- 1Department of Urology, Tianjin Medical University General Hospital, Tianjin, China
- 2Department of Urology, Central Hospital Affiliated to Shandong First Medical University, Jinan, China
- 3Center for Reproductive Medicine, Central Hospital Affiliated to Shandong First Medical University, Jinan, China
Introduction: Semen quality is decreasing worldwide, leading to increased male infertility. This study analyzed the microbiota of the gut, semen, and urine in individuals with semen abnormalities to identify potential probiotics and pathogenic bacteria that affect semen parameters and help develop new methods for the diagnosis and treatment of patients with semen abnormalities.
Methods: We recruited 12 individuals with normal semen parameters (control group), 12 with asthenospermia but no semen hyperviscosity (Group_1), 6 with oligospermia (Group_2), 9 with severe oligospermia or azoospermia (Group_3), and 14 with semen hyperviscosity only (Group_4). The semen, gut, and urine microbiota were examined by analyzing the 16S ribosomal RNA gene sequence using next-generation sequencing.
Results: The gut microbes were clustered into the highest number of operational taxonomic units, followed by urine and semen. Furthermore, the α-diversity of gut microbes was highest and significantly different from that of urine and semen microbiota. The microbiota of the gut, urine, and semen were all significantly different from each other in terms of β-diversity. The gut abundance of Collinsella was significantly reduced in groups 1, 3, and 4. Furthermore, the gut abundance of Bifidobacterium and Blautia was significantly decreased in Group_1, while that of Bacteroides was significantly increased in Group_3. The abundance of Staphylococcus was significantly increased in the semen of groups 1 and 4. Finally, Lactobacillus abundance was significantly reduced in the urine of groups 2 and 4.
Discussion: This study comprehensively describes the differences in intestinal and genitourinary tract microbiota between healthy individuals and those with abnormal semen parameters. Furthermore, our study identified Collinsella, Bifidobacterium, Blautia, and Lactobacillus as potential probiotics. Finally, the study identified Bacteroides in the gut and Staphylococcus in semen as potential pathogenic bacteria. Our study lays the foundation of a new approach to the diagnosis and treatment of male infertility.
1. Introduction
Studies have shown that semen quality is severely declined worldwide (Carlsen et al., 1992; Levine et al., 2017; Sengupta et al., 2018), which is associated with increased male infertility (Said et al., 2004; Dohle et al., 2005). However, the specific causes for this decline require further exploration and research. Genitourinary tract infections are thought to be involved in reducing semen quality (Jequier, 1985; Urata et al., 2001; Oghbaei et al., 2020); however, the role of specific bacteria might have been underestimated as these previous studies have used traditional bacterial culture methods, which can identify less than 2% of all known bacteria (Wade, 2002). Fortunately, 16S ribosomal RNA (rRNA) gene sequencing can provide a detailed and reliable classification of microbes present in various body compartments.
Previous studies have shown that the gut microbiota or its products can affect multiple organs other than the gut, including the male reproductive system. Gut microbiota transplantation has beneficial effects on pediatric autism (Kang et al., 2017, 2019) and ameliorates fertility in male mice (Zhang et al., 2021; Hao et al., 2022a,b). Gut flora can also influence the permeability of the blood–brain barrier (BBB) (Braniste et al., 2014; Hoyles et al., 2021). The bidirectional regulation of the microbiome-gut-brain axis plays an important role in regulating immune and metabolic processes (Zhang et al., 2015), promoting androgen production, and regulating gonadal development and testicular immunity (Cai et al., 2022). Gut microbiota dysbiosis can lead to increased permeability of the blood-testis barrier (BTB) and affect serum follicle-stimulating hormone (FSH), luteinizing hormone (LH), and testosterone levels in mice (Al-Asmakh et al., 2014). Perturbations in the gut microbiota impair testicular functions in mice and rats and reduce semen utilization rate in Duroc boars (Guo et al., 2020; Zhao et al., 2021; Liu et al., 2022). Amino acids play an important role in regulating reproductive processes such as spermatogenesis (Dai et al., 2015; Zhao et al., 2021). Numerous studies have shown that gut bacteria play an important role in amino acid metabolism and recycling (Fuller and Reeds, 1998; Dai et al., 2011; Neis et al., 2015; Dodd et al., 2017; Liu et al., 2020; Agus et al., 2021; Zhao et al., 2021). Therefore, it is essential to study the relationship between gut microbiota and semen quality. Furthermore, several studies have demonstrated that the normal human semen (Rodin et al., 2003; Hou et al., 2013; Baud et al., 2019; Yang et al., 2020; Lundy et al., 2021) and testicular microenvironment is non-sterile (Alfano et al., 2018; Molina et al., 2021) and testicular/epididymal microbiome is present in the human semen (Lundy et al., 2021). Studies have reported that the paternal semen microbiome can influence the health of the offspring (Rando and Simmons, 2015; Feng and Liu, 2022). The offspring of the high-protein diet-fed male rats exhibit enhanced production of short-chain fatty acids (SFCAs) and increased abundance of Bifidobacterium in the gut (Chleilat et al., 2021). This finding suggests that the health of the father can influence the health of the offspring. An ecologically normal microbiota in semen is likely necessary for normal sperm production and function, and the good health of the offspring. However, little is known about the effects of semen, gut, and urine microbiomes on semen parameters. Herein, we performed 16S rRNA gene sequencing to identify the specific flora of the gut, semen, and urine. Furthermore, we also tried to identify the potentially pathogenic bacteria that might be associated with abnormal semen parameters. The outcomes of this study lay the groundwork for a new approach to diagnosing and treating men with reduced semen quality.
2. Materials and methods
2.1. Study participants
Fifty-three participants from the Central Hospital Affiliated to Shandong First Medical University were recruited. The participants were divided into the healthy control group and four abnormal semen groups. The healthy control group had normal semen parameters. We recruited 12 individuals with normal semen parameters (control group), 12 with asthenospermia (proportion of forward motile sperm <32%) but no semen hyperviscosity (Group_1), 6 with oligospermia (sperm concentration < 15 million/mL) (Group_2), 9 with severe oligospermia or azoospermia (sperm concentration < 2 million/mL) (Group_3), and 14 with semen hyperviscosity only (Semen still forms a thread of more than 2 cm after 1 h of liquefaction) (Group_4). Semen abnormalities were defined according to the fifth edition of the World Health Organization. All participants met the following requirements: no antibiotic use within 3 months, no history of genitourinary surgery within 1 year, no long-term exposure to toxic and harmful substances, no history of tumor, no testosterone use within 2 years, no history of hypertension, and no history of diabetes. All individuals volunteered to participate in this clinical study and signed an informed consent form.
2.2. Ethics statement
The Ethics Committee of Shandong First Medical University approved this study with ethics approval no. (R202203030053).
2.3. Sample collection
All participants abstained from sex and masturbation for 2–7 days before semen collection. The detailed aseptic procedure for sample collection was explained to all participants. Before semen collection, all participants washed their hands thrice with soap. The penis, glans, and coronal sulcus were washed three times with soap and dried with sterile gauze. Finally, semen was collected directly into a sterile container. Similarly, before collecting urine, the penis, glans, and coronal sulcus were washed three times with soap and dried with sterile gauze. Subsequently, 10 mL of midstream urine was collected in a sterile container. Approximately 1 g of fresh feces was collected with a sterile spoon in a 5 mL sterile fecal collection container. The collected fecal and urine samples were stored at −80°C. After semen collection, a routine semen examination was performed, and the remaining semen samples were transferred to sterile cryotubes and stored at −80°C. All participants had their samples collected within 24 h of participating in the study. The order was semen, followed by urine and finally feces. This order was followed by all participants. The collection of all samples was done in the hospital.
2.4. DNA extraction
DNA was extracted from the three samples using the PowerSoil DNA Isolation Kit (MoBio Laboratories, Carlsbad, CA, United States) and Omega Stool DNA Kit following the manufacturer’s instructions. Samples that passed the DNA quality and concentration tests were stored at −80°C for subsequent experiments.
2.5. 16S ribosomal RNA gene sequencing
The primers 338F (5′-ACTCCTACGGGAGGCAGCAG-3′) and 806R (5′-GGACTACNNGGGTATCTAAT-3′) (Munyaka et al., 2015) were used to amplify the V3-V4 hypervariable region of the bacterial 16S rRNA gene. An 8 bp barcode sequence was added at the 5′ end of each upstream and downstream primers to distinguish between different samples. Amplicons were separated on 2% agarose gels, purified using the AxyPrep DNA Gel Extraction Kit according to the manufacturer’s instructions, and quantified using QuantiFluor-ST. The purified amplicons were pooled in equimolar concentrations and paired-end sequenced (2 × 250) on the Illumina platform according to standard protocols. PCR reaction mixture (25 μL) contained 12.5 μL 2xTaq Plus Master Mix, 3 μL BSA (2 ng/μL), 1 μL Forward Primer (5 μM), 1 μL Reverse Primer (5 μM), 2 μL template DNA, and 5.5 μL ddH2O. Reaction parameters were as follows: pre-denaturation at 95°C for 5 min followed by 28 cycles of denaturation at 95°C for 45 s, annealing at 55°C for 50 s, and extension at 72°C for 45 s, and a final step of extension at 72°C for 10 min. The PCR products were detected by 1% agarose gel electrophoresis to determine the size of the amplified target bands, which were then purified using the Agencourt AMPure XP Nucleic Acid Purification Kit.
2.6. MiSeq sequencing
The PCR products were used to construct a microbial diversity sequencing library and paired-end sequencing was performed. The raw data were uploaded to the SRA database of NCBI (accession number: PRJNA900680).
2.7. Data analysis and processing
The raw data were split using the QIIME1 (v1.8.0) software based on the barcode sequences, and the data were filtered and spliced using the Pear (v0.9.6) software. To obtain high-quality clean reads: (a) reads containing >10% of unknown nucleotides (N) were removed and (b) reads containing <80% of bases with quality (Q-value) >20 were filtered out. The minimum overlap was set to 10 bp and the mismatch rate was set to 1%. After splicing, sequences less than 230 bp in length were removed using the Vsearch (v2.7.1) software, and chimeric sequences were removed by comparing with the Gold Database using the UCHIME method. Effective tags were clustered into operational taxonomic units (OTUs) with ≥ 97% similarity using the MOTHUR pipeline. The tag sequence with the highest abundance was selected as the representative sequence for each cluster. OTU clustering was performed using the Vsearch (v2.7.1) software. UPARSE algorithm was used to obtain quality sequences with a sequence similarity threshold of 97% (Edgar, 2013). The Ribosomal Database Project (RDP) Classifier tool was used to classify all sequences into different taxonomic groups against the SILVA database (release 128) (Cole et al., 2009). Representative sequences were classified into organisms based on the naïve Bayesian model using the RDP classifier (V.2.2) based on Greengenes (V.gg_13_5). QIIME (v1.8.0) was used to calculate richness and diversity indices based on the OTU information. To compare the composition and structure of microbial communities in different samples, a heat map of the top 20 OTUs was generated using Mothur (Jami et al., 2013). Non-metric multidimensional scaling (NMDS) was performed and data was plotted using QIIME (v1.8.0) and ggplot2 3.3.2. Stamp analysis was performed using the STAMP 2.1.3 software and comparisons between the two groups were made using Welch’s t-test.
2.8. Statistical analysis
Continuous data were tested for normal distribution using the Shapiro–Wilk test. If normally distributed, the data were expressed as mean (standard deviation), and comparisons between groups were performed using the analysis of variance (ANOVA) while Bonferroni’s method was used for two-way comparisons. The non-normally distributed data were expressed as median with interquartile ranges (25th–75th percentiles) (IQR), and the overall comparison between groups was performed using the Kruskal-Wallis test. Two-way comparisons were performed using the Kruskal-Wallis one-way ANOVA. Categorical data were expressed as numbers and proportions, and the overall comparison between groups was performed using the corrected chi-square test. Differences in the abundance of various bacterial species were analyzed using Welch’s t-test. The relationship between selected differentially abundant genera and abnormal semen and demographic parameters was analyzed using a generalized linear model. When analyzing the gut microbiota sequencing data, we performed a two-tailed Wilcoxon rank-sum test using the R Project. A value of p < 0.05 was considered statistically significant.
3. Results
3.1. Demographic characteristics and semen parameters
To minimize, as much as possible, the effects of relevant confounding factors, we selected individuals with similar age, body mass index (BMI), smoking status, and alcohol consumption in each study group (Table 1).
3.2. Microbiota composition of the gut, semen, and urine
One participant of Group_3 failed to provide the stool sample while the genomic DNA extraction and amplification from the semen of another participant was unsuccessful, thus, both of these samples were excluded and 157 samples were finally analyzed. These 157 samples yielded 12,393,226 raw sequences and 11,140,437 clean sequences, of which, high-quality sequences were mainly distributed in the range of 400–440 bp. The total reads were clustered into 9,612 OTUs, which included 6,270 OTUs in the gut, 5,920 in urine, and 3,547 in semen, for a total of 1,058 OTUs for all three kinds of samples (Figure 1A). The α-diversity of the gut microbes was higher and significantly different from that of urine and semen microbiota; however, no significant difference was observed between urine and semen (Figure 1B). The gut, urine, and semen microbiota were all significantly different from each other in terms of β-diversity (Figure 1C). The phylum of the top four gut and urine are the same including Firmicutes, Actinobacteriota, Bacteroidetes, and Proteobacteria (Supplementary Figure S1). Firmicutes, Bacteroidetes, unidentified, and Actinobacteriota are the four main phylum in semen (Supplementary Figure S1). At the genus level, Bifidobacterium, Blautia, Bacteroides, Faecalibacterium, and Prevotella were predominant in the gut, Staphylococcus, Streptococcus, Prevotella, Finegoldia, and Corynebacterium were predominant in urine, and Lactobacillus, Prevotella, Finegoldia, Staphylococcus, Streptococcus, Ureaplasma, and other unidentified bacteria were predominant in semen (Figure 1D).
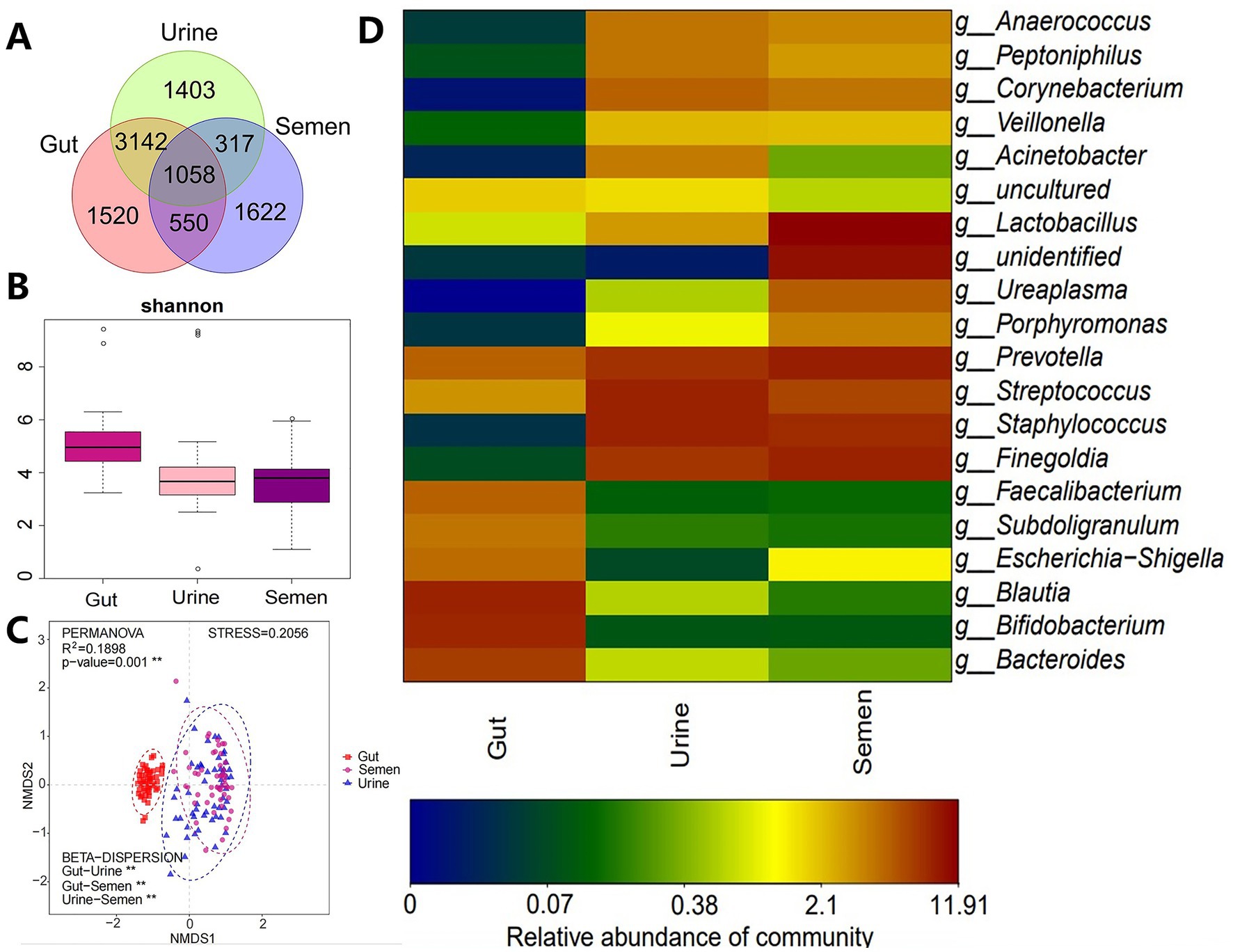
Figure 1. (A) Veen diagram showing OTUs enriched in the gut, urine, and semen. (B) Shannon index was used to estimate the α-diversity. (C) Graph showing β-diversity. **p < 0.001. (D) Heatmap showing the relative abundance of the topmost differentially abundant OTUs in the gut, urine, and semen.
The α-diversity of the gut microbiota was not significantly different between the control and abnormal semen groups (Figure 2A). While the β-diversity of the control group was significantly different from that of groups 1 and 3 only (Figure 2B). In Group_1, the gut abundance of Collinsella, Blautia, and Bifidobacterium was significantly decreased (Figure 3A). In Group_2, the gut abundance of Clostridium sensu stricto 1, the Christensenellaceae R-7 group, and Intestinibacter was significantly decreased (Figure 3B). In Group_3, there was a significant decrease in the gut abundance of Collinsella and a significant increase in that of Bacteroides (Figure 3C). In Group_4, there was a significant decrease in the gut Collinsella abundance (Figure 3D).
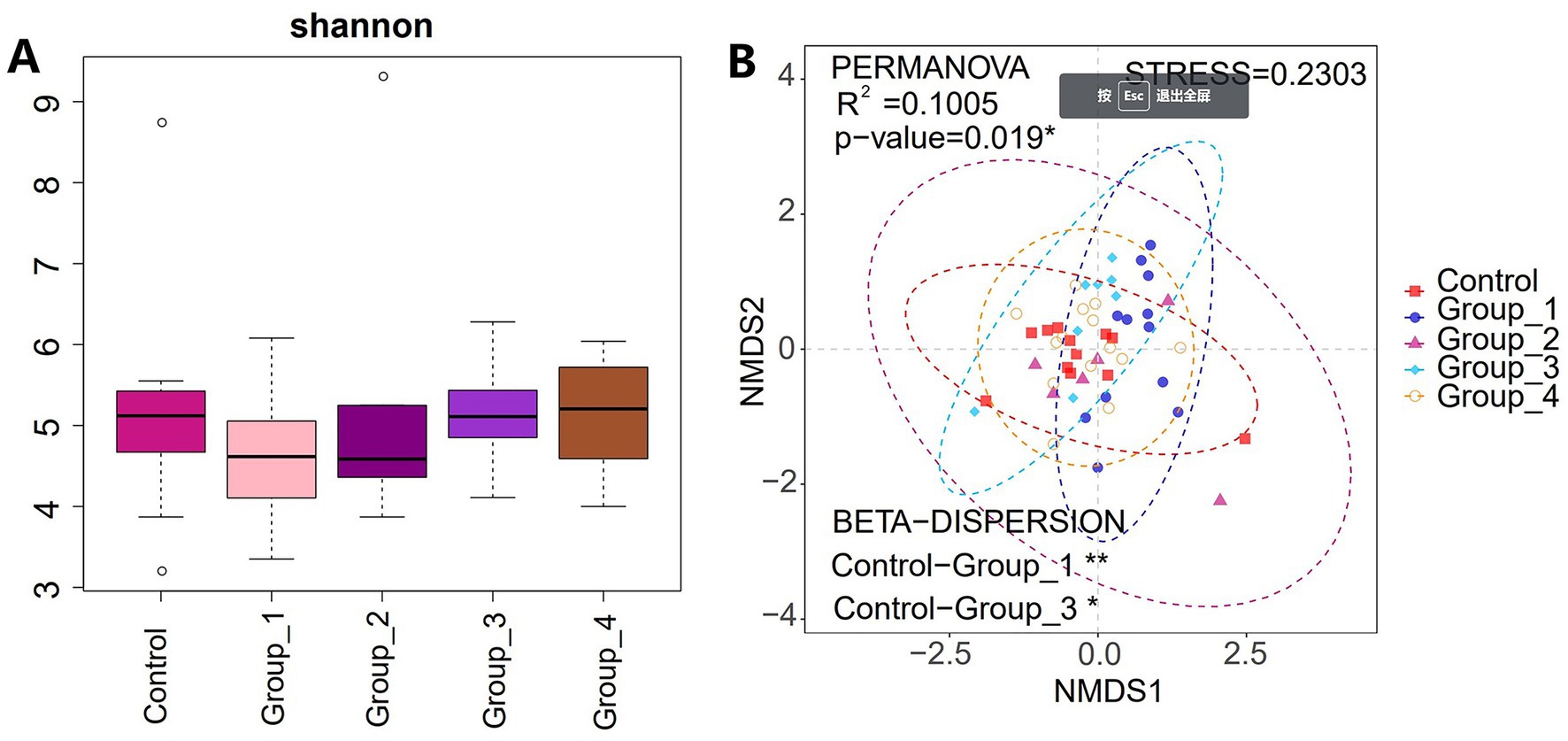
Figure 2. (A) Shannon index was used to estimate the α-diversity of the gut microbiota. (B) Graph showing β-diversity of the gut microbes.
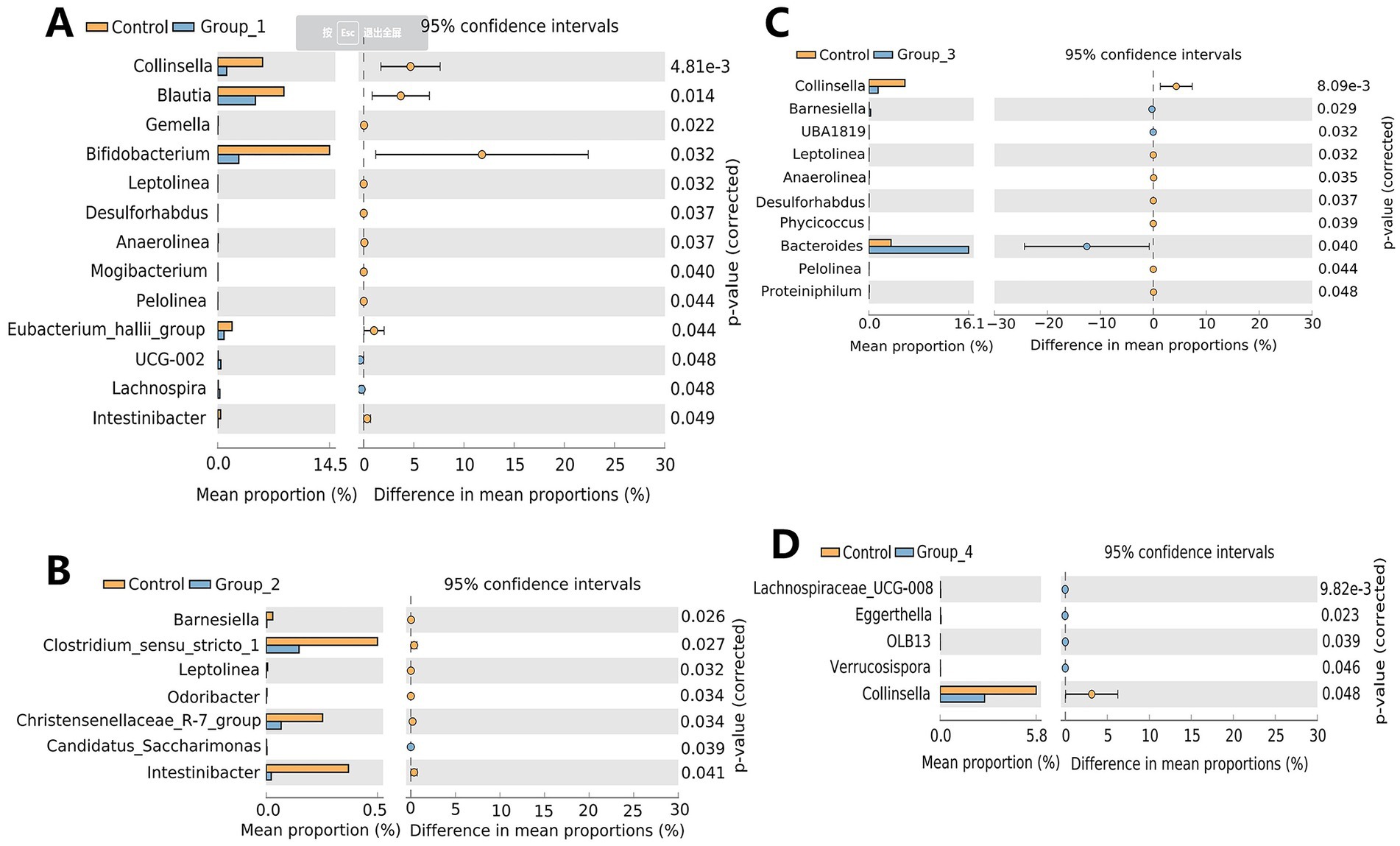
Figure 3. Stamp analysis showing differences in the gut microbiota between the control group, Group_1 (A), Group_2 (B), Group_3 (C), and Group_4 (D).
The α- and β-diversities of the seminal microbiota were not significantly different between the control and abnormal semen groups (Figure 4). However, groups 1 and 4 showed a significant increase in seminal Staphylococcus abundance (Figures 5A,D).
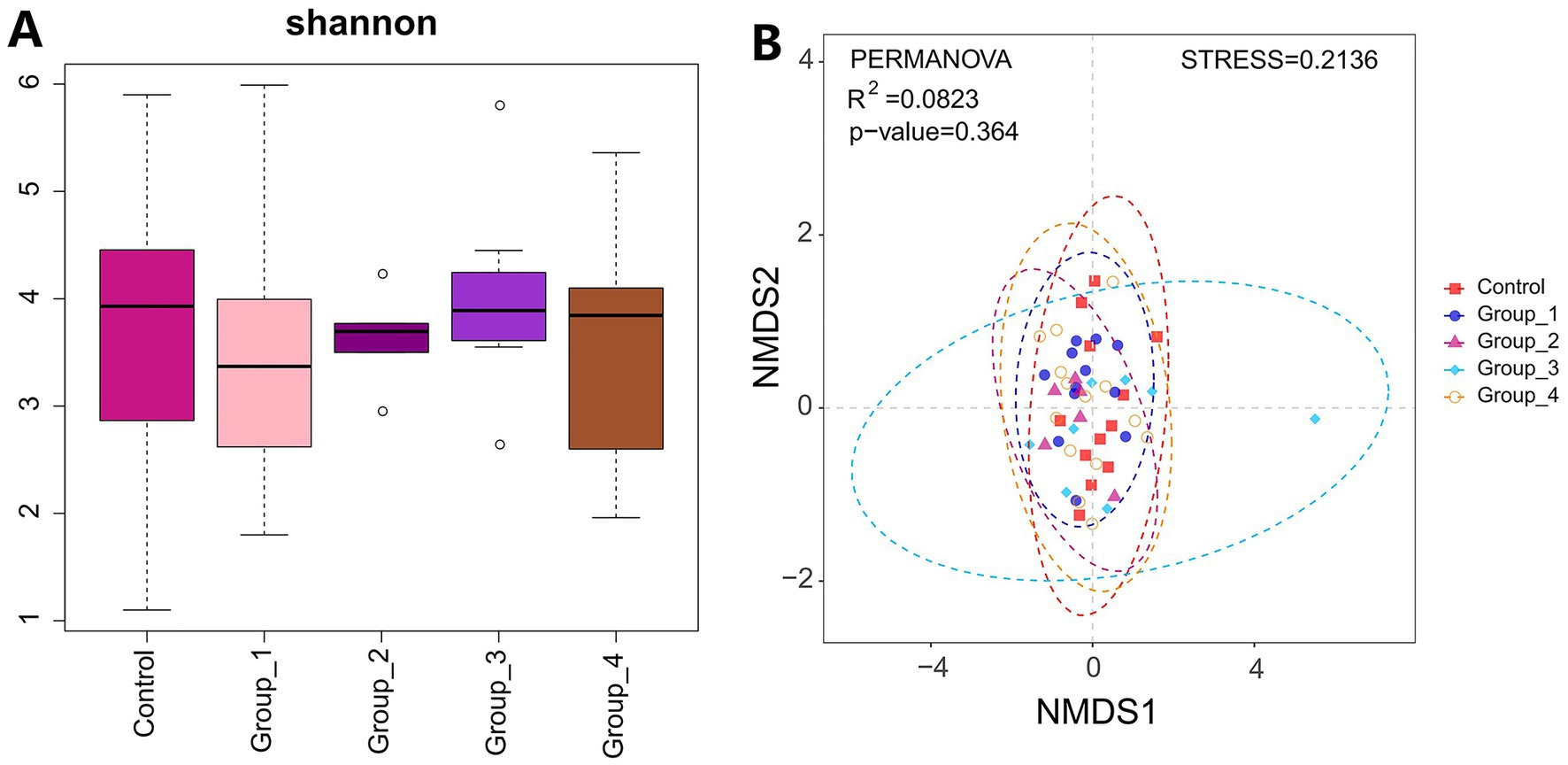
Figure 4. (A) Shannon index was used to estimate the α-diversity of semen microbiota. (B) Graph showing β-diversity of semen microbiota.
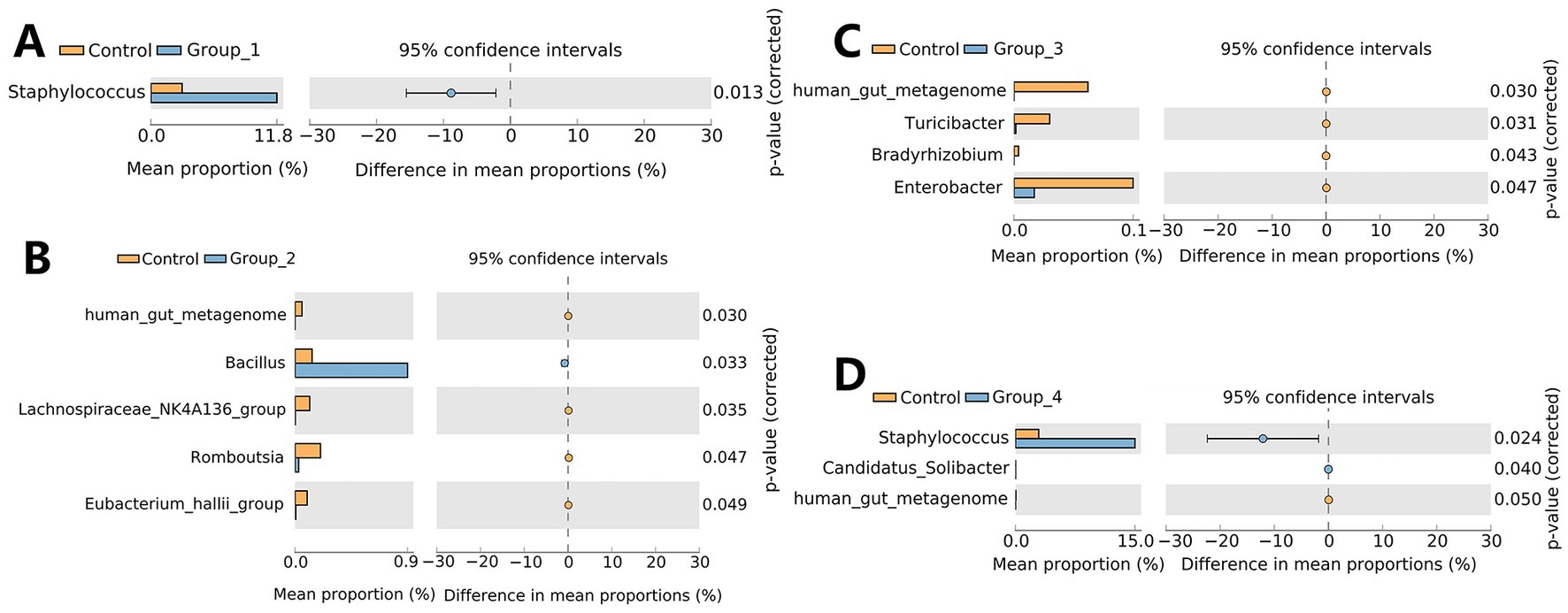
Figure 5. Stamp analysis showing differences in semen microbiota between the control group, Group_1 (A), Group_2 (B), Group_3 (C), and Group_4 (D) at the genus level.
Regarding urinary microbiota, no significant difference in the α- and β-diversities was observed between the control and abnormal semen groups (Figure 6). In Group_1, the urinary abundance of the Tissierellia bacterium S7-1-4 was significantly reduced (Figure 7A). Group_2 showed a significant decrease in the urinary abundance of Lactobacillus and the Tissierellia bacterium S7-1-4 (Figure 7B). There were no significant differences between Group_3 and the control group. Group_4 exhibited a significant decrease in urinary Lactobacillus abundance (Figure 7C).
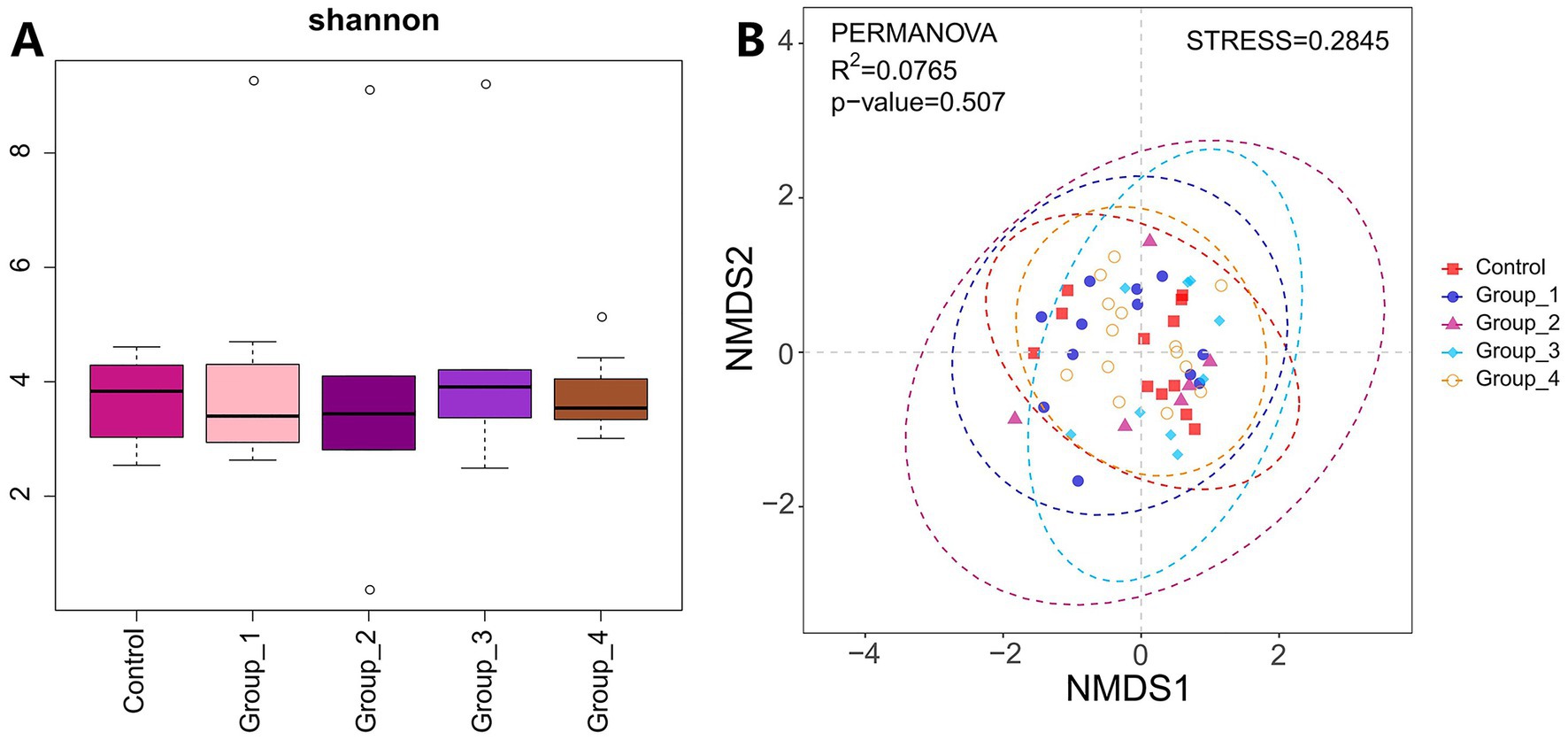
Figure 6. (A) Shannon index was used to estimate the α-diversity of microbes present in urine. (B) Graph showing β-diversity of urine microbiota.
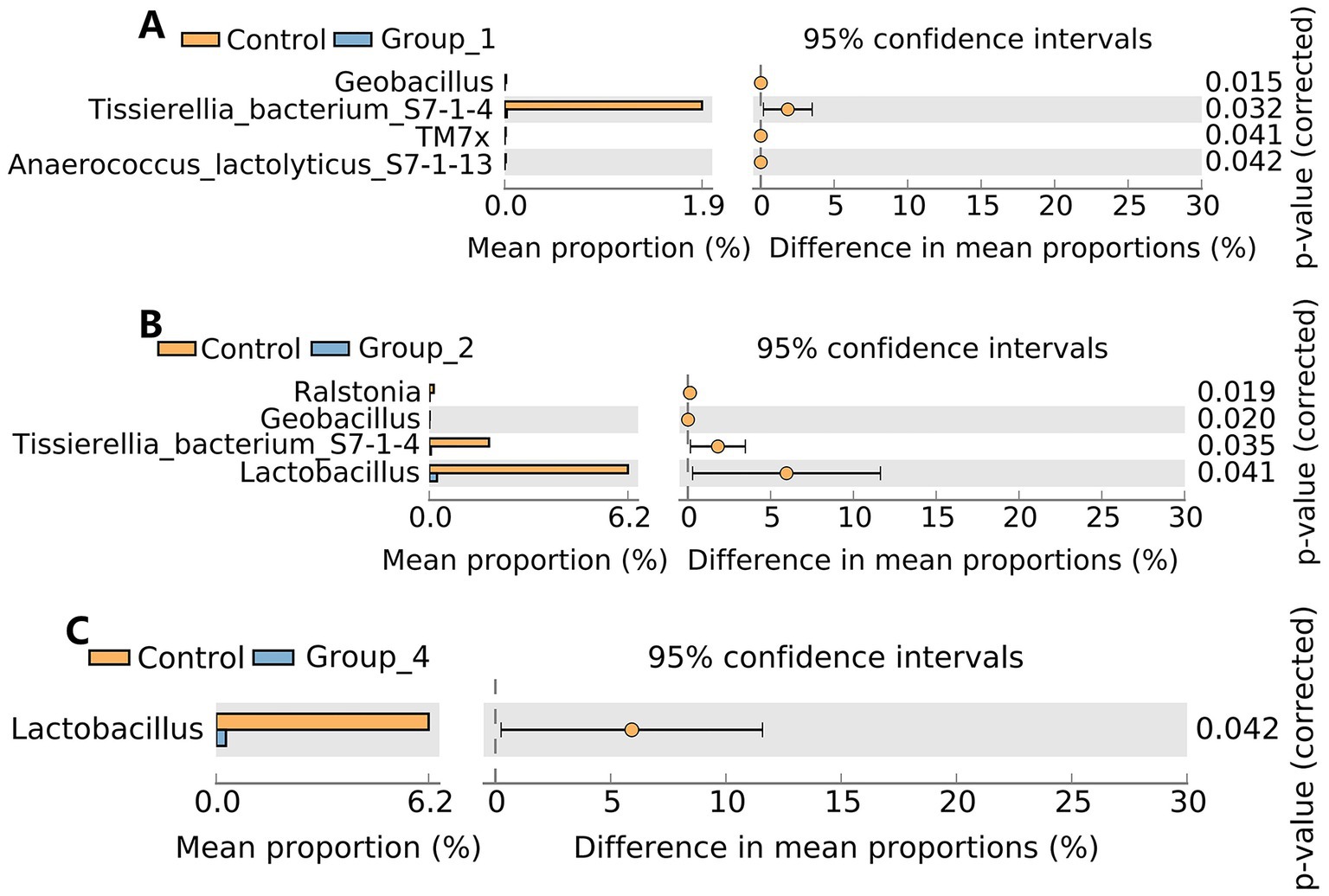
Figure 7. Stamp analysis showing differences in urine microbiota between the control group, Group_1 (A), Group_2 (B), and Group_4 (C) at the genus level. There was no significant difference between the control group and Group_3.
3.3. Generalized linear models for selected genera and key clinical characteristics
Generalized linear model analysis showed that Lactobacillus and Collinsella are positively correlated with sperm concentration and total sperm count. Moreover, Collinsella and Bifidobacterium were positively correlated with forward motile sperms. Bacteroides, Prevotella, and Alicycliphilus were negatively correlated with sperm concentration and total sperm count, and Bacteroides were positively correlated with age (Figure 8).
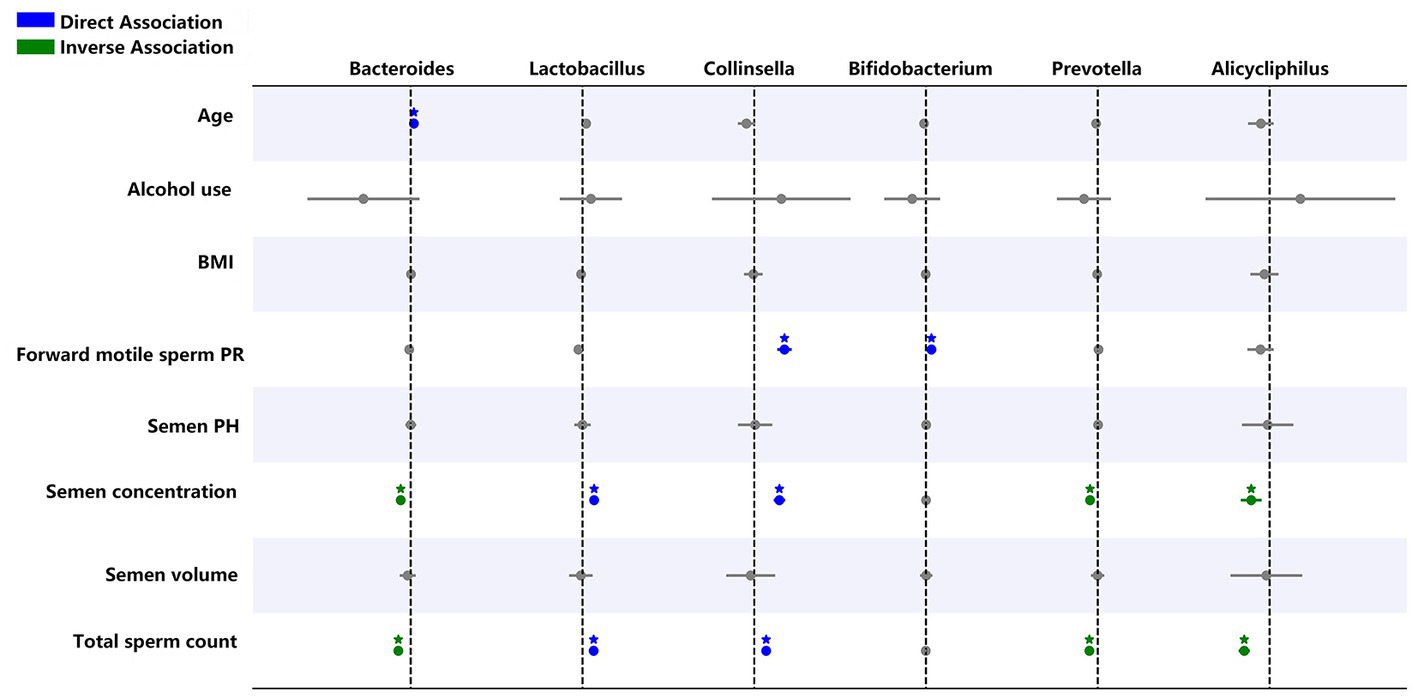
Figure 8. Generalized linear regression models showing the relationship between selected genera, semen abnormality parameters, and demographic parameters. Blue indicates a direct relationship while green shows an inverse relationship. *p < 0.05.
4. Discussion
This study investigated the relationship between the microbiota of the gut and urogenital tract and abnormalities in semen parameters. We found that the gut microbiota could be clustered into most OTUs, followed by urine and semen microbiota. The gut microbiota had a higher α-diversity and was significantly different from that of urine and semen. Furthermore, the β-diversity of the microbes was significantly different among the gut, semen, and urine. The composition of the urine and semen microbiota was similar but different from that of the gut (Figures 1B,D). These results are similar to those of a previous study (Lundy et al., 2021). Our study is the first showing that at the genus level, Bifidobacterium, Blautia, Bacteroides, Faecalibacterium, and Prevotella are predominant in the gut, Staphylococcus, Streptococcus, Prevotella, Finegoldia, and Corynebacterium are predominant in urine, and Lactobacillus, Prevotella, Finegoldia, Staphylococcus, Streptococcus, Ureaplasma, and other unidentified bacteria are predominant in semen (Figure 1D). A significant reduction in the semen abundance of Staphylococcus after vasectomy has also been reported (Lundy et al., 2021). Our study showed no significant differences in the α-diversity of the gut, semen, and urine microbiota between groups, which is consistent with previous reports (Baud et al., 2019; Garcia-Segura et al., 2022). However, few studies have reported differences in the α-diversity of semen microbes (Chen et al., 2018; Lundy et al., 2021; Yao et al., 2022). This might be due to differences in study participants as well as differences in their geographic location and the surrounding environment; however, this hypothesis needs to be further investigated.
Regarding the gut microbiota, our study showed that the abundance of Collinsella is significantly reduced in groups 1, 3, and 4 compared to that in the control group. This indicates that gut Collinsella has a beneficial effect on sperm motility, sperm count, and semen viscosity. Hirayama et al. (2021) showed that ursodeoxycholate produced by Collinsella prevents SARS-CoV-2 infection and improves acute respiratory distress syndrome in patients with COVID-19. Therefore, Collinsella not only exerts a beneficial effect on semen quality but also plays an important role in the control of COVID-19. The gut abundance of Blautia and Bifidobacterium was significantly reduced in the asthenospermia group compared to the control group. Bifidobacterium is a probiotic that protects against other harmful bacteria, improves gastrointestinal barrier function, inhibits pro-inflammatory cytokine production (Xue et al., 2017), improves memory functions (Azad et al., 2018), and alleviates food allergies (Fu et al., 2017). Supplementation with Bifidobacterium can improve sperm motility (Valcarce et al., 2017). It has been shown that cultured gut Bifidobacterium produces γ-aminobutyric acid (GABA) (Cai et al., 2022), which is important for fertilization. GABA not only promotes the acrosome reaction (Kurata et al., 2019) but also facilitates sperm capacitation by promoting tyrosine phosphorylation of the sperm proteins (Cai et al., 2022). Blautia can also inhibit pathogenic bacterial colonization in the gut by producing bacteriocins, which inhibit the proliferation of Clostridium perfringens and vancomycin-resistant enterococci (Liu et al., 2021). The oligospermatozoa group (Group_2) had a low gut abundance of differential bacteria, mainly Clostridium sensu stricto 1, the Christensenellaceae R-7 group, and Intestinibacter. Butyric acid is an important component of SCFAs. Butyrate plays an important role in male fertility by improving testicular endocrine functions and BTB permeability (Al-Asmakh et al., 2014). Supplementation with butyrate increases sperm count, sperm motility, and testosterone levels in adult roosters (Alhaj et al., 2018). Blautia, Bifidobacterium, Lactobacillus, and the Christensenellaceae R-7 group can produce SCFAs (Kim et al., 2014; Calderon-Perez et al., 2020; Markowiak-Kopec and Slizewska, 2020; Hajar-Azhari et al., 2021). Blautia could also prevent the development of obesity-related diseases (Kimura et al., 2013). Intestinibacter can reduce the risk of type I diabetes, making it a potential probiotic (Russell et al., 2019). The gut abundance of Bacteroides was significantly increased in Group_3, and the generalized linear model analysis showed that gut Bacteroides are positively correlated with age and negatively correlated with semen sperm concentration and total sperm count. A previous cohort study also showed a higher proportion of Bacteroides in the intestinal flora of older adults (Claesson et al., 2011). Our study suggests that Bacteroides are potentially pathogenic bacteria.
A significant increase in the abundance of Staphylococcus was observed in the semen of asthenospermia and seminal hyperviscosity only groups compared to the control group. Studies have shown that Staphylococcus may impair the secretory capacity of the prostate, seminal vesicles, and epididymis and may even reduce semen quality (Marconi et al., 2009). Consistent with our results, a previous study in bovines showed that an increase in the semen abundance of Staphylococcus reduces sperm viability and induces oxidative stress, leading to sperm DNA damage (Duracka et al., 2021). The OTUs that were differentially represented in Group_2 and Group_3 were very few, and mainly included Bacillus and Enterobacter (Figures 5B,C), whose abundance was increased and decreased, respectively. This indicates that semen flora has very little effect on sperm count.
Lactobacillus is a known probiotic that has positive effects on several physiological functions of the human body. Previous studies have shown that the presence of Lactobacillus in semen improves its quality (Weng et al., 2014; Monteiro et al., 2018; Baud et al., 2019; Alqawasmeh et al., 2022; Garcia-Segura et al., 2022) and boosts the success rate of assisted reproduction in infertile women (Moreno et al., 2022). Studies have also shown that supplementation with Lactobacillus reuteri can improve sperm motility (Valcarce et al., 2017) and increase testosterone levels in aged male mice (Poutahidis et al., 2014). Supplementation with L. casei rhamnosus Döderleini improves sperm immunogenicity and might be helpful for couples suffering from recurrent immunological spontaneous abortion (Rafiee et al., 2022). Oral administration of the three commensal Lactobacillus spp. can enhance sperm quality in dogs (Mahiddine et al., 2022). In addition, supplementation with L. salivarius CECT5713 and L. gasseri CECT5714 improves the symptoms of lactational mastitis caused by Staphylococcus in women (Jimenez et al., 2008). In summary, our study showed that the urinary abundance of Lactobacillus was significantly reduced in the oligospermia and seminal hyperviscosity only groups, suggesting that Lactobacillus could be a potential probiotic with beneficial effects on semen quality. In addition, the urinary abundance of the Tissierellia bacterium S7-1-4 was significantly reduced in the asthenospermia group.
Previous studies have shown that Prevotella is associated with abnormal semen parameters (Weng et al., 2014; Baud et al., 2019; Farahani et al., 2021). Similarly, generalized linear models in the present study also showed that Prevotella and Alicycliphilus are negatively correlated with sperm concentration and total sperm count. Moreover, we found that Lactobacillus and Collinsella are positively correlated with sperm concentration and total sperm count. Therefore, these microbiotas could be important factors for assessing semen quality.
However, our study has some limitations. This study was a single-center study with a small sample size. First, mid -stream urination and masturbation both contain urethral microbiomes, which are present in the urethra. Catheterization and seminal vesicle aspiration are more appropriate; however, catheterization and seminal vesicle aspiration are unlikely to be acceptable and appropriate for the volunteers. In moreover, neither negative control along the sampling process nor decontamination software were used to reduce potential contamination. Second, future multi-omics approaches such as metabolomics need to be combined to further elucidate the mechanisms of semen quality decline. Repeat sequencing rather than single sequencing can improve the accuracy of results. In addition, semen parameters such as sperm DNA damage, viability and mitochondrial membrane potential in relation to microbiota need to be explored in the future. Finally, fecal microbiota transplantation on experimental animals is needed to further validate the observations of this study, and studies with larger numbers of participants are needed to draw conclusions.
5. Conclusion
This study describes the flora of the intestinal and genitourinary tracts in healthy individuals and those with abnormal semen parameters. Our study suggests that Collinsella, Bifidobacterium, Blautia, and Lactobacillus can be used as probiotics. Furthermore, Bacteroides in the gut and Staphylococcus in semen are potentially pathogenic bacteria. The number and the proportion of differentially abundant bacteria were higher in the asthenospermia and seminal hyperviscosity only groups in all three kinds of samples analyzed. These results suggest that microbiota dysbiosis is closely associated with asthenospermia and seminal hyperviscosity. The differential abundance of OTUs between the healthy control and abnormal semen parameter groups was in the order of gut > semen > urine. This suggests that gut flora has the greatest influence on semen quality, followed by semen flora, while urinary flora plays a smaller role.
Data availability statement
The datasets presented in this study can be found in online repositories. The names of the repository/repositories and accession number(s) can be found below: https://www.ncbi.nlm.nih.gov/, PRJNA900680.
Ethics statement
The studies involving human participants were reviewed and approved by The Ethics Committee of Shandong First Medical University approved this study with ethics approval no. (R202203030053). The patients/participants provided their written informed consent to participate in this study.
Author contributions
TC, SW, XL, and FG: experimental design. TC: experimental data collection and writing of the manuscript. BW, YZ, YW, and JT: semen analysis. TC, YP, SW, and QX: data analysis. XL: funded and revised the manuscript. All authors contributed to the article and approved the submitted version.
Funding
This study was funded by National Natural Science Funds of China (82171594) and Zhao Yi-Cheng Medical Science Foundation (ZYYFY2018031).
Conflict of interest
The authors declare that the research was conducted in the absence of any commercial or financial relationships that could be construed as a potential conflict of interest.
Publisher’s note
All claims expressed in this article are solely those of the authors and do not necessarily represent those of their affiliated organizations, or those of the publisher, the editors and the reviewers. Any product that may be evaluated in this article, or claim that may be made by its manufacturer, is not guaranteed or endorsed by the publisher.
Supplementary material
The Supplementary material for this article can be found online at: https://www.frontiersin.org/articles/10.3389/fmicb.2023.1182320/full#supplementary-material
Supplementary Figure S1 | Barplot showing the phylum in the gut, urine, and semen.
References
Agus, A., Clement, K., and Sokol, H. (2021). Gut microbiota-derived metabolites as central regulators in metabolic disorders. Gut 70, 1174–1182. doi: 10.1136/gutjnl-2020-323071
Al-Asmakh, M., Stukenborg, J. B., Reda, A., Anuar, F., Strand, M. L., Hedin, L., et al. (2014). The gut microbiota and developmental programming of the testis in mice. PLoS One 9:e103809. doi: 10.1371/journal.pone.0103809
Alfano, M., Ferrarese, R., Locatelli, I., Ventimiglia, E., Ippolito, S., Gallina, P., et al. (2018). Testicular microbiome in azoospermic men-first evidence of the impact of an altered microenvironment. Hum. Reprod. 33, 1212–1217. doi: 10.1093/humrep/dey116
Alhaj, H. W., Li, Z., Shan, T., Dai, P., Zhu, P., Li, Y., et al. (2018). Effects of dietary sodium butyrate on reproduction in adult breeder roosters. Anim. Reprod. Sci. 196, 111–119. doi: 10.1016/j.anireprosci.2018.07.002
Alqawasmeh, O., Fok, E., Yim, H., Li, T., Chung, J., and Chan, D. (2022). The microbiome and male infertility: looking into the past to move forward. Hum. Fertil. (Camb.), 1–13. doi: 10.1080/14647273.2022.2098540
Azad, M. A. K., Sarker, M., Li, T., and Yin, J. (2018). Probiotic species in the modulation of gut microbiota: an overview. Biomed. Res. Int. 2018:9478630. doi: 10.1155/2018/9478630
Baud, D., Pattaroni, C., Vulliemoz, N., Castella, V., Marsland, B. J., and Stojanov, M. (2019). Sperm microbiota and its impact on semen parameters. Front. Microbiol. 10:234. doi: 10.3389/fmicb.2019.00234
Braniste, V., Al-Asmakh, M., Kowal, C., Anuar, F., Abbaspour, A., Toth, M., et al. (2014). The gut microbiota influences blood-brain barrier permeability in mice. Sci. Transl. Med. 6:263ra158. doi: 10.1126/scitranslmed.3009759
Cai, H., Cao, X., Qin, D., Liu, Y., Liu, Y., Hua, J., et al. (2022). Gut microbiota supports male reproduction via nutrition, immunity, and signaling. Front. Microbiol. 13:977574. doi: 10.3389/fmicb.2022.977574
Calderon-Perez, L., Gosalbes, M. J., Yuste, S., Valls, R. M., Pedret, A., Llaurado, E., et al. (2020). Gut metagenomic and short chain fatty acids signature in hypertension: a cross-sectional study. Sci. Rep. 10:6436. doi: 10.1038/s41598-020-63475-w
Carlsen, E., Giwercman, A., Keiding, N., and Skakkebaek, N. E. (1992). Evidence for decreasing quality of semen during past 50 years. BMJ 305, 609–613. doi: 10.1136/bmj.305.6854.609
Chen, H., Luo, T., Chen, T., and Wang, G. (2018). Seminal bacterial composition in patients with obstructive and non-obstructive azoospermia. Exp. Ther. Med. 15, 2884–2890. doi: 10.3892/etm.2018.5778
Chleilat, F., Schick, A., Deleemans, J. M., Ma, K., Alukic, E., Wong, J., et al. (2021). Paternal high protein diet modulates body composition, insulin sensitivity, epigenetics, and gut microbiota intergenerationally in rats. FASEB J. 35:e21847. doi: 10.1096/fj.202100198RR
Claesson, M. J., Cusack, S., O'Sullivan, O., Greene-Diniz, R., de Weerd, H., Flannery, E., et al. (2011). Composition, variability, and temporal stability of the intestinal microbiota of the elderly. Proc. Natl. Acad. Sci. U. S. A. 108, 4586–4591. doi: 10.1073/pnas.1000097107
Cole, J. R., Wang, Q., Cardenas, E., Fish, J., Chai, B., Farris, R. J., et al. (2009). The ribosomal database project: improved alignments and new tools for rRNA analysis. Nucleic Acids Res. 37, D141–D145. doi: 10.1093/nar/gkn879
Dai, Z., Wu, Z., Hang, S., Zhu, W., and Wu, G. (2015). Amino acid metabolism in intestinal bacteria and its potential implications for mammalian reproduction. Mol. Hum. Reprod. 21, 389–409. doi: 10.1093/molehr/gav003
Dai, Z. L., Wu, G., and Zhu, W. Y. (2011). Amino acid metabolism in intestinal bacteria: links between gut ecology and host health. Front. Biosci. (Landmark Ed) 16, 1768–1786. doi: 10.2741/3820
Dodd, D., Spitzer, M. H., Van Treuren, W., Merrill, B. D., Hryckowian, A. J., Higginbottom, S. K., et al. (2017). A gut bacterial pathway metabolizes aromatic amino acids into nine circulating metabolites. Nature 551, 648–652. doi: 10.1038/nature24661
Dohle, G. R., Colpi, G. M., Hargreave, T. B., Papp, G. K., Jungwirth, A., Weidner, W., et al. (2005). EAU guidelines on male infertility. Eur. Urol. 48, 703–711. doi: 10.1016/j.eururo.2005.06.002
Duracka, M., Husarcikova, K., Jancov, M., Galovicova, L., Kacaniova, M., Lukac, N., et al. (2021). Staphylococcus-induced Bacteriospermia in vitro: consequences on the bovine spermatozoa quality, extracellular calcium and magnesium content. Animals (Basel) 11:3309. doi: 10.3390/ani11113309
Edgar, R. C. (2013). UPARSE: highly accurate OTU sequences from microbial amplicon reads. Nat. Methods 10, 996–998. doi: 10.1038/nmeth.2604
Farahani, L., Tharakan, T., Yap, T., Ramsay, J. W., Jayasena, C. N., and Minhas, S. (2021). The semen microbiome and its impact on sperm function and male fertility: a systematic review and meta-analysis. Andrology 9, 115–144. doi: 10.1111/andr.12886
Feng, T., and Liu, Y. (2022). Microorganisms in the reproductive system and probiotic's regulatory effects on reproductive health. Comput. Struct. Biotechnol. J. 20, 1541–1553. doi: 10.1016/j.csbj.2022.03.017
Fu, L., Song, J., Wang, C., Fu, S., and Wang, Y. (2017). Bifidobacterium infantis potentially alleviates shrimp tropomyosin-induced allergy by tolerogenic dendritic cell-dependent induction of regulatory T cells and alterations in gut microbiota. Front. Immunol. 8:1536. doi: 10.3389/fimmu.2017.01536
Fuller, M. F., and Reeds, P. J. (1998). Nitrogen cycling in the gut. Annu. Rev. Nutr. 18, 385–411. doi: 10.1146/annurev.nutr.18.1.385
Garcia-Segura, S., Del Rey, J., Closa, L., Garcia-Martinez, I., Hobeich, C., Castel, A. B., et al. (2022). Seminal microbiota of idiopathic infertile patients and its relationship with sperm DNA integrity. Front. Cell Dev. Biol. 10:937157. doi: 10.3389/fcell.2022.937157
Guo, L., Wu, Y., Wang, C., Wei, H., Tan, J., Sun, H., et al. (2020). Gut microbiological disorders reduce semen utilization rate in Duroc boars. Front. Microbiol. 11:581926. doi: 10.3389/fmicb.2020.581926
Hajar-Azhari, S., Hafiz Abd Rahim, M., Razid Sarbini, S., Muhialdin, B. J., Olusegun, L., and Saari, N. (2021). Enzymatically synthesised fructooligosaccharides from sugarcane syrup modulate the composition and short-chain fatty acid production of the human intestinal microbiota. Food Res. Int. 149:110677. doi: 10.1016/j.foodres.2021.110677
Hao, Y., Feng, Y., Yan, X., Chen, L., Ma, X., Tang, X., et al. (2022a). Gut microbiota-testis Axis: FMT mitigates high-fat diet-diminished male fertility via improving systemic and testicular Metabolome. Microbiol. Spectr. 10:e0002822. doi: 10.1128/spectrum.00028-22
Hao, Y., Feng, Y., Yan, X., Chen, L., Zhong, R., Tang, X., et al. (2022b). Gut microbiota-testis axis: FMT improves systemic and testicular micro-environment to increase semen quality in type 1 diabetes. Mol. Med. 28:45. doi: 10.1186/s10020-022-00473-w
Hirayama, M., Nishiwaki, H., Hamaguchi, T., Ito, M., Ueyama, J., Maeda, T., et al. (2021). Intestinal collinsella may mitigate infection and exacerbation of COVID-19 by producing ursodeoxycholate. PLoS One 16:e0260451. doi: 10.1371/journal.pone.0260451
Hou, D., Zhou, X., Zhong, X., Settles, M. L., Herring, J., Wang, L., et al. (2013). Microbiota of the seminal fluid from healthy and infertile men. Fertil. Steril. 100, 1261–1269. doi: 10.1016/j.fertnstert.2013.07.1991
Hoyles, L., Pontifex, M. G., Rodriguez-Ramiro, I., Anis-Alavi, M. A., Jelane, K. S., Snelling, T., et al. (2021). Regulation of blood-brain barrier integrity by microbiome-associated methylamines and cognition by trimethylamine N-oxide. Microbiome 9:235. doi: 10.1186/s40168-021-01181-z
Jami, E., Israel, A., Kotser, A., and Mizrahi, I. (2013). Exploring the bovine rumen bacterial community from birth to adulthood. ISME J. 7, 1069–1079. doi: 10.1038/ismej.2013.2
Jequier, A. M. (1985). Obstructive azoospermia: a study of 102 patients. Clin. Reprod. Fertil. 3, 21–36.
Jimenez, E., Fernandez, L., Maldonado, A., Martin, R., Olivares, M., Xaus, J., et al. (2008). Oral administration of Lactobacillus strains isolated from breast milk as an alternative for the treatment of infectious mastitis during lactation. Appl. Environ. Microbiol. 74, 4650–4655. doi: 10.1128/AEM.02599-07
Kang, D. W., Adams, J. B., Coleman, D. M., Pollard, E. L., Maldonado, J., McDonough-Means, S., et al. (2019). Long-term benefit of microbiota transfer therapy on autism symptoms and gut microbiota. Sci. Rep. 9:5821. doi: 10.1038/s41598-019-42183-0
Kang, D. W., Adams, J. B., Gregory, A. C., Borody, T., Chittick, L., Fasano, A., et al. (2017). Microbiota transfer therapy alters gut ecosystem and improves gastrointestinal and autism symptoms: an open-label study. Microbiome 5:10. doi: 10.1186/s40168-016-0225-7
Kim, C. H., Park, J., and Kim, M. (2014). Gut microbiota-derived short-chain fatty acids, T cells, and inflammation. Immune Netw. 14, 277–288. doi: 10.4110/in.2014.14.6.277
Kimura, I., Ozawa, K., Inoue, D., Imamura, T., Kimura, K., Maeda, T., et al. (2013). The gut microbiota suppresses insulin-mediated fat accumulation via the short-chain fatty acid receptor GPR43. Nat. Commun. 4:1829. doi: 10.1038/ncomms2852
Kurata, S., Hiradate, Y., Umezu, K., Hara, K., and Tanemura, K. (2019). Capacitation of mouse sperm is modulated by gamma-aminobutyric acid (GABA) concentration. J. Reprod. Dev. 65, 327–334. doi: 10.1262/jrd.2019-008
Levine, H., Jorgensen, N., Martino-Andrade, A., Mendiola, J., Weksler-Derri, D., Mindlis, I., et al. (2017). Temporal trends in sperm count: a systematic review and meta-regression analysis. Hum. Reprod. Update 23, 646–659. doi: 10.1093/humupd/dmx022
Liu, J.-B., Chen, K., Li, Z.-F., Wang, Z.-Y., and Wang, L. (2022). Glyphosate-induced gut microbiota dysbiosis facilitates male reproductive toxicity in rats. Sci. Total Environ. 805:150368. doi: 10.1016/j.scitotenv.2021.150368
Liu, Y., Hou, Y., Wang, G., Zheng, X., and Hao, H. (2020). Gut microbial metabolites of aromatic amino acids as signals in host-microbe interplay. Trends Endocrinol. Metab. 31, 818–834. doi: 10.1016/j.tem.2020.02.012
Liu, X., Mao, B., Gu, J., Wu, J., Cui, S., Wang, G., et al. (2021). Blautia-a new functional genus with potential probiotic properties? Gut Microbes 13, 1–21. doi: 10.1080/19490976.2021.1903826
Lundy, S. D., Sangwan, N., Parekh, N. V., Selvam, M. K. P., Gupta, S., McCaffrey, P., et al. (2021). Functional and taxonomic dysbiosis of the gut, urine, and semen microbiomes in male infertility. Eur. Urol. 79, 826–836. doi: 10.1016/j.eururo.2021.01.014
Mahiddine, F. Y., You, I., Park, H., and Kim, M. J. (2022). Commensal lactobacilli enhance sperm qualitative parameters in dogs. Front. Vet. Sci. 9:888023. doi: 10.3389/fvets.2022.888023
Marconi, M., Pilatz, A., Wagenlehner, F., Diemer, T., and Weidner, W. (2009). Impact of infection on the secretory capacity of the male accessory glands. Int. Braz. J. Urol. 35, 299–309. Discussion 308-299. doi: 10.1590/S1677-55382009000300006
Markowiak-Kopec, P., and Slizewska, K. (2020). The effect of probiotics on the production of short-chain fatty acids by human intestinal microbiome. Nutrients 12:1107. doi: 10.3390/nu12041107
Molina, N. M., Plaza-Diaz, J., Vilchez-Vargas, R., Sola-Leyva, A., Vargas, E., Mendoza-Tesarik, R., et al. (2021). Assessing the testicular sperm microbiome: a low-biomass site with abundant contamination. Reprod. Biomed. Online 43, 523–531. doi: 10.1016/j.rbmo.2021.06.021
Monteiro, C., Marques, P. I., Cavadas, B., Damiao, I., Almeida, V., Barros, N., et al. (2018). Characterization of microbiota in male infertility cases uncovers differences in seminal hyperviscosity and oligoasthenoteratozoospermia possibly correlated with increased prevalence of infectious bacteria. Am. J. Reprod. Immunol. 79:e12838. doi: 10.1111/aji.12838
Moreno, I., Garcia-Grau, I., Perez-Villaroya, D., Gonzalez-Monfort, M., Bahceci, M., Barrionuevo, M. J., et al. (2022). Endometrial microbiota composition is associated with reproductive outcome in infertile patients. Microbiome 10:1. doi: 10.1186/s40168-021-01184-w
Munyaka, P. M., Eissa, N., Bernstein, C. N., Khafipour, E., and Ghia, J. E. (2015). Antepartum antibiotic treatment increases offspring susceptibility to experimental colitis: a role of the gut microbiota. PLoS One 10:e0142536. doi: 10.1371/journal.pone.0142536
Neis, E. P., Dejong, C. H., and Rensen, S. S. (2015). The role of microbial amino acid metabolism in host metabolism. Nutrients 7, 2930–2946. doi: 10.3390/nu7042930
Oghbaei, H., Rastgar Rezaei, Y., Nikanfar, S., Zarezadeh, R., Sadegi, M., Latifi, Z., et al. (2020). Effects of bacteria on male fertility: spermatogenesis and sperm function. Life Sci. 256:117891. doi: 10.1016/j.lfs.2020.117891
Poutahidis, T., Springer, A., Levkovich, T., Qi, P., Varian, B. J., Lakritz, J. R., et al. (2014). Probiotic microbes sustain youthful serum testosterone levels and testicular size in aging mice. PLoS One 9:e84877. doi: 10.1371/journal.pone.0084877
Rafiee, M., Sereshki, N., Alipour, R., Ahmadipanah, V., Pashoutan Sarvar, D., and Wilkinson, D. (2022). The effect of probiotics on immunogenicity of spermatozoa in couples suffering from recurrent spontaneous abortion. BMC Immunol. 23:32. doi: 10.1186/s12865-022-00506-3
Rando, O. J., and Simmons, R. A. (2015). I'm eating for two: parental dietary effects on offspring metabolism. Cells 161, 93–105. doi: 10.1016/j.cell.2015.02.021
Rodin, D. M., Larone, D., and Goldstein, M. (2003). Relationship between semen cultures, leukospermia, and semen analysis in men undergoing fertility evaluation. Fertil. Steril. 79, 1555–1558. doi: 10.1016/S0015-0282(03)00340-6
Russell, J. T., Roesch, L. F. W., Ordberg, M., Ilonen, J., Atkinson, M. A., Schatz, D. A., et al. (2019). Genetic risk for autoimmunity is associated with distinct changes in the human gut microbiome. Nat. Commun. 10:3621. doi: 10.1038/s41467-019-11460-x
Said, T. M., Paasch, U., Glander, H. J., and Agarwal, A. (2004). Role of caspases in male infertility. Hum. Reprod. Update 10, 39–51. doi: 10.1093/humupd/dmh003
Sengupta, P., Borges, E. Jr., Dutta, S., and Krajewska-Kulak, E. (2018). Decline in sperm count in European men during the past 50 years. Hum. Exp. Toxicol. 37, 247–255. doi: 10.1177/0960327117703690
Urata, K., Narahara, H., Tanaka, Y., Egashira, T., Takayama, F., and Miyakawa, I. (2001). Effect of endotoxin-induced reactive oxygen species on sperm motility. Fertil. Steril. 76, 163–166. doi: 10.1016/S0015-0282(01)01850-7
Valcarce, D. G., Genoves, S., Riesco, M. F., Martorell, P., Herraez, M. P., Ramon, D., et al. (2017). Probiotic administration improves sperm quality in asthenozoospermic human donors. Benef. Microbes 8, 193–206. doi: 10.3920/BM2016.0122
Wade, W. (2002). Unculturable bacteria – the uncharacterized organisms that cause oral infections. J. R. Soc. Med. 95, 81–83. doi: 10.1177/014107680209500207
Weng, S. L., Chiu, C. M., Lin, F. M., Huang, W. C., Liang, C., Yang, T., et al. (2014). Bacterial communities in semen from men of infertile couples: metagenomic sequencing reveals relationships of seminal microbiota to semen quality. PLoS One 9:e110152. doi: 10.1371/journal.pone.0110152
Xue, L., He, J., Gao, N., Lu, X., Li, M., Wu, X., et al. (2017). Probiotics may delay the progression of nonalcoholic fatty liver disease by restoring the gut microbiota structure and improving intestinal endotoxemia. Sci. Rep. 7:45176. doi: 10.1038/srep45176
Yang, H., Zhang, J., Xue, Z., Zhao, C., Lei, L., Wen, Y., et al. (2020). Potential pathogenic Bacteria in seminal microbiota of patients with different types of Dysspermatism. Sci. Rep. 10:6876. doi: 10.1038/s41598-020-63787-x
Yao, Y., Qiu, X. J., Wang, D. S., Luo, J. K., Tang, T., Li, Y. H., et al. (2022). Semen microbiota in normal and leukocytospermic males. Asian J. Androl. 24, 398–405. doi: 10.4103/aja202172
Zhang, Y. J., Li, S., Gan, R. Y., Zhou, T., Xu, D. P., and Li, H. B. (2015). Impacts of gut bacteria on human health and diseases. Int. J. Mol. Sci. 16, 7493–7519. doi: 10.3390/ijms16047493
Zhang, C., Xiong, B., Chen, L., Ge, W., Yin, S., Feng, Y., et al. (2021). Rescue of male fertility following faecal microbiota transplantation from alginate oligosaccharide-dosed mice. Gut 70, 2213–2215. doi: 10.1136/gutjnl-2020-323593
Keywords: microbiota, semen, urine, gut, 16S rRNA, semen abnormalities
Citation: Cao T, Wang S, Pan Y, Guo F, Wu B, Zhang Y, Wang Y, Tian J, Xing Q and Liu X (2023) Characterization of the semen, gut, and urine microbiota in patients with different semen abnormalities. Front. Microbiol. 14:1182320. doi: 10.3389/fmicb.2023.1182320
Edited by:
Muhammad Yasir, King Abdulaziz University, Saudi ArabiaReviewed by:
Tarique Hussain, Nuclear Institute for Agriculture and Biology, PakistanAlberto Sola-Leyva, University of Granada, Spain
Copyright © 2023 Cao, Wang, Pan, Guo, Wu, Zhang, Wang, Tian, Xing and Liu. This is an open-access article distributed under the terms of the Creative Commons Attribution License (CC BY). The use, distribution or reproduction in other forums is permitted, provided the original author(s) and the copyright owner(s) are credited and that the original publication in this journal is cited, in accordance with accepted academic practice. No use, distribution or reproduction is permitted which does not comply with these terms.
*Correspondence: Xiaoqiang Liu, eGlhb3FpYW5nbGl1MUAxNjMuY29t