- 1College of Agronomy, Guizhou University, Guiyang, China
- 2Science and Technology Innovation Development Center of Bijie City, Bijie, China
- 3Institute of Crop Science, Chinese Academy of Agriculture Science, Beijing, China
- 4School of Agriculture and Biology, Shanghai Jiao Tong University, Shanghai, China
Trichoderma is mainly used to control soil-borne diseases as well as some leaf and panicle diseases of various plants. Trichoderma can not only prevent diseases but also promotes plant growth, improves nutrient utilization efficiency, enhances plant resistance, and improves agrochemical pollution environment. Trichoderma spp. also behaves as a safe, low-cost, effective, eco-friendly biocontrol agent for different crop species. In this study, we introduced the biological control mechanism of Trichoderma in plant fungal and nematode disease, including competition, antibiosis, antagonism, and mycoparasitism, as well as the mechanism of promoting plant growth and inducing plant systemic resistance between Trichoderma and plants, and expounded on the application and control effects of Trichoderma in the control of various plant fungal and nematode diseases. From an applicative point of view, establishing a diversified application technology for Trichoderma is an important development direction for its role in the sustainable development of agriculture.
Introduction
In the traditional crop cultivation process, the excessive use of pesticides and chemical fertilizers, as well as the long-term large-scale planting of a single crop, has led to the destruction of the farmland ecological environment, plant diseases, insect pest problems, crop pesticide residues, and soil and water environment pollution (Bardin et al., 2015). With green agricultural development, people are urgently seeking safe, effective, and environmentally friendly plant disease control measures. Biological control is mainly used to control harmful organisms in plants through beneficial organisms and their products to control plant diseases and effectively reduce the application of chemical fertilizers and pesticides (Harman et al., 2021). Trichoderma, a biological fungus widely used for plant pest control, mainly exists in the soil, air, plant surface, and other ecological environments and can effectively control a variety of plant diseases (Haouhach et al., 2020; Zheng et al., 2021; Wang R. et al., 2022). Trichoderma is mainly used to control soil-borne diseases in various plants and some leaf and spike diseases (Samuels et al., 2006; Vicente et al., 2020; Abbas et al., 2022). Trichoderma can prevent disease, promote plant growth, improve nutrient utilization efficiency, enhance plant resistance, and repair agrochemical pollution (Tilocca et al., 2020; Fontana et al., 2021; Sánchez-Montesinos et al., 2021; Al-Surhanee, 2022; Tyśkiewicz et al., 2022).
Trichoderma belonging to Eumycota, Deuteromycotina, Hyphomycetes, Hyphomycetales, and Moniliaceae (Kubicek et al., 2019). Its sexual stage includes the Ascomycota, Sordariomycetes, Hypocreales, Hypocreaceae, and Trichoderma ssp. (Sun et al., 2012). There are more than 370 Trichoderma spp. including T. harzianum, T. viride, T. asperellum, T. hamatum, T. atroviride, T. koningii, T. longibrachiatum, and T. aureoviride (Sánchez-Montesinos et al., 2021; Sun et al., 2022). Trichoderma has been used in biological control research, including T. harzianum, T. hamatum, T. longibrachiatum, T. koningii, T. viride, T. polysporum, and T. asperellum (Di Marco et al., 2022). Many studies have shown that most Trichoderma spp. can produce bioactive substances and have antagonistic effects on plant-pathogenic fungi and plant-pathogenic nematodes (Druzhinina et al., 2018). These bioactive substances, including secondary metabolites and cell wall-degrading enzymes, can effectively improve crop resistance, reduce plant diseases, and promote plant growth (Kubicek et al., 2019). Professor Harman of Cornell University isolated and purified T. harzianum T22 strain and systematically studied its application in biological control of plant pests and commercial development of biological control technology (Harman, 2000). This study systematically and comprehensively elaborated on the research progress on Trichoderma spp. and its role in plant disease control, its application as a biological control and its mechanism, as well as preliminarily discussed the problems and prospects of Trichoderma as a biological control agent, providing a reference for future research and application.
Application and mechanism of action of Trichoderma in plant fungal disease control
Application of Trichoderma in biological control of plant fungal diseases
Trichoderma is a biocontrol fungus widely distributed worldwide. Trichoderma has a huge application value and potential in the field of biological control of plant diseases (Tyśkiewicz et al., 2022). Research on the use of Trichoderma to control plant diseases has been reported worldwide. T. viride and T. harzianum have different degrees of inhibitory effects on 29 species of plant pathogenic fungi belonging to 18 genera, including Botrytis, Fusarium, and Rhizoctonia. Trichoderma has control effects on a variety of plant pathogenic fungi, such as Rhizoctonia solani, Pythium ultimum, Fusarium oxysporum, Sclerotinia sclerotiorum, Botrytis cinerea, Pseudocercospora spp. and Colletotrichum spp. (Tian et al., 2016, 2018; Saravanakumar et al., 2017; Debbi et al., 2018; Li et al., 2018; Bubici et al., 2019; Filizola et al., 2019; Herrera-Téllez et al., 2019; Álvarez-García et al., 2020; Andrade-Hoyos et al., 2020; Carro-Huerga et al., 2020; Damodaran et al., 2020; Zhang et al., 2020, 2021; Al-Askar et al., 2021; Chen et al., 2021; Degani and Dor, 2021; Dugassa et al., 2021; Intana et al., 2021; Zhang C. et al., 2022; Zhang Y. et al., 2022). Trichoderma has been widely used for the biological control of cotton verticillium wilt, crop gray mold, tomato gray mold, melon wilt, potato dry rot, tobacco root rot, and other plant diseases (Rashmi et al., 2016; Andrade-Hoyos et al., 2020; Alfiky and Weisskopf, 2021; Lazazzara et al., 2021; Leal et al., 2021; Manganiello et al., 2021; Degani et al., 2021a; Pollard-Flamand et al., 2022; Rees et al., 2022; Risoli et al., 2022). T. longibrachiatum T6 biocontrol agent has a good control effect on pepper damping off and can effectively control the spread of pepper disease (Girma, 2022). The control effect was up to 54.8%, which is 12.5% higher than that of the chemical pesticide carbendazim (Yuan et al., 2019; Al-Askar et al., 2022). T. harzianum has a good control effect on pepper and potato Phytophthora blight. It can inhibit the growth of Phytophthora blight in soil, reduce the number of pathogenic fungi, and effectively reduce the rate of dead seedlings and disease index of plants (Guzmán-Guzmán et al., 2017; Kappel et al., 2020; Mahmoud et al., 2021; Liu Y. et al., 2022). The control effect of 50× T. asperellum fungal fluid on apple canker reached 88.24%, which was significantly higher than that of benziothiazolinone (Ruangwong et al., 2021a). T. asperellum has different effects on different pathogenic fungi, among which its inhibitory effect on the pathogen causing corn leaf spot is the best, at up to 77.91%, followed by Pythium and Fusarium; and the worst inhibition effect is on corn sheath blight (Guo et al., 2019; Intana et al., 2022). Therefore, using Trichoderma to prevent and control plant diseases can not only inhibit the growth of pathogenic fungi, which is conducive to plant growth but can also reduce the use of chemical pesticides, which is conducive to protecting the ecological environment.
Storage resistance and processing technology of Trichoderma products
The commercial application of biocontrol Trichoderma depends to a large extent on the stress resistance (such as high temperature, drying, ultraviolet radiation, etc.) and storage resistance (more than 1 year at normal temperature) of the Trichoderma preparation (Alfiky and Weisskopf, 2021). At present, there are two main technologies: on the one hand, reducing acidity and regulating oxygen utilization to induce Trichoderma to produce stress-resistant chlamydospores; on the other hand, some chemical additives (such as copper) are added to the preparation. Monfil and Casas-Flores (2014) increased the resistance of Trichoderma to high temperature (35 ~ 40°C) and ultraviolet radiation by adding trehalose to Trichoderma. Monfil and Casas-Flores (2014) added glycerin to the Trichoderma preparation as a humectant to prolong its shelf life. Special packaging design, vacuum drying, and low-density polyethylene packaging materials can extend the shelf-life to 15 months. In the field of Trichoderma preparation form processing, Chen et al. (2021) developed the Trichoderma conidia powder agent and obtained a patented technology for inducing Trichoderma to produce chlamydospores. With the increasingly mature biological control technology, the types of commercial preparations for Trichoderma spp. are also becoming diverse. There are four main categories: (1) Wettable powders, which are made by mixing conidia powder, powdery carriers, and humectant. (2) Granules are made by mixing and stirring conidia and carrier. (3) A mixture consisting of spore powder and chemical fungicides mixed in proportion on a suitable carrier. (4) Suspenso-emulsion is prepared by suspending conidia in a lotion composed of vegetable oil, mineral oil, emulsifier, etc. In the current market for Trichoderma biological agents, T. Harzianum is the largest, followed by T. viride and T. koningii. Trichoderma agents widely used in plant disease control mainly include Trichodex (Makhteshim Chemical Works Ltd., Israel), a commercial preparation of T. harzianum T-39; RootShield (Bioworks, USA), a commercial preparation of T. harzianum T-22; Binab TF (Binab Bio Innovation AB, Sweden), a mixed-agent of T. harzianum and T. polyspora; Sentinel (Novozymes, Denmark), a commercial preparation of T. atrovilide; And Supervivit (Borregaard Bioplant, Denmark), a commercial preparation of T. harzianum.
Mechanism of Trichoderma-induced endophytic microbiome synergistically stimulating plant immune response
Competitive role of Trichoderma
Trichoderma are saprophytic fungi with fast mycelial growth and strong adaptability to the environment. It can seize the invasive part of the pathogenic fungi in the root of a plant, thus hindering the invasion of the pathogen fungi. It can also rapidly absorb the nutrients required for the growth of the pathogen fungi, resulting in nutrient deficiency and inhibiting the growth and reproduction of the pathogen fungi (Guo et al., 2019; Bazghaleh et al., 2020; Halifu et al., 2020; Figure 1A). Trichoderma has strong adaptability to the environment (Köhl et al., 2019; Morán-Diez et al., 2019; Pescador et al., 2022; Xu et al., 2022). Through its rapid growth and reproduction, it can seize nutrients and space near the plant rhizosphere, consume oxygen in the air, and weaken the growth of plant pathogenic fungi (Basińska-Barczak et al., 2020; Oszust et al., 2020; Panchalingam et al., 2022). The growth rate of Trichoderma is much faster than that of plant-pathogenic fungi; therefore, it can effectively inhibit the growth of plant-pathogenic fungi (Mohiddin et al., 2021). After entering the soil for 24 h, Trichoderma can quickly adsorb to the roots of crops for propagation, and the hyphae quickly wrap the roots of crops to form a protective layer, protect the roots of crops from the invasion of pathogens, and kill the nearby pathogens. Risoli et al. (2022) found that the growth rate of T. harzianum was 2.0 to 4.2 times faster than that of B. cinerea. Trichoderma mycelium competed with Fusarium graminearum by clinging, twining, inter-penetration, and other mechanisms, which caused the mycelium of F. graminearum to deform and eventually disappear (Dugassa et al., 2021). Trichoderma can capture water and nutrients, occupy space, and consume oxygen, etc. through rapid growth and reproduction, to weaken and exclude the gray mold pathogen in the same habitat (Herrera-Téllez et al., 2019).
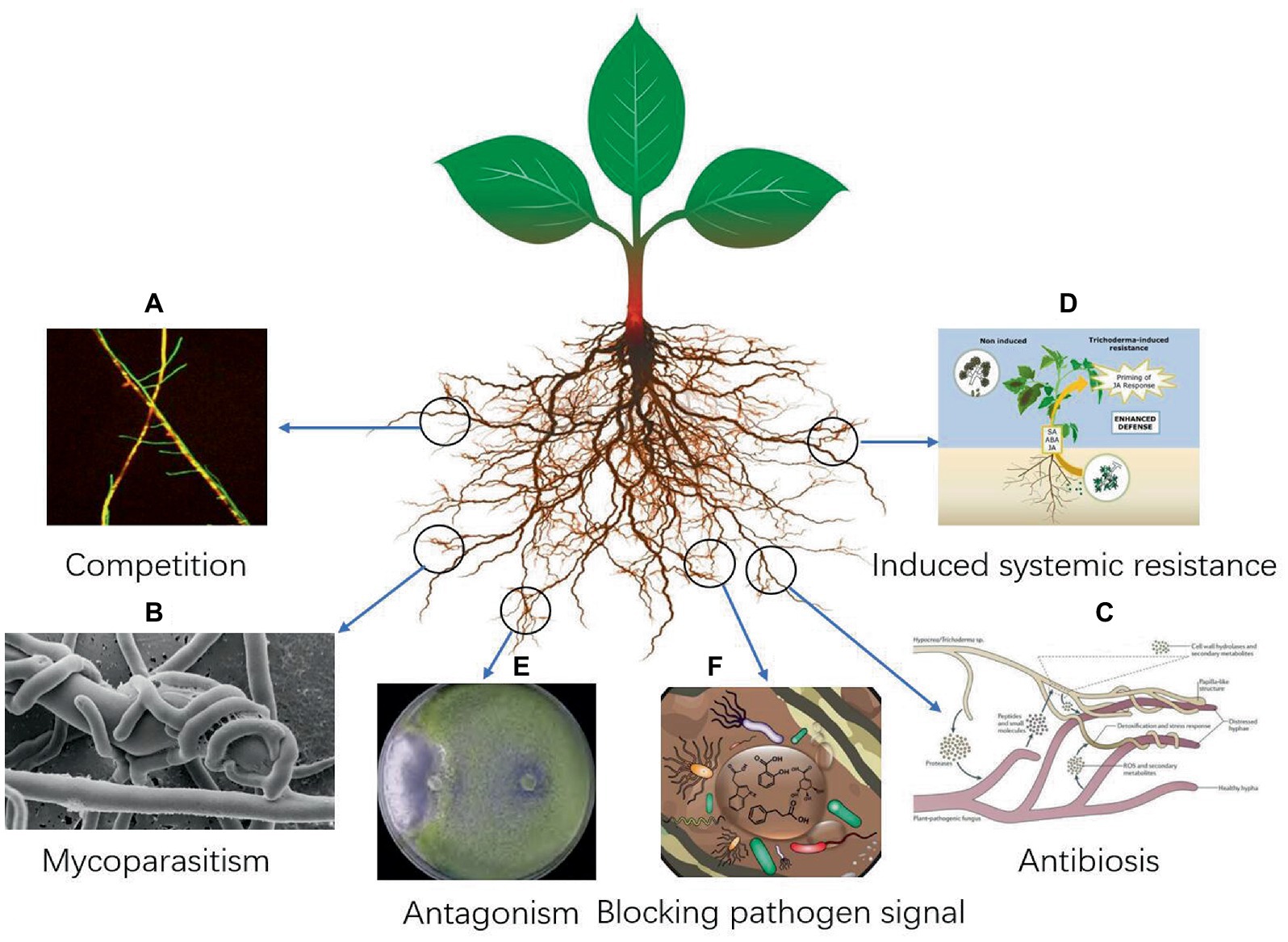
Figure 1. (A–F) Schematic diagram of the mechanism of action of Trichoderma in plant fungal disease control (Martínez-Medina et al., 2013; Lamdan et al., 2015; Morán-Diez et al., 2020; Manzar et al., 2022; Tamizi et al., 2022).
Mycoparasitism of Trichoderma
Mycoparasitism is one of the important mechanisms in the biological control of Trichoderma (Figure 1B). Trichoderma can parasitize about 18 genera of Pythium, Phytophthora, Rhizoctonia, and Peronospora. They directly invade or wound the mycelium, causing the pathogen cells to expand, deform, shorten, become round, shrink the protoplasm, and break the cell wall. Trichoderma TM can invade the hyphae of Sclerotinia sclerotiorum, attach to and wrap around the hyphae of pathogenic fungi, and break the hyphae of S. sclerotiorum until it disintegrates (Shaw et al., 2016). Risoli et al. (2022) found that Trichoderma can form putrescence in a specific environment, which has a mycoparasitic effect on Botrytis cinerea. It forms a large number of branches and sexual structures after entering the host hyphae, thus inhibiting the appearance of grape B. cinerea symptoms (Aswani et al., 2022). Trichoderma can degrade the cell wall of pathogenic fungi by secreting chitin-degrading enzymes, so as to better invade the interior of pathogenic fungi. Trichoderma mycelium hyperparasitized Fusarium graminearum by clinging, twining, inter-penetration, and other mechanisms, which caused the mycelium of F. graminearum to deform and eventually disappear (Tian et al., 2018). Chitinase secreted by T. harzianum plays an important role in promoting cell wall dissolution, mycelial autolysis, chitin assimilation, fungal parasitism, and inhibiting spore germination, mycelial growth, and spore formation (Saravanakumar et al., 2017). T. koningiopsis can invade the hyphae of Sclerotinia sclerotiorum, attach to and wrap around the hyphae of pathogenic fungi, and break the hyphae of S. sclerotiorum until it disintegrates (Shaw et al., 2016).
Antibiosis effect of Trichoderma
Antibiosis mainly refers to the ability of Trichoderma to inhibit the growth of plant pathogenic fungi by secreting antagonistic substances (Kottb et al., 2015; Izquierdo-García et al., 2020; Morán-Diez et al., 2020; Shobha et al., 2020; El-Hasan et al., 2022; Figure 1C). Trichoderma can produce hundreds of antimicrobial secondary metabolites, including trichomycin, gelatinomycin, chlorotrichomycin, and antibacterial peptides (Maruyama et al., 2020). These secondary metabolites can act as antibacterial agents, promote plant growth, and provide rich materials for the development of agricultural antibiotics (Nawrocka et al., 2018). Naglot et al. (2015) found that the metabolites of T. viride had a significant inhibitory effect on the wilt-specific form of F. oxysporum, with an inhibition rate of 54.81%. Manganiello et al. (2018) found that the volatile secondary metabolites secreted by T. viride TG050 609 can cause the mycelium of P. nicotianae to grow irregularly, break, or even dissolve, proving that T. viride has an antibiosis effect on P. nicotianae. In addition, most Trichoderma strains can produce antimicrobial substances such as pentaibols, which can inhibit a variety of plant pathogenic fungi and can also cooperate with cell wall-degrading enzymes on pathogenic fungi to effectively inhibit their growth (Debode et al., 2018; Mayo-Prieto et al., 2019; Kovács et al., 2021; Martínez-Salgado et al., 2021; Tamizi et al., 2022). Some studies have shown that some Trichoderma spp. can produce volatile metabolites, which can inhibit the growth of colonies to varying degrees, and some of them can inhibit the growth of colonies by more than 80% (Navazio et al., 2007; Vos et al., 2015; Samuelian et al., 2016; Marik et al., 2019; Thambugala et al., 2020; Kong et al., 2022; Li M. et al., 2022).
In recent years, research on the genome, transcriptome, proteome, and metabolome of Trichoderma has developed rapidly (Zhang Y. et al., 2022). Genome and EST sequencing, and microarray and microarray based expression profiling have become important tools for exploring Trichoderma genes and studying the mechanism of action (Tamizi et al., 2022). In genomics research, a cDNA library of T. harzianum EST has been constructed, and multiple new genes have been identified (Ferreira Filho et al., 2020). The researchers completed the genome sequencing of T. reesei, T. virens, and T. atroviride. Rubio et al. (2014) used high-density oligonucleotide (HDO) microarray technology and bioinformatics analysis to detect and analyze: after 20 h of interaction between T. hamatum T7 and tomato, there were 200 differentially expressed genes, of which 166 were up-regulated and 34 were down-regulated; 43.14% of genes are related to molecular function, 56.86% are related to biological processes, and 32.0% are related to cell component formation. Shoresh and Harman (2010) identified the changes of 27 endochitinase genes and 4 exochitinase genes in maize after interaction between T. harzianum T22 and maize using proteomic methods and EST libraries and discovered a new specific chitinase. Chen et al. (2021) used proteomic techniques to identify proteins related to resistance to root rot in maize, among which chitinase, SOD, isoflavone reductase, and PR protein are associated with resistance to root rot in maize seedlings.
Induced systemic resistance of Trichoderma
Trichoderma can induce host plants to produce defense responses. While inhibiting the growth and reproduction of pathogenic fungi, it can also induce crops to produce self-defense systems to obtain local or systemic disease resistance (Figure 1D). Trichoderma-induced plant disease resistance is achieved through two approaches: one is to regulate the plant disease resistance response by regulating elicitors or effectors; second, the cell wall-degrading enzyme produced by Trichoderma releases oligosaccharides that can induce plant resistance (Gomes et al., 2015). At present, there are more than 10 elicitors of Trichoderma that induce plant resistance, including Sm1, QID74 hydrophobic protein, chitin-degrading enzyme, MRSP1, xylanase, cellulase, endopolygalacturonase, sucrase, and antibacterial peptides. These substances are mainly derived from five Trichoderma species: T. asperellum, T. viride, T. atroviride, and T. harzianum (Karimi Aghcheh et al., 2013; Lamdan et al., 2015; Ngo et al., 2021; Matas-Baca et al., 2022; Zaid et al., 2022; Zhu et al., 2022). Saravanakumar et al. (2016) found that the activities of peroxidase (POD) and phenylalanine ammonia lyase (PAL) of corn seeds coated with Trichoderma increased significantly, and the plants were resistant to curvularia leaf spot of corn.
Antagonism of Trichoderma
The antagonism of Trichoderma is often considered the result of simultaneous or sequential action of more than two mechanisms (Saravanakumar et al., 2016; Sui et al., 2022; Figures 1E,F). Based on multiple mechanisms, Trichoderma has synergistic capabilities (Alonso-Ramírez et al., 2014; Moreno-Ruiz et al., 2020; Stracquadanio et al., 2020; Alukumbura et al., 2022; Chung et al., 2022; Kappel et al., 2022). Jogaiah et al. (2018) found that the synergistic use of T. harzianum and fungicides can effectively inhibit tomato gray mold, and the inhibition rate was higher than that of both fungicides alone. Zhang et al. (2017) found that the fermentation metabolites of T. viride CCTCC-SWB0199 and brassinolide in a certain proportion had a higher effect on the control of tomato gray mold than when the two were applied separately. Jogaiah et al. (2018) found that the biocontrol effect of Trichoderma spp. against plant pathogens fungi are often the result of a combination of multiple mechanisms, and different strains have different emphasis on biocontrol mechanisms. Monfil and Casas-Flores (2014) used transcriptology and metabolomics to study the tripartite interactions of Arabidopsis, Trichoderma, and Pseudomonas syringae tomato varieties. The results showed that the treatment of Arabidopsis roots with Trichoderma for 48 h induced more than 300 gene expression changes in the roots, but the changes in leaf genes were different from those in the roots (Monfil and Casas-Flores, 2014). Trichoderma induces the differential expression of host plant genes, mainly at the level of quantity (Viterbo et al., 2005; Malmierca et al., 2012; Park et al., 2019). A metabolomics study found that 27 compounds were related to induced resistance in Arabidopsis thaliana (Monfil and Casas-Flores, 2014). The biocontrol effect of Trichoderma on plant pathogenic fungi is often the result of multiple mechanisms, and different strains have different biocontrol mechanisms (De Zotti et al., 2020; Cai et al., 2021; Ji et al., 2021; Ruangwong et al., 2021b; Figure 1).
Application and mechanism of action of Trichoderma in plant nematode disease control
Application of Trichoderma in the control of plant nematodes
At present, the reported Trichoderma with nematicidal activity mainly includes T. longibrachiatum, T. viride, T. harzianum, T. Hamatum, T. atroviride, and T. koningii (Zhu et al., 2022). The fermentation broth of T. longibrachiatum T6 has a strong lethal effect on the eggs and second-instar larvae of cereal cyst nematodes in wheat (Zhu et al., 2022). The relative inhibition rate of the two concentrated fermentation broths on egg hatching was 46.47%, and the corrected mortality rate for the second-instar larvae was 44.45% (Sokhandani et al., 2016). Microscopic observation showed that the fermentation liquid of T. longibrachiatum T6 could digest the contents of nematode eggs and body cavities of the second instar larvae (Zhu et al., 2022). Khan et al. (2020) used inducers to make T. koningiopsis UFSMQ40 produced fermentation broth containing a large amount of chitinase, and its lethal rate to root-knot nematodes of South China and Java was 90.4 and 63.2%, respectively. Baazeem et al. (2021) analyzed the transcriptional activity of chi18-5 and chi18-12 genes of T. harzianum FB10 in Trichoderma egg parasitism. Compared to the control, the expression of chi18-5 and chi18-12 genes during parasitism increased significantly, indicating that the chitinase content increased, which could provide favorable conditions for egg cleavage (Baazeem et al., 2021).
Mechanism of Trichoderma resistance to nematode disease
The mechanism by which Trichoderma inhibits nematode disease remains unclear. Some studies suggest that the serine protease pr1 of Trichoderma has similar biochemical characteristics to the protein Pr1 of nematicidal fungi, so it has a certain nematode inhibition effect (Forghani and Hajihassani, 2020). The antimicrobial peptides produced by Trichoderma also have nematicidal effects (Fan et al., 2020).
Mycoparasitism effect of Trichoderma
Trichoderma mycoparasitism is an important mechanism for controlling nematodes and includes identification, contact, entanglement, penetration, and parasitism (Li et al., 2020; Figure 2). Trichoderma mycelium penetrates the eggshell or cuticle of larvae and adults of nematodes, colonizes, absorbs nutrients from nematodes, and causes nematode death (Marraschi et al., 2019). Trichoderma mycoparasitic nematode processes involve the production and co-secretion of various degrading enzymes (Moo-Koh et al., 2022). The induction of Trichoderma activities of β-1, 3-glucanase, chitinase, and protease are increased, which can enhance the immunity of plants to nematodes (Poveda et al., 2020). The hyperparasitic process is mainly regulated by heterotrimeric G protein, cAMP, and MAPK motif signals, and secretes extracellular chitinase, glucanase, xylanase, cellulase, and protease, among which chitinase and protease are particularly important and can degrade the cyst, egg, larva, and adult body wall of nematodes (Li et al., 2020). Trichoderma secretes glucosidases NAG1 and NAG2, which act on the extracellular and self-cell walls, respectively, and their main function is to degrade chitin so that they can protect their cell walls from degradation during the process of hyperparasitism (Poveda et al., 2020).
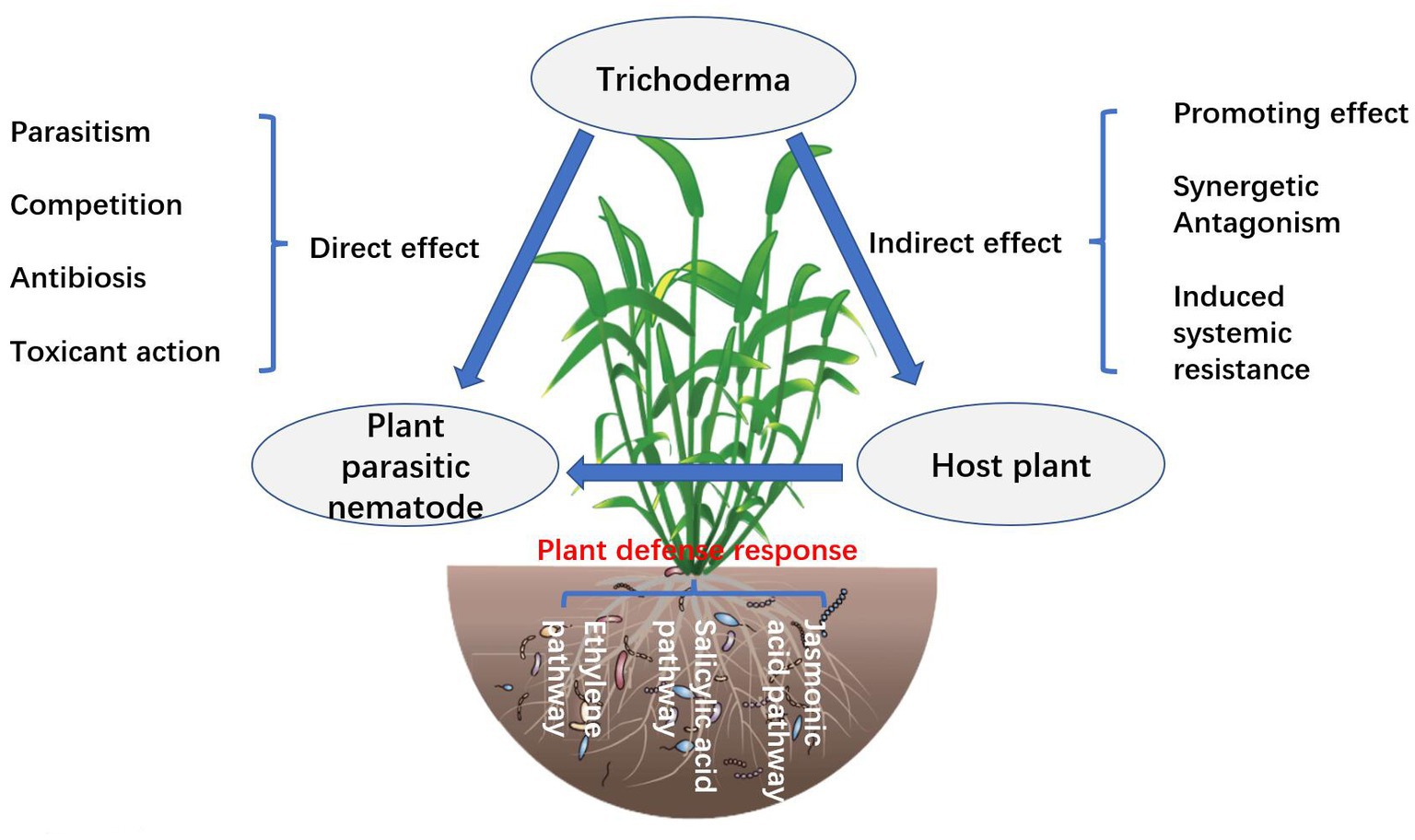
Figure 2. Schematic diagram of the mechanism of action of Trichoderma in plant nematode disease control (Sokhandani et al., 2016; Forghani and Hajihassani, 2020; Khan et al., 2020).
Antibiosis effect of Trichoderma
Trichoderma can inhibit the growth and reproduction of nematodes by secreting antagonistic substances (Moo-Koh et al., 2022). Trichoderma produces a variety of secondary metabolites, including trichomycin, gliotoxin, viridin, antibacterial peptide, β-1, 3-glucanase, chitinase, polypeptides, polyketones, butyrolactones, sesquiterpene heptadecarboxylic acid, terpenes, and some volatile substances (hydrocarbons, alcohols, furans, aldehydes, alkanes, olefins, esters, aromatic compounds, heterocyclic compounds, and various terpenoids) (Kappel et al., 2020). Contina et al. (2017) reported for the first time that T. harzianum ThzID1-M3 labeled with GFP significantly inhibited the reproduction of potato cyst nematodes, with a cyst decline rate of 60%. The inhibition rates of the fermentation broth of T. hamatum HZ-9 and T. virens HZ-L9 on the hatching of soybean cyst nematode eggs were 80.6 and 79.4%, respectively (Contina et al., 2017). The secondary metabolites produced by the same Trichoderma species in different media have different effects on nematode resistance (Forghani and Hajihassani, 2020). The inhibition rate of T. viride secondary metabolites on wheat medium and solid medium on egg hatching of southern root knot nematode was 71.6 and 67.3%, respectively (Baazeem et al., 2021). Baazeem et al. (2021) detected and analyzed the T. hamatum FB10 secondary metabolite nematicidal active ingredient; thirteen kinds of chemical substances were obtained, including 6-amyl-α-pyranone. The inhibition rate of egg hatching of Meloidogyne incognita was 78.26%.
Toxicity effect of Trichoderma
Trichoderma produces toxic secondary metabolites that directly come into contact with nematodes, which is an important direct biological control mechanism (Khan et al., 2020). It has been found that the toxic secondary metabolites produced by Trichoderma are divided into two categories, one is small molecules and volatile substances, including aromatic compounds, polyketides, butenolactones, and terpenoids, etc.; the other is macromolecular metabolites, including peptides, enzymes, etc. (Khan et al., 2020). It has been reported that the main nematicidal substances isolated from Trichoderma are trichodermin, acetic acid, gliotoxin, and peptide cyclosporin A (Fan et al., 2020). Meanwhile, Moo-Koh et al. (2022) used GC–MS to detect and analyze the nematicidal active components of Trichoderma TUV-13 strain and obtained more than 40 chemical components, among which of which the main alkanes are the most, in addition to organic acids, esters, ketones, steroids, and other organic compounds. Li et al. (2020) summarized and analyzed the secondary metabolites and activities of 20 species of Trichoderma, including T. aureoviride, T. arundinaceum, T. brevicompactum, T. citrinoviride, T. gamsii, T. polysporum, T. saturnisporum, T. spirale, T. cremeum, T. pesudokoningii, and T. lignorum. There were 390 non-volatile secondary metabolites, among which wickerol A, harziandione, trichodermin, and cyclonerodiol exhibited nematicidal activity (Khan et al., 2020). The T. virens B3 fermentation broth has strong toxic activity against cereal cyst nematodes, and the killing rate is as high as 86.2% (Forghani and Hajihassani, 2020). The fermentation broth can maintain good stability for a long time. The fermentation broth of T. citrinoviride,T. harzianum,T. acroviride, and T. koningiopsis had a strong toxic effect on the southern root-knot nematode J2, with a mortality rate of more than 85% (Du et al., 2020).
Induced resistance effect of Trichoderma
Induced resistance is the response of plants to stress, which is stimulated by external factors. Trichoderma-colonized plant roots cause physiological and metabolic changes and produce a variety of secondary metabolites that act as elicitors (Al-Hazmi and TariqJaveed, 2016). At present, there are more than 20 elicitors produced by Trichoderma that induce plant resistance, including antitoxins, polypeptides, lipopeptides, cellulases, hydrophobic proteins, non-toxic gene proteins, terpenoids, phenol derivatives, glycosidic ligands, and flavonoids (Pocurull et al., 2020). These secondary metabolites induce plant defense responses and promote plant growth. The interaction between Trichoderma and plants increases the synthesis of defense-related enzymes and substances. T. hamatum can induce the activities of phenylalanine ammonia lyase (PAL), polyphenol oxidase (PPO), and peroxidase (POD), which are related to tobacco defense reaction, to increase significantly (Al-Hazmi and TariqJaveed, 2016). In tomatoes treated with T. harzianum, the control effect against M. incognita was 61.88%. Further studies have shown that the levels of reactive oxygen species (ROS), superoxide (O2−), hydrogen peroxide (H2O2), and malondialdehyde (MDA) in tomatoes were significantly increased, and the defense-related genes PAL, C4H, 4CL, CAD, LPO, CCOMT, Tpx1, and G6PDH were upregulated, thus inducing the defense response of tomatoes to M. incognita (Pocurull et al., 2020). Plant-induced resistance mainly involves signal transduction pathways such as those of salicylic acid (SA), jasmonic acid (JA), and ethylene (ET). In the interaction between Trichoderma and Arabidopsis, tomato, and cucumber, JA, SA, and ET contents increased to varying degrees, indirectly improving plant resistance (Hinterdobler et al., 2021). This process is also related to activating chitinase and glucanase activities and inhibiting the plant antioxidant enzyme system. The expression of ETR1 and LOX1 genes of jasmonic acid and the ethylene signal pathway increased significantly in T. asperellum DQ-1 irrigated tomato, which enhanced tomato resistance (Agbessenou et al., 2022). Some volatile secondary metabolites of Trichoderma are important elicitors that induce plant resistance (Al-Hazmi and TariqJaveed, 2016). The volatile substances produced by T. harzianum and T. asperellum act as elicitors to stimulate the up-regulated expression of Arabidopsis-induced resistance-related transcription factor MYB72, which triggers a JA-regulated defense response (Agbessenou et al., 2022). At present, the interaction mechanisms and signal transduction pathways between Trichoderma volatile secondary metabolites and plants have not been thoroughly studied. The active substances produced by Trichoderma are recognized by plants, thus activating the signal transduction pathway and inducing the production of plant system resistance. The microbial determinants recognized by microorganisms are called microbe-associated molecular patterns (MAMPs) (Baazeem et al., 2021). After Trichoderma infects plant roots, it releases a variety of MAMPs to activate immune response (MTI), thus inducing plant systemic resistance (ISR) (Li X. et al., 2022). Abdelkhalek et al. (2022) showed that T. hamatum strain Th23 promotes tomato growth and induces systemic resistance against tobacco mosaic virus.
Application and mechanism of action of Trichoderma in promoting crop growth and repairing environment
Application of Trichoderma in promoting plant growth and repairing environment
Trichoderma can produce plant growth stimulators, such as indoleacetic acid (IAA) and harzianolide, to promote the development and growth of plant roots by secreting phytase and ferritin to promote the absorption of P and Fe by plants; decomposes soil organic matter; increases the supply of soil nutrients; improves crop photosynthetic efficiency; improves plant height, stem diameter, and other agronomic traits; and increases production (Lombardi et al., 2020a). Many studies have shown that most Trichoderma spp. can produce bioactive substances and have antagonistic effects on plant-pathogenic fungi and plant-pathogenic nematodes (Şesan et al., 2020; Abdelkhalek et al., 2022; Organo et al., 2022; Rao et al., 2022). Bioactive substances, including secondary metabolites and cell wall-degrading enzymes, can effectively improve the resistance of crops, reduce plant diseases, and promote plant growth (Domínguez et al., 2016; Viriyasuthee et al., 2019; Jaiswal et al., 2020; Tseng et al., 2020).
Trichoderma can improve soil nutrient availability and utilization efficiency. The aboveground biomass of cucumber seedlings inoculated with Trichoderma MF-2 increased by 39.07%, with a significant growth-promoting effect, and an increased number of beneficial microorganisms in the soil (Singh et al., 2019; Ye et al., 2020). Different Trichoderma strains had different degrees of antagonism to F. oxysporum, and the combination of Trichoderma wettable powder treatment significantly increased banana yield (Samuelian, 2016; Bubici et al., 2019; Damodaran et al., 2020). Li et al. (2020) found that the biocontrol agent Trichoderma GYXM-1p1 strain had a strong growth-promoting effect through pot cultivation. After treatment with this strain, the root length, plant height, root fresh weight, dry weight, total fresh weight, and total dry weight of cabbage plants were significantly improved compared to the water control (p < 0.05), and the total fresh weight and total dry weight of cabbage plants were increased by 417. 60% and 762. 69%, respectively, compared with water control. Ruan et al. (Intana et al., 2021; Nuangmek et al., 2021) found that the application of nitrogen fertilizer with Trichoderma promoted the quality of muskmelon. After the application of Trichoderma, the soluble sugar content of muskmelon fruit increases significantly, improving the quality of muskmelon. The application of Trichoderma can increase the SPAD value of chlorophyll in peanut leaves, improve the main agronomic traits of peanuts, significantly increase the activity of protective enzymes in peanut roots, stems, and leaves, and reduce the content of MDA (Kovács et al., 2021; Al-Askar et al., 2022). When 1.5 kg/666.7 m−2 was applied, the number of pods per plant, pod weight, seed kernel weight, 100 fruit quality, 100 fruit kernel quality, and yield per plant of peanut increased by 24.63, 20.22, 14.10, 4.86, 7.63, and 12.85%, respectively, compared with the control (Al-Askar et al., 2022).
Mechanism of Trichoderma in promoting plant growth and repairing environment
Trichoderma can promote plant growth, produce substances that can promote plant growth, improve the solubility of nutrients in the soil, and improve plant rhizosphere microecology, thereby promoting plant absorption and growth (Karuppiah et al., 2019a; Kakabouki et al., 2021; Marra et al., 2021; Figure 3). Trichoderma plant interactions can not only induce resistance but also improve the resistance of plants to abiotic stress factors (salt, high temperature, UV). Treatment of cucumber seeds with T. asperellum T203 improved the plant’s salt tolerance, and the activities of Mn/Cu SOD and catalase (CAT), and significantly reduced ascorbic acid in the plant (Illescas et al., 2022). Trichoderma can significantly enhance the Na+ efflux from the root system of Lycium barbarum and its transport to the upper ground, ensure K+ absorption and maintain the ion balance in the plant, thus reducing the damage of PSII caused by ion toxicity and oxidative stress, protecting photosynthetic pigments, maintaining the photosynthetic performance of L. barbarum under salt stress, and reducing biomass loss (Brotman et al., 2013).
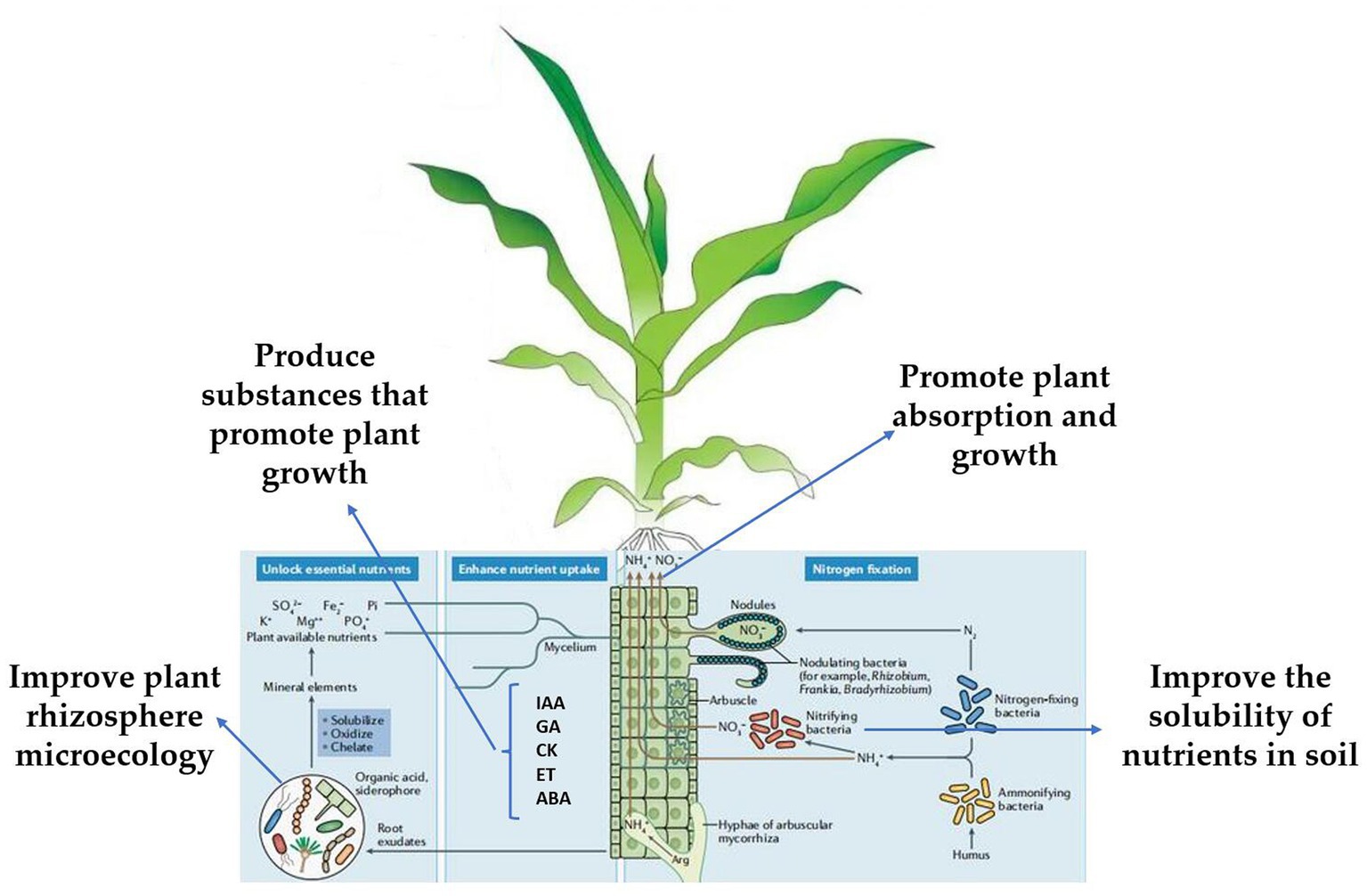
Figure 3. Schematic diagram of the mechanism of action of Trichoderma in promoting crop growth and repairing the environment (Jaiswal et al., 2020; Lombardi et al., 2020a; Şesan et al., 2020; Karuppiah et al., 2021).
The synthesis of plant growth hormones, such as IAA, ABA, ET, GA, and CK is the main mechanism of Trichoderma (Karuppiah et al., 2019b; Wang et al., 2021; Degani et al., 2021b; Agbessenou et al., 2022). T. asperellum induced cucumber to produce IAA, GA, and ABA to promote growth (Liu H. et al., 2022). The height, stem diameter, soluble sugar content, and absorption rate of available nitrogen of tomato seedlings treated with T. asperellum were significantly increased, and the expression of tomato hormone signal transduction-related genes JAR1, MYC2, NPR1, PR1, and GH3 was significantly increased (Rawal et al., 2022). Another study showed that T. asperellum can upregulate the expression of xylanase genes in poplar and has a significant growth-promoting effect (Karuppiah et al., 2019b). T. harzianum regulates tricarboxylic acid cycle (TAC) and hexose monophosphate pathway (HMP) to promote tomato growth by enhancing succinate dehydrogenase and glucose-6-phosphate dehydrogenase activities (Manganiello et al., 2018). Trichoderma produces acidic substances that can dissolve insoluble trace elements in soil and provide more nutrition to plants (Samuelian, 2016). T. asperellum can transform insoluble phosphate in the soil into effective phosphorus and promote the absorption and utilization of cucumbers (Karuppiah et al., 2021; Figure 3). T. koningiopsis can produce organic acids that dissolve insoluble tricalcium phosphate under high alkaline stress and can also produce alkaline phosphatase under drought stress to solubilize phosphorus and improve the utilization of nutrients by plants (You et al., 2022). Many experiments have verified that Trichoderma promotes plant growth (Mayo et al., 2015; Bononi et al., 2020; Lombardi et al., 2020b; Swain et al., 2021; Velasco et al., 2021; Bridžiuvienė et al., 2022; Joo and Hussein, 2022; Li X. et al., 2022); under complex field production conditions, the mechanism by which Trichoderma promotes plant growth requires more systematic research.
Conclusion and future perspectives
At present, chemical control is the main method used for plant disease control and is achieved by spraying pesticides and fungicides. Although the effect of chemical control is good and helpful in increasing agricultural production, the unscientific use of chemical pesticides has caused serious pollution to the environment and enhanced pathogens’ resistance to and chemical pesticides. Several experiments have proven that Trichoderma has good biological control effects and can reduce the use of chemical pesticides. However, there are still few biocontrol agents against Trichoderma on the market, and more effective and suitable strains need to be found to join the biocontrol team (Nieto-Jacobo et al., 2017; Fiorentino et al., 2018; López et al., 2019; Nawrocka et al., 2019; Poveda et al., 2019; Cabral-Miramontes et al., 2022). Although Trichoderma has great prospects for agricultural applications, there are still some problems in the development and utilization of Trichoderma (Rubio et al., 2014; Zhang et al., 2018; Phoka et al., 2020; Santos et al., 2020; Wang H. et al., 2022). Because the spore preparation of Trichoderma is generally a living fungal preparation, which is often affected by various natural factors such as humidity, temperature, soil acidity, alkalinity, and the soil microbial community when it is applied in the field, the field test performance is unstable, and the biological control effect is weakened. In addition, the shelf life of biological control agents is relatively short, and some microorganisms must be stored at low temperatures to ensure the concentration of live microorganisms when they are applied.
There are still several problems to be solved in the application of Trichoderma in the biological control of plant diseases (Caruso et al., 2020; He et al., 2020; Boamah et al., 2021; González-López et al., 2021). The first is to explore and produce efficient strains, which can be screened through genetic engineering technology to produce Trichoderma biocontrol-engineered strains that are resistant to chemical pesticides and low temperatures. At the same time, it is necessary to develop effective Trichoderma agents suitable for use with various application methods to enhance the control effect and improve the processing technology of Trichoderma agents to extend the shelf life of biological control agents. Second, exploring the combined effects of Trichoderma spp. and other microorganisms is necessary. The development of Pesticides with volatile and non-volatile secondary metabolites secreted by Trichoderma as the main active ingredient will be the focus of future research and the development of new Pesticides. To improve the quality of Trichoderma biopesticides, in addition to monitoring traditional indicators such as pH, dissolved oxygen, and temperature in the fermentation process, it is also necessary to monitor its correlation with the yield of antagonistic substances at the level of the cell metabolic flow, genome, proteome, and metabolome (Mulatu et al., 2021). However, it is necessary to establish more scientific quality standards for Trichoderma products in vivo, such as increasing the content of antagonistic substances or activity indicators (Niu et al., 2020). The development of new dosage forms, such as cell microcapsules, water-in-oil emulsions, and other protective dosage forms, should be strengthened, and the molecular mechanism of chlamydospore production should be further studied (Table 1).
With further study of transgenic Trichoderma, a prospective study on the biological and environmental safety of transgenic Trichoderma should be conducted (Li et al., 2021). At present, the balance regulation of Trichoderma colonizing host and plant immune response, long-distance and trans-growth period transduction mechanism of systematically induced plant disease resistance and its defense signals, identification of Trichoderma elicitors to recognize plant targets or receptors, and mechanism of Trichoderma-induced plant endophytic microbiome to synergistically stimulate plant immune response has become an international research topic of interest. Studies on miRNA regulation of Trichoderma colonization host process and plant immune response, and the regulation mechanism of cross-border miRNA transduction between Trichoderma, plants, and pathogenic microorganisms are emerging. The combination design or co-culture technology of Trichoderma and other microorganisms has become key for tapping new metabolites with specific functions of microorganisms, broadening the target spectrum of microbial metabolites, and developing new biopesticides and biostimulants based on metabolites (Wang Y. et al., 2022). It is expected to become a new direction for the development of macromolecular biopesticides by molecular construction or modification of the Trichoderma multi-stimulator fusion protein and the development of new plant immune-activating protein pesticides. At present, it is urgent to reveal the synergistic interactions among Trichoderma, plants, and pathogenic microorganisms in induced disease resistance on a cross-genome scale, develop Trichoderma and other microbial symbiotic agents that can cure both diseases and pests, and develop new biostimulator products based on Trichoderma metabolites.
Compared with single-life biocontrol fungi, compound biocontrol fungi can better avoid the problems of weak adaptability to the environment, narrow range of disease resistance, and insufficient control effect. At present, there are many preparations containing different kinds of Trichoderma, which are being used in sustainable agricultural crops, but the application of these preparations is still expensive, and not available to all farmers. The application of compatible or affinity multiple microorganisms for compounding has become a trend in the development of biocontrol agents. Trichoderma can form alliances with a variety of microorganisms such as bacteria and fungi to directly or indirectly improve the ability of plants to prevent and control diseases. The following aspects may be the main research focus of Trichoderma as a biocontrol fungus in the future: the molecular mechanism of the specific interaction between Trichoderma and plants; Molecular basis of plant immunity induced by Trichoderma; Improvement of Trichoderma fermentation process; Establishing diversified application technology models of Trichoderma. The commercial application of biocontrol Trichoderma depends largely on the stress resistance (such as high temperature, drying, ultraviolet radiation, etc.) and storage resistance (more than 1 year at normal temperature) of the Trichoderma agent. At present, there are two main technologies. One is to reduce the acidity and regulate the utilization of oxygen to induce Trichoderma to produce stress-resistant chlamydospores, and the other is to add some chemical additives (such as copper) to the inoculum. How the effectors produced by Trichoderma interact with plant cell receptors has become the key to revealing the mechanism of Trichoderma inducing plant immunity. With the deepening of the research on transgenic Trichoderma, prospective research on the biological and environmental safety of transgenic Trichoderma should be carried out.
Author contributions
MZ and XY: software. KZ and MZ: data curation. XY: writing – original draft preparation. JR, JC, and KZ: writing, review, and editing. All authors read and agreed to the published version of the manuscript.
Funding
This research was supported by the National Science Foundation of China (32161143005 and 32160669) and the Guizhou Science and Technology Support Program ([2020]1Y125).
Conflict of interest
The authors declare that the research was conducted in the absence of any commercial or financial relationships that could be construed as a potential conflict of interest.
Publisher’s note
All claims expressed in this article are solely those of the authors and do not necessarily represent those of their affiliated organizations, or those of the publisher, the editors and the reviewers. Any product that may be evaluated in this article, or claim that may be made by its manufacturer, is not guaranteed or endorsed by the publisher.
References
Abbas, A., Mubeen, M., Zheng, H., Sohail, M. A., Shakeel, Q., Solanki, M. K., et al. (2022). Trichoderma spp. genes involved in the biocontrol activity against Rhizoctonia solani. Front. Microbiol. 13:884469. doi: 10.3389/fmicb.2022.884469
Abdelkhalek, A., Al-Askar, A. A., Arishi, A. A., and Behiry, S. I. (2022). Trichoderma hamatum strain Th23 promotes tomato growth and induces systemic resistance against tobacco mosaic virus. J. Fungi 8:228. doi: 10.3390/jof8030228
Agbessenou, A., Akutse, K. S., Yusuf, A. A., and Khamis, F. M. (2022). The endophyte Trichoderma asperellum M2RT4 induces the systemic release of methyl salicylate and (Z)-jasmone in tomato plant affecting host location and herbivory of Tuta absoluta. Front. Plant Sci. 13:860309. doi: 10.3389/fpls.2022.860309
Al-Askar, A. A., Rashad, E. M., Moussa, Z., Ghoneem, K. M., Mostafa, A. A., Al-Otibi, F. O., et al. (2022). A novel endophytic Trichoderma longibrachiatum WKA55 with biologically active metabolites for promoting germination and reducing mycotoxinogenic fungi of peanut. Front. Microbiol. 13:772417. doi: 10.3389/fmicb.2022.772417
Al-Askar, A. A., Saber, W., Ghoneem, K. M., Hafez, E. E., and Ibrahim, A. A. (2021). Crude citric acid of Trichoderma asperellum: tomato growth promotor and suppressor of Fusarium oxysporum f. sp. lycopersici. Plants 10:222. doi: 10.3390/plants10020222
Alfiky, A., and Weisskopf, L. (2021). Deciphering Trichoderma-plant-pathogen interactions for better development of biocontrol applications. J. Fungi 7:61. doi: 10.3390/jof7010061
Al-Hazmi, A. S., and TariqJaveed, M. (2016). Effects of different inoculum densities of Trichoderma harzianum and Trichoderma viride against Meloidogyne javanica on tomato. Saudi J. Biol. Sci. 23, 288–292. doi: 10.1016/j.sjbs.2015.04.007
Alonso-Ramírez, A., Poveda, J., Martín, I., Hermosa, R., Monte, E., and Nicolás, C. (2014). Salicylic acid prevents Trichoderma harzianum from entering the vascular system of roots. Mol. Plant Pathol. 15, cc doi: 10.1111/mpp.12141
Al-Surhanee, A. A. (2022). Protective role of antifusarial eco-friendly agents (Trichoderma and salicylic acid) to improve resistance performance of tomato plants. Saudi J. Biol. Sci. 29, 2933–2941. doi: 10.1016/j.sjbs.2022.01.020
Alukumbura, A. S., Bigi, A., Sarrocco, S., Fernando, W., Vannacci, G., Mazzoncini, M., et al. (2022). Minimal impacts on the wheat microbiome when Trichoderma gamsii T6085 is applied as a biocontrol agent to manage fusarium head blight disease. Front. Microbiol. 13:972016. doi: 10.3389/fmicb.2022.972016
Álvarez-García, S., Mayo-Prieto, S., Gutiérrez, S., and Casquero, P. A. (2020). Self-inhibitory activity of Trichoderma soluble metabolites and their antifungal effects on Fusarium oxysporum. J. Fungi 6:176. doi: 10.3390/jof6030176
Andrade-Hoyos, P., Silva-Rojas, H. V., and Romero-Arenas, O. (2020). Endophytic Trichoderma species isolated from Persea americana and Cinnamomum verum roots reduce symptoms caused by Phytophthora cinnamomi in avocado. Plan. Theory 9:1220. doi: 10.3390/plants9091220
Aswani, R., Roshmi, T., and Radhakrishnan, E. K. (2022). Induction of plant defense response by endophytic microorganisms. In Biocontrol Mechanisms of Endophytic Microorganisms. Academic Press. 89–115.
Baazeem, A., Almanea, A., Manikandan, P., Alorabi, M., Vijayaraghavan, P., and Abdel-Hadi, A. (2021). In vitro antibacterial, antifungal, nematocidal and growth promoting activities of Trichoderma hamatum FB10 and its secondary metabolites. J. Fungi 7:331. doi: 10.3390/jof7050331
Bardin, M., Ajouz, S., Comby, M., Lopez-Ferber, M., Graillot, B., Siegwart, M., et al. (2015). Is the efficacy of biological control against plant diseases likely to be more durable than that of chemical pesticides? Front. Plant Sci. 6:566. doi: 10.3389/fpls.2015.00566
Basińska-Barczak, A., Błaszczyk, L., and Szentner, K. (2020). Plant cell wall changes in common wheat roots as a result of their interaction with beneficial fungi of Trichoderma. Cells 9:2319. doi: 10.3390/cells9102319
Bazghaleh, N., Prashar, P., Woo, S., and Vandenberg, A. (2020). Effects of lentil genotype on the colonization of beneficial Trichoderma species and biocontrol of aphanomyces root rot. Microorganisms 8:1290. doi: 10.3390/microorganisms8091290
Batista, K. O. M., Silva, D. V., Nascimento, V. L., and de Souza, D. J. (2021). Effects of Trichoderma strigosellum in eucalyptus urophylla development and leaf-cutting ant behavior. J Fungi 8:15.
Boamah, S., Zhang, S., Xu, B., Li, T., and Calderón-Urrea, A. (2021). Trichoderma longibrachiatum (TG1) enhances wheat seedlings tolerance to salt stress and resistance to Fusarium pseudograminearum. Front. Plant Sci. 12:741231. doi: 10.3389/fpls.2021.741231
Bononi, L., Chiaramonte, J. B., Pansa, C. C., Moitinho, M. A., and Melo, I. S. (2020). Phosphorus-solubilizing Trichoderma spp. from Amazon soils improves soybean plant growth. Sci. Rep. 10:2858. doi: 10.1038/s41598-020-59793-8
Bridžiuvienė, D., Raudonienė, V., Švedienė, J., Paškevičius, A., Baužienė, I., Vaitonis, G., et al. (2022). Impact of soil chemical properties on the growth promotion ability of Trichoderma ghanense, T. tomentosum and their complex on Rye in different land-use systems. J. Fungi 8:85. doi: 10.3390/jof8010085
Brotman, Y., Landau, U., Cuadros-Inostroza, Á., Tohge, T., Fernie, A. R., Chet, I., et al. (2013). Trichoderma-plant root colonization: escaping early plant defense responses and activation of the antioxidant machinery for saline stress tolerance. PLoS Pathog. 9:e1003221. doi: 10.1371/journal.ppat.1003221
Bubici, G., Kaushal, M., Prigigallo, M. I., Gómez-Lama Cabanás, C., and Mercado-Blanco, J. (2019). Biological control agents against Fusarium wilt of Banana. Front. Microbiol. 10:616. doi: 10.3389/fmicb.2019.00616
Cabral-Miramontes, J. P., Olmedo-Monfil, V., Lara-Banda, M., Zúñiga-Romo, E. R., and Aréchiga- Carvajal, E. T. (2022). Promotion of plant growth in arid zones by selected Trichoderma spp. strains with adaptation plasticity to alkaline pH. Biology 11:1206. doi: 10.3390/biology11081206
Cai, X., Zhao, H., Liang, C., Li, M., and Liu, R. (2021). Effects and mechanisms of symbiotic microbial combination agents to control tomato Fusarium crown and root rot disease. Front. Microbiol. 12:629793. doi: 10.3389/fmicb.2021.629793
Carro-Huerga, G., Compant, S., Gorfer, M., Cardoza, R. E., Schmoll, M., Gutiérrez, S., et al. (2020). Colonization of Vitis vinifera L. by the endophyte Trichoderma sp. strain T154: biocontrol activity against Phaeoacremonium minimum. Front. Plant Sci. 11:1170. doi: 10.3389/fpls.2020.01170
Caruso, G., El-Nakhel, C., Rouphael, Y., Comite, E., Lombardi, N., Cuciniello, A., et al. (2020). Diplotaxis tenuifolia (L.) DC. Yield and quality as influenced by cropping season, protein hydrolysates, and Trichoderma applications. Plan. Theory 9:697. doi: 10.3390/plants9060697
Chen, J., Zhou, L., Din, I. U., Arafat, Y., Li, Q., Wang, J., et al. (2021). Antagonistic activity of Trichoderma spp. against Fusarium oxysporum in rhizosphere of radix pseudostellariae triggers the expression of host defense genes and improves its growth under long-term monoculture system. Front. Microbiol. 12:579920. doi: 10.3389/fmicb.2021.781826
Chung, D., Kwon, Y. M., Lim, J. Y., Bae, S. S., Choi, G., and Lee, D. S. (2022). Characterization of chitinolytic and antifungal activities in marine-derived Trichoderma bissettii strains. Mycobiology 50, 244–253. doi: 10.1080/12298093.2022.2105509
Contina, J. B., Dandurand, L. M., and Knudsen, G. R. (2017). Use of GFP-tagged Trichoderma harzianum as a tool to study the biological control of the potato cyst nematode Globodera pallida. Appl. Soil Ecol. 115, 31–37. doi: 10.1016/j.apsoil.2017.03.010
Coppola,. , Diretto, G., Digilio, M. C., Woo, S. L., Giuliano, G., Molisso, D., et al. (2019). Transcriptome and metabolome reprogramming in tomato plants by Trichoderma harzianum strain T22 primes and enhances defense responses against aphids. Front. Physiol. 10:745.
Damodaran, T., Rajan, S., Muthukumar, M., Gopal, R., Yadav, K., Kumar, S., et al. (2020). Biological management of banana Fusarium wilt caused by Fusarium oxysporum f. sp. cubense tropical race 4 using antagonistic fungal isolate CSR-T-3 (Trichoderma reesei). Front. Microbiol. 11:595845. doi: 10.3389/fmicb.2020.595845
Daza, F. F. F., Roman, G. R., Rodriguez, M. V., Vargas, I. A. G., Heano, H. C., Cereda, M. P., et al. (2019). Spores of Beauveria bassiana and Trichoderma lignorum as a bioinsecticide for the control of atta cephalotes. Biol Res. 52:51.
De Zotti, M., Sella, L., Bolzonello, A., Gabbatore, L., Peggion, C., Bortolotto, A., et al. (2020). Targeted amino acid substitutions in a Trichoderma Peptaibol confer activity against fungal plant pathogens and protect host tissues from Botrytis cinerea infection. Int. J. Mol. Sci. 21:7521. doi: 10.3390/ijms21207521
Debbi, A., Boureghda, H., Monte, E., and Hermosa, R. (2018). Distribution and genetic variability of Fusarium oxysporum associated with tomato diseases in Algeria and a biocontrol strategy with indigenous Trichoderma spp. Front. Microbiol. 9:282. doi: 10.3389/fmicb.2018.00282
Debode, J., De Tender, C., Cremelie, P., Lee, A. S., Kyndt, T., Muylle, H., et al. (2018). Trichoderma- inoculated miscanthus straw can replace peat in strawberry cultivation, with beneficial effects on disease control. Front. Plant Sci. 9:213. doi: 10.3389/fpls.2018.00213
Degani, O., and Dor, S. (2021). Trichoderma biological control to protect sensitive maize hybrids against late wilt disease in the field. J. Fungi 7:315. doi: 10.3390/jof7040315
Degani, O., Khatib, S., Becher, P., Gordani, A., and Harris, R. (2021a). Trichoderma asperellum secreted 6-pentyl-α-pyrone to control Magnaporthiopsis maydis, the maize late wilt disease agent. Biology 10:897. doi: 10.3390/biology10090897
Degani, O., Rabinovitz, O., Becher, P., Gordani, A., and Chen, A. (2021b). Trichoderma longibrachiatum and Trichoderma asperellum confer growth promotion and protection against late wilt disease in the field. J. Fungi 7:444. doi: 10.3390/jof7060444
Di Marco, S., Metruccio, E. G., Moretti, S., Nocentini, M., Carella, G., Pacetti, A., et al. (2022). Activity of Trichoderma asperellum strain ICC 012 and Trichoderma gamsii strain ICC 080 toward diseases of esca complex and associated pathogens. Front. Microbiol. 12:813410. doi: 10.3389/fmicb.2021.813410
Domínguez, S., Rubio, M. B., Cardoza, R. E., Gutiérrez, S., Nicolás, C., Bettiol, W., et al. (2016). Nitrogen metabolism and growth enhancement in tomato plants challenged with Trichoderma harzianum expressing the Aspergillus nidulans acetamidase amdS gene. Front. Microbiol. 7:1182. doi: 10.3389/fmicb.2016.01182
Druzhinina, I. S., Chenthamara, K., Zhang, J., Atanasova, L., Yang, D., Miao, Y., et al. (2018). Massive lateral transfer of genes encoding plant cell wall-degrading enzymes to the mycoparasitic fungus Trichoderma from its plant- associated hosts. PLoS Genet. 14:e1007322. doi: 10.1371/journal.pgen.1007322
Du, F. Y., Ju, G. L., Xiao, L., Zhou, Y. M., and Wu, X. (2020). Sesquiterpenes and cyclodepsipeptides from marine-derived fungus Trichoderma longibrachiatum and their antagonistic activities against soil-borne pathogens. Mar. Drugs 18:165. doi: 10.3390/md18030165
Dugassa, A., Alemu, T., and Woldehawariat, Y. (2021). In-vitro compatibility assay of indigenous Trichoderma and Pseudomonas species and their antagonistic activities against black root rot disease (Fusarium solani) of faba bean (Vicia faba L.). BMC Microbiol. 21:115. doi: 10.1186/s12866-021-02181-7
El-Hasan, A., Walker, F., Klaiber, I., Schöne, J., Pfannstiel, J., and Voegele, R. T. (2022). New approaches to manage Asian soybean rust (Phakopsora pachyrhizi) using Trichoderma spp. or their antifungal secondary metabolites. Meta 12:507. doi: 10.3390/metabo12060507
Fan, H., Yao, M., Wang, H., Zhao, D., Zhu, X., Wang, Y., et al. (2020). Isolation and effect of Trichoderma citrinoviride Snef1910 for the biological control of root-knot nematode, Meloidogyne incognita. BMC Microbiol. 20:299. doi: 10.1186/s12866-020-01984-4
Ferreira Filho, J. A., Horta, M. A. C., Dos Santos, C. A., Almeida, D. A., Murad, N. F., Mendes, J. S., et al. (2020). Integrative genomic analysis of the bioprospection of regulators and accessory enzymes associated with cellulose degradation in a filamentous fungus (Trichoderma harzianum). BMC Genomics 21:757. doi: 10.1186/s12864-020-07158-w
Filizola, P., Luna, M., de Souza, A. F., Coelho, I. L., Laranjeira, D., and Campos-Takaki, G. M. (2019). Biodiversity and phylogeny of novel Trichoderma isolates from mangrove sediments and potential of biocontrol against Fusarium strains. Microb. Cell Factories 18:89. doi: 10.1186/s12934-019-1108-y
Fiorentino, N., Ventorino, V., Woo, S. L., Pepe, O., De Rosa, A., Gioia, L., et al. (2018). Trichoderma-based biostimulants modulate rhizosphere microbial populations and improve N uptake efficiency, yield, and nutritional quality of leafy vegetables. Front. Plant Sci. 9:743. doi: 10.3389/fpls.2018.00743
Fontana, D. C., de Paula, S., Torres, A. G., de Souza, V., Pascholati, S. F., Schmidt, D., et al. (2021). Endophytic fungi: biological control and induced resistance to phytopathogens and abiotic stresses. Pathogens 10:570. doi: 10.3390/pathogens10050570
Forghani, F., and Hajihassani, A. (2020). Recent advances in the development of environmentally benign treatments to control root-knot nematodes. Front. Plant Sci. 11:1125. doi: 10.3389/fpls.2020.01125
Girma, A. (2022). In vitro biocontrol evaluation of some selected Trichoderma strains against the root pathogen Fusarium oxysporum of hot pepper (Capsicum annum L.) in Bure Woreda, Ethiopia. Int. J. Microbiol. 2022:1664116. doi: 10.1155/2022/1664116
Gomes, E. V., Costa, M., de Paula, R. G., de Azevedo, R. R., da Silva, F. L., Noronha, E. F., et al. (2015). The Cerato-Platanin protein Epl-1 from Trichoderma harzianum is involved in mycoparasitism, plant resistance induction and self-cell wall protection. Sci. Rep. 5:17998. doi: 10.1038/srep17998
González-López, M., Jijón-Moreno, S., Dautt-Castro, M., Ovando-Vázquez, C., Ziv, T., Horwitz, B. A., et al. (2021). Secretome analysis of Arabidopsis-Trichoderma atroviride interaction unveils new roles for the plant glutamate: Glyoxylate aminotransferase GGAT1 in plant growth induced by the fungus and resistance against Botrytis cinerea. Int. J. Mol. Sci. 22:6804. doi: 10.3390/ijms22136804
Guo, Y., Ghirardo, A., Weber, B., Schnitzler, J. P., Benz, J. P., and Rosenkranz, M. (2019). Trichoderma species differ in their volatile profiles and in antagonism toward ectomycorrhiza Laccaria bicolor. Front. Microbiol. 10:891. doi: 10.3389/fmicb.2019.00891
Guzmán-Guzmán, P., Alemán-Duarte, M. I., Delaye, L., Herrera-Estrella, A., and Olmedo-Monfil, V. (2017). Identification of effector- like proteins in Trichoderma spp. and role of a hydrophobin in the plant-fungus interaction and mycoparasitism. BMC Genet. 18:16. doi: 10.1186/s12863-017-0481-y
Halifu, S., Deng, X., Song, X., Song, R., and Liang, X. (2020). Inhibitory mechanism of Trichoderma virens ZT05 on Rhizoctonia solani. Plan. Theory 9:912. doi: 10.3390/plants9070912
Haouhach, S., Karkachi, N., Oguiba, B., Sidaoui, A., Chamorro, I., Kihal, M., et al. (2020). Three new reports of Trichoderma in Algeria: T. atrobrunneum, (South) T. longibrachiatum (South), and T. afroharzianum (Northwest). Microorganisms 8:1455. doi: 10.3390/microorganisms8101455
Harman, G. E. (2000). Myths and dogmas of biocontrol changes in perceptions derived from research on Trichoderma harzinum T-22. Plant Dis. 84, 377–393. doi: 10.1094/PDIS.2000.84.4.377
Harman, G., Khadka, R., Doni, F., and Uphoff, N. (2021). Benefits to plant health and productivity from enhancing plant microbial symbionts. Front. Plant Sci. 11:610065. doi: 10.3389/fpls.2020.610065
He, C., Wang, W., and Hou, J. (2020). Plant performance of enhancing licorice with dual inoculating dark septate endophytes and Trichoderma viride mediated via effects on root development. BMC Plant Biol. 20:325. doi: 10.1186/s12870-020-02535-9
Hernández,. , Cazapal-Monteiro, C. F., Arroyo, F. L., Silva, M. I., Palomero, A. M., Paz-Silva, A., et al. (2018). Biological control of soil transmitted helminths (STHs) in a zoological park by using saprophytic fungi. Biol Control, 122, 24–30.
Herrera-Téllez, V. I., Cruz-Olmedo, A. K., Plasencia, J., Gavilanes-Ruíz, M., Arce-Cervantes, O., Hernández-León, S., et al. (2019). The protective effect of Trichoderma asperellum on tomato plants against Fusarium oxysporum and Botrytis cinerea diseases involves inhibition of reactive oxygen species production. Int. J. Mol. Sci. 20:2007. doi: 10.3390/ijms20082007
Hinterdobler, W., Li, G., Spiegel, K., Basyouni-Khamis, S., Gorfer, M., and Schmoll, M. (2021). Trichoderma reesei isolated from Austrian soil with high potential for biotechnological application. Front. Microbiol. 12:552301. doi: 10.3389/fmicb.2021.552301
Illescas, M., Morán-Diez, M. E., Martínez de Alba, Á. E., Hermosa, R., and Monte, E. (2022). Effect of Trichoderma asperellum on wheat plants' biochemical and molecular responses, and yield under different water stress conditions. Int. J. Mol. Sci. 23:6782. doi: 10.3390/ijms23126782
Intana, W., Kheawleng, S., and Sunpapao, A. (2021). Trichoderma asperellum T76-14 released volatile organic compounds against postharvest fruit rot in muskmelons (Cucumis melo) caused by Fusarium incarnatum. J. Fungi 7:46. doi: 10.3390/jof7010046
Intana, W., Wonglom, P., Suwannarach, N., and Sunpapao, A. (2022). Trichoderma asperelloides PSU-P1 induced expression of pathogenesis-related protein genes against gummy stem blight of muskmelon (Cucumis melo) in field evaluation. J. Fungi 8:156. doi: 10.3390/jof8020156
Izquierdo-García, L. F., González-Almario, A., Cotes, A. M., and Moreno-Velandia, C. A. (2020). Trichoderma virens Gl006 and Bacillus velezensis Bs006: a compatible interaction controlling Fusarium wilt of cape gooseberry. Sci. Rep. 10:6857. doi: 10.1038/s41598-020-63689-y
Jaiswal, A. K., Mengiste, T. D., Myers, J. R., Egel, D. S., and Hoagland, L. A. (2020). Tomato domestication attenuated responsiveness to a beneficial soil microbe for plant growth promotion and induction of systemic resistance to foliar pathogens. Front. Microbiol. 11:604566. doi: 10.3389/fmicb.2020.604566
Ji, S., Liu, Z., and Wang, Y. (2021). Trichoderma-induced ethylene responsive factor MsERF105 mediates defense responses in Malus sieversii. Front. Plant Sci. 12:708010. doi: 10.3389/fpls.2021.708010
Jogaiah, S., Abdelrahman, M., Tran, L. P., and Ito, S. I. (2018). Different mechanisms of Trichoderma virens-mediated resistance in tomato against Fusarium wilt involve the jasmonic and salicylic acid pathways. Mol. Plant Pathol. 19, 870–882. doi: 10.1111/mpp.12571
Joo, J. H., and Hussein, K. A. (2022). Biological control and plant growth promotion properties of volatile organic compound-producing antagonistic Trichoderma spp. Front. Plant Sci. 13:897668. doi: 10.3389/fpls.2022.897668
Kakabouki, I., Tataridas, A., Mavroeidis, A., Kousta, A., Karydogianni, S., Zisi, C., et al. (2021). Effect of colonization of Trichoderma harzianum on growth development and CBD content of hemp (Cannabis sativa L.). Microorganisms 9:518. doi: 10.3390/microorganisms9030518
Kappel, L., Kosa, N., and Gruber, S. (2022). The multilateral efficacy of chitosan and Trichoderma on sugar beet. J. Fungi 8:137. doi: 10.3390/jof8020137
Kappel, L., Münsterkötter, M., Sipos, G., Escobar Rodriguez, C., and Gruber, S. (2020). Chitin and chitosan remodeling defines vegetative development and Trichoderma biocontrol. PLoS Pathog. 16:e1008320. doi: 10.1371/journal.ppat.1008320
Karimi Aghcheh, R., Druzhinina, I. S., and Kubicek, C. P. (2013). The putative protein methyltransferase LAE1 of Trichoderma atroviride is a key regulator of asexual development and mycoparasitism. PLoS One 8:e67144. doi: 10.1371/journal.pone.0067144
Karuppiah, V., Sun, J., Li, T., Vallikkannu, M., and Chen, J. (2019a). Co-cultivation of Trichoderma asperellum GDFS1009 and Bacillus amyloliquefaciens 1841 causes differential gene expression and improvement in the wheat growth and biocontrol activity. Front. Microbiol. 10:1068. doi: 10.3389/fmicb.2019.01068
Karuppiah, V., Vallikkannu, M., Li, T., and Chen, J. (2019b). Simultaneous and sequential based co-fermentations of Trichoderma asperellum GDFS1009 and Bacillus amyloliquefaciens 1841: a strategy to enhance the gene expression and metabolites to improve the bio-control and plant growth promoting activity. Microb. Cell Fact. 18:185. doi: 10.1186/s12934-019-1233-7
Karuppiah, V., Zhixiang, L., Liu, H., Vallikkannu, M., and Chen, J. (2021). Co-culture of Vel1-over- expressed Trichoderma asperellum and Bacillus amyloliquefaciens: an eco-friendly strategy to hydrolyze the lignocellulose biomass in soil to enrich the soil fertility, plant growth and disease resistance. Microb. Cell Factories 20:57. doi: 10.1186/s12934-021-01540-3
Khan, R., Najeeb, S., Mao, Z., Ling, J., Yang, Y., Li, Y., et al. (2020). Bioactive secondary metabolites from Trichoderma spp. against phytopathogenic bacteria and root-knot nematode. Microorganisms 8:401. doi: 10.3390/microorganisms8030401
Köhl, J., Kolnaar, R., and Ravensberg, W. J. (2019). Mode of action of microbial biological control agents against plant diseases: relevance beyond efficacy. Front. Plant Sci. 10:845. doi: 10.3389/fpls.2019.00845
Kong, W. L., Ni, H., Wang, W. Y., and Wu, X. Q. (2022). Antifungal effects of volatile organic compounds produced by Trichoderma koningiopsis T2 against Verticillium dahliae. Front. Microbiol. 13:1013468. doi: 10.3389/fmicb.2022.1013468
Kottb, M., Gigolashvili, T., Großkinsky, D. K., and Piechulla, B. (2015). Trichoderma volatiles effecting Arabidopsis: from inhibition to protection against phytopathogenic fungi. Front. Microbiol. 6:995. doi: 10.3389/fmicb.2015.00995
Kovács, C., Csótó, A., Pál, K., Nagy, A., Fekete, E., Karaffa, L., et al. (2021). The biocontrol potential of endophytic Trichoderma fungi isolated from Hungarian grapevines. Part I. isolation, identification and in vitro studies. Pathogens 10:1612. doi: 10.3390/pathogens10121612
Kubicek, C. P., Steindorff, A. S., Chenthamara, K., Manganiello, G., Henrissat, B., Zhang, J., et al. (2019). Evolution and comparative genomics of the most common Trichoderma species. BMC Genomics 20:485. doi: 10.1186/s12864-019-5680-7
Lamdan, N. L., Shalaby, S., Ziv, T., Kenerley, C. M., and Horwitz, B. A. (2015). Secretome of Trichoderma interacting with maize roots: role in induced systemic resistance. Mol. Cell. Proteomics 14, 1054–1063. doi: 10.1074/mcp.M114.046607
Lazazzara, V., Vicelli, B., Bueschl, C., Parich, A., Pertot, I., Schuhmacher, R., et al. (2021). Trichoderma spp. volatile organic compounds protect grapevine plants by activating defense-related processes against downy mildew. Physiol. Plant. 172, 1950–1965. doi: 10.1111/ppl.13406
Leal, C., Richet, N., Guise, J. F., Gramaje, D., Armengol, J., Fontaine, F., et al. (2021). Cultivar contributes to the beneficial effects of Bacillus subtilis PTA-271 and Trichoderma atroviride SC1 to protect grapevine against Neofusicoccum parvum. Front. Microbiol. 12:726132. doi: 10.3389/fmicb.2021.726132
Li, N., Alfiky, A., Wang, W., Islam, M., Nourollahi, K., Liu, X., et al. (2018). Volatile compound- mediated recognition and inhibition between Trichoderma biocontrol agents and Fusarium oxysporum. Front. Microbiol. 9:2614. doi: 10.3389/fmicb.2018.02614
Li, X., Leng, J., Yu, L., Bai, H., Li, X., Wisniewski, M., et al. (2022). Efficacy of the biocontrol agent Trichoderma hamatum against Lasiodiplodia theobromae on macadamia. Front. Microbiol. 13:994422. doi: 10.3389/fmicb.2022.994422
Li, W. C., Lin, T. C., Chen, C. L., Liu, H. C., Lin, H. N., Chao, J. L., et al. (2021). Complete genome sequences and genome-wide characterization of Trichoderma biocontrol agents provide new insights into their evolution and variation in genome organization, sexual development, and fungal-plant interactions. Microbiol. Spectr. 9:e0066321. doi: 10.1128/Spectrum.00663-21
Li, J., Philp, J., Li, J., Wei, Y., Li, H., Yang, K., et al. (2020). Trichoderma harzianum inoculation reduces the incidence of Clubroot disease in Chinese cabbage by regulating the rhizosphere microbial community. Microorganisms 8:1325. doi: 10.3390/microorganisms8091325
Li, M., Ren, Y., He, C., Yao, J., Wei, M., and He, X. (2022). Complementary effects of dark septate endophytes and Trichoderma strains on growth and active ingredient accumulation of Astragalus mongholicus under drought stress. J. Fungi 8:920. doi: 10.3390/jof8090920
Liu, H., Hao, D., Li, Y., Wang, X., and Chen, J. (2022). Approaches for the establishment of optimized co-culture system of multiple Trichoderma strains for culture metabolites highly effective in cucumber growth promotion. Front. Microbiol. 13:1020077. doi: 10.3389/fmicb.2022.1020077
Liu, Y., He, P., He, P., Munir, S., Ahmed, A., Wu, Y., et al. (2022). Potential biocontrol efficiency of Trichoderma species against oomycete pathogens. Front. Microbiol. 13:974024. doi: 10.3389/fmicb.2022.974024
Lombardi, N., Caira, S., Troise, A. D., Scaloni, A., Vitaglione, P., Vinale, F., et al. (2020a). Trichoderma applications on strawberry plants modulate the physiological processes positively affecting fruit production and quality. Front. Microbiol. 11:1364. doi: 10.3389/fmicb.2020.01364
Lombardi, N., Salzano, A. M., Troise, A. D., Scaloni, A., Vitaglione, P., Vinale, F., et al. (2020b). Effect of Trichoderma bioactive metabolite treatments on the production, quality, and protein profile of strawberry fruits. J. Agric. Food Chem. 68, 7246–7258. doi: 10.1021/acs.jafc.0c01438
López, A. C., Alvarenga, A. E., Zapata, P. D., Luna, M. F., and Villalba, L. L. (2019). Trichoderma spp. from Misiones, Argentina: effective fungi to promote plant growth of the regional crop Ilex paraguariensis St. Hil. Mycology 10, 210–221. doi: 10.1080/21501203.2019.1606860
Mahmoud, G. A., Abdel-Sater, M. A., Al-Amery, E., and Hussein, N. A. (2021). Controlling Alternaria cerealis MT808477 tomato phytopathogen by Trichoderma harzianum and tracking the plant physiological changes. Plan. Theory 10:1846. doi: 10.3390/plants10091846
Malmierca, M. G., Cardoza, R. E., Alexander, N. J., McCormick, S. P., Hermosa, R., Monte, E., et al. (2012). Involvement of Trichoderma trichothecenes in the biocontrol activity and induction of plant defense-related genes. Appl. Environ. Microbiol. 78, 4856–4868. doi: 10.1128/AEM.00385-12
Manganiello, G., Nicastro, N., Caputo, M., Zaccardelli, M., Cardi, T., and Pane, C. (2021). Functional hyperspectral imaging by high-related vegetation indices to track the wide-spectrum Trichoderma biocontrol activity against soil-borne diseases of baby-leaf vegetables. Front. Plant Sci. 12:630059. doi: 10.3389/fpls.2021.630059
Manganiello, G., Sacco, A., Ercolano, M. R., Vinale, F., Lanzuise, S., Pascale, A., et al. (2018). Modulation of tomato response to Rhizoctonia solani by Trichoderma harzianum and its secondary metabolite harzianic acid. Front. Microbiol. 9:1966. doi: 10.3389/fmicb.2018.01966
Manzar, N., Kashyap, A. S., Goutam, R. S., Rajawat, M. V. S., Sharma, P. K., Sharma, S. K., et al. (2022). Trichoderma: advent of versatile biocontrol agent, its secrets and insights into mechanism of biocontrol potential. Sustainability 14:12786. doi: 10.3390/su141912786
Marik, T., Tyagi, C., Balázs, D., Urbán, P., Szepesi, Á., Bakacsy, L., et al. (2019). Structural diversity and bioactivities of peptaibol compounds from the longibrachiatum clade of the filamentous fungal genus Trichoderma. Front. Microbiol. 10:1434. doi: 10.3389/fmicb.2019.01434
Marra, R., Lombardi, N., Piccolo, A., Bazghaleh, N., Prashar, P., Vandenberg, A., et al. (2021). Mineral biofortification and growth stimulation of lentil plants inoculated with Trichoderma strains and metabolites. Microorganisms 10:87. doi: 10.3390/microorganisms10010087
Marraschi, R., Ferreira, A., da Silva Bueno, R. N., Leite, J., Lucon, C., Harakava, R., et al. (2019). A protocol for selection of Trichoderma spp. to protect grapevine pruning wounds against Lasiodiplodia theobromae. Braz. J. Microbiol. 50, 213–221. doi: 10.1007/s42770-018-0029-y
Martínez-Medina, A., Fernández, I., Sánchez-Guzmán, M. J., Jung, S. C., Pascual, J. A., and Pozo, M. J. (2013). Deciphering the hormonal signalling network behind the systemic resistance induced by Trichoderma harzianum in tomato. Front. Plant Sci. 4:206. doi: 10.3389/fpls.2013.00206
Martínez-Salgado, S. J., Andrade-Hoyos, P., Parraguirre Lezama, C., Rivera-Tapia, A., Luna-Cruz, A., and Romero-Arenas, O. (2021). Biological control of charcoal rot in peanut crop through strains of Trichoderma spp., in Puebla, Mexico. Plan. Theory 10:2630. doi: 10.3390/plants10122630
Maruyama, C. R., Bilesky-José, N., de Lima, R., and Fraceto, L. F. (2020). Encapsulation of Trichoderma harzianum preserves enzymatic activity and enhances the potential for biological control. Front. Bioeng. Biotechnol. 8:225. doi: 10.3389/fbioe.2020.00225
Matas-Baca, M. Á., Urías García, C., Pérez-Álvarez, S., Flores-Córdova, M. A., Escobedo- Bonilla, C. M., Magallanes-Tapia, M. A., et al. (2022). Morphological and molecular characterization of a new autochthonous Trichoderma sp. isolate and its biocontrol efficacy against Alternaria sp. Saudi. J. Biol. Sci. 29, 2620–2625. doi: 10.1016/j.sjbs.2021.12.052
Mayo, S., Gutiérrez, S., Malmierca, M. G., Lorenzana, A., Campelo, M. P., Hermosa, R., et al. (2015). Influence of Rhizoctonia solani and Trichoderma spp. in growth of bean (Phaseolus vulgaris L.) and in the induction of plant defense-related genes. Front. Plant Sci. 6:685. doi: 10.3389/fpls.2015.00685
Mayo-Prieto, S., Marra, R., Vinale, F., Rodríguez-González, Á., Woo, S. L., Lorito, M., et al. (2019). Effect of Trichoderma velutinum and Rhizoctonia solani on the metabolome of bean plants (Phaseolus vulgaris L.). Int. J. Mol. Sci. 20:549. doi: 10.3390/ijms20030549
Mohiddin, F. A., Padder, S. A., Bhat, A. H., Ahanger, M. A., Shikari, A. B., Wani, S. H., et al. (2021). Phylogeny and optimization of Trichoderma harzianum for Chitinase production: evaluation of their antifungal behaviour against the prominent soil borne Phyto-pathogens of temperate India. Microorganisms 9:1962. doi: 10.3390/microorganisms9091962
Monfil, V. O., and Casas-Flores, S. (2014). Molecular mechanisms of biocontrol in Trichoderma spp. and their applications in agriculture. Biotechnol. Biol. Trichoderma 8:447. doi: 10.1016/B978-0-444-59576-8.00032-1
Moo-Koh, F. A., Cristóbal-Alejo, J., Andrés, M. F., Martín, J., Reyes, F., Tun-Suárez, J. M., et al. (2022). In vitro assessment of organic and residual fractions of nematicidal culture filtrates from thirteen tropical Trichoderma strains and metabolic profiles of most-active. J. Fungi 8:82. doi: 10.3390/jof8010082
Morán-Diez, M. E., Carrero-Carrón, I., Rubio, M. B., Jiménez-Díaz, R. M., Monte, E., and Hermosa, R. (2019). Transcriptomic analysis of Trichoderma atroviride overgrowing plant-wilting Verticillium dahliae reveals the role of a new M14 metallocarboxypeptidase CPA1 in biocontrol. Front. Microbiol. 10:1120. doi: 10.3389/fmicb.2019.01120
Morán-Diez, M. E., Tranque, E., Bettiol, W., Monte, E., and Hermosa, R. (2020). Differential response of tomato plants to the application of three Trichoderma species when evaluating the control of Pseudomonas syringae populations. Plan. Theory 9:626. doi: 10.3390/plants9050626
Moreno-Ruiz, D., Lichius, A., Turrà, D., Di Pietro, A., and Zeilinger, S. (2020). Chemotropism assays for plant symbiosis and mycoparasitism related compound screening in Trichoderma atroviride. Front. Microbiol. 11:601251. doi: 10.3389/fmicb.2020.601251
Mulatu, A., Alemu, T., Megersa, N., and Vetukuri, R. R. (2021). Optimization of culture conditions and production of bio-fungicides from Trichoderma species under solid-state fermentation using mathematical modeling. Microorganisms 9:1675. doi: 10.3390/microorganisms9081675
Naglot, A., Goswami, S., Rahman, I., Shrimali, D. D., Yadav, K. K., Gupta, V. K., et al. (2015). Antagonistic potential of native Trichoderma viride strain against potent tea fungal pathogens in north East India. Plant Pathol. J. 31, 278–289. doi: 10.5423/PPJ.OA.01.2015.0004
Navazio, L., Baldan, B., Moscatiello, R., Zuppini, A., Woo, S. L., Mariani, P., et al. (2007). Calcium- mediated perception and defense responses activated in plant cells by metabolite mixtures secreted by the biocontrol fungus Trichoderma atroviride. BMC Plant Biol. 7:41. doi: 10.1186/1471-2229-7-41
Nawrocka, J., Gromek, A., and Małolepsza, U. (2019). Nitric oxide as a beneficial signaling molecule in Trichoderma atroviride TRS25- induced systemic defense responses of cucumber plants against Rhizoctonia solani. Front. Plant Sci. 10:421. doi: 10.3389/fpls.2019.00421
Nawrocka, J., Małolepsza, U., Szymczak, K., and Szczech, M. (2018). Involvement of metabolic components, volatile compounds, PR proteins, and mechanical strengthening in multilayer protection of cucumber plants against Rhizoctonia solani activated by Trichoderma atroviride TRS25. Protoplasma 255, 359–373. doi: 10.1007/s00709-017-1157-1
Ngo, M. T., Nguyen, M. V., Han, J. W., Park, M. S., Kim, H., and Choi, G. J. (2021). In vitro and in vivo antifungal activity of Sorbicillinoids produced by Trichoderma longibrachiatum. J. Fungi 7:428. doi: 10.3390/jof7060428
Nieto-Jacobo, M. F., Steyaert, J. M., Salazar-Badillo, F. B., Nguyen, D. V., Rostás, M., Braithwaite, M., et al. (2017). Environmental growth conditions of Trichoderma spp. affects Indole acetic acid derivatives, volatile organic compounds, and plant growth promotion. Front. Plant Sci. 8:102. doi: 10.3389/fpls.2017.00102
Niu, B., Wang, W., Yuan, Z., Sederoff, R. R., Sederoff, H., Chiang, V. L., et al. (2020). Microbial interactions within multiple-strain biological control agents impact soil-borne plant disease. Front. Microbiol. 11:585404. doi: 10.3389/fmicb.2020.585404
Nuangmek, W., Aiduang, W., Kumla, J., Lumyong, S., and Suwannarach, N. (2021). Evaluation of a newly identified endophytic fungus, Trichoderma phayaoense for plant growth promotion and biological control of gummy stem blight and wilt of muskmelon. Front. Microbiol. 12:634772. doi: 10.3389/fmicb.2021.634772
Organo, N. D., Granada, S., Pineda, H., Sandro, J. M., Nguyen, V. H., and Gummert, M. (2022). Assessing the potential of a Trichoderma-based compost activator to hasten the decomposition of incorporated rice straw. Sci. Rep. 12:448. doi: 10.1038/s41598-021-03828-1
Oszust, K., Cybulska, J., and Frąc, M. (2020). How do Trichoderma genus fungi win a nutritional competition battle against soft fruit pathogens? A report on niche overlap nutritional potentiates. Int. J. Mol. Sci. 21:4235. doi: 10.3390/ijms21124235
Panchalingam, H., Powell, D., Adra, C., Foster, K., Tomlin, R., Quigley, B. L., et al. (2022). Assessing the various antagonistic mechanisms of Trichoderma strains against the brown root rot pathogen Pyrrhoderma noxium infecting heritage fig trees. J. Fungi 8:1105. doi: 10.3390/jof8101105
Park, Y. H., Chandra Mishra, R., Yoon, S., Kim, H., Park, C., Seo, S. T., et al. (2019). Endophytic Trichoderma citrinoviride isolated from mountain-cultivated ginseng (Panax ginseng) has great potential as a biocontrol agent against ginseng pathogens. J. Ginseng Res. 43, 408–420. doi: 10.1016/j.jgr.2018.03.002
Pescador, L., Fernandez, I., Pozo, M. J., Romero-Puertas, M. C., Pieterse, C., and Martínez- Medina, A. (2022). Nitric oxide signalling in roots is required for MYB72-dependent systemic resistance induced by Trichoderma volatile compounds in Arabidopsis. J. Exp. Bot. 73, 584–595. doi: 10.1093/jxb/erab294
Phoka, N., Suwannarach, N., Lumyong, S., Ito, S. I., Matsui, K., Arikit, S., et al. (2020). Role of volatiles from the endophytic fungus Trichoderma asperelloides PSU-P1 in biocontrol potential and in promoting the plant growth of Arabidopsis thaliana. J. Fungi 6:341. doi: 10.3390/jof6040341
Pocurull, M., Fullana, A. M., Ferro, M., Valero, P., Escudero, N., Saus, E., et al. (2020). Commercial formulates of Trichoderma induce systemic plant resistance to Meloidogyne incognita in tomato and the effect is additive to that of the Mi-1.2 resistance gene. Front. Microbiol. 10:3042. doi: 10.3389/fmicb.2019.03042
Pollard-Flamand, J., Boulé, J., Hart, M., and Úrbez-Torres, J. R. (2022). Biocontrol activity of Trichoderma species isolated from grapevines in British Columbia against botryosphaeria dieback fungal pathogens. J. Fungi 8:409. doi: 10.3390/jof8040409
Poveda, J., Abril-Urias, P., and Escobar, C. (2020). Biological control of plant-parasitic nematodes by filamentous fungi inducers of resistance: Trichoderma, Mycorrhizal and Endophytic Fungi. Front. Microbiol. 11:992. doi: 10.3389/fmicb.2020.00992
Poveda, J., Hermosa, R., Monte, E., and Nicolás, C. (2019). Trichoderma harzianum favours the access of arbuscular mycorrhizal fungi to non-host Brassicaceae roots and increases plant productivity. Sci. Rep. 9:11650. doi: 10.1038/s41598-019-48269-z
Rao, Y., Zeng, L., Jiang, H., Mei, L., and Wang, Y. (2022). Trichoderma atroviride LZ42 releases volatile organic compounds promoting plant growth and suppressing Fusarium wilt disease in tomato seedlings. BMC Microbiol. 22:88. doi: 10.1186/s12866-022-02511-3
Rashmi, S., Maurya, S., and Upadhyay, R. S. (2016). The improvement of competitive saprophytic capabilities of Trichoderma species through the use of chemical mutagens. Braz. J. Microbiol. 47, 10–17. doi: 10.1016/j.bjm.2015.11.003
Rawal, R., Scheerens, J. C., Fenstemaker, S. M., Francis, D. M., Miller, S. A., and Benitez, M. S. (2022). Novel Trichoderma isolates alleviate water deficit stress in susceptible tomato genotypes. Front. Plant Sci. 13:869090. doi: 10.3389/fpls.2022.869090
Rees, H. J., Drakulic, J., Cromey, M. G., Bailey, A. M., and Foster, G. D. (2022). Endophytic Trichoderma spp. can protect strawberry and privet plants from infection by the fungus Armillaria mellea. PLoS One 17:e0271622. doi: 10.1371/journal.pone.0271622
Risoli, S., Cotrozzi, L., Sarrocco, S., Nuzzaci, M., Pellegrini, E., and Vitti, A. (2022). Trichoderma-induced resistance to Botrytis cinerea in Solanum species: a meta-analysis. Plan. Theory 11:180. doi: 10.3390/plants11020180
Ruangwong, O. U., Pornsuriya, C., Pitija, K., and Sunpapao, A. (2021a). Biocontrol mechanisms of Trichoderma koningiopsis PSU3-2 against postharvest anthracnose of chili pepper. J. Fungi 7:276. doi: 10.3390/jof7040276
Ruangwong, O. U., Wonglom, P., Suwannarach, N., Kumla, J., Thaochan, N., Chomnunti, P., et al. (2021b). Volatile organic compound from Trichoderma asperelloides TSU1: impact on plant pathogenic fungi. J. Fungi 7:187. doi: 10.3390/jof7030187
Rubio, M. B., Quijada, N. M., Pérez, E., Domínguez, S., Monte, E., and Hermosa, R. (2014). Identifying beneficial qualities of Trichoderma parareesei for plants. Appl. Environ. Microbiol. 80, 1864–1873. doi: 10.1128/AEM.03375-13
Samuelian, S. (2016). Potential of Trichoderma harzianum for control of banana leaf fungal pathogens when applied with a food source and an organic adjuvant. 3 Biotech 6:8. doi: 10.1007/s13205-015-0327-0
Samuels, G. J., Dodd, S. L., Lu, B. S., Petrini, O., Schroers, H. J., and Druzhinina, I. S. (2006). The Trichoderma koningii aggregate species. Stud. Mycol. 56, 67–133. doi: 10.3114/sim.2006.56.03
Sánchez-Montesinos, B., Santos, M., Moreno-Gavíra, A., Marín-Rodulfo, T., Gea, F. J., and Diánez, F. (2021). Biological control of fungal diseases by Trichoderma aggressivum f. europaeum and its compatibility with fungicides. J. Fungi 7:598. doi: 10.3390/jof7080598
Santos, M., Santos, L., Costa, D., Vieira, T. A., and Lustosa, D. C. (2020). Trichoderma spp. on treatment of Handroanthus serratifolius seeds: effect on seedling germination and development. Heliyon 6:e04044. doi: 10.1016/j.heliyon.2020.e04044
Saravanakumar, K., Fan, L., Fu, K., Yu, C., Wang, M., Xia, H., et al. (2016). Cellulase from Trichoderma harzianum interacts with roots and triggers induced systemic resistance to foliar disease in maize. Sci. Rep. 6:35543. doi: 10.1038/srep35543
Saravanakumar, K., Li, Y., Yu, C., Wang, Q. Q., Wang, M., Sun, J., et al. (2017). Effect of Trichoderma harzianum on maize rhizosphere microbiome and biocontrol of Fusarium stalk rot. Sci. Rep. 7:1771. doi: 10.1038/s41598-017-01680-w
Şesan, T. E., Oancea, A. O., Ştefan, L. M., Mănoiu, V. S., Ghiurea, M., Răut, I., et al. (2020). Effects of foliar treatment with a Trichoderma plant biostimulant consortium on Passiflora caerulea L. yield and quality. Microorganisms 8:123. doi: 10.3390/microorganisms8010123
Shaw, S., Le Cocq, K., Paszkiewicz, K., Moore, K., Winsbury, R., de Torres Zabala, M., et al. (2016). Transcriptional reprogramming underpins enhanced plant growth promotion by the biocontrol fungus Trichoderma hamatum GD12 during antagonistic interactions with Sclerotinia sclerotiorum in soil. Mol. Plant Pathol. 17, 1425–1441. doi: 10.1111/mpp.12429
Shobha, B., Lakshmeesha, T. R., Ansari, M. A., Almatroudi, A., Alzohairy, M. A., Basavaraju, S., et al. (2020). Mycosynthesis of ZnO nanoparticles using Trichoderma spp. isolated from rhizosphere soils and its synergistic antibacterial effect against Xanthomonas oryzae pv. oryzae. J. Fungi 6:181. doi: 10.3390/jof6030181
Shoresh, M., and Harman, G. E. (2010). Differential expression of maize chitinases in the presence or absence of Trichoderma harzianum strain T22 and indications of a novel exo-endo-heterodimeric chitinase activity. BMC Plant Biol. 10, 136–111. doi: 10.1186/1471-2229-10-136
Singh, B. N., Dwivedi, P., Sarma, B. K., Singh, G. S., and Singh, H. B. (2019). A novel function of N-signaling in plants with special reference to Trichoderma interaction influencing plant growth, nitrogen use efficiency, and cross talk with plant hormones. 3 Biotech 9:109. doi: 10.1007/s13205-019-1638-3
Sokhandani, Z., Moosavi, M. R., and Basirnia, T. (2016). Optimum concentrations of Trichoderma longibrachiatum and cadusafos for controlling Meloidogyne javanica on Zucchini plants. J. Nematol. 48, 54–63. doi: 10.21307/jofnem-2017-009
Stracquadanio, C., Quiles, J. M., Meca, G., and Cacciola, S. O. (2020). Antifungal activity of bioactive metabolites produced by Trichoderma asperellum and Trichoderma atroviride in liquid medium. J. Fungi 6:263. doi: 10.3390/jof6040263
Sui, L., Li, J., Philp, J., Yang, K., Wei, Y., Li, H., et al. (2022). Trichoderma atroviride seed dressing influenced the fungal community and pathogenic fungi in the wheat rhizosphere. Sci. Rep. 12:9677. doi: 10.1038/s41598-022-13669-1
Sun, J., Karuppiah, V., Li, Y., Pandian, S., Kumaran, S., and Chen, J. (2022). Role of cytochrome P450 genes of Trichoderma atroviride T23 on the resistance and degradation of dichlorvos. Chemosphere 290:133173. doi: 10.1016/j.chemosphere.2021.133173
Sun, R. Y., Liu, Z. C., Fu, K., Fan, L., and Chen, J. (2012). Trichoderma biodiversity in China. J. Appl. Genet. 53, 343–354. doi: 10.1007/s13353-012-0093-1
Swain, H., Adak, T., Mukherjee, A. K., Sarangi, S., Samal, P., Khandual, A., et al. (2021). Seed biopriming with Trichoderma strains isolated from tree bark improves plant growth, antioxidative defense system in Rice and enhance straw degradation capacity. Front. Microbiol. 12:633881. doi: 10.3389/fmicb.2021.633881
Tamizi, A. A., Mat-Amin, N., Weaver, J. A., Olumakaiye, R. T., Akbar, M. A., Jin, S., et al. (2022). Genome sequencing and analysis of Trichoderma (Hypocreaceae) isolates exhibiting antagonistic activity against the papaya dieback pathogen, Erwinia mallotivora. J. Fungi 8:246. doi: 10.3390/jof8030246
Thambugala, K. M., Daranagama, D. A., Phillips, A., Kannangara, S. D., and Promputtha, I. (2020). Fungi vs. fungi in biocontrol: an overview of fungal antagonists applied against fungal plant pathogens. Front. Cell. Infect. Microbiol. 10:604923. doi: 10.3389/fcimb.2020.604923
Tian, Y., Tan, Y., Liu, N., Yan, Z., Liao, Y., Chen, J., et al. (2016). Detoxification of deoxynivalenol via glycosylation represents novel insights on antagonistic activities of Trichoderma when confronted with Fusarium graminearum. Toxins 8:335. doi: 10.3390/toxins8110335
Tian, Y., Tan, Y., Yan, Z., Liao, Y., Chen, J., De Boevre, M., et al. (2018). Antagonistic and detoxification potentials of Trichoderma isolates for control of zearalenone (ZEN) producing Fusarium graminearum. Front. Microbiol. 8:2710. doi: 10.3389/fmicb.2017.02710
Tilocca, B., Cao, A., and Migheli, Q. (2020). Scent of a killer: microbial volatilome and its role in the biological control of plant pathogens. Front. Microbiol. 11:41. doi: 10.3389/fmicb.2020.00041
Tseng, Y. H., Rouina, H., Groten, K., Rajani, P., Furch, A., Reichelt, M., et al. (2020). An endophytic Trichoderma strain promotes growth of its hosts and defends against pathogen attack. Front. Plant Sci. 11:573670. doi: 10.3389/fpls.2020.573670
Tyśkiewicz, R., Nowak, A., Ozimek, E., and Jaroszuk-Ściseł, J. (2022). Trichoderma: the current status of its application in agriculture for the biocontrol of fungal phytopathogens and stimulation of plant growth. Int. J. Mol. Sci. 23:2329. doi: 10.3390/ijms23042329
Velasco, P., Rodríguez, V. M., Soengas, P., and Poveda, J. (2021). Trichoderma hamatum increases productivity, glucosinolate content and antioxidant potential of different leafy brassica vegetables. Plan. Theory 10:2449. doi: 10.3390/plants10112449
Vicente, I., Baroncelli, R., Morán-Diez, M. E., Bernardi, R., Puntoni, G., Hermosa, R., et al. (2020). Combined comparative genomics and gene expression analyses provide insights into the terpene synthases inventory in Trichoderma. Microorganisms 8:1603. doi: 10.3390/microorganisms8101603
Viriyasuthee, W., Jogloy, S., Saksirirat, W., Saepaisan, S., Gleason, M. L., and Chen, R. S. (2019). Biological control of Alternaria leaf spot caused by Alternaria spp. in Jerusalem artichoke (Helianthus tuberosus L.) under two fertilization regimes. Plan. Theory 8:463. doi: 10.3390/plants8110463
Viterbo, A., Harel, M., Horwitz, B. A., Chet, I., and Mukherjee, P. K. (2005). Trichoderma mitogen-activated protein kinase signaling is involved in induction of plant systemic resistance. Appl. Environ. Microbiol. 71, 6241–6246. doi: 10.1128/AEM.71.10.6241-6246.2005
Vos, C. M., De Cremer, K., Cammue, B. P., and De Coninck, B. (2015). The toolbox of Trichoderma spp. in the biocontrol of Botrytis cinerea disease. Mol. Plant Pathol. 16, 400–412. doi: 10.1111/mpp.12189
Wang, Y., Chen, H., Ma, L., Gong, M., Wu, Y., Bao, D., et al. (2022). Use of CRISPR-Cas tools to engineer Trichoderma species. Microb. Biotechnol. 15, 2521–2532. doi: 10.1111/1751-7915.14126
Wang, R., Liu, C., Jiang, X., Tan, Z., Li, H., Xu, S., et al. (2022). The newly identified Trichoderma harzianum partitivirus (ThPV2) does not diminish spore production and biocontrol activity of its host. Viruses 14:1532. doi: 10.3390/v14071532
Wang, H., Zhang, R., Duan, Y., Jiang, W., Chen, X., Shen, X., et al. (2021). The endophytic strain Trichoderma asperellum 6S-2: an efficient biocontrol agent against apple replant disease in China and a potential plant-growth-promoting fungus. J. Fungi 7:1050. doi: 10.3390/jof7121050
Wang, H., Zhang, R., Mao, Y., Jiang, W., Chen, X., Shen, X., et al. (2022). Effects of Trichoderma asperellum 6S-2 on apple tree growth and replanted soil microbial environment. J. Fungi 8:63. doi: 10.3390/jof8010063
Xu, H., Yan, L., Zhang, M., Chang, X., Zhu, D., Wei, D., et al. (2022). Changes in the density and composition of rhizosphere pathogenic Fusarium and beneficial Trichoderma contributing to reduced root rot of intercropped soybean. Pathogens 11:478. doi: 10.3390/pathogens11040478
Ye, L., Zhao, X., Bao, E., Li, J., Zou, Z., and Cao, K. (2020). Bio-organic fertilizer with reduced rates of chemical fertilization improves soil fertility and enhances tomato yield and quality. Sci. Rep. 10:177. doi: 10.1038/s41598-019-56954-2
You, J., Li, G., Li, C., Zhu, L., Yang, H., Song, R., et al. (2022). Biological control and plant growth promotion by volatile organic compounds of Trichoderma koningiopsis T-51. J. Fungi 8:131. doi: 10.3390/jof8020131
Yuan, M., Huang, Y., Ge, W., Jia, Z., Song, S., Zhang, L., et al. (2019). Involvement of jasmonic acid, ethylene and salicylic acid signaling pathways behind the systemic resistance induced by Trichoderma longibrachiatum H9 in cucumber. BMC Genomics 20:144. doi: 10.1186/s12864-019-5513-8
Zaid, R., Koren, R., Kligun, E., Gupta, R., Leibman-Markus, M., Mukherjee, P. K., et al. (2022). Gliotoxin, an immunosuppressive fungal metabolite, primes plant immunity: evidence from Trichoderma virens-tomato interaction. MBio 13:e0038922. doi: 10.1128/mbio.00389-22
Zhang, J., Chen, J., Liu, Z., Guan, S., Li, J., Zhang, C., et al. (2017). Evaluation of the combined use of Trichoderma atroviride metabolite and brassinolide in the promotion of vegetable growth and control of Botrytis cinere. J. Shanghai Jiaotong Univ. 35, 1–7. doi: 10.3969/J.ISSN.1671-9964.2017.05.001
Zhang, S., Gan, Y., Liu, J., Zhou, J., and Xu, B. (2020). Optimization of the fermentation media and parameters for the bio-control potential of Trichoderma longibrachiatum T6 against nematodes. Front Microbiol. 11:574601.
Zhang, F., Huo, Y., Cobb, A. B., Luo, G., Zhou, J., Yang, G., et al. (2018). Trichoderma biofertilizer links to altered soil chemistry, altered microbial communities, and improved grassland biomass. Front. Microbiol. 9:848. doi: 10.3389/fmicb.2018.00848
Zhang, C., Wang, W., Hu, Y., Peng, Z., Ren, S., Xue, M., et al. (2022). A novel salt-tolerant strain Trichoderma atroviride HN082102.1 isolated from marine habitat alleviates salt stress and diminishes cucumber root rot caused by Fusarium oxysporum. BMC Microbiol. 22:67. doi: 10.1186/s12866-022-02479-0
Zhang, C., Wang, W., Xue, M., Liu, Z., Zhang, Q., Hou, J., et al. (2021). The combination of a biocontrol agent Trichoderma asperellum SC012 and hymexazol reduces the effective fungicide dose to control Fusarium wilt in cowpea. J. Fungi 7:685. doi: 10.3390/jof7090685
Zhang, Y., Xiao, J., Yang, K., Wang, Y., Tian, Y., and Liang, Z. (2022). Transcriptomic and metabonomic insights into the biocontrol mechanism of Trichoderma asperellum M45a against watermelon Fusarium wilt. PLoS One 17:e0272702. doi: 10.1371/journal.pone.0272702
Zheng, H., Qiao, M., Lv, Y., Du, X., Zhang, K. Q., and Yu, Z. (2021). New species of Trichoderma isolated as endophytes and saprobes from Southwest China. J. Fungi 7:467. doi: 10.3390/jof7060467
Zhou, D., Huang, X. F., Guo, J., Dos-Santos, M. L., and Vivanco,. (2018). Trichoderma gamsii affected herbivore feeding behaviour on arabidopsis thaliana by modifying the leaf metabolome and phytohormones. Microb Biotechnol. 11, 1195–1206.
Keywords: Trichoderma , plant diseases, biological control, growth promotion, action mechanism
Citation: Yao X, Guo H, Zhang K, Zhao M, Ruan J and Chen J (2023) Trichoderma and its role in biological control of plant fungal and nematode disease. Front. Microbiol. 14:1160551. doi: 10.3389/fmicb.2023.1160551
Edited by:
Mina Salehi, Tarbiat Modares University, IranReviewed by:
Rosa E. Cardoza, Universidad de León, SpainPranab Dutta, Central Agricultural University, India
Copyright © 2023 Yao, Guo, Zhang, Zhao, Ruan and Chen. This is an open-access article distributed under the terms of the Creative Commons Attribution License (CC BY). The use, distribution or reproduction in other forums is permitted, provided the original author(s) and the copyright owner(s) are credited and that the original publication in this journal is cited, in accordance with accepted academic practice. No use, distribution or reproduction is permitted which does not comply with these terms.
*Correspondence: Jingjun Ruan, jjruan@gzu.edu.cn; Jie Chen, jiechen59@sjtu.edu.cn
†These authors have contributed equally to this work