- 1Hygiene and Zoonoses Department, Faculty of Veterinary Medicine, Mansoura University, Mansoura, Egypt
- 2Poultry Microbiological Safety and Processing Research Unit, US National Poultry Research Center, USDA-ARS, Athens, GA, United States
- 3Department of Food Hygiene and Control, Faculty of Veterinary Medicine, Mansoura University, Mansoura, Egypt
- 4Department of Microbiology and Immunology, Faculty of Pharmacy, Kafrelsheikh University, Kafr El-Sheikh, Egypt
- 5Evolutionary Biology, Institute for Biology, Freie Universität Berlin, Berlin, Germany
- 6Animal Health Research Institute, Agriculture Research Center, Cairo, Egypt
- 7Department of Food Hygiene and Control, Faculty of Veterinary Medicine, Benha University, Tukh, Qalyubia, Egypt
- 8Department of Bacteriology, Immunology and Mycology, Faculty of Veterinary Medicine, Suez Canal University, Ismailia, Egypt
The similarity of the Listeria innocua genome with Listeria monocytogenes and their presence in the same niche may facilitate gene transfer between them. A better understanding of the mechanisms responsible for bacterial virulence requires an in-depth knowledge of the genetic characteristics of these bacteria. In this context, draft whole genome sequences were completed on five L. innocua isolated from milk and dairy products in Egypt. The assembled sequences were screened for antimicrobial resistance and virulence genes, plasmid replicons and multilocus sequence types (MLST); phylogenetic analysis of the sequenced isolates was also performed. The sequencing results revealed the presence of only one antimicrobial resistance gene, fosX, in the L. innocua isolates. However, the five isolates carried 13 virulence genes involved in adhesion, invasion, surface protein anchoring, peptidoglycan degradation, intracellular survival, and heat stress; all five lacked the Listeria Pathogenicity Island 1 (LIPI-1) genes. MLST assigned these five isolates into the same sequence type (ST), ST-1085; however, single nucleotide polymorphism (SNP)-based phylogenetic analysis revealed 422–1,091 SNP differences between our isolates and global lineages of L. innocua. The five isolates possessed an ATP-dependent protease (clpL) gene, which mediates heat resistance, on a rep25 type plasmids. Blast analysis of clpL-carrying plasmid contigs showed approximately 99% sequence similarity to the corresponding parts of plasmids of L. monocytogenes strains 2015TE24968 and N1-011A previously isolated from Italy and the United States, respectively. Although this plasmid has been linked to L. monocytogenes that was responsible for a serious outbreak, this is the first report of L. innocua containing clpL-carrying plasmids. Various genetic mechanisms of virulence transfer among Listeria species and other genera could raise the possibility of the evolution of virulent strains of L. innocua. Such strains could challenge processing and preservation protocols and pose health risks from dairy products. Ongoing genomic research is necessary to identify these alarming genetic changes and develop preventive and control measures.
Introduction
Food animals are considered a major reservoir for human infection with foodborne pathogens such as non-typhoidal Salmonella, Campylobacter species, Escherichia coli, and Listeria spp. (Heredia and García, 2018). Genus Listeria includes different species that are either pathogenic or non-pathogenic. Listeria monocytogenes and L. ivanovii, two pathogenic species belonging to Listeria sensu strictu, are the primary agents that cause listeriosis in humans and animals, respectively (Schardt et al., 2017). The pathogenicity of these two species is linked to an approximately 9 kb virulence gene cluster (Schmid et al., 2005). Other Listeria species, L. innocua, L. seeligeri, L. welshimeri, L. marthii, and L. grayi are thought to be likely non-pathogenic Listeria species (Liu, 2013). Except for L. grayi, which belongs to the Listeria sensu lato group, all of the presumed non-pathogenic species were classified as Listeria sensu strictu and are considered saprophytes (Chiara et al., 2015).
The patterns of genome evolution in Listeria sensu strictu and sensu lato isolates were investigated in previous sequencing papers (Schmid et al., 2005; den Bakker et al., 2010; Chiara et al., 2015). Surprisingly, these papers documented the acquisition of virulence factors that were lacking in non-pathogenic species of Listeria sensu strictu and sensu lato at the time they were identified. Gene duplication, gene divergence, and lateral gene transfer—most often from sources outside of Listeria—are all common throughout the genus and are presumed to be the underlying mechanisms of bacterial evolution (Chiara et al., 2015).
Despite the fact that L. innocua is typically nonhemolytic, a previous study documented the existence of an atypical hemolytic L. innocua isolate, which contained every member of the L. monocytogenes prfA-regulated virulence gene cluster (Listeria pathogenicity island 1) but was avirulent in the mouse pathogenicity test (Johnson et al., 2004). Nevertheless, cases of animal listeriosis caused by L. innocua which shared a close genetic relationship with L. monocytogenes have been reported (Matto et al., 2022). Therefore, ongoing characterization of atypically pathogenic Listeria species, as well as changes in their taxonomy, are critical for development and testing of preservation and processing conditions that prevent their growth and spread in the food industry (Orsi and Wiedmann, 2016).
Whole-genome sequencing (WGS) is now recognized as the most effective approach for genetic characterization of pathogens compared to time-consuming and labor-intensive conventional molecular diagnostic techniques that include isolation, identification, and molecular confirmation of the microbe (Lüth et al., 2018; Uelze et al., 2020). WGS can be used to uncover traits such as those responsible for pathogenicity/virulence, antibiotic resistance, and dissemination of mobile genetic elements. WGS can further be applied to provide a more precise description of the taxonomic differences and phylogenetic relatedness between pathogens through multilocus sequence typing (MLST), clonal complex (CC) determination, core genome MLST (cgMLST) and single nucleotide polymorphism (SNP)-based phylogeny (Ramadan et al., 2021; Stessl et al., 2021; Parra-Flores et al., 2022).
Non-pathogenic Listeria spp. particularly L. innocua, have been reported in different studies from Egypt from different sources (El-Shenawy et al., 2011; Ismaiel et al., 2014; Dahshan et al., 2016), yet there is a lack of information about its genomic characterization. We aimed in the present study to characterize five L. innocua isolates from milk and dairy products. Our goal was to test the emergence of virulence factors and genetic components that enable bacteria to survive, spread, and cause listeriosis.
Materials and methods
Listeria innocua isolates for whole-genome sequencing
Five L. innocua isolates (four from raw milk and one from yoghurt) were chosen from our previous study (Youssef et al., 2020) and subjected to WGS for further genomic characterization. Isolates were recovered from raw milk and dairy products (yoghurt and ice cream) purchased from local supermarkets and retail stores in Mansoura City, Egypt during the period between 2014 and 2018. A 25 mL sample from raw milk or 25 g from dairy products were homogenized with 225 mL of trypticase soy broth (TSB), and the sample-TSB mixture was then processed for culturing on Listeria selective media as previously described (Youssef et al., 2020). Listeria spp. isolates were submitted to the Bacterial Epidemiology and Antimicrobial Resistance (BEAR) Research Unit, United States Department of Agriculture (USDA), Athens, Georgia, United States, through a Material Transfer Research Agreement between Mansoura University and USDA (Agreement No. 58-6040-0-001-F). Isolates were revived by culture in brain heart infusion (BHI) broth (Becton Dickinson, Sparks, MD, United States) which was incubated for 24 h at 35°C. From incubated BHI broth, 10 μL were streaked onto Modified Oxford (MOX) agar with selective supplement (Oxoid, Basingstoke, Hampshire, United Kingdom) and plates were then incubated at 37°C for 24 h. Characteristic small black Listeria spp. colonies were picked from MOX agar and plated onto nutrient agar plates for further biochemical identification using Vitek (BioMérieux, Durham, NC, United States).
Genomic DNA extraction, DNA library preparation and whole-genome sequencing
Genomic DNA (gDNA) was extracted from Listeria isolates using the blood and tissue genomic DNA extraction kit (Qiagen, Germantown, MD, United States); purity of extracted gDNA was assessed using a NanoDrop™ spectrophotometer. The concentration of gDNA was determined using a Qubit® double-stranded DNA (dsDNA) high-sensitivity (HS) assay kit (Life Technologies Inc., Carlsbad, CA, United States) on an Invitrogen Qubit 2.0 Fluorometer (Thermo Fisher Scientific, Waltham, MA, United States) according to manufacturer’s instructions. Sequencing libraries were prepared using Nextera™ XT DNA Sample Preparation Kit and Nextera™ XT Index Kit (Illumina Inc., San Diego, CA, United States). Libraries were sequenced on an Illumina MiSeq platform using a MiSeq v2 reagent kit (Illumina Inc., San Diego, CA, United States) with 500 cycles to generate a paired-end read length of 2 × 250 bp. Quality check of raw reads was determined using FastQC tool,1 and low quality reads were trimmed using trimmomatic with the following parameters: leading: 10, trailing: 10, sliding window: 4:20, and minlen: 40. Obtained reads were then de novo assembled using A5-miseq assembler (Coil et al., 2015) and assembly statistics that included genome length, number of contigs, coverage, GC% and N50 were determined.
Bioinformatics analysis
Assembled fasta files were uploaded into Galaxy server2 and screened against different databases: ResFinder (Zankari et al., 2012), virulence factor database (VFDB) (Chen et al., 2016), and PlasmidFinder (Carattoli et al., 2014) using the ABRicate (version 1.0.1) tool3 for identification of resistance and virulence genes and plasmid replicons, respectively. Further in silico analysis was performed using Plasmid SPAdes and PLACNETw tools to separate plasmid contigs from WGS of the examined isolates (Vielva et al., 2017). The reconstructed plasmid sequences were then blasted against the National Center for Biotechnology Information (NCBI) database to determine the closely matched plasmids. A genetic comparison was performed between the reconstructed L. innocua plasmids from this study and plasmid sequences retrieved from NCBI using the BLAST Ring Image Generator (BRIG) tool.4 Linear comparison to determine the genetic environment of resistance and/or virulence genes located on L. innocua plasmids was also determined using the Easyfig5 tool (Sullivan et al., 2011). Other mobile genetic elements such as insertion sequences (IS) were searched for using the ISFinder6 tool.
To determine MLST types for the examined Listeria isolates, the assembled sequences were blasted against the Listeria sequence typing database available on BIGSdb.7 To put our isolates into context with global lineages of L. innocua, a single nucleotide polymorphism (SNP)-based phylogenetic analysis was performed using Snippy v4.4.48 with the following variant calling parameters: minimum base quality 60, minimum read coverage 10 and minimum proportion for variant evidence 0.9. Our five isolates were compared to publicly available genomes (n = 260) of L. innocua recovered from different sources in the NCBI database (updated April 10th, 2021), and all the enrolled isolates were mapped to the reference L. innocua ATCC 33091 genome. Metadata for L. innocua sequences retrieved from NCBI database are listed in Supplementary Tables S1, S2. Output files from Snippy that determined SNPs variant calling were combined using Snippy core into a core SNPs alignment. Using the Randomized Accelerated Maximum Likelihood (RAxML) tool, maximum likelihood phylogenetic trees were generated from SNPs alignment, and the trees were then visualized with iTOL (Letunic and Bork, 2016).
Results
In this study, the 5 L. innocua isolates from milk and dairy products were subjected to WGS. Assembly statistics of the sequences of L. innocua are listed in Table 1. The genome size was 2.96 Mbp with GC% ranging from 37.2 to 37.3, which is consistent with average genome size and GC% of the complete genome of L. innocua. Draft genome sequences of the examined isolates were assembled into an average of 16 contigs (14 contigs for LI-36, 15 for L-I33 and LI-35, 16 for LI-34 and 18 for LI-32), with N50 of 544,812 bp for isolate LI-32, 544,816 bp for isolates LI-34 and LI-35 and 545,607 bp for isolates LI-33 and LI-36.
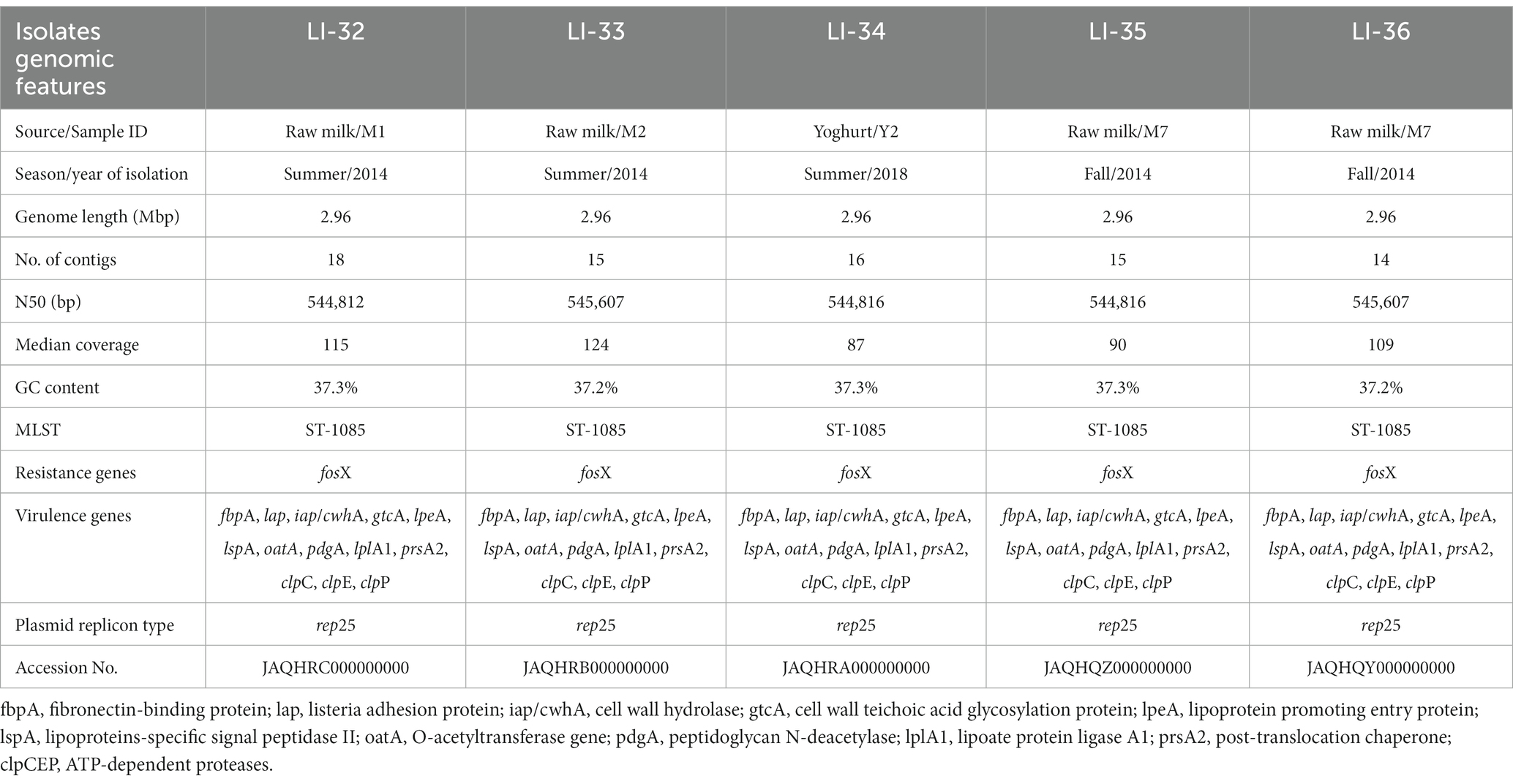
Table 1. Assembly statistics, multilocus sequence typing (MLST), resistance and virulence genes and plasmid replicons of clpL-producing Listeria innocua recovered from milk and dairy products from Egypt.
ResFinder analysis of the examined 5 L. innocua revealed absence of antimicrobial resistance genes; only fosX responsible for intrinsic resistance of Listeria spp. to fosfomycin was identified. Screening L. innocua contigs using the ABRicate tool in Galaxy against the virulence factor database (VFDB), with a minimum 80% identity and coverage showed the presence of multiple virulence genes belonging to adherence (fbpA, and lap), invasion (iap/cwhA, gtcA, and lpeA), surface protein anchoring (lspA), peptidoglycan modification (oatA, and pdgA), intracellular survival (lplA1, and prsA2) and heat shock proteins (clpC, clpE, and clpP). PlasmidFinder results exhibited the presence of a single plasmid replicon type (rep25) in the examined isolates (Table 1).
Using plasmid SPAdes and PLACNETw tools, plasmid contigs were distinguished from the draft genome sequences of the examined isolates. Blast analysis of the reconstructed plasmids from our L. innocua isolates showed high sequence similarity with plasmids of L. monocytogenes: clinical strain 2015TE24968 (accession no. CP015985.1) isolated from an outbreak of invasive listeriosis in Italy in 2015 and environmental strain N1-011A (accession no. CP006611.1) isolated from the United States. Noticeably, the reconstructed L. innocua plasmids (pLI-32, pLI-33, pLI-34, pLI-35, pLI-36) carried the clpL gene which mediates heat resistance (Figure 1). Blast analysis of clpL carrying contigs from our isolates showed approximately 99% sequence similarity to the corresponding parts of plasmids of L. monocytogenes strain 2015TE24968 and strain N1-011A. Genetic context of clpL in our L. innocua and the retrieved NCBI sequences of L. monocytogenes revealed the presence of insertion sequence ISLmo8 and ISLmo9 downstream and upstream to the clpL gene, respectively (Figure 2).
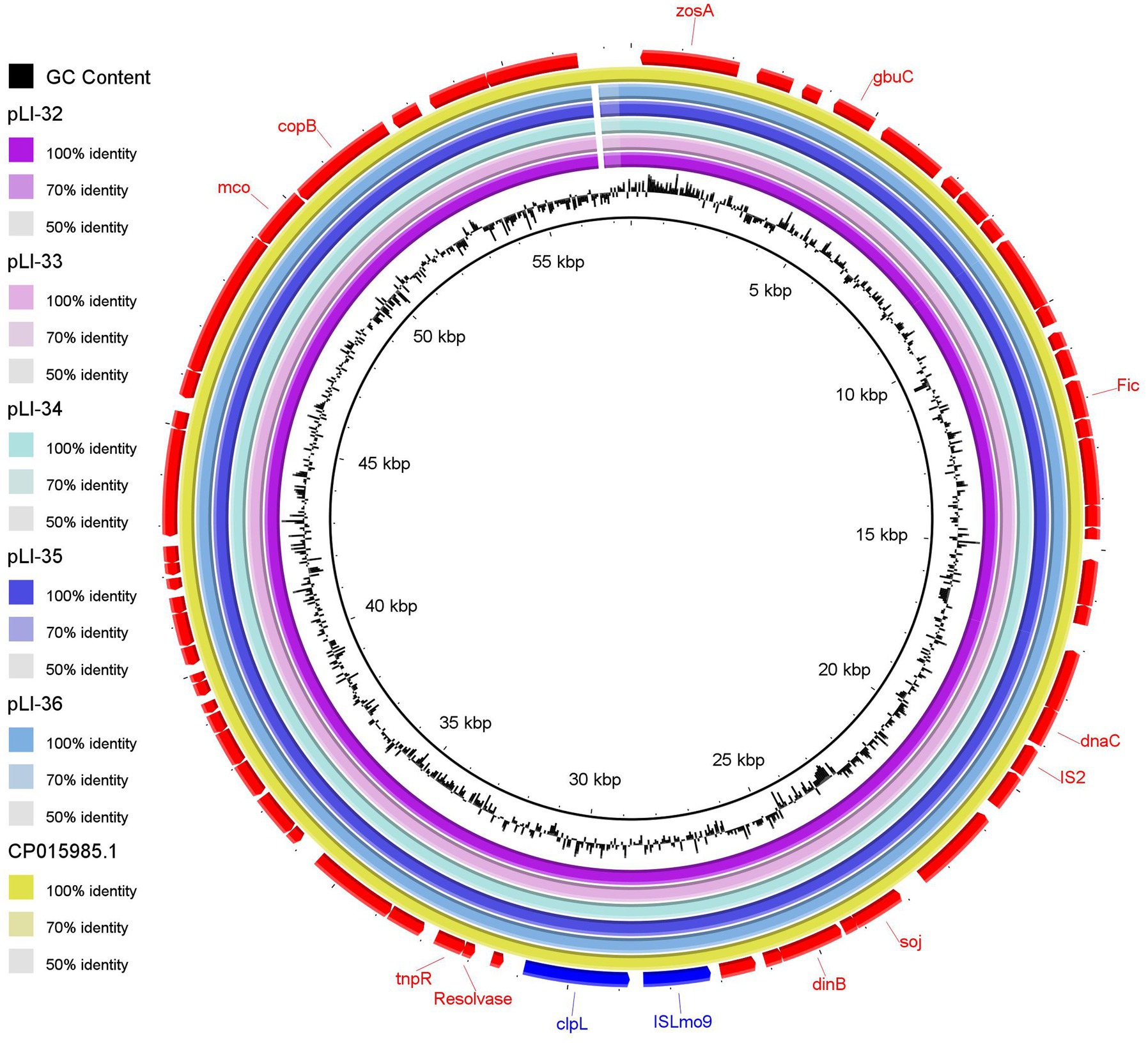
Figure 1. Comparative sequence analysis of the reconstructed plasmids carrying the clpL gene from the whole genome sequences of the examined Listeria innocua isolates. The reconstructed plasmids pLI-32, pLI-33, pLI-34, pLI-35, and pLI-36 from isolates, were represented as circles from inside to outside. The out-layer circle (red color) represents the reference plasmid (accession no. CP015985.1) used for sequence comparisons. The figure was generated using BLAST Ring Image Generator (BRIG) tool (http://sourceforge.net/projects/brig).
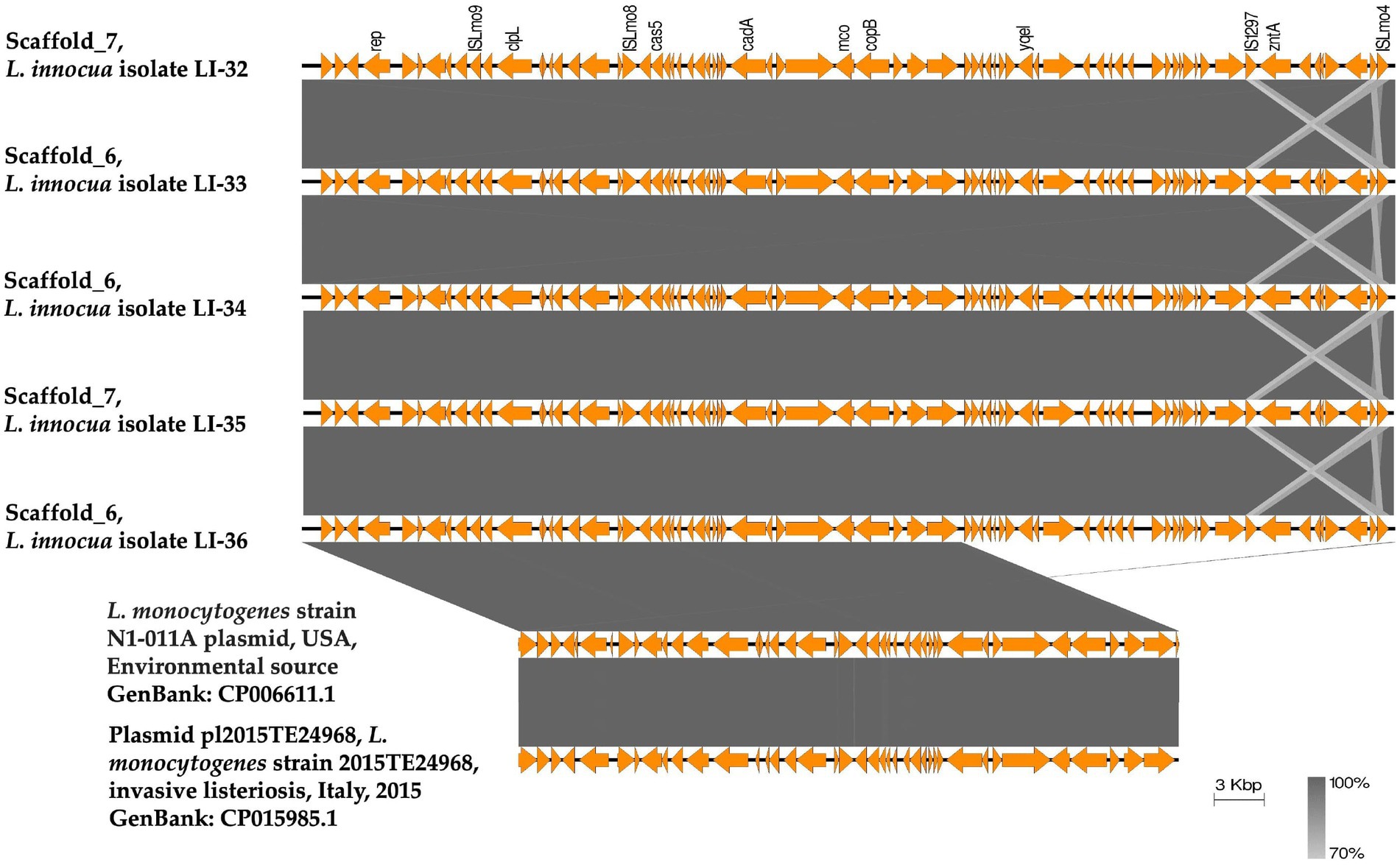
Figure 2. Linear comparison of the plasmid contigs carrying the heat shock clpL gene identified from the draft genome sequences of L. innocua strains analyzed in this study with a similar plasmid detected from L. monocytogenes strain N1-011 in the United States from an environmental source (GenBank: CP006611.1) and plasmid pl2015TE24968 detected from L. monocytogenes strain 2015TE24968 isolated from an outbreak of invasive listeriosis in Italy in 2015 (GenBank: CP015985.1). A BLASTN search showed that the plasmid contig carrying the clpL gene of the examined L. innocua isolates have 99.97% identity with 74% query coverage to the plasmid detected from L. monocytogenes strain N1-011, and 99.91% identity with 74% query coverage to plasmid pl2015TE24968. The figure was drawn using the EasyFig tool (http://mjsull.github.io/Easyfig/).
The allelic profile for the seven housekeeping genes retrieved from MLST 2.0 was determined as follows: abcZ, 188; bglA, 157; cat, 182; dapE, 223; dat, 136; ldh, 353; lhkA, 148 that assigned the 5 L. innocua isolates, regardless of isolate source, into the same sequence type ST-1085. We performed a SNP-based phylogenetic analysis to compare our isolates with the global lineages of L. innocua. Findings of SNP-based phylogeny revealed that no specific trends have been observed for clustering isolates based on their source. This was observed from the clustering of examined isolates in a clade with L. innocua isolates from different sources, food, and environment (Figure 3A). To zoom in on the differences among isolates within this clade, isolates were separately subjected to a SNP-based phylogeny (Figure 3B). No more than 10 SNP differences were identified among this study isolates, indicating that the five isolates from milk and yoghurt are clonal. The number of SNPs between our isolates and closely related L. innocua isolates (SAMN12370801, SAMN12374550, SAMN14487858, SAMN10075820, SAMN17265727, SAMN17153928, and SAMN12374702) ranged from 422 to 1,091 (Supplementary Table S3); where all isolates were assigned to the same ST type (ST-1085) and were sourced from food except isolate SAMN14487858 that was recovered from the environment.
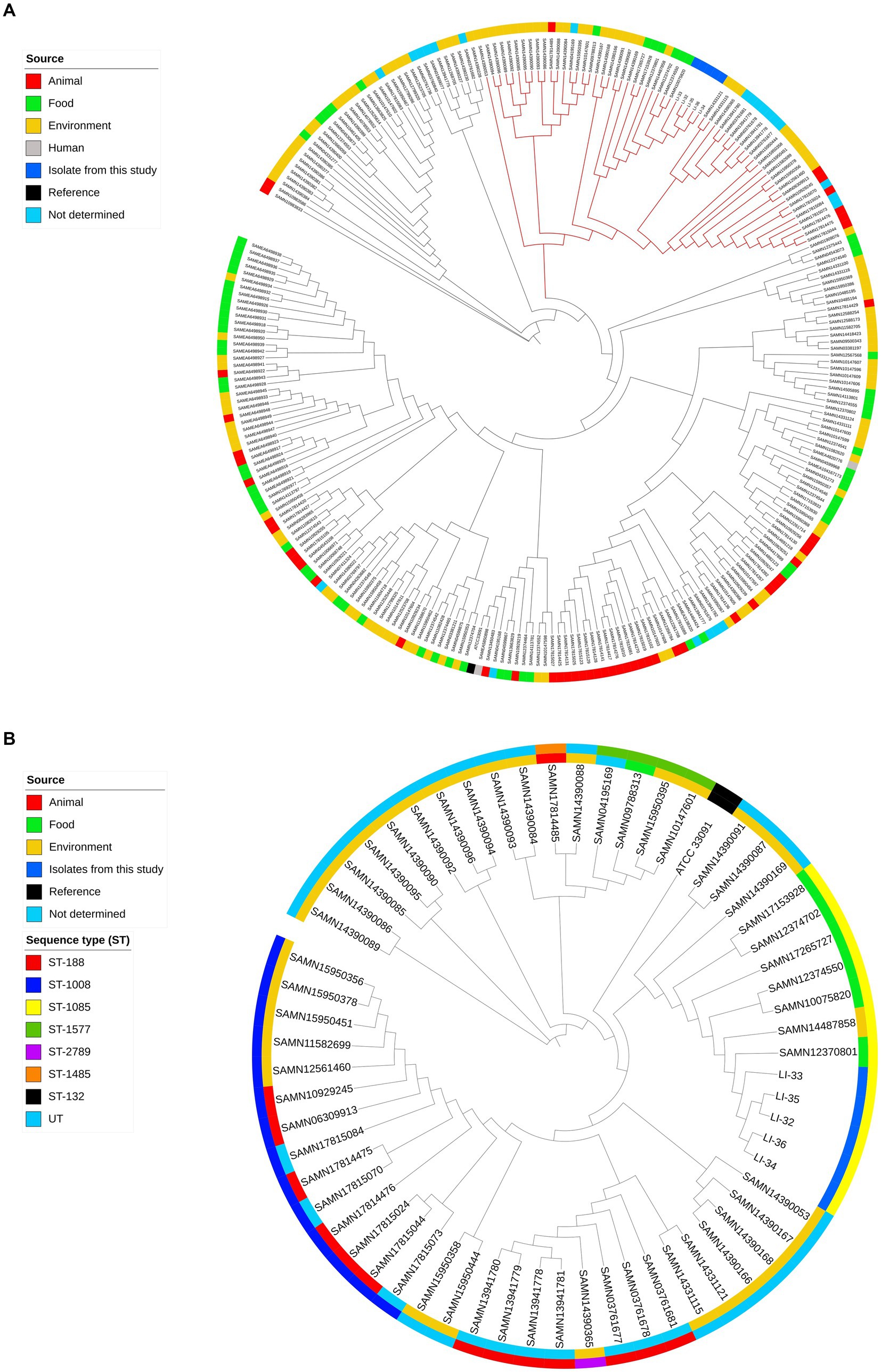
Figure 3. (A) Phylogenomic analysis of Listeria innocua isolates and publicly available genomes (n = 260) of L. innocua recovered from different sources in the NCBI database (updated April 10th, 2021); all the enrolled isolated were mapped to the reference L. innocua ATCC 33091 genome. The clade with branches in red denotes clustering of closely related NCBI L. innocua (n = 56) to our isolates. (B) Isolates in this clade were further subjected to SNP-based phylogeny for understanding closer genetic relationships among these isolates. From inside-out, the first circle indicates the source of isolates. The second circle indicates the sequence types (STs).
Discussion
Listeria infection is a significant foodborne disease that can be transmitted to humans primarily via the food chain. Many food sources are implicated in Listeria foodborne infections, including milk and milk products, an important source of protein available to many groups of consumers (Bintsis, 2017; Kim et al., 2018). The pathogenic potential of L. monocytogenes is explained by its possession of certain virulence genes that increases bacterial fitness and survivability (Abdelhamed et al., 2022; Osek et al., 2022); however, the pathogenic potentiality of some non-pathogenic Listeria spp. that are isolated at a high rate requires further research to explain. Here, we performed the comparative genome sequence analysis of L. innocua recovered from dairy and dairy products in Egypt.
As indicated from WGS, we observed absence of antimicrobial resistance genes except FosX. This gene that confers resistance to fosfomycin has been identified in previous studies from Listeria spp. (Scortti et al., 2018; Wilson et al., 2018; Parra-Flores et al., 2022). In both pathogenic and non-pathogenic Listeria spp., a FosX enzyme that is expressed by the fosX gene confers intrinsic fosfomycin resistance. However, when infected with pathogenic L. monocytogenes, the host signals activate prfA virulence regulons, hpt, and prfA and epistatically promote greater fosfomycin influx into the bacterial cell, suppressing fosX-mediated resistance (Scortti et al., 2018). Current whole genome sequenced L. innocua isolates showed absence of both prfA virulence regulons, hpt and prfA, indicating that fosfomycin treatment would not be effective under infection circumstances. ResFinder results also revealed absence of other antimicrobial resistance genes in isolates from this study. The few antimicrobial resistance genes found in these L. innocua isolates were generally consistent with past findings (Hof, 1991; Charpentier and Courvalin, 1999), and the most recent study conducted in the United States between 2010 and 2021 (Jorgensen et al., 2021; Hanes and Huang, 2022). As reported in these studies, most L. monocytogenes as well as strains of other Listeria spp., were found to be susceptible to a wide range of antimicrobials and there was no increase in antimicrobial resistance genes except for cephalosporin, fosfomycin, lincosamide and tetracycline resistance genes. Despite the few occurrences of antimicrobial resistance genes among the examined L. innocua isolates, which is considered a good sign for infection treatment, the spread of antimicrobial resistance among different bacterial species remains a major problem in Egyptian dairy farms (Tartor et al., 2021; Badawy et al., 2022). Further studies on larger sets would be helpful to determine the state of antimicrobial resistance in Listeria from milk and dairy products, since high antimicrobial resistances among different bacterial species from dairy farms in Egypt prevail.
L. monocytogenes pathogenesis requires the coordinated expression of six genes, namely prfA, plcA, hly, mpl, actA, and plcB, which are primarily assembled in the 9 kb Listeria Pathogenicity Island 1 (LIPI-1) (Vázquez-Boland et al., 2001). The prfA gene encodes a transcriptional activator, prfA, which directly or indirectly induces the transcription of over 140 genes, including the other five genes found in LIPI-1 (Paramithiotis et al., 2014). Both plcA and plcB encode phospholipases C (phosphatidylinositol and phosphatidylcholine), which, in conjunction with listeriolysin O (LLO), protect Listeria from cytoplasmic phagosomes (Schlüter et al., 1998). The mpl encodes a zinc-metalloprotease required for pro-plcB maturation (Raveneau et al., 1992), and actA is a multifunctional virulence factor (Travier et al., 2013). Our findings revealed the existence of 13 virulence genes among the examined isolates, belonging to adherence (fbpA, and lap), invasion (iap/cwhA, gtcA, and lpeA), surface protein anchoring (lspA), peptidoglycan modification (oatA, and pdgA), intracellular survival (lplA1, and prsA2) and heat shock proteins (clpC, clpE, and clpP). These genes that encode for minor or accessory virulence factors, were detected in most L. innocua strains. Nevertheless, L. innocua isolates carrying the above virulence profile were found associated with clinical cases in different hosts such as ruminants (Matto et al., 2022), and birds (Moura et al., 2019). Fortunately, none of the important LIPI-1 genes were found in the currently studied L. innocua. Virulence genes such as hly, which is responsible for the β-hemolysis associated with L. monocytogenes and some atypical hemolytic L. innocua (Johnson et al., 2004), were not identified in these L. innocua isolates.
Listeria spp. strains might acquire genes via mobile genetic elements, enhancing their capacity to endure and colonize a variety of food processing environments. These resistance and adaptation genes can increase tolerance of Listeria to a variety of stressors, such as sanitizers, antimicrobials, and environmental toxins, as well as extremes in salinity, acidity, and temperature. Transfer of such genes may also explain the widespread nature of Listeria in both natural and agricultural settings (Korsak and Szuplewska, 2016; Hingston et al., 2017; Pöntinen et al., 2017). Several genes that are responsible for the survivability and pathogenicity of Listeria spp., are plasmid mediated which can be transferred within and between Listeria spp. (Kuenne et al., 2010; Schmitz-Esser et al., 2021). Based on their replication protein repA, Listeria plasmids mostly belong to groups 1 and 2 which are commonly known in plasmid database (PLSDB) as rep25 and rep26, respectively. The examined L. innocua isolates were found possessing plasmids of rep25 replicon type; this replicon type was previously identified in L. monocytogenes strain 2015TE24968, causing a severe listeriosis outbreak in Central Italy (Orsini et al., 2018) and environmental strain N1-011A (GenBank accession number CP006611.1) isolated in the United States. The ATP-dependent protease (clpL), which increases heat resistance, was initially discovered in L. monocytogenes harboring plasmid-borne clpL. It was revealed that clpL gene introduction into a naturally heat-sensitive strain of L. monocytogenes greatly boosted the recipient strain’s heat resistance, but plasmid-borne clpL deletion resulted in dramatically lower heat resistance in the wild-type strain (Pöntinen et al., 2017). To our knowledge, this is the first time that plasmid-borne clpL has been reported in L. innocua. The presence of such plasmid-borne clpL in L. innocua isolated from dairy products may allow them to survive at high processing temperatures, necessitating a stricter food product control protocol. It is also critical to understand the role of these plasmid-mediated genes in the pathogenicity and persistence of Listeria in challenging and in vitro environments. Fortunately, due to the similarities in ecology, genome, and physiological traits between both L. monocytogenes and L. innocua, researchers are able to control L. monocytogenes by using L. innocua surrogates and identifying hidden metabolomic tools for surviving stressors (He et al., 2021; Wu et al., 2021). Considering this, further research into the metabolic changes caused by the plasmid-borne clpL gene in L. innocua will be required.
WGS has become a powerful tool used for routine epidemiological surveillance of infectious diseases, outbreak investigations, and tracing transmission routes (Oakeson et al., 2018; Brown et al., 2021). MLST and SNP-based phylogeny are frequently used for bacterial subtyping, that enables precise source attribution and identification of the origin of pathogen associated-outbreaks (Stessl et al., 2021). In the present study, the 5 L. innocua isolates sourced from milk and yoghurt were assigned to the same sequence type, ST-1085. SNP-based phylogeny confirmed the MLST findings of the sequenced isolates, and few SNP differences (less than 10 SNPs) were identified among isolates indicating the circulation of the same L. innocua clone in raw milk and dairy product. Comparing our isolates to the global lineages of L. innocua, our isolates showed close similarity with L. innocua isolates (422-1,091 SNPs) that were recovered from food and the environment. This highlights the importance of WGS-based phylogeny for monitoring the potential sources of foodborne pathogens.
In conclusion, the sequencing results revealed that current isolates of L. innocua lacked the Listeria Pathogenicity Island 1 (LIPI-1) gene, the hly gene, and any antimicrobial resistance genes, except for the fosX gene. However, the absence of fosX gene suppressing regulons, hpt, and prfA suggests that fosfomycin treatment would be ineffective under infection conditions. Furthermore, the 5 L. innocua isolates possessed 13 virulence genes involved in adhesion, invasion, surface protein anchoring, peptidoglycan degradation, intracellular survival, and heat stress. Though these genes encode for minor or accessory virulence factors, the close genetic relationship with L. monocytogenes and the potential for horizontal virulence gene transfer raises the possibility of evolution of virulent L. innocua strains. The ATP-dependent protease (clpL), mediating elevated heat resistance of strains which carry it, was reported for the first time in the currently studied L. innocua, specifically that clpL was carried by plasmids. The first documentation of clpL-carrying plasmids in L. innocua, along with the common virulence and antimicrobial resistance genes, and the ongoing various genetic mechanisms of virulence transfer among Listeria and other genus, could endanger current industrial processing and preservation protocols and present health risks from dairy products. Therefore, ongoing genomic studies are necessary to identify these alarming genetic changes and research is needed to develop and test preventive and control measures.
Data availability statement
The datasets presented in this study can be found in online repositories. The names of the repository/repositories and accession number(s) can be found at: https://www.ncbi.nlm.nih.gov/, Bioproject ID. PRJNA921817.
Author contributions
HR, MA-A, and CJ conceived and designed the study. HR performed the microbiology work and whole-genome sequencing of isolates, analyzed the genome data, and wrote the original draft of the manuscript. AS and ME participated to the analysis of WGS data. IS contributed to the data analysis and manuscript drafting. MY and LH participated to microbiology work. AA contributed to the data analysis. HR, MA-A, MB, JF, and CJ secured funding and provided project administration. HR, MA-A, AS, ME, IS, AA, MB, JF, and CJ reviewed and edited the manuscript. All authors contributed to the article and approved the submitted version.
Funding
This work has been funded by the U.S. Department of Agriculture (USDA) project 6040-32000-079-00-D.
Acknowledgments
The authors acknowledge the technical help of Eric Adams, USDA, Athens, GA.
Conflict of interest
The authors declare that the research was conducted in the absence of any commercial or financial relationships that could be construed as a potential conflict of interest.
Publisher’s note
All claims expressed in this article are solely those of the authors and do not necessarily represent those of their affiliated organizations, or those of the publisher, the editors and the reviewers. Any product that may be evaluated in this article, or claim that may be made by its manufacturer, is not guaranteed or endorsed by the publisher.
Supplementary material
The Supplementary material for this article can be found online at: https://www.frontiersin.org/articles/10.3389/fmicb.2023.1160244/full#supplementary-material
Footnotes
1. ^https://www.bioinformatics.babraham.ac.uk/projects/fastqc/
3. ^https://github.com/tseemann/abricate
4. ^http://sourceforge.net/projects/brig
5. ^http://mjsull.github.io/Easyfig/
6. ^https://www-is.biotoul.fr/blast.php
References
Abdelhamed, H., Ramachandran, R., Narayanan, L., Islam, S., Ozan, O., Freitag, N., et al. (2022). Role of FruR transcriptional regulator in virulence of Listeria monocytogenes and identification of its regulon. PLoS One 17:e0274005. doi: 10.1371/journal.pone.0274005
Badawy, B., Elafify, M., Farag, A. M. M., Moustafa, S. M., Sayed-Ahmed, M. Z., Moawad, A. A., et al. (2022). Ecological distribution of virulent multidrug-resistant Staphylococcus aureus in livestock, environment, and dairy products. Antibiotics 11:1651. doi: 10.3390/antibiotics11111651
Bintsis, T. (2017). Foodborne pathogens. AIMS Microbiol. 3, 529–563. doi: 10.3934/microbiol.2017.3.529
Brown, B., Allard, M., Bazaco, M. C., Blankenship, J., and Minor, T. (2021). An economic evaluation of the whole genome sequencing source tracking program in the U.S. PLoS One 16:e0258262. doi: 10.1371/journal.pone.0258262
Carattoli, A., Zankari, E., García-Fernández, A., Voldby Larsen, M., Lund, O., Villa, L., et al. (2014). In silico detection and typing of plasmids using PlasmidFinder and plasmid multilocus sequence typing. Antimicrob. Agents Chemother. 58, 3895–3903. doi: 10.1128/aac.02412-14
Charpentier, E., and Courvalin, P. (1999). Antibiotic resistance in Listeria spp. Antimicrob. Agents Chemother. 43, 2103–2108. doi: 10.1128/aac.43.9.2103
Chen, L., Zheng, D., Liu, B., Yang, J., and Jin, Q. (2016). VFDB 2016: hierarchical and refined dataset for big data analysis—10 years on. Nucleic Acids Res. 44, D694–D697. doi: 10.1093/nar/gkv1239
Chiara, M., Caruso, M., D’Erchia, A. M., Manzari, C., Fraccalvieri, R., Goffredo, E., et al. (2015). Comparative genomics of Listeria sensu lato: genus-wide differences in evolutionary dynamics and the progressive gain of complex, potentially pathogenicity-related traits through lateral gene transfer. Genome Biol. Evol. 7, 2154–2172. doi: 10.1093/gbe/evv131
Coil, D., Jospin, G., and Darling, A. E. (2015). A5-MISEQ: an updated pipeline to assemble microbial genomes from illumina MiSeq data. Bioinformatics 31, 587–589. doi: 10.1093/bioinformatics/btu661
Dahshan, H., Merwad, A. M. A., and Mohamed, T. S. (2016). Listeria species in broiler poultry farms: potential public health hazards. J. Microbiol. Biotechnol. 26, 1551–1556. doi: 10.4014/jmb.1603.03075
den Bakker, H. C., Bundrant, B. N., Fortes, E. D., Orsi, R. H., and Wiedmann, M. (2010). A population genetics-based and phylogenetic approach to understanding the evolution of virulence in the genus Listeria. Appl. Environ. Microbiol. 76, 6085–6100. doi: 10.1128/AEM.00447-10
El-Shenawy, M., El-Shenawy, M., Mañes, J., and Soriano, J. M. (2011). Listeria spp. in street-vended ready-to-eat foods. Interdiscip. Perspect Infect. Dis. 2011:968031. doi: 10.1155/2011/968031
Hanes, R. M., and Huang, Z. (2022). Investigation of antimicrobial resistance genes in Listeria monocytogenes from 2010 through to 2021. Int. J. Environ. Res. Public Health 19:5506. doi: 10.3390/ijerph19095506
He, Y., Zhao, X., Chen, L., Zhao, L., and Yang, H. (2021). Effect of electrolysed water generated by sodium chloride combined with sodium bicarbonate solution against Listeria innocua in broth and on shrimp. Food Control 127:108134. doi: 10.1016/j.foodcont.2021.108134
Heredia, N., and García, S. (2018). Animals as sources of food-borne pathogens: a review. Anim. Nutr. 4, 250–255. doi: 10.1016/j.aninu.2018.04.006
Hingston, P., Chen, J., Dhillon, B. K., Laing, C., Bertelli, C., Gannon, V., et al. (2017). Genotypes associated with Listeria monocytogenes isolates displaying impaired or enhanced tolerances to cold, salt, acid, or desiccation stress. Front. Microbiol. 8:369. doi: 10.3389/fmicb.2017.00369
Hof, H. (1991). Therapeutic activities of antibiotics in listeriosis. Infection 19, S229–S233. doi: 10.1007/bf01644039
Ismaiel, A. A.-R., Ali, A. E.-S., and Enan, G. (2014). Incidence of Listeria in Egyptian meat and dairy samples. Food Sci. Biotechnol. 23, 179–185. doi: 10.1007/s10068-014-0024-5
Johnson, J., Jinneman, K., Stelma, G., Smith, B. G., Lye, D., Messer, J., et al. (2004). Natural atypical Listeria innocua strains with Listeria monocytogenes pathogenicity island 1 genes. Appl. Environ. Microbiol. 70, 4256–4266. doi: 10.1128/aem.70.7.4256-4266.2004
Jorgensen, J., Bland, R., Waite-Cusic, J., and Kovacevic, J. (2021). Diversity and antimicrobial resistance of Listeria spp. and L. monocytogenes clones from produce handling and processing facilities in the Pacific northwest. Food Control 123:107665. doi: 10.1016/j.foodcont.2020.107665
Kim, S. W., Haendiges, J., Keller, E. N., Myers, R., Kim, A., Lombard, J. E., et al. (2018). Genetic diversity and virulence profiles of Listeria monocytogenes recovered from bulk tank milk, milk filters, and milking equipment from dairies in the United States (2002 to 2014). PLoS One 13:e0197053. doi: 10.1371/journal.pone.0197053
Korsak, D., and Szuplewska, M. (2016). Characterization of nonpathogenic Listeria species isolated from food and food processing environment. Int. J. Food Microbiol. 238, 274–280. doi: 10.1016/j.ijfoodmicro.2016.08.032
Kuenne, C., Voget, S., Pischimarov, J., Oehm, S., Goesmann, A., Daniel, R., et al. (2010). Comparative analysis of plasmids in the genus Listeria. PLoS One 5:e12511. doi: 10.1371/journal.pone.0012511
Letunic, I., and Bork, P. (2016). Interactive tree of life (iTOL) v3: an online tool for the display and annotation of phylogenetic and other trees. Nucleic Acids Res. 44, W242–W245. doi: 10.1093/nar/gkw290
Liu, D. (2013). Molecular approaches to the identification of pathogenic and nonpathogenic Listeriae. Microbiol. Insights 6, 59–69. doi: 10.4137/mbi.S10880
Lüth, S., Kleta, S., and Al Dahouk, S. (2018). Whole genome sequencing as a typing tool for foodborne pathogens like Listeria monocytogenes – the way towards global harmonisation and data exchange. Trends Food Sci. Technol. 73, 67–75. doi: 10.1016/j.tifs.2018.01.008
Matto, C., D’Alessandro, B., Mota, M. I., Braga, V., Buschiazzo, A., Gianneechini, E., et al. (2022). Listeria innocua isolated from diseased ruminants harbour minor virulence genes of L. monocytogenes. Veter. Med. Sci. 8, 735–740. doi: 10.1002/vms3.710
Moura, A., Disson, O., Lavina, M., Thouvenot, P., Huang, L., Leclercq, A., et al. (2019). Atypical hemolytic Listeria innocua isolates are virulent, albeit less than Listeria monocytogenes. Infect. Immun. 87:e00758. doi: 10.1128/iai.00758-18
Oakeson, K. F., Wagner, J. M., Rohrwasser, A., and Atkinson-Dunn, R. (2018). Whole-genome sequencing and bioinformatic analysis of isolates from foodborne illness outbreaks of campylobacter jejuni and Salmonella enterica. J. Clin. Microbiol. 56, e00161–e00118. doi: 10.1128/JCM.00161-18
Orsi, R. H., and Wiedmann, M. (2016). Characteristics and distribution of Listeria spp., including Listeria species newly described since 2009. Appl. Microbiol. Biotechnol. 100, 5273–5287. doi: 10.1007/s00253-016-7552-2
Orsini, M., Cornacchia, A., Patavino, C., Torresi, M., Centorame, P., Acciari, V. A., et al. (2018). Whole-genome sequences of two Listeria monocytogenes Serovar 1/2a strains responsible for a severe Listeriosis outbreak in Central Italy. Genome Announc. 6:e00236. doi: 10.1128/genomeA.00236-18
Osek, J., Lachtara, B., and Wieczorek, K. (2022). Listeria monocytogenes – how this pathogen survives in food-production environments? Front. Microbiol. 13:866462. doi: 10.3389/fmicb.2022.866462
Paramithiotis, S., Hadjilouka, A., and Drosinos, E. H. (2014). “Listeria Pathogenicity Island 1. Structure and function” in Listeria Monocytogenes: food sources, prevalence and management strategies. ed. E. C. Hambrick, 265–282.
Parra-Flores, J., Holý, O., Bustamante, F., Lepuschitz, S., Pietzka, A., Contreras-Fernández, A., et al. (2022). Virulence and antibiotic resistance genes in Listeria monocytogenes strains isolated from ready-to-eat foods in Chile. Front. Microbiol. 12:796040. doi: 10.3389/fmicb.2021.796040
Pöntinen, A., Aalto-Araneda, M., Lindström, M., and Korkeala, H. (2017). Heat resistance mediated by pLM58 plasmid-borne clpL in Listeria monocytogenes. mSphere 2:e00364. doi: 10.1128/mSphere.00364-17
Ramadan, H., Soliman, A. M., Hiott, L. M., Elbediwi, M., Woodley, T. A., Chattaway, M. A., et al. (2021). Emergence of multidrug-resistant Escherichia coli producing CTX-M, MCR-1, and FosA in retail food from Egypt. Front. Cell. Infect. Microbiol. 11:681588. doi: 10.3389/fcimb.2021.681588
Raveneau, J., Geoffroy, C., Beretti, J. L., Gaillard, J. L., Alouf, J. E., and Berche, P. (1992). Reduced virulence of a Listeria monocytogenes phospholipase-deficient mutant obtained by transposon insertion into the zinc metalloprotease gene. Infect. Immun. 60, 916–921. doi: 10.1128/iai.60.3.916-921.1992
Schardt, J., Jones, G., Müller-Herbst, S., Schauer, K., D’Orazio, S. E. F., and Fuchs, T. M. (2017). Comparison between Listeria sensu stricto and Listeria sensu lato strains identifies novel determinants involved in infection. Sci. Rep. 7:17821. doi: 10.1038/s41598-017-17570-0
Schlüter, D., Domann, E., Buck, C., Hain, T., Hof, H., Chakraborty, T., et al. (1998). Phosphatidylcholine-specific phospholipase C from Listeria monocytogenes is an important virulence factor in murine cerebral listeriosis. Infect. Immun. 66, 5930–5938. doi: 10.1128/iai.66.12.5930-5938.1998
Schmid, M. W., Ng, E. Y., Lampidis, R., Emmerth, M., Walcher, M., Kreft, J., et al. (2005). Evolutionary history of the genus Listeria and its virulence genes. Syst. Appl. Microbiol. 28, 1–18. doi: 10.1016/j.syapm.2004.09.005
Schmitz-Esser, S., Anast, J. M., and Cortes, B. W. (2021). A large-scale sequencing-based survey of plasmids in Listeria monocytogenes reveals global dissemination of plasmids. Front. Microbiol. 12:653155. doi: 10.3389/fmicb.2021.653155
Scortti, M., Han, L., Alvarez, S., Leclercq, A., Moura, A., Lecuit, M., et al. (2018). Epistatic control of intrinsic resistance by virulence genes in Listeria. PLoS Genet. 14:e1007525. doi: 10.1371/journal.pgen.1007525
Stessl, B., Wagner, M., and Ruppitsch, W. (2021). Multilocus sequence typing (MLST) and whole genome sequencing (WGS) of Listeria monocytogenes and Listeria innocua. Methods Mol. Biol. 2220, 89–103. doi: 10.1007/978-1-0716-0982-8_7
Sullivan, M. J., Petty, N. K., and Beatson, S. A. (2011). Easyfig: a genome comparison visualizer. Bioinformatics 27, 1009–1010. doi: 10.1093/bioinformatics/btr039
Tartor, Y. H., Gharieb, R. M. A., Abd El-Aziz, N. K., El Damaty, H. M., Enany, S., Khalifa, E., et al. (2021). Virulence determinants and plasmid-mediated Colistin resistance MCR genes in gram-negative bacteria isolated from bovine milk. Front. Cell. Infect. Microbiol. 11:761417. doi: 10.3389/fcimb.2021.761417
Travier, L., Guadagnini, S., Gouin, E., Dufour, A., Chenal-Francisque, V., Cossart, P., et al. (2013). ActA promotes Listeria monocytogenes aggregation, intestinal colonization and carriage. PLoS Pathog. 9:e1003131. doi: 10.1371/journal.ppat.1003131
Uelze, L., Grützke, J., Borowiak, M., Hammerl, J. A., Juraschek, K., Deneke, C., et al. (2020). Typing methods based on whole genome sequencing data. One Health Outlook 2:3. doi: 10.1186/s42522-020-0010-1
Vázquez-Boland, J. A., Kuhn, M., Berche, P., Chakraborty, T., Domínguez-Bernal, G., Goebel, W., et al. (2001). Listeria pathogenesis and molecular virulence determinants. Clin. Microbiol. Rev. 14, 584–640. doi: 10.1128/cmr.14.3.584-640.2001
Vielva, L., de Toro, M., Lanza, V. F., and de la Cruz, F. (2017). PLACNETw: a web-based tool for plasmid reconstruction from bacterial genomes. Bioinformatics 33, 3796–3798. doi: 10.1093/bioinformatics/btx462
Wilson, A., Gray, J., Chandry, P. S., and Fox, E. M. (2018). Phenotypic and genotypic analysis of antimicrobial resistance among Listeria monocytogenes isolated from Australian food production chains. Genes 9:80. doi: 10.3390/genes9020080
Wu, J., Zhao, L., Lai, S., and Yang, H. (2021). NMR-based metabolomic investigation of antimicrobial mechanism of electrolysed water combined with moderate heat treatment against Listeria monocytogenes on salmon. Food Control 125:107974. doi: 10.1016/j.foodcont.2021.107974
Youssef, M., Ramadan, H., and Al-Ashmawy, M. (2020). Prevalence of Listeria species in raw milk, ice cream and yogurt and effect of selected natural herbal extract on its survival. Mansoura Veter. Med. J. 21, 99–106. doi: 10.21608/mvmj.2020.21.317
Keywords: Listeria innocua, whole-genome sequencing, genetic context, clpL gene, phylogenetic analysis, milk, Egypt
Citation: Ramadan H, Al-Ashmawy M, Soliman AM, Elbediwi M, Sabeq I, Yousef M, Algammal AM, Hiott LM, Berrang ME, Frye JG and Jackson CR (2023) Whole-genome sequencing of Listeria innocua recovered from retail milk and dairy products in Egypt. Front. Microbiol. 14:1160244. doi: 10.3389/fmicb.2023.1160244
Edited by:
Fereidoun Forghani, IEH Laboratories and Consulting Group, United StatesReviewed by:
Laurel S. Burall, United States Food and Drug Administration, United StatesHongshun Yang, Jiangnan University (Shaoxing) Industrial Technology Research Institute, China
Shaoting Li, University of Georgia, United States
Copyright © 2023 Ramadan, Al-Ashmawy, Soliman, Elbediwi, Sabeq, Yousef, Algammal, Hiott, Berrang, Frye and Jackson. This is an open-access article distributed under the terms of the Creative Commons Attribution License (CC BY). The use, distribution or reproduction in other forums is permitted, provided the original author(s) and the copyright owner(s) are credited and that the original publication in this journal is cited, in accordance with accepted academic practice. No use, distribution or reproduction is permitted which does not comply with these terms.
*Correspondence: Hazem Ramadan, aGF6ZW1faGFzc2FuQG1hbnMuZWR1LmVn; Charlene R. Jackson, Y2hhcmxlbmUuamFja3NvbkB1c2RhLmdvdg==