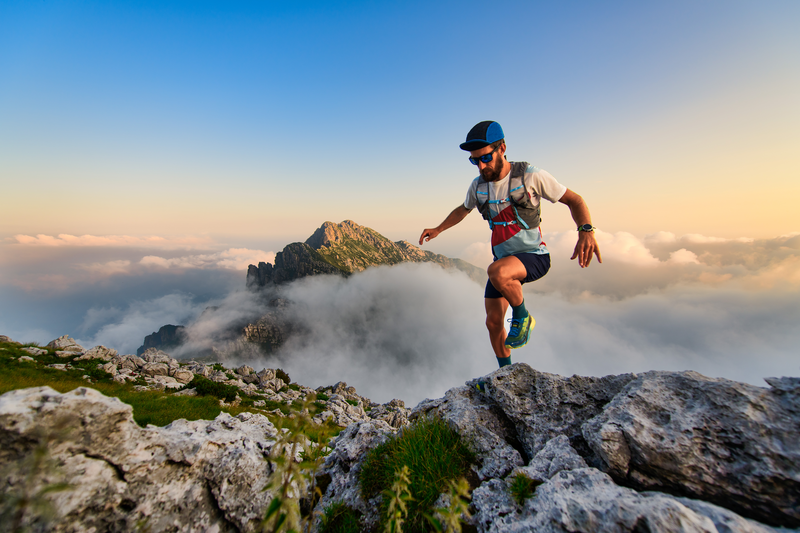
95% of researchers rate our articles as excellent or good
Learn more about the work of our research integrity team to safeguard the quality of each article we publish.
Find out more
ORIGINAL RESEARCH article
Front. Microbiol. , 23 August 2023
Sec. Microorganisms in Vertebrate Digestive Systems
Volume 14 - 2023 | https://doi.org/10.3389/fmicb.2023.1182460
This article is part of the Research Topic Women in Microorganisms in Vertebrate Digestive Systems: 2023 View all 17 articles
Background: Growing evidence supports the role of gut microbiota in obesity, yet exact associations remain largely unknown. Specifically, very little is known about this association in the Emirati population.
Methods: We explored differences in gut microbiota composition, particularly the Firmicutes/Bacteroidetes (F/B) ratio, between 43 obese and 31 lean adult Emirate counterparts, and its association with obesity markers, by using V3-V4 regions of 16 S ribosomal RNA gene sequencing data. Furthermore, we collected anthropometric and biochemical data.
Results: The two major phyla in obese and lean groups were Firmicutes and Bacteroidetes. We observed a significantly lower alpha diversity (Shannon index) in obese subjects and a significant difference in beta diversity and phylum and genus levels between the two groups. The obese group had higher abundances of Verrucomicrobia and Saccharibacteira and lower abundances of Lentisphaerae. Acidaminococcus and Lachnospira were more abundant in obese subjects and positively correlated with adiposity markers. No correlations were found between the gut microbiota and biochemical variables, such as fasting blood sugar, total cholesterol, HDL cholesterol, LDL cholesterol, and triglycerides.
Conclusion: We reveal significant differences in the gut microbiota between obese and lean adult Emiratis and an association between certain microbial genera of the gut microbiota and obesity. A better understanding of the interactions between gut microbes, diet, lifestyle, and health is warranted.
Obesity is a multifactorial disease (Cannon and Kumar, 2009), declared by the World Health Organization (WHO) in 2016 as a pandemic (World Health Organization, 2016). Long-standing factors involved in obesity include excessive caloric intake and a sedentary lifestyle (Morgen and Sørensen, 2014). More recently, obesity was also found to present a complicated disequilibrium of the diversity, richness, and evenness of the bacterial component of the gut microbiota (Aguirre and Venema, 2015), known as dysbiosis (Adlerberth and Wold, 2009; Round and Mazmanian, 2009). The latter has been proposed as part of the etiology of obesity (Davis, 2016) through its effect on digestion, regulation of metabolism, adipose tissue composition, short-chain fatty acid (SCFA) production, modulation of the production of gut peptides and hormones, among others (Corfe et al., 2015; Patrone et al., 2016). The human gut microbiota is primarily composed of Firmicutes and Bacteroidetes, which make up about 90% of all bacterial species, in addition to Actinobacteria, Fusobacteria, Proteobacteria, and Verrucomicrobia (Abenavoli et al., 2019; Rinninella et al., 2019). Nevertheless, substantial differences in composition and function in the microbiota between obese and healthy individuals have been established (Turnbaugh and Gordon, 2009; Tremaroli and Bäckhed, 2012; Pinart et al., 2021). In some studies, obesity in humans is typically characterized by high intestinal concentrations of Firmicutes and low concentrations of Bacteroidetes (Seganfredo et al., 2017; Crovesy et al., 2020); whereby the higher Firmicutes-to-Bacteroidetes ratio (F/B) among obese (Armougom et al., 2009; Furet et al., 2010; Kong et al., 2013) is potentially associated with a greater energy harvest from host diet (Turnbaugh et al., 2006). However, other studies show the opposite F/B ratio in obesity (Schwiertz et al., 2010), or no relation at all (Duncan et al., 2008) and thus, the cause-and-effect relationship between F/B and obesity is still to be elucidated.
Improvements in bacterial DNA sequencing allowed researchers to understand the gut microbiota and its composition and explore its complex relationship with health and disease (Gordon et al., 2007; Duncan et al., 2008). However, there are still gaps in our understanding of the role of the gut microbiota in the etiology of obesity and the effectiveness of interventions targeting gut microbiota for preventing and managing obesity (Tehrani et al., 2012), “e.g., it is still not entirely clear whether differences in the microbiota cause obesity, or whether obesity causes a difference in microbiota composition.” Primarily, this is because most studies have used rodent models, which have different gut microbiota composition, fermentation process, and dietary practices than humans (Heinritz et al., 2013; Nguyen et al., 2015). Moreover, inconsistencies in human studies might be attributed to the different approaches to analyzing the composition of the gut microbiota, as well as the recruitment of subjects with ethnic differences and inter-individual dissimilarities in genetics, diets, and lifestyles which can shape the composition of the gut microbiota (Seganfredo et al., 2017; Crovesy et al., 2020; Pinart et al., 2021; Zeng et al., 2021). The characteristics of the gut microbiota in subjects with obesity are highlighted. Nevertheless, little is known about the association between gut microbiota and clinical variables (fasting blood sugar, total cholesterol, HDL cholesterol, LDL cholesterol, triglycerides, and anthropometrics) linked to obesity in humans. This warrants scientific investigations to explore microbial patterns associated with obesity markers.
In the Gulf countries, the prevalence of obesity is steadily increasing. Specifically, in the United Arab Emirates (UAE), the prevalence of overweight and obesity doubled between 1989 and 2017 (Radwan et al., 2018), and there are 27.8% of adults obese, according to the National Health Survey 2017–2018 (Sulaiman et al., 2017; Ministry of Health and Prevention, 2023). In this work, we aimed to profile the gut microbiota and its association with clinical variables and explore differences (through a comparative analysis) between obese and lean Emirate subjects. Exploring the gut microbiome of Emiratis adds a piece of the puzzle to our understanding of obesity, with the ultimate goal of uncovering preventive and interventional measures tailored to the Emirati population aiming to curb the obesity epidemic in the country.
This is a cross-sectional study conducted among Emirati subjects living in the UAE. The present study is part of a pre-post study registered at clinicaltrials.gov (NCT04200521) and performed in the UAE.
Ethical approval was granted by the Research Ethics Committee of the Ministry of Health and Prevention of UAE (MOHP/DXB-REC-52/2018), the Dubai Health Care Regulatory Research Ethics Committee (DHCR-REC), and the Zayed University Ethical Committee Board (ZU19_51_F). The study followed the Declaration of Helsinki. Written informed consent was collected from all study subjects.
We determined the sample size by following standard calculations based on normal distributions. The sample size was calculated based on the change in F/B from pre- to post-bariatric surgery (Brant, 2015), based on the study by Damms-Machado et al. (2015). In that study, the mean(SD) fecal F/B increased significantly from 5.9 (2.1) to 10.4 (1.4) in 3 months post-bariatric surgery (Damms-Machado et al., 2015). To detect a similarly significant effect, two patients were needed to achieve 80% power at a two-sided alpha level of 5%. To allow for the high expected dropout rate, the minimum sample size was multiplied by 15. Hence, we targeted 30 Emirati obese adults residing in the UAE of both sex and planning to undergo the bariatric procedure. In addition, we recruited a sample of 30 age and sex-matched control subjects (lean adults living in the UAE).
Emirati residents of UAE, aged between 18 and 60 years, of either sex, free of antibiotics for at least 3 months, falling into one of the two body mass index (BMI) categories were included: (1) obese with a BMI of ≥35 kg/m2 and willing to undergo bariatric surgery; (2) lean counterparts with a BMI of 18.5–24.9 kg/m2 and consenting to participate in the study.
Obese participants were recruited from one hospital in Dubai and another in Sharjah. In contrast, lean subjects were recruited from community settings in Dubai through word-of-mouth and flyer postings. Recruitment took place from October 2019 till March 2021.
Individuals who consumed alcohol exceeding two drinks per day for men and one drink per day for women (Snetselaar et al., 2021), were pregnant at the time of the study, experienced significant weight loss (≥5%) in the past 3 months, or were not willing to consent for the study were excluded.
General demographic characteristics collected from the patient included age and sex. Furthermore, health-related information was collected using a subjective screening questionnaire, including the presence of any chronic diseases such as dyslipidemia, diabetes, and hypertension, surgical history in the past 5 years, weight loss of at least 5% in the past 3 months, current intake of medication including antibiotics, and current consumption of prebiotic/probiotic/fiber supplement and probiotic foods, consumption of other nutrition supplements, and engagement in a physically active lifestyle and exercise.
Physical activity level was analyzed using two questions from our initial screening questionnaire. A “yes” or “no” answer was required for the following two questions: “Do you integrate physical activity in your daily routine” and “Do you incorporate any exercise program.” Sedentary was denoted if “no” was answered to both questions; light physical activity if “yes” was only answered to the first question; moderate physical activity if “yes” was only responded to the second question; and high physical activity if “yes” was answered to both questions.
For each participant, anthropometric measurements, lipid profile, fasting blood sugar (FBS), and microbiota analyses were done.
Anthropometric data were collected by trained research assistants and dietitians.
Body weight (kg): measured via portable Seca 762 scale (Vogel & Halke, Hamburg, Germany), following best practices, i.e., with light clothes and without shoes. Height (cm): measured using a portable stadiometer attached to the weighing scale following best practices, i.e., to the nearest 0.1 cm, without shoes, with the participant stretching to the maximum height while having the head positioned in the Frankfort plane. BMI (kg/m2): calculated according to the standard formula by dividing weight in kilograms by squared height in meters. Waist circumference (WC) (cm): measured using a Seca 201 ergonomic circumference measuring tape, at the mid-point between the right iliac crest and the lower costal region, to the nearest 0.1 cm (Van der Kooy and Seidell, 1993). WC was categorized as appropriate (<94 cm in men and < 80 cm in women) or increased ( 94 cm for men and 80 cm for women; Ford, 2005).
Waist-to-height ratio (WHtR): calculated by dividing the WC (cm) by height (cm), whereby abdominal obesity was defined as a WHtR 0.5 (Ashwell and Gibson, 2016). Fat mass (FM) and Percent Body Fat (PBF): measured via bioelectrical impedance analyzer (BC-420 MA, Tanita Corporation, Tokyo, Japan) with the participants being well hydrated, without drinking caffeine for 12 h, not participating in excessive physical activity 24 h before the analysis, and wearing comfortable clothes. Elevated PBF was defined as PBF ≥ 25% and ≥ 35% in men and women, respectively (Romero-Corral et al., 2008).
Total cholesterol (TC), low-density lipoprotein cholesterol (LDL-C), high-density lipoprotein cholesterol (HDL-C), triglycerides (TG), and FBS: measured after 12 h of fasting via portable Lux Meter Blood Test (Biochemical Systems International, S.p.A; Arezzo, Italy) following the manufacturer’s instructions.
Stool samples were collected in Zymo DNA/RNA Shield fecal collection tubes and stored at room temperature. Two samples were collected from each participant, one of which was analyzed, and the second was held for accuracy verification. Sample collection followed standard protocols and regulations. Collection tubes (with a spoon attached to the cap for collecting 1 gram of feces) were pre-filled with DNA/RNA Shield™ (9 mL). The nucleic acids (DNA & RNA) in the samples are preserved at room temperature (DNA > 1 year, RNA up to 1 month).
DNA isolation and sequencing of the barcoded amplicons of the V3–V4 region of the 16S rRNA gene was done as per the protocols of Illumina (Illumina, Eindhoven, The Netherlands; Surono et al., 2022). The sequencing was performed using the Illumina MiSeq system (San Diego, CA, United States) using barcodes and the 2 × 300 bp protocol. We used Quantitative Insights Into Microbial Ecology: QIIME 21 software to analyze raw sequences; the latter were classified using the Silva database (version 132) as a reference 16S rRNA gene database. Reads were filtered, and only those present in at least 20% of the samples were taken.
α Diversity indices: observed-OTUs, Chao1 index, Phylogenetic diversity (Faith’s PD), Pielou evenness, and Shannon diversity index, which were calculated with QIIME 2 and shown using the R software [R (4.1.x) (R Core Team)]2 in RStudio. The rarefaction curves were generated using the ggplot2 package for R. Rarefication depth was set at 3800.
β Diversity indices were visualized in a Principal Coordinate Analysis (PCoA) based on Bray–Curtis dissimilarity, Jaccard distance, and weighted and unweighted UniFrac using QIIME2 software.
The statistical significance of β diversity differences between the two groups was determined using Permutational Multivariate Analysis of Variance (PERMANOVA). The value of p was calculated through the Pairwise permanova method to compare β diversity between each category in all samples. Differences between obesity and lean samples (at phyla and genus levels) were analyzed via the non-parametric Kruskal–Wallis test corrected with the Benjamini–Hochberg false discovery rate (FDR) for multiple comparisons in Rstudio (the software package R (4.1.x), R Core Team, footnote 2).
The composition of the two groups was visualized using the Krona tool.3
Linear discriminant analysis (LDA) effect size (LEfSe) was used to explore microbial taxa with differential abundance between lean and obese (version 1.0).4 Taxa showing LDA values above two at a value of p <0.05 were considered enriched taxa in each group.
The non-parametric Spearman’s rank-order correlations between the Amplicon Sequence Variant (ASVs) and continuous variables (BMI, WC, WHtR, PBF, FM, LDL, HDL, TC, TG, TC/HDL, and FBS) were calculated. Q-values, i.e., adjusted value of ps after the FDR, were considered significantly different at a cut-off of <0.05. The F/B ratio was calculated by dividing the relative abundances of Firmicutes and Bacteroidetes.
A heatmap of Spearman correlation analysis was generated in R software (ggplot2 package).
We analyzed ‘participants’ characteristics using the Statistical Package for the Social Sciences software version 21 (SPSS Inc. Chicago, IL, United States). We assessed the normality of the data using Skewness and Kurtosis. Normal data were summarized as mean (M) ± standard deviation (SD) and skewed data as median (Mdn) and interquartile range (IQR). Between-group differences were explored using independent samples t-test for normally distributed continuous variables, Mann–Whitney U-test for skewed continuous variables, and Chi-square for categorical values. A value of p < 0.05 was used to denote statistical significance.
The characteristics of participant groups are presented in Table 1. Forty-three obese subjects were included (37.2% men; mean ± SD age of 29.95 ± 9.13 years), whereas 31 lean subjects participated (38.7% men; mean ± SD age 29.67 ± 10.73 years). No significant differences in gender and age were observed between the groups. The obese group had higher anthropometric (BMI, WC, WHtR, and PBF) and biochemical parameters (FBS, LDL, TC, and TC/HDL-c ratio) than the lean group, as expected. There was no significant difference in lifestyle factors between the two groups (prebiotic, probiotic, fiber, alcohol consumption, and smoking). The level of physical activity in the obese group was mainly light and significantly lower than that of the lean group (Supplementary Table S1).
Table 1. Demographic, anthropometric, and biochemical parameters between lean (n = 31) and obese participants (n = 43).
After filtering for taxa that were present in at least 20% of the samples, the microbiota was composed of eight phyla and 109 genera.
At the phylum level, Firmicutes (50% in lean vs. 47% in obese) and Bacteroidetes (44% in lean vs. 49% in obese) were the two most abundant bacterial phyla in the gut of obese and lean subjects. The obese group had significantly higher abundances of the phyla Verrucomicrobia (0.5% in obese vs. 0.3% in lean, Benjamini-Hochberg correction, q = 0.048), and Saccharibacteira (0.003% in obese vs. 0.0009% in lean, q = 0.0002) than the lean group and significantly lower abundances of the phyla Lentisphaerae (0.03% in obese vs. 0.08% in lean, q = 0.004). However, there was no significant difference in the relative abundance of Bacteroidetes (q = 0.12), Firmicutes (q = 0.32), Fusobacteria (q = 0.2), Actinobacteria (q = 0.54), and Proteobacteria (q = 0.85) among the two groups. Furthermore, there was no significant difference in the F/B ratio between the obese (0.95) and lean group (1.1); p = 0.315 (Supplementary Figures S1A,B; Table 2).
Table 2. The gut microbial composition (relative abundance in %) of obese and lean participants (phylum level).
Taxonomic assignment was also performed at the genus level and revealed a total of 21 major genera with a significant difference between the obese and lean group, where 16 of these belonged to Firmicutes, as depicted in Table 3.
Table 3. The difference in bacterial composition (relative abundance in %) between obese and lean subjects (genus level).
Of the 21 genera, Acidaminococcus and Lachnospira (belonging to the Firmicutes phylum) were higher in the obese group, while the remaining 19 were lower, including (a) from the Firmicutes phylum, an uncharacterized taxon in the Clostridiales.vadinBB60.group (q = 0.001), Anaerotruncus (q = 0.001), an uncharacterized Ruminococcaceae (q = 0.003), Ruminococcaceae.UCG.014 (q = 0.004), Ruminococcaceae.UCG.010 (q = 0.008), Family.XIII.UCG.001 (of the Clostridiales order, Family XIII; q = 0.006), an uncharacterized Erysipelotrichaceae (q = 0.007), Christensenellaceae.R.7.group (q = 0.008), Lachnospiraceae.UCG.010 (q = 0.013), Ruminococcaceae.UCG.005 (q = 0.013), Ruminococcaceae.UCG.002 (q = 0.02), Ruminiclostridium.6 (q = 0.02), an uncharacterized Lachnospiraceae (q = 0.02), Lachnospiraceae.NK4A136.group (q = 0.04), and (b) from the Bacteroidetes phylum, Alistipes (q = 0.04), Odoribacter (q = 0.03), Barnesiella (q = 0.03), (c) from the Proteobacteria phylum, an uncharacterized Rhodospirillaceae (q = 0.001), and (d) from the Verrucomicrobia phylum, Victivallis (q = 0.002; Table 3; Figures 1A,B; Supplementary Figures 2A,B).
Figure 1. (A) Comparison of relative abundance of Acidaminococcus (genus level); (B) Comparison of relative abundance of Lachnospira (genus level) between lean and obese participants. *q < 0.05.
We performed a Linear Discriminant Analysis Effect Size (LEfSe) on the taxa from the obese and lean groups. A total of 26 genera showed significantly different abundances between the two groups with LDA scores >2. In this case, 23 genera were higher in the lean group, and three genera were higher in the obese group, as shown in Figure 2.
Figure 2. LEfSe analysis between the obese and lean groups (LDA score > 2). The LDA score (log10) for the more prevalent genera in the obese group is represented on a positive scale (green), and the LDA score for the more prevalent genera in the lean group is defined on a negative scale (red).
Of the three genera in the obese group, two belonged to Firmicutes (Lachnospira and Acidaminococcus) and one to Verrucomicrobia (Akkermansia). In the Kruskal-Wallis test, the latter was not statistically significant. In contrast, 17, 3, one, one, and one of the 23 genera for the lean group belonged to Firmicutes, Bacteroidetes, Actinobacteria, and Verrucumictobia phyla.
According to the α -diversity metrics (Figure 3), obese adults showed significantly lower diversity (Shannon index, p = 0.031), coinciding with the lower observed OTUs (p = 0.006) and lower Chao1 richness estimator (p = 0.008). Furthermore, the obese group showed lower “Faith’s phylogenetic diversity (PD; p = 0.069) and lower evenness (‘Pielou’s evenness, p = 0.53). However, the latter two were not statistically significant.
Figure 3. Obese individuals tend to have lower microbiome α-diversity. α-diversity was calculated using: (A) Shannon diversity; (B) Pielou’s Evenness; (C) Observed_OTUs; (D) Choa1 diversity; (E) Faith’s PD.
Principal coordinate Analysis (PCoA) based on unweighted UniFrac, weighted UniFrac, Bray–Curtis, and Jaccard showed a marked separation between the gut microbiota community of obese and lean groups, confirmed by the pairwise permanova analysis that indicated a significant difference in β-diversity between the two groups (Figure 4; p = 0.009; p = 0.034; p = 0.029; p = 0.002, respectively).
Figure 4. Principal coordinate Analysis (PCoA) based on: (A) Unweighted UniFrac; (B) Weighted UniFrac; (C) Bray–Curtis and (D) Jaccard; Obese (blue spheres) and lean participants (red spheres).
Certain microbes were positively or negatively correlated with BMI, WC, WHtR, PBF, and FM. Statistically significant correlations between microbes and anthropometric measurements are provided in (Supplementary Table S2) and the heat map (Figure 5).
Figure 5. Heatmap of Spearman correlation analysis between gut microbiota (at genus level) and clinical variables.
Several taxa that were significantly lower in obese compared with lean using Kruskal–Wallis or LEfSe were negatively correlated with BMI, WC, WHtR, PBF, and FM (uncharacterized Rhodospirillaceae), (uncharacterized Clostridiales.vadinBB60.group), BMI, WHtR (Victivallis), BMI, WC and FM (uncharacterized Ruminococcaceae), BMI, WC, WHtR and FM (uncharacterized Erysipelotrichaceae), BMI & FM (Lachnospiraceae.UCG.010), BMI, WHtR and FM (Christensenellaceae.R.7.group, Ruminococcaceae.UCG.005, Ruminococcaceae.UCG.010, Anaerotruncus), BMI, WHtR, PBF and FM (Ruminococcaceae.UCG.014); BMI, PBF and FM (Family.XIII.UCG.001), WHtR (Ruminococcaceae.UCG.002). On the other hand, the taxa which were higher in the obese group presented positive correlations with BMI and FM (Acidaminococcus), and WHtR (Lachnospira); except for Akkermansia, which was prevalent in the obese group (LEfSe) was found to be negatively correlated with WHtR.
No correlations were found between the gut microbiota at the genus level and the following clinical variables: FBS, total cholesterol, HDL cholesterol, LDL cholesterol, and triglycerides. Furthermore, There were no correlations with age or gender.
Recent research on the human gut microbiota has revealed its various roles in health and disease, with obesity being among the many conditions to which changes in the gut microbiota have been linked (Jinatham et al., 2018). Research on gut microbiota and obesity has mainly focused on Western populations, with limited studies on Arabs and almost none on Emiratis. This study pioneered in contrasting the fecal microbial composition of obese and lean Emirati people using 16S rRNA gene sequencing (V3-V4 region) and exploring its association with clinical parameters. It is difficult to compare this with previous studies in Western populations, because also diet, which differs between the Arabic and Western culture, also significantly affects microbiota composition.
To the best of our knowledge, the present cross-sectional study is first to provide insights into the relationships between clinical variables and the gut microbiota in obese and lean Emirati subjects. The α- diversity richness was significantly lower in the obese than the lean Emirate without significant difference in evenness. These data are in line with the majority of available studies (Pinart et al., 2021). Conversely, β-diversity, denoting dissimilarities in gut microbiota composition, showed significant differences between obese and lean; this also agrees with most available studies. Thus, the microbiota in the obese group presented fewer bacterial taxa, and lean samples showed a more diverse gut microbiota profile than obese samples based on Shannon index results. Controversy regarding relative abundance at genus and phylum level in individuals with obesity, compared with lean subjects, was observed.
Moreover, higher genera in the obese group correlated positively with BMI and FM (Acidaminococcus) and WHtR (Lachnospira). The exception was Akkermansia which was more prevalent in the obese group but was negatively associated with WHtR. This is in contrast to other studies (Gao X. et al., 2018; Jinatham et al., 2018), in different populations. On the other hand, genera higher in the lean group correlated negatively with adiposity (BMI, WC, WHtR, and FM).
The structure, function, and diversity of the gut microbiota in subjects with obesity differ from those of lean people (Cheng et al., 2019). In general, people with obesity show lower richness and biodiversity (Turnbaugh et al., 2009; Cotillard et al., 2013; Le Chatelier et al., 2013; Isolauri, 2017) similar to our findings, while Kocełak et al. (2013) and Kasai et al. (2015) found greater diversity richness in individuals with obesity. Le Chatelier et al. reported that subjects with obesity who have low bacterial richness present more overall adiposity, dyslipidemia, insulin resistance, and higher inflammation compared with obese individuals who have an elevated gut bacterial richness (Le Chatelier et al., 2013; Crovesy et al., 2020). In line with the literature, the present study revealed that Firmicutes and Bacteroidetes were the two most abundant bacterial phyla in the gut of obese and lean subjects without significant differences noted at the level of Firmicutes, Bacteroidetes (Pinart et al., 2021) nor F/B ratio (Castaner et al., 2018; Jinatham et al., 2018) between the two groups. It is well known that Firmicutes and Bacteroidetes predominate the gut microbiome globally (Castaner et al., 2018). Scarce data on the gut microbiota of people in the Arabian Peninsula describe the dominance of Firmicutes and Actinobacteria as phyla in individuals from Saudi Arabia and the preponderance of either Firmicutes or Bacteroidetes in individuals from Kuwait (Plummer et al., 2020).
Of interest, it was believed in early reports that the ratio of Firmicutes and Bacteroidetes differs between lean and obese people, with a high ratio observed in the US (Plummer et al., 2020). However, this was later refuted, and the majority of evidence does not show significant differences in the relative abundance of Firmicutes (Pinart et al., 2021), whereas contradictory findings in the relative abundance of Bacteroidetes persist (Crovesy et al., 2020; Pinart et al., 2021).
The usefulness of a high F/B ratio as an indicator of obesity is still debatable and more complicated than a mere difference in the relative abundance between phyla (Turnbaugh and Gordon, 2009; Venema, 2010; Jinatham et al., 2018). The critical impact of some bacteria on human metabolism may be due to the number of less abundant bacteria or the higher abundance of other ones (Sonnenburg and Backhed, 2016). Gut microbiota geared towards the production of SCFA, rather than a simple increase in F/B ratio, may be associated with an increased energy harvest from ‘one’s diet leading to obesity. Investigating the association between energy harvest related to bacterial SCFA production and obesity is warranted (Venema, 2010; Jinatham et al., 2018). Furthermore, the F/B ratio may be strongly associated with metabolic markers of obesity, such as insulin resistance and metabolic syndrome, instead of obesity (Louis et al., 2016). Based on our results, a high F/B ratio may not be a valid global biomarker of obesity (Houtman et al., 2022). It is more likely that differences at the genus (and even species) level, compared with the phylum level, could be related to changes in metabolic function (Bauer et al., 2016). While significantly higher Lentisphaerae in lean individuals and Saccharibacteria and Verrucomicrobia in the obese group were reported, no differences in the relative abundance of Fusobacteria, Actinobacteria, and Proteobacteria are observed. The issue of differences between phyla in obese and lean individuals remains controversial in the literature (Pinart et al., 2021).
Similarly, differences between the obese and lean groups at the genus level remain controversial (Crovesy et al., 2020). Interestingly, in our study, the genus Lachnospiraceae NK4A136 was significantly higher in the lean group. Studies in mice suggest that this genus has a protective and anti-inflammatory effect being a potential butyrate producer (Companys et al., 2021). Furthermore, Companys et al. (2021) first identified the genus Lachnospiraceae NK4A136 as a biomarker of leanness in humans. Furthermore, in our study, as shown elsewhere (Gao R. et al., 2018), butyrate-producing Ruminococcus was higher in the lean group, denoting a more diverse microbiome profile.
Moreover, Christensenellaceae.group.7 (Firmicutes phylum) was significantly higher in the lean group. It is usually associated with a low BMI (Castaner et al., 2018), and when transplanted to mice, it promotes a lean host phenotype and positively impacts the diversity of the community (Aguirre and Venema, 2015). The abundance of Alistipes was higher in the lean group; this new genus is significantly elevated in high-fat-diet-fed mice than in controls (Yang et al., 2022); individuals with obesity have a higher abundance of Alistipes (Crovesy et al., 2020). This aberrant finding may be related to the fact that Emirate adults could have a distinct gut microbiota composition given their varying dietary factors and lifestyle. In UAE, there is a transition towards energy-dense diets high in animal protein, total and saturated fatty acids, and simple sugars (Cheikh Ismail et al., 2020). High-fat diets are linked to considerable changes in the gut microbiota composition at the phylum and genus levels (Rosenbaum et al., 2015).
Our finding of a negative association between the genus Lachnospiraceae and PBF and BMI is also reported by Lippert et al. in older adults in Austria (Lippert et al., 2017), but not in a study with human stool from Ghanaian volunteers (Dugas et al., 2018). The negative correlation between bacterial taxa that were dominant in the lean group and the adiposity markers (BMI, WC, WHtR, FM, PBF) is unexpected, considering that most of these taxa belong to the Firmicutes phylum.
In contrast, genera that were higher in obese subjects positively correlated with BMI and FM (Acidaminococcus) as well as WHtR (Lachnospira), suggesting them as potential microbiota biomarkers of obesity (Companys et al., 2021). In addition, levels of Acidamonicoccus positively correlated with type 2 diabetes in the Saudi population (Al-Muhanna et al., 2022). Contrary to our results, a study in overweight adults showed that reduced Lachnospira abundance was associated with weight gain (Companys et al., 2021). These conflicting findings may have been attained because the two genera comprise various species whose role in obesity has not been thoroughly studied; such issues are still debatable (Aguirre and Venema, 2015).
Some of the identified microbial taxa correlated with adiposity but not other biochemical parameters, i.e., glucose and lipid homeostasis markers. It is tempting to assume that taxa showing negative correlations with adiposity are appropriate markers of metabolic health. Nevertheless, larger studies are required to ascertain such relationships (Palmas et al., 2021).
The presence of divergent results between this study and other studies in the literature might be attributed to the geographical location of the studies, given dietary and environmental variations known to affect gut microbiota (Al-Muhanna et al., 2022) or to many other factors, such as the population under study, gender, physical activity, drugs, ethnicity, and the season, as well as methods used to analyze the gut microbiota, such as different DNA extraction techniques, different areas of the 16S rRNA gene sequenced and primers used. The composition of the gut microbiota can be easily influenced, and all factors that affect it are difficult to control (Crovesy et al., 2020). The majority of available studies did not evaluate factors that can affect the gut microbiota, such as diet, physical activity, smoking, stress, and sleep, which might explain the possible differences found between studies (Rosenbaum et al., 2015; Aljazairy et al., 2022). Amongst these, the diet is likely to greatly impact the gut microbiota composition (Crovesy et al., 2020).
This study provides meaningful insight into the relationship between gut microbiota and obesity, specifically in Emirati adults, and is a useful addition to the international microbiome reference dataset. Yet, this study is limited by its relatively small sample size. Furthermore, we did not adjust for a potential confounding factor such as dietary intake, hindering our ability to link unique gut microbiota profiles with specific diets. Finally, our results should be interpreted cautiously as they are based on observational data.
We revealed significant differences in microbiota between obese and lean subjects at phylum and genus levels. Remarkably, our study showed no significant differences in Firmicutes and Bacteroidetes or F/B ratio, suggesting that F/B cannot be used as a universal predictor biomarker for obesity in adults. Genera that were higher in obese subjects correlated positively with BMI and FM (Acidaminococcus) as well as WHtR (Lachnospira) and are genera that might serve as microbiota biomarkers of obesity in Emerati. In contrast, genera higher in the lean group correlated negatively with adiposity. Future studies are needed to confirm our results and to understand better the interplay between diet, lifestyle, gut microbiota, and health. Whether changes in the gut microbial composition are a cause or a consequence of obesity remains an open question.
The raw sequences and corresponding metadata have been archived in the Sequence Read Archive (SRA) repository at the NCBI under accession number PRJNA962831: http://www.ncbi.nlm.nih.gov/bioproject/962831.
The studies involving human participants were reviewed and approved by Ethical approval was granted by the Research Ethics Committee of the Ministry of Health and Prevention of UAE (MOHP/DXB-REC-52/2018), the Dubai Health Care Regulatory Research Ethics Committee (DHCR-REC), and the Zayed University Ethical Committee Board (ZU19_51_F). The study followed the Declaration of Helsinki. Written informed consent was collected from all study subjects. The patients/participants provided their written informed consent to participate in this study. The studies were conducted in accordance with the local legislation and institutional requirements. The participants provided their written informed consent to participate in this study.
MA, MK, and KV: conceptualization, methodology, and data curation. MA and KV: software and supervision. MK, KV, and CM: validation. MA: formal analysis, investigation, and writing – original draft preparation. MK, KV, CM, GW, and TM: resources and writing – review and editing. MA, MK, KV, CM, GW, and TM: visualization. MK and KV: project administration. CA and KV: funding acquisition. All authors contributed to the article and approved the submitted version.
This research was funded by Zayed University, Dubai, UAE (grant number R19054) and the Dutch Province of Limburg with a grant to the Centre for Healthy Eating and Food Innovation (HEFI) of Maastricht University – Campus Venlo (grant number HEFI-1).
We thank the research assistants and students from the College of Natural and Health Sciences department at Zayed University in Dubai campus for their assistance in data collection, Dr. Sulaiman al Habib Hospital (Dubai, UAE), Al Qassimi Hospital (Sharjah, UAE), and the Research Institute for Medical and Health Sciences (Sharjah, UAE) for their collaboration and involvement in the study.
The authors declare that the research was conducted without any commercial or financial relationships that could be construed as a potential conflict of interest.
All claims expressed in this article are solely those of the authors and do not necessarily represent those of their affiliated organizations, or those of the publisher, the editors and the reviewers. Any product that may be evaluated in this article, or claim that may be made by its manufacturer, is not guaranteed or endorsed by the publisher.
The Supplementary material for this article can be found online at: https://www.frontiersin.org/articles/10.3389/fmicb.2023.1182460/full#supplementary-material
Abenavoli, L., Scarpellini, E., Colica, C., Boccuto, L., Salehi, B., Sharifi-Rad, J., et al. (2019). Gut microbiota and obesity: a role for probiotics. Nutrients 11:2690. doi: 10.3390/nu11112690
Adlerberth, I., and Wold, A. (2009). Establishment of the gut microbiota in Western infants. Acta Paediatr. 98, 229–238. doi: 10.1111/j.1651-2227.2008.01060.x
Aguirre, M., and Venema, K. (2015). Does the gut microbiota contribute to obesity? Going beyond the gut feeling. Microorganisms 3, 213–235. doi: 10.3390/microorganisms3020213
Aljazairy, E. A. A., Al-Musharaf, S., Abudawood, M., Almaarik, B., Hussain, S. D., Alnaami, A. M., et al. (2022). Influence of adiposity on the gut microbiota composition of Arab women: a case-control study. Biology 11:1586. doi: 10.3390/biology11111586
Al-Muhanna, F. A., Dowdell, A. K., Al Eleq, A. H., Albaker, W. I., Brooks, A. W., Al-Sultan, A. I., et al. (2022). Gut microbiota analyses of Saudi populations for type 2 diabetes-related phenotypes reveals significant association. BMC Microbiol. 22, 1–9. doi: 10.1186/s12866-022-02714-8
Armougom, F., Henry, M., Vialettes, B., Raccah, D., and Raoult, D. (2009). Monitoring bacterial community of human gut microbiota reveals an increase in Lactobacillus in obese patients and methanogens in anorexic patients. PLoS One 4:e7125. doi: 10.1371/journal.pone.0007125
Ashwell, M., and Gibson, S. (2016). Waist-to-height ratio as an indicator of 'early health 'risk': simpler and more predictive than using a 'matrix' based on BMI and waist circumference. BMJ Open 6:e010159. doi: 10.1136/bmjopen-2015-010159
Bauer, P. V., Hamr, S. C., and Duca, F. A. (2016). Regulation of energy balance by a gut–brain axis and involvement of the gut microbiota. Cell. Mol. Life Sci. 73, 737–755. doi: 10.1007/s00018-015-2083-z.pdf
Brant, R. (2015). Inference for Means: Comparing Two Independent Samples. Web-Based Sample Size Calculators. Available at: http://newton.Stat.Ubc.Ca/~rollin/stats/ssize(2). (Accessed March 1, 2023).
Cannon, C. P., and Kumar, A. (2009). Treatment of overweight and obesity: lifestyle, pharmacologic, and surgical options. Clin. Cornerstone 9, 55–71. doi: 10.1016/S1098-3597(09)80005-7
Castaner, O., Goday, A., Park, Y. M., Lee, S. H., Magkos, F., Shiow, S. T. E., et al. (2018). The gut microbiome profile in obesity: a systematic review. Int. J. Endocrinol. 2018:4095789. doi: 10.1155/2018/4095789
Cheikh Ismail, L., Osaili, T. M., Mohamad, M. N., Al Marzouqi, A., Jarrar, A. H., Abu Jamous, D. O., et al. (2020). Eating habits and lifestyle during COVID-19 lockdown in the United Arab Emirates: a cross-sectional study. Nutrients 12:3314. doi: 10.3390/nu12113314
Cheng, L., Chen, Y., Zhang, X., Zheng, X., Cao, J., Wu, Z., et al. (2019). A metagenomic analysis of the modulatory effect of Cyclocarya paliurus flavonoids on the intestinal microbiome in a high-fat diet-induced obesity mouse model. J. Sci. Food Agric. 99, 3967–3975. doi: 10.1002/jsfa.9622
Companys, J., Gosalbes, M. J., Pla-Pagà, L., Calderón-Pérez, L., Llauradó, E., Pedret, A., et al. (2021). Gut microbiota profile and its association with clinical variables and dietary intake in overweight/obese and lean subjects: a cross-sectional study. Nutrients 13:2032. doi: 10.3390/nu13062032
Corfe, B. M., Harden, C. J., Bull, M., and Garaiova, I. (2015). The multifactorial interplay of diet, the microbiome and appetite control: current knowledge and future challenges. Proc. Nutr. Soc. 74, 235–244. doi: 10.1017/S0029665114001670
Cotillard, A., Kennedy, S. P., Kong, L. C., Prifti, E., Pons, N., Le Chatelier, E., et al. (2013). Dietary intervention impact on gut microbial gene richness. Nature 500, 585–588. doi: 10.1038/nature12480
Crovesy, L., Masterson, D., and Rosado, E. L. (2020). Profile of the gut microbiota of adults with obesity: a systematic review. Eur. J. Clin. Nutr. 74, 1251–1262. doi: 10.1038/s41430-020-0607-6
Damms-Machado, A., Mitra, S., Schollenberger, A. E., Kramer, K. M., Meile, T., Konigsrainer, A., et al. (2015). Effects of surgical and dietary weight loss therapy for obesity on gut microbiota composition and nutrient absorption. Biomed. Res. Int. 2015:806248. doi: 10.1155/2015/806248
Davis, C. D. (2016). The gut microbiome and its role in obesity. Nutr. Today 51, 167–174. doi: 10.1097/nt.0000000000000167
Dugas, L. R., Bernabé, B. P., Priyadarshini, M., Fei, N., Park, S. J., Brown, L., et al. (2018). Decreased microbial co-occurrence network stability and SCFA receptor level correlates with obesity in African-origin women. Sci. Rep. 8:17135. doi: 10.1038/s41598-018-35230-9
Duncan, S. H., Lobley, G., Holtrop, G., Ince, J., Johnstone, A., Louis, P., et al. (2008). Human colonic microbiota associated with diet, obesity and weight loss. Int. J. Obes. 32, 1720–1724. doi: 10.1038/ijo.2008.155
Ford, E. S. (2005). Prevalence of the metabolic syndrome defined by the international diabetes federation among adults in the US. Diabetes Care 28, 2745–2749. doi: 10.2337/diacare.28.11.2745
Furet, J. P., Kong, L. C., Tap, J., Poitou, C., Basdevant, A., Bouillot, J. L., et al. (2010). Differential adaptation of human gut microbiota to bariatric surgery-induced weight loss: links with metabolic and low-grade inflammation markers. Diabetes 59, 3049–3057. doi: 10.2337/db10-0253
Gao, X., Zhang, M., Xue, J., Huang, J., Zhuang, R., Zhou, X., et al. (2018). Body mass index differences in the gut microbiota are gender specific. Front. Microbiol. 9:1250. doi: 10.3389/fmicb.2018.01250
Gao, R., Zhu, C., Li, H., Yin, M., Pan, C., Huang, L., et al. (2018). Dysbiosis signatures of gut microbiota along the sequence from healthy, young patients to those with overweight and obesity. Obesity (Silver Spring) 26, 351–361. doi: 10.1002/oby.22088
Gordon, J. I., Turnbaugh, P., Ley, R., Hamady, M., Fraser-Liggett, C., and Knight, R. (2007). The human microbiome project. Nature 449, 804–810. doi: 10.1038/nature06244
Heinritz, S. N., Mosenthin, R., and Weiss, E. (2013). Use of pigs as a potential model for research into dietary modulation of the human gut microbiota. Nutr. Res. Rev. 26, 191–209. doi: 10.1017/S0954422413000152
Houtman, T. A., Eckermann, H. A., Smidt, H., and de Weerth, C. (2022). Gut microbiota and BMI throughout childhood: the role of firmicutes, bacteroidetes, and short-chain fatty acid producers. Sci. Rep. 12:3140. doi: 10.1038/s41598-022-07176-6
Isolauri, E. (2017). Microbiota and obesity. Nestle Nutr Inst Workshop Ser 88, 95–105. doi: 10.1159/000455217
Jinatham, V., Kullawong, N., Kespechara, K., Gentekaki, E., and Popluechai, S. (2018). Comparison of gut microbiota between lean and obese adult Thai individuals. Microbiol Biotechnol Lett 46, 277–287. doi: 10.4014/mbl.1711.11003
Kasai, C., Sugimoto, K., Moritani, I., Tanaka, J., Oya, Y., Inoue, H., et al. (2015). Comparison of the gut microbiota composition between obese and non-obese individuals in a Japanese population, as analyzed by terminal restriction fragment length polymorphism and next-generation sequencing. BMC Gastroenterol. 15:100. doi: 10.1186/s12876-015-0330-2
Kocełak, P., Zak-Gołąb, A., Zahorska-Markiewicz, B., Aptekorz, M., Zientara, M., Martirosian, G., et al. (2013). Resting energy expenditure and gut microbiota in obese and normal weight subjects. Eur. Rev. Med. Pharmacol. Sci. 17, 2816–2821.
Kong, L. C., Tap, J., Aron-Wisnewsky, J., Pelloux, V., Basdevant, A., Bouillot, J. L., et al. (2013). Gut microbiota after gastric bypass in human obesity: increased richness and associations of bacterial genera with adipose tissue genes. Am. J. Clin. Nutr. 98, 16–24. doi: 10.3945/ajcn.113.058743
Le Chatelier, E., Nielsen, T., Qin, J., Prifti, E., Hildebrand, F., Falony, G., et al. (2013). Richness of human gut microbiome correlates with metabolic markers. Nature 500, 541–546. doi: 10.1038/nature12506
Lippert, K., Kedenko, L., Antonielli, L., Kedenko, I., Gemeier, C., Leitner, M., et al. (2017). Gut microbiota dysbiosis associated with glucose metabolism disorders and the metabolic syndrome in older adults. Benefic. Microbes 8, 545–556. doi: 10.3920/BM2016.0184
Louis, S., Tappu, R.-M., Damms-Machado, A., Huson, D. H., and Bischoff, S. C. (2016). Characterization of the gut microbial community of obese patients following a weight-loss intervention using whole metagenome shotgun sequencing. PLoS One 11:e0149564. doi: 10.1371/journal.pone.0149564
Ministry of Health and Prevention. (2023). MoHAP Ramps Up Efforts to Improve Results of National Indicator on Prevalence of Obesity. Available at: https://mohap.gov.ae/en/media-center/news/4/3/2022/mohap-ramps-up-efforts-to-improve-results-of-national-indicator-on-prevalence-of-obesity (Accessed February 26, 2023).
Morgen, C. S., and Sørensen, T. I. (2014). Global trends in the prevalence of overweight and obesity. Nat. Rev. Endocrinol. 10, 513–514. doi: 10.1038/nrendo.2014.124
Nguyen, T. L. A., Vieira-Silva, S., Liston, A., and Raes, J. (2015). How informative is the mouse for human gut microbiota research? Dis. Model. Mech. 8, 1–16. doi: 10.1242/dmm.017400
Palmas, V., Pisanu, S., Madau, V., Casula, E., Deledda, A., Cusano, R., et al. (2021). Gut microbiota markers associated with obesity and overweight in Italian adults. Sci. Rep. 11, 1–14. doi: 10.1038/s41598-021-84928-w
Patrone, V., Vajana, E., Minuti, A., Callegari, M. L., Federico, A., Loguercio, C., et al. (2016). Postoperative changes in fecal bacterial communities and fermentation products in obese patients undergoing Bilio-intestinal bypass. Front. Microbiol. 7:200. doi: 10.3389/fmicb.2016.00200
Pinart, M., Dötsch, A., Schlicht, K., Laudes, M., Bouwman, J., Forslund, S. K., et al. (2021). Gut microbiome composition in obese and non-obese persons: a systematic review and meta-analysis. Nutrients 14:12. doi: 10.3390/nu14010012
Plummer, E., Bulach, D., Carter, G., and Albert, M. J. (2020). Gut microbiome of native Arab Kuwaitis. Gut Pathogens 12, 1–9. doi: 10.1186/s13099-020-00351-y
Radwan, H., Ballout, R. A., Hasan, H., Lessan, N., Karavetian, M., and Rizk, R. (2018). The epidemiology and economic burden of obesity and related cardiometabolic disorders in the United Arab Emirates: a systematic review and qualitative synthesis. J. Obes. 2018, 1–23. doi: 10.1155/2018/2185942
Rinninella, E., Raoul, P., Cintoni, M., Franceschi, F., Miggiano, G. A. D., Gasbarrini, A., et al. (2019). What is the healthy gut microbiota composition? A changing ecosystem across age, environment, diet, and diseases. Microorganisms 7:14. doi: 10.3390/microorganisms7010014
Romero-Corral, A., Somers, V. K., Sierra-Johnson, J., Thomas, R. J., Collazo-Clavell, M., Korinek, J., et al. (2008). Accuracy of body mass index in diagnosing obesity in the adult general population. Int. J. Obes. 32, 959–966. doi: 10.1038/ijo.2008.11
Rosenbaum, M., Knight, R., and Leibel, R. L. (2015). The gut microbiota in human energy homeostasis and obesity. Trends Endocrinol. Metab. 26, 493–501. doi: 10.1016/j.tem.2015.07.002
Round, J. L., and Mazmanian, S. K. (2009). The gut microbiota shapes intestinal immune responses during health and disease. Nat. Rev. Immunol. 9, 313–323. doi: 10.1038/nri2515
Schwiertz, A., Taras, D., Schäfer, K., Beijer, S., Bos, N. A., Donus, C., et al. (2010). Microbiota and SCFA in lean and overweight healthy subjects. Obesity 18, 190–195. doi: 10.1038/oby.2009.167
Seganfredo, F., Blume, C., Moehlecke, M., Giongo, A., Casagrande, D., Spolidoro, J., et al. (2017). Weight-loss interventions and gut microbiota changes in overweight and obese patients: a systematic review. Obes. Rev. 18, 832–851. doi: 10.1111/obr.12541
Snetselaar, L. G., de Jesus, J. M., DeSilva, D. M., and Stoody, E. E. (2021). Dietary guidelines for Americans, 2020–2025: understanding the scientific process, guidelines, and key recommendations. Nutr. Today 56, 287–295. doi: 10.1097/nt.0000000000000512
Sonnenburg, J. L., and Backhed, F. (2016). Diet-microbiota interactions as moderators of human metabolism. Nature 535, 56–64. doi: 10.1038/nature18846
Sulaiman, N., Elbadawi, S., Hussein, A., Abusnana, S., Madani, A., Mairghani, M., et al. (2017). Prevalence of overweight and obesity in United Arab Emirates expatriates: the UAE national diabetes and lifestyle study. Diabetol. Metab. Syndr. 9, 1–9. doi: 10.1186/s13098-017-0287-0
Surono, I. S., Simatupang, A., Kusumo, P. D., Waspodo, P., Verbruggen, S., Verhoeven, J., et al. (2022). Effect of different functional food supplements on the gut microbiota of Prediabetic Indonesian individuals during weight loss. Nutrients 14:781. doi: 10.3390/nu14040781
Tehrani, A. B., Nezami, B. G., Gewirtz, A., and Srinivasan, S. (2012). Obesity and its associated disease: a role for microbiota? Neurogastroenterol Motility 24, 305–311. doi: 10.1111/j.1365-2982.2012.01895.x
Tremaroli, V., and Bäckhed, F. (2012). Functional interactions between the gut microbiota and host metabolism. Nature 489, 242–249. doi: 10.1038/nature11552
Turnbaugh, P. J., and Gordon, J. I. (2009). The core gut microbiome, energy balance and obesity. J. Physiol. 587, 4153–4158. doi: 10.1113/jphysiol.2009.174136
Turnbaugh, P. J., Hamady, M., Yatsunenko, T., Cantarel, B. L., Duncan, A., Ley, R. E., et al. (2009). A core gut microbiome in obese and lean twins. Nature 457, 480–484. doi: 10.1038/nature07540
Turnbaugh, P. J., Ley, R. E., Mahowald, M. A., Magrini, V., Mardis, E. R., and Gordon, J. I. (2006). An obesity-associated gut microbiome with increased capacity for energy harvest. Nature 444, 1027–1031. doi: 10.1038/nature05414
Van der Kooy, K., and Seidell, J. C. (1993). Techniques for the measurement of visceral fat: a practical guide. Int. J. Obes. 17, 187–196.
Venema, K. (2010). Role of gut microbiota in the control of energy and carbohydrate metabolism. Curr Opin Clin Nutr Metab Care 13, 432–438. doi: 10.1097/MCO.0b013e32833a8b60
World Health Organization. (2016). Obesity and Overweight. Available at: https://www.who.int/news-room/fact-sheets/detail/obesity-and-overweight (Accessed March 5, 2023).
Yang, J., Wei, H., Zhou, Y., Szeto, C.-H., Li, C., Lin, Y., et al. (2022). High-fat diet promotes colorectal tumorigenesis through modulating gut microbiota and metabolites. Gastroenterology 162, 135–149.e2. doi: 10.1053/j.gastro.2021.08.041
Keywords: gut microbiota, obesity, firmicutes, bacteroidetes, 16S rRNA, United Arab Emirates
Citation: Ahmad MA, Karavetian M, Moubareck CA, Wazz G, Mahdy T and Venema K (2023) Association of the gut microbiota with clinical variables in obese and lean Emirati subjects. Front. Microbiol. 14:1182460. doi: 10.3389/fmicb.2023.1182460
Received: 10 March 2023; Accepted: 01 August 2023;
Published: 23 August 2023.
Edited by:
Rebeca Martín, INRAE Centre Jouy-en-Josas, FranceReviewed by:
Vinod Kumar Gupta, Mayo Clinic, United StatesCopyright © 2023 Ahmad, Karavetian, Moubareck, Wazz, Mahdy and Venema. This is an open-access article distributed under the terms of the Creative Commons Attribution License (CC BY). The use, distribution or reproduction in other forums is permitted, provided the original author(s) and the copyright owner(s) are credited and that the original publication in this journal is cited, in accordance with accepted academic practice. No use, distribution or reproduction is permitted which does not comply with these terms.
*Correspondence: Koen Venema, ay52ZW5lbWFAbWFhc3RyaWNodHVuaXZlcnNpdHkubmw=
Disclaimer: All claims expressed in this article are solely those of the authors and do not necessarily represent those of their affiliated organizations, or those of the publisher, the editors and the reviewers. Any product that may be evaluated in this article or claim that may be made by its manufacturer is not guaranteed or endorsed by the publisher.
Research integrity at Frontiers
Learn more about the work of our research integrity team to safeguard the quality of each article we publish.