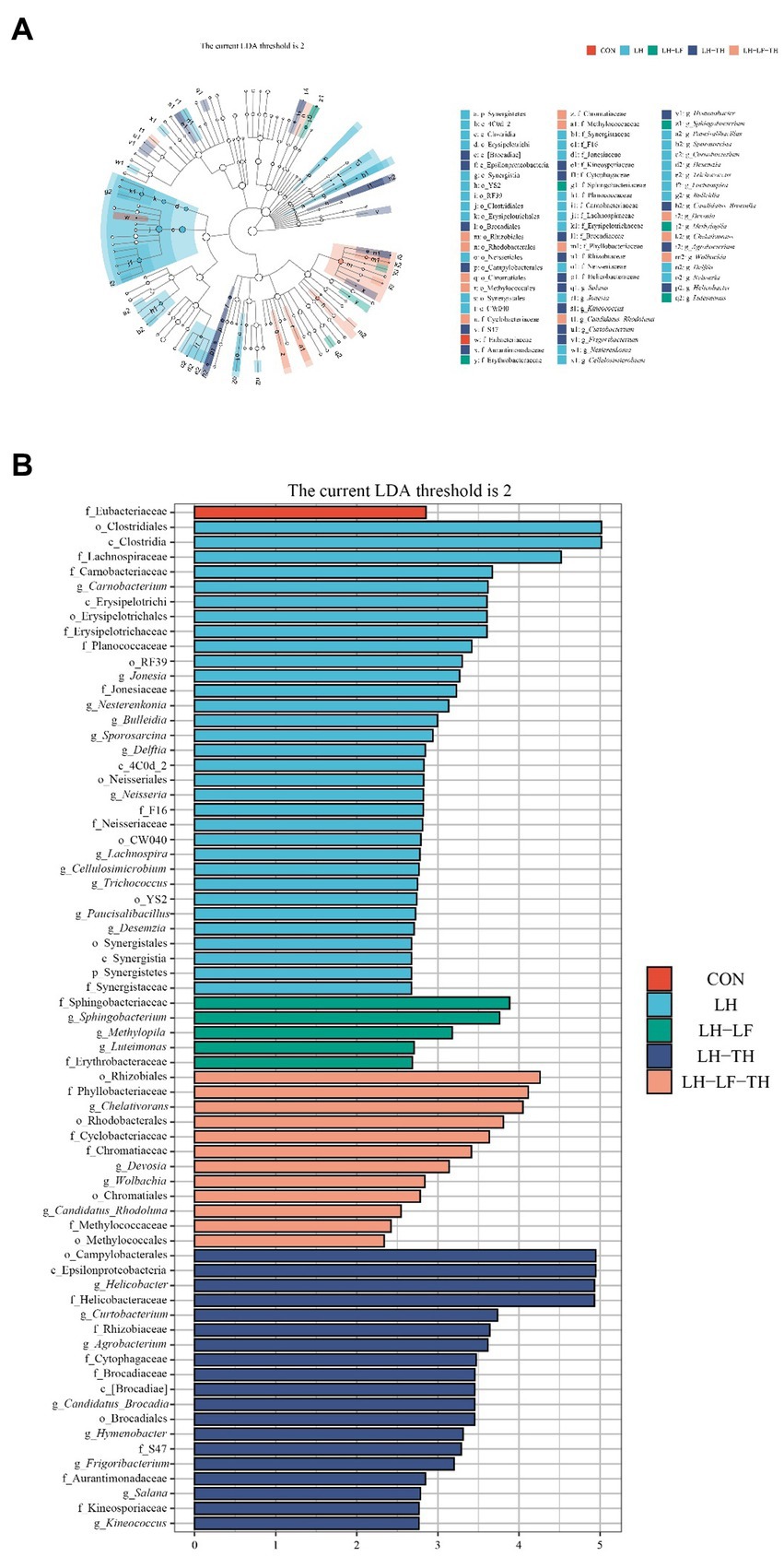
Figure 8. Effects of dietary Chinese herb ultrafine powder supplementation on differential bacterial taxa in the jejunum of laying hens (n = 7–8). (A) Taxonomic cladogram and (B) histogram of the distribution of LDA values for different species identified by the LEfSe algorithm. Species with significant difference have an LDA score greater than the estimated value (2.0). CON, control group, fed a basal diet; LH group, basal diet supplemented with 0.5% Leonuri herba; LF group, basal diet supplemented with 0.25% Ligustri lucidi fructus; TH group, basal diet supplemented with 0.25% Taraxaci herba; LH-LF group, basal diet supplemented with 0.5% LH + 0.25% LF; LH-TH group, basal diet supplemented with 0.5% LH + 0.25% TH; LF-TH group, basal diet supplemented with 0.25% LF + 0.25% TH; LH-LF-TH group, basal diet supplemented with 0.5% LH + 0.25% LF + 0.25% TH.
Effects of dietary Chinese herb ultrafine powder on function prediction of jejunal microbiota in laying hens
The functional abundances of jejunal microbial communities predicted by the PICRUSt2 are shown in Figure 9. Dietary LH supplementation increased (p < 0.05) the relative abundances of microbial genes involved in the ansamycins, tetracycline biosynthesis, β-lactam resistance, β-alanine metabolism, butirosin and neomycin biosynthesis, and polyketide sugar unit biosynthesis compared with the control group (Figure 9A). Dietary LF supplementation increased (p < 0.05) the relative abundances of microbial genes involved in the biosynthesis of ansamycins compared with the control group (Figure 9B). Compared with the control group, dietary TH supplementation increased (p < 0.05) the relative abundances of microbial genes involved in the ubiquinone and other terpenoid quinone biosynthesis, styrene degradation, and biosynthesis of siderophore group nonribosomal peptides (Figure 9C). The relative abundances of microbial genes involved in the polycyclic aromatic hydrocarbon degradation and ascorbate/aldarate metabolism were enhanced (p < 0.05) in the LH-LF group compared with the control group (Figure 9D). Diets supplemented with LH-TH increased (p < 0.05) the relative abundances of microbial genes involved in the atrazine degradation and ethylbenzene degradation (Figure 9E). As shown in Figure 9F, dietary LF-TH supplementation increased (p < 0.05) the relative abundances of microbial genes involved in the ascorbate and aldarate metabolism compared with the control group. Compared with the control group, dietary LH-LF-TH supplementation increased (p < 0.05) the relative abundances of microbial genes involved in the retinol metabolism and aminobenzoate degradation (Figure 9G). Moreover, the relative abundances of microbial genes involved in the D-arginine and D-ornithine metabolism were increased (p < 0.05) in the LH and TH groups, whereas steroid biosynthesis, limonene and pinene degradation, and polyketide sugar unit biosynthesis were increased (p < 0.05) in the LH-LF, LH-TH, and LH-LF-TH groups, as well as the microbial genes involved in the ascorbate and aldarate metabolism and polyketide sugar unit biosynthesis in the TH and LF-TH groups.
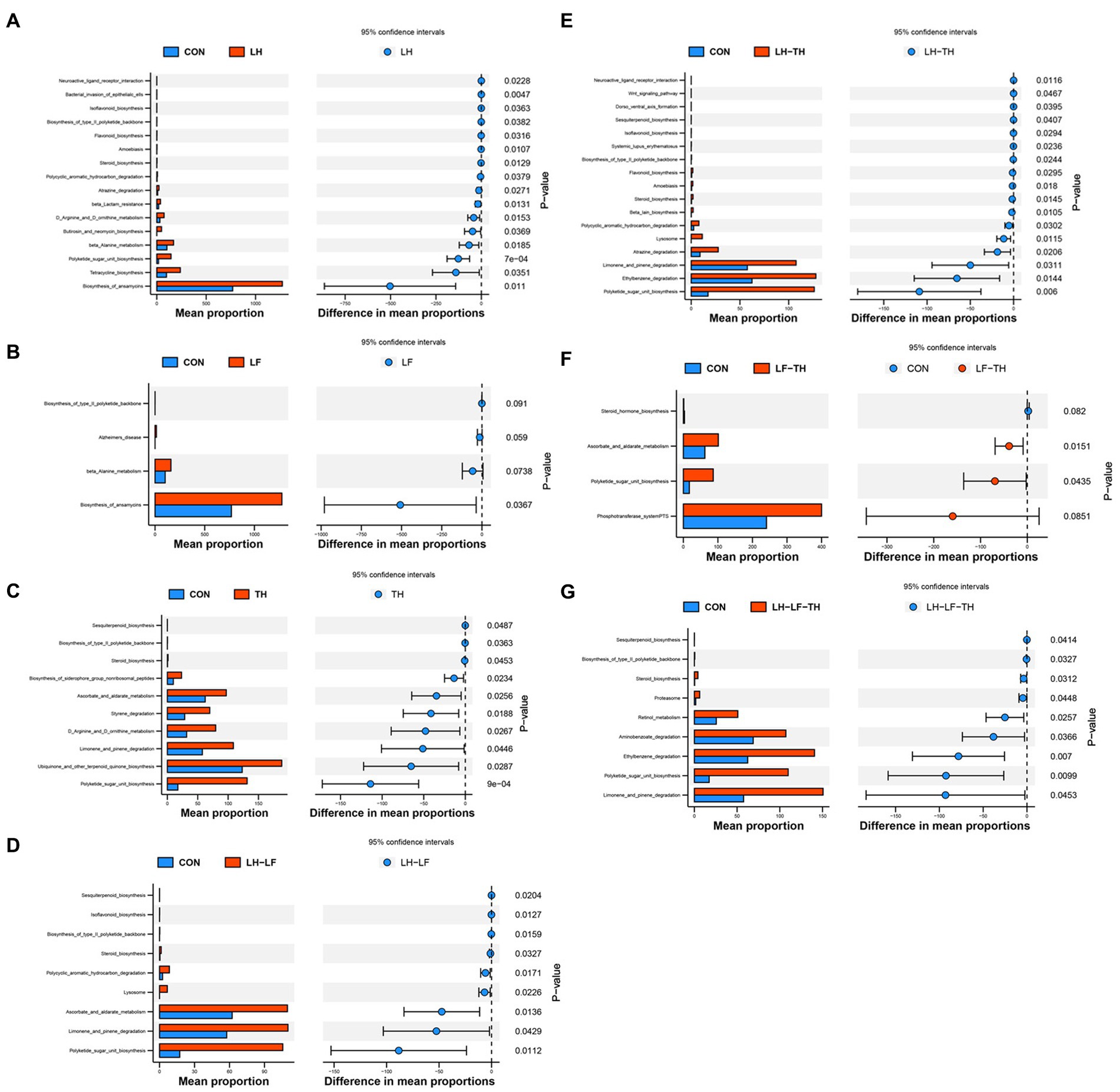
Figure 9. Differences in metabolic functions based on PICRUSt2 analysis of jejunal microbiota of laying hens. (A) CON vs. LH, (B) CON vs. LF, (C) CON vs. TH, (D) CON vs. LH-LF, (E) CON vs. LH-TH, (F) CON vs. LF-TH, and (G) CON vs. LH-LF-TH. CON, control group, fed a basal diet; LH group, basal diet supplemented with 0.5% Leonuri herba; LF group, basal diet supplemented with 0.25% Ligustri lucidi fructus; TH group, basal diet supplemented with 0.25% Taraxaci herba; LH-LF group, basal diet supplemented with 0.5% LH + 0.25% LF; LH-TH group, basal diet supplemented with 0.5% LH + 0.25% TH; LF-TH group, basal diet supplemented with 0.25% LF + 0.25% TH; LH-LF-TH group, basal diet supplemented with 0.5% LH + 0.25% LF + 0.25% TH.
Correlation between microbiota abundances and morphology and physical barrier function of jejunum in laying hens
The Spearman’s correlation analysis was performed to explore the differential abundances of jejunal microbiota associated with jejunal morphology and physical barrier function-related gene expressions (Figure 10). The VH was positively correlated (p < 0.05) with the relative abundances of Firmicutes, Subdoligranulum, Carnobacteriaceae, and Carnobacterium, while negatively (p < 0.05) correlated with WPS2. The CD was positively correlated (p < 0.05) with the relative abundances of Spirochaetes, Treponema, Deferribacteres, and Elusimicrobia, while negatively correlated (p < 0.05) with Acinetobacter. Moreover, the VCR was negatively correlated (p < 0.05) with the relative abundances of Proteobacteria, Spirochaetes, Treponema, Deferribacteres, and Elusimicrobia, whereas positively correlated (p < 0.05) with Staphylococcus, Moraxellaceae, Chloroflexi, GN04, and SBR1093. Claudin-1 expression was positively correlated (p < 0.05) with the relative abundances of Erysipelotrichaceae, Clostridiaceae, Planococcaceae, and WS6. Claudin-5 expression was negatively correlated (p < 0.05) with the relative abundances of Helicobacteraceae, Helicobacter, Staphylococcaceae, and Euryarchaeota. Occludin expression was negatively correlated (p < 0.05) with the relative abundances of Atopobium and OP11. MUC2 expression was positively correlated (p < 0.05) with the relative abundances of Erysipelotrichaceae, Clostridiaceae, Subdoligranulum, Clostridium, and Planococcaceae, while negatively correlated (p < 0.05) with Coriobacteriaceae, Atopbium, and WPS2. Moreover, ZO-1 expression was positively correlated (p < 0.05) with the relative abundance of Micrococcaceae and negatively correlated (p < 0.05) with WPS2.
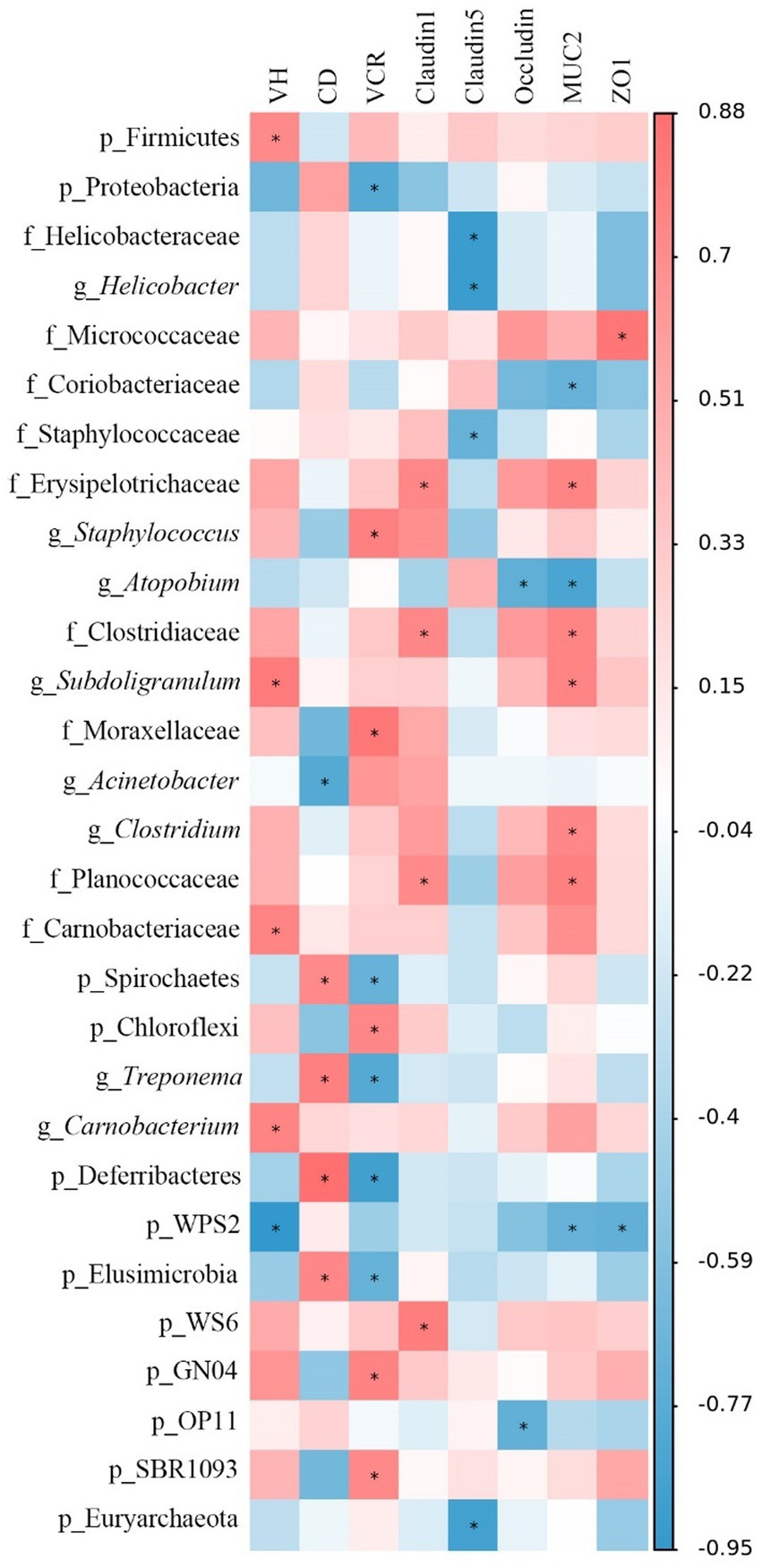
Figure 10. Spearman’s correlation analysis between jejunal morphology, gene expression level, and the microbiota abundances. Red represents a significant positive correlation, and blue represents a significant negative correlation. *p < 0.05. VH, villus height; CD, crypt depth; VCR, VH to CD ratio; MUC-2, mucin-2; ZO-1, zonula occludens-1.
Discussion
During the late laying period, the laying performance, immune, and metabolic function of laying hens are decreased, and the egg quality is severely affected. Intestinal health is crucial to the health of the body and is closely related to the intestinal barrier function and microbiota. Therefore, the present study explored the effects of dietary CHUP supplementation on intestinal morphology, physical barrier functions, and microbial community composition in laying hens during the late laying period. The findings indicate that dietary CHUP improved the jejunal morphology and physical barrier function and increased the relative abundances of SCFAs and bacteriocins-producing bacteria and metabolic processes in the jejunum of laying hens.
The intestinal morphology reflects the intestinal function and health of animals (Liao and Nyachoti, 2017). The improvement of the bird’s nutrient absorption capacity mainly depends on the intestinal surface area, which is mainly associated with the intestinal mucosal structure (Gong et al., 2021). The intestinal epithelium is composed of abundant crypt-villus units. The absorptive surface area in villi could mediate nutrient transport (Clevers, 2013). In the present study, dietary LH-LF and LH-LF-TH supplementation increased the jejunal VCR, suggesting that these CHUP additives improved the intestinal nutrient absorption function of laying hens. A previous study demonstrated that dietary supplementation with leonurine hydrochloride at 120 mg/kg increased the VH and decreased the CD in the jejunum of 1-d-old male Ross broilers (Yang et al., 2019). However, dietary LH or TH had no impacts on the jejunal morphology of laying hens in the present study, probably because the combination compounds were more effective than the single Chinese herb. However, further studies are needed to elucidate the possible causes of such improvement.
Tight junction (TJ) proteins include claudins, occludin, and ZO-1 (Jian et al., 2015). Claudins play an indispensable role in maintaining the epidermal barrier (Furuse et al., 2002), and claudin-1 and claudin-5 are members of the claudins (Garcia-Hernandez et al., 2017). The down-regulation of ZOs and claudins (except claudin-2) expressions was found to be involved with the impairment of intestinal barrier function in the jejunal tissues of broilers (Yang et al., 2022). In the present study, dietary LH supplementation up-regulated the jejunal claudin-1 expression, whereas dietary LF-TH up-regulated the claudin-5 expression, suggesting that LH and LF-TH could improve the jejunal physical barrier function of laying hens. In addition, dietary CHUP supplementation down-regulated the jejunal occludin expression compared with the control group. Kuo et al. (2022) suggested that the down-regulation of occludin expression may be an adaptive response that limits tissue injury under inflammatory conditions. Furthermore, dietary LH, LF, and TH have anti-inflammatory characteristics, and the down-regulation of jejunal occludin expression might be one of the potential mechanisms of these Chinese herbs. The specific mechanism of these CHUP to improve the physical barrier of the intestine remains unclear and warrants further studies.
The intestinal microbiota is an important regulator of host metabolism (Tremaroli and Bäckhed, 2012). The correlation between microbiota composition or the relative abundances of bacterial species and physiological health has been previously reported (Hills et al., 2019). Previous studies reported that the improvement of feed conversion was related to the enhancement of intestinal bacteria diversity in laying hens (Zhou J. M. et al., 2021). In the present study, 16S rRNA sequencing results showed that dietary LH supplementation increased α-diversity indices of the jejunal microbiota compared to the LF-TH group. Moreover, the β-diversity analysis indicated significant separations between the control group and the LH-LF, LH-TH, and LH-LF-TH groups. These findings indicate that there were different effects on the richness and diversity of the intestinal microbiota between compounds and single Chinese herb, and the increased microbial richness in the jejunum might be beneficial for the growth of laying hens.
The intestinal microbiota, especially beneficial bacteria, regulates host health in various ways (Ding et al., 2021). Several intestinal bacteria, such as Enterococcus, Bacteroides, and Feacalibacterium, contribute to the body weight gain and egg-laying performance of hens (Han et al., 2022). Blautia is considered as a potential probiotic bacteria and is widely present in the intestines of mammals (Liu et al., 2021). In the present study, the relative abundances of Blautia, Clostridia, Erysipelotrichaceae, and Carnobacterium were enhanced by dietary LH supplementation. In recent years, metabolites produced by intestinal microbiota have attracted much attention due to their ability to regulate intestinal homeostasis (Sittipo et al., 2019). Blautia and Carnobacterium can produce bacteriocins with bactericidal activity and have non-bacteriolytic properties (Pilet et al., 1995). Bacteriocins are antimicrobial peptides that can inhibit pathogenic bacteria (Zou et al., 2018). Moreover, Blautia, Clostridium (Zhu et al., 2022), and Erysipelotrichaceae (Zhou J. et al., 2021) have been found to produce SCFAs, such as butyrate and propionate, which could improve the integrity of the intestinal epithelial and mucosal barrier. These bacteria species also positively affect the immune response and intestinal microbiota diversity (Peng et al., 2022). Collectively, these findings suggest that dietary LH may prevent the infection of antibiotic-resistant pathogens and would be beneficial for maintaining intestinal barrier integrity in laying hens. In addition, dietary LH-LF supplementation increased the abundances of several natural antimicrobial probiotics (i.e., Enterococcus and Aeriscardovia) and decreased some SCFAs-producing bacteria (i.e., Feacalibacterium and Ruminococcus). Research evidence reported that the active ingredients of Chinese herbs could be converted into bioactive metabolites after oral administration, which could affect intestinal microbiota composition (Lin et al., 2021). Therefore, the decreased SCFAs-producing bacteria may be related to the interaction of bioactive ingredients of the Chinese herbs with intestinal metabolites.
The interactions between intestinal microbiota and host nutrient metabolism have recently become a research hotspot (Zhang et al., 2020). Accumulating research evidence suggested that tetracycline, butirosin, and neomycin are important antibiotics that inhibit protein synthesis by binding to the bacterial ribosome (Kudo and Eguchi, 2009), and tetracycline has oxygen radical scavenging and anti-inflammatory properties (Griffin et al., 2010). In the present study, dietary LH supplementation enriched the abundances of microbial genes related to the biosynthesis of tetracycline, butirosin, and neomycin. This may be related to the increased bacteriocins-producing bacteria (Blautia and Carnobacterium) in the present study. In addition, the abundances of microbial genes related to the D-arginine and D-ornithine metabolism were enriched by dietary LH supplementation. Previous studies reported that D-arginine and D-ornithine metabolism in the intestine was associated with improved intestinal morphology and function by synthesizing polyamines and nitric oxide. Polyamines were found in the differentiation and proliferation of intestinal cells (Cynober, 1994). These findings indicate that the up-regulated claudin-1 expression by dietary LH supplementation may be related to the regulation of D-arginine and D-ornithine metabolism in the jejunum of laying hens. Moreover, the present study also found that the abundances of jejunal microbial genes responsible for the limonene and pinene degradation were higher in the TH, LH-LF, LH-TH, and LH-LF-TH groups. Terpenoids are widely presented in Chinese herbs (Matkowski et al., 2013), and limonene and pinene are the intermediate products of terpenoids; suggesting that terpenoids present in Chinese herbs were effectively absorbed by laying hens after administration.
The Spearman’s correlation analysis revealed that jejunal Erysipelotrichaceae, Clostridiaceae, and Planococcaceae abundances were positively correlated with jejunal claudin-1 expression. The positive correlation of these bacterial abundances may be explained by the fact that dietary LH up-regulated the jejunal claudin-1 expression by increasing the abundance of jejunal SCFAs-producing bacteria. Subdoligranulum is a butyrate-producing bacteria (Qin et al., 2020). Zuo et al. (2020) reported that sodium butyrate could increase the VH and VCR in the small intestine of weaned lambs. The increased VH may be related to the production of sodium butyrate by the Subdoligranulum. These findings suggest that the effects of dietary LH and LH-LF-TH supplementation on jejunal morphology and physical barrier function may be partly responsible for the enrichment of potential probiotic bacteria species.
Conclusion
In summary, dietary CHUP supplementation during the late laying period promoted jejunal physical barrier function in laying hens, which may be partly mediated by increasing the abundance of SCFAs and bacteriocins-producing bacteria and metabolic processes in the jejunum. Considering the beneficial effects of these CHUP on the jejunal morphology, gene expressions related to physical barrier function, and jejunal microbiota, dietary LH-LF, LF-TH, and LH-LF-TH supplementation had better effects, which could be potential dietary additives in poultry production.
Data availability statement
The datasets presented in this study can be found in online repositories. The names of the repository/repositories and accession number(s) can be found at: https://www.scidb.cn/s/6VjuMz, 10.57760/sciencedb.07486.
Ethics statement
The animal study was reviewed and approved by The Ethics Committee of the Institute of Subtropical Agriculture, Chinese Academy of Science.
Author contributions
XK, YC, and JH designed the experiments. JG, WLi, CM, XH, and WLa conducted the experiments. JG, MA, and XK wrote and revised the paper. All authors contributed to the article and approved the submitted version.
Funding
This study was supported by the City-School Cooperation Project of the Special Funds of Science and Technology in Fuyang City undertaken by Fuyang Normal University (SXHZ2020007) and the Special Funds of Construction of Innovative Provinces in Hunan Province (2019RS3022).
Conflict of interest
The authors declare that the research was conducted in the absence of any commercial or financial relationships that could be construed as a potential conflict of interest.
Publisher’s note
All claims expressed in this article are solely those of the authors and do not necessarily represent those of their affiliated organizations, or those of the publisher, the editors and the reviewers. Any product that may be evaluated in this article, or claim that may be made by its manufacturer, is not guaranteed or endorsed by the publisher.
Supplementary material
The Supplementary material for this article can be found online at: https://www.frontiersin.org/articles/10.3389/fmicb.2023.1185806/full#supplementary-material
References
Abd El-Hack, M. E., El-Saadony, M. T., Shafi, M. E., Qattan, S. Y. A., Batiha, G. E., Khafaga, A. F., et al. (2020). Probiotics in poultry feed: a comprehensive review. J. Anim. Physiol. Anim. Nutr. 104, 1835–1850. doi: 10.1111/jpn.13454
Ashour, E. A., El-Kholy, M. S., Alagawany, M., Abd El-Hack, M. E., Mohamed, L. A., Taha, A. E., et al. (2020). Effect of dietary supplementation with Moringa oleifera leaves and/or seeds powder on production, egg characteristics, hatchability and blood chemistry of laying Japanese quails. Sustainability 12:2463. doi: 10.3390/su12062463
Bedford, M. R., and Cowieson, A. J. (2012). Exogenous enzymes and their effects on intestinal microbiology. Anim. Feed Sci. Technol. 173, 76–85. doi: 10.1016/j.anifeedsci.2011.12.018
Chang, E.B., and Martinez-Guryn, K. (2019) Small intestinal microbiota: the neglected stepchild needed for fat digestion and absorption. Gut Microbes 10, 235–240. doi: 10.1080/19490976.2018.1502539
Chen, F., Wen, Q., Jiang, J., Li, H. L., Tan, Y. F., Li, Y. H., et al. (2016). Could the gut microbiota reconcile the oral bioavailability conundrum of traditional herbs? J. Ethnopharmacol. 179, 253–264. doi: 10.1016/j.jep.2015.12.031
Cheng, G., Hao, H., Xie, S., Wang, X., Dai, M., Huang, L., et al. (2014). Antibiotic alternatives: the substitution of antibiotics in animal husbandry? Front. Microbiol. 5:217. doi: 10.3389/fmicb.2014.00217
Chinese Pharmacopoeia Commission. (2020). Pharmacopoeia of the People’s republic of China: Edition Volume I Beijing: China Medical Science Press.
Clevers, H. (2013). The intestinal crypt, a prototype stem cell compartment. Cells 154, 274–284. doi: 10.1016/j.cell.2013.07.004
Cynober, L. (1994). Can arginine and ornithine support gut functions? Gut 35, S42–S45. doi: 10.1136/gut.35.1_suppl.s42
Ding, S. J., Yan, W. X., Ma, Y., and Fang, J. (2021). The impact of probiotics on gut health via alternation of immune status of monogastric animals. Anim. Nutr. 7, 24–30. doi: 10.1016/j.aninu.2020.11.004
Dong, X. Y., Azzam, M. M. M., and Zou, X. T. (2017). Effects of dietary threonine supplementation on intestinal barrier function and gut microbiota of laying hens. Poult. Sci. 96, 3654–3663. doi: 10.3382/ps/pex185
Furuse, M., Hata, M., Furuse, K., Yoshida, Y., Haratake, A., Sugitani, Y., et al. (2002). Claudin-based tight junctions are crucial for the mammalian epidermal barrier: a lesson from claudin-1-deficient mice. J. Cell Biol. 156, 1099–1111. doi: 10.1083/jcb.200110122
Gao, J., Xu, K., Liu, H., Liu, G., Bai, M., Peng, C., et al. (2018). Impact of the gut microbiota on intestinal immunity mediated by tryptophan metabolism. Front. Cell. Infect. Microbiol. 8:13. doi: 10.3389/fcimb.2018.00013
Garcia-Hernandez, V., Quiros, M., and Nusrat, A. (2017). Intestinal epithelial claudins: expression and regulation in homeostasis and inflammation. Ann. N. Y. Acad. Sci. 1397, 66–79. doi: 10.1111/nyas.13360
Gong, H., Yang, Z., Celi, P., Yan, L., Ding, X., Bai, S., et al. (2021). Effect of benzoic acid on production performance, egg quality, intestinal morphology, and cecal microbial community of laying hens. Poult. Sci. 100, 196–205. doi: 10.1016/j.psj.2020.09.065
Griffin, M. O., Fricovsky, E., Ceballos, G., and Villarreal, F. (2010). Tetracyclines: a pleitropic family of compounds with promising therapeutic properties. Am. J. Physiol. Cell Physiol. 299, C539–C548. doi: 10.1152/ajpcell.00047.2010
Han, H., Sun, Y., Fan, Y., Zhang, H., Yang, J., Chi, R., et al. (2022). Microbial diversity and community composition of duodenum microbiota of high and low egg-yielding taihang chickens identified using 16S rRNA amplicon sequencing. Life 12:1262. doi: 10.3390/life12081262
Han, H., Xu, B., Amin, A., Li, H., Yu, X., Gong, M., et al. (2019). Quercetin-3-O-α-L-rhamnopyranoside derived from the leaves of Lindera aggregata (Sims) Kosterm. Evokes the autophagy-induced nuclear factor erythroid 2-related factor 2 antioxidant pathway in human umbilical vein endothelial cells. Int. J. Mol. Med. 43, 461–474. doi: 10.3892/ijmm.2018.3976
Hills, R. D. Jr., Pontefract, B. A., Mishcon, H. R., Black, C. A., Sutton, S. C., and Theberge, C. R. (2019). Gut microbiome: profound implications for diet and disease. Nutrients 11:1613. doi: 10.3390/nu11071613
Huang, P., Zhang, Y., Xiao, K., Jiang, F., Wang, H., Tang, D., et al. (2018). The chicken gut metagenome and the modulatory effects of plant-derived benzylisoquinoline alkaloids. Microbiome 6:211. doi: 10.1186/s40168-018-0590-5
Jian, Y. K., Chen, C. Q., Li, B., and Tian, X. B. (2015). Delocalized claudin-1 promotes metastasis of human osteosarcoma cells. Biochem. Biophys. Res. Commun. 466, 356–361. doi: 10.1016/j.bbrc.2015.09.028
Kudo, F., and Eguchi, T. (2009). Biosynthetic enzymes for the aminoglycosides butirosin and neomycin. Methods Enzymol. 459, 493–519. doi: 10.1016/s0076-6879(09)04620-5
Kuo, W. T., Odenwald, M. A., Turner, J. R., and Zuo, L. (2022). Tight junction proteins occludin and ZO-1 as regulators of epithelial proliferation and survival. Ann. N. Y. Acad. Sci. 1514, 21–33. doi: 10.1111/nyas.14798
Li, L., Liu, Z., Fang, B., Xu, J., Dong, X., Yang, L., et al. (2022). Effects of vitamin a and K-3 on immune function and intestinal antioxidant capacity of aged laying hens. Braz. J. Poult. Sci. 24:eRBCA-2021-1572. doi: 10.1590/1806-9061-2021-1572
Liao, S. F., and Nyachoti, M. (2017). Using probiotics to improve swine gut health and nutrient utilization. Anim. Nutr. 3, 331–343. doi: 10.1016/j.aninu.2017.06.007
Lin, T. L., Lu, C. C., Lai, W. F., Wu, T. S., Lu, J. J., Chen, Y. M., et al. (2021). Role of gut microbiota in identification of novel TCM-derived active metabolites. Protein Cell 12, 394–410. doi: 10.1007/s13238-020-00784-w
Liu, X. M., Mao, B. Y., Gu, J. Y., Wu, J. Y., Cui, S. M., Wang, G., et al. (2021). Blautia-a new functional genus with potential probiotic properties? Gut Microbes 13:e1875796. doi: 10.1080/19490976.2021.1875796
Ma, W. Q., Gu, Y., Lu, J. Y., Yuan, L. F., and Zhao, R. Q. (2014). Effects of chromium propionate on egg production, egg quality, plasma biochemical parameters, and egg chromium deposition in late-phase laying hens. Biol. Trace Elem. Res. 157, 113–119. doi: 10.1007/s12011-013-9875-5
Ma, L., Ni, Y., Wang, Z., Tu, W., Ni, L., Zhuge, F., et al. (2020). Spermidine improves gut barrier integrity and gut microbiota function in diet-induced obese mice. Gut Microbes 12:e1832857. doi: 10.1080/19490976.2020.1832857
Matkowski, A., Jamiolkowska-Kozlowska, W., and Nawrot, I. (2013). Chinese medicinal herbs as source of antioxidant compounds-where tradition meets the future. Curr. Med. Chem. 20, 984–1004. doi: 10.2174/0929867311320080003
Nii, T. (2022). Relationship between mucosal barrier function of the oviduct and intestine in the productivity of laying hens. J. Poult. Sci. 59, 105–113. doi: 10.2141/jpsa.0210090
Peng, K. X., Xia, S. H., Xiao, S. Q., and Yu, Q. (2022). Short-chain fatty acids affect the development of inflammatory bowel disease through intestinal barrier, immunology, and microbiota: a promising therapy? J. Gastroenterol. Hepatol. 37, 1710–1718. doi: 10.1111/jgh.15970
Pilet, M. F., Dousset, X., Barré, R., Novel, G., Desmazeaud, M., and Piard, J. C. (1995). Evidence for two bacteriocins produced by Carnobacterium piscicola and Carnobacterium divergens isolated from fish and active against Listeria monocytogenes. J. Food Prot. 58, 256–262. doi: 10.4315/0362-028x-58.3.256
Qin, S. M., Zhang, K. Y., Applegate, T. J., Ding, X. M., Bai, S. P., Luo, Y. H., et al. (2020). Dietary administration of resistant starch improved caecal barrier function by enhancing intestinal morphology and modulating microbiota composition in meat duck. Br. J. Nutr. 123, 172–181. doi: 10.1017/s0007114519002319
Rao, T., Gong, Y. F., Peng, J. B., Wang, Y. C., He, K., Zhou, H. H., et al. (2019). Comparative pharmacokinetic study on three formulations of Astragali Radix by an LC-MS/MS method for determination of formononetin in human plasma. Biomed. Chromatogr. 33:e4563. doi: 10.1002/bmc.4563
Rao, X., Huang, X., Zhou, Z., and Lin, X. (2013). An improvement of the 2ˆ(−delta delta CT) method for quantitative real-time polymerase chain reaction data analysis. Biostat. Bioinforma. Biomath. 3, 71–85.
Shu, Z. J., Cao, Y., and Halmurat, U. (2011). Gut flora may offer new therapeutic targets for the traditional Chinese medicine enteric dialysis. Expert Opin. Ther. Targets 15, 1147–1152. doi: 10.1517/14728222.2011.614234
Sittipo, P., Shim, J. W., and Lee, Y. K. (2019). Microbial metabolites determine host health and the status of some diseases. Int. J. Mol. Sci. 20:5296. doi: 10.3390/ijms20215296
Tremaroli, V., and Bäckhed, F. (2012). Functional interactions between the gut microbiota and host metabolism. Nature 489, 242–249. doi: 10.1038/nature11552
Wang, W. W., Wang, J., Zhang, H. J., Wu, S. G., and Qi, G. H. (2019). Transcriptome analysis reveals mechanism underlying the differential intestinal functionality of laying hens in the late phase and peak phase of production. BMC Genomics 20:970. doi: 10.1186/s12864-019-6320-y
Wang, Y., Xu, L., Sun, X., Wan, X., Sun, G., Jiang, R., et al. (2020). Characteristics of the fecal microbiota of high- and low-yield hens and effects of fecal microbiota transplantation on egg production performance. Res. Vet. Sci. 129, 164–173. doi: 10.1016/j.rvsc.2020.01.020
Wei, X., Tao, J., Xiao, S., Jiang, S., Shang, E., Zhu, Z., et al. (2018). Xiexin Tang improves the symptom of type 2 diabetic rats by modulation of the gut microbiota. Sci. Rep. 8:3685. doi: 10.1038/s41598-018-22094-2
Yang, L., Liu, G., Lian, K. X., Qiao, Y. J., Zhang, B. J., Zhu, X. Q., et al. (2019). Dietary leonurine hydrochloride supplementation attenuates lipopolysaccharide challenge-induced intestinal inflammation and barrier dysfunction by inhibiting the NF-κB/MAPK signaling pathway in broilers. J. Anim. Sci. 97, 1679–1692. doi: 10.1093/jas/skz078
Yang, S. Q., Xiong, Z. H., Xu, T. F., Peng, C. C., Hu, A. M., Jiang, W. J., et al. (2022). Compound probiotics alleviate cadmium-induced intestinal dysfunction and microbiota disorders in broilers. Ecotoxicol. Environ. Saf. 234:113374. doi: 10.1016/j.ecoenv.2022.113374
Zhang, H. Y., Tian, J. X., Lian, F. M., Li, M., Liu, W. K., Zhen, Z., et al. (2021). Therapeutic mechanisms of traditional Chinese medicine to improve metabolic diseases via the gut microbiota. Biomed. Pharmacother. 133:110857. doi: 10.1016/j.biopha.2020.110857
Zhang, H., Xu, R., Su, Y., and Zhu, W. (2020). A review: gut microbiota in monogastric animals. Chin. J. Anim. Nutr. 32, 4674–4685. doi: 10.3969/j.issn.1006-267x.2020.10.019
Zhang, B., Yue, R., Chen, Y., Yang, M., Huang, X., Shui, J., et al. (2019). Gut microbiota, a potential new target for Chinese herbal medicines in treating diabetes mellitus. Evid. Based Complement. Alternat. Med. 2019:2634898. doi: 10.1155/2019/2634898
Zhou, J., Luo, J., Yang, S. M., Xiao, Q. L., Wang, X. L., Zhou, Z. T., et al. (2021). Different responses of microbiota across intestinal tract to Enterococcus faecium HDRsEf1 and their correlation with inflammation in weaned piglets. Microorganisms 9:1767. doi: 10.3390/microorganisms9081767
Zhou, J. M., Wu, S. G., Qi, G. H., Fu, Y., Wang, W. W., Zhang, H. J., et al. (2021). Dietary supplemental xylooligosaccharide modulates nutrient digestibility, intestinal morphology, and gut microbiota in laying hens. Anim. Nutr. 7, 152–162. doi: 10.1016/j.aninu.2020.05.010
Zhu, Z. M., Zhu, L. Y., and Jiang, L. (2022). Dynamic regulation of gut Clostridium-derived short-chain fatty acids. Trends Biotechnol. 40, 266–270. doi: 10.1016/j.tibtech.2021.10.005
Zou, J., Jiang, H., Cheng, H., Fang, J. H., and Huang, G. R. (2018). Strategies for screening, purification and characterization of bacteriocins. Int. J. Biol. Macromol. 117, 781–789. doi: 10.1016/j.ijbiomac.2018.05.233
Keywords: Chinese herb, intestinal barrier function, microbiota, ultrafine powder, Xinyang black-feather hens
Citation: Gui J, Azad MAK, Lin W, Meng C, Hu X, Cui Y, Lan W, He J and Kong X (2023) Dietary supplementation with Chinese herb ultrafine powder improves intestinal morphology and physical barrier function by altering jejunal microbiota in laying hens. Front. Microbiol. 14:1185806. doi: 10.3389/fmicb.2023.1185806
Edited by:
Jiajia Ni, Guangdong Academy of Science, ChinaReviewed by:
Jie Cai, Zhejiang University, ChinaLonglong Li, Nanjing Agricultural University, China
Copyright © 2023 Gui, Azad, Lin, Meng, Hu, Cui, Lan, He and Kong. This is an open-access article distributed under the terms of the Creative Commons Attribution License (CC BY). The use, distribution or reproduction in other forums is permitted, provided the original author(s) and the copyright owner(s) are credited and that the original publication in this journal is cited, in accordance with accepted academic practice. No use, distribution or reproduction is permitted which does not comply with these terms.
*Correspondence: Xiangfeng Kong, bm5reGZAaXNhLmFjLmNu