- 1School of Pharmacy, Zunyi Medical University, Zunyi, China
- 2School of Public Health, Zunyi Medical University, Zunyi, China
- 3Centre of Excellence in Fungal Research, Mae Fah Luang University, Chiang Rai, Thailand
Background: The antibiotic resistance in various bacteria is consistently increasing and is posing a serious threat to human health, prompting the need for the discovery of novel structurally featured natural products with promising biological activities in drug research and development. Endolichenic microbes have been proven to be a fertile source to produce various chemical components, and therefore these microbes have been on a prime focus for exploring natural products. In this study, to explore potential biological resources and antibacterial natural products, the secondary metabolites of an endolichenic fungus have been investigated.
Methods: The antimicrobial products were isolated from the endolichenic fungus using various chromatographic methods, and the antibacterial and antifungal activities of the compounds were evaluated by the broth microdilution method under in vitro conditions. The antimicrobial mechanism has been discussed with measuring the dissolution of nucleic acid and protein, as well as the activity of alkaline phosphatase (AKP) in preliminary manner. Chemical synthesis of the active product compound 5 was also performed, starting from commercially available 2,6-dihydroxybenzaldehyde through a sequence of transformations that included methylation, the addition of propylmagnesium bromide on formyl group, the oxidation of secondary alcohol, and the deprotection of methyl ether motif.
Results: Among the 19 secondary metabolites of the endolichenic fungus, Daldinia childiae (compound 5) showed attractive antimicrobial activities on 10 of the 15 tested pathogenic strains, including Gram-positive bacteria, Gram-negative bacteria, and fungus. The Minimum Inhibitory Concentration (MIC) of compound 5 for Candida albicans 10213, Micrococcus luteus 261, Proteus vulgaris Z12, Shigella sonnet, and Staphylococcus aureus 6538 was identified as 16 μg/ml, whereas the Minimum Bactericidal Concentration (MBC) of other strains was identified as 64 μg/ml. Compound 5 could dramatically inhibit the growth of S. aureus 6538, P. vulgaris Z12, and C. albicans 10213 at the MBC, likely affecting the permeability of the cell wall and cell membrane. These results enriched the library of active strains and metabolites resources of endolichenic microorganisms. The chemical synthesis of the active compound was also performed in four steps, providing an alternative pathway to explore antimicrobial agents.
1. Introduction
The antibiotic resistance in various bacteria is consistently increasing and is posing a serious threat to human health. Due to the overuse or abuse of antibiotics, pathogenic bacteria have developed resistance to a variety of antibacterial drugs, posing a serious threat to global public health and economic stability. Drug resistance has become one of the most pressing global public health issues, making it imperative to find new antibacterial agents (Seukepa et al., 2022; Shinu et al., 2022). To provide chemical entities for the current rigorous drug research and development, new natural products with novel structures and significant activity are urgently needed. Antibiotics such as penicillin, streptomycin, erythromycin, and many others were discovered from microorganisms. According to the literature, 12 out of the 22 antibacterial drugs marketed after 2,000 originated from microbial secondary metabolites. In the global drug market, approximately 70% of antibiotics are derived from microorganisms (Rao et al., 2020). Microbial natural products have always been the main source of biological drug innovation, and are important resources for developing clinical antibacterial, anti-tumor, immunosuppressant, and other drugs which are also widely used in agriculture, food, manufacturing industry, and other fields (Anderson et al., 2022).
However, the problem of repeated screening of microbial resources has become increasingly prominent as the intense research on secondary metabolites from microorganisms (Maliehe et al., 2022). Obviously, the probability of the discovery of novel structural active substances from microbes in conventional environments has gradually declined. It is crucial to actively search for new microbial resources to advance the research and development of natural product drugs (Nursyam, 2017; Shevkar et al., 2022).
Lichens are considered as stable complex organisms formed by the symbiosis of fungi and algae. Through the long process of biological evolution, mycobionts, photobionts, and endolichenic fungi have developed a special symbiotic relationship. Due to their distinctive ecological environments, it is widely believed that lichens and endophytes contain a diverse array of unique active metabolites (Zhao et al., 2022). Progressively more novel and bioactive compounds have been reported from endolichenic fungi in recent years (Lin et al., 2021; Luo et al., 2022). Guizhou Province of Southwestern China is a typical mountainous region with a high level of species diversity owing to its unique Karst terrain and humid climate.
The endolichenic fungus, D. childiae, from Punticelia sp. isolated by our research team showed strong- and broad-spectrum antibacterial activities on Staphylococcus aureus 25923 and 6538, Staphylococcus epidermidis Z12, Bacillus subtilis 163, and so on (Zhou et al., 2021a). In this fungus, a new compound and several first-isolated natural products were discovered (Zhou et al., 2020, 2021b). Moreover, it was exciting to observe that a product 1-(2,6-dihydroxyphenyl) butane-1-one (5) exhibited inhibitory activity on 10 pathogenic bacteria among 15 strains provided in clinical and food fields. In this study, the antibacterial, antifungi activities, and anti-microorganism mechanisms were investigated. Furthermore, in this study, the chemical synthesis was explored. The results from this study indicate that endolichenic fungi are valuable and potential resources for exploiting new antibacterial substances as the candidates in the clinical application and in preserving the food safety.
2. Materials and methods
2.1. General experimental procedures
The Nuclear Magnetic Resonance (NMR) data were detected by the DD2400-MR Nuclear Magnetic Resonance Instrument (Agilent, Santa Clara, CA, USA). Preparative High-Performance Liquid Chromatography (Preparative HPLC) was performed on the LC3000N Liquid Chromatography System, equipped with a C18 (5 μm 10 × 250 mm, HPLC semipreparative column) (Column Company, Seattle, WA, USA) and a UV3000 Ultraviolet–Visible Light Detector. The absorbance of the substances was detected by a Microplate Reader (MK3 Thermo Company, Pleasanton, CA, USA). Sterile conditions were provided by an Aseptic ultra-clean bench (Suzhou Purification Equipment Co., Ltd., Suzhou, China). The thermostatic shaker is manufactured by Shanghai Tiancheng Technology Co., Ltd. (Shanghai, China). Concentrations in the suspension of the strain were identified by Bacterial Turbidity Meter (WGZ-2XJ, Shanghai, China). Mueller–Hinton (MH) broth was purchased from Beijing Solabao Technology Co., Ltd., (Beijing, China) while yeast extract and peptone were purchased from Guangzhou Lu Cheng Biotechnology Co., Ltd. (Guangzhou, China). Other analytical-grade solvents were purchased from Tianjin Fuyu Fine Chemical Co., Ltd., Tianjin, China.
2.2. Fungal materials
The fungal strain D. childiae isolated from the Punticelia was identified by the Internal Transcribed Spacer (ITS) sequence (GenBank No. MT139887) and deposited in China General Microbiological Culture Collection Center (CGMCC No. 17469). A total of 15 pathogenic bacterial and fungal strains including Gram-positive bacteria, Gram-negative bacteria, and fungi were provided. Listeria monocytogenes CMCC 54008, Salmonella typhimurium CMCC 50071, Vibrio parahaemolyticus ATCC 17802, Pseudomonas aeruginosa, Enterobacter aerogenes, Vibrio vulnificus, Proteus mirabilis, Shigella sonnei, Shigella sonnet were provided by School of Public Health, Zunyi Medical University, Guizhou, China. Brucella sp. B103, Proteus vulgaris Z12, B. subtilis 163 and Micrococcus luteus 261 were provided by Affiliated Hospital, Zunyi Medical University, Guizhou, China. S. aureus 6538 and Candida albicans 10213 were provided by the School of Pharmacy, Zunyi Medical University, Guizhou, China.
2.3. The isolation of compound 5 and inhibitory activity on pathogenic strains
Compound 5 was isolated from the rice solid fermentation extract obtained from ethyl acetate through silica gel column chromatography (Zhou et al., 2021b). The antibacterial activity of compound 5 was evaluated by the filter paper method.
2.3.1. Preparation of culture media
Mueller–Hinton liquid media (9.125 g MHA powder in 250 ml distilled water) and Luria-Bertani (LB) liquid media (yeast extract, NaCl, and peptone in the ratio of 1 g: 1 g:2 g in 200 ml distilled water) were prepared and the solid media were formed with addition with 20-g ager into 1 L liquid media.
2.3.2. Activation of test strains
The 15 tested strains were inoculated on the sterile LB solid media and incubated at 37°C for 12 h to obtain a single colony. Then the single colony was picked to add the LB liquid media and incubated at 37°C at 150 rpm for another 12 h.
2.3.3. Screening of the active compounds on tested strains
Nineteen isolated compounds were screened for their antibacterial and antifungal activities using the Kirby–Bauer method. Fifteen test strains of bacteria and fungi were inoculated on MHA media, and drug-sensitivity papers were placed on the plates. Then, 1.5 mg of the compound was dissolved in 500 μl of methanol and 5 μl of the solution of each compound was placed on the filter paper, with methanol as the blank control. The treated strains were incubated at 37°C with three replicates. After 18 h, the inhibition zones were measured to evaluate the antibacterial and anti-fungal effects of the isolated secondary metabolites.
2.4. MIC and MBC of compound 5 by microdilution method
2.4.1. Preparation of solutions of compound 1 and pathogenic strains
An amount of 2 mg of compound 5 was added to 1 ml of methanol in a 1.5 ml Eppendorf (EP) tube and mixed thoroughly. Then, 224 μl of the resulting solution was added to 126 μl of broth and further diluted to a concentration of 1,280 μg/ml. The concentration of the final solution was achieved by continuous dilution to 1.25 μg/ml.
The bacterial solution was diluted to 0.5 Maxwell’s turbidity with the turbidimetric tube as a reference, which is about 1 × 108 CFU/ml by the broth. Subsequently, 950 μl of MH culture media and 50 μl of bacterial solution with 0.5 Maxwell’s turbidity were mixed in a new EP pipette to make the bacterial suspension with an approximate concentration of 1 × 107 CFU/ml.
2.4.2. Determination of MIC
The MICs of the compounds were determined by the broth microdilution method (Clarke et al., 2020). Initially, 180 μl of broth culture media were added to the holes 1–10 in each row, while 190 μl of broth culture media were added to the holes 11–12 as the control in a sterile 96-well plate. Subsequently, 10 μl of bacterial solution was added to the holes 1–11 and 10 μl of the prepared drug solution was added to the holes 1–10 of each row. Additionally, 10 μl of the minimum concentration solution was added to the hole 12. The tests were repeated 3 times in parallel. All 96-well plates were incubated for 24–36 h at 37°C. The MIC of the drug was determined as the one with a clear and transparent solution and in the other holes, it was observed with a black background, while that of the negative control was observed as turbid liquid.
2.4.3. Determination of MBC
Based on the MIC, 10 μl of culture solution in which the test bacteria did not grow from a 96-well plate were incubated on the MHA media without drug solution at 37°C for 24–36 h after coating. According to Liu et al. (2015), the concentration of the compound without the growth of test bacteria is considered as the MBC value.
2.5. Determination of growth curve of test bacteria
2.5.1. Activation of test bacteria
First, three strains including Gram-positive bacteria, S. aureus 6538; Gram-negative bacteria, P. vulgaris Z12; and fungus, C. albicans 10213, were activated from the stored plate at –20°C on the LB solid media for 12 h at 37°C. The single colony was picked out and put into the LB liquid media for another 12 h at 37°C in a shaker at 150 rpm.
2.5.2. Determination of growth curve
According to the method by Wu et al. (2017), the activated test bacterial solution was diluted with MH broth to a concentration of 107 CFU/ml, and then 200 μl of the diluted test bacterial solution was taken into a flask containing 20 ml sterile nutrient broth. To achieve a final concentration of 1 × MBC, compound 5 was dissolved in methanol and added to the solution. The control group was set up with MH broth that did not contain the compound but contained the solvent and the bacterial solution being tested. All the bacterial solutions were incubated at 37°C and 120 rpm, and the growth of the test bacteria was monitored every 2 h under OD600 on the enzyme-labeled instrument.
2.6. The content of nucleic acid
2.6.1. Preparation of PBS buffer solution
A total amount of 17.4170 g of K2HPO4 and 13.609 g of KH2PO4 were added into a 100 ml volumetric flask to make the solutions. Then a 50.3 ml of KH2PO4 and 49.7 ml of K2HPO4 were mixed in a new 250 ml triangular flask. The PBS buffer solution with pH 6.8 was prepared after sterilization of the mixed liquids at 121°C for 15 min.
2.6.2. Determination of the content of nucleic acid
The content of nucleic acid was determined by the improved method used by Zhang et al. (2020). The test bacterial suspension with a concentration of 107 CFU/ml was inoculated into 100 ml MH broth into the logarithmic phase. Then 10 ml of bacterial suspension was taken into a 50 ml centrifuge tube and centrifuged at 2,700 g for 30 min and the bacterial suspension was washed 3 times with PBS buffer solution. To obtain a final concentration of 1 × MBC, 100 μl of compound 5 was dissolved in methanol and was added to the solution, followed by the addition of MH broth to bring the final volume to 10 ml. After 3 h at 37°C, the bacterial suspension was centrifuged at 2,700 g for 15 min. To measure the concentration of the extracted nucleic acid, 200 μl of the supernatant was transferred to a 96-well plate, and the absorbance at OD260 was recorded using a microplate reader. The control group was set up with only methanol but without the compound. The phosphate-buffered saline (PBS) buffer solution and MH broth were used for blank correction, and the determination was performed in triplicate.
2.7. Determination of the content of protein
The supernatant was obtained according to the method of determination of nucleic acid, and the content of protein was determined by the method by Chen et al. (2020). For the bicinchoninic acid (BCA) determination, the working solution and diluted BSA standard solution were prepared according to the instructions suggested by the assay kit used (Solarbio, China). The standard curve was drawn based on the value of OD562 with the enzyme-labeled instrument. All the measurements were repeated 3 times in parallel and the protein concentration was calculated by the standard curve. The control group was treated in the same manner as the compound group except for the addition of the compound. Both the compound group and the control group were subjected to the same experimental conditions to ensure any observed effects, due to the presence or absence of the compound, were solely being tested.
2.8. Determination of content of AKP
The content of AKP was determined by the modified method (He et al., 2018). A compound background group was set to deduct the interference of the compound itself in the experiment. The supernatant was obtained by the same method followed in the determination of nucleic acid and measured at optical density (OD520) on the microplate reader according to the instructions of the kit. The measurements were repeated 3 times in parallel and then the AKP in the liquid was calculated according to the following formula:
Definition: A volume of 100 ml liquid reacts with the matrix for 15 min to produce 1 mg of phenol at 37°C, which is defined as 1 Guinness unit.
2.9. Statistical analysis
The Microsoft Excel 2019 and SPSS 17 software were used for the statistical analysis of the data. The results were expressed in [± standard deviation (SD)], and p < 0.05 was statistically significant.
2.10. Synthesis of 2-hydroxy-6-methoxybenzaldehyde (compound 2)
To a stirred mixture of 2,6-dihydroxybenzaldehyde (690.6 mg, 5 mmol, 1.0 equiv.), and K2CO3 (2.764 g, 20 mmol, 4.0 equiv.) in tetrahydrofuran (THF) (30 ml), CH3I (2.280 g, 1 ml, 15 mmol, 2 equiv.) was added slowly dropwise at room temperature. When the 2,6-dihydroxybenzaldehyde was consumed completely, which was detected by thin-layer chromatography (TLC) (petroleum ether: ethyl acetate in the ratio of 15:1, v/v), the resulting mixture was quenched with saturated aqueous NH4Cl (5 ml). Reaction conditions, including reaction temperature, reaction time, and solvents, are presented in Table 1. Then, the mixture was filtered, and extracted with ethyl acetate (5 ml × 3). The combined organic phases were dried over anhydrous Na2SO4, filtered, and concentrated under reduced pressure. The residue was purified by column chromatography on silica gel with petroleum ether: ethyl acetate in the ratio of 30:1 (v/v) to afford 538.6 mg white solid of compound 2 in 78% yield. 1H NMR (400 MHz, CDCl3) (Supplementary Figure 1): δ 11.85 (s, 1H), 10.31 (s, 1H), 7.38 (t, J = 8.4 Hz, 1H), 6.49 (d, 1H), and 6.36 (d, J = 8.3, 0.9 Hz, 1H), 3.86 (s, 3H).
2.11. Synthesis of 1 (2-hydroxy, 6-methoxyphenyl) butanol (compound 3)
Under the argon atmosphere, in an oven-dried 100 ml two-neck flask equipped with a magnetic stir bar, 2-hydroxy, 6-methoxybenzaldehyde (634 mg, 3.83 mmol) and anhydrous tetrahydrofuran (5 ml) were added. The reaction mixture was cooled to 0°C with ice–water bath. Then an 8 ml propylmagnesium bromide (8.0142 g, 7.66 mmol) was added into the reaction mixture dropwise and stirred at 0°C for further 6 h. The mixture was quenched with saturated NH4Cl solution. Reaction conditions, including reaction temperature, reaction time, and solvents, are presented in Table 2. Then, the resulting mixture was extracted with ethyl acetate (5 ml × 3). The combined organic phases were dried over anhydrous Na2SO4, filtered, and concentrated under reduced pressure. The residue was purified by column chromatography using petroleum ether: ethyl acetate in the ratio of 10:1 (v/v) as eluent to afford 443.8 mg light yellow oil in 70% yield. 1H NMR (400 MHz, CDCl3) (Supplementary Figure 2): δ 8.72 (s, 1H), 7.08 (t, J = 8.2 Hz, 1H), 6.49 (d, J = 8.2 Hz, 1H), 6.38 (d, J = 8.2 Hz, 1H), 5.33 (td, J = 5.8, 5.3, 2.8 Hz, 1H), 3.73 (s, 3H), 2.91 (s,1H), 2.33—2.31 (m, 2H), 1.69 (m, 2H), and 0.93 (t, J = 7.4 Hz, 3H). 13C NMR (100 MHz, CDCl3) (Supplementary Figure 3): δ 156.97, 156.63, 128.70, 116.07, 110.21, 102.13, 70.14, 55.59, 38.60, 18.87, 13.98.
2.12. Synthesis of 1-(2-hydroxy-6-methoxyphenyl) butanone (compound 4)
In an oven-dried 100 ml round-bottomed flask equipped with a magnetic stir bar, 1-(2-hydroxy-6-methoxyphenyl)butanol (69 mg, 0.35 mmol) was dissolved in anhydrous dichloromethane (10.0 ml). Then Pyridine and CrO3 complex salt (PCC) (in hydrochloric acid solution) (75.5 mg, 0.35 mmol) was added, and the mixture was stirred at room temperature for 2 h. After the transformation was completed, the reaction mixture was filtered and concentrated under reduced pressure. Purification of the residue through column chromatography on silica gel using a gradient mixture of petroleum ether: ethyl acetate (in the ratio ranging from 80:1 to 50:1, v/v) and afforded 20.7 mg white solid 1-(2-hydroxy, 6-methoxyphenyl) butanone in 30% yield. 1H NMR (400 MHz, CDCl3) (Supplementary Figure 4): δ 13.30 (s, 1H), 7.33 (t, J = 8.3 Hz, 1H), 6.57 (d, J = 8.9 Hz, 1H), 6.39 (d, J = 8.3 Hz, 1H), 3.90 (s, 3H), 3.03 (t, J = 7.3 Hz, 2H), 1.71 (m, J = 7.4 Hz, 2H), and 0.99 (t, J = 7.4 Hz, 3H). Reaction conditions, including reaction temperature, reaction time, and solvents, are presented in Table 3.
2.13. Synthesis of 1-(2,6-dihydroxyphenyl) butane-1-one (compound 5)
In an oven-dried 100 ml two-necked flask equipped with a magnetic stir bar, 1 (2-hydroxy6-methoxyphenyl)butanone (40 mg, 0.2 mmol, 1.0 equiv.) was dissolved in dry dichloromethane (2.0 ml) under an argon atmosphere. The solution was cooled to 0°C with an ice–water bath and BBr3 (50 μl, 0.6 mmol, 3 equiv.) was dropwise added into the solution. Then, the reaction mixture was stirred for 10 h at the same temperature. After that, the reaction solution was warmed to room temperature, diluted with dichloromethane (10 ml), and poured into ice water. The resulting mixture was extracted with dichloromethane (5 ml × 3). The combined organic phases were dried over anhydrous Na2SO4, filtered, and concentrated under reduced pressure. The residue was purified by column chromatography on silica gel using petroleum ether: ethyl acetate in the ratio of 10:1 (v/v) as eluent to afford 20.5 mg white solid of 1-(2,6-dihydroxyphenyl)butane-1-one in 51% yield. 1H NMR (400 MHz, CDCl3) (Supplementary Figure 5): δ 9.82 (s, 2H), 7.22 (t, J = 8.2 Hz, 1H), 6.40 (d, J = 8.1 Hz, 2H), 3.13 (t, J = 14.6 Hz, 2H), 1.75 (m, 2H), and 1.01 (t, J = 7.4 Hz, 3H). 13C NMR (100 MHz, CDCl3) (Supplementary Figure 6): δ 209.24, 160.45, 129.39, 112.02, 110.50, 105.90, 59.90, 45.87, 18.27, 14.37. Reaction conditions, including reaction temperature, reaction time, and solvents, are presented in Table 4.
3. Results
3.1. The inhibitory activity on pathogenic strains of compound 5
The crude ethyl acetate extract of D. childiae showed strong activity on the tested pathogenic strains. The 19 secondary metabolites isolated from endolichenic fungus were further investigated for antimicrobial activity. However, in this study, only compound 5, namely, 1-(2,6-dihydroxyphenyl) butane-1-one exhibited the antibacterial activity. Moreover, the compound showed the broad-spectrum antimicrobial activity.
Compound 5 demonstrated inhibitory effects on 10 out of the 15 tested strains including Gram-positive bacteria, Gram-negative bacteria, and fungus. As shown in Figure 1, compound 5 shows strong inhibition activity against fungus C. albicans 10213 with an inhibition zone of more than 16.5 mm (Figure 1A). The inhibitory effects are moderate on S. aureus 6538, M. luteus 261, B. subtilis 163, and P. vulgaris Z12 with the inhibition zone of 11, 13, 13, and 13 mm, respectively, (Figures 1B–E) but the weak effect on S. sonnet, Brucella sp. B103, E. aerogenes, P. mirabilis, and S. typhimurium CMCC 50071 with an inhibition zone of 7.0–10.5 mm (Figures 1F–J).
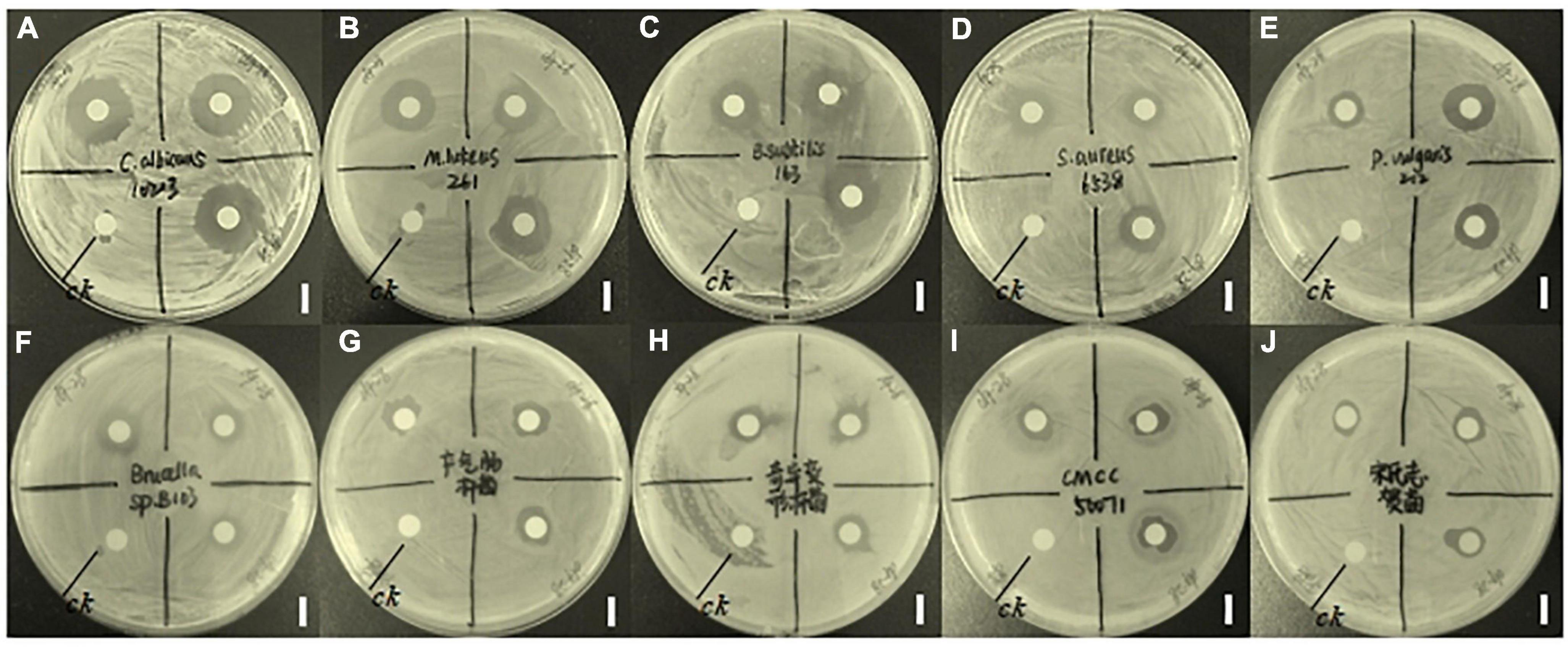
Figure 1. Inhibitory effects of compound 5 on 10 tested strains. The CK represents a blank control and the bar scale is 9 mm. Panel (A) represents the inhibitory effect of compound 5 on C. albicans 10213. Panel (B) represents the inhibitory effect of compound 5 on M. luteus 261. Panel (C) represents the inhibitory effects of compound 5 on B. subtilis 163. Panel (D) represents the inhibitory effect of compound 5 on S. aureus 6538. Panel (E) represents the inhibitory effect of compound 5 on P. vulgaris Z12. Panel (F) represents the inhibitory effect of compound 5 on Brucella sp. B103. Panel (G) represents the inhibitory effect of compound 5 on E. aerogenes. Panel (H) represents the inhibitory effect of compound 5 on P. mirabilis. Panel (I) represents the inhibitory effect of compound 5 on S. typhimurium CMCC 50071. Panel (J) represents the inhibitory effect of compound 5 on S. sonnet.
3.2. MIC and MBC of compound 5 in vitro conditions
The minimum inhibitory concentration (MIC) and minimum bactericidal concentration (MBC) in vitro conditions were further measured to evaluate the antibacterial activities of compound 5. It was found that MIC of C. albicans 10213, M. luteus 261, P. vulgaris Z12, S. sonnet, S. aureus 6538, Brucella sp. B103, B. subtilis 163 were 16, 16, 16, 16, 64, 64, and 64 μg/ml, respectively. Furthermore, MBC of C. albicans 10213, M. luteus 261, P. vulgaris Z12, S. sonnet, S. aureus 6538, Brucella sp. B103, B. subtilis 163 are 64, 128, 64, 64, 64, 64, 128 μg/ml, respectively, as shown in Figure 2.
3.3. The growth curve of three strain
In this study, to study the antimicrobial mechanism of compound 5 in this experiment, three strains of Gram-positive bacteria S. aureus 6538, Gram-negative bacteria P. vulgaris Z12, and fungus C. albicans 10213 were selected. The growth curves of S. aureus 6538, P. vulgaris Z12, and C. albicans 10213 have been determined as shown in Figures 3A–C. It was found that under normal conditions, the three tested strains entered the logarithmic growth period after 10 h of culture, and the OD600 increased exponentially and became stable after 20 h. However, after the addition of compound 5 with MBC concentration, the OD600 did not change significantly and tended to increase by “0,” indicating that compound 5 could obviously inhibit the growth of the three pathogenic bacteria.
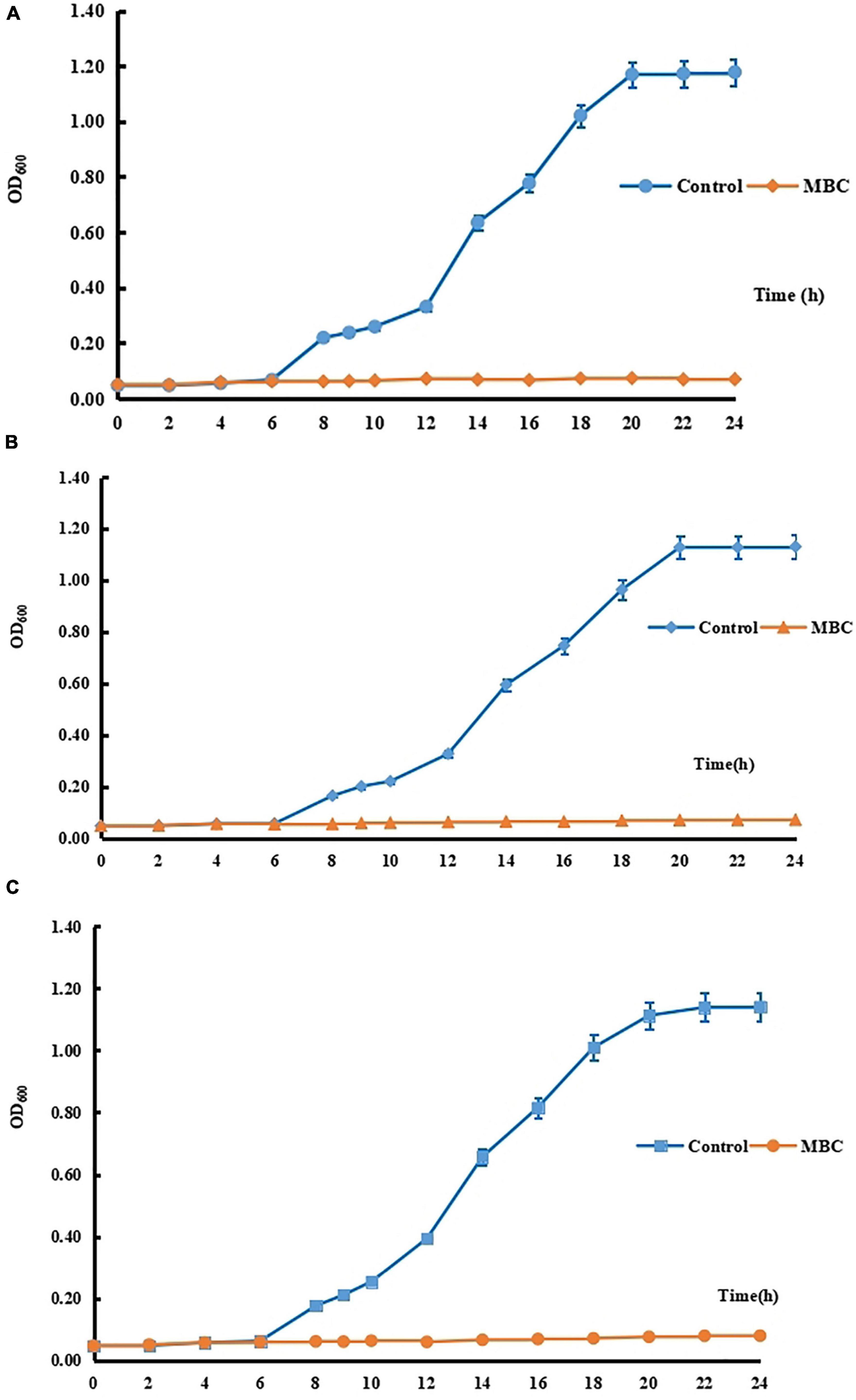
Figure 3. (A) The growth curve of C. albicans 10213. (B) The growth curve of P. vulgaris Z12. (C) The growth curve of S. aureus 6538.
3.4. The content of nucleic acid
When the cell wall or cell membrane of microbes is damaged, the nucleic acid will permeate to the outside of the cell, leading to a higher content of nucleic acid. The effects of compound 5 with a concentration equal to the MBC on the permeability of the cell wall and cell membrane of S. aureus 6538, P. vulgaris Z12, and C. albicans 10213 were explored. Compared to the control group, the contents of nucleic acid in the test groups adding compound 5 are higher, as shown in Figure 4 and Table 5, which was statistically significant (p < 0.05). Compound 5 was effective at inhibiting the growth of all three strains, with a concentration of MBC. The results suggested that the ability of compound 5 to inhibit the growth of tested strains may be due to its ability to cause damage to the cell membrane and cell wall. This damage led to an increase in the permeability of the three tested strains, which in turn caused more nucleic acid to leak out of the cells.
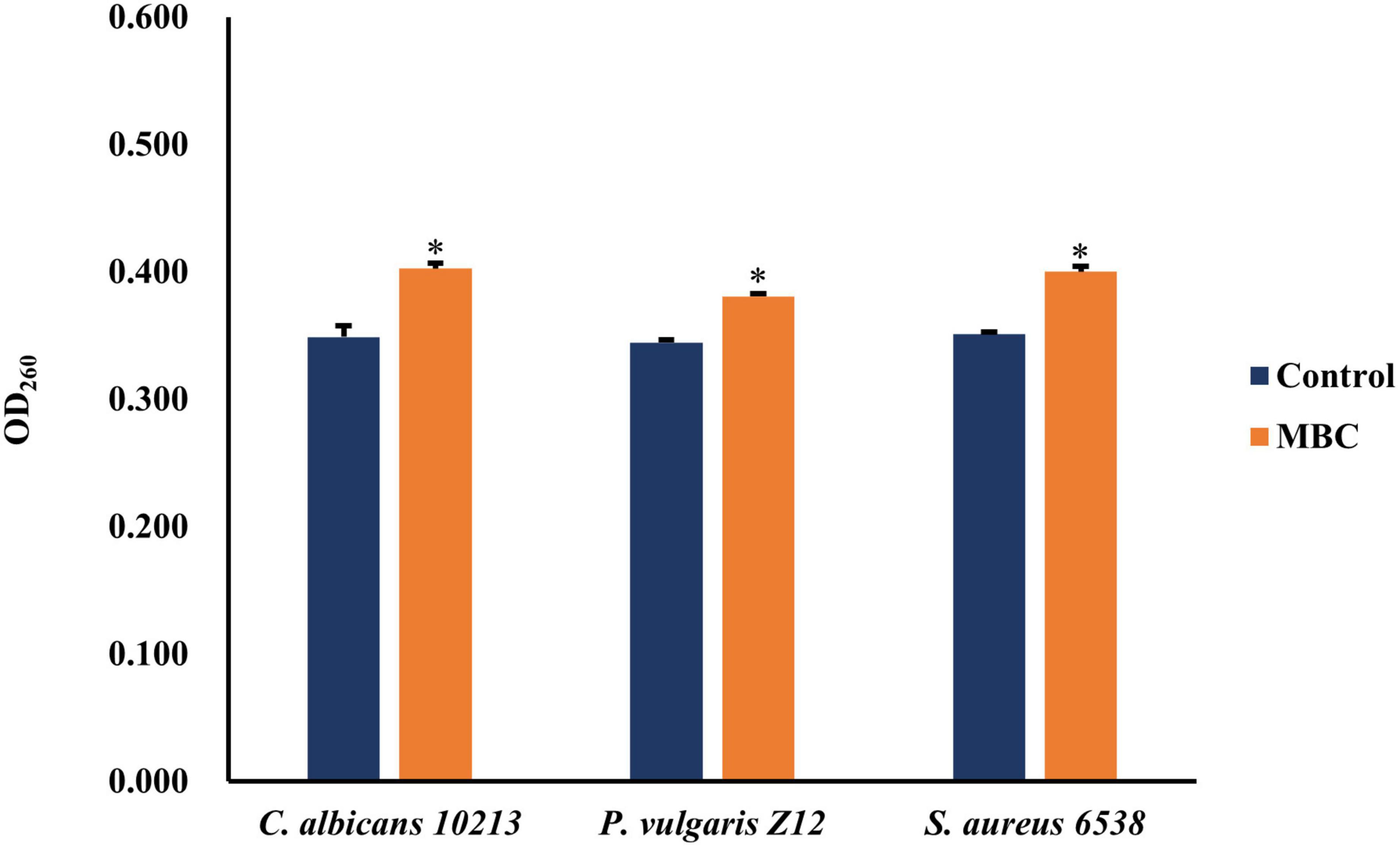
Figure 4. The effect of compound 5 on leakage of nucleic acid from tested strains. *Indicates a significant difference compared to the control group.
3.5. Determination of the content of protein
3.5.1. The standard curve of bovine albumin (BSA)
A series of concentrations of BSA ranging from 0.05 mg/ml to 0.50 mg/ml was used as the ordinate, and the standard curve of standard BSA was formed with the corresponding OD562 value as the abscissa. There is a linear correlation between the concentration and the absorbance as depicted in Figure 5, as evidenced by the linear regression equation of y = 1.734x—0.003, with R2 = 0.990.
The content of protein in pathogenic strains was determined by the BCA method. In alkaline conditions, the Cu2+ will be reduced to Cu+ by protein. The Cu+ and BCA reagent formed a purple complex, with two molecular BCA chelating one Cu+. The content of protein measured could be calculated by the standard curve of BSA. After adding compound 5 of MBC concentration, the OD value of S. aureus 6538, P. vulgaris Z12, and C. albicans 10213 increases as shown in Figure 6 and Table 6. The OD value of the three test strains at 562 nm was higher than that of the control group (p < 0.05), which was statistically significant. Compared to the control group, the content of protein in the test groups increased, indicating that the permeability of the cell membrane and cell wall of the three strains may have been damaged by compound 5, leading to protein leakage and increased content. These results were consistent with the influence on the nucleic acid.
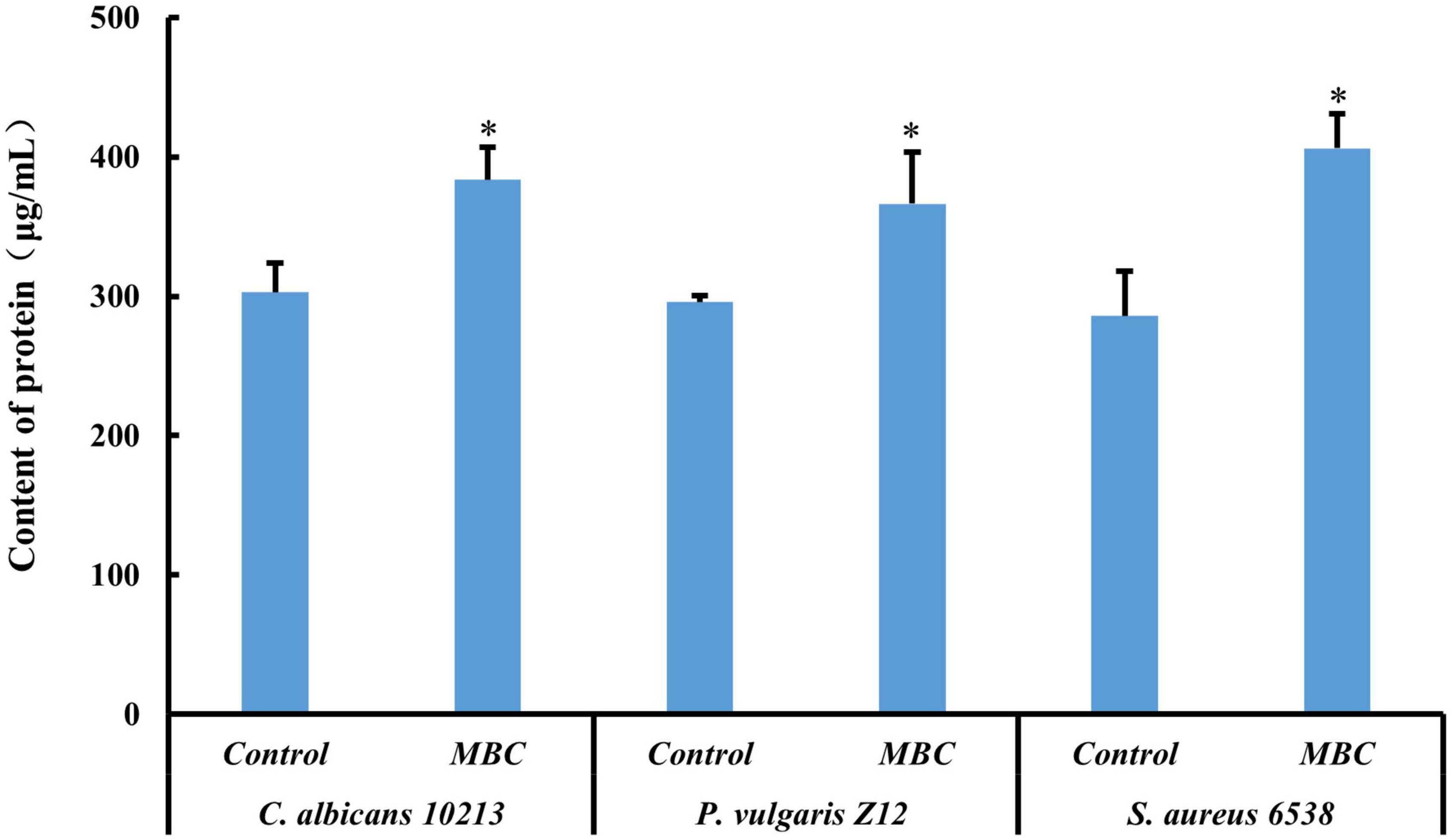
Figure 6. Effect of compound 5 on leakage of protein from the tested strains. *Indicates a significant difference compared to the control group.
3.6. Determination of content of AKP
Alkaline phosphatase is a glycoprotein containing zinc. In an alkaline environment, AKP catalyzes the conversion of disodium diphenyl phosphate to free phenols, which is then oxidized with 4-aminoantipyrine by potassium ferricyanide to form red quinone derivatives with a characteristic light absorption at 520 nm. As shown in Table 7, after adding compound 5 with MBC concentration, the OD value of the three kinds of tested strains, Gram-positive bacteria S. aureus 6538, Gram-negative bacteria P. vulgaris Z12 and fungus C. albicans 10213 at 520 nm is found to be higher than that of the control group (p < 0.05), which is statistically significant. Combined with the calculation formula, as shown in Figure 7, the AKP activity of the MBC group is significantly stronger than that of the control group, indicating that under MBC concentration, compound 5 may have damaged the cell walls and cell membranes of the three tested strains, leading to the dissolution of AKP and the enhancement of its activity.
3.7. Synthesis of 2-hydroxy-6-methoxybenzaldehyde
The substrate 2,6-dihydroxybenzaldehyde and K2CO3 reacted for 3 and 6 h, respectively, at room temperature, 68 and 80°C in THF solvent. The highest yield of 2-hydroxy, 6-methoxybenzaldehyde was 78% at room temperature for 6 h.
Treatment of 2,6-dihydroxybenzaldehyde with CH3I in the presence of K2CO3 at room temperature in THF provided the desired product 2-hydroxy-6-methoxybenzaldehyde in 78% yield.
3.8. Synthesis of 1-(2-hydroxy -6-methoxyphenyl) butanol
The nucleophilic addition reaction of 2-hydroxy- 6-methoxybenzaldehyde with Grignard reagent propylmagnesium bromide produced the desired product 1-(2-hydroxy-6-methoxyphenyl) butanol in 70% yield under the optimal reaction conditions (compound 3).
3.9. Synthesis of 1-(2-hydroxy-6-methoxyphenyl) butanone
The variation of reaction conditions for secondary alcohol oxidation was carried out using different oxidants, such as PCC, IBX (2-iodoxybenzoic acid), and DMP (1,1,1-triacetoxy-1,1-dihydro-1,2-benziodoxol-3 (1H)-One). Among these screened oxidants, PPC was found to be the best for the reaction.
3.10. Synthesis of 1-(2,6-dihydroxyphenyl) butane-1-one
The methoxy group at C6 on the benzene ring of compound 4 was catalyzed into the hydroxyl group by oxidant BBr3 through nucleophilic substitution reaction to generate the target compound 5. At room temperature, the yield was higher than −20 and 0°C which reached 51%.
Deprotection of the methyl with BBr3 in anhydrous CH2Cl2 at room temperature afforded the desired product 5 in a yield of 51%.
4. Discussion
In recent years, due to the irrational use of antibiotics, the phenomenon of antibiotic resistance in various bacteria is consistently increasing and has become increasingly serious to human health, so it is important to explore new antibacterial active ingredients. Mycobionts not only coexist with photobionts but also with a large number of endophytes, which are considered as the hidden microbial resources. Due to the special habitat, endolichenic fungi could produce many secondary metabolites with novel structures or with significant activities, which is of great significance to provide a basis for exploring potential active compounds. Nineteen compounds were isolated from an endophytic fungus with antimicrobial activity, and only compound 5 showed strong and broad-spectrum antimicrobial activity.
According to the literature (Shen et al., 2015; Luo et al., 2019), if the cell membrane and cell wall of the test bacteria are damaged, it will lead to the leakage of nucleic acid, protein, and other substances, which will inhibit the growth of the test bacteria, which cause even death. In our study, compound 5 at MBC concentration could significantly inhibit the growth of S. aureus 6538, P. vulgaris Z12, and fungus C. albicans 10213. The increased dissolution of nucleic acid and protein, along with the enhancement of AKP activity, indicated that compound 5 inhibited the pathogenic microbes by affecting the permeability of the cell membrane and cell wall. These findings suggest that compound 5 may have the potential as an antimicrobial agent that can target a broad range of microorganisms.
Many natural products are highly favored due to their good biological activity, but the low content limits their further application. The chemical synthesis of natural products provided an alternative method. In this study, the target compound has been successfully synthesized through four steps of a chemical reaction. Oxidants PCC, 2-Iodoxybenzoic acid (IBX), and Dess Martin were screened in the synthesis of 1-(2-hydroxy, 6-methoxyphenyl) butanone (compound 4). PCC is a mild and selective oxidant. However, as the reaction proceeded, dark brown oily residues were generated that could wrap the products and led to a reduction in the yield of compound 4. IBX is another mild, highly selective, environmentally friendly, widely used, and selective oxidant but has a better solubility in dimethyl sulfoxide (DMSO). However, the boiling point of the solvent DMSO is higher while 1-(2, hydroxy, 6-methoxyphenyl) butanol is a reactant with a low boiling point. Moreover, the reaction needed to be carried out at room temperature which led to the low solubility of IBX in DMSO and low yield of compound 4 as the oxidant. Besides, Dess Martin could also be an efficient oxidant to oxidize 1-(2,hydroxy,6-methoxyphenyl) butanol into compound 3 at room temperature (Dess Martin, which is an oxidant, can oxidize primary alcohols to aldehydes). However, this oxidant is hydrophobic and needs to be added drop by drop at low temperatures with troublesome post-treatment that led to poor products. Herein, PCC was selected in the present study. In the final synthesis of the target product, BBr3 is used for demethylation reaction. One of the biggest disadvantages of BBr3 is that it is overly sensitive to air. Moreover, a large number of aerosols with pungent smells will affect the rate of aerosol deposition, leading to an incomplete reaction. Additionally, the pH of the extraction also had an impact on the yield of the products.
5. Conclusion
Compound 5, a secondary metabolite with broad-spectrum antimicrobial activity isolated from the endolichenic fungus from Guizhou province, showed inhibitory effects on 10 of the 15 tested strains, including Gram-positive bacteria, Gram-negative bacteria, and fungus. The MICs of compound 5 for C. albicans 10213, M. luteus 261, P. vulgaris Z12, S. sonnet, and S. aureus 6538 was 16 μg/ml, while the MBCs of C. albicans 10213, P. vulgaris Z12, S. aureus 6538, and Brucella sp. B103 were 64 μg/ml. Compound 5 could obviously inhibit the growth of S. aureus 6538, Proteus sp., and C. albicans 10213 at the concentration of MBC, likely by affecting the permeability of the cell wall and membrane. The results enriched the library of active strains and metabolites resources of endolichenic fungi. Moreover, the exploration of the chemical synthesis of active compounds was also achieved by four steps for the first time, providing new alternative methods for the excavation of antimicrobial agents.
Data availability statement
The datasets presented in this study can be found in online repositories. The names of the repository/repositories and accession number(s) can be found in the article/Supplementary material.
Author contributions
S-BF designed the experiment, supervised the study, and corrected the manuscript. XZ and M-YW performed the experiments. XZ, Q-FM, Q-PC, ZY, and S-BF analyzed the data. Q-FM and S-BF wrote the manuscript. All authors contributed to the article and approved the submitted version.
Funding
This study was funded by the Project of Science and the Technology Support of Guizhou Province [QKHZC(2021)249], the Project of Science and Technology of Zunyi [HZ(2021)189], and College Students’ the Innovation and Entrepreneurship Project (S202110661023 and ZYD2020013).
Conflict of interest
The authors declare that the research was conducted in the absence of any commercial or financial relationships that could be construed as a potential conflict of interest.
Publisher’s note
All claims expressed in this article are solely those of the authors and do not necessarily represent those of their affiliated organizations, or those of the publisher, the editors and the reviewers. Any product that may be evaluated in this article, or claim that may be made by its manufacturer, is not guaranteed or endorsed by the publisher.
Supplementary material
The Supplementary Material for this article can be found online at: https://www.frontiersin.org/articles/10.3389/fmicb.2023.1168386/full#supplementary-material
References
Anderson, V. M., Wendt, K. L., Caughron, J. B., Matlock, H. P., Rangu, N., Najar, F. Z., et al. (2022). Assessing microbial metabolic and biological diversity to natural product library assembly. J. Nat. Prod. 85, 1079–1088. doi: 10.1021/acs.jnatprod.1c01197
Chen, J. L., Tang, C. L., Zhang, R. F., Ye, S. X., Zhao, Z. M., Huang, Y. Q., et al. (2020). Metabolomics analysis to evaluate the antibacterial activity of the essential oil from the leaves of Cinnamomum camphora (Linn.) Presl. J. Ethnopharmaco. 253:112652. doi: 10.1016/jjep.2020.112652
Clarke, K., Roy, P., Facey, P., Petrea, F., Trevor, Y., and Camille, T. R. (2020). Chemical composition and biological activities of Pimenta richardii. Flavour Frag J. 36, 272–279. doi: 10.1002/ffj.3642
He, N., Juan, Z., Miyun, H., Mang, C. Y., and Kang, W. Y. (2018). The mechanism of antibacterial activity of corylifolinin against three clinical bacteria from Psoralen corylifolia L. Open Chem. 16, 882–889. doi: 10.1515/chem-2018-0091
Lin, L. B., Gao, Y. Q., Han, R., Xiao, J., Wang, Y. M., Zhang, Q., et al. (2021). Alkylated salicylaldehydes and prenylated indole alkaloids from the endolichenic fungus Aspergillus chevalieri and their bioactivities. J. Agric. Food Chem. 69, 6524–6534. doi: 10.1021/acs.jafc.1c01148
Liu, J. X., Li, Y., Lian, L. F., and Zhang, W. P. (2015). Study on the in vitro antibacterial activity of n-butanol extract from the root of Euphorbia odorifera. Lishizhen Med. Mater. Med. Res. 26, 1849–1850.
Luo, M. A., Chang, S. S., Li, Y. H., Xi, X. M., Chen, M. H., He, N., et al. (2022). Molecular networking-based screening led to the discovery of a cyclic heptadepsipeptide from an endolichenic Xylaria sp. J. Nat. Prod. 85, 972–979. doi: 10.1021/acs.jnatprod.1c01108
Luo, Z. P., Pan, L. W., and Li, L. (2019). Antimicrobial activity of extracts from Clerodendrum japonicum and its antibacterial mechanism on Staphylococcus aureus. J. Southern Agric. 50, 2778–2786. doi: 10.3969/j.issn.2095-1191.2019.12.21
Maliehe, T. S., Mbambo, M., Nqotheni, M. I., Senzo, N. S., and Shandu, E. J. S. (2022). Antibacterial effect and mode of action of secondary metabolites from fungal endophyte associated with Aloe ferox Mill. Microbiol. Res. 13, 90–101. doi: 10.3390/microbiolres13010007
Nursyam, H. (2017). Antibacterial activity of metabolites products of vibrio alginolyticus isolated from sponge Haliclona sp. against Staphylococcus aureus. Ital. J. Food Saf. 6:6237. doi: 10.4081/ijfs.2017.6237
Rao, C., Yun, X., Yu, Y., and Deng, Z. X. (2020). Recent progress of synthetic biology applications in microbial pharmaceuticals research. Syn. Bio. J. 1, 92–102.
Seukepa, A. J., Nembua, N. E., Mbuntchab, H. G., and Kueteb, V. (2022). Bacterial drug resistance towards natural products. Adv. Bot. Res. 106, 21–45. doi: 10.1016/bs.abr.2022.08.002
Shen, S. X., Zhang, T. H., Yuan, Y. Y., Lin, S. Y., Xu, L. Y., and Ye, H. Q. (2015). Effects of cinnamaldehyde on Escherichia coli and Staphylococcus aureus membrane. Food Control. 47, 196–202. doi: 10.1016/j.foodcont.2014.07.003
Shevkar, C., Pradhan, P., Armarkar, A., Pandey, K., Kalia, K., Paranagama, P., et al. (2022). Exploration of potent cytotoxic molecules from fungi in recent past to discover plausible anticancer scaffolds. Chem. Biodivers. 19, 1–17. doi: 10.1002/cbdv.202100976
Shinu, P., Mouslem, A. K., Nair, A. B., Venugopala, K. N., Attimarad, M., Singh, V. A., et al. (2022). Progress report: Antimicrobial drug discovery in the resistance era. Pharmaceuticals 15:413. doi: 10.3390/ph15040413
Wu, Y. P., Bai, J. R., Zhong, K., Huang, Y. A., and Gao, P. (2017). A dual antibacterial mechanism involved in membrane disruption and DNA binding of 2R, 3R-dihydromyricetin from pine needles of Cedrus deodara against Staphylococcus aureus. Food Chem. 218, 463–470. doi: 10.1016/j.foodchem.2016.07.090
Zhang, C. H., Zhao, M. M., Zou, H. J., Zou, H. J., Zhang, X. M., Sheng, R. T., et al. (2020). An enhanced antibacterial nanoflowers AgPW@PDA@Nisin constructed from polyoxometalate and nisin. J. Inorg. Biochem. 212:111212. doi: 10.1016/j.jinorgbio.2020.111212
Zhao, L., Kim, J. C., and Hur, J. S. (2022). 7-Hydroxy-2-octenoic acid-ethyl ester mixture as an UV protectant secondary metabolite of an endolichenic fungus isolated from Menegazzia terebrata. Arch. Microbiol. 204:395. doi: 10.1007/s00203-022-02997-5
Zhou, X., Yang, C. L., Meng, Q. F., Liu, L., and Fu, S. B. (2020). A new alkanol from the endolichenic fungus Daldinia childiae. J. Chin. Chem. Soc. 68, 678–681. doi: 10.1002/jccs.202000274
Zhou, X., Yang, C. L., Meng, Q. F., Liu, L., and Fu, S. B. (2021a). An endolichenic fungus inhibiting clinical pathogenic bacteria. Mycosystema 40, 87–94. doi: 10.13346/j.mycosystema.200059
Keywords: natural product, endolichenic fungus, anti-microorganism activity, mechanism, chemical synthesis
Citation: Zhou X, Wang M-Y, Cao Q-P, Yang Z, Meng Q-F and Fu S-B (2023) Chemical synthesis and mechanism of a natural product from endolichenic fungus with a broad-spectrum anti microorganism activity. Front. Microbiol. 14:1168386. doi: 10.3389/fmicb.2023.1168386
Received: 17 February 2023; Accepted: 28 March 2023;
Published: 05 May 2023.
Edited by:
Peng Zhang, Chinese Academy of Agricultural Sciences, ChinaReviewed by:
Da-Le Guo, Chengdu University of Traditional Chinese Medicine, ChinaChenyang Huang, Chinese Academy of Agricultural Sciences, China
Copyright © 2023 Zhou, Wang, Cao, Yang, Meng and Fu. This is an open-access article distributed under the terms of the Creative Commons Attribution License (CC BY). The use, distribution or reproduction in other forums is permitted, provided the original author(s) and the copyright owner(s) are credited and that the original publication in this journal is cited, in accordance with accepted academic practice. No use, distribution or reproduction is permitted which does not comply with these terms.
*Correspondence: Shao-Bin Fu, c2JmdUB6bXUuZWR1LmNu; Qing-Feng Meng, cWZtZW5nQDEyNi5jb20=
†These authors have contributed equally to this work