- Department of Applied Biosciences, Graduate School of Bioagricultural Sciences, Nagoya University, Nagoya, Aichi, Japan
Among the genes involved in the biosynthesis of trichothecene (Tri genes), Tri6 and Tri10 encode a transcription factor with unique Cys2His2 zinc finger domains and a regulatory protein with no consensus DNA-binding sequences, respectively. Although various chemical factors, such as nitrogen nutrients, medium pH, and certain oligosaccharides, are known to influence trichothecene biosynthesis in Fusarium graminearum, the transcriptional regulatory mechanism of Tri6 and Tri10 genes is poorly understood. Particularly, culture medium pH is a major regulator in trichothecene biosynthesis in F. graminearum, but it is susceptible to metabolic changes posed by nutritional and genetic factors. Hence, appropriate precautions should be considered to minimize the indirect influence of pH on the secondary metabolism while studying the roles of nutritional and genetic factors on trichothecene biosynthesis regulation. Additionally, it is noteworthy that the structural changes of the trichothecene gene cluster core region exert considerable influence over the normal regulation of Tri gene expression. In this perspective paper, we consider a revision of our current understanding of the regulatory mechanism of trichothecene biosynthesis in F. graminearum and share our idea toward establishing a regulatory model of Tri6 and Tri10 transcription.
Introduction
Fusarium graminearum and related species cause Fusarium head blight (FHB), a devastating disease of small grain cereals, such as wheat and barley (Goswami and Kistler, 2004). In addition to the yield loss, they accumulate trichothecene mycotoxins in the maturing seeds, thereby posing serious threats to the safety of grains and their commodities. As deoxynivalenol (DON) and its acetylated precursors, 3-acetyldeoxynivalenol and 15-acetyldeoxynivalenol (15-ADON), are the most prevalent trichothecenes associated with FHB, permissible limits were set for DON level in foods and feeds by administrative organizations in many countries. For the development of appropriate measures to reduce the risk of mycotoxin contamination, understanding the transcriptional regulatory mechanism of trichothecene biosynthesis genes (Tri genes) is important. Trichothecene biosynthesis in F. graminearum is known to be regulated by various nutritional and environmental factors, including the pH of the culture media (Gardiner et al., 2009b), water activity (Ramirez et al., 2006), nitrogen source (Min et al., 2012; Giese et al., 2013), oligosaccharide inducers containing an α-(1 → 2) (glucosyl/xylosyl)-fructosyl linkage (Nakajima et al., 2016), and oxidative stress (Ponts et al., 2006).
Most genes involved in trichothecene biosynthesis assemble in the gene cluster, of which the core region is comprised of four Tri genes, Tri4–Tri6–Tri5–Tri10, that are sufficient to build up the toxic trichothecene skeleton of isotrichodermol (Kimura et al., 2007; Desjardins, 2009; McCormick et al., 2013). Among the four minimal Tri genes, Tri6 and Tri10 are regulatory genes. Tri6 encodes a transcription factor with three unique Cys2His2 zinc finger motifs for DNA binding to the minimum sequence of YNAGGCC (Hohn et al., 1999), and the protein is indispensable for the transcription of pathway Tri genes (Proctor et al., 1995). Tri10 encodes a protein without any known DNA-binding motifs, which plays a pivotal role in the transcriptional activation of Tri genes (Tag et al., 2001). The remaining two genes, Tri5 and Tri4, are required for the cyclization of farnesyl pyrophosphate to trichodiene (Hohn and Beremand, 1989) and oxygenations of trichodiene to isotrichotriol (McCormick et al., 2006; Tokai et al., 2007), respectively; of which the isotrichotriol is subsequently cyclized to isotrichodermol non-enzymatically under acidic conditions.
The molecular genetic study of trichothecene biosynthesis was initially started in the late 1980s by the research group of USDA-ARS (United States Department of Agriculture, Agricultural Research Service, Peoria, IL) using the T-2 toxin-producing Fusarium sporotrichioides NRRL 3299 as a model strain (Desjardins et al., 1993). Distinct from other Fusarium species such as F. graminearum and F. venenatum, the trichothecene biosynthesis is not tightly regulated in F. sporotrichioides. Indeed, T-2 toxin accumulates in various media under different culture conditions where F. graminearum fails to produce trichothecenes. With increasing availability of genomic information and molecular tools in the 2000s, research interest was shifted to the regulatory mechanism of trichothecene biosynthesis in F. graminearum, which is the primary causal agent of FHB (Merhej et al., 2011). Up to now, several regulatory models of Tri gene expression have been proposed in previous studies (Seong et al., 2009; Nasmith et al., 2011; Hou et al., 2015; Jiang et al., 2016), but a definitive model remains to be established.
While interpreting the influence of a certain nutritional or genetic factor on a biological phenotype, a “cause-and-effect” relationship should not be established unless other factors that have critical impacts on the phenotype are consistent with the control condition. Therefore, it is important to set up experimental designs so that the “effect” is not determined by such critical factors. For example, to elucidate the genetic basis of trichothecene biosynthesis regulation in F. graminearum, each mutant should be evaluated considering the pH, which is a major regulatory factor, to be essentially the same as that of the wild-type (Nakajima et al., 2020). In this perspective paper, we discuss the underlying issues associated with the use of various culture systems and exemplify the possible approaches to cope with the challenges. The overlooked impact of perturbing the structure of the trichothecene gene cluster core region is also highlighted, as well as the future direction of studies from methodological and strategic aspects.
Choice of appropriate culture systems and conditions is essential for the study of the regulatory mechanism of trichothecene biosynthesis in F. graminearum
The pH of a medium is critical and has a decisive influence on the regulation of the secondary metabolism. Exposure to an extremely low pH bypasses the requirement of certain factors that are involved in the activation of trichothecene biosynthesis in F. graminearum. In addition to chemical factors, less well known are physical factors that mediate trichothecene biosynthesis. Mycelia within a submerged shake culture exist either in dispersed or aggregate forms under a certain physical condition (determined by multiple factors; e.g., culture volume, vessel size and shape, and agitation speed), which affects trichothecene biosynthesis regulation (Nakajima et al., 2014). Furthermore, the trichothecene induction is associated with mycelial morphology. For example, F. graminearum produces trichothecenes in aerial hyphae grown on YG medium (0.5% [w/v] yeast extract, 2% [w/v] glucose) solidified by agar, despite its inability to produce trichothecenes in submerged hyphae on liquid YG medium (Ochiai et al., 2007). Other culture conditions or components, such as salt concentrations, may also exert influence over the expression of Tri genes, and thus, subsequent trichothecene biosynthesis. However, on liquid media with gyratory shaking, small increases in salt concentration, needed for pH adjustments of the culture to assess the impact of agmatine (Nakajima et al., 2020), marginally affected toxin production. In this section, we show the importance of choosing appropriate culture systems and conditions for the study of the regulatory mechanism of trichothecene biosynthesis, while highlighting instances in which erroneous conclusions tend to be made and/or obvious facts could be overlooked.
Advantages and disadvantages associated with the use of various types of culture media
Numerous dispensable and indispensable factors are involved in the induction of trichothecene biosynthesis, and their combined effects determine the amount of the mycotoxin accumulation in F. graminearum. Both complex and defined media (synthetic media) have been used to evaluate the role of a certain factor on trichothecene induction.
The advantage of using complex media for trichothecene biosynthesis regulatory studies is the relatively mild acidic conditions during culture and generally strong induction of toxin synthesis. On the other hand, acidic culture conditions below pH 3.7 (e.g., acidification of the medium by fungal catabolism) are prerequisites for activation of trichothecene production if defined media are used (Nakajima et al., 2020). Although the ingredients differ significantly among products, yeast extract is often used as a source of nitrogen and micronutrients for the preparation of complex media. When a good manufacturing lot of yeast extract product is used, trichothecene biosynthesis is induced more strongly than with the use of defined media, despite the culture pH remaining at higher conditions. Specifically, F. graminearum produces trichothecenes on liquid YS_60 medium (0.1% [w/v] yeast extract, 6% [w/v] sucrose) with a pH above 3.7 (Nakajima et al., 2014). However, a disadvantage is that the mycotoxin-inducing activity differs considerably among the brands and lots of the yeast extract products. Complex media prepared with certain yeast extract products cannot induce trichothecenes by F. graminearum, posing difficulty in their utilization for biosynthesis regulation studies (Sørensen and Sondergaard, 2014; Tanaka et al., 2019). Interestingly, extraction of the complex media with ethyl acetate, followed by removal of the solvent fraction, results in increased trichothecene accumulation. Hence, several ethyl acetate-extractable substances in yeast extract negatively affects trichothecene production (Tanaka et al., 2019).
Utilization of defined media provides an advantage over the complex media in that their ingredients are consistent with each preparation, which is important for the experimental reproducibility of results. However, the pH of defined media generally tends to change more drastically than that of complex media. A key challenge in the utilization of defined media optimized for trichothecene production (Gardiner et al., 2009a) is the extreme acidification of the culture associated with fungal growth, which could misrepresent the involvement of factors that determine the culture pH, such as the catabolism of polyamines, in trichothecene induction (Nakajima et al., 2020). Hence, carefully worked-out plans will be necessary to evaluate the role of chemical and biological factors, whose direct impacts on trichothecene regulation are much less significant than their indirect and non-specific effects via pH.
Example 1: Polyamines are not inducers of trichothecene production in axenic culture
Polyamines are aliphatic amines that are involved in stress responses in plants (Alcázar et al., 2006). A previous study has reported that trichothecene production in vitro was significantly lower compared to in planta, which led to the hypothesis that there are signals from host plants, such as polyamines, that act as inducers of trichothecene biosynthesis (Gardiner et al., 2009a). During the culture of F. graminearum under different amino acids and amines as the sole nitrogen sources, agmatine and putrescine drastically activated Tri5 expression and trichothecene production, which motivated Gardiner and co-workers to speculate that such polyamines from plant polyamine biosynthetic pathways act as in planta signals for induction of trichothecene biosynthesis (Gardiner et al., 2009a).
However, when agmatine and putrescine were added in micromolar concentrations to F. graminearum JCM 9873 (15-ADON chemotype) on liquid YG_60 medium (0.1% [w/v] yeast extract, 6% [w/v] glucose), trichothecene biosynthesis was not induced (Nakajima et al., 2020). In contrast, other known trichothecene biosynthesis activators, such as sucrose (Nakajima et al., 2016) and NPD12671 chemical compound (Maeda et al., 2018), were effective in induction at micromolar concentrations in the same complex medium, demonstrating that such polyamines are not inducers (Nakajima et al., 2020). Additionally, in media containing either agmatine or L-Gln as the sole nitrogen source, 15-ADON production levels were similar between these two nitrogen sources when the pH of the L-Gln culture was frequently adjusted to the pH of the agmatine culture (Nakajima et al., 2020). Furthermore, the agmatine culture could not induce trichothecene biosynthesis when liquid cultures were maintained at a pH above 4 (Nakajima et al., 2020). Consequently, the natural pH decrease in the agmatine culture appears to be the factor responsible for inducing trichothecene biosynthesis. Together with the fact that the polyamines agmatine and putrescine are unable to induce trichothecene production at micromolar amounts, polyamines are not suitable to be defined as inducers unless their inducing abilities are proven under carefully controlled and considered culture conditions.
Example 2: FgAreA, a nitrogen regulatory gene, is not essential for trichothecene biosynthesis
AreA is a GATA family transcription factor involved in the regulation of the utilization of secondary nitrogen sources (Tudzynski et al., 1999), and the ortholog FgAreA was reported to be indispensable for the utilization of most amino acids by F. graminearum strain PH-1(Giese et al., 2013). To study the effect of FgAreA deletion on trichothecene biosynthesis, wild-type (JCM 9873) and ΔFgareA mutant strains were cultured on liquid L-Gln medium with gyratory shaking. In accordance with previous reports on the role of FgAreA in secondary metabolism in strains PH-1 (Giese et al., 2013) and GZ3639 (Min et al., 2012), trichothecene was not detected in ΔFgareA cultures (Nakajima et al., 2020). Thus, FgAreA appears to be essential for trichothecene production. However, the time-dependent pH profiles of the liquid cultures were considerably different between the strains due to variations in growth rates, and the pH profiles of the ΔFgareA culture could not be superimposed on that of the wild-type by increasing the inoculum size (Nakajima et al., 2020). In fact, the pH of the ΔFgareA culture never fell below pH 3.7, a condition which we observe to be necessary to trigger trichothecene biosynthesis in defined media. When the experiment was performed with an L-Gln liquid medium constantly maintained at pH 2.5, trichothecene production by the ΔFgareA strain was comparable to that of the wild-type (Nakajima et al., 2020). The results showed that FgAreA is dispensable for trichothecene production and that the contribution of FgAreA to biosynthesis regulation depends on the pH of the cell culture medium. Since low pH affects trichothecene biosynthesis to a greater extent than FgAreA, when studying the roles of FgAreA, pH profiles must be considered during the experimental design and interpretation of results.
Example 3: Overexpression experiment of the WOR1 ortholog Fgp1 under certain culture conditions could mislead its role in trichothecene biosynthesis
WOR1 is a master transcriptional regulator of the white-opaque switching in Candida albicans (Zhang et al., 2014), and its homologs in various plant pathogenic fungi play pleiotropic roles, such as regulation of pathogenicity, conidiation, secondary metabolism, and other biological processes (Michielse et al., 2009, 2011; Jonkers et al., 2012; Santhanam and Thomma, 2013; Chen et al., 2014; Okmen et al., 2014). The WOR1 ortholog in F. graminearum PH-1, Fgp1, was demonstrated to be essential for pathogenicity and trichothecene biosynthesis; revealing it plays a regulatory role in the expression of Tri genes (Jonkers et al., 2012). There is a conserved threonine-67 (T67) residue within the putative protein kinase A (Pka) phosphorylation site of Fgp1. As mutants containing a T67A mutation exhibit dramatically impaired virulence similar to the deletion mutant, phosphorylation of this residue is suggested to be important in the activation of Fgp1 (Yu et al., 2015). To further analyze and elucidate the role of Fgp1, we constructed an Fgp1 overexpressor (ΔFgp1 + PGPD::Fgp1; Fgp1 connected to a glyceraldehyde 3-phosphate dehydrogenase [GPD] promoter) strain using an Fgp1 deletion (ΔFgp1) host strain derived from JCM 9873, in addition to the complemented (ΔFgp1 + Fgp1) and phosphorylation site mutant (ΔFgp1 + Fgp1T67A) strains (Supplementary Figure 1).
When cultured axenically on liquid defined media with 3% (w/v) glucose and at a starting pH of 4 (see Supplementary Table 1 for medium composition), none of the strains produced 15-ADON (Figure 1A; panels on the first column). In the presence of sucrose in exchange for glucose, the wild-type and complemented strains produced 15-ADON, whereas the overexpressor did not (Figure 1A; panels on the second column). Interestingly, the pH profile of the overexpressor remarkably deviated from those of the other strains (Figure 1B; upper graphs); the Fgp1 overexpressor showed a higher pH (above 4.2) as the growth reached the stationary phase and the pH never decreased below 3.7 over the entire growth phase. As WOR1 homologs play pleiotropic roles, the large amount of Fgp1 present could result in metabolism that differed greatly from that of the wild-type on this particular defined medium and subsequent deviation in the pH profile of the culture. Since the pH of the culture medium has a significant impact on trichothecene biosynthesis in F. graminearum (Gardiner et al., 2009b), it is essential to observe trichothecene biosynthesis in all tested strains under the same pH condition. Thus, all four strains were then tested in media at a pH of 2.5 instead, where the pH profiles of all four strains were almost identical without any adjustment (Figure 1B; lower graphs). Under gyratory shaking on glucose medium, production of 15-ADON was marginal in the wild-type and complemented strains, while the overexpressor exhibited a marked increase in 15-ADON production (Figure 1A; panels on the third column). In the sucrose media, the wild-type, complemented, and overexpressor strains all exhibited high 15-ADON production levels (Figure 1A; panels on the fourth column), which was expected of due to the highly inducing conditions from the combination of low pH and presence of the inducer molecule sucrose. In agreement with the previous report on the functional importance of the T67 residue (Yu et al., 2015), no 15-ADON production by the T67A mutant was observed under all four culture conditions (Figure 1A). To confirm that the increased production of 15-ADON by the overexpressor is not limited to the extremely acidic culture conditions, the fungal strains were grown in YS_60 medium with higher culture pH. The results showed that the Fgp1 overexpressor produced a greater amount of 15-ADON when the pH profile was similar to that of the wild-type (Supplementary Figure 2). Thus, we speculate that Fgp1 may play a role in leading to an active euchromatin structure around the trichothecene gene cluster, which subsequently triggers transcription of the cluster Tri genes.
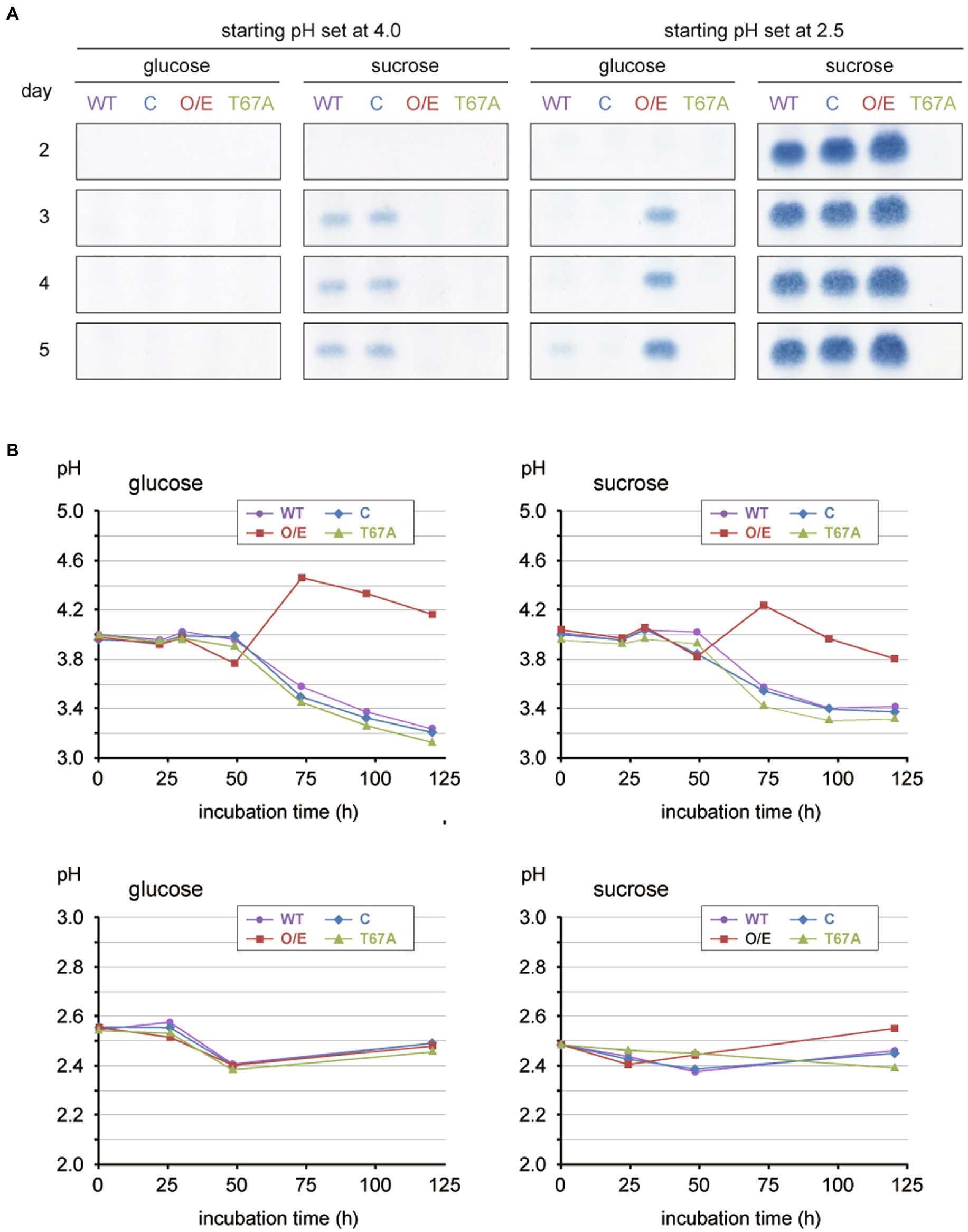
Figure 1. Toxin production assay of the various Fgp1 mutant strains with two independent parameters, carbon source (glucose and sucrose) and initial pHs (4 and 2.5), cultured on 30 mL of medium in a 100-mL Erlenmeyer flask with gyratory shaking (135 rpm) at 25°C. (A) Each of the four strains tested (wild-type, WT; complemented, C; overexpressor, O/E; phosphorylation site mutant, T67A) was cultured on the defined media with amino acids mixture nitrogen source (Supplementary Table 1), and the carbon source and initial pH as indicated at the top. Each culture was sampled on days 2, 3, 4 and 5 of incubation. TLC panels show the spots of 15-ADON extracted from 500 μL of the medium with ethyl acetate. (B) Time course of pH changes of each fungal culture. When cultured at initial pH of 4, the wild-type, complemented, and T67A strains exhibited similar pH profiles, while the overexpressor displayed an increase in pH and deviated from the other three strains (upper graphs). When cultured at initial pH of 2.5, all four strains exhibited similar pH profiles (lower graphs).
If the effect of overexpressing Fgp1 was interpreted based solely on the amount of the trichothecene produced, a conclusion on the effect of high Fgp1 expression and subsequently the role of Fgp1 could be erroneously made. Our results therefore showed the importance of choosing appropriate experimental conditions to keep other factors that have a significant impact on trichothecene biosynthesis consistent, so that the effects of the factor studied can be observed more accurately.
Example 4: Culturing of F. graminearum in a 24-well plate under gyratory shaking reveals the inducer role of sucrose in trichothecene biosynthesis
In liquid media, the size and shape of the culture vessel determine the morphological features of growing F. graminearum under gyratory shaking. Trichothecene biosynthesis is more strongly induced in flat mycelial flocs obtained by culturing in a 24-well plate than in dispersed mycelia obtained by culturing on the same medium in an Erlenmeyer flask (Nakajima et al., 2016). Notably, the trichothecene-inducing activity of oligosaccharides containing α-(1 → 2) (glucosyl/xylosyl)-fructosyl linkages, such as sucrose and kestose, could be observed only by culturing in 24-well plates (1 mL medium per well) (Nakajima et al., 2016). Although sucrose was previously believed to be required as a carbon source for the induction of trichothecene biosynthesis (Jiao et al., 2008; Kawakami et al., 2014), the use of a 24-well culture plate revealed that sucrose contributes even at lower concentrations of 100 μM. Consequently, this concentration is sufficiently low to be regarded as a specific inducer, rather than as a carbon source (Nakajima et al., 2016). Interestingly, if complex media are prepared by using a good yeast extract lot with the elimination of the ethyl acetate-extractable fraction, sucrose is unnecessary for trichothecene production, and F. graminearum cultured on liquid YF_60 medium (0.1% [w/v] yeast extract, 6% [w/v] fructose) accumulates a considerable amount of trichothecenes under gyratory shaking in a 24-well plate (Tanaka et al., 2019). Such a strong trichothecene-inducing culture condition is not appropriate for studying the inducer role of sucrose. Therefore, it is important to establish appropriate culture systems and conditions so that the specific role of sucrose in trichothecene biosynthesis can be observed.
Mutational analyses of Tri genes in the trichothecene gene cluster core region
To date, the functional characterization of the Tri genes has mostly been investigated by conventional gene disruption techniques using vectors carrying the cloned homologous regions and a positive selection marker gene cassette. Both single and double crossover homologous recombination methods have been applied to knockout the Tri genes.
When the middle portion of a Tri gene is cloned into the vector and used for Fusarium transformation, two dysfunctional gene copies truncated at the 5′ and 3′ ends arise via single crossover homologous integration (method 1). Consequently, the distance between the upstream and downstream Tri genes is extended by the size of the gene disruption vector. The disruption vector can also be designed by inserting a marker cassette between the 5′ and 3′ regions of the Tri gene to be disrupted (or deleted if the homologous sequences do not include the gene-coding regions). The disruption vector backbone is eliminated via double crossover homologous integration, and the target Tri gene is replaced with the marker cassette (method 2). Thus, the length of the intergenic region in the trichothecene gene cluster may be changed. As discussed below, the extension of the cluster core region (refer to the Introduction section for the definition of the core region) remarkably affects Tri gene expression, presumably through changes in chromatin structure.
Deregulation of trichothecene biosynthesis by structural changes of the cluster core region
While F. sporotrichioides produces a considerable amount of trichothecene on a liquid shake culture with glucose as the carbon source, F. graminearum produces a limited amount of trichothecene under the same culture condition (Nakajima et al., 2016). In an attempt to boost trichothecene production, Chen et al. (2000) transformed F. graminearum with a series of vectors containing a promoter of F. graminearum Tri5 (P Tri5 ), a β-D-glucuronidase (GUS) reporter gene, and/or a transcription unit of F. sporotrichioides Tri6 (FsTri6) (Supplementary Figure 3A). The result revealed that trichothecene production is increased in either transformant, in which the Tri5–Tri6 intergenic region was extended via homologous integration of the vector through the P Tri5 sequence (Figure 2A). This study represents the first finding of a disordered regulation of trichothecene biosynthesis by changing the length of the cluster core region. Therefore, molecular genetic studies on the regulatory mechanisms of Tri gene expression should be performed without perturbating the core region of the gene cluster.
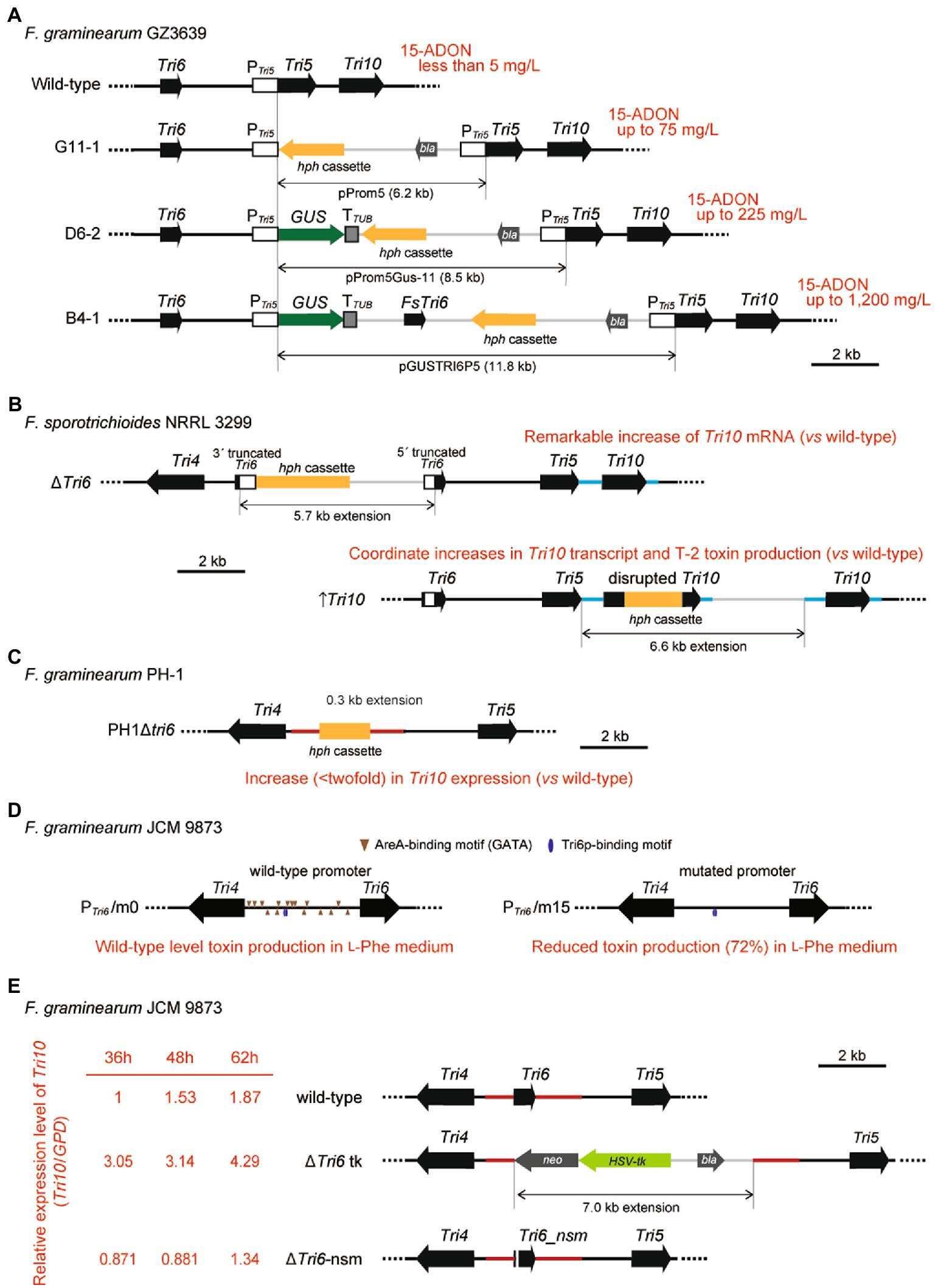
Figure 2. Genetic manipulations of the Tri4–Tri6–Tri5–Tri10 locus in Fusarium. Deregulation of Tri6 and Tri10 occurs in cases when the targeted gene disruptions based on homologous recombination change the length of the core region of the trichothecene gene cluster. Vector integrations via single or double crossover homologous recombination for each subfigure are described in detail in Supplementary Figure 3. (A) Genetically engineered cluster core region of 15-ADON chemotype F. graminearum GZ3639 (Chen et al., 2000). The structure of the cluster core region is illustrated. Transformants, G11-1, D6-2, and B4-1, were obtained by homologous integration of vectors pProm5 (containing PTri5 alone), pProm5GUS-11 (containing PTri5 fused to GUS; PTri5::GUS), and pGUSTRI6P5 (containing both PTri5::GUS and FsTri6), respectively. Integration of pProm5, pProm5GUS-11, and pGUSTRI6P5 through the Tri5 promoter region extends the Tri5–Tri6 intergenic region by 6.2 kb (G11-1), 8.5 kb (D6-2), and 11.8 kb (B4-1), respectively. While the wild-type GZ3639 accumulates less than 5 mg/L of trichothecenes by shake culture on liquid medium with glucose, elongation of the cluster core region resulted in increased production of 15-ADON (up to 75 mg/L by G11-1, up to 225 mg/L by D6-2, and up to 1,200 mg/L by B4-1), irrespective of the presence or absence of FsTri6 on the vector. Ectopic integration of pGUSTRI6P5 (e.g., up to 54 mg/l by strain B9-1; not shown in the subfigure) did not yield 15-ADON above the amount obtained by strain G11-1 lacking FsTri6. Notably, compared with strain B9-1, much stronger GUS activity was detected from strain B4-1 (50- to 100-fold increase), in which PTri5::GUS is located at the cluster core region. (B) Genetically engineered cluster core region of F. sporotrichioides strain NRRL 3299 (Tag et al., 2001). The structure of the cluster core region of each derived mutant strain is illustrated. The resulting ΔTri6 strain contains a 3′-truncated Tri6 gene followed by an hph cassette and then a 5′-truncated Tri6 gene, and is expected to have a 5.7 kb extension at the Tri6 region. The ↑Tri10 and ΔTri10 strains were constructed from the same plasmid pTri10-1 which could undergo single or double crossover (Continued)FIGURE 2 (Continued)homologous recombination events at the upstream and/or downstream regions, respectively. The ↑Tri10 strain contains a disrupted copy of Tri10 followed by a functional copy of Tri10 downstream of Tri5 with a 6.6 kb extension, while the ΔTri10 strain contains only a disrupted copy of Tri10 with a 1.6 kb extension in the same region (a ΔTri10 strain described in Supplementary Figure 3B only). (C) Disrupted Tri6 in F. graminearum strain NRRL 31084 (PH-1) (Seong et al., 2009). The PH1Δtri6 strain was generated by replacing the Tri6 region with an hph cassette, resulting in a slight perturbation within the cluster core region. The region is extended by 0.3 kb in PH1Δtri6. (D) Wild-type and mutated Tri6 promoters without structural perturbation of the cluster core region in F. graminearum strain JCM 9873 (Nakajima et al., 2020). PTri6/m0 (complemented) and PTri6/m15 (mutated) strains were designed by applying a two-step transformation process involving double crossover homologous recombination events. All fifteen 5′-GATA-3′ AreA-binding consensus sequences were mutated to 5′-GGTG-3′ in the wild-type Tri4–Tri6 bidirectional promoter in PTri6/m15. Both PTri6/m0 and PTri6/m15 strains are marker free and introduce no perturbation within the core cluster region. (E) Two types of Tri6 disrupted mutants of F. graminearum strain JCM 9873. Both ΔTri6 tk (Nakajima et al., 2014) and ΔTri6-nsm strains were generated to study the effect of Tri6 deletion. The ΔTri6 tk strain was generated using a one-step transformation process and contains a selection marker cassette replacing the Tri6 coding region, which results in a 7.0 kb extension in the cluster. The ΔTri6-nsm strain was generated using a two-step transformation process and contains a dysfunctional copy of the Tri6 gene containing a nonsense mutation, which does not result in perturbation within the cluster region. The relative expression level of Tri10 (represented as Tri10/GPD) was determined using the expression of GPD as an endogenous reference in the same RNA samples. The Tri10/GPD ratio only increased in ΔTri6 tk, while that in ΔTri6-nsm was similar to the expression level in the wild-type.
Similarly, in molecular genetic studies of F. sporotrichioides, Beremand’s group reported a deregulated expression of Tri genes in the cluster core region. When a 6.6 kb vector pTri10-1 was integrated into the Tri5–Tri10 region via single crossover homologous recombination (Supplementary Figure 3B), the resulting transformants showed coordinate increases in Tri10 transcript and T-2 toxin production over the wild-type levels (Figure 2B; ↑Tri10) (Tag et al., 2001). In addition, a remarkable increase of Tri10 mRNA was observed on the northern blot of the Tri6 disruption mutant (Figure 2B; strain ΔTri6 created by method 1 (Proctor et al., 1995)), in which the cluster core region was extended by 5.7 kb via single crossover homologous integration of the pTX6T-1 disruption vector at the Tri6 locus (Supplementary Figure 3B). This observation led them to assume that transcription of Tri10 is negatively regulated by Tri6p. Similarly, Kistler and co-workers reported an increase (<twofold) in Tri10 expression (Figure 2C; PH1Δtri6) in their Tri6 disruption mutant of F. graminearum (Supplementary Figure 3C; Seong et al., 2009), although smaller than that observed by Beremand’s group. The PH1Δtri6 mutant created by double crossover homologous recombination (method 2) contains a Tri5–Tri10 region elongated by 0.3 kb, which is much shorter than that of the F. sporotrichioides ΔTri6 mutant. Thus, the degree of extension of the cluster core region appears to affect the increase in Tri10 expression level. Although the presence of Tri6p-binding motifs in the Tri10 coding region (Supplementary Figure 6) led the researchers to hypothesize that they could be utilized to effectively block Tri10 expression (Tag et al., 2001), the observed Tri10 overexpression phenotype of the Tri6 disruption mutants appears to be caused mainly by elongation of the Tri5–Tri10 region, rather than Tri6 inactivation (see next section).
Introducing mutations into Tri genes at their native locus
Based on the issues described above, the structural organization of the trichothecene gene cluster should not be altered during the mutational analysis of the Tri4–Tri6–Tri5–Tri10 region. To maintain the location and size of the genetic elements that reside in the cluster core region, mutations should be introduced into the native locus where the target genes are originally located at. Consequently, we use a two-step transformation process involving double crossover homologous recombination (Khang et al., 2005); in which self-cloning strains are generated by replacing the wild-type genetic elements with mutated alleles. In this process, marker-free mutants are obtained by positive selection and subsequent conditional negative selection against the hph::tk cassette, which contains a hygromycin B phosphotransferase gene (hph) that is translationally fused to a herpes simplex virus thymidine kinase gene (tk) (Maeda and Ohsato, 2017). This self-cloning strategy enabled the correct evaluation of the effect of mutations introduced at the native locus on the regulation of trichothecene biosynthesis. Using this strategy, our group mutated all fifteen 5′-GATA-3′ AreA-binding consensus sequences to 5′-GGTG-3′ in the wild-type Tri4–Tri6 bidirectional promoter (Supplementary Figure 3D), and assessed precisely the degree of contribution of catabolizing L-Phe used as the sole nitrogen source of culture medium on trichothecene biosynthesis (Figure 2D; Nakajima et al., 2020). When generating knock-out strains, this strategy is also useful as the native gene can be replaced with a gene containing a nonsense mutation, instead of conventionally replacing the target gene with a selection marker cassette that is often of different lengths.
Given the altered trichothecene production caused by structural perturbation of the gene cluster core region (Chen et al., 2000), we question previous models on the roles of Tri6 and Tri10 in the transcriptional activation of Tri genes. As mentioned previously, several studies have reported that Tri10 expression was elevated in Tri6 deletion strains, thus concluding that Tri10 was negatively regulated by Tri6 (Tag et al., 2001; Seong et al., 2009). However, our pilot study comparing the Tri10 expression between two types of Tri6 disruptant strains, Tri6 replaced with either a selection cassette (strain ΔTri6 tk) or Tri6_nsm containing a nonsense mutation (strain ΔTri6-nsm) (Supplementary Figure 3E), revealed that Tri10 expression was elevated only in the ΔTri6 tk strain, whereas that in ΔTri6-nsm was similar to that in the wild-type (Figure 2E; see fold change of Tri10/GPD). This shows that the increase in Tri10 expression in Tri6 deletion mutants reported in previous studies (Tag et al., 2001; Seong et al., 2009) was likely due to the structural extension in the core gene cluster upstream of Tri10 (Figures 2B,C), and the model which suggests that Tri6 negatively regulates Tri10 requires revision. Another group suggested that Tri10p and FgAreAp interact with each other and this interaction is possibly important for the activation of other Tri genes (Hou et al., 2015). To further examine whether FgAreA and Tri10 deletion would have the same extent of effect due to the loss of such interaction, we investigated 15-ADON production in these mutant strains. At low pH of 2.5, the ΔFgareA strain (Nakajima et al., 2020) produced a considerable amount of 15-ADON, while the ΔTri10-nsm strain, carrying Tri10_nsm with a nonsense mutation and without perturbing the gene cluster core region, produced no 15-ADON (Supplementary Figure 7). The result suggests that Tri10 is much more important than FgAreA in the regulation of trichothecene biosynthesis and that the physical interaction between FgAreAp and Tri10p (Hou et al., 2015) is unessential.
Thus far, several studies have overlooked the impact of extension in the trichothecene gene cluster, thus, there is a need for revisions to improve our present knowledge of the regulatory mechanism of Tri gene expression. In addition, future studies must consider these effects. Genome editing using Clustered Regularly Interspaced Short Palindromic Repeats (CRISPR)-associated (Cas) nucleases may be a promising tool for genetic manipulation of F. graminearum without perturbations in the cluster core region, but in the absence of selection, the recovery of mutants is relatively infrequent and poses a problem for efficient research currently (Gardiner and Kazan, 2018). Finally, we close this perspective paper by presenting a novel idea that could lead to a deeper understanding of the complex regulation of Tri6.
Future perspectives
Results from the studies performed by our group suggest that Tri6 transcription is under two types of regulation as follows: Tri6p-independent initial activation and subsequent Tri6p-dependent feedback activation by itself (Maeda et al., 2018). For molecular genetic studies to discriminate between the two modes of regulation, we have constructed a strain that contains a copy of Tri6_nsm at the native locus and a copy of highly-expressing synonymous Tri6 gene at an ectopic locus (ΔTri6-nsm/synTri6) by using the ΔTri6-nsm strain (described in the above subsection; see also Supplementary Figure 3E) as the parent. In the ΔTri6-nsm strain, no Tri6p is produced; thus, Tri6p-dependent activation cannot occur. Further we established that nonsense-mediated Tri6_nsm mRNA decay (Wen and Brogna, 2010) does not occur in ΔTri6-nsm, as suggested by the observation of similar levels of Tri6p-independent transcription of mutated Tri6, made from the native locus in mutant strains with dysfunctional zinc finger domains. A copy of the synonymous Tri6 gene, which has a different DNA sequence from that of the native Tri6 gene but encodes the same amino acid sequence, is highly expressed from an ectopic locus. By comparing the expression level of Tri6_nsm at the native locus in both the ΔTri6-nsm and ΔTri6-nsm/synTri6 strains, the Tri6p-independent initial activation and subsequent Tri6p-dependent activation could be differentiated while studying the effects of the associated factors, thus, providing further clarity and insight into the regulatory mechanism of trichothecene biosynthesis.
Data availability statement
The original contributions presented in the study are included in the article/Supplementary material, further inquiries can be directed to the corresponding author.
Author contributions
MXXL, YN, and MK conceived the study. MXXL, YN, KM, and NK performed the wet experiments and analyzed data. MXXL and MK wrote and elaborated the manuscript. MXXL, YN, KM, and MK designed the figures. All authors read and approved the final manuscript.
Funding
This work was supported by a grant from the JSPS KAKENHI, Grant-in-Aid for Scientific Research C (22 K05379), and a research grant from IFO, the Institute for Fermentation, Osaka (G-2021-2-098).
Acknowledgments
We thank Adeeb Qunash for making suggestions in English phrases and expressions.
Conflict of interest
The authors declare that the research was conducted in the absence of any commercial or financial relationships that could be construed as a potential conflict of interest.
Publisher’s note
All claims expressed in this article are solely those of the authors and do not necessarily represent those of their affiliated organizations, or those of the publisher, the editors and the reviewers. Any product that may be evaluated in this article, or claim that may be made by its manufacturer, is not guaranteed or endorsed by the publisher.
Supplementary material
The Supplementary material for this article can be found online at: https://www.frontiersin.org/articles/10.3389/fmicb.2023.1148771/full#supplementary-material
References
Alcázar, R., Marco, F., Cuevas, J. C., Patron, M., Ferrando, A., Carrasco, P., et al. (2006). Involvement of polyamines in plant response to abiotic stress. Biotechnol. Lett. 28, 1867–1876. doi: 10.1007/s10529-006-9179-3
Chen, L., McCormick, S. P., and Hohn, T. M. (2000). Altered regulation of 15-acetyldeoxynivalenol production in Fusarium graminearum. Appl. Environ. Microbiol. 66, 2062–2065. doi: 10.1128/AEM.66.5.2062-2065.2000
Chen, Y., Zhai, S., Zhang, H., Zuo, R., Wang, J., Guo, M., et al. (2014). Shared and distinct functions of two Gti1/Pac2 family proteins in growth, morphogenesis and pathogenicity of Magnaporthe oryzae. Environ. Microbiol. 16, 788–801. doi: 10.1111/1462-2920.12204
Desjardins, A. E. (2009). From yellow rain to green wheat: 25 years of trichothecene biosynthesis research. J. Agric. Food Chem. 57, 4478–4484. doi: 10.1021/jf9003847
Desjardins, A. E., Hohn, T. M., and McCormick, S. P. (1993). Trichothecene biosynthesis in Fusarium species: chemistry, genetics, and significance. Microbiol. Rev. 57, 595–604. doi: 10.1128/mr.57.3.595-604.1993
Gardiner, D. M., and Kazan, K. (2018). Selection is required for efficient Cas9-mediated genome editing in Fusarium graminearum. Fungal Biol. 122, 131–137. doi: 10.1016/j.funbio.2017.11.006
Gardiner, D. M., Kazan, K., and Manners, J. M. (2009a). Nutrient profiling reveals potent inducers of trichothecene biosynthesis in Fusarium graminearum. Fungal Genet. Biol. 46, 604–613. doi: 10.1016/j.fgb.2009.04.004
Gardiner, D. M., Osborne, S., Kazan, K., and Manners, J. M. (2009b). Low pH regulates the production of deoxynivalenol by Fusarium graminearum. Microbiology 155, 3149–3156. doi: 10.1099/mic.0.029546-0
Giese, H., Sondergaard, T. E., and Sørensen, J. L. (2013). The AreA transcription factor in Fusarium graminearum regulates the use of some nonpreferred nitrogen sources and secondary metabolite production. Fungal Biol. 117, 814–821. doi: 10.1016/j.funbio.2013.10.006
Goswami, R. S., and Kistler, H. C. (2004). Heading for disaster: Fusarium graminearum on cereal crops. Mol. Plant Pathol. 5, 515–525. doi: 10.1111/j.1364-3703.2004.00252.x
Hohn, T. M., and Beremand, P. D. (1989). Isolation and nucleotide sequence of a sesquiterpene cyclase gene from the trichothecene-producing fungus Fusarium sporotrichioides. Gene 79, 131–138. doi: 10.1016/0378-1119(89)90098-X
Hohn, T. M., Krishna, R., and Proctor, R. H. (1999). Characterization of a transcriptional activator controlling trichothecene toxin biosynthesis. Fungal Genet. Biol. 26, 224–235. doi: 10.1006/fgbi.1999.1122
Hou, R., Jiang, C., Zheng, Q., Wang, C., and Xu, J. R. (2015). The AreA transcription factor mediates the regulation of deoxynivalenol (DON) synthesis by ammonium and cyclic adenosine monophosphate (cAMP) signalling in Fusarium graminearum. Mol. Plant Pathol. 16, 987–999. doi: 10.1111/mpp.12254
Jiang, C., Zhang, C., Wu, C., Sun, P., Hou, R., Liu, H., et al. (2016). TRI6 and TRI10 play different roles in the regulation of deoxynivalenol (DON) production by cAMP signalling in Fusarium graminearum. Environ. Microbiol. 18, 3689–3701. doi: 10.1111/1462-2920.13279
Jiao, F., Kawakami, A., and Nakajima, T. (2008). Effects of different carbon sources on trichothecene production and tri gene expression by Fusarium graminearum in liquid culture. FEMS Microbiol. Lett. 285, 212–219. doi: 10.1111/j.1574-6968.2008.01235.x
Jonkers, W., Dong, Y., Broz, K., and Kistler, H. C. (2012). The Wor1-like protein Fgp1 regulates pathogenicity, toxin synthesis and reproduction in the phytopathogenic fungus Fusarium graminearum. PLoS Pathog. 8:e1002724. doi: 10.1371/journal.ppat.1002724
Kawakami, A., Nakajima, T., and Hirayae, K. (2014). Effects of carbon sources and amines on induction of trichothecene production by Fusarium asiaticum in liquid culture. FEMS Microbiol. Lett. 352, 204–212. doi: 10.1111/1574-6968.12386
Khang, C. H., Park, S.-Y., Lee, Y.-H., and Kang, S. (2005). A dual selection based, targeted gene replacement tool for Magnaporthe grisea and Fusarium oxysporum. Fungal Genet. Biol. 42, 483–492. doi: 10.1016/j.fgb.2005.03.004
Kimura, M., Tokai, T., Takahashi-Ando, N., Ohsato, S., and Fujimura, M. (2007). Molecular and genetic studies of Fusarium trichothecene biosynthesis: pathways, genes, and evolution. Biosci. Biotechnol. Biochem. 71, 2105–2123. doi: 10.1271/bbb.70183
Maeda, K., Ichikawa, H., Nakajima, Y., Motoyama, T., Ohsato, S., Kanamaru, K., et al. (2018). Identification and characterization of small molecule compounds that modulate trichothecene production by Fusarium graminearum. ACS Chem. Biol. 13, 1260–1269. doi: 10.1021/acschembio.8b00044
Maeda, K., and Ohsato, S. (2017). Molecular genetic characterization of Fusarium graminearum genes identified as encoding a precocene II-binding protein. JSM Mycotoxins 67, 1–3. doi: 10.2520/myco.67-1-3
McCormick, S. P., Alexander, N. J., and Proctor, R. H. (2006). Fusarium Tri4 encodes a multifunctional oxygenase required for trichothecene biosynthesis. Can. J. Microbiol. 52, 636–642. doi: 10.1139/w06-011
McCormick, S. P., Alexander, N. J., and Proctor, R. H. (2013). “Trichothecene triangle: toxins, genes, and plant disease” in Recent advances in Phytochemistry (phytochemicals, plant growth, and the environment). ed. D. R. Gang (London: Springer International Publishing AG)
Merhej, J., Richard-Forget, F., and Barreau, C. (2011). Regulation of trichothecene biosynthesis in Fusarium: recent advances and new insights. Appl. Microbiol. Biotechnol. 91, 519–528. doi: 10.1007/s00253-011-3397-x
Michielse, C. B., Becker, M., Heller, J., Moraga, J., Collado, I. G., and Tudzynski, P. (2011). The Botrytis cinerea Reg1 protein, a putative transcriptional regulator, is required for pathogenicity, conidiogenesis, and the production of secondary metabolites. Mol. Plant-Microbe Interact. 24, 1074–1085. doi: 10.1094/MPMI-01-11-0007
Michielse, C. B., van Wijk, R., Reijnen, L., Manders, E. M., Boas, S., Olivain, C., et al. (2009). The nuclear protein Sge1 of Fusarium oxysporum is required for parasitic growth. PLoS Pathog. 5:e1000637. doi: 10.1371/journal.ppat.1000637
Min, K., Shin, Y., Son, H., Lee, J., Kim, J. C., Choi, G. J., et al. (2012). Functional analyses of the nitrogen regulatory gene areA in Gibberella zeae. FEMS Microbiol. Lett. 334, 66–73. doi: 10.1111/j.1574-6968.2012.02620.x
Nakajima, Y., Akasaka, M., Shiobara, T., Kitou, Y., Maeda, K., Kanamaru, K., et al. (2020). Impact of nitrogen metabolism-associated culture pH changes on regulation of Fusarium trichothecene biosynthesis: revision of roles of polyamine agmatine and transcription factor AreA. Curr. Genet. 66, 1179–1190. doi: 10.1007/s00294-020-01102-x
Nakajima, Y., Maeda, K., Jin, Q., Takahashi-Ando, N., Kanamaru, K., Kobayashi, T., et al. (2016). Oligosaccharides containing an α-(1→2) (glucosyl/xylosyl)-fructosyl linkage as inducer molecules of trichothecene biosynthesis for Fusarium graminearum. Int. J. Food Microbiol. 238, 215–221. doi: 10.1016/j.ijfoodmicro.2016.09.011
Nakajima, Y., Tokai, T., Maeda, K., Tanaka, A., Takahashi-Ando, N., Kanamaru, K., et al. (2014). A set of heterologous promoters useful for investigating gene functions in Fusarium graminearum. JSM Mycotoxins 64, 147–152. doi: 10.2520/myco.64.147
Nasmith, C. G., Walkowiak, S., Wang, L., Leung, W. W. Y., Gong, Y., Johnston, A., et al. (2011). Tri6 is a global transcription regulator in the phytopathogen Fusarium graminearum. PLoS Pathog. 7:e1002266. doi: 10.1371/journal.ppat.1002266
Ochiai, N., Tokai, T., Takahashi-Ando, N., Fujimura, M., and Kimura, M. (2007). Genetically engineered Fusarium as a tool to evaluate the effects of environmental factors on initiation of trichothecene biosynthesis. FEMS Microbiol. Lett. 275, 53–61. doi: 10.1111/j.1574-6968.2007.00869.x
Okmen, B., Collemare, J., Griffiths, S., van der Burgt, A., Cox, R., and de Wit, P. J. (2014). Functional analysis of the conserved transcriptional regulator CfWor1 in Cladosporium fulvum reveals diverse roles in the virulence of plant pathogenic fungi. Mol. Microbiol. 92, 10–27. doi: 10.1111/mmi.12535
Ponts, N., Pinson-Gadais, L., Verdal-Bonnin, M. N., Barreau, C., and Richard-Forget, F. (2006). Accumulation of deoxynivalenol and its 15-acetylated form is significantly modulated by oxidative stress in liquid cultures of Fusarium graminearum. FEMS Microbiol. Lett. 258, 102–107. doi: 10.1111/j.1574-6968.2006.00200.x
Proctor, R. H., Hohn, T. M., McCormick, S. P., and Desjardins, A. E. (1995). Tri6 encodes an unusual zinc finger protein involved in regulation of trichothecene biosynthesis in Fusarium sporotrichioides. Appl. Environ. Microbiol. 61, 1923–1930. doi: 10.1128/aem.61.5.1923-1930.1995
Ramirez, M. L., Chulze, S., and Magan, N. (2006). Temperature and water activity effects on growth and temporal deoxynivalenol production by two Argentinean strains of Fusarium graminearum on irradiated wheat grain. Int. J. Food Microbiol. 106, 291–296. doi: 10.1016/j.ijfoodmicro.2005.09.004
Santhanam, P., and Thomma, B. P. (2013). Verticillium dahliae Sge1 differentially regulates expression of candidate effector genes. Mol. Plant-Microbe Interact. 26, 249–256. doi: 10.1094/MPMI-08-12-0198-R
Seong, K.-Y., Pasquali, M., Zhou, X., Song, J., Hilburn, K., McCormick, S., et al. (2009). Global gene regulation by Fusarium transcription factors Tri6 and Tri10 reveals adaptations for toxin biosynthesis. Mol. Microbiol. 72, 354–367. doi: 10.1111/j.1365-2958.2009.06649.x
Sørensen, J. L., and Sondergaard, T. E. (2014). The effects of different yeast extracts on secondary metabolite production in Fusarium. Int. J. Food Microbiol. 170, 55–60. doi: 10.1016/j.ijfoodmicro.2013.10.024
Tag, A. G., Garifullina, G. F., Peplow, A. W., Ake, C., Phillips, T. D., Hohn, T. M., et al. (2001). A novel regulatory gene, Tri10, controls trichothecene toxin production and gene expression. Appl. Environ. Microbiol. 67, 5294–5302. doi: 10.1128/AEM.67.11.5294-5302.2001
Tanaka, Y., Nakajima, Y., Maeda, K., Matsuyama, M., Kanamaru, K., Kobayashi, T., et al. (2019). Inhibition of Fusarium trichothecene biosynthesis by yeast extract components extractable with ethyl acetate. Int. J. Food Microbiol. 289, 24–29. doi: 10.1016/j.ijfoodmicro.2018.08.026
Tokai, T., Koshino, H., Takahashi-Ando, N., Sato, M., Fujimura, M., and Kimura, M. (2007). Fusarium Tri4 encodes a key multifunctional cytochrome P450 monooxygenase for four consecutive oxygenation steps in trichothecene biosynthesis. Biochem. Biophys. Res. Commun. 353, 412–417. doi: 10.1016/j.bbrc.2006.12.033
Tudzynski, B., Homann, V., Feng, B., and Marzluf, G. A. (1999). Isolation, characterization and disruption of the areA nitrogen regulatory gene of Gibberella fujikuroi. Mol. Gen. Genet. 261, 106–114. doi: 10.1007/s004380050947
Wen, J., and Brogna, S. (2010). Splicing-dependent NMD does not require the EJC in Schizosaccharomyces pombe. EMBO J. 29, 1537–1551. doi: 10.1038/emboj.2010.48
Yu, F. W., Zhang, X. P., Yu, M. H., Yin, Y. N., and Ma, Z. H. (2015). The potential protein kinase a (Pka) phosphorylation site is required for the function of FgSge1 in Fusarium graminearum. World J. Microbiol. Biotechnol. 31, 1419–1430. doi: 10.1007/s11274-015-1894-2
Keywords: complex media and defined media (synthetic media), nitrogen regulatory gene AreA, structural changes of the secondary metabolite gene cluster core region, trichothecene mycotoxin, Tri6 transcription under two types of regulation, WOR1 ortholog Fgp1, zinc finger transcription factor
Citation: Liew MXX, Nakajima Y, Maeda K, Kitamura N and Kimura M (2023) Regulatory mechanism of trichothecene biosynthesis in Fusarium graminearum. Front. Microbiol. 14:1148771. doi: 10.3389/fmicb.2023.1148771
Edited by:
Laurent Dufossé, Université de la Réunion, FranceReviewed by:
Jun-ichi Maruyama, The University of Tokyo, JapanSusan McCormick, United States Department of Agriculture (USDA), United States
Copyright © 2023 Liew, Nakajima, Maeda, Kitamura and Kimura. This is an open-access article distributed under the terms of the Creative Commons Attribution License (CC BY). The use, distribution or reproduction in other forums is permitted, provided the original author(s) and the copyright owner(s) are credited and that the original publication in this journal is cited, in accordance with accepted academic practice. No use, distribution or reproduction is permitted which does not comply with these terms.
*Correspondence: Makoto Kimura, bWtpbXVyYUBhZ3IubmFnb3lhLXUuYWMuanA=